- 1Pharmacy sterilization department, Nouvel Hopital Civil, Strasbourg University Hospital, Strasbourg, France
- 2Pulmonology department, Nouvel Hopital Civil, Strasbourg University Hospital, Strasbourg, France
- 3Laboratory Streinth (STress REsponse and INnovative THerapy against cancer), Inserm Unité Mixte de Recherche (UMR_S 1113), Interface de Recherche Fondamentale et Appliquée en Cancérologie (IRFAC), Université de Strasbourg, Instituts Thématiques Interdisciplinaires (ITI) InnoVec, Strasbourg, France
Introduction: Gut microbiota can significantly affect the effectiveness of immune checkpoint inhibitors (ICIs) in cancer patients. Recently, antibiotics were shown to decrease survival rate of patients treated by ICIs. Proton pump inhibitors (PPIs) can indeed modulate microbiota’s diversity, therefore altering ICIs response. A meta-analysis was performed based on published data to verify this hypothesis.
Methods: In this study, over 41 publications, exploring the impact of concomitant PPI treatment on outcomes of ICI-treated patients, were analyzed. Evaluated endpoints were overall survival (OS) and progression-free survival (PFS). Pooled hazard ratios (HRs) with a 95% confidence interval (CI) were reported in ICIs in PPI users versus non-PPI users. Subgroup analyses were performed to minimize the impact of study heterogeneity and to investigate the influence of PPI on the different groups of interest. There was no evidence of publication bias for OS and PFS analysis in subgroup analysis.
Results: Forty-one studies were included in the meta-analysis, including a total of 20,042 patients. OS of patients receiving ICIs was negatively correlated in patients concomitantly treated with PPI (HR=1.37; 95%CI, 1.23–1.52). PFS of cancer patients receiving ICIs was also negatively correlated with PPI treatment (HR=1.28; 95%CI, 1.15–1.42). PPI and ICI use was associated with worst OS and PFS not only for non-small-cell lung cancer (NSCLC) or urothelial cancer patients but also for patients treated with anti PD-1 (OS) and anti PD-L1 (OS and PFS) immunotherapies when administered in non-first line and when PPI was received as baseline treatment or in 60 days before ICI initiation. PPI and ICI use also showed the worst OS and PFS for patients from Europe and Asia.
Conclusion: This meta-analysis suggests that PPI treatment leads to significantly worse outcomes in advanced cancer patients treated by ICIs in terms of PFS and OS.
1 Introduction
Proton pump inhibitors (PPIs) are one of the most prescribed therapeutic classes in the world (1). The indications of PPIs are the treatment of gastroesophageal reflux disease (GERD) and esophagitis reflux. PPIs are also particularly efficient to treat patients at risk of gastrointestinal lesions by non-steroidal anti-inflammatories (and their prevention) and are used to treat gastroduodenal ulcer and eradicate Helicobacter pylori with concomitant antibiotics (2). However, PPIs are also often used off-label and sometimes for longer than recommended (3). PPIs also have multiple side effects. Possible short-term use side effects include rash, headache, dizziness, flatulence, abdominal pain, nausea, constipation and diarrhea, while possible long-term use may lead to enteric infection (particularly Clostridium difficile infection), peritonitis, liver diseases, pneumonia, ions and vitamins deficiencies (calcium, magnesium, iron, and vitamin B12), kidney disease, acute kidney injury, and finally dementia. Unnecessary or too long exposure can therefore present some risks for patients (4).
At the same time, the use of immune checkpoint inhibitors (ICIs) have revolutionized cancer patients’ treatment, particularly for non-small cell lung cancer (NSCLC) (5), melanoma (6), and urothelial carcinoma (7). The growing use of immunotherapy highlights the importance and risks of pharmacodynamics drug interactions. For example, recent publications showed the decrease in both the progression-free survival (PFS) and overall survival (OS) for patients with NSCLC treated by corticosteroids (8, 9). Such effect can be explained not only by the pharmacodynamics of corticosteroids and the inhibition of the inflammatory response and immune system homeostasis but also by their immunosuppressive proprieties in chronic uses, which is totally in opposition with action mechanism of ICIs.
In addition, antibiotics could be linked with poorer outcomes in cancer patients treated by ICIs. Several studies have shown a decrease in OS (10–13), PFS (12, 13), and disease control (10). Such pharmacodynamic interactions may be explained by the alteration of gut microbiota and a decrease in bacterial diversity by antibiotics treatment. In fact, ICI responses are closely related to the gut microbiome composition (14) because bacteria types or bacteria metabolites modulate the antitumor immunity and inflammation (15).
Other therapies can also modulate gut microbiota and therefore alter responses to ICIs therapies. Among those, PPIs are frequently prescribed in cancer patients, and several publications have shown that PPIs may be associated with poor outcome when used concomitantly with ICIs. However, this impact is still debated and a meta-analysis was therefore conducted to evaluate the role of the use of PPIs concomitantly with ICIs on the outcome of cancer patients.
2 Materials and methods
2.1 Identification of the publication for aggregation
For this systematic review of the literature on the role of PPIs on outcome with ICIs for cancer patients, the search for relevant publications was performed in both PubMed and Cochrane library database. Additional web searches were also performed to find other studies. The keywords and Medical Subject Headings (MeSH) used were “immunotherapy,” “immune checkpoint inhibitor,” “PDL-1 antibody,” “PD1-antibody,” “CTLA-4 inhibitor,” “pembrolizumab,” “atezolizumab,” “ipilimumab,” “nivolumab” “durvalumab,” “proton pump inhibitor,” “omeprazole,” “pantoprazole,” “rabeprazole,” “esomeprazole,” “lansoprazole,” “dexlansoprazole,” “concomitant medication,” “chronic medication,” “survival,” “overall survival,” and “progression free survival.”
Studies with various associations of previously cited keywords were included. Research strategy was as follows: (immunotherapy OR immune checkpoint inhibitor OR PDL-1 antibody OR PD1-antibody OR CTLA-4 inhibitor OR pembrolizumab OR atezolizumab OR ipilimumab OR nivolumab OR durvalumab) AND (proton pump inhibitor OR omeprazole OR pantoprazole OR rabeprazole OR esomeprazole OR lansoprazole OR dexlansoprazole OR concomitant medication OR chronic medication) AND (survival OR overall survival OR progression-free survival). Only studies with the following criteria were considered: 1) include patients diagnosed for advanced malignant tumors (any types of cancer) treated with ICIs, 2) ICIs could be administered alone or in combination with other anticancer drugs regardless of the therapeutic line, 3) potential association between PPI use and outcomes (OS and/or PFS) when co-prescribed with ICI needed to be assessed by comparing a PPI group and PPI-free group based on their historic use, and finally, 4) statistical data, in particular hazard ratio (HR), with 95% CI for OS and PFS were required.
Publications not responding to the previous criteria were discarded together with case reports and animal experiments. Reviews were not included in the analysis but considered by the authors to find potential missing publications. Studies that did not report informations of patients or did not present sufficient data on survival were excluded. Only full papers and abstracts with sufficient data were selected. Studies not yet fully published were not included in the meta-analysis. All reference lists from assessed articles were examined to identify additional potential articles of interest. The Preferred Reporting Items for Systematic Review and Meta-Analysis (PRISMA) guidelines were used to assess publications. Full papers published until November 2022 were included.
2.2 Quality evaluation
The Newcastle Ottawa quality assessment Scale (NOS) (16) was used for quality evaluation of the publications included in the meta-analysis. Each study was evaluated on three aspects: selection of groups (representativeness of the exposed cohort, selection of the non-exposed cohort, ascertainment of exposure, demonstration that the outcome of interest was not present at the start of the study), and group’s comparability and ascertainment of outcomes (assessment of outcome, sufficient follow-up for the occurrence of outcomes, and adequacy of follow-up cohort). Two investigators (SL and MK) graded independently all the studies with a maximum of 9 points. The selection of patients, assessment of outcomes, and comparability were assessed. The studies with less than six stars were excluded from the meta-analysis. The two investigators were used to score studies independently to avoid any bias. When discordant scores were obtained, the study was revalued, and a consensus was found after discussion between the authors.
2.3 Data extraction
Two reviewers independently assessed the eligibility of the studies included in the meta-analysis. One investigator (LP), with experience in statistical analysis, extracted the following data from the studies: first author, publication year, number of PPI and non-PPI users, hazard ratio (HR), and 95%CI for PFS and/or OS between PPI users and non-users. Another investigator (SL) was responsible for extracting the following data: type of studies, number of patients included, patients age and sex, Eastern Cooperative Group performance status (ECOG PS), region, cancer type, treatment line, ICI treatment used, type of PPI treatment, PPI use window, and outcomes.
2.4 Statistical analysis
The overall HRs and 95%CI for PFS and OS were calculated to compare the impact of PPI use on ICI treatment. An HR > 1.0 indicated a better outcome in the no-PPI-treated arm. On the other hand, an HR < 1.0 implied a greater treatment effect in the PPI-treated arm and therefore a favorable effect of PPI use on ICI treatment. Statistical significance was determined using the Cochran’s chi-square test. p-values of <0.05 were considered statistically significant.
The heterogeneity of the included studies was estimated using Cochrane’s Q test and I² statistics. For the Q test, p<0.10 represented statistically significant heterogeneity. When I² was higher than 50%, substantial heterogeneity was considered between studies. For low heterogeneity studies (p<0.10 and I² ≤50%), a fixed-effects model was used, while for high-heterogeneity studies (p<0.10 and I²>50%), a random-effects model was used. Subgroup analyses were performed in order to minimize the impact of study heterogeneity and therefore to investigate whether the influence of PPI use varied between different groups of interest (region, type of cancer, type of ICI, treatment line, and PPI-use window).
Sensitivity analyses were performed using the “one-study removed” approach to assess its effect on the pooled outcome hazard ratio.
Funnel plots with Egger’s regression tests were used to examine publication bias across studies.
All statistical analyses and forest plots were conducted using Review Manager (RevMan 5.4; the Cochrane Collaboration, Oxford, United Kingdom).
3 Results
3.1 Search results
A total of 2,995 publications were collected from the primary publication search, from which 2,919 were immediately discarded because they dealt with different topics or animal studies. A total of 71 studies were included in the full text analyze. Of these, 30 were excluded for the following reasons: 10 were reviews and/or meta-analysis, 11 were not relevant because of insufficient data on the outcomes or because hazard ratio was not available, 8 were non-PPI studies, and 1 study included the same patient cohort as another publication. Finally, 41 publications were eligible and were included in the meta-analysis (17, 18, 19; 20, 21, 10, 22-25, 26; 27-38, 39; 40-43, 44; 45-55, 13).
The PRISMA flow diagram of articles identification and selection is shown in Figure 1.
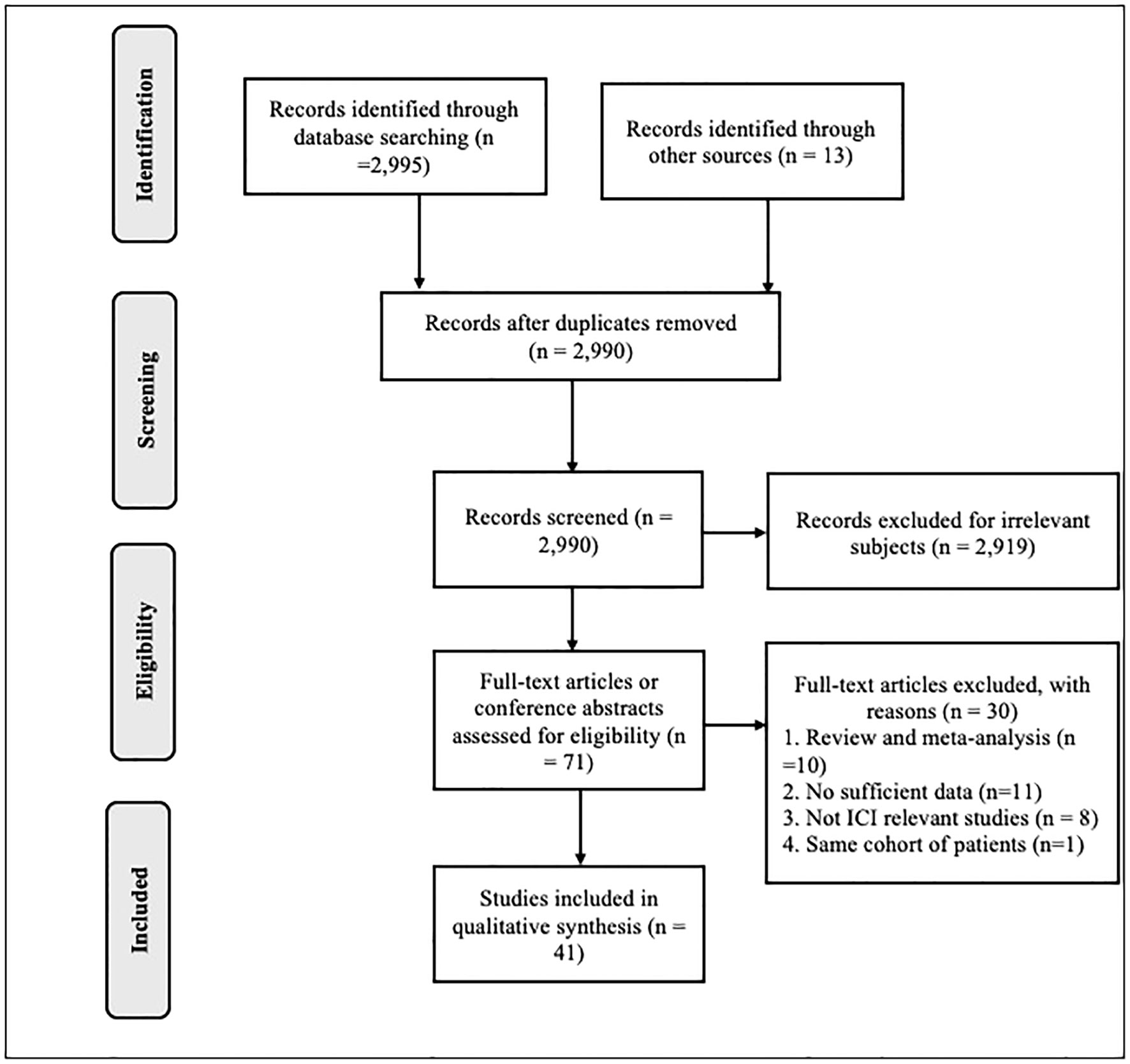
Figure 1 Preferred Reporting Items for Systematic Review and Meta-Analysis (PRISMA) flow diagram of articles identification and selection. PPI, proton pump inhibitor.
3.2 Baselines characteristics of the included publications
Baseline characteristics of the included publications are shown in Table 1. All of them were retrospective studies (Table 2), and they included 20,042 patients in total. Among these patients, 8,647 (43,1%) had taken PPI before (30, 60, or 90 days prior to initiate ICIs), during, and/or after (30–60 days) the immunotherapy treatment. The most common cancer observed in these studies was NSCLC with 11,555 cases (57,7%). All the publications assessed the impact of PPI use on OS and/or PFS, with 2 studies showing a positive impact, 21 a negative impact, and 18 no significant effect of PPI. All HR values were extracted from either univariate or multivariate analysis (if available). The agents used for immunotherapy treatments were anti-PD-1, anti-PD-L1, and anti-CTLA-4 for first line, second line, or beyond.
3.3 Quality assessment
All publications were graded with six to eight stars, on a maximum of 9 points, and no studies were excluded from the meta-analysis. The studies and their scores are shown in Table 2.
3.4 Effect of concomitant use of PPI on overall survival and progression-free survival
Among the 41 publications selected for the meta-analysis, 42 cohorts (the study from Homicsko et al. including three cohorts) provided data for OS (n=19,972 patients), and 31 cohorts provided data for PFS (n=11,086 patients).
A statistically significant association between PPI and ICI use and shorter OS was observed in 19 cohorts. No difference in OS was observed for patients treated with ICI whether they received PPI or not in 22 studies. Only Peng et al. showed a longer OS among patients with PPI (HR=1.22; 95%CI, 0.80–1.96). Overall, results showed that not using PPI significantly increased the OS of patients treated with ICI (HR=1.37; 95% CI, 1.23–1.52) (Figure 2A).
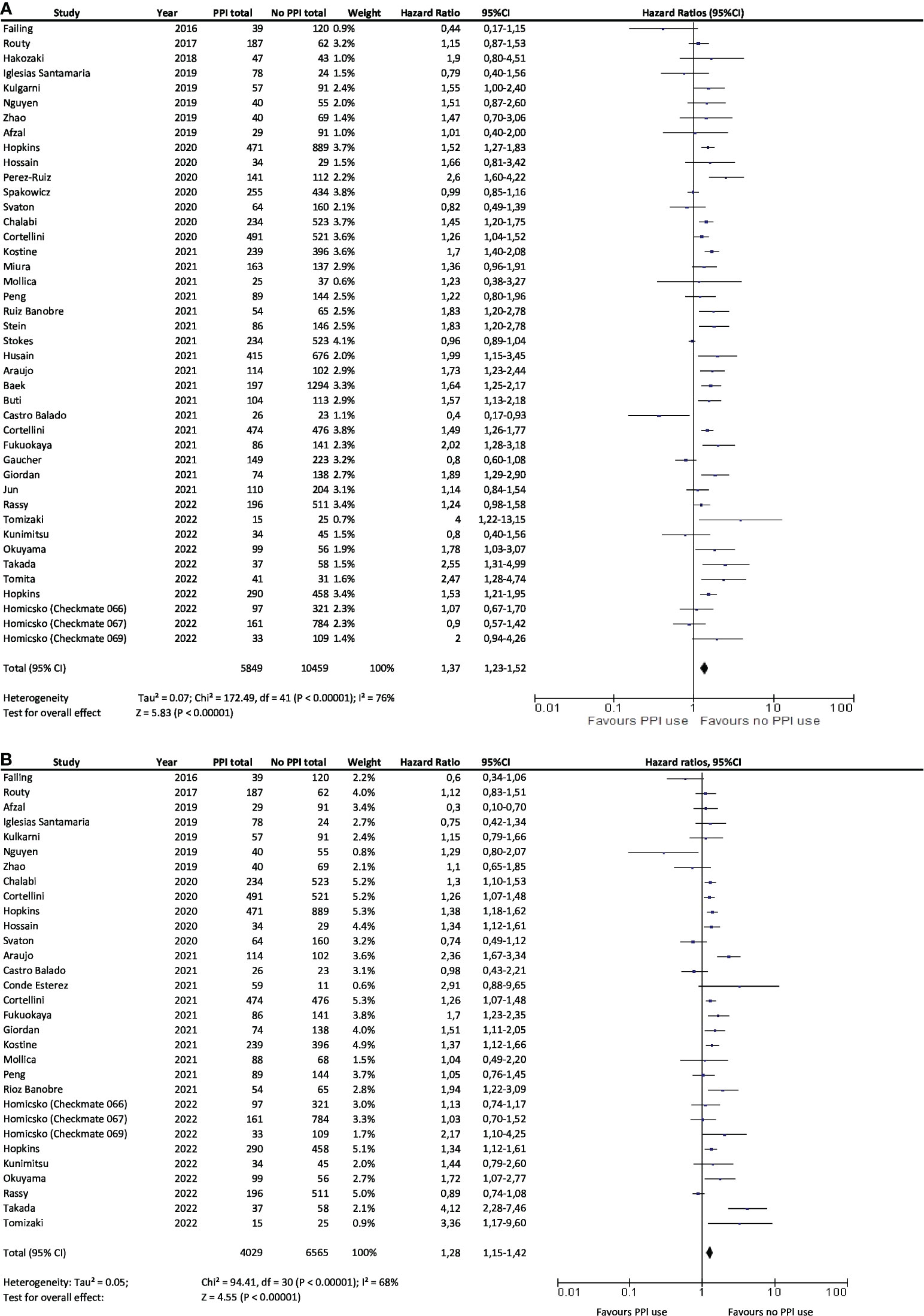
Figure 2 Association between PPI use and overall survival (A) and progression-free survival (B) in cancer patients treated with immunotherapy. PPI, proton pump inhibitor; CI, confidence interval.
A statistically significant association was observed in 15 studies between PPI and ICI use and shorter PFS. However, in 15 studies, there was no difference in PFS whether patients treated with ICI received PPI or not. Only one study showed a benefit in PFS for patients treated with ICI and receiving PPI (HR=0.30; 95%CI, 0.10–0.70). Overall, results also showed that PFS was significantly and negatively associated with the use of PPIs with ICI treatment (HR=1.28; 95%CI, 1.15–1.42; Figure 2B).
The between-study heterogeneity was moderate, with I² = 76% for OS and I² = 68% for PFS. Pooled HRs with 95% CIs were therefore calculated using random-effects models. The pooled HRs for OS were not significantly modified after excluding one study at a time in the sensitivity analysis. The pooled HRs for PFS did not significantly differ either in the sensitivity analysis (Supplementary Tables S1, S2). However, this did not necessarily seem relevant to analyze given the large number of studies included. There was no evidence of publication bias for pooled HR for OS and PFS analysis considering the funnel plots (Figures 3A, B).
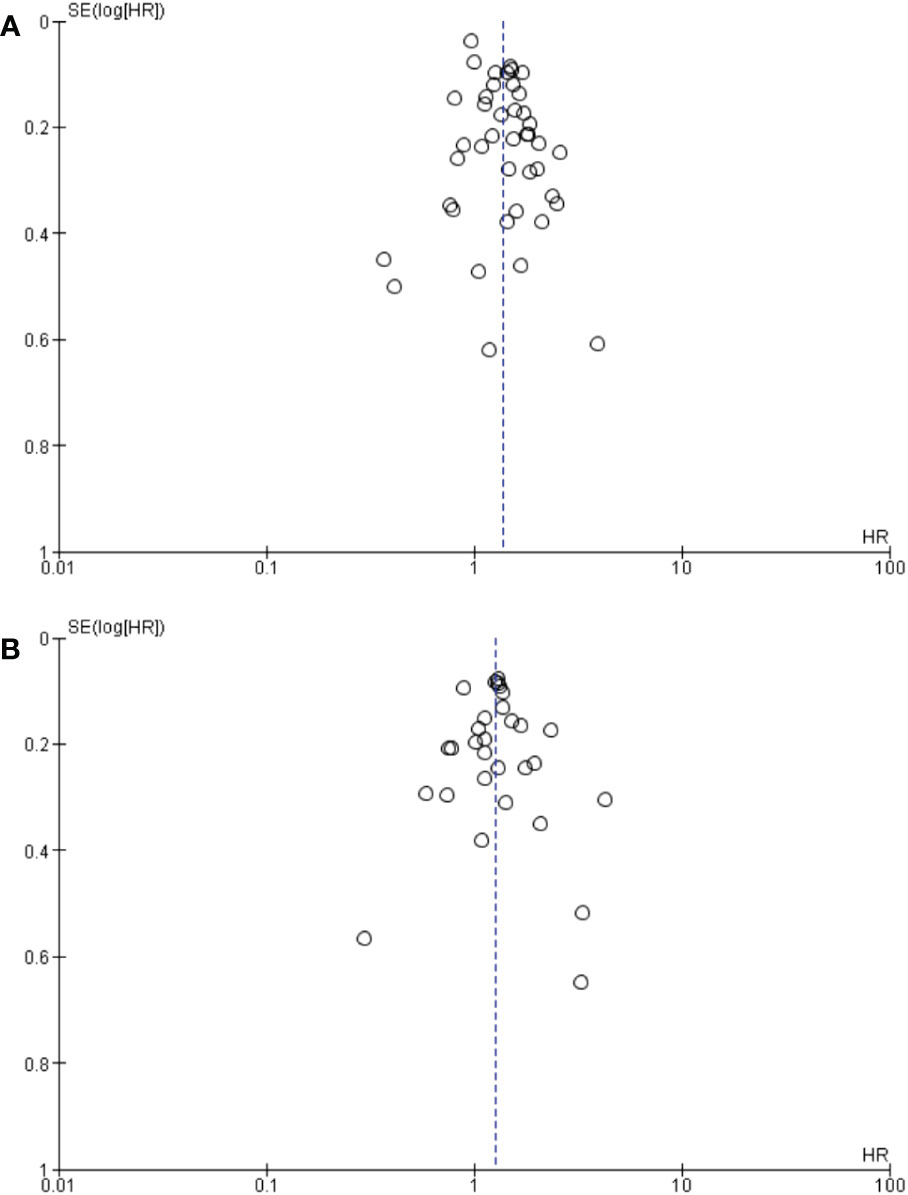
Figure 3 Begg’s funnel plot of HR ratios of (A) OS and (B) PFS. HR, hazard ratio; SE, standard error.
Subgroup analysis showed that worst OS was associated with PPI and ICI use for NSCLC or urothelial cancer patients (HR=1.33, 95%CI 1.13–1.57 and HR=1.61, 95%CI 1.29–2.01; Figure 4A), and for patients treated with anti PD-1 and anti PD-L1 immunotherapies (HR=1.33, 95%CI 1.09–1.62 and HR=1.31, 95%CI 1.11–1.54, Figure 4B) when administered in non-first line (HR=1.44; 95%CI, 1.24–1.67; Figure 4C), and when PPIs was received as baseline treatment or in 60 days before ICIs initiation (HR=1.35, 95%CI 1.18–1.53 and HR=1.35, 95%CI 1.19–1.54; Figure 4D). OS was also worst for patients originating from Europe (HR=1.35; 95%CI, 1.15–1.58; Figure 4E) and Asia (HR=1.59; 95%CI, 1.30–1.94; Figure 4E). The lowest PFS were also associated with PPI and ICI use for NSCLC (HR=1.29; 95%CI, 1.10–1.51; Figure 5A) and urothelial carcinoma patients (HR=1.50; 95%CI, 1.32–1.70; Figure 5A), for patients treated with anti-PD-L1 (HR=1.32; 95%CI, 1.20–1.45; Figure 5B) when administered in non-first line (HR=1.47; 95%CI, 1.07–2.02; Figure 5C), and when PPIs were received in 60 days before ICIs initiation (HR=1.34; 95%CI, 1.21–1.49; Figure 5D). PFS was worst for Asian and European patients (HR=1.78, 95%CI 1.30–2.43 and HR=1.19, 95%CI 1.02–1.37; Figure 5E).
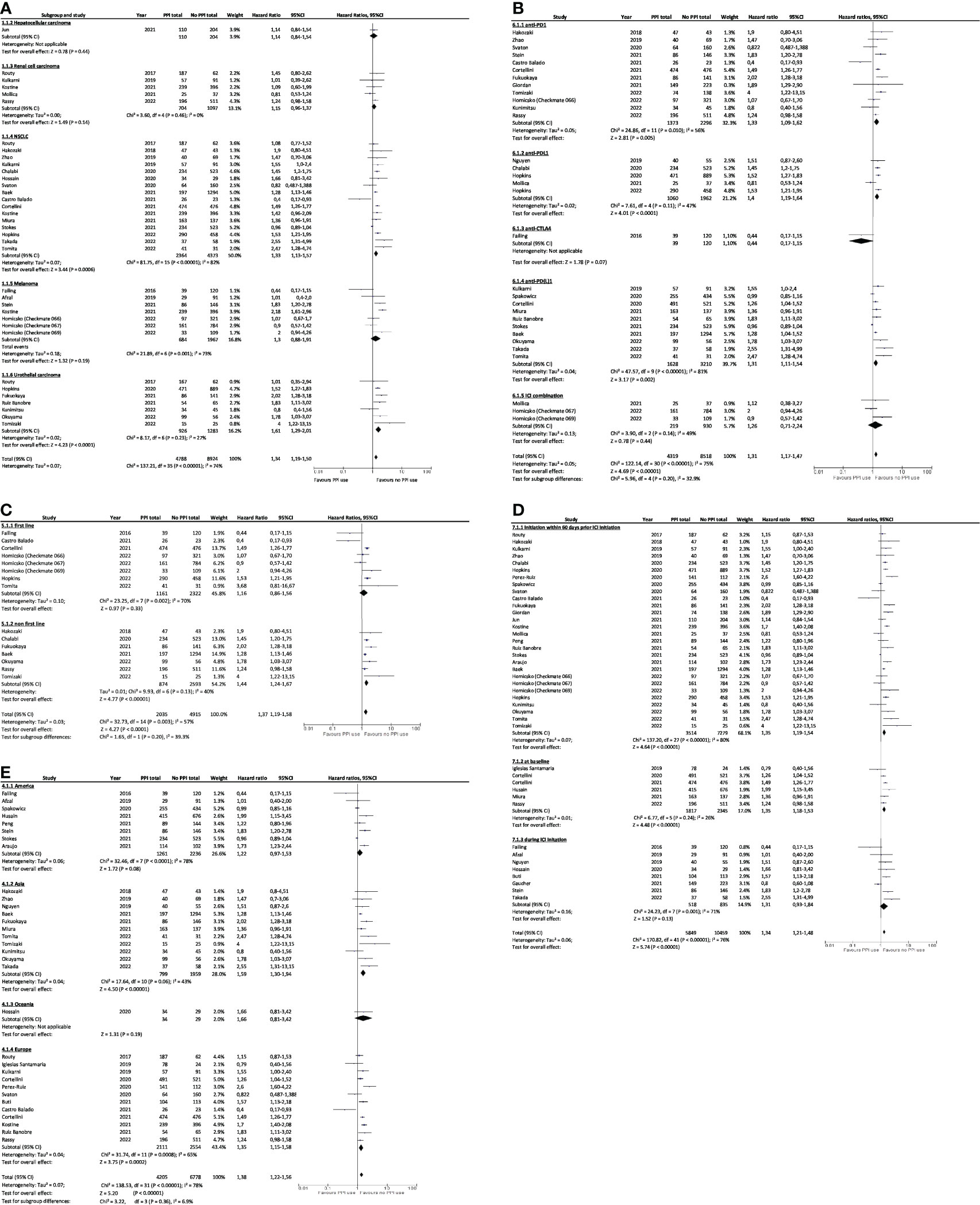
Figure 4 Subgroup analysis of association between PPI use and overall survival by (A) cancer type, (B) type of immunotherapy, (C) treatment line, (D) PPIs use window, and (E) continents. PPI, proton pump inhibitor; CI, confidence interval; NSCLC, non-small cell lung cancer; ICI, immune checkpoint inhibitor.
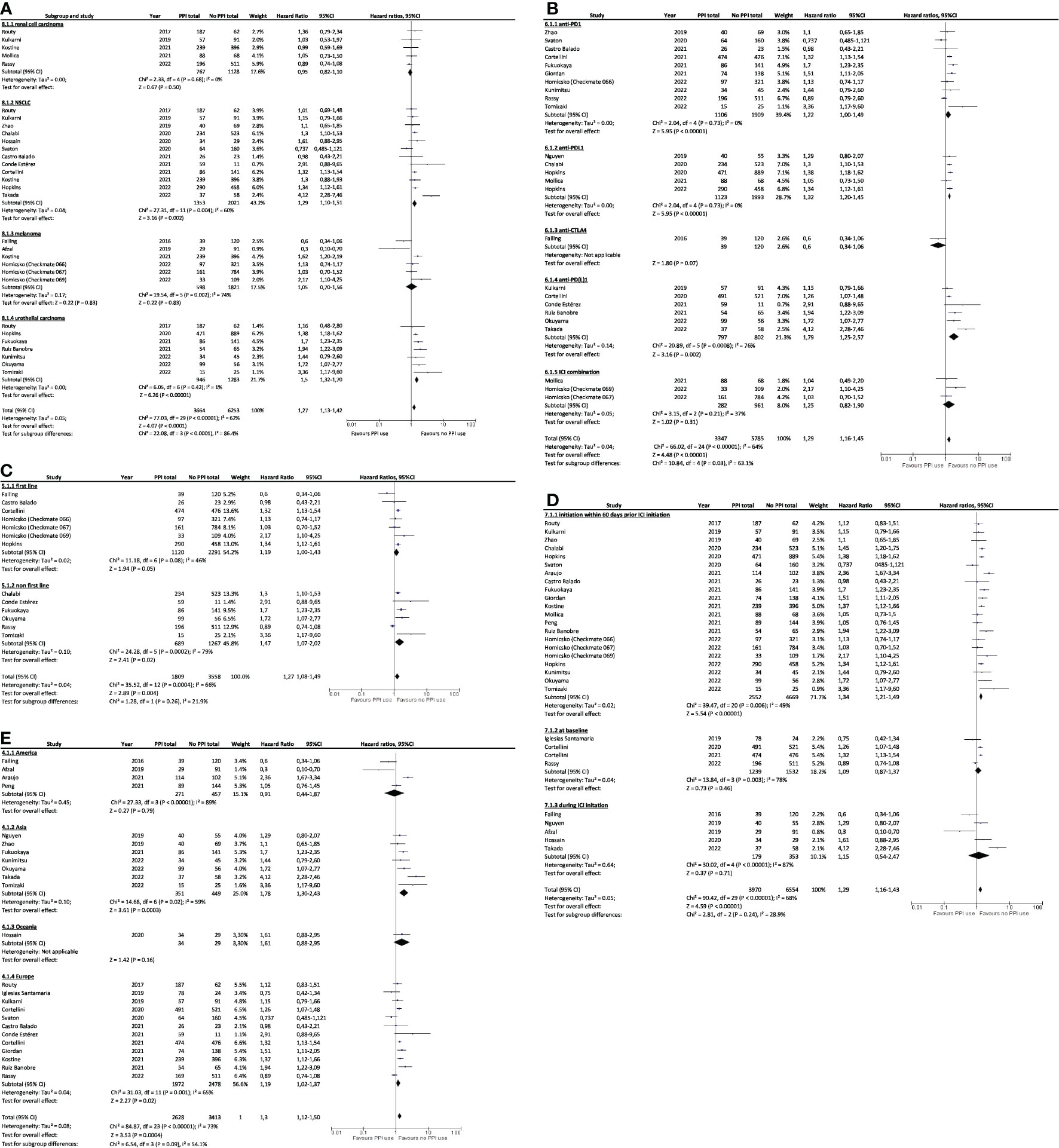
Figure 5 Subgroup analysis of association between PPI use and progression-free survival by (A) cancer type, (B) type of immunotherapy, (C) treatment line, (D) PPIs use window, and (E) by continents. PPI, proton pump inhibitor; CI, confidence interval; NSCLC, non-small cell lung cancer; ICI, immune checkpoint inhibitor.
4 Discussion
Despite the revolution of cancer immunotherapies, the response rate of cancer patients to ICIs remains approximately 30% (56). Identifying predictive factors could contribute to improve patient selection for ICI treatment and is currently the topic of many ongoing research projects worldwide. Some predictive factors have already been identified but remain insufficient for patient selection in practice (e.g., PD-L1 expression, mutations, interferon signature). The present meta-analysis, which included 20,042 patients from 41 retrospective studies, suggested that concomitant PPI treatment was significantly associated with poorer OS and PFS in advanced solid cancer patients treated by ICIs. These results were in good agreement with the meta-analysis of Deng et al. (n = 16,147 patients, 30 publications) (57) and Chen et al. (n = 15,957 patients, 33 studies) (58). Two other meta-analyses showed no association between PPI consumption and survival outcomes in ICI patients (n=1,167 patients, five publications and 1,392 patients, seven studies, respectively) (59, 60), but a small sample size may have affected the results of the association between PPI use and ICI effectiveness.
Several studies showed that intestinal microbiota had a significant impact on immune system and ICI responses (61). Bifidobacterium sp., and in particular B. breve, B. adolescentis and B. longum, were associated with response to ICIs in mice models (14). Matson et al. (62) confirmed these data and observed other species in responders’ patients. In another study, Routy et al. (47) highlighted the abundance of Akkermansia muciniphila, Ruminococcus sp., Alistipes sp. in responders to ICIs. The oral supplementation of A. muciniphila, alone and/or with Enterococcus hirae, contributed to the restoration of the response to ICIs in mouse (47). However, a recent study showed that the best survival was achieved only when A. muciniphila was present in small quantities (median survival of 27.7 months compared to 7.8 months when present in large quantities and to 15.5 months when absent from the gastrointestinal tract) (63). In a Japanese study, Clostridium butyrium supplementations improved ICI efficacy for a cohort of NSCLC patients (54). Firmicutes, Faecalibaterium prausnitzii, Streptococcus parasanguinis, Bacteroides caccae, and high alpha diversity also appeared to be associated with patient’s response to ICIs, while Bacteroidetes, low alpha diversity, and Escherichia coli were associated with non-responder patients (64–66). Finally, T-cell response specific for Bacteroides fragilis was significantly associated with the response to anti CTLA-4 (67). The influence of the intestinal microbiome on the anticancer immune response varies depending on the species. Some bacteria found on patient’s responders treated with ICIs also showed different types of immune modulations, such as B. fragilis, which activated Th1 cells and cross-reactivity between bacterial antigens and tumor antigens (68). Bifidobacterium enhances interferon gamma (IFN-γ) production by TCD8 cells and the tumor infiltration, whereas Akkermansia muciniphila induces interleukin (IL)-12 (47). Microbiota was also shown to be involved in the activation of intratumoral and splenic dendritic cells (DC) (14). Gut microbiota microbial- or pathogen-associated molecular patterns (MAMPs or PAMPs) also participate to the immunomodulation of the tumor microenvironment. For example, lipopolysaccharides (LPS) improve adoptive T-cell activity (69), and bacterial DNAs modulate the balance of regulatory T/effective T cells (70). Finally, microbial metabolites can also be involved in the modulation of immune system (71). For example, short-chain fatty acids were shown to impact cytokines production, DC function (72, 73), and B-cell class switching and to facilitate Treg differentiation (74, 75).
PPI can also induce gut dysbiosis through their direct mechanism of action on HK/ATPase pump, which in turn reduces gastric acidity (76). More specifically, the population of bacteria associated with response to ICIs, including Bifidobacterium sp (76–78), Ruminococcaceae (76–78), Akkermansia muciniphila (79, 80), and Alistipes sp (77), were found to be decreased by PPI treatment. Alpha diversity was also negatively impacted by PPIs (75, 76, 81). On the contrary, the population of bacteria associated with resistance to ICIs, such as Bacteroidetes (79) and Escherichia coli (76, 77), increased with PPI treatment.
Obesity is a defined risk factor of GERD, and PPI are prescribed for GERD treatment. However, obesity seems to be correlated to better OS in patients treated by ICIs. In 2018, McQuade et al. showed a positive impact of high body mass index (BMI) on OS and PFS for metastatic melanoma patients treated by ICIs (82) but not for the chemotherapy group. In a 2022 retrospective study, Lee et al. showed similar results on OS for melanoma (83). In another study, Cortellini et al. (84) reported that PFS and OS were longer for patients with advanced cancers in BMI>25 group. Finally, in NSCLC patients treated by atezolizumab, survival was improved in the high BMI group (85). Several hypotheses to explain this association have been raised. First, obesity may cause low systemic inflammation and impaired immune response, which could induce exhausted T-cell (which expressed PD-1) and lymphocytes dysfunction (85). Leptin is more secreted, which could increase PD-1 expression too (83). Second, gut microbiota is modified in obese patients. Further research would, however, be necessary to verify these hypotheses. In previous cited publications, no possible confounder, such as concomitant treatment, was proposed, and it is therefore not clear if the population with high BMI had more or less PPIs than the normal BMI group. Similarly, retrospective studies that were investigating the association between PPIs and survival did not provide any information regarding BMI in PPI and PPI-free groups. BMI therefore seems to be a predictive factor of response to ICIs, but it is not possible to conclude on concomitant PPI treatment in the obese group. Further studies are recommended to more precisely evaluate the impact of PPI on this population.
Usually, patients in poor general condition may have more comedications, including PPI, which may explain the negative effect on OS or PFS. However, in the studies included in this meta-analysis, no difference in terms of ECOG score in PPI and PPI-free groups was observed. However, some other factors, such as comorbidities (e.g., cardiovascular, psychiatric, and gastrointestinal) or the number and sites of metastasis, were inconstantly presented, which could have induced some bias. Including these various factors would require a prospective study.
Results presented in this article are promising for better treatment strategies; however, meta-analyses present some limitations that somewhat reduce the scope of these results. First, the included publications were retrospective studies that could induce a selection bias and lead to some missing information. For example, the type of PPI use, dosage, duration, and timing of initiation were not available. In some studies, the concomitant medications were also not available, even though antibiotics are expected to have a deleterious impact on ICI outcomes. Some factors that may impact OS and/or PFS were also missing in several studies, such as PD-L1 expression, tumor mass, or LIPI score in lung cancer. Despite these few limitations, this meta-analysis was based on a strong conceptual framework, and the robustness of the main results were confirmed using sensitivity analyses. In addition, Higgins’s I² test was 72% for PFS and 65% for OS, indicating a substantial heterogeneity of the studies included in this meta-analysis. This heterogeneity can partly be explained by the different types of studies used for this meta-analysis, since post-hoc analyses of prospective studies, and retrospective studies and abstracts, were included. This was a voluntary choice to increase the number of patients included. Indeed, data on the subject are limited, and some of them lead to totally opposite conclusions without having any prospective study available to be able to conclude. Associating all the available data on the subject was therefore deemed interesting, even though this automatically resulted in the increase in the global heterogeneity. Consequently, the type of immunotherapy (monotherapy versus dual therapy) and associated treatment [such as anti-vascular endothelial growth factor (anti-VEGF)] and the type of PPI used could vary from one study to another. The studies may also have involved patients of different ethnicities, the time between the introduction of PPI and immunotherapy may have varied from one study to another, and the studies involved various types of cancers (such as NSCLC, melanoma, and urothelial), which prognosis varies with immunotherapy treatment independently of concomitant treatments. However, when available, PFS and OS data for bi-immunotherapy were always preferred to monotherapy data, and NSCLC cancer data were chosen over other cancer types in case of post-hoc analyses to reduce the heterogeneity. Similarly, multivariate data were systematically chosen over univariate data. While most of the studies included in this meta-analysis independently concluded that PPIs had a detrimental effect on survival, some showed opposite results (i.e., a beneficial effect on OS or in PFS) (17, 25). These studies all have in common that they were conducted on a relatively small sample, with <100 patients for each treatment arm and low statistical weight in the global meta-analysis. Subgroup analysis was therefore conducted to estimate whether the influence of PPI on different groups of interest (region, type of cancer, type of ICI, treatment line, and timeframe of PPI exposure). In the cancer-type subgroup, PPI use was significantly associated with a significant decrease in OS and PFS for NSCLC and urothelial carcinoma. On the opposite, PPI use was associated (yet not statistically significantly) with improved PFS among patients with melanoma. This observation could (at least partially) be explained by the fact that patients with melanoma are often treated with anti-CTLA4 and that in the ICI type subgroup, patients treated with anti-CTLA4 had greater PFS and OS outcome when concomitantly treated with PPIs. However, only one study was included in the CTLA4 subgroup (Failing 2016), so more retrospective studies are needed to clarify the role of each subgroup on the correlation between PPIs and ICIs. In addition, the plan was initially to additionally investigate the effect of ECOG status and age, but there were too many missing data to conclude.
In conclusion, the meta-analysis conducted in this research suggested that concomitant PPI treatment was significantly associated with poorer OS and PFS for advanced solid cancer patients treated with ICIs. The evaluation of PPI necessity and indication by clinicians is therefore strongly recommended at the initiation of anticancer immune therapies. PPI deprescription should be conducted whenever possible, following deprescribing protocols (86, 87). Information concerning the type of PPI use, the posology, the duration, and the moment of their initiation should systematically be reported to improve future retrospective analyses. Larger prospective studies adjusting for cofounding factors are needed to determine the reel impact of PPI on survival outcomes in patients treated by ICIs and to evaluate the time limit of initiation and posology impact. A follow-up of microbiota changes in patients treated concomitantly with PPIs and ICIs would also be useful to determine the moment when negative impact of PPIs may appear.
Data availability statement
The original contributions presented in the study are included in the article/Supplementary Material. Further inquiries can be directed to the corresponding author.
Author contributions
There are two first authors in this manuscript, SL and LP, who worked equally to this project. LP was responsible of statistical analyses. MK and SL granted the quality assessment of the included studies. SL and LP were responsible for data analysis and writing the article. CM, AD, BM, and BG were responsible for the design of the project. All authors contributed to the article and approved the submitted version.
Conflict of interest
CM was employed by Roche, AstraZeneca, MSD, Kephren, Janssens, Bristol-Myers Squibb, Pfizer, Takeda, Sanofi, Boehringer Ingelheim, Novartis, and Amgen. BG was employed by MSD, Sobi, and Pfizer. AD was employed by Novartis, Amgen, Takeda, and Pfizer.
The remaining authors declare that the research was conducted in the absence of any commercial or financial relationships that could be construed as a potential conflict of interest.
Publisher’s note
All claims expressed in this article are solely those of the authors and do not necessarily represent those of their affiliated organizations, or those of the publisher, the editors and the reviewers. Any product that may be evaluated in this article, or claim that may be made by its manufacturer, is not guaranteed or endorsed by the publisher.
Supplementary material
The Supplementary Material for this article can be found online at: https://www.frontiersin.org/articles/10.3389/fimmu.2023.1070076/full#supplementary-material
References
1. Forgacs I, Loganayagam A. Overprescribing proton pump inhibitors. BMJ (2008) 336(7634):2–3. doi: 10.1136/bmj.39406.449456.BE
2. Lassalle M, Le Tri T, Bardou M, Biour M, Kirchgesner J, Rouby F, et al. Use of proton pump inhibitors in adults in France: a nationwide drug utilization study. Eur J Clin Pharmacol (2020) 76(3):449–57. doi: 10.1007/s00228-019-02810-1
3. Esteves M, Rollason V, Grosgurin O. Surprescription des inhibiteurs de la pompe à protons [Proton pump inhibitors overprescription]. Rev Med Suisse (2017) 13(579):1782–6.
4. Haastrup PF, Thompson W, Søndergaard J, Jarbøl DE. Side effects of long-term proton pump inhibitor use: A review. Basic Clin Pharmacol Toxicol (2018) 123(2):114–21. doi: 10.1111/bcpt.13023
5. Gadgeel S, Rodriguez-Abreu D, Speranza G, Esteban E, Felip E, Domine M, et al. Updated analysis from KEYNOTE-189: pembrolizumab or placebo plus pemetrexed and platinum for previously untreated metastatic nonsquamous non-Small-Cell lung cancer. J Clin Oncol (2020) 38(14):1505–17. doi: 10.1200/JCO.19.03136
6. McDermott D, Haanen J, Chen TT, Lorigan P, O’Day S, MDX010-20 investigators. Efficacy and safety of ipilimumab in metastatic melanoma patients surviving more than 2 years following treatment in a phase III trial (MDX010-20). Ann Oncol (2013) 24(10):2694–8. doi: 10.1093/annonc/mdt291
7. Balar AV, Castellano D, O’Donnell PH, Griva P, Vuky J, Powles T, et al. First-line pembrolizumab in cisplatin-ineligible patients with locally advanced and unresectable or metastatic urothelial cancer (KEYNOTE-052): a multicenter, single-arm, phase 2 study. Lancet Oncol (2017) 18(11):1483–92. doi: 10.1016/S1470-2045(17)30616-2
8. Arbour KC, Mezquita L, Long N, Rizvi H, Auclin E, Ni A, et al. Impact of baseline steroids on efficacy of programmed cell death-1 and programmed death-ligand 1 blockade in patients with non-Small-Cell lung cancer. J Clin Oncol (2018) 36(28):2872–8. doi: 10.1200/JCO.2018.79.0006
9. Fucà G, Galli G, Poggi M, Lo Russo G, Proto C, Imbimbo M, et al. Modulation of peripheral blood immune cells by early use of steroids and its association with clinical outcomes in patients with metastatic non-small cell lung cancer treated with immune checkpoint inhibitors. ESMO Open (2019) 4(1):e000457. doi: 10.1136/esmoopen-2018-000457
10. Chalabi M, Cardona A, Nagarkar DR, Dhawahir Scala A, Gandara DR, Rittmeyer A, et al. Efficacy of chemotherapy and atezolizumab in patients with non-small-cell lung cancer receiving antibiotics and proton pump inhibitors: pooled post hoc analyses of the OAK and POPLAR trials. Ann Oncol (2020) 31(4):525–31. doi: 10.1016/j.annonc.2020.01.006
11. Sen S, Carmagnani Pestana R, Hess K, Viola GM, Subbiah V. Impact of antibiotic use on survival in patients with advanced cancers treated on immune checkpoint inhibitor phase I clinical trials. Ann Oncol (2018) 29(12):2396–8. doi: 10.1093/annonc/mdy453
12. Kim H, Lee JE, Hong SH, Lee MA, Kang JH, Kim IH. The effect of antibiotics on the clinical outcomes of patients with solid cancers undergoing immune checkpoint inhibitor treatment: a retrospective study. BMC Cancer (2019) 19(1):1100. doi: 10.1186/s12885-019-6267-z
13. Zhao S, Gao G, Li W, Li X, Zhao C, Jiang T, et al. Antibiotics are associated with attenuated efficacy of anti-PD-1/PD-L1 therapies in Chinese patients with advanced non-small cell lung cancer. Lung Cancer (2019) 130:10–7. doi: 10.1016/j.lungcan.2019.01.017
14. Sivan A, Corrales L, Hubert N, Williams JB, Aquino-Michaels K, Earley ZM, et al. Commensal bifidobacterium promotes antitumor immunity and facilitates anti-PD-L1 efficacy. Science (2015) 350(6264):1084–9. doi: 10.1126/science.aac4255
15. Belkaid Y, Hand TW. Role of the microbiota in immunity and inflammation. Cell (2014) 157(1):121–41. doi: 10.1016/j.cell.2014.03.011
16. Wells GA, Shea B, O’connell D, Peterson J, Welch V, Losos M, et al. The Newcastle-Ottawa scale (NOS) for assessing the quality if nonrandomized studies in meta-analyses. (2009).
17. Afzal MZ, Shirai K. What impact do the proton pump inhibitors have on the efficacy of immune check point inhibitors in metastatic malignant melanoma? J Clin Oncol (2019) 37:(15_suppl). doi: 10.1200/JCO.2019.37.15_suppl.e21040
18. Araujo H, Moniz CMV, Braghiroli OFM, Mak MP, Uratani LF, Dahmer Tiecher R, et al. Proton pump inhibitors and antibiotics impact on toxicities and clinical outcomes in cancer patients treated with immunotherapy. J Clin Oncol (2021) 39(15_suppl):2652–. doi: 10.1200/JCO.2021.39.15_suppl.2652
19. Baek YH, Kang EJ, Hong S, Park S, Kim JH, Shin JY. Survival outcomes of patients with nonsmall cell lung cancer concomitantly receiving proton pump inhibitors and immune checkpoint inhibitors. Int J Cancer (2022) 150(8):1291–300. doi: 10.1002/ijc.33892
20. Buti S, Bersanelli M, Perrone F, Tiseo M, Tucci M, Adamo V, et al. Effect of concomitant medications with immune-modulatory properties on the outcomes of patients with advanced cancer treated with immune checkpoint inhibitors: development and validation of a novel prognostic index. Eur J Cancer (2021) 142:18–28. doi: 10.1016/j.ejca.2020.09.033
21. Castro Balado A, Tourís Lores M, Álvarez Sánchez R, Bernardez Ferran B, Lopez Montero E, Mosquera Torre A, et al. 4CPS-301 association of antibiotics and proton pump inhibitors on clinical activity of firstline pembrolizumab for non-small cell lung cancer: 2 years of real world data. Eur J Hosp Pharm (2021) 28:A65.
22. Conde-Estévez D, Monge-Escartín I, Ríos-Hoyo A, Monzonis X, Echeverría-Esnal D, Moliner L, et al. Prognostic factors and effect on survival of immune-related adverse events in patients with non-small-cell lung cancer treated with immune checkpoint blockage. J Chemother (2021) 33(1):32–9. doi: 10.1080/1120009X.2020.1849488
23. Cortellini A, Tucci M, Adamo V, Stucci LS, Russo A, Tanda ET, et al. Integrated analysis of concomitant medications and oncological outcomes from PD-1/PD-L1 checkpoint inhibitors in clinical practice. J Immunother Cancer (2020) 8(2):e001361. doi: 10.1136/jitc-2020-001361
24. Cortellini A, Di Maio M, Nigro O, Leonetti A, Cortinovis DL, Aerts JG, et al. Differential influence of antibiotic therapy and other medications on oncological outcomes of patients with non-small cell lung cancer treated with first-line pembrolizumab versus cytotoxic chemotherapy. J Immunother Cancer (2021) 9(4):e002421. doi: 10.1136/jitc-2021-002421
25. Failing JJ, Finnes HD, Kottschade LA, Allred JB, Markovic SN. Effects of commonly used chronic medications on the outcomes of ipilimumab therapy in patients with metastatic melanoma. Melanoma Res (2016) 26(6):609–15. doi: 10.1097/CMR.0000000000000299
26. Fukuokaya W, Kimura T, Komura K, Uchimoto T, Nishimura K, Yanagisawa T, et al. Effectiveness of pembrolizumab in patients with urothelial carcinoma receiving proton pump inhibitors. Urol Oncol (2022) 40(7):346.e1–8. doi: 10.1016/j.urolonc.2022.02.020
27. Gaucher L, Adda L, Séjourné A, Joachim C, Guillaume C, Poulet C, et al. Associations between dysbiosis-inducing drugs, overall survival and tumor response in patients treated with immune checkpoint inhibitors. Ther Adv Med Oncol (2021) 13:17588359211000591. doi: 10.1177/17588359211000591
28. Giordan Q, Salleron J, Vallance C, Moriana C, Clement-Duchene C. Impact of antibiotics and proton pump inhibitors on efficacy and tolerance of anti-PD-1 immune checkpoint inhibitors. Front Immunol (2021) 12:716317. doi: 10.3389/fimmu.2021.716317
29. Hakozaki T, Okuma Y, Omori M, Hosomi Y. Impact of prior antibiotic use on the efficacy of nivolumab for non-small cell lung cancer. Oncol Lett (2019) 17(3):2946–52. doi: 10.3892/ol.2019.9899
30. Homicsko K, Dummer R, Hoeller C, Wolchok JD, Hodi FS, Larkin J, et al. Proton pump inhibitor use and efficacy of nivolumab and ipilimumab in advanced melanoma. Cancers (Basel) (2022) 14(9):2300. doi: 10.3390/cancers14092300
31. Hopkins AM, Kichenadasse G, Karapetis CS, Rowland A, Sorich MJ. Concomitant proton pump inhibitor use and survival in urothelial carcinoma treated with atezolizumab. Clin Cancer Res (2020) 26(20):5487–93. doi: 10.1158/1078-0432.CCR-20-1876
32. Hopkins AM, Kichenadasse G, McKinnon RA, Abuhelwa AY, Logan JM, Badaoui S, et al. Efficacy of first-line atezolizumab combination therapy in patients with non-small cell lung cancer receiving proton pump inhibitors: post hoc analysis of IMpower150. Br J Cancer (2022) 126(1):42–7. doi: 10.1038/s41416-021-01606-4
33. Hossain T, Htut S and Pathmanathan S. Immunotherapy efficacy and concomitant proton pump inhibitor use in non-small cell lung cancer. Asia Pac J Clin Oncol (2020) 16:37.
34. Husain M, Xu M, Patel S, Johns A, Grogan M, Li M, et al. Proton pump inhibitor use (PPI) in patients treated with immune checkpoint inhibitors (ICI) for advanced cancer: Survival and prior therapy. J Clin Oncol (2021) 39(15_suppl):2633. doi: 10.1200/JCO.2021.39.15_suppl.2633
35. Iglesias-Santamaría A. Impact of antibiotic use and other concomitant medications on the efficacy of immune checkpoint inhibitors in patients with advanced cancer. Clin Transl Oncol (2020) 22(9):1481–90. doi: 10.1007/s12094-019-02282-w
36. Jun T, Ozbek U, Dharmapuri S, Hardy-Abeloos C, Zhu H, Lin JY, et al. Antacid exposure and immunotherapy outcomes among patients with advanced hepatocellular carcinoma. Ther Adv Med Oncol (2021) 13:17588359211010937. doi: 10.1177/17588359211010937
37. Kostine M, Mauric E, Tison A, Barnetche T, Barre A, Nikolski M, et al. Baseline co-medications may alter the anti-tumoural effect of checkpoint inhibitors as well as the risk of immune-related adverse events. Eur J Cancer (2021) 157:474–84. doi: 10.1016/j.ejca.2021.08.036
38. Kulkarni A, Kumar M, Pease DF, Yang Y, DeFor TE, Patel M, et al. Impact of antibiotics and proton pump inhibitors on clinical outcomes of immune check point blockers in advanced non-small cell lung cancers and metastatic renal cell cancer. J Clin Oncol (2019) 37:e20520–0. doi: 10.1200/JCO.2019.37.15_suppl.e20520
39. Kunimitsu Y, Morio K, Hirata S, Yamamoto K, Omura T, Hara T, et al. Effects of proton pump inhibitors on survival outcomes in patients with metastatic or unresectable urothelial carcinoma treated with pembrolizumab. Biol Pharm Bull (2022) 45(5):590–5. doi: 10.1248/bpb.b21-00939
40. Miura K, Sano Y, Niho S, Kawasumi K, Mochizuki N, Yoh K, et al. Impact of concomitant medication on clinical outcomes in patients with advanced non-small cell lung cancer treated with immune checkpoint inhibitors: A retrospective study. Thorac Cancer (2021) 12(13):1983–94. doi: 10.1111/1759-7714.14001
41. Mollica V, Santoni M, Matrana MR, Basso U, De Giorgi U, Rizzo A, et al. Concomitant proton pump inhibitors and outcome of patients treated with nivolumab alone or plus ipilimumab for advanced renal cell carcinoma. Target Oncol (2022) 17(1):61–8. doi: 10.1007/s11523-021-00861-y
42. Nguyen QP, Nomura M, Matsumoto S, Muto M. The effect of proton pump inhibitors on the efficacy of nivolumab monotherapy in different types of cancer. Ann Oncol (2019) 30:vi115. doi: 10.1093/annonc/mdz338.107
43. Okuyama Y, Hatakeyama S, Numakura K, Narita T, Tanaka T, Miura Y, et al. Prognostic impact of proton pump inhibitors for immunotherapy in advanced urothelial carcinoma. BJUI Compass (2021) 3(2):151–61. doi: 10.1002/bco2.118
44. Peng K, Chen K, Teply BA, Yee GC, Farazi PA, Lyden ER. Impact of proton pump inhibitor use on the effectiveness of immune checkpoint inhibitors in advanced cancer patients. Ann Pharmacother (2022) 56(4):377–86. doi: 10.1177/10600280211033938
45. Pérez-Ruiz E, Jiménez-Castro J, Berciano-Guerrero MA, Valdivia J, Estalella-Mendoza S, Toscano F, et al. Impact of intestinal dysbiosis-related drugs on the efficacy of immune checkpoint inhibitors in clinical practice. Clin Transl Oncol (2020) 22(10):1778–85. doi: 10.1007/s12094-020-02315-9
46. Rassy E, Dalban C, Colomba E, Derosa L, Alves Costa Silva C, Negrier S, et al. Efficacy and safety of concomitant proton pump inhibitor and nivolumab in renal cell carcinoma: Results of the GETUG-AFU 26 NIVOREN multicenter phase II study. Clin Genitourin Cancer (2022) 20(5):488–94. doi: 10.1016/j.clgc.2022.07.003
47. Routy B, Le Chatelier E, Derosa L, Duong CPM, Alou MT, Daillère R, et al. Gut microbiome influences efficacy of PD-1-based immunotherapy against epithelial tumors. Science (2018) 359(6371):91–7. doi: 10.1126/science.aan3706
48. Ruiz-Bañobre J, Molina-Díaz A, Fernández-Calvo O, Fernández-Núñez N, Medina-Colmenero A, Santomé L, et al. Rethinking prognostic factors in locally advanced or metastatic urothelial carcinoma in the immune checkpoint blockade era: a multicenter retrospective study. ESMO Open (2021) 6(2):100090. doi: 10.1016/j.esmoop.2021.100090
49. Spakowicz D, Hoyd R, Muniak M, Husain M, Bassett JS, Wang L, et al. Inferring the role of the microbiome on survival in patients treated with immune checkpoint inhibitors: causal modeling, timing, and classes of concomitant medications. BMC Cancer (2020) 20(1):383. doi: 10.1186/s12885-020-06882-6
50. Stein C, Burtey S, Mancini J, Pelletier M, Sallée M, Brunet P, et al. Acute kidney injury in patients treated with anti-programmed death receptor-1 for advanced melanoma: a real-life study in a single-centre cohort. Nephrol Dial Transplant (2021) 36(9):1664–74. doi: 10.1093/ndt/gfaa137
51. Stokes WA, Behera M, Jiang R, Gutman D, Giuste F, Burns A, et al. Association of proton pump inhibitors with survival in veterans with non-small cell lung cancer receiving immunotherapy. J Clin Oncol (2021) 39(15_suppl):e18729. doi: 10.1200/JCO.2021.39.15_suppl.e18729
52. Svaton M, Zemanova M, Zemanova P, Kultan J, Fischer O, Skrickova J, et al. Impact of concomitant medication administered at the time of initiation of nivolumab therapy on outcome in non-small cell lung cancer. Anticancer Res (2020) 40(4):2209–17. doi: 10.21873/anticanres.14182
53. Takada K, Shimokawa M, Takamori S, Shimamatsu S, Hirai F, Ono Y, et al. The clinical impact of concomitant medication use on the outcome of postoperative recurrent non-small-cell lung cancer in patients receiving immune checkpoint inhibitors. PLoS One (2022) 17(2):e0263247. doi: 10.1371/journal.pone.0263247
54. Tomita Y, Goto Y, Sakata S, Imamura K, Minemura A, Oka K, et al. Clostridium butyricum therapy restores the decreased efficacy of immune checkpoint blockade in lung cancer patients receiving proton pump inhibitors. Oncoimmunology (2022) 11(1):2081010. doi: 10.1080/2162402X.2022.2081010
55. Tomisaki I, Harada M, Minato A, Nagata Y, Kimuro R, Higashijima K, et al. Impact of the use of proton pump inhibitors on pembrolizumab effectiveness for advanced urothelial carcinoma. Anticancer Res (2022) 42(3):1629–34. doi: 10.21873/anticanres.15638
56. Kfoury M, Disdero V, Vicier C, Le Saux O, Gougis P, Sajous C, et al. Immune checkpoints inhibitors: Recent data from ASCO’s meeting and perspecives. Bull du Cancer (2018) 150:686–95. doi: 10.1016/j.bulcan.2018.04.011
57. Deng R, Zhang H, Li Y, Shi Y. Effect of antacid use on immune checkpoint inhibitors in advanced solid cancer patients: A systematic review and meta-analysis. J Immunother (2022). doi: 10.1097/CJI.0000000000000442
58. Chen B, Yang C, Dragomir MP, Chi D, Chen W, Horst D, et al. Association of proton pump inhibitor use with survival outcomes in cancer patients treated with immune checkpoint inhibitors: a systematic review and meta-analysis. Ther Adv Med Oncol (2022) 14:17588359221111703. doi: 10.1177/17588359221111703
59. Li C, Xia Z, Li A, Meng J. The effect of proton pump inhibitor uses on outcomes for cancer patients treated with immune checkpoint inhibitors: a meta-analysis. Ann Transl Med (2020) 8(24):1655. doi: 10.21037/atm-20-7498
60. Li M, Zeng C, Yao J, Ge Y, An G. The association between proton pump inhibitors use and clinical outcome of patients receiving immune checkpoint inhibitors therapy. Int Immunopharmacol (2020) 88:106972. doi: 10.1016/j.intimp.2020.106972
61. Fessler J, Matson V, Gajewski TF. Exploring the emerging role of the microbiome in cancer immunotherapy. J Immunother Cancer (2019) 7(1):108. doi: 10.1186/s40425-019-0574-4
62. Matson V, Fessler J, Bao R, Chongsuwat T, Zha Y, Alegre ML, et al. The commensal microbiome is associated with anti-PD-1 efficacy in metastatic melanoma patients. Science (2018) 359(6371):104–8. doi: 10.1126/science.aao3290
63. Derosa L, Routy B, Thomas AM, Iebba V, Zalcman G, Friard S, et al. Intestinal akkermansia muciniphila predicts clinical response to PD-1 blockade in patients with advanced non-small-cell lung cancer. Nat Med 1 févr (2022) 28(2):315–24. doi: 10.1038/s41591-021-01655-5
64. Chaput N, Lepage P, Coutzac C, Soularue E, Le Roux K, Monot C, et al. Baseline gut microbiota predicts clinical response and colitis in metastatic melanoma patients treated with ipilimumab. Ann Oncol (2017) 28(6):1368–79. doi: 10.1093/annonc/mdx108
65. Frankel AE, Coughlin LA, Kim J, Froehlich TW, Xie Y, Frenkel EP, et al. Metagenomic shotgun sequencing and unbiased metabolomic profiling identify specific human gut microbiota and metabolites associated with immune checkpoint therapy efficacy in melanoma patients. Neoplasia (2017) 19(10):848–55. doi: 10.1016/j.neo.2017.08.004
66. Gopalakrishnan V, Spencer CN, Nezi L, Reuben A, Andrews MC, Karpinets TV, et al. Gut microbiome modulates response to anti-PD-1 immunotherapy in melanoma patients. Science (2018) 359(6371):97–103. doi: 10.1126/science.aan4236
67. Vétizou M, Pitt JM, Daillère R, Lepage P, Waldschmitt N, Flament C, et al. Anticancer immunotherapy by CTLA-4 blockade relies on the gut microbiota. Science (2015) 350(6264):1079–84. doi: 10.1126/science.aad1329
68. Viaud S, Saccheri F, Mignot G, Yamazaki T, Daillère R, Hannani D, et al. The intestinal microbiota modulates the anticancer immune effects of cyclophosphamide. Science (2013) 342(6161):971–6. doi: 10.1126/science.1240537
69. Paulos CM, Wrzesinski C, Kaiser A, Hinrichs CS, Chieppa M, Cassard L, et al. Microbial translocation augments the function of adoptively transferred self/tumor-specific CD8+ T cells via TLR4 signaling. J Clin Invest (2007) 117(8):2197–204. doi: 10.1172/JCI32205
70. Hall JA, Bouladoux N, Sun CM, Wohlfert EA, Blank RB, Zhu Q, et al. Commensal DNA limits regulatory T cell conversion and is a natural adjuvant of intestinal immune responses. Immunity (2008) 29(4):637–49. doi: 10.1016/j.immuni.2008.08.009
71. Rooks MG, Garrett WS. Gut microbiota, metabolites and host immunity. Nat Rev Immunol (2016) 16(6):341–52. doi: 10.1038/nri.2016.42
72. Iraporda C, Errea A, Romanin DE, Cayet D, Pereyra E, Pignataro O, et al. Lactate and short chain fatty acids produced by microbial fermentation downregulate proinflammatory responses in intestinal epithelial cells and myeloid cells. Immunobiology (2015) 220(10):1161–9. doi: 10.1016/j.imbio.2015.06.004
73. Gurav A, Sivaprakasam S, Bhutia YD, Boettger T, Singh N, Ganapathy V. Slc5a8, a na+-coupled high-affinity transporter for short-chain fatty acids, is a conditional tumour suppressor in colon that protects against colitis and colon cancer under low-fibre dietary conditions. Biochem J (2015) 469(2):267–78. doi: 10.1042/BJ20150242
74. White CA, Pone EJ, Lam T, Tat C, Hayama KL, Li G, et al. Histone deacetylase inhibitors upregulate b cell microRNAs that silence AID and blimp-1 expression for epigenetic modulation of antibody and autoantibody responses. J Immunol (2014) 193(12):5933–50. doi: 10.4049/jimmunol.1401702
75. Arpaia N, Campbell C, Fan X, Dikiy S, van der Veeken J, deRoos P, et al. Metabolites produced by commensal bacteria promote peripheral regulatory T-cell generation. Nature (2013) 504(7480):451–5. doi: 10.1038/nature12726
76. Bruno G, Zaccari P, Rocco G, Scalese G, Panetta C, Porowska B, et al. Proton pump inhibitors and dysbiosis: Current knowledge and aspects to be clarified. World J Gastroenterol (2019) 25(22):2706–19. doi: 10.3748/wjg.v25.i22.2706
77. Imhann F, Bonder MJ, Vich Vila A, Fu J, Mujagic Z, Vork L, et al. Proton pump inhibitors affect the gut microbiome. Gut (2016) 65(5):740–8. doi: 10.1136/gutjnl-2015-310376
78. Vich Vila A, Collij V, Sanna S, Sinha T, Imhann F, Bourgonje AR, et al. Impact of commonly used drugs on the composition and metabolic function of the gut microbiota. Nat Commun (2020) 11(1):362. doi: 10.1038/s41467-019-14177-z
79. Sands SA, Tsau S, Yankee TM, Parker BL, Ericsson AC, LeVine SM. The effect of omeprazole on the development of experimental autoimmune encephalomyelitis in C57BL/6J and SJL/J mice. BMC Res Notes (2014) 7:605. doi: 10.1186/1756-0500-7-605
80. Davis JA, Collier F, Mohebbi M, Stuart AL, Loughman A, Pasco JA, et al. Obesity, akkermansia muciniphila, and proton pump inhibitors: Is there a link? Obes Res Clin Pract (2020) 14(6):524–30. doi: 10.1016/j.orcp.2020.10.006
81. Jackson MA, Goodrich JK, Maxan ME, Freedberg DE, Abrams JA, Poole AC, et al. Proton pump inhibitors alter the composition of the gut microbiota. Gut (2016) 65(5):749–56. doi: 10.1136/gutjnl-2015-310861
82. McQuade JL, Daniel CR, Hess KR, Mak C, Wang DY, Rai RR, et al. Association of body mass index and outcomes in patients with metastatic melanoma treated with targeted therapy, immunotherapy, or chemotherapy: a retrospective, multicohort analysis. Lancet Oncol (2018) 19(3):310–22. doi: 10.1016/S1470-2045(18)30078-0
83. Lee JH, Hyung S, Lee J, Choi SH. Visceral adiposity and systemic inflammation in the obesity paradox in patients with unresectable or metastatic melanoma undergoing immune checkpoint inhibitor therapy: a retrospective cohort study. J Immunother Cancer (2022) 10(8):e005226. doi: 10.1136/jitc-2022-005226
84. Cortellini A, Bersanelli M, Buti S, Cannita K, Santini D, Perrone F, et al. A multicenter study of body mass index in cancer patients treated with anti-PD-1/PD-L1 immune checkpoint inhibitors: when overweight become favorable. J Immunother Cancer (2019) 7(1):57. doi: 10.1186/s40425-019-0527-y
85. Kichenadasse G, Miners JO, Mangoni AA, Rowland A, Hopkins AM, Sorich MJ. Association between body mass index and overall survival with immune checkpoint inhibitor therapy for advanced non-small cell lung cancer. JAMA Oncol (2020) 6(4):512–8. doi: 10.1001/jamaoncol.2019.5241
86. Farrell B, Pottie K, Rojas-Fernandez CH, Bjerre LM, Thompson W, Welch V. Methodology for developing deprescribing guidelines: Using evidence and GRADE to guide recommendations for deprescribing. PLoS One (2016) 11(8):e0161248. doi: 10.1371/journal.pone.0161248
87. Valette S, Dory A, Gourieux B, Weber JC. Évaluation de l’implantation d’un processus de dé-prescription des inhibiteurs de la pompe à protons (IPP) à l’aide d’un algorithme au sein d’un service de médecine interne [Evaluation of the implantation of a de-prescribing process for proton pump inhibitor (PPI) using an algorithm within an internal medicine department]. Rev Med Interne (2021) 42(8):535–40. doi: 10.1016/j.revmed.2020.11.014
Keywords: immune checkpoint inhibitors, proton pump inhibitors, survival, solid cancer, meta – analysis
Citation: Lopes S, Pabst L, Dory A, Klotz M, Gourieux B, Michel B and Mascaux C (2023) Do proton pump inhibitors alter the response to immune checkpoint inhibitors in cancer patients? A meta-analysis. Front. Immunol. 14:1070076. doi: 10.3389/fimmu.2023.1070076
Received: 14 October 2022; Accepted: 09 January 2023;
Published: 26 January 2023.
Edited by:
Alvaro Francisco Lopes Sousa, Hospital Sirio Libanes, BrazilReviewed by:
Baoqing Chen, Sun Yat-sen University Cancer Center (SYSUCC), ChinaReidar Fossmark, Norwegian University of Science and Technology, Norway
Jean-Luc Raoul, Institut de Cancérologie de l’Ouest (ICO), France
Copyright © 2023 Lopes, Pabst, Dory, Klotz, Gourieux, Michel and Mascaux. This is an open-access article distributed under the terms of the Creative Commons Attribution License (CC BY). The use, distribution or reproduction in other forums is permitted, provided the original author(s) and the copyright owner(s) are credited and that the original publication in this journal is cited, in accordance with accepted academic practice. No use, distribution or reproduction is permitted which does not comply with these terms.
*Correspondence: Céline Mascaux, Y2VsaW5lLm1hc2NhdXhAY2hydS1zdHJhc2JvdXJnLmZy
†These authors contributed equally to this work and share first authorship