- 1Center for Public Health Research, Medical School of Nanjing University, Nanjing, China
- 2Department of Burn and Plastic Surgery, Affiliated Hospital of Zunyi Medical University, Zunyi, China
- 3Hôpital Maisonneuve-Rosemont, School of Medicine, University of Montreal, Montréal, Canada
- 4State Key Laboratory of Analytical Chemistry for Life Science, Nanjing University, Nanjing, China
- 5Jiangsu Key Laboratory of Molecular Medicine, Medical School, Nanjing University, Nanjing, China
Interleukin-25 (IL-25), also known as IL-17E, is a recently identified cytokine of the IL-17 family. Numerous studies illustrated that the expression of IL-25 is regulated by multiple pathogens, including parasitic, viral, and bacterial infections. IL-25 has a dual function in infectious diseases. On the one hand, IL-25 activates type 2 immunity via the relevant cytokines, including IL-4, IL-5, and IL-13, which are associated with the development of pathogenic infection-related allergic diseases. On the other hand, IL-25 involves in the recruitment of group 2 innate lymphoid cells (ILC2) to enhanced T helper 2 (Th2) cell differentiation, which are important to the clearance of pathogens. However, the precise roles of IL-25 in infectious diseases remain largely unknown. Thus, the current review will shed light on the pivotal roles of IL-25 in infectious diseases.
Introduction
Interleukin-17 (IL-17) was discovered and described in 1993 (1). IL-17 family consists of six members including IL-17A-F (2). IL-17E was also named interleukin-25 (IL-25), which was initially identified by sequence alignment from human genomic DNA sequence information in 2001 and located on the q-arm of chromosome 14 (14q11.2) with two exons and encodes 161 amino acids (3). Il-25 possesses approximately 16% to 20% homology with other IL-17 family members, and binds to specific homologous IL-17 receptors to transmit signals (4). Murine Il-25 is located on chromosome 7 and encodes 160 amino acids, which has 80% sequence homology with the human Il-25 (5).
Since the finding of IL-25, the exploration of the cellular origin of IL-25 has been dedicated. Initially, Fort et al. reported that IL-25 is mainly produced by T helper 2 (Th2) cells (4); subsequently, IKeda et al. found that mast cells may produce IL-25 by enhancing Th2-type immune response (6); and Kang et al. successfully used TiO2 to induce the secretion of IL-25 in alveolar macrophages in vitro (7); further studies identified tuft cells in the intestinal system also contributed to the expression of IL-25 (8, 9); Wang et al. found that human chorionic gonadotropin promoted the expression of IL-25 in decidual stromal cells (10); furthermore, IL-25 is also produced by activated eosinophils, basophils, liver cells, kidney cells, lung cells, innate immune cells, fibroblasts, and endothelial cells (11). In light of the above, IL-25 is widely secreted and expressed in various tissues and systems.
In fact, IL-25 has a dual role in regulating immune responses in different diseases. On the one hand, IL-25 is a driver of multiple allergic diseases (12). IL-25 is an amplifier of Th2 immune responses and binds to its receptor composed of interleukin 17 receptor A (IL-17RA) and IL-17RB for signal transduction (13). IL-25 activates nuclear factor of activated T cells c1 (NFATc1) and JunB transcription factors to induce the expression of interleukin 4 (IL-4), which enhances Th2 cell differentiation (2, 14, 15). Upon meeting with the stimulation of IL-25, nuclear transcription factor kappa B activator 1 (Act 1) binds to IL-17RB and then mediates the secretion of IL-5 and IL-13, which participates in the development of allergic diseases, via recruiting and activating eosinophils and stimulating the production of immunoglobulin E (IgE) (16–20). On the other hand, IL-25 also has positive effects. One of the characteristics of inflammatory bowel disease (IBD) is a low level of IL-25, and IL-25 treatment inhibits Toll-like receptors (TLRs)-induced inflammation and further alleviates the symptoms of IBD (21, 22). IL-25 suppresses IL-22-induced osteoclastogenesis via activation of signal transducer and activator of transcription 3 (STAT3) and p38 mitogen-Activated Protein Kinase (MAPK) pathway, participating in arthritic anti-inflammatory responses (19). Sonobe et al. reported that IL-25 down regulated the expression of junction adhesion molecule claudin 5 to maintain blood-brain barrier (BBB) in multiple sclerosis (23). Furthermore, IL-25 may also have anti-inflammatory effects in parasites infection, type 1 diabetes and systemic lupus erythematosus (2, 24).
IL-25 can be induced by a variety of factors, and the most common agents are allergens (25). IL-25 is highly expressed in the murine model of asthma established by ovalbumin (OVA) or house dust mite (26, 27). Pollen-allergic patients or pollen-challenged mice have an enhanced expression of IL-25 in the respiratory tract (28, 29). IL-25 can also be induced by environmental pollutants such as detergents, tobacco, ozone, particulate matter, diesel exhaust, nanoparticles and microplastic in the air or water, which damage epithelial cells and induce the secretion of IL-25 (30). What is of great interest is that pathogenic infection is also reported to be one of the factors that induce IL-25 secretion. It has reported that IL-25 is up-regulated during some parasitic, viral, and bacterial infections (31–33). Notably, the biofunction of IL-25 in infectious diseases is still unclear. Thus, in this review, we aim to briefly summarize the recent research progress on the functions of IL-25 in infectious diseases and probe into the potential therapeutic effects.
The roles of IL-25 in parasitic infections
Helminth infection
Helminths are multicellular organisms, and most of them are parasites including digenean flukes (trematodes), tapeworms (cestodes), and Nematoda (roundworms), which account for millions of infections worldwide (34). Helminths could disturb the host immune system, and the studies on helminths-host interactions focus on the fields of allergy and autoimmunity (35). Immunologically, chronic helminth infections are characterized by a skewing towards Th2 response, in which IL-25 plays a critical role (36, 37). IL-25 was strongly induced after multiple helminths infection in intestinal epithelial cells, including Heligmosoides polygyrus (H. polygyrus), Trichuris muris (T. muris), Nippostrongylus brasiliensis (N. brasiliensis), Ascaris lumbricoides (A. lumbricoides), and Schistosoma chinensis (S. chinensis) (37, 38). As one of the main sources of IL-25 in human intestinal, tuft cells are rapidly increased during helminth infection (39). It is widely thought that IL-25 is an ‘alarmin’ for the helminth infection (40). Therefore, the biological function of IL-25 in the helminth infection is intriguing.
First of all, IL-25 is crucial for the clearance of helminth infections. Previous studies showed that Il-25 deficient mice exhibited an increased burden of helminth infection (41). Il-17rb deficient mice could not expel helminths in case of IL-25 overexpression (42). Group 2 innate lymphoid cells (ILC2) is considered as the innate counterpart of Th2 cells and participates in the host response against helminths (43). Fallon et al. clarified the protective role of ILC2 in preventing helminth colonization (44). Recombinant IL-25 (rIL-25) protein treatment promoted the expansion of ILC2s in helminths-infected-mice (45). IL-25-mediated-ILC2 activation resulted in the cooperation of Th2 and ILC2 and further promoted the secretion of Th2 cytokines, such as IL-4, IL-5, and IL-13 (36). These cytokines are involved in the recruitment of mast cells and eosinophil, which are important for the clearance of helminths (46). In short, the IL-25/ILC2/Th2 axis may be a potential therapeutic target for helminth infections, as shown in Figure 1. We perceive that the IL-25/ILC2/Th2 axis is responsible for the positive effect of the Th2 response in helminth infection related diseases.
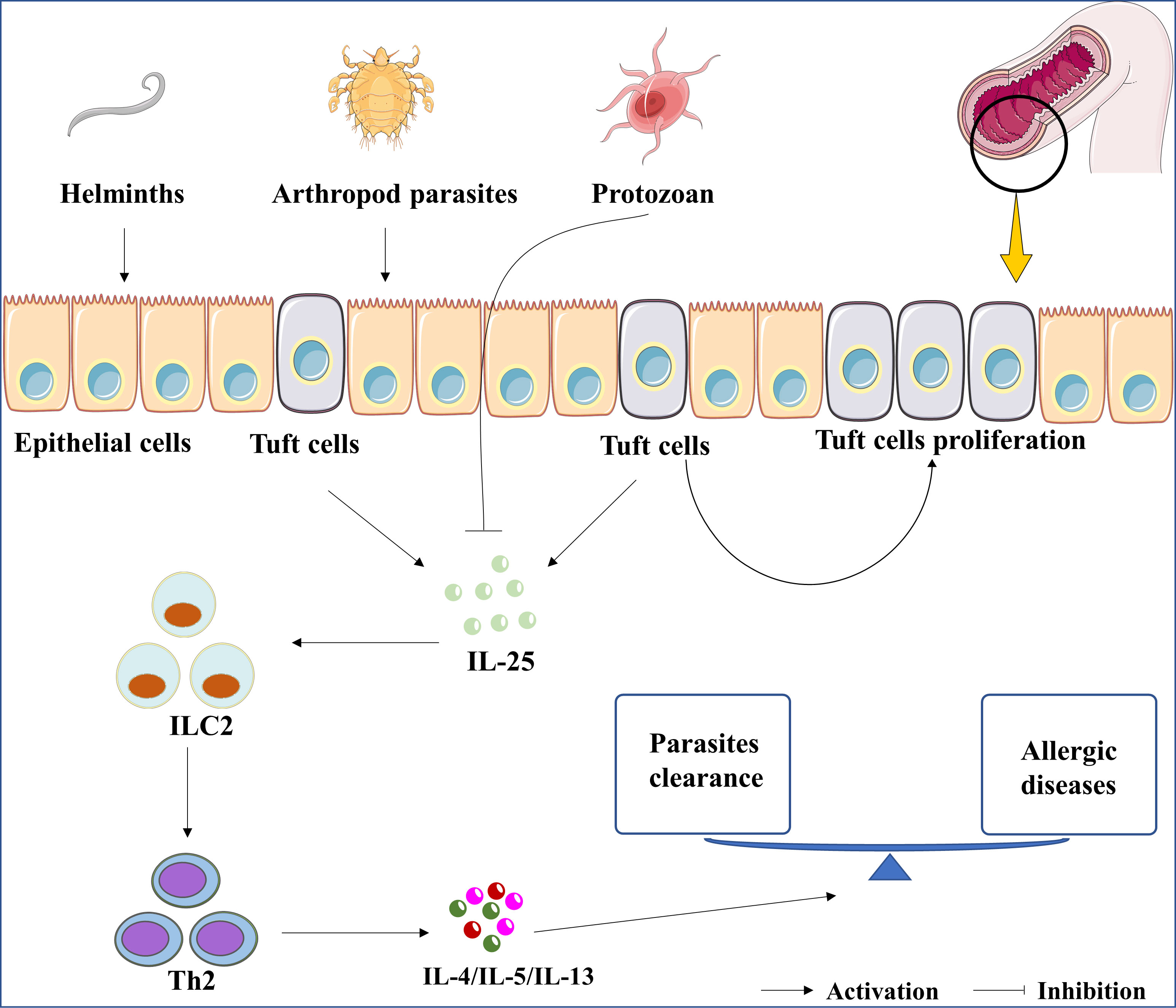
Figure 1 The roles of IL-25 in parasitic infection. The up-regulation of IL-25 was induced by the infection of parasites, including helminths and arthropod parasites. In contrast, IL-25 was downregulated by protozoan infections. Whatever, parasites-induced IL-25 or rIL-25 promotes the proliferation of tuft cells. Subsequently, the cytokines of IL-4, IL-5, and IL-13 are induced by IL-25/ILC2/Th2 axis to clean the parasite. Lastly, the expression of IL-4, IL-5, and IL-13 are associated with the development of asthma, allergic rhinitis, and atopic dermatitis.
Secondly, IL-25 also suppresses the inflammation caused by helminth infection. It is reported that Il-25 deficient mice developed an exaggerated intestinal inflammation via increasing interferon gamma (IFN-γ) expression following T. muris infection (47). Overexpression of IL-25 could reduce IFNs expression (48). The cytokines of Th1 response, such as IL-1, IL-6, and tumor necrosis factor α (TNF-α), can be induced by the most of helminth infections (49–52), however, they can be reduced by IL-25 (53, 54). Kleinschek et al. discovered an elevated expression of TNF-α in Il-25 deficient mice, and the administration of rIL-25 suppressed IL-1β and IL-6 expression in dendritic cells in mice (55), whose suppression will expand the response of Th2 cells.
Finally, IL-25 may also be involved in the pathogenic process of helminths. For instance, Toxocara spp larvae migrate to the lung and causing asthma-like symptoms, while IL-25 expression is one of the key cytokines for the development of asthma (56, 57). Vannella et al. reported that IL-25 induced by helminth infection was associated with the progression of pulmonary fibrosis (58). The development of cancer was also associated with helminth infections (59, 60). IL-25-activated ILC2s created an innate cancer-permissive microenvironment (61). However, there has been no direct evidence to verify that helminth infection-induced IL-25 is associated with tumorigenesis, which is a promising area that deserves us to explore in depth in the future.
Protozoan infection
Protozoan parasites are single-celled organisms. Diarrheal diseases caused by intestinal protozoan parasites is a major food-borne public health problem worldwide (62). The infection induced by a protozoan parasite can reduce IL-25 expression while the administration of rIL-25 protein can help control amebiasis in mice (63). Similar to the helminth infection, IL-25 recruits ILC2, which mediates the clearance of protozoan parasites (64). In addition, IL-25 was downregulated in mice infected with Plasmodium berghei (P. berghei), and the incidence of parasitemia in the Il-25 depleted mice were higher than that in the wild-type mice (65). Unfortunately, the underlying mechanism is unknown and this study ignored the therapeutic effect of rIL-25 on P.berghei. Given the previous evidence that IL-25 is extensively downregulated in the infection of protozoans and the supplement of IL-25 is beneficial for treating protozoan infections, more studies are needed to better elucidate the mechanism of IL-25 in protozoan infections.
Arthropod parasites
Arthropod parasites are usually ectoparasitic and commonly include dust mites, ticks, and fleas (66). It is well known that house dust mite (HDMs) is a major factor for allergic diseases, such as atopic dermatitis, allergic rhinitis, and asthma (67). HDM-specific immunoglobulins in serum are positively correlated with IL-25 expression, and IL-25 involved in the development of atopic dermatitis, allergic rhinitis, and asthma sensitized to HDM (68). For atopic dermatitis, intelectin (ITLN) is a key factor for the expression of IL-25 in airway epithelial cells and aggravates allergic airway inflammation (69). The expression of IL-25 is up-regulated in nasal tissues from patients with allergic rhinitis (AR) (70); stimulation of Th2 cells with IL-25 locally promotes IL-13 and IL-9 production, which contributes to the pathology of allergic asthma (71). So far, the roles of IL-25 contributing to the pathogenesis of arthropod parasites infection are not fully understood, and increased attention is requisite.
The roles of IL-25 in viral infections
Respiratory syncytial virus (RSV)
RSV, as a contagious virus, can bring about acute respiratory tract infections, which is the primary cause of infant hospitalization worldwide (72). Although RSV infection usually manifests as a mild illness in healthy adults, it causes severe illness in the elderly or immunocompromised patients (73). RSV may infect the lower respiratory tract, resulting in an elevated risk of developing asthma (74). A previous study reported that Il-17rb deficient mice were protected from asthma aggravation deriving from RSV infection (75), establishing that the IL-17 family plays an important role in the pathogenesis of RSV-induced asthma. Meantime, after the RSV challenge, administration of anti-IL-25 antibody in mice prevents some pivotal features of asthma (76). It has been clarified that IL-25 acts on the progression of asthma and has been considered as a biomarker for the prognosis (77). Nevertheless, the way how IL-25 serves as RSV infection therapeutic remains unknown.
Hepatitis C virus (HCV)
The infection of hepatitis viruses causes viral hepatitis. Five liver-specific viruses (A to E) exist, all of which have their own unique epidemiology, risk of liver complications, and responsiveness to antiviral therapies (78). HCV infection is one of the major causes of severe liver diseases such as chronic hepatitis, liver cirrhosis, and hepatocellular carcinoma (79). According to the World Health Organization (WHO), about 71 million people are infected with HCV worldwide, with at least 400,000 death annually (80). IL-17RA is expressed nearly in all types of liver cells (81). IL-25 was up-regulated in the serum of patients with HCV infection, and the levels of IL-25 in the serum were mainly associated with higher aspartate transaminase (AST) and alanine transaminase (ALT) (82). However, Cabral et al. reported that IL-25 could not be induced by the stimulation of HCV antigens in peripheral blood mononuclear cells (83), implying that IL-25 is not directly induced by HCV infection. IL-25 is also reported to be involved in the oncogenic effects of HCV (84). Tumor-associated macrophages (TAM), especially type 2 macrophages (M2), and their related cytokines are closely linked with the progression of hepatic cancer (85–87). Previous studies reported that IL-25 could induce M2 macrophage polarization, which was associated with the progression of hepatic cell carcinoma (HCC) (88, 89). However, the expression level of IL-25 in liver tissues with HCV infection is still not explored in the present decades. In addition, the mechanism of IL-25 in inflammation and chronic liver diseases has not been extensively studied. Thus, more studies are needed to better elucidate the effects of IL-25 in the development of viral hepatitis.
Herpes simplex virus-1 (HSV-1)
HSV-1 is one of the most well-known members of the herpesviruses family, and about 70% of the population are infected with HSV-1 all over the world (90). About 3% of the patients with atopic dermatitis (AD), the most common chronic inflammatory skin disease in the world, are infected with HSV-1 and got eczema herpeticum (EH) (91). In addition to symptoms such as itching and soreness, HSV-1 infection poses a potentially life-threatening risk for AD patients (92). Previous study reported that the concentration of IL-25 in serum of AD patients was higher than control group (93), and IL-25 was involved in the pathogenesis of AD (94). IL-25 promoted the infection of HSV-1 among AD patients by suppressing the expression of interferon-gamma (IFN-γ), a key factor for the host to defend against viral infection (92, 95, 96). Subsequently, IL-25 treatment in the cell culture medium enhanced the replication of HSV-1 in keratinocytes (95). Ultimately, Salimi et al. confirm that IL-25-induced-ILC2s were present in mouse skin and exacerbated AD-like inflammation (97). The above evidences suggest that IL-25 is a key factor driving the process of AD following HSV-1 infection.
Herpes simplex keratitis (HSK) is a disease of the cornea caused by HSV-1 infection, and mice lacking Il-17ra showed a decreased severity of the lesion during HSV-1 infection (98), as well as a negligible damage to the epithelial layer, little fibrosis, and decreased infiltration of CD4+ T cells (99). Notably, Il-17ra deficiency makes a mouse unable to respond to any IL-17 family members. Until now, there are still lacking studies on the relationship between the IL-17 family and HSK, and the effect of IL-25/IL-17RA on HSK is still unclear, more studies are needed to elucidate it.
Other viruses
IL-25 is also involved in the pathogenic process of multiple viruses. Beale et al. demonstrated that IL-25 was significantly induced in epithelial cells with rhinovirus (RHV) infection (100), and a higher level of IL-25 expression is associated with mucous metaplasia in RHV-infected infants and immature mice (101, 102). Haiyu et al. found that influenza virus (IAV) more powerfully induced the expression of IL-25 in vitro, and IL-25 positively correlated with the load of IAV (103). A respiratory allergic reaction caused by cytomegalovirus (CMV) is also associated with IL-25 secretion (104). IL-25 treatment exacerbates mouse intestinal West Nile virus (WNV) infection (105). A recent study showed that IL-25 blockade improved antiviral immunity during respiratory viral infection, and exogenous IL-25 treatment increased viral loads including rhinovirus and coronavirus (106). The evidence implies that IL-25 participates in the progression of many viral infectious diseases, as shown in Figure 2. However, the mechanisms of IL-25 associated with infectious diseases call for an urgent need to investigate.
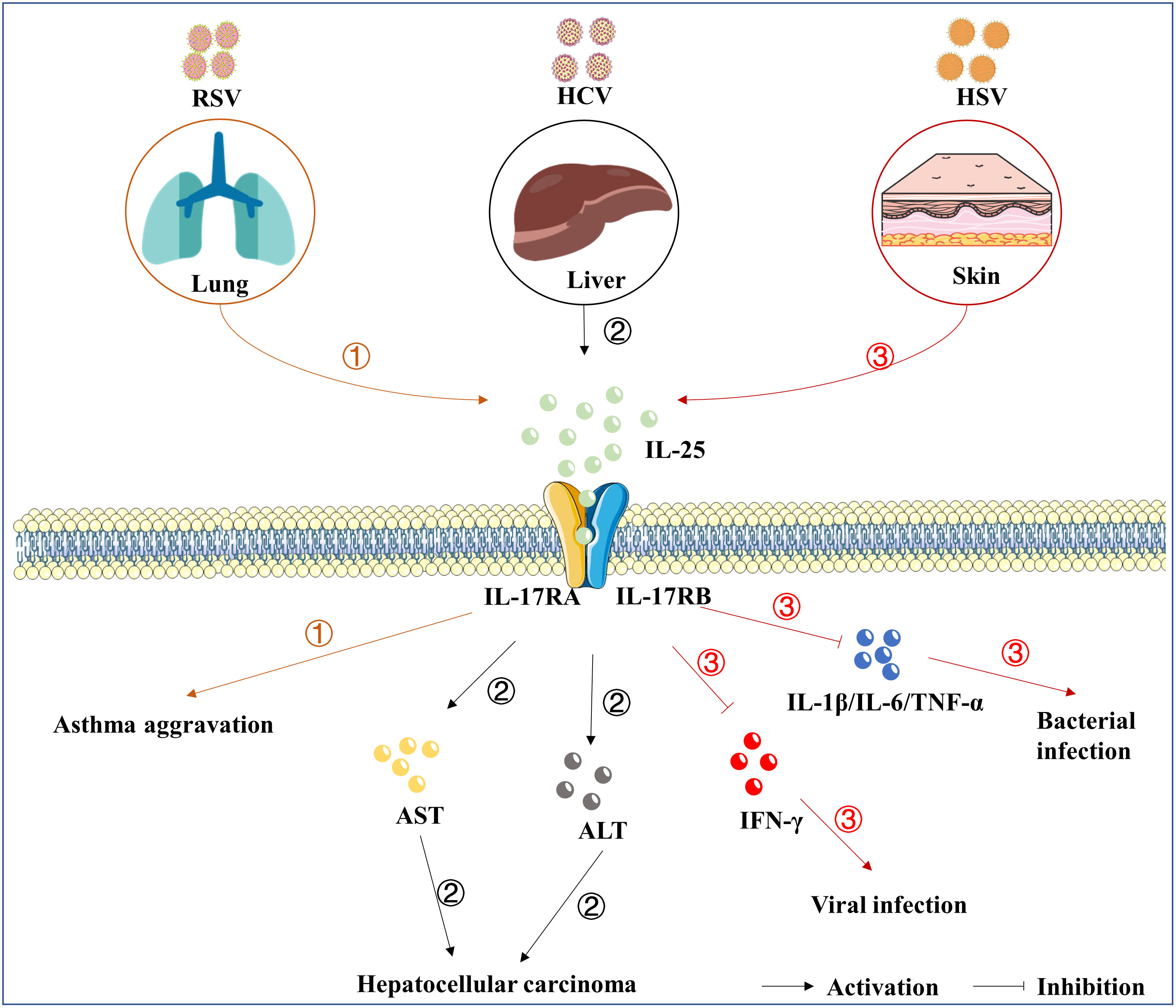
Figure 2 The roles of IL-25 in viral infection. IL-25 is up-regulated following the infection of RSV ①, HCV ②, and HSV-1 ③. Subsequently, IL-25 goes through the downstream signaling cascades by mediating the complex of receptors, consisting of IL-17RA and IL-17RB. IL-25 also induces the expression of IL-4/IL-5/IL-13, which are associated with the development of asthma. Furthermore, IL-25 overexpression was related to elevated AST and ALT involving chronic hepatitis, liver cirrhosis, and hepatocellular carcinoma. In addition, the up-regulation of IL-25 suppresses the cytokines of IFN-γ, IL-1β, IL-6, and TNF-α. However, direct evidence that IL-25 causes pathological damage is lacking.
The roles of IL-25 in bacterial infections
Bacterial-derived metabolites or structural components are a strong activator of immune signaling pathways (107). IL-25 is one of the typical examples. The expression of IL-25 is regulated by pathogenic or commensal bacteria. Francisella tularensis (Ft) is a Gram-negative bacterium that causes tularemia (108). IL-25 strongly activates ILC2, which rapidly secretes IL-25 and results in a positive feed-back loop upon Ft infection, and the administration of IL-25 notably increases IgM production in mice; antibody-mediated depletion of ILC2 mainly supports the source of IL-5, which is required for IgM production; therefore, discovering the IL-25-ILC2-IL-5 axis is a novel strategy to improve vaccination depending on the IL-17RB signal pathway (109). Clostridium difficile (C. difficile) is a Gram-positive, spore-forming, anaerobic bacillus (110). C. difficile is widely distributed in the intestinal tract of humans and animals, and in the environment (111). Antibiotic-induced dysbiosis is the primary cause of the frequency and severity of C. difficile infection (CDI), which has become one of the most common hospital-acquired infections (112). Antibiotic-induced dysbiosis reduced colonic expression of IL-25, and fecal microbiota transplantation (FMT) could recover IL -25 expression by suppressing the expression of inflammatory genes (113). In addition, rIL-25 could also reduce the host mortality and the tissue pathology during the activating state of CDI infection (114). Staphylococcus aureus (S. aureus) strains infecting human brains may develop brain abscesses with the characteristics of inflammatory and septic lesions surrounded by fibrotic cysts (115). S. aureus can induce the release of IL-25 by epithelial cells (33). Compared with WT mice, Il-17ra deficient mice displayed a higher burdens of S. aureus in the brain; the influx of γ and δ T cells was increased in Il-17ra deficient mice following S. aureus infection; subsequently, the high infiltration of natural killer (NK) cells were absent in the brain abscesses in Il-17ra deficient mice, implying that IL-25 signaling played an important role in the regulation of adaptive immunity with the infection of S. aureus (116). However, IL-17A and IL-17F, which share IL-17RA with IL-25, play major roles in the host against bacterial infections (117). Il-17ra deficiency also makes a mouse unable to respond to IL-17A and IL-17F. Therefore, we should not ignore the effects of other members of the IL-17 family in the host to defend against bacterial infections.
Alterations in the composition of intestinal commensal bacteria are associated with enhanced susceptibility to multiple inflammatory diseases (118). The infection of intestinal commensal bacteria up-regulates the expression of IL-25 by intestinal epithelial cells and limits the expansion of Th17 cells in the intestine via inhibiting the expression of macrophage-derived IL-23, indicating that commensal bacteria influence intestinal immune homeostasis via direct regulation of the IL-25-IL-23-IL-17 axis (119). In summary, for bacterial infectious diseases, IL-25 is involved in the regulation of adaptive immunity and anti-inflammatory effect and influences the homeostasis of the intestinal immune through Th2 cells. In addition, IL-25 may have a potential therapeutic effect on treating bacterial infectious diseases, as shown in Figure 3, whereas the current studies are insufficient to give a definitive answer.
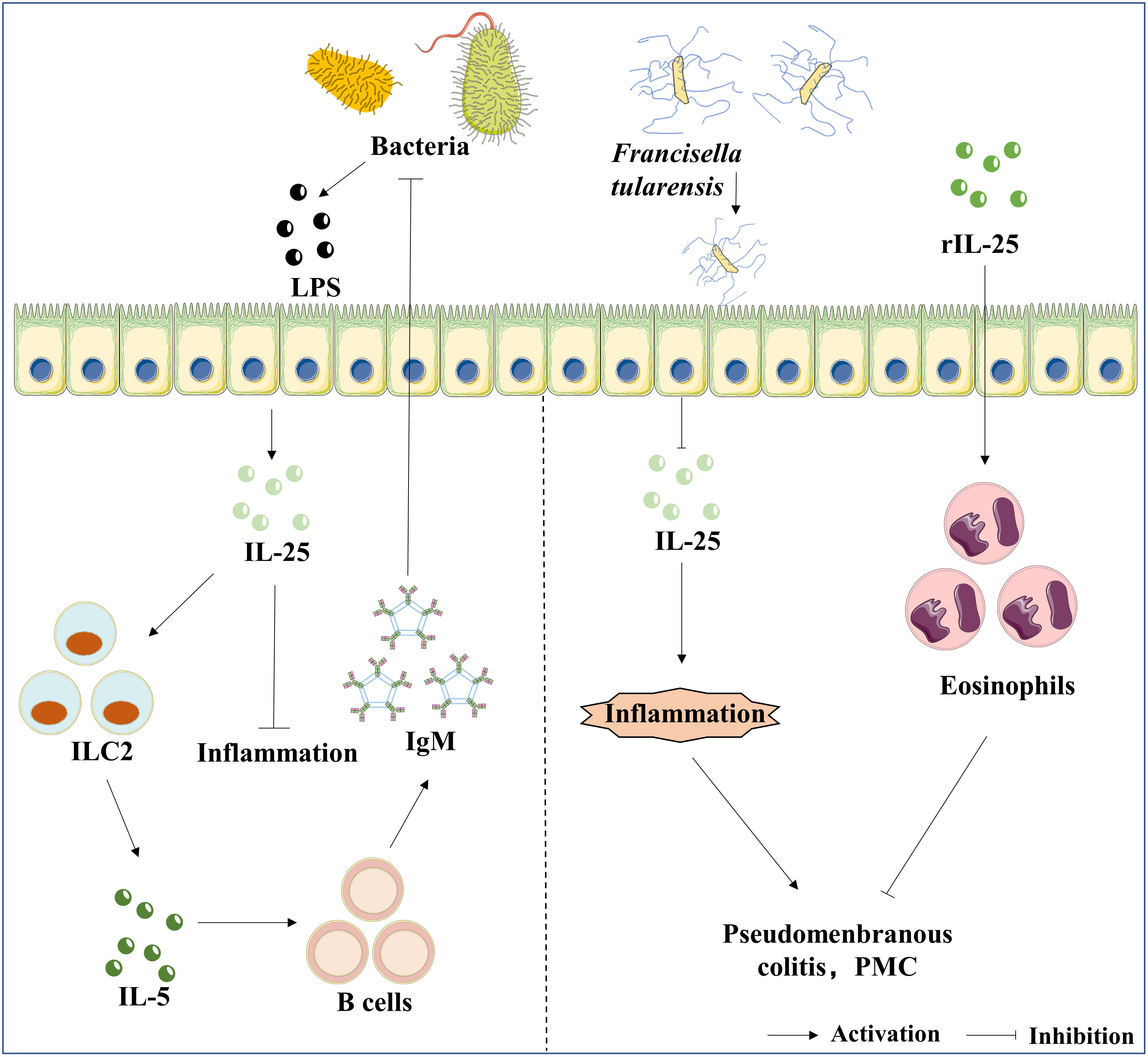
Figure 3 The roles of IL-25 in bacterial infection. LPS is the bacteria’s component, an inducer of IL-25 expression in epithelial cells. Furthermore, the axis of IL-25/ILC2/IL-5 mediates the production of IgM, and IgM exhibits a protective effect against bacterial infection. In contrast, Ft infection suppresses the expression of IL-25, which further results in pseudomembranous colitis (PMC). The administration of rIL-25 reduces the inflammatory storm caused by the infection of Ft.
Conclusions
IL-25 is a cytokine with a dual function in infectious diseases. In certain circumstances, IL-25 involves in the development of allergic diseases caused by the pathogenic infection. Most of pathogenic infection induces the up-regulation of IL-25. And then, IL-25 recruits ILC2 cells to promote the activation of Th2 cells. Th2 response up-regulates the expression of IL-4, IL-5, and IL-13, which are essential for allergic diseases caused by the pathogenic infection. Meanwhile, IL-25 plays an important role in the clearance of pathogens. Mechanically, IL-4, IL-5, and IL-13-induced by the expression of IL-25 to enhance the differentiation of Th2 cell and stimulate the production of IgE and IgM by B cells, as well as recruit and activate eosinophils, mast cells and basophils to perform the function of the clearance of pathogens. Therefore, it’s feasible to use IL-25 as a novel therapeutic target for infectious diseases. However, IL-25 treatment inhibits the expression of IFN-γ, which is beneficial for the infection of HSV-1. Thus, further investigations are necessary to explore the different mechanisms induced by IL-25 expression in different infectious diseases. Remarkably, given the circumstance Il-17ra deficiency also makes a mouse unable to respond to many IL-17 family members, any interpretation of IL-25 based on Il-17ra deficiency in this review should be made with caution in the future.
Author contributions
DC and JW contributed to the conception and design of the current review article. JW was responsible for drafting the manuscript. JW and FZ revised the manuscript. FZ, HT, and WN helped to draw the figures in the paper. ZW and DC performed the manuscript review and writing-review and editing. ZW provided the funding to support the review. All authors contributed to the article and approved the submitted version.
Funding
This work was supported by grants from the National Natural Science Foundation of China (Grant Number: 81900823 to DC and 31970149 to ZW).
Conflict of interest
The authors declare that the research was conducted in the absence of any commercial or financial relationships that could be construed as a potential conflict of interest.
Publisher’s note
All claims expressed in this article are solely those of the authors and do not necessarily represent those of their affiliated organizations, or those of the publisher, the editors and the reviewers. Any product that may be evaluated in this article, or claim that may be made by its manufacturer, is not guaranteed or endorsed by the publisher.
References
1. McGeachy MJ, Cua DJ, Gaffen SL. The IL-17 family of cytokines in health and disease. Immunity (2019) 50:892–906. doi: 10.1016/j.immuni.2019.03.021
2. Deng C, Peng N, Tang Y, Yu N, Wang C, Cai X, et al. Roles of IL-25 in type 2 inflammation and autoimmune pathogenesis. Front Immunol (2021) 12:691559. doi: 10.3389/fimmu.2021.691559
3. Gowhari Shabgah A, Amir A, Gardanova ZR, Olegovna Zekiy A, Thangavelu L, Ebrahimi Nik M, et al. Interleukin-25: New perspective and state-of-the-art in cancer prognosis and treatment approaches. Cancer Med (2021) 10:5191–202. doi: 10.1002/cam4.4060
4. Fort MM, Cheung J, Yen D, Li J, Zurawski SM, Lo S, et al. IL-25 induces IL-4, IL-5, and IL-13 and Th2-associated pathologies in vivo. Immunity (2001) 15:985–95. doi: 10.1016/S1074-7613(01)00243-6
5. Liu Y, Shao Z, Shangguan G, Bie Q, Zhang B. Biological properties and the role of IL-25 in disease pathogenesis. J Immunol Res (2018) 2018:6519465. doi: 10.1155/2018/6519465
6. Ikeda K, Nakajima H, Suzuki K, Kagami S, Hirose K, Suto A, et al. Mast cells produce interleukin-25 upon fc epsilon RI-mediated activation. Blood (2003) 101:3594–6. doi: 10.1182/blood-2002-09-2817
7. Kang CM, Jang AS, Ahn MH, Shin JA, Kim JH, Choi YS, et al. Interleukin-25 and interleukin-13 production by alveolar macrophages in response to particles. Am J Respir Cell Mol Biol (2005) 33:290–6. doi: 10.1165/rcmb.2005-0003OC
8. Reynolds JM, Lee YH, Shi Y, Wang X, Angkasekwinai P, Nallaparaju KC, et al. Interleukin-17B antagonizes interleukin-25-Mediated mucosal inflammation. Immunity (2015) 42:692–703. doi: 10.1016/j.immuni.2015.03.008
9. Gerbe F, Sidot E, Smyth DJ, Ohmoto M, Matsumoto I, Dardalhon V, et al. Intestinal epithelial tuft cells initiate type 2 mucosal immunity to helminth parasites. Nature (2016) 529:226–30. doi: 10.1038/nature16527
10. Wang Y, Zhang Y, Li MQ, Fan DX, Wang XH, Li DJ, et al. Interleukin-25 induced by human chorionic gonadotropin promotes the proliferation of decidual stromal cells by activation of JNK and AKT signal pathways. Fertil Steril (2014) 102:257–63. doi: 10.1016/j.fertnstert.2014.03.025
11. Valizadeh A, Khosravi A, Zadeh LJ, Parizad EG. Role of IL-25 in immunity. J Clin Diagn Res (2015) 9:Oe01–4. doi: 10.7860/JCDR/2015/12235.5814
12. Omata Y, Frech M, Saito T, Schett G, Zaiss MM, Tanaka S. Inflammatory arthritis and bone metabolism regulated by type 2 innate and adaptive immunity. Int J Mol Sci (2022) 23:1104. doi: 10.3390/ijms23031104
13. Amatya N, Garg AV, Gaffen SL. IL-17 signaling: The yin and the yang. Trends Immunol (2017) 38:310–22. doi: 10.1016/j.it.2017.01.006
14. Bredo G, Storie J, Shrestha Palikhe N, Davidson C, Adams A, Vliagoftis H, et al. Interleukin-25 initiates Th2 differentiation of human CD4(+) T cells and influences expression of its own receptor. Immun Inflammation Dis (2015) 3:455–68. doi: 10.1002/iid3.87
15. KleinJan A. Airway inflammation in asthma: key players beyond the Th2 pathway. Curr Opin Pulm Med (2016) 22:46–52. doi: 10.1097/MCP.0000000000000224
16. Claudio E, Sønder SU, Saret S, Carvalho G, Ramalingam TR, Wynn TA, et al. The adaptor protein CIKS/Act1 is essential for IL-25-mediated allergic airway inflammation. J Immunol (2009) 182:1617–30. doi: 10.4049/jimmunol.182.3.1617
17. Swaidani S, Bulek K, Kang Z, Liu C, Lu Y, Yin W, et al. The critical role of epithelial-derived Act1 in IL-17- and IL-25-mediated pulmonary inflammation. J Immunol (2009) 182:1631–40. doi: 10.4049/jimmunol.182.3.1631
18. Maezawa Y, Nakajima H, Suzuki K, Tamachi T, Ikeda K, Inoue J, et al. Involvement of TNF receptor-associated factor 6 in IL-25 receptor signaling. J Immunol (2006) 176:1013–8. doi: 10.4049/jimmunol.176.2.1013
19. Min HK, Won JY, Kim BM, Lee KA, Lee SJ, Lee SH, et al. Interleukin (IL)-25 suppresses IL-22-induced osteoclastogenesis in rheumatoid arthritis via STAT3 and p38 MAPK/IκBα pathway. Arthritis Res Ther (2020) 22:222. doi: 10.1186/s13075-020-02315-8
20. Pan S, Zhang Z, Li C, Yang D. Interleukin-25 regulates matrix metalloproteinase-2 and -9 expression in periodontal fibroblast cells through ERK and P38MAPK pathways. Cell Biol Int (2020) 44:2220–30. doi: 10.1002/cbin.11430
21. Caruso R, Sarra M, Stolfi C, Rizzo A, Fina D, Fantini MC, et al. Interleukin-25 inhibits interleukin-12 production and Th1 cell-driven inflammation in the gut. Gastroenterology (2009) 136:2270–9. doi: 10.1053/j.gastro.2009.02.049
22. Su J, Chen T, Ji XY, Liu C, Yadav PK, Wu R, et al. IL-25 downregulates Th1/Th17 immune response in an IL-10-dependent manner in inflammatory bowel disease. Inflammation Bowel Dis (2013) 19:720–8. doi: 10.1097/MIB.0b013e3182802a76
23. Sonobe Y, Takeuchi H, Kataoka K, Li H, Jin S, Mimuro M, et al. Interleukin-25 expressed by brain capillary endothelial cells maintains blood-brain barrier function in a protein kinase cepsilon-dependent manner. J Biol Chem (2009) 284:31834–42. doi: 10.1074/jbc.M109.025940
24. Xu M, Dong C. IL-25 in allergic inflammation. Immunol Rev (2017) 278:185–91. doi: 10.1111/imr.12558
25. Boonpiyathad T, Sözener ZC, Satitsuksanoa P, Akdis CA. Immunologic mechanisms in asthma. Semin Immunol (2019) 46:101333. doi: 10.1016/j.smim.2019.101333
26. Li Y, Chen S, Chi Y, Yang Y, Chen X, Wang H, et al. Kinetics of the accumulation of group 2 innate lymphoid cells in IL-33-induced and IL-25-induced murine models of asthma: a potential role for the chemokine CXCL16. Cell Mol Immunol (2019) 16:75–86. doi: 10.1038/s41423-018-0182-0
27. Unno H, Arae K, Matsuda A, Ikutani M, Tamari M, Motomura K, et al. Critical role of IL-33, but not IL-25 or TSLP, in silica crystal-mediated exacerbation of allergic airway eosinophilia. Biochem Biophys Res Commun (2020) 533:493–500. doi: 10.1016/j.bbrc.2020.09.046
28. Kouzaki H, Kikuoka H, Matsumoto K, Kato T, Tojima I, Shimizu S, et al. A mechanism of interleukin-25 production from airway epithelial cells induced by Japanese cedar pollen. Clin Immunol (2018) 193:46–51. doi: 10.1016/j.clim.2018.01.009
29. van Rijt LS, Logiantara A, Canbaz D, van Ree R. Birch pollen-specific subcutaneous immunotherapy reduces ILC2 frequency but does not suppress IL-33 in mice. Clin Exp Allergy (2018) 48:1402–11. doi: 10.1111/cea.13254
30. Celebi Sözener Z, Cevhertas L, Nadeau K, Akdis M, Akdis CA. Environmental factors in epithelial barrier dysfunction. J Allergy Clin Immunol (2020) 145:1517–28. doi: 10.1016/j.jaci.2020.04.024
31. Chang SH, Dong C. Signaling of interleukin-17 family cytokines in immunity and inflammation. Cell Signalling (2011) 23:1069–75. doi: 10.1016/j.cellsig.2010.11.022
32. Nakagome K, Nagata M. Innate immune responses by respiratory viruses, including rhinovirus, during asthma exacerbation. Front Immunol (2022) 13:865973. doi: 10.3389/fimmu.2022.865973
33. Chung EJ, Luo CH, Thio CL, Chang YJ. Immunomodulatory role of staphylococcus aureus in atopic dermatitis. Pathog (2022) 11:422. doi: 10.3390/pathogens11040422
34. van Riet E, Hartgers FC, Yazdanbakhsh M. Chronic helminth infections induce immunomodulation: Consequences and mechanisms. Immunobiology (2007) 212:475–90. doi: 10.1016/j.imbio.2007.03.009
35. Cattadori IM, Pathak AK, Ferrari MJ. External disturbances impact helminth-host interactions by affecting dynamics of infection, parasite traits, and host immune responses. Ecol Evol (2019) 9:13495–505. doi: 10.1002/ece3.5805
36. Maggi L, Mazzoni A, Capone M, Liotta F, Annunziato F, Cosmi L. The dual function of ILC2: From host protection to pathogenic players in type 2 asthma. Mol Aspects Med (2021) 80:100981. doi: 10.1016/j.mam.2021.100981
37. Oyesola OO, Früh SP, Webb LM, Tait Wojno ED. Cytokines and beyond: Regulation of innate immune responses during helminth infection. Cytokine (2020) 133:154527. doi: 10.1016/j.cyto.2018.08.021
38. Yasuda K, Nakanishi K. Host responses to intestinal nematodes. Int Immunol (2018) 30:93–102. doi: 10.1093/intimm/dxy002
39. von Moltke J, Ji M, Liang HE, Locksley RM. Tuft-cell-derived IL-25 regulates an intestinal ILC2-epithelial response circuit. Nature (2016) 529:221–5. doi: 10.1038/nature16161
40. Bouchery T, Le Gros G, Harris N. ILC2s-trailblazers in the host response against intestinal helminths. Front Immunol (2019) 10:623. doi: 10.3389/fimmu.2019.00623
41. Zaiss MM, Maslowski KM, Mosconi I, Guenat N, Marsland BJ, Harris NL. IL-1β suppresses innate IL-25 and IL-33 production and maintains helminth chronicity. PloS Pathog (2013) 9:e1003531. doi: 10.1371/journal.ppat.1003531
42. Smith KA, Löser S, Varyani F, Harcus Y, McSorley HJ, McKenzie AN, et al. Concerted IL-25R and IL-4Rα signaling drive innate type 2 effector immunity for optimal helminth expulsion. Elife (2018) 7:e38269. doi: 10.7554/eLife.38269
43. Shimokawa C, Kanaya T, Hachisuka M, Ishiwata K, Hisaeda H, Kurashima Y, et al. Mast cells are crucial for induction of group 2 innate lymphoid cells and clearance of helminth infections. Immunity (2017) 46:863–874.e4. doi: 10.1016/j.immuni.2017.04.017
44. Fallon PG, Ballantyne SJ, Mangan NE, Barlow JL, Dasvarma A, Hewett DR, et al. Identification of an interleukin (IL)-25-dependent cell population that provides IL-4, IL-5, and IL-13 at the onset of helminth expulsion. J Exp Med (2006) 203:1105–16. doi: 10.1084/jem.20051615
45. Hepworth MR, Maurer M, Hartmann S. Regulation of type 2 immunity to helminths by mast cells. Gut Microbes (2012) 3:476–81. doi: 10.4161/gmic.21507
46. Ayelign B, Akalu Y, Teferi B, Molla MD, Shibabaw T. Helminth induced immunoregulation and novel therapeutic avenue of allergy. J Asthma Allergy (2020) 13:439–51. doi: 10.2147/JAA.S273556
47. Owyang AM, Zaph C, Wilson EH, Guild KJ, McClanahan T, Miller HR, et al. Interleukin 25 regulates type 2 cytokine-dependent immunity and limits chronic inflammation in the gastrointestinal tract. J Exp Med (2006) 203:843–9. doi: 10.1084/jem.20051496
48. Zhang Y, Wang Y, Li MQ, Duan J, Fan DX, Jin LP. IL-25 promotes Th2 bias by upregulating IL-4 and IL-10 expression of decidual γδT cells in early pregnancy. Exp Ther Med (2018) 15:1855–62. doi: 10.3892/etm.2017.5638
49. Amor D, Santos LN, Silva ES, de Santana MBR, Belitardo E, Sena FA, et al. Toxocara canis extract fractions promote mainly the production of Th1 and regulatory cytokines by human leukocytes in vitro. Acta Trop (2022) 234:106579. doi: 10.1016/j.actatropica.2022.106579
50. de Oliveira Nóbrega CG, do Nascimento WRC, Santos PDA, de Lorena VMB, Medeiros D, Costa VMA, et al. Schistosoma mansoni infection is associated with decreased risk of respiratory allergy symptoms and low production of CCL2. Trop Med Int Health (2021) 26:1098–109. doi: 10.1111/tmi.13639
51. Lin K, Zhou D, Li M, Meng J, He F, Yang X, et al. Echinococcus granulosus cyst fluid suppresses inflammatory responses by inhibiting TRAF6 signalling in macrophages. Parasitology (2021) 148:887–94. doi: 10.1017/S0031182021000548
52. M’Bondoukwé NP, Moutongo R, Gbédandé K, Ndong Ngomo JM, Hountohotegbé T, Adamou R, et al. IL-10, and TNF-alpha and IL-10/IL-6 and IL-10/TNF-alpha ratio profiles of polyparasitized individuals in rural and urban areas of gabon. PloS Negl Trop Dis (2022) 16:e0010308. doi: 10.1371/journal.pntd.0010308
53. Fina D, Franzè E, Rovedatti L, Corazza GR, Biancone L, Sileri PP, et al. Interleukin-25 production is differently regulated by TNF-α and TGF-β1 in the human gut. Mucosal Immunol (2011) 4:239–44. doi: 10.1038/mi.2010.68
54. Xu M, Wang C, Tian Y, Kijlstra A, Yang P. Inhibition of proinflammatory cytokine by IL-25 in vogt-Koyanagi-Harada syndrome. Ocul Immunol Inflammation (2014) 22:294–9. doi: 10.3109/09273948.2013.854391
55. Kleinschek MA, Owyang AM, Joyce-Shaikh B, Langrish CL, Chen Y, Gorman DM, et al. IL-25 regulates Th17 function in autoimmune inflammation. J Exp Med (2007) 204:161–70. doi: 10.1084/jem.20061738
56. Pinelli E, Aranzamendi C. Toxocara infection and its association with allergic manifestations. Endocr Metab Immune Disord Drug Targets (2012) 12:33–44. doi: 10.2174/187153012799278956
57. Angkasekwinai P, Park H, Wang YH, Wang YH, Chang SH, Corry DB, et al. Interleukin 25 promotes the initiation of proallergic type 2 responses. J Exp Med (2007) 204:1509–17. doi: 10.1084/jem.20061675
58. Vannella KM, Ramalingam TR, Borthwick LA, Barron L, Hart KM, Thompson RW, et al. Combinatorial targeting of TSLP, IL-25, and IL-33 in type 2 cytokine-driven inflammation and fibrosis. Sci Transl Med (2016) 8:337ra65. doi: 10.1126/scitranslmed.aaf1938
59. Leija-Montoya AG, González-Ramírez J, Martínez-Coronilla G, Mejía-León ME, Isiordia-Espinoza M, Sánchez-Muñoz F, et al. Roles of microRNAs and long non-coding RNAs encoded by parasitic helminths in human carcinogenesis. Int J Mol Sci (2022) 23:8173. doi: 10.3390/ijms23158173
60. Quinteros SL, O’Brien B, Donnelly S. Exploring the role of macrophages in determining the pathogenesis of liver fluke infection. Parasitology (2022) 149:1–10. doi: 10.1017/S0031182022000749
61. Ham J, Shin JW, Ko BC, Kim HY. Targeting the epithelium-derived innate cytokines: From bench to bedside. Immune Netw (2022) 22:e11. doi: 10.4110/in.2022.22.e11
62. Xie SC, Dick LR, Gould A, Brand S, Tilley L. The proteasome as a target for protozoan parasites. Expert Opin Ther Targets (2019) 23:903–14. doi: 10.1080/14728222.2019.1685981
63. Noor Z, Watanabe K, Abhyankar MM, Burgess SL, Buonomo EL, Cowardin CA, et al. Role of eosinophils and tumor necrosis factor alpha in interleukin-25-Mediated protection from amebic colitis. mBio (2017) 8:e02329–16. doi: 10.1128/mBio.02329-16
64. Hendel SK, Kellermann L, Hausmann A, Bindslev N, Jensen KB, Nielsen OH. Tuft cells and their role in intestinal diseases. Front Immunol (2022) 13:822867. doi: 10.3389/fimmu.2022.822867
65. Shibui A, Takamori A, Tolba MEM, Nambu A, Shimura E, Yamaguchi S, et al. IL-25, IL-33 and TSLP receptor are not critical for development of experimental murine malaria. Biochem Biophysics Rep (2016) 5:191–5. doi: 10.1016/j.bbrep.2015.12.007
66. Heath A. Climate change and its potential for altering the phenology and ecology of some common and widespread arthropod parasites in new Zealand. N Z Vet J (2021) 69:5–19. doi: 10.1080/00480169.2020.1787276
67. Yasuda Y, Nagano T, Kobayashi K, Nishimura Y. Group 2 innate lymphoid cells and the house dust mite-induced asthma mouse model. Cells (2020) 9:1178. doi: 10.3390/cells9051178
68. Park JY, Choi JH, Lee SN, Cho HJ, Ahn JS, Kim YB, et al. Protein arginine methyltransferase 1 contributes to the development of allergic rhinitis by promoting the production of epithelial-derived cytokines. J Allergy Clin Immunol (2021) 147:1720–31. doi: 10.1016/j.jaci.2020.12.646
69. Yi L, Cheng D, Zhang K, Huo X, Mo Y, Shi H, et al. Intelectin contributes to allergen-induced IL-25, IL-33, and TSLP expression and type 2 response in asthma and atopic dermatitis. Mucosal Immunol (2017) 10:1491–503. doi: 10.1038/mi.2017.10
70. Kim DW, Kim DK, Eun KM, Bae JS, Chung YJ, Xu J, et al. IL-25 could be involved in the development of allergic rhinitis sensitized to house dust mite. Mediators Inflammation (2017) 2017:3908049. doi: 10.1155/2017/3908049
71. Claudio E, Wang H, Kamenyeva O, Tang W, Ha HL, Siebenlist U. IL-25 orchestrates activation of Th cells via conventional dendritic cells in tissue to exacerbate chronic house dust mite-induced asthma pathology. J Immunol (2019) 203:2319–27. doi: 10.4049/jimmunol.1900254
72. Openshaw PJM, Chiu C, Culley FJ, Johansson C. Protective and harmful immunity to RSV infection. Annu Rev Immunol (2017) 35:501–32. doi: 10.1146/annurev-immunol-051116-052206
73. Nam HH, Ison MG. Respiratory syncytial virus infection in adults. Bmj (2019) 366:l5021. doi: 10.1136/bmj.l5021
74. Griffiths C, Drews SJ, Marchant DJ. Respiratory syncytial virus: Infection, detection, and new options for prevention and treatment. Clin Microbiol Rev (2017) 30:277–319. doi: 10.1128/CMR.00010-16
75. Petersen BC, Dolgachev V, Rasky A, Lukacs NW. IL-17E (IL-25) and IL-17RB promote respiratory syncytial virus-induced pulmonary disease. J Leukoc Biol (2014) 95:809–15. doi: 10.1189/jlb.0913482
76. Siegle JS, Hansbro N, Dong C, Angkasekwinai P, Foster PS, Kumar RK. Blocking induction of T helper type 2 responses prevents development of disease in a model of childhood asthma. Clin Exp Immunol (2011) 165:19–28. doi: 10.1111/j.1365-2249.2011.04392.x
77. Wang J, Lu H, Yu L, Cheng W, Yan W, Jing X. Aggravation of airway inflammation in RSV-infected asthmatic mice following infection-induced alteration of gut microbiota. Ann Palliative Med (2021) 10:5084–97. doi: 10.21037/apm-20-2052
78. Stella L, Santopaolo F, Gasbarrini A, Pompili M, Ponziani FR. Viral hepatitis and hepatocellular carcinoma: From molecular pathways to the role of clinical surveillance and antiviral treatment. World J Gastroenterol (2022) 28:2251–81. doi: 10.3748/wjg.v28.i21.2251
79. Bassendine MF, Sheridan DA, Bridge SH, Felmlee DJ, Neely RD. Lipids and HCV. Semin Immunopathol (2013) 35:87–100. doi: 10.1007/s00281-012-0356-2
80. Roger S, Ducancelle A, Le Guillou-Guillemette H, Gaudy C, Lunel F. HCV virology and diagnosis. Clin Res Hepatol Gastroenterol (2021) 45:101626. doi: 10.1016/j.clinre.2021.101626
81. Wang B, Zhao XP, Fan YC, Zhang JJ, Zhao J, Wang K. IL-17A but not IL-22 suppresses the replication of hepatitis b virus mediated by over-expression of MxA and OAS mRNA in the HepG2. 2.15 Cell Line Antiviral Res (2013) 97:285–92. doi: 10.1016/j.antiviral.2012.12.018
82. Askoura M, Abbas HA, Al Sadoun H, Abdulaal WH, Abu Lila AS, Almansour K, et al. Elevated levels of IL-33, IL-17 and IL-25 indicate the progression from chronicity to hepatocellular carcinoma in hepatitis c virus patients. Pathogens (2022) 11:57. doi: 10.3390/pathogens11010057
83. Cabral MS, Santos TPS, Santos PL, Schinoni MI, Oliveira IS, Pereira AB, et al. Immune response of Th17-associated cytokines by peripheral blood mononuclear cells from patients with chronic hepatitis c virus infection. Cytokine (2018) 102:200–5. doi: 10.1016/j.cyto.2017.09.015
84. Shen J, Wu H, Peng N, Cai J. An eight cytokine signature identified from peripheral blood serves as a fingerprint for hepatocellular cancer diagnosis. Afr Health Sci (2018) 18:260–6. doi: 10.4314/ahs.v18i2.9
85. Mantovani A, Allavena P, Sica A, Balkwill F. Cancer-related inflammation. Nature (2008) 454:436–44. doi: 10.1038/nature07205
86. Khalifa A, Lewin DN, Sasso R, Rockey DC. The utility of liver biopsy in the evaluation of liver disease and abnormal liver function tests. Am J Clin Pathol (2021) 156:259–67. doi: 10.1093/ajcp/aqaa225
87. Feng J, Li L, Ou Z, Li Q, Gong B, Zhao Z, et al. IL-25 stimulates M2 macrophage polarization and thereby promotes mitochondrial respiratory capacity and lipolysis in adipose tissues against obesity. Cell Mol Immunol (2018) 15:493–505. doi: 10.1038/cmi.2016.71
88. Li Q, Ma L, Shen S, Guo Y, Cao Q, Cai X, et al. Intestinal dysbacteriosis-induced IL-25 promotes development of HCC via alternative activation of macrophages in tumor microenvironment. J Exp Clin Cancer Research: CR (2019) 38:303. doi: 10.1186/s13046-019-1271-3
89. Tsai ML, Tsai YG, Lin YC. IL-25 induced ROS-mediated M2 macrophage polarization via AMPK-associated mitophagy. Int J Mol Sci (2021) 23:3. doi: 10.3390/ijms23010003
90. Arduino PG, Porter SR. Herpes simplex virus type 1 infection: overview on relevant clinico-pathological features. J Oral Pathol Med (2008) 37:107–21. doi: 10.1111/j.1600-0714.2007.00586.x
91. Damour A, Garcia M, Seneschal J, Lévêque N, Bodet C. Eczema herpeticum: Clinical and pathophysiological aspects. Clin Rev Allergy Immunol (2020) 59:1–18. doi: 10.1007/s12016-019-08768-3
92. Traidl S, Roesner L, Zeitvogel J, Werfel T. Eczema herpeticum in atopic dermatitis. Allergy (2021) 76:3017–27. doi: 10.1111/all.14853
93. Basałygo M, Śliwińska J, Żbikowska-Gotz M, Lis K, Socha E, Nowowiejska L, et al. Evaluation of the effect of the interleukin-25 serum concentration on the intensity of the symptoms of atopic dermatitis and epidermal barrier. Postepy Dermatol Alergol (2021) 38:1071–7. doi: 10.5114/ada.2021.109686
94. Ong PY. New insights in the pathogenesis of atopic dermatitis. Pediatr Res (2014) 75:171–5. doi: 10.1038/pr.2013.196
95. Kim BE, Bin L, Ye YM, Ramamoorthy P, Leung DYM. IL-25 enhances HSV-1 replication by inhibiting filaggrin expression, and acts synergistically with Th2 cytokines to enhance HSV-1 replication. J Invest Dermatol (2013) 133:2678–85. doi: 10.1038/jid.2013.223
96. Leung DY, Gao PS, Grigoryev DN, Rafaels NM, Streib JE, Howell MD, et al. Human atopic dermatitis complicated by eczema herpeticum is associated with abnormalities in IFN-γ response. J Allergy Clin Immunol (2011) 127:965–73.e1-5. doi: 10.1016/j.jaci.2011.02.010
97. Salimi M, Barlow JL, Saunders SP, Xue L, Gutowska-Owsiak D, Wang X, et al. A role for IL-25 and IL-33-driven type-2 innate lymphoid cells in atopic dermatitis. J Exp Med (2013) 210:2939–50. doi: 10.1084/jem.20130351
98. Suryawanshi A, Veiga-Parga T, Rajasagi NK, Reddy PB, Sehrawat S, Sharma S, et al. Role of IL-17 and Th17 cells in herpes simplex virus-induced corneal immunopathology. J Immunol (2011) 187:1919–30. doi: 10.4049/jimmunol.1100736
99. Molesworth-Kenyon SJ, Yin R, Oakes JE, Lausch RN. IL-17 receptor signaling influences virus-induced corneal inflammation. J Leukoc Biol (2008) 83:401–8. doi: 10.1189/jlb.0807571
100. Beale J, Jayaraman A, Jackson DJ, Macintyre JDR, Edwards MR, Walton RP, et al. Rhinovirus-induced IL-25 in asthma exacerbation drives type 2 immunity and allergic pulmonary inflammation. Sci Transl Med (2014) 6:256ra134. doi: 10.1126/scitranslmed.3009124
101. Han M, Rajput C, Hong JY, Lei J, Hinde JL, Wu Q, et al. The innate cytokines IL-25, IL-33, and TSLP cooperate in the induction of type 2 innate lymphoid cell expansion and mucous metaplasia in rhinovirus-infected immature mice. J Immunol (2017) 199:1308–18. doi: 10.4049/jimmunol.1700216
102. Hong JY, Bentley JK, Chung Y, Lei J, Steenrod JM, Chen Q, et al. Neonatal rhinovirus induces mucous metaplasia and airways hyperresponsiveness through IL-25 and type 2 innate lymphoid cells. J Allergy Clin Immunol (2014) 134:429–39. doi: 10.1016/j.jaci.2014.04.020
103. Hong H, Tan KS, Yan Y, Chen F, Ong HH, Oo Y, et al. Induction of IL-25 expression in human nasal polyp epithelium by influenza virus infection is abated by interferon-alpha pretreatment. J Inflammation Res (2021) 14:2769–80. doi: 10.2147/JIR.S304320
104. Reddehase MJ. Adverse immunological imprinting by cytomegalovirus sensitizing for allergic airway disease. Med Microbiol Immunol (2019) 208:469–73. doi: 10.1007/s00430-019-00610-z
105. Desai P, Janova H, White JP, Reynoso GV, Hickman HD, Baldridge MT, et al. Enteric helminth coinfection enhances host susceptibility to neurotropic flaviviruses via a tuft cell-IL-4 receptor signaling axis. Cell (2021) 184:1214–1231.e16. doi: 10.1016/j.cell.2021.01.051
106. Williams TC, Loo SL, Nichol KS, Reid AT, Veerati PC. IL-25 blockade augments antiviral immunity during respiratory virus infection. Commun Biol (2022) 5:415. doi: 10.1038/s42003-022-03367-z
107. Galli G, Saleh M. Immunometabolism of macrophages in bacterial infections. Front Cell Infect Microbiol (2020) 10:607650. doi: 10.3389/fcimb.2020.607650
108. Bahuaud O, Le Brun C, Lemaignen A. Host immunity and francisella tularensis: A review of tularemia in immunocompromised patients. Microorganisms (2021) 9:2539. doi: 10.3390/microorganisms9122539
109. Barbosa CHD, Lantier L, Reynolds J, Wang J, Re F. Critical role of IL-25-ILC2-IL-5 axis in the production of anti-francisella LPS IgM by B1 b cells. PloS Pathog (2021) 17:e1009905. doi: 10.1371/journal.ppat.1009905
110. Liu XW, Yang YJ, Qin Z, Li SH, Bai LX, Ge WB, et al. Isobavachalcone from Cullen corylifolium presents significant antibacterial activity against clostridium difficile through disruption of the cell membrane. Front Pharmacol (2022) 13:914188. doi: 10.3389/fphar.2022.914188
111. Sehgal K, Cifu AS, Khanna S. Treatment of clostridioides difficile infection. JAMA (2022). doi: 10.1001/jama.2022.12251
112. Czepiel J, Dróżdż M, Pituch H, Kuijper EJ, Perucki W, Mielimonka A, et al. Clostridium difficile infection: Review. Eur J Clin Microbiol Infect Dis (2019) 38:1211–21. doi: 10.1007/s10096-019-03539-6
113. Jan N, Hays RA, Oakland DN, Kumar P, Ramakrishnan G, Behm BW, et al. Fecal microbiota transplantation increases colonic IL-25 and dampens tissue inflammation in patients with recurrent clostridioides difficile. mSphere (2021) 6:e0066921. doi: 10.1128/mSphere.00669-21
114. Buonomo EL, Cowardin CA, Wilson MG, Saleh MM, Pramoonjago P, Petri WA Jr. Microbiota-regulated IL-25 increases eosinophil number to provide protection during clostridium difficile infection. Cell Rep (2016) 16:432–43. doi: 10.1016/j.celrep.2016.06.007
115. Brook I. Microbiology and treatment of brain abscess. J Clin Neurosci (2017) 38:8–12. doi: 10.1016/j.jocn.2016.12.035
116. Vidlak D, Kielian T. Differential effects of interleukin-17 receptor signaling on innate and adaptive immunity during central nervous system bacterial infection. J Neuroinflamm (2012) 9:128. doi: 10.1186/1742-2094-9-128
117. Ishigame H, Kakuta S, Nagai T, Kadoki M, Nambu A, Komiyama Y, et al. Differential roles of interleukin-17A and -17F in host defense against mucoepithelial bacterial infection and allergic responses. Immunity (2009) 30:108–19. doi: 10.1016/j.immuni.2008.11.009
118. Federici S, Kredo-Russo S, Valdés-Mas R, Kviatcovsky D, Weinstock E, Matiuhin Y, et al. Targeted suppression of human IBD-associated gut microbiota commensals by phage consortia for treatment of intestinal inflammation. Cell (2022) 185:2879–2898.e24. doi: 10.1016/j.cell.2022.07.003
Keywords: IL-17 family, IL-25, Th2, inflammation, infectious diseases
Citation: Wu J, Zhang F, Tao H, Nawaz W, Chen D and Wu Z (2022) The potential roles of interleukin-25 in infectious diseases. Front. Immunol. 13:986118. doi: 10.3389/fimmu.2022.986118
Received: 04 July 2022; Accepted: 18 August 2022;
Published: 02 September 2022.
Edited by:
Kari Ann Shirey, University of Maryland, United StatesReviewed by:
Rafael Toledo, University of Valencia, SpainMohan Tulapurkar, University of Maryland, Baltimore, United States
Copyright © 2022 Wu, Zhang, Tao, Nawaz, Chen and Wu. This is an open-access article distributed under the terms of the Creative Commons Attribution License (CC BY). The use, distribution or reproduction in other forums is permitted, provided the original author(s) and the copyright owner(s) are credited and that the original publication in this journal is cited, in accordance with accepted academic practice. No use, distribution or reproduction is permitted which does not comply with these terms.
*Correspondence: Deyan Chen, Y2hlbmRleWFuQG5qdS5lZHUuY24=; Zhiwei Wu, d3pod0BuanUuZWR1LmNu
†These authors have contributed equally to this work