- 1Laboratory of Translational Bioinformatics, Moscow Institute of Physics and Technology, Dolgoprudny, Russia
- 2PathoBiology Group, European Organization for Research and Treatment of Cancer (EORTC), Brussels, Belgium
- 3Group for Genomic Analysis of Cell Signaling, Shemyakin-Ovchinnikov Institute of Bioorganic Chemistry of the Russian Academy of Sciences, Moscow, Russia
- 4Laboratory for Clinical Genomic Bioinformatics, Sechenov First Moscow State Medical University, Moscow, Russia
- 5Gene Immunooncotherapy Group, Shemyakin-Ovchinnikov Institute of Bioorganic Chemistry of the Russian Academy of Sciences, Moscow, Russia
- 6Laboratory of human genes structure and functions, Shemyakin-Ovchinnikov Institute of Bioorganic Chemistry of the Russian Academy of Sciences, Moscow, Russia
- 7Gene oncotherapy sector, Institute of Molecular Genetics of National Research Centre (Kurchatov Institute), Moscow, Russia
- 8Laboratory of Epigenetics, Institute of Oncogynecology and Mammology, National Medical Research Center for Obstetrics, Gynecology and Perinatology Named after Academician V.I. Kulakov, Ministry of Healthcare of the Russian Federation, Moscow, Russia
Treatment of metastatic disease remains among the most challenging tasks in oncology. One of the early events that predicts a poor prognosis and precedes the development of metastasis is the occurrence of clusters of cancer cells in the blood flow. Moreover, the presence of heterogeneous clusters of cancerous and noncancerous cells in the circulation is even more dangerous. Review of pathological mechanisms and biological molecules directly involved in the formation and pathogenesis of the heterotypic circulating tumor cell (CTC) clusters revealed their common properties, which include increased adhesiveness, combined epithelial-mesenchymal phenotype, CTC-white blood cell interaction, and polyploidy. Several molecules involved in the heterotypic CTC interactions and their metastatic properties, including IL6R, CXCR4 and EPCAM, are targets of approved or experimental anticancer drugs. Accordingly, analysis of patient survival data from the published literature and public datasets revealed that the expression of several molecules affecting the formation of CTC clusters predicts patient survival in multiple cancer types. Thus, targeting of molecules involved in CTC heterotypic interactions might be a valuable strategy for the treatment of metastatic cancers.
1 Introduction
One of the cancer hallmarks is cancer cells dissemination and metastasis which is a leading cause of cancer associated death (1). Metastasis develops as a consequence of changes within cancer cells that lead to an ability to move through the tissue, survive in the circulation, attach and grow in the distal site, meanwhile escaping immune surveillance (2). Research of the last decades revealed that a key factor which determines the ability of cancer cells to metastasize is pathological interactions with neighboring non-cancerous cells such as fibroblasts, mesenchymal and immune cells, so called cells of tumor microenvironment (TME).
Therefore, development of drugs targeting key molecules involved in the TME interactions that can suppress metastasis is a hot theme of current investigations (3–7).
Cancer associated stromal cells as well as circulating exosomes migrate from the primary tumor to distal sites and change local microenvironment forming so-called pre-metastatic niche permissive for the cancer cells recruitment and growth (8–12). At the same time, cancer cells might disseminate from the primary tumor in the circulation in clusters with cancer associated cells (13–15). These clusters are thought to be relatively rare in the cancer patient population (14, 16, 17), although they have strong metastatic potential (16, 18), and their presence is associated with metastasis and worse prognosis in breast (13, 14, 19), lung cancers (20–22), renal cell carcinoma (23), colorectal cancer (24–26), and others (27).
Our review of current literature revealed that cells involved in the metastasis-promoting heterotypic CTC interactions include platelets, cancer associated fibroblast (CAFs), white blood cells (WBCs), specific population of tumor-associated macrophages, neutrophils and polymorphonuclear myeloid-derived suppressor cells (PMN-MDSCs).
A number of investigations identified several key molecules involved in the heterotypic cell interactions such as IL1R1 (16), IL6, NODA, NOTCH1 (17), CD44 (14), CXCR4 (4), TGFBR2 (4), CDH1 (4, 28), EPCAM (29), ICAM1 (30), CCR1 (31) (Table 1). Their expression promotes formation of CTC clusters and metastasis by inducing adhesion (4, 30, 33), proliferation (16), by metabolic adaptation to oxidative stress (17, 34), and through the epithelial-mesenchymal transition (31, 35). Quite intriguing, in lung cancer most CTCs interacting with WBCs were polyploid (21) thus, implying repression of the mitotic checkpoint, induction of cell survival and migration (36–38).
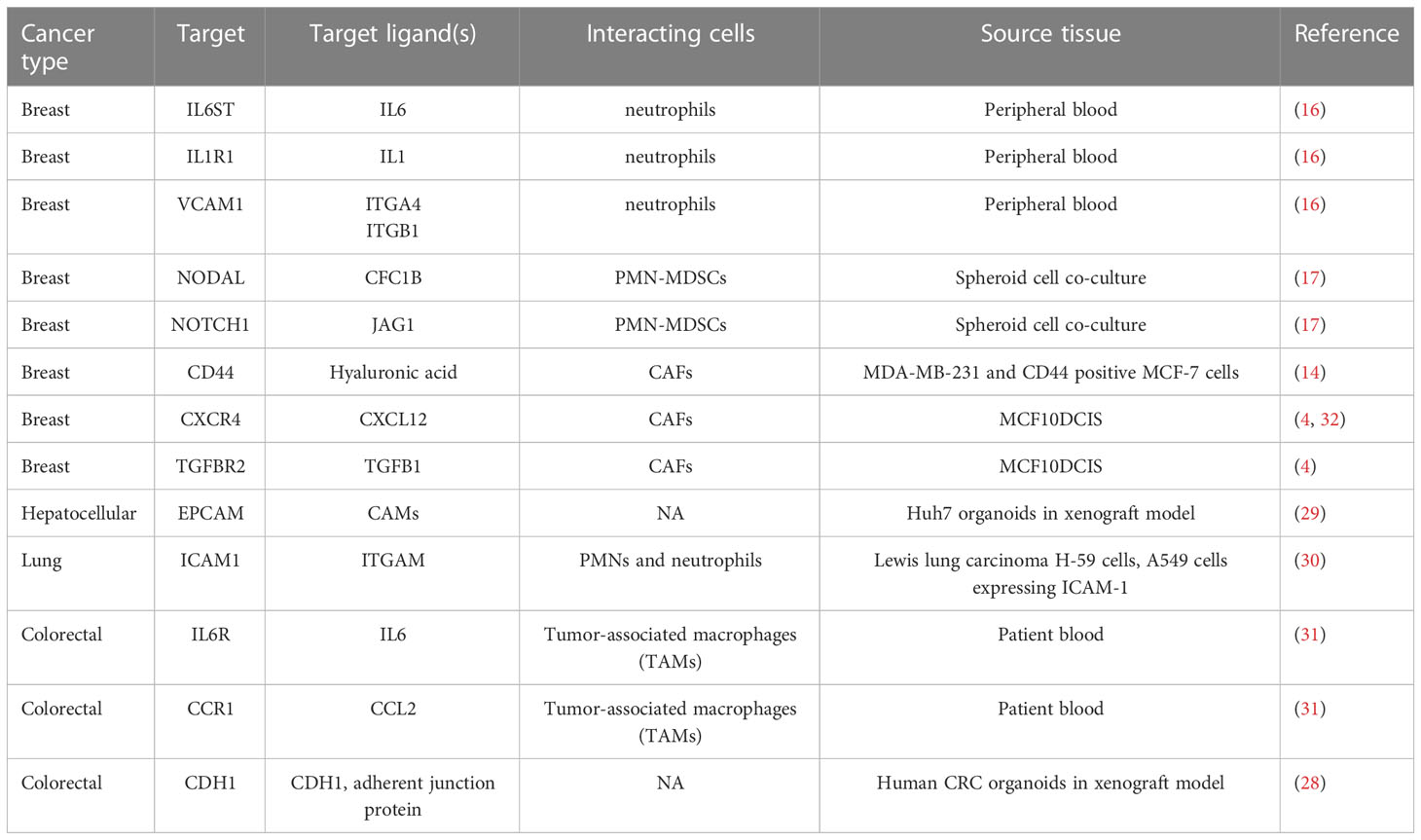
Table 1 Genes involved in cancer cell-stromal cell interaction promoting CTC clustering and metastasis.
The analysis of literature and public databases revealed that expression of some genes affecting CTC clusters and metastasis predicts prognosis in many cancer types. Some of these molecules are targeted by the approved or experimental anti-cancer drugs (such as plerixafor for CXCR4 or tocilizumab for IL6R). Altogether, our review suggests the existence of common and cancer tissue specific mechanisms of CTC complex formation with implication for drug development and cancer treatment.
2 Tumor microenvironment promotes formation of the CTC clusters and metastasis
2.1 Interactions with white blood cells
The CTCs can interact with a variety of WBCs in the circulation such as neutrophils (39), PMN-MDSC (17, 40, 41), platelets (31), macrophages (35), and lymphocytes (16).
2.1.1 Interaction with neutrophils
One of the mechanisms of neutrophil mediated metastasis is formation of the neutrophil extracellular traps (NETs) consisting of neutrophil DNA (39). As NETs interact with and provide a niche for CTCs, blocking NET formation by DNAse, e.g. coated with nanoparticles inhibits lung metastasis (39).
Using in vivo metastasis models, Spicer et al. have demonstrated a novel role of neutrophils in the early adhesive steps of liver metastasis in the Lewis lung carcinoma mice model (30). Their findings suggest that neutrophils promote cancer cell adhesion within liver sinusoids, thus influencing metastasis. The neutrophil ITGAM/ICAM-1 mediated the adhesion of lipopolysaccharide-activated neutrophils to the cancer cells (30).
In breast cancer, CTCs interact with WBCs and in out of 70 investigated patients with invasive disease, CTCs were found in 34 (49%) patients. Among them, homotypic CTC clusters were found in 14 (20%) patients, out of which 6 (9%) also had CTC-WBC clusters and 4 (6%) had CTC-WBC clusters only (16). On average, about 2 CTCs were found in the CTC-WBC clusters that represented about 10% of all circulating CTCs (16). Most of these WBCs (75%) were myeloid cells, specifically neutrophils and T-cells.
The neutrophil-CTC interactions detected in blood were associated with worse prognosis of patients (16). Neutrophil-CTC clusters promoted cancer cell proliferation in vitro and were characterized by higher metastatic potential in mice upon tail vein injection. Analysis of gene expression from either CTC alone or in a complex with neutrophils revealed 41 upregulated genes involved in the DNA replication and cell cycle progression. Further analysis of genes dysregulated in cancer associated neutrophils revealed that TNF-α, Oncostatin M (OSM), IL-1β and IL-6 cytokines are expressed in the neutrophils with corresponding expression of their receptors in CTCs. Reciprocal experiment detected cytokines granulocyte colony-stimulating factor (G-CSF), TGF-β3 and IL-15 in the CTCs with corresponding expression of the receptors in neutrophils. CRISPR-Cas9 mediated knockout of IL6ST and IL1R1 in cancer cells suppressed the growth advantage of the neutrophil-CTC clusters without effect on their frequency (16). In addition, vascular cell adhesion molecule (VCAM1) was identified in a CRISPR-Cas9 screen in the CTC as a molecule required for formation of the neutrophil-CTC clusters (16). Neutrophil recruitment to the primary site and metastasis was dependent on expression of CXCL1/2 in 4T1 breast cancer cells. Among molecules that block cancer cell invasion mediated by neutrophils were also NADPH oxidase, neutrophil elastase inhibitors, and DNAse (39).
2.1.2 Interaction with PMN-MDSCs
Another type of myeloid cell - PMN-MDSCs normally function as suppressors of the immune response and have profound pro-carcinogenic properties promoting angiogenesis, formation of the pre-metastatic niche and cell proliferation (42–45),
It was predicted that PMN-MDSCs interact with CTCs and it was hypothesized (yet to be proven) that PMN-MDSCs shield CTCs from the T-cell mediated destruction (46). At that time, CTCs were usually isolated as CD45 negative cells, thereby clusters of CTC with leukocytes (including PMN-MDSCs) were missed from the analysis.
Indeed, PMN-MDSC clusters with circulating tumor cells were detected in patients with melanoma or breast cancer (17). It was reported that the ratio of cancer and non-cancerous cells in the clusters varied in the range 1:1 to 1:4 in six out of eight patients tested (17).
Interestingly, a previous paper from the same group revealed that aggressive triple negative breast and melanoma cancers overexpress Nodal, an embryonic morphogen of the TGF-β family (47) and a a putative Notch/RBPJ signaling pathway target (48). The patients with aggressive breast cancer had higher levels of Nodal in serum and PMN-MDSCs could promote survival of the CTCs in culture by activating reactive oxygen species (ROS) and Jagged2 response (17). Accordingly, CTCs promote differentiation of the PMN-MDSCs in pro-cancerous “type-2” phenotype by the Nodal signaling (17).
Arnoletti et al. investigated the effect of interactions between the CTCs, MDSCs and T-cells extracted from the portal blood of pancreatic adenocarcinoma patients on CTC and T-cell proliferation, apoptosis and activation. It was demonstrated that MDSCs tended to cooperate with CTCs by repressing T-cells proliferation, although no significant effects on activation and anergy were reported (49).
The mathematical modeling and direct measurements of genomic aberrations in breast cancer CTC clusters isolated by filtration revealed that the fraction of cancer cells in the clusters is in the range of 8%-48% (50). In contrast, isolation of multicellular clusters from the blood of breast cancer patients followed by single cell RNA-seq analysis identified genes associated specifically with clusters, in comparison to single cells, but failed to identify other cell types except platelets (18). In agreement with other studies, cell clusters contributed to metastasis 23 times more actively than the single cells and the presence of clusters in breast and prostate cancers was associated with significantly worse prognosis (18).
The differences in CTC isolation protocols might lead to the differences in cell populations detected within CTC clusters. The latter study (18) utilized HBCTC-Chip coated with cocktail of EPCAM, EGFR and HER2 antibodies (18), whereas Parsortix microfluidic device using Cell Separation Cassettes (GEN3D6.5, ANGLE) was used in the subsequent study that characterized neutrophils-CTC (16), whereas PMN-MDSC-CTCs clusters were isolated by FACS (17, 40, 41).
2.1.3 Interaction with tumor associated macrophages
Interaction of CTCs with tumor associated macrophage (TAMs) seems to promote metastasis. Nanomechanical characterization of tumor associated macrophage-CTC clusters isolated from blood of prostate cancer patients revealed that contact with the macrophages softens and promotes adhesiveness of CTCs, which corresponds to mixed epithelial - mesenchymal phenotype (35). Notably, previous publication of the same group reported softness, deformability, and adhesiveness of single CTCs as markers of aggressive metastatic prostate cancer (51). The presence of TAMs in the invasive front was associated with the mesenchymal phenotype of CTCs and poor prognosis in colorectal cancer (31). Mechanistically, the Il-6 produced by the TAMs induced JAK2/STAT3/miR-506-3p/FoxQ1 signaling in cancer cells, thus promoting epithelial mesenchymal transition (EMT), metastasis and further attraction of macrophages by secretion of CCl2 (31).
2.1.4 Interaction with lymphocytes
We found only one report that mentions interaction of CTCs with lymphocytes (16). However, CTCs are associated with impairments of adaptive immunity. The quantity of CTCs correlates with the presence in peripheral blood of the CD95(FAS)-positive T-helper cells and stage 3 breast cancer as well as with lower percentage of the CD8+ T-cells with activated T-cell receptor (52, 53), the absence of tumor associated antigen specific TCRs and low TCR heterogeneity (54), and positively associated with intratumoral populations of T-regs (55).
2.2 Interactions with cancer associated fibroblasts
Aside from single CTCs and cancer associated fibroblasts (CAFs), the presence of homotypic and heterotypic clusters of CTCs and CAFs was reported in patients with stages 1-4 of breast cancer (14). In their study, Sharma et al. detected CTCs in 90% and circulating CAFs (cCAFs) in 80% of patients; homotypic CTC clusters were found in 50% and heterotypic - in 25% of patients in treatment naive stages 2-3. Interestingly, only 25% of patients in stage 4 had homotypic clusters and 25% had heterotypic CTC-CAF clusters. The number of cCAFs and CTCs was much higher in patient blood with metastatic breast cancer in comparison to localized cancers whereas nothing was detected in the control group. The effect of cancer treatment on these clusters was not yet addressed (14).
Using MDA-MB-231 cells and CD44-enriched MCF7 cells, authors have been able to demonstrate involvement of the stem cell marker CD44 in the heterotypic clustering and that heterotypic clusters metastasize more efficiently (14). Accordingly, it was shown that tumor suppressor Rb represses CD44 dependent collective invasion, release of breast cancer cells in circulation and lung metastasis (3).
Circulating CAFs and CTCs were also detected in small groups of colorectal and prostate cancer patients (13). Consistent with others, the paper shows images of the distinct multicellular CTC clusters with CAF and with leukocytes, which were obtained by the negative filtration through 10 µm filter (13).
2.3 Interaction with platelets
Activation of the coagulation cascade and formation of platelet-rich thrombus around tumor cells in the vasculature have both been proposed to play major roles in physically shielding CTCs from the stress of blood flow and from lysis by the Natural killer cells (56–58). One of the mechanisms is substitution of cancer cell MHC1 by platelets-derived MHC1 carrying normal peptides thereby protecting cancer cells from both NK and T-cell recognition (59).
Analysis of the single cell gene expression of the CTCs in the pancreatic cancer mouse model revealed that 32% of the circulating cells interact with platelets leading to suppression of epithelial markers and expression changes of many other genes (60).
Accordingly, direct interaction with platelets promotes EMT in cancer cells and either inhibition of NF-kB in cancer cells or inhibition of TGF-β in platelets was sufficient to protect against lung metastasis (61). In turn, disruption of platelets interactions with cancer cell by S-nitrosocaptopril (CapNO) inhibits adhesion to endothelial cells and lung cancer metastasis in immunocompetent mouse models through multiple mechanisms including reduction of Sialyl-Lewis X (Slex) levels in cancer cells and ADP-induced P-selectin in platelets, IL-1b induced VCAM1, ICAM-1, and E-selectin by HUVECs (33).
3 Polyploidy and epithelial-to-mesenchymal transition in CTC clusters
As it is discussed in the previous sections, interaction with TAM (31) or platelets (61) induced metastasis promoting EMT in cancer cells (62). EMT is associated with cancer progression and metastasis (63). During EMT epithelial cells lose contact with epithelial or endothelial cells, change their cytoskeleton and consequentially, become less rigid, acquiring an ability to move (51, 64). In addition, EMT induces stem cell properties in cancer, regulates and is regulated by immunosuppressive cancer microenvironment (65, 66). Notably, cancer stem cells are characterized by mixed epithelial – mesenchymal phenotype (67).
Interestingly, interaction with white blood cells also correlates with mixed Epithelial-mesenchymal phenotype and cancer cells polyploidy (21, 68, 69) that play a key role in cancer resistance to treatment and metastasis (37, 70–72).
The presence of CTC-WBC clusters was associated with worse prognosis in lung (21, 22), breast cancers (19), and hepatocellular carcinoma (73, 74). Remarkably, in lung cancer, CTCs in complex with WBCs were exclusively polyploid (21).
In turn, in glioblastoma, examination of ploidy together with expression of endothelial marker CD31 revealed that pre-operative small triploid CD31 negative CTCs were predictive of inferior prognosis (68).
A recent paper employed the iFISH method combining FISH DNA staining and immunofluorescence (21, 22) to create Atlas of Circulating Rare Cells (69). High throughput imaging analysis of circulating rare cells (CRCs) purified by WBC subtraction categorized cells into 71 subtypes based on the CD45 leukocyte staining, cell size, chromosome 8 ploidy and the presence of a few tumor cell markers including PD-L1 (EPCAM/CK18/PD-L1/AFP/HER2/CA19-9), endothelial CD31, mesenchymal Vimentin and stem cell CD133 markers (69).
Authors presented a set of cell images with polyploid chromosome 8. There were cells double positive for CD31 and Vimentin staining with abnormal chromosomes which can coincide with cytokeratin CK18, and even CD45-/EPCAM+/CD31+/Vim+ “aneuploid mesenchymal epithelial-endothelial fusion clusters” were detected. These observations are consistent with the previous data generated by iFISH linking polyploidy with EMT (21, 75, 76). The presence of CD45 positive cells was detected in the clusters with polyploid or multinuclear cancer cells (21, 69).
Quite importantly, comparison of the total count of CTCs and/or circulating tumor endothelial cells between 31 conditions revealed that CTCs are present in multiple cancers, however, the highest frequency of “CTCs” is observed within the group of non-neoplastic infectious diseases, suggesting that the pure presence of cells with these markers could not be used as a diagnostic test itself (69).
Consistent with the Atlas of Circulating Rare Cells (69), sequencing of CTC clusters and individual circulating cancer cells revealed the mixed epithelial-mesenchymal markers in hepatocellular carcinoma (Vimentin, epithelial: CDH1, EPCAM, ASGR2, Keratin 8, stemness: CD133, POU5F1, NOTCH1 and STAT3) (62) prostate cancer (EPCAM, keratins, E-cad, Vimentin, CD44) (77) and Vimentin in lung cancer (78).
However, two major conceptual questions here currently remain not sufficiently addressed:
(i) How heterotypic interactions of cancer cells with WBCs promote polyploidy?
(ii) How does the combination of ploidy and mesenchymal phenotype enhance metastasis?
Mechanisms of how heterotypic interactions promote mobility and mesenchymal phenotype are described in the subsequent section.
4 Heterotypic interactions within tumor microenvironment are pivotal for CTC cluster formation
Interactions with cells of cancer microenvironment promote EMT, formation of CTC clusters and metastasis (4, 31). Classically, EMT is accompanied by decrease of E-cadherin/N-cadherin ratio (79). A recent publication highlighted a novel role of the E-cadherin (E-cad, encoded by CDH1 gene) expressing cells in breast cancer metastasis (80, 81). It turned out that when cancer cells grow in the presence of CAFs there is a gradient of the E-cad from low at the trailing edge of the invading cancer cells to high E-cad behind it (4). Furthermore, another paper demonstrated that in breast cancer spheroid model stem cells lead the collective invasion co-expressing mesenchymal and epithelial marks (82).
Dermal implants of CAFs with MCF10 cells with low intrinsic metastatic potential promoted this low-high E-cad gradient, the CTC cell clustering and metastasis (4). High throughput RNA expression profiles revealed induction of carcinoembryonic antigen-related cell adhesion molecule 5 (CEACAM5; CAM5) and CEACAM6 (CAM6) in the presence of CAFs. This experiment revealed overexpression of 44 CAF-induced genes, whose expression is associated with poor prognosis in breast cancer. Mechanistically, E-cad, CAM5 and CAM6 interact with each other forming an adherent junction complex on the cell surface. Functional shRNA studies revealed attenuation of lung metastasis upon E-cad, CAM5, or CAM6 depletion. Other excellent functional investigations reported in this paper revealed that CAF produced SDF-1(encoded by CXCL12 gene) and TGF-β that through their cognate receptors CXCR4 and TGFBRII activate SRC kinase phosphorylation/Zeb1 axis altogether mediating tumor cell cluster formation that are also detected as CTC clusters. The caveat of this report for our purposes is that we do not know if fibroblasts travel in the bloodstream with cancer cells. However, this paper clearly demonstrates stromal-cancer cell molecular interactions that regulate the ability of cancer to metastasize (4). Importantly, CRCX4 mediates immunosuppressive tumor microenvironment not only in cancer cells, but also in the SMA positive stromal cells including myofibroblasts and pericytes (32). CRE-Lox mediated knockout of CRCX4 in SMA expressing cells improved survival in mice with breast cancer, and pharmacological inhibition of CRCX4 potentiated activity of immune checkpoint inhibitors in the nude mice bearing human metastatic breast cancer (32).
Similarly, to observation in breast cancer, cells of the collective invasion packs were E-cad positive in lung adenocarcinoma (5). The role of CAFs in the metastasis was demonstrated by the fact that only surrounding CAFs express vimentin and in the vimentin knockout mice, the CAFs motility decreases in vitro and in vivo. Vimentin was required for the heterotypic cancer cell - CAFs interaction, collective invasion, and lung adenocarcinoma metastasis (5).
Thus, formation of Epithelial-mesenchymal gradient during collective invasion is mediated by cancer cell – stromal cell interaction and pivotal for CTC formation and metastasis (4, 5).
We schematized major findings on CTC interactions and their molecular physiological effects on Figures 1, 2.
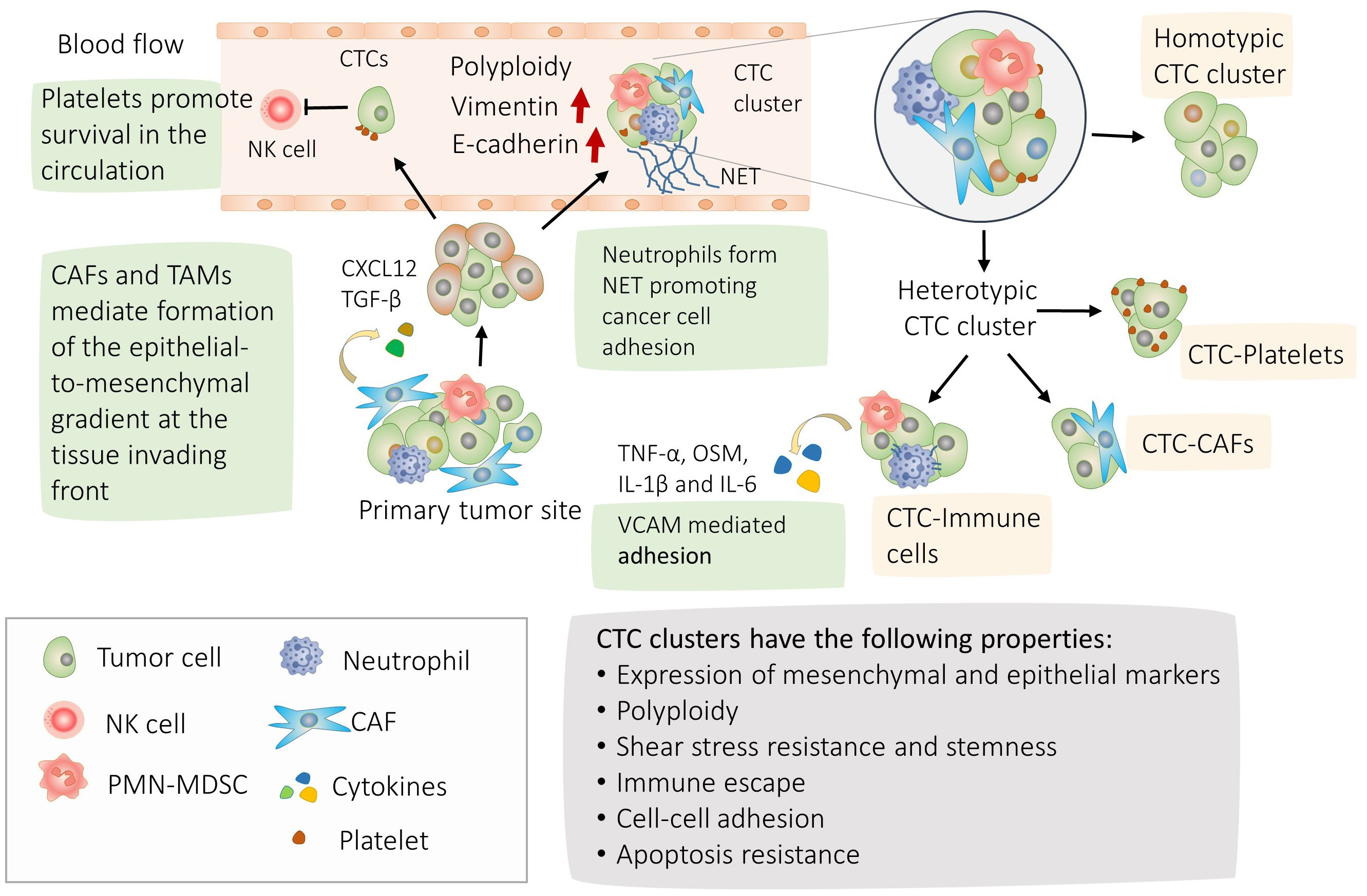
Figure 1 Mechanisms of circulating tumor cells (CTC) cluster formation and their properties. Highlighted in green, the process of CTC clusters formation; Tumor cells can separate from adjacent cells with more mobile mesenchymal cells at the leading edge of the invasion and cells with more epithelial properties behind (4, 5). Accordingly, mixed epithelial mesenchymal phenotype and polyploidy are frequently observed in the CTC clusters (21, 22). CTCs and CTC clusters are able to withstand the shear stress in the blood circulation and escape natural killer (NK). CTCs can form homotypic clusters or interact with CAFs, neutrophils, PMN-MDSCs, Tumor associated macrophages (TAM) or platelets forming heterotypic clusters (14, 16, 17). CAFs circulate in the bloodstream in heterotypic CTC clusters and promote cancer cell clustering by secreting CXCL12 and TGF-β (4, 13, 20). During transit and metastasis, platelet-rich thrombus form around CTCs providing protection from shear stress and against lysis by NK cells (56–58). CTC-associated neutrophils express TNF-α, OSM, IL-1β and IL-6 cytokines and their receptors are expressed correspondingly in CTCs. The interaction between CTCs and neutrophils is mediated by VCAM1, whereas TNF-α, OSM, IL-1β, and IL-6 promote proliferation of CTCs (16). In addition, neutrophils promote metastasis by releasing their DNA forming neutrophil extracellular traps (NET) (39). In turn, interaction with polymorphonuclear myeloid derived suppressor cells promote survival of the CTC clusters (17).
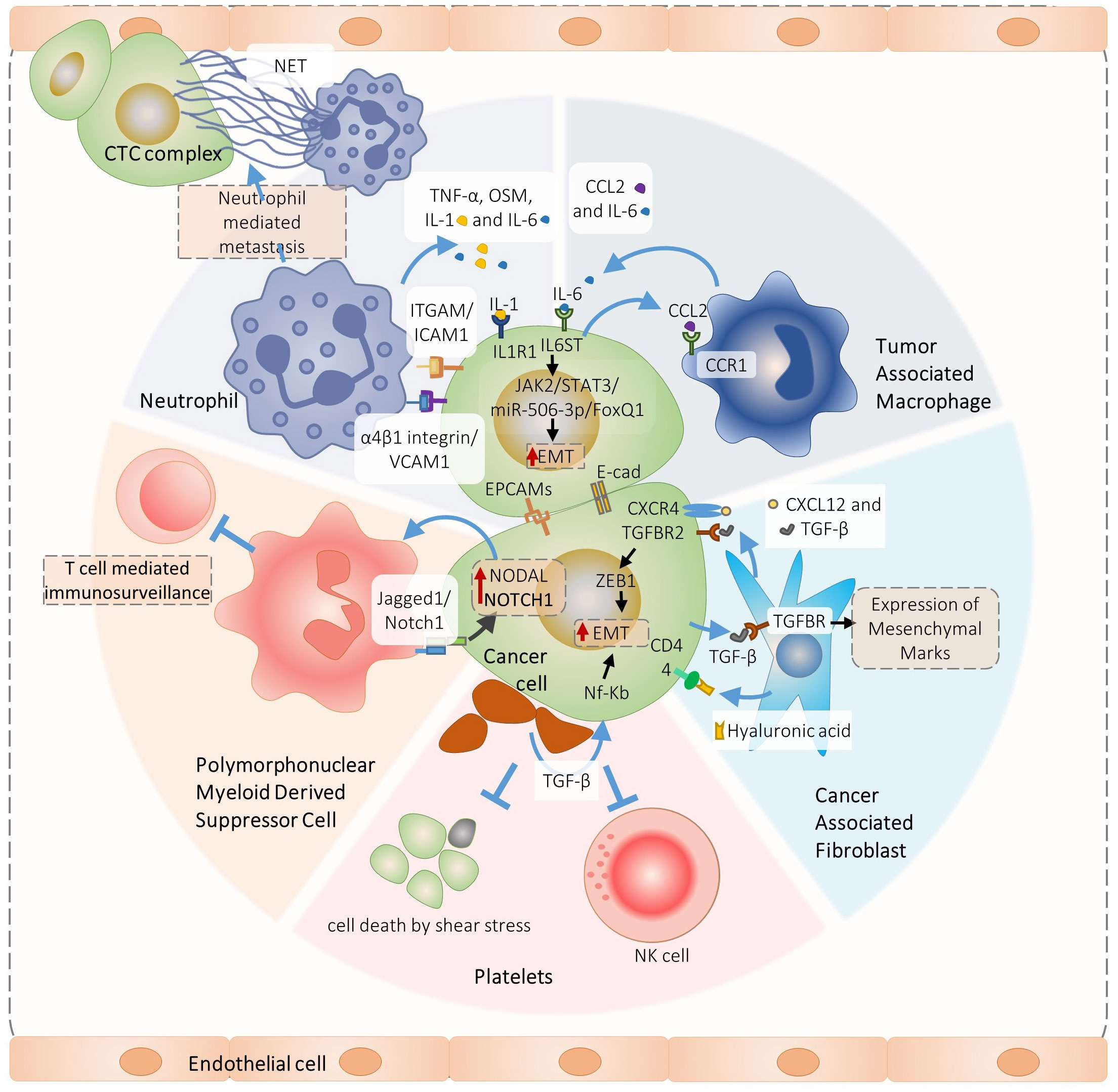
Figure 2 Overview of signaling pathways involved in the heterotypic cancer cell interactions pivotal for circulating tumor cells complex formation and metastasis. Specifically, PMN-MDSC-cancer cell interaction promotes ROS-induces Jagged1/Notch1/Nodal signaling that induces CTC cluster formation and metastasis (17), In turn, neutrophil interact with cancer cells via ITGAM/ICAM-1 adhesion facilitating interaction with liver sinusoids and metastasis (30). In addition, VCAM1 is required for the neutrophil-CTC cluster formation (29). Neutrophils produce IL1 and IL-6 that promote growth of neutrophils-CTC clusters via IL6ST and IL1R1 receptors (16), The Il-6 is also produced by the tumor associated macrophages which induce JAK2/STAT3/miR-506-3p/FoxQ1 signaling in cancer cells promoting epithelial mesenchymal transition (EMT), metastasis and further attraction of macrophages by the CCl2 secretion (31). A similar positive feedback loop is organized by the cancer-associated fibroblasts (CAFs) and cancer cells interactions. The CAFs produce TGF-β and CXCL12 that interact with TGFBR2 and CXCR4 receptors, inducing cancer cell EMT, CTC clusters and metastasis (4). In turn, cancer cells produce TGF-β and induce CAFs myofibroblast differentiation (83).
5 Expression of molecules involved in the CTC cluster formation and metastasis correlate with cancer survival
As it is discussed in the previous sections, the formation of the CTC clusters and metastasis in particular cancers depend on IL1R1 (16), IL6, NODAL, NOTCH1 (17), CD44 (14), CXCR4 (4), TGFBR2 (4), CDH1 (4, 28), EPCAM (29), ICAM1 (30), and CCR1 (31). Theoretically, these molecules can impact cancer metastasis with little to no information on the mechanisms involved in CTC cluster formation. To address this possibility, we interrogated a publicly available The Cancer Genome Atlas project (TCGA) database and research papers to examine if high or low expression of molecules that are functionally important for the formation of CTC clusters may characterize patient survival in multiple cancers. For example, it was demonstrated that IL1R1 protein induces CTC proliferation in breast cancer (BRCA) (16), and high IL1R gene expression corresponds to inferior prognosis in the TCGA-BRCA cohort (Figure 3A) as well as in many other cancers (Figures 3B, C; Table 2). In turn, high expression of CXCR4 in BRCA corresponds to better prognosis, smaller yet significant difference between Kaplan-Meier curves predicting better prognosis was observed for lung adenocarcinoma (LUAD) and thyroid cancer (THCA) (Figure 3B, Table 2), whereas no difference was observed in lung squamous cell carcinoma (LUSC).
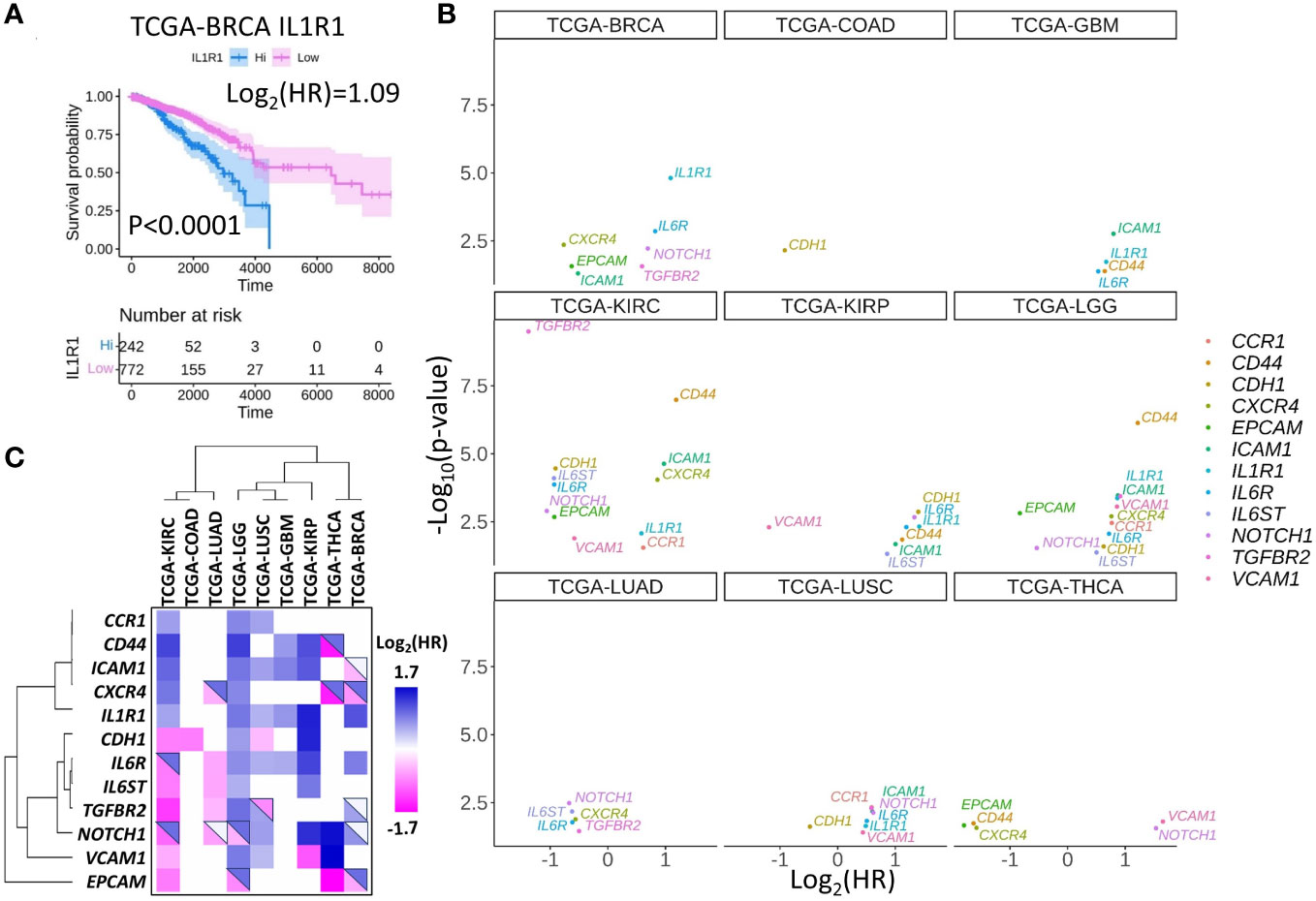
Figure 3 Set of selected CTC-associated genes differentially predict survival in several human cancer types. (A) An example of Kaplan-Meier curve for breast cancer (BRCA) patients stratified by high or low IL1R1 expression in tumors. Shaded areas represent 95% confidence intervals. Time is shown in days. (B) Hazard ratios (HR) and significance of the differences between Kaplan-Meier curves for patients stratified by the expression of indicated genes for the panel of solid tumors in TCGA database. Only genes with p<0.05 are shown. Note that log2(HR)<0 for good prognosis corresponding to high gene expression and log2(HR)>0 for bad prognosis. (C) Hazard ratio clustering for gene - cancer combinations revealed two major gene clusters. Top-right triangles depict approximations of the HR collected from the literature. Blue colors represent genes or gene products whose expression is associated with worse prognosis, pink colors represent genes or gene products whose expression is associated with good prognosis while white colors represent cases where the data is controversial.
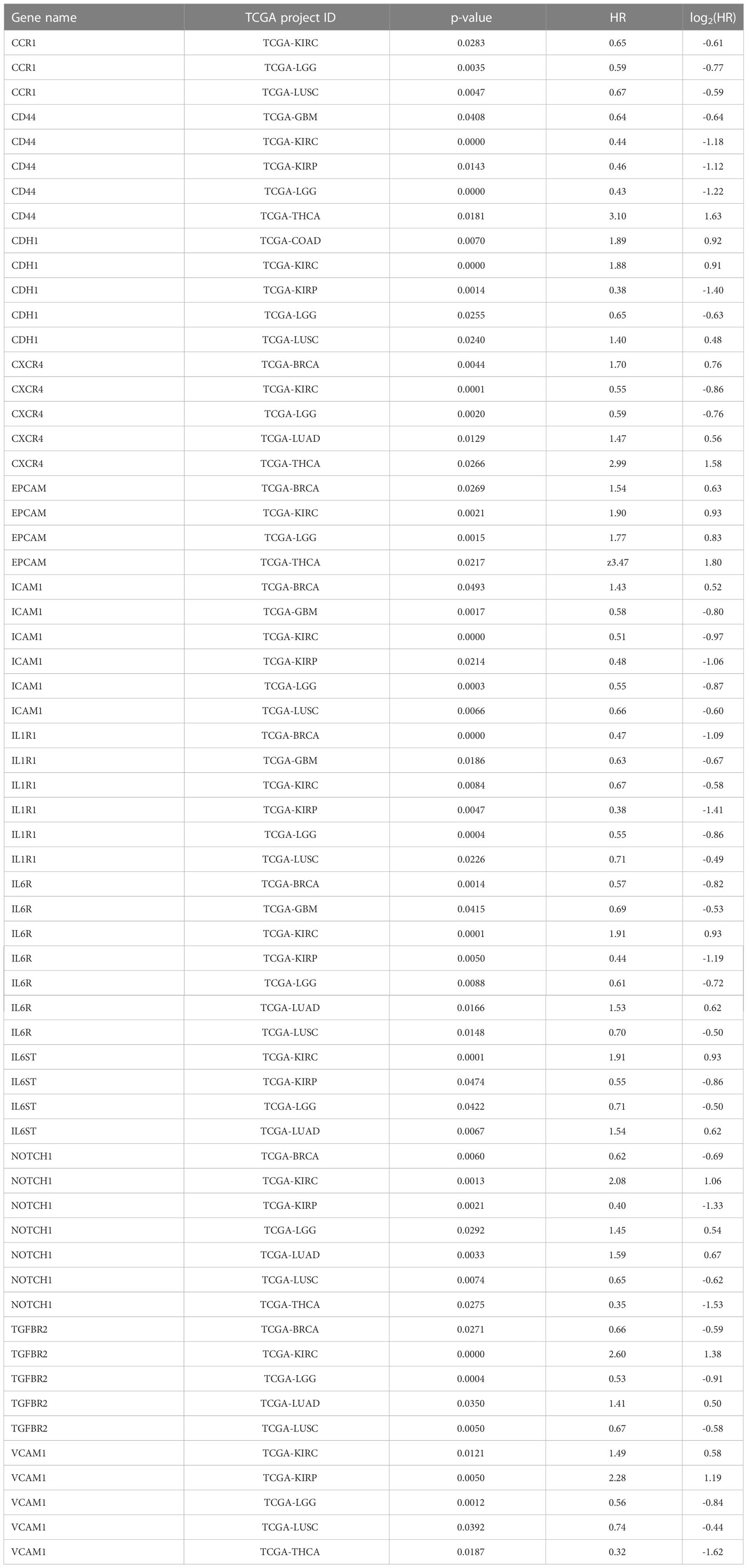
Table 2 p-values and Hazard ratio (HR) levels for survival of patients with high gene expression in tumors depicted in Figures 3B, C.
The clustering analysis separated CTC marker genes into two major groups: (i) CD44, CXCR4, ICAM1, CCR1, and IL1R1 where high expression correlated with poor survival for low grade gliomas (LGG), kidney renal clear cell carcinoma (KIRC), for glioblastoma (GBM), kidney renal papillary cell carcinoma (KIRP) or lung squamous cell carcinoma (LUSC) (Figure 3C).
The second gene cluster (ii) includes TGFBR2, IL6ST, IL6R, CDH1, and IGFBP5. In this group we observed a correlation between high expression and better prognosis in KIRC and worse prognosis in KIRP and LGG.
As discussed in the previous sections, molecules included in the analysis promote CTC cluster formation or metastasis in functional studies. Indeed, the results of clustering analysis suggest that high expression of genes from the first cluster predicts a rapid disease progression in multiple cancers. Conversely, the second cluster contains more genes whose expression promotes cancer progression in a cancer specific manner.
However, in some cases focused investigations contradict prediction of patient survival based on the TCGA dataset (Table 3, upper right triangles in Figure 3B depict approximates for HR collected from the literature). Specifically, high expression of stem cell marker CD44 corresponded to poor prognosis in kidney cancers (KIRC, KIRP) in TCGA data and, accordingly, high CD44 and b-catenin immunostaining correlated with advanced stage, although no significant correlation with survival could be observed in a specific focused study (117). However, other reports communicated a correlation between high CD44 levels and decrease of progression free survival in renal cell carcinoma after treatment with multi-targeted tyrosine kinase inhibitor (84). Consistent with the literature, high CD44 expression predicts inferior prognosis in LGG and GBM TCGA cohorts (85, 86). The only case of association between CD44 expression and positive thyroid cancer prognosis contradicts to the literature (87) (Figure 3B).
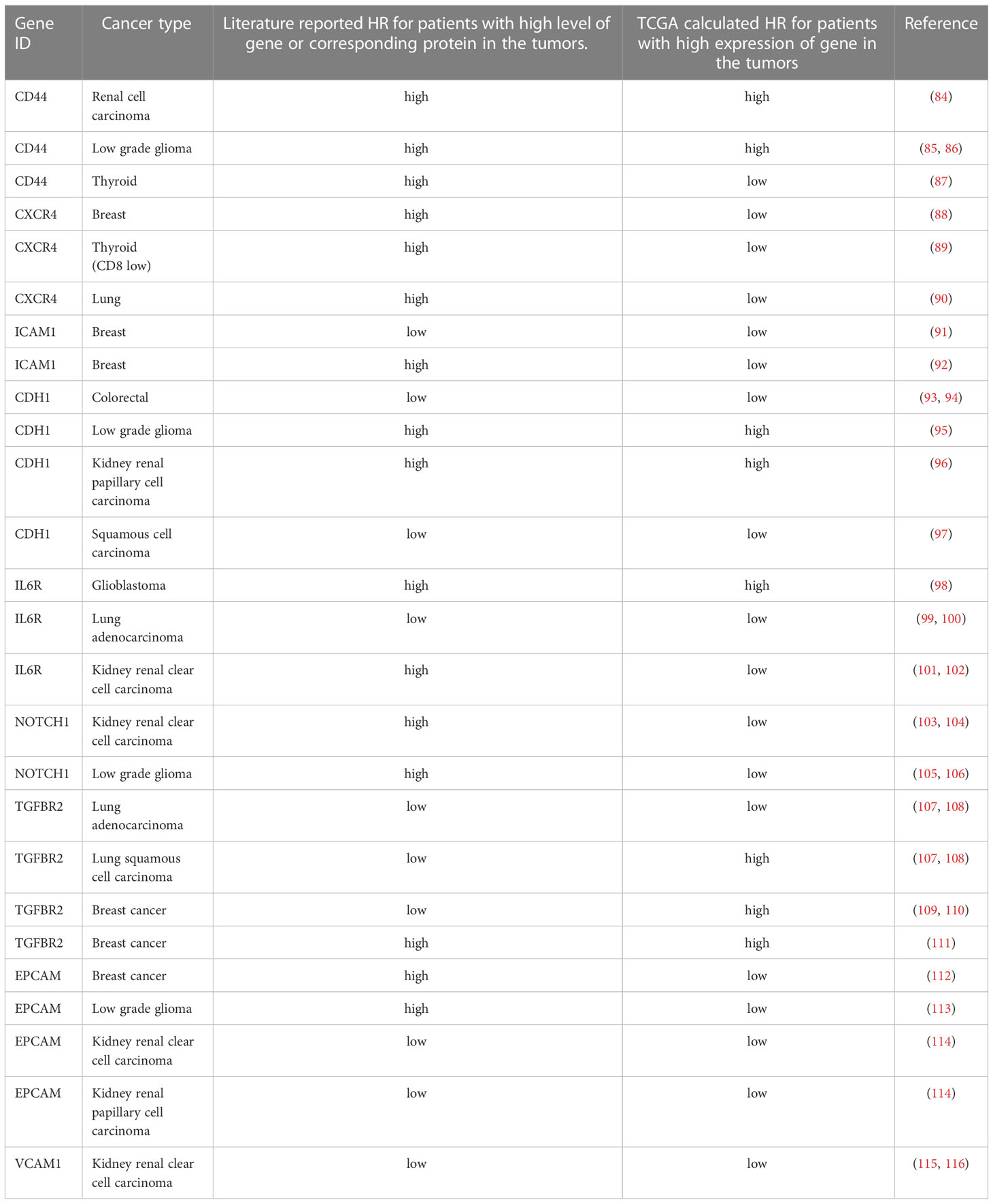
Table 3 Comparison between prediction of patient survival based on TCGA dataset and review of published literature.
It was reported that high CXCR4 expression corresponds to bad prognosis for breast (88), lung (90) and colorectal (118) cancers contradicting TCGA-based findings (Figures 3B, C). It was recently reported that in advanced CD8 negative thyroid cancer, high expression of CXCR4 and its ligand CXCL12 (SDF-1) correlates with bad prognosis, thus contradicting to TCGA data (89).
In contrast, ICAM1 expression is associated with favorable prognosis in the breast cancer TCGA cohort, consistent with similar survival analysis of NCBI GEO dataset and repression of the lung metastasis in spontaneous breast cancer metastasis model (91) and contradicting another paper reporting pivotal role of the ICAM1 in the CTC cluster formation, trans-endothelial migration and metastasis in breast cancer (92).
Thus, the positive associations between expression of CD44, CXCR4 and ICAM1 for thyroid, lung and breast cancers in TCGA dataset are not consistent with the literature suggesting that the first cluster is indeed represents genes whose high expression correlates with inferior prognosis consistent with their role in the CTCs biology.
Further we compared TCGA prediction with the literature for a few genes from the second cluster to address the question if they have more tissue specific roles in cancer metastasis.
One of such genes is CDH1 (E-cad protein) whose high expression was a predictor of better prognosis for colorectal cancer in agreement with TCGA data (93, 94). Again, consistent with TCGA data, high protein staining of E-cad in kidney renal papillary cell carcinoma was associated with worse prognosis, and no association was detected for kidney renal clear cell carcinoma (96). Recent analysis of E-cad in the cohort of NSCLC with 66% cases representing squamous cell carcinoma identifies E-cad as a positive prognostic factor consistent with TCGA data (97).
When astrocytomas, oligodendrogliomas and oligoastrocytomas were analyzed, the loss of E-cad immunostaining and hypermethylation of its promoter were associated with worse prognosis contradicting TCGA data, although, gene expression analysis was not performed (95). In contrast, consistent with TCGA data, a positive association between higher E-cad expression and worse prognosis was reported in the low-grade gliomas and in glioblastoma (119, 120).
IL6 receptors IL6ST and IL6R are involved in the CTC heterotypic interactions in breast (16) and colorectal cancers (31). Consistent with the literature, expression of IL6R has strong prognostic value in glioblastoma (98) and in lung adenocarcinoma (99, 100). In contrast, in kidney clear cell carcinoma we found a contradiction between the literature and TCGA data concerning the biomarker potential of IL6R expression: good predictor according to the literature (101, 102), and poor predictor according to TCGA data. Thus, the role of IL6R expression in cancer can be considered tissue specific.
The member of TGFb receptor family - TGFBR2 is a tumor suppressor in lung cancer, and the loss of TGFBR2 expression is associated with worse prognosis of both squamous cell cancer and adenocarcinoma (107, 108). Accordingly, TGFBR2 mutation predicts lung cancer resistance to checkpoint inhibitors (121). Thus, the literature supports prediction of TCGA dataset regarding the role of TGFBR2 in LUAD progression and contradicts association of high TGFBR2 with negative prognosis in LUSC. In breast cancer, reduced expression of TGFBR2 is associated with worse prognosis contradicting the TCGA data (109) especially in ER positive patients (110), while the report by Gao and coauthors is in line with the TCGA data (111). Theoretically, these contradictions might be connected with the presence of TGFBR2 mutations which were not investigated in these published reports. Little is known about the influence of the TGFBR2 on glioma survival, however TCGA prediction of the negative association might be valuable since TGBFR2 compensates for inhibition of PDGFR, thereby promoting survival (122).
NOTCH1 activation as measured by the immunostaining against NOTCH intracellular domain correlates with poor prognosis of kidney renal clear cell carcinoma (KIRC) (123). In turn, high total NOTCH1 immunostaining is associated with progression of kidney renal clear cell carcinoma contradicting TCGA prediction (103, 104). Likewise, in contrast to TCGA data, literature suggests association of NOTCH1 expression and glioma progression by modulating CXCL12/CXCR4 (105, 124).
In contrast, a recent meta-analysis revealed that NOTCH1 expression does not correlate with overall survival in adenocarcinoma, although DLL4 and HES1 were associated with worse prognosis (125).
Measurements of VCAM1 in KIRC revealed association of high expression with good prognosis consistent with TCGA data analysis (115, 116). In turn, for KIRP we found no published data that can validate the association of VCAM1 high expression with good prognosis observed for the TCGA dataset.
EPCAM expression was associated with favorable prognosis of breast cancer in TCGA data, however immunohistochemical analysis has shown that it is associated with worse prognosis specifically in the basal-like and luminal B HER2+ subtypes (113). However, in the HER2+ subtype, EPCAM was also reported to be associated with worse prognosis (112). Again, in LGG the protein level of EPCAM was associated with poor prognosis, which contradicts to the TCGA trends (113). In thyroid cancers, the presence of EPCAM cleavage product was associated with more aggressive disease progression, although gene expression was not measured in this report (126). Finally, in agreement with the TCGA dataset, high EPCAM expression was associated with better prognosis in kidney cancers (114).
Overall, after comparison of TCGA data with the literature, it is possible to conclude that genes of the first cluster (top, Figure 3B) are mostly predictors of poor prognosis, whereas genes of the second cluster (bottom, Figure 3B) predict survival in a cancer type-specific manner (Figure 3B).
For interrogation of TCGA expression and survival data, we used standard analytic tools from the TCGA project portal GDC (127, 128). The discrepancies between results of TCGA data analysis and the literature could originate from different experimental methods used to assess gene expression, or different cohorts of patients and different treatment regimens among others. Thus, results of positive or negative gene association with patient survival require independent verification to identify or to confirm reliable biomarkers of disease progression and potential targets for drug development.
6 Molecules involved in the CTC heterotypic interaction and known drug targets
Analysis of the TCGA data and the literature revealed that high expression of molecules involved in the CTC heterotypic interactions predicts survival in many cancer types. Accordingly, as it is discussed in the previous sections, these molecules are pivotal for metastasis and therefore sometimes represent targets of clinically approved or experimental cancer drugs. Specifically, results of TCGA dataset analysis suggest poor prognosis for IL6 overexpressing low grade gliomas and glioblastomas. Indeed, pre-clinical data demonstrated that IL6 blockade combined with CD40 stimulation sensitized glioblastoma to immune checkpoint inhibitors and improved survival (129, 130). Likewise, pre-clinical investigations revealed that targeting of the IL6 signaling might be beneficial for other cancers as well, where bad prognosis is associated with high IL6 level such as renal cell carcinoma (131, 132), non-small cell lung cancer (133), and breast cancer (134). We found a single, currently suspended clinical trial of the IL6R antibody tocilizumab for gliomas and glioblastoma treatment (NCT04729959), trials for metastatic breast cancer (NCT03135171), non-small lung cancer among others (NCT04940299, Table 4). Targeting of IL-6 improves immunotherapy outcome in mice models (155, 156). However, IL6-specific antibody siltuximab demonstrated no efficiency against renal cell carcinoma (157) and prostate cancer (158).
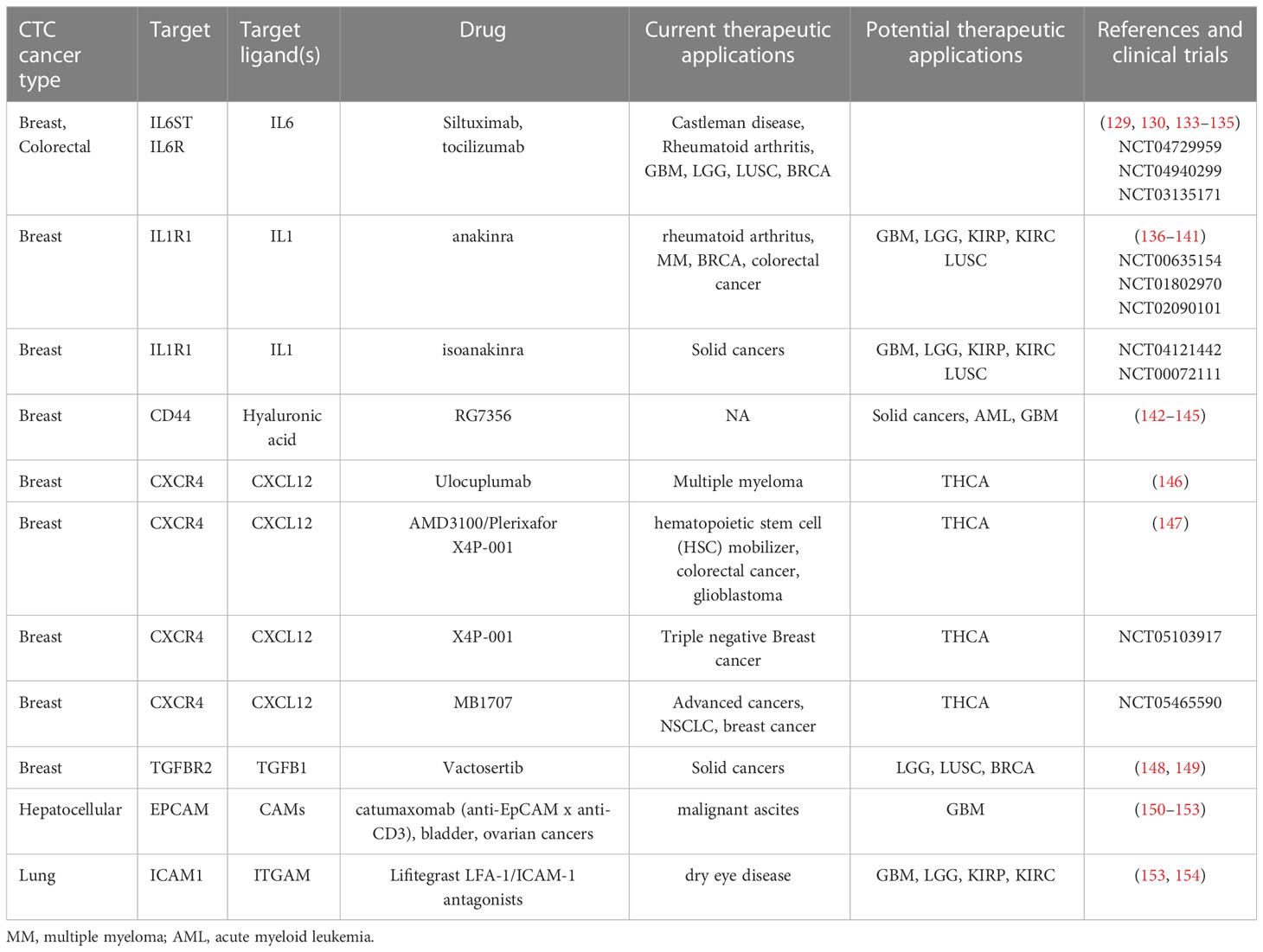
Table 4 Potential off-label applications of drugs targeting molecules involved in the heterotypic CTCs interactions.
IL1R1 expression predicts poor survival in nearly the same set of cancer types as IL6R. There are multiple clinical trials testing IL1R agonist an anti-rheumatoid arthritis drug anakinra against multiple myeloma (136), metastatic breast cancer, and colorectal cancer (159), listed in Table 4. However, we didn’t find any specific records for gliomas, lung or kidney cancers. Still, several preclinical investigations have shown that targeting of IL1 signaling in GBM (137, 138), LGG (139) kidney (140) and lung cancer (141) suggest its potential clinical usefulness.
Expression of adhesion molecule ICAM1 also predicts poor prognosis for several cancer types, closely mimicking the effects observed for the IL1R1 and IL6R genes. Specifically, low ICAM1 expression corresponds to better survival in GBM. Indeed, bispecific CAR-T cells against EPCAM and ICAM1 elicited good response in GBM mice model (153), consistent with other preclinical studies (160, 161). Similarly, CAR-T cells targeted against ICAM1 were successfully tested in mice models of gastric (162), thyroid (163, 164), and triple negative breast cancer (165).
ICAM-1 conjugated with a cytotoxic drug was extensively tested for multiple myeloma (166) and another bispecific anti-CD38-ICAM-1 drug for multiple myeloma is under development (167). The vaccine targeting ICAM-1 is also at the early stage of clinical investigation against ICAM-1 overexpressing bladder cancers (168) or lung cancer (NCT02043665). However, so far, we did not find reports on ICAM-1 targeted therapies clinically tested against gliomas and kidney cancers.
A stem cell marker CD44 predicts poor prognosis in renal cancers and in gliomas. The CD44-specific antibody RG7356 in clinical trials showed moderate efficiency in solid tumors (142) and in acute myeloid leukemia (169). There is also multiple evidence suggesting potential efficiency of CD44 targeting for the treatment of GBM, although additional clinical validation is clearly needed (143–145).
Catumaxomab (genetically engineered bivalent anti-EPCAM and anti-CD3 antibody) is approved for the treatment of malignant ascites (150) and it has been also used experimentally for the treatment of bladder (151) and ovarian (152) cancers. Bispecific CAR-T simultaneously targeting EPCAM and ICAM-1 demonstrated promising results in mice models of gastric and pancreatic cancers (153).
Anti-CXCR4 antibody demonstrated efficiency in multiple myeloma in combination with lenalidomide or bortezomib plus dexamethasone (146), and several related clinical trials are ongoing. A CXCR4 inhibitor AMD3100/Plerixafor was approved by FDA as a hematopoietic stem cell mobilizer and it was recently tested in humans against pancreatic and colorectal cancers as the potential inducer of the immune response (147). Also, preclinical studies showed that inhibition of the CXCR4 might be potentially efficient against other cancers including GBM (170, 171), and the first human clinical trial of plerixafor as an adjunct to combined chemoradiotherapy was conducted in newly diagnosed GBM patients (172) achieving median overall survival of ~21 months. This is a significant improvement over ~17 months period characteristic for the standard chemoradiotherapy (173).
Finally, gamma secretase inhibitors showed therapeutic effects only in CNS tumors and desmoids (174). Targeting of TGF-β receptor is also in development and in clinical trials (148). In turn, anti-VCAM antibodies dramatically reduced pancreatic ductal adenocarcinoma progression in mice models (175, 176).
In Table 4, we summarized drugs targeting molecules involved in the CTC heterotypic interactions.
7 Conclusions
Analysis of the literature describing factors leading to formation of CTC clusters revealed three major features. First - the presence of either heterotypic or homotypic CTC aggregates often means unfavorable prognosis and predicts metastasis in many cancer types. Targeting the formation of such clusters is a valuable strategy for metastasis suppression (4, 6, 17). Second - cells carry mesenchymal (Vimentin) and epithelial (E-cad) markers together, which is a hallmark of intermediate epithelial associated with stemness of cancer cells (177, 178). Third - in turn, intermediate Mesenchymal- Epithelial state frequently coincides with polyploidy as it was shown in lung and colorectal cancers (179, 180). In lung cancer, polyploidy was accompanied by the interaction with WBCs, which were identified as neutrophils or PMN-MDSCs.
It is well established that both polyploidy/mixed EMT phenotype and immunosuppressive PMN-MDSC and TAM contribute to cancer progression, however, how the interaction between them mediates metastatic advantage is yet to be investigated.
Taken together, these findings highlight common mechanisms of metastasis with implication for drug development and cancer treatment.
Author contributions
JMR wrote the text, edited figures and performed data analysis, TM wrote the text and prepared figures, AAB conceptualization and text writing and editing, JMR, AAB and TM These authors contributed equally to this work and share first authorship. OAR, DAD, VVP, and IVA collected related literature, compiled data and wrote the manuscript. All authors contributed to the article and approved the submitted version.
Funding
This study was supported by Russian Scienсе Foundation (project No. 22-15-00483), https://rscf.ru/en/project/22-15-00483/.
Conflict of interest
The authors declare that the research was conducted in the absence of any commercial or financial relationships that could be construed as a potential conflict of interest.
Publisher’s note
All claims expressed in this article are solely those of the authors and do not necessarily represent those of their affiliated organizations, or those of the publisher, the editors and the reviewers. Any product that may be evaluated in this article, or claim that may be made by its manufacturer, is not guaranteed or endorsed by the publisher.
References
1. Hanahan D. Hallmarks of cancer: New dimensions. Cancer Discovery (2022) 12(1):31–46. doi: 10.1158/2159-8290.CD-21-1059
2. Blomberg OS, Spagnuolo L, de Visser KE. Immune regulation of metastasis: Mechanistic insights and therapeutic opportunities. Dis Model Mech (2018) 11(10). doi: 10.1242/dmm.036236
3. Kim K-J, Godarova A, Seedle K, Kim M-H, Ince TA, Wells SI, et al. Rb Suppresses collective invasion, circulation and metastasis of breast cancer cells in CD44-dependent manner. PloS One (2013) 8(12):e80590. doi: 10.1371/journal.pone.0080590
4. Matsumura Y, Ito Y, Mezawa Y, Sulidan K, Daigo Y, Hiraga T, et al. Stromal fibroblasts induce metastatic tumor cell clusters via epithelial-mesenchymal plasticity. Life Sci Alliance (2019) 2(4). doi: 10.26508/lsa.201900425
5. Richardson AM, Havel LS, Koyen AE, Konen JM, Shupe J, Wiles WG, et al. Vimentin is required for lung adenocarcinoma metastasis via heterotypic tumor cell-Cancer-Associated fibroblast interactions during collective invasion. Clin Cancer Res (2018) 24(2):420–32. doi: 10.1158/1078-0432.CCR-17-1776
6. Gkountela S, Castro-Giner F, Szczerba BM, Vetter M, Landin J, Scherrer R, et al. Circulating tumor cell clustering shapes DNA methylation to enable metastasis seeding. Cell (2019) 176(1–2):98–112.e14. doi: 10.1016/j.cell.2018.11.046
7. Rozenberg JM, Zvereva S, Dalina A, Blatov I, Zubarev I, Luppov D, et al. Dual role of p73 in cancer microenvironment and DNA damage response. Cells (2021) 10(12). doi: 10.3390/cells10123516
8. Herrera M, Galindo-Pumariño C, García-Barberán V, Peña C. A snapshot of the tumor microenvironment in colorectal cancer: The liquid biopsy. Int J Mol Sci (2019) 20(23). doi: 10.3390/ijms20236016
9. Nakamura M, Suetsugu A, Hasegawa K, Satake T, Kunisada T, Shimizu M, et al. Color-coded imaging distinguishes cancer cells, stromal cells, and recombinant cancer-stromal cells in the tumor microenvironment during metastasis. Anticancer Res (2018) 38(8):4417–23. doi: 10.21873/anticanres.12743
10. Shtam T, Naryzhny S, Kopylov A, Petrenko E, Samsonov R, Kamyshinsky R, et al. Functional properties of circulating exosomes mediated by surface-attached plasma proteins. J Hematol (2018) 7(4):149–53. doi: 10.14740/jh412w
11. Shtam T, Naryzhny S, Samsonov R, Karasik D, Mizgirev I, Kopylov A, et al. Plasma exosomes stimulate breast cancer metastasis through surface interactions and activation of FAK signaling. Breast Cancer Res Treat (2019) 174(1):129–41. doi: 10.1007/s10549-018-5043-0
12. Costa-Silva B, Aiello NM, Ocean AJ, Singh S, Zhang H, Thakur BK, et al. Pancreatic cancer exosomes initiate pre-metastatic niche formation in the liver. Nat Cell Biol (2015) 17(6):816–26. doi: 10.1038/ncb3169
13. Ao Z, Shah SH, Machlin LM, Parajuli R, Miller PC, Rawal S, et al. Identification of cancer-associated fibroblasts in circulating blood from patients with metastatic breast cancer. Cancer Res (2015) 75(22):4681–7. doi: 10.1158/0008-5472.CAN-15-1633
14. Sharma U, Medina-Saenz K, Miller PC, Troness B, Spartz A, Sandoval-Leon A, et al. Heterotypic clustering of circulating tumor cells and circulating cancer-associated fibroblasts facilitates breast cancer metastasis. Breast Cancer Res Treat (2021) 189(1):63–80. doi: 10.1007/s10549-021-06299-0
15. Chen Q, Zou J, He Y, Pan Y, Yang G, Zhao H, et al. A narrative review of circulating tumor cells clusters: A key morphology of cancer cells in circulation promote hematogenous metastasis. Front Oncol (2022) 12:944487. doi: 10.3389/fonc.2022.944487
16. Szczerba BM, Castro-Giner F, Vetter M, Krol I, Gkountela S, Landin J, et al. Neutrophils escort circulating tumour cells to enable cell cycle progression. Nature (2019) 566(7745):553–7. doi: 10.1038/s41586-019-0915-y
17. Sprouse ML, Welte T, Boral D, Liu HN, Yin W, Vishnoi M, et al. PMN-MDSCs enhance CTC metastatic properties through reciprocal interactions via ROS/Notch/Nodal signaling. Int J Mol Sci (2019) 20(8). doi: 10.3390/ijms20081916
18. Aceto N, Bardia A, Miyamoto DT, Donaldson MC, Wittner BS, Spencer JA, et al. Circulating tumor cell clusters are oligoclonal precursors of breast cancer metastasis. Cell (2014) 158(5):1110–22. doi: 10.1016/j.cell.2014.07.013
19. Jansson S, Bendahl P-O, Larsson A-M, Aaltonen KE, Rydén L. Prognostic impact of circulating tumor cell apoptosis and clusters in serial blood samples from patients with metastatic breast cancer in a prospective observational cohort. BMC Cancer. (2016) 16:433. doi: 10.1186/s12885-016-2406-y
20. Duda DG, Duyverman AMMJ, Kohno M, Snuderl M, Steller EJA, Fukumura D, et al. Malignant cells facilitate lung metastasis by bringing their own soil. Proc Natl Acad Sci USA (2010) 107(50):21677–82. doi: 10.1073/pnas.1016234107
21. Li Z, Fan L, Wu Y, Niu Y, Zhang X, Wang B, et al. Analysis of the prognostic role and biological characteristics of circulating tumor cell-associated white blood cell clusters in non-small cell lung cancer. J Thorac Dis (2022) 14(5):1544–55. doi: 10.21037/jtd-22-423
22. Zhu H-H, Liu Y-T, Feng Y, Zhang L-N, Zhang T-M, Dong G-L, et al. Circulating tumor cells (CTCs)/circulating tumor endothelial cells (CTECs) and their subtypes in small cell lung cancer: Predictors for response and prognosis. Thorac Cancer. (2021) 12(20):2749–57. doi: 10.1111/1759-7714.14120
23. Guan Y, Xu F, Tian J, Gao K, Wan Z, Wang Y, et al. The prognostic value of circulating tumour cells (CTCs) and CTC white blood cell clusters in patients with renal cell carcinoma. BMC Cancer. (2021) 21(1):826. doi: 10.1186/s12885-021-08463-7
24. Xu Y, Zhang Q, Xu Z, Xie Q, Ding W, Liu H, et al. Association of circulating tumor cell-white blood cell clusters with survival outcomes in patients with colorectal cancer after curative intent surgery. Res Sq. (2022). doi: 10.21203/rs.3.rs-1969451/v1
25. Chu H-Y, Yang C-Y, Yeh P-H, Hsu C-J, Chang L-W, Chan W-J, et al. Highly correlated recurrence prognosis in patients with metastatic colorectal cancer by synergistic consideration of circulating tumor Cells/Microemboli and tumor markers CEA/CA19-9. Cells (2021) 10(5). doi: 10.3390/cells10051149
26. Kozuka M, Battaglin F, Jayachandran P, Wang J, Arai H, Soni S, et al. Clinical significance of circulating tumor cell induced epithelial-mesenchymal transition in patients with metastatic colorectal cancer by single-cell RNA-sequencing. Cancers (Basel) (2021) 13(19). doi: 10.3390/cancers13194862
27. Herath S, Razavi Bazaz S, Monkman J, Ebrahimi Warkiani M, Richard D, O’Byrne K, et al. Circulating tumor cell clusters: Insights into tumour dissemination and metastasis. Expert Rev Mol Diagn. (2020) 20(11):1139–47. doi: 10.1080/14737159.2020.1846523
28. Mizukoshi K, Okazawa Y, Haeno H, Koyama Y, Sulidan K, Komiyama H, et al. Metastatic seeding of human colon cancer cell clusters expressing the hybrid epithelial/mesenchymal state. Int J Cancer. (2020) 146(9):2547–62. doi: 10.1002/ijc.32672
29. Hu C-L, Zhang Y-J, Zhang X-F, Fei X, Zhang H, Li C-G, et al. 3D culture of circulating tumor cells for evaluating early recurrence and metastasis in patients with hepatocellular carcinoma. Onco Targets Ther (2021) 14:2673–88. doi: 10.2147/OTT.S298427
30. Spicer JD, McDonald B, Cools-Lartigue JJ, Chow SC, Giannias B, Kubes P, et al. Neutrophils promote liver metastasis via mac-1-mediated interactions with circulating tumor cells. Cancer Res (2012) 72(16):3919–27. doi: 10.1158/0008-5472.CAN-11-2393
31. Wei C, Yang C, Wang S, Shi D, Zhang C, Lin X, et al. Crosstalk between cancer cells and tumor associated macrophages is required for mesenchymal circulating tumor cell-mediated colorectal cancer metastasis. Mol Cancer. (2019) 18(1):64. doi: 10.1186/s12943-019-0976-4
32. Chen IX, Chauhan VP, Posada J, Ng MR, Wu MW, Adstamongkonkul P, et al. Blocking CXCR4 alleviates desmoplasia, increases T-lymphocyte infiltration, and improves immunotherapy in metastatic breast cancer. Proc Natl Acad Sci USA (2019) 116(10):4558–66. doi: 10.1073/pnas.1815515116
33. Lu Y, Lian S, Ye Y, Yu T, Liang H, Cheng Y, et al. S-nitrosocaptopril prevents cancer metastasis in vivo by creating the hostile bloodstream microenvironment against circulating tumor cells. Pharmacol Res (2019) 139:535–49. doi: 10.1016/j.phrs.2018.10.020
34. Giesing M, Suchy B, Driesel G, Molitor D. Clinical utility of antioxidant gene expression levels in circulating cancer cell clusters for the detection of prostate cancer in patients with prostate-specific antigen levels of 4-10 ng/mL and disease prognostication after radical prostatectomy. BJU Int (2010) 105(7):1000–10. doi: 10.1111/j.1464-410X.2009.08920.x
35. Osmulski PA, Cunsolo A, Chen M, Qian Y, Lin C-L, Hung C-N, et al. Contacts with macrophages promote an aggressive nanomechanical phenotype of circulating tumor cells in prostate cancer. Cancer Res (2021) 81(15):4110–23. doi: 10.1158/0008-5472.CAN-20-3595
36. Alhaddad L, Pustovalova M, Blokhina T, Chuprov-Netochin R, Osipov AN, Leonov S. IR-surviving NSCLC cells exhibit different patterns of molecular and cellular reactions relating to the multifraction irradiation regimen and p53-family proteins expression. Cancers (Basel) (2021) 13(11). doi: 10.3390/cancers13112669
37. Zhao Q, Zhang K, Li Z, Zhang H, Fu F, Fu J, et al. High migration and invasion ability of pgccs and their daughter cells associated with the nuclear localization of S100A10 modified by sumoylation. Front Cell Dev Biol (2021) 9:696871. doi: 10.3389/fcell.2021.696871
38. Rozenberg JM, Zvereva S, Dalina A, Blatov I, Zubarev I, Luppov D, et al. The p53 family member p73 in the regulation of cell stress response. Biol Direct. (2021) 16(1):23. doi: 10.1186/s13062-021-00307-5
39. Park J, Wysocki RW, Amoozgar Z, Maiorino L, Fein MR, Jorns J, et al. Cancer cells induce metastasis-supporting neutrophil extracellular DNA traps. Sci Transl Med (2016) 8(361):361ra138. doi: 10.1126/scitranslmed.aag1711
40. Vishnoi M, Peddibhotla S, Yin W, T Scamardo A, George GC, Hong DS, et al. The isolation and characterization of CTC subsets related to breast cancer dormancy. Sci Rep (2015) 5:17533. doi: 10.1038/srep17533
41. Vishnoi M, Boral D, Liu H, Sprouse ML, Yin W, Goswami-Sewell D, et al. Targeting USP7 identifies a metastasis-competent state within bone marrow-resident melanoma CTCs. Cancer Res (2018) 78(18):5349–62. doi: 10.1158/0008-5472.CAN-18-0644
42. Zeng X, Liao G, Li S, Liu H, Zhao X, Li S, et al. Eliminating METTL1-mediated accumulation of PMN-MDSCs prevents hepatocellular carcinoma recurrence after radiofrequency ablation. Hepatology (2022). doi: 10.1002/hep.32585
43. Lu X, Lu X. Enhancing immune checkpoint blockade therapy of genitourinary malignancies by co-targeting PMN-MDSCs. Biochim Biophys Acta Rev Cancer. (2022) 1877(3):188702. doi: 10.1016/j.bbcan.2022.188702
44. Zhou J, Nefedova Y, Lei A, Gabrilovich D. Neutrophils and PMN-MDSC: Their biological role and interaction with stromal cells. Semin Immunol (2018) 35:19–28. doi: 10.1016/j.smim.2017.12.004
45. Gabrilovich DI. Myeloid-derived suppressor cells. Cancer Immunol Res (2017) 5(1):3–8. doi: 10.1158/2326-6066.CIR-16-0297
46. Liu Q, Liao Q, Zhao Y. Myeloid-derived suppressor cells (MDSC) facilitate distant metastasis of malignancies by shielding circulating tumor cells (CTC) from immune surveillance. Med Hypotheses. (2016) 87:34–9. doi: 10.1016/j.mehy.2015.12.007
47. Boral D, Vishnoi M, HN L, Yin W, Sprouse ML, Scamardo A, et al. Molecular characterization of breast cancer CTCs associated with brain metastasis. Nat Commun (2017) 8(1):196. doi: 10.1038/s41467-017-00196-1
48. Raya A, Kawakami Y, Rodriguez-Esteban C, Buscher D, Koth CM, Itoh T, et al. Notch activity induces nodal expression and mediates the establishment of left-right asymmetry in vertebrate embryos. Genes Dev (2003) 17(10):1213–8. doi: 10.1101/gad.1084403
49. Arnoletti JP, Fanaian N, Reza J, Sause R, Almodovar AJ, Srivastava M, et al. Pancreatic and bile duct cancer circulating tumor cells (CTC) form immune-resistant multi-cell type clusters in the portal venous circulation. Cancer Biol Ther (2018) 19(10):887–97. doi: 10.1080/15384047.2018.1480292
50. Silvestri M, Reduzzi C, Feliciello G, Vismara M, Schamberger T, Köstler C, et al. Detection of genomically aberrant cells within circulating tumor microemboli (CTMs) isolated from early-stage breast cancer patients. Cancers (Basel) (2021) 13(6). doi: 10.3390/cancers13061409
51. Osmulski P, Mahalingam D, Gaczynska ME, Liu J, Huang S, Horning AM, et al. Nanomechanical biomarkers of single circulating tumor cells for detection of castration resistant prostate cancer. Prostate (2014) 74(13):1297–307. doi: 10.1002/pros.22846
52. Gruber I, Landenberger N, Staebler A, Hahn M, Wallwiener D, Fehm T. Relationship between circulating tumor cells and peripheral T-cells in patients with primary breast cancer. Anticancer Res (2013) 33(5):2233–8.
53. Mego M, Gao H, Cohen EN, Anfossi S, Giordano A, Sanda T, et al. Circulating tumor cells (CTC) are associated with defects in adaptive immunity in patients with inflammatory breast cancer. J Cancer. (2016) 7(9):1095–104. doi: 10.7150/jca.13098
54. Muraro E, Del Ben F, Turetta M, Cesselli D, Bulfoni M, Zamarchi R, et al. Clinical relevance of the combined analysis of circulating tumor cells and anti-tumor T-cell immunity in metastatic breast cancer patients. Front Oncol (2022) 12:983887. doi: 10.3389/fonc.2022.983887
55. Xue D, Xia T, Wang J, Chong M, Wang S, Zhang C. Role of regulatory T cells and CD8+ T lymphocytes in the dissemination of circulating tumor cells in primary invasive breast cancer. Oncol Lett (2018) 16(3):3045–53. doi: 10.3892/ol.2018.8993
56. Palumbo JS, Talmage KE, Massari JV, La Jeunesse CM, Flick MJ, Kombrinck KW, et al. Platelets and fibrin(ogen) increase metastatic potential by impeding natural killer cell-mediated elimination of tumor cells. Blood (2005) 105(1):178–85. doi: 10.1182/blood-2004-06-2272
57. Erpenbeck L, Schön MP. Deadly allies: the fatal interplay between platelets and metastasizing cancer cells. Blood (2010) 115(17):3427–36. doi: 10.1182/blood-2009-10-247296
58. Contursi A, Sacco A, Grande R, Dovizio M, Patrignani P. Platelets as crucial partners for tumor metastasis: from mechanistic aspects to pharmacological targeting. Cell Mol Life Sci (2017) 74(19):3491–507. doi: 10.1007/s00018-017-2536-7
59. Placke T, Örgel M, Schaller M, Jung G, Rammensee H-G, Kopp H-G, et al. Platelet-derived MHC class I confers a pseudonormal phenotype to cancer cells that subverts the antitumor reactivity of natural killer immune cells. Cancer Res (2012) 72(2):440–8. doi: 10.1158/0008-5472.CAN-11-1872
60. Ting DT, Wittner BS, Ligorio M, Vincent Jordan N, Shah AM, Miyamoto DT, et al. Single-cell RNA sequencing identifies extracellular matrix gene expression by pancreatic circulating tumor cells. Cell Rep (2014) 8(6):1905–18. doi: 10.1016/j.celrep.2014.08.029
61. Labelle M, Begum S, Hynes RO. Direct signaling between platelets and cancer cells induces an epithelial-mesenchymal-like transition and promotes metastasis. Cancer Cell (2011) 20(5):576–90. doi: 10.1016/j.ccr.2011.09.009
62. Chen VL, Huang Q, Harouaka R, Du Y, Lok AS, Parikh ND, et al. A dual-filtration system for single-cell sequencing of circulating tumor cells and clusters in HCC. Hepatol Commun (2022) 6(6):1482–91. doi: 10.1002/hep4.1900
63. Teeuwssen M, Fodde R. Cell heterogeneity and phenotypic plasticity in metastasis formation: The case of colon cancer. Cancers (Basel) (2019) 11(9). doi: 10.3390/cancers11091368
64. Zeisberg M, Neilson EG. Biomarkers for epithelial-mesenchymal transitions. J Clin Invest. (2009) 119(6):1429–37. doi: 10.1172/JCI36183
65. Guen VJ, Chavarria TE, Kröger C, Ye X, Weinberg RA, Lees JA. EMT programs promote basal mammary stem cell and tumor-initiating cell stemness by inducing primary ciliogenesis and hedgehog signaling. Proc Natl Acad Sci USA (2017) 114(49):E10532–9. doi: 10.1073/pnas.1711534114
66. Taki M, Abiko K, Ukita M, Murakami R, Yamanoi K, Yamaguchi K, et al. Tumor immune microenvironment during epithelial-mesenchymal transition. Clin Cancer Res (2021) 27(17):4669–79. doi: 10.1158/1078-0432.CCR-20-4459
67. Majc B, Sever T, Zarić M, Breznik B, Turk B, Lah TT. Epithelial-to-mesenchymal transition as the driver of changing carcinoma and glioblastoma microenvironment. Biochim Biophys Acta Mol Cell Res (2020) 1867(10):118782. doi: 10.1016/j.bbamcr.2020.118782
68. Li M, Gao F, Ren X, Dong G, Chen H, Lin AY, et al. Nonhematogenic circulating aneuploid cells confer inferior prognosis and therapeutic resistance in gliomas. Cancer Sci (2022) 113(10):3535–46. doi: 10.1111/cas.15516
69. Hu B, Gong Y, Wang Y, Xie J, Cheng J, Huang Q. Comprehensive atlas of circulating rare cells detected by SE-iFISH and image scanning platform in patients with various diseases. Front Oncol (2022) 12:821454. doi: 10.3389/fonc.2022.821454
70. Mirzayans R, Andrais B, Murray D. Roles of polyploid/multinucleated giant cancer cells in metastasis and disease relapse following anticancer treatment. Cancers (Basel) (2018) 10(4). doi: 10.3390/cancers10040118
71. Fei F, Zhang D, Yang Z, Wang S, Wang X, Wu Z, et al. The number of polyploid giant cancer cells and epithelial-mesenchymal transition-related proteins are associated with invasion and metastasis in human breast cancer. J Exp Clin Cancer Res (2015) 34:158. doi: 10.1186/s13046-015-0277-8
72. Alhaddad L, Chuprov-Netochin R, Pustovalova M, Osipov AN, Leonov S. Polyploid/Multinucleated giant and slow-cycling cancer cell enrichment in response to X-ray irradiation of human glioblastoma multiforme cells differing in radioresistance and TP53/PTEN status. Int J Mol Sci (2023) 24(2). doi: 10.3390/ijms24021228
73. Luo Q, Wang C, Peng B, Pu X, Cai L, Liao H, et al. Circulating tumor-Cell-Associated white blood cell clusters in peripheral blood indicate poor prognosis in patients with hepatocellular carcinoma. Front Oncol (2020) 10:1758. doi: 10.3389/fonc.2020.01758
74. Wang C, Luo Q, Huang W, Zhang C, Liao H, Chen K, et al. Correlation between circulating tumor cell DNA genomic alterations and mesenchymal CTCs or CTC-associated white blood cell clusters in hepatocellular carcinoma. Front Oncol (2021) 11:686365. doi: 10.3389/fonc.2021.686365
75. Lin PP, Gires O, Wang DD, Li L, Wang H. Comprehensive in situ co-detection of aneuploid circulating endothelial and tumor cells. Sci Rep (2017) 7(1):9789. doi: 10.1038/s41598-017-10763-7
76. Ye Z, Ding Y, Chen Z, Li Z, Ma S, Xu Z, et al. Detecting and phenotyping of aneuploid circulating tumor cells in patients with various malignancies. Cancer Biol Ther (2019) 20(4):546–51. doi: 10.1080/15384047.2018.1538000
77. Boya M, Ozkaya-Ahmadov T, Swain BE, Chu C-H, Asmare N, Civelekoglu O, et al. High throughput, label-free isolation of circulating tumor cell clusters in meshed microwells. Nat Commun (2022) 13(1):3385. doi: 10.1038/s41467-022-31009-9
78. Hou J-M, Krebs M, Ward T, Sloane R, Priest L, Hughes A, et al. Circulating tumor cells as a window on metastasis biology in lung cancer. Am J Pathol (2011) 178(3):989–96. doi: 10.1016/j.ajpath.2010.12.003
79. Mendonsa AM, Na T-Y. Gumbiner BM. E-cadherin contact inhibition cancer. Oncogene. (2018) 37(35):4769–80. doi: 10.1038/s41388-018-0304-2
80. Padmanaban V, Krol I, Suhail Y, Szczerba BM, Aceto N, Bader JS, et al. E-cadherin is required for metastasis in multiple models of breast cancer. Nature (2019) 573(7774):439–44. doi: 10.1038/s41586-019-1526-3
81. Na T-Y, Schecterson L, Mendonsa AM, Gumbiner BM. The functional activity of e-cadherin controls tumor cell metastasis at multiple steps. Proc Natl Acad Sci USA (2020) 117(11):5931–7. doi: 10.1073/pnas.1918167117
82. Quan Q, Wang X, Lu C, Ma W, Wang Y, Xia G, et al. Cancer stem-like cells with hybrid epithelial/mesenchymal phenotype leading the collective invasion. Cancer Sci (2020) 111(2):467–76. doi: 10.1111/cas.14285
83. Tan H-X, Cao Z-B, He T-T, Huang T, Xiang C-L, Liu Y. TGFβ1 is essential for MSCs-CAFs differentiation and promotes HCT116 cells migration and invasion via JAK/STAT3 signaling. Onco Targets Ther (2019) 12:5323–34. doi: 10.2147/OTT.S178618
84. Sekino Y, Takemoto K, Murata D, Babasaki T, Kobatake K, Kitano H, et al. CD44 is involved in sunitinib resistance and poor progression-free survival after sunitinib treatment of renal cell carcinoma. Anticancer Res (2021) 41(10):4875–83. doi: 10.21873/anticanres.15301
85. Dong Q, Li Q, Wang M, Hu J, Dai J, Niu L, et al. Elevated CD44 expression predicts poor prognosis in patients with low-grade glioma. Oncol Lett (2019) 18(4):3698–704. doi: 10.3892/ol.2019.10728
86. Si D, Yin F, Peng J, Zhang G. High expression of CD44 predicts a poor prognosis in glioblastomas. Cancer Manag Res (2020) 12:769–75. doi: 10.2147/CMAR.S233423
87. Kim HM, Koo JS. Immunohistochemical analysis of cancer stem cell marker expression in papillary thyroid cancer. Front Endocrinol (Lausanne). (2019) 10:523. doi: 10.3389/fendo.2019.00523
88. Mukherjee D, Zhao J. The role of chemokine receptor CXCR4 in breast cancer metastasis. Am J Cancer Res (2013) 3(1):46–57.
89. Wilhelm A, Lemmenmeier I, Lalos A, Posabella A, Kancherla V, Piscuoglio S, et al. The prognostic significance of CXCR4 and SDF-1 in differentiated thyroid cancer depends on CD8+ density. BMC Endocr Disord (2022) 22(1):292. doi: 10.1186/s12902-022-01204-2
90. Qiu L, Xu Y, Xu H, Yu B. The clinicopathological and prognostic value of CXCR4 expression in patients with lung cancer: A meta-analysis. BMC Cancer. (2022) 22(1):681. doi: 10.1186/s12885-022-09756-1
91. Regev O, Kizner M, Roncato F, Dadiani M, Saini M, Castro-Giner F, et al. ICAM-1 on breast cancer cells suppresses lung metastasis but is dispensable for tumor growth and killing by cytotoxic T cells. Front Immunol (2022) 13:849701. doi: 10.3389/fimmu.2022.849701
92. Taftaf R, Liu X, Singh S, Jia Y, Dashzeveg NK, Hoffmann AD, et al. ICAM1 initiates CTC cluster formation and trans-endothelial migration in lung metastasis of breast cancer. Nat Commun (2021) 12(1):4867. doi: 10.1038/s41467-021-25189-z
93. Gao M, Zhang X, Li D, He P, Tian W, Zeng B. Expression analysis and clinical significance of eIF4E, VEGF-c, e-cadherin and MMP-2 in colorectal adenocarcinoma. Oncotarget (2016) 7(51):85502–14. doi: 10.18632/oncotarget.13453
94. Chang K, Jiang L, Sun Y, Li H. Effect of e-cadherin on prognosis of colorectal cancer: A meta-analysis update. Mol Diagn Ther (2022) 26(4):397–409. doi: 10.1007/s40291-022-00593-3
95. D’Urso PI, D’Urso OF, Storelli C, Catapano G, Gianfreda CD, Montinaro A, et al. Retrospective protein expression and epigenetic inactivation studies of CDH1 in patients affected by low-grade glioma. J Neurooncol. (2011) 104(1):113–8. doi: 10.1007/s11060-010-0481-5
96. Burandt E, Lübbersmeyer F, Gorbokon N, Büscheck F, Luebke AM, Menz A, et al. E-cadherin expression in human tumors: a tissue microarray study on 10,851 tumors. biomark Res (2021) 9(1):44. doi: 10.1186/s40364-021-00299-4
97. Gkogkou P, Peponi E, Ntaskagiannis D, Murray S, Demou A, Sainis I, et al. E-cadherin and syndecan-1 expression in patients with advanced non-small cell lung cancer treated with chemoradiotherapy. In Vivo. (2020) 34(1):453–9. doi: 10.21873/invivo.11795
98. Jiang Y, Han S, Cheng W, Wang Z, Wu A. NFAT1-regulated IL6 signalling contributes to aggressive phenotypes of glioma. Cell Commun Signal (2017) 15(1):54. doi: 10.1186/s12964-017-0210-1
99. Sun G, Wang T, Shi M, Zhou H, Wang J, Huang Z, et al. Low expression of IL6R predicts poor prognosis for lung adenocarcinoma. Ann Transl Med (2021) 9(13):1057. doi: 10.21037/atm-21-36
100. Xu B, Chen Q, Yue C, Lan L, Jiang J, Shen Y, et al. Prognostic value of IL-6R mRNA in lung adenocarcinoma and squamous cell carcinoma. Oncol Lett (2018) 16(3):2935–48. doi: 10.3892/ol.2018.9044
101. Fu Q, Chang Y, An H, Fu H, Zhu Y, Xu L, et al. Prognostic value of interleukin-6 and interleukin-6 receptor in organ-confined clear-cell renal cell carcinoma: A 5-year conditional cancer-specific survival analysis. Br J Cancer (2015) 113(11):1581–9. doi: 10.1038/bjc.2015.379
102. Pilskog M, Bostad L, Edelmann RJ, Akslen LA, Beisland C, Straume O. Tumour cell expression of interleukin 6 receptor α is associated with response rates in patients treated with sunitinib for metastatic clear cell renal cell carcinoma. J Pathol Clin Res (2018) 4(2):114–23. doi: 10.1002/cjp2.96
103. Zhuang Z, Lin J, Huang Y, Lin T, Zheng Z, Ma X. Notch 1 is a valuable therapeutic target against cell survival and proliferation in clear cell renal cell carcinoma. Oncol Lett (2017) 14(3):3437–44. doi: 10.3892/ol.2017.6587
104. Ai Q, Ma X, Huang Q, Liu S, Shi T, Zhang C, et al. High-level expression of Notch1 increased the risk of metastasis in T1 stage clear cell renal cell carcinoma. PloS One (2012) 7(4):e35022. doi: 10.1371/journal.pone.0035022
105. Yi L, Zhou X, Li T, Liu P, Hai L, Tong L, et al. Notch1 signaling pathway promotes invasion, self-renewal and growth of glioma initiating cells via modulating chemokine system CXCL12/CXCR4. J Exp Clin Cancer Res (2019) 38(1):339. doi: 10.1186/s13046-019-1319-4
106. Li J, Cui Y, Gao G, Zhao Z, Zhang H, Wang X. Notch1 is an independent prognostic factor for patients with glioma. J Surg Oncol (2011) 103(8):813–7. doi: 10.1002/jso.21851
107. Malkoski SP, Haeger SM, Cleaver TG, Rodriguez KJ, Li H, Lu S-L, et al. Loss of transforming growth factor beta type II receptor increases aggressive tumor behavior and reduces survival in lung adenocarcinoma and squamous cell carcinoma. Clin Cancer Res (2012) 18(8):2173–83. doi: 10.1158/1078-0432.CCR-11-2557
108. Nolan K, Tippimanchai D, Verzosa G, Zhao W, Li H, Phillip S, et al. Reduced TGFBR2 expression in NSCLC drives IL-17A dependent inflammation via γδT cells. J Thorac Oncol (2017) 12(8):S1537. doi: 10.1016/j.jtho.2017.06.023
109. Wei C-Y, Tan Q-X, Zhu X, Qin Q-H, Zhu F-B, Mo Q-G, et al. Expression of CDKN1A/p21 and TGFBR2 in breast cancer and their prognostic significance. Int J Clin Exp Pathol (2015) 8(11):14619–29.
110. Busch S, Sims AH, Stål O, Fernö M, Landberg G. Loss of tgfβ receptor type 2 expression impairs estrogen response and confers tamoxifen resistance. Cancer Res (2015) 75(7):1457–69. doi: 10.1158/0008-5472.CAN-14-1583
111. Gao N, Zhai Q, Li Y, Huang K, Bian D, Wang X, et al. Clinical implications of tβrii expression in breast cancer. PloS One (2015) 10(11):e0141412. doi: 10.1371/journal.pone.0141412
112. Soysal SD, Muenst S, Barbie T, Fleming T, Gao F, Spizzo G, et al. EpCAM expression varies significantly and is differentially associated with prognosis in the luminal b HER2(+), basal-like, and HER2 intrinsic subtypes of breast cancer. Br J Cancer. (2013) 108(7):1480–7. doi: 10.1038/bjc.2013.80
113. Chen X, Ma W-Y, Xu S-C, Liang Y, Fu Y-B, Pang B, et al. The overexpression of epithelial cell adhesion molecule (EpCAM) in glioma. J Neurooncol. (2014) 119(1):39–47. doi: 10.1007/s11060-014-1459-5
114. Zimpfer A, Maruschke M, Rehn S, Kundt G, Litzenberger A, Dammert F, et al. Prognostic and diagnostic implications of epithelial cell adhesion/activating molecule (EpCAM) expression in renal tumours: A retrospective clinicopathological study of 948 cases using tissue microarrays. BJU Int (2014) 114(2):296–302. doi: 10.1111/bju.12487
115. Labrousse-Arias D, Martínez-Alonso E, Corral-Escariz M, Bienes-Martínez R, Berridy J, Serrano-Oviedo L, et al. VHL promotes immune response against renal cell carcinoma via NF-κB-dependent regulation of VCAM-1. J Cell Biol (2017) 216(3):835–47. doi: 10.1083/jcb.201608024
116. Shioi K, Komiya A, Hattori K, Huang Y, Sano F, Murakami T, et al. Vascular cell adhesion molecule 1 predicts cancer-free survival in clear cell renal carcinoma patients. Clin Cancer Res (2006) 12(24):7339–46. doi: 10.1158/1078-0432.CCR-06-1737
117. Agrawal A, Datta C, Panda CK, Pal DK. Association of beta-catenin and CD44 in the development of renal cell carcinoma. Urologia (2021) 88(2):125–9. doi: 10.1177/0391560320980672
118. Ottaiano A, Santorsola M, Del Prete P, Perri F, Scala S, Caraglia M, et al. Prognostic significance of CXCR4 in colorectal cancer: An updated meta-analysis and critical appraisal. Cancers (Basel) (2021) 13(13):3284. doi: 10.3390/cancers13133284
119. Bourkoula E, Mangoni D, Ius T, Pucer A, Isola M, Musiello D, et al. Glioma-associated stem cells: a novel class of tumor-supporting cells able to predict prognosis of human low-grade gliomas. Stem Cells (2014) 32(5):1239–53. doi: 10.1002/stem.1605
120. Lewis-Tuffin LJ, Rodriguez F, Giannini C, Scheithauer B, Necela BM, Sarkaria JN, et al. Misregulated e-cadherin expression associated with an aggressive brain tumor phenotype. PloS One (2010) 5(10):e13665. doi: 10.1371/journal.pone.0013665
121. Li T, Wang H, Xu J, Li C, Zhang Y, Wang G, et al. TGFBR2 mutation predicts resistance to immune checkpoint inhibitors in patients with non-small cell lung cancer. Ther Adv Med Oncol (2021) 13:17588359211038476. doi: 10.1177/17588359211038477
122. Song K, Yuan Y, Lin Y, Wang Y-X, Zhou J, Gai Q-J, et al. ERBB3, IGF1R, and TGFBR2 expression correlate with PDGFR expression in glioblastoma and participate in PDGFR inhibitor resistance of glioblastoma cells. Am J Cancer Res (2018) 5:792–809.
123. An H, Zhu Y, Xu L, Chen L, Lin Z, Xu J. Notch1 predicts recurrence and survival of patients with clear-cell renal cell carcinoma after surgical resection. Urology (2015) 85(2):483.e9–483.e14. doi: 10.1016/j.urology.2014.10.022
124. D’Amico M, De Amicis F. Aberrant notch signaling in gliomas: a potential landscape of actionable converging targets for combination approach in therapies resistance. Cancer Drug Resist (2022) 5(4):939–53. doi: 10.20517/cdr.2022.46
125. Yuan X, Wu H, Xu H, Han N, Chu Q, Yu S, et al. Meta-analysis reveals the correlation of notch signaling with non-small cell lung cancer progression and prognosis. Sci Rep (2015) 5:10338. doi: 10.1038/srep10338
126. Ralhan R, Cao J, Lim T, Macmillan C, Freeman JL, Walfish PG. EpCAM nuclear localization identifies aggressive thyroid cancer and is a marker for poor prognosis. BMC Cancer. (2010) 10:331. doi: 10.1186/1471-2407-10-331
127. Love MI, Huber W, Anders S. Moderated estimation of fold change and dispersion for RNA-seq data with DESeq2. Genome Biol (2014) 15(12):550. doi: 10.1186/s13059-014-0550-8
128. Page RDM. Tree view: An application to display phylogenetic trees on personal computers. Bioinformatics (1996) 12(4):357–8. doi: 10.1093/bioinformatics/12.4.357
129. Yang F, He Z, Duan H, Zhang D, Li J, Yang H, et al. Synergistic immunotherapy of glioblastoma by dual targeting of IL-6 and CD40. Nat Commun (2021) 12(1):3424. doi: 10.1038/s41467-021-23832-3
130. Lamano JB, Lamano JB, Li YD, DiDomenico JD, Choy W, Veliceasa D, et al. Glioblastoma-derived IL6 induces immunosuppressive peripheral myeloid cell PD-L1 and promotes tumor growth. Clin Cancer Res (2019) 25(12):3643–57. doi: 10.1158/1078-0432.CCR-18-2402
131. Ishibashi K, Koguchi T, Matsuoka K, Onagi A, Tanji R, Takinami-Honda R, et al. Interleukin-6 induces drug resistance in renal cell carcinoma. Fukushima J Med Sci (2018) 64(3):103–10. doi: 10.5387/fms.2018-15
132. Gudbrandsdottir G, Aarstad HH, Bostad L, Hjelle KM, Aarstad HJ, Bruserud Ø, et al. Serum levels of the IL-6 family of cytokines predict prognosis in renal cell carcinoma (RCC). Cancer Immunol Immunother. (2021) 70(1):19–30. doi: 10.1007/s00262-020-02655-z
133. Ogawa H, Koyanagi-Aoi M, Otani K, Zen Y, Maniwa Y, Aoi T. Interleukin-6 blockade attenuates lung cancer tissue construction integrated by cancer stem cells. Sci Rep (2017) 7(1):12317. doi: 10.1038/s41598-017-12017-y
134. Chung AW, Kozielski AJ, Qian W, Zhou J, Anselme AC, Chan AA, et al. Tocilizumab overcomes chemotherapy resistance in mesenchymal stem-like breast cancer by negating autocrine IL-1A induction of IL-6. NPJ Breast Cancer. (2022) 8(1):30. doi: 10.1038/s41523-021-00371-0
135. Li L, Li Z, Lu C, Li J, Zhang K, Lin C, et al. Ibrutinib reverses IL-6-induced osimertinib resistance through inhibition of laminin α5/FAK signaling. Commun Biol (2022) 5(1):155. doi: 10.1038/s42003-022-03111-7
136. Lust JA, Lacy MQ, Zeldenrust SR, Witzig TE, Moon-Tasson LL, Dinarello CA, et al. Reduction in c-reactive protein indicates successful targeting of the IL-1/IL-6 axis resulting in improved survival in early stage multiple myeloma. Am J Hematol (2016) 91(6):571–4. doi: 10.1002/ajh.24352
137. Chen Z, Giotti B, Kaluzova M, Herting CJ, Pinero G, Vallcorba MP, et al. A paracrine circuit of IL-1β/IL-1R1 between myeloid and tumor cells drives glioblastoma progression. BioRxiv (2022). doi: 10.1101/2022.04.03.486888
138. Tarassishin L, Lim J, Weatherly DB, Angeletti RH, Lee SC. Interleukin-1-induced changes in the glioblastoma secretome suggest its role in tumor progression. J Proteomics. (2014) 99:152–68. doi: 10.1016/j.jprot.2014.01.024
139. Tarassishin L, Casper D, Lee SC. Aberrant expression of interleukin-1β and inflammasome activation in human malignant gliomas. PloS One (2014) 9(7):e103432. doi: 10.1371/journal.pone.0103432
140. Aggen DH, Ager CR, Obradovic AZ, Chowdhury N, Ghasemzadeh A, Mao W, et al. Blocking IL1 beta promotes tumor regression and remodeling of the myeloid compartment in a renal cell carcinoma model: Multidimensional analyses. Clin Cancer Res (2021) 27(2):608–21. doi: 10.1158/1078-0432.CCR-20-1610
141. Zhang J, Veeramachaneni N. Targeting interleukin-1β and inflammation in lung cancer. biomark Res (2022) 10(1):5. doi: 10.1186/s40364-021-00341-5
142. Menke-van der Houven van Oordt CW, Gomez-Roca C, van Herpen C, Coveler AL, Mahalingam D, Verheul HMW, et al. First-in-human phase I clinical trial of RG7356, an anti-CD44 humanized antibody, in patients with advanced, CD44-expressing solid tumors. Oncotarget (2016) 7(48):80046–58. doi: 10.18632/oncotarget.11098
143. Yeh M, Wang Y-Y, Yoo JY, Oh C, Otani Y, Kang JM, et al. MicroRNA-138 suppresses glioblastoma proliferation through downregulation of CD44. Sci Rep (2021) 11(1):9219. doi: 10.1038/s41598-021-88615-8
144. Xiao Y, Yang K, Wang Z, Zhao M, Deng Y, Ji W, et al. CD44-mediated poor prognosis in glioma is associated with M2-polarization of tumor-associated macrophages and immunosuppression. Front Surg (2021) 8:775194. doi: 10.3389/fsurg.2021.775194
145. Lubanska D, Alrashed S, Mason GT, Nadeem F, Awada A, DiPasquale M, et al. Impairing proliferation of glioblastoma multiforme with CD44+ selective conjugated polymer nanoparticles. Sci Rep (2022) 12(1):12078. doi: 10.1038/s41598-022-15244-0
146. Ghobrial IM, Liu C-J, Redd RA, Perez RP, Baz R, Zavidij O, et al. A phase Ib/II trial of the first-in-Class anti-CXCR4 antibody ulocuplumab in combination with lenalidomide or bortezomib plus dexamethasone in relapsed multiple myeloma. Clin Cancer Res (2020) 26(2):344–53. doi: 10.1158/1078-0432.CCR-19-0647
147. Biasci D, Smoragiewicz M, Connell CM, Wang Z, Gao Y, Thaventhiran JED, et al. CXCR4 inhibition in human pancreatic and colorectal cancers induces an integrated immune response. Proc Natl Acad Sci USA (2020) 117(46):28960–70. doi: 10.1073/pnas.2013644117
148. Kim B-G, Malek E, Choi SH, Ignatz-Hoover JJ, Driscoll JJ. Novel therapies emerging in oncology to target the TGF-β pathway. J Hematol Oncol (2021) 14(1):55. doi: 10.1186/s13045-021-01053-x
149. Teixeira AF, Ten Dijke P, Zhu H-J. On-target anti-TGF-β therapies are not succeeding in clinical cancer treatments: What are remaining challenges? Front Cell Dev Biol (2020) 8:605. doi: 10.3389/fcell.2020.00605
150. Jäger M, Schoberth A, Ruf P, Hess J, Hennig M, Schmalfeldt B, et al. Immunomonitoring results of a phase II/III study of malignant ascites patients treated with the trifunctional antibody catumaxomab (anti-EpCAM x anti-CD3). Cancer Res (2012) 72(1):24–32. doi: 10.1158/0008-5472.CAN-11-2235
151. Ruf P, Bauer HW, Schoberth A, Kellermann C, Lindhofer H. First time intravesically administered trifunctional antibody catumaxomab in patients with recurrent non-muscle invasive bladder cancer indicates high tolerability and local immunological activity. Cancer Immunol Immunother. (2021) 70(9):2727–35. doi: 10.1007/s00262-021-02930-7
152. Sehouli J, Reinthaller A, Marth C, Reimer D, Reimer T, Stummvoll W, et al. Intra- and postoperative catumaxomab in patients with epithelial ovarian cancer: Safety and two-year efficacy results from a multicentre, single-arm, phase II study. Br J Cancer. (2014) 111(8):1519–25. doi: 10.1038/bjc.2014.443
153. Yang Y, McCloskey JE, Yang H, Puc J, Alcaina Y, Vedvyas Y, et al. Bispecific CAR T cells against EpCAM and inducible ICAM-1 overcome antigen heterogeneity and generate superior antitumor responses. Cancer Immunol Res (2021) 9(10):1158–74. doi: 10.1158/2326-6066.CIR-21-0062
154. Haber SL, Benson V, Buckway CJ, Gonzales JM, Romanet D, Scholes B. Lifitegrast: a novel drug for patients with dry eye disease. Ther Adv Ophthalmol (2019) 11:2515841419870366. doi: 10.1177/2515841419870366
155. Hailemichael Y, Johnson DH, Abdel-Wahab N, Foo WC, Bentebibel S-E, Daher M, et al. Interleukin-6 blockade abrogates immunotherapy toxicity and promotes tumor immunity. Cancer Cell (2022) 40(5):509–523.e6. doi: 10.1016/j.ccell.2022.04.004
156. Bent EH, Millán-Barea LR, Zhuang I, Goulet DR, Fröse J, Hemann MT. Microenvironmental IL-6 inhibits anti-cancer immune responses generated by cytotoxic chemotherapy. Nat Commun (2021) 12(1):6218. doi: 10.1038/s41467-021-26407-4
157. Rossi JF, Négrier S, James ND, Kocak I, Hawkins R, Davis H, et al. A phase I/II study of siltuximab (CNTO 328), an anti-interleukin-6 monoclonal antibody, in metastatic renal cell cancer. Br J Cancer (2010) 103(8):1154–62. doi: 10.1038/sj.bjc.6605872
158. Fizazi K, De Bono JS, Flechon A, Heidenreich A, Voog E, Davis NB, et al. Randomised phase II study of siltuximab (CNTO 328), an anti-IL-6 monoclonal antibody, in combination with mitoxantrone/prednisone versus mitoxantrone/prednisone alone in metastatic castration-resistant prostate cancer. Eur J Cancer (2012) 48(1):85–93. doi: 10.1016/j.ejca.2011.10.014
159. Gottschlich A, Endres S, Kobold S. Therapeutic strategies for targeting IL-1 in cancer. Cancers (Basel) (2021) 13(3):477. doi: 10.3390/cancers13030477
160. Piao Y, Henry V, Tiao N, Park SY, Martinez-Ledesma J, Dong JW, et al. Targeting intercellular adhesion molecule-1 prolongs survival in mice bearing bevacizumab-resistant glioblastoma. Oncotarget (2017) 8(57):96970–83. doi: 10.18632/oncotarget.18859
161. Yoo K-C, Kang J-H, Choi M-Y, Suh Y, Zhao Y, Kim M-J, et al. Soluble ICAM-1 a pivotal communicator between tumors and macrophages, promotes mesenchymal shift of glioblastoma. Adv Sci (Weinh). (2022) 9(2):e2102768. doi: 10.1002/advs.202102768
162. Jung M, Yang Y, McCloskey JE, Zaman M, Vedvyas Y, Zhang X, et al. Chimeric antigen receptor T cell therapy targeting ICAM-1 in gastric cancer. Mol Ther Oncolytics. (2020) 18:587–601. doi: 10.1016/j.omto.2020.08.009
163. Min IM, Shevlin E, Vedvyas Y, Zaman M, Wyrwas B, Scognamiglio T, et al. CAR T therapy targeting ICAM-1 eliminates advanced human thyroid tumors. Clin Cancer Res (2017) 23(24):7569–83. doi: 10.1158/1078-0432.CCR-17-2008
164. Gray KD, McCloskey JE, Vedvyas Y, Kalloo OR, Eshaky SE, Yang Y, et al. PD1 blockade enhances ICAM1-directed CAR T therapeutic efficacy in advanced thyroid cancer. Clin Cancer Res (2020) 26(22):6003–16. doi: 10.1158/1078-0432.CCR-20-1523
165. Wei H, Wang Z, Kuang Y, Wu Z, Zhao S, Zhang Z, et al. Intercellular adhesion molecule-1 as target for CAR-T-Cell therapy of triple-negative breast cancer. Front Immunol (2020) 11:573823. doi: 10.3389/fimmu.2020.573823
166. Sherbenou DW, Su Y, Behrens CR, Aftab BT, Perez de Acha O, Murnane M, et al. Potent activity of an anti-ICAM1 antibody-drug conjugate against multiple myeloma. Clin Cancer Res (2020) 26(22):6028–38. doi: 10.1158/1078-0432.CCR-20-0400
167. Chen X, Wong OK, Post L. CD38 x ICAM1 bispecific antibody is a novel approach for treating multiple myeloma and lymphoma. Blood (2021) 138(Supplement 1):2413–3. doi: 10.1182/blood-2021-146041
168. Annels NE, Mansfield D, Arif M, Ballesteros-Merino C, Simpson GR, Denyer M, et al. Phase I trial of an ICAM-1-Targeted immunotherapeutic-coxsackievirus A21 (CVA21) as an oncolytic agent against non muscle-invasive bladder cancer. Clin Cancer Res (2019) 25(19):5818–31. doi: 10.1158/1078-0432.CCR-18-4022
169. Vey N, Delaunay J, Martinelli G, Fiedler W, Raffoux E, Prebet T, et al. Phase I clinical study of RG7356, an anti-CD44 humanized antibody, in patients with acute myeloid leukemia. Oncotarget (2016) 7(22):32532–42. doi: 10.18632/oncotarget.8687
170. Cornelison RC, Brennan CE, Kingsmore KM, Munson JM. Convective forces increase CXCR4-dependent glioblastoma cell invasion in GL261 murine model. Sci Rep (2018) 8(1):17057. doi: 10.1038/s41598-018-35141-9
171. Daniele S, La Pietra V, Piccarducci R, Pietrobono D, Cavallini C, D’Amore VM, et al. CXCR4 antagonism sensitizes cancer cells to novel indole-based MDM2/4 inhibitors in glioblastoma multiforme. Eur J Pharmacol (2021) 897:173936. doi: 10.1016/j.ejphar.2021.173936
172. Thomas RP, Nagpal S, Iv M, Soltys SG, Bertrand S, Pelpola JS, et al. Macrophage exclusion after radiation therapy (MERT): A first in human phase I/II trial using a CXCR4 inhibitor in glioblastoma. Clin Cancer Res (2019) 25(23):6948–57. doi: 10.1158/1078-0432.CCR-19-1421
173. Brown NF, Ottaviani D, Tazare J, Gregson J, Kitchen N, Brandner S, et al. Survival outcomes and prognostic factors in glioblastoma. Cancers (Basel) (2022) 14(13):3161. doi: 10.3390/cancers14133161
174. McCaw TR, Inga E, Chen H, Jaskula-Sztul R, Dudeja V, Bibb JA, et al. Gamma secretase inhibitors in cancer: A current perspective on clinical performance. Oncologist (2021) 26(4):e608–21. doi: 10.1002/onco.13627
175. Sano M, Takahashi R, Ijichi H, Ishigaki K, Yamada T, Miyabayashi K, et al. Blocking VCAM-1 inhibits pancreatic tumour progression and cancer-associated thrombosis/thromboembolism. Gut (2021) 70(9):1713–23. doi: 10.1136/gutjnl-2020-320608
176. Takahashi R, Ijichi H, Sano M, Miyabayashi K, Mohri D, Kim J, et al. Soluble VCAM-1 promotes gemcitabine resistance via macrophage infiltration and predicts therapeutic response in pancreatic cancer. Sci Rep (2020) 10(1):21194. doi: 10.1038/s41598-020-78320-3
177. Grosse-Wilde A, Fouquier d’Hérouël A, McIntosh E, Ertaylan G, Skupin A, Kuestner RE, et al. Stemness of the hybrid Epithelial/Mesenchymal state in breast cancer and its association with poor survival. PloS One (2015) 10:e0126522. doi: 10.1371/journal.pone.0126522
178. Kuburich NA, den Hollander P, Pietz JT, Mani SA. Vimentin and cytokeratin: Good alone, bad together. Semin Cancer Biol (2022) 86(Pt 3):816–26. doi: 10.1016/j.semcancer.2021.12.006
179. Rohnalter V, Roth K, Finkernagel F, Adhikary T, Obert J, Dorzweiler K, et al. A multi-stage process including transient polyploidization and EMT precedes the emergence of chemoresistent ovarian carcinoma cells with a dedifferentiated and pro-inflammatory secretory phenotype. Oncotarget (2015) 6(37):40005–25. doi: 10.18632/oncotarget.5552
Keywords: cancer, metastasis, circulating cancer cell, tumor microenvironment, heterotypic cell interactions
Citation: Rozenberg JM, Buzdin AA, Mohammad T, Rakitina OA, Didych DA, Pleshkan VV and Alekseenko IV (2023) Molecules promoting circulating clusters of cancer cells suggest novel therapeutic targets for treatment of metastatic cancers. Front. Immunol. 14:1099921. doi: 10.3389/fimmu.2023.1099921
Received: 16 November 2022; Accepted: 28 February 2023;
Published: 15 March 2023.
Edited by:
Silvia Pesce, University of Genoa, ItalyReviewed by:
Abhijit Aithal, University of Nebraska Medical Center, United StatesSabrina Giglio, University of Cagliari, Italy
Roberto Piñeiro, Health Research Institute of Santiago de Compostela (IDIS), Spain
Copyright © 2023 Rozenberg, Buzdin, Mohammad, Rakitina, Didych, Pleshkan and Alekseenko. This is an open-access article distributed under the terms of the Creative Commons Attribution License (CC BY). The use, distribution or reproduction in other forums is permitted, provided the original author(s) and the copyright owner(s) are credited and that the original publication in this journal is cited, in accordance with accepted academic practice. No use, distribution or reproduction is permitted which does not comply with these terms.
*Correspondence: Anton Buzdin, YnV6ZGluQG9uY29ib3guY29t
†These authors have contributed equally to this work