- Engineering and Technology Research Center for Transplantation Medicine of National Health Comission, Third Xiangya Hospital, Central South University, Changsha, China
Ischemia-reperfusion injury (IRI) is a common complication of surgery, which can cause rapid deterioration of the liver function, increase the risk of graft rejection, and seriously affect the prognosis of patients. The signal transducer and activator of transcription 3 (STAT3) protein has been implicated in pathogenesis of IRI. STAT3 influences the mitochondria through multiple pathways and is also involved in apoptosis and other forms of programmed cell death. STAT3 is associated with Janus kinase (JAK), phosphoinositide-3 kinase (PI3K), and heme oxygenase-1 (HO-1) in liver IRI. The STAT3 pathway plays a dual role in IRI as it can also regulate lipid metabolism which may have potential for treating IRI fatty liver. In this review, we summarize research on the function of STAT3 in liver IRI to provide references for its application in the clinic.
Ischemia-reperfusion injury (IRI) is a common complication after liver surgery (such as surgery for liver cancer or liver transplantation). The interruption of the oxygen supply during ischemia causes hepatic sinusoidal stenosis as well as secondary microcirculation disorders (1, 2). Various factors such as tissue hypoxia, nutrient deficiencies, and metabolic disruption during ischemia can lead to hepatocyte injury. Inflammatory factors, apoptotic pathways, and reactive oxygen species (ROS), which are activated during reperfusion, can result in a rapid deterioration of the liver function, which also increases the risk of rejection and can adversely affect patient prognosis (3, 4). IRI is usually classified as warm IRI in vivo and cold IRI in vitro. Although both are primarily caused by hypoxia and the consumption of substrates caused by ischemia, the treatment methods differ due to the differences in the temperature and cell metabolic energy (5). Liver IRI is still a major problem in liver surgery, and no effective treatments are currently available. Signal transducer and activator of transcription (STAT) proteins are a class of transcription factors present in the cytoplasm, and mainly function to transmit signals from cell-surface receptors to the nucleus. The STAT family consists of seven distinct members, namely, STAT1, STAT2, STAT3, STAT4, STAT5A, STAT5B, and STAT 6. These STAT proteins contain between 750 and 850 amino acids and have similar structures and functions. STAT3 is composed of six different functional regions, namely, the N-domain/STAT protein interaction domain, coiled-coil domain (CCD), DNA-binding domain (DBD), linker domain, the SH2 domain, and the Transcriptional Activation Domain (TAD) (6, 7). There are prior reviews about the involvement of STAT3 in many organs. However, the role of STAT3-related signaling pathways in liver IRI has not been systematically summarized. Therefore, we have analyzed the relevant literature with keywords such as STAT3, ischemia reperfusion injury, and liver, amongst others. This paper aimed to review the various studies of STAT3 in liver IRI and discuss its associated pathways and different roles, to provide a reference for further research.
1 Structure and function of STAT3 and the relationship between STAT3 and the mitochondria
The human STAT3 gene is located on chromosome 17q21 and encodes an 89-kDa protein (8). STAT3 mainly consists of three different isoforms, namely STAT3α, STAT3β, and STAT3γ, of which the first is the most common. STAT3α can bind to IL-6 and IL-10 secreted by macrophages. STAT3β can inhibit the synthesis of inflammatory factors and plays an anti-inflammatory role while STAT3γ is mainly produced by the degradation of STAT3α and is activated by differentiated neutrophils (9, 10).
Moreover, based on in vitro and in vivo experiments, Lucy Xi Lou showed that STAT3 knockout resulted in increased expression of transaminase and inflammatory indicators suggesting that endogenous STAT3 plays a protective role in IRI (11). However, the upstream and downstream pathways associated with STAT3 were not investigated in this study, and the specific mechanism requires confirmation by subsequent experiments. Endogenous negative regulators of STAT3, such as suppressor of cytokine-induced STAT signaling (SOCs), can bind to activated receptors and interact with Janus kinase (JAK), which in turn inhibits the activation of the STAT pathway (12). In addition, there are nuclear factors that can bind to phosphorylated STAT, commonly known as PIAS (protein inhibitors of activated STATs), of which PIAS3 is a specific inhibitor of STAT3. It can block dimerization of the STAT3 monomer or promote the dissociation of dimerized STAT3, thus inhibiting STAT3 activation (13). STAT3 has two different phosphorylation sites, namely, Tyrosine 705 (Y705) and Serine 727 (S727). STAT3 phosphorylated at Y705 dimerizes and translocates to the nucleus, while phosphorylation at S727 leads to translocation to the mitochondria (14, 15). P-STAT3 regulates the activity of the electron transport chain (ETC) through S727 (16). Mitochondria are the main sites for ROS production, and the ETC is the most important source of ATP (17). In IR, excessive ROS and Ca2+ can cause the opening of the mitochondrial permeability transfer pore (MPTP) and adversely affect the mitochondrial membrane potential. This, in turn, can lead to peroxidation of the mitochondrial membrane, the release of cytochrome c, the inhibition of ATP synthesis, and, finally, irreversible cell death caused by mitochondrial membrane peroxidation (18, 19). ROS can activate STAT3 during IRI (20). STAT3 can inhibit MPTP opening caused by ROS production (Figure 1), thereby reducing mitochondrial damage (21). The levels of P-STAT3 in the mitochondria increase rapidly during reperfusion, while the P-STAT3 level in the cytosol decreases rapidly (22). Phosphorylated STAT3 (P-STAT3) is usually present in mitochondrial inner membrane adjacent to the matrix and is important for maintaining mitochondrial integrity. GRIM-19, the main component of mitochondrial complex I, promotes the entry of P-STAT3 into the mitochondria (23).The binding of P-STAT3 to the respiratory chain increases the membrane potential and increases ATP production. STAT3 knockdown can inhibit the rate of mitochondrial respiratory chain and complex I, II activity, which can then lead to the release of excess cytochrome C, thereby aggravating IRI (24).
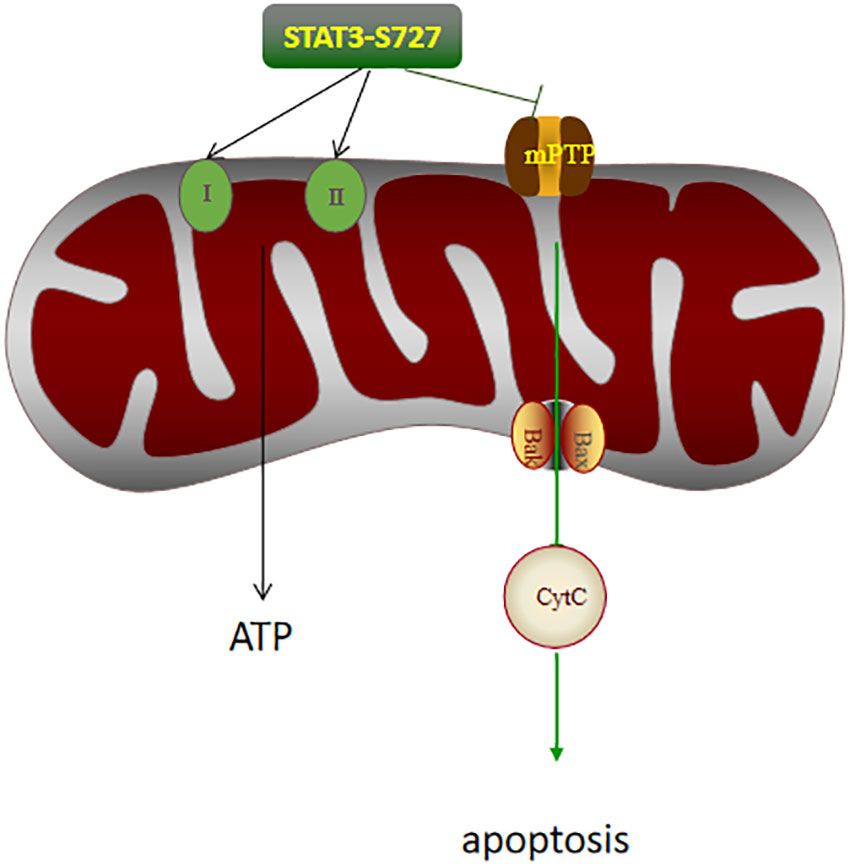
Figure 1 The effects of P-STAT3 on the mitochondria in hepatic IRI. P-STAT3 can promote ATP synthesis by increasing the activity of respiratory chain complex I and II. P-STAT3 also inhibited the opening of mPTP, thereby inhibiting the expression of Bax and the release of CytC, and finally alleviating apoptosis.
2 STAT3-related modes of death
Liver IRI is associated with various forms of cell death, including necrosis, apoptosis, autophagy, and ferroptosis, but those most associated with are apoptosis and autophagy. Apoptosis is a form of programmed cell death responsible for the maintenance of homeostasis in multicellular organisms (25). A number of studies have reported that 50-70% of endothelial cells and 40-60% of hepatocytes undergo apoptosis during reperfusion (26, 27). STAT3 can inhibit apoptosis in two distinct ways. First, STAT3 can play a direct anti-apoptotic role by upregulating the expression of the anti-apoptotic protein Bcl-2 and downregulating the expression of the pro-apoptotic protein Bax (28–30). Second, STAT3 can also inhibit MPTP formation to stabilize the mitochondrial membrane potential ΔΨm and thereby reduce ROS production, both of which can simultaneously inhibit the release of apoptosis-related cytokines, suppress caspase-related death pathways, attenuate the fragmentation of genetic material DNA, and ultimately inhibit apoptosis (31, 32). Autophagy is a process involved in the degradation of proteins and organelles in cells. Autophagy-related 5 (ATG5) and Microtubule-associated protein 1 Light BII (LC3BII) are two important autophagy-related proteins in IR. Yufang Han found that STAT3 was able to activate ATG5-mediated autophagy, thereby attenuating IRI (33). Shipeng Li reported that microRNA-17 (mir-17) promoted the expression of autophagy protein LC3BII by inhibiting the expression of STAT3, and ultimately aggravated liver IRI (34). Therefore, the potential relationship between STAT3 and liver IRI reported during autophagy needs to be further explored.
3 STAT3 and liver cells
The liver is the largest parenchymal organ in the human body, and contains non-inflammatory cells such as hepatocytes and endothelial cells as well as inflammatory cells such as Kupffer cells and lymphocytes.
3.1 STAT3 and non-inflammatory cells
Hepatocytes account for 80% of the liver tissue maintaining its main metabolic functions. In the carbon tetrachloride and alcohol models of acute liver injury, the inflammatory index was found to be lower in STAT3-knockout mice, while the inflammatory index was higher in the ConA-induced hepatitis and LPS-induced models of STAT3-knockout mice. STAT3 may inhibit inflammation by inhibiting STAT1, so STAT3 can inhibit the activation of the pro-inflammatory factor STAT1 in ConA-induced and LPS-induced hepatitis (35–38). STAT3 has a dual role in hepatocytes. Model differences are one of the important reasons, which need to be further explored in other models in the future. Some researchers have found that the degree of apoptosis and increased inflammatory response in mice with endothelial-cell STAT3 knockout in the alcoholic liver model, but the specific mechanism has not been explored. There are few studies on STAT3 in endothelial cells, and further exploration of its actions is needed in the future (39).
3.2 STAT3 and inflammatory cells
Hepatic macrophages are termed Kupffer cells (KCs). KCs account for 20% to 35% of all the non-parenchymal cells in the liver and are an important component of the immune cell compartment. KCs can generate oxidative stress through regulating different pathways and stimulating the production of TNF-α and other inflammatory factors, thereby aggravating IRI (40). KCs act mainly through the recognition of Toll-like receptors (TLRs), which are important receptors involved in the inflammatory cascade (41, 42). TLR4 is the most important member of the TLR family, and STAT3 is one of its important ligands. TLR4-deficient mice have significantly reduced IRI. KCs are activated by two mechanisms (Figure 2), M1 and M2. M1 can release various inflammatory factors and cause tissue damage, whereas M2, in contrast to M1, has anti-inflammatory effects (43, 44). SS-31 is a novel antioxidant targeting mitochondria, whose main effects include promoting the production of ATP and inhibiting ROS production (45, 46). Longcheng Shang reported that SS-31 could inhibit the production of mitochondrial ROS, thereby reducing the phosphorylation of STAT1 and STAT3. This can suppress the polarization of M1 macrophages, inhibit the release of inflammatory factors such as TNF-α and IL-1β, and ultimately alleviate liver IRI (47). Dexmedetomidine is a selective α2 adrenergic receptor agonist used for sedation and anesthesia in surgical patients. Haoming Zhou found that dexmedetomidine could activate the peroxisome proliferator-activated receptor-γ (PPARγ)/STAT3 pathway, thereby promoting the activation of M2 macrophages, suppressing the release of TNF-α and other inflammatory factors, and ultimately alleviating liver IRI (48–50). Zhuqing Rao reported that hyperglycemia could aggravate liver IRI by inhibiting the polarization of M2 macrophages and IL-10 activation by inhibiting STAT3 through CCAAT/enhancer-binding protein(C/EBP) protein-mediated ER stress (51). Roquin-1 is an E3 ubiquitin ligase originally identified in a mutated gene in SLE mice (52). Lei Zheng found that Roquin-1 effectively inhibited the polarization of M1 macrophages and promoted the activation of M2 macrophages, which inhibited AMP-activated protein kinase a (AMPKa) activity and promoted the activation of mammalian target of rapamycin (mTOR) and STAT3, which, in turn, led to the reduced production of related inflammatory factors and ultimately alleviated hepatic IRI (53). Tammy M found that proteolysis inducing factor (PIF) may activate STAT3 in human Kupffer cells, thereby inducing the inflammatory response. To improve the condition of patients with cachexia, inhibitors of this pathway should be further investigated (54). Lara Campana found that the STAT3-IL10-IL6 pathway promotes phenotypic transformation of human macrophages, thereby alleviating acute liver injury (55). Ozturk Akcora STAT3 inhibitor BWP1066 inhibits the release of inflammatory cytokines from human macrophages, thereby alleviating acute liver injury (56). STAT3 is essential for the growth and development of B lymphocytes, and IL-21 secreted by T cells promotes the transformation of CD19+B cell precursors into plasma cells that secrete IgG. Leptin can promote the secretion of IL-6 and TNF-α by human B cells through activation of the JAK2/STAT3 pathway and thus aggravate the inflammatory response (57–59). STAT3 in CD8+T lymphocytes is closely related to IL-21. In CD8+T lymphocytes, IL-6 can promote the expression of STAT3, which promotes the production of IL-21 and ultimately stimulates the production of CD8+ memory cells (60). STAT3 plays an important role in Th17 cells. STAT3 promoted the secretion of the anti-inflammatory factors TGF-β1 and IL-10 by CD4+T lymphocytes, inducing more Th3 cells (61).
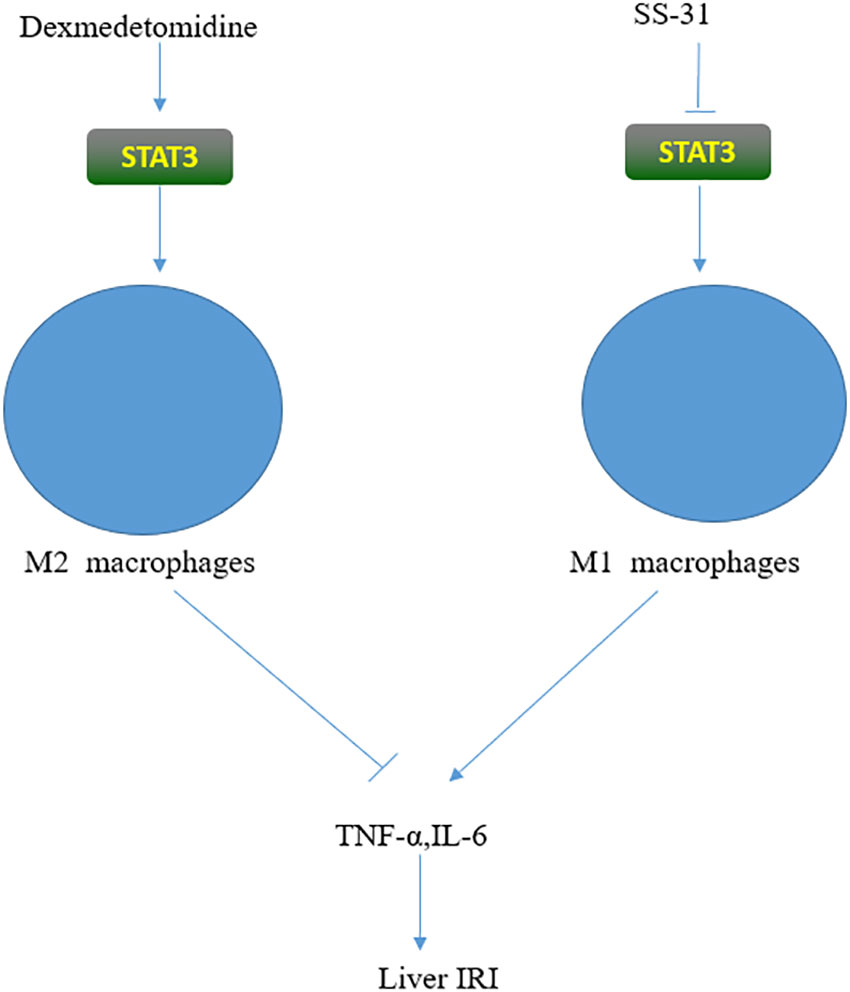
Figure 2 STAT3 has opposing effects on macrophages. On the one hand, it can promote the release of inflammatory factors and aggravate IRI by activating M1; on the other hand, it can activate M2, inhibit the release of inflammatory factors, thus alleviating IRI. The different effect depends on the activator of STAT3.
4 STAT3 and upstream inflammatory cytokines
KCs can secrete several inflammatory cytokines. Many inflammatory factors such as the interleukin family (ILs) can act as ligands to influence STAT3 activation. ILs that function as ligands mainly include IL-6, IL-11, and IL-22. For example, Heng Zhou reported that after vagotomy, the expression of IL-22 was decreased, and then the expression of STAT3 was reduced, inflammatory cell infiltration was increased, and IRI was aggravated. Exogenous IL-22 supplementation can promote the phosphorylation of STAT3, thereby promoting the expression of the cyclin D1 gene, and ultimately reversing liver IRI; however the mechanisms through which cyclin D1 can potentially reverse IRI in this model needs to be further explored (62). Paul J Chestovich found that IL-22 could effectively promote the phosphorylation of STAT3, inhibit the production of inflammatory factors, and ultimately reduce liver IRI but the endogenous IL-22 content was significantly increased after 24 hours of reperfusion (63). Bai, Y reported that IL-22 could activate STAT3, inhibit apoptosis and oxidative stress, and alleviate biliary IRI after liver transplantation (64). Wanzhen Li found that Ac2-26, a derivative of the endogenous inflammatory inhibitor Annexin A1 (AnxA1), can inhibit hepatocyte apoptosis induced by the mitochondrial pathway through activation of the IL-22/STAT3 pathway. Ac2-26 can also protect ATP and the mitochondrial membrane potential (MMP), inhibit MDA and ROS production, thus reducing IRI (65, 66). Nicolas Melin found that the TLR5 agonist CBLB502 can attenuate hepatic IRI by binding to the TLR5 receptor and stimulating IL-22 production by affecting the different immune cells through activation of STAT3 (67). These findings suggest that IL-22 can act as an important inflammatory factor and associate closely with STAT3, which is worthy of further study in the future. Miao Zhu showed that IL-11 could inhibit the phosphorylation of STAT3, thereby suppressing the activation of inflammatory factors such as TNF-α and IL-10, and thus attenuating liver IRI (68). Some relevant inflammatory factors play an important role in chronic liver injury, and IL-17A is a key factor in liver fibrosis. Xiao Wei Zhang found that activation of the IL-17A/STAT3 pathway can inhibit autophagy in liver cells, thus aggravating liver fibrosis, while an IL-17A inhibitor could reverse the development of fibrosis (69). Hongwei Tang added IL-6 rs1800796 into human L02 cells to activate the IL-6/STAT3 pathway, inhibit the expression of autophagy proteins, and thus reduce IRI. Recombinant human IL-6 can be a therapeutic target for hepatic IRI (70). Kun Xie found that exosomal mir-1246 derived from human umbilical cord blood mesenchymal stem cells can regulate the balance of helper/modulator T cells through the mir-1246-mediated IL-6-gp130 (IL-6 receptor)-STAT3 axis, which ultimately could attenuate liver IRI (71). Matsumoto pointed out that ischemic preconditioning (IPC) can significantly reduce hepatic IRI through activation of the IL-6-GP130-STAT3 axis, but the specific mechanisms require further exploration (72). Dayoub R found that the IL-6-STAT3-thrombopoietin (TPO) pathway can stimulate the production of megakaryocytes in the spleen and bone marrow and play a hemostatic role after acute liver injury (73). Rania Dayoub found that exogenous the acute phase response (ALR) can inhibit the IL-6/STAT3 pathway in L-02 cells, inhibit acute phase proteins (APPs) and thus ultimately inhibit inflammatory response. However, endogenous ALR activates the IL-6/STAT3 pathway and enhances the inflammatory response, so ALR has a dual role. However, the relationship between ALR and STAT3 phosphorylation needs further investigation (74). IL-6 is pleiotropic factor. As a ligand, it can play a positive role by activating the various downstream proteins. On the other hand, persistent release of IL-6 in has been implicated in various diseases. Thus, the key challenge remains to effectively balance the physiological and pathological functions of IL-6 in cells.
5 JAK - STAT3 pathway
The JAK-STAT pathway was originally discovered by Darnell et al. It is known to be an important intracellular signal transduction pathway, and has been implicated in the regulation of growth, differentiation, apoptosis, and development of various cells (Figure 3). It can promote the phosphorylation and activation of diverse proteins with tyrosine residues, generate a cascade reaction of kinase activation, and transduce the activated signal to other molecules, such as STAT, thereby triggering a series of genetic and protein changes (75, 76). In mammals, more than 40 cytokines can potentially activate the JAK -STAT pathway to influence hepatic IRI (77–79). JAK is a tyrosine kinase widely present in different types of cells, and can be divided into four types, namely, JAK1, JAK2, JAK3, and TYK2 (80). When the ligand binds to its surface receptor, it causes changes in the cytosolic part of the receptor, thus promoting the phosphorylation of the JAK protein. STAT3 and JAK are closely bound together in the resting state. When JAK is phosphorylated, STAT3 and JAK are separated to promote the phosphorylation of STAT3, and the activated STAT3 can undergo heterodimerization followed by the translocation to the nucleus, which can promote the transcriptional expression of the various genes (80–82). The STAT3 TAD contains three different sites, namely Y701, Y705, and Ser 727. Since TAD contains binding sites for its own dimer, it is quickly activated once stimulated in the cell. Exposure of Tyr705 can accelerate the process of heterodimerization, and it has been documented that increased phosphorylation of STAT3 at Tyr705 in the nucleus can reverse the inhibition of STAT3 phosphorylation at 727 during IRI (20, 83, 84).
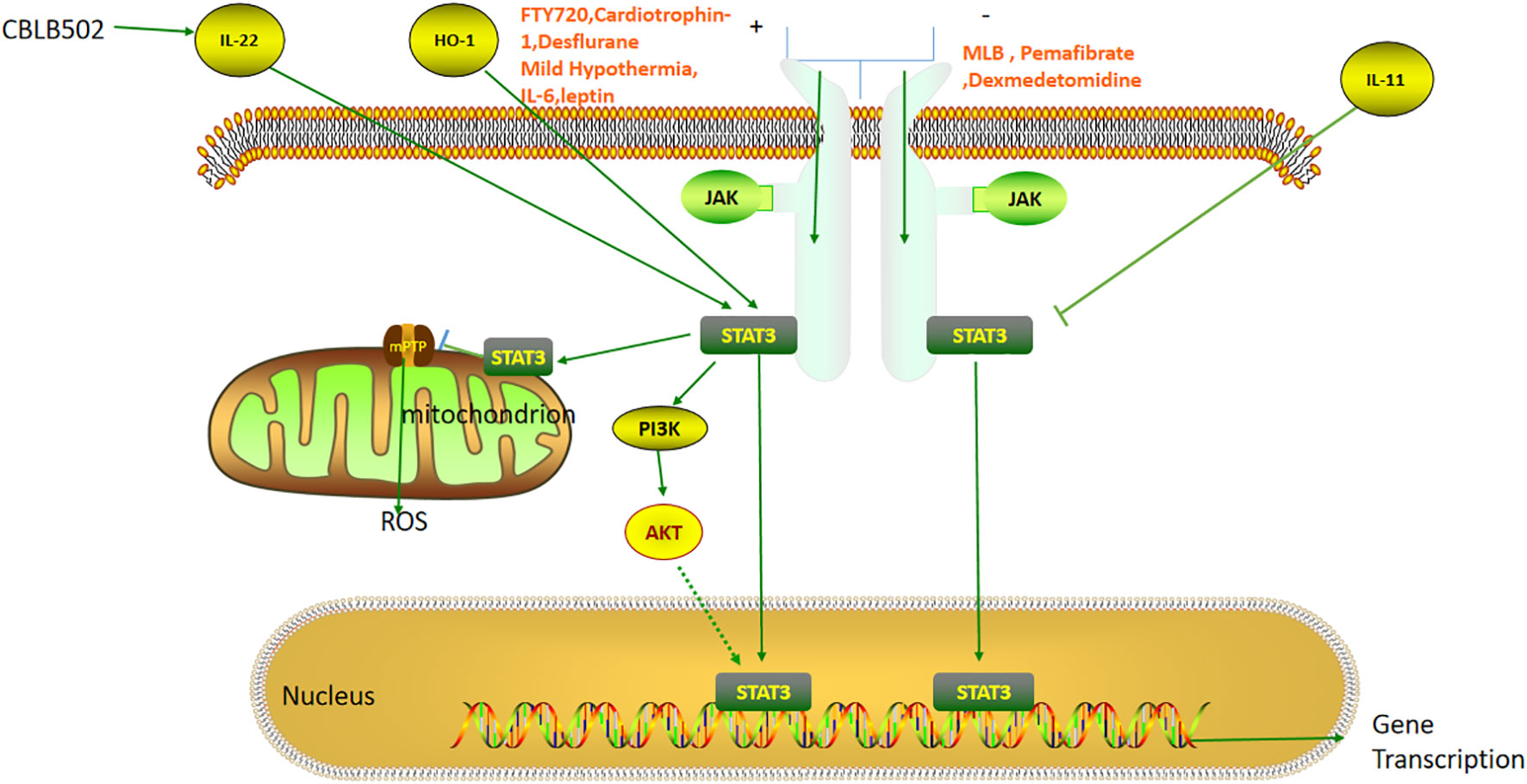
Figure 3 The role of the STAT3 pathway in liver ischemia-reperfusion injury. FTY720, Cardiotrophin-1, Desflurane, Mild Hypothermia, IL-6, and Leptin reduce hepatic ischemia-reperfusion injury by activating JAK-STAT3 pathway. MLB, Pemafibrate, and Dexmedetomidine can attenuate the injury by inhibiting the JAK-STAT3 pathway. IL-22, HO-1 and IL-11 can directly activate and inhibit STAT3 respectively. STAT3 can also attenuate ischemia-reperfusion injury by inhibiting mPTP opening and thereby reducing ROS release.
JAK-STAT3 activation can attenuate liver IRI. For instance, Mahmoud AR has found that coenzyme Q10 (CoQ10), which forms part of the mitochondrial respiratory chain in hepatocytes, was able to suppress apoptosis and oxidative stress by activating the JAK1/STAT3 pathway (85). Fingolimod (FTY720) is an inhibitor of the Sphingosine-1-phosphate (S1P) receptor with diverse anti-inflammatory effects (86). Xiangmin He demonstrated that Fingolimod (FTY720) could activate the JAK2/STAT3 pathway, thereby inhibiting hepatic IRI induced by acetaminophen (APAP) (87). Relevant studies have confirmed that STAT3 in hepatocytes can promote liver regeneration after hepatectomy. However, Feng D found that STAT3 had no effect after 6 h of APAP-induced ALI (88, 89) while Nishina T observed that STAT3 was still functional after 24 h; thus the specific relationship between APAP and STAT3 requires further investigation (90).The authors also reported that P-JAK2/P-STAT3 expression decreased after ischemia-reperfusion alone, which was inconsistent with previous results. The authors explained that it was related to time, and the times of IRI in this model were 1 h and 6 h, both of which were significantly shorter than those used in previous studies (91). Therefore, it is necessary to further study the activation of the pathway at the different time points in the future. Heng Chao Yu found that the Notch pathway could also activate JAK2/STAT3, promote the expression of manganese superoxide dismutase (MnSOD), inhibit ROS and apoptosis, and ultimately attenuate hepatic IRI (92). Cardiotrophin-1 was originally used as a drug to promote cardiac hypertrophy (93). Maria Iniguez reported that the myocardial nutrient cardiotrophin-1 could attenuate hepatic IPI by activating the JAK/STAT3 pathway, but the specific mechanism requires further study (94). Mengxia Zhong found that desflurane inhibited mir-135b-5p, thereby stimulating JAK2-STAT3 activation, inhibiting apoptosis, and ultimately alleviating liver IRI (95). However, there are also several reports that support the opposite conclusion. L Xiong found that mir-93 could inhibit the JAK/STAT3 pathway, thereby suppressing the production of apoptosis, inflammatory factors and transaminases, and leading to the alleviation of liver IRI (96). Ziqi Cheng reported that pemafibrate, a selective inhibitor of PPARα, could inhibit the release of inflammatory factors produced by Kupffer cells, attenuate the JAK2/STAT3β/PPARα pathway, suppress cell apoptosis as well as autophagy, and ultimately attenuate liver IRI (97). Ning Zhang found that Magnesium Lithospermate B, a traditional Chinese medicine, could markedly inhibit the production of inflammatory factors such as TNF-α and IL-6 by inhibiting the JAK2/STAT3 pathway, thereby attenuating hepatic IRI (98). Maria Cecilia S. Freitas reported that the JAK2 inhibitor AG490 could negatively regulate JAK-STAT signaling, thereby reducing the production of inflammatory factors, inhibiting apoptosis, and ultimately reducing IRI. It was also found that STAT1 activation was more likely to cause IRI than STAT3 (99). Y X Zhu found that dexmedetomidine can inhibit JAK/STAT3 signaling, apoptosis and the inflammatory response, as well as oxygen-glucose deprivation (OGD)-mediated human hepatic IRI (100). STAT1 and STAT3 have been reported to exert opposite effects on cell proliferation, differentiation, and apoptosis, which deserves further study in the future (101).
6 STAT3 and PI3K/AKT
The phosphoinositide-3 kinase (PI3K)/protein kinase B (PKB/AKT) is an important pathway involved in protein synthesis and is closely related to the regulation of redox reactions in mitochondria. PI3K/AKT can alleviate hepatic IRI by inhibiting the release of inflammatory factors and cell apoptosis while promoting autophagy (102). Bibo Ke reported that heme oxygenase-1 (HO-1) could promote the phosphorylation of STAT3, activate PI3K/AKT, inhibit the release of TNF-α and IL-10 induced by TLR-4, leading to reduced IRI (103). Bibo Ke found that STAT3 could activate PI3K/AKT by activating β-catenin, inhibiting IL-12 and Bax, and ultimately attenuating liver IRI (104). Huang J found that the nuclear factor E2-related factor 2 (Nrf2)-HO-1 axis could activate the Notch1/Hairy and enhancer of split homolog-1(Hes1)/STAT3 pathway, promote the macrophage differentiation and PI3K/AKT pathway activation, inhibit apoptosis, and ultimately reduce liver IRI (105). Therefore, the STAT3/PI3K/AKT pathway can play a key role in acute injury but the specific downstream mechanism of PI3K/AKT needs to be further explored.
7 STAT3 and lipid metabolism
Given the increasing number of patients with nonalcoholic fatty liver disease (NAFLD), the number of patients with fatty liver disease requiring organ transplantation has increased. Fatty liver is susceptible to IRI and two different hypotheses have been proposed to account for this, namely, impaired hepatic microcirculation and mitochondrial dysfunction (Figure 4). The volume of the steatotic hepatocytes becomes larger, squeezing and narrowing the perisinusoidal space, thus increasing the resistance of hepatic microcirculation. Fatty liver can also cause mitochondrial dysfunction through promoting the production of ROS, thereby interfering with cellular energy metabolism in the liver (106, 107). STAT3 activates peroxisome proliferator-activated receptor (PPAR)γ, then up-regulates the transcription of C/EBP, and promotes the transformation of preadipocytes into adipocytes. STAT3 knockout mice showed weight gain due to hypertrophy of adipocytes, suggesting that STAT3 plays a role in lipid degradation. JAK2-STAT3 promotes lipid degradation by inhibiting the expression of fatty acid synthase and acetyl-CoA carboxylase (108, 109). In several adipose models, STAT3 knockdown in the hepatocytes was observed to aggravate steatosis (35, 110, 111). Sterol regulatory element-binding protein-1 (SREBP-1) is a transcription factor that can regulate liver lipid metabolism. STAT3 can inhibit hepatic fat accumulation by suppressing SREBP-1, and ultimately reduce hepatic steatosis (110, 112). Marco Carbone reported that the addition of leptin to the preservation solution could activate STAT3 and reduce the degree of apoptosis, thereby attenuating the development of cold IRI (113). However, the specific mechanism of STAT3 in liver cold preservation needs to be further explored. Renalase is a ubiquitous pan-xanthine dinucleotide amine oxidase found in many organs (114). Tao Zhang reported that renalase could activate the STAT3-SIRT1 pathway and inhibit IRI in fatty liver (17). Zhihui Jiao found that the secretory proteome of adipose-derived mesenchymal stem cells could inhibit the expression of SOC3 and the negative feedback effect of SOC3 on STAT3 can lead to increase the expression of P-STAT3, and reduce IL-6, TNF-α and other related inflammatory factors, thereby alleviating liver IRI (115). Euno Choi found that P-STAT3 might aggravate liver steatosis and inflammatory injury, which was the first time for P-STAT3 to be explored in specimens of patients with fatty liver disease. The authors did not clarify whether this effect was related to the leptin pathway, and further research is needed to explore the relationship (116).Therefore, STAT3 plays different roles in regulating the process of lipid metabolism, and future studies to investigate its role in fatty liver are warranted.
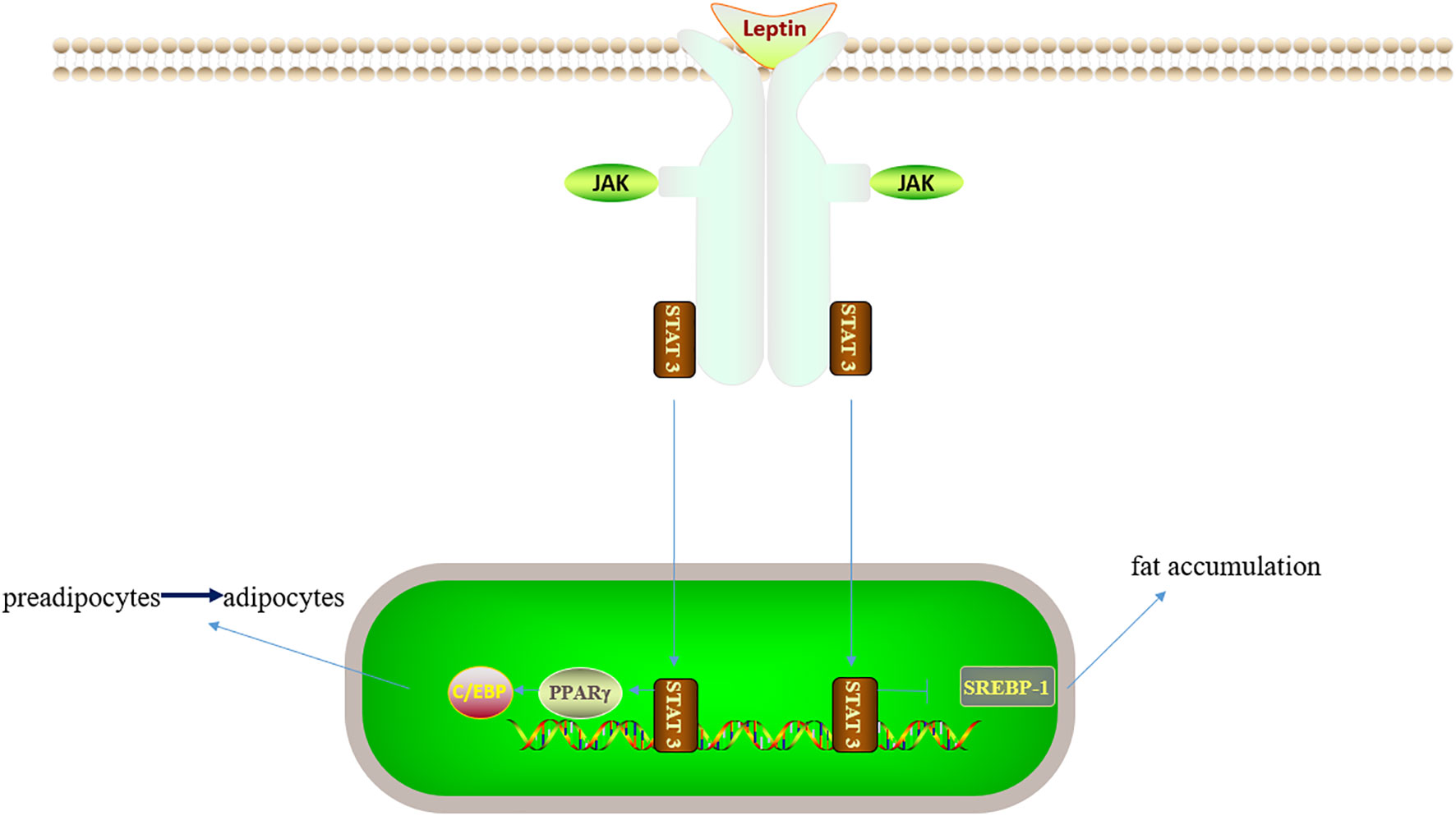
Figure 4 Leptin/JAK/STAT3 related lipid metabolic pathway. On the one hand, STAT3 can activate PPARγ, C/EBP and inhibit liver adipocyte maturation; on the other hand, STAT3 can inhibit SREBP-1 and thus inhibit fat accumulation. Therefore, STAT3 has different roles in lipid metabolism.
8 Conclusion
In hepatic IRI, STAT3 usually binds to the mitochondria to regulate programmed cell death. STAT3 plays a role in many hepatic cells. The STAT3-associated IR pathway includes upstream cytokines, and JAK, and downstream PI3K/AKT. The role of STAT3 in liver IRI is controversial (Table 1). On the one hand, STAT3 can play a protective role through the modulation of various proteins, inflammatory factors, and cells while on the other hand, it can aggravate IRI. The reason can be partly attributed to the fact that that P-Janus kinase (P-JAK) can activate both STAT3 and STAT1, and STAT3 can inhibit apoptosis whereas STAT1 can promote apoptosis. The JAK-specific inhibitor AG490 can inhibit both, thus producing different effects, but these are closely related to the length of the model time (117). The same protein may have different effects at different times and the same inflammatory factors can play diverse roles. STAT3 can also play a dual role in the regulation of lipid metabolism. On the one hand, STAT3 can promote the maturation of adipocytes while, on the other hand, it can promote lipolysis. STAT3 may also affect the microcirculation and energy metabolism by influencing fat accumulation. Therefore, STAT3 has an important effect on IRI in fatty liver. The incidence of fatty liver is increasing and it is necessary to further explore the functions of STAT3 in adipocyte maturation and lipolysis. Thus, further analysis of STAT3-related pathways in liver IRI is needed to provide a foundation for clinical treatment.
Author contributions
HY, YZ, PZ contributed to manuscript writing and editing. HY, YZ, PZ, QW, KC conducted a critical revision of the manuscript. All authors contributed to the article and approved the submitted version.
Funding
This study was supported by Hunan Provincial Natural Science Foundation of China (2022JJ40759 to PPZ,2022JJ30911 to YZ) and National Natural Science Foundation of China (82100695 to PZ)
Conflict of interest
The authors declare that the research was conducted in the absence of any commercial or financial relationships that could be construed as a potential conflict of interest.
Publisher’s note
All claims expressed in this article are solely those of the authors and do not necessarily represent those of their affiliated organizations, or those of the publisher, the editors and the reviewers. Any product that may be evaluated in this article, or claim that may be made by its manufacturer, is not guaranteed or endorsed by the publisher.
References
1. Peralta C, Closa D, Hotter G, Gelpí E, Prats N, Roselló-Catafau J. Liver ischemic preconditioning is mediated by the inhibitory action of nitric oxide on endothelin. Biochem Biophys Res Commun (1996) 229(1):264–70. doi: 10.1006/bbrc.1996.1790
2. Peralta C, Jiménez-Castro MB, Gracia-Sancho J. Hepatic ischemia and reperfusion injury: effects on the liver sinusoidal milieu. J Hepatol (2013) 59(5):1094–106. doi: 10.1016/j.jhep.2013.06.017
3. Zhou Y, Zhang J, Lei B, Liang W, Gong J, Zhao C, et al. DADLE improves hepatic ischemia/reperfusion injury in mice via activation of the Nrf2/HO−1 pathway. Mol Med Rep (2017) 16(5):6214–21. doi: 10.3892/mmr.2017.7393
4. Liu Z, Zhang X, Xiao Q, Ye S, Lai CH, Luo J, et al. Pretreatment donors after circulatory death with simvastatin alleviates liver ischemia reperfusion injury through a KLF2-dependent mechanism in rat. Oxid Med Cell Longev (2017) 2017:3861914. doi: 10.1155/2017/3861914
5. Bao WM, Guo YZ, Li L, Tang YM, Yang JH. Effects of cold/hot ischemia-reperfusion injury on energy status, apoptosis and expansion of liver cells in rats. Chin J Exp Surg (2005) 22(3):302–4. doi: 10.3760/j.issn:1001-9030.2005.03.015
6. Wang W, Kagaya Y, Asaumi Y, Fukui S, Takeda M, Shimokawa H. Protective effects of recombinant human erythropoietin against pressure overload-induced left ventricular remodeling and premature death in mice. Tohoku J Exp Med (2011) 225(2):131–43. doi: 10.1620/tjem.225.131
7. Ma Y, Yuan X, Deng L, Xu W, Zheng Y, Yue C, et al. Imbalanced frequencies of Th17 and treg cells in acute coronary syndromes are mediated by IL-6-STAT3 signaling. PloS One (2013) 8(8):e72804. doi: 10.1371/journal.pone.0072804
8. La Sala G, Michiels C, Kükenshöner T, Brandstoetter T, Maurer B, Koide A, et al. Selective inhibition of STAT3 signaling using monobodies targeting the coiled-coil and n-terminal domains. Nat Commun (2020) 11(1):4115. doi: 10.1038/s41467-020-17920-z
9. Yoo JY, Huso DL, Nathans D, Desiderio S. Specific ablation of Stat3beta distorts the pattern of Stat3-responsive gene expression and impairs recovery from endotoxic shock. Cell (2002) 108(3):331–44. doi: 10.1016/S0092-8674(02)00636-0
10. Hevehan DL, Miller WM, Papoutsakis ET. Differential expression and phosphorylation of distinct STAT3 proteins during granulocytic differentiation. Blood (2002) 99(5):1627–37. doi: 10.1182/blood.V99.5.1627
11. Lou LX, Uemura T, Mani H, Yang C, Li W, Kadry Z, et al. Endogenous signal transducer and activator of transcription 3 is required for the protection of hepatocytes against warm ischemia/reperfusion injury. Liver Transpl (2013) 19(10):1078–87. doi: 10.1002/lt.23693
12. Yasukawa H, Sasaki A, Yoshimura A. Negative regulation of cytokine signaling pathways. Annu Rev Immunol (2000) 18:143–64. doi: 10.1146/annurev.immunol.18.1.143
13. Chung CD, Liao J, Liu B, Rao X, Jay P, Berta P, et al. Specific inhibition of Stat3 signal transduction by PIAS3. Sci (1997) 278(5344):1803–5. doi: 10.1126/science.278.5344.1803
14. Heusch G, Musiolik J, Gedik N, Skyschally A. Mitochondrial STAT3 activation and cardioprotection by ischemic postconditioning in pigs with regional myocardial ischemia/reperfusion. Circ Res (2011) 109(11):1302–8. doi: 10.1161/CIRCRESAHA.111.255604
15. Wegrzyn J, Potla R, Chwae YJ, Sepuri NB, Zhang Q, Koeck T, et al. Function of mitochondrial Stat3 in cellular respiration. Sci (2009) 323(5915):793–7. doi: 10.1126/science.1164551
16. Gough DJ, Corlett A, Schlessinger K, Wegrzyn J, Larner AC, Levy DE. Mitochondrial STAT3 supports ras-dependent oncogenic transformation. Sci (2009) 324(5935):1713–6. doi: 10.1126/science.1171721
17. Zhang T, Gu J, Guo J, Chen K, Li H, Wang J. Renalase attenuates mouse fatty liver Ischemia/Reperfusion injury through mitigating oxidative stress and mitochondrial damage via activating SIRT1. Oxid Med Cell Longev (2019) 2019:7534285. doi: 10.1155/2019/7534285
18. Fernandez-Sanz C, Ruiz-Meana M, Castellano J, Miro-Casas E, Nuñez E, Inserte J, et al. Altered FoF1 ATP synthase and susceptibility to mitochondrial permeability transition pore during ischaemia and reperfusion in aging cardiomyocytes. Thromb Haemost (2015) 113(3):441–51. doi: 10.1160/TH14-10-0901
19. Wang Y, Yuan Y, Wang X, Wang Y, Cheng J, Tian L, et al. Tilianin post-conditioning attenuates myocardial Ischemia/Reperfusion injury via mitochondrial protection and inhibition of apoptosis. Med Sci Monit (2017) 23:4490–9. doi: 10.12659/MSM.903259
20. Wang M, Zhang W, Crisostomo P, Markel T, Meldrum KK, Fu XY, et al. Endothelial STAT3 plays a critical role in generalized myocardial proinflammatory and proapoptotic signaling. Am J Physiol Heart Circ Physiol (2007) 293(4):H2101–8. doi: 10.1152/ajpheart.00125.2007
21. Lemoine S, Zhu L, Legallois D, Massetti M, Manrique A, Hanouz JL. Atorvastatin-induced cardioprotection of human myocardium is mediated by the inhibition of mitochondrial permeability transition pore opening via tumor necrosis factor-α and janus kinase/signal transducers and activators of transcription pathway. Anesthesiol (2013) 118(6):1373–84. doi: 10.1097/ALN.0b013e31828a7039
22. Skyschally A, Gent S, Amanakis G, Schulte C, Kleinbongard P, Heusch G. Across-species transfer of protection by remote ischemic preconditioning with species-specific myocardial signal transduction by reperfusion injury salvage kinase and survival activating factor enhancement pathways. Circ Res (2015) 117(3):279–88. doi: 10.1161/CIRCRESAHA.117.306878
23. Tammineni P, Anugula C, Mohammed F, Anjaneyulu M, Larner AC, Sepuri NB. The import of the transcription factor STAT3 into mitochondria depends on GRIM-19, a component of the electron transport chain. J Biol Chem (2013) 288(7):4723–32. doi: 10.1074/jbc.M112.378984
24. Yamada Y, Kobayashi H, Iwasa M, Sumi S, Ushikoshi H, Aoyama T, et al. Postinfarct active cardiac-targeted delivery of erythropoietin by liposomes with sialyl Lewis X repairs infarcted myocardium in rabbits. Am J Physiol Heart Circ Physiol (2013) 304(8):H1124–33. doi: 10.1152/ajpheart.00707.2012
25. Bi J, Zhang J, Ren Y, Du Z, Li Q, Wang Y, et al. Irisin alleviates liver ischemia-reperfusion injury by inhibiting excessive mitochondrial fission, promoting mitochondrial biogenesis and decreasing oxidative stress. Redox Biol (2019) 20:296–306. doi: 10.1016/j.redox.2018.10.019
26. Gao W, Bentley RC, Madden JF, Clavien PA. Apoptosis of sinusoidal endothelial cells is a critical mechanism of preservation injury in rat liver transplantation. Hepatol (1998) 27(6):1652–60. doi: 10.1002/hep.510270626
27. Kohli V, Selzner M, Madden JF, Bentley RC, Clavien PA. Endothelial cell and hepatocyte deaths occur by apoptosis after ischemia-reperfusion injury in the rat liver. Transplantation (1999) 67(8):1099–105. doi: 10.1097/00007890-199904270-00003
28. Wu J, Yang C, Liu J, Chen J, Huang C, Wang J, et al. Betulinic acid attenuates T-2-Toxin-Induced testis oxidative damage through regulation of the JAK2/STAT3 signaling pathway in mice. Biomolecules (2019) 9(12). doi: 10.3390/biom9120787
29. Zhang X, Zhou J, Hu Q, Liu Z, Chen Q, Wang W, et al. The role of janus Kinase/Signal transducer and activator of transcription signalling on preventing intestinal Ischemia/Reperfusion injury with dexmedetomidine. J Nanosci Nanotechnol (2020) 20(5):3295–302. doi: 10.1166/jnn.2020.16416
30. Qaed E, Wang J, Almoiliqy M, Song Y, Liu W, Chu P, et al. Phosphocreatine improves cardiac dysfunction by normalizing mitochondrial respiratory function through JAK2/STAT3 signaling pathway In vivo and in vitro. Oxid Med Cell Longev (2019) 2019:6521218. doi: 10.1155/2019/6521218
31. Boengler K, Hilfiker-Kleiner D, Heusch G, Schulz R. Inhibition of permeability transition pore opening by mitochondrial STAT3 and its role in myocardial ischemia/reperfusion. Basic Res Cardiol (2010) 105(6):771–85. doi: 10.1007/s00395-010-0124-1
32. Szczepanek K, Chen Q, Derecka M, Salloum FN, Zhang Q, Szelag M, et al. Mitochondrial-targeted signal transducer and activator of transcription 3 (STAT3) protects against ischemia-induced changes in the electron transport chain and the generation of reactive oxygen species. J Biol Chem (2011) 286(34):29610–20. doi: 10.1074/jbc.M111.226209
33. Han YF, Zhao YB, Li J, Li L, Li YG, Li SP, et al. Stat3-Atg5 signal axis inducing autophagy to alleviate hepatic ischemia-reperfusion injury. J Cell Biochem (2018) 119(4):3440–50. doi: 10.1002/jcb.26516
34. Li S, Zhang J, Wang Z, Wang T, Yu Y, He J, et al. MicroRNA-17 regulates autophagy to promote hepatic ischemia/reperfusion injury via suppression of signal transductions and activation of transcription-3 expression. Liver Transpl (2016) 22(12):1697–709. doi: 10.1002/lt.24606
35. Horiguchi N, Wang L, Mukhopadhyay P, Park O, Jeong WI, Lafdil F, et al. Cell type-dependent pro- and anti-inflammatory role of signal transducer and activator of transcription 3 in alcoholic liver injury. Gastroenterol (2008) 134(4):1148–58. doi: 10.1053/j.gastro.2008.01.016
36. Horiguchi N, Lafdil F, Miller AM, Park O, Wang H, Rajesh M, et al. Dissociation between liver inflammation and hepatocellular damage induced by carbon tetrachloride in myeloid cell-specific signal transducer and activator of transcription 3 gene knockout mice. Hepatol (2010) 51(5):1724–34. doi: 10.1002/hep.23532
37. Sakamori R, Takehara T, Ohnishi C, Tatsumi T, Ohkawa K, Takeda K, et al. Signal transducer and activator of transcription 3 signaling within hepatocytes attenuates systemic inflammatory response and lethality in septic mice. Hepatol (2007) 46(5):1564–73. doi: 10.1002/hep.21837
38. Hong F, Jaruga B, Kim WH, Radaeva S, El-Assal ON, Tian Z, et al. Opposing roles of STAT1 and STAT3 in T cell-mediated hepatitis: regulation by SOCS. J Clin Invest (2002) 110(10):1503–13. doi: 10.1172/JCI0215841
39. Miller AM, Wang H, Park O, Horiguchi N, Lafdil F, Mukhopadhyay P, et al. Anti-inflammatory and anti-apoptotic roles of endothelial cell STAT3 in alcoholic liver injury. Alcohol Clin Exp Res (2010) 34(4):719–25. doi: 10.1111/j.1530-0277.2009.01141.x
40. Rude MK, Watson R, Crippin JS. Recurrent hepatic epithelioid hemangioendothelioma after orthotopic liver transplantation. Hepatol (2014) 59(5):2050–2. doi: 10.1002/hep.26891
41. Zhang B, Li M, Zhang WF. The role of kupffer cells in ischemia-reperfusion injury after liver transplantation and its research status. Adv Physiol Sci (2015) 1):73–6.
42. Stewart RK, Dangi A, Huang C, Murase N, Kimura S, Stolz DB, et al. A novel mouse model of depletion of stellate cells clarifies their role in ischemia/reperfusion- and endotoxin-induced acute liver injury. J Hepatol (2014) 60(2):298–305. doi: 10.1016/j.jhep.2013.09.013
43. Wan J, Benkdane M, Teixeira-Clerc F, Bonnafous S, Louvet A, Lafdil F, et al. M2 kupffer cells promote M1 kupffer cell apoptosis: a protective mechanism against alcoholic and nonalcoholic fatty liver disease. Hepatol (2014) 59(1):130–42. doi: 10.1002/hep.26607
44. Yue S, Rao J, Zhu J, Busuttil RW, Kupiec-Weglinski JW, Lu L, et al. Myeloid PTEN deficiency protects livers from ischemia reperfusion injury by facilitating M2 macrophage differentiation. J Immunol (2014) 192(11):5343–53. doi: 10.4049/jimmunol.1400280
45. Zhao K, Zhao GM, Wu D, Soong Y, Birk AV, Schiller PW, et al. Cell-permeable peptide antioxidants targeted to inner mitochondrial membrane inhibit mitochondrial swelling, oxidative cell death, and reperfusion injury. J Biol Chem (2004) 279(33):34682–90. doi: 10.1074/jbc.M402999200
46. Szeto HH, Liu S, Soong Y, Wu D, Darrah SF, Cheng FY, et al. Mitochondria-targeted peptide accelerates ATP recovery and reduces ischemic kidney injury. J Am Soc Nephrol (2011) 22(6):1041–52. doi: 10.1681/ASN.2010080808
47. Shang L, Ren H, Wang S, Liu H, Hu A, Gou P, et al. SS-31 protects liver from ischemia-reperfusion injury via modulating macrophage polarization. Oxid Med Cell Longev (2021) 2021:6662156. doi: 10.1155/2021/6662156
48. Zhou H, Sun J, Zhong W, Pan X, Liu C, Cheng F, et al. Dexmedetomidine preconditioning alleviated murine liver ischemia and reperfusion injury by promoting macrophage M2 activation via PPARγ/STAT3 signaling. Int Immunopharmacol (2020) 82:106363. doi: 10.1016/j.intimp.2020.106363
49. Paris A, Mantz J, Tonner PH, Hein L, Brede M, Gressens P. The effects of dexmedetomidine on perinatal excitotoxic brain injury are mediated by the alpha2A-adrenoceptor subtype. Anesth Analg (2006) 102(2):456–61. doi: 10.1213/01.ane.0000194301.79118.e9
50. McCutcheon CA, Orme RM, Scott DA, Davies MJ, McGlade DP. A comparison of dexmedetomidine versus conventional therapy for sedation and hemodynamic control during carotid endarterectomy performed under regional anesthesia. Anesth Analg (2006) 102(3):668–75. doi: 10.1213/01.ane.0000197777.62397.d5
51. Rao Z, Sun J, Pan X, Chen Z, Sun H, Zhang P, et al. Hyperglycemia aggravates hepatic ischemia and reperfusion injury by inhibiting liver-resident macrophage M2 polarization via C/EBP homologous protein-mediated endoplasmic reticulum stress. Front Immunol (2017) 8:1299. doi: 10.3389/fimmu.2017.01299
52. Athanasopoulos V, Barker A, Yu D, Tan AH, Srivastava M, Contreras N, et al. The ROQUIN family of proteins localizes to stress granules via the ROQ domain and binds target mRNAs. FEBS J (2010) 277(9):2109–27. doi: 10.1111/j.1742-4658.2010.07628.x
53. Zheng L, Ling W, Zhu D, Li Z, Kong L. Roquin-1 regulates macrophage immune response and participates in hepatic ischemia-reperfusion injury. J Immunol (2020) 204(5):1322–33. doi: 10.4049/jimmunol.1900053
54. Watchorn TM, Dowidar N, Dejong CH, Waddell ID, Garden OJ, Ross JA. The cachectic mediator proteolysis inducing factor activates NF-kappaB and STAT3 in human kupffer cells and monocytes. Int J Oncol (2005) 27(4):1105–11.
55. Campana L, Starkey Lewis PJ, Pellicoro A, Aucott RL, Man J, O'Duibhir E, et al. The STAT3-IL-10-IL-6 pathway is a novel regulator of macrophage efferocytosis and phenotypic conversion in sterile liver injury. J Immunol (2018) 200(3):1169–87. doi: 10.4049/jimmunol.1701247
56. Öztürk Akcora B, Vassilios Gabriël A, Ortiz-Perez A, Bansal R. Pharmacological inhibition of STAT3 pathway ameliorates acute liver injury in vivo via inactivation of inflammatory macrophages and hepatic stellate cells. FASEB Bioadv (2020) 2(2):77–89. doi: 10.1096/fba.2019-00070
57. Chou WC, Levy DE, Lee CK. STAT3 positively regulates an early step in b-cell development. Blood (2006) 108(9):3005–11. doi: 10.1182/blood-2006-05-024430
58. Esashi E, Wang YH, Perng O, Qin XF, Liu YJ, Watowich SS. The signal transducer STAT5 inhibits plasmacytoid dendritic cell development by suppressing transcription factor IRF8. Immunity (2008) 28(4):509–20. doi: 10.1016/j.immuni.2008.02.013
59. Fornek JL, Tygrett LT, Waldschmidt TJ, Poli V, Rickert RC, Kansas GS. Critical role for Stat3 in T-dependent terminal differentiation of IgG b cells. Blood (2006) 107(3):1085–91. doi: 10.1182/blood-2005-07-2871
60. Yang R, Masters AR, Fortner KA, Champagne DP, Yanguas-Casás N, Silberger DJ, et al. IL-6 promotes the differentiation of a subset of naive CD8+ T cells into IL-21-producing b helper CD8+ T cells. J Exp Med (2016) 213(11):2281–91. doi: 10.1084/jem.20160417
61. Kinjyo I, Inoue H, Hamano S, Fukuyama S, Yoshimura T, Koga K, et al. Loss of SOCS3 in T helper cells resulted in reduced immune responses and hyperproduction of interleukin 10 and transforming growth factor-beta 1. J Exp Med (2006) 203(4):1021–31. doi: 10.1084/jem.20052333
62. Zhou H, Xu J, Huang S, He Y, He X, Guo L, et al. Blocking the hepatic branch of the vagus aggravates hepatic ischemia-reperfusion injury via inhibiting the expression of IL-22 in the liver. J Immunol Res (2021) 2021:6666428. doi: 10.1155/2021/6666428
63. Chestovich PJ, Uchida Y, Chang W, Ajalat M, Lassman C, Sabat R, et al. Interleukin-22: implications for liver ischemia-reperfusion injury. Transplantation (2012) 93(5):485–92. doi: 10.1097/TP.0b013e3182449136
64. Bai Y, Wu H, Zhang J, Zhang S, Zhang Z, Wang H, et al. IL-22 protects against biliary ischemia-reperfusion injury after liver transplantation via activating STAT3 and reducing apoptosis and oxidative stress levels In vitro and in vivo. Oxid Med Cell Longev (2022) 2022:9635075. doi: 10.1155/2022/9635075
65. Li W, Jiang H, Bai C, Yu S, Pan Y, Wang C, et al. Ac2-26 attenuates hepatic ischemia-reperfusion injury in mice via regulating IL-22/IL-22R1/STAT3 signaling. PeerJ (2022) 10:e14086. doi: 10.7717/peerj.14086
66. Perretti M, Chiang N, La M, Fierro IM, Marullo S, Getting SJ, et al. Endogenous lipid- and peptide-derived anti-inflammatory pathways generated with glucocorticoid and aspirin treatment activate the lipoxin A4 receptor. Nat Med (2002) 8(11):1296–302. doi: 10.1038/nm786
67. Melin N, Sánchez-Taltavull D, Fahrner R, Keogh A, Dosch M, Büchi I, et al. Synergistic effect of the TLR5 agonist CBLB502 and its downstream effector IL-22 against liver injury. Cell Death Dis (2021) 12(4):366. doi: 10.1038/s41419-021-03654-3
68. Zhu M, Lu B, Cao Q, Wu Z, Xu Z, Li W, et al. IL-11 attenuates liver Ischemia/Reperfusion injury (IRI) through STAT3 signaling pathway in mice. PloS One (2015) 10(5):e0126296. doi: 10.1371/journal.pone.0126296
69. Zhang XW, Mi S, Li Z, Zhou JC, Xie J, Hua F, et al. Antagonism of interleukin-17A ameliorates experimental hepatic fibrosis by restoring the IL-10/STAT3-suppressed autophagy in hepatocytes. Oncotarget (2017) 8(6):9922–34. doi: 10.18632/oncotarget.14266
70. Tang H, Fang H, Guo W, Cao S, Guo D, Zhang H, et al. Single nucleotide polymorphisms in interleukin-6 attenuates hepatocytes injury in hypoxia/re-oxygenation via STAT3 signal pathway mediated autophagy. Mol Biol Rep (2021) 48(2):1687–95. doi: 10.1007/s11033-020-06090-2
71. Xie K, Liu L, Chen J, Liu F. Exosomal miR-1246 derived from human umbilical cord blood mesenchymal stem cells attenuates hepatic ischemia reperfusion injury by modulating T helper 17/regulatory T balance. IUBMB Life (2019) 71(12):2020–30. doi: 10.1002/iub.2147
72. Matsumoto T, O'Malley K, Efron PA, Burger C, McAuliffe PF, Scumpia PO, et al. Interleukin-6 and STAT3 protect the liver from hepatic ischemia and reperfusion injury during ischemic preconditioning. Surgery (2006) 140(5):793–802. doi: 10.1016/j.surg.2006.04.010
73. Reusswig F, Fazel Modares N, Brechtenkamp M, Wienands L, Krüger I, Behnke K, et al. Efficiently restored thrombopoietin production by ashwell-morell receptor and IL-6R induced janus kinase 2/Signal transducer and activator of transcription signaling early after partial hepatectomy. Hepatol (2021) 74(1):411–27. doi: 10.1002/hep.31698
74. Dayoub R, Buerger L, Ibrahim S, Melter M, Weiss TS. Augmenter of liver regeneration (ALR) exhibits a dual signaling impact on hepatic acute-phase response. Exp Mol Pathol (2017) 102(3):428–33. doi: 10.1016/j.yexmp.2017.05.011
75. Darnell JE Jr., Kerr IM, Stark GR. Jak-STAT pathways and transcriptional activation in response to IFNs and other extracellular signaling proteins. Sci (1994) 264(5164):1415–21. doi: 10.1126/science.8197455
76. Wang JR, Bo XZ. Stats: transcriptional control and biological impact. Nat Rev Mol Cell Biol (2007) 29(2):140–3. doi: 10.1038/nrm909
77. Coorey NJ, Shen W, Zhu L, Gillies MC. Differential expression of IL-6/gp130 cytokines, jak-STAT signaling and neuroprotection after müller cell ablation in a transgenic mouse model. Invest Ophthalmol Vis Sci (2015) 56(4):2151–61. doi: 10.1167/iovs.14-15695
78. Fan C, Dai Y, Zhang L, Rui C, Wang X, Luan T, et al. Aerobic vaginitis induced by escherichia coli infection during pregnancy can result in adverse pregnancy outcomes through the IL-4/JAK-1/STAT-6 pathway. Front Microbiol (2021) 12:651426. doi: 10.3389/fmicb.2021.651426
79. Liu G, Wang B, Chen Q, Li Y, Li B, Yang N, et al. Interleukin (IL)-21 promotes the differentiation of IgA-producing plasma cells in porcine peyer's patches via the JAK-STAT signaling pathway. Front Immunol (2020) 11:1303. doi: 10.3389/fimmu.2020.01303
80. Rane SG, Reddy EP. Janus kinases: Components of multiple signaling pathways. Oncogene (2000) 19(49):5662–79. doi: 10.1038/sj.onc.1203925
81. Kelly RF, Lamont KT, Somers S, Hacking D, Lacerda L, Thomas P, et al. Ethanolamine is a novel STAT-3 dependent cardioprotective agent. Basic Res Cardiol (2010) 105(6):763–70. doi: 10.1007/s00395-010-0125-0
82. Ricke-Hoch M, Bultmann I, Stapel B, Condorelli G, Rinas U, Sliwa K, et al. Opposing roles of akt and STAT3 in the protection of the maternal heart from peripartum stress. Cardiovasc Res (2014) 101(4):587–96. doi: 10.1093/cvr/cvu010
83. Narimatsu M, Maeda H, Itoh S, Atsumi T, Ohtani T, Nishida K, et al. Tissue-specific autoregulation of the stat3 gene and its role in interleukin-6-induced survival signals in T cells. Mol Cell Biol (2001) 21(19):6615–25. doi: 10.1128/MCB.21.19.6615-6625.2001
84. Levy DE, Darnell JE Jr. Stats: transcriptional control and biological impact. Nat Rev Mol Cell Biol (2002) 3(9):651–62. doi: 10.1038/nrm909
85. Mahmoud AR, Ali FEM, Abd-Elhamid TH, Hassanein EHM. Coenzyme Q(10) protects hepatocytes from ischemia reperfusion-induced apoptosis and oxidative stress via regulation of Bax/Bcl-2/PUMA and nrf-2/FOXO-3/Sirt-1 signaling pathways. Tissue Cell (2019) 60:1–13. doi: 10.1016/j.tice.2019.07.007
86. Wang Z, Kawabori M, Houkin K. FTY720 (Fingolimod) ameliorates brain injury through multiple mechanisms and is a strong candidate for stroke treatment. Curr Med Chem (2020) 27(18):2979–93. doi: 10.2174/0929867326666190308133732
87. He X, Kang K, Pan D, Sun Y, Chang B. FTY720 attenuates APAP−induced liver injury via the JAK2/STAT3 signaling pathway. Int J Mol Med (2022) 49(5). doi: 10.3892/ijmm.2022.5123
88. Li W, Liang X, Kellendonk C, Poli V, Taub R. STAT3 contributes to the mitogenic response of hepatocytes during liver regeneration. J Biol Chem (2002) 277(32):28411–7. doi: 10.1074/jbc.M202807200
89. Feng D, Wang Y, Wang H, Weng H, Kong X, Martin-Murphy BV, et al. Acute and chronic effects of IL-22 on acetaminophen-induced liver injury. J Immunol (2014) 193(5):2512–8. doi: 10.4049/jimmunol.1400588
90. Nishina T, Komazawa-Sakon S, Yanaka S, Piao X, Zheng DM, Piao JH, et al. Interleukin-11 links oxidative stress and compensatory proliferation. Sci Signal (2012) 5(207):ra5. doi: 10.1126/scisignal.2002056
91. Wang W, Hu X, Xia Z, Liu Z, Zhong Z, Lu Z, et al. Mild hypothermia attenuates hepatic ischemia-reperfusion injury through regulating the JAK2/STAT3-CPT1a-Dependent fatty acid β-oxidation. Oxid Med Cell Longev (2020) 2020:5849794. doi: 10.1155/2020/5849794
92. Yu HC, Qin HY, He F, Wang L, Fu W, Liu D, et al. Canonical notch pathway protects hepatocytes from ischemia/reperfusion injury in mice by repressing reactive oxygen species production through JAK2/STAT3 signaling. Hepatol (2011) 54(3):979–88. doi: 10.1002/hep.24469
93. Pennica D, King KL, Shaw KJ, Luis E, Rullamas J, Luoh SM, et al. Expression cloning of cardiotrophin 1, a cytokine that induces cardiac myocyte hypertrophy. Proc Natl Acad Sci U S A (1995) 92(4):1142–6. doi: 10.1073/pnas.92.4.1142
94. Iñiguez M, Berasain C, Martinez-Ansó E, Bustos M, Fortes P, Pennica D, et al. Cardiotrophin-1 defends the liver against ischemia-reperfusion injury and mediates the protective effect of ischemic preconditioning. J Exp Med (2006) 203(13):2809–15. doi: 10.1084/jem.20061421
95. Zhong M, Che L, Du M, Liu K, Wang D. Desflurane protects against liver ischemia/reperfusion injury via regulating miR-135b-5p. J Chin Med Assoc (2021) 84(1):38–45. doi: 10.1097/JCMA.0000000000000427
96. Xiong L, Yu KH, Zhen SQ. MiR-93 blocks STAT3 to alleviate hepatic injury after ischemia-reperfusion. Eur Rev Med Pharmacol Sci (2018) 22(16):5295–304. doi: 10.26355/eurrev_201808_15729
97. Cheng Z, Guo C. Pemafibrate pretreatment attenuates apoptosis and autophagy during hepatic ischemia-reperfusion injury by modulating JAK2/STAT3β/PPARα pathway. PPAR Res (2021) 2021:6632137. doi: 10.1155/2021/6632137
98. Zhang N, Han L, Xue Y, Deng Q, Wu Z, Peng H, et al. The protective effect of magnesium lithospermate b on hepatic Ischemia/Reperfusion via inhibiting the Jak2/Stat3 signaling pathway. Front Pharmacol (2019) 10:620. doi: 10.3389/fphar.2019.00620
99. Freitas MC, Uchida Y, Zhao D, Ke B, Busuttil RW, Kupiec-Weglinski JW. Blockade of janus kinase-2 signaling ameliorates mouse liver damage due to ischemia and reperfusion. Liver Transpl (2010) 16(5):600–10. doi: 10.1002/lt.22036
100. Zhu YX, Zhou JH, Li GW, Zhou WY, Ou SS, Xiao XY. Dexmedetomidine protects liver cell line l-02 from oxygen-glucose deprivation-induced injury by down-regulation of microRNA-711. Eur Rev Med Pharmacol Sci (2018) 22(19):6507–16. doi: 10.26355/eurrev_201810_16065
101. Stephanou A, Latchman DS. Opposing actions of STAT-1 and STAT-3. Growth Factors (2005) 23(3):177–82. doi: 10.1080/08977190500178745
102. Carter AN, Born HA, Levine AT, Dao AT, Zhao AJ, Lee WL, et al. Wortmannin attenuates seizure-induced hyperactive PI3K/Akt/mTOR signaling, impaired memory, and spine dysmorphology in rats. eNeuro (2017) 4(3). doi: 10.1523/ENEURO.0354-16.2017
103. Ke B, Shen XD, Ji H, Kamo N, Gao F, Freitas MC, et al. HO-1-STAT3 axis in mouse liver ischemia/reperfusion injury: regulation of TLR4 innate responses through PI3K/PTEN signaling. J Hepatol (2012) 56(2):359–66. doi: 10.1016/j.jhep.2011.05.023
104. Ke B, Shen XD, Kamo N, Ji H, Yue S, Gao F, et al. β-catenin regulates innate and adaptive immunity in mouse liver ischemia-reperfusion injury. Hepatol (2013) 57(3):1203–14. doi: 10.1002/hep.26100
105. Huang J, Shen XD, Yue S, Zhu J, Gao F, Zhai Y, et al. Adoptive transfer of heme oxygenase-1 (HO-1)-modified macrophages rescues the nuclear factor erythroid 2-related factor (Nrf2) antiinflammatory phenotype in liver ischemia/reperfusion injury. Mol Med (2014) 20(1):448–55. doi: 10.2119/molmed.2014.00103
106. Goldaracena N, Cullen JM, Kim DS, Ekser B, Halazun KJ. Expanding the donor pool for liver transplantation with marginal donors. Int J Surg (2020) 82s:30–5. doi: 10.1016/j.ijsu.2020.05.024
107. Seifalian AM, Chidambaram V, Rolles K, Davidson BR. In vivo demonstration of impaired microcirculation in steatotic human liver grafts. Liver Transpl Surg (1998) 4(1):71–7. doi: 10.1002/lt.500040110
108. Cernkovich ER, Deng J, Bond MC, Combs TP, Harp JB. Adipose-specific disruption of signal transducer and activator of transcription 3 increases body weight and adiposity. Endocrinol (2008) 149(4):1581–90. doi: 10.1210/en.2007-1148
109. Li YC, Zheng XL, Liu BT, Yang GS. Regulation of ATGL expression mediated by leptin in vitro in porcine adipocyte lipolysis. Mol Cell Biochem (2010) 333(1-2):121–8. doi: 10.1007/s11010-009-0212-4
110. Inoue H, Ogawa W, Ozaki M, Haga S, Matsumoto M, Furukawa K, et al. Role of STAT-3 in regulation of hepatic gluconeogenic genes and carbohydrate metabolism in vivo. Nat Med (2004) 10(2):168–74. doi: 10.1038/nm980
111. Kroy DC, Beraza N, Tschaharganeh DF, Sander LE, Erschfeld S, Giebeler A, et al. Lack of interleukin-6/glycoprotein 130/signal transducers and activators of transcription-3 signaling in hepatocytes predisposes to liver steatosis and injury in mice. Hepatol (2010) 51(2):463–73. doi: 10.1002/hep.23322
112. Fukushima A, Loh K, Galic S, Fam B, Shields B, Wiede F, et al. T-Cell protein tyrosine phosphatase attenuates STAT3 and insulin signaling in the liver to regulate gluconeogenesis. Diabetes (2010) 59(8):1906–14. doi: 10.2337/db09-1365
113. Carbone M, Campagnolo L, Angelico M, Tisone G, Almerighi C, Telesca C, et al. Leptin attenuates ischemia-reperfusion injury in the rat liver. Transpl Int (2012) 25(12):1282–8. doi: 10.1111/j.1432-2277.2012.01555.x
114. Xu J, Li G, Wang P, Velazquez H, Yao X, Li Y, et al. Renalase is a novel, soluble monoamine oxidase that regulates cardiac function and blood pressure. J Clin Invest (2005) 115(5):1275–80. doi: 10.1172/JCI24066
115. Jiao Z, Ma Y, Zhang Q, Wang Y, Liu T, Liu X, et al. The adipose-derived mesenchymal stem cell secretome promotes hepatic regeneration in miniature pigs after liver ischaemia-reperfusion combined with partial resection. Stem Cell Res Ther (2021) 12(1):218. doi: 10.1186/s13287-021-02284-y
116. Choi E, Kim W, Joo SK, Park S, Park JH, Kang YK, et al. Expression patterns of STAT3, ERK and estrogen-receptor α are associated with development and histologic severity of hepatic steatosis: a retrospective study. Diagn Pathol (2018) 13(1):23. doi: 10.1186/s13000-018-0698-8
Keywords: signal transducers and activators of transcription3 (STAT3), ischemia-reperfusion injury (IRI), mitochondria, cell apoptosis, liver
Citation: Yang H, Zhang P, Wang Q, Cheng K and Zhao Y (2023) The research development of STAT3 in hepatic ischemia-reperfusion injury. Front. Immunol. 14:1066222. doi: 10.3389/fimmu.2023.1066222
Received: 10 October 2022; Accepted: 10 January 2023;
Published: 24 January 2023.
Edited by:
Sandra Sacre, Brighton and Sussex Medical School, United KingdomReviewed by:
Guandou Yuan, The First Affiliated Hospital of Guangxi Medical University, ChinaNhat Tu Le, Houston Methodist Research Institute, United States
Copyright © 2023 Yang, Zhang, Wang, Cheng and Zhao. This is an open-access article distributed under the terms of the Creative Commons Attribution License (CC BY). The use, distribution or reproduction in other forums is permitted, provided the original author(s) and the copyright owner(s) are credited and that the original publication in this journal is cited, in accordance with accepted academic practice. No use, distribution or reproduction is permitted which does not comply with these terms.
*Correspondence: Yujun Zhao, zyjdoc@aliyun.com