- 1Sino-Norway Joint Lab on Fish Gut Microbiota, Institute of Feed Research, Chinese Academy of Agricultural Sciences, Beijing, China
- 2Norway-China Joint Lab on Fish Gastrointestinal Microbiota, Institute of Biology, Norwegian University of Science and Technology, Trondheim, Norway
- 3Laboratory of Gene Therapy, Department of Biochemistry, College of Life Sciences, Shaanxi Normal University, Xi’an, China
- 4Norwegian College of Fisheries Science, Faculty of Biosciences, Fisheries and Economics, University of Tromsø (UiT) The Arctic University of Norway, Tromsø, Norway
- 5Key Laboratory for Feed Biotechnology of the Ministry of Agriculture and Rural Affairs, Institute of Feed Research, Chinese Academy of Agricultural Sciences, Beijing, China
Spring viremia of carp virus (SVCV) can cause high mortality of fish. The aim of this study was to investigate the effects of Lactobacillus rhamnosus GCC-3 exopolysaccharides (GCC-3 EPS) on zebrafish (Danio rerio) infected with SVCV and elucidate the underlying mechanisms. Zebrafish were fed with a control diet or diet supplemented with 0.5% and 1% of GCC-3 EPS for 2 weeks. The results showed that supplementation of GCC-3 EPS significantly improved the survival rate of zebrafish compared with the control group. In addition, dietary 0.5% and 1% GCC-3 EPS significantly up-regulated the expression of genes related to type I interferon (IFN) antiviral immunity. Consistent with in vivo results, GCC-3 EPS significantly inhibited SVCV replication in zebrafish embryonic fibroblast (ZF4) cells while significantly increased the expression of type I IFN signaling pathway related genes. Furthermore, knocking down TANK-binding kinase 1 significantly blocked the antiviral effect of GCC-3 EPS. Dietary GCC-3 EPS improved gut microbiota, and the culture supernatant of GCC-3 EPS-associated microbiota significantly inhibited SVCV replication in ZF4 cells compared with the control-microbiota counterpart. In conclusion, our results indicate that dietary GCC-3 EPS can improve the resistance of zebrafish against SVCV infection, and the mechanism may involve enhanced type I interferon signaling.
Introduction
In recent years, the aquaculture industry has been affected by the outbreak of viral diseases (1, 2). Spring viremia of carp is caused by spring viremia of carp virus (SVCV) of a single-stranded RNA virus belonging to a member of the genus Sprivivirus of the family Rhabdoviridae and results in high mortality of juvenile carps with no effective commercial therapy (3–5). Therefore, the World Organization for Animal Health identifies SVCV as the pathogen of infectious diseases that must be reported (2, 6). Interferons (IFN) are widely expressed cytokines with antiviral effects and are the first line of defense against viral infections (7). To date, large numbers of IFN have been discovered, which can be classified into three subgroups as type I IFN, type II IFN, and type III IFN (8). Type I IFN system plays an important role in the antiviral immune response of fish (9). Consistent with the function in mammals, type I IFN in fish can directly inhibit the replication of virus by inducing the expression of antiviral proteins (9–11).
Gut microbiota has been proved to interact with the host during virus infection (12, 13). Studies have indicated that the removal of gut microbiota increases viral infection in antibiotic-treated or germ-free mice, suggesting the importance of commensal microbiota in anti-viral immunity (13–15). Consistent with these findings in mammals, Galindo-Villegas et al. found that conventional zebrafish (Danio rerio) had higher resistance to spring viremia of carp virus (SVCV) infection than germ-free counterparts, supporting that the gut microbiota contributed to fish antiviral capabilities (16). A recent study reported that the predominant commensal bacterium Cetobacterium somerae improved the antiviral immunity of zebrafish after SVCV infection and the underlying mechanism may involve type I IFN signaling (4), suggesting the contribution of commensal bacterium to the antiviral effect of microbiota. Moreover, some studies show that probiotics can significantly improve the survival rate after the virus challenge (13). For example, Bacillus subtilis 7k has been shown to be able to inhibit iridovirus infection in grouper (Epinephelus fuscoguttatus × E. lanceolatus) (17). In addition, Clostridium butyricum can protect gibel carp (Carassius auratus gibelio) from Carassius auratus herpesvirus infection (18). Lactobacillus comprises an important group of probiotics for both humans and animals. The antiviral effect of Lactobacillus has been reported in mammals (19–22). Similarly, some studies showed that dietary Lactobacillus can protect fish against virus infection (23, 24). The exopolysaccharides (EPS) of Lactobacillus have been found to play an important role in the antiviral function in many studies in mammals (25–29). However, there is little information about the antiviral effect of Lactobacillus EPS in fish.
In this study, we investigated the effects of dietary supplementation of EPS from Lactobacillus rhamnosus GCC-3 on zebrafish against SVCV infection. In addition, GCC-3 EPS was added to ZF4 cells at different concentrations to evaluate its effect on SVCV replication in vitro, and the effect of GCC-3 EPS on the type I IFN signaling pathway was investigated. The gut microbiota of zebrafish fed with GCC-3 EPS was measured by 16S ribosomal RNA (16S rRNA) sequencing and the effect of gut microbiota-mediated antiviral effect was investigated. To our knowledge, this is the first study about the effects of Lactobacillus EPS on the antiviral immunity of fish. Our findings demonstrated that dietary GCC-3 EPS improved the resistance of zebrafish against SVCV infection, which involved enhanced anti-viral IFN immunity after viral infection.
Materials and methods
Lactobacillus rhamnosus GCC-3 exopolysaccharides (GCC-3 EPS)
Lactobacillus rhamnosus GCC-3 was purchased from the China Center of Industrial Culture Collection and preserved by the China-Norway Joint Lab of Fish Gastrointestinal Microbiota of the Chinese Academy of Agricultural Sciences. The culture of GCC-3 and extraction and purification of GCC-3 EPS were conducted according to previous protocols (26).
Fish husbandry and feeding
Zebrafish were provided by China Zebrafish Resource Center. All zebrafish were housed in the laboratory for 2 weeks to reduce stress responses before the start of the experiment. All experimental protocols of zebrafish were agreed the Institute of Feed Research of the Chinese Academy of Agricultural Sciences chaired by the China Council for Animal Care (No. 2018-AF-FRI-CAAS-001). A total of 180 2-month-old zebrafish with the same initial weight were randomly allocated into three groups, each with three repeating tanks and 20 fish. The control diet and GCC-3 EPS diet (0.5% and 1% of GCC-3 EPS) were fed at 6% of body weight every day. The feed formula is shown in Table 1. Water quality was monitored every day with a temperature of 28.5 ± 0.5 °C, and a pH of 6.6 - 7.1. The dissolved oxygen was not less than 6.05 mg O2/L, and the nitrate level was less than 0.11 mg N/L.
Cell lines and virus
Zebrafish embryonic fibroblast (ZF4) cells were purchased from American Type Culture Collection (ATCC number CRL-2050). Epithelioma papulosum cyprini (EPC) cells and SVCV (ATCC: VR-1390) were gifts by professor Jun-Fa Yuan (Huazhong agricultural university, Wuhan, Hubei, China). The SVCV was propagated in EPC cells at 28°C. The virus production was conducted according to previously described protocols (30). ZF4 and EPC cells were cultured at 28 °C in a 5% CO2 incubator in DMEM/F-12 medium (Corning, USA) and MEM medium (Corning, USA) with 10% fetal bovine serum (Gibco, Australia), respectively.
SVCV challenge
SVCV challenge was conducted by the previous description (30). Briefly, fish were transferred to the incubator at 22°C to adapt before the SVCV challenge. The concentration of SVCV bath immersion was 106 TCID50/ml. The death of zebrafish was recorded, and the survival rate was calculated for 14 days. On the 4th day after the challenge, zebrafish were anesthetized with MS222, then the spleen was sampled and RNA was extracted, and the expression of antiviral genes of zebrafish was detected. The primer sequences are listed in Table 2.
Construction of SVCV standard plasmid and detection of whole fish viral load
The SVCV target fragment of PCR amplified was recovered by MiniBEST Agarose Gel DNA Extraction Kit (Takara). The amplified target fragment was inserted into the pLB vector (Tiangen) to construct the pLB-SVCV-FR plasmid, which was used as a standard plasmid to construct a calibration curve for estimating the copies of SVCV. Multiplex real-time quantitative RT-PCR (mqRT-PCR) was used to detect the viral load of whole fish. The concentration of pLB-SVCV-FR plasmid copies was adjusted to 1010 copies/μL. Then 10-109 pLB-SVCV-FR plasmid copies were obtained by continuous dilution. The primer sequence of SVCV G protein was shown in Table 2. The sequence of SVCV G protein P primer is as follows: 5′-FAM-ATGAAGARGAGTAAACKGCCTGCAACAGA-TAMRA-3′. RNA was extracted from the whole zebrafish after the challenge. The cDNA synthesis was performed with the Fast-King gDNA Dispelling RT SuperMix (TIANGEN, KR118) using 1 μg of RNA and following the manufacturer’s indications. The standard plasmid was used to establish the standard curve of virus concentration. The PCR conditions were as follows: 95°C for 2 min, 40 cycles (95°C 3 s and 55°C 30 s). The standard curve was drawn with the CT value and the standard plasmid, and then the viral load was calculated according to the CT value obtained by different groups of whole fish cDNA.
Detection of SVCV resistance of GCC-3 EPS in ZF4 cells
ZF4 cells with a concentration of 105/well were inoculated into a 12-well plate and cultured at 28°C. GCC-3 EPS at the doses of 5, 10, and 15 μg/ml was added to the cell culture and incubated for 12 h. Subsequently, cells were infected with SVCV (0.1 MOI) for 24 h. Afterwards, the supernatant was collected to evaluate the TCID50 of SVCV as described previously (30). The total RNA of cells was extracted, and qPCR was used to determine the expression of the N protein of SVCV. The primer sequence of SVCV N is listed in Table 2.
TBK1 gene silencing with small interfering RNA
In a 12-well plate, ZF4 cells in the logarithmic growth phase were inoculated. The reagent Lipofectamine RNAiMAX Transfection (Invitrogen) was used to transfect scrambled small interfering RNA (siRNA; negative control) and TBK1 siRNA into the plate according to the kit procedure. The sequence of TBK1 siRNA is as follows: Sense: GCGACAUCUUACACCGCAUTT; Antisense: AUGCGGUGUAAGAUGUCGCTT. After 24 h, 5 μg/ml GCC-3 EPS was added. SVCV at a concentration of 0.1MOI was administered after 6 h. qPCR was used to determine knocking down the efficacy of the siRNA therapy as well as the expression of the SVCV N protein.
16S rRNA gene sequencing
After a 2-week feeding, the intestinal contents of zebrafish were collected from each treatment group 4 h after the last feeding. Samples from six fish per tank were pooled as a replicate. DNA extraction, sequencing, and data analysis were conducted as previously described (26). Microbiota sequencing data in this study are available from the National Center for Biotechnology Information (NCBI) under accession number PRJNA841809.
Culture of gut microbiota in vitro
The zebrafish were anesthetized with MS222 6 h after the last feeding. The intestinal contents of 5 fish per group were collected, pooled, and incubated in BHI liquid medium (Haibo, China) in an anaerobic incubator (Electrotek, England) containing 7% oxygen for 24 h and 48 h. The culture supernatants of the microbiota from control and EPS-fed zebrafish were collected by centrifugation and were then filtered through a 0.22 μm sterile tube top filter (Corning Inc., Corning). 30 μL supernatant was added to the ZF4 cell culture system and incubated for 24 h. Then cells were infected with SVCV (0.1 MOI) for 24 h. Afterwards, the cells were collected, and RNA extracted. The expression of the N protein of SVCV was quantified by qPCR to evaluate virus replication.
Data analysis
Data were analyzed by GraphPad Prism 8.0. The Student’s t-test was used to compare the differences between the two groups. The survival rate after the SVCV challenge was analyzed by Log-rank (Mantel-Cox). Data were presented as mean ± SEMs. When P values were less than 0.05, the difference was considered significant.
Results
Effects of GCC-3 EPS on the antiviral ability of zebrafish
As shown in Figure 1, compared with the control group, dietary supplementation of 0.5% and 1% GCC-3 EPS significantly improved the survival rate of zebrafish post SVCV challenge after a 14-day feeding (Figure 1, P < 0.05). On the 5th and 6th days after the SVCV challenge, the viral load of the whole fish was detected. It was found that the load of SVCV in the whole fish of 0.5% and 1% GCC-3 EPS groups was significantly decreased (Supplemental Figure 1, P < 0.05).
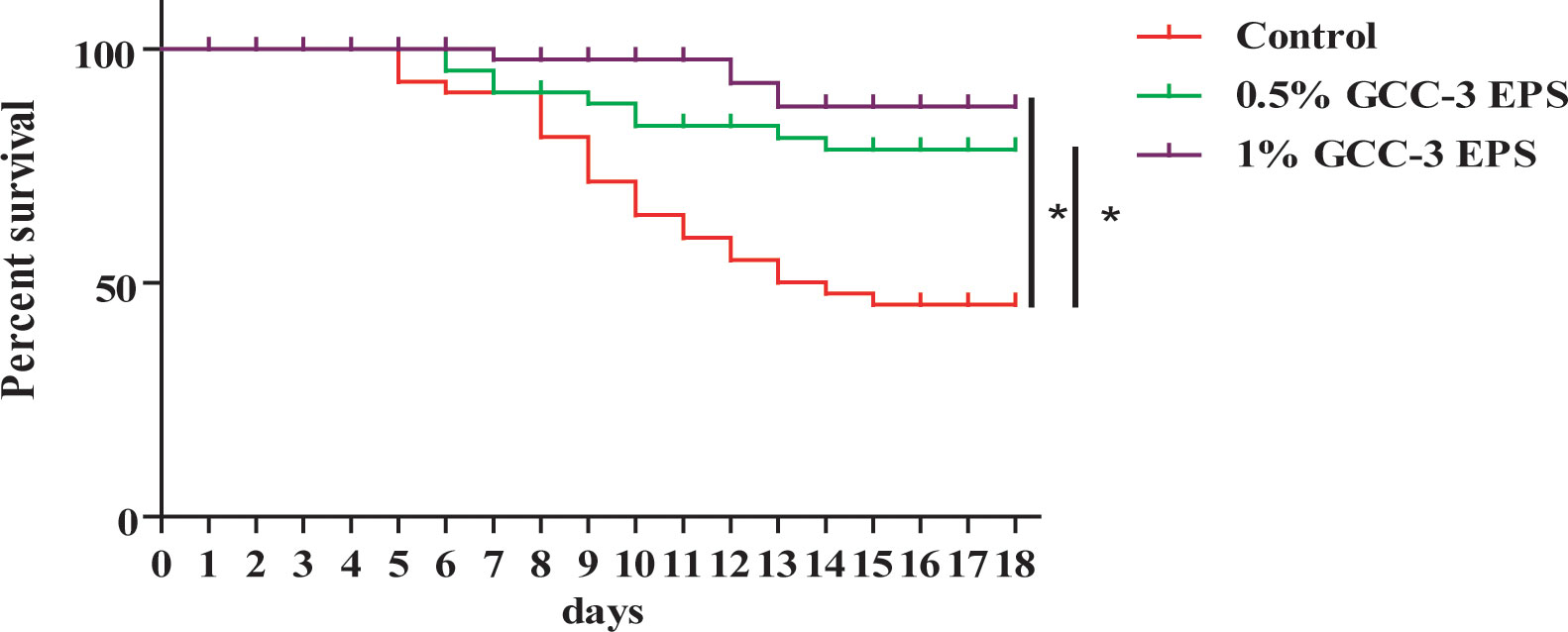
Figure 1 Effects of GCC-3 EPS supplementation on survival rate after SVCV infection. *, P < 0.05 compared to the control group. Log-rank (Mantel-Cox) test for survival rate.
Effects of GCC-3 EPS on the antiviral immune response of zebrafish
As shown in Figure 2, dietary 0.5% and 1% GCC-3 EPS significantly up-regulated the expression of type I IFN genes in the spleen including ifn-φ 1、ifn-φ 2、ifn-φ 3 and the expression of IFN-stimulated genes including mxb and mxc (P < 0.05). In addition, 0.5% and 1% GCC-3 EPS supplementation significantly increased the expression of virus recognition receptors related genes including tlr7 and rig-I as well as the expression of downstream genes of IFN pathway such as mavs, irf3 and irf7 (Figure 2, P < 0.05).
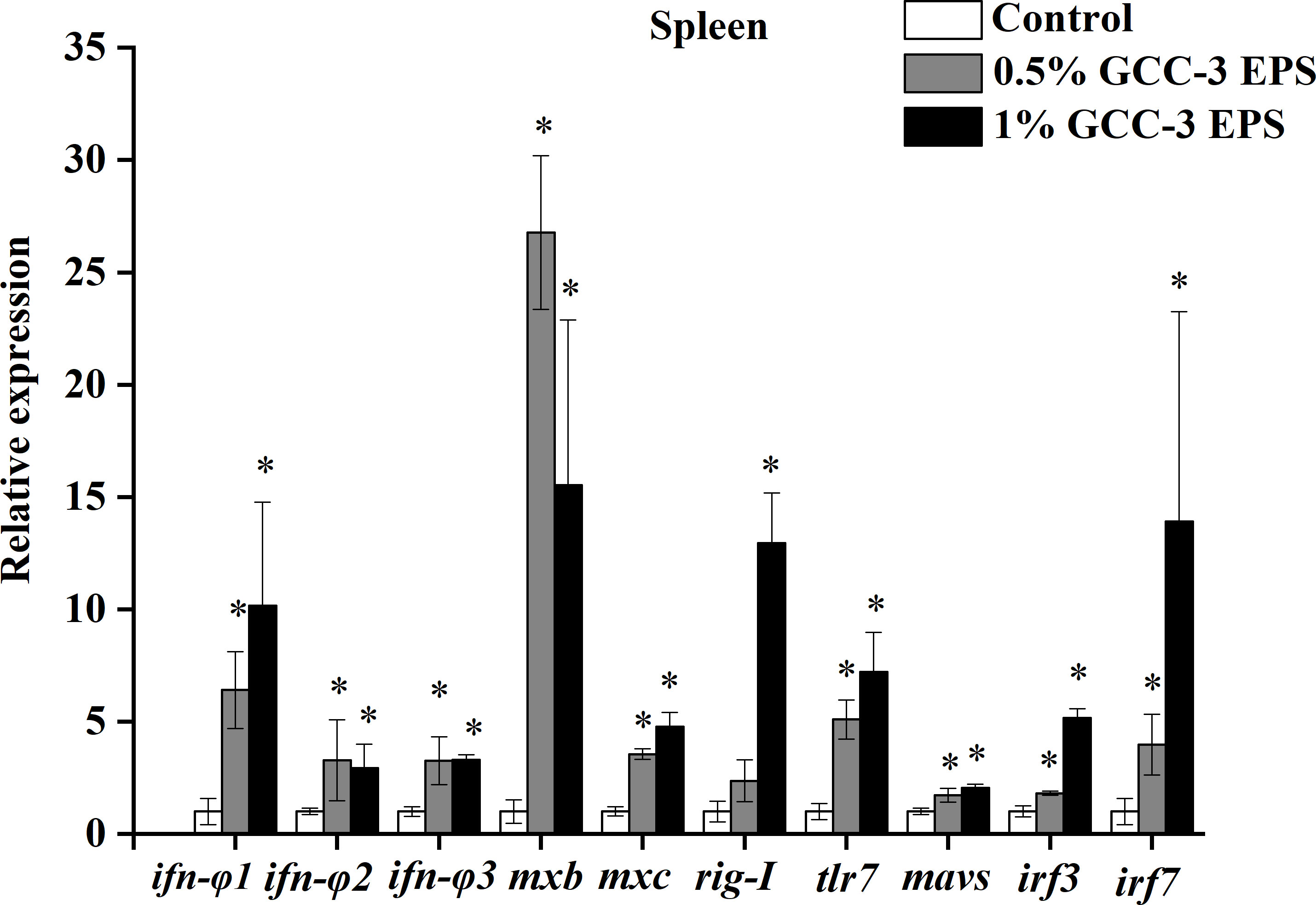
Figure 2 Relative mRNA expression of genes related to antiviral immunity in the spleen of zebrafish fed GCC-3 EPS and control diets after SVCV challenge. Data were expressed as the means ± SEMs (n = 6 biological replicates). *, P < 0.05 compared to the control group. (Student’s t test).
Effects of GCC-3 EPS on antiviral ability in ZF4 cells
GCC-3 EPS significantly reduced the expression of SVCV N protein at the three doses, indicating an inhibitory effect on viral replication (Figure 3A, P < 0.05). The expression of SVCV N protein in the 5 μg/mL GCC-3 EPS group was the lowest and therefore was selected for subsequent experiments. Consistent with the qPCR results, 5 μg/mL GCC-3 EPS significantly reduced SVCV titer in the supernatant of infected ZF4 cells (Figure 3B, P < 0.05).
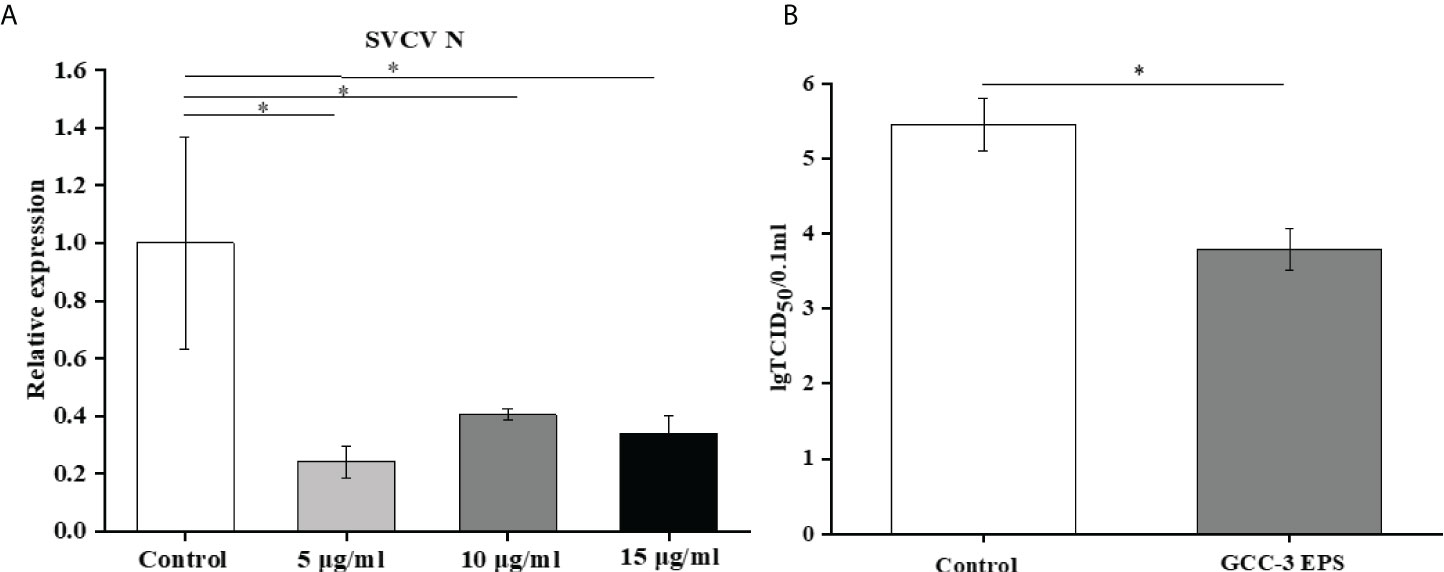
Figure 3 GCC-3 EPS suppressed SVCV replication in cells (A) and viral titer in supernatant of infected zebrafish embryonic fibroblast cells (B). Data were expressed as the means ± SEMs (n = 5~6 biological replicates). *, P < 0.05 compared to the control group.
The antiviral function of GCC-3 EPS depends on the type I interferon signaling pathway
To eliminate the confounding effect of viral replication on antiviral gene expression, viral RNA mimics polyriboinosinic polyribocytidylic acid (poly (I: C)) (10 μg/ml) was transfected into ZF4 cells after GCC-3 EPS treatment, and the expression of genes related to the type I IFN signaling pathway was detected. Results showed that 5 μg/mL GCC-3 EPS treatment significantly increased the expression of ifn-φ 1、ifn-φ 2、ifn-φ 3、mxb and mxc after poly (I:C) stimulation compared with control, supporting that GCC-3 EPS can stimulate the type I IFN antiviral immunity (Figure 4A, P < 0.05). Therefore, GCC-3 EPS might exert antiviral effect by activating the IFN signaling pathway. To verify this hypothesis, siRNA of TBK1 was used to knock down the TBK1 gene in ZF4 cells (Figure 4B). After SVCV infection, the expression of SVCV N protein was detected to evaluate SVCV replication. Knocking down TBK1 significantly enhanced the expression of SVCV N protein in ZF4 cells versus control siRNA counterpart groups (Figure 4C), indicating the importance of IFN signaling pathway in suppressing SVCV infection. Interestingly, we found that after knocking down TBK1, there was no significant difference in SVCV replication between GCC-3 EPS group and control (Figure 4C, P > 0.05), supporting that the antiviral effect of GCC-3 EPS was mediated by type I IFN signaling pathway.
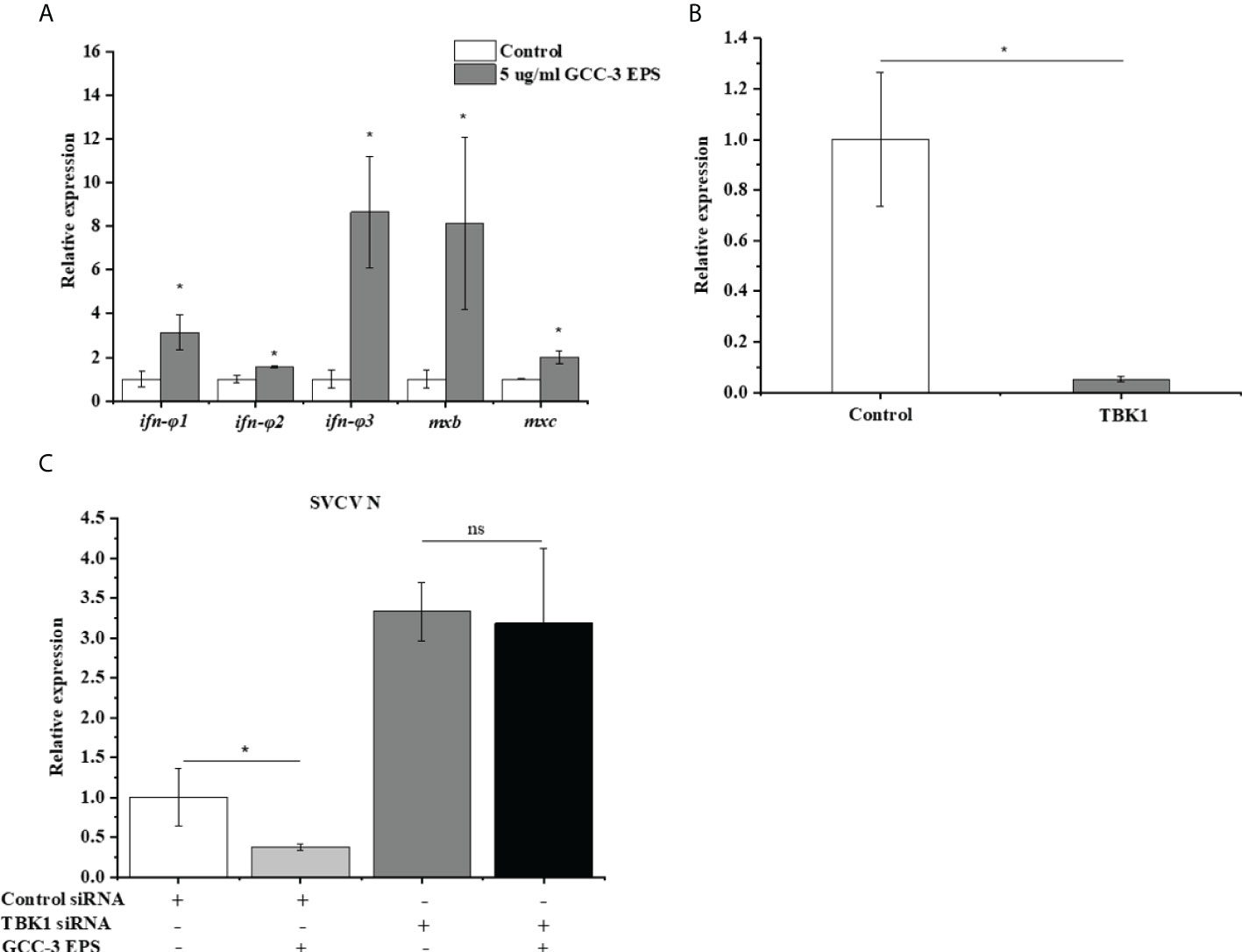
Figure 4 GCC-3 EPS activated the expression of genes related to type I interferon signaling pathway in zebrafish embryonic fibroblast cells (A). Effect of small interfering RNA (siRNA) targeting TANK-binding kinase 1 (TBK1) on the expression of TBK1 (B). Knocking down TBK1 abrogated the antiviral effect of GCC-3 EPS (C) in zebrafish embryonic fibroblast cells. Data were expressed as the means ± SEMs (n = 5~6 biological replicates). *, P < 0.05 compared to the control group.
Effects of GCC-3 EPS on the gut microbiota of zebrafish
Compared to the control group, dietary GCC-3 EPS decreased the relative abundance of Proteobacteria and Actinobacteria while increased Firmicutes abundance at the phylum level (Figure 5A). At the genus level, the addition of GCC-3 EPS increased the abundance of Lactobacillus and Bifidobacterium (Figure 5B). Principal coordinate analysis (PCoA) analysis showed that there was a significant difference in the composition of gut microbiota between the control group and the GCC-3 EPS group at the phylum and genus level (Figure 6).
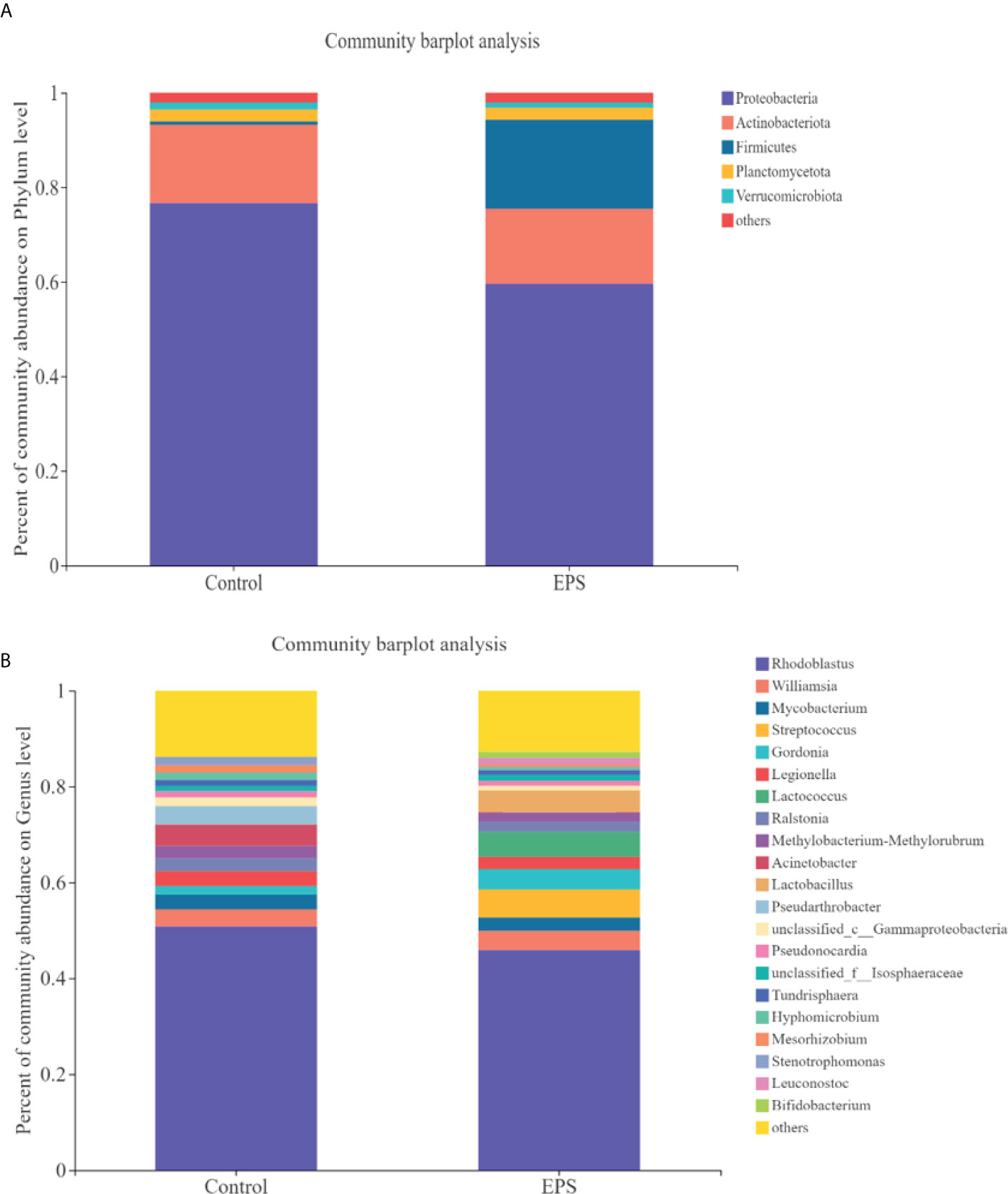
Figure 5 Effects of GCC-3 EPS on the composition of gut microbiota of zebrafish. Staked bar chart of relative abundance of the intestinal microbiota at the phylum level (A) and genus level (B). Data were expressed as the means ± SEMs (n = 6 biological replicates). EPS represents the group of 1% GCC-3 EPS.
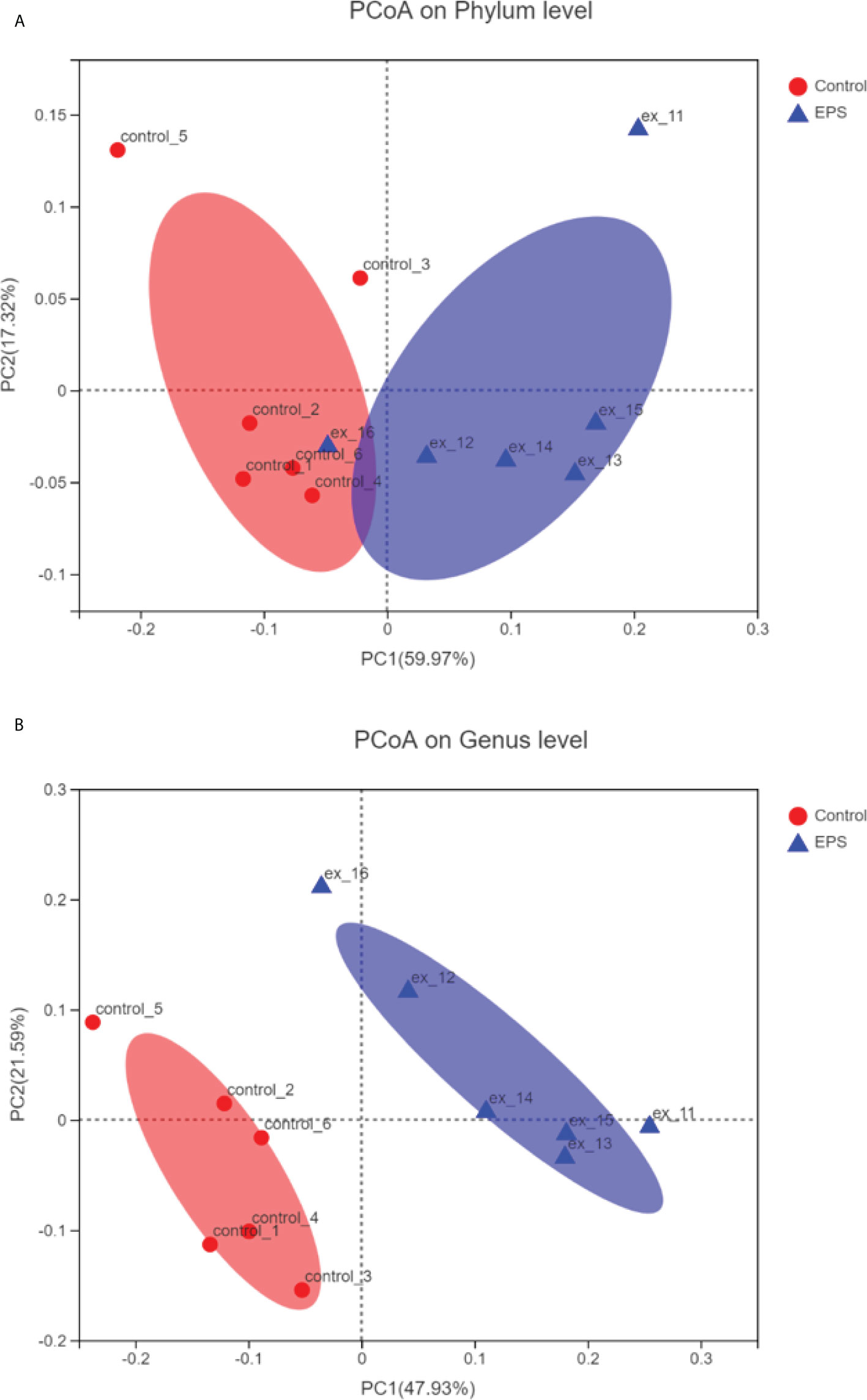
Figure 6 Principal coordinate analysis (PCoA) of control- and GCC-3 EPS-associated microbiota at the phylum level (A) and genus level (B). Data were expressed as the means ± SEMs (n = 6 biological replicates). EPS represents the group of 1% GCC-3 EPS.
Effects of culture supernatant of GCC-3 EPS-associated microbiota on SVCV replication
The GCC-3 EPS-microbiota and control microbiota was suspended in BHI medium and were cultured in an anaerobic incubator containing 7% oxygen for 24 h and 48 h. The culture supernatants were added to ZF4 cells and the expression of SVCV N protein was detected after SVCV addition for 24 h. Results showed that the culture supernatant of GCC-3 EPS-associated microbiota significantly inhibited the expression of SVCV N protein of SVCV in ZF4 cells compared with the counterpart of control microbiota, suggesting that the GCC-3 EPS microbiota contributed to the antiviral effect (Figure 7, P < 0.05).
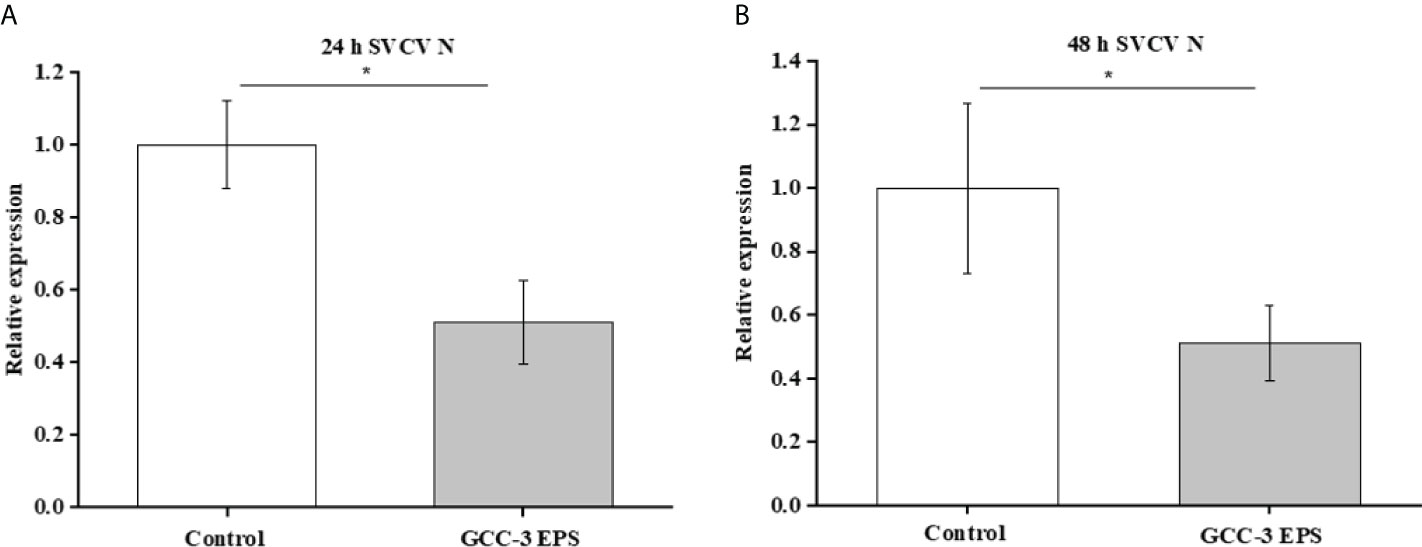
Figure 7 In vitro culture supernatant of gut microbiota of 1% GCC-3 EPS fed zebrafish suppressed SVCV replication in zebrafish embryonic fibroblast cells versus control microbiota counterpart. (A) 24 h culture supernatant, (B) 48 h culture supernatant. Data were expressed as the means ± SEMs (n = 6 biological replicates). *, P < 0.05 compared to the control group.
Discussion
Dietary Lactobacillus EPS is known to have beneficial effects on the function of immune regulation (25–29). However, the effect of Lactobacillus-derived EPS on the antiviral ability of fish has not been reported. In the present work, 0.5% and 1% GCC-3 EPS were added to the diet of zebrafish, followed by SVCV challenge after a 14-day feeding. Results showed dietary 0.5% and 1% GCC-3 EPS improved the survival rate of zebrafish. Spleen is an important immune organ of fish (31). SVCV has been reported to attack the spleen after entering fish (2). Therefore, the spleen was collected to detect the expression of antiviral genes. We found that dietary 0.5% and 1% GCC-3 EPS increased the expression of type I IFN genes and IFN-stimulated genes in the spleen. IFN system is critical in combating viruses and can control the majority of viral infections in the absence of adaptive immunity (32, 33). Furthermore, dietary 0.5% and 1% GCC-3 EPS also up-regulated the expression of virus recognition receptors related genes including tlr7 and rig-I and downstream genes of virus recognition receptors activation including mavs, irf3 and irf7 in the spleen. rig-I and tlr7 are important cytoplasmic pattern-recognition receptors that stimulate the production of type I IFN in host innate immunity (34, 35). After activation, they can activate the expression of downstream genes including mavs, irf3 and irf7 (36). Taken together, these results showed that GCC-3 EPS enhanced the antiviral immune response of zebrafish, and the type I interferon signaling pathway might play an important role.
To further investigate the underlying mechanisms of the antiviral effect of GCC-3 EPS, we conducted experiments in ZF4 cells. Consistent with the in vivo results, we found that the addition of GCC-3 EPS reduced the expression of SVCV N protein, and the dose of 5 μg/mL exhibited the best effect. Poly(I:C) has been shown to be the most effective inducer of type I IFN (37, 38). The present results further demonstrated that GCC-3 EPS induced higher expression of type I IFN signaling pathway related genes after poly (I:C) stimulation versus control, indicating that GCC-3 EPS can activate the type I IFN signaling pathway at the cellular level. TANK-binding kinase 1 (TBK1) is a critical protein kinase in the synthesis of type I IFN and is important in antiviral innate immune response (39, 40). TBK1 can be activated by the mitochondrial antiviral signaling protein (mavs) signaling pathway mediated by the retinoic acid-inducible gene-I (rig-I) receptor in the cytoplasm (41). The activation of TBK1 affects the phosphorylation of IFN regulatory factor 3 and 7 (IRF3 and IRF7), which can induce the expression of type I IFN (42, 43). Notably, knockdown of the TBK1 gene blocked the effect of GCC-3 EPS on antiviral ability, indicating that the type I IFN signaling pathway is essential for the inhibitory effect of GCC-3 EPS against SVCV infection (44).
EPS is used as the source of energy and nutrients available to the gut microbiota and its addition can alter the composition of gut microbiota (45). Studies have shown that gut microbiota plays an important role in virus infection (13, 17, 46). In the present study, dietary GCC-3 EPS improved the composition of gut microbiota of zebrafish by increasing Firmicutes abundance and decreasing the relative abundance of Proteobacteria and Actinobacteria. PCoA analysis showed that there was a big difference in the composition of gut microbiota between the control group and the GCC-3 EPS group. Previous studies indicated that some bacterial species belonging to Firmicutes can protect fish against viral infection (17, 23, 24), suggesting that the higher abundance of Firmicutes might lead to an enhanced antiviral effect of EPS-associated microbiota. To initially evaluate whether GCC-3 EPS-associated microbiota contributes to the antiviral effect, we added the culture supernatant of GCC-3 EPS-associated microbiota to ZF4 cells and found the replication of SVCV in ZF4 cells was reduced as compared with the control microbiota culture supernatant group. These findings suggest that GCC-3 EPS-associated microbiota might contribute to the prevention of SVCV infection. However, the associated effectors need further investigation.
In conclusion, the present work indicates that dietary GCC-3 EPS can improve the antiviral ability of zebrafish. Studies using ZF4 cells showed that the antiviral function of GCC-3 EPS depended on the type I interferon signaling pathway. Further results showed that GCC-3 EPS altered the microbiota, and the EPS-associated microbiota might contribute to the antiviral effect. As a whole, our results provide novel insights into the antiviral effect of dietary supplemented Lactobacillus EPS against SVCV in zebrafish.
Data availability statement
The datasets presented in this study can be found in online repositories. The names of the repository/repositories and accession number(s) can be found below: https://www.ncbi.nlm.nih.gov/, PRJNA841809.
Ethics statement
The animal study was reviewed and approved by the Institute of Feed Research of the Chinese Academy of Agricultural Sciences chaired by the China Council for Animal Care (No. 2018-AF-FRI-CAAS-001).
Author contributions
MX: wrote the paper and conducted the research; YL: conducted the research, formal analysis, and data curation; RO: resources and methodology; ER: resources and methodology; YY: analyzed the data; ZZ: analyzed the data; CR: research design, data curation, funding acquisition, and revising the draft; ZGZ: research design, data curation, funding acquisition, and revising the draft. All authors contributed to the article and approved the submitted version.
Funding
This study was funded by National Key R&D Program of China (2018YFD0900400) and National Natural Science Foundation of China (31925038, 32122088).
Conflict of interest
The authors declare that the research was conducted in the absence of any commercial or financial relationships that could be construed as a potential conflict of interest.
Publisher’s note
All claims expressed in this article are solely those of the authors and do not necessarily represent those of their affiliated organizations, or those of the publisher, the editors and the reviewers. Any product that may be evaluated in this article, or claim that may be made by its manufacturer, is not guaranteed or endorsed by the publisher.
Supplementary material
The Supplementary Material for this article can be found online at: https://www.frontiersin.org/articles/10.3389/fimmu.2022.968348/full#supplementary-material
References
1. Ke F, Zhang QY. Aquatic animal viruses mediated immune evasion in their host. Fish Shellfish Immunol (2019) 86:1096–105. doi: 10.1016/j.fsi.2018.12.027
2. Zhu F. A review on the application of herbal medicines in the disease control of aquatic animals. Aquaculture (2020) 526:735422. doi: 10.1016/j.aquaculture.2020.735422
3. Wang Y, Zhang H, Lu Y, Wang F, Liu L, Liu J, et al. Comparative transcriptome analysis of zebrafish (Danio rerio) brain and spleen infected with spring viremia of carp virus (SVCV). Fish Shellfish Immunol (2017) 69:35–45. doi: 10.1016/j.fsi.2017.07.055
4. Xie M, Xie Y, Li Y, Zhou W, Zhang Z, Yang Y, et al. Stabilized fermentation product of Cetobacterium somerae improves gut and liver health and antiviral immunity of zebrafish. Fish Shellfish Immunol (2022) 120:56–66. doi: 10.1016/j.fsi.2021.11.017
5. Ahne W, Bjorklund HV, Essbauer S, Fijan N, Kurath G, Winton JR. Spring viremia of carp (SVC). Dis Aquat Organ (2002) 52(3):261–72. doi: 10.3354/dao052261
6. Basic A, Schachner O, Bilic I, Hess M. Phylogenetic analysis of spring viraemia of carp virus isolates from Austria indicates the existence of at least two subgroups within genogroup id. Dis Aquat Organ (2009) 85(1):31–40. doi: 10.3354/dao02069
7. Platanias LC. Mechanisms of type-i- and type-II-interferon-mediated signaling. Nat Rev Immunol (2005) 5(5):375–86. doi: 10.1038/nri1604
8. Guan XL, Zhang BC, Sun L. Pol-miR-194a of Japanese flounder (Paralichthys olivaceus) suppresses type I interferon response and facilitates Edwardsiella tarda infection. Fish Shellfish Immunol (2019) 87:220–5. doi: 10.1016/j.fsi.2019.01.017
9. Robertsen B. The interferon system of teleost fish. Fish Shellfish Immunol (2006) 20(2):172–91. doi: 10.1016/j.fsi.2005.01.010
10. Gan Z, Chen SN, Huang B, Zou J, Nie P. Fish type I and type II interferons: composition, receptor usage, production and function. Rev Aquac (2020) 12(2):773–804. doi: 10.1111/raq.12349
11. Langevin C, Aleksejeva E, Passoni G, Palha N, Levraud JP, Boudinot P. The antiviral innate immune response in fish: evolution and conservation of the IFN system. J Mol Biol (2013) 425(24):4904–20. doi: 10.1016/j.jmb.2013.09.033
12. Mazel-Sanchez B, Yildiz S, Schmolke M. Ménage à trois: Virus, host, and microbiota in experimental infection models. Trends Microbiol (2019) 27(5):440–52. doi: 10.1016/j.tim.2018.12.004
13. Ran C, Li Y, Ma X, Xie Y, Xie M, Zhang Y, et al. Interactions between commensal bacteria and viral infection: insights for viral disease control in farmed animals. Sci China Life Sci (2021) 64(9):1437–48. doi: 10.1007/s11427-020-1721-5
14. Ichinohe T, Pang IK, Kumamoto Y, Peaper DR, Ho JH, Murray TS, et al. Microbiota regulates immune defense against respiratory tract influenza a virus infection. Proc Natl Acad Sci U S A. (2011) 108(13):5354–9. doi: 10.1073/pnas.1019378108
15. Steed AL, Christophi GP, Kaiko GE, Sun L, Goodwin VM, Jain U, et al. The microbial metabolite desaminotyrosine protects from influenza through type I interferon. Sci (New York N.Y.) (2017) 357(6350):498–502. doi: 10.1126/science.aam5336
16. Galindo-Villegas J, García-Moreno D, de Oliveira S, Meseguer J, Mulero V. Regulation of immunity and disease resistance by commensal microbes and chromatin modifications during zebrafish development. Proc Natl Acad Sci U S A. (2012) 109:E2605–14. doi: 10.1073/pnas.1209920109
17. Zhou S, Song D, Zhou X, Mao X, Zhou X, Wang S, et al. Characterization of Bacillus subtilis from gastrointestinal tract of hybrid hulong grouper (Epinephelus fuscoguttatus × E. lanceolatus) and its effects as probiotic additives. Fish Shellfish Immunol (2019) 84:1115–24. doi: 10.1016/j.fsi.2018.10.058
18. Li T, Ke F, Gui JF, Zhou L, Zhang XJ, Zhang QY. Protective effect of clostridium butyricum against Carassius auratus herpesvirus in gibel carp. Aquacult Int (2019) 27:905–14. doi: 10.1007/s10499-019-00377-3
19. Jung YJ, Lee YT, Ngo VL, Cho YH, Ko EJ, Hong SM, et al. Heat-killed Lactobacillus casei confers broad protection against influenza a virus primary infection and develops heterosubtypic immunity against future secondary infection. Sci Rep (2017) 7(1):17360. doi: 10.1038/s41598-017-17487-8
20. Tukenmez U, Aktas B, Aslim B, Yavuz S. The relationship between the structural characteristics of lactobacilli-EPS and its ability to induce apoptosis in colon cancer cells in vitro. Sci Rep (2019) 9(1):1–14. doi: 10.1038/s41598-019-44753-8
21. Takahashi E, Sawabuchi T, Kimoto T, Sakai S, Kido H. Lactobacillus delbrueckii ssp. bulgaricus OLL1073R-1 feeding enhances humoral immune responses, which are suppressed by the antiviral neuraminidase inhibitor oseltamivir in influenza a virus-infected mice. J Dairy Sci (2019) 102(11):9559–69. doi: 10.3168/jds.2019-16268
22. Waki N, Yajima N, Suganuma H, Buddle BM, Luo D, Heiser A, et al. Oral administration of Lactobacillus brevis KB290 to mice alleviates clinical symptoms following influenza virus infection. Lett Appl Microbiol (2014) 58(1):87–93. doi: 10.1111/lam.12160
23. Harikrishnan R, Balasundaram C, Heo MS. Effect of probiotics enriched diet on paralichthys olivaceus infected with lymphocystis disease virus (LCDV). Fish Shellfish Immunol (2010) 29:868–74. doi: 10.1016/j.fsi.2010.07.031
24. Son VM, Chang CC, Wu MC, Guu YK, Chiu CH, Cheng W. Dietary administration of the probiotic, Lactobacillus plantarum, enhanced the growth, innate immune responses, and disease resistance of the grouper epinephelus coioides. Fish Shellfish Immunol (2009) 26:691–8. doi: 10.1016/j.fsi.2009.02.018
25. Kim K, Lee G, Thanh HD, Kim JH, Konkit M, Yoon S, et al. Exopolysaccharide from Lactobacillus plantarum LRCC5310 offers protection against rotavirus-induced diarrhea and regulates inflammatory response. J Dairy Sci (2018) 101(7):5702–12. doi: 10.3168/jds.2017-14151
26. Zhang Z, Ran C, Ding QW, Liu HL, Xie MX, Yang YL, et al. Ability of prebiotic polysaccharides to activate a HIF1α-antimicrobial peptide axis determines liver injury risk in zebrafish. Commun Biol (2019) 2:274. doi: 10.1038/s42003-019-0526-z
27. Zhang Z, Zhou Z, Li Y, Zhou L, Ding Q, Xu L. Isolated exopolysaccharides from Lactobacillus rhamnosus GG alleviated adipogenesis mediated by TLR2 in mice. Sci Rep (2016) 6:36083. doi: 10.1038/srep36083
28. Kanmani P, Albarracin L, Kobayashi H, Iida H, Komatsu R, Humayun Kober A, et al. Exopolysaccharides from Lactobacillus delbrueckii OLL1073R-1 modulate innate antiviral immune response in porcine intestinal epithelial cells. Mol Immunol (2018) 93:253–65. doi: 10.1016/j.molimm.2017.07.009
29. Nagai T, Makino S, Ikegami S, Itoh H, Yamada H. Effects of oral administration of yogurt fermented with Lactobacillus delbrueckii ssp. bulgaricus OLL1073R-1 and its exopolysaccharides against influenza virus infection in mice. Int Immunopharmacol (2011) 11(12):2246–50. doi: 10.1016/j.intimp.2011.09.012
30. Li Y, Ran C, Wei K, Xie Y, Xie M, Zhou W, et al. The effect of astragalus polysaccharide on growth, gut and liver health, and anti-viral immunity of zebrafish. Aquaculture (2021) 540:736677. doi: 10.1016/j.aquaculture.2021.736677
31. Zou J, Carrington A, Collet B, Dijkstra JM, Yoshiura Y, Bols N, et al. Identification and bioactivities of IFN-gamma in rainbow trout Oncorhynchus mykiss: the first Th1-type cytokine characterized functionally in fish. J Immunol (2005) 175(4):2484–94. doi: 10.4049/jimmunol.175.4.2484
32. Randall RE, Goodbourn S. Interferons and viruses: an interplay between induction, signalling, antiviral responses and virus countermeasures. J Gen Virol (2008) 89(Pt 1):1–47. doi: 10.1099/vir.0.83391-0
33. Varela M, Figueras A, Novoa B. Modelling viral infections using zebrafish: Innate immune response and antiviral research. Antiviral Res (2017) 139:59–68. doi: 10.1016/j.antiviral.2016.12.013
34. Hornung V, Ellegast J, Kim S, Brzózka K, Jung A, Kato H, et al. 5'-triphosphate RNA is the ligand for RIG-I. Science (2006) 314(5801):994–7. doi: 10.1126/science.1132505
35. Yoneyama M, Kikuchi M, Natsukawa T, Shinobu N, Imaizumi T, Miyagishi M, et al. The RNA helicase RIG-I has an essential function in double-stranded RNA-induced innate antiviral responses. Nat Immunol (2004) 5(7):730–7. doi: 10.1038/ni1087
36. Zou PF, Chang MX, Li Y, Zhang SH, Fu JP, Chen SN, et al. Higher antiviral response of RIG-I through enhancing RIG-I/MAVS-mediated signaling by its long insertion variant in zebrafish. Fish Shellfish Immunol (2015) 43(1):13–24. doi: 10.1016/j.fsi.2014.12.001
37. Field A, Tytell A, Lampson G, Hilleman M. Inducers of interferon and host resistance. II. multistranded synthetic polynucleotide complexes. Proc Natl Acad Sci U.S.A. (1967) 58(3):1004. doi: 10.1073/pnas.58.3.1004
38. Matsumoto M, Seya T. TLR3: interferon induction by double-stranded RNA including poly (I: C). Adv Drug Delivery Rev (2008) 60(7):805–12. doi: 10.1016/j.addr.2007.11.005
39. Matsui K, Kumagai Y, Kato H, Sato S, Kawagoe T, Uematsu S, et al. Cutting edge: Role of TANK-binding kinase 1 and inducible IkappaB kinase in IFN responses against viruses in innate immune cells. J Immunol (Baltimore Md.: 1950) (2006) 177(9):5785–9. doi: 10.4049/jimmunol.177.9.5785
40. Zhao C, Zhao W. TANK-binding kinase 1 as a novel therapeutic target for viral diseases. Expert Opin Ther Targets (2019) 23(5):437–46. doi: 10.1080/14728222.2019.1601702
41. Li S, Lu LF, Wang ZX, Chen DD, Zhang YA. Fish IRF6 is a positive regulator of IFN expression and involved in both of the MyD88 and TBK1 pathways. Fish Shellfish Immunol (2016) 57:262–8. doi: 10.1016/j.fsi.2016.08.059
42. Fitzgerald KA, McWhirter SM, Faia KL, Rowe DC, Latz E, Golenbock DT, et al. IKKepsilon and TBK1 are essential components of the IRF3 signaling pathway. Nat Immunol (2003) 4(5):491–6. doi: 10.1038/ni921
43. Liu S, Cai X, Wu J, Cong Q, Chen X, Li T, et al. Phosphorylation of innate immune adaptor proteins MAVS, STING, and TRIF induces IRF3 activation. Sci (New York N.Y.) (2015) 347(6227):aaa2630. doi: 10.1126/science.aaa2630
44. Chen H, Jiang Z. The essential adaptors of innate immune signaling. Protein Cell (2013) 4(1):27–39. doi: 10.1007/s13238-012-2063-0
45. Ma C, Guo H, Chang H, Huang S, Jiang S, Huo D, et al. The effects of exopolysaccharides and exopolysaccharide-producing Lactobacillus on the intestinal microbiome of zebrafish (Danio rerio). BMC Microbiol (2020) 20(1):1–11. doi: 10.1186/s12866-020-01990-6
Keywords: GCC-3 EPS, gut microbiota, spring viremia of carp virus, type I IFN, zebrafish
Citation: Xie M, Li Y, Olsen RE, Ringø E, Yang Y, Zhang Z, Ran C and Zhou Z (2022) Dietary supplementation of exopolysaccharides from Lactobacillus rhamnosus GCC-3 improved the resistance of zebrafish against spring viremia of carp virus infection. Front. Immunol. 13:968348. doi: 10.3389/fimmu.2022.968348
Received: 15 June 2022; Accepted: 12 July 2022;
Published: 05 August 2022.
Edited by:
Ming Xian Chang, Institute of Hydrobiology (CAS), ChinaCopyright © 2022 Xie, Li, Olsen, Ringø, Yang, Zhang, Ran and Zhou. This is an open-access article distributed under the terms of the Creative Commons Attribution License (CC BY). The use, distribution or reproduction in other forums is permitted, provided the original author(s) and the copyright owner(s) are credited and that the original publication in this journal is cited, in accordance with accepted academic practice. No use, distribution or reproduction is permitted which does not comply with these terms.
*Correspondence: Zhigang Zhou, emhvdXpoaWdhbmcwM0BjYWFzLmNu; Chao Ran, cmFuY2hhb0BjYWFzLmNu
†These authors have contributed equally to this work and share first authorship