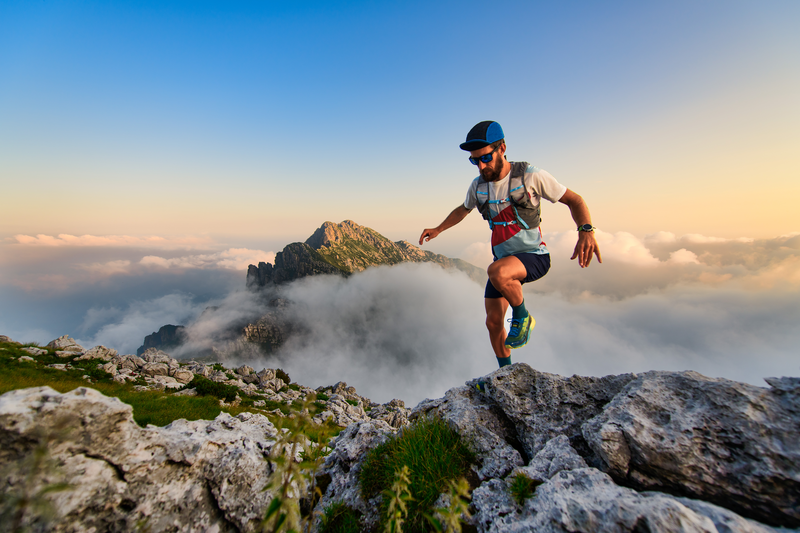
94% of researchers rate our articles as excellent or good
Learn more about the work of our research integrity team to safeguard the quality of each article we publish.
Find out more
CASE REPORT article
Front. Immunol. , 08 November 2022
Sec. Alloimmunity and Transplantation
Volume 13 - 2022 | https://doi.org/10.3389/fimmu.2022.963445
This article is part of the Research Topic Expert Opinions and Perspectives in Hematopoietic Cell Transplantation: 2022 View all 5 articles
Acute respiratory distress syndrome (ARDS) is a life-threatening lung disease. It may occur during the pancytopenia phase following allogeneic hematopoietic cell transplantation (HCT). ARDS is rare following HCT. Mesenchymal stromal cells (MSCs) have strong anti-inflammatory effect and first home to the lung following intravenous infusion. MSCs are safe to infuse and have almost no side effects. During the Covid-19 pandemic many patients died from ARDS. Subsequently MSCs were evaluated as a therapy for Covid-19 induced ARDS. We report three patients, who were treated with MSCs for ARDS following HCT. Two were treated with MSCs derived from the bone marrow (BM). The third patient was treated with MSCs obtained from the placenta, so-called decidua stromal cells (DSCs). In the first patient, the pulmonary infiltrates cleared after infusion of BM-MSCs, but he died from multiorgan failure. The second patient treated with BM-MSCs died of aspergillus infection. The patient treated with DSCs had a dramatic response and survived. He is alive after 7 years with a Karnofsky score of 100%. We also reviewed experimental and clinical studies using MSCs or DSCs for ARDS. Several positive reports are using MSCs for sepsis and ARDS in experimental animals. In man, two prospective randomized placebo-controlled studies used adipose and BM-MSCs, respectively. No difference in outcome was seen compared to placebo. Some pilot studies used MSCs for Covid-19 ARDS. Positive results were achieved using umbilical cord and DSCs however, optimal source of MSCs remains to be elucidated using randomized trials.
Acute respiratory distress syndrome (ARDS) is a life-threatening lung condition with a mortality rate that ranges between 25-50% (1–4). ARDS is characterized by acute hypoxic respiratory failure with bilateral pulmonary infiltrates that may be caused by septicemia, pneumonia, shock, following aspiration of gastric contents, or severe trauma (3, 4). ARDS may independently be caused by blood transfusion (5). ARDS is characterized by inflammation, neutrophil accumulation, a systemic and local inflammatory reaction, and alveolar injury. The damage induces protein-rich pulmonary edema, which leads to hypoxemia and impaired carbon dioxide (CO2) elimination. ARDS is classified as mild, moderate, and severe, based on the degree of hypoxemia. ARDS is used if the patient´s PaO2/FiO2 was less than 300 mmHg (6).
Diffuse alveolar damage (DAD) leading to high permeability followed by pulmonary edema is considered the histopathologic hallmark of ARDS. It usually happens when the patient already is critically ill or has had significant injuries (7, 8). ARDS causes the deaths of approximately 75.000 people in the United States annually (2, 7). In the Scandinavian countries, there are almost 170 patients/million inhabitants/year of ARDS with a mortality rate of 40% (9). Apart from substantial mortality, ARDS is associated with high morbidity rate and high costs on health systems. Patients with ARDS need ventilator support and are treated in intensive care units (ICUs) which is cumbersome and costly. Mechanical ventilation is required to keep good oxygenation and adequate CO2 elimination but may also lead to lung injury by rupturing healthy alveoli and triggering a secondary inflammatory response (1, 10).
Standard therapy for ARDS includes mechanical ventilation and fluid management therapy (11). There is no specific or targeted therapy against the underlying pathophysiological process of ARDS shown to be beneficial. For instance, β-2 agonist treatment and simvastatin were used for ARDS with no beneficial effects (12, 13).
Infection with the novel coronavirus, SARS-CoV-2, causing the ongoing Covid-19-pandemic, can lead to massive pro-inflammatory responses contributing to the development of ARDS and a concomitant cytokine storm reaction (14). The cytokine storm was studied in more detail however there is no consensus on a detailed definition (15, 16). Moreover, coagulopathy in these patients could possibly worsen the clinical outcome (17). Several ongoing trials investigate immunomodulatory therapies in Covid-19, including e.g. interleukin-6 inhibitors.
Various pulmonary problems are common following allogeneic hematopoietic cell transplantation (HCT) and include idiopathic pneumonia, viral- bacterial and fungal pneumonia, obstructive bronchiolitis and alveolar hemorrhage etc (18). ARDS is rare after HCT but is associated with almost 100% mortality.
The rationale to use mesenchymal stromal cells (MSCs) stems from the very potent cellular immunomodulatory and anti-inflammatory effects (19–21). When MSCs are infused intravenously, they first home to the lungs (22–25). A study in mice showed that around 80% of the MSCs were found in the lungs within a few minutes after injection (26). MSC may not migrate beyond the lungs after intravenous infusion (27). We were the first group to treat ARDS using MSCs in a patient in 2004 (28).
In this article, we describe three patients treated with MSCs for ARDS following HCT and summarizes the immunomodulatory and anti-inflammatory effects of MSCs. We also review the experimental and clinical experience of treating ARDS with MSCs and future perspectives.
MSCs were first described by Friedenstein et al. (29). They described an adherent, fibroblast-like cell population in the bone marrow that could regenerate rudiments of bone in vivo (30, 31). MSCs were found in all tissues of the body and have been estimated to be 1/10.000 nucleated cells (32–34). Because MSCs can differentiate into several cells of mesenchymal cell lineages including bone, cartilage, tendons, cardiomyocytes, bone cells, and fat, they have raised interest in regenerative medicine (35, 36). There is no specific marker for MSCs, but they stain positive for CD29, CD73, CD90, CD105 and CD166 (32, 33, 37). They are negative for hematopoietic markers CD34, CD45, and CD14. MSCs have immunomodulatory effects and inhibit T-cell alloreactivity induced in mixed lymphocyte cultures (MLC) (20). MSCs suppression of alloreactivity is independent of the HLA system. HLA compatibility between MSCs and target lymphocytes are not necessary for immunosuppression to occur. This suggests the possibility to use third-party MSCs (21). Furthermore, the infusion of MSCs in humans was shown to be safe (38). Skin allograft survival was prolonged in baboons (19). These findings inspired us to treat a 9-year-old boy, with life-threatening grade IV acute graft-versus-host disease (GVHD), with MSCs. He had a most dramatic response and MSCs were also used in a pilot study by us including 8 patients (39, 40). Subsequently, MSCs are used to treat a wide range of inflammatory and autoimmune disorders such as Crohn’s disease, ulcerous colitis, multiple sclerosis, and ARDS among others (41–44).
MSCs have multiple effects on the immune system. MSCs increase the number of both CD4+ and CD8+ regulatory T cells and IL-10 production (45–49). MSCs decrease markers for activated T cells, CD25, CD69 and CD38 (50, 51). Furthermore, dendritic cells decrease TNF-α and IL-12 when co-cultured with MSCs (46). MSCs also increase IL-10 secretion by LPS-stimulated dendritic cells, and CD4+ cells had decreased IL-5 secretion.
Type 1 helper T cells (Th1) IFN-γ production was significantly decreased by MSCs. Type 2 helper T cells (Th2) increased IL-4 secretion in the presence of MSCs. MSCs inhibition of alloreactivity in mixed lymphocyte cultures and subsequent development of cytotoxic T cells was induced by a soluble factor. Several immunoinhibitory soluble factors are produced by MSCs, among them are prostaglandin E2 (46), HLA-G5 (52). The immunoinhibitory effect by MSCs is augmented by IFN-γ (53, 54). MSCs can also be activated by stimulation of their Toll-like receptors (55). The T-cell inhibitory enzyme indoleamine-2,3 dioxygenase (IDO), is induced by IFN-γ, which catalyzes the conversion from tryptophan to kynurenine and inhibits T cell responses (56, 57). IDO reduced by MSCs is involved in the induction of regulatory T cells and the inhibition of T helper 17 (Th17) differentiation (58, 59). IDO-produced MSCs can also promote the differentiation of macrophages towards the M2 phenotype (60). MSCs have been reported to induce macrophage reprogramming through an anti-inflammatory/immunosuppressive profile (61, 62). MSCs may also inhibit reactivity by T cell inhibitory molecule PD-L1 (60). MSCs may use adenosine-mediated suppression via CD39 and CD73 to suppress activated T cells (63). Inhibition and apoptosis of T cells may also be mediated by galectin induced by MSCs (64). It has been proposed that MSCs decrease B cell proliferation when used in a high concentration of MSCs (65). In contrast, lower doses of MSCs stimulated B cell antibody secretion in human spleen cells (66). MSCs decreased anti-inflammatory gene 6 protein (TSG-6) also stimulated by human tumor necrosis factor α (TNF-α), to induce an anti-inflammatory effect (67). MSCs immunosuppressive activity also involves the engagement of regulatory T cells (48, 49).
Activated MSCs can modulate adaptive immune cells through contact-dependent mechanisms. It includes activation of the PD-1 pathway (60), FAS-mediated T cell apoptosis (68) engagement of VCAM-1 and ICAM-1 (69), and through upregulation of CD39 and increasing adenosine production (63). Direct contact is also important for MSCs induced immunosuppression in mice (70). In a murine model of GVHD, it was demonstrated that MSCs are actively induced to undergo perforin-dependent apoptosis by recipient cytotoxic T cells and that this process is essential to initiate MSCs-induced immunosuppression (71). After infusion recipient phagocytes engulf apoptotic MSCs and produce indoleamine 2,3-dioxinease, which is necessary for inducing immunosuppression. A recent study in NSG mice demonstrated that shortly after intravenous administration, MSC become apoptotic in the lungs and are eliminated locally by several phagocyte subsets. Efferocytosis by alveolar macrophages is critical for the therapeutic effect of MSC (72). In addition, administration of apoptotic human umbilical cord blood-derived MSC exerts a beneficial effect in acute lung injury in rats (73). The importance of MSCs apoptosis and efferocytosis for MSC immunosuppression was also found by de Witte et. al (74).
MSCs were thought to be immune-privileged cells (53, 75). However, in a baboon model, it was shown that alloantibodies were formed after infusion of allogeneic MSCs (76). Furthermore, MSCs are rejected in allogeneic mice (77). We did not detect any anti-HLA antibodies in GVHD patients treated with MSCs or DSCs in patients who have undergone HCT (78, 79). However, a small girl diagnosed with epidermolysis bullosa developed multi-specific anti-HLA antibodies after repeated infusion of DSCs.
After infusion in human blood, MSCs were susceptible to complement activation (80), which resulted in cell death (81). Coagulation factors are activated when MSCs are infused into the blood (82).
Exosomes and macrovesicles derived from MSCs were shown to protect from acute kidney injuries (83) myocardial ischemia (84), and pulmonary hypotension (85) in various animal models. MSC-derived exosomes have also been used in ARDS/ALI (86–88).
Infusion of MSCs seems safe and there are few side-effects reported (89). In a meta-analysis from more than 1000 patients who were treated for various disorders such as GVHD among others, the toxicity of allogeneic MSCs were limited to transient fever (90).
The most commonly used source of MSCs is the bone marrow (BM) from which it is renewable (38, 91). Adipose tissue is commonly used as a source of MSCs (92). Other sources of MSCs include the umbilical cord and placenta (34, 93, 94).
The most common indication for MSCs therapy in HCT patients is acute GVHD with numerous encouraging pilot studies (40, 95–98). Other indications following HCT are chronic GVHD, hemorrhagic cystitis, pneumomediastinum (99), and in a few patients reported here ARDS. The immunomodulatory capacity of MSCs has also been used to treat autoimmune inflammatory disorders such as multiple sclerosis (100). Other immune disorders treated by MSCs include rheumatoid arthritis (41), inflammatory bowel disorders (42), and Systemic Lupus Erythematosus (43). MSCs are used to repair several mesodermal tissues including bone, tendon, cartilage, and fat. They were also used to repair cardiac damage (44). Furthermore, they have been used to prevent aging (101).
There is no specific treatment for sepsis and ARDS and the therapy is mainly supportive. However, there is growing evidence of the potential of cell-based therapies for the treatment of sepsis and ARDS. Worldwide more than 5 million people die every year due to sepsis (102).
Symptomatic therapy for ARDS include low-tidal volume ventilation (103), neuromuscular blockage (104), prone position (105), and extracorporeal membrane oxygenation (106).
For a decade MSCs have been studied in several experimental models of sepsis and ARDS. In a mouse model, it was demonstrated that MSCs attenuated septicemia and the effect was dependent on MSCs-derived prostaglandin E2, which reprogrammed macrophages in septic lines to produce anti-inflammatory IL-10 (61). Similarly, MSCs also promote recovery and repair lung injury in rats (107, 108). In a lung contusion injury model followed by hemorrhagic shock in rats, MSCs decreased injury in part by increasing the proportion of regulatory T cells (109). Human MSCs also reduced bacteremia and mortality in mice with gram-negative sepsis (110). In this model, MSCs altered the polarization of alveolar macrophages to an M2-like anti-inflammatory phenotype. The phagocytic ability of macrophages was also enhanced by MSCs in mice who had pneumonia and peritoneal sepsis (111). Similar effects were also seen in a human lung injury model (112).
Devaney et al. also showed that human MSCs improved lung injury induced by E.coli in rats by decreasing levels of alveolar neutrophil inflammation and increased levels of IL-10 and subsequently enhanced function of macrophages (113).
In a mouse model of endotoxin-induced ALI, it was shown that intrapulmonary infusion of BM-MSCs improved survival and attenuated lung injury (114). In mice, it was shown that MSCs resolution of endotoxin-induced lung injury partly was mediated by lipoxin-A4, derived from arachidonic acid (115). Condor and colleagues demonstrated in a sepsis-induced multi-organ failure model that human MSCs increased production of prostaglandin E2 and IL-10 and improved survival (116). Several investigators showed in mice models that administration of human MSCs from various origins i.v. to mice, had positive effects on ALI such as decreased IL-1β, lung edema, decreased IL-1β in bronchoalveolar lavage (BAL), reduced inflammation, and enhanced anti-apoptotic effects (117). Similarly, human umbilical cord-derived MSCs (UC-MSCs) reduced ARDS in rats and improved survival (118). In most of these studies, lung injury was induced by lipopolysaccharide (LPS). Various strategies were also used to enhance the effects of MSCs in the treatment of experimental ARDS. In an LPS model of lung injury in mice, Wang et al. used MSCs overexpressing IL-10 which increased B- and T-cell production of IL-10 and decreased TNF-α, and improved survival (119). The toll-like receptor 3 ligands, poly (I:C), led to increased prostaglandin E2 production and enhanced effect by MSCs on macrophage function in a sepsis model (120). Methods to potentiate the effects of MSCs in experimental ARDS include overexpressing soluble ST2 via lentiviral transfection (121), MSCs transfused with the E-prostanoid 2 receptor (122), overexpression of receptor tyrosine kinase (ROR2) (123), overexpression of platelet-derived growth factor receptor (124), overexpression of ANG-1 (125), overexpression of angiotensin-converting enzyme-2 (126), and overexpression of the gene Angiotensin-converting enzyme growth factor-2 (127). The sphingosine-1 phosphate analog FTY720 was affected together with human MSCs in ARDS in mice (128). Exosomes from MSCs were also beneficial to treat ARDS in mice (88). However, a study showed a better response of lung injury using cells as compared to secretomes (129).
Pulmonary complications are common in patients, following HCT (18, 130, 131). Pulmonary disorders following HCT include, idiopathic pneumonia syndrome, interstitial pneumonitis, diffuse alveolar hemorrhage, peri-engraftment ARDS, noncardiogenic capillary leak syndrome, bronchiolitis obliterans and broncho-pneumonia. ARDS is not so common, but a severe condition post HCT. In a study including 3920 HCT patients, 4,5% developed ARDS, with a one-year mortality of 70% (132). At the end stage of respiratory distress syndrome, the patients may present with hypoxic respiratory failure, meeting the full criteria for ARDS according to the Berlin definition. Among 2635 patients following allogeneic or autologous HCT, 133 cases 5,0% developed ARDS (133). One year mortality was 67%.
Patients who develop ARDS following HCT have a very poor outcome. In the 1990th mortality approached 100% in the intubated patients (134). In intubated patients with multiple organ failure (MOF) after HCT, mortality was 94-100%. With such a dismal outlook, palliative care was commonly chosen, in those days.
Improvements in intensive care in more recent years, as using ventilation in a prone position, restrictive fluid therapy, new diagnostic methods, and possibility to treat pathogens, have resulted in improved survival rate in this group. Extracorporeal membrane oxygenation (ECMO) was used to treat ARDS in 37 adult patients at a median of 147 days after HCT (135). Overall, 7(19%) of the patients survived until discharge. Patients treated more than 240 days after HCT had a better survival 6/13(46%) as opposed to 1/24(4%) among those treated early(p<0.001). In pediatric patients with ARDS, survival was reported to be 24,6% (136). MSCs were often used to treat severe acute GVHD following HCT (98). However, MSCs were rarely used to treat ARDS after HCT. Therefore, these case reports are of interest.
There is so far limited experience using MSCs in patients compared to the multiple studies in experimental models of ALI/ARDS. Adipose-derived MSCs were used in a small prospective randomized placebo-controlled study including 12 patients with ARDS (137). Intravenous Adipose-MSCs 1x106 cells/kg vs placebo was given and there was no difference in outcome in patients treated with Adipose-MSCs versus placebo. In a phase I study, 3 patients each with ARDS received 1x106, 5x106, or 10x106 MSCs/kg as a single intravenous dose for ARDS (138). There were no MSC infusion-related hemodynamic, or respiratory adverse events reported. The treatment was well tolerated and the dose of 10x106 cells/kg was selected for further study. A randomized phase 2 safety trial using 10x106 BM-MSC/kg showed no statistical difference in survival between the BM-MSC groups (n=40) and the placebo group (n=20) (139),. The primary endpoint was safety, and it was shown that it was safe to infuse 10x106 BM-MSC/kg in patients with moderate to severe ARDS. There were no MSCs infusion-related hemodynamic, or respiratory adverse events reported. In a separate study it was found that compared with placebo, MSC treatment significantly reduced airspace total protein, angiopoietin-2 (Ang-2), IL-6, and soluble TNF receptor-1 concentrations (140). In conclusion from these randomized studies in ARDS patients, adipose or BM-MSCs were safe to infuse, but did not improve survival compared to placebo.
Several trials are registered to use MSCs to treat Covid-19-induced ARDS (141). A study showed that MSCs are resistant to SARS-CoV-2-infection and retain their immunomodulation potential, supporting their applicability for Covid-19-induced ARDS (142). Five patients were given 1x106 UC-MSCs/kg for Covid-19 ARDS. After infusion of UC-MSCs, there was a rise of PaO2/FiO2 in all patients, three patients survived. In a German study 5 out of 23 patients with severe Covid-19 were treated with 1x106 MSCs/kg (143). Four of the five BM-MSC-treated patients got ECMO to support vs 9/18 of the controls. Four of the 5 BM-MSCs-treated patients survived to discharge compared to 8/18 of the controls. A study used UC-MSCs in six and placenta-derived MSCs in 5 patients (144). The patients received fixed doses, 200x106 MSCs infused every other day for 3 doses. No side effects were seen and 6 of the 11 patients survived (144). UC-MSCs were used in a double-blind pilot study including 24 Covid-19 ARDS patients (145). Two fixed doses of 100x106 UC-MSCs were given to 12 patients. UC-MSCs infusions were safe. Survival was 91% in the UC-MSC group compared to 42% among the controls (p=0.015) (145). A Chinese study compared 26 patients treated for Covid-19 for ARDS with 3 doses of MSCs from menstrual blood with 18 control patients (146). Safety was the same between the two groups. Mortality was 7.69% in the MSCs group as opposed to 33.33% among the controls (p=0.048). During the Covid-19 pandemic several groups conducted small phase 1 trials using MSCs (147–154). Monsel et al. did not find an improved outcome following 3 infusions of UC-MSC compared to the control group (151). Dilogo et al. randomized 20 patients to UC-MSC and 20 patients to placebo. 10 patients in the UC arm survived compared to 4 patients in the placebo arm (P<0.05). Length of stay in ICU and ventilator usage did not differ between the two groups (153). In a prospective randomized study, enrolling 101 patients, who were given UC-MSCs or placebo, the UC-MSC group, the UC-MSC group had a trend for numerically improvement in whole lung lesion volume on day 28 (P<0.08) (152). Gregoire and colleagues used BM-MSCs in a phase I/II trial and treated 8 intensive care patients with Covid-19 induced ARDS with three i.v. infusions at three-day intervals with 1.5-3x10-6 cells/kg (155). All MSCs treated patients were alive at 60 days which was significantly higher compared to 70.8% in 24 well-matched control patients, who were not given MSCs (p<0.0082).
DSCs were given to l0 ARDS patients with Covid-19 disease in Tehran (156). Pulmonary infiltrates disappeared in all patients. Seven patients survived and were discharged. To conclude, several trials used MSCs from different origin to treat Covid-19 induced ARDS. Some promising outcomes were seen using UC-MSCs and DSCs.
Following HCT three patients developed ALI/ARDS and were treated with BM-MSCs in two cases and DSCs in the third case (28). This is a case study, conducted in an academic setting. ARDS is defined according to the Berlin criteria (157). ALI have similar clinical features without fulfilling all ARDS criteria according to the Berlin definition (158).
The first patient was a 22-year-old male with acute lymphoblastic leukemia in second remission, who was given a cord blood graft (Table 1). The second patient was a 13-year-old boy with acute lymphoblastic leukemia (ALL) with a graft from an unrelated donor. A 33-year-old man with chronic myeloid leukemia, resistant to Nilotinib, received a graft from an HLA-identical unrelated donor (Table 1). The first two patients were conditioned before transplant with cyclophosphamide and fractionated total body irradiation (159). The third patient was conditioned with cyclophosphamide and busulfan. Prophylaxis against GVHD was methotrexate and cyclosporine. The transplantation procedure was previously published in detail (160, 161) (Table 1).
The culture method and expansion of MSCs and DSCs was previously published in detail (21, 162). The stromal cells expressed CD166, CD105, CD73, CD44, and CD29 but not hematopoietic markers CD34, CD14, and CD45. The cells were cultured, and control samplings were negative for bacteria, mycoplasma, and fungi during expansion and before infusion. The stromal cells were cultured and expanded in a good manufacturing process condition, at the Department of Clinical Immunology, Karolinska University Hospital, Huddinge, Sweden.
Initially we used BM-MSCs for immunomodulation in HCT patients (40, 99). Due to insufficient clinical effect using BM-MSCs and a better immunomodulatory effect by DSCs, compare to BM-MSCs, we later switched to DSCs (94, 163, 164).
The MSCs and DSCs were stored in liquid nitrogen, thawed, and suspended in cliniMACS PBS/EDTA buffer, supplemented with 10% AB plasma for MSCs and 5% albumin for DSCs. The cells were washed three times and resuspended in NaCl and 10% AB-serum (MSCs) or 5% albumin (DSCs) (164, 165). The infusion solution was then filtered through a 70μM cell strainer (BD Bioscience, Franklin Lakrs, NI). It was then transferred to a heparinized syringe at 2x106 cells/ml. The MSCs and DSCs were infused i.v. via a central venous line. Dosing aimed at 1x106 MSCs/kg, due to the number of cells in frozen vials and following thawing, the final dose given to the three patients varied from 1.0-1.4 x106 cell/kg in this report. The line was flushed with 2-5 ml NaCl containing 50 IE heparin/ml in the two adults and 25 IE heparin/ml in the boy.
Patient 1 underwent HCT in 2004. Following initial acute gastrointestinal GVHD with diarrhea, he developed hemorrhagic cystitis and was treated with 0.7x106 MSCs/kg on day 81 after HCT. A week before MSCs infusion he required 8 units of erythrocyte concentrates, 14 units of fresh frozen plasma, and 9 platelet transfusions. The week following MSCs infusion he only required 2 platelet transfusions and no erythrocyte transfusions. On day +93 he worsened and developed multiorgan failure. Pulmonary X-ray revealed ALI/ARDS. On day +96 he was given a second dose of 1.4x106/kg of HLA haploidentical MSCs from his mother (Table 2). Following this, a new X-ray was performed and showed clear lungs with no signs of ALI/ARDS (Table 2). Despite this, the patient deteriorated and died on day +104 due to multi-organ failure. Patient developed renal, liver, and cardiac failure, which was the cause of death (Table 2). Autopsy revealed that the bladder was healed.
Patient 2 was a thirteen-year-old boy with ALL and prolonged neutropenia after HCT. Due to this, he was treated with granulocyte transfusions to treat his bacterial infection. Following this, he had deteriorated pulmonary function and an X-ray showed bilateral pulmonary infiltrates (Table 2). This was thought to be due to granulocyte transfusion. Due to the picture of ALI/ARDS, he was treated with 1x106 MSCs/kg. Following this, he required ventilatory assistance and died of massive aspergillosis pneumonia as found at autopsy (Table 2). The reason of death was pulmonary failure. The role of BM-MSCs in exacerbating invasive fungal infection is discussed in the discussion (second paragraph).
Patient 3 was a thirty-three-year-old man with chronic myeloid leukemia who underwent a transplant in 2014 and developed septicemia during the neutropenic phase after HCT. On day +8 he had a fever (40°C) and was treated with high doses of piperacillin/tazobactam. Chest radiography was normal. However, the fever continued, and the maximum pulse increased to 155/min. Blood culture showed alpha streptococci. Hemoglobin decreased from 100 to 60g/l. Weight increased by 5 kg. On day +10 he was short of breath and chest radiography suggested ARDS (Table 2). He required oxygen supply, and this was increased to 15L/min. He was given large doses of albumin and furosemide. Oxygen saturation varied between 92-96%. The patient was completely exhausted and needed ventilatory support. From a compassionate basis and according to the Declaration of Helsinki, he was given 1x106 DSCs/kg on day +11. Oxygen saturation instantly increased from 88-92% to 98% (and was thereby stabilized). Oxygen supply, which was successively decreased, was discontinued five days later. Chest radiography improved and was normal on day +22 after HCT when the patient was discharged from the hospital (Table 2). He had elevated granulocyte colony-stimulating factor, IL-6, IL-8, and MCP-1 which all decreased 3 hours after DSC infusion and normalized one week after infusion. The patient is alive and well after 7 years.
The reason we used MSCs for the treatment of ARDS was that these cells first home in the lungs when given i.v (22, 24, 25). and they have a very potent immunomodulatory and anti-inflammatory effect (19, 20, 166). The hope was that they would turn off the ARDS-induced cytokine storm in the lungs. Recently Wick KD et al. showed that MSCs infusion locally decrease the inflammatory cytokine level compared to the control group (140). In our first patient, the pulmonary infiltrates cleared (Table 2). However, he suffered from multiple medical problems and finally died from multi-organ failure.
The second patient died from invasive aspergillosis. An invasive fungal infection may be a problem after MSC therapy (163). This could be due to local immunosuppression following MSCs infusion, paving the way for invasive fungal infection (140). We also experienced an increased risk of invasive fungal infection and death by pneumonia in HCT patients given MSCs (167). Therefore, antifungal prophylaxis should be considered in patients treated with MSC following HCT (28).
The third patient responded dramatically to DSCs with a very rapid recovery. Reasons for this better response compared to BM-MSCs may be the more potent immunosuppressive and anti-inflammatory effects by DSCs as opposed to BM-MSCs seen in vitro and in vivo for the treatment of acute GVHD (94, 164). Although BM-MSCs and DSCs have similar surface markers, such as CD105, CD166, CD73, CD90, and CD29, there are several differences between BM-MSCs and DSCs apart from a more potent immunosuppressive effect by DSCs (162, 168). DSCs are half the size of BM-MSCs and do not differentiate well to fat and cartilage (169).
In the ARDS patient who was successfully treated by DSCs, cytokines/chemokines G-CSF, IL-6, IL-8, MCP1, and TNF-α all decreased dramatically in blood. In addition, DSCs also decrease IFN-γ and IL-17 (94). All these cytokines contribute to the cytokine storm during ARDS (16, 170).
Furthermore, DSCs compared to BM-MSCs, have a stronger expression of CD49b, a marker for homing to inflamed tissue. This may be of importance in the context of ARDS. The data presented here must of course be taken with a lot of caution because these are only three anecdotal cases and performed over ten years. In fact, ARDS is relatively rare following HCT. The more promising immunomodulatory and anti-inflammatory response using DSCs as opposed to BM-MSCs includes also patients treated for acute GVHD (n=38) and hemorrhagic cystitis (n=11) (164, 171). Although these studies suggest that DSCs have a better immunosuppressive effect as opposed to BM-MSCs (164), well-designed prospective randomized trials are necessary to prove the superior efficacy of DSCs.
In the literature, numerous preclinical studies are supporting the effects and rationale to use MSCs for sepsis and ARDS, some of which are referred to in this review, and there are more published (172–174). Concerning GVHD, MSCs from different sources do not work very well to reverse this disorder in mice in contrast to humans (175).
In mice BM-MSCs need to be licensed by various methods, like treatment with IFN-γ, insertion of IL-10 promoting genes, and more, to be effective to reverse acute GVHD. In a similar way, several methods to enhance the anti-inflammatory effect of MSCs were also used for ARDS and sepsis in several animal models. Several of these modalities are previously described in detail (176).
In the clinic and for manufacturing of MSCs, it may be somewhat more problematic if gene-manipulation is needed to make MSCs useful for therapy.
In the limited clinical experience using MSCs, the two randomized ARDS studies so far, did not show any difference in outcome comparing MSCs from adipose or BM and placebo (137, 139). Although there were no significant differences in survival between the MSC patients and the placebo controls, there was a numerically increased mortality in the patients in the MSC group. This was due to the fact that the patients in the MSC group had numerically higher severity scores. A concern with this trial may be that viability of the MSC at infusion was rather poor 36%-85%. In our clinically responding DSC-treated patients, DSC viability was a median of 95% (164). Although it has been shown that MSCs immunosuppressive function in the body comes after apoptosis (71, 72), but it is important that we inject live cells rather than dead cells. When the cell dies outside the body, they might not have the same apoptotic or efferocytosis effects in vivo. The high numbers of BM-MSC 10x106/kg compared to 1x106 DSC/kg in our DSC-treated patient did not seem to compensate for poor cell viability.
In all clinical studies so far, it appears safe to inject MSCs and there were no serious adverse events reported. This is also in line with two meta-analyses regarding the safety of MSC therapy (89, 90). MSCs appear safe and side-effects seem to be limited to a slight fever. There is much less experience with DSCs. However, safety data in rats and mice as well as from the clinic suggest that they are as safe to inject as MSCs from BM (165, 177). In the clinical experience, side-effects were limited to three cases at the time of infusion and were spontaneously reversible without the need for any medical intervention.
Even if there is limited clinical experience using MSC to treat ALI/ARDS, it is worth pursuing this potentially effective therapy. As stated in this review and some other reviews, MSC therapy is demonstrated to be effective in several preclinical models of sepsis and ARDS (108, 172–174). In the clinic, several questions need to be addressed before we have an optimal stromal cell therapy for ARDS. What is the optimal source, bone marrow, adipose, UC, or placenta-derived? What is the optimal dose, and what is the best route of administration, i.v. or intratracheal? Intramuscular is probably less effective for ARDS because you want to shut off the cytokine storm in the lung. Are soluble factors and exosomes more or less useful than stromal cells (178)? Will priming with e.g., IFN-γ, TNF-α, IL-1α or -β or IL-17A be beneficial (176)? There are several unanswered questions to be studied before this promising therapy can move forward and be an established therapy for sepsis and ARDS. Technically allogeneic MSCs should be used for ARDS, because they can be prepared and taken “off the shelf”. Autologous MSCs in not an option because it takes several weeks to expand them to a meaningful number for clinical use.
With the global threats of the new coronavirus, Covid-19 pandemic, mortality noted in 3.7% of the infected patients seems to be due to ARDS in most (88%) of the patients (179). Many of the COVID-19 patients are in a hypercoagulable procoagulant state and at high risk for disseminated intravascular coagulation, thromboembolism, and thrombotic multi-organ failure (17). When choosing a therapeutic approach with or without cell based therapy we should consider this condition.
Therefore, it is an urgent need for effective therapy of ARDS. The burden of ARDS patients needing ventilator assistance is tremendous, and hopefully, stromal cell therapy may be used successfully to limit the burden on intensive care units worldwide.
In ARDS induced by bacterial sepsis, there are most effective antibiotics to be used, like in our HCT case treated for ARDS by DSCs. For Covid-19, there is no effective drug to inhibit viral replication and subsequent dissemination to the heart, kidneys, and intestine. In some cases, it may be sufficient to turn off the cytokine storm and the alveolar damage. In others, this may not be enough. There is limited clinical experience using various sources of MSCs for Covid-19-induced ARDS. So far, MSCs from UC and DCSs hold some promise (145, 156).
In conclusion, MSCs from various tissues, like BM, adipose, UC, and placental tissues, have taken the step from experimental animals to clinical trials. We have added three rare HCT cases to the so far limited clinical experience. One of them had a most dramatic clinical response to DSCs treatment. There are too few HCT patients with ARDS, so large prospective studies have to be performed in other patients’ groups. There are increasing numbers of pilot studies reporting on the use of various sources of MSCs for Covid-19-induced ARDS. Well-designed prospective placebo-controlled studies are needed to prove efficacy.
We had ethical approval to harvest MSCs from volunteer donors and to treat the patients against GVHD and other toxicities following HCT (Dnr 328/03). We also had ethical permission to harvest DSCs from Caesarean section placentas and use them for the treatment of GVHD and other toxic conditions after HCT (2009/418-31-34 and 2010/2061-32, 2010/452-31/4 and 2014/2132-32). The procedure to use DSCs was also approved by the Central Ethical Review Board in Sweden (Dnr 011-2016). Bone marrow (BM) was aspirated from volunteer donors. The methods for clinical culture of DSCs were previously approved by the Swedish Product Agency (Dnr 6.1.3 – 42994/2013). Written informed consent was obtained from the individuals and, regarding the pediatric patient, both parents gave informed consent for the publication of any potentially identifiable images or data included in this article.
OR wrote the first draft of the article, complemented, and revised by BS, BG, and MC. All authors contributed to the article and approved the submitted version.
The study was supported with grants from the Swedish Cancer Foundation (CAN 2018, 419) and the Cancer Society in Stockholm (111293). OR was the recipient of a Distinguished Professor Award from the Karolinska Institutet. BG was recipient of grant (PR2020-0058) from Swedish children cancer society (Barncancerfonden).
We thank Gunilla Tillinger for the excellent typing of this manuscript.
The authors declare that the research was conducted in the absence of any commercial or financial relationships that could be construed as a potential conflict of interest.
All claims expressed in this article are solely those of the authors and do not necessarily represent those of their affiliated organizations, or those of the publisher, the editors and the reviewers. Any product that may be evaluated in this article, or claim that may be made by its manufacturer, is not guaranteed or endorsed by the publisher.
1. Phua J, Badia JR, Adhikari NK, Friedrich JO, Fowler RA, Singh JM, et al. Has mortality from acute respiratory distress syndrome decreased over time?: A systematic review. Am J Respir Crit Care Med (2009) 179(3):220–7. doi: 10.1164/rccm.200805-722OC
2. Rubenfeld GD, Caldwell E, Peabody E, Weaver J, Martin DP, Neff M, et al. Incidence and outcomes of acute lung injury. N Engl J Med (2005) 353(16):1685–93. doi: 10.1056/NEJMoa050333
3. Ashbaugh DG, Bigelow DB, Petty TL, Levine BE. Acute respiratory distress in adults. Lancet (1967) 2(7511):319–23. doi: 10.1016/S0140-6736(67)90168-7
4. Matthay MA, Zemans RL. The acute respiratory distress syndrome: pathogenesis and treatment. Annu Rev Pathol (2011) 6:147–63. doi: 10.1146/annurev-pathol-011110-130158
5. Zilberberg MD, Carter C, Lefebvre P, Raut M, Vekeman F, Duh MS, et al. Red blood cell transfusions and the risk of acute respiratory distress syndrome among the critically ill: a cohort study. Crit Care (2007) 11(3):R63. doi: 10.1186/cc5934
6. Ferguson ND, Fan E, Camporota L, Antonelli M, Anzueto A, Beale R, et al. The Berlin definition of ARDS: an expanded rationale, justification, and supplementary material. Intensive Care Med (2012) 38(10):1573–82. doi: 10.1007/s00134-012-2682-1
7. Tomashefski JF Jr.. Pulmonary pathology of the adult respiratory distress syndrome. Clin Chest Med (1990) 11(4):593–619. doi: 10.1016/S0272-5231(21)00758-9
8. Bellani G, Laffey JG, Pham T, Fan E, Brochard L, Esteban A, et al. Epidemiology, Patterns of Care, and Mortality for Patients With Acute Respiratory Distress Syndrome in Intensive Care Units in 50 Countries. Jama (2016) 315(8):788–800. doi: 10.1001/jama.2016.0291
9. Luhr OR, Antonsen K, Karlsson M, Aardal S, Thorsteinsson A, Frostell CG, et al. Incidence and mortality after acute respiratory failure and acute respiratory distress syndrome in Sweden, Denmark, and Iceland. ARF Study Group Am J Respir Crit Care Med (1999) 159(6):1849–61. doi: 10.1164/ajrccm.159.6.9808136
10. Angus DC, Musthafa AA, Clermont G, Griffin MF, Linde-Zwirble WT, Dremsizov TT, et al. Quality-adjusted survival in the first year after the acute respiratory distress syndrome. Am J Respir Crit Care Med (2001) 163(6):1389–94. doi: 10.1164/ajrccm.163.6.2005123
11. Thompson BT, Chambers RC, Liu KD. Acute Respiratory Distress Syndrome. N Engl J Med (2017) 377(6):562–72. doi: 10.1056/NEJMra1608077
12. Gao Smith F, Perkins GD, Gates S, Young D, McAuley DF, Tunnicliffe W, et al. Effect of intravenous β-2 agonist treatment on clinical outcomes in acute respiratory distress syndrome (BALTI-2): a multicentre, randomised controlled trial. Lancet (2012) 379(9812):229–35. doi: 10.1016/S0140-6736(11)61623-1
13. McAuley DF, Laffey JG, O'Kane CM, Perkins GD, Mullan B, Trinder TJ, et al. Simvastatin in the acute respiratory distress syndrome. N Engl J Med (2014) 371(18):1695–703. doi: 10.1056/NEJMoa1403285
14. Felsenstein S, Herbert JA, McNamara PS, Hedrich CM. COVID-19: Immunology and treatment options. Clin Immunol (2020) 215:108448. doi: 10.1016/j.clim.2020.108448
15. Fajgenbaum DC, June CH. Cytokine Storm. N Engl J Med (2020) 383(23):2255–73. doi: 10.1056/NEJMra2026131
16. Chousterman BG, Swirski FK, Weber GF. Cytokine storm and sepsis disease pathogenesis. Semin Immunopathol (2017) 39(5):517–28. doi: 10.1007/s00281-017-0639-8
17. Moll G, Drzeniek N, Kamhieh-Milz J, Geissler S, Volk HD, Reinke P. MSC Therapies for COVID-19: Importance of Patient Coagulopathy, Thromboprophylaxis, Cell Product Quality and Mode of Delivery for Treatment Safety and Efficacy. Front Immunol (2020) 11:1091. doi: 10.3389/fimmu.2020.01091
18. John R, Wingard DAG, Leather HL, Snyder E, Zbigniew M. Szczepiorkowski. In: Wingard JR, editor. Hematopoietic Stem Cell Transplantation: A Handbook for Clinicians (USA: American Association of Blood Banks (AABB)) (2015) 493–512.
19. Bartholomew A, Sturgeon C, Siatskas M, Ferrer K, McIntosh K, Patil S, et al. Mesenchymal stem cells suppress lymphocyte proliferation in vitro and prolong skin graft survival in vivo. Exp Hematol (2002) 30(1):42–8. doi: 10.1016/S0301-472X(01)00769-X
20. Di Nicola M, Carlo-Stella C, Magni M, Milanesi M, Longoni PD, Matteucci P, et al. Human bone marrow stromal cells suppress T-lymphocyte proliferation induced by cellular or nonspecific mitogenic stimuli. Blood (2002) 99(10):3838–43. doi: 10.1182/blood.V99.10.3838
21. Le Blanc K, Tammik L, Sundberg B, Haynesworth SE, Ringdén O. Mesenchymal stem cells inhibit and stimulate mixed lymphocyte cultures and mitogenic responses independently of the major histocompatibility complex. Scand J Immunol (2003) 57(1):11–20. doi: 10.1046/j.1365-3083.2003.01176.x
22. Gao J, Dennis JE, Muzic RF, Lundberg M, Caplan AI. The dynamic in vivo distribution of bone marrow-derived mesenchymal stem cells after infusion. Cells Tissues Organs (2001) 169(1):12–20. doi: 10.1159/000047856
23. Devine SM, Cobbs C, Jennings M, Bartholomew A, Hoffman R. Mesenchymal stem cells distribute to a wide range of tissues following systemic infusion into nonhuman primates. Blood (2003) 101(8):2999–3001. doi: 10.1182/blood-2002-06-1830
24. Leibacher J, Henschler R. Biodistribution, migration and homing of systemically applied mesenchymal stem/stromal cells. Stem Cell Res Ther (2016) 7:7. doi: 10.1186/s13287-015-0271-2
25. Erkers T, Kaipe H, Nava S, Mollden P, Gustafsson B, Axelsson R, et al. Treatment of severe chronic graft-versus-host disease with decidual stromal cells and tracing with (111)indium radiolabeling. Stem Cells Dev (2015) 24(2):253–63. doi: 10.1089/scd.2014.0265
26. Lee RH, Pulin AA, Seo MJ, Kota DJ, Ylostalo J, Larson BL, et al. Intravenous hMSCs improve myocardial infarction in mice because cells embolized in lung are activated to secrete the anti-inflammatory protein TSG-6. Cell Stem Cell (2009) 5(1):54–63. doi: 10.1016/j.stem.2009.05.003
27. Eggenhofer E, Benseler V, Kroemer A, Popp FC, Geissler EK, Schlitt HJ, et al. Mesenchymal stem cells are short-lived and do not migrate beyond the lungs after intravenous infusion. Front Immunol (2012) 3:297. doi: 10.3389/fimmu.2012.00297
28. Ringdén O. Mesenchymal stem (stromal) cells for treatment of acute respiratory distress syndrome. Lancet Respir Med (2015) 3(4):e12. doi: 10.1016/S2213-2600(15)00047-8
29. Friedenstein AJ, Petrakova KV, Kurolesova AI, Frolova GP. Heterotopic of bone marrow. Anal precursor Cells osteogenic hematopoietic tissues. Transplant (1968) 6(2):230–47.
30. Friedenstein AJ. Precursor cells of mechanocytes. Int Rev Cytol (1976) 47:327–59. doi: 10.1016/S0074-7696(08)60092-3
31. Friedenstein AJ, Chailakhyan RK, Gerasimov UV. Bone marrow osteogenic stem cells: in vitro cultivation and transplantation in diffusion chambers. Cell Tissue Kinet (1987) 20(3):263–72. doi: 10.1111/j.1365-2184.1987.tb01309.x
32. Pittenger MF, Mackay AM, Beck SC, Jaiswal RK, Douglas R, Mosca JD, et al. Multilineage potential of adult human mesenchymal stem cells. Science (1999) 284(5411):143–7. doi: 10.1126/science.284.5411.143
33. Prockop DJ. Marrow stromal cells as stem cells for nonhematopoietic tissues. Science (1997) 276(5309):71–4. doi: 10.1126/science.276.5309.71
34. In 't Anker PS, Scherjon SA, Kleijburg-van der Keur C, de Groot-Swings GM, Claas FH, Fibbe WE, et al. Isolation of mesenchymal stem cells of fetal or maternal origin from human placenta. Stem Cells (2004) 22(7):1338–45. doi: 10.1634/stemcells.2004-0058
36. Toma C, Pittenger MF, Cahill KS, Byrne BJ, Kessler PD. Human mesenchymal stem cells differentiate to a cardiomyocyte phenotype in the adult murine heart. Circulation (2002) 105(1):93–8. doi: 10.1161/hc0102.101442
37. Barry F, Boynton R, Murphy M, Haynesworth S, Zaia J. The SH-3 and SH-4 antibodies recognize distinct epitopes on CD73 from human mesenchymal stem cells. Biochem Biophys Res Commun (2001) 289(2):519–24. doi: 10.1006/bbrc.2001.6013
38. Koç ON, Gerson SL, Cooper BW, Dyhouse SM, Haynesworth SE, Caplan AI, et al. Rapid hematopoietic recovery after coinfusion of autologous-blood stem cells and culture-expanded marrow mesenchymal stem cells in advanced breast cancer patients receiving high-dose chemotherapy. J Clin Oncol (2000) 18(2):307–16. doi: 10.1200/JCO.2000.18.2.307
39. Le Blanc K, Rasmusson I, Sundberg B, Gotherstrom C, Hassan M, Uzunel M, et al. Treatment of severe acute graft-versus-host disease with third party haploidentical mesenchymal stem cells. Lancet (2004) 363(9419):1439–41. doi: 10.1016/S0140-6736(04)16104-7
40. Ringden O, Uzunel M, Rasmusson I, Remberger M, Sundberg B, Lonnies H, et al. Mesenchymal stem cells for treatment of therapy-resistant graft-versus-host disease. Transplantation (2006) 81(10):1390–7. doi: 10.1097/01.tp.0000214462.63943.14
41. Wang L, Wang L, Cong X, Liu G, Zhou J, Bai B, et al. Human umbilical cord mesenchymal stem cell therapy for patients with active rheumatoid arthritis: safety and efficacy. Stem Cells Dev (2013) 22(24):3192–202. doi: 10.1089/scd.2013.0023
42. Duijvestein M, Vos AC, Roelofs H, Wildenberg ME, Wendrich BB, Verspaget HW, et al. Autologous bone marrow-derived mesenchymal stromal cell treatment for refractory luminal Crohn's disease: results of a phase I study. Gut (2010) 59(12):1662–9. doi: 10.1136/gut.2010.215152
43. Alexander T, Thiel A, Rosen O, Massenkeil G, Sattler A, Kohler S, et al. Depletion of autoreactive immunologic memory followed by autologous hematopoietic stem cell transplantation in patients with refractory SLE induces long-term remission through de novo generation of a juvenile and tolerant immune system. Blood (2009) 113(1):214–23. doi: 10.1182/blood-2008-07-168286
44. Hatzistergos KE, Quevedo H, Oskouei BN, Hu Q, Feigenbaum GS, Margitich IS, et al. Bone marrow mesenchymal stem cells stimulate cardiac stem cell proliferation and differentiation. Circ Res (2010) 107(7):913–22. doi: 10.1161/CIRCRESAHA.110.222703
45. Rasmusson I, Ringdén O, Sundberg B, Le Blanc K. Mesenchymal stem cells inhibit lymphocyte proliferation by mitogens and alloantigens by different mechanisms. Exp Cell Res (2005) 305(1):33–41. doi: 10.1016/j.yexcr.2004.12.013
46. Aggarwal S, Pittenger MF. Human mesenchymal stem cells modulate allogeneic immune cell responses. Blood (2005) 105(4):1815–22. doi: 10.1182/blood-2004-04-1559
47. Maccario R, Podestà M, Moretta A, Cometa A, Comoli P, Montagna D, et al. Interaction of human mesenchymal stem cells with cells involved in alloantigen-specific immune response favors the differentiation of CD4+ T-cell subsets expressing a regulatory/suppressive phenotype. Haematologica (2005) 90(4):516–25. doi: 10.3324/%25x
48. English K, Ryan JM, Tobin L, Murphy MJ, Barry FP, Mahon BP. Cell contact, prostaglandin E(2) and transforming growth factor beta 1 play non-redundant roles in human mesenchymal stem cell induction of CD4+CD25(High) forkhead box P3+ regulatory T cells. Clin Exp Immunol (2009) 156(1):149–60. doi: 10.1111/j.1365-2249.2009.03874.x
49. Prevosto C, Zancolli M, Canevali P, Zocchi MR, Poggi A. Generation of CD4+ or CD8+ regulatory T cells upon mesenchymal stem cell-lymphocyte interaction. Haematologica (2007) 92(7):881–8. doi: 10.3324/haematol.11240
50. Le Blanc K, Rasmusson I, Götherström C, Seidel C, Sundberg B, Sundin M, et al. Mesenchymal stem cells inhibit the expression of CD25 (interleukin-2 receptor) and CD38 on phytohaemagglutinin-activated lymphocytes. Scand J Immunol (2004) 60(3):307–15. doi: 10.1111/j.0300-9475.2004.01483.x
51. Rasmusson I, Ringdén O, Sundberg B, Le Blanc K. Mesenchymal stem cells inhibit the formation of cytotoxic T lymphocytes, but not activated cytotoxic T lymphocytes or natural killer cells. Transplantation (2003) 76(8):1208–13. doi: 10.1097/01.TP.0000082540.43730.80
52. Selmani Z, Naji A, Gaiffe E, Obert L, Tiberghien P, Rouas-Freiss N, et al. HLA-G is a crucial immunosuppressive molecule secreted by adult human mesenchymal stem cells. Transplantation (2009) 87(9 Suppl):S62–6. doi: 10.1097/TP.0b013e3181a2a4b3
53. Le Blanc K, Tammik C, Rosendahl K, Zetterberg E, Ringdén O. HLA expression and immunologic properties of differentiated and undifferentiated mesenchymal stem cells. Exp Hematol (2003) 31(10):890–6. doi: 10.1016/S0301-472X(03)00110-3
54. Krampera M, Cosmi L, Angeli R, Pasini A, Liotta F, Andreini A, et al. Role for interferon-gamma in the immunomodulatory activity of human bone marrow mesenchymal stem cells. Stem Cells (2006) 24(2):386–98. doi: 10.1634/stemcells.2005-0008
55. Opitz CA, Litzenburger UM, Lutz C, Lanz TV, Tritschler I, Köppel A, et al. Toll-like receptor engagement enhances the immunosuppressive properties of human bone marrow-derived mesenchymal stem cells by inducing indoleamine-2,3-dioxygenase-1 via interferon-beta and protein kinase R. Stem Cells (2009) 27(4):909–19. doi: 10.1002/stem.7
56. Munn DH, Zhou M, Attwood JT, Bondarev I, Conway SJ, Marshall B, et al. Prevention of allogeneic fetal rejection by tryptophan catabolism. Science (1998) 281(5380):1191–3. doi: 10.1126/science.281.5380.1191
57. Meisel R, Zibert A, Laryea M, Göbel U, Däubener W, Dilloo D. Human bone marrow stromal cells inhibit allogeneic T-cell responses by indoleamine 2,3-dioxygenase-mediated tryptophan degradation. Blood (2004) 103(12):4619–21. doi: 10.1182/blood-2003-11-3909
58. Ge W, Jiang J, Arp J, Liu W, Garcia B, Wang H. Regulatory T-cell generation and kidney allograft tolerance induced by mesenchymal stem cells associated with indoleamine 2,3-dioxygenase expression. Transplantation (2010) 90(12):1312–20. doi: 10.1097/TP.0b013e3181fed001
59. Tatara R, Ozaki K, Kikuchi Y, Hatanaka K, Oh I, Meguro A, et al. Mesenchymal stromal cells inhibit Th17 but not regulatory T-cell differentiation. Cytotherapy (2011) 13(6):686–94. doi: 10.3109/14653249.2010.542456
60. Augello A, Tasso R, Negrini SM, Amateis A, Indiveri F, Cancedda R, et al. Bone marrow mesenchymal progenitor cells inhibit lymphocyte proliferation by activation of the programmed death 1 pathway. Eur J Immunol (2005) 35(5):1482–90. doi: 10.1002/eji.200425405
61. Németh K, Leelahavanichkul A, Yuen PS, Mayer B, Parmelee A, Doi K, et al. Bone marrow stromal cells attenuate sepsis via prostaglandin E(2)-dependent reprogramming of host macrophages to increase their interleukin-10 production. Nat Med (2009) 15(1):42–9. doi: 10.1038/nm.1905
62. Melief SM, Schrama E, Brugman MH, Tiemessen MM, Hoogduijn MJ, Fibbe WE, et al. Multipotent stromal cells induce human regulatory T cells through a novel pathway involving skewing of monocytes toward anti-inflammatory macrophages. Stem Cells (2013) 31(9):1980–91. doi: 10.1002/stem.1432
63. Saldanha-Araujo F, Ferreira FI, Palma PV, Araujo AG, Queiroz RH, Covas DT, et al. Mesenchymal stromal cells up-regulate CD39 and increase adenosine production to suppress activated T-lymphocytes. Stem Cell Res (2011) 7(1):66–74. doi: 10.1016/j.scr.2011.04.001
64. Gieseke F, Böhringer J, Bussolari R, Dominici M, Handgretinger R, Müller I. Human multipotent mesenchymal stromal cells use galectin-1 to inhibit immune effector cells. Blood (2010) 116(19):3770–9. doi: 10.1182/blood-2010-02-270777
65. Corcione A, Benvenuto F, Ferretti E, Giunti D, Cappiello V, Cazzanti F, et al. Human mesenchymal stem cells modulate B-cell functions. Blood (2006) 107(1):367–72. doi: 10.1182/blood-2005-07-2657
66. Rasmusson I, Le Blanc K, Sundberg B, Ringdén O. Mesenchymal stem cells stimulate antibody secretion in human B cells. Scand J Immunol (2007) 65(4):336–43. doi: 10.1111/j.1365-3083.2007.01905.x
67. Choi H, Lee RH, Bazhanov N, Oh JY, Prockop DJ. Anti-inflammatory protein TSG-6 secreted by activated MSCs attenuates zymosan-induced mouse peritonitis by decreasing TLR2/NF-κB signaling in resident macrophages. Blood (2011) 118(2):330–8. doi: 10.1182/blood-2010-12-327353
68. Akiyama K, Chen C, Wang D, Xu X, Qu C, Yamaza T, et al. Mesenchymal-stem-cell-induced immunoregulation involves FAS-ligand-/FAS-mediated T cell apoptosis. Cell Stem Cell (2012) 10(5):544–55. doi: 10.1016/j.stem.2012.03.007
69. Ren G, Zhao X, Zhang L, Zhang J, L'Huillier A, Ling W, et al. Inflammatory cytokine-induced intercellular adhesion molecule-1 and vascular cell adhesion molecule-1 in mesenchymal stem cells are critical for immunosuppression. J Immunol (2010) 184(5):2321–8. doi: 10.4049/jimmunol.0902023
70. Ren G, Zhang L, Zhao X, Xu G, Zhang Y, Roberts AI, et al. Mesenchymal stem cell-mediated immunosuppression occurs via concerted action of chemokines and nitric oxide. Cell Stem Cell (2008) 2(2):141–50. doi: 10.1016/j.stem.2007.11.014
71. Galleu A, Riffo-Vasquez Y, Trento C, Lomas C, Dolcetti L, Cheung TS, et al. Apoptosis in mesenchymal stromal cells induces in vivo recipient-mediated immunomodulation. Sci Transl Med (2017) 9(416):1–19. doi: 10.1126/scitranslmed.aam7828
72. Pang SHM, D'Rozario J, Mendonca S, Bhuvan T, Payne NL, Zheng D, et al. Mesenchymal stromal cell apoptosis is required for their therapeutic function. Nat Commun (2021) 12(1):6495. doi: 10.1038/s41467-021-26834-3
73. Liu FB, Lin Q, Liu ZW. A study on the role of apoptotic human umbilical cord mesenchymal stem cells in bleomycin-induced acute lung injury in rat models. Eur Rev Med Pharmacol Sci (2016) 20(5):969–82.
74. de Witte SFH, Luk F, Sierra Parraga JM, Gargesha M, Merino A, Korevaar SS, et al. Immunomodulation By Therapeutic Mesenchymal Stromal Cells (MSC) Is Triggered Through Phagocytosis of MSC By Monocytic Cells. Stem Cells (2018) 36(4):602–15. doi: 10.1002/stem.2779
75. Tse WT, Pendleton JD, Beyer WM, Egalka MC, Guinan EC. Suppression of allogeneic T-cell proliferation by human marrow stromal cells: implications in transplantation. Transplantation (2003) 75(3):389–97. doi: 10.1097/01.TP.0000045055.63901.A9
76. Beggs KJ, Lyubimov A, Borneman JN, Bartholomew A, Moseley A, Dodds R, et al. Immunologic consequences of multiple, high-dose administration of allogeneic mesenchymal stem cells to baboons. Cell Transplant (2006) 15(8-9):711–21. doi: 10.3727/000000006783981503
77. Eliopoulos N, Stagg J, Lejeune L, Pommey S, Galipeau J. Allogeneic marrow stromal cells are immune rejected by MHC class I- and class II-mismatched recipient mice. Blood (2005) 106(13):4057–65. doi: 10.1182/blood-2005-03-1004
78. Sundin M, Ringdén O, Sundberg B, Nava S, Götherström C, Le Blanc K. No alloantibodies against mesenchymal stromal cells, but presence of anti-fetal calf serum antibodies, after transplantation in allogeneic hematopoietic stem cell recipients. Haematologica (2007) 92(9):1208–15. doi: 10.3324/haematol.11446
79. Kazemi S, Parivar K, Hayati Roudbari N, Yaghmaei P, Sadeghi B. Growth kinetic comparison of Human Mesenchymal Stem Cells from Bone Marrow, Adipose Tissue and Decidua. Med Sci (2020) 24(101):223–34.
80. Moll G, Jitschin R, von Bahr L, Rasmusson-Duprez I, Sundberg B, Lönnies L, et al. Mesenchymal stromal cells engage complement and complement receptor bearing innate effector cells to modulate immune responses. PloS One (2011) 6(7):e21703. doi: 10.1371/journal.pone.0021703
81. Li Y, Lin F. Mesenchymal stem cells are injured by complement after their contact with serum. Blood (2012) 120(17):3436–43. doi: 10.1182/blood-2012-03-420612
82. Moll G, Rasmusson-Duprez I, von Bahr L, Connolly-Andersen AM, Elgue G, Funke L, et al. Are therapeutic human mesenchymal stromal cells compatible with human blood? Stem Cells (2012) 30(7):1565–74. doi: 10.1002/stem.1111
83. Bruno S, Grange C, Collino F, Deregibus MC, Cantaluppi V, Biancone L, et al. Microvesicles derived from mesenchymal stem cells enhance survival in a lethal model of acute kidney injury. PloS One (2012) 7(3):e33115. doi: 10.1371/journal.pone.0033115
84. Lai RC, Arslan F, Lee MM, Sze NS, Choo A, Chen TS, et al. Exosome secreted by MSC reduces myocardial ischemia/reperfusion injury. Stem Cell Res (2010) 4(3):214–22. doi: 10.1016/j.scr.2009.12.003
85. Lee C, Mitsialis SA, Aslam M, Vitali SH, Vergadi E, Konstantinou G, et al. Exosomes mediate the cytoprotective action of mesenchymal stromal cells on hypoxia-induced pulmonary hypertension. Circulation (2012) 126(22):2601–11. doi: 10.1161/CIRCULATIONAHA.112.114173
86. Kordelas L, Rebmann V, Ludwig AK, Radtke S, Ruesing J, Doeppner TR, et al. MSC-derived exosomes: a novel tool to treat therapy-refractory graft-versus-host disease. Leukemia (2014) 28(4):970–3. doi: 10.1038/leu.2014.41
87. Dutra Silva J, Su Y, Calfee CS, Delucchi KL, Weiss D, McAuley DF, et al. Mesenchymal stromal cell extracellular vesicles rescue mitochondrial dysfunction and improve barrier integrity in clinically relevant models of ARDS. Eur Respir J (2021) 58(1):1–18. doi: 10.1183/13993003.02978-2020
88. Zhu YG, Feng XM, Abbott J, Fang XH, Hao Q, Monsel A, et al. Human mesenchymal stem cell microvesicles for treatment of Escherichia coli endotoxin-induced acute lung injury in mice. Stem Cells (2014) 32(1):116–25. doi: 10.1002/stem.1504
89. Thompson M, Mei SHJ, Wolfe D, Champagne J, Fergusson D, Stewart DJ, et al. Cell therapy with intravascular administration of mesenchymal stromal cells continues to appear safe: An updated systematic review and meta-analysis. EClinicalMedicine (2020) 19:100249. doi: 10.1016/j.eclinm.2019.100249
90. Lalu MM, McIntyre L, Pugliese C, Fergusson D, Winston BW, Marshall JC, et al. Safety of cell therapy with mesenchymal stromal cells (SafeCell): a systematic review and meta-analysis of clinical trials. PloS One (2012) 7(10):e47559. doi: 10.1371/journal.pone.0047559
91. Lazarus HM, Koc ON, Devine SM, Curtin P, Maziarz RT, Holland HK, et al. Cotransplantation of HLA-identical sibling culture-expanded mesenchymal stem cells and hematopoietic stem cells in hematologic malignancy patients. Biol Blood Marrow Transplant (2005) 11(5):389–98. doi: 10.1016/j.bbmt.2005.02.001
92. Anderson P, Souza-Moreira L, Morell M, Caro M, O'Valle F, Gonzalez-Rey E, et al. Adipose-derived mesenchymal stromal cells induce immunomodulatory macrophages which protect from experimental colitis and sepsis. Gut (2013) 62(8):1131–41. doi: 10.1136/gutjnl-2012-302152
93. Romanov YA, Svintsitskaya VA, Smirnov VN. Searching for alternative sources of postnatal human mesenchymal stem cells: candidate MSC-like cells from umbilical cord. Stem Cells (2003) 21(1):105–10. doi: 10.1634/stemcells.21-1-105
94. Karlsson H, Erkers T, Nava S, Ruhm S, Westgren M, Ringden O. Stromal cells from term fetal membrane are highly suppressive in allogeneic settings in vitro. Clin Exp Immunol (2012) 167(3):543–55. doi: 10.1111/j.1365-2249.2011.04540.x
95. Le Blanc K, Frassoni F, Ball L, Locatelli F, Roelofs H, Lewis I, et al. Mesenchymal stem cells for treatment of steroid-resistant, severe, acute graft-versus-host disease: a phase II study. Lancet (2008) 371(9624):1579–86. doi: 10.1016/S0140-6736(08)60690-X
96. Kebriaei P, Isola L, Bahceci E, Holland K, Rowley S, McGuirk J, et al. Adult human mesenchymal stem cells added to corticosteroid therapy for the treatment of acute graft-versus-host disease. Biol Blood Marrow Transplant (2009) 15(7):804–11. doi: 10.1016/j.bbmt.2008.03.012
97. Kurtzberg J, Prockop S, Teira P, Bittencourt H, Lewis V, Chan KW, et al. Allogeneic human mesenchymal stem cell therapy (remestemcel-L, Prochymal) as a rescue agent for severe refractory acute graft-versus-host disease in pediatric patients. Biol Blood Marrow Transplant (2014) 20(2):229–35. doi: 10.1016/j.bbmt.2013.11.001
98. Hashmi S, Ahmed M, Murad MH, Litzow MR, Adams RH, Ball LM, et al. Survival after mesenchymal stromal cell therapy in steroid-refractory acute graft-versus-host disease: systematic review and meta-analysis. Lancet Haematol (2016) 3(1):e45–52. doi: 10.1016/S2352-3026(15)00224-0
99. Ringden O, Uzunel M, Sundberg B, Lonnies L, Nava S, Gustafsson J, et al. Tissue repair using allogeneic mesenchymal stem cells for hemorrhagic cystitis, pneumomediastinum and perforated colon. Leukemia (2007) 21(11):2271–6. doi: 10.1038/sj.leu.2404833
100. Connick P, Kolappan M, Crawley C, Webber DJ, Patani R, Michell AW, et al. Autologous mesenchymal stem cells for the treatment of secondary progressive multiple sclerosis: an open-label phase 2a proof-of-concept study. Lancet Neurol (2012) 11(2):150–6. doi: 10.1016/S1474-4422(11)70305-2
101. Zhu Y, Ge J, Huang C, Liu H, Jiang H. Application of mesenchymal stem cell therapy for aging frailty: from mechanisms to therapeutics. Theranostics (2021) 11(12):5675–85. doi: 10.7150/thno.46436
102. McNicholas BA, Rooney GM, Laffey JG. Lessons to learn from epidemiologic studies in ARDS. Curr Opin Crit Care (2018) 24(1):41–8. doi: 10.1097/MCC.0000000000000473
103. Serpa Neto A, Cardoso SO, Manetta JA, Pereira VG, Espósito DC, Pasqualucci Mde O, et al. Association between use of lung-protective ventilation with lower tidal volumes and clinical outcomes among patients without acute respiratory distress syndrome: a meta-analysis. Jama (2012) 308(16):1651–9. doi: 10.1001/jama.2012.13730
104. Papazian L, Forel JM, Gacouin A, Penot-Ragon C, Perrin G, Loundou A, et al. Neuromuscular blockers in early acute respiratory distress syndrome. N Engl J Med (2010) 363(12):1107–16. doi: 10.1056/NEJMoa1005372
105. Guérin C, Reignier J, Richard JC, Beuret P, Gacouin A, Boulain T, et al. Prone positioning in severe acute respiratory distress syndrome. N Engl J Med (2013) 368(23):2159–68. doi: 10.1056/NEJMoa1214103
106. Peek GJ, Mugford M, Tiruvoipati R, Wilson A, Allen E, Thalanany MM, et al. Efficacy and economic assessment of conventional ventilatory support versus extracorporeal membrane oxygenation for severe adult respiratory failure (CESAR): a multicentre randomised controlled trial. Lancet (2009) 374(9698):1351–63. doi: 10.1016/S0140-6736(09)61069-2
107. Curley GF, Hayes M, Ansari B, Shaw G, Ryan A, Barry F, et al. Mesenchymal stem cells enhance recovery and repair following ventilator-induced lung injury in the rat. Thorax (2012) 67(6):496–501. doi: 10.1136/thoraxjnl-2011-201059
108. Hayes M, Masterson C, Devaney J, Barry F, Elliman S, O'Brien T, et al. Therapeutic efficacy of human mesenchymal stromal cells in the repair of established ventilator-induced lung injury in the rat. Anesthesiology (2015) 122(2):363–73. doi: 10.1097/ALN.0000000000000545
109. Gore AV, Bible LE, Song K, Livingston DH, Mohr AM, Sifri ZC. Mesenchymal stem cells increase T-regulatory cells and improve healing following trauma and hemorrhagic shock. J Trauma Acute Care Surg (2015) 79(1):48–52. doi: 10.1097/TA.0000000000000681
110. Krasnodembskaya A, Samarani G, Song Y, Zhuo H, Su X, Lee JW, et al. Human mesenchymal stem cells reduce mortality and bacteremia in gram-negative sepsis in mice in part by enhancing the phagocytic activity of blood monocytes. Am J Physiol Lung Cell Mol Physiol (2012) 302(10):L1003–13. doi: 10.1152/ajplung.00180.2011
111. Krasnodembskaya A, Song Y, Fang X, Gupta N, Serikov V, Lee JW, et al. Antibacterial effect of human mesenchymal stem cells is mediated in part from secretion of the antimicrobial peptide LL-37. Stem Cells (2010) 28(12):2229–38. doi: 10.1002/stem.544
112. Lee JW, Krasnodembskaya A, McKenna DH, Song Y, Abbott J, Matthay MA. Therapeutic effects of human mesenchymal stem cells in ex vivo human lungs injured with live bacteria. Am J Respir Crit Care Med (2013) 187(7):751–60. doi: 10.1164/rccm.201206-0990OC
113. Devaney J, Horie S, Masterson C, Elliman S, Barry F, O'Brien T, et al. Human mesenchymal stromal cells decrease the severity of acute lung injury induced by E. coli in the rat. Thorax (2015) 70(7):625–35. doi: 10.1136/thoraxjnl-2015-206813
114. Gupta N, Su X, Popov B, Lee JW, Serikov V, Matthay MA. Intrapulmonary delivery of bone marrow-derived mesenchymal stem cells improves survival and attenuates endotoxin-induced acute lung injury in mice. J Immunol (2007) 179(3):1855–63. doi: 10.4049/jimmunol.179.3.1855
115. Fang X, Abbott J, Cheng L, Colby JK, Lee JW, Levy BD, et al. Human Mesenchymal Stem (Stromal) Cells Promote the Resolution of Acute Lung Injury in Part through Lipoxin A4. J Immunol (2015) 195(3):875–81. doi: 10.4049/jimmunol.1500244
116. Cóndor JM, Rodrigues CE, Sousa Moreira R, Canale D, Volpini RA, Shimizu MH, et al. Treatment With Human Wharton's Jelly-Derived Mesenchymal Stem Cells Attenuates Sepsis-Induced Kidney Injury, Liver Injury, and Endothelial Dysfunction. Stem Cells Transl Med (2016) 5(8):1048–57. doi: 10.5966/sctm.2015-0138
117. Zhang S, Jiang W, Ma L, Liu Y, Zhang X, Wang S. Nrf2 transfection enhances the efficacy of human amniotic mesenchymal stem cells to repair lung injury induced by lipopolysaccharide. J Cell Biochem (2018) 119(2):1627–36. doi: 10.1002/jcb.26322
118. Lee FY, Chen KH, Wallace CG, Sung PH, Sheu JJ, Chung SY, et al. Xenogeneic human umbilical cord-derived mesenchymal stem cells reduce mortality in rats with acute respiratory distress syndrome complicated by sepsis. Oncotarget (2017) 8(28):45626–42. doi: 10.18632/oncotarget.17320
119. Wang C, Lv D, Zhang X, Ni ZA, Sun X, Zhu C. Interleukin-10-Overexpressing Mesenchymal Stromal Cells Induce a Series of Regulatory Effects in the Inflammatory System and Promote the Survival of Endotoxin-Induced Acute Lung Injury in Mice Model. DNA Cell Biol (2018) 37(1):53–61. doi: 10.1089/dna.2017.3735
120. Zhao X, Liu D, Gong W, Zhao G, Liu L, Yang L, et al. The toll-like receptor 3 ligand, poly(I:C), improves immunosuppressive function and therapeutic effect of mesenchymal stem cells on sepsis via inhibiting MiR-143. Stem Cells (2014) 32(2):521–33. doi: 10.1002/stem.1543
121. Martínez-González I, Roca O, Masclans JR, Moreno R, Salcedo MT, Baekelandt V, et al. Human mesenchymal stem cells overexpressing the IL-33 antagonist soluble IL-1 receptor-like-1 attenuate endotoxin-induced acute lung injury. Am J Respir Cell Mol Biol (2013) 49(4):552–62. doi: 10.1165/rcmb.2012-0406OC
122. Han J, Lu X, Zou L, Xu X, Qiu H. E-Prostanoid 2 Receptor Overexpression Promotes Mesenchymal Stem Cell Attenuated Lung Injury. Hum Gene Ther (2016) 27(8):621–30. doi: 10.1089/hum.2016.003
123. Cai SX, Liu AR, Chen S, He HL, Chen QH, Xu JY, et al. The Orphan Receptor Tyrosine Kinase ROR2 Facilitates MSCs to Repair Lung Injury in ARDS Animal Model. Cell Transplant (2016) 25(8):1561–74. doi: 10.3727/096368915X689776
124. Wang S, Mo M, Wang J, Sadia S, Shi B, Fu X, et al. Platelet-derived growth factor receptor beta identifies mesenchymal stem cells with enhanced engraftment to tissue injury and pro-angiogenic property. Cell Mol Life Sci (2018) 75(3):547–61. doi: 10.1007/s00018-017-2641-7
125. Mei SH, McCarter SD, Deng Y, Parker CH, Liles WC, Stewart DJ. Prevention of LPS-induced acute lung injury in mice by mesenchymal stem cells overexpressing angiopoietin 1. PloS Med (2007) 4(9):e269. doi: 10.1371/journal.pmed.0040269
126. Min F, Gao F, Li Q, Liu Z. Therapeutic effect of human umbilical cord mesenchymal stem cells modified by angiotensin-converting enzyme 2 gene on bleomycin-induced lung fibrosis injury. Mol Med Rep (2015) 11(4):2387–96. doi: 10.3892/mmr.2014.3025
127. He H, Liu L, Chen Q, Liu A, Cai S, Yang Y, et al. Mesenchymal Stem Cells Overexpressing Angiotensin-Converting Enzyme 2 Rescue Lipopolysaccharide-Induced Lung Injury. Cell Transplant (2015) 24(9):1699–715. doi: 10.3727/096368914X685087
128. Natarajan V, Dudek SM, Jacobson JR, Moreno-Vinasco L, Huang LS, Abassi T, et al. Sphingosine-1-phosphate, FTY720, and sphingosine-1-phosphate receptors in the pathobiology of acute lung injury. Am J Respir Cell Mol Biol (2013) 49(1):6–17. doi: 10.1165/rcmb.2012-0411TR
129. Hayes M, Curley GF, Masterson C, Devaney J, O'Toole D, Laffey JG. Mesenchymal stromal cells are more effective than the MSC secretome in diminishing injury and enhancing recovery following ventilator-induced lung injury. Intensive Care Med Exp (2015) 3(1):29. doi: 10.1186/s40635-015-0065-y
130. Chi AK, Soubani AO, White AC, Miller KB. An update on pulmonary complications of hematopoietic stem cell transplantation. Chest (2013) 144(6):1913–22. doi: 10.1378/chest.12-1708
131. Wieruszewski PM, Herasevich S, Gajic O, Yadav H. Respiratory failure in the hematopoietic stem cell transplant recipient. World J Crit Care Med (2018) 7(5):62–72. doi: 10.5492/wjccm.v7.i5.62
132. Herasevich S, Frank RD, Bo H, Alkhateeb H, Hogan WJ, Gajic O, et al. Pretransplant Risk Factors Can Predict Development of Acute Respiratory Distress Syndrome after Hematopoietic Stem Cell Transplantation. Ann Am Thorac Soc (2021) 18(6):1004–12. doi: 10.1513/AnnalsATS.202004-336OC
133. Yadav H, Nolan ME, Bohman JK, Cartin-Ceba R, Peters SG, Hogan WJ, et al. Epidemiology of Acute Respiratory Distress Syndrome Following Hematopoietic Stem Cell Transplantation. Crit Care Med (2016) 44(6):1082–90. doi: 10.1097/CCM.0000000000001617
134. Ramirez-Sarmiento A, Orozco-Levi M, Walter EC, Au MA, Chien JW. Influence of pretransplantation restrictive lung disease on allogeneic hematopoietic cell transplantation outcomes. Biol Blood Marrow Transplant (2010) 16(2):199–206. doi: 10.1016/j.bbmt.2009.09.016
135. Wohlfarth P, Beutel G, Lebiedz P, Stemmler HJ, Staudinger T, Schmidt M, et al. Characteristics and Outcome of Patients After Allogeneic Hematopoietic Stem Cell Transplantation Treated With Extracorporeal Membrane Oxygenation for Acute Respiratory Distress Syndrome. Crit Care Med (2017) 45(5):e500–7. doi: 10.1097/CCM.0000000000002293
136. Rowan CM, Smith LS, Loomis A, McArthur J, Gertz SJ, Fitzgerald JC, et al. Pediatric Acute Respiratory Distress Syndrome in Pediatric Allogeneic Hematopoietic Stem Cell Transplants: A Multicenter Study. Pediatr Crit Care Med (2017) 18(4):304–9. doi: 10.1097/PCC.0000000000001061
137. Zheng G, Huang L, Tong H, Shu Q, Hu Y, Ge M, et al. Treatment of acute respiratory distress syndrome with allogeneic adipose-derived mesenchymal stem cells: a randomized, placebo-controlled pilot study. Respir Res (2014) 15(1):39. doi: 10.1186/1465-9921-15-39
138. Wilson JG, Liu KD, Zhuo H, Caballero L, McMillan M, Fang X, et al. Mesenchymal stem (stromal) cells for treatment of ARDS: a phase 1 clinical trial. Lancet Respir Med (2015) 3(1):24–32. doi: 10.1016/S2213-2600(14)70291-7
139. Matthay MA, Calfee CS, Zhuo H, Thompson BT, Wilson JG, Levitt JE, et al. Treatment with allogeneic mesenchymal stromal cells for moderate to severe acute respiratory distress syndrome (START study): a randomised phase 2a safety trial. Lancet Respir Med (2019) 7(2):154–62. doi: 10.1016/S2213-2600(18)30418-1
140. Wick KD, Leligdowicz A, Zhuo H, Ware LB, Matthay MA. Mesenchymal stromal cells reduce evidence of lung injury in patients with ARDS. JCI Insight (2021) 6(12):1–11. doi: 10.1172/jci.insight.148983
141. Hamdan H, Hashmi SK, Lazarus H, Gale RP, Qu W, El Fakih R. Promising role for mesenchymal stromal cells in coronavirus infectious disease-19 (COVID-19)-related severe acute respiratory syndrome? Blood Rev (2021) 46:100742. doi: 10.1016/j.blre.2020.100742
142. Schäfer R, Spohn G, Bechtel M, Bojkova D, Baer PC, Kuçi S, et al. Human Mesenchymal Stromal Cells Are Resistant to SARS-CoV-2 Infection under Steady-State, Inflammatory Conditions and in the Presence of SARS-CoV-2-Infected Cells. Stem Cell Rep (2021) 16(3):419–27. doi: 10.1016/j.stemcr.2020.09.003
143. Haberle H, Magunia H, Lang P, Gloeckner H, Korner A, Koeppen M, et al. Mesenchymal Stem Cell Therapy for Severe COVID-19 ARDS. J Intensive Care Med (2021) 681–88. doi: 10.1177/0885066621997365
144. Hashemian SR, Aliannejad R, Zarrabi M, Soleimani M, Vosough M, Hosseini SE, et al. Mesenchymal stem cells derived from perinatal tissues for treatment of critically ill COVID-19-induced ARDS patients: a case series. Stem Cell Res Ther (2021) 12(1):91. doi: 10.1186/s13287-021-02165-4
145. Lanzoni G, Linetsky E, Correa D, Messinger Cayetano S, Alvarez RA, Kouroupis D, et al. Umbilical cord mesenchymal stem cells for COVID-19 acute respiratory distress syndrome: A double-blind, phase 1/2a, randomized controlled trial. Stem Cells Transl Med (2021) 10(5):660–73. doi: 10.1002/sctm.20-0472
146. Xu X, Jiang W, Chen L, Xu Z, Zhang Q, Zhu M, et al. Evaluation of the safety and efficacy of using human menstrual blood-derived mesenchymal stromal cells in treating severe and critically ill COVID-19 patients: An exploratory clinical trial. Clin Transl Med (2021) 11(2):e297. doi: 10.1002/ctm2.297
147. Meng F, Xu R, Wang S, Xu Z, Zhang C, Li Y, et al. Human umbilical cord-derived mesenchymal stem cell therapy in patients with COVID-19: a phase 1 clinical trial. Signal Transduct Target Ther (2020) 5(1):172. doi: 10.1038/s41392-020-00286-5
148. Guo Z, Chen Y, Luo X, He X, Zhang Y, Wang J. Administration of umbilical cord mesenchymal stem cells in patients with severe COVID-19 pneumonia. Crit Care (2020) 24(1):420. doi: 10.1186/s13054-020-03142-8
149. Iglesias M, Butrón P, Torre-Villalvazo I, Torre-Anaya EA, Sierra-Madero J, Rodriguez-Andoney JJ, et al. Mesenchymal Stem Cells for the Compassionate Treatment of Severe Acute Respiratory Distress Syndrome Due to COVID 19. Aging Dis (2021) 12(2):360–70. doi: 10.14336/AD.2020.1218
150. Ercelen NO, Pekkoc-Uyanik KC, Alpaydin N, Gulay GR, Simsek M. Clinical experience on umbilical cord mesenchymal stem cell treatment in 210 severe and critical COVID-19 cases in Turkey. Stem Cell Rev Rep (2021) 17(5):1917–25. doi: 10.1007/s12015-021-10214-x
151. Monsel A, Hauw-Berlemont C, Mebarki M, Heming N, Mayaux J, Nguekap Tchoumba O, et al. Treatment of COVID-19-associated ARDS with mesenchymal stromal cells: a multicenter randomized double-blind trial. Crit Care (2022) 26(1):48. doi: 10.1186/s13054-022-03930-4
152. Shi L, Huang H, Lu X, Yan X, Jiang X, Xu R, et al. Effect of human umbilical cord-derived mesenchymal stem cells on lung damage in severe COVID-19 patients: a randomized, double-blind, placebo-controlled phase 2 trial. Signal Transduct Target Ther (2021) 6(1):58. doi: 10.1038/s41392-021-00488-5
153. Dilogo IH, Aditianingsih D, Sugiarto A, Burhan E, Damayanti T, Sitompul PA, et al. Umbilical cord mesenchymal stromal cells as critical COVID-19 adjuvant therapy: A randomized controlled trial. Stem Cells Transl Med (2021) 10(9):1279–87. doi: 10.1002/sctm.21-0046
154. Shu L, Niu C, Li R, Huang T, Wang Y, Huang M, et al. Treatment of severe COVID-19 with human umbilical cord mesenchymal stem cells. Stem Cell Res Ther (2020) 11(1):361. doi: 10.1186/s13287-020-01875-5
155. Gregoire C, Layios N, Lambermont B, Lechanteur C, Briquet A, Bettonville V, et al. Bone Marrow-Derived Mesenchymal Stromal Cell Therapy in Severe COVID-19: Preliminary Results of a Phase I/II Clinical Trial. Front Immunol (2022) 13:932360. doi: 10.3389/fimmu.2022.932360
156. Sadeghi B, Roshandel E, Pirsalehi A, Kazemi S, Sankanian G, Majidi M, et al. Conquering the cytokine storm in COVID-19-induced ARDS using placenta-derived decidua stromal cells. J Cell Mol Med (2021) 25(22):10554–64. doi: 10.1111/jcmm.16986
157. Force ADT, Ranieri VM, Rubenfeld GD, Thompson BT, Ferguson ND, Caldwell E, et al. Acute respiratory distress syndrome: the Berlin Definition. JAMA (2012) 307(23):2526–33. doi: 10.1001/jama.2012.5669
158. Johnson ER, Matthay MA. Acute lung injury: epidemiology, pathogenesis, and treatment. J Aerosol Med Pulm Drug Delivery (2010) 23(4):243–52. doi: 10.1089/jamp.2009.0775
159. Ringdén O, Remberger M, Ruutu T, Nikoskelainen J, Volin L, Vindeløv L, et al. Increased risk of chronic graft-versus-host disease, obstructive bronchiolitis, and alopecia with busulfan versus total body irradiation: long-term results of a randomized trial in allogeneic marrow recipients with leukemia. Nordic Bone Marrow Transplant Group Blood (1999) 93(7):2196–201. doi: 10.1182/blood.V93.7.2196
160. Ringden O, Remberger M, Persson U, Ljungman P, Aldener A, Andstrom E, et al. Similar incidence of graft-versus-host disease using HLA-a, -b and -DR identical unrelated bone marrow donors as with HLA-identical siblings. Bone Marrow Transplant (1995) 15(4):619–25.
161. Forslöw U, Mattsson J, Ringden O, Klominek J, Remberger M. Decreasing mortality rate in early pneumonia following hematopoietic stem cell transplantation. Scand J Infect Dis (2006) 38(11-12):970–6. doi: 10.1080/00365540600786481
162. Ringden O, Erkers T, Nava S, Uzunel M, Iwarsson E, Conrad R, et al. Fetal membrane cells for treatment of steroid-refractory acute graft-versus-host disease. Stem Cells (2013) 31(3):592–601. doi: 10.1002/stem.1314
163. Remberger M, Ringden O. Treatment of severe acute graft-versus-host disease with mesenchymal stromal cells: a comparison with non-MSC treated patients. Int J Hematol (2012) 96(6):822–4. doi: 10.1007/s12185-012-1218-3
164. Ringden O, Baygan A, Remberger M, Gustafsson B, Winiarski J, Khoein B, et al. Placenta-Derived Decidua Stromal Cells for Treatment of Severe Acute Graft-Versus-Host Disease. Stem Cells Transl Med (2018) 7(4):325–31. doi: 10.1002/sctm.17-0167
165. Sadeghi B, Moretti G, Arnberg F, Samen E, Kohein B, Catar R, et al. Preclinical Toxicity Evaluation of Clinical Grade Placenta-Derived Decidua Stromal Cells. Front Immunol (2019) 10:2685. doi: 10.3389/fimmu.2019.02685
166. Le Blanc K, Ringden O. Immunomodulation by mesenchymal stem cells and clinical experience. J Intern Med (2007) 262(5):509–25. doi: 10.1111/j.1365-2796.2007.01844.x
167. Forslow U, Blennow O, LeBlanc K, Ringden O, Gustafsson B, Mattsson J, et al. Treatment with mesenchymal stromal cells is a risk factor for pneumonia-related death after allogeneic hematopoietic stem cell transplantation. Eur J Haematol (2012) 89(3):220–7. doi: 10.1111/j.1600-0609.2012.01824.x
168. Moll G, Ignatowicz L, Catar R, Luecht C, Sadeghi B, Hamad O, et al. Different Procoagulant Activity of Therapeutic Mesenchymal Stromal Cells Derived from Bone Marrow and Placental Decidua. Stem Cells Dev (2015) 24(19):2269–79. doi: 10.1089/scd.2015.0120
169. Erkers T, Nava S, Yosef J, Ringden O, Kaipe H. Decidual stromal cells promote regulatory T cells and suppress alloreactivity in a cell contact-dependent manner. Stem Cells Dev (2013) 22(19):2596–605. doi: 10.1089/scd.2013.0079
170. Teijaro JR. Cytokine storms in infectious diseases. Semin Immunopathol (2017) 39(5):501–3. doi: 10.1007/s00281-017-0640-2
171. Aronsson-Kurttila W, Baygan A, Moretti G, Remberger M, Khoein B, Moll G. Placenta-Derived Decidua Stromal Cells for Hemorrhagic Cystitis after Stem Cell Transplantation. Acta Haematol (2018) 139(2):106–14. doi: 10.1159/000485735
172. Laffey JG, Matthay MA. Fifty Years of Research in ARDS. Cell-based Therapy for Acute Respiratory Distress Syndrome. Biology and Potential Therapeutic Value. Am J Respir Crit Care Med (2017) 196(3):266–73. doi: 10.1164/rccm.201701-0107CP
173. Wang J, Loh SW, Lee JH. Paediatric acute respiratory distress syndrome: progress over the past decade. J Emergency Crit Care Med (2018) 2(2):1–13. doi: 10.21037/jeccm.2018.02.04
174. Horie S, Gonzalez HE, Laffey JG, Masterson CH. Cell therapy in acute respiratory distress syndrome. J Thorac Dis (2018) 10(9):5607–20. doi: 10.21037/jtd.2018.08.28
175. Sadeghi ,B, Ringden O. Mesenchymal stem cells for graft-versus-host disease in experimental animal models, in Stem Cell- Dependent Therapies-Mesenchymal Stem Cells in Chronic Inflammatory Disorders. Gross; G, Haupl T, editors. (Germany: Walter de Gruyter GmbH) (2013) p. 125–42.
176. Noronha NC, Mizukami A, Caliári-Oliveira C, Cominal JG, Rocha JLM, Covas DT, et al. Priming approaches to improve the efficacy of mesenchymal stromal cell-based therapies. Stem Cell Res Ther (2019) 10(1):131. doi: 10.1186/s13287-019-1224-y
177. Baygan A, Aronsson-Kurttila W, Moretti G, Tibert B, Dahllof G, Klingspor L, et al. Safety and Side Effects of Using Placenta-Derived Decidual Stromal Cells for Graft-versus-Host Disease and Hemorrhagic Cystitis. Front Immunol (2017) 8:795. doi: 10.3389/fimmu.2017.00795
178. Shah TG, Predescu D, Predescu S. Mesenchymal stem cells-derived extracellular vesicles in acute respiratory distress syndrome: a review of current literature and potential future treatment options. Clin Transl Med (2019) 8(1):25. doi: 10.1186/s40169-019-0242-9
Keywords: mesenchymal stromal cells (MSCs), decidua stromal cells (DSCs), hematopoietic cell transplantation, acute respiratory distress syndrome (ARDS), cell therapy
Citation: Sadeghi B, Ringdén O, Gustafsson B and Castegren M (2022) Mesenchymal stromal cells as treatment for acute respiratory distress syndrome. Case Reports following hematopoietic cell transplantation and a review. Front. Immunol. 13:963445. doi: 10.3389/fimmu.2022.963445
Received: 07 June 2022; Accepted: 18 October 2022;
Published: 08 November 2022.
Edited by:
Antoine Toubert, Université Paris Cité, FranceReviewed by:
Philippe Saas, INSERM U1098 Interactions Hôte-Greffon-Tumeur & Ingénierie Cellulaire et Génique, FranceCopyright © 2022 Sadeghi, Ringdén, Gustafsson and Castegren. This is an open-access article distributed under the terms of the Creative Commons Attribution License (CC BY). The use, distribution or reproduction in other forums is permitted, provided the original author(s) and the copyright owner(s) are credited and that the original publication in this journal is cited, in accordance with accepted academic practice. No use, distribution or reproduction is permitted which does not comply with these terms.
*Correspondence: Behnam Sadeghi, YmVobmFtLnNhZGVnaGlAa2kuc2U=
Disclaimer: All claims expressed in this article are solely those of the authors and do not necessarily represent those of their affiliated organizations, or those of the publisher, the editors and the reviewers. Any product that may be evaluated in this article or claim that may be made by its manufacturer is not guaranteed or endorsed by the publisher.
Research integrity at Frontiers
Learn more about the work of our research integrity team to safeguard the quality of each article we publish.