- 1Immune Tolerance Network, Benaroya Research Institute at Virginia Mason, Seattle, WA, United States
- 2Department of Medicine, Harvard Medical School, the Division of Allergy and Clinical Immunology, Brigham and Women’s Hospital, Boston, MA, United States
- 3Immune Tolerance Network, University of California San Francisco, San Francisco, CA, United States
Immunotherapy for food-allergic patients has been effective in inducing desensitization in some populations, but long-term tolerance has remained an elusive target. A challenge facing our field is how to differentiate immune markers that are impacted by immunotherapy from those that are critical biomarkers of tolerance. Data from recent clinical trials have identified several biomarkers and mechanisms for achieving tolerance. These biomarkers include younger age, lower food-specific IgE, lower food component-specific IgE, specific linear epitope profiles, and subsets of food-specific CD4+ T cells. Additional biomarkers under investigation for their relevance in tolerance induction include TCR repertoires, gastrointestinal and skin microbiome, and local tissue immunity. This mini-review highlights recent advances in understanding biomarkers and mechanisms of tolerance induction in food immunotherapy and how these are influencing clinical trial development.
Introduction
Global estimates of the rate of food allergy are as high as 11%, with higher prevalence amongst children and in Western countries (1–3). Despite the frequency of food allergies, the most common clinical management strategy is to avoid the offending food and carry an epinephrine autoinjector. Multiple small trials of peanut oral immunotherapy (OIT) conducted from the 1990s to 2010s and assessed in a meta-analysis suggested that the threshold of reactivity to peanut could be improved through OIT (4). The landmark Peanut Allergy Oral Immunotherapy Study of AR101 for Desensitization in Children and Adults (PALISADE) trial, a phase 3 trial of OIT with AR101, a peanut-derived powder, provided the confirmatory evidence in a large clinical trial that we could modulate the immune system of a large population of peanut-allergic patients and prevent their reactions to peanut consumption (5). In 2020, the FDA approved the first OIT product for peanut allergy based on this trial (6). Importantly, the primary outcome of the PALISADE trial was desensitization, defined as the ability to consume a food without allergic reaction while still on therapy. Subsequent trials have aimed to achieve tolerance, which is defined as the ability to consume a food without reaction even after stopping therapy. Numerous terms synonymous with tolerance are used in the literature including sustained unresponsiveness and remission.
Achievement of tolerance has proven to be relatively elusive, leading to the investigation of new approaches including altering the route of immunotherapy (e.g. sublingual, epicutaneous), adding a biologic or adjuvant to immunotherapy, vaccines, and peptide therapy. Mechanistic studies have been and are being performed in association with clinical trials to elucidate the immune mechanisms necessary to achieve desensitization and subsequent tolerance. Improving our understanding of these mechanisms is essential to moving this field forward. The aim of this mini-review is to discuss known mechanisms to achieve desensitization and/or tolerance with an eye on how this can inform design of future clinical trials.
Immunologic response to immunotherapy
Innate immune response
Examination of the innate immune response to immunotherapy (IT) has revealed novel mechanisms of desensitization and tolerance. Dendritic cells play an important role in the pathophysiology of atopy through antigen presentation, pro-inflammatory cytokine production, and preferential promotion of Th2 differentiation over Treg differentiation (7). Peanut OIT, SLIT, and milk SLIT all decrease TLR-induced pro-inflammatory cytokine production of monocytic dendritic cells (8, 9). Peanut OIT has also been shown to lead to a partial improvement in impaired IFN-α secretion by plasmacytoid dendritic cells (7, 8, 10). It is likely that dendritic cells have roles in tolerance, but replication of the above findings and additional clarification of pro-tolerogenic roles is needed.
Cytokine response
Atopy, and food allergy specifically, is known to be mediated primarily by the Th2 axis of the adaptive immune system. Most early studies of food immunotherapy have shown that Th2 cytokines are decreased by OIT. Specific cytokines for which food immunotherapy causes decreases in peripheral blood levels include IL-5 (11–14), IL-4 (13), and IL-13 (12, 14). However, IL-5 levels have also been shown to increase in response to peanut OIT (15).
While mouse studies have shown that either maintenance of adequate levels or increased levels of Th1 cytokines and the regulatory cytokine IL-10 in OIT may be important for tolerance development, there are few studies looking at this in humans (16, 17). One trial of peanut SLIT found no changes in IL-10 or IFN-γ in participants who received SLIT (11). Some trials of peanut OIT found a reduction in IL-10 (13, 14), IFN-γ (14), and TNF-α (14), but one trial of peanut OIT had conflicting findings of an increase in IL-10, IFN-γ, and TNF-α (15). The role of these cytokines in tolerance requires further study and is becoming increasingly relevant in an era of targeted biologics.
Humoral immune response
It is well-established that food-specific IgE levels increase transiently early in treatment with food IT but are thereafter decreased (11, 18–21). IgE to food-specific components are also decreased in response to immunotherapy (21–24). While lower baseline food-specific IgE has been identified as a biomarker of tolerance development (discussed below), no trials have found that decreasing food or component-specific IgE below a particular threshold led to tolerance.
Food and component-specific IgG4 are increased by food IT (11, 12, 18, 20, 22, 23), but again, neither changes in IgG4 levels nor the ratio of food-specific IgG4 to food-specific IgE have been associated with tolerance development. It is also possible that IgG4 doesn’t correlate with tolerance because it is a reflection of exposure to the allergen prior to IT initiation and is thus reflective of less severe allergy at baseline. Food immunotherapy trials have largely not measured other IgG subclasses (25), though in vitro studies suggest that IgG2 & IgG3 are also relevant to blocking mast cell degranulation (26, 27). Additional studies are needed to measure IgG1, 2, 3, and 4 levels and relate them to food immunotherapy outcomes.
Immunotherapy has also been shown to alter IgE binding to sequential (linear) peanut epitopes. In a trial of milk OIT, significant changes were induced in 73% of IgE-binding and 91% of IgG4-binding epitopes and epitope diversity was significantly decreased for IgE but not IgG4-specific epitopes (28). Overall, OIT-induced changes in epitope diversity were not different between participants who achieved desensitization without tolerance compared to those who achieved desensitization and tolerance (28).
Studies of B cells in food IT are limited in part due to their low frequency in peripheral blood (29). One study using tetramer-based approach found that peanut OIT led to an early increase in affinity matured, somatically hypermutated, oligoclonal Ara h 2 specific memory B cells (30). Another study utilized sorting of Ara h 1 or 2 reactive B cells followed by deep sequencing of the B cell repertoire and discovered that immunotherapy induces somatic mutations in IgG4 (29). A potential implication of this finding is that OIT may induce IgG4 with a higher affinity to block IgE-mediated mast cell degranulation. With recent technological advances, including single cell omics and use of tetramers, we may be better able to understand the role of B cells in response to immunotherapy.
T cell response
Antigen-specific Th2 CD4+ cells most critical to food allergy pathogenesis and induction of tolerance are defined differently across studies and it appears that there is heterogeneity within this population of cells (21, 31–33). Regardless of whether these cells are defined as IL-4 and/or IL-13 producing antigen-specific CD4+ cells, as antigen-specific Th2A cells (CD4+CD45RO+CD27-CD45RBloCRTH2+CD49d+CD161+), or by their transcriptomic signature, they exhibit a sharp decrease early (by 12 weeks) in IT and a continued slower decrease thereafter (20, 21, 33). These cells also drop to lower frequencies in patients who go on to develop desensitization (32), implying that depletion of this cell population may be a crucial step in the pathway to tolerance. However, whether deletion, anergy, or exhaustion is required is still under investigation. There are two studies to date that suggest development of an anergic cluster of T cells is necessary for desensitization to food allergens (33, 34), but studies in mice and/or of aeroallergen desensitization in humans provide mixed data about whether deletion, anergy, or exhaustion is required (31, 35–38). One study of peanut OIT also suggests that transient changes in antigen-specific Th2 CD4+ cells may also be relevant for tolerance (39).
Conceptually, it would make sense that Treg cells are important in tolerance development. However, data from immunotherapy (IT) studies on Treg frequency differ with some studies finding that IT leads to increases in Tregs (12, 40), others finding the opposite (21), and still others finding no impact of IT on Tregs (11, 21). This may be in part due to differences in how Tregs are defined or which subtypes are evaluated in each study.
A recently identified subset of T follicular helper cells (Tfh), Tfh13 cells, has been shown to have a key role in the pathogenesis of food allergy through production of high-affinity IgE (41). Unfortunately, evidence is again conflicting as to whether these cell populations are altered by IT and whether modulation of these cells is needed for tolerance (33, 39).
Basophil response
The basophil activation test (BAT) is a laboratory assay whereby a participant’s basophils are stimulated with the relevant allergen and measures of basophil activation (reactivity) are recorded (42). BAT is useful in the diagnosis of food allergy and is often measured in clinical trials of food IT (9, 14, 20, 22, 40, 42–46). It has been well-established that oral immunotherapy (OIT), sublingual immunotherapy (SLIT), and epicutaneous immunotherapy (EPIT) all result in decreases in BAT (14, 20, 43, 45). Lower basophil reactivity can be seen as early as the build-up phase of immunotherapy and either continues to decrease or remains stable during maintenance (22, 43, 45). Several studies, including studies of peanut OIT and milk OIT/SLIT have found that early decreases in BAT, during or at the end of immunotherapy build-up, predict later development of tolerance (43, 44). In the Peanut Oral Immunotherapy Study: Safety, Efficacy and Discovery (POISED) trial, lower basophil activation throughout the trial correlated with development of tolerance (45). Notably, decreases in basophil reactivity have been identified to be transient, often rebounding after immunotherapy is discontinued (9, 22, 45). Despite the rebound, participants who achieved clinical tolerance in POISED and another trial, the Peanut Oral Immunotherapy in Children (IMPACT) trial, had less increase in basophil reactivity after discontinuation of IT than did participants who did not achieve tolerance (22, 45). Together, these data suggest that decreasing basophil reactivity is necessary to achieve clinical desensitization and tolerance. It is possible that we achieve low rates of tolerance in food immunotherapy clinical trials in part because we have not yet achieved a sufficient degree of basophil blocking and/or suppression or the mechanism by which we accomplish basophil blocking and/or suppression is not permanent.
Baseline biomarkers that predict desensitization and tolerance
In addition to understanding mechanisms by which tolerance can be achieved, there is utility in recognizing biomarkers that are predictive of tolerance prior to initiation of immunotherapy. Baseline biomarkers identified in clinical trials include food-specific antibodies, basophil activation tests, and T cell subsets. Clarification of such biomarkers would provide clinicians with tools to select and appropriately counsel patients and their families regarding likelihood of achieving a good clinical response to immunotherapy. Clinical trials targeting biomarkers of poor response with specific adjuvants to immunotherapy may be a worthwhile approach to improve tolerance outcomes.
Age as a predictor of desensitization or tolerance
Age has garnered much discussion in relation to food immunotherapy outcomes. The Learning Early About Peanut Allergy (LEAP) study, a primary prevention study of peanut allergy in high-risk infants, provided the first evidence that peanut allergy can be largely prevented through early peanut introduction (47). Multiple peanut OIT studies have shown that tolerance can best be induced in patients with known peanut allergy at younger ages (22, 48), however, trials in older children did not find age impacted outcomes (24, 49). This suggests an early immune malleability that disappears with age.
Specific IgE as a predictor of desensitization or tolerance
The most well-studied mechanistic biomarker of tolerance has been food-specific IgE, and multiple trials of milk, egg, and peanut OIT have shown that lower baseline IgE correlates with better outcomes (18, 22, 24, 48, 50, 51) with rare exception (21). Other immune globulins or immune globulin components likely play a role as well. For example, in a trial of peanut OIT, a lower total IgE and a higher peanut-specific IgG4:IgE ratio independently associated with higher rates of tolerance (24). Lower IgE to food components including ovalbumin, casein, and Ara h 1, 2, 3 and 6 have also been identified as potential biomarkers of desensitization and tolerance with varying significance (18, 21–24, 50, 51).
IgE binding to sequential (linear) epitopes has been shown to have a high diagnostic accuracy for diagnosis of peanut, milk, and egg allergy (52–54). Further, the pattern of IgE-binding epitopes identified at baseline for a cohort of participants undergoing milk OIT differentiated those who achieved desensitization only, desensitization and tolerance, or failed therapy (55).
Basophil activation as a predictor of desensitization or tolerance
While BAT has demonstrated utility for predicting outcomes during OIT, data regarding whether BAT prior to initiation of immunotherapy is a useful biomarker of subsequent tolerance are mixed, possibly due to variation in specimen type, processing, or assay protocol (9, 21, 40, 42, 43, 45, 46). Standardization is needed before we can determine the clinical utility of baseline BAT in predicting tolerance outcomes.
T cell subsets as predictors of desensitization or tolerance
Interrogation of Th2 cell subsets has led to correlation of specific subsets with atopy (32) and ability to achieve tolerance in OIT trials (21). In CoFAR7, a lower number of peanut-specific T cells expressing IL-4 or IL-13 separated those who were able to achieve desensitization or tolerance from treatment failures. Other baseline assessments including peanut-specific T cells expressing IL-10 or IFN-γ did not correlate with clinical outcomes (21). Alternatively, Monian et al. found that expression of Th2 gene signature was not associated with tolerance, but that lower expression of gene module defined by T cell activation and effector response (OX40, OX40L, Th17 function, STAT1, and GPR15), lower Th1-conv, and lower Th17 cells were associated with tolerance (33). Limited data have been published on Tregs as predictors of OIT outcome. A study of egg OIT, baseline Treg frequency did not correlate with outcomes (21). Additional studies are needed to determine whether it is the quantity of or function of T cell subsets at baseline that relate to achieving tolerance.
Newer biomarkers/mechanisms of tolerance under investigation
In addition to continuing investigation of the mechanisms of immunotherapy and biomarkers of tolerance discussed above, knowledge and technological advances are allowing us to expand research into other potential mechanisms of tolerance. These include TCR repertoires, the microbiome, and the role of the epithelial barrier.
The TCR repertoire can now be reliably measured after antigen stimulation to isolate food antigen-specific T cells (56, 57). In one study of 27 peanut-allergic individuals, there were TCRβ sequences shared across individuals suggesting that these sequences could be important in the pathogenesis of peanut allergy and specific epitope recognition (57). A follow-up study examined the TCR repertoire in response to peanut OIT and found that the repertoire was not dramatically impacted by OIT (33).
The microbiome from the skin and gut are skewed in individuals with food allergy. Specific gut microbes have been correlated with the development of food allergy and recent studies have suggested that these microbes relate to alterations in the metabolome and subsequent immune deviation (58–65). This is also an area that could be a potential therapeutic target, as peanut OIT may expand the diversity of the gut microbiome (66).
The gut epithelial barrier is also known to play a role in the pathogenesis of food allergy, largely through antigen uptake and cytokine production (TSLP, IL-33, and IL-15). The gut epithelium is also home to a many types of immune cells that are postulated to be relevant to food allergy development and tolerance induction. To date, only one clinical trial has examined gut epithelial biopsies during food OIT and they identified that tissue eosinophilia often develops during OIT but is usually transient (67).
How biomarkers influence future clinical trial and therapeutic drug development
In the current era of targeted drugs and biologics, one tactic for tolerance induction is to add a targeted therapeutic medication alongside OIT. Table 1 summarizes biomarkers of tolerance and biologics that have preliminary evidence suggesting they may be useful for targeting of a specific biomarker. Ongoing and future clinical trials will be needed to elucidate how well the biologics, when used in combination with IT, actually influence a particular biomarker and tolerance overall.
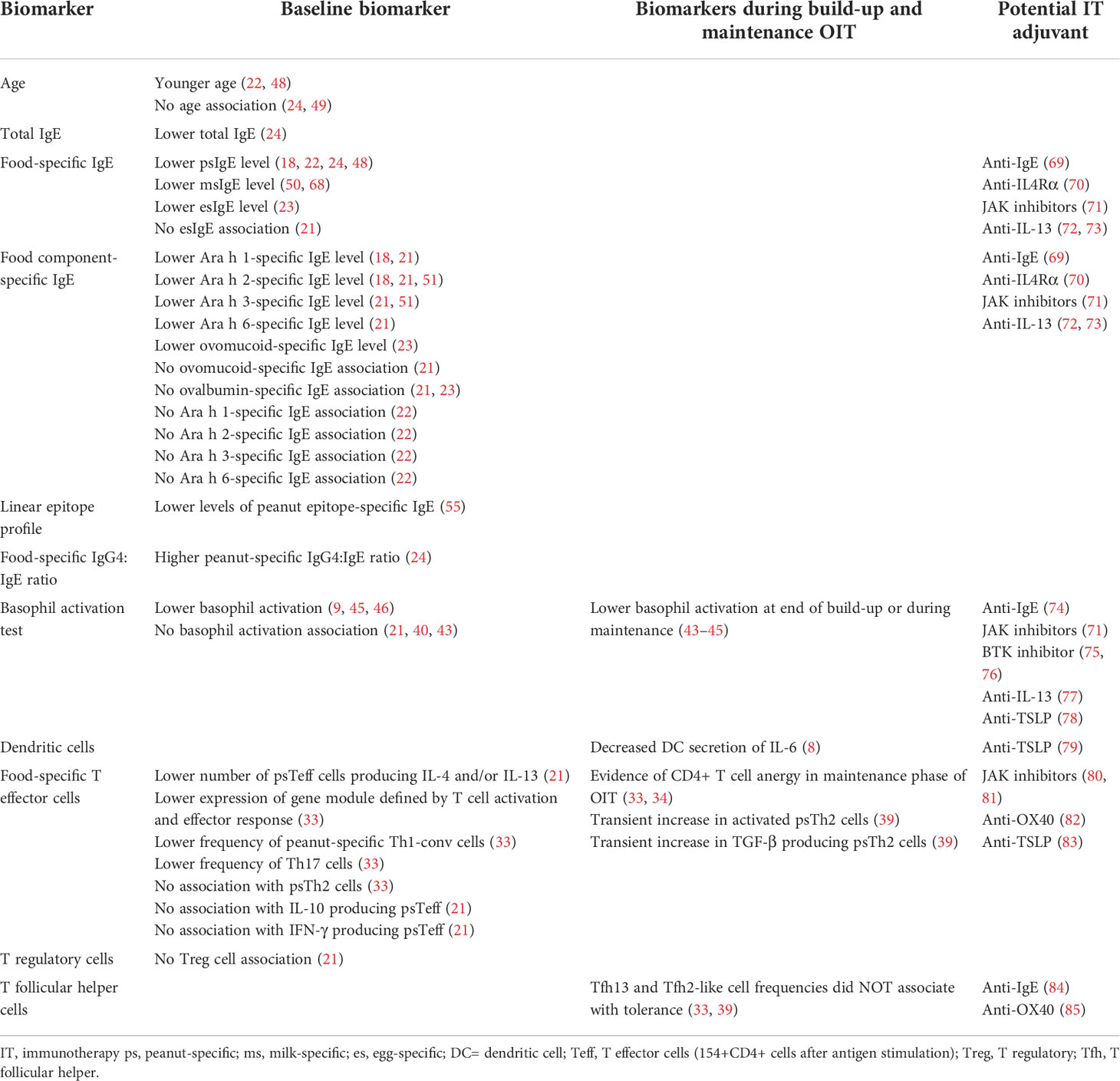
Table 1 Biomarkers of tolerance in food allergy IT studies and potential corresponding IT adjuvants.
The most well-studied example of this is OIT plus omalizumab. Studies have shown that adding omalizumab, thereby blocking IgE from binding to mast cells, has been helpful for either reducing adverse reactions during OIT up-dosing or for allowing expedited up-dosing (50, 86, 87). It is not clear that this approach leads to increased rates of tolerance, but additional trials are still underway (NCT03881696, NCT04045301). A broader approach of immune modulation or an approach targeted to another aspect of the allergic immune response may be required to improve rates of tolerance.
Additional OIT plus trials include the Adjuvant Treatment with abatacept to Promote Remission During Peanut Oral Immunotherapy (ATARI) trial (NCT04872218) and a phase 2a trial of dupilumab plus Palforzia (NCT03682770). Abatacept is a CTLA-4-immunoglobulin fusion protein that binds to CD80 and CD86 on antigen presenting cells thus preventing T cells from receiving the activating signal they need to respond to antigen presentation (88). Without this signal, the balance of T effector, regulatory, and helper cells could be restored and in studies of autoimmunity, abatacept has been shown to decrease T follicular helper cells and T regulatory cells (88). Dupilumab is an IL-4 receptor alpha antagonist and blocks signaling of both IL-4 and IL-13. In atopic individuals, dupilumab leads to a reduction in a number of biomarkers of type 2 inflammation, including serum and/or plasma levels of thymus and activation-regulated chemokine (TARC), eotaxin-3, periostin, and total IgE, which could provide an immune environment that is more conducive to the induction of tolerance (70).
Ongoing trials of biologics as monotherapy for food allergy are also paving the way for subsequent OIT plus studies and include a pilot of abrocitinib, a selective JAK inhibitor, for adults with peanut allergy (NCT05069831). A number of cytokines relevant to food allergy signal through JAK1 including TSLP, IL-4, IL-13, and IL-9 (80). JAK inhibitors have already been shown to modulate these cytokines in atopic dermatitis trials (81) and by blocking signaling of these cytokines abrocitinib has the potential to decrease Th2 induction, induce Tregs, inhibit IgE class-switching, and inhibit mast cell expansion.
Potential future candidates for OIT plus trials include BTK inhibitors, Anti-OX40 antibody, anti-IL-13 antibodies, anti-TSLP antibody, and other JAK inhibitors.
Conclusions
Performing mechanistic studies in conjunction with food immunotherapy clinical trials has taught us about mechanisms of tolerance and has helped to identify biomarkers of tolerance. This information is guiding the development of current and future clinical trials. It is imperative that we continue to perform mechanistic assays to improve our understanding of tolerance if we want to move beyond inducing only desensitization and tolerance to only one bite of a food with immunotherapy.
Author contributions
Draft manuscript preparation: CB, MF, and TL. All authors contributed to the article and approved the submitted version.
Funding
The work of the authors was supported by the National Institute of Allergy and Infectious Diseases of the National Institutes of Health under Award Number UM1AI109565.
Acknowledgments
The authors would like to acknowledge the investigators and their teams who performed the trials and the mechanistic assays. We would additionally like to acknowledge the patients and families who participated in the clinical trials discussed here. We thank the Immune Tolerance Network (ITN) for the unique opportunity to study mechanisms of tolerance across different clinical trials in atopy.
Conflict of interest
The authors declare that the research was conducted in the absence of any commercial or financial relationships that could be construed as a potential conflict of interest.
Publisher’s note
All claims expressed in this article are solely those of the authors and do not necessarily represent those of their affiliated organizations, or those of the publisher, the editors and the reviewers. Any product that may be evaluated in this article, or claim that may be made by its manufacturer, is not guaranteed or endorsed by the publisher.
Author disclaimer
The content is solely the responsibility of the authors and does not necessarily represent the official views of the National Institutes of Health.
References
1. Gupta RS, Warren CM, Smith BM, Blumenstock JA, Jiang J, Davis MM, et al. The public health impact of parent-reported childhood food allergies in the united states. Pediatrics (2018) 142(6):e20181235. doi: 10.1542/peds.2018-1235
2. Gupta RS, Warren CM, Smith BM, Jiang J, Blumenstock JA, Davis MM, et al. Prevalence and severity of food allergies among US adults. JAMA Netw Open (2019) 2(1):e185630. doi: 10.1001/jamanetworkopen.2018.5630
3. Loh W, Tang MLK. The epidemiology of food allergy in the global context. Int J Environ Res Public Health (2018) 15(9):2043. doi: 10.3390/ijerph15092043
4. Nurmatov U, Dhami S, Arasi S, Pajno GB, Fernandez-Rivas M, Muraro A, et al. Allergen immunotherapy for IgE-mediated food allergy: A systematic review and meta-analysis. Allergy (2017) 72(8):1133–47. doi: 10.1111/all.13124
5. Investigators PGoC, Vickery BP, Vereda A, Casale TB, Beyer K, du Toit G, et al. AR101 oral immunotherapy for peanut allergy. N Engl J Med (2018) 379(21):1991–2001. doi: 10.1056/NEJMoa1812856
6. Caccomo S. FDA Approves first drug for treatment of peanut allergy for children (2020). Available at: https://www.fda.gov/news-events/press-announcements/fda-approves-first-drug-treatment-peanut-allergy-children.
7. Gorelik M, Frischmeyer-Guerrerio PA. Innate and adaptive dendritic cell responses to immunotherapy. Curr Opin Allergy Clin Immunol (2015) 15(6):575–80. doi: 10.1097/ACI.0000000000000213
8. Frischmeyer-Guerrerio PA, Keet CA, Guerrerio AL, Chichester KL, Bieneman AP, Hamilton RG, et al. Modulation of dendritic cell innate and adaptive immune functions by oral and sublingual immunotherapy. Clin Immunol (2014) 155(1):47–59. doi: 10.1016/j.clim.2014.08.006
9. Gorelik M, Narisety SD, Guerrerio AL, Chichester KL, Keet CA, Bieneman AP, et al. Suppression of the immunologic response to peanut during immunotherapy is often transient. J Allergy Clin Immunol (2015) 135(5):1283–92. doi: 10.1016/j.jaci.2014.11.010
10. Tversky JR, Bieneman AP, Chichester KL, Hamilton RG, Schroeder JT. Subcutaneous allergen immunotherapy restores human dendritic cell innate immune function. Clin Exp Allergy (2010) 40(1):94–102. doi: 10.1111/j.1365-2222.2009.03388.x
11. Kim EH, Bird JA, Kulis M, Laubach S, Pons L, Shreffler W, et al. Sublingual immunotherapy for peanut allergy: Clinical and immunologic evidence of desensitization. J Allergy Clin Immunol (2011) 127(3):640–6.e1. doi: 10.1016/j.jaci.2010.12.1083
12. Varshney P, Jones SM, Scurlock AM, Perry TT, Kemper A, Steele P, et al. A randomized controlled study of peanut oral immunotherapy: Clinical desensitization and modulation of the allergic response. J Allergy Clin Immunol (2011) 127(3):654–60. doi: 10.1016/j.jaci.2010.12.1111
13. Blumchen K, Trendelenburg V, Ahrens F, Gruebl A, Hamelmann E, Hansen G, et al. Efficacy, safety, and quality of life in a multicenter, randomized, placebo-controlled trial of low-dose peanut oral immunotherapy in children with peanut allergy. J Allergy Clin Immunol Pract (2019) 7(2):479–91.e10. doi: 10.1016/j.jaip.2018.10.048
14. Kulis M, Yue X, Guo R, Zhang H, Orgel K, Ye P, et al. High- and low-dose oral immunotherapy similarly suppress pro-allergic cytokines and basophil activation in young children. Clin Exp Allergy (2019) 49(2):180–9. doi: 10.1111/cea.13256
15. Jones SM, Pons L, Roberts JL, Scurlock AM, Perry TT, Kulis M, et al. Clinical efficacy and immune regulation with peanut oral immunotherapy. J Allergy Clin Immunol (2009) 124(2):292–300.e1-97. doi: 10.1016/j.jaci.2009.05.022
16. Tordesillas L, Mondoulet L, Blazquez AB, Benhamou PH, Sampson HA, Berin MC. Epicutaneous immunotherapy induces gastrointestinal LAP(+) regulatory T cells and prevents food-induced anaphylaxis. J Allergy Clin Immunol (2017) 139(1):189–201.e4. doi: 10.1016/j.jaci.2016.03.057
17. Dioszeghy V, Mondoulet L, Puteaux E, Dhelft V, Ligouis M, Plaquet C, et al. Differences in phenotype, homing properties and suppressive activities of regulatory T cells induced by epicutaneous, oral or sublingual immunotherapy in mice sensitized to peanut. Cell Mol Immunol (2017) 14(9):770–82. doi: 10.1038/cmi.2016.14
18. Vickery BP, Scurlock AM, Kulis M, Steele PH, Kamilaris J, Berglund JP, et al. Sustained unresponsiveness to peanut in subjects who have completed peanut oral immunotherapy. J Allergy Clin Immunol (2014) 133(2):468–75. doi: 10.1016/j.jaci.2013.11.007
19. Jones SM, Burks AW, Keet C, Vickery BP, Scurlock AM, Wood RA, et al. Long-term treatment with egg oral immunotherapy enhances sustained unresponsiveness that persists after cessation of therapy. J Allergy Clin Immunol (2016) 137(4):1117–27.e10. doi: 10.1016/j.jaci.2015.12.1316
20. Jones SM, Sicherer SH, Burks AW, Leung DY, Lindblad RW, Dawson P, et al. Epicutaneous immunotherapy for the treatment of peanut allergy in children and young adults. J Allergy Clin Immunol (2017) 139(4):1242–52.e9. doi: 10.1016/j.jaci.2016.08.017
21. Berin MC, Agashe C, Burks AW, Chiang D, Davidson WF, Dawson P, et al. Allergen-specific T cells and clinical features of food allergy: Lessons from CoFAR immunotherapy cohorts. J Allergy Clin Immunol (2022) 149(4):1373–82.e12. doi: 10.1016/j.jaci.2021.09.029
22. Jones SM, Kim EH, Nadeau KC, Nowak-Wegrzyn A, Wood RA, Sampson HA, et al. Efficacy and safety of oral immunotherapy in children aged 1-3 years with peanut allergy (the immune tolerance network IMPACT trial): A randomised placebo-controlled study. Lancet (2022) 399(10322):359–71. doi: 10.1016/S0140-6736(21)02390-4
23. Wright BL, Kulis M, Orgel KA, Burks AW, Dawson P, Henning AK, et al. Component-resolved analysis of IgA, IgE, and IgG4 during egg OIT identifies markers associated with sustained unresponsiveness. Allergy (2016) 71(11):1552–60. doi: 10.1111/all.12895
24. Chinthrajah RS, Purington N, Andorf S, Long A, O'Laughlin KL, Lyu SC, et al. Sustained outcomes in oral immunotherapy for peanut allergy (POISED study): A large, randomised, double-blind, placebo-controlled, phase 2 study. Lancet (2019) 394(10207):1437–49. doi: 10.1016/S0140-6736(19)31793-3
25. MacGlashan D Jr., Alvarez-Arango S, Tversky J. Subclasses of allergen-specific IgG: Serum IgG2 and IgG3 levels are not predicted by IgG1/IgG4 levels. Clin Exp Allergy (2021) 51(8):1093–5. doi: 10.1111/cea.13977
26. Dodev TS, Bowen H, Shamji MH, Bax HJ, Beavil AJ, McDonnell JM, et al. Inhibition of allergen-dependent IgE activity by antibodies of the same specificity but different class. Allergy (2015) 70(6):720–4. doi: 10.1111/all.12607
27. MacGlashan D Jr., Hamilton RG. Parameters determining the efficacy of CD32 to inhibit activation of FcepsilonRI in human basophils. J Allergy Clin Immunol (2016) 137(4):1256–8.e11. doi: 10.1016/j.jaci.2015.10.043
28. Savilahti EM, Kuitunen M, Valori M, Rantanen V, Bardina L, Gimenez G, et al. Use of IgE and IgG4 epitope binding to predict the outcome of oral immunotherapy in cow's milk allergy. Pediatr Allergy Immunol (2014) 25(3):227–35. doi: 10.1111/pai.12186
29. Hoh RA, Joshi SA, Liu Y, Wang C, Roskin KM, Lee JY, et al. Single b-cell deconvolution of peanut-specific antibody responses in allergic patients. J Allergy Clin Immunol (2016) 137(1):157–67. doi: 10.1016/j.jaci.2015.05.029
30. Patil SU, Ogunniyi AO, Calatroni A, Tadigotla VR, Ruiter B, Ma A, et al. Peanut oral immunotherapy transiently expands circulating ara h 2-specific b cells with a homologous repertoire in unrelated subjects. J Allergy Clin Immunol (2015) 136(1):125–34.e12. doi: 10.1016/j.jaci.2015.03.026
31. Wambre E, DeLong JH, James EA, LaFond RE, Robinson D, Kwok WW. Differentiation stage determines pathologic and protective allergen-specific CD4+ T-cell outcomes during specific immunotherapy. J Allergy Clin Immunol (2012) 129(2):544–51, 51.e1-7. doi: 10.1016/j.jaci.2011.08.034
32. Wambre E, Bajzik V, DeLong JH, O'Brien K, Nguyen QA, Speake C, et al. A phenotypically and functionally distinct human TH2 cell subpopulation is associated with allergic disorders. Sci Transl Med (2017) 9(401):eaam9171. doi: 10.1126/scitranslmed.aam9171
33. Monian B, Tu AA, Ruiter B, Morgan DM, Petrossian PM, Smith NP, et al. Peanut oral immunotherapy differentially suppresses clonally distinct subsets of T helper cells. J Clin Invest (2022) 132(2):e150634. doi: 10.1172/JCI150634
34. Ryan JF, Hovde R, Glanville J, Lyu SC, Ji X, Gupta S, et al. Successful immunotherapy induces previously unidentified allergen-specific CD4+ T-cell subsets. Proc Natl Acad Sci U S A (2016) 113(9):E1286–95. doi: 10.1073/pnas.1520180113
35. Okano M, Otsuki N, Azuma M, Fujiwara T, Kariya S, Sugata Y, et al. Allergen-specific immunotherapy alters the expression of b and T lymphocyte attenuator, a co-inhibitory molecule, in allergic rhinitis. Clin Exp Allergy (2008) 38(12):1891–900. doi: 10.1111/j.1365-2222.2008.03116.x
36. Guerra F, Carracedo J, Solana-Lara R, Sanchez-Guijo P, Ramirez R. TH2 lymphocytes from atopic patients treated with immunotherapy undergo rapid apoptosis after culture with specific allergens. J Allergy Clin Immunol (2001) 107(4):647–53. doi: 10.1067/mai.2001.112263
37. Ciepiela O, Zawadzka-Krajewska A, Kotula I, van Overveld F, Kulus M, Demkow U. Sublingual immunotherapy for asthma: Affects T-cells but does not impact basophil activation. Pediatr Allergy Immunol Pulmonol (2014) 27(1):17–23. doi: 10.1089/ped.2014.0328
38. Burton BR, Britton GJ, Fang H, Verhagen J, Smithers B, Sabatos-Peyton CA, et al. Sequential transcriptional changes dictate safe and effective antigen-specific immunotherapy. Nat Commun (2014) 5:4741. doi: 10.1038/ncomms5741
39. Wang W, Lyu SC, Ji X, Gupta S, Manohar M, Dhondalay GKR, et al. Transcriptional changes in peanut-specific CD4+ T cells over the course of oral immunotherapy. Clin Immunol (2020) 219:108568. doi: 10.1016/j.clim.2020.108568
40. Syed A, Garcia MA, Lyu SC, Bucayu R, Kohli A, Ishida S, et al. Peanut oral immunotherapy results in increased antigen-induced regulatory T-cell function and hypomethylation of forkhead box protein 3 (FOXP3). J Allergy Clin Immunol (2014) 133(2):500–10. doi: 10.1016/j.jaci.2013.12.1037
41. Gowthaman U, Chen JS, Zhang B, Flynn WF, Lu Y, Song W, et al. Identification of a T follicular helper cell subset that drives anaphylactic IgE. Science (2019) 365(6456):eaaw6433. doi: 10.1126/science.aaw6433
42. Paranjape A, Tsai M, Mukai K, Hoh RA, Joshi SA, Chinthrajah RS, et al. Oral immunotherapy and basophil and mast cell reactivity in food allergy. Front Immunol (2020) 11:602660. doi: 10.3389/fimmu.2020.602660
43. Keet CA, Frischmeyer-Guerrerio PA, Thyagarajan A, Schroeder JT, Hamilton RG, Boden S, et al. The safety and efficacy of sublingual and oral immunotherapy for milk allergy. J Allergy Clin Immunol (2012) 129(2):448–55, 55.e1-5. doi: 10.1016/j.jaci.2011.10.023
44. Patil SU, Steinbrecher J, Calatroni A, Smith N, Ma A, Ruiter B, et al. Early decrease in basophil sensitivity to ara h 2 precedes sustained unresponsiveness after peanut oral immunotherapy. J Allergy Clin Immunol (2019) 144(5):1310–9.e4. doi: 10.1016/j.jaci.2019.07.028
45. Tsai M, Mukai K, Chinthrajah RS, Nadeau KC, Galli SJ. Sustained successful peanut oral immunotherapy associated with low basophil activation and peanut-specific IgE. J Allergy Clin Immunol (2020) 145(3):885–96.e6. doi: 10.1016/j.jaci.2019.10.038
46. Goldberg MR, Nachshon L, Appel MY, Elizur A, Levy MB, Eisenberg E, et al. Efficacy of baked milk oral immunotherapy in baked milk-reactive allergic patients. J Allergy Clin Immunol (2015) 136(6):1601–6. doi: 10.1016/j.jaci.2015.05.040
47. Du Toit G, Roberts G, Sayre PH, Bahnson HT, Radulovic S, Santos AF, et al. Randomized trial of peanut consumption in infants at risk for peanut allergy. N Engl J Med (2015) 372(9):803–13. doi: 10.1056/NEJMoa1414850
48. Vickery BP, Berglund JP, Burk CM, Fine JP, Kim EH, Kim JI, et al. Early oral immunotherapy in peanut-allergic preschool children is safe and highly effective. J Allergy Clin Immunol (2017) 139(1):173–81.e8. doi: 10.1016/j.jaci.2016.05.027
49. Burks AW, Jones SM, Wood RA, Fleischer DM, Sicherer SH, Lindblad RW, et al. Oral immunotherapy for treatment of egg allergy in children. N Engl J Med (2012) 367(3):233–43. doi: 10.1056/NEJMoa1200435
50. Wood RA, Kim JS, Lindblad R, Nadeau K, Henning AK, Dawson P, et al. A randomized, double-blind, placebo-controlled study of omalizumab combined with oral immunotherapy for the treatment of cow's milk allergy. J Allergy Clin Immunol (2016) 137(4):1103–10.e11. doi: 10.1016/j.jaci.2015.10.005
51. Burk CM, Kulis M, Leung N, Kim EH, Burks AW, Vickery BP. Utility of component analyses in subjects undergoing sublingual immunotherapy for peanut allergy. Clin Exp Allergy (2016) 46(2):347–53. doi: 10.1111/cea.12635
52. Suarez-Farinas M, Suprun M, Kearney P, Getts R, Grishina G, Hayward C, et al. Accurate and reproducible diagnosis of peanut allergy using epitope mapping. Allergy (2021) 76(12):3789–97. doi: 10.1111/all.14905
53. Jarvinen KM, Chatchatee P, Bardina L, Beyer K, Sampson HA. IgE and IgG binding epitopes on alpha-lactalbumin and beta-lactoglobulin in cow's milk allergy. Int Arch Allergy Immunol (2001) 126(2):111–8. doi: 10.1159/000049501
54. Suprun M, Sicherer SH, Wood RA, Jones SM, Leung DYM, Burks AW, et al. Mapping sequential IgE-binding epitopes on major and minor egg allergens. Int Arch Allergy Immunol (2022) 183(3):249–61. doi: 10.1159/000519618
55. Suarez-Farinas M, Suprun M, Chang HL, Gimenez G, Grishina G, Getts R, et al. Predicting development of sustained unresponsiveness to milk oral immunotherapy using epitope-specific antibody binding profiles. J Allergy Clin Immunol (2019) 143(3):1038–46. doi: 10.1016/j.jaci.2018.10.028
56. Tu AA, Gierahn TM, Monian B, Morgan DM, Mehta NK, Ruiter B, et al. TCR sequencing paired with massively parallel 3' RNA-seq reveals clonotypic T cell signatures. Nat Immunol (2019) 20(12):1692–9. doi: 10.1038/s41590-019-0544-5
57. Smith NP, Ruiter B, Virkud YV, Tu AA, Monian B, Moon JJ, et al. Identification of antigen-specific TCR sequences based on biological and statistical enrichment in unselected individuals. JCI Insight (2021) 6(13):e140028. doi: 10.1172/jci.insight.140028
58. Henrick BM, Rodriguez L, Lakshmikanth T, Pou C, Henckel E, Arzoomand A, et al. Bifidobacteria-mediated immune system imprinting early in life. Cell (2021) 184(15):3884–98.e11. doi: 10.1016/j.cell.2021.05.030
59. Tan J, McKenzie C, Vuillermin PJ, Goverse G, Vinuesa CG, Mebius RE, et al. Dietary fiber and bacterial SCFA enhance oral tolerance and protect against food allergy through diverse cellular pathways. Cell Rep (2016) 15(12):2809–24. doi: 10.1016/j.celrep.2016.05.047
60. Savage JH, Lee-Sarwar KA, Sordillo J, Bunyavanich S, Zhou Y, O'Connor G, et al. A prospective microbiome-wide association study of food sensitization and food allergy in early childhood. Allergy (2018) 73(1):145–52. doi: 10.1111/all.13232
61. Lee KH, Guo J, Song Y, Ariff A, O'Sullivan M, Hales B, et al. Dysfunctional gut microbiome networks in childhood IgE-mediated food allergy. Int J Mol Sci (2021) 22(4):2079. doi: 10.3390/ijms22042079
62. Bao R, Hesser LA, He Z, Zhou X, Nadeau KC, Nagler CR. Fecal microbiome and metabolome differ in healthy and food-allergic twins. J Clin Invest (2021) 131(2):e141935. doi: 10.1172/JCI141935
63. Bunyavanich S, Shen N, Grishin A, Wood R, Burks W, Dawson P, et al. Early-life gut microbiome composition and milk allergy resolution. J Allergy Clin Immunol (2016) 138(4):1122–30. doi: 10.1016/j.jaci.2016.03.041
64. Lee-Sarwar K, Kelly RS, Lasky-Su J, Moody DB, Mola AR, Cheng TY, et al. Intestinal microbial-derived sphingolipids are inversely associated with childhood food allergy. J Allergy Clin Immunol (2018) 142(1):335–8.e9. doi: 10.1016/j.jaci.2018.04.016
65. Jang H, Kim EG, Kim M, Kim SY, Kim YH, Sohn MH, et al. Metabolomic profiling revealed altered lipid metabolite levels in childhood food allergy. J Allergy Clin Immunol (2022) 149(5):1722–31.e9. doi: 10.1016/j.jaci.2021.10.034
66. He Z, Vadali VG, Szabady RL, Zhang W, Norman JM, Roberts B, et al. Increased diversity of gut microbiota during active oral immunotherapy in peanut-allergic adults. Allergy (2021) 76(3):927–30. doi: 10.1111/all.14540
67. Wright BL, Fernandez-Becker NQ, Kambham N, Purington N, Cao S, Tupa D, et al. Gastrointestinal eosinophil responses in a longitudinal, randomized trial of peanut oral immunotherapy. Clin Gastroenterol Hepatol (2021) 19(6):1151–9.e14. doi: 10.1016/j.cgh.2020.05.019
68. Keet CA, Seopaul S, Knorr S, Narisety S, Skripak J, Wood RA. Long-term follow-up of oral immunotherapy for cow's milk allergy. J Allergy Clin Immunol (2013) 132(3):737–9.e6. doi: 10.1016/j.jaci.2013.05.006
69. Kaplan AP, Gimenez-Arnau AM, Saini SS. Mechanisms of action that contribute to efficacy of omalizumab in chronic spontaneous urticaria. Allergy (2017) 72(4):519–33. doi: 10.1111/all.13083
70. Hamilton JD, Harel S, Swanson BN, Brian W, Chen Z, Rice MS, et al. Dupilumab suppresses type 2 inflammatory biomarkers across multiple atopic, allergic diseases. Clin Exp Allergy (2021) 51(7):915–31. doi: 10.1111/cea.13954
71. Yamaki K, Yoshino S. Remission of food allergy by the janus kinase inhibitor ruxolitinib in mice. Int Immunopharmacol (2014) 18(2):217–24. doi: 10.1016/j.intimp.2013.11.029
72. Punnonen J, Yssel H, de Vries JE. The relative contribution of IL-4 and IL-13 to human IgE synthesis induced by activated CD4+ or CD8+ T cells. J Allergy Clin Immunol (1997) 100(6 Pt 1):792–801. doi: 10.1016/S0091-6749(97)70276-8
73. Stokes J, Casale TB. Rationale for new treatments aimed at IgE immunomodulation. Ann Allergy Asthma Immunol (2004) 93(3):212–7. quiz 7-9, 71. doi: 10.1016/S1081-1206(10)61490-1
74. Poddighe D, Vangelista L. Effects of omalizumab on basophils: Potential biomarkers in asthma and chronic spontaneous urticaria. Cell Immunol (2020) 358:104215. doi: 10.1016/j.cellimm.2020.104215
75. Dispenza MC, Krier-Burris RA, Chhiba KD, Undem BJ, Robida PA, Bochner BS. Bruton's tyrosine kinase inhibition effectively protects against human IgE-mediated anaphylaxis. J Clin Invest (2020) 130(9):4759–70. doi: 10.1172/JCI138448
76. Xing Y, Chu KA, Wadhwa J, Chen W, Zhu J, Bradshaw JM, et al. Preclinical mechanisms of topical PRN473, a bruton tyrosine kinase inhibitor, in immune-mediated skin disease models. Immunohorizons (2021) 5(7):581–9. doi: 10.4049/immunohorizons.2100063
77. Kasaian MT, Tan XY, Jin M, Fitz L, Marquette K, Wood N, et al. Interleukin-13 neutralization by two distinct receptor blocking mechanisms reduces immunoglobulin e responses and lung inflammation in cynomolgus monkeys. J Pharmacol Exp Ther (2008) 325(3):882–92. doi: 10.1124/jpet.108.136515
78. Noti M, Kim BS, Siracusa MC, Rak GD, Kubo M, Moghaddam AE, et al. Exposure to food allergens through inflamed skin promotes intestinal food allergy through the thymic stromal lymphopoietin-basophil axis. J Allergy Clin Immunol (2014) 133(5):1390–9, 9.e1-6. doi: 10.1016/j.jaci.2014.01.021
79. Liu YJ. TSLP in epithelial cell and dendritic cell cross talk. Adv Immunol (2009) 101:1–25. doi: 10.1016/S0065-2776(08)01001-8
80. Damsky W, Peterson D, Ramseier J, Al-Bawardy B, Chun H, Proctor D, et al. The emerging role of janus kinase inhibitors in the treatment of autoimmune and inflammatory diseases. J Allergy Clin Immunol (2021) 147(3):814–26. doi: 10.1016/j.jaci.2020.10.022
81. Pavel AB, Song T, Kim HJ, Del Duca E, Krueger JG, Dubin C, et al. Oral janus kinase/SYK inhibition (ASN002) suppresses inflammation and improves epidermal barrier markers in patients with atopic dermatitis. J Allergy Clin Immunol (2019) 144(4):1011–24. doi: 10.1016/j.jaci.2019.07.013
82. Fukushima A, Yamaguchi T, Ishida W, Fukata K, Yagita H, Ueno H. Roles of OX40 in the development of murine experimental allergic conjunctivitis: exacerbation and attenuation by stimulation and blocking of OX40. Invest Ophthalmol Vis Sci (2006) 47(2):657–63. doi: 10.1167/iovs.05-1064
83. Liu YJ, Soumelis V, Watanabe N, Ito T, Wang YH, Malefyt Rde W, et al. TSLP: an epithelial cell cytokine that regulates T cell differentiation by conditioning dendritic cell maturation. Annu Rev Immunol (2007) 25:193–219. doi: 10.1146/annurev.immunol.25.022106.141718
84. Bergantini L, d'Alessandro M, Cameli P, Pianigiani T, Fanetti M, Sestini P, et al. Follicular T helper and breg cell balance in severe allergic asthma before and after omalizumab therapy. Mol Diagn Ther (2021) 25(5):593–605. doi: 10.1007/s40291-021-00545-3
85. Fu Y, Lin Q, Zhang Z, Zhang L. Therapeutic strategies for the costimulatory molecule OX40 in T-cell-mediated immunity. Acta Pharm Sin B (2020) 10(3):414–33. doi: 10.1016/j.apsb.2019.08.010
86. Brandstrom J, Vetander M, Sundqvist AC, Lilja G, Johansson SGO, Melen E, et al. Individually dosed omalizumab facilitates peanut oral immunotherapy in peanut allergic adolescents. Clin Exp Allergy (2019) 49(10):1328–41. doi: 10.1111/cea.13469
87. MacGinnitie AJ, Rachid R, Gragg H, Little SV, Lakin P, Cianferoni A, et al. Omalizumab facilitates rapid oral desensitization for peanut allergy. J Allergy Clin Immunol (2017) 139(3):873–81.e8. doi: 10.1016/j.jaci.2016.08.010
Keywords: peanut allergy, food allergy, tolerance, allergen immunotherapy (AIT), oral immunotherapy (OIT)
Citation: Baloh CH, Huffaker MF and Laidlaw T (2022) Biomarkers and mechanisms of tolerance induction in food allergic patients drive new therapeutic approaches. Front. Immunol. 13:972103. doi: 10.3389/fimmu.2022.972103
Received: 17 June 2022; Accepted: 12 September 2022;
Published: 03 October 2022.
Edited by:
Amy Rosenberg, EpiVax, United StatesReviewed by:
Scott P. Commins, University of North Carolina at Chapel Hill, United StatesAlessandro Fiocchi, Bambino Gesù Children’s Hospital, (IRCCS), Italy
Copyright © 2022 Baloh, Huffaker and Laidlaw. This is an open-access article distributed under the terms of the Creative Commons Attribution License (CC BY). The use, distribution or reproduction in other forums is permitted, provided the original author(s) and the copyright owner(s) are credited and that the original publication in this journal is cited, in accordance with accepted academic practice. No use, distribution or reproduction is permitted which does not comply with these terms.
*Correspondence: Carolyn H. Baloh, cbaloh@immunetolerance.org