- 1Department of Oncology, The First Affiliated Hospital of Anhui Medical University, Hefei, China
- 2Inflammation and Immune Mediated Diseases Laboratory of Anhui Province, Hefei, China
Alcoholic liver disease (ALD) is a leading chronic liver disease in which immune cells play a vital role. Myeloid cells have been extensively studied in ALD, including granulocytes, macrophages, monocytes, and dendritic cells, which are involved in the occurrence and progression of steatosis, inflammation, fibrosis, and eventual cirrhosis. These cells can be popularly targeted and regulated by factors from different sources, including cytokines secreted by other cells, extracellular vesicles, and substances in serum—for example, infiltration of monocytes or neutrophils, activation of Kupffer cells, and polarization of macrophages. These processes can affect and change the function and phenotype of myeloid cells. Here we mainly review the key mediators that affect the infiltration and function of mainly myeloid cells in ALD as well as their regulatory mechanisms on target cells, which may provide novel immunotherapeutic approaches. The single-cell multimodal omics of myeloid cells is also discussed to help transform them into basic research or therapeutic strategy of ALD clinically.
Introduction
Liver diseases are a serious global health burden, causing two million demises worldwide annually (1). There is a far-flung range of liver diseases, including diversiform chronic or acute events such as simplex steatosis, alcoholic liver disease (ALD), drug hepatitis and steatohepatitis, and so on. Chronic liver diseases diagnosed clinically mainly include non-alcoholic fatty liver disease (NAFLD) and ALD, with mild clinical symptoms (2). Nevertheless, dramatically increased alcohol intake in a short-term is associated with an acute inflammatory manifestation of alcoholic hepatitis (AH) in ALD. The persistence of chronic or acute liver lesions frequently leads to fibrosis, cirrhosis, and even hepatocellular carcinoma (HCC). Besides this, the mechanisms of these pathological processes involve the drive of in situ liver innate immune cells and the pro-inflammatory cascade activated in circulation (3). Under the influence of the nature or stage of the disease, the liver immune environment of patients is more intricate and untoward to decipher.
Myeloid cells are derived from myeloid progenitor cells which commonly exist in bone marrow (4). This lineage includes monocytes, granulocytes, erythrocytes, and platelets and serves as a master component of the innate immune system and the first block of defense against infection. According to our previous work, this review is principally focused on macrophages and neutrophils. Macrophages pertain to a multitudinous and heterogeneous population rooted in the transient but malleable monocyte precursors (5). In addition, there is the special resident Kupffer cells (KCs) in the liver, which is differentiated by the adhesion of blood monocytes to the hepatic sinusoid wall. Divertingly, neutrophilic percolation is also a sign of AH in patients with chronic alcoholism or in mice (6). The lethal effect of liver neutrophils may be achieved by the generation of reactive oxygen species (ROS) and vast inflammatory substances (7). Extensive mouse and human research have shown that neutrophils and macrophages exert a pivotal part in the foundation, development, and reversion of liver diseases, including in guiding tissue remodeling. With the progress of technology, more and more myeloid cell subtypes have been identified and located by single-cell multimodal omics (8–12). Each myeloid type acts as a unique individual participating in the immune response. Identifying and quantifying the existence of each myeloid subtype is momentous to comprehend the patterns in which different cell clusters are activated by certain pathogens and to promote the regression of immune stress.
Alcoholic liver diseases
ALD are a concerning part of global chronic liver diseases and can be a consequence of the chronic abuse of alcohol (13). Normally, the broad spectrum of ALD includes simple steatosis, AH, fibrosis, cirrhosis, and HCC (14). Ample evidence is provided showing that the progress in ALD is chiefly related with the scale and sustainment of alcohol consumption. The inchoate pathophysiological response to chronic alcohol consumption is reflected in fat amassment in hepatocytes, which appears to be reversible (15). Nevertheless, when hepatocytes are damaged, the release of damage-associated molecular patterns tends to attract the infiltration of the surrounding immune cells toward it. Myeloid cells (especially macrophages and neutrophils) follow and promote liver inflammation as well as the incidence of alcoholic liver fibrosis and cirrhosis (16). Cirrhosis ratio was enriched in throng with added alcohol consumption and is higher in people with AH. About 3–12% of patients with AH progress to cirrhosis yearly (1). Data from 2009 to 2016 in the United States show that people in the 25–34 age group experienced the highest annual increase in liver cirrhosis-related mortality, driven entirely by ALD (17). Roughly 75% of ALD patients were currently diagnosed after decompensated cirrhosis, which disqualified them from the first-rank pharmaceutical treatment for alcohol use disorders (18). Emerging reports expounded multifarious omics and biomarkers for ALD diagnosis gradually (19, 20). Niu et al. established a machine learning model based on proteomics, constructed a diagnostic model superior to the existing clinical analysis, and discovered novel circulating protein markers on the basis of confirming the previous diagnostic markers, which provided potential protein targets with diagnostic, prognostic, and therapeutic value for ALD (21). Currently, the treatment of ALD still mainly relies on abstinence and nutritional support but also affected by the lack of advanced and effective therapeutic breakthroughs (22). Divertingly, based on the inherent ability of KCs to absorb most nanomaterials efficiently and non-specifically and the importance of KCs in the process of ALD, it may be possible to target myeloid cells, especially KCs, in the treatment of ALD by nano-drugs. Similarly, the therapeutic effect of liver macrophage-targeted nanoparticles in the NASH model has also been effectively confirmed recently (23).
Myeloid cells
Myeloid cells have been diffusely discussed in liver diseases and are involved in the occurrence and development of ALD to varying degrees. The myeloid population is capable of recognizing, ingesting, and degrading cellular debris, foreign bodies, or pathogens as the first preventer to antagonize infection (24). Their studies in the liver tend to focus on macrophages, monocytes, granulocytes, and dendritic cells (DCs). Based on our forepassed research, this paper focuses on the mononuclear system, inherent KCs, and neutrophils, besides their mechanisms and frontier orientations in ALD and cirrhosis.
Neutrophils
Neutrophils (the most enriched granulocytes) are first-line responders to inflammation and infection and can attack and eliminate invasive microbes by phagocytosis, which play a vital role in immune and inflammatory reaction (25). During liver infection and damage, neutrophils patrolling the hepatic sinusoids are rapidly recruited to the site of injury through diversified means, including phagocytosis, ROS generation, degranulation, cytokine and chemokine production, and neutrophil extracellular trap promotion to clear the pathogens and maintain tissue homeostasis (26).
There is growing evidence that the quantity of hepatic neutrophils is related to the ponderance of ALD (27, 28). CXCL1 is a key factor leading to neutrophil infiltration during alcoholic liver injury. It is eminently raised in the liver of patients with AH; besides this, its corresponding receptor CXCR2 is also highly expressed in neutrophils, which, in turn, induces neutrophil infiltration (29). Lipocalin (LCN2)—also called neutrophil gelatinase-associated lipocalin—is a secretory glycoprotein primarily localized to neutrophils, which participates in innate immunity. The expression of LCN2 is increased in humans and mice with alcoholic liver disease. More importantly, LCN2-/- mice possessed lessened neutrophil permeation, liver injury, and hepatic steatosis in contrast to wild-type controls. Furthermore, antibody-mediated LCN2 blockade was also protective to confront with alcohol-induced liver injury, suggesting that LCN2 may be an underlying therapeutic target in ALD (30, 31).
Sirtuin1 (SIRT1), an NAD+-dependent histone deacetylase, is concerned with the regulation of senium. Neutrophilic SIRT1 expression is reduced in patients with acute alcohol consumption, and the deficiency of SIRT1 gene in myeloid cells accelerates liver injury and inflammation caused by ethanol and downregulates the neutrophil miR-223 level, leading to increased secretion of IL1β, TNFα, CXCL1, and ROS (32). Notably, miR-223 exerts a protective role in diversiform liver inflammatory diseases as one of the amplest miRNAs in neutrophils. Especially in ALD, miR-223 can reduce ROS production in neutrophils by directly inhibiting IL-6 expression and inhibiting the expression of phagocytosis oxidase (phox) p47phox (33). Interestingly, a recent study found that neutrophils can also interact with bile duct cells in patients with AH. Specifically, cell adhesion molecules on neutrophils bind to ITGB1 in cholangiocytes, triggering RAC1-induced JNK activation and resulting in c-JUN-mediated reduction of ITPR3 in cholangiocytes, thereby exacerbating bile in patients with alcoholic hepatitis siltation (34).
Axel Périanin et al. report that the reduced neutrophil peroxidase liberates, and bactericidal activity observed in patients with decompensated alcoholic cirrhosis is mainly correlated with impaired activation of AKT, p38-MAP-kinase and ERK1/2 signaling, NOX2 degradation, and lack of mTOR-dependent translation mechanisms on neutrophils (35). Although some studies have demonstrated the negative role of neutrophils in AH, many challenges remain in targeting neutrophils as therapeutic targets for ALD considering the complex functions and regulatory mechanisms of neutrophils.
Macrophages
Hepatic macrophages are universally composed of tissue-resident macrophages and infiltrating macrophages (36, 37). Macrophages are usually present in the liver sinusoids, and when a liver infection occurs, macrophages infiltrate the liver in large numbers. Contraposing to capture hepatic macrophages, liver CD45+ cells were selected by fluorescence-activated cell sorting, in which F4/80hiCD11bint cells were derived from resident KCs and macrophages, whereas F4/80intCD11bhi cells were identified as infiltrating monocytes (38). Infiltrating monocytes give rise to two macrophage subtypes, including Ly6Clo-derived monocytes with anti-inflammatory and protective phenotypes and Ly6Chi-derived monocytes exhibiting pro-inflammatory and tissue-damaging phenotypes. The increase of Ly6Clo/Ly6Chi cell ratio was considered to antagonize alcohol-induced liver injury in Gao-binge-fed mice (39). Macrophages are also plastic cells that can change their phenotype into pro-inflammatory cells (M1-like macrophages) or anti-inflammatory cells (M2-like macrophages) in response to a different signaling phage (40). Confrontationally, the IL10 released by M2 KCs promoted the death of M1 KCs and protected the liver injury of mice exposed to alcohol (41). Otherwise, the phagocytosis of macrophages, especially KCs, is a vital part of liver ecology in situ. A proximate study has found that the repair of phagocytosis of macrophages also contributes to resist alcoholic liver injury (42). Until now, numerous studies have increasingly shown the vital function of macrophages in hepatic steatosis, injury, inflammation, fibrosis, and cirrhosis.
Gyongyi Szabo et al. reported enhancive macrophages and an increased expression of chemokine receptors (CCR2 and CCR5) in the liver of patients with ALD, with corresponding increases in circulating chemokine CCL2 and CCL5 levels. Importantly, disposing with the dual CCR2/5 inhibitor cenicriviroc significantly reduced the increase in infiltrating macrophages and production of pro-inflammatory macrophages of the liver in alcohol-fed mice (43). In addition, studies have shown that the heat shock protein 96 (GP96) is extremely expressed in human and mouse ALD liver. Compared with WT mice, alcohol-fed GP96KO mice had significantly reduced extents of steatosis, serum endotoxins, and pro-inflammatory cytokines. In vitro, the pharmacological inhibition of GP96 or knockdown of small interfering RNA attenuated the inflammatory response of primary macrophages, suggesting that the targeted inhibition of GP96 may be a promising macrophage-based therapy for ALD (44).
Extracellular vesicles (EVs) may also mediate macrophage infiltration and activation during ALD—for example, it has been shown that ALD mice have increased total circulating EVs and that ALD-EVs can cause changes in hepatocyte function and a pro-inflammatory macrophage phenotype in naive recipient mice after injection by binding to Hsp90 (45). Furthermore, a study showed that alcohol treatment resulted in the production of mtdsRNA-rich exosomes in human and mouse hepatocytes, which were transported to adjacent KCs, resulting in TLR3 activation and IL-1β production by KCs. The subsequent activation of KCs further promoted the recruitment of γδ T cells and the level of IL-17A in the inchoate stages of ALD (46).
Our recent study found that TLR2 and TLR3 deficiency ameliorated and exacerbated alcoholic liver injury, respectively, and importantly, we found that gallocatechin gallate can directly interact with TLR2/3 in KCs to induce the production of IL-10 to regulate the progression of ALD through NF-κB signal, suggesting an innovative strategy for the treatment of ALD (47).
Moreover, according to single-cell sequencing and RNA-seq data from Adam Kim and Argemi et al., we recovered the changes of Mp1-Mp5 (LYZ+MARCO-) and non-inflammatory macrophages (MARCO+) in normal subjects, early alcohol-associated hepatitis, sAH with emergency liver transplants, and severe alcohol-associated hepatitis (8) (Figure 1A). Distinct macrophage subtypes from the liver in situ and in circulation have been described to perform different cell proportions with the evolution of ALD, indicating the research-based significance for disparate myeloid subtypes.
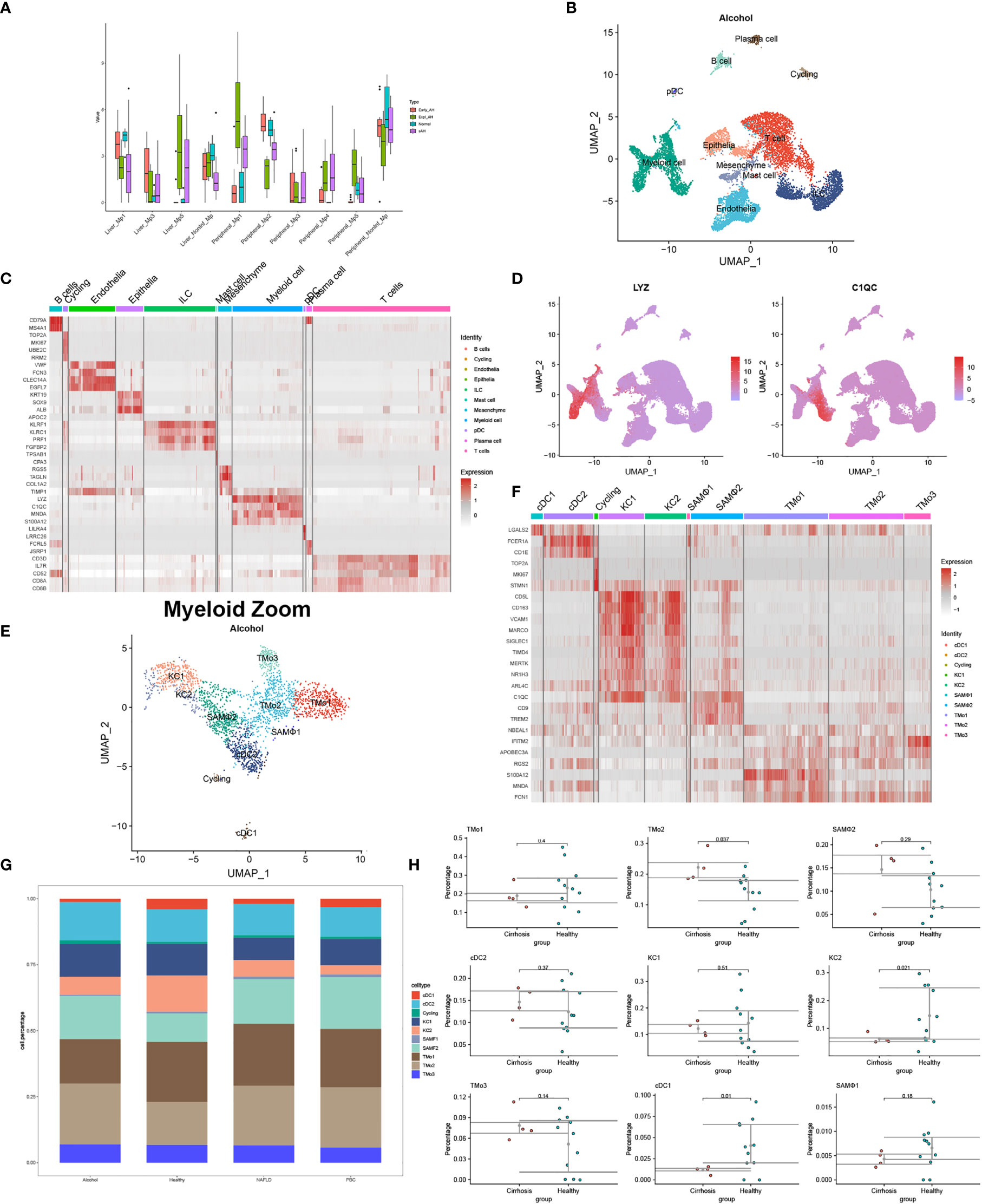
Figure 1 Identification of myeloid landscape in human alcoholic liver disease (ALD) and liver cirrhosis. (A) Changes of macrophages in the progression of ALD come from the mapping of single-cell sequencing data to RNA-seq. (B) Clustering 12382 cells from two cirrhotic human livers caused by alcohol and cell lineage as inferred from expression of marker gene signatures. ILC, innate lymphoid cell; pDC, plasmacytoid dendritic cell. (C) Heat map: cell types and marker genes. (D) Expression of LYZ and C1QC mRNA. (E) Myeloid cells were isolated from (B) and re-clustered with Seurat_4.1.0. (F) Heat map: myeloid cell types and marker genes. (G) The proportion of myeloid cells among normal patients and liver cirrhosis caused by alcohol, non-alcoholic fatty liver disease, and primary biliary cirrhosis. (H) Percentages of myeloid subpopulations in five healthy versus two cirrhotic livers, with a significant difference in the proportion of TMo2, scar-associated macrophages, KC2, and cDC1 between the two groups (t-test).
Dendritic cells
DCs are capable of ingesting and processing antigens and expressing MHC molecules, which subsequently migrate to lymphoid organs to mediate the activation of naive T cells and secrete cytokines to initiate adaptive immunity and are powerful antigen-presenting cells (48). Classical DCs comprise two subtypes: type 1 cDCs (interacting with CD8+ T cells primarily via MHC-I) and type 2 cDCs (cDC2s) (presenting MHC-II-bound antigens to CD4+ T cells) (48). The function of DCs in hepatic steatosis, inflammation, and fibrosis remains controversial. In a thioacetamide- and leptin-induced liver fibrosis model, DCs activated hepatic stellate cells, NKT cells, and T cells by producing TNF-α, thus promoting the progression of liver fibrosis (36). However, although DCs promoted the mild activation of hepatic stellate cells, the depletion of DCs did not impact how liver fibrosis evolved in the carbon tetrachloride-induced liver fibrosis model (37).
The impact of alcohol intake on dendritic cell function has been of interest for nearly 20 years, but not much attention has been highlighted on the effect of DCs in the liver. In 2006, AH Lau et al. first reported that alcohol treatment impaired DC cell differentiation and function within the liver in in vitro experiments. However, in vivo, liver DCs were significantly less affected than spleen DCs after alcohol diet feeding in C57BL/6 mice (49). Earlier, Thomson et al. indicated that chronic ethanol intake impacts the in vivo migration of hepatic DCs to secondary lymphoid tissues (50). Comparatively interesting is a recent study by Alharshawi et al. They expounded that 12 weeks of alcohol consumption increased the hepatic plasmacytoid dendritic cells in female mice and that this increase was sex and organ specific. Furthermore, the mRNA expression level of CCR2 in the liver pDCs of female mice was significantly increased, and CCR2 controlled the pDC egress from the bone marrow at steady state and upon alcohol exposure, but not liver pDC recruitment (51). These studies suggest that DCs act as a momentous part in the progression of ALD and may be able to explain, to some extent, the population heterogeneity in the effect of alcohol intake on the liver.
Vista
Although the subject of this review highlights myeloid cells, the liver serves as a complex multi-functional organ and constitutes a variety of cells from endoderm and mesoderm, including parenchyma cells and non-parenchyma cells. In disparate physiological and pathological conditions, including natural development, metabolism, aging, acute and chronic inflammation, scar formation, and other courses, myeloid cells showed different functions, accompanied by changes in cell interaction. The single co-culture experiment in liver research still has application defects in spatial and temporal backgrounds. With the progress of technology, organoids research is gradually emerging, which contains a variety of cell types to better simulate organ surroundings. Wang et al. established a novel model for the study of alcoholic liver disease through expandable hepatic organoids derived from human ESC, and Guan et al. used a human multi-lineage hepatic organoid model for the study of liver fibrosis (52, 53). Nevertheless, these studies were largely based on parenchymal cells, which would assuredly lead to a mature direction of organoid for myeloid cells in the liver.
Different from whole-tissue RNA sequencing analysis, scRNA-seq, spatial transcriptomics, and emerging single-cell multimodal omics endowed the study of transcriptional activity at the single-cell or spatial level, further broadening our understanding of tissue–cell interaction in situ—for example, under physiological conditions, scRNA-seq analyzed the livers of mice at 1, 3, 7, 21, and 56 days after birth and identified myeloid cell subsets at different time points, including a unique source of Dcn+ Mac from day 7 (11). At these periods, there was a stable Wnt signal in the interaction between KCs and hepatocytes, but a unique NOV-NOTCH1 signal interaction was produced in D7. We used GSE136103 (2 × NAFLD, 2 × ALD, and 1 × PBC) to reconstruct the single-cell landscape of two alcoholic liver cirrhosis samples and annotated the liver CD45- and CD45+ cells as well as the types of myeloid cells according to the method provided by P Ramachandran et al. (9) (Figures 1B–H). Prominent differences in myeloid cells such as tissue monocytes (TMo), scar-associated macrophages, KCs, and DCs were observed between cirrhotic patients and normal controls. Interestingly, alcoholic cirrhosis showed a different proportion of myeloid subsets from other disease states, showing more cDC2 and TMo3 and less TMo1 (Figure 1G). It presumed that myeloid subtypes may come into a different play, manifesting the value of exploration on myeloid subtypes in alcoholic liver cirrhosis or other liver diseases extended. Another scRNA-seq study found that the proportion and absolute number of Mp1–Mp5 and non-inflammatory macrophages in the peripheral blood of patients with sAH increased to varying degrees, although the article did not probe into the heterogeneity between these myeloid subtypes (8). Differently from the single cell study of alcoholic liver diseases, in livers of mice and human with NASH, researchers combined single-cell CITEseq, single-nuclei sequencing, spatial transcriptomics, and spatial proteomics to identify and locate lipid-associated macrophages derived from bile ducts and to determine the key axis in the development of KCs (10). Until now, there is no study pertaining to single-cell multimodal omics in ALD and cirrhosis ever yet, especially the role of rare myeloid-derived subtypes. This may be due to the limitations of technology and computing, the complexity of data integration, the high cost of application, and so on. The application of single-cell multi-group technology in more groups of layers requires methods that can integrate three or more types of data to effectively characterize the regulatory relationship between different groups of layers.
The study of myeloid subtypes possesses instructive significance in deciphering the involute immune environment and cellular interaction in the liver (Figure 2).
Author contributions
HW and WX contributed to the work design, article construction and key revisions, and graphics design. WX, MW, and BC drafted the manuscript and reviewed the related articles. WX, MW, and BC contributed to analysis and final approval. All authors contributed to the article and approved the submitted version.
Conflict of interest
The authors declare that the research was conducted in the absence of any commercial or financial relationships that could be construed as a potential conflict of interest.
Publisher’s note
All claims expressed in this article are solely those of the authors and do not necessarily represent those of their affiliated organizations, or those of the publisher, the editors and the reviewers. Any product that may be evaluated in this article, or claim that may be made by its manufacturer, is not guaranteed or endorsed by the publisher.
References
1. Asrani SK, Devarbhavi H, Eaton J, Kamath PS. Burden of liver diseases in the world. J Hepatol (2019) 70(1):151–71. doi: 10.1016/j.jhep.2018.09.014
2. de Carvalho Ribeiro M, Szabo G. Role of the inflammasome in liver disease. Annu Rev Pathol (2022) 17:345–65. doi: 10.1146/annurev-pathmechdis-032521-102529
3. Del Campo JA, Gallego P, Grande L. Role of inflammatory response in liver diseases: Therapeutic strategies. World J Hepatol (2018) 10(1):1–7. doi: 10.4254/wjh.v10.i1.1
4. Singh S, Zhang XHF, Rosen JM. TIME is a great healer-targeting myeloid cells in the tumor immune microenvironment to improve triple-negative breast cancer outcomes. Cells (2020) 10(1):11. doi: 10.3390/cells10010011
5. Krenkel O, Hundertmark J, Abdallah AT, Kohlhepp M, Puengel T, Roth T, et al. Myeloid cells in liver and bone marrow acquire a functionally distinct inflammatory phenotype during obesity-related steatohepatitis. Gut (2020) 69(3):551–63. doi: 10.1136/gutjnl-2019-318382
6. Bertola A, Park O, Gao B. Chronic plus binge ethanol feeding synergistically induces neutrophil infiltration and liver injury in mice: a critical role for e-selectin. Hepatology (2013) 58(5):1814–23. doi: 10.1002/hep.26419
7. Gao B, Ahmad MF, Nagy LE, Tsukamoto H. Inflammatory pathways in alcoholic steatohepatitis. J Hepatol (2019) 70(2):249–59. doi: 10.1016/j.jhep.2018.10.023
8. Kim A, Wu X, Allende DS, Nagy LE. Gene deconvolution reveals aberrant liver regeneration and immune cell infiltration in alcohol-associated hepatitis. Hepatology (2021) 74(2):987–1002. doi: 10.1002/hep.31759
9. Ramachandran P, Dobie R, Wilson-Kanamori JR, Dora EF, Henderson BEP, Luu NT, et al. Resolving the fibrotic niche of human liver cirrhosis at single-cell level. Nature (2019) 575(7783):512–8. doi: 10.1038/s41586-019-1631-3
10. Guilliams M, Bonnardel J, Haest B, Vanderborght B, Wagner C, Remmerie A, et al. Spatial proteogenomics reveals distinct and evolutionarily conserved hepatic macrophage niches. Cell (2022) 185(2):379–96 e38. doi: 10.1016/j.cell.2021.12.018
11. Liang Y, Kaneko K, Xin B, Lee J, Sun X, Zhang K, et al. Temporal analyses of postnatal liver development and maturation by single-cell transcriptomics. Dev Cell (2022) 57(3):398–414 e5. doi: 10.1016/j.devcel.2022.01.004
12. Ben-Moshe S, Veg T, Manco R, Dan S, Papinutti D, Lifshitz A, et al. The spatiotemporal program of zonal liver regeneration following acute injury. Cell Stem Cell (2022) 29(6):973–89 e10. doi: 10.1016/j.stem.2022.04.008
13. Seitz HK, Bataller R, Cortez-Pinto H, Gao B, Gual A, Lackner C, et al. Alcoholic liver disease. Nat Rev Dis Primers (2018) 4(1):16. doi: 10.1038/s41572-018-0014-7
14. Gao B, Bataller R. Alcoholic liver disease: pathogenesis and new therapeutic targets. Gastroenterology (2011) 141(5):1572–85. doi: 10.1053/j.gastro.2011.09.002
15. You M, Arteel GE. Effect of ethanol on lipid metabolism. J Hepatol (2019) 70(2):237–48. doi: 10.1016/j.jhep.2018.10.037
16. Roerecke M, Vafaei A, Hasan OSM, Chrystoja BR, Cruz M, Lee R, et al. Alcohol consumption and risk of liver cirrhosis: A systematic review and meta-analysis. Am J Gastroenterol (2019) 114(10):1574–86. doi: 10.14309/ajg.0000000000000340
17. Lucey MR. Alcohol-associated cirrhosis. Clin Liver Dis (2019) 23(1):115–26. doi: 10.1016/j.cld.2018.09.013
18. Nilsson E, Anderson H, Sargenti K, Lindgren S, Prytz H. Clinical course and mortality by etiology of liver cirrhosis in Sweden: a population based, long-term follow-up study of 1317 patients. Aliment Pharmacol Ther (2019) 49(11):1421–30. doi: 10.1111/apt.15255
19. Hardesty J, Day L, Warner J, Warner D, Gritsenko M, Asghar A, et al. Hepatic protein and phosphoprotein signatures of alcohol-associated cirrhosis and hepatitis. Am J Pathol (2022) 192(7):1066–82. doi: 10.1016/j.ajpath.2022.04.004
20. Wu T, Cooper SA, Shah VH. Omics and AI advance biomarker discovery for liver disease. Nat Med (2022) 28(6):1131–2. doi: 10.1038/s41591-022-01853-9
21. Niu L, Thiele M, Geyer PE, Rasmussen DN, Webel HE, Santos A, et al. Noninvasive proteomic biomarkers for alcohol-related liver disease. Nat Med (2022) 28(6):1277–87. doi: 10.1038/s41591-022-01850-y
22. Szabo G, Thursz M, Shah VH. Therapeutic advances in alcohol-associated hepatitis. J Hepatol (2022) 76(6):1279–90. doi: 10.1016/j.jhep.2022.03.025
23. Zhou JE, Sun L, Liu L, Jia Y, Han Y, Shao J, et al. Hepatic macrophage targeted siRNA lipid nanoparticles treat non-alcoholic steatohepatitis. J Control Release (2022) 343:175–86. doi: 10.1016/j.jconrel.2022.01.038
24. Weston CJ, Zimmermann HW, Adams DH. The role of myeloid-derived cells in the progression of liver disease. Front Immunol (2019) 10:893. doi: 10.3389/fimmu.2019.00893
25. Liew PX, Kubes P. The neutrophil's role during health and disease. Physiol Rev (2019) 99(2):1223–48. doi: 10.1152/physrev.00012.2018
26. Papayannopoulos V. Neutrophil extracellular traps in immunity and disease. Nat Rev Immunol (2018) 18(2):134–47. doi: 10.1038/nri.2017.105
27. Jiang Y, Xi Y, Li Y, Zuo Z, Zeng C, Fan J, et al. Ethanol promoting the upregulation of c-X-C motif chemokine ligand 1CXCL1 and c-X-C motif chemokine ligand 6CXCL6 in models of early alcoholic liver disease. Bioengineered (2022) 13(3):4688–701. doi: 10.1080/21655979.2022.2030557
28. Kusumanchi P, Liang T, Zhang T, Ross RA, Han S, Chandler K, et al. Stress-responsive gene FK506-binding protein 51 mediates alcohol-induced liver injury through the hippo pathway and chemokine (C-X-C motif) ligand 1 signaling. Hepatology (2021) 74(3):1234–50. doi: 10.1002/hep.31800
29. Roh YS, Zhang B, Loomba R, Seki E. TLR2 and TLR9 contribute to alcohol-mediated liver injury through induction of CXCL1 and neutrophil infiltration. Am J Physiol Gastrointest Liver Physiol (2015) 309(1):G30–41. doi: 10.1152/ajpgi.00031.2015
30. Dubuquoy L. Lipocalin 2 highlights the complex role of neutrophils in alcoholic liver disease. J Hepatol (2016) 64(4):770–2. doi: 10.1016/j.jhep.2016.01.020
31. Wieser V, Tymoszuk P, Adolph TE, Grander C, Grabherr F, Enrich B, et al. Lipocalin 2 drives neutrophilic inflammation in alcoholic liver disease. J Hepatol (2016) 64(4):872–80. doi: 10.1016/j.jhep.2015.11.037
32. Ren R, He Y, Ding D, Cui A, Bao H, Ma J, et al. Aging exaggerates acute-on-chronic alcohol-induced liver injury in mice and humans by inhibiting neutrophilic sirtuin 1-C/EBPalpha-miRNA-223 axis. Hepatology (2022) 75(3):646–60. doi: 10.1002/hep.32152
33. Li M, He Y, Zhou Z, Ramirez T, Gao Y, Gao Y, et al. MicroRNA-223 ameliorates alcoholic liver injury by inhibiting the IL-6-p47(phox)-oxidative stress pathway in neutrophils. Gut (2017) 66(4):705–15. doi: 10.1136/gutjnl-2016-311861
34. Takeuchi M, Vidigal PT, Guerra MT, Hundt MA, Robert ME, Olave-Martinez M, et al. Neutrophils interact with cholangiocytes to cause cholestatic changes in alcoholic hepatitis. Gut (2021) 70(2):342–56. doi: 10.1136/gutjnl-2020-322540
35. Boussif A, Rolas L, Weiss E, Bouriche H, Moreau R, Perianin A. Impaired intracellular signaling, myeloperoxidase release and bactericidal activity of neutrophils from patients with alcoholic cirrhosis. J Hepatol (2016) 64(5):1041–8. doi: 10.1016/j.jhep.2015.12.005
36. Daemen S, Gainullina A, Kalugotla G, He L, Chan MM, Beals JW, et al. Dynamic shifts in the composition of resident and recruited macrophages influence tissue remodeling in NASH. Cell Rep (2021) 34(2):108626. doi: 10.1016/j.celrep.2020.108626
37. Wang M, You Q, Lor K, Chen F, Gao B, Ju C. Chronic alcohol ingestion modulates hepatic macrophage populations and functions in mice. J Leukoc Biol (2014) 96(4):657–65. doi: 10.1189/jlb.6A0114-004RR
38. Daemen S, Chan MM, Schilling JD. Comprehensive analysis of liver macrophage composition by flow cytometry and immunofluorescence in murine NASH. STAR Protoc (2021) 2(2):100511. doi: 10.1016/j.xpro.2021.100511
39. Sanz-Garcia C, Poulsen KL, Bellos D, Wang H, McMullen MR, Li X, et al. The non-transcriptional activity of IRF3 modulates hepatic immune cell populations in acute-on-chronic ethanol administration in mice. J Hepatol (2019) 70(5):974–84. doi: 10.1016/j.jhep.2019.01.021
40. Mantovani A, Sozzani S, Locati M, Allavena P, Sica A. Macrophage polarization: tumor-associated macrophages as a paradigm for polarized M2 mononuclear phagocytes. Trends Immunol (2002) 23(11):549–55. doi: 10.1016/s1471-4906(02)02302-5
41. Wan J, Benkdane M, Teixeira-Clerc F, Bonnafous S, Louvet A, Lafdil F, et al. M2 kupffer cells promote M1 kupffer cell apoptosis: a protective mechanism against alcoholic and nonalcoholic fatty liver disease. Hepatology (2014) 59(1):130–42. doi: 10.1002/hep.26607
42. Wu X, Fan X, McMullen MR, Miyata T, Kim A, Pathak V, et al. Macrophage-derived MLKL in alcohol-associated liver disease: regulation of phagocytosis. Hepatology (2022) 1–17. doi: 10.1002/hep.32612
43. Ambade A, Lowe P, Kodys K, Catalano D, Gyongyosi B, Cho Y, et al. Pharmacological inhibition of CCR2/5 signaling prevents and reverses alcohol-induced liver damage, steatosis, and inflammation in mice. Hepatology (2019) 69(3):1105–21. doi: 10.1002/hep.30249
44. Ratna A, Lim A, Li Z, Argemi J, Bataller R, Chiosis G, et al. Myeloid endoplasmic reticulum resident chaperone GP96 facilitates inflammation and steatosis in alcohol-associated liver disease. Hepatol Commun (2021) 5(7):1165–82. doi: 10.1002/hep4.1713
45. Saha B, Momen-Heravi F, Furi I, Kodys K, Catalano D, Gangopadhyay A, et al. Extracellular vesicles from mice with alcoholic liver disease carry a distinct protein cargo and induce macrophage activation through heat shock protein 90. Hepatology (2018) 67(5):1986–2000. doi: 10.1002/hep.29732
46. Lee JH, Shim YR, Seo W, Kim MH, Choi WM, Kim HH, et al. Mitochondrial double-stranded RNA in exosome promotes interleukin-17 production through toll-like receptor 3 in alcohol-associated liver injury. Hepatology (2020) 72(2):609–25. doi: 10.1002/hep.31041
47. Luo P, Wang F, Wong NK, Lv Y, Li X, Li M, et al. Divergent roles of kupffer cell TLR2/3 signaling in alcoholic liver disease and the protective role of EGCG. Cell Mol Gastroenterol Hepatol (2020) 9(1):145–60. doi: 10.1016/j.jcmgh.2019.09.002
48. Anderson DA 3rd, Dutertre CA, Ginhoux F, Murphy KM. Genetic models of human and mouse dendritic cell development and function. Nat Rev Immunol (2021) 21(2):101–15. doi: 10.1038/s41577-020-00413-x
49. Lau AH, Abe M, Thomson AW. Ethanol affects the generation, cosignaling molecule expression, and function of plasmacytoid and myeloid dendritic cell subsets in vitro and in vivo. J Leukoc Biol (2006) 79(5):941–53. doi: 10.1189/jlb.0905517
50. Lau AH, Thomson AW, Colvin BL. Chronic ethanol exposure affects in vivo migration of hepatic dendritic cells to secondary lymphoid tissue. Hum Immunol (2007) 68(7):577–85. doi: 10.1016/j.humimm.2007.03.008
51. Alharshawi K, Fey H, Vogle A, Klenk T, Kim M, Aloman C. Sex specific effect of alcohol on hepatic plasmacytoid dendritic cells. Int Immunopharmacol (2021) 90:107166. doi: 10.1016/j.intimp.2020.107166
52. Wang S, Wang X, Tan Z, Su Y, Liu J, Chang M, et al. Human ESC-derived expandable hepatic organoids enable therapeutic liver repopulation and pathophysiological modeling of alcoholic liver injury. Cell Res (2019) 29(12):1009–26. doi: 10.1038/s41422-019-0242-8
Keywords: myeloid cell, immunity, alcoholic liver disease, cell-cell communication, single-cell ‘omics
Citation: Xu W, Wu M, Chen B and Wang H (2022) Myeloid cells in alcoholic liver diseases: Mechanism and prospect. Front. Immunol. 13:971346. doi: 10.3389/fimmu.2022.971346
Received: 16 June 2022; Accepted: 19 July 2022;
Published: 10 August 2022.
Edited by:
Jinhang Gao, Sichuan University, ChinaReviewed by:
Zhengsheng Zou, Fifth Medical Center of the PLA General Hospital, ChinaXiaoqin Wu, Cleveland Clinic, United States
Copyright © 2022 Xu, Wu, Chen and Wang. This is an open-access article distributed under the terms of the Creative Commons Attribution License (CC BY). The use, distribution or reproduction in other forums is permitted, provided the original author(s) and the copyright owner(s) are credited and that the original publication in this journal is cited, in accordance with accepted academic practice. No use, distribution or reproduction is permitted which does not comply with these terms.
*Correspondence: Hua Wang, wanghua@ahmu.edu.cn
†These authors have contributed equally to this work and share first authorship