- 1School of Agriculture, Ludong University, Yantai, China
- 2College of Life Sciences, Yantai University, Yantai, China
Sepia esculenta is a popular economic cephalopod with high yield, delicious meat, and rich nutrition. With the rapid development of heavy industry and medical industry, a large amount of waste has been released into the ocean recklessly in recent years, inducing a significant increase in the content of heavy metals, especially cadmium (Cd) and copper (Cu), in the ocean. This phenomenon significantly affects the growth and development of S. esculenta, causing a serious blow to its artificial breeding. In this study, transcriptome analysis is used to initially explore immune response mechanisms of Cd and Cu co-exposed juvenile S. esculenta. The results show that 1,088 differentially expressed genes (DEGs) are identified. And DEGs functional enrichment analysis results suggests that co-exposure may promote inflammatory and innate immune responses in juvenile S. esculenta. Fifteen key genes that might regulate the immunity of S. esculenta are identified using protein-protein interaction (PPI) network and KEGG enrichment analyses, of which the three genes with the highest number of interactions or involve in more KEGG pathways are identified as hub genes that might significantly affect the immune response processes. Comprehensive analysis of PPI network and KEGG signaling pathway is used for the first time to explore co-exposed S. esculenta juvenile immune response processes. Our results preliminarily reveal immune response mechanisms of cephalopods exposed to heavy metals and provide a valuable resource for further understanding of mollusk immunity.
1 Introduction
Heavy metals were metallic elements which were difficult to be degraded and easy to accumulate in organisms (1, 2). Due to high toxicity, strong persistence, wide sources, and strong destructive of heavy metals, heavy metal pollution, especially in the ocean, has attracted attention of various countries (3, 4). In recent years, more and more effluent and waste were discharged into oceans with the rapid development of petrochemical industry, fishery, medical industry, and agriculture, resulting in the continuous increase of heavy metal content, especially Cu and Cd, in nearshore oceans, inducing a large amount of heavy metals to accumulate in marine organisms, whether they are caught or cultured, and ultimately have a certain impact on human health (5–8). Organisms living in oceans with heavy metals would accumulate large amounts of heavy metals, which significantly affected the growth, immunity, metabolism, and other processes (4, 9–11). For instance, Sfakianakis et al. found that heavy metal accumulation would induce fish deformities, which had a devastating impact on the growth and survival of fish (12). And Ivanina et al. indicated that heavy metal exposure significantly affected the immune, inflammation, metabolic, and oxidation processes of oyster (13). Cadmium (Cd) and copper (Cu) were toxic heavy metals found in the world’s oceans. They could accumulate in aquatic organisms and could significantly affect the growth, development, movement, and reproduction of them after certain accumulation levels (12, 14–19). For cephalopods, previous studies have shown that Cu can induce octopus tissue peroxidation to induce oxidative damage (20); and Cd was found to accumulate abundantly in digestive glands and induce toxic responses (21). Meanwhile, the immune responses of organisms exposed to Cd or Cu alone were significantly affected. Xie and Wen et al. found that Cd exposure suppressed expressions of immune-related genes in fish, thereby inhibiting the immune response processes (22, 23). And Sheir et al. indicated that Cd could inhibit the innate immune response of shellfish (24). Meanwhile, Cu has been reported in previous research to significantly inhibit the functions of immune cells, and inhibit lysozyme activity and phagocytosis, resulting in immune system disorders (25–27). Hitherto, the effects of Cd and Cu co-exposure on immune response mechanisms have been rarely studied in marine organisms and have not been studied in cephalopods.
Golden cuttlefish (Sepia esculenta) was an important economic cephalopod in the world, mainly distributed in the surrounding waters of eastern China, South Korea and the Philippines (28–31). It was loved by people because of its rich nutrition and valuable medicinal value (31, 32). Because of overfishing and harsh ocean conditions, the number of S. esculenta wild stocks has declined sharply, and it would even become an endangered species in recent years (33, 34). Under natural conditions, juvenile S. esculenta was spawned and hatched in the shallow water near the coast. Juveniles were vulnerable to heavy metal stress, which significantly affected their biological processes and reduced their hatchability and survival rates (35). Therefore, in order to ensure that wild resources would not be exhausted, it was urgent to study the effects of heavy metals on S. esculenta juvenile biological processes. Previous studies have shown that Cd and Cu inhibited the growth and development of mollusks and affected their immune response processes (24–26). However, immune response mechanisms of co-exposure on S. esculenta have not been widely studied and needed to be further explored.
High-throughput RNA-sequencing (RNA-Seq) was a significant technique for exploring differences between samples at the molecular level. And it was used to study the molecular functions of organisms exposed to heavy metals in recent years. For instance, Zhou et al. found that the processes of endoplasmic reticulum stress, protein modification and apoptosis of Pocillopora damicornis changed significantly after Cd exposure through transcriptome (36). And Zhao et al. indicated that Cu exposure induced the oxidative stress in the testis of Procambarus clarkii (37). Similarly, it could also be used to explore how Cd and Cu affected the immune response processes of S. esculenta at the molecular level.
In our research, we carried out transcriptome sequencing of primary hatching juvenile S. esculenta with 24 h Cd and Cu co-exposure. GO, KEGG, and protein-protein interaction (PPI) network analyses based on DEGs were used to explore key genes and pathways affecting juvenile biological functions. We first studied S. esculenta juvenile immune response mechanisms after co-exposure based on comprehensive analysis of KEGG and PPI network. Our results laid a function for exploring the effects of heavy metal pollution on biological processes of cephalopods, and further deepen researchers’ understanding of changes in immune response processes of mollusks after environmental stress.
2 Materials and methods
2.1 S. esculenta breeding and sample collection
Sepia esculenta parents in spawning period were caught in the Yellow Sea in mid-July. After a short transport, S. esculenta were temporarily reared in a breeding pond with temperature of 21 ± 1°C and salinity of 30.6 ± 0.2 to adapt to the environment. In the meantime, frozen shrimps were used to feed S. esculenta three times a day. Sepia esculenta laid eggs after a week. These eggs were collected and temporarily reared in perforated plastic pots (dissolved oxygen: 5.6 mg/L) that floated on the surface of flowing water (pH: 8.2; temperature: 20.7 ± 0.6°C; salinity: 30.5 ± 0.2) until hatching. The eggs hatched after 29 days. Two hundred primary hatching juvenile S. esculenta (38) were collected equally in two 100 L breeding barrels, respectively. Among these, one hundred juveniles were grown in suitable seawater for 24 h (C), and another 100 juveniles were exposed for 24 h to seawater with both Cd and Cu concentrations of 50 ug/L (CuCd). Finally, these juveniles were collected at 0, 4, and 24 h, respectively, and stored at -80°C.
2.2 RNA preparation, library construction, and RNA-Seq
In each group, nine juveniles at each time points were randomly selected, and their RNA were extracted using TRIzol method: normal growth for 0 h (C_0h), normal growth for 4 h (C_4h), normal growth for 24 h (C_24h), Cd and Cu co-exposure for 4 h (CuCd_4h), and Cd and Cu co-exposure for 24 h (CuCd_24h). At each time points, equal molar mass of RNA from three juveniles were pooled as the first replicate for RNA-Seq library construction; and another six juveniles were pooled as the second and the third replicates. The remaining juvenile RNA was used for quantitative RT-PCR (qRT-PCR) verification.
In our research, we constructed library using NEBNext® Ultra™ RNA Library Prep Kit for Illumina®. First, we purified mRNA from total RNA using poly-T oligo-attached magnetic beads. Secondly, mRNA broke at high temperatures, and first-strand cDNA was synthesized using the M-MuLV Reverse Transcriptase (RNase H-) based on a template of fragment mRNA and primers of random oligonucleotides. Then, second-strand cDNA was synthesized from dNTPs using DNA polymerase I and RNase H. After purification, double-stranded cDNA was end joining repaired, spliced with poly-A, and connected to the sequencing adaptor. cDNA fragments of preferentially 250~300 bp in length were selected using AMPure XP system (Beckman Coulter, Beverly, USA), and PCR was used to amplified these fragments. In the end, PCR products were purified (AMPure XP system), and the library quality was assessed on the Agilent Bioanalyzer 2100 system. Juvenile S. esculenta were sequenced by Illumina NovaSeq 6000 (Illumina, USA).
2.3 Data quality control, mapping, and differential expression analysis
First, some raw reads were removed including adapter reads, reads containing more than 10% of undetermined bases, and low-quality reads. Then, HISAT2 software (39) was used to map clean reads to reference S. esculenta genome (unpublished). And these mapped genes were compared to the NR, NT, SwissProt, KO, KOG, and Pfam databases to find their functions and used for functional enrichment analyses. FPKM was used to analyze expression level and abundance of genes. Finally, DESeq2 was used to identify differentially expressed genes (DEGs) with the criteria p-value ≤ 0.05 and fold change ≥ 1.5 (40). And the union of DEGs at each time points was used for functional analyses.
2.4 Enrichment analyses and PPI network analyses
DAVID v6.8 was used to enrich DEGs into the GO terms and KEGG signaling pathways (41). All annotated genes were used as the background gene set, and DEGs were used as a validation set to analyze the functional differences between the control and exposed groups. Then, DEGs were enriched into KEGG pathways and GO terms of biological process, molecular function, and cellular component. Finally, significantly enriched terms and pathways were identified to explore S. esculenta juvenile immune response mechanisms after co-exposure. And STRING v11.5 with default parameters was used to construct protein-protein interaction networks using DEGs enriched in the screened significant KEGG signaling pathways (42).
2.5 Quantitative RT-PCR assay
Gene-specific primers were designed using Primer Premier 5.0 (Table 1) (43). Three reference genes were identified the stability in S. esculenta tissues and embryo development stages. And β-actin was selected based on its more stable expression level. The specific method of qRT-PCR was described by Li et al. (44).
3 Results
3.1 Transcriptome sequencing and mapping
Healthy and Cd and Cu co-exposed juvenile S. esculenta was sequenced using RNA-Seq method. A total of 669,380,246 raw reads were generated from juvenile S. esculenta, and 660,384,274 (98.66%) clean reads were identified after removing reads that contained adapters, low quality reads, and reads with more than 10% uncertain bases (Table 2). On average, the Q20 of clean reads was 97.42%, the Q30 of clean reads was 92.99%, and the GC of clean reads was 39.63%. An average of 87.73% clean reads were mapped to our reference genome.
3.2 DEGs expression
Compared with the control group (C), 276 (188 up-regulated and 88 down-regulated) and 876 (441 up-regulated and 435 down-regulated) DEGs were identified at 4 and 24 h, respectively, after co-exposure (Figure 1). Venn diagram showed that a total of 1,088 DEGs were differentially expressed, of which 64 DEGs were differentially expressed at both 4 and 24 h (Figure 2). And DEGs clustering distribution were visualized in a heatmap (Figure 3).
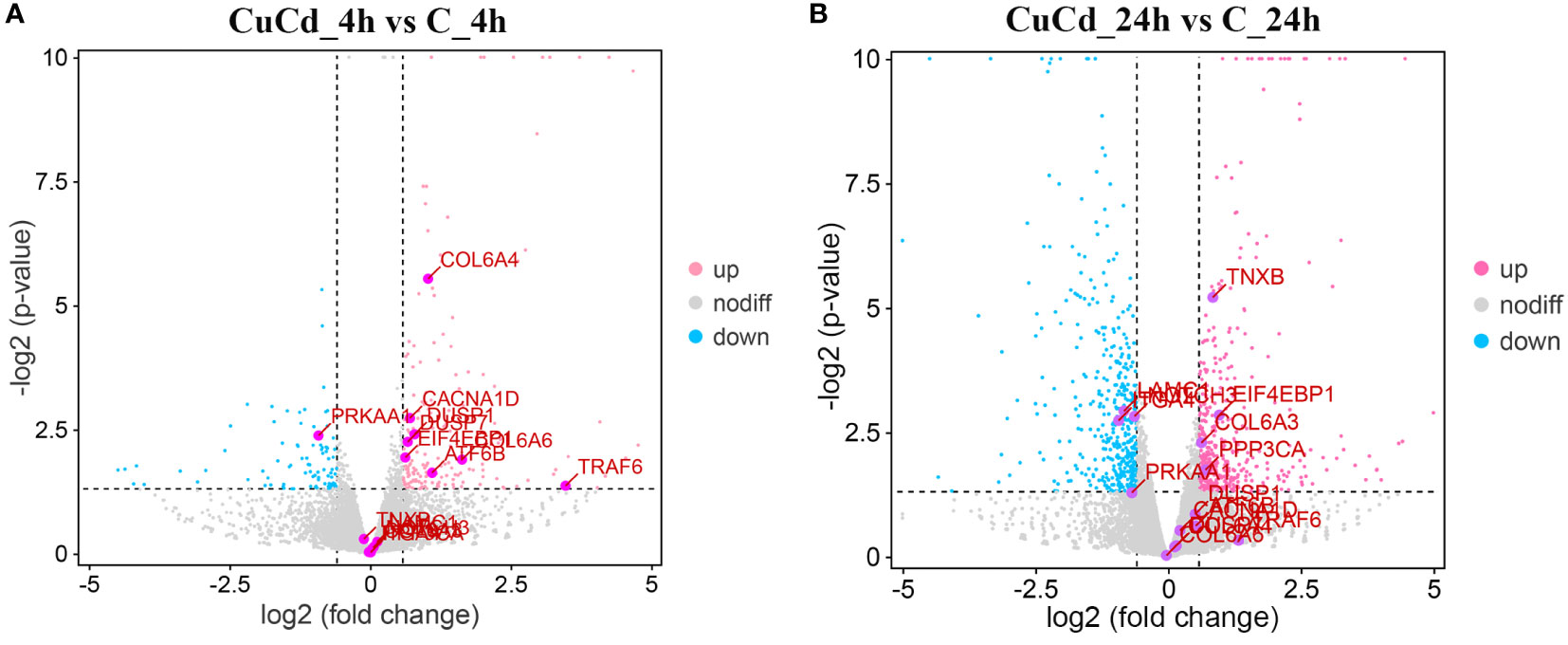
Figure 1 DEGs expression distributions. (A) DEGs expression distributions between CuCd_4h and C_4h. Up-regulated DEGs are represented as pink dots; down-regulated DEGs are expressed as blue dots; and non-regulated DEGs are indicated by grey dots. (B) DEGs expression distributions between CuCd_24h and C_24h.
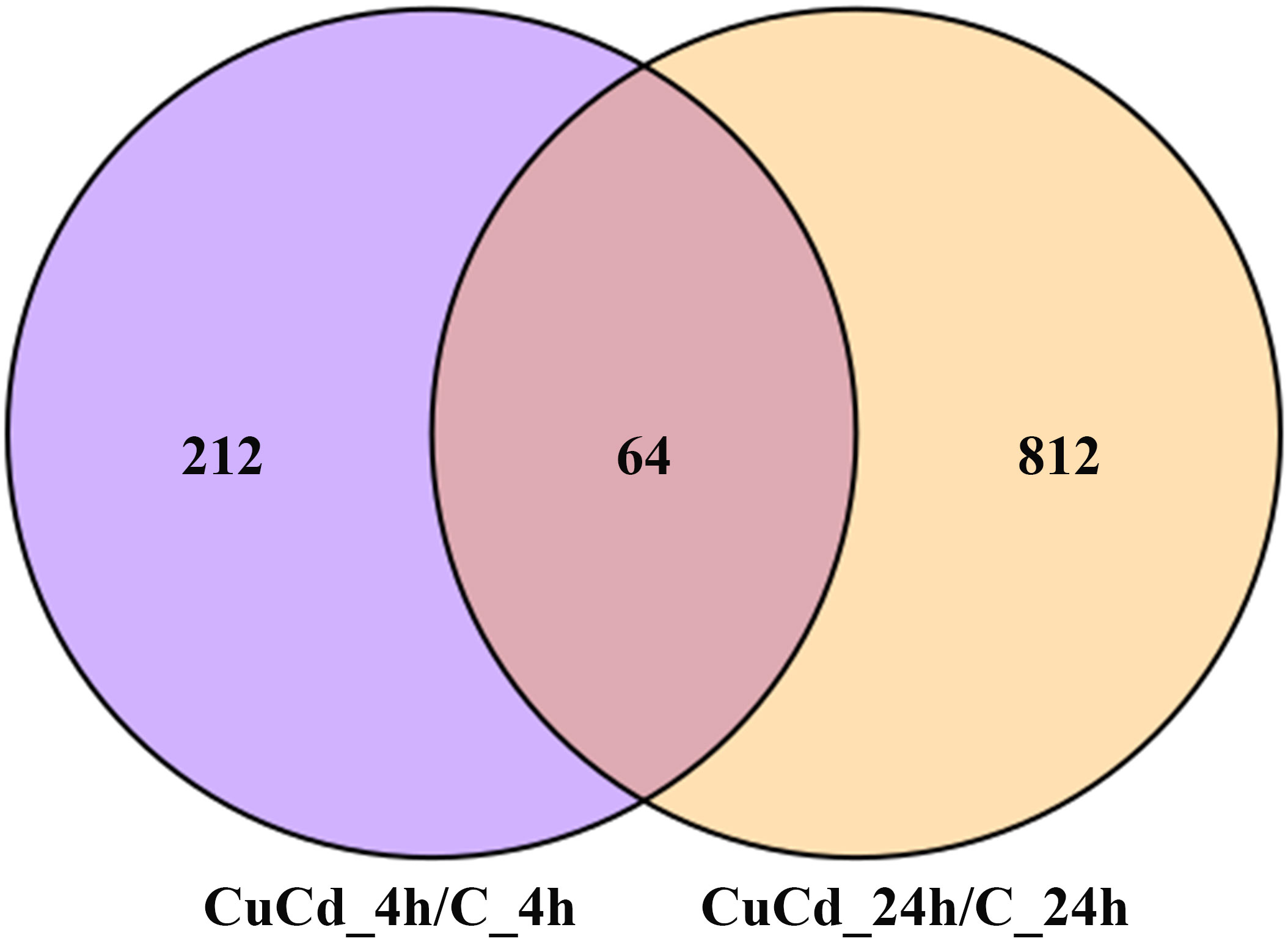
Figure 2 DEGs distributions between two time point. 1,088 DEGs are differential expressed at 4 and 24 h after exposure. 212 DEGs are differential expressed only at 4 h; 812 DEGs are differential expressed only at 24 h; 64 DEGs are differential expressed at both 4 and 24 h.
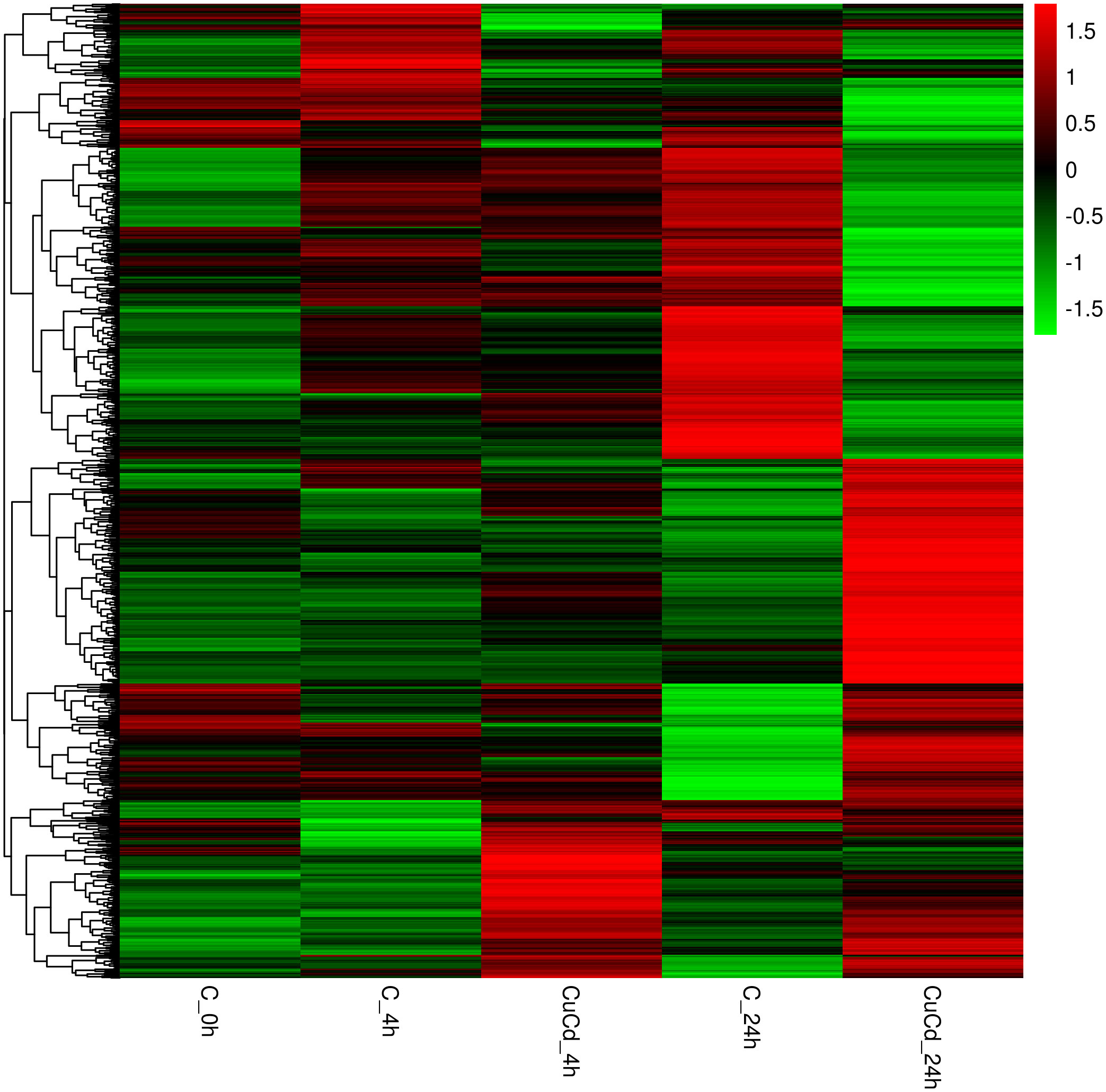
Figure 3 DEGs expression clustering. The gene expressions in different groups are shown in a row; the gene expressions in each group are represented in a column.
3.3 Functional enrichment analyses of DEGs
DEG functions were analyzed using GO and KEGG enrichment analyses. A total of 137 GO terms (p-value ≤ 0.05) containing three clusters (biological process, cellular components, and molecular function) were significantly enriched in our study. And the top 10 terms in each cluster are shown in Figure 4. Among these GO terms, response to interleukin-1, leukocyte migration, and cell adhesion terms in biological process cluster suggested that Cd and Cu co-exposure might induce a series of immune defense processes. The KEGG enrichment analysis results indicated that multiple DEGs were enriched to immune-related level-2 KEGG signaling pathways, including immune system, infectious diseases, and immune disease (Figure 5). And nine immune-related KEGG signaling pathways were significantly enriched (Table 3). Among them, the enrichment of immune-related pathways such as PI3K-Akt signaling pathway and MAPK signaling pathway suggested that multiple immune cells were activated, and key immune-related genes might be highly expressed after co-exposure.
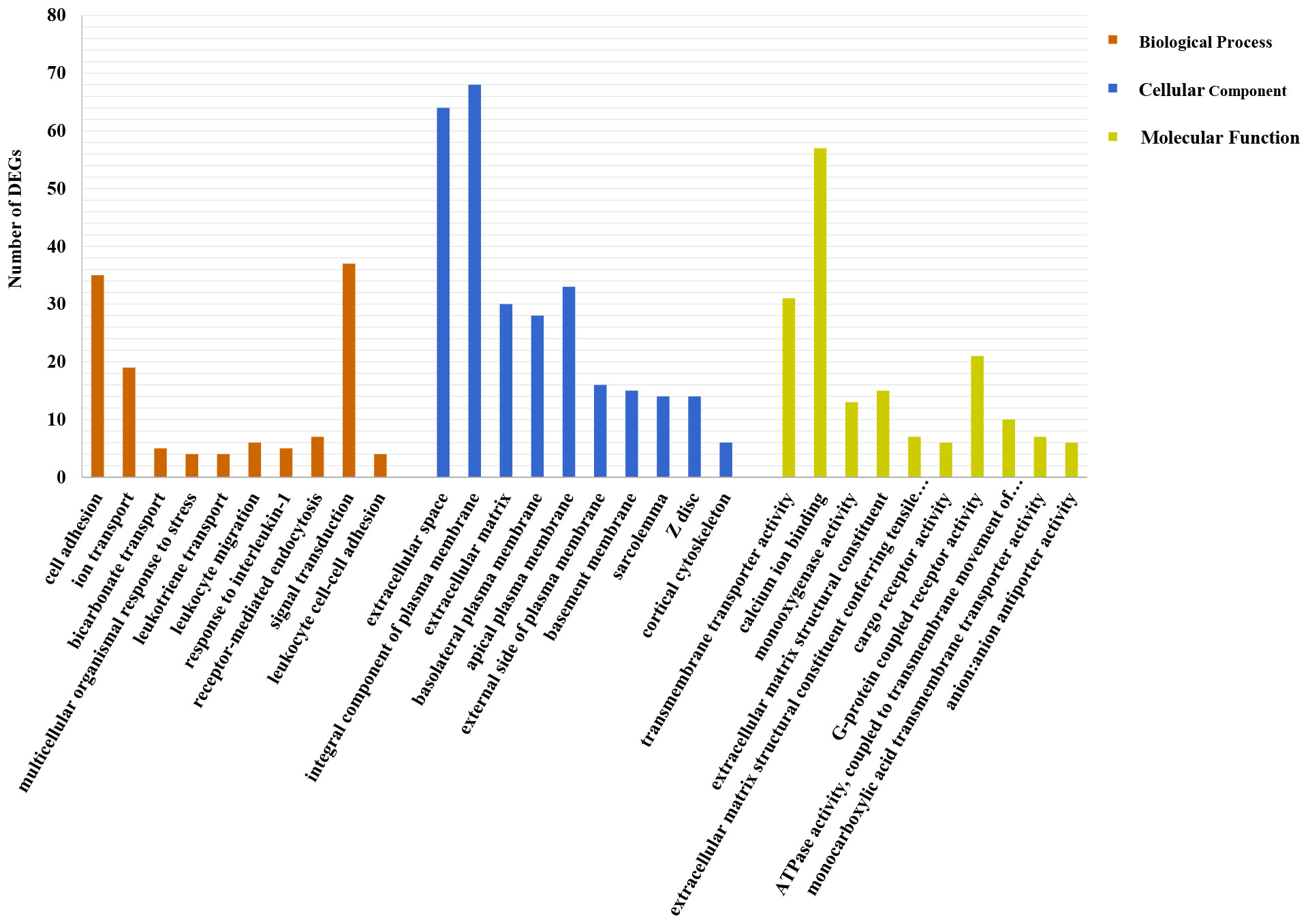
Figure 4 Top 10 significantly enriched GO terms in each cluster. The number of DEGs is shown as the ordinate; specific names of terms are displayed as the abscissa.
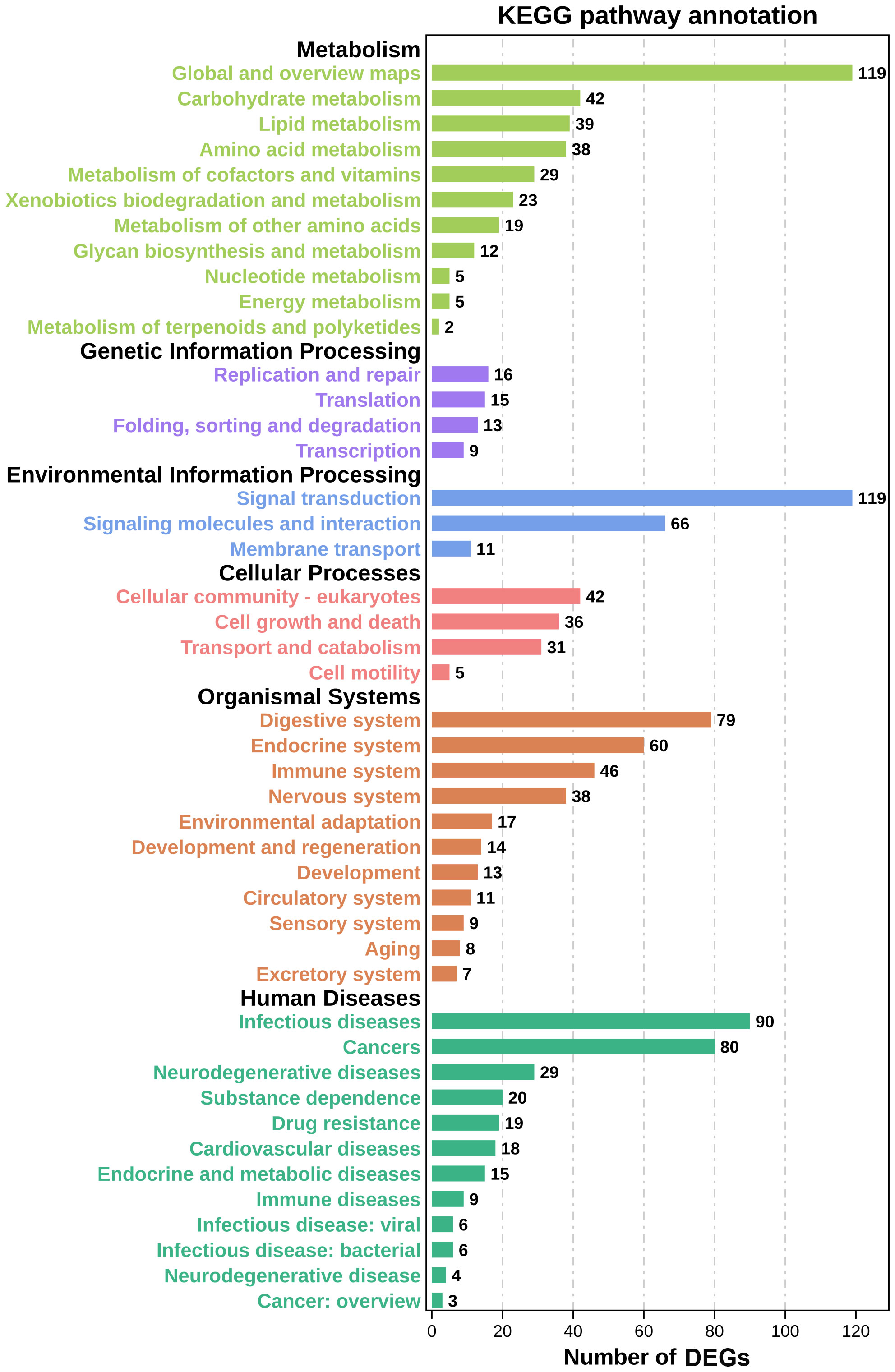
Figure 5 Level-2 KEGG signaling pathways results. The abscissa represents the number of DEGs in the pathway; the ordinate indicates the specific name of these pathways.
3.4 Screening and validation of key and hub DEGs
A total of 38 DEGs enriched in KEGG signaling pathways in Table 3 were used to construct a PPI network (Figure 6). And the network parameters are shown in Table 4. Above genes have significant interactions, and the average node degree was 4.55. The network clustering coefficient was 0.547, and the p-value was ≤ 8.44E-4. Fifteen DEGs with higher interaction numbers or higher KEGG signaling pathway participation numbers were identified and listed in Table 5. Among these genes, NOTCH3, PRKAA1, and ITGA4 interacted with more genes, which were identified as hub genes; and other 12 DEGs with high interaction numbers were defined as key genes. Previous research had shown that these genes were involved in the activation of immune cells and immune signaling pathways and regulated expressions of immune genes. Analysis of above gene functions in juvenile S. esculenta would help to further understand immune response mechanisms of S. esculenta.
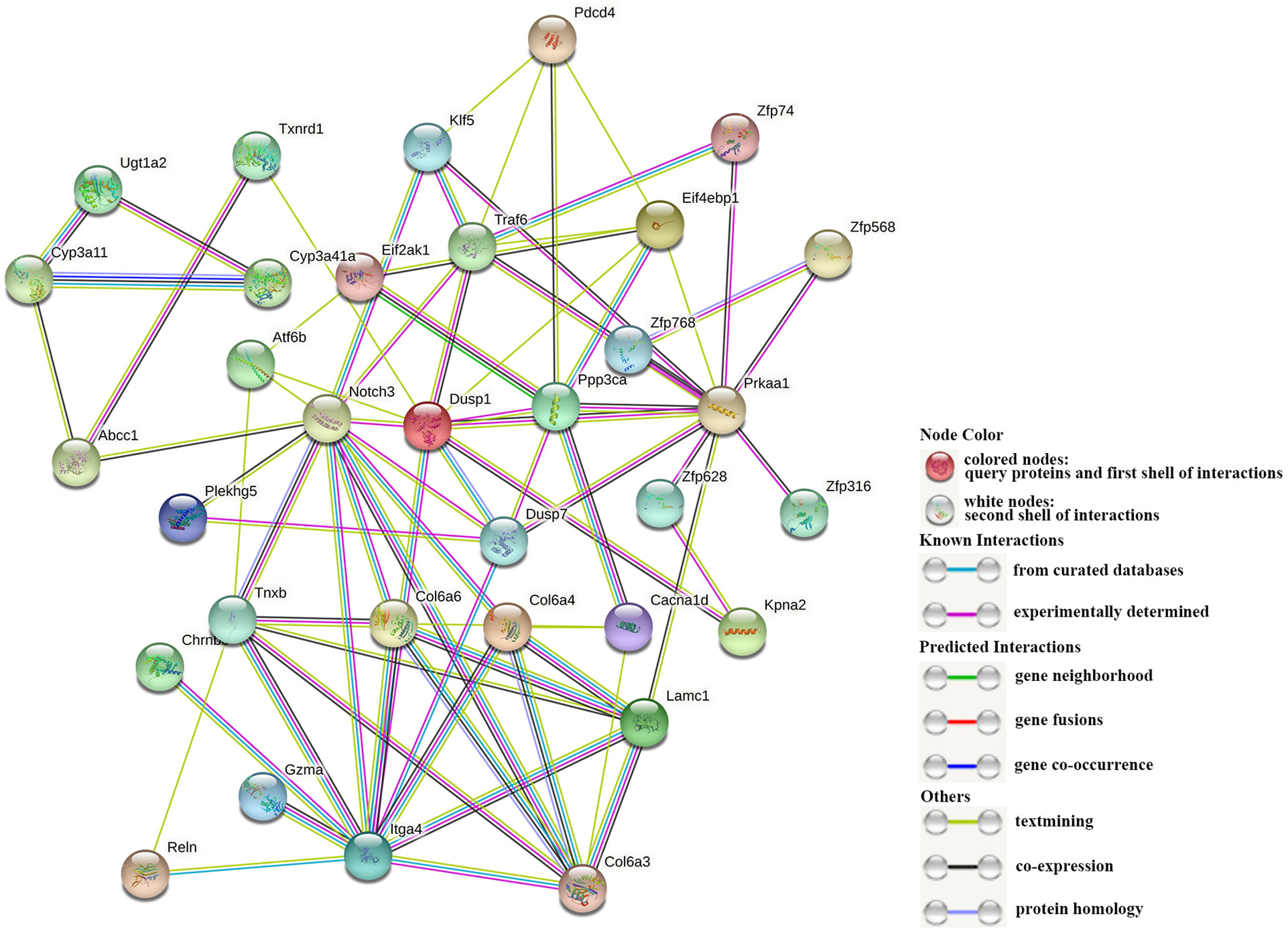
Figure 6 The interaction of DEGs. Each dot represents a protein, and their names are shown next to them. The different lines between dots represent different interactions.
The qRT-PCR was used to verify the accuracy of above gene expressions. The results showed that all DEGs measured were single products. And compared to gene expression profiles of RNA-Seq results, qRT-PCR results showed the same trend, suggesting that the qRT-PCR results were accurate (Figure 7).
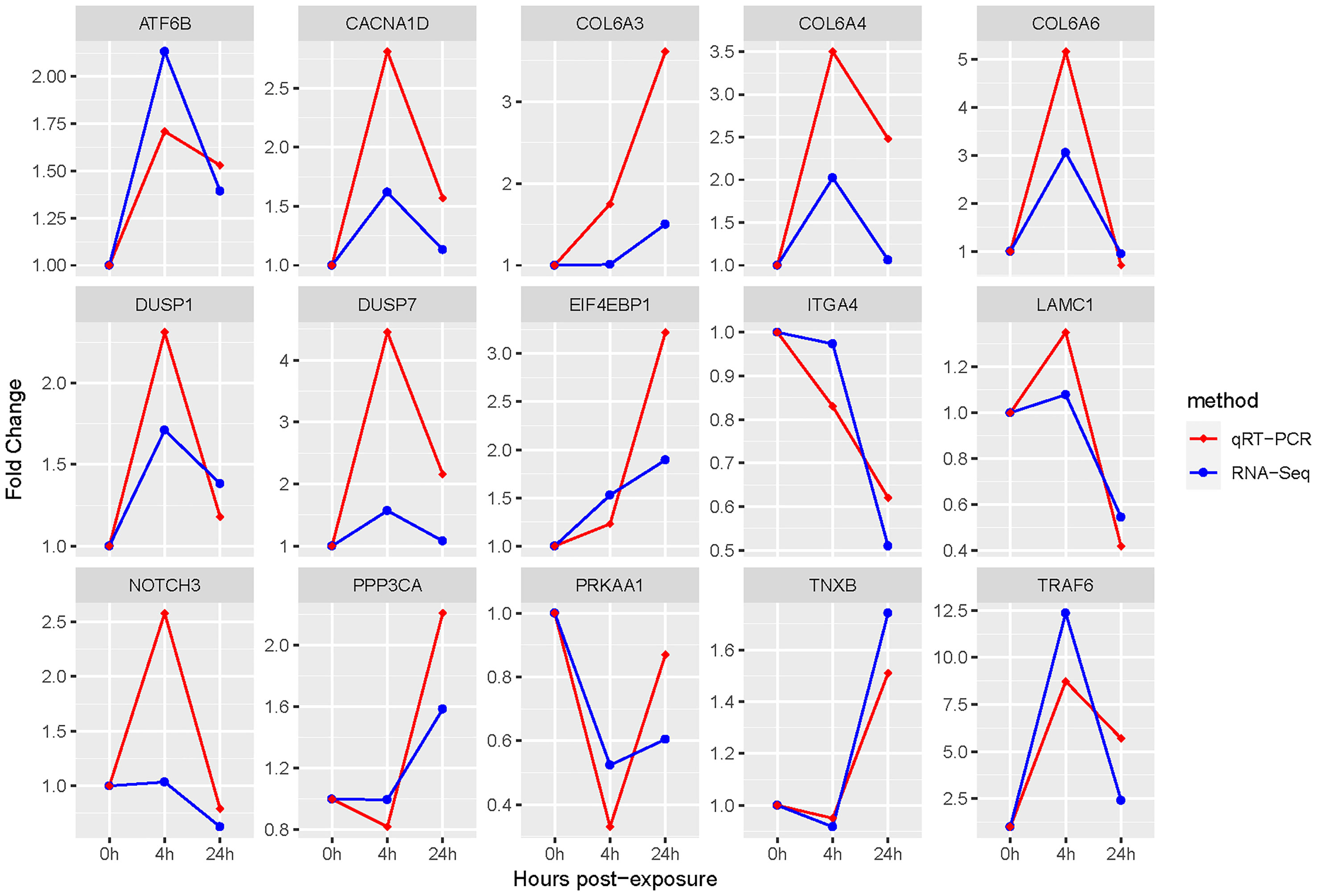
Figure 7 qRT-PCR and RNA-Seq results of key DEGs. The β-actin gene is used to normalize the gene expressions of qRT-PCR and RNA-Seq. The abscissa stands for the exposed time of Cd and Cu; the ordinate indicates fold change.
4 Discussion
4.1 Cd and Cu-induced immune response in mollusk
As common and high concentrations of heavy metals in the ocean, Cd and Cu could easily accumulate in mollusks and induce immune response processes (24, 45, 46). The effects of Cd and Cu exposure on immunity of shellfish have been studied extensively in the past 10 years. For instance, Cd could suppress immune responses in Tegillarca granosa by regulating Ca2+ transport and Ca2+-related apoptosis processes (47). Meanwhile, it could induce immune cell damage in Perna canaliculus and destroy the immune system, thereby reducing disease resistance (48). Cu could inhibit the apoptosis, phagocytosis, and adhesion functions of hemocytes, thereby reducing the immune resistance of Crassostrea rivularis and Crassostrea virginica (49, 50). In addition, Cu exposure has been reported in previous research to inhibit expressions of TLRs and NLRs and inhibit innate immune responses in Mizuhopecten yessoensis (51). Cd and Cu-induced immune responses have not been widely studied in cephalopods. This study was designed to preliminarily explore immune response mechanisms of juvenile S. esculenta after co-exposure at the molecular level, and laid a foundation for further study cephalopod immunity.
4.2 Analyses of DEGs expression
DEGs expression analyses indicated that multiple genes were differently expressed after Cd and Cu co-exposure. The volcano plot results implied that more immune-related genes might be differentially up-regulated with increasing co-exposed time. The Venn diagram analysis revealed that 64 DEGs containing PDCD4, ETV7, and ARG2 were significantly differentially expressed at both 4 and 24 h. These genes have been reported in previous studies to regulate the activation of immune cells and induce multiple immune response processes including inflammatory responses (52–55). We speculated that these might be key genes regulating juvenile immunity after co-exposure, and their specific immune functions in S. esculenta needed to be further studied. The results of the heatmap indicated that gene expressions were significantly different after co-exposure and might severely affect S. esculenta juvenile immune response processes.
4.3 Immune-related functional enrichment analyses
GO enrichment analysis results showed that most of top 10 GO terms of biological processes cluster were significantly related to immune response. The enrichment of response to interleukin-1 term indicated that co-exposure might affect the innate immune response of S. esculenta by regulating the expression of IL-1 to regulate TLRs and Toll-like receptor signaling pathways. This result was consistent with previous studies in Biomphalaria glabrata and Mytilus coruscus (56, 57). The enrichment of cell adhesion term suggested that co-exposure might inhibit S. esculenta immune cell adhesion and reduce immune cell activity (58, 59). According to the results of GO enrichment analysis, we preliminarily speculated that immune response of co-exposed juvenile S. esculenta might be significantly affected.
Nine immune-related KEGG pathways were significantly enriched in our study. These have been reported to regulate the activation, proliferation, and differentiation of immune cells and immune signal transduction in previous studies, indicating that co-exposure might significantly affect the immune response processes of S. esculenta (60–62). Among them, three KEGG signaling pathways identified by previous research as most likely to significantly affect the immune response after co-exposure such as pathways in cancer signaling pathway, MAPK signaling pathway, and PI3K-Akt signaling pathway were screened, identified, and analyzed to further explore S. esculenta immune response mechanisms after co-exposure.
4.3.1 Pathways in cancer signaling pathway analysis
Cancer was the deadliest disease in recent decades, and there were few cures (63, 64). After being infected with cancer, some cells grew uncontrollably and proliferated malignantly to form tumor cells (65). And tissue bleeding, decreased vitality, loss of appetite, and other adverse phenomena have also appeared one after another (66, 67). At the same time, immune cells such as T cells and NK cells were activated to suppress and destroy tumor cells (68, 69). Previous studies have shown that pathways in cancer signaling pathway activated WNT signaling pathway, toll-like receptor signaling pathway, and other signaling pathways during cancer and expressed HSPs to regulate immune responses (70–72). Based on our results, it was speculated that co-exposure might induce malignant proliferation of some cells and activated immune signals to promote the processes of S. esculenta juvenile immune response. However, cancer has only been initially studied in fish and has not been explored in other aquatic organisms, especially mollusks (73–75). Therefore, the exact immune response mechanisms of juvenile S. esculenta in response to cancer were unclear and required further study.
4.3.2 MAPK signaling pathway analysis
MAPK signaling pathway was an important immune regulatory signaling pathway for organisms to induce immune defense responses after biotic and abiotic stresses and played a significant role in the innate immunity of mollusks (76, 77). Previous studies have shown that it was involved in various cellular processes such as proliferation, differentiation, and migration of intestinal immune cells and promoted innate immune responses by activating phagocytic immune responses (78–80). Meanwhile, MAPK signaling pathway has also been found to regulate the production of pro-inflammatory factors such as IL-1β, TNF-α, and TFN-γ to promote inflammatory responses (79, 81, 82). In this study, MAPK signaling pathway was significantly enriched after Cd and Cu co-exposure, indicating that co-exposure might induce the proliferation and differentiation of S. esculenta immune cells and promoted inflammatory and innate immune response processes. Three key DEGs, including TRAF6, DUSP1, and DUSP7 were enriched in this pathway, and their expression levels were significantly up-regulated after co-exposure. These have been reported to involved in and regulate MAPK, PI3K, NF-κB, and other signaling pathways to regulate immune responses (83–85), suggesting that juvenile S. esculenta might defend against heavy metal stress by regulating innate immune signaling pathways.
4.3.3 PI3K-Akt signaling pathway analysis
As everyone knows that the PI3K-Akt signaling pathway is the core pathway that regulates the immune response and plays a significant part in the proliferation, differentiation, and migration of immune cells (86, 87). It regulates the activation of multiple immune signals such as TLR and NF-κB to promote immune response. At the same time, it can promote inflammatory response by regulating the release of inflammatory factors (88–90). In mollusks, the PI3K-Akt signaling pathway involved in and regulated many physiological and pathological processes of innate immunity (91, 92). For instance, it could regulate phagocytosis to promote innate immune response processes (93). And it can regulate and promote the apoptosis and growth of immune cells and induce immune defense responses after environmental stress (94, 95). In our study, multiple immune-related DEGs such as ATF6B and EIF4EBP1 were enriched into PI3K-Akt signaling pathway, and their expression levels were significantly up-regulated after co-exposure. The above results suggested that the PI3K-Akt signaling pathway might play a crucial role in the immune response processes after co-exposure. The immune response mechanisms of the PI3K-Akt signaling pathway in cephalopods has not been studied. We preliminarily speculated that it might regulate the proliferation and differentiation of S. esculenta juvenile immune cells and promote the activation of inflammatory responses and immune signaling factors.
4.4 Hub genes functional analysis
In this research, a comprehensive analysis of PPI network and KEGG signaling pathway is used to explore immune functions of juvenile S. esculenta co-exposed to Cu and Cd. Three DEGs with highest number of protein interactions or highest KEGG signaling pathway participation numbers including NOTCH3, PRKAA1, and ITGA4 were identified as hub genes most likely to regulate juvenile immunity after Cu and Cd co-exposure. NOTCH3 was a key regulator of cellular function and played a significant role in immune and inflammatory responses (96, 97). Previous studies have shown that NOTCH3 involved in and regulated leukocyte migration and adhesion, tumorigenesis, M2 macrophage infiltration, antigen presentation, activation of cytokines and integrins, tissue inflammation, and multiple immune response processes (98–101). And multiple immune signaling pathways such as PI3K-Akt signaling pathway and rap1 signaling pathway were activated and regulated by NOTCH3 (97). AMPK was a key enzyme widely expressed in cells that regulated various physiological functions such as cell proliferation and autophagy, tumorigenesis, and energy homeostasis (102). And it played an important role in innate immune and inflammation responses (103). As a subunit of AMPK, PRKAA1 played a similar role as AMPK. It promoted the expression of immune genes such as TLR4 and TNF-α to regulate innate immune response (104, 105). Meanwhile, PRKAA1 activated TAK1 and NF-κB to induce inflammatory responses (106). Integrins were an important family of cellular receptors that regulated cell growth, survival, and migration (107, 108). They promoted immune responses primarily by mediating cell adhesion processes (109). ITGA4 was an important integrin subunit efficiently regulating leukocyte adhesion and migration in blood (110). And another function of ITGA4 was recently reported that regulated the infiltration of immune cells such as macrophages, dendritic cells, and neutrophils to induce immune responses (111, 112). In conclusion, we understood that these three genes were closely related to immune and inflammatory responses. However, these have not been studied in cephalopods up to now. An interesting result was that in addition to NOTCH3, which was slightly up-regulated and then down-regulated within 24 h co-exposure, the expression levels of other two genes were continuously down-regulated after co-exposure. Based on above results, we preliminarily speculated that the down-regulation of these genes might inhibit the malignant proliferation of cells to inhibit the generation of tumor cells and prevent excessive inflammatory responses.
4.5 Other key DEGs and pathways analyses
In addition to the above three hub genes and three signaling pathways, other identified genes and signaling pathways are also significantly related to immune responses. For instance, COL6A3 is involved in and regulates immune cell infiltration and inflammatory responses (113). And natural killer cell mediated cytotoxicity signaling pathway can regulate the proliferation and apoptosis of NK cells to promote the immune response processes (114, 115). These results further illustrate that co-exposure may significantly affect S. esculenta juvenile immunity. Immune response mechanisms of these genes and pathways in co-exposed juvenile S. esculenta are unclear until now, and needs to be further studied.
5 Conclusion
This study preliminarily explores immune response mechanisms of juvenile S. esculenta after Cd and Cu co-exposure using transcriptome analysis. A large number of DEGs suggest that co-exposure may affect juvenile molecular and physiological functions. The results of functional enrichment and PPI network analyses indicate that co-exposure may significantly promote the innate immune and inflammatory responses of S. esculenta. In conclusion, co-exposure may significantly affect cuttlefish juvenile immunity, and the results lay a foundation for further understanding of cephalopod immunity after heavy metal exposure.
Data availability statement
The data presented in the study are deposited in the NCBI repository, accession number SRR19578101, SRR19578102, SRR19578103, SRR19578104, SRR19578105, SRR19578106, SRR19578107, SRR19578113, SRR19578114, SRR20545806, SRR20545807, SRR20545808, SRR20545809, SRR20545814, SRR20545815 at the following link: https://www.ncbi.nlm.nih.gov/Traces/study/?acc=PRJNA844162&o=library_name_s%3Aa.
Ethics statement
The animal study was reviewed and approved by the Institutional Animal Care and Use Committee of the Ludong University (protocol number LDU-IRB20210308NXY) and the China Government Principles for the Utilization and Care of Invertebrate Animals Used in Testing, Research, and Training (State Science and Technology Commission of the People’s Republic of China for No. 2, October 31, 1988.
Author contributions
ZL and JY designed and supervised the study. XB, YF, XX, GS and BL prepared the samples. XB, WW, XC, XX and XL analyzed all sequencing data. XB and ZL wrote the manuscript. All authors have read and approved the final manuscript.
Funding
This research was supported by China Agriculture Research System of MOF and MARA, the National Natural Science Foundation of China (No. 42006077), and the Natural Science Foundation of Shandong Province (No. ZR2019BC052).
Conflict of interest
The authors declare that the research was conducted in the absence of any commercial or financial relationships that could be construed as a potential conflict of interest.
Publisher’s note
All claims expressed in this article are solely those of the authors and do not necessarily represent those of their affiliated organizations, or those of the publisher, the editors and the reviewers. Any product that may be evaluated in this article, or claim that may be made by its manufacturer, is not guaranteed or endorsed by the publisher.
References
1. Rahman Z. An overview on heavy metal resistant microorganisms for simultaneous treatment of multiple chemical pollutants at co-contaminated sites, and their multipurpose application. J Hazard Mater (2020) 396:122682. doi: 10.1016/j.jhazmat.2020.122682
2. Tchounwou PB, Yedjou CG, Patlolla AK, Sutton DJ. Heavy metal toxicity and the environment. Exp Suppl (2012) 101:133–64. doi: 10.1007/978-3-7643-8340-4_6
3. Rainbow PS, Luoma SN. Metal toxicity, uptake and bioaccumulation in aquatic invertebrates–modelling zinc in crustaceans. Aquat Toxicol (2011) 105(3-4):455–65. doi: 10.1016/j.aquatox.2011.08.001
4. Wang SL, Xu XR, Sun YX, Liu JL, Li HB. Heavy metal pollution in coastal areas of south China: A review. Ma pollut Bull (2013) 76(1–2):7–15. doi: 10.1016/j.marpolbul.2013.08.025
5. Deng GP, Yang WQ, Zhou GY, Li Y, Liu SL. Heavy metals and polycyclic aromatic hydrocarbons in sediments from the shenzhen river, south China. Environ Sci pollut Res Int (2014) 21(18):10594–600. doi: 10.1007/s11356-014-2995-4
6. Ekere N, Yakubu N, Ihedioha J. Ecological risk assessment of heavy metals and polycyclic aromatic hydrocarbons in sediments of rivers Niger and benue confluence, lokoja, central Nigeria. Environ Sci pollut Res Int (2017) 24(23):18966–78. doi: 10.1007/s11356-017-9511-6
7. Ceci A, Pinzari F, Russo F, Persiani AM, Gadd GM. Roles of saprotrophic fungi in biodegradation or transformation of organic and inorganic pollutants in co-contaminated sites. Appl Microbio Biotechnol (2019) 103(1):53–68. doi: 10.1007/s00253-018-9451-1
8. Rahman Z, Singh VP. Bioremediation of toxic heavy metals (THMs) contaminated sites: concepts, applications and challenges. Environ Sci pollut Res Int (2020) 27(22):27563–81. doi: 10.1007/s11356-020-08903-0
9. Zhang WY, Zhi HY, Sun H. Effects of heavy metal pollution on fitness and swimming performance of Bufo raddei tadpole. Bull Environ Contam Toxicol (2020) 105(3):387–92. doi: 10.1007/s00128-020-02953-3
10. Rahman Z, Singh VP. The relative impact of toxic heavy metals (THMs) (arsenic (As), cadmium (Cd), chromium (Cr)(VI), mercury (Hg), and lead (Pb)) on the total environment: an overview. Environ Monit Assess. (2019) 191(7):419. doi: 10.1007/s10661-019-7528-7
11. Zhang Y, Li ZY, Kholodkevich S, Sharov A, Feng YJ, Ren NQ, et al. Cadmium-induced oxidative stress, histopathology, and transcriptome changes in the hepatopancreas of freshwater crayfish (Procambarus clarkii). Sci Total Environ (2019) 666:944–55. doi: 10.1016/j.scitotenv.2019.02.159
12. Sfakianakis DG, Renieri E, Kentouri M, Tsatsakis AM. Effect of heavy metals on fish larvae deformities: A review. Environ Res (2015) 137:246–55. doi: 10.1016/j.envres.2014.12.014
13. Ivanina AV, Hawkins C, Sokolova IM. Immunomodulation by the interactive effects of cadmium and hypercapnia in marine bivalves Crassostrea virginica and Mercenaria mercenaria. Fish Shellfish Immunol (2014) 37(2):299–312. doi: 10.1016/j.fsi.2014.02.016
14. Zhang ZW, Zheng Z, Cai JZ, Liu Q, Yang J, Gong YF, et al. Effect of cadmium on oxidative stress and immune function of common carp (Cyprinus carpio l.) by transcriptome analysis. Aquat Toxicol (2017) 192:171–7. doi: 10.1016/j.aquatox.2017.09.022
15. Jin XW, Liu F, Wang YY, Zhang LS, Li Z, Wang ZJ, et al. Probabilistic ecological risk assessment of copper in Chinese offshore marine environments from 2005 to 2012. Mar pollut Bull (2015) 94(1–2):96–102. doi: 10.1016/j.marpolbul.2015.03.005
16. Malhotra N, Ger T, Uapipatanakul B, Huang JC, Chen KH, Hsiao C. Review of copper and copper nanoparticle toxicity in fish. Nanomaterials (2020) 10(6):1126. doi: 10.3390/nano10061126
17. Ivanina AV, Hawkins C, Sokolova IM. Interactive effects of copper exposure and environmental hypercapnia on immune functions of marine bivalves Crassostrea virginica and Mercenaria mercenaria. Fish Shellfish Immunol (2016) 49:54–65. doi: 10.1016/j.fsi.2015.12.011
18. Simpson SL, Yverneau H, Cremazy A, Jarolimek CV, Price HL, Jolley DF. DGT-induced copper flux predicts bioaccumulation and toxicity to bivalves in sediments with varying properties. Environ Sci Technol (2012) 46(16):9038–46. doi: 10.1021/es301225d
19. Myint UM, Tyler PA. Effects of temperature, nutritive and metal stressors on the reproductive biology of Mytilus edulis. Mar Biol (1982) 67(2):209–23. doi: 10.1007/BF00401287
20. Semedo M, Reis-Henriques MA, Rey-Salgueiro L, Oliveira M, Delerue-Matos C, Morais S, et al. Mental accumulation and oxidative stress biomarkers in octopus (Octopus vulgaris) from Northwest Atlantic. Sci Total Environ (2012) 433:230–7. doi: 10.1016/j.scitotenv.2012.06.058
21. Tanaka M, Yamaguchi Y, Harada Y, Tsuchiya K, Takaku Y. As, cd and Hg in the organs of Todarodes pacificus, Sepia longipes and Sepia madokai in the East China Sea. Ecotoxicol Environ Saf (2017) 145:103–10. doi: 10.1016/j.ecoenv.2017.06.054
22. Xie DM, Li YW, Liu ZH, Chen QL. Inhibitory effect of cadmium exposure on digestive activity, antioxidant capacity and immune defense in the intestine of yellow catfish (Pelteobagrus fulvidraco). Comp Biochem Physiol C Toxicol Pharmacol (2019) 222:65–73. doi: 10.1016/j.cbpc.2019.04.012
23. Wen B, Jin SR, Chen ZZ, Gao JZ, Liu YN, Liu JH, et al. Single and combined effects of microplastics and cadmium on the cadmium accumulation, antioxidant defence and innate immunity of the discus fish (Symphysodon aequifasciatus). Environ pollut (2018) 243:462–71. doi: 10.1016/j.envpol.2018.09.029
24. Sheir SK, Handy RD. Tissue injury and cellular immune responses to cadmium chloride exposure in the common mussel Mytilus edulis: modulation by lipopolysaccharide. Arch Environ Contam Toxicol (2010) 73(6):1338–44. doi: 10.1007/s00244-010-9502-9
25. Hoseini SM, Sinha R, Fazel A, Khosraviani K, Hosseinpour DF, Arghideh M, et al. Histopathological damage and stress- and immune-related genes’ expression in the intestine of common carp, Cyprinus carpio exposed to copper and polyvinyl chloride microparticle. J Exp Zool A Ecol Integr Physiol (2022) 337(2):181–90. doi: 10.1002/jez.2555
26. Lee H, Kim JH, Park HJ, Kang JC. Toxic effects of dietary copper and EGCG on bioaccumulation, antioxidant enzyme and immune response of Korean bullhead. Pseudobagrus fulvidraco Fish Shellfish Immunol (2021) 111:119–26. doi: 10.1016/j.fsi.2021.01.010
27. Gopi N, Vijayakumar S, Thaya R, Govindarajan M, Alharbi NS, Kadaikunnan S, et al. Chronic exposure of Oreochromis niloticus to sub-lethal copper concentrations: Effects on growth, antioxidant, non-enzymatic antioxidant, oxidative stress and non-specific immune responses. J Trace Elem Med Biol (2019) 55:170–9. doi: 10.1016/j.jtemb.2019.06.011
28. Cl L, FZ Z, Yan JP, CS L, SW L, Chen SQ. Transcriptome sequencing and De novo assembly of golden cuttlefish Sepia esculenta Hoyle. Int J Mol Sci (2016) 17(10):1479. doi: 10.3390/ijms17101749
29. Ivanina AV, Taylor C, Sokolova IM. Effects of elevated temperature and cadmium exposure on stress protein response in eastern oysters Crassostrea virginica (Gmelin). Aquat Toxicol (2009) 91(3):245–54. doi: 10.1016/j.aquatox.2008.11.016
30. Qiu LM, Chen H, Zhou Z, Zhang H, Liu R, Yi QL, et al. Transcriptomic profile of oyster Crassostrea gigas hemocyte after short-term cadmium exposure and bacteria stimulation. Fish Shellfish Immunol (2019) 98:138–46. doi: 10.1016/j.fsi.2019.12.083
31. Liu YX, Liu YM. Indoor artificial breeding technology of cuttlefish (Sepia esculenta). Sci Fish Farm (2019) 11:60–2. doi: 10.14184/j.cnki.issn1004-843x.2019.11.032
32. Wang XQ, Shen Y, Cui CH, Cao M, Wang L, Ding XF, et al. Effects of low salinity stress on the survival, immune enzyme activity and gene expression of Sepia esculenta. J Jiangsu Ocean Univ (2020) 29(2):6–14. doi: 10.3969/j.issn.2096-8248.2020.02.002
33. Bai QQ, Li JT, Xu XW, Zhang ZH, Li WT, Zhang XM. Effects of social hierarchy on the behavioral phenotype and energy metabolism of Sepia esculenta in the reproductive period. Acta Ecologica Sinica (2020) 40(15):5408–17. doi: 10.5846/stxb201905090947
34. Bian L, Liu CL, Chen SQ, Zhao FZ, Ge JL, Tan J. Transcriptome analysis of gene expression patterns during embryonic development in golden cuttlefish (Sepia esculenta). Genes Genomics (2017) 40(1):1–11. doi: 10.1007/s13258-017-0588-6
35. Lacoue-Labarthe T, Bustamante P, Hörlin E, Luna-Acosta A, Bado-Nilles A, Thomas-Guyon H. Phenoloxidase activation in the embryo of the common cuttlefish Sepia officinalis and responses to the Ag and Cu exposure. Fish Shellfish Immunol (2009) 27(3):516–21. doi: 10.1016/j.fsi.2009.07.002
36. Zhou Z, Yu XP, Tang J, Wu YB, Wang LG, Huang B. Systemic response of the stony coral Pocillopora damicornis against acute cadmium stress. Aquat Toxicol (2018) 194:132–9. doi: 10.1016/j.aquatox.2017.11.013
37. Zhao D, Zhang XN, Li XF, Ru SG, Wang YW, Yin JB, et al. Oxidative damage induced by copper in testis of the red swamp crayfish Procambarus clarkii and its underlying mechanisms. Aquat Toxicol (2018) 207:120–31. doi: 10.1016/j.aquatox.2018.12.006
38. Chen SQ, Liu CL, Zhuang ZM, Jia WP, Sun JM, Yu GC, et al. Observations on the embryonic development of Sepia esculenta Hoyle. Prog Fish Sci (2010) 31(5):1–7. doi: 10.3969/j.issn.1000-7075.2010.05.001
39. Kim D, Paggi JM, Park C, Bennett C, Salzberg SL. Graph-based genome alignment and genotyping with HISAT2 and HISAT-genotype. Nat Biotechnol (2019) 37(8):907–15. doi: 10.1038/s41587-019-0201-4
40. Love MI, Huber W, Anders S. Moderated estimation of fold change and dispersion for RNA-seq data with DESeq2. Genome Biol (2014) 15(12):550. doi: 10.1186/s13059-014-0550-8
41. Jiao X, Sherman BT, Huang DW, Stephens R, Baseler MW, Lane HC, et al. DAVID-WS: a stateful web service to facilitate gene/protein list analysis. Bioinformatics (2012) 28(13):1805–6. doi: 10.1093/bioinformatics/bts251
42. Szklarczyk D, Gable AL, Lyon D, Junge A, Wyder S, Huerta-Cepas J, et al. STRING v11: protein-protein association networks with increased coverage, supporting functional discovery in genome-wide experimental datasets. Nucleic Acids Res (2019) 47:607–13. doi: 10.1093/nar/gky1131
43. Ren L, Zhu B, Zhang Y, Wang H, Li C, Su Y, et al. The research of applying primer primer 5.0 to design PCR primer. J Jinzhou Med Coll (2004) 25(6):43–6. doi: 10.3969/j.issn.1674-0424.2004.06.015
44. Liu XM, Li Z, Wu WZ, Liu YX, Liu JX, He Y, et al. Sequencing-based network analysis provides a core set of gene resource for understanding kidney immune response against Edwardsiella tarda infection in Japanese flounder. Fish Shellfish Immunol (2017) 67:643–54. doi: 10.1016/j.fsi.2017.06.051
45. Perez DG, Fontanetti CS. Hemocitical responses to environmental stress in invertebrates: a review. Environ Monit Assess. (2011) 177(1-4):437–47. doi: 10.1007/s10661-010-1645-7
46. Carballal MJ, Lopez C, Azevedo C, Villalba A. Enzymes involved in defense functions of hemocytes of mussel Mytilus galloprovincialis. J Invertebr Pathol (1997) 70(2):96–105. doi: 10.1006/jipa.1997.4670
47. Shi W, Guan XF, Han Y, Guo C, Rong JH, Su WH, et al. Waterborne Cd2+ weakens the immune responses of blood clam through impacting Ca2+ signaling and Ca2+ related apoptosis pathways. Fish Shellfish Immunol (2018) 77:208–13. doi: 10.1016/j.fsi.2018.03.055
48. Chandurvelan R, Marsden ID, Gaw S, Glover CN. Waterborne cadmium impacts immunocytotoxic and cytogenotoxic endpoints in green-lipped mussel, perna canaliculus. Aquat Toxicol (2013) 142–143(66):283–93. doi: 10.1016/j.aquatox.2013.09.002
49. Huang XZ, Jiang XY, Sun M, Dupont S, Huang W, Hu MH, et al. Effects of copper on hemocyte parameters in the estuarine oyster Crassostrea rivularis under low pH conditions. Aquat Toxicol (2018) 203:61–8. doi: 10.1016/j.aquatox.2018.08.003
50. Foster B, Grewal S, Graves O, Hughes FM, Sokolova IM. Copper exposure affects hemocyte apoptosis and Perkinsus marinus infection in eastern oysters Crassostrea virginica (Gmelin). Fish Shellfish Immunol (2011) 31(2):341–9. doi: 10.1016/j.fsi.2011.05.024
51. Meng XL, Tian X, Nie GX, Wang JL, Liu M, Jiang KY, et al. The transcriptomic response to copper exposure in the digestive gland of Japanese scallops (Mizuhopecten yessoensis). Fish Shellfish Immunol (2015) 46(2):161–7. doi: 10.1016/j.fsi.2015.05.022
52. Yin YH, Pham TL, Shin J, Shin N, Kang DW, Lee SY, et al. Arginase 2 deficiency promotes neuroinflammation and pain behaviors following nerve injury in mice. J Clin Med (2020) 9(2):305. doi: 10.3390/jcm9020305
53. Qu H, Zhao H, Zhang X, Liu Y, Li F, Sun YY, et al. Integrated analysis of the ETS family in melanoma reveals a regulatory role of ETV7 in the immune microenvironment. Front Immunol (2020) 11:612784. doi: 10.3389/fimmu.2020.612784
54. Tran TT, Rane CK, Zito CR, Weiss SA, Jessel S, Lucca L, et al. Clinical significance of PDCD4 in melanoma by subcellular expression and in tumor-associated immune cells. Cancers (2021) 13(5):1049. doi: 10.3390/cancers13051049
55. Braun C, Katholnig K, Kaltenecker C, Linke M, Sukhbaatar N, Hengstschläger M, et al. p38 regulates the tumor suppressor PDCD4 via the TSC-mTORC1 pathway. Cell Stress. (2021) 5(12):176–82. doi: 10.15698/cst2021.12.260
56. Liu SJ, Ge DL, Long ZH, Chi CF, Lv ZM, Liu HH. Molecular features of interleukin-1 receptor-associated kinase-b and a in Mytilus coruscus, regulating their function by infection of aquatic pathogens and the expression of their serine/threonine protein kinase functional domains. Fish Shellfish Immunol (2020) 102:469–79. doi: 10.1016/j.fsi.2020.05.006
57. Granath WO, Connors VA, Tarleton RL. Interleukin 1 activity in haemolymph from strains of the snail biomphalaria glabrata varying in susceptibility to the human blood fluke, schistosoma mansoni: presence, differential expression, and biological function. Cytokine (1994) 6(1):21–7. doi: 10.1016/1043-4666(94)90003-5
58. Saco A, Rey-Campos M, Novoa B, Figueras A. Transcriptomic response of mussel gills after a Vibrio splendidus infection demonstrates their role in the immune response. Front Immunol (2020) 11:615580. doi: 10.3389/fimmu.2020.615580
59. Park E, Lee J, Huh KM, Lee SH, Lee H. Toxicity-attenuated glycol chitosan adhesive inspired by mussel adhesion mechanisms. Adv Healthc Mater (2019) 8(14):1900275. doi: 10.1002/adhm.201900275
60. Wang JK, Wang Z, Li GD. MicroRNA-125 in immunity and cancer. Cancer Lett (2019) 454:134–45. doi: 10.1016/j.canlet.2019.04.015
61. Kwon J, Bakhoum SF. The cytosolic DNA-sensing cGAS–sting pathway in cancer. Cancer Discovery (2020) 10(1):26–39. doi: 10.1158/2159-8290.CD-19-0761
62. Prager I, Watzl C. Mechanisms of natural killer cell-mediated cellular cytotoxicity. J Leukoc Biol (2019) 105(6):1319–29. doi: 10.1002/JLB.MR0718-269R
63. Roy PS, Saikia BJ. Cancer and cure: A critical analysis. Indian J Cancer (2016) 53(3):441–2. doi: 10.4103/0019-509X.200658
64. Ikeda H, Togashi Y. Aging, cancer, and antitumor immunity. Int J Clin Oncol (2021) 27(2):316–22. doi: 10.1007/s10147-021-01913-z
65. Ferber S, Gonzalez RJ, Cryer AM, Andrian UH, Artzi N. Immunology-guided biomaterial design for mucosal cancer vaccines. Adv Mater (2019) 32(13):1903847. doi: 10.1002/adma.201903847
66. Karsch-Bluman A, Feiglin A, Arbib E, Stern T, Shoval H, Schwob O, et al. Tissue necrosis and its role in cancer progression. Oncogene (2019) 38(11):1920–35. doi: 10.1038/s41388-018-0555-y
67. Hariyanto TI, Kurniawan A. Appetite problem in cancer patients: Pathophysiology, diagnosis, and treatment. Cancer Treat Res Commun (2021) 27:100336. doi: 10.1016/j.ctarc.2021.100336
68. Liu GN, Zhu MT, Zhao X, Nie GJ. Nanotechnology-empowered vaccine delivery for enhancing CD8 + T cells-mediated cellular immunity. Adv Drug Delivery Rev (2021) 176:113889. doi: 10.1016/j.addr.2021.113889
69. Cooper MA, Fehniger TA, Colonna M. Is there natural killer cell memory and can it be harnessed by vaccination? vaccination strategies based on NK cell and ILC memory. Cold Spring Harb Perspect Biol (2017) 10(10):a029512. doi: 10.1101/cshperspect.a029512
70. Srivastava PK. Heat shock proteins in immune response to cancer: The fourth paradigm. Experientia (1994) 50(11-12):1054–60. doi: 10.1007/BF01923461
71. Taciak B, Pruszynska I, Kiraga L, Bialasek M, Krol M. Wnt signaling pathway in development and cancer. J Physiol Pharmacol (2018) 69(2):185–96. doi: 10.26402/jpp.2018.2.07
72. Moghadam SO, Nowroozi MR. Toll-like receptors: The role in bladder cancer development, progression and immunotherapy. Scand J Immunol (2019) 90(6):e12818. doi: 10.1111/sji.12818
73. Astin JW, Crosier PS. Lymphatics, cancer and zebrafish. Adv Exp Med Biol (2016) 916:199–218. doi: 10.1007/978-3-319-30654-4_9
74. Astell KR, Sieger D. Zebrafish in vivo models of cancer and metastasis. Cold Spring Harb Perspect Med (2019) 10(8):a037077. doi: 10.1101/cshperspect.a037077
75. Schartl M, Walter RB. Xiphophorus and medaka cancer models. Adv Exp Med Biol (2016) 916:531–52. doi: 10.1007/978-3-319-30654-4_23
76. Lang JL, Colcombet J. Sustained incompatibility between MAPK signaling and pathogen effectors. Int J Mol Sci (2020) 21(21):7954. doi: 10.3390/ijms21217954
77. Ciacci C, Betti M, Canonico B, Citterio B, Roch P, Canesi L. Specificity of anti-vibrio immune response through p38 MAPK and PKC activation in the hemocytes of the mussel Mytilus galloprovincialis. J Invertebr Pathol (2010) 105(1):49–55. doi: 10.1016/j.jip.2010.05.010
78. Wang J, Han CH, Lu Z, Ge PP, Cui Y, Zhao DD, et al. Simulated microgravity suppresses MAPK pathway-mediated innate immune response to bacterial infection and induces gut microbiota dysbiosis. FASEB J (2020) 34(11):14631–44. doi: 10.1096/fj.202001428R
79. Arthur JS, Ley SC. Mitogen-activated protein kinases in innate immunity. Nat Rev Immunol (2013) 13(9):679–92. doi: 10.1038/nri3495
80. Perez-Lopez A, Behnsen J, Nuccio SP, Raffatellu M. Mucosal immunity to pathogenic intestinal bacteria. Nat Rev Immunol (2016) 16(3):135–48. doi: 10.1038/nri.2015.17
81. Pham TT, Ban J, Hong YJ, Lee J, Vu TH, Truong AD, et al. MicroRNA gga-miR-200a-3p modulates immune response via MAPK signaling pathway in chicken afflicted with necrotic enteritis. Vet Res (2020) 51(1):8. doi: 10.1186/s13567-020-0736-x
82. Wang L, Zhang XM, Wu GY, Qi YH, Zhang JH, Yang J, et al. Streptococcus pneumoniae aminopeptidase n contributes to bacterial virulence and elicits a strong innate immune response through MAPK and PI3K/AKT signaling. J Microbiol (2020) 58(4):1–10. doi: 10.1007/s12275-020-9538-0
83. Walsh MC, Lee J, Choi Y. Tumor necrosis factor receptor- associated factor 6 (TRAF6) regulation of development, function, and homeostasis of the immune system. Immunol Rev (2015) 266(1):72–92. doi: 10.1111/imr.12302
84. Zhang YM, Liu QQ, Yin H, Min YH, Li S. Selenium deficiency causes immune damage by activating the DUSP1/NF-κB pathway and endoplasmic reticulum stress in chicken spleen. Food Funct (2020) 11(7):6467–75. doi: 10.1039/d0fo00394h
85. Ham JE, Oh EK, Kim DH, Choi SH. Differential expression profiles and roles of inducible DUSPs and ERK1/2-specific constitutive DUSP6 and DUSP7 in microglia. Biochem Biophys Res Commun (2015) 467(2):254–60. doi: 10.1016/j.bbrc.2015.09.180
86. Vergadi E, Ieronymaki E, Lyroni K, Vaporidi K, Tsatsanis C. Akt signaling pathway in macrophage activation and M1/M2 polarization. J Immunol (2017) 198(3):1006–14. doi: 10.4049/jimmunol.1601515
87. Song G, Ouyang GL, Bao SD. The activation of Akt/PKB signaling pathway and cell survival. J Cell Mol Med (2005) 9(1):59–71. doi: 10.1111/j.1582-4934.2005.tb00337.x
88. López-Peláez M, Soria-Castro I, Boscá L, Fernández M, Alemany S. Cot/tpl2 activity is required for TLR-induced activation of the akt p70 S6k pathway in macrophages: Implications for NO synthase 2 expression. Eur J Immunol (2011) 41(6):1733–41. doi: 10.1002/eji.201041101
89. Fukao T, Koyasu S. PI3K and negative regulation of TLR signaling. Trends Immunol (2003) 24(7):358–63. doi: 10.1016/s1471-4906(03)00139-x
90. Troutman TD, Bazan JF, Pasare C. Toll-like receptors, signaling adapters and regulation of the pro-inflammatory response by PI3K. Cell Cycle (2012) 11(19):3559–67. doi: 10.4161/cc.21572
91. Canesi L, Betti M, Ciacci C, Scarpato A, Citterio B, Pruzzo C, et al. Signaling pathways involved in the physiological response of mussel hemocytes to bacterial challenge: the role of stress-activated p38 MAP kinases. Dev Comp Immunol (2002) 36(4):325–34. doi: 10.1016/s0145-305x(01)00078-7
92. Canesi L, Gallo G, Gavioli M, Pruzzo C. Bacteria-hemocyte interactions and phagocytosis in marine bivalves. Microsc Res Tech. (2002) 57(6):469–76. doi: 10.1002/jemt.10100
93. Sun YL, Zhang X, Wang GD, Lin S, Zeng XY, Wang YL, et al. PI3K-AKT signaling pathway is involved in hypoxia/thermal-induced immunosuppression of small abalone Haliotis diversicolor. Fish Shellfish Immunol (2016) 59:492–508. doi: 10.1016/j.fsi.2016.11.011
94. Wang YH, Zhou SS, Liu TY, Chen MY, Zhang XM. De novo transcriptome analysis of stressed blood clam (Anadara broughtonii) and identification of genes associated with hemoglobin. Genes Genomics (2019) 42(2):189–202. doi: 10.1007/s13258-019-00887-7
95. Yu JJ, Wang HX, Yue X, Liu BZ. Dynamic immune and metabolism response of clam Meretrix petechialis to Vibrio challenge revealed by a time series of transcriptome analysis. Fish Shellfish Immunol (2019) 94:17–26. doi: 10.1016/j.fsi.2019.08.057
96. Cui YH, Li Q, Li W, Wang Y, Lv F, Shi XY, et al. NOTCH3 is a prognostic factor and is correlated with immune tolerance in gastric cancer. Front Oncol (2021) 10:574937. doi: 10.3389/fonc.2020.574937
97. Xue DY, Li D, Dou C, Li JS. A comprehensive bioinformatic analysis of NOTCH pathway involvement in stomach adenocarcinoma. Dis Markers (2021) 2021:4739868. doi: 10.1155/2021/4739868
98. Wei K, Korsunsky I, Marshall JL, Gao AQ, Watts GFM, Major T, et al. Notch signalling drives synovial fibroblast identity and arthritis pathology. Nature (2020) 582(7811):259–64. doi: 10.1038/s41586-020-2222-z
99. Brandt S, Ballhause TM, Bernhardt A, Becker A, Salaru D, Le-Deffge HM, et al. Fibrosis and immune cell infiltration are separate events regulated by cell-specific receptor Notch3 expression. J Am Soc Nephrol (2020) 31(11):2019121289. doi: 10.1681/ASN.2019121289
100. Breitkopf DM, Jankowski V, Ohl K, Hermann J, Hermert D, Tenbrock K, et al. The YB-1: Notch-3 axis modulates immune cell responses and organ damage in systemic lupus erythematosus. Kidney Int (2019) 97(2):289–303. doi: 10.1016/j.kint.2019.09.031
101. Yan KX, Wang YT, Lu YX, Yan ZY. Coexpressed genes that promote the infiltration of M2 macrophages in melanoma can evaluate the prognosis and immunotherapy outcome. J Immunol Res (2021) 2021(3):6664791. doi: 10.1155/2021/6664791
102. Steinberg GR, Schertzer JD. AMPK promotes macrophage fatty acid oxidative metabolism to mitigate inflammation: implications for diabetes and cardiovascular disease. Immunol Cell Biol (2014) 92(4):340–5. doi: 10.1038/icb.2014.11
103. Yuan J, Zhang Y, Yan FT, Zheng X. Association of PRKAA1 gene polymorphisms with chronic hepatitis b virus infection in Chinese han population. Braz J Infect Dis (2016) 20(6):564–8. doi: 10.1016/j.bjid.2016.08.003
104. Yang ZG, Kahn BB, Shi H, Xue BZ. Macrophage α1 AMP-activated protein kinase (α1AMPK) antagonizes fatty acid-induced inflammation through SIRT1. J Biol Chem (2010) 285(25):19051–9. doi: 10.1074/jbc.M110.123620
105. Trillo-Tinoco J, Sierra RA, Mohamed E, Cao Y, Mingo-Pulido Á, Gilvary DL, et al. AMPK alpha-1 intrinsically regulates the function and differentiation of tumor myeloid-derived suppressor cells. Cancer Res (2019) 79(19):5034–47. doi: 10.1158/0008-5472.CAN-19-0880
106. Kim SY, Jeong S, Jung E, Baik KH, Chang MH, Kim SA, et al. AMP-activated protein kinase-α1 as an activating kinase of TGF-β-activated kinase 1 has a key role in inflammatory signals. Cell Death Dis (2012) 3(7):e357. doi: 10.1038/cddis.2012.95
107. Ginsberg MH. Integrin activation. BMB Rep (2004) 117(5):657–66. doi: 10.5483/bmbrep.2014.47.12.241
108. Nolte M, Margadant C. Controlling immunity and inflammation through integrin-dependent regulation of TGF-β. Trends Cell Biol (2020) 30(1):49–59. doi: 10.1016/j.tcb.2019.10.002
109. Giancotti FG, Ruoslahti E. Integrin signaling. Science (1999) 285(5430):1028–32. doi: 10.1126/science.285.5430.1028
110. Zhou Z, Ferdous F, Montagner P, Luchini DN, Corrêa MN, Loor JJ. Methionine and choline supply during the peripartal period alter polymorphonuclear leukocyte immune response and immunometabolic gene expression in Holstein cows. J Dairy Sci (2018) 101(11):10374–82. doi: 10.3168/jds.2018-14972
111. Rojas K, Baliu-Piqué M, Manzano A, Saiz-Ladera C, García-Barberán V, Cimas FJ, et al. In silico transcriptomic mapping of integrins and immune activation in basal-like and HER2+ breast cancer. Cell Oncol (2021) 44(3):569–80. doi: 10.1007/s13402-020-00583-9
112. Nurzat Y, Su WJ, Min P, Li K, Xu H, Zhang YX. Identification of therapeutic targets and prognostic biomarkers among integrin subunits in the skin cutaneous melanoma microenvironment. Front Oncol (2021) 11:751875. doi: 10.3389/fonc.2021.751875
113. Hu Y, Li JJ, Luo HF, Song WL, Yang JY. Differential expression of COL1A1, COL1A2, COL6A3, and SULF1 as prognostic biomarkers in gastric cancer. Int J Gen Med (2021) 14:5835–43. doi: 10.2147/IJGM.S321265
114. Wu SY, Fu T, Jiang YZ, Shao ZM. Natural killer cells in cancer biology and therapy. Mol Cancer (2020) 19(1):120. doi: 10.1186/s12943-020-01238-x
Keywords: Cd and Cu co-exposure, heavy metals, immunity, protein-protein interaction network, Sepia esculenta, transcriptome
Citation: Bao X, Wang W, Chen X, Feng Y, Xu X, Sun G, Li B, Liu X, Li Z and Yang J (2022) Exploration of immune response mechanisms in cadmium and copper co-exposed juvenile golden cuttlefish (Sepia esculenta) based on transcriptome profiling. Front. Immunol. 13:963931. doi: 10.3389/fimmu.2022.963931
Received: 08 June 2022; Accepted: 01 September 2022;
Published: 23 September 2022.
Edited by:
Linlin Zhang, Chinese Academy of Sciences (CAS), ChinaReviewed by:
Tongtong Li, Zhejiang University of Technology, ChinaYuewen Deng, Guangdong Ocean University, China
Copyright © 2022 Bao, Wang, Chen, Feng, Xu, Sun, Li, Liu, Li and Yang. This is an open-access article distributed under the terms of the Creative Commons Attribution License (CC BY). The use, distribution or reproduction in other forums is permitted, provided the original author(s) and the copyright owner(s) are credited and that the original publication in this journal is cited, in accordance with accepted academic practice. No use, distribution or reproduction is permitted which does not comply with these terms.
*Correspondence: Zan Li, bGl6YW5seG1AMTYzLmNvbQ==; Jianmin Yang, bGFkZGVydXBAMTI2LmNvbQ==