- Department of Hematology, The Third Affiliated Hospital, Sun Yat-sen University, Guangzhou, China
Cytomegalovirus (CMV) infection remains a frequent complication after hematopoietic stem cell transplantation (HSCT) and causes significant morbidity and mortality in transplantation recipients. In this review, we highlight the role of major risk factors that are associated with the incidence of CMV infection. Advances in immunosurveillance may predict CMV infection, allowing early interventions to prevent severe infection. Furthermore, numerous therapeutic strategies against CMV infection after HSCT are summarized. A comprehensive understanding of the current situation of CMV treatment may provide a hint for clinical practice and even promote the development of novel strategies for precision medicine.
1 Introduction
Allogeneic hematopoietic stem cell transplantation (allo-HSCT) is considered to be the strongest curative treatment for malignant hematologic diseases. It begins with a conditioning regimen that destroys cancer cells and the immune system, then allows the recipient to accept an immune system-reconstituting infusion of donor stem cells. Before the hematopoiesis and immune reconstitution, allo-HSCT recipients are susceptible to infection by bacteria, fungi, or viruses, which affect the outcome after allo-HSCT. Among those pathogens, cytomegalovirus (CMV) reactivation is considered one of the most serious challenges during HSCT.
CMV, classified as the beta-human-herpesvirus type 5 (HHV-5), is prevalent globally, with seroprevalences ranging from 30% to over 90% according to age and geographical factors (1–3). Primary CMV infection typically progresses to latency in human epithelial tissue, polymorphonuclear cells, myeloid progenitors, and T lymphocytes, for it is normally inhibited by the host’s immune system. In those who have received HSCT, latent CMV is often reactivated in the early stages of immune recovery, usually within 3-4 months after HSCT (4). Early CMV infection before day 100 after HSCT leads to numerous end-organ diseases, increases the risk of acute or chronic graft-versus-host disease (GVHD), enhances invasive fungal infection, contributes to graft failure and fatal outcomes (5, 6). In post-transplant patients, early infection was also mentioned to be associated with increased non-relapse mortality, which appears to be linked to complex effects on the post-transplant CMV-specific T cell recovery (7–9). Thus, in this review, we focus on major risk factors, diagnosis, and management of early CMV infection after HSCT.
2 Risk factors for CMV infection
As suggested by current studies, there are multiple risk factors (Table 1) that predispose a patient to CMV infection, such as the states of both donors and recipients, and the strategies during HSCT. Among all the risk factors mentioned above, four of them are considered major: CMV serostatus, acute or chronic GVHD, type of donor, and in-vivo/ex-vivo T cell depletion (10, 11).
2.1 CMV donor and recipient serology
In allo-HSCT recipients, the most important risk factor for CMV infection is the CMV serological status of both donors and recipients (12). CMV seropositive recipients (R+) have the highest incidence of reactivation, especially when they were transplanted from seronegative donors (D−) (13–15). The D−/R− have the lowest risk of CMV infection (16). In D+/R+ patients, reactivation may derive from endogenous latent CMV in the R+ and/or from latently infected cells transferred within the D+ stem cell graft. D−/R+ experience the incidence of invasive CMV disease, which could be a consequence of delayed CMV-specific immune recovery owing to the lack of pre-existing CMV-specific memory T cells in the graft (14, 15). The CMV-specific memory T cells transferred with D+ grafts facilitate antigen-driven amplification in the recipient early after transplantation and contribute to early control of the reactivation (14, 15). As a result, whenever possible, attempts are made to match serostatus in donors and recipients in current clinical practice. However, there are debates on the benefits of transferred CMV-specific T cell (17, 18). Some believed that the benefit is very limited for the number of CMV-specific memory T cells transferred is small. Moreover, HSCT recipients are routinely treated with immunosuppressive agents to prevent GVHD, which will also inhibit the function of CMV-specific T cells.
2.2 GVHD
The incidence of GVHD increases the risk of CMV infection, especially with its treatment by steroids (19, 20). Similar to immunosuppressive drugs, steroids suppress the immune system by inhibiting CMV-specific T cell activation. In return, CMV infection may also increase the risk of GVHD. As reported, CMV-infected cells promote the production of IL-6 and lead to GVHD (21, 22). Years ago, Styczynski’s project showed that CMV infection occurred nearly twice as much in patients with acute GVHD as in those without acute GVHD [P<0.0001, 60.1% (885/1472) vs 32.1% (892/2780)] (23). Moreover, enhanced transplant-related mortality and decreased overall survival were reported in those R+ recipients with acute GVHD (19).
2.3 Type of donor
Recipients of transplants from different types of donors have been analyzed in multiple studies, including match-related donors (MRD), match-unrelated donors (MUD), haploids, and umbilical cord blood (UCB). Accordingly, CMV infection occurred in less than 40% of MRD transplant recipients (24–28), while in more than half of MUD (24, 25, 27) and haploid cases (25–28), respectively. Clearly, the risk of CMV infection was higher in MUD and haploid transplants than that in MRD (OR 1.96, 95% 1.76-2.20, P<0.0001). One of the reasons is that recipients from HLA mismatched donors are prone to develop GVHD. Alloimmune responses mediated by GVHD impair thymopoiesis, which delays the reconstitution of CMV-specific T cells (29). Moreover, recipients of UCB transplantation have the highest incidence of CMV infection, with an infection rate of 74.5% (246/330) (30–33). The T cells in cord blood grafts are naive and have impaired functional recovery, which contributes to prolonged reconstitution of antigen-specific immunity and increased risks of viral infection (34). Yet recipients from mismatch-unrelated donors (MMUD) were seldom reported.
2.4 In-vivo/ex-vivo T cell depletion (TCD)
To prevent severe acute and chronic GVHD (aGVHD and cGVHD), both in-vivo and ex-vivo TCD are used as part of a myeloablative conditioning regimen prior to allo-HSCT in malignant diseases. However, these approaches are associated with delayed immune reconstitution and an increased risk of infection (26). Compared to in-vivo TCD, traditional ex-vivo TCD experiences a higher incidence of infectious complications, and relapse (35). Thus in the current clinical practice, in-vivo TCD, such as post-transplant cyclophosphamide (PTCy) and anti-thymocyte (ATG), is the most frequently used method to prevent GVHD. With the beneficial effect on severe aGVHD and cGVHD, PTCy resulted in a higher incidence of CMV infection than other in-vivo TCD approaches (26). ATG is generally considered to deplete T cells effectively and allow great expansion of regulatory T cells, which release inflammatory factors (such as IL-10 and TGF-β) to inhibit GVHD (36). Compared with patients not receiving ATG, this TCD was reported to slow down the recipient’s reconstitution of CD4+ and CD8+ T cells in the peripheral blood (37, 38). Different ATG regimens are applied in current studies, and CMV infections vary, but many support that a lower dose decreases the risk of CMV infection (39–41).
In conclusion, CMV infection should be regarded as a consequence of the single or multiple risk factors mentioned above. Optimization of these factors helps to achieve a lower viral activation rate.
3 Post-HSCT immune recovery after CMV infection
After the transplantation, neutrophils are the first cell line to reconstitute, followed by monocytes, natural killer (NK) cells, and T cells by day 100, and B cells take 1 to 2 years (42–44). During the first 100 days after HSCT, NK cells and T cells are reported to be the major immune cells that protect the recipient from CMV infection (Figure 1). NK cells are the first lymphocytes to recover in HSCT recipients, but are functionally impaired. When CMV reactivates, it stimulates and expands a distinctive NK cell population that is able to secrete cytokines and be cytotoxic. These activated NK cells express numerous activating and inhibitory receptors on their surface, matching with the corresponding ligands on healthy cells (45). One group of the inhibitory receptors, namely killer cell immunoglobulin-like receptors (KIRs), specifically recognizes class I HLA, an inhibitory ligand expressed on normal cells (45, 46). The balance of receptors and ligands is essential to keep healthy cells alive. However, class I HLA is down-regulated in CMV-infected cells, breaking down the balance. Both the undermined inhibitory effect and the superiority of activating receptors-ligands signals initiate cytotoxicity of activated NK cells (45, 47). These NK cells also secrete cytokines, including tumor necrosis factor-a (TNF-a) and interferon-g (IFN-g), which likely contribute to the early control of the CMV infection (45). Moreover, activated NK cells provide an early source of IFN-g, promoting T helper cell type 1 (Th1) responses, which further enhances CD4+ T cell against CMV infection by secreting IFN-γ and TNF-α (48).
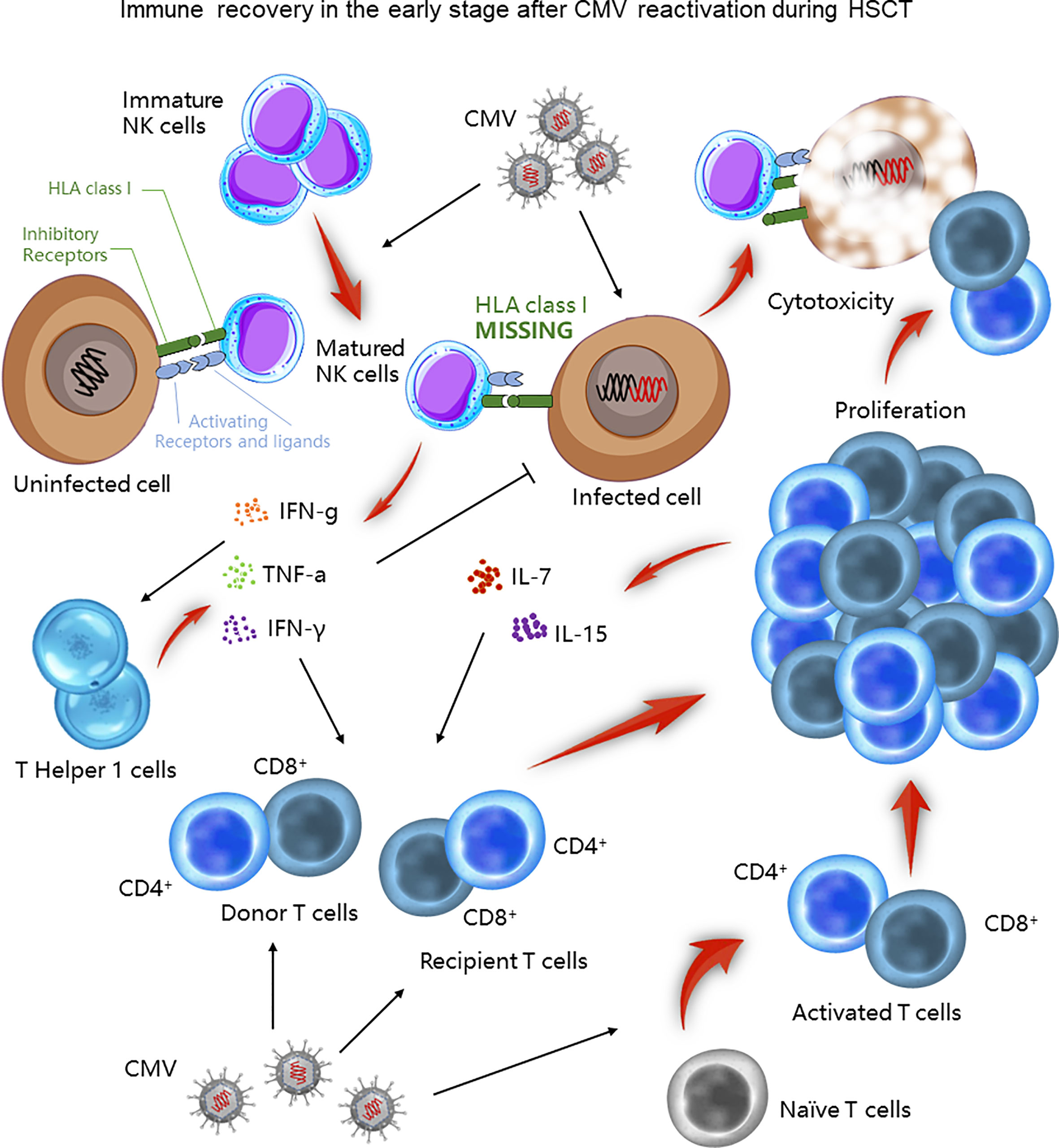
Figure 1 Immune recovery in the early stage after CMV infection during HSCT. During the first 100 days after HSCT, NK and T cells play a crucial role in controlling CMV infection. Matured NK cells directly lyse CMV infected cells or indirectly facilitate Th1 responses to control CMV infection. T cells also exert their cytotoxic function through both existing and newly generated populations.
On the other hand, CMV-specific CD4+ and CD8+ T cells also play an important role against CMV infection. The early post‐transplant period is characterized by the peripheral expansion of either donor T cells in the graft or recipient T cells that survived conditioning. Their memory feature urges them to react and proliferate rapidly soon after CMV infection, thereby killing the infected cells. In response to lymphopenia, proliferated T cells also secrete interleukin (IL) -7 and IL-15 to promote their reconstitution. Yet, these T cells are with restricted T cell receptor (TCR) repertoire, limited antigen specificity, and are alloreactive, which is capable to cause GVHD (29). In contrast, complete reconstitution of the TCR relies on bone-marrow-derived and thymus-matured naive T cells (29). Compared to the early period, this broadens the TCR diversity of a de novo group of T cells. Intriguingly, this virus was reported to directly infiltrate the thymic epithelium, activating cytotoxic T-cell responses toward the thymus, and therefore impairing this complete immune reconstitution (49).
4 Early detection for CMV infection
To include and benefit as many patients as possible, particularly for those with inevitable high risks, monitoring viral DNA is believed to be another crucial approach. Rapid confirmation of CMV infection will alert the practitioners, and clinical strategies will be made in time to reduce CMV-related mortality early after transplantation. With the development of technology, the ways of monitoring CMV status vary from time to time.
4.1 Traditional methods for clinical diagnosis
Over the years, several diagnostic methods have been developed to detect CMV infection. The most commonly used methods are detections of the CMV phosphoprotein (pp65) antigen by antigenemia assay and DNA viral load using CMV Quantitative Nucleic Acid Test (QNAT) (50).
4.1.1 CMV antigen
Detecting this viral structural protein (51) was once a feasible way to diagnose CMV infection for its operational and economic advantages. However, it has been gradually replaced by CMV QNAT, another detective approach that aims specifically at CMV DNA.
4.1.2 CMV QNAT
The application of CMV QNAT has been increased in recent years given its high sensitivity and quantification of viral load that conveys important prognostic information of patients (52, 53). Yet, there are some limitations. For instance, the results vary depending on the specimen types. Most CMV DNA in plasma consists of small fragments, which increases the measured values and causes bias. Studies suggested that most viral load values in plasma are about 10-fold higher than that in the whole blood (52), for both cell-free and intracellular virus DNA can be detected. Hence, a fixed sample type is recommended for serial viral load monitoring. Moreover, there is no universal standard for viral load, due to the lack of standardization among various commercial and laboratory-developed assays. Although the W.H.O. expert committee established an International Standard (IS) for CMV QNAT in October 2010, some variability in the test results still remains (54). Therefore, most transplant centers have to establish their own threshold for preemptive therapy (55, 56).
4.2 Novel methods for prediction
The pathogenesis of CMV infection is complex, and it interacts with the immune system in many ways, mainly through cellular immunity and humoral immunity. As recently reported, cytokines produced by CMV-specific CD4+ and CD8+ T helper cells, as well as CMV-specific antibodies produced by the humoral immune response can be used to predict and determine the CMV infection (57–60).
4.2.1 CMV-specific antibodies
Recipient CMV seropositivity is a known risk factor for CMV infection after transplantation, some studies have reported that CMV-IgG titers before transplantation can predict the risk of CMV infection (57, 58, 61). However, the results were contradictory in HSCT and solid organ transplantation (SOT). In SOT, Bruminhent’s team proposed a lower risk of CMV infection in patients with higher CMV-IgG titers (57). Yet, Leonardo et al. argued that a higher recipient pre-transplantation CMV-IgG titer was significantly associated with the development of the CMV infection (58). The same result was observed in another project (61). It retrospectively analyzed 309 HSCT patients and found that CMV infection occurred most frequently in the high-titer group. Based on the observation of clinic practices, a higher CMV-IgG titer might indicate humoral immunity against CMV itself in organ transplantation and increased viral load of latently infected CMV in HSCT recipients. The mechanism is still unclear, and more projects should be carried out to identify the role of CMV-specific antibodies in CMV infection.
4.2.2 CD4+/CD8+ specific T cell response
Until recent years, people start to be aware that the different efficacy in antiviral treatment among recipients is closely related to the CMV-specific T cell immune response. Assessment of CMV-specific T cells may help evaluate the risk of CMV infection, or monitoring of CMV-specific T cells during the onset of CMV infection may help adjust the strategy of antiviral therapy. Thus, attempts have been made to measure CMV-specific-cell-mediated immunity (CMI) in HSCT recipients. In current assays, CMV-CMI is mainly acquired by indirectly quantifying cytokines (TNF α, IFN γ, IL-2, etc.) or directly measuring the number of those CMV-specific T cells.
QuantiFERON, an ELISA-based assay indirectly measures CMV-specific IFN-γ (62). The stimuli in this test include 23 CMV peptide epitopes, which allows it to cover a wide spectrum of CMV subtypes (63). And its testing efficacy has been well illustrated by multiple prospective studies (63–65). Another IFN-γ–based assay, ELISPOT, measures the cytokine of mononuclear cells in peripheral blood following ex vivo stimulation of CMV antigens (IE-1 and pp65). Its better concordance with CMV infection in patients with a low CMV-CMI made it a sensitive approach (66, 67). However, it failed to show a difference in the project of Barron et al. (68), therefore the efficacy is still debatable. Flow cytometry with intracellular cytokine staining (ICS) can not only measure multiple CMV-specific cellular markers, but also the number of CMV-specific T cells (69). However, it lacks standardization. In addition, clinicians may obtain different results depending on the method selected to measure CMV-specific CMI and large-scale prospective studies are insufficient.
Despite the limitation, CMV-CMI seems to affect the development and prognosis of CMV infection. Cellular immunity mediated by T cells is known to be responsible for controlling CMV replication, and the absence or delayed recovery of CMV-specific T cells causes CMV infection and CMV diseases (70–72). Even though more studies are necessary before their practical applications, monitoring CMV-specific antibodies and CMV-specific cell responses, may help in determining the duration of prophylaxis and treatment, thereby minimizing drug exposure.
5 Current therapeutic strategies for CMV infection in HSCT
5.1 Preemptive treatment
Preemptive treatment is the most widely used strategy for the prevention of CMV infection after HSCT, which is initiated right after the early detection of CMV infection.
5.1.1 Antiviral agents for treatment
Antiviral agents serve as the backbone for CMV treatment in transplant recipients (73): the first-line anti-CMV medication includes intravenous ganciclovir or oral valganciclovir, while foscarnet and cidofovir are considered second-line agents that are reserved for treatment of resistant and refractory CMV. Antiviral agents have been reported to achieve CMV elimination in up to 70% of cases, which greatly reduces the incidence of CMV disease, one of the most dangerous post-transplant complications, whose current morbidity is nearly10% of HSCT patients (23, 50). However, routine usage of preemptive therapy also shows significant side effects including granulopenia/agranulocytosis (ganciclovir and valganciclovir) and renal dysfunction (foscarnet and cidofovir) (52, 74). Maribavir, a novel antiviral agent for CMV, has been brought up recently. It is a benzimidazole antiviral that inhibits viral replication by inhibiting the viral protein kinase UL97 (64). After successful Phase 1 and 2 trials, maribavir failed to show efficacy in the initial Phase 3 clinical trial (75). Although maribavir is not routinely used as a first-line preemptive treatment, it is recommended as a second-line treatment for resistant CMV infection, because its therapeutic efficacy has been proved in more than half of the refractory and drug-resistant cases in Robin K Avery’s study (76). Recently, drug resistance has been reported with the emergence of the mutations in the UL97 kinase, particularly the virus strains that resist ganciclovir and its pro-drug valganciclovir, since they have been used as first-line drugs in approximately 90% of patients (77). However, resistance can develop with all antiviral drugs used for CMV prophylaxis and therapy. Therefore, the resistance must be considered in patients whose CMV load still increases even though they are on appropriately dosed therapy for 2 weeks.
5.1.2 CMV intravenous immunoglobulin (CMV-IVIG) for treatment
CMV-IVIG functions to prevent CMV from entering the host cells. Besides, this CMV-specific antibody facilitates cytotoxicity, phagocytosis, and complement-mediated cytolysis (78–80). With these capabilities, strategies combining CMV-IVIG and antiviral agents have been proposed for CMV treatment: some found that, for preemptive treatment, this IVIG reduced the high risk of CMV infection from 62% to 36% (81–83). Compared to antiviral agents, CMV-IVIG offers an alternative option for CMV treatment without renal and bone marrow impairment. However, according to the 2017 European Conference on Infections in Leukaemia (ECIL 7) guideline, the addition of CMV-IVIG to second-line or third-line treatment is not recommended, except for the CMV pneumonia (1), for there is still a lack of solid evidence.
5.1.3 Adopted T cell therapy
Since cellular immunity is essential for the control of CMV infection, the use of CMV special cytotoxic lymphocytes (CTLs) becomes an attractive emerging therapy. There are various ways to generate CMV-specific T cells, which involve stimulation of CMV-specific cells by using the viral peptides, protein, lysate, or antigen-presenting cells. Then, CMV-specific T cells can be expanded in vitro or by direct infusion into the recipients, thus proliferating under a physiological environment in vivo (84). As reported, adoptive CMV special T cells therapy can be useful in controlling resistant and refractory CMV infection. In a prospective multicenter clinical trial, HSCT patients with refractory CMV infection received ex vivo CMV-specific T cells, were all detected CMV epitope CMV-specific T cells (85). Another study from a single center examined the CMV-specific T cells expansion of 32 HSCT patients with refractory CMV infection and showed that 27 of 32 (84.4%) patients had a resolution of CMV infection within 4 weeks after adoptive T-cell transfer, and did not experience further viral recurrence (86). However, long-term in vivo persistence of transferred CMV-specific T cells required the development of an endogenous CMV-specific T-helper response. Therefore, the limitation restrains these strategies from being popularized in the clinical practice (87). Moreover, current studies that used adoptive special T cells for the treatment of resistant and refractory CMV infection were limited by small numbers and lacked comparison groups in the setting of randomized controlled trials (85, 86, 88, 89). More studies are needed to assess its efficacy.
Though preemptive treatment greatly reduces the risk of CMV infection, several studies have demonstrated that CMV infection after HSCT was associated with increased overall and all-cause mortality, and the risk increases with the viral load (9, 90), independent of the use of preemptive therapy (90, 91). Therefore, prevention of viral replication, rather than surveillance-based preemptive therapy, is considered a more suitable choice for allogenic transplant recipients at high risk of CMV infection.
5.2 General prophylaxis
General prophylaxis usually refers to the usage of antiviral agents to prevent CMV infection prior to HSCT. The antiviral agent is administered shortly before transplantation, and continued for at least 3 months (92, 93).
5.2.1 Antiviral agents for prophylaxis
In the 1980s and 1990s, anti-CMV prophylaxis with high-dose acyclovir or valacyclovir was brought up for the first time owing to the efficacy of suppressing the CMV infection (94). For decades, effective agents to control CMV infection were limited to those with significant toxicity, such as ganciclovir, foscarnet, and cidofovir. And ganciclovir is the only drug that has been evaluated as prophylaxis in randomized trials (17, 95, 96). However, it failed to improve the overall survival due to severe neutropenia, as well as secondary bacterial and fungal infections (1, 18, 97). Thus, antiviral prophylaxis has not been considered a routine or common approach for most centers.
Recently, clinical trials on novel antiviral agents for CMV prophylaxis have been studied. Letermovir, a novel antiviral agent that inhibits the viral terminase complex without major toxic effects, is recently approved to decrease CMV infection after HSCT in phase 3, randomized, placebo-controlled trial (98). After the result of this trial, many centers have adopted the use of letermovir prophylaxis to prevent CMV infection after HSCT. The result of this trial has been replicated in the real world too (99, 100). Despite its favorable clinical efficacy, there appeared to be an increased rate of late CMV events after the discontinuation of the letermovir prophylaxis (98). A prospective study of 56 allogenic transplant recipients who received letermovir as prophylaxis, compared to those who received PCR-guide preemptive therapy, showed that letermovir may delay CMV-specific T cell reconstitution, which is possibly related to decreased CMV antigen exposure (101). Moreover, mutations in CMV UL56 terminase leading to letermovir resistance in HSCT recipients have been reported (102), yet further evaluation is still necessary. In addition to the drug resistance, false-positive cases were also reported (103). Letermovir inhibits the terminal phase of CMV replication by targeting the CMV terminase complex. As a result, a very late stage of CMV replication is inhibited and the long DNA concatenator is unable to split into individual viral subunits, producing noninfectious long DNA molecules, which can be detected by the current highly sensitive CMV QNAT method in the blood sample. Therefore, the results of CMV QNAT during letermovir prophylaxis may be misinterpreted.
Another novel antiviral agent, brincidofovir, is a lipid conjugate prodrug of cidofovir, which reduces the nephrotoxic and marrow toxic side effects of cidofovir. A phase 3 randomized controlled trial that compared brincidofovir to placebo for CMV prophylaxis in HSCT patients failed to show a reduction in clinically significant CMV infection at 24 weeks. The agent was also associated with an increased incidence of GVHD (104), and its use was not approved for this indication. In addition, current antiviral agents do not target CMV during latency, but viral replication. This highlights the potential for clinically relevant recurrence of CMV infection following therapy cessation.
5.2.2 CMV-IVIG for prophylaxis
After HSCT, the deficiencies of cellular and humoral immunity give chances to viral infections. Owing to the immune deprivation before immunological reconstitution or persistent immune dysfunction in post-HSCT, several studies started to focus on CMV-IVIG in preventing CMV infection and disease in the past decades. There was evidence from the 1980s to 1990s indicating that prophylactic use of CMV-IVIG was associated with reduced incidence of CMV infection and improved disease condition (105, 106). Following these studies, randomized controlled trials and large meta-analyses refuted the role of CMV-IVIG in preventing CMV infection, for it found no significant effect on CMV infections or overall mortality (107, 108). As a result, CMV-IVIG was not included in the updated guidelines for CMV prevention after HSCT. However, with the development of HSCT technology including reduced-intensity conditioning and the elder population of patients receiving HSCT, present clinical practice in HSCT may differ from the previous one (107). In addition, recent technical development of immunoglobulin production has improved intact CMV-IVIG preparations with normal half-lives, effector functions, higher pathogen safety, and fewer coagulating factors (109). Taken together, the previously available data may not be adequate to inform current HSCT practices. Accordingly, several recent clinical trials have suggested that prophylactic CMV-IVIG showed a significant reduction in CMV infection (83, 110, 111)(Table 2). Given the well-established mechanisms and its safety profile as well as less toxicity, CMV-IVIG has a promising future as prophylaxis in selective patients who are susceptible to CMV infection. Likewise, further randomized controlled trials also are needed.
5.2.3 Adopted T-cell therapy
CMV CTLs immunotherapy has been used more frequently in HSCT. Some centers have investigated its use as a prophylactic measure to prevent CMV infection in HSCT recipients, and showed that adoptive T cell therapy is beneficial to prevent CMV infection (112, 113). In one study, prophylactic adoptive transfer of ex vivo CTLs was given to nine R+ HSCT recipients. Two R+ developed CMV viremia, while none of them required antiviral treatment and were cured (112). Another study showed that infusions of low-dose donor memory T-lymphocytes may lead to the expansion of CMV CTLs, and therefore prevent CMV infection in HSCT transplantation (113).
However, these studies were limited by insufficient sample sizes and the lack of comparison groups in the setting of randomized controlled trials. Before clinical practice, additional large-scale studies are necessary to further evaluate its efficacy and side effects.
5.2.4 CMV vaccines
The development of an effective and safe vaccine against CMV remains an important medical priority. Few vaccine trials for prophylaxis of CMV infection are currently underway (114, 115). ASP0113, the DNA vaccine, is the most studied in HSCT recipients. ASP0113 contains two types of plasmids encoding the CMV antigens tegument pp65 and gB: the former is a major structural protein of CMV, and it is also a dominant CMV antigen recognized by CD4+ and CD8+ T cells (116); the latter is a surface protein that is neutralized by antibodies (117). Therefore after being vaccinated, pp65 induces T cell-mediated responses, while gB causes the release of viral-specific antibodies, representing the activation of host cellular and humoral immunity respectively (118). The prophylactic effectiveness of this CMV vaccine has been proved in a phase 2 study (115), yet its phase 3 study is being still carried on. Even though CMV vaccine development is promising, it appears that the translation to clinical practice may still take years.
5.3 CMV disease treatment during early CMV infection
Since the prevalence of therapeutic strategies against CMV infection, the incidence of CMV disease in the early stages of HSCT has been reduced, and the current morbidity rate is nearly 3% (119). The most common CMV disease has switched from CMV pneumonia to CMV gastrointestinal disease. Despite the rareness, the mortality of CMV pneumonia remains high. Once the diagnosis is confirmed, a combination of intravenous ganciclovir and high-dose IVIG is standardly recommended (120–122). However, given the non-randomized setting of these studies, the efficacy of IVIG is still debatable. For other CMV diseases, ganciclovir is the first-line choice. When the toxic effects or drug resistance restrain the usage of ganciclovir, foscarnet and cidofovir can be chosen as substitutes (1). Maribavir is recommended as a second-line treatment for resistant CMV infection (76).
6 Summary
CMV is a highly prevalent, opportunistic pathogen that continues to cause CMV infection and CMV disease, whose increased morbidity and mortality are considered as a big challenge for HSCT. To better prevent and control its infection, further understanding of the conditions that enable its susceptibility to infection is required. Future strategies of diagnosis might involve plasmatic metabolomics profiling to predict the emergence of CMV infection. Though current treatment against CMV has been summarized (Table 3), novel therapies for CMV infection are actively being pursued and these will benefit the HSCT recipients in the near future.
Author contributions
BL and XL offered direction and helped on the whole project. JC, KZ, and YS drafted the manuscript. XZ and RW modified the manuscript. BL review the manuscript and made significant revisions on the manuscript. All authors contributed to the article and approved the submitted version.
Funding
This work was supported by the National Natural Science Foundation of China (81700149), Guangdong Basic and Applied Basic Research Foundation (No. 2020A1515010199), and the Fundamental Research Funds for the Central Universities (No. 20ykpy30).
Conflict of interest
The authors declare that the research was conducted in the absence of any commercial or financial relationships that could be construed as a potential conflict of interest.
Publisher’s note
All claims expressed in this article are solely those of the authors and do not necessarily represent those of their affiliated organizations, or those of the publisher, the editors and the reviewers. Any product that may be evaluated in this article, or claim that may be made by its manufacturer, is not guaranteed or endorsed by the publisher.
References
1. Ljungman P, Camara R, Robin C, Crocchiolo R, Einsele H, Hill J A, et al. Guidelines for the management of cytomegalovirus infection in patients with haematological malignancies and after stem cell transplantation from the 2017 European conference on infections in leukaemia (ECIL 7). Lancet Infect Dis (2019) 19(8):e260–72. doi: 10.1016/S1473-3099(19)30107-0
2. Litjens NHR, van der Wagen L, Kuball J, Kwekkeboom J. Potential beneficial effects of cytomegalovirus infection after transplantation. Front Immunol (2018) 9:389. doi: 10.3389/fimmu.2018.00389
3. Cho SY, Lee DG, Kim HJ. Cytomegalovirus infections after hematopoietic stem cell transplantation: Current status and future immunotherapy. Int J Mol Sci (2019) 20(11):2666. doi: 10.3390/ijms20112666
4. Jang JE, Kim SJ, Cheong JW, Hyun SY, Kim YD, Kim YR, et al. Early CMV replication and subsequent chronic GVHD have a significant anti-leukemic effect after allogeneic HSCT in acute myeloid leukemia. Ann Hematol (2015) 94(2):275–82. doi: 10.1007/s00277-014-2190-1
5. Teira P, Battiwalla M, Ramanathan M, Barrett AJ, Ahn KW, Chen M, et al. Early cytomegalovirus reactivation remains associated with increased transplant-related mortality in the current era: a CIBMTR analysis. Blood (2016) 127(20):2427–38. doi: 10.1182/blood-2015-11-679639
6. Chan ST, Logan AC. The clinical impact of cytomegalovirus infection following allogeneic hematopoietic cell transplantation: Why the quest for meaningful prophylaxis still matters. Blood Rev (2017) 31(3):173–83. doi: 10.1016/j.blre.2017.01.002
7. Leserer S, Bayraktar E, Trilling M, Bogdanov R, Arrieta‐Bolaños E, Tsachakis‐Mück N, et al. Cytomegalovirus kinetics after hematopoietic cell transplantation reveal peak titers with differential impact on mortality, relapse and immune reconstitution. Am J Hematol (2021) 96(4):436–45. doi: 10.1002/ajh.26094
8. Sahin U, Toprak SK, Atilla PA, Atilla E, Demirer T. An overview of infectious complications after allogeneic hematopoietic stem cell transplantation. J Infect Chemother (2016) 22(8):505–14. doi: 10.1016/j.jiac.2016.05.006
9. Green ML, Leisenring W, Xie H, Mast TC, Cui Y, Sandmaier BM, et al. Cytomegalovirus viral load and mortality after haemopoietic stem cell transplantation in the era of pre-emptive therapy: a retrospective cohort study. Lancet Haematol (2016) 3(3):e119–27. doi: 10.1016/S2352-3026(15)00289-6
10. Risk factors for cytomegalovirus infection after allogeneic hematopoietic cell transplantation in malignancies: Proposal for classification. Anticancer Res (2017) 37(12):6551–6. doi: 10.21873/anticanres.12111
11. Bonifazi F, Rubio MT, Bacigalupo A, Boelens JJ, Finke J, Greinix H, et al. Rabbit ATG/ATLG in preventing graft-versus-host disease after allogeneic stem cell transplantation: consensus-based recommendations by an international expert panel. Bone Marrow Transplant (2020) 55(6):1093–102. doi: 10.1038/s41409-020-0792-x
12. Withers B, Clancy L, Burgess J, Simms R, Brown R, Micklethwaite K, et al. Establishment and operation of a third-party virus-specific T cell bank within an allogeneic stem cell transplant program. Biol Blood Marrow Transplant (2018) 24(12):2433–42. doi: 10.1016/j.bbmt.2018.08.024
13. Ljungman P, Brand R, Hoek J, de la Camara R, Cordonnier C, Einsele H, et al. Donor cytomegalovirus status influences the outcome of allogeneic stem cell transplant: a study by the European group for blood and marrow transplantation. Clin Infect Dis (2014) 59(4):473–81. doi: 10.1093/cid/ciu364
14. Zhou W, Longmate J, Lacey SF, Palmer JM, Gallez-Hawkins G, Thao L, et al. Impact of donor CMV status on viral infection and reconstitution of multifunction CMV-specific T cells in CMV-positive transplant recipients. Blood (2009) 113(25):6465–76. doi: 10.1182/blood-2009-02-203307
15. Ganepola S, Gentilini C, Hilbers U, Lange T, Rieger K, Hofmann J, et al. Patients at high risk for CMV infection and disease show delayed CD8+ T-cell immune recovery after allogeneic stem cell transplantation. Bone Marrow Transplant (2007) 39(5):293–9. doi: 10.1038/sj.bmt.1705585
16. Nichols WG, Corey L, Gooley T, Davis C, Boeckh M. High risk of death due to bacterial and fungal infection among cytomegalovirus (CMV)-seronegative recipients of stem cell transplants from seropositive donors: evidence for indirect effects of primary CMV infection. J Infect Dis (2002) 185(3):273–82. doi: 10.1086/338624
17. Goodrich JM, Mori M, Gleaves CA, Mond Du C, Cays M, Ebeling DF, et al. Early treatment with ganciclovir to prevent cytomegalovirus disease after allogeneic bone marrow transplantation. N Engl J Med (1991) 325(23):1601–7. doi: 10.1056/NEJM199112053252303
18. Robin C, Hemery F, Dindorf C, Thillard J, Cabanne L, Redjoul R, et al. Economic burden of preemptive treatment of CMV infection after allogeneic stem cell transplantation: a retrospective study of 208 consecutive patients. BMC Infect Dis (2017) 17(1):747. doi: 10.1186/s12879-017-2854-2
19. Cantoni N, Hirsch HH, Khanna N, Gerull S, Buser A, Bucher C, et al. Evidence for a bidirectional relationship between cytomegalovirus replication and acute graft-versus-host disease. Biol Blood Marrow Transplant (2010) 16(9):1309–14. doi: 10.1016/j.bbmt.2010.03.020
20. Melendez-Munoz R, Marchalik R, Jerussi T, Dimitrova D, Nussenblatt V, Beri A, et al. Cytomegalovirus infection incidence and risk factors across diverse hematopoietic cell transplantation platforms using a standardized monitoring and treatment approach: A comprehensive evaluation from a single institution. Biol Blood Marrow Transplant (2019) 25(3):577–86. doi: 10.1016/j.bbmt.2018.10.011
21. Visseren FLJ, Verkerk MSA, Bouter KP, Diepersloot RJA, Erkelens DW. Interleukin-6 production by endothelial cells after infection with influenza virus and cytomegalovirus. J Lab Clin Med (1999) 134(6):623–30. doi: 10.1016/S0022-2143(99)90103-8
22. Chen X, Das R, Komorowski R, Beres A, Hessner MJ, Mihara M, et al. Blockade of interleukin-6 signaling augments regulatory T-cell reconstitution and attenuates the severity of graft-versus-host disease. Blood (2009) 114(4):891–900. doi: 10.1182/blood-2009-01-197178
23. Styczynski J. Who is the patient at risk of CMV recurrence: A review of the current scientific evidence with a focus on hematopoietic cell transplantation. Infect Dis Ther (2018) 7(1):1–16. doi: 10.1007/s40121-017-0180-z
24. Takenaka K, Nishida T, Asano-Mori Y, Oshima K, Ohashi K, Mori T, et al. Cytomegalovirus reactivation after allogeneic hematopoietic stem cell transplantation is associated with a reduced risk of relapse in patients with acute myeloid leukemia who survived to day 100 after transplantation: The Japan society for hematopoietic cell transplantation transplantation-related complication working group. Biol Blood Marrow Transplant (2015) 21(11):2008–16. doi: 10.1016/j.bbmt.2015.07.019
25. Lin CH, Su YJ, Hsu CY, Wang PN, Teng CJ. Haploidentical allogeneic hematopoietic stem cell transplantation increases the risk of cytomegalovirus infection in adult patients with acute leukemia. Transpl Infect Dis (2019) 21(4):e13096. doi: 10.1111/tid.13096
26. Goldsmith SR, Abid MB, Auletta JJ, Bashey A, Beitinjaneh A, Castillo P, et al. Posttransplant cyclophosphamide is associated with increased cytomegalovirus infection: a CIBMTR analysis. Blood (2021) 137(23):3291–305. doi: 10.1182/blood.2020009362
27. Oltolini C, Greco R, Galli L, Clerici D, Lorentino F, Xue E, et al. Infections after allogenic transplant with post-transplant cyclophosphamide: Impact of donor HLA matching. Biol Blood Marrow Transplant (2020) 26(6):1179–88. doi: 10.1016/j.bbmt.2020.01.013
28. Gao XN, Lin J, Wang LJ, Li F, Li HH, Wang SH, et al. Risk factors and associations with clinical outcomes of cytomegalovirus reactivation after haploidentical versus matched-sibling unmanipulated PBSCT in patients with hematologic malignancies. Ann Hematol (2020) 99(8):1883–93. doi: 10.1007/s00277-020-04156-6
29. Velardi E, Tsai JJ, van den Brink MRM. T Cell regeneration after immunological injury. Nat Rev Immunol (2020) 21(5):277–91. doi: 10.1038/s41577-020-00457-z
30. Milano F, Pergam SA, Xie H, Leisenring WM, Gutman JA, Riffkin I, et al. Intensive strategy to prevent CMV disease in seropositive umbilical cord blood transplant recipients. Blood (2011) 118(20):5689–96. doi: 10.1182/blood-2011-06-361618
31. Dahi PB, Perales MA, Devlin SM, Olson A, Lubin M, Gonzales AM, et al. Incidence, nature and mortality of cytomegalovirus infection after double-unit cord blood transplant. Leuk Lymphoma (2015) 56(6):1799–805. doi: 10.3109/10428194.2014.963079
32. Tong J, Sun Z, Liu H, Geng L, Zheng C, Tang B, et al. Risk factors of CMV infection in patients after umbilical cord blood transplantation: a multicenter study in China. Chin J Cancer Res (2013) 25(6):695–703. doi: 10.3978/j.issn.1000-9604.2013.11.08
33. Hill JA, Zamora D, Xie H, Thur LA, Delaney C, Dahlberg A, et al. Delayed-onset cytomegalovirus infection is frequent after discontinuing letermovir in cord blood transplant recipients. Blood Adv (2021) 5(16):3113–9. doi: 10.1182/bloodadvances.2021004362
34. Komanduri KV, St John LS, de Lima M, McMannis J, Rosinski S, McNiece I, et al. Delayed immune reconstitution after cord blood transplantation is characterized by impaired thymopoiesis and late memory T-cell skewing. Blood (2007) 110(13):4543–51. doi: 10.1182/blood-2007-05-092130
35. Gooptu M, Antin JH. GVHD prophylaxis 2020. Front Immunol (2021) 12. doi: 10.3389/fimmu.2021.605726
36. Feng X, Kajigaya S, Solomou EE, Keyvanfar K, Xu X, Raghavachari N, et al. Rabbit ATG but not horse ATG promotes expansion of functional CD4+CD25highFOXP3+ regulatory T cells in vitro. Blood (2008) 111(7):3675–83. doi: 10.1182/blood-2008-01-130146
37. Chen X, Wei J, Huang Y, He Y, Yang D, Zhang R, et al. Effect of antithymocyte globulin source on outcomes of HLA-matched sibling allogeneic hematopoietic stem cell transplantation for patients with severe aplastic anemia. Biol Blood Marrow Transplant (2018) 24(1):86–90. doi: 10.1016/j.bbmt.2017.10.007
38. Servais S, Menten-Dedoyart C, Beguin Y, Seidel L, Gothot A, Daulne C, et al. Impact of pre-transplant anti-T cell globulin (ATG) on immune recovery after myeloablative allogeneic peripheral blood stem cell transplantation. PloS One (2015) 10(6):e0130026. doi: 10.1371/journal.pone.0130026
39. Walker I, Panzarella T, Couban S, Couture F, Devins G, Elemary M, et al. Pretreatment with anti-thymocyte globulin versus no anti-thymocyte globulin in patients with haematological malignancies undergoing haemopoietic cell transplantation from unrelated donors: a randomised, controlled, open-label, phase 3, multicentre trial. Lancet Oncol (2016) 17(2):164–73. doi: 10.1016/S1470-2045(15)00462-3
40. Soiffer RJ, Kim HT, McGuirk J, Horwitz ME, Johnston L, Patnaik MM, et al. Prospective, randomized, double-blind, phase III clinical trial of anti-T-Lymphocyte globulin to assess impact on chronic graft-Versus-Host disease-free survival in patients undergoing HLA-matched unrelated myeloablative hematopoietic cell transplantation. J Clin Oncol (2017) 35(36):4003–11. doi: 10.1200/JCO.2017.75.8177
41. Locatelli F, Bernardo ME, Bertaina A, Rognoni C, Comoli P, Rovelli A, et al. Efficacy of two different doses of rabbit anti-t-lymphocyte globulin to prevent graft-versus-host disease in children with haematological malignancies transplanted from an unrelated donor: a multicentre, randomised, open-label, phase 3 trial. Lancet Oncol (2017) 18(8):1126–36. doi: 10.1016/S1470-2045(17)30417-5
42. de Koning C, Langenhorst J, van Kesteren C, Lindemans CA, Huitema ADR, Nierkens S, et al. Innate immune recovery predicts CD4(+) T cell reconstitution after hematopoietic cell transplantation. Biol Blood Marrow Transplant (2019) 25(4):819–26. doi: 10.1016/j.bbmt.2018.10.013
43. Velardi E, Tsai JJ, van den Brink MRM. T cell regeneration after immunological injury. Nat Rev Immunol (2021) 21:277–91. doi: 10.1038/s41577-020-00457-z.
44. Chang YJ, Zhao XY, Huang XJ. Immune reconstitution after haploidentical hematopoietic stem cell transplantation. Biol Blood Marrow Transplant (2014) 20(4):440–9. doi: 10.1016/j.bbmt.2013.11.028
45. Cooley S, Parham P, Miller JS. Strategies to activate NK cells to prevent relapse and induce remission following hematopoietic stem cell transplantation. Blood (2018) 131(10):1053–62. doi: 10.1182/blood-2017-08-752170
46. Vilches C, Parham P. KIR: diverse, rapidly evolving receptors of innate and adaptive immunity. Annu Rev Immunol (2002) 20:217–51. doi: 10.1146/annurev.immunol.20.092501.134942
47. Ljunggren H-G, Kärre K. In search of the ‘missing self’: MHC molecules and NK cell recognition. Immunol Today (1990) 11:237–44. doi: 10.1016/0167-5699(90)90097-S
48. Martín-Fontecha A, Thomsen LL, Brett S, Gerard C, Lipp M, Lanzavecchia A, et al. Induced recruitment of NK cells to lymph nodes provides IFN-γ for TH1 priming. Nat Immunol (2004) 5(12):1260–5. doi: 10.1038/ni1138
49. Suessmuth Y, Mukherjee R, Watkins B, Koura DT, Finstermeier K, Desmarais C, et al. CMV reactivation drives posttransplant T-cell reconstitution and results in defects in the underlying TCRbeta repertoire. Blood (2015) 125(25):3835–50. doi: 10.1182/blood-2015-03-631853
50. Pollack M, Heugel J, Xie H, Leisenring W, Storek J, Young JA, et al. An international comparison of current strategies to prevent herpesvirus and fungal infections in hematopoietic cell transplant recipients. Biol Blood Marrow Transplant (2011) 17(5):664–73. doi: 10.1016/j.bbmt.2010.07.026
51. Grefte JM, van der Gun BT, Schmolke S, van der Giessen M, van Son WJ, Plachter B, et al. The lower matrix protein pp65 is the principal viral antigen present in peripheral blood leukocytes during an active cytomegalovirus infection. J Gen Virol (1992) 73(Pt 11):2923–32. doi: 10.1099/0022-1317-73-11-2923
52. Lisboa LF, Asberg A, Kumar D, Pang X, Hartmann A, Preiksaitis JK, et al. The clinical utility of whole blood versus plasma cytomegalovirus viral load assays for monitoring therapeutic response. Transplantation (2011) 91(2):231–6. doi: 10.1097/TP.0b013e3181ff8719
53. Gerna G, Lilleri D, Caldera D, Furione M, Bragotti Zenone L, Alessandrino EP. Validation of a DNAemia cutoff for preemptive therapy of cytomegalovirus infection in adult hematopoietic stem cell transplant recipients. Bone Marrow Transplant (2008) 41(10):873–9. doi: 10.1038/sj.bmt.1705986
54. Hayden RT, Sun Y, Tang L, Procop GW, Hillyard DR, Pinsky BA, et al. Progress in quantitative viral load testing: Variability and impact of the WHO quantitative international standards. J Clin Microbiol (2017) 55(2):423–30. doi: 10.1128/JCM.02044-16
55. Razonable RR, Brown RA, Wilson J, Groettum C, Kremers W, Espy M, et al. The clinical use of various blood compartments for cytomegalovirus (CMV) DNA quantitation in transplant recipients with CMV disease. Transplantation (2002) 73(6):968–73. doi: 10.1097/00007890-200203270-00025
56. Boeckh M, Boivin G. Quantitation of cytomegalovirus: methodologic aspects and clinical applications. Clin Microbiol Rev (1998) 11(3):533–54. doi: 10.1128/CMR.11.3.533
57. Bruminhent J, Thongprayoon C, Dierkhising RA, Kremers WK, Theel ES, Razonable RR. Risk factors for cytomegalovirus reactivation after liver transplantation: can pre-transplant cytomegalovirus antibody titers predict outcome? Liver Transpl (2015) 21(4):539–46. doi: 10.1002/lt.24078
58. Arcuri LJ, Schirmer M, Colares M, Maradei S, Tavares R, Moreira MCR, et al. Impact of anti-CMV IgG titers and CD34 count prior to hematopoietic stem cell transplantation from alternative donors on CMV reactivation. Biol Blood Marrow Transplant (2020) 26(11):e275–9. doi: 10.1016/j.bbmt.2020.07.034
59. Cantisan S, Lara R, Montejo M, Redel J, Rodriguez-Benot A, Gutierrez-Aroca J, et al. Pretransplant interferon-gamma secretion by CMV-specific CD8+ T cells informs the risk of CMV replication after transplantation. Am J Transplant (2013) 13(3):738–45. doi: 10.1111/ajt.12049
60. Lopez-Oliva MO, Martinez V, Buitrago A, Jimenez C, Rivas B, Escuin F, et al. Pretransplant CD8 T-cell response to IE-1 discriminates seropositive kidney recipients at risk of developing CMV infection posttransplant. Transplantation (2014) 97(8):839–45. doi: 10.1097/01.TP.0000438025.96334.eb
61. Kawamura S, Nakasone H, Takeshita J, Kimura S-I, Nakamura Y, Kawamura M, et al. Prediction of cytomegalovirus reactivation by recipient cytomegalovirus-IgG titer before allogeneic hematopoietic stem cell transplantation. Transplant Cell Ther (2021) 27(8):683.e1–7. doi: 10.1016/j.jtct.2021.04.024
62. Egli A, Humar A, Kumar D. State-of-the-art monitoring of cytomegalovirus-specific cell-mediated immunity after organ transplant: a primer for the clinician. Clin Infect Dis (2012) 55(12):1678–89. doi: 10.1093/cid/cis818
63. Tey SK, Kennedy GA, Cromer D, Davenport MP, Walker S, Jones LI, et al. Clinical assessment of anti-viral CD8+ T cell immune monitoring using QuantiFERON-CMV(R) assay to identify high risk allogeneic hematopoietic stem cell transplant patients with CMV infection complications. PloS One (2013) 8(10):e74744. doi: 10.1371/journal.pone.0074744
64. Yong MK, Cameron PU, Slavin M, Morrissey CO, Bergin K, Spencer A, et al. Identifying cytomegalovirus complications using the quantiferon-CMV assay after allogeneic hematopoietic stem cell transplantation. J Infect Dis (2017) 215(11):1684–94. doi: 10.1093/infdis/jix192
65. Krawczyk A, Ackermann J, Goitowski B, Trenschel R, Ditschkowski M, Timm J, et al. Assessing the risk of CMV reactivation and reconstitution of antiviral immune response post bone marrow transplantation by the QuantiFERON-CMV-assay and real time PCR. J Clin Virol (2018) 99-100:61–6. doi: 10.1016/j.jcv.2018.01.002
66. Chemaly RF, El Haddad L, Winston DJ, Rowley SD, Mulane KM, Chandrasekar P, et al. Cytomegalovirus (CMV) cell-mediated immunity and CMV infection after allogeneic hematopoietic cell transplantation: The REACT study. Clin Infect Dis (2020) 71(9):2365–74. doi: 10.1093/cid/ciz1210
67. Nesher L, Shah DP, Ariza-Heredia EJ, Azzi JM, Siddiqui HK, Ghantoji SS, et al. Utility of the enzyme-linked immunospot interferon-gamma-Release assay to predict the risk of cytomegalovirus infection in hematopoietic cell transplant recipients. J Infect Dis (2016) 213(11):1701–7. doi: 10.1093/infdis/jiw064
68. Barron MA, Gao D, Springer KL, Patterson JA, Brunvand MW, McSweeney PA, et al. Relationship of reconstituted adaptive and innate cytomegalovirus (CMV)–specific immune responses with CMV viremia in hematopoietic stem cell transplant recipients. Clin Infect Dis (2009) 49(12):1777–83. doi: 10.1086/648423
69. Navarro D, Amat P, de la Camara R, Lopez J, Vazquez L, Serrano D, et al. Efficacy and safety of a preemptive antiviral therapy strategy based on combined virological and immunological monitoring for active cytomegalovirus infection in allogeneic stem cell transplant recipients. Open Forum Infect Dis (2016) 3(2):ofw107. doi: 10.1093/ofid/ofw107
70. Ljungman P, Hakki M, Boeckh M. Cytomegalovirus in hematopoietic stem cell transplant recipients. Hematol Oncol Clin North Am (2011) 25(1):151–69. doi: 10.1016/j.hoc.2010.11.011
71. Blyth E, Withers B, Clancy L, Gottlieb D. CMV-specific immune reconstitution following allogeneic stem cell transplantation. Virulence (2016) 7(8):967–80. doi: 10.1080/21505594.2016.1221022
72. Perez-Martinez A, Lilleri D, Gerna G, Zelini P, Chiesa A, Rognoni V, et al. Monitoring of human cytomegalovirus and virus-specific T-cell response in young patients receiving allogeneic hematopoietic stem cell transplantation. PloS One (2012) 7(7):e41648. doi: 10.1371/journal.pone.0041648
73. Lazzarotto T, Chiereghin A, Piralla A, Piccirilli G, Girello A, Campanini G, et al. Cytomegalovirus and Epstein-Barr virus DNA kinetics in whole blood and plasma of allogeneic hematopoietic stem cell transplantation recipients. Biol Blood Marrow Transplant (2018) 24(8):1699–706. doi: 10.1016/j.bbmt.2018.03.005
74. Meesing A, Razonable RR. New developments in the management of cytomegalovirus infection after transplantation. Drugs (2018) 78(11):1085–103. doi: 10.1007/s40265-018-0943-1
75. Marty FM, Ljungman P, Papanicolaou GA, Winston DJ, Chemaly RF, Strasfeld L, et al. Maribavir prophylaxis for prevention of cytomegalovirus disease in recipients of allogeneic stem-cell transplants: a phase 3, double-blind, placebo-controlled, randomised trial. Lancet Infect Dis (2011) 11(4):284–92. doi: 10.1016/S1473-3099(11)70024-X
76. Papanicolaou GA, Silveira FP, Langston AA, Pereira MR, Avery RK, Uknis M, et al. Maribavir for refractory or resistant cytomegalovirus infections in hematopoietic-cell or solid-organ transplant recipients: A randomized, dose-ranging, double-blind, phase 2 study. Clin Infect Dis (2019) 68(8):1255–64. doi: 10.1093/cid/ciy706
77. Boeckh M, Ljungman P. How we treat cytomegalovirus in hematopoietic cell transplant recipients. Blood (2009) 113(23):5711–9. doi: 10.1182/blood-2008-10-143560
78. Bonaros NE, Kocher A, Dunkler D, Grimm M, Zuckermann A, Ankersmit J, et al. Comparison of combined prophylaxis of cytomegalovirus hyperimmune globulin plus ganciclovir versus cytomegalovirus hyperimmune globulin alone in high-risk heart transplant recipients. Transplantation (2004) 77(6):890–7. doi: 10.1097/01.TP.0000119722.37337.DC
79. Grossi P, et al. Cytomegalovirus immunoglobulin after thoracic transplantation: An overview. Transplantation (2016) 100 Suppl 3:S1–4. doi: 10.1097/TP.0000000000001094
80. Kotton CN, Kumar D, Caliendo AM, Asberg A, Chou S, Danziger-Isakov L, et al. Updated international consensus guidelines on the management of cytomegalovirus in solid-organ transplantation. Transplantation (2013) 96(4):333–60. doi: 10.1097/TP.0b013e31829df29d
81. Alexander BT, Hladnik LM, Augustin KM, Casabar E, McKinnon PS, Reichley RM, et al. Use of cytomegalovirus intravenous immune globulin for the adjunctive treatment of cytomegalovirus in hematopoietic stem cell transplant recipients. Pharmacotherapy (2010) 30(6):554–61. doi: 10.1592/phco.30.6.554
82. Alsuliman T, Kitel C, Dulery R, Guillaume T, Larosa F, Cornillon J, et al. Cytotect(R)CP as salvage therapy in patients with CMV infection following allogeneic hematopoietic cell transplantation: a multicenter retrospective study. Bone Marrow Transplant (2018) 53(10):1328–35. doi: 10.1038/s41409-018-0166-9
83. Malagola M, Greco R, Santarone S, Natale A, Iori AP, Quatrocchi L, et al. CMV management with specific immunoglobulins: A multicentric retrospective analysis on 92 allotransplanted patients. Mediterr J Hematol Infect Dis (2019) 11(1):e2019048. doi: 10.4084/mjhid.2019.048
84. Kaeuferle T, Krauss R, Blaeschke F, Willier S, Feuchtinger T. Strategies of adoptive T -cell transfer to treat refractory viral infections post allogeneic stem cell transplantation. J Hematol Oncol (2019) 12(1):13. doi: 10.1186/s13045-019-0701-1
85. Neuenhahn M, Albrecht J, Odendahl M, Schlott F, Dossinger G, Schiemann M, et al. Transfer of minimally manipulated CMV-specific T cells from stem cell or third-party donors to treat CMV infection after allo-HSCT. Leukemia (2017) 31(10):2161–71. doi: 10.1038/leu.2017.16
86. Pei XY, Zhao XY, Chang YJ, Liu J, Xu LP, Wang Y, et al. Cytomegalovirus-specific T-cell transfer for refractory cytomegalovirus infection after haploidentical stem cell transplantation: The quantitative and qualitative immune recovery for cytomegalovirus. J Infect Dis (2017) 216(8):945–56. doi: 10.1093/infdis/jix357
87. Einsele H, Roosnek E, Rufer N, Sinzger C, Riegler S, Loffler J, et al. Infusion of cytomegalovirus (CMV)-specific T cells for the treatment of CMV infection not responding to antiviral chemotherapy. Blood (2002) 99(11):3916–22. doi: 10.1182/blood.V99.11.3916
88. Tzannou I, Papadopoulou A, Naik S, Leung K, Martinez CA, Ramos CA, et al. Off-the-Shelf virus-specific T cells to treat BK virus, human herpesvirus 6, cytomegalovirus, Epstein-Barr virus, and adenovirus infections after allogeneic hematopoietic stem-cell transplantation. J Clin Oncol (2017) 35(31):3547–57. doi: 10.1200/JCO.2017.73.0655
89. Withers B, Blyth E, Clancy LE, Yong A, Fraser C, Burgess J, et al. Long-term control of recurrent or refractory viral infections after allogeneic HSCT with third-party virus-specific T cells. Blood Adv (2017) 1(24):2193–205. doi: 10.1182/bloodadvances.2017010223
90. Camargo JF, Kimble E, Rosa R, Shimose LA, Bueno MX, Jeyakumar N, et al. Impact of cytomegalovirus viral load on probability of spontaneous clearance and response to preemptive therapy in allogeneic stem cell transplantation recipients. Biol Blood Marrow Transplant (2018) 24(4):806–14. doi: 10.1016/j.bbmt.2017.11.038
91. Boeckh M, Leisenring W, Riddell SR, Bowden RA, Huang ML, Myerson D, et al. Late cytomegalovirus disease and mortality in recipients of allogeneic hematopoietic stem cell transplants: importance of viral load and T-cell immunity. Blood (2003) 101(2):407–14. doi: 10.1182/blood-2002-03-0993
92. Goodrich JM, Bowden RA, Fisher L, Keller C, Schoch G, Meyers JD. Ganciclovir prophylaxis to prevent cytomegalovirus disease after allogeneic marrow transplant. Ann Intern Med (1993) 118(3):173–8. doi: 10.7326/0003-4819-118-3-199302010-00003
93. Humar A, Lebranchu Y, Vincenti F, Blumberg EA, Punch JD, Limaye AP, et al. The efficacy and safety of 200 days valganciclovir cytomegalovirus prophylaxis in high-risk kidney transplant recipients. Am J Transplant (2010) 10(5):1228–37. doi: 10.1111/j.1600-6143.2010.03074.x
94. Ljungman P, de La Camara R, Milpied N, Volin L, Russell CA, Crisp A, et al. Randomized study of valacyclovir as prophylaxis against cytomegalovirus reactivation in recipients of allogeneic bone marrow transplants. Blood (2002) 99(8):3050–6. doi: 10.1182/blood.V99.8.3050
95. Boeckh M, Gooley TA, Myerson D, Cunningham T, Schoch G, Bowden RA. Cytomegalovirus pp65 antigenemia-guided early treatment with ganciclovir versus ganciclovir at engraftment after allogeneic marrow transplantation: a randomized double-blind study. Blood (1996) 88(10):4063–71. doi: 10.1182/blood.V88.10.4063.bloodjournal88104063
96. Reusser P, Einsele H, Lee J, Volin L, Rovira M, Engelhard D, et al. Randomized multicenter trial of foscarnet versus ganciclovir for preemptive therapy of cytomegalovirus infection after allogeneic stem cell transplantation. Blood (2002) 99(4):1159–64. doi: 10.1182/blood.V99.4.1159
97. Yong MK, Ananda-Rajah M, Cameron PU, Morrissey CO, Spencer A, Ritchie D, et al. Cytomegalovirus reactivation is associated with increased risk of late-onset invasive fungal disease after allogeneic hematopoietic stem cell transplantation: A multicenter study in the current era of viral load monitoring. Biol Blood Marrow Transplant (2017) 23(11):1961–7. doi: 10.1016/j.bbmt.2017.07.025
98. Marty FM, Ljungman P, Chemaly RF, Maertens J, Dadwal SS, Duarte RF, et al. Letermovir prophylaxis for cytomegalovirus in hematopoietic-cell transplantation. N Engl J Med (2017) 377(25):2433–44. doi: 10.1056/NEJMoa1706640
99. Anderson A, Raja M, Vazquez N, Morris M, Komanduri K, Camargo J. Clinical “real-world” experience with letermovir for prevention of cytomegalovirus infection in allogeneic hematopoietic cell transplant recipients. Clin Transplant (2020) 34(7):e13866. doi: 10.1111/ctr.13866
100. Lin A, Maloy M, Su Y, Bhatt V, DeRespiris L, Griffin M, et al. Letermovir for primary and secondary cytomegalovirus prevention in allogeneic hematopoietic cell transplant recipients: Real-world experience. Transpl Infect Dis (2019) 21(6):e13187. doi: 10.1111/tid.13187
101. Zamora D, Duke ER, Xie H, Edmison BC, Akoto B, Kiener R, et al. Cytomegalovirus-specific T-cell reconstitution following letermovir prophylaxis after hematopoietic cell transplantation. Blood (2021) 138(1):34–43. doi: 10.1182/blood.2020009396
102. El Chaer F, Shah DP, Chemaly RF. How I treat resistant cytomegalovirus infection in hematopoietic cell transplantation recipients. Blood (2016) 128(23):2624–36. doi: 10.1182/blood-2016-06-688432
103. Cassaniti I, Colombo AA, Bernasconi P, Malagola M, Russo D, Iori AP, et al. Positive HCMV DNAemia in stem cell recipients undergoing letermovir prophylaxis is expression of abortive infection. Am J Transplant (2021) 21(4):1622–8. doi: 10.1111/ajt.16450
104. Marty FM, Winston DJ, Chemaly RF, Mullane KM, Shore TB, Papanicolaou GA, et al. A randomized, double-blind, placebo-controlled phase 3 trial of oral brincidofovir for cytomegalovirus prophylaxis in allogeneic hematopoietic cell transplantation. Biol Blood Marrow Transplant (2019) 25(2):369–81. doi: 10.1016/j.bbmt.2018.09.038
105. Sullivan KM, Kopecky KJ, Jocom J, Fisher L, Buckner CD, Meyers JD, et al. Immunomodulatory and antimicrobial efficacy of intravenous immunoglobulin in bone marrow transplantation. N Engl J Med (1990) 323(11):705–12. doi: 10.1056/NEJM199009133231103
106. Winston DJ, Ho WG, Lin CH, Bartoni K, Budinger MD, Gale RP, et al. Intravenous immune globulin for prevention of cytomegalovirus infection and interstitial pneumonia after bone marrow transplantation. Ann Intern Med (1987) 106(1):12–8. doi: 10.7326/0003-4819-106-1-12
107. Raanani P, Gafter-Gvili A, Paul M, Ben-Bassat I, Leibovici L, Shpilberg O, et al. Immunoglobulin prophylaxis in hematopoietic stem cell transplantation: systematic review and meta-analysis. J Clin Oncol (2009) 27(5):770–81. doi: 10.1200/JCO.2008.16.8450
108. Cordonnier C, Chevret S, Legrand M, Rafi H, Dhedin N, Lehmann B, et al. Should immunoglobulin therapy be used in allogeneic stem-cell transplantation? a randomized, double-blind, dose effect, placebo-controlled, multicenter trial. Ann Intern Med (2003) 139(1):8–18. doi: 10.7326/0003-4819-139-1-200307010-00007
109. Radosevich M, Burnouf T. Intravenous immunoglobulin G: trends in production methods, quality control and quality assurance. Vox Sang (2010) 98(1):12–28. doi: 10.1111/j.1423-0410.2009.01226.x
110. Goldstein G, Rutenberg TF, Mendelovich SL, Hutt D, Oikawa MT, Toren A, et al. The role of immunoglobulin prophylaxis for prevention of cytomegalovirus infection in pediatric hematopoietic stem cell transplantation recipients. Pediatr Blood Cancer (2017) 64(7):e26420. doi: 10.1002/pbc.26420
111. Zamora D, Krantz EM, Green ML, Joncas-Schronce L, Blazevic R, Edmison BC, et al. Cytomegalovirus humoral response against epithelial cell entry-mediated infection in the primary infection setting after hematopoietic cell transplantation. J Infect Dis (2020) 221(9):1470–9. doi: 10.1093/infdis/jiz596
112. Micklethwaite K, Hansen A, Foster A, Snape E, Antonenas V, Sartor M, et al. Ex vivo expansion and prophylactic infusion of CMV-pp65 peptide-specific cytotoxic T-lymphocytes following allogeneic hematopoietic stem cell transplantation. Biol Blood Marrow Transplant (2007) 13(6):707–14. doi: 10.1016/j.bbmt.2007.02.004
113. Maschan M, Blagov S, Shelikhova L, Shekhovtsova Z, Balashov D, Starichkova J, et al. Low-dose donor memory T-cell infusion after TCR alpha/beta depleted unrelated and haploidentical transplantation: results of a pilot trial. Bone Marrow Transplant (2018) 53(3):264–73. doi: 10.1038/s41409-017-0035-y
114. Wloch MK, Smith LR, Boutsaboualoy S, Reyes L, Han C, Kehler J, et al. Safety and immunogenicity of a bivalent cytomegalovirus DNA vaccine in healthy adult subjects. J Infect Dis (2008) 197(12):1634–42. doi: 10.1086/588385
115. Kharfan-Dabaja MA, Boeckh M, Wilck MB, Langston AA, Chu AH, Wloch MK, et al. A novel therapeutic cytomegalovirus DNA vaccine in allogeneic haemopoietic stem-cell transplantation: a randomised, double-blind, placebo-controlled, phase 2 trial. Lancet Infect Dis (2012) 12(4):290–9. doi: 10.1016/S1473-3099(11)70344-9
116. Sylwester AW, Mitchell BL, Edgar JB, Taormina C, Pelte C, Ruchti F, et al. Broadly targeted human cytomegalovirus-specific CD4+ and CD8+ T cells dominate the memory compartments of exposed subjects. J Exp Med (2005) 202(5):673–85. doi: 10.1084/jem.20050882
117. Cranage MP, Kouzarides T, Bankier AT, Satchwell S, Weston K, Tomlinson P, et al. Identification of the human cytomegalovirus glycoprotein b gene and induction of neutralizing antibodies via its expression in recombinant vaccinia virus. EMBO J (1986) 5(11):3057–63. doi: 10.1002/j.1460-2075.1986.tb04606.x
118. Kharfan-Dabaja MA, Nishihori T. Vaccine therapy for cytomegalovirus in the setting of allogeneic hematopoietic cell transplantation. Expert Rev Vaccines (2015) 14(3):341–50. doi: 10.1586/14760584.2015.989990
119. Einsele H, Ljungman P, Boeckh M. How I treat CMV reactivation after allogeneic hematopoietic stem cell transplantation. Blood (2020) 135(19):1619–29. doi: 10.1182/blood.2019000956
120. Emanuel D, Cunningham I, Jules-Elysee K, Brochstein JA, Kernan NA, Laver J, et al. Cytomegalovirus pneumonia after bone marrow transplantation successfully treated with the combination of ganciclovir and high-dose intravenous immune globulin. Ann Intern Med (1988) 109(10):777–82. doi: 10.7326/0003-4819-109-10-777
121. Ljungman P, Engelhard D, Link H, Biron P, Brandt L, Brunet S, et al. Treatment of interstitial pneumonitis due to cytomegalovirus with ganciclovir and intravenous immune globulin: experience of European bone marrow transplant group. Clin Infect Dis (1992) 14(4):831–5. doi: 10.1093/clinids/14.4.831
122. Reed EC, Bowden RA, Dandliker PS, Lilleby KE, Meyers JD. Treatment of cytomegalovirus pneumonia with ganciclovir and intravenous cytomegalovirus immunoglobulin in patients with bone marrow transplants. Ann Intern Med (1988) 109(10):783–8. doi: 10.7326/0003-4819-109-10-783
Keywords: diagnosis, treatment, CMV, HSCT, immune recovery
Citation: Cui J, Zhao K, Sun Y, Wen R, Zhang X, Li X and Long B (2022) Diagnosis and treatment for the early stage of cytomegalovirus infection during hematopoietic stem cell transplantation. Front. Immunol. 13:971156. doi: 10.3389/fimmu.2022.971156
Received: 16 June 2022; Accepted: 05 September 2022;
Published: 23 September 2022.
Edited by:
Susan Prockop, Boston Children’s Hospital and Harvard Medical School, United StatesReviewed by:
Luca Castagna, Azienda Ospedaliera Ospedali Riuniti Villa Sofia Cervello, ItalyIoannis Politikos, Memorial Sloan Kettering Cancer Center, United States
Copyright © 2022 Cui, Zhao, Sun, Wen, Zhang, Li and Long. This is an open-access article distributed under the terms of the Creative Commons Attribution License (CC BY). The use, distribution or reproduction in other forums is permitted, provided the original author(s) and the copyright owner(s) are credited and that the original publication in this journal is cited, in accordance with accepted academic practice. No use, distribution or reproduction is permitted which does not comply with these terms.
*Correspondence: Bing Long, longb3@ mail.sysu.edu.cn; Xudong Li, 13631359815@139.com
†These authors have contributed equally to this work