- 1Cancer Biology Program, Holden Comprehensive Cancer Center, The University of Iowa, Iowa City, IA, United States
- 2Department of Microbiology and Immunology, The University of Iowa, Iowa City, IA, United States
- 3Free Radical and Radiation Biology Program, Department of Radiation Oncology, Holden Comprehensive Cancer Center, The University of Iowa, Iowa City, IA, United States
- 4Human Immunology Core & Holden Comprehensive Cancer Center, The University of Iowa, Iowa City, IA, United States
- 5Department of Radiology, Holden Comprehensive Cancer Center, The University of Iowa, Iowa City, IA, United States
- 6Division of Hematology, Oncology, and Blood & Marrow Transplantation, Department of Internal Medicine, Holden Comprehensive Cancer Center, The University of Iowa, Iowa City, IA, United States
- 7Department of Surgery, Holden Comprehensive Cancer Center, The University of Iowa, Iowa City, IA, United States
Pharmacological ascorbate (i.e., intravenous infusions of vitamin C reaching ~ 20 mM in plasma) is under active investigation as an adjuvant to standard of care anti-cancer treatments due to its dual redox roles as an antioxidant in normal tissues and as a prooxidant in malignant tissues. Immune checkpoint inhibitors (ICIs) are highly promising therapies for many cancer patients but face several challenges including low response rates, primary or acquired resistance, and toxicity. Ascorbate modulates both innate and adaptive immune functions and plays a key role in maintaining the balance between pro and anti-inflammatory states. Furthermore, the success of pharmacological ascorbate as a radiosensitizer and a chemosensitizer in pre-clinical studies and early phase clinical trials suggests that it may also enhance the efficacy and expand the benefits of ICIs.
1 Introduction
Immune evasion is one of the cancer hallmarks, defined as a mechanism by which cancer cells either suppress the immune response or mask themselves from immune detection and destruction (1, 2). Anti-tumor immunity is crucial during cancer initiation, progression, and metastasis. Tumors develop multiple mechanisms to evade anti-tumor immunity depending on the level of immune infiltration in the tumor microenvironment that defines it canonically as “cold” or “hot” (3). Cold tumors are considered “immune deserts” characterized by low immune cell infiltration (3). The decreased immunogenicity of cold tumors enables them to evade immune destruction by decreasing levels of tumor antigens, inhibiting maturation of dendritic cells, and suppressing T cell activation, infiltration, and migration (3). Hot tumors are highly infiltrated by immune cells; however, they evade anti-tumor immunity through impaired T cell tumor recognition, increased immune suppressor cell activation, and increased expression of inhibitory immune checkpoint molecules such as programmed death-ligand 1 (PD-L1) (3).
The work of James P. Allison and Tasuku Honjo in the 1990s and early 2000s led to the discovery of immune checkpoint inhibitors (ICIs). ICIs are drugs or antibodies that bind immune checkpoint proteins and prevent the activation of their immune-suppressive function, subsequently leading to increased immune activity. In 2011, Ipilimumab (anti-cytotoxic T-lymphocyte antigen 4 or anti CTLA-4) was the first ICI to receive FDA approval after demonstrating positive clinical outcomes in melanoma patients (4). In 2014, the programmed death protein 1 (anti PD-1) Nivolumab and Pembrolizumab received FDA approval following successful clinical trials in metastatic melanoma (5).
ICIs have since expanded from melanoma to multiple malignancies such as lung, bladder, breast, kidney, and head and neck cancers, etc. (6). However, their application in clinical settings faces numerous challenges such as primary and acquired resistance, toxicity, and limited response in some patient populations. Therefore, it is essential to develop new approaches that increase the sensitivity of tumors to ICIs to meet the clinical need. One approach to overcome these ICI limitations is pharmacological ascorbate (intravenous infusions of vitamin C resulting in ~ 20 mM concentrations in plasma (7)).
Ascorbate (L-ascorbic acid, C6H8O6-) is a six-carbon small molecule antioxidant with two ionizable hydroxyl groups and a molecular mass of 176.12 grams discovered by Szent-Györgyi and colleagues in the 1930s (8, 9). Commonly known as vitamin C, ascorbate is an essential micronutrient for humans who need to meet a daily intake of 100 – 200 mg via consuming fruits and vegetables (10). Humans are not capable of ascorbate biosynthesis due to a mutation that prevents the conversion of L-gulonolactone to L-ascorbic acid (11, 12). Without adequate ascorbate intake, the homeostasis of multiple physiological functions is disturbed. Ascorbate is a donor antioxidant capable of undergoing two one-electron oxidations; this function plays a central role in collagen biosynthesis and elasticity, redox regulation, enzyme function (as a cofactor), hormone regulation and biosynthesis, and amino acid metabolism (13). As a cofactor, ascorbate functions as an epigenetic regulator for enzymes such as Ten-eleven translocation (TET) proteins and Jumonji C domain-containing histone demethylases (JHDMs) (14–16). By reducing Fe3+ to Fe2+ ascorbate promotes DNA demethylation, thus epigenetically modifying gene expression in multiple cell populations including cancer cells (as an anticancer effector) and immune cells (14–22).
At a physiological state with adequate dietary vitamin C intake, ascorbate concentrations in the plasma are ≤ 0.08 mM (23). With intravenous administration, ascorbate has unique pharmacokinetics that affects its final plasma concentration and urinary excretion. Oral ascorbate administration with doses up to 6 g/day yields plasma concentrations ≤ 0.22 mM due to the presence of tight physiologic control that leads to urinary excretion of excess ascorbate (23–25). Interestingly, administering ascorbate intravenously can yield plasma concentrations of 15-20 mM temporarily bypassing the strict ascorbate transporter regulation plasma concentrations (7, 23, 25). A remarkable outcome of these unique ascorbate pharmacokinetics is the selective cancer cell killing millimolar plasma concentrations, whilst sparing non-malignant cells, making it an emerging potential anticancer therapy with a plethora of clinical applications (23, 25, 26).
This review discusses the potential of pharmacological ascorbate as a sensitizer for cancer immunotherapy. We discuss the role of ascorbate in the context of immune function and immune regulation, review the most recent findings combining pharmacological ascorbate with ICIs, and highlight potential future directions for this promising approach.
2 Ascorbate and immune regulation
Because of ascorbate’s potential to enhance ICIs in cancer therapy, it is imperative to evaluate the effects of ascorbate on the immune function. However, experimental evidence regarding the effects of pharmacological dosing of ascorbate on immune function remains limited but a great deal of information can be learned from previous studies of the effects of ascorbic acid on cells of innate and the adaptive immune systems and how it orchestrates immune function by acting on their proliferation, differentiation, and cytokine secretion.
2.1 Ascorbate and the innate immune system
2.1.1 Macrophages and monocytes
Macrophages are phagocytic tissue-differentiated monocytes essential for innate immune defense (27). The main functions of macrophages include phagocytosis, cytokine secretion, and professional antigen presentation to the adaptive immune system (28). In the tumor microenvironment, the role of macrophages can be profoundly influenced by their polarization status which determines their effector functions. Based on their polarization, tumor-associated macrophages (TAMs) can be broadly categorized into M1 and M2 macrophages. M1 macrophages are pro-inflammatory and exert anti-tumor effects by secreting IL-1β, TNF, IL-12, and IL-18 while the several subtypes of M2 macrophages are anti-inflammatory and promote tumor progression by secreting IL-4, IL-10, IL-13, and TGF-β (28–30). Monocytes and macrophages accumulate high ascorbate concentrations (4-6 mM) by upregulating the ascorbate transporter, SVCT2 (31–35). In these cells, ascorbate is a cofactor for the hydroxylase enzyme responsible for regulating the hypoxia-inducible factors (HIF) family (22) whose activation impacts the polarization of TAMs towards the M2 phenotype that suppresses anti-tumor immunity and the associated T cell cytotoxicity (36–39). Additionally, some evidence in macrophages pointed at TET proteins and Jumonji C domain-containing histone demethylases that use ascorbate (21, 22) suggesting that ascorbate may play a role in macrophage function and polarization through epigenetic regulation.
Generally, ascorbate plays a pro-phagocytic role that promotes monocyte survival, protects against reactive oxygen species (ROS), and enhances phagocytosis (32, 40). A study by Oberritter and colleagues showed that ascorbate levels in macrophages decrease from baseline after phagocytosis, indicating that it is required to protect macrophages from ROS generated during phagocytosis (41). Monocytes treated with ascorbate (4-20 mM) were protected from FAS-induced cell death, thus promoting survival and differentiation (42). Ascorbate can also exert an anti-inflammatory effect on macrophages. In an in vitro study, ascorbate reduced the secretion of the pro-inflammatory cytokines, TNF, and IL-6 (43). Thus, ascorbate may selectively drive macrophage activity in either a pro or anti-inflammatory direction. A potential explanation for this duality is maintaining the balance between pro and anti-inflammatory responses and protecting normal tissues; however, more research is needed to elucidate the complex relationship between ascorbate and macrophage differentiation.
2.1.2 Neutrophils
Neutrophils make up to 70% of total leukocytes, and they are the most abundant immune cells in circulation produced at approximately 1011 cells per day (44). Neutrophils are the first responders of the innate immune system. They can carry out phagocytosis or degradation of pathogens by ROS and reactive nitrogen species (RNS) production (superoxide, hydrogen peroxide, and nitric oxide) or release their cytotoxic granules. The function of neutrophils has been well-established in innate immune function and antimicrobial activity, and recently, interest in neutrophils as regulators of the tumor microenvironment has developed. Neutrophils have been linked to tumor suppression -via hydrogen peroxide and nitric oxide production- and metastasis promotion (by inducing ROS-mediated DNA damage, IL-1 secretion, and other mechanisms) (45).
Neutrophils maintain high concentrations of ascorbate that can reach up to 14 mM (46). Motility is vital to neutrophils as they must travel to the primary site of infection to eliminate the pathogen or damaged tissue, and exposure to ROS can significantly hinder their motility. In vitro and in vivo work by Anderson demonstrated that the antioxidant action of ascorbate can rescue neutrophils from ROS exposure and stimulate migration by inhibiting the peroxidase-H2O2-halide system (47). These high levels of ascorbate promote chemotaxis and stimulate neutrophil superoxide and hydrogen peroxide production, thus allowing them to carry out their phagocytic and killing function once they are at the site of infection (32). In vitro, neutrophils treated with 0.1-0.3 mM ascorbate showed a significant increase in ROS production (48). Similarly, human studies with ascorbate supplementation demonstrated improved phagocytic activity and increased ROS production in neutrophils (49, 50).
Neutrophil extracellular traps (NETs) are large web-like extensions of decondensed chromatin with cytosolic and granule proteins that neutrophils form upon stimulation by ROS and the subsequent downstream activation of neutrophil elastase (NE) and protein-arginine deiminase type 4 (PAD4) in a process known as NETosis (51). NETs can also lead to neutrophil death by causing a loss of the nuclear envelope and plasma membrane degradation (51). NETs can promote tumor proliferation, cancer metastasis, and increase the infiltration of immunosuppressive T regulatory lymphocytes into the tumor microenvironment (45, 51, 52). Treating human neutrophils with ascorbate resulted in a decrease in phorbol ester-induced NETosis in vitro. In ascorbate deficient mice, NETosis was increased (53). Recently, an ex vivo study showed that ascorbate reduced sepsis-induced NETosis in a dose-dependent manner. At 1 mM, it induced a reduction in NETosis, while concentrations > 10 mM increased NETosis (54). These findings suggest that ascorbate is a potential regulator of NETosis which may have beneficial implications for cancer therapy.
2.1.3 Natural killer cells
Natural killer (NK) cells are innate immune granular lymphocytes that kill pathogen infected cells and tumor cells via cytotoxic action and produce cytokines such as IFN-γ (55). NK cell depletion is linked to cancer development and a poor prognosis (55). NKs have a unique ability to recognize cancer cells that escape T lymphocytes. 40-90% of human tumors downregulate major histocompatibility complex 1 (MHC-I) molecules used for antigen recognition by CD8+ T lymphocytes needed for an immune response (56). However, NK cells can recognize cancer cells regardless of their MHC-I status as they can recognize other surface markers on tumor cells (55, 57), highlighting their importance in anti-tumor immunity and as targets for immunotherapy.
Treating NKs with ascorbate (0.28 mM) increased cell proliferation and promoted the expansion and differentiation of cytokine-stimulated cultures containing NK progenitors without hindering the cytotoxic capacity of the differentiated NKs against leukemia cells under normoxic and hypoxic conditions [46]. In a human toxicology study where NK function was dramatically compromised by toxic chemical exposure (e.g., formaldehyde), 78% of individuals who received ascorbate (0.06 g/Kg body weight dose) showed over a ten-fold enhancement in NK function compared to untreated subjects (58). Similarly, treating functionally-impaired NKs derived from patients with β-thalassemia with 1 mM ascorbate resulted in a significant rescue of NK function compared to untreated controls (59). These data suggest that ascorbate supports NK survival and function. The susceptibility of NKs to oxidative stress (60) – a classic feature of the tumor microenvironment- suggests that ascorbate functions as an antioxidant that can protect NKs in the tumor microenvironment and enable them to carry out their anti-tumor function.
2.2 Ascorbate and the adaptive immune system
2.2.1 B lymphocytes
B lymphocytes carry out the humoral immune response. They differentiate into antibody-secreting plasma cells and memory B-cells that play a role in a prolonged immune response. Ascorbate accumulation has been described in B lymphocytes suggesting its functional relevance in humoral immunity (61). Anti-µ-primed spleen-derived B lymphocytes showed enhanced differentiation into plasma cells represented by increased IgM production and increased viability upon treatment with ≥ 0.5 mM 2-O-α-D-glucopyranosyl-L-ascorbic acid (a stable derivative of ascorbate) compared to untreated controls (62). A more recent study demonstrated that treating B-lymphocytes with 0.002-0.01 mM ascorbate promotes plasma cell differentiation by enhancing TET2/3-Mediated DNA demethylation; a similar response was reproduced in animals receiving 4 mg/g body weight ascorbate (18). In a study by Woo et. al, activated B-cells treated with 0.0625 mM – 1 mM ascorbate demonstrated no changes in proliferation but apoptosis was induced in a dose-dependent manner in vitro (63).
In human studies, evidence of ascorbate’s role in B cell function is conflicting. Vallance, Prinz, and colleagues demonstrated that vitamin C supplementation (1 g daily) increased IgG and IgM plasma levels suggesting an association between ascorbate and B cell differentiation and function (64, 65). In contrast, Kennes and colleagues found that ascorbate (0.5 g daily) did not affect immunoglobulin levels (66). However, Kennes used a lower dose of ascorbate compared to Prinz and tested an older patient population, which could justify the contradictory findings between the two studies. Hence, more investigation is needed to elucidate the complex role of ascorbate in mediating humoral immunity.
2.2.2 T lymphocytes
T-lymphocytes are responsible for cellular immune response through the action of CD4+ T helper cells (Th) and CD8+ cytotoxic T cells (Tc) (67). CD4+ T cells differentiate into different subsets such as Th1, Th2, Th17, and T Regulatory cells (Treg) (67). Th1 cells drive a pro-inflammatory cell mediated immune response by secreting IFN-γ, IL-2, TNF, and Th2 cells carry out an inflammatory immune response mediated by the cytokines IL4, IL-5, IL-6, IL-10, and IL-13 (67). Th17 cells are pro-inflammatory cells that produce IL-17 and IL-22 and some studies indicate that they may play a role in tumorigenesis (67, 68). Treg cells regulate immune cells by a number of mechanisms including secreting suppressive cytokines such as IL-10 and TGF- β, inducing apoptosis, or using cytokine deprivation to directly suppress other CD4+ and CD8+ T cells to downregulate cell-mediated immune response (67, 69). Tc cells secrete pro-inflammatory cytokines TNF and IFN-γ that have cytotoxic effects on tumor cells, further, Tc cells can directly kill cells through secretion of perforin and granzyme B that generate pores in the plasma membrane and activate cellular caspases, respectively (67, 70). All T cell subsets and their respective cytokines play essential roles in tumor immunity (67, 70), and major cancer-killing role of T lymphocytes has made them the target of the majority of immunotherapies such as CAR-T (chimeric antigen receptor) cell therapy and ICIs that target PD-1 and CTLA-4 on T cells (71).
Like macrophages, neutrophils, and B cells, T cells accumulate high millimolar levels of ascorbate (72, 73). Ascorbate is vital for numerous cellular functions in the life of a T cell, from development and maturation, proliferation and differentiation, to activation and regulation. When it comes to thymic T cell maturation and development, Manning and colleagues treated T cells with 0.03 mM ascorbate and observed increased T cell maturation, enhanced T cell receptor selection, and CD8 coreceptor upregulation. Similarly, ascorbate-deficient mice showed impaired T cell maturation compared to mice that accumulate ascorbate (73). The Huijskens group treated T cell progenitors with 0.095 mM ascorbate and found it induced T cell maturation and promoted differentiation of double-positive (CD4+CD8+) thymocytes in the absence of stromal cells, and that ascorbate is required for T cell development when stromal cells are present (74).
As for peripheral T cells, in vitro, ascorbate acted as an inhibitor of multiple forms of T cell apoptosis, such as growth factor withdrawal, spontaneous apoptosis, and steroid-induced death (75). Some evidence suggests a potential dose-dependent effect of ascorbate on T cell viability and proliferation. Low ascorbate concentrations (0–0.125 mM) had no effect on T cell viability and proliferation while higher concentrations (0.25-0.5 mM) decreased both (76, 77). Ascorbate polarizes Th cells toward the Th1 phenotype (78, 79). Similarly, naïve T cells had a preference towards differentiating into Th1 cells in the presence of IL-12 produced by dendritic cells treated with 0.08 – 2 mM ascorbate (80). Moreover, dendritic cells treated with 0 – 2 mM ascorbate had elevated IL-12 production that to led increased effector CD8+ differentiation and activation causing improved tumor killing in vivo (81). With regard to Treg cells, studies show that ascorbate may promote the conversion of effector γδ T cells into Treg cells t through the TET-mediated epigenetic regulation of Foxp3 expression through DNA demethylation, suggesting increased immune-suppressive functions (82, 83). In contrast, Oyarce and colleagues reported that although ascorbate promotes the differentiation of Tregs, their ability to suppress immune response against skin allograft was diminished (84). The impaired Treg function observed by Oyarce is consistent with suppressed Treg function and increased effector T cell activation with ascorbate supplementation in ascorbate-deficient mice as reported by Maeng and colleagues (85).
The first evidence on pharmacological ascorbate as a modulator of anti-tumor immunity in humans was reported in a clinical trial where NSCLC patients received pharmacological ascorbate with carboplatin and paclitaxel, and those with progression-free survival ≥ 6 months showed enhanced CD8+ activation by 4.2 fold compared to 1.6 fold in patients with progression-free survival < 6 months (86). Through its role in regulating T cell maturation, differentiation, and activation, ascorbate is not only an immune modulator, but also a very promising regulator T cell mediated antitumor immunity. More investigation is needed in this area in order to elucidate the multifaceted function of ascorbate in immune response and clearly define the role of dose and timing on immune cell proliferation and activation.
3 Ascorbate and cancer therapy
Cameron and Pauling lead clinical trials on ascorbate and cancer in the 1970s. Terminal patients were treated with high dose intravenous and oral ascorbate and it was found that ascorbate supplementation prolonged survival by 210-300 days and 22% of ascorbate-treated patients had greatly increased survival time (over 2.4 years) compared to matched controls (87, 88). Subsequent clinical trials with oral ascorbate in patients with advanced colorectal, lung, stomach, liver, and pancreatic cancer failed to reproduce Cameron and Pauling’s findings (89, 90). Unfortunately, this decreased the interest in ascorbate as an anti-cancer therapy. A pivotal criticism of these trials is that they did not use intravenous ascorbate which was later shown to have a pharmacokinetic ability to increase plasma ascorbate concentrations to the tens of milli-molar range (91). Multiple studies by Levine and colleagues then confirmed that for cancer cell killing to be accomplished, pharmacological doses of ascorbate are required as they lead to the generation of toxic concentrations of hydrogen peroxide in the extracellular space in the tumor but not in the blood leading to selective killing (92–94). These findings initiated a new wave of ascorbate research in cancer therapy in the early 2000s (7, 95–100).
A growing body of evidence shows that ascorbate can selectively kill cancer cells by acting as a prooxidant by generating high fluxes of hydrogen peroxide that can react with intracellular labile iron via Fenton chemistry while it is believed to simultaneously protect normal tissue by acting as a donor antioxidant (26, 93). Chen and colleagues showed that ten cancer cell lines had an EC50 of < 4 mM with ascorbate while the non-malignant cell lines could survive ascorbate concentrations up to 20 mM (93).
These findings were confirmed in vivo in xenograft models of ovarian, pancreatic, and glioblastoma (GBM) cancers where pharmacological ascorbate significantly reduced tumor growth (93). These findings inspired the investigation of the potential of ascorbate as a sensitizing agent in combination with chemotherapy and radiation. In pancreatic cancer, pharmacological ascorbate was a radiosensitizer in vitro and in a xenograft mouse model (95). Concordantly, clinical trial data suggests that pharmacological ascorbate increases overall survival in patients with pancreatic cancer receiving concurrent chemotherapy (97). As a chemosensitizer, pharmacological ascorbate exhibited a synergistic effect when combined with gemcitabine in vitro and pre-clinical pancreatic cancer models (101). Consistent with these results, subsequent early phase clinical trials showed that pharmacological ascorbate is safe with promising clinical outcomes when combined with gemcitabine (102, 103). Additional early phase clinical trials in GBM, non-small cell lung carcinoma (NSCLC), and ovarian cancer also found pharmacological ascorbate to be safe with promising clinical outcomes (96, 98, 104, 105). In addition to enhancing radiation and chemotherapy effectiveness, pharmacological ascorbate has also significantly reduced chemotherapy induced normal tissue toxicity as reported by Ma and colleagues in ovarian cancer, and radiation-induced normal tissue injury as reported by Alexander and colleagues in pancreatic cancer (97, 104). Taken together, data from all the aforementioned in vitro, pre-clinical, and clinical studies demonstrate its excellent capacity to kill cancer cells, improve response to conventional therapy -even in cancers known for poor response and poor prognosis such as pancreatic cancer-, and minimize the normal tissue damage associated with chemotherapy and radiation. With our current understanding of the importance of tumor immunology, ascorbate may increase the efficacy of ICIs.
4 Ascorbate as a sensitizer for cancer immunotherapy
While multiple basic and clinical studies focused on ascorbate and its clinical application in the context of chemotherapy and radiotherapy, very few studies have explored its potential as a sensitizer for cancer immunotherapy. The roles that ascorbate plays in immune regulation previously discussed in this review, along with its prooxidant action against cancer and antioxidant protective action in normal tissues, suggest pharmacological ascorbate may enhance the response of cancer cells to ICIs and protect against ICI-induced normal tissue injury such as pneumonitis.
Luchtel and colleagues evaluated pharmacological ascorbate (1.5 M) in a mouse lymphoma model in combination with anti-PD-1. This study showed an increase in lymphoma immunogenicity represented by a 15%- 21% increase in Tc-mediated cell killing (106). Another finding from this study was that combining high doses of ascorbate with ani PD-1 significantly increased Tc and macrophage infiltration in the tumor microenvironment and increased IL-12 production, increased Tc and NK activation, and increased granzyme B production (106). Lastly, this group showed that combining pharmacological ascorbate (4g/kg) with anti-PD-1 has a synergistic effect that significantly decreased tumor growth compared to untreated controls or treatment with single agents (106). Magrì, A., et al. evaluated mouse models of melanoma, breast, pancreatic, and colorectal cancers to test the effect of ascorbate in combination with anti-PD-1 and anti-CTLA-4. First, they examined the effect of ascorbate on anti-tumor immune response and found it is primarily a Tc mediated process emphasizing the potential of ascorbate sensitizing against anti-PD-1 and anti-CTLA-4 (107). Consistent with Luchtel’s group, they reported significantly increased Tc infiltration in the tumor microenvironment and reduced tumor growth when combining ascorbate with anti-PD-1 and anti-CTLA-4 and (107). Tumor volume was significantly reduced in breast, colorectal, and pancreatic cancers by the ascorbate anti-PD-1 and anti-CTLA-4 combinations (107). Mice that showed complete response exhibited progression-free survival for up to a year (107). Most recently, a study reported a synergistic effect in a mouse model of renal cell carcinoma treated with high dose (0.5 g/kg) ascorbate combined with anti PD-L1 (17). Compared to untreated controls, high dose ascorbate alone, or anti PD-L1 alone, the combination showed the highest levels of CD4+ and CD8+ infiltration in the tumors, in addition to elevated IFN-γ and IFN-γ-induced CXCL9, CXCL10 and CXCL11 (17). Interestingly, the expression levels of PD-L1 were significantly increased by high dose ascorbate, suggesting that it can potentially make tumors more responsive to checkpoint blockade (17). These initial findings describing pharmacological ascorbate and cancer immunotherapy, ICIs in particular, are promising and warrant further investigation.
5 Discussion and future directions
Through its antioxidant activity and epigenetic regulation, ascorbate is a crucial modulator of immune function and the anti-tumor immune response (Figure 1). Moreover, as a small molecule antioxidant, it protects normal tissue against ROS. Metabolic changes in cancer cells, such as their reduced ability to metabolize hydrogen peroxide and their increased iron accumulation, make them susceptible to the prooxidant action of ascorbate. As a result, pharmacological ascorbate has been recently exploited in multiple clinical trials as a chemo- and radiosensitizer, but the field of ascorbate and cancer immunotherapy has yet to be explored significantly. Three studies showed pharmacological ascorbate combinations with ICIs: a) increase Tc infiltration in the tumor microenvironment and b) exert synergistic effects on tumor suppression. These findings emphasize the importance of this novel approach to cancer therapy. Future studies should include other malignancies and clinically relevant combinations such as using ICIs with chemotherapy and radiation. Additionally, future studies should address how pharmacological ascorbate affects primary and acquired resistance to ICIs, and whether or not it protects normal tissue from adverse effects associated with ICIs such as pneumonitis and colitis. Mechanistic studies that elucidate the route of action of ascorbate as a sensitizer to ICIs are also critical. A noteworthy potential mechanism that can be studied in this context is the epigenetic regulation of TET2; prior research suggests that ascorbate promotes TET2 activation and leads to the expression of genes associated with anti-tumor immunity (19). Peng and colleagues explored this mechanism in their study and showed that with the loss of TET, high dose ascorbate lost the synergistic effect it had with anti PD-L1 in a mouse model of renal cell carcinoma, thus emphasizing the importance of epigenetic regulation as a focus for mechanistic studies on ascorbate and immunotherapy (17). There are many questions that need to be answered about ascorbate and cancer immunotherapy. Answering these questions will help expedite the establishment of clinical trials that will create a new and potentially improved standard of care regimens for cancer patients.
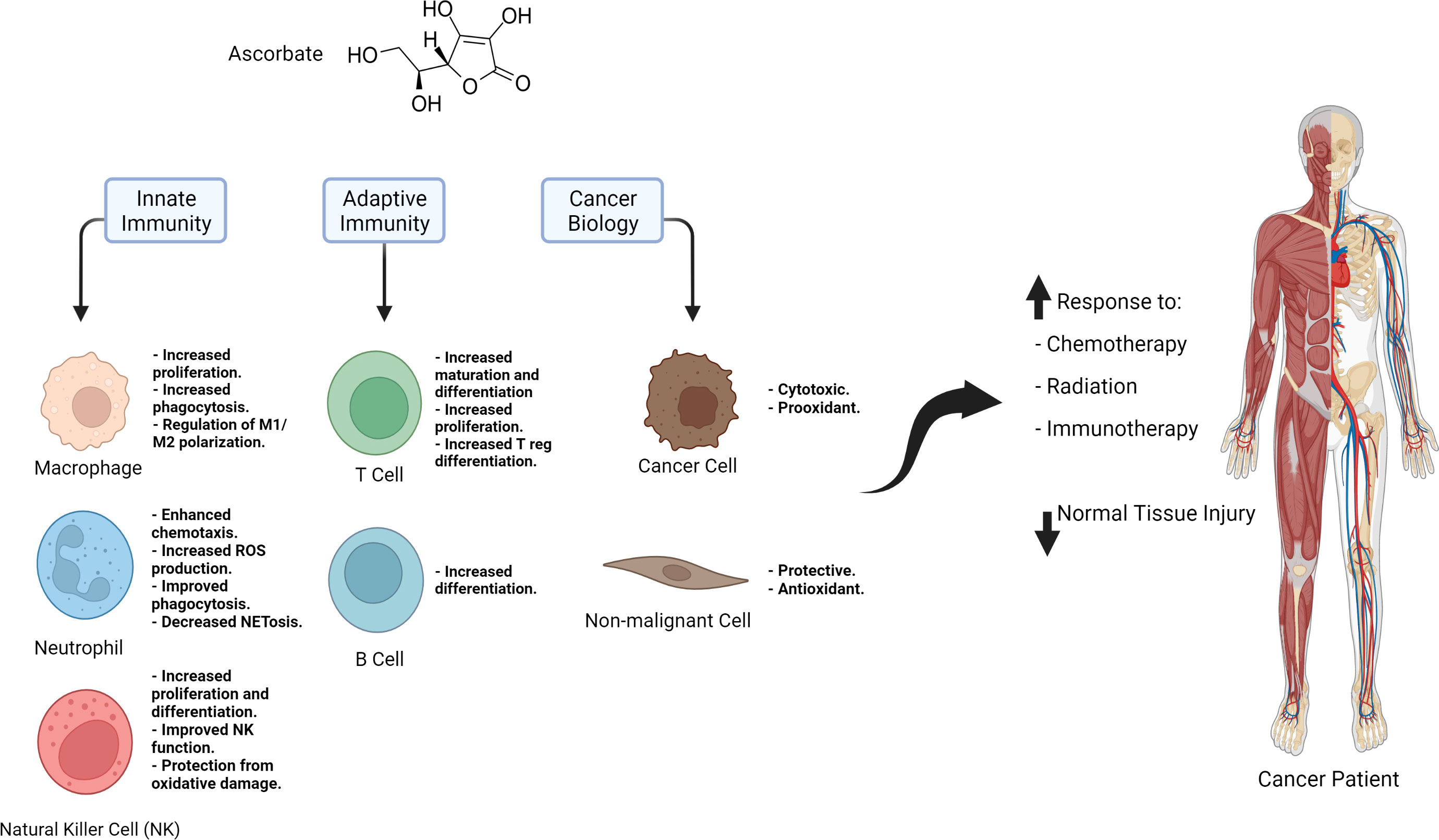
Figure 1 Summary of the immune regulatory effects of ascorbate, the role it plays it cancer and non-malignant cells, and its potential applications in cancer therapy.
Author contributions
AZ and BA wrote the manuscript. LS, AM, SH, JS, MP, ZZ, TW, VM, JC, MF, JH, SV, and DS provided expertise and feedback. BA, DS, MF, SV, and JH provided funding. All authors contributed to the article and approved the submitted version.
Funding
This work was supported by NCI P30CA086862, Gateway for Cancer Research award G-17-1500T32 GM007337, UIHC-CCOM Iowa Aging Initiative, NIAID R21AI157121, NIH/NCI T32CA078586, NCI P01CA217797, and the Holden Comprehensive Cancer Center Gift Funds.
Conflict of interest
The authors declare that the research was conducted in the absence of any commercial or financial relationships that could be construed as a potential conflict of interest.
Publisher’s note
All claims expressed in this article are solely those of the authors and do not necessarily represent those of their affiliated organizations, or those of the publisher, the editors and the reviewers. Any product that may be evaluated in this article, or claim that may be made by its manufacturer, is not guaranteed or endorsed by the publisher.
References
1. Hanahan D, Weinberg RA. Hallmarks of cancer: The next generation. Cell (2011) 144(5):646–74. doi: 10.1016/j.cell.2011.02.013
2. Hanahan D. Hallmarks of cancer: New dimensions. Cancer Discov (2022) 12(1):31–46. doi: 10.1158/2159-8290.CD-21-1059
3. Kim SK, Cho SW. The evasion mechanisms of cancer immunity and drug intervention in the tumor microenvironment. Front Pharmacol (2022) 13. doi: 10.3389/fphar.2022.868695
4. Hodi FS, O'Day SJ, McDermott DF, Weber RW, Sosman JA, Haanen JB, et al. Improved survival with ipilimumab in patients with metastatic melanoma. New Engl J Med (2010) 363(8):711–23. doi: 10.1056/NEJMoa1003466
5. Dobosz P, Dzieciątkowski T. The intriguing history of cancer immunotherapy. Front Immunol (2019) 10:2965. doi: 10.3389/fimmu.2019.02965
6. Vaddepally RK, Kharel P, Pandey R, Garje R, Chandra AB. Review of indications of FDA-approved immune checkpoint inhibitors per NCCN guidelines with the level of evidence. Cancers (Basel) (2020) 12(3):738. doi: 10.3390/cancers12030738
7. Schoenfeld JD, Alexander MS, Waldron TJ, Sibenaller ZA, Spitz DR, Buettner GR, et al. Pharmacological ascorbate as a means of sensitizing cancer cells to radio-chemotherapy while protecting normal tissue. Semin Radiat Oncol (2019) 29(1):25–32. doi: 10.1016/j.semradonc.2018.10.006
8. Buettner GR, Schafer FQ. Special feature: vitamin c identification. Biochemist (2006) 28(5):31–3. doi: 10.1111/odi.12446
9. Cox EG, Hirst EL, Reynolds RJW. Hexuronic acid as the antiscorbutic factor. Nature (1932) 130(3293):888–. doi: 10.1038/130888a0
10. Levine M. Criteria and recommendations for vitamin c intake. JAMA (1999) 281(15):1415. doi: 10.1001/jama.281.15.1415
11. Burns JJ. Missing step in man, monkey and Guinea pig required for the biosynthesis of l-ascorbic acid. Nature (1957) 180(4585):553–. doi: 10.1038/180553a0
12. Chatterjee IB, Majumder AK, Nandi BK, Subramanian N. Synthesis and some major functions of vitamin c in animals. Ann New York Acad Sci (1975) 258:24–47. doi: 10.1111/j.1749-6632.1975.tb29266.x
13. Jacob RA, Sotoudeh G. Vitamin c function and status in chronic disease. Nutr Clin Care (2002) 5(2):66–74. doi: 10.1046/j.1523-5408.2002.00005.x
14. Yin R, Mao S-Q, Zhao B, Chong Z, Yang Y, Zhao C, et al. Ascorbic acid enhances tet-mediated 5-methylcytosine oxidation and promotes DNA demethylation in mammals. J Am Chem Society (2013) 135(28):10396–403. doi: 10.1021/ja4028346
15. Tsukada Y-I, Fang J, Erdjument-Bromage H, Warren ME, Borchers CH, Tempst P, et al. Histone demethylation by a family of JmjC domain-containing proteins. Nature (2006) 439(7078):811–6. doi: 10.1038/nature04433
16. Klose RJ, Yamane K, Bae Y, Zhang D, Erdjument-Bromage H, Tempst P, et al. The transcriptional repressor JHDM3A demethylates trimethyl histone H3 lysine 9 and lysine 36. Nature (2006) 442(7100):312–6. doi: 10.1038/nature04853
17. Peng D, He A, He S, Ge G, Wang S, Ci W, et al. Ascorbic acid induced TET2 enzyme activation enhances cancer immunotherapy efficacy in renal cell carcinoma. Int J Biol Sci (2022) 18(3):995–1007. doi: 10.7150/ijbs.67329
18. Qi T, Sun M, Zhang C, Chen P, Xiao C, Chang X. Ascorbic acid promotes plasma cell differentiation through enhancing TET2/3-mediated DNA demethylation. Cell Rep (2020) 33(9):108452. doi: 10.1016/j.celrep.2020.108452
19. Xu Y-P, Lv L, Liu Y, Smith MD, Li W-C, Tan X-M, et al. Tumor suppressor TET2 promotes cancer immunity and immunotherapy efficacy. J Clin Invest (2019) 129(10):4316–31. doi: 10.1172/JCI129317
20. Shenoy N, Bhagat T, Nieves E, Stenson M, Lawson J, Choudhary GS, et al. Upregulation of TET activity with ascorbic acid induces epigenetic modulation of lymphoma cells. Blood Cancer J (2017) 7(7):e587–e. doi: 10.1038/bcj.2017.65
21. Lee Chong T, Ahearn EL, Cimmino L. Reprogramming the epigenome with vitamin c. Front Cell Dev Biol (2019) 7. doi: 10.3389/fcell.2019.00128
22. Ang A, Pullar JM, Currie MJ, Vissers MCM. Vitamin c and immune cell function in inflammation and cancer. Biochem Soc Trans (2018) 46(5):1147–59. doi: 10.1042/BST20180169
23. Padayatty SJ, Sun H, Wang Y, Riordan HD, Hewitt SM, Katz A, et al. Vitamin c pharmacokinetics: implications for oral and intravenous use. Ann Intern Med (2004) 140(7):533–7. doi: 10.7326/0003-4819-140-7-200404060-00010
24. Levine M, Conry-Cantilena C, Wang Y, Welch RW, Washko PW, Dhariwal KR, et al. Vitamin c pharmacokinetics in healthy volunteers: evidence for a recommended dietary allowance. Proc Natl Acad Sci U S A. (1996) 93(8):3704–9. doi: 10.1073/pnas.93.8.3704
25. Levine M, Violet P-C. Data triumph at c. Cancer Cell (2017) 31(4):467–9. doi: 10.1016/j.ccell.2017.03.008
26. Chen Q, Espey MG, Krishna MC, Mitchell JB, Corpe CP, Buettner GR, et al. Pharmacologic ascorbic acid concentrations selectively kill cancer cells: Action as a pro-drug to deliver hydrogen peroxide to tissues. Proc Natl Acad Sci (2005) 102(38):13604–9. doi: 10.1073/pnas.0506390102
27. Wynn TA, Chawla A, Pollard JW. Macrophage biology in development, homeostasis and disease. Nature (2013) 496(7446):445–55. doi: 10.1038/nature12034
28. Murray PJ, Wynn TA. Protective and pathogenic functions of macrophage subsets. Nat Rev Immunol (2011) 11(11):723–37. doi: 10.1038/nri3073
29. Chávez-Galán L, Olleros ML, Vesin D, Garcia I. Much more than M1 and M2 macrophages, there are also CD169+ and TCR+ macrophages. Front Immunol (2015) 6. doi: 10.3389/fimmu.2015.00263
30. Boutilier AJ, Elsawa SF. Macrophage polarization states in the tumor microenvironment. Int J Mol Sci (2021) 22(13):6995. doi: 10.3390/ijms22136995
31. Mousavi S, Bereswill S, Heimesaat MM. Immunomodulatory and antimicrobial effects of vitamin c. Eur J Microbiol Immunol (2019) 9(3):73–9. doi: 10.1556/1886.2019.00016
32. Jafari D, Esmaeilzadeh A, Mohammadi-Kordkhayli M, Rezaei N. Vitamin c and the immune system. Denmark: Springer International Publishing (2019) p. 81–102.
33. Qiao H, May JM. Macrophage differentiation increases expression of the ascorbate transporter (SVCT2). Free Radical Biol Med (2009) 46(8):1221–32. doi: 10.1016/j.freeradbiomed.2009.02.004
34. Bergsten P, Amitai G, Kehrl J, Dhariwal KR, Klein HG, Levine M. Millimolar concentrations of ascorbic acid in purified human mononuclear leukocytes. Depletion Reaccumulation J Biol Chem (1990) 265(5):2584–7. doi: 10.1016/S0021-9258(19)39841-2
35. Evans RM, Currie L, Campbell A. The distribution of ascorbic acid between various cellular components of blood, in normal individuals, and its relation to the plasma concentration. Br J Nutr (1982) 47(3):473–82. doi: 10.1079/BJN19820059
36. Imtiyaz HZ, Williams EP, Hickey MM, Patel SA, Durham AC, Yuan L-J, et al. Hypoxia-inducible factor 2α regulates macrophage function in mouse models of acute and tumor inflammation. J Clin Invest (2010) 120(8):2699–714. doi: 10.1172/JCI39506
37. Colegio OR, Chu N-Q, Szabo AL, Chu T, Rhebergen AM, Jairam V, et al. Functional polarization of tumour-associated macrophages by tumour-derived lactic acid. Nature (2014) 513(7519):559–63. doi: 10.1038/nature13490
38. Noman MZ, Desantis G, Janji B, Hasmim M, Karray S, Dessen P, et al. PD-L1 is a novel direct target of HIF-1α, and its blockade under hypoxia enhanced MDSC-mediated T cell activation. J Exp Med (2014) 211(5):781–90. doi: 10.1084/jem.20131916
39. Corzo CA, Condamine T, Lu L, Cotter MJ, Youn J-I, Cheng P, et al. HIF-1α regulates function and differentiation of myeloid-derived suppressor cells in the tumor microenvironment. J Exp Med (2010) 207(11):2439–53. doi: 10.1084/jem.20100587
40. Wintergerst ES, Maggini S, Hornig DH. Immune-enhancing role of vitamin c and zinc and effect on clinical conditions. Ann Nutr Metab (2006) 50(2):85–94. doi: 10.1159/000090495
41. Oberritter H, Glatthaar B, Moser U, Schmidt KH. Effect of functional stimulation on ascorbate content in phagocytes under physiological and pathological conditions. Int Arch Allergy Appl Immunol (1986) 81(1):46–50. doi: 10.1159/000234106
42. Perez-Cruz I, Carcamo JM, Golde DW. Vitamin c inhibits FAS-induced apoptosis in monocytes and U937 cells. Blood (2003) 102(1):336–43. doi: 10.1182/blood-2002-11-3559
43. Hartel C. Effects of vitamin c on intracytoplasmic cytokine production in human whole blood monocytes and lymphocytes. Cytokine (2004) 27(4-5):101–6. doi: 10.1016/j.cyto.2004.02.004
44. Dancey JT, Deubelbeiss KA, Harker LA, Finch CA. Neutrophil kinetics in man. J Clin Invest (1976) 58(3):705–15. doi: 10.1172/JCI108517
45. Xiong S, Dong L, Cheng L. Neutrophils in cancer carcinogenesis and metastasis. J Hematol Oncol (2021) 14(1):173. doi: 10.1186/s13045-021-01187-y
46. Washko P, Wang Y, Levine M. Ascorbic acid recycling in human neutrophils. J Biol Chem (1993) 268(21):15531–5. doi: 10.1016/S0021-9258(18)82289-X
47. Anderson R. Ascorbate-mediated stimulation of neutrophil motility and lymphocyte transformation by inhibition of the peroxidase/H2O2/halide system in vitro and in vivo. Am J Clin Nutr (1981) 34(9):1906–11. doi: 10.1093/ajcn/34.9.1906
48. Sharma P, Raghavan SAV, Saini R, Dikshit M. Ascorbate-mediated enhancement of reactive oxygen species generation from polymorphonuclear leukocytes: modulatory effect of nitric oxide. J Leukocyte Biol (2004) 75(6):1070–8. doi: 10.1189/jlb.0903415
49. Levy R, Schlaeffer F. Successful treatment of a patient with recurrent furunculosis by vitamin c: improvement of clinical course and of impaired neutrophil functions. Int J Dermatol (1993) 32(11):832–4. doi: 10.1111/j.1365-4362.1993.tb02780.x
50. Bozonet S, Carr A, Pullar J, Vissers M. Enhanced human neutrophil vitamin c status, chemotaxis and oxidant generation following dietary supplementation with vitamin c-rich SunGold kiwifruit. Nutrients (2015) 7(4):2574–88. doi: 10.3390/nu7042574
51. Papayannopoulos V. Neutrophil extracellular traps in immunity and disease. Nat Rev Immunol (2018) 18(2):134–47. doi: 10.1038/nri.2017.105
52. Wang H, Zhang H, Wang Y, Brown ZJ, Xia Y, Huang Z, et al. Regulatory T-cell and neutrophil extracellular trap interaction contributes to carcinogenesis in non-alcoholic steatohepatitis. J Hepatol (2021) 75(6):1271–83. doi: 10.1016/j.jhep.2021.07.032
53. Mohammed BM, Fisher BJ, Kraskauskas D, Farkas D, Brophy DF, Fowler AA 3rd, et al. Vitamin c: a novel regulator of neutrophil extracellular trap formation. Nutrients (2013) 5(8):3131–51. doi: 10.3390/nu5083131
54. Sae-Khow K, Tachaboon S, Wright HL, Edwards SW, Srisawat N, Leelahavanichkul A, et al. Defective neutrophil function in patients with sepsis is mostly restored by ex vivo ascorbate incubation. J Inflamm Res (2020) 13:263–74. doi: 10.2147/JIR.S252433
55. Vivier E, Tomasello E, Baratin M, Walzer T, Ugolini S. Functions of natural killer cells. Nat Immunol (2008) 9(5):503–10. doi: 10.1038/ni1582
56. Bubeník J. Tumour MHC class I downregulation and immunotherapy. Oncol Rep (2003) 10(6):2005–8. doi: 10.3892/or.10.6.2005
57. Wu S-Y, Fu T, Jiang Y-Z, Shao Z-M. Natural killer cells in cancer biology and therapy. Mol Cancer (2020) 19(1):120. doi: 10.1186/s12943-020-01238-x
58. Heuser G, Vojdani A. Enhancement of natural killer cell activity and T and b cell function by buffered vitamin c in patients exposed to toxic chemicals: The role of protein kinase-c. Immunopharmacol Immunotoxicol (1997) 19(3):291–312. doi: 10.3109/08923979709046977
59. Atasever B, Ertan NZ, Erdem-Kuruca S, Karakas Z. In vitro effects of vitamin c and selenium on nk activity of patients with β -thalassemia major. Pediatr Hematol Oncol (2006) 23(3):187–97. doi: 10.1080/08880010500506420
60. Nakamura K, Matsunaga K. Susceptibility of natural killer (NK) cells to reactive oxygen species (ROS) and their restoration by the mimics of superoxide dismutase (SOD). Cancer Biother Radiopharm (1998) 13(4):275–90. doi: 10.1089/cbr.1998.13.275
61. Bergsten P, Yu R, Kehrl J, Levine M. Ascorbic acid transport and distribution in human b lymphocytes. Arch Biochem Biophys (1995) 317(1):208–14. doi: 10.1006/abbi.1995.1155
62. Ichiyama K, Mitsuzumi H, Zhong M, Tai A, Tsuchioka A, Kawai S. Promotion of IL-4- and IL-5-dependent differentiation of anti-mu-primed b cells by ascorbic acid 2-glucoside. Immunol Lett (2009) 122(2):219–26. doi: 10.1016/j.imlet.2009.01.007
63. Woo A, Kim J, Jeong Y, Maeng H, Lee Y, Kang J. Vitamin c acts indirectly to modulate isotype switching in mouse b cells. Anat Cell Biol (2010) 43(1):25–35. doi: 10.5115/acb.2010.43.1.25
64. Vallance S. Relationships between ascorbic acid and serum proteins of the immune system. Br Med J (1977) 2(6084):437–8. doi: 10.1136/bmj.2.6084.437
65. Prinz W, Bortz R, Bregin B, Hersch M. The effect of ascorbic acid supplementation on some parameters of the human immunological defence system. Int J Vitam Nutr Res (1977) 47(3):248–57.
66. Kennes B, Dumont I, Brohee D, Hubert C, Neve P. Effect of vitamin c supplements on cell-mediated immunity in old people. Gerontology (1983) 29(5):305–10. doi: 10.1159/000213131
67. Broere F, Van Eden W. T Cell subsets and T cell-mediated immunity. Switzerland: Springer International Publishing (2019) p. 23–35.
68. Tesmer LA, Lundy SK, Sarkar S, Fox DA. Th17 cells in human disease. Immunol Rev (2008) 223:87–113. doi: 10.1111/j.1600-065X.2008.00628.x
69. Kondělková K, Vokurková D, Krejsek J, Borská L, Fiala Z, Andrýs C. Regulatory T cells (Treg) and their roles in immune system with respect to immunopathological disorders. Acta Med (2010) 53(2):73–7. doi: 10.14712/18059694.2016.63
70. St Paul M, Ohashi PS. The roles of CD8(+) T cell subsets in antitumor immunity. Trends Cell Biol (2020) 30(9):695–704. doi: 10.1016/j.tcb.2020.06.003
71. Waldman AD, Fritz JM, Lenardo MJ. A guide to cancer immunotherapy: from T cell basic science to clinical practice. Nat Rev Immunol (2020) 20(11):651–68. doi: 10.1038/s41577-020-0306-5
72. Hong JM, Kim JH, Kang JS, Lee WJ, Hwang YI. Vitamin c is taken up by human T cells via sodium-dependent vitamin c transporter 2 (SVCT2) and exerts inhibitory effects on the activation of these cells in vitro. Anat Cell Biol (2016) 49(2):88–98. doi: 10.5115/acb.2016.49.2.88
73. Manning J, Mitchell B, Appadurai DA, Shakya A, Pierce LJ, Wang H, et al. Vitamin c promotes maturation of T-cells. Antioxidants Redox Signaling (2013) 19(17):2054–67. doi: 10.1089/ars.2012.4988
74. Huijskens MJ, Walczak M, Koller N, Briedé JJ, Senden-Gijsbers BL, Schnijderberg MC, et al. Technical advance: ascorbic acid induces development of double-positive T cells from human hematopoietic stem cells in the absence of stromal cells. J Leukoc Biol (2014) 96(6):1165–75. doi: 10.1189/jlb.1TA0214-121RR
75. Campbell JD, Cole M, Bunditrutavorn B, Vella AT. Ascorbic acid is a potent inhibitor of various forms of T cell apoptosis. Cell Immunol (1999) 194(1):1–5. doi: 10.1006/cimm.1999.1485
76. Eylar E, Báez I, Navas J, Mercado C. Sustained levels of ascorbic acid are toxic and immunosuppressive for human T cells. P R Health Sci J (1996) 15(1):21–6.
77. Maeng HG, Lim H, Jeong YJ, Woo A, Kang JS, Lee WJ, et al. Vitamin c enters mouse T cells as dehydroascorbic acid in vitro and does not recapitulate in vivo vitamin c effects. Immunobiology (2009) 214(4):311–20. doi: 10.1016/j.imbio.2008.09.003
78. Noh K, Lim H, Moon SK, Kang JS, Lee WJ, Lee D, et al. Mega-dose vitamin c modulates T cell functions in balb/c mice only when administered during T cell activation. Immunol Lett (2005) 98(1):63–72. doi: 10.1016/j.imlet.2004.10.012
79. Chang HH, Chen CS, Lin JY. High dose vitamin c supplementation increases the Th1/Th2 cytokine secretion ratio, but decreases eosinophilic infiltration in bronchoalveolar lavage fluid of ovalbumin-sensitized and challenged mice. J Agric Food Chem (2009) 57(21):10471–6. doi: 10.1021/jf902403p
80. Jeong YJ, Hong SW, Kim JH, Jin DH, Kang JS, Lee WJ, et al. Vitamin c-treated murine bone marrow-derived dendritic cells preferentially drive naïve T cells into Th1 cells by increased IL-12 secretions. Cell Immunol (2011) 266(2):192–9. doi: 10.1016/j.cellimm.2010.10.005
81. Jeong YJ, Kim JH, Hong JM, Kang JS, Kim HR, Lee WJ, et al. Vitamin c treatment of mouse bone marrow-derived dendritic cells enhanced CD8(+) memory T cell production capacity of these cells in vivo. Immunobiology (2014) 219(7):554–64. doi: 10.1016/j.imbio.2014.03.006
82. Sasidharan Nair V, Song MH, Oh KI. Vitamin c facilitates demethylation of the Foxp3 enhancer in a tet-dependent manner. J Immunol (2016) 196(5):2119–31. doi: 10.4049/jimmunol.1502352
83. Kouakanou L, Peters C, Sun Q, Floess S, Bhat J, Huehn J, et al. Vitamin c supports conversion of human γδ T cells into FOXP3-expressing regulatory cells by epigenetic regulation. Sci Rep (2020) 10(1):6550. doi: 10.1038/s41598-020-63572-w
84. Oyarce K, Campos-Mora M, Gajardo-Carrasco T, Pino-Lagos K. Vitamin c fosters the In vivo differentiation of peripheral CD4(+) Foxp3(-) T cells into CD4(+) Foxp3(+) regulatory T cells but impairs their ability to prolong skin allograft survival. Front Immunol (2018) 9:112. doi: 10.3389/fimmu.2018.00112
85. Maeng HG, Kang JS, Kim HM, Lee NE, Kong JM, Bae SY, et al. Effect of vitamin c on the activation of T cell in gulo(-/-) mice (33.23). J Immunol (2009) 182(1 Supplement):33.23.
86. Furqan M, Abu-Hejleh T, Stephens LM, Hartwig SM, Mott SL, Pulliam CF, et al. Pharmacological ascorbate improves the response to platinum-based chemotherapy in advanced stage non-small cell lung cancer. Redox Biol (2022) 53:102318. doi: 10.1016/j.redox.2022.102318
87. Cameron E, Pauling L. Supplemental ascorbate in the supportive treatment of cancer: Prolongation of survival times in terminal human cancer. Proc Natl Acad Sci U S A. (1976) 73(10):3685–9. doi: 10.1073/pnas.73.10.3685
88. Cameron E, Pauling L. Supplemental ascorbate in the supportive treatment of cancer: reevaluation of prolongation of survival times in terminal human cancer. Proc Natl Acad Sci USA. (1978) 75(9):4538–42. doi: 10.1073/pnas.75.9.4538
89. Creagan ET, Moertel CG, O'Fallon JR, Schutt AJ, O'Connell MJ, Rubin J, et al. Failure of high-dose vitamin c (ascorbic acid) therapy to benefit patients with advanced cancer. a controlled trial. N Engl J Med (1979) 301(13):687–90. doi: 10.1056/NEJM197909273011303
90. Moertel CG, Fleming TR, Creagan ET, Rubin J, O'Connell MJ, Ames MM. High-dose vitamin c versus placebo in the treatment of patients with advanced cancer who have had no prior chemotherapy. A Randomized Double-blind Comparison N Engl J Med (1985) 312(3):137–41. doi: 10.1056/NEJM198501173120301
91. Padayatty SJ, Levine M. Reevaluation of ascorbate in cancer treatment: emerging evidence, open minds and serendipity. J Am Coll Nutr (2000) 19(4):423–5. doi: 10.1080/07315724.2000.10718941
92. Levine M, Espey MG, Chen Q. Losing and finding a way at c: new promise for pharmacologic ascorbate in cancer treatment. Free Radic Biol Med (2009) 47(1):27–9. doi: 10.1016/j.freeradbiomed.2009.04.001
93. Chen Q, Espey MG, Sun AY, Pooput C, Kirk KL, Krishna MC, et al. Pharmacologic doses of ascorbate act as a prooxidant and decrease growth of aggressive tumor xenografts in mice. Proc Natl Acad Sci (2008) 105(32):11105–9. doi: 10.1073/pnas.0804226105
94. Chen Q, Espey MG, Sun AY, Lee J-H, Krishna MC, Shacter E, et al. Ascorbate in pharmacologic concentrations selectively generates ascorbate radical and hydrogen peroxide in extracellular fluid in vivo. Proc Natl Acad Sci (2007) 104(21):8749–54. doi: 10.1073/pnas.0702854104
95. Du J, Cieslak JA, Welsh JL, Sibenaller ZA, Allen BG, Wagner BA, et al. Pharmacological ascorbate radiosensitizes pancreatic cancer. Cancer Res (2015) 75(16):3314–26. doi: 10.1158/0008-5472.CAN-14-1707
96. Schoenfeld JD, Sibenaller ZA, Mapuskar KA, Wagner BA, Cramer-Morales KL, Furqan M, et al. O 2 ·– and h 2 O 2 -mediated disruption of fe metabolism causes the differential susceptibility of NSCLC and GBM cancer cells to pharmacological ascorbate. Cancer Cell (2017) 31(4):487–500.e8. doi: 10.1016/j.ccell.2017.02.018
97. Alexander MS, Wilkes JG, Schroeder SR, Buettner GR, Wagner BA, Du J, et al. Pharmacologic ascorbate reduces radiation-induced normal tissue toxicity and enhances tumor radiosensitization in pancreatic cancer. Cancer Res (2018) 78(24):6838–51. doi: 10.1158/0008-5472.CAN-18-1680
98. O'Leary BR, Houwen FK, Johnson CL, Allen BG, Mezhir JJ, Berg DJ, et al. Pharmacological ascorbate as an adjuvant for enhancing radiation-chemotherapy responses in gastric adenocarcinoma. Radiat Res (2018) 189(5):456–65. doi: 10.1667/RR14978.1
99. O'Leary BR, Alexander MS, Du J, Moose DL, Henry MD, Cullen JJ. Pharmacological ascorbate inhibits pancreatic cancer metastases via a peroxide-mediated mechanism. Sci Rep (2020) 10(1):17649. doi: 10.1038/s41598-020-74806-2
100. Mehdi Z, Petronek MS, Stolwijk JM, Mapuskar KA, Kalen AL, Buettner GR, et al. Utilization of pharmacological ascorbate to enhance hydrogen peroxide-mediated radiosensitivity in cancer therapy. Int J Mol Sci (2021) 22(19):10880. doi: 10.3390/ijms221910880
101. Espey MG, Chen P, Chalmers B, Drisko J, Sun AY, Levine M, et al. Pharmacologic ascorbate synergizes with gemcitabine in preclinical models of pancreatic cancer. Free Radical Biol Med (2011) 50(11):1610–9. doi: 10.1016/j.freeradbiomed.2011.03.007
102. Monti DA, Mitchell E, Bazzan AJ, Littman S, Zabrecky G, Yeo CJ, et al. Phase I evaluation of intravenous ascorbic acid in combination with gemcitabine and erlotinib in patients with metastatic pancreatic cancer. PloS One (2012) 7(1):e29794. doi: 10.1371/journal.pone.0029794
103. Welsh J, Wagner B, Van’t Erve T, Zehr P, Berg D, Halfdanarson T, et al. Pharmacological ascorbate with gemcitabine for the control of metastatic and node-positive pancreatic cancer (PACMAN): results from a phase I clinical trial. Cancer Chemother Pharmacol (2013) 71(3):765–75. doi: 10.1007/s00280-013-2070-8
104. Ma Y, Chapman J, Levine M, Polireddy K, Drisko J, Chen Q. High-dose parenteral ascorbate enhanced chemosensitivity of ovarian cancer and reduced toxicity of chemotherapy. Sci Trans Med (2014) 6(222):222ra18–ra18. doi: 10.1126/scitranslmed.3007154
105. Schoenfeld JD, Sibenaller ZA, Mapuskar KA, Bradley MD, Wagner BA, Buettner GR, et al. Redox active metals and H2O2 mediate the increased efficacy of pharmacological ascorbate in combination with gemcitabine or radiation in pre-clinical sarcoma models. Redox Biol (2018) 14:417–22. doi: 10.1016/j.redox.2017.09.012
106. Luchtel RA, Bhagat T, Pradhan K, Jacobs WR, Levine M, Verma A, et al. High-dose ascorbic acid synergizes with anti-PD1 in a lymphoma mouse model. Proc Natl Acad Sci (2020) 117(3):1666–77. doi: 10.1073/pnas.1908158117
Keywords: pharmacological ascorbate, immune regulation, anti-PD-1, cancer immunotherapy, immune checkpoint inhibitors, antioxidant therapy, prooxidant therapy
Citation: Zaher A, Stephens LM, Miller AM, Hartwig SM, Stolwijk JM, Petronek MS, Zacharias ZR, Wadas TJ, Monga V, Cullen JJ, Furqan M, Houtman JCD, Varga SM, Spitz DR and Allen BG (2022) Pharmacological ascorbate as a novel therapeutic strategy to enhance cancer immunotherapy. Front. Immunol. 13:989000. doi: 10.3389/fimmu.2022.989000
Received: 07 July 2022; Accepted: 03 August 2022;
Published: 22 August 2022.
Edited by:
Huashan Shi, Sichuan University, ChinaReviewed by:
Qi Chen, University of Kansas Medical Center, United StatesYang Wang, Sichuan University, China
Copyright © 2022 Zaher, Stephens, Miller, Hartwig, Stolwijk, Petronek, Zacharias, Wadas, Monga, Cullen, Furqan, Houtman, Varga, Spitz and Allen. This is an open-access article distributed under the terms of the Creative Commons Attribution License (CC BY). The use, distribution or reproduction in other forums is permitted, provided the original author(s) and the copyright owner(s) are credited and that the original publication in this journal is cited, in accordance with accepted academic practice. No use, distribution or reproduction is permitted which does not comply with these terms.
*Correspondence: Bryan G. Allen, YnJ5YW4tYWxsZW5AdWlvd2EuZWR1