- 1Department of Biomedical Sciences, Seoul National University College of Medicine, Seoul, Republic of Korea
- 2Department of Microbiology and Immunology, Seoul National University College of Medicine, Seoul, Republic of Korea
- 3Center for Integrative Rheumatoid Transcriptomics and Dynamics, The Catholic University of Korea, Seoul, Republic of Korea
- 4Department of Biomedicine & Health Sciences, The Catholic University of Korea, Seoul, Republic of Korea
- 5Department of Clinical Pharmacology and Therapeutics, Seoul National University College of Medicine, Seoul, Republic of Korea
- 6School of Biological Sciences, Seoul National University, Seoul, Republic of Korea
- 7Institute for Basic Science, Seoul, Republic of Korea
- 8Department of Molecular Bioscience, College of Biomedical Science, Kangwon National University, Chuncheon, Republic of Korea
- 9Department of Microbiology, College of Medicine, The Catholic University of Korea, Seoul, Republic of Korea
- 10Division of Rheumatology, Department of Internal Medicine, the Catholic University of Korea, Seoul, Republic of Korea
- 11Institute of Endemic Diseases, Seoul National University Medical Research Center, Seoul, Republic of Korea
Objectives: To investigate whether and how inflammatory disease in the intestine influences the development of arthritis, considering that organ-to-organ communication is associated with many physiological and pathological events.
Methods: First, mice were given drinking water containing dextran sodium sulfate (DSS) and then subjected to inflammatory arthritis. We compared the phenotypic symptoms between the cohoused and separately-housed mice. Next, donor mice were divided into DSS-treated and untreated groups and then cohoused with recipient mice. Arthritis was then induced in the recipients. The fecal microbiome was analyzed by 16S rRNA amplicon sequencing. We obtained type strains of the candidate bacteria and generated propionate-deficient mutant bacteria. Short-chain fatty acids were measured in the bacterial culture supernatant, serum, feces, and cecum contents using gas chromatography-mass spectrometry. Mice fed with candidate and mutant bacteria were subjected to inflammatory arthritis.
Results: Contrary to expectations, the mice treated with DSS exhibited fewer symptoms of inflammatory arthritis. Intriguingly, the gut microbiota contributes, at least in part, to the improvement of colitis-mediated arthritis. Among the altered microorganisms, Bacteroides vulgatus and its higher taxonomic ranks were enriched in the DSS-treated mice. B. vulgatus, B. caccae, and B. thetaiotaomicron exerted anti-arthritic effects. Propionate production deficiency further prevented the protective effect of B. thetaiotaomicron on arthritis.
Conclusions: We suggest a novel relationship between the gut and joints and an important role of the gut microbiota as communicators. Moreover, the propionate-producing Bacteroides species examined in this study may be a potential candidate for developing effective treatments for inflammatory arthritis.
Introduction
Organ-to-organ communication has evolved concomitantly in multicellular organisms to adjust to the external environment and maintain homeostasis (1). The interplay between organs is involved in many physiological and pathological events in the body, such as the adaptive response to external factors, maintenance of energy homeostasis, and migration of cells to target sites (1). The gastrointestinal system influences bodily functions that extend beyond traditional digestive functions (2, 3). The condition of the intestines directly influences the optimal function of other organs, which has yielded the concept of organ-to-organ communication, such as that between the gut and brain, lungs, and heart (2, 4, 5). The communication is mediated by hormones, cytokines, chemokines, and adhesion molecules (1, 4, 5). The gut microorganisms residing in the intestinal tract are involved directly and indirectly in interactions with other organs through various routes including the immune system, tryptophan metabolism, and vagus nerve and enteric nervous system (4–7). Gut microbes and their metabolites, such as short-chain fatty acids (SCFAs), branched-chain amino acids, and peptidoglycans, may contribute to the crosstalk between the gut and other organs by migrating to other parts of the body. This yields beneficial or pathological effects in target organs (2, 7, 8). Despite the increasing interest in organ-to-organ communications, the various forms and their mechanisms remain to be elucidated.
Concomitant diseases, in which each disease develops in different organs and tissues, can have a positive or negative relationship with each other (1, 9) but may result in poor organ-to-organ communication. For example, 36–46% of patients with inflammatory bowel disease (IBD) have at least one extraintestinal symptom, and rheumatic manifestations are the most frequent and comprise peripheral and axial joint involvement (10, 11). Peripheral arthritis is frequently found in Crohn’s disease (CD) and ulcerative colitis (UC), with a prevalence ranging from 17% to 20%. It mainly affects the large joints of the lower limbs, and less frequently those of the upper limbs (10). Axial involvement is more prevalent in patients with CD than in those with UC. It can include symptomatic or asymptomatic sacroiliitis with or without ankylosing spondylitis (10, 11).
Several genetic and nongenetic factors related to the development of colitis may contribute to the risk of inflammatory arthritis (9). The leaky gut of patients with IBD may allow the entry of gut microbiota from the lumen into the host and cause other problems in disseminated organs, such as joints, spine, skin, and eye (12). In addition, certain gut microbes are involved in the initiation and progression of inflammation-driven arthritis (13–15). However, the pathogenesis of rheumatic manifestations in patients with IBD has not yet been clarified. There is not yet direct evidence on the causality between IBD and inflammatory arthritis; thus, it remains unclear whether IBD and its associated changes in the microbiome accelerate or decelerate the progression of arthritis. Here, we investigated the influence of gut conditions on disease pathogenesis in joints using a colitis model, DSS-induced colitis, which is the most popular model in IBD research (16). We also used two animal models of inflammatory arthritis. Next, we examined whether the gut microbiota was involved in the influence of colitis on inflammatory arthritis. To identify bacterial candidates and their underlying mechanisms involved in the communication between the gut and joints, we performed 16S rRNA amplicon next generation sequencing (NGS) and in vivo experiments using mutant and type strains of bacteria.
Materials and methods
Mice
C57BL/6 female mice were purchased from Orientbio (Korea) and housed under specific pathogen free (SPF) or semi-SPF conditions in animal facilities at Seoul National University College of Medicine and the Catholic University of Korea. The mice were randomly allocated and raised in their appointed cages. All animal experiments were performed in accordance with protocols approved by the Institutional Animal Care and Use Committee at Seoul National University (SNU-181128-2-6, SNU-201008-2-2, and SNU-211129-2-2), and the Catholic University of Korea (CUMS-2016-0227-03, CUMS-2018-0318-01, and CUMS-2021-0080-20).
Culture and preparation of B. vulgatus, B. caccae, and B. thetaiotaomicron
Type strains of B. vulgatus (NCTC 11154, cat. # ATCC 8482), B. caccae (VPI 3452A, cat# ATCC 8482) and B. thetaiotaomicron (VPI 5482, cat# KCTC 5723) were purchased or obtained from the American Type Culture Collection (Manassas, VA, USA) and Korean Collection for Type Cultures (Korea). Bacteroides were grown on brain heart infusion (BHI; BD Difco) agar plates supplemented with 5% sheep blood (cat# MB-S1876, MBcell, Korea) or in BHI broth supplemented (BHIS) with 5 mg/mL of hemin, 1 g/L of L-cysteine, 1 mg/L of resazurin and 1 g/L of NaHCO3 (Sigma). A single colony on the culture plate was transferred to 5 mL of BHIS broth and incubated in an anaerobic chamber (COY) for 16 h at 37 °C (17). These stationary phase cultures were diluted to 1:50 (B. vulgatus and B. caccae) or 1:20 (B. thetaiotaomicron) in 5 mL of fresh BHIS broth and then cultured anaerobically at 37 °C until the bacteria were at a concentration of 1 × 1010 CFU/mL (B. vulgatus and B. caccae) or 1 × 1011 CFU/mL (B. thetaiotaomicron). The bacterial culture supernatants obtained by centrifugation were used to analyze SCFAs, and the bacterial pellets were washed and resuspended in phosphate-buffered saline (PBS; Welgene, Korea) for the animal experiments.
Generation of a B. thetaiotaomicron propionate-deficient mutant
The BT1686-1689 genes of B. thetaiotaomicron putatively encoding the four subunits of methyl malonyl-CoA transcarboxylase were deleted to block conversion of succinate into propionate (18). This was achieved with pLGB30 vectors according to a previously described deletion strategy (19). The upstream and downstream regions of the target locus were PCR-amplified using Phusion Hot Start II High-fidelity PCR Master Mix (cat.#F565, Thermo Fisher Scientific) and the following primer sets: BT1686-89_upstream, 5 ′-GCA TTA TGA GTG GAT CCC CCC ACA TGA ACA GCA TGC TTA TC-3′ (forward) and 5′-TAT TTT GTC CGA GTT CTT GTT GTT TTT TTA GAG TTT ATG-3′ (reverse); BT1686-89_downstream, 5′-ACA AGA ACT CGG ACA AAA TAT TAG GTG CGG-3′ (forward) and 5′-ATA TCG AAT TCC TGC AGC CCA TAG CTG ATA CCC AGA TAA TAA CAG-3′ (reverse). The PCR products were cloned into the SmaI site of the suicide vector pLGB30 using NEBuilder HiFi DNA Assembly (#E2621, NEB) according to the manufacturer’s instructions. The recombinant plasmid was transformed into the E. coli strain S17-λpir for conjugal transfer into B. thetaiotaomicron. The deletions of the target genes were confirmed by a PCR analysis of bacterial genomic DNA.
DSS-induced colitis and CFA (complete freund’s adjuvant)-induced arthritis model
Mice were ad libitum given fresh water (vehicle group) or water bottles containing DSS (DSS group) for 5 days (Figure S1). Then, the water bottles were replaced with fresh water and, after anesthetizing the mice, 20 μL of CFA (containing 2 mg/mL of heat-killed Mycobacterium tuberculosis; Chondrex) and IFA (Chondrex) were subcutaneously injected into each footpad (Figure S1). The swelling of the footpads and ankles was measured using calipers at indicated time points. After euthanization, the CFA-injected limbs were used to obtain macroscopic and hematoxylin and eosin (H&E)-staining images. In addition, body weights and stool states were monitored until the end of the study. The disease activity index (DAI) was calculated by scoring body weight loss, bloody stool, and stool consistency (Table S1).
DSS-induced colitis and mBSA/IL-1β-induced arthritis model
Mice were ad libitum provided with fresh water (vehicle group) or 2.5% DSS water bottles (DSS group) for 5 days. Then, the water bottles were replaced with fresh water and, after anesthetizing the mice, 200 μg of methylated bovine serum albumin (mBSA, Sigma) was intra-articularly injected into the bilateral knee joints. The next day, recombinant human IL-1β (250 ng; R&D Systems) was subcutaneously administered into the left hind footpads, and the injection was repeated on the following two days. Seven days after the mBSA injections, the mice were euthanized, and tissues containing the knee joints were collected for H&E staining.
Comparison of cohousing and separate housing
Mice were provided with fresh water bottles (vehicle group) or water bottles containing DSS (DSS group) for 5 days. Then, the water bottles were replaced with fresh water and, after anesthetizing the mice, CFA and IFA were subcutaneously injected into each footpad of all the mice, and the control and colitis groups were raised in the same cages or separately raised in divided cages until the end of the study (Figure S2A). Footpad and ankle swellings were measured using calipers at indicated time points.
Transfer of gut microbes using coprophagy
The mice were divided into donor and recipient groups (Figure S2B). To reduce the number of preexisting gut microbes, the recipient group was provided with water bottles containing an antibiotic cocktail (1 g/L of ampicillin, 1 g/L of neomycin, 1 g/L of metronidazole, and 0.5 g/L of vancomycin) for 2 weeks before cohousing. The donor mice were divided into control and colitis groups. To transfer gut microbes from the donor to recipient mice, the mice in each donor group were cohoused with the recipient mice after 5 days of the DSS treatment (Figure S2B). Two days later, CFA and IFA were injected into the footpads of the recipient mice. Footpad and ankle swellings in the CFA-injected limbs were measured, and feces were collected at indicated time points (Figure S2B).
Gavage experiment using Bacteroides
The mice were provided with water bottles containing an antibiotic cocktail ad libitum for 14 days. After ceasing the antibiotics, the mice were repeatedly administered Bacteroides species (1 × 109 CFU/mouse) or PBS (200 μL; vehicle) at indicated time points. Four days after ceasing the antibiotics, CFA and IFA were subcutaneously injected into each footpad. Footpad and ankle swellings in the CFA-injected limbs were measured, and feces were collected at indicated time points.
Hematoxylin and eosin staining
The CFA-injected hind limbs and mBSA/IL-1β-induced knee joints were fixed in 4% paraformaldehyde (Biosesang, Korea), decalcified in a decalcifying solution (Sigma) for 36 h, and embedded in paraffin. Sections (4-μm thick) were cut in the sagittal plane and stained with H&E. The slides were scanned using a Pannoramic MIDI digital slide scanner (3DHISTECH). Images were extracted using the Case Viewer software (3DHISTECH). The joint sections were cut at five depths that were approximately 100 μm apart. The slides were qualitatively evaluated using the following parameters: synovial hyperplasia, inflammation, and bone destruction. Disease severity was graded histologically from 0 (normal) to 3 (severe) by an investigator blinded to the experimental groups (20).
Microbiome analysis
Total bacterial genomic DNA was extracted from feces using a stool DNA kit (Omega Bio-Tek). The Illumina 16S metagenomic sequencing library preparation protocol was then used to prepare 16S ribosomal RNA amplicons. The library was sequenced using the Illumina MiSeq system on a 250 bp paired-end platform. The obtained data were analyzed using QIIME 2 (version 2020.8). Linear discriminant analysis [LDA] effect size (LEfSe) analysis was performed using the online-based Galaxy server (https://huttenhower.sph.harvard.edu/galaxy/).
Quantitative analysis of short-chain fatty acids
The concentrations of SCFAs were analyzed in the feces, cecum, and bacterial culture supernatant samples as previously described (21). In brief, water was added to the feces and cecum at a 50 mg: 500 µL ratio, after which it was homogenized by vortexing for 20 min. The mixture was centrifuged for 5 min at 18,341 × g and 4°C, and the supernatants were transferred into new plastic tubes. Next, 10 µL of 1.0 M hydrochloric acid and 20 µL of internal standard solution (500 µg/mL acetic acid-d4 and 30 µg/mL butyric acid-d7) were added to each 100 µL of fecal homogenate, cecal homogenate and bacterial supernatant. SCFAs were extracted with 200 µL of methyl tert-butyl ether (MTBE). The MTBE phase was then transferred into an autosampler vial and analyzed by gas chromatography-mass spectrometry (Agilent 7890B gas chromatography coupled with Agilent 7000B mass spectrometer, Agilent Technologies Inc., Santa Clara, CA, USA). Ions at 60.0 m/z were monitored for acetic acid, butyric acid, isovaleric acid and valeric acid; 63.0 m/z for acetic acid-d4 and butyric acid-d7; 73.0 m/z for isobutyric acid; and 74.0 m/z for propionic acid. A calibration curve was constructed to quantify the SCFAs by plotting the peak area ratio of each SCFA to the corresponding internal standard (acetic acid-d4 for acetic acid and butyric acid-d7 for propionic acid, butyric acid, valeric acid, isobutyric acid, and isovaleric acid) versus the concentration of each SCFA. Data were acquired and analyzed using the Masshunter quantitative program B.06.00 (Agilent Technologies Inc., Santa Clara, CA, USA).
IL-10 expression in the spleen and mesenteric lymph nodes
Splenocytes and lymphocytes were collected by mashing the spleen and mesenteric lymph nodes using a 70-μm cell strainer (BD Falcon). Red blood cells (RBCs) were lysed following the addition of cold RBC Lysis Buffer (eBiosciences) for 5 min. Splenocytes and lymphocytes (1 × 106 cells/well in a 96 well-plate) were treated with 50 ng/mL of Phorbol myristate acetate (PMA; Sigma) and 1 μg/mL of ionomycin (Sigma). After 24 h, the supernatants were collected, and the levels of IL-10 were measured using an ELISA kit (R&D Systems) according to the manufacturer’s instructions.
Data access
The 16S rRNA amplicon raw data were deposited in the SRA database (accession code PRJNA869574).
Statistical analyses
General statistical analyses were performed using the Prism software (GraphPad Software, version 8.02). We examined differences in the results between groups of individual animals using the Mann–Whitney test (between two groups) and Kruskal–Wallis test, followed by Dunn’s multiple comparisons test (between three groups). No samples or animals were excluded from the analyses, except for dead individuals. Differences were considered statistically significant at P < 0.05.
Results
Colitis protects from inflammatory arthritis
To investigate the effects of colitis on inflammatory arthritis, the mice were given water bottles with or without DSS ad libitum. CFA and IFA were then subcutaneously injected into their footpads, and the thickness of their footpads and ankles was monitored (Figure S1). Contrary to our expectations, the footpads and ankles of the DSS-pretreated mice were less swollen in the CFA-injected limbs than those of the control group (Figure 1A). To confirm the protective effects of colitis on arthritic symptoms, the mice were divided into 0, 2, 2.5 and 3% DSS-treated groups. All groups were administered CFA and IFA. Edema at the footpads and ankles following the CFA injections was ameliorated by DSS in a dose-dependent manner and most distinctly suppressed by high doses of DSS (Figure 1B). Moreover, the arthritic symptoms of the individual mice were inversely proportional to the severity of their colitis (Figure 1C). DSS-induced colitis therefore exerts a preventive effect on the development of inflammatory arthritis. Next, we switched the order of the induction of colitis and arthritis. Post-treatment with DSS significantly suppressed the CFA-induced swelling of the footpads and ankles, suggesting that colitis exerts a therapeutic effect on arthritic symptoms (Figure 1D). To examine whether the protective effects of colitis were limited to a specific model of CFA-induced arthritis, we used the mBSA/IL-1β-induced arthritis model. Similar to the results in the CFA-induced arthritis model, colitis ameliorated the histopathological severity of knee joint arthritis caused by mBSA and recombinant IL-1β (Figure S3). Inducing colitis can therefore ameliorate the symptoms of inflammatory arthritis.
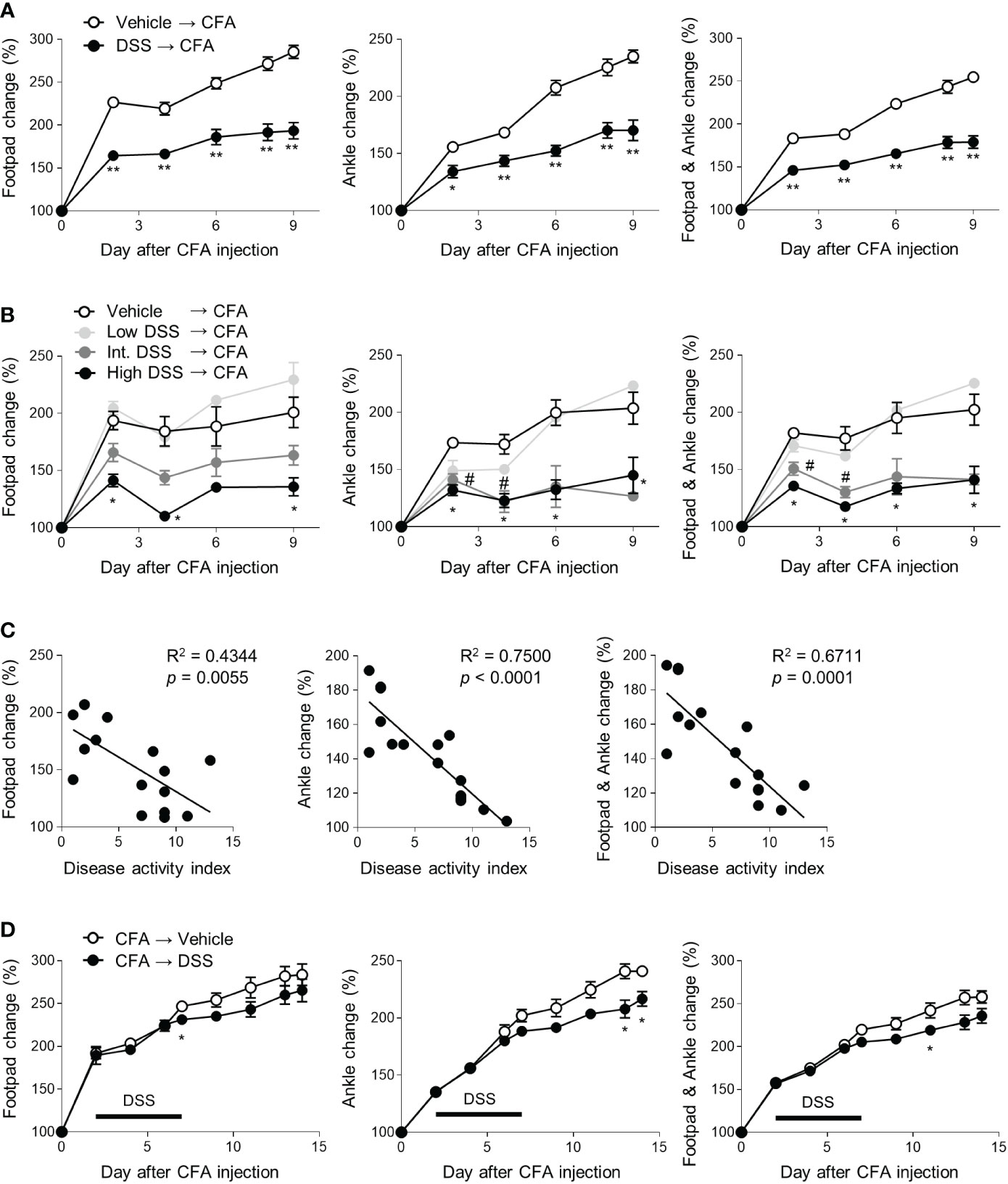
Figure 1 Protective effects of colitis on CFA-induced arthritis. (A–C) Mice (n = 5 or 4 per group) were provided with drinking water with or without DSS for 5 days. CFA and IFA (20 μL) were then subcutaneously injected into each footpad. The swelling at the footpads and ankles was measured at indicated time points after the injections (C) The correlation between the DAI score and swelling at footpads and ankles of the mice on day 9 among data in the (B). (D) Mice (n = 5 per group) were subcutaneously treated with CFA and IFA, and 2 days later, given drinking water containing DSS or fresh water for 5 days (marked as a bar). The concentration of DSS was 2.5% (A, D) and Low DSS, Int. DSS, and High DSS indicated 2, 2.5, and 3% concentration, respectively (B). The results are representative of at least two independent experiments. The data are shown as means ± SEM. *P < 0.05 and **P < 0.01 with the Mann–Whitney test (A, D). *P < 0.05 (between the vehicle and DSS groups or high DSS groups) and #P < 0.05 (between the vehicle and intermediate DSS groups) with the Mann–Whitney test (B).
Gut microbiota altered by colitis ameliorates inflammatory arthritis
Given the coprophagy observed in rodents and other animals, cohousing transfers the gut microbiota from one mouse to the others in the same cage, which is widely accepted as a method for normalizing microbial communities (22). To examine the role of gut microbiota in colitis-induced protection against arthritis, we utilized cohousing. First, we compared arthritic symptoms when the DSS-treated and untreated mice were raised in separate and the same cages (Figure S2A). In the cohoused mice, the protective effects of colitis on inflammatory arthritis decreased compared with those in the separately-housed mice (Figure 2A). To further confirm the importance of gut microbiota, we divided the mice into two donor and two recipient groups (Figures S2B, 2B). The donor mice were provided water bottles with or without DSS for 5 days and then cohoused with each recipient group that had been pretreated with an antibiotic cocktail for 2 weeks before cohousing (Figures S2B, 2B). After 2 days of cohousing, CFA and IFA were injected into the footpads of the recipients. The thickness of the footpads and ankles was then measured, and feces were collected (Figures S2B, 2B). As expected, the beta-diversity, which describes differences in the gut microbiota between each pair of samples showed that DSS altered the gut microbiota and that the gut microbial compositions were similar within the donor and recipient mice raised in the same cage (Figure 2C). Consistent with the results from the comparison of cohousing and separate-housing, recipients cohoused with DSS-treated mice during the initial period of arthritis induction displayed less swelling at the footpads and ankles than recipients cohoused with the control (Figures 2D, E). Gut microbiota may, therefore at least partly, contribute to the beneficial effects of colitis on inflammatory arthritis.
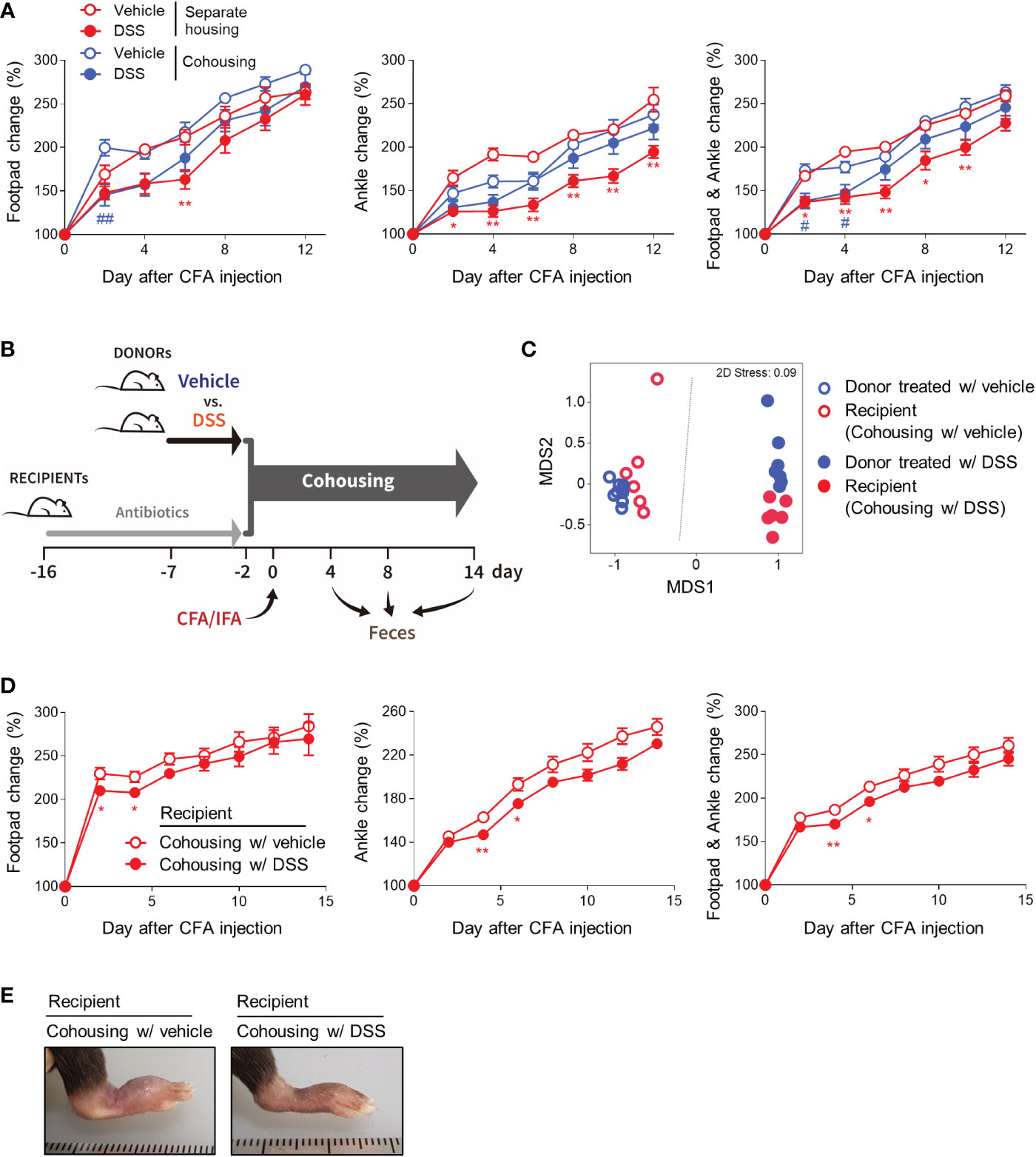
Figure 2 Gut microbiota contributes to colitis-mediated protection from arthritis. (A) Mice (n = 5 per group) were provided with fresh water or 2.5% DSS water bottles for 5 days. CFA and IFA were then subcutaneously injected into the footpads of all the mice. The control and colitis groups were mixed in the same cages or raised separately in the divided cages. The swelling at the footpads and ankles was then assessed. (B–E) Recipient mice (n = 6 per group) were given water bottles containing an antibiotic cocktail for 2 weeks before cohousing. Donor mice (n = 8 per group) were provided with fresh water or 2.5% DSS water bottles for 5 days before cohousing. The donor mice were cohoused with the recipient mice and, 2 days later, CFA and IFA were subcutaneously injected into each footpad of the recipient mice. (B) Experimental scheme. (C) Beta-diversity (non-metric multidimensional scaling [NMDS] plot) of the microbial communities on day 4. Each dot represents an individual mouse. (D) The swelling at the footpads and ankles of the recipient mice. (E) Representative macroscopic images of the CFA-injected limbs. The results are representative of at least two independent experiments. The data are shown as means ± SEM. *P < 0.05 and **P < 0.01 (between separately-housed groups) or #P < 0.05 and ##P < 0.01 (between cohoused groups) with the Mann–Whitney test (A). *P < 0.05 and **P < 0.01 with the Mann–Whitney test (D).
B. vulgatus is one of the gut microorganisms altered by DSS-induced colitis
To identify bacteria that exert protective effects against inflammatory arthritis, feces obtained from the recipient mice on days 4, 8, and 14 after cohousing were analyzed (Figure 2B). There was no difference in the alpha-diversity of the microbial communities, as indicated by the Chao1, Simpson, and Shannon indices, on days 4 and 8 (Figure S4A). The control recipients on day 14 had more species, however, and fewer differences in the abundance of each species than the recipients cohoused with the DSS-treated donors (Figure S4A). Consistent with the data in Figure 2C, the recipients cohoused with the DSS-treated donors had different gut microbiomes compared to the recipients cohoused with the control, regardless of the collection time points (Figure 3A). After comparing the bacterial abundance in the recipients on day 8, the genera Muribaculaceae and Akkermansia were found to be abundant in the control recipients cohoused with vehicle donors, while the genera Bacteroides and Lactobacillus were enriched in the recipients cohoused with DSS-treated donors (Figure 3B). Additionally, an LEfSe analysis of the amplicon sequence variants (ASVs) revealed that the family Muribaculaceae, genera Desulfovibrio and Prevotellaceae uncultured group 001, and their associated taxonomic ranks were more abundant in the control recipients, while the family Bacteroidaceae, genus Bacteroides, and species Bacteroides vulgatus and Clostridiales bacterium were enriched in the recipients cohoused with DSS-treated donors (Figure 3C). Likewise, an increase in the genus Bacteroides and decrease in the family Muribaculaceae were found in fecal samples on days 4 and 14 (Figures S4B–D). Among the distinguishing bacteria between the recipient groups, the family Bacteroidaceae, genus Bacteroides, and species B. vulgatus increased substantially in the recipients cohoused with DSS-treated donors (Figure 3D), suggesting that B. vulgatus may be one of the bacterial candidates involved in colitis-induced protection from inflammatory arthritis.
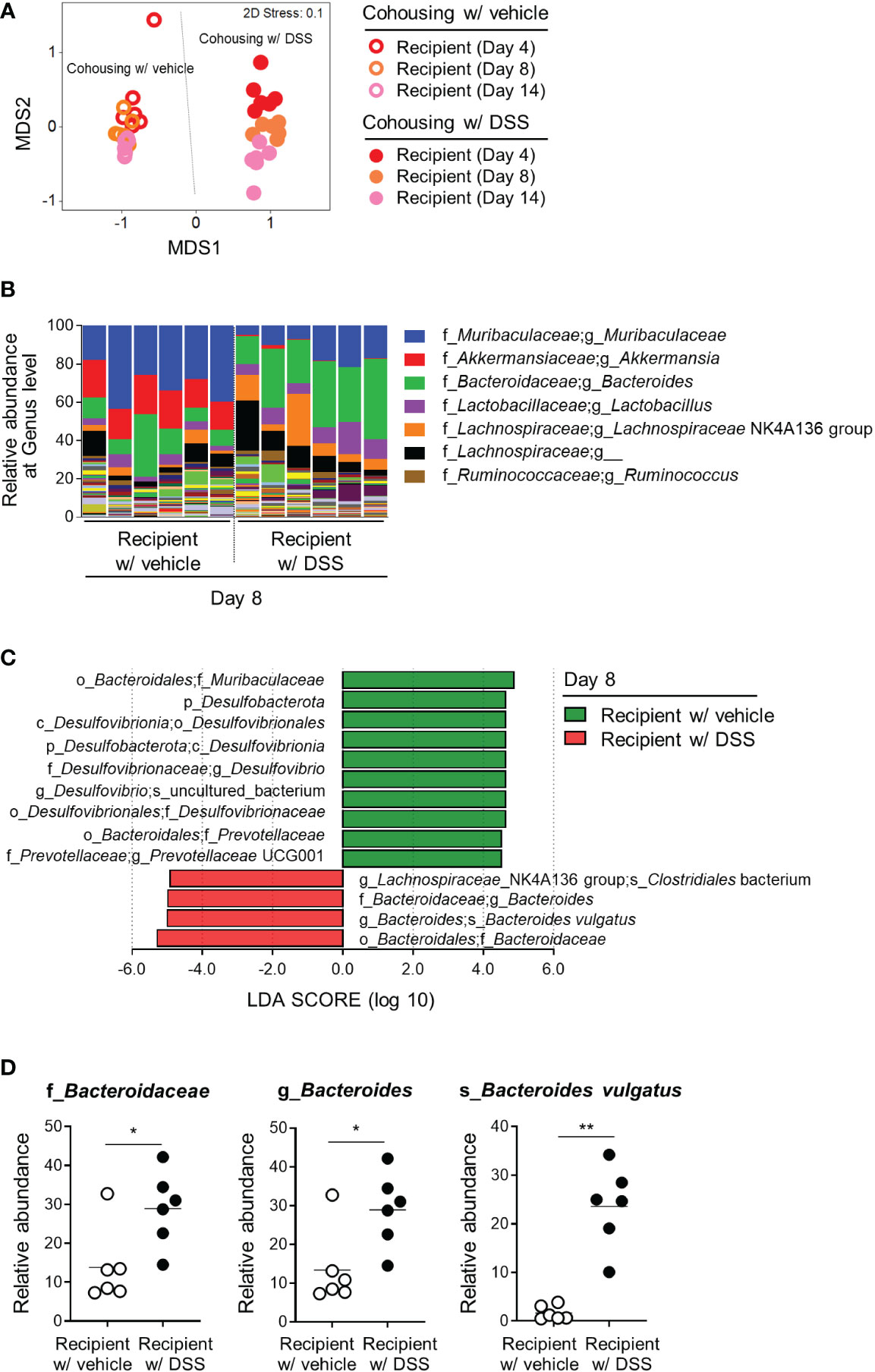
Figure 3 Gut microbial candidates involved in the colitis-mediated protection from arthritis. The gut microbiome obtained from the recipients in Figure 2B was analyzed. (A) Beta-diversity (NMDS plot) of the microbial communities of the recipient mice. Each dot represents an individual mouse. (B) Relative abundance of bacterial taxa at the genus level. The top seven taxa are listed in the right legend. (C) LEfSe analysis of the recipients cohoused with the vehicle and DSS-induced colitis groups (logarithmic LDA score > 4). Significant differences in taxa were obtained using the Kruskal–Wallis test (α < 0.05). (D) Among the bacterial taxa selected from the LEfSe analysis, the relative abundance of the family Bacteroidaceae, genus Bacteroides, and species Bacteroides vulgatus in each taxonomic rank are shown. Each dot represents an individual mouse and the means are displayed as a line. *P < 0.05 and **P < 0.01 with the Mann-Whitney test.
B. vulgatus prevents inflammatory arthritis
To examine whether B. vulgatus is beneficial in the pathogenesis of arthritis, the mice were repeatedly fed with a type strain of B. vulgatus or PBS (Vehicle) at indicated time points, and CFA and IFA were subcutaneously injected into each footpad (Figure 4A). B. vulgatus significantly suppressed the swelling of the ankles and footpads compared to the PBS-fed vehicle group (Figures 4B, C). Consistent with macroscopic pedal edema, the number of infiltrated inflammatory cells was reduced in the B. vulgatus-administered mice compared to that in the limbs of the vehicle group (Figure 4D). Thus, B. vulgatus may be an important contributor to colitis-mediated protection from inflammatory arthritis.
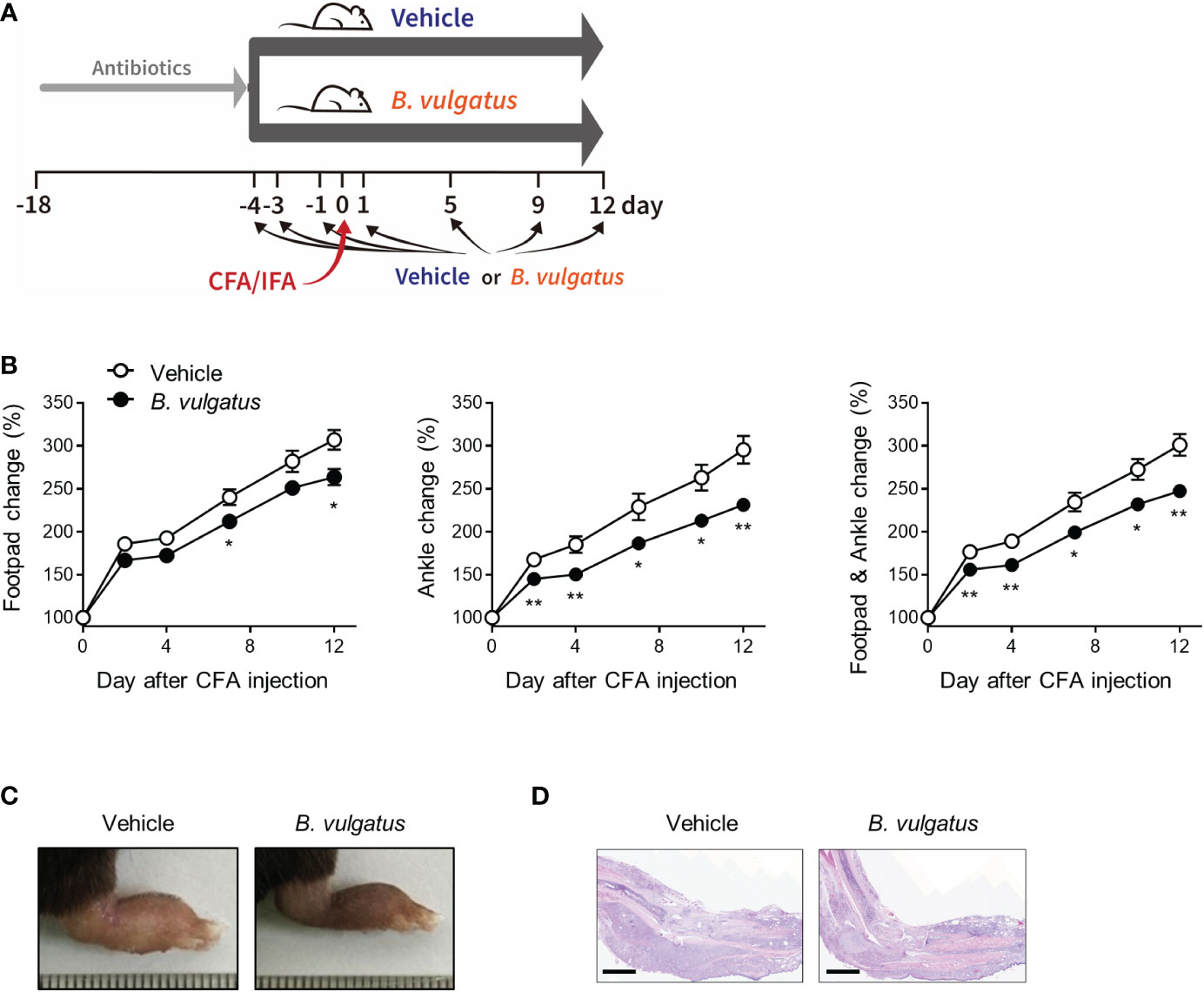
Figure 4 B. vulgatus protects from CFA-induced arthritis. Mice (n = 9 or 10 per group) were given drinking water containing an antibiotic cocktail for 14 days. After the cessation of the antibiotics, the mice were repeatedly administered B. vulgatus at indicated time points. Four days after the cessation of the antibiotics, CFA and IFA were injected into each footpad. (A) Experimental scheme. (B) The swelling at the footpads and ankles. The data are shown as means ± SEM. Representative macroscopic images (C) and H&E staining image (D) of CFA-injected limbs. Scale bar, 2 mm. The results are representative of at least two independent experiments. *P < 0.05 and **P< 0.01 with the Mann–Whitney test.
DSS treatment and B. vulgatus induce the production of propionate
To understand the mechanisms underlying the beneficial effects of B. vulgatus and DSS-induced colitis in remote joint tissue, we assessed the expression of the anti-inflammatory cytokine IL-10. This was not only because IL-10 is an important contributor to immune homeostasis, but also because B. fragilis, another Bacteroides species, induces IL-10-secreting T cells (23). However, there were no differences in IL-10 expression in the splenocytes and mesenteric lymph node cells between B. vulgatus-administered and control mice, indicating that IL-10 may not be involved in the anti-inflammatory effects of B. vulgatus (Figure S5).
SCFAs derived from the microbial fermentation of dietary fibers exert immune regulatory properties (24). Serum SCFAs correlate with the progression of arthritis in individuals at risk of rheumatoid arthritis (RA), and SCFA treatment inhibits the pathogenesis of experimental arthritis (25, 26). Therefore, we examined the level of SCFAs in the culture media of B. vulgatus and the sera, feces, and cecum contents of DSS-treated mice. B. vulgatus produced propionate and acetate, but butyrate, isobutyrate, isovalerate, and valerate were not detected (Figure 5A and data not shown). Moreover, DSS gradually increased the concentration of propionate in the feces and decreased the levels of isovalerate (Figure 5B). Similar changes were observed in the sera of the DSS-treated mice (Figure 5C). Other SCFAs, including acetate, butyrate, and isobutyrate, in the sera and feces did not show a consistent trend (Figures 5B, C). There were no significant changes in the SCFA levels in the cecum contents (Figure S6). Bacteria-derived propionate may therefore mediate the beneficial effects of colitis and B. vulgatus on inflammatory arthritis.
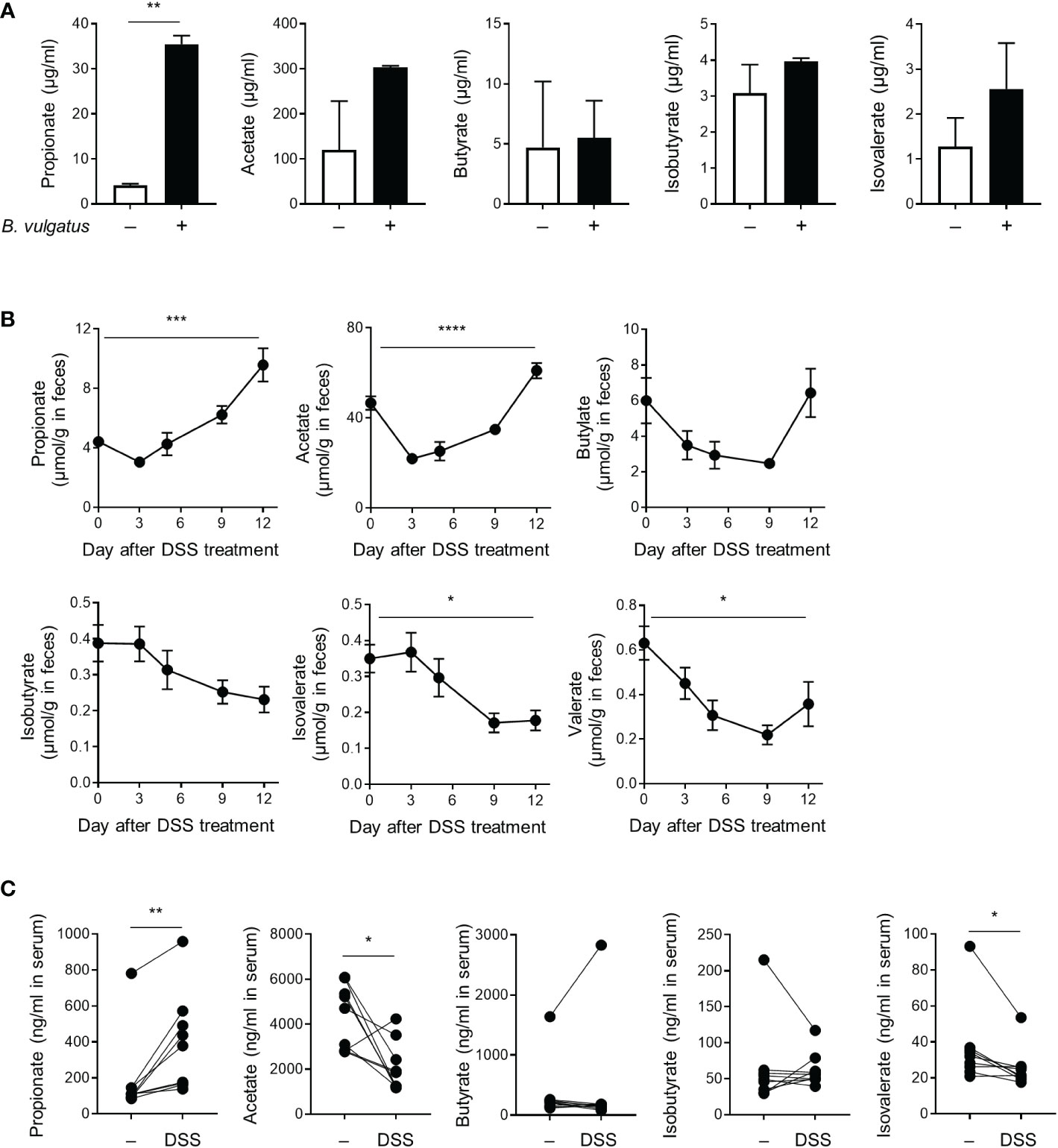
Figure 5 Production of short-chain fatty acids. (A) The amount of SCFAs secreted by B. vulgatus (2 × 109 CFU/mL) during cultivation in BHIS broth. **P < 0.01 by an unpaired t-test. (B, C) Mice (n = 9) were provided with drinking water with DSS for 5 days, which was then replaced with fresh water. (B) The amount of SCFAs in the feces collected at indicated time points. The data are shown as means ± SEM. *P < 0.05, ***P < 0.001 and ****P < 0.0001 by a one-way RM ANOVA. (C) The amount of SCFAs in the serum collected before DSS-treatment and after 12 days of DSS-treatment. Each dot represents an individual mouse. *P < 0.05 and **P < 0.01 with a paired t-test.
Propionate-producing bacteria play a critical role in the protection from inflammatory arthritis
Bacteroides are among the largest contributors to propionate formation within the human gut (27, 28). To investigate whether other Bacteroides and their propionate protect against inflammatory arthritis, we obtained other Bacteroides species, including B. caccae and B. thetaiotaomicron, and produced a mutant form (Δ1686-1689) of B. thetaiotaomicron with a defect in propionate production. As expected, B. caccae and B. thetaiotaomicron produced propionate, acetate, and isovalerate, but not butyrate or valerate, to varying extents (Figures 6A, B and data not shown). In contrast, the propionate-producing ability of B. thetaiotaomicron disappeared in the mutant form, despite the unabated production of acetate and isovalerate (Figure 6B). Similar to B. vulgatus, B. caccae and B. thetaiotaomicron significantly ameliorated edema at the footpads and ankles compared to the control (Figure 6C). However, the beneficial effects of B. thetaiotaomicron on arthritis disappeared in the mutant form of B. thetaiotaomicron (Figures 6C, S7). Bacteroides-producing propionate may therefore be responsible for the colitis-induced amelioration of inflammatory arthritis.
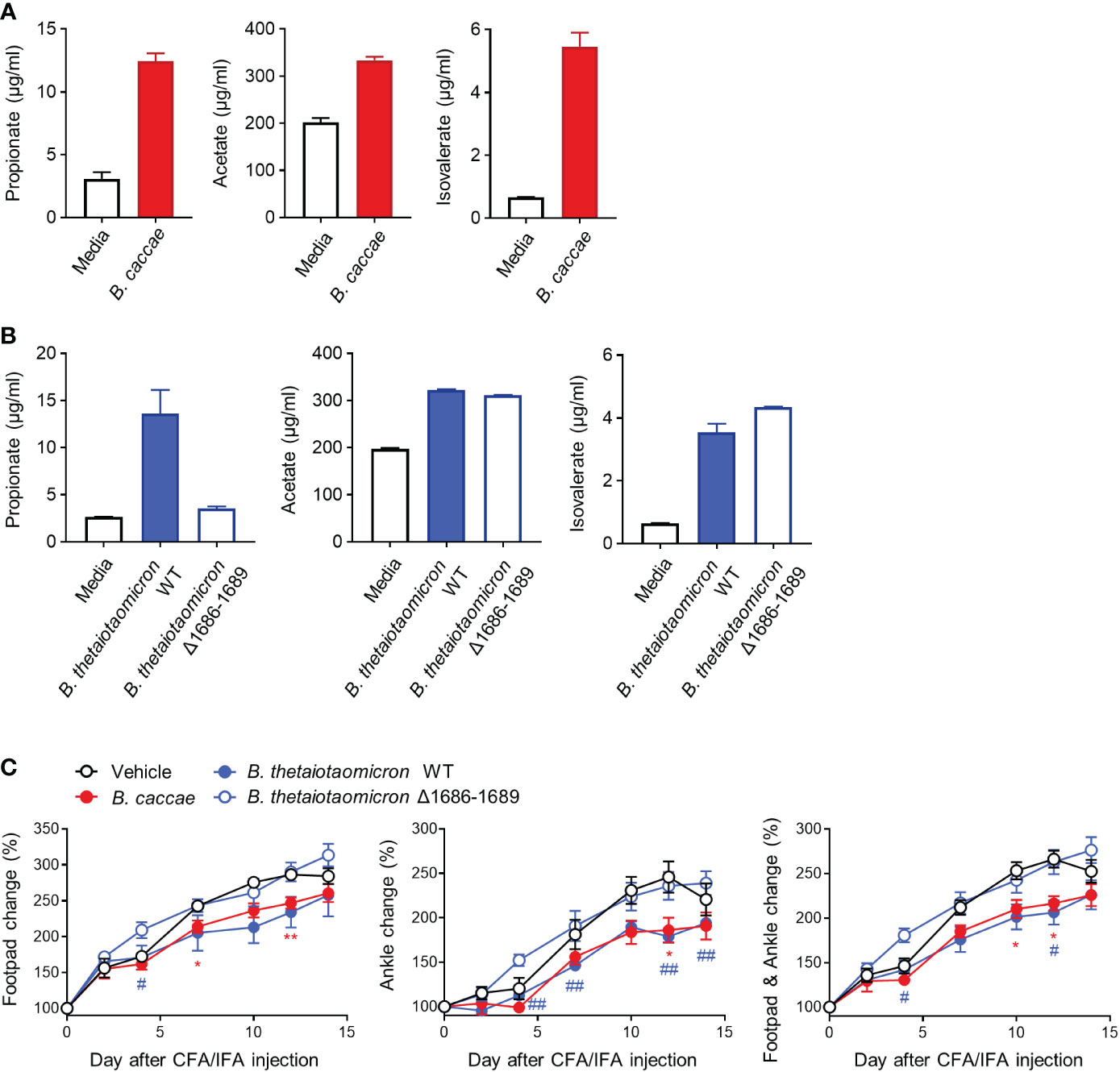
Figure 6 Other propionate-producing Bacteroides and mutant form and their protective effects on CFA-induced arthritis. The amount of SCFAs secreted by B. caccae (A) and B. thetaiotaomicron (WT and mutant Δ1686-1689) (B) (2 × 109 CFU/mL) during cultivation in BHIS broth. (C) Mice (n = 5 per group) were intragastrically administered B. caccae and B. thetaiotaomicron (WT and mutant Δ1686-1689) and treated with CFA/IFA according to the same scheme in Figure 4B. The data are shown as means ± SEM. *P < 0.05 and **P < 0.01 (B. caccae to vehicle) or #P < 0.05 and ##P < 0.01 (B. thetaiotaomicron WT to vehicle) with the Mann–Whitney test.
Discussion
Organ-to-organ communication has been suggested as a gatekeeper of metabolic health (1) and regulator of lipid metabolism, tissue homeostasis, and protection (29–31). We explored whether IBD in the intestines directly worsens inflammatory arthritis in the joints and, if so, assessed potential messengers which mediate the interaction between the gut and joints. To accomplish this, we used DSS-induced colitis, CFA-induced arthritis, and mBSA/IL-1β-induced arthritis mouse models. Contrary to our expectations, DSS-induced colitis was negatively correlated with the severity of arthritic symptoms in our animal models. Thus, our first hypothesis was reconsidered. HLA-B27-associated human disorders and transgenic rats expressing HLA-B27 and human β2m have shown that B27 is indispensable for several concomitant diseases, such as ankylosing spondylitis, reactive arthritis, psoriatic arthritis, IBD, and uveitis (32). Similarly, many joint diseases associated with gastrointestinal complications can be attributed to genetic factors. The protective effects of colitis on arthritis may therefore not be an unusual phenotype, but rather a new finding, regardless of common genetic factors.
Most of the microorganisms that inhabit the body exist in the gastrointestinal tract (3). There is accumulating data on the signaling of gut microbes to extraintestinal organs that influence health and disease (3, 33). Gut microbiota plays a crucial role in the pathogenesis of inflammatory arthritis. For example, arthritic symptoms spontaneously develop in K/BxN, IL1rn−/−, and SKG mice under specific pathogen-free conditions but not under germ-free (GF) conditions (13, 34, 35). In addition, the gut microbial composition of patients with RA differs from that of healthy individuals (13, 36). Various commensal bacteria, including segmented filamentous bacteria, Lactobacillus bifidus and Prevotella copri, contribute to the development and severity of arthritis, whereas others, including Prevotella histicola, ameliorate the severity of arthritis (13, 14, 34, 36). Here, we used the coprophagy of the mice to demonstrate that the gut microorganisms altered by IBD were attributable to the amelioration of inflammatory arthritis, indicating that the gut microbes act as messengers in the communication between the gut and joints. The altered microbiota further helped to maintain homeostasis in the joints, which may have resulted from the coevolution of host-microbiome system to protect the host from excessive inflammation. Microbiota can manipulate rapid phenotypic changes in hosts, which may be important for attuning to unpredictable or fluctuating environments, such as in the defense against pathogens (37). These interactions may be based on reciprocal selection between the host and its microbiota. However, further evidence is needed to corroborate whether and at what scale such selection can cause coevolution (37).
Identifying bacterial species or their metabolites is of even greater interest because it is difficult to precisely adjust the whole bacterial community, and treatment with antibiotics may result in adverse effects (38). We observed an increase in the abundance of B. vulgatus and its higher taxonomic ranks in the DSS-treated mice. Members of the genus Bacteroides are major commensals in healthy humans and gram-negative obligate anaerobes that colonize the colon (39). As commensals, Bacteroides generally play beneficial roles in the gut, such as inhibiting pathogen colonization and supplying nutrients to other commensal residents (39). Conversely, Bacteroides may act as pathobionts in genetic and environmental contexts (40). To our knowledge, however, Bacteroides-dominated feces or B. vulgatus have not previously been implicated in the pathogenesis of inflammatory arthritis, although a Bacteroides-dominated group has been found among patients with new-onset RA (13).
In this study, the mice were repeatedly administered pure cultures of B. vulgatus, B. caccae, and B. thetaiotaomicron, which led to reduced edema in their footpads and ankles. Several strains of probiotics, including Lactobacillus and Bifidobacteria, have been tested for their benefits in patients with RA (41). Although probiotic supplements can boost the effects of concomitant pharmacological treatments, evidence remains insufficient to make definite recommendations (41). Future studies should further investigate whether the beneficial effects of Bacteroides on RA are better than those of probiotics. However, because commensal Bacteroides are prevalent in the colon (39), administering Bacteroides species may be favorable for colonizing the intestinal tract, which affects their abundance in the gut. Moreover, because probiotic supplementation seems to have no clinically significant adverse effects (41), there is little concern regarding the side effects of Bacteroides. Thus, the three Bacteroides species examined in this study may serve as potential probiotics for the prevention and treatment of inflammatory arthritis.
SCFAs, which are primary gut bacterial products derived from dietary fibers, are beneficial for various physiological processes in the body and exhibit health-promoting properties in many inflammatory disorders, including colitis and arthritis (42, 43). Bacteria-derived SCFAs allow communication with peripheral organs and tissues in the host via multiple mechanisms, including histone deacetylase inhibition, G-protein-coupled receptor signaling, and energy source supplies (8, 43). Small amounts of SCFAs (mostly acetate and possibly propionate) move to target organs at remote sites and yield direct beneficial effects (43). Although a wide range of pre-clinical evidence has supported the role of SCFAs as modulators of joint inflammation, there are no reports of using SCFAs for arthritis in clinical trials. Instead, high-fiber dietary intervention has been found to increase SCFA levels and decrease the levels of pro-inflammatory cytokines and bone erosion markers in patients with RA (42, 44). A high-fiber diet leads to a predominance of the phylum Bacteroidetes in mice with collagen-induced arthritis and promotes the concomitant production of propionate, resulting in the improvement of arthritis and bone erosion (45). In addition, propionate suppresses the induction of inflammatory mediators and induces cellular senescence in synovial fibroblasts (46). The addition of propionate to drinking water improves arthritic symptoms in mice with collagen- and mBSA/MSU-induced arthritis (45, 46). Here, we found that DSS increased propionate levels in the blood and feces, whereas the B. thetaiotaomicron mutant with a defect in propionate production lost its beneficial effects on inflammatory arthritis. Thus, propionate production may be an important mechanism underlying the improvement of inflammatory arthritis upon treatment with DSS or Bacteroides. Further studies are required to elucidate the molecular mechanistic gaps between propionate production and joint inflammation.
Despite our findings, this study had several limitations. First, here, we did not consider the gut microbial composition in the process of epithelial repair and wound healing after ceasing DSS-treatment. Because their effect on the inflammatory arthritis may differ from our results, more exquisite experimental design would be needed in the further study. Second, commensal species and mechanisms that were not identified in this study may also contribute to the observed effects on the progression of inflammatory arthritis. Third, our results do not support a bidirectional relationship between the gut and joints. Future studies should therefore investigate whether the conditions of the joints influence the state of the intestines. Finally, because numerous studies have shown that the beneficial effects of probiotics depend on the strain (41), we should attempt to identify more effective stains than the type strains of the three Bacteroides species for the treatment of inflammatory arthritis.
In conclusion, our study provides novel insights into the gut-joint axis and highlights the importance of the gut microbiota as nongenetic factors related to the pathogenesis of arthritis and messengers between the gut and joints. Moreover, the protective effects of Bacteroides and propionate noted in this study may provide a basis for developing potent probiotics and postbiotics for the prevention or treatment of inflammatory arthritis.
Data availability statement
The datasets presented in this study can be found in online repositories. The names of the repository/repositories and accession number(s) can be found below: https://www.ncbi.nlm.nih.gov/bioproject/PRJNA869574/.
Ethics statement
The animal study was reviewed and approved by Institutional Animal Care and Use Committee (IACUC) at Seoul National University: IACUC at the Catholic University of Korea.
Author contributions
DK contributed Conceptualization, Formal analysis, Investigation, Writing – Original Draft, Writing – Review & Editing, Visualization, Supervision, Project administration and Funding acquisition. W-UK contributed Conceptualization, Writing – Review & Editing, Supervision, Project administration and Funding acquisition. H-JS contributed Methodology, Validation, Investigation and Writing – Original Draft. Y-MK contributed Methodology, Investigation, Validation, Writing – Original Draft, and Funding acquisition. KK analyzed Investigation, Validation, and Writing – Original Draft. J-OC contributed Validation and Investigation. S-HC contributed Investigation. SA contributed Investigation. S-HP contributed Investigation. Y-JC contributed Software, Data Curation, and Writing - Original Draft. J-HP contributed Writing – Original Draft and Supervision. S-US contributed Investigation. J-YC contributed Writing - Original Draft and Supervision. All authors contributed to the article and approved the submitted version.
Funding
This work was supported by National Research Foundation of Korea grants funded by the Ministry of Science, ICT and Future Planning (Grant 2015R1A3A2032927 to W-UK), the Ministry of Science and ICT (Grant 2022R1A2C1010356 to DK), the Ministry of Education (Grant 2017R1D1A1B04033009 to DK, and 2018R1A6A3A01013428 and 2020R1I1A2069425 to Y-MK), and the Technology Innovation Program funded by the Ministry of Trade, Industry & Energy (MOTIE, Korea; Grant 20013712 to J-YC), and the Liu Inkyung Memorial Endowment Fund and Creative-Pioneering Researchers Program through Seoul National University.
Acknowledgments
We thank for scholarships from the BK21 FOUR education program (H-JS, J-OC, and SA).
Conflict of interest
The authors declare that the research was conducted in the absence of any commercial or financial relationships that could be construed as a potential conflict of interest.
Publisher’s note
All claims expressed in this article are solely those of the authors and do not necessarily represent those of their affiliated organizations, or those of the publisher, the editors and the reviewers. Any product that may be evaluated in this article, or claim that may be made by its manufacturer, is not guaranteed or endorsed by the publisher.
Supplementary material
The Supplementary Material for this article can be found online at: https://www.frontiersin.org/articles/10.3389/fimmu.2023.1064900/full#supplementary-material
References
1. Castillo-Armengol J, Fajas L, Lopez-Mejia IC. Inter-organ communication: a gatekeeper for metabolic health. EMBO Rep (2019) 20(9):e47903. doi: 10.15252/embr.201947903
2. Gebrayel P, Nicco C, Al Khodor S, Bilinski J, Caselli E, Comelli EM, et al. Microbiota medicine: towards clinical revolution. J Transl Med (2022) 20(1):111. doi: 10.1186/s12967-022-03296-9
3. Kim D, Zeng MY, Núñez G. The interplay between host immune cells and gut microbiota in chronic inflammatory diseases. Exp Mol Med (2017) 49(5):e339. doi: 10.1038/emm.2017.24
4. Bravo JA, Julio-Pieper M, Forsythe P, Kunze W, Dinan TG, Bienenstock J, et al. Communication between gastrointestinal bacteria and the nervous system. Curr Opi Pharmacol (2012) 12(6):667–72. doi: 10.1016/j.coph.2012.09.010
5. Carabotti M, Scirocco A, Maselli MA, Severi C. The gut-brain axis: interactions between enteric microbiota, central and enteric nervous systems. Ann Gastroenterol (2015) 28(2):203–9.
6. Cryan JF, O'Riordan KJ, Cowan CSM, Sandhu KV, Bastiaanssen TFS, Boehme M, et al. The microbiota-Gut-Brain axis. Physiol Rev (2019) 99(4):1877–2013. doi: 10.1152/physrev.00018.2018
7. Ge Y, Wang X, Guo Y, Yan J, Abuduwaili A, Aximujiang K, et al. Gut microbiota influence tumor development and alter interactions with the human immune system. J Exp Clin Cancer Res (2021) 40(1):42. doi: 10.1186/s13046-021-01845-6
8. Kim CH. Control of lymphocyte functions by gut microbiota-derived short-chain fatty acids. Cell Mol Immunol (2021) 18(5):1161–71. doi: 10.1038/s41423-020-00625-0
9. Zhang X, Guarin D, Mohammadzadehhonarvar N, Chen X, Gao X. Parkinson’s disease and cancer: a systematic review and meta-analysis of over 17 million participants. BMJ Open (2021) 11(7):e046329. doi: 10.1136/bmjopen-2020-046329
10. Peluso R, Di Minno MND, Iervolino S, Manguso F, Tramontano G, Ambrosino P, et al. Enteropathic spondyloarthritis: from diagnosis to treatment. Clin Dev Immunol (2013) 2013:631408–. doi: 10.1155/2013/631408
11. Rodríguez-Reyna TS, Martínez-Reyes C, Yamamoto-Furusho JK. Rheumatic manifestations of inflammatory bowel disease. World J Gastroenterol (2009) 15(44):5517–24. doi: 10.3748/wjg.15.5517
12. Mu Q, Kirby J, Reilly CM, Luo XM. Leaky gut as a danger signal for autoimmune diseases. Front Immunol (2017) 8:598. doi: 10.3389/fimmu.2017.00598
13. Maeda Y, Kurakawa T, Umemoto E, Motooka D, Ito Y, Gotoh K, et al. Dysbiosis contributes to arthritis development via activation of autoreactive T cells in the intestine. Arthritis Rheumatol (2016) 68(11):2646–61. doi: 10.1002/art.39783
14. Balakrishnan B, Luckey D, Bodhke R, Chen J, Marietta E, Jeraldo P, et al. Prevotella histicola protects from arthritis by expansion of allobaculum and augmenting butyrate production in humanized mice. Front Immunol (2021) 12:609644. doi: 10.3389/fimmu.2021.609644
15. Chen J, Wright K, Davis JM, Jeraldo P, Marietta EV, Murray J, et al. An expansion of rare lineage intestinal microbes characterizes rheumatoid arthritis. Genome Med (2016) 8(1):43. doi: 10.1186/s13073-016-0299-7
16. Eichele DD, Kharbanda KK. Dextran sodium sulfate colitis murine model: An indispensable tool for advancing our understanding of inflammatory bowel diseases pathogenesis. World J Gastroenterol (2017) 23(33):6016–29. doi: 10.3748/wjg.v23.i33.6016
17. Bacic MK, Smith CJ. Laboratory maintenance and cultivation of Bacteroides species. Curr Protoc Microbiol (2008) 9(1):13C.1.1–C.1.21. doi: 10.1002/9780471729259.mc13c01s9
18. Kovatcheva-Datchary P, Nilsson A, Akrami R, Lee YS, De Vadder F, Arora T, et al. Dietary fiber-induced improvement in glucose metabolism is associated with increased abundance of prevotella. Cell Metab (2015) 22(6):971–82. doi: 10.1016/j.cmet.2015.10.001
19. García-Bayona L, Comstock LE. Streamlined genetic manipulation of diverse Bacteroides and Parabacteroides isolates from the human gut microbiota. mBio (2019) 10(4):e01762–19. doi: 10.1128/mBio.01762-19
20. Bischof RJ, Zafiropoulos D, Hamilton JA, Campbell IK. Exacerbation of acute inflammatory arthritis by the colony-stimulating factors CSF-1 and granulocyte macrophage (GM)-CSF: evidence of macrophage infiltration and local proliferation. Clin Exp Immunol (2000) 119(2):361–7. doi: 10.1046/j.1365-2249.2000.01125.x
21. Kim KS, Lee Y, Chae W, Cho JY. An improved method to quantify short-chain fatty acids in biological samples using gas chromatography-mass spectrometry. Metabolites (2022) 12(6):525. doi: 10.3390/metabo12060525
22. Caruso R, Ono M, Bunker ME, Núñez G, Inohara N. Dynamic and asymmetric changes of the microbial communities after cohousing in laboratory mice. Cell Rep (2019) 27(11):3401–12.e3. doi: 10.1016/j.celrep.2019.05.042
23. Ramakrishna C, Kujawski M, Chu H, Li L, Mazmanian SK, Cantin EM. Bacteroides fragilis polysaccharide a induces IL-10 secreting b and T cells that prevent viral encephalitis. Nat Comm (2019) 10(1):2153. doi: 10.1038/s41467-019-09884-6
24. Parada Venegas D, de la Fuente MK, Landskron G, González MJ, Quera R, Dijkstra G, et al. Short chain fatty acids (SCFAs)-mediated gut epithelial and immune regulation and its relevance for inflammatory bowel diseases. Front Immunol (2019) 10:277. doi: 10.3389/fimmu.2019.00277
25. Tajik N, Frech M, Schulz O, Schälter F, Lucas S, Azizov V, et al. Targeting zonulin and intestinal epithelial barrier function to prevent onset of arthritis. Nat Comm (2020) 11(1):1995. doi: 10.1038/s41467-020-15831-7
26. Martinsson K, Dürholz K, Schett G, Zaiss MM, Kastbom A. Higher serum levels of short-chain fatty acids are associated with non-progression to arthritis in individuals at increased risk of RA. Ann Rheumatic Dis (2022) 81(3):445–7. doi: 10.1136/annrheumdis-2021-221386
27. Aguirre M, Eck A, Koenen ME, Savelkoul PHM, Budding AE, Venema K. Diet drives quick changes in the metabolic activity and composition of human gut microbiota in a validated in vitro gut model. Res Microbiol (2016) 167(2):114–25. doi: 10.1016/j.resmic.2015.09.006
28. Salonen A, Lahti L, Salojärvi J, Holtrop G, Korpela K, Duncan SH, et al. Impact of diet and individual variation on intestinal microbiota composition and fermentation products in obese men. ISME J (2014) 8(11):2218–30. doi: 10.1038/ismej.2014.63
29. Ito M, Adachi-Akahane S. Inter-organ communication in the regulation of lipid metabolism: focusing on the network between the liver, intestine, and heart. J Pharmacol Sci (2013) 123(4):312–7. doi: 10.1254/jphs.13R09CP
30. Veiga-Fernandes H, Artis D. Neuronal-immune system cross-talk in homeostasis. Science (2018) 359(6383):1465–6. doi: 10.1126/science.aap9598
31. Huh JR, Veiga-Fernandes H. Neuroimmune circuits in inter-organ communication. Nat Rev Immunol (2020) 20(4):217–28. doi: 10.1038/s41577-019-0247-z
32. Hammer RE, Maika SD, Richardson JA, Tang J-P, Taurog JD. Spontaneous inflammatory disease in transgenic rats expressing HLA-B27 and human β2m: An animal model of HLA-B27-associated human disorders. Cell (1990) 63(5):1099–112. doi: 10.1016/0092-8674(90)90512-D
33. Schroeder BO, Bäckhed F. Signals from the gut microbiota to distant organs in physiology and disease. Nat Med (2016) 22(10):1079–89. doi: 10.1038/nm.4185
34. Ivanov II, Atarashi K, Manel N, Brodie EL, Shima T, Karaoz U, et al. Induction of intestinal Th17 cells by segmented filamentous bacteria. Cell (2009) 139(3):485–98. doi: 10.1016/j.cell.2009.09.033
35. Abdollahi-Roodsaz S, Joosten LA, Koenders MI, Devesa I, Roelofs MF, Radstake TR, et al. Stimulation of TLR2 and TLR4 differentially skews the balance of T cells in a mouse model of arthritis. J Clin Invest (2008) 118(1):205–16. doi: 10.1172/JCI32639
36. Scher JU, Sczesnak A, Longman RS, Segata N, Ubeda C, Bielski C, et al. Expansion of intestinal Prevotella copri correlates with enhanced susceptibility to arthritis. eLife (2013) 2:e01202. doi: 10.7554/eLife.01202
37. Koskella B, Bergelson J. The study of host-microbiome (co)evolution across levels of selection. Philos Trans R Soc Lond B Biol Sci (2020) 375(1808):20190604. doi: 10.1098/rstb.2019.0604
38. Willing BP, Russell SL, Finlay BB. Shifting the balance: antibiotic effects on host–microbiota mutualism. Nat Rev Microbiol (2011) 9(4):233–43. doi: 10.1038/nrmicro2536
39. Zafar H, Saier MH Jr. Gut Bacteroides species in health and disease. Gut Microbes (2021) 13(1):1–20. doi: 10.1080/19490976.2020.1848158
40. Bloom SM, Bijanki VN, Nava GM, Sun L, Malvin NP, Donermeyer DL, et al. Commensal Bacteroides species induce colitis in host-genotype-specific fashion in a mouse model of inflammatory bowel disease. Cell Host Microbe (2011) 9(5):390–403. doi: 10.1016/j.chom.2011.04.009
41. Ferro M, Charneca S, Dourado E, Guerreiro CS, Fonseca JE. Probiotic supplementation for rheumatoid arthritis: A promising adjuvant therapy in the gut microbiome era. Front Pharmacol (2021) 12:711788. doi: 10.3389/fphar.2021.711788
42. Dürholz K, Hofmann J, Iljazovic A, Häger J, Lucas S, Sarter K, et al. Dietary short-term fiber interventions in arthritis patients increase systemic SCFA levels and regulate inflammation. Nutrients (2020) 12(10):3207. doi: 10.3390/nu12103207
43. Koh A, De Vadder F, Kovatcheva-Datchary P, Bäckhed F. From dietary fiber to host physiology: Short-chain fatty acids as key bacterial metabolites. Cell (2016) 165(6):1332–45. doi: 10.1016/j.cell.2016.05.041
44. Häger J, Bang H, Hagen M, Frech M, Träger P, Sokolova MV, et al. The role of dietary fiber in rheumatoid arthritis patients: A feasibility study. Nutrients (2019) 11(10):3207. doi: 10.3390/nu11102392
45. Bai Y, Li Y, Marion T, Tong Y, Zaiss MM, Tang Z, et al. Resistant starch intake alleviates collagen-induced arthritis in mice by modulating gut microbiota and promoting concomitant propionate production. J Autoimmun (2021) 116:102564. doi: 10.1016/j.jaut.2020.102564
Keywords: arthritis, colitis, gut microbiota, Bacteroides, propionate
Citation: Shon H-J, Kim Y-M, Kim KS, Choi J-O, Cho S-H, An S, Park S-H, Cho Y-J, Park J-H, Seo S-U, Cho J-Y, Kim W-U and Kim D (2023) Protective role of colitis in inflammatory arthritis via propionate-producing Bacteroides in the gut. Front. Immunol. 14:1064900. doi: 10.3389/fimmu.2023.1064900
Received: 10 October 2022; Accepted: 16 January 2023;
Published: 30 January 2023.
Edited by:
Jennifer M. Monk, University of Guelph, CanadaReviewed by:
Sang Il Lee, Gyeongsang National University, Republic of KoreaCoen Govers, Wageningen University and Research, Netherlands
Copyright © 2023 Shon, Kim, Kim, Choi, Cho, An, Park, Cho, Park, Seo, Cho, Kim and Kim. This is an open-access article distributed under the terms of the Creative Commons Attribution License (CC BY). The use, distribution or reproduction in other forums is permitted, provided the original author(s) and the copyright owner(s) are credited and that the original publication in this journal is cited, in accordance with accepted academic practice. No use, distribution or reproduction is permitted which does not comply with these terms.
*Correspondence: Donghyun Kim, YmlvbG9nb2tpbUBzbnUuYWMua3I=; Wan-Uk Kim, d2FuNzI1QGNhdGhvbGljLmFjLmty
†These authors have contributed equally to this work and share first authorship