- 1State Key Laboratory of Animal Nutrition, Beijing Engineering Technology Research Center of Raw Milk Quality and Safety Control, College of Animal Science and Technology, China Agricultural University, Beijing, China
- 2Faculty of Veterinary and Animal Sciences, The University of Agriculture, Dera Ismail Khan, Pakistan
- 3Anhui Province Key Laboratory of Embryo Development and Reproduction Regulation, Anhui Province Key Laboratory of Environmental Hormone and Reproduction, School of Biological and Food Engineering, Fuyang Normal University, Fuyang, China
- 4Genome Analysis Laboratory of the Ministry of Agriculture, Agricultural Genomics Institute at Shenzhen, Chinese Academy of Agricultural Sciences, Shenzhen, China
Bovine mastitis, the inflammation of the mammary gland, is a contagious disease characterized by chemical and physical changes in milk and pathological changes in udder tissues. Depressed immunity and higher expression of inflammatory cytokines with an elevated milk somatic cell count can be observed during mastitis in dairy cattle. The use of somatic cell count (SCC) and somatic cell score (SCS) as correlated traits in the indirect selection of animals against mastitis resistance is in progress globally. Traditional breeding for mastitis resistance seems difficult because of the low heritability (0.10-0.16) of SCC/SCS and clinical mastitis. Thus, genetic-marker-selective breeding to improve host genetics has attracted considerable attention worldwide. Moreover, genomic selection has been found to be an effective and fast method of screening for dairy cattle that are genetically resistant and susceptible to mastitis at a very early age. The current review discusses and summarizes the candidate gene approach using polymorphisms in immune- and inflammation-linked genes (CD4, CD14, CD46, TRAPPC9, JAK2, Tf, Lf, TLRs, CXCL8, CXCR1, CXCR2, C4A, C5, MASP2, MBL1, MBL2, LBP, NCF1, NCF4, MASP2, A2M, and CLU, etc.) and their related signaling pathways (Staphylococcus aureus infection signaling, Toll-like receptor signaling, NF-kappa B signaling pathway, Cytokine-cytokine receptor, and Complement and coagulation cascades, etc.) associated with mastitis resistance and susceptibility phenotypic traits (IL-6, interferon-gamma (IFN-γ), IL17, IL8, SCS, and SCC) in dairy cattle.
1. Background
Normally, the mammary gland performs three functions, including the provision of nutrition to offspring in the form of milk, the transfer of immunity from mother to offspring through immunoglobulins in milk, and the provision of protection against microbes (1, 2). Bovine mastitis, the inflammation of mammary glands, occurs when the udder is exposed to any physical injury or pathogenic microorganisms (3). Bacterial pathogens, upon entry, compromise the immunity of the mammary gland and trigger the abnormal regulation of the immune system, followed by inflammatory changes, resulting in mastitis (4).
Based on clinical signs, mastitis can be divided into two types, i.e., clinical and sub-clinical mastitis (5–8). Clinical mastitis is characterized by visible signs of inflammation in the udder and microbiological physical, and chemical changes in the milk (9–11). Escherichia coli is one of the major bacteria responsible for acute or clinical mastitis, while subclinical mastitis is caused by the gram-positive Staphylococcus aureus (12–14), which is characterized by a marked decrease in milk quantity and quality (15). The other signs of subclinical mastitis are the increase in milk SCC level, which shows the level of leucocytes and epithelial cells in milk (16). Elevated levels of leukocytes are the primary indicator of mammary infection.
An SCC of more than 200,000 cells/mL is an indication of mammary gland infections, while less than 100,000 cells/mL indicates that the cow is uninfected (17). Thus, increased levels of SCC are considered a sign of mastitis. The SCC and/or log-transformed SCC (somatic cell score, SCS) are widely targeted as early indicators of mastitis (3) because of a strong positive genetic correlation (0.6 to 0.90) between mastitis and milk SCC (18–20). The SCC and SCS have comparatively higher heritability than mastitis (21) and are therefore widely targeted in mastitis control by selecting cows with low SCS/SCC (22). However, increased SCC in early lactation can signify the presence of intra-mammary infection, and in many countries, indirect selection against mastitis using milk SCC is practiced (23). However, in the early phases of infectivity, the neutrophil, including the level of inflammatory cytokines, increases more rapidly than the total SCC (24). Moreover, resistance to the pathogenesis of mastitis is a complicated biological mechanism involving various molecules, cells, and pathways (25). That’s why, nowadays, people are more interested in the increasing cells and cytokine levels in milk and blood rather than just the overall SCC, which may show the status of udder health at an earlier stage (26).
The epithelial cells of the inner surface of the mammary gland play a key role in recognizing mastitis-causing pathogens by synthesizing toll-like receptors (TLR2 & TLR4) (27, 28). Upon activation, TLRs further regulate nuclear factor-κB, which translocates into the nucleus and causes the mediation of pro-inflammatory signaling molecules (tumor necrosis factor-α (TNF-α), IL-1β and IL-6, and IL-8) that are essential for the animal’s local and systemic immune reactions (27, 29–31). It has been well-studied that serum cytokines, such as interferon, tumor necrosis factor, IL17, IL6, and IL4, have a key role in inflammatory circumstances, which suggests their possible role in bovine mastitis (32–35). Furthermore, previous studies have also suggested that in addition to SCC and SCS, serum cytokines could also be considered indirect parameters in the control strategies against bovine mastitis (36–38).
The control of mastitis using traditional selection methods is quite challenging due to the low heritability of indirect mastitis resistance phenotypic traits (SCC, SCS, and inflammatory cytokines) and host genetics (39). The use of milk SCC as a surrogate trait for raising mastitis resistance in cattle has achieved limited outcomes (36), thus, the information on molecular markers for mastitis susceptibility/resistance is valuable in identifying genetically mastitis-resistant cattle (40, 41). Due to the limited and slow progress in improving udder health using conventional selection procedures with indirect traits, demand has increased for information on molecular markers to enhance host genetics against mastitis in cattle breeding (4, 42).
Usually, two types of candidate gene approaches (direct and indirect) are used while looking for genetically mastitis-resistant cattle (43–45). The first approach is the use of linked markers (indirect), which are markers that are closed to the gene or QTL that has a significant role in mastitis. The second is the use of functional or direct genetic markers, in which the polymorphisms in genes associated with variation in mastitis resistance phenotypic traits are targeted (43, 46). It is well documented that many genes control mastitis, and all of them contribute considerably to either mastitis resistance or development, so, it is necessary to consider the combination of genes for mastitis resistance because some genes have little effect. It has been documented that the immune and inflammatory response to bacteria is usually regulated by inflammation- and immune-associated genes (47).
So far, several genetic polymorphisms in inflammation- and immune-related genes have been identified for their possible association with mastitis resistance phenotypic traits (33, 34, 37, 40, 41, 46, 48–50). In the current review, we discuss all the polymorphisms in immune- and inflammation-associated genes that are linked to mastitis resistance phenotypic traits (SCC, SCS, IL-6, IL8, IL17, and IFN-gamma). Based on the current review, we suggest that all the highlighted SNPs of the genes discussed could be considered potential genetic markers for mastitis resistance in dairy cattle.
2. Methods for collection of literature studies
For this review, we used Google Scholar, Web of Science, and PubMed. Furthermore, we used NCBI to verify the information regarding the genes, such as the number of exons and chromosome location. We only considered data published in the English language and in SCI journals. In addition, we used data that was published between 2000 and 2022. Only the polymorphisms located in exonic regions, promoters, or 5 or 3 untranslated regions of genes were utilized in the current study. Finally, we used the online software, DAVID for biological signaling pathways. The keywords, such as SCS, SCC, inflammatory cytokines, immune- and inflammation-associated genes, and polymorphisms were considered while collecting literature for the current review.
3. Genetic polymorphisms of genes associated with mastitis resistance/susceptibility in dairy cattle
The detection of single nucleotide polymorphisms (SNPs) in genes regulating the mammary gland’s innate immunity in response to pathogens has attracted considerable attention within the field of genetic markers in mastitis control research (51–55). Several immune- and inflammation-associated genes and their polymorphisms have been discovered for their association with bovine mastitis susceptibility/resistance (33, 56–60). All the reported polymorphisms in immune- and inflammation-associated genes are summarized in Table 1.
3.1. Innate immune-compartment-associated genes
3.1.1. Bovine peptidoglycan recognition protein 1 (PGLYRP1)
Bovine peptidoglycan recognition protein 1 located on chromosome 18, having 3 exons, has a significant role in regulating inflammation, response to infection, and post-infection healing (97). Because PGLYRP1 works as a receptor for murein peptidoglycans (PGN) of gram-positive and gram-negative bacteria, it is considered a key player in the activation of innate immunity (92). Considering its important role in immunity and inflammation, PGLYRP1 has been widely targeted in bovine mastitis research (92, 97). A higher expression of PGLYRP1 has been documented in cows with mastitis (36, 97–99). Consequently, the polymorphisms (G + 102C, T -12G) in PGLYRP1 and SNP-C+4867T in PGLYRP2 in the exonic region showed an association with milk SCS in dairy cattle (100, 101). Moreover, a study reported that the polymorphism rs68268284 at exon 1 of PGLYRP1 correlated with milk SCC (92), as mentioned in Table 1.
3.1.2. Calcium channel, voltage-dependent, alpha-2/delta subunit 1 (CACNA2D1)
Calcium channel, voltage-dependent, alpha-2/delta subunit 1 residing on chromosome 4, containing 42 exons, is another key genetic signature with an important role in clinical mastitis. It has been documented that SNP-G519663A at exon 18 (80), SNP-G519663A, and SNP-A526745G at exon 24 in the bovine CACNA2D1 gene were significantly linked with lower milk SCS levels and mastitis resistance in dairy cattle (82, 102). Moreover, it has also been noticed that SNP G38819398A at exon 18 (81), variant-T38826986G at exon 19 (103), and SNP-C367284A of CACNA2D1 (104) were positively linked with milk SCS in Sahiwal and Karan Fries. The above findings show that CACNA2D1 could be a potential genetic marker against mastitis resistance in dairy cattle.
3.1.3. Mannose-binding lectin (MBL)
Mannose-binding lectin is a critical player belonging to the collectin protein family that attaches to a variety of microorganisms and regulates the innate immunity lectin-complement pathway (69). MBL-A and MBL-C proteins are encoded by the MBL1 and MBL2 genes respectively. The reduced level of MBL may expose dairy cattle to various infections, including mastitis (66). Similarly, a study reported a strong correlation of SNP g.2651G>A in the MBL gene with milk SCS, suggesting its possible role in mastitis resistance (65). In addition, other studies have also reported the significant association of SNPs in the MBL with milk SCS, an essential phenotypic indicator of bovine mastitis (66, 70).
3.1.4. Mannose-binding lectin-associated serine protease 2 (MASP2)
Mannose-binding lectin-associated serine protease 2 which is located on chromosome 16 and contains 11 exons, is considered the key protease of the complement system. The MASP2 gene plays an important role in innate immunity and creates resistance to infections of the mammary gland (105) and is therefore widely studied for its link with mastitis in dairy cattle (83, 105). The polymorphism G553A in MASP2 was found to be associated with mastitis resistance and lower milk SCC in Chinese Holsteins (83). Similarly, another study also reported that the polymorphisms (g.14047A > C, g.14248T > C, and g.14391C > T) in MASP2 had a significant correlation with lower milk SCC and mastitis resistance in dairy cattle (105), as shown in Table 1.
3.1.5. Lactoferrin (Lf)
Lactoferrin is an important, iron-binding glycoprotein member of the serum-transferring protein family, which is produced by the mammary gland and immune cells and distributed in external secretions, such as milk, tears, and polymorphonuclear neutrophil (PMN) cells and plays a crucial role in eliminating bacterial load in certain organs. The Lf has several biological functions, including immunity and bacteriostatic activities, which protect the udder from pathogenic infections (106). A review article comprehensively highlighted the role of lactoferrin in bovine mastitis (107). The increased mRNA expression of Lf and the elevated level of SCC in the mammary gland with mastitis indicate their role in acute phase response in the mammary gland during mastitis (108). Similarly, another study reported a higher Lf expression during mammary gland infection (109). Recently, several reports have proven the association of genetic polymorphisms in the bovine Lf gene with mastitis susceptibility (53, 110–116). One study documented that SNPs (SNP −190 G>A, −270 T > C, and −190 G >A) in the bovine lactoferrin gene were associated with milk SCS. Furthermore, it has been demonstrated that a higher expression of this gene may render dairy cows susceptible to mastitis (78).
3.1.6. Transferrin (Tf)
Transferrin is a β-globulin protein involved in iron ion transportation and plays a significant role in the regulation of innate immunity against microbial pathogens and blocked pathogenic access to iron (117, 118). Being a key player in immunity, Tf has been studied for its association with mastitis resistance in dairy cattle (79). In addition, it has been documented that cows with SNP g.13942T>C in Tf show the lowest milk SCS level. Moreover, they noticed a higher expression of Tf in the mammary glands of cows with mastitis, which suggests its crucial role in mastitis resistance (79).
3.1.7. Neutrophil cytosolic factor 4 (NCF4)
Calcium channel, voltage-dependent, alpha-2/delta subunit 1 is located on bovine chromosome 5 and contains 9 exons. Being a key component of the nicotinamide dinucleotide phosphate (NADPH) oxidase complex, NCF4 plays an important role in the regulation of biochemical pathways and innate immune responses against microbial infection (119). The role of the NCF4 has been well studied in bovine mastitis research (76, 77). Ju et al. reported that the polymorphism g.18174 A>G on exon 9 of NCF4 showed a noteworthy relationship with high milk SCC levels and is responsible for the mastitis susceptibility of Chinese Holsteins (77). Another study found an SNP on g.18475 A>G in the 3′ UTR of NCF4, which was linked with higher levels of milk SCC, suggesting its critical role in mastitis susceptibility (76). It has been well established that, upon challenge with bacteria, the microRNAs (miRNAs) were significantly expressed in the mammary gland, which shows their role in the regulation of host immunity (120). Several studies have documented the role of miRNAs in bovine mastitis and host immune regulation against pathogenic infections (76, 96, 121–125). Consequently, a study reported that the miRNAs-mRNA interaction significantly regulates the expression of NCF4, which is linked with mastitis susceptibility and host immunity (76). Similarly, the SNP g.10766 T>C in NCF1 caused the aberrant splice variant NCF1-TV1 production, which showed an association with high milk SCS in dairy cattle (126).
3.1.8. Bovine Alpha-2-macroglobulin (A2M) gene
Bovine Alpha-2-macroglobulin gene has 38 exons and is located on chromosome 5. By binding with proteases, alpha-2-macroglobulin (A2M) in the plasma and tissues of vertebrates and invertebrates acts as a defense barrier against pathogens (127). Recently, a study briefly explored the role of the A2M gene in immunity, inflammation, and infectious diseases (128). As mastitis is characterized by immune depression followed by inflammatory changes, making it a key player in immunity and inflammation, A2M has been widely studied in mastitis susceptibility in dairy cattle research (127, 129). The SNP c.3535A> T at exon 29 of the A2M gene caused the aberrant splice variant A2M-AS4 production, which exposes dairy cattle mastitis (127). The Bta-miR-2898 was found to be up-regulated in cows with mastitis when compared with healthy cows. It was experimentally proven that polymorphism at point c.4659_4661delC of the A2M gene significantly influences the target bta-miR-2898 binding affinity. This shows that it might be possible that A2M has a significant association with mastitis susceptibility (129).
3.1.9. Cluster of differentiation 4 (CD4) gene
During mastitis, the inflammatory cells are recruited to the site of infection, where T cells, particularly CD4 cells, were predominantly observed (130). Polymorphisms in CD4 and STAT5B genes and their link with mastitis resistance phenotypic traits have been well studied (49). The polymorphism in CD4 at loci g.13598C>T has been identified for its significant association with SCS, which is a crucial indicator of mastitis. Additionally, the study reported that although polymorphisms in STAT5B did not show any link with mastitis, when a combination analysis was conducted with CD4 gene polymorphisms, it was noticed that the combination of both genes showed a considerable effect on SCS (49). In addition, a study has reported a significant association of polymorphisms at point T104010752C and C104028410T in CD4 and LAG3 genes, respectively, with milk SCC (131). Based on the published studies, it can be concluded that CD4 might be a valuable addition to the genetic markers for mastitis resistance in dairy cattle.
3.2. Genes encoding pathogen recognition receptors
3.2.1. Toll-like receptors
Toll-like receptors, the family of recognition patterns, are associated with the regulation of innate immunity (132, 133). Recently, various reports have been published on the role of TLRs in mastitis (134–141). The toll-like receptors on mammary epithelial cells show early expression during mammary infection because of interaction with microbial pathogens (142). Upon activation, TLR regulates the expression of several chemokines and pro-inflammatory cytokines, which further facilitate the recruitment of neutrophils and activate innate and acquired immune responses (53). The SNPs within the pattern recognition receptors (PRR) may alter the host response to pathogens and lead to either mastitis resistance or susceptibility (71).
Bovine TLR4, which is located on chromosome 8, contains 4 exons, and is a type I transmembrane protein and member of the TLR family, has been identified as a key pattern recognition receptor (PRR) (143). The TLR4 regulates innate immune system cells when stimulated by pathogen-associated molecular patterns (PAMPs) of foreign microorganisms, including viruses, fungi, and bacteria (144). Furthermore, Wang et al. (143) reported that the LPS, which is considered an essential PAMPS, could be found in most gram-negative and some gram-positive bacteria that interact with TLR4 in mammary epithelial cells, which is why the higher expression of TLR4 has been noticed during mastitis in dairy cattle (143). A study reported that SNP- rs8193069 in the TLR4 gene was significantly associated with higher milk SCC (145, 146). Similarly, another study found that the SNP- 1,397-C-T at exon 3 of TLR4 correlated with milk SCS in Chinese Holstein, Sanhe cattle, and Chinese Simmental (145, 147). Furthermore, Mesquita et al. (71) documented that SNP at point rs8193060 G>A in TLR4 was significantly associated with the lowest milk SCS level in Brazilian Holsteins. From the above studies, we concluded that TLR4 has a critical role in mastitis development, and it might, therefore, be considered a potential genetic marker for mastitis resistance.
The polymorphism (SNP -79 T > G) in bovine TLR1 predisposes dairy cattle to mammary infection due to the excessive activation of NF-κB signaling and poor immune response to pathogens (148). Furthermore, it has been revealed that mutation in TLR1 also influences the expression of CXCL8 (IL-8) (149), TLR2, and IL-6 (34), and high expression of these genes has been reported in the mammary epithelial cells of cows with mastitis. Besides, TLR1 can establish heterodimers with TLR2 (150) to widen the recognized ligands. Pant et al. revealed that mutations in TLR2 and caspase recruitment domain 15 (CARD15) are associated with milk SCS and increase the susceptibility of cattle to mastitis. TLR2 and CARD15 are key pattern recognition receptors that play an essential role in the stimulation of inflammatory and immune response (151, 152). A study documented that SNP T385G at exon 2 in the TLR2 gene is linked with high milk SCS and mastitis susceptibility (72).
3.2.2. Lipopolysaccharide-binding protein (LBP)
Lipopolysaccharide-binding protein is another critical gene that has a vital role to play in the innate immune recognition of gram-negative bacteria in dairy cattle. The gram-negative bacteria has been documented to have an essential role in mastitis (87). LBP is the critical protein that combines with LPS, a primary plotter from gram-negative bacteria with the ability to activate inflammation in animals. Furthermore, by binding with LPS, the LBP is presented to CD14 + cells, resulting in the regulation of the TLR4 pathway. The TLR4 pathway further activates the pro-inflammatory response caused by tumor necrosis factor-a (TNF-a), interleukin (IL) -1, or IL-6. In addition, with the help of the soluble form of CD14, the LPS-LBP complex also activates the CD14 cell inflammatory response, which shows that LBP could significantly contribute to blocking the inflammatory cascade before the release of inflammatory cytokines. Cheng et al. (87) reported that mutations in the LBP gene have a significant association with mastitis in dairy cattle, as shown in Table 1.
3.3. Cytokines encoded genes and their receptors associated with mastitis
The inflammatory cytokines and their associated genes have attracted considerable attention in mastitis research (38). Interleukin-8 receptor α (IL-8RA), coded by the chemokine receptor CXCR1, is located on the surface of neutrophil and connects the pro-inflammatory IL-8 with high affinity and is, therefore, targeted widely as a potential marker in mastitis control research (153). Due to the immunosuppressive nature of S. aureus-mastitis, IL8RA plays an important role in the control of bovine mastitis by enhancing immunity (154). IL8, also called CXC chemokine ligand 8 (CXCL8), is located on chromosome 6 and has 4 exons. IL8 is considered a potent mediator of inflammation and is also involved in the recruitment of leukocytes to sites of infection (155). The polymorphisms in the IL8 gene have been the focus of some mastitis research (156, 157). A polymorphism at +472 A>G in IL8 was reported to be associated with high milk SCC in S. aureus mastitis-infected dairy cattle (153). Moreover, the SNP -105G>A in IL8 has been found to be associated with high milk SCS, immunity enhancement, and mastitis resistance (85). It has been documented that the mutation at point +735 G>C could change the amino acid glutamine to histidine in the amino chain of CXCR2, which is linked to calcium signaling and G-protein interaction and has a key role in mastitis (158).
The CXCR1 gene, which coded interleukin 8 receptor α (IL8RA) and has the potential to bind IL8 with high affinity, is located on the surface of neutrophil surface (159, 160). The role of CXCR1 has been well-studied in mastitis resistance in dairy cattle research (158). Bacteria activate the CXCR1 after interaction with TLR4, which further regulates the NF-κB signaling. The NF-κB translocates to the nucleus, binds with DNA, and causes the expression of the CXCR1 gene. Furthermore, the interaction of CXCR1 or CXCR2 with IL-8 brings changes in neutrophils, which allow their chemotaxis toward the infection in the mammary gland (161), mediates the cell survival and migration, and increases the activity of phagocytosis (162, 163). Several polymorphisms in CXCR genes have been identified so far for their possible role in mastitis resistance. Polymorphisms (SNPs -1830AA, -1768TT, and -344TT) (164) and mutation at point (SNP -1768T>A) (165) in the CXCR1 gene were linked with high milk SCC in dairy cattle. Similarly, another study also reported the significant association of SNP c.337A>G and c.365C>T in CXCR1 with the milk SCC, suggesting their role in the host response against mastitis (166). A study found that the CXCR1+472 variant was significantly linked to milk SCS and increases the susceptibility to S. aureus-mastitis in dairy cattle (153). Similarly, other studies have also reported a strong link between CXCR1 mutations c.980AG, c.735C>G, CXCR1+472, CXCR1+777, and CXCR1−1768 at position 5′ upstream region with milk SCC in dairy cattle (153, 165, 167–169). Based on published research, it can be concluded that CXCR1 and its reported polymorphisms might be considered potential markers for mastitis resistance/susceptibility in dairy cattle.
The bovine IL-17 located on chromosome 23, containing 3 exons, is another critical gene studied for its important role in immunity and inflammation pathology (170). Comprehensive reviews have been published on the role of IL-17 in the mediation of immunity and inflammation (171, 172). Furthermore, it has been demonstrated that the members of the IL-17 family have a key function in acute and chronic inflammation and have been associated with enhancing the host’s defense against microbial organisms (173, 174). Hu et al. reported that the expression of IL-17 was significantly up-regulated upon challenging the mammary glands of mice with lipopolysaccharide (LPS). The elevated level of IL-17 is also associated with the regulation of nuclear factor-κB (NF-κB) signaling, which is crucial in mastitis susceptibility. The blockage of IL-17 with the anti-IL-17A antibody has been shown to protect dairy cows from LPS-induced mastitis by suppressing the pro-inflammatory cytokine levels, myeloperoxidase activity, and neutrophil infiltration and NF-κB signaling pathway (175). In 2017, Usman and his co-workers found polymorphism (24392436C/T) in IL-17F and mutation (24345410 A > G) in IL-17A, which shows an association between the regulation of immunity and inflammation signaling and high milk SCS in both Chinese Holstein and Sanhe cattle (38). Furthermore, the polymorphism (1578A>G) in IL17A regulated the milk SCC and, thus, their expression might be a potential marker for mastitis susceptibility (91). The IL-17A production was documented during S. uberis mastitis (176), and slightly increased expression was also noticed in S. aureus-infected cows’ somatic cells (177). Furthermore, an in-vitro study illustrated that the IL-17A reinforces the ability of mammary epithelial cells (MEC) to resist the consequences of S. aureus (178). Additionally, it has been found that IL-17F and IL-17A are significantly regulated in mammary tissue in response to E.coli (179). Moreover, Roussel and his co-workers experimentally proved that IL-17 F and IL-17A could play an essential role in regulating host-pathogen relations during mastitis development (179). In addition, a study reported that IL-17 positively regulates CD4+ T cells to facilitate the immune system against pathogenic infection (180).
3.4. The association of genetic polymorphisms in JAK-STAT pathway genes with mastitis
The JAK-STAT has been widely studied for its critical role in immunity and inflammation (181, 182), and evidence indicates that persistent activation of this pathway might lead to many immunity- (183) and inflammation-related diseases (184, 185). Due to its significant role in immunity, cell proliferation, cell differentiation, and inflammation, the JAK-STAT pathway has been widely targeted for therapeutic purposes in several diseases (186). Furthermore, playing a critical role in mammary gland development, any abnormal regulation of the JAK-STAT pathway may disturb normal function, resulting in impairment of mammary gland development and exposure to mammary infections. As mastitis is an immunity and inflammatory-related disease, the JAK-STAT pathway should be explored in mastitis control research (187). JAK2 and STAT5A & B are the key parts of JAK-STAT signaling, which have been recently studied for their association with mastitis resistance (32, 34, 37, 48, 95). Furthermore, it has been noticed that the polymorphism at point 39630048C/T in JAK2 was associated with interleukin-17 (IL-17) (34), IL-6 and IFN-γ (32). In addition, the SNPs (39652267A/G, 39631175T/C) in the JAK2 gene were documented for their significant links with milk SCC, IL-6, and IFN-γ (32, 34). The mutation (39631044G/A) in the Jak2 gene was noticed to be significantly associated with milk SCS in Chinese Holstein (34). Moreover, the variation at point 39645396C/T in the JAK2 gene was linked to milk SCC, IL-6, and IFN-γ (37), while SNP-39631044G/A in JAK2 was associated with milk SCS (34). Consequently, it is predicted that the SNP (39645396C/T), which regulates the production of inflammatory cytokines, is responsible for changing the amino acid from lysine to asparagines and can, therefore, be targeted as a functional candidate marker for mastitis resistance (37). Other studies also reported a higher expression of IL-6 in Plasma cell mastitis (PCM), which indicates that the IL-6/STAT3 pathway could play a vital role in the pathogenesis of PCM (188, 189).
A variety of cytokines and growth factors activate STATs, which are a family of latent transcription factors. Members of the STATs family are involved in growth, differentiation, survival, and apoptosis. Among the seven members of the STATs family (STAT1-4, 5a, 5b, and 6) in mammalian cells, STAT5A and STAT5B are the most closely linked and are the result of duplication (190). STAT5, a primary gene of the JAK/STAT inflammation signaling pathway, has an essential role to play in prolactin-induced mammary gland development and is associated with mammary gland development in transgenic mice (191). A few studies noticed a significant association of polymorphism in the STAT5A and STAT5B genes with mastitis resistance phenotypic traits (34, 37). They documented that polymorphism in STAT5A (43046497A/C) was associated with IL-6 and also changed the amino acid isoleucine to valine (34). Similarly, mutation at point 43673888A>G in the STAT5B gene was significantly linked to mastitis resistance phenotypic traits (IL-4 and SCC) (37). Bochniarz et al. reported the elevated level of IL-6 and the reduced level of IL-4 in the milk and serum of cows infected with S. aureus (192). Khatib et al. noticed that variant 12195T/C in STAT5A was significantly linked to a decrease in milk fat and protein percentages as well as levels of SCS in dairy cattle (193). Based on the above-published data, it can be concluded that JAK-STAT signaling plays a key role in immunity and inflammation; thus, the polymorphisms in the genes of JAK-STAT signaling might be valuable additions to the genetic markers for increasing genetic mastitis resistance in dairy cattle.
4. Bioinformatic analysis to find the biological signaling pathways for the above-mentioned genes
Bioinformatics analysis was performed to discover the significantly regulated biological signaling pathways of the above-mentioned genes. For this purpose, we collected all the genes (mentioned in Supplementary Table 1) with their Ensembl IDs (already discussed in the current review article) and uploaded them to the online DAVID software (https://david.ncifcrf.gov/tools.jsp) (194). Through bioinformatics analysis, we reported several immunity and inflammatory signaling pathways (Supplementary Table 2), of which, staphylococcus aureus infection, chemokine signaling pathway, toll-like receptor signaling pathway, complement and coagulation cascades, cytokine-cytokine receptor interaction, and NF-kappa B signaling pathway were found for their involvement in mastitis (Table 2). In addition, the regulation mechanisms of staphylococcus aureus infection, chemokine signaling pathway, and toll-like receptors signaling pathways are shown in Figures 1–3 respectively.
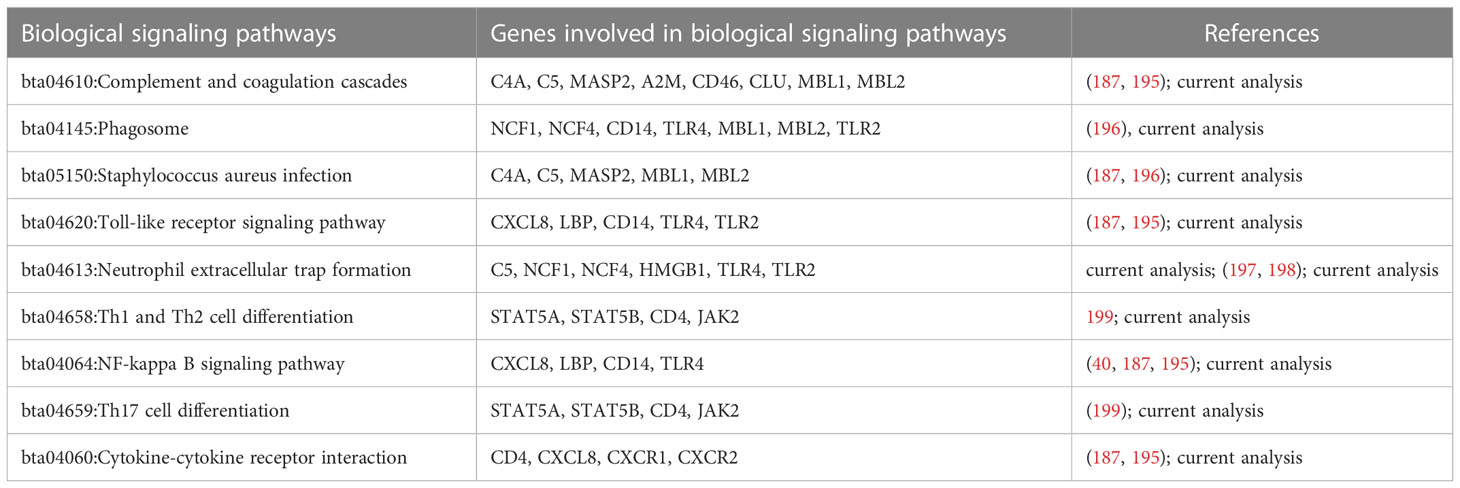
Table 2 Immune- and inflammation-associated genes and their biological signaling pathways linked with bovine mastitis.
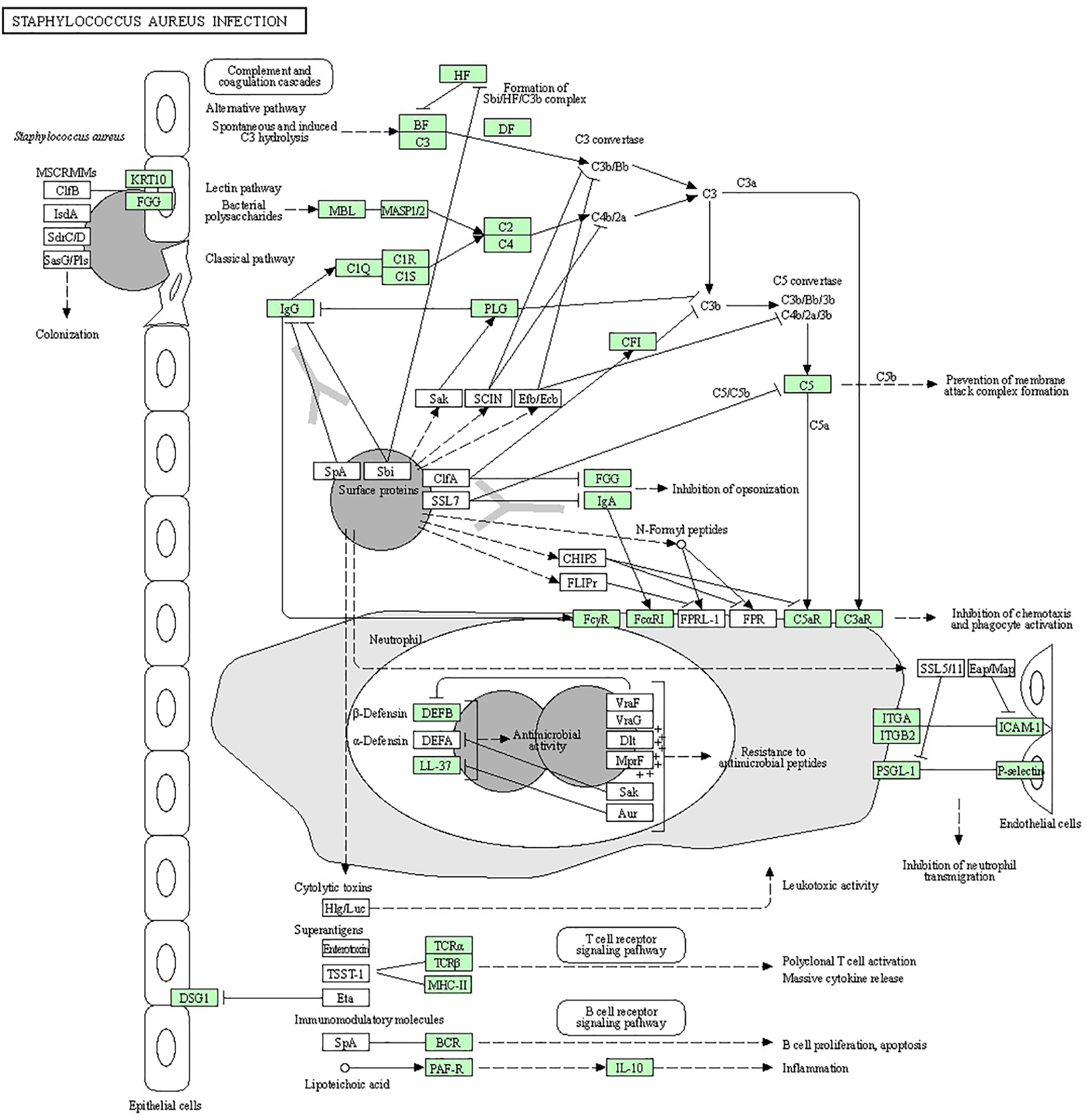
Figure 1 Staphylococcus aureus can cause multiple forms of infections ranging from superficial skin infections to food poisoning and life-threatening infections. The organism has several ways to divert the effectiveness of the immune system: secreting immune-modulating proteins that inhibit complement activation and neutrophil chemotaxis or lysis, modulating sensitivity to cationic antimicrobial peptides (such as defensin) by increasing the positive net charge of its cytoplasmic membrane, and the expression of superantigens that prevent the development of a normal immune response or cause an emetic response when ingested.
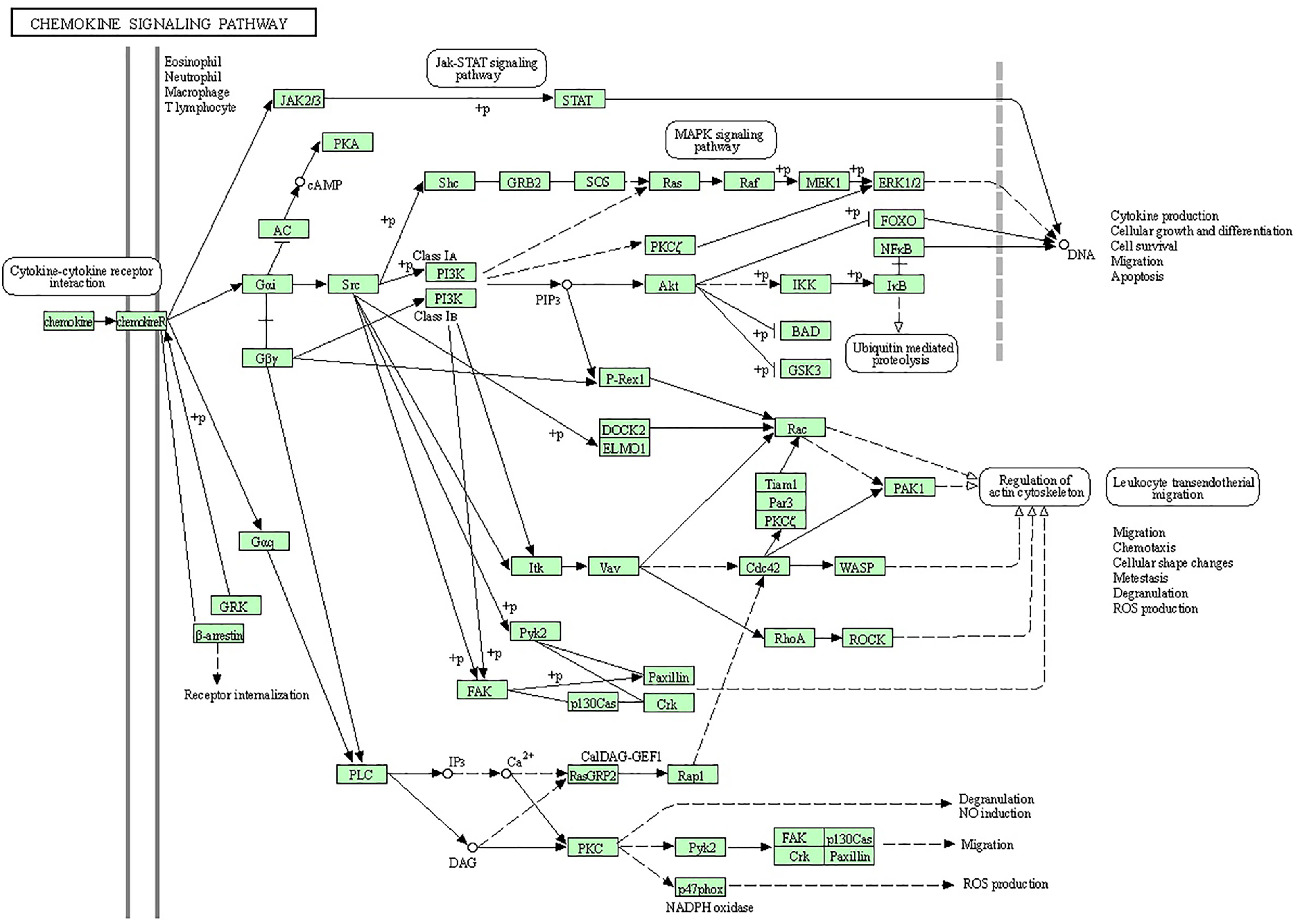
Figure 2 Inflammatory immune response requires the recruitment of leukocytes to the site of inflammation upon foreign insult. Chemokines are small chemoattractant peptides that provide directional cues for cell trafficking and are therefore vital for protective host response. In addition, chemokines regulate a plethora of biological processes of hematopoietic cells, leading to cellular activation, differentiation, and survival. The chemokine signal is transduced by chemokine receptors (G-protein coupled receptors) expressed on the immune cells. After receptor activation, the alpha- and beta-gamma-subunits of G protein dissociate to activate diverse downstream pathways, resulting in cellular polarization and actin reorganization. Various members of small GTPases are involved in this process. The induction of nitric oxide and the production of reactive oxygen species are also regulated by the chemokine signal via calcium mobilization and diacylglycerol production.
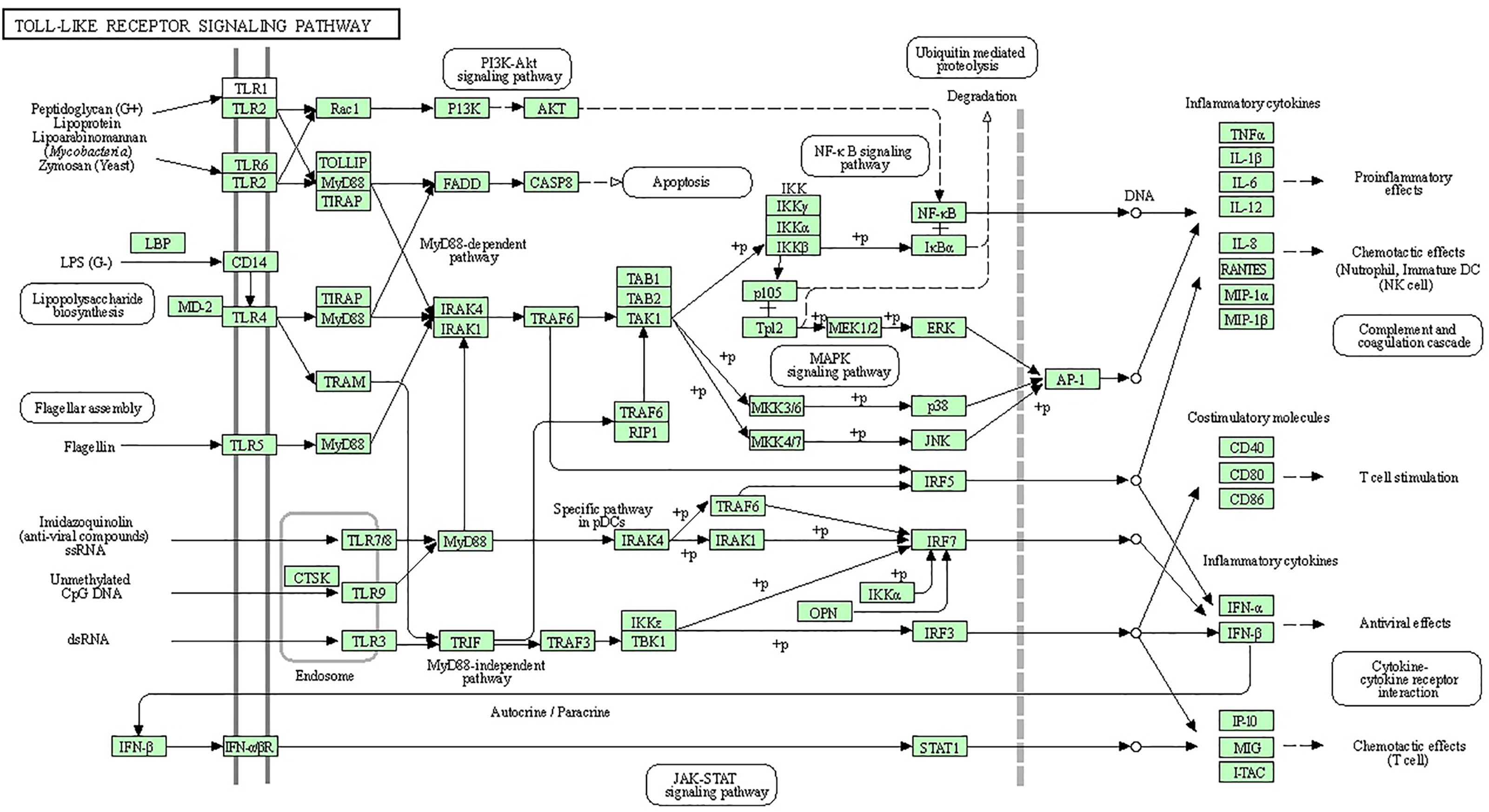
Figure 3 Specific families of pattern recognition receptors are responsible for detecting microbial pathogens and generating innate immune responses. Toll-like receptors (TLRs) are membrane-bound receptors identified as homologs of Toll in Drosophila. Mammalian TLRs are expressed on innate immune cells, such as macrophages and dendritic cells, and respond to the membrane components of Gram-positive or Gram-negative bacteria. Pathogen recognition by TLRs provokes the rapid activation of innate immunity by inducing the production of proinflammatory cytokines and the upregulation of costimulatory molecules. TLR signaling pathways are separated into two groups: a MyD88-dependent pathway that leads to the production of proinflammatory cytokines with the quick activation of NF-KB and MAPK and a MyD88-independent pathway associated with the induction of IFN-beta and IFN-inducible genes and the maturation of dendritic cells with slow activation of NF-KB and MAPK.
5. Conclusion
Altogether, we concluded that an appropriate approach to identifying genetically resistant animals would be to study the association of host genetics with mastitis susceptibility and resistance, thus, genetic marker selection was determined as the best approach to screening genetically mastitis-resistant cattle. In the current review, we have highlighted potential genetic polymorphisms in inflammation- and immune-associated gene markers that are significantly associated with mastitis resistance/susceptibility in dairy cattle. The highlighted polymorphisms in immune- and inflammation-associated genes could be considered potential biomarkers in bovine mastitis control research.
Author contributions
MZK, JW, SL and ZC designed the study and wrote the manuscript; ZC and SL supervised the manuscript; MM, QU, AK, YM, JW, TC, IMK, MZK and SL helped in the collection of data resources and editing of final version of the manuscript. All authors contributed to the article and approved the submitted version.
Funding
This work was supported by the grants from National Key Research and Development Program of China (2021YFF1000703-03), the 2115 Talent Development Program of China Agricultural University (2115) and the Key Research and Development Program of Ningxia Hui Autonomous Region (2022BBF02018-01). The funders had no role in study design, data collection, and analysis, decision to publish, or preparation of the manuscript.
Acknowledgments
We acknowledged the support provided by the National Key Research and Development Program of China (2021YFF1000703-03). We are thankful to the 2115 Talent Development Program of China Agricultural University (Beijing, P. R. China), the Key Research and Development Program of Ningxia Hui Autonomous Region (2022BBF02018-01) and China Animal Husbandry Group (DR201905) for their financial support.
Conflict of interest
The authors declare that the research was conducted in the absence of any commercial or financial relationships that could be construed as a potential conflict of interest.
Publisher’s note
All claims expressed in this article are solely those of the authors and do not necessarily represent those of their affiliated organizations, or those of the publisher, the editors and the reviewers. Any product that may be evaluated in this article, or claim that may be made by its manufacturer, is not guaranteed or endorsed by the publisher.
Supplementary material
The Supplementary Material for this article can be found online at: https://www.frontiersin.org/articles/10.3389/fimmu.2023.1082144/full#supplementary-material
References
1. Vorbach C, Capecchi MR, Penninger JM. Evolution of the mammary gland from the innate immune system? Bioessays (2006) 28(6):606–16. doi: 10.1002/bies.20423
2. Rainard P, Gilbert FB, Germon P. Immune defenses of the mammary gland epithelium of dairy ruminants. Front Immunol (2022) 13:6294. doi: 10.3389/fimmu.2022.1031785
3. Wang L, Yang F, Wei XJ, Luo YJ, Guo WZ, Zhuo XZ, et al. Prevalence and risk factors of subclinical mastitis in lactating cows in Northwest China. Isr J Vet Med (2019) 74:17–22.
4. Martin P, Barkema HW, Brito LF, Narayana SG, Miglior F. Symposium review: novel strategies to genetically improve mastitis resistance in dairy cattle. J dairy Sci (2018) 101(3):2724–36. doi: 10.3168/jds.2017-13554
5. Jing XQ, Zhao YQ, Shang CC, Yao YL, Tian TT, Li J, et al. Dynamics of cytokines associated with IL-17 producing cells in serum and milk in mastitis of experimental challenging with staphylococcus aureus and escherichia coli in dairy goats. J Anim Vet Adv (2012) 11:475–9. doi: 10.3923/javaa.2012.475.479
6. Jensen K, Günther J, Talbot R, Petzl W, Zerbe H, Schuberth HJ, et al. Escherichia coli- and staphylococcus aureus-induced mastitis differentially modulate transcriptional responses in neighbouring uninfected bovine mammary gland quarters. BMC Genom (2013) 14:36. doi: 10.1186/1471-2164-14-36
7. Pumipuntu N, Kulpeanprasit S, Santajit S, Tunyong W, Kong-Ngoen T, Hinthong W, et al. Screening method for staphylococcus aureus identification in subclinical bovine mastitis from dairy farms. Vet World (2017) 10:721–6. doi: 10.14202/vetworld.2017.721-726
8. Santman-Berends IMGA, Lam TJGM, Keurentjes J, Van Schaik G. An estimation of the clinical mastitis incidence per 100 cows per year based on routinely collected herd data. J Dairy Sci (2015) 98(10):6965–77. doi: 10.3168/jds.2015-9642
9. Pilla R, Malvisi M, Snel GGM, Schwarz D, König S, Czerny CP, et al. Differential cell count as an alternative method to diagnose dairy cow mastitis. J Dairy Sci (2013) 96:1653–60. doi: 10.3168/jds.2012-6298
10. Santman-Berends IMGA, Swinkels JM, Lam TJGM, Keurentjes J, Van Schaik G. Evaluation of udder health parameters and risk factors for clinical mastitis in Dutch dairy herds in the context of a restricted antimicrobial usage policy. J Dairy Sci (2016) 99(4):2930–9. doi: 10.3168/jds.2015-10398
11. Tijs SHW, Holstege MMC, Scherpenzeel CGM, Santman-Berends IMGA, Velthuis AGJ, Lam TJGM. Effect of selective dry cow treatment on udder health and antimicrobial usage on Dutch dairy farms. J Dairy Sci (2022) 105(6):5381–92. doi: 10.3168/jds.2021-21026
12. Hagiwara S, Mori K, Okada H, Oikawa S, Nagahata H. Acute escherichia coli mastitis in dairy cattle: Diagnostic parameters associated with poor prognosis. J Vet Med Sci (2014) 76:1431–6. doi: 10.1292/jvms.13-0610
13. Biswas S, Chakravarti S, Barui A. Emergence of coagulase positive methicillin resistant staphylococcus aureus isolated from buffalo mastitis milk samples. Explor Anim Med Res (2018) 8:190–6.
14. Li T, Lu H, Wang X, Gao Q, Dai Y, Shang J, et al. Molecular characteristics of staphylococcus aureus causing bovine mastitis between 2014 and 2015. Front Cell infect Microbiol (2017) 7:127. doi: 10.3389/fcimb.2017.00127
15. Gilbert FB, Cunha P, Jensen K, Glass EJ, Foucras G, Robert-Granié C, et al. Differential response of bovine mammary epithelial cells to staphylococcus aureus or escherichia coli agonists of the innate immune system. Vet Res (2013) 44(1):40. doi: 10.1186/1297-9716-44-40
16. Li N, Richoux R, Boutinaud M, Martin P, Gagnaire V. Role of somatic cells on dairy processes and products: A review. Dairy Sci Technol (2014) 94:517–38. doi: 10.1007/s13594-014-0176-3
17. Pighetti GM, Elliott AA. Gene polymorphisms: the keys for marker assisted selection and unraveling core regulatory pathways for mastitis resistance. J Mammary Gland Biol Neoplasia (2011) 16(4):421–32. doi: 10.1007/s10911-011-9238-9
18. Carlen E, Strandberg E, Roth A. Genetic parameters for clinical mastitis, somatic cell score, and production in the first three lactations of Swedish Holstein cows. J dairy Sci (2004) 87(9):3062–70. doi: 10.3168/jds.S0022-0302(04)73439-6
19. Yuan Z, Li J, Li J, Gao X, Xu S. SNPs identification and its correlation analysis with milk somatic cell score in bovine MBL1 gene. Mol Biol Rep (2013) 40(1):7–12. doi: 10.1007/s11033-012-1934-z
20. Chu MX, Ye SC, Qiao L, Wang JX, Huang DW, Cao GL, et al. Polymorphism of exon 2 of BoLA-DRB3 gene and its relationship with somatic cell score in Beijing Holstein cows. Mol Biol Rep (2012) 39, 2909–14. doi: 10.1007/s11033-011-1052-3
21. Hu HC, Wang HM, Li JB, Wang CF, Lai SJ, Li QL, et al. Genetic polymorphism of Nramp1 gene and correlation with mastitis in Holstein cattle. Hereditas (2009) 31:57–2. doi: 10.3724/sp.j.1005.2009.00057
22. Rupp R, Hernandez A, Mallard BA. Association of bovine leukocyte antigen (BoLA) DRB3. 2 with immune response, mastitis, and production and type traits in Canadian Holsteins. J Dairy Sci (2007) 90(2):1029–38. doi: 10.3168/jds.S0022-0302(07)71589-8
23. Weigel KA, Shook GE. Genetic selection for mastitis resistance. Vet Clin North Am - Food Anim Pract (2018) 34:457–72. doi: 10.1016/j.cvfa.2018.07.001
24. Pilla R, Schwarz D, König S, Piccinini R. Microscopic differential cell counting to identify inflammatory reactions in dairy cow quarter milk samples. J Dairy Sci (2012) 95:4410–20. doi: 10.3168/jds.2012-5331
25. El-Halawany N, Abd-El-Monsif AS, Al-Tohamy AF, Abdel-Latif MA, Abdel-Shafy H, Ghazi YA, et al. Effect of complement component 5 polymorphisms on mastitis resistance in Egyptian buffalo and cattle. Res vet Sci (2018) 119:45–51. doi: 10.1016/j.rvsc.2018.05.015
26. Schwarz D, Rivas AL, König S, Diesterbeck US, Schlez K, Zschöck M, et al. CD2/CD21 index: A new marker to evaluate udder health in dairy cows. J Dairy Sci (2013) 96:5106–19. doi: 10.3168/jds.2013-6804
27. Rainard P, Riollet C. Innate immunity of the bovine mammary gland. Vet Res (2006) 37:369–400. doi: 10.1051/vetres:2006007
28. Moyes KM, Drackley JK, Morin DE, Loor JJ. Greater expression of TLR2, TLR4, and IL6 due to negative energy balance is associated with lower expression of HLA-DRA and HLA-a in bovine blood neutrophils after intramammary mastitis challenge with streptococcus uberis. Funct Integr Genomics (2010) 10:53–61. doi: 10.1007/s10142-009-0154-7
29. Zhang Y, Lu Y, Ma L, Cao X, Xiao J, Chen J, et al. Activation of vascular endothelial growth factor receptor-3 in macrophages restrains TLR4-NF-κB signaling and protects against endotoxin shock. Immunity (2014) 40(4):501–14. doi: 10.1016/j.immuni.2014.01.013
30. Griesbeck-Zilch B, Osman M, Kühn C, Schwerin M, Bruckmaier RH, Pfaffl MW, et al. Analysis of key molecules of the innate immune system in mammary epithelial cells isolated from marker-assisted and conventionally selected cattle. J dairy Sci (2009) 92(9):4621–33. doi: 10.3168/jds.2008-1954
31. Schukken YH, Gunther J, Fitzpatrick J, Fontaine MC, Goetze L, Holst O, et al. Host-response patterns of intramammary infections in dairy cows. Vet Immunol Immunopathol (2011) 144:270–89. doi: 10.1016/j.vetimm.2011.08.022
32. Khan MZ, Wang D, Liu L, Usman T, Wen H, Zhang R, et al. Significant genetic effects of JAK2 and DGAT1 mutations on milk fat content and mastitis resistance in holsteins. J Dairy Res (2019) 86(4):388–93. doi: 10.1017/S0022029919000682
33. Khan MZ, Dari G, Khan A, Yu Y. Genetic polymorphisms of TRAPPC9 and CD4 genes and their association with milk production and mastitis resistance phenotypic traits in Chinese Holstein. Front Vet Sci (2022) 23:9. doi: 10.3389/fvets.2022.1008497
34. Usman T, Yu Y, Liu C, Wang X, Zhang Q, Wang Y. Genetic effects of single nucleotide polymorphisms in JAK2 and STAT5A genes on susceptibility of Chinese holsteins to mastitis. Mol Biol Rep (2014) 41:8293–301. doi: 10.1007/s11033-014-3730-4
35. Liu Y, Zhang J, Zhou YH, Zhang HM, Wang K, Ren Y, et al. Activation of the IL-6/JAK2/STAT3 pathway induces plasma cell mastitis in mice. Cytokine (2018) 110:150–8. doi: 10.1016/j.cyto.2018.05.002
36. Karthikeyan A, Radhika G, Aravindhakshan TV, Anilkumar K. Expression profiling of innate immune genes in milk somatic cells during subclinical mastitis in crossbred dairy cows. Anim Biotechnol (2016) 27(4):303–9. doi: 10.1080/10495398.2016.1184676
37. Usman T, Wang Y, Liu C, Wang X, Zhang Y, Yu Y. Association study of single nucleotide polymorphisms in JAK2 and STAT5B genes and their differential mRNA expression with mastitis susceptibility in Chinese Holstein cattle. Anim Genet (2015) 46:371–80. doi: 10.1111/age.12306
38. Usman T, Wang Y, Liu C, He Y, Wang X, Dong Y, et al. Novel SNPs in IL-17F and IL-17A genes associated with somatic cell count in Chinese Holstein and inner-Mongolia sanhe cattle. J Anim Sci Biotechnol (2017b) 8:1–9. doi: 10.1186/s40104-016-0137-1
39. Heringstad B, Chang YM, Andersen-Ranberg IM, Gianola D. Genetic analysis of number of mastitis cases and number of services to conception using a censored threshold model. J Dairy Sci (2006) 89:4042–8. doi: 10.3168/jds.S0022-0302(06)72447-X
40. Khan MZ, Khan A, Xiao J, Ma J, Ma Y, Chen T, et al. Overview of research development on the role of NF-κB signaling in mastitis. Animals (2020) 10(9):1625. doi: 10.3390/ani10091625
41. Khan MZ, Khan A, Xiao J, Ma Y, Ma J, Gao J, et al. Role of the JAK-STAT pathway in bovine mastitis and milk production. Animals (2020) 10(11):2107. doi: 10.3390/ani10112107
42. Wiggans GR, Sonstegard TS, VanRaden PM, Matukumalli LK, Schnabel RD, Taylor JF, et al. Selection of single-nucleotide polymorphisms and quality of genotypes used in genomic evaluation of dairy cattle in the united states and Canada. J Dairy Sci (2009) 92:3431–6. doi: 10.3168/jds.2008-1758
43. Tabor HK, Risch NJ, Myers RM. Candidate-gene approaches for studying complex genetic traits: practical considerations. Nat Rev Genet (2002) 3(5):391–7. doi: 10.1038/nrg796
44. Zhu M, Zhao S. Candidate gene identification approach: Progress and challenges. Int J Biol Sci (2007) 3(7):420. doi: 10.7150/ijbs.3.420
45. Wiggans GR, Van Raden PM, Cooper TA. The genomic evaluation system in the united states: past, present, future. J Dairy Sci (2011) 94:3202–11. doi: 10.3168/jds.2010-3866
46. Sender G, Korwin-Kossakowska A, Pawlik A, Hameed KGA, Oprzadek J. Genetic basis of mastitis resistance in dairy cattle-a review. Ann Anim Sci (2013) 13(4):663. doi: 10.2478/aoas-2013-0043
47. Shelley S, Hill AVS. Genetic susceptibility to infectious disease. Trends Microbiol (2003) 11:445–8. doi: 10.1016/S0966-842X(03)00207-5
48. Usman T, Ali N, Wang Y, Yu Y. Association of aberrant DNA methylation level in the CD4 and JAK-STAT-Pathway-Related genes with mastitis indicator traits in Chinese Holstein dairy cattle. Animals (2022) 12:65. doi: 10.3390/ani12010065
49. He Y, Chu Q, Ma P, Wang Y, Zhang Q, Sun D, et al. Association of bovine CD4 and STAT5b single nucleotide polymorphisms with somatic cell scores and milk production traits in Chinese holsteins. J Dairy Res (2011) 78:242–9. doi: 10.1017/S0022029911000148
50. Rupp R, Boichard D. Genetics of resistance to mastitis in dairy cattle. Vet Res (2003) 34(5):671–88. doi: 10.1051/vetres:2003020
51. Skelding A, Schenkel FS, Sharma BS, Verschoor C, Pant S, Biggar G, et al. Identification of single nucleotide polymorphisms in the bovine interleukin-12 and interleukin-23 receptor genes and their associations with health and production traits in Holstein cows. J Dairy Sci (2010) 93:4860–71. doi: 10.3168/jds.2009-2392
52. Beecher C, Daly M, Childs S, Berry DP, Magee DA, McCarthy TV, et al. Polymorphisms in bovine immune genes and their associations with somatic cell count and milk production in dairy cattle. BMC Genet (2010) 11(1):1–9. doi: 10.1186/1471-2156-11-99
53. Carvajal AM, Huircan P, Lepori A. Single nucleotide polymorphisms in immunity-related genes and their association with mastitis in Chilean dairy cattle. Genet Mol Res (2013) 12(3):2702–11. doi: 10.4238/2013.July.30.8
54. Quirion MR, Gregory GD, Umetsu SE, Winandy S, Brown MA. Cutting edge: Ikaros is a regulator of Th2 cell differentiation. J Immunol (2009) 182:741–5. doi: 10.4049/jimmunol.182.2.741
55. Yang Y, Li Q, Ju Z, Huang J, Zhou L, Li R, et al. Three novel single-nucleotide polymorphisms of complement component 4 gene (C4A) in Chinese Holstein cattle and their associations with milk performance traits and CH50. Vet Immunol immunopathol (2012) 145(1-2):223–32. doi: 10.1016/j.vetimm.2011.11.010
56. Sharma BS, Leyva I, Schenkel F, Karrow NA. Association of toll-like receptor 4 polymorphisms with somatic cell score and lactation persistency in Holstein bulls. J Dairy Sci (2006) 89:3626–35. doi: 10.3168/jds.S0022-0302(06)72402-X
57. Bhattarai D, Chen X, Zia R, Xingjie H, Farman U, Rahim D, et al. Association of MAP4K4 gene single nucleotide polymorphism with mastitis and milk traits in Chinese Holstein cattle. J Dairy Res (2017) 84(1):76–9. doi: 10.1017/S0022029916000832
58. Liu YX, Xu CH, Gao TY, Sun Y. Polymorphisms of the ATP1A1 gene associated with mastitis in dairy cattle. Genet Mol Res (2012) 11(1):651–60. doi: 10.4238/2012.March.16.3
59. Ma Y, Khan MZ, Xiao J, Alugongo GM, Chen X, Chen T, et al. Genetic markers associated with milk production traits in dairy cattle. Agriculture (2021) 11(10):1018. doi: 10.3390/agriculture11101018
60. Chen R, Yang Z, Ji D, Mao Y, Chen Y, Zhang Y, et al. SNPs of CXCR1 gene and its associations with somatic cell score in Chinese Holstein cattle. Anim Biotechnol (2011) 22(3):133–42.
61. Yuan Z, Li J, Li J, Zhang L, Gao X, Gao HJ, et al. Investigation on BRCA1 SNPs and its effects on mastitis in Chinese commercial cattle. Gene (2012) 505(1):190–4. doi: 10.1016/j.gene.2012.05.010
62. Yuan Z, Chu G, Dan Y, Li J, Zhang L, Gao X, et al. BRCA1: a new candidate gene for bovine mastitis and its association analysis between single nucleotide polymorphisms and milk somatic cell score. Mol Biol Rep (2012) 39(6):6625–31. doi: 10.1007/s11033-012-1467-5
63. Magotra A, Gupta ID, Ahmad T, Alex R. Polymorphism in DNA repair gene BRCA1 associated with clinical mastitis and production traits in indigenous dairy cattle. Res Vet Sci (2020) 133:194–201. doi: 10.1016/j.rvsc.2020.09.013
64. Yang D, Khan S, Sun Y, Hess K, Shmulevich I, Sood AK, et al. Association of BRCA1 and BRCA2 mutations with survival, chemotherapy sensitivity, and gene mutator phenotype in patients with ovarian cancer. Jama (2011) 306(14):1557–65. doi: 10.1001/jama.2011.1456
65. Wang C, Liu M, Li Q, Ju Z, Huang J, Li J, et al. Three novel single-nucleotide polymorphisms of MBL1 gene in Chinese native cattle and their associations with milk performance traits. Vet Immunol immunopathol (2011) 139(2-4):229–36. doi: 10.1016/j.vetimm.2010.10.023
66. Liu J, Ju Z, Li Q, Huang J, Li R, Li J, et al. Mannose-binding lectin 1 haplotypes influence serum MBL-a concentration, complement activity, and milk production traits in Chinese Holstein cattle. Immunogenetics (2011) 63(11):727–42. doi: 10.1007/s00251-011-0548-2
67. Magotra A, Pander BL, Dalal DS, Malik BS, Garg AR, Malik A. Evaluation of candidate genotype of immune gene MBL1 associated with udder health and performance traits in dairy cattle and buffalo of India. Trop Anim Health Product (2021) 53(4):1–8. doi: 10.1007/s11250-021-02865-2
68. Baghel M, Sharma D, Singh SP, Tiwari M, Kumar A. Single nucleotide polymorphisms in MBL1 gene of cattle and their association with milk production traits and somatic cell score. Indian J Anim Sci (2022) 92(2):208–14. doi: 10.56093/ijans.v92i2.122095
69. Wang X, Ju Z, Huang J, Hou M, Zhou L, Qi C, et al. The relationship between the variants of the bovine MBL2 gene and milk production traits, mastitis, serum MBL-c levels and complement activity. Vet Immunol Immunopathol (2012a) 148(3-4):311–9. doi: 10.1016/j.vetimm.2012.06.017
70. Zhao ZL, Wang CF, Li QL, Ju ZH, Huang JM, Li JB, et al. Novel SNPs of the mannan-binding lectin 2 gene and their association with production traits in Chinese holsteins. Genet Mol Res (2012) 11(4):3744–54. doi: 10.4238/2012.October.15.6
71. Mesquita A, Rezende C, Albenones M, Jardim E. Kipnis, A.Association of TLR4 polymorphisms with subclinical mastitis in Brazilian holsteins. Braz J Microbiol (2012) 43(2):692–7. doi: 10.1590/S1517-83822012000200034
72. Zhang LP, Gan QF, Ma TH, Li HD, Wang XP, Li JY, et al. Toll-like receptor 2 gene polymorphism and its relationship with SCS in dairy cattle. Anim Biotechnol (2009) 20(3):87–95. doi: 10.1080/10495390902873096
73. Chen R, Yang Z, Ji D, Mao Y, Chen Y, Zhang Y, et al. SNPs of CXCR1Gene and its associations with somatic cell score in Chinese Holstein cattle. Anim Biotechnol (2011a) 22(3):133–42. doi: 10.1080/10495398.2011.582804
74. Li J, Wang Q, Chen F, Wang H, Chen J, Wang Z, et al. SNPs of CD14 change the mastitis morbidity of Chinese Holstein. Mol Med Rep (2017) 16(6):9102–10. doi: 10.3892/mmr.2017.7727
75. Wang X, Zhong J, Gao Y, Ju Z, Huang J. A SNP in intron 8 of CD46 causes a novel transcript associated with mastitis in holsteins. BMC Genomics (2014) 15(1):1–11. doi: 10.1186/1471-2164-15-630
76. Ju Z, Wang C, Wang X, Yang C, Zhang Y, Sun Y, et al. The effect of the SNP g. 18475 a> G in the 3′ UTR of NCF4 on mastitis susceptibility in dairy cattle. Cell Stress chaperones (2018) 23(3):385–91. doi: 10.1007/s12192-017-0848-z
77. Ju Z, Wang C, Wang X, Yang C, Sun Y, Jiang Q, et al. Role of an SNP in alternative splicing of bovine NCF4 and mastitis susceptibility. PloS One (2015) 10(11):e0143705. doi: 10.1371/journal.pone.0143705
78. Mao Y, Zhu X, Xing S, Zhang M, Zhang H, Wang X, et al. Polymorphisms in the promoter region of the bovine lactoferrin gene influence milk somatic cell score and milk production traits in Chinese Holstein cows. Res Vet Sci (2015) 103:107–12. doi: 10.1016/j.rvsc.2015.09.021
79. Ju ZH, Li QL, Huang JM, Hou MH, Li RL, Li JB, et al. Three novel SNPs of the bovine tf gene in Chinese native cattle and their associations with milk production traits. Genet Mol Res (2011) 10:340–52. doi: 10.4238/vol10-1gmr1038
80. Deb R, Singh U, Kumar S, Kumar A, Singh R, Sengar G, et al. Genotypic to expression profiling of bovine calcium channel, voltage-dependent, alpha-2/delta subunit 1 gene, and their association with bovine mastitis among frieswal (HFX sahiwal) crossbred cattle of Indian origin. Anim Biotechnol (2014) 25(2):128–38. doi: 10.1080/10495398.2013.836106
81. Magotra A, Gupta ID, Verma A, Alex R, Mr V, Ahmad T. Candidate SNP of CACNA2D1 gene associated with clinical mastitis and production traits in sahiwal (Bos taurus indicus) and karan fries (Bos taurus taurus× bos taurus indicus). Anim Biotechnol (2019) 30(1):75–81. doi: 10.1080/10495398.2018.1437046
82. Yuan Z, Li J, Zhang L, Zhang L, Chen C, Chen X, et al. Novel SNPs polymorphism of bovine CACNA2D1 gene and their association with somatic cell score. Afr J Biotechnol (2011a) 10(10):1789–93.
83. Wu J, Bai JY, Li L, Huang S, Li CM, Wang GL. Genetic polymorphisms of the BMAP-28 and MASP-2 genes and their correlation with the somatic cell score in Chinese Holstein cattle. Genet Mol Res (2015) 14(1):1–8. doi: 10.4238/2015.January.15.1
84. Ji D, Chen R, Yang Z, Zhu G, Mao Y, Chen Y, et al. IL-8 gene SNP-180A/G affects significantly somatic cell score in Chinese Holstein cow. Sci Agric Sin (2012) 45(7):1387–91. doi: 10.3892/mmr.2017.7727
85. Chen R, Wang Z, Yang Z, Zhu X, Ji D, Mao Y. Association of IL8-105G/A with mastitis somatic cell score in Chinese Holstein dairy cows. Animal Biotechnol (2015) 26(2):143–7. doi: 10.1080/10495398.2014.939657
86. Chen L, Han Y, Chen Y, Li Z, Wang H, Liu Y, et al. Eight SNVs in NF-κB pathway genes and their different performances between subclinical mastitis and mixed Chinese Holstein cows. Gene (2015) 555(2):242–9. doi: 10.1016/j.gene.2014.11.011
87. Cheng J, Li J, Zhang W, Cai Y, Wang G. Mutations in lipopolysaccharide-binding protein (LBP) gene change the susceptibility to clinical mastitis in Chinese Holstein. Mol Biol Rep (2012) 39(10):9601–12. doi: 10.1007/s11033-012-1824-4
88. Worku D, Gowane GR, Mukherjee A, Alex R, Joshi P, Verma A. Associations between polymorphisms of LAP3 and SIRT1 genes with clinical mastitis and milk production traits in sahiwal and karan fries dairy cattle. Vet Med Sci Vet Med Sci (2022) 2022:1–12. doi: 10.1002/vms3.924
89. Kowalewska-Łuczak I, Kulig H. Polymorphism of the FAM13A, ABCG2, OPN, LAP3, HCAP-G, PPARGC1A genes and somatic cell count of Jersey cows–preliminary study. Res vet Sci (2013) 94(2):252–5. doi: 10.1016/j.rvsc.2012.08.006
90. Alain K, Karrow NA, Thibault C, St-Pierre J, Lessard M, Bissonnette N. Osteopontin: an early innate immune marker of escherichia coli mastitis harbors genetic polymorphisms with possible links with resistance to mastitis. BMC Genomics (2009) 10(1):1–17. doi: 10.1186/1471-2164-10-444
91. Ghulam Mohyuddin S, Liang Y, Ni W, Adam Idriss Arbab A, Zhang H, Li M, et al. Polymorphisms of the IL-17A gene influence milk production traits and somatic cell score in Chinese Holstein cows. Bioengineering (2022) 9(9):448. doi: 10.3390/bioengineering9090448
92. Zabolewicz T, Puckowska P, Brym P, Oleński K, Kamiński S. Relationship between polymorphism within peptidoglycan recognition protein 1 gene (PGLYRP1) and somatic cell counts in milk of Holstein cows. Ann Anim Sci (2022) 22(2):593–9. doi: 10.2478/aoas-2021-0067
93. Wang Z, Huang J, Zhong J, Wang G. Molecular cloning, promoter analysis, SNP detection of clusterin gene and their associations with mastitis in Chinese Holstein cows. Mol Biol Rep (2012c) 39:2439–45. doi: 10.1007/s11033-011-0994-9
94. Li Z, Zhai M, Wang H, Chen L, Wang L, Ru C, et al. Identification of splice variants, expression analysis and single nucleotide polymorphisms of the PRMT2 gene in dairy cattle. Gene (2014) 539(1):37–43. doi: 10.1016/j.gene.2014.01.065
95. Ali N, Niaz S, Khan NU, Gohar A, Khattak I, Dong Y, et al. Polymorphisms in JAK2 gene are associated with production traits and mastitis resistance in dairy cattle. Ann Anim Sci (2020) 20:409–23. doi: 10.2478/aoas-2019-0082
96. Li L, Huang J, Zhang X, Ju Z, Qi C, Zhang Y, Li Q, et al. One SNP in the 3′-UTR of HMGB1 gene affects the binding of target bta-miR-223 and is involved in mastitis in dairy cattle. Immunogenetics (2012) 64:817–24. doi: 10.1007/s00251-012-0641-1
97. McConnel CS, Crisp SA, Biggs TD, Ficklin SP, Parrish LM, Trombetta SC, et al. A fixed cohort field study of gene expression in circulating leukocytes from dairy cows with and without mastitis. Front vet Sci (2020) 7:559279. doi: 10.3389/fvets.2020.559279
98. Chen Z, Xia H, Shen H, Xu X, Arbab AAI, Li M, et al. Pathological features of staphylococcus aureus induced mastitis in dairy cows and isobaric-tags-for-relative-and-absolute-quantitation proteomic analyses. J Agric Food Chem (2018) 66(15):3880–90. doi: 10.1021/acs.jafc.7b05461
99. Jayashankar MR, Nagaraja R, Yathiraj S, Rajeshwari YB, Isloor S. Genetic polymorphism of PGLYRP-1 gene and its association with mastitis in deoni and Holstein friesian crossbred cows. Ruminant Sci (2018) 7(2):199–202.
100. Wang HL, Li ZX, Wang LJ, He H, Yang J, Chen L, et al. Polymorphism in PGLYRP-1 gene by PCR-RFLP and its association with somatic cell score in Chinese Holstein. Res vet Sci (2013) 95(2):508–14. doi: 10.1016/j.rvsc.2013.06.005
101. Wang HL, Li ZX, Chen L, Yang J, Wang LJ, He H, et al. Polymorphism in PGLYRP-2 gene by PCR-RFLP and its association with somatic cell score and percentage of fat in Chinese Holstein. Genet Mol Res (2013) 12:6743–51. doi: 10.4238/2013.December.13.7
102. Yuan ZR, Li J, Liu L, Zhang LP, Zhang LM, Chen C, et al. Single nucleotide polymorphism of CACNA2D1 gene and its association with milk somatic cell score in cattle. Mol Biol Rep (2011b) 38(8):5179–83. doi: 10.1007/s11033-010-0667-0
103. Magotra A, Gupta ID, Verma A, Chaudhari MV, Arya A, Vineeth MR, et al. Characterization and validation of point mutation in exon 19 of CACNA2D1 gene in karan fries (Bos taurus x bos indicus) cattle. Indian J Anim Res (2017) 51(2):227–30. doi: 10.0.73.117/ijar.5668"10.18805/ijar.5668
104. Deng GX, Yuan ZR, Gao X, Li JY, Chen JB, Gao HJ, et al. Identification mutation of the CACNA2D1 gene and its effect on somatic cell score in cattle. J Appl Anim Res (2011) 39(1):15–8. doi: 10.1080/09712119.2011.558616
105. Zhang H, Wei Y, Zhang F, Liu Y, Li Y, Li G, et al. Polymorphisms of MASP2 gene and its relationship with mastitis and milk production in Chinese Holstein cattle. Biotechnol Biotechnol Equip (2019) 33(1):589–96. doi: 10.1080/13102818.2019.1596755
106. Lacasse P, Lauzon K, Diarra MS, Petitclerc D. Utilization of lactoferrin to fight antibiotic-resistant mammary gland pathogens. J Anim Sci (2008) 86:66–71. doi: 10.2527/jas.2007-0216
107. Shimazaki KI, Kawai K. Advances in lactoferrin research concerning bovine mastitis. Biochem Cell Biol (2017) 95(1):69–75. doi: 10.1139/bcb-2016-0044
108. Griesbeck-Zilch B, Meyer HH, Kühn CH, Schwerin M, Wellnitz O. Staphylococcus aureus and escherichia coli cause deviating expression profiles of cytokines and lactoferrin messenger ribonucleic acid in mammary epithelial cells. J Dairy Sci (2008) 91:2215–222. doi: 10.3168/jds.2007-0752
109. Bruckmaier RM. Gene expression of factors related to the immune reaction in response to intramammary escherichia coli lipopolysaccharide challenge. J Dairy Res (2005) 72:120–4. doi: 10.1017/S0022029905001159
110. Li GH, Zhang Y, Sun DX, Li N. Study on the polymorphism of bovine lactoferrin gene and its relationship with mastitis. Anim Biotechnol (2004) 15:67–76. doi: 10.1081/ABIO-120037899
111. Rahmani SF, Jayashankar MR, Nagaraja CS, Bhaskaran R, Byregowda SM, Satyanarayan K. Association of genotypes at promoter region of lactoferrin gene with mastitis in crossbred cattle. Indian J Anim Sci (2012) 82:718–20.
112. Huang J, Wang H, Wang C, Li J, Li Q, Hou M, et al. Single nucleotide polymorphisms, haplotypes and combined genotypes of lactoferrin gene and their associations with mastitis in Chinese Holstein cattle. Mol Biol Rep (2010) 37:477–83. doi: 10.1007/s11033-009-9669-1
113. Zhao C, He G, Wang Y, Zhang Z. Polymorphism of lactoferrin gene with PCR – RFLP and its association with subclinical mastitis in dairy cows. Modern Appl Sci (2009) 3:144–8. doi: 10.5539/mas.v3n2p144
114. Chopra A, Gupta ID, Verma A, Vohra V. Detection of allelic variants in lactoferrin gene promoter using created restriction site PCR-RFLP and its association with mastitis. Indian J Anim Sci (2014) 84:1068–70.
115. Chopra A, Gupta ID, Verma A, Chakravarty AK, Vohra V. Lactoferrin gene promoter variants and their association with clinical and subclinical mastitis in indigenous and crossbred cattle. Polish J Vet Sci (2015) 18(3):465–71. doi: 10.1515/pjvs-2015-0061
116. Dinesh K, Verma A, Gupta ID, Thakur YP, Verma N, Arya A. Identification of polymorphism in exons 7 and 12 of lactoferrin gene and its association with incidence of clinical mastitis in murrah buffalo. Trop Anim Health Product (2015) 47(4):643–7. doi: 10.1007/s11250-015-0765-z
117. Ali A, Rehman MU, Ahmad SM, Mehraj T, Hussain I, Nadeem A, et al. In silico tools for analysis of single-nucleotide polymorphisms in the bovine transferrin gene. Animals (2022) 12:693. doi: 10.3390/ani12060693
118. Chaneton L, Tirante L, Maito J, Chaves J, Bussmann LE. Relationship between milk lactoferrin and etiological agent in the mastitic bovine mammary gland. J Dairy Sci (2008) 91:1865–73. doi: 10.3168/jds.2007-0732
119. Ellson CD, Davidson K, Ferguson GJ, O'Connor R, Stephens LR, Hawkins PT. Neutrophils from p40phox-/- mice exhibit severe defects in NADPH oxidase regulation, oxidant-dependent bacterial killing. J Exp Med (2006) 203:1927–37. doi: 10.1084/jem.20052069
120. Jin W, Ibeagha-Awemu EM, Liang G, Beaudoin F, Zhao X, Guan LL. Transcriptome microRNA profiling of bovine mammary epithelial cells challenged with escherichia coli or staphylococcus aureus bacteria reveals pathogen directed microRNA expression profiles. BMC Genomics (2014) 15:181. doi: 10.1186/1471-2164-15-181
121. Lawless N, Foroushani AB, McCabe MS, O’Farrelly C, Lynn DJ. Next generation sequencing reveals the expression of a unique miRNA profile in response to a gram-positive bacterial infection. PloS One (2013) 8:e57543. doi: 10.1371/journal.pone.0057543
122. Lawless N, Vegh P, O’Farrelly C, Lynn DJ. The role of microRNAs in bovine infection and immunity. Front Immunol (2014) 5:611. doi: 10.3389/fimmu.2014.00611
123. Li L, Huang J, Ju Z, Li Q, Wang C, Qi C, et al. Multiple promoters and targeted microRNAs direct the expressions of HMGB3 gene transcripts in dairy cattle. Anim Genet (2013) 44:241–50. doi: 10.1111/age.12007
124. Ha M, Kim VN. Regulation of microRNA biogenesis. Nat Rev Mol Cell Biol (2014) 15:509–24. doi: 10.1038/nrm3838
125. Hou Q, Huang J, Ju Z, Li Q, Li L, Wang C, et al. Identification of splice variants, targeted microRNAs and functional single nucleotide polymorphisms of the BOLA-DQA2 gene in dairy cattle. DNA Cell Biol (2012) 31:739–44. doi: 10.1089/dna.2011.1402
126. Zhang Z, Wang X, Li R, Ju Z, Qi C, Zhang Y, et al. Genetic mutations potentially cause two novel NCF1 splice variants up-regulated in the mammary gland, blood and neutrophil of cows infected by escherichia coli. Microbiol Res (2015) 174:24–32. doi: 10.1016/j.micres.2015.03.005
127. Wang X, Huang J, Zhao L, Wang C, Ju Z, Li Q, et al. The exon 29 c. 3535A> T in the alpha-2-macroglobulin gene causing aberrant splice variants is associated with mastitis in dairy cattle. Immunogenetics (2012) 64(11):807–16. doi: 10.1007/s00251-012-0639-8
128. Vandooren J, Itoh Y. Alpha-2-macroglobulin in inflammation, immunity and infections. Front Immunol (2021) 12. doi: 10.3389/fimmu.2021.803244
129. Wang XG, Huang JM, Feng MY, Ju ZH, Wang CF, Yang GW, et al. Regulatory mutations in the a 2M gene are involved in the mastitis susceptibility in dairy cows. Anim Genet (2014) 45(1):28–37. doi: 10.1111/age.12099
130. Mehrzad J, Zhao X. T Lymphocyte proliferative capacity and CD4+/CD8+ ratio in primiparous and pluriparous lactating cows. J Dairy Res (2008) 75:457–65. doi: 10.1017/S0022029908003439
131. Usman T, Wang Y, Song M, Wang X, Dong Y, Liu C, et al. Novel polymorphisms in bovine CD4 and LAG-3 genes associated with somatic cell counts of clinical mastitis cows. Genet Mol Res (2017a) 17(1). doi: 10.4238/gmr16039859
132. Opsal MA, Våge DI, Hayes B, Berget I, Lien S. Genomic organization and transcript profiling of the bovine toll-like receptor gene cluster TLR6-TLR1-TLR10. Gene (2006) 384:45–50. doi: 10.1016/j.gene.2006.06.027
133. Kumar H, Kawai T, Akira S. Pathogen recognition by the innate immune system. Int Rev Immunol (2011) 30(1):16–34. doi: 10.3109/08830185.2010.529976
134. Bhattarai D, Worku T, Dad R, Rehman ZU, Gong X, Zhang S. Mechanism of pattern recognition receptors (PRRs) and host pathogen interplay in bovine mastitis. Microbial Pathogen (2018) 120:64–70. doi: 10.1016/j.micpath.2018.04.010
135. He X, Wei Z, Zhou E, Chen L, Kou J, Wang J, et al. Baicalein attenuates inflammatory responses by suppressing TLR4 mediated NF-κB and MAPK signaling pathways in LPS-induced mastitis in mice. Internat Immunopharmacol (2015) 28:470–47. doi: 10.1016/j.intimp.2015.07.012
136. Li J, Yin P, Gong P, Lv A, Zhang Z, Liu F. 8-methoxypsoralen protects bovine mammary epithelial cells against lipopolysaccharide-induced inflammatory injury via suppressing JAK/STAT and NF-κB pathway. Microbiol Immunol (2019) 63:427–37. doi: 10.1111/1348-0421.12730
137. Sun L, Chen L, Wang F, Zheng X, Yuan C, Niu Q, et al. Exogenous hydrogen sulfide prevents lipopolysaccharide-induced inflammation by blocking the TLR4/NF-κB pathway in MAC-T cells. Gene (2019) 710:114–21. doi: 10.1016/j.gene.2019.05.033
138. Yu S, Liu X, Yu D, Changyong E, Yang J. Morin protects LPS-induced mastitis via inhibiting NLRP3 inflammasome and NF-κB signaling pathways. Inflammation (2020) 43:1293–303. doi: 10.1007/s10753-020-01208-x
139. Zhan K, Yang T, Feng B, Zhu X, Chen Y, Huo Y, et al. The protective roles of tea tree oil extracts in bovine mammary epithelial cells and polymorphonuclear leukocytes. J AnimSci Biotechnol (2020) 11:62. doi: 10.1186/s40104-020-00468-9
140. Akhtar M, Guo S, Guo Y, Zahoor A, Shaukat A, Chen Y, et al. Upregulated-gene expression of pro-inflammatory cytokines (TNF-α, IL-1β and IL-6) via TLRs following NF-κB and MAPKs in bovine mastitis. Acta Tropica (2020) 207:105458. doi: 10.1016/j.actatropica.2020.105458
141. Wang H, Bi C, Wang Y, Sun J, Meng X, Li J. Selenium ameliorates staphylococcus aureus-induced inflammation in bovine mammary epithelial cells by inhibiting activation of TLR2, NF-KB and MAPK signaling pathways. BMC Vet Res (2018) 14:1–8. doi: 10.1186/s12917-018-1508-y
142. Oviedo-Boyso J, Valdez-Alarcón JJ, Cajero-Juárez M, Ochoa-Zarzosa A, López-Meza JE, Bravo-Patiño A, et al. Innate immune response of bovine mammary gland to pathogenic bacteria responsible for mastitis. J Infect (2007) 54(4):399–409. doi: 10.1016/j.jinf.2006.06.010
143. Wang M, Song H, Zhu X, Xing S, Zhang M, Zhang H, et al. Toll-like receptor 4 gene polymorphisms influence milk production traits in Chinese Holstein cows. J Dairy Res (2018) 85(4):407–11. doi: 10.1017/S0022029918000535
144. Lu Y-C, Yeh W-C, Ohashi PS. LPS/TLR4 signal transduction pathway. Cytokine (2008) 42(2):145–51. doi: 10.1016/j.cyto.2008.01.006
145. Wang XP, Xu SZ, Gao X, Li JY, Ren HY, Luoren ZM. Cloning and SNP screening of the TLR4 gene and the association between its polymorphism and somatic cell score in dairy cattle. South Afr J Anim Sci (2008) 38(2):101–9.
146. Chen H, Liu C, Xiang M, Yu J, Xia Y, Hu X, et al. Contribution of the mutation rs8193069 in TLR4 to mastitis resistance and performance in Holstein cows in southern China. Vet Med Sci (2022) 8(1):357–66. doi: 10.1002/vms3.671
147. Wang X, Xu S, Gao X, Ren H, Chen J. Genetic polymorphism of TLR4 gene and correlation with mastitis in cattle. J Genet Genomics (2007) 34:406–12. doi: 10.1016/S1673-8527(07)60044-7
148. Russell CD, Widdison S, Leigh JA, Coffey TJ. Identification of single nucleotide polymorphisms in the bovine toll-like receptor 1 gene and association with health traits in cattle. Vet Res (2012) 43(1):1–12. doi: 10.1186/1297-9716-43-17
149. Lee JW, Bannerman DD, Paape MJ, Huang MK, Zhao X. Characterization of cytokine expression in milk somatic cells during intramammary infections with escherichia coli or staphylococcus aureus by real-time PCR. Vet Res (2006) 37(2):219–29. doi: 10.1051/vetres:2005051
150. Takeuchi O, Sato S, Horiuchi T, Hoshino K, Takeda K, Dong Z, et al. Cutting edge: role of toll-like receptor 1 in mediating immune response to microbial lipoproteins. J Immunol (2002) 169(1):10–4. doi: 10.4049/jimmunol.169.1.10
151. Pant SD, Schenkel FS, Leyva-Baca I, Sharma BS, Karrow NA. Identification of single nucleotide polymorphisms in bovine CARD15 and their associations with health and production traits in Canadian holsteins. BMC Genomics (2007) 8(1):421. doi: 10.1186/1471-2164-8-421
152. Pant SD, Schenkel FS, Leyva-Baca I, Sharma BS, Karrow NA. Identification of polymorphisms in bovine TLR2 and CARD15, associations between CARD15 polymorphisms and milk somatic cell score in Canadian holsteins, and functional relevance of SNP c. 3020A>; T. In: Animal genomics for animal health, vol. 132. Karger Publishers (2008). p. 247–53.
153. Pawlik A, Sender G, Kapera M, Korwin-Kossakowska A. Association between interleukin 8 receptor α gene (CXCR1) and mastitis in dairy cattle. Cent Eur J Immunol (2015) 40(2):153–8. doi: 10.5114/ceji.2015.52828
154. Alluwaimi AM. IL8 transcriptional activity in s. aureus mastitis. Int J Diary Sci (2005) 1:18–20. doi: 10.1016/j.vetimm.2016.09.006
155. Stojkovic B, McLoughlin RM, Meade KG. In vivo relevance of polymorphic interleukin 8 promoter haplotype for the systemic immune response to LPS in Holstein-friesian calves. Vet Immunol Immunopathol (2016) 182:1–10. doi: 10.1016/j.vetimm.2016.09.006
156. De Matteis G, Scatà MC, Grandoni F, Crisà A, O’Brien MB, Meade KG, et al. Effect of IL8 haplotype on immunological traits in periparturient dairy cows. Vet Immunol Immunopathol (2021) 238:110288. doi: 10.1016/j.vetimm.2021.110288
157. De Matteis G, Scatà MC, Catillo G, Grandoni F, Rossi E, Zilio DM, et al. Comparison of metabolic, oxidative and inflammatory status of simmental× Holstein crossbred with parental breeds during the peripartal and early lactation periods. J Dairy Res (2021) 88(3):253–60. doi: 10.1017/S0022029921000650
158. Youngerman SM, Saxton AM, Oliver SP, Pighetti GM. Association of CXCR2 polymorphisms with subclinical and clinical mastitis in dairy cattle. J Dairy Sci (2004) 87(8):2442–8. doi: 10.3168/jds.S0022-0302(04)73367-6
159. Han ZJ, Li YB, Yang LX, Cheng HJ, X. and Chen H. Roles of the CXCL8-CXCR1/2 axis in the tumor microenvironment andimmunotherapy. Molecules (2021) 27(1):137. doi: 10.3390/molecules27010137
160. Leyva-Baca I, Schenkel F, Sharma BS, Jansen GB, Karrow NA. Identification of single nucleotide polymorphisms in the bovine CCL2, IL8, CCR2 and IL8RA genes and their association with health and production in Canadian holsteins. Anim Genet (2007) 38(3):198–202. doi: 10.1111/j.1365-2052.2007.01588.x
161. Olson TS, Ley K. Chemokines and chemokine receptors in leukocyte trafficking. Am J Physiol Regul Integr Comp Physiol (2002) 283:R7–R28. doi: 10.1152/ajpregu.00738.2001
162. Mitchell GB, Albright BN, Casewell JL. Effect of interleukin-8 and granulocyte colony stimulating factor on priming and activation of bovine neutrophils. Infect Immun (2003) 71:1643–9. doi: 10.1128/IAI.71.4.1643-1649.2003
163. Lahouassa H, Rainard P, Caraty A, Riollet C. Identification and characterization of a new interleukin-8 receptor in bovine species. Mol Immunol (2008) 45:1153–64. doi: 10.1016/j.molimm.2007.07.011
164. Chen R, Yang Z, Ji D, Mao Y, Chen Y, Li Y, et al. Polymorphisms of the IL8 gene correlate with milking traits, SCS and mRNA level in Chinese Holstein. Mol Biol Rep (2011b) 38:4083–8. doi: 10.1007/s11033-010-0528-x
165. Leyva-Baca I, Schenkel F, Martin J, Karrow NA. Polymorphisms in the 5′ upstream region of the CXCR1 chemokine receptor gene, and their association with somatic cell score in Holstein cattle in Canada. J Dairy Sci (2008) 91(1):407–17. doi: 10.3168/jds.2007-0142
166. Zhou L, Wang HM, Ju ZH, Zhang Y, Huang JM, Qi C, et al. Association of novel single nucleotide polymorphisms of the CXCR1 gene with the milk performance traits of Chinese native cattle. Genet Mol Res (2013) 12(3):2725–39. doi: 10.4238/2013.July.30.10
167. Goertz I, Baes C, Weimann C, Reinsch N, Erhardt G. Association between single nucleotide polymorphisms in the CXCR1 gene and somatic cell score in Holstein dairy cattle. J dairy Sci (2009) 92(8):4018–22. doi: 10.3168/jds.2008-1536
168. Verbeke J, Van Poucke M, Peelman L, Piepers S, De Vliegher S. Associations between CXCR1 polymorphisms and pathogen-specific incidence rate of clinical mastitis, test-day somatic cell count, and test-day milk yield. J dairy Sci (2014) 97(12):7927–39. doi: 10.3168/jds.2014-8216
169. Verbeke J, Piccart K, Piepers S, Van Poucke M, Peelman L, De Visscher A, et al. Somatic cell count and milk neutrophil viability of dairy heifers with specific CXCR1 genotypes following experimental intra-mammary infection with staphylococcus chromogenes originating from milk. Vet J (2015) 204(3):322–6. doi: 10.1016/j.tvjl.2015.04.010
170. Miossec P, Kolls KJ. Targeting IL-17 and TH17 cells in chronic inflammation. Nat Rev Drug Discovery (2012) 11(10):763–76. doi: 10.1038/nrd3794
171. Zenobia C, Hajishengallis G. Basic biology and role of interleukin-17 in immunity and inflammation. Periodontol 2000 (2015) 69(1):142–59. doi: 10.1111/prd.12083
172. Abusleme L, Moutsopoulos NM. IL-17: overview and role in oral immunity and microbiome. Oral Dis (2017) 23(7):854–65. doi: 10.1111/odi.12598
173. Milner JD. IL-17 producing cells in host defense and atopy. Curr Opin Immunol (2011) 23:784–8. doi: 10.1016/j.coi.2011.09.006
174. Pappu R, Ramirez-Carrozzi V, Sambandam A. The interleukin-17 cytokine family. critical players in host defence and inflammatory diseases. Immunology (2011) 134:8–16. doi: 10.1111/j.1365-2567.2011.03465.x
175. Hu X, He Z, Jiang P, Wang K, Guo J, Zhao C, et al. Neutralization of interleukin-17A attenuates lipopolysaccharide-induced mastitis by inhibiting neutrophil infiltration and the inflammatory response. J Interferon Cytokine Res (2019) 39(9):577–84. doi: 10.1089/jir.2019.0069
176. Tassi R, McNeilly TN, Fitzpatrick JL, Fontaine MC, Reddick D, Ramage C, et al. Strain-specific pathogenicity of putative host-adapted and nonadapted strains of streptococcus uberis in dairy cattle. J Dairy Sci (2013) 96:5129–45. doi: 10.3168/jds.2013-6741
177. Tao W, Mallard B. Differentially expressed genes associated with staphylococcus aureus mastitis of Canadian Holstein cows. Vet Immunol Immunopathol (2007) 120:201–11. doi: 10.1016/j.vetimm.2007.06.019
178. Bougarn S, Cunha P, Gilbert FB, Harmache A, Foucras G, Rainard P. Staphylococcal-associated molecular patterns enhance expression of immune defense genes induced by IL-17 in mammary epithelial cells. Cytokine (2011) 56:749–59. doi: 10.1016/j.cyto.2011.09.020
179. Roussel P, Cunha P, Porcherie A, Petzl W, Gilbert FB, Riollet C, et al. Investigating the contribution of IL-17A and IL-17F to the host response during escherichia coli mastitis. Vet Res (2015) 46(1):1–14. doi: 10.1186/s13567-015-0201-4
180. Chung Y, Yang X, Chang SH, Ma L, Tian Q, Dong C. Expression and regulation of IL-22 in the IL-17-producing CD4+ T lymphocytes. Cell Res (2006) 16:902–7. doi: 10.1038/sj.cr.7310106
181. Zhou GY, Yi YX, Jin LX, Lin W, Fang PP, Lin XZ, et al. The protective effect of juglanin on fructose-induced hepatitis by inhibiting inflammation and apoptosis through TLR4 and JAK2/STAT3 signaling pathways in fructose-fed rats. BioMed Pharmacother (2016) 81:318–28. doi: 10.1016/j.biopha.2016.04.013
182. Zhang C, Jun L, Changqing Y, Qiuxia J, Daiyun C, Han Z, et al. JAK2/STAT3 is associated with the inflammatory process in periapical granuloma. Int J Clin Exp Pathol (2019) 12:190–7.
183. Shuai K, Liu B. Regulation of JAK–STAT signaling in the immune system. Nat Rev Immunol (2003) 3:900–11. doi: 10.1038/nri1226
184. Xin P, Xiaoyun X, Chengjie D, Shuang L, Youzhi W, Xuegang Z, et al. The role of JAK/STAT signaling pathway and its inhibitors in diseases. Int Immunopharmacol (2020) 80:106210. doi: 10.1016/j.intimp.2020.106210
185. Villarino VA, Yuka K, John JOS. Mechanisms of Jak/STAT signaling in immunity and disease. J Immunol (2015) 194:21–7. doi: 10.4049/jimmunol.1401867
186. Kiu H, Sandra EN. Biology and significance of the JAK/STAT signalling pathways. Growth Factors (2012) 30:88–106. doi: 10.3109/08977194.2012.660936
187. Khan MZ, Zhang Z, Liu L, Wang D, Mi S, Liu X, et al. Folic acid supplementation regulates key immunity-associated genes and pathways during the periparturient period in dairy cows. Asian-Australasian J Anim Sci (2020) 33(9):1507. doi: 10.5713/ajas.18.0852
188. Furth PA, Nakles RE, Millman S, Diaz-Cruz ES, Cabrera MC. Signal transducer and activator of transcription 5 as a key signaling pathway in normal mammary gland developmental biology and breast cancer. Breast Cancer Res (2011) 13:1–4. doi: 10.1186/bcr2921
189. Liu Y, Zhang J, Zhou YH, Jiang YN, Zhang W, Tang XJ, et al. IL-6/STAT3 signaling pathway is activated in plasma cell mastitis. Int J Clin Exp Pathol (2015) 8(10):12541.
190. Liu Y, Sun Y, Zhou Y, Tang X, Wang K, Ren Y, et al. Sinomenine hydrochloride inhibits the progression of plasma cell mastitis by regulating IL-6/JAK2/STAT3 pathway. Int Immunopharmacol (2020) 81:106025. doi: 10.1016/j.intimp.2019.106025
191. Vafaizadeh V, Ab Klemmt P, Groner B. Stat5 assumes distinct functions in mammary gland development and mammary tumor formation. Front Bioscience-Landmark (2012) 17(4):1232–50. doi: 10.2741/3983
192. Bochniarz M, Zdzisińska B, Wawron W, Szczubiał M, Dąbrowski R. Milk and serum IL-4, IL-6, IL-10, and amyloid a concentrations in cows with subclinical mastitis caused by coagulase-negative staphylococci. J Dairy Sci (2017) 100:9674–80. doi: 10.3168/jds.2017-13552
193. Khatib H, Monson RL, Schutzkus V, Kohl DM, Rosa GJM, Rutledge JJ. Mutations in the STAT5A gene are associated with embryonic survival and milk composition in cattle. J Dairy Sci (2008) 91:784–93. doi: 10.3168/jds.2007-0669
194. Huang DW, Sherman BT, Lempicki RA. Systematic and integrative analysis of large gene lists using DAVID bioinformat-ics resources. Nat Protocol (2009) 4:44–57. doi: 10.1038/nprot.2008.211
195. Fang L, Hou Y, An J, Li B, Song M, Wang X, et al. Genome-wide transcriptional and post-transcriptional regulation of innate immune and defense responses of bovine mammary gland to staphylococcus aureus. Front Cell infect Microbiol (2016) 6:193. doi: 10.3389/fcimb.2016.00193
196. Wang D, Liu L, Augustino S, Duan T, Hall TJ, MacHugh DE, et al. Identification of novel molecular markers of mastitis caused by staphylococcus aureus using gene expression profiling in two consecutive generations of Chinese Holstein dairy cattle. J Anim Sci Biotechnol (2020) 11(1):1–7. doi: 10.1186/s40104-020-00494-7
197. Li M, Gao Y, Wang Z, Wu B, Zhang J, Xu Y, et al. Taurine inhibits streptococcus uberis-induced NADPH oxidase-dependent neutrophil extracellular traps via TAK1/MAPK signaling pathways. Front Immunol (2022) 13:927215. doi: 10.3389/fimmu.2022.927215
198. Lippolis JD, Reinhardt TA, Goff JP, Horst RL. Neutrophil extracellular trap formation by bovine neutrophils is not inhibited by milk. Vet Immunol immunopathol (2006) 113(1-2):248–55. doi: 10.1016/j.vetimm.2006.05.004
Keywords: bovine mastitis, immunity and inflammation, genetic markers, polymorphisms, SCS, SCC, inflammatory cytokines
Citation: Khan MZ, Wang J, Ma Y, Chen T, Ma M, Ullah Q, Khan IM, Khan A, Cao Z and Liu S (2023) Genetic polymorphisms in immune- and inflammation-associated genes and their association with bovine mastitis resistance/susceptibility. Front. Immunol. 14:1082144. doi: 10.3389/fimmu.2023.1082144
Received: 27 October 2022; Accepted: 06 January 2023;
Published: 23 February 2023.
Edited by:
Jagadeesh Bayry, Indian Institute of Technology Palakkad, IndiaReviewed by:
Kecheng Zhu, South China Sea Fisheries Research Institute (CAFS), ChinaBianca Castiglioni, National Research Council (CNR), Italy
Copyright © 2023 Khan, Wang, Ma, Chen, Ma, Ullah, Khan, Khan, Cao and Liu. This is an open-access article distributed under the terms of the Creative Commons Attribution License (CC BY). The use, distribution or reproduction in other forums is permitted, provided the original author(s) and the copyright owner(s) are credited and that the original publication in this journal is cited, in accordance with accepted academic practice. No use, distribution or reproduction is permitted which does not comply with these terms.
*Correspondence: Shuai Liu, bGl1c2h1YWljYXVAY2F1LmVkdS5jbg==
†These authors have contributed equally to this work and share first authorship