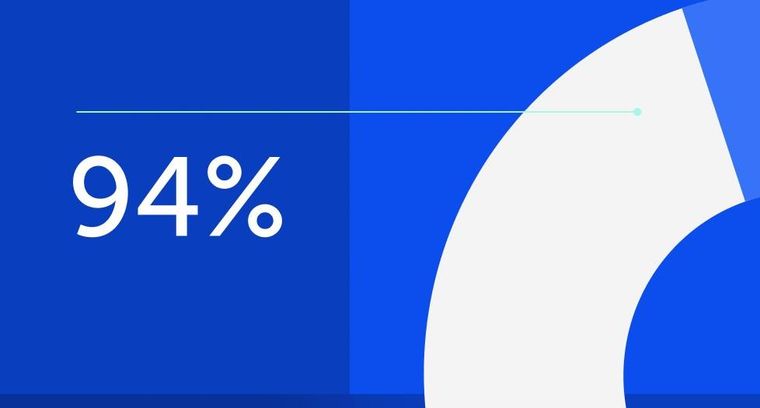
94% of researchers rate our articles as excellent or good
Learn more about the work of our research integrity team to safeguard the quality of each article we publish.
Find out more
MINI REVIEW article
Front. Immunol., 16 August 2022
Sec. Viral Immunology
Volume 13 - 2022 | https://doi.org/10.3389/fimmu.2022.974088
This article is part of the Research TopicHuman T cell Leukemia Virus-1 (HTLV-1) infection, associated pathology and response of the hostView all 20 articles
Human T-cell leukemia virus-1 (HTLV-1) is a retrovirus that persistently infects CD4+ T-cells, and is the causative agent of adult T-cell leukemia/lymphoma (ATLL), tropical spastic paraparesis/HTLV-1-associated myelopathy (TSP/HAM) and several inflammatory diseases. T-cell transformation by HTLV-1 is driven by multiple interactions between viral regulatory proteins and host cell pathways that govern cell proliferation and survival. Studies performed over the last decade have revealed alterations in the expression of many microRNAs in HTLV-1-infected cells and ATLL cells, and have identified several microRNA targets with roles in the viral life cycle and host cell turnover. This review centers on miR-150-5p, a microRNA whose expression is temporally regulated during lymphocyte development and altered in several hematological malignancies. The levels of miR-150-5p are reduced in many HTLV-1-transformed- and ATLL-derived cell lines. Experiments in these cell lines showed that downregulation of miR-150-5p results in activation of the transcription factor STAT1, which is a direct target of the miRNA. However, data on miR-150-5p levels in freshly isolated ATLL samples are suggestive of its upregulation compared to controls. These apparently puzzling findings highlight the need for more in-depth studies of the role of miR-150-5p in HTLV-1 infection and pathogenesis based on knowledge of miR-150-5p-target mRNA interactions and mechanisms regulating its function in normal leukocytes and hematologic neoplasms.
Human T-cell leukemia virus-1 (HTLV-1), the first retrovirus identified as pathogenic in humans (1), infects 5-10 million persons worldwide (2). HTLV-1 is the causative agent of adult T-cell leukemia/lymphoma (ATLL), tropical spastic paraparesis/HTLV-1-associated myelopathy (TSP/HAM) and several inflammatory diseases (3). The unique characteristics of HTLV-1 replication and persistence have thus far impeded the development of effective strategies to control infection and treat HTLV-1-associated diseases [reviewed by (4, 5)].
HTLV-1 codes for the regulatory/accessory proteins Tax, Rex, p30, p13, p12, and HBZ in addition to the Gag, Env and Pol genes common to all retroviruses [reviewed by (6, 7)]. Tax and Rex are essential for productive viral infection, with Tax driving transcription of the plus-strand genome [reviewed by (8)] and Rex functioning as an RNA escort that facilitates exit of plus-strand viral transcripts from the nucleus [reviewed by (9)].
CD4+ CD25+ T-cells are the main targets of HTLV-1 infection and transformation in vivo (10). In the current model of ATLL pathogenesis, Tax is considered to be the primary driver of neoplastic transformation while HBZ is essential for maintaining the transformed cell population [reviewed by (11, 12)].
microRNAs (miRNAs) are small RNAs of about 22 nt that induce degradation and/or block translation of target mRNAs after binding to complementary sequences in the mRNA’s 3’UTR. miRNA-mRNA interactions thus contribute to the precise control of gene expression in physiological and pathological settings including cancer [reviewed by (13)].
The role of miRNAs in HTLV-1 infection and disease has been investigated using PBMC from ATLL patients and asymptomatic carriers, cell lines and clones stabilized from ATLL cells or infected PBMC, and HTLV-1-transformed cell lines generated by cocultivating normal cells with patients’ infected cells. Control cells included normal PBMC, isolated CD4+ T-cells and the T-cell acute lymphoblastic leukemia (T-ALL)-derived cell line Jurkat. Results of these studies yielded many up-and downregulated miRNAs [reviewed by (14)].
Both Tax and HBZ directly influence the expression of specific miRNAs [reviewed by (15)]. Tax and HBZ also have general disruptive effects on miRNA expression through Tax-mediated ubiquitination and degradation of Drosha (16) and HBZ-mediated repression of Dicer expression (17). Dicer activity is also reduced through interactions with Rex (18).
Only a few miRNA-mRNA interactions have been described in the context of HTLV-1-transformed cells. miR-93 and miR-130b, identified as upregulated in ATLL samples and ATLL-derived cell lines, target the mRNA coding for the pro-apoptotic protein TP53INP1 (19). Two miRNAs upregulated by HBZ, miR-17 and miR-21, repress expression of the DNA-binding protein OBFC2A (gene symbol NABP1) (20). miR-34a-5p is overexpressed in HTLV-1-transformed cell lines and ATLL samples (19, 21); its targets identified in HTLV-1-transformed cell lines include the protein deacetylase SIRT1, the pro-apoptotic protein BAX, and the anti-apoptotic protein BIRC5 (Survivin) (21). miRNA profiling of a large panel of ATLL samples and normal CD4+ T-cell controls revealed downregulation of many miRNAs in ATLL cells (22). The most dramatically downregulated miRNA, miR-31, is silenced by genetic deletions and Polycomb-mediated repression. Downregulation of miR-31 increases levels of its target MAP3K14 (NIK), a positive regulator of the noncanonical NF-ĸB pathway (22). Bellon et al. showed that miR-124a is downregulated in ATLL samples, ATLL-derived cell lines and chronically infected cell lines compared to normal PBMC due to promoter methylation, and identified STAT3 as a miR-124a target (23).
miR-150-5p, one of the most highly expressed miRNAs in normal naïve CD4+ T-cells (see below), stands out among the many miRNAs that have been identified as deregulated by HTLV-1, as it was initially reported to be downregulated in HTLV-1-transformed cell lines and ATLL-derived cell lines but upregulated in ATLL samples compared to normal PBMC (19) and CD4+ T-cells (24). On the other hand, the 2012 study by Yamagishi et al. (22) did not identify miR-150 as up- or downregulated in ATLL samples vs. CD4+ controls using a stringent > 5-fold cutoff for differential expression.
RNAseq analyses confirmed significant downregulation of miR-150-5p in the HTLV-1-transformed cell lines C91PL and MT-2 compared to normal CD4+ T-cells (25). miRBase (version 22.1; https://www.mirbase.org) indicates a strong bias for expression of miR-150-5p compared to miR-150-3p; the text below refers to miR-150-5p as miR-150.
In an analysis of miR-150 and miR-223 in additional HTLV-1-transformed cell lines and ATLL cell lines (IL-2-independent or IL-2-dependent) compared to Jurkat cells, Moles et al. (26) detected reduced levels of both miRNAs in the transformed cell lines and the IL-2-independent ATLL cell lines, while the IL-2-dependent ATLL cell lines showed reduced levels of miR-223, but increased levels of miR-150. Analyses of the cell lines cultured with or without IL-2 indicated a link between miR-150 expression and IL-2 stimulation/dependence. Functional assays showed that miR-150 and miR-223 directly target STAT1. Ectopic expression of miR-150 or miR-223 interfered with proliferation of an HTLV-1-transformed cell line, indicating a tumor suppressor function (26).
Recent RNAseq analyses of PBMC from asymptomatic HTLV-1-infected patients (ASP) and control PBMC identified many differentially expressed small RNAs, including upregulated miR-150, in the ASP samples (27). Additional studies that included PBMC from ATLL patients detected higher levels of miR-150 in ATLL vs. both ASP and control PBMC (28). These findings would be strengthened by comparison of miRNAs in purified CD4+ cells, since PBMC preparations from ATLL samples contain a preponderance of infected CD4+ neoplastic cells compared to ASP or control PBMC samples.
Given the importance of miR-150 in normal lymphocyte development and its altered expression in a variety of neoplasms (29, 30), the expression of miR-150 and its mRNA targets in the context of HTLV-1 infection and disease merit further investigation. The following sections provide information on the role of miR-150 in normal CD4+ T-cells and selected hematologic malignancies that could help guide the design of further investigations of this miRNA as a potential actor in HTLV-1 replication and pathogenesis.
miR-150 levels are temporally regulated during B- and T-cell maturation. The first detailed miRNA profiling analyses of murine lymphocyte populations revealed abundant levels of miR-150 in mature B-cells and CD4+ T-cells compared to pro-B cells and thymocytes (31). In vitro activation of mature naïve CD4+ T-cells and CD8+ T-cells is accompanied by substantial alterations in the pattern of miRNA expression, including a rapid decline in miR-150 levels (31–33).
HTLV-1-infected cells and ATLL cells frequently express markers of regulatory T-cells (Tregs) including GITR (34), CD45RO (35) and FOXP3 (36). Early studies of murine lymphocytes showed that miR-150 is much less abundant in Tregs compared to helper CD4+ T-cells, and that forced expression of FOXP3 In CD4+ T-cells leads to downregulation of miR-150 (32).
Forced premature expression of miR-150 in hematopoietic stem/progenitor cells blocks B-cell development at the pro-B stage without overt disruption of T- and myeloid populations (37), while miR-150-knockout mice develop an expanded population of the B-cell subtype B1 without substantial effects on other B-cell subtypes or T-lymphocytes (38). The studies of murine B-cell maturation led to the identification of the transcription factor MYB (c-Myb) as a target of miR-150 (38). MYB plays a key role in the regulation of hematopoietic cell development and turnover, and it is deregulated in cancer cells [reviewed by (39)].
Recent miR-150-RNA-pull-down experiments in resting and in vitro-activated human CD4+ T-cells yielded the MYB transcript and many additional bound mRNAs, including PIK3R1 (phosphoinositide-3-kinase regulatory subunit 1), HNRNPAB (heterogeneous nuclear ribonucleoprotein AB), and PDAP1 (PDGFA-associated protein 1) (40). Results of CRISPR/Cas-9 editing experiments verified the relevance of miR-150, MYB and PDAP1 in regulating the activation state of CD4+ T-cells (40).
Although the potential for miR-150 to regulate MYB in the context of HTLV-1 has not been investigated, MYB function and expression are known to be altered in HTLV-1-infected cells through the actions of Tax and HBZ, with contrasting effects: while Tax represses expression of MYB (41, 42) and competes with MYB for binding to the transcription coactivators CBP/p300 (43), HBZ displaces Tax from p300/CBP and promotes MYB-CBP/p300 binding (44).
MYB is upregulated in ATLL samples compared to CD4+ T-cell controls (45). ATLL samples exhibit very high expression of MYB and in particular the splicing variant MYB-9A, a shorter protein isoform that is more active than full-length MYB. Both MYB and MYB-9A were shown to activate the NF-κB pathway, and knockdown of total MYB or MYB-9A alone induces apoptosis of ATLL cells (45). As depicted in Figure 1A, the predicted 3’UTR region of the MYB-9A mRNA lacks 2 functionally verified binding sites for miR-150 that are present in the full-length MYB transcript (38), suggesting that the MYB-9A mRNA in ATLL cells might bypass negative regulation by miR-150.
Figure 1 miR-150 binding site predictions for MYB-9A and NOTCH1. Panel (A) The alternatively spliced transcript coding for full-length MYB (640 amino acids) contains 15 exons. TargetScan (46) predicts 3 binding sites for miR-150 in the MYB 3’UTR (ENST00000367814.4, 1193 nt). The second and third sites were found to be functionally relevant by Xiao et al. (38). The 3’UTR of MYB-9A (402 amino acids) has not been experimentally verified. The red line indicates a predicted 3’UTR based on the presence of a consensus sequence for polyadenylation (47) downstream of the MYB-9A stop codon. The miRNA target prediction tools STarMir (48) and RNA22 (49) do not identify miR-150-5p binding sites in this sequence. Panel (B) An NCBI BLAST search of the region spanning the functionally relevant miR-150 binding site in the murine NOTCH1 3’UTR characterized by Deng et al. (50) identified a highly similar sequence in the human NOTCH1 3’UTR. This segment contains a potential binding site for miR-150-5p according to TargetScan analysis of the NOTCH1 3’UTR (ENST00000277541.6, 1627 nt). TargetScan Release 8.0: https://www.targetscan.org/vert_80/, STarMir: http://sfold.wadsworth.org/, RNA22 version 2.0: http://cm.jefferson.edu/rna22/Interactive/.
An analysis of miRNA expression during human thymocyte maturation identified miR-150 among the most strongly upregulated miRNAs in the transition from CD4+CD8+ double-positive (DP) cells to mature CD4+ or CD8+ single-positive (SP) cells, and confirmed its sustained expression in mature circulating CD4+ and CD8+ SP populations (51). Integration of gene expression data obtained for thymocyte populations with miRNA target predictions led to the identification of NOTCH3 as a miR-150 target. Forced expression of miR-150 in T-ALL cell lines reduced the levels of NOTCH3, slowed cell proliferation and induced apoptotic death (51).
The NOTCH family proteins (NOTCH1-4) are cell-surface, transmembrane proteins that, after interacting with a NOTCH ligand (DLL1, DLL3, DLL4, JAG1, JAG2), undergo proteolytic cleavage of their cytoplasmic tails. The resulting Notch intracellular domain (NICD) peptide transfers to the nucleus, where it regulates transcription of target genes. NOTCH signaling regulates development and cell fate, including that of T- and B-cells, and is frequently deregulated in hematologic- and solid cancers [reviewed by (52)].
The NOTCH pathway is activated through diverse mechanisms in ATLL cells, including oncogenic mutations in NOTCH1 (53, 54), overexpression of JAG1 (55), a Tax-mediated increase in the half-life of the NICD (56), inactivating mutations of FBXW7, a ubiquitin ligase that directs NICD degradation (57), and mutations in NOTCH pathway-regulating genes ATXN1 and ZFP36L2 (54).
A recent study of pathways involved in the activation of murine macrophages demonstrated that miR-150 directly targets NOTCH1 (50). As shown in Figure 1B, the human NOTCH1 3’UTR contains a predicted binding site for miR-150 that is highly similar to the murine sequence. The possible impact of miR-150 on NOTCH signaling in HTLV-1-infected cells through its repression of NOTCH1 and/or NOTCH3 thus merits investigation.
The extent of miR-150 downregulation upon stimulation of naïve T-cells and downstream effects depend on the activating stimulus. Analyses of the response of CD4+ T-cells to stimulation with either anti-CD3/CD28 or anti-CD3/CD46 antibodies revealed a more pronounced downregulation of miR-150 in CD3/CD46 stimulated cells, which was paralleled by a more substantial increase in MYB levels (58). Further characterization of the activated cells identified the glucose transporter SLC2A1 (GLUT1) as a miR-150 target (58). These findings are of interest in the context of HTLV-1 infection, as GLUT1 plays a key role in the binding/entry phase of HTLV-1 infection (59). The observation that GLUT1 is needed to set up a metabolic profile that supports HIV-1 replication in CD4+ T-cells (60) suggests that regulation of the miR-150/GLUT1 balance might influence HTLV-1 replication at both the entry and post-entry phases.
miR-150 regulates the maturation and activity of NK cells (61, 62), and is frequently downregulated in NK/T lymphomas (63, 64). Forced expression of miR-150 in NK/T lymphoma cells results in reduced cell proliferation, increased apoptosis and a senescent phenotype. These effects were attributed in part to miR-150-mediated targeting of DKC1 (dyskerin pseudouridine synthase 1) and AKT2 (AKT serine/threonine kinase 2), both of which regulate telomerase activity (63). A subsequent study identified both AKT2 and AKT3 as miR-150 targets and showed that reintroduction of the miRNA into NK/T lymphoma cells increases their sensitivity to killing by ionizing radiation (64).
AKT is activated in ATLL cells and HTLV-1-transformed cell lines (65). Activated AKT feeds into the mTOR signaling network, which plays a central role in nutrient sensing, metabolism, and cell growth, and is frequently deregulated in cancer (66). Studies of T-ALL cells indicated a role for the AKT/mTOR pathway in downregulating miR-150 (67). Treatment of the T-ALL cell line Jurkat with the mTORC1-inhibitor rapamycin resulted in an increase in the levels of miR-150 and a block in the cell cycle; forced expression of miR-150 plus rapamycin augmented the anti-proliferative effect (67). Experiments performed in the myeloid leukemia cell line K562 led to the identification of FOXO4, TET3, PRKCA and EIF4B as direct miR-150 targets (68). The fact that EIF4B is an important downstream participant in mTOR signaling suggests that mTOR and miR-150 functionally antagonize each other. These observations are of interest, as both Tax and HBZ stimulate the mTOR pathway (69, 70). HTLV-1-transformed cell lines and ATLL samples respond to rapamycin and its analogue everolimus with growth arrest (65, 71), with long-term everolimus treatment inducing a senescent phenotype followed by apoptosis (71). It would thus be interesting to evaluate the effects of mTOR inhibition on miR-150 expression in ATLL/HTLV-1-infected cells and the impact of combining mTOR inhibitors with a miR-150 mimic on cell senescence/death.
Integration of gene expression data from rapamycin-treated/control Jurkat cells and miRNA target predictions suggested a role for miR-150 in cell cycle regulation, and led to the identification of CDK2 (cyclin-dependent kinase 2) as a direct miR-150 target (67). It is noteworthy that CDK2 was found to be significantly upregulated in a panel of ATLL-derived and HTLV-1-transformed cell lines compared to activated T-cell controls (72); low miR-150 levels might thus contribute to sustain growth of HTLV-1-infected cells by relaxing control of CDK2 expression.
Cutaneous T-cell lymphoma (CTCL) defines a variety of neoplasms characterized by the accumulation of mature T-cells in the skin, and includes ATLL cases with cutaneous homing [reviewed by (73)]. An analysis of a panel of CTCL cell lines and primary CTCL samples (including ATLL lymph node biopsies) revealed significant downregulation of miR-150 in CTCL cells compared to normal CD4+ T-cells (74). Ectopic expression of miR-150 in CTCL cells reduced their growth in immunodeficient mice and interfered with their migration/dissemination properties. These effects were linked to miR-150-mediated downregulation of CCR6, a chemokine receptor recognized by CCL20 (74). Treatment with histone deacetylase inhibitors resulted in a decline in CCR6 levels accompanied by changes in expression of many miRNAs, including upregulation of miR-150 (75). The survival of CTCL-inoculated mice was prolonged by systemic treatment with a miR-150 mimic or a siRNA against CCR6, suggesting a possible therapeutic application for miR-150 (75). These findings are of interest, as an earlier study of inflammatory signals associated with HTLV-1 pathogenesis had shown that HTLV-1-transformed cell lines express high levels of both CCR6 and its ligand, CCL20, which is upregulated by Tax through the NF-κB pathway (76). Consistent with these observations, expression of CCR6 was found to be elevated in PBMC from TSP/HAM patients and asymptomatic carriers compared to healthy controls (77). Taken together, these observations suggest that low miR-150 levels may contribute to sustain CCR6 expression in HTLV-1-infected cells.
Cellular miRNAs can potentially influence the retroviral life cycle by directly targeting viral transcripts. miR-150, together with miR-28, miR-125b, miR-223, and miR-382, contribute to maintain HIV-1 latency in resting CD4+ T cells by binding to the viral 3’ UTR, resulting in repression of viral gene expression (78). A search for interactions between miRNAs and HTLV-1 transcript showed that miR-28-3p binds to the unspliced viral RNA (genomic and coding for Gag-Pro-Pol) and singly spliced Env mRNA (79). This interaction blocks viral replication at the reverse transcription step (79). The HTLV-1 plus- and minus strand primary transcripts contain many additional predicted binding sites for cellular miRNAs, including 2 predicted sites for miR-150 on the minus-strand transcript coding for HBZ (80); the potential for miR-150 to regulate HBZ expression remains to be explored.
Mechanisms known to repress miR-150 expression/function include promoter methylation, interference with miRNA precursor processing, inhibition by lncRNAs (long noncoding RNAs), and release from the cell.
The miR-150 stem-loop is coded on the minus strand of chromosome 19q13.33 (chr19: 49,500,785-49,500,868 [-]). An investigation of a ~ 500-bp sequence spanning this region identified several CpG dinucleotides that are targets for methylation by DNMT1 and mediate miR-150 silencing in anaplastic large-cell lymphoma cells (81).
Early studies of miRNA regulation showed that MYC represses expression of many miRNAs, including miR-150 (82). Further investigations revealed a control circuit in which MYC induces expression of LIN28, an RNA-binding protein that interferes with miRNA maturation, including that of miR-150. This leads to deregulation of oncogenes normally targeted by miR-150, including MYB and FLT3 (83, 84). The impact of MYC on miR-150 may be cell-context dependent, as MYC was shown to repress miR-150 expression independently of LIN28 in follicular lymphoma cells (85). The role of MYC in miR-150 transcription/processing may be relevant in HTLV-1 infection, given the findings that MYC expression and function are increased through the activities of the viral regulatory proteins HBZ and p30, respectively (86, 87).
The multiple functions of lncRNAs include direct miRNA binding, which ‘sponges’ the miRNA from its 3’UTR targets. An investigation of lncRNAs in a panel of HTLV-1-transformed- and ATLL cell lines revealed expression of AVRIL, HOTAIR H19, TUSC7, MALAT1 and SAF lncRNAs and demonstrated roles for AVRIL in NF-κB signaling and repression of CDKN1A expression (88). MALAT1 is also a good candidate for further study, as it was recently shown to act as a sponge for miR-150 in non-Hodgkin lymphoma (89).
Many miRNAs, including miR-150, are released into the extracellular environment as cargo in extracellular vesicles (EVs) or in association with Argonaute proteins or high-density lipoproteins (HDL) [reviewed by (90)]. Extracellular release of miRNAs can serve as a mechanism to rapidly eliminate them from the cell, or to target them to recipient cells.
Studies of CD4+ T-cells indicated that the rapid decline in miR-150 levels induced by in vitro stimulation is associated with its active export in EVs and upregulation of MYB expression (91). Tregs also actively export miR-150 in EVs which can in turn induce downregulation of MYB in recipient cells and reduce the proliferation of in vitro-stimulated CD4+ T-cells (92).
HTLV-1-infected cell lines are known to release EVs that contain Tax protein, a subset of viral mRNAs, and cell signaling molecules [reviewed by (93)]. These EVs can have activating effects on recipient cells and promote cell-cell contact, thereby facilitating virus transmission (94). It would be interesting to determine whether HTLV-1-infected cells release miR-150 as a means of depleting their intracellular pools or to provide signals to surrounding cells.
Future studies aimed at defining the expression pattern of miR-150 and its targets in HTLV-1-infected- and ATLL cells will help complete the picture of the interplay between the miRNA regulatory network and HTLV-1 infection and pathogenesis. An important task will be to extend miR-150 expression analyses to primary ATLL samples and PBMC from asymptomatic patients to specific populations enriched for CD4+, infected cells.
The strong downregulation of miR-150 observed in many HTLV-1-transformed- and ATLL-derived cell lines makes these lines useful models for discovering miR-150 targets that are relevant to T-cells and the virus, STAT1 being the first such example (26) (see Figure 2). An in vitro-co-cultivation system could be employed to verify some of the mRNA targets described above, e.g. GLUT1, and to test the effects of forced expression/silencing of miR-150 on HTLV-1 infection and immortalization. The results of these studies will be instrumental to assess the potential relevance of miR-150 as a biomarker and possible therapeutic target in HTLV-1 infection and disease.
Figure 2 miR-150-mRNA interactions in HTLV-1 infection/transformation: one confirmed, many to explore. miR-150 is known to repress expression of STAT1 (highlighted in green) when overexpressed in ATLL cell lines (24). As depicted here and described in the text, many other genes of potential relevance to HTLV-1 infection and pathogenesis are known to be controlled by miR-150 in normal and neoplastic T-cells and other leukocyte populations.
DD’A, VR, MS-B, and VC wrote the manuscript. MS-B and DD’A prepared Figure 1. VC and DD’A prepared Figure 2. All authors contributed to the article and approved the submitted version.
Investigator Grant #24935, from AIRC (VC) and BIRD #204551/20 from the Department of Biomedical Sciences, University of Padova (DD’A).
The authors declare that the research was conducted in the absence of any commercial or financial relationships that could be construed as a potential conflict of interest.
All claims expressed in this article are solely those of the authors and do not necessarily represent those of their affiliated organizations, or those of the publisher, the editors and the reviewers. Any product that may be evaluated in this article, or claim that may be made by its manufacturer, is not guaranteed or endorsed by the publisher.
1. Gallo RC, Willems L, Tagaya Y. Time to go back to the original name. Front Microbiol (2017) 8:1800. doi: 10.3389/fmicb.2017.01800
2. Gessain A, Cassar O. Epidemiological aspects and world distribution of HTLV-1 infection. Front Microbiol (2012) 3:388. doi: 10.3389/fmicb.2012.00388
3. Futsch N, Mahieux R, Dutartre H. HTLV-1, the other pathogenic yet neglected human retrovirus: From transmission to therapeutic treatment. Viruses (2017) 10(1):1. doi: 10.3390/v0010001.
4. Hirons A, Khoury G, Purcell DFJ. Human T-cell lymphotropic virus type-1: a lifelong persistent infection, yet never truly silent. Lancet Infect Dis (2021) 21(1):e2–e10. doi: 10.1016/S1473-3099(20)30328-5
5. Willems L, Hasegawa H, Accolla R, Bangham C, Bazarbachi A, Bertazzoni U, et al. Reducing the global burden of HTLV-1 infection: An agenda for research and action. Antiviral Res (2017) 137:41–8. doi: 10.1016/j.antiviral.2016.10.015
6. Cavallari I, Rende F, D'Agostino DM, Ciminale V. Converging strategies in expression of human complex retroviruses. Viruses (2011) 3(8):1395–414. doi: 10.3390/v3081395
7. Ratner L. Molecular biology of human T cell leukemia virus. Semin Diagn Pathol (2020) 37(2):104–9. doi: 10.1053/j.semdp.2019.04.003
8. Pluta A, Jaworski JP, Douville RN. Regulation of expression and latency in BLV and HTLV. Viruses (2020) 12(10):1079. doi: 10.3390/v12101079
9. D'Agostino DM, Cavallari I, Romanelli MG, Ciminale V. Post-transcriptional regulation of HTLV gene expression: Rex to the rescue. Front Microbiol (2019) 10:1958. doi: 10.3389/fmicb.2019.01958
10. Yamano Y, Cohen CJ, Takenouchi N, Yao K, Tomaru U, Li HC, et al. Increased expression of human T lymphocyte virus type I (HTLV-I) Tax11-19 peptide-human histocompatibility leukocyte antigen A*201 complexes on CD4+ CD25+ T cells detected by peptide-specific, major histocompatibility complex-restricted antibodies in patients with HTLV-i-associated neurologic disease. J Exp Med (2004) 199(10):1367–77. doi: 10.1084/jem.20032042
11. Mohanty S, Harhaj EW. Mechanisms of oncogenesis by HTLV-1 tax. Pathogens (2020) 9(7):543. doi: 10.3390/pathogens9070543
12. Matsuoka M, Mesnard JM. HTLV-1 bZIP factor: the key viral gene for pathogenesis. Retrovirology (2020) 17(1):2. doi: 10.1186/s12977-020-0511-0
13. Hayes J, Peruzzi PP, Lawler S. MicroRNAs in cancer: biomarkers, functions and therapy. Trends Mol Med (2014) 20(8):460–9. doi: 10.1016/j.molmed.2014.06.005
14. Machado CB, da Cunha LS, Maués JHDS, Pessoa FMCP, de Oliveira MB, Ribeiro RM, et al. Role of miRNAs in human T cell leukemia virus type 1 induced T cell leukemia: A literature review and bioinformatics approach. Int J Mol Sci (2022) 23(10):5486. doi: 10.3390/ijms23105486
15. Fochi S, Ciminale V, Trabetti E, Bertazzoni U, D'Agostino DM, Zipeto D, et al. NF-κB and MicroRNA deregulation mediated by HTLV-1 tax and HBZ. Pathogens (2019) 8(4):290. doi: 10.3390/pathogens8040290
16. Van Duyne R, Guendel I, Klase Z, Narayanan A, Coley W, Jaworski E, et al. Localization and sub-cellular shuttling of HTLV-1 tax with the miRNA machinery. PloS One (2012) 7(7):e40662. doi: 10.1371/journal.pone.0040662
17. Gazon H, Belrose G, Terol M, Meniane JC, Mesnard JM, Cesaire R, et al. Impaired expression of DICER and some microRNAs in HBZ expressing cells from acute adult T-cell leukemia patients. Oncotarget (2016) 7(21):30258–75. doi: 10.18632/oncotarget.7162
18. Abe M, Suzuki H, Nishitsuji H, Shida H, Takaku H. Interaction of human T-cell lymphotropic virus type I Rex protein with dicer suppresses RNAi silencing. FEBS lett (2010) 584(20):4313–8. doi: 10.1016/j.febslet.2010.09.031
19. Yeung ML, Yasunaga J, Bennasser Y, Dusetti N, Harris D, Ahmad N, et al. Roles for microRNAs, miR-93 and miR-130b, and tumor protein 53-induced nuclear protein 1 tumor suppressor in cell growth dysregulation by human T-cell lymphotrophic virus 1. Cancer Res (2008) 68(21):8976–85. doi: 10.1158/0008-5472.CAN-08-0769
20. Vernin C, Thenoz M, Pinatel C, Gessain A, Gout O, Delfau-Larue MH, et al. HTLV-1 bZIP factor HBZ promotes cell proliferation and genetic instability by activating OncomiRs. Cancer Res (2014) 74(21):6082–93. doi: 10.1158/0008-5472.CAN-13-3564
21. Sharma VK, Raimondi V, Ruggero K, Pise-Masison CA, Cavallari I, Silic-Benussi M, et al. Expression of miR-34a in T-cells infected by human T-lymphotropic virus 1. Front Microbiol (2018) 9:832. doi: 10.3389/fmicb.2018.00832
22. Yamagishi M, Nakano K, Miyake A, Yamochi T, Kagami Y, Tsutsumi A, et al. Polycomb-mediated loss of miR-31 activates NIK-dependent NF-κB pathway in adult T cell leukemia and other cancers. Cancer Cell (2012) 21(1):121–35. doi: 10.1016/j.ccr.2011.12.015
23. Bellon M, Lu L, Nicot C. Constitutive activation of Pim1 kinase is a therapeutic target for adult T-cell leukemia. Blood (2016) 127(20):2439–50. doi: 10.1182/blood-2015-11-685032
24. Bellon M, Lepelletier Y, Hermine O, Nicot C. Deregulation of microRNA involved in hematopoiesis and the immune response in HTLV-I adult T-cell leukemia. Blood (2009) 113(20):4914–7. doi: 10.1182/blood-2008-11-189845
25. Ruggero K, Guffanti A, Corradin A, Sharma VK, De Bellis G, Corti G, et al. Small noncoding RNAs in cells transformed by human T-cell leukemia virus type 1: A role for a tRNA fragment as a primer for reverse transcriptase. J Virol (2014) 88(7):3612–22. doi: 10.1128/JVI.02823-13
26. Moles R, Bellon M, Nicot C. STAT1: A novel target of miR-150 and miR-223 is involved in the proliferation of HTLV-I-Transformed and ATL cells. Neoplasia (2015) 17(5):449–62. doi: 10.1016/j.neo.2015.04.005
27. Valadão de Souza DR, Pessôa R, Nascimento A, Nukui Y, Pereira J, Casseb J, et al. Small RNA profiles of HTLV-1 asymptomatic carriers with monoclonal and polyclonal rearrangement of the T-cell antigen receptor γ-chain using massively parallel sequencing: A pilot study. Oncol Lett (2020) 20(3):2311–21. doi: 10.3892/ol.2020.11803
28. Nascimento A, Valadão de Souza DR, Pessôa R, Pietrobon AJ, Nukui Y, Pereira J, et al. Global expression of noncoding RNome reveals dysregulation of small RNAs in patients with HTLV-1-associated adult T-cell leukemia: A pilot study. Infect Agent Cancer (2021) 16(1):4. doi: 10.1186/s13027-020-00343-2
29. Wang F, Ren X, Zhang X. Role of microRNA-150 in solid tumors. Oncol Lett (2015) 10(1):11–6. doi: 10.3892/ol.2015.3170
30. He Y, Jiang X, Chen J. The role of miR-150 in normal and malignant hematopoiesis. Oncogene (2014) 33(30):3887–93. doi: 10.1038/onc.2013.346
31. Monticelli S, Ansel KM, Xiao C, Socci ND, Krichevsky AM, Thai TH, et al. MicroRNA profiling of the murine hematopoietic system. Genome Biol (2005) 6(8):R71. doi: 10.1186/gb-2005-6-8-r71
32. Cobb BS, Hertweck A, Smith J, O'Connor E, Graf D, Cook T, et al. A role for dicer in immune regulation. J Exp Med (2006) 203(11):2519–27. doi: 10.1084/jem.20061692
33. Wu H, Neilson JR, Kumar P, Manocha M, Shankar P, Sharp PA, et al. miRNA profiling of naïve, effector and memory CD8 T cells. PloS One (2007) 2(10):e1020. doi: 10.1371/journal.pone.0001020
34. Bal HP, Cheng J, Murakami A, Tallarico AS, Wang W, Zhou D, et al. GITR overexpression on CD4+CD25+ HTLV-1 transformed cells: Detection by massively parallel signature sequencing. Biochem Biophys Res Commun (2005) 332(2):569–84. doi: 10.1016/j.bbrc.2005.04.162
35. Richardson JH, Edwards AJ, Cruickshank JK, Rudge P, Dalgleish AG. In vivo cellular tropism of human T-cell leukemia virus type 1. J Virol (1990) 64(11):5682–7. doi: 10.1128/JVI.64.11.5682-5687.1990
36. Karube K, Ohshima K, Tsuchiya T, Yamaguchi T, Kawano R, Suzumiya J, et al. Expression of FoxP3, a key molecule in CD4CD25 regulatory T cells, in adult T-cell leukaemia/lymphoma cells. Br J Haematol (2004) 126(1):81–4. doi: 10.1111/j.1365-2141.2004.04999.x.
37. Zhou B, Wang S, Mayr C, Bartel DP, Lodish HF. miR-150, a microRNA expressed in mature b and T cells, blocks early b cell development when expressed prematurely. Proc Natl Acad Sci U S A. (2007) 104(17):7080–5. doi: 10.1073/pnas.0702409104
38. Xiao C, Calado DP, Galler G, Thai TH, Patterson HC, Wang J, et al. MiR-150 controls b cell differentiation by targeting the transcription factor c-myb. Cell (2007) 131(1):146–59. doi: 10.1016/j.cell.2007.07.021
39. Wang X, Angelis N, Thein SL. MYB - a regulatory factor in hematopoiesis. Gene (2018) 665:6–17. doi: 10.1016/j.gene.2018.04.065
40. Chirichella M, Bianchi N, Džafo E, Foli E, Gualdrini F, Kenyon A, et al. RFX transcription factors control a miR-150/PDAP1 axis that restrains the proliferation of human T cells. PloS Biol (2022) 20(2):e3001538. doi: 10.1371/journal.pbio.3001538
41. Nicot C, Opavsky R, Mahieux R, Johnson JM, Brady JN, Wolff L, et al. Tax oncoprotein trans-represses endogenous b-myb promoter activity in human T cells. AIDS Res Hum Retroviruses (2000) 16(16):1629–32. doi: 10.1089/08892220050193065
42. Nicot C, Mahieux R, Pise-Masison C, Brady J, Gessain A, Yamaoka S, et al. Human T-cell lymphotropic virus type 1 tax represses c-myb-dependent transcription through activation of the NF-kappaB pathway and modulation of coactivator usage. Mol Cell Biol (2001) 21(21):7391–402. doi: 10.1128/MCB.21.21.7391-7402.2001
43. Colgin MA, Nyborg JK. The human T-cell leukemia virus type 1 oncoprotein tax inhibits the transcriptional activity of c-myb through competition for the CREB binding protein. J Virol (1998) 72(11):9396–9. doi: 10.1128/JVI.72.11.9396-9399.1998
44. Cook PR, Polakowski N, Lemasson I. HTLV-1 HBZ protein deregulates interactions between cellular factors and the KIX domain of p300/CBP. J Mol Biol (2011) 409(3):384–98. doi: 10.1016/j.jmb.2011.04.003
45. Nakano K, Uchimaru K, Utsunomiya A, Yamaguchi K, Watanabe T. Dysregulation of c-myb pathway by aberrant expression of proto-oncogene MYB provides the basis for malignancy in adult T-cell leukemia/lymphoma cells. Clin Cancer Res (2016) 22(23):5915–28. doi: 10.1158/1078-0432.CCR-15-1739
46. Agarwal V, Bell GW, Nam JW, Bartel DP. Predicting effective microRNA target sites in mammalian mRNAs. Elife (2015) 4:1. doi: 10.7554/eLife.05005
47. Proudfoot NJ. Ending the message: poly(A) signals then and now. Genes Dev (2011) 25(17):1770–82. doi: 10.1101/gad.17268411
48. Rennie W, Liu C, Carmack CS, Wolenc A, Kanoria S, Lu J, et al. STarMir: a web server for prediction of microRNA binding sites. Nucleic Acids Res (2014) 42(Web Server issue):W114–8. doi: 10.1093/nar/gku376
49. Miranda KC, Huynh T, Tay Y, Ang YS, Tam WL, Thomson AM, et al. A pattern-based method for the identification of MicroRNA binding sites and their corresponding heteroduplexes. Cell (2006) 126(6):1203–17. doi: 10.1016/j.cell.2006.07.031
50. Deng X, Lin Z, Zuo C, Fu Y. Upregulation of miR-150-5p alleviates LPS-induced inflammatory response and apoptosis of RAW264.7 macrophages by targeting Notch1. Open Life Sci (2020) 15(1):544–52. doi: 10.1515/biol-2020-0058
51. Ghisi M, Corradin A, Basso K, Frasson C, Serafin V, Mukherjee S, et al. Modulation of microRNA expression in human T-cell development: Targeting of NOTCH3 by miR-150. Blood (2011) 117(26):7053–62. doi: 10.1182/blood-2010-12-326629
52. Gragnani L, Lorini S, Marri S, Zignego AL. Role of notch receptors in hematologic malignancies. Cells (2020) 10(1):16. doi: 10.3390/cells10010016
53. Pancewicz J, Taylor JM, Datta A, Baydoun HH, Waldmann TA, Hermine O, et al. Notch signaling contributes to proliferation and tumor formation of human T-cell leukemia virus type 1-associated adult T-cell leukemia. Proc Natl Acad Sci U S A. (2010) 107(38):16619–24. doi: 10.1073/pnas.1010722107
54. Kataoka K, Nagata Y, Kitanaka A, Shiraishi Y, Shimamura T, Yasunaga J, et al. Integrated molecular analysis of adult T cell leukemia/lymphoma. Nat Genet (2015) 47(11):1304–15. doi: 10.1038/ng.3415
55. Bellon M, Moles R, Chaib-Mezrag H, Pancewicz J, Nicot C. JAG1 overexpression contributes to Notch1 signaling and the migration of HTLV-1-transformed ATL cells. J Hematol Oncol (2018) 11(1):119. doi: 10.1186/s13045-018-0665-6
56. Cheng W, Zheng T, Wang Y, Cai K, Wu W, Zhao T, et al. Activation of Notch1 signaling by HTLV-1 tax promotes proliferation of adult T-cell leukemia cells. Biochem Biophys Res Commun (2019) 512(3):598–603. doi: 10.1016/j.bbrc.2019.03.094
57. Yeh CH, Bellon M, Pancewicz-Wojtkiewicz J, Nicot C. Oncogenic mutations in the FBXW7 gene of adult T-cell leukemia patients. Proc Natl Acad Sci U S A. (2016) 113(24):6731–6. doi: 10.1073/pnas.1601537113
58. King BC, Esguerra JL, Golec E, Eliasson L, Kemper C, Blom AM. CD46 activation regulates miR-150-Mediated control of GLUT1 expression and cytokine secretion in human CD4+ T cells. J Immunol (2016) 196(4):1636–45. doi: 10.4049/jimmunol.1500516
59. Manel N, Kim FJ, Kinet S, Taylor N, Sitbon M, Battini JL. The ubiquitous glucose transporter GLUT-1 is a receptor for HTLV. Cell (2003) 115(4):449–59. doi: 10.1016/s0092-8674(03)00881-x
60. Loisel-Meyer S, Swainson L, Craveiro M, Oburoglu L, Mongellaz C, Costa C, et al. Glut1-mediated glucose transport regulates HIV infection. Proc Natl Acad Sci U S A. (2012) 109(7):2549–54. doi: 10.1073/pnas.1121427109
61. Bezman NA, Chakraborty T, Bender T, Lanier LL. miR-150 regulates the development of NK and iNKT cells. J Exp Med (2011) 208(13):2717–31. doi: 10.1084/jem.20111386
62. Kim N, Kim M, Yun S, Doh J, Greenberg PD, Kim TD, et al. MicroRNA-150 regulates the cytotoxicity of natural killers by targeting perforin-1. J Allergy Clin Immunol (2014) 134(1):195–203. doi: 10.1016/j.jaci.2014.02.018
63. Watanabe A, Tagawa H, Yamashita J, Teshima K, Nara M, Iwamoto K, et al. The role of microRNA-150 as a tumor suppressor in malignant lymphoma. Leukemia (2011) 25(8):1324–34. doi: 10.1038/leu.2011.81
64. Wu SJ, Chen J, Wu B, Wang YJ, Guo KY. MicroRNA-150 enhances radiosensitivity by inhibiting the AKT pathway in NK/T cell lymphoma. J Exp Clin Cancer Res (2018) 37(1):18. doi: 10.1186/s13046-017-0639-5
65. Ikezoe T, Nishioka C, Bandobashi K, Yang Y, Kuwayama Y, Adachi Y, et al. Longitudinal inhibition of PI3K/Akt/mTOR signaling by LY294002 and rapamycin induces growth arrest of adult T-cell leukemia cells. Leuk Res (2007) 31(5):673–82. doi: 10.1016/j.leukres.2006.08.001
66. Cargnello M, Tcherkezian J, Roux PP. The expanding role of mTOR in cancer cell growth and proliferation. Mutagenesis (2015) 30(2):169–76. doi: 10.1093/mutage/geu045
67. Podshivalova K, Wang EA, Hart T, Salomon DR. Expression of the miR-150 tumor suppressor is restored by and synergizes with rapamycin in a human leukemia T-cell line. Leuk Res (2018) 11:74:1–9. doi: 10.1016/j.leukres.2018.09.009
68. Fang ZH, Wang SL, Zhao JT, Lin ZJ, Chen LY, Su R, et al. miR-150 exerts antileukemia activity in vitro and in vivo through regulating genes in multiple pathways. Cell Death Dis (2016) 7(9):e2371. doi: 10.1038/cddis.2016.256
69. Yoshita M, Higuchi M, Takahashi M, Oie M, Tanaka Y, Fujii M. Activation of mTOR by human T-cell leukemia virus type 1 tax is important for the transformation of mouse T cells to interleukin-2-independent growth. Cancer Sci (2012) 103(2):369–74. doi: 10.1111/j.1349-7006.2011.02123.x
70. Mukai R, Ohshima T. HTLV-1 HBZ positively regulates the mTOR signaling pathway via inhibition of GADD34 activity in the cytoplasm. Oncogene (2014) 33(18):2317–28. doi: 10.1038/onc.2013.181
71. Darwiche N, Sinjab A, Abou-Lteif G, Chedid MB, Hermine O, Dbaibo G, et al. Inhibition of mammalian target of rapamycin signaling by everolimus induces senescence in adult T-cell leukemia/lymphoma and apoptosis in peripheral T-cell lymphomas. Int J Cancer (2011) 129(4):993–1004. doi: 10.1002/ijc.25742
72. Pise-Masison CA, Radonovich M, Mahieux R, Chatterjee P, Whiteford C, Duvall J, et al. Transcription profile of cells infected with human T-cell leukemia virus type I compared with activated lymphocytes. Cancer Res (2002) 62(12):3562–71.
73. Patil K, Kuttikrishnan S, Khan AQ, Ahmad F, Alam M, Buddenkotte J, et al. Molecular pathogenesis of cutaneous T cell lymphoma: Role of chemokines, cytokines, and dysregulated signaling pathways. Semin Cancer Biol (2021). doi: 10.1016/j.semcancer.2021.12.003
74. Ito M, Teshima K, Ikeda S, Kitadate A, Watanabe A, Nara M, et al. MicroRNA-150 inhibits tumor invasion and metastasis by targeting the chemokine receptor CCR6, in advanced cutaneous T-cell lymphoma. Blood (2014) 123(10):1499–511. doi: 10.1182/blood-2013-09-527739
75. Abe F, Kitadate A, Ikeda S, Yamashita J, Nakanishi H, Takahashi N, et al. Histone deacetylase inhibitors inhibit metastasis by restoring a tumor suppressive microRNA-150 in advanced cutaneous T-cell lymphoma. Oncotarget (2017) 8(5):7572–85. doi: 10.18632/oncotarget.13810
76. Imaizumi Y, Sugita S, Yamamoto K, Imanishi D, Kohno T, Tomonaga M, et al. Human T cell leukemia virus type-I tax activates human macrophage inflammatory protein-3 alpha/CCL20 gene transcription via the NF-kappa b pathway. Int Immunol (2002) 14(2):147–55. doi: 10.1093/intimm/14.2.147
77. Rafatpanah H, Felegari M, Azarpazhooh MR, Vakili R, Rajaei T, Hampson I, et al. Altered expression of CXCR3 and CCR6 and their ligands in HTLV-1 carriers and HAM/TSP patients. J Med Virol (2017) 89(8):1461–8. doi: 10.1002/jmv.24779
78. Huang J, Wang F, Argyris E, Chen K, Liang Z, Tian H, et al. Cellular microRNAs contribute to HIV-1 latency in resting primary CD4+ T lymphocytes. Nat Med (2007) 13(10):1241–7. doi: 10.1038/nm1639
79. Bai XT, Nicot C. miR-28-3p is a cellular restriction factor that inhibits human T cell leukemia virus, type 1 (HTLV-1) replication and virus infection. J Biol Chem (2015) 290(9):5381–90. doi: 10.1074/jbc.M114.626325
80. Ruggero K, Corradin A, Zanovello P, Amadori A, Bronte V, Ciminale V, et al. Role of microRNAs in HTLV-1 infection and transformation. Mol Aspects Med (2010) 31(5):367–82. doi: 10.1016/j.mam.2010.05.001
81. Hoareau-Aveilla C, Valentin T, Daugrois C, Quelen C, Mitou G, Quentin S, et al. Reversal of microRNA-150 silencing disadvantages crizotinib-resistant NPM-ALK(+) cell growth. J Clin Invest (2015) 125(9):3505–18. doi: 10.1172/JCI78488
82. Chang TC, Yu D, Lee YS, Wentzel EA, Arking DE, West KM, et al. Widespread microRNA repression by myc contributes to tumorigenesis. Nat Genet (2008) 40(1):43–50. doi: 10.1038/ng.2007.30
83. Chang TC, Zeitels LR, Hwang HW, Chivukula RR, Wentzel EA, Dews M, et al. Lin-28B transactivation is necessary for myc-mediated let-7 repression and proliferation. Proc Natl Acad Sci U S A. (2009) 106(9):3384–9. doi: 10.1073/pnas.0808300106
84. Jiang X, Huang H, Li Z, Li Y, Wang X, Gurbuxani S, et al. Blockade of miR-150 maturation by MLL-fusion/MYC/LIN-28 is required for MLL-associated leukemia. Cancer Cell (2012) 22(4):524–35. doi: 10.1016/j.ccr.2012.08.028
85. Musilova K, Mraz M. MicroRNAs in b-cell lymphomas: How a complex biology gets more complex. Leukemia (2015) 29(5):1004–17. doi: 10.1038/leu.2014.351
86. Nakagawa M, Shaffer AL, Ceribelli M, Zhang M, Wright G, Huang DW, et al. Targeting the HTLV-I-Regulated BATF3/IRF4 transcriptional network in adult T cell Leukemia/Lymphoma. Cancer Cell (2018) 34(2):286–97. doi: 10.1016/j.ccell.2018.06.014
87. Romeo MM, Ko B, Kim J, Brady R, Heatley HC, He J, et al. Acetylation of the c-MYC oncoprotein is required for cooperation with the HTLV-1 p30(II) accessory protein and the induction of oncogenic cellular transformation by p30(II)/c-MYC. Virology (2015) 476:271–88. doi: 10.1016/j.virol.2014.12.008
88. Song Z, Wu W, Chen M, Cheng W, Yu J, Fang J, et al. Long noncoding RNA ANRIL supports proliferation of adult T-cell leukemia cells through cooperation with EZH2. J Virol (2018) 92(24):e00909-18. doi: 10.1128/JVI.00909-18
89. Fernandes M, Marques H, Teixeira AL, Medeiros R. ceRNA network of lncRNA/miRNA as circulating prognostic biomarkers in non-Hodgkin lymphomas: Bioinformatic analysis and assessment of their prognostic value in an NHL cohort. Int J Mol Sci (2021) 23(1):201. doi: 10.3390/ijms23010201
90. Makarova J, Turchinovich A, Shkurnikov M, Tonevitsky A. Extracellular miRNAs and cell-cell communication: Problems and prospects. Trends Biochem Sci (2021) 46(8):640–51. doi: 10.1016/j.tibs.2021.01.007
91. de Candia P, Torri A, Gorletta T, Fedeli M, Bulgheroni E, Cheroni C, et al. Intracellular modulation, extracellular disposal and serum increase of MiR-150 mark lymphocyte activation. PloS One (2013) 8(9):e75348. doi: 10.1371/journal.pone.0075348
92. Torri A, Carpi D, Bulgheroni E, Crosti MC, Moro M, Gruarin P, et al. Extracellular MicroRNA signature of human helper T cell subsets in health and autoimmunity. J Biol Chem (2017) 292(7):2903–15. doi: 10.1074/jbc.M116.769893
93. Al Sharif S, Pinto DO, Mensah GA, Dehbandi F, Khatkar P, Kim Y, et al. Extracellular vesicles in HTLV-1 communication: The story of an invisible messenger. Viruses (2020) 12(12):1422. doi: 10.3390/v12121422
Keywords: HTLV-1, miR-150, T-cells, microRNA, adult T-cell leukemia/lymphoma, leukemia, MYB
Citation: D’Agostino DM, Raimondi V, Silic-Benussi M and Ciminale V (2022) MiR-150 in HTLV-1 infection and T-cell transformation. Front. Immunol. 13:974088. doi: 10.3389/fimmu.2022.974088
Received: 20 June 2022; Accepted: 25 July 2022;
Published: 16 August 2022.
Edited by:
Roberto S Accolla, University of Insubria, ItalyReviewed by:
Jean-Marie Peloponese, UMR9004 Institut de Recherche en Infectiologie de Montpellier (IRIM), FranceCopyright © 2022 D’Agostino, Raimondi, Silic-Benussi and Ciminale. This is an open-access article distributed under the terms of the Creative Commons Attribution License (CC BY). The use, distribution or reproduction in other forums is permitted, provided the original author(s) and the copyright owner(s) are credited and that the original publication in this journal is cited, in accordance with accepted academic practice. No use, distribution or reproduction is permitted which does not comply with these terms.
*Correspondence: Donna M. D’Agostino, ZG0uZGFnb3N0aW5vQHVuaXBkLml0; Vincenzo Ciminale, di5jaW1pbmFsZUB1bmlwZC5pdA==
Disclaimer: All claims expressed in this article are solely those of the authors and do not necessarily represent those of their affiliated organizations, or those of the publisher, the editors and the reviewers. Any product that may be evaluated in this article or claim that may be made by its manufacturer is not guaranteed or endorsed by the publisher.
Research integrity at Frontiers
Learn more about the work of our research integrity team to safeguard the quality of each article we publish.