- 1Department of Microbiology and Immunology, Geisel School of Medicine at Dartmouth, Lebanon, NH, United States
- 2Thayer School of Engineering, Dartmouth College, Hanover, NH, United States
The fetal/neonatal period represents both a unique window of opportunity for interventions as well as vulnerability to a number of viral infections. While Herpesviruses such as herpes simplex virus (HSV) are highly prevalent and typically of little consequence among healthy adults, they are among the most consequential infections of early life. Despite treatment with antiviral drugs, neonatal HSV (nHSV) infections can still result in significant mortality and lifelong neurological morbidity. Fortunately, newborns in our pathogen-rich world inherit some of the protection provided by the maternal immune system in the form of transferred antibodies. Maternal seropositivity, resulting in placental transfer of antibodies capable of neutralizing virus and eliciting the diverse effector functions of the innate immune system are associated with dramatically decreased risk of nHSV. Given this clear epidemiological evidence of reduced risk of infection and its sequelae, we present what is known about the ability of monoclonal antibody therapies to treat or prevent HSV infection and explore how effective antibody-based interventions in conjunction with antiviral therapy might reduce early life mortality and long-term morbidity.
Introduction
The World Health Organization estimates that around the world over 3.7 billion people have oral herpes infections, and that approximately half a billion people experience genital herpes (1). Herpes simplex virus (HSV) infects the host for their lifetime by infecting neurons of the peripheral nervous system or central nervous system (CNS), the virus can then reactivate asymptomatically or cause cutaneous lesions (2). Whereas current antivirals (acyclovir and its derivatives) decrease the duration and severity of symptoms for millions of adult individuals living with HSV, infections in early life result in substantial morbidity and mortality despite therapy (3–7), therefore adjunct therapy with distinct mechanisms from those employed by small molecule antivirals could provide additive or synergistic benefits. Human and animal model data support that antibodies can provide robust protection in the setting of primary HSV infection. This review will describe the therapeutic prospects of antibody (Ab) mediated protection in primary and recurrent HSV infections and the pipeline to develop monoclonal antibody (mAb) therapy with a focus on neo/perinatal HSV (nHSV) infection.
Maternal and neonatal HSV infections
Incidence
Approximately 2 – 4% of women acquire HSV during pregnancy (8). While both HSV serotypes can result in nHSV, HSV-1 predominates in the Americas, Europe and the Western Pacific, and HSV-2 predominates in Africa, South East Asia, and the Eastern Mediterranean (9). Maternal infection during pregnancy is managed with anti-viral therapy and can resolve without severe outcomes in both mother and child (10). Strong epidemiological evidence as to the importance of antibodies in preventing HSV infection comes from the dramatic influence of maternal seropositivity on nHSV risk (Figure 1). Primary maternal infections acquired during late gestation present a significant risk (25 - 50%) of transmitting HSV to neonates when compared to women with recurring genital infections (< 3%) (11, 12). The basis for this reduction is believed to be derived from the development and transfer of protective maternal IgG antibodies, which cannot be achieved if infection takes place close to parturition. Thus, whereas an effective HSV vaccine to prevent adult-to adult spread remains a challenging goal (13, 14), accomplishment of nHSV protection, with its defined and short period of risk, may be readily achievable.
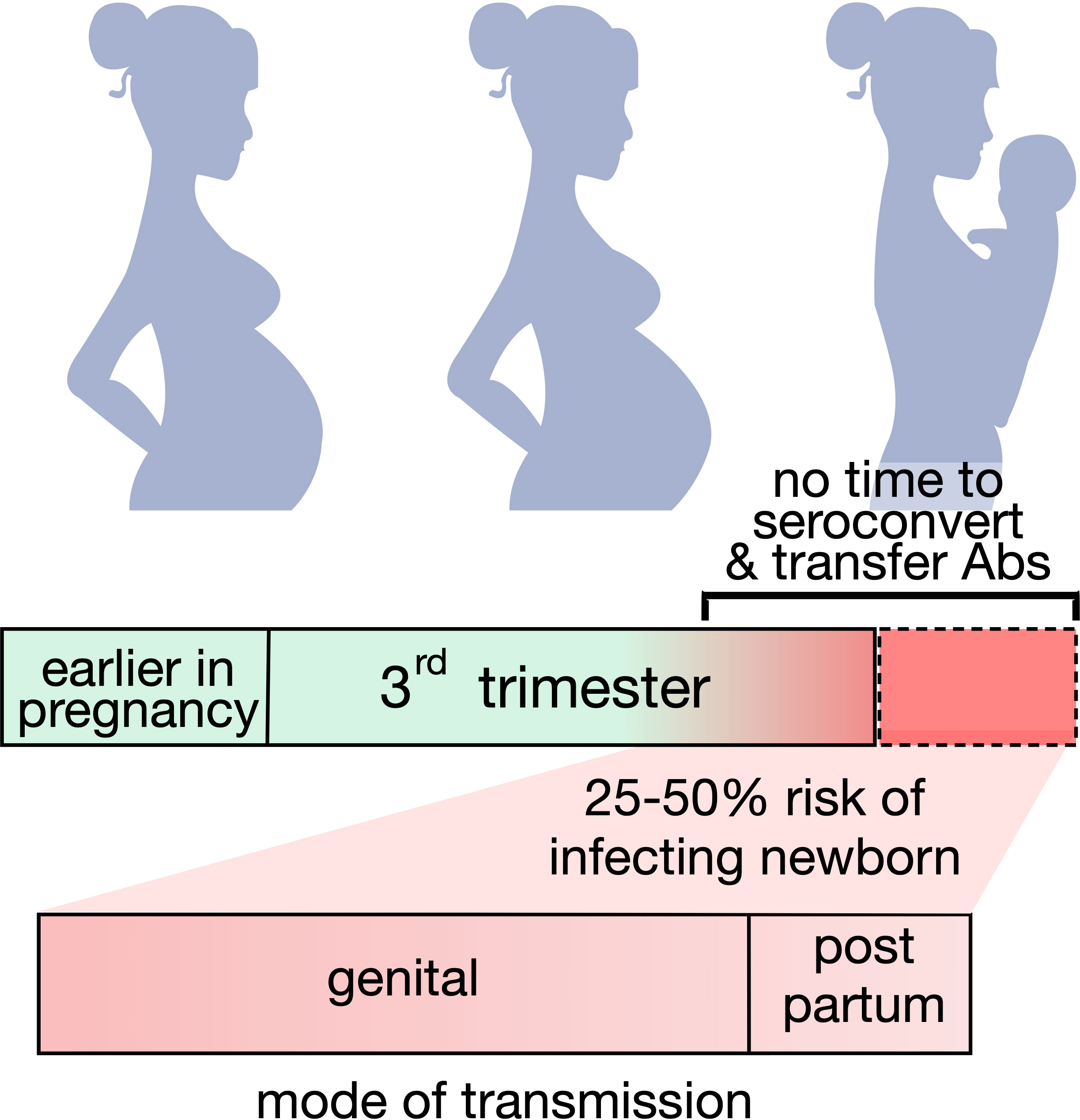
Figure 1 Maternal seroconversion and nHSV infection risk. Graphic representing the potential risk of transmitting neonatal HSV when seroconversion is not achieved or achieved too close to delivery. The majority of infants acquire disease from infected mothers during birth.
Timing and outcomes
In utero infections are rare, representing 5% of nHSV infections. The majority of nHSV infections, 85%, are acquired during parturition, while the remaining 10% of infectious take place post-partum via close contact. Most vertically transmitted nHSV results from asymptomatic viral shedding of a pregnant parent, as symptomatic maternal infections often result in birth via cesarean section, which significantly reduces the risk of transmission (8, 11, 15).
Congenital HSV infections may result in skin vesicles or scarring, eye involvement, microcephaly, and hydranencephaly that are associated with severe neurological morbidity, and blindness. Vertical transmission of HSV due to placental hematogenous spread of infection, as well as amniotic infection, have both been reported. Despite the low numbers of congenital infections, detection of HSV DNA in the placenta is more common than one would expect. In a recent study, 37% of placentas (n = 160) assessed were positive for HSV-1 viral DNA, with previous reports ranging from 4 – 28% (16), while HSV-2 DNA was detected in 9% of placentas (17). Both studies also reported the presence of HSV DNA in neonatal cord blood, however neither study had appropriate follow up to determine if clinically evident neonatal HSV infections took place following the detection of HSV DNA (16, 17).
Intrapartum infections tend to be less severe than congenital infections, but can also result in significant morbidity and mortality, often presenting as skin, eye and mouth (SEM) disease, CNS-associated infection, and disseminated viral infection in visceral organs with or without CNS involvement (Figure 2). SEM disease typically presents with pathognomonic skin vesicles. If untreated, SEM disease can progress to more severe CNS and disseminated disease. Since the implementation of acyclovir (ACV) therapy and improvements in dosing strategies, more and more cases initially presenting as SEM are resolved before progression to more severe disease (7). However, despite antiviral treatment with ACV, >50% of CNS-associated disease survivors have neurological morbidity, and disseminated disease results in ~40% mortality (7).
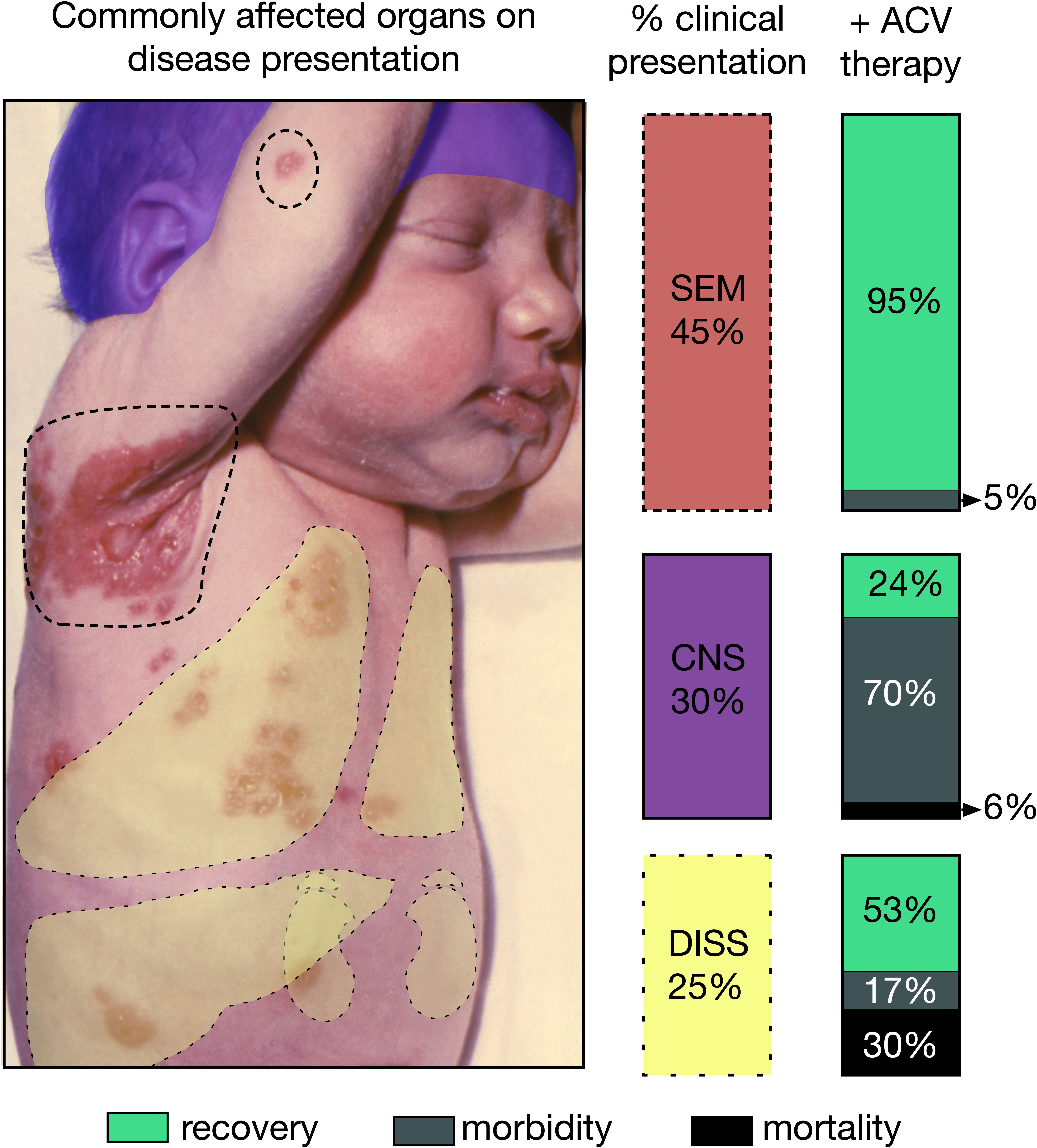
Figure 2 Consequences of neonatal herpes simplex virus (nHSV). Graphic depicting the different presentations and their respective estimated occurrence in the clinical setting. Outcomes of each disease presentation when treated with ACV are also displayed for each presentation to the right of color-coded columns. Skin, eye and mouth (SEM) disease often shows characteristic lesions, some of which are highlighted with dotted lines. Disseminated (DISS) can often affect lungs, liver, kidneys, and adrenals depicted as yellow overlays. Central nervous system (CNS) disease depicted as a purple overlay over affected infant’s head. Infant’s image courtesy of K.L. Herrmann, Centers of Disease Control public image library.
Additionally, ACV-resistant viruses are recalcitrant to therapy. The documented mechanisms of ACV resistance involve defects in the virally-encoded thymidine kinase (TK) accounting for ~95% of isolated mutants (18). Generally, TK mutations decrease the fitness of the virus, hindering reactivation and replication (19), thus in the immunocompetent host, TK mutant viruses are rarely isolated (0.3%), and are often expediently cleared (20). For immunocompromised individuals, who rely exclusively on the therapeutic effect of ACV for viral clearance, ACV resistance is a major challenge, and alternatives to ACV are not as readily tolerated and have increased toxicity (18, 20). To date, ACV resistance has not been documented in a neonatal case, but it remains a broader public health issue, especially in bone marrow transplant recipients. Thus, while antiviral therapy has undoubtedly reduced the morbidity and mortality of devastating neonatal infections, additional therapeutic interventions are urgently needed to save and improve the quality of life of infected neonates (4, 12, 21).
Polyclonal Ab-based protection
Primary disease
The role of polyclonal Abs (pAbs) in protecting humans from primary HSV infection is better defined than its role in reactivated HSV disease. The most compelling evidence for Ab-mediated protection in the setting of HSV disease is the protection afforded to neonates via the placental transfer of antibodies (8, 11, 21–23). Specifically, high titers of neutralizing and antibody-dependent cellular cytotoxicity (ADCC) inducing Abs in mothers and infants are associated with the absence of disseminated nHSV (24).
Protection across serotypes
This protection, however, can be HSV serotype specific, as HSV-1 seropositive mothers who acquired first time genital HSV-2 infections close to parturition provided less protection against nHSV. On the other hand, infants born to mothers who were already seropositive for genital HSV-2 and acquired a new genital HSV-1 infection were protected (8). It is not completely understood if this is owed to the anatomical site of infection (orolabial vs. genital) or to serotype-specific differences. It has also been noted however, that people who are HSV-1 seropositive often experience a milder or asymptomatic course of genital HSV-2 infection (25).
Further evidence of type specific infection was also observed during the HerpeVac clinical trial for young women (ClinicalTrials.gov Identifier: NCT00057330). This HSV-2 subunit vaccine targeting glycoprotein D (gD) protected women from genital HSV-1 acquisition, though not from HSV-2 (26). Together, clinical studies and observations all support the notion that Abs offer protection from primary infection, and highlight gaps in our knowledge regarding HSV serotype specific differences in protection.
Recurring or reactivated disease
While passively transferred Abs clearly provide significant protection in early life, it is also clear that the presence of HSV-specific antibodies do not prevent HSV reactivation in seropositive individuals. Individuals with reactivating HSV disease have been noted to have high binding and neutralizing Ab titers, likely due to repeated antigen exposure (27, 28). Serological and functional analysis of seropositive symptomatic and asymptomatic individuals have proposed that Ab specificity (29) or that naturally occurring Fcγ Receptor and Ab polymorphisms (30) may contribute to viral control. Larger follow up studies are necessary to better understand the clinical impact of these observations and to determine how they may relate to nHSV associated with recurrent/reactivated disease.
In animal models
Preclinical evidence for Ab-mediated protection during primary infection is extensive and has allowed rigorous and well-controlled evaluation of the role of antibodies in affording protection. In agreement with the clinical studies, pre-existing maternal infections (31), or vaccination with live-attenuated (32, 33), and trivalent-subunit (34) vaccines show protection from nHSV disease. Additionally, these studies have established in both animal models and humans that maternally derived HSV-specific Abs can access the nervous system (35), and can decrease neurological behavioral associated with neonatal HSV infection in animal models (32, 36).
Furthermore, administration of purified HSV-specific IgG to pregnant dams is also protective (32). Together, these findings demonstrate that neonatal protection is mediated via passive vaccination, specifically through Ab transfer. Similar evidence is available in the context of adult infection, in a guinea pig model of passive vaccination with HSV-antiserum 24 hours post-infection had significant reduction in vaginal lesions, as well as disease reactivation and latent genome copy numbers (37). Abs that depress the spread of infection within the nervous system via passive immunization have protected the mouse eye, and skin, and have also restricted the number of affected sensory ganglia (38–40).
Towards therapeutic application of mAbs
Lessons from the past
The reliable isolation of monoclonal antibodies (mAbs) from hybridomas (41) has expanded the experimental tool box to better understand and more reliably dissect the role of Abs and their functional properties in HSV infection (Table 1). Antibodies recognizing diverse viral glycoproteins and their various subdomains have been isolated and screened in vitro for neutralization, complement-dependent cytotoxicity (CDC) or ADCC activity and in vivo for preventing infection. While these activities tend to be considered in isolation, they can be synergistic, as demonstrated by the ability of complement to enhance neutralization and ADCC (57, 58). Several important observations relating to protective mAb properties arose from these studies: 1) Different glycoprotein targets could confer protection, however, not all epitopes within or antibodies recognizing the same glycoprotein protected equally. 2) Protection was highly specific, as single point mutant viruses could abolish mAb efficacy. 3) Protection could be derived from neutralization, though most studies reported superior protection via ADCC mechanisms. 4) mAbs displayed variability in their protective capacity depending on when and how they and viral challenges were administered.
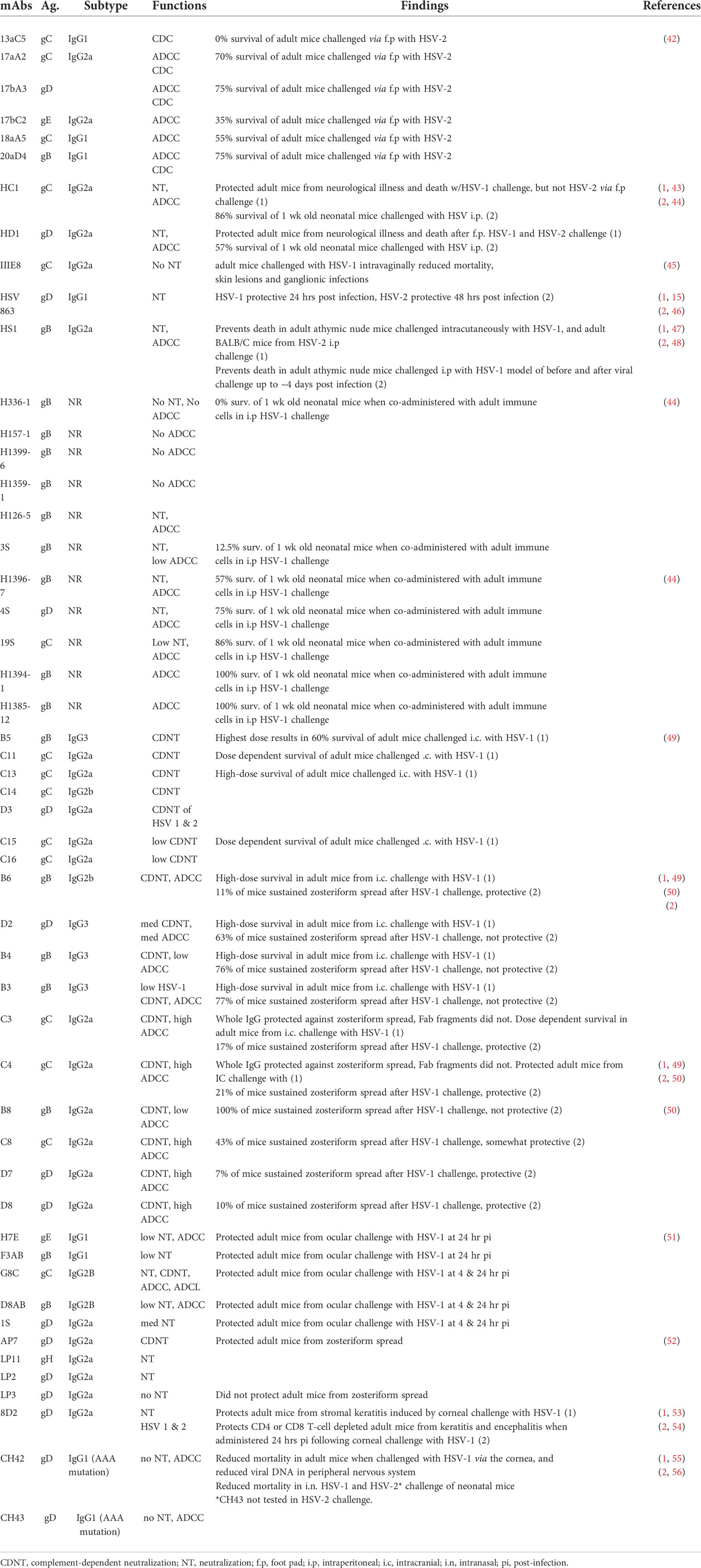
Table 1 Summary of studies investigating the role of antibodies in herpes simplex virus infection. .
Neutralization continues to be a benchmark for therapeutic efficacy in the setting of diverse viral infections. In the past this therapeutic strategy has proven fruitful, as Palivizumab, a respiratory syncytial virus (RSV)-neutralizing mAb, has been a highly successful intervention in neonates in RSV infection in high risk infants (56, 59). Prophylactically administered Palivizumab (MEDI-493) reduced hospitalization due to RSV disease by 55% when compared to placebo (60). Both direct and indirect antiviral activities can be readily achieved by a single mAb. For several decades, however, the importance of neutralization-independent, Fc-mediated functions have been noted in humans as well as in animal models. A recent review (61) highlighted the protective effect of FcγR-mediated effector functions in protection from adult HSV. Here we will focus on remaining questions regarding the role of Ab Fc in neonates and neonatal models of infection.
The age-dependent susceptibility of neonates to HSV motivates further understanding of Ab-protective mechanisms of action in this specific population. Earlier studies demonstrated that co-infusion of neonatal mice with human IgG, interferon, and immune cells could protect these mice from lethal challenge (62), therefore it was of interest to determine if Abs to specific epitopes and with defined ADCC and neutralizing activities could also protect neonatal mice. While pAbs reactive with gD or gB peptides protected against low-dose viral challenge model, adult mouse macrophages, which are strong mediators of ADCC, were also required to protect against high dose challenge. Similar results were observed for mAbs specific for gB, gC, and gD, which were shown to be protective when co-administered with macrophages and when they had high ADCC activity (44). Therefore, these studies demonstrated that both neutralization and ADCC activity were associated with protection, in agreement with clinical studies in which high neutralizing or ADCC-inducing Ab titers were independently associated with the absence of disseminated disease in neonates (24).
Thus, while these studies supported the notion that ADCC mediated by macrophages is a protective mechanism of action against HSV infection in mice, they also suggested that neonatal immune cells may not be sufficient to elicit a protective response in the setting of a high dose challenge. In the clinic, however, neonates born to mothers with high neutralizing or ADCC antibody titers are protected from disseminated viral disease, suggesting that neonatal immune cells are functionally capable of protecting neonates, or high neutralizing titers can compensate for low ADCC function, which is typically mediated by NK cells in humans, or vice versa. Alternatively, these mothers may shed less virus as a result of their own immunological response. An additional caveat is that the mAbs used in previous animal studies were not sufficiently efficacious to prevent disease in newborn mice. While these remain open questions, recent work suggests that diverse mAbs can protect from infection and sequelae in the neonatal mouse model (63), suggesting that clinical administration of systemic or local biologics could emulate and/or improve upon the observed maternal Ab-based protection seen in naturally infected mothers.
mAbs in clinical trials to treat HSV disease
Given the significant number of individuals affected by recurrent/reactivating HSV, a number of human mAbs tested for efficacy in preventing HSV disease in small animal models are transitioning from bench to bedside (Table 2), and may present new options in the prevention and treatment of nHSV infection. Therapeutic mAbs that recognize conserved epitopes required for viral entry may present a particular advantage as these targets are decoupled from the most common mechanisms of small molecule antiviral resistance, and can act at an earlier point in the infection and replication continuum.
2c
2c targets gB and was isolated from mice immunized with HSV-1 strain 342 hv (45). This mAb binds a discontinuous epitope in domain I of gB (71) necessary for infectivity (72) and is able to neutralize virus with or without complement, and can carry out ADCC. In 1991, Eis-Hubinger et al. described the efficacy of mAb 2c in preventing viral shedding at mucous membranes after intravaginal challenge, and protection from subsequent viral spread to neural tissues and death in C57B6 mice (45). This mAb was later humanized and assessed for efficacy in preventing disease with drug resistant HSV-1 in NOD/SCID mice (70). Humanized 2c (h2c) is able to prevent mortality following intravaginal challenge with a multidrug resistant HSV-1 clinical strain (70, 71), and also protected mice from developing herpetic stromal keratitis in a corneal scarification model (70, 73). The h2c mAb may present an important therapeutic option for immunocompromised patients who are at high risk if infected with resistant strains. Clinical trials in Germany and the United States are currently underway with this mAb under the biological identifier HDIT101. A topical preparation of h2c will be tested for efficacy in preventing orolabial lesions in participants infected with HSV-1 (NCT04539483), while prevention of anogenital lesions due to HSV-2 infections will also be assessed via intravenous infusion (NCT04165122).
HSV8
HSV8 is a glycoprotein D-specific human IgG1 mAb isolated from an Ab library via phage display, with the capability of neutralizing HSV-1 and HSV-2 (74). Topical application of HSV8 prevented genital HSV disease, and systemic delivery prevented death in athymic nude mice challenged via the cornea or flank model (68, 75). When added to human cervicovaginal mucus, HSV8 is able to trap HSV, limiting viral movement, presenting an exciting additional effector property to be explored in the setting of sexually transmitted infections (76). In human clinical trials, this mAb has been assessed for local delivery via the MB66 vaginal film, in which HSV8 is co-delivered with a broadly neutralizing HIV-specific antibody. The film was well tolerated and allowed for local biologically functional concentrations of mAbs as tested in vitro (69). In addition, this mAb protects two-day old mice from HSV-1 and HSV-2 induced mortality (63).
UB-621/E317
E317 is a gD-specific human IgG1 mAb isolated from a single chain variable fragment (scFv) library via phage display that can neutralize HSV-1 and HVS-2 (66). The crystal structure of the E317 Fab binding gD has been solved, and this mAb is able to disrupt gD interactions with both Nectin and HVEM receptors (64). Systemic administration of E317 can protect adult SCID mice from lethal viral infection with HSV-1 when administered before (100% survival) or after infection (75% survival) (66). The clinical grade product of this antibody, UB-621, is currently in clinical trials for prevention of orolabial and genital disease (NCT02346760, NCT03595995, NCT04714060, NCT04979975). UB-621 has shown to be protective from HSV-1 and HSV-2 induced mortality in a neonatal mouse model of infection (63).
Limitations
Barriers to the development and use of mAbs are cost, storage requirements, and route of administration of the drug. While convenient subcutaneous mAb administration is becoming more common, and consumer prices for mAbs vary widely depending on application (77), distribution in resource-limited settings is likely to be highly challenging. Additionally, the timely identification of infected neonates or at-risk pregnancies poses challenges to successful practical deployment based on limited maternal testing. Delays in initiating ACV therapy increase the risk of in-hospital death (78), therefore it is likely that early and/or prophylactic mAb administration would have the best therapeutic outcomes. It is estimated that 3849 women need to be screened in order to prevent one nHSV case that results in severe morbidity or mortality. While screening was effective in reducing the rate of HSV transmission, and also reduced the number of cesarean deliveries, the associated costs were significant (79). We remain optimistic that decreased costs in emerging testing platforms could, in the future, decrease the barriers to implementation of maternal HSV screening.
Conclusions
The significant mortality and morbidity observed in nHSV infection despite small molecule antiviral therapy demonstrates a critical clinical need for new interventions. Whereas long-term prevention of recurrent reactivation or initial infection in adult populations remains a challenge to modern vaccine development efforts, rich preclinical and epidemiological evidence supports the potential value of antibodies in prevention and treatment of nHSV infection. With prevention of RSV infection via palivizumab serving as a model of effective early life antibody therapy and several HSV-specific mAbs in clinical development for adult populations, the evidence reviewed here provides a strong scientific rationale to assess mAbs in human clinical trials for nHSV. Two out of three HSV-specific mAbs in human clinical trials have been tested and shown protection in a neonatal mouse of model of HSV-1 and HSV-2 infection, further bolstering the promise of this approach. Trials for rare diseases, such as nHSV, represent a considerable but worthwhile effort, especially to reduce not just the mortality, but the significant morbidity associated with this devastating neonatal infection.
Author contributions
IB wrote the initial draft and all authors contributed to manuscript revision. All authors contributed to the article and approved the submitted version.
Funding
This review was supported in part by NIH NIAID R21 AI147714, P01 AI098681, R01 09083, and U19AI145825.
Conflict of interest
Authors IB, DL, and MA have received UB-621 and HSV8 from companies involved in their clinical development and have filed provisional patents related to antibody-based prevention and therapy of nHSV infection.
Publisher’s note
All claims expressed in this article are solely those of the authors and do not necessarily represent those of their affiliated organizations, or those of the publisher, the editors and the reviewers. Any product that may be evaluated in this article, or claim that may be made by its manufacturer, is not guaranteed or endorsed by the publisher.
References
1. James C, Harfouche M, Welton NJ, Turner KM, Abu-Raddad LJ, Gottlieb SL, et al. Herpes simplex virus: global infection prevalence and incidence estimates, 2016. Bull World Health Organ (2020) 98:315–29. doi: 10.2471/BLT.19.237149
2. Fields BN, Knipe DM, Howley PM eds. DNA Viruses. seventh edition. In: New York Philadelphia Baltimore London Buenos Aires Hong Kong Sydney Tokyo, vol. 739 . Wolters Kluwer: Lippincot Williams & Wilkins.
3. Whitley R, Arvin A, Prober C, Burchett S, Corey L, Powell D, et al. A controlled trial comparing vidarabine with acyclovir in neonatal herpes simplex virus infection. infectious diseases collaborative antiviral study group. N Engl J Med (1991) 324:444–9. doi: 10.1056/NEJM199102143240703
4. Kimberlin DW, Lin C-Y, Jacobs RF, Powell DA, Corey L, Gruber WC, et al. Safety and efficacy of high-dose intravenous acyclovir in the management of neonatal herpes simplex virus infections. PEDIATRICS (2001) 108:230–8. doi: 10.1542/peds.108.2.230
5. Sheffield JS, Hill JB, Hollier LM, Laibl VR, Roberts SW, Sanchez PJ, et al. Valacyclovir prophylaxis to prevent recurrent herpes at delivery: A randomized clinical trial. Obstet Gynecol (2006) 108:141–7. doi: 10.1097/01.AOG.0000219749.96274.15
6. Kimberlin DW, Whitley RJ, Wan W, Powell DA, Storch G, Ahmed A, et al. Oral acyclovir suppression and neurodevelopment after neonatal herpes. N Engl J Med (2011) 365:1284–92. doi: 10.1056/NEJMoa1003509
7. Melvin AJ, Mohan KM, Vora SB, Selke S, Sullivan E, Wald A. Neonatal herpes simplex virus infection: Epidemiology and outcomes in the modern era. J Pediatr Infect Dis Soc (2022) 11(3):94–101. doi: 10.1093/jpids/piab105
8. Brown ZA, Selke S, Zeh J, Kopelman J, Maslow A, Ashley RL, et al. The acquisition of herpes simplex virus during pregnancy. N Engl J Med (1997) 337:509–16. doi: 10.1056/NEJM199708213370801
9. Looker KJ, Magaret AS, May MT, Turner KME, Vickerman P, Newman LM, et al. First estimates of the global and regional incidence of neonatal herpes infection. Lancet Glob Health (2017) 5:e300–9. doi: 10.1016/S2214-109X(16)30362-X
10. ACOG. Management of genital herpes in pregnancy: ACOG practice bulletin, number 220. Obstet Gynecol (2020) 135:e193. doi: 10.1097/AOG.0000000000003840
11. Brown ZA, Wald A, Morrow RA, Selke S, Zeh J, Corey L. Effect of serologic status and cesarean delivery on transmission rates of herpes simplex virus from mother to infant. JAMA (2003) 289:203–9. doi: 10.1001/jama.289.2.203
12. Corey L, Wald A. Maternal and neonatal herpes simplex virus infections. N Engl J Med (2009) 361:1376–85. doi: 10.1056/NEJMra0807633
13. Egan K, Hook LM, LaTourette P, Desmond A, Awasthi S, Friedman HM. Vaccines to prevent genital herpes. Transl Res J Lab Clin Med (2020) 220:138–52. doi: 10.1016/j.trsl.2020.03.004
14. Krishnan R, Stuart PM. Developments in vaccination for herpes simplex virus. Front Microbiol (2021) 12:798927. doi: 10.3389/fmicb.2021.798927
15. Kimberlin DW. Herpes simplex virus infections of the newborn. Semin Perinatol (2007) 31:19–25. doi: 10.1053/j.semperi.2007.01.003
16. Avila EC, Finger-Jardim F, Gonçalves CV, da Hora VP, Soares MA, de Martínez AMB. High incidence of herpes simplex virus-1 in cord blood and placenta infection of women in southern Brazil. Rev Bras Ginecol E Obstetrícia RBGO Gynecol Obstet (2020) 42:005–11. doi: 10.1055/s-0039-1700794
17. Finger-Jardim F, Teixeira LO, de Oliveira GR, Barral MFM, da Hora VP, Gonçalves CV, et al. Herpes simplex virus: prevalence in placental tissue and incidence in neonatal cord blood samples. J Med Virol (2014) 86:519–24. doi: 10.1002/jmv.23817
18. Jiang Y-C, Feng H, Lin Y-C, Guo X-R. New strategies against drug resistance to herpes simplex virus. Int J Oral Sci (2016) 8:1–6. doi: 10.1038/ijos.2016.3
19. Coen DM. Acyclovir-resistant, pathogenic herpesviruses. Trends Microbiol (1994) 2:481–5. doi: 10.1016/0966-842X(94)90652-1
20. Bacon TH, Levin MJ, Leary JJ, Sarisky RT, Sutton D. Herpes simplex virus resistance to acyclovir and penciclovir after two decades of antiviral therapy. Clin Microbiol Rev (2003) 16:114–28. doi: 10.1128/CMR.16.1.114-128.2003
21. Whitley R, Arvin A, Prober C, Corey L, Burchett S, Plotkin S, et al. Predictors of morbidity and mortality in neonates with herpes simplex virus infections. the national institute of allergy and infectious diseases collaborative antiviral study group. N Engl J Med (1991) 324:450–4. doi: 10.1056/NEJM199102143240704
22. Whitley RJ, Nahmias AJ, Visintine AM, Fleming CL, Alford CA, Yeager A, et al. The natural history of herpes simplex virus infection of mother and newborn. Pediatrics (1980) 66:489–94. doi: 10.1542/peds.66.4.489
23. Yeager AS, Arvin AM, Urbani LJ, Kemp JA. Relationship of antibody to outcome in neonatal herpes simplex virus infections. Obstet Gynecol Surv (1981) 36:191–2. doi: 10.1097/00006254-198104000-00012
24. Kohl S, West MS, Prober CG, Sullender WM, Loo LS, Arvin AM. Neonatal antibody-dependent cellular cytotoxic antibody levels are associated with the clinical presentation of neonatal herpes simplex virus infection. J Infect Dis (1989) 160:770–6. doi: 10.1093/infdis/160.5.770
25. Wald A, Zeh J, Selke S, Warren T, Ryncarz AJ, Ashley R, et al. Reactivation of genital herpes simplex virus type 2 infection in asymptomatic seropositive persons. N Engl J Med (2000) 342:844–50. doi: 10.1056/NEJM200003233421203
26. Belshe RB, Leone PA, Bernstein DI, Wald A, Levin MJ, Stapleton JT, et al. Efficacy results of a trial of a herpes simplex vaccine. N Engl J Med (2012) 366:34–43. doi: 10.1056/NEJMoa1103151
27. Douglas RG, Couch RB. A prospective study of chronic herpes simplex virus infection and recurrent herpes labialis in humans. J Immunol (1970) 104:289–95.
28. Spruance SL, Evans TG, McKeough MB, Thai L, Araneo BA, Daynes RA, et al. Th1/Th2-like immunity and resistance to herpes simplex labialis. Antiviral Res (1995) 28:39–55. doi: 10.1016/0166-3542(95)00037-m
29. Dasgupta G, Chentoufi AA, Kalantari M, Falatoonzadeh P, Chun S, Lim CH, et al. Immunodominant “Asymptomatic” herpes simplex virus 1 and 2 protein antigens identified by probing whole-ORFome microarrays with serum antibodies from seropositive asymptomatic versus symptomatic individuals. J Virol (2012) 86:4358–69. doi: 10.1128/JVI.07107-11
30. Moraru M, Black LE, Muntasell A, Portero F, López-Botet M, Reyburn HT, et al. NK cell and ig interplay in defense against herpes simplex virus type 1: Epistatic interaction of CD16A and IgG1 allotypes of variable affinities modulates antibody-dependent cellular cytotoxicity and susceptibility to clinical reactivation. J Immunol (2015) 195:1676–84. doi: 10.4049/jimmunol.1500872
31. Jiang Y. Viral corruption of immune privilege: Immunity and pathology in the cornea and nervous system after HSV-1 infection. [Ph.D.] (2017). United States – New Hampshire: Dartmouth College. Available at: http://www.proquest.com/pqdtlocal1006600/docview/1953259642/abstract/7FCB83C114D84094PQ/3 (Accessed September 22, 2021).
32. Patel CD, Backes IM, Taylor SA, Jiang Y, Marchant A, Pesola JM, et al. Maternal immunization confers protection against neonatal herpes simplex mortality and behavioral morbidity. Sci Transl Med (2019) 11:eaau6039. doi: 10.1126/scitranslmed.aau6039
33. Kao CM, Goymer J, Loh LN, Mahant A, Aschner CB, Herold BC. Murine model of maternal immunization demonstrates protective role for antibodies that mediate antibody-dependent cellular cytotoxicity in protecting neonates from herpes simplex virus type 1 and type 2. J Infect Dis (2019) 221(5):729–38. doi: 10.1093/infdis/jiz521
34. Patel CD, Taylor SA, Mehrbach J, Awasthi S, Friedman HM, Leib DA. Trivalent glycoprotein subunit vaccine prevents neonatal herpes simplex virus mortality and morbidity. J Virol (2020) 94:e02163–19. doi: 10.1128/JVI.02163-19
35. Jiang Y, Patel CD, Manivanh R, North B, Backes IM, Posner DA, et al. Maternal antiviral immunoglobulin accumulates in neural tissue of neonates to prevent HSV neurological disease. mBio (2017) 8:e00678–17. doi: 10.1128/mBio.00678-17
36. Patel CD. Harnessing maternal humoral immunity to prevent neonatal herpes neurological sequela. [Ph.D.]. United States – New Hampshire: Dartmouth College (2020). p. 188.
37. Bourne N, Pyles RB, Bernstein DI, Stanberry LR. Modification of primary and recurrent genital herpes in guinea pigs by passive immunization. J Gen Virol (2002) 83:2797–801. doi: 10.1099/0022-1317-83-11-2797
38. Shimeld C, Hill TJ, Blyth WA, Easty DL. Passive immunization protects the mouse eye from damage after herpes simplex virus infection by limiting spread of virus in the nervous system. J Gen Virol (1990) 71(Pt 3):681–7. doi: 10.1099/0022-1317-71-3-681
39. Walz N, Mühlberger A, Pauli P. A human open field test reveals thigmotaxis related to agoraphobic fear. Biol Psychiatry (2016) 80:390–7. doi: 10.1016/j.biopsych.2015.12.016
40. Kino Y, Hayashi Y, Hayashida I, Mori R. Dissemination of herpes simplex virus in nude mice after intracutaneous inoculation and effect of antibody on the course of infection. J Gen Virol (1982) 63:475–9. doi: 10.1099/0022-1317-63-2-475
41. Köhler G, Milstein C. Continuous cultures of fused cells secreting antibody of predefined specificity. Nature (1975) 256:495–7. doi: 10.1038/256495a0
42. Balachandran N, Bacchetti S, Rawls WE. Protection against lethal challenge of BALB/c mice by passive transfer of monoclonal antibodies to five glycoproteins of herpes simplex virus type 2. Infect Immun (1982) 37:1132–7. doi: 10.1128/IAI.37.3.1132-1137.1982
43. Dix RD, Pereira L, Baringer JR. Use of monoclonal antibody directed against herpes simplex virus glycoproteins to protect mice against acute virus-induced neurological disease. Infect Immun (1981) 34:192–9. doi: 10.1128/iai.34.1.192-199.1981
44. Kohl S, Strynadka NC, Hodges RS, Pereira L. Analysis of the role of antibody-dependent cellular cytotoxic antibody activity in murine neonatal herpes simplex virus infection with antibodies to synthetic peptides of glycoprotein d and monoclonal antibodies to glycoprotein b. J Clin Invest (1990) 86:273–8. doi: 10.1172/JCI114695
45. Eis-Hübinger AM, Mohr K, Schneweis KE. Different mechanisms of protection by monoclonal and polyclonal antibodies during the course of herpes simplex virus infection. Intervirology (1991) 32:351–60. doi: 10.1159/000150219
46. Harfeldt E, Lake P, Nottage B, Ostberg LG. Monoclonal antibody to herpes simplex virus and cell line producing same (1997). Available at: https://patents.google.com/patent/US5646041/en?oq=HSV+863 (Accessed December 6, 2021).
47. Kino Y, Eto T, Ohtomo N, Hayashi Y, Yamamoto M, Mori R. Passive immunization of mice with monoclonal antibodies to glycoprotein gB of herpes simplex virus. Microbiol Immunol (1985) 29:143–9. doi: 10.1111/j.1348-0421.1985.tb00812.x
48. Yamamoto M, Tang J-L, Kumano Y, Mori R, Kino Y. Ability of monoclonal antibody to herpes simplex virus glycoprotein gB to promote healing of herpetic skin lesions in nude mice. Antiviral Res (1986) 6:223–31. doi: 10.1016/0166-3542(86)90003-3
49. Kümel G, Kaerner HC, Levine M, Schröder CH, Glorioso JC. Passive immune protection by herpes simplex virus-specific monoclonal antibodies and monoclonal antibody-resistant mutants altered in pathogenicity. J Virol (1985) 56:930–7. doi: 10.1128/JVI.56.3.930-937.1985
50. Mester JC, Glorioso JC, Rouse BT. Protection against zosteriform spread of herpes simplex virus by monoclonal antibodies. J Infect Dis (1991) 163:263–9. doi: 10.1093/infdis/163.2.263
51. Rector JT, Lausch RN, Oakes JE. Use of monoclonal antibodies for analysis of antibody-dependent immunity to ocular herpes simplex virus type 1 infection. Infect Immun (1982) 38:168–74. doi: 10.1128/iai.38.1.168-174.1982
52. Simmons A, Nash A. Role of antibody in primary and recurrent herpes simplex virus infection. J Virol (1985) 53:944–8. doi: 10.1128/jvi.53.3.944-948.1985
53. Lausch RN, Oakes JE, Metcalf JF, Scimeca JM, Smith LA, Robertson SM. Quantitation of purified monoclonal antibody needed to prevent HSV-1 induced stromal keratitis in mice. Curr Eye Res (1989) 8:499–506. doi: 10.3109/02713688909000030
54. Staats HF, Oakes JE, Lausch RN. Anti-glycoprotein d monoclonal antibody protects against herpes simplex virus type 1-induced diseases in mice functionally depleted of selected T-cell subsets or asialo GM1+ cells. J Virol (1991) 65:6008–14. doi: 10.1128/JVI.65.11.6008-6014.1991
55. Wang K, Tomaras GD, Jegaskanda S, Moody MA, Liao H-X, Goodman KN, et al. Monoclonal antibodies, derived from humans vaccinated with the RV144 HIV vaccine containing the HVEM binding domain of herpes simplex virus (HSV) glycoprotein d, neutralize HSV infection, mediate antibody-dependent cellular cytotoxicity, and protect mice from ocular challenge with HSV-1. J Virol (2017) 91:e00411-17. doi: 10.1128/JVI.00411-17
56. Buchwald AG, Graham BS, Traore A, Haidara FC, Chen M, Morabito K, et al. Respiratory syncytial virus (RSV) neutralizing antibodies at birth predict protection from RSV illness in infants in the first 3 months of life. Clin Infect Dis (2021) 73:e4421–7. doi: 10.1093/cid/ciaa648
57. Leu RW, Zhou AQ, Rummage JA, Kennedy MJ, Shannon BJ. Exogenous C1q reconstitutes resident but not inflammatory mouse peritoneal macrophages for fc receptor-dependent cellular cytotoxicity and phagocytosis. relationship to endogenous C1q availability. J Immunol (1989) 143:3250–7.
58. Ackerman M, Nimmerjahn F. Antibody Fc : Linking adaptive and innate immunity (2013). Amsterdam: Academic Press. Available at: http://dartmouth.idm.oclc.org/login?url=https://search.ebscohost.com/login.aspx?direct=true&AuthType=ip,url,uid&db=e000xna&AN=486009&site=ehost-live&scope=site (Accessed December 23, 2021).
59. Glezen WP, Paredes A, Allison JE, Taber LH, Frank AL. Risk of respiratory syncytial virus infection for infants from low-income families in relationship to age, sex, ethnic group, and maternal antibody level. J Pediatr (1981) 98:708–15. doi: 10.1016/S0022-3476(81)80829-3
60. The IMpact-RSV Study Group. Palivizumab, a humanized respiratory syncytial virus monoclonal antibody, reduces hospitalization from respiratory syncytial virus infection in high-risk infants. Pediatrics (1998) 102:531–7. doi: 10.1542/peds.102.3.531
61. Jenks JA, Goodwin ML, Permar SR. The roles of host and viral antibody fc receptors in herpes simplex virus (HSV) and human cytomegalovirus (HCMV) infections and immunity. Front Immunol (2019) 10:2110. doi: 10.3389/fimmu.2019.02110
62. Kohl S, Loo LS, Greenberg SB. Protection of newborn mice from a lethal herpes simplex virus infection by human interferon, antibody, and leukocytes. J Immunol (1982) 128:1107–11.
63. Backes IM, Byrd BK, Patel CD, Taylor SA, Garland CR, MacDonald SW, et al. Maternally transferred monoclonal antibodies protect neonatal mice from herpes simplex virus-induced mortality and morbidity. bioRxiv (2022). doi: 10.1101/2022.01.12.476098
64. Lee C-C, Lin L-L, Chan W-E, Ko T-P, Lai J-S, Wang AH-J. Structural basis for the antibody neutralization of Herpes simplex virus. Acta Crystallogr D Biol Crystallogr (2013) 69:1935–45. doi: 10.1107/S0907444913016776
65. Lai J-S, Lin Y-S, Chan W-E. Anti-herpes simplex virus antibodies (2010). Available at: https://patents.google.com/patent/WO2010087813A1/en (Accessed May 10, 2021).
66. Lai J-S, Chan W-E. Anti-herpes simplex virus antibodies and methods of use thereof (2013). Available at: https://patents.google.com/patent/US8431118/en (Accessed May 1, 2020).
67. Clementi N, Criscuolo E, Cappelletti F, Burioni R, Clementi M, Mancini N. Novel therapeutic investigational strategies to treat severe and disseminated HSV infections suggested by a deeper understanding of in vitro virus entry processes. Drug Discovery Today (2016) 21:682–91. doi: 10.1016/j.drudis.2016.03.003
68. Sanna PP, De logu A, Williamson RA, Hom Y-L, Straus SE, Bloom FE, et al. Protection of nude mice by passive immunization with a type-common human recombinant monoclonal antibody against HSV. Virology (1996) 215:101–6. doi: 10.1006/viro.1996.0011
69. Politch JA, Cu-Uvin S, Moench TR, Tashima KT, Marathe JG, Guthrie KM, et al. Safety, acceptability, and pharmacokinetics of a monoclonal antibody-based vaginal multipurpose prevention film (MB66): A phase I randomized trial. PLoS Med (2021) 18:e1003495. doi: 10.1371/journal.pmed.1003495
70. Krawczyk A, Arndt MAE, Grosse-Hovest L, Weichert W, Giebel B, Dittmer U, et al. Overcoming drug-resistant herpes simplex virus (HSV) infection by a humanized antibody. Proc Natl Acad Sci (2013) 110:6760–5. doi: 10.1073/pnas.1220019110
71. Krawczyk A, Krauss J, Eis-Hübinger AM, Däumer MP, Schwarzenbacher R, Dittmer U, et al. Impact of valency of a glycoprotein b-specific monoclonal antibody on neutralization of herpes simplex virus. J Virol (2011) 85:1793–803. doi: 10.1128/JVI.01924-10
72. Däumer MP, Schneider B, Giesen DM, Aziz S, Kaiser R, Kupfer B, et al. Characterisation of the epitope for a herpes simplex virus glycoprotein b-specific monoclonal antibody with high protective capacity. Med Microbiol Immunol (Berl) (2011) 200:85–97. doi: 10.1007/s00430-010-0174-x
73. Krawczyk A, Dirks M, Kasper M, Buch A, Dittmer U, Giebel B, et al. Prevention of herpes simplex virus induced stromal keratitis by a glycoprotein b-specific monoclonal antibody. PoS One (2015) 10:e0116800. doi: 10.1371/journal.pone.0116800
74. Burioni R, Williamson RA, Sanna PP, Bloom FE, Burton DR. Recombinant human fab to glycoprotein d neutralizes infectivity and prevents cell-to-cell transmission of herpes simplex viruses 1 and 2. vitro Proc Natl Acad Sci (1994) 91:355–9. doi: 10.1073/pnas.91.1.355
75. Zeitlin L, Whaley KJ, Sanna PP, Moench TR, Bastidas R, Logu AD, et al. Topically applied human recombinant monoclonal IgG1Antibody and its fab and F(ab′)2Fragments protect mice from vaginal transmission of HSV-2. Virology (1996) 225:213–5. doi: 10.1006/viro.1996.0589
76. Schroeder HA, Nunn KL, Schaefer A, Henry CE, Lam F, Pauly MH, et al. Herpes simplex virus-binding IgG traps HSV in human cervicovaginal mucus across the menstrual cycle and diverse vaginal microbial composition. Mucosal Immunol (2018) 11:1477–86. doi: 10.1038/s41385-018-0054-z
77. Hernandez I, Bott SW, Patel AS, Wolf CG, Hospodar AR, Sampathkumar S, et al. Pricing of monoclonal antibody therapies: higher if used for cancer? Am J Manag Care (2018) 24:109–12.
78. Shah SS, Aronson PL, Mohamad Z, Lorch SA. Delayed acyclovir therapy and death among neonates with herpes simplex virus infection. PEDIATRICS (2011) 128:1153–60. doi: 10.1542/peds.2011-0177
Keywords: IgG, herpes simplex virus (HSV) infection, monoclonal Ab, neonatal infection, effector function
Citation: Backes IM, Leib DA and Ackerman ME (2022) Monoclonal antibody therapy of herpes simplex virus: An opportunity to decrease congenital and perinatal infections. Front. Immunol. 13:959603. doi: 10.3389/fimmu.2022.959603
Received: 01 June 2022; Accepted: 20 July 2022;
Published: 09 August 2022.
Edited by:
Wen Shi Lee, The University of Melbourne, AustraliaReviewed by:
Patrick Michael Stuart, Saint Louis University, United StatesMadavaraju Krishnaraju, University of Illinois at Chicago, United States
Copyright © 2022 Backes, Leib and Ackerman. This is an open-access article distributed under the terms of the Creative Commons Attribution License (CC BY). The use, distribution or reproduction in other forums is permitted, provided the original author(s) and the copyright owner(s) are credited and that the original publication in this journal is cited, in accordance with accepted academic practice. No use, distribution or reproduction is permitted which does not comply with these terms.
*Correspondence: David A. Leib, ZGF2aWQuYS5sZWliQGRhcnRtb3V0aC5lZHU=; Margaret E. Ackerman, bWFyZ2FyZXQuZS5hY2tlcm1hbkBkYXJ0bW91dGguZWR1