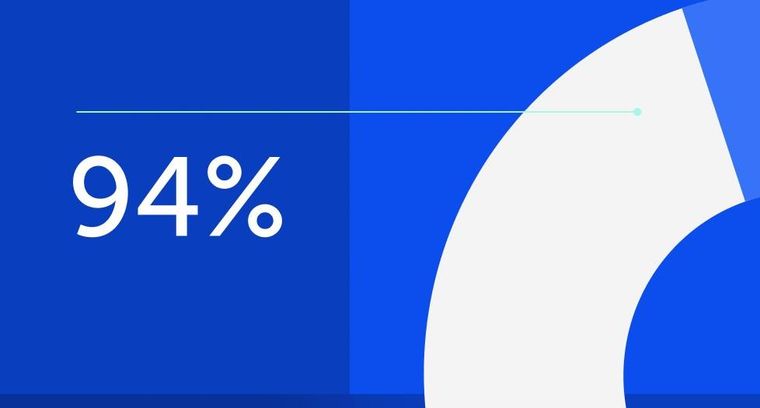
94% of researchers rate our articles as excellent or good
Learn more about the work of our research integrity team to safeguard the quality of each article we publish.
Find out more
REVIEW article
Front. Immunol., 20 March 2023
Sec. Viral Immunology
Volume 14 - 2023 | https://doi.org/10.3389/fimmu.2023.1070077
This article is part of the Research TopicImmune Response to SARS-CoV-2 and Implications for Clinical OutcomeView all 30 articles
The T cell response plays an indispensable role in the early control and successful clearance of SARS-CoV-2 infection. However, several important questions remain about the role of cellular immunity in COVID-19, including the shape and composition of disease-specific T cell repertoires across convalescent patients and vaccinated individuals, and how pre-existing T cell responses to other pathogens—in particular, common cold coronaviruses—impact susceptibility to SARS-CoV-2 infection and the subsequent course of disease. This review focuses on how the repertoire of T cell receptors (TCR) is shaped by natural infection and vaccination over time. We also summarize current knowledge regarding cross-reactive T cell responses and their protective role, and examine the implications of TCR repertoire diversity and cross-reactivity with regard to the design of vaccines that confer broader protection against SARS-CoV-2 variants.
The clinical manifestations and subsequent immune response to SARS-CoV-2 infection are diverse, with patients exhibiting a wide range of disease severity and susceptibility to future reinfections. T cells are crucial for early control and successful clearance of viral infections alongside the humoral response. The involvement of CD4+ and CD8+ T cells in the immune response reduces the severity of disease (1–3), and the presence of pre-existing SARS-CoV-2-specific T cells can prevent the development of COVID-19 (4, 5) and decrease the risk of reinfection (6). Accordingly, it has been shown that a subgroup of seronegative patients was partially protected from infection by T cells (7, 8). On the other hand, anergy of T cells is associated with a poor prognosis (9).
However, it is not only the magnitude of the T cell response but also its diversity that ultimately influences the outcome of infection (10, 11). Recently, researchers have focused on analyzing the dynamics of the TCR repertoire as an indicator of the immune response in autoimmune diseases such as multiple sclerosis (12) and rheumatoid arthritis (10), viral infections (13), and cancer (14). TCR repertoire analysis is also proving useful as a biomarker of the response to immunotherapy (15). The TCR repertoire can provide insights into immunodominance, functionality, and the protective effects of the T cell response (16, 17). Even though some conclusions may be ambiguous due to different approaches and consideration of both antigen-specific and non-antigen-specific data such as characteristics of overall TCR repertoire, TCR repertoire analysis offers a valuable tool for understanding the parameters of T cell-mediated immune responses to SARS-CoV-2 and the impact of viral mutations on immunological protection against newly-emerging SARS-CoV-2 variants.
The ability of the adaptive immune system to protect host organisms from a wide variety of pathogens is facilitated by the production of T cells that collectively express a large and diverse repertoire of unique TCRs. Naïve TCR diversity is generated by random rearrangement of the V and J segments of the TCR alpha (TCRα) genes and V, D, and J segments of the TCR beta (TCRβ) genes in maturing T cells within the thymus. But the ultimate structure of memory repertoire is shaped by interactions of these naïve cells with various pathogens over the course of a lifetime. The size, frequency, and publicity of individual clonotypes within a TCR repertoire can reveal both successful and failed immune responses, and recent studies have shown that the SARS-CoV-2-specific repertoire not only has its own architecture (18), but also differs depending on the severity of the disease and can change over time (19).
The SARS-CoV-2 proteome comprises at least 29 proteins (20), and as such, the number of potential epitopes is huge. However, the immunogenic regions of this proteome are unevenly distributed. ORF1 is the largest SARS-CoV-2 protein, and makes the largest contribution to T cell recognition, although the much-smaller ORF3 and Spike (S) proteins have a higher density of immunogenic epitopes compared to ORF1 (21). About 25% of the overall antigen-specific T cell response is accounted for S protein response (22). Other structural proteins are highly recognized as well and accounted for roughly 55% of CD4+ and CD8+ T cell response (22, 23). Many immunogenic epitopes in SARS-CoV-2 have been identified (24–27). However, most of these do not achieve 100% immunogenicity in convalescent donors (5) and T cells of each individual recognize 30 to 40 different CD4+ and CD8+ epitopes (22). This can be explained by the fact that the structure of the TCR repertoire is mostly determined by the presence of specific HLA alleles (28, 29), as well as the fact that epitopes compete for antigen presentation (30). Moreover, different TCRs have different probabilities of formation during the recombination process, such that the frequency of naïve cells with such TCRs may vary (28, 31). Lastly, methods of assessing immunogenicity can differ in their sensitivity and specificity, and the structure of the repertoire may vary in different studies. One particularly important factor is the time of sampling: at the peak of infection, more than 10% of total CD8+ T cells may be specific to a single SARS-CoV-2 epitope (21, 26). A month after infection, the frequency of most epitope-specific T cell populations is typically < 1% of total CD8+ T cells (24, 32–35).
The abundance of a given memory T cell clonotype in blood does not correlate with the immunodominance of its corresponding epitope (32, 35, 36). For example, studies have shown that CD8+ T cells specific to the highly immunogenic epitope YLQ are present at very low levels in the blood of convalescent donors (36, 37).
Limited diversity of T cell repertoire seems to be associated with severe disease (38, 39), whereas higher diversity is more likely to result in successful elimination of the virus. Multiple studies have found that the overall diversity of non-antigen-specific TCRs in blood samples taken from patients with COVID-19 is lower than that of healthy donors (40, 41), and is even lower in patients with severe disease. For example, a cohort of patients with pneumonia had a slightly less diverse overall TCR repertoire compared to those with mild disease (38) presumably due to expansion of SARS-CoV-2 specific T to defend against the infection cells in symptomatic and hospitalized individuals (42) On the other hand, low repertoire diversity may be a prognostic factor and explain the higher risk of serious illness and death in elderly patients (43–47), as it is well known that TCR repertoire diversity declines with aging, and this is also known to affect the antiviral response to other pathogens, such as the human influenza A virus (48, 49).
In general, peripheral selection and expansion of antigen-specific clonotypes driven by persistent pathogens leads to a higher proportion of shared clones among abundant clonotypes (50). The overlap of the overall non-antigen specific TCR repertoire between individuals is significantly higher in COVID-19 patients than in healthy individuals (41), and this is primarily because some epitopes of SARS-CoV-2 tend to give rise to shared, public clonotypes (35, 36, 51). Public clonotypes tend to have short CDR3 regions and arise from specific V(D)J-rearrangement events that occur with higher probability (28). Such clonotypes are thought to play a crucial role in establishing an effective pathogen-specific response and infection control of other pathogens like Cytomegalovirus (CMV), Epstein-Barr virus (EBV) and Adenovirus (52, 53).
Numerous studies have shown that the TCR repertoire in patients with mild COVID-19 infection remains relatively diverse within CDR3 central region, with high generation probability compare to severe patients (38). This leads to a broad range of SARS-CoV-2-specific sequences observed in mild disease, with many public CDR3 sequences (19, 36, 38, 54) This potentially explains why pneumonia patients have TCRs with longer CDR3 regions arising from lower-probability V(D)J-rearrangement events relative to the SARS-CoV-2-associated TCR repertoires in patients with mild disease, which also tend to prominently feature public clonotypes (38, 40). TCR repertoire profiles in asymptomatic infection is similar to mild disease (36).
It was demonstrated that preferential usage of V-, D-, and J- genes is significantly different in different viral infections including COVID-19 (55). Moreover, few studies demonstrated overrepresentation or underrepresentation of particular V-, D- and J-segments in patients with different COVID-19 clinical picture (55). Asymptomatic patients had overrepresentation of TRAV (TRAV17, TRAV12-1, TRAV19, TRAV35, and TRAV41), TRBV (TRBV12-5 and TRBV19) (56), TRAJ16 and TRBJ2-1 (57) genes compared to patients with symptoms. Frequency of TRAV2, TRAJ8, TRAJ40, TRBV3-1 and TRBV5-1 were the highest in symptomatic patients (57) while four TCBV gene segments (TRBV5-6, TRBV14, TRBV13 and TRBV24-1) were found to be overrepresented in severe patients with little Jβ gene-segment skewing (58). Another 25 sequences of the central part of the CDR3 region were found predictive for severe infection (54). The data suggest distinct clonal expansion influence on the disease progression.
During SARS-CoV-2 infection, the diversity and clonality of the antigen-specific TCR repertoire peaks within 8–14 days, then contracts slightly (25, 59) before returning to basal levels within one week after virus elimination (60). A SARS-CoV-2 specific TCR repertoire can be detected in the vast majority of convalescent patients, persisting for up to 15 months after viral clearance with a slight decrease (35, 42, 59) or even increase of clonal diversity (61). Moreover, SARS-CoV-2 epitope-specific T cells are able to proliferate in individuals who were vaccinated after infection (42) or in the re-detectable positive cases (Y. 62). Notably, SARS-CoV-1 specific T cells have demonstrated an impressive ability to persist for long periods of time, with one study detecting such clones up to 17 years after infection (63).
The durability of an antigen-specific response is determined by characteristics such as the publicity, diversity, and clonality of clonotypes recognizing that antigen (35, 51). It has been shown that long-term immunity is principally mediated by the clonal diversity of the antigen-specific T cell response (35, 61), whereas clonality does not appear to play a significant role (35). In some cases, however, dominant clones in the acute phase coincide with those found in the recovery phase (59). Numerous studies offer clear evidence that a highly diverse repertoire protects against a wide range of antigens of CMV, EBV and Human Immunodeficiency Virus-1 (HIV-1) (64, 65), and it is quite likely that such repertoires are associated with a higher level of avidity, affinity, and overall functionality (66, 67).
Despite numerous attempts to predict the longevity of virus-specific T cell immune response based on repertoire characteristics (35, 51, 68, 69), Bensouda Koraichi et al. study surmised that TCR clonotypes dynamic can be described by geometric Brownian motion. The model includes random unstimulated T cell proliferation and death, as well as asymptomatic or weakly symptomatic antigenic stimulation. However, the actual longevity of response varies from individual to individual, and in young individuals, the repertoire changes faster than in older individuals (70). Thus, at the moment, TCR clonotypes cannot be considered as the sole reliable predictor of the strength and effectiveness of the immune response.
The high levels of mortality and morbidity associated with COVID-19 have prompted a massive, global vaccine development effort. At the time of writing this review, more than 170 vaccines have been developed, according to the World Health Organization (WHO) (https://www.who.int/publications/m/item/draft-landscape-of-covid-19-candidate-vaccines). Nearly a dozen of these are now in clinical use, and most demonstrate high efficiency in terms of protection (71, 72) and induce an immune response closely resembling that induced by infection in terms of immunophenotype, magnitude of CD4+ response and antibody levels (73–75). However, vaccine-induced CD8+ T cell expansion seems to be relatively weaker and with fewer distinct clonotype clusters compared to those induced by natural infection (76).
Only a small subset of vaccines consists of inactivated viral particles or mixtures of different viral proteins. Instead, the vast majority are aimed at inducing an immune response to the S protein (77). This approach produces a skewed T cell response that is enhanced against immunodominant epitopes (51) while also being targeted at less-dominant S-derived epitopes in vaccine recipients compared to convalescent individuals (78). In the aftermath of natural infection, the resulting CD8+ T cell clones are likely to recognize a broader set of viral epitopes that are not encountered in vaccines (76), and this T cell repertoire also demonstrates a higher rate of cross-recognition of epitopes from common-cold coronaviruses (79). Nevertheless, the repertoire induced by S protein-based vaccines is generally capable of protecting against existing variants as well as emerging variants of concern (VOCs) (80–82).
The antigen-specific TCR repertoire induced by both the virus and vaccines undergoes significant clonal contraction over time (79), along with an overall decrease in immune response (35), and the only way to increase protection over the long term may be booster vaccination (78).
Over time, SARS-CoV-2-primed T cells transition to a memory phenotype, and the diversity of the SARS-CoV-2-specific TCR repertoire decreases alongside the humoral response in convalescent individuals (35). Since T cells and antibodies provide effective antiviral protection, the exhaustion of any of them leads to a decrease in protection properties, which was shown in the large-scale prospective study (8). However, the existence of a pool of memory cells is important for fending off the virus in future encounters, and the complete absence of SARS-CoV-2-specific antibodies and T cells may lead to reinfection, although neutralizing antibodies play more important role in protection from reinfection (83)
Vaccination offers a way to boost previously primed immunity, and it has been shown that the vaccine-induced cellular response is more robust in convalescent donors. Most convalescent individuals demonstrate the same level of T cell and humoral response as previously-unexposed individuals after one shot of mRNA vaccine (84). Because the antigen-specific CD8+ T cell response develops more slowly than the CD4+ T cell response after natural infection and primary vaccination (5), it reaches its maximum only after administration of the second vaccine dose (84).
Vaccine response patterns may differ due to the difficulty of involving naïve CD8+ precursors in the immune response. While the vaccine-induced response of CD4+ T cells includes both the recruitment of memory cells and the proliferation of new, unique S protein-specific CD4+ T cells (79) in convalescent individuals, a rapid boost of S protein-specific CD8+ T cells is predominantly provided by persisting early memory S protein-specific CD38-CD8+ T cells (85, 86). Moreover, the overall magnitude of the S protein-specific CD8+ T cell response to vaccination in convalescent individuals is the same as in previously unexposed individuals due to the involvement of the memory compartment. However, it has been shown that vaccination selectively stimulates the expansion of S protein-specific clones and the contraction of clonotypes with non-S-protein specificity in convalescent donors (51).
SARS-CoV-2 mutations can reduce recognition of the virus by the CD8+ T cell compartment, possibly due to escape from HLA binding (87), although the T cell response is generally capable of effectively responding to mutant viral strains (88, 89). Vaccine-induced T cell response was also preserved across different SARS-CoV-2 variants while B cell and neutralizing antibodies recognition was significantly reduced (90). Moreover T cell response may be enhanced with booster vaccination (78, 91). which substantially increase effectiveness of protection against reinfection from 24.7% with previous infection up to 41.8% with combination of infection and vaccination. However, the most important thing is that vaccination after infection is much more effective against hospital admission or severe disease than infection alone: the effectiveness of protection increased from 74.6% to 97.4% with vaccination (92).
Nevertheless, there remains a need for further vaccine optimization and the incorporation of more immunogenic epitopes (93) that can elicit more broadly protective T cell responses (81) even in the face of the emergence of new SARS-CoV-2 variants. This is especially important for the protection of immunocompromised individuals and elderly people, and despite a greater proportion of pre-existing memory T cells in the elderly compared to the young, booster vaccination has been shown to be less effective in older individuals due to the minimal contribution of memory clonotypes in supporting high-quality T cell responses (94).
A more robust T cell response is also conferred by their capacity for cross-reactivity. A single T cell can cross-react to up to 106–107 foreign peptides (95), and this has been shown to be an essential feature of the T cell response (96–98). For some individuals who remain asymptomatic and seronegative even after close contact with COVID-19 patients (5), it has been shown that T cell-mediated protection may arise from cross-reactivity to T cells that target self-antigens and epitopes derived from various other pathogens including CMV, influenza A, EBV and HIV-1 (99, 100). Some parts of SARS-CoV-2 are very highly conserved relative to other ‘common cold’ human coronaviruses (HCoVs) (101, 102), and pre-existing protective T cells most likely originate from memory T cells derived after exposure to viruses such as HCoV-OC43, HCoV-HKU1, HCoV-NL63 and HCoV-229E (4), which circulate widely in the human population (63, 103, 104).
Some studies have shown that more than 20% of pre-pandemic samples contained SARS-CoV-2-reactive T cells (63, 103, 104), which protect patients from developing severe illness (105). But other research has failed to confirm such strong cross-reactivity, and has instead revealed that these cross-reactive T cells from pre-pandemic samples have a predominantly naïve phenotype, which means that they did not develop from an immune response to HCoVs (32, 77). This difference in results may be attributable to the choice of peptides used in the study. T cells specific to peptides that are conserved among coronaviruses are more abundant and tend to have a memory phenotype compared to those which recognize unique SARS-CoV-2 peptides. Notably, CD8+ T cells that cross-react to these conserved epitopes are much more plentiful in patients with mild COVID-19 versus those with severe illness, suggesting a protective role (105). Moreover, TCR repertoires that recognize the same conserved peptides were similar in unexposed donors and convalescent individuals (106). Other studies have suggested that pre-existing T cells that react to SARS-CoV-2 RNA polymerase may also be associated with asymptomatic disease (4, 107).
One of the most cross-reactive epitopes in unexposed individuals is SPRWYFYYLN105-113 (SPR) restricted in HLA-B*07:02 (77, 108). The immunodominance of SPR originates from a high frequency of naïve precursors in pre-pandemic samples. Many naïve SPR progenitors arise from a highly diverse TCRαß repertoire (77, 109), and a diverse SPR-specific CD8+ T cell response with high functional avidity and antiviral effector functions has been detected in patients with mild disease compared to individuals with severe COVID-19 (108). Interestingly to note that only one SPR homologous epitope from HCoVs, LPRWYFYYL, has also demonstrated the ability to elicit a cross-reactive response (109). Other highly immunodominant epitopes, like KPRQKRTATN257-265 (KPR), YLQPRTFLLS269-277 (YLQ), and QYIKWPWYIS1208-1217 (QYI), have been shown to be abundant in pre-existing naïve T cell repertoires (32, 77, 108).
The importance of cross-reactive T cell response for protection against newly-emerging mutant strains is well established (110). New VOCs may be less susceptible to neutralizing antibodies (80, 111, 112), while T cells retain their protective capabilities (89, 113, 114). This protective capacity is shaped by the wide variety of epitopes recognized in different people (21). Nevertheless, the emergence of non-synonymous mutations in some T cell epitopes can lead to a decrease in peptide and MHC binding or a reduced ability to activate T cells (115, 116). However, such mutations are rarely found in VOCs, and it is likely that an epitope that evades presentation by one HLA allele will become presentable by the other (87). This mechanism may explain why the magnitude of T cell response to new variants is typically decreased by only 20–30% (89, 117).
It should also be noted that cross-recognition does not always provide protection, and can also be associated with worse disease outcomes; this suggests that other mechanisms are coming into play, including age-related differences in the involvement of different cell populations in the immune response (118). Several studies have examined the potential protective effects of Bacille Calmette–Guérin (BCG) immunization due to the presence of epitopes that resemble epitopes from SARS-CoV-2 (119, 120), but clinical trials have offered no evidence for such protection (121).
The severity of COVID-19 can vary from asymptomatic to lethal disease, and many different factors contribute to the outcome of infection, most notably including gender, ethnicity, health, and age (46, 47). However, demographics only partially explain the differences in mortality rates between countries (122), and numerous studies strongly point to the influence of the TCR repertoire on the ultimate course of infection. It has been shown that TCR repertoire diversity and clonality might determine the success of the immune response to both the virus and the vaccine, and several machine learning-based tools have been developed and applied in order to distinguish between convalescent and naive individuals (18) and predict disease outcomes (19, 54, 123) based on TCR repertoire. Despite high hopes and numerous studies of cross-reactive responses from pre-existing immunity to other HCoVs and other pathogens, protectiveness of cross-recognition is still debating (124). The studies to date have shown that protectiveness of cross-recognition in the context of prior infection with SARS-CoV-2 against reinfection is relatively low and waned to 24·7% at 12 months, but may be significantly improved with vaccination (92). Against this backdrop, the ongoing spread of SARS-CoV-2 and emergence of new, potentially immune-escaping VOCs reinforces the urgency of further optimizing the composition of vaccines based on insights derived from research into the T cell response against SARS-CoV-2.
AB, and KZ contributed to conception of the review. KZ wrote the first draft of the manuscript. KZ, SS, AR, and RI wrote sections of the manuscript. SS drew the graphical abstract. All authors contributed to manuscript revision, read, and approved the submitted version. All authors contributed to the article and approved the submitted version.
Russian Science Foundation grant 20-15-00395.
The authors declare that the research was conducted in the absence of any commercial or financial relationships that could be construed as a potential conflict of interest.
All claims expressed in this article are solely those of the authors and do not necessarily represent those of their affiliated organizations, or those of the publisher, the editors and the reviewers. Any product that may be evaluated in this article, or claim that may be made by its manufacturer, is not guaranteed or endorsed by the publisher.
1. Liao M, Liu Y, Yuan J, Wen Y, Xu G, Zhao J, et al. Single-cell landscape of bronchoalveolar immune cells in patients with COVID-19. Nat Med (2020) 26(6):842–4. doi: 10.1038/s41591-020-0901-9
2. Rydyznski Moderbacher C, Ramirez SI, Dan JM, Grifoni A, Hastie KM, Weiskopf D, et al. Antigen-specific adaptive immunity to SARS-CoV-2 in acute COVID-19 and associations with age and disease severity. Cell (2020) 183(4):996–1012.e19. doi: 10.1016/j.cell.2020.09.038
3. Tan M, Liu Y, Zhou R, Deng X, Li F, Liang K, et al. Immunopathological characteristics of coronavirus disease 2019 cases in guangzhou, China. Immunology (2020) 160(3):261–8. doi: 10.1111/imm.13223
4. Swadling L, Diniz MO, Schmidt NM, Amin OE, Chandran A, Shaw E, et al. Pre-existing polymerase-specific T cells expand in abortive seronegative SARS-CoV-2. Nature (2022) 601(7891):110–7. doi: 10.1038/s41586-021-04186-8
5. Titov A, Shaykhutdinova R, Shcherbakova OV, Serdyuk YV, Sheetikov SA, Zornikova KV, et al. Immunogenic epitope panel for accurate detection of non-cross-reactive T cell response to SARS-CoV-2. JCI Insight (2022) 7(9). doi: 10.1172/jci.insight.157699
6. Wyllie D, Jones HE, Mulchandani R, Trickey A, Taylor-Phillips S, Brooks T, et al. SARS-CoV-2 responsive T cell numbers and anti-Spike IgG levels are both associated with protection from COVID-19: A prospective cohort study in keyworkers. MedRxiv (2021). doi: 10.1101/2020.11.02.20222778
7. Marasco V, Carniti C, Guidetti A, Farina L, Magni M, Miceli R, et al. T-Cell immune response after mRNA SARS-CoV-2 vaccines is frequently detected also in the absence of seroconversion in patients with lymphoid malignancies. Br J Haematol (2022) 196(3):548–58. doi: 10.1111/bjh.17877
8. Molodtsov IA, Kegeles E, Mitin AN, Mityaeva O, Musatova OE, Panova AE, et al. Severe acute respiratory syndrome coronavirus 2 (SARS-CoV-2)–specific T cells and antibodies in coronavirus disease 2019 (COVID-19) protection: A prospective study. Clin Infect Dis (2022) 75(1):e1–9. doi: 10.1093/cid/ciac278
9. Renner K, Schwittay T, Chaabane S, Gottschling J, Müller C, Tiefenböck C, et al. Severe T cell hyporeactivity in ventilated COVID-19 patients correlates with prolonged virus persistence and poor outcomes. Nat Commun (2021) 12(1):3006. doi: 10.1038/s41467-021-23334-2
10. Chang C-M, Hsu Y-W, Wong HS-C, Wei JC-C, Liu X, Liao H-T, et al. Characterization of T-cell receptor repertoire in patients with rheumatoid arthritis receiving biologic therapies. Dis Markers (2019) 2019:1–12. doi: 10.1155/2019/2364943
11. Wang F, Hou H, Yao Y, Wu S, Huang M, Ran X, et al. Systemically comparing host immunity between survived and deceased COVID-19 patients. Cellular & Molecular Immunology (2020) 17(8):875–7. doi: 10.1038/s41423-020-0483-y
12. Alves Sousa A, de P, Johnson KR, Ohayon J, Zhu J, Muraro PA, et al. Comprehensive analysis of TCR-β repertoire in patients with neurological immune-mediated disorders. Sci Rep (2019) 9(1):344. doi: 10.1038/s41598-018-36274-7
13. Krummey SM, Morris AB, Jacobs JR, McGuire DJ, Ando S, Tong KP, et al. CD45RB status of CD8+ T cell memory defines T cell receptor affinity and persistence. Cell Rep (2020) 30(5):1282–1291.e5. doi: 10.1016/j.celrep.2020.01.016
14. Reuben A, Zhang J, Chiou S-H, Gittelman RM, Li J, Lee W-C, et al. Comprehensive T cell repertoire characterization of non-small cell lung cancer. Nat Commun (2020) 11(1):603. doi: 10.1038/s41467-019-14273-0
15. Valpione S, Galvani E, Tweedy J, Mundra PA, Banyard A, Middlehurst P, et al. Immune awakening revealed by peripheral T cell dynamics after one cycle of immunotherapy. Nat Cancer (2020) 1(2):210–21. doi: 10.1038/s43018-019-0022-x
16. Messaoudi I, Patiño JAG, Dyall R, LeMaoult J, Nikolich-Žugich J. Direct link between mhc polymorphism, T cell avidity, and diversity in immune defense. Science (2002) 298(5599):1797–800. doi: 10.1126/science.1076064
17. Ndhlovu ZM, Kamya P, Mewalal N, Kløverpris HN, Nkosi T, Pretorius K, et al. Magnitude and kinetics of CD8+ T cell activation during hyperacute HIV infection impact viral set point. Immunity (2015) 43(3):591–604. doi: 10.1016/j.immuni.2015.08.012
18. Shoukat MS, Foers AD, Woodmansey S, Evans SC, Fowler A, Soilleux EJ. Use of machine learning to identify a T cell response to SARS-CoV-2. Cell Rep Med (2021) 2(2):100192. doi: 10.1016/j.xcrm.2021.100192
19. Schultheiß C, Paschold L, Simnica D, Mohme M, Willscher E, von Wenserski L, et al. Next-generation sequencing of T and b cell receptor repertoires from COVID-19 patients showed signatures associated with severity of disease. Immunity (2020) 53(2):442–455.e4. doi: 10.1016/j.immuni.2020.06.024
20. Rauti R, Shahoha M, Leichtmann-Bardoogo Y, Nasser R, Paz E, Tamir R, et al. Effect of SARS-CoV-2 proteins on vascular permeability. ELife (2021) 10. doi: 10.7554/eLife.69314
21. Saini SK, Hersby DS, Tamhane T, Povlsen HR, Hernandez SPA, Nielsen M, et al. SARS-CoV-2 genome-wide T cell epitope mapping reveals immunodominance and substantial CD8 + T cell activation in COVID-19 patients. Sci Immunol (2021) 6(58). doi: 10.1126/sciimmunol.abf7550
22. Tarke A, Sidney J, Kidd CK, Dan JM, Ramirez SI, Yu ED, et al. Comprehensive analysis of T cell immunodominance and immunoprevalence of SARS-CoV-2 epitopes in COVID-19 cases. Cell Rep Med (2021) 2(2):100204. doi: 10.1016/j.xcrm.2021.100204
23. Li L, Chen Q, Han X, Shen M, Hu C, Chen S, et al. T Cell immunity evaluation and immunodominant epitope T cell receptor identification of severe acute respiratory syndrome coronavirus 2 spike glycoprotein in COVID-19 convalescent patients. Front Cell Dev Biol (2021) 9:696662. doi: 10.3389/fcell.2021.696662
24. Ferretti AP, Kula T, Wang Y, Nguyen DMV, Weinheimer A, Dunlap GS, et al. Unbiased screens show CD8+ T cells of COVID-19 patients recognize shared epitopes in SARS-CoV-2 that largely reside outside the spike protein. Immunity (2020) 53(5):1095–1107.e3. doi: 10.1016/j.immuni.2020.10.006
25. Snyder TM, Gittelman RM, Klinger M, May DH, Osborne EJ, Taniguchi R, et al. Magnitude and dynamics of the T-cell response to SARS-CoV-2 infection at both individual and population levels. MedRxiv (2020). doi: 10.1101/2020.07.31.20165647
26. Gangaev A, Ketelaars SLC, Isaeva OI, Patiwael S, Dopler A, Hoefakker K, et al. Identification and characterization of a SARS-CoV-2 specific CD8+ T cell response with immunodominant features. Nat Commun (2021) 12(1):2593. doi: 10.1038/s41467-021-22811-y
27. Schulien I, Kemming J, Oberhardt V, Wild K, Seidel LM, Killmer S, et al. Characterization of pre-existing and induced SARS-CoV-2-specific CD8+ T cells. Nat Med (2021) 27(1):78–85. doi: 10.1038/s41591-020-01143-2
28. Pogorelyy MV, Fedorova AD, McLaren JE, Ladell K, Bagaev DV, Eliseev AV, et al. Exploring the pre-immune landscape of antigen-specific T cells. Genome Med (2018) 10(1):68. doi: 10.1186/s13073-018-0577-7
29. Fischer JC, Schmidt AG, Bölke E, Uhrberg M, Keitel V, Feldt T, et al. Association of HLA genotypes, AB0 blood type and chemokine receptor 5 mutant CD195 with the clinical course of COVID-19. Eur J Med Res (2021) 26(1):107. doi: 10.1186/s40001-021-00560-4
30. Adorini L, Nagy ZA. Peptide competition for antigen presentation. Immunol Today (1990) 11:21–4. doi: 10.1016/0167-5699(90)90006-U
31. Jackson KJL, Kidd MJ, Wang Y, Collins AM. The shape of the lymphocyte receptor repertoire: Lessons from the b cell receptor. Front Immunol (2013) 4:263. doi: 10.3389/fimmu.2013.00263
32. Habel JR, Nguyen THO, van de Sandt CE, Juno JA, Chaurasia P, Wragg K, et al. Suboptimal SARS-CoV-2–specific CD8 + T cell response associated with the prominent HLA-A*02:01 phenotype. Proc Natl Acad Sci (2020) 117(39):24384–91. doi: 10.1073/pnas.2015486117
33. Peng Y, Mentzer AJ, Liu G, Yao X, Yin Z, Dong D, et al. Broad and strong memory CD4+ and CD8+ T cells induced by SARS-CoV-2 in UK convalescent individuals following COVID-19. Nat Immunol (2020) 21(11):1336–45. doi: 10.1038/s41590-020-0782-6
34. Kared H, Redd AD, Bloch EM, Bonny TS, Sumatoh H, Kairi F, et al. SARS-CoV-2–specific CD8+ T cell responses in convalescent COVID-19 individuals. J Clin Invest (2021) 131(5). doi: 10.1172/JCI145476
35. Zornikova KV, Khmelevskaya A, Sheetikov SA, Kiryukhin DO, Shcherbakova OV, Titov A, et al. Clonal diversity predicts persistence of SARS-CoV-2 epitope-specific T-cell response. Commun Biol (2022) 5(1):1351. doi: 10.1038/s42003-022-04250-7
36. Shomuradova AS, Vagida MS, Sheetikov SA, Zornikova K, Kiryukhin D, Titov A, et al. SARS-CoV-2 epitopes are recognized by a public and diverse repertoire of human T cell receptors. Immunity (2020) 53(6):1245–1257.e5. doi: 10.1016/j.immuni.2020.11.004
37. Nguyen THO, Rowntree LC, Petersen J, Chua BY, Hensen L, Kedzierski L, et al. CD8+ T cells specific for an immunodominant SARS-CoV-2 nucleocapsid epitope display high naive precursor frequency and TCR promiscuity. Immunity (2021) 54(5):1066–1082.e5. doi: 10.1016/j.immuni.2021.04.009
38. Chang C-M, Feng P, Wu T-H, Alachkar H, Lee K-Y, Chang W-C. Profiling of T cell repertoire in SARS-CoV-2-Infected COVID-19 patients between mild disease and pneumonia. J Clin Immunol (2021) 41(6):1131–45. doi: 10.1007/s10875-021-01045-z
39. Salomé B, Horowitz A. Impaired CD4 T-cell response to SARS-CoV-2: Rationale for PD-1 blockade in patients with cancer and COVID-19? Cancer Discovery (2021) 11(8):1877–8. doi: 10.1158/2159-8290.CD-21-0613
40. Park JJ, Lee KA, Lam SZ, Moon KS, Fang Z, Chen S. Machine learning identifies T cell receptor repertoire signatures associated with COVID-19 severity. Commun Biol (2023) 6(1):76. doi: 10.1038/s42003-023-04447-4
41. Wang G, Wang Y, Jiang S, Fan W, Mo C, Gong W, et al. Comprehensive analysis of TCR repertoire of COVID-19 patients in different infected stage. Genes Genomics (2022) 44(7):813–22. doi: 10.1007/s13258-022-01261-w
42. Gittelman RM, Lavezzo E, Snyder TM, Zahid HJ, Carty CL, Elyanow R, et al. Longitudinal analysis of T cell receptor repertoires reveals shared patterns of antigen-specific response to SARS-CoV-2 infection. JCI Insight (2022) 7(10). doi: 10.1172/jci.insight.151849
43. Grasselli G, Zangrillo A, Zanella A, Antonelli M, Cabrini L, Castelli A, et al. Baseline characteristics and outcomes of 1591 patients infected with SARS-CoV-2 admitted to ICUs of the Lombardy region, Italy. JAMA (2020) 323(16):1574. doi: 10.1001/jama.2020.5394
44. Gutierrez L, Beckford J, Alachkar H. Deciphering the TCR repertoire to solve the COVID-19 mystery. Trends Pharmacol Sci (2020) 41(8):518–30. doi: 10.1016/j.tips.2020.06.001
45. Ruan Q, Yang K, Wang W, Jiang L, Song J. Clinical predictors of mortality due to COVID-19 based on an analysis of data of 150 patients from wuhan, China. Intensive Care Med (2020) 46(5):846–8. doi: 10.1007/s00134-020-05991-x
46. Wu Z, McGoogan JM. Characteristics of and important lessons from the coronavirus disease 2019 (COVID-19) outbreak in China. JAMA (2020) 323(13):1239. doi: 10.1001/jama.2020.2648
47. Zhou F, Yu T, Du R, Fan G, Liu Y, Liu Z, et al. Clinical course and risk factors for mortality of adult inpatients with COVID-19 in wuhan, China: a retrospective cohort study. Lancet (2020) 395(10229):1054–62. doi: 10.1016/S0140-6736(20)30566-3
48. Gil A, Yassai MB, Naumov YN, Selin LK. Narrowing of human influenza a virus-specific T cell receptor α and β repertoires with increasing age. J Virol (2015) 89(8):4102–16. doi: 10.1128/JVI.03020-14
49. Nguyen THO, Sant S, Bird NL, Grant EJ, Clemens EB, Koutsakos M, et al. Perturbed CD8+ T cell immunity across universal influenza epitopes in the elderly. J Leukocyte Biol (2018) 103(2):321–39. doi: 10.1189/jlb.5MA0517-207R
50. Chandran SS, Paria BC, Srivastava AK, Rothermel LD, Stephens DJ, Dudley ME, et al. Persistence of CTL clones targeting melanocyte differentiation antigens was insufficient to mediate significant melanoma regression in humans. Clin Cancer Res (2015) 21(3):534–43. doi: 10.1158/1078-0432.CCR-14-2208
51. Minervina AA, Pogorelyy MV, Kirk AM, Crawford JC, Allen EK, Chou C-H, et al. SARS-CoV-2 antigen exposure history shapes phenotypes and specificity of memory CD8+ T cells. Nat Immunol (2022) 23(5):781–90. doi: 10.1038/s41590-022-01184-4
52. Zhao Y, Nguyen P, Ma J, Wu T, Jones LL, Pei D, et al. Preferential use of public TCR during autoimmune encephalomyelitis. J Immunol (2016) 196(12):4905–14. doi: 10.4049/jimmunol.1501029
53. Huisman W, Hageman L, Leboux DAT, Khmelevskaya A, Efimov GA, Roex MCJ, et al. Public T-cell receptors (TCRs) revisited by analysis of the magnitude of identical and highly-similar TCRs in virus-specific T-cell repertoires of healthy individuals. Front Immunol (2022) 13:851868. doi: 10.3389/fimmu.2022.851868
54. Sidhom J-W, Baras AS. Deep learning identifies antigenic determinants of severe SARS-CoV-2 infection within T-cell repertoires. Sci Rep (2021) 11(1):14275. doi: 10.1038/s41598-021-93608-8
55. Wang P, Jin X, Zhou W, Luo M, Xu Z, Xu C, et al. Comprehensive analysis of TCR repertoire in COVID-19 using single cell sequencing. Genomics (2021) 113(2):456–62. doi: 10.1016/j.ygeno.2020.12.036
56. Sen K, Datta S, Ghosh A, Jha A, Ahad A, Chatterjee S, et al. Single-cell immunogenomic approach identified SARS-CoV-2 protective immune signatures in asymptomatic direct contacts of COVID-19 cases. Front Immunol (2021) 12:733539. doi: 10.3389/fimmu.2021.733539
57. Bai H, Ma J, Mao W, Zhang X, Nie Y, Hao J, et al. Identification of TCR repertoires in asymptomatic COVID-19 patients by single-cell T-cell receptor sequencing. Blood Cells Molecules Dis (2022) 97:102678. doi: 10.1016/j.bcmd.2022.102678
58. Cheng MH, Zhang S, Porritt RA, Noval Rivas M, Paschold L, Willscher E, et al. Superantigenic character of an insert unique to SARS-CoV-2 spike supported by skewed TCR repertoire in patients with hyperinflammation. Proc Natl Acad Sci (2020) 117(41):25254–62. doi: 10.1073/pnas.2010722117
59. Adamo S, Michler J, Zurbuchen Y, Cervia C, Taeschler P, Raeber ME, et al. Signature of long-lived memory CD8+ T cells in acute SARS-CoV-2 infection. Nature (2022) 602(7895):148–55. doi: 10.1038/s41586-021-04280-x
60. Luo L, Liang W, Pang J, Xu G, Chen Y, Guo X, et al. Dynamics of TCR repertoire and T cell function in COVID-19 convalescent individuals. Cell Discovery (2021) 7(1):89. doi: 10.1038/s41421-021-00321-x
61. Bilich T, Nelde A, Heitmann JS, Maringer Y, Roerden M, Bauer J, et al. T Cell and antibody kinetics delineate SARS-CoV-2 peptides mediating long-term immune responses in COVID-19 convalescent individuals. Sci Trans Med (2021) 13(590). doi: 10.1126/scitranslmed.abf7517
62. Li Y, Hu J, Wang Y, Liu D, Shi Y, Zhang J, et al. T-Cell repertoire characteristics of asymptomatic and re-detectable positive COVID-19 patients. Front Immunol (2022) 12:769442. doi: 10.3389/fimmu.2021.769442
63. le Bert N, Tan AT, Kunasegaran K, Tham CYL, Hafezi M, Chia A, et al. SARS-CoV-2-specific T cell immunity in cases of COVID-19 and SARS, and uninfected controls. Nature (2020) 584(7821):457–62. doi: 10.1038/s41586-020-2550-z
64. Miconnet I, Marrau A, Farina A, Taffé P, Vigano S, Harari A, et al. Large TCR diversity of virus-specific CD8 T cells provides the mechanistic basis for massive TCR renewal after antigen exposure. J Immunol (2011) 186(12):7039–49. doi: 10.4049/jimmunol.1003309
65. Dash P, Fiore-Gartland AJ, Hertz T, Wang GC, Sharma S, Souquette A, et al. Quantifiable predictive features define epitope-specific T cell receptor repertoires. Nature (2017) 547(7661):89–93. doi: 10.1038/nature22383
66. Price DA, Brenchley JM, Ruff LE, Betts MR, Hill BJ, Roederer M, et al. Avidity for antigen shapes clonal dominance in CD8+ T cell populations specific for persistent DNA viruses. J Exp Med (2005) 202(10):1349–61. doi: 10.1084/jem.20051357
67. Zehn D, Lee SY, Bevan MJ. Complete but curtailed T-cell response to very low-affinity antigen. Nature (2009) 458(7235):211–4. doi: 10.1038/nature07657
68. Sant S, Grzelak L, Wang Z, Pizzolla A, Koutsakos M, Crowe J, et al. Single-cell approach to influenza-specific CD8+ T cell receptor repertoires across different age groups, tissues, and following influenza virus infection. Front Immunol (2018) 9:1453. doi: 10.3389/fimmu.2018.01453
69. Attaf M, Roider J, Malik A, Rius Rafael C, Dolton G, Prendergast AJ, et al. Cytomegalovirus-mediated T cell receptor repertoire perturbation is present in early life. Front Immunol (2020) 11:1587. doi: 10.3389/fimmu.2020.01587
70. Bensouda Koraichi M, Ferri S, Walczak AM, Mora T. Inferring the T cell repertoire dynamics of healthy individuals. Proc Natl Acad Sci (2023) 120(4). doi: 10.1073/pnas.2207516120
71. Fiolet T, Kherabi Y, MacDonald C-J, Ghosn J, Peiffer-Smadja N. Comparing COVID-19 vaccines for their characteristics, efficacy and effectiveness against SARS-CoV-2 and variants of concern: a narrative review. Clin Microbiol Infection (2022) 28(2):202–21. doi: 10.1016/j.cmi.2021.10.005
72. Ssentongo P, Ssentongo AE, Voleti N, Groff D, Sun A, Ba DM, et al. SARS-CoV-2 vaccine effectiveness against infection, symptomatic and severe COVID-19: a systematic review and meta-analysis. BMC Infect Dis (2022) 22(1):439. doi: 10.1186/s12879-022-07418-y
73. Keech C, Albert G, Cho I, Robertson A, Reed P, Neal S, et al. Phase 1–2 trial of a SARS-CoV-2 recombinant spike protein nanoparticle vaccine. New Engl J Med (2020) 383(24):2320–32. doi: 10.1056/NEJMoa2026920
74. Krammer F. SARS-CoV-2 vaccines in development. Nature (2020) 586(7830):516–27. doi: 10.1038/s41586-020-2798-3
75. Ewer KJ, Barrett JR, Belij-Rammerstorfer S, Sharpe H, Makinson R, Morter R, et al. T Cell and antibody responses induced by a single dose of ChAdOx1 nCoV-19 (AZD1222) vaccine in a phase 1/2 clinical trial. Nat Med (2021) 27(2):270–8. doi: 10.1038/s41591-020-01194-5
76. Sureshchandra S, Lewis SA, Doratt BM, Jankeel A, Coimbra Ibraim I, Messaoudi I. Single-cell profiling of T and b cell repertoires following SARS-CoV-2 mRNA vaccine. JCI Insight (2021) 6(24). doi: 10.1172/jci.insight.153201
77. Nguyen THO, Cohen CA, Rowntree LC, Bull MB, Hachim A, Kedzierska K, et al. T Cells targeting SARS-CoV-2: By infection, vaccination, and against future variants. Front Med (2021) 8:793102. doi: 10.3389/fmed.2021.793102
78. Lang-Meli J, Luxenburger H, Wild K, Karl V, Oberhardt V, Salimi Alizei E, et al. SARS-CoV-2-specific T-cell epitope repertoire in convalescent and mRNA-vaccinated individuals. Nat Microbiol (2022) 7(5):675–9. doi: 10.1038/s41564-022-01106-y
79. Dykema AG, Zhang B, Woldemeskel BA, Garliss CC, Rashid R, Westlake T, et al. SARS-CoV-2 vaccination diversifies the CD4+ spike-reactive T cell repertoire in patients with prior SARS-CoV-2 infection. EBioMedicine (2022) 80:104048. doi: 10.1016/j.ebiom.2022.104048
80. Alter G, Yu J, Liu J, Chandrashekar A, Borducchi EN, Tostanoski LH, et al. Immunogenicity of Ad26.COV2.S vaccine against SARS-CoV-2 variants in humans. Nature (2021) 596(7871):268–72. doi: 10.1038/s41586-021-03681-2
81. Tarke A, Sidney J, Methot N, Yu ED, Zhang Y, Dan JM, et al. Impact of SARS-CoV-2 variants on the total CD4+ and CD8+ T cell reactivity in infected or vaccinated individuals. Cell Rep Med (2021) 2(7):100355. doi: 10.1016/j.xcrm.2021.100355
82. Ballesteros-Sanabria L, Pelaez-Prestel HF, Ras-Carmona A, Reche PA. Resilience of spike-specific immunity induced by COVID-19 vaccines against SARS-CoV-2 variants. Biomedicines (2022) 10(5):996. doi: 10.3390/biomedicines10050996
83. Cromer D, Steain M, Reynaldi A, Schlub TE, Wheatley AK, Juno JA, et al. Neutralising antibody titres as predictors of protection against SARS-CoV-2 variants and the impact of boosting: a meta-analysis. Lancet Microbe (2022) 3(1):e52–61. doi: 10.1016/S2666-5247(21)00267-6
84. Painter MM, Mathew D, Goel RR, Apostolidis SA, Pattekar A, Kuthuru O, et al. Rapid induction of antigen-specific CD4+ T cells is associated with coordinated humoral and cellular immunity to SARS-CoV-2 mRNA vaccination. Immunity (2021) 54(9):2133–2142.e3. doi: 10.1016/j.immuni.2021.08.001
85. Mazzoni A, di Lauria N, Maggi L, Salvati L, Vanni A, Capone M, et al. First-dose mRNA vaccination is sufficient to reactivate immunological memory to SARS-CoV-2 in subjects who have recovered from COVID-19. J Clin Invest (2021) 131(12). doi: 10.1172/JCI149150
86. Oberhardt V, Luxenburger H, Kemming J, Schulien I, Ciminski K, Giese S, et al. Rapid and stable mobilization of CD8+ T cells by SARS-CoV-2 mRNA vaccine. Nature (2021) 597(7875):268–73. doi: 10.1038/s41586-021-03841-4
87. Nersisyan S, Zhiyanov A, Shkurnikov M, Tonevitsky A. T-CoV: a comprehensive portal of HLA-peptide interactions affected by SARS-CoV-2 mutations. Nucleic Acids Res (2022) 50(D1):D883–7. doi: 10.1093/nar/gkab701
88. GeurtsvanKessel CH, Geers D, Schmitz KS, Mykytyn AZ, Lamers MM, Bogers S, et al. Divergent SARS-CoV-2 omicron–reactive T and b cell responses in COVID-19 vaccine recipients. Sci Immunol (2022) 7(69). doi: 10.1126/sciimmunol.abo2202
89. Keeton R, Tincho MB, Ngomti A, Baguma R, Benede N, Suzuki A, et al. T Cell responses to SARS-CoV-2 spike cross-recognize omicron. Nature (2022) 603(7901):488–92. doi: 10.1038/s41586-022-04460-3
90. Tarke A, Coelho CH, Zhang Z, Dan JM, Yu ED, Methot N, et al. SARS-CoV-2 vaccination induces immunological T cell memory able to cross-recognize variants from alpha to omicron. Cell (2022) 185(5):847–859.e11. doi: 10.1016/j.cell.2022.01.015
91. Naranbhai V, Nathan A, Kaseke C, Berrios C, Khatri A, Choi S, et al. T Cell reactivity to the SARS-CoV-2 omicron variant is preserved in most but not all individuals. Cell (2022) 185(6):1041–1051.e6. doi: 10.1016/j.cell.2022.01.029
92. Bobrovitz N, Ware H, Ma X, Li Z, Hosseini R, Cao C, et al. Protective effectiveness of previous SARS-CoV-2 infection and hybrid immunity against the omicron variant and severe disease: a systematic review and meta-regression. Lancet Infect Dis (2023). doi: 10.1016/S1473-3099(22)00801-5
93. Nathan A, Rossin EJ, Kaseke C, Park RJ, Khatri A, Koundakjian D, et al. Structure-guided T cell vaccine design for SARS-CoV-2 variants and sarbecoviruses. Cell (2021) 184(17):4401–4413.e10. doi: 10.1016/j.cell.2021.06.029
94. Saggau C, Martini GR, Rosati E, Meise S, Messner B, Kamps A-K, et al. The pre-exposure SARS-CoV-2-specific T cell repertoire determines the quality of the immune response to vaccination. Immunity (2022) 55(10):1924–39.e5. doi: 10.1016/j.immuni.2022.08.003
95. Mason D. A very high level of crossreactivity is an essential feature of the T-cell receptor. Immunol Today (1998) 19(9):395–404. doi: 10.1016/S0167-5699(98)01299-7
96. Sewell AK. Why must T cells be cross-reactive? Nat Rev Immunol (2012) 12(9):669–77. doi: 10.1038/nri3279
97. Wooldridge L, Ekeruche-Makinde J, van den Berg HA, Skowera A, Miles JJ, Tan MP, et al. A single autoimmune T cell receptor recognizes more than a million different peptides. J Biol Chem (2012) 287(2):1168–77. doi: 10.1074/jbc.M111.289488
98. Lee CH, Salio M, Napolitani G, Ogg G, Simmons A, Koohy H. Predicting cross-reactivity and antigen specificity of T cell receptors. Front Immunol (2020) 11:565096. doi: 10.3389/fimmu.2020.565096
99. Mahajan S, Kode V, Bhojak K, Karunakaran C, Lee K, Manoharan M, et al. Immunodominant T-cell epitopes from the SARS-CoV-2 spike antigen reveal robust pre-existing T-cell immunity in unexposed individuals. Sci Rep (2021) 11(1):13164. doi: 10.1038/s41598-021-92521-4
100. Buckley PR, Lee CH, Pereira Pinho M, Ottakandathil Babu R, Woo J, Antanaviciute A, et al. HLA-dependent variation in SARS-CoV-2 CD8 + T cell cross-reactivity with human coronaviruses. Immunology (2022) 166(1):78–103. doi: 10.1111/imm.13451
101. Tan CCS, Owen CJ, Tham CYL, Bertoletti A, van Dorp L, Balloux F. Pre-existing T cell-mediated cross-reactivity to SARS-CoV-2 cannot solely be explained by prior exposure to endemic human coronaviruses. Infection Genet Evol (2021) 95:105075. doi: 10.1016/j.meegid.2021.105075
102. Zhang B, Tian J, Zhang Q, Xie Y, Wang K, Qiu S, et al. Comparing the nucleocapsid proteins of human coronaviruses: Structure, immunoregulation, vaccine, and targeted drug. Front Mol Biosci (2022) 9:761173. doi: 10.3389/fmolb.2022.761173
103. Grifoni A, Weiskopf D, Ramirez SI, Mateus J, Dan JM, Moderbacher CR, et al. Targets of T cell responses to SARS-CoV-2 coronavirus in humans with COVID-19 disease and unexposed individuals. Cell (2020) 181(7):1489–1501.e15. doi: 10.1016/j.cell.2020.05.015
104. Weiskopf D, Schmitz KS, Raadsen MP, Grifoni A, Okba NMA, Endeman H, et al. Phenotype and kinetics of SARS-CoV-2–specific T cells in COVID-19 patients with acute respiratory distress syndrome. Sci Immunol (2020) 5(48). doi: 10.1126/sciimmunol.abd2071
105. Mallajosyula V, Ganjavi C, Chakraborty S, McSween AM, Pavlovitch-Bedzyk AJ, Wilhelmy J, et al. CD8 + T cells specific for conserved coronavirus epitopes correlate with milder disease in patients with COVID-19. Sci Immunol (2021) 6(61). doi: 10.1126/sciimmunol.abg5669
106. Hu W, He M, Wang X, Sun Q, Kuang M. Specific CD8+ TCR repertoire recognizing conserved antigens of SARS-CoV-2 in unexposed population: A prerequisite for broad-spectrum CD8+ T cell immunity. Vaccines (2021) 9(10):1093. doi: 10.3390/vaccines9101093
107. Nesterenko PA, McLaughlin J, Tsai BL, Burton Sojo G, Cheng D, Zhao D, et al. HLA-A∗02:01 restricted T cell receptors against the highly conserved SARS-CoV-2 polymerase cross-react with human coronaviruses. Cell Rep (2021) 37(13):110167. doi: 10.1016/j.celrep.2021.110167
108. Peng Y, Felce SL, Dong D, Penkava F, Mentzer AJ, Yao X, et al. An immunodominant NP105–113-B*07:02 cytotoxic T cell response controls viral replication and is associated with less severe COVID-19 disease. Nat Immunol (2022) 23(1):50–61. doi: 10.1038/s41590-021-01084-z
109. Lineburg KE, Grant EJ, Swaminathan S, Chatzileontiadou DSM, Szeto C, Sloane H, et al. CD8+ T cells specific for an immunodominant SARS-CoV-2 nucleocapsid epitope cross-react with selective seasonal coronaviruses. Immunity (2021) 54(5):1055–1065.e5. doi: 10.1016/j.immuni.2021.04.006
110. Goel RR, Painter MM, Apostolidis SA, Mathew D, Meng W, Rosenfeld AM, et al. mRNA vaccines induce durable immune memory to SARS-CoV-2 and variants of concern. Science (2021) 374(6572). doi: 10.1126/science.abm0829
111. Cele S, Gazy I, Jackson L, Hwa S-H, Tegally H, Lustig G, et al. Escape of SARS-CoV-2 501Y.V2 from neutralization by convalescent plasma. Nature (2021) 593(7857):142–6. doi: 10.1038/s41586-021-03471-w
112. Tegally H, Wilkinson E, Giovanetti M, Iranzadeh A, Fonseca V, Giandhari J, et al. Detection of a SARS-CoV-2 variant of concern in south Africa. Nature (2021) 592(7854):438–43. doi: 10.1038/s41586-021-03402-9
113. Geers D, Shamier MC, Bogers S, den Hartog G, Gommers L, Nieuwkoop NN, et al. SARS-CoV-2 variants of concern partially escape humoral but not T cell responses in COVID-19 convalescent donors and vaccine recipients. Sci Immunol (2021) 6(59). doi: 10.1126/sciimmunol.abj1750
114. Riou C, Keeton R, Moyo-Gwete T, Hermanus T, Kgagudi P, Baguma R, et al. Escape from recognition of SARS-CoV-2 variant spike epitopes but overall preservation of T cell immunity. Sci Trans Med (2022) 14(631). doi: 10.1126/scitranslmed.abj6824
115. Agerer B, Koblischke M, Gudipati V, Montaño-Gutierrez LF, Smyth M, Popa A, et al. SARS-CoV-2 mutations in MHC-i-restricted epitopes evade CD8 + T cell responses. Sci Immunol (2021) 6(57). doi: 10.1126/sciimmunol.abg6461
116. Wu D, Kolesnikov A, Yin R, Guest JD, Gowthaman R, Shmelev A, et al. Structural assessment of HLA-A2-restricted SARS-CoV-2 spike epitopes recognized by public and private T-cell receptors. Nat Commun (2022) 13(1):19. doi: 10.1038/s41467-021-27669-8
117. Gao Y, Cai C, Grifoni A, Müller TR, Niessl J, Olofsson A, et al. Ancestral SARS-CoV-2-specific T cells cross-recognize the omicron variant. Nat Med (2022) 28(3):472–6. doi: 10.1038/s41591-022-01700-x
118. Jo N, Zhang R, Ueno H, Yamamoto T, Weiskopf D, Nagao M, et al. Aging and CMV infection affect pre-existing SARS-CoV-2-Reactive CD8+ T cells in unexposed individuals. Front Aging (2021) 2:719342. doi: 10.3389/fragi.2021.719342
119. Tomita Y, Sato R, Ikeda T, Sakagami T. BCG Vaccine may generate cross-reactive T cells against SARS-CoV-2: In silico analyses and a hypothesis. Vaccine (2020) 38(41):6352–6. doi: 10.1016/j.vaccine.2020.08.045
120. Eggenhuizen PJ, Ng BH, Chang J, Fell AL, Cheong RMY, Wong WY, et al. BCG Vaccine derived peptides induce SARS-CoV-2 T cell cross-reactivity. Front Immunol (2021) 12:692729. doi: 10.3389/fimmu.2021.692729
121. Upton CM, van Wijk RC, Mockeliunas L, Simonsson USH, McHarry K, van den Hoogen G, et al. Safety and efficacy of BCG re-vaccination in relation to COVID-19 morbidity in healthcare workers: A double-blind, randomised, controlled, phase 3 trial. EClinicalMedicine (2022) 48:101414. doi: 10.1016/j.eclinm.2022.101414
122. O’Driscoll M, Ribeiro Dos Santos G, Wang L, Cummings DAT, Azman AS, Paireau J, et al. Age-specific mortality and immunity patterns of SARS-CoV-2. Nature (2021) 590(7844):140–5. doi: 10.1038/s41586-020-2918-0
123. Liu Y, Wu Y, Liu B, Zhang Y, San D, Chen Y, et al. Biomarkers and immune repertoire metrics identified by peripheral blood transcriptomic sequencing reveal the pathogenesis of COVID-19. Front Immunol (2021) 12:677025. doi: 10.3389/fimmu.2021.677025
Keywords: T cell receptors, T cell immune response, SARS-CoV-2, T cell repertoire, T cell repertoire dynamic
Citation: Zornikova KV, Sheetikov SA, Rusinov AY, Iskhakov RN and Bogolyubova AV (2023) Architecture of the SARS-CoV-2-specific T cell repertoire. Front. Immunol. 14:1070077. doi: 10.3389/fimmu.2023.1070077
Received: 14 October 2022; Accepted: 08 March 2023;
Published: 20 March 2023.
Edited by:
Martijn van Griensven, Maastricht University, NetherlandsReviewed by:
Yu Nee Lee, Sheba Medical Center, IsraelCopyright © 2023 Zornikova, Sheetikov, Rusinov, Iskhakov and Bogolyubova. This is an open-access article distributed under the terms of the Creative Commons Attribution License (CC BY). The use, distribution or reproduction in other forums is permitted, provided the original author(s) and the copyright owner(s) are credited and that the original publication in this journal is cited, in accordance with accepted academic practice. No use, distribution or reproduction is permitted which does not comply with these terms.
*Correspondence: Apollinariya V. Bogolyubova, YXBvbGxpbmFyaXlhLmJvZ29seXVib3ZhQGdtYWlsLmNvbQ==
Disclaimer: All claims expressed in this article are solely those of the authors and do not necessarily represent those of their affiliated organizations, or those of the publisher, the editors and the reviewers. Any product that may be evaluated in this article or claim that may be made by its manufacturer is not guaranteed or endorsed by the publisher.
Research integrity at Frontiers
Learn more about the work of our research integrity team to safeguard the quality of each article we publish.