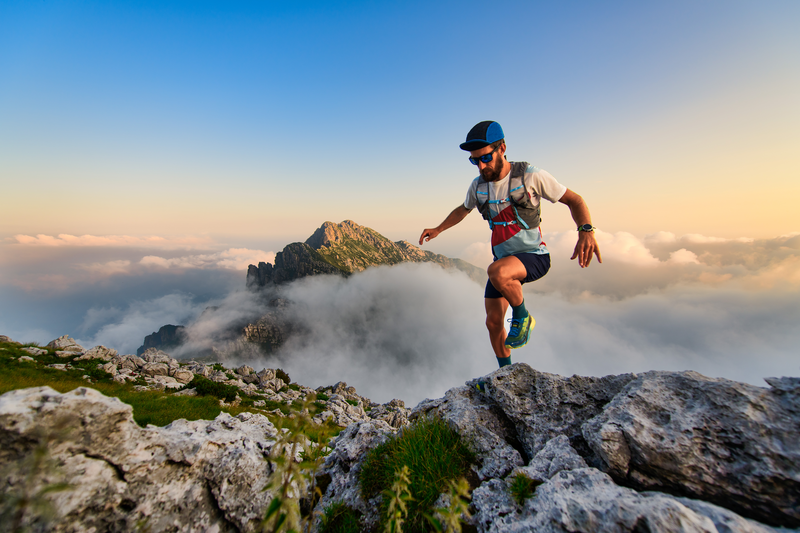
95% of researchers rate our articles as excellent or good
Learn more about the work of our research integrity team to safeguard the quality of each article we publish.
Find out more
REVIEW article
Front. Immunol. , 11 January 2023
Sec. Cytokines and Soluble Mediators in Immunity
Volume 13 - 2022 | https://doi.org/10.3389/fimmu.2022.991433
This article is part of the Research Topic Insights into the immunological microenvironment within neoplastic and inflammatory skin conditions View all 7 articles
The use of immune checkpoint inhibitors (ICIs) has evolved rapidly with unprecedented treatment benefits being obtained for cancer patients, including improved patient survival. However, over half of the patients experience immune related adverse events (irAEs) or toxicities, which can be fatal, affect the quality of life of patients and potentially cause treatment interruption or cessation. Complications from these toxicities can also cause long term irreversible organ damage and other chronic health conditions. Toxicities can occur in various organ systems, with common observations in the skin, rheumatologic, gastrointestinal, hepatic, endocrine system and the lungs. These are not only challenging to manage but also difficult to detect during the early stages of treatment. Currently, no biomarker exists to predict which patients are likely to develop toxicities from ICI therapy and efforts to identify robust biomarkers are ongoing. B cells and antibodies against autologous antigens (autoantibodies) have shown promise and are emerging as markers to predict the development of irAEs in cancer patients. In this review, we discuss the interplay between ICIs and toxicities in cancer patients, insights into the underlying mechanisms of irAEs, and the involvement of the humoral immune response, particularly by B cells and autoantibodies in irAE development. We also provide an appraisal of the progress, key empirical results and advances in B cell and autoantibody research as biomarkers for predicting irAEs. We conclude the review by outlining the challenges and steps required for their potential clinical application in the future.
An understanding of the tumor immune response and the role of immune checkpoints in immune tolerance paved the way for the development of a new generation of treatments for cancer patients. The development of immune checkpoint inhibitors (ICIs) has in particular revolutionized the melanoma treatment landscape, with the attainment of durable treatment response and patient survival benefits (1). ICIs re-activate T cells to recognize and destroy cancer cells by blocking the inhibitory signaling pathways necessary for maintaining immune tolerance (2). However, as a consequence of their mechanism of action, ICIs can also cause highly variable non-specific autoinflammation and other tissue directed autoimmune manifestations known as checkpoint toxicities or immune related adverse events (irAEs) (3).
Several ICI clinical trials have outlined a profile of toxicities involving various organ systems, including dermatological, such as maculopapular rash and pruritus (4), pneumonitis in the lungs, gastrointestinal including diarrhea and colitis (5) and hepatic toxicity, which presents with increases in circulatory liver enzymes such as aspartate (AST) and alanine transaminases (ALT) (5). Endocrine toxicities such as thyroiditis and hypophysitis (6), rheumatologic toxicities including arthralgia and arthritis (7) and other non-organ specific events such as fatigue (8) have also been reported (Figure 1). IrAEs are graded and managed according to the common terminology criteria for adverse events (CTCAE), and patients may be classified based on mild (grade 1 and 2) or severe (grade 3-5) irAEs. Among melanoma patients, more than 50% who undergo treatment with ICIs experience severe grade (grade 3-5) irAEs (9).
Although irreversible complications can occur, irAEs are generally reversible and can be managed with immunosuppressive drugs and corticosteroids (10). However, this is largely dependent on the early recognition, and prompt treatment of the toxicity. In this context, biomarkers that are predictive of which patients are likely to suffer irAEs prior to or early during treatment have gained importance to support the early irAE detection and its management.
In an effort to identify biomarkers for the prediction of irAEs, numerous biomarkers have been pursued (11). Herein, we focus on the role of the humoral immune response involving B cells and autoantibodies (AAbs). In this review, we discuss ICIs and irAEs in various cancers, the underlying mechanisms of irAEs, and the involvement of AAbs and B cells in irAE development. We also discuss the evidence that supports the validity of AAbs and B cells as biomarkers of irAEs and address the challenges encountered for their clinical utilization and new avenues for research.
We relied on original published articles and limited our search in NCBI PubMed to the following keywords “autoantibodies as biomarkers of checkpoint toxicities”, “immune related adverse events”, “checkpoint toxicities”, “autoantibodies and B cells as biomarkers of immune related adverse events”, “B cells in immune related adverse events’’, “B cell profiling in cancer”, “autoantibody profiling in cancer” and “side effects of immune checkpoint inhibition”.
The contribution of ICIs to both controlling tumor growth and causing irAEs, highlights a dynamic interplay that exists between this treatment outcomes (Figure 2). The physiologic function of immune checkpoints is to maintain immune tolerance (10). Unlike cytotoxic T-lymphocyte-associated antigen 4 (CTLA-4) which suppresses T cell activation at the initial priming phase of T cell activation (12, 13), engagement of programmed cell death 1 (PD-1) with its ligands (programmed death-ligand 1 (PD-L1) and also PD-L2) inhibits the T cell effector phase. This results in the failure in the proliferation of effector T cells and the production of cytokines such as interleukin-2 (IL-2), tumor necrosis factor-α (TNF-α) and interferon gamma (IFN)-γ (14). During ICI therapy, this inhibitory control mechanism is interrupted which enables the immune recognition and destruction of cancer cells. But because this process is not antigen specific, ICIs may also reactivate the immune system to self-antigens, capable of causing irAEs (Figure 3).
IrAEs are thought to be primarily mediated by an overactivation of the immune system. However, the immune components that drive them are heterogenous and may be affected by other factors such as the gut microbiome dysregulation, pre-existing autoimmune disease, host genetics and environmental cues (15). Here, we describe the mechanisms that have been implicated in the current literature (Figure 3).
The expression of immune checkpoints in normal tissues has been implicated in the development of organ-specific irAEs (16, 17) (Figure 3). An example is hypophysitis, which is rarely seen following anti-PD-1 therapy. The modelling of hypophysitis in C57BL/6J mice provided evidence to support the off-target effects of monoclonal antibodies as a potential mechanism for ICI toxicity (16). In this study, CTLA-4 expressed in the hypothalamic and pituitary tissues in mice, was reported to serve as a target for monoclonal antibody (anti-CTLA-4)-mediated injury, complement activation and subsequent organ damage of the pituitary (16). Caturegli et al. (17) also analyzed the pituitary expression of CTLA-4 in 6 cancer patients treated with anti-CTLA-4. They found that CTLA-4 expression was highest in severe hypophysitis patients compared to other pituitary glands. A higher CTLA-4 expression was associated with extensive destruction of the pituitary via immune mechanisms including T cell infiltration, antibody-dependent complement fixation and phagocytosis. They showed that higher levels of CTLA-4 in the pituitary can result in hypophysitis through T cell and antibody dependent immune mechanisms, during CTLA-4 blockade (17).
The chronic exposure of antigens to infiltrated T cells (TILs) in the tumor microenvironment (TME) can also result in an exhausted T cell phenotype (14), characterized by the expression of multiple inhibitory cell surface checkpoint molecules (such as PD-1), changes in the metabolic and epigenetic profiles of T cells (18) and a loss of T cell effector function (19). This expansion of exhausted T cells (with upregulated expression of immune checkpoints) results in limited damage to cancer cells (19), while preventing any autoimmune damage to the patient (3). CTLA-4 also reduces immune system overactivation, by binding to B7 proteins (CD80 and CD86) present on antigen presenting cells (13). This attenuates the MHC-TCR signaling, restraining T cells from overactivation. During cancer therapy, ICIs counteract these immune checkpoints in the TME to reinvigorate the immune system for cancer cell recognition and attack. The re-activation of the immune system to fight cancer cells by altering this highly important regulatory network, has been shown to present with an unspecific and unpredictable inflammatory feedback that worsens systemic autoimmunity and the occurrence of irAEs.
The capability of a T cell receptor (TCR) to bind more than one peptide has also been shown to be very important in autoimmune responses (20). Peptides can activate autoreactive T cells due to the structural similarity between seemingly unrelated peptide-loaded major histocompatibility complexes (pMHC) and cause autoimmune diseases (21–23). Several studies suggests that the off target T cell recognition of tumoral antigens on healthy cells can cause adverse events in patients treated with ICIs (24) (Figure 3). Studies have reported tumoral T cell infiltration into healthy tissues (25), identical TCR sequences in both tumor and healthy tissue (26) and similar shared antigen recognition in tumor and affected organs in the occurrence of irAEs (27–30). In these studies, epitope spreading was shown to be one of the mechanisms, that drive the off-target T cells (31, 32). Treatment with anti-CTLA-4 was shown to broaden the peripheral TCR repertoire in melanoma patients, that differed for those with irAEs and those without (33). Similar early T cell diversification of the TCR repertoire by CTLA-4 blockade was reported by Oh et al. (34). In these studies, the expansion of the TCR repertoire was shown to correlate with the occurrence of irAEs. Altogether, these studies demonstrate that ICIs can trigger irAEs by re-activating and de-novo inducing T cell clones, some of which not only recognize tumor antigens but also self-antigens.
IrAEs can also occur as a consequence of a downregulation of regulatory T cell (Treg) function. A subset of Tregs express CTLA-4, and maintain immune tolerance by immune response suppression, either by producing inhibitory cytokines, mediating T cell metabolic disruption, or by modulation of dendritic-cell (DC) maturation or function (35). CTLA-4 blockade was shown to impair Treg cell function and survival (36, 37), which was shown to increase the ratio of effector T cells to Treg cells (Teffs/Tregs) (37). An altered balance between Tregs and T effector cells (Teffs) was also shown to result in a loss of peripheral tolerance (38), that can lead to the development of autoimmune disease in various cancers (39).
A randomized controlled trial in cancer patients, reported decreased numbers of circulating Treg cells in patients who experienced irAEs on anti-CTLA-4 treatment (40). However, another study (41) did not report intratumoral depletion of Treg cells in cancer patients treated with anti-CTLA-4 therapies.
Despite this, it is plausible that targeting of regulatory T cells by ICI therapy can lead to an enhanced T cell effector proliferation and survival capable of killing tumor cells and also cause autoimmune side effects. PD-1 and PD-L1 also play a role in the development and survival of Treg cells (42, 43) and their inhibition has been shown to result in decreased Treg cell function that can cause irAEs.
A range of studies suggest that the release of cytokines during ICI therapy may also play a role in the development of irAEs. Cytokines regulate the immune system activity (44, 45) and provide an enabling environment for the function of other immune cells. They mediate several cell signaling pathways for T cell activation, cytotoxicity and survival (46, 47) and B cell differentiation into antibody producing plasma cells (48, 49). However, proinflammatory cytokines can trigger a systemic inflammatory response, which can lead to the occurrence of irAEs. For example, CTLA-4 blockade to promote T cell activation was shown to release pro-inflammatory cytokines in circulation (50). Melanoma patients who were treated with anti-CTLA-4, showed increased levels in the IL-17 producing CD4+ Th17 cells following treatment (51). IL-17 has been shown to mediate irAEs (2) and has been implicated in several autoimmune diseases (52). In one study, increased baseline circulation of IL-17 was found to correlate with the occurrence of severe colitis among advanced melanoma patients receiving neoadjuvant ipilimumab (50). Lim and colleagues (53) also reported elevated circulatory levels of pro-inflammatory cytokines in metastatic melanoma patients who experienced irAEs on combination therapy. Patients who developed irAEs also showed lower serum levels of C-X-C motif chemokine ligand 9 (CXCL9), CXCL10, CXCL11 and CXCL19 at baseline and higher levels of CXCL9 and CXCL10 post treatment in patients who did not develop any irAEs (54). Several studies have demonstrated IL-6 association with several irAEs (55–57). IL-6 is associated with the polarization of CD4+T cells to Th17 cells (46). IL-6 is also associated with an increase in the acute phase proteins such as C-reactive protein (CRP), which is also a marker of inflammation. Reduction in serum IL-6 levels was correlated with resolution of ICI-induced colitis, in response to corticosteroids and reduction of infiltrating Th17 cells in the inflamed sections of the colon (58).
Cytokine inhibitors such as infliximab (anti-TNF-a), tocilizumab (anti-IL-6R) and secukinumab (anti-IL-17a) that are administered secondary to corticosteroids in severe irAEs, have also demonstrated efficacy in resolving irAEs (59, 60). Tocilizumab binds to IL-6 receptors, and inhibits the IL-6 receptor complex signaling that can cause systemic and local inflammation (46) and also B cell differentiation (49). Recently, inhibition of IL-17A was shown to significantly reduce the development of thyroid irAE in ICI-treated tumor-bearing mice (61). Altogether, these findings suggest an important role of cytokines in the occurrence of irAEs.
The contribution of the gut microbiome to autoimmune diseases has gained momentum in recent years and the gut microbiome appear to also regulate toxicities that arise from the use of ICIs (62, 63) In addition to their key roles in metabolism and absorption, gut microorganisms are involved in the maintenance of the host immune homeostasis (such as by bacteroidetes which can stimulate the differentiation of Treg cells) (63). Dysregulation of the composition of the gut microbiome has been implicated in the occurrence of irAEs and two published studies provided evidence to support this suggestion. Chaput et al. (57), investigated the baseline composition of the gut microbiome of metastatic melanoma patients on ipilimumab, and identified two microbial populations which were associated with colitis. They identified a lower population of Bacteroidetes and a higher abundance of Firmicutes which was significantly associated with the occurrence of colitis. Dubin et al. (64) also reported an increase in fecal Bacteroidetes in baseline samples of metastatic melanoma patients who did not develop any colitis on ipilimumab treatment.
A study by Andrews et al. (62) also found a higher abundance of Bacteroides intestinalis in metastatic melanoma patients who developed severe grade irAEs on combination therapy. In this study, an upregulation of mucosal IL-1β (which is a key mediator of autoinflammatory responses) was reported in patient samples of colitis and in pre-clinical models. The successful treatment of ICI-induced colitis with fecal transplantation (a method that enables the modulation of the microbial composition in the gut) also lends support to the role of the gut microbiota in the occurrence of irAEs (65). Despite the possible association between the dysregulation of the gut microbiome and the development of irAEs, further studies are required to determine their underlying mechanisms.
Breakdown of self-tolerance mechanisms against autoantigens results in the initiation of antibody effects (66, 67) and it is becoming clear that AAbs can mediate irAEs by causing systemic inflammation that results in cellular and tissue injury (67) (Figure 3). AAbs may also cause autoimmune phenotypes by inhibiting the function of their target proteins either by stimulating protein receptors or blocking stimulation by its natural ligand (67). In Graves’ disease, for example, the binding of AAbs against the thyroid stimulating hormone receptor on thyroid cells was shown to cause the abnormal production of thyroid hormones which can lead to hyperthyroidism in patients (68). In a study by Osorio et al. (69), antithyroid antibodies were frequently found in patients who developed ICI-induced thyroid dysfunction.
Other possible pathogenic roles include activating the complement system and causing organ damage via the formation and deposition of immune complexes (67). In another retrospective study in advanced NSCLC patients treated with anti-PD-1, patients positive for any AAbs were significantly more likely to develop irAEs than those who were AAb‐negative (70).
In metastatic melanoma patients who were treated with ICIs, increased levels of pre-existing AAbs were shown to preceed the development of severe grade irAEs (71). 4 out of 5 cancer patients who had pre-existing antiacetylcholine receptor AAbs, were also shown to develop myositis on anti-PD-L1 treatment (72).
The presence of pre-existing AAbs is characteristic of many autoimmune diseases including rheumatoid arthritis and systemic lupus erythematosus and cancer patients with pre-existing autoimmune disease may also be prone to the development of irAEs or an autoimmune disease flare (73–75). A systematic review of cancer patients with autoimmune disease showed that, 75% of patients who were treated with ICIs had exacerbation of their pre-existing autoimmune disease, development of a de novo irAE or both (75). In this study, patients who were receiving treatment for pre-existing autoimmune disease when ICI therapy started had fewer irAEs compared to those who were not receiving any treatment (75). An increase in autoimmune disease flare was also reported by Menzies et al. (76) among patients with active autoimmune disease compared with patients with inactive disease. Altogether, suggesting an underlying predisposition to the occurrence of irAEs.
Success with B cell depleting agents such as rituximab (anti-CD20) (77, 78), also support the concept that B cells and antibodies may play a role in the occurrence of irAEs. Rituximab reduces B cell differentiation, and has been useful in the management of both classical autoimmune diseases (77) and irAEs (78). While focus of most of the published literature has been on assessing IgGs in checkpoint toxicities, a brief case report by Zaenker, Prentice and Ziman (79) reported higher levels of tropomyosin IgA AAbs which was associated with the occurrence of ICI-induced myositis in a uveal melanoma patient. Serum IgA has been implicated in autoimmunity and inflammation, and their aggregation can be detrimental, capable of inducing inflammatory diseases (80, 81). Overall, while the mechanisms underlying the role of AAbs in the development of irAEs remains unclear, their presence has been implicated in irAEs which can suggest some important pathology element. Nevertheless, whether AAbs are causal, or just correlative of an unspecific B cell activation from ICI therapy is still not clear.
Studies have showed that ICIs can impact other immune cells (82), which can also explain the extent of adverse events associated with ICIs. Here we describe the expression of CTLA-4 and PD-1/PD-L1 proteins in B cells and the role of ICI in this immune cell populations in the occurrence of irAEs.
Yang et al. (83) demonstrated the expression of CTLA-4 in mouse B-1a cells and showed that deletion of CTLA-4 from B cells results in mice that develop AAbs, T follicular helper (Tfh) cells, germinal centers in the spleen, and autoimmune outcomes. This impaired immune homeostasis was demonstrated to be as a result of B cell dysfunction upon loss of CTLA-4 (83). This aligns with earlier studies implicating CTLA-4 in the control of B cell responses and antibody production by modulating T follicular helper (Tfh) cells (84). A study published in 2014 by Sage et al. (84) showed that, a deletion or dysfunction of CTLA-4 in mice increased Tfh and T follicular regulatory (Tfr) cell numbers with improved B cell responses. The loss of CTLA-4 on the Tfh cells increased B cell responses, whereas loss of CTLA-4 on Tfr cells resulted in increased antigen-specific antibody responses.
Thibult et al. (85), also demonstrated the expression of PD-1 on major human B-cells subsets. They showed that blockade of the PD-1 pathways increased the proliferation and activation of B cells and the production of inflammatory cytokines. Indeed, since its discovery, PD-1 has been shown to play a role in the negative regulation of B cell proliferation and differentiation (86).
In patients with melanoma treated with combination ICI therapy, changes in B cell populations was shown to increase the likelihood of irAEs (87). An overall decrease in circulatory B cells and an increase in plasmablasts and CD21lo PD-1+ B cells was observed (87). Another study also reported an enhanced increase in circulating plasmablasts in cancer patients on anti-CTLA-4 (88) who experienced various autoimmune outcomes. In this study, sequencing of immunoglobulins produced by the plasmablasts showed evidence of somatic hypermutation, clonal expansion and class switching. In humans with germline mutations in CTLA-4 who experienced autoimmune disease, increases in CD21lo B cells were also found in circulation with an overall decrease in total B cells (89).
The recognition of PD-1 and CTLA-4 as regulators of B cell activation provide clues as to how ICIs may affect the balance of B cells and AAb production in the occurrence of irAEs.
Generally, an ideal biomarker of irAEs will need to be highly sensitive, specific, and precise to distinguish between toxicity groups. It should be robust for accurate prediction, allowing for early irAE diagnosis, determining severity and risk prior to therapy. Predicting the onset of toxicities prior to therapy has become an important clinical need to support patient selection and personalized monitoring. However, history of autoimmune disease remains the clinical risk stratification parameter, for ICI toxicities in cancer patients. Although other routine clinical laboratory assays, that assesses blood enzymes and hormones, may be useful, they often reflect end organ-toxicity, and may not provide predictive value. Here, we provide a summary of the extent of research on AAbs and B cells as toxicity biomarkers in cancer.
Antibodies have been found that recognize several tumor and self-antigens, including overexpressed or aberrantly expressed antigens, modified proteins and intracellular molecules, and neoantigens (originating from mutations and alternative splicing events) that can drive humoral responses (66). Serum AAb levels against tumor-associated and self-antigens have been proposed as biomarkers for cancer detection (90), prognosis (91), ICI response and toxicities (reviewed here, Table 1).
For example, in a group of 5 NSCLC patients undergoing treatment with nivolumab, Maekura et al. (92) identified baseline levels of anti-thyroid peroxidase (TPO) and anti-thyroglobulin (Tg) that were positive for the occurrence of hypothyroidism. Similar findings were recorded by Kimbara et al. (93) in melanoma and NSCLC patients, and Kurimoto et al. (98) in various cancer patients on anti-CTLA-4, anti-PD-1 and anti-CTLA-1/PD-1 combination therapy. Another study conducted by Toi et al. (70) also identified pre-existing rheumatological antibodies particularly, rheumatoid factor (RF), antinuclear antibodies (ANA), anti-Tg and anti-TPO, that were independently associated with various irAEs in a multivariate analysis. In this study, skin and thyroid disorders were frequently found in patients with pre-existing RF and antithyroid antibodies respectively. Zhang et al. (102) also found an association between pre-existing ANAs (at a titer ≥1:320) and various irAEs, and an increase in adverse skin reactions among ANA-positive patients compared to negative patients.
Campochiaro et al. (105), examined a panel of rheumatological AAbs prior to glucocorticoid use and at follow-up in a group of cancer patients, who presented with rheumatic irAEs following ICI therapy. In this study, AAb positivity was the only factor that was associated with the need for an add-on therapy (immunosuppressants) during follow-up. By considering the requirement of an add-on therapy as a surrogate for disease severity, the findings of this study suggested the utility of rheumatological AAbs to predict disease severity or a high disease activity, even though none of the patients were tested for AAbs prior to the start of ICI therapy.
At the time of this review, only one retrospective study in a small group of cancer patients had demonstrated the diagnostic performance (accuracy, sensitivity and specificity) of a classical rheumatological AAb battery to predict toxicities (100). Despite the study limitation of small sample size, classical AAbs composed of ANA, antineutrophil cytoplasmic antibody (ANCA), RF and antithyroid antibodies (ATA) measured after first dose of anti-PD-1(nivolumab), showed a diagnostic accuracy of 80.8% with a sensitivity and specificity of 87.5 and 70% for toxicity prediction (100).
While these findings may be encouraging, conflicting results exist in the utility of prototypical rheumatological AAbs as predictors of irAEs. Ghosh et al. (101) examined the expression of several rheumatological AAbs (ANA, RF and anti-CCP (cyclic citrullinated peptide)) among advanced melanoma patients on combination (ipilimumab/nivolumab) therapy using a customized microarray at baseline and 6 weeks during therapy. Although AAb levels increased after 6 weeks of treatment in patients with irAEs, baseline levels of ANA, RF and anti-CCP, were not predictive of specific irAEs. ANA, RF and anti-CCP did not show any significant difference (p = 0.13) between serological negative and positive patients for irAE development, onset, severity or survival (101). Similarly, although De Moel et al. (106) also recorded an increase in rheumatologic AAbs from baseline in late-stage melanoma patients (who were antibody negative pre-treatment), no association with any irAEs was observed. Several reports have also found no significant association between pre-existing ANAs in various cancer patients prior to ICI therapy, with the occurrence of irAEs (97, 99, 103, 104). Despite the temporal resemblance of classic autoimmune diseases with irAEs, it appears that their mechanisms might be different and whether an increase or decrease in classic rheumatological AAbs is beneficial for the prediction of irAEs remains controversial and requires further investigation.
Several other studies involving various analytical approaches have identified antibody targets that correlate with irAEs. Duarte et al. (71) identified an increase in a repertoire of AAb targets in a cohort of melanoma patients on ipilimumab, that correlated with the occurrence of irAEs. Interestingly, increase in serum AAbs from baseline, preceded the development of irAEs. Using a high throughput protein microarray, Gowen et al. (94) also identified differential pre-treatment AAb proteomic profiles that predicted the occurrence of irAEs among late-stage melanoma patients with an accuracy of >90%. Findings from this study provided further evidence to suggest the existence of a subclinical autoimmune profile in a subset of ICI-treated patients, that renders them susceptible to the development of irAEs. Other case observations and organ related AAb targets have been identified that correlate with irAEs. Tahir et al. (96) screened baseline plasma samples from various cancer patients who developed ICI-induced hypophysitis and pneumonitis against a cDNA expression library of the brain and lungs. The authors identified increased pre-treatment anti-guanine nucleotide-binding protein G subunit alpha (anti-GNAL) which was associated with hypophysitis (OR = 2.66; 95% CI 1,14-7.29 p = 0.02, AUC = 0.79), and anti-CD74 levels with pneumonitis (OR = 1.25; 95% CI 1.03-1.52 p = 0.03, AUC = 0.76). GNAL has been shown to play a significant role in the activation of the cAMP signaling pathway; a pathway important in cell proliferation, hormone synthesis and secretion in the pituitary (107).
As observed in other studies (98, 101, 106), an increase in fold change expression of both GNAL and CD74 from pre-treatment to post treatment was recorded, which discriminated toxicity groups (healthy vs irAEs) with an AUC = 1 for both markers. In addition, post treatment levels of both markers (i.e anti-GNAL and anti-CD74) discriminated toxicity groups with an AUC of 0.92 and 0.95 respectively. Hassan et al. (95) also demonstrated the co-expression of the B cell targeted antigen bullous pemphigoid 180 (BP180) between healthy skin and NSCLC tissues and identified elevated anti-BP180 IgG at baseline that correlated with skin toxicities (p = 0.04), therapy response (p = 0.01) and overall survival (p = 0.04). While the exact roles of toxicity-associated antibodies remain elusive, a subset of AAbs may be involved in irAEs, and their measurements could be useful for the prediction of ICI toxicities.
Recent work on the role of B cells have led to the recognition of their importance in the immune surveillance process and as cancer biomarkers (108–110). While research is ongoing on the exact roles of B cells in the tumor environment, several studies have demonstrated their potential as biomarkers of response and survival in various cancers (111–114). Based on various user-defined immune phenotypes (markers), various B cell subsets including activated B cells, germinal center B cells, plasmablasts, plasma cells, transitional and memory B cells have been found in cancer patients treated with ICIs with various predictive roles (108, 112, 115).
Recently, Barth et al. (116) identified an increase in peripheral switched memory B-cells which was associated with reduced odds for disease control rate (DCR) (OR = 0.06, 95% CI = 0.01-0.70, p = 0.025) and an increase in naïve B-cells which was associated with an improved DCR (OR =12.31, 95% CI = 1.13-134.22, p =0.039) in cancer patients on ICI treatments.
Despite the progress made in the understanding of the B cell role in cancer and as predictive biomarkers, studies on the role of B cells as biomarkers of toxicities may be lacking. At the time of this review, one study by Das et al. (87) had reported an association between ICI-induced changes in circulating B cells and high grade irAEs (87). In this study, circulating B cells were analyzed in 39 advanced melanoma patients before and after first cycle of treatment with anti PD-1, anti-CTLA4 or combination therapy. They found a reduction in total circulating B cells (mean fold change, 0.7; p ≤ 0.0001) after one cycle of combination therapy (anti-PD-1/anti-CTLA-4) together with enrichment of plasmablasts (CD19+CD27+CD38h), an increase in plasma CXCL13, and CD21lo PD-1+ B cell subset (enriched in PD-1 expression). CD21lo PD-1+ B cells also expressed CXCR4 and CXCR5 at lower levels compared to CD21hi B cells. Although B cell decline was not recorded in monotherapy (anti-PD-1 and anti-CTLA-4), combination therapy induced changes preceded and correlated with the frequency, timing and maximum grade of toxicity. Combination therapy also resulted in a proliferation of CD4+ and CD8+ T cells in this study. However, no correlation with risk of irAEs was observed. A similar increase in CD21lo B cells after the first cycle of combination therapy, was reported in 23 renal cell carcinoma patients who experienced irAEs (117). Liver cancer patients treated with anti-PD-1 and tyrosine kinase inhibitors also showed decrease B cells in severe irAE patients (118).
A longitudinal assessment of blood in an advanced lung cancer patient who developed late-onset irAEs on PD-1 inhibition, also reported increases in plasmablasts in circulation (119). Using functional ex vivo assays and mass cytometry analysis, defects in the regulatory B cell repertoire was found to predispose NSCLC patients to the development of immune related toxicity following anti-PD-1(L1) blockade (120). Notably, an attenuated presence of circulatory immunosuppressive B cell subsets (IL-10+, TGF-B+ PDL-1+) was observed in patients that developed toxicities compared to those that did not. Overall, while there is some evidence to support the utility of circulatory B cells as biomarkers of toxicity, more studies need to be conducted to address issues such as the small sample sizes, and to investigate whether B cell changes occur for specific ICI therapy for toxicity prediction. Other studies could also address the dynamics of circulatory and tumor infiltrated B cells as toxicity biomarkers and the mechanistic understanding of these changes.
One important consideration in any further development of AAbs and B cells as toxicity biomarkers is the need to validate the emergence of potential markers. Currently, many interesting studies have sought to assess differential features among cancer patients with toxicities and those without with encouraging discriminatory benefit. However, this potential has since not been validated for use in the clinical settings. Increased funding for well-developed clinical validation studies will need to be considered to advance this field of research. Also, considering the occurrence of delayed onset irAEs (i.e irAEs that occur > 6-12 months following ICI treatment) (121, 122), there is a limitation of a true control group, to test differential expression of AAb markers in patients (101). Hence, longitudinal studies or landmark analyses will need to be considered in any further studies for a better discrimination of toxicity groups.
High grade irAEs (grade 3-5) present with increased complications as compared to grade 1 and 2 toxicities. Therefore, biomarker discovery studies should be preferably towards markers associated with and of clinical significance for these toxicities. Future studies will need to be well powered or sub-analyzed, with a focus on identifying AAb biomarkers for higher grade irAEs. Currently, it is unlikely that one single AAb marker will be specific or sensitive enough to accurately predict irAEs. Single targets do not possess the sensitivity and specificity to be used effectively as screening biomarkers (123). Fortunately, the emergence of novel proteome microarrays such as the Huprot™ microarray, allow for the detection of a large number of AAb targets at the same time, covering about 81% of the human proteome. By leveraging these technologies, differences in circulating proteins can be identified and validated using multiplex assays or focused arrays for toxicity prediction.
The application of artificial intelligence and robust statistical analysis will also be critical to address the complexity of data generated particularly from proteomics as these techniques have been very useful in the prediction of various diseases (124). AAb markers for anti-PD-1(L1) related irAEs may also be different from anti-CTLA-4 markers. The continuous appreciation of the diverse mechanism of PD-1(L1) and CTLA-4 inhibition in antitumor activity and toxicities, provides enough justification for the biological possibility of this suggestion (125, 126). An impetus for further future direction has been provided by Gowen et al. (94) who reported differential expression of AAb markers of toxicity among patients on anti-PD-1, anti-CTLA-4 and combination therapy.
Many cancer patients that undergo treatments with ICIs may have received prior treatments such as with chemotherapy or radiation therapy which can affect B cells (127, 128). For example, a longitudinal assessment of B cell populations in patients with solid malignancies showed decreases in total and specific B-cell subsets following chemotherapy (128). Hence, studies assessing B cell changes as biomarkers of toxicity will need to consider the possible effects of these prior therapies. While studies on the effect of prior therapies on the risk of irAEs in cancer patients treated with ICIs remain scarce, the sequential treatment of cancer patients with ICIs (3 months following radiotherapy), showed no increased risk for the occurrence of irAEs (129). Whether these therapies can affect B cells and AAb changes for the prediction of ICI-toxicities, will need to be well studied.
It is also possible that organs involved in irAEs may express organ specific AAbs, and therefore sufficiently powered studies in these separate populations should be encouraged. Furthermore, whether AAbs are functionally linked to the development of irAEs or are just bystanders also needs to be determined.
Finally, assessing the kinetics of AAb titers and B cells as a diagnostic adjunct for patients suspected of irAEs, would help clinicians determine whether to continue or hold treatments following irAE onset. B cells and serum AAbs have clinical applications in this view and studies will need to be performed to test this approach. More studies will also be required to identify B cell alterations and to understand the mechanisms of B cell hyperactivity and tolerance imbalance for toxicity prediction.
In conclusion, given the advances in the research in this field, there is some air of optimism regarding the identification of AAbs and B cells for the prediction and monitoring of irAEs. To achieve this, large clinical studies are required to determine patients who can maximize the benefits of ICIs while minimizing toxicities. There is also the opportunity to assist with clinical surveillance in order to avoid the occurrence of severe, potentially life-threatening side effects. In the future, the focus should be on the development and validation of AAb and B cell biomarkers that are capable of translation into the clinical setting.
JT, PZ and EG conceived the idea of this review. JT wrote the draft. PZ, EG and AG reviewed the manuscript and provided insights. All authors contributed to the article and approved the submitted version.
JT is supported by the ECU Vice chancellor research fellow PhD scholarship. PZ is supported by the ECU Vice chancellor research fellowship. EG is supported by fellowships from the Cancer Council Western Australia. AG was supported by the Western Australia Cancer and Palliative Care Network (WACPCN) fellowship.
We would like to thank Professor Michael Millward for his insights on this work.
The authors declare that the research was conducted in the absence of any commercial or financial relationships that could be construed as a potential conflict of interest.
All claims expressed in this article are solely those of the authors and do not necessarily represent those of their affiliated organizations, or those of the publisher, the editors and the reviewers. Any product that may be evaluated in this article, or claim that may be made by its manufacturer, is not guaranteed or endorsed by the publisher.
1. Carlino MS, Larkin J, Long GV. Immune checkpoint inhibitors in melanoma. Lancet (2021) 398(10304):1002–14. doi: 10.1016/S0140-6736(21)01206-X
2. Weinmann SC, Pisetsky DS. Mechanisms of immune-related adverse events during the treatment of cancer with immune checkpoint inhibitors. Rheumatol Oxf Engl (2019) 58(Suppl 7):vii59–67. doi: 10.1093/rheumatology/kez308
3. Khan S, Gerber DE. Autoimmunity, checkpoint inhibitor therapy and immune-related adverse events: A review. Semin Cancer Biol (2020) 64:93–101. doi: 10.1016/j.semcancer.2019.06.012
4. Geisler AN, Phillips GS, Barrios DM, Wu J, Leung DYM, Moy AP, et al. Immune checkpoint inhibitor-related dermatologic adverse events. J Am Acad Dermatol (2020) 83(5):1255–68. doi: 10.1016/j.jaad.2020.03.132
5. Grover S, Rahma OE, Hashemi N, Lim RM. Gastrointestinal and hepatic toxicities of checkpoint inhibitors: Algorithms for management. Am Soc Clin Oncol Educ Book Am Soc Clin Oncol Annu Meet (2018) 38:13–9. doi: 10.1200/EDBK_100013
6. Joshi MN, Whitelaw BC, Palomar MTP, Wu Y, Carroll PV. Immune checkpoint inhibitor-related hypophysitis and endocrine dysfunction: Clinical review. Clin Endocrinol (Oxf) (2016) 85(3):331–9. doi: 10.1111/cen.13063
7. Calabrese LH, Calabrese C, Cappelli LC. Rheumatic immune-related adverse events from cancer immunotherapy. Nat Rev Rheumatol (2018) 14(10):569–79. doi: 10.1038/s41584-018-0074-9
8. Cortellini A, Vitale MG, De Galitiis F, Di Pietro FR, Berardi R, Torniai M, et al. Early fatigue in cancer patients receiving PD-1/PD-L1 checkpoint inhibitors: An insight from clinical practice. J Transl Med (2019) 17(1):376. doi: 10.1186/s12967-019-02132-x
9. Larkin J, Chiarion-Sileni V, Gonzalez R, Grob JJ, Rutkowski P, Lao CD, et al. Five-year survival with combined nivolumab and ipilimumab in advanced melanoma. N Engl J Med (2019) 381(16):1535–46. doi: 10.1056/NEJMoa1910836
10. Postow MA, Sidlow R, Hellmann MD. Immune-related adverse events associated with immune checkpoint blockade. N Engl J Med (2018) 378(2):158–68. doi: 10.1056/NEJMra1703481
11. Hommes JW, Verheijden RJ, Suijkerbuijk KPM, Hamann D. Biomarkers of checkpoint inhibitor induced immune-related adverse events-a comprehensive review. Front Oncol (2020) 10:585311. doi: 10.3389/fonc.2020.585311
12. Carreno BM, Bennett F, Chau TA, Ling V, Luxenberg D, Jussif J, et al. CTLA-4 (CD152) can inhibit T cell activation by two different mechanisms depending on its level of cell surface expression. J Immunol Baltim Md 1950 (2000) 165(3):1352–6. doi: 10.4049/jimmunol.165.3.1352
13. Sansom DM. CD28, CTLA-4 and their ligands: Who does what and to whom? Immunology (2000) 101(2):169–77. doi: 10.1046/j.1365-2567.2000.00121.x
14. Zhang Z, Liu S, Zhang B, Qiao L, Zhang Y, Zhang Y. T Cell dysfunction and exhaustion in cancer. Front Cell Dev Biol (2020) 8:17. doi: 10.3389/fcell.2020.00017
15. Johnson DB, Nebhan CA, Moslehi JJ, Balko JM. Immune-checkpoint inhibitors: Long-term implications of toxicity. Nat Rev Clin Oncol (2022) 19(4):254–67. doi: 10.1038/s41571-022-00600-w
16. Iwama S, De Remigis A, Callahan MK, Slovin SF, Wolchok JD, Caturegli P. Pituitary expression of CTLA-4 mediates hypophysitis secondary to administration of CTLA-4 blocking antibody. Sci Transl Med (2014) 6(230):230ra45. doi: 10.1126/scitranslmed.3008002
17. Caturegli P, Di Dalmazi G, Lombardi M, Grosso F, Larman HB, Larman T, et al. Hypophysitis secondary to cytotoxic T-Lymphocyte-Associated protein 4 blockade: Insights into pathogenesis from an autopsy series. Am J Pathol (2016) 186(12):3225–35. doi: 10.1016/j.ajpath.2016.08.020
18. Franco F, Jaccard A, Romero P, Yu YR, Ho PC. Metabolic and epigenetic regulation of T-cell exhaustion. Nat Metab (2020) 2(10):1001–12. doi: 10.1038/s42255-020-00280-9
19. Jiang W, He Y, He W, Wu G, Zhou X, Sheng Q, et al. Exhausted CD8+T cells in the tumor immune microenvironment: New pathways to therapy. Front Immunol (2020) 11:622509. doi: 10.3389/fimmu.2020.622509
20. Wucherpfennig KW, Sethi D. T Cell receptor recognition of self and foreign antigens in the induction of autoimmunity. Semin Immunol (2011) 23(2):84–91. doi: 10.1016/j.smim.2011.01.007
21. Petrova G, Ferrante A, Gorski J. Cross-reactivity of T cells and its role in the immune system. Crit Rev Immunol (2012) 32(4):349–72. doi: 10.1615/CritRevImmunol.v32.i4.50
22. Slansky JE, Nakayama M. Peptide mimotopes alter T cell function in cancer and autoimmunity. Semin Immunol (2020) 47:101395. doi: 10.1016/j.smim.2020.101395
23. Wooldridge L, Ekeruche-Makinde J, van den Berg HA, Skowera A, Miles JJ, Tan MP, et al. A single autoimmune T cell receptor recognizes more than a million different peptides. J Biol Chem (2012) 287(2):1168–77. doi: 10.1074/jbc.M111.289488
24. Michot JM, Bigenwald C, Champiat S, Collins M, Carbonnel F, Postel-Vinay S, et al. Immune-related adverse events with immune checkpoint blockade: a comprehensive review. Eur J Cancer Oxf Engl 1990 (2016) 54:139–48. doi: 10.1016/j.ejca.2015.11.016
25. Johnson DB, Balko JM, Compton ML, Chalkias S, Gorham J, Xu Y, et al. Fulminant myocarditis with combination immune checkpoint blockade. N Engl J Med (2016) 375(18):1749–55. doi: 10.1056/NEJMoa1609214
26. Berner F, Bomze D, Diem S, Ali OH, Fässler M, Ring S, et al. Association of checkpoint inhibitor-induced toxic effects with shared cancer and tissue antigens in non-small cell lung cancer. JAMA Oncol (2019) 5(7):1043–7. doi: 10.1001/jamaoncol.2019.0402
27. Larsabal M, Marti A, Jacquemin C, Rambert J, Thiolat D, Dousset L, et al. Vitiligo-like lesions occurring in patients receiving anti-programmed cell death-1 therapies are clinically and biologically distinct from vitiligo. J Am Acad Dermatol (2017) 76(5):863–70. doi: 10.1016/j.jaad.2016.10.044
28. Darnell RB, Posner JB. Paraneoplastic syndromes involving the nervous system. N Engl J Med (2003) 349(16):1543–54. doi: 10.1056/NEJMra023009
29. Graus F, Dalmau J. Paraneoplastic neurological syndromes in the era of immune-checkpoint inhibitors. Nat Rev Clin Oncol (2019) 16(9):535–48. doi: 10.1038/s41571-019-0194-4
30. Yshii LM, Gebauer CM, Pignolet B, Mauré E, Quériault C, Pierau M, et al. CTLA4 blockade elicits paraneoplastic neurological disease in a mouse model. Brain J Neurol (2016) 139(11):2923–34. doi: 10.1093/brain/aww225
31. Vanderlugt CL, Miller SD. Epitope spreading in immune-mediated diseases: implications for immunotherapy. Nat Rev Immunol (2002) 2(2):85–95. doi: 10.1038/nri724
32. Kwek SS, Dao V, Roy R, Hou Y, Alajajian D, Simko JP, et al. Diversity of antigen-specific responses induced in vivo with CTLA-4 blockade in prostate cancer patients. J Immunol Baltim Md 1950 (2012) 189(7):3759–66. doi: 10.4049/jimmunol.1201529
33. Robert L, Tsoi J, Wang X, Emerson R, Homet B, Chodon T, et al. CTLA4 blockade broadens the peripheral T-cell receptor repertoire. Clin Cancer Res Off J Am Assoc Cancer Res (2014) 20(9):2424–32. doi: 10.1158/1078-0432.CCR-13-2648
34. Oh DY, Cham J, Zhang L, Fong G, Kwek SS, Klinger M, et al. Immune toxicities elicted by CTLA-4 blockade in cancer patients are associated with early diversification of the T-cell repertoire. Cancer Res (2017) 77(6):1322–30. doi: 10.1158/0008-5472.CAN-16-2324
35. Vignali DAA, Collison LW, Workman CJ. How regulatory T cells work. Nat Rev Immunol (2008) 8(7):523–32. doi: 10.1038/nri2343
36. Romano E, Kusio-Kobialka M, Foukas PG, Baumgaertner P, Meyer C, Ballabeni P, et al. Ipilimumab-dependent cell-mediated cytotoxicity of regulatory T cells ex vivo by nonclassical monocytes in melanoma patients. Proc Natl Acad Sci U.S.A. (2015) 112(19):6140–5. doi: 10.1073/pnas.1417320112
37. Selby MJ, Engelhardt JJ, Quigley M, Henning KA, Chen T, Srinivasan M, et al. Anti-CTLA-4 antibodies of IgG2a isotype enhance antitumor activity through reduction of intratumoral regulatory T cells. Cancer Immunol Res (2013) 1(1):32–42. doi: 10.1158/2326-6066.CIR-13-0013
38. Buckner JH. Mechanisms of impaired regulation by CD4(+)CD25(+)FOXP3(+) regulatory T cells in human autoimmune diseases. Nat Rev Immunol (2010) 10(12):849–59. doi: 10.1038/nri2889
39. Jacobs JFM, Nierkens S, Figdor CG, de Vries IJM, Adema GJ. Regulatory T cells in melanoma: The final hurdle towards effective immunotherapy? Lancet Oncol (2012) 13(1):e32–42. doi: 10.1016/S1470-2045(11)70155-3
40. de Coaña YP, Poschke I, Gentilcore G, Mao Y, Nyström M, Hansson J, et al. Ipilimumab treatment results in an early decrease in the frequency of circulating granulocytic myeloid-derived suppressor cells as well as their Arginase1 production. Cancer Immunol Res (2013) 1(3):158–62. doi: 10.1158/2326-6066.CIR-13-0016
41. Sharma A, Subudhi SK, Blando J, Scutti J, Vence L, Wargo J, et al. Anti-CTLA-4 immunotherapy does not deplete FOXP3+ regulatory T cells (Tregs) in human cancers. Clin Cancer Res Off J Am Assoc Cancer Res (2019) 25(4):1233–8. doi: 10.1158/1078-0432.CCR-18-0762
42. Gianchecchi E, Fierabracci A. Inhibitory receptors and pathways of lymphocytes: The role of PD-1 in treg development and their involvement in autoimmunity onset and cancer progression. Front Immunol (2018) 9:2374. doi: 10.3389/fimmu.2018.02374
43. Fife BT, Pauken KE. The role of the PD-1 pathway in autoimmunity and peripheral tolerance. Ann N Y Acad Sci (2011) 1217:45–59. doi: 10.1111/j.1749-6632.2010.05919.x
44. Harlin H, Meng Y, Peterson AC, Zha Y, Tretiakova M, Slingluff C, et al. Chemokine expression in melanoma metastases associated with CD8+ T-cell recruitment. Cancer Res (2009) 69(7):3077–85. doi: 10.1158/0008-5472.CAN-08-2281
45. Peng W, Liu C, Xu C, Lou Y, Chen J, Yang Y, et al. PD-1 blockade enhances T-cell migration to tumors by elevating IFN-γ inducible chemokines. Cancer Res (2012) 72(20):5209–18. doi: 10.1158/0008-5472.CAN-12-1187
46. Kang JH, Bluestone JA, Young A. Predicting and preventing immune checkpoint inhibitor toxicity: Targeting cytokines. Trends Immunol (2021) 42(4):293–311. doi: 10.1016/j.it.2021.02.006
47. Li B, Jones LL, Geiger TL. IL-6 promotes T cell proliferation and expansion under inflammatory conditions in association with low-level RORγt expression. J Immunol Baltim Md 1950 (2018) 201(10):2934–46. doi: 10.4049/jimmunol.1800016
48. Maeda K, Mehta H, Drevets DA, Coggeshall KM. IL-6 increases b-cell IgG production in a feed-forward proinflammatory mechanism to skew hematopoiesis and elevate myeloid production. Blood (2010) 115(23):4699–706. doi: 10.1182/blood-2009-07-230631
49. Kang S, Tanaka T, Narazaki M, Kishimoto T. Targeting interleukin-6 signaling in clinic. Immunity (2019) 50(4):1007–23. doi: 10.1016/j.immuni.2019.03.026
50. Tarhini AA, Zahoor H, Lin Y, Malhotra U, Sander C, Butterfield LH, et al. Baseline circulating IL-17 predicts toxicity while TGF-β1 and IL-10 are prognostic of relapse in ipilimumab neoadjuvant therapy of melanoma. J Immunother Cancer (2015) 3:39. doi: 10.1186/s40425-015-0081-1
51. von Euw E, Chodon T, Attar N, Jalil J, Koya RC, Comin-Anduix B, et al. CTLA4 blockade increases Th17 cells in patients with metastatic melanoma. J Transl Med (2009) 7:35. doi: 10.1186/1479-5876-7-35
52. Weaver CT, Elson CO, Fouser LA, Kolls JK. The Th17 pathway and inflammatory diseases of the intestines, lungs and skin. Annu Rev Pathol (2013) 8:477–512. doi: 10.1146/annurev-pathol-011110-130318
53. Lim SY, Lee JH, Gide TN, Menzies AM, Guminski A, Carlino MS, et al. Circulating cytokines predict immune-related toxicity in melanoma patients receiving anti-PD-1-Based immunotherapy. Clin Cancer Res Off J Am Assoc Cancer Res (2019) 25(5):1557–63. doi: 10.1158/1078-0432.CCR-18-2795
54. Khan S, Khan SA, Luo X, Fattah FJ, Saltarski J, Gloria-McCutchen Y, et al. Immune dysregulation in cancer patients developing immune-related adverse events. Br J Cancer (2019) 120(1):63–8. doi: 10.1038/s41416-018-0155-1
55. Valpione S, Pasquali S, Campana LG, Piccin L, Mocellin S, Pigozzo J, et al. Sex and interleukin-6 are prognostic factors for autoimmune toxicity following treatment with anti-CTLA4 blockade. J Transl Med (2018) 16(1):94. doi: 10.1186/s12967-018-1467-x
56. Tanaka R, Okiyama N, Okune M, Ishitsuka Y, Watanabe R, Furuta J, et al. Serum level of interleukin-6 is increased in nivolumab-associated psoriasiform dermatitis and tumor necrosis factor-α is a biomarker of nivolumab recativity. J Dermatol Sci (2017) 86(1):71–3. doi: 10.1016/j.jdermsci.2016.12.019
57. Chaput N, Lepage P, Coutzac C, Soularue E, Le Roux K, Monot C, et al. Baseline gut microbiota predicts clinical response and colitis in metastatic melanoma patients treated with ipilimumab. Ann Oncol Off J Eur Soc Med Oncol (2019) 30(12):2012. doi: 10.1093/annonc/mdz224
58. Yoshino K, Nakayama T, Ito A, Sato E, Kitano S. Severe colitis after PD-1 blockade with nivolumab in advanced melanoma patients: potential role of Th1-dominant immune response in immune-related adverse events: Two case reports. BMC Cancer (2019) 19(1):1019. doi: 10.1186/s12885-019-6138-7
59. Stroud CR, Hegde A, Cherry C, Naqash AR, Sharma N, Addepalli S, et al. Tocilizumab for the management of immune mediated adverse events secondary to PD-1 blockade. J Oncol Pharm Pract Off Publ Int Soc Oncol Pharm Pract (2019) 25(3):551–7. doi: 10.1177/1078155217745144
60. Horisberger A, La Rosa S, Zurcher JP, Zimmermann S, Spertini F, Coukos G, et al. A severe case of refractory esophageal stenosis induced by nivolumab and responding to tocilizumab therapy. J Immunother Cancer (2018) 6(1):156. doi: 10.1186/s40425-018-0481-0
61. Lechner MG, Cheng MI, Patel AY, Hoang AT, Yakobian N, Astourian M, et al. Inhibition of IL-17A protects against thyroid immune-related adverse events while preserving checkpoint inhibitor antitumor efficacy. J Immunol Baltim Md 1950 (2022) 209(4):696–709. doi: 10.4049/jimmunol.2200244
62. Andrews MC, Duong CPM, Gopalakrishnan V, Iebba V, Chen WS, Derosa L, et al. Gut microbiota signatures are associated with toxicity to combined CTLA-4 and PD-1 blockade. Nat Med (2021) 27(8):1432–41. doi: 10.1038/s41591-021-01406-6
63. Clavel T, Gomes-Neto JC, Lagkouvardos I, Ramer-Tait AE. Deciphering interactions between the gut microbiota and the immune system via microbial cultivation and minimal microbiomes. Immunol Rev (2017) 279(1):8–22. doi: 10.1111/imr.12578
64. Dubin K, Callahan MK, Ren B, Khanin R, Viale A, Ling L, et al. Intestinal microbiome analyses identify melanoma patients at risk for checkpoint-blockade-induced colitis. Nat Commun (2016) 7:10391. doi: 10.1038/ncomms10391
65. Wang Y, Wiesnoski DH, Helmink BA, Gopalakrishnan V, Choi K, DuPont HL, et al. Fecal microbiota transplantation for refractory immune checkpoint inhibitor-associated colitis. Nat Med (2018) 24(12):1804–8. doi: 10.1038/s41591-018-0238-9
66. Zaenker P, Gray ES, Ziman MR. Autoantibody production in cancer–the humoral immune response toward autologous antigens in cancer patients. Autoimmun Rev (2016) 15(5):477–83. doi: 10.1016/j.autrev.2016.01.017
67. Ludwig RJ, Vanhoorelbeke K, Leypoldt F, Kaya Z, Bieber K, McLachlan SM, et al. Mechanisms of autoantibody-induced pathology. Front Immunol (2017) 8:603. doi: 10.3389/fimmu.2017.00603
68. Ando T, Latif R, Davies TF. Thyrotropin receptor antibodies: new insights into their actions and clinical relevance. Best Pract Res Clin Endocrinol Metab (2005) 19(1):33–52. doi: 10.1016/j.beem.2004.11.005
69. Osorio JC, Ni A, Chaft JE, Pollina R, Kasler MK, Stephens D, et al. Antibody-mediated thyroid dysfunction during T-cell checkpoint blockade in patients with non-small-cell lung cancer. Ann Oncol Off J Eur Soc Med Oncol (2017) 28(3):583–9. doi: 10.1093/annonc/mdw640
70. Toi Y, Sugawara S, Sugisaka J, Ono H, Kawashima Y, Aiba T, et al. Profiling preexisting antibodies in patients treated with anti-PD-1 therapy for advanced non-small cell lung cancer. JAMA Oncol (2019) 5(3):376–83. doi: 10.1001/jamaoncol.2018.5860
71. Da Gama Duarte J, Parakh S, Andrews MC, Woods K, Pasam A, Tutuka C, et al. Autoantibodies may predict immune-related toxicity: Results from a phase I study of intralesional bacillus calmette–guérin followed by ipilimumab in patients with advanced metastatic melanoma. Front Immunol (2018) 9:411. doi: 10.3389/fimmu.2018.00411
72. Mammen AL, Rajan A, Pak K, Lehky T, Casciola-Rosen L, Donahue RN, et al. Pre-existing antiacetylcholine receptor autoantibodies and b cell lymphopaenia are associated with the development of myositis in patients with thymoma treated with avelumab, an immune checkpoint inhibitor targeting programmed death-ligand 1. Ann Rheum Dis (2019) 78(1):150–2. doi: 10.1136/annrheumdis-2018-213777
73. Leonardi GC, Gainor JF, Altan M, Kravets S, Dahlberg SE, Gedmintas L, et al. Safety of programmed death-1 pathway inhibitors among patients with non-Small-Cell lung cancer and preexisting autoimmune disorders. J Clin Oncol Off J Am Soc Clin Oncol (2018) 36(19):1905–12. doi: 10.1200/JCO.2017.77.0305
74. Brown LJ, Weppler A, Bhave P, Allayous C, Patrinely JR, Ott P, et al. Combination anti-PD1 and ipilimumab therapy in patients with advanced melanoma and pre-existing autoimmune disorders. J Immunother Cancer (2021) 9(5):e002121. doi: 10.1136/jitc-2020-002121
75. Abdel-Wahab N, Shah M, Lopez-Olivo MA, Suarez-Almazor ME. Use of immune checkpoint inhibitors in the treatment of patients with cancer and preexisting autoimmune disease: A systematic review. Ann Intern Med (2018) 168(2):121–30. doi: 10.7326/M17-2073
76. Menzies AM, Johnson DB, Ramanujam S, Atkinson VG, Wong ANM, Park JJ, et al. Anti-PD-1 therapy in patients with advanced melanoma and preexisting autoimmune disorders or major toxicity with ipilimumab. Ann Oncol Off J Eur Soc Med Oncol (2017) 28(2):368–76. doi: 10.1093/annonc/mdw443
77. Hofmann K, Clauder AK, Manz RA. Targeting b cells and plasma cells in autoimmune diseases. Front Immunol (2018) 9:835. doi: 10.3389/fimmu.2018.00835
78. Brahmer JR, Lacchetti C, Schneider BJ, Atkins MB, Brassil KJ, Caterino JM, et al. Management of immune-related adverse events in patients treated with immune checkpoint inhibitor therapy: American society of clinical oncology clinical practice guideline. J Clin Oncol Off J Am Soc Clin Oncol (2018) 36(17):1714–68. doi: 10.1200/JCO.2017.77.6385
79. Zaenker P, Prentice D, Ziman M. Tropomyosin autoantibodies associated with checkpoint inhibitor myositis. Oncoimmunology (2020) 9(1):1804703. doi: 10.1080/2162402X.2020.1804703
80. Monteiro RC. Role of IgA and IgA fc receptors in inflammation. J Clin Immunol (2010) 30(1):1–9. doi: 10.1007/s10875-009-9338-0
81. Ben Mkaddem S, Rossato E, Heming N, Monteiro RC. Anti-inflammatory role of the IgA fc receptor (CD89): From autoimmunity to therapeutic perspectives. Autoimmun Rev (2013) 12(6):666–9. doi: 10.1016/j.autrev.2012.10.011
82. Kuske M, Haist M, Jung T, Grabbe S, Bros M. Immunomodulatory properties of immune checkpoint inhibitors-more than boosting T-cell responses? Cancers (2022) 14(7):1710. doi: 10.3390/cancers14071710
83. Yang Y, Li X, Ma Z, Wang C, Yang Q, Byrne-Steele M, et al. CTLA-4 expression by b-1a b cells is essential for immune tolerance. Nat Commun (2021) 12(1):525. doi: 10.1038/s41467-020-20874-x
84. Sage PT, Paterson AM, Lovitch SB, Sharpe AH. The coinhibitory receptor CTLA-4 controls b cell responses by modulating T follicular helper, T follicular regulatory, and T regulatory cells. Immunity (2014) 41(6):1026–39. doi: 10.1016/j.immuni.2014.12.005
85. Thibult ML, Mamessier E, Gertner-Dardenne J, Pastor S, Just-Landi S, Xerri L, et al. PD-1 is a novel regulator of human b-cell activation. Int Immunol (2013) 25(2):129–37. doi: 10.1093/intimm/dxs098
86. Nishimura H, Minato N, Nakano T, Honjo T. Immunological studies on PD-1 deficient mice: Implication of PD-1 as a negative regulator for b cell responses. Int Immunol (1998) 10(10):1563–72. doi: 10.1093/intimm/10.10.1563
87. Das R, Bar N, Ferreira M, Newman AM, Zhang L, Bailur JK, et al. Early b cell changes predict autoimmunity following combination immune checkpoint blockade. J Clin Invest (2018) 128(2):715–20. doi: 10.1172/JCI96798
88. DeFalco J, Harbell M, Manning-Bog A, Baia G, Scholz A, Millare B, et al. Non-progressing cancer patients have persistent b cell responses expressing shared antibody paratopes that target public tumor antigens. Clin Immunol Orlando Fla (2018) 187:37–45. doi: 10.1016/j.clim.2017.10.002
89. Kuehn HS, Ouyang W, Lo B, Deenick EK, Niemela JE, Avery DT, et al. Immune dysregulation in human subjects with heterozygous germline mutations in CTLA4. Science (2014) 345(6204):1623–7. doi: 10.1126/science.1255904
90. Zaenker P, Lo J, Pearce R, Cantwell P, Cowell L, Lee M, et al. A diagnostic autoantibody signature for primary cutaneous melanoma. Oncotarget (2018) 9(55):30539–51. doi: 10.18632/oncotarget.25669
91. Sexauer D, Gray E, Zaenker P. Tumour- associated autoantibodies as prognostic cancer biomarkers- a review. Autoimmun Rev (2022) 21(4):103041. doi: 10.1016/j.autrev.2022.103041
92. Maekura T, Naito M, Tahara M, Ikegami N, Kimura Y, Sonobe S, et al. Predictive factors of nivolumab-induced hypothyroidism in patients with non-small cell lung cancer. In Vivo (2017) 31(5):1035–9. doi: 10.21873/invivo.11166
93. Kimbara S, Fujiwara Y, Iwama S, Ohashi K, Kuchiba A, Arima H, et al. Association of antithyroglobulin antibodies with the development of thyroid dysfunction induced by nivolumab. Cancer Sci (2018) 109(11):3583–90. doi: 10.1111/cas.13800
94. Gowen MF, Giles KM, Simpson D, Tchack J, Zhou H, Moran U, et al. Baseline antibody profiles predict toxicity in melanoma patients treated with immune checkpoint inhibitors. J Transl Med (2018) 16(1):82. doi: 10.1186/s12967-018-1452-4
95. Hasan Ali O, Bomze D, Ring SS, Berner F, Fässler M, Diem S, et al. BP180-specific IgG is associated with skin adverse events, therapy response, and overall survival in non-small cell lung cancer patients treated with checkpoint inhibitors. J Am Acad Dermatol (2020) 82(4):854–61. doi: 10.1016/j.jaad.2019.08.045
96. Tahir SA, Gao J, Miura Y, Blando J, Tidwell RSS, Zhao H, et al. Autoimmune antibodies correlate with immune checkpoint therapy-induced toxicities. Proc Natl Acad Sci (2019) 116(44):22246–51. doi: 10.1073/pnas.1908079116
97. Yoneshima Y, Tanaka K, Shiraishi Y, Hata K, Watanabe H, Harada T, et al. Safety and efficacy of PD-1 inhibitors in non-small cell lung cancer patients positive for antinuclear antibodies. Lung Cancer Amst Neth (2019) 130:5–9. doi: 10.1016/j.lungcan.2019.01.014
98. Kurimoto C, Inaba H, Ariyasu H, Iwakura H, Ueda Y, Uraki S, et al. Predictive and sensitive biomarkers for thyroid dysfunctions during treatment with immune-checkpoint inhibitors. Cancer Sci (2020) 111(5):1468–77. doi: 10.1111/cas.14363
99. Sakakida T, Ishikawa T, Chihara Y, Harita S, Uchino J, Tabuchi Y, et al. Safety and efficacy of PD-1/PD-L1 blockade in patients with preexisting antinuclear antibodies. Clin Transl Oncol Off Publ Fed Span Oncol Soc Natl Cancer Inst Mex (2020) 22(6):919–27. doi: 10.1007/s12094-019-02214-8
100. Les I, Martínez M, Narro A, Pérez I, Sánchez C, Puntí L, et al. Association of immune-related adverse events induced by nivolumab with a battery of autoantibodies. Ann Med (2021) 53(1):762–9. doi: 10.1080/07853890.2021.1931956
101. Ghosh N, Postow M, Zhu C, Jannat-Khah D, Li QZ, Vitone G, et al. Lower baseline autoantibody levels are associated with immune-related adverse events from immune checkpoint inhibition. J Immunother Cancer (2022) 10(1):e004008. doi: 10.1136/jitc-2021-004008
102. Zhang D, Shi Y, Liu X, Liu J, Xu Y, Zhao J, et al. Safety and efficacy of immune checkpoint inhibitors in non-small cell lung cancer patients with preexisting antinuclear antibodies: A retrospective cohort study. Transl Lung Cancer Res (2022) 11(7):1420–33. doi: 10.21037/tlcr-22-464
103. Tang H, Geng R, Xu X, Wang Y, Zhou J, Zhang S, et al. Safety and efficacy of PD-1/PD-L1 inhibitors in cancer patients with preexisting autoantibodies. Front Immunol (2022) 13:893179. doi: 10.3389/fimmu.2022.893179
104. Barth DA, Stanzer S, Spiegelberg J, Bauernhofer T, Absenger G, Posch F, et al. Evaluation of autoantibodies as predictors of treatment response and immune-related adverse events during the treatment with immune checkpoint inhibitors: A prospective longitudinal pan-cancer study. Cancer Med (2022) 11(16):3074–83. doi: 10.1002/cam4.4675
105. Campochiaro C, Farina N, Tomelleri A, Ferrara R, Viola S, Lazzari C, et al. Autoantibody positivity predicts severity of rheumatic immune-related adverse events to immune-checkpoint inhibitors. Eur J Intern Med (2022) S0953-6205(22):95–99. doi: 10.1016/j.ejim.2022.07.005
106. de Moel EC, Rozeman EA, Kapiteijn EH, Verdegaal EME, Grummels A, Bakker JA, et al. Autoantibody development under treatment with immune-checkpoint inhibitors. Cancer Immunol Res (2019) 7(1):6–11. doi: 10.1158/2326-6066.CIR-18-0245
107. Pertuit M, Barlier A, Enjalbert A, Gérard C. Signalling pathway alterations in pituitary adenomas: Involvement of gsalpha, cAMP and mitogen-activated protein kinases. J Neuroendocrinol (2009) 21(11):869–77. doi: 10.1111/j.1365-2826.2009.01910.x
108. Selitsky SR, Mose LE, Smith CC, Chai S, Hoadley KA, Dittmer DP, et al. Prognostic value of b cells in cutaneous melanoma. Genome Med (2019) 11(1):36. doi: 10.1186/s13073-019-0647-5
109. Germain C, Gnjatic S, Tamzalit F, Knockaert S, Remark R, Goc J, et al. Presence of b cells in tertiary lymphoid structures is associated with a protective immunity in patients with lung cancer. Am J Respir Crit Care Med (2014) 189(7):832–44. doi: 10.1164/rccm.201309-1611OC
110. Sautès-Fridman C, Petitprez F, Calderaro J, Fridman WH. Tertiary lymphoid structures in the era of cancer immunotherapy. Nat Rev Cancer (2019) 19(6):307–25. doi: 10.1038/s41568-019-0144-6
111. Helmink BA, Reddy SM, Gao J, Zhang S, Basar R, Thakur R, et al. B cells and tertiary lymphoid structures promote immunotherapy response. Nature (2020) 577(7791):549–55. doi: 10.1038/s41586-019-1922-8
112. Petitprez F, de Reyniès A, Keung EZ, Chen TWW, Sun CM, Calderaro J, et al. B cells are associated with survival and immunotherapy response in sarcoma. Nature (2020) 577(7791):556–60. doi: 10.1038/s41586-019-1906-8
113. Cabrita R, Lauss M, Sanna A, Donia M, Skaarup Larsen M, Mitra S, et al. Tertiary lymphoid structures improve immunotherapy and survival in melanoma. Nature (2020) 577(7791):561–5. doi: 10.1038/s41586-019-1914-8
114. Wouters MCA, Nelson BH. Prognostic significance of tumor-infiltrating b cells and plasma cells in human cancer. Clin Cancer Res Off J Am Assoc Cancer Res (2018) 24(24):6125–35. doi: 10.1158/1078-0432.CCR-18-1481
115. Griss J, Bauer W, Wagner C, Simon M, Chen M, Grabmeier-Pfistershammer K, et al. B cells sustain inflammation and predict response to immune checkpoint blockade in human melanoma. Nat Commun (2019) 10(1):4186. doi: 10.1038/s41467-019-12160-2
116. Barth DA, Stanzer S, Spiegelberg JA, Bauernhofer T, Absenger G, Szkandera J, et al. Patterns of peripheral blood b-cell subtypes are associated with treatment response in patients treated with immune checkpoint inhibitors: A prospective longitudinal pan-cancer study. Front Immunol (2022) 13:840207. doi: 10.3389/fimmu.2022.840207
117. Nishimura K, Konishi T, Ochi T, Watanabe R, Noda T, Fukumoto T, et al. CD21lo b cells could be a potential predictor of immune-related adverse events in renal cell carcinoma. J Pers Med (2022) 12(6):888. doi: 10.3390/jpm12060888
118. Yu Y, Wang S, Su N, Pan S, Tu B, Zhao J, et al. Increased circulating levels of CRP and IL-6 and decreased frequencies of T and b lymphocyte subsets are associated with immune-related adverse events during combination therapy with PD-1 inhibitors for liver cancer. Front Oncol (2022) 12:906824. doi: 10.3389/fonc.2022.906824
119. Gonugunta AS, von IMS, Mu-Mosley H, Fattah F, Farrar JD, Mobely A, et al. Humoral and cellular correlates of a novel immune-related adverse event and its treatment. J Immunother Cancer (2021) 9(12):e003585. doi: 10.1136/jitc-2021-003585
120. Patel AJ, Willsmore ZN, Khan N, Richter A, Naidu B, Drayson MT, et al. Regulatory b cell repertoire defects predispose lung cancer patients to immune-related toxicity following checkpoint blockade. Nat Commun (2022) 13(1):3148. doi: 10.1038/s41467-022-30863-x
121. Owen CN, Bai X, Quah T, Lo SN, Allayous C, Callaghan S, et al. Delayed immune-related adverse events with anti-PD-1-based immunotherapy in melanoma. Ann Oncol Off J Eur Soc Med Oncol (2021) 32(7):917–25. doi: 10.1016/j.annonc.2021.03.204
122. Khan S, von Itzstein MS, Lu R, Bermas BL, Karp DR, Khan SA, et al. Late-onset immunotherapy toxicity and delayed autoantibody changes: Checkpoint inhibitor-induced raynaud’s-like phenomenon. Oncologist (2020) 25(5):e753–7. doi: 10.1634/theoncologist.2019-0666
123. Pedersen JW, Wandall HH. Autoantibodies as biomarkers in cancer. Lab Med (2011) 42(10):623–8. doi: 10.1309/LM2T3OU3RZRTHKSN
124. Patel AJ, Tan TM, Richter AG, Naidu B, Blackburn JM, Middleton GW. A highly predictive autoantibody-based biomarker panel for prognosis in early-stage NSCLC with potential therapeutic implications. Br J Cancer (2022) 126(2):238–46. doi: 10.1038/s41416-021-01572-x
125. Wei SC, Anang NAAS, Sharma R, Andrews MC, Reuben A, Levine JH, et al. Combination anti-CTLA-4 plus anti-PD-1 checkpoint blockade utilizes cellular mechanisms partially distinct from monotherapies. Proc Natl Acad Sci U S A (2019) 116(45):22699–709. doi: 10.1073/pnas.1821218116
126. Wei SC, Levine JH, Cogdill AP, Zhao Y, Anang NAAS, Andrews MC, et al. Distinct cellular mechanisms underlie anti-CTLA-4 and anti-PD-1 checkpoint blockade. Cell (2017) 170(6):1120–33.e17. doi: 10.1016/j.cell.2017.07.024
127. Franiak-Pietryga I, Miyauchi S, Kim SS, Sanders PD, Sumner W, Zhang L, et al. Activated b cells and plasma cells are resistant to radiation therapy. Int J Radiat Oncol Biol Phys (2022) 112(2):514–28. doi: 10.1016/j.ijrobp.2021.08.037
128. Waidhauser J, Schuh A, Trepel M, Schmälter AK, Rank A. Chemotherapy markedly reduces b cells but not T cells and NK cells in patients with cancer. Cancer Immunol Immunother CII (2020) 69(1):147–57. doi: 10.1007/s00262-019-02449-y
129. Anscher MS, Arora S, Weinstock C, Amatya A, Bandaru P, Tang C, et al. Association of radiation therapy with risk of adverse events in patients receiving immunotherapy: A pooled analysis of trials in the US food and drug administration database. JAMA Oncol (2022) 8(2):232–40. doi: 10.1001/jamaoncol.2021.6439
Keywords: autoantibodies, B cells, immune related adverse events, blood-based biomarkers, immune checkpoint toxicities, risk prediction
Citation: Taylor J, Gandhi A, Gray E and Zaenker P (2023) Checkpoint inhibitor immune-related adverse events: A focused review on autoantibodies and B cells as biomarkers, advancements and future possibilities. Front. Immunol. 13:991433. doi: 10.3389/fimmu.2022.991433
Received: 11 July 2022; Accepted: 07 December 2022;
Published: 11 January 2023.
Edited by:
Luca Quartuccio, University of Udine, ItalyReviewed by:
Corrado Campochiaro, San Raffaele Hospital (IRCCS), ItalyCopyright © 2023 Taylor, Gandhi, Gray and Zaenker. This is an open-access article distributed under the terms of the Creative Commons Attribution License (CC BY). The use, distribution or reproduction in other forums is permitted, provided the original author(s) and the copyright owner(s) are credited and that the original publication in this journal is cited, in accordance with accepted academic practice. No use, distribution or reproduction is permitted which does not comply with these terms.
*Correspondence: John Taylor, am9obi50YXlsb3JAZWN1LmVkdS5hdQ==
Disclaimer: All claims expressed in this article are solely those of the authors and do not necessarily represent those of their affiliated organizations, or those of the publisher, the editors and the reviewers. Any product that may be evaluated in this article or claim that may be made by its manufacturer is not guaranteed or endorsed by the publisher.
Research integrity at Frontiers
Learn more about the work of our research integrity team to safeguard the quality of each article we publish.