- 1Laboratory of Phage Molecular Biology, Hirszfeld Institute of Immunology and Experimental Therapy, Polish Academy of Sciences, Wroclaw, Poland
- 2Research and Development Centre, Regional Specialist Hospital, Wroclaw, Poland
- 3Institute for Bioscience and Biotechnology Research, University of Maryland, Rockville, MD, United States
Bacteriolytic enzymes are promising antibacterial agents, but they can cause a typical immune response in vivo. In this study, we used a targeted modification method for two antibacterial endolysins, Pal and Cpl-1. We identified the key immunogenic amino acids, and designed and tested new, bacteriolytic variants with altered immunogenicity. One new variant of Pal (257-259 MKS → TFG) demonstrated decreased immunogenicity while a similar mutant (257-259 MKS → TFK) demonstrated increased immunogenicity. A third variant (280-282 DKP → GGA) demonstrated significantly increased antibacterial activity and it was not cross-neutralized by antibodies induced by the wild-type enzyme. We propose this variant as a new engineered endolysin with increased antibacterial activity that is capable of escaping cross-neutralization by antibodies induced by wild-type Pal. We show that efficient antibacterial enzymes that avoid cross-neutralization by IgG can be developed by epitope scanning, in silico design, and substitutions of identified key amino acids with a high rate of success. Importantly, this universal approach can be applied to many proteins beyond endolysins and has the potential for design of numerous biological drugs.
1 Introduction
Bacteriophage (or phage)-encoded cell wall hydrolases, termed endolysins, are currently being developed as novel therapeutic tools for treating infections by Gram-positive pathogens. Endolysins are normally active late in the phage infection cycle, rapidly cleaving bonds necessary for peptidoglycan stability in order to facilitate bacterial lysis and release of progeny phage. When applied directly to susceptible bacteria in a purified form in the absence of bacteriophage, endolysins act “from without” to hydrolyze the peptidoglycan, resulting in osmotic lysis of the organism (1, 2). Significantly, due to their rapid lytic actions, endolysins are not susceptible to efflux pumps, penicillin-binding proteins, alterations of metabolic pathways, or other mechanisms of resistance seen with traditional antibiotics, making them ideal alternative therapeutics to treat multi-drug resistant organisms (3, 4). Additionally, endolysins are species-specific and, as such, represent a potential narrow-spectrum antimicrobial therapeutic that exploits the targeted killing capability of phage. Indeed, the US FDA granted “Breakthrough Therapy” designation status to endolysins in 2020 and several biotechnology/pharmaceutical companies have been conducting human clinical trials on endolysins, thus validating endolysin technologies as antimicrobial biologics, as reviewed (5).
Among the most notable endolysins in pre-clinical development are Pal, derived from Streptococcus phage Dp-1, and Cpl-1, derived from Streptococcus phage Cp-1. Streptococcus pneumoniae, the target of Cpl-1 and Pal, is the most common cause of bacteremia, pneumonia, meningitis, and otitis media in children - despite a successful vaccine campaign against pneumococcal disease over the past two decades, a Global Burden of Disease Study suggested that over 500,000 deaths still occur annually due to S. pneumoniae infection (6). Thus, pneumococcal endolysins represent a high potential for therapeutic application.
In spite of being promising antibacterial agents, endolysins are prokaryotic proteins that induce a normal immune response in vivo (1, 7, 8). It has been demonstrated for lysostaphin, which is also a peptidoglycan-targeting antibacterial enzyme, that reduction of its immunogenicity translated into improved efficacy in vivo (9). An engineered lysostaphin variant provided protection against repeated challenges of methicillin-resistant Staphylococcus aureus (MRSA), whereas the wild-type (WT) enzyme was efficacious only against the initial MRSA infection, but failed to clear subsequent bacterial challenges that were coincident with escalating anti-drug antibody titers. As demonstrated, endolysin-specific serum from immunized mice also reduced the rate of antibacterial activity of these enzymes (7, 10). However, only general observations about the immunogenicity of endolysins have been made to date. Particularly, molecular features (i.e. epitopes defined by amino acid composition) that contribute to this effect remain unknown.
Although many investigators have developed improved endolysins with the aim of achieving better efficacy, these investigations only focus on improvements of endolysin stability or alterations of their bacterial host range or catalytic action. While these features are of great importance, they only relate to the basic activity that the enzyme exhibits in vitro. For in vivo applications, antibacterial drugs must overcome specific conditions and pressure from the living system, including the prominent effects of the immune system. In this study, we seek to understand how immune-reactive epitopes are distributed within endolysin proteins, and what modifications result in variants capable of escaping from the specific immune response induced by the WT enzymes. We apply true-epitope identification using the EndoScan platform technology derived from the innovative VirScan technology developed for identification of viral antigenic epitopes by Xu et al. (11). This technology allows us to identify linear B cell epitopes that have elicited specific antibodies either in animals or in humans. Our approach applies, for the first time, a high-throughput method of linear B cell epitope identification in endolysins. As such, it validates a novel, highly efficient method for improvement and adaptation of many other protein-based biological drugs and extends the existing methods of protein de-immunization by engineering identified antigenic determinants.
2 Materials and methods
2.1 Epitope analysis
Identification of amino acids interacting with specific IgGs was performed in accordance with the protocol published by Xu et al. and adapted for our research using coding sequences of the investigated endolysins as the source for library design (11). The peptide library was created by selecting 56 amino acid (aa) long fragments tailing through the Cpl-1 (acc. no.: CAA87744.1) and Pal (acc. no: YP_004306947.1) endolysins, starting every 10 aa from the first amino acid, or where necessary, shorter oligopeptides near the end of the protein. In addition to the oligopeptides representing wild-type peptides, oligopeptides with mutations (alanine substitutions) were introduced. Alanine residues in the original sequences were substituted by glycine. Thus, our library contains single-, double- and triple-substitutions for amino acids at each position in the protein sequence. The total number of (oligopeptides) were: 31 natural and over 2,500 mutated for Cpl-1 and 27 natural and almost 2,200 mutated for Pal.
The peptide library was reverse-translated into DNA sequences using codons optimized for expression in E. coli. The oligonucleotide library was synthesized using the SurePrint technology for nucleotide printing (Agilent). These oligonucleotides were used to create a phage library representing all oligopeptides using the T7Select 415-1 Cloning Kit (Merck Millipore). Immunoprecipitation of the library was performed in accordance with a previously published (11, 12). Briefly, the phage library was amplified in a standard culture, purified by PEG precipitation, and dialyzed against Phage Extraction Buffer (20 mM Tris-HCl, pH 8.0, 100 mM NaCl, 6 mM MgSO4). All plastic equipment used for immunoprecipitation, including Eppendorf tubes and 96-well plates, were prepared by blocking with 3% bovine serum albumin (BSA) in TBST buffer overnight on a rotator (400 rpm, 4°C). A sample representing an average of 105 copies of each clone was mixed with 2 µL of serum with high levels of anti-endolysin IgGs (see: specific sera induction in mice) or human serum from healthy volunteers. This mix was prepared in 250 µL phage extraction buffer and incubated overnight at 4°C on a rotator (400 rpm). A 40 μL aliquot of a 1:1 mixture of Protein A and Protein G Dynabeads (Invitrogen) was added and incubated for an additional 4 h at 4°C on a rotator (400 rpm). Liquid in all wells was separated from Dynabeads on a magnetic stand and removed. Beads were washed 3 times with 150 µL of a wash buffer (50 mM Tris-HCl, pH 7.5, 150 mM NaCl, 0.1% Tween-20) and beads were resuspended in 60 µL of water.
The immunoprecipitated part of the library was then used for amplification of the insert region according to the manufacturer’s instructions with a Phusion Blood Direct PCR Kit (Thermo Fisher Scientific). The primers T7_Endo_Lib_LONG_FOR GCCCTCTGTGTGAATTCT and T7_Endo_Lib_LONG_REV GTCACCGACACAAGCTTA were used and a second round of PCR was carried out with the IDT for Illumina UD indexes (Illumina Corp.) to add adapter tags. Sequencing of the amplicons in accordance with Illumina next generation sequencing (NGS) technology was outsourced (Genomed, Warszawa).
2.2 Sequencing data analysis
Sequenced amplicons were mapped to the original nucleotide library sequences by the bowtie2 software as published by Xu et al. (11, 13). NGS sequencing reads were mapped by the bowtie2 software package with use of the file with list of oligonucleotide sequences synthesised originally to create a library as indexes (local mode) (13, 14). The number of hits that mapped to each reference sequence (highest score) was counted (count, c).
The signal in each sample was calculated according to a formula (1).
s – signal of i-th sequence in j-th serum sample and m-th technical replicate;
c – number of reads mapped to i-th sequence in m-th technical replicate of j-th serum sample;
I – set of all reference sequences (used as indexes to in mapping by the bowtie2 software).
The vast majority of clones were washed away and were not enriched, if detected at all. Thus, a zero-inflated negative binomial model was used to evaluate the probability of each signal to be random. Noteworthy, this model fitted control data better than zero-inflated Poisson distribution proposed by Xu et al. (11). Next, all p-values of oligopeptides detected in the sample were adjusted for multiple hypotheses (false discovery rate method) (15). Input sample: sample of phage library before immunoprecipitation representing starting levels of oligopeptides in the immunoprecipitation procedure. Relative signal (enrichment): an average signal in technical replicates of the sample divided by the average signal in ‘input samples’ of the same sequence is a signal ratio (relative signal). A series of t-tests evaluated the significance of differences in signals for each oligopeptide separately between input samples (one group) and the tested sera.
2.3 Variant design
Every detected immunogenic amino acid (that after being substituted with alanine/glycine caused an oligopeptide to not be recognized by IgG antibodies) was substituted in silico with every other possible amino acid and the differences in free folding energy (ΔΔG) was calculated using the FoldX software (16). The three-dimensional structure of Cpl-1 was downloaded from the PDB (Code: 2IXU) (17) and a model of the Pal structure was created using the I-Tasser software since its crystal structure has not previously been determined (18). Structural variants were then chosen based on the following assumptions:
a) amino acids that differed from WT in their electrostatic charge and chemical structure were selected to disrupt epitope interaction with specific antibodies;
b) the lowest energy state of the folded variant was preferred;
c) smaller amino acids were preferred for the substitution due to lower steric tensions.
2.4 Protein expression
The endolysins Pal (acc. no. YP_004306947) (Pal WT), derived from Streptococcus phage Dp-1, and Cpl-1 (acc. no. CAA87744) (Cpl-1 WT), derived from Streptococcus phage Cp-1, were used in this study. The Cpl-1 and Pal coding sequences were cloned into pBAD24 with a C-terminal 6xHis tag. Vectors with genes coding variants of Pal WT were synthesized de novo and cloned into the pBAD_HisA plasmid (BioCat GmbH, Germany).
Cpl-1 WT and Cpl variant 4 (v4) (see Table 1) were synthesized de novo and additional mutations were introduced by PCR with primers coding for the mutagenized site and ligated with Liga5 (A&A Biotechnology). Vector inserts were sequenced by Sanger sequencing to confirm validity of the cloning procedure. These vectors were transformed into E. coli B834(DE3) cells (EMD) and grown at 37°C with shaking in Luria-Bertani (LB) broth (10 g/L tryptone, 10 g/L NaCl, 5 g/L yeast extract) supplemented with ampicillin (50 mg/L) (Sigma-Aldrich, Europe), until the OD600 reached 1.0. Then, protein expression was induced by the addition of arabinose at a final concentration of 2.5 g/L (0.25%). The culture was incubated overnight at 22°C with intensive shaking.
2.5 Protein purification
Bacteria were harvested using centrifugation (7 000 x g, 5 min) and suspended in PBS (140 mM NaCl, 2.68 mM KCl, 1.47 mM KH2PO4, 6.46 mM Na2HPO4, pH 7.2), which was supplemented with PMSF (1 mM) and lysozyme (0.5 mg/mL). The slurry was incubated for 6–7 h on ice and lysed using the freeze-thaw method. Mg2+ (up to 0.25 mM), DNAse (up to 20 μg/mL), and RNAse (up to 40 μg/mL) were then added to the extract and incubated on ice for 3 h. The fractions were separated using centrifugation (12 000 x g, 30 min, 4°C) and the soluble fraction (supernatant) was collected.
For proteins to be used for mouse immunization studies, the crude supernatant was then incubated with NiNTA agarose (Qiagen) at room temperature, washed with PBS (5× volume of the agarose), and subsequently washed with an increasing concentration of imidazole (20 mM, 100 mM, 250 mM, and 500 mM). The 100 mM and 250 mM fractions containing the eluted endolysins were dialyzed against PBS at 4°C and they were further purified using gel filtration (fast protein liquid chromatography) on a Superdex 75 10/300 GL column (GE Healthcare Life Sciences). The final step was LPS removal, which was performed with an EndoTrap Blue column (Hyglos GmbH, Munich, Germany). Purified, endotoxin-free protein samples were dialyzed against PBS and filtered through sterile 0.22-μm polyvinylidene difluoride filters (Millipore). Endotoxin levels were monitored by EndoZyme (bioMeriux) and shown to be lower than 1 EU per mouse (in vivo).
For proteins used in all other experiments, Cpl-1 and Pal (both WT and variants) were supplemented in PBS up to 75 mM before incubation with NiNTA agarose. Then the slurry was washed with 10x of 85 mM imidazole in PBS and the active protein was eluted with 250 mM imidazole and dialyzed three times against a 100-fold excess of PBS at 4°C (3,000 kDa molecular weight cut-off, Spectra/Por, Repligen). Finally, samples were filtered through sterile 0.22-μm polyvinylidene difluoride filters (Millipore). Samples were monitored by SDS-PAGE at all stages. Protein concentrations were determined by the Bradford assay (Sigma-Aldrich, Europe) following the manufacturer’s instructions.
2.6 Testing activity, fluorometric assay
The Sytox Green solution from the ViaGram Red+ Bacterial Gram Stain and Viability Kit (Thermo Fisher Scientific) was used to measure bacteriolytic activity via a fluorescence plate reader in accordance to a previously published protocol (19). In short, mixtures of bacteria, endolysin, and Sytox™ Green (ThermoFisher Scientific) were prepared. Immediately after mixing, changes in the fluorescence signal of Sytox™ Green were measured for 15 min. The raw data from the fluorometric assay was normalized to the progress of the lysis with values between 0 (no lysis) and 1.0 (complete lysis). Progress values were used to calculate lytic activity as part of the total bacterial sample lysed per unit of time [min-1] using a linear regression model for Cpl-1 and a one-phase association model for Pal as previously described. Lytic activity is defined as the highest rate of lysis per unit of time for the linear part of the curve (for Cpl-1) or the beginning of the reaction (for Pal) before the reaction slows down due to diminishing bacterial substrate in the sample as described (19).
2.7 Specific sera induction in mice
C57BL/6J normal female mice (N=6 or 7) were obtained from Mossakowski Medical Research Centre Polish Academy of Sciences in Warsaw and housed in the Animal Breeding Centre of the Hirszfeld Institute of Immunology and Experimental Therapy (HIIET) in an isolated area in specific pathogen free conditions. To produce endolysin-specific serum, C57BL6/J mice were challenged intraperitoneally (i.p.) with Pal, Cpl-1, or their variants (0.1 mg per mouse, endotoxin level below 1 EU per mouse) on day 0; no adjuvants were used. Murine blood was collected into clotting tubes under anesthesia from the tail vein on day 28. Serum was separated from the blood using double centrifugation (2,250 x g for 5 min and then 10,000 x g for 10 min). The sera were stored at −20°C.
For comparison the immunogenicity of Pal and its variants, blood was collected as above every 3–5 days until at least day 50. Similarly, to compare immunogenicity of Cpl-1 and its variants, mice were challenged twice, on day 0 and at least 30 days later, since overall immunogenicity of Cpl-1 is lower than that of Pal (7).
2.8 Measurement of specific IgG antibody levels
MaxiSorp flat-bottom 96-well plates (Nunc, Thermo Scientific) were coated in sterile conditions overnight at 4°C with endolysins or PBS as a control using 100 μL per well at a concentration of 10 μg/mL, except for Pal v1 that demonstrated lower signal unless used at concentration 80 μg/mL (Supplementary Figure S1). Subsequently, wells were washed five times with PBS and blocked for 30 min with SuperBlock Blocking buffer (Life Technologies Europe BV, Bleiswijk, Netherlands) at 150 μL per well and at room temperature. The solution was removed and the plates were washed five times with 0.05% Tween-20 (AppliChem GmbH, Darmstadt, Germany) in PBS at 100 μL per well. One hundred microliters per well of diluted serum (1:350 in PBS) was applied to the wells coated with endolysins. Each sample was investigated in five repeats. The plates were incubated at 37°C for 2 h, after which serum was removed and the plates were washed five times with 0.05% Tween-20 in PBS at 200 μL per well. One hundred microliters per well of diluted detection antibody (peroxidase-conjugated goat anti-mouse IgG (Jackson ImmunoResearch Laboratories) was applied to the plates and incubated for 1 h at room temperature in the dark. The antibody solution was removed and the plates were washed with PBS with 0.05% Tween 20 five times at 100 μL per well. TMB (50 μL) was used as a substrate reagent for peroxidase according to the manufacturer’s instructions (R&D Systems) and incubated for 30 min in the dark. Twenty-five microliters of 2N H2SO4 was added to stop the reaction and the absorbance was measured at 450 nm (main reading) and normalized by subtracting the background absorbance at 550 nm.
2.9 Protein concentration measurement
Protein concentration was determined with use of the Bradford assay (Sigma) according to manufacturer’s manual if not explicitly mentioned otherwise. In short: 200 µl of Bradford reagent was put in a well of a 96-well plate and 6.66 µl of the protein sample was added. After three min, the sample was mixed by pipetting and the absorbance was measured at 590 nm and 450 nm. The ratio was compared to a calibration curve (BSA in concentrations from 62.5 µg/mL to 1000 µg/mL) and the concentration of the protein in the sample was calculated from a linear regression of the standards. If the concentration of the protein was too high, the samples were diluted 4-fold.
2.10 Ethics approval statement
All human subjects gave informed consent for inclusion before they participated in the study. The study was conducted in accordance with the Declaration of Helsinki, and the protocol was approved by the Bioethics Committee of the Regional Specialist Hospital in Wrocław (Project identification code: KB/nr 2/2017). All animal experiments were performed according to EU directive 2010/63/EU for animal experiments and were approved by the 1st Local Committee for Experiments with the Use of Laboratory Animals, Wrocław, Poland (project no. 76/2011). The authors followed the ARRIVE (Animal Research: Reporting of in vivo Experiments) guidelines.
3 Results
3.1 EndoScan: identification of antigenic epitopes in Pal and Cpl-1 endolysins
Identification of antigenic epitopes was done by epitope scanning (EndoScan), a new approach proposed herein for protein epitope identification, derived from the innovative VirScan technology developed by Xu et al. (11). The EndoScan approach is presented in Figure 1. Briefly, a phage display library of all epitopes in the endolysins was constructed with a nucleotide-printed library of oligopeptides representing overlapping sequences of the investigated endolysins. This library was incubated with (i) murine sera containing high levels of anti-Pal or anti-Cpl-1 IgG induced by specific challenge, or (ii) human sera representing the normal population of healthy volunteers.
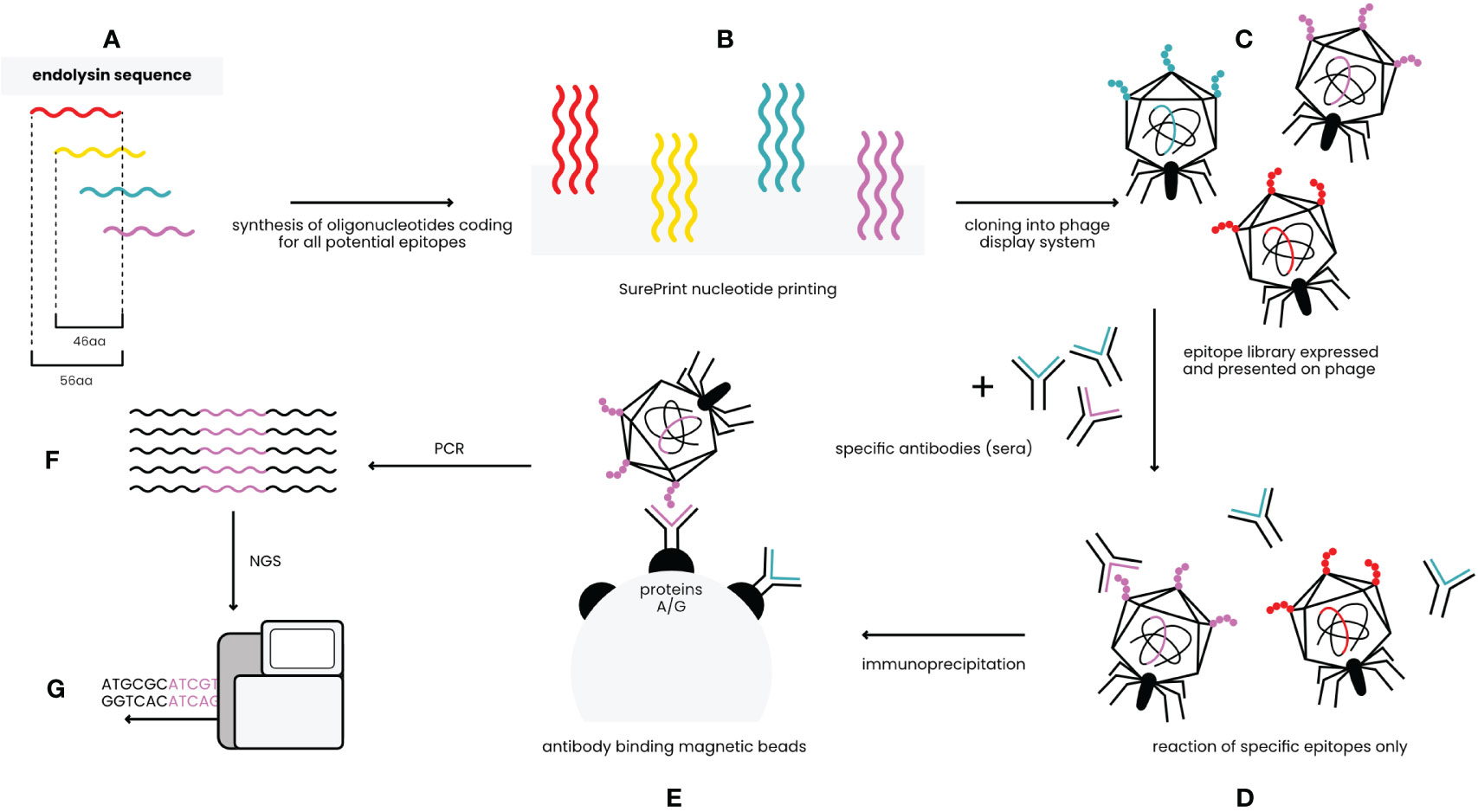
Figure 1 EndoScan technology – an overview (modified from Xu et al.) (11). (A) In silico design of peptides covering sequences of Pal and Cpl-1. (B) Synthesis of oligonucleotides coding for the peptides. (C) Constructing the phage display library of endolysin-derived peptides. (D) Reaction of the library with endolysin-specific sera. (E) Immunoprecipitation with magnetic beads binding Fc fragments of antibodies. (F) Amplification by PCR reaction. (G) NGS sequencing.
The investigated sera were immunoprecipitated with IgG-specific magnetic beads. Thus, a fraction of the phage display library that exposed endolysin epitopes reactive to IgG present in sera was isolated. Since the library contains whole phage particles, each phage that displays a specific epitope contains a sequence coding for this epitope in its genome. Thus, NGS sequencing of the relevant region in the library genomes was used to reveal which epitopes were effectively recognized by specific IgG (Figure 1).
Oligopeptides used in the library were 56 aa long and they overlapped each other by 46 aa, thus creating up to 6-fold coverage for any given residue, to avoid splitting epitopes or loosing interactions with surrounding peptide structures. Two types of these libraries were used to allow for complete analysis of immunogenic epitopes. The first library allowed for general screening and detection of immunoreactive regions within the endolysins; the same regions were identified in a mouse model and in a human population (Figure 2 and Supplementary Table S1).
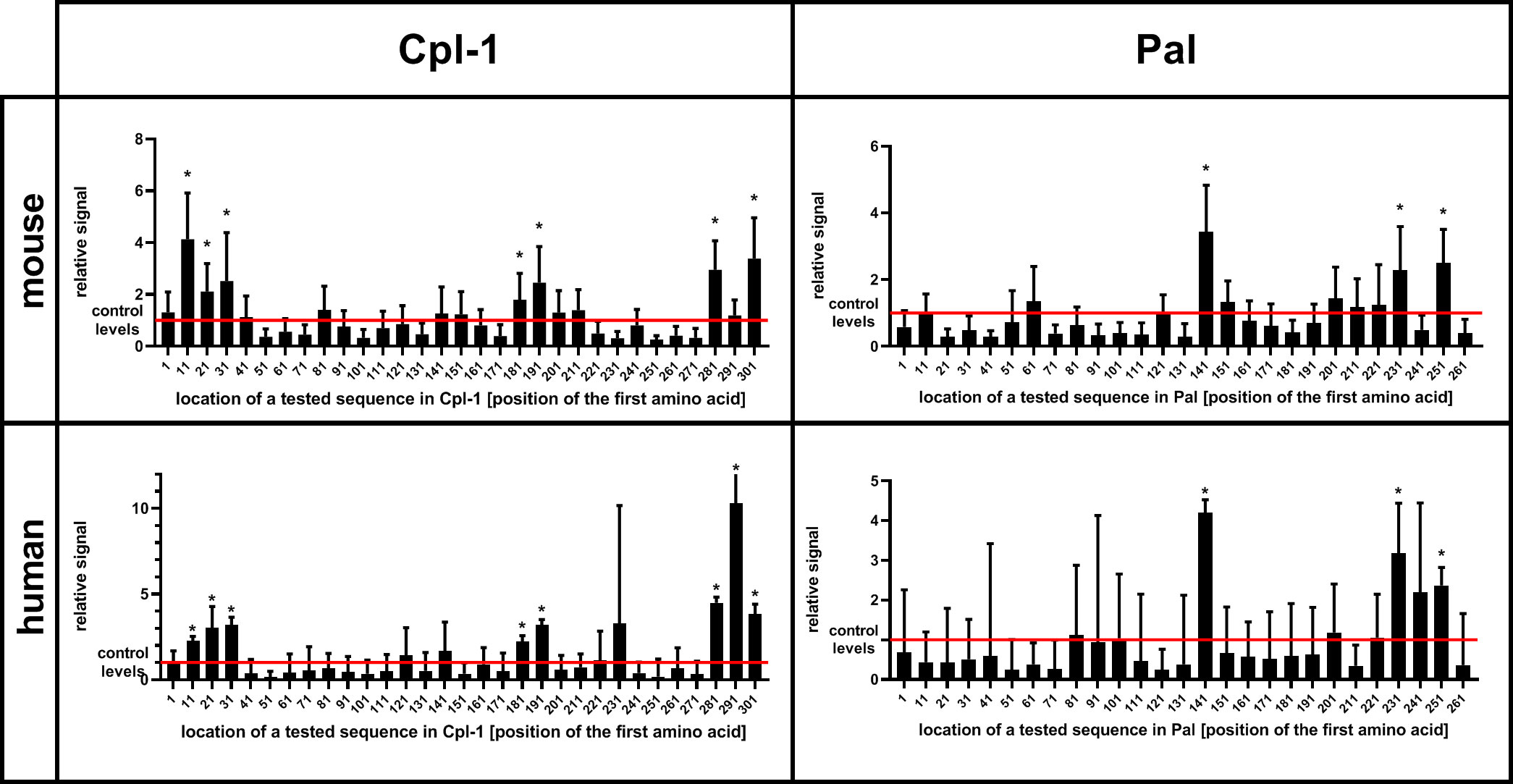
Figure 2 Immunoreactivity of short oligopeptides representing whole sequences of Cpl-1 and Pal endolysins in humans and mice. Oligopeptides used in the library were 56 aa long each and they overlapped by 46 aa (10 aa shift). An increase in relative signal (signal to control ratio) demonstrates interactions between IgG and the oligopeptide. X-axis represents sequence of an endolysin from N-terminus (left) to C-terminus (right). Bars represent relative signal (signal to control ratio) of each measured oligopeptide. Oligopeptides were expressed as a phage display library, immunoprecipitated with sera from mice or humans representing a normal population of healthy volunteers. * adj. p value<0.05 between relative signal (ratio) of a measured oligopeptide after immunoprecipitation and before (control level in input sample, red line), Kruskal-Wallis test (two-sided), GraphPad Prism 9. Red line – levels for control group, a library before immunoprecipitation (input sample), data represent 7 (mouse) or 56 (human) biological replicates. Bars and whiskers represent mean value and standard deviation.
The second library was designed to define key amino acids forming the epitope sites. Specifically, when key amino acids are substituted, the variants fail to react with specific antibody (Supplementary Figure S2). Thus, the second library contained a set of oligonucleotides coding for the same fragments of the endolysins, but bearing alanine substitutions in the sequence. Where the original sequence contained alanine, a glycine was used as the substitution. Further, to reduce the risk of false positive and false negative responses, variants with double substitutions and triple substitutions (targeted amino acid plus neighboring amino acids) were also included, resulting in 165 variants for each oligopeptide. The mouse sera were used for identification of amino acids that contributed to the Pal and Cpl-1-specific responses. The EndoScan technology as described above allowed for identification of 41 amino acids in Pal and 21 amino acids in Cpl-1 (Figure 3).
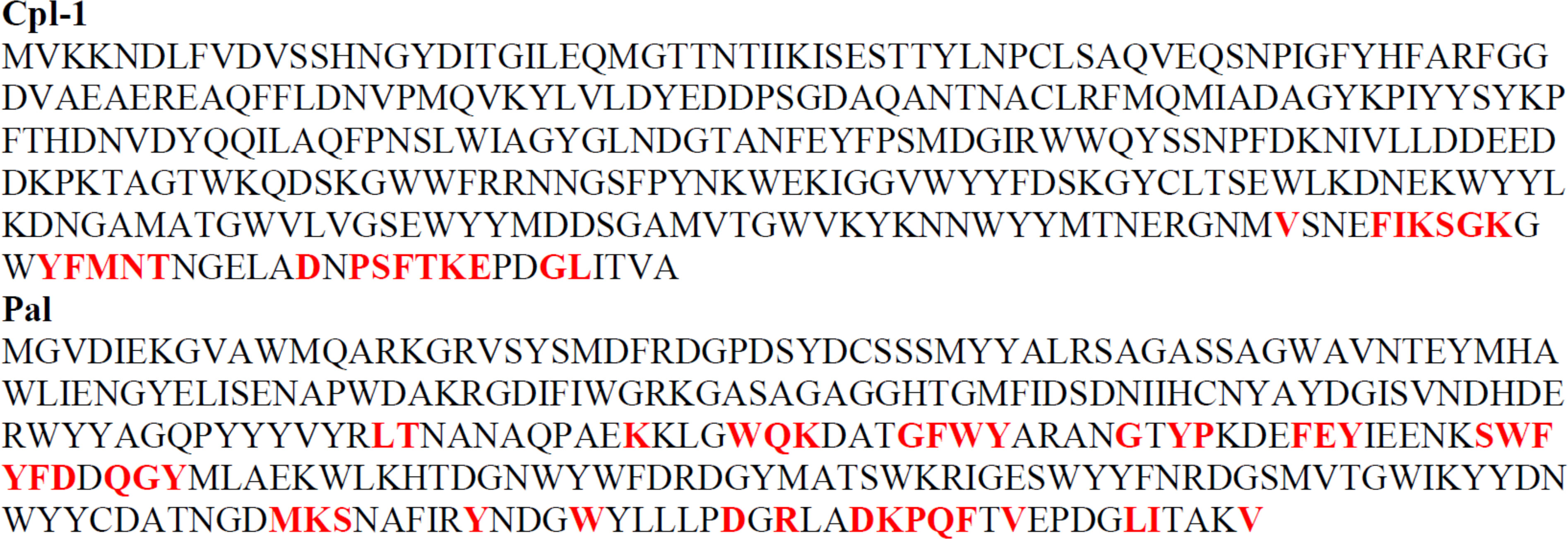
Figure 3 Key immunogenic amino acids (in red and bold) forming immunoreactive epitopes within Pal and Cpl-1 endolysins as defined by the EndoScan technology. Each amino acid in detected immunoreactive fragments was substituted with alanine (alanine with a glycine). If an oligopeptide after alanine substitution lost its previous immunoreactivity, such amino acid was designated as a key one for immunoreactivity of a protein.
3.2 Pal and Cpl-1 variants with engineered immunoreactive epitopes
Engineering Pal and Cpl-1 to escape recognition by epitope-specific antibodies was conducted manually with 3D protein models by amino acid substitutions with the following assumptions:
● each substitution was complemented with amino acids with different charge and chemical type of its substituents to disturb the epitope match with antibodies;
● the change in a folding energy of the protein (ΔΔG) calculated after substitution should be as low as possible for stabilization of the protein tertiary structure;
● smaller amino acids were preferred to larger amino acids to minimize steric tensions.
Nine possible variants of Pal were designed, but expression in the E. coli system yielded only three that were expressed as soluble proteins: Pal v1, Pal v3, and Pal v9. For Cpl-1, five possible variants were designed and they all yielded satisfactory expression of soluble enzymes (Table 1, full sequences are presented in Supplementary Figure S3). Our variants were restricted to residues located in the C-terminus, in part because this region was the most immunoreactive in both Pal and Cpl-1, and in part because we wanted to avoid modifications to the catalytic domain, which occupies an N-terminal location in both endolysins.
All expressed variants of Pal and Cpl-1 were tested for their antibacterial activity against pneumococci. This testing was completed over a range of enzyme concentrations and revealed that all selected variants had antibacterial activity, although the antibacterial activity in variants v1 and v9 of Pal and variants v2, v4, and v5 of Cpl-1 was significantly lower than in the WT enzymes (Figure 4). Variants Cpl-1 v1 and Cpl-1 v3 were active similarly to the WT enzyme, and Pal v3 was significantly more active against sensitive bacteria than the WT enzyme (Figure 4). Since all variants demonstrated at least partial antibacterial activity, they were all used in further immunological studies.
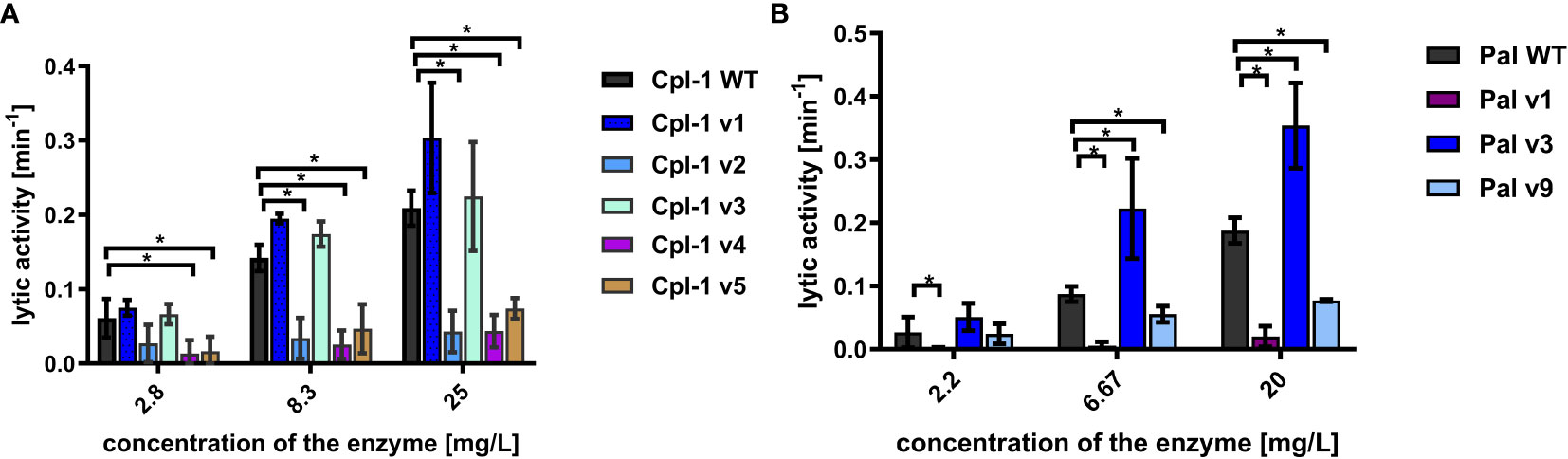
Figure 4 Antibacterial activity of variants in PBS. (A) Antibacterial activity of endolysins Cpl-1 and its variants with engineered epitopes. (B) Antibacterial activity of endolysins Pal and its variants with engineered epitopes. Lytic activity represents a part of the sample that is being lysed in one minute at the peak activity. Bars and whiskers represent mean and SD of 3 experiments (two replicates each). * adj. p<0.05, unpaired, two-sided t-test with p value adjusted using the Holm-Sidak method, GraphPad Prism 9.
3.3 Immune responses to Pal and Cpl-1 variants with engineered immunoreactive epitopes
Biologically active proteins can be prone to non-specific inactivation by the complex serum matrix. This might change the variants’ applicability in vivo, independent of their possible improved performance in the presence of antibodies targeting the WT endolysin. Endolysin variants were therefore tested for antibacterial activity ex vivo in naïve sera from unchallenged animals. In the WT enzymes, as well as most of the tested variants, sera were found to significantly decrease lytic activity (Figure 5). Three Cpl-1 variants, Cpl-1 v2, Cpl-1 v4 and Cpl-1 v5 demonstrated severely impaired overall activity, even though the Cpl-1 v4 did not demonstrate a significant difference in its activity with or without serum. Pal v1, also with intrinsic poor activity, demonstrated improved activity in the presence of serum, but it was still lower than that of the WT protein (Figure 5).
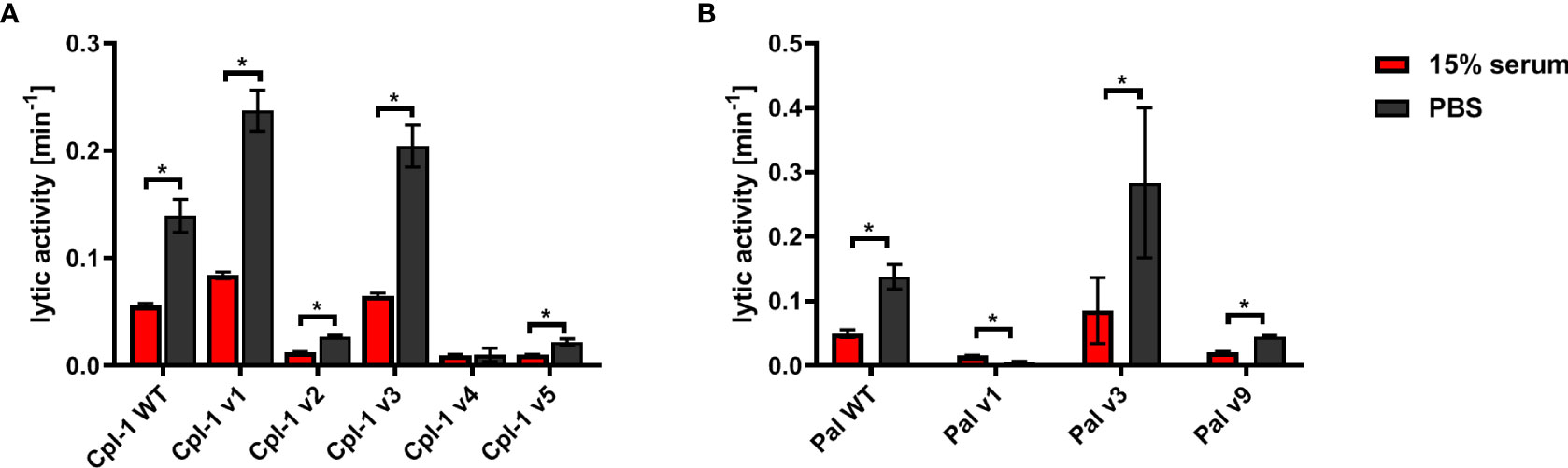
Figure 5 Effect of naïve (non-specific) murine serum on antibacterial activities of endolysins Cpl-1 and Pal. (A) Comparison of activity in non-specific serum (15%) and PBS of Cpl-1 endolysin (10 µg/mL) and its variants. (B) Comparison of activity in non-specific serum (15%) and PBS of Pal endolysin (10 µg/mL) and its variants. Lytic activity represents a part of the sample that is being lysed in one minute at the peak activity. Bars and whiskers represent mean and SD of 3 experiments (two replicates each). * adj. p<0.05, unpaired, two-sided t-test with p value adjusted using the Holm-Sidak method, GraphPad Prism 9.
Pal variants were further investigated for their in vivo immunogenicity in an animal model. Immunogenicity herein is considered as an induction of serum levels of specific IgG. Seroconversion of animals immunized with all proteins (Pal WT, and its variants v1, v3, v9) occurred during the second-third week, and then it drops in Pal WT, Pal v3, and Pal v9 (Figure 6). In the case of Pal v9, its specific IgG level was significantly decreased in comparison to Pal WT, but only on days 40 and 50 after challenge (p<0.001). In contrast, Pal v1-specific IgG maintained similar concentration until week 7, and thus it was significantly higher on days 40 and 50 after challenge (p<0.001) than Pal WT-specific IgG (Figure 6). Thus, the overall ability of the variants to induce specific antibodies in comparison to WT enzymes was decreased only in one case, and this variant demonstrated considerably lower antibacterial activity than the WT endolysin.
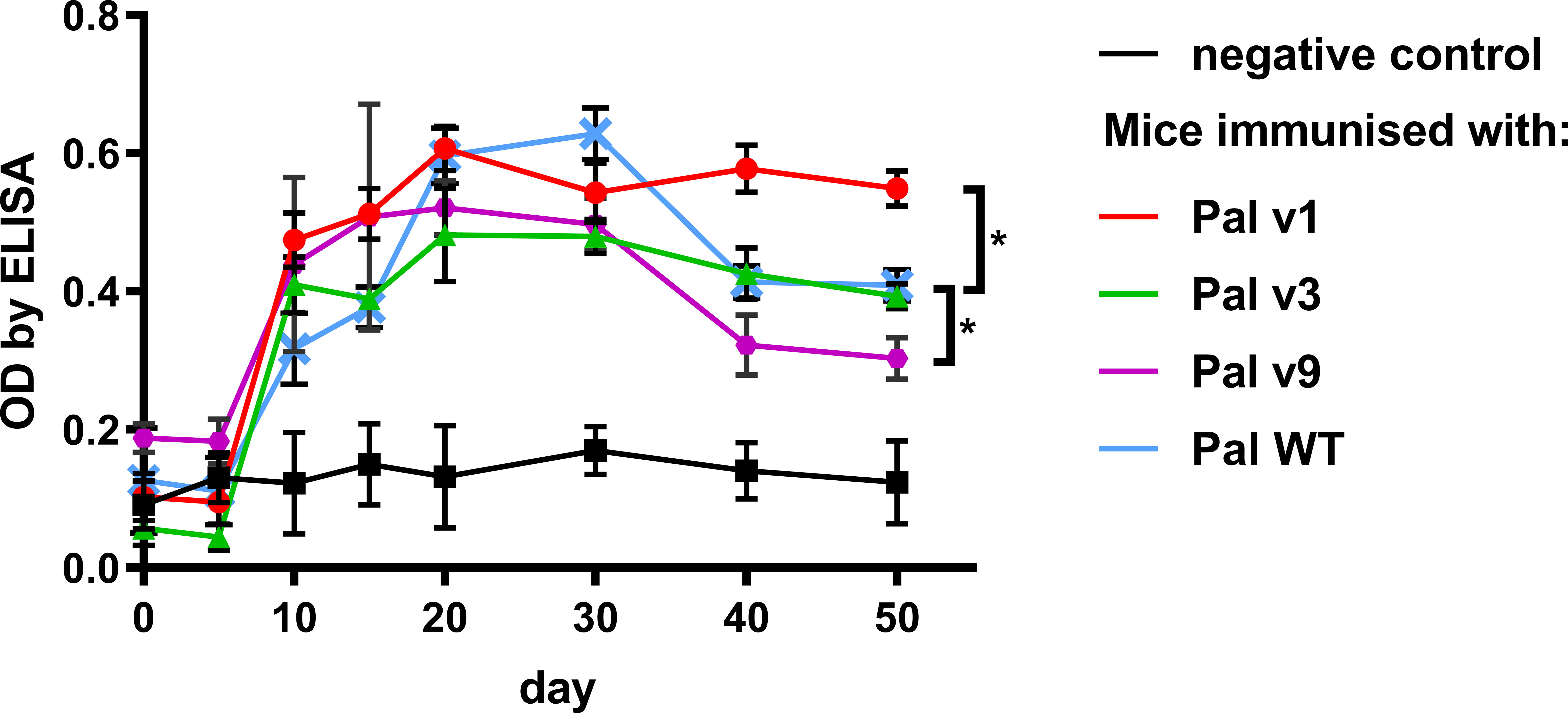
Figure 6 Serum levels of specific IgG induced by Pal and its variants with engineered epitopes identified by EndoScan. Mice were challenged with Pal or its variants on day 0 and serum levels of specific IgG antibodies on following days are presented as measured by ELISA tests. Points and whiskers represent mean and standard deviation of 6 biological replicates in a group, * - p<0.05 between indicated groups at a day 50 (biological replicates in each group, n=6), unpaired, two-sided t-test with p value adjusted using the Holm-Sidak method, GraphPad Prism 9.
Specific antibodies induced by an active protein can potentially limit success of this protein in situations of repeated use. Here, in most cases, we did not achieve overall deimmunization. Nonetheless, variants with the same overall immunogenicity but different immunogenic epitopes may escape cross-reactions with antibodies induced by the WT protein, thus being useful in repeat treatments, particularly in difficult drug resistant infections. Therefore, we investigated by ELISA cross-reactions of Pal and Cpl-1 variants with IgG induced by the WT enzymes. In all variants, cross-reactivity was weaker than the reactivity of WT Pal or Cpl-1, with the decrease of the Pal variants’ cross-reactivity being more marked (Figure 7). Therefore, we next tested ex vivo how the Pal variants escaped cross-neutralization by specific serum induced by WT Pal. The lytic activity of Pal WT, and its variants v3 and v9, was compared in the reaction with Pal-specific serum used as a blocking agent. Pal v1 was not investigated due to its overall significantly weakened antibacterial activity. This testing revealed that Pal v3 demonstrated significantly stronger antibacterial activity in Pal-specific serum than the WT Pal enzyme (p<0.05). Specifically, two effects contributed to this advantage: Pal v3 was not neutralized by Pal-specific serum, and it demonstrated intrinsic higher activity than WT Pal (Figure 8). Thus, Pal v3 is a variant of the Pal endolysin that escapes cross-neutralization with specific antibodies induced by the WT Pal, and it has overall improved antibacterial efficacy.
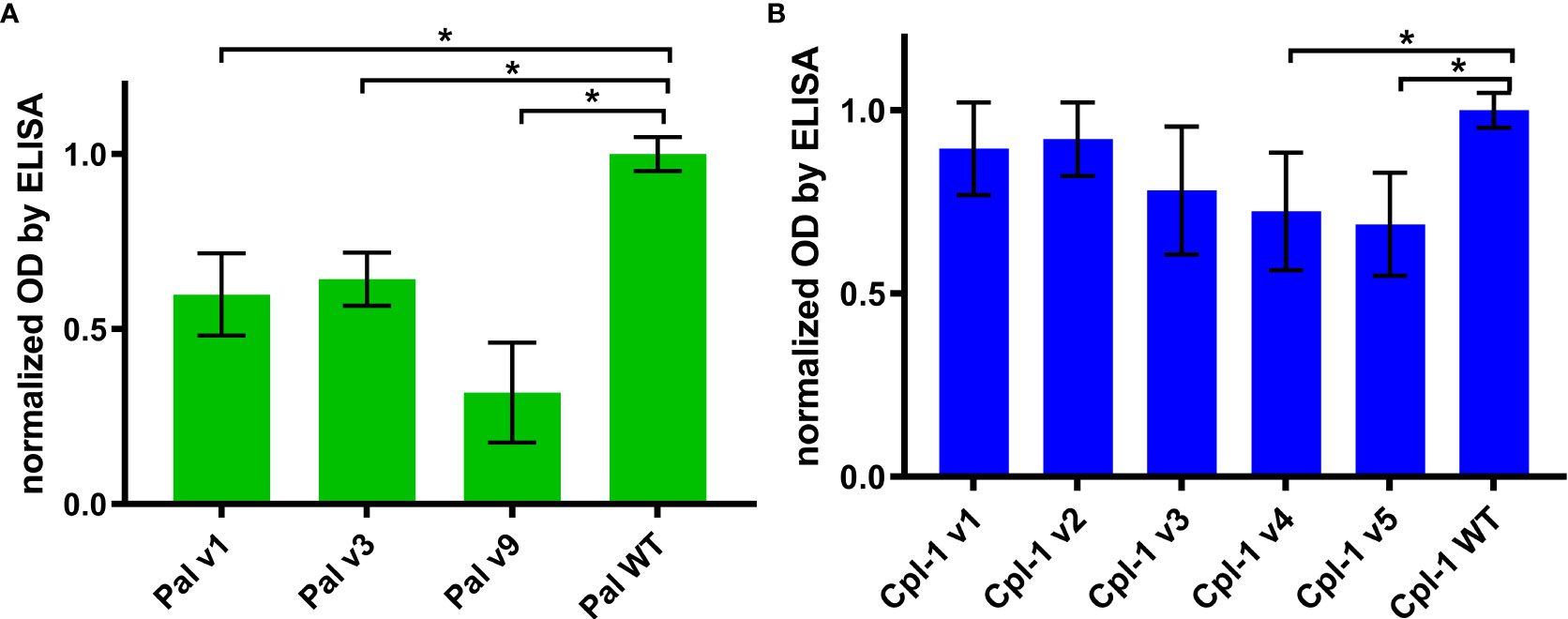
Figure 7 Cross-reactivity of serum with IgG specific to WT Pal and Cpl-1 to its variants. (A) Cross-reactivity of WT Pal specific IgG. (B) Cross-reactivity of WT Cpl-1 specific IgG. Bars and whiskers represent mean and standard deviation of 6 biological replicates per group. * adj. p<0.01, unpaired, one-sided t-test with p value adjusted using the Benjamini & Hochberg method (detailed information on statistics with p values is given in Supplementary Table S2).
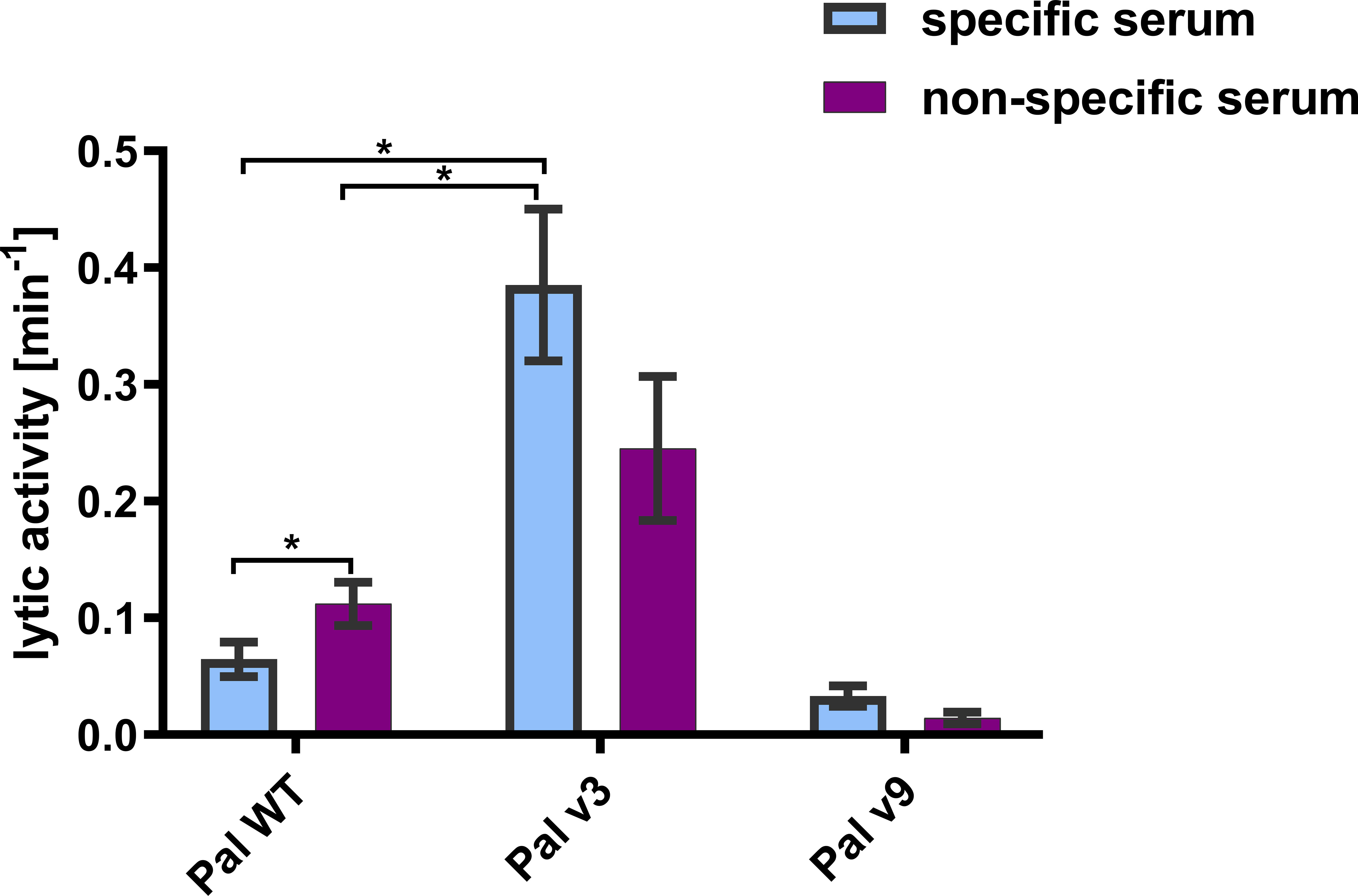
Figure 8 Lytic activity of WT Pal and its variants v3 and v9 in specific and non-specific serum. Lytic activities in specific and non-specific serum is presented. The tested concentration of endolysins and all variants was 20 µg/mL. Serum concentration was 15%. Bar and whiskers represent mean and SD of 3 measurements (two technical replicates each). * adj. p<0.05, unpaired t-test with p value adjusted using the Holm-Sidak method, GraphPad Prism 9.
4 Discussion
In spite of being promising antibacterial agents, endolysins are prokaryotic proteins that have been demonstrated to induce a normal immune response in vivo, including induction of specific antibodies (7, 8). Importantly, antibodies targeting a protein do not enter random interactions with the proteins surface. Rather, they bind only selected sites that constitute antigenic epitopes that mediate protein immunogenicity (20). In this study, we applied a modified approach of antigenic epitope scanning adapted from Xu et al. for identification of antigenic epitopes within two antibacterial enzymes, the endolysins Pal and Cpl-1 (Figure 2) (11). This approach identifies real (physical) epitope-antibody interaction, therefore extending and validating any theoretical predictions potentially available for immunogenic epitopes. Herein, scanning included both a mouse model and human sera. Animal sera provided a source of IgG specifically targeting the investigated endolysins, developed by challenge with WT Pal or Cpl-1. Human sera allowed for identification of endolysin epitopes frequently recognized by IgG in the human population. In some individuals, a relatively increased reactivity can be observed (Supplementary Figure S4). It is not clear whether this reactivity is due to natural contact with endolysins or it results from cross-reactions of antibodies induced by other unspecified antigens. We hypothesize that in addition to the already demonstrated and common induction of antibodies by structural proteins of phage virions, non-structural phage proteins produced in phage life cycles may challenge humans due to release of these proteins in bacterial cell lysis (21, 22). This may have significant implications for therapeutic use of phage-derived enzymes, since some individuals may demonstrate preexisting, natural antibodies, even when no previous treatment was conducted.
In this study, specific antigenic epitopes were identified in Pal and Cpl-1 endolysins (Figure 3). However, some important limitations of the study should be considered: (i) B cell linear epitopes were targeted by this technology, not conformational epitopes or T cell epitopes, and (ii) the animal challenge was conducted with His-tagged proteins, thus the tag could affect the immunogenicity of a protein. Nevertheless, the epitopes identified with human sera (natural) were to some extent the same as those targeted by specific IgG developed in the animal model, particularly epitopes in the C-terminal region of Cpl-1. Therefore, we propose this identification as the strongest one. Other epitopes were not equally represented in both the human and mouse sera (Figure 2) and these differences could be explained either by (i) molecular differences in human and murine immune responses mechanisms, or by (ii) the fact that human antibodies targeting the investigated endolysins are natural, that is they have not been achieved by a specific challenge. Instead, they may represent complex cross-reactions of antibodies induced by similar, or even very distant antigens that affect human immunity in the natural environment. The first possibility is related to the fact that the production of specific IgG is dependent on class switching of the B cell induced by the interaction with a matching T cell. T cell activation is dependent on epitope presentation bound to MHC II, which is highly polymorphic and varies between individuals and different species. Differences observed herein can be linked to these differences. The second possibility is driven by the multitude of microbial and other antigens to which humans are constantly exposed in their life. We propose that the clinically relevant detection of immunoreactivity should include sera from patients treated with the investigated endolysins, hence our reliance on the mouse model sera rather than the human sera for the identification of epitopes. This identification approach could be beneficial in future therapeutic trials, either for Pal and Cpl-1, or for other enzymes.
Identification of immunoreactive regions up to specific amino acids allowed herein for in silico design of new variants of the Pal and Cpl-1 endolysins with the aim to disrupt their antigenic activity. Our protein engineering approach was based on amino acid substitutions or deletion. Specifically, among 19 possible amino acid substitutions, amino acids with different (to WT) electrostatic charge were selected to disrupt matching of interactions between epitope-paratope amino acid residues (23). Also, possible increases in the folding energy of a variant in comparison to WT was avoided to prevent disrupting protein tertiary structure. If more than one amino acid met both conditions, the smallest one was chosen to minimize steric tensions within the epitope. Further, based on the structural data, amino acids in the enzyme catalytic site were avoided minimize effects on the enzymatic activity. In addition to the enzymatic functions, molecular engineering may negatively affect physicochemical properties of a protein. Particularly, decreased stability or solubility can be observed.
Active enzymes can be prone to non-specific inactivation by serum components, like many other biological drugs that demonstrate insufficient serum half-life (24). If so, in vivo applicability of variants would be limited independent of their possible improved performance in the presence of specific antibodies. Thus, we first tested variants designed herein for their antibacterial activity ex vivo in naïve sera from unchallenged animals to identify potential non-specific inactivation. Notably, both WT enzymes were partially inactivated by non-specific serum and similar results were noted for the variants, with the activity of all enzymes in serum approximately 40% of their baseline activity in buffer. Interestingly, three variants, Pal v3, Cpl-1 v1, and Cpl-1 v3, retained higher overall activity than the WT enzymes, even in serum (Figure 5), indicating their improved applicability in vivo. These observations suggest endolysins could be further improved by developing modifications or molecular engineering that make these enzymes resistant to non-specific serum inactivation. Notably, CF-301, an anti-staphylococcal endolysin, was shown to have 32-fold higher activity in serum compared to media, as measured by the minimal inhibitory concentration, and this difference was attributable to endolysin interactions with serum albumin (25). Consistent with this premise, fusions of albumin binding domains to LysK, another anti-staphylococcal endolysin, significantly extended its half-life in serum, although tradeoffs in activity were also noted (26). Taken together, future engineering studies on Pal, Cpl-1 and other endolysins for optimal activity and half-life in serum are warranted and feasible for translational development.
In addition to the goal of engineering reduced immunogenicity for Pal and Cpl-1, we were also interested in whether the variants displayed less cross-reactivity with IgGs induced by the WT enzymes. If so, such variants would be useful for long-term repeated treatments or recurrent infections. Notably, no decrease of Pal v3 activity in the presence of WT Pal-specific serum was noted in comparison to its activity in naïve serum. Furthermore, the overall antibacterial activity of Pal v3 in the presence of WT Pal-specific serum was significantly higher than that of WT Pal in the same conditions despite relatively small change in its structure (Figures 8, 9). These findings are noteworthy because many approved biological therapeutics lose efficacy due to issues with immunogenicity and alternatives must be sought as follows: (i) Since the 1970’s, L-asparaginase derived from Escherichia coli has been considered the first-line therapy to deplete serum asparagine levels in acute lymphoblastic leukemia patients. However, up to 30% of patients develop anti-asparaginase antibodies that limit the efficacy of treatment. As a solution, asparaginase derived from Erwinia chrysanthemi, which exhibits no cross-reactivity with the E. coli derived asparaginase, is used clinically for these patients (27); (ii) Patients with hemophilia A disease, a genetic deficiency of clotting Factor VIII, must receive regular prophylactic infusions of Factor VIII as replacement therapy. Although Factor VIII is purified from pooled human serum, up to a third of hemophilia patients do not recognize the protein as “self” due to their genetic deficiency and the inability to acquire immune tolerance during ontogeny (28). Strategies aimed at immune tolerance include Factor VIII chimeric fusions with Fc, engineering regulatory T cells, and similar to what we have done for Pal and Cpl-1, protein engineering to “de-immunize” the Factor VIII sequence and (iii) Thrombolytics work by converting plasminogen to plasmin, which can itself break down fibrin clots (29, 30). As such, thrombolytics are widely used for the treatment of acute myocardial infarction, pulmonary embolism, and acute ischemic stroke. The human-based enzymes, tissue plasminogen activator (tPA) and urokinase, are susceptible to endogenous inhibitors of the serpin family. Non-human-based enzymes, streptokinase and staphylokinase, are susceptible to immunogenicity since they are foreign proteins. Toward this end, protein engineering techniques have been applied to both streptokinase and staphylokinase to de-immunize these proteins. In particular, combinatorial mutagenesis applied to immunodominant regions located on the staphylokinse surface decreased the immunogenicity to less than 30% of the WT enzyme (31). Thus, our findings of a Pal variant that displays little cross reactivity to antibodies specific for WT Pal suggests a solution for future immunogenicity issues and further validates the EndoScan approach.
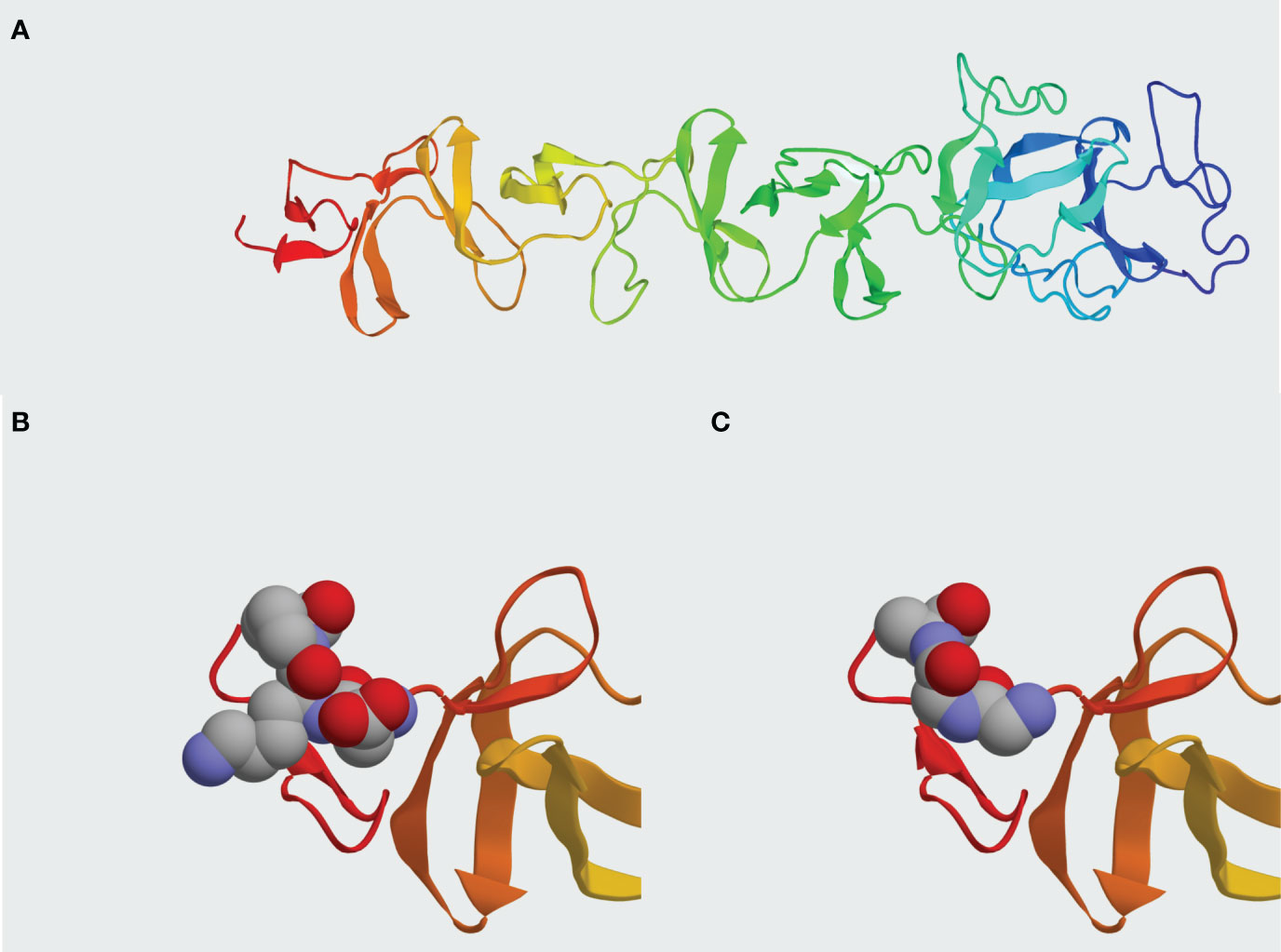
Figure 9 Comparison between Pal WT and Pal v3 (280-282: DKP→GGA) molecular structures. (A) In silico model of Pal WT developed in I-TASSER. (B) Presentation of epitope DKP in Pal WT. (C) Presentation of epitope GGA replacing DKP in Pal v3. Atoms within the epitopes are represented by spheres: grey – carbon, red – oxygen, blue – nitrogen. Representation generated by Protean 3D software.
This study demonstrates a new efficient approach using EndoScan for the identification of antigenic epitopes in proteins. EndoScan identifies physical interaction between protein-induced antibodies and the targeted protein, which may complement epitope identification in silico. Of note, the EndoScan technology focuses on linear B cell epitopes, thus conformational epitopes or T cell epitopes are not subjected to specific verification here. In recent years, a very interesting and promising approach for deimmunization of bacteriolytic proteins was demonstrated by Griswold and co-workers (9, 32), who used a computational method to design depletion of T cell epitopes in lysostaphin, an anti-staphylococcal enzyme. They demonstrated new variants of lysostaphin that could evade a specific immune response, but remain active against Staphylococcus. Possibly an efficient extension of the EndoScan method could employ T cell epitope identification to further increase the efficacy and accuracy of epitope identification. Here, we selectively modified epitopes identified with EndoScan in streptococcal endolysins and proposed new variants to decrease the immunoreactivity of these enzymes without losing antibacterial activity. To some extent, the immunogenicity of a protein can be decreased (as in Pal v9), or its cross-reactions with WT-induced antibodies can be minimized (as in Pal v3). Thus, EndoScan can be used for optimization of active enzymes designed for therapeutic applications, by modifying the immunogenicity of a protein without sacrificing its activity, with a relatively low number of tested proteins. Importantly, this approach is universal and can be applied to many active proteins, so its utility reaches far beyond endolysins and has potential in the design of numerous biological drugs.
5 Conclusions
● Antigenic epitopes were identified in two endolysins, Pal and Cpl-1, by epitope scanning (EndoScan).
● Substitutions of key amino acids within the identified epitopes results in changes of protein immunogenicity, including identification of variants with lower immunogenicity.
● Substitutions of key amino acids within the identified epitopes may result in changes of protein cross-reactivity to specific IgG induced by the WT proteins.
● We propose Pal variant v3 (280-282: DKP→GGA) as a new engineered endolysin with higher antibacterial activity than WT Pal. Further, while variant-specific antibodies are eventually induced, this variant is not cross-neutralized by antibodies induced by WT Pal. This suggests the variant may have applicability in situations of long-term administration of enzyme or for recurrent infections where WT antibodies may be present.
Data availability statement
The data presented in the study are deposited in the ENA repository, accession number PRJEB65416 (https://www.ebi.ac.uk/ena/browser/view/PRJEB65416).
Ethics statement
All human subjects gave informed consent for inclusion before they participated in the study. The study was conducted in accordance with the Declaration of Helsinki, and the protocol was approved by the Bioethics Committee of the Regional Specialist Hospital in Wrocław (Project identification code: KB/nr 2/2017). The studies were conducted in accordance with the local legislation and institutional requirements. The participants provided their written informed consent to participate in this study. All animal experiments were performed according to EU directive 2010/63/EU for animal experiments and were approved by the 1st Local Committee for Experiments with the Use of Laboratory Animals, Wrocław, Poland (project no. 76/2011). The authors followed the ARRIVE (Animal Research: Reporting of in vivo Experiments) guidelines. The study was conducted in accordance with the local legislation and institutional requirements.
Author contributions
MH - executed majority of the experiments, including library design, cloning, expression, purification, immunoprecipitation and analysis of immunoreactive epitopes, endolysin expression and purification, ELISA testing, and Sytox assay for endolysin activity, he conducted statistical analysis and participated in concluding results. KG, IR, ZK, PM, and JM – helped in endolysin production and phage display library construction, ELISA assays, Sytox assay, and in animal experiments. WB - contributed with graphics. DN - supervised all aspects of endolysin biology and biotechnology, participated in study design and in writing the manuscript. BO –helped in purification of samples for animal experimentation. KD- conceived and designed the study, executed and supervised animal studies and immunological aspects, analysed and concluded results, drafted the manuscript. All authors contributed to the article and approved the submitted version.
Funding
This work was supported by the National Science Centre in Poland, grant no. UMO-2015/18/M/NZ6/00412 (granted to KD) and grant no. UMO-2019/35/N/NZ6/02564 (granted to MH).
Acknowledgments
This manuscript appeared online in the form of a preprint in bioRxiv, ‘The Preprint Server For Biology’ (33) https://www.biorxiv.org/content/10.1101/2022.10.06.511193v1.full-text.
Conflict of interest
The authors declare that the research was conducted in the absence of any commercial or financial relationships that could be construed as a potential conflict of interest.
Publisher’s note
All claims expressed in this article are solely those of the authors and do not necessarily represent those of their affiliated organizations, or those of the publisher, the editors and the reviewers. Any product that may be evaluated in this article, or claim that may be made by its manufacturer, is not guaranteed or endorsed by the publisher.
Supplementary material
The Supplementary Material for this article can be found online at: https://www.frontiersin.org/articles/10.3389/fimmu.2023.1075774/full#supplementary-material
References
1. Schmelcher M, Loessner MJ. Bacteriophage endolysins — extending their application to tissues and the bloodstream. Curr Opin Biotechnol [Internet]. (2021) 68:51–9. doi: 10.1016/j.copbio.2020.09.012
2. Nelson DC, Schmelcher M, Rodriguez-Rubio L, Klumpp J, Pritchard DG, Dong S, et al. Endolysins as antimicrobials. In: Advances in virus research, vol. 83. Amsterdam: Elsevier Inc (2012). p. 299–365. p. 1st ed.
3. Gutiérrez D, Fernández L, Rodríguez A, García P. Are phage lytic proteins the secret weapon to kill staphylococcus aureus? MBio. (2018) 9(1):10–1128 HERE. doi: 10.1128/mBio.01923-17
4. Oliveira H, São-José C, Azeredo J. Phage-derived peptidoglycan degrading enzymes: Challenges and future prospects for in vivo therapy. Viruses (2018) 10(6):292. doi: 10.3390/v10060292
5. Murray E, Draper LA, Ross RP, Hill C. The advantages and challenges of using endolysins in a clinical setting. Viruses. (2021) 13(4):1–22. doi: 10.3390/v13040680
6. Naghavi M, Wang H, Lozano R, Davis A, Liang X, Zhou M, et al. Global, regional, and national age-sex specific all-cause and cause-specific mortality for 240 causes of death, 1990-2013: A systematic analysis for the Global Burden of Disease Study 2013. Lancet [Internet] (2015) 385(9963):117–71. doi: 10.1016/S0140-6736(14)61682-2
7. Harhala M, Nelson DC, Miernikiewicz P, Heselpoth RD, Brzezicka B, Majewska J, et al. Safety studies of pneumococcal endolysins Cpl-1 and Pal. Viruses (2018) 10(11):638. doi: 10.3390/v10110638
8. Jun SY, Jang IJ, Yoon S, Jang K, Yu KS, Cho JY, et al. Pharmacokinetics and Tolerance of the Phage endolysin-based candidate drug SAL200 after a single intravenous administration among healthy volunteers. Antimicrob Agents Chemother (2017) 61(6):10–128. doi: 10.1128/AAC.02629-16
9. Zhao H, Verma D, Li W, Choi Y, Ndong C, Fiering SN, et al. Depletion of T cell epitopes in lysostaphin mitigates anti-drug antibody response and enhances antibacterial efficacy in vivo. Chem Biol [Internet] (2015) 22(5):629–39. doi: 10.1016/j.chembiol.2015.04.017
10. Rashel M, Uchiyama J, Ujihara T, Uehara Y, Kuramoto S, Sugihara S, et al. Efficient elimination of multidrug-resistant Staphylococcus aureus by cloned lysin derived from bacteriophage φMR11. J Infect Dis (2007) 196(8):1237–47. doi: 10.1086/521305
11. Xu GJ, Kula T, Xu Q, Li MZ, Vernon SD, Ndung’u T, et al. Comprehensive serological profiling of human populations using a synthetic human virome. Sci (80- ) (2015) 348(6239):6239. doi: 10.1126/science.aaa0698
12. Harhala M, Gembara K, Nelson DC, Miernikiewicz P, Dabrowska K. Immunogenicity of endolysin plyC. Basel, Switzerland: Antibiotics, (2022). pp. 1–12.
13. Langmead B, Salzberg SL. Fast gapped-read alignment with Bowtie 2. Nat Methods (2012) 9(4):357–9. doi: 10.1038/nmeth.1923
14. Langmead B, Trapnell C, Pop M, Salzberg SL. Ultrafast and memory-efficient alignment of short DNA sequences to the human genome. Genome Biol (2009) 10(3):1–10. doi: 10.1186/gb-2009-10-3-r25
15. Benjamini Y, Hochberg Y. Controlling the false discovery rate: a practical and powerful approach to multiple testing. J R Stat Soc Ser B [Internet]. (1995) 57(1):289–300. doi: 10.1111/j.2517-6161.1995.tb02031.x
16. Schymkowitz J, Borg J, Stricher F, Nys R, Rousseau F, Serrano L. The FoldX web server: An online force field. Nucleic Acids Res (2005) ;33(SUPPL. 2):382–8. doi: 10.1093/nar/gki387
17. Pérez-Dorado I, Campillo NE, Monterroso B, Hesek D, Lee M, Páez JA, et al. Elucidation of the molecular recognition of bacterial cell wall by modular pneumococcal phage endolysin CPL-1. J Biol Chem (2007) 282(34):24990–9. doi: 10.1074/jbc.M704317200
18. Roy A, Kucukural A, Zhang Y. I-TASSER: a unified platform for automated protein structure and function prediction. Nat Protoc (2005) 5(4):45–67. doi: 10.1038/nprot.2010.5
19. Harhala M, Gembara K, Miernikiewicz P, Owczarek B, Kaźmierczak Z, Majewska J, et al. DNA dye sytox green in detection of bacteriolytic activity: high speed, precision and sensitivity demonstrated with endolysins. Front Microbiol (2021) 12(October):1–10. doi: 10.3389/fmicb.2021.752282
20. Janeway, Charles AJ, Travers P, Walport M, Shlomchik MJ. Immunobiology: the immune system in health and disease. New York: Garland Science (2001).
21. Gembara K, Dabrowska K. Phage-specific antibodies. Curr Opin Biotechnol (2021), 16–8. doi: 10.1016/j.copbio.2020.11.011
22. Majewska J, Kaźmierczak Z, Lahutta K, Lecion D, Szymczak A, Miernikiewicz P, et al. Induction of Phage-Specific Antibodies by Two Therapeutic Staphylococcal Bacteriophages Administered per os. Front Immunol (2019) 10:467826. doi: 10.3389/fimmu.2019.02607
23. Stave JW, Lindpaintner K. Antibody and antigen contact residues define epitope and paratope size and structure. J Immunol (2013), 13–7. doi: 10.4049/jimmunol.1203198
24. Kontermann RE. Strategies for extended serum half-life of protein therapeutics. Curr Opin Biotechnol [Internet]. (2011) 22(6):868–76. doi: 10.1016/j.copbio.2011.06.012
25. Indiani C, Sauve K, Raz A, Abdelhady W, Xiong YQ, Cassino C, et al. The antistaphylococcal lysin, CF-301, activates key host factors in human blood to potentiate methicillin-resistant staphylococcus aureus bacteriolysis. Antimicrob Agents Chemother (2019) 63(4):10–128. doi: 10.1128/AAC.02291-18
26. Seijsing J, Sobieraj AM, Keller N, Shen Y, Zinkernagel AS, Loessner MJ, et al. Improved biodistribution and extended serum half-life of a bacteriophage endolysin by albumin binding domain fusion. Front Microbiol (2018) 9(November):1–9. doi: 10.3389/fmicb.2018.02927
27. Egler RA, Ahuja SP, Matloub Y. L − asparaginase in the treatment of patients with acute lymphoblastic leukemia. J Pharmacol Pharmacother. (2016) 7:62–71. doi: 10.4103/0976-500X.184769
28. Scott DW, Pratt KP. Factor VIII : perspectives on immunogenicity and tolerogenic strategies. Front Immunol (2020) 10(January). doi: 10.3389/fimmu.2019.03078
29. Pratt KP. Engineering less immunogenic and antigenic FVIII proteins. Cell Immunol (2016) 301:12. doi: 10.1016/j.cellimm.2015.10.008
30. Lacroix-desmazes S, Voorberg J, Lillicrap D, Scott DW, Pratt KP. Tolerating factor VIII : recent progress. Front Immunol (2020) 10(January):1–20. doi: 10.3389/fimmu.2019.02991
31. Mican J, Toul M, Bednar D, Damborsky J. Structural biology and protein engineering of thrombolytics. Comput Struct Biotechnol J [Internet]. (2019) 17:917–38. doi: 10.1016/j.csbj.2019.06.023
32. Zhao H, Brooks SA, Eszterhas S, Heim S, Li L, Xiong YQ, et al. Globally deimmunized lysostaphin evades human immune surveillance and enables highly efficacious repeat dosing. Sci Adv (2020) 6(36):1–12. doi: 10.1126/sciadv.abb9011
Keywords: deimmunization, endolysin, PAL, Cpl-1, epitope engineering, VirScan
Citation: Harhala MA, Gembara K, Rybicka I, Kaźmierczak ZM, Miernikiewicz P, Majewska JM, Budziar W, Nasulewicz-Goldeman A, Nelson DC, Owczarek B and Dąbrowska K (2023) Immunogenic epitope scanning in bacteriolytic enzymes Pal and Cpl-1 and engineering Pal to escape antibody responses. Front. Immunol. 14:1075774. doi: 10.3389/fimmu.2023.1075774
Received: 20 October 2022; Accepted: 16 August 2023;
Published: 15 September 2023.
Edited by:
Jai Rudra, Washington University in St. Louis, United StatesCopyright © 2023 Harhala, Gembara, Rybicka, Kaźmierczak, Miernikiewicz, Majewska, Budziar, Nasulewicz-Goldeman, Nelson, Owczarek and Dąbrowska. This is an open-access article distributed under the terms of the Creative Commons Attribution License (CC BY). The use, distribution or reproduction in other forums is permitted, provided the original author(s) and the copyright owner(s) are credited and that the original publication in this journal is cited, in accordance with accepted academic practice. No use, distribution or reproduction is permitted which does not comply with these terms.
*Correspondence: Krystyna Dąbrowska, ZGFicm93c2thQGhpcnN6ZmVsZC5wbA==