- 1Thoracic Oncology Ward, Cancer Center, West China Hospital, Sichuan University, Chengdu, Sichuan, China
- 2State Key Laboratory of Biotherapy, Sichuan University, Chengdu, Sichuan, China
- 3Clinical Trial Center, National Medical Products Administration Key Laboratory for Clinical Research and Evaluation of Innovative Drugs, West China Hospital, Sichuan University, Chengdu, Sichuan, China
Although breakthroughs in cancer treatment have been achieved, immunotherapy yields only modest benefits in most patients. There is still a gap in clarifying the immune evasiveness and immune-resistance mechanisms. Identifying other candidate targets for cancer immunotherapy is therefore a clear unmet clinical need. The complement system, a pillar of innate immunity, has recently entered the limelight due to its immunoregulatory functions in the tumor microenvironment (TME). In particular, gC1qR, a receptor for globular heads of C1q, serves as a promising new target and has attracted more attention. gC1qR, also named P32/C1qBP/HABP1, is a multifunctional protein that is overexpressed in various cancers and holds prognostic value. It regulates the tumorigenic, progression and metastatic properties of tumor cells through several downstream signaling pathways, including the Wnt/β-catenin, PKC–NF-κB and Akt/PKB pathways. A few preclinical experiments conducted through gC1qR interventions, such as monoclonal antibody, chimeric antigen receptor T‐cell (CAR‐T) therapy, and tumor vaccination, have shown encouraging results in anticancer activity. The efficacy may rely on the regulatory role on the TME, induction of tumor cells apoptosis and antiangiogenic activity. Nevertheless, the current understanding of the relationship between cancer immunotherapy and gC1qR remains elusive and often contradictory, posing both opportunities and challenges for therapeutic translation in the clinic. In this review, we focus on the current understanding of gC1qR function in cancer immunology and highlight the vital roles in regulating the TME. We also examines the rationale behind targeting gC1qR and discusses the potential for translating into clinical practice.
Introduction
Immune checkpoint inhibitors (ICIs) therapy targeting cytotoxic T lymphocyte antigen 4 (CTLA4) or programmed cell death-1 (PD-1) and its ligand PD-L1 has become the backbone of treatment for many cancers (1–3). Immune checkpoints are mainly expressed on the immune cells and tumor cells and inhibit the activation of immune system and help tumors escape from the host immune attack (4, 5). ICIs, therefore, could block the negative signaling on T-cell activation from immune checkpoints and potentiate antitumor T-cell activity (6, 7). However, the majority of patients exhibit poor or limited responses to these therapies even in combination, do not derive clinical benefit regardless of the cancer type, which highlights the necessity for finding alternative targets to gain optimal patient benefits (8, 9). The complement system is an integral part of innate immunity that consists of a network of plasma and membrane proteins (10). Complement could protect against nonself material, such as aberrant endogenous proteins and bacteria, by orchestrating the immune response through opsonization, recruitment of immune cells to the site of infection and causing cell lysis (11, 12). Recent studies have revealed the immunoregulatory functions of complement in cancer immunotherapy (13, 14). Complement regulates signaling pathway of tumor cells, thus promoting tumor growth, invasion and metastasis. Moreover, the complement system also correlates with angiogenesis, stromal composition and immune responses (15).
gC1qR, also named P32/C1qBP/HABP1, a receptor for globular heads of C1q, has begun to garner significant interest in the immune-oncology field as a novel potential target (16, 17). This protein bound with high affinity to the globular heads of C1q under physiological ionic strength (18). Under normal physiology, the gC1qR engages in a wide range of physiological activities, including mitochondrial metabolism and dynamics, apoptosis, splicing, immunological response, and inflammation (18). It binds to a plethora of proteins found in plasma, on the cell surface and on pathogenic microorganisms. In contrast, in cancer conditions, gC1qR played a vital role in cancer progression and correlated with patient prognosis (19). gC1qR could reshape the tumor microenvironment (TME) by modulating immune cells and cancer cells, resulting in poor anti-tumor efficacy. The activity of targeting gC1qR has been explored in CAR‐T therapy, monoclonal antibodies and cancer which showed effective anti-tumour immune responses (20, 21). Here, we focus on recent advances in the understanding of gC1qR in cancer, review results supporting the role of gC1qR in cancer immunology and illustrate its potential for translation into clinical practice.
Structure, expression, and function of gC1qR
gC1qR has several names because it was discovered by three distinct groups independently. HABP1 was first identified as a glycoprotein containing sialic acid by D’Souza and Datta in 1985 (22). Subsequently, studies have established that the HABP1 was involved in many regulatory processes related to hyaluronan (HA), such as adhesive function and regulatory role in reproduction (23). In 1991, Krainer and collaborators described that P32, which co-purified with the splicing factor SF2, had the same cDNA sequence as HABP1 (24). gC1q-R is a highly conserved, acidic protein (pI=4.15), which was first isolated from membranes of the lymphoblastoid cell line Raji and identified as a receptor for globular heads of C1q (25, 26). The amino acid sequences of HABP1, P32, and gC1qR were identical, and they were all codified by the same gene. The gC1qR gene is located on chromosome 17p13.3 in humans, and chromosome 11 in mice. The gene is highly conserved and the cDNA sequence between the human and rodent genes is almost identical (~89.9%) (27).
The molecular weight of gC1qR in SDS-PAGE is about 33 kDa. Under non-denaturing and non-dissociating conditions, it is a doughnut-shaped trimer of 3 identical chains with 97.2kDa (18). The formation of multimers may be crucial to enhancing its affinity for multivalent ligands, such as C1q and high molecular weight kininogen (HK) (28). The doughnut-shaped quaternary structure has two sides. The solution side contains a high distribution of negatively charged residues, whereas the membrane face has a more or less neutral net charge (29). The protein is synthesized as a prepro-protein of 282 amino acid residues and then becomes a mature protein of 209 residues through a site-specific cleavage and removal during post-translational processing (30). It has one Cys at residue 186 and thus does not have any intrachain disulfide bonding (29). Research on the translated amino acid sequence reveals there is no conventional consensus motif or glycosylphosphatidylinositols (GPIs) anchor present (31). Hence, it may transfer signals through the association with partner transmembrane proteins (18). As research has progressed, gC1qR has been identified as a multicompartmental and multifunctional protein (32). gC1qR not only presents in the mitochondrial matrix but also localizes at all compartments of the cell, including the extracellular cell surface and nucleus (33).
The ubiquitous distribution of gC1qR suggests that it may be involved in a wide range of biological responses. In addition to combining with C1q, gC1qR could bind multiple ligands including thrombin, vitronectin, HK and factor XII (HF) (34–36). The interplay between gC1qR and its binding partners leads to the classical complement pathway activation, cell adhesion, and activation of the kinin system (37, 38). Furthermore, it regulates the homeostatic and thrombotic events (39). The contact system proteins HF, prekallikrein (PK), and HK comprise the initiators of the so-called intrinsic blood coagulation system (40). gC1qR could interact with HK and HF, and then activate intrinsic coagulation and kinin pathways (39, 41, 42). gC1qR also plays a critical role in the maintenance of phosphorylation (43, 44). The disruption of the p30 gene (homolog of p32 in yeast) impaired mitochondrial ATP synthesis, which could be restored by introducing human p32 cDNA. gC1qR is necessary for functional mitoribosome formation to synthesize proteins within mitochondria and induce mitochondria-dependent cell death (45, 46). In addition, gC1qR was correlated with cell apoptosis. The overexpression of gC1qR in fibroblast cells induces inhibited cell growth, extensive vacuolation, restricted entry to the S-phase, and finally leading to apoptosis (47).
Moreover, a growing number of studies have shown the link between gC1qR and virus, such as respiratory syncytial virus (RSV), hepatitis C virus (HCV) and human immunodeficiency virus (HIV-1). The gC1qR protein was present as a key factor for production of RSV and the mitochondrial localized gC1qR contributes to RSV infection (48). gC1qR has been identified as an HCV core-binding protein. The combination of gC1qR on T cells and HCV core protein suppressed the T-cell proliferation, thus further impacting the human cell–mediated immune response (49). And the inhibition of gC1qR could reverse the T-cell responsiveness. The gC1qR-mediated immune suppression in HCV also confirmed in other studies, the interaction between gC1qR and HCV core protein also influenced the Th1 differentiation of CD4+ T cells by inhibiting the dendritic cell IL-12 production (50). In addition, the gC1qR also participated in the HIV-1 pathogenesis (51). These results highlight the critical role gC1qR plays in a growing list of diseases.
gC1qR in cancer
The role of gC1qR in cancer diagnosis, prognosis, and putative signaling pathways
Recent researches have shown that gC1qR contributes to cancer progression, invasion and metastasis, and correlates with clinicopathological features of tumors (Figure 1) (23, 52–55). Its overexpression was found in multiple cancer cells, including breast, ovarian, prostate, melanoma, lung, pancreatic, colon cancer, and malignant pleural mesothelioma (55–58). It has been reported the expression level of gC1qR was correlated with tumor stage, grade, tumor size, and clinical outcome (59, 60). In breast cancer, gC1qR overexpression was significantly related to distant metastasis, higher TNM stages, increased tumor size, axillary node metastasis and poor survival (23, 60). A poor outcome was also found in patients with ovarian cancer with gC1qR overexpression (61). Similar observations were reported in patients with gastric cancer (62) and endometrial cancer (63). Additionally, it is involved in cancer cell chemotaxis and metabolism. gC1qR modulates the cancer cell chemotaxis through binding to protein kinase C ζ (64, 65). Active caspase-1 cleaves gC1qR and then promotes aerobic glycolysis in tumor cells and boosts carcinogenesis (66). However, its roles may vary according to different cancer types. In renal cell carcinoma (RCC), higher expression levels of Y-box-binding protein 1 (YBX1) and lower expression of gC1qR were found in tumor tissues (67). And the gC1qR negatively regulates the activation of YBX1 which is closely associated with tumorigenesis.
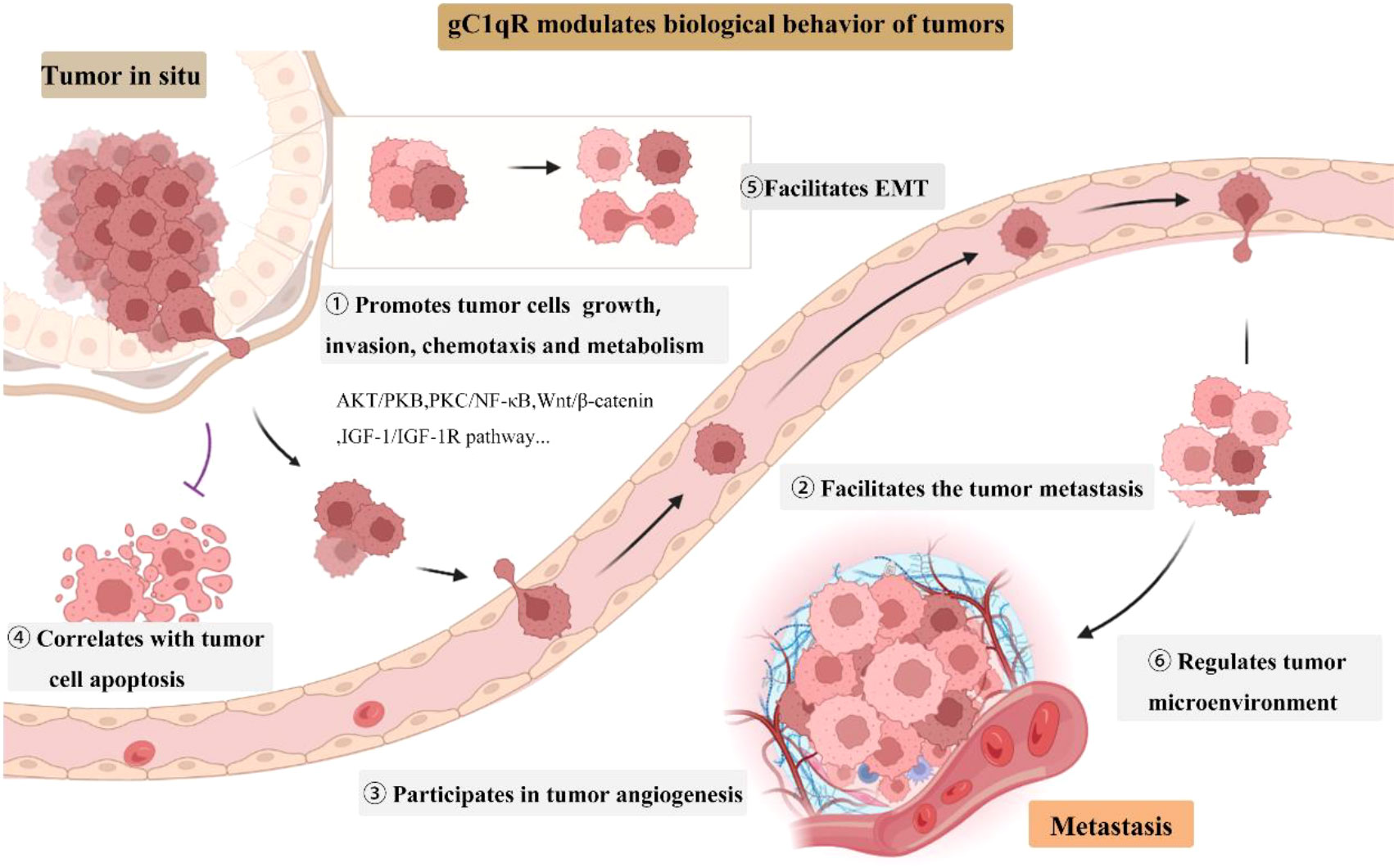
Figure 1 gC1qR roles in tumor growth, invasion, and progression. EMT, epithelial to mesenchymal transition.
In addition to tumor cellular gC1qR expression, soluble gC1qR (sgC1qR) was also discovered. The researchers observed an increased level of soluble gC1qR in metastatic pancreatic cancer patients were noted with disease progression (68). Soluble gC1qR was also detected in malignant pleural and peritoneal effusions and the level was higher in malignant effusions than serum. Another finding demonstrated that soluble gC1qR may serve as an autocrine growth signal for cancer cell proliferation (69).
gC1qR is involved in multiple signaling pathways to modulate the biological behavior of tumors. In HepG2 cells, gC1qR overexpression boosts the cell proliferation through up-regulation of cyclin D1 in AKT-dependent pathway (70). Sinha S et al. revealed the gC1qR regulates cell proliferation, migration, and invasion in melanoma by regulating AKT/PKB signaling and altering oncogenes as well as epithelial to mesenchymal transition (EMT) markers in both mouse and human melanoma (71). In addition, gC1qR also induced the NF-kappa B dependent MMP-2 activation through integrin αvβ3 interaction to regulate the cell migration and tumor development (72). Depletion of gC1qR in triple-negative breast cancer (TNBC) inhibits hypoxia-induced activation of the PKC/NF-KB/VCAM-1 signaling pathway, resulting in cancer cell metastasis blocking (73). The Wnt/β-catenin pathway also correlated with gC1qR. Circular RNA MTCL1 could regulate the Wnt/β-catenin pathway through interacting with gC1qR and cause the laryngeal squamous cell carcinoma progression (74). Moreover, gC1qR mediates hepatic metastasis of pancreatic cancer via IGF-1/IGF-1R signaling (57). However, another contradictory study indicated that gC1qR may serve as a tumor suppresser by modulating the p-GSK3/β-Catenin/L1CAM expression in RCC (75).
To summarize, gC1qR plays a pivotal role in the growth, survival, and metastasis of tumor cells. It also serves as a novel marker for cancer prognosis and diagnosis, provides new opportunities for cancer therapy although the regulatory mechanisms of gC1qR in various cancers have not yet been elucidated.
gC1qR and the tumor microenvironment
TME, which consists of immune cells, non-tumorigenic stromal cells, and tumor cells, is the key to carcinogenesis and associates with immune resistance as well as immune evasion (76). Despite some conflicting conclusions, accumulating evidence suggests that gC1qR may serve as a potential regulator of TME interactions.
gC1qR has been proved to be essential for CD8+ T cell survival, proliferation, and anti-tumor immune function (20, 77). It regulates the epigenetic program and promotes the dynamic transcriptional program of effector CD8+ T cells. gC1qR increases T cell proliferation through regulating the AKT‐mTORC1 signaling pathway and improving T cell survival by recruiting anti-apoptotic proteins such as Bcl2 and BclXL, thereby inhibiting caspase3 cleavage and PARP inactivation (20). gC1qR deficiency impedes T cell proliferation, hinders the CD4+ and CD8+ T cell infiltration and aggravates tumor infiltrating T cell exhaustion (20). Additionally, gC1qR knockdown aggravated the exhausted phenotype of CD4+ and CD8+ T cells through increasing co–inhibitory molecules such as PD‐1, Tim‐3, and LAG‐3. gC1qR knockdown also impaired the efficacy of CAR-T cells (20). gC1qR has been shown to be required for the dendritic cells (DCs) metabolism and maturation (78). But the relationship between DCs and gC1qR in cancer immunotherapy has not been elucidated. Fogal et al. also demonstrated that the cell-surface gC1qR could serve as the marker for tumor-associated macrophages/myeloid cells. They found gC1qR is the receptor for LyP-1 and the p32/LyP-1 positive cells were also positive for macrophage/myeloid cell markers (79). gC1qR also acts on the macrophages and leukocytes infiltration in TME and gC1qR knockdown inhibits the infiltration of these cells (71).
Angiogenesis, a key component of the TME, is necessary for tumor invasion and metastasis. Abnormal tumor blood vessels lead to hypoxia and contribute to inhibitory TME (80). gC1qR was identified to be expressed on the cell surface of activated (angiogenic) endothelial cells and evidence showed that the gC1qR may participate in tumor angiogenesis, thereby modulating the TME (71, 81, 82). The neutralization of cell-surface gC1qR with antibody inhibits vascular endothelial growth factor (VEGF) signaling and prevents angiogenesis in human umbilical vein endothelial cells (HUVECs) (81). Another study also revealed gC1qR-silenced tumors showed decreased proliferation and angiogenesis compared to control tumors in melanoma (71). Those results suggested the gC1qR may function as an angiogenic-related protein and serve as a potential therapeutic target for blocking tumor angiogenesis. Hypoxia is a widely established factor of the TME that could upregulate the hypoxia-inducible factor-1α (HIF-1α) and PD-L1 expression, and promote immune escape and immune resistance (83). Studies showed that the expression of gC1qR was enhanced by HIF-1α (73) and increased expression of gC1qR was found in hypoxia area of tumor (79). gC1qR, also a novel receptor of Hyaluronic acid (HA), could interact with HA and regulated HA-mediated cellular event as well as affect behavior of tumor cells. The extracellular matrix (ECM) is a dynamic network that serves as a highly active part of the TME (84). HA is one of major ECM components which relates to the cell–cell contacts such as adhesion, motility, and differentiation and cancer metastasis (85).
These results serve to illustrate the conflicting role of gC1qR on TME. gC1qR knockdown may modulate T cells’ mitochondrial fitness to decrease the infiltration and anti-tumor function, but the blockage of cell-surface gC1qR could decrease the blood supply of tumor. Although the exact role of gC1qR in TME has not been fully validated, it shows potential to regulate tumor cells, immune cells, angiogenesis and other components of the TME.
Potential for translating into clinic
Advances in preclinical research
The complex function of gC1qR in tumor regulation and immunity poses opportunities for therapeutic translation in cancer immunotherapy. Studies on preclinical mouse tumor models have indicated that gC1qR can be considered as a suitable target for different cancer therapy approaches, such as monoclonal antibody therapy, small molecules, CAR-T technology, and tumor vaccination (Figure 2).
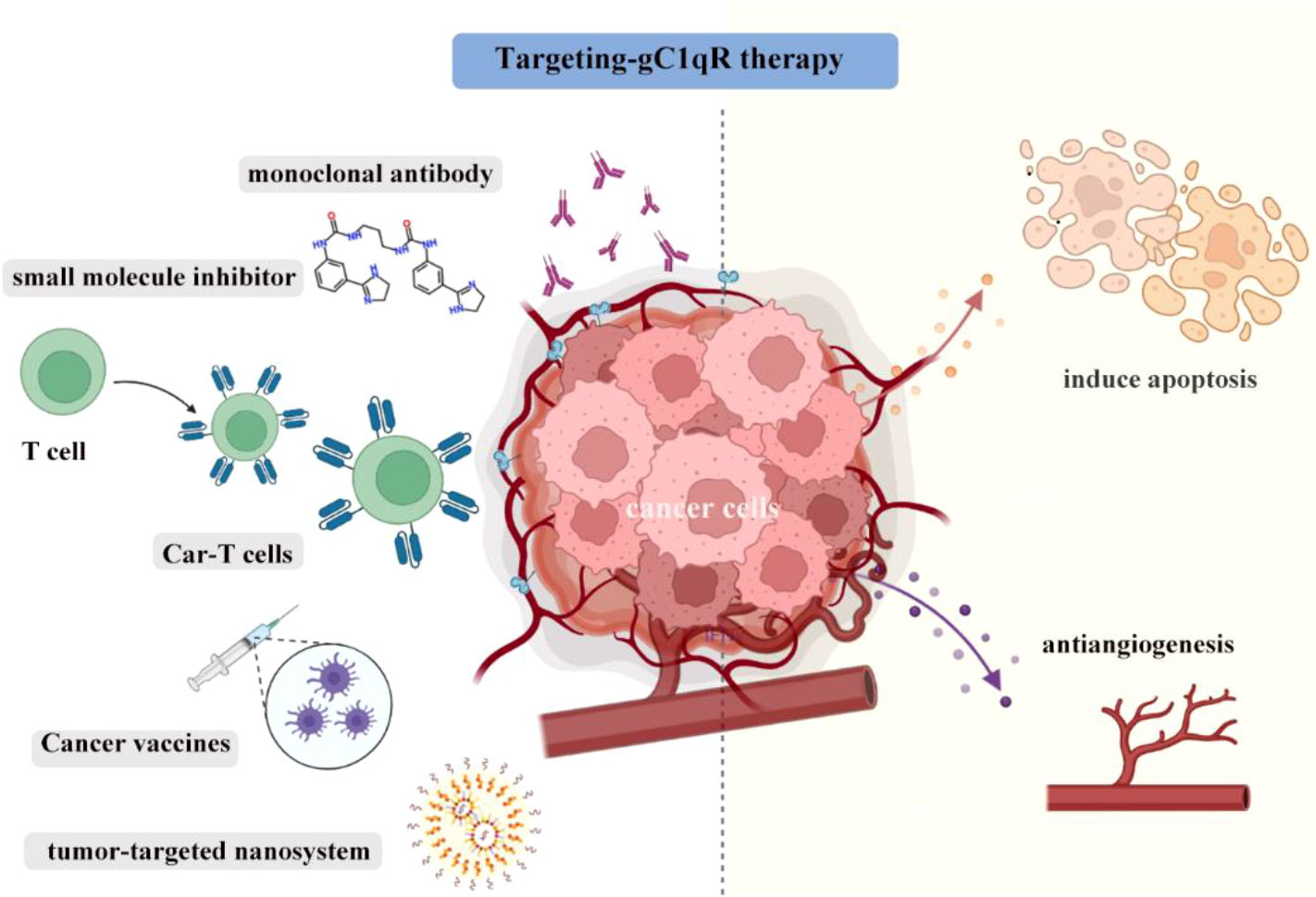
Figure 2 The gC1qR blockade can be largely classified into monoclonal antibody therapy, highly selective small molecules, CAR-T therapy, tumor vaccination and used in nanosystem to kill tumors. Induction of tumor cells apoptosis and antiangiogenic activity are key element in successful and efficient gC1qR targeting.
In TNBC, gC1qR monoclonal antibody 60.11-treated mice have smaller tumors than control mice. Mechanistically, mice in treatment group had higher expression levels of apoptosis-related markers and decreased CD31 (angiogenesis-related marker) expression (21). In other words, the antibody targeting gC1qR promoted tumor cell apoptosis and decreased angiogenesis. Potential toxicity was also evaluated and the results showed that there was no evidence of tissue damage observed on vital organs. As gC1qR was overexpressed in malignant pleural mesothelioma and mesothelioma (MSTO) cells lines, thus the antitumor efficacy gC1qR monoclonal antibody 60.11 was also assessed in MSTO mice models. The results indicated that gC1qR monoclonal antibody 60.11 reduced mesothelioma tumor volume via increasing apoptosis and reducing neovascularization (19). Additionally, the gC1qR-neutralizing antibody 3D9 could prevent the cells migration of plenty of cancer cell lines, including A549, MCF7 and MDA-MB-231. It also inhibits the growth factor-stimulated lamellipodia formation and receptor tyrosine kinases (RTKs) signaling (81). More importantly, 3D9 also had an anti-angiogenic effect and prevented lamellipodia formation, cell migration and VEGF signaling in HUVECs. In vivo, 3D9 significantly inhibited the tumor growth in A549 tumor-bearing nude mice (81). Above results confirmed the anti-tumor efficacy of gC1qR monoclonal antibody in cancer immunotherapy, but it is worth noting that in some specific tumors such as the glioblastoma, the blood–brain barrier may decrease the antitumor activity. To solve this problem and to specifically target the mitochondrial-localized gC1qR, Yenugonda et al. identified a highly selective and brain-penetrant small molecule M36 (86). M36 was selective for gC1qR overexpressing cells. It effectively impaired the growth of glioma cells, and sensitized the tumor cells to glucose depletion.
CAR-T technology has developed rapidly in recent years and is one of the most promising strategies in cancer therapy (87). Rousso-Noori et al. designed gC1qR CAR T cells in glioma after confirming that gC1qR was expressed in mice and human glioma. The novel gC1qR CAR-T therapy significantly induces tumor regression in both syngeneic and xenograft models, and extended the overall survival of mice (88). Consistent with the above findings, the gC1qR CAR T cells also had antiangiogenic activity in this study.
Cancer vaccines hold promise as an immunotherapeutic modality and it could induce long-term immunological memory for antitumor responses (89, 90). Dehghan-Manshadi et al. designed vaccine based on peptides derived from mouse gC1qR protein and the results showed that compared with the control group, mice in the vaccinated group had higher levels of IFN-γ and perforin, lower tumor size and longer survival time (91). Additionally, they also found that forkhead box P3 (Foxp3) gene was significantly down-regulated and Fas ligand (FasL) gene expression was up-regulated in splenocytes vaccinated mice group (91).
Nanotechnology has recently attracted significant attention worldwide for cancer treatment (92). Previous research revealed the gC1qR was the receptor for tumor-homing peptide LyP-1 which could specifically bind to tumor lymphatics and tumor cells (79, 93, 94). LyP-1 has been used to deliver nanoparticles to breast cancer with gC1qR overexpression and effectively reduced tumor growth in vivo (86, 95). Sharma S et al. also described a tumor-penetrating nanosystem that strongly inhibits breast tumor growth in mice, in which a homing component targets tumor vessels through binding gC1qR (82). In this context, targeting gC1qR seems to be an increasingly promising strategy in the field of cancer therapy.
Questions and challenges
Considerable insight into its function and therapeutic potential have been gained until now. However, key questions remain regarding fundamental gC1qR biology and translational application. First, anti-gC1qR monotherapy has shown anti-tumor activity in pre-clinical murine models, but such activity in humans is still unknown. As animal models cannot mimic the complex environment of human body, thus, well designed clinical trials are essential to demonstrate the efficacy and safety of anti-gC1qR in human beings. Moreover, monotherapy often displays important drawbacks and given the promise of ICI-based combination therapy (96, 97), the efficacy of ICIs and anti-gC1qR therapy also demand investigation. Second, the exact role of gC1qR in the regulation of the TME is still not sufficiently elucidated and current knowledge on this target is not enough. Determination of gC1qR expression in human T-cell subsets especially tumor-infiltrating lymphocytes and comprehensive validation of related functional mechanisms in immune cells are prerequisites for the development of anti-gC1qR therapeutics. Additionally, gC1qR is also expressed on the normal cells (98), the safety of targeting gC1qR should be assessed carefully in human body, although no obvious side effects were revealed in mice. Finally, targeting gC1qR has just started as a new field within cancer immunotherapy and further in vitro and in vivo studies are still needed. Based on abundant and solid evidence, clinical trials are the next step directions and targeting gC1qR might benefit selected patient populations.
Conclusion
Despite ICIs having led to unprecedented breakthroughs in cancer treatment, most patients do not respond well to ICIs. Such results pave the way for targeting other promising immune checkpoints. In this review, we provide a strong foundation for targeting the gC1qR to be a novel anticancer therapeutic approach and hold promise for translate into the clinic, although there still have no related clinical trial to date. Accumulating evidence has shown that gC1qR modulates tumor growth, invasion, and progression. It participates in multiple signaling pathways and regulates the TME. The gC1qR blockade can be largely classified into monoclonal antibody therapy, highly selective small molecules, CAR-T therapy, tumor vaccination and nanoparticle strategies for cancer therapy. Considering the characteristics as presented here, it seems that the regulation role on TME, induces the apoptosis of tumor cells and antiangiogenic activity are key elements in successful and efficient gC1qR targeting. However, the complex function of gC1qR poses both opportunities and challenges for therapeutic translation in clinic. More efforts should be devoted to accelerating the development of gC1qR inhibitors for clinical use.
Author contributions
YW: Conceptualization. YL, XL: Original draft preparation. all authors contributed to the article and approved the submitted version.
Funding
This work was supported by the China Postdoctoral Science Foundation (No. 2021M702346), the Post-Doctor Research Project, West China Hospital, Sichuan University(2021HXBH092) and the National Natural Science Foundation of China (Grant No. 82073369, 8227102577).
Conflict of interest
The authors declare that the research was conducted in the absence of any commercial or financial relationships that could be construed as a potential conflict of interest.
Publisher’s note
All claims expressed in this article are solely those of the authors and do not necessarily represent those of their affiliated organizations, or those of the publisher, the editors and the reviewers. Any product that may be evaluated in this article, or claim that may be made by its manufacturer, is not guaranteed or endorsed by the publisher.
References
1. Wang DR, Wu XL, Sun YL. Therapeutic targets and biomarkers of tumor immunotherapy: response versus non-response. Signal Transduct Target Ther (2022) 7(1):331. doi: 10.1038/s41392-022-01136-2
2. Billan S, Kaidar-Person O, Gil Z. Treatment after progression in the era of immunotherapy. Lancet Oncol (2020) 21(10):e463–76. doi: 10.1016/S1470-2045(20)30328-4
3. Liu M, Guo F. Recent updates on cancer immunotherapy. Precis Clin Med (2018) 1(2):65–74. doi: 10.1093/pcmedi/pby011
4. Pardoll DM. The blockade of immune checkpoints in cancer immunotherapy. Nat Rev Cancer (2012) 12(4):252–64. doi: 10.1038/nrc3239
5. Gajewski TF. The next hurdle in cancer immunotherapy: Overcoming the non–T-Cell–Inflamed tumor microenvironment. Semin Oncol (2015) 42(4):663–71. doi: 10.1053/j.seminoncol.2015.05.011
6. Bagchi S, Yuan R, Engleman E. Immune checkpoint inhibitors for the treatment of cancer: Clinical impact and mechanisms of response and resistance. Annu Rev Pathol (2021) 16:223–49. doi: 10.1146/annurev-pathol-042020-042741
8. Li T, Qiao T. Unraveling tumor microenvironment of small-cell lung cancer: Implications for immunotherapy. Semin Cancer Biol (2022) 86(Pt 2):117–25. doi: 10.1016/j.semcancer.2022.09.005
9. Kim TK, Vandsemb EN, Herbst RS, Chen L. Adaptive immune resistance at the tumour site: mechanisms and therapeutic opportunities. Nat Rev Drug Discovery (2022) 21(7):529–40. doi: 10.1038/s41573-022-00493-5
10. Dunkelberger JR, Song WC. Complement and its role in innate and adaptive immune responses. Cell Res (2010) 20(1):34–50. doi: 10.1038/cr.2009.139
11. Leslie JD, Mayor R. Complement in animal development: Unexpected roles of a highly conserved pathway. Semin Immunol (2013) 25(1):39–46. doi: 10.1016/j.smim.2013.04.005
12. Ricklin D, Hajishengallis G, Yang K, Lambris JD. Complement: A key system for immune surveillance and homeostasis. Nat Immunol (2010) 11(9):785–97. doi: 10.1038/ni.1923
13. Bordron A, Bagacean C, Tempescul A, Berthou C, Bettacchioli E, Hillion S, et al. Complement system: a neglected pathway in immunotherapy. Clin Rev Allergy Immunol (2020) 58(2):155–71. doi: 10.1007/s12016-019-08741-0
14. Kolev M, Markiewski M. Targeting complement-mediated immunoregulation for cancer immunotherapy. Semin Immunol (2018) 37:85–97. doi: 10.1016/j.smim.2018.02.003
15. Senent Y, Tavira B, Pio R, Ajona D. The complement system as a regulator of tumor-promoting activities mediated by myeloid-derived suppressor cells. Cancer Lett (2022) 549:215900. doi: 10.1016/j.canlet.2022.215900
16. Egusquiza-Alvarez C, Robles-Flores M. An approach to p32/gC1qR/HABP1: a multifunctional protein with an essential role in cancer. J Cancer Res Clin Oncol (2022) 148(8):1831–54. doi: 10.1007/s00432-022-04001-5
17. Sánchez-Martín D, Cuesta AM, Fogal V, Ruoslahti E, Alvarez-Vallina L. The multicompartmental p32/gClqR as a new target for antibody-based tumor targeting strategies. J Biol Chem (2011) 286(7):5197–203. doi: 10.1074/jbc.M110.161927
18. Ghebrehiwet B, Geisbrecht BV, Xu X, Savitt AG, Peerschke EIB. The C1q receptors: Focus on gC1qR/p33 (C1qBP, p32, HABP-1)(1). Semin Immunol (2019) 45:101338. doi: 10.1016/j.smim.2019.101338
19. Peerschke E, Stier K, Li X, Kandov E, de Stanchina E, Chang Q, et al. gC1qR/HABP1/p32 is a potential new therapeutic target against mesothelioma. Front Oncol (2020) 10:1413. doi: 10.3389/fonc.2020.01413
20. Tian H, Wang G, Wang Q, Zhang B, Jiang G, Li H, et al. Complement C1q binding protein regulates T cells' mitochondrial fitness to affect their survival, proliferation, and anti-tumor immune function. Cancer Sci (2022) 113(3):875–90. doi: 10.1111/cas.15261
21. Peerschke EI, Stanchina E, Chang Q, Manova-Todorova K, Barlas A, Savitt AG, et al. Anti gC1qR/p32/HABP1 antibody therapy decreases tumor growth in an orthotopic murine xenotransplant model of triple negative breast cancer. Antibodies (Basel) (2020) 9(4). doi: 10.3390/antib9040051
22. D'souza M, Datta K. Evidence for naturally occurring hyaluronic acid binding protein in rat liver. Biochem Int (1985) 10(1):43–51.
23. Saha P, Datta K. Multi-functional, multicompartmental hyaluronan-binding protein 1 (HABP1/p32/gC1qR): implication in cancer progression and metastasis. Oncotarget (2018) 9(12):10784–807. doi: 10.18632/oncotarget.24082
24. Krainer AR, Mayeda A, Kozak D, Binns G. Functional expression of cloned human splicing factor SF2: homology to RNA-binding proteins, U1 70K, and drosophila splicing regulators. Cell (1991) 66(2):383–94. doi: 10.1016/0092-8674(91)90627-B
25. Ghebrehiwet B. C1q inhibitor (C1qINH): functional properties and possible relationship to a lymphocyte membrane-associated C1q precipitin. J Immunol (1981) 126(5):1837–42. doi: 10.4049/jimmunol.126.5.1837
26. Van Den Berg RH, Faber-Krol MC, Van De Klundert JA, Van Es LA, Daha MR. Inhibition of the hemolytic activity of the first component of complement C1 by an escherichia coli C1q binding protein. J Immunol (1996) 156(11):4466–73. doi: 10.4049/jimmunol.156.11.4466
27. Lynch NJ, Reid KB, Van Den Berg RH, Daha MR, Leigh LA, Ghebrehiwet B, et al. Characterisation of the rat and mouse homologues of gC1qBP, a 33 kDa glycoprotein that binds to the globular 'heads' of C1q. FEBS Lett (1997) 418(1-2):111–4. doi: 10.1016/S0014-5793(97)01348-3
28. Ghebrehiwet B, Jesty J, Peerschke EIB. gC1q-R/p33: Structure-function predictions from the crystal structure. Immunobiology (2002) 205(4):421–32. doi: 10.1078/0171-2985-00143
29. Ghebrehiwet B, Jesty J, Vinayagasundaram R, Vinayagasundaram U, Ji Y, Valentino A, et al. Targeting gC1qR domains for therapy against infection and inflammation. In: Lambris JD, Holers VM, Ricklin D, editors. Complement therapeutics. New York, NY: Springer US (2013). p. 97–110.
30. Kitazawa S, Takenaka A, Kondo T, Mizoguchi A, Kitazawa R. Protruding disordered loop of gC1qR is specifically exposed and related to antiapoptotic property in germ cell lineage. Histochem Cell Biol (2006) 126(6):665–77. doi: 10.1007/s00418-006-0225-y
31. Ghebrehiwet B, Lim BL, Peerschke EI, Willis AC, Reid KB. Isolation, cDNA cloning, and overexpression of a 33-kD cell surface glycoprotein that binds to the globular "heads" of C1q. J Exp Med (1994) 179(6):1809–21. doi: 10.1084/jem.179.6.1809
32. Ghebrehiwet B, Hosszu KK, Valentino A, Ji Y, Peerschke EI. Monocyte expressed macromolecular C1 and C1q receptors as molecular sensors of danger: Implications in SLE. Front Immunol (2014) 5:278. doi: 10.3389/fimmu.2014.00278
33. Ghebrehiwet B, Lim BL, Kumar R, Feng X, Peerschke EI. gC1q-R/p33, a member of a new class of multifunctional and multicompartmental cellular proteins, is involved in inflammation and infection. Immunol Rev (2001) 180:65–77. doi: 10.1034/j.1600-065X.2001.1800106.x
34. Lim BL, Reid KB, Ghebrehiwet B, Peerschke EI, Leigh LA, Preissner KT. The binding protein for globular heads of complement C1q, gC1qR. functional expression and characterization as a novel vitronectin binding factor. J Biol Chem (1996) 271(43):26739–44. doi: 10.1074/jbc.271.43.26739
35. Joseph K, Ghebrehiwet B, Peerschke EI, Reid KB, Kaplan AP. Identification of the zinc-dependent endothelial cell binding protein for high molecular weight kininogen and factor XII: identity with the receptor that binds to the globular "heads" of C1q (gC1q-r). Proc Natl Acad Sci U.S.A. (1996) 93(16):8552–7. doi: 10.1073/pnas.93.16.8552
36. Ghebrehiwet B, Jesty J. Peerschke e I.gC1q-R/p33: structure-function predictions from the crystal structure. Immunobiology (2002) 205(4-5):421–32.
37. Ghebrehiwet B, Cebadamora C, Tantral L, Jesty J, Peerschke EI. gC1qR/p33 serves as a molecular bridge between the complement and contact activation systems and is an important catalyst in inflammation. (2006) 586:95–105. doi: 10.1007/0-387-34134-X_7
38. Biswas AK, Hafiz A, Banerjee B, Kim KS, Datta K, Chitnis CE, et al. Plasmodium falciparum uses gC1qR/HABP1/p32 as a receptor to bind to vascular endothelium and for platelet-mediated clumping. PloS Pathog (2007) 3(9):1271–80. doi: 10.1371/journal.ppat.0030130
39. Peerschke EI, Jesty J, Reid KB, Ghebrehiwet B. The soluble recombinant form of a binding protein/receptor for the globular domain of C1q (gC1qR) enhances blood coagulation. Blood Coagul Fibrinolysis (1998) 9(1):29–37. doi: 10.1097/00001721-199801000-00004
40. Schmaier AH, Emsley J, Feener EP, Gailani D, Govers-Riemslag JWP, Kaplan AP, et al. Nomenclature of factor XI and the contact system. J Thromb Haemost (2019) 17(12):2216–9. doi: 10.1111/jth.14595
41. Lim BL, White RA, Hummel GS, Schwaeble W, Lynch NJ, Peerschke EI, et al. Characterization of the murine gene of gC1qBP, a novel cell protein that binds the globular heads of C1q, vitronectin, high molecular weight kininogen and factor XII. Gene (1998) 209(1-2):229–37. doi: 10.1016/S0378-1119(98)00055-9
42. Kaira BG, Slater A, Mccrae KR, Dreveny I, Sumya U, Mutch NJ, et al. Factor XII and kininogen asymmetric assembly with gC1qR/C1QBP/P32 is governed by allostery. Blood (2020) 136(14):1685–97. doi: 10.1182/blood.2020004818
43. Muta T, Kang D, Kitajima S, Fujiwara T, Hamasaki N. p32 protein, a splicing factor 2-associated protein, is localized in mitochondrial matrix and is functionally important in maintaining oxidative phosphorylation*. J Biol Chem (1997) 272(39):24363–70. doi: 10.1074/jbc.272.39.24363
44. Mcgee AM, Baines CP. Complement 1q-binding protein inhibits the mitochondrial permeability transition pore and protects against oxidative stress-induced death. Biochem J (2011) 433(1):119–25. doi: 10.1042/BJ20101431
45. Yagi M, Uchiumi T, Takazaki S, Okuno B, Nomura M, Yoshida S, et al. p32/gC1qR is indispensable for fetal development and mitochondrial translation: importance of its RNA-binding ability. Nucleic Acids Res (2012) 40(19):9717–37. doi: 10.1093/nar/gks774
46. Hu M, Crawford SA, Henstridge DC, Ng IH, Boey EJ, Xu Y, et al. p32 protein levels are integral to mitochondrial and endoplasmic reticulum morphology, cell metabolism and survival. Biochem J (2013) 453(3):381–91. doi: 10.1042/BJ20121829
47. Kamal A, Datta K. Upregulation of hyaluronan binding protein 1 (HABP1/p32/gC1qR) is associated with cisplatin induced apoptosis. Apoptosis (2006) 11(5):861–74. doi: 10.1007/s10495-006-5396-4
48. Hu M, Li HM, Bogoyevitch MA, Jans DA. Mitochondrial protein p32/HAPB1/gC1qR/C1qbp is required for efficient respiratory syncytial virus production. Biochem Biophys Res Commun (2017) 489(4):460–5. doi: 10.1016/j.bbrc.2017.05.171
49. Kittlesen DJ, Chianese-Bullock KA, Yao ZQ, Braciale TJ, Hahn YS. Interaction between complement receptor gC1qR and hepatitis c virus core protein inhibits T-lymphocyte proliferation. J Clin Invest (2000) 106(10):1239–49. doi: 10.1172/JCI10323
50. Waggoner SN, Hall CH, Hahn YS. HCV core protein interaction with gC1q receptor inhibits Th1 differentiation of CD4+ T cells via suppression of dendritic cell IL-12 production. J Leukoc Biol (2007) 82(6):1407–19. doi: 10.1189/jlb.0507268
51. Pednekar L, Pandit H, Paudyal B, Kaur A, Al-Mozaini MA, Kouser L, et al. Complement protein C1q interacts with DC-SIGN via its globular domain and thus may interfere with HIV-1 transmission. Front Immunol (2016) 7:600. doi: 10.3389/fimmu.2016.00600
52. Kim KB, Yi JS, Nguyen N, Lee JH, Kwon YC, Ahn BY, et al. Cell-surface receptor for complement component C1q (gC1qR) is a key regulator for lamellipodia formation and cancer metastasis. J Biol Chem (2011) 286(26):23093–101. doi: 10.1074/jbc.M111.233304
53. Peerschke EI, Ghebrehiwet B. cC1qR/CR and gC1qR/p33: observations in cancer. Mol Immunol (2014) 61(2):100–9. doi: 10.1016/j.molimm.2014.06.011
54. Saha SK, Kim KE, Islam SMR, Cho SG, Gil M. Systematic multiomics analysis of alterations in C1QBP mRNA expression and relevance for clinical outcomes in cancers. J Clin Med (2019) 8(4). doi: 10.3390/jcm8040513
55. Egusquiza-Alvarez CA, Castañeda-Patlán MC, Albarran-Gutierrez S, Gonzalez-Aguilar H, Moreno-Londoño AP, Maldonado V, et al. Overexpression of multifunctional protein p32 promotes a malignant phenotype in colorectal cancer cells. Front Oncol (2021) 11:642940. doi: 10.3389/fonc.2021.642940
56. Rubinstein DB, Stortchevoi A, Boosalis M, Ashfaq R, Ghebrehiwet B, Peerschke EI, et al. Receptor for the globular heads of C1q (gC1q-r, p33, hyaluronan-binding protein) is preferentially expressed by adenocarcinoma cells. Int J Cancer (2004) 110(5):741–50. doi: 10.1002/ijc.20105
57. Shi H, Fang W, Liu M, Fu D. Complement component 1, q subcomponent binding protein (C1QBP) in lipid rafts mediates hepatic metastasis of pancreatic cancer by regulating IGF-1/IGF-1R signaling. Int J Cancer (2017) 141(7):1389–401. doi: 10.1002/ijc.30831
58. Li X, Eguchi T, Aly RG, Chintala NK, Tan KS, Zauderer MG, et al. Globular C1q receptor (gC1qR/p32/HABP1) is overexpressed in malignant pleural mesothelioma and is associated with increased survival in surgical patients treated with chemotherapy. Front Oncol (2019) 9:1042. doi: 10.3389/fonc.2019.01042
59. Matsumoto K, Bay BH. Role of C1QBP/p32 and its therapeutic potential in breast carcinoma and other cancers. Curr Med Chem (2021) 28(25):5048–65. doi: 10.2174/0929867328666201231124038
60. Chen YB, Jiang CT, Zhang GQ, Wang JS, Pang D. Increased expression of hyaluronic acid binding protein 1 is correlated with poor prognosis in patients with breast cancer. J Surg Oncol (2009) 100(5):382–6. doi: 10.1002/jso.21329
61. Yu G, Wang J. Significance of hyaluronan binding protein (HABP1/P32/gC1qR) expression in advanced serous ovarian cancer patients. Exp Mol Pathol (2013) 94(1):210–5. doi: 10.1016/j.yexmp.2012.06.007
62. Gao H, Yao Q, Lan X, Li S, Wu J, Zeng G, et al. Elevated HABP1 protein expression correlates with progression and poor survival in patients with gastric cancer. Onco Targets Ther (2016) 9:6711–8. doi: 10.2147/OTT.S114756
63. Zhao J, Liu T, Yu G, Wang J. Overexpression of HABP1 correlated with clinicopathological characteristics and unfavorable prognosis in endometrial cancer. Tumour Biol (2015) 36(2):1299–306. doi: 10.1007/s13277-014-2761-8
64. Zhang X, Zhang F, Guo L, Wang Y, Zhang P, Wang R, et al. Interactome analysis reveals that C1QBP (complement component 1, q subcomponent binding protein) is associated with cancer cell chemotaxis and metastasis. Mol Cell Proteomics (2013) 12(11):3199–209. doi: 10.1074/mcp.M113.029413
65. Dang CV. p32 (C1QBP) and cancer cell metabolism: is the warburg effect a lot of hot air? Mol Cell Biol (2010) 30(6):1300–2. doi: 10.1128/MCB.01661-09
66. Sünderhauf A, Raschdorf A, Hicken M, Schlichting H, Fetzer F, Brethack AK, et al. GC1qR cleavage by caspase-1 drives aerobic glycolysis in tumor cells. Front Oncol (2020) 10:575854. doi: 10.3389/fonc.2020.575854
67. Wang Y, Yue D, Xiao M, Qi C, Chen Y, Sun D, et al. C1QBP negatively regulates the activation of oncoprotein YBX1 in the renal cell carcinoma as revealed by interactomics analysis. J Proteome Res (2015) 14(2):804–13. doi: 10.1021/pr500847p
68. Peerschke EI, Brandwijk RJ, Dembitzer FR, Kinoshita Y, Ghebrehiwet B. Soluble gC1qR in blood and body fluids: Examination in a pancreatic cancer patient cohort. Int J Cancer Res Mol Mech (2015) 1(3). doi: 10.16966/2381-3318.110
69. Kandov E, Kaur A, Kishore U, Ji P, Williams J, Peerschke E, et al. C1q and C1q receptors (gC1qR and cC1qR) as potential novel targets for therapy against breast cancer. Curr Trends Immunol (2018) 19:59–76.
70. Kaul R, Saha P, Saradhi M, Prasad RL, Chatterjee S, Ghosh I, et al. Overexpression of hyaluronan-binding protein 1 (HABP1/p32/gC1qR) in HepG2 cells leads to increased hyaluronan synthesis and cell proliferation by up-regulation of cyclin D1 in AKT-dependent pathway. J Biol Chem (2012) 287(23):19750–64. doi: 10.1074/jbc.M111.266270
71. Sinha S, Singh SK, Jangde N, Ray R, Rai V. p32 promotes melanoma progression and metastasis by targeting EMT markers, Akt/PKB pathway, and tumor microenvironment. Cell Death Dis (2021) 12(11):1012. doi: 10.1038/s41419-021-04311-5
72. Prakash M, Kale S, Ghosh I, Kundu GC, Datta K. Hyaluronan-binding protein 1 (HABP1/p32/gC1qR) induces melanoma cell migration and tumor growth by NF-kappa b dependent MMP-2 activation through integrin α(v)β(3) interaction. Cell Signal (2011) 23(10):1563–77. doi: 10.1016/j.cellsig.2011.04.009
73. Wu H, Chu Y, Sun S, Li G, Xu S, Zhang X, et al. Hypoxia-mediated complement 1q binding protein regulates metastasis and chemoresistance in triple-negative breast cancer and modulates the PKC-NF-κB-VCAM-1 signaling pathway. Front Cell Dev Biol (2021) 9:607142.
74. Wang Z, Sun A, Yan A, Yao J, Huang H, Gao Z, et al. Circular RNA MTCL1 promotes advanced laryngeal squamous cell carcinoma progression by inhibiting C1QBP ubiquitin degradation and mediating beta-catenin activation. Mol Cancer (2022) 21(1):92. doi: 10.1186/s12943-022-01570-4
75. Wang Y, Fu D, Su J, Chen Y, Qi C, Sun Y, et al. C1QBP suppresses cell adhesion and metastasis of renal carcinoma cells. Sci Rep (2017) 7(1):999.
76. Xiao Y, Yu D. Tumor microenvironment as a therapeutic target in cancer. Pharmacol Ther (2021) 221:107753. doi: 10.1016/j.pharmthera.2020.107753
77. Zhai X, Liu K, Fang H, Zhang Q, Gao X, Liu F, et al. Mitochondrial C1qbp promotes differentiation of effector CD8(+) T cells via metabolic-epigenetic reprogramming. Sci Adv (2021) 7(49):eabk0490. doi: 10.1126/sciadv.abk0490
78. Gotoh K, Morisaki T, Setoyama D, Sasaki K, Yagi M, Igami K, et al. Mitochondrial p32/C1qbp is a critical regulator of dendritic cell metabolism and maturation. Cell Rep (2018) 25(7):1800–1815.e4. doi: 10.1016/j.celrep.2018.10.057
79. Fogal V, Zhang L, Krajewski S, Ruoslahti E. Mitochondrial/Cell-surface protein p32/gC1qR as a molecular target in tumor cells and tumor stroma. Cancer Res (2008) 68(17):7210–8. doi: 10.1158/0008-5472.CAN-07-6752
80. García-Caballero M, Sokol L, Cuypers A, Carmeliet P. Metabolic reprogramming in tumor endothelial cells. Int J Mol Sci (2022) 23(19). doi: 10.3390/ijms231911052
81. Kim BC, Hwang HJ, An HT, Lee H, Park JS, Hong J, et al. Antibody neutralization of cell-surface gC1qR/HABP1/SF2-p32 prevents lamellipodia formation and tumorigenesis. Oncotarget (2016) 7(31):49972–85. doi: 10.18632/oncotarget.10267
82. Sharma S, Kotamraju VR, Mölder T, Tobi A, Teesalu T, Ruoslahti E, et al. Tumor-penetrating nanosystem strongly suppresses breast tumor growth. Nano Lett (2017) 17(3):1356–64. doi: 10.1021/acs.nanolett.6b03815
83. Kheshtchin N, Hadjati J. Targeting hypoxia and hypoxia-inducible factor-1 in the tumor microenvironment for optimal cancer immunotherapy. J Cell Physiol (2022) 237(2):1285–98. doi: 10.1002/jcp.30643
84. Karlsson S, Nyström H. The extracellular matrix in colorectal cancer and its metastatic settling - alterations and biological implications. Crit Rev oncology/hematology (2022) 175:103712. doi: 10.1016/j.critrevonc.2022.103712
85. Yu H, Liu Q, Xin T, Xing L, Dong G, Jiang Q, et al. Elevated expression of hyaluronic acid binding protein 1 (HABP1)/P32/C1QBP is a novel indicator for lymph node and peritoneal metastasis of epithelial ovarian cancer patients. Tumour Biol J Int Soc Oncodevelopmental Biol Med (2013) 34(6):3981–7. doi: 10.1007/s13277-013-0986-6
86. Yenugonda V, Nomura N, Kouznetsova V, Tsigelny I, Fogal V, Nurmemmedov E, et al. A novel small molecule inhibitor of p32 mitochondrial protein overexpressed in glioma. J Transl Med (2017) 15(1):210. doi: 10.1186/s12967-017-1312-7
87. Huynh D, Winter P, Märkl F, Endres S, Kobold S. Beyond direct killing-novel cellular immunotherapeutic strategies to reshape the tumor microenvironment. Semin immunopathology (2022). doi: 10.1007/s00281-022-00962-4
88. Rousso-Noori L, Mastandrea I, Talmor S, Waks T, Globerson Levin A, Haugas M, et al. P32-specific CAR T cells with dual antitumor and antiangiogenic therapeutic potential in gliomas. Nat Commun (2021) 12(1):3615. doi: 10.1038/s41467-021-23817-2
89. Liu J, Liew S, Wang J, Pu K. Bioinspired and biomimetic delivery platforms for cancer vaccines. Advanced materials (Deerfield Beach Fla.) (2022) 34(1):e2103790. doi: 10.1002/adma.202103790
90. Hu X, Zhou W, Pi R, Zhao X, Wang W. Genetically modified cancer vaccines: Current status and future prospects. Medicinal Res Rev (2022) 42(4):1492–517. doi: 10.1002/med.21882
91. Dehghan-Manshadi M, Nikpoor AR, Hadinedoushan H, Zare F, Sankian M, Fesahat F, et al. Protective immune response against P32 oncogenic peptide-pulsed PBMCs in mouse models of breast cancer. Int Immunopharmacol (2021) 93:107414. doi: 10.1016/j.intimp.2021.107414
92. Dessale M, Mengistu G, Mengist H. Nanotechnology: A promising approach for cancer diagnosis, therapeutics and theragnosis. Int J nanomedicine (2022) 17:3735–49. doi: 10.2147/IJN.S378074
93. Timur SS, Yalçın G, Çevik Ö, Andaç C, Gürsoy RN. Molecular dynamics, thermodynamic, and mutational binding studies for tumor-specific LyP-1 in complex with p32. J Biomol Struct Dyn (2018) 36(5):1134–44. doi: 10.1080/07391102.2017.1313779
94. Laakkonen P, Porkka K, Hoffman JA, Ruoslahti E. A tumor-homing peptide with a targeting specificity related to lymphatic vessels. Nat Med (2002) 8(7):751–5. doi: 10.1038/nm720
95. Paasonen L, Sharma S, Braun GB, Kotamraju VR, Chung TD, She ZG, et al. New p32/gC1qR ligands for targeted tumor drug delivery. Chembiochem (2016) 17(7):570–5. doi: 10.1002/cbic.201500564
96. Patel S, Minn A. Combination cancer therapy with immune checkpoint blockade: Mechanisms and strategies. Immunity (2018) 48(3):417–33. doi: 10.1016/j.immuni.2018.03.007
97. Kumari S, Advani D, Sharma S, Ambasta R, Kumar P. Combinatorial therapy in tumor microenvironment: Where do we stand? Biochim Biophys Acta Rev Cancer (2021) 1876(2):188585. doi: 10.1016/j.bbcan.2021.188585
Keywords: gC1qR, cancer, immunotherapy, tumor microenvironment, antiangiogenic
Citation: Lei Y, Li X, Qin D, Zhang Y and Wang Y (2023) gC1qR: A New Target for Cancer Immunotherapy. Front. Immunol. 14:1095943. doi: 10.3389/fimmu.2023.1095943
Received: 11 November 2022; Accepted: 02 January 2023;
Published: 26 January 2023.
Edited by:
Anne Grey Savitt, Stony Brook Medicine, United StatesReviewed by:
Abhijit Aithal, University of Nebraska Medical Center, United StatesCopyright © 2023 Lei, Li, Qin, Zhang and Wang. This is an open-access article distributed under the terms of the Creative Commons Attribution License (CC BY). The use, distribution or reproduction in other forums is permitted, provided the original author(s) and the copyright owner(s) are credited and that the original publication in this journal is cited, in accordance with accepted academic practice. No use, distribution or reproduction is permitted which does not comply with these terms.
*Correspondence: Yongsheng Wang, d2FuZ3lzQHNjdS5lZHUuY24=
†These authors have contributed equally to this work