- 1Department of Anesthesia and Intensive Care Medicine, Division of Emergencies and Critical Care, Oslo University Hospital, Oslo, Norway
- 2Institute of Clinical Medicine, University of Oslo, Oslo, Norway
- 3Department of Research & Development, Division of Emergencies and Critical Care, Oslo University Hospital, Oslo, Norway
- 4Department of Transplantation Medicine, Section of Transplantation Surgery, Oslo University Hospital, Oslo, Norway
- 5Research Laboratory, Nordland Hospital, Bodø, Norway
- 6Department of Transplantation Medicine, Section of Nephrology, Oslo University Hospital, Oslo, Norway
- 7Department of Immunology, Oslo University Hospital, and University of Oslo, Oslo, Norway
- 8Centre of Molecular Inflammation Research, Norwegian University of Science and Technology, Trondheim, Norway
- 9Department of Intensive Care Nursing, Lovisenberg University College, Oslo, Norway
Background: Pancreas transplant alone (PTA) recipients are more affected by pancreas graft thrombosis, and graft loss compared to simultaneous pancreas-kidney (SPK) recipients. The pathophysiology is unknown, but an increased immune response has been suggested in the PTA recipients. In this observational study, we compared perioperative thromboinflammation between PTA (n=32) and SPK (n=35) recipients, and between PTA recipients with (n=14) versus without (n=18) early graft thrombosis.
Methods: We measured C-reactive protein (CRP), plasma markers of activated coagulation and complement, and cytokines preoperatively and daily during the first postoperative week.
Results: Preoperatively, coagulation and complement activation markers were comparable between PTA and SPK recipients, while cytokine concentrations were higher in SPK recipients (TNF, IL-8, IP-10, MCP-1, MIP-1α; all p<0.05). On the first postoperative day, PTA recipients had higher coagulation activation, measured as thrombin-antithrombin complex (TAT), than SPK recipients (p=0.008). In the first postoperative week, PTA recipients showed higher relative cytokine release (IL-6, IL-8, G-CSF, IP-10, MCP-1, and MIP-1α; all p<0.05) while SPK recipients showed higher absolute cytokine concentrations (TNF, IL-1ra, IL-8, MIP-1α, and IL-4; all p<0.05). PTA and SPK recipients showed similar terminal complement complex (TCC, sC5b-9) activation. On the first postoperative day, TCC (OR 1.2 [95% CI 1.0-1.5] for 0.1 CAU/ml increase, p=0.02) and CRP (OR 1.2 [95% CI 1.0-1.3] for 10 mg/L increase, p=0.04) were associated with an increased risk of early graft thrombosis. TCC was specific for graft thrombosis, while CRP increased with several complications. PTA recipients with compared to those without graft thrombosis had higher TCC pre- (p=0.04) and postoperatively (p=0.03).
Conclusion: The relative increase in postoperative thromboinflammatory response was more pronounced in PTA recipients. Complement activation was associated with an increased risk of graft thrombosis. This study indicates that innate immune activation rather than elevated levels may affect early postoperative pancreas graft thrombosis.
Clinical trial registration: https://clinicaltrials.gov/ct2/show/NCT01957696, identifier NCT01957696
Introduction
Pancreas transplantation is currently the only definite treatment for type 1 diabetes mellitus, restoring the body’s glycemic control. The indication is brittle diabetes, and transplantation is performed either as a pancreas transplant alone (PTA) in patients with preserved kidney function or more commonly, as a simultaneous pancreas-kidney (SPK) transplant in patients with a concurrent end-stage renal failure (1).
SPK recipients have approximately 90% 1-year and 80% 5-year graft survival, compared to 75% 1-year and 50% 5-year graft survival for PTA recipients (2–5). The pathophysiology behind this survival gap is poorly understood, but a more active immune system in PTA recipients and a lack of pancreas-specific rejection markers have been suggested (6, 7). Graft thrombosis, the most common cause of early graft loss, is reported to be more frequent in PTA recipients (5, 8, 9). Many risk factors for pancreas graft thrombosis have been identified, but the pathogenesis remains to be explained (9, 10). In the transplantation setting, the ischemia-reperfusion injury triggers a response involving an interplay between several plasma cascades, including complement, coagulation, and fibrinolytic systems, leading to thromboinflammation (11).
C-reactive protein (CRP), one of the most commonly measured markers of inflammation, has been demonstrated to predict several pancreas graft-related complications (12). To explore the thromboinflammatory response further, activation markers of different plasma cascades must be measured. The thrombin-antithrombin (TAT) complex, can be used as a marker of activated coagulation. It is formed to regulate blood coagulation when large amounts of thrombin are generated; thrombin is a potent platelet agonist that cleaves fibrinogen to form a fibrin clot (13). For the complement cascade, C3 is an early marker in the common cascade that can be activated by any of three major activation pathways. C3 in turn activates C5, leading to the formation of the highly pro-inflammatory anaphylatoxin C5a, and the end product, the terminal C5b-9 complement complex (TCC) is shown to be a robust marker of complement activation (14). There are several examples of crosstalk between the coagulation and complement systems (15–17). The complement system, being a part of the innate immune system, plays a major role in the defense against infections, but can also activate coagulation factors and stimulate platelets leading to thrombosis formation (11, 18, 19). The thromboinflammatory response includes the release of cytokines, and the activation of leukocytes, platelets, and endothelial cells (20). After surgery, cytokines are necessary for the defense against infections and for wound healing (21). However, a dysregulation can lead to tissue damage, and an imbalance between pro- and anti-inflammatory cytokines may be involved in the pathophysiology of venous thromboembolism (22).
The nature of the thromboinflammatory response after a solid organ pancreas transplantation, its involvement in pancreas graft thrombosis, and potential differences between PTA and SPK recipients are not yet known. We hypothesized that the perioperative systemic thromboinflammatory response is more pronounced in PTA versus SPK recipients, and between PTA recipients with versus without early graft thrombosis.
Materials and methods
Study design, patients, and ethics
We conducted a prospective, observational, single-center study. During a pre-specified 3-year period, from April 2015 to March 2018, all patients (>18 years) admitted for solid-organ pancreas transplantation, as PTA or SPK transplantation, to the Oslo University Hospital in Norway were eligible for study inclusion. Because of very few pancreas after kidney (PAK) transplantations, these were excluded. The study presented is a sub-study of the Norwegian pancreas transplantation study (clinicaltrials.gov NCT01957696, South-Eastern Norwegian ethical committee 2012/2278). Written informed consent was obtained from all participants and we report according to the STROBE checklist (Figure S1).
The primary outcome was perioperative differences in thromboinflammatory plasma markers between PTA and SPK recipients. Secondary outcomes were differences in thromboinflammatory plasma markers in PTA recipients with and without graft thrombosis. To describe the postoperative course of our cohort, we registered clinically verified pancreas graft complications (graft thrombi, hematomas/bleedings, and pancreatic leakages) within 30 days, and pancreas graft failure, defined as the need for exogenous insulin or explantation of the transplanted organ, within one-year post-transplantation.
Patient treatment: Surgery, anticoagulation, and immunosuppression
Pancreas grafts were transplanted in a right retrocolic standing position, with a duodenoduodenostomy and vessel anastomoses to the right common iliac artery and the inferior vena cava.
All study participants received identical treatment, apart from a modification in the clinical protocol for anticoagulation. The first 34 patients (18 SPK, 16 PTA) received intraoperative 30 IU/kg unfractionated heparin intravenously (IV) and 2500 IU low molecular weight heparin (LMWH) subcutaneously (SC) 6 hours postoperatively. This was followed by 2500-5000 IU for SPK and 5000-7500 IU for PTA recipients daily from postoperative day (POD) 1. Acetylsalicylic acid 75 mg per orally (PO) was introduced from POD 7. The remaining 33 patients (17 SPK, 16 PTA) received identical intraoperative heparin, but with the addition of 500 mL of dextran 40, 100 mg/mL intraoperatively and on POD 1. LMWH was administered daily, 5000 IU SC, in both PTA and SPK recipients alike, and acetylsalicylic acid, 75 mg PO, was started from POD 3.
Perioperative immunosuppression consisted of methylprednisolone IV (250 mg, 350 mg if >90kg), mycophenolate mofetil IV (1g twice daily), and anti-thymocyte globulin IV (2mg/kg). Additional doses of anti-thymocyte globulin (1mg/kg) were administered during the first ten postoperative days depending on T-cell count, up to a maximum total dose of 6.5 mg/kg. Maintenance immunosuppression consisted of tacrolimus PO aimed at trough plasma concentrations of 10-12 ng/L during the first 8 postoperative weeks and 6-10 ng/L thereafter, mycophenolate PO (1g twice daily), and a daily dose of prednisolone tapered from 20 mg on POD 1 to 7.5 mg once daily on week 8, then subsequently to 5 mg once daily 6 months postoperatively. Immunosuppressive therapies were the same for PTA and SPK recipients.
Clinical post-operative evaluations
The study protocol included Doppler ultrasound evaluation of the pancreas graft four hours after reperfusion, with a computed tomography if the ultrasound proved inconclusive. In addition, Doppler ultrasound imaging and contrast-enhanced computed tomography (Iomeron, Bracco, Milan, Italy) were performed on POD 5. Additional imaging was performed on clinical indication. All hematomas/bleedings, pancreatic leakages, and venous thrombi, including both occluding and non-occluding thrombi, are reported in this paper, regardless of their need of intervention. Pancreas graft thromboses were evaluated by a senior radiologist according to the CT-grading system proposed by Hakeem (23) as peripheral thrombosis (grade 1), intermediate non-occlusive thrombosis (grade 2), and central occlusive thrombosis (grade 3). A grade 3 was also assigned in case of ultrasound-based diagnosis of an occlusive thrombus and thus unnecessity of CT-examination.
Plasma samples
Blood samples were obtained preoperatively and daily for the first seven postoperative days. Blood was collected in 4-mL tubes containing ethylenediaminetetraacetic acid (EDTA). Samples were kept on ice for up to 2 hours before they were centrifuged with 3000 x g, for 10 min at 4°C. The isolated plasma was thereafter immediately frozen at -70°C until analysis.
Analyses of inflammatory markers
Acute phase protein CRP was analyzed routinely by the Division of Laboratory Medicine at Oslo University Hospital by immune turbidimetry.
Coagulation marker thrombin-antithrombin complex (TAT) was quantified using enzyme-linked immunosorbent assay (ELISA) (Siemens Healthineers, Marburg, Germany). Reference range (97.5th percentile) from healthy adults, as reported by the manufacturer.
Complement activation products C3bc and sC5b-9, terminal complement complex (TCC), were analyzed with ELISA, in-house assays, with reference range defined based on healthy blood donors and expressed in complement arbitrary units (CAU)/ml as previously described by Bergseth et al. (24).
Cytokines were analyzed using a multiplex cytokine assay (Bio-Plex Human Cytokine 27-Plex Panel; Bio-Rad Laboratories Inc., Hercules, CA, USA) containing interleukins, chemokines, interferons, and growth factors: interleukin (IL)-1β, IL-1 receptor antagonist (IL-1ra), IL-2, IL-4, IL-5, IL-6, IL-7, IL-8, IL-9, IL-10, IL-12 (p70), IL-13, IL-15, IL-17A, eotaxin, basic fibroblast growth factor (bFGF), granulocyte colony-stimulating factor (G-CSF), granulocyte-macrophage colony stimulating-factor (GM-CSF), interferon-gamma (IFN-γ), interferon-inducible protein (IP-10), monocyte chemotactic protein (MCP-1), macrophage inflammatory protein (MIP)-1α, MIP-1β, platelet-derived growth factor-BB (PDGF-BB), regulated upon activation T cell expressed and secreted (RANTES), tumor necrosis factor (TNF), and vascular endothelial growth factor (VEGF). The samples were analyzed on a Multiplex Analyzer (Bio-Rad Laboratories) according to instructions from the manufacturer. For cytokines, we used previously published data from healthy blood donors for reference values (25).
Thirteen of the cytokines, IFN- γ, IL-1β, IL-2, IL-9, IL-12, IL-13, IL-17A, PDGF-BB, RANTES, bFGF, eotaxin, GM-CSF and VEGF, were detectable but low, and within the reference for healthy humans, or demonstrated only minor to no variation during the first postoperative week. These were regarded as negative, and no further analyses were performed.
Statistics
Patient characteristics between groups were compared with the Mann-Whitney U-test for continuous, and the Chi-squared test for categorical parameters. The outcome between groups was compared with the Fisher’s exact test. All inflammatory biomarkers were non-normally distributed. The Mann-Whitney U-test was used to compare the preoperative inflammatory markers between groups. Fold changes were calculated on each postoperative day as ratios compared to preoperative values. Differences in fold changes at POD 1 compared to preoperative values were evaluated with the Sign’s test separately in PTA and SPK groups and compared between groups with the Mann Whitney U-test. Sums of fold changes from postoperative days 1-7, reflecting relative changes over the first postoperative week, were compared between PTA and SPK recipients with the Mann Whitney U-test.
We used log-10 transformed data in mixed model analyses comparing the time courses of the inflammatory markers between PTA and SPK recipients during the first postoperative week. For the whole study sample, four separate linear mixed models with fixed effects of either time (days), group (PTA/SPK), the interaction of time and group, and all three variables combined were performed with patient identification as a random effect. The effect of time described the change in concentration of the inflammatory marker over the first postoperative week for all patients, independent of group effect. The effect of groups described differences between the SPK and PTA recipients but did not examine variations between groups over time. The interaction between group and time, investigated the variation of the inflammatory marker over time in the two groups giving information if changes over time were different between the two groups. Including all dependent variables (time, group, and interaction thereof) in one model enabled post-estimation of estimated marginal means and thus comparison of inflammatory markers at specific time points between groups and within groups. In PTA recipients only, a subgroup analysis was performed comparing the time courses of the inflammatory markers, and kidney function markers, between recipients with and without graft thrombosis. A significant contribution of the explanatory variable (time/group/interaction thereof) was evaluated by the Wald Chi-Squared test in every model. Due to the change in the anticoagulation regime, the effect of the groups (PTA/SPK) was also assessed separately for the first 34 and the following 33 patients.
Univariate logistic regression was used to determine the odds of developing thrombosis for increased concentrations of any of the inflammatory markers preoperatively or on the first postoperative day. This was done in all recipients together, and separately in PTA recipients. Markers associated with graft thrombosis identified in the logistic regression were further analyzed in mixed model analyses to investigate their abilities to distinguish non-event patients from graft thrombosis, pancreatic leakage, and hematoma/bleeding. Univariate logistic regressions and multivariable logistic regressions including group (PTA/SPK) as an independent variable were also performed to investigate the odds for thrombosis. In addition to inflammatory markers, Body Mass Index (BMI), hemoglobin, and pancreas artery flow were included as these factors may constitute potential confounding factors for the development of graft thrombosis. Probability level p<0.05 was considered statistically significant.
We used the following software for the statistical analyses and graphical presentation: R (Core Team (2020). R: A language and environment for statistical computing. R Foundation for Statistical Computing, Vienna, Austria. URL https://www.R-project.org/), StataCorp. 2019. Stata Statistical Software: Release 16. College Station, TX: StataCorp LLC and GraphPad Prism version 8.0.0 for Windows, GraphPad Software, San Diego, California USA, https://www.graphpad.com.
Results
Study cohort and clinical outcome
Sixty-seven patients were included, 32 PTA and 35 SPK, providing a total of 473 plasma samples. One patient who did not consent due to language difficulties was excluded (Figure 1). Patient characteristics are displayed in Table 1. Venous graft thrombosis was diagnosed in 44% of PTA (n=14, 2 peripheral (grade 1), 5 non-occlusive (grade 2), and 7 occlusive (grade 3)), compared to 11% of SPK recipients (n=4, 0 peripheral (grade 1), 1 non-occlusive (grade 2), and 3 occlusive (grade 3) (p=0.005, Table 2). Graft losses within the first postoperative year were significantly higher in PTA than in SPK recipients (p=0.029, Table 2). Out of 13 lost grafts, 8 (6 PTA and 2 SPK) had an early graft thrombosis. Arterial pancreas graft thrombi, hematomas/bleedings, and pancreatic leakages did not differ between PTA and SPK recipients (Table 2). All arterial pancreas graft thrombi were deemed clinically insignificant and occurred in patients with concurrent venous graft thrombosis. Eight patients (5 SPK, 3 PTA) underwent relaparotomy during the first postoperative week. None of these patients suffered early graft thrombosis.
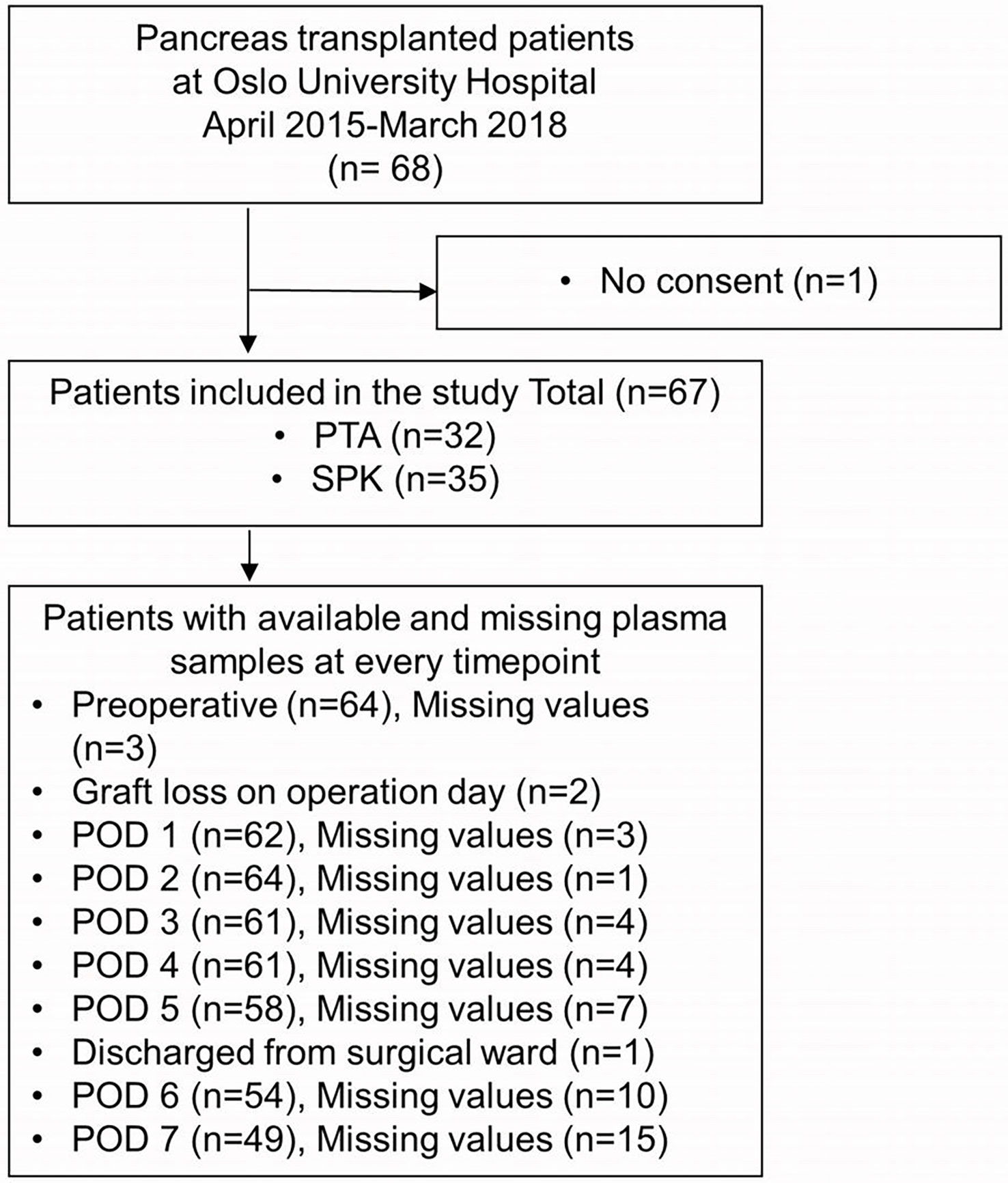
Figure 1 Inclusion of pancreas transplanted patients and available plasma samples throughout the study. PTA, pancreas transplant alone; SPK, simultaneous pancreas-kidney transplantation; POD, postoperative day.
Preoperative differences in plasma inflammatory parameters between PTA and SPK recipients
Coagulation marker TAT and complement activation products C3bc and TCC did not differ between PTA and SPK recipients preoperatively. Six of the investigated cytokines: TNF, IL-8, IP-10, MCP-1, MIP-1α and IL-4, were significantly higher in SPK compared to PTA recipients (Table 3).
Time course of plasma inflammatory parameters over the first postoperative week for PTA and SPK recipients
CRP, coagulation marker TAT and complement markers C3bc and TCC increased significantly (p<0.001 for all) from preoperative values to POD 1 (Figures 2A–D, Table S1). During the first postoperative week, CRP, TAT, and C3bc decreased, demonstrating considerable time and group-by-time effects (p<0.001 for all). TCC remained elevated compared to preoperative values in both groups (Figure 2D). The changes over time of these markers did not differ between PTA and SPK recipients (Tables S1, S2). TAT, however, was significantly (p=0.008) higher on POD 1 (Table S3), and summed fold changes for TCC from POD 1 to POD 7 (p=0.031) in PTA compared to SPK recipients (Figure 3, Table S4).
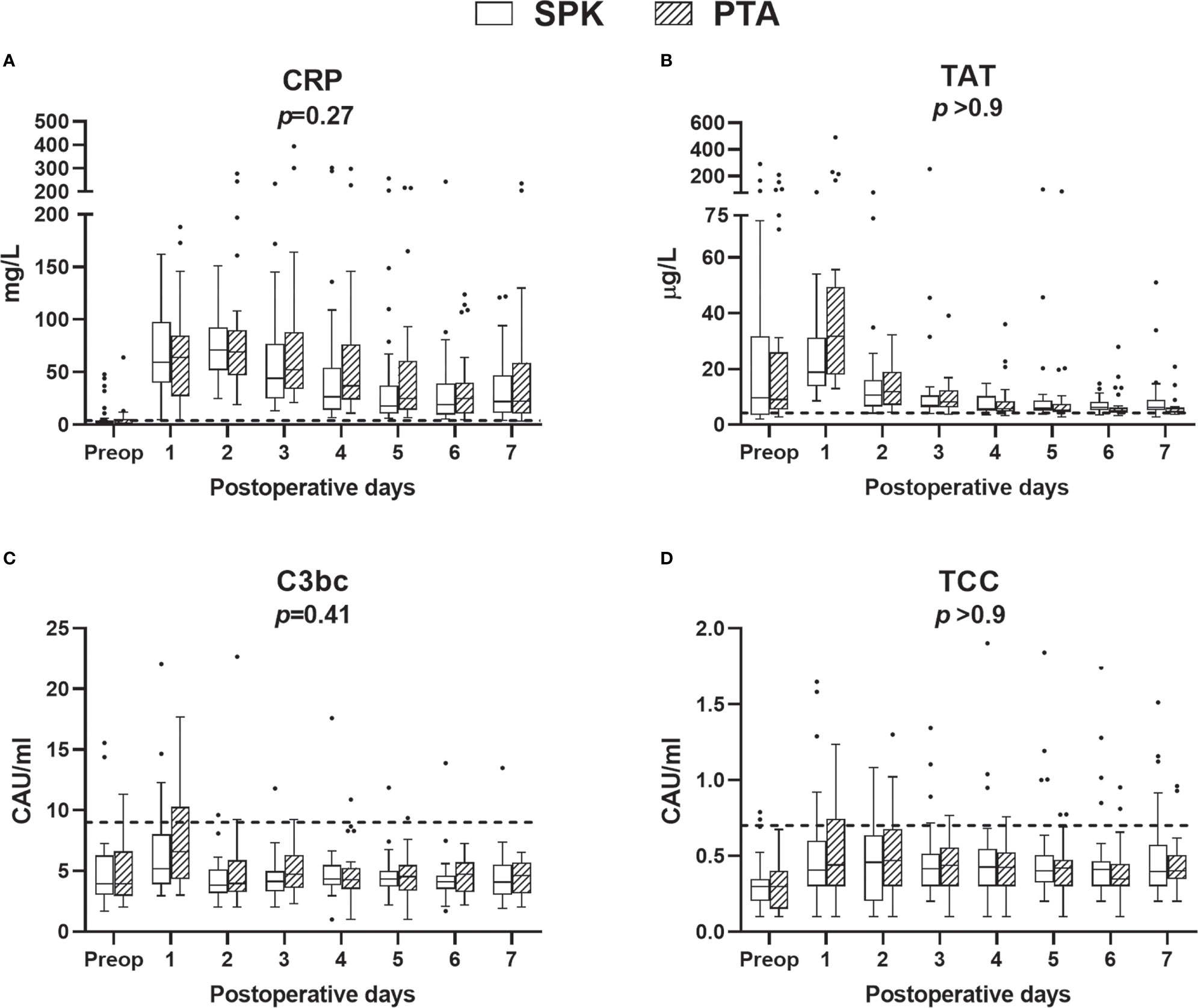
Figure 2 Plasma concentrations of C-reactive protein (CRP) (A), coagulation (B), and complement markers (C, D), preoperatively (preop) and during the first postoperative week, for simultaneous pancreas-kidney transplantation (SPK) (n=35) and pancreas transplant alone (PTA) (n=32) patients. Data are presented as boxplots (line, median; box, interquartile range) with whiskers (25th and 75th percentiles -/+ 1.5 x interquartile range). Dashed lines represent upper reference limits defined previously in samples from healthy blood donors and are given for reference only. Two outliers are not shown, C3bc value 34 and TCC value 3.8, in PTA patients on postoperative day 1. P-values represent the-postoperative overall group effect (Wald Chi-Squared test), excluding the preoperative samples, in a linear mixed model. CAU, complement arbitrary unit; CRP, C-reactive protein; TAT, thrombin-antithrombin complex; TCC, terminal complement complex.
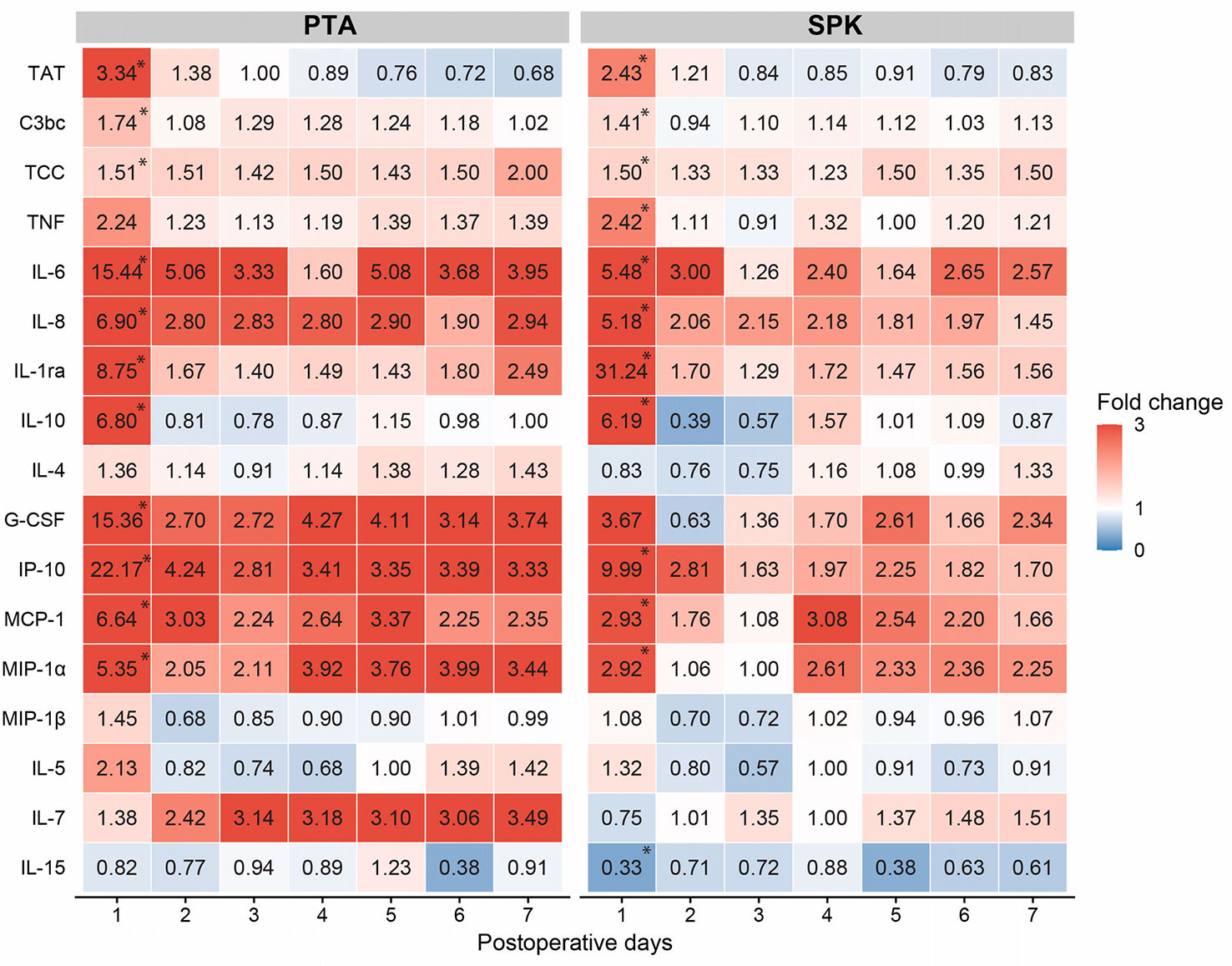
Figure 3 Median fold changes of inflammatory markers for postoperative days 1-7 compared to preoperative values (day=0) for pancreas transplant alone (PTA) and simultaneous pancreas-kidney (SPK) recipients. The red color indicates increased fold change and the blue color decreased fold change compared to preoperative median concentration. *Indicates significantly (p<0.05) increased fold change from preoperative values to the first postoperative day (Sign test) within PTA and SPK groups. Fold changes on POD 1 and sums of fold changes POD1-7 were compared between SPK and PTA recipients (Mann Whitney U-test) and presented in Table S4. CRP, C-reactive protein; G-CSF, granulocyte colony-stimulating factor; IL, interleukin; IL-1ra; interleukin-1 receptor antagonist; IP-10, interferon gamma-induced protein 10; MCP-1, monocyte chemoattractant protein 1; MIP, macrophage inflammatory protein; TAT, thrombin-antithrombin complex; TCC, terminal complement complex; TNF, tumor necrosis factor.
Cytokines were grouped according to their main function and subgroups (Figures 4, 5). From preoperative values to POD 1, eight of the fourteen investigated cytokines increased significantly in both PTA and SPK recipients: IL-6, IL-8, IL-1ra, IL-10, G-CSF, IP-10, MCP-1, and MIP-1α (p ≤ 0.031 for all, Table S1). TNF increased significantly (p=0.02) only in SPK recipients (Table S1). All cytokines demonstrating an initial increase decreased significantly from POD 1 to POD 7 (p ≤ 0.036 for all, Figures 4, 5, Table S1). IL-7 increased significantly over the first postoperative week (p ≤ 0.017), while there were no discernible time patterns for MIP-1α, IL-4, IL-5, and IL-15 (Figures 4, 5, Table S1). Except for IL-15, all cytokines demonstrated postoperative time and time-by-group effects (p<0.001 for all, Table S2). Five cytokines were elevated in SPK compared to PTA recipients: TNF, IL-8, IL-1ra, MIP1α, and IL-4 (p ≤ 0.031, Table S2). The trend with higher inflammatory markers in SPK recipients was seen both before and after the change in the anticoagulation regime (Table S4). Three cytokines had higher concentrations on POD 1 in SPK versus PTA recipients: TNF, IL-8, and IL-1ra (p ≤ 0.034 for all, Table S3). Fold changes from preoperative values to POD 1 were significantly higher in PTA than in SPK recipients for IL-6, IL-4, IP-10, and MCP-1 (p ≤ 0.03 for all, Figure 3, Table S5). The changes over the first postoperative week, reflected by summed fold changes from POD 1 to POD 7, were significantly higher in PTA than in SPK recipients for eight of the cytokines: IL-6, IL-8, IL-4, G-CSF, IP-10, MCP-1, MIP-1α and IL-7 (p<0.05 for all, Figure 3, Table S5).
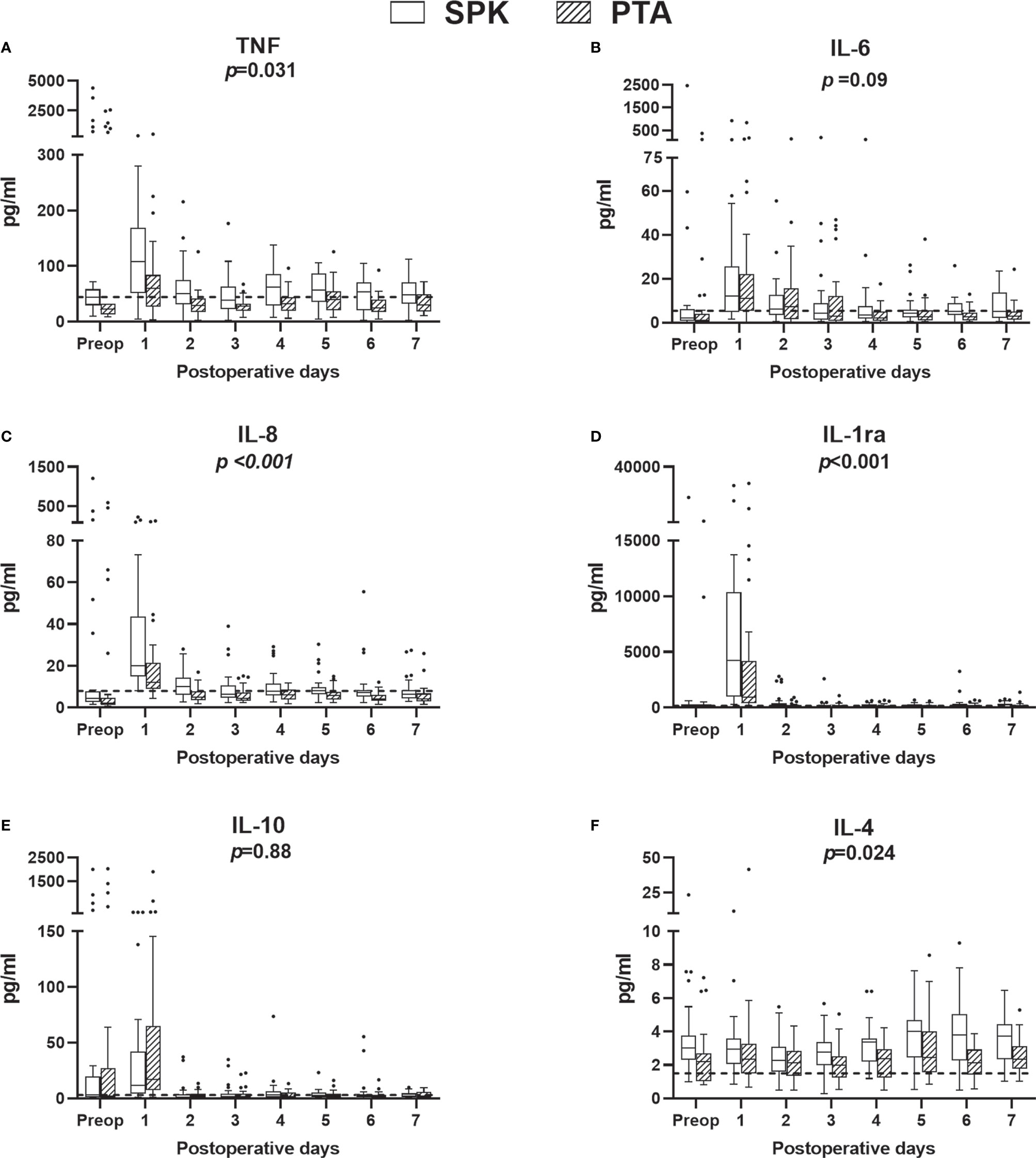
Figure 4 Plasma concentrations of proinflammatory (A–C) and anti-inflammatory (D–F) cytokine preoperatively (preop) and during the first postoperative week, for simultaneous pancreas-kidney (SPK) (n=35) transplantation and pancreas transplant alone (PTA) (n=32) patients. Boxplots, reference lines, and overall effects of transplantation group are calculated and presented as in Figure 2. IL, interleukin; IL-1ra; interleukin-1 receptor antagonist; pg, picogram; TNF, tumor necrosis factor.
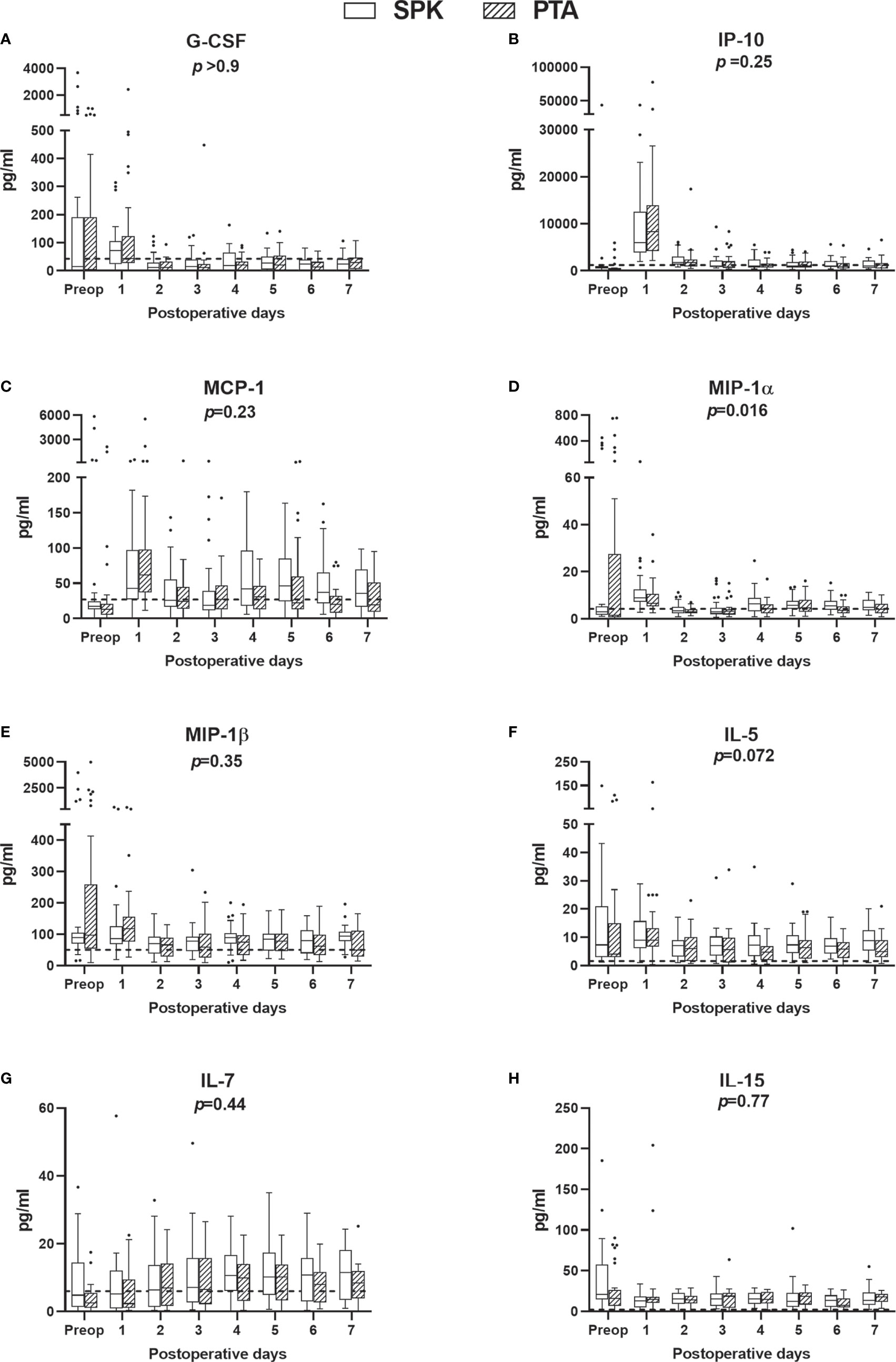
Figure 5 (A) Growth factor, chemokines (B–E), and pleiotropic (F–H) cytokine plasma concentrations preoperatively (preop) and during the first postoperative week, for simultaneous pancreas-kidney (SPK) (n=35) transplantation and pancreas transplant alone (PTA) (n=32). Boxplots, reference lines, and overall effects of transplantation group were calculated and presented as in Figure 2. G-CSF, granulocyte colony-stimulating factor; IL, interleukin; pg, picogram.; IP-10, interferon gamma-induced protein 10; MCP-1, monocyte chemoattractant protein 1; MIP, macrophage inflammatory protein.
Risk for thrombotic complications
PTA was associated with a higher risk for venous graft thrombosis formation within 30 days postoperatively, compared to SPK transplantation (OR 6.0 [95% CI 1.7-21.2], p<0.001). Of the investigated inflammatory markers, only CRP (OR 1.16 [95% CI 1.01-1.34] for 10 mg/L increase, p=0.042) and TCC (OR 1.23 [95% CI 1.04-1.47] for 0.1 CAU/ml increase, p=0.019) at the first postoperative day were associated with an increased risk of graft thrombosis (Table S6). CRP was significantly increased in patients with early graft thrombosis (p=0.008), pancreatic leakage (p=0.015), and bleeding/hematoma (p=0.007) compared to patients with no events. TCC was significantly increased in patients with graft thrombosis (p=0.012), but not in patients with pancreatic leakage (p=0.32) or bleeding/hematoma (p=0.09).
In univariate logistic regression analyses the potential confounding factors, BMI, hemoglobin, and pancreas artery flow, were all associated with graft thrombosis (Table S7). However, BMI, but neither hemoglobin nor pancreas artery flow was associated with an increased risk of graft thrombosis when adjusted for the type of transplantation (SPK/PTA) (Table S7). The association between increased TCC on POD 1 with increased risk for graft thrombosis remained when adjusted for the type of transplantation (OR 1.3[1.1-1.5], p=0.029) and BMI (OR 1.5[1.0-2.2], p=0.009, Table S8).
Subgroup analysis of PTA recipients for thrombotic complication
As most thrombi were detected in PTA recipients, we performed a subgroup analysis to investigate differences between recipients with (n=14) and without graft thrombosis (n=18). The complement activation product TCC was significantly higher preoperatively (p=0.038), on POD 1 (p<0.001), and demonstrated a significant group effect (p=0.03), with overall higher concentrations in thrombotic recipients (Figure 6, Tables S9-S11). CRP was significantly higher in thrombotic recipients on POD 1 (p=0.031). TCC (OR 1.4 [95% CI 1.0-2.0], for 0.1 CAU/ml increase, p=0.029) and CRP (OR 1.4 [95% CI 1.1-1.8] for 10 mg/ml increase, p=0.021) on POD 1 were associated with an increased risk of thrombosis formation in PTA recipients (Table S12). Furthermore, IL-6 (p=0.011) was significantly elevated on POD 1, and MIP-1α (p=0.016) and IL-5 (p=0.035) demonstrated significant group effects in thrombotic recipients (Table S10, S11). Pre- and postoperative kidney function measured as creatinine and urea, and perioperative pancreas artery flow did not differ between PTA recipients with and without pancreas graft thrombosis (all p>0.1).
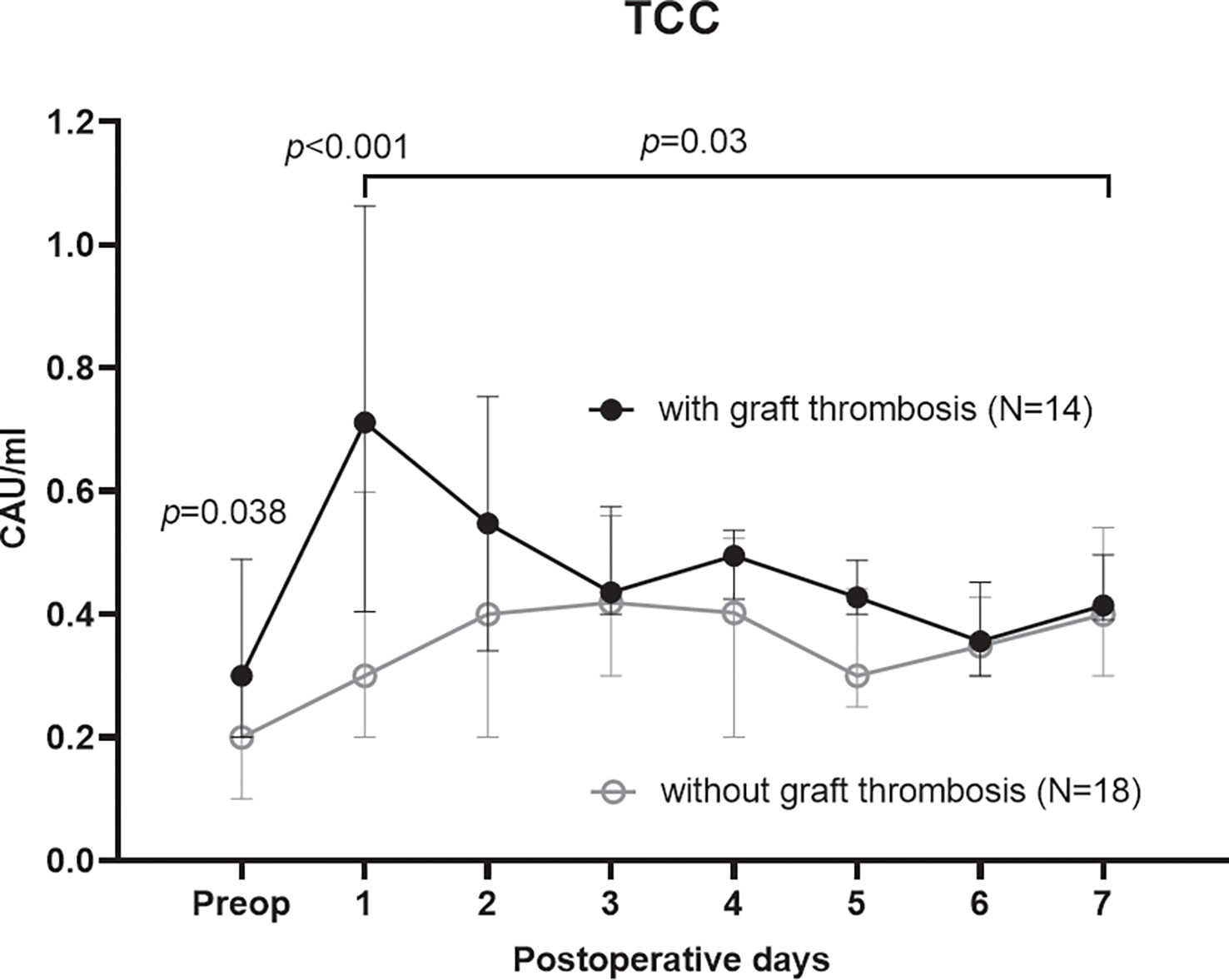
Figure 6 Terminal complement complex (TCC), as a marker of complement activation, in the subgroup analysis of PTA recipients with and without an early postoperative graft thrombosis. TCC was significantly higher in PTA patients with a graft thrombosis both preoperatively (Mann Whitney U-test), on the first postoperative day (mixed model analysis), and as a group effect over the first postoperative week (mixed model analysis).
Discussion
To our knowledge, this prospective observational study is the first to compare thromboinflammation, including complement activation, between PTA and SPK recipients. PTA recipients demonstrated an increased coagulation activation early postoperatively, and a relatively higher cytokine release, while the absolute cytokine concentrations were higher in SPK recipients both pre- and postoperatively. Notably, CRP and complement activation as detected by TCC early postoperatively, were associated with an increased risk of pancreas graft thrombosis.
Pancreas graft thrombosis is associated with early graft loss and our total incidence of 27% diagnosed graft thrombosis within the first postoperative month is comparable with previous reports including both partial and complete thrombi (23, 26, 27). Our study cohort had 48% of pancreas transplantations performed as PTA, while most centers report less than 10% (7, 28). The number of graft thrombi leading to early graft loss and the significantly lower 1-year graft survival in PTA than in SPK recipients observed in this study were comparable to internationally published data (5, 7–9, 29).
Preoperatively, TAT and cytokine concentrations were overall higher than reference values, which may be explained by the hypercoagulable state and hyperglycemia-related low-grade chronic inflammation related to diabetes (30, 31). With similar preoperative coagulation and complement activation markers in PTA and SPK recipients, a pre-existing increased thrombogenicity in PTA compared to SPK recipients appears unlikely, even if the platelet dysfunction associated with uremia could be protective for SPK recipients (32). The higher absolute cytokine concentrations found in SPK compared to PTA recipients can be caused by chronic inflammation precipitated by chronic kidney disease and hemodialysis, together with a decreased renal clearance (33, 34).
After transplantation, CRP, the coagulation and complement activation markers, and most cytokines increased in all recipients, most probably due to the ischemia-reperfusion injury (35) and the surgical trauma (36). The PTA recipients demonstrated a more prominent coagulation activation with higher TAT levels on the first postoperative day and higher relative increases of several cytokines, which could correspond to previous suggestions of a “healthier” immune system in the PTA versus the SPK recipients (6, 7). Transplantation of two organs in SPK recipients did not lead to a larger relative increase of cytokines compared to PTA patients, which might be due to uremia-mediated immune dysfunction (37). These differences in the thromboinflammatory response between SPK and PTA recipients warrants further investigations into the potential role of thromboinflammation for the outcome.
Increased postoperative CRP and TCC were the only markers investigated in our study that were associated with an increased risk of early pancreas graft thrombosis. We did not discover any associations between graft thrombosis and increased concentrations of postoperative TAT nor any of the cytokines. Thus, it remains to be proven that short-term TAT increases result in an increased risk of thrombosis in non-cancer patients (38). Cytokines have been reported to regulate local formation and resolution of thrombosis (22) and closer monitoring of cytokines near the graft might thus be necessary to prove this association in pancreas transplantation. CRP has previously been associated with thrombosis formation and an increased risk of thromboembolism in other settings (39–41), but is a non-specific marker that increases with several complications. Our results correspondingly demonstrated increased CRP with graft thrombosis, pancreatic leakage, and bleeding during the first postoperative week, while TCC only increased with graft thrombosis, indicating that complement activation is more specific for graft thrombosis compared to CRP early postoperatively. Complement activation has previously been associated with graft thrombosis in liver transplantation (42), and venous thromboembolism in the general population (43). The association between complement activation and graft thrombosis supports the concept of complement-coagulation cascade cross-talk, but whether the increased complement activation contributes to thrombosis formation, is caused by thrombosis formation or both cannot be answered by our observational study design (11, 17, 19). However, the notion that PTA recipients with graft thrombosis had already pretransplant increased TCC concentrations and that early postoperative increase in TCC was higher in patients with graft thrombosis at any time-point indicate that TCC might be considered as a risk factor for graft thrombosis.
Through the subgroup analysis of PTA recipients, we eliminated potential confounders like uremia-induced inflammation and anticoagulatory effects, which occur more frequently in SPK recipients. Here, we found significantly higher both pre- and postoperative complement activation and elevated postoperative cytokines (IL-6, IL-1ra, and MIP-1β) in PTA recipients with versus without early graft thrombosis. This pro-inflammatory pattern may indicate an amplified thromboinflammation in patients experiencing graft thrombosis. However, we cannot conclude if this pattern is causative or caused by venous thrombosis, but it supports the notion that inflammation plays a central role in venous thrombosis (44).
Current immunosuppressive therapies used in transplantation are mainly designed to affect the adaptive and not the innate immune response and thus lead to reduced production of pro- and anti-inflammatory cytokines, but do not inhibit the secretion of readily produced cytokines nor complement activation (45, 46). Complement inhibition has been suggested as a therapeutic option for ischemia-reperfusion injury in transplantation (47). Treatment with eculizumab, a complement blocker, that blocks cleavage of C5 and thus prevents C5a and C5b-9 generation, has reduced thrombosis rates in patients with paroxysmal nocturnal hematuria (48) and atypical hemolytic uremic syndrome (49). These conditions are chronic and require life-long treatment, while the transplantation setting represents a more temporary imbalance in complement system regulation. Presumably, a short complement inhibition may therefore reduce ischemia/reperfusion injury induced inflammation and risk of thrombosis.
This study has several limitations. Relatively few patients were included, especially for the subgroup analyses, which affects the power. On the other hand, this study included a high proportion of PTA recipients, and allowed for a comparison in the thromboinflammatory response between SPK and PTA recipients, which to our knowledge has not previously been performed. This is a single center-study with well-known limitations as to the generalizability of the results, but with the advantage that similar conditions for surgical technique, treatment, and follow-up were applied to all patients. We acknowledge that the graft thrombosis rate in this study is high. This most likely reflects the postoperative imaging protocol leading to an increased detection of partial graft thrombi that otherwise would have gone unnoticed. However, it enabled us to correlate inflammatory markers to any thrombotic event, rather than limiting the study to only include occluding thrombi.
We have some potential confounding factors. Anticoagulation was intensified during the study period, which may have affected thromboinflammation (50–52). However, to what extent is uncertain, and we demonstrated similar trends in inflammatory response between PTA and SPK recipients both before and after the anticoagulation change. Relaparotomy could increase thromboinflammation. However, no patients with early graft thrombosis underwent relaparotomy. The PTA and SPK populations differed pre- and perioperatively, which might have affected the postoperative outcome. PTA recipients had higher BMI and lower pancreas artery flow, factors that are putative risk factors for pancreas graft thrombosis (10). Indeed, BMI was identified as a risk factor for graft thrombosis. However, TCC concentrations on the first postoperative day remained a contributing risk factor when adjusted for BMI highlighting the importance of thromboinflammation. In addition, the higher hemoglobin concentration and lower pancreas artery flow observed in PTA recipients could increase the risk of thrombosis (53) but did not seem to be a contributing factor in our cohort when adjusted for the effect of transplantation recipient type (SPK/PTA). The study is descriptive and the results and their relevance for clinical outcomes need to be confirmed by further studies.
Conclusion
Postoperatively, we found a relatively higher increase in the thromboinflammatory response in PTA recipients and higher absolute cytokine concentrations in SPK recipients.
CRP-increase and complement activation were associated with an increased risk of graft thrombosis in the early postoperative phase. TCC was more specific for graft thrombosis compared to CRP. Based on these findings, we suggest that the thromboinflammatory response in general, and complement activation, in particular, could be considered as contributing or as synergetic risk factors for early pancreas graft thrombosis.
Data availability statement
The full datasets generated and analyzed in the current study are not publicly available due to Norwegian National Legislation prohibiting the publishing of information that could compromise research participant privacy. The collected data are of such nature that it falls under the provision of the Norwegian Health Research Act. However, an anonymized dataset of the inflammatory markers are available upon request to the corresponding authors. Additional data are available from Oslo University hospital’s data protection officer for research upon reasonable request. Researchers who meet the criteria for access to confidential data should make contact viacGVyc29udmVybkBvdXMtaGYubm8=to receive access. Requests to access the datasets should be directed to Tor Åsmund Martinsen, cGVyc29udmVybkBvdXMtaGYubm8=.
Ethics statement
The studies involving human participants were reviewed and approved by South-Eastern Norwegian ethical committee. The patients/participants provided their written informed consent to participate in this study.
Author contributions
All authors contributed substantially and followed the guidelines of the International Committee of Medical Journal Editors. KR, TM, HH, and SP participated in the study conception and research design, statistical analysis and interpretation and writing of the article. KR, GK, and HH collected the data. JK and TM performed the analyses. GK, RH, JK, TJ, P-DL, and TT reviewed and edited the manuscript. KR, SP, and HH participated in visualization. SP, HH, TT, and TM participated in supervision. HH, SP, TT, and TM provided resources. All authors were responsible for final approval and accountability for all aspects of the work. All authors contributed to the article and approved the submitted version.
Funding
This work was supported by a PhD grant from the South Eastern Norwegian Health Government (HSØ 2016028).
Acknowledgments
The authors would like to thank Researcher (Statistician); Ph.D., Are Hugo Pripp for statistical advice, Data Scientist; Ph.D., Mattias Rydenfelt for support with R, MD; senior consultant in radiology; Ph.D., Audun Elnaes Berstad for grading the severity of pancreas allograft thromboses, and senior engineer Camilla Schjalm at the Department of Immunology for help with handling the plasma samples and for giving insight into the practical work with the analyses.
Conflict of interest
The authors declare that the research was conducted in the absence of any commercial or financial relationships that could be construed as a potential conflict of interest.
Publisher’s note
All claims expressed in this article are solely those of the authors and do not necessarily represent those of their affiliated organizations, or those of the publisher, the editors and the reviewers. Any product that may be evaluated in this article, or claim that may be made by its manufacturer, is not guaranteed or endorsed by the publisher.
Supplementary material
The Supplementary Material for this article can be found online at: https://www.frontiersin.org/articles/10.3389/fimmu.2023.1044444/full#supplementary-material
Glossary
References
1. Dholakia S, Oskrochi Y, Easton G, Papalois V. Advances in pancreas transplantation. J R Soc Med (2016) 109(4):141–6. doi: 10.1177/0141076816636369
2. Knight S, Vogel T, Friend P. Pancreas transplantation. Surg (Oxford) (2020) 38(7):418–24. doi: 10.1016/j.mpsur.2020.04.007
3. Lindahl JP, Horneland R, Nordheim E, Hartmann A, Aandahl EM, Grzyb K, et al. Outcomes in pancreas transplantation with exocrine drainage through a duodenoduodenostomy versus duodenojejunostomy. Am J Transplant (2018) 18:154–62. doi: 10.1111/ajt.14420
4. Gruessner AC, Gruessner RW. Long-term outcome after pancreas transplantation: A registry analysis. Curr Opin Organ Transplant (2016) 21(4):377–85. doi: 10.1097/MOT.0000000000000331
5. Gruessner AC, Gruessner RWG. The current state of pancreas transplantation in the USA–a registry report. Curr Transplant Rep (2018) 5(4):304–14. doi: 10.1007/s40472-018-0213-x
6. Gruessner RW, Gruessner AC. Pancreas transplant alone: A procedure coming of age. Diabetes Care (2013) 36(8):2440–7. doi: 10.2337/dc12-2195
7. Niederhaus SV. Pancreas transplant alone. Curr Opin Organ Transplant (2015) 20(1):115–20. doi: 10.1097/MOT.0000000000000157
8. Ramessur Chandran S, Kanellis J, Polkinghorne KR, Saunder AC, Mulley WR. Early pancreas allograft thrombosis. Clin Transplant (2013) 27(3):410–6. doi: 10.1111/ctr.12105
9. Farney AC, Rogers J, Stratta RJ. Pancreas graft thrombosis: Causes, prevention, diagnosis, and intervention. Curr Opin Organ Transplant (2012) 17(1):87–92. doi: 10.1097/MOT.0b013e32834ee717
10. Blundell J, Shahrestani S, Lendzion R, Pleass HJ, Hawthorne WJ. Risk factors for early pancreatic allograft thrombosis following simultaneous pancreas-kidney transplantation: A systematic review. Clin Appl Thromb Hemost (2020) 26:1–14. doi: 10.1177/1076029620942589
11. Ekdahl KN, Teramura Y, Hamad OA, Asif S, Duehrkop C, Fromell K, et al. Dangerous liaisons: Complement, coagulation, and Kallikrein/Kinin cross-talk act as a linchpin in the events leading to thromboinflammation. Immunol Rev (2016) 274(1):245–69. doi: 10.1111/imr.12471
12. Wullstein C, Drognitz O, Woeste G, Schareck WD, Bechstein WO, Hopt UT, et al. High levels of c-reactive protein after simultaneous pancreas-kidney transplantation predict pancreas graft-related complications and graft survival. Transplantation (2004) 77(1):60–4. doi: 10.1097/01.TP.0000100683.92689.27
13. Lippi G, Cervellin G, Franchini M, Favaloro EJ. Biochemical markers for the diagnosis of venous thromboembolism: The past, present and future. J Thromb Thrombolysis (2010) 30(4):459–71. doi: 10.1007/s11239-010-0460-x
14. Harboe M, Thorgersen EB, Mollnes TE. Advances in assay of complement function and activation. Advanced Drug Del Rev (2011) 63(12):976–87. doi: 10.1016/j.addr.2011.05.010
15. Dzik S. Complement and coagulation: Cross talk through time. Transfus Med Rev (2019) 33(4):199–206. doi: 10.1016/j.tmrv.2019.08.004
16. Oikonomopoulou K, Oikonomopoulou K, Ricklin D, Ricklin D, Ward PA, Ward PA, et al. Interactions between coagulation and complement–their role in inflammation. Semin Immunopathol (2012) 34(1):151–65. doi: 10.1007/s00281-011-0280-x
17. Markiewski MM, Nilsson B, Nilsson Ekdahl K, Mollnes TE, Lambris JD. Complement and coagulation: Strangers or partners in crime? Trends Immunol (2007) 28(4):184–92. doi: 10.1016/j.it.2007.02.006
18. Eriksson O, Mohlin C, Nilsson B, Ekdahl KN. The human platelet as an innate immune cell: Interactions between activated platelets and the complement system. Front Immunol (2019) 10:1590(1590). doi: 10.3389/fimmu.2019.01590
19. Rawish E, Sauter M, Sauter R, Nording H, Langer HF. Complement, inflammation and thrombosis. Br J Pharmacol (2021) 178(14):2892–904. doi: 10.1111/bph.15476
20. Saghazadeh A, Hafizi S, Rezaei N. Inflammation in venous thromboembolism: Cause or consequence? Int Immunopharmacol (2015) 28(1):655–65. doi: 10.1016/j.intimp.2015.07.044
21. Hsing C-H, Wang J-J. Clinical implication of perioperative inflammatory cytokine alteration. Acta Anaesthesiol Taiwan (2015) 53(1):23–8. doi: 10.1016/j.aat.2015.03.002
22. Najem MY, Couturaud F, Lemarié CA. Cytokine and chemokine regulation of venous thromboembolism. J Thromb Haemost (2020) 18(5):1009–19. doi: 10.1111/jth.14759
23. Hakeem A, Chen J, Iype S, Clatworthy MR, Watson CJE, Godfrey EM, et al. Pancreatic allograft thrombosis: Suggestion for a ct grading system and management algorithm. Am J Transplant (2018) 18(1):163–79. doi: 10.1111/ajt.14433
24. Bergseth G, Ludviksen JK, Kirschfink M, Giclas PC, Nilsson B, Mollnes TE. An international serum standard for application in assays to detect human complement activation products. Mol Immunol (2013) 56(3):232–9. doi: 10.1016/j.molimm.2013.05.221
25. Hennø LT, Storjord E, Christiansen D, Bergseth G, Ludviksen JK, Fure H, et al. Effect of the anticoagulant, storage time and temperature of blood samples on the concentrations of 27 multiplex assayed cytokines – consequences for defining reference values in healthy humans. Cytokine (2017) 97:86–95. doi: 10.1016/j.cyto.2017.05.014
26. Harbell JW, Morgan T, Feldstein VA, Roll GR, Posselt A, Kang SM, et al. Splenic vein thrombosis following pancreas transplantation: Identification of factors that support conservative management. Am J Transplant (2017) 17(11):2955–62. doi: 10.1111/ajt.14428
27. Kopp WH, van Leeuwen CAT, Lam HD, Huurman VAL, de Fijter JW, Schaapherder AF, et al. Retrospective study on detection, treatment, and clinical outcome of graft thrombosis following pancreas transplantation. Transpl Int (2019) 32(4):410–7. doi: 10.1111/tri.13384
28. Gruessner AC, Gruessner RWG. Pancreas transplantation of us and non-us cases from 2005 to 2014 as reported to the united network for organ sharing (Unos) and the international pancreas transplant registry (Iptr). Rev Diabetes Stud (2016) 13(1):35–58. doi: 10.1900/RDS.2016.13.35
29. Durlik M, Baumgart-Gryn K. Almost 200 pancreas transplantations: A single-center experience. Transplant Proc (2018) 50(7):2124–7. doi: 10.1016/j.transproceed.2018.02.097
30. Carr ME. Diabetes mellitus: A hypercoagulable state. J Diabetes Complications (2001) 15(1):44–54. doi: 10.1016/s1056-8727(00)00132-x
31. Chatzigeorgiou A, Harokopos V, Mylona-Karagianni C, Tsouvalas E, Aidinis V, Kamper EF. The pattern of Inflammatory/Anti-inflammatory cytokines and chemokines in type 1 diabetic patients over time. Ann Med (2010) 42(6):426–38. doi: 10.3109/07853890.2010.495951
32. Boccardo P, Remuzzi G, Galbusera M. Platelet dysfunction in renal failure. Semin Thromb Hemost (2004) 30(5):579–89. doi: 10.1055/s-2004-835678
33. Silva RE, Santos EC, Justino PBI, Santos MP, Galdino G, Goncalves RV, et al. Cytokines and chemokines systemic levels are related to dialysis adequacy and creatinine clearance in patients with end-stage renal disease undergoing hemodialysis. Int Immunopharmacol (2021) 100:108154. doi: 10.1016/j.intimp.2021.108154
34. Akchurin OM, Kaskel F. Update on inflammation in chronic kidney disease. Blood Purif (2015) 39(1-3):84–92. doi: 10.1159/000368940
35. Eltzschig HK, Eckle T. Ischemia and reperfusion–from mechanism to translation. Nat Med (2011) 17(11):1391–401. doi: 10.1038/nm.2507
36. Shankar Hari M, Summers C. Major surgery and the immune system: From pathophysiology to treatment. Curr Opin Crit Care (2018) 24(6):588–93. doi: 10.1097/MCC.0000000000000561
37. Cohen G. Immune dysfunction in uremia 2020. Toxins (2020) 12(7):439. doi: 10.3390/toxins12070439
38. Lundbech M, Krag AE, Christensen TD, Hvas A-M. Thrombin generation, thrombin-antithrombin complex, and prothrombin fragment F1+2 as biomarkers for hypercoagulability in cancer patients. Thromb Res (2020) 186:80–5. doi: 10.1016/j.thromres.2019.12.018
39. Grimnes G, Isaksen T, Tichelaar YIGV, Brox J, Brækkan SK, Hansen J-B. C-reactive protein and risk of venous thromboembolism: Results from a population-based case-crossover study. Haematologica (2018) 103(7):1245–50. doi: 10.3324/haematol.2017.186957
40. Fay WP. Linking inflammation and thrombosis: Role of c-reactive protein. World J Cardiol (2010) 2(11):365. doi: 10.4330/wjc.v2.i11.365
41. Park D-W, Yun S-C, Park S-J, Lee J-Y, Kim W-J, Kang S-J, et al. C-reactive protein and the risk of stent thrombosis and cardiovascular events after drug-eluting stent implantation. Circulation (2009) 120(20):1987–95. doi: 10.1161/CIRCULATIONAHA.109.876763
42. Haugaa H, Thorgersen EB, Pharo A, Boberg KM, Foss A, Line PD, et al. Inflammatory markers sampled by microdialysis catheters distinguish rejection from ischemia in liver grafts. Liver Transpl (2012) 18(12):1421–9. doi: 10.1002/lt.23503
43. Høiland II, Liang RA, Brækkan SK, Pettersen K, Ludviksen JK, Latysheva N, et al. Complement activation assessed by the plasma terminal complement complex and future risk of venous thromboembolism. J Thromb Haemost (2019) 17(6):934–43. doi: 10.1111/jth.14438
44. Branchford BR, Carpenter SL. The role of inflammation in venous thromboembolism. Front Pediatr (2018) 6:142. doi: 10.3389/fped.2018.00142
45. Zaza G, Leventhal J, Signorini L, Gambaro G, Cravedi P. Effects of antirejection drugs on innate immune cells after kidney transplantation. Front Immunol (2019) 10:2978. doi: 10.3389/fimmu.2019.02978
46. Grafals M, Thurman JM. The role of complement in organ transplantation. Front Immunol (2019) 10:2380. doi: 10.3389/fimmu.2019.02380
47. Garred P, Tenner AJ, Mollnes TE. Therapeutic targeting of the complement system: From rare diseases to pandemics. Pharmacol Rev (2021) 73(2):792–827. doi: 10.1124/pharmrev.120.000072
48. Hillmen P, Muus P, Dührsen U, Risitano AM, Schubert J, Luzzatto L, et al. Effect of the complement inhibitor eculizumab on thromboembolism in patients with paroxysmal nocturnal hemoglobinuria. Blood (2007) 110(12):4123–8. doi: 10.1182/blood-2007-06-095646
49. Cofiell R, Kukreja A, Bedard K, Yan Y, Mickle AP, Ogawa M, et al. Eculizumab reduces complement activation, inflammation, endothelial damage, thrombosis, and renal injury markers in ahus. Blood (2015) 125(21):3253–62. doi: 10.1182/blood-2014-09-600411
50. Innes A, Tingle S, Ibrahim I, Thompson E, Bates L, Manas D, et al. Use of dextran 40 after pancreas transplant may reduce early inflammation and significant bleeding compared to a heparin-based protocol. Transplant Proc (2021) 53(2):712–5. doi: 10.1016/j.transproceed.2020.10.020
51. Yu H, Munoz E, Edens R, Linhardt R. Kinetic studies on the interactions of heparin and complement proteins using surface plasmon resonance. Biochim Biophys Acta (BBA) - Gen Subj (2005) 1726(2):168–76. doi: 10.1016/j.bbagen.2005.08.003
52. Litov L, Petkov P, Rangelov M, Ilieva N, Lilkova E, Todorova N, et al. Molecular mechanism of the anti-inflammatory action of heparin. Int J Mol Sci (2021) 22(19):10730. doi: 10.3390/ijms221910730
Keywords: thromboinflammation, pancreas transplant alone, simultaneous pancreas-kidney transplantation, pancreas graft thrombosis, complement, cytokines, coagulation
Citation: Rydenfelt K, Kjøsen G, Horneland R, Ludviksen J K, Jenssen TG, Line P-D, Tønnessen TI, Mollnes TE, Haugaa H and Pischke SE (2023) Thromboinflammatory response is increased in pancreas transplant alone versus simultaneous pancreas-kidney transplantation and early pancreas graft thrombosis is associated with complement activation. Front. Immunol. 14:1044444. doi: 10.3389/fimmu.2023.1044444
Received: 14 September 2022; Accepted: 14 March 2023;
Published: 29 March 2023.
Edited by:
Christian Morath, Heidelberg University, GermanyReviewed by:
Cheng Yang, Fudan University, ChinaMohammad Golriz, Heidelberg University Hospital, Germany
Palmina Petruzzo, University of Cagliari, Italy
Copyright © 2023 Rydenfelt, Kjøsen, Horneland, Ludviksen, Jenssen, Line, Tønnessen, Mollnes, Haugaa and Pischke. This is an open-access article distributed under the terms of the Creative Commons Attribution License (CC BY). The use, distribution or reproduction in other forums is permitted, provided the original author(s) and the copyright owner(s) are credited and that the original publication in this journal is cited, in accordance with accepted academic practice. No use, distribution or reproduction is permitted which does not comply with these terms.
*Correspondence: Kristina Rydenfelt, a3JpcnlkQG91cy1oZi5ubw==; Søren Erik Pischke, cy5lLnBpc2Noa2VAbWVkaXNpbi51aW8ubm8=