- 1Department of Tissue Engineering and Cell Therapy, School of Advanced Technologies in Medicine, Shiraz University of Medical Sciences, Shiraz, Iran
- 2Student Research Committee, Shiraz University of Medical Sciences, Shiraz, Iran
- 3Department of Tissue Engineering and Applied Cell Science, School of Advanced Technologies in Medicine, Shaheed Beheshti University of Medical Sciences, Tehran, Iran
- 4Department of Ophthalmology School of Medicine, Shiraz University of Medical Sciences, Shiraz, Iran
- 5Department of Pathology, University of Toronto, Toronto, ON, Canada
- 6Transplant Research Center, Shiraz University of Medical Sciences, Shiraz, Iran
Extracellular vesicles include exosomes, microvesicles, and apoptotic bodies. Their cargos contain a diverse variety of lipids, proteins, and nucleic acids that are involved in both normal physiology and pathology of the ocular system. Thus, studying extracellular vesicles may lead to a more comprehensive understanding of the pathogenesis, diagnosis, and even potential treatments for various diseases. The roles of extracellular vesicles in inflammatory eye disorders have been widely investigated in recent years. The term “inflammatory eye diseases” refers to a variety of eye conditions such as inflammation-related diseases, degenerative conditions with remarkable inflammatory components, neuropathy, and tumors. This study presents an overview of extracellular vesicles’ and exosomes’ pathogenic, diagnostic, and therapeutic values in inflammatory eye diseases, as well as existing and potential challenges.
1 Introduction
Globally, at least 2.2 billion individuals are affected by visual impairment or have an underlying visual condition that will eventually result in blindness (1). Epidemiological studies have demonstrated that the incidence of Inflammatory eye diseases (IEDs) and autoimmune ocular diseases has increased significantly over the past two decades (2). All-cause mortality is considerably higher in individuals with visual impairment compared with individuals with normal vision (3). IEDs are responsible for a significant proportion of presentations to ophthalmology clinics (4). IED is characterized by a broad spectrum of clinical manifestations, from relatively benign to potentially sight-threatening conditions. IEDs can variably affect all ocular parts; the inflammation can involve only the eyes or can present as a part of systemic inflammatory disorders (4). Various factors, including infections, allergies, autoimmune diseases, and trauma to the globe, eyelids, and surrounding tissues, can cause IEDs (5).
To avoid excessive immune responses and inflammations, various regulators are present in the eye (6, 7). These regulators activate tolerogenic antigen-presenting cells and regulatory T cells, suppress inflammatory activity, and produce anti-inflammatory cytokines (8, 9). Inflammation may lead to the breakdown of ocular immune privilege and progress to autoimmunity through improper activation of effector immune cells (e.g., T cells and B cells) and overexpression of proinflammatory mediators (10, 11).
Generally, IEDs are classified based on both the mechanism of injury and the affected location. Based on injury mechanism, IEDs are categorized as: (a) inflammation-related diseases, (b) degenerative conditions with remarkable inflammatory components, (c) neuropathy, and (d) tumors (12, 13). Typical examples of ocular degenerations are Dry Eye Syndrome (DES), glaucoma, Diabetic Retinopathy (DR), and Age-related Macular Degeneration (AMD). According to anatomical location, ocular inflammations are classified into two types: (a) extraocular (affecting the conjunctiva, cornea, and sclera) and (b) intraocular (primarily affecting the uvea and retina) such as conjunctivitis, keratitis, and uveitis (14, 15).
Although IEDs’ primary triggers may not be immune-related, chronic inflammation significantly influences the initiation, progression, and outcome of IEDs (14). The complicated and multifactorial nature of IEDs has led to multiple hypotheses regarding underlying mechanisms and the course of progression. Therefore, novel diagnostic and therapeutic strategies consider multiple mechanisms rather than focusing on a single one. Consequently, it is suggested to consider the immunoregulatory effects of Mesenchymal stem cells (MSCs) and cell-derived exosomes (Exos) in novel therapeutic approaches to IED (16–20).
The value of various extracellular vesicles (EVs) and cell-derived Exos has been extensively investigated in diagnostic and therapeutic studies of several ocular disorders (21–23). Recent reports propose exosomes as valuable diagnostic biomarkers of eye disorders (16, 24). Likewise, cell-derived Exos have gained scientific interest due to their promising therapeutic values. Cell-derived Exos are safer than intravitreal MSCs injections regarding complications such as blindness following hemorrhage or retinal detachment (25). Moreover, exosomes could be stored for a long time under certain conditions without sustaining noticeable damage to their RNA content (26). This study aims to review the evidence on the EVs’ and Exos’ pathogenic, diagnostic, and therapeutic values for IEDs and discuss the existing and potential challenges of clinical application.
EVs are secreted vesicles by cells to facilitate intercellular communication(27). EVs differ in terms of their size, surface proteins, and internal cargo and are classified based on these characteristics (28). Three main classifications of EVs are Exos (30-150 nm), microvesicles (100-1000 nm), and apoptotic bodies (>1000 nm) (28). Exosomes are formed during the maturation process of early endosomes to late endosomes due to the cargo loading into the inward budding of the multivesicular membrane (29). Microvesicles are generated through the plasma membrane’s outward budding (30). Apoptotic bodies are vesicles secreted by cells undergoing apoptosis, which consequently summon macrophages to remove the debris left behind by the cell’s death (31). Due to insufficient evidence on the therapeutic effects of apoptotic bodies on eye diseases, this review skips this topic. On the other hand, exosomes have been widely explored for their therapeutic effects on various inflammatory disorders (such as myocardial infarction, inflammatory bowel diseases, asthma, and systemic inflammations) (32–35).
Exosomes contain nucleic acids (DNA, RNA, microRNAs (miRNAs), and long noncoding RNA), metabolites, lipids, proteins, and peptides (36, 37). Exosome cargos are complicated compositions influenced by originated cell type and state (38), depending on the secretory cell and the triggering mechanism of exosome release. Exosomes can paradoxically both regulate and initiate inflammasome activation (38). Accordingly, stem cell-secreted Exos have immunosuppressive effects (by inhibiting inflammasome activation), whereas immune cell-secreted exosomes intensify inflammation (through promoting inflammasome activity). Therefore, EV loading occurs through a selective, specific, and active process. As a result, exosomes can play different roles in antigen presentation, extracellular matrix modulation, and immune regulation (39–43). Hence, the multivalent nature of exosomes proposes them as diagnostic biomarkers and promising multifactorial treatments for many diseases, including IEDs.
2 Inflammation-related diseases
2.1 Autoimmune uveitis
The term uveitis, or uveoretinitis, refers to a group of conditions characterized by intraocular inflammation involving the uvea and retina. Uveitis is responsible for approximately 10% of blindness cases (1). Depending on the anatomical location of the inflammation, it can be classified as anterior, intermediate, posterior, or pan-uveitis if it affects both the anterior and posterior parts of the eye (2). Additionally, according to the causative agent, uveitis is classified into two categories, infectious and non-infectious. The underlying cause of non-infectious uveitis is believed to be an autoimmune or immune-mediated process (3).
In some cases, autoimmune uveitis (AU) is accompanied by systemic autoimmune syndromes affecting organs other than the eyes, such as Behçet’s disease, systemic sarcoidosis, and Vogt-Koyanagi-Harada disease. On the other hand, isolated involvement of the eyes is not uncommon. This condition can be detected in some disorders, such as sympathetic ophthalmia, birdshot retinochoroidopathy, and idiopathic uveitis (3).
During AU, aside from the involvement of the uvea tract, other anatomical structures of the eye can also serve as transport pathways for inflammatory cells to spread to other ocular tissues. This permeability to inflammatory cells can facilitate the inflammatory process and increase the damage to other healthy tissues (4). A blood-retinal barrier (BRB) and a blood-aqueous barrier (BAB) are responsible for preventing the entry of large protein molecules and cells into the eye (5, 44). The uvea barrier, however, breaks down as a result of inflammation. Neutrophils predominantly enter the eye during acute uveitis, while mononuclear cells enter in chronic uveitis (6).
Currently, the treatments available for these patients are divided into two general categories: corticosteroids and immune modulatory drugs (45). Antimetabolites, alkylating agents, calcineurin inhibitors, and biological response modifiers are four classifications of immune modulatory medications used in treating AU (7). Even with these treatments, the uveitis prognosis is still poor; it may even result in blindness (8). Due to these conditions, there is still a need for more effective drugs with fewer side effects.
2.1.1 The potential of EVs in the pathogenesis of autoimmune uveitis
The inappropriate immune response mediated by T-cells can result in AU (46, 47). Furthermore, different inflammatory cytokines are involved in the process (48). A recent study on plasma-derived Exos of patients with Vogt-Koyanagi-Harada syndrome, a common type of AU, showed that they are involved in the inflammatory process of the disease (49). In this study, exosome proteins were analyzed and found to be involved in platelet activation, phagosome function, focal adhesion, actin cytoskeleton regulation, and migration of leukocytes across endothelial cells (49). Furthermore, the analysis revealed that two proteins present in exosomes were more abundant in patients at an active stage of inflammation as compared with controls. Therefore, the author suggested that the level of carbonic anhydrase 2(CA2) and Ras-related protein Rap-1b(RAP1B) in exosomes may be used as biomarkers of early inflammation attacks in patients (49). Exosome pathogenesis and biomarkers of AU are the subjects of limited research, and more studies are needed to uncover their underlying mechanisms (Table 1).
2.1.2 The potential of EVs in the treatment of autoimmune uveitis
Considering the disease’s pathogenesis, the treatment goals should be to suppress inflammation, prevent recurrences, and protect vision while reducing side effects; in this regard, applying EVs-based therapeutic approaches are among promising treatments (80). A recent study demonstrated that human umbilical cord MSC-derived Exos improved experimental autoimmune uveitis (EAU) in rat models by restricting the infiltration of inflammatory cells (81). Also, they demonstrated that MSC-Exo treatment inhibited the chemo-attractive effects of C–C motif chemokine ligands 2 (CCL2) and C–C motif chemokine ligand 21 (CCL21) on inflammatory cells, in addition to protecting retinal structure and function (81) Moreover, another study indicated that retinal pigment epithelium (RPE)-derived EVs of patients with non-infectious uveitis could inhibit T-cell proliferation and have anti-inflammatory effects on monocytes several studies (17). Furthermore, Kang et al. reported that Ex-vivo-generated interleukin-35-producing regulatory B-cells(i35-Bregs) secreted exosomes containing interleukin-35 (i35-Exos) could protect retinal function against uveitis by inhibiting Th-17 responses in (EAU) mice model (82). In another study with EAU mice, MSC-Exo was shown to prevent disruptions of the photoreceptor layer, inhibit antigen-presenting cells (APCs) activity, inhibit T-helper cell development (Th-1 and Th-17), increase the level of interleukin-10(IL-10) expression, and reduce proinflammatory cytokines transcription (interleukin-2(IL-2), interleukin-1b(IL-1b), interferon-gamma (IFN-g), interleukin-17A(IL-17A), interleukin-6(IL-6), and interleukin-12A(IL-12A)). However, the effectiveness of MSC-derived Exos on inhibition of T cell proliferation remains doubtful (18, 81) and more research is needed to clarify the issue. Exosomes derived from MSCs and some other sources may have anti-inflammatory and immunosuppressive properties. Consequently, they have the potential to be used as therapeutic agents.(Table 2)
2.2 Corneal diseases
The human cornea, transparency, and integrity are crucial to vision (5). Corneal stroma consists of keratocytes and a highly organized collagen matrix constricting almost 90% of corneal thickness. It plays a crucial role in maintaining corneal transparency (91, 94). Corneal injury can be caused by infections, mechanical traumas, chemical or thermal burns, and ocular and systemic disorders and, consequently, may lead to inflammation, neovascularization, ulceration, scar formation, and eventually visual impairment (95, 96).
Treating corneal diseases is still challenging for healthcare providers as corneal wounds heal through a complicated and multi-step process that includes apoptosis, cell proliferation, and migration (70, 91, 92). Current treatments such as topical antibiotics, corticosteroids, and non-steroidal anti-inflammatory drugs are mainly supportive care and do not entirely prevent corneal scars (91, 92). Therefore, corneal transplantation (keratoplasty) has been suggested as an effective therapeutic strategy that can restore vision and corneal integrity (64, 94). However, the risk of several complications (particularly immunological rejection) still remains and threatens graft acceptance (71).
2.2.1 The potential role of EVs in the pathogenesis of corneal diseases
Studies demonstrated that EVs are secreted in the wounded cornea and are able to communicate between the corneal stroma and epithelium (56, 95, 97). Also, Han et al. showed that mouse corneal epithelial cell-derived Exos might induce stromal fibroblast differentiation into myofibroblasts and play an essential role in corneal wound healing (70). Epithelial-derived Exos carry proteins like thrombospondin-2, latent-transforming growth factor beta-binding protein 1, C–X–C motif chemokine 5, and C-C motif chemokine 2, which are involved in wound healing and neovascularization (70, 71). Moreover, corneal fibroblasts secrete Exos that contain matrix metalloproteinase 14 (MMP14), which enhances corneal angiogenesis via degenerating vascular endothelial growth factor (VEGF) receptor 1 and VEGF-induced proliferation and migration of endothelial cells (72, 73). Corneal stromal stem cells (CSSCs)-derived and corneal MSCs-derived EVs modulate inflammatory responses during corneal wound healing through decreasing early neutrophil infiltration, changing macrophage phenotypes, and diminishing the production of inflammatory cytokines (91, 95, 98, 99) (Figure 1, Table 1).
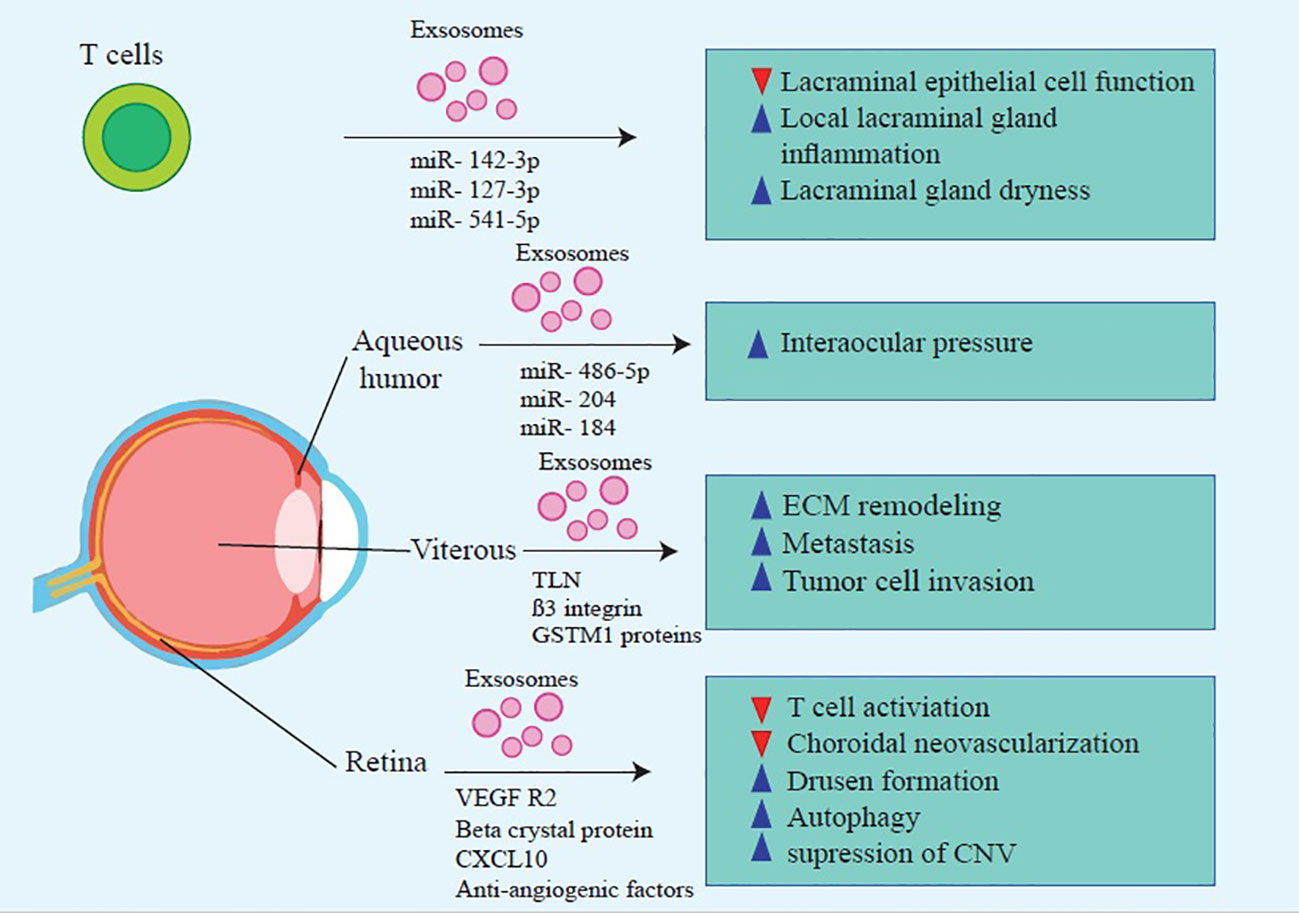
Figure 1 Molecular mechanisms that contribute to MSC-Exos’ beneficial effects on treating inflammatory ocular diseases.
2.2.2 Potential role of EVs in the treatment of corneal diseases
Cell-free EV-based treatments might be a promising treatment method for corneal diseases (56, 93). Exos derived from human corneal MSCs considerably improve corneal wound closure (91, 92). Besides, CSSC-derived EVs decrease stromal scarring and regenerate normal corneal collagen (91, 92). Also, adipose-derived MSC-derived Exos enhance corneal stromal cell proliferation, inhibit apoptosis, and are involved in extracellular matrix remodeling (87, 93).
Additionally, different studies showed that exosomes mediated adaptive immune responses and participated in corneal allograft rejection (100). MSC-derived Exos prolong corneal graft survival by prohibiting the Th1 signaling pathway, reducing chemokines like CXCL9, CXCL10, and CXCL11, and upregulating regulatory T cells (87, 101). Therefore, MSC-derived Exos might be potential therapeutic agents for refractory graft-versus-host disease and corneal transplant rejection (102, 103).
Various investigations indicated that MSC-derived EVs decrease the production of inflammatory cytokines, apoptosis, and corneal epithelial defects, consequently increasing corneal wound repair and stromal cell proliferation, propounding EVs as promising candidates for corneal disease therapy (95). However, there are still concerns about potency, pharmacokinetics, safety, route of administration, stability, and storage conditions (91). Therefore, further studies are warranted to address these concerns (Figure 2, Table 2).
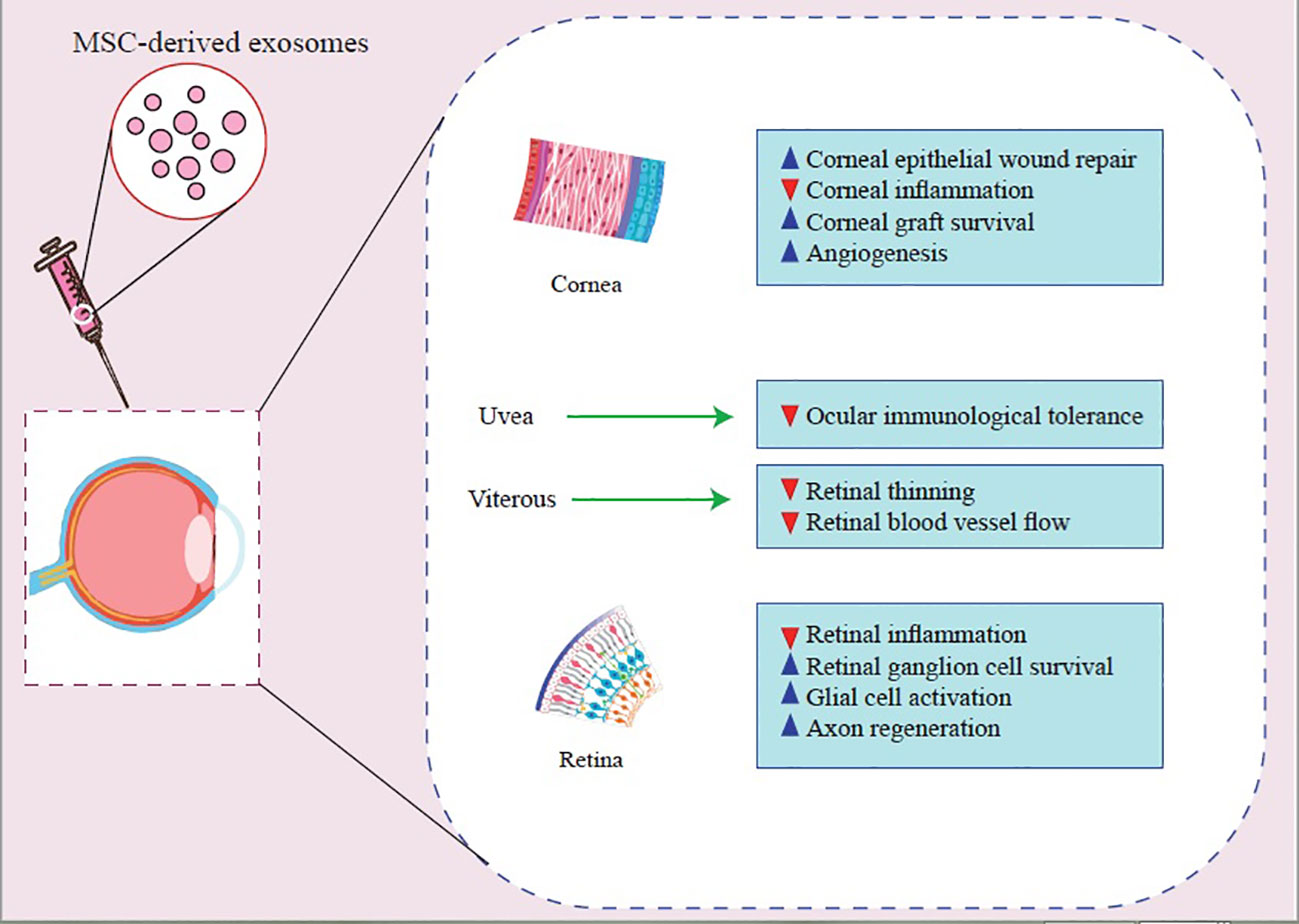
Figure 2 Molecular mechanisms that contribute to MSC-Exos’ beneficial effects on treating inflammatory ocular diseases.
3 Degenerative conditions
3.1 Sjogren’s syndrome
SS is a multifactorial autoimmune disorder characterized by infiltrating lymphocytes into exocrine glands resulting in impairment of their function (104). The dysfunction of the salivary glands and the lacrimal glands causes dryness in the mouth and the eye (105). In dry eye disease, tear production reduces and leads to inflammation of the orbital surface (106). While dry eye is associated with several diseases, it is generally more severe in patients with SS (107). There are two types of SS: solitary (primary (pSS)) or in association with another underlying autoimmune disorder (secondary) (108, 109). Even though the exact pathophysiology of pSS remains unclear, genetic predisposition, environmental factors, factors affecting the autonomic nervous system, hormonal factors, and the immune system (both innate and adoptive), in particular autoimmunity, are thought to be involved (110–112). In vulnerable individuals, a primary insult to the gland may trigger a series of events leading to the development of SS; this insult may be viral or non-viral (113, 114).
The revised criteria of American–European consensus group (AECG) For pSS diagnosis include (1):oral symptoms, (2) ocular symptoms, (3) ocular signs, (4) salivary gland involvement, and (5) histopathology and (6) autoantibodies; Autoantibodies which involved are Anti-Sjogren’s Syndrome A(Anti-SS-A) and anti-Sjogren’s syndrome type B (anti-SS-B) (115). The prevalence rate depends on the classification criteria; Based on AEGC criteria, the prevalence was reported between 0.09% to 0.72% (116–118).
Treatment options for SS include tear and saliva substitutes, immunosuppressants, and systemic secretagogues (119–121). While these medications are effective in treating SS, long-term use may result in adverse side effects (113, 120, 122). The treatment of SS is based on disease activity and extra glandular manifestations (123); however, there is increasing evidence that exosome may play a role in the treatment of SS (16, 24, 124).
3.1.1 The potential of EVs in the pathogenesis of SS
Kapsogeorgou et al. reported the first detection of SS-autoantigens in exosomes (containing: Ro/SSA, La/SSB, and Sm ribonucleoproteins (RNPs)), indicating that exosomes play an essential role in developing SS by delivering their contents to autoreactive lymphocytes (50). A recent study revealed that patients with pSS, compared to healthy individuals, contain a higher level of Epstein-Barr virus (EBV)-miR-BART13-3p, encoded by the virus. EBV-infected B lymphocytes transferred miRNA via exosomes to epithelial cells of salivary glands, resulting in a reduction in Aquaporin 5 (AQP5) and Stromal interaction molecule 1 (STIM1) protein level and calcium signaling impairment (51). Furthermore, another study demonstrated that activated T-lymphocytes secrete Exos that are transferred to glandular cells in patients suffering from pSS (52). These Exos contain miR-142-3p, which targets three proteins (sarcoplasmic reticulum Ca2+ ATPase2b (SERCA2B), adenylate cyclase 9, and ryanodine receptor 2). Consequently, T cell-derived Exos may impair calcium signaling, inhibits cyclic AMP (cAMP) production, and reduces salivary gland protein secretion (52). Moreover, an analysis of the tear fluid of patients with SS revealed the presence of 14 miRNAs that may be involved in the pathogenesis of the disease (53).
In several studies, the expression of miRNAs in salivary gland epithelial cells differed between patients and healthy individuals. Thus, they could serve as diagnostic biomarkers (125, 126). In patients with SS, EVs from saliva and tears were found to contain some components that may serve as new biomarkers for diseases that affect the salivary and lacrimal glands. These biomarkers include Copine-1 (CPNE1), Neutrophil gelatinase-associated lipocalin-2(LCN2), and Adipocyte plasma membrane-associated protein (APMAP), which are involved in Tumour necrosis factor α (TNFα) signaling, involved in innate immunity and apoptosis and adipocyte differentiation (127). Another study compared Exos from oral rinse samples from SS patients and healthy individuals. The results indicated that miR-1290, let-7b-5p, miR-3648, and miR-34a-5p were upregulated in patients with SS oral rinse-derived Exos compared to healthy ones. Therefore, miRNAs derived from oral rinse samples could be used to screen and diagnose SS non-invasively (23) (Table 1).
3.1.2 The potential of EVs in the treatment of SS
Despite the existing treatments, there is still a need for more effective treatments; using exosomes may be the promising treatment for SS treatment. Utilizing exosomes containing miRNAs from Chromosome19 Micro RNA Cluster (C19MC) analogs derived from the placenta could be a potential treatment for autoimmune diseases such as SS by targeting immune cells and suppressing their function (83). Li et al. treated mouse models of SS with Labial gland-derived MSCs (LGMSCs)-derived Exos; They reported that LGMSCs and their derived Exos decrease inflammatory cells infiltration to salivary glands and reserve their secretory function in non-obese diabetic (NOD) mice, raise regulatory T cells proliferation and inhibit T helper-cell 17 differentiation in NOD mice and SS patients invitro with increase Tcell secretions of IL-10 and transforming growth factor beta(TGF-β) and decreased cytokine levels(IL-6, IL-17, Interferon-gamma) (85). Also, another study has reported that injection of olfactory recto-mesenchymal stem cell-derived Exos (OE-MSC-Exos) into experimental Sjogren’s syndrome (ESS) mice models increased saliva flow rate and decreased tissue damage (84). OE-MSC-Exos increase Nitric Oxide (NO) and Reactive Oxygen Species (ROS) level and upregulate arginase expression; All these together lead to improve myeloid-derived suppressor cells (MDSCs) suppressive function (84). Also MDSCs found to be increased in mice model with ESS, however its suppressive function decreased with disease progression (128, 129). Additionally, exosome-secreted IL-6 activates Signal transducers and activators of transcription 3 (STAT3), while exosome-releasing S100A4 links to MDSCsTLR4 and triggers IL-6 production; Together, these factors contribute to MDSC’s immunosuppressive properties (84). All these together suggest that exosome therapy could be a promising candidate for SS treatment (Table 2).
3.2 Age-related macular degeneration
AMD is one of the leading causes of visual loss in the elderly population. AMD is a multifactorial disease which its pathogenesis is not only considered as degenerative but also as inflammatory and immune-mediated (130, 131). AMD has two phenotypes: (a) early non-exudative (dry), which is characterized by drusen (extracellular deposit) formation and macular atrophy (132, 133), and (b) late exudative (wet) that is marked by abnormal choroidal neovascularization (CNV) (133, 134). Unfortunately, current therapeutic methods such as drugs, radiation and photodynamic therapy, and vitreous surgery are not entirely effective (134, 135). Although anti-VEGFs are the most recent treatment options for AMD (134, 136), yet, they have not been shown to have promising effects in a proportion of patients with late wet AMD (135).
3.2.1 Potential role of EVs in the pathogenesis of AMD
Aged retinal pigment epithelial cells (RPE)-derived Exos are probably responsible for drusen formation (63) since their markers (LAMP2, CD63, and CD81) were found in the drusen that have been collected from AMD patients (48, 63). Moreover, the increased exocytotic activity of aged RPE causes extracellular protein release through exosomes (63). Oxidative stress, one of the main risk factors of AMD, stimulates the secretion of RPE cell-derived Exos, which transfer stress messages to healthy RPE cells resulting in RPE dysfunction (137). Human RPE cell lines (ARPE-19) under oxidative stress released Exos with overexpressed apoptotic protease activating factor 1 (Apaf1) (62). Apaf1, a key molecule in the mitochondrial apoptotic pathway, increases cell apoptosis and inflammatory response through Caspase-9 signaling pathway activation (62). Additionally, Zhang et al. demonstrated that photo-oxidative blue-light stimulation in RPE cells promotes the secretion of nod–like receptor protein 3 (NLRP3) inflammasome mRNA-containing Exos (89). Exo-associated inflammasome activation in RPE cells is involved in AMD pathogenesis and may be a potential therapeutic target for AMD treatment (89). Activating NLRP3 inflammasome stimulates the maturation of inflammatory cytokines (like IL-1β and IL-18) (48, 61). Recent findings suggested that exosomes derived from RPE cells under pathologic conditions promoted endothelial cell migration and tube formation, resulting in angiogenesis and CNV development (138). Besides, stressed RPE cells release exosomes with high expression of VEGFR2 that contribute to new blood vessel formation (63).(Table 1)
3.2.2 Potential role of EVs in the diagnosis and treatment of AMD
Exo cargos, especially miRNAs and proteins, may act as biomarkers for the diagnosis of AMD (72). Previous studies have identified proteins secreted from RPE cells-derived Exos, involved in the autophagy-lysosomal pathway and epithelial-mesenchymal transition, such as cathepsin D, cytokeratin 8, actin, myosin-9 and heat shock protein (Hsp70) (64, 139). These proteins were found in individuals with AMD’s aqueous humor (AH) and might be potential biomarkers and therapeutic targets for AMD diagnosis and treatment (64, 139). Results of a recent study revealed that Exo miRNAs correlate with apoptosis and angiogenesis; particularly, miR-410 and miR-19-a are related to the VEGF signaling pathway, which is a pivotal factor in CNV development (65). Chen et al. found that miRNA-410 reduces VEGF expression, and eye drops containing miRNA-410 inhibits VEGF expression and retinal angiogenesis in mice models of oxygen-induced retinopathy (64, 66). Retinal astrocytes (RACs)-released Exos have anti-angiogenic properties and inhibit laser-induced CNV (87, 88). Human umbilical cord mesenchymal stem cell-derived Exos ameliorate laser-induced retinal injury by down-regulation of VEGF-A in rats (140). Moreover, MSC-derived Exos regulate macrophage polarization and diminish VEGF secretion, two known triggering mechanisms of CNV in AMD; thus, they are able to regulate abnormal neovascularization (141, 142). EVs’ diagnostic and therapeutic applications in AMD are still in the early preclinical stages. Further investigations and trials are substantial to determine their safety, efficacy, and generalizability (Table 2).
3.3 Glaucoma
Glaucoma is a visual disorder characterized by elevated intraocular pressure (IOP), causing optic neuropathy and leading to vision loss. IOP is regulated based on the equilibrium between the inflow and outflow of AH through the trabecular meshwork (TM). The main risk factor for glaucoma is the aqueous drainage reduction, which raises the IOP causing mechanical compression with subsequent ischemia of the optic nerve (143–145). Approximately 3.5 percent of the world’s population suffers from glaucoma, and the prevalence is on the rise (146).
Various subtypes of glaucoma are classified as primary and secondary and open-angle glaucoma (OAG) and angle closure glaucoma (ACG). Primary glaucoma results from congenital or anatomical defects within the eye, while the complications of another underlying disease or pathology, such as trauma or neovascularization, cause secondary glaucoma. The term ACG also refers to the closure of the angle causing impairing drainage from TM. Also, OAG refers to the normal-appearing angle of the anterior chamber with ocular hypertension (147, 148).
However, there are adequate drug therapies, like prostaglandins, carbonic anhydrase inhibitors, and β-blockers, and surgical approaches for decreasing the IOP of glaucoma patients. The retinal ganglion cells (RGCs) are susceptible to injury, which necessitates innovative therapeutics. A novel therapeutic approach should enhance neuroprotection and regeneration. Strategies such as gene therapy and novel drug delivery methods could reduce glaucoma complications in the future (149, 150).
3.3.1 Potential role of EVs in the pathogenesis of glaucoma
Exosomes participate in various biological processes during nerve injury associated with glaucoma, including regenerative and pathological features (132). For example, AH exosomes contain miR-486-5p, miR-204, and miR-184, which regulate AH inflow and outflow (54). Additionally, TM cells produce myocilin (MYOC) that carries exosomes in response to pathologic changes in the eye. Although there is a lack of understanding regarding MYOC’s exact role (55), there was shown that mutations in MYOC can increase the IOP (56). This could be attributed to the reduction of AH drainage (57).
It has also been proposed that exosomes can be used to regulate intraocular pressure in a non-TM-mediated manner (58). Tabak et al. found that non-pigmented ciliary epithelial cells (NPCE)-derived exosomes reduce the levels of phosphorylated glycogen synthase kinase 3 (GSK3) and β-catenin in the TM by triggering Wnt signaling. Among the components of the extracellular matrix, cadherin can increase the size of the TM pore, which leads to an increase in AH outflow resistance and a rise the intraocular pressure (59, 60). NPCE-derived Exos contain a high concentration of negative regulators of Wnt signal transduction, such as miR-29b (58). Upon increased IOP, exosomes released from immature microglial cells increase proinflammatory cytokines, enhance phagocytosis, and generate ROS to modify the number of RGCs (151) (Table 1).
3.3.2 Potential role of EVs in the treatment of glaucoma
Increased IOP is associated with dysfunction and loss of many optic cells. Therefore, patients with glaucoma can benefit from strategies that reduce IOP (152). The exosomes may act as a communication medium between the producer and drainer cells of AH (143). Using exosomes for delivery can also reduce the risk of fibrosis following glaucoma surgery (19). Also, MSC-derived Exos containing brain-derived neurotrophic factor (BDNF) may protect retinal precursor cells from hypoxia-induced damage (48).
Consequently, MSC-derived Exos prevent glaucoma-related blindness caused by the loss of RGCs and their axons. As nanoscale particles, exosomes can also reach RGCs rapidly and offer neurotrophic proteins with saturable binding to vitreous fluids. The release of exosomes by MSCs enhances RGC survival and axon regeneration and, to some extent, avoids RGC axon loss and dysfunction (20, 86). MSC-derived exosomes significantly improve functional recovery in mice with retinal ischemia while decreasing neuroinflammation and apoptosis. Furthermore, exosomes can be seen in vitreous fluids for up to four weeks following treatment (86). Exosomes generated from human Bone marrow stromal cells (BMSCs) were also an efficient neuroprotective therapy. This neuroprotection feature could sustain for one year and maintain the number of RGCs. On the other hand, exosomes of BMSCs showed inhibitory effects on axial mutations in a model of chronic ocular hypertension (153). These modifications on RGCs were partially explained by miRNA-dependent mechanisms (153). Furthermore, exosomes from umbilical cord MSCs (UMSCs) increased RGC survival and glial cell activation, suggesting that they could be employed for the therapy of glaucoma injuries (60). However, UMSCs could not induce axonal regeneration like BMSCs (72). Exosome therapy looks to be an efficient, stable, and durable way in comparison with other drug delivery systems. Besides, Exos derived from MSCs could be a suggestive way as a cell-free therapy for glaucoma (Table 2).
3.4 Diabetic retinopathy
DR is the most prevalent complication of diabetes mellitus (DM) and suffers one-third of the patients with DM (154, 155). This condition could result in blindness and permanent visual impairment (156). In patients with DM, the presence of DR is a risk factor for cognitive and cardiovascular disorders (156).
As a DM microvascular complication, DR may lead to retinal ischemia. The resulting hypoxia and subsequent reperfusion induce oxidative stress, ROS, and increased inflammatory reactions. This condition is called retinal ischemia-reperfusion injury (IRI) (157, 158). Lastly, IRI advances into regional cell necrosis, apoptosis, and autophagy resulting in neuron loss, specially RGCs (159).
The DR development chain involves two phases: (a) the non-proliferative and (b) the proliferative phase. During the non-proliferate phase, because of high glucose levels, patients develop microaneurysms, micro infarctions, flame-shaped hemorrhages, and capillary widening, which are followed by the proliferative phase. During the proliferative phase, neovascularization happens, which may lead to several complications, such as hemorrhages, tractional retinal detachment, and neovascular glaucoma (160).
Despite DR’s widespread prevalence among the human population, there are limited therapeutic options for the late stages (161). Treatment options include photocoagulation with laser, vitrectomy, glucocorticoids, and VEGF neutralizing agents; however, neither of these options are capable of reversing the clinical progression and injuries (162). Therefore, novel treatments are needed (162).
3.4.1 Potential role of EVs in the pathogenesis of DR
Intercellular communication, which EVs facilitate, is an essential biological component of retinal homeostasis (163). Therefore, it is hypothesized that during DM, EVs and their contents alter, which could be accounted for vascular complications (164). During DM, early retinal endothelial damage can alter BRB permeability (165). The altered BRB permeability allows EVs to accumulate at the injury site (163). Accumulated EVs containing circular RNA (circRNA cPWW2P2A) could be transferred into endothelial resulting in dysfunction of retinal vascularization (67). CircRNAs also regulate neo-angiogenesis, inflammatory response, and death in retinal cells.
Exosomes containing circRNAs are promising therapeutic options for DR (68). Also, MCS-derived EVs could cause retinal vascular destabilization through pericyte detachment (166). Another pathogenesis contributing to retinal vascular injury is the activation of the complement system, which results in the formation of Membrane Attack Complexes (MACs) (163). This process may be triggered by the increased production of IgG-laden antibody-contained Exos (167).
miRNAs play an important role in the pathogenesis of DR. They regulate several DR-related mechanisms, such as inflammation, neurodegeneration, angiogenesis, autophagy, and oxidative stress (168). It has been shown that in mice models, during DR, several miRNAs become dysregulated; these miRNAs include miR-381–3p, miR-206–3p, miR-106a-5p, miR-27a-5p, miR-27b-3p, miR-20a-5p, miR-20a-3p and miR-20b (169). Also, high glucose concentrations can increase VEGF levels and trigger angiogenic processes. Moreover, high glucose levels suppress the expression of miRNA inhibitors of VEGF, such as miR-20a-3p, miR-20a-5p, miR-106a-5p, and miR-20b, resulting in the presence of VEGF angiogenic characteristics (170). Other miRNAs contribute to DR in additional ways, such as miR-874, which reduces inflammation, ROS levels, and apoptosis in the retina (171). MiR-145 also attenuate oxidative stress caused by high glucose level through regulating toll-like receptor 4 (TLR4)/nuclear factor kappa light chain enhancer of activated B cells (NF-κB) signaling (172). On the other hand, it had been found that exosomes containing miR-15a that secrets from pancreatic β-cells can induce pathologies caused by DM. This miRNA could contribute in pathogenesis of DR (69) (Figure 1, Table 1).
3.4.2 Potential role of EVs in the treatment of DR
EVs-including Exos, are promising vehicles for DR treatment because of their potential for transporting many factors (22). Also, Exos could deliver gene editing factors for genetic manipulation or non-drug biological components that could change the efficacy of drugs or non-coding RNAs. These features made EVs practical tools in novel drug therapies (173).
MSC-derived Exos, which contain miRNA-126, can attenuate hyperglycemia-induced retinal inflammation by downregulating the expression of the high-mobility group box 1 (HMGB1). Therefore Exos containing miRNA-126 may serve as novel carriers for MSC-based therapeutics (16, 89). Additionally, it has been demonstrated that intravitreal administration of human MSC-derived Exos, cultured under hypoxia conditions, reduces retinal ischemia (174). Moreover, Exos secreted by retinal pigmented cells containing miR-202-5p have been shown to reduce the complications of proliferative DR. miR-202-5p inhibits cell growth, migration, and cell transition (90).
Exos from platelet-rich plasma (PRP) regulate hyperglycemic retinal damage by upregulating components of the TLR4 signaling pathway via C-X-C motif chemokine ligand 10 (CXCL10). Blocking CXCL10 with a CXCL10 neutralizing antibody significantly downregulates the TLR4 signaling, which can reduce the inflammation caused by TLR4 signaling (16, 89, 175).
Peroxisome proliferator-activated receptor y (PPARy) plays a significant role in DR by suppressing multiple cytokines, chemokines, growth factors, and inflammatory factors. The PPARy agonists’ pioglitazone, rosiglitazone, and troglitazone have anti-proliferative and anti-inflammatory properties, which aid in ameliorating proliferative DR. These drugs can be delivered to the retina via EVs for practical application (176, 177). As proposed, EVs could be used to deliver miRNAs and medications to the retinal area, which could ameliorate and reduce the pathogenesis induced by DM. Further studies could discover many other benefits of EVs, including clinical usage of EVs in DR (Figure 2, Table 2).
4 Neuropathy and tumors
4.1 Optic neuropathy
Optic neuropathy is a condition characterized by damage to the optic nerve, composed of axons of neural cells known as RGCs (178). Optic neuropathy is commonly associated with optic neuritis and ischemic optic neuropathy, which may lead to permanent visual loss (179). An optic nerve injury may be caused by traumatic, ischemic, demyelinating, and inflammatory events, such as anterior and posterior ischemic optic neuropathy (AION and PION, respectively) and direct and indirect trauma. The function of the optic nerve cannot be completely restored after the injury to the optic nerve. This lack of complete regeneration is because of the anatomical position and microenvironmental factors that made the optic nerve (180–182). RGCs can’t regenerate themselves, but inducing them to activate the intrinsic growth state could partially help reverse the optic neuropathy damages. However, there are signaling molecules in the extracellular space of RGCs that could prevent the regeneration of RGCs (183).
Due to the multifaceted nature of optic neuropathy, different treatment approaches are used (184); like in traumatic optic neuropathy (TON), high-dose corticosteroids and decompressing surgeries are the main treatment options for reducing the effect of injury on the optic nerve. However, there is not enough evidence that surgery could benefit the patient in the case of visual loss (185). Also, optic neuropathy can happen due to infections. The treatment of infections causing optic neuropathy concentrated on anti-infectious and anti-inflammatory drugs to prevent further damage to the optic nerve (186). In the ischemic types of optic neuropathy, reperfusion and resolving the underlying factors that cause ischemia is essential. For example, glucocorticoids are helpful in vasculitis-induced optic neuropathy caused by giant cell arteritis (187). Considering the sensitivity of the optic nerve, advances in optic nerve injuries and precise therapies are required (184).
4.1.1 Potential role of EVs in the treatment of optic neuropathy
Using exosomes in remission of RGCs after optic neuropathy is a novel approach (180). Recent research on bone marrow-derived-MSC (BMSC)-derived Exos has shown that intravitreal injections of BMSC Exos promote the regeneration of RGC axons and protect the optic nerve after crush injury (20). BMSC-derived Exos replicated this result in other glaucoma models as well (21). Although umbilical cord-derived MSCs could increase the survival of RGCs, they could not promote their regeneration (60). Generally, MSCs have anti-inflammatory properties, which may be attributed to their carrying different miRNAs. The immune suppressive effects of MSCs may contribute to their beneficial impact on RGCs (21).
The miRNAs are essential factors that regulate the regeneration and protection of RGCs and the induction of inflammation (178). Furthermore, secretomes derived from stem cells and amnion cells showed promising effects on optic neuropathies (188–190). The EV may serve as a vehicle for delivering these beneficial components to the optic nerve since anatomical difficulties prevent the delivery of many therapeutics to the optic nerve (191) (Table 2).
4.2 Eye tumor
Despite being rare, eye cancers are one of the life-threatening orbital disorders. There are two types of intraocular cancers: 1-primary, which originates from the eyeball, and 2-secondary, which occurs due to metastasis from different organs to the orbit. Among the primary intraocular malignancies, uveal melanoma (UM) and retinoblastoma (RB) are the most common (192–194). Although treatment measures have improved considerably, metastasis is still a cause of death; Therefore, finding the underlying tumorigenesis mechanism could help to improve treatment strategies (195–197).
Based on emerging research, exosomes have a substantial role in mediating cancer pathways related to Epithelial-to-Mesenchymal-Transition (EMT), angiogenesis, and metastasis (5, 198, 199); Exosomes have potential uses in the diagnosis and prognosis of ocular cancers like RB and UM. Since there is limited evidence on the correlation between exosomes and eye cancers, this article will focus only on RB and UM.
4.2.1 The potential of EVs in the pathogenesis of ocular tumor
RB is the most common eye cancer in children, caused by loss of function mutation in the RB1 gene located in chromosome 13 (13q1–4) (200, 201). The tumor is fast-growing and is almost fatal if it remains untreated (192, 202). The current therapeutic options for RB treatment include chemotherapy, radiotherapy, and surgery; However, metastasis and tumor invasion remain major concerns (195).
Due to the life-threatening nature of metastases, the evaluation of circulating biomarkers should be considered a non-invasive screening tool for these patients. A potential diagnostic target for RB could be exosomal biomarkers, as tumor-derived Exos infiltrate the microenvironment and cause tumor progression (5, 77, 203). Chen et al. showed miRNAs s(miR-17, miR-129a, miR-20a, and miR-92a), thrombospondin-1(TSP-1), C-X-C chemokine receptor type 4 (CXCR4) were found in RB cells-derived Exos (77); They play a role in tumor-associated macrophages (TAMs) recruitment and proliferation (204, 205). TAM can potentially increase the programming invasion, metastasis, and angiogenesis of neoplastic tissues (206–209). Furthermore, another study has shown that despite the upregulation of hsa-miR-216b-5p and hsa-miR-301b-3p expression in RB tissue, serum exosomes containing these miRNAs did not change, suggesting that serum exosomal miRNA may not be a reliable biomarker for RB prognosis (78). According to a new study, exosomal proteins involved in RB vitreous seeding (RBVS) (ß3 integrin, Talin-1, Glutathione S-Transferase Mu) were involved in a variety of mechanisms, including anoikis resistance, glucose, amino acid metabolism, and extracellular matrix remodeling, all of which are responsible for tumor invasion and metastasis (79). As a result, RBVS analysis can be regarded as a promising non-invasive diagnostic technique (Table 1).
UM is the most common primary intraocular neoplasm in adults within the eye uveal tract (192). UM is highly metastatic and mainly affects the liver, with an average survival of 4-5 months (196, 210–212). There has been no significant improvement in the survival rate of UM patients despite advances in the available methods for treatment and diagnosis (196). A possible explanation is the occurrence of metastasis at the early stages of the disease, as circulating malignant cells (CMCs) can be detectable in the bloodstream at the time of diagnosis (213).
Several studies have demonstrated that Exos can participate in various UM stages; The findings of these studies emphasize the importance of understanding exosomes to diagnose diseases and to understand how they participate in the pathogenesis process (74–76, 214–217). Tsering et al. exhibited that the transformation of UM-derived EVs to human breast Cancer gene 1(BRCA1)-deficient fibroblasts (Fibro-BKO) and then inoculation of these cells in Severe combined immunodeficiency (SCID) mice would result in the development of UM with liver metastasis. Analysis of this study exhibited that UM-EVs are involved in endocytosis, PI3K-AKT signaling pathway, and adhesion (focal and cell to cell); They also reported that integrin V, HSP70, and HSP90 were highly expressed in UM-EVs (76). Moreover, a recent study demonstrated that upregulated proteins in UM-EVs, such as GNAQ, GNA11, and integrin αV, are linked to UM tumorigenesis and liver metastasis (76, 218). Ragusa et al. also showed that miR-146a levels were upregulated in the vitreous humor (VH) and serum of UM patients, which could have a regulatory role in UM melanocyte surveillance (75). Therefore, it could be a potential diagnostic circulatory biomarker (75). In another study on UM proteins secreted from cancer cells, it was demonstrated that exosomal proteins (such as Synaptosomal-associated protein 23(SNAP23), Complement C1s subcomponent GN = C1S) that could involve in ECM remodeling, cancer cell migration, and invasion and metastasis (216, 219). A recent analysis of hepatic perfusate-derived Exos in 12 UM patients with liver metastasis revealed that UM patients’ circulating exosome levels were significantly higher compared with healthy controls; additionally, the miRNA composition of liver perfusate exosomal cargo is different from other neoplastic cargo’s composition (74). These results indicated that exosomal miRNA might be a possible specific marker for UM diagnosis (75).
UM-EVs have principal roles in cancer cell proliferation, migration, invasion, and metastasis (64). While various clinical trials analyze exosomes as diagnostic biomarkers for non-ophthalmic cancers, studies on exosomes as a biomarker for ocular cancers are still at preclinical levels (64). Consequently, more studies are essential to explore the potential and prospects of EVs in ocular malignancies (Table 1).
5 Conclusion
Incidence, prevalence, morbidity, and mortality of inflammatory eye diseases have increased over the past decades. However, current therapeutic and diagnostic approaches lack favorable efficiency. Therefore, novel, effective, and safer measures are required to reach sufficient medical care for these patients. Extracellular vesicles mediate a variety of extra- and intracellular activities in the visual organs and are potential candidates for diagnostic and therapeutic agents; however, data that support such evidence are limited. Moreover, there are several challenges regarding the production, isolation, purification, safety, potency, and contamination susceptibility of extracellular vesicles. Therefore, a complete understanding of extracellular vesicles and their cargo is essential.
Author contributions
All authors listed have made a substantial, direct, and intellectual contribution to the work and approved it for publication.
Conflict of interest
The authors declare that the research was conducted in the absence of any commercial or financial relationships that could be construed as a potential conflict of interest.
Publisher’s note
All claims expressed in this article are solely those of the authors and do not necessarily represent those of their affiliated organizations, or those of the publisher, the editors and the reviewers. Any product that may be evaluated in this article, or claim that may be made by its manufacturer, is not guaranteed or endorsed by the publisher.
References
1. WHO. Blindness and vision impairment: World health organization (WHO) (2022). Available at: https://www.who.int/news-room/fact-sheets/detail/blindness-and-visual-impairment#:~:text=Prevalence,has%20yet%20to%20be%20addressed.
2. Ji J, Sundquist J, Sundquist K. Gender-specific incidence of autoimmune diseases from national registers. J Autoimmun (2016) 69:102–6. doi: 10.1016/j.jaut.2016.03.003
3. Ehrlich JR, Ramke J, Macleod D, Burn H, Lee CN, Zhang JH, et al. Association between vision impairment and mortality: a systematic review and meta-analysis. Lancet Glob Health (2021) 9(4):e418–e30. doi: 10.1016/S2214-109X(20)30549-0
4. Miller JR, Hanumunthadu D. Inflammatory eye disease: An overview of clinical presentation and management. Clin Med (London England) (2022) 22(2):100–3. doi: 10.7861/clinmed.2022-0046
5. Klingeborn M, Dismuke WM, Bowes Rickman C, Stamer WD. Roles of exosomes in the normal and diseased eye. Prog retinal eye Res (2017) 59:158–77. doi: 10.1016/j.preteyeres.2017.04.004
6. Benhar I, London A, Schwartz M. The privileged immunity of immune privileged organs: the case of the eye. Front Immunol (2012) 3:296. doi: 10.3389/fimmu.2012.00296
7. de Andrade FA, Fiorot SHS, Benchimol EI, Provenzano J, Martins VJ, Levy RA. The autoimmune diseases of the eyes. Autoimmun Rev (2016) 15(3):258–71. doi: 10.1016/j.autrev.2015.12.001
8. Taylor AW. Ocular immune privilege and transplantation. Front Immunol (2016) 7:37. doi: 10.3389/fimmu.2016.00037
9. Keino H, Horie S, Sugita S. Immune privilege and eye-derived T-regulatory cells. J Immunol Res (2018) 2018. doi: 10.1155/2018/1679197
10. Caspi RR. Ocular autoimmunity: the price of privilege? Immunol Rev (2006) 213(1):23–35. doi: 10.1111/j.1600-065X.2006.00439.x
11. Stern M, Schaumburg C, Dana R, Calonge M, Niederkorn J, Pflugfelder S. Autoimmunity at the ocular surface: pathogenesis and regulation. Mucosal Immunol (2010) 3(5):425–42. doi: 10.1038/mi.2010.26
12. Kaur G, Singh NK. Inflammation and retinal degenerative diseases. Neural regeneration Res (2023) 18(3):513–8. doi: 10.4103/1673-5374.350192
13. Gordon LK. Orbital inflammatory disease: a diagnostic and therapeutic challenge. Eye (2006) 20(10):1196–206. doi: 10.1038/sj.eye.6702383
14. Xu H, Rao N. Grand challenges in ocular inflammatory diseases. Front Ophthalmol (2022) 2:756689. doi: 10.3389/fopht.2022.756689
15. Zhang Y, Amin S, Lung KI, Seabury S, Rao N, Toy BC. Incidence, prevalence, and risk factors of infectious uveitis and scleritis in the united states: A claims-based analysis. PloS One (2020) 15(8):e0237995. doi: 10.1371/journal.pone.0237995
16. Zhang W, Wang Y, Kong Y. Exosomes derived from mesenchymal stem cells modulate miR-126 to ameliorate hyperglycemia-induced retinal inflammation via targeting HMGB1. Invest Ophthalmol Visual Sci (2019) 60(1):294–303. doi: 10.1167/iovs.18-25617
17. Knickelbein JE, Liu B, Arakelyan A, Zicari S, Hannes S, Chen P, et al. Modulation of immune responses by extracellular vesicles from retinal pigment epithelium. Invest Ophthalmol Vis Sci (2016) 57(10):4101–7. doi: 10.1167/iovs.15-18353
18. Shigemoto-Kuroda T, Oh JY, Kim DK, Jeong HJ, Park SY, Lee HJ, et al. MSC-derived extracellular vesicles attenuate immune responses in two autoimmune murine models: Type 1 diabetes and uveoretinitis. Stem Cell Rep (2017) 8(5):1214–25. doi: 10.1016/j.stemcr.2017.04.008
19. Lin QY, Li XJ, Leng Y, Zhu XM, Tang M, Lin Y, et al. Exosome-mediated aptamer S58 reduces fibrosis in a rat glaucoma filtration surgery model. Int J Ophthalmol (2022) 15(5):690–700. doi: 10.18240/ijo.2022.05.02
20. Mead B, Tomarev S. Bone marrow-derived mesenchymal stem cells-derived exosomes promote survival of retinal ganglion cells through miRNA-dependent mechanisms. Stem Cells Trans Med (2017) 6(4):1273–85. doi: 10.1002/sctm.16-0428
21. Mead B, Ahmed Z, Tomarev S. Mesenchymal stem cell-derived small extracellular vesicles promote neuroprotection in a genetic DBA/2J mouse model of glaucoma. Invest Ophthalmol Vis Sci (2018) 59(13):5473–80. doi: 10.1167/iovs.18-25310
22. Safwat A, Sabry D, Ragiae A, Amer E, Mahmoud R, Shamardan R. Adipose mesenchymal stem cells–derived exosomes attenuate retina degeneration of streptozotocin-induced diabetes in rabbits. J Circulating Biomarkers (2018) 7. doi: 10.1177/1849454418807827
23. Yamashiro K, Hamada T, Mori K, Nishi K, Nakamura M, Beppu M, et al. Exosome-derived microRNAs from mouthrinse have the potential to be novel biomarkers for sjögren syndrome. J Pers Med (2022) 12(9). doi: 10.3390/jpm12091483
24. Wassmer S, Carvalho L, Gy örgy B, Vandenberghe LH, Maguire CA. Exosome-associated AAV2 vector mediates robust gene delivery into the murine retina upon intravitreal injection. Sci Rep (2017) 7:45329. doi: 10.1038/srep45329
25. Kuriyan AE, Albini TA, Townsend JH, Rodriguez M, Pandya HK, Leonard RE 2nd, et al. Vision loss after intravitreal injection of autologous "Stem cells" for AMD. New Engl J Med (2017) 376(11):1047–53. doi: 10.1056/NEJMoa1609583
26. Kusuma GD, Barabadi M, Tan JL, Morton DAV, Frith JE, Lim R. To protect and to preserve: Novel preservation strategies for extracellular vesicles. Front Pharmacol (2018) 9:1199. doi: 10.3389/fphar.2018.01199
27. Fujimoto S, Fujita Y, Kadota T, Araya J, Kuwano K. Intercellular communication by vascular endothelial cell-derived extracellular vesicles and their MicroRNAs in respiratory diseases. Front Mol Biosci (2021) 7:619697. doi: 10.3389/fmolb.2020.619697
28. Gould SJ, Raposo G. As we wait: coping with an imperfect nomenclature for extracellular vesicles. J extracellular vesicles (2013) 2. doi: 10.3402/jev.v2i0.20389
29. Minciacchi VR, Freeman MR, Di Vizio D. Extracellular vesicles in cancer: exosomes, microvesicles and the emerging role of large oncosomes. Semin Cell Dev Biol (2015) 40:41–51. doi: 10.1016/j.semcdb.2015.02.010
30. Raposo G, Stoorvogel W. Extracellular vesicles: exosomes, microvesicles, and friends. J Cell Biol (2013) 200(4):373–83. doi: 10.1083/jcb.201211138
31. Santavanond JP, Rutter SF, Atkin-Smith GK, Poon IKH. Apoptotic bodies: Mechanism of formation, isolation and functional relevance. Sub-cellular Biochem (2021) 97:61–88. doi: 10.1007/978-3-030-67171-6_4
32. Sun C, Li W, Li Y, Chen J, An H, Zeng G, et al. MiR-182-5p mediated by exosomes derived from bone marrow mesenchymal stem cell attenuates inflammatory responses by targeting TLR4 in a mouse model of myocardial infraction. Immune network (2022) 22(6):e49. doi: 10.4110/in.2022.22.e49
33. Guo J, Wang F, Hu Y, Luo Y, Wei Y, Xu K, et al. Exosome-based bone-targeting drug delivery alleviates impaired osteoblastic bone formation and bone loss in inflammatory bowel diseases. Cell Rep Med (2023) 4(1):100881. doi: 10.1016/j.xcrm.2022.100881
34. Yuan JY, Wang XY, Tong ZY, Dong YC, Zhao JY, Zhang Y, et al. Promising therapeutic functions of bone marrow mesenchymal stem cells derived-exosome in asthma. Can Respir J (2022) 2022:1485719. doi: 10.1155/2022/1485719
35. Eshghi F, Tahmasebi S, Alimohammadi M, Soudi S, Khaligh SG, Khosrojerdi A, et al. Study of immunomodulatory effects of mesenchymal stem cell-derived exosomes in a mouse model of LPS induced systemic inflammation. Life Sci (2022) 310:120938. doi: 10.1016/j.lfs.2022.120938
36. Akers JC, Gonda D, Kim R, Carter BS, Chen CC. Biogenesis of extracellular vesicles (EV): exosomes, microvesicles, retrovirus-like vesicles, and apoptotic bodies. J neuro-oncology (2013) 113(1):1–11. doi: 10.1007/s11060-013-1084-8
37. Skotland T, Sagini K, Sandvig K, Llorente A. An emerging focus on lipids in extracellular vesicles. Advanced Drug delivery Rev (2020) 159:308–21. doi: 10.1016/j.addr.2020.03.002
38. Noonin C, Thongboonkerd V. Exosome-inflammasome crosstalk and their roles in inflammatory responses. Theranostics (2021) 11(9):4436–51. doi: 10.7150/thno.54004
39. Gangoda L, Boukouris S, Liem M, Kalra H, Mathivanan S. Extracellular vesicles including exosomes are mediators of signal transduction: are they protective or pathogenic? Proteomics (2015) 15(2-3):260–71. doi: 10.1002/pmic.201400234
40. Bowes Rickman C, Farsiu S, Toth CA, Klingeborn M. Dry age-related macular degeneration: mechanisms, therapeutic targets, and imaging. Invest Ophthalmol Vis Sci (2013) 54(14):Orsf68–80. doi: 10.1167/iovs.13-12757
41. Greening DW, Gopal SK, Xu R, Simpson RJ, Chen W. Exosomes and their roles in immune regulation and cancer. Semin Cell Dev Biol (2015) 40:72–81. doi: 10.1016/j.semcdb.2015.02.009
42. Roy Chowdhury U, Hann CR, Stamer WD, Fautsch MP. Aqueous humor outflow: dynamics and disease. Invest Ophthalmol Vis Sci (2015) 56(5):2993–3003. doi: 10.1167/iovs.15-16744
43. Mittelbrunn M, Gutiérrez-Vázquez C, Villarroya-Beltri C, González S, Sánchez-Cabo F, González M, et al. Unidirectional transfer of microRNA-loaded exosomes from T cells to antigen-presenting cells. Nat Commun (2011) 2:282. doi: 10.1038/ncomms1285
44. Horai R, Caspi RR. Microbiome and Autoimmune Uveitis. Front Immunol (2019) 10:232. doi: 10.3389/fimmu.2019.00232
45. Castiblanco C, Foster CS. Review of Systemic Immunosuppression for Autoimmune Uveitis. Ophthalmol Ther (2014) 3(1-2):17–36. doi: 10.1007/s40123-014-0023-x
46. Barry RJ, Nguyen QD, Lee RW, Murray PI, Denniston AK. Pharmacotherapy for uveitis: current management and emerging therapy. Clin Ophthalmol (2014) 8:1891–911. doi: 10.2147/OPTH.S47778
47. Krishna U, Ajanaku D, Denniston AK, Gkika T. Uveitis: a sight-threatening disease which can impact all systems. Postgrad Med J (2017) 93(1106):766–73. doi: 10.1136/postgradmedj-2017-134891
48. Zhang Z, Mugisha A, Fransisca S, Liu Q, Xie P, Hu Z. Emerging role of exosomes in retinal diseases. Front Cell Dev Biol (2021) 9:643680. doi: 10.3389/fcell.2021.643680
49. Zheng H, Yang F, Ea V, Zhou L, Wu L, Zhao G, et al. Proteomics profiling of plasma exosomes in VKH patients. Curr Mol Med (2021) 21(8):675–89. doi: 10.2174/1566524020666200719021653
50. Kapsogeorgou EK, Abu-Helu RF, Moutsopoulos HM, Manoussakis MN. Salivary gland epithelial cell exosomes: A source of autoantigenic ribonucleoproteins. Arthritis Rheum (2005) 52(5):1517–21. doi: 10.1002/art.21005
51. Gallo A, Jang SI, Ong HL, Perez P, Tandon M, Ambudkar I, et al. Targeting the Ca(2+) sensor STIM1 by exosomal transfer of ebv-miR-BART13-3p is associated with sjogren's syndrome. EBioMedicine (2016) 10:216–26. doi: 10.1016/j.ebiom.2016.06.041
52. Cortes-Troncoso J, Jang SI, Perez P, Hidalgo J, Ikeuchi T, Greenwell-Wild T, et al. T Cell exosome-derived miR-142-3p impairs glandular cell function in sjögren's syndrome. JCI Insight (2020) 5(9). doi: 10.1172/jci.insight.133497
53. Kim YJ, Yeon Y, Lee WJ, Shin YU, Cho H, Sung YK, et al. Comparison of MicroRNA expression in tears of normal subjects and sjögren syndrome patients. Invest Ophthalmol Vis Sci (2019) 60(14):4889–95. doi: 10.1167/iovs.19-27062
54. Dismuke WM, Challa P, Navarro I, Stamer WD, Liu Y. Human aqueous humor exosomes. Exp eye Res (2015) 132:73–7. doi: 10.1016/j.exer.2015.01.019
55. Hardy KM, Hoffman EA, Gonzalez P, McKay BS, Stamer WD. Extracellular trafficking of myocilin in human trabecular meshwork cells. J Biol Chem (2005) 280(32):28917–26. doi: 10.1074/jbc.M504803200
56. Yeung V, Boychev N, Farhat W, Ntentakis DP, Hutcheon AE, Ross AE, et al. Extracellular vesicles in corneal Fibrosis/Scarring. Int J Mol Sci (2022) 23(11):5921. doi: 10.3390/ijms23115921
57. Perkumas KM, Hoffman EA, McKay BS, Allingham RR, Stamer WD. Myocilin-associated exosomes in human ocular samples. Exp Eye Res (2007) 84(1):209–12. doi: 10.1016/j.exer.2006.09.020
58. Lerner N, Schreiber-Avissar S, Beit-Yannai E. Extracellular vesicle-mediated crosstalk between NPCE cells and TM cells result in modulation of wnt signalling pathway and ECM remodelling. J Cell Mol Med (2020) 24(8):4646–58. doi: 10.1111/jcmm.15129
59. Tabak S, Schreiber-Avissar S, Beit-Yannai E. Extracellular vesicles have variable dose-dependent effects on cultured draining cells in the eye. J Cell Mol Med (2018) 22(3):1992–2000. doi: 10.1111/jcmm.13505
60. Pan D, Chang X, Xu M, Zhang M, Zhang S, Wang Y, et al. UMSC-derived exosomes promote retinal ganglion cells survival in a rat model of optic nerve crush. J Chem Neuroanat (2019) 96:134–9. doi: 10.1016/j.jchemneu.2019.01.006
61. Kim Y, Tarallo V, Kerur N, Yasuma T, Gelfand BD, Bastos-Carvalho A, et al. DICER1/Alu RNA dysmetabolism induces Caspase-8–mediated cell death in age-related macular degeneration. Proc Natl Acad Sci (2014) 111(45):16082–7. doi: 10.1073/pnas.1403814111
62. Ke Y, Fan X, Rui H, Xinjun R, Dejia W, Chuanzhen Z, et al. Exosomes derived from RPE cells under oxidative stress mediate inflammation and apoptosis of normal RPE cells through Apaf1/caspase-9 axis. J Cell Biochem (2020) 121(12):4849–61. doi: 10.1002/jcb.29713
63. Wang AL, Lukas TJ, Yuan M, Du N, Tso MO, Neufeld AH. Autophagy and exosomes in the aged retinal pigment epithelium: possible relevance to drusen formation and age-related macular degeneration. PloS One (2009) 4(1):e4160. doi: 10.1371/journal.pone.0004160
64. Manukonda R, Attem J, Yenuganti VR, Kaliki S, Vemuganti GK. Exosomes in the visual system: New avenues in ocular diseases. Tumor Biol (2022) 44(1):129–52. doi: 10.3233/TUB-211543
65. ElShelmani H, Wride MA, Saad T, Rani S, Kelly DJ, Keegan D. The role of deregulated microRNAs in age-related macular degeneration pathology. Trans Vision Sci Technol (2021) 10(2):12–. doi: 10.1167/tvst.10.2.12
66. Chen N, Wang J, Hu Y, Cui B, Li W, Xu G, et al. MicroRNA-410 reduces the expression of vascular endothelial growth factor and inhibits oxygen-induced retinal neovascularization. PloS One (2014) 9(4):e95665. doi: 10.1371/journal.pone.0095665
67. Liu C, Ge HM, Liu BH, Dong R, Shan K, Chen X, et al. Targeting pericyte-endothelial cell crosstalk by circular RNA-cPWWP2A inhibition aggravates diabetes-induced microvascular dysfunction. Proc Natl Acad Sci U.S.A. (2019) 116(15):7455–64. doi: 10.1073/pnas.1814874116
68. He M, Zhou R, Liu S, Cheng W, Wang W. Circular RNAs: potential star molecules involved in diabetic retinopathy. Curr Eye Res (2021) 46(3):277–83. doi: 10.1080/02713683.2020.1812086
69. Kamalden TA, Macgregor-Das AM, Kannan SM, Dunkerly-Eyring B, Khaliddin N, Xu Z, et al. Exosomal MicroRNA-15a transfer from the pancreas augments diabetic complications by inducing oxidative stress. Antioxid Redox Signal (2017) 27(13):913–30. doi: 10.1089/ars.2016.6844
70. Han K-Y, Tran JA, Chang J-H, Azar DT, Zieske JD. Potential role of corneal epithelial cell-derived exosomes in corneal wound healing and neovascularization. Sci Rep (2017) 7(1):40548. doi: 10.1038/srep40548
71. Ma S, Liu X, Yin J, Hao L, Diao Y, Zhong J. Exosomes and autophagy in ocular surface and retinal diseases: new insights into pathophysiology and treatment. Stem Cell Res Ther (2022) 13(1):1–11. doi: 10.1186/s13287-022-02854-8
72. Liu J, Jiang F, Jiang Y, Wang Y, Li Z, Shi X, et al. Roles of exosomes in ocular diseases. Int J Nanomedicine (2020) 15:10519–38. doi: 10.2147/IJN.S277190
73. Han K-Y, Dugas-Ford J, Seiki M, Chang J-H, Azar DT. Evidence for the involvement of MMP14 in MMP2 processing and recruitment in exosomes of corneal fibroblasts. Invest Ophthalmol Visual Sci (2015) 56(9):5323–9. doi: 10.1167/iovs.14-14417
74. Eldh M, Olofsson Bagge R, Lässer C, Svanvik J, Sjöstrand M, Mattsson J, et al. MicroRNA in exosomes isolated directly from the liver circulation in patients with metastatic uveal melanoma. BMC Cancer (2014) 14:962. doi: 10.1186/1471-2407-14-962
75. Ragusa M, Barbagallo C, Statello L, Caltabiano R, Russo A, Puzzo L, et al. miRNA profiling in vitreous humor, vitreal exosomes and serum from uveal melanoma patients: Pathological and diagnostic implications. Cancer Biol Ther (2015) 16(9):1387–96. doi: 10.1080/15384047.2015.1046021
76. Tsering T, Laskaris A, Abdouh M, Bustamante P, Parent S, Jin E, et al. Uveal melanoma-derived extracellular vesicles display transforming potential and carry protein cargo involved in metastatic niche preparation. Cancers (Basel) (2020) 12(10). doi: 10.3390/cancers12102923
77. Chen S, Chen X, Qiu J, Chen P, Han X, Wu Y, et al. Exosomes derived from retinoblastoma cells enhance tumour deterioration by infiltrating the microenvironment. Oncol Rep (2021) 45(1):278–90. doi: 10.3892/or.2020.7858
78. Ravishankar H, Mangani AS, Phoebe Moses GL, Mani SP, Parameswaran S, Khetan V, et al. Serum exosomal miRNA as biomarkers for retinoblastoma. Exp Eye Res (2020) 199:108184. doi: 10.1016/j.exer.2020.108184
79. Galardi A, Colletti M, Lavarello C, Di Paolo V, Mascio P, Russo I, et al. Proteomic profiling of retinoblastoma-derived exosomes reveals potential biomarkers of vitreous seeding. Cancers (Basel) (2020) 12(6). doi: 10.3390/cancers12061555
80. Hassan M, Karkhur S, Bae JH, Halim MS, Ormaechea MS, Onghanseng N, et al. New therapies in development for the management of non-infectious uveitis: A review. Clin Exp Ophthalmol (2019) 47(3):396–417. doi: 10.1111/ceo.13511
81. Bai L, Shao H, Wang H, Zhang Z, Su C, Dong L, et al. Effects of mesenchymal stem cell-derived exosomes on experimental autoimmune uveitis. Sci Rep (2017) 7(1):4323. doi: 10.1038/s41598-017-04559-y
82. Kang M, Choi JK, Jittayasothorn Y, Egwuagu CE. Interleukin 35-producing exosomes suppress neuroinflammation and autoimmune uveitis. Front Immunol (2020) 11:1051. doi: 10.3389/fimmu.2020.01051
83. Bullerdiek J, Flor I. Exosome-delivered microRNAs of "chromosome 19 microRNA cluster" as immunomodulators in pregnancy and tumorigenesis. Mol Cytogenet (2012) 5(1):27. doi: 10.1186/1755-8166-5-27
84. Rui K, Hong Y, Zhu Q, Shi X, Xiao F, Fu H, et al. Olfactory ecto-mesenchymal stem cell-derived exosomes ameliorate murine sjogren's syndrome by modulating the function of myeloid-derived suppressor cells. Cell Mol Immunol (2021) 18(2):440–51. doi: 10.1038/s41423-020-00587-3
85. Li B, Xing Y, Gan Y, He J, Hua H. Labial gland-derived mesenchymal stem cells and their exosomes ameliorate murine sjögren's syndrome by modulating the balance of treg and Th17 cells. Stem Cell Res Ther (2021) 12(1):478. doi: 10.1186/s13287-021-02541-0
86. Mathew B, Ravindran S, Liu X, Torres L, Chennakesavalu M, Huang C-C, et al. Mesenchymal stem cell-derived extracellular vesicles and retinal ischemia-reperfusion. Biomaterials (2019) 197:146–60. doi: 10.1016/j.biomaterials.2019.01.016
87. Zhang Z, Liang X, Zhou J, Meng M, Gao Y, Yi G, et al. Exosomes in the pathogenesis and treatment of ocular diseases. Exp Eye Res (2021) 209:108626. doi: 10.1016/j.exer.2021.108626
88. Hajrasouliha AR, Jiang G, Lu Q, Lu H, Kaplan HJ, Zhang H-G, et al. Exosomes from retinal astrocytes contain antiangiogenic components that inhibit laser-induced choroidal neovascularization. J Biol Chem (2013) 288(39):28058–67. doi: 10.1074/jbc.M113.470765
89. Zhang W, Ma Y, Zhang Y, Yang J, He G, Chen S. Photo-oxidative blue-light stimulation in retinal pigment epithelium cells promotes exosome secretion and increases the activity of the NLRP3 inflammasome. Curr eye Res (2019) 44(1):67–75. doi: 10.1080/02713683.2018.1518458
90. Gu S, Liu Y, Zou J, Wang W, Wei T, Wang X, et al. Retinal pigment epithelial cells secrete miR-202-5p-containing exosomes to protect against proliferative diabetic retinopathy. Exp Eye Res (2020) 201:108271. doi: 10.1016/j.exer.2020.108271
91. Deng SX, Dos Santos A, Gee S. Therapeutic potential of extracellular vesicles for the treatment of corneal injuries and scars. Trans Vision Sci Technol (2020) 9(12):1. doi: 10.1167/tvst.9.12.1
92. Samaeekia R, Rabiee B, Putra I, Shen X, Park YJ, Hematti P, et al. Effect of human corneal mesenchymal stromal cell-derived exosomes on corneal epithelial wound healing. Invest Ophthalmol Visual Sci (2018) 59(12):5194–200. doi: 10.1167/iovs.18-24803
93. Shen T, Zheng Q-Q, Shen J, Li Q-S, Song X-H, Luo H-B, et al. Effects of adipose-derived mesenchymal stem cell exosomes on corneal stromal fibroblast viability and extracellular matrix synthesis. Chin Med J (2018) 131(06):704–12. doi: 10.4103/0366-6999.226889
94. Yu B, Li X-R, Zhang X-M. Mesenchymal stem cell-derived extracellular vesicles as a new therapeutic strategy for ocular diseases. World J Stem Cells (2020) 12(3):178. doi: 10.4252/wjsc.v12.i3.178
95. McKay TB, Yeung V, Hutcheon AE, Guo X, Zieske JD, Ciolino JB. Extracellular vesicles in the cornea: Insights from other tissues. Analytical Cell Pathol (2021) 2021. doi: 10.1155/2021/9983900
96. Mansoor H, Ong HS, Riau AK, Stanzel TP, Mehta JS, Yam GH-F. Current trends and future perspective of mesenchymal stem cells and exosomes in corneal diseases. Int J Mol Sci (2019) 20(12):2853. doi: 10.3390/ijms20122853
97. Zieske JD, Hutcheon AE, Guo X. Extracellular vesicles and cell–cell communication in the cornea. Anatomical Rec (2020) 303(6):1727–34. doi: 10.1002/ar.24181
98. Shojaati G, Khandaker I, Funderburgh ML, Mann MM, Basu R, Stolz DB, et al. Mesenchymal stem cells reduce corneal fibrosis and inflammation via extracellular vesicle-mediated delivery of miRNA. Stem Cells Trans Med (2019) 8(11):1192–201. doi: 10.1002/sctm.18-0297
99. Yu C, Chen P, Xu J, Liu Y, Li H, Wang L, et al. hADSCs derived extracellular vesicles inhibit NLRP3inflammasome activation and dry eye. Sci Rep (2020) 10(1):1–12. doi: 10.1038/s41598-020-71337-8
100. Aiello S, Rocchetta F, Longaretti L, Faravelli S, Todeschini M, Cassis L, et al. Extracellular vesicles derived from T regulatory cells suppress T cell proliferation and prolong allograft survival. Sci Rep (2017) 7(1):1–19. doi: 10.1038/s41598-017-08617-3
101. Jia Z, Li F, Zeng X, Lv Y, Zhao S. The effects of local administration of mesenchymal stem cells on rat corneal allograft rejection. BMC Ophthalmol (2018) 18(1):1–10. doi: 10.1186/s12886-018-0802-6
102. Li N, Zhao L, Wei Y, Ea VL, Nian H, Wei R. Recent advances of exosomes in immune-mediated eye diseases. Stem Cell Res Ther (2019) 10(1):1–10. doi: 10.1186/s13287-019-1372-0
103. Kordelas L, Rebmann V, Ludwig A, Radtke S, Ruesing J, Doeppner T, et al. MSC-derived exosomes: a novel tool to treat therapy-refractory graft-versus-host disease. Leukemia (2014) 28(4):970–3. doi: 10.1038/leu.2014.41
104. Qin B, Wang J, Yang Z, Yang M, Ma N, Huang F, et al. Epidemiology of primary sjögren's syndrome: a systematic review and meta-analysis. Ann Rheum Dis (2015) 74(11):1983–9. doi: 10.1136/annrheumdis-2014-205375
105. Hayashi T. Dysfunction of lacrimal and salivary glands in sjögren's syndrome: nonimmunologic injury in preinflammatory phase and mouse model. J BioMed Biotechnol (2011) 2011:407031. doi: 10.1155/2011/407031
106. Kassan SS, Moutsopoulos HM. Clinical manifestations and early diagnosis of sjögren syndrome. Arch Intern Med (2004) 164(12):1275–84. doi: 10.1001/archinte.164.12.1275
107. Tsubota K, Toda I, Yagi Y, Ogawa Y, Ono M, Yoshino K. Three different types of dry eye syndrome. Cornea (1994) 13(3):202–9. doi: 10.1097/00003226-199405000-00002
108. Bloch KJ, Buchanan WW, Wohl MJ, Bunim JJ. Sjoegren's syndrome. a clinical, pathological, and serological study of sixty-two cases. Med (Baltimore) (1965) 44:187–231. doi: 10.1097/00005792-196505000-00001
109. Feltkamp TE. Sjögren's syndrome in relation to other autoimmune diseases. Neth J Med (1992) 40(3-4):105–7.
110. Nair JJ, Singh TP. Sjogren's syndrome: Review of the aetiology, pathophysiology & potential therapeutic interventions. J Clin Exp Dent (2017) 9(4):e584–e9. doi: 10.4317/jced.53605
111. Nikolov NP, Illei GG. Pathogenesis of sjögren's syndrome. Curr Opin Rheumatol (2009) 21(5):465–70. doi: 10.1097/BOR.0b013e32832eba21
112. Moutsopoulos HM. Sjögren's syndrome: autoimmune epithelitis. Clin Immunol Immunopathol (1994) 72(2):162–5. doi: 10.1006/clin.1994.1123
114. Fox RI, Stern M, Michelson P. Update in sjögren syndrome. Curr Opin Rheumatol (2000) 12(5):391–8. doi: 10.1097/00002281-200009000-00007
115. Vitali C, Bombardieri S, Jonsson R, Moutsopoulos HM, Alexander EL, Carsons SE, et al. Classification criteria for sjögren's syndrome: a revised version of the European criteria proposed by the American-European consensus group. Ann Rheum Dis (2002) 61(6):554–8. doi: 10.1136/ard.61.6.554
116. Patel R, Shahane A. The epidemiology of sjögren's syndrome. Clin Epidemiol (2014) 6:247–55. doi: 10.2147/CLEP.S47399
117. Alamanos Y, Tsifetaki N, Voulgari PV, Venetsanopoulou AI, Siozos C, Drosos AA. Epidemiology of primary sjögren's syndrome in north-west Greece, 1982-2003. Rheumatol (Oxford) (2006) 45(2):187–91. doi: 10.1093/rheumatology/kei107
118. Kabasakal Y, Kitapcioglu G, Turk T, Oder G, Durusoy R, Mete N, et al. The prevalence of sjögren's syndrome in adult women. Scand J Rheumatol (2006) 35(5):379–83. doi: 10.1080/03009740600759704
119. Mavragani CP. Mechanisms and new strategies for primary sjögren's syndrome. Annu Rev Med (2017) 68:331–43. doi: 10.1146/annurev-med-043015-123313
120. Ramos-Casals M, Brito-Zeron P, Bombardieri S, Bootsma H, De Vita S, Dorner T, et al. EULAR recommendations for the management of sjogren's syndrome with topical and systemic therapies. Ann Rheum Dis (2020) 79(1):3–18. doi: 10.1136/annrheumdis-2019-216114
121. Ramos-Casals M, Tzioufas AG, Stone JH, Sisó A, Bosch X. Treatment of primary sjögren syndrome: a systematic review. Jama (2010) 304(4):452–60. doi: 10.1001/jama.2010.1014
122. Huang Y, Li R, Ye S, Lin S, Yin G, Xie Q. Recent advances in the use of exosomes in sjögren's syndrome. Front Immunol (2020) 11:1509. doi: 10.3389/fimmu.2020.01509
123. Stefanski AL, Tomiak C, Pleyer U, Dietrich T, Burmester GR, Dörner T. The diagnosis and treatment of sjögren's syndrome. Dtsch Arztebl Int (2017) 114(20):354–61. doi: 10.3238/arztebl.2017.0354
124. Li N NH, Zhao L, Wei Y, Wu Y, Wei R. Regulation of human umbilical cord mesenchymal stem cells derived exosomes on peripheral blood macrophages from rabbit autoimmune dry eye. Chin J Exp Ophthalmol (2019) 12:854–62.
125. Alevizos I, Alexander S, Turner RJ, Illei GG. MicroRNA expression profiles as biomarkers of minor salivary gland inflammation and dysfunction in sjögren's syndrome. Arthritis Rheum (2011) 63(2):535–44. doi: 10.1002/art.30131
126. Michael A, Bajracharya SD, Yuen PS, Zhou H, Star RA, Illei GG, et al. Exosomes from human saliva as a source of microRNA biomarkers. Oral Dis (2010) 16(1):34–8. doi: 10.1111/j.1601-0825.2009.01604.x
127. Aqrawi LA, Galtung HK, Vestad B, Øvstebø R, Thiede B, Rusthen S, et al. Identification of potential saliva and tear biomarkers in primary sjögren's syndrome, utilising the extraction of extracellular vesicles and proteomics analysis. Arthritis Res Ther (2017) 19(1):14. doi: 10.1186/s13075-017-1228-x
128. Cripps JG, Gorham JD. MDSC in autoimmunity. Int Immunopharmacol (2011) 11(7):789–93. doi: 10.1016/j.intimp.2011.01.026
129. Tian J, Rui K, Hong Y, Wang X, Xiao F, Lin X, et al. Increased GITRL impairs the function of myeloid-derived suppressor cells and exacerbates primary sjögren syndrome. J Immunol (2019) 202(6):1693–703. doi: 10.4049/jimmunol.1801051
130. Perez VL, Caspi RR. Immune mechanisms in inflammatory and degenerative eye disease. Trends Immunol (2015) 36(6):354–63. doi: 10.1016/j.it.2015.04.003
131. Wong WL, Su X, Li X, Cheung CMG, Klein R, Cheng C-Y, et al. Global prevalence of age-related macular degeneration and disease burden projection for 2020 and 2040: a systematic review and meta-analysis. Lancet Global Health (2014) 2(2):e106–e16. doi: 10.1016/S2214-109X(13)70145-1
132. Li SF, Han Y, Wang F, Su Y. Progress in exosomes and their potential use in ocular diseases. Int J Ophthalmol (2020) 13(9):1493–8. doi: 10.18240/ijo.2020.09.23
133. Pennington KL, DeAngelis MM. Epidemiology of age-related macular degeneration (AMD): associations with cardiovascular disease phenotypes and lipid factors. Eye Vision (2016) 3(1):1–20. doi: 10.1186/s40662-016-0063-5
134. Tong Y, Zhou Y-L, Wang Y-X, Zhao P-Q, Wang Z-Y. Retinal pigment epithelium cell-derived exosomes: Possible relevance to CNV in wet-age related macular degeneration. Med Hypotheses (2016) 97:98–101. doi: 10.1016/j.mehy.2016.10.027
135. Mitchell P. A systematic review of the efficacy and safety outcomes of anti-VEGF agents used for treating neovascular age-related macular degeneration: comparison of ranibizumab and bevacizumab. Curr Med Res Opin (2011) 27(7):1465–75. doi: 10.1185/03007995.2011.585394
136. Naga SHA, Dithmer M, Chitadze G, Kabelitz D, Lucius R, Roider J, et al. Intracellular pathways following uptake of bevacizumab in RPE cells. Exp eye Res (2015) 131:29–41. doi: 10.1016/j.exer.2014.12.010
137. Shah N, Ishii M, Brandon C, Ablonczy Z, Cai J, Liu Y, et al. Extracellular vesicle-mediated long-range communication in stressed retinal pigment epithelial cell monolayers. Biochim Biophys Acta (BBA)-Molecular Basis Dis (2018) 1864(8):2610–22. doi: 10.1016/j.bbadis.2018.04.016
138. Fukushima A, Takahashi E, Saruwatari J, Tanihara H, Inoue T. The angiogenic effects of exosomes secreted from retinal pigment epithelial cells on endothelial cells. Biochem biophysics Rep (2020) 22:100760. doi: 10.1016/j.bbrep.2020.100760
139. Kang G-Y, Bang JY, Choi AJ, Yoon J, Lee W-C, Choi S, et al. Exosomal proteins in the aqueous humor as novel biomarkers in patients with neovascular age-related macular degeneration. J Proteome Res (2014) 13(2):581–95. doi: 10.1021/pr400751k
140. He G-H, Zhang W, Ma Y-X, Yang J, Chen L, Song J, et al. Mesenchymal stem cells-derived exosomes ameliorate blue light stimulation in retinal pigment epithelium cells and retinal laser injury by VEGF-dependent mechanism. Int J Ophthalmol (2018) 11(4):559. doi: 10.18240/ijo.2018.04.04
141. Rad LM, Yumashev AV, Hussen BM, Jamad HH, Ghafouri-Fard S, Taheri M, et al. Therapeutic potential of microvesicles in cell therapy and regenerative medicine of ocular diseases with an especial focus on mesenchymal stem cells-derived microvesicles. Front Genet (2022) 13:570. doi: 10.3389/fgene.2022.847679
142. Li J, Xue H, Li T, Chu X, Xin D, Xiong Y, et al. Exosomes derived from mesenchymal stem cells attenuate the progression of atherosclerosis in ApoE–/-mice via miR-let7 mediated infiltration and polarization of M2 macrophage. Biochem Biophys Res Commun (2019) 510(4):565–72. doi: 10.1016/j.bbrc.2019.02.005
143. Lerner N, Avissar S, Beit-Yannai E. Extracellular vesicles mediate signaling between the aqueous humor producing and draining cells in the ocular system. PloS One (2017) 12(2):e0171153. doi: 10.1371/journal.pone.0171153
144. Vidal-Sanz M, Salinas-Navarro M, Nadal-Nicolás FM, Alarcón-Martínez L, Valiente-Soriano FJ, de Imperial JM, et al. Understanding glaucomatous damage: anatomical and functional data from ocular hypertensive rodent retinas. Prog retinal eye Res (2012) 31(1):1–27. doi: 10.1016/j.preteyeres.2011.08.001
145. Sambhara D, Aref AA. Glaucoma management: relative value and place in therapy of available drug treatments. Ther Adv chronic Dis (2014) 5(1):30–43. doi: 10.1177/2040622313511286
146. Tham YC, Li X, Wong TY, Quigley HA, Aung T, Cheng CY. Global prevalence of glaucoma and projections of glaucoma burden through 2040: a systematic review and meta-analysis. Ophthalmology (2014) 121(11):2081–90. doi: 10.1016/j.ophtha.2014.05.013
147. Casson RJ, Chidlow G, Wood JP, Crowston JG, Goldberg I. Definition of glaucoma: clinical and experimental concepts. Clin Exp Ophthalmol (2012) 40(4):341–9. doi: 10.1111/j.1442-9071.2012.02773.x
148. Foster PJ, Buhrmann R, Quigley HA, Johnson GJ. The definition and classification of glaucoma in prevalence surveys. Br J Ophthalmol (2002) 86(2):238–42. doi: 10.1136/bjo.86.2.238
149. Fogagnolo P, Rossetti L. Medical treatment of glaucoma: present and future. Expert Opin Investig Drugs (2011) 20(7):947–59. doi: 10.1517/13543784.2011.579901
150. Lusthaus J, Goldberg I. Current management of glaucoma. Med J Aust (2019) 210(4):180–7. doi: 10.5694/mja2.50020
151. Aires ID, Ribeiro-Rodrigues T, Boia R, Catarino S, Girão H, Ambrósio AF, et al. Exosomes derived from microglia exposed to elevated pressure amplify the neuroinflammatory response in retinal cells. Glia (2020) 68(12):2705–24. doi: 10.1002/glia.23880
152. Jeong D, Jo W, Yoon J, Kim J, Gianchandani S, Gho YS, et al. Nanovesicles engineered from ES cells for enhanced cell proliferation. Biomaterials (2014) 35(34):9302–10. doi: 10.1016/j.biomaterials.2014.07.047
153. Mead B, Amaral J, Tomarev S. Mesenchymal stem cell–derived small extracellular vesicles promote neuroprotection in rodent models of glaucoma. Invest Ophthalmol Visual Sci (2018) 59(2):702–14. doi: 10.1167/iovs.17-22855
154. Cheung N, Mitchell P, Wong TY. Diabetic retinopathy. Lancet (2010) 376(9735):124–36. doi: 10.1016/S0140-6736(09)62124-3
155. Wang W, Lo ACY. Diabetic retinopathy: Pathophysiology and treatments. Int J Mol Sci (2018) 19(6). doi: 10.3390/ijms19061816
156. Vujosevic S, Aldington SJ, Silva P, Hernández C, Scanlon P, Peto T, et al. Screening for diabetic retinopathy: new perspectives and challenges. Lancet Diabetes Endocrinol (2020) 8(4):337–47. doi: 10.1016/S2213-8587(19)30411-5
157. Walker R. Diabetic retinopathy: protecting the vision of people with diabetes. Br J Community Nurs (2004) 9(12):545–7. doi: 10.12968/bjcn.2004.9.12.17245
158. Mu H, Wang Y, Wei H, Lu H, Feng Z, Yu H, et al. Collagen peptide modified carboxymethyl cellulose as both antioxidant drug and carrier for drug delivery against retinal ischaemia/reperfusion injury. J Cell Mol Med (2018) 22(10):5008–19. doi: 10.1111/jcmm.13768
159. Liu L, Sun Q, Wang R, Chen Z, Wu J, Xia F, et al. Methane attenuates retinal ischemia/reperfusion injury via anti-oxidative and anti-apoptotic pathways. Brain Res (2016) 1646:327–33. doi: 10.1016/j.brainres.2016.05.037
160. Kollias AN, Ulbig MW. Diabetic retinopathy: Early diagnosis and effective treatment. Dtsch Arztebl Int (2010) 107(5):75–83. doi: 10.3238/arztebl.2010.0075
161. Lechner J, O'Leary OE, Stitt AW. The pathology associated with diabetic retinopathy. Vision Res (2017) 139:7–14. doi: 10.1016/j.visres.2017.04.003
162. Whitehead M, Wickremasinghe S, Osborne A, Van Wijngaarden P, Martin KR. Diabetic retinopathy: a complex pathophysiology requiring novel therapeutic strategies. Expert Opin Biol Ther (2018) 18(12):1257–70. doi: 10.1080/14712598.2018.1545836
163. Martins B, Amorim M, Reis F, Ambrósio AF, Fernandes R. Extracellular vesicles and MicroRNA: Putative role in diagnosis and treatment of diabetic retinopathy. Antioxidants (Basel) (2020) 9(8). doi: 10.3390/antiox9080705
164. Zhou F, Huang L, Qu SL, Chao R, Yang C, Jiang ZS, et al. The emerging roles of extracellular vesicles in diabetes and diabetic complications. Clin Chim Acta (2019) 497:130–6. doi: 10.1016/j.cca.2019.07.032
165. Santiago AR, Boia R, Aires ID, Ambrósio AF, Fernandes R. Sweet stress: Coping with vascular dysfunction in diabetic retinopathy. Front Physiol (2018) 9:820. doi: 10.3389/fphys.2018.00820
166. Beltramo E, Lopatina T, Berrone E, Mazzeo A, Iavello A, Camussi G, et al. Extracellular vesicles derived from mesenchymal stem cells induce features of diabetic retinopathy in vitro. Acta Diabetol (2014) 51(6):1055–64. doi: 10.1007/s00592-014-0672-1
167. Huang C, Fisher KP, Hammer SS, Navitskaya S, Blanchard GJ, Busik JV. Plasma exosomes contribute to microvascular damage in diabetic retinopathy by activating the classical complement pathway. Diabetes (2018) 67(8):1639–49. doi: 10.2337/db17-1587
168. Li X, Yu ZW, Wang Y, Fu YH, Gao XY. MicroRNAs: Potential targets in diabetic retinopathy. Horm Metab Res (2020) 52(3):142–8. doi: 10.1055/a-1107-2943
169. Platania CBM, Maisto R, Trotta MC, D'Amico M, Rossi S, Gesualdo C, et al. Retinal and circulating mi RNA expression patterns in diabetic retinopathy: An in silico and in vivo approach. Br J Pharmacol (2019) 176(13):2179–94. doi: 10.1111/bph.14665
170. Maisto R, Trotta MC, Petrillo F, Izzo S, Cuomo G, Alfano R, et al. Resolvin D1 modulates the intracellular VEGF-related miRNAs of retinal photoreceptors challenged with high glucose. Front Pharmacol (2020) 11. doi: 10.3389/fphar.2020.00235
171. Li R, Yuan H, Zhao T, Yan Y, Liu Z, Cai J, et al. miR-874 ameliorates retinopathy in diabetic rats by NF-κB signaling pathway. Adv Clin Exp Med (2021) 30(4):421–30. doi: 10.17219/acem/130602
172. Hui Y, Yin Y. MicroRNA-145 attenuates high glucose-induced oxidative stress and inflammation in retinal endothelial cells through regulating TLR4/NF-κB signaling. Life Sci (2018) 207:212–8. doi: 10.1016/j.lfs.2018.06.005
173. Xu YX, Pu SD, Li X, Yu ZW, Zhang YT, Tong XW, et al. Exosomal ncRNAs: Novel therapeutic target and biomarker for diabetic complications. Pharmacol Res (2022) 178:106135. doi: 10.1016/j.phrs.2022.106135
174. Moisseiev E, Anderson JD, Oltjen S, Goswami M, Zawadzki RJ, Nolta JA, et al. Protective effect of intravitreal administration of exosomes derived from mesenchymal stem cells on retinal ischemia. Curr Eye Res (2017) 42(10):1358–67. doi: 10.1080/02713683.2017.1319491
175. Zhang W, Dong X, Wang T, Kong Y. Exosomes derived from platelet-rich plasma mediate hyperglycemia-induced retinal endothelial injury via targeting the TLR4 signaling pathway. Exp eye Res (2019) 189:107813. doi: 10.1016/j.exer.2019.107813
176. Katome T, Namekata K, Mitamura Y, Semba K, Egawa M, Naito T, et al. Expression of intraocular peroxisome proliferator-activated receptor gamma in patients with proliferative diabetic retinopathy. J Diabetes its Complications (2015) 29(2):275–81. doi: 10.1016/j.jdiacomp.2014.10.010
177. Pershadsingh HA, Moore DM. PPARγ agonists: potential as therapeutics for neovascular retinopathies. PPAR Res (2008) 2008. doi: 10.1155/2008/164273
178. Mead B, Tomarev S. The role of miRNA in retinal ganglion cell health and disease. Neural Regener Res (2022) 17(3):516–22. doi: 10.4103/1673-5374.320974
179. Cui Y, Liu C, Huang L, Chen J, Xu N. Protective effects of intravitreal administration of mesenchymal stem cell-derived exosomes in an experimental model of optic nerve injury. Exp Cell Res (2021) 407(1):112792. doi: 10.1016/j.yexcr.2021.112792
180. Li H, Su Y, Wang F, Tao F. Exosomes: a new way of protecting and regenerating optic nerve after injury. Hum Cell (2022) 35(3):771–8. doi: 10.1007/s13577-022-00688-3
181. Hayreh SS. Ischemic optic neuropathy. Prog Retin Eye Res (2009) 28(1):34–62. doi: 10.1016/j.preteyeres.2008.11.002
182. Bastakis GG, Ktena N, Karagogeos D, Savvaki M. Models and treatments for traumatic optic neuropathy and demyelinating optic neuritis. Dev Neurobiol (2019) 79(8):819–36. doi: 10.1002/dneu.22710
183. Benowitz LI, Yin Y. Optic nerve regeneration. Arch Ophthalmol (2010) 128(8):1059–64. doi: 10.1001/archophthalmol.2010.152
184. Bennett JL, Costello F, Chen JJ, Petzold A, Biousse V, Newman NJ, et al. Optic neuritis and autoimmune optic neuropathies: advances in diagnosis and treatment. Lancet Neurol (2023) 22(1):89–100. doi: 10.1016/S1474-4422(22)00187-9
185. Oh HJ, Yeo DG, Hwang SC. Surgical treatment for traumatic optic neuropathy. Korean J Neurotrauma (2018) 14(2):55–60. doi: 10.13004/kjnt.2018.14.2.55
186. Kahloun R, Abroug N, Ksiaa I, Mahmoud A, Zeghidi H, Zaouali S, et al. Infectious optic neuropathies: a clinical update. Eye Brain (2015) 7:59. doi: 10.2147/EB.S69173
187. Biousse V, Newman NJ. Ischemic optic neuropathies. New Engl J Med (2015) 372(25):2428–36. doi: 10.1056/NEJMra1413352
188. Sanie-Jahromi F, Mahmoudi A, Khalili MR, Nowroozzadeh MH. A review on the application of stem cell secretome in the protection and regeneration of retinal ganglion cells; a clinical prospect in the treatment of optic neuropathies. Curr Eye Res (2022) 47(11):1463–71. doi: 10.1080/02713683.2022.2103153
189. Khan RS, Dine K, Bauman B, Lorentsen M, Lin L, Brown H, et al. Intranasal delivery of a novel amnion cell secretome prevents neuronal damage and preserves function in a mouse multiple sclerosis model. Sci Rep (2017) 7(1):41768. doi: 10.1038/srep41768
190. Grinblat GA, Khan RS, Dine K, Wessel H, Brown L, Shindler KS. RGC neuroprotection following optic nerve trauma mediated by intranasal delivery of amnion cell secretome. Invest Ophthalmol Vis Sci (2018) 59(6):2470–7. doi: 10.1167/iovs.18-24096
191. Mead B, Tomarev S. Extracellular vesicle therapy for retinal diseases. Prog retinal eye Res (2020) 79:100849. doi: 10.1016/j.preteyeres.2020.100849
192. Eagle RC Jr. The pathology of ocular cancer. Eye (Lond) (2013) 27(2):128–36. doi: 10.1038/eye.2012.237
193. Kleinstein RN, Lehman HF. Incidence and prevalence of eye cancer. Am J Optom Physiol Opt (1977) 54(1):49–51. doi: 10.1097/00006324-197701000-00008
194. Lande K, Gupta J, Ranjan R, Kiran M, Torres Solis LF, Solis Herrera A, et al. Exosomes: Insights from retinoblastoma and other eye cancers. Int J Mol Sci (2020) 21(19). doi: 10.3390/ijms21197055
195. Abramson DH, Shields CL, Munier FL, Chantada GL. Treatment of retinoblastoma in 2015: Agreement and disagreement. JAMA Ophthalmol (2015) 133(11):1341–7. doi: 10.1001/jamaophthalmol.2015.3108
196. Kaliki S, Shields CL, Shields JA. Uveal melanoma: estimating prognosis. Indian J Ophthalmol (2015) 63(2):93–102. doi: 10.4103/0301-4738.154367
197. Xu L, Li W, Shi Q, Wang M, Li H, Yang X, et al. MicroRNA−936 inhibits the malignant phenotype of retinoblastoma by directly targeting HDAC9 and deactivating the PI3K/AKT pathway. Oncol Rep (2020) 43(2):635–45. doi: 10.3892/or.2020.7456
198. Atienzar-Aroca S, Serrano-Heras G, Freire Valls A, Ruiz de Almodovar C, Muriach M, Barcia JM, et al. Role of retinal pigment epithelium-derived exosomes and autophagy in new blood vessel formation. J Cell Mol Med (2018) 22(11):5244–56. doi: 10.1111/jcmm.13730
199. Park JE, Tan HS, Datta A, Lai RC, Zhang H, Meng W, et al. Hypoxic tumor cell modulates its microenvironment to enhance angiogenic and metastatic potential by secretion of proteins and exosomes. Mol Cell Proteomics (2010) 9(6):1085–99. doi: 10.1074/mcp.M900381-MCP200
200. Rao R, Honavar SG. Retinoblastoma. Indian J Pediatr (2017) 84(12):937–44. doi: 10.1007/s12098-017-2395-0
201. Rodriguez-Galindo C, Orbach DB, VanderVeen D. Retinoblastoma. Pediatr Clin North Am (2015) 62(1):201–23. doi: 10.1016/j.pcl.2014.09.014
202. Abramson DH, Schefler AC, Beaverson KL, Rollins IS, Ruddat MS, Kelly CJ. Rapid growth of retinoblastoma in a premature twin. Arch Ophthalmol (2002) 120(9):1232–3. doi: 10.1001/archopht.120.9.1232
203. Quail DF, Joyce JA. Microenvironmental regulation of tumor progression and metastasis. Nat Med (2013) 19(11):1423–37. doi: 10.1038/nm.3394
204. Hirschberger S, Hinske LC, Kreth S. MiRNAs: dynamic regulators of immune cell functions in inflammation and cancer. Cancer Lett (2018) 431:11–21. doi: 10.1016/j.canlet.2018.05.020
205. Mathiyalagan P, Liang Y, Kim D, Misener S, Thorne T, Kamide CE, et al. Angiogenic mechanisms of human CD34(+) stem cell exosomes in the repair of ischemic hindlimb. Circ Res (2017) 120(9):1466–76. doi: 10.1161/CIRCRESAHA.116.310557
206. Pollard JW. Trophic macrophages in development and disease. Nat Rev Immunol (2009) 9(4):259–70. doi: 10.1038/nri2528
207. Qian BZ, Pollard JW. Macrophage diversity enhances tumor progression and metastasis. Cell (2010) 141(1):39–51. doi: 10.1016/j.cell.2010.03.014
208. Takase N, Koma Y, Urakawa N, Nishio M, Arai N, Akiyama H, et al. NCAM- and FGF-2-mediated FGFR1 signaling in the tumor microenvironment of esophageal cancer regulates the survival and migration of tumor-associated macrophages and cancer cells. Cancer Lett (2016) 380(1):47–58. doi: 10.1016/j.canlet.2016.06.009
209. Esbona K, Yi Y, Saha S, Yu M, Van Doorn RR, Conklin MW, et al. The presence of cyclooxygenase 2, tumor-associated macrophages, and collagen alignment as prognostic markers for invasive breast carcinoma patients. Am J Pathol (2018) 188(3):559–73. doi: 10.1016/j.ajpath.2017.10.025
210. Kujala E, Makitie T, Kivela T. Very long-term prognosis of patients with malignant uveal melanoma. Invest Ophthalmol Vis Sci (2003) 44:4651–9. doi: 10.1167/iovs.03-0538
211. Diener-West M, Reynolds SM, Agugliaro DJ, Caldwell R, Cumming K, Earle JD, et al. Development of metastatic disease after enrollment in the COMS trials for treatment of choroidal melanoma: Collaborative ocular melanoma study group report no. 26. Arch Ophthalmol (2005) 123(12):1639–43. doi: 10.1001/archopht.123.12.1639
212. Albert D., Diener-West M., McCaffrey L., Mo C.S., Scully R.Assessment of metastatic disease status at death in 435 patients with large choroidal melanoma in the collaborative ocular melanoma study (COMS): COMS report no. 15. Arch Ophthalmol (2001) 119(5):670–6. doi: 10.1001/archopht.119.5.670
213. Callejo SA, Antecka E, Blanco PL, Edelstein C, Burnier MN Jr. Identification of circulating malignant cells and its correlation with prognostic factors and treatment in uveal melanoma. a prospective longitudinal study. Eye (Lond) (2007) 21(6):752–9. doi: 10.1038/sj.eye.6702322
214. Kilic E, Smit K, van Poppelen N, Lunavat T, Derks K, Vaarwater J, et al. miRNA profiling of uveal melanoma exosomes as a metastatic risk biomarker. Acta Ophthalmol (2017). doi: 10.1111/j.1755-3768.2017.03642
215. Surman MSen E, Przybyło M. Melanoma-derived extracellular vesicles: Focus on their proteome. Proteomes (2019) 7. doi: 10.3390/proteomes7020021
216. Angi M, Kalirai H, Prendergast S, Simpson D, Hammond DE, Madigan MC, et al. In-depth proteomic profiling of the uveal melanoma secretome. Oncotarget (2016) 7:49623–35. doi: 10.18632/oncotarget.10418
217. Pardo M, Garcia Á., Antrobus R, Blanco MJ, Dwek RA, Zitzmann N. Biomarker discovery from uveal melanoma secretomes: Identification of gp100 and cathepsin d in patient serum. J Proteome Res (2007) 6:2802–11. doi: 10.1021/pr070021t
218. Hoshino A, Costa-Silva B, Shen TL, Rodrigues G, Hashimoto A, Tesic Mark M, et al. Tumour exosome integrins determine organotropic metastasis. Nature (2015) 527(7578):329–35. doi: 10.1038/nature15756
Keywords: inflammation, autoimmune, infection, extracellular vesicles, exosome, eye, mesenchymal stem cells
Citation: Habibi A, Zarei-Behjani Z, Falamarzi K, Malekpour M, Ebrahimi F, Soleimani M, Nejabat M, Khosravi A, Moayedfard Z, Pakbaz S, Dehdari Ebrahimi N and Azarpira N (2023) Extracellular vesicles as a new horizon in the diagnosis and treatment of inflammatory eye diseases: A narrative review of the literature. Front. Immunol. 14:1097456. doi: 10.3389/fimmu.2023.1097456
Received: 13 November 2022; Accepted: 14 February 2023;
Published: 09 March 2023.
Edited by:
Amin Tamadon, PerciaVista R&D Co., IranReviewed by:
João Alfredo Moraes, Federal University of Rio de Janeiro, BrazilAfshin Zare, Bushehr University of Medical Sciences, Iran
Copyright © 2023 Habibi, Zarei-Behjani, Falamarzi, Malekpour, Ebrahimi, Soleimani, Nejabat, Khosravi, Moayedfard, Pakbaz, Dehdari Ebrahimi and Azarpira. This is an open-access article distributed under the terms of the Creative Commons Attribution License (CC BY). The use, distribution or reproduction in other forums is permitted, provided the original author(s) and the copyright owner(s) are credited and that the original publication in this journal is cited, in accordance with accepted academic practice. No use, distribution or reproduction is permitted which does not comply with these terms.
*Correspondence: Negar Azarpira, negarazarpira@gmail.com; Niloofar Dehdari Ebrahimi, niloofar.dehdari6021@gmail.com