- 1Department of Hepatobiliary and Pancreatic Surgery, The First Affiliated Hospital of Zhengzhou University, Zhengzhou, China
- 2Department of Colorectal Surgery, The First Affiliated Hospital of Zhengzhou University, Zhengzhou, China
- 3Department of Radiotherapy, Affiliated Cancer Hospital of Zhengzhou University, Henan Cancer Hospital, Zhengzhou, China
Cell death is generally classified into two categories: regulated cell death (RCD) and accidental cell death (ACD). In particular, RCD is a kind of genetically controlled process, including programmed apoptotic death and programmed necrotic death. Pyroptosis, an inflammatory form of programmed necrotic death, causes inflammation in cells. The influence of pyroptosis on tumor is complicated. On the one hand, pyroptosis triggers antitumor response. On the other hand, pyroptosis may induce carcinogenesis. Pyroptosis is initiated by various factors, especially non-coding RNAs. In this review, we discuss the effects of ncRNAs on pyroptosis and the mechanisms by which ncRNAs initiate pyroptosis. Moreover, we introduce the influence of ncRNA on tumor resistance via pyroptosis. Additionally, we summarize how ncRNA-associated pyroptosis modulates the tumor microenvironment (TME) and thereafter triggers antitumor immune response. Finally, pyroptosis-related ncRNAs are promising diagnostic and immunotherapeutic biomarkers and therapeutic targets
Highlights
● NcRNA-regulated pyroptosis is a double-edged sword for tumor development.
● NcRNAs regulate tumor resistance via pyroptosis and could be potential therapeutic targets to overcome tumor resistance.
● NcRNAs trigger pyroptosis, causing fierce inflammatory response and remodeling TME.
● Pyroptosis-related ncRNAs are expected to serve as biomarkers of diagnoses and immunotherapeutic efficiency.
Open questions
● The influence of ncRNA-regulated pyroptosis is complex on tumor and requires further experimentations.
● There is still a lack of a precise pyroptosis-related drug delivery platform to specifically trigger pyroptosis in extracellular milieu in tumor sites.
● The factors leading to the expression change of pyroptosis-associated ncRNAs within a cancerous context are unknown.
● The mechanisms by which circRNAs are sensed in the pyroptotic pathway are unclear.
Pyroptosis
The history and characteristics of pyroptosis
In 1992, Lindgren, S. W. firstly described pyroptosis in Shigella flexneri-infected macrophages (1). Pyroptosis is the inflammatory programmed necrotic death that is characterized by cell lysis, cell content release, membrane rupture, DNA fragmentation, and an intact nucleus (2–7). Rapid death speed and huge morphological change distinguish pyroptotic cells from apoptotic cells (8).
Programmed necrotic death is generally categorized as necroptosis, ferroptosis, and pyroptosis. Evolutionarily, viruses attained the ability to evade their host’s defense and the immune system (9, 10). Necroptosis is thought to be conserved as an MLKL-mediated necrosis to defend viruses during evolution (11). Ferroptosis is a kind of iron-dependent necrosis by lipid peroxidation. Unlike necroptosis and pyroptosis, ferroptosis is not induced by classic signaling, such as through a death receptor or a DNA sensor. Additionally, it is not comprehensively clarified how the plasma membrane might rupture in ferroptosis. Furthermore, this necrotic-type cell death, ferroptosis, occurs in a non-cell-autonomous manner referred to as synchronized regulated necrosis (11). Given pyroptosis, it is a gasdermin-mediated necrosis to amplify inflammasome action and eventually trigger fierce inflammatory response (12–18).
The roles of pyroptosis in human diseases have emerged. Triggering pyroptosis contributes to various diseases, including infection such as L. pneumophila (19, 20), autoinflammatory genetic diseases such as Familial Mediterranean Fever (FMF) (21, 22), inflammation such as cytokine release syndrome (CRS) (23, 24), noninfectious diseases such as alcoholic hepatitis (25, 26), and cancers such as non-small cell lung cancer (NSCLC) (27, 28). Notably, pyroptosis is a double-edged sword for tumorigenesis and progression. LINC00958 inhibited miR-4306 levels to trigger AIM2-mediated pyroptosis pathway and promoted cancer cell survival (29). By contrast, Liu et al. reported that pyroptosis attenuates cancer cell viability and proliferation in glioma (30). Therefore, the mechanisms of pyroptosis in tumor are attracting increasing attention.
The pathways of pyroptosis
Dying cells caused by therapeutic regimens, including chemotherapy, radiotherapy, targeted therapy, and immune therapy, activate pyroptosis in cancer (17, 31). In addition, alcohol accumulation also triggers pyroptosis in the distal esophagus (32). However, it is still unclear whether ultrasound irradiation could activate pyroptosis in extracellular matrix.
Pyroptotic cell death is mainly triggered by two pathways. In the GSDMD-mediated canonical pathway, the danger-associated molecular patterns (DAMPs) and pathogen-associated molecular patterns (PAMPs) induce and activate caspase-1 inflammasome. Thereafter, the caspase-1 inflammasome cleave GSDMD and pro-IL-1 family. GSDMD-N terminal (GSDMD-NT), the active state of GSDMD, forms a pore in cell membrane, leading to the liberation of cellular contents, including mature IL-1β, IL-18, ATP, HMGB1, and chemokines (33–35). In addition to caspase-1, caspase-4, -5, and -11 also are critical for pyroptosis activation. Caspase-4, -5, and -11 directly recognize bacterial lipopolysaccharide (35), whereas caspase-11-activating inflammasomes only lead to GSDMD cleavage without maturation of the cytokines (11, 16). In the GSDME-mediated noncanonical pathway, activated caspase-3 cleaves GSDME, which converts noninflammatory apoptosis to inflammatory pyroptosis. GSDME-NT eventually generates cell membrane pores (36, 37).
There are other GSDM family members mediating pyroptosis. GSDMA, for example, is the first GSDM family member being identified (38). However, GSDMA polymorphisms have been linked to autoimmune (39) and chronic inflammatory diseases (40). The understanding of the impact of GSDMA on cancers needs to be further explored. In addition, the overexpression of GSDMB has been found in many cancers, such as cervical, breast, gastrointestinal, and hepatic cancers, which is associated with the poor prognosis (41–44). Furthermore, GSDMC was first clarified in melanoma as a marker for progression. It was worth noting that GSDMC can be induced in breast cancer by hypoxia-triggered STAT2 phosphorylation and its complexation with nuclear-translocated programmed cell death ligand 1 (PDL1) (45).
Taken together, pyroptosis was firstly observed in infectious diseases. Furthermore, GSDM family members are the key executors of pyroptosis. As research on pyroptosis accumulated, the effects of pyroptosis were clarified deeply and comprehensively, especially on cancers. Additionally, the regulatory networks of pyroptosis were also demonstrated, among which ncRNAs have a pronounced impact on cancer cell pyroptosis and the tumor microenvironment (TME).
Effects of ncRNAs on tumor pyroptosis
For decades, in the field of cancer biology, researchers focused on the involvement of protein-coding genes. However, recently, it was discovered that an entire class of molecules, termed non-coding RNA (ncRNA), plays crucial regulatory roles in shaping cellular activity (46). Accumulating lines of evidence suggest that ncRNAs could initiate pyroptosis in cancers (47). In particular, the regulatory functions and molecular mechanisms of ncRNAs on tumor cell pyroptosis are attracting growing attention with the development of high-throughput sequencing technology (48, 49). In this section, we reviewed the influence of ncRNAs, including microRNA, lncRNA, and circRNA, on tumor pyroptotic cell death (Figure 1).
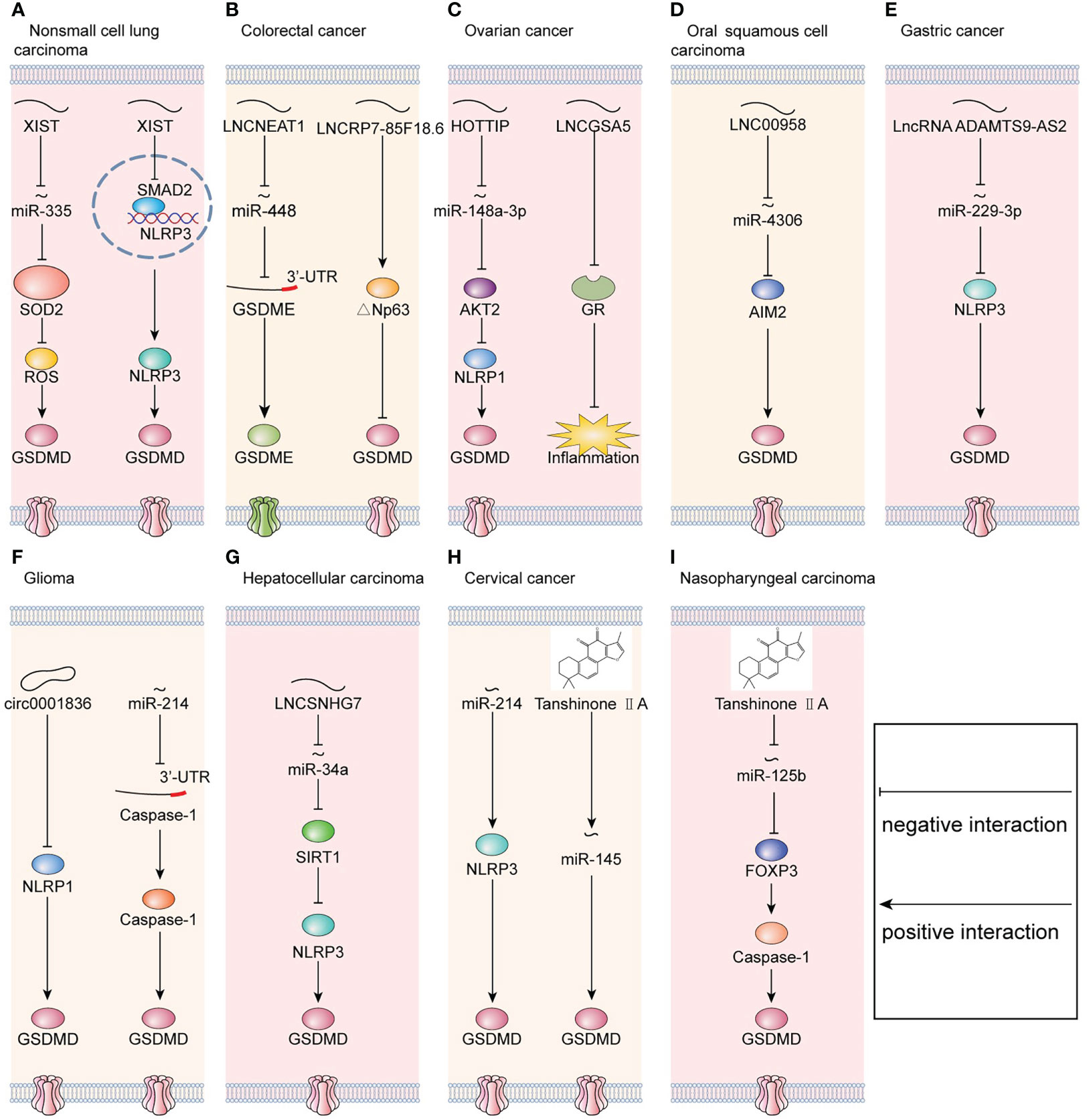
Figure 1 Molecular mechanisms that regulate pyroptosis in cancers. (A) In non-small cell lung carcinoma, LNCXIST regulates pyroptosis via the miR-335/SOD2 axis and SMAD2/NLRP3 axis simultaneously. (B) In colorectal cancer, LNCNEAT1 regulates pyroptosis via the miR-448/GSDME axis, and LNCRP7-85F18.6 regulates pyroptosis via △Np63. (C) In ovarian cancer, LNCHOTTIP regulates pyroptosis via the miR-148a-3p/AKT2/NLRP1 axis. Meanwhile, LNCGSA5 interferes with glucocorticoid receptor (GR), which blocks the upregulation of anti-inflammatory proteins and eventually promotes inflammasome formation. (D) In oral squamous cell carcinoma, LNC00958 regulates pyroptosis via the miR-4306/AIM2 axis. (E) In gastric cancer, LncRNA ADAMTS9-AS2 regulates pyroptosis via the miR-229-3p/NLRP3 axis. (F) In glioma, downregulation of circ0001836 promotes pyroptosis via epigenetically upregulating NLRP1. Meanwhile, miR-214 directly binds to the 3’-UTR of caspase-1 and consequently inhibits pyroptosis. (G) In hepatocellular carcinoma, LNCSNHG7 regulates pyroptosis via the miR-34a/SIRT1 axis. (H) In cervical cancer, miR-214 regulates pyroptosis via targeting to NLRP3. Moreover, tanshinone IIA promotes pyroptosis via upregulating miR-145. (I) In nasopharyngeal carcinoma, tanshinone IIA regulates pyroptosis via the miR-125b/FOXP3 axis.
MicroRNAs
Numerous studies have found that the dysregulation of microRNAs is part of the pathological processes of cancers, including pyroptosis. MicroRNAs bind to a protein-coding mRNA and thereafter decrease target gene expression post-transcriptionally (50). In glioma, miR-214 suppresses cell proliferation and migration via caspase-1-mediated pyroptosis. Furthermore, miR-214 bind to the 3’-untranslated region (3’-UTR) of caspase-1 (51). Nevertheless, in cervical cancer, miR-214 targets NLRP3 and thereafter induces pyroptosis in cervical cancer (52). Therefore, in distinct cancers, miR-214 has different targeted genes. Additionally, miR-145/GSDMD signaling is regulated by tanshinone IIA, a lipophilic pharmacologically active compound extracted from Salvia miltiorrhiza Bunge (Danshen) (53). Similarly, tanshinone IIA enhances the pyroptosis in nasopharyngeal carcinoma (NPC) and suppresses the progression of NPC via miR−125b/foxp3/caspase−1/GSDMD signaling (54). Moreover, in enterovirus A71 (EV-A71)-infected human neuroblastoma, miR-195 inhibits pyroptosis by targeting NLRX1. Therefore, these mechanisms by which microRNAs initiate pyroptosis in cancers provide the antitumor therapy with a novel direction.
LncRNAs
In addition to mRNAs, complementarity between lncRNA and microRNA has been identified to be part of the regulation of pyroptosis. In colorectal cancer (CRC), lncRNA nuclear paraspeckle assembly transcript 1 (NEAT1) enhances the expression of GSDME and then promotes pyroptosis in CRC by binding to miR-448 (55). Similarly, lncRNA small nucleolar host gene 7 (SNHG7) expression was increased in hepatocellular carcinoma (HCC). Furthermore, SNHG7 inhibited NLR family pyrin domain containing 3 (NLRP3)-dependent pyroptosis serving as a competing endogenous RNA of miR−34a (56). In oral squamous cell carcinoma (OSCC), LINC00958 decreases the levels of miR-4306 to activate the AIM2-dependent pyroptosis (29). In ovarian cancer (OC), lncRNA HOTTIP significantly enhanced NLRP1 inflammasome-mediated pyroptosis via the miR-148a-3p/AKT2 axis (57). Therefore, lncRNAs targeting microRNAs are the critical regulators of pyroptosis in cancers and have the potential of therapeutic targets.
Apart from sponging microRNA, lncRNA could influence gene expression directly. For example, in NSCLC, lncRNA X inactive-specific transcript (LNCXIST) suppressed pyroptosis by inhibiting the SOD2/ROS signal pathway (27). In ovarian cancer, long noncoding RNA growth arrest-specific transcript 5 (lncRNA GAS5) functions as a suppressor of tumor progression by inducing inflammasome formation (58). Meanwhile, lncRNA GAS5 blocks the upregulation of gene expression by activating glucocorticoid receptor (GR) (59, 60). Furthermore, the activated GR complex could increase the level of anti-inflammatory proteins in the nucleus or inhibit the proinflammatory proteins in the cytosol (58).
Collectively, lncRNAs initiate pyroptosis in TME by acting as competing endogenous RNAs (ceRNAs) indirectly or influencing targeting gene expression directly.
CircRNAs
Circular RNAs are a group of different non-coding RNAs with a covalently closed loop structure (61). CircRNAs are abundantly expressed in tumor cells and initiate the pyroptosis-related gene expression (62, 63). On the one hand, circRNAs initiated DNA methylation of pyroptosis-associated genes (64). For example, in glioma cells, circRNA-0001836 promotes the expression of NLRP1 via DNA demethylation, leading to the activation of pyroptosis (30). In vivo, knockdown of circRNA-0001836 suppressed tumorigenesis by activating NLRP1/GSDMD signaling (30). On the other hand, circRNAs unlock the inhibitory effects of microRNAs on downstream targets serving as microRNA sponges (65–67). For instance, in lung adenocarcinoma (LUAD), circNEIL3 initiates pyroptosis by directly binding to miR-1184 (68). However, the mechanisms by which circRNAs are sensed in the pyroptotic pathway are still vague, which needs to be further explored in the future.
In summary, microRNA, lncRNA, and circRNA initiate cancer cell pyroptosis by sponging upstream or downstream molecules. Understanding these mechanisms has pronounced effects on the development of diagnostic biomarkers and therapeutic targets in clinics. In the future, screening the comprehensive spatial and temporal RNA-expressing profiles of each cancer is needed to expand the utilization of pyroptosis for cancer therapy.
The influences of pyroptosis-related ncRNAs on tumor resistance
Although overall survival has been prolonged significantly with various treatments, tumor resistance remains an obstacle in clinics (69). Tumor resistance is complex and multifaceted. In 2020, David M. Hyman defined and separated the key determinants of tumor resistance, including tumor burden, growth kinetics, tumor heterogeneity, physical barriers, undruggable cancer drivers, application of therapeutic pressures, immune system, and microenvironment (70). Pyroptosis, an inflammatory PCD, has attracted notable interest within the cancer research community for its potential to address tumor resistance by remodeling immunosuppressive TME.
Recently, it has been illustrated that ncRNAs may regulate tumor resistance via pyroptosis (71, 72). For example, lncRNA X inactive-specific transcript (LNCXIST) is upregulated in cisplatin [cis-diamminedichloroplatinum (II); DDP]-treated NSCLC. In vitro, LNCXIST knockdown enhances DDP chemosensitivity via pyroptosis (71). Furthermore, LNCXIST binds to the TGF-β effector SMAD2, inhibiting its translocation to nucleus. The SMAD2 in nucleus prevented the transcription of NLRP3 (71). Eventually, the DDP chemoresistance is promoted by LNCXIST via pyroptosis. In vivo, similarly, LNCXIST mediates DDP chemoresistance by inhibiting pyroptosis (71). Additionally, the expression of miR-556-5p is significantly upregulated in the cisplatin-resistant NSCLC (CR-NSCLC) than the cisplatin-sensitive NSCLC (CS-NSCLC) (28). Knockdown of miR-556-5p triggered pyroptotic cell death in cisplatin-treated CR-NSCLC cells via upregulating NLRP3 (28). Moreover, the expression of lncRNA ADAMTS9-AS2 was downregulated, and miR223-3p was upregulated in cisplatin-resistant gastric cancer (CR-GC) (73). Furthermore, the overexpression of lncRNA ADAMTS9-AS2 enhanced the cytotoxic effects of cisplatin on CR-GC with the upregulation of NLRP3 inflammasome through targeting miR-223-3p (73). Therefore, these identifications suggest that the expression of LNCXIST, lncRNA ADAMTS9-AS2, miR-556-5p, and miR-223-3p may serve as promising biomarkers to predict DDP treatment efficacy, and may help in the design of new therapies to circumvent DDP chemoresistance in NSCLC and other tumor types.
NcRNAs remodel tumor microenvironment via pyroptosis
The ncRNA-regulated pyroptosis releases various cellular contents, such as inflammatory caspase, IL-1 family, cleaved GSDMD, and chemokines (74), most of which must not be liberated normally. These cellular contents cause unexpected reactions in TME.
The effects of pyroptosis-related ncRNAs on chronic inflammation
The chronic inflammation caused by ncRNA-regulated pyroptosis promotes tumorigenesis (75), progression, angiogenesis, and metastasis (76–78). For example, in oral squamous cellular carcinoma (OSCC), LINC00958 activates AIM-mediated pyroptosis pathway, which released caspase-1 and IL-1 family into TME and eventually caused chronic inflammation in TME (29). Moreover, the tumor-promoting effects of inflammation were confirmed in the CANTOS trial, in which anti-IL-1 antibody significantly prolonged overall survival and reduced the incidence of lung cancer (79). Therefore, the ncRNA-regulated pyroptosis may have strong tumor-promoting effects in the early stage of tumors.
The effects of ncRNAs on antitumor immunity
The tumor with low levels of tumor-infiltrating lymphocytes (TILs) was named “cold tumor”, which is considered as non-responsive to immune checkpoint inhibitors (ICIs) (80). In addition to “cold tumor”, many tumors possess acquired resistance to ICI therapy (80), which remarkably constrains the efficiency of ICIs. MiR-214 elicits robust antitumor immune response in TME via promoting pyroptosis with the elevation of IL-1β, IL-18, and caspase-1 (51). After being liberated into TME, IL-1β promotes the maturation of dendritic cells (DCs), activates antigen-specific cytotoxic CD8+ T cells, recruits Th1 CD4+ T cells, and represses the differentiation of immunosuppressive Treg cells (81, 82). Additionally, IL-18 polarizes Th1 cells, recruits and activates natural killer (NK) cells, and produces adhesion molecules, chemokine, and nitric oxide (83) (Figure 2).
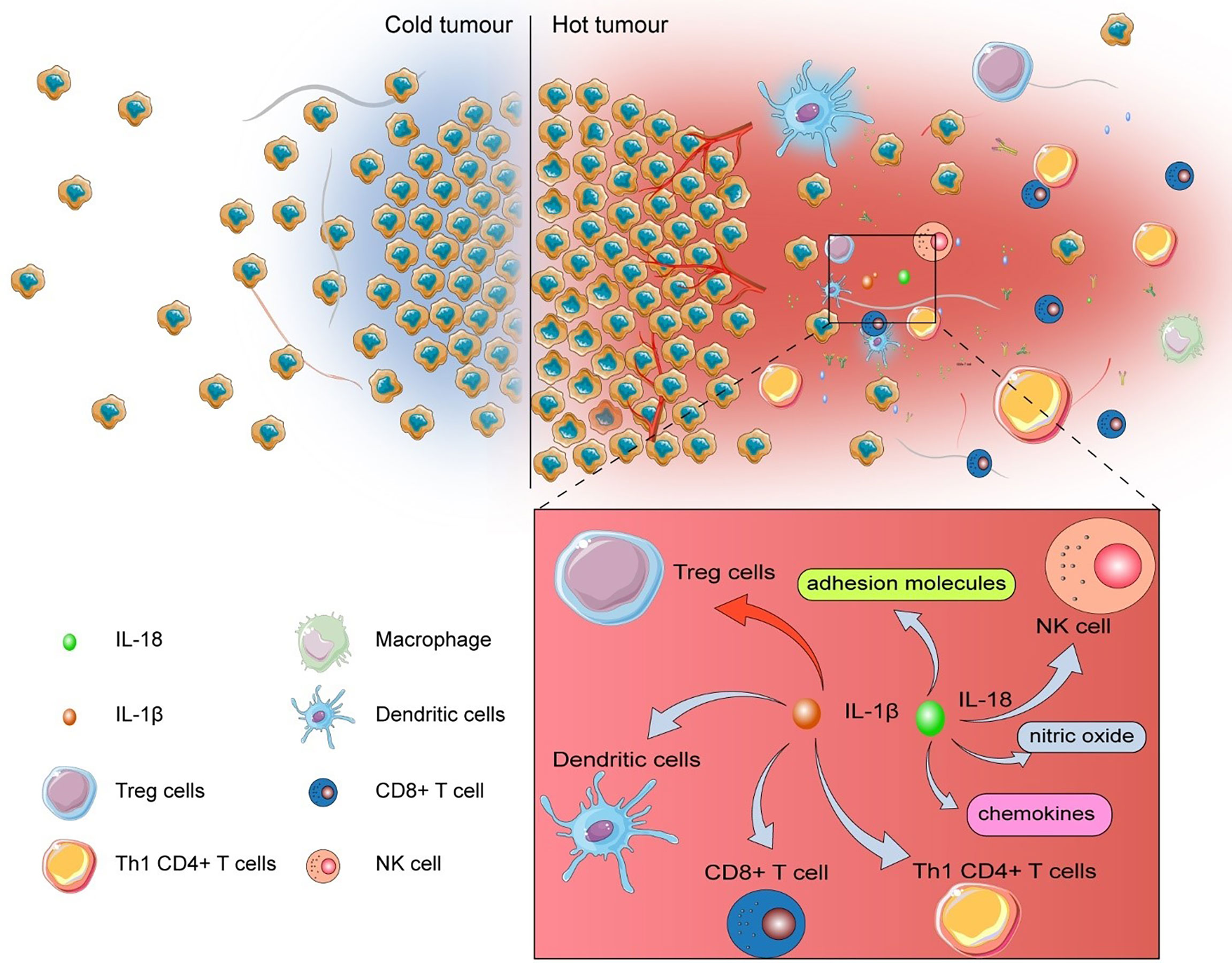
Figure 2 The influence of pyroptosis on TME.After being released into TME, IL-1β escalates the maturation of dendritic cells (DCs), activates antigen-specific cytotoxic CD8+ T cells, recruits Th1 CD4+ T cells, but suppresses the differentiation of immunosuppressive Treg cells. IL-18 polarizes Th1 cells, recruits and activates natural killer (NK) cells, and produces adhesion molecules, chemokine, and nitric oxide.
Therefore, these inflammatory cytokines and caspase not only cause the innate immune response, but also recruit adaptive immune cells, promote antigen presentation, and activate toll-like receptor (TLR) (82, 84, 85). Consequently, ncRNA-regulated pyroptosis enhances the efficiency of immunotherapy. In the future, understanding the underlying mechanisms of pyroptosis-produced inflammatory mediators in TME and the methods to manipulate the inflammatory condition via ncRNA-regulated pyroptosis is critical to increase the clinical effect of ICIs on patients.
Collectively, ncRNA-regulated pyroptosis not only converts “cold tumor” to “hot tumor”, but also unleashes various inflammatory mediators to reboot immune response in TME. Therefore, screening ncRNA-expression profiles spatiotemporally is meaningful to expand the clinical application of pyroptosis.
The clinical value of ncRNAs in pyroptotic cancer cells
Numerous studies have verified that ncRNAs are involved in cell pyroptosis and regulate the content of TME. Hence, converting these studies to clinics is rational and feasible.
Pyroptosis-associated ncRNA as diagnostic and immunotherapeutic biomarkers
Liquid biopsy is characterized by minimal invasion, which is non-invasive and accessible for cancer diagnosis and monitoring (86). As the mechanisms by which ncRNAs initiate the pathogenesis of cancers were found, the potential diagnostic biomarkers have been emerging. In ovarian cancer, the levels of lncRNA GAS5 was downregulated, while lncRNA HOTTIP was upregulated, indicating that lncRNA GAS5 and HOTTIP may be the futuristic diagnostic biomarkers (57, 58). Similarly, the expression of lncRNA RP1−85F18.6 and ΔNp63 increased and GSDMD cleavage was suppressed in primary CRC, which suggests that the levels of lncRNA RP1−85F18.6, ΔNp63, and GSDMD-N domain should be highlighted diagnostically. Additionally, cancer immunotherapy has gained success in prolonging the survival of patients. However, only a small proportion of patients achieved satisfactory clinical outcomes after immunotherapy (87). Therefore, identifying more precise biomarkers in liquid biopsy for predicting the efficiency of immunotherapy is urgent. For example, it was observed that LNCXIST (27, 71) and miR-556-5p (28) were upregulated in NSCLC, whereas pyroptosis was suppressed. The pyroptosis-generated inflammation evokes a switch from cold tumor to hot tumor, which promotes immunotherapy response. Therefore, LNCXIST and miR-556-5p are the feasible biomarkers for predicting whether patients with NSCLC could respond to immunotherapy effectively.
Pyroptosis-associated ncRNAs as novel chemo- and immunotherapeutic direction
Tumor resistance has significantly constrained the efficiency of current drugs such as cisplatin (28, 73), sorafenib (88, 89), and pembrolizumab (90, 91). One way to overcome tumor resistance is to develop novel anticancer drugs. For instance, in glioma, the validity of circ_0001836 (30) and caspase-1 (51) serving as therapeutic targets for the treatment of gliomas has been proven. Because there is a conserved binding site for miR-214 in the 3’UTR of the caspase 1 gene. By binding to the the 3’UTR, miR-214 could inhibit cell proliferation and migration in glioma. Meanwhile, circ_0001836 ablation suppresses tumorigenesis in the xenograft model by triggering pyroptosis via epigenetically upregulating NLRP1. Nevertheless, the innovation, design, and validation of a new anticancer drug require extraordinary investment and effort. Therefore, natural products have been reviewed, and it has been identified that natural products are a type of potent noncanonical cell death inducers in antitumor therapy (92). For example, tanshinone IIA, a major component of S. miltiorrhiza, suppresses cell proliferation and promotes pyroptosis in nasopharyngeal carcinoma by targeting the miR-125b/foxp3/caspase-1 axis, and it is believed that tanshinone IIA is a potent molecule to exert anticancer effects (53, 54). Additionally, in cervical cancer, tanshinone II A was found to regulate tumor growth via the miR-145/GSDMD signaling pathway. The expression of GSDMD and miR-145 was dramatically increased after tanshinone II A administration (53). However, one big obstacle to utilizing ncRNAs clinically is the precise delivery of ncRNAs to specific tissues (93). Accumulating lines of evidence have demonstrated that engineered exosome is expected to be a new generation of bioinspired nanoscale drug delivery systems (DDS), which could release chemotherapeutic drugs, mRNAs, regulatory ncRNAs, lipids, and proteins specifically (94–96). For example, engineered exosomes loaded with miR-449a specifically suppress the growth of homologous NSCLC in vitro and in vivo (97). Although there are no reports about the engineered exosomes’ loaded ncRNAs regulating pyroptosis in cancers, engineered exosomes could act as DDS to modulate pyroptosis in tumor sites precisely. With the precise delivery platforms, pyroptosis-associated ncRNA drugs have the ability to attain stronger anticancer effects and less unexpected side effects. Therefore, pyroptosis-related ncRNAs offer clinicians a novel direction for either developing new drugs or identifying potent natural products.
Collectively, the tissue-specific characteristics of ncRNAs make it possible to diagnose primary cancer and predict the efficiency of immunotherapy. Engineered exosomes loaded with pyroptosis-related ncRNAs may manipulate tumor-specific pyroptosis for chemo- and immunotherapy precisely.
Conclusion
Taken together, this article reviews the effects of pyroptosis-associated ncRNAs in cancers. Furthermore, we summarize the mechanisms by which ncRNAs initiate pyroptosis. Meanwhile, we outline the influence of ncRNAs on tumor resistance and TME via pyroptosis. Finally, the promising biomarkers and therapeutic targets of pyroptosis-associated ncRNAs are discussed. In the future, the increasingly new advanced analytical techniques and nanotechnology are expected to provide new insights into ncRNA-based biomarkers and drugs.
However, the influence of ncRNA-regulated pyroptosis on tumor resistance and microenvironment is complex. There still exist many problems about the regulation of ncRNAs on pyroptosis, for example, whether there are other ncRNAs that initiate pyroptosis and how ncRNA-regulated pyroptosis modulates tumor resistance and immunity spatiotemporally. Additionally, it is also vague what leads to the expression change of pyroptosis-associated ncRNAs within a cancerous context and how circRNAs are sensed in the pyroptotic pathway. In the future, more experiments are needed to resolve the above questions.
Author contributions
MZ finished the manuscript and designed the figures. PD collected the related papers. YL, BQ, and ZS gave constructive guidance and made critical revisions. All authors contributed to the article and approved the submitted version.
Funding
This study was supported by the National Natural Science Foundation of China (81972663, 82173055, and U2004112), the Excellent Youth Science Project of Henan Natural Science Foundation (212300410074), the Key Scientific Research Project of Henan Higher Education Institutions (20A310024), the Youth Talent Innovation Team Support Program of Zhengzhou University (32320290), the Provincial and Ministry co-constructed key projects of Henan Medical Science and Technology (SBGJ202102134), the Key Scientific and Technological Research Projects of Henan Provincial Department of Science and Technology (212102310117), the Henan Provincial Health Commission and Ministry of Health Co-construction Project, and the Henan Provincial Health and Health Commission Joint Construction Project (LHGJ20200158).
Conflict of interest
The authors declare that the research was conducted in the absence of any commercial or financial relationships that could be construed as a potential conflict of interest.
Publisher’s note
All claims expressed in this article are solely those of the authors and do not necessarily represent those of their affiliated organizations, or those of the publisher, the editors and the reviewers. Any product that may be evaluated in this article, or claim that may be made by its manufacturer, is not guaranteed or endorsed by the publisher.
Abbreviations
PCDs, programmed cell deaths; GSDMs, gasdermins; TME, tumor microenvironment; ncRNAs, non-coding RNAs; sncRNA, short ncRNA; lncRNA, long ncRNA; circRNA, circular ncRNA; microRNA, microRNA; GC, gastric cancer; NSCLC, non-small cell lung cancer; CRC, colorectal cancer; DAMPs, danger-associated molecular patterns; PAMPs, pathogen-associated molecular patterns; CC, cervical cancer; GR, glucocorticoid receptor; SBE, SMAD-binding elements; IR, ionizing radiation; HDAC, histone deacetylase; ASC, apoptosis-associated speck-like protein; TIL, tumor-infiltrating lymphocytes; ICIs, immune checkpoint inhibitors; TLR, toll-like receptor; DCs, dendritic cells; NK cells, natural killer cells; AEs, adverse effects; APF, antigen-presenting function.
References
1. Zychlinsky A, Prevost MC, Sansonetti PJ. Shigella flexneri induces apoptosis in infected macrophages. Nature (1992) 358:167–9. doi: 10.1038/358167a0
2. Medzhitov R, Janeway CA Jr. Decoding the patterns of self and nonself by the innate immune system. Science (2002) 296:298–300. doi: 10.1126/science.1068883
3. Jorgensen I, Miao EA. Pyroptotic cell death defends against intracellular pathogens. Immunol Rev (2015) 265:130–42. doi: 10.1111/imr.12287
4. Bergsbaken T, Cookson BT. Macrophage activation redirects yersinia-infected host cell death from apoptosis to caspase-1-dependent pyroptosis. PloS Pathog (2007) 3:e161. doi: 10.1371/journal.ppat.0030161
5. Mariathasan S, Weiss DS, Dixit VM, Monack DM. Innate immunity against francisella tularensis is dependent on the ASC/caspase-1 axis. J Exp Med (2005) 202:1043–9. doi: 10.1084/jem.20050977
6. Xu YJ, Zheng L, Hu YW, Wang Q. Pyroptosis and its relationship to atherosclerosis. Clin Chim Acta (2018) 476:28–37. doi: 10.1016/j.cca.2017.11.005
7. Fernandes-Alnemri T, Wu J, Yu JW, Datta P, Miller B, Jankowski W. The pyroptosome: a supramolecular assembly of ASC dimers mediating inflammatory cell death via caspase-1 activation. Cell Death Differ (2007) 14:1590–604. doi: 10.1038/sj.cdd.4402194
8. Liu X, Lieberman J. Knocking 'em dead: Pore-forming proteins in immune defense. Annu Rev Immunol (2020) 38:455–85. doi: 10.1146/annurev-immunol-111319-023800
9. Kaiser WJ, Upton JW, Mocarski ES. Viral modulation of programmed necrosis. Curr Opin Virol (2013) 3:296–306. doi: 10.1016/j.coviro.2013.05.019
10. Mocarski ES, Upton JW, Kaiser WJ. Viral infection and the evolution of caspase 8-regulated apoptotic and necrotic death pathways. Nat Rev Immunol (2011) 12:79–88. doi: 10.1038/nri3131
11. Tonnus W, Belavgeni A, Beuschlein F, Eisenhofer G, Fassnacht M, Kroiss M. The role of regulated necrosis in endocrine diseases. Nat Rev Endocrinol (2021) 17:497–510. doi: 10.1038/s41574-021-00499-w
12. Broz P, Dixit VM. Inflammasomes: mechanism of assembly, regulation and signalling. Nat Rev Immunol (2016) 16:407–20. doi: 10.1038/nri.2016.58
13. Guo H, Callaway JB, Ting JP. Inflammasomes: mechanism of action, role in disease, and therapeutics. Nat Med (2015) 21:677–87. doi: 10.1038/nm.3893
14. Man SM. Inflammasomes in the gastrointestinal tract: infection, cancer and gut microbiota homeostasis. Nat Rev Gastroenterol Hepatol (2018) 15:721–37. doi: 10.1038/s41575-018-0054-1
15. Rathinam VA, Fitzgerald KA. Inflammasome complexes: Emerging mechanisms and effector functions. Cell (2016) 165:792–800. doi: 10.1016/j.cell.2016.03.046
16. Shi J, Gao W, Shao F. Pyroptosis: Gasdermin-mediated programmed necrotic cell death. Trends Biochem Sci (2017) 42:245–54. doi: 10.1016/j.tibs.2016.10.004
17. Tan Y, Chen Q, Li X, Zeng Z, Xiong W, Li G, et al. Pyroptosis: A new paradigm of cell death for fighting against cancer. J Exp Clin Cancer Res CR (2021) 40:153. doi: 10.1186/s13046-021-01959-x
18. Yu P, Zhang X, Liu N, Tang L, Peng C, Chen X. Pyroptosis: mechanisms and diseases. Signal transduction targeted Ther (2021) 6:128. doi: 10.1038/s41392-021-00507-5
19. Dubois H, Sorgeloos F, Sarvestani ST, Martens L, Saeys Y, Mackenzie JM, et al. Nlrp3 inflammasome activation and gasdermin d-driven pyroptosis are immunopathogenic upon gastrointestinal norovirus infection. PloS Pathog (2019) 15:e1007709. doi: 10.1371/journal.ppat.1007709
20. Liu T, Zhou YT, Wang LQ, Li LY, Bao Q, Tian S, et al. NOD-like receptor family, pyrin domain containing 3 (NLRP3) contributes to inflammation, pyroptosis, and mucin production in human airway epithelium on rhinovirus infection. J Allergy Clin Immunol (2019) 144:777–787.e779. doi: 10.1016/j.jaci.2019.05.006
21. Kanneganti A, Malireddi RKS, Saavedra PHV, Vande Walle L, Van Gorp H, Kambara H, et al. GSDMD is critical for autoinflammatory pathology in a mouse model of familial Mediterranean fever. J Exp Med (2018) 215:1519–29. doi: 10.1084/jem.20172060
22. O'Neill LA. The interleukin-1 receptor/Toll-like receptor superfamily: signal transduction during inflammation and host defense. Sci STKE (2000) 2000(44):re1. doi: 10.1126/stke.442000re1
23. Evavold CL, Ruan J, Tan Y, Xia S, Wu H, Kagan JC, et al. The pore-forming protein gasdermin d regulates interleukin-1 secretion from living macrophages. Immunity (2018) 48:35–44.e36. doi: 10.1016/j.immuni.2017.11.013
24. Heilig R, Dick MS, Sborgi L, Meunier E, Hiller S, Broz P, et al. The gasdermin-d pore acts as a conduit for IL-1β secretion in mice. Eur J Immunol (2018) 48:584–92. doi: 10.1002/eji.201747404
25. Xu B, Jiang M, Chu Y, Wang W, Chen D, Li X, et al. Gasdermin d plays a key role as a pyroptosis executor of non-alcoholic steatohepatitis in humans and mice. J Hepatol (2018) 68:773–82. doi: 10.1016/j.jhep.2017.11.040
26. Khanova E, Wu R, Wang W, Yan R, Chen Y, French SW, et al. Pyroptosis by caspase11/4-gasdermin-D pathway in alcoholic hepatitis in mice and patients. Hepatology (2018) 67:1737–53. doi: 10.1002/hep.29645
27. Liu J, Yao L, Zhang M, Jiang J, Yang M, Wang Y, et al. Downregulation of LncRNA-XIST inhibited development of non-small cell lung cancer by activating miR-335/SOD2/ROS signal pathway mediated pyroptotic cell death. Aging-Us (2019) 11:7830–46. doi: 10.18632/aging.102291
28. Shi F, Zhang L, Liu X, Wang Y. Knock-down of microRNA miR-556-5p increases cisplatin-sensitivity in non-small cell lung cancer (NSCLC) via activating NLR family pyrin domain containing 3 (NLRP3)-mediated pyroptotic cell death. Bioengineered (2021) 12:6332–42. doi: 10.1080/21655979.2021.1971502
29. Jiang L, Ge W, Cui Y, Wang X. The regulation of long non-coding RNA 00958 (LINC00958) for oral squamous cell carcinoma (OSCC) cells death through absent in melanoma 2 (AIM2) depending on microRNA-4306 and Sirtuin1 (SIRT1) in vitro. Bioengineered (2021) 12:5085–98. doi: 10.1080/21655979.2021.1955561
30. Tan C, Liu W, Zheng ZH, Wan XG. LncRNA HOTTIP inhibits cell pyroptosis by targeting miR-148a-3p/AKT2 axis in ovarian cancer. Cell Biol Int (2021) 45:1487–97. doi: 10.1002/cbin.11588
31. Tang R, Xu J, Zhang B, Liu J, Liang C, Hua J, et al. Ferroptosis, necroptosis, and pyroptosis in anticancer immunity. J Hematol Oncol (2020) 13:110. doi: 10.1186/s13045-020-00946-7
32. Wang F, Li G, Ning J, Chen L, Xu H, Kong X, et al. Alcohol accumulation promotes esophagitis via pyroptosis activation. Int J Biol Sci (2018) 14:1245–55. doi: 10.7150/ijbs.24347
33. Li S, Wu Y, Yang D, Wu C, Ma C, Liu X, et al. Gasdermin d in peripheral myeloid cells drives neuroinflammation in experimental autoimmune encephalomyelitis. J Exp Med (2019) 216:2562–81. doi: 10.1084/jem.20190377
34. Kayagaki N, Stowe IB, Lee BL, O'Rourke K, Anderson K, Warming S, et al. Caspase-11 cleaves gasdermin d for non-canonical inflammasome signalling. Nature (2015) 526:666–71. doi: 10.1038/nature15541
35. Shi J, Zhao Y, Wang K, Shi X, Wang Y, Huang H, et al. Cleavage of GSDMD by inflammatory caspases determines pyroptotic cell death. Nature (2015) 526:660–5. doi: 10.1038/nature15514
36. Wang Y, Gao W, Shi X, Ding J, Liu W, He H, et al. Chemotherapy drugs induce pyroptosis through caspase-3 cleavage of a gasdermin. Nature (2017) 547:99–103. doi: 10.1038/nature22393
37. Rogers C, Fernandes-Alnemri T, Mayes L, Alnemri D, Cingolani G, Alnemri ES, et al. Cleavage of DFNA5 by caspase-3 during apoptosis mediates progression to secondary necrotic/pyroptotic cell death. Nat Commun (2017) 8:14128. doi: 10.1038/ncomms14128
38. Sato H, Koide T, Masuya H, Wakana S, Sagai T, Umezawa A, et al. A new mutation Rim3 resembling re(den) is mapped close to retinoic acid receptor alpha (Rara) gene on mouse chromosome 11. Mamm Genome (1998) 9:20–5. doi: 10.1007/s003359900673
39. Terao C, Kawaguchi T, Dieude P, Varga J, Kuwana M, Hudson M, et al. Transethnic meta-analysis identifies GSDMA and PRDM1 as susceptibility genes to systemic sclerosis. Ann Rheum Dis (2017) 76:1150–8. doi: 10.1136/annrheumdis-2016-210645
40. Yu J, Kang MJ, Kim BJ, Kwon JW, Song YH, Choi WA, et al. Polymorphisms in GSDMA and GSDMB are associated with asthma susceptibility, atopy and BHR. Pediatr Pulmonol (2011) 46:701–8. doi: 10.1002/ppul.21424
41. Carl-McGrath S, Schneider-Stock R, Ebert M, Röcken C. Differential expression and localisation of gasdermin-like (GSDML), a novel member of the cancer-associated GSDMDC protein family, in neoplastic and non-neoplastic gastric, hepatic, and colon tissues. Pathology (2008) 40:13–24. doi: 10.1080/00313020701716250
42. Hergueta-Redondo M, Sarrio D, Molina-Crespo Á, Vicario R, Bernadó-Morales C, Martínez L, et al. Gasdermin-b promotes invasion and metastasis in breast cancer cells. PloS One (2014) 9:e90099. doi: 10.1371/journal.pone.0090099
43. Hergueta-Redondo M, Sarrió D, Molina-Crespo Á, Megias D, Mota A, Rojo-Sebastian A, et al. Gasdermin b expression predicts poor clinical outcome in HER2-positive breast cancer. Oncotarget (2016) 7:56295–308. doi: 10.18632/oncotarget.10787
44. Sun Q, Yang J, Xing G, Sun Q, Zhang L, He F, et al. Expression of GSDML associates with tumor progression in uterine cervix cancer. Transl Oncol (2008) 1:73–83. doi: 10.1593/tlo.08112
45. Hou J, Zhao R, Xia W, Chang CW, You Y, Hsu JM, et al. PD-L1-mediated gasdermin c expression switches apoptosis to pyroptosis in cancer cells and facilitates tumour necrosis. Nat Cell Biol (2020) 22:1264–75. doi: 10.1038/s41556-020-0575-z
46. Slack FJ, Chinnaiyan AM. The role of non-coding RNAs in oncology. Cell (2019) 179:1033–55. doi: 10.1016/j.cell.2019.10.017
47. Schwarzer R, Laurien L, Pasparakis M. New insights into the regulation of apoptosis, necroptosis, and pyroptosis by receptor interacting protein kinase 1 and caspase-8. Curr Opin Cell Biol (2020) 63:186–93. doi: 10.1016/j.ceb.2020.02.004
48. Pon JR, Marra MA. Driver and passenger mutations in cancer. Annu Rev Pathol (2015) 10:25–50. doi: 10.1146/annurev-pathol-012414-040312
49. Shen P, Jing Y, Zhang R, Cai MC, Ma P, Chen H, et al. Comprehensive genomic profiling of neuroendocrine bladder cancer pinpoints molecular origin and potential therapeutics. Oncogene (2018) 37:3039–44. doi: 10.1038/s41388-018-0192-5
50. Guo H, Ingolia NT, Weissman JS, Bartel DP. Mammalian microRNAs predominantly act to decrease target mRNA levels. Nature (2010) 466:835–40. doi: 10.1038/nature09267
51. Jiang Z, Yao L, Ma H, Xu P, Li Z, Guo M, et al. miRNA-214 inhibits cellular proliferation and migration in glioma cells targeting caspase 1 involved in pyroptosis. Oncol Res (2017) 25:1009–19. doi: 10.3727/096504016X14813859905646
52. Yu S, Zhao N, He M, Zhang K, Bi X. MiRNA-214 promotes the pyroptosis and inhibits the proliferation of cervical cancer cells via regulating the expression of NLRP3. Cell Mol Biol (2020) 66:59–64. doi: 10.14715/cmb/2020.66.6.11
53. Tong W, Guo J, Yang C. Tanshinone II a enhances pyroptosis and represses cell proliferation of HeLa cells by regulating miR-145/GSDMD signaling pathway. Biosci Rep (2020) 40:BSR20200259. doi: 10.1042/BSR20200259
54. Wang Y, Jin W, Wang J. Tanshinone IIA regulates microRNA-125b/foxp3/caspase-1 signaling and inhibits cell viability of nasopharyngeal carcinoma. Mol Med Rep (2021) 23:371. doi: 10.3892/mmr.2021.12010
55. Su F, Duan J, Zhu J, Fu H, Zheng X, Ge C, et al. Long non-coding RNA nuclear paraspeckle assembly transcript 1 regulates ionizing radiation-induced pyroptosis via microRNA-448/gasdermin e in colorectal cancer cells. Int J Oncol (2021) 59:79. doi: 10.3892/ijo.2021.5259
56. Chen Z, He M, Chen J, Li C, Zhang Q. Long non-coding RNA SNHG7 inhibits NLRP3-dependent pyroptosis by targeting the miR-34a/SIRT1 axis in liver cancer. Oncol Lett (2020) 20:893–901. doi: 10.3892/ol.2020.11635
57. Tan C, Liu W, Zheng Z-H, Wan X-G. LncRNA HOTTIP inhibits cell pyroptosis by targeting miR-148a-3p/AKT2 axis in ovarian cancer. Cell Biol Int (2021) 45:1487–97. doi: 10.1002/cbin.11588
58. Li J, Yang C, Li Y, Chen A, Li L, You Z, et al. LncRNA GAS5 suppresses ovarian cancer by inducing inflammasome formation. Biosci Rep (2018) 38:BSR20171150. doi: 10.1042/bsr20171150
59. Garabedian MJ, Logan SK. Glucocorticoid receptor DNA binding decoy is a gas. Sci Signal (2010) 3:pe5. doi: 10.1126/scisignal.3108pe5
60. Kino T, Hurt DE, Ichijo T, Nader N, Chrousos GP. Noncoding RNA gas5 is a growth arrest- and starvation-associated repressor of the glucocorticoid receptor. Sci Signal (2010) 3:ra8. doi: 10.1126/scisignal.2000568
61. Kristensen LS, Andersen MS, Stagsted LVW, Ebbesen KK, Hansen TB, Kjems J, et al. The biogenesis, biology and characterization of circular RNAs. Nat Rev Genet (2019) 20:675–91. doi: 10.1038/s41576-019-0158-7
62. Gao JN, Chen X, Wei P, Wang Y, Li P, Shao K, et al. Regulation of pyroptosis in cardiovascular pathologies: Role of noncoding RNAs. Mol Ther-Nucleic Acids (2021) 25:220–36. doi: 10.1016/j.omtn.2021.05.016
63. Memczak S, Jens M, Elefsinioti A, Torti F, Krueger J, Rybak A, et al. Circular RNAs are a large class of animal RNAs with regulatory potency. Nature (2013) 495:333–8. doi: 10.1038/nature11928
64. Chen JB, Dong SS, Yao S, Duan YY, Hu WX, Chen H, et al. Modeling circRNA expression pattern with integrated sequence and epigenetic features demonstrates the potential involvement of H3K79me2 in circRNA expression. Bioinformatics (2020) 36:4739–48. doi: 10.1093/bioinformatics/btaa567
65. Cheng Z, Yu C, Cui S, Wang H, Jin H, Wang C, et al. circTP63 functions as a ceRNA to promote lung squamous cell carcinoma progression by upregulating FOXM1. Nat Commun (2019) 10:3200. doi: 10.1038/s41467-019-11162-4
66. Luan J, Jiao C, Kong W, Fu J, Qu W, Chen Y, et al. circHLA-c plays an important role in lupus nephritis by sponging miR-150. Mol Ther Nucleic Acids (2018) 10:245–53. doi: 10.1016/j.omtn.2017.12.006
67. Wang J, Zhao X, Wang Y, Ren F, Sun D, Yan Y, et al. circRNA-002178 act as a ceRNA to promote PDL1/PD1 expression in lung adenocarcinoma. Cell Death Dis (2020) 11:32. doi: 10.1038/s41419-020-2230-9
68. Zhang T, Wu DM, Luo PW, Liu T, Han R, Deng SH, et al. CircNEIL3 mediates pyroptosis to influence lung adenocarcinoma radiotherapy by upregulating PIF1 through miR-1184 inhibition. Cell Death Dis (2022) 13:167. doi: 10.1038/s41419-022-04561-x
69. Ganoth A, Merimi KC, Peer D. Overcoming multidrug resistance with nanomedicines. Expert Opin Drug Delivery (2015) 12:223–38. doi: 10.1517/17425247.2015.960920
70. Vasan N, Baselga J. & Hyman, d. m. a view on drug resistance in cancer. Nature (2019) 575:299–309. doi: 10.1038/s41586-019-1730-1
71. Xu X, Zhou X, Chen Z, Gao C, Zhao L, Cui Y, et al. Silencing of lncRNA XIST inhibits non-small cell lung cancer growth and promotes chemosensitivity to cisplatin. Aging-Us (2020) 12:4711–26. doi: 10.18632/aging.102673
72. Ren N, Jiang T, Wang C, Xie S, Xing Y, Piao D, et al. LncRNA ADAMTS9-AS2 inhibits gastric cancer (GC) development and sensitizes chemoresistant GC cells to cisplatin by regulating miR-233-3p/NLRP3 axis. Aging-Us (2020) 12:11025–41. doi: 10.18632/aging.103314
73. Ren N, Jiang T, Wang C, Xie S, Xing Y, Piao D, et al. LncRNA ADAMTS9-AS2 inhibits gastric cancer (GC) development and sensitizes chemoresistant GC cells to cisplatin by regulating miR-223-3p/NLRP3 axis. Aging (2020) 12:11025–41. doi: 10.18632/aging.103314
74. Chen X, He WT, Hu L, Li J, Fang Y, Wang X, et al. Pyroptosis is driven by non-selective gasdermin-d pore and its morphology is different from MLKL channel-mediated necroptosis. Cell Res (2016) 26:1007–20. doi: 10.1038/cr.2016.100
75. Looi CK, Hii LW, Chung FF, Mai CW, Lim WM, Leong CO, et al. Roles of inflammasomes in Epstein-Barr virus-associated nasopharyngeal cancer. Cancers (2021) 13:1786. doi: 10.3390/cancers13081786
76. Wang Z, Fan P, Zhou J, Weng J, Feng B. Role of macrophages in angiogenesis. Chin J Immunol (2021) 37:2710–4.
77. Tahergorabi Z, Khazaei M. The relationship between inflammatory markers, angiogenesis, and obesity. ARYA Atheroscl (2013) 9:247–53.
78. Bian XW, Chen JH, Jiang XF, Bai JS, Wang QL, Zhang X, et al. Angiogenesis as an immunopharmacologic target in inflammation and cancer. Int Immunopharmacol (2004) 4:1537–47. doi: 10.1016/j.intimp.2004.07.017
79. Zhang Z, Zhang Y, Lieberman J. Lighting a fire: Can we harness pyroptosis to ignite antitumor immunity? Cancer Immunol Res (2021) 9:2–7. doi: 10.1158/2326-6066.Cir-20-0525
80. Rosenbaum SR, Wilski NA, Aplin AE. Fueling the fire: Inflammatory forms of cell death and implications for cancer immunotherapy. Cancer Discovery (2021) 11:266–81. doi: 10.1158/2159-8290.Cd-20-0805
81. Ben-Sasson SZ, Hogg A, Hu-Li J, Wingfield P, Chen X, Crank M, et al. IL-1 enhances expansion, effector function, tissue localization, and memory response of antigen-specific CD8 T cells. J Exp Med (2013) 210:491–502. doi: 10.1084/jem.20122006
82. Jain A, Song R, Wakeland EK, Pasare C. T Cell-intrinsic IL-1R signaling licenses effector cytokine production by memory CD4 T cells. Nat Commun (2018) 9:3185. doi: 10.1038/s41467-018-05489-7
83. Dinarello CA. Overview of the IL-1 family in innate inflammation and acquired immunity. Immunol Rev (2018) 281:8–27. doi: 10.1111/imr.12621
84. Tian J, Avalos AM, Mao SY, Chen B, Senthil K, Wu H, et al. Toll-like receptor 9-dependent activation by DNA-containing immune complexes is mediated by HMGB1 and RAGE. Nat Immunol (2007) 8:487–96. doi: 10.1038/ni1457
85. Yu M, Wang H, Ding A, Golenbock DT, Latz E, Czura CJ, et al. HMGB1 signals through toll-like receptor (TLR) 4 and TLR2. Shock (2006) 26:174–9. doi: 10.1097/01.shk.0000225404.51320.82
86. Yu D, Li Y, Wang M, Gu J, Xu W, Cai H, et al. Exosomes as a new frontier of cancer liquid biopsy. Mol Cancer (2022) 21:56. doi: 10.1186/s12943-022-01509-9
87. Hamid O, Robert C, Daud A, Hodi FS, Hwu WJ, Kefford R, et al. Five-year survival outcomes for patients with advanced melanoma treated with pembrolizumab in KEYNOTE-001. Ann Oncol (2019) 30:582–8. doi: 10.1093/annonc/mdz011
88. Dong J, Zhai B, Sun W, Hu F, Cheng H, Xu J, et al. Activation of phosphatidylinositol 3-kinase/AKT/snail signaling pathway contributes to epithelial-mesenchymal transition-induced multi-drug resistance to sorafenib in hepatocellular carcinoma cells. PloS One (2017) 12:e0185088. doi: 10.1371/journal.pone.0185088
89. Wang H, Qian Z, Zhao H, Zhang X, Che S, Zhang H, et al. CSN5 silencing reverses sorafenib resistance of human hepatocellular carcinoma HepG2 cells. Mol Med Rep (2015) 12:3902–8. doi: 10.3892/mmr.2015.3871
90. Robert C, Ribas A, Wolchok JD, Hodi FS, Hamid O, Kefford R, et al. Anti-programmed-death-receptor-1 treatment with pembrolizumab in ipilimumab-refractory advanced melanoma: a randomised dose-comparison cohort of a phase 1 trial. Lancet (2014) 384:1109–17. doi: 10.1016/s0140-6736(14)60958-2
91. Zaretsky JM, Garcia-Diaz A, Shin DS, Escuin-Ordinas H, Hugo W, Hu-Lieskovan S, et al. Mutations associated with acquired resistance to PD-1 blockade in melanoma. N Engl J Med (2016) 375:819–29. doi: 10.1056/NEJMoa1604958
92. Greco G, Catanzaro E, Fimognari C. Natural products as inducers of non-canonical cell death: A weapon against cancer. Cancers (2021) 13:64. doi: 10.3390/cancers13020304
93. Shibata C, Otsuka M, Kishikawa T, Ohno M, Yoshikawa T, Takata A, et al. Diagnostic and therapeutic application of noncoding RNAs for hepatocellular carcinoma. World J Hepatol (2015) 7:1–6. doi: 10.4254/wjh.v7.i1.1
94. Luan X, Sansanaphongpricha K, Myers I, Chen H, Yuan H, Sun D, et al. Engineering exosomes as refined biological nanoplatforms for drug delivery. Acta Pharmacol Sin (2017) 38:754–63. doi: 10.1038/aps.2017.12
95. Hood JL. Post isolation modification of exosomes for nanomedicine applications. Nanomed (Lond) (2016) 11:1745–56. doi: 10.2217/nnm-2016-0102
96. Vader P, Mol EA, Pasterkamp G, Schiffelers RM. Extracellular vesicles for drug delivery. Adv Drug Delivery Rev (2016) 106:148–56. doi: 10.1016/j.addr.2016.02.006
Keywords: pyroptosis, non-coding RNA, cancer, tumor resistance, tumor immunity
Citation: Zhang M, Dang P, Liu Y, Qiao B and Sun Z (2022) Noncoding RNAs in pyroptosis and cancer progression: Effect, mechanism, and clinical application. Front. Immunol. 13:982040. doi: 10.3389/fimmu.2022.982040
Received: 30 June 2022; Accepted: 20 July 2022;
Published: 17 August 2022.
Edited by:
Michal Amit Rahat, Technion-Israel Institute of Technology, IsraelReviewed by:
Yuanliang Yan, Xiangya Hospital, Central South University, ChinaCopyright © 2022 Zhang, Dang, Liu, Qiao and Sun. This is an open-access article distributed under the terms of the Creative Commons Attribution License (CC BY). The use, distribution or reproduction in other forums is permitted, provided the original author(s) and the copyright owner(s) are credited and that the original publication in this journal is cited, in accordance with accepted academic practice. No use, distribution or reproduction is permitted which does not comply with these terms.
*Correspondence: Zhenqiang Sun, ZmNjc3VuenFAenp1LmVkdS5jbg==; Bingbing Qiao, ZmNjcWlhb2JiQHp6dS5lZHUuY24=; Yang Liu, emx5eWxpdXlhbmcxNDQwQHp6dS5lZHUuY24=