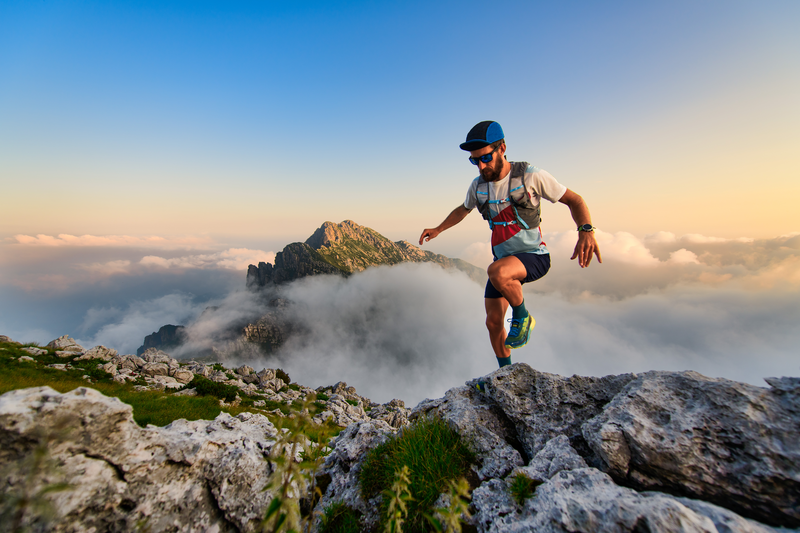
95% of researchers rate our articles as excellent or good
Learn more about the work of our research integrity team to safeguard the quality of each article we publish.
Find out more
SYSTEMATIC REVIEW article
Front. Immunol. , 13 March 2023
Sec. Cancer Immunity and Immunotherapy
Volume 14 - 2023 | https://doi.org/10.3389/fimmu.2023.1065510
This article is part of the Research Topic Mechanism and Application of Synergistic Effect of Radiotherapy and Immunotherapy View all 7 articles
Background: It is now widely accepted that radiotherapy (RT) can provoke a systemic immune response, which gives a strong rationale for the combination of RT and immune checkpoint inhibitors (ICIs). However, RT is a double-edged sword that not only enhances systemic antitumor immune response, but also promotes immunosuppression to some extent. Nevertheless, many aspects regarding the efficacy and safety of this combination therapy remain unknown. Therefore, a systematic review and meta-analysis was performed in order to assess the safety and efficacy of RT/chemoradiotherapy (CRT) and ICI combination therapy for non-small cell lung cancer (NSCLC) patients.
Methods: PubMed and several other databases were searched (according to specific criteria) to find relevant studies published prior to the 28th of February 2022.
Results: 3,652 articles were identified for screening and 25 trials containing 1,645 NSCLC patients were identified. For stage II-III NSCLC, the one- and two-year overall survival (OS) was 83.25% (95% confidence interval (CI): 79.42%-86.75%) and 66.16% (95% CI: 62.3%-69.92%), respectively. For stage IV NSCLC, the one- and two-year OS was 50% and 25%. In our study, the pooled rate of grade 3-5 adverse events (AEs) and grade 5 AEs was 30.18% (95% CI: 10.04%-50.33%, I2: 96.7%) and 2.03% (95% CI: 0.03%-4.04%, I2: 36.8%), respectively. Fatigue (50.97%), dyspnea (46.06%), dysphagia (10%-82.5%), leucopenia (47.6%), anaemia (5%-47.6%), cough (40.09%), esophagitis (38.51%), fever (32.5%-38.1%), neutropenia (12.5%-38.1%), alopecia (35%), nausea (30.51%) and pneumonitis (28.53%) were the most common adverse events for the combined treatment. The incidence of cardiotoxicity (0%-5.00%) was low, but it was associated with a high mortality rate (0%-2.56%). Furthermore, the incidence of pneumonitis was 28.53% (95% CI: 19.22%-38.88%, I2: 92.00%), grade ≥ 3 pneumonitis was 5.82% (95% CI: 3.75%-8.32%, I2: 57.90%) and grade 5 was 0%-4.76%.
Conclusion: This study suggests that the addition of ICIs to RT/CRT for NSCLC patients may be both safe and feasible. We also summarize details of different RT combinations with ICIs to treat NSCLC. These findings may help guide the design of future trials, the testing of concurrent or sequential combinations for ICIs and RT/CRT could be particularly useful to guide the treatment of NSCLC patients.
Non‐small cell lung cancer (NSCLC) is the leading cause of cancer mortality in the world (1). Immune Checkpoint Inhibitors (ICI) for NSCLC have become a common treatment strategy as numerous clinical trials have demonstrated their clinical benefits (2–4). Radiotherapy (RT) has been the predominant conventional local treatment for both locally advanced and metastasis NSCLC patients. It can be used for either curative or palliative purposes. Palliative RT can not only improve progression-free survival (PFS) and overall survival (OS) for metastasis NSCLC patients but also improve the patient’s quality of life (QOL) by relieving or avoiding the occurrence of symptoms (5–7). On the other hand, more aggressive treatments can avoid symptoms from local progression or delay initiation of a new systemic therapy (8). Mole (9) reported the abscopal effect in 1953, where radiation at one site may lead to tumor regression in both distal and distal non-irradiated sites. In recent years, RT has been described to increase the effects of ICIs on systemic antitumor responses by affecting almost all steps of the cancer-immunity cycle. RT can convert “cold” tumors that are typified by low immune cell infiltration into “hot” tumors with lymphocytic infiltration (10). The immunomodulatory effects associated with RT provide the premise underlying ICI response and a combination therapeutic strategy. Indeed, RT and ICI (RT&ICI) combination therapies have been proven to successfully treat patients with many kinds of cancers (11–13). Shaverdian et al. (14) found that patients with advanced NSCLC had longer PFS and OS when administered a therapeutic regimen that combined RT&ICIs. Similarly, Formenti et al. (15) found that patients with NSCLC that was previously unresponsive to ICI therapy experienced longer OS when treated with a RT&ICI combination.
RT can prove to be a double-edged sword that not only enhances systemic antitumor immune responses; but can also lead to the promotion of immunosuppression. For example, RT can promote immune response efficacy via T cell activation (16); yet it can cause lymphopenia and thereby reduce the efficacy of combination therapies (17). On one hand, RT may induce tumor-associated neutrophils to exhibit antitumor properties through interferon-β; on the other hand, pro-tumor properties may be induced via transforming growth factor-β (10). Some studies have also found that RT may increase myeloid-derived suppressor cell abundance, which can contribute to tumor progression in certain human organs or tissues (18). However, other studies have found that RT may promote dendritic cell mediated immune system efficacy (10, 19).
The potential for RT&ICI associated toxicity is also noteworthy. The most serious adverse effects include radiation-induced lung injury and radiation-induced heart disease, the latter of which can present with cardiomyopathy, conduction system abnormalities and coronary artery disease. The incidence of potentially lethal ICI-associated pneumonitis and cardiotoxicity is 3% to 5% (20) and 3.1% (21), respectively. Moreover, some preclinical studies have reported increased toxicity (especially pulmonary toxicity) when ICIs were combined with RT (22, 23). In a post-hoc analysis of the phase I KEYNOTE-001 trial, patients who had received prior chest RT developed more pulmonary toxicities when compared to patients without prior chest RT (13% vs. 1%, P=0.046) (14). Botticella et al. (24) also observed that patients receiving combination therapy were more likely to develop grade ≥3 pneumonitis when compared to patients receiving ICIs alone (16.7% vs. 2.4%, P=<0.001). Additionally, radiation recall pneumonitis localized within a previously irradiated area may occur when using ICIs (25). Hence, the aforementioned adverse events raise substantial concerns for the overlapping toxicity associated with RT&ICIs.
The efficacy and safety of RT/chemoradiotherapy (CRT) when combined with ICIs remains controversial, with the selection of ICIs needing further exploration and optimization. This is also true for aspects regarding the accompanying RT, including: ICI agent selection, treatment sequencing, RT dose, fractionation and irradiated site applicability. Therefore, a systematic review and meta-analysis to elucidate RT&ICI combination therapy efficacy and toxicity for NSCLC was performed and described herein.
This systematic review and meta-analysis was conducted using the Preferred Reporting Items for Systematic Review and Meta-analysis statement (26). Ethical approval was not required for this study since the data included was obtained exclusively from previously published sources.
PubMed, Web of Science and the Cochrane Library were searched to identify literature in English language journals published prior to the 28th of February 2022, without a lower date boundary. The following search terms were used: 1) “non-small cell lung neoplasm (s)/cancer (s)/carcinoma (s)” or “NSCLC”. 2) “radiotherapy” or “radiation therapy” or “radiation treatment” or “irradiation” or “SABR” or “stereotactic ablative radiotherapy” or “SBRT” or “stereotactic body radiation therapy” or “SRS” or “stereotactic radiosurgery” or “SRT” or “stereotactic radiotherapy” or “radio-chemotherapy” or “chemoradiotherapy”. 3) “immunotherapy” or “immune checkpoint inhibitors” or “checkpoint inhibitor “ or “checkpoint blockade” or “programmed cell death 1 receptor” or “programmed cell death 1 ligand 1” or “programmed death-1” or “PD-1” or “programmed death ligand-1” or “PD-L1” or “cytotoxic T lymphocyte-associated antigen-4 (CTLA-4) antigen” or “anti-CTLA-4” or “anti-PD-1” or “anti-PD-L1” or “CTLA-4” or “Durvalumab” or “Imfinzi” or “ MEDI-4736” or “Atezolizumab” or “ MPDL3280A” or “Tecentriq” or “RO5541267” or “RG7446” or “Pembrolizumab” or “Lambrolizumab” or “Keytruda” or “SCH 900475” or “MK-3475” or “Nivolumab” or “Opdivo” or “Ono-4538” or “MDX-1106” or “BMS-936558” or “Nivo” or “Avelumab” or “Barvencik” or “MSB0010718C” or “Toripalima” or “Tislelizumab” or “Camrelizumab” or “Sintilimab” or “Tremelimumab” or “Ipilimumab” or “Cemiplimab” or “Libtayo” or “PDCD1” or “CD274”.The comprehensive search string is available as Supplementary Materials Table 1.
Studies that met the following criteria were included in the meta-analysis: 1) describe participants with histologically confirmed lung cancer; 2) original papers describing human clinical trials that reported the outcomes for CRT/RT combined with ICIs; 3) prospective or retrospective study; 4) outcomes included treatment safety and efficacy; 5) published in English.
Conference abstracts, case reports, comments, reviews, animal studies, and mechanistic studies were excluded. Studies that did not have sufficient data (missing clinical safety data and efficacy data) or unclear descriptions (poorly described trials or did not accurately describe trial results) were also excluded. When articles described the same study population, only the most recent or complete analysis was included. Disagreements related to article selection were resolved in a discussion with all the authors of this study.
The data was extracted by two independent investigators, including: first author name, time of publication, country, number of cases, pathological subgroups, type of study, treatment regimens, radiotherapy type and dose, treatment characteristics including timing of ICI therapy and rates of pneumonitis and treatment related deaths. Any discrepancies were resolved through group discussions until a consensus was reached. The revised Cochrane Risk of Bias tool for randomized trials (27) and Risk of Bias in Non-randomized Studies of Interventions tool (28) were conducted to appraise the quality of randomized controlled trials and non-randomized trials, respectively.
The random effects model was used for the meta-analysis with the “meta” package implemented in R (version 4.1.2, R Foundation for Statistical Computing). A 95% confidence interval (CI) was used, and Cochran’s Q and I2 statistics were used to assess heterogeneity. 0%, 25%, 50% and 75% of I2 represented no, low, moderate and high heterogeneity, respectively. Meta-regression was considered inappropriate due to the insufficient number of studies (<10). Subgroup and sensitivity analyses were performed to explore study heterogeneity and to determine the impact of each individual study. Publication bias was estimated using the Begg’s and Egger’s test. P=<0.05 was used as the threshold for considering statistical significance.
The search process (Figure 1) enabled the identification of twenty-five trials (Table 1) for this systematic review and meta-analysis (11, 14, 29–51). The twenty-five trials consisted of twenty-four trials non-randomized trials (11, 14, 29, 31–51) and one randomized controlled trial (30). Thirteen trials were conducted in North America (11, 14, 29, 31, 33, 34, 39, 43, 45, 46, 49–51), nine in Asia (32, 35–38, 40–42, 44), and three in Europe (30, 47, 48). Of the twenty-five studies included, nine were prospective studies (14, 29, 30, 39, 45, 46, 48–50) and sixteen were retrospective studies (11, 31–38, 40–44, 47, 51). Two trials utilized conventional RT and stereotactic body radiotherapy (SBRT) (46, 51), two focused upon SBRT (11, 50) and twenty-one trials used conventional RT (14, 29–45, 47–49). Durvalumab (30–38, 47), pembrolizumab (14, 29, 46, 49), nivolumab (40, 41, 48), ipilimumab (45), and atezolizumab (39) were used in nine, four, three, one and one study (ies), respectively; pembrolizumab and nivolumab (43, 44, 50) were used in three studies. ICIs were administered after RT in eighteen studies (11, 14, 29–38, 40–44, 50), prior to RT in one study (45), concurrently with CRT in three studies (11, 46, 50), whereas two studies examined both concurrent and sequential administration of RT with ICIs (48, 49). A total radiation dose of < 60 Gy (35, 40, 46, 49) was administered in four studies and a total radiation dose of >=60 Gy (34, 39, 48) was prescribed in three studies. Both randomized (30) and non-randomized trials (11, 14, 29, 31–51) were judged to be at moderate risk of bias (Supplementary Materials Table 2, Table 3 and Figures 1, 2). A total of 1,645 patients were included in the aforementioned trials.
Figure 2 Meta-analysis and forest plots for non-small cell lung cancer patients treated with radiotherapy/chemoradiotherapy and immune checkpoint inhibitors who experienced grade 3-5 adverse events.
The trials included in this study had different primary efficacy variables, which prevented the performance of an efficacy based meta-analysis. The objective response rate (ORR) was 25.8%-73.4% for II-III stage NSCLC and 45%-45.9% for IV stage NSCLC. The one-year to five-year PFS and OS for II-III stage NSCLC and IV stage NSCLC were shown in Table 2.
All adverse events are described in Table 3. The pooled rate for grade 3-5 adverse reactions was 30.18% (95% CI: 10.04%-50.33%, I2: 96.7%) and 2.03% (95% CI: 0.03%-4.04%, I2: 36.8%) for grade 5 adverse events (Figures 2, 3). The most common adverse events included: fatigue (50.97%), dyspnea (46.06%), dysphagia (10%-82.5%), leucopenia (47.6%), anaemia (5%-47.6%), cough (40.09%), esophagitis (38.51%), fever (32.50%-38.10%), neutropenia (12.5%-38.1%), alopecia (35%), nausea (30.51%) and pneumonitis (28.53%). Most events were grade 1-2. The most common grade 3-5 events included: inflection (0.2%-20%), cough (0%-15%), gastric hemorrhage (2.5%-8.1%), elevated liver enzymes (7.64%), neutropenia (2.5%-9.5%), dehydration (0%-7.5%), pneumonitis (5.82%) and adrenal insufficiency (5.40%). Of the patients with elevated liver enzymes, adrenal insufficiency and gastric hemorrhage, all the events were categorized as grade 3-5. The most common grade 5 adverse reactions were pneumonitis (0%-4.76%), gastric hemorrhage (0%-2.70%) and myocardial infarction (0.21%-2.56%). For pulmonary adverse events, the grade 1-5 adverse reactions accounted for 0.21%-46.06%, and the most common effects included: dyspnea (46.06%), cough (40.09%), esophagitis (38.51%) and pneumonitis (28.53%). The grade 3-5 adverse reactions accounted for 0%-15.00%, and the most common side effects included: pneumonitis (5.82%) and cough (0%-15.00%). The grade 5 adverse reactions included: pneumonitis (0%-4.76%), emphysema and respiratory failure (0.2%-0.21%), hemoptysis (0%-0.2%) and dyspnea (0%-0.2%). The incidence of hemoptysis, pulmonary embolism, emphysema and respiratory failure, was relatively low; but were grade 3-5 events. The mortality associated with hemoptysis, pulmonary embolism, emphysema and respiratory failure was 0%-0.21%. It is worth noting that whilst the incidence of cardiotoxicity (0%-5%) was low, it was associated with a high mortality rate (0%-2.56%). The grade 3-5 adverse reactions accounted for 0%-2.7%, with the most common effects including: acute coronary syndrome (2.5%), heart failure (2.5%), atrial fibrillation (0%-2.70%) and myocardial infarction (0.21%-2.56%). The grade 5 adverse reactions observed during the trials were: myocardial infarction (0.21%-2.56%), cardiac arrest (0.42%), cardiomyopathy (0.21%), cardiopulmonary failure (0.21%), aortic dissection (0.21%) and right ventricular failure (0.21%). We identified that myocardial infarction was adverse event associated with the highest risk of mortality.
Table 3 Adverse events experienced by non-small cell lung cancer patients following treatment with ICIs and RT.
Figure 3 Meta-analysis and forest plots for non-small cell lung cancer patients treated with radiotherapy/chemoradiotherapy and immune checkpoint inhibitors who experienced grade 5 adverse events.
The pooled rate of grade 1-5 pneumonitis was 28.53% (95% CI: 19.22%-38.88%, I2: 92.00%). For conventional RT, the incidence of grade 1-5 pneumonitis was 29.92% (95% CI: 19.89%-41.04%, I2: 91.70%), and 12.79% (95% CI: 3.70%-26.25%, I2: 71.80%) for SBRT (Figure 4).
Figure 4 Meta-analysis and forest plots for non-small cell lung cancer patients treated with radiotherapy/chemoradiotherapy and immune checkpoint inhibitors who experienced grade 1-5 pneumonitis.
The pooled rate of grade 3-5 pneumonitis was 5.82% (95% CI: 3.75%-8.32%, I2: 57.90%). For conventional RT the occurrence of grade 3-5 pneumonitis was 5.10% (95% CI: 3.40%-7.13%, I2: 46.00%), and 11.62% (95% CI: 2.56%-26.00%, I2: 81.40%) for SBRT (Figure 5).
Figure 5 Meta-analysis and forest plots for non-small cell lung cancer patients treated with radiotherapy/chemoradiotherapy and immune checkpoint inhibitors who experienced grade 3-5 pneumonitis.
The statistical analysis for grade 5 pneumonitis analysis was not reported in this study due to the detection of publication bias. Nevertheless, the rate of grade 5 pneumonitis was 0%-4.76% overall, with 0%-4.76% for conventional RT and 0% for SBRT.
Grade 1-5 pneumonitis was higher in studies that used a total radiation dose >=60 Gy when compared to those that limited the total radiation dose to <60 Gy. With a grade 1-5 rate for pneumonitis of 33.70% versus 15.98% (>=60 vs. <60: 95%, CI: 28.56%-39.05%, I2: 35.1% and 95% CI: 4.59%-27.37%, I2: 62.50%, respectively) (Figure 6). A subgroup analysis was not performed for SBRT alone because only two articles were available.
Figure 6 Subgroup analysis of meta-analysis and forest plot for non-small cell lung cancer patients treated with radiotherapy/chemoradiotherapy and immune checkpoint inhibitors who experienced grade 1-5 pneumonitis.
In our study, only one trial (42) explored the relationship between tumor location and pneumonitis; there was no increased risk of pneumonitis in the lower lobe when compared to the middle/upper lobe. Moreover, only one trial explored the effects of different radiotherapy techniques (combined with ICIs) on pneumonitis (51); for which no difference was identified, perhaps due to the low number of patients included.
The pooled rate of grade 1-5 pneumonitis for patients treated with ICIs programmed cell death-ligand 1 (PD-L1) was 35.09% (95% CI: 21.99%-49.45%, I2: 89.0%) which was higher than those targeting programmed cell death protein-1 (PD-1) (29.11%; 95% CI: 17.19%-49.31%, I2: 94.00%). The rate of grade 3-5 pneumonitis for PD-L1 was 5.07% (95% CI: 2.84%-7.88%, I2: 49.4%) and 5.45% (95% CI: 2.87%-8.03%, I2: 1.4%) for PD-1 (Figures 6, 7). A subgroup analysis was not performed for SBRT alone because only two articles were available.
Figure 7 Subgroup analysis of meta-analysis and forest plot for non-small cell lung cancer patients treated with radiotherapy/chemoradiotherapy and immune checkpoint inhibitors who experienced grade 3-5 pneumonitis.
When ICIs were administered for more than one year, the rate of grade 1-5 and grade 3-5 pneumonitis was 26.57% (95% CI: 15.70%-37.44%, I2: 89.1%) and 4.79% (95% CI: 3.26%-6.32%, I2: 0.6%), respectively. The rate of grade 1-5 and 3-5 pneumonitis for patients administered ICIs for less than one year, was 0%-17.24% and 0%-6.90%, respectively.
Pneumonitis was identified at a higher rate in retrospective studies than prospective studies at both grade 1-5 (36.73% vs. 20.84%; 95% CI: 23.20%-51.42%, I2: 92.80 and 95% CI: 9.96%-31.73%, I2: 93.50%) and grade 3-5 (5.37% vs. 4.57%; 95% CI: 2.73%-8.83%, I2: 55.6% and 95% CI: 3.06%-6.09%, I2: 0.0%) (Figures 6, 7). A subgroup analysis was not performed for SBRT alone because only two articles were available.
The Begg’s and Egger’s tests are enumerated in Supplementary Materials Table 4.
The study included herein provides an overview of published NSCLC trials focusing on the use of RT with ICIs. This review and meta-analysis systematically, quantitatively and comprehensively analyzed the efficacy and toxicity of RT when combined with ICIs for 1,645 NSCLC patients from 25 studies.
The efficacy of RT when combined with ICIs (ORR, 45-45.9%) appeared to be superior for patients that had previously received treatment for metastatic NSCLC. The efficacy (ORR) for nivolumab or pembrolizumab against pretreated metastatic NSCLC ranged from 19 to 36% in the Checkmate 017, Checkmate 057 and Keynote 011 trials (52, 53). The one-year OS for metastatic NSCLC patients was 50% and the two-year OS was 25%, which was consistent with the aforementioned studies (one-year OS:42%-58%, two-year OS:23%-29%).
While concurrent CRT has been the standard treatment for inoperable cancer; durvalumab has been widely used as maintenance therapy following CRT, which was based upon evidence from the PACIFIC study (30). In our study, the pooled 1-year PFS was 56.39% (95% CI: 50.66%-62.03%, I2:39.4%), one-year OS was 83.25% (95% CI: 79.42%-86.75%, I2:17.6%) and two-year OS was 66.16% (95% CI: 62.30%-69.92%, I2:0.0%). When viewed in relation to other recent reports, the one-year PFS was 48% in both the 60 Gy group from RTOG 0617 (54) and the cisplatin-pemetrexed group from PROCLAIM (55), and 55.9% in the durvalumab group of PACIFIC. The one-year OS for RTOG 0617, PROCLAIM and PACIFIC was 78%, 76%, 83.1%, respectively. Therefore, RT/CRT when combined with ICIs may improve OS, PFS, and tumor response rates in NSCLC patients, although further study may be needed.
Zhou et al. (56), found that the overall incidence of grade 3-5 adverse events (AEs) ranged from 35% to 40% in PD-1 or PD-L1 monotherapy arms, while a rate of 40% to 50% AEs was observed when ICIs were combined with CRT (for 23,322 patients from 52 randomized controlled trials). In our study, the pooled rate of grade 3-5 AEs was 30.18% (95% CI: 10.04%-50.33%, I2: 96.7%) and 2.03% (95% CI: 0.03%-4.04%, I2: 36.8%) for grade 5 AEs, which was lower than the aforementioned study.
Cardiotoxicity is a rare but potentially fatal adverse effect of ICI therapy, for NSCLC patients, the overall incidence of cardiotoxicity is low (0%-5%); but the mortality associated with cardiotoxicity is high (0%-2.56%). We found that the most common form of lethal cardiotoxicity was myocardial infarction (0.21%-2.56%). In our study, the incidence of grade 1-5 myocarditis was 0.76%, which is within the range found by previous studies (0.05%-1.14%) (57, 58). RT&ICIs did not appear to increase the rate of myocarditis. However, Mahmood et al. (58), found that myocarditis (early during treatment) was more common following ICI treatment with ICI occurred, thus further research may be warranted.
Overall, the most common grade 5 adverse event was pneumonitis (0%-4.76%). Previous meta-analyses have demonstrated that the incidence of radiation pneumonitis is 1.5%–40%, with a 1.1%-6.6% incidence of grade ≥ 3 pneumonitis and 0%-3.1% for grade 5 (59, 60). In our study, the pooled incidence of pneumonitis was 28.53% (95% CI: 19.22%-38.88%, I2: 92.00%), with a 5.82% (95% CI: 3.75%-8.32%, I2: 57.90%) incidence of grade ≥ 3 pneumonitis and 0%-4.76% for grade 5. Thus, the incidence of pneumonitis following RT&ICIs is similar to RT alone, and likely safe for use in NSCLC patients.
There remain concerns among researchers in the field that the RT&ICI prospective studies may not reflect the real-world clinical application. For example, patients with a history of interstitial lung disease were excluded from prospective trials. This notion is supported by Suresh et al. (61), who found a higher incidence of immune-associated pneumonia in real-world setting. It is notable that we found that the incidence of grade 1-5 and grade 3-5 pneumonitis to be higher in retrospective studies than in the prospective studies (36.73% vs. 20.84% and 5.37% vs. 4.57%, respectively).
Only one trial (42) included in this study explored the relationship between tumor location and pneumonitis, and no increased risk of pneumonitis was observed in the lower lobe compared with the middle/upper lobe. However, further research is warranted since Zhang et al. (62) found that tumors located in the lower lobe were associated with a significantly increased probability of grade ≥2 radiation-pneumonitis.
Elective node irradiation is performed for NSCLC patients to treat lymph nodes that are not known to contain metastases, including the bilateral hilar, mediastinum, and even supraclavicular areas. Grills et al. (63), has suggested that elective node irradiation would increase the volume of the radiotherapy target, lead to toxicity and make it hard to improve the therapeutic dose. The administration of immunotherapy further complicates the choice between involved-field and elective node irradiation. Previous studies have demonstrated that direct irradiation of lymph nodes can partially explain lymphopenia (64, 65). Additionally, there is increasing evidence that lymphocyte depletion in NSCLC is associated with a poorer prognosis, due to the lower likelihood of a robust immunotherapeutic response (66). It is possible that involved-field irradiation may have greater synergy with ICIs, and form a more a powerful RT strategy in the era of immunotherapy.
In addition to involved-field irradiation, clinical target volume (CTV) omission is another strategy that has been used in conjunction with immunotherapy. CTV is defined as the volume of tissue containing gross tumor volume and subclinical microscopic malignant lesions. A retrospective study of 105 stage III NSCLC patients treated with (n=50) or without (n=55) CTV did not experience a significantly different rate of local recurrence, distant metastasis, PFS or OS. Notably, the incidence of grade 3–4 radiation-pneumonitis was significantly lower in group that did not receive CTV (P=0.044) (67). Kilburn et al. (68), found that only 1.8% of patients (2/110) experienced CTV (planning target volumes expanded 1 cm) failures, suggesting that CTV omission appears to be a viable strategy, further study may be warranted.
We found higher rates of grade 1-5 pneumonitis in studies where a total radiation dose of >=60 Gy (vs. < 60 Gy) was administered (33.70% vs. 15.98%). Prior to the advent ICIs, the RTOG 7301 trial established that the standard radiation dose for unresectable NSCLC was 60–63 Gy in 1.8–2 Gy daily fractions (69). Subsequently, the RTOG 0617 trial identified that dose escalation from 60 to 74 Gy resulted in worse OS when compared to 60 Gy (70). In addition to dose escalation, there has been considerable interest in the potential benefits of unconventional fractionated radiotherapy for NSCLC. A meta-analysis has shown that hyper-fractionated and accelerated radiotherapy can yield a modest survival benefit (compared to conventional regimens) for NSCLC patients (71).
A number of immune system effects have been associated with RT, for example Crittenden et al. (72) demonstrated that immunosuppressive tumor-associated macrophages occur in the presence of high-dose irradiation (60 Gy). A significant reduction in myeloid-derived suppressor cells has also been observed 7–14 days after single high-dose RT for colonic tumors in mice (73). Other studies also found that single dose of 8 to 10 Gy is more immunogenic than a traditional grading protocol (74–76). Therefore, hypo-fractionated radiation therapy (HFRT) has been suggested as being more likely to activate immune effects. A pooled analysis of the PEMBRO-RT and MDACC trials found that ablative radiotherapy combined with pembrolizumab had a significantly (P < 0.05) better ORR (48% and 54% for 24Gy/3 and 50Gy/4, respectively) than both non-ablative radiotherapy (18% ORR with 45Gy/15 fractions) or pembrolizumab (20% ORR) alone (77). Additionally, low-dose radiation has been suggested as a means of producing a systemic immune effect (78). Several studies have explored the use of low-dose radiotherapy (LDRT) (0.5 to 2.0 Gy, with 1 or a few fractions) to enhance the abscopal response of distant tumors and improve the immunogenicity of “cold tumors” (79, 80). Limei Yin et al. (81) demonstrated that patients with stage IV NSCLC have a better systemic antitumor with a triple treatment consisting of LDRT, HFRT and ICIs, partial response was achieved for three patients and stable disease for two patients. Taken together, these findings suggest that different dose-fractionation regimens may have different effects on the immune system and dose-fractionation schedules should be re-evaluated to determine the optimal RT&ICI regimen.
Radiotherapy dose distribution also plays an important role, according to results from the phase III RTOG 0617 clinical trial, a V20 dose of less than 35% can minimize the risk of pneumonitis (70). Shaikh et al. (82) suggested that a V5 of <65% and V20 of <25% reduced the risk of grade 2 radiation-pneumonitis. Additionally, the dose constraints were tightened when employing ICIs in combination with CRT. Landman et al. (32) found that a V5 of <55%, V20 <23% and mean lung dose <14.8 Gy were the thresholds for pneumonitis when combined with ICIs. Jang et al. (42) identified that a mean lung dose of 16 Gy, V30 and V40 could significantly predict the occurrence of radiation-pneumonitis following ICI treatment; and in the non-ICI group, only V20 could significantly predict occurrence of radiation-pneumonitis. They also found that V40 had the largest area under the curve among various parameters in the ICI group. Hassanzadeh et al (34), found that pneumonitis-free survival rate was correlated with lung V5; while lung V20 and mean lung dose were not significantly correlated with pneumonitis. Schoenfeld et al. (83) also suggested that low dose radiation exposure (represented by V5) may be more important in pneumonitis risk when consolidation ICIs are used. Further studies of dosimetric parameters and their association with pneumonitis are warranted.
Our study identified only one trial that investigated the effects on pneumonitis for different radiotherapy techniques in combination with ICIs (51). However, few patients were included and no differences were found. Advances in radiotherapy technology could be particularly important when paired with ICI therapy. The shift from two-dimensional radiotherapy to advanced three-dimensional-based radiation techniques, including 3-dimensional conformal radiotherapy and intensity-modulated radiotherapy, allows for more precise radiation delivery and reduced exposure to adjacent critical structures. Recently, intensity-modulated radiotherapy was found to provide similar outcomes (OS and PFS) with a significantly lower risk of radiation pneumonitis when compared to 3-dimensional conformal radiotherapy (3.5% versus 7.9%, respectively) (84). There are few studies on the application of new radiotherapy techniques with ICIs.
An analysis of two prospective metastatic NSCLC trials reported that the PFS associated with SBRT when combined with anti-PD1 treatment was significantly better than anti-CTLA4; although the efficacy was not statistically different (11). Gu et al. (85) found an increased likelihood of hypothyroidism after PD-1 inhibition against lung cancer, and renal injury for PD-L1. Pneumonitis was more common following PD-1 treatment, but hepatitis, rash and lipase elevation were more common for PD-L1. In our study, we found grade 3-5 pneumonitis was higher for patients treated with PD-1 inhibitors than PD-L1 inhibitors (5.45% vs. 5.07%), which is consistent with other studies (56, 85–87). Inhibition of PD-1 and blockage of PD-1-PD-L2 may contribute to cytokine release and proliferation of autoreactive T cells, leading to an enhanced antitumor effect and AEs (88). We also found that grade 1-5 pneumonitis was lower for PD-1 inhibitors than PD-L1 inhibitors (29.11% vs. 35.09%). However, many retrospective studies focused upon the use of PD-L1 in our study and further research is necessary. Li et al. (87) suggested that RT may be the leading factor for these differences, rather than the ICIs themselves. This would account for the similar incidence of AEs following treatment using PD-1 and PD-L1 inhibitors when combined with RT, which indicates that selection of ICIs should be mainly based upon their efficacy rather than toxicity.
We also found that the administration of ICIs for more than one year was associated with a 26.57% (95% CI: 15.70%-37.44%, I2: 89.1%) rate of grade 1-5 pneumonitis and a 4.79% (95% CI: 3.26%-6.32%, I2: 0.6%) rate of grade 3-5 pneumonitis. When the ICI treatment lasted for less than one year, the rate of grade 1-5 and grade 3-5 pneumonitis was 0%-17.24% and 0%-6.90%, respectively. Sukari et al. (89) found the duration of PD1 was significantly associated with the development of pneumonitis, and Shankar et al. (90) also found that longer ICI treatment was associated with a higher incidence of immune-related AEs (and a greater therapeutic effect). Therefore, the increased potential for AEs should be considered when prolonged extended treatment regimens.
No definitive conclusions can be made regarding the optimal schedule for the administration of RT/CRT with ICIs. A retrospective analysis from a prospective study showed patients treated with pembrolizumab (and prior RT) showed improved PFS and OS (14); another study reached the opposite conclusion, that prior RT was associated with poorer survival (91). One study found that metastatic cancer (80% lung cancer and 20% other cancers) patients who received (SBRT/SRT) after completion of immunotherapy (3.6 months) had significantly worse OS (P=0.01) than those who received SRT prior to or concurrent with immunotherapy (13.0 months) (92). However, Lesueur et al. suggested that there may be no OS or PFS differences for metastatic NSCLC patients treated with RT before or during/after ICI administration (93). Interestingly, some preclinical studies have suggested that the optimal timing may depend on the type of ICI. Anti-CTLA-4 therapies were found to be most effective when administered before RT; whereas the optimal timing of anti-OX40 delivery was one day following RT, during the increased antigen presentation post-radiation window (94).
The PACIFIC trial (95) found that consolidative durvalumab reduced the risk of progression more effectively and improved the survival rate when initiated following concurrent CRT within two weeks or less (PFS: hazard ratio (HR), 0.42 [CI, 0.29–0.61], OS: HR, 0.53 [CI, 0.35–0.79]), compared to more than two weeks (PFS: HR, 0.65 [CI, 0.52–0.81], OS: HR, 0.78 [CI, 0.61–0.99]). Bassanelli et al. (96) suggested that the first ≤60 days prior to the completion of RT (mOS, 22.4 months vs. 8.6 months, p = 0.005) was the most appropriate window for ICI administration. However, Bryant et al. (97) found that initiation of durvalumab ≤14 days after RT was not associated with improvement in PFS or OS. Denault et al. (98) also found no significant difference in efficacy or toxicity between two and four weeks durvalumab therapy for patients treated with CRT. Recently, Anscher et al. (99) identified that RT administered within 90 days prior to ICI did not increase the risk of serious AEs using data for 16,835 patients from 68 prospective trials. Patients who were administered ICIs and RT within ≤90 days had slightly higher rates of fatigue, endocrine disorders and pneumonitis than those without RT. These differences were due to low-grade (grade 1-2) AEs, thus ICI administration within 90 days of RT appears to be safe.
Some difficulties remain for the identification of patients that will gain the greatest survival benefit from combination therapy or those who are more likely to have adverse effects. PD-L1 and tumor mutation burden (TMB) were widely used in clinical stratification of ICI patients, but their predictive effects are still controversial. Many other markers based upon tumor tissue, peripheral blood, and radiological images have been investigated, including: cell surface markers, various immune cells, immune related gene and imaging biomarkers (100). However, they showed limited ability to distinguish non-responders from responders following RT combined with ICI therapy.
It is important to note that most of the studies included in this meta-analysis were single-arm clinical trials, which prevents the comparison of the advantages and disadvantages associated with CRT/RT + ICIs and CRT/RT based upon a balanced baseline. Whilst the results from these trials may contain a high amount of statistical heterogeneity, subgroup analyses were performed to identify sources of study heterogeneity. Finally, due to data availability for lung cancer patients in this field, we could not explore certain details regarding the efficacy and safety of RT/CRT plus ICIs, including: the influence of different radiotherapy doses/fractionations, irradiation target volumes, chemotherapy regimen.
In conclusion, this comprehensive meta-analysis of 1,645 non-small cell lung cancer patients (from 25 studies) systemically and quantitatively explored the clinical efficacy and safety of immune checkpoint inhibitors when combined with radiotherapy/chemoradiotherapy. Based upon the studies presented herein, the combination of (chemo)radiotherapy and immune checkpoint inhibitors appears to be a both safe and feasible mode of treatment. These findings may help clinicians in the design of future trials testing concurrent or sequential combinations of immune checkpoint inhibitors and radiotherapy/chemoradiotherapy for the treatment of patients with non-small cell lung cancer.
The original contributions presented in the study are included in the article/Supplementary Material. Further inquiries can be directed to the corresponding authors.
YuZ and YaZ conceptualized the study. JW, RD, YL, CF and TN collected the data. JW, TN, QinZ, FT, QiZ and YX analyzed the data. JW and YZ wrote the manuscript. All authors contributed to the article and approved the submitted version.
This work was supported by the: (1) Science and Technology Fund Project of Guizhou Health Commission (Grant no. gzwjkj2020-1-032); (2) Lian Yun Gang Shi Hui Lan Public Foundation (Grant no. HL-HS2020-33); (3) Supported by Guizhou Provincial Science and Technology Projects (Grant no. ZK [2021] General 455); (4) Health Commission Science and Technology Foundation of Guizhou Province (Grant no. gzwkj 294 2022-028); (5) Youth Fund of Guizhou Provincial People’s Hospital (Grant no. GZSYQN [2021]03); (6) Youth Fund of Guizhou Provincial People’s Hospital (Grant no. GZSYQN [2022]09); (7) Doctor Foundation of Guizhou Provincial People's Hospital (Grant no. GZSYBS [2022]09).
This is a short text to acknowledge the contributions of specific colleagues, institutions, or agencies that aided the efforts of the authors.
The authors declare that the research was conducted in the absence of any commercial or financial relationships that could be construed as a potential conflict of interest.
All claims expressed in this article are solely those of the authors and do not necessarily represent those of their affiliated organizations, or those of the publisher, the editors and the reviewers. Any product that may be evaluated in this article, or claim that may be made by its manufacturer, is not guaranteed or endorsed by the publisher.
The Supplementary Material for this article can be found online at: https://www.frontiersin.org/articles/10.3389/fimmu.2023.1065510/full#supplementary-material
1. Sung H, Ferlay J, Siegel RL, Laversanne M, Soerjomataram I, Jemal A, et al. Global cancer statistics 2020: Globocan estimates of incidence and mortality worldwide for 36 cancers in 185 countries. CA: Cancer J Clin (2021) 71(3):209–49. doi: 10.3322/caac.21660
2. Herbst RS, Baas P, Kim DW, Felip E, Pérez-Gracia JL, Han JY, et al. Pembrolizumab versus docetaxel for previously treated, pd-L1-Positive, advanced non-Small-Cell lung cancer (Keynote-010): A randomised controlled trial. Lancet (London England) (2016) 387(10027):1540–50. doi: 10.1016/s0140-6736(15)01281-7
3. Rittmeyer A, Barlesi F, Waterkamp D, Park K, Ciardiello F, von Pawel J, et al. Atezolizumab versus docetaxel in patients with previously treated non-Small-Cell lung cancer (Oak): A phase 3, open-label, multicentre randomised controlled trial. Lancet (London England) (2017) 389(10066):255–65. doi: 10.1016/s0140-6736(16)32517-x
4. Antonia SJ, Villegas A, Daniel D, Vicente D, Murakami S, Hui R, et al. Durvalumab after chemoradiotherapy in stage iii non-Small-Cell lung cancer. New Engl J Med (2017) 377(20):1919–29. doi: 10.1056/NEJMoa1709937
5. Gomez DR, Blumenschein GR Jr., Lee JJ, Hernandez M, Ye R, Camidge DR, et al. Local consolidative therapy versus maintenance therapy or observation for patients with oligometastatic non-Small-Cell lung cancer without progression after first-line systemic therapy: A multicentre, randomised, controlled, phase 2 study. Lancet Oncol (2016) 17(12):1672–82. doi: 10.1016/s1470-2045(16)30532-0
6. Gomez DR, Tang C, Zhang J, Blumenschein GR Jr., Hernandez M, Lee JJ, et al. Local consolidative therapy vs. maintenance therapy or observation for patients with oligometastatic non-Small-Cell lung cancer: Long-term results of a multi-institutional, phase ii, randomized study. J Clin Oncol (2019) 37(18):1558–65. doi: 10.1200/jco.19.00201
7. Lutz ST, Jones J, Chow E. Role of radiation therapy in palliative care of the patient with cancer. J Clin Oncol (2014) 32(26):2913–9. doi: 10.1200/jco.2014.55.1143
8. Petrelli F, Ghidini A, Cabiddu M, Tomasello G, De Stefani A, Bruschieri L, et al. Addition of radiotherapy to the primary tumour in oligometastatic nsclc: A systematic review and meta-analysis. Lung Cancer (Amsterdam Netherlands) (2018) 126:194–200. doi: 10.1016/j.lungcan.2018.11.017
9. Mole RH. Whole body irradiation; radiobiology or medicine? Br J Radiol (1953) 26(305):234–41. doi: 10.1259/0007-1285-26-305-234
10. Zhang Z, Liu X, Chen D, Yu J. Radiotherapy combined with immunotherapy: The dawn of cancer treatment. Signal transduct targeted Ther (2022) 7(1):258. doi: 10.1038/s41392-022-01102-y
11. Chen D, Menon H, Verma V, Guo C, Ramapriyan R, Barsoumian H, et al. Response and outcomes after anti-Ctla4 versus anti-Pd1 combined with stereotactic body radiation therapy for metastatic non-small cell lung cancer: Retrospective analysis of two single-institution prospective trials. J Immunother Cancer (2020) 8(1):e000492. doi: 10.1136/jitc-2019-000492
12. Jiang H, Yu K, Cui Y, Ren X, Li M, Yang C, et al. Combination of immunotherapy and radiotherapy for recurrent malignant gliomas: Results from a prospective study. Front Immunol (2021) 12:632547. doi: 10.3389/fimmu.2021.632547
13. Lin Z, Cai M, Zhang P, Li G, Liu T, Li X, et al. Phase ii, single-arm trial of preoperative short-course radiotherapy followed by chemotherapy and camrelizumab in locally advanced rectal cancer. J Immunother Cancer (2021) 9(11):e003554. doi: 10.1136/jitc-2021-003554
14. Shaverdian N, Lisberg AE, Bornazyan K, Veruttipong D, Goldman JW, Formenti SC, et al. Previous radiotherapy and the clinical activity and toxicity of pembrolizumab in the treatment of non-Small-Cell lung cancer: A secondary analysis of the keynote-001 phase 1 trial. Lancet Oncol (2017) 18(7):895–903. doi: 10.1016/s1470-2045(17)30380-7
15. Formenti SC, Rudqvist NP, Golden E, Cooper B, Wennerberg E, Lhuillier C, et al. Radiotherapy induces responses of lung cancer to ctla-4 blockade. Nat Med (2018) 24(12):1845–51. doi: 10.1038/s41591-018-0232-2
16. Heylmann D, Rödel F, Kindler T, Kaina B. Radiation sensitivity of human and murine peripheral blood lymphocytes, stem and progenitor cells. Biochim Biophys Acta (2014) 1846(1):121–9. doi: 10.1016/j.bbcan.2014.04.009
17. Cho Y, Park S, Byun HK, Lee CG, Cho J, Hong MH, et al. Impact of treatment-related lymphopenia on immunotherapy for advanced non-small cell lung cancer. Int J Radiat oncol biol Phys (2019) 105(5):1065–73. doi: 10.1016/j.ijrobp.2019.08.047
18. Zhao Y, Wu T, Shao S, Shi B, Zhao Y. Phenotype, development, and biological function of myeloid-derived suppressor cells. Oncoimmunology (2016) 5(2):e1004983. doi: 10.1080/2162402x.2015.1004983
19. Hilligan KL, Ronchese F. Antigen presentation by dendritic cells and their instruction of Cd4+ T helper cell responses. Cell Mol Immunol (2020) 17(6):587–99. doi: 10.1038/s41423-020-0465-0
20. Naidoo J, Wang X, Woo KM, Iyriboz T, Halpenny D, Cunningham J, et al. Pneumonitis in patients treated with anti-programmed death-1/Programmed death ligand 1 therapy. J Clin Oncol (2017) 35(7):709–17. doi: 10.1200/jco.2016.68.2005
21. Rubio-Infante N, Ramírez-Flores YA, Castillo EC, Lozano O, García-Rivas G, Torre-Amione G. Cardiotoxicity associated with immune checkpoint inhibitor therapy: A meta-analysis. Eur J Heart Failure (2021) 23(10):1739–47. doi: 10.1002/ejhf.2289
22. Li M, Gan L, Song A, Xue J, Lu Y. Rethinking pulmonary toxicity in advanced non-small cell lung cancer in the era of combining anti-Pd-1/Pd-L1 therapy with thoracic radiotherapy. Biochim Biophys Acta Rev Cancer (2019) 1871(2):323–30. doi: 10.1016/j.bbcan.2019.02.004
23. Bang A, Wilhite TJ, Pike LRG, Cagney DN, Aizer AA, Taylor A, et al. Multicenter evaluation of the tolerability of combined treatment with pd-1 and ctla-4 immune checkpoint inhibitors and palliative radiation therapy. Int J Radiat oncol biol Phys (2017) 98(2):344–51. doi: 10.1016/j.ijrobp.2017.02.003
24. Botticella A, Ibrahim T, Mezquita L, Hendriks L, Le Pavec J, Ferrara R, et al. P1.01-07 immune-related pneumonitis in nsclc patients treated with immune checkpoint inhibitors (Ici): Impact of previous thoracic radiotherapy. J Thorac Oncol (2018) 13(10):S461–2. doi: 10.1016/j.jtho.2018.08.563
25. De Giglio A, Scorsetti M, Franceschini D, Massari F, Ardizzoni A. Bilateral radiation recall pneumonitis during immunotherapy for an advanced renal cell carcinoma: A challenging case enhances the need for a multidisciplinary approach. Eur J Cancer (Oxford Engl 1990) (2021) 143:75–7. doi: 10.1016/j.ejca.2020.10.024
26. Moher D, Liberati A, Tetzlaff J, Altman DG. Preferred reporting items for systematic reviews and meta-analyses: The prisma statement. PloS Med (2009) 6(7):e1000097. doi: 10.1371/journal.pmed.1000097
27. Sterne JAC, Savović J, Page MJ, Elbers RG, Blencowe NS, Boutron I, et al. Rob 2: A revised tool for assessing risk of bias in randomised trials. BMJ (Clinical Res ed) (2019) 366:l4898. doi: 10.1136/bmj.l4898
28. Sterne JA, Hernán MA, Reeves BC, Savović J, Berkman ND, Viswanathan M, et al. Robins-I: A tool for assessing risk of bias in non-randomised studies of interventions. BMJ (Clinical Res ed) (2016) 355:i4919. doi: 10.1136/bmj.i4919
29. Shukla NA, Althouse S, Meyer Z, Hanna N, Durm G. Association of immune-related adverse events and efficacy outcomes with consolidation pembrolizumab after chemoradiation in patients with inoperable stage iii non-Small-Cell lung cancer. Clin Lung Cancer (2021) 22(4):274–81. doi: 10.1016/j.cllc.2020.12.014
30. Faivre-Finn C, Spigel DR, Senan S, Langer C, Perez BA, Özgüroğlu M, et al. Impact of prior chemoradiotherapy-related variables on outcomes with durvalumab in unresectable stage iii nsclc (Pacific). Lung Cancer (Amsterdam Netherlands) (2021) 151:30–8. doi: 10.1016/j.lungcan.2020.11.024
31. LeClair JN, Merl MY, Cohenuram M, Luon D. Real-world incidence of pneumonitis in patients receiving durvalumab. Clin Lung Cancer (2022) 23(1):34–42. doi: 10.1016/j.cllc.2021.08.006
32. Landman Y, Jacobi O, Kurman N, Yariv O, Peretz I, Rotem O, et al. Durvalumab after concurrent chemotherapy and high-dose radiotherapy for locally advanced non-small cell lung cancer. Oncoimmunology (2021) 10(1):1959979. doi: 10.1080/2162402x.2021.1959979
33. Aredo JV, Mambetsariev I, Hellyer JA, Amini A, Neal JW, Padda SK, et al. Durvalumab for stage iii egfr-mutated nsclc after definitive chemoradiotherapy. J Thorac Oncol (2021) 16(6):1030–41. doi: 10.1016/j.jtho.2021.01.1628
34. Hassanzadeh C, Sita T, Savoor R, Samson PP, Bradley J, Gentile M, et al. Implications of pneumonitis after chemoradiation and durvalumab for locally advanced non-small cell lung cancer. J Thorac Dis (2020) 12(11):6690–700. doi: 10.21037/jtd-20-1792
35. Miura Y, Mouri A, Kaira K, Yamaguchi O, Shiono A, Hashimoto K, et al. Chemoradiotherapy followed by durvalumab in patients with unresectable advanced non-small cell lung cancer: Management of adverse events. Thorac Cancer (2020) 11(5):1280–7. doi: 10.1111/1759-7714.13394
36. Jung HA, Noh JM, Sun JM, Lee SH, Ahn JS, Ahn MJ, et al. Real world data of durvalumab consolidation after chemoradiotherapy in stage iii non-Small-Cell lung cancer. Lung Cancer (Amsterdam Netherlands) (2020) 146:23–9. doi: 10.1016/j.lungcan.2020.05.035
37. Inoue H, Ono A, Kawabata T, Mamesaya N, Kawamura T, Kobayashi H, et al. Clinical and radiation dose-volume factors related to pneumonitis after treatment with radiation and durvalumab in locally advanced non-small cell lung cancer. Invest New Drugs (2020) 38(5):1612–7. doi: 10.1007/s10637-020-00917-2
38. Chu CH, Chiu TH, Wang CC, Chang WC, Huang AC, Liu CY, et al. Consolidation treatment of durvalumab after chemoradiation in real-world patients with stage iii unresectable non-small cell lung cancer. Thorac Cancer (2020) 11(6):1541–9. doi: 10.1111/1759-7714.13426
39. Lin SH, Lin Y, Yao L, Kalhor N, Carter BW, Altan M, et al. Phase ii trial of concurrent atezolizumab with chemoradiation for unresectable nsclc. J Thorac Oncol (2020) 15(2):248–57. doi: 10.1016/j.jtho.2019.10.024
40. Yamaguchi O, Kaira K, Hashimoto K, Mouri A, Miura Y, Shiono A, et al. Radiotherapy is an independent prognostic marker of favorable prognosis in non-small cell lung cancer patients after treatment with the immune checkpoint inhibitor, nivolumab. Thorac Cancer (2019) 10(4):992–1000. doi: 10.1111/1759-7714.13044
41. Tamiya A, Tamiya M, Nakahama K, Taniguchi Y, Shiroyama T, Isa SI, et al. Correlation of radiation pneumonitis history before nivolumab with onset of interstitial lung disease and progression-free survival of patients with pre-treated advanced non-small cell lung cancer. Anticancer Res (2017) 37(9):5199–205. doi: 10.21873/anticanres.11943
42. Jang JY, Kim SS, Song SY, Kim YJ, Kim SW, Choi EK. Radiation pneumonitis in patients with non-Small-Cell lung cancer receiving chemoradiotherapy and an immune checkpoint inhibitor: A retrospective study. Radiat Oncol (London England) (2021) 16(1):231. doi: 10.1186/s13014-021-01930-2
43. Barrón F, Sánchez R, Arroyo-Hernández M, Blanco C, Zatarain-Barrón ZL, Catalán R, et al. Risk of developing checkpoint immune pneumonitis and its effect on overall survival in non-small cell lung cancer patients previously treated with radiotherapy. Front Oncol (2020) 10:570233. doi: 10.3389/fonc.2020.570233
44. Amino Y, Kitazono S, Uematsu S, Hasegawa T, Yoshizawa T, Uchibori K, et al. Efficacy of anti-Pd-1 therapy for recurrence after chemoradiotherapy in locally advanced nsc lc. Int J Clin Oncol (2020) 25(1):67–73. doi: 10.1007/s10147-019-01537-4
45. Boyer MJ, Gu L, Wang X, Kelsey CR, Yoo DS, Onaitis MW, et al. Toxicity of definitive and post-operative radiation following ipilimumab in non-small cell lung cancer. Lung Cancer (Amsterdam Netherlands) (2016) 98:76–8. doi: 10.1016/j.lungcan.2016.05.014
46. Welsh J, Menon H, Chen D, Verma V, Tang C, Altan M, et al. Pembrolizumab with or without radiation therapy for metastatic non-small cell lung cancer: A randomized phase I/Ii trial. J Immunother Cancer (2020) 8(2):e001001. doi: 10.1136/jitc-2020-001001
47. Bruni A, Scotti V, Borghetti P, Vagge S, Cozzi S, D'Angelo E, et al. A real-world, multicenter, observational retrospective study of durvalumab after concomitant or sequential chemoradiation for unresectable stage iii non-small cell lung cancer. Front Oncol (2021) 11:744956. doi: 10.3389/fonc.2021.744956
48. Peters S, Felip E, Dafni U, Tufman A, Guckenberger M, Álvarez R, et al. Progression-free and overall survival for concurrent nivolumab with standard concurrent chemoradiotherapy in locally advanced stage iiia-b nsclc: Results from the European thoracic oncology platform nicolas phase ii trial (European thoracic oncology platform 6-14). J Thorac Oncol (2021) 16(2):278–88. doi: 10.1016/j.jtho.2020.10.129
49. Jabbour SK, Berman AT, Decker RH, Lin Y, Feigenberg SJ, Gettinger SN, et al. Phase 1 trial of pembrolizumab administered concurrently with chemoradiotherapy for locally advanced non-small cell lung cancer: A nonrandomized controlled trial. JAMA Oncol (2020) 6(6):848–55. doi: 10.1001/jamaoncol.2019.6731
50. Bestvina CM, Pointer KB, Karrison T, Al-Hallaq H, Hoffman PC, Jelinek MJ, et al. A phase 1 trial of concurrent or sequential ipilimumab, nivolumab, and stereotactic body radiotherapy in patients with stage iv nsclc study. J Thorac Oncol (2022) 17(1):130–40. doi: 10.1016/j.jtho.2021.08.019
51. Voong KR, Hazell SZ, Fu W, Hu C, Lin CT, Ding K, et al. Relationship between prior radiotherapy and checkpoint-inhibitor pneumonitis in patients with advanced non-Small-Cell lung cancer. Clin Lung Cancer (2019) 20(4):e470–e9. doi: 10.1016/j.cllc.2019.02.018
52. Horn L, Spigel DR, Vokes EE, Holgado E, Ready N, Steins M, et al. Nivolumab versus docetaxel in previously treated patients with advanced non-Small-Cell lung cancer: Two-year outcomes from two randomized, open-label, phase iii trials (Checkmate 017 and checkmate 057). J Clin Oncol (2017) 35(35):3924–33. doi: 10.1200/jco.2017.74.3062
53. Kurata T, Nakagawa K, Satouchi M, Seto T, Sawada T, Han S, et al. Phase 1 study of pembrolizumab plus chemotherapy as first-line treatment in Japanese patients with advanced nsclc. Cancer Treat Res Commun (2021) 29:100458. doi: 10.1016/j.ctarc.2021.100458
54. Bradley JD, Hu C, Komaki RR, Masters GA, Blumenschein GR, Schild SE, et al. Long-term results of nrg oncology rtog 0617: Standard- versus high-dose chemoradiotherapy with or without cetuximab for unresectable stage iii non-Small-Cell lung cancer. J Clin Oncol (2020) 38(7):706–14. doi: 10.1200/jco.19.01162
55. Senan S, Brade A, Wang LH, Vansteenkiste J, Dakhil S, Biesma B, et al. Proclaim: Randomized phase iii trial of pemetrexed-cisplatin or etoposide-cisplatin plus thoracic radiation therapy followed by consolidation chemotherapy in locally advanced nonsquamous non-Small-Cell lung cancer. J Clin Oncol (2016) 34(9):953–62. doi: 10.1200/jco.2015.64.8824
56. Zhou C, Li M, Wang Z, An D, Li B. Adverse events of immunotherapy in non-small cell lung cancer: A systematic review and network meta-analysis. Int Immunopharmacol (2022) 102:108353. doi: 10.1016/j.intimp.2021.108353
57. Nso N, Antwi-Amoabeng D, Beutler BD, Ulanja MB, Ghuman J, Hanfy A, et al. Cardiac adverse events of immune checkpoint inhibitors in oncology patients: A systematic review and meta-analysis. World J Cardiol (2020) 12(11):584–98. doi: 10.4330/wjc.v12.i11.584
58. Mahmood SS, Fradley MG, Cohen JV, Nohria A, Reynolds KL, Heinzerling LM, et al. Myocarditis in patients treated with immune checkpoint inhibitors. J Am Coll Cardiol (2018) 71(16):1755–64. doi: 10.1016/j.jacc.2018.02.037
59. Palma DA, Senan S, Tsujino K, Barriger RB, Rengan R, Moreno M, et al. Predicting radiation pneumonitis after chemoradiation therapy for lung cancer: An international individual patient data meta-analysis. Int J Radiat Oncol Biol Phys (2013) 85(2):444–50. doi: 10.1016/j.ijrobp.2012.04.043
60. Or M, Liu B, Lam J, Vinod S, Xuan W, Yeghiaian-Alvandi R, et al. A systematic review and meta-analysis of treatment-related toxicities of curative and palliative radiation therapy in non-small cell lung cancer. Sci Rep (2021) 11(1):5939. doi: 10.1038/s41598-021-85131-7
61. Suresh K, Voong KR, Shankar B, Forde PM, Ettinger DS, Marrone KA, et al. Pneumonitis in non-small cell lung cancer patients receiving immune checkpoint immunotherapy: Incidence and risk factors. J Thorac Oncol (2018) 13(12):1930–9. doi: 10.1016/j.jtho.2018.08.2035
62. Zhang XJ, Sun JG, Sun J, Ming H, Wang XX, Wu L, et al. Prediction of radiation pneumonitis in lung cancer patients: A systematic review. J Cancer Res Clin Oncol (2012) 138(12):2103–16. doi: 10.1007/s00432-012-1284-1
63. Grills IS, Yan D, Martinez AA, Vicini FA, Wong JW, Kestin LL. Potential for reduced toxicity and dose escalation in the treatment of inoperable non-Small-Cell lung cancer: A comparison of intensity-modulated radiation therapy (Imrt), 3d conformal radiation, and elective nodal irradiation. Int J Radiat Oncol Biol Phys (2003) 57(3):875–90. doi: 10.1016/s0360-3016(03)00743-0
64. Venkatesulu BP, Mallick S, Lin SH, Krishnan S. A systematic review of the influence of radiation-induced lymphopenia on survival outcomes in solid tumors. Crit Rev oncol/hematol (2018) 123:42–51. doi: 10.1016/j.critrevonc.2018.01.003
65. Tang C, Liao Z, Gomez D, Levy L, Zhuang Y, Gebremichael RA, et al. Lymphopenia association with gross tumor volume and lung V5 and its effects on non-small cell lung cancer patient outcomes. Int J Radiat oncol biol Phys (2014) 89(5):1084–91. doi: 10.1016/j.ijrobp.2014.04.025
66. Karantanos T, Karanika S, Seth B, Gignac G. The absolute lymphocyte count can predict the overall survival of patients with non-small cell lung cancer on nivolumab: A clinical study. Clin Trans Oncol (2019) 21(2):206–12. doi: 10.1007/s12094-018-1908-2
67. Liang X, Yu H, Yu R, Xu G, Zhu G. Efficacy of the smaller target volume for stage iii non-small cell lung cancer treated with intensity-modulated radiotherapy. Mol Clin Oncol (2015) 3(5):1172–6. doi: 10.3892/mco.2015.588
68. Kilburn JM, Lucas JT, Soike MH, Ayala-Peacock DN, Blackstock AW, Hinson WH, et al. Is a clinical target volume (Ctv) necessary in the treatment of lung cancer in the modern era combining 4-d imaging and image-guided radiotherapy (Igrt)? Cureus (2016) 8(1):e466. doi: 10.7759/cureus.466
69. Perez CA, Stanley K, Rubin P, Kramer S, Brady L, Perez-Tamayo R, et al. A prospective randomized study of various irradiation doses and fractionation schedules in the treatment of inoperable non-Oat-Cell carcinoma of the lung. preliminary report by the radiation therapy oncology group. Cancer (1980) 45(11):2744–53. doi: 10.1002/1097-0142(19800601)45:11<2744::aid-cncr2820451108>3.0.co;2-u
70. Bradley JD, Paulus R, Komaki R, Masters G, Blumenschein G, Schild S, et al. Standard-dose versus high-dose conformal radiotherapy with concurrent and consolidation carboplatin plus paclitaxel with or without cetuximab for patients with stage iiia or iiib non-small-cell lung cancer (Rtog 0617): A randomised, two-by-Two factorial phase 3 study. Lancet Oncol (2015) 16(2):187–99. doi: 10.1016/s1470-2045(14)71207-0
71. Mauguen A, Le Péchoux C, Saunders MI, Schild SE, Turrisi AT, Baumann M, et al. Hyperfractionated or accelerated radiotherapy in lung cancer: An individual patient data meta-analysis. J Clin Oncol (2012) 30(22):2788–97. doi: 10.1200/jco.2012.41.6677
72. Wang N, Liang H, Zen K. Molecular mechanisms that influence the macrophage M1-M2 polarization balance. Front Immunol (2014) 5:614. doi: 10.3389/fimmu.2014.00614
73. Xu J, Escamilla J, Mok S, David J, Priceman S, West B, et al. Csf1r signaling blockade stanches tumor-infiltrating myeloid cells and improves the efficacy of radiotherapy in prostate cancer. Cancer Res (2013) 73(9):2782–94. doi: 10.1158/0008-5472.Can-12-3981
74. Morisada M, Moore EC, Hodge R, Friedman J, Cash HA, Hodge JW, et al. Dose-dependent enhancement of T-lymphocyte priming and ctl lysis following ionizing radiation in an engineered model of oral cancer. Oral Oncol (2017) 71:87–94. doi: 10.1016/j.oraloncology.2017.06.005
75. Golden EB, Frances D, Pellicciotta I, Demaria S, Helen Barcellos-Hoff M, Formenti SC. Radiation fosters dose-dependent and chemotherapy-induced immunogenic cell death. Oncoimmunology (2014) 3:e28518. doi: 10.4161/onci.28518
76. Morisada M, Clavijo PE, Moore E, Sun L, Chamberlin M, Van Waes C, et al. Pd-1 blockade reverses adaptive immune resistance induced by high-dose hypofractionated but not low-dose daily fractionated radiation. Oncoimmunology (2018) 7(3):e1395996. doi: 10.1080/2162402x.2017.1395996
77. Welsh JW, Chen D, Baas P, Chang JY, Verma V, Comeaux N, et al. Radiotherapy to augment pembrolizumab responses and outcomes in metastatic non-small cell lung cancer: Pooled analysis of two randomized trials. J Clin Oncol (2020) 38(15_suppl):9548–. doi: 10.1200/JCO.2020.38.15_suppl.9548
78. Herrera FG, Romero P, Coukos G. Lighting up the tumor fire with low-dose irradiation. Trends Immunol (2022) 43(3):173–9. doi: 10.1016/j.it.2022.01.006
79. Klug F, Prakash H, Huber PE, Seibel T, Bender N, Halama N, et al. Low-dose irradiation programs macrophage differentiation to an Inos+/M1 phenotype that orchestrates effective T cell immunotherapy. Cancer Cell (2013) 24(5):589–602. doi: 10.1016/j.ccr.2013.09.014
80. Shin SC, Lee KM, Kang YM, Kim K, Kim CS, Yang KH, et al. Alteration of cytokine profiles in mice exposed to chronic low-dose ionizing radiation. Biochem Biophys Res Commun (2010) 397(4):644–9. doi: 10.1016/j.bbrc.2010.05.121
81. Yin L, Xue J, Li R, Zhou L, Deng L, Chen L, et al. Effect of low-dose radiation therapy on abscopal responses to hypofractionated radiation therapy and anti-Pd1 in mice and patients with non-small cell lung cancer. Int J Radiat oncol biol Phys (2020) 108(1):212–24. doi: 10.1016/j.ijrobp.2020.05.002
82. Shaikh T, Churilla TM, Monpara P, Scott WJ, Cohen SJ, Meyer JE. Risk of radiation pneumonitis in patients receiving taxane-based trimodality therapy for locally advanced esophageal cancer. Pract Radiat Oncol (2016) 6(6):388–94. doi: 10.1016/j.prro.2016.02.004
83. Schoenfeld JD, Nishino M, Severgnini M, Manos M, Mak RH, Hodi FS. Pneumonitis resulting from radiation and immune checkpoint blockade illustrates characteristic clinical, radiologic and circulating biomarker features. J immunother Cancer (2019) 7(1):112. doi: 10.1186/s40425-019-0583-3
84. Chun SG, Hu C, Choy H, Komaki RU, Timmerman RD, Schild SE, et al. Impact of intensity-modulated radiation therapy technique for locally advanced non-Small-Cell lung cancer: A secondary analysis of the nrg oncology rtog 0617 randomized clinical trial. J Clin Oncol (2017) 35(1):56–62. doi: 10.1200/jco.2016.69.1378
85. Gu Y, Zhang H, Liu Z, Xia Y, Liang B, Liang L. Different patterns of treatment-related adverse events of programmed cell death-1 and its ligand-1 inhibitors in different cancer types: A meta-analysis and systemic review of clinical trials. Asia-Pacific J Clin Oncol (2020) 16(5):e160–e78. doi: 10.1111/ajco.13385
86. Khunger M, Rakshit S, Pasupuleti V, Hernandez AV, Mazzone P, Stevenson J, et al. Incidence of pneumonitis with use of programmed death 1 and programmed death-ligand 1 inhibitors in non-small cell lung cancer: A systematic review and meta-analysis of trials. Chest (2017) 152(2):271–81. doi: 10.1016/j.chest.2017.04.177
87. Li B, Jiang C, Pang L, Zou B, Ding M, Sun X, et al. Toxicity profile of combining pd-1/Pd-L1 inhibitors and thoracic radiotherapy in non-small cell lung cancer: A systematic review. Front Immunol (2021) 12:627197. doi: 10.3389/fimmu.2021.627197
88. Latchman Y, Wood CR, Chernova T, Chaudhary D, Borde M, Chernova I, et al. Pd-L2 is a second ligand for pd-1 and inhibits T cell activation. Nat Immunol (2001) 2(3):261–8. doi: 10.1038/85330
89. Sukari A, Nagasaka M, Alhasan R, Patel D, Wozniak A, Ramchandren R, et al. Cancer site and adverse events induced by immune checkpoint inhibitors: A retrospective analysis of real-life experience at a single institution. Anticancer Res (2019) 39(2):781–90. doi: 10.21873/anticanres.13175
90. Shankar B, Zhang J, Naqash AR, Forde PM, Feliciano JL, Marrone KA, et al. Multisystem immune-related adverse events associated with immune checkpoint inhibitors for treatment of non-small cell lung cancer. JAMA Oncol (2020) 6(12):1952–6. doi: 10.1001/jamaoncol.2020.5012
91. Owen DH, Wei L, Bertino EM, Edd T, Villalona-Calero MA, He K, et al. Incidence, risk factors, and effect on survival of immune-related adverse events in patients with non-Small-Cell lung cancer. Clin Lung Cancer (2018) 19(6):e893–900. doi: 10.1016/j.cllc.2018.08.008
92. Ju AW, Woody S, Hegde AM, Arastu HH, Walker P. Survival is worse in patients completing immunotherapy prior to Sbrt/Srs compared to those receiving it concurrently or after. Int J Radiat OncologyBiologyPhysics (2020) 108(3):e174–e5. doi: 10.1016/j.ijrobp.2020.07.1377
93. Lesueur P, Escande A, Thariat J, Vauléon E, Monnet I, Cortot A, et al. Safety of combined pd-1 pathway inhibition and radiation therapy for non-Small-Cell lung cancer: A multicentric retrospective study from the gfpc. Cancer Med (2018) 7(11):5505–13. doi: 10.1002/cam4.1825
94. Young KH, Baird JR, Savage T, Cottam B, Friedman D, Bambina S, et al. Optimizing timing of immunotherapy improves control of tumors by hypofractionated radiation therapy. PloS One (2016) 11(6):e0157164. doi: 10.1371/journal.pone.0157164
95. Faivre-Finn C, Vicente D, Kurata T, Planchard D, Paz-Ares L, Vansteenkiste JF, et al. Four-year survival with durvalumab after chemoradiotherapy in stage iii nsclc-an update from the pacific trial. J Thorac Oncol (2021) 16(5):860–7. doi: 10.1016/j.jtho.2020.12.015
96. Bassanelli M, Ricciuti B, Giannarelli D, Cecere FL, Roberto M, Giacinti S, et al. Systemic effect of radiotherapy before or after nivolumab in lung cancer: An observational, retrospective, multicenter study. Tumori (2022) 108(3):250–7. doi: 10.1177/03008916211004733
97. Bryant AK, Sankar K, Strohbehn GW, Zhao L, Elliott D, Daniel V, et al. Timing of adjuvant durvalumab initiation is not associated with outcomes in stage iii non-small cell lung cancer. Int J Radiat oncol biol Phys (2022) 113(1):60–5. doi: 10.1016/j.ijrobp.2021.12.176
98. Denault MH, Kuang S, Shokoohi A, Leung B, Liu M, Berthelet E, et al. Comparison of 2-weekly versus 4-weekly durvalumab consolidation for locally advanced nsclc treated with chemoradiotherapy: A brief report. JTO Clin Res Rep (2022) 3(5):100316. doi: 10.1016/j.jtocrr.2022.100316
99. Anscher MS, Arora S, Weinstock C, Amatya A, Bandaru P, Tang C, et al. Association of radiation therapy with risk of adverse events in patients receiving immunotherapy: A pooled analysis of trials in the us food and drug administration database. JAMA Oncol (2022) 8(2):232–40. doi: 10.1001/jamaoncol.2021.6439
Keywords: immune checkpoint inhibitors, radiation therapy, non-small cell lung cancer, efficacy, safety, meta-analysis
Citation: Wu J, Ni T, Deng R, Li Y, Zhong Q, Tang F, Zhang Q, Fang C, Xue Y, Zha Y and Zhang Y (2023) Safety and efficacy of radiotherapy/chemoradiotherapy combined with immune checkpoint inhibitors for non-small cell lung cancer: A systematic review and meta-analysis. Front. Immunol. 14:1065510. doi: 10.3389/fimmu.2023.1065510
Received: 09 October 2022; Accepted: 27 February 2023;
Published: 13 March 2023.
Edited by:
Jing Yu, Zhongnan Hospital, Wuhan University, ChinaReviewed by:
Ruiguang Zhang, Huazhong University of Science and Technology, ChinaCopyright © 2023 Wu, Ni, Deng, Li, Zhong, Tang, Zhang, Fang, Xue, Zha and Zhang. This is an open-access article distributed under the terms of the Creative Commons Attribution License (CC BY). The use, distribution or reproduction in other forums is permitted, provided the original author(s) and the copyright owner(s) are credited and that the original publication in this journal is cited, in accordance with accepted academic practice. No use, distribution or reproduction is permitted which does not comply with these terms.
*Correspondence: Yu Zhang, c2t5bGluZV96eXVAMTYzLmNvbQ==; Yan Zha, Y2hheWFuQGd6NTA1NS5jb20=
†These authors have contributed equally to this work
Disclaimer: All claims expressed in this article are solely those of the authors and do not necessarily represent those of their affiliated organizations, or those of the publisher, the editors and the reviewers. Any product that may be evaluated in this article or claim that may be made by its manufacturer is not guaranteed or endorsed by the publisher.
Research integrity at Frontiers
Learn more about the work of our research integrity team to safeguard the quality of each article we publish.