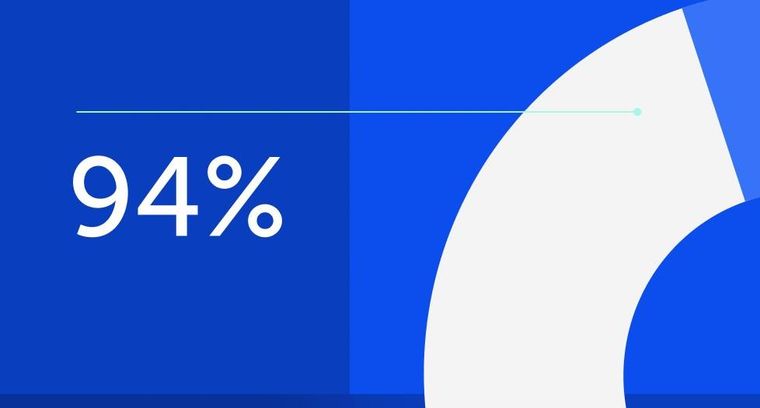
94% of researchers rate our articles as excellent or good
Learn more about the work of our research integrity team to safeguard the quality of each article we publish.
Find out more
ORIGINAL RESEARCH article
Front. Immunol., 11 January 2023
Sec. Nutritional Immunology
Volume 13 - 2022 | https://doi.org/10.3389/fimmu.2022.994706
This article is part of the Research TopicThe Nutritional Immunological Effects and Mechanisms of Chemical Constituents from the Homology of Medicine and FoodView all 11 articles
The isolation of Stropharia rugosoannulata polysaccharide (SRP) by three-phase extraction was optimized, and its structure and biological activities were identified. The optimal extraction conditions were: mass fraction of ammonium sulfate, 20%; volume ratio of sample solution to t-butanol, 1:1.5; extraction temperature, 35°C. Under these conditions, the yield of SRP was 6.85% ± 0.13%. SRP was found to be composed of glucose (35.79%), galactose (26.80%), glucuronic acid (9.92%), fructose (8.65%), xylose (7.92%), fucose (4.19%), arabinose (3.46%) and rhamnose (3.26%), with the molecular weight of 27.52 kDa. The results of DPPH, hydroxyl, ABTS+ radical scavenging and reducing power tests showed that SRP had good antioxidant capacities. SRP had no cytotoxic effect on RAW264.7 macrophages at the concentrations of 25-200 μg/mL, and could significantly promote phagocytosis activity and cell migration according to CCK-8 assay, phagocytosis assay and cell scratch experiment. SRP can significantly stimulate the transcript expression levels of TNF-α, IL-1β and IL-6, as determined by RT-PCR and Western blot assays. SRP activated the TLR4/NF-κB signaling pathway, and autophagy also occurred. These results suggest that SRP is a safe antioxidant and immunomodulator, and that it can be used in the development of functional foods and/or pharmaceuticals.
Stropharia rugosoannulata Farlow (SR) is a mushroom, also known as wine cap stropharia. It is one of the mushrooms recommended by the Food and Agriculture Organization of the United Nations (FAO) for cultivating, so it is now grown in many developing countries (1). The fruiting body of SR has been reported to include multiple beneficial components, such as flavonoids, vitamins, polysaccharides, and protein. Thus it has potential medicinal value (2).
Fungal polysaccharides have been isolated from fruiting bodies or from fungal mycelia in fermentation broth. Researches have demonstrated that fungal polysaccharides have anti-virus (3), anti-tumor (4), immunomodulatory and anti-aging activities (5). Many have already been developed into a variety of drugs and functional food additives (6). Recently, fungal polysaccharides and their complexes have attracted more attention. S. rugosoannulata polysaccharide is one of many fungal polysaccharides and its medicinal value has been gradually recognized. Many studies have shown that polysaccharides from mushrooms have immunomodulatory activity and are good natural immunomodulatory adjuvants (3, 5).
The method of extracting polysaccharides from fungal mycelia affects not only yield but also the structure and biological activities of the polysaccharides extracted. At present, the conventional extraction method is water extraction followed by ethanol precipitation (7). This method has the shortcomings of long time and low efficiency. Three-phase extraction is a new method to extract active ingredients. It has the advantages of high efficiency and environmental sustainability. Recently, this method has been applied to extract polysaccharides from animal material (e.g., shrimp shell (8); Corbicula fluminea (9)), plant material (e.g., aloe (10)), and microorganisms. However, there are few reports of using it to isolate polysaccharides from edible or medicinal fungi.
In this research, the polysaccharides of S. rugosoannulata were extracted by three-phase extraction. Physicochemical properties such as molecular weight and monosaccharide composition were determined. Scanning electron microscopy (SEM) was used for morphological studies. Antioxidant and immunomodulatory properties were evaluated comprehensively through in vitro antioxidant experiments and cell models. The results provide information for the development of SRP as a functional component in foods or medicine.
S. rugosoannulata was collected from a field in Yucheng County, Henan Province, China. Trypsin and RPMI1640 were purchased from Gibco (Grand Island, NY, United States). The Cell counting kit-8 (CCK-8) was purchased from Han Heng Biotechnology Co., Ltd. (Shanghai, China). Lipopolysaccharide (LPS) was purchased from Sigma Chemical Co. (St. Louis, MO, USA). The remaining chemicals or reagents were all of laboratory or analytical quality.
Referring to the method of Yan (9), we made minor modifications. The fruiting body of SR was dried at 45°C in the air-drying box and then ground into powder with a grinder. Then, 95% ethanol (1:200, g/mL) was refluenced 5 hours, repeated 3 times, which was designed to skim and decolorize. Distilled water was added according to the ratio of 1:30 (w/v), and extracted by stirring in a water bath at 100°C for 2 hours. After continuous extraction for two times, the filtrate was merged for two times and concentrated. Added 15-35% (NH4)2SO4 (w/v) to SR powder and vortexed gently. The suspension was then diluted with t-butanol at a ratio of 1.5:1 to 1:2.5 (v/v). Kept the combination at 20-40°C for an hour. Then, the mixture was placed in a centrifuge at 4000 RPM/separation center for 10 minutes to accelerate three-phase extraction. After centrifugation, the three phases formed were carefully separated. The lower phase is mainly ammonium sulfate and polysaccharides, and polysaccharides were freeze-dried after removing inorganic salts by molecular dialysis (3500 Da).
The basic components of carbohydrate, protein and polyphenol were detected according to our previous methods (11, 12).
Gel permeability chromatography (GPC) was used to determine the molecular weight distribution of SRP using a Sugar KS805 column fitted with an Agilent refractive index detector.
Using high-performance anion exchange chromatography (HPAEC) coupled with a pulse ammeter detector, we determined the monosaccharide composition of SRP. Gas chromatography (GC) was used to determine the monosaccharide composition of SRP following the procedure described by Yang (13). 5 mg of SRP was dissolved in 1mL 2.5 M trifluoroacetic, and was mixed by the vortex. The sample was placed in the oven for 1.5 hours at 121°C, during which the sample was vortexed every 30 minutes. Once the SRP were hydrolyzed, they were diluted, filtered, and injected into an HPAEC system (Dionex, ICS-5000+, USA) using an ASAP automatic sampler and a calcium carbonate PA-20 column (3×150 mm, Dionex). The monosaccharides in SRP were determined by eight different monosaccharides.
Empyrean X-ray diffractometer (panalit LTD., Netherlands) was used to record the diffraction patterns of SRP at 40kV and 15 mA. The scanning speed was 3°/min, the step size was 0.01, and the 2 range was 2 to 40°.
An SEM Model S-4800 II FESEM (Hitachi, Japan) was used to study the molecular morphologies of SRP. A layer of gold foil was laid on the sample and then placed on the substrate. Under a high vacuum and 10.0 kV voltage, the image was observed by a magnification of 100× or 500× fold.
The DPPH radicals scavenging activity of SRP was determined according to the method of Wang et al. (11). 500 μL different concentrations of SRP (0.05, 0.1, 0.5, 1, 2, 4, 6, 8, 10 mg/mL) were mixed with the newly configured 2-diphenyl-1-picrylhydrazyl (DPPH) solution (dissolved in ethanol) in equal volume, and the reaction solution was left in a dark place at 26°C for half an hour. The blank group consisted of the same volume of distilled water as the sample solution, and the positive control had the same concentration of Vc. The absorbance of the reaction mixture was determined at 517 nm. Scavenging activity (percent) against DPPH was calculated by using the following equation:
Where A0 was the absorbance of the blank control; AX was the absorbance of the sample solution; AX0 was the background absorbance of the sample solution.
Added 400 μL FeSO4 solution to 400 μL different concentrations of SRP (0.05, 0.1, 0.5, 1, 2, 4, 6, 8, 10 mg/mL). After adding 800 μL of H2O2 solution, the reaction was started and the solution was left to react at 37°C for 30 minutes. The blank group consisted of the same amount of distilled water that was used to replace the sample solution as the positive control had the same concentration of Vc. The 510 nm wavelength of the reaction solution was measured. To determine the hydroxyl scavenging capacity (in percent), the following equation was used:
Where A0 was the absorbance of the blank control; AX was the absorbance of the sample solution; AX0 was the background absorbance of the sample solution.
ABTS+ radicals removing capacity of SRP was according to the work of Qiu et al. (14). The ABTS+ working solution was made by reacting 7 mmol/L of ABTS+ solution with 2.45 mmol/L of K2S2O8 solution at 26°C for 16 hours in the dark. When using, adjust the absorbance of the ABTS+ working solution at 734 nm. Then, took 100 μL different concentrations of SRP (0.05, 0.5, 0.1, 0.5, 1, 2, 4, 6, 8, 10 mg/mL), added 0.6 mL ABTS+ solution, mixed evenly and placed at 26°C for 10 minutes. The same concentration of Vc solution as the positive group. The reaction solution’s absorbance at 734 nm was measured. Using these formulae, we were able to determine the ABTS+ scavenging efficiency (in percentage):
Where A0 was the absorbance of the blank control; AX was the absorbance of the sample solution; AX0 was the background absorbance of the sample solution.
The method of Gao et al. (15) was used for sample reduction force detection, and some modifications were made. In brief, 200 μL different concentrations of SRP (0.05, 0.1, 0.5, 1, 2, 4, 6, 8, 10 mg/mL) were taken and 500 μL PBS and 100 μL 1% (w/v) iron oxide clock solution was added, then the reaction system was incubated in 50°C for 20 minutes. After quick cooling, it was combined with a 500 μL 10 percent (w/v) trichloroacetic acid solution, and then centrifuged. Next, took 500 μL supernatant, added 500 μL distilled water and 100 μL 0.1% (w/v) FeCl3 solution successively, then mixed them evenly at 26°C. The absorbance of the reaction solution was measured at 700 nm and a standard solution with the same quantity of Vc was employed as the positive control. The sample’s reducing power is proportional to its absorbance value, which measures the reaction system’s efficiency.
RAW 264.7 macrophages were grown in an incubator with 5% CO2 at 37°C in RPMI1640 media supplemented with 10% fetal bovine serum, 100 U/mL penicillin, and 100 g/mL streptomycin. Every 2-3 days, new media will be added.
The cell viability of SRP was detected by the CCK-8 method (16). RAW 264.7 macrophages were placed onto a 96-well plate with 100 μL/well cell suspension and cultured in the cell incubator for 24 hours. 10 μL varying quantities of SRP (25, 50, 100, 200, 400, 600, 800 μg/mL) and LPS (1 μg/mL) were set as normal group and posititive group, each group was set up with 4 multiple wells, and blank medium group was put in a 37°C, 5 percent CO2 cell incubator for a length of time. Then, CCK-8 with 1/10 volume of cell culture media was added to it, and the absorbance of the reaction solution was measured at 450 nm after incubation in a constant temperature incubator.
When the confluence of RAW264.7 macrophages reached 80%, the medium was used to dilute the cell density to 1×104 cells/mL. A 96-well plate containing a cell suspension of RAW 264.7 macrophages at a concentration of 100 μL/well was placed in a cell incubator for 24 hours. 100 μL varied doses of SRP (25, 50, 100, 200 μg/mL) and LPS (1 μg/mL) were added and cultured in a cell incubator for 24 hours, adding 100 μL 0.1 percent of the neutral red toμ each well, and the final concentration of neutral red in each well is 1 μg/mL. After washing with PBS, 100 μL cell lysis solution was added to each well and gently shaken for 15 seconds, then left the plate at 37°C for 2 hours. The absorbance of the reaction solution was measured at 540 nm.
Cell scratch experiment was detected and the method was according to the report of Zubair et al. (17). RAW264.7 macrophages were seeded at a density of 1 ×106 cells per well and cultured in a 6-well plate for 24 hours before being scratched with the tip of a 200 μL pistol to ensure even damage. Then washing with PBS for 3 times to remove the scratched cells, and the medium with different concentrations of SRP (25, 50, 100, 200 μg/mL) and LPS (1 μg/mL) were added and incubated for 24 hours. The medium without drugs was used as the blank control group. The samples were taken and the migration of cells at the same location was recorded.
Table 1 displayed primer sequences that were developed using real-time PCR primer design concepts. Total RNA was isolated using the Trizol procedure, and its concentration and quality were evaluated using a Nanodrop 8000. Then, the obtained RNA were used as templates, cDNA were synthesized by reverse transcription using qPCR (+gDNA) of HiScriptIII RT SuperMix. The fluorescence quantitative reaction system was prepared according to Taq Pro Universal SYBR qPCR Master Mix. After the reaction, the relative gene expression changes were recorded by the 2-ΔΔCT method.
Total cell protein was isolated using a protein lysate. Protein was quantified using a kit (Bicinchoninic acid, BCA method), transferred to a PVDF membrane using SDS-PAGE gel electrophoresis, blocked in skim milk for 2 hours, and then incubated at 4°C overnight with a diluted primary antibody. The next day, we washed away the residual primary antibody with TBST solution, added the appropriate secondary antibody, and incubated everything at 26°C for 2 hours. After three washes in TBST buffer solution, any lingering secondary antibodies were removed, and an ECL luminous reagent was poured into an automated chemiluminescence imager. Image J was used to save and examine the data.
SPSS 21.0 was used to conduct the statistical analysis, and one-way analysis of variance (ANOVA) was employed for the multiple comparisons. In this study, significant differences were defined as those with a probability level of less than 0.05 (represented as “mean ± standard deviation”).
As shown in Figure 1A, as the concentration of ammonium sulfate rose from 15%-35%, the yield of SRP increased first and then decreased. When the mass fraction of ammonium sulfate was 20%, the yield reached the maximum value of 7.20%. The extraction yield of polysaccharides was diminished because of the high salt concentration’s potential to disrupt the hydrogen-bond network between SRP and water molecules. Similar to our study, Wang et al. also showed that the extraction efficiency of rice bran polysaccharides decreased with the increase of the concentration of ammonium sulfate (18).
Figure 1 Effect of different factors on SRP yield. (A) Concentration of ammonium sulphate; (B) Slurry to t-butanol ratio; (C) Temperature.
As shown in Figure 1B, the yield of SRP increased gradually with the increasing proportion of t-butanol volume up to a certain point; this was presumably due to increased interaction between increased t-butanol and ammonium sulfate (19). When the ratio of sample solution to t-butanol was 1:1.5 (v/v), the yield reached the maximum value of 7.0%. With further increase of t-butanol, however, the yield of SRP was decreased, thus reducing the overall extraction yield of SRP. This reduction may be due to a lack of sufficient water to hydrate sulfate ions adequately (11).
As shown in Figure 1C, the yield of SRP increased gradually with the increase of extraction temperature from 20-30°C; above 30°C, the yield of SRP showed a downward trend. This may be because high temperature accelerated the thermal movement of molecules in the three-phase extraction system, and thus enhanced the hydrophilicity of polysaccharides (20). Therefore, the yield of SRP reached its highest value at the tested temperature, 30°C.
Using the single factor investigation results, an orthogonal test was used to optimize the three extraction parameters of mass fraction of ammonium sulfate, t-butanol volume ratio and extraction temperature, and three levels were set for each factor (Table 2). Without considering interaction, an L9(33) orthogonal experiment was conducted to optimize the extraction.
As can be seen from the R-value in the Table 3, the influence of these three parameters on the yield of polysaccharides is as follows: B (t-butanol volume ratio) > C (extraction temperature) > A (ammonium sulfate mass fraction). On this basis, the optimum extraction conditions were determined as follows: mass fraction of ammonium sulfate, 20%; volume ratio of sample solution to t-butanol, 1:1.5; extraction temperature, 35°C.
As shown in Table 4, the extraction yield of SRP was 6.85%, and the carbohydrate content of SRP was 56.18%. The protein content was 6.78%, and the polyphenol content was 1.73%. The XRD pattern showed a small diffraction peak at 20°, which indicated that SRP has an amorphous structure. Its crystallinity was 18.21%. The crystal structure is directly determined by properties such as elasticity and swelling (21).
Molecular weight is an essential physicochemical parameter closely related to the biological activity of polysaccharides. The weight average molecular weight (Mw) of SRP was 27.52 kDa, and its number average molecular weight (Mn) of SRP was 26.07 kDa, which was similar to Chen (22). The polymer dispersion index Mw/Mn of SRP was 1.06, and the closer the dispersion index was to 1, the more evenly the polysaccharide was distributed.
Monosaccharide composition results (Table 5) showed that the SRP sample was composed of fucose, rhamnose, arabinose, galactose, glucose, xylose, fructose and glucuronic acid in a molar ratio of 4.19: 3.46: 3.26: 26.80: 35.79: 7.92: 8.65: 9.92. Galactose and glucose were the most abundant. The results showed that SRP was heteropolysaccharide. Liu et al. (2) identified mannose, galactose and glucose as the main monosaccharide components in SRP. Similarly, Diego Morales et al. found that the monosaccharide components of Lentinula edodes polysaccharides are mainly glucose, galactose and mannose (23). Different sources of raw materials and preparation conditions may be responsible for the differences.
The morphology of SRP was characterized by SEM. SRP had a rough surface, irregular flake and massive distribution, and pores on the surface after magnification (Figure 2). These microstructure characteristics may be caused by the destruction of cell wall structure and the decomposition of polysaccharide aggregates during the three-phase extraction processes. The surface topographic characteristics and microstructure change of SRP may be related to its physicochemical properties and antioxidant activity (24). SRP obtained by the three-phase extraction method is due to the joint action of ammonium sulfate and tert-butanol, which is affected by various forces and forms an obvious flake structure.
The antioxidant potential of polysaccharides may be easily guaranteed by measuring their capacity to scavenge DPPH free radicals. In general, the eliminated ability of polysaccharides to DPPH free radicals relies on the hydrogen supply ability of the antioxidant (25). Figure 3A displayed SRP’s capacity to scavenge DPPH free radicals. The scavenging activities of SRP reached 88.60 percent when the SRP reached 2 mg/mL. The result indicated that SRP had the ability to clear DPPH, which was similar to Wang et al. (26).
Figure 3 Antioxidant activity of SRP (A-D) DPPH, Hydroxyl, ABTS+ radical scavenging ability and reducing power.
Excessive levels of hydroxyl radical, one of the most powerful free radicals in the body, may disrupt the delicate equilibrium of the body. Upon contact with nearby biomolecules, hydroxyl radicals may cause extensive damage, and in extreme cases, even cell death (27). Figure 3B displayed SRP’s ability to quench hydroxyl radicals. Generally, SRP had potential scavenging activity on hydroxyl radical. SRP’s scavenging activity rose to 94.46 percent at 6 mg/mL. When the SRP concentration was 10 mg/mL, its scavenging efficiency was 98.06%. SRP was shown to have a clear capacity to scavenge hydroxyl radicals, as shown by the findings. SRP had the potential to remove hydroxyl radical because it has a better scavenging power on hydroxyl radical.
The antioxidant potential of polysaccharides may be easily neutralized using the ABTS+ free radical technique (28). ABTS+ free radical scavenging experiments are based on electron transfer from antioxidants to ABTS+ free radicals. SRP’s capacity to scavenge ABTS+ radicals were shown in Figure 3C. As a positive control, the scavenging power of Vc remained stable between 88.13% and 99.83% in the range of experimental concentrations. At 2 mg/mL, SRP showed an 89.44 percent increase in scavenging activities. These results showed that SRP had the ability to scavenge ABTS+ radical.
In Figure 3D, the lowering power of SRP compared to the positive control, Vc. The concentration of SRP has a positive correlation with the reducing power. SRP had an absorbance of 0.66 at 700 nm at a dosage of 10 mg/mL. These findings demonstrated that SRP has some degree of astringent activity.
The results of testing the viability of cells treated with SRP were shown in Figure 4A. By comparing with the blank group, different concentrations of SRP had different effects on RAW264.7 macrophages activity. When SRP ≤ 200 µg/mL, SRP can significantly promote cell growth (p<0.05) indicating that SRP had no toxicity to cells. Concentrations greater than or equal to 400 µg/mL inhibited cell proliferation, indicating some toxicity.
Figure 4 Cell viability and phagocytic capacity of the SRP. (A) Cell viability; (B) Phagocytic capacity. Significant differences with the control group: *p<0.05 and **p<0.01.
Therefore, based on these results, a concentration between 25-200 µg/mL could be used for experimental treatment of cells, specifically for evaluating the immune regulatory effect of SRP on RAW264.7 macrophages.
The effect of SRP on phagocytosis was detected by macrophage uptake of neutral red (29). The results were shown in Figure 4B. When the concentration of SRP was between 25-200 μg/mL, all samples significantly promoted the uptake of neutral red by RAW264.7 macrophages compared with the blank control group (p<0.05). The results showed that SRP significantly promoted the uptake of neutral red by macrophages, thus further regulating immune activity.
The most direct method to detect cell migration is the scratch test (30). As shown in Figure 5, RAW264.7 macrophages were treated with LPS (1 μg/mL) and different concentrations of SRP (25-200 μg/mL), and the percent of cell scratch healing on the macrophages was observed. The results showed that SRP significantly improved cell scratch healing at all tested concentrations between 25-200 μg/mL compared with the blank group (p<0.05), and the scratch healing rate of the RAW264.7 macrophages at 25-50 μg/mL SRP was higher than that at 100-200 μg/mL.
Figure 5 Results of the macrophages RAW264.7 scratch experiment. (A) Image of the cell scratch: a-f indicates the control, LPS, SRP (25, 50, 100, 200 μg/mL) groups; (B) Percent of cell scratch healing. Significant differences with the control group: *p< 0.05 and **p< 0.01.
Cytokines are produced by activated immune cells and are considered to be major immune mediators (31). The transcription levels of the three cytokine genes IL-1β, IL-6 and TNF-α were detected by RT-PCR. Figure 6 showed that transcription levels of all three were significantly increased within the limits of SRP (25-50 μg/mL) or LPS (1 μg/mL) groups compared with the blank group (p<0.05). The result was similar to the results reported by Liu on the immunomodulatory activity of Sinonovacula constricta polysaccharide (32).
Figure 6 Effects of SRP on the expression of cytokine genes in RAW264.7 macrophages. (A-C) Expression of TNF-α, IL-1β and IL-6 mRNA. Significant differences with the control group: *p< 0.05 and **p< 0.01.
NF-κB plays a crucial role in immune regulation and inflammatory responses (33). TLR4 is a significant member of the Toll-like receptor family, and breakthroughs have been made in research on TLR4. A variety of signaling pathways have been confirmed to be related to mediating immune responses (34). To determine whether the immune activity of SRP is mediated by the TLR4/NF-κB signaling pathway, we investigated western blot analysis was performed to analyze the effect of SRP on the expression levels of key proteins in the TLR4/ NF-κB signaling pathway in RAW 264.7 cells.
Figure 7 showed the influence of SRP on the expression levels of the key proteins TLR4, MyD88 and NF-κB in the TLR4/NF-κB signaling pathway (β-actin as internal reference). Compared with the positive control group, SRP groups showed decreased the protein expression levels. Compared with the blank group, SRP (25-50 μg/mL) and LPS groups showed significantly increased expression of TLR4, MyD88 and NF-κB protein (p<0.05). We speculated that SRP activates RAW264.7 macrophages by up-regulating the expression of key proteins in the TLR4/NF-κB pathway.
Figure 7 Effect of SRP on the protein expression levels of the TLR4/NF-κB pathway. (A) Western blot analysis of protein bands; (B) TLR4; (C) MyD88; (D) NF-κB protein expression. Significant differences with the control group: *p< 0.05 and **p< 0.01.
Autophagy is a cellular mechanism of self-degradation, transformation and energy production. Autophagy is closely related to inflammation. The activation of pattern recognition Toll-like receptor can induce autophagy, and autophagy can regulate the inflammatory response. Defective autophagy can induce inflammation (35). LC3 is a hallmark protein of autophagy. p62 is a substrate for autophagy degradation; it plays an important role in the recognition and encapsulation of degraded substrates. Beclin 1 over expression promotes autophagy in mammalian cells, and the expression increases with the enhancement of autophagy. LC3, Beclin 1 and p62 are all used as indicators of autophagy (36).
Autophagic protein expression results were shown in Figure 8. The expression of autophagic protein in the positive control group was higher than in the blank group. Compared with the positive control group, the protein expression levels of LC3 and Beclin 1 increased and the protein expression levels of p62 decreased in SRP with different mass concentrations. Compared with the blank group, the protein expression levels of LC3, Beclin 1 and p62 in different mass concentrations of SRP were significantly increased (p<0.05), which indicated that SRP can promote autophagy in cells.
Figure 8 Expression of autophagic proteins. (A) Western blot analysis of protein bands; (B-D) LC3, Beclin1 and p62 protein expression. Significant differences with the control group: *p< 0.05 and **p< 0.01.
Recently, many studies have been carried out on the extraction of polysaccharides from S. rugosoannulata, which due to its better biological. The main extraction methods are solvent extraction, ultrasonic extraction, alkali-assisted extraction (2), etc. However, these extraction methods are complex, typically requiring a long processing time.
As a new protein separation and purification technology, three-phase extraction is a safe, effective, and environmentally friendly green technology, including salting out, isoelectric point precipitation and solvent precipitation (37). However, most researchers mainly focus on the protein phase (38). It is rarely reported that polysaccharides are isolated and purified from edible fungi by three-phase extraction. Ours may be the first attempt to isolate SRP by three-phase extraction. Three-phase extraction is relatively simple, safe, and environmentally friendly compared to conventional extraction methods. Furthermore, the method preserves the biological activity of the polysaccharides better than the existing method.
In this study, single factor and multiple factor orthogonal tests were used to obtain the optimal extraction conditions for extracting polysaccharides. These conditions were: mass fraction of ammonium sulfate, 20%; volume ratio of sample solution to t-butanol, 1:1.5; and extraction temperature, 35°C. Under these conditions, the yield of SRP was 6.85% ± 0.13%, which was higher than that of the traditional method of water extraction and alcohol precipitation (39).
The physiological functions of polysaccharides are closely related to structure. Some scholars have studied the structure and function of polysaccharides of S. rugosoannulata. Chen et al. (22) obtained a polysaccharide by hot water extraction, and proved this structure was mainly composed of five monosaccharides, predominaatly xylose, galactose and glucose, with a relative molecular weight of about 22 kDa. Liu et al. (2) isolated two components, SRP-1 and SRP-2 from the fruiting body of S. rugosoannulata. Both components contained glucose and galactose, but SRP-2 also contained ribose and uronic acid. We speculate that these differences in monosaccharide compositions of S. rugosoannulata were due to differences in the source material.
In our study, the polysaccharide from S. rugosoannulata was extracted by three-phase extraction. According to our analysis, the polysaccharide was heteropolysaccharide composed of 8 monosaccharides, and the molecular weight was 27.52 kDa, which was larger than that polysaccharides obtained by hot water extraction (22). The molecular mass of SRP was bigger, the monosaccharide composition was richer, and its physiological activity was stronger. At the same time, this method also had a positive effect on the structure modification, which can interrupt the long molecular chain of polysaccharides and the change of molecular aggregation state, so that the structure of polysaccharides can be changed. Finally, three-phase extraction affects the biological activity and chemical and physical properties, such as improving the antioxidant activity of the polysaccharides (40).
Polysaccharides can improve the immune activity of cells in vitro. MOP-3, a novel polysaccharide extracted from Moringa oleifera leaves, can enhance the ability of macrophages to secrete reactive oxygen species (ROS), nitric oxide (NO), interleukin-6 (IL-6) and tumor necrosis factor α (TNF-α), indicating that the polysaccharide can activate macrophages to produce cytokines, thus achieving the purpose of fighting pathogens (41). In this experiment, compared with blank control group, the the SRP-treated group showed increased proliferation, phagocytosis and cell migration of 25-200 μg/mL.
Numerous studies have shown that polysaccharides can activate intracellular signaling pathways through TLR4 receptor-mediated macrophages, promote the release of related cytokines, and play immunomodulatory roles. For example, mushroom polysaccharides can activate peritoneal macrophages through the TLR4/NF-κB pathway, and significantly enhance the secretion of cytokines (42). Our study results showed that SRP significantly enhanced the protein expression of TLR4, MyD88 and NF-κB in the range of 25-50 μg/mL, and activated the TLR4-mediated MyD88 dependent pathway, which confirmed that TLR4/NF-κB signaling pathway is one of the important pathways through which polysaccharides exert immunomodulatory effects.
Autophagy is a highly conserved metabolic pathway in organisms. It can selectively degrade intracellular harmful components and play an important role in regulating immunity (35). At present, no articles related to SRP and autophagy have been found. So, this study confirmed that SRP can up-regulate autophagy related proteins LC3 and Beclin1, and down-regulate the expression of p62 protein to induce increased autophagy. This indicates that the mechanism of SRP’s effect on inflammation is related to autophagy induction. Moreover, the expression levels of related proteins and autophagy proteins in the TLR4/NF-κB signaling pathway were significantly increased in the macrophage SRP groups treated with 25-50 μg/mL, suggesting that SRP can activate the TLR4/NF-κB signaling pathway and autophagy.
In this study, a polysaccharide was extracted from the mushroom Stropharia rgosoanmulata, using a new method, three-phase extraction. Extraction parameters were optimized as follows; mass fraction of ammonium sulfate, 20%; volume ratio of sample solution to t-butanol, 1:1.5; and the extraction temperature, 35°C. With these parameters, the yield of polysaccharides was 6.85% ± 0.13%. SRP had the molecular weight of 27.52 kDa. SEM showed that SRP surface was rough and flakey. XRD analysis showed that it had both crystalline and amorphous structures. The results of antioxidation experiments showed that SRP has antioxidant activity. The results of CCK-8 method showed that the polysaccharide concentration in the range of 25-200 μg/mL promoted cell proliferation and had no cytotoxicity. This means that SRP is safe for clinical applications. RT-PCR results confirmed that SRP can significantly promote the release of cytokines such as IL-6 and TNF-α from peritoneal macrophages in the range of 25-100 μg/mL, and the purpose of immune enhancement by enhancing the activity of cytokines. Western blot results showed that SRP induced macrophages RAW264.7 to activate the TLR4/NF-κB signaling pathway of autophagy.
In conclusion, S. rugosoannulata has antioxidant and immunomodulatory activities. It appears to be safe. Thus, S. rugosoannulata has a broad prospect for further development as a new immunomodulator and antioxidant..
The datasets presented in this study can be found in online repositories. The names of the repository/repositories and accession number(s) can be found in the article/Supplementary Material.
XL, ZZ: Research concept, Methodology, Data extraction, Analysis, Draft writing. XM: Resource searching, Verification, Formal analysis, Supervision, Manuscript reviewing and editing. YW: Funding sponsorship. LW, HZ, YJ: Resources, Methodology, Project administration, Supervision, Manuscript reviewing and editing. BM, JL: Resource searching, Manuscript reviewing and editing. All authors contributed to the article and approved the submitted version.
The present work was funded by the science and technology correspondent of Henan Province (2022, No.1963), the National Natural Science Foundation of China (Grant Nos.31602098 and 32072906), the Scientific and technological project of Henan province (Grant No. 192102110188 and 192102110184), the Special Fund for Henan Agriculture Research System (Grant No. HARS-22-16-Z1), the Science and Technology Project of Henan province (Grant No. 222102110063).
We would especially like to thank all those involved in the study.
Author JL was employed by Yucheng Jinlong Mushroom Co., LTD. Author YW was employed by GeneGenieDx Corporation.
The remaining authors declare that the research was conducted in the absence of any commercial or financial relationships that could be construed as a potential conflict of interest.
All claims expressed in this article are solely those of the authors and do not necessarily represent those of their affiliated organizations, or those of the publisher, the editors and the reviewers. Any product that may be evaluated in this article, or claim that may be made by its manufacturer, is not guaranteed or endorsed by the publisher.
The Supplementary Material for this article can be found online at: https://www.frontiersin.org/articles/10.3389/fimmu.2022.994706/full#supplementary-material
1. Jing W, Suzuki T, Choi JH, Yasuda N, Kawagishi H. An unusual sterol from the mushroom Stropharia rugosoannulata. Tetrahedron Lett (2013) 54(36):4900–2. doi: 10.1016/j.tetlet.2013.06.142
2. Liu Y, Hu CF, Feng X, Cheng L, Huang W. Isolation, characterization and antioxidant of polysaccharides from Stropharia rugosoannulata. Int J Biol Macromol (2019) 155(19):883-889. doi: 10.1016/j.ijbiomac.2019.11.045
3. He X, Fang J, Guo Q, Wang M, Huang L. Advances in antiviral polysaccharides derived from edible and medicinal plants and mushrooms. Carbohydr Polym (2019) 229:115548. doi: 10.1016/j.carbpol.2019.115548
4. Zhang S, Li Y, Li Z, Liu W, Zhang H, Ohizumi Y, et al. Structure, anti-tumor activity, and potential anti-tumor mechanism of a fungus polysaccharide from Fomes officinalis. Carbohydr Polym (2022) 295:119794. doi: 10.1016/j.carbpol.2022.119794
5. Ma X, Guo D, Peterson EC, Dun Y, Li DY. Structural characterization and anti-aging activity of a novel extracellular polysaccharide from fungus Phellinus sp. in a mammalian system. Food Funct (2016) 7(8):3468–79. doi: 10.1039/C6FO00422A
6. Guo Q, Liang SM, Xiao ZC, Ge CR. Research progress on extraction technology and biological activity of polysaccharides from edible fungi: A review. Food Rev Int (2022) 3:1–32. doi: 10.1080/87559129.2022.2039182
7. Hui HP, Gao WJ. Physicochemical features and antioxidant activity of polysaccharides from herba Patriniae by gradient ethanol precipitation. Arabian J Chem (2022) 15(5):103770. doi: 10.1016/j.arabjc.2022.103770
8. Coimbra C, Lopes CE, Calazans G. Three-phase partitioning of hydrolyzed levan bioresource technology. Bioresource Technol (2010) 101(12):4725–8. doi: 10.1016/j.biortech.2010.01.091
9. Yan JK, Wang YY, Qiu WY, Shao N. Three-phase partitioning for efficient extraction and separation of polysaccharides from Corbicula fluminea. Carbohydr Polym (2017) 163:10–9. doi: 10.1016/j.carbpol.2017.01.021
10. Tan ZJ, Wang CY, Yi YJ, Wang HY, Zhou WL. Three phase partitioning for simultaneous purification of aloe polysaccharide and protein using a single-step extraction. Process Biochem (2015) 50(3):482–6. doi: 10.1016/j.procbio.2015.01.004
11. Wang L, Liu HM, Qin GY. Structure characterization and antioxidant activity of polysaccharides from Chinese quince seed meal. Food Chem (2017) 234:314. doi: 10.1016/j.foodchem.2017.05.002
12. Wang L, Zhao ZW, Zhao HQ, Liu M, Lin C, Li LZ, et al. Pectin polysaccharide from Flos magnoliae (Xin yi, Magnolia biondii pamp. flower buds): Hot-compressed water extraction, purification and partial structural characterization. Food Hydrocolloids (2022) 122:107061. doi: 10.1016/j.foodhyd.2021.107061
13. Yang WJ, Pei F, Shi Y, Zhao LY, Fang Y, Hu QH. Purification, characterization and anti-proliferation activity of polysaccharides from Flammulina velutipes. Carbohydr Polym (2012) 88(2):474–80. doi: 10.1016/j.carbpol.2011.12.018
14. Qiu JQ, Zhang H, Wang ZY. Ultrasonic degradation of polysaccharides from Auricularia auricula and the antioxidant activity of their degradation products. LWT (2019) 113:108266. doi: 10.1016/j.lwt.2019.108266
15. Gao CJ, Wang YH, Wang CY, Wang ZY. Antioxidant and immunological activity in vitro of polysaccharides from Gomphidius rutilus mycelium. Carbohydr Polym (2013) 92(2):2187–92. doi: 10.1016/j.carbpol.2012.12.011
16. Zhang ZQ, Li D, Ma X, Li XH, Guo ZH, Liu YL, et al. Carboxylated nanodiamond-mediated NH2-PLGA nanoparticle-encapsulated fig polysaccharides for strongly enhanced immune responses in vitro and in vivo. Int J Biol Macromol (2020) 165:1331–45. doi: 10.1016/j.ijbiomac.2020.10.010
17. Zubair M, Ekholm A, Nybom H, Renvert S, Widen C, Rumpunen K. Effects of Plantago major l. leaf extracts on oral epithelial cells in a scratch assay. J Ethnopharmacol (2012) 141(3):825–30. doi: 10.1016/j.jep.2012.03.016
18. Wang H, Geng HY, Chen J, Wang X, Li D, Wang T, et al. Three phase partitioning for simultaneous extraction of oil, protein and polysaccharide from rice bran. Innovative Food Sci Emerg Technol (2020) 65:102447. doi: 10.1016/j.ifset.2020.102447
19. Khataei MM, Yamini Y, Nazaripour A, Karimi M. Novel generation of deep eutectic solvent as an acceptor phase in three-phase hollow fiber liquid phase microextraction for extraction and preconcentration of steroidal hormones from biological fluids. Talanta (2018) 178:473–80. doi: 10.1016/j.talanta.2017.09.068
20. Krisna C, Duong-Ly SB. Gabelli, chapter seven-salting out of proteins using ammonium sulfate precipitation. Methods Enzymol (2014) 541:85–94. doi: 10.1016/B978-0-12-420119-4.00007-0
21. Ktari N, Feki A, Trabelsi I, Triki M, Maalej H, Slima SB, et al. Structure, functional and antioxidant properties in Tunisian beef sausage of a novel polysaccharide from Trigonella foenum-graecumin seeds. Int J Biol Macromol (2017) 98:169–81. doi: 10.1016/j.jep.2012.03.016
22. Chen JC, Weng MJ, Lai PF. Distribution of Stropharia rugoso-annulata polysaccharides molecular weight and component sugar. China Agric Sci (2011) 44(10):2109–17. doi: 10.3846/j.issn.0578-175.2011.10.016
23. Morales D, Smiderle FR, Villalva M, Abreu H, Rico C, Santoyo S, et al. Testing the effect of combining innovative extraction technologies on the biological activities of obtained β-glucan-enriched fractions from Lentinula edodes. J Funct Foods (2019) 60:103446–6. doi: 10.1016/j.jff.2019.103446
24. Yang Y, Li C, Ni S, Zhang H, Dong C. Ultrastructure and development of acanthocytes, specialized cells in Stropharia rugosoannulata, revealed by scanning electron microscopy (SEM) and cryo-SEM. Mycologia (2021) 113(1):65–77. doi: 10.1080/00275514.2020.1823184
25. Li WA, Pzz A, Jws B, Yyq A, Miao LA, Yuan RA, et al. Physicochemical properties and bioactivities of original and Se-enriched polysaccharides with different molecular weights extracted from Pleurotus ostreatus. Int J Biol Macromol (2019) 141(C):150–60. doi: 10.1016/j.ijbiomac.2019.03.168
26. Wang Q, Zhao Y, Feng X, Ibrahim SA, Liu Y. Effects of drying on the structural characteristics and antioxidant activities of polysaccharides from Stropharia rugosoannulata. J Food Sci Technol Mysore (2021) 1):1–10. doi: 10.1007/s13197-021-05120-6
27. Yan JK, Ding ZC, Gao XL, Wang YY, Yang Y, Zhang HN. Comparative study of physicochemical properties and bioactivity of Hericium erinaceus polysaccharides at different solvent extractions. Carbohydr Polym: Sci Technol Aspects Industrially Important Polysaccharides (2018) 193:373–82. doi: 10.1016/j.carbpol.2018.04.019
28. Liu XG, Bian J, Li DQ, Liu CF, Xu SS, Zhang GL, et al. Structural features, antioxidant and acetylcholinesterase inhibitory activities of polysaccharides from stem of Physalis alkekengi l. Ind Crops Prod (2019) 129:654–61. doi: 10.1016/j.indcrop.2018.12.047
29. Wang HL, Ma CY, Sun-Waterhouse DX, Wang JM, Waterhouse GIN, Kang WY. Immunoregulatory polysaccharides from Apocynum venetum l. flowers stimulate phagocytosis and cytokine expression via activating the NF-κB/MAPK signaling pathways in RAW264. 7 cells. Food Sci Hum Wellness (2022) 11(4):806–14. doi: 10.1016/j.fshw.2022.03.012
30. Bobadilla AVP, Arévalo J, Sarró E, Byrne HM, Maini PK, Carraro T, et al. In vitro cell migration quantification method for scratch assays. J R Soc Interface (2019) 16(151):20180709. doi: 10.1098/rsif.2018.0709
31. Cao HY, Liu JX, Shen P, Cai JP, Han YC, Zhu KP, et al. Protective effect of naringin on DSS-induced ulcerative colitis in mice. J Agric Food Chem (2018) 66(50):13133–40. doi: 10.1021/acs.jafc.8b03942
32. Liu ZD, Liu ZF, Li LH, Zhang JJ, Zhao QC, Lin N, et al. Immunomodulatory effects of the polysaccharide from Sinonovacula constricta on RAW264.7 macrophage cells. Food Sci Nutr (2022) 10(4):1093–1102. doi: 10.1002/fsn3.2735
33. Ren DY, Lin DH, Alim A, Zheng Q, Yang XB. Chemical characterization of a novel polysaccharide ASKP-1 from Artemisia sphaerocephala krasch seed and its macrophage activation via MAPK, PI3k/Akt and NF-κB signaling pathways in RAW264.7 cells. Food Funct (2017) 8(3):1299–312. doi: 10.1039/C6FO01699E
34. Chen GP, Yan F, Wei W, Wang FF, Wang ZR, Nie JH, et al. CD38 deficiency protects the retina from ischaemia/reperfusion injury partly via suppression of TLR4/MyD88/NF-κB signalling. Exp Eye Res (2022) 219:109058. doi: 10.1016/j.exer.2022.109058
35. Cui B, Lin H, Yu JM, Yu JJ, Hu ZW. Autophagy and the immune response. Autophagy: Biol Dis (2019) 1206:595–634. doi: 10.1007/978-981-15-0602-4_27
36. Maiuri MC, Criollo A, Kroemer G. Crosstalk between apoptosis and autophagy within the beclin 1 interactome. EMBO J (2010) 29(3):515–6. doi: 10.1038/emboj.2009.377
37. Alves A, Sousa RA, Reis RL. A practical perspective on ulvan extracted from green algae. J Appl Phycol (2013) 25(2):407–24. doi: 10.1007/s10811-012-9875-4
38. Waghmare AG, Salve MK, LeBlanc JG, Arya SS. Concentration and characterization of microalgae proteins from Chlorella pyrenoidosa. Biores Bioprocessing (2016) 3(1):1–11. doi: 10.1186/s40643-016-0094-8
39. Lu QJ, Xue S, Yang DS, Wang H, Li L. Effects of three extraction methods on antioxidant properties of crude polysaccharides from Stropharia rugoso. Food Sci Technol (2021) 46(11):171–8. doi: 10.13684/j.cnki.spkj.2021.11.027
40. Ji XL, Hou CY, Shi MM, Yan YZ, Liu YQ. An insight into the research concerning Panax ginseng CA Meyer polysaccharides: a review. Food Rev Int (2020) 227:1149–65. doi: 10.1080/87559129.2020.1771363
41. Li C, Dong ZP, Zhang B, Huang Q, Liu G, Fu X. Structural characterization and immune enhancement activity of a novel polysaccharide from Moringa oleifera leaves. Carbohydr Polym (2020) 234:115897. doi: 10.1016/j.carbpol.2020.115897
Keywords: Stropharia rugosoannulata, polysaccharide, three-phase extraction, structure, bioactivities
Citation: Li X, Zhang Z, Wang L, Zhao H, Jia Y, Ma X, Li J, Wang Y and Ma B (2023) Three-phase extraction of polysaccharide from Stropharia rugosoannulata: Process optimization, structural characterization and bioactivities. Front. Immunol. 13:994706. doi: 10.3389/fimmu.2022.994706
Received: 15 July 2022; Accepted: 23 August 2022;
Published: 11 January 2023.
Edited by:
Yi Wu, Nanjing Agricultural University, ChinaReviewed by:
Yun peng Fan, Northwest A&F University, ChinaCopyright © 2023 Li, Zhang, Wang, Zhao, Jia, Ma, Li, Wang and Ma. This is an open-access article distributed under the terms of the Creative Commons Attribution License (CC BY). The use, distribution or reproduction in other forums is permitted, provided the original author(s) and the copyright owner(s) are credited and that the original publication in this journal is cited, in accordance with accepted academic practice. No use, distribution or reproduction is permitted which does not comply with these terms.
*Correspondence: Li Wang, d2FuZ2xpaHVpbmFAMTYzLmNvbQ==; Xia Ma, bWF4aWE4MDEwMTBAMTI2LmNvbQ==; Bingji Ma, bWJqMTIzQHNpbmEuY29t
†These authors have contributed equally to this work
Disclaimer: All claims expressed in this article are solely those of the authors and do not necessarily represent those of their affiliated organizations, or those of the publisher, the editors and the reviewers. Any product that may be evaluated in this article or claim that may be made by its manufacturer is not guaranteed or endorsed by the publisher.
Research integrity at Frontiers
Learn more about the work of our research integrity team to safeguard the quality of each article we publish.