- 1Division of Medical Oncology, National Cancer Centre Singapore, Singapore, Singapore
- 2Lymphoma Genomic Translational Research Laboratory, Division of Cellular and Molecular Research, National Cancer Centre Singapore, Singapore, Singapore
Peripheral T-cell lymphoma (PTCL) and natural killer/T-cell lymphoma (NKTCL) are rare subtypes of non-Hodgkin’s lymphoma that are typically associated with poor treatment outcomes. Contemporary first-line treatment strategies generally involve the use of combination chemoimmunotherapy, radiation and/or stem cell transplant. Salvage options incorporate a number of novel agents including epigenetic therapies (e.g. HDAC inhibitors, DNMT inhibitors) as well as immune checkpoint inhibitors. However, validated biomarkers to select patients for individualized precision therapy are presently lacking, resulting in high treatment failure rates, unnecessary exposure to drug toxicities, and missed treatment opportunities. Recent advances in research on the tumor and microenvironmental factors of PTCL and NKTCL, including alterations in specific molecular features and immune signatures, have improved our understanding of these diseases, though several issues continue to impede progress in clinical translation. In this Review, we summarize the progress and development of the current predictive biomarker landscape, highlight potential knowledge gaps, and discuss the implications on novel therapeutics development in PTCL and NKTCL.
Introduction
The peripheral T cell lymphomas (PTCL) consist of several uncommon, heterogeneous subgroups of non-Hodgkin lymphomas classically associated with an aggressive clinical course and dismal survival outcomes (1). PTCL originates from post-thymic lymphocytes and span across a broad range of morphologic and immunophenotypic variability, having since been classified by the World Health Organization into 27 distinct subtypes based on clinicopathologic features and immunohistochemistry. Its most recent 2016 Fourth Edition classification of lymphoid neoplasms aids in further establishing homogeneity within these entities with the addition of recent literature (2).
Out of the PTCL subgroups, the commonest subtypes include peripheral T cell lymphoma, not otherwise specified (PTCL-NOS), anaplastic large cell lymphoma (ALCL), angioimmunoblastic T cell lymphoma (AITL), and extranodal natural killer T-cell lymphoma, nasal type (NKTCL). This classification aims to stratify disease based on the principles of its individual cell biology as well as ensuring homogeneity in the diagnostic, prognostic and therapeutic implications of disease within its overarching entity (3).
Patients with PTCL often present with advanced disease at diagnosis staged according to the Lugano classification for non-Hodgkin’s lymphoma, which is based upon the Ann Arbor system (4). Studies have shown that the majority of disease presents with stage III or IV at diagnosis, with low 5-year overall survival (OS) rates and dismal risk scores on various prognostic indices (1, 5–7). These patients also often exhibit resistance to standard chemotherapy regimens, and frequently become refractory to standard combinations indicated for the general subset of non-Hodgkin lymphomas (7, 8). When this occurs, this substantial subset of recurrent/relapsed disease subsequently fare unfavorably on second line or salvage therapy under their respective clinical trials, of which there may be no standard of care available (9, 10).
With the influx of targeted therapies in this age of molecular therapeutics, an exploration into novel treatment modalities via the identification of exploitable biomarkers offers a glimpse into future avenues for therapy in a landscape historically scathed by a paucity of effective therapeutic options. Despite the advent of methods such as gene expression profiling and the breakthroughs in high-throughput immunophenotyping, the identification of feasible targets remains elusive. In this Review, we thus summarize the progress and development of the current predictive biomarker landscape, highlight potential knowledge gaps, and discuss the implications on novel therapeutics development in PTCL and NKTCL.
Search terms
In this Review, we incorporated a literature review on the MEDLINE and EMBASE databases. Search terms incorporating ‘Peripheral T Cell Lymphoma’ and its related variants, alongside ‘biomarkers’ and its related variants were used and standardized for both databases. A further in-depth search query was then applied for every identified biomarker target in conjunction with ‘Peripheral T Cell Lymphoma’. Search filters were applied to the results to include only English papers and to restrict search to articles within the last fifteen years to minimize the utilization of outdated data.
We placed an increased focus on newer papers and literature which specific respect to predictive novel biomarkers which have been identified as an exploitable immune marker for the specific treatment of peripheral T cell lymphoma. A reference of the exact search terms utilized can be found under Annex A.
Current clinical systems for risk stratification
Risk stratification is an integral part of cancer management which guides principles of therapy and informs medical providers of prognosis for the purposes of highlighting at-risk patients for increased supervision and for end-of-life planning.
The first indicator on prognosis is conveyed right at the time of diagnosis. The exact PTCL histotype itself inherently confers prognostic information, given that certain subgroups such as ALK-positive ALCL have been shown to confer better outcomes, whilst others such as NKTCL perform considerably worse (11). With further workup, phenotypic cell markers have historically acted as surrogates for the prediction of mortality risk. One such marker used for prognostication involves Ki-67, an indicator for cell proliferation, with a higher Ki-67 staining at variable cut-offs reported by several studies to be predictive of poor outcomes (12–14). A high serum lactate dehydrogenase (LDH) level, as well as the presence of tumor Epstein-Barr Virus (EBV) infection assessed by EBV-encoded RNA (EBER) positivity, had also been identified to be predictors of worse prognosis (13, 15, 16). Interim PET/CT imaging, typically performed in the setting of Hodgkin lymphoma, has also been applied in the setting of T cell lymphomas with optimistic results on its prognostic value. Several early studies have reported that PET positivity after 2 cycles of chemotherapy indicates significantly poorer prognosis (17–19). Most recently, POD24 negativity, defined as a patient not experiencing disease progression within 24 months of front-line chemoimmunotherapy, was demonstrated to be a powerful predictor of good clinical outcome in AITL (20, 21). A common understanding, however, is that such individual factors are often too variable and lack sufficient accuracy to be utilized as sole predictors of prognosis.
Given the limitations of individual prognostic factors, clinical scoring systems have been devised to improve the accuracy and quality of analysis, composing of multiple prognostic indicators interpreted as a whole. The International Prognostic Index (IPI), initially developed for the general prognostic grading of all non-Hodgkin lymphomas, was found to be valid when applied to PTCL (11, 22). In particular, the IPI was found to be predictive of disease outcomes and each independent variable in the scoring system was shown to have significant prognostic value (23, 24). We must, however, bear in mind that this prognostic scoring system was not devised with the specifics of the PTCL cohort, and thus inevitably suffers from inaccuracy when applied to PTCL subgroups with especially optimistic or poor prognostic outcomes such as cutaneous ALCL or extranasal NKTCL, respectively (24, 25). Another subsequent limitation of the use of the IPI scoring system in PTCL is that the IPI only accurately delineated outcomes with statistical significance when stratified into two groups (simplified 2-class IPI) which simply reflected subsets of lower and higher risk (13, 24).
To better define the clinical outcomes of PTCLs as a unique entity with notable differences to other non-Hodgkin lymphomas, a separate prognostic model has been created specifically to rate outcomes of this uncommon disease. Gallamini et al. devised the Prognostic Index for PTCL (PIT) model which stratified patients into four risk categories, which held superior predictive capacity to the IPI when considering all four PIT risk classes as well as when using a simplified 2-class PIT which grouped scores of 1-2 and 3-4 into low risk and high risk groups, respectively (26). Went et al. further extrapolated on this study and proposed a modified version of PIT (m-PIT) which was the first scoring system to include the tumor-specific molecular factor Ki-67 instead of relying on the presence of bone marrow involvement, of which this modified system indeed fared better on statistical review (13). Recent additions of newer prognostic scoring systems such as the International Peripheral T-Cell Lymphoma Project Score (IPTCLP score) (14) and the T-cell score (27) which aim to identify novel prognostic markers have also contributed to the literature. These scoring systems (IPI, PIT, mPIT, IPTCLP) were each recognized to have utility in independently predicting the risk of early death within each system’s definition of intermediate- and high-risk patients derived from a cohort of predominantly Caucasian patients. Within these systems, the IPTCLP scoring system was identified to be the most valuable in predicting OS (28). We however also note that an analysis of PTCL outcomes as part of the “Intercontinental cooperative non-Hodgkin T-cell lymphoma prospective registry study in Asia” (ICT study) comprising of an Asian population yielded no significance for any of the four scoring systems in predicting survival outcomes, suggesting possible variability in the disease characteristics within PTCL of Asian origin (29).
With the influx of clinical prognostic scoring systems, further studies have also led to the creation of prognostic indices relevant to specific subtypes of PTCL itself, resulting in further specialized scoring systems. The Prognostic Index for AITL (PIAI) (30) and AITL score (31) were created to further reflect and categorize outcomes across the dismal survival outcomes of the AITL landscape; similarly, the Korean Prognostic Index (KPI) (32), Prognostic Index of Natural Killer Lymphoma (PINK/PINK-E (33), with ‘E’ representing the addition of EBV positivity data) were created for similar purposes while highlighting the varying differences in the NKTCL population. Novel prognostic models have also been suggested in recent years for NKTCL, such as the NABS score featuring the inclusion of a high peripheral blood neutrophil-to-lymphocyte ratio (NLR) of >3.5 as a scoring criterion with validated independent significance for prediction of OS and progression-free survival (PFS) (34). High initial SUVmax of the most FDG-avid lesion on primary FDG-PET/CT scanning and post-treatment Deauville scores of 4-5 which precluded a PET complete metabolic response were both additionally assessed to be associated with worse OS and PFS; a further subgroup analysis in this study further reflected that the NABS score accurately predicted survival outcomes in cohorts irrespective of the attainment of a complete metabolic response as indicated by post-treatment Deauville scores of 1-3 (35). A consolidated view of the criteria used for each prognostic index can be found below for PTCL indices and specialized indices in Tables 1, 2 respectively.
The wealth of scoring systems currently available and the improvements in accuracy at predicting clinical outcomes of patients with PTCL is certainly valuable to the field. The key issue at hand, however, is that all of them are currently only validated for prognostication purposes and have yet to be fully applied or extrapolated to a predictive setting. While prognostication is indeed an important requirement in the initial workup of lymphoma, the end goal is to apply these clinical parameters towards better stratification of patients for targeted and personalized therapy, with the hope of utilizing scoring systems to guide the feasibility of treatment options such as dose intensification in the context of each individual patient to improve treatment outcomes. These scoring systems additionally do not incorporate molecular or immunopathological features, which could be instrumental in furthering the utility of such systems in the predictive context.
The genomic and immune landscape of peripheral T cell lymphoma
PTCL is recognized as an uncommon non-Hodgkin’s lymphoma which frequently expresses pan-T cell markers such as CD2, CD3 and CD5 (6). Despite years of study, the diagnosis of specific T cell lymphoma remains challenging due to numerous overlying similarities, often requiring a consolidated review of clinical, laboratory, and immunohistological findings and expert analysis for categorization. Modern technological options such as the utility of gene expression signatures via transcriptomic analysis have also been studied as novel means of increasing the accuracy of diagnosing each subtype of PTCL, including further substratification of PTCL-NOS into its GATA3- or TBX21-expressing subgroups (36). This categorization was found to possibly even trump expert consensus by pathological analysis in certain cases, whereby two patients in the study were diagnosed by transcriptomic signature expression to have adult T cell lymphoma/leukemia that was previously misdiagnosed as PTCL-NOS by experts via pathology. Ultimately, a robust molecular classification of PTCL may be incorporated into routine clinical classification systems for improved diagnosis.
Peripheral T cell lymphoma, not otherwise specified (PTCL-NOS)
PTCL-NOS is the most common subtype of PTCL in Western countries, constituting of a general header that categorizes T cell lymphomas that are not otherwise able to be categorized into the more distinct subtypes. As a result, PTCL-NOS as a subgroup comprises of a highly heterogeneous group of T cell lymphomas with an incompletely characterized immunophenotype and a lack of distinct clinical features. PTCL-NOS often presents as a nodal lymphoma with the potential for extranodal involvement, particularly of the cutaneous and gastrointestinal systems (37).
A gene expression model proposed by Iqbal et al. (38) has shed insight on a dichotomy in gene expression within the heterogeneity of PTCL-NOS – an expression of transcription factors T-box-21 (TBX21) and GATA 3 binding protein (GATA3) which drive differentiation of mutant T lymphocytes into Th1 and Th2 subtypes, respectively. With particular reference to the PTCL-GATA3 subgroup, the higher burden of chromosomal abnormalities as well as enrichment of MYC gene signatures related to proliferation (CCR4, IL18RA, CXCR7, IK), mTOR (PI3K), and marginal enrichment of β-catenin gene signature was associated with poor survival; conversely, the PTCL-TBX21 group showed significant enhancement of IFNγ-related gene signatures and NF-κB gene signatures and association with a favorable clinical outcome (38, 39). These PTCL subcategories are postulated to have evolved through distinct genetic pathways and provided biological rationale for therapeutic targets that may be exploited.
Mutations in various signaling pathways such as T cell receptors, PTEN-PI3K, NOTCH1 signaling and ITK-SYK have been postulated as progenitors of this oncogenic signaling cascade (38, 40, 41). The NF-κB pathway has also been implicated in cases of PTCL-NOS via identification of signals such as FYN-TRAF3IP2 (42). Mutations in DNMT3A within PTCL-TBX21 cases were associated with significant enrichment of activated CD8+ T-cell cytotoxic gene signatures and resulted in worse overall survival outcomes (43).
Angioimmunoblastic T cell lymphoma (AITL)/PTCL-T follicular helper (PTCL-TFH)
A subgroup of PTCL-NOS cases was recently subclassified into a distinct subgroup as PTCL-TFH, consisting of lymphomas which were observed to manifest a T follicular helper cell phenotype including TFH-related antigens such as BCL6, CCR5, CD10. As AITL cells originate from the follicular T helper (TFH) cell, these lymphomas share considerable similarities in pathogenesis and gene expression with the AITL subgroup of PTCL (44, 45). The 2016 WHO revision acknowledged this overlap by creating ‘AITL and other nodal lymphomas of TFH origin’ as an umbrella category specifically to highlight the spectrum of lymphomas which share a TFH phenotype, given the similarities of the genetic abnormalities within these two groups, particularly that of identified pan-TFH antigens including CD279/PD1, CD10, BCL6, CXCL13, ICOS, SAP, and CCR5. The 2016 WHO classification further designates that PTCL of a TFH subtype should express at least 2 or 3 of these markers for diagnosis (2). The overarching AITL subgroup was associated with a unique spectrum of mutations consisting of TET2, IDH2, DNMT3A, RHOA, CD28, as well as gene fusions such as ITK-SYK or CTLA4-CD28. TET2 mutations were present in 76% of AITL, and patients harboring DNMT3A mutations were found in a study to be invariably associated with the presence of a concurrent TET2 mutation (46). RHOA mutations encoding a Gly17Val alteration affecting GTPase activity and inhibiting the proposed tumor suppressor function of active RHOA was identified in 53-68% of AITL cases and was similarly identified to coexist with the presence of a TET2 mutation (47–49). In the subset of tumors harboring an IDH2 mutation, an overwhelming majority of patients additionally presented with concurrent RHOA and TET2 mutations, suggesting some interlink between the genetic pathways underlying these four mutations in AITL oncogenesis. This is further characterized by TFH lymphocyte derivation and the presence of FYN, CXCL13, PD1 and vascular endothelial growth factor (VEGF) expression in recognized AITL, along with descriptions of a proliferation of follicular dendritic cells and malignant TFH cells within close proximity high endothelial venules in pathological samples (12, 49, 50). In PTCL-TFH, RHOA mutations were also frequently detected, albeit at lower frequency than AITL (51).
Anaplastic large cell lymphoma (ALCL)
ALCL is a subset of PTCL derived from Th17 cells with the characteristic expression of IL-17A and IL-17F, and is uniformly CD30-positive in nature (52, 53). The first important dichotomy in addressing systemic ALCL involves histology. ALCL is distinctly split into ALK-positive and ALK-negative subtypes with differing epidemiology, pathogenetic origin, and crucial differences in clinical disease pattern (54). ALK positivity has been recognized to be associated with significantly improved prognosis and response to first-line chemotherapy, typically anthracycline-containing regimens, additionally opening up the possibilities for novel therapeutics involving ALK-targeting agents (23). This ALK-positive subtype also expresses a unique epidemiology, characteristically presenting in pediatric patients and young adults (55). At the molecular level, ALK-positive ALCL contains a genomic rearrangement of the ALK gene on chromosome 2 with one of several partner genes – the most common being t(2;5)(p23;q35), in which ALK is fused with the NPM1 gene on chromosome 5 (56).
On the other side of the equation concerning ALK-negative ALCL, a further stratification into distinct subgroups based on rearrangements of DUSP22 and TP63 has been associated with significant effects on prognostication. DUSP22 rearrangement has been identified as an excellent predictor of clinical outcome similar to ALK-positive ALCL, whereas TP63 rearrangement is instead less favorable (53). These two mutations are typically mutually exclusive and are only present in the subset of ALK-negative ALCL, whereby DUSP22 rearrangements make up 13-30% of the cohort and TP63 rearrangements make up 2-27% (57, 58). The remainder of ALCL that does not present with any of these alterations are subsequently dubbed as that of a ‘triple negative’ subtype with intermediate prognosis.
DUSP22 rearrangement conferred superior five-year OS rates at 40-90% which is equivalent or even better than the previously documented ALK-positive subtype, whereas TP63 rearrangement resulted in dismal five-year OS rates of 0-17%. The remaining ‘triple-negative’ cases retained a five-year OS of 33-42%, roughly comparable with the survival for ALK-negative ALCL before stratification into DUSP22 and TP63 positive subgroups (58–60). Unfortunately, these targets have not yet been exploited for targeted therapeutics, though further workup of the downstream signaling regulated by these genes could very well generate suitable targeted agents in the future.
Extranodal NK/T cell lymphoma, nasal type (NKTCL)
Extranodal NK/T cell lymphoma, nasal type is a subset of PTCL with an aggressive clinical course and a predominance for Asian and South American populations, derived from NK or γδT cells and further characterized by frequent expansion of CD56+ and cytoCD3+ lymphocytes (2, 61). These are often generated via numerous pathways, of which mutations located in the DDX3X gene regulating RNA helicase, along with tumor suppressor genes such as TP53 and involvement of the JAK-STAT, NF-κB, and PD-1/PD-L1 pathways stand out (62, 63). NKTCL is also always linked to positive EBV infection, expressing EBV-encoded RNA (EBER) and EBV-related proteins which could potentially be used for diagnosis and as therapeutic targets (64).
Clinically, the presence of the DDX3X mutation has been identified to be associated with poorer prognosis in patients treated with CHOP-based therapy (65). Given the heavy involvement of EBV in many cases of NKTCL, EBER positivity and EBV-encoded proteins such as latent membrane proteins (LMP), LMP1, and LMP2A have also been identified as characteristic of NKTCL and have potential prognostic value for NKTCL patients (66, 67). Lee et al. recently also identified EGR1 as a regulator of genes including GAS1, CD59, CXCR7, and RAMP3 in NKTCL, which is often present in localized and low-risk patients and which may thus present as a tool useful for stratifying NKTCL patients who are likely to perform better (68).
Other PTCLs
Enteropathy-associated T cell lymphoma (EATL) is classically associated with celiac disease and has been largely documented within the European continent. Its inherent association with longstanding celiac disease has caused EATL to be recognized as a complication of longstanding celiac disease, which can occur directly from a baseline of celiac disease or from a precursor, low-grade precursor intraepithelial lesion stemming from refractory celiac disease (RCD) which conveys poorer prognosis (69). RCD itself has been stratified into two distinct subtypes, with type I displaying no atypia in intraepithelial lymphocytes, T cell receptors, CD3 and CD8 expressions by intraepithelial T lymphocytes, alongside a polyclonal pattern identifiable on T cell receptor gene arrangement studies. Type II RCD (RCDII) instead displays clonal rearrangements of T cell receptors, often monoclonal, and importantly is the only subset of RCDs which present with the ability to transform into EATL (70).
Monomorphic epitheliotropic intestinal T-cell lymphoma (MEITL) was previously labelled under the header of EATL but was later revised given its non-association with celiac disease and resultant prevalence within Asia whereby celiac disease is less encountered. Mutation analyses on MEITL samples have identified involvement within SETD2, TP53, STAT5B, GNAI2, MYC, and JAK3 of the JAK/STAT pathway (71, 72). CHOP-based chemoregimens were identified to be ineffective in achieving response, and thus other regimens such as ICE (ifosfamide, carboplatin, etoposide) or IVAC (ifosfamide, etoposide, high-dose cytarabine) have been pushed to the forefront of treatment for this disease. Notably, autologous stem cell transplantation (ASCT) consolidation therapy has been shown to be associated with favorable outcomes following induction chemotherapy, and has since been suggested as a current standard of care in some centers (71, 73).
Hepatosplenic T cell lymphoma (HSTL) is described as a neoplasm of mature gamma and delta T cells which infiltrate the spleen, liver, and bone marrow sinusoids of diseased individuals, often presenting with dismal survival outcomes and a predilection for a younger age of onset. Studies of HSTL specimens subjected to whole exome sequencing identified the chromatin modifying gene SETD2 as a common mutated gene in HSTL as well, with other involved chromatin modifier genes such as INO80, TET3, SMARCA2 and signaling pathway mutation genes such as STAT5B, STAT3, PIK3CD, and TP53 being implicated. A STAT5B inhibitor (CAS 285986-31-4) was identified to significantly reduce cellular proliferation in vitro in HSTL tissue, and the addition of the PI-3 kinase inhibitor idelalisib further reduced cellular viability in cells (74). CHOP and CHOP-like regimens have displayed lacking outcomes and for this reason non-CHOP induction followed by stem cell transplantation consolidation is the current recommendation, but the data regarding clinical trials is still presently limited in this department (75).
Daniels et al. (76) performed genome-wide sequencing on a population of primary cutaneous gamma-delta T cell lymphomas (PCGDTL), shedding insight on the nature of the origin cells of various subsets of this group of lymphomas. The PCGDTL group of cancers has been stratified into two groups originating from two distinct cancer origin cells, Vδ1, which originates from cells superficially in the epidermis or dermis and Vδ2, originating from deeper cells within the subcutaneous tissue layer. These distinct groups inform about clinical phenotype, given that Vδ1 origin T lymphomas (which includes γδ MF phenotype, γδ MF phenotype with PCGDTL-like progression, and PCGDTL phenotypes) present with much better survivals as compared to Vδ2 origin T lymphomas with a median survival of 89 months and 12.75 months respectively and present with less tumor lesions and B symptoms in patients at diagnosis; individuals with cells of origin from the epidermis (Vδ1) had significantly better survivals (179 months) as compared to those in the dermis (Vδ1, 31 months) and subcutaneous tissue layer (Vδ2, 12.75 months). The specific γδ MF phenotype only found in Vδ1 T lymphomas were also associated with better prognosis and response to therapy, though progression involving a phenotypic switch to PCGDTL resulted in a nullification of the previous survival benefits of the γδ MF phenotype subgroup.
Present-day treatment landscape of PTCL and NKTCL
The treatment landscape of PTCL has not greatly evolved over the past decades and remains an area of unmet clinical need. In the upfront setting, the majority of patients receive CHOP-like regimens, though the exact benefit of anthracyclines remains controversial (7, 11, 77). The addition of etoposide to CHOP has been suggested to improve event-free survival in younger patients of age up to 60 years (16, 23). Outcomes of patients treated with these CHOP-based regimens are generally poor, with 5-year OS rates of less than 50% (7, 24, 77). Most trials evaluating the addition of various agents to the CHOP backbone have been disappointing, except for the use of the CD30-targeted antibody drug conjugate brentuximab vedotin in CD30+ PTCL in the pivotal ECHELON-2 trial (78). Till date, the role of consolidation with autologous stem cell transplantation remains highly debatable, though it may potentially provide benefit in patients who successfully achieve complete remission following frontline induction therapy, as well as in the subgroup of patients with AITL, advanced stage disease and intermediate to high risk IPI scores (79, 80).
In NKTCL, L-asparaginase-containing treatment regimens such as SMILE (dexamethasone, methotrexate, ifosfamide, L-asparaginase, etoposide) (81, 82), P-GEMOX (pegaspargase, gemcitabine, and oxaliplatin) (83), DDGP (dexamethasone, cisplatin, gemcitabine, and pegaspargase) (84), and AspaMetDex (pegaspargase, methotrexate, and dexamethasone) (85) have been regarded as the preferred first-line therapy for advanced disease (67). Similar to PTCL, the role of hematopoietic stem cell transplant in NKTCL is highly controversial (29, 86). Despite improvements in survival outcomes with the incorporation of these newer chemotherapeutics in the management of NKTCL, a significant proportion of patients still relapse or remain refractory to treatment. Moreover, most of these multi-agent regimens frequently risk significant adverse side effects including severe myelosuppression, infections, and hypersensitivity reactions. An optimized approach to prognostication and risk stratification, as well as the discovery of predictive biomarkers are strongly desired so as to achieve the best survival outcomes while reducing adverse effects.
In the setting of relapsed/refractory PTCL and/or NKTCL, the efficacy of contemporary salvage therapeutic options using platinum-based or gemcitabine-based regimens is typically dismal, often with response rates below 50% (29, 86). Newer chemotherapeutic agents such as bendamustine (87) and pralatrexate (8) provide only modest benefit, and strategies directed at novel targets, immune checkpoints and epigenetic pathways have been explored.
Emerging therapeutic strategies
Cell-surface based targeted therapeutics
CD52
Cell surface antigens have been long recognized as an exploitable target for various targeted therapeutics, regulating oncogenic cell signaling pathways via the enhancement or blockade of various cell surface molecules or utilizing them as vectors for the administration of cytotoxic material to induce tumor cell death. CD52 is a cell surface marker found on the surface of mature lymphocytes and expressed in 35-100% of PTCL-NOS, 40-100% of AITL, 25-47% of NKTCL and 0-22% of systemic ALCL patients (88–92). This receptor was first exploited by alemtuzumab, a monoclonal antibody for CD52, postulated to cause anti-tumorigenic effects through the activation of antibody-dependent cell-mediated cytotoxicity (ADCC). Whilst primarily indicated for the treatment of chronic lymphocytic leukaemia (CLL) and multiple sclerosis (MS), alemtuzumab has been explored by Gallamini et al. (93) who suggested the feasibility of first-line combination chemotherapy with a CHOP regimen combined with alemtuzumab (A-CHOP), attaining an ORR of 75%. The ACT-1 and ACT-2 trials additionally supplemented this with randomized phase III data comparing CHOP with A-CHOP in treatment naïve PTCL patients, establishing superior ORR to control although noting no significance on survival outcomes, largely attributed to treatment toxicity (94, 95). Dhanda et al. (96) also presented an observation pertaining to the change of PTCL immunophenotype from CD52+ to CD52- after alemtuzumab treatment, a phenomenon which closely alludes to the documented loss of CD20 expression after rituximab in diffuse large B-cell lymphoma (DLBCL), which is a significant cause of rituximab resistance (97). The significant toxicity and high rate of severe toxicity events preclude alemtuzumab therapy to date, and further investigations have since moved on to other therapeutic targets.
CCR4
CCR4 is a surface protein extensively expressed on T lymphocytes, particularly that of T-helper and T-reg cells, which as a member of the chemokine receptor family aids in cellular migration (chemotaxis) to tissue sites of inflammation (98). In diseased states, the chemokine system can be abused by tumor cells to facilitate metastasis to distant sites (99), and can directly modulate its tumor environment to enhance immune evasion (98). CCR4 was identified to be expressed in several PTCL subtypes, notably in ALCL ALK-, PTCL-NOS and AITL (100). Mogamulizumab, an anti-CCR4 antibody, targets CCR4 and induces an ADCC reaction leading to tumor cell death and lysis. A review published by Remer et al. (98) in 2014 succinctly summarized the efficacy of mogamulizumab, highlighting its feasibility in CCR4+ PTCL obtaining an ORR of up to 50%, but emphasized the high frequency of adverse events in treated populations specifically pertaining to neutropenia and lymphopenia. A multicenter phase II trial for mogamulizumab conducted in Japan further identified an ORR of 34% in their cohort of PTCL treated with the anti-CCR4 antibody, but reported no significant correlation between CCR4 expression levels and response rates to mogamulizumab. The significant frequency of severe neutropenia and lymphopenia events were further recognized by this trial, with 19% and 73% of the cohort experiencing these adverse effects respectively (101). The significant toxicity of mogamulizumab as a therapeutic option precluded its utility in achieving survival benefits and it has since fallen out of favor.
CD30
Brentuximab vedotin (BV) is an antibody-drug conjugate targeting CD30 as a means of internalizing the antimitotic agent monomethyl auristatin E (MMAE) for oncolysis (102). CD30 is expressed in approximately 32-64% of PTCL-NOS, 43-63% of AITL, 100% of systemic ALCL and 46-80% of NKTCL, reflecting a biomarker with significant prevalence and thus utility in the population of PTCL (14, 103–105). In part, trials evaluating the efficacy of BV together with a combination chemotherapy protocol incorporating cyclophosphamide, doxorubicin and prednisolone (BV-CHP) in CD30+ PTCL (defined as PTCL with >10% CD30 expression) has shown impressive clinical outcomes, particularly championed by the ECHELON-2 landmark trial which boasted a hazard ratio of 0.66 and 0.71 against standard CHOP therapy in terms of OS and PFS respectively, together with a reported 70.1% and 51.4% 5-year OS and PFS (78, 106). The notable outcomes and responses to this regimen has resulted in the establishment of a new standard of care for CD30+ PTCL, and has reinvigorated the field towards a cautious but optimistic search for future novel predictive biomarkers and therapeutic targets. Ongoing trials are evaluating frontline treatment using BV-CHP in PTCL with less than 10% CD30 expression (NCT04569032), as well as a similar BV-CHEP regimen which includes the addition of etoposide (NCT05006664).
CD25
CD25, also known as the interleukin-2 receptor α-chain or IL-2R, is positively expressed in 40-50% of PTCL (107, 108). Denileukin diftitox, a fusion protein linking diphtheria toxin to IL-2, elicited an ORR of 65% when used in combination with CHOP chemotherapy in a cohort of newly diagnosed PTCL alongside well-tolerated side effect profiles (109); applied to a cohort of R/R PTCL, it was able to elicit an ORR of 61.5% in CD25+ patients and 45.5% in CD25- patients (110). This was furthered by a phase II trial of E7777 in Japan, which features a similar mechanism of action and drug profile to denileukin difitox albeit with claims of improved purity – this new drug featured an ORR of 41.2% with one patient in the study obtaining a complete response (111). In particular, patients with less than 20% observed CD25+ cells were still able to attain an ORR of 25.0% against a ORR of 41.7% in the subgroup of patients with >20% CD25+ cells, implying that while CD25 positivity might factor in as a predictive biomarker for therapeutic response to anti-CD25 pharmacological agents, the lack of CD25 expression does not necessarily preclude E7777 as a therapeutic option in these patients. A further phase I trial of camidanlumab tesirine, an antibody-drug conjugate exploiting CD25 to deliver cytotoxic crosslinking of cellular DNA, whereby preliminary results similarly suggest correlation of CD25 expression to the drug’s therapeutic efficacy (112).
Immune checkpoint inhibitors
Another initial area of exploration in the field of immunotherapy involves the usage of immune checkpoint inhibitors which block pathways that downregulate the immunological surveillance and induce a state of anergy in T cells. The blockade of these inhibitory signals re-activates T cells, thus mounting an immunologic response against cancer cells and inducing a phenomenon of adaptive oncologic clearance (113). Two pathways have been studied extensively pertaining to this underlying principle.
PD-1/PD-L1
One of the major immune checkpoint pathways involves PD-1, expressed on T regulatory (T-reg) and activated effector (CD4 and CD8) T cells and its ligand PD-L1, often expressed on evading cancer cells. When activated, PD-1/PD-L1 binding suppresses native T cell activation and by extension, adaptive T cell mediated cancer cell clearance (113). The activation of the PD-1/PD-L1 pathway has also been proposed to be mediated by the JAK-STAT oncogenic pathway activation in a subset of patients (114). High levels of PD-1 expression measured on T-reg cells in PTCL were thus identified to be associated with poorer prognosis in a group of patients (115) and recently, PD-L1 expression alongside V-domain immunoglobulin suppressor of T cell activation (VISTA) in NKTCL has been found to act as synergistic negative predictors of prognosis, further establishing the role of T cell suppression in oncologic immune evasion (116, 117).
Various immune checkpoint inhibitors have since been commissioned targeting this immune pathway. Pembrolizumab, an anti-PD-1 antibody, has been increasingly used in the literature surrounding malignancies such as melanoma and other non-Hodgkin lymphomas and has been explored in recurrent/relapsed (R/R) mature PTCL with an ORR of 33% (118). Geptanolimab and nivolumab, which are also anti-PD-1 antibodies, have been studied in R/R PTCL with an ORR of 40.4% and 33%, respectively (119, 120). In NKTCL, blockade of the PD-1/PD-L1 axis has emerged as a promising treatment strategy as well (121–124). Recent studies also noted that chidamide, a histone deacetylase (HDAC) inhibitor, seemed to leverage upon anti-tumor effects provided within PD-1+ cells to enhance the expression of genes associated with chemokine and chemotaxis activity, exposing the possibility of synergistic effects when combined with standard PD-1 blockade therapy (125, 126).
CTLA-4
Another major immune checkpoint pathway related to the principle of T cell anergy concerns the CTLA-4 ligand which acts as a direct and complete blockade of co-stimulation in T cells via its stronger affinity for B7 (CD80, CD86) as compared to the normal activator CD28 (127). CTLA-4 inhibitors thus function by inhibiting CTLA-4, allowing CD28-B7 crosslinking and activation of T cells for its activity against tumor cells. A study of PTCL via Sanger sequencing identified that CTLA-4/CD28 fusion genes occurred in approximately 30% of PTCL samples, and up to 58% in AITL (128). Despite the similarities that it shares with the PD-1/PD-L1 pathways, CTLA-4 inhibitors have not yet been adequately studied in the population of PTCL within clinical trials despite there being pharmacological agents such as ipilimumab available in the market for the treatment of other malignancies (128). However, a case series performed on PTCL tumors had found substantial CTLA-4 mutations in various subtypes of PTCL, notably in that of AITL and PTCL-NOS, suggesting possible room for therapeutics against the CTLA-4 surface marker in future studies (129).
Epigenetic targeted agents
Epigenetic therapeutics involve the targeting of changes which occur in the cancer epigenome, whereby oncogenesis often is derived from changes in expression of proteins through complex factors involving the transcription and translation of genetic material in vivo. Two key mechanisms are understood to play key roles in the genesis of cancer through this route, histone modification and DNA methylation, which have been targeted through HDAC inhibitors and DNMT inhibitors as respective pharmacological agents.
Histone deacetylases (HDAC) are a family of enzymes which are involved in the modification of histones, predominantly deacetylating them, resulting in increased binding of DNA to histones which results in silencing and suppression of DNA transcription. HDAC inhibitors therefore aid to induce cell cycle arrest, differentiation, or apoptosis as valuable effectors of oncolysis (130). Within this class of drugs, romidepsin, belinostat and chidamide have since been trialed as monotherapy agents in the setting of R/R PTCL. They have been demonstrated in various phase II trials to induce modest responses in some patients with generally tolerable side effect profiles, with romidepsin, belinostat and chidamide exhibiting an ORR of 25%, 26%, 28% and inducing CR in 15%, 11%, and 14% of patients respectively (131–133). Interestingly, chidamide was identified to exhibit more durable responses in the population of AITL, achieving an ORR of 50% and a CR of 40% in this particular subgroup. Most recently, romidepsin was trialed together with oral 5-azacytidine in a population which included both treatment-naïve and R/R PTCL with an ORR of 61% and CR of 43%; in the subgroup analyses of treatment-naïve patients and PTCL-TFH an even higher response rate was observed, with ORRs of 70% and 80% respectively (134). However, a recent phase III study by Bachy et al. failed to find any significant improvement in PFS, OS, nor overall response rates in a comparison between romidepsin and CHOP versus CHOP-only therapy, whilst the romidepsin and CHOP group exhibited an increased incidence in severe treatment-related adverse events (135). This perhaps highlight the heterogeneity of PTCL and suggests the need for clinical trials specifically evaluating epigenetic-targeted agents in AITL/PTCL-TFH.
DNA methyltransferases (DNMTs) are a family of enzymes involved in epigenetic methylation of the human genome, which is known to result in the silencing of tumor suppressor genes; DNMT inhibitors have thus been utilized to induce hypomethylation of the genome for therapeutic benefit in cancer treatment (136). Due to the frequent involvement of the TET2, IDH2 and DNMT3A genes in AITL subtypes which are associated with cytosine methylation and hydroxymethylation, DNMT inhibitors such as 5-azacytidine have been evaluated in AITL with reported significant and durable responses (137–139). Despite this, the recently published final results of the ORACLE phase III study comparing oral 5-azacytidine to investigator’s choice in R/R AITL failed to achieve significance in the primary endpoint of PFS with a median PFS in the 5-azacytidine group of 5.6 months versus 2.8 months in the standard arm (p = 0.0421) (140). Overall survival, however, was associated with significantly improved OS in the 5-azacytidine arm, with a median OS of 18.4 months in the 5-azacytidine group versus 10.3 months in the standard arm (HR 0.557, 95CI 0.323-0.961). Another important takeaway was that the study found no significance between the presence of TET2, RHOA, DNMT3A, and IDH2 mutations and PFS or OS during subgroup analysis. The authors concluded that errors made in view of their overly optimistic hypothesis of PFS improvement might have resulted in an underpowering of the study; despite this, the favorable safety profile of oral 5-azacytidine and attainment of OS endpoints suggest utility in the treatment of AITL.
Other immunoregulatory agents
Lenalidomide is a thalidomide analogue which has immunomodulatory activity via the induction of apoptosis of tumor cells and anti-angiogenic effects and is a therapeutic option often used in multiple myeloma and non-Hodgkin lymphoma. Lenalidomide has been evaluated as a possible therapeutic option in PTCL with highly variable reports of its efficacy. Multiple phase II trials of lenalidomide monotherapy in populations of PTCL involving PTCL-NOS, ALCL, and AITL yielded ORR rates of 22-26%, though there was significant heterogeneity of the treatment population consisting of both R/R PTCL and untreated PTCL patients who were not candidates for combination chemotherapy (141, 142). In AITL, the study by Morschhauser et al. reported an ORR of 31%. In recent years, combination therapy involving lenalidomide has been further explored with a combination of lenalidomide with vorinostat and dexamethasone yielding an ORR of 25% in R/R PTCL (143) and a combination of lenalidomide and CHOEP (cyclophosphamide, doxorubicin, vincristine, etoposide, and prednisone) (len-CHOEP) yielding an ORR of 68% with a CR rate of 48%, although the len-CHOEP regimen had a 38% prevalence of associated serious adverse events (144). Lenalidomide combination therapy with CHOP in AITL was also explored in one study to be associated with an ORR of 47.4% and a CR rate of 43.6% (145).
Bortezomib is a proteasome inhibitor exhibiting anti-tumoral activity through inhibition of intracellular degradation of pro-apoptotic factors such as the P53 protein and hence activation of programmed cell death, which has also been used mainly in the setting of multiple myeloma. Overexpression of microRNA-187 in PTCL-NOS has been proposed to be a potential mediator in chemoresistance whilst conversely informing of susceptibility to proteasome inhibitor therapy (146). Bortezomib monotherapy has been trialed in the setting of cutaneous T cell lymphoma with an ORR of 67% (147), as well as in numerous combination therapy regimens. Bortezomib in combination with pralatrexate had an ORR of 20% in a small series of five PTCL cases (148) and bortezomib in combination with panobinostat yielded an ORR of 43% in a study of 23 PTCL cases (149). In this latter study, the AITL population yielded the best response rates as well with an ORR of 50%. Bortezomib in combination with CHOP chemotherapy was also investigated in several trials, with an ORR of 87% in the subgroup of PTCL-NOS, AITL, and ALCL in the study led by Kim et al., which was observed to be higher than that of responses to CHOP-only therapy within the literature (150). The study yielded low ORR of 40% in the subgroup of extranodal NKTCL, which was attributed by the authors to potentially be due to the frequent expression of a multidrug-resistant p-glycoprotein in extranodal NKTCL. Of note, a newer generation of orally administrated proteasome inhibitor, ixazomib, was also investigated as a monotherapy regimen in a single phase II trial which only yielded one case of response within a population of 13 patients (151). The authors concluded that further detailed studies focusing on the mechanisms conferring susceptibility or resistance to novel therapeutic strategies, including proteasome inhibition, should be considered for future personalized treatment in this domain.
Though controversial, stem cell transplantation (SCT) is often used as consolidation therapy and autologous SCT has since been regarded by some as the mainstay of therapy for optimal outcomes in PTCL following high-dose inductive chemotherapy (152–154). The principle of treatment is for the usage of high dose chemotherapy regimens on patients with subsequent rescue via autologous SCT, and such a consolidation therapy utilized on first complete remission (known as ‘upfront autologous SCT’) has been validated to be associated with increased OS in all stages of disease and in patients with intermediate-to-high IPI scores (80, 155). However, in the setting of R/R PTCL, the choice of autologous versus allogenic SCT is less well understood; allogenic SCT is associated with increased side effects such as transplant-related mortality (TRM) and non-relapse mortality (NRM) in transplant patients. On this topic, a systematic review and meta-analysis by Du et al. (156) comparing outcomes between autologous and allogenic SCT in R/R PTCL has since revealed no significant difference in 5-year OS and 3-year PFS between autologous SCT and allogenic SCT group used in R/R PTCL, though the 3-year OS was significantly higher in the allogenic SCT group and especially so in the subpopulation of patients who did not attain CR before transplantation. The allogenic SCT group, as expected, also attained significantly higher rates of TRM at both the 3-year and 5-year marks. The current consensus is thus that allogenic SCT yields outcomes comparable to autologous SCT in the setting of R/R PTCL with the downside of increased transfusion-related effects, although allogenic SCT could potentially remain an appropriate option in fitter or younger patients with better baselines and less comorbidities. Autologous SCT, however, should not be routinely recommended as salvage therapy later than as second-line therapy if patients have already undergone prior multiline treatment as the associated prognosis is likely to be worse (157).
Emerging biomarker landscape in PTCL and NKTCL
The interaction between PD-1 and PD-L1 remains a complex entity as of yet not fully understood but holds considerable importance and potential in paving the way for immunotherapeutics in PTCL (158). Studies have been conducted in datasets of solid tumors, particularly those of melanoma and NSCLC types, which identified a reasonable correlation between PD-L1 expression on immunohistochemistry and response to PD-1/PD-L1 antagonistic agents (159). In these solid tumors, the expression of markers such as HLA-DR, CTLA-4, CD56 and CD45RO, with lower amounts of CD3, CD27 and CD28 were identified in responders to PD-1/PD-L1 inhibitors (160). However, no such study has been conducted in a population of lymphomas, nor has any analysis been conducted to suggest that the similar pattern is upheld in lymphomas.
We also identify JAK mutations as common shared mediators of tumorigenesis across the spectrum of PTCL which remain important exploitable targets for targeted therapeutics, such as the like of JAK inhibitors which generate anti-tumoral effects via inhibition of the JAK-STAT signaling pathway for tumor suppression. A phase II biomarker-driven study by Moskowitz et al. (161) recently demonstrated the efficacy of the JAK inhibitor ruxolitinib across various patients with R/R PTCL in attaining a primary endpoint of clinical benefit rate (CBR), defined as the percentage of the cohort which demonstrated a complete response, partial response, or stable disease lasting at least 6 months. They crucially elucidated a meaningful association between CBR and the presence of JAK/STAT mutations and STAT3 expression, with the subgroup with identified JAK/STAT mutations achieving a CBR of 53% and the subgroup with pSTAT3 expression ≥30% assessed via immunohistochemistry achieving a CBR of 45%, significantly elevated over the subgroup which did not fulfil the criteria for either of the two categories with a CBR of 13% (p = 0.02). JAK/STAT mutations and expression thus appear to be a prospective biomarker which could readily inform clinicians on the choice of therapeutic options in R/R patients, especially as we gravitate towards the setting of personalized medicine.
PTCL-NOS
DNMT3A is a gene that has been documented as a progenitor of PTCL oncogenesis, whereby its involvement in the PTCL-NOS subtype is characterized by mutations within its functional domains, featuring mutations skewed towards its MTase domain and a R882H/C hotspot mutation identified within roughly 30% of the PTCL-NOS cohort (43). In extension of the previously recognized PTCL-NOS subgroups of PTCL-TBX21 and PTCL-GATA3, DNMT3A-mutant patients with a concurrent PTCL-TBX21 subtype were identified to have significantly inferior OS as compared to DNMT3A-wildtype PTCL-TBX21 patients; this finding was not reproduced in the cohort of PTCL-GATA3 patients. This poor prognostic significance of DNMT3A-mutant PTCL-TBX21 patients was further observed to negate the survival benefits of the PTCL-TBX21 subtype over that of the PTCL-GATA3 subgroup, resulting in similar survival curves observed between these two subgroups.
AITL
AITL is characterized by specific epigenetic mutations such as TET2, DNMT3A, IDH2, and RHOA, which have been identified as genes which regulate functionality in the sequence of events required for DNA demethylation (162, 163). As a result, hypomethylating agents have been hypothesized to be able to play a significant role in the management of AITL by directly opposing the background DNA hypermethylation that occurs as a result of these characteristic mutations, thus controlling oncogenic drive and disease. It is further theorized that hypomethylating agents such as 5-azacytidine would be effective in TET2-mutated cancers given the significantly increased efficacy of 5-azacytidine in TET2 mutant subgroups in the treatment of myelodysplastic syndrome (164). Treatment with the hypomethylating agent 5-azacytidine was found to induce a sustained response, achieving 75% ORR in a cohort of 12 patients with 6 patients achieving CR, although no relationship was found between the number of TET2/DNM3A/IDH2/RHOA mutations and response to treatment in this cohort (137). A more recent retrospective study on the efficacy of 5-azacytidine as salvage therapy was recently published as well and reported 60.0% ORR in patients who were able to tolerate the optimal dose of therapy (139). The identified RHOA-VAV1 signaling in AITL may also be amenable to dasatinib, a multikinase inhibitor, with a promising outcomes observed from a preliminary phase I trial in AITL (165, 166).
AITL tumors are also identified to overexpress CXCL12 in over 50% of cases. Preliminary results of a phase two study showed that tipifarnib, a selective inhibitor of the farnesyltransferase enzyme which aids CXCL12 secretion which directs T cell homing and chemotaxis, was found to have a 43% ORR and 73% clinical benefit rate (CBR) in AITL (167). In particular, the presence of the KIR3DL2 gene variants C336R/Q386E predicted complete response to tipifarnib therapy and improved outcomes, postulated to be due to the variants’ association with low levels of CXCL5, reducing possible resistance to tipifarnib. Finally, we appraise new data identifying AITL to express a higher quantity of PD-1 and PD-L1 in both tumor cells and the surrounding immune microenvironment, along with an association of increased PD-1 and PD-L1 expression to poorer prognosis (168). Given the current enthusiasm for PD-1 inhibitors such as pembrolizumab and nivolumab, we anticipate further data on the utility of PD-1/PD-L1 expression in predicting response to these immune checkpoint inhibitors and their novel combination regimens in AITL, although recent data suggests modest activity and risk of hyperprogression in patients treated with nivolumab alone (120).
ALCL
ALCLs, independent of their ALK status, express near homogeneous CD30 positivity which renders this subtype of PTCL amenable to therapy with the CD30-targeting antibody drug conjugate brentuximab vedotin (169).
The presence of ALK rearrangement in ALCL predicts sensitivity to conventional anthracycline-based chemotherapeutic regimens, and thus often present with superior survival outcomes amongst PTCLs (23). The unique subset of ALK-positive ALCL patients additionally presents with the potential for targeted therapeutics involving small molecule inhibitors against ALK, such as crizotinib, ceritinib and alectinib. Crizotinib has been explored in the treatment of pediatric ALK+ ALCL patients with positive efficacy and safety profiles (170), with results demonstrating its utility in inducing complete remissions in the setting of advanced, relapsed ALK-positive ALCL cases (171, 172). In a phase two study involving 12 patients with R/R ALK+ ALCL, crizotinib monotherapy resulted in a remarkable ORR of 83.3%, with 7 patients (58.3%) achieving complete response (173). Likewise, ceritinib was demonstrated to induce durable complete remission on a singular case report (174). Fukano et al. further showed a similarly high response rate of 80% in a phase two study evaluating alectinib on 10 patients (175).
In addition, the subgroup of ALK+ ALCL boasts a unique phenotype in which PD-L1 expression was detected at high rates in the tumor and microenvironment of these lymphomas, although this has not yet been correlated to response rates to PD-1/PD-L1 targeting agents (176). The PD-L1 expression on tumor cells and tumor-infiltrating immune cells were further found to have no significance on prognosis when independently evaluated as factors, although the specific subset of ALK+ ALCL with both high tumor cell PD-L1 and high tumor environment immune cell PD-L1 yielded significantly lower 5-year PFS (177). Further studies in this regard should help evaluate the role of the extended tumor microenvironment as a biomarker in PTCL, which might present a differing point of view for further analysis apart from simply targeting tumoral cells for analysis.
NKTCL
PD-1/PD-L1 has been explored in the setting of NKTCL, with reports of PD-L1 expression in various cohorts to range between 38 to 93% (68, 178), and the usage of PD-1 inhibitors such as pembrolizumab has shown promising results in the setting of R/R NKTCL (121, 122). Unfortunately, the expression of PD-L1 has not yet shown to be correlated to treatment response to PD-L1/PD-1 inhibitors in NKTCL; subsequent papers have also suggested that EBV latency factors such as LMP1 could induce the expression of PD-L1 in NKTCL (179), and that induced PD-L1 could be a factor of resistance to immune checkpoint blockade instead, thus explaining an apparent lack of correlation between PD-L1 expression and response to PD-1/PD-L1 inhibitors (180).
In a recent study by Cho et al. (181), immunohistochemistry was performed to stratify NKTCL cases into four subgroups, separated according to tumor immune microenvironments, through quantifying the expression of CD68, CD163, CD8 and FoxP3. These four subgroups, separated into immune-tolerant, immune evasion (A and B), and immune-silenced groups, was suggested to predict response to pembrolizumab, although the dataset was too small for definitive conclusions. Sensitivity to PD-1/PD-L1 checkpoint inhibitors has also been found to be adequately predicted by exploring a 3’-UTR mutation of PD-L1 (PD-L1MUT) identified via whole-genome sequencing (WGS) in R/R NKTCL (182). All patients whose tumor harbored PD-L1MUT (5/20 cases) achieved complete response to PD-1 checkpoint inhibitor pembrolizumab, demonstrating sensitivity of 100% as a biomarker of response. Interestingly, all these patients have survived for more than 3 years which is uncommon for this group of patients. The small cohorts in these studies however, present room for further analysis of these predictive biomarkers in future, larger populations.
A study analyzing a combination of anti-PD-1 antibody therapy with pegaspargase, gemcitabine, and oxaliplatin (P-GEMOX) by Cai et al. also hypothesized that the P-GEMOX regimen might aid in sensitizing tumors to anti-PD-1 immunotherapy, alongside the DDX3X mutation leading to anti-PD-1 drug resistance as a negative predictive biomarker (183). V-domain immunoglobulin suppressor of T cell activation (VISTA) has also been explored as a factor which predicted negative response to the usage of PD-1 inhibitors in NKTCL, and presents as a target for future therapeutics whereby VISTA blockade could be used as a tool for increasing sensitivity to anti-PD-1 options (117).
CD38 expression status was also identified to be an independent adverse prognostic factor in patients with NKTCL, with a cohort studied by Wang et al. predicting significantly reduced PFS intervals (184). The antibody to CD38, daratumumab, has also been trialed in this group of patients in a phase two study, achieving a modest ORR of 25% (185, 186). Interestingly, the ratio of CD38 to complement inhibitory proteins (CIP) was subsequently demonstrated to better correlate with antitumor efficacy of daratumumab than using either CD38 or CIP expression alone (187). The inherent positivity of CD56 in NKTCL has also been observed; although no therapeutic target to CD56 currently exists, a study in solid tumors identified that higher expression of CD56 were observed in responders to anti-PD-1/PD-L1 therapy (160). Given the high expression rates of CD56 in NKTCL, further investigation might be warranted into the clinical significance of CD56 in therapeutics.
In terms of prognostic indices, a new-generation genomic-augmented multivariate prognostic model was recently developed via the identification of 13 mutated genes which offered significant prognostic significance in terms of reduced OS and PFS in a cohort of 210 NKTCL tumors, of which including BCOR, KRAS, JAK3, DCC, FAS, NOTCH1, BIRC3, etc. and implicating 39% of the total NKTCL samples used in generation of the model (178). These prognostic genes had mild correlation with high-risk features as denoted via previous models such as the IPI, PINK, and PINK-E, and an augmentation of these prognostic indices via the addition of a point in the presence of one of the 13 recognized mutations resulted in improvements in the prognostic significance of the three indices for both PFS and OS. These models were rescored and renamed as IPI-G, PINK-G, and PINK-E-G respectively. Another multivariate analysis identified a 7-SNP-based classifier which was also able to predict PFS and OS between high-risk and low-risk cohorts, with the additional benefit of having clinical predictive significance in Ann Arbor stage I patients, whereby patients classified as of high-risk were documented to receive increased OS and PFS when treated with chemotherapy in addition to baseline radiotherapy (188).
The final point is a discussion on studies pertaining to biomarkers involving the JAK-STAT signaling pathways. In particular, the expression of JAK3/STAT3 signatures and the downregulation of tumor suppressor positive regulatory domain containing I (PRDM1) was identified as a prognostic biomarker, with PRDM1-/STAT3+ phenotypes expressing poor survival outcomes (189). Most recently, Dong et al. showed that PRDM1 loss and STAT3 mutation cooperates to promote NK cell growth (190). JAK inhibitors such as ruxolitinib, tofacitinib, or novel small-molecule STAT3 inhibitors such as Stattic might thus pose to be a potential agent for this emerging biomarker.
EBV-associated T cell lymphomas
Epstein-Barr virus (EBV) infection has since been recognized as a major player in the pathology of numerous B lymphomas and our understanding of its role in PTCL is steadily emerging. NKTCLs are inherently characterized by EBV involvement, whereby EBV-infected cells proliferate, undergo clonal expansion and achieve immortalization of the clonal species through an of yet unknown mechanism (191). The virally encoded latent membrane protein 1 (LMP1) has been postulated to interact with the tumor necrosis factor (TNF) family of receptors, as well as inducing gene expressions which are relevant to cell growth and the cell cycle (192). In the particular setting of NKTCL, EBV is believed to present with a type II latency pattern, which encompasses EBNA1+ and LMP1+ and other EBV-related genes (193). The role of EBV in AITL however is more controversial. EBV positive B-cells have been observed in 85-95% of AITL, although its specific role in AITL pathology and oncogenesis is still unclear (194). The presence of EBV in AITL cases were largely of EBV-1 origin, expressed genes from all stages of the EBV lytic cycle, but predominantly exhibited a type II latency pattern similarly to that observed in NKTCL (194, 195). EBV infection may therefore be exploited in the treatment of PTCL and NKTCL.
In the wake of type II latency EBV positivity in infected cells, we understand that EBNA, LMP1 and LMP2 proteins are often present (196). Kalra et al. goes further to characterize the presence of BARF1, an EBV type II and III latency-associated antigen with MHC class I and II epitopes, which might present as a useful future target for immunotherapy involving CD4 and CD8 T effector cells (197). With the underlying principle of targeting EBV as a means to eradicate the source of cancer, recent studies regarding tenofovir disoproxil fumarate (TDF) and tenofovir alafenamide (TAF) show significant antiviral activity against EBV (198). HDAC inhibitors have also been identified to induce EBV reactivation, pushing EBV cells out of their latency phase into a lytic phase, which might be postulated to aid in the prevention of immune evasion and to induce the clearance of EBV from the body (199). Certainly, whilst these present attractive possible avenues for therapeutics, many more studies will have to be performed to confirm the validity of these mechanisms.
Treatment with autologous EBV-specific cytotoxic T lymphocytes (CTLs) has been explored with a subset of patients with EBV positivity. A case series involving 10 NKTCL patients successfully utilized in vitro expanded antigen-specific CTLs against the EBV proteins LMP-1 and LMP-2a in a cohort of patients with lymphomas expressing EBV latent proteins, including patients with NKTCL (200). This concept was extended to a cohort which had achieved complete response post-first-line chemotherapy in an effort to prolong remission post-response (201). It also remains to be seen if the basis of targeting EBV-infected tumor cells via expanded autologous EBV-specific CTLs would continue to hold true in a subset such as AITL whereby the role of EBV in oncogenesis is less understood.
Finally, Myint Wai et al. (202) recently described a unique group of PTCLs which resembled extranodal NKTCL but held sufficient differences in clinical outcomes and immune signaling pathways to warrant further consideration as a subgroup distinct from that of currently defined NKTCL. This unique group of patients are presently labelled as ‘primary Epstein-Barr virus (EBV)-positive nodal T/NK-cell lymphoma’ (PTCL-EBV) and were identified via clinicopathological features such as the primary presentation of nodal disease where the bulk of tumor is localized, lack of nasal involvement, and/or expression of a CD8+/CD56– phenotype. Notably, these lymphomas had poorer clinical outcomes, low genomic instability, an upregulation of immune pathways involving NF-κB, IFNγ, and IL6-JAK-STAT3, and downregulation of EBV miRNA when compared to classical NKTCL. Tumors classified under PTCL-EBV exhibited significantly shorter median OS (4.6 months versus 14.7 months in NKTCL, p = 0.001), and PD-L1 was also identified to be significantly upregulated in PTCL-EBV likely from upstream pathways such as NK-κB and IFNγ which induce PD-L1 expression, presenting a potential biomarker for targeted therapy. The glaring discrepancy between the aggressiveness of PTCL-EBV tumors and its low genomic instability was proposed to be due to suppression of instability by underlying NF-κB activation amidst a paucity of TP53 mutations, which might contribute towards the low genomic instability but high aggressiveness of this tumor.
Other PTCLs
A study by Cording et al. (203) identified mutations in the JAK1-STAT3 pathway in 100% of RCDII samples subjected to whole exome sequencing and comparative genomic hybridization. Mutations in the NF-κB pathway were subsequently identified in 90% of the samples, implicating the JAK1-STAT3 pathway and NF-κB activating mutations in RCDII lesions; these findings were further correlated with frozen biopsy analysis via targeted next-generation sequencing and targeted amplicon sequencing as well as the identification of increased cytokine responsiveness through these pathways. With a comparison of EATL and RCDII-transformed-EATL displaying similarities in the involvement of the JAK1-STAT3 pathway in particular, JAK inhibitors such as ruxolitinib and abrocitinib were investigated in RCDII cell lines with an inhibition of proliferation and induction of apoptosis, presenting with the possibility of some utility of JAK inhibitors in inhibiting progression of RCDII to full-fledged EATL. The proteasome inhibitor bortezomib additionally displayed STAT3 phosphorylation and growth inhibition in RCDII cell lines, opening up possibilities regarding the therapeutic value of prevention of RCDII progression in the pathogenesis of RCDII-induced-EATL.
Huang et al. (72) created a MEITL patient-derived xenograft (PDX) model which was subsequently utilized in the performance of an AI-driven digital medicine tool, the Quadratic Phenotypic Optimization Platform (QPOP), in generating predictions of response to combinations of therapeutic regimens. The combination of pimozide and romidepsin yielded high predictive efficacy against the MEITL model and further utility of this combination could be evaluated in human trials, with reductions in dose proposed to mediate the side effect profile associated with romidepsin toxicity.
Tumor microenvironment and immune cell function
In addition to the exploration of key cellular, immunological, and genetic features as covered above, we hope to round up our holistic discussion by considering a further look at the wider tumoral microenvironment (TME) and the complex role of different groups of microenvironmental immune cells in the interplay between new generation pharmacological agents and observed clinical responses. Treg cells have been identified to loosely conform to four main functional groups in the setting of lymphomas, predominantly that of suppressor Treg, malignant Treg, direct tumor-killing Treg, and incompetent Treg, of which this classification matters as the first two groups of Treg correlate higher circulating numbers of Treg cells with a poorer prognosis whereas the last two groups correlate higher numbers of Treg cells with an improved prognosis (204). In PTCL-NOS specifically, Treg cells were more frequently associated with suppressor cell function, directly contributing to the aggressiveness of the disease.
Tumor-associated macrophages (TAMs) are also key players in the lymphoma tumor microenvironment and have been postulated to create an immunosuppressive TME through the production of cytokines, growth factors, and inhibitory immune checkpoints, leading to cancer initiation and promotion, immune regulation and distant metastasis (205, 206). Whilst not yet extrapolated to the specific subset of PTCLs, paclitaxel was identified as an agent in non-Hodgkin lymphoma which was able to potentiate phagocytic capabilities of macrophages such as TAMs in CD47-targeting macrophage therapy (207). This potentially presents us with a peek into the possibility of utilizing TAMs to remodel the TME in lymphomas to induce tumoricidal effects and oncological clearance.
We finally turn to the activity of myeloid-derived suppressor cells (MDSCs) in the TME and its role in potentiating an immunosuppressive TME. MDSCs are pathologically activated neutrophils and monocytes with inherent immunosuppressive activity via upregulation of STAT3 expression, induction of endoplasmic reticulum stress, as well as utilization or reactive oxygen species (ROS), prostaglandin E2, and immunosuppressive cytokines (205, 208). In particular, MDSCs immunosuppressive activity downregulates pivotal signaling pathways, interrupting exploitative pathways utilized by PD-1 immune checkpoint inhibitors via FATP2 ROS-mediated immunosuppression and upregulated expression of PD-1/PD-L1 secondary to inflammation and T cell exhaustion, which could potentially be reversed via MDSC blockade (209–211). Epigenetic modifiers such as HDAC inhibitors have thus been identified to promote sensitization of tumor cells to PD-1/PD-L1 immune checkpoint inhibitors via reversal of MDSC’s immunosuppressive effect on the cancer milieu, demonstrating synergistic effects with immunotherapeutic options in solid tumors (212). We observe further agents such as artemisinin used in MDSC suppression, which improved the efficacy of anti-PD-L1 blockade therapy in mice with T cell lymphoma (213). The presence of a deficiency in the MDSC-polarizing TIPE2 gene additionally informed of MDSC activity and thus delayed tumor progression in mice (214). Though these current studies are of yet relatively removed from the field of PTCL, we believe that these developments can inform clinicians of future aspects for investigation in the field of PTCL surrounding epigenetic and immune checkpoint blockade therapy.
Conclusions and prospects
The literature surrounding biomarkers in the exploration of therapeutics in PTCL has come a long way in the last few years with the advent of numerous new trials and studies investigating biomarkers at both the genome and epigenome level. For reference, a consolidated figure delineating the most important biomarkers covered in our Review can be found below under Figure 1. Despite this, upon taking a closer look at the literature, we understand that the current landscape of biomarkers includes many that are prognostic in nature, but few and little present further utility as predictive markers to guide real-world clinical therapeutic decisions. A list of specific molecular features with direct effects on patient clinical outcomes and prognosis which can potentially inform clinicians on prognosis and survival into Table 3 below for reference at a glance.
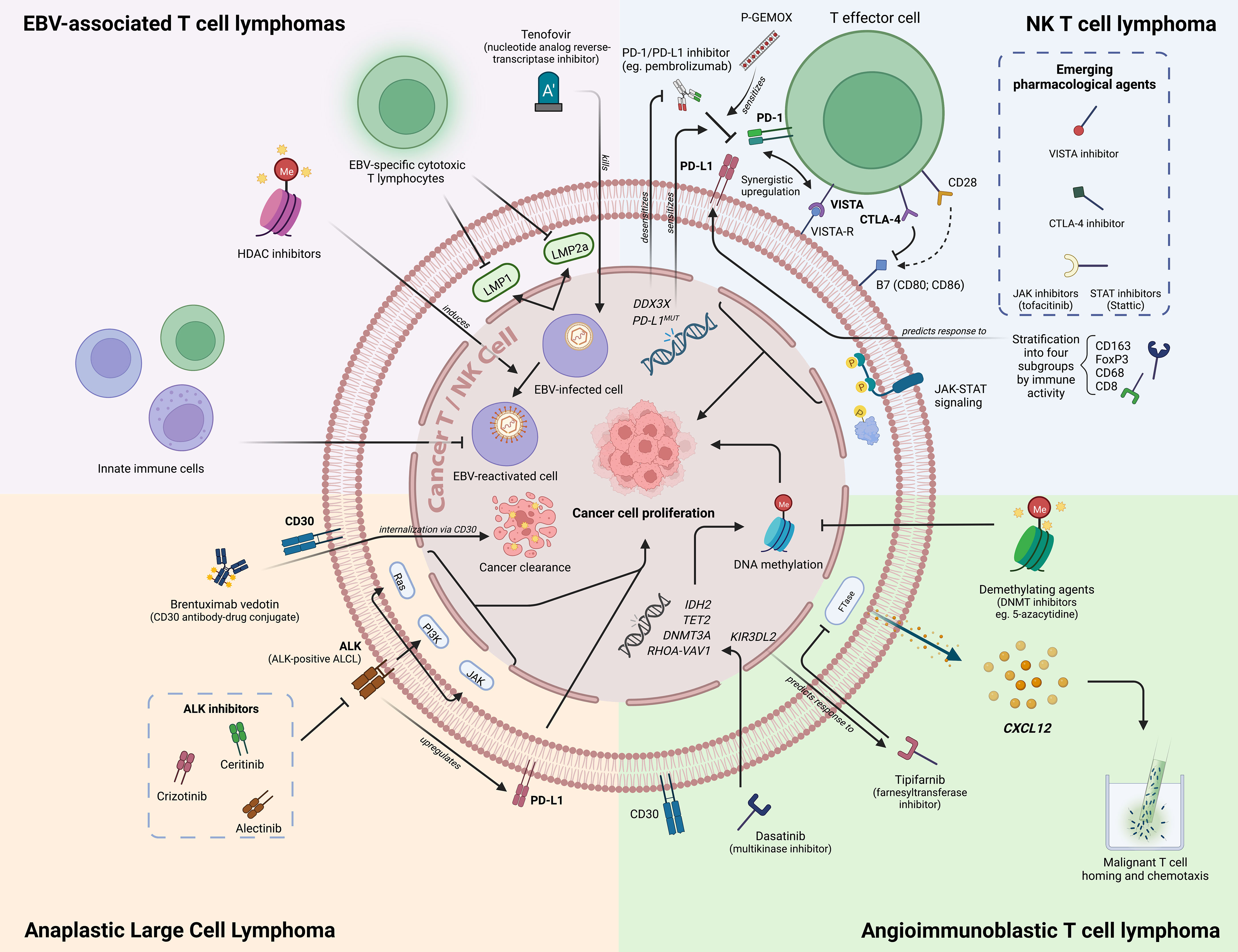
Figure 1 An overview of novel biomarkers in PTCL/NKTCL. Figure created with BioRender.com.
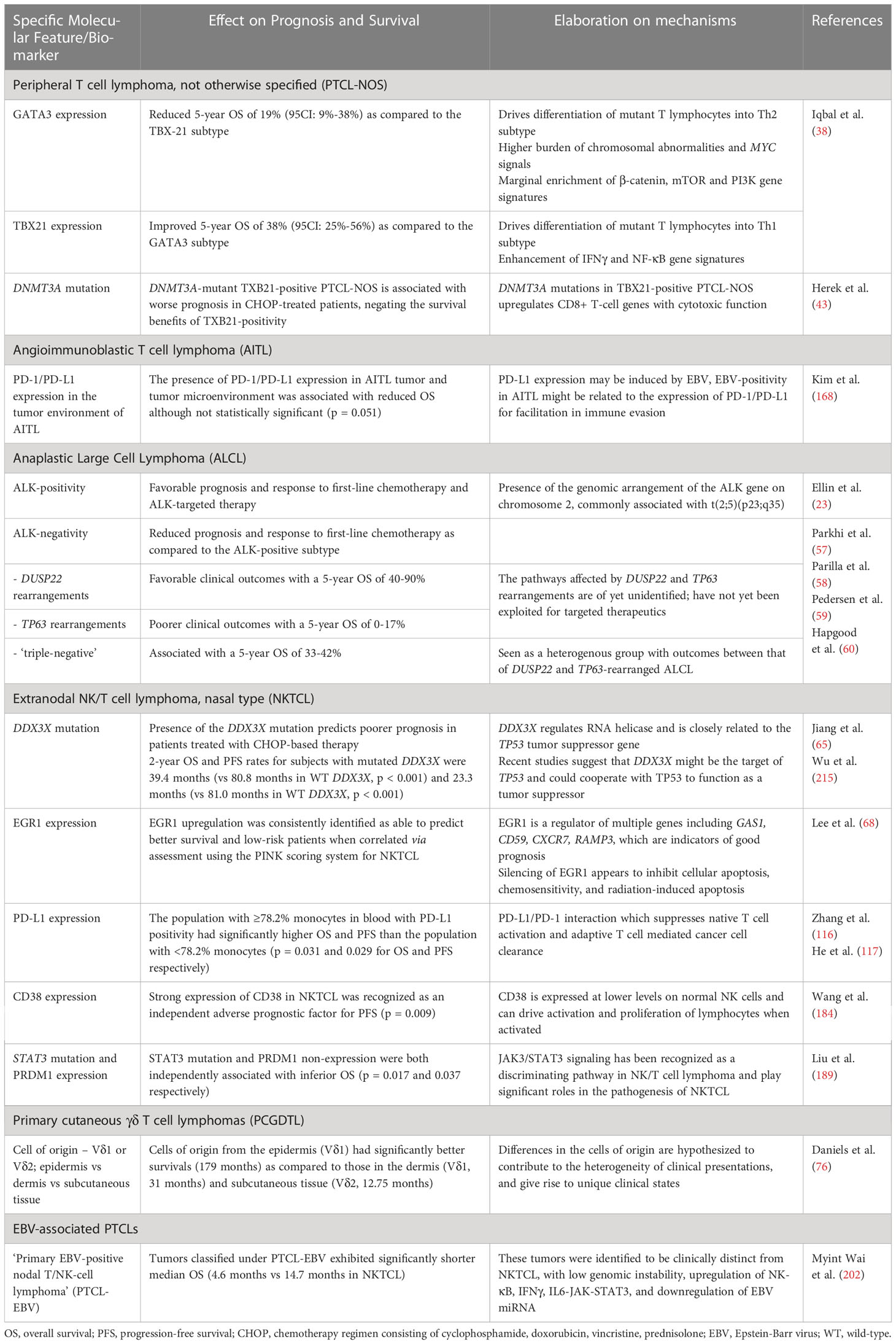
Table 3 Summary of the effect of specific molecular features and biomarkers on prognosis and survival.
A consolidated table of current novel therapeutics and their efficacies can also be viewed in Table 4. Whilst the current climate in the treatment for PTCL sparks optimism in its avid generation of numerous pathways for exploitation via novel therapeutic options, the current direction lacks a purpose and an efficiency that can only be matched via a better understanding of the efficacy of therapeutics in each individualized patient, guided by predictive biomarkers. There is thus a growing importance to move towards personalized treatment options and individualized targeted therapy as a means of improving treatment outcomes and minimizing mortality profiles. This includes an expansion of the role of prognostic indices to guide decisions in patient selection, along with further updating of our prognostic indices with new data that we have unearthed with respect to the role of molecular and immunological phenotypes in disease prognosis. With this, we can follow up with patient stratification based on assessed immunophenotypic subtypes and profiles for personalized treatment and management.
While we work on the identification of relevant tumor markers, we would also do well to continue our investigation into the utility of new molecular techniques. Chimeric antigen receptor (CAR) T cells have been developed as vessels for the deployment of immunologically active treatment towards targeting of malignant T cells in PTCL and show promising activity. Current agents in the market include that of tisagenlecleucel or axicabtagene ciloleucel which are specifically targeted against CD19, but these options have only been evaluated in the setting of B-cell lymphomas (216); CAR-T targeting CD30 has also been trialed in the subset of ALCL in an early phase I study (217). We also consider the possibility of cancer vaccines – vaccines against oncogenic infections such as EBV, but to date no efficacious vaccine development for prophylaxis of EBV infection has been pioneered (218).
Finally, after addressing the above lapses, we would do well to take a slight step back to assess the present roadblocks hindering the feasibility of personalized medicine as a whole. Issues at hand pertaining to the cost of detailed investigations require comprehensive cost-benefit analyses which should inform decision making via the stratification of patients into subgroups which predict the utility of further testing. These subgroups should be based on preliminary identification of tumor biology and the selection of high-yield biomarkers for testing, and consistently cross-referenced against present and developing literature. As the throughput for personalized medicine rises, non-invasive techniques such as liquid biopsies could present an important quality of life consideration, utilizing ctDNA as a means for planning for precision therapeutics and serial monitoring of tumor characteristics and biomarker availability. In time, we also envision a role for multiplex methods such as spatial profiling amidst a clinical landscape whereby hundreds of important biomarkers are routinely considered, providing clinicians and researchers alike with the ability to explore disease profiles across space and time. We thus look towards these exciting prospects on the horizon as we continue our work in developing this field towards a refinement of personalized and precision medicine.
Author contributions
Conceptualization DY, CO, and JC; original draft preparation, DY and JC; writing, review and editing, DY, JL, DH, CO, and JC. All authors contributed to the article and approved the submitted version.
Funding
This work was supported by the Tanoto Foundation Professorship in Medical Oncology, New Century Foundation Limited, Ling Foundation, Singapore Ministry of Health’s National Medical Research Council Research Transition Award (TA21jun-0005), Large Collaborative Grant (OFLCG18May-0028), and Collaborative Centre Grant (TETRAD II). The funders were not involved in the study design, collection, analysis, and interpretation of data, the writing of this article or the decision to submit it for publication.
Conflict of interest
The authors declare that the research was conducted in the absence of any commercial or financial relationships that could be construed as a potential conflict of interest.
Publisher’s note
All claims expressed in this article are solely those of the authors and do not necessarily represent those of their affiliated organizations, or those of the publisher, the editors and the reviewers. Any product that may be evaluated in this article, or claim that may be made by its manufacturer, is not guaranteed or endorsed by the publisher.
Supplementary material
The Supplementary Material for this article can be found online at: https://www.frontiersin.org/articles/10.3389/fimmu.2023.1068662/full#supplementary-material
References
1. Armitage JO. The aggressive peripheral T-cell lymphomas: 2015. Am J Hematol (2015) 90:665–73. doi: 10.1002/ajh.24076
2. Swerdlow SH, Campo E, Pileri SA, Harris NL, Stein H, Siebert R, et al. The 2016 revision of the world health organization classification of lymphoid neoplasms. Blood (2016) 127:2375–90. doi: 10.1182/blood-2016-01-643569
3. Campo E, Swerdlow SH, Harris NL, Pileri S, Stein H, Jaffe ES. The 2008 WHO classification of lymphoid neoplasms and beyond: evolving concepts and practical applications. Blood (2011) 117:5019–32. doi: 10.1182/blood-2011-01-293050
4. Cheson BD, Fisher RI, Barrington SF, Cavalli F, Schwartz LH, Zucca E, et al. Recommendations for initial evaluation, staging, and response assessment of Hodgkin and non-Hodgkin lymphoma: The lugano classification. J Clin Oncol (2014) 32:3059–67. doi: 10.1200/JCO.2013.54.8800
5. Vose JM. Peripheral T-cell non-hodgkin’s lymphoma. Hematol Oncol Clin North Am (2008) 22:997–1005. doi: 10.1016/j.hoc.2008.07.010
6. Foss FM, Zinzani PL, Vose JM, Gascoyne RD, Rosen ST, Tobinai K. Peripheral T-cell lymphoma. Blood (2011) 117:6756–67. doi: 10.1182/blood-2010-05-231548
7. AbouYabis AN, Shenoy PJ, Sinha R, Flowers CR, Lechowicz MJ. A systematic review and meta-analysis of front-line anthracycline-based chemotherapy regimens for peripheral T-cell lymphoma. ISRN Hematol (2011) 2011:623924. doi: 10.5402/2011/623924
8. O’Connor OA, Pro B, Pinter-Brown L, Bartlett N, Popplewell L, Coiffier B, et al. Pralatrexate in patients with relapsed or refractory peripheral T-cell lymphoma: Results from the pivotal PROPEL study. J Clin Oncol (2011) 29:1182–9. doi: 10.1200/JCO.2010.29.9024
9. Bellei M, Foss FM, Shustov AR, Horwitz SM, Marcheselli L, Kim WS, et al. The outcome of peripheral T-cell lymphoma patients failing first-line therapy: a report from the prospective, international T-cell project. Haematologica (2018) 103:1191–7. doi: 10.3324/haematol.2017.186577
10. Lansigan F, Horwitz SM, Pinter-Brown LC, Rosen ST, Pro B, Hsi ED, et al. Outcomes for relapsed and refractory peripheral T-cell lymphoma patients after front-line therapy from the COMPLETE registry. Acta Haematol (2020) 143:40–50. doi: 10.1159/000500666
11. International T-Cell Lymphoma Project. International peripheral T-cell and natural Killer/T-cell lymphoma study: Pathology findings and clinical outcomes. J Clin Oncol (2008) 26:4124–30. doi: 10.1200/JCO.2008.16.4558
12. Agostinelli C, Piccaluga PP, Went P, Rossi M, Gazzola A, Righi S, et al. Peripheral T cell lymphoma, not otherwise specified: the stuff of genes, dreams and therapies. J Clin Pathol (2008) 61:1160–7. doi: 10.1136/jcp.2008.055335
13. Went P, Agostinelli C, Gallamini A, Piccaluga PP, Ascani S, Sabattini E, et al. Marker expression in peripheral T-cell lymphoma: A proposed clinical-pathologic prognostic score. J Clin Oncol (2006) 24:2472–9. doi: 10.1200/JCO.2005.03.6327
14. Weisenburger DD, Savage KJ, Harris NL, Gascoyne RD, Jaffe ES, MacLennan KA, et al. Peripheral T-cell lymphoma, not otherwise specified: a report of 340 cases from the international peripheral T-cell lymphoma project. Blood (2011) 117:3402–8. doi: 10.1182/blood-2010-09-310342
15. Dupuis J, Emile JF, Mounier N, Gisselbrecht C, Martin-Garcia N, Petrella T, et al. Prognostic significance of Epstein-Barr virus in nodal peripheral T-cell lymphoma, unspecified: a groupe d’Etude des lymphomes de l’Adulte (GELA) study. Blood (2006) 108:4163–9. doi: 10.1182/blood-2006-04-017632
16. Schmitz N, Trümper L, Ziepert M, Nickelsen M, Ho AD, Metzner B, et al. Treatment and prognosis of mature T-cell and NK-cell lymphoma: an analysis of patients with T-cell lymphoma treated in studies of the German high-grade non-Hodgkin lymphoma study group. Blood (2010) 116:3418–25. doi: 10.1182/blood-2010-02-270785
17. Qian L, Yan M, Zhang W, Zhou D, Zhang Y, Huo L, et al. Prognostic value of interim 18F-FDG PET/CT in T-cell lymphomas. Leuk Lymphoma. (2020) 61:927–33. doi: 10.1080/10428194.2019.1697815
18. Malecek MK, Mehta-Shah N. Prognosis and risk stratification of peripheral T-cell lymphomas. Semin Hematol (2021) 58:70–7. doi: 10.1053/j.seminhematol.2021.02.001
19. Aldin A, Umlauff L, Estcourt LJ, Collins G, Moons KG, Engert A, et al. Interim PET-results for prognosis in adults with Hodgkin lymphoma: a systematic review and meta-analysis of prognostic factor studies. Cochrane Database Syst Rev (2020) 2020(1):CD012643. doi: 10.1002/14651858.CD012643.pub2
20. Casulo C, Dixon JG, Le-Rademacher J, Hoster E, Hochster HS, Hiddemann W, et al. Validation of POD24 as a robust early clinical end point of poor survival in FL from 5225 patients on 13 clinical trials. Blood (2022) 139:1684–93. doi: 10.1182/blood.2020010263
21. Skrypets T, Civallero M, Manni M, Pileri S, D Weisenburger D, Hyeh Ko Y, et al. TCL-233: Angioimmunoblastic T-cell lymphoma: Report on 282 cases from the prospective international T-cell lymphoma project. Clin Lymphoma Myeloma Leuk. (2020) 20:S253–4. doi: 10.1016/S2152-2650(20)30851-X
22. International Non-Hodgkin's Lymphoma Prognostic Factors Project. A predictive model for aggressive non-hodgkin’s lymphoma. N Engl J Med (1993) 329:987–94. doi: 10.1056/NEJM199309303291402
23. Ellin F, Landström J, Jerkeman M, Relander T. Real-world data on prognostic factors and treatment in peripheral T-cell lymphomas: a study from the Swedish lymphoma registry. Blood (2014) 124:1570–7. doi: 10.1182/blood-2014-04-573089
24. Savage KJ, Chhanabhai M, Gascoyne RD, Connors JM. Characterization of peripheral T-cell lymphomas in a single north American institution by the WHO classification. Ann Oncol (2004) 15:1467–75. doi: 10.1093/annonc/mdh392
25. Mulvey E, Ruan J. Biomarker-driven management strategies for peripheral T cell lymphoma. J Hematol OncolJ Hematol Oncol (2020) 13:59. doi: 10.1186/s13045-020-00889-z
26. Gallamini A, Stelitano C, Calvi R, Bellei M, Mattei D, Vitolo U, et al. Peripheral T-cell lymphoma unspecified (PTCL-u): a new prognostic model from a retrospective multicentric clinical study. Blood (2004) 103:2474–9. doi: 10.1182/blood-2003-09-3080
27. Federico M, Bellei M, Marcheselli L, Schwartz M, Manni M, Tarantino V, et al. Peripheral T cell lymphoma, not otherwise specified (PTCL-NOS). a new prognostic model developed by the international T cell project network. . Br J Haematol (2018) 181:760–9. doi: 10.1111/bjh.15258
28. Gutiérrez-García G, García-Herrera A, Cardesa T, Martínez A, Villamor N, Ghita G, et al. Comparison of four prognostic scores in peripheral T-cell lymphoma. Ann Oncol (2011) 22:397–404. doi: 10.1093/annonc/mdq359
29. Yoon SE, Song Y, Kim SJ, Yoon DH, Chen TY, Koh Y, et al. Comprehensive analysis of peripheral T-cell and natural killer/T-cell lymphoma in Asian patients: A multinational, multicenter, prospective registry study in Asia. Lancet Reg Health - West Pac. (2021) 10:100126. doi: 10.1016/j.lanwpc.2021.100126
30. Federico M, Rudiger T, Bellei M, Nathwani BN, Luminari S, Coiffier B, et al. Clinicopathologic characteristics of angioimmunoblastic T-cell lymphoma: Analysis of the international peripheral T-cell lymphoma project. J Clin Oncol (2013) 31:240–6. doi: 10.1200/JCO.2011.37.3647
31. Advani RH, Skrypets T, Civallero M, Spinner MA, Manni M, Kim WS, et al. Outcomes and prognostic factors in angioimmunoblastic T-cell lymphoma: final report from the international T-cell project. Blood (2021) 138:213–20. doi: 10.1182/blood.2020010387
32. Lee J, Suh C, Park YH, Ko YH, Bang SM, Lee JH, et al. Extranodal natural killer T-cell lymphoma, nasal-type: A prognostic model from a retrospective multicenter study. J Clin Oncol (2006) 24:612–8. doi: 10.1200/JCO.2005.04.1384
33. Kim SJ, Yoon DH, Jaccard A, Chng WJ, Lim ST, Hong H, et al. A prognostic index for natural killer cell lymphoma after non-anthracycline-based treatment: a multicentre, retrospective analysis. Lancet Oncol (2016) 17:389–400. doi: 10.1016/S1470-2045(15)00533-1
34. Tan KM, Chia B, Lim JQ, Khoo LP, Cheng CL, Tan L, et al. A clinicohaematological prognostic model for nasal-type natural killer/T-cell lymphoma: A multicenter study. Sci Rep (2019) 9:14961. doi: 10.1038/s41598-019-51522-0
35. Huang HL, Ngam PI, Tan KM, Ng DCE, Lim ST, Chan JY. The exact deauville score, NABS score and high SUVmax predicts outcome in extranodal natural killer/T-cell lymphoma. Ann Nucl Med (2021) 35:557–68. doi: 10.1007/s12149-021-01598-4
36. Amador C, Bouska A, Wright G, Weisenburger DD, Feldman AL, Greiner TC, et al. Gene expression signatures for the accurate diagnosis of peripheral T-cell lymphoma entities in the routine clinical practice. J Clin Oncol (2022). 40:4262–75. doi: 10.1200/JCO.21.02707
37. Zain JM, Hanona P. Aggressive T-cell lymphomas: 2021 updates on diagnosis, risk stratification and management. Am J Hematol (2021) 96:1027–46. doi: 10.1002/ajh.26270
38. Iqbal J, Wright G, Wang C, Rosenwald A, Gascoyne RD, Weisenburger DD, et al. Gene expression signatures delineate biological and prognostic subgroups in peripheral T-cell lymphoma. Blood (2014) 123:2915–23. doi: 10.1182/blood-2013-11-536359
39. Heavican TB, Bouska A, Yu J, Lone W, Amador C, Gong Q, et al. Genetic drivers of oncogenic pathways in molecular subgroups of peripheral T-cell lymphoma. Blood (2019) 133:1664–76. doi: 10.1182/blood-2018-09-872549
40. Oluwasanjo A, Kartan S, Johnson W, Alpdogan O, Gru A, Mishra A, et al. Peripheral T-cell lymphoma, not otherwise specified (PTCL-NOS). Cancer Treat Res (2019) 176:83–98. doi: 10.1007/978-3-319-99716-2_4
41. Gao X, Wang C, Abdelrahman S, Kady N, Murga-Zamalloa C, Gann P, et al. Notch signaling promotes mature T-cell lymphomagenesis. Cancer Res (2022). 82:3763–73. doi: 10.1158/0008-5472.CAN-22-1215
42. Debackere K, Marcelis L, Demeyer S, Vanden Bempt M, Mentens N, Gielen O, et al. Fusion transcripts FYN-TRAF3IP2 and KHDRBS1-LCK hijack T cell receptor signaling in peripheral T-cell lymphoma, not otherwise specified. Nat Commun (2021) 12:3705. doi: 10.1038/s41467-021-24037-4
43. Herek TA, Bouska A, Lone WG, Sharma S, Amador C, Heavican-Foral TB, et al. DNMT3A mutations define a unique biological and prognostic subgroup associated with cytotoxic T-cells in PTCL-NOS. Blood (2022). 140:1278–90. doi: 10.1182/blood.2021015019
44. Chiba S, Sakata-Yanagimoto M. Advances in understanding of angioimmunoblastic T-cell lymphoma. Leukemia (2020) 34:2592–606. doi: 10.1038/s41375-020-0990-y
45. de Leval L, Rickman DS, Thielen C, de Reynies A, Huang YL, Delsol G, et al. The gene expression profile of nodal peripheral T-cell lymphoma demonstrates a molecular link between angioimmunoblastic T-cell lymphoma (AITL) and follicular helper T (TFH) cells. Blood (2007) 109:4952–63. doi: 10.1182/blood-2006-10-055145
46. Odejide O, Weigert O, Lane AA, Toscano D, Lunning MA, Kopp N, et al. A targeted mutational landscape of angioimmunoblastic T-cell lymphoma. Blood (2014) 123:1293–6. doi: 10.1182/blood-2013-10-531509
47. Yoo HY, Sung MK, Lee SH, Kim S, Lee H, Park S, et al. A recurrent inactivating mutation in RHOA GTPase in angioimmunoblastic T cell lymphoma. Nat Genet (2014) 46:371–5. doi: 10.1038/ng.2916
48. Sakata-Yanagimoto M, Enami T, Yoshida K, Shiraishi Y, Ishii R, Miyake Y, et al. Somatic RHOA mutation in angioimmunoblastic T cell lymphoma. Nat Genet (2014) 46:171–5. doi: 10.1038/ng.2872
49. Palomero T, Couronné L, Khiabanian H, Kim MY, Ambesi-Impiombato A, Perez-Garcia A, et al. Recurrent mutations in epigenetic regulators, RHOA and FYN kinase in peripheral T cell lymphomas. Nat Genet (2014) 46:166–70. doi: 10.1038/ng.2873
50. Lunning MA, Vose JM. Angioimmunoblastic T-cell lymphoma: the many-faced lymphoma. Blood (2017) 129:1095–102. doi: 10.1182/blood-2016-09-692541
51. Rodríguez M, Alonso-Alonso R, Tomás-Roca L, Rodríguez-Pinilla SM, Manso-Alonso R, Cereceda L, et al. Peripheral T-cell lymphoma: molecular profiling recognizes subclasses and identifies prognostic markers. Blood Adv (2021) 5:5588–98. doi: 10.1182/bloodadvances.2021005171
52. Iqbal J, Weisenburger DD, Greiner TC, Vose JM, McKeithan T, Kucuk C, et al. Molecular signatures to improve diagnosis in peripheral T-cell lymphoma and prognostication in angioimmunoblastic T-cell lymphoma. Blood (2010) 115:1026–36. doi: 10.1182/blood-2009-06-227579
53. Shustov A, Soma L. Anaplastic Large cell lymphoma: Contemporary concepts and optimal management. T-Cell NK-Cell Lymphomas (2019). 176:127–44. doi: 10.1007/978-3-319-99716-2_6
54. Leventaki V, Bhattacharyya S, Lim MS. Pathology and genetics of anaplastic large cell lymphoma. Semin Diagn Pathol (2020) 37:57–71. doi: 10.1053/j.semdp.2019.12.002
55. Adams SV, Newcomb PA, Shustov AR. Racial patterns of peripheral T-cell lymphoma incidence and survival in the united states. J Clin Oncol (2016) 34:963–71. doi: 10.1200/JCO.2015.63.5540
56. Amador C, Feldman AL. How I diagnose anaplastic Large cell lymphoma. Am J Clin Pathol (2021) 155:479–97. doi: 10.1093/ajcp/aqab012
57. Parkhi M, Bal A, Das A, Kashyap D, Bhardwaj S, Prakash G, et al. ALK-negative anaplastic Large cell lymphoma (ALCL): Prognostic implications of molecular subtyping and JAK-STAT pathway. Appl Immunohistochem Mol Morphol. (2021) 29:648–56. doi: 10.1097/PAI.0000000000000936
58. Parrilla Castellar ER, Jaffe ES, Said JW, Swerdlow SH, Ketterling RP, Knudson RA, et al. ALK-negative anaplastic large cell lymphoma is a genetically heterogeneous disease with widely disparate clinical outcomes. Blood (2014) 124:1473–80. doi: 10.1182/blood-2014-04-571091
59. Pedersen MB, Hamilton-Dutoit SJ, Bendix K, Ketterling RP, Bedroske PP, Luoma IM, et al. DUSP22 and TP63 rearrangements predict outcome of ALK-negative anaplastic large cell lymphoma: a Danish cohort study. Blood (2017) 130:554–7. doi: 10.1182/blood-2016-12-755496
60. Hapgood G, Ben-Neriah S, Mottok A, Lee DG, Robert K, Villa D, et al. Identification of high-risk DUSP22-rearranged ALK-negative anaplastic large cell lymphoma. Br J Haematol (2019) 186:e28–31. doi: 10.1111/bjh.15860
61. Nagata H, Konno A, Kimura N, Zhang Y, Kimura M, Demachi A, et al. Characterization of novel natural killer (NK)–cell and γδ T-cell lines established from primary lesions of nasal T/NK-cell lymphomas associated with the Epstein-Barr virus. Blood (2001) 97:708–13. doi: 10.1182/blood.V97.3.708
62. Tse E, Kwong YL. How I treat NK/T-cell lymphomas. Blood (2013) 121:4997–5005. doi: 10.1182/blood-2013-01-453233
63. de Mel S, Hue SSS, Jeyasekharan AD, Chng WJ, Ng SB. Molecular pathogenic pathways in extranodal NK/T cell lymphoma. J Hematol OncolJ Hematol Oncol (2019) 12:33. doi: 10.1186/s13045-019-0716-7
64. Wang H, Fu B-b, Gale RP, Liang Y. NK-/T-cell lymphomas. Leukemia (2021) 35:2460–8. doi: 10.1038/s41375-021-01313-2
65. Jiang L, Gu ZH, Yan ZX, Zhao X, Xie YY, Zhang ZG, et al. Exome sequencing identifies somatic mutations of DDX3X in natural killer/T-cell lymphoma. Nat Genet (2015) 47:1061–6. doi: 10.1038/ng.3358
66. Xiong J, Cui BW, Wang N, Dai YT, Zhang H, Wang CF, et al. Genomic and transcriptomic characterization of natural killer T cell lymphoma. Cancer Cell (2020) 37:403–419.e6. doi: 10.1016/j.ccell.2020.02.005
67. Chan JY, Lim JQ, Ong CK. Towards next generation biomarkers in natural Killer/T-cell lymphoma. Life (2021) 11:838. doi: 10.3390/life11080838
68. Lee JY, Kim JH, Bang H, Cho J, Ko YH, Kim SJ, et al. EGR1 as a potential marker of prognosis in extranodal NK/T-cell lymphoma. Sci Rep (2021) 11:10342. doi: 10.1038/s41598-021-89754-8
69. Malamut G, Chandesris O, Verkarre V, Meresse B, Callens C, Macintyre E, et al. Enteropathy associated T cell lymphoma in celiac disease: A large retrospective study. Dig Liver Dis (2013) 45:377–84. doi: 10.1016/j.dld.2012.12.001
70. Malamut G, Cellier C. Refractory celiac disease. Gastroenterol Clin North Am (2019) 48:137–44. doi: 10.1016/j.gtc.2018.09.010
71. Veloza L, Cavalieri D, Missiaglia E, Ledoux-Pilon A, Bisig B, Pereira B, et al. Monomorphic epitheliotropic intestinal T-cell lymphoma comprises morphologic and genomic heterogeneity impacting outcome. Haematologica (2022). 108:181–85. doi: 10.3324/haematol.2022.281226
72. Huang D, Lim JQ, Cheah DMZ, Kahliab KHBM, Laurensia Y, Pang JWL, et al. Whole-genome sequencing reveals potent therapeutic strategy for monomorphic epitheliotropic intestinal T-cell lymphoma. Blood Adv (2020) 4:4769–74. doi: 10.1182/bloodadvances.2020001782
73. Yi JH, Lee GW, Do YR, Jung HR, Hong JY, Yoon DH, et al. Multicenter retrospective analysis of the clinicopathologic features of monomorphic epitheliotropic intestinal T-cell lymphoma. Ann Hematol (2019) 98:2541–50. doi: 10.1007/s00277-019-03791-y
74. McKinney M, Moffitt AB, Gaulard P, Travert M, De Leval L, Nicolae A, et al. The genetic basis of hepatosplenic T cell lymphoma. Cancer Discovery (2017) 7:369–79. doi: 10.1158/2159-8290.CD-16-0330
75. Pro B, Allen P, Behdad A. Hepatosplenic T-cell lymphoma: a rare but challenging entity. Blood (2020) 136:2018–26. doi: 10.1182/blood.2019004118
76. Daniels J, Doukas PG, Escala MEM, Ringbloom KG, Shih DJH, Yang J, et al. Cellular origins and genetic landscape of cutaneous gamma delta T cell lymphomas. Nat Commun (2020) 11:1806. doi: 10.1038/s41467-020-15572-7
77. Briski R, Feldman AL, Bailey NG, Lim MS, Ristow K, Habermann TM, et al. The role of front-line anthracycline-containing chemotherapy regimens in peripheral T-cell lymphomas. Blood Cancer J (2014) 4:e214–4. doi: 10.1038/bcj.2014.34
78. Horwitz S, O’Connor OA, Pro B, Illidge T, Fanale M, Advani R, et al. Brentuximab vedotin with chemotherapy for CD30-positive peripheral T-cell lymphoma (ECHELON-2): a global, double-blind, randomised, phase 3 trial. Lancet Lond Engl (2019) 393:229–40. doi: 10.1016/S0140-6736(18)32984-2
79. Yin J, Wei J, Xu JH, Xiao Y, Zhang YC. Autologous stem cell transplantation as the first-line treatment for peripheral T cell lymphoma: Results of a comprehensive meta-analysis. Acta Haematol (2014) 131:114–25. doi: 10.1159/000353778
80. Park SI, Horwitz SM, Foss FM, Pinter-Brown LC, Carson KR, Rosen ST, et al. The role of autologous stem cell transplantation in patients with nodal peripheral T-cell lymphomas in first complete remission: Report from COMPLETE, a prospective, multicenter cohort study. Cancer (2019) 125:1507–17. doi: 10.1002/cncr.31861
81. Yamaguchi M, Kwong YL, Kim WS, Maeda Y, Hashimoto C, Suh C, et al. Phase II study of SMILE chemotherapy for newly diagnosed stage IV, relapsed, or refractory extranodal natural killer (NK)/T-cell lymphoma, nasal type: The NK-cell tumor study group study. J Clin Oncol (2011) 29:4410–6. doi: 10.1200/JCO.2011.35.6287
82. Kwong YL, Kim WS, Lim ST, Kim SJ, Tang T, Tse E, et al. SMILE for natural killer/T-cell lymphoma: analysis of safety and efficacy from the Asia lymphoma study group. Blood (2012) 120:2973–80. doi: 10.1182/blood-2012-05-431460
83. Wang JH, Wang L, Liu CC, Xia ZJ, Huang HQ, Lin TY, et al. Efficacy of combined gemcitabine, oxaliplatin and pegaspargase (P-gemox regimen) in patients with newly diagnosed advanced-stage or relapsed/refractory extranodal NK/T-cell lymphoma. Oncotarget (2016) 7:29092–101. doi: 10.18632/oncotarget.8647
84. Wang X, Zhang L, Liu X, Li X, Li L, Fu X, et al. Efficacy and safety of a pegasparaginase-based chemotherapy regimen vs an l-asparaginase–based chemotherapy regimen for newly diagnosed advanced extranodal natural Killer/T-cell lymphoma: A randomized clinical trial. JAMA Oncol (2022) 8:1035–41. doi: 10.1001/jamaoncol.2022.1968
85. Jaccard A, Gachard N, Marin B, Rogez S, Audrain M, Suarez F, et al. Efficacy of l-asparaginase with methotrexate and dexamethasone (AspaMetDex regimen) in patients with refractory or relapsing extranodal NK/T-cell lymphoma, a phase 2 study. Blood (2011) 117:1834–9. doi: 10.1182/blood-2010-09-307454
86. Chan JY, Lim ST. Novel findings from the Asian lymphoma study group: focus on T and NK-cell lymphomas. Int J Hematol (2018) 107:413–9. doi: 10.1007/s12185-018-2406-6
87. Damaj G, Gressin R, Bouabdallah K, Cartron G, Choufi B, Gyan E, et al. Results from a prospective, open-label, phase II trial of bendamustine in refractory or relapsed T-cell lymphomas: The BENTLY trial. J Clin Oncol (2013) 31:104–10. doi: 10.1200/JCO.2012.43.7285
88. Piccaluga PP, Agostinelli C, Righi S, Zinzani PL, Pileri SA. Expression of CD52 in peripheral T-cell lymphoma. Haematologica (2007) 92:566–7. doi: 10.3324/haematol.10767
89. Jiang L, Yuan C, Hubacheck J, Janik JE, Wilson W, Morris JC, et al. Variable CD52 expression in mature T cell and NK cell malignancies: Implications for alemtuzumab therapy. Br J Haematol (2009) 145:173–9. doi: 10.1111/j.1365-2141.2009.07606.x
90. Rodig SJ, Abramson JS, Pinkus GS, Treon SP, Dorfman DM, Dong HY, et al. Heterogeneous CD52 expression among hematologic neoplasms: Implications for the use of alemtuzumab (CAMPATH-1H). Clin Cancer Res (2006) 12:7174–9. doi: 10.1158/1078-0432.CCR-06-1275
91. Geissinger E, Bonzheim I, Roth S, Rosenwald A, Müller-Hermelink HK, Rüdiger T. CD52 expression in peripheral T-cell lymphomas determined by combined immunophenotyping using tumor cell specific T-cell receptor antibodies. Leuk Lymphoma. (2009) 50:1010–6. doi: 10.1080/10428190902926981
92. Chang ST, Lu CL, Chuang SS. CD52 expression in non-mycotic T- and NK/T-cell lymphomas. Leuk Lymphoma. (2007) 48:117–21. doi: 10.1080/10428190601016167
93. Gallamini A, Zaja F, Patti C, Billio A, Specchia MR, Tucci A, et al. Alemtuzumab (Campath-1H) and CHOP chemotherapy as first-line treatment of peripheral T-cell lymphoma: results of a GITIL (Gruppo italiano terapie innovative nei linfomi) prospective multicenter trial. Blood (2007) 110:2316–23. doi: 10.1182/blood-2007-02-074641
94. d’Amore F, Leppä S, Silva MGDA, Relander T, Lauritzsen GF, Brown PDN, et al. Final analysis of the front-line phase III randomized ACT-1 trial in younger patients with systemic peripheral T-cell lymphoma treated with CHOP chemotherapy with or without alemtuzumab and consolidated by autologous hematopoietic stem cell transplant. Blood (2018) 132:998. doi: 10.1182/blood-2018-99-110429
95. Wulf GG, Altmann B, Ziepert M, D’Amore F, Held G, Greil R, et al. Alemtuzumab plus CHOP versus CHOP in elderly patients with peripheral T-cell lymphoma: the DSHNHL2006-1B/ACT-2 trial. Leukemia (2021) 35:143–55. doi: 10.1038/s41375-020-0838-5
96. Dhandha MM, Sufficool KE, Vidal CI, Robbins KJ, Fesler MJ, Batanian JR, et al. Immunophenotype expression change from CD52+ to CD52– on erythrodermic peripheral T-cell lymphoma, not otherwise specified after treatment with alemtuzumab. Am J Dermatopathol. (2018) 40:547–50. doi: 10.1097/DAD.0000000000001000
97. Maeshima AM, Taniguchi H, Fujino T, Saito Y, Ito Y, Hatta S, et al. Immunohistochemical CD20-negative change in b-cell non-Hodgkin lymphomas after rituximab-containing therapy. Ann Hematol (2020) 99:2141–8. doi: 10.1007/s00277-019-03853-1
98. Remer M, Al-Shamkhani A, Glennie M, Johnson P. Mogamulizumab and the treatment of CCR4-positive T-cell lymphomas. Immunotherapy (2014) 6:1187–206. doi: 10.2217/imt.14.94
99. Balkwill F, Mantovani A. Inflammation and cancer: back to virchow? Lancet (2001) 357:539–45. doi: 10.1016/S0140-6736(00)04046-0
100. Ishida T, Inagaki H, Utsunomiya A, Takatsuka Y, Komatsu H, Iida S, et al. CXC chemokine receptor 3 and CC chemokine receptor 4 expression in T-cell and NK-cell lymphomas with special reference to clinicopathological significance for peripheral T-cell lymphoma, unspecified. Clin Cancer Res (2004) 10:5494–500. doi: 10.1158/1078-0432.CCR-04-0371
101. Ogura M, Ishida T, Hatake K, Taniwaki M, Ando K, Tobinai K, et al. Multicenter phase II study of mogamulizumab (KW-0761), a defucosylated anti-CC chemokine receptor 4 antibody, in patients with relapsed peripheral T-cell lymphoma and cutaneous T-cell lymphoma. J Clin Oncol (2014) 32:1157–63. doi: 10.1200/JCO.2013.52.0924
102. Alperovich A, Younes A. Targeting CD30 using brentuximab vedotin in the treatment of Hodgkin lymphoma. Cancer J Sudbury Mass. (2016) 22:23–6. doi: 10.1097/PPO.0000000000000168
103. Maura F, Dodero A, Carniti C, Bolli N. Biology of peripheral T cell lymphomas – not otherwise specified: Is something finally happening? Pathogenesis (2016) 3:9–18. doi: 10.1016/j.pathog.2016.02.002
104. Bossard C, Dobay MP, Parrens M, Lamant L, Missiaglia E, Haioun C, et al. Immunohistochemistry as a valuable tool to assess CD30 expression in peripheral T-cell lymphomas: high correlation with mRNA levels. Blood (2014) 124:2983–6. doi: 10.1182/blood-2014-07-584953
105. Sabattini E, Pizzi M, Tabanelli V, Baldin P, Sacchetti CS, Agostinelli C, et al. CD30 expression in peripheral T-cell lymphomas. Haematologica (2013) 98:e81–2. doi: 10.3324/haematol.2013.084913
106. Horwitz S, O’Connor OA, Pro B, Trümper L, Iyer S, Advani R, et al. The ECHELON-2 trial: 5-year results of a randomized, phase III study of brentuximab vedotin with chemotherapy for CD30-positive peripheral T-cell lymphoma☆. Ann Oncol (2022) 33:288–98. doi: 10.1016/j.annonc.2021.12.002
107. Strauchen JA, Breakstone BA. IL-2 receptor expression in human lymphoid lesions. immunohistochemical study of 166 cases. Am J Pathol (1987) 126:506–12.
108. Karube K, Aoki R, Nomura Y, Yamamoto K, Shimizu K, Yoshida S, et al. Usefulness of flow cytometry for differential diagnosis of precursor and peripheral T-cell and NK-cell lymphomas: Analysis of 490 cases. Pathol Int (2008) 58:89–97. doi: 10.1111/j.1440-1827.2007.02195.x
109. Foss FM, Sjak-Shie N, Goy A, Jacobsen E, Advani R, Smith MR, et al. A multicenter phase II trial to determine the safety and efficacy of combination therapy with denileukin diftitox and cyclophosphamide, doxorubicin, vincristine and prednisone in untreated peripheral T-cell lymphoma: the CONCEPT study. Leuk Lymphoma. (2013) 54:1373–9. doi: 10.3109/10428194.2012.742521
110. Dang NH, Pro B, Hagemeister FB, Samaniego F, Jones D, Samuels BI, et al. Phase II trial of denileukin diftitox for relapsed/refractory T-cell non-Hodgkin lymphoma. Br J Haematol (2007) 136:439–47. doi: 10.1111/j.1365-2141.2006.06457.x
111. Kawai H, Ando K, Maruyama D, Yamamoto K, Kiyohara E, Terui Y, et al. Phase II study of E7777 in Japanese patients with relapsed/refractory peripheral and cutaneous T-cell lymphoma. Cancer Sci (2021) 112:2426–35. doi: 10.1111/cas.14906
112. Hamadani M, Collins GP, Caimi PF, Samaniego F, Spira A, Davies A, et al. Camidanlumab tesirine in relapsed/refractory lymphoma: A phase 1, multicenter, open-label, dose-escalation, dose-expansion study. Lancet Haematol (2021) 8:e433–45. doi: 10.1016/S2352-3026(21)00103-4
113. Ai L, Xu A, Xu J. Roles of PD-1/PD-L1 pathway: Signaling, cancer, and beyond. Regul Cancer Immune Checkp (2020), 1248:33–59. doi: 10.1007/978-981-15-3266-5_3
114. Song TL, Nairismägi ML, Laurensia Y, Lim JQ, Tan J, Li ZM, et al. Oncogenic activation of the STAT3 pathway drives PD-L1 expression in natural killer/T-cell lymphoma. Blood (2018) 132:1146–58. doi: 10.1182/blood-2018-01-829424
115. Zuo M, Shen H, Yin J, Wang W, Zhang Y, Zhou DB, et al. Expression of PD-1 on peripheral blood treg cells is related to the diagnosis, prognosis and treatment of T cell non-Hodgkin lymphoma. Leuk Res (2018) 70:56–61. doi: 10.1016/j.leukres.2018.05.008
116. Zhang XW, Bi XW, Liu PP, Liu ZL, Nie M, Yang H, et al. Expression of PD-L1 on monocytes is a novel predictor of prognosis in natural Killer/T-cell lymphoma. Front Oncol (2020) 10. doi: 10.3389/fonc.2020.01360
117. He HX, Gao Y, Fu JC, Zhou QH, Wang XX, Bai B, et al. VISTA and PD-L1 synergistically predict poor prognosis in patients with extranodal natural killer/T-cell lymphoma. OncoImmunology (2021) 10:1907059. doi: 10.1080/2162402X.2021.1907059
118. Barta SK, Zain J, MacFarlane AW, Smith SM, Ruan J, Fung HC, et al. Phase II study of the PD1-inhibitor pembrolizumab for the treatment of relapsed or refractory mature T-cell lymphoma. Clin Lymphoma Myeloma Leuk. (2019) 19:356–364.e3. doi: 10.1016/j.clml.2019.03.022
119. Shi Y, Wu J, Wang Z, Zhang L, Wang Z, Zhang M, et al. Efficacy and safety of geptanolimab (GB226) for relapsed or refractory peripheral T cell lymphoma: an open-label phase 2 study (Gxplore-002). J Hematol OncolJ Hematol Oncol (2021) 14:12. doi: 10.1186/s13045-021-01033-1
120. Bennani NN, Kim HJ, Pederson LD, Atherton PJ, Micallef IN, Thanarajasingam G, et al. Nivolumab in patients with relapsed or refractory peripheral T-cell lymphoma: modest activity and cases of hyperprogression. J Immunother Cancer. (2022) 10:e004984. doi: 10.1136/jitc-2022-004984
121. Kwong YL, Chan TSY, Tan D, Kim SJ, Poon LM, Mow B, et al. PD1 blockade with pembrolizumab is highly effective in relapsed or refractory NK/T-cell lymphoma failing l-asparaginase. Blood (2017) 129:2437–42. doi: 10.1182/blood-2016-12-756841
122. Li X, Cheng Y, Zhang M, Yan J, Li L, Fu X, et al. Activity of pembrolizumab in relapsed/refractory NK/T-cell lymphoma. J Hematol OncolJ Hematol Oncol (2018) 11:15. doi: 10.1186/s13045-018-0559-7
123. Kim SJ, Lim JQ, Laurensia Y, Cho J, Yoon SE, Lee JY, et al. Avelumab for the treatment of relapsed or refractory extranodal NK/T-cell lymphoma: an open-label phase 2 study. Blood (2020) 136:2754–63. doi: 10.1182/blood.2020007247
124. Tao R, Fan L, Song Y, Hu Y, Zhang W, Wang Y, et al. Sintilimab for relapsed/refractory extranodal NK/T cell lymphoma: a multicenter, single-arm, phase 2 trial (ORIENT-4). Signal Transduct Target Ther (2021) 6:1–7. doi: 10.1038/s41392-021-00768-0
125. Zhang W, Shen H, Zhang Y, Wang W, Hu S, Zou D, et al. Circulating PD-1 (+) cells may participate in immune evasion in peripheral T-cell lymphoma and chidamide enhance antitumor activity of PD-1 (+) cells. Cancer Med (2019) 8:2104–13. doi: 10.1002/cam4.2097
126. Wei C, Hu S, Luo M, Chen C, Wang W, Zhang W, et al. A novel mechanism of action of histone deacetylase inhibitor chidamide: Enhancing the chemotaxis function of circulating PD-1(+) cells from patients with PTCL. Front Oncol (2021) 11:682436. doi: 10.3389/fonc.2021.682436
127. Stamper CC, Zhang Y, Tobin JF, Erbe DV, Ikemizu S, Davis SJ, et al. Crystal structure of the B7-1/CTLA-4 complex that inhibits human immune responses. Nature (2001) 410:608–11. doi: 10.1038/35069118
128. Chen X, Wu W, Wei W, Zou L. Immune checkpoint inhibitors in peripheral T-cell lymphoma. Front Pharmacol (2022) 13:869488. doi: 10.3389/fphar.2022.869488
129. Yoo HY, Kim P, Kim WS, Lee SH, Kim S, Kang SY, et al. Frequent CTLA4-CD28 gene fusion in diverse types of T-cell lymphoma. Haematologica (2016) 101:757–63. doi: 10.3324/haematol.2015.139253
130. Zhao LM, Zhang JH. Histone deacetylase inhibitors in tumor immunotherapy. Curr Med Chem (2019) 26:2990–3008. doi: 10.2174/0929867324666170801102124
131. Coiffier B, Pro B, Prince HM, Foss F, Sokol L, Greenwood M, et al. Results from a pivotal, open-label, phase II study of romidepsin in relapsed or refractory peripheral T-cell lymphoma after prior systemic therapy. J Clin Oncol (2012) 30:631–6. doi: 10.1200/JCO.2011.37.4223
132. O’Connor OA, Horwitz S, Masszi T, Van Hoof A, Brown P, Doorduijn J, et al. Belinostat in patients with relapsed or refractory peripheral T-cell lymphoma: Results of the pivotal phase II BELIEF (CLN-19) study. J Clin Oncol (2015) 33:2492–9. doi: 10.1200/JCO.2014.59.2782
133. Shi Y, Dong M, Hong X, Zhang W, Feng J, Zhu J, et al. Results from a multicenter, open-label, pivotal phase II study of chidamide in relapsed or refractory peripheral T-cell lymphoma. Ann Oncol (2015) 26:1766–71. doi: 10.1093/annonc/mdv237
134. Falchi L, Ma H, Klein S, Lue JK, Montanari F, Marchi E, et al. Combined oral 5-azacytidine and romidepsin are highly effective in patients with PTCL: a multicenter phase 2 study. Blood (2021) 137:2161–70. doi: 10.1182/blood.2020009004
135. Bachy E, Camus V, Thieblemont C, Sibon D, Casasnovas RO, Ysebaert L, et al. Romidepsin plus CHOP versus CHOP in patients with previously untreated peripheral T-cell lymphoma: Results of the ro-CHOP phase III study (Conducted by LYSA). J Clin Oncol (2022) 40(3):242–51. doi: 10.1200/JCO.21.01815
136. Giri AK, Aittokallio T. DNMT inhibitors increase methylation in the cancer genome. Front Pharmacol (2019) 10:385. doi: 10.3389/fphar.2019.00385
137. Lemonnier F, Dupuis J, Sujobert P, Tournillhac O, Cheminant M, Sarkozy C, et al. Treatment with 5-azacytidine induces a sustained response in patients with angioimmunoblastic T-cell lymphoma. Blood (2018) 132:2305–9. doi: 10.1182/blood-2018-04-840538
138. Gregory GP, Dickinson M, Yannakou CK, Wong J, Blombery P, Corboy G, et al. Rapid and durable complete remission of refractory AITL with azacitidine treatment in absence of TET2 mutation or concurrent MDS. HemaSphere (2019) 3:e187. doi: 10.1097/HS9.0000000000000187
139. Yoon SE, Cho J, Kim YJ, Kim SJ, Kim WS. Real-world efficacy of 5-azacytidine as salvage chemotherapy for angioimmunoblastic T-cell lymphoma. Clin Lymphoma Myeloma Leuk. (2022) 0:e972–80. doi: 10.1016/j.clml.2022.07.009
140. Lemonnier F. Oral azacytidine in patients with Relapsed/Refractory angioimmunoblastic T-cell lymphoma: Final analysis of the oracle phase III study. New Orleans, USA: ASH (2022). Available at: https://ash.confex.com/ash/2022/webprogram/Paper156789.html.
141. Toumishey E, Prasad A, Dueck G, Chua N, Finch D, Johnston J, et al. Final report of a phase 2 clinical trial of lenalidomide monotherapy for patients with T-cell lymphoma. Cancer (2015) 121:716–23. doi: 10.1002/cncr.29103
142. Morschhauser F, Fitoussi O, Haioun C, Thieblemont C, Quach H, Delarue R, et al. A phase 2, multicentre, single-arm, open-label study to evaluate the safety and efficacy of single-agent lenalidomide (Revlimid®) in subjects with relapsed or refractory peripheral T-cell non-Hodgkin lymphoma: The EXPECT trial. Eur J Cancer. (2013) 49:2869–76. doi: 10.1016/j.ejca.2013.04.029
143. Hopfinger G, Nösslinger T, Lang A, Linkesch W, Melchardt T, Weiss L, et al. Lenalidomide in combination with vorinostat and dexamethasone for the treatment of relapsed/refractory peripheral T cell lymphoma (PTCL): report of a phase I/II trial. Ann Hematol (2014) 93:459–62. doi: 10.1007/s00277-014-2009-0
144. Lunning MA, Horwitz SM, Advani R, Vose JM, Lee HJ, Mehta-Shah N, et al. Phase I/II study of CHOEP plus lenalidomide as initial therapy for patients with stage II-IV peripheral T-cell lymphoma: Phase II results. Blood (2018) 132:2899. doi: 10.1182/blood-2018-99-114110
145. Lemonnier F, Safar V, de Leval L, Cottereau AS, Pelletier L, Robe C, et al. Lenalidomide in combination with CHOP in patients with angioimmunoblastic T-cell lymphoma (AITL): Final analysis of clinical and molecular data of a phase 2 lysa study. Blood (2018) 132:999. doi: 10.1182/blood-2018-99-110354
146. Yan ZX, Wu LL, Xue K, Zhang QL, Guo Y, Romero M, et al. MicroRNA187 overexpression is related to tumor progression and determines sensitivity to bortezomib in peripheral T-cell lymphoma. Leukemia (2014) 28:880–7. doi: 10.1038/leu.2013.291
147. Zinzani PL, Musuraca G, Tani M, Stefoni V, Marchi E, Fina M, et al. Phase II trial of proteasome inhibitor bortezomib in patients with relapsed or refractory cutaneous T-cell lymphoma. J Clin Oncol (2007) 25:4293–7. doi: 10.1200/JCO.2007.11.4207
148. Lee SS, Jung SH, Ahn JS, Kim YK, Cho MS, Jung SY, et al. Pralatrexate in combination with bortezomib for relapsed or refractory peripheral T cell lymphoma in 5 elderly patients. J Korean Med Sci (2016) 31:1160–3. doi: 10.3346/jkms.2016.31.7.1160
149. Tan D, Phipps C, Hwang WYK, Tan SY, Yeap CH, Chan YH, et al. Panobinostat in combination with bortezomib in patients with relapsed or refractory peripheral T-cell lymphoma: an open-label, multicentre phase 2 trial. Lancet Haematol (2015) 2:e326–33. doi: 10.1016/S2352-3026(15)00097-6
150. Kim SJ, Yoon DH, Kang HJ, Kim JS, Park SK, Kim HJ, et al. Bortezomib in combination with CHOP as first-line treatment for patients with stage III/IV peripheral T-cell lymphomas: A multicentre, single-arm, phase 2 trial. Eur J Cancer. (2012) 48:3223–31. doi: 10.1016/j.ejca.2012.06.003
151. Boonstra PS, Avery P, Brown N, Hristov AC, Bailey NG, Kaminski MS, et al. A single center phase II study of ixazomib in patients with relapsed or refractory cutaneous or peripheral T-cell lymphomas. Am J Hematol (2017) 92:1287–94. doi: 10.1002/ajh.24895
152. Domingo-Domènech E, Boumendil A, Climent F, Sengeloev H, Wahlin B, Wattad W, et al. Autologous hematopoietic stem cell transplantation for relapsed/refractory systemic anaplastic large cell lymphoma. a retrospective analysis of the lymphoma working party (LWP) of the EBMT. Bone Marrow Transplant. (2020) 55:796–803. doi: 10.1038/s41409-019-0734-7
153. Marchi E, O’Connor OA. The rapidly changing landscape in mature T-cell lymphoma (MTCL) biology and management. CA Cancer J Clin (2020) 70:47–70. doi: 10.3322/caac.21589
154. Allen PB, Pro B. Therapy of peripheral T cell lymphoma: Focus on nodal subtypes. Curr Oncol Rep (2020) 22:44. doi: 10.1007/s11912-020-00902-1
155. d’Amore F, Relander T, Lauritzsen GF, Jantunen E, Hagberg H, Anderson H, et al. Up-front autologous stem-cell transplantation in peripheral T-cell lymphoma: NLG-T-01. J Clin Oncol (2012) 30:3093–9. doi: 10.1200/JCO.2011.40.2719
156. Du J, Yu D, Han X, Zhu L, Huang Z. Comparison of allogeneic stem cell transplant and autologous stem cell transplant in refractory or relapsed peripheral T-cell lymphoma: A systematic review and meta-analysis. JAMA Netw Open (2021) 4:e219807. doi: 10.1001/jamanetworkopen.2021.9807
157. Mamez AC, Dupont A, Blaise D, Chevallier P, Forcade E, Ceballos P, et al. Allogeneic stem cell transplantation for peripheral T cell lymphomas: a retrospective study in 285 patients from the société francophone de greffe de moelle et de thérapie cellulaire (SFGM-TC). J Hematol OncolJ Hematol Oncol (2020) 13:56. doi: 10.1186/s13045-020-00892-4
158. Lee JY, Kannan B, Lim BY, Li Z, Lim AH, Loh JW, et al. The multi-dimensional biomarker landscape in cancer immunotherapy. Int J Mol Sci (2022) 23:7839. doi: 10.3390/ijms23147839
159. Patel SP, Kurzrock R. PD-L1 expression as a predictive biomarker in cancer immunotherapy. Mol Cancer Ther (2015) 14:847–56. doi: 10.1158/1535-7163.MCT-14-0983
160. Krieg C, Nowicka M, Guglietta S, Schindler S, Hartmann FJ, Weber LM, et al. High-dimensional single-cell analysis predicts response to anti-PD-1 immunotherapy. Nat Med (2018) 24:144–53. doi: 10.1038/nm.4466
161. Moskowitz AJ, Ghione P, Jacobsen E, Ruan J, Schatz JH, Noor S, et al. A phase 2 biomarker-driven study of ruxolitinib demonstrates effectiveness of JAK/STAT targeting in T-cell lymphomas. Blood (2021) 138:2828–37. doi: 10.1182/blood.2021013379
162. Tari G, Lemonnier F, Morschhauser F. Epigenetic focus on angioimmunoblastic T-cell lymphoma: pathogenesis and treatment. Curr Opin Oncol (2021) 33:400–5. doi: 10.1097/CCO.0000000000000773
163. Tulstrup M, Soerensen M, Hansen JW, Gillberg L, Needhamsen M, Kaastrup K, et al. TET2 mutations are associated with hypermethylation at key regulatory enhancers in normal and malignant hematopoiesis. Nat Commun (2021) 12:6061. doi: 10.1038/s41467-021-26093-2
164. Lin Y, Lin Z, Cheng K, Fang Z, Li Z, Luo Y, et al. Prognostic role of TET2 deficiency in myelodysplastic syndromes: A meta-analysis. Oncotarget (2017) 8:43295–305. doi: 10.18632/oncotarget.17177
165. Fujisawa M, Sakata-Yanagimoto M, Nishizawa S, Komori D, Gershon P, Kiryu M, et al. Activation of RHOA–VAV1 signaling in angioimmunoblastic T-cell lymphoma. Leukemia (2018) 32:694–702. doi: 10.1038/leu.2017.273
166. Nguyen TB, Sakata-Yanagimoto M, Fujisawa M, Nuhat ST, Miyoshi H, Nannya Y, et al. Dasatinib is an effective treatment for angioimmunoblastic T-cell lymphoma. Cancer Res (2020) 80:1875–84. doi: 10.1158/0008-5472.CAN-19-2787
167. Witzig TE, Sokol L, Foss FM, Kim WS, Jacobsen E, de la Cruz M de F, et al. Proof of concept for tipifarnib in relapsed or refractory angioimmunoblastic T-cell lymphoma (AITL) and CXCL12+ peripheral T-cell lymphoma (PTCL): Preliminary results from an open-label, phase 2 study. Blood (2019) 134:468. doi: 10.1182/blood-2019-128513
168. Kim S, Kwon D, Koh J, Nam SJ, Kim YA, Kim TM, et al. Clinicopathological features of programmed cell death-1 and programmed cell death-ligand-1 expression in the tumor cells and tumor microenvironment of angioimmunoblastic T cell lymphoma and peripheral T cell lymphoma not otherwise specified. Virchows Arch (2020) 477:131–42. doi: 10.1007/s00428-020-02790-z
169. Pro B, Advani R, Brice P, Bartlett NL, Rosenblatt JD, Illidge T, et al. Five-year results of brentuximab vedotin in patients with relapsed or refractory systemic anaplastic large cell lymphoma. Blood (2017) 130:2709–17. doi: 10.1182/blood-2017-05-780049
170. Mossé YP, Voss SD, Lim MS, Rolland D, Minard CG, Fox E, et al. Targeting ALK with crizotinib in pediatric anaplastic Large cell lymphoma and inflammatory myofibroblastic tumor: A children’s oncology group study. J Clin Oncol (2017) 35:3215–21. doi: 10.1200/JCO.2017.73.4830
171. Gambacorti-Passerini C, Messa C, Pogliani EM. Crizotinib in anaplastic Large-cell lymphoma. N Engl J Med (2011) 364:775–6. doi: 10.1056/NEJMc1013224
172. Gambacorti Passerini C, Farina F, Stasia A, Redaelli S, Ceccon M, Mologni L, et al. Crizotinib in advanced, chemoresistant anaplastic lymphoma kinase–positive lymphoma patients. JNCI J Natl Cancer Inst (2014) 106:djt378. doi: 10.1093/jnci/djt378
173. Bossi E, Aroldi A, Brioschi FA, Steidl C, Baretta S, Renso R, et al. Phase two study of crizotinib in patients with anaplastic lymphoma kinase (ALK)-positive anaplastic large cell lymphoma relapsed/refractory to chemotherapy. Am J Hematol (2020) 95:E319–21. doi: 10.1002/ajh.25967
174. Subbiah V, Kuravi S, Ganguly S, Welch DR, Vivian CJ, Mushtaq MU, et al. Precision therapy with anaplastic lymphoma kinase inhibitor ceritinib in ALK-rearranged anaplastic large cell lymphoma. ESMO Open (2021) 6:100172. doi: 10.1016/j.esmoop.2021.100172
175. Fukano R, Mori T, Sekimizu M, Choi I, Kada A, Saito AM, et al. Alectinib for relapsed or refractory anaplastic lymphoma kinase-positive anaplastic large cell lymphoma: An open-label phase II trial. Cancer Sci (2020) 111:4540–7. doi: 10.1111/cas.14671
176. Manso R, Rodríguez-Perales S, Torres-Ruiz R, Santonja C, Rodríguez-Pinilla SM. PD-L1 expression in peripheral T-cell lymphomas is not related to either PD-L1 gene amplification or rearrangements. Leuk Lymphoma. (2021) 62:1648–56. doi: 10.1080/10428194.2021.1881511
177. Iwafuchi H, Nakazawa A, Sekimizu M, Mori T, Osumi T, Iijima-Yamashita Y, et al. Clinicopathological features and prognostic significance of programmed death ligand 1 in pediatric ALK-positive anaplastic large cell lymphoma: results of the ALCL99 treatment in Japan. Hum Pathol (2021) 116:112–21. doi: 10.1016/j.humpath.2021.07.011
178. Lim JQ, Huang D, Chan JY, Laurensia Y, Wong EKY, Cheah DMZ, et al. A genomic-augmented multivariate prognostic model for the survival of natural-killer/T-cell lymphoma patients from an international cohort. Am J Hematol (2022) 97:1159–69. doi: 10.1002/ajh.26636
179. Bi XW, Wang H, Zhang WW, Wang JH, Liu WJ, Xia ZJ, et al. PD-L1 is upregulated by EBV-driven LMP1 through NF-κB pathway and correlates with poor prognosis in natural killer/T-cell lymphoma. J Hematol OncolJ Hematol Oncol (2016) 9:109. doi: 10.1186/s13045-016-0341-7
180. Ribas A, Hu-Lieskovan S. What does PD-L1 positive or negative mean? J Exp Med (2016) 213:2835–40. doi: 10.1084/jem.20161462
181. Cho J, Kim SJ, Park WY, Kim J, Woo J, Kim G, et al. Immune subtyping of extranodal NK/T-cell lymphoma: a new biomarker and an immune shift during disease progression. Mod Pathol (2020) 33:603–15. doi: 10.1038/s41379-019-0392-8
182. Lim JQ, Huang D, Tang T, Tan D, Laurensia Y, Peng RJ, et al. Whole-genome sequencing identifies responders to pembrolizumab in relapse/refractory natural-killer/T cell lymphoma. Leukemia (2020) 34:3413–9. doi: 10.1038/s41375-020-1000-0
183. Cai J, Liu P, Huang H, Li Y, Ma S, Zhou H, et al. Combination of anti-PD-1 antibody with p-GEMOX as a potentially effective immunochemotherapy for advanced natural killer/T cell lymphoma. Signal Transduct Target Ther (2020) 5:289. doi: 10.1038/s41392-020-00331-3
184. Wang L, Wang H, Li PF, Lu Y, Xia ZJ, Huang HQ, et al. CD38 expression predicts poor prognosis and might be a potential therapy target in extranodal NK/T cell lymphoma, nasal type. Ann Hematol (2015) 94:1381–8. doi: 10.1007/s00277-015-2359-2
185. Huang H, Zhu J, Yao M, Kim TM, Yoon DH, Cho SG, et al. Daratumumab monotherapy for patients with relapsed or refractory natural killer/T-cell lymphoma, nasal type: an open-label, single-arm, multicenter, phase 2 study. J Hematol OncolJ Hematol Oncol (2021) 14:25. doi: 10.1186/s13045-020-01020-y
186. Hari P, Raj RV, Olteanu H. Targeting CD38 in refractory extranodal natural killer cell–T-Cell lymphoma. N Engl J Med (2016) 375:1501–2. doi: 10.1056/NEJMc1605684
187. Mustafa N, Nee AHF, Chooi JY, Toh SHM, Chung TH, Selvarajan V, et al. Determinants of response to daratumumab in Epstein-Barr virus-positive natural killer and T-cell lymphoma. J Immunother Cancer. (2021) 9:e002123. doi: 10.1136/jitc-2020-002123
188. Tian XP, Ma SY, Young KH, Ong CK, Liu YH, Li ZH, et al. A composite single-nucleotide polymorphism prediction signature for extranodal natural killer/T-cell lymphoma. Blood (2021) 138:452–63. doi: 10.1182/blood.2020010637
189. Liu J, Liang L, Li D, Nong L, Zheng Y, Huang S, et al. JAK3/STAT3 oncogenic pathway and PRDM1 expression stratify clinicopathologic features of extranodal NK/T−cell lymphoma, nasal type. Oncol Rep (2019) 41:3219–32. doi: 10.3892/or.2019.7112
190. Dong G, Liu X, Wang L, Yin W, Bouska A, Gong Q, et al. Genomic profiling identifies distinct genetic subtypes in extra-nodal natural killer/T-cell lymphoma. Leukemia (2022) 36:2064–75. doi: 10.1038/s41375-022-01623-z
191. Pope JH, Scott W, Moss DJ. Human lymphoid cell transformation by Epstein-Barr virus. Nat New Biol (1973) 246:140–1. doi: 10.1038/newbio246140a0
192. Pagano JS, Blaser M, Buendia MA, Damania B, Khalili K, Raab-Traub N, et al. Infectious agents and cancer: criteria for a causal relation. Semin Cancer Biol (2004) 14:453–71. doi: 10.1016/j.semcancer.2004.06.009
193. Piccaluga PP, Gazzola A, Agostinelli C, Bacci F, Sabattini E, Pileri SA. Pathobiology of Epstein-Barr virus-driven peripheral T-cell lymphomas. Semin Diagn Pathol (2011) 28:234–44. doi: 10.1053/j.semdp.2011.02.007
194. Bahri R, Boyer F, Halabi MA, Chaunavel A, Feuillard J, Jaccard A, et al. Epstein-Barr Virus (EBV) is mostly latent and clonal in angioimmunoblastic T cell lymphoma (AITL). Cancers (2022) 14:2899. doi: 10.3390/cancers14122899
195. Bayda N, Tilloy V, Chaunavel A, Bahri R, Halabi MA, Feuillard J, et al. Comprehensive Epstein-Barr virus transcriptome by RNA-sequencing in angioimmunoblastic T cell lymphoma (AITL) and other lymphomas. Cancers (2021) 13:610. doi: 10.3390/cancers13040610
196. Hutzinger R, Feederle R, Mrazek J, Schiefermeier N, Balwierz PJ, Zavolan M, et al. Expression and processing of a small nucleolar RNA from the Epstein-Barr virus genome. PloS Pathog (2009) 5:e1000547. doi: 10.1371/journal.ppat.1000547
197. Kalra M, Gerdemann U, Luu JD, Ngo MC, Leen AM, Louis CU, et al. Epstein-Barr Virus (EBV)-derived BARF1 encodes CD4- and CD8-restricted epitopes as targets for T-cell immunotherapy. Cytotherapy (2019) 21:212–23. doi: 10.1016/j.jcyt.2018.08.001
198. Drosu NC, Edelman ER, Housman DE. Tenofovir prodrugs potently inhibit Epstein–Barr virus lytic DNA replication by targeting the viral DNA polymerase. Proc Natl Acad Sci (2020) 117:12368–74. doi: 10.1073/pnas.2002392117
199. Tsai PF, Lin SJ, Weng PL, Tsai SC, Lin JH, Chou YC, et al. Interplay between PKCδ and Sp1 on histone deacetylase inhibitor-mediated Epstein-Barr virus reactivation. J Virol (2011) 85:2373–85. doi: 10.1128/JVI.01602-10
200. Bollard CM, Gottschalk S, Torrano V, Diouf O, Ku S, Hazrat Y, et al. Sustained complete responses in patients with lymphoma receiving autologous cytotoxic T lymphocytes targeting Epstein-Barr virus latent membrane proteins. J Clin Oncol (2014) 32:798–808. doi: 10.1200/JCO.2013.51.5304
201. Cho SG, Kim N, Sohn HJ, Lee SK, Oh ST, Lee HJ, et al. Long-term outcome of extranodal NK/T cell lymphoma patients treated with postremission therapy using EBV LMP1 and LMP2a-specific CTLs. Mol Ther (2015) 23:1401–9. doi: 10.1038/mt.2015.91
202. Wai CMM, Chen S, Phyu T, Fan S, Leong SM, Zheng W, et al. Immune pathway upregulation and lower genomic instability distinguish EBV-positive nodal T/NK-cell lymphoma from ENKTL and PTCL-NOS. Haematologica (2022) 107:1864–79. doi: 10.3324/haematol.2021.280003
203. Cording S, Lhermitte L, Malamut G, Berrabah S, Trinquand A, Guegan N, et al. Oncogenetic landscape of lymphomagenesis in coeliac disease. Gut (2022) 71:497–508. doi: 10.1136/gutjnl-2020-322935
204. Wang J, Ke XY. The four types of tregs in malignant lymphomas. J Hematol OncolJ Hematol Oncol (2011) 4:50. doi: 10.1186/1756-8722-4-50
205. Engblom C, Pfirschke C, Pittet MJ. The role of myeloid cells in cancer therapies. Nat Rev Cancer. (2016) 16:447–62. doi: 10.1038/nrc.2016.54
206. Lin Y, Xu J, Lan H. Tumor-associated macrophages in tumor metastasis: biological roles and clinical therapeutic applications. J Hematol OncolJ Hematol Oncol (2019) 12:76. doi: 10.1186/s13045-019-0760-3
207. Cao X, Wang Y, Zhang W, Zhong X, Gunes EG, Dang J, et al. Targeting macrophages for enhancing CD47 blockade–elicited lymphoma clearance and overcoming tumor-induced immunosuppression. Blood (2022) 139:3290–302. doi: 10.1182/blood.2021013901
208. Veglia F, Sanseviero E, Gabrilovich DI. Myeloid-derived suppressor cells in the era of increasing myeloid cell diversity. Nat Rev Immunol (2021) 21:485–98. doi: 10.1038/s41577-020-00490-y
209. Law AMK, Valdes-Mora F, Gallego-Ortega D. Myeloid-derived suppressor cells as a therapeutic target for cancer. Cells (2020) 9:561. doi: 10.3390/cells9030561
210. Adeshakin AO, Liu W, Adeshakin FO, Afolabi LO, Zhang M, Zhang G, et al. Regulation of ROS in myeloid-derived suppressor cells through targeting fatty acid transport protein 2 enhanced anti-PD-L1 tumor immunotherapy. Cell Immunol (2021) 362:104286. doi: 10.1016/j.cellimm.2021.104286
211. Adeshakin AO, Yan D, Zhang M, Wang L, Adeshakin FO, Liu W, et al. Blockade of myeloid-derived suppressor cell function by valproic acid enhanced anti-PD-L1 tumor immunotherapy. Biochem Biophys Res Commun (2020) 522:604–11. doi: 10.1016/j.bbrc.2019.11.155
212. Adeshakin AO, Adeshakin FO, Yan D, Wan X. Regulating histone deacetylase signaling pathways of myeloid-derived suppressor cells enhanced T cell-based immunotherapy. Front Immunol (2022) 13:781660. doi: 10.3389/fimmu.2022.781660
213. Zhang M, Wang L, Liu W, Wang T, De Sanctis F, Zhu L, et al. Targeting inhibition of accumulation and function of myeloid-derived suppressor cells by artemisinin via PI3K/AKT, mTOR, and MAPK pathways enhances anti-PD-L1 immunotherapy in melanoma and liver tumors. J Immunol Res (2022) 2022:2253436. doi: 10.1155/2022/2253436
214. Yan D, Wang J, Sun H, Zamani A, Zhang H, Chen W, et al. TIPE2 specifies the functional polarization of myeloid-derived suppressor cells during tumorigenesis. J Exp Med (2019) 217:e20182005. doi: 10.1084/jem.20182005
215. Wu DW, Lee MC, Wang J, Chen CY, Cheng YW, Lee H. DDX3 loss by p53 inactivation promotes tumor malignancy via the MDM2/Slug/E-cadherin pathway and poor patient outcome in non-small-cell lung cancer. Oncogene (2014) 33:1515–26. doi: 10.1038/onc.2013.107
216. AlDallal SM. Yescarta: A new era for non-Hodgkin lymphoma patients. Cureus (2020) 12:e11504. doi: 10.7759/cureus.11504
217. Ramos CA, Ballard B, Zhang H, Dakhova O, Gee AP, Mei Z, et al. Clinical and immunological responses after CD30-specific chimeric antigen receptor–redirected lymphocytes. J Clin Invest. (2017) 127:3462–71. doi: 10.1172/JCI94306
Keywords: tumor microenvironment, genomics, checkpoint immunotherapy, precision oncology, targeted therapy
Citation: Yap DRY, Lim JQ, Huang D, Ong CK and Chan JY (2023) Emerging predictive biomarkers for novel therapeutics in peripheral T-cell and natural killer/T-cell lymphoma. Front. Immunol. 14:1068662. doi: 10.3389/fimmu.2023.1068662
Received: 13 October 2022; Accepted: 09 January 2023;
Published: 26 January 2023.
Edited by:
Rachel E. Crossland, Newcastle University, United KingdomReviewed by:
Lijie Han, First Affiliated Hospital of Zhengzhou University, ChinaDehong Yan, Shenzhen Institutes of Advanced Technology (CAS), China
Copyright © 2023 Yap, Lim, Huang, Ong and Chan. This is an open-access article distributed under the terms of the Creative Commons Attribution License (CC BY). The use, distribution or reproduction in other forums is permitted, provided the original author(s) and the copyright owner(s) are credited and that the original publication in this journal is cited, in accordance with accepted academic practice. No use, distribution or reproduction is permitted which does not comply with these terms.
*Correspondence: Jason Yongsheng Chan, jason.chan.y.s@singhealth.com.sg; Choon Kiat Ong, cmrock@nccs.com.sg