- 1Department of Respiratory and Critical Care Medicine, Shandong Provincial Hospital affiliated to Shandong First Medical University, Jinan, China
- 2Shandong Key Laboratory of Infectious Respiratory Disease, Jinan, China
- 3Medical Science and Technology Innovation Center, Shandong First Medical University & Shandong Academy of Medical Sciences, Jinan, China
- 4Department of Respiratory and Critical Care Medicine, Shanghai Ninth People's Hospital, School of Medicine, Shanghai Jiao Tong University, Shanghai, China
- 5Department of Thoracic Surgery, Shandong Provincial Hospital Affiliated to Shandong First Medical University, Jinan, China
- 6Department of Thoracic Surgery, Fudan University Shanghai Cancer Center, Shanghai, China
Immune checkpoint inhibitors (ICIs), such as programmed death-1 (PD-1), programmed death-ligand 1 (PD-L1), cytotoxic T lymphocyte antigen 4 (CTLA-4) antibodies, etc, have revolutionized cancer treatment strategies, including non-small cell lung cancer (NSCLC). While these immunotherapy agents have achieved durable clinical benefits in a subset of NSCLC patients, they bring in a variety of immune-related adverse events (irAEs), which involve cardiac, pulmonary, gastrointestinal, endocrine and dermatologic system damage, ranging from mild to life-threatening. Thus, there is an urgent need to better understand the occurrence of irAEs and predict patients who are susceptible to those toxicities. Herein, we provide a comprehensive review of what is updated about the clinical manifestations, mechanisms, predictive biomarkers and management of ICI-associated toxicity in NSCLC. In addition, this review also provides perspective directions for future research of NSCLC-related irAEs.
1. Introduction
Checkpoint inhibitor-based immunotherapy has changed the landscape of cancer treatment in the past decade. Unlike other treatments, immune checkpoint inhibitors (ICIs) are monoclonal antibodies designed to block negative regulators of T-cell function, including programmed death-1 (PD-1), PD-ligand 1 (PD-L1) and cytotoxic T lymphocyte antigen 4 (CTLA-4), etc, to activate anti-tumor immunity (1). These ICI agents have improved the clinical outcomes in a subset of advanced NSCLC patients. For example, in a study of patients with advanced NSCLC who had received chemotherapy plus concurrent radiation therapy, progression-free survival (PFS) was 16.8 months in patients treated with durvalumab compared to 5.6 months in the placebo group (2). Patients with advanced NSCLC have a 5-fold increase in overall survival (OS) after nivolumab treatment compared to the chemotherapy group, with no severe adverse events (3). Similarly, ipilimumab showed a better potential in extending PFS in NSCLC (4). Specifically, there are seven US Food and Drug Administration (FDA)-approved ICIs for the first-line or second-line treatment of NSCLC, including pembrolizumab, nivolumab, cemiplimab, atezolizumab, durvalumab, tremelimumab and ipilimumab (5–7).
Despite the durable anti-tumor responses, those ICIs also causes many complications in normal tissues, which are referred to as immune-related adverse events (irAEs). Clinical trial data and literatures suggest that irAEs in NSCLC immunotherapy mainly involve the cardiac, pulmonary, gastrointestinal, endocrine, dermatologic and nervous system damage (8–11). While the majority of cases are mild and tolerable, some of them can present with moderate to severe toxicities, which are related to decreased organic function, as well as poor quality of life (12–14). A study showed that the overall incidence of irAEs of any grade in patients with NSCLC was 30%, with a severe grade of 6% (15). However, the clinical features of some early-onset, low-grade immunotoxicity are not easily identified, and it is difficult to determine whether they are associated with ICI therapy. In addition, the detailed mechanisms of irAEs have not been fully elucidated. Therefore, close attention must be paid to better understand the occurrence of irAEs and to screen populations who are most likely to experience irAEs, in order to reduce the toxic effects of immunotherapy. In this review, we will summarize the clinical manifestations of irAEs according to different ICIs and explore possible mechanisms and potential predictive biomarkers of irAEs in NSCLC immunotherapy. At last, we also provide a comprehensive review of current therapies for irAE treatment in NSCLC. This article may have implications for future research.
2. Clinical manifestations of irAEs in NSCLC immunotherapy
CTLA-4 and PD-1 are immune checkpoints capable of downregulating T-cell immune functions, but their roles are fundamentally different. Both CTLA-4 and PD-1 have been shown to suppress T cells by blocking CD28-mediated cellular metabolism (16). However, CTLA-4 downregulates T-cell proliferation in the early phase of immune responses, while PD-1 is thought to suppress T-cell immune functions primarily in peripheral tissues in the late stage of immune responses (17). ICI-associated toxicities in NSCLC immunotherapy involve multiple organs throughout the body (Table 1). Despite that commonalities in immunotoxicity exist among ICIs, there are differences in specific irAEs, organs involved, as well as clinical manifestations (87).
2.1. Anti-CTLA-4
CTLA-4 plays an essential role in attenuating T-cell responses, preventing immune deregulation, inducing immune tolerance, and controlling autoimmunity (88). CTLA-4 is expressed on the surface of activated T cells, while its ligands, CD80/CD86, is predominantly expressed on antigen-presenting cells (APCs), including dendritic cells (DCs) and macrophages. In the early phase of T-cell activation, CTLA-4 competes with CD28 for binding to CD80/CD86 in APCs (89, 90). Additionally, CTLA-4 expressed on regulatory T cells (Tregs) can reduce the availability of CD80/CD86 expressed on DCs through trans-endocytosis (91), and induce the production of broadspectrum, immunosuppressive cytokines, such as transforming growth factor-β (TGF-β) and interleukin-10 (IL-10) (92, 93), leading to an attenuated antigen-presenting activity of APCs and a suppression of T-cell activation. While the lack of CTLA-4 gene can result in multi-organ lymphocytic infiltration, Treg cell defect and autoantibody production, CTLA-4 blockade did not induce substantial autoimmunity (90, 94, 95).
The most frequently reported irAEs in NSCLC patients receiving anti-CTLA-4 therapy were diarrhea, rash and nausea/vomiting (Table 1). The incidence of gastrointestinal system adverse events was relatively high, particularly diarrhea, hepatic injury and nausea/vomiting, with the clinical data showing an incidence of approximately 30% for diarrhea, 5%-40% for hepatic impairment, and 4%-8% for enteritis (Table 1). And the incidence of skin toxicity is also high where the incidence of rash is about 17%-41% (Table 1). As shown in Table 1, the incidence of adrenal insufficiency in NSCLC receiving anti-CTLA-4 was significantly higher than that of patients treated with anti-PD-1/PD-L1 drugs. The occurrence of checkpoint inhibitor pneumonitis (CIP) was relatively rare in patients treated with anti-CTLA-4 compared with other ICIs; however, its true incidence may be underestimated (96).
At present, anti-CTLA-4 drugs for the treatment of NSCLC are ipilimumab and tremelimumab; however, there are clear differences with regard to the toxicity of these two drugs in different organs. Clinical trials suggested that common adverse reactions to ipilimumab for NSCLC are diarrhea (27%-30%), rash (17%-28%), nausea/vomiting (8%-18%), hepatic impairment (5%-42%), arthralgia (7%-14%), and pituitary inflammation (1%) (Table 1). Moreover, ipilimumab treatment can be associated with atrial fibrillation, uveitis, pancreatic toxicity, and peripheral neuropathy. As for tremelimumab, gastrointestinal and skin toxicity are the most common adverse events in patients with NSCLC, with nausea and vomiting at 10%, diarrhea at 30%, hepatic impairment at 8.3%-30%, and skin rash at 13%-41% (Table 1).
2.2. Anti-PD-1/PD-L1
PD-1 is expressed on activated T cells, B cells, natural killer (NK) cells, and cells of the myeloid lineage. PD-L1 is one of the ligand which can be expressed on a variety of immune and non-immune cells, including tumor cells, while the expression of another ligand of PD-1, PD-L2, is limited to APCs (97, 98). Compared to CTLA-4, PD-1 is involved in a broader range of immune regulation and mainly suppresses the effector phase of activated T cells (99). The binding of PD-1 to its ligands impairs T-cell proliferation and cytokine production by abrogating the cascade response activity of two signals, namely the PI3K/Akt and Ras/MEK/Erk pathways (16, 100–102). Notably, the binding of PD-1 to different ligands leads to distinct biological effects (103). The binding of PD-1 with PD-L2 leads to an enhanced Th2 activity, whereas PD-1/PD-L1 binding inhibits T-cell responses (104, 105). Anti-PD-1/PD-L1 antibodies are also known as “immune normalizers”, which are intended to “normalize” T-cell immunity in tumor microenvironment (106). IrAEs are manifestations of immune imbalance when anti-PD-1/PD-L1 antibodies enhance T cell-mediated immune responses in normal tissues.
Gastrointestinal toxicity is the most common adverse event in NSCLC receiving anti-PD-1/PD-L1 antibodies, followed by pulmonary, neurological, cutaneous, endocrine and musculoskeletal toxicities (Table 1). Clinical data showed that the incidence of gastrointestinal adverse events is the highest, with diarrhea (1%-48%), nausea/vomiting (2%-17.2%) and hepatitis (1%-23%) being the most commonly reported (Table 1). This was followed by CIP (1%-30%), and the presence of severe pneumonia can severely compromise the efficacy of ICI therapy (107, 108). The incidence of peripheral nerve injury (1%-38%) is high; however, mild neurological toxicity may be easily overlooked (Table 1). In addition, all ICIs have a high incidence of skin toxicity, with anti-PD-1/PD-L1 having the highest incidence of rash (3%-27%) and maculopapular rash (1%-15%) (Table 1). Overall, the incidence of major endocrine toxicities associated with anti-PD-1/PD-L1 therapy is generally low, with the exception of hypothyroidism (1%-28%) (Table 1). Other adverse events with a relatively high incidence were arthralgia (2%-26%) and muscle pain (3%-20%) (Table 1). As reported, diarrhea, rash, maculopapular rash and renal injury were significantly higher in anti-PD-1 than in anti-PD-L1. Conversely, the incidence of myocarditis, stomatitis and peripheral neuropathy were higher in anti-PD-L1 than in anti-PD-1 (Table 1).
On the other hand, the incidence of adverse events differs significantly between different anti-PD-1/PD-L1 antibodies. The higher incidence of irAEs with nivolumab in the treatment of NSCLC are rash (5.7%-27%), diarrhea (8.9%-24%), arthralgia (5%-26%), and myalgia (4%-20%) (Table 1). Events such as pneumonia, interstitial pneumonia, nausea/vomiting, enteritis, hepatic impairment, hypothyroidism, hyperthyroidism, pituitary inflammation and hyperalgesia were also frequently reported. Hypothyroidism (6.7%-28.8%), pneumonia (4.4%-23%), rash (7%-22%), nausea/vomiting (2%-17.2%) and hyperthyroidism (4%-11%) are the irAEs of a higher incidence with pembrolizumab (Table 1). Gastrointestinal toxicity such as diarrhea (5%-24%) was the highest and most frequently reported adverse event in cemiplimab therapy (Table 1). Adverse events involving the respiratory and circulatory systems were frequently reported with cemiplimab, but not at a high incidence rate. Adverse events of a high incidence with durvalumab are diarrhea (4%-20%), pneumonia (1.6%-16.7%), hypothyroidism (10.5%-15.2%), rash (3.2%-15%), hepatic impairment (13%), hyperthyroidism (6.3%-12.1%) and pancreatitis (2%-12.1%) (Table 1). The frequently reported adverse events with atezolizumab were diarrhea (6.2%-20.6%), hepatic impairment (1%-23%), nausea/vomiting (7.7%-14.2%), hypothyroidism (5%-14.2%), arthralgia (2%-16.8%), myalgia (13.5%) and rash (5%-11%) (Table 1). Avelumab had a significantly lower incidence of adverse reactions than durvalumab or atezolizumab, with the commonly reported adverse events being arthralgia (5%) and rash (3.2%) (Table 1).
2.3. Other ICIs
Recently, the conversion of other immune checkpoints, such as lymphocyte activation gene-3 (LAG-3), T cell immunoglobulin and ITIM domain (TIGIT) and T cell immunoglobulin and mucin-domain containing-3 (TIM-3), B7-H3 and NKG2A, into clinical next-generation immunomodulatory targets has been increasingly investigated (109). The binding of LAG-3 to corresponding ligands, including major histocompatibility complex II (MHC-II), liver sinusoidal endothelial cell lectin (LSECtin) and hepatic fibrinogen-like protein 1 (FGL-1), induces immune cell depletion and reduces cytokine secretion (110–114). On the other hand, LAG-3 deficiency leads to an increased autoimmune susceptibility in mice (115, 116). REGN3767 is a human LAG-3 monoclonal antibody. In clinical trials of REGN3767 monotherapy for advanced malignancies, the most frequent adverse reaction was nausea (22.2%) (117). Whether LAG-3 potentiates irAEs of known checkpoint blockers or generates new irAEs still needs to be further explored. TIGIT is an inhibitory receptor that is widely expressed on lymphocytes (118, 119). The interaction of TIGIT with its ligands (CD155, CD112 and CD113) can impair DC-triggered T-cell responses and inhibit the cytotoxicity of CD8+ T cells (120, 121). IrAEs associated with anti-TIGIT drugs (vibostolimab) were rash (24%), pneumonia (18%), arthralgia (18%), nausea/vomiting (15%), myalgia (6%), and enteritis (3%) (Table 1). TIM-3 is expressed in tumor cells and immune cells. The interaction of TIM-3 with its ligand induces T-cell suppression (122). TIM-3 is highly upregulated on infiltrating CD4+ and CD8+ T cells from human lung cancer tissues, and high TIM-3 expression may be associated with tumor progression (123). Phase I clinical trials found that the most frequent adverse events with anti-TIM-3 monotherapy (cobolimab) in advanced solid tumors (NSCLC and melanoma) were fatigue (13.0%) and nausea (8.7%) (60). B7-H3, also called CD276, is a member of the B7 family, which was also recognized as a co-stimulatory molecule. Although its ligand has not been well studied, certain treatments targeting B7-H3 are undergoing clinical trials. Inhibitors of immune checkpoints such as VISTA, ICOS and BTLA are currently in clinical trials for the treatment of advanced solid tumors.
2.4. Combined immunotherapies
The most common combination regimens are anti-CTLA-4 plus anti-PD-1/PD-L1, anti-PD-1/PD-L1 plus anti-TIGIT, anti-PD-1 plus anti-B7-H3, anti-PD-L1 plus anti-NKG2A, anti-PD-1 plus anti-LAG-3, anti-PD-1 plus anti-TIM-3, and anti-PD-1 plus anti-B7-H3. Compared with the application of one checkpoint inhibitor or chemotherapy, the combination of ICIs for NSCLC was associated with an increased incidence of adverse reactions and high-grade immunotoxicities (Grade ≥ 3) in patients while prolonging the PFS (23, 79, 124). Gastrointestinal toxicities (10%-50%) and endocrine toxicities (1%-20%) are significantly higher in combined immunotherapy than in monotherapy, such as diarrhea (6.4%-57%), nausea/vomiting (1.2%-42.9%), hypothyroidism (10%-22%), hyperthyroidism (8.7%-10%), hypothyroidism and glucose abnormalities (1%-10.2%) (Table 1). Elsewhere, the incidence of maculopapular rash (5%-14%) and uveitis (9%) was relatively higher in combined immunotherapy than in monotherapy (Table 1). The differences in cardiac and pulmonary toxicity were not significant (Table 1). Adverse events with higher rates in ipilimumab plus nivolumab are rash (10%-20%), diarrhea (6.4%-20%), hypothyroidism (11%-16%), maculopapular rash (5%-14%), pancreatitis (2%-10.4%), hyperthyroidism (8.7%), pneumonia (3%-7%), enterocolitis (1%-6%), hepatic impairment (1%-6%), and hyperaldosteronism (1%-4.7%) (Table 1). The incidence of enterocolitis (10%-26%) and arrhythmias (3%) with tremelimumab plus durvalumab treatment was significantly higher than other combination regimens (Table 1). The most prominent adverse events with ipilimumab plus pembrolizumab were pneumonia (7%-17.2%) (Table 1). In contrast, the overall respiratory, gastrointestinal and endocrine system toxicity was higher with anti-PD-1/PD-L1 in combination with other ICIs for NSCLC than with anti-CTLA-4 plus anti-PD-1/PD-L1 (Table 1).
3. Mechanism of irAEs
The underlying mechanism of irAEs in NSCLC immunotherapy remains largely unknown. Here, we summarized three main factors that may be involved in the pathogenesis of irAEs: pre-existing autoimmunity, loss of tolerance, and presentation of self-antigens (Figure 1). Currently, accumulating evidence suggested that genetic predisposition or subclinical autoimmunity contributes to the development of irAEs. Specific HLA alleles may enhance the autoimmune response and be associated with the production of autoantibodies (125, 126) (Figure 1). For example, the presence of HLA-DRB1 allele is associated with the specific autoantibody, antithyroglobulin (127, 128). HLA allele DRB1*04:05 is associated with the development of arthritis in ICI-treated patients (129). A case series study revealed that HLA-DR4, a genetic predictor for type 1 diabetes, is enriched in ICI-related diabetes, which can also be considered as a complication of autoimmune pancreatitis induced by ICIs (130). Another study demonstrated that in advanced NSCLC patients with subclinical autoimmune disease, the pre-existing antibodies were positively correlated with the occurrence of irAEs after anti-PD-1 treatment (131). Loss of immune tolerance due to the HLA allele and other genes results in the production of heterogeneous autoantibodies and inflammatory cytokines that may be present for months or even years (Figure 1). In response to appropriate environmental factors, auto-reactive cells are activated and recruited to target tissues (128, 132) (Figure 1).
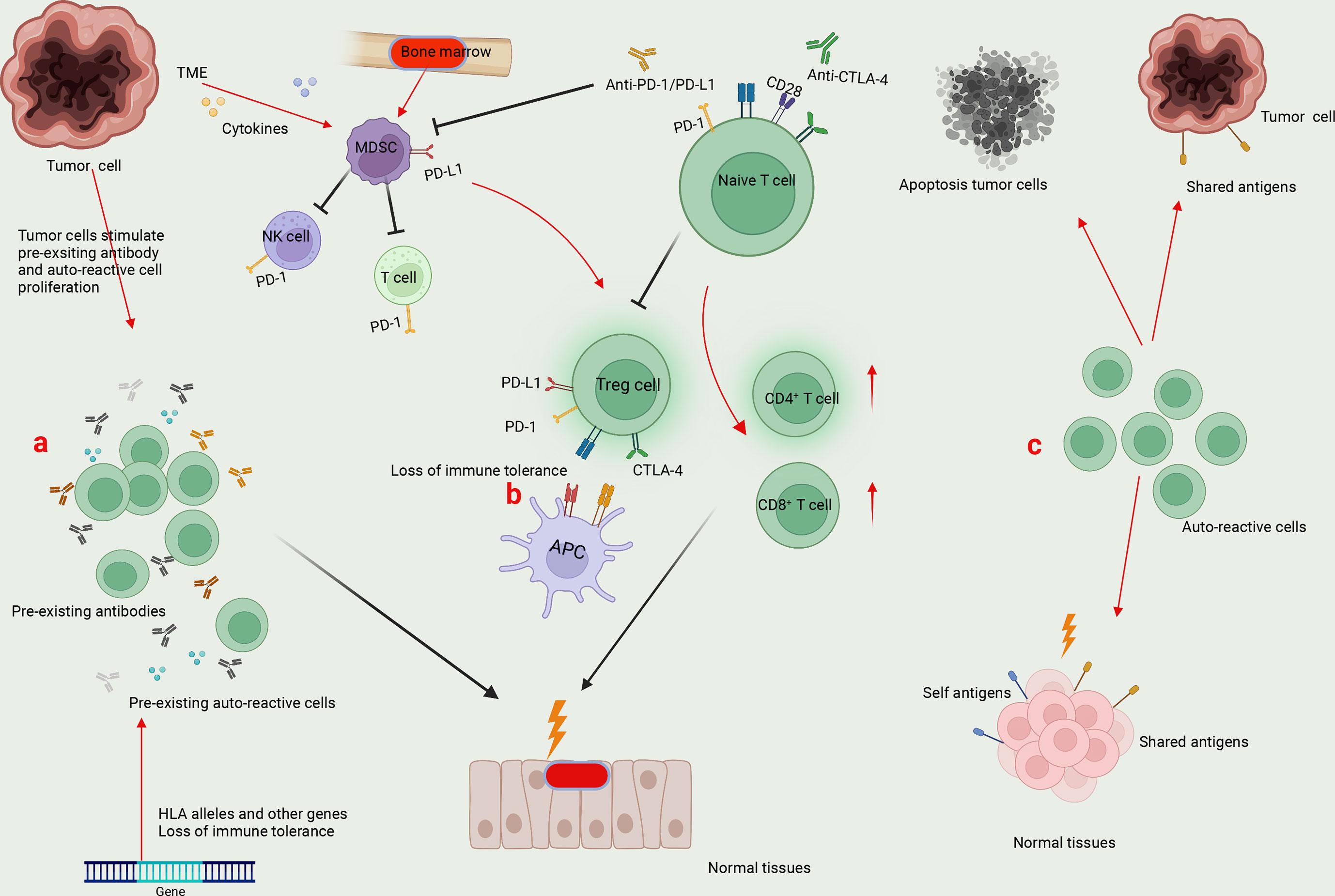
Figure 1 Mechanisms underlying irAEs. (A) Specific HLA alleles and other genes result in the generation of pre-existing auto-reactive cells and antibodies in cancer patients. The enhanced auto-reactive cells by checkpoint inhibitors further promotes the production of autoantibodies, leading to the attack of normal tissues. (B) Tumor microenvironment (TME) favors the generation of myeloid-derived suppressor cells (MDSCs), which directly suppress antitumor immunity by expressing PD-L1 and indirectly induce Treg differentiation to inhibit T-cell function. On one hand, the application of ICIs to block PD-1/PD-L1 and CTLA-4-mediated immune suppression may affect the function of MDSCs and Tregs, leading to the loss of immune tolerance. On the other hand, by promoting the effector function of CD4+ T and CD8+ T cells, ICI treatment enhances the potential immune responses against normal tissues. (C) Tumor cells can express shared antigens with normal tissues. Meanwhile, tumor cell death releases self and tumor antigens. The auto-reactive T cells can recognize shared or self antigens, leading to the collapse of immune tolerance and normal tissue damage.
Except for activated T cells, CTLA-4 and PD-1 are constitutively expressed by Tregs and some myeloid cells, which play a vital role in maintaining immune tolerance (133, 134) (Figure 1). A canonical theory of irAEs is that treatment with ICIs leads to the loss of tolerance in cancer patients, paving ways for auto-reactive T-cell activation and the overwhelming humoral immunity (Figure 1). For instance, a previous study dissected a significant accumulation of CD8+ T cells with highly cytotoxic and proliferative status in ICI-associated colitis, a substantial fraction of which were found to originate from tissue-resident populations in TCR sequence analysis (135). It has been recognized that the most common irAE tends to occur in organs that are highly dependent on peripheral tolerance to maintain immune homeostasis (136).
Furthermore, tumor cells can express shared antigens with normal tissues, potentializing the excessive T-cell activity upon ICI treatment, which is thought to be another primer factor in the development of irAEs (Figure 1). In immune-related skin toxicity in NSCLC patients, nine T-cell shared antigens between tumor and skin tissues were identified (137). In CIP of NSCLC patients, a significant overlap of T-cell repertoire was detected via gene sequencing between tumor-infiltrating lymphocytes (TILs) and CIP-infiltrating lymphocytes, rather than T cells from either the secondary lymphoid organs or peripheral blood (138). Consistently, ipilimumab treatment led to a greater diversification of T-cell repertoire, a clear indicator of auto-reactivity to multiple antigens, in cancer patients with irAEs compared with those without irAEs (139). Thus, it has been realized that ICI-induced epitope spreading represents a newly specific mechanism accounting for this broadening of T-cell repertoire and cross-reactivity in cancer patients.
Other factors, such as non-autoimmune inflammation and gut microbiome, may also play a critical role in the development of irAEs (140, 141). Ultimately, as irAEs become a limitation in anti-tumor immunotherapy, more efforts are required in future to learn the pathophysiology of irAEs.
4. Predictive biomarkers
In the past few years, biomarkers have been investigated to predict adverse effects during cancer immunotherapy. To date, the potential biomarkers reported mainly include immune cells, cytokines/chemokines, autoantibodies, genetics and other factors (Figure 2).
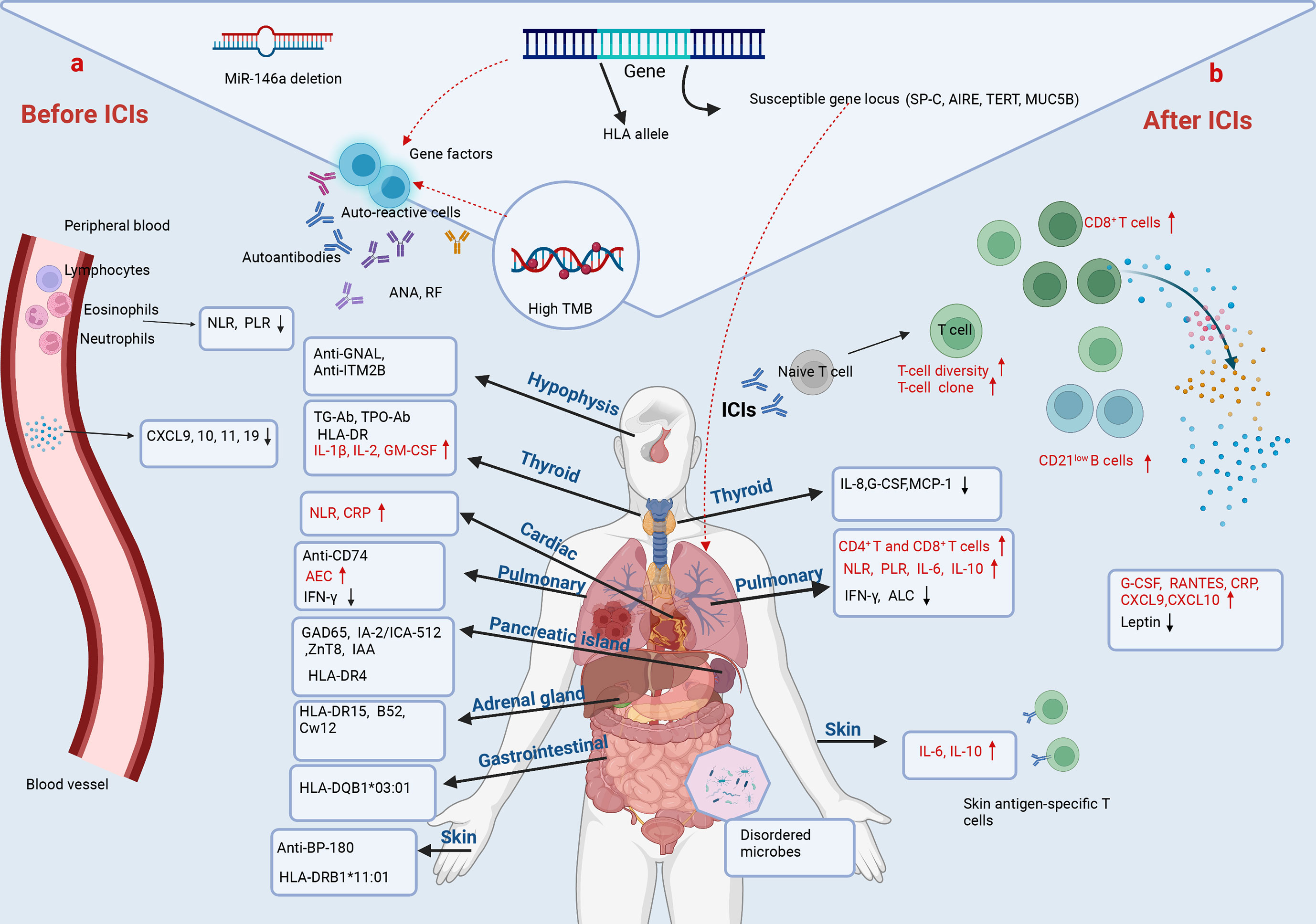
Figure 2 Predictive biomarkers of irAEs in NSCLC. (A) Before ICIs. The baseline cytokines, chemokines, peripheral-blood cell counts, and pre-existing antibodies and genetic alterations are associated with irAEs in NSCLC. Lower baseline levels of NLR, PLR, CXCL9, CXCL10, CXCL11 and CXCL19 correlate with irAEs. MiR-146a deletion even predicts the occurrence of severe irAEs. However, the baseline NLR and CRP are increased in NSCLC with immune-related cardiotoxicities. The pre-existing anti-GNAL and anti-ITM2B in plasma predict immune-related hypophysitis. ICI-related thyroid dysfunction is associated with pre-existing TG-Ab, TPO-Ab, HLA-DR, and higher baseline value of IL-1β, IL-2 and GM-CSF. Anti-CD274, a higher baseline level of AEC and lower baseline IFN-γ and ALC are associated with CIP. HLA alleles and autoantibodies as indicated are related to immune-related diabetes, colitis, adrenal and skin adverse events. Age, BMI and past medical history have also been shown to be strongly associated with irAEs. (B) After ICIs. An increased diversification of peripheral T-cell repertoire, early expansion of CD8+ T-cell clones (≥ 55), and increased circulating CD21low B cells characterized by higher PD-L1 expression and greater clonality after immunotherapy are associated with irAEs. Higher post-treatment levels of serum G-CSF, RANTES, CRP, CXCL9 and CXCL10, and lower post-treatment leptin correlate with irAEs. Changes of cytokines during ICI treatment predict organ-specific adverse events. Decreased IL-8, G-CSF and MCP-1 predict immune-related thyroid dysfunction. Increased IL-6 and IL-10, and decreased IFN-γ predict CIP. Increased IL-6 and IL-8, and the presence of skin antigen-specific T cells in blood after ICIs predict skin toxicities. In addition, disturbances in gut microbes are associated with gastrointestinal toxicities.
4.1. Immune cells
Considering the central role in mediating the toxicities of ICI therapy, immune cells have long been recognized as attractive cellular biomarkers in the prediction of irAEs. Research has focused on using immune signatures in peripheral blood as candidate biomarkers given the ease of access and minimal invasiveness. In agreement with the pathogenesis of irAEs, skin antigen-specific T cells were found in blood samples of anti-PD-1-treated NSCLC patients who developed autoimmune skin toxicities, implying a potential predictive value of this immune cell subset for skin-related irAEs (137) (Figure 2). Similarly, an early expansion of CD8+ T cell clones (≥ 55) in peripheral blood within two weeks of ICI starting therapy indicates the forthcoming occurrence of severe irAEs in prostate cancers receiving ipilimumab (142) (Figure 2). Significant increases in CD4+ T and CD8+ T cells were observed in biopsies from cancer patients treated with PD-1/PD-L1 inhibitors resulting in mechanical pneumonia, and in bronchoalveolar lavage (BAL) samples from patients with CIP (143, 144) (Figure 2). An early and greater diversification of peripheral T-cell repertoire after checkpoint blockade was observed in cancer patients with irAEs than in those without irAEs, indicating its predictive value for irAE (139) (Figure 2). Moreover, recent studies also suggested the utility of humoral immune cells in irAE identification. Das et al. reported that an early increase in circulating CD21low B cells, characterized by a higher expression of PD-L1 and a greater clonality than CD21high B subsets, correlated with severe irAEs after anti-PD-1 and anti-CTLA-4 combined therapy (145) (Figure 2).
4.2. Cytokines and chemokines
Cytokine release syndrome (CRS) is a systemic inflammatory disorder characterized by a massive release of cytokines, which may occur in cancer patients receiving ICIs treatment (146). Since the storm of T cell-activating cytokines induces broad over-activity of T cells targeting self-tissues, a series of symptoms, ranging from mild to life-threating, can accompany with CRS. In accordance, recent studies have evaluated the baseline and changes of cytokines and chemokines in cancer patients to predict adverse reactions of ICIs therapy. In a longitudinal study, higher post-treatment levels of serum G-CSF and RANTES, as well as a lower level of leptin after treatment, were observed in irAE NSCLC patients, compared with non-irAE NSCLC patients (147) (Figure 2). Another study, which included 53 lung cancer patients, indicated that the subjects who developed irAEs possessed lower baseline levels of CXCL9, CXCL10, CXCL11 and CXCL19, as well as a greater increase in CXCL9 and CXCL10 at post-treatment, compared with those without irAEs (148) (Figure 2).
As for organ-specific irAEs, Lin et al. reported that increased levels of serum IL-6 and IL-10 during ICI treatment may act as useful biomarkers to predict CIP in lung cancer patients (149) (Figure 2). Another study of NSCLC even showed that both a low baseline level of IFN-γ and decrease of IFN-γ after ICI therapy were correlated with the development of CIP (150) (Figure 2). Additionally, higher baseline levels of IL-1β, IL-2 and GM-CSF, as well as early decrease of IL-8, G-CSF and MCP-1 were demonstrated to be associated with ICI-related thyroid dysfunction (151) (Figure 2). Phillips et al. retrospectively reviewed patients with immune-related cutaneous adverse events (ircAEs), and suggested that increased serum IL-6 and IL-10 may act as potential biomarkers for ircAEs (152) (Figure 2).
4.3. Autoantibodies
Cancer patients with co-existing autoimmune diseases are at a higher risk for the development of irAEs. This notion is supported by the following studies, suggesting that pre-existing autoantibodies in the body of cancer patients are predictive of irAEs following ICI treatment. It has been reported that autoantibodies, including anti-GNAL, anti-ITM2B and anti-CD74, both in pre-treatment and on-treatment plasma samples of cancer patients correlate with the development of immune-related hypophysitis and pneumonitis after ICI therapy, respectively (153) (Figure 2). The presence of anti-thyroid peroxidase and anti-thyroglobulin antibodies at baseline were associated with a higher risk of thyroid dysfunction during immunotherapy (154–157) (Figure 2). And an elevated anti-BP-180 antibody at baseline correlated with a higher probability to develop skin adverse events in NSCLC during anti-PD-1/PD-L1 therapy (158) (Figure 2). In addition, insulin-dependent diabetes occurred in nearly 1% of solid cancer patients treated with anti-PD-1/PD-L1 antibodies, of which 40% had at least one positive auto-antibody and 21% had two or more, including glutamic acid decarboxylase (GAD65), islet antigen2 (IA-2/ICA-512), zinc transporter8 (ZnT8) and insulin autoantibodies (IAA) (159) (Figure 2). Toi et al. profiled the pre-existing autoimmune markers in advanced NSCLC patients with subclinical autoimmune disease who underwent anti-PD-1 therapy, and found that the presence of pre-existing antinuclear antibody (ANA), rheumatoid factor (RF), anti-thyroglobulin (TG) and anti-thyroid peroxidase (TPO) were associated with the development of irAEs, as well as with clinical benefits to PD-1 inhibitors (131) (Figure 2). Mechanistically, this correlation can be explained that the enhanced auto-reactive T cells can induce the production of auto-antibodies in B cells following ICIs treatment, which eventually cause irAEs (160).
However, not all antibody positivity leads to adverse effects in ICI treatment. A recent study showed that patients with ICI-induced inflammatory arthritis were more likely to be autoantibody-negative for RF and anti-cyclic citrullinated peptide (CCP) antibodies (129). Importantly, it should be noteworthy that cancer patients with pre-existing autoimmune disorders were often excluded from access to immunotherapy-based, anti-cancer clinical trials and only small-scale retrospective cohort studies are currently available to determine the correlation between autoantibodies and irAEs. Thus, further well-designed larger prospective studies are needed to validate these findings and investigate whether a specific autoimmune framework predicts different irAEs.
4.4. Genetic alterations
Recently, genetic pre-disposition to irAEs has attracted increasing attention. Hoefsmit et al. reported that susceptible genetic loci of immune-related genes, including surfactant protein C (SP-C), autoimmune regulator (AIRE), telomerase reverse transcriptase (TERT) and mucin 5B oligomeric mucus/gel-forming (MUC5B), are linked to the development of CIP in cancer patients (161) (Figure 2). A recent database study reported a positive correlation between tumor mutational burden (TMB) and anti-PD-1-induced irAEs across 18 cancer types (162) (Figure 2). In addition, certain HLA types were also found to be associated with the development of specific irAEs. A retrospective study of cancer patients by Danae et al. found an increased expression of HLA-DR on the surface of monocytes in patients with thyroid toxicity caused by anti-PD-1 inhibitor (pembrolizumab) (163) (Figure 2). Studies on the treatment of advanced cancer patients including NSCLC by ICIs showed that HLA-DR15, B52, and Cw12 may be related to the occurrence and development of adrenal insufficiency (164) (Figure 2). A prospective observational clinical study dissected associations between HLA-DRB1*11:01 and pruritus, and between HLA-DQB1*03:01 and colitis in metastatic NSCLC and melanoma patients receiving anti-PD-1, anti-CTLA4, or both (165) (Figure 2). Stamatouli and colleagues identified a predominance of HLA-DR4 in 76% of cancer patients with autoimmune, insulin-dependent diabetes caused by ICIs, suggesting the potential of HLA-DR4 in identifying those at a higher risk to develop this kind of irAE (159) (Figure 2). In addition, preclinical data showed that microRNA-146a (miR-146a) deletion was associated with the occurrence of severe irAE (166) (Figure 2). However, despite this progress, the predictive value of genetic alterations for irAEs remains less well-defined. Larger association studies are required to extend the usage of genetic determinants in irAEs.
4.5. Other factors
Even more creative strategies to predict irAEs have been considered. Until now, there are some other emerging irAE biomarkers on the rise, such as demographic information, body mass index (BMI), past medical history, peripheral-blood cell count, gut microbiome and omics information. A retrospective study of cancer patients receiving pembrolizumab treatment found that an increase in BMI was associated with an increased risk of irAEs (167). It has been reported that patients older than 70 years or with a smoking history are more likely to develop CIP during ICIs treatment (168, 169). Notably, the past medical history was found to be closely associated with the occurrence of organ-specific irAEs. Recent studies found that patients undergoing cancer immunotherapy with pre-existing interstitial lung disease or asthma experienced a greater incidence of CIP than those without these diseases (168, 169). Similarly, accumulating data highlighted the contribution and prediction of specific gut microbes in the development of ICI-induced intestinal irAEs (170) (Figure 2). In addition, a higher baseline absolute eosinophil count (AEC) was observed in patients with CIP than in those without CIP, which could act as a potential biomarker (171) (Figure 2). Pavan et al. investigated the predictive value of the neutrophil-to-lymphocyte ratio (NLR) and platelet-to-lymphocyte ratio (PLR) for irAEs in advanced NSCLC treated with ICIs (172). Patients with a lower baseline level of NLR or PLR had a higher risk of irAEs (Figure 2). However, results from a retrospective study showed that increased NLR and PLR, and decreased absolute lymphocyte count (ALC) during ICI therapy were associated with the development of CIP in lung cancer patients (149) (Figure 2). A study of immune-related cardiotoxicity showed a significant increase in NLR and C-reactive protein (CRP) compared with the baseline value in patients with NSCLC treated with ICIs (173) (Figure 2). As a peripheral blood inflammation biomarker, NLR is relatively easy-to-obtain to predict irAEs. However, there is still no consistent point of view. In future, we need a large-sample study on the relationship between baseline NLR levels and irAEs in different cancer types to guide clinical work. Recently, people have developed a new strategy to combine pharmacovigilance and multi-omics data, and a bivariate regression model of LCP1 and ADPGK was established to predict irAEs across different cancer types (174).
5. Treatment modalities for irAEs
Treatment of irAEs, which involve multiple organ systems throughout the body and carry a risk of death in severe cases, is critical for cancer patients. For mild to moderate adverse events, suspension or discontinuation of ICIs or treatment with corticosteroid represents the most prevalent and primary treatment modality. A retrospective study of advanced NSCLC showed that glucocorticoids were effective in mitigating mild irAEs and did not affect the efficacy of ICIs (175). Meanwhile, various second-line therapies have been used with some success in patients with corticosteroid-refractory toxicity and serious adverse events. For example, immunoglobulin and plasma replacement therapy can be effective in relieving symptoms of neurogenic immunotoxicity (176, 177). Other immunomodulatory agents also play an important role in the treatment of immunotoxicity. For example, TNF antagonists (infliximab), inosine monophosphate dehydrogenase (IMPDH) inhibitors (mycophenolate), and anti-integrin α4β7 antibodies (vedolizumab) are effective in ameliorating steroid-refractory gastrointestinal toxicity (178–180). As the mechanisms of irAEs have been investigated, cytokine inhibitors are used to ameliorate the symptoms of immunotoxicity. A patient with NSCLC who developed psoriasis flares after treatment with pembrolizumab was treated with an IL-17 inhibitor (secukinumab), which was found to reduce toxicity but did not affect tumor control by pembrolizumab (181). IL-6 inhibitors (tocilizumab) also demonstrated treatment efficiency in patients with irAEs (182). Antibodies against B cells (rituximab) can effectively treat these ICI-induced toxic reactions and some autoimmune toxicities (160, 183). Some other cytokine inhibitors, such as IL-1 inhibitors and IL-23 inhibitors, are effective in relieving clinical symptoms when used in cancer patients presenting with organ-specific irAEs (184). Acupuncture significantly improved neurological symptoms in a patient with advanced lung cancer who developed Guillain-Barre syndrome after receiving immunotherapy (176). There are still many patients with irAEs who still have refractory or immunosuppressive toxicities. A more precise understanding of the pathophysiology of specific irAEs is urgently needed to guide the treatment of severe irAEs.
6. Discussion
At present, our understanding of cancer immunotherapy is still at an early stage. Although immunotherapy has significantly improved the survival rate and quality of life in cancer patients as compared to conventional chemotherapy, irAEs caused by immunotherapy pose a great threat to the lives and property of patients. The lack of consensus on the mechanism of irAEs also poses a great challenge to further clinical treatment. Therefore, we still need to learn more about how to apply ICIs more safely and effectively, and how to deal with adverse events caused by ICIs. The mechanism of irAEs and the causes of different adverse reactions caused by different immune drugs need to be further studied. Whether it is possible to screen the high-risk population of irAEs and explore the biomarkers related to the early response and safety of immune drugs remains an essential challenge to address in future. In addition, how to achieve individualized management of immunotoxicity in different organs and at different degrees without compromising immunotherapy is crucial. This article summarized the incidence and mechanism of irAEs, as well as biomarkers to predict immunotoxicity in NSCLC ICI-based immunotherapy. With the further study of predictive markers of immunotoxicity, it was shown that cytokine inhibitors and immunomodulators against autoantibodies have provided a better therapeutic efficacy for organ-specific immunotoxicities. Further research is needed to determine whether organs or patients at risk for irAEs can be pretreated based on immunotoxicity biomarkers.
Author contributions
WY and SJ designed and conceived the study. XH and LW carried out the literature search and wrote the manuscript draft. BS, JW and JS developed the table. BL and LS prepared the figures. WY critically revised the manuscript. SJ reviewed the manuscript. All authors contributed to the article and approved the submitted version.
Funding
This work was supported in part by grant 81902325 from the National Natural Science Foundation of China, grants ZR2020MH005 and ZR2021ZD35 from the Shandong Provincial Natural Science Foundation (China), grants 202019201 and 202225051 from the Jinan Science and Technology Plan Project (China), grant 2019GSF107051 from the Key Research and Development Plan of Shandong Province (China), and grant 2021GXRC018 from Jinan Talent Development Special Foundation (China).
Conflict of interest
The authors declare that the research was conducted in the absence of any commercial or financial relationships that could be construed as a potential conflict of interest.
Publisher’s note
All claims expressed in this article are solely those of the authors and do not necessarily represent those of their affiliated organizations, or those of the publisher, the editors and the reviewers. Any product that may be evaluated in this article, or claim that may be made by its manufacturer, is not guaranteed or endorsed by the publisher.
Glossary
References
1. Ramos-Casals M, Brahmer JR, Callahan MK, Flores-Chávez A, Keegan N, Khamashta MA, et al. Immune-related adverse events of checkpoint inhibitors. Nat Rev Dis Primers (2020) 6(1):38. doi: 10.1038/s41572-020-0160-6
2. Antonia SJ, Villegas A, Daniel D, Vicente D, Murakami S, Hui R, et al. Durvalumab after chemoradiotherapy in stage III non-Small-Cell lung cancer. N Engl J Med (2017) 377(20):1919–29. doi: 10.1056/NEJMoa1709937
3. Borghaei H, Gettinger S, Vokes EE, Chow LQM, Burgio MA, de Castro Carpeno J, et al. Five-year outcomes from the randomized, phase III trials checkmate 017 and 057: Nivolumab versus docetaxel in previously treated non-Small-Cell lung cancer. J Clin Oncol (2021) 39(7):723–33. doi: 10.1200/jco.20.01605
4. Tomasini P, Khobta N, Greillier L, Barlesi F. Ipilimumab: Its potential in non-small cell lung cancer. Ther Adv Med Oncol (2012) 4(2):43–50. doi: 10.1177/1758834011431718
5. Akinboro O, Larkins E, Pai-Scherf LH, Mathieu LN, Ren Y, Cheng J, et al. FDA Approval summary: Pembrolizumab, atezolizumab, and cemiplimab-rwlc as single agents for first-line treatment of Advanced/Metastatic PD-L1-High NSCLC. Clin Cancer Res (2022) 28(11):2221–8. doi: 10.1158/1078-0432.Ccr-21-3844
6. Glode AE, May MB. Immune checkpoint inhibitors: Significant advancements in non-small cell lung cancer treatment. Am J Health Syst Pharm (2021) 78(9):769–80. doi: 10.1093/ajhp/zxab041
7. Keam SJ. Tremelimumab: First approval. Drugs (2023) 83(1):93–102. doi: 10.1007/s40265-022-01827-8
8. Genova C, Rossi G, Rijavec E, Biello F, Barletta G, Tagliamento M, et al. Releasing the brake: Safety profile of immune check-point inhibitors in non-small cell lung cancer. Expert Opin Drug Saf (2017) 16(5):573–85. doi: 10.1080/14740338.2017.1313228
9. Vaddepally R, Doddamani R, Sodavarapu S, Madam NR, Katkar R, Kutadi AP, et al. Review of immune-related adverse events (irAEs) in non-Small-Cell lung cancer (NSCLC)-their incidence, management, multiorgan irAEs, and rechallenge. Biomedicines (2022) 10(4):790. doi: 10.3390/biomedicines10040790
10. Nishijima TF, Shachar SS, Nyrop KA, Muss HB. Safety and tolerability of PD-1/PD-L1 inhibitors compared with chemotherapy in patients with advanced cancer: A meta-analysis. Oncologist (2017) 22(4):470–9. doi: 10.1634/theoncologist.2016-0419
11. Xu C, Chen YP, Du XJ, Liu JQ, Huang CL, Chen L, et al. Comparative safety of immune checkpoint inhibitors in cancer: Systematic review and network meta-analysis. Bmj (2018) 363:k4226. doi: 10.1136/bmj.k4226
12. Zhao H, Ning J, Gu Y, Zhang X, Yu W, Chen T, et al. Consecutive severe immune-related adverse events after PD-1 inhibitor induction and surgery in locally advanced non-small cell lung cancer: A case report. Transl Lung Cancer Res (2021) 10(8):3682–8. doi: 10.21037/tlcr-21-603
13. Leitinger M, Varosanec MV, Pikija S, Wass RE, Bandke D, Weis S, et al. Fatal necrotizing encephalopathy after treatment with nivolumab for squamous non-small cell lung cancer: Case report and review of the literature. Front Immunol (2018) 9:108. doi: 10.3389/fimmu.2018.00108
14. Li D, He C, Xia Y, Du Y, Zhang J. Pembrolizumab combined with stereotactic body radiotherapy in a patient with human immunodeficiency virus and advanced non-small cell lung cancer: A case report. J Med Case Rep (2018) 12(1):104. doi: 10.1186/s13256-018-1667-2
15. Yan YD, Cui JJ, Fu J, Su YJ, Chen XY, Gu ZC, et al. A network comparison on safety profiling of immune checkpoint inhibitors in advanced lung cancer. Front Immunol (2021) 12:760737. doi: 10.3389/fimmu.2021.760737
16. Parry RV, Chemnitz JM, Frauwirth KA, Lanfranco AR, Braunstein I, Kobayashi SV, et al. CTLA-4 and PD-1Receptors inhibit T-cell activation by distinct mechanisms. Mol Cell Biol (2005) 25(21):9543–53. doi: 10.1128/mcb.25.21.9543-9553.2005
17. Keir ME, Butte MJ, Freeman GJ, Sharpe AH. PD-1 and its ligands in tolerance and immunity. Annu Rev Immunol (2008) 26:677–704. doi: 10.1146/annurev.immunol.26.021607.090331
18. Mok TSK, Wu YL, Kudaba I, Kowalski DM, Cho BC, Turna HZ, et al. Pembrolizumab versus chemotherapy for previously untreated, PD-L1-Expressing, locally advanced or metastatic non-Small-Cell lung cancer (Keynote-042): A randomised, open-label, controlled, phase 3 trial. Lancet (2019) 393(10183):1819–30. doi: 10.1016/s0140-6736(18)32409-7
19. Nosaki K, Saka H, Hosomi Y, Baas P, de Castro G Jr., Reck M, et al. Safety and efficacy of pembrolizumab monotherapy in elderly patients with PD-L1-Positive advanced non-Small-Cell lung cancer: Pooled analysis from the keynote-010, keynote-024, and keynote-042 studies. Lung Cancer (2019) 135:188–95. doi: 10.1016/j.lungcan.2019.07.004
20. Felip E, Altorki N, Zhou C, Csőszi T, Vynnychenko I, Goloborodko O, et al. Adjuvant atezolizumab after adjuvant chemotherapy in resected stage IB-IIIA non-Small-Cell lung cancer (Impower010): A randomised, multicentre, open-label, phase 3 trial. Lancet (2021) 398(10308):1344–57. doi: 10.1016/s0140-6736(21)02098-5
21. Mazieres J, Rittmeyer A, Gadgeel S, Hida T, Gandara DR, Cortinovis DL, et al. Atezolizumab versus docetaxel in pretreated patients with NSCLC: Final results from the randomized phase 2 poplar and phase 3 oak clinical trials. J Thorac Oncol (2021) 16(1):140–50. doi: 10.1016/j.jtho.2020.09.022
22. Aggarwal C, Prawira A, Antonia S, Rahma O, Tolcher A, Cohen RB, et al. Dual checkpoint targeting of B7-H3 and PD-1 with enoblituzumab and pembrolizumab in advanced solid tumors: Interim results from a multicenter phase I/II trial. J Immunother Cancer (2022) 10(4):e004424. doi: 10.1136/jitc-2021-004424
23. Gettinger SN, Redman MW, Bazhenova L, Hirsch FR, Mack PC, Schwartz LH, et al. Nivolumab plus ipilimumab vs nivolumab for previously treated patients with stage IV squamous cell lung cancer: The lung-map S1400i phase 3 randomized clinical trial. JAMA Oncol (2021) 7(9):1368–77. doi: 10.1001/jamaoncol.2021.2209
24. Hellmann MD, Ciuleanu TE, Pluzanski A, Lee JS, Otterson GA, Audigier-Valette C, et al. Nivolumab plus ipilimumab in lung cancer with a high tumor mutational burden. N Engl J Med (2018) 378(22):2093–104. doi: 10.1056/NEJMoa1801946
25. Sezer A, Kilickap S, Gümüş M, Bondarenko I, Özgüroğlu M, Gogishvili M, et al. Cemiplimab monotherapy for first-line treatment of advanced non-Small-Cell lung cancer with PD-L1 of at least 50%: A multicentre, open-label, global, phase 3, randomised, controlled trial. Lancet (2021) 397(10274):592–604. doi: 10.1016/s0140-6736(21)00228-2
26. Seto T, Nosaki K, Shimokawa M, Toyozawa R, Sugawara S, Hayashi H, et al. Phase II study of atezolizumab with bevacizumab for non-squamous non-small cell lung cancer with high PD-L1 expression (Be study). J Immunother Cancer (2022) 10(2):e004025. doi: 10.1136/jitc-2021-004025
27. Antonia SJ, Borghaei H, Ramalingam SS, Horn L, De Castro Carpeño J, Pluzanski A, et al. Four-year survival with nivolumab in patients with previously treated advanced non-Small-Cell lung cancer: A pooled analysis. Lancet Oncol (2019) 20(10):1395–408. doi: 10.1016/s1470-2045(19)30407-3
28. Planchard D, Reinmuth N, Orlov S, Fischer JR, Sugawara S, Mandziuk S, et al. Arctic: Durvalumab with or without tremelimumab as third-line or later treatment of metastatic non-Small-Cell lung cancer. Ann Oncol (2020) 31(5):609–18. doi: 10.1016/j.annonc.2020.02.006
29. Stratigos AJ, Sekulic A, Peris K, Bechter O, Prey S, Kaatz M, et al. Cemiplimab in locally advanced basal cell carcinoma after hedgehog inhibitor therapy: An open-label, multi-centre, single-arm, phase 2 trial. Lancet Oncol (2021) 22(6):848–57. doi: 10.1016/s1470-2045(21)00126-1
30. Leighl NB, Redman MW, Rizvi N, Hirsch FR, Mack PC, Schwartz LH, et al. Phase II study of durvalumab plus tremelimumab as therapy for patients with previously treated anti-PD-1/PD-L1 resistant stage IV squamous cell lung cancer (Lung-MAP substudy S1400f, NCT03373760). J Immunother Cancer (2021) 9(8):e002973. doi: 10.1136/jitc-2021-002973
31. Schoenfeld JD, Giobbie-Hurder A, Ranasinghe S, Kao KZ, Lako A, Tsuji J, et al. Durvalumab plus tremelimumab alone or in combination with low-dose or hypofractionated radiotherapy in metastatic non-Small-Cell lung cancer refractory to previous PD(L)-1 therapy: An open-label, multicentre, randomised, phase 2 trial. Lancet Oncol (2022) 23(2):279–91. doi: 10.1016/s1470-2045(21)00658-6
32. Yang CJ, McSherry F, Mayne NR, Wang X, Berry MF, Tong B, et al. Surgical outcomes after neoadjuvant chemotherapy and ipilimumab for non-small cell lung cancer. Ann Thorac Surg (2018) 105(3):924–9. doi: 10.1016/j.athoracsur.2017.09.030
33. Gettinger S, Rizvi NA, Chow LQ, Borghaei H, Brahmer J, Ready N, et al. Nivolumab monotherapy for first-line treatment of advanced non-Small-Cell lung cancer. J Clin Oncol (2016) 34(25):2980–7. doi: 10.1200/jco.2016.66.9929
34. Moreno V, Garrido P, Papadopoulos KP, De Miguel Luken MJ, Gil-Martin M, Aljumaily R, et al. Tolerability and antitumor activity of cemiplimab, a human monoclonal anti-PD-1, as monotherapy in patients with pretreated non-small cell lung cancer (NSCLC): Data from the phase 1 NSCLC expansion cohort. Lung Cancer (2021) 155:151–5. doi: 10.1016/j.lungcan.2021.02.034
35. Herbst RS, Majem M, Barlesi F, Carcereny E, Chu Q, Monnet I, et al. Coast: An open-label, phase II, multidrug platform study of durvalumab alone or in combination with oleclumab or monalizumab in patients with unresectable, stage III non-Small-Cell lung cancer. J Clin Oncol (2022) 40(29):3383–93. doi: 10.1200/jco.22.00227
36. Rothschild SI, Zippelius A, Eboulet EI, Savic Prince S, Betticher D, Bettini A, et al. Sakk 16/14: Durvalumab in addition to neoadjuvant chemotherapy in patients with stage IIIA(N2) non-Small-Cell lung cancer-a multicenter single-arm phase II trial. J Clin Oncol (2021) 39(26):2872–80. doi: 10.1200/jco.21.00276
37. Niu J, Maurice-Dror C, Lee DH, Kim DW, Nagrial A, Voskoboynik M, et al. First-in-Human phase 1 study of the anti-TIGIT antibody vibostolimab as monotherapy or with pembrolizumab for advanced solid tumors, including non-Small-Cell lung cancer. Ann Oncol (2022) 33(2):169–80. doi: 10.1016/j.annonc.2021.11.002
38. Cho BC, Abreu DR, Hussein M, Cobo M, Patel AJ, Secen N, et al. Tiragolumab plus atezolizumab versus placebo plus atezolizumab as a first-line treatment for PD-L1-Selected non-Small-Cell lung cancer (CITYSCAPE): Primary and follow-up analyses of a randomised, double-blind, phase 2 study. Lancet Oncol (2022) 23(6):781–92. doi: 10.1016/s1470-2045(22)00226-1
39. Govindan R, Szczesna A, Ahn MJ, Schneider CP, Gonzalez Mella PF, Barlesi F, et al. Phase III trial of ipilimumab combined with paclitaxel and carboplatin in advanced squamous non-Small-Cell lung cancer. J Clin Oncol (2017) 35(30):3449–57. doi: 10.1200/jco.2016.71.7629
40. Rizvi NA, Mazières J, Planchard D, Stinchcombe TE, Dy GK, Antonia SJ, et al. Activity and safety of nivolumab, an anti-PD-1 immune checkpoint inhibitor, for patients with advanced, refractory squamous non-Small-Cell lung cancer (CheckMate 063): A phase 2, single-arm trial. Lancet Oncol (2015) 16(3):257–65. doi: 10.1016/s1470-2045(15)70054-9
41. Rizvi NA, Hellmann MD, Brahmer JR, Juergens RA, Borghaei H, Gettinger S, et al. Nivolumab in combination with platinum-based doublet chemotherapy for first-line treatment of advanced non-Small-Cell lung cancer. J Clin Oncol (2016) 34(25):2969–79. doi: 10.1200/jco.2016.66.9861
42. Felip E, Ardizzoni A, Ciuleanu T, Cobo M, Laktionov K, Szilasi M, et al. Checkmate 171: A phase 2 trial of nivolumab in patients with previously treated advanced squamous non-small cell lung cancer, including ecog ps 2 and elderly populations. Eur J Cancer (2020) 127:160–72. doi: 10.1016/j.ejca.2019.11.019
43. Ikeda S, Kato T, Kenmotsu H, Ogura T, Iwasawa S, Iwasawa T, et al. A phase II study of atezolizumab for pretreated Advanced/Recurrent non-small cell lung cancer with idiopathic interstitial pneumonias: Rationale and design for the TORG1936/AMBITIOUS study. Ther Adv Med Oncol (2020) 12:1758835920922022. doi: 10.1177/1758835920922022
44. Herbst RS, Giaccone G, de Marinis F, Reinmuth N, Vergnenegre A, Barrios CH, et al. Atezolizumab for first-line treatment of PD-L1-Selected patients with NSCLC. N Engl J Med (2020) 383(14):1328–39. doi: 10.1056/NEJMoa1917346
45. Peters S, Gettinger S, Johnson ML, Jänne PA, Garassino MC, Christoph D, et al. Phase II trial of atezolizumab as first-line or subsequent therapy for patients with programmed death-ligand 1-selected advanced non-Small-Cell lung cancer (BIRCH). J Clin Oncol (2017) 35(24):2781–9. doi: 10.1200/jco.2016.71.9476
46. Reck M, Wehler T, Orlandi F, Nogami N, Barone C, Moro-Sibilot D, et al. Safety and patient-reported outcomes of atezolizumab plus chemotherapy with or without bevacizumab versus bevacizumab plus chemotherapy in non-Small-Cell lung cancer. J Clin Oncol (2020) 38(22):2530–42. doi: 10.1200/jco.19.03158
47. Leighl NB, Hellmann MD, Hui R, Carcereny E, Felip E, Ahn MJ, et al. Pembrolizumab in patients with advanced non-Small-Cell lung cancer (KEYNOTE-001): 3-year results from an open-label, phase 1 study. Lancet Respir Med (2019) 7(4):347–57. doi: 10.1016/s2213-2600(18)30500-9
48. Garassino MC, Gadgeel S, Esteban E, Felip E, Speranza G, Domine M, et al. Patient-reported outcomes following pembrolizumab or placebo plus pemetrexed and platinum in patients with previously untreated, metastatic, non-squamous non-Small-Cell lung cancer (KEYNOTE-189): A multicentre, double-blind, randomised, placebo-controlled, phase 3 trial. Lancet Oncol (2020) 21(3):387–97. doi: 10.1016/s1470-2045(19)30801-0
49. Gulley JL, Rajan A, Spigel DR, Iannotti N, Chandler J, Wong DJL, et al. Avelumab for patients with previously treated metastatic or recurrent non-Small-Cell lung cancer (JAVELIN solid tumor): Dose-expansion cohort of a multicentre, open-label, phase 1b trial. Lancet Oncol (2017) 18(5):599–610. doi: 10.1016/s1470-2045(17)30240-1
50. Barlesi F, Vansteenkiste J, Spigel D, Ishii H, Garassino M, de Marinis F, et al. Avelumab versus docetaxel in patients with platinum-treated advanced non-Small-Cell lung cancer (JAVELIN lung 200): An open-label, randomised, phase 3 study. Lancet Oncol (2018) 19(11):1468–79. doi: 10.1016/s1470-2045(18)30673-9
51. Park K, Özgüroğlu M, Vansteenkiste J, Spigel D, Yang JCH, Ishii H, et al. Avelumab versus docetaxel in patients with platinum-treated advanced NSCLC: 2-year follow-up from the JAVELIN lung 200 phase 3 trial. J Thorac Oncol (2021) 16(8):1369–78. doi: 10.1016/j.jtho.2021.03.009
52. Boyer M, Şendur MAN, Rodríguez-Abreu D, Park K, Lee DH, Çiçin I, et al. Pembrolizumab plus ipilimumab or placebo for metastatic non-Small-Cell lung cancer with PD-L1 tumor proportion score ≥ 50%: Randomized, double-blind phase III KEYNOTE-598 study. J Clin Oncol (2021) 39(21):2327–38. doi: 10.1200/jco.20.03579
53. Gubens MA, Sequist LV, Stevenson JP, Powell SF, Villaruz LC, Gadgeel SM, et al. Pembrolizumab in combination with ipilimumab as second-line or later therapy for advanced non-Small-Cell lung cancer: KEYNOTE-021 cohorts d and h. Lung Cancer (2019) 130:59–66. doi: 10.1016/j.lungcan.2018.12.015
54. Antonia S, Goldberg SB, Balmanoukian A, Chaft JE, Sanborn RE, Gupta A, et al. Safety and antitumour activity of durvalumab plus tremelimumab in non-small cell lung cancer: A multicentre, phase 1b study. Lancet Oncol (2016) 17(3):299–308. doi: 10.1016/s1470-2045(15)00544-6
55. Lu S, Wang J, Cheng Y, Mok T, Chang J, Zhang L, et al. Nivolumab versus docetaxel in a predominantly Chinese patient population with previously treated advanced non-small cell lung cancer: 2-year follow-up from a randomized, open-label, phase 3 study (CheckMate 078). Lung Cancer (2021) 152:7–14. doi: 10.1016/j.lungcan.2020.11.013
56. Paz-Ares LG, Ramalingam SS, Ciuleanu TE, Lee JS, Urban L, Caro RB, et al. First-line nivolumab plus ipilimumab in advanced NSCLC: 4-year outcomes from the randomized, open-label, phase 3 CheckMate 227 part 1 trial. J Thorac Oncol (2022) 17(2):289–308. doi: 10.1016/j.jtho.2021.09.010
57. O’Byrne KJ, Lee KH, Kim SW, Park K, Nishio M, Sakai H, et al. First-line nivolumab + ipilimumab in advanced NSCLC: CheckMate 227 subpopulation analyses in Asian patients. ESMO Open (2022) 7(1):100394. doi: 10.1016/j.esmoop.2022.100394
58. Lynch TJ, Bondarenko I, Luft A, Serwatowski P, Barlesi F, Chacko R, et al. Ipilimumab in combination with paclitaxel and carboplatin as first-line treatment in stage IIIB/IV non-Small-Cell lung cancer: Results from a randomized, double-blind, multicenter phase II study. J Clin Oncol (2012) 30(17):2046–54. doi: 10.1200/jco.2011.38.4032
59. Socinski MA, Jotte RM, Cappuzzo F, Orlandi F, Stroyakovskiy D, Nogami N, et al. Atezolizumab for first-line treatment of metastatic nonsquamous NSCLC. N Engl J Med (2018) 378(24):2288–301. doi: 10.1056/NEJMoa1716948
60. Falchook GS, Ribas A, Davar D, Eroglu Z, Wang JS, Luke JJ, et al. Phase 1 trial of TIM-3 inhibitor cobolimab monotherapy and in combination with PD-1 inhibitors nivolumab or dostarlimab (Amber). J Clin Oncol (2022) 40(16_suppl):2504. doi: 10.1200/JCO.2022.40.16_suppl.2504
61. Carbone DP, Reck M, Paz-Ares L, Creelan B, Horn L, Steins M, et al. First-line nivolumab in stage IV or recurrent non-Small-Cell lung cancer. N Engl J Med (2017) 376(25):2415–26. doi: 10.1056/NEJMoa1613493
62. Provencio M, Nadal E, Insa A, García-Campelo MR, Casal-Rubio J, Dómine M, et al. Neoadjuvant chemotherapy and nivolumab in resectable non-Small-Cell lung cancer (NADIM): An open-label, multicentre, single-arm, phase 2 trial. Lancet Oncol (2020) 21(11):1413–22. doi: 10.1016/s1470-2045(20)30453-8
63. Leighl NB, Laurie SA, Goss GD, Hughes BGM, Stockler M, Tsao MS, et al. CCTG BR34: A randomized phase 2 trial of durvalumab and tremelimumab with or without platinum-based chemotherapy in patients with metastatic NSCLC. J Thorac Oncol (2022) 17(3):434–45. doi: 10.1016/j.jtho.2021.10.023
64. Verschraegen CF, Jerusalem G, McClay EF, Iannotti N, Redfern CH, Bennouna J, et al. Efficacy and safety of first-line avelumab in patients with advanced non-small cell lung cancer: Results from a phase ib cohort of the JAVELIN solid tumor study. J Immunother Cancer (2020) 8(2):e001064. doi: 10.1136/jitc-2020-001064
65. Riudavets M, Naigeon M, Texier M, Dorta M, Barlesi F, Mazieres J, et al. Gefitinib plus tremelimumab combination in refractory non-small cell lung cancer patients harbouring EGFR mutations: The GEFTREM phase I trial. Lung Cancer (2022) 166:255–64. doi: 10.1016/j.lungcan.2021.11.018
66. Arrieta O, Barrón F, Ramírez-Tirado LA, Zatarain-Barrón ZL, Cardona AF, Díaz-García D, et al. Efficacy and safety of pembrolizumab plus docetaxel vs docetaxel alone in patients with previously treated advanced non-small cell lung cancer: The PROLUNG phase 2 randomized clinical trial. JAMA Oncol (2020) 6(6):856–64. doi: 10.1001/jamaoncol.2020.0409
67. Pujol JL, Greillier L, Audigier-Valette C, Moro-Sibilot D, Uwer L, Hureaux J, et al. A randomized non-comparative phase II study of anti-programmed cell death-ligand 1 atezolizumab or chemotherapy as second-line therapy in patients with small cell lung cancer: Results from the IFCT-1603 trial. J Thorac Oncol (2019) 14(5):903–13. doi: 10.1016/j.jtho.2019.01.008
68. Clay TD, Majem M, Felip E, Doger B, Carcereny Costa E, Forster M, et al. Results from a phase II study of eftilagimod alpha (Soluble lag-3 protein) and pembrolizumab in patients with PD-L1 unselected metastatic non-small cell lung carcinoma. J Clin Oncol (2021) 39(15_suppl):9046. doi: 10.1200/JCO.2021.39.15_suppl.9046
69. Baas P, Scherpereel A, Nowak AK, Fujimoto N, Peters S, Tsao AS, et al. First-line nivolumab plus ipilimumab in unresectable malignant pleural mesothelioma (CheckMate 743): A multicentre, randomised, open-label, phase 3 trial. Lancet (2021) 397(10272):375–86. doi: 10.1016/s0140-6736(20)32714-8
70. Hellmann MD, Paz-Ares L, Bernabe Caro R, Zurawski B, Kim SW, Carcereny Costa E, et al. Nivolumab plus ipilimumab in advanced non-Small-Cell lung cancer. N Engl J Med (2019) 381(21):2020–31. doi: 10.1056/NEJMoa1910231
71. Rizvi NA, Cho BC, Reinmuth N, Lee KH, Luft A, Ahn MJ, et al. Durvalumab with or without tremelimumab vs standard chemotherapy in first-line treatment of metastatic non-small cell lung cancer: The mystic phase 3 randomized clinical trial. JAMA Oncol (2020) 6(5):661–74. doi: 10.1001/jamaoncol.2020.0237
72. Paz-Ares L, Luft A, Vicente D, Tafreshi A, Gümüş M, Mazières J, et al. Pembrolizumab plus chemotherapy for squamous non-Small-Cell lung cancer. N Engl J Med (2018) 379(21):2040–51. doi: 10.1056/NEJMoa1810865
73. Reck M, Rodríguez-Abreu D, Robinson AG, Hui R, Csőszi T, Fülöp A, et al. Updated analysis of KEYNOTE-024: Pembrolizumab versus platinum-based chemotherapy for advanced non-Small-Cell lung cancer with PD-L1 tumor proportion score of 50% or greater. J Clin Oncol (2019) 37(7):537–46. doi: 10.1200/jco.18.00149
74. Chang J, Wu YL, Lu S, Wang J, Mok T, Zhang L, et al. Three-year follow-up and patient-reported outcomes from CheckMate 078: Nivolumab versus docetaxel in a predominantly Chinese patient population with previously treated advanced non-small cell lung cancer. Lung Cancer (2021) 165:71–81. doi: 10.1016/j.lungcan.2021.12.009
75. Gandhi L, Rodríguez-Abreu D, Gadgeel S, Esteban E, Felip E, De Angelis F, et al. Pembrolizumab plus chemotherapy in metastatic non-Small-Cell lung cancer. N Engl J Med (2018) 378(22):2078–92. doi: 10.1056/NEJMoa1801005
76. Reck M, Ciuleanu TE, Cobo M, Schenker M, Zurawski B, Menezes J, et al. First-line nivolumab plus ipilimumab with two cycles of chemotherapy versus chemotherapy alone (Four cycles) in advanced non-Small-Cell lung cancer: Checkmate 9LA 2-year update. ESMO Open (2021) 6(5):100273. doi: 10.1016/j.esmoop.2021.100273
77. Herbst RS, Baas P, Kim DW, Felip E, Pérez-Gracia JL, Han JY, et al. Pembrolizumab versus docetaxel for previously treated, PD-L1-Positive, advanced non-Small-Cell lung cancer (KEYNOTE-010): A randomised controlled trial. Lancet (2016) 387(10027):1540–50. doi: 10.1016/s0140-6736(15)01281-7
78. Pinto JA, Raez LE, Oliveres H, Rolfo CC. Current knowledge of ipilimumab and its use in treating non-small cell lung cancer. Expert Opin Biol Ther (2019) 19(6):509–15. doi: 10.1080/14712598.2019.1610380
79. Ready N, Hellmann MD, Awad MM, Otterson GA, Gutierrez M, Gainor JF, et al. First-line nivolumab plus ipilimumab in advanced non-Small-Cell lung cancer (CheckMate 568): Outcomes by programmed death ligand 1 and tumor mutational burden as biomarkers. J Clin Oncol (2019) 37(12):992–1000. doi: 10.1200/jco.18.01042
80. Cascone T, William WN Jr., Weissferdt A, Leung CH, Lin HY, Pataer A, et al. Neoadjuvant nivolumab or nivolumab plus ipilimumab in operable non-small cell lung cancer: The phase 2 randomized NEOSTAR trial. Nat Med (2021) 27(3):504–14. doi: 10.1038/s41591-020-01224-2
81. Fujimoto D, Yomota M, Sekine A, Morita M, Morimoto T, Hosomi Y, et al. Nivolumab for advanced non-small cell lung cancer patients with mild idiopathic interstitial pneumonia: A multicenter, open-label single-arm phase II trial. Lung Cancer (2019) 134:274–8. doi: 10.1016/j.lungcan.2019.06.001
82. Haratani K, Hayashi H, Chiba Y, Kudo K, Yonesaka K, Kato R, et al. Association of immune-related adverse events with nivolumab efficacy in non-Small-Cell lung cancer. JAMA Oncol (2018) 4(3):374–8. doi: 10.1001/jamaoncol.2017.2925
83. Saka H, Nishio M, Hida T, Nakagawa K, Sakai H, Nogami N, et al. Five-year follow-up results from phase II studies of nivolumab in Japanese patients with previously treated advanced non-small cell lung cancer: Pooled analysis of the ONO-4538-05 and ONO-4538-06 studies. Jpn J Clin Oncol (2021) 51(1):106–13. doi: 10.1093/jjco/hyaa157
84. Rijavec E, Genova C, Barletta G, Burrafato G, Biello F, Dal Bello MG, et al. Ipilimumab in non-small cell lung cancer and small-cell lung cancer: New knowledge on a new therapeutic strategy. Expert Opin Biol Ther (2014) 14(7):1007–17. doi: 10.1517/14712598.2014.907786
85. Paz-Ares L, Ciuleanu TE, Cobo M, Schenker M, Zurawski B, Menezes J, et al. First-line nivolumab plus ipilimumab combined with two cycles of chemotherapy in patients with non-Small-Cell lung cancer (Checkmate 9LA): An international, randomised, open-label, phase 3 trial. Lancet Oncol (2021) 22(2):198–211. doi: 10.1016/s1470-2045(20)30641-0
86. Wong DJ, Bauer TM, Gordon MS, Bene-Tchaleu F, Zhu J, Zhang X, et al. Safety and clinical activity of atezolizumab plus ipilimumab in locally advanced or metastatic non-small cell lung cancer: Results from a phase 1b trial. Clin Lung Cancer (2022) 23(3):273–81. doi: 10.1016/j.cllc.2021.07.002
87. Khoja L, Day D, Wei-Wu Chen T, Siu LL, Hansen AR. Tumour- and class-specific patterns of immune-related adverse events of immune checkpoint inhibitors: A systematic review. Ann Oncol (2017) 28(10):2377–85. doi: 10.1093/annonc/mdx286
88. Takahashi T, Tagami T, Yamazaki S, Uede T, Shimizu J, Sakaguchi N, et al. Immunologic self-tolerance maintained by CD25(+)CD4(+) regulatory T cells constitutively expressing cytotoxic T lymphocyte-associated antigen 4. J Exp Med (2000) 192(2):303–10. doi: 10.1084/jem.192.2.303
89. Buchbinder EI, Desai A. CTLA-4 and PD-1 pathways: Similarities, differences, and implications of their inhibition. Am J Clin Oncol (2016) 39(1):98–106. doi: 10.1097/coc.0000000000000239
90. Rowshanravan B, Halliday N, Sansom DM. CTLA-4: A moving target in immunotherapy. Blood (2018) 131(1):58–67. doi: 10.1182/blood-2017-06-741033
91. Qureshi OS, Zheng Y, Nakamura K, Attridge K, Manzotti C, Schmidt EM, et al. Trans-endocytosis of CD80 and CD86: A molecular basis for the cell-extrinsic function of CTLA-4. Science (2011) 332(6029):600–3. doi: 10.1126/science.1202947
92. Wang CJ, Kenefeck R, Wardzinski L, Attridge K, Manzotti C, Schmidt EM, et al. Cutting edge: Cell-extrinsic immune regulation by CTLA-4 expressed on conventional T cells. J Immunol (2012) 189(3):1118–22. doi: 10.4049/jimmunol.1200972
93. Kong KF, Fu G, Zhang Y, Yokosuka T, Casas J, Canonigo-Balancio AJ, et al. Protein kinase C-H controls CTLA-4-Mediated regulatory T cell function. Nat Immunol (2014) 15(5):465–72. doi: 10.1038/ni.2866
94. Klocke K, Sakaguchi S, Holmdahl R, Wing K. Induction of autoimmune disease by deletion of CTLA-4 in mice in adulthood. Proc Natl Acad Sci U.S.A. (2016) 113(17):E2383–92. doi: 10.1073/pnas.1603892113
95. Wing K, Onishi Y, Prieto-Martin P, Yamaguchi T, Miyara M, Fehervari Z, et al. CTLA-4 control over Foxp3+ regulatory T cell function. Science (2008) 322(5899):271–5. doi: 10.1126/science.1160062
96. Mahmood SS, Fradley MG, Cohen JV, Nohria A, Reynolds KL, Heinzerling LM, et al. Myocarditis in patients treated with immune checkpoint inhibitors. J Am Coll Cardiol (2018) 71(16):1755–64. doi: 10.1016/j.jacc.2018.02.037
97. Marzec M, Zhang Q, Goradia A, Raghunath PN, Liu X, Paessler M, et al. Oncogenic kinase NPM/ALK induces through STAT3 expression of immunosuppressive protein CD274 (PD-L1, B7-H1). Proc Natl Acad Sci U.S.A. (2008) 105(52):20852–7. doi: 10.1073/pnas.0810958105
98. Taube JM, Anders RA, Young GD, Xu H, Sharma R, McMiller TL, et al. Colocalization of inflammatory response with B7-H1 expression in human melanocytic lesions supports an adaptive resistance mechanism of immune escape. Sci Transl Med (2012) 4(127):127ra37. doi: 10.1126/scitranslmed.3003689
99. Akbari O, Stock P, Singh AK, Lombardi V, Lee WL, Freeman GJ, et al. PD-L1 and PD-L2 modulate airway inflammation and iNKT-Cell-Dependent airway hyperreactivity in opposing directions. Mucosal Immunol (2010) 3(1):81–91. doi: 10.1038/mi.2009.112
100. Okazaki T, Maeda A, Nishimura H, Kurosaki T, Honjo T. PD-1 immunoreceptor inhibits b cell receptor-mediated signaling by recruiting src homology 2-Domain-Containing tyrosine phosphatase 2 to phosphotyrosine. Proc Natl Acad Sci U.S.A. (2001) 98(24):13866–71. doi: 10.1073/pnas.231486598
101. Kuang DM, Zhao Q, Peng C, Xu J, Zhang JP, Wu C, et al. Activated monocytes in peritumoral stroma of hepatocellular carcinoma foster immune privilege and disease progression through PD-L1. J Exp Med (2009) 206(6):1327–37. doi: 10.1084/jem.20082173
102. Appleman LJ, van Puijenbroek AA, Shu KM, Nadler LM, Boussiotis VA. CD28 costimulation mediates down-regulation of p27kip1 and cell cycle progression by activation of the Pi3K/PKB signaling pathway in primary human T cells. J Immunol (2002) 168(6):2729–36. doi: 10.4049/jimmunol.168.6.2729
103. Sheppard KA, Fitz LJ, Lee JM, Benander C, George JA, Wooters J, et al. PD-1 inhibits T-cell receptor induced phosphorylation of the ZAP70/CD3zeta signalosome and downstream signaling to pkctheta. FEBS Lett (2004) 574(1-3):37–41. doi: 10.1016/j.febslet.2004.07.083
104. Huber S, Hoffmann R, Muskens F, Voehringer D. Alternatively activated macrophages inhibit T-cell proliferation by Stat6-dependent expression of PD-L2. Blood (2010) 116(17):3311–20. doi: 10.1182/blood-2010-02-271981
105. Butte MJ, Keir ME, Phamduy TB, Sharpe AH, Freeman GJ. Programmed death-1 ligand 1 interacts specifically with the B7-1 costimulatory molecule to inhibit T cell responses. Immunity (2007) 27(1):111–22. doi: 10.1016/j.immuni.2007.05.016
106. Sanmamed MF, Chen L. A paradigm shift in cancer immunotherapy: From enhancement to normalization. Cell (2018) 175(2):313–26. doi: 10.1016/j.cell.2018.09.035
107. Khunger M, Rakshit S, Pasupuleti V, Hernandez AV, Mazzone P, Stevenson J, et al. Incidence of pneumonitis with use of programmed death 1 and programmed death-ligand 1 inhibitors in non-small cell lung cancer: A systematic review and meta-analysis of trials. Chest (2017) 152(2):271–81. doi: 10.1016/j.chest.2017.04.177
108. Pérol M. Multidisciplinary approach of immune checkpoint inhibitor-related pneumonitis: A key to address knowledge and management gaps. J Thorac Oncol (2020) 15(8):1261–4. doi: 10.1016/j.jtho.2020.05.007
109. Andrews LP, Marciscano AE, Drake CG, Vignali DA. LAG3 (CD223) as a cancer immunotherapy target. Immunol Rev (2017) 276(1):80–96. doi: 10.1111/imr.12519
110. Johnson AM, Bullock BL, Neuwelt AJ, Poczobutt JM, Kaspar RE, Li HY, et al. Cancer cell-intrinsic expression of MHC class II regulates the immune microenvironment and response to anti-PD-1 therapy in lung adenocarcinoma. J Immunol (2020) 204(8):2295–307. doi: 10.4049/jimmunol.1900778
111. Wang J, Sanmamed MF, Datar I, Su TT, Ji L, Sun J, et al. Fibrinogen-like protein 1 is a major immune inhibitory ligand of LAG-3. Cell (2019) 176(1-2):334–47.e12. doi: 10.1016/j.cell.2018.11.010
112. Yan J, Yu Y, Wang N, Chang Y, Ying H, Liu W, et al. LFIRE-1/HFREP-1, a liver-specific gene, is frequently downregulated and has growth suppressor activity in hepatocellular carcinoma. Oncogene (2004) 23(10):1939–49. doi: 10.1038/sj.onc.1207306
113. Nagasaki J, Togashi Y, Sugawara T, Itami M, Yamauchi N, Yuda J, et al. The critical role of CD4+ T cells in PD-1 blockade against MHC-II-Expressing tumors such as classic Hodgkin lymphoma. Blood Adv (2020) 4(17):4069–82. doi: 10.1182/bloodadvances.2020002098
114. Hemon P, Jean-Louis F, Ramgolam K, Brignone C, Viguier M, Bachelez H, et al. MHC class II engagement by its ligand LAG-3 (CD223) contributes to melanoma resistance to apoptosis. J Immunol (2011) 186(9):5173–83. doi: 10.4049/jimmunol.1002050
115. Bettini M, Szymczak-Workman AL, Forbes K, Castellaw AH, Selby M, Pan X, et al. Cutting edge: Accelerated autoimmune diabetes in the absence of LAG-3. J Immunol (2011) 187(7):3493–8. doi: 10.4049/jimmunol.1100714
116. Jha V, Workman CJ, McGaha TL, Li L, Vas J, Vignali DA, et al. Lymphocyte activation gene-3 (LAG-3) negatively regulates environmentally-induced autoimmunity. PLoS One (2014) 9(8):e104484. doi: 10.1371/journal.pone.0104484
117. Papadopoulos KP, Lakhani NJ, Johnson ML, Park H, Wang D, Yap TA, et al. First-in-Human study of Regn3767 (R3767), a human LAG-3 monoclonal antibody (mAb), ± cemiplimab in patients (Pts) with advanced malignancies. J Clin Oncol (2019) 37(15_suppl):2508. doi: 10.1200/JCO.2019.37.15_suppl.2508
118. Liu S, Zhang H, Li M, Hu D, Li C, Ge B, et al. Recruitment of Grb2 and Ship1 by the ITT-like motif of TIGIT suppresses granule polarization and cytotoxicity of nk cells. Cell Death Differ (2013) 20(3):456–64. doi: 10.1038/cdd.2012.141
119. Li M, Xia P, Du Y, Liu S, Huang G, Chen J, et al. T-Cell immunoglobulin and ITIM domain (TIGIT) Receptor/Poliovirus receptor (PVR) ligand engagement suppresses interferon-Γ production of natural killer cells Via B-arrestin 2-mediated negative signaling. J Biol Chem (2014) 289(25):17647–57. doi: 10.1074/jbc.M114.572420
120. Yu X, Harden K, Gonzalez LC, Francesco M, Chiang E, Irving B, et al. The surface protein TIGIT suppresses T cell activation by promoting the generation of mature immunoregulatory dendritic cells. Nat Immunol (2009) 10(1):48–57. doi: 10.1038/ni.1674
121. Stanietsky N, Simic H, Arapovic J, Toporik A, Levy O, Novik A, et al. The interaction of TIGIT with PVR and PVRL2 inhibits human NK cell cytotoxicity. Proc Natl Acad Sci U S A (2009) 106(42):17858–63. doi: 10.1073/pnas.0903474106
122. Gao X, Zhu Y, Li G, Huang H, Zhang G, Wang F, et al. TIM-3 expression characterizes regulatory T cells in tumor tissues and is associated with lung cancer progression. PLoS One (2012) 7(2):e30676. doi: 10.1371/journal.pone.0030676
123. Zhang Y, Cai P, Liang T, Wang L, Hu L. TIM-3 is a potential prognostic marker for patients with solid tumors: A systematic review and meta-analysis. Oncotarget (2017) 8(19):31705–13. doi: 10.18632/oncotarget.15954
124. Goldman JW, Dvorkin M, Chen Y, Reinmuth N, Hotta K, Trukhin D, et al. Durvalumab, with or without tremelimumab, plus platinum-etoposide versus platinum-etoposide alone in first-line treatment of extensive-stage small-cell lung cancer (Caspian): Updated results from a randomised, controlled, open-label, phase 3 trial. Lancet Oncol (2021) 22(1):51–65. doi: 10.1016/s1470-2045(20)30539-8
125. Allcock RJ. The major histocompatibility complex: A paradigm for studies of the human genome. Methods Mol Biol (2012) 882:1–7. doi: 10.1007/978-1-61779-842-9_1
126. Hofstetter AR, Sullivan LC, Lukacher AE, Brooks AG. Diverse roles of non-diverse molecules: MHC class ib molecules in host defense and control of autoimmunity. Curr Opin Immunol (2011) 23(1):104–10. doi: 10.1016/j.coi.2010.09.009
127. Kanga U, Tandon N, Marwaha RK, Khanna R, Bhattacharya B, Singh S, et al. Immunogenetic association and thyroid autoantibodies in juvenile autoimmune thyroiditis in north India. Clin Endocrinol (Oxf) (2006) 64(5):573–9. doi: 10.1111/j.1365-2265.2006.02511.x
128. Perricone C, Agmon-Levin N, Ceccarelli F, Valesini G, Anaya JM, Shoenfeld Y. Genetics and autoantibodies. Immunol Res (2013) 56(2-3):206–19. doi: 10.1007/s12026-013-8396-9
129. Cappelli LC, Dorak MT, Bettinotti MP, Bingham CO, Shah AA. Association of HLA-DRB1 shared epitope alleles and immune checkpoint inhibitor-induced inflammatory arthritis. Rheumatol (Oxford) (2019) 58(3):476–80. doi: 10.1093/rheumatology/key358
130. Marchand L, Disse E, Dalle S, Reffet S, Vouillarmet J, Fabien N, et al. The multifaceted nature of diabetes mellitus induced by checkpoint inhibitors. Acta Diabetol (2019) 56(12):1239–45. doi: 10.1007/s00592-019-01402-w
131. Toi Y, Sugawara S, Sugisaka J, Ono H, Kawashima Y, Aiba T, et al. Profiling preexisting antibodies in patients treated with anti-PD-1 therapy for advanced non-small cell lung cancer. JAMA Oncol (2019) 5(3):376–83. doi: 10.1001/jamaoncol.2018.5860
132. Ma WT, Chang C, Gershwin ME, Lian ZX. Development of autoantibodies precedes clinical manifestations of autoimmune diseases: A comprehensive review. J Autoimmun (2017) 83:95–112. doi: 10.1016/j.jaut.2017.07.003
133. Chen L, Flies DB. Molecular mechanisms of T cell Co-stimulation and Co-inhibition. Nat Rev Immunol (2013) 13(4):227–42. doi: 10.1038/nri3405
134. Nam S, Lee A, Lim J, Lim JS. Analysis of the expression and regulation of PD-1 protein on the surface of myeloid-derived suppressor cells (MDSCs). Biomol Ther (Seoul) (2019) 27(1):63–70. doi: 10.4062/biomolther.2018.201
135. Luoma AM, Suo S, Williams HL, Sharova T, Sullivan K, Manos M, et al. Molecular pathways of colon inflammation induced by cancer immunotherapy. Cell (2020) 182(3):655–71.e22. doi: 10.1016/j.cell.2020.06.001
136. Byrne EH, Fisher DE. Immune and molecular correlates in melanoma treated with immune checkpoint blockade. Cancer (2017) 123(S11):2143–53. doi: 10.1002/cncr.30444
137. Berner F, Bomze D, Diem S, Ali OH, Fässler M, Ring S, et al. Association of checkpoint inhibitor-induced toxic effects with shared cancer and tissue antigens in non-small cell lung cancer. JAMA Oncol (2019) 5(7):1043–7. doi: 10.1001/jamaoncol.2019.0402
138. Läubli H, Koelzer VH, Matter MS, Herzig P, Dolder Schlienger B, Wiese MN, et al. The T cell repertoire in tumors overlaps with pulmonary inflammatory lesions in patients treated with checkpoint inhibitors. Oncoimmunology (2018) 7(2):e1386362. doi: 10.1080/2162402x.2017.1386362
139. Oh DY, Cham J, Zhang L, Fong G, Kwek SS, Klinger M, et al. Immune toxicities elicted by CTLA-4 blockade in cancer patients are associated with early diversification of the T-cell repertoire. Cancer Res (2017) 77(6):1322–30. doi: 10.1158/0008-5472.Can-16-2324
140. Nishino M, Giobbie-Hurder A, Hatabu H, Ramaiya NH, Hodi FS. Incidence of programmed cell death 1 inhibitor-related pneumonitis in patients with advanced cancer: A systematic review and meta-analysis. JAMA Oncol (2016) 2(12):1607–16. doi: 10.1001/jamaoncol.2016.2453
141. Chaput N, Lepage P, Coutzac C, Soularue E, Le Roux K, Monot C, et al. Baseline gut microbiota predicts clinical response and colitis in metastatic melanoma patients treated with ipilimumab. Ann Oncol (2019) 30(12):2012. doi: 10.1093/annonc/mdz224
142. Subudhi SK, Aparicio A, Gao J, Zurita AJ, Araujo JC, Logothetis CJ, et al. Clonal expansion of CD8 T cells in the systemic circulation precedes development of ipilimumab-induced toxicities. Proc Natl Acad Sci U.S.A. (2016) 113(42):11919–24. doi: 10.1073/pnas.1611421113
143. Naidoo J, Cottrell TR, Lipson EJ, Forde PM, Illei PB, Yarmus LB, et al. Chronic immune checkpoint inhibitor pneumonitis. J Immunother Cancer (2020) 8(1):e000840. doi: 10.1136/jitc-2020-000840
144. Suresh K, Naidoo J, Zhong Q, Xiong Y, Mammen J, de Flores MV, et al. The alveolar immune cell landscape is dysregulated in checkpoint inhibitor pneumonitis. J Clin Invest (2019) 129(10):4305–15. doi: 10.1172/jci128654
145. Das R, Bar N, Ferreira M, Newman AM, Zhang L, Bailur JK, et al. Early b cell changes predict autoimmunity following combination immune checkpoint blockade. J Clin Invest (2018) 128(2):715–20. doi: 10.1172/jci96798
146. Gödel P, Shimabukuro-Vornhagen A, von Bergwelt-Baildon M. Understanding cytokine release syndrome. Intensive Care Med (2018) 44(3):371–3. doi: 10.1007/s00134-017-4943-5
147. Oyanagi J, Koh Y, Sato K, Mori K, Teraoka S, Akamatsu H, et al. Predictive value of serum protein levels in patients with advanced non-small cell lung cancer treated with nivolumab. Lung Cancer (2019) 132:107–13. doi: 10.1016/j.lungcan.2019.03.020
148. Khan S, Khan SA, Luo X, Fattah FJ, Saltarski J, Gloria-McCutchen Y, et al. Immune dysregulation in cancer patients developing immune-related adverse events. Br J Cancer (2019) 120(1):63–8. doi: 10.1038/s41416-018-0155-1
149. Lin X, Deng H, Yang Y, Wu J, Qiu G, Li S, et al. Peripheral blood biomarkers for early diagnosis, severity, and prognosis of checkpoint inhibitor-related pneumonitis in patients with lung cancer. Front Oncol (2021) 11:698832. doi: 10.3389/fonc.2021.698832
150. Hirashima T, Kanai T, Suzuki H, Yoshida H, Matsushita A, Kawasumi H, et al. The levels of interferon-gamma release as a biomarker for non-Small-Cell lung cancer patients receiving immune checkpoint inhibitors. Anticancer Res (2019) 39(11):6231–40. doi: 10.21873/anticanres.13832
151. Kurimoto C, Inaba H, Ariyasu H, Iwakura H, Ueda Y, Uraki S, et al. Predictive and sensitive biomarkers for thyroid dysfunctions during treatment with immune-checkpoint inhibitors. Cancer Sci (2020) 111(5):1468–77. doi: 10.1111/cas.14363
152. Phillips GS, Wu J, Hellmann MD, Postow MA, Rizvi NA, Freites-Martinez A, et al. Treatment outcomes of immune-related cutaneous adverse events. J Clin Oncol (2019) 37(30):2746–58. doi: 10.1200/jco.18.02141
153. Tahir SA, Gao J, Miura Y, Blando J, Tidwell RSS, Zhao H, et al. Autoimmune antibodies correlate with immune checkpoint therapy-induced toxicities. Proc Natl Acad Sci U S A (2019) 116(44):22246–51. doi: 10.1073/pnas.1908079116
154. Maekura T, Naito M, Tahara M, Ikegami N, Kimura Y, Sonobe S, et al. Predictive factors of nivolumab-induced hypothyroidism in patients with non-small cell lung cancer. In Vivo (2017) 31(5):1035–9. doi: 10.21873/invivo.11166
155. Luongo C, Morra R, Gambale C, Porcelli T, Sessa F, Matano E, et al. Higher baseline tsh levels predict early hypothyroidism during cancer immunotherapy. J Endocrinol Invest (2021) 44(9):1927–33. doi: 10.1007/s40618-021-01508-5
156. Brilli L, Danielli R, Campanile M, Secchi C, Ciuoli C, Calabrò L, et al. Baseline serum tsh levels predict the absence of thyroid dysfunction in cancer patients treated with immunotherapy. J Endocrinol Invest (2021) 44(8):1719–26. doi: 10.1007/s40618-020-01480-6
157. Yoon JH, Hong AR, Kim HK, Kang HC. Characteristics of immune-related thyroid adverse events in patients treated with PD-1/PD-L1 inhibitors. Endocrinol Metab (Seoul) (2021) 36(2):413–23. doi: 10.3803/EnM.2020.906
158. Hasan Ali O, Bomze D, Ring SS, Berner F, Fässler M, Diem S, et al. BP180-specific IgG is associated with skin adverse events, therapy response, and overall survival in non-small cell lung cancer patients treated with checkpoint inhibitors. J Am Acad Dermatol (2020) 82(4):854–61. doi: 10.1016/j.jaad.2019.08.045
159. Stamatouli AM, Quandt Z, Perdigoto AL, Clark PL, Kluger H, Weiss SA, et al. Collateral damage: Insulin-dependent diabetes induced with checkpoint inhibitors. Diabetes (2018) 67(8):1471–80. doi: 10.2337/dbi18-0002
160. Williams TJ, Benavides DR, Patrice KA, Dalmau JO, de Ávila AL, Le DT, et al. Association of autoimmune encephalitis with combined immune checkpoint inhibitor treatment for metastatic cancer. JAMA Neurol (2016) 73(8):928–33. doi: 10.1001/jamaneurol.2016.1399
161. Hoefsmit EP, Rozeman EA, Haanen J, Blank CU. Susceptible loci associated with autoimmune disease as potential biomarkers for checkpoint inhibitor-induced immune-related adverse events. ESMO Open (2019) 4(4):e000472. doi: 10.1136/esmoopen-2018-000472
162. Bomze D, Hasan Ali O, Bate A, Flatz L. Association between immune-related adverse events during anti-PD-1 therapy and tumor mutational burden. JAMA Oncol (2019) 5(11):1633–5. doi: 10.1001/jamaoncol.2019.3221
163. Delivanis DA, Gustafson MP, Bornschlegl S, Merten MM, Kottschade L, Withers S, et al. Pembrolizumab-induced thyroiditis: Comprehensive clinical review and insights into underlying involved mechanisms. J Clin Endocrinol Metab (2017) 102(8):2770–80. doi: 10.1210/jc.2017-00448
164. Yano S, Ashida K, Sakamoto R, Sakaguchi C, Ogata M, Maruyama K, et al. Human leucocyte antigen Dr15, a possible predictive marker for immune checkpoint inhibitor-induced secondary adrenal insufficiency. Eur J Cancer (2020) 130:198–203. doi: 10.1016/j.ejca.2020.02.049
165. Hasan Ali O, Berner F, Bomze D, Fässler M, Diem S, Cozzio A, et al. Human leukocyte antigen variation is associated with adverse events of checkpoint inhibitors. Eur J Cancer (2019) 107:8–14. doi: 10.1016/j.ejca.2018.11.009
166. Marschner D, Falk M, Javorniczky NR, Hanke-Müller K, Rawluk J, Schmitt-Graeff A, et al. Microrna-146a regulates immune-related adverse events caused by immune checkpoint inhibitors. JCI Insight (2020) 5(6):e132334. doi: 10.1172/jci.insight.132334
167. Eun Y, Kim IY, Sun JM, Lee J, Cha HS, Koh EM, et al. Risk factors for immune-related adverse events associated with anti-PD-1 pembrolizumab. Sci Rep (2019) 9(1):14039. doi: 10.1038/s41598-019-50574-6
168. Cho JY, Kim J, Lee JS, Kim YJ, Kim SH, Lee YJ, et al. Characteristics, incidence, and risk factors of immune checkpoint inhibitor-related pneumonitis in patients with non-small cell lung cancer. Lung Cancer (2018) 125:150–6. doi: 10.1016/j.lungcan.2018.09.015
169. Galant-Swafford J, Troesch A, Tran L, Weaver A, Doherty TA, Patel SP. Landscape of immune-related pneumonitis in cancer patients with asthma being treated with immune checkpoint blockade. Oncology (2020) 98(2):123–30. doi: 10.1159/000503566
170. Helmink BA, Khan MAW, Hermann A, Gopalakrishnan V, Wargo JA. The microbiome, cancer, and cancer therapy. Nat Med (2019) 25(3):377–88. doi: 10.1038/s41591-019-0377-7
171. Chu X, Zhao J, Zhou J, Zhou F, Jiang T, Jiang S, et al. Association of baseline peripheral-blood eosinophil count with immune checkpoint inhibitor-related pneumonitis and clinical outcomes in patients with non-small cell lung cancer receiving immune checkpoint inhibitors. Lung Cancer (2020) 150:76–82. doi: 10.1016/j.lungcan.2020.08.015
172. Pavan A, Calvetti L, Dal Maso A, Attili I, Del Bianco P, Pasello G, et al. Peripheral blood markers identify risk of immune-related toxicity in advanced non-small cell lung cancer treated with immune-checkpoint inhibitors. Oncologist (2019) 24(8):1128–36. doi: 10.1634/theoncologist.2018-0563
173. Moey MYY, Tomdio AN, McCallen JD, Vaughan LM, O’Brien K, Naqash AR, et al. Characterization of immune checkpoint inhibitor-related cardiotoxicity in lung cancer patients from a rural setting. JACC CardioOncol (2020) 2(3):491–502. doi: 10.1016/j.jaccao.2020.07.005
174. Jing Y, Liu J, Ye Y, Pan L, Deng H, Wang Y, et al. Multi-omics prediction of immune-related adverse events during checkpoint immunotherapy. Nat Commun (2020) 11(1):4946. doi: 10.1038/s41467-020-18742-9
175. Skribek M, Rounis K, Afshar S, Grundberg O, Friesland S, Tsakonas G, et al. Effect of corticosteroids on the outcome of patients with advanced non-small cell lung cancer treated with immune-checkpoint inhibitors. Eur J Cancer (2021) 145:245–54. doi: 10.1016/j.ejca.2020.12.012
176. Li J, Xu D, Liu Y, Cao Y, He J, Liao M. Acupuncture treatment of Guillain-Barré syndrome after using immune checkpoint inhibitors: A case report. Front Neurol (2022) 13:908282. doi: 10.3389/fneur.2022.908282
177. Touat M, Talmasov D, Ricard D, Psimaras D. Neurological toxicities associated with immune-checkpoint inhibitors. Curr Opin Neurol (2017) 30(6):659–68. doi: 10.1097/wco.0000000000000503
178. Andreyev J, Ross P, Donnellan C, Lennan E, Leonard P, Waters C, et al. Guidance on the management of diarrhoea during cancer chemotherapy. Lancet Oncol (2014) 15(10):e447–60. doi: 10.1016/s1470-2045(14)70006-3
179. Luo J, Beattie JA, Fuentes P, Rizvi H, Egger JV, Kern JA, et al. Beyond steroids: Immunosuppressants in steroid-refractory or resistant immune-related adverse events. J Thorac Oncol (2021) 16(10):1759–64. doi: 10.1016/j.jtho.2021.06.024
180. Bergqvist V, Hertervig E, Gedeon P, Kopljar M, Griph H, Kinhult S, et al. Vedolizumab treatment for immune checkpoint inhibitor-induced enterocolitis. Cancer Immunol Immunother (2017) 66(5):581–92. doi: 10.1007/s00262-017-1962-6
181. Monsour EP, Pothen J, Balaraman R. A novel approach to the treatment of pembrolizumab-induced psoriasis exacerbation: A case report. Cureus (2019) 11(10):e5824. doi: 10.7759/cureus.5824
182. Stroud CR, Hegde A, Cherry C, Naqash AR, Sharma N, Addepalli S, et al. Tocilizumab for the management of immune mediated adverse events secondary to PD-1 blockade. J Oncol Pharm Pract (2019) 25(3):551–7. doi: 10.1177/1078155217745144
183. Burak KW, Swain MG, Santodomingo-Garzon T, Lee SS, Urbanski SJ, Aspinall AI, et al. Rituximab for the treatment of patients with autoimmune hepatitis who are refractory or intolerant to standard therapy. Can J Gastroenterol (2013) 27(5):273–80. doi: 10.1155/2013/512624
Keywords: non-small cell lung cancer, immune-related toxicity, combined immunotherapies, predictive biomarker, immune checkpoint inhibitor
Citation: Hu X, Wang L, Shang B, Wang J, Sun J, Liang B, Su L, You W and Jiang S (2023) Immune checkpoint inhibitor-associated toxicity in advanced non-small cell lung cancer: An updated understanding of risk factors. Front. Immunol. 14:1094414. doi: 10.3389/fimmu.2023.1094414
Received: 10 November 2022; Accepted: 20 February 2023;
Published: 06 March 2023.
Edited by:
Gaurisankar Sa, Bose Institute, IndiaReviewed by:
Yukihiro Toi, Sendai Kousei Hospital, JapanMichael Shafique, Moffitt Cancer Center, United States
Copyright © 2023 Hu, Wang, Shang, Wang, Sun, Liang, Su, You and Jiang. This is an open-access article distributed under the terms of the Creative Commons Attribution License (CC BY). The use, distribution or reproduction in other forums is permitted, provided the original author(s) and the copyright owner(s) are credited and that the original publication in this journal is cited, in accordance with accepted academic practice. No use, distribution or reproduction is permitted which does not comply with these terms.
*Correspondence: Wenjie You, youwenjie655@163.com; Shujuan Jiang, docjiangshujuan@163.com
†These authors have contributed equally to this work and share first authorship
‡ORCID: Wenjie You, https://orcid.org/0000-0002-8044-3914
Shujuan Jiang, https://orcid.org/0000-0003-3073-1931