- 1Department of Biology, Faculty of Science, Ferdowsi University of Mashhad, Mashhad, Iran
- 2Stem Cells and Regenerative Medicine Research Group, Academic Center for Education, Culture and Research (ACECR)-Khorasan Razavi, Mashhad, Iran
- 3Department of Medical and Surgical Sciences for Children & Adults, University Hospital of Modena, Modena, Italy
- 4Novel Diagnostics and Therapeutics Research Group, Institute of Biotechnology, Ferdowsi University of Mashhad, Mashhad, Iran
- 5Department of Stem Cells and Developmental Biology, Cell Science Research Center, Royan Institute for Stem Cell Biology and Technology, ACECR, Tehran, Iran
- 6Advanced Therapy Medicinal Product Technology Development Center (ATMP-TDC), Cell Sciences Research Center, Royan Institute for Stem Cell Biology and Technology, ACECR, Tehran, Iran
- 7School of Biomedical Engineering, University of Technology Sydney, Sydney, NSW, Australia
- 8Department of Obstetrics and Gynaecology, Monash University, Clayton VIC, Australia
- 9Cancer Prevention Research Center, Shahroud University of Medical Sciences, Shahroud, Iran
- 10Industrial Biotechnology Research Group, Institute of Biotechnology, Ferdowsi University of Mashhad, Mashhad, Iran
- 11Blood Borne Infections Research Center, Academic Center for Education, Culture and Research (ACECR)-Khorasan Razavi, Mashhad, Iran
The conventional therapeutic approaches to treat autoimmune diseases through suppressing the immune system, such as steroidal and non-steroidal anti-inflammatory drugs, are not adequately practical. Moreover, these regimens are associated with considerable complications. Designing tolerogenic therapeutic strategies based on stem cells, immune cells, and their extracellular vesicles (EVs) seems to open a promising path to managing autoimmune diseases’ vast burden. Mesenchymal stem/stromal cells (MSCs), dendritic cells, and regulatory T cells (Tregs) are the main cell types applied to restore a tolerogenic immune status; MSCs play a more beneficial role due to their amenable properties and extensive cross-talks with different immune cells. With existing concerns about the employment of cells, new cell-free therapeutic paradigms, such as EV-based therapies, are gaining attention in this field. Additionally, EVs’ unique properties have made them to be known as smart immunomodulators and are considered as a potential substitute for cell therapy. This review provides an overview of the advantages and disadvantages of cell-based and EV-based methods for treating autoimmune diseases. The study also presents an outlook on the future of EVs to be implemented in clinics for autoimmune patients.
1 Introduction
An ever-increasing number of people worldwide suffer from some form of autoimmune disease. These are clinical conditions collectively defined by the loss of tolerance/immunological inertness (1, 2), which is mainly accompanied by a simultaneous failure of regenerative mechanisms (1, 3). Losing central or peripheral tolerance could be resulted from genetic mutations, environmental factors, and stochastic or epigenetic phenomena, with possible devastating immunological consequences (4–6). Upon the activation of innate immune responses, adaptive immune responses are also triggered, which are significantly involved in determining the “extension” and “persistence” of autoimmune diseases (7). Furthermore, it was proposed that abnormal inflammatory responses are correlated with chronic autoimmune diseases (8). Activated T and B cells, inappropriately responding to self-antigens, are responsible for cell death during autoimmune diseases (1, 3).
According to some epidemiological studies, autoimmune diseases affect approximately 5–8% of the world population (9). They are associated with 80 chronic conditions (10). Autoimmune diseases are classified as either organ-specific or systemic (11). Autoimmune diseases could be considered monogenic, polygenic, or mixed patterns and may originate from deregulations in innate and adaptive immune responses (12, 13). It is unclear whether these classifications will affect the treatment strategy or outcomes, especially in cell-based therapeutics. However, these features could be considered critical in deciding which cell source or the administration route would be more suitable.
Immunosuppression is among the most common therapeutic strategies to manage autoimmunity. However, the main barrier in this approach is the complexity of the immune system and its exact fine-tuning under different circumstances. Although over-activity of the immune system is not desirable, some level of function is essential for efficient defence against pathogens. Hence, a broad-spectrum of the immune suppressants are undesirable as their long-term use may result in serious side effects ranging from susceptibility to infections to malignancies (11). Drug toxicity and low penetration capacity are other obstacles limiting the efficacy of routine therapeutic strategies (14, 15). Some pharmaceutical and even biological strategies, applied to manage autoimmune diseases in a target-specific mode, were reported to trigger new autoimmune diseases and have significant side effects (16). As a general trend, despite the cancer cases in which immunostimulatory features are desirable to control immune-scape mechanisms, in regenerative medicine, immunosuppressive features are the primary therapeutic modality to manage exhaustive tissue inflammation for the benefit of fundamental regenerative mechanisms (17, 18).
2 Cell-based therapies for autoimmune diseases
Cell-based therapies have been delegated to overcome the limits of conventional medicine in managing autoimmune disorders, perhaps because they affect cell-cell interactions as fundamental players of the immune responses. Understanding the molecular mechanisms and signaling pathways responsible for the pathogenesis of autoimmune diseases has provided a basis for employing cells with diverse abilities to compensate for various functional defects of the immune system. The current scope of cell-based therapies for managing autoimmune diseases has been previously discussed (19). An overview of clinical trials registered in ClinicalTrials.gov for leading prevalent autoimmune diseases indicates that four main cell types have been assessed. These include i) hematopoietic stem cells (± immune ablation); ii) mesenchymal stem/stromal cells (bone marrow, adipose, umbilical cord, placenta, Wharton’s Jelly); iii) tolerogenic dendritic cells (TolDCs), and iv) T regulatory cells (Treg) (Figure 1). Other cell sources/products were also reported, such as peripheral blood CD34+ stem cells, allogeneic lymphocytes, bone marrow aspirates, lipo-aspirates, adipose stem cells secretome, MSC conditioned media, the stromal vascular fraction (SVF) cells/vascular fraction from the adipose tissue, adipose-derived regenerative cells, autologous centrifuged adipose tissue, mesenchymal trophic factors (MTFs) from the umbilical cord, autologous apoptotic cells, and third party antigen-specific T-cells (ClinicalTrials.gov). A closer look shows that in addition to the four main cell types, some tissue components or cell suspension combinations have also been tested, containing one of these cell types or their derivatives. Different administration routes and the autologous vs. allogeneic application of the cells are other variations observed among previously registered clinical trials. First, it is necessary to highlight how much these disorders threaten global health to better describe the significance of cells and their derivatives in managing autoimmune diseases.
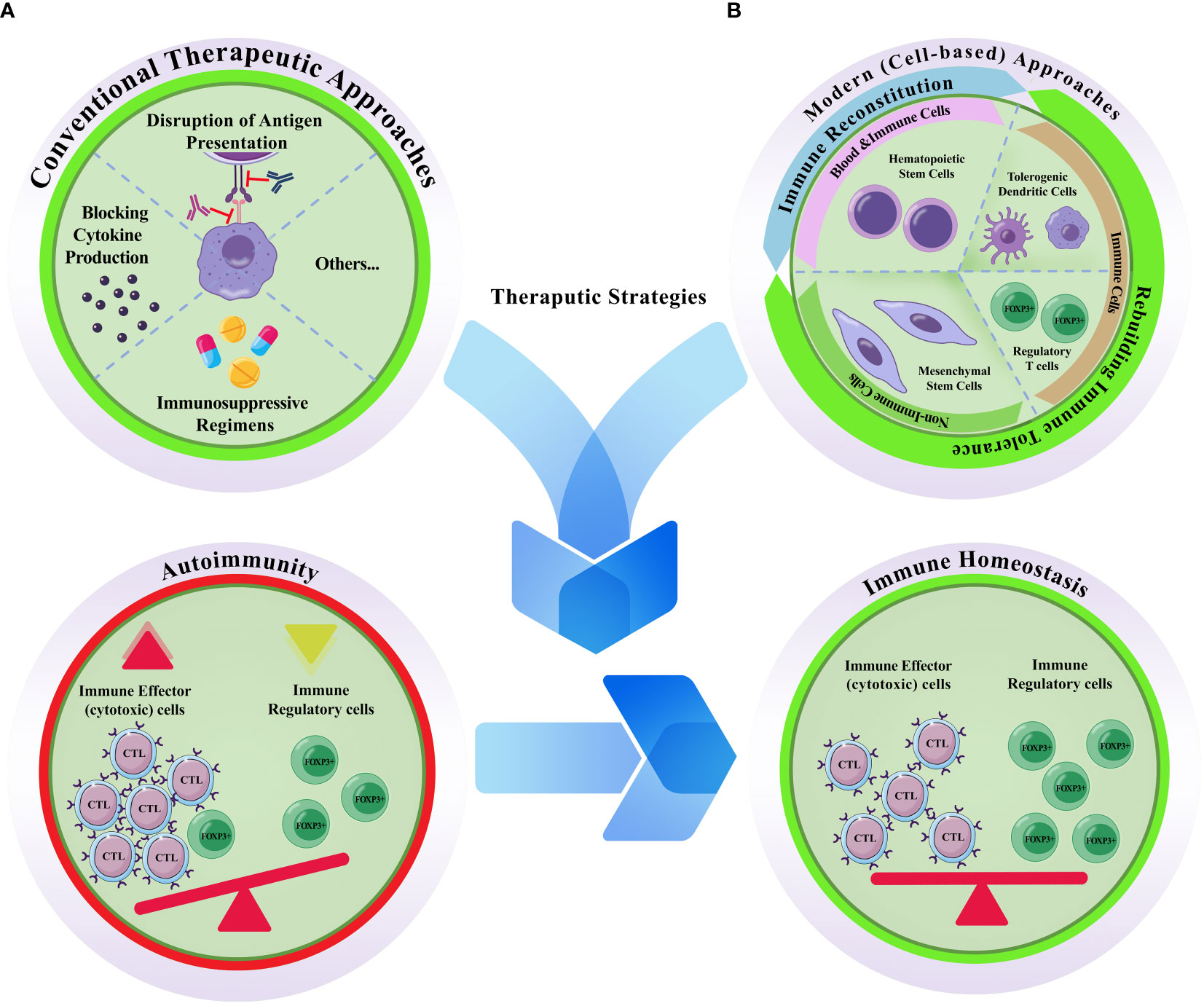
Figure 1 Conventional vs. modern cell based therapeutic approaches for autoimmune diseases. (A) Conventional therapeutic approaches are mostly depend on disruption of antigen presentation, blocking cytokine production, and/or the prescription of immunosuppressive regimens. (B) Modern cell-based therapeutics. Hematopoietic stem cells, tolerogenic dendritic cells, regulatory T cells, and mesenchymal stem cells are the four main cellular sources that have been proposed to treat autoimmune diseases. Autoimmunity is accompanied by abnormal accumulation of activated immune effector cells (mostly cytotoxic T lymphocytes; CTLs) and concurrent decrease/functional deficiency of the inhibitory/regulatory cells (FOXP3+) of the immune system; otherwise it is defined as the failure of the self-tolerance. Hence, effective therapeutic strategies are those with the capacity to re-induce the homeostatic state of the immune system. While hematopoietic stem cell transplantation can reconstitute the immune system, the effects of the other three cell types are attributed to their capacity for rebuilding autoimmune tolerance. Unlike the conventional methods which are typically temporary, and are accompanied by harsh side effects, cellular toxicity, and single-effect mechanism of action, cell-based methods are provide the patients with a long-lasting alleviative effects.
2.1 Hematopoietic stem cells
Hematopoietic stem cell transplantation (HSCT) is one of the primary efficient cell-based therapies utilized to treat autoimmune diseases (20, 21). They operate based on eliminating autoreactive immune cells and reconstituting the self-tolerant immune system. Although HSCT is an intensive one-off procedure, it brings a treatment-free remission for the patients upon success. With more than 3300 auto-immune patients registered for HSCT, the European Society for Blood and Marrow Transplantation (EBMT) Autoimmune Diseases Working Party (ADWP) has made significant progress in the field (22, 23). HSCT is currently the therapeutic modality for multiple sclerosis, systemic sclerosis, and Crohn’s disease by the professional stem cell transplant societies of America, Europe, and Brazil (24). The rationale and mode of action of HSCT were reviewed by another group (22). Given the prerequisites of this type of treatment, allogeneic HSCT is still considered a high-risk strategy that is only prescribed for patients in the very severe stages of autoimmune diseases (19, 25–27).
2.2 Tolerogenic DCs
Dendritic cells (DCs) are antigen-presenting cells (APCs) that regulate innate and acquired immune systems, balancing antigen-specific immunity and promoting immune tolerance. Based on their subtypes, DCs can play different and sometimes opposite roles. DCs act as pro- or anti-inflammation inducers upon external application. For instance, in the context of autoimmune diseases, they can contribute to the worsening of the disease through the production of pro-inflammatory cytokines and the activation of autologous antigen-reactive T cells (28). In the context of organ transplantation, donor DC-derived EVs can promote undesirable allograft-targeting immune responses (29). At the same time, tolerogenic DCs comprise a specific type of APCs with immunoregulatory function (30, 31).
The function of DCs depends on several factors, such as pattern recognition receptors (PPRs), specific cytokines and metabolites, some TLRs agonists, dexamethasone, and vitamin A or vitamin D3, which could be applied to maintain tolerogenic DCs stable and to enhance their lymph node-homing capacity (32, 33). The situation seems to be fine-tuned by their maturation status. Studies show that immature and semi-mature DCs demonstrate tolerogenic features (34) through different mechanisms (35). In experimental models, immature DC-derived EVs that carry tolerogenic content were applied successfully in the context of rheumatoid arthritis (36–38). DC-derived EVs maintain their producer cells’ features. Hence they could be considered proper cell replacements (39). These EVs may be enriched with either immunoregulatory or immunostimulatory content to target a particular subset of the immune cells, resulting in their suppression, reprogramming or activation. As well-known anti-inflammatory molecules, EVs with tailored anti-inflammatory content are shown to be produced by DCs, modified for the benefit of IDO1, TGF-β1, IL-10, IL-4, Fas-L, and CTLA-4 (40).
2.3 Regulatory T cells
Regulatory T cells (Tregs) actively maintain peripheral immune tolerance (41). Two major types of Treg cells (polyclonal and antigen-specific Tregs) have been investigated based on their immunomodulatory capacities. Pre-clinical studies indicate that antigen-specific Tregs have superior tolerogenic properties compared to polyclonal Tregs as they have more defined specificity (42). Potential applications, large-scale production, safety and efficacy of Tregs produced via good manufacturing practice (GMP) were recently described (43, 44).
An alternative approach to deploying tailored Tregs is via their genetic engineering, known as chimeric antigen receptors (CAR)-Tregs. Tregs are engineered as an improved version of the cells, which facilitates the design of advanced therapeutic procedures to induce immune-tolerogenic responses (43, 45, 46). The biology of Treg cells, advantages, challenges, and some technical details were discussed by Raffin and Bluestone (47). The possible role of these cells in effectively managing autoimmune diseases remains to be elucidated.
2.4 Mesenchymal stem/stromal cells
MSCs possess high regenerative capacities and special immunoregulatory functions affecting all types of innate and adaptive immune cells (48). MSCs, trans-differentiated to a macrophage-like profile in vivo, were shown to carry secretory capabilities that resembled phagosomes. This functional shift in the injury site is accompanied by the overexpression of CD45 and major histocompatibility complex (MHC) class II while maintaining their MSC-specific surface marker expression (49).
Due to their unique features, MSCs are currently widely applied in the late phases of clinical trials. Previous clinical studies regarding the application of MSCs for immune-related diseases are classified based on criteria, including disease type, donors, and tissue sources (50). A brief timeline for significant events in studying the immunosuppressive effects of MSCs and the progress in their clinical applications was discussed in detail by Wu et al., confirming their safety and efficacy (51).
The consequences of autologous/allogeneic MSC therapy are heavily investigated in clinical trials to treat autoimmune and inflammatory diseases along with transplant rejection, including graft versus host disease (GvHD), acute respiratory distress syndrome (ARDS), acute lung injury (ALI), ankylosing spondylitis, autoimmune hepatitis, type 1 and 2 diabetes, refractory autoimmune thrombocytopenia, skin diseases, inflammatory bowel disease (IBD), MS, CD, Stevens-Johnson syndrome (SJS), systemic lupus erythematosus (SLE), rheumatoid arthritis (RA), osteoarthritis (OA), and even in coronavirus disease of 2019 (COVID-19) as an immune-dysregulating infectious disease. As dysfunctional MSCs were proposed to be involved in the pathogenesis of some autoimmune diseases, such as SLE or immune thrombocytopenia (ITP), the utilization of MSC-based therapeutics could be considered more seriously as an effective treatment strategy (52–55).
In addition to the intrinsic capacity for migration towards inflammatory tissues, MSCs possess different immunomodulatory properties, including the inhibition of apoptosis, induction/maintenance of immune tolerance, and immunosuppression (10). The beneficial properties of MSCs, which play a role in modulating inflammatory responses and infiltration processes, have been attributed to a synergy by i) MSC-released signaling molecules, ii) the reaction of immune cells and other target cells to these signaling molecules, and iii) feedback in the MSC-molecule-target cell axis (56). However, the precise way MSCs act is still under investigation.
MSCs produce and release extracellular vesicles (EVs) enriched with immunomodulatory factors as long as their cellular resources are kept in proper and defined conditions (57). Furthermore, they can induce tolerogenic Treg cells and even tolerogenic DCs following their administration (58, 59). The immunomodulatory, pro-angiogenic, and tissue-tropic activities of MSCs-secretome and -EVs are comparable to the producer cells, and they were proposed as valuable sources for treating inflammatory disease (60, 61). Hence, based on their paracrine effects, the potential to alter the function of immune cells, and considerable potential for EV production, we focus on these cells and their EV counterparts. The potential of MSCs for large-scale production of EVs with immunomodulatory content was compared with HEK293, ESCs, iPSCs, and ESC/iPSC-derived MSCs from different aspects in a previous publication (62). We highlight some open issues regarding the differences between cells and EVs to compare their applications.
3 Extracellular vesicles, a new therapeutic paradigm for autoimmune diseases
The application of cell-free products secreted by the stem and progenitor cells has been proposed to lower the risks of direct cell injection while maintaining good efficacy (63). Among several cell-free products, EVs are gaining closer attention as novel anti-inflammatory therapeutics (64). These vesicles are involved in key innate and adaptive immunity processes, including but not limited to antigen presentation, inflammation, anti-microbial defence, development and activation of B- and T-cells, and allergic, autoimmune or anti-tumor responses (65). EVs have raised a good deal of promise for the efficient treatment of autoimmune diseases, as they carry the analogous advantages of their producer cells (66). While EVs from CD3+, activated CD8+, or engineered T-cells, natural killer cells, and M1 macrophages are mostly considered immune-active nano-vesicles, EVs from regulatory T-cells, MSCs, and M2 macrophages, as well as erythrocytes, neutrophils, platelets or cancerous cells are reported to have immunosuppressive features. The key consideration is that they exert immunosuppressive or immune-active properties in a producer- and target-cell-dependent manner (67).
3.1 The biological properties of EVs
EVs of 30 nm to 1 µm in diameter are described as “heterogeneous bilayer membranous vesicles lacking a functional nucleus” and as “multi-signal messengers” which are enriched with a variety of biomolecules (68, 69). The biogenesis, intracellular trafficking, and secretion of EVs are tightly regulated by specific proteins, including the RAB family of small GTPases (70) and lipids, and need the molecular motors- (myosin, kinases, dynein, and GTPases) mediated cytoskeleton rearrangement (68, 71). These vesicles share characteristics regarding their membrane organizers, lipid content, cell adhesion molecules, intracellular trafficking mediators, enzymes, signal transduction, biogenesis factors, chaperones, and nucleic acids, while they are different in some others (72). Identifying these commonalities and differences between EV types will help to better understand their biology and function.
Different classification criteria and nomenclature have been applied to define various types of vesicles (73), including exosomes, microvesicles/ectosomes, apoptotic bodies, migrasomes, and oncosomes that are released by the cells under normal physiological and pathological conditions (74–76), amongst exosomes are the most well-known cellular nano-vesicles (77)(Figure 2). The most recently introduced member of the secreted nano-particles family includes exomeres [with a dot-shaped morphology], which are smaller than 50 nm and are enriched with metabolic pathways [glycolysis and mTORC1 signaling regulatory proteins such as β-galactoside α2, 6-sialyltransferase 1 (ST6Gal-I) and the EGFR ligand, amphiregulin (AREG) (76, 78, 79). Supermeres are the other new class of extracellular particles, indicating a different protein and RNA cargo than EVs and exomeres. Although similar to EVs and exomeres, they have the potential to carry extracellular RNA in a protected mode and play a role during chronic disease conditions (80–82). It was proposed that supermeres may be functionally involved in the immune supervision of cell-death-derived ribonucleoprotein complexes (83).
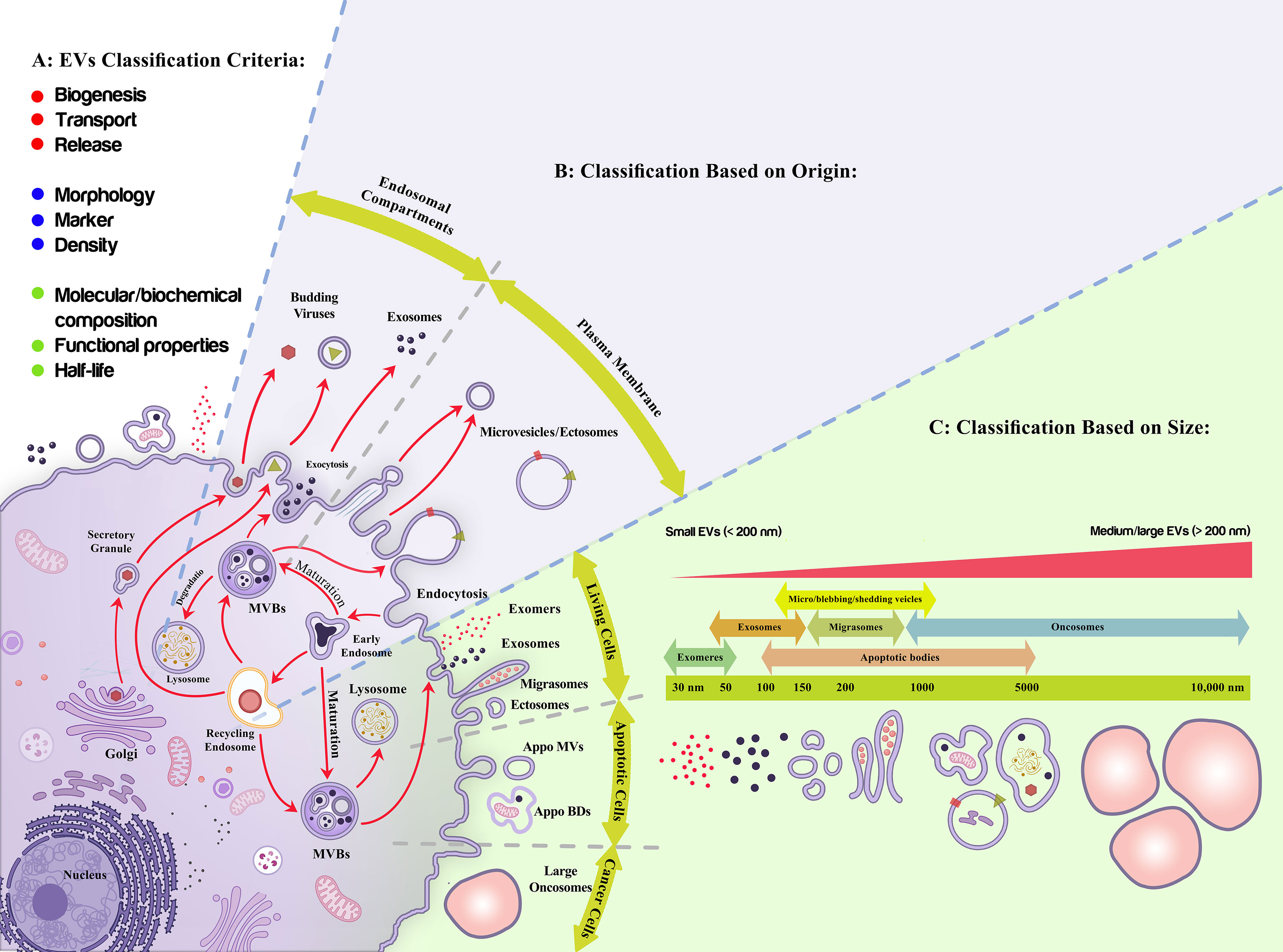
Figure 2 Extracellular vesicles classification. (A) Different criteria have been proposed to classify Extracellular vesicles (EVs) including production mechanism (biogenesis, transport, release), morphology, markers, density, half-life, molecular composition, and functional properties; among which their origin (B), and size (C) are more applicable due to their quantitative modality and functional consequences, respectively. (B) As it is shown, exosomes are the nano-entities released upon the exocytosis of multivesicular bodies while ectosomes/microvesicles are produced upon the assembly of plasma membrane into the membranous vesicles. (C) Live cells, apoptotic cells, and cancerous cells produce nano-size extracellular vesicles with different characteristics. These vesicles have an overlapping size distribution, gradually increased from exomeres to oncosomes. EVs are also classified as small (<200 nm) and large EVs (>200 nm).
However, the isolation steps or the characterization methods for a definite type of vesicles are not convincing enough. For instance, essential controls for the different subtypes are not considered while comparing the functional immune-related properties. On the other hand, it was shown by experimental studies that their immunomodulatory characteristic is prominently diminished upon removing EVs from MSC-secretome. Although several subtypes of nano-vesicles are currently recognized, due to the recent bibliographic studies (2002-2021), two terms, “exosomes” and “extracellular vesicles”, are yet the most frequent keywords applied to describe the role of these cell-derived membranous nano-vesicles in autoimmune diseases (84, 85). Despite the initial trend, it is now evidenced that the impact of EV subtypes and their versatility is so critical compared to what it inferred at first (86, 87) and should not be underestimated during designing and performing future pre-clinical experiments and clinical trials. Here, following the International Society for Extracellular Vesicles (ISEV) consensus recommendation (88), we apply the term “extracellular vesicles” as the representative for the nano-sized natural and non-replicative particles focusing on membranous exosomes and ectosomes, which are produced by almost all cell types (68, 76, 89–92).
Isolation (93), characterization (94), labeling, and quantification strategies of different EV subtypes are constantly evolving (95). It should be noted that the various isolation methods of EVs end with different characteristics and potencies in terms of application (96, 97). It was shown that EVs from 3D cultures are functionally different and are more similar to the patient-derived EVs compared to those obtained from traditional 2D-culture conditions (98–100). These imply that spending time for optimization of isolation method/s (101, 102) to reach pure EVs with higher efficiency (103) in a reproducible manner (104) is of great importance. This way, it would be possible to compare the results more accurately (102).
EVs’ biogenesis, biological properties, and contents have been comprehensively discussed over the last few years and shown that they are essential for their function and clinical applications (68). The quality of endogenous EV preparations (105), from body fluids, tissues, or intracellular spaces (40), is affected by the circadian clock (106). In addition, isolation method, purity, storage protocol (107), time and temperature (108), presence or absence of anti-coagulants, isotonic buffers, other reagents such as cryoprotectants (109), pH, and ionic strength (110–112) are the other influencing factors. The concentration and content of EVs are influenced by demographic factors, including sex, age and race to some extent (113) and more than that, the EV cargo could be deliberately engineered through microenvironment modifications (114).
EVs are considered a powerful means for short- and long-distance communications (juxtacrine, autocrine, paracrine, and endocrine modes (65)) of the cells (115–117). These cross-talks have remained conserved throughout evolution and play a role in “inter-kingdom” mutual communications (118). This way, EVs can mediate (attenuate or promote) cell death, inflammation, and immune responses in a context-dependent mode (119, 120). Extracellular matrix-bound EVs are a recently-introduced subset of EVs with predicted roles in tissue repair and regeneration, which may be mediated by their active or passive tissue traversing or extracellular matrix (ECM) remodeling capacity (121). While cells are changing entities, EVs do not change following their release. Their biological function could, however, be impaired upon exposure to hostile microenvironments (122). In other words, EVs are membranous packages that carry various DNA-, RNA-, protein-, lipid cargoes and metabolites to their target cells (69, 123–125). They transfer active components to their target cells via different mechanisms, including receptors (integrins, tetraspanins, lectins, proteoglycans)-mediated binding, plasma membrane fusion, macropinocytosis, and lipid raft-mediated endocytosis, clathrin-mediated endocytosis, phagocytosis, and caveolae-dependent endocytosis, and trigger certain downstream events.
Tissue-derived EVs (Ti-EVs) reflect the tissue of origin microenvironment and cell-to-cell intercommunications and are critically important in studying and monitoring some developmental events. Like cell-culture-derived EVs, optimized and standardized protocols should be applied for efficient isolation and enrichment of Ti-EVs (126). Examples of the importance and usability of Ti-EVs have been reported in the pathogenesis, diagnosis, and treatment of Alzheimer’s disease (127, 128). EVs from individuals diagnosed with AD possess a specific protein content and miRNAs profiles which could be applied for early detection of the disease. In a recent experiment, NMDAR2A was identified as a CNS-specific EV surface marker, and it was shown in a multicenter study, including both discovery and validation cohorts, that the number of plasma EVs with NMDAR2A and L1CAM expression is lower in patients with AD in comparison to the healthy controls (129). On the other hand, as EVs are responsible for the “spread of tau pathology”, their secretion inhibition could be proposed as a novel targeted therapeutic strategy for patients with Alzheimer’s disease (126, 130, 131). Bone marrow MSC-EVs can decrease BACE-1 and Aβ, increase sphingosine-1-phosphate and exert amenable results in the context of Alzheimer’s disease (66, 132, 133).
Preparing qualified endogenous EVs from bio-fluids as the primary source is more complex, as several factors interfere with efficient EV isolation and purification (134). The quality of the final sample will be determined as a consequence of donor-related factors in addition to the biophysical and chemical properties of the fluid. The material of the sample collection tube, the application of coagulant or anticoagulants, the initial volume of the sample, mixing or agitation steps, the duration of pre-processing or pre-storage steps, and hemolysis degree or other depletion strategies are among the essential determinants of the quality of EVs. It is also essential to manage possible contaminations (111, 135).
Among their broad applications, EVs are sometimes utilized based on their nano-sized features and ability to pass through blood barriers (136). In such cases, EVs could be applied as a new paradigm with amenable priorities (137, 138). EVs can achieve most features of the synthetic nano-carriers (i.e., liposomes and nano-particles). Moreover, they have higher biocompatibility, chemical stability, longer-distance intercellular communications, and cell fusion capacity (139). As non-toxic nano-carriers, EVs demonstrated better pharmacokinetics and pharmacodynamics features (absorption, distribution, metabolism, and excretion) than synthetic nano-carriers (140, 141). However, there are controversies regarding their application as drug delivery systems (DDSs) like nano-shuttles (142). Some technical limitations have been raised regarding their flexibility in applying different reagents, preparation procedures, and surface functionalization strategies (139). Also, they have widely been recognized as natural and valuable means for diagnosing and monitoring various disease conditions and novel therapeutic agents (111, 143).
3.2 Therapeutic potential of EVs
Therapeutic applications of EVs in managing different inflammatory and autoimmune diseases and their immunomodulatory features and composition were discussed in recently published articles (144–146). Extensive parallel research during the last decade has revealed new aspects in the biology of MSCs, leading to changes in our orientation regarding their applications. These changes highlighted the importance of the paracrine effects of MSCs and proposed the possibility of replacing the cells with their cell-free products, especially in the allogeneic context (147). “Secretome” is the common term that represents all substances released by MSCs, including free-soluble factors (various growth factors, chemokines, and cytokines) and insoluble EVs (148–150). Different aspects of the pleiotropic therapeutic effects of MSCs and their paracrine action were discussed in a recent publication, emphasizing the active components of the MSCs’ secretome (116). The paracrine strength of these cells is so significant that it has been suggested that the name of these cells would be changed to the medicinal signaling cells (151, 152). Munoz-Perez et al. reviewed the latest trends regarding the potential of MSC-secretome to treat immune-encountered inflammatory diseases (153).
There is a growing tendency toward local administration of EVs employing diverse scaffolds from acellular tissues to solid patches and hydrogels (154–158). One good reason favoring the local administration of EVs is the lower amount required, making their utilization much more cost-effective. Furthermore, their gradual and sustained release following the administration is a positive point (87). Moreover, the application of scaffolds/biomaterials increases the bioavailability, homing, and/or biocompatibility of EVs at the site of injection, while they are also effective in avoiding the side effects of the injection procedure (159). This preference may be pronounced when dealing with local inflammatory conditions or tissue-specific autoimmune diseases.
It was confirmed that MSC-EVs are therapeutically efficient in 30 animal models mimicking various human diseases (111). While different roles were described for EVs during the pathogenesis/immunopathology of Rheumatoid arthritis and joint impairment of RA patients, the therapeutic impact of MSCs- and non-MSCs-EVs (dendritic cells, polymorphonuclear neutrophils, myeloid-derived suppressor cells) were confirmed in animal studies (160). The first case report regarding the benefits of the MSC-EVs was related to a patient with GvHD (161). The high significance of MSC-EVs, as a novel therapeutic for managing Alzheimer’s disease, has been thoroughly discussed recently in mice and human cases, emphasizing the mechanisms of the disease progression (66).
Similar to their producer cells, MSC-EVs eventually found their place in pre-clinical and clinical studies (162). However, each step of scalable EV manufacturing under GMP compliance is still exposed to several challenges (163, 164). These challenges exist not only in upstream and downstream stages (165, 166) of EV production and isolation, such as cell culture and purification steps, respectively, but also during EV storage (108), quality control (167), and functional evaluation steps (148, 168).
EVs were previously enriched from the conditioned media of different immune cells, including dendritic cells, T regulatory cells, M1/M2 macrophages, and CD4+ T cells (169–171), and their potential was evaluated in the context of autoimmune diseases (44). However, despite the initial trend, it is now evident that these vesicles, regardless of their producer cell type, are produced with different biological content under different circumstances (172), and their application is accompanied by several challenges and complications (173–175). One remaining question could be: How can we learn from the long-term and extensive research on their producer cells to make EVs-based therapeutics safe and efficient in the frame of translational medicine? Finally, the main questions considered to be addressed by this review are the level of superiority of EVs to intact cells in reducing patients’ symptoms of autoimmune disorders and the maturity of techniques for preparing clinical-grade EVs (176).
4 MSC-EVs vs. MSCs: Open issues
4.1 Interaction with the immune system
MSCs have immunosuppressive and immune privilege properties; hence, their interactions with the immune system are expected to benefit immune homeostasis and restore immune tolerance. However, there is concern regarding their allogeneic and repeated administration (177). MSC-EVs, like their original cell sources (MSCs), are believed to have low immunogenicity as well as lower carcinogenic risk (178). They do not release certain inflammatory factors (IL-1, IL-6, and IL-8), which may be vastly released from dead cells following cell injection procedures, especially in tissues lacking adequate blood/nutrients (179). In addition, EVs demonstrate enhanced circulation stability due to the immune system’s evasion (39). Although similar to allogeneic cells, these particles may potentially carry some immunogenic proteins (e.g., MHC molecules), it is open to question whether these proteins are transferred from the vesicles to other cells in communication and if they can trigger alloimmune responses (Table 1) (29, 177, 180).
MSCs‐EVs exert their immunomodulation through a similar mechanism known for MSCs‐associated tolerance. This process is mediated by programmed death ligand‐1, galectin‐1, and TGF-β (206). They impose immunomodulation instead of immunosuppression, leading to reduced immune reactions and homeostasis (188, 189). MSC-EVs contain many parental biomolecules, including chemokines, cytokines, and growth factors (207). Suh et al. described the mechanisms responsible for the therapeutic effects of EVs in the context of different inflammatory diseases. Similar to the cells, the mechanisms responsible for the therapeutic effects of the EVs in the context of different inflammatory diseases are reducing the microglia/macrophage activation, oxidative stress, pro-inflammatory cytokine and chemokine release, T-cell activation, tissue fibrosis, viral infectivity, immune cell infiltration, and apoptosis/necrosis (146). Moreover, EVs regulate M1/M2 macrophage polarization for the benefit of M2 cells, induce collagen regeneration, and prevent scar generation (181).
Despite similar immunomodulatory properties, differences in the two entities’ level and mechanism of action have been reported. The way EVs communicate with the effector cells of the immune cells is not as simple as it sounds and the mediators of MSC-EV-based immune modulation are yet remained incompletely described (182). In comparison to MSCs, EVs have less cell-dependent and independent mechanisms to exert their immunomodulatory properties upon exposure to various immune system cells (183–187). On the other hand, EVs can penetrate deeper into the tissues (208, 209), so they may have a higher chance of regulating the immune cells (183). In addition, the therapeutic anti-inflammatory properties of EVs could be more pronounced due to their capacity for passing through blood barriers (210). Also, the immune rejection rate of EVs is considerably low compared to the producer cells, as they have a low-level expression of membrane histocompatibility molecules (187). EVs have been shown to carry, mediate, and regulate cytokine transport from cells to members of the immune system (190).
Unlike the cells, MSC-EVs cannot impact the production of CD3+ T cells. Allogeneic EVs could bind to T cells, but they do not stimulate these cells during in vitro experiments unless in the presence of APCs in the culture media. In addition, EVs do not change the ratio of B cells and plasma cells following their co-culture with human peripheral blood mononuclear cells (PBMCs) in vitro (182, 191–193). Allogeneic EVs would activate T cells following their in vivo administration in mice only if delivered into an inflammatory microenvironment (196). MSC-EVs can interfere with the feed-forward loop between complements and neutrophils via the inhibition of the complement activation in a CD59-mediated manner. Hence, MSC-EVs are influential players able to modulate inflammatory status spatially and temporally (194). While MSCs and their cell-free counterparts can increase the ratio of FOXP3+CD25+CD4+ T regulatory cells, EVs are less functional against DCs (185, 195).
The therapeutic effects of extracellular vesicles as new biologicals for immune regulation have been supported by pre-clinical studies. Native or modified MSC-EVs were used to regulate the immune-associated cells while focusing on their mechanism of action (211, 212). There is almost no doubt that MSC-EVs can trigger anti-inflammatory responses based on restoring the balance action in the milieu or immune cells. This could be attributed to their miRNAs, other non-coding RNAs, or protein cargo; otherwise, surface EV-associated proteins may pave the role by activating the downstream intracellular pathways in recipient cells (211, 213). The heterogeneous nature of producer cells, diverse EV isolation, quantification and standardization strategies and the critical role of disease-specific pathways are considered the main challenges to introducing unique regulatory mechanisms (212, 214). Moreover, the clinical practice of EVs is challenged by their heterogeneity to some extent. Optimization of current digital assays has been proposed to do single-vesicle-resolution studies, especially during the clinical analysis of liquid biopsies (215). Immune biocompatibility of EVs is the other criterion to be investigated in autoimmune diseases. It was shown in a previous study that although both autologous and allogeneic small EVs may have efficient therapeutic effects, autologous EVs are more viable and impactful in damaged tissues (216, 217).
Recent updates unravel the crucial role of EVs and vesicular trafficking in the immune system (65) and during the pathogenesis of immune-based diseases, in addition to their therapeutic perspectives (218). An accurate understanding of the mechanisms by which tumors or other pathological statuses induce immune cell dysfunction via their extracellular particles will allow a better understanding of how vesicles interact with the cells of the immune system. These data would potentially facilitate developing novel methods to face the overactive immune system and the associated diseases.
4.2 MSC-EVs vs. MSCs: Homing capabilities
The migratory capacities of MSCs towards ischemic and damaged sites have received substantial consideration for treating diffuse and localized inflammatory and degenerative conditions (219). However, low homing efficiency is a significant drawback associated with temporary therapeutic benefits (220). Hence, diverse approaches have been employed to enhance the entrapment rate of systemically infused MSCs (221). EVs must also follow the route to the affected areas as membrane-bound cell-derived structures. Various cell-to-cell communication functions are ascribed to EVs, including autocrine and paracrine missions (222). Sung et al. discovered that EVs could even contribute to directional cell migration. They confirmed that malignant cells could find their path toward EV deposits, resulting in EVs’ endocytosis (223). In specific migration paths, cells use almost the same delivery process as depicted for growth factors and peptides, in addition to their active migration (224). Here, we raise some open issues to compare MSCs and EVs concerning their homing capabilities.
When systemically administered, exogenous MSCs imitate the delivery route via which endogenous MSCs reach their destination (225). As tissue injuries often coincide with inflammation or ischemia, inflammatory factors could provide cues to mobilize cells towards the damaged tissues. The expression of homing receptors on the cell surface is proven to mediate MSCs’ migration towards their ligands at the ischemic target microenvironment (226). Upon arriving at the damaged site, circulating MSCs or their bioactive components need to leave the vasculature and transmigrate across the endothelium to get into the stromal region, where they exert their primary function both in passive and active manners (220, 227). Paracrine interactions of culture-expanded cells and the target milieu necessitate reciprocating various bioactive determinants (228). Furthermore, the bio-distribution of exogenous MSCs has always been challenging, as many infused MSCs are entrapped in other organs, particularly the lungs, liver, and spleen (220).
4.2.1 Are EVs capable of finding their targets too?
The capability of MSCs to desirably home in insulted tissues is of substantial cell-based treatment advantages. It has been shown that some migratory axes, such as chemokines at the site of injury and chemokine receptors on the surface of cells, namely the SDF-1/CXCR4 axis, orchestrate the stem cells’ homing process (229, 230). Regarding the selective EV uptake, proteins and glycoproteins on the surface of EV and the recipient cell mediate EV-cell interactions. Hence, most strategies that were applied to increase the tissue specificity of EVs are trying to reconfigure the surface glycoproteins of the vesicles (231). Through various targeting mechanisms, EVs interact with recipient cells to transmit selective biological information. Superficial cytokines on EVs could act as barcodes recognized by the recipient cells’ cytokine receptors (197). On the contrary, some studies suggest that EV uptake is not cell-specific (198).
4.2.2 How do the uptake process mechanism and homing, about extravasation and transmigration of vesicles, differ from that of stem cells?
Homing of stem cells is described as the arrest of cells within the vasculature of a tissue followed by transmigration across the endothelium, usually in response to a chemokine gradient in the target region. The leading role of receptor/ligand axes, e.g., VLA-4/VCAM-1, is highlighted in its contribution to tethering, rolling, firm adhesion, and transmigration of the cells (220).
EVs were reported to demonstrate cell and tissue-specific autonomous targeting capabilities (139, 215). The interactions between EVs and the neighboring or distant acceptor cells occur through diverse mechanisms. EVs’ size and surface components affect their recognition and capture by the acceptor cells (115). The literature is inconsistent on whether EVs can target specific tissues, mainly due to the diverse tracking methods. It is proposed that MSC-EVs have the same receptors of MSCs on their membrane, which could be why MSC-EVs could find the injury site, perhaps through a similar mechanism (199, 232). While some studies depict no specific in vivo biodistribution of EVs, others show they tend to accumulate in tumors or injured tissues, which could explain EVs’ therapeutic effects (233).
The receptor-mediated binding of EVs to cells could stimulate a signaling cascade to transmit information without delivering their content. Upon the fusion of vesicles with the cell membrane, EVs content could be randomly released into the cytoplasm. Additionally, acceptor cells may internalize vesicles to intracellular specific molecular targets. Clathrin-dependent, clathrin-independent pathways such as caveolin-mediated uptake, macropinocytosis, phagocytosis, and cholesterol-rich lipid rafts mechanisms are among the numerous endocytosis processes outlined in the literature (203).
Clathrin-mediated endocytosis requires adaptins, which connect membrane cargo to clathrin, forming a polyhedral lattice surrounding the vesicle. Clathrin-mediated endocytosis involves the assimilation of receptors based on their ligands in clathrin-coated pits on the plasma membrane, collapsing into a vesicular bud and forming clathrin-coated vesicles. The subsequent intracellular vesicle goes through clathrin uncoating and then integrates with the endosome to deposit its contents. Some treatment approaches, e.g., cancer, prevent clathrin-coated pits, resulting in decreased EVs’ uptake (222). Caveolae-mediated endocytosis evolved from the oligomerization capacity of caveolin proteins. Oligomerization of caveolins mediates the formation of caveolin-rich rafts in the plasma membrane. Caveolae are tiny cave-like introversions in the cell membrane that can become internalized into the cell (203, 222).
4.2.3 How do EVs communicate with their target microenvironment?
To segregate EVs from soluble mediators, MISEV-2018 has suggested that cell-cell-contact could be regarded as necessary for signaling, but it may also happen by exchanging plasma membrane-enclosed signals between EV-donor and EV-recipient cells (91). These vesicles harbor their cargo from enzymatic degradation while trafficking through the extracellular milieu (203). EVs, are not the same as their parent cells but contribute to altering the microenvironment. EVs are shown to manage specific paracrine intercommunication in the tumor microenvironment (234). As tumor progression starts to develop, the surrounding microenvironment performs anti-tumor immunity and aims to subside tumorigenesis. Once the tumorigenesis progresses, the microenvironment evolves into tumor-conducive. Cancer cells take advantage of EVs’ paracrine intercommunication through conversion from a normal milieu to a tumor microenvironment. EVs are used to preserve normal tissues then support tumorigenesis, provide nutrition, and facilitate immune escape (204). It is possible for EVs to acquire soluble proteins, and hence new biological functions, from the surrounding extracellular milieu. The main determinants are the physical and biochemical characteristics of their surface and protein concentration-based environmental changes. For example, during the pathogenesis of autoimmune diseases and innate and acquired immune responses, autoantigens may be packaged in EVs prior to or post-release (200–202).
4.3 Shifting from MSCs to MSC-EVs regarding their application
Differences between cells and their extracellular descendants have put a debate regarding their potential applications (see Table 2 for a detailed comparison). The preparation of characterized EVs and their standardization needs more interdisciplinary knowledge, as they are nano-sized entities (273). In the case of cells, there is less concern regarding the preparation of a determined number, while in the case of EVs, it is not as easy due to the differences in the availability and sensitivity of the quantification methods (274). Different criteria have been applied to quantify EVs, including the number of producer cells in the culture, the protein content of EV preparations, and the number of EVs/ml of the final preparation. This puts a big hurdle in comparing the results of different experiments (185). Unlike cells that need vascular structures to receive essential nutrients and survive, EVs could be administered in tissues with a lower capillary network, such as intervertebral discs. Also, as EVs do not release toxic or harmful metabolites, there is no concern regarding their utilization in such tissues (179).
Having mentioned these differences, EVs generally have some clinically relevant superiorities to cell therapy (185, 264, 265). The immune rejection rate of EVs is considerably low compared to the producer cells (181), so they have an increased half-life and are more stable in circulation (39). EVs demonstrate an innate tropism to specific tissues (76) and exert cell-targeting properties (111). Unlike cells, there is no concern regarding the possibility of necrosis or their abnormal differentiation (181). They do not show self-replicative and tumor-formation properties (139) and are not seriously affected by the surrounding inflammatory microenvironment (186). They reach deeper into injured tissue layers (183). Moreover, EVs have a more straightforward pre-banking capacity, are less sensitive to low temperatures, and are more stable during freeze-thaw cycles. EVs can also be combined with existing compositions or drug delivery methods (66). These vesicles would provide the scientists with a suitable manipulative platform (269).
5 EVs from modified cells and EV modification strategies
EV secretion is likely affected by different pharmacologic and/or environmental insults that target producer cells’ cytoskeleton (115, 275). Furthermore, another specific priming/stimulating strategy may be applied as one of the most convenient strategies to persuade the cells to produce and secrete EVs with desirable features/contents (276–278). In a hybrid approach, to facilitate the large-scale production of EVs with determined properties, Gomzikova et al. evaluated the immunomodulatory properties of the cytochalasin B-induced membrane vesicles (CIMVs). They demonstrated that human MSC-EVs prepared with this approach could inhibit the activation and proliferation of human PBMCs (279). Yuan et al. demonstrated the beneficial consequences of a 3D dynamic culture of aggregated hMSCs for the efficient generation of 3D-hMSC-EVs regarding their size, concentration (EVs/Cell/2 days), common exosomal markers (CD63, Alix, Flotillin-2, and CD81), and immunomodulatory capacity (IDO activity) in the presence or absence of interferon-gamma (280).
EV modification strategies could also be utilized to enhance the accumulation of the particles in desirable target organs, change their surface properties, or reduce their phagocytosis and endocytosis by macrophages (62). To reduce immune cell recognition, polyethylene glycol (PEG) is a practical approach to increase the circulation time of EVs (178). It was shown that preparing engineered EVs (EEVs) with surface expression of CD47, due to their bio-inertness and immune-evasive properties (178), will provide us with vesicles that are less prone to systemic clearance (281). Engineered EVs were also innovatively equipped with receptors that adsorb pro-inflammatory cytokine IL-6 from diseased muscle tissue with chronic inflammatory status (282).
A successful example of engineered EVs as autoimmune disease therapeutics was proposed by Zampieri et al. in a pre-clinical study. To induce immune tolerance via molecular farming, they designed plant virus nano-particles displaying recombinant peptides associated with autoimmune diabetes and rheumatoid arthritis. They demonstrated that the virus structure could play carrier roles for the recombinant peptide and adjuvant, as tomato bushy stunt virus (TBSV) demonstrated intrinsic immunomodulatory properties. However, to a lesser extent, the viral particles carry the recombinant peptides (283).
Cargo pre-loading and post-loading could be considered a primary classification for EV modification (284–286)(Figure 3). Natural or specific packaging could be applied to pre-load EVs with target cargo. The producer cells are loaded with the molecules of interest during natural packaging through cell conditioning, cell and cargo incubation, or common cell modification methods, such as transfection, transduction, and electroporation (124, 287). Moreover, exogenous materials could be introduced to the EVs based on liposome or micelle-mediated mechanisms. For more efficient drug delivery, EV bilayer membranes are commonly permeabilized to allow the dynamic loading of the vesicles (288, 289). In specific loading, the basis of cargo enrichment lies in protein-protein interactions, the fusion of proteins, protein-ubiquitin, and protein-RNA interactions. EV post-loading is achievable via physical and chemical modifications, which are the two major ways for transfering the cargo of interest to EVs. Incubating EVs with the cargo of interest (passive loading) is a convenient and effective way, which was applied to load EVs with nucleic acids, proteins or peptides, drugs, and nano-materials (205, 290, 291). Different methods are proposed for direct loading of EVs with cargos of interest, such as the application of transfection reagents, electroporation, incubation, sonication, freeze/thaw cycles, saponin, extrusion and dialysis (205, 270). The loading efficiency of EVs depends on several factors, mainly the quality of the starting material, the physical and biochemical properties of cargo, and its stability and functional maintenance during the loading process (286). Covalent and non-covalent interactions are proposed as active chemistry method subsets to modify the inner or outer surface of EVs, each of which includes a variety of methods, gradually updated based on recent innovations (292).
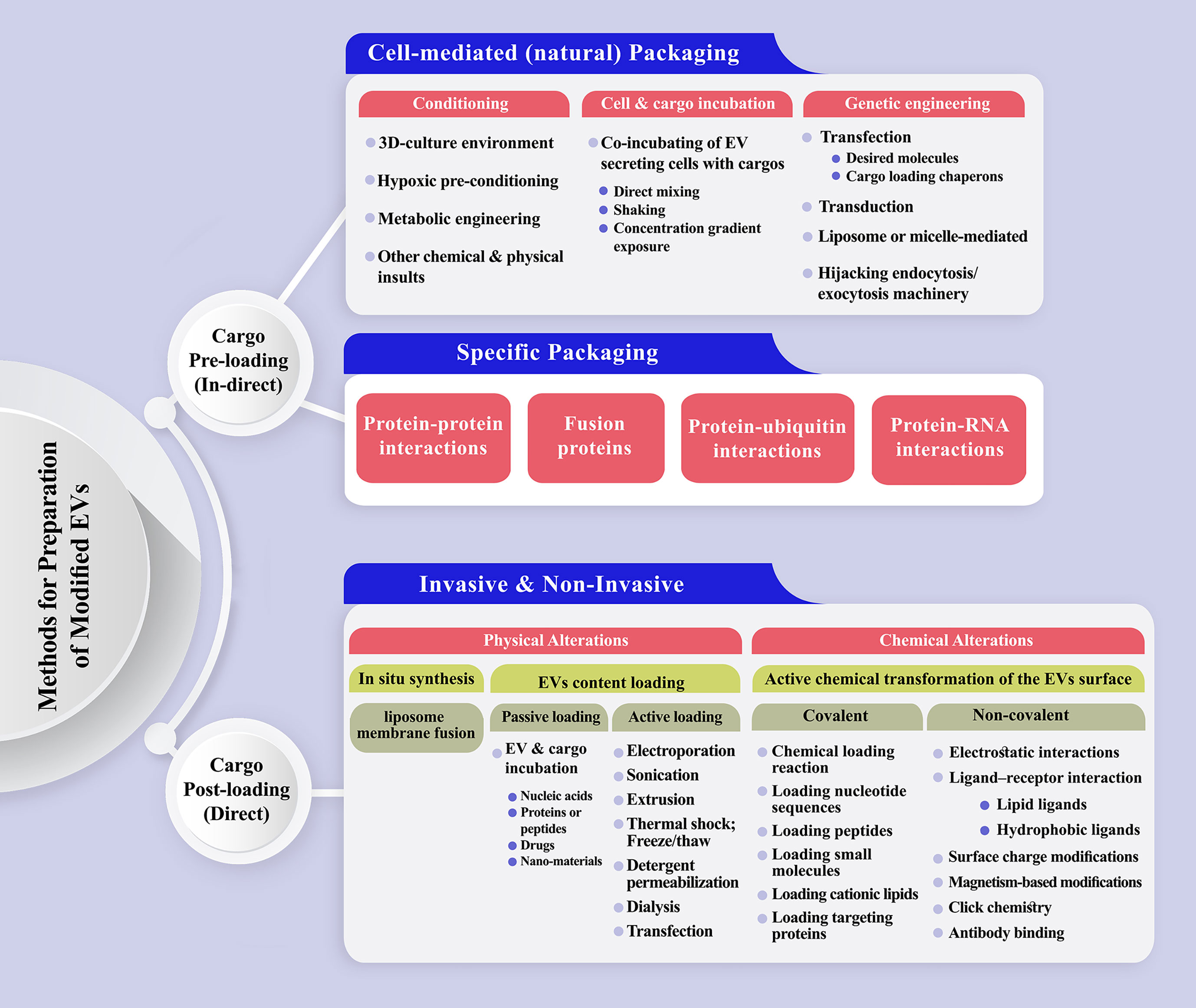
Figure 3 A schematic representation of the methods has been proposed to prepare engineered extracellular vesicles. (Top) Cargo pre-loading (in-direct) path is subdivided to cell-mediated and specific packaging fashion. The latter one could be designed based on protein-protein, protein-RNA, protein-ubiquitin interactions or the production of fusion proteins. (Bottom) Direct EV post-loading is achievable via physical and chemical modifications which are the two major ways for transferring the cargo of interest to EVs. Physical alterations mostly include passive and active loading techniques. Incubating EVs with the cargo of interest (passive loading) is a convenient and effective way, which was applied to load EVs with nucleic acids, proteins or peptides, drugs, and nano-materials. Electroporation, sonication, extrusion, thermal shock, detergent permeabilization, dialysis, and transfection are the main active loading techniques for intensifying the content of EVs in the benefit of desirable molecule/s. Covalent and non-covalent interactions are proposed as active chemistry methods to modify the inner or outer surface of EVs.
Three models were proposed as the primary cell-mediated packaging of EVs with desirable nucleic acids (293): induced packaging, cellular protein-assisted packaging, and engineered protein-mediated packaging. To reach therapeutic-grade EVs, they are prepared from specific producer cells (294). Otherwise, modified molecules (proteins or chemical compounds) with organ-specific tendencies are introduced to the EV surfaces (295). Various interfering mechanisms also play significant roles, including intracellular calcium level, external stress, cytoskeletal blocking, and the consequences of specific gene expression could be highlighted (124). Following the entry to target cells, EVs, enriched with coding or non-coding oligonucleotides, trigger gene expression pathways or regulate specific gene transcription procedures. However, the risk of EV internalization in the endosomal compartment, degradation, and re-release of intact vesicles should be considered (296).
Protein-loaded EVs are mainly administered as fluorescent reporters or targeting moieties (288). Regardless of the engineering or priming strategy applied to prepare modified EVs, one should consider the immunogenic potential of surface-engineered EVs. For example, tumor-targeting antigenic (TTA) peptide glycoprotein 100 (gp100) engineered EVs are rapidly internalized by APCs and are highly immunogenic for stimulating cytokine production (297). Engineered EVs were applied in pre-clinical studies and have found their way into engineered EV-based therapeutics (269, 298).
A deep molecular understanding of various biogenesis pathways and structural properties is essential for designing desirable EVs. Further, we should consider the variety of molecules that are involved in the specific sorting of various cargoes to the small and large EVs, including Ras-related (RAB) GTPases in the brain, glycosphingolipids, flotillins, chaperone HSC70, and small integral membrane proteins of the lysosome/late endosome (SIMPLE) (299–301).
Clinical translation of the EVs is affected by different issues, including isolation, purification, standardization, yield, and functional heterogeneity (287, 302). Accordingly, a field for EV engineering has emerged to augment their natural properties (165) and recapitulate their function in semi-synthetic and synthetic EVs. In recent work, Xu et al. introduced a novel peptide-equipped EV platform to enhance the efficiency of EV penetration and oligonucleotide loading capacities (303). More translational examples of the application of EVs to facilitate personalized cancer therapeutic methods were provided by a previous publication (304). Also, the other recent review summarized most of the available examples regarding the administration of native and engineered EVs from various sources, including MSCs, immune, and tissue-specific cells for inflammation therapy in different tissues such as brain, eye, lung, heart, liver, bowel, bone, and skin (181).
6 Safety and risk factors associated with cells or EV administration
The administration of cells or EVs should be considered rigorously according to many associated risk factors. All ethical considerations, scientific aspects, and guidelines proposed for cell-based therapies should be considered before EV application (305). In this piece, we try to highlight some issues to sensitize and promote more efforts in evaluating the safety of cells or EV-based therapies.
6.1 Contaminations
Both cells and EVs can be contaminated with bioactive components, including but not limited to viruses, bacteria, and endotoxins, that should be checked before any administration. Considering similarities between viruses and exosomes (306–309), viral contaminations for EV preparations are one of the main concerns. Usually, the size of bacteria and fungi are larger than EVs; however, infections specifically by intracellular contaminations such as mycoplasma can change both cell and EVs properties (310–313).
6.2 Proliferation
The risk of uncontrolled proliferation of cells is associated with cell-based therapies. Unlike cells, EVs cannot proliferate, and multiple administrations are usually required to reach comparable results. However, more studies are required to show if EVs have machinery like viruses to propagate.
6.3 Transfer of genetic materials
Similar to cells, EVs carry DNA (314–316) and mitochondria content (317–319). Recently, it has been shown that genetic materials can also be incorporated into the EV bio-corona (63, 319–321). Therefore, safety concerns are associated with administering both cells and EVs regarding their genetic materials.
6.4 Cross-species contaminations
Considering the danger of cross-species contaminations, cells are cultured using human-derived components in xeno-free culture systems. The same issues for EVs should be considered even more rigorously. Firstly, cells can uptake materials from the medium during the culturing process and re-packing them into their EVs derivatives. Second, EVs or particles originating from bovine-derived serum supplements cannot be efficiently removed based on current methods (321–324). Therefore, the risk of cross-species contaminations should be assessed in both cells and EV administration.
6.5 Passing through the barriers
Unlike cells, EVs can cross barriers, including but not limited to the blood-brain barrier (325–327). The risk of passing through the barriers is another issue that should be considered explicitly in the EV administration.
The issues raised about the enclosed risk of EV administration belong to unmodified EVs. In cases where there is a need to expose the cells to different physical or chemical agents or to engineer the producer cells or EVs, more details should be taken into account (328). Maybe that is why a debate is being opened about whether exosomes could be considered a medicinal product rather than a biological product similar to their producer cells. The point here remains to be done is the release of internationally accepted guidelines, designed explicitly for EVs and their clinical applications.
7 Prospective future: MSC-EVs vs. MSCs
Immunosuppression is among the most favorable therapeutic strategies for managing autoimmune diseases (329–332). Stem cells have been proposed as promising biologicals with superior properties compared to conventional methods (1, 11, 333). Mesenchymal stem cells (MSCs) could be considered one of the safest and most commonly used advanced therapy medicinal products (ATMPs) (334, 335) due to their high regenerative capacities and special immunoregulatory functions (336, 337) affecting all types of innate and adaptive immune cells (338). However, some reviews and meta-analyses questioned why translational outcomes were not as efficient as we expected throughout these years (339). In most cases, short-term effects and the heterogeneous responses of patients to treatment are considered the main challenges. Despite current concerns, MSCs have found their way to treat autoimmune diseases by alleviating the symptoms.
Extensive parallel researches during the last decade has highlighted the importance of MSCs’ paracrine effects (85) and proposed the possibility of replacing the cells with their cell-free products, even in the allogeneic context (147). Here, we debated the potential applications and limitations of extracellular vesicles (EVs) compared to their producer cells, especially in relation to autoimmune diseases. Despite the initial trend, it is now proposed that several challenges and complexities accompany these vesicles (174, 340). Some critical challenges are defining the optimal culture conditions, large-scale production, reproducibility of the isolation procedures, homogenous content of the final prep, efficient and aim-specific functional characterizations, storage, and standardization (148, 341–347). One of the most crucial concerns regarding EVs application is a possible viral infection (348, 349). In addition, viral components can affect EV biogenesis, composition, and secretion of unknown “off-target” side interferences (350).
The other criteria that highlighted the potential of EVs for future therapeutic applications are their ability to be engineered or modified (269, 271, 272, 351, 352). They could be loaded with unexpected target/s or encapsulated with different bio-scaffolds upon the urgent need (237, 353). Furthermore, EVs could be recovered easily following their freeze-thaw cycles (109). These features facilitate their application as a good platform for cellular products in emergencies. Moreover, recent progress in the fields has made their room temperature storage, characterization and maintenance more straightforward and friendly than before, and therefore a significant step closer to their extended application, pharmaceuticalization, and commercialization (163, 354, 355). As it was inferred from the literature, EVs have a broad spectrum of applications. Some applications focus on their ability to pass through blood barriers or other unique properties versus the cells or other synthetic nano-carriers (261, 356). As non-toxic nano-carriers, EVs demonstrated better pharmacokinetics and pharmacodynamics features (absorption, distribution, metabolism, and excretion) than synthetic nano-carriers (140, 141, 357). This may pave the way for application of EVs as the smart carrier for conventional drugs in the context of autoimmune diseases (267).
Although EVs are reported to be efficient in dampening the symptoms of different immunological and non-immunological diseases, sometimes with a completely different mechanism of action (182, 242), they may not seem as efficient as their parent cells. We noted that it is not already possible to assess the exact amount of EVs which are functionally equal and comparable to a specific number of transplanted cells. Nevertheless, EVs have the potential for repeated rounds and off-the-shelf applications. To increase the efficiency of the treatments, in the case of some acute or chronic diseases, including autoimmune disorders, the simultaneous application of cell- and EV-therapy procedures were proposed and are currently under clinical investigation (ClinicalTrials.gov Identifiers: NCT05387278, NCT05520125). It is assumed that the combinatorial strategy may provide the patients with enhanced and prolonged effectiveness of the biological treatments (358, 359). Future research will determine whether EVs are efficient enough or if we still need the cells to reach the proper efficiency.
Regardless of the safety, the central morality of the EV application is of the same importance. The efficiency of the treatments and the cost-benefit aspect of EVs administration for patients is critical when physicians allocate patients to new treatment strategies. Many questions need to be addressed in this regard. Whether this method is applied as an effective treatment or only as a palliative method? Does the patient have enough knowledge and a good idea about this treatment method and its effectiveness? Undoubtedly, having their consent is necessary when participating in a clinical trial.
As we focused on autoimmune diseases in the current piece, we should emphasize the pathogenesis of the disease, the current status of the patients, the disease status, and the availability of strong support from previous pre-clinical and clinical studies are essential before referring the patients. Fortunately, among different diseases and conditions, clinicians are provided with more valid data from pre-clinical studies (360–363), clinical trials (50, 364–366), and comprehensive reviews (257, 367, 368) on the efficiency of EVs in the frame of different autoimmune diseases (369). Although it is inferred from pre-clinical and clinical data that MSC-EVs are safe and efficient (51, 370), and great promise is accompanied by their future applications for treating life-threatening autoimmune diseases, this is of particular importance that we avoid generalization and prejudice regarding their overall efficiency. Other than personalized medicine aspects, it should be considered that each disease has its unique pathogenesis, grading, and mechanisms of spread/progression, which is crucial when we decide to apply EVs for their treatment. Moreover, our current knowledge is not equivalent in the case of different autoimmune diseases regarding the consequences of MSC-EVs administration. Further, although, based on the current data, small extracellular vesicles (sEVs) could be enriched with immunomodulatory components (371, 372), it should be confirmed practically in proper autoimmune disease models and human cases that it has a real functional impact. Despite increasing data that EVs are potent immunomodulators in mimicking their producer cells (146, 207, 373), it is yet to be confirmed whether EVs are superior to their producer cells and, if so, which subtype of EVs is preferred for different autoimmune diseases. Upon defining the GMP-compatible protocols and standardization, the next step will be designing the procedures for preparing modified disease-specific EVs with ideal functional properties (374). These EVs may carry a higher level of immunoregulatory genes, RNAs, miRNAs, lipids, and proteins or target specific molecules, cells, or tissues.
Author contributions
AH (PhD): Literature review, designing, writing the manuscript, drafting tables and figures, and approval the final version of the manuscript. HB (MD): Conceptualization, drafting, critical editing, and approval the final version of the manuscript. MMa (PhD): Drafting, contribution to design, critical editing, and approval the final version of the manuscript. FS (PhD): Drafting, contribution to design, critical editing, and approval the final version of the manuscript. ME (PhD): Editing, and approval the final version of the manuscript. RL (PhD): Editing, and approval the final version of the manuscript. NA (PhD): Drafting, contribution to design, critical editing, and approval the final version of the manuscript. MMi (PhD candidate): Graphical design of figures, editing, and approval the final version of the manuscript. AB (PhD): Conceptualization, drafting, supervising, funding acquisition, and approval the final version of the manuscript. HB (MD, PhD): Conceptualization, drafting, supervising, funding acquisition, and approval the final version of the manuscript. All authors contributed to the article and approved the submitted version.
Funding
AH was partially supported by a grant from the Ferdowsi University of Mashhad (No. FUM-14002794075).
Acknowledgments
The present work was supported by ACECR-Khorasan Razavi and Ferdowsi University of Mashhad. We thank Hojjatollah Nazari from University of Technology Sydney for his valuable contribution to the technical editing.
Conflict of interest
The authors declare that the research was conducted in the absence of any commercial or financial relationships that could be construed as a potential conflict of interest.
Publisher’s note
All claims expressed in this article are solely those of the authors and do not necessarily represent those of their affiliated organizations, or those of the publisher, the editors and the reviewers. Any product that may be evaluated in this article, or claim that may be made by its manufacturer, is not guaranteed or endorsed by the publisher.
Glossary
References
1. Luo X, Miller SD, Shea LD. Immune tolerance for autoimmune disease and cell transplantation. Annu Rev BioMed Eng. (2016) 18:181–205. doi: 10.1146/annurev-bioeng-110315-020137
2. Takahashi H, Iriki H, Mukai M, Kamata A, Nomura H, Yamagami J, et al. Autoimmunity and immunological tolerance in autoimmune bullous diseases. Int Immunol (2019) 31(7):431–7. doi: 10.1093/intimm/dxz030
3. Zakrzewski JL, van den Brink MR, Hubbell JA. Overcoming immunological barriers in regenerative medicine. Nat Biotechnol (2014) 32(8):786–94. doi: 10.1038/nbt.2960
4. Amaya-Uribe L, Rojas M, Azizi G, Anaya JM, Gershwin ME. Primary immunodeficiency and autoimmunity: A comprehensive review. J Autoimmun (2019) 99:52–72. doi: 10.1016/j.jaut.2019.01.011
5. Mazzone R, Zwergel C, Artico M, Taurone S, Ralli M, Greco A, et al. The emerging role of epigenetics in human autoimmune disorders. Clin Epigenetics (2019) 11(1):34. doi: 10.1186/s13148-019-0632-2
6. Wang YF, Zhang Y, Lin Z, Zhang H, Wang TY, Cao Y, et al. Identification of 38 novel loci for systemic lupus erythematosus and genetic heterogeneity between ancestral groups. Nat Commun (2021) 12(1):772. doi: 10.1038/s41467-021-21049-y
7. Juarranz Y. Molecular and cellular basis of autoimmune diseases. Cells (2021) 10(2):474. doi: 10.3390/cells10020474
8. Duan L, Rao X, Sigdel KR. Regulation of inflammation in autoimmune disease. J Immunol Res (2019) 2019:7403796. doi: 10.1155/2019/7403796
9. Júnior DST. Environmental and individual factors associated with protection and predisposition to autoimmune diseases. Int J Health Sci (Qassim) (2020) 14(6):13–23.
10. Popis M, Konwerska A, Partyka M, Wieczorkiewicz M, Ciesiółka S, Stefańska K, et al. Mesenchymal stem cells and their secretome -candidates for safe and effective therapy for systemic lupus erythematosus. Med J Cell Biol (2021) 9(3):110–22. doi: 10.2478/acb-2021-0016
11. Fugger L, Jensen LT, Rossjohn J. Challenges, progress, and prospects of developing therapies to treat autoimmune diseases. Cell. (2020) 181(1):63–80. doi: 10.1016/j.cell.2020.03.007
12. Langan D, Rose NR, Moudgil KD. Common innate pathways to autoimmune disease. Clin Immunol (2020) 212:108361. doi: 10.1016/j.clim.2020.108361
13. Saferding V, Blüml S. Innate immunity as the trigger of systemic autoimmune diseases. J Autoimmun (2020) 110:102382. doi: 10.1016/j.jaut.2019.102382
14. Soualmia F, El Amri C. Serine protease inhibitors to treat inflammation: a patent review (2011-2016). Expert Opin Ther Pat. (2018) 28(2):93–110. doi: 10.1080/13543776.2018.1406478
15. Tahamtan A, Teymoori-Rad M, Nakstad B, Salimi V. Anti-inflammatory MicroRNAs and their potential for inflammatory diseases treatment. Front Immunol (2018) 9:1377. doi: 10.3389/fimmu.2018.01377
16. Patil S, Gs V, Sarode GS, Sarode SC, Khurayzi TA, Mohamed Beshir SE, et al. Exploring the role of immunotherapeutic drugs in autoimmune diseases: A comprehensive review. J Oral Biol Craniofac Res (2021) 11(2):291–6. doi: 10.1016/j.jobcr.2021.02.009
17. Kou M, Huang L, Yang J, Chiang Z, Chen S, Liu J, et al. Mesenchymal stem cell-derived extracellular vesicles for immunomodulation and regeneration: a next generation therapeutic tool? Cell Death Dis (2022) 13(7):580. doi: 10.1038/s41419-022-05034-x
18. Li M, Fang F, Sun M, Zhang Y, Hu M, Zhang J. Extracellular vesicles as bioactive nanotherapeutics: An emerging paradigm for regenerative medicine. Theranostics. (2022) 12(11):4879–903. doi: 10.7150/thno.72812
19. Wang Z, Liu X, Cao F, Bellanti JA, Zhou J, Zheng SG. Prospects of the use of cell therapy to induce immune tolerance. Front Immunol (2020) 11:792. doi: 10.3389/fimmu.2020.00792
20. Chan AY, Leiding JW, Liu X, Logan BR, Burroughs LM, Allenspach EJ, et al. Hematopoietic cell transplantation in patients with primary immune regulatory disorders (PIRD): A primary immune deficiency treatment consortium (PIDTC) survey. Front Immunol (2020) 11:239. doi: 10.3389/fimmu.2020.00239
21. Snowden JA, Badoglio M, Alexander T. The rise of autologous HCT for autoimmune diseases: what is behind it and what does it mean for the future of treatment? an update on behalf of the EBMT autoimmune diseases working party. Expert Rev Clin Immunol (2019) 15(10):981–5. doi: 10.1080/1744666X.2019.1656526
22. Alexander T, Greco R, Snowden JA. Hematopoietic stem cell transplantation for autoimmune disease. Annu Rev Med (2021) 72:215–28. doi: 10.1146/annurev-med-070119-115617
23. Snowden JA, Sánchez-Ortega I, Corbacioglu S, Basak GW, Chabannon C, de la Camara R, et al. Indications for haematopoietic cell transplantation for haematological diseases, solid tumours and immune disorders: current practice in Europe, 2022. Bone Marrow Transplant (2022), 1–23.
24. Oliveira MC, Elias JB, Moraes DA, Simões BP, Rodrigues M, Ribeiro AAF, et al. A review of hematopoietic stem cell transplantation for autoimmune diseases: multiple sclerosis, systemic sclerosis and crohn's disease. position paper of the Brazilian society of bone marrow transplantation. Hematol Transfus Cell Ther (2021) 43(1):65–86. doi: 10.1016/j.htct.2020.03.002
25. Greco R, Labopin M, Badoglio M, Veys P, Furtado Silva JM, Abinun M, et al. Allogeneic HSCT for autoimmune diseases: A retrospective study from the EBMT ADWP, IEWP, and PDWP working parties. Front Immunol (2019) 10:1570. doi: 10.3389/fimmu.2019.01570
26. Sullivan KM, Sarantopoulos S. Allogeneic HSCT for autoimmune disease: a shared decision. Nat Rev Rheumatol (2019) 15(12):701–2. doi: 10.1038/s41584-019-0306-7
27. Yanir AD, Hanson IC, Shearer WT, Noroski LM, Forbes LR, Seeborg FO, et al. High incidence of autoimmune disease after hematopoietic stem cell transplantation for chronic granulomatous disease. Biol Blood Marrow Transplant (2018) 24(8):1643–50. doi: 10.1016/j.bbmt.2018.03.029
28. Ganguly D, Haak S, Sisirak V, Reizis B. The role of dendritic cells in autoimmunity. Nat Rev Immunol (2013) 13(8):566–77. doi: 10.1038/nri3477
29. Liu Q, Rojas-Canales DM, Divito SJ, Shufesky WJ, Stolz DB, Erdos G, et al. Donor dendritic cell-derived exosomes promote allograft-targeting immune response. J Clin Invest (2016) 126(8):2805–20. doi: 10.1172/JCI84577
30. Lan Q, Zhou X, Fan H, Chen M, Wang J, Ryffel B, et al. Polyclonal CD4+Foxp3+ treg cells induce TGFβ-dependent tolerogenic dendritic cells that suppress the murine lupus-like syndrome. J Mol Cell Biol (2012) 4(6):409–19. doi: 10.1093/jmcb/mjs040
31. Ramalingam R, Larmonier CB, Thurston RD, Midura-Kiela MT, Zheng SG, Ghishan FK, et al. Dendritic cell-specific disruption of TGF-β receptor II leads to altered regulatory T cell phenotype and spontaneous multiorgan autoimmunity. J Immunol (2012) 189(8):3878–93. doi: 10.4049/jimmunol.1201029
32. Iberg CA, Hawiger D. Natural and induced tolerogenic dendritic cells. J Immunol (2020) 204(4):733–44. doi: 10.4049/jimmunol.1901121
33. Wang C, Börger V, Sardari M, Murke F, Skuljec J, Pul R, et al. Mesenchymal stromal cell-derived small extracellular vesicles induce ischemic neuroprotection by modulating leukocytes and specifically neutrophils. Stroke. (2020) 51(6):1825–34. doi: 10.1161/STROKEAHA.119.028012
34. Bell GM, Anderson AE, Diboll J, Reece R, Eltherington O, Harry RA, et al. Autologous tolerogenic dendritic cells for rheumatoid and inflammatory arthritis. Ann Rheum Dis (2017) 76(1):227–34. doi: 10.1136/annrheumdis-2015-208456
35. García-González P, Ubilla-Olguín G, Catalán D, Schinnerling K, Aguillón JC. Tolerogenic dendritic cells for reprogramming of lymphocyte responses in autoimmune diseases. Autoimmun Rev (2016) 15(11):1071–80. doi: 10.1016/j.autrev.2016.07.032
36. Bianco NR, Kim SH, Ruffner MA, Robbins PD. Therapeutic effect of exosomes from indoleamine 2,3-dioxygenase-positive dendritic cells in collagen-induced arthritis and delayed-type hypersensitivity disease models. Arthritis Rheumatol (2009) 60(2):380–9. doi: 10.1002/art.24229
37. Kim SH, Bianco N, Menon R, Lechman ER, Shufesky WJ, Morelli AE, et al. Exosomes derived from genetically modified DC expressing FasL are anti-inflammatory and immunosuppressive. Mol Ther (2006) 13(2):289–300. doi: 10.1016/j.ymthe.2005.09.015
38. Kim SH, Bianco NR, Shufesky WJ, Morelli AE, Robbins PD. Effective treatment of inflammatory disease models with exosomes derived from dendritic cells genetically modified to express IL-4. J Immunol (2007) 179(4):2242–9. doi: 10.4049/jimmunol.179.4.2242
39. Di Bella MA. Overview and update on extracellular vesicles: Considerations on exosomes and their application in modern medicine. Biology. (2022) 11(6):804. doi: 10.3390/biology11060804
40. Elashiry M, Elsayed R, Cutler CW. Exogenous and endogenous dendritic cell-derived exosomes: Lessons learned for immunotherapy and disease pathogenesis. Cells. (2021) 11(1):115. doi: 10.3390/cells11010115
41. Sakaguchi S. Regulatory T cells: history and perspective. Methods Mol Biol (2011) 707:3–17. doi: 10.1007/978-1-61737-979-6_1
42. Janssens I, Cools N. Regulating the regulators: Is introduction of an antigen-specific approach in regulatory T cells the next step to treat autoimmunity? Cell Immunol (2020) 358:104236. doi: 10.1016/j.cellimm.2020.104236
43. Ferreira LMR, Muller YD, Bluestone JA, Tang Q. Next-generation regulatory T cell therapy. Nat Rev Drug Discovery (2019) 18(10):749–69. doi: 10.1038/s41573-019-0041-4
44. Terry LV, Oo YH. The next frontier of regulatory T cells: Promising immunotherapy for autoimmune diseases and organ transplantations. Front Immunol (2020) 11:565518. doi: 10.3389/fimmu.2020.565518
45. Mohseni YR, Tung SL, Dudreuilh C, Lechler RI, Fruhwirth GO, Lombardi G. The future of regulatory T cell therapy: Promises and challenges of implementing CAR technology. Front Immunol (2020) 11:1608. doi: 10.3389/fimmu.2020.01608
46. Rosado-Sánchez I, Levings MK. Building a CAR-treg: Going from the basic to the luxury model. Cell Immunol (2020) 358:104220. doi: 10.1016/j.cellimm.2020.104220
47. Raffin C, Vo LT, Bluestone JA. T(reg) cell-based therapies: challenges and perspectives. Nat Rev Immunol (2020) 20(3):158–72. doi: 10.1038/s41577-019-0232-6
48. Caplan H, Olson SD, Kumar A, George M, Prabhakara KS, Wenzel P, et al. Mesenchymal stromal cell therapeutic delivery: Translational challenges to clinical application. Front Immunol (2019) 10:1645. doi: 10.3389/fimmu.2019.01645
49. Hakim R, Covacu R, Zachariadis V, Frostell A, Sankavaram SR, Brundin L, et al. Mesenchymal stem cells transplanted into spinal cord injury adopt immune cell-like characteristics. Stem Cell Res Ther (2019) 10(1):115. doi: 10.1186/s13287-019-1218-9
50. Wang S, Zhu R, Li H, Li J, Han Q, Zhao RC. Mesenchymal stem cells and immune disorders: from basic science to clinical transition. Front Med (2019) 13(2):138–51. doi: 10.1007/s11684-018-0627-y
51. Wu X, Jiang J, Gu Z, Zhang J, Chen Y, Liu X. Mesenchymal stromal cell therapies: immunomodulatory properties and clinical progress. Stem Cell Res Ther (2020) 11(1):345. doi: 10.1186/s13287-020-01855-9
52. Fathollahi A, Gabalou NB, Aslani S. Mesenchymal stem cell transplantation in systemic lupus erythematous, a mesenchymal stem cell disorder. Lupus. (2018) 27(7):1053–64. doi: 10.1177/0961203318768889
53. He Y, Xu LL, Feng FE, Wang QM, Zhu XL, Wang CC, et al. Mesenchymal stem cell deficiency influences megakaryocytopoiesis through the TNFAIP3/NF-κB/SMAD pathway in patients with immune thrombocytopenia. Br J Haematol (2018) 180(3):395–411. doi: 10.1111/bjh.15034
54. Sun LY, Zhang HY, Feng XB, Hou YY, Lu LW, Fan LM. Abnormality of bone marrow-derived mesenchymal stem cells in patients with systemic lupus erythematosus. Lupus. (2007) 16(2):121–8. doi: 10.1177/0961203306075793
55. Zhu Y, Feng X. Genetic contribution to mesenchymal stem cell dysfunction in systemic lupus erythematosus. Stem Cell Res Ther (2018) 9(1):149. doi: 10.1186/s13287-018-0898-x
56. Markov A, Thangavelu L, Aravindhan S, Zekiy AO, Jarahian M, Chartrand MS, et al. Mesenchymal stem/stromal cells as a valuable source for the treatment of immune-mediated disorders. Stem Cell Res Ther (2021) 12(1):192. doi: 10.1186/s13287-021-02265-1
57. Zhu D, Fang H, Kusuma GD, Schwab R, Barabadi M, Chan ST, et al. Impact of chemically defined culture media formulations on extracellular vesicle production by amniotic epithelial cells. Proteomics (2021) 21(13-14):e2000080. doi: 10.1002/pmic.202000080
58. Arabpour M, Saghazadeh A, Rezaei N. Anti-inflammatory and M2 macrophage polarization-promoting effect of mesenchymal stem cell-derived exosomes. Int Immunopharmacol (2021) 97:107823. doi: 10.1016/j.intimp.2021.107823
59. You DG, Lim GT, Kwon S, Um W, Oh BH, Song SH, et al. Metabolically engineered stem cell-derived exosomes to regulate macrophage heterogeneity in rheumatoid arthritis. Sci Adv (2021) 7(23):eabe0083. doi: 10.1126/sciadv.abe0083
60. Edelmann MJ, Kima PE. Current understanding of extracellular vesicle homing/tropism. Zoonoses (Burlingt) (2022) 2:14. doi: 10.15212/ZOONOSES-2022-0004
61. Harrell CR, Jovicic N, Djonov V, Arsenijevic N, Volarevic V. Mesenchymal stem cell-derived exosomes and other extracellular vesicles as new remedies in the therapy of inflammatory diseases. Cells. (2019) 8(12):1605. doi: 10.3390/cells8121605
62. Johnson J, Shojaee M, Mitchell Crow J, Khanabdali R. From mesenchymal stromal cells to engineered extracellular vesicles: A new therapeutic paradigm. Front Cell Dev Biol (2021) 9:705676. doi: 10.3389/fcell.2021.705676
63. Georgy DS, Peter PN, Zhanna AA, Pavel IM, Anastasia Yu E. “Cell-free therapeutics” from components secreted by mesenchymal stromal cells as a novel class of biopharmaceuticals. In: Ming-Kung Y, Yuan-Chuan C, editors. Biopharmaceuticals. Rijeka: IntechOpen (2018). p. 3.
64. Hwang HS, Kim H, Han G, Lee JW, Kim K, Kwon IC, et al. Extracellular vesicles as potential therapeutics for inflammatory diseases. Int J Mol Sci (2021) 22(11):5487. doi: 10.3390/ijms22115487
65. Buzas EI. The roles of extracellular vesicles in the immune system. Nat Rev Immunol (2022), 1–15. doi: 10.1038/s41577-022-00763-8
66. Meldolesi J. News about therapies of alzheimer’s disease: Extracellular vesicles from stem cells exhibit advantages compared to other treatments. Biomedicines. (2022) 10(1):105. doi: 10.3390/biomedicines10010105
67. Zhang E, Phan P, Zhao Z. Cellular nanovesicles for therapeutic immunomodulation: A perspective on engineering strategies and new advances. Acta Pharm Sin B (2022). doi: 10.1016/j.apsb.2022.08.020
68. Gurunathan S, Kang MH, Kim JH. A comprehensive review on factors influences biogenesis, functions, therapeutic and clinical implications of exosomes. Int J Nanomed (2021) 16:1281–312. doi: 10.2147/IJN.S291956
69. O'Brien K, Breyne K, Ughetto S, Laurent LC, Breakefield XO. RNA Delivery by extracellular vesicles in mammalian cells and its applications. Nat Rev Mol Cell Biol (2020) 21(10):585–606. doi: 10.1038/s41580-020-0251-y
70. Luo L, Wu Z, Wang Y, Li H. Regulating the production and biological function of small extracellular vesicles: current strategies, applications and prospects. J Nanobiotech (2021) 19(1):422. doi: 10.1186/s12951-021-01171-1
71. Kwok ZH, Wang C, Jin Y. Extracellular vesicle transportation and uptake by recipient cells: A critical process to regulate human diseases. Processes (Basel) (2021) 9(2):273. doi: 10.3390/pr9020273
72. van Niel G, D'Angelo G, Raposo G. Shedding light on the cell biology of extracellular vesicles. Nat Rev Mol Cell Biol (2018) 19(4):213–28. doi: 10.1038/nrm.2017.125
73. Hua Y, Chang X, Fang L, Wang Z. Subgroups of extracellular vesicles: Can they be defined by "Labels?". DNA Cell Biol (2022) 41(3):249–56. doi: 10.1089/dna.2021.0488
74. Cocozza F, Grisard E, Martin-Jaular L, Mathieu M, Théry C. SnapShot: Extracellular vesicles. Cell. (2020) 182(1):262.e1. doi: 10.1016/j.cell.2020.04.054
75. Théry C, Ostrowski M, Segura E. Membrane vesicles as conveyors of immune responses. Nat Rev Immunol (2009) 9(8):581–93. doi: 10.1038/nri2567
76. Zhang H, Freitas D, Kim HS, Fabijanic K, Li Z, Chen H, et al. Identification of distinct nanoparticles and subsets of extracellular vesicles by asymmetric flow field-flow fractionation. Nat Cell Biol (2018) 20(3):332–43. doi: 10.1038/s41556-018-0040-4
77. Ganey T, Temple HT, Hunter CW. Exosomes. In: Hunter CW, Davis TT, DePalma MJ, editors. Regenerative medicine : A complete guide for musculoskeletal and spine disorders. Cham: Springer International Publishing (2023). p. 89–100.
78. Anand S, Samuel M, Mathivanan S. Exomeres: A new member of extracellular vesicles family. Subcell Biochem (2021) 97:89–97. doi: 10.1007/978-3-030-67171-6_5
79. Zhang Q, Higginbotham JN, Jeppesen DK, Yang YP, Li W, McKinley ET, et al. Transfer of functional cargo in exomeres. Cell Rep (2019) 27(3):940–54.e6. doi: 10.1016/j.celrep.2019.01.009
80. Jeppesen DK, Fenix AM, Franklin JL, Higginbotham JN, Zhang Q, Zimmerman LJ, et al. Reassessment of exosome composition. Cell. (2019) 177(2):428–45.e18. doi: 10.1016/j.cell.2019.02.029
81. Rondelli V, Helmy S, Passignani G, Parisse P, Di Silvestre D. Integrated strategies for a holistic view of extracellular vesicles. ACS Omega (2022) 7(23):19058–69. doi: 10.1021/acsomega.2c01003
82. Zhang Q, Jeppesen DK, Higginbotham JN, Graves-Deal R, Trinh VQ, Ramirez MA, et al. Supermeres are functional extracellular nanoparticles replete with disease biomarkers and therapeutic targets. Nat Cell Biol (2021) 23(12):1240–54. doi: 10.1038/s41556-021-00805-8
83. Tosar JP, Cayota A, Witwer K. Exomeres and supermeres: Monolithic or diverse? J Extracellular Biol (2022) 1(6):e45. doi: 10.1002/jex2.45
84. Wu F, Gao J, Kang J, Wang X, Niu Q, Liu J, et al. Knowledge mapping of exosomes in autoimmune diseases: A bibliometric analysis (2002-2021). Front Immunol (2022) 13:939433. doi: 10.3389/fimmu.2022.939433
85. Zhang X, Lu Y, Wu S, Zhang S, Li S, Tan J. An overview of current research on mesenchymal stem cell-derived extracellular vesicles: A bibliometric analysis from 2009 to 2021. Front Bioeng Biotechnol (2022) 10:910812. doi: 10.3389/fbioe.2022.910812
86. Eisenstein M. Eavesdropping on extracellular vesicles. Nat Methods (2022) 19(12):1518–23. doi: 10.1038/s41592-022-01698-7
87. Williams T, Salmanian G, Burns M, Maldonado V, Smith E, Porter RM, et al. Versatility of mesenchymal stem cell-derived extracellular vesicles in tissue repair and regenerative applications. Biochimie (2022) 207:33–48. doi: 10.1016/j.biochi.2022.11.011
88. Witwer KW, Goberdhan DC, O'Driscoll L, Théry C, Welsh JA, Blenkiron C, et al. Updating MISEV: Evolving the minimal requirements for studies of extracellular vesicles. J Extracell Vesicles (2021) 10(14):e12182. doi: 10.1002/jev2.12182
89. Nieuwland R, Falcón-Pérez JM, Théry C, Witwer KW. Rigor and standardization of extracellular vesicle research: Paving the road towards robustness. J Extracell Vesicles (2020) 10(2):e12037. doi: 10.1002/jev2.12037
90. Racchetti G, Meldolesi J. Extracellular vesicles of mesenchymal stem cells: Therapeutic properties discovered with extraordinary success. Biomedicines. (2021) 9(6):667. doi: 10.3390/biomedicines9060667
91. Théry C, Witwer KW, Aikawa E, Alcaraz MJ, Anderson JD, Andriantsitohaina R, et al. Minimal information for studies of extracellular vesicles 2018 (MISEV2018): a position statement of the international society for extracellular vesicles and update of the MISEV2014 guidelines. J Extracell Vesicles (2018) 7(1):1535750. doi: 10.1080/20013078.2018.1535750
92. Witwer KW, Théry C. Extracellular vesicles or exosomes? on primacy, precision, and popularity influencing a choice of nomenclature. J Extracell Vesicles (2019) 8(1):1648167. doi: 10.1080/20013078.2019.1648167
93. Staubach S, Bauer FN, Tertel T, Börger V, Stambouli O, Salzig D, et al. Scaled preparation of extracellular vesicles from conditioned media. Adv Drug Delivery Rev (2021) 177:113940. doi: 10.1016/j.addr.2021.113940
94. Imanbekova M, Suarasan S, Lu Y, Jurchuk S, Wachsmann-Hogiu S. Recent advances in optical label-free characterization of extracellular vesicles. Nanophotonics. (2022) 11(12):2827–63. doi: 10.1515/nanoph-2022-0057
95. De Sousa KP, Rossi I, Abdullahi M, Ramirez MI, Stratton D, Inal JM. Isolation and characterization of extracellular vesicles and future directions in diagnosis and therapy. WIREs Nanomed Nanobiotechnology (2023) 15(1):e1835. doi: 10.1002/wnan.1835
96. Ter-Ovanesyan D, Norman M, Lazarovits R, Trieu W, Lee JH, Church GM, et al. Framework for rapid comparison of extracellular vesicle isolation methods. Elife (2021) 10:e70725. doi: 107554/eLife.70725
97. Veerman RE, Teeuwen L, Czarnewski P, Güclüler Akpinar G, Sandberg A, Cao X, et al. Molecular evaluation of five different isolation methods for extracellular vesicles reveals different clinical applicability and subcellular origin. J Extracell Vesicles (2021) 10(9):e12128. doi: 10.1002/jev2.12128
98. Kyykallio H, Faria AVS, Hartmann R, Capra J, Rilla K, Siljander PR. A quick pipeline for the isolation of 3D cell culture-derived extracellular vesicles. J Extracell Vesicles (2022) 11(10):e12273. doi: 10.1002/jev2.12273
99. Thippabhotla S, Zhong C, He M. 3D cell culture stimulates the secretion of in vivo like extracellular vesicles. Sci Rep (2019) 9(1):13012. doi: 10.1038/s41598-019-49671-3
100. Villasante A, Marturano-Kruik A, Ambati SR, Liu Z, Godier-Furnemont A, Parsa H, et al. Recapitulating the size and cargo of tumor exosomes in a tissue-engineered model. Theranostics. (2016) 6(8):1119–30. doi: 10.7150/thno.13944
101. Akbar A, Malekian F, Baghban N, Kodam SP, Ullah M. Methodologies to isolate and purify clinical grade extracellular vesicles for medical applications. Cells. (2022) 11(2):186. doi: 10.3390/cells11020186
102. Zhao AG, Shah K, Cromer B, Sumer H. Comparative analysis of extracellular vesicles isolated from human mesenchymal stem cells by different isolation methods and visualisation of their uptake. Exp Cell Res (2022) 414(2):113097. doi: 10.1016/j.yexcr.2022.113097
103. Busatto S, Yang Y, Iannotta D, Davidovich I, Talmon Y, Wolfram J. Considerations for extracellular vesicle and lipoprotein interactions in cell culture assays. J Extracell Vesicles (2022) 11(4):e12202. doi: 10.1002/jev2.12202
104. Nieuwland R, Siljander PR, Falcón-Pérez JM, Witwer KW. Reproducibility of extracellular vesicle research. Eur J Cell Biol (2022) 101(3):151226. doi: 10.1016/j.ejcb.2022.151226
105. Liguori GL, Kisslinger A. Quality management tools on the stage: Old but new allies for rigor and standardization of extracellular vesicle studies. Front Bioeng Biotechnol (2022) 10:826252. doi: 10.3389/fbioe.2022.826252
106. Yeung CC, Dondelinger F, Schoof EM, Georg B, Lu Y, Zheng Z, et al. Circadian regulation of protein cargo in extracellular vesicles. Sci Adv (2022) 8(14):eabc9061. doi: 10.1126/sciadv.abc9061
107. Zeng Y, Qiu Y, Jiang W, Shen J, Yao X, He X, et al. Biological features of extracellular vesicles and challenges. Front Cell Dev Biol (2022) 10. doi: 10.3389/fcell.2022.816698
108. Görgens A, Corso G, Hagey DW, Jawad Wiklander R, Gustafsson MO, Felldin U, et al. Identification of storage conditions stabilizing extracellular vesicles preparations. J Extracell Vesicles (2022) 11(6):e12238. doi: 10.1002/jev2.12283
109. Sivanantham A, Jin Y. Impact of storage conditions on EV Integrity/Surface markers and cargos. Life (Basel) (2022) 12(5):697. doi: 10.3390/life12050697
110. Bahr MM, Amer MS, Abo-El-Sooud K, Abdallah AN, El-Tookhy OS. Preservation techniques of stem cells extracellular vesicles: a gate for manufacturing of clinical grade therapeutic extracellular vesicles and long-term clinical trials. Int J Vet Sci Med (2020) 8(1):1–8. doi: 10.1080/23144599.2019.1704992
111. Soekmadji C, Li B, Huang Y, Wang H, An T, Liu C, et al. The future of extracellular vesicles as theranostics - an ISEV meeting report. J Extracell Vesicles (2020) 9(1):1809766. doi: 10.1080/20013078.2020.1809766
112. Trenkenschuh E, Richter M, Heinrich E, Koch M, Fuhrmann G, Friess W. Enhancing the stabilization potential of lyophilization for extracellular vesicles. Adv Healthc Mater (2021) 11(5):e2100538. doi: 10.1002/adhm.202100538
113. Noren Hooten N, Byappanahalli AM, Vannoy M, Omoniyi V, Evans MK. Influences of age, race, and sex on extracellular vesicle characteristics. Theranostics. (2022) 12(9):4459–76. doi: 10.7150/thno.72676
114. Kusuma GD, Carthew J, Lim R, Frith JE. Effect of the microenvironment on mesenchymal stem cell paracrine signaling: Opportunities to engineer the therapeutic effect. Stem Cells Dev (2017) 26(9):617–31. doi: 10.1089/scd.2016.0349
115. Mathieu M, Martin-Jaular L, Lavieu G, Théry C. Specificities of secretion and uptake of exosomes and other extracellular vesicles for cell-to-cell communication. Nat Cell Biol (2019) 21(1):9–17. doi: 10.1038/s41556-018-0250-9
116. Merimi M, El-Majzoub R, Lagneaux L, Moussa Agha D, Bouhtit F, Meuleman N, et al. The therapeutic potential of mesenchymal stromal cells for regenerative medicine: Current knowledge and future understandings. Front Cell Dev Biol (2021) 9:661532. doi: 10.3389/fcell.2021.661532
117. Verweij FJ, Revenu C, Arras G, Dingli F, Loew D, Pegtel DM, et al. Live tracking of inter-organ communication by endogenous exosomes in vivo. Dev Cell (2019) 48(4):573–89.e4. doi: 10.1016/j.devcel.2019.01.004
118. Correa R, Caballero Z, De León LF, Spadafora C. Extracellular vesicles could carry an evolutionary footprint in interkingdom communication. Front Cell Infect Microbiol (2020) 10:76. doi: 10.3389/fcimb.2020.00076
119. Dixson AC, Dawson TR, Di Vizio D, Weaver AM. Context-specific regulation of extracellular vesicle biogenesis and cargo selection. Nat Rev Mol Cell Biol (2023). doi: 10.1038/s41580-023-00576-0
120. Sanwlani R, Gangoda L. Role of extracellular vesicles in cell death and inflammation. Cells. (2021) 10(10):2663. doi: 10.3390/cells10102663
121. Al Halawani A, Mithieux SM, Yeo GC, Hosseini-Beheshti E, Weiss AS. Extracellular vesicles: Interplay with the extracellular matrix and modulated cell responses. Int J Mol Sci (2022) 23(6):3389. doi: 10.3390/ijms23063389
122. Zinger A, Brozovich A, Pasto A, Sushnitha M, Martinez JO, Evangelopoulos M, et al. Bioinspired extracellular vesicles: Lessons learned from nature for biomedicine and bioengineering. Nanomaterials (Basel) (2020) 10(11):2172. doi: 10.3390/nano10112172
123. Kurian TK, Banik S, Gopal D, Chakrabarti S, Mazumder N. Elucidating methods for isolation and quantification of exosomes: A review. Mol Biotechnol (2021) 63(4):249–66. doi: 10.1007/s12033-021-00300-3
124. Liu C, Su C. Design strategies and application progress of therapeutic exosomes. Theranostics. (2019) 9(4):1015–28. doi: 10.7150/thno.30853
125. Mashouri L, Yousefi H, Aref AR, Ahadi AM, Molaei F, Alahari SK. Exosomes: composition, biogenesis, and mechanisms in cancer metastasis and drug resistance. Mol Canc (2019) 18(1):75. doi: 10.1186/s12943-019-0991-5
126. Li SR, Man QW, Gao X, Lin H, Wang J, Su FC, et al. Tissue-derived extracellular vesicles in cancers and non-cancer diseases: Present and future. J Extracell Vesicles (2021) 10(14):e12175. doi: 10.1002/jev2.12175
127. Gabrielli M, Prada I, Joshi P, Falcicchia C, D'Arrigo G, Rutigliano G, et al. Microglial large extracellular vesicles propagate early synaptic dysfunction in alzheimer's disease. Brain (2022) 145(8):2849–68. doi: 10.1093/brain/awac083
128. Garcia-Contreras M, Thakor AS. Extracellular vesicles in alzheimer's disease: from pathology to therapeutic approaches. Neural Regener Res (2023) 18(1):18–22. doi: 10.4103/1673-5374.343882
129. Tian C, Stewart T, Hong Z, Guo Z, Aro P, Soltys D, et al. Blood extracellular vesicles carrying synaptic function- and brain-related proteins as potential biomarkers for alzheimer's disease. Alzheimers Dement (2022). doi: 10.1002/alz.12723
130. Cheng L, Vella LJ, Barnham KJ, McLean C, Masters CL, Hill AF. Small RNA fingerprinting of alzheimer's disease frontal cortex extracellular vesicles and their comparison with peripheral extracellular vesicles. J Extracell Vesicles (2020) 9(1):1766822. doi: 10.1080/20013078.2020.1766822
131. Muraoka S, DeLeo AM, Sethi MK, Yukawa-Takamatsu K, Yang Z, Ko J, et al. Proteomic and biological profiling of extracellular vesicles from alzheimer's disease human brain tissues. Alzheimers Dement (2020) 16(6):896–907. doi: 10.1002/alz.12089
132. Wang X, Yang G. Bone marrow mesenchymal stem cells-derived exosomes reduce aβ deposition and improve cognitive function recovery in mice with alzheimer's disease by activating sphingosine kinase/sphingosine-1-phosphate signaling pathway. Cell Biol Int (2021) 45(4):775–84. doi: 10.1002/cbin.11522
133. Xiang C, Yang K, Liang Z, Wan Y, Cheng Y, Ma D, et al. Sphingosine-1-phosphate mediates the therapeutic effects of bone marrow mesenchymal stem cell-derived microvesicles on articular cartilage defect. Transl Res (2018) 193:42–53. doi: 10.1016/j.trsl.2017.12.003
134. Martins TS, Vaz M, Henriques AG. A review on comparative studies addressing exosome isolation methods from body fluids. Anal Bioanal Chem (2023) 415(7):1239–63. doi: 10.1007/s00216-022-04174-5
135. Huang Y, Cheng L, Turchinovich A, Mahairaki V, Troncoso JC, Pletniková O, et al. Influence of species and processing parameters on recovery and content of brain tissue-derived extracellular vesicles. J Extracell Vesicles (2020) 9(1):1785746.
136. Zhou L, Kodidela S, Godse S, Thomas-Gooch S, Kumar A, Raji B, et al. Targeted drug delivery to the central nervous system using extracellular vesicles. Pharm (Basel) (2022) 15(3):358. doi: 10.3390/ph15030358
137. Murphy DE, de Jong OG, Brouwer M, Wood MJ, Lavieu G, Schiffelers RM, et al. Extracellular vesicle-based therapeutics: natural versus engineered targeting and trafficking. Exp Mol Med (2019) 51(3):1–12. doi: 10.1038/s12276-019-0223-5
138. Takayama Y, Kusamori K, Nishikawa M. Mesenchymal stem/stromal cells as next-generation drug delivery vehicles for cancer therapeutics. Expert Opin Drug Delivery (2021) 18(11):1627–42. doi: 10.1080/17425247.2021.1960309
139. Wei W, Ao Q, Wang X, Cao Y, Liu Y, Zheng SG, et al. Mesenchymal stem cell-derived exosomes: A promising biological tool in nanomedicine. Front Pharmacol (2020) 11:590470. doi: 10.3389/fphar.2020.590470
140. Kim DH, Kothandan VK, Kim HW, Kim KS, Kim JY, Cho HJ, et al. Noninvasive assessment of exosome pharmacokinetics In vivo: A review. Pharmaceutics (2019) 11(12):649. doi: 10.3390/pharmaceutics11120649
141. Perets N, Hertz S, London M, Offen D. Intranasal administration of exosomes derived from mesenchymal stem cells ameliorates autistic-like behaviors of BTBR mice. Mol Autism (2018) 9:57. doi: 10.1186/s13229-018-0240-6
142. Burnouf T, Agrahari V, Agrahari V. Extracellular vesicles as nanomedicine: Hopes and hurdles in clinical translation. Int J Nanomed (2019) 14:8847–59. doi: 10.2147/IJN.S225453
143. Yáñez-Mó M, Siljander PR, Andreu Z, Zavec AB, Borràs FE, Buzas EI, et al. Biological properties of extracellular vesicles and their physiological functions. J Extracell Vesicles (2015) 4:27066. doi: 10.3402/jev.v4.27066
144. Cheng L, Hill AF. Therapeutically harnessing extracellular vesicles. Nat Rev Drug Discovery (2022) 21(5):379–99. doi: 10.1038/s41573-022-00410-w
145. Ryan ST, Hosseini-Beheshti E, Afrose D, Ding X, Xia B, Grau GE, et al. Extracellular vesicles from mesenchymal stromal cells for the treatment of inflammation-related conditions. Int J Mol Sci (2021) 22(6):3023. doi: 10.3390/ijms22063023
146. Suh JH, Joo HS, Hong EB, Lee HJ, Lee JM. Therapeutic application of exosomes in inflammatory diseases. Int J Mol Sci (2021) 22(3):1144. doi: 10.3390/ijms22031144
147. Gentile P, Sterodimas A, Pizzicannella J, Dionisi L, De Fazio D, Calabrese C, et al. Systematic review: Allogenic use of stromal vascular fraction (SVF) and decellularized extracellular matrices (ECM) as advanced therapy medicinal products (ATMP) in tissue regeneration. Int J Mol Sci (2020) 21(14):4982. doi: 10.3390/ijms21144982
148. Bari E, Ferrarotti I, Di Silvestre D, Grisoli P, Barzon V, Balderacchi A, et al. Adipose mesenchymal extracellular vesicles as alpha-1-Antitrypsin physiological delivery systems for lung regeneration. Cells (2019) 8(9):965. doi: 10.3390/cells8090965
149. Eleuteri S, Fierabracci A. Insights into the secretome of mesenchymal stem cells and its potential applications. Int J Mol Sci (2019) 20(18):4597. doi: 10.3390/ijms20184597
150. Nagelkerke A, Ojansivu M, van der Koog L, Whittaker TE, Cunnane EM, Silva AM, et al. Extracellular vesicles for tissue repair and regeneration: Evidence, challenges and opportunities. Adv Drug Delivery Rev (2021) 175:113775. doi: 10.1016/j.addr.2021.04.013
151. Caplan AI. Mesenchymal stem cells: Time to change the name! Stem Cells Transl Med (2017) 6(6):1445–51. doi: 10.1002/sctm.17-0051
152. Hosseiniyan Khatibi SM, Kheyrolahzadeh K, Barzegari A, Rahbar Saadat Y, Zununi Vahed S. Medicinal signaling cells: A potential antimicrobial drug store. J Cell Physiol (2020) 235(11):7731–46. doi: 10.1002/jcp.29728
153. Munoz-Perez E, Gonzalez-Pujana A, Igartua M, Santos-Vizcaino E, Hernandez RM. Mesenchymal stromal cell secretome for the treatment of immune-mediated inflammatory diseases: Latest trends in isolation, content optimization and delivery avenues. Pharmaceutics. (2021) 13(11):1802. doi: 10.3390/pharmaceutics13111802
154. Berger A, Araújo-Filho I, Piffoux M, Nicolás-Boluda A, Grangier A, Boucenna I, et al. Local administration of stem cell-derived extracellular vesicles in a thermoresponsive hydrogel promotes a pro-healing effect in a rat model of colo-cutaneous post-surgical fistula. Nanoscale. (2021) 13(1):218–32. doi: 10.1039/D0NR07349K
155. Chabria Y, Duffy GP, Lowery AJ, Dwyer RM. Hydrogels: 3D drug delivery systems for nanoparticles and extracellular vesicles. Biomedicines. (2021) 9(11). doi: 10.3390/biomedicines9111694
156. Hernandez MJ, Gaetani R, Pieters VM, Ng NW, Chang AE, Martin TR, et al. Decellularized extracellular matrix hydrogels as a delivery platform for MicroRNA and extracellular vesicle therapeutics. Adv Ther (Weinh) (2018) 1(3):1800032. doi: 10.1002/adtp.201800032
157. Liu X, Yang Y, Li Y, Niu X, Zhao B, Wang Y, et al. Integration of stem cell-derived exosomes with in situ hydrogel glue as a promising tissue patch for articular cartilage regeneration. Nanoscale. (2017) 9(13):4430–8. doi: 10.1039/C7NR00352H
158. Xie Y, Guan Q, Guo J, Chen Y, Yin Y, Han X. Hydrogels for exosome delivery in biomedical applications. Gels. (2022) 8(6):328. doi: 10.3390/gels8060328
159. Khazaei F, Rezakhani L, Alizadeh M, Mahdavian E, Khazaei M. Exosomes and exosome-loaded scaffolds: Characterization and application in modern regenerative medicine. Tissue Cell (2022) 80:102007. doi: 10.1016/j.tice.2022.102007
160. Miao HB, Wang F, Lin S, Chen Z. Update on the role of extracellular vesicles in rheumatoid arthritis. Expert Rev Mol Med (2022) 24:e12. doi: 10.1017/erm.2021.33
161. Kordelas L, Rebmann V, Ludwig AK, Radtke S, Ruesing J, Doeppner TR, et al. MSC-derived exosomes: a novel tool to treat therapy-refractory graft-versus-host disease. Leukemia. (2014) 28(4):970–3. doi: 10.1038/leu.2014.41
162. Dabrowska S, Andrzejewska A, Janowski M, Lukomska B. Immunomodulatory and regenerative effects of mesenchymal stem cells and extracellular vesicles: Therapeutic outlook for inflammatory and degenerative diseases. Front Immunol (2020) 11:591065. doi: 10.3389/fimmu.2020.591065
163. Silva AKA, Morille M, Piffoux M, Arumugam S, Mauduit P, Larghero J, et al. Development of extracellular vesicle-based medicinal products: A position paper of the group "Extracellular vesicle translatiOn to clinicaL perspectiVEs - EVOLVE france". Adv Drug Delivery Rev (2021) 179:114001. doi: 10.1016/j.addr.2021.114001
164. Wiest EF, Zubair AC. Challenges of manufacturing mesenchymal stromal cell-derived extracellular vesicles in regenerative medicine. Cytotherapy. (2020) 22(11):606–12. doi: 10.1016/j.jcyt.2020.04.040
165. de Almeida Fuzeta M, Gonçalves PP, Fernandes-Platzgummer A, Cabral JMS, Bernardes N, da Silva CL. From promise to reality: Bioengineering strategies to enhance the therapeutic potential of extracellular vesicles. Bioengineering (Basel) (2022) 9(11):675. doi: 10.3390/bioengineering9110675
166. Paolini L, Monguió-Tortajada M, Costa M, Antenucci F, Barilani M, Clos-Sansalvador M, et al. Large-Scale production of extracellular vesicles: Report on the “massivEVs” ISEV workshop. J Extracellular Biol (2022) 1(10):e63. doi: 10.1002/jex2.63
167. Ha DH, Kim HK, Lee J, Kwon HH, Park GH, Yang SH, et al. Mesenchymal Stem/Stromal cell-derived exosomes for immunomodulatory therapeutics and skin regeneration. Cells (2020) 9(5):1157. doi: 10.3390/cells9051157
168. Gimona M, Brizzi MF, Choo ABH, Dominici M, Davidson SM, Grillari J, et al. Critical considerations for the development of potency tests for therapeutic applications of mesenchymal stromal cell-derived small extracellular vesicles. Cytotherapy. (2021) 23(5):373–80. doi: 10.1016/j.jcyt.2021.01.001
169. Jahromi LP, Shahbazi MA, Maleki A, Azadi A, Santos HA. Chemically engineered immune cell-derived microrobots and biomimetic nanoparticles: Emerging biodiagnostic and therapeutic tools. Adv Sci (Weinh) (2021) 8(8):2002499. doi: 10.1002/advs.202002499
170. Shpigelman J, Lao FS, Yao S, Li C, Saito T, Sato-Kaneko F, et al. Generation and application of a reporter cell line for the quantitative screen of extracellular vesicle release. Front Pharmacol (2021) 12:668609. doi: 10.3389/fphar.2021.668609
171. Wen H, Peng L, Chen Y. The effect of immune cell-derived exosomes in the cardiac tissue repair after myocardial infarction: Molecular mechanisms and pre-clinical evidence. J Cell Mol Med (2021) 25(14):6500-10. doi: 10.1111/jcmm.16686
172. Budden CF, Gearing LJ, Kaiser R, Standke L, Hertzog PJ, Latz E. Inflammasome-induced extracellular vesicles harbour distinct RNA signatures and alter bystander macrophage responses. J Extracell Vesicles (2021) 10(10):e12127. doi: 10.1002/jev2.12127
173. Liu CJ, Xie GY, Miao YR, Xia M, Wang Y, Lei Q, et al. EVAtlas: a comprehensive database for ncRNA expression in human extracellular vesicles. Nucleic Acids Res (2021) 25(14):6500-10.
174. Perocheau D, Touramanidou L, Gurung S, Gissen P, Baruteau J. Clinical applications for exosomes: Are we there yet? Br J Pharmacol (2021) 178(12):2375-92. doi: 10.1111/bph.15432
175. Phillips W, Willms E, Hill AF. Understanding extracellular vesicle and nanoparticle heterogeneity: Novel methods and considerations. Proteomics (2021) 21(13-14):e2000118. doi: 10.1002/pmic.202000118
176. Enderle D, Noerholm M. Are extracellular vesicles ready for the clinical laboratory? Journal of Laboratory Medicine (2022) 46(4):273–82. doi: 10.1515/labmed-2022-0064
177. Lohan P, Treacy O, Griffin MD, Ritter T, Ryan AE. Anti-donor immune responses elicited by allogeneic mesenchymal stem cells and their extracellular vesicles: Are we still learning? Front Immunol (2017) 8:1626. doi: 10.3389/fimmu.2017.01626
178. Wang Z, Mo H, He Z, Chen A, Cheng P. Extracellular vesicles as an emerging drug delivery system for cancer treatment: Current strategies and recent advances. Biomed Pharmacother (2022) 153:113480. doi: 10.1016/j.biopha.2022.113480
179. Xia Y, Yang R, Hou Y, Wang H, Li Y, Zhu J, et al. Application of mesenchymal stem cell-derived exosomes from different sources in intervertebral disc degeneration. Front Bioeng Biotechnol (2022) 10:1019437. doi: 10.3389/fbioe.2022.1019437
180. Park JA, Sharif AS, Tschumperlin DJ, Lau L, Limbrey R, Howarth P, et al. Tissue factor-bearing exosome secretion from human mechanically stimulated bronchial epithelial cells in vitro and in vivo. J Allergy Clin Immunol (2012) 130(6):1375–83. doi: 10.1016/j.jaci.2012.05.031
181. Ma X, Liu B, Fan L, Liu Y, Zhao Y, Ren T, et al. Native and engineered exosomes for inflammatory disease. Nano Res (2022). doi: 10.1007/s12274-022-5275-5
182. Allan D, Tieu A, Lalu M, Burger D. Mesenchymal stromal cell-derived extracellular vesicles for regenerative therapy and immune modulation: Progress and challenges toward clinical application. Stem Cells Transl Med (2020) 9(1):39–46. doi: 10.1002/sctm.19-0114
183. Long Q, Upadhya D, Hattiangady B, Kim DK, An SY, Shuai B, et al. Intranasal MSC-derived A1-exosomes ease inflammation, and prevent abnormal neurogenesis and memory dysfunction after status epilepticus. Proc Natl Acad Sci U S A (2017) 114(17):E3536–e45. doi: 10.1073/pnas.1703920114
184. Farzamfar S, Hasanpour A, Nazeri N, Razavi H, Salehi M, Shafei S, et al. Extracellular micro/nanovesicles rescue kidney from ischemia-reperfusion injury. J Cell Physiol (2019) 234(8):12290–300. doi: 10.1002/jcp.27998
185. Lai P, Weng J, Guo L, Chen X, Du X. Novel insights into MSC-EVs therapy for immune diseases. biomark Res (2019) 7:6. doi: 10.1186/s40364-019-0156-0
186. Li X, Wang S, Zhu R, Li H, Han Q, Zhao RC. Lung tumor exosomes induce a pro-inflammatory phenotype in mesenchymal stem cells via NFκB-TLR signaling pathway. J Hematol Oncol (2016) 9:42. doi: 10.1186/s13045-016-0269-y
187. Reis M, Ogonek J, Qesari M, Borges NM, Nicholson L, Preußner L, et al. Recent developments in cellular immunotherapy for HSCT-associated complications. Front Immunol (2016) 7:500. doi: 10.3389/fimmu.2016.00500
188. Giebel B, Hermann DM. Identification of the right cell sources for the production of therapeutically active extracellular vesicles in ischemic stroke. Ann Transl Med (2019) 7(9):188. doi: 10.21037/atm.2019.03.49
189. Zhang B, Yeo RWY, Lai RC, Sim EWK, Chin KC, Lim SK. Mesenchymal stromal cell exosome-enhanced regulatory T-cell production through an antigen-presenting cell-mediated pathway. Cytotherapy. (2018) 20(5):687–96. doi: 10.1016/j.jcyt.2018.02.372
190. Holtzman J, Lee H. Emerging role of extracellular vesicles in the respiratory system. Exp Mol Med (2020) 52(6):887–95. doi: 10.1038/s12276-020-0450-9
191. Conforti A, Scarsella M, Starc N, Giorda E, Biagini S, Proia A, et al. Microvescicles derived from mesenchymal stromal cells are not as effective as their cellular counterpart in the ability to modulate immune responses in vitro. Stem Cells Dev (2014) 23(21):2591–9. doi: 10.1089/scd.2014.0091
192. Del Fattore A, Luciano R, Pascucci L, Goffredo BM, Giorda E, Scapaticci M, et al. Immunoregulatory effects of mesenchymal stem cell-derived extracellular vesicles on T lymphocytes. Cell Transplant (2015) 24(12):2615–27. doi: 10.3727/096368915X687543
193. Meisel R, Zibert A, Laryea M, Göbel U, Däubener W, Dilloo D. Human bone marrow stromal cells inhibit allogeneic T-cell responses by indoleamine 2,3-dioxygenase-mediated tryptophan degradation. Blood. (2004) 103(12):4619–21. doi: 10.1182/blood-2003-11-3909
194. Loh JT, Zhang B, Teo JKH, Lai RC, Choo ABH, Lam KP, et al. Mechanism for the attenuation of neutrophil and complement hyperactivity by MSC exosomes. Cytotherapy. (2022) 24(7):711–9. doi: 10.1016/j.jcyt.2021.12.003
195. Chen W, Huang Y, Han J, Yu L, Li Y, Lu Z, et al. Immunomodulatory effects of mesenchymal stromal cells-derived exosome. Immunol Res (2016) 64(4):831–40. doi: 10.1007/s12026-016-8798-6
196. Prunevieille A, Babiker-Mohamed MH, Aslami C, Gonzalez-Nolasco B, Mooney N, Benichou G. T Cell antigenicity and immunogenicity of allogeneic exosomes. Am J Transplant (2021) 21(7):2583–89. doi: 10.1111/ajt.16591
197. Margolis L, Sadovsky Y. The biology of extracellular vesicles: The known unknowns. PloS Biol (2019) 17(7):e3000363. doi: 10.1371/journal.pbio.3000363
198. Svensson KJ, Christianson HC, Wittrup A, Bourseau-Guilmain E, Lindqvist E, Svensson LM, et al. Exosome uptake depends on ERK1/2-heat shock protein 27 signaling and lipid raft-mediated endocytosis negatively regulated by caveolin-1. J Biol Chem (2013) 288(24):17713–24. doi: 10.1074/jbc.M112.445403
199. Grange C, Tapparo M, Bruno S, Chatterjee D, Quesenberry PJ, Tetta C, et al. Biodistribution of mesenchymal stem cell-derived extracellular vesicles in a model of acute kidney injury monitored by optical imaging. Int J Mol Med (2014) 33(5):1055–63. doi: 10.3892/ijmm.2014.1663
200. Cloutier N, Tan S, Boudreau LH, Cramb C, Subbaiah R, Lahey L, et al. The exposure of autoantigens by microparticles underlies the formation of potent inflammatory components: the microparticle-associated immune complexes. EMBO Mol Med (2013) 5(2):235–49. doi: 10.1002/emmm.201201846
201. Graham DK, DeRyckere D, Davies KD, Earp HS. The TAM family: phosphatidylserine sensing receptor tyrosine kinases gone awry in cancer. Nat Rev Canc (2014) 14(12):769–85. doi: 10.1038/nrc3847
202. Ramos AP, Sebinelli HG, Ciancaglini P, Rosato N, Mebarek S, Buchet R, et al. The functional role of soluble proteins acquired by extracellular vesicles. J Extracellular Biol (2022) 1(1):e34. doi: 10.1002/jex2.34
203. Mulcahy LA, Pink RC, Carter DR. Routes and mechanisms of extracellular vesicle uptake. J Extracell Vesicles (2014) 3. doi: 10.3402/jev.v3.24641
204. Tao SC, Guo SC. Role of extracellular vesicles in tumour microenvironment. Cell Commun Signal (2020) 18(1):163. doi: 10.1186/s12964-020-00643-5
205. Fu S, Wang Y, Xia X, Zheng JC. Exosome engineering: Current progress in cargo loading and targeted delivery. NanoImpact. (2020) 20:100261. doi: 10.1016/j.impact.2020.100261
206. Mokarizadeh A, Delirezh N, Morshedi A, Mosayebi G, Farshid AA, Mardani K. Microvesicles derived from mesenchymal stem cells: potent organelles for induction of tolerogenic signaling. Immunol Lett (2012) 147(1-2):47–54. doi: 10.1016/j.imlet.2012.06.001
207. Silvestro S, Chiricosta L, Gugliandolo A, Pizzicannella J, Diomede F, Bramanti P, et al. Extracellular vesicles derived from human gingival mesenchymal stem cells: A transcriptomic analysis. Genes (Basel) (2020) 11(2):118. doi: 10.3390/genes11020118
208. Banks WA, Sharma P, Bullock KM, Hansen KM, Ludwig N, Whiteside TL. Transport of extracellular vesicles across the blood-brain barrier: Brain pharmacokinetics and effects of inflammation. Int J Mol Sci (2020) 21(12):4407. doi: 10.3390/ijms21124407
209. Hong Y, Nam G-H, Koh E, Jeon S, Kim GB, Jeong C, et al. Exosome as a vehicle for delivery of membrane protein therapeutics, PH20, for enhanced tumor penetration and antitumor efficacy. Advanced Funct Materials (2018) 28(5):1703074. doi: 10.1002/adfm.201703074
210. Wu X, Xu X, Xiang Y, Fan D, An Q, Yue G, et al. Exosome-mediated effects and applications in inflammatory diseases of the digestive system. Eur J Med Res (2022) 27(1):163. doi: 10.1186/s40001-022-00792-y
211. Martinez-Arroyo O, Ortega A, Forner MJ, Cortes R. Mesenchymal stem cell-derived extracellular vesicles as non-coding RNA therapeutic vehicles in autoimmune diseases. Pharmaceutics. (2022) 14(4):733. doi: 10.3390/pharmaceutics14040733
212. Soler-Botija C, Monguió-Tortajada M, Munizaga-Larroudé M, Gálvez-Montón C, Bayes-Genis A, Roura S. Mechanisms governing the therapeutic effect of mesenchymal stromal cell-derived extracellular vesicles: A scoping review of preclinical evidence. BioMed Pharmacother (2022) 147:112683. doi: 10.1016/j.biopha.2022.112683
213. Toh WS, Lai RC, Zhang B, Lim SK. MSC exosome works through a protein-based mechanism of action. Biochem Soc Trans (2018) 46(4):843–53. doi: 10.1042/BST20180079
214. Almeria C, Kreß S, Weber V, Egger D, Kasper C. Heterogeneity of mesenchymal stem cell-derived extracellular vesicles is highly impacted by the tissue/cell source and culture conditions. Cell Biosci (2022) 12(1):51. doi: 10.1186/s13578-022-00786-7
215. Morales RT, Ko J. Future of digital assays to resolve clinical heterogeneity of single extracellular vesicles. ACS Nano (2022) 16(8):11619-45. doi: 10.1021/acsnano.2c04337
216. Al-Masawa ME, Alshawsh MA, Ng CY, Ng AMH, Foo JB, Vijakumaran U, et al. Efficacy and safety of small extracellular vesicle interventions in wound healing and skin regeneration: A systematic review and meta-analysis of animal studies. Theranostics. (2022) 12(15):6455–508. doi: 10.7150/thno.73436
217. Lu M, Peng L, Ming X, Wang X, Cui A, Li Y, et al. Enhanced wound healing promotion by immune response-free monkey autologous iPSCs and exosomes vs. their allogeneic counterparts. EBioMedicine. (2019) 42:443–57. doi: 10.1016/j.ebiom.2019.03.011
218. Ortega MA, Fraile-Martinez O, Garcia-Montero C, Alvarez-Mon MA, Gomez-Lahoz AM, Albillos A, et al. An updated view of the importance of vesicular trafficking and transport and their role in immune-mediated diseases: Potential therapeutic interventions. Membranes. (2022) 12(6):552. doi: 10.3390/membranes12060552
219. Damasceno PKF, de Santana TA, Santos GC, Orge ID, Silva DN, Albuquerque JF, et al. Genetic engineering as a strategy to improve the therapeutic efficacy of mesenchymal Stem/Stromal cells in regenerative medicine. Front Cell Dev Biol (2020) 8:737. doi: 10.3389/fcell.2020.00737
220. Karp JM, Leng Teo GS. Mesenchymal stem cell homing: the devil is in the details. Cell Stem Cell (2009) 4(3):206–16. doi: 10.1016/j.stem.2009.02.001
221. Naderi-Meshkin H, Bahrami AR, Bidkhori HR, Mirahmadi M, Ahmadiankia N. Strategies to improve homing of mesenchymal stem cells for greater efficacy in stem cell therapy. Cell Biol Int (2015) 39(1):23–34. doi: 10.1002/cbin.10378
222. Corbeil D, Santos MF, Karbanová J, Kurth T, Rappa G, Lorico A. Uptake and fate of extracellular membrane vesicles: Nucleoplasmic reticulum-associated late endosomes as a new gate to intercellular communication. Cells. (2020) 9(9):1931. doi: 10.3390/cells9091931
223. Sung BH, von Lersner A, Guerrero J, Krystofiak ES, Inman D, Pelletier R, et al. A live cell reporter of exosome secretion and uptake reveals pathfinding behavior of migrating cells. Nat Commun (2020) 11(1):2092. doi: 10.1038/s41467-020-15747-2
224. Danielyan L, Schwab M, Siegel G, Brawek B, Garaschuk O, Asavapanumas N, et al. Cell motility and migration as determinants of stem cell efficacy. EBioMedicine. (2020) 60:102989. doi: 10.1016/j.ebiom.2020.102989
225. Sorrell JM, Caplan AI. Topical delivery of mesenchymal stem cells and their function in wounds. Stem Cell Res Ther (2010) 1(4):30. doi: 10.1186/scrt30
226. Eggenhofer E, Luk F, Dahlke MH, Hoogduijn MJ. The life and fate of mesenchymal stem cells. Front Immunol (2014) 5:148. doi: 10.3389/fimmu.2014.00148
227. Bateman ME, Strong AL, Gimble JM, Bunnell BA. Concise review: Using fat to fight disease: A systematic review of nonhomologous adipose-derived Stromal/Stem cell therapies. Stem Cells (2018) 36(9):1311–28. doi: 10.1002/stem.2847
228. Ma S, Xie N, Li W, Yuan B, Shi Y, Wang Y. Immunobiology of mesenchymal stem cells. Cell Death Differ (2014) 21(2):216–25. doi: 10.1038/cdd.2013.158
229. Honczarenko M, Le Y, Swierkowski M, Ghiran I, Glodek AM, Silberstein LE. Human bone marrow stromal cells express a distinct set of biologically functional chemokine receptors. Stem Cells (2006) 24(4):1030–41. doi: 10.1634/stemcells.2005-0319
230. Ullah M, Liu DD, Thakor AS. Mesenchymal stromal cell homing: Mechanisms and strategies for improvement. iScience. (2019) 15:421–38. doi: 10.1016/j.isci.2019.05.004
231. Zheng W, He R, Liang X, Roudi S, Bost J, Coly PM, et al. Cell-specific targeting of extracellular vesicles though engineering the glycocalyx. J Extracell Vesicles (2022) 11(12):e12290. doi: 10.1002/jev2.12290
232. Granger E, McNee G, Allan V, Woodman P. The role of the cytoskeleton and molecular motors in endosomal dynamics. Semin Cell Dev Biol (2014) 31(100):20–9. doi: 10.1016/j.semcdb.2014.04.011
233. Choi H, Lee DS. Illuminating the physiology of extracellular vesicles. Stem Cell Res Ther (2016) 7(1):55. doi: 10.1186/s13287-016-0316-1
234. Maas SLN, Breakefield XO, Weaver AM. Extracellular vesicles: Unique intercellular delivery vehicles. Trends Cell Biol (2017) 27(3):172–88. doi: 10.1016/j.tcb.2016.11.003
235. Parayath NN, Padmakumar S, Amiji MM. Extracellular vesicle-mediated nucleic acid transfer and reprogramming in the tumor microenvironment. Cancer Lett (2020) 482:33–43. doi: 10.1016/j.canlet.2020.04.009
236. Mishra VK, Shih HH, Parveen F, Lenzen D, Ito E, Chan TF, et al. Identifying the therapeutic significance of mesenchymal stem cells. Cells (2020) 9(5):1145. doi: 10.3390/cells9051145
237. Fröhlich E. Therapeutic potential of mesenchymal stem cells and their products in lung diseases-intravenous administration versus inhalation. Pharmaceutics (2021) 13(2):232. doi: 10.3390/pharmaceutics13020232
238. Lara P, Chan AB, Cruz LJ, Quest AFG, Kogan MJ. Exploiting the natural properties of extracellular vesicles in targeted delivery towards specific cells and tissues. Pharmaceutics. (2020) 12(11):1022. doi: 10.3390/pharmaceutics12111022
239. Lawson C, Kovacs D, Finding E, Ulfelder E, Luis-Fuentes V. Extracellular vesicles: Evolutionarily conserved mediators of intercellular communication. Yale J Biol Med (2017) 90(3):481–91.
240. Pistono C, Bister N, Stanová I, Malm T. Glia-derived extracellular vesicles: Role in central nervous system communication in health and disease. Front Cell Dev Biol (2020) 8:623771. doi: 10.3389/fcell.2020.623771
241. Bruno S, Chiabotto G, Favaro E, Deregibus MC, Camussi G. Role of extracellular vesicles in stem cell biology. Am J Physiol Cell Physiol (2019) 317(2):C303–c13. doi: 10.1152/ajpcell.00129.2019
242. Sancho-Albero M, Navascués N, Mendoza G, Sebastián V, Arruebo M, Martín-Duque P, et al. Exosome origin determines cell targeting and the transfer of therapeutic nanoparticles towards target cells. J Nanobiotech (2019) 17(1):16. doi: 10.1186/s12951-018-0437-z
243. Ludwig N, Whiteside TL, Reichert TE. Challenges in exosome isolation and analysis in health and disease. Int J Mol Sci (2019) 20(19):4684. doi: 10.3390/ijms20194684
244. Vallant N, Sandhu B, Hamaoui K, Prendecki M, Pusey C, Papalois V. Immunomodulatory properties of mesenchymal stromal cells can vary in genetically modified rats. Int J Mol Sci (2021) 22(3):1181. doi: 10.3390/ijms22031181
245. Kronstadt SM, Van Heyningen LH, Aranda A, Jay SM. Assessment of anti-inflammatory bioactivity of extracellular vesicles is susceptible to error via media component contamination. Cytotherapy (2022) 2022:08.25.505301. 10.1016/j.jcyt.2022.12.002
246. Ullah M, Qiao Y, Concepcion W, Thakor AS. Stem cell-derived extracellular vesicles: role in oncogenic processes, bioengineering potential, and technical challenges. Stem Cell Res Ther (2019) 10(1):347. doi: 10.1186/s13287-019-1468-6
247. Andjus P, Kosanović M, Milićević K, Gautam M, Vainio SJ, Jagečić D, et al. Extracellular vesicles as innovative tool for diagnosis, regeneration and protection against neurological damage. Int J Mol Sci (2020) 21(18):6859. doi: 10.3390/ijms21186859
248. Shaimardanova AA, Solovyeva VV, Chulpanova DS, James V, Kitaeva KV, Rizvanov AA. Extracellular vesicles in the diagnosis and treatment of central nervous system diseases. Neural Regener Res (2020) 15(4):586–96. doi: 10.4103/1673-5374.266908
249. Eitan E, Green J, Bodogai M, Mode NA, Bæk R, Jørgensen MM, et al. Age-related changes in plasma extracellular vesicle characteristics and internalization by leukocytes. Sci Rep (2017) 7(1):1342. doi: 10.1038/s41598-017-01386-z
250. Agliardi C, Clerici M. Blood extracellular vesicles (EVs) of central nervous system origin: a window into the brain. Neural Regener Res (2020) 15(1):55–6. doi: 10.4103/1673-5374.264454
251. Davidson SM. Benefit of extracellular vesicles at the blood-brain barrier. Arterioscler Thromb Vasc Biol (2021) 41(3):1146–8. doi: 10.1161/ATVBAHA.121.315833
252. Kahmini FR, Shahgaldi S. Therapeutic potential of mesenchymal stem cell-derived extracellular vesicles as novel cell-free therapy for treatment of autoimmune disorders. Exp Mol Pathol (2021) 118:104566. doi: 10.1016/j.yexmp.2020.104566
253. Saint-Pol J, Gosselet F, Duban-Deweer S, Pottiez G, Karamanos Y. Targeting and crossing the blood-brain barrier with extracellular vesicles. Cells. (2020) 9(4):851. doi: 10.3390/cells9040851
254. Sil S, Dagur RS, Liao K, Peeples ES, Hu G, Periyasamy P, et al. Strategies for the use of extracellular vesicles for the delivery of therapeutics. J Neuroimmune Pharmacol (2020) 15(3):422–42. doi: 10.1007/s11481-019-09873-y
255. Warnecke A, Prenzler N, Harre J, Köhl U, Gärtner L, Lenarz T, et al. First-in-human intracochlear application of human stromal cell-derived extracellular vesicles. J Extracellular Vesicles (2021) 10(8):e12094. doi: 10.1002/jev2.12094
256. Sagini K, Costanzi E, Emiliani C, Buratta S, Urbanelli L. Extracellular vesicles as conveyors of membrane-derived bioactive lipids in immune system. Int J Mol Sci (2018) 19(4):1227. doi: 10.3390/ijms19041227
257. Seo Y, Kim HS, Hong IS. Stem cell-derived extracellular vesicles as immunomodulatory therapeutics. Stem Cells Int (2019) 2019:5126156. doi: 10.1155/2019/5126159
258. Kalluri R, LeBleu VS. The biology, function, and biomedical applications of exosomes. Science. (2020) 367(6478):eaau6977. doi: 10.1126/science.aau6977
259. Bulut Ö, GÜrsel İ. Mesenchymal stem cell derived extracellular vesicles: promising immunomodulators against autoimmune, autoinflammatory disorders and SARS-CoV-2 infection. Turk J Biol (2020) 44(3):273–82. doi: 10.3906/biy-2002-79
260. Giunti D, Marini C, Parodi B, Usai C, Milanese M, Bonanno G, et al. Role of miRNAs shuttled by mesenchymal stem cell-derived small extracellular vesicles in modulating neuroinflammation. Sci Rep (2021) 11(1):1740. doi: 10.1038/s41598-021-81039-4
261. Yi YW, Lee JH, Kim SY, Pack CG, Ha DH, Park SR, et al. Advances in analysis of biodistribution of exosomes by molecular imaging. Int J Mol Sci (2020) 21(2):665. doi: 10.3390/ijms21020665
262. Frangioni JV, Hajjar RJ. In vivo tracking of stem cells for clinical trials in cardiovascular disease. Circulation. (2004) 110(21):3378–83. doi: 10.1161/01.CIR.0000149840.46523.FC
263. Simon T, Kumaran A, Veselu DF, Giamas G. Three method-combination protocol for improving purity of extracellular vesicles. Int J Mol Sci (2020) 21(9):3071. doi: 10.3390/ijms21093071
264. Giebel B, Kordelas L, Börger V. Clinical potential of mesenchymal stem/stromal cell-derived extracellular vesicles. Stem Cell Investig (2017) 4:84. doi: 10.21037/sci.2017.09.06
265. Lener T, Gimona M, Aigner L, Börger V, Buzas E, Camussi G, et al. Applying extracellular vesicles based therapeutics in clinical trials - an ISEV position paper. J Extracell Vesicles (2015) 4:30087. doi: 10.3402/jev.v4.30087
266. Chen H, Luo C, Yang M, Li J, Ma P, Zhang X. Intracellular uptake of and sensing with SERS-active hybrid exosomes: insight into a role of metal nanoparticles. Nanomed (Lond) (2020) 15(9):913–26. doi: 10.3390/v12050486
267. Kumar S, Zhi K, Mukherji A, Gerth K. Repurposing antiviral protease inhibitors using extracellular vesicles for potential therapy of COVID-19. Viruses. (2020) 12(5).
268. Alagesan S, Brady J, Byrnes D, Fandiño J, Masterson C, McCarthy S, et al. Enhancement strategies for mesenchymal stem cells and related therapies. Stem Cell Res Ther (2022) 13(1):75. doi: 10.1186/s13287-022-02747-w
269. Jafari D, Shajari S, Jafari R, Mardi N, Gomari H, Ganji F, et al. Designer exosomes: A new platform for biotechnology therapeutics. BioDrugs. (2020) 34(5):567–86. doi: 10.1007/s40259-020-00434-x
270. Zhang F, Guo J, Zhang Z, Duan M, Wang G, Qian Y, et al. Application of engineered extracellular vesicles for targeted tumor therapy. J Biomed Scie (2022) 29(1):14. doi: 10.1186/s12929-022-00798-y
271. Villa F, Quarto R, Tasso R. Extracellular vesicles as natural, safe and efficient drug delivery systems. Pharmaceutics. (2019) 11(11):557. doi: 10.3390/pharmaceutics11110557
272. Walker S, Busatto S, Pham A, Tian M, Suh A, Carson K, et al. Extracellular vesicle-based drug delivery systems for cancer treatment. Theranostics. (2019) 9(26):8001–17. doi: 10.7150/thno.37097
273. Picciolini S, Rodà F, Bedoni M, Gualerzi A. Advances in the field of micro- and nanotechnologies applied to extracellular vesicle research: Take-home message from ISEV2021. Micromachines. (2021) 12(12):1563. doi: 10.3390/mi12121563
274. Zhang P, Zhou X, He M, Shang Y, Tetlow AL, Godwin AK, et al. Ultrasensitive detection of circulating exosomes with a 3D-nanopatterned microfluidic chip. Nat BioMed Eng. (2019) 3(6):438–51. doi: 10.1038/s41551-019-0356-9
275. Gurunathan S, Kang MH, Qasim M, Khan K, Kim JH. Biogenesis, membrane trafficking, functions, and next generation nanotherapeutics medicine of extracellular vesicles. Int J Nanomed (2021) 16:3357–83. doi: 10.2147/IJN.S310357
276. Ciullo A, Biemmi V, Milano G, Bolis S, Cervio E, Fertig ET, et al. Exosomal expression of CXCR4 targets cardioprotective vesicles to myocardial infarction and improves outcome after systemic administration. Int J Mol Sci (2019) 20(3):476. doi: 10.3390/ijms20030468
277. Joo HS, Suh JH, Lee HJ, Bang ES, Lee JM. Current knowledge and future perspectives on mesenchymal stem cell-derived exosomes as a new therapeutic agent. Int J Mol Sci (2020) 21(3):727. doi: 10.3390/ijms21030727
278. Kim ES, Kida K, Mok J, Seong Y, Jo SY, Kanaki T, et al. Cellhesion VP enhances the immunomodulating potential of human mesenchymal stem cell-derived extracellular vesicles. Biomaterials. (2021) 271:120742. doi: 10.1016/j.biomaterials.2021.120742
279. Gomzikova MO, Kletukhina SK, Kurbangaleeva SV, Neustroeva OA, Vasileva OS, Garanina EE, et al. Mesenchymal stem cell derived biocompatible membrane vesicles demonstrate immunomodulatory activity inhibiting activation and proliferation of human mononuclear cells. Pharmaceutics (2020) 12(6):577. doi: 10.3390/pharmaceutics12060577
280. Yuan X, Sun L, Jeske R, Nkosi D, York SB, Liu Y, et al. Engineering extracellular vesicles by three-dimensional dynamic culture of human mesenchymal stem cells. J Extracell Vesicles (2022) 11(6):e12235. doi: 10.1002/jev2.12235
281. Kamerkar S, LeBleu VS, Sugimoto H, Yang S, Ruivo CF, Melo SA, et al. Exosomes facilitate therapeutic targeting of oncogenic KRAS in pancreatic cancer. Nature. (2017) 546(7659):498–503. doi: 10.1038/nature22341
282. Conceição M, Forcina L, Wiklander OPB, Gupta D, Nordin JZ, Vrellaku B, et al. Engineered extracellular vesicle decoy receptor-mediated modulation of the IL6 trans-signalling pathway in muscle. Biomaterials. (2021) 266:120435. doi: 10.1016/j.biomaterials.2020.120435
283. Zampieri R, Brozzetti A, Pericolini E, Bartoloni E, Gabrielli E, Roselletti E, et al. Prevention and treatment of autoimmune diseases with plant virus nanoparticles. Sci Adv (2020) 6(19):eaaz0295. doi: 10.1126/sciadv.aaz0295
284. Hettich BF, Bader JJ, Leroux JC. Encapsulation of hydrophilic compounds in small extracellular vesicles: Loading capacity and impact on vesicle functions. Adv Healthc Mater (2021) 11(5):e2100047. doi: 10.1002/adhm.202100047
285. Massaro C, Sgueglia G, Frattolillo V, Baglio SR, Altucci L, Dell'Aversana C. Extracellular vesicle-based nucleic acid delivery: Current advances and future perspectives in cancer therapeutic strategies. Pharmaceutics. (2020) 12(10):980. doi: 10.3390/pharmaceutics12100980
286. Rankin-Turner S, Vader P, O'Driscoll L, Giebel B, Heaney LM, Davies OG. A call for the standardised reporting of factors affecting the exogenous loading of extracellular vesicles with therapeutic cargos. Adv Drug Delivery Rev (2021) 173:479–91. doi: 10.1016/j.addr.2021.04.012
287. Chen J, Liu R, Huang T, Sun H, Jiang H. Adipose stem cells-released extracellular vesicles as a next-generation cargo delivery vehicles: a survey of minimal information implementation, mass production and functional modification. Stem Cell Res Ther (2022) 13(1):182. doi: 10.1186/s13287-022-02849-5
288. Armstrong JP, Holme MN, Stevens MM. Re-engineering extracellular vesicles as smart nanoscale therapeutics. ACS Nano (2017) 11(1):69–83. doi: 10.1021/acsnano.6b07607
289. Man K, Brunet MY, Jones MC, Cox SC. Engineered extracellular vesicles: Tailored-made nanomaterials for medical applications. Nanomaterials (Basel) (2020) 10(9):1838. doi: 10.3390/nano10091838
290. Han Y, Jones TW, Dutta S, Zhu Y, Wang X, Narayanan SP, et al. Overview and update on methods for cargo loading into extracellular vesicles. Processes (Basel) (2021) 9(2):356. doi: 10.3390/pr9020356
291. Rajput A, Varshney A, Bajaj R, Pokharkar V. Exosomes as new generation vehicles for drug delivery: Biomedical applications and future perspectives. Molecules. (2022) 27(21):7289. doi: 10.3390/molecules27217289
292. Luan X, Sansanaphongpricha K, Myers I, Chen H, Yuan H, Sun D. Engineering exosomes as refined biological nanoplatforms for drug delivery. Acta Pharmacol Sin (2017) 38(6):754–63. doi: 10.1038/aps.2017.12
293. Shrivastava S, Morris KV. The multifunctionality of exosomes; from the garbage bin of the cell to a next generation gene and cellular therapy. Genes (Basel) (2021) 12(2):173. doi: 10.3390/genes12020173
294. You B, Xu W, Zhang B. Engineering exosomes: a new direction for anticancer treatment. Am J Cancer Res (2018) 8(8):1332–42.
295. Komuro H, Kawai-Harada Y, Aminova S, Pascual N, Malik A, Contag CH, et al. Engineering extracellular vesicles to target pancreatic tissue in vivo. Nanotheranostics (2021) 5(4):378–90. doi: 10.7150/ntno.54879
296. O'Brien K, Ughetto S, Mahjoum S, Nair AV, Breakefield XO. Uptake, functionality, and re-release of extracellular vesicle-encapsulated cargo. Cell Rep (2022) 39(2):110651. doi: 10.1016/j.celrep.2022.110651
297. Zhao Z, McGill J, Gamero-Kubota P, He M. Microfluidic on-demand engineering of exosomes towards cancer immunotherapy. Lab Chip (2019) 19(10):1877–86. doi: 10.1039/c8lc01279b
298. Butreddy A, Kommineni N, Dudhipala N. Exosomes as naturally occurring vehicles for delivery of biopharmaceuticals: Insights from drug delivery to clinical perspectives. Nanomaterials. (2021) 11(6):1481. doi: 10.3390/nano11061481
299. Blanc L, Vidal M. New insights into the function of rab GTPases in the context of exosomal secretion. Small GTPases (2018) 9(1-2):95–106. doi: 10.1080/21541248.2016.1264352
300. Gao Y, Qin Y, Wan C, Sun Y, Meng J, Huang J, et al. Small extracellular vesicles: A novel avenue for cancer management. Front Oncol (2021) 11:638357. doi: 10.3389/fonc.2021.638357
301. Juan T, Fürthauer M. Biogenesis and function of ESCRT-dependent extracellular vesicles. Semin Cell Dev Biol (2018) 74:66–77. doi: 10.1016/j.semcdb.2017.08.022
302. Allelein S, Medina-Perez P, Lopes ALH, Rau S, Hause G, Kölsch A, et al. Potential and challenges of specifically isolating extracellular vesicles from heterogeneous populations. Sci Rep (2021) 11(1):11585. doi: 10.1038/s41598-021-91129-y
303. Xu H, Liao C, Liang S, Ye BC. A novel peptide-equipped exosomes platform for delivery of antisense oligonucleotides. ACS Appl Mater Interfaces (2021) 13(9):10760–7. doi: 10.1021/acsami.1c00016
304. Harishkumar M, Radha M, Yuichi N, Muthukalianan GK, Kaoru O, Shiomori K, et al. Designer exosomes: Smart nano-communication tools for translational medicine. Bioengineering. (2021) 8(11):158. doi: 10.3390/bioengineering8110158
305. Börger V, Weiss DJ, Anderson JD, Borràs FE, Bussolati B, Carter DRF, et al. International society for extracellular vesicles and international society for cell and gene therapy statement on extracellular vesicles from mesenchymal stromal cells and other cells: considerations for potential therapeutic agents to suppress coronavirus disease-19. Cytotherapy. (2020) 22(9):482–5. doi: 10.1016/j.jcyt.2020.05.002
306. Gulfaraz K, Waqar A, Pretty SP. Exosomes and their role in viral infections. In: Jin W, editor. Novel implications of exosomes in diagnosis and treatment of cancer and infectious diseases. Rijeka: IntechOpen (2017). p. 4.
307. Nolte-'t Hoen E, Cremer T, Gallo RC, Margolis LB. Extracellular vesicles and viruses: Are they close relatives? Proc Natl Acad Sci U.S.A. (2016) 113(33):9155–61. doi: 10.1073/pnas.1605146113
308. Jia X, Yin Y, Chen Y, Mao L. The role of viral proteins in the regulation of exosomes biogenesis. Front Cell Infection Microbiol (2021) 11:671625. doi: 10.3389/fcimb.2021.671625
309. Rezaie J, Aslan C, Ahmadi M, Zolbanin NM, Kashanchi F, Jafari R. The versatile role of exosomes in human retroviral infections: from immunopathogenesis to clinical application. Cell Bioscie (2021) 11(1):19. doi: 10.1186/s13578-021-00537-0
310. Yang C, Chalasani G, Ng YH, Robbins PD. Exosomes released from mycoplasma infected tumor cells activate inhibitory b cells. PloS One (2012) 7(4):e36138. doi: 10.1371/journal.pone.0036138
311. Cronemberger-Andrade A, Xander P, Soares RP, Pessoa NL, Campos MA, Ellis CC, et al. Trypanosoma cruzi-infected human macrophages shed proinflammatory extracellular vesicles that enhance host-cell invasion via toll-like receptor 2. Front Cell Infect Microbiol (2020) 10:99. doi: 10.3389/fcimb.2020.00099
312. Quah BJ, O'Neill HC. Mycoplasma contaminants present in exosome preparations induce polyclonal b cell responses. J Leukoc Biol (2007) 82(5):1070–82. doi: 10.1189/jlb.0507277
313. Young L, Sung J, Stacey G, Masters JR. Detection of mycoplasma in cell cultures. Nat Protoc (2010) 5(5):929–34. doi: 10.1038/nprot.2010.43
314. Elzanowska J, Semira C, Costa-Silva B. DNA In extracellular vesicles: biological and clinical aspects. Mol Oncol (2021) 15(6):1701–14. doi: 10.1002/1878-0261.12777
315. Thakur BK, Zhang H, Becker A, Matei I, Huang Y, Costa-Silva B, et al. Double-stranded DNA in exosomes: a novel biomarker in cancer detection. Cell Res (2014) 24(6):766–9. doi: 10.1038/cr.2014.44
316. Malkin EZ, Bratman SV. Bioactive DNA from extracellular vesicles and particles. Cell Death disease (2020) 11(7):584. doi: 10.1038/s41419-020-02803-4
317. D'Acunzo P, Pérez-González R, Kim Y, Hargash T, Miller C, Alldred MJ, et al. Mitovesicles are a novel population of extracellular vesicles of mitochondrial origin altered in down syndrome. Sci Adv (2021) 7(7):eabe5085. doi: 10.1126/sciadv.abe5085
318. Amari L, Germain M. Mitochondrial extracellular vesicles – origins and roles. Front Mol Neurosci (2021) 14. doi: 10.3389/fnmol.2021.767219
319. Ranches G, Zeidler M, Kessler R, Hoelzl M, Hess MW, Vosper J, et al. Exosomal mitochondrial tRNAs and miRNAs as potential predictors of inflammation in renal proximal tubular epithelial cells. Mol Ther - Nucleic Acids (2022) 28:794–813. doi: 10.1016/j.omtn.2022.04.035
320. Amari L, Germain M. Mitochondrial extracellular vesicles - origins and roles. Front Mol Neurosci (2021) 14:767219. doi: 10.3389/fnmol.2021.767219
321. Lehrich BM, Liang Y, Khosravi P, Federoff HJ, Fiandaca MS. Fetal bovine serum-derived extracellular vesicles persist within vesicle-depleted culture media. Int J Mol Sci (2018) 19(11):3538. doi: 10.3390/ijms19113538
322. Shelke GV, Lässer C, Gho YS, Lötvall J. Importance of exosome depletion protocols to eliminate functional and RNA-containing extracellular vesicles from fetal bovine serum. J Extracell Vesicles (2014) 3. doi: 10.3402/jev.v3.24783
323. Kornilov R, Puhka M, Mannerström B, Hiidenmaa H, Peltoniemi H, Siljander P, et al. Efficient ultrafiltration-based protocol to deplete extracellular vesicles from fetal bovine serum. J Extracell Vesicles (2018) 7(1):1422674. doi: 10.1080/20013078.2017.1422674
324. Tosar JP, Cayota A, Eitan E, Halushka MK, Witwer KW. Ribonucleic artefacts: are some extracellular RNA discoveries driven by cell culture medium components? J Extracell Vesicles (2017) 6(1):1272832. doi: 10.1080/20013078.2016.1272832
325. Ramos-Zaldívar HM, Polakovicova I, Salas-Huenuleo E, Corvalán AH, Kogan MJ, Yefi CP, et al. Extracellular vesicles through the blood–brain barrier: a review. Fluids Barriers CNS (2022) 19(1):60. doi: 10.1186/s12987-022-00359-3
326. Heidarzadeh M, Gürsoy-Özdemir Y, Kaya M, Eslami Abriz A, Zarebkohan A, Rahbarghazi R, et al. Exosomal delivery of therapeutic modulators through the blood–brain barrier; promise and pitfalls. Cell Bioscie (2021) 11(1):142. doi: 10.1186/s13578-021-00650-0
327. Mustapic M, Eitan E, Werner JK Jr., Berkowitz ST, Lazaropoulos MP, Tran J, et al. Plasma extracellular vesicles enriched for neuronal origin: a potential window into brain pathologic processes. Front Neurosci (2017) 11:278. doi: 10.3389/fnins.2017.00278
328. Yang Y, Hong Y, Cho E, Kim GB, Kim IS. Extracellular vesicles as a platform for membrane-associated therapeutic protein delivery. J Extracell Vesicles (2018) 7(1):1440131. doi: 10.1080/20013078.2018.1440131
329. Haustein UF. Theoretical basis of immunosuppression in autoimmune diseases. Dermatol Monatsschr (1972) 158(8):547–71.
330. Pezzilli R. Immunosuppressive drugs for the treatment of autoimmune pancreatitis. Immunotherapy. (2014) 6(4):477–83. doi: 10.2217/imt.14.20
331. Richard-Eaglin A, Smallheer BA. Immunosuppressive/Autoimmune disorders. Nurs Clin North Am (2018) 53(3):319–34. doi: 10.1016/j.cnur.2018.04.002
332. Rossert J, Druet P. Immunosuppressive drugs and autoimmune diseases. Ann Biol Clin (Paris) (1991) 49(6):327–37.
333. El-Kadiry AE, Rafei M, Shammaa R. Cell therapy: Types, regulation, and clinical benefits. Front Med (Lausanne) (2021) 8:756029. doi: 10.3389/fmed.2021.756029
334. Mönch D, Reinders MEJ, Dahlke MH, Hoogduijn MJ. How to make sense out of 75,000 mesenchymal stromal cell publications? Cells. (2022) 11(9):1419. doi: 10.3390/cells11091419
335. Muthu S, Jeyaraman M, Kotner MB, Jeyaraman N, Rajendran RL, Sharma S, et al. Evolution of mesenchymal stem cell therapy as an advanced therapeutic medicinal product (ATMP)-an Indian perspective. Bioengineering (Basel) (2022) 9(3):111. doi: 10.3390/bioengineering9030111
336. Huang Y, Wu Q, Tam PKH. Immunomodulatory mechanisms of mesenchymal stem cells and their potential clinical applications. Int J Mol Sci (2022) 23(17):10023. doi: 10.3390/ijms231710023
337. Joseph J, John A. 7 - immunomodulatory properties of mesenchymal stem cells and hematopoietic stem cells–potential therapeutic target for COVID-19. In: Sharma CP, Agrawal DK, Thankam FG, editors. Stem cells and COVID-19. (United States: Academic Press) (2022). p. 95–109.
338. Jovic D, Yu Y, Wang D, Wang K, Li H, Xu F, et al. A brief overview of global trends in MSC-based cell therapy. Stem Cell Rev Rep (2022) 18(5):1525–45. doi: 10.1007/s12015-022-10369-1
339. García-Bernal D, García-Arranz M, Yáñez RM, Hervás-Salcedo R, Cortés A, Fernández-García M, et al. The current status of mesenchymal stromal cells: Controversies, unresolved issues and some promising solutions to improve their therapeutic efficacy. Front Cell Dev Biol (2021) 9:650664. doi: 10.3389/fcell.2021.650664
340. Jeppesen DK, Zhang Q, Franklin JL, Coffey RJ. Extracellular vesicles and nanoparticles: emerging complexities. Trends Cell Biol (2023), s0962-8924(23)00005-3. doi: 10.1016/j.tcb.2023.01.002
341. Claridge B, Lozano J, Poh QH, Greening DW. Development of extracellular vesicle therapeutics: Challenges, considerations, and opportunities. Front Cell Dev Biol (2021) 9:734720. doi: 10.3389/fcell.2021.734720
342. Gandham S, Su X, Wood J, Nocera AL, Alli SC, Milane L, et al. Technologies and standardization in research on extracellular vesicles. Trends Biotechnol (2020) 38(10):1066–98. doi: 10.1016/j.tibtech.2020.05.012
343. Gelibter S, Marostica G, Mandelli A, Siciliani S, Podini P, Finardi A, et al. The impact of storage on extracellular vesicles: A systematic study. J Extracell Vesicles (2022) 11(2):e12162. doi: 10.1002/jev2.12162
344. Jakl V, Ehmele M, Winkelmann M, Ehrenberg S, Eiseler T, Friemert B, et al. A novel approach for large-scale manufacturing of small extracellular vesicles from bone marrow-derived mesenchymal stromal cells using a hollow fiber bioreactor. Front Bioeng Biotechnol (2023) 11:1107055. doi: 10.3389/fbioe.2023.1107055
345. Miclau K, Hambright WS, Huard J, Stoddart MJ, Bahney CS. Cellular expansion of MSCs: Shifting the regenerative potential. Aging Cell (2023) 22(1):e13759. doi: 10.1111/acel.13759
346. Nelson BC, Maragh S, Ghiran IC, Jones JC, DeRose PC, Elsheikh E, et al. Measurement and standardization challenges for extracellular vesicle therapeutic delivery vectors. Nanomed (Lond) (2020) 15(22):2149–70. doi: 10.2217/nnm-2020-0206
347. Swatler J, Dudka W, Piwocka K. Isolation and characterization of extracellular vesicles from cell culture conditioned medium for immunological studies. Curr Protoc Immunol (2020) 129(1):e96. doi: 10.1002/cpim.96
348. McNamara RP, Dittmer DP. Modern techniques for the isolation of extracellular vesicles and viruses. J Neuroimmune Pharmacol (2020) 15(3):459–72. doi: 10.1007/s11481-019-09874-x
349. Moulin C, Crupi MJF, Ilkow CS, Bell JC, Boulton S. Extracellular vesicles and viruses: Two intertwined entities. Int J Mol Sci (2023) 24(2):1036. doi: 10.3390/ijms24021036
350. Ratajczak MZ, Ratajczak J. Extracellular microvesicles/exosomes: discovery, disbelief, acceptance, and the future? Leukemia (2020) 34(12):3126–35. doi: 10.1038/s41375-020-01041-z
351. Cauwels A, Tavernier J. Tolerizing strategies for the treatment of autoimmune diseases: From ex vivo to in vivo strategies. Front Immunol (2020) 11:674. doi: 10.3389/fimmu.2020.00674
352. Sadeghi S, Tehrani FR, Tahmasebi S, Shafiee A, Hashemi SM. Exosome engineering in cell therapy and drug delivery. Inflammopharmacology (2023), 1–25. doi: 10.1007/s10787-022-01115-7
353. Öztürk S, Elçin AE, Koca A, Elçin YM. Therapeutic applications of stem cells and extracellular vesicles in emergency care: Futuristic perspectives. Stem Cell Rev Rep (2021) 17(2):390–410. doi: 10.1007/s12015-020-10029-2
354. Ng CY, Kee LT, Al-Masawa ME, Lee QH, Subramaniam T, Kok D, et al. Scalable production of extracellular vesicles and its therapeutic values: A review. Int J Mol Sci (2022) 23(14):7986. doi: 10.3390/ijms23147986
355. Tsuchiya A, Terai S, Horiguchi I, Homma Y, Saito A, Nakamura N, et al. Basic points to consider regarding the preparation of extracellular vesicles and their clinical applications in Japan. Regener Ther (2022) 21:19–24. doi: 10.1016/j.reth.2022.05.003
356. Murphy DE, de Jong OG, Evers MJW, Nurazizah M, Schiffelers RM, Vader P. Natural or synthetic RNA delivery: A stoichiometric comparison of extracellular vesicles and synthetic nanoparticles. Nano Lett (2021) 21(4):1888–95. doi: 10.1021/asc.nanolett.1c00094
357. Bahmani L, Ullah M. Different sourced extracellular vesicles and their potential applications in clinical treatments. Cells. (2022) 11(13):1989. doi: 10.3390/cells11131989
358. Cho WJ, Ahn J, Lee M, Choi H, Park S, Cha KY, et al. Combinatorial effect of mesenchymal stem cells and extracellular vesicles in a hydrogel on cartilage regeneration. Tissue Eng Regener Med (2023) 20(1):143–54. doi: 10.1007/s13770-022-00509-6
359. Jarrige M, Frank E, Herardot E, Martineau S, Darle A, Benabides M, et al. The future of regenerative medicine: Cell therapy using pluripotent stem cells and acellular therapies based on extracellular vesicles. Cells (2021) 10(2):240. doi: 10.3390/cells10020240
360. Aheget H, Mazini L, Martin F, Belqat B, Marchal JA, Benabdellah K. Exosomes: Their role in pathogenesis, diagnosis and treatment of diseases. Cancers (Basel) (2020) 13(1):84. doi: 10.3390/cancers13010084
361. Shekari F, Nazari A, Assar Kashani S, Hajizadeh-Saffar E, Lim R, Baharvand H. Pre-clinical investigation of mesenchymal stromal cell-derived extracellular vesicles: a systematic review. Cytotherapy. (2021) 23(4):277–84. doi: 10.1016/j.jcyt.2020.12.009
362. Tieu A, Lalu MM, Slobodian M, Gnyra C, Fergusson DA, Montroy J, et al. An analysis of mesenchymal stem cell-derived extracellular vesicles for preclinical use. ACS Nano (2020) 14(8):9728–43. doi: 10.1021/acsnano.0c01363
363. Tieu A, Slobodian M, Fergusson DA, Montroy J, Burger D, Stewart DJ, et al. Methods and efficacy of extracellular vesicles derived from mesenchymal stromal cells in animal models of disease: a preclinical systematic review protocol. Syst Rev (2019) 8(1):322. doi: 10.1186/s13643-019-1242-y
364. Lui PPY, Leung YT. Practical considerations for translating mesenchymal stromal cell-derived extracellular vesicles from bench to bed. Pharmaceutics. (2022) 14(8):1684. doi: 10.3390/pharmaceutics14081684
365. Rezaie J, Feghhi M, Etemadi T. A review on exosomes application in clinical trials: perspective, questions, and challenges. Cell Commun Signal (2022) 20(1):145. doi: 10.1186/s12964-022-00959-4
366. Sanz-Ros J, Mas-Bargues C, Romero-García N, Huete-Acevedo J, Dromant M, Borrás C. Extracellular vesicles as therapeutic resources in the clinical environment. Int J Mol Sci (2023) 24(3):2344. doi: 10.3390/ijms24032344
367. Draguet F, Bouland C, Dubois N, Bron D, Meuleman N, Stamatopoulos B, et al. Potential of mesenchymal stromal cell-derived extracellular vesicles as natural nanocarriers: Concise review. Pharmaceutics (2023) 15(2):558. doi: 10.3390/pharmaceutics15020558
368. Dwivedi M, Ghosh D, Saha A, Hasan S, Jindal D, Yadav H, et al. Biochemistry of exosomes and their theranostic potential in human diseases. Life Sci (2023) 315:121369. doi: 10.1016/j.lfs.2023.121369
369. Karnas E, Dudek P, Zuba-Surma EK. Stem cell- derived extracellular vesicles as new tools in regenerative medicine - immunomodulatory role and future perspectives. Front Immunol (2023) 14:1120175. doi: 10.3389/fimmu.2023.1120175
370. Gomzikova MO, James V, Rizvanov AA. Therapeutic application of mesenchymal stem cells derived extracellular vesicles for immunomodulation. Front Immunol (2019) 10:2663. doi: 10.3389/fimmu.2019.02663
371. Lee I, Choi Y, Shin D-U, Kwon M, Kim S, Jung H, et al. Small extracellular vesicles as a new class of medicines. Pharmaceutics (2023) 15(2):325. doi: 10.3390/pharmaceutics15020325
372. Zhang W, Lin J, Shi P, Su D, Cheng X, Yi W, et al. Small extracellular vesicles derived from MSCs have immunomodulatory effects to enhance delivery of ASO-210 for psoriasis treatment. Front Cell Dev Biol (2022) 10:842813. doi: 10.3389/fcell.2022.842813
373. Cai H, Guo H. Mesenchymal stem cells and their exocytotic vesicles. Int J Mol Sci (2023) 24(3):2085. doi: 10.3390/ijms24032085
Keywords: autoimmune diseases (AIDs), immunological tolerance and regulation, cellular therapy, extracellular vesicles (EVs), mesenchymal stem/stromal cells (MSCs)
Citation: Haghighitalab A, Dominici M, Matin MM, Shekari F, Ebrahimi Warkiani M, Lim R, Ahmadiankia N, Mirahmadi M, Bahrami AR and Bidkhori HR (2023) Extracellular vesicles and their cells of origin: Open issues in autoimmune diseases. Front. Immunol. 14:1090416. doi: 10.3389/fimmu.2023.1090416
Received: 05 November 2022; Accepted: 20 February 2023;
Published: 08 March 2023.
Edited by:
Amy Rosenberg, EpiVax, United StatesReviewed by:
Steven Bauer, Wake Forest University, United StatesSherri L. Christian, Memorial University of Newfoundland, Canada
Copyright © 2023 Haghighitalab, Dominici, Matin, Shekari, Ebrahimi Warkiani, Lim, Ahmadiankia, Mirahmadi, Bahrami and Bidkhori. This is an open-access article distributed under the terms of the Creative Commons Attribution License (CC BY). The use, distribution or reproduction in other forums is permitted, provided the original author(s) and the copyright owner(s) are credited and that the original publication in this journal is cited, in accordance with accepted academic practice. No use, distribution or reproduction is permitted which does not comply with these terms.
*Correspondence: Ahmad Reza Bahrami, ar-bahrami@um.ac.ir; Hamid Reza Bidkhori, bidkhori@acecr.ac.ir