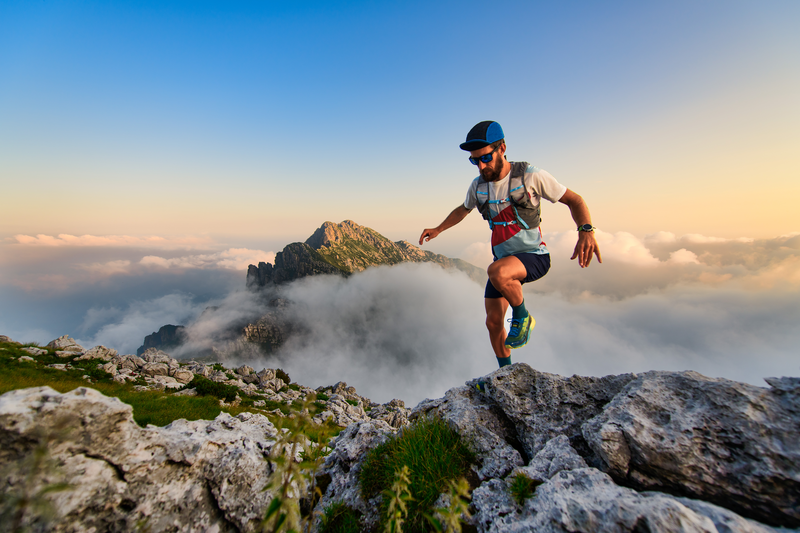
94% of researchers rate our articles as excellent or good
Learn more about the work of our research integrity team to safeguard the quality of each article we publish.
Find out more
REVIEW article
Front. Immunol. , 31 August 2022
Sec. Autoimmune and Autoinflammatory Disorders
Volume 13 - 2022 | https://doi.org/10.3389/fimmu.2022.980079
This article is part of the Research Topic Pathogenesis and Target-Treatments of Systemic Lupus Erythematosus View all 18 articles
Treatment of systemic lupus erythematosus (SLE) currently employs agents with relatively unselective immunosuppressive properties. However, two target-specific biological drugs have been approved: belimumab (anti-B-cell-activating factor/BAFF) and anifrolumab (anti-interferon alpha receptor-1/IFNAR1). Here, we performed a comparative risk-benefit assessment for both drugs based on the role of BAFF and IFNAR1 in host defense and the pathogenesis of SLE and by considering the available data on safety and efficacy. Due to differences in target expression sites, anti-IFNAR1, but not anti-BAFF, might elicit organ-specific effects, consistent with clinical efficacy data. The IFNAR1 is specifically involved in innate and adaptive antiviral immunity in most cells of the body. Consistent with this observation, the available safety data obtained from patients negatively selected for LN and neuropsychiatric SLE, primary immunodeficiencies, splenectomy and chronic HIV, HBV, HCV infections suggest an increased risk for some viral infections such as varicella zoster and perhaps influenza. In contrast, BAFF is mainly involved in adaptive immune responses in lymphoid tissues, thus anti-BAFF therapy modulates SLE activity and prevents SLE flares without interfering with local innate host defense mechanisms and should only marginally affect immune memory to previous pathogen exposures consistent with the available safety data from SLE patients without chronic HIV, HBV or HCV infections. When using belimumab and anifrolumab, careful patient stratification and specific precautions may minimize risks and maximize beneficial treatment effects for patients with SLE.
Systemic lupus erythematosus (SLE) is a chronic systemic autoimmune disorder presenting with a wide spectrum of clinical manifestations that relate either to systemic inflammation or to autoimmune tissue inflammation and injury (1). Uncontrolled disease activity leads to accumulating tissue injury-related disability and potentially to organ failure (2). Immunosuppressive agents (ISA) can often suppress the aberrant immune response and limit tissue injury. However, drug toxicity remains an important concern (1–3). All currently available drugs for SLE are associated with adverse effects (Table 1); thus, developing more specific agents with better safety profiles remains a critical unmet medical need in SLE (3).
To date, the U.S. Food and Drug Administration has approved two biological drugs for SLE: the anti-B-cell-activating factor IgG belimumab (anti-BAFF) and the anti-interferon alpha-receptor 1 IgG anifrolumab (anti-IFNAR1). The FDA approved belimumab for the treatment of moderate-severe lupus in patients over 18 years old in 2011, patients with systemic lupus over 5 years old in 2019, and patients with lupus nephritis in 2020. Hence, a considerable amount of efficacy and safety data has accumulated (4). In 2021, the FDA approval of anifrolumab sparked the discussion as to how best to implement this new drug option into the current treatment landscape of SLE (5).
Because head-to-head comparisons between drugs remain scarce, specific recommendations on the preferential use of drugs remain difficult (6). In this article, we discuss the potential risks and benefits of anti-BAFF and anti-IFNAR1 based on their different roles in host defense and the pathogenesis of SLE. We further elaborate on how the ongoing pandemic of coronavirus disease (COVID)-19 may affect risk-benefit assessments for belimumab and anifrolumab.
BAFF and the IFNAR1 are both elements of the immune system and, thus, their primary function in human physiology relates to host defense (7, 8). Only the IFNAR1 is broadly expressed as part of the tissue`s innate immune system (Figure 1) (8). The innate immune system supports host defense in all plants and animals (9) (10) and all immune cells express the IFNAR1 to mediate the numerous biological effects of the type I IFNs (IFN-I, Figure 1) (11). The IFNAR1 is a key element of the danger alert system that converts local danger recognition into systemic inflammation and induction of danger resilience at sites distant from pathogen entry (8). In this process, IFNAR1 signaling is linked to the sensing of viral components (12). In tissue macrophages, type I IFNs induce a pro-inflammatory M1 phenotype (13). In addition, IFNAR1 signaling induces the maturation of antigen-presenting cells and, therefore, triggers the initiation and persistence of adaptive immunity (8, 14). In addition, the vast majority of non-immune cells express the IFNAR1 to activate innate and adaptive host defense mechanisms.
Figure 1 BAFF and the IFNAR1 in host defense. All classes of pathogens release pathogen-associated molecular patterns (PAMP) that can activate pattern recognition receptors in myeloid cells to upregulate the expression and release of B cell activating factor (BAFF) and/or interferon-alpha and -beta (IFNα/β). Viruses are particularly potent in inducing IFNα/β as their viral nucleic acid activate specific nucleic acid sensors in intracellullar compartments such as phagolysosomes inside the cytosol. BAFF mediates its biological effects via the BAFF receptor (BAFF-R) on T1 B cells in the bone marrow, blood, and spleen, on B1B cells in the peritoneal cavity, and on follicular B cells and marginal zone B cells in the spleen. BAFF has a specific role in the induction and maintenance of adaptive immune responses. In addition, tissue macrophages express BAFF-R and IFNAR1 and BAFF and IFNAR1 ligation both induce a M1 proinflammatory macrophage phenotype, an innate mechanism of host defense. In addition, nearly all cells of the body express the IFNAR1. Activation of the IFNAR1 activates immune and tissue cells to induce several hundred genes important in antiviral host defense.
In contrast, the expression of BAFF receptors is restricted to certain maturation stages of B cells, dendritic cells and tissue macrophages (Figure 1) (7). BAFF supports the maturation, differentiation, and survival of B-lymphocytes (7), which serve two central roles in the adaptive immune system: (i) B cells are antigen-presenting cells and therefore key initiators and drivers of antigen-specific T cell immunity (15, 16). and (ii) B cells are effector cells of the adaptive immune system and produce immunoglobulins as they undergo maturation into plasma cells (17). Both functions of B cells are critical for the development of long-lasting antigen-specific immune memory (18, 19), which limits the severity of recurrent infections and accounts for relapsing chronic autoimmune diseases such as SLE (16, 20). Thus, in contrast to the IFNAR1, targeting BAFF is associated with greater selectivity.
BAFF and IFNAR1 in anti-bacterial host defense. Antibacterial host defense involves all aspects of innate and adaptive immunity. Toll-like and other pathogen recognition receptors detect a wide spectrum of bacterial components and trigger a diverse range of cytotoxic mechanisms (9). The system is highly redundant, leaving many specific genetic defects without clinical consequences (21). Indeed, severe bacterial infections relate more to profound and diffuse immunodeficiencies, such as in the setting of hereditary or acquired immunoglobulin deficiencies, treatment with glucocorticoids (GC), nonselective ISA or advanced organ failure (heart, kidney, liver) (21). Bacterial products activate BAFF secretion from myeloid cells; hence, during infection, BAFF levels increase in biological fluids. BAFF deficiency increases the susceptibility of mice to certain bacterial strains but BAFF does not seem to have a non-redundant role in innate host defense against bacteria in humans (22). In contrast, BAFF is necessary for priming adaptive immunity during de novo exposure to a new bacterial pathogen. IFN-I and the IFNAR1 partially contribute to antibacterial host defense by sensing bacterial CpG-DNA and potentially de-methylated nucleic acids released from infected cells (23).
BAFF and IFNAR1 in antiviral host defense. The sensing and priming of antiviral immunity differ significantly from antibacterial immunity. Viral nucleic acids play an important role in sensing viral infections and involve a set of intracellular nucleic acid sensors that all trigger the secretion of type I/III IFNs as the central cytokine signals of antiviral immunity. IFN-I activate the IFNAR1, which not only induces the release of nucleases and viral transcription inhibitors to limit viral spreading throughout the body but results also in systemic symptoms such as malaise, fatigue, fever, arthralgia or myalgia. In addition, IFNAR1 activation-related BAFF release from immune cells supports antigen-presentation and the expansion of viral antigen-specific B cells. This applies especially for de novo exposures to previously unknown viruses. Thus, the risk for viral infections could be higher with therapeutic IFNAR1 inhibition compared to therapeutic BAFF inhibition. BAFF released from neutrophils and dendritic cells contributes to antiviral immunity (24), but accelerates gamma herpesvirus infection (25). Both therapeutics might affect the priming of immune memory to novel viruses or vaccines.
BAFF in host defense to plasmodia. In human malaria infection, BAFF levels increase and IgD (–)CD38(low)CD21(-)CD27(-) atypical B cells temporarily expand (26). An interesting line of evidence suggests that BAFF plays an important role in the host defense to plasmodia falciparum. The population of Sardinia has an unusually high prevalence of SLE in association with a genetic BAFF variant, referred to as “BAFF-var” (27). BAFF-var is an insertion-deletion, which ultimately increases BAFF levels likely accounting for the unique high prevalence of SLE in Sardinia compared to the rest of Europe (27). An extraordinarily high prevalence of certain genetic variants frequently relates to distinct infectious selection pressures such as apolipoprotein-1 variants that confer some protection from severe trypanosomiasis or hemoglobin variants that protect from severe malaria (28, 29). Indeed, malaria was endemic in Sardinia until the 1950s, and BAFF-var-related BAFF overexpression enhances the mucosal immune response against plasmodia falciparum (30). Hence, therapeutic BAFF inhibition might increase the risk for parasite infections such as malaria, a specific exposure risk, underrepresented in the recent clinical trials.
Anti-BAFF. Since its initial approval by the U.S. Food and Drug administration (FDA) in March of 2011, belimumab has consistently demonstrated an overall reassuring safety profile in comparison to standard of care (SOC) therapy in both adult and pediatric SLE patients (Table 2). One study analyzed pooled data from 1458 adult participants treated with placebo or belimumab at doses of 1 mg/kg, 4 mg/kg, or 10 mg/kg on background standard of care therapy from the phase II, BLISS-52 phase III, and BLISS-76 phase III trials (41). The trial durations ranged from 52-76 weeks. All treatment groups had similar rates of overall adverse events (AEs), treatment-related AEs, serious AEs, and AEs resulting in treatment discontinuation. The rates of infectious AEs were balanced among the treatment groups. Of note, three opportunistic infections occurred in two participants in the belimumab groups. One participant in the 10 mg/kg group developed acinetobacter bacteremia and later disseminated cytomegalovirus infection (both resolved) and one participant in the 1 mg/kg group developed Acinetobacter iwolfii pneumonia on study day 1 (resolved). Rates of serious psychiatric disorder and depression were higher in the belimumab groups and there were two completed suicides, one each in the 1mg/kg and 10mg/kg groups.
In terms of laboratory parameters, more participants in the belimumab groups experienced reductions in IgG, IgM, and IgA levels below the lower limit of normal. However, there was not an observed association between infectious AEs and reduction in immunoglobulin levels.
The longest study of belimumab completed to date is the open-label continuation study of the phase II, double-blind trial (42). All participants received the licensed dose of belimumab 10mg/kg IV every 4 weeks in addition to standard background therapy. 298/476 (63%) entered the continuation study after completing the phase II trial. 96 (32%) participants remained in the study until the end at year 13. Over the course of the study, 44 (14.9%) discontinued therapy or withdrew from the study because of an AE. The rates of serious infections were stable over the course of the study. There were no cases of progressive multifocal leukoencephalopathy, although several such cases were reported in patients treated with belimumab outside clinical trials (43).
BASE was a 52- week multicenter, double-blind, randomized, placebo-controlled phase IV trial required by both the FDA and the European Medicines Agency to investigate the rates of all-cause mortality and adverse events of special interest in belimumab versus placebo on background standard of care (44). The as-treated population included 2002 belimumab-treated participants and 2001 placebo-treated participants. The incidence of all-cause mortality was similar between the two groups, 0.5% and 0.4% in the belimumab and placebo groups, respectively. Serious infections occurred in 3.7% in the belimumab group and 4.1% in the placebo group. There was no significant difference in the frequency of opportunistic infections, infections of special interest, and malignancies between the two groups. More belimumab-treated participants experienced serious depression than those treated with placebo (0.35% versus 0.05%).
Lastly, in the PLUTO trial of participants with childhood SLE, belimumab was well-tolerated and the safety profile was similar to the experience in adult SLE patients described above (35). Together, even long-term inhibition of BAFF does not seem to be associated with significant increases in the rates of infections, although exposures to plasmodium falciparum or other important (sub-)tropical parasites may have been rare in these studies. Interestingly, COVID-19 outcomes have been more favorable in the small subset of patients with SLE treated with belimumab (45, 46).
Anti-IFNAR1. Anifrolumab was approved by the FDA as the 2nd biologic available for the management of moderate-severe systemic lupus in patients over 18 years old in 2021. Anifrolumab was approved based on data from three randomized double-blind, placebo controlled, 52-week clinical trials: the MUSE phase II and the phase III TULIP 1 and 2 clinical trials (37–39). These trials excluded patients with LN and neuropsychiatric SLE, primary immunodeficiencies, splenectomy and positive serologies for HIV, HBV, HCV. Patients with history of severe herpes [disseminated HZ involving ≥3 dermatomes, herpes encephalitis, ophthalmic herpes, or recurrent HZ (2 episodes within 2 years)] were also excluded. In addition, patients with a history of recent severe opportunistic infections and recent chronic infections (i.e., osteomyelitis; bronchiectasis) were excluded. Pooled safety data from all 3 trials were analyzed by Tummala et al. (47). The safety analysis focused on the comparison of 459 patients that received the FDA approved 300 mg dose with 466 patients that had placebo. The anifrolumab 300 mg and placebo groups were well balanced regarding demographics, comorbidities, treatment with GC, antimalarials, and ISA. More patients in the anifrolumab 300 mg group (87%) had ≥1 AE compared to those on placebo (79%). AEs more common in the anifrolumab 300 mg group than in placebo were nasopharyngitis (16% vs 9%), URTI (16% vs 10%), bronchitis (10% vs 4%), and HZ (6.1% vs 1.3%). Most were mild or moderate. Severe AE (SAE) occurred in 11.8% and 16.7% receiving anifrolumab 300 vs placebo. Among SAE, infection rates were 22 (4.8%) versus 26 (5.6%). There were 2 deaths due to pneumonia in the anifrolumab 300 mg group and 1 in the placebo group due to encephalitis. There was also one death in the anifrolumab 1000 mg group due to acute colitis and macrophage activation syndrome. There were two severe opportunistic infections: one on anifrolumab 300 with mycobacterium complex infection (treatment was discontinued), and one on placebo with cryptococcal meningitis. There were no active tuberculosis cases but four (0.9%) and one (0.2%) patient, respectively, with latent tuberculosis. HZ occurred in 28 patients (6.1%) in the anifrolumab 300 group and 6 patients on placebo (1.3%). Only two HZ cases (both on anifrolumab 300) were SAEs: 1 in MUSE trial had transverse myelitis with positive PCR for the virus in the cerebrospinal fluid but no cutaneous symptoms. The patient discontinued treatment and fully recovered with antivirals and high dose GC. All cases received antiviral treatment (except 2 in the placebo group) and all resolved. In the TULIP studies (dermatome involvement data were available), 4/28 cases were disseminated: 3/23 in the anifrolumab 300 and 1/5 in the placebo group. Of note, the rate of HZ was higher in the anifrolumab 300 group who received ISA (n=17, 9.8%) than those who did not (n=6, 3.2%). In the MUSE trial, the HZ rate was higher for the anifrolumab 1000 group (9.5%) compared to anifrolumab 300 (5.1%) and placebo (2%) groups (37).
The TULIP-LN, phase II double-blind randomized trial of anifrolumab in patients with active class III/IV lupus nephritis (LN) was recently published (40). In this study 147 patients were randomized to receive the monthly anifrolumab basic regimen (BR, 300 mg, n=45), an intensified anifrolumab regimen (IR, 900 mg X3 followed by 300 mg, n=51), or placebo (n=49), for 52 weeks. Eligible patients could enter a 2nd year of treatment. Only the first-year data were published. AE occurred in 90 (94%) of patients in the combined anifrolumab group compared to 44 (90%) in placebo, but were only mild/moderate in intensity,. SAE occurred in 20% of anifrolumab and 16% of placebo groups. AEs more common in anifrolumab than placebo included HZ (17% vs. 8%), influenza (8% vs. 2%), and UTI (17% vs. 10%). Of the 16 HZ cases in the combined anifrolumab group, 6 were serious but only cutaneous and resolved with antiviral treatment.
The longest anifrolumab safety data are available from the MUSE open-label 3-year extension study (48). Of the 246 patients who completed the MUSE trial, 218 (88.6%) enrolled in this study and 139 (63.8%) completed 3 years of treatment. Frequency and patterns of SAE and AESI over 3 years were consistent with those reported for one year in patients on anifrolumab in the parent study. HZ occurred in 11 patients (5%; 2 were disseminated, but not serious) in 3 years compared to 15 (7.4%) in the parent trial. There were 6 patients (2.8%) with latent tuberculosis compared to 2 (1%) in MUSE. A further placebo-controlled long-term observation extension study of patients enrolled in TULIP1 and 2 trials is ongoing.
While these studies suggest that anifrolumab does not increase serious adverse events in SLE, there was an increase in the frequency of upper respiratory tract infections, nasopharyngeal, bronchitis and HZ (49). Limitations in these data include the relatively short follow-up available and the selection of patients at lower risk for HZ and other infections. In addition, the numerically higher incidence of latent tuberculosis and influenza infections in some of these trials needs further study.
BAFF and IFNAR1 are both involved in the early stages of the pathogenesis of SLE. Because the diagnosis of SLE is founded on the presence of antinuclear antibodies (50), loss-of-tolerance and priming of autoreactive lymphocyte clones has already occurred at the time of diagnosis. Importantly, the presence of antinuclear antibodies is usually long-lasting, implying persistent memory T cells and long-lived plasma cells (51). To address these factors, we have chosen to discuss the role of BAFF and the IFNAR1 in relation to the level of SLE disease activity.
BAFF and IFNAR1 in SLE disease activity. The central element of the pathogenesis of SLE is the loss of tolerance to chromatin (1). Monogenic forms of SLE provide clues for key triggers of this process such as the hereditary overproduction of IFN-I, hereditary defects in apoptosis, opsonins or chromatin clearance defects all promoting the priming of anti-chromatin immunity (52). In this context, IFNAR1 signaling is the central element of monogenic interferonopathy-related SLE and critically involved in the innate sensing of (viral particle-like) self-chromatin as well as the priming of adaptive anti-chromatin immunity (Figure 2) (53). For example, Toll-like receptor 7, a viral RNA recognition receptor sensing also lupus autoantigens, is a key driver of murine and human SLE and mediates the resistance of SLE activity to, e.g., glucocorticoids (54–59). Thus, the IFNAR1 mediates SLE activity whenever chromatin release into the extracellular space boosts anti-chromatin immunity, e.g., cell death related to sunburns, trauma or NET release during infection (14). In addition, viral infection-related flares of SLE likely involve the IFNAR1, while infections related to other microbes likely trigger disease flares via alternative signaling pathways not necessarily involving the IFNAR1, e.g., Toll-like receptors-2, -4, and -5 for gram-negative and gram-positive bacteria (60). However, there are not yet data demonstrating that blocking the IFNAR1 with anifrolumab reduces SLE flare rates. In contrast, belimumab attenuates the frequency and severity of SLE flares (61, 62), even if the role of BAFF is limited to the adaptive immune system. Obviously, BAFF-related B cell functions including antigen-presentation, production of autoantibodies (Figure 2), and circulating immune complexes predominate as drivers of human SLE activity, while the role of the innate immune system had been demonstrated by animal studies (63, 64). Thus, flares of SLE activity involve BAFF-dependent B cell functions such as autoantigen-presentation, expansion of autoreactive T and B cell clones (65).
Figure 2 BAFF and IFNAR1 in the pathogenesis and disease activity of SLE. Lupus autoantigens stimulate antigen-presenting cells to secrete interferon-alpha and -beta (IFNα/β) just like viral particles, a process that triggers an antiviral-like adaptive immune response against lupus autoantigens. Lupus activity largely depends on the size and activity of the autoreactive T and B cell clones and IFNAR1 signaling is centrally involved in clone size regulation triggered by extracellular lupus autoantigens but not necessarily by drug- or bacterial infection-related lupus flares. B cell-activating factor (BAFF) is specifically involved in B cell maturation towards plasma cells but may also endorse B cell-mediated T cell priming. DC, dendritic cell.
BAFF and IFNAR1 in specific organ manifestations of SLE. SLE is a systemic disorder with much of its pathophysiology localizing to the lymphoid organs, i.e., spleen and lymph nodes (1). Thus, organ-specific features may be associated with factors acting in the periphery, such as the IFNAR1, but not to factors acting preferentially in the lymphoid organs such as BAFF (Figure 2). Thus, certain organ manifestations in SLE may relate to local IFNAR1 activation, e.g., in the skin, the synovium, central nervous system, kidneys and blood vessels, either due to infection, UV light (in skin), or plasmacytoid dendritic cell migration to these sites. Evidence for IFNAR1 activation in skin (66, 67), CNS (68), synovium (69), kidneys (67), and endothelial cells has been described (70). In addition, high systemic type I IFN activity associates with active class III/IV LN (71).
Viral infections are frequent in the respiratory tract, while persistent viruses reside in the skin, the nervous system, the liver and the immune system (72). Indeed, a comparative single cell transcriptome analysis found strong IFN signaling in lupus skin (67).
In summary, while BAFF should be involved in all forms of flaring SLE activity, the IFNAR1 could be specifically involved in flares triggered by viral infections and release of endogenous chromatin antigens (73, 74). The expression of the IFNAR1 in peripheral tissues may involve IFNAR1 differently in the various SLE manifestations, which is less likely for BAFF.
Anti-BAFF. Belimumab received FDA approval in 2011 for treatment of moderate-severe systemic lupus in patients over 18 years old based upon efficacy demonstrated in BLISS-52 (n=577) and BLISS-76 (n=548) (31, 32). In BLISS-52, subjects were randomized to receive standard of care plus 1 mg/kg, 10 mg/kg belimumab or placebo every 4 weeks with a loading dose administered at week 2. After 52 weeks, subjects receiving belimumab 10 mg/kg were statistically more likely to achieve an SRI-4 response compared to those receiving placebo (58% vs. 44%, p<0.001). Similarly, in BLISS-72, subjects randomized to intravenous belimumab 10 mg/kg achieved an SRI-4 response statistically more frequently than those receiving placebo infusions (43% vs. 34%, p=0.021). Evaluation of a subcutaneous route of administration (200 mg/week) in an 839 subject trial showed achievement of an SRI-4 in 61% of subjects receiving belimumab compared to 48% in those receiving placebo (p=0.0006) and led to the approval of subcutaneous belimumab in 2017 for active autoantibody positive SLE. A subsequent study in Asia (n=707) confirmed the superior efficacy of intravenous belimumab compared to placebo (SRI-4 54.3 vs 40.1 p<0.00001) in this population. In 2019, Belimumab was approved for use by pediatric patients with SLE following an international study (PLUTO) in which 93 subjects, aged 5-17 years, were randomized to receive belimumab (10mg/kg) or placebo. Numerically greater numbers of subjects receiving belimumab achieved a SRI-4 (52.8% vs 43.6%; OR 1.49 (95% CI 0.64 to 3.46)) (35). These studies have consistently shown a clinical effect differentiating belimumab treatment from placebo after 16 weeks of therapy, sustained through the 52-week endpoint. Additionally, compared to placebo, patients treated with belimumab have lower frequencies of severe flare and prolonged median times to flare. GC tapering was not mandatory and left to the investigator’s discretion in these studies.
In BLISS-52, patients receiving belimumab were significantly more likely to achieve a 50% reduction in GC exposure, while trends for decreased GC exposure have been demonstrated in the other studies. Post-hoc examination of the pooled BLISS-52 and BLISS-76 studies showed that a greater number of subjects receiving belimumab had a decrease in their GC dose, and a lower number had increases. Furthermore, GC dose was lower in subjects receiving belimumab (75). Additional post-hoc studies of the pooled BLISS-52 and BLISS-76 results demonstrated that improvement occurs across multiple domains and is not restricted to a single organ (76). Patients with high disease activity (i.e., a Safety of Estrogens in Lupus National Assessment–Systemic Lupus Erythematosus Disease Activity Index (SELENA-SLEDAI) ≥10], hypocomplementemia, anti-dsDNA positivity and/or use of prednisone at baseline are the most likely individuals to attain a clinical response compared to SOC (77). Subsequently, studies of long-term use of belimumab have demonstrated that its use is associated with a reduction in accrual of damage (78–80).
Belimumab, both intravenous and subcutaneous, received FDA approval for the treatment of active lupus nephritis following the 104-week BLISS-LN study (36). In this Phase III trial, 448 patients with Class III, IV (with or without Class V) or Class V LN, received either intravenous belimumab 10 mg/kg or placebo along with standard of care (cyclophosphamide/azathioprine or mycophenolate mofetil). Patients receiving belimumab were significantly more likely to achieve a primary efficacy renal response (PERR) at week 104. PERR included a urinary protein to creatinine ratio of ≤0.7, an estimated glomerular filtration rate [eGFR], which could not be ≥ 20% below the pre-renal flare value or ≥60 ml per minute per 1.73 m2 of body-surface area, and no use of rescue therapy). Complete renal response was defined as a urinary protein to creatinine ratio of <0.5, an eGFR, which could not be ≥10% below the pre-renal flare value or ≥90 ml per minute per 1.73 m2 of body-surface area, and no use of rescue therapy. Belimumab and placebo achieved, 43% vs. 32% (p=0.03) and 30% vs. 20% (p=0.02) for PERR and CR, respectively.
Anti-IFNAR1. Anifrolumab was approved for the treatment of SLE based on consistent efficacy data from the phase IIb MUSE and phase III TULIP 1 and 2 trials (81). In MUSE, 305 patients were randomized to receive IV anifrolumab 300 mg (n=99), 1000 mg (n=104), or placebo (n=102), every 4 weeks for 48 weeks (37). The primary endpoint was the percentage of patients achieving an SRI-4 at week 24 with concurrent sustained reduction of oral GC to <10 mg/day and not higher than the baseline dose. GC taper was encouraged but not required by the protocol. More patients treated with anifrolumab 300 mg (34.3%) and 1000 mg (28.8%) responded compared to placebo (17.6%). P values were 0.014 and 0.063 respectively for the two experimental groups vs placebo. Improved responses were also seen for SRI-4, and BICLA responses at 52 weeks. The two phase III anifrolumab trials had a similar design, except that TULIP 1 also included a lower anifrolumab dose (150 mg) group. There was a requirement for patients that received baseline GC doses ≥ 10 mg/day to attempt to taper the dose to ≤ 7.5 mg/day from weeks 8-40.
TULIP 1 randomized 457 patients to IV anifrolumab 300 mg (n = 180), 150 mg (n = 93), or placebo (n = 184) every 4 weeks for 48 weeks (38). The primary endpoint was the SRI-4 at week 52 and there was no difference between the two groups. However, after amending the NSAID use responder rules, post hoc analysis showed all outcome measures improved, although the primary endpoint was still not significant. Of note, BICLA responses at week 52 were in favor of anifrolumab 300 mg (46%) versus placebo (30%). Pharmacodynamic assessment of IFN-High patients showed neutralization of IFN-I 21 gene panel to a level of 12.6% as early as week 12 and throughout the study period for the anifrolumab 300 mg group but not for the placebo group. The experience from the TULIP-1 study regarding NSAID restriction rules and BICLA vs SRI-4 responses, informed a protocol amendment of the twin study TULIP 2, before that data were unblinded (39). The primary endpoint was changed from SRI-4 to BICLA response at week 52. Flares were defined as ≥ 1 new BILAG-2004 A item or ≥ 2 new BILAG-2004 B items, as compared with the previous visit. TULIP 2 randomized 362 patients to anifrolumab (n=180) or placebo (n=182). The study reached its primary endpoint, as 47.8% patients on anifrolumab compared to 31.5% of the placebo group responded according to BICLA). Regarding key secondary outcomes, this was also true for the IFN-I-High group (48% versus 30.7%; p=0.002), sustained GC reduction (51.5% versus 30.2%; p=0.01), and ≥50% reduction in CLASI at week 12 (49% versus 25%; p=0.04).
The data from the two TULIP trials have been pooled and analyzed (82, 83). There were 360 patients in the anifrolumab 300 group and 366 in the placebo group. Two thirds of the patients were white, 13% AA, 10.5% Asians, and 8.4% other. About 52% were on prednisone ≥10 mg/day at baseline, 70% were on antimalarials, and about 48% on ISA (mycophenolate mofetil ≤ 2g/day, mycophenolic acid ≤ 1.44 g/day, azathioprine ≤ 200 mg/day, methotrexate ≤ 25 mg/week, or mizoribine ≤ 150 mg/day). 70% had SLEDAI-2K≥10, and 59% had ≥1 abnormal serologic markers at baseline (high levels of anti-dsDNA, or low levels of C3 or C4). About 28% had a CLASI-activity ≥10 and 41% had ≥ 6 tender and ≥6 swollen joints. More patients in the anifrolumab 300 mg group achieved BICLA response compared to the placebo group (47.5% vs 30.8; difference 16.6%; nominal p<0.001) (83). Similarly, more patients in the anifrolumab 300 mg group achieved SRI-4 responses (52.2% vs 40.1%), sustained GC taper (50.5% vs 31.8%), ≥50% reduction in CLASI-activity (46% vs 24.9%), ≥50% reduction in active joints (49.4% vs 36.8%) compared to PLB treated patients; all with significant nominal p values. Pooled analysis of the combined TULIP data with emphasis on lupus flares, is also available (82). Most flares were in the mucocutaneous (24.8%) and musculoskeletal (22.5%) systems, and much fewer flares in the renal (6.2%), cardiorespiratory (2.5%) and other systems. The anifrolumab 300 mg group had a lower annualized flare rate (AFR) compared to placebo (0.51 versus 0.67; rate ratio 0.75; P=0.017), prolonged median time to first flare (140 days versus 119 days; hazard ratio 0.70; P = 0.003), and fewer patients with ≥1 flare (33.6% vs 42.9%; difference -9.3%; p=0.009). AFR favored the anifrolumab group for patients with BMI ≤ 28 kg/m2, patients on GC ≥10 mg/day, baseline SLEDAI-2K≥10, active baseline serology (≥1 marker), and IFN-I-High patients. Of note, flare rates were similar (~34%) for all placebo-treated patients, but higher for placebo-treated IFN-I High patients (45%). Importantly, among patients who achieved sustained GC reductions more remained flare-free with anifrolumab (40%) versus placebo (17%).
The primary outcome of the TULIP-LN trial was change in 24-hour urinary protein creatinine ratio (UPCR) at week 52 for the combined anifrolumab (BR and IR) groups vs placebo (40). Secondary endpoint was complete renal response (CRR), defined as 24-hour UPCR ≤ 0.7 mg/mg, eGFR≥60 ml/min/1.73 m2, or no decrease ≥20% from baseline, no investigational product discontinuation, and no use of restricted medications. There was no difference in the primary endpoint between the combined experimental and placebo groups. However, there were numerical improvements in CRR attained by the IR group versus placebo (45.5% vs 31.1%), as well as in more stringent CRR definitions, and in sustained GC dose reductions (55.6% vs 33.3%). Such improvements were not seen in the BR group vs placebo. Due to proteinuria, serum concentrations were higher in the anifrolumab IR group but suboptimal in the BR group (below those expected in similarly treated non-renal SLE patients) limiting this group’s exposure to anifrolumab and any potential benefit.
Collectively these data confirm the efficacy of anifrolumab on many clinical outcomes, including BICLA and SRI-4, mucocutaneous and musculoskeletal outcomes, lower flare rates, successful GC taper to ≤7.5 mg/day for those on baseline doses ≥ 10 mg/day, increases in C3 levels, as well as in functional measures. Interestingly, the data suggest a preferred beneficial effect in patients with IFN-I pathway activation, patients with active mucocutaneous disease, higher serologic activity, and Asians. Pharmacodynamic neutralization of the IFN-I pathway was consistently seen in the IFN-I High patients treated with anifrolumab 300 mg which corroborates the efficacy findings.
Both anti-BAFF and anti-IFNAR1 have demonstrated efficacy in controlling SLE activity. A recent indirect treatment comparison analysis suggested that patients with moderate-to-severe SLE are more likely to achieve an improvement in disease activity with anifrolumab (84), but such types of analysis have to be handled with caution due to the many confounding differences between the respective trials. In addition, such comparisons do not inform about which drug to choose in which patient.
Drug safety profiles largely guide treatment decisions, and indeed, the safety profiles of belimumab and anifrolumab are quite different. More patients treated with belimumab developed serious depression, treatment-emergent suicidality, sponsor-adjudicated serious suicide, or self-injury events (44). On the other hand, severe COVID-19 outcomes in individuals with SLE were favorable with belimumab (45, 46). Unlike anti-BAFF, anti-IFNAR1 significantly increased the rates of certain viral infections such as HZ and perhaps influenza, a finding that connects well with its mechanism-of-action that blocks a key element of antiviral immunity. How anti-IFNAR1 therapy affects the risk of COVID-19 and its complications is not known, but numerous data support that lacking capacity to rapidly induce a IFN-I response is a crucial risk factor for severe COVID-19 (85), although type III IFN also seem to be important (86). Moreover, the presence of autoantibodies against type I IFNs predisposes patients to life-threatening COVID-19 (87). Thus, although we do not have definite data, it remains possible that treatment with anti-IFNAR1 will have a similar effect. In view of these potential infectious risks, full vaccination against HZ and SARS-Cov-2 (avoiding live vaccines) is strongly recommended before starting anti-IFNAR1 therapy for SLE and is probably advisable for any other biological therapy or ISA. Modern antiviral drugs or even passive immunization with SARS-CoV-2 antibodies can protect from severe COVID-19 in patients at risk (88, 89). Caution should be used when considering anti-IFNAR1 treatment in patients with a history of chronic or recurrent infections or at high risk for infections. Prompt identification and treatment of HZ is recommended.
Economic considerations may influence individual patient treatment decisions. We made the deliberate decision not to discuss financial issues in this review. Belimumab and anifrolumab are both biological drugs with relatively higher costs than conventional drugs, and reimbursement rates vary among geographical regions and insurance carriers.
The FDA approved belimumab and anifrolumab for the treatment of moderate-severe SLE because both drugs convincingly demonstrated efficacy beyond standard-of-care. Anti-BAFF blocks a key element of the adaptive immune system involved in SLE without interfering with innate host defense, which explains why anti-BAFF is not associated with much of an increased risk of infections. In contrast, the molecular target of anti-IFNAR1 is a key element of innate and adaptive antiviral immunity, which suggests why anti-IFNAR1 therapy is associated with a higher risk for certain viral infections such as HZ. Long-term studies are needed to evaluate, whether reactivation of other persisting viruses such as CMV, EBV or HPV are associated with related vascular disorders, lymphoma or cervical and epidermal cancers, respectively. Therefore, the use of anti-IFNAR in patients with concomitant risk factors for viral infections including a known primary or secondary immunodeficiency requires caution.
In addition, the ongoing COVID-19 pandemic implies an important need to prime adaptive immunity against a novel virus, a process potentially impaired by all drugs that interfere with the adaptive immune system. How anti-BAFF and anti-IFNAR1 compare in this context is still uncertain but given the important role of type I IFN in antiviral immunity remains important gather more long-term data how anti-IFNAR1 therapy affects the risk for severe COVID-19 severe HZ and its consequences such as post-herpetic neuralgia or other viral infections.
The availability of belimumab and anifrolumab for the treatment of SLE has improved the quality of life of patients with SLE. Individual risk-benefit assessments and shared decision-making are important when considering which therapy is appropriate for which patient.
All authors researched data, wrote parts of the manuscript, and edited and approved the final version.
H-JA received research funding from the Deutsche Forschungsgemeinschaft (AN372/14-4, 20-2, 27-1, 30-1) and from the Volkswagen Foundation (97–744).
H-JA received consultancy or lecture fees from Bayer, GSK, AstraZeneca, Novartis, Otsuka, Janssen, Kezar, Lilly, Sanofi, and PreviPharma. MD’E received consultancy fees from GSK, AstraZeneca, Aurinia, Biogen, and Amgen. KK received consultancy fees from Aurinia. CA has received research support from GSK and consultancy fees from GSK, AstraZeneca, BristolMeyerSqibb and Kezar Inc.
The reviewer JH-Y declared a shared affiliation with the author CA to the handling editor at the time of review.
All claims expressed in this article are solely those of the authors and do not necessarily represent those of their affiliated organizations, or those of the publisher, the editors and the reviewers. Any product that may be evaluated in this article, or claim that may be made by its manufacturer, is not guaranteed or endorsed by the publisher.
1. Kaul A, Gordon C, Crow MK, Touma Z, Urowitz MB, van Vollenhoven R, et al. Systemic lupus erythematosus. Nat Rev Dis Primers (2016) 2:16039. doi: 10.1038/nrdp.2016.39
2. Anders HJ, Saxena R, Zhao MH, Parodis I, Salmon JE, Mohan C. Lupus nephritis. Nat Rev Dis Primers (2020) 6(1):7. doi: 10.1038/s41572-019-0141-9
3. Durcan L, O’Dwyer T, Petri M. Management strategies and future directions for systemic lupus erythematosus in adults. Lancet (2019) 393(10188):2332–43. doi: 10.1016/s0140-6736(19)30237-5
4. Levy RA, Gonzalez-Rivera T, Khamashta M, Fox NL, Jones-Leone A, Rubin B, et al. 10 years of belimumab experience: What have we learnt? Lupus (2021) 30(11):1705–21. doi: 10.1177/09612033211028653
5. Deeks ED. Anifrolumab: First approval. Drugs (2021) 81(15):1795–802. doi: 10.1007/s40265-021-01604-z
6. Kent DM, Steyerberg E, van Klaveren D. Personalized evidence based medicine: predictive approaches to heterogeneous treatment effects. Bmj (2018) 363:k4245. doi: 10.1136/bmj.k4245
7. Smulski CR, Eibel H. BAFF and BAFF-receptor in b cell selection and survival. Front Immunol (2018) 9:2285. doi: 10.3389/fimmu.2018.02285
8. Mesev EV, LeDesma RA, Ploss A. Decoding type I and III interferon signalling during viral infection. Nat Microbiol (2019) 4(6):914–24. doi: 10.1038/s41564-019-0421-x
9. Fitzgerald KA, Kagan JC. Toll-like receptors and the control of immunity. Cell (2020) 180(6):1044–66. doi: 10.1016/j.cell.2020.02.041
10. Bottazzi B, Doni A, Garlanda C, Mantovani A. An integrated view of humoral innate immunity: pentraxins as a paradigm. Annu Rev Immunol (2010) 28:157–83. doi: 10.1146/annurev-immunol-030409-101305
11. Schreiber G. The molecular basis for differential type I interferon signaling. J Biol Chem (2017) 292(18):7285–94. doi: 10.1074/jbc.R116.774562
12. Wu J, Chen ZJ. Innate immune sensing and signaling of cytosolic nucleic acids. Annu Rev Immunol (2014) 32:461–88. doi: 10.1146/annurev-immunol-032713-120156
13. Günthner R, Anders HJ. Interferon-regulatory factors determine macrophage phenotype polarization. Mediators Inflammation (2013) 2013:731023. doi: 10.1155/2013/731023
14. Crow MK, Olferiev M, Kirou KA. Type I interferons in autoimmune disease. Annu Rev Pathol (2019) 14:369–93. doi: 10.1146/annurev-pathol-020117-043952
15. Ghosh D, Jiang W, Mukhopadhyay D, Mellins ED. New insights into b cells as antigen presenting cells. Curr Opin Immunol (2021) 70:129–37. doi: 10.1016/j.coi.2021.06.003
16. Rawlings DJ, Metzler G, Wray-Dutra M, Jackson SW. Altered b cell signalling in autoimmunity. Nat Rev Immunol (2017) 17(7):421–36. doi: 10.1038/nri.2017.24
17. Nutt SL, Hodgkin PD, Tarlinton DM, Corcoran LM. The generation of antibody-secreting plasma cells. Nat Rev Immunol (2015) 15(3):160–71. doi: 10.1038/nri3795
18. Inoue T, Moran I, Shinnakasu R, Phan TG, Kurosaki T. Generation of memory b cells and their reactivation. Immunol Rev (2018) 283(1):138–49. doi: 10.1111/imr.12640
19. Cyster JG, Allen CDC. B cell responses: Cell interaction dynamics and decisions. Cell (2019) 177(3):524–40. doi: 10.1016/j.cell.2019.03.016
20. Hiepe F, Dörner T, Hauser AE, Hoyer BF, Mei H, Radbruch A. Long-lived autoreactive plasma cells drive persistent autoimmune inflammation. Nat Rev Rheumatol. (2011) 7(3):170–8. doi: 10.1038/nrrheum.2011.1
21. Notarangelo LD, Bacchetta R, Casanova JL, Su HC. Human inborn errors of immunity: An expanding universe. Sci Immunol. (2020) 5(49):eabb1662. doi: 10.1126/sciimmunol.abb1662
22. Sakai J, Akkoyunlu M. The role of BAFF system molecules in host response to pathogens. Clin Microbiol Rev (2017) 30(4):991–1014. doi: 10.1128/cmr.00046-17
23. Boxx GM, Cheng G. The roles of type I interferon in bacterial infection. Cell Host Microbe (2016) 19(6):760–9. doi: 10.1016/j.chom.2016.05.016
24. Giordano D, Kuley R, Draves KE, Roe K, Holder U, Giltiay NV, et al. BAFF produced by neutrophils and dendritic cells is regulated differently and has distinct roles in antibody responses and protective immunity against West Nile virus. J Immunol (2020) 204(6):1508–20. doi: 10.4049/jimmunol.1901120
25. Frederico B, May JS, Efstathiou S, Stevenson PG. BAFF receptor deficiency limits gammaherpesvirus infection. J Virol (2014) 88(8):3965–75. doi: 10.1128/jvi.03497-13
26. Scholzen A, Teirlinck AC, Bijker EM, Roestenberg M, Hermsen CC, Hoffman SL, et al. BAFF and BAFF receptor levels correlate with b cell subset activation and redistribution in controlled human malaria infection. J Immunol (2014) 192(8):3719–29. doi: 10.4049/jimmunol.1302960
27. Steri M, Orrù V, Idda ML, Pitzalis M, Pala M, Zara I, et al. Overexpression of the cytokine BAFF and autoimmunity risk. N Engl J Med (2017) 376(17):1615–26. doi: 10.1056/NEJMoa1610528
28. Genovese G, Friedman DJ, Ross MD, Lecordier L, Uzureau P, Freedman BI, et al. Association of trypanolytic ApoL1 variants with kidney disease in African americans. Science (2010) 329(5993):841–5. doi: 10.1126/science.1193032
29. Richer J, Chudley AE. The hemoglobinopathies and malaria. Clin Genet (2005) 68(4):332–6. doi: 10.1111/j.1399-0004.2005.00503.x
30. Lodde V, Floris M, Beerman I, Munk R, Guha R, Steri M, et al. Evolutionarily selected overexpression of the cytokine BAFF enhances mucosal immune response against p. falciparum. Front Immunol (2020) 11:575103. doi: 10.3389/fimmu.2020.575103
31. Navarra SV, Guzmán RM, Gallacher AE, Hall S, Levy RA, Jimenez RE, et al. Efficacy and safety of belimumab in patients with active systemic lupus erythematosus: a randomised, placebo-controlled, phase 3 trial. Lancet (2011) 377(9767):721–31. doi: 10.1016/s0140-6736(10)61354-2
32. Furie R, Petri M, Zamani O, Cervera R, Wallace DJ, Tegzová D, et al. Randomized, placebo-controlled study of belimumab, a monoclonal antibody that inhibits b lymphocyte stimulator, in patients with systemic lupus erythematosus. Arthritis Rheum. (2011) 63(12):3918–30. doi: 10.1002/art.30613
33. Stohl W, Schwarting A, Okada M, Scheinberg M, Doria A, Hammer AE, et al. Efficacy and safety of subcutaneous belimumab in systemic lupus erythematosus: A fifty-Two-Week randomized, double-blind, placebo-controlled study. Arthritis Rheumatol. (2017) 69(5):1016–27. doi: 10.1002/art.40049
34. Zhang F, Bae SC, Bass D, Chu M, Egginton S, Gordon D, et al. A pivotal phase III, randomised, placebo-controlled study of belimumab in patients with systemic lupus erythematosus located in China, Japan and south Korea. Ann Rheum. Dis (2018) 77(3):355–63. doi: 10.1136/annrheumdis-2017-211631
35. Brunner HI, Abud-Mendoza C, Viola DO, Calvo Penades I, Levy D, Anton J, et al. Safety and efficacy of intravenous belimumab in children with systemic lupus erythematosus: results from a randomised, placebo-controlled trial. Ann Rheum. Dis (2020) 79(10):1340–8. doi: 10.1136/annrheumdis-2020-217101
36. Furie R, Rovin BH, Houssiau F, Malvar A, Teng YKO, Contreras G, et al. Two-year, randomized, controlled trial of belimumab in lupus nephritis. N Engl J Med (2020) 383(12):1117–28. doi: 10.1056/NEJMoa2001180
37. Furie R, Khamashta M, Merrill JT, Werth VP, Kalunian K, Brohawn P, et al. Anifrolumab, an anti-interferon-α receptor monoclonal antibody, in moderate-to-Severe systemic lupus erythematosus. Arthritis Rheumatol. (2017) 69(2):376–86. doi: 10.1002/art.39962
38. Furie RA, Morand EF, Bruce IN, Manzi S, Kalunian KC, Vital EM, et al. Investigators: Type I interferon inhibitor anifrolumab in active systemic lupus erythematosus (TULIP-1): a randomised, controlled, phase 3 trial. Lancet Rheumatol. (2019) 1(4):E208–19. doi: 10.1016/S2665-9913(19)30076-1. o. b. o. t. T.-s.
39. Morand EF, Furie R, Tanaka Y, Bruce IN, Askanase AD, Richez C, et al. Trial of anifrolumab in active systemic lupus erythematosus. N Engl J Med (2020) 382(3):211–21. doi: 10.1056/NEJMoa1912196
40. Jayne D, Rovin B, Mysler EF, Furie RA, Houssiau FA, Trasieva T, et al. Phase II randomised trial of type I interferon inhibitor anifrolumab in patients with active lupus nephritis. Ann Rheum. Dis (2022) 81(4):496–506. doi: 10.1136/annrheumdis-2021-221478
41. Wallace DJ, Navarra S, Petri MA, Gallacher A, Thomas M, Furie R, et al. Safety profile of belimumab: pooled data from placebo-controlled phase 2 and 3 studies in patients with systemic lupus erythematosus. Lupus (2013) 22(2):144–54. doi: 10.1177/0961203312469259
42. Wallace DJ, Ginzler EM, Merrill JT, Furie RA, Stohl W, Chatham WW, et al. Safety and efficacy of belimumab plus standard therapy for up to thirteen years in patients with systemic lupus erythematosus. Arthritis Rheumatol. (2019) 71(7):1125–34. doi: 10.1002/art.40861
43. Raisch DW, Rafi JA, Chen C, Bennett CL. Detection of cases of progressive multifocal leukoencephalopathy associated with new biologicals and targeted cancer therapies from the FDA’s adverse event reporting system. Expert Opin Drug Saf (2016) 15(8):1003–11. doi: 10.1080/14740338.2016.1198775
44. Sheikh SZ, Scheinberg M, Wei JC-C, Tegzova D, Stohl W, Acayaba de Toledo R, et al. Mortality and adverse events of special interest with intravenous belimumab for adults with active, autoantibody-positive systemic lupus erythematosus (BASE): a multicentre, double-blind, randomised, placebo-controlled, phase 4 trial. Lancet Rheumatol. (2021) 3(2):E122–30. doi: 10.1016/S2665-9913(20)30355-6
45. Ugarte-Gil MF, Alarcón GS, Izadi Z, Duarte-García A, Reátegui-Sokolova C, Clarke AE, et al. Characteristics associated with poor COVID-19 outcomes in individuals with systemic lupus erythematosus: data from the COVID-19 global rheumatology alliance. Ann Rheum. Dis (2022) 81(7):970–8. doi: 10.1136/annrheumdis-2021-221636
46. Mehta P, Gasparyan AY, Zimba O, Kitas GD. Systemic lupus erythematosus in the light of the COVID-19 pandemic: infection, vaccination, and impact on disease management. Clin Rheumatol. 1-18 (2022) 81(7):970–978. doi: 10.1007/s10067-022-06227-7
47. Tummala R, Abreu G, Pineda L, Michaels MA, Kalyani RN, Furie RA, et al. Safety profile of anifrolumab in patients with active SLE: an integrated analysis of phase II and III trials. Lupus Sci Med (2021) 8(1):e000464. doi: 10.1136/lupus-2020-000464
48. Chatham WW, Furie R, Saxena A, Brohawn P, Schwetje E, Abreu G, et al. Long-term safety and efficacy of anifrolumab in adults with systemic lupus erythematosus: Results of a phase II open-label extension study. Arthritis Rheumatol. (2021) 73(5):816–25. doi: 10.1002/art.41598
49. Koh JWH, Ng CH, Tay SH. Biologics targeting type I interferons in SLE: A meta-analysis and systematic review of randomised controlled trials. Lupus (2020) 29(14):1845–53. doi: 10.1177/0961203320959702
50. Aringer M, Costenbader K, Daikh D, Brinks R, Mosca M, Ramsey-Goldman R, et al. 2019 European League against Rheumatism/American college of rheumatology classification criteria for systemic lupus erythematosus. Arthritis Rheumatol. (2019) 71(9):1400–12. doi: 10.1002/art.40930
51. Hoyer BF, Manz RA, Radbruch A, Hiepe F. Long-lived plasma cells and their contribution to autoimmunity. Ann N Y Acad Sci (2005) 1050:124–33. doi: 10.1196/annals.1313.014
52. Omarjee O, Picard C, Frachette C, Moreews M, Rieux-Laucat F, Soulas-Sprauel P, et al. Monogenic lupus: Dissecting heterogeneity. Autoimmun. Rev (2019) 18(10):102361. doi: 10.1016/j.autrev.2019.102361
53. Lee-Kirsch MA. The type I interferonopathies. Annu Rev Med (2017) 68:297–315. doi: 10.1146/annurev-med-050715-104506
54. Anders HJ, Krug A, Pawar RD. Molecular mimicry in innate immunity? the viral RNA recognition receptor TLR7 accelerates murine lupus. Eur J Immunol (2008) 38(7):1795–9. doi: 10.1002/eji.200838478
55. Brown GJ, Cañete PF, Wang H, Medhavy A, Bones J, Roco JA, et al. TLR7 gain-of-function genetic variation causes human lupus. Nature (2022) 605(7909):349–56. doi: 10.1038/s41586-022-04642-z
56. Guiducci C, Gong M, Xu Z, Gill M, Chaussabel D, Meeker T, et al. TLR recognition of self nucleic acids hampers glucocorticoid activity in lupus. Nature (2010) 465(7300):937–41. doi: 10.1038/nature09102
57. Lau CM, Broughton C, Tabor AS, Akira S, Flavell RA, Mamula MJ, et al. RNA-Associated autoantigens activate b cells by combined b cell antigen receptor/Toll-like receptor 7 engagement. J Exp Med (2005) 202(9):1171–7. doi: 10.1084/jem.20050630
58. Pawar RD, Ramanjaneyulu A, Kulkarni OP, Lech M, Segerer S, Anders HJ. Inhibition of toll-like receptor-7 (TLR-7) or TLR-7 plus TLR-9 attenuates glomerulonephritis and lung injury in experimental lupus. J Am Soc Nephrol (2007) 18(6):1721–31. doi: 10.1681/asn.2006101162
59. Savarese E, Steinberg C, Pawar RD, Reindl W, Akira S, Anders HJ, et al. Requirement of toll-like receptor 7 for pristane-induced production of autoantibodies and development of murine lupus nephritis. Arthritis Rheum. (2008) 58(4):1107–15. doi: 10.1002/art.23407
60. Lorenz G, Lech M, Anders HJ. Toll-like receptor activation in the pathogenesis of lupus nephritis. Clin Immunol (2017) 185:86–94. doi: 10.1016/j.clim.2016.07.015
61. Iaccarino L, Bettio S, Reggia R, Zen M, Frassi M, Andreoli L, et al. Effects of belimumab on flare rate and expected damage progression in patients with active systemic lupus erythematosus. Arthritis Care Res (Hoboken) (2017) 69(1):115–23. doi: 10.1002/acr.22971
62. Rovin BH, Furie R, Teng YKO, Contreras G, Malvar A, Yu X, et al. A secondary analysis of the belimumab international study in lupus nephritis trial examined effects of belimumab on kidney outcomes and preservation of kidney function in patients with lupus nephritis. Kidney Int (2022) 101(2):403–13. doi: 10.1016/j.kint.2021.08.027
63. Allam R, Anders HJ. The role of innate immunity in autoimmune tissue injury. Curr Opin Rheumatol. (2008) 20(5):538–44. doi: 10.1097/BOR.0b013e3283025ed4
64. Fillatreau S, Manfroi B, Dörner T. Toll-like receptor signalling in b cells during systemic lupus erythematosus. Nat Rev Rheumatol. (2021) 17(2):98–108. doi: 10.1038/s41584-020-00544-4
65. Möckel T, Basta F, Weinmann-Menke J, Schwarting A. B cell activating factor (BAFF): Structure, functions, autoimmunity and clinical implications in systemic lupus erythematosus (SLE). Autoimmun. Rev (2021) 20(2):102736. doi: 10.1016/j.autrev.2020.102736
66. Turnier JL, Kahlenberg JM. The role of cutaneous type I IFNs in autoimmune and autoinflammatory diseases. J Immunol (2020) 205(11):2941–50. doi: 10.4049/jimmunol.2000596
67. Der E, Suryawanshi H, Morozov P, Kustagi M, Goilav B, Ranabothu S, et al. Tubular cell and keratinocyte single-cell transcriptomics applied to lupus nephritis reveal type I IFN and fibrosis relevant pathways. Nat Immunol (2019) 20(7):915–27. doi: 10.1038/s41590-019-0386-1
68. Aw E, Zhang Y, Carroll M. Microglial responses to peripheral type 1 interferon. J Neuroinflamm (2020) 17(1):340. doi: 10.1186/s12974-020-02003-z
69. Nzeusseu Toukap A, Galant C, Theate I, Maudoux AL, Lories RJ, Houssiau FA, et al. Identification of distinct gene expression profiles in the synovium of patients with systemic lupus erythematosus. Arthritis Rheum. (2007) 56(5):1579–88. doi: 10.1002/art.22578
70. Ding X, Xiang W, Yi R, Huang X, Lin Q, He X. Neutralizing interferon-α blocks inflammation-mediated vascular injury via PI3K and AMPK in systemic lupus erythematosus. Immunology (2021) 164(2):372–85. doi: 10.1111/imm.13379
71. Iwamoto T, Dorschner JM, Selvaraj S, Mezzano V, Jensen MA, Vsetecka D, et al. High systemic type I interferon activity is associated with active class III/IV lupus nephritis. J Rheumatol. (2022) 49(4):388–97. doi: 10.3899/jrheum.210391
72. Beltra JC, Decaluwe H. Cytokines and persistent viral infections. Cytokine (2016) 82:4–15. doi: 10.1016/j.cyto.2016.02.006
73. Joo YB, Lim YH, Kim KJ, Park KS, Park YJ. Association of influenza infection with hospitalisation-related systemic lupus erythematosus flares: a time series analysis. Clin Exp Rheumatol. (2021) 39(5):1056–62. doi: 10.55563/clinexprheumatol/fmkp4b
74. Nasser N, Kurban M, Abbas O. Plasmacytoid dendritic cells and type I interferons in flares of systemic lupus erythematosus triggered by COVID-19. Rheumatol. Int (2021) 41(5):1019–20. doi: 10.1007/s00296-021-04825-3
75. van Vollenhoven RF, Petri M, Wallace DJ, Roth DA, Molta CT, Hammer AE, et al. Cumulative corticosteroid dose over fifty-two weeks in patients with systemic lupus erythematosus: Pooled analyses from the phase III belimumab trials. Arthritis Rheumatol. (2016) 68(9):2184–92. doi: 10.1002/art.39682
76. Manzi S, Sánchez-Guerrero J, Merrill JT, Furie R, Gladman D, Navarra SV, et al. Effects of belimumab, a b lymphocyte stimulator-specific inhibitor, on disease activity across multiple organ domains in patients with systemic lupus erythematosus: combined results from two phase III trials. Ann Rheum. Dis (2012) 71(11):1833–8. doi: 10.1136/annrheumdis-2011-200831
77. van Vollenhoven RF, Petri MA, Cervera R, Roth DA, Ji BN, Kleoudis CS, et al. Belimumab in the treatment of systemic lupus erythematosus: high disease activity predictors of response. Ann Rheum. Dis (2012) 71(8):1343–9. doi: 10.1136/annrheumdis-2011-200937
78. Urowitz MB, Ohsfeldt RL, Wielage RC, Kelton KA, Asukai Y, Ramachandran S. Organ damage in patients treated with belimumab versus standard of care: a propensity score-matched comparative analysis. Ann Rheum. Dis (2019) 78(3):372–9. doi: 10.1136/annrheumdis-2018-214043
79. Furie RA, Wallace DJ, Aranow C, Fettiplace J, Wilson B, Mistry P, et al. Long-term safety and efficacy of belimumab in patients with systemic lupus erythematosus: A continuation of a seventy-Six-Week phase III parent study in the united states. Arthritis Rheumatol. (2018) 70(6):868–77. doi: 10.1002/art.40439
80. van Vollenhoven RF, Navarra SV, Levy RA, Thomas M, Heath A, Lustine T, et al. Long-term safety and limited organ damage in patients with systemic lupus erythematosus treated with belimumab: a phase III study extension. Rheumatol (Oxford) (2020) 59(2):281–91. doi: 10.1093/rheumatology/kez279
81. Tanaka Y, Tummala R. Anifrolumab, a monoclonal antibody to the type I interferon receptor subunit 1, for the treatment of systemic lupus erythematosus: an overview from clinical trials. Mod. Rheumatol. (2021) 31(1):1–12. doi: 10.1080/14397595.2020.1812201
82. Furie R, Morand EF, Askanase AD, Vital EM, Merrill JT, Kalyani RN, et al. Anifrolumab reduces flare rates in patients with moderate to severe systemic lupus erythematosus. Lupus (2021) 30(8):1254–63. doi: 10.1177/09612033211014267
83. Vital EM, Merrill JT, Morand EF, Furie RA, Bruce IN, Tanaka Y, et al. Anifrolumab efficacy and safety by type I interferon gene signature and clinical subgroups in patients with SLE: post hoc analysis of pooled data from two phase III trials. Ann Rheum. Dis (2022) 81(7):951–61. doi: 10.1136/annrheumdis-2021-221425
84. Bruce IN, Golam S, Steenkamp J, Wang P, Worthington E, Desta B, et al. Indirect treatment comparison of anifrolumab efficacy versus belimumab in adults with systemic lupus erythematosus. J Comp Eff Res (2022) 11(10):765–77. doi: 10.2217/cer-2022-0040
85. Acharya D, Liu G, Gack MU. Dysregulation of type I interferon responses in COVID-19. Nat Rev Immunol (2020) 20(7):397–8. doi: 10.1038/s41577-020-0346-x
86. Jafarzadeh A, Nemati M, Saha B, Bansode YD, Jafarzadeh S. Protective potentials of type III interferons in COVID-19 patients: Lessons from differential properties of type I- and III interferons. Viral Immunol (2021) 34(5):307–20. doi: 10.1089/vim.2020.0076
87. Bastard P, Rosen LB, Zhang Q, Michailidis E, Hoffmann HH, Zhang Y, et al. Casanova: Autoantibodies against type I IFNs in patients with life-threatening COVID-19. Science (2020) 370(6515):eabd4585. doi: 10.1126/science.abd4585
88. Rommasi F, Nasiri MJ, Mirsaiedi M. Antiviral drugs proposed for COVID-19: action mechanism and pharmacological data. Eur Rev Med Pharmacol Sci (2021) 25(11):4163–73. doi: 10.26355/eurrev_202106_26060
Keywords: glucocorticoids, viral infection, belimumab, anifrolumab, systemic lupus
Citation: Kirou KA, Dall`Era M, Aranow C and Anders H-J (2022) Belimumab or anifrolumab for systemic lupus erythematosus? A risk-benefit assessment. Front. Immunol. 13:980079. doi: 10.3389/fimmu.2022.980079
Received: 28 June 2022; Accepted: 11 August 2022;
Published: 31 August 2022.
Edited by:
Changhai Ding, Southern Medical University, ChinaReviewed by:
David P. D'Cruz, Guy’s and St. Thomas’ NHS Foundation Trust, United KingdomCopyright © 2022 Kirou, Dall`Era, Aranow and Anders. This is an open-access article distributed under the terms of the Creative Commons Attribution License (CC BY). The use, distribution or reproduction in other forums is permitted, provided the original author(s) and the copyright owner(s) are credited and that the original publication in this journal is cited, in accordance with accepted academic practice. No use, distribution or reproduction is permitted which does not comply with these terms.
*Correspondence: Hans-Joachim Anders, hjanders@med.uni-muenchen.de
Disclaimer: All claims expressed in this article are solely those of the authors and do not necessarily represent those of their affiliated organizations, or those of the publisher, the editors and the reviewers. Any product that may be evaluated in this article or claim that may be made by its manufacturer is not guaranteed or endorsed by the publisher.
Research integrity at Frontiers
Learn more about the work of our research integrity team to safeguard the quality of each article we publish.