- 1Department of Interventional Radiology, The First Affiliated Hospital of Zhengzhou University, Zhengzhou, China
- 2Interventional Institute of Zhengzhou University, Zhengzhou, China
- 3Interventional Treatment and Clinical Research Center of Henan Province, Zhengzhou, China
- 4Department of Colorectal Surgery, The First Affiliated Hospital of Zhengzhou University, Zhengzhou, China
- 5Department of Cardiology, The First Affiliated Hospital of Zhengzhou University, Zhengzhou, China
- 6Department of Respiratory and Critical Care Medicine, The First Affiliated Hospital of Zhengzhou University, Zhengzhou, China
Metastatic dissemination represents a hallmark of cancer that is responsible for the high mortality rate. Recently, emerging evidence demonstrates a time-series event—pre-metastatic niche (PMN) has a profound impact on cancer metastasis. Exosomes, cell-free DNA (cfDNA), circulating tumor cells (CTC), and tumor microenvironment components, as critical components in PMN establishment, could be monitored by liquid biopsy. Intensive studies based on the molecular profile of liquid biopsy have made it a viable alternative to tissue biopsy. Meanwhile, the complex molecular mechanism and intercellular interaction are great challenges for applying liquid biopsy in clinical practice. This article reviews the cellular and molecular components involved in the establishment of the PMN and the promotion of metastasis, as well as the mechanisms of their interactions. Better knowledge of the characteristics of the PMN may facilitate the application of liquid biopsy for clinical diagnosis, prognosis, and treatment.
Introduction
Distant metastasis was the terminal stage of tumor progression and the crucial cause of tumor death (1, 2). The immaturity of early diagnostic techniques and drug resistance indirectly promoted distant metastasis, leading to a high mortality rate of cancer (3, 4). Cancer progression is a dynamic process. Metastasis is an organ-selective and multi-stepping complex process that requires in-depth study, in order to find a better approach to diagnosis and treatment (5). The early perspective was that tumor cells migrated out of the primary site into the lymphatics or the bloodstream, survived in the circulation, and extravasated into the tissue, eventually forming metastasis (6). However, this theory was not enough to guide tumor-specific diagnosis and treatment. Stephen et al. first proposed the concept of “seed and soil” theory, which emphasized the importance of the microenvironment and revealed the organotropism of metastasis. The secondary site has established an abnormal, tumor growth-favoring microenvironment before tumor cells arrive (7). These predetermined soil microenvironments were termed “pre-metastatic niches”, which actively attracted the colonization of tumor cells (8). With the characteristics of inflammation, immunosuppression, angiogenesis/vascular permeability, reprogramming, organotropism, and lymphangiogenesis, the indispensable role of the PMN in metastasis has attracted increasing attention in recent years (9–13) (Figure 1).
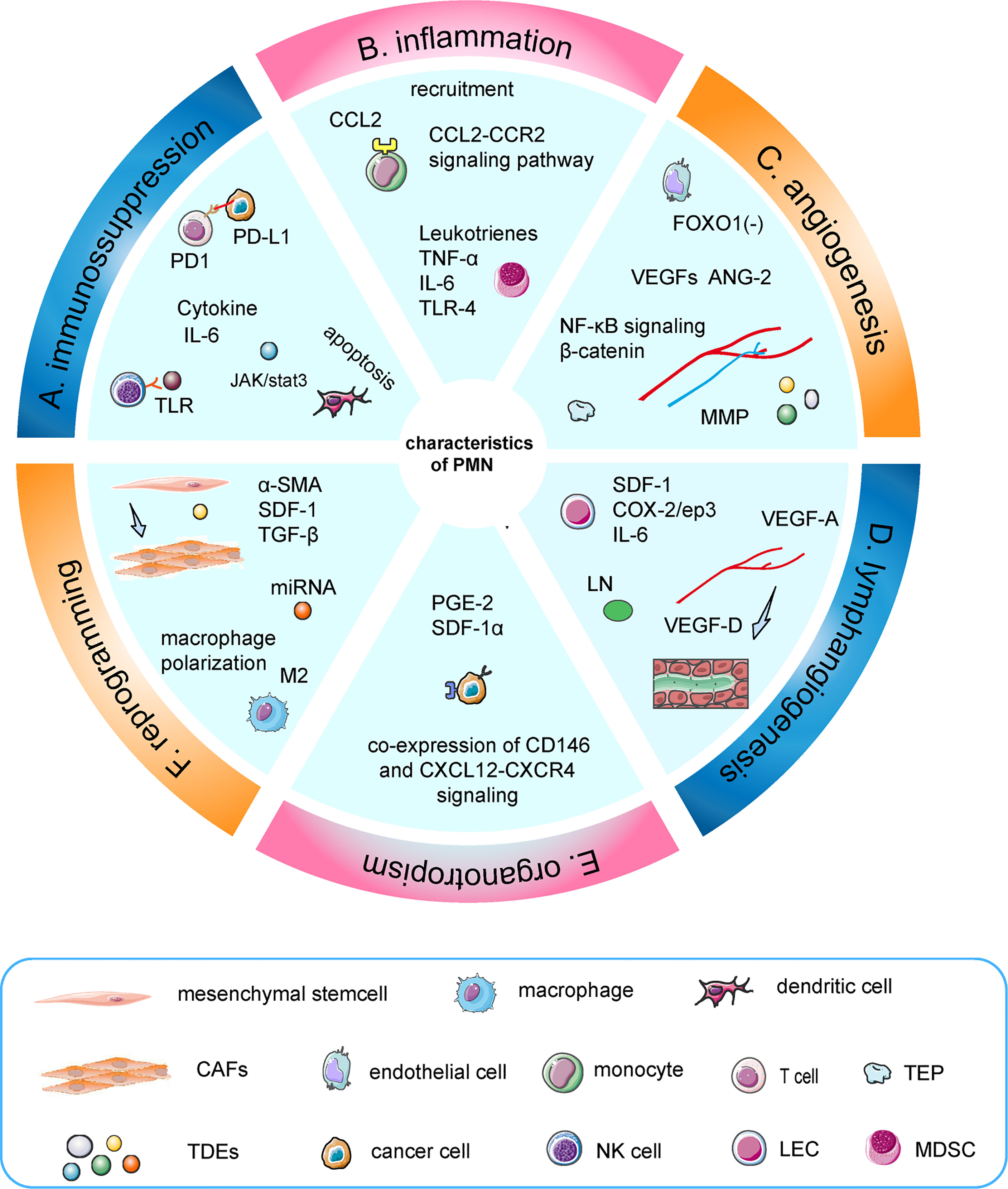
Figure 1 Characteristics of the PMN. (A) TDEs induced apoptosis of dendritic cells, increased secretion of IL-6, and inhibited the function of T cells and NK cells. (B) TNF-α induced S100A8-SAA3-TLR4 signaling and maintained an inflammatory state, which was mediated by MDSC. Similarly, IL-6, leukotrienes, and CCL2–CCR2 signaling pathways were involved. (C) Upregulation of angiopoietin-2 and VEGFs could regulate the angiogenic switch, in which TEPs and MMPs had a synergistic effect. Moreover, miR-135b promotes angiogenesis by inhibiting FOXO1 expression in endothelial cells. (D) VEGF-A and VEGF-D are crucial factors in the induction of premetastatic lymphangiogenesis. Furthermore, dendritic cells induce a PMN during LN metastasis through COX-2/ep3-dependent induction of SDF-1. (E) Tumor cells interacted with resident mesenchymal stem cells/pericytes around the surrounding blood vessels to achieve organotropism through co-expressed CD146 and Sdf-1/CXCL12-CXCR4 signaling. (F) Exosomes induced the transformation of mesenchymal stem cells into CAFs and macrophage M2-like polarization.
Nowadays, tissue biopsy, as the gold standard of cancer identification, remains the first-line clinical mean (14). However, conventional tissue biopsies are invasive and sometimes only small samples can be obtained (15), making it impossible to characterize tumor heterogeneity or dynamically monitor tumor progression (16, 17). Moreover, it is restricted by tissue excision site, adverse accuracy and sensitivity, and high procedural expenses (18–20). Therefore, a novel diagnostic method has emerged—liquid biopsy. It is a neoteric skill to identify tumor markers among the accessible samples, such as cfDNA or RNA, circulating tumor DNA (ctDNA), CTCs, exosomes, circulating tumor-derived endothelial cells (CTECs), tumor-educated blood platelets (TEPs), and protein molecules (21–25). Biomarkers for liquid biopsies can be derived from cerebrospinal fluid (CSF), saliva, blood, ascites, urine, stool, and pleural fluid (26–28). Better than tissue biopsy, liquid biopsy is non-invasive, easier to repeat, and could better overcome tumor heterogeneity due to the wide range of samples (16, 29). In accord with the patient’s will not only from the macro perspective but also from the micro mechanism analysis, the progress of gene detection technology supports the development of the clinical application. Whole-exome sequencing or whole-genome sequencing data analysis explores the changes of genes and tumor burden in the course of patients (30), carries out comprehensive dynamic monitoring at the molecular level in a non-invasive manner, predicts tumor progression, and provides support for the formulation of subsequent precise treatment programs (31). Fortunately, cellular and molecular components such as exosomes, CTCs, and TEPs, which promote the formation of the PMN, are also widely present in plasma, urine, ascites, and other body fluids, making the application of liquid biopsy feasible (32, 33).
In this review, we focus on the critical molecular and cellular components that could be used in liquid biopsy at various stages of tumor metastasis niche formation and explain their clinical applications in prediction, prognosis, and treatment.
The Evolution of PMN and the Process of Promoting Metastasis
PMNs evolve in phased, sequential, and distinct ways, with each stage contributing to the metastasis process in its way (8, 9). Complex molecular and cellular changes have taken place in PMN to support the growth of metastatic tumors in the future (34, 35). Some molecules or cells could serve as markers of liquid biopsy. To simplify the complex development of time series events and more clearly explain the mechanism of liquid biopsy, the formation and metastasis promotion process of PMN can be divided into the following four temporal phases in sequence (9) (Figure 2).
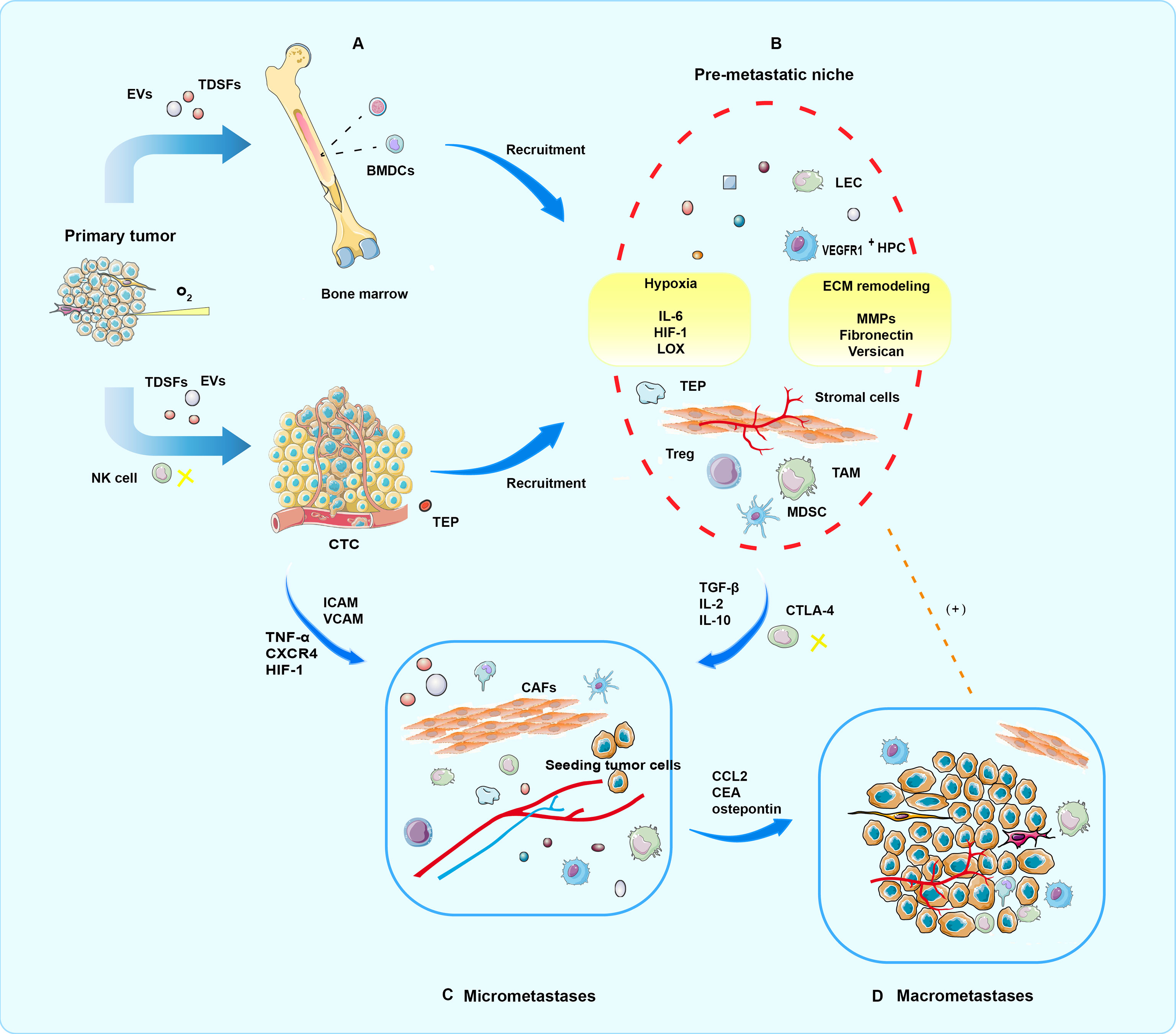
Figure 2 Four periods of PMN formation. (A) In the priming phase, produced by primary tumor cells, TDSFs, EVs, and other molecular components trigger an immature PMN formation in the secondary organ site or the same organ outside the primary tumor. (B) In the licensing phase, BMDC, regulatory, and suppressive immune cells involved in ECM remodeling are mobilized and recruited into the secondary sites. Finally, a mature PMN prepared well for potential seeding and colonization of CTCs. In addition, hypoxia is a critical condition for progress. (C) In the initiation phase, partial CTCs arrive and survive at the fertile PMN, where tumor cell seeding, colonization, and outgrowth occur, eventually resulting in micrometastases. (D) In the progression phase, PMNs attract more tumor cells to colonize. Moreover, mutual promotion of tumor cells and PMN is locked in a vicious circle, which culminates in macrometastases.
Priming
During the priming period, when the primary tumor proliferates uncontrollably, hypoxia and inflammation are produced, which induces the secretion of extracellular vesicles (EVs), tumor-derived soluble factors (TDSFs), and other molecular components (36). Exosomes secreted by cancer cells under hypoxic conditions may remodel distant PMN. Exosomes were isolated from human prostate cancer cells under normoxic and hypoxic conditions, and their effects on key biomarkers associated with PMN in nude mice were observed. It was found that the exosomes produced under hypoxic conditions increased the levels of matrix metalloproteinases (MMP) and extracellular matrix proteins (fibronectin and collagen) as well as enhanced the number of CD11b+ cells at selective PMN sites (37). In addition, abnormal glycolysis and high lactate production may reduce tissue pH, which has been verified in the tumor mouse model. The average pH of the tumor stroma was prominently lower than the surrounding tissue.
Moreover, acidifying the tumor microenvironment is not conducive to roving immune cells, establishing an anergic state in human tumor-specific CD8+ T lymphocytes, and facilitating immune escape (38, 39). Acidic conditions also alter key phenotypes of malignancies and determine the type and quantity of exosomes released from tumor cells. Likewise, in prostate cancer, the microenvironment acidity exerts selective pressure to induce an upregulation of nanovesicle production, expressing both the exosome biomarker CD81 and prostate-specific antigen (PSA). The ratio of PSA-expressing exosomes in the plasma of cancer patients is significantly higher compared to benign prostatic hyperplasia and healthy individuals (40). Therefore, as a tool for early diagnosis and screening, PSA cancer exosomes are considered novel and non-invasive.
Pervasively, TDSFs would serve as tumor precursors for organ-specific preparation of PMNs prior to CTC colonization. TDSFs, mainly including cytokines, chemokines, and growth factors, can contribute to the recruitment and activation of inflammatory cells (41). Many types of cancer are characterized by aberrant IL-6/JAK/STAT3 activation, which strongly inhibits the antitumor immune response (42). Besides IL-6, TNF-α and TGF-β also affect the myeloid-derived suppressor cell (MDSC) recruitment in the premetastatic lung, which is modulated by the generation of inflammatory chemoattractants S100A9 and S100A8 (43). In breast cancer, with CCR2 (the chemokine receptor for CCL2) expressed, inflammatory monocytes are more promising to accumulate in the pulmonary metastases than in primary tumors. Metastatic seeding is also suppressed by inhibition of the CCL2–CCR2 pathway and exhaustion of tumor-derived CCL2. All these suggest that a CCL2 overexpression improves macrophage infiltration and worsens the prognosis of human breast cancer (44). Moreover, CXCR2-positive MDSCs are attracted to metastatic sites by CXCL1 derived from tumor-associated macrophages (TAM) due to the stimulation of VEGFA released from primary tumor cells (45). From these results, it can be seen that hypoxia and acidic conditions are two key factors that lay the foundation for remodeling the matrix microenvironment, while also regulating tumor progression, recruitment, and activation of inflammatory cells, as well as facilitating the production of bioactive molecules.
Licensing
In the licensing phase, under the action of TDSFs and EVs, a continuous flow of regulatory/suppressive immune cells and bone marrow-derived cells (BMDCs) is activated and mobilized into the transfer sites, which start to re-educate the stromal environment of the distant secondary sites to form a soil environment suitable for CTC colonization. Exosomes play a profound role in this stage (46). Extracellular vesicles, messengers between primary tumor sites and metastatic sites, are indispensable in forming PMN. Studies have shown that extracellular vesicles are small membrane vesicles from cells during activation and apoptosis. They can be divided into at least three types: apoptotic bodies, microvesicles, and exosomes with a size ranging from 40 to 100 nm (47, 48).
Interestingly, the biological functions of exosomes are different according to their sources (49). Tumor-derived exosomes (TDEs) carry a variety of bioactive components (nucleic acid, protein, and lipid), which are effective tools for intercellular communication. Exosomal microRNAs are the major messengers to execute these functions among these bioactive components. For instance, microRNA can establish an immunosuppressive environment by changing the phenotype and function of various immune cells. It is reported that MDSCs mediate the immunosuppressive environment, and hypoxia promotes the secretion of TDEs. MDSCs ingest TDEs, and tumor exogenous miR-9 and miR-181a targeted SOCS3 and PIAS3 to activate the JAK/STAT signal pathway, thus affecting the differentiation and activation of MDSCs and enhancing its immunosuppressive effect (50, 51). TDEs could not only directly inhibit NK cells’ toxicity and T cells’ proliferation but also achieve immunosuppression by enhancing the immune tolerance mediated by regulatory T cells. In terms of matrix remodeling, TDEs trigger the differentiation of mesenchymal stem cells (MSCs) to carcinoma-associated fibroblasts (CAFs) via the TGF-β/Smad pathway (52, 53). CAFs can modify the surrounding ECM to generate an ecological niche supporting cancer cell invasion, as well as facilitate cancer cell migration and invasion so as to promote tumor occurrence, metastasis, and diffusion to malignancy (35).
Furthermore, hypoxia stimulates hypersecretion of TDEs, enhances the recruitment of TDE to macrophages, and promotes M2-like polarization. In gastric cancer, miR-135b accelerates angiogenesis due to the inhibition of FOXO1 expression from endothelial cells (54). Notably, the critical role of exosomal proteins in promoting the production of PMN and as markers of liquid biopsy should not be ignored. For example, exosomes containing metalloproteinases promote angiogenesis, exosomal PD-L1 enhances immunosuppression, and exosome-induced T-cell inhibition depends on ICAM-1-mediated adhesion (55–57). From the above description, it could be demonstrated that exosomes are reasonable and practical as liquid biopsy biomarkers.
During this period, lymphangiogenesis is critical in the PMN, in addition to immunosuppression, angiogenesis, and matrix remodeling. Lymph node (LN) metastasis is a pivotal prognostic indicator of disease stage; thereby, the lymphatic system is considered to be a vital route of metastatic spread except for hematogenous metastasis (58). Partial tumors secrete lymphatic growth factors, which induce lymphangiogenesis and act on lymphatic vessels to boost metastasis. Namely, in the interior of the primary tumor or metastatic sites, such as sentinel LN (59), the original blood vessels form new lymphatic vessels and participate in regulating the immune response to the tumor under the action of TDSFs (60, 61). Clinical evidence indicates that tumor-derived VEGF-D and VEGF-A are key factors in the induction of premetastatic lymphangiogenesis in sentinel LN.
Moreover, since VEGF-D is associated with higher LN metastasis, it may be a potential predictor of positive LN metastasis in patients (62). Furthermore, when LN metastasis occurs, dendritic cells induce a PMN through COX-2/ep3-dependent SDF-1, suggesting that restraining this signaling axis may be an available measure to inhibit PMN formation and LN metastasis (63). Crucially, immunosuppression is facilitated by the presence of lymphatic vessels in the PMN, through which exosomes can reach mouse and human melanoma. In LN, exosomes preferentially bind CD169+ macrophages, destroy them to escape immune recognition, invade the LN cortex, and disrupt humoral immunity via interacting with B cells (64). Lymphatic endothelial cells (LECs), a composition of lymphatic vessels in the PMN, are regulated by TDSFs. Mechanistically, IL-6 induces the expression of HIF-1α, CCL5, and VEGF via the phosphorylation pathway to facilitate the recruitment, extravasation, and colonization of CCR5+ tumor cells in the niche, which lays the foundation for the smooth progress of the next stage.
Additionally, there is a self-reinforcing paracrine loop between cancer cells and LECs (65). Stromal lymphatic vessels are the primary route of metastasis in some cancers (66); moreover, the presence of LN metastasis worsens the prognosis (67). Consequently, an in-depth study on the molecular and cellular matrix and identifying critical factors in lymphangiogenesis may contribute to discovering new targets, which could be used in liquid biopsy to decrease tumor dissemination and improve prognosis.
Initiation
In the initiation phase, through epithelial–mesenchymal transformation (EMT) (68, 69), tumor cells become CTCs and infiltrate from the blood vessels of the primary site. Some of them arrive and survive at the fertile PMN, where sowing, colonization, and proliferation of tumor cells occur, eventually leading to micrometastases. Nevertheless, before the arrival of CTCs, BMDCs migrated to distant sites induced by factors of the primary tumor, such as TNF-α, TGF-β, and TDEs. BMDCs contribute to tumor vascularization and neoplastic cell migration, which are latent promoters of CTC extravasation with organotropism (70).
Tumor cells migrating from the primary site to the PMN need to go through several critical stages: intravasation, intravascular survival, extravasation, and colonization of secondary sites. CTCs acquire enhanced migratory and invasive abilities through EMT (71), which is a transient, reversible process of cell differentiation. TAMs usually play a protumoral role, providing conditions for PMN and promoting extravasation, survival, and the continuous growth of tumor cells (72). EMT, a vital sign of solid tumors, has recently been shown to be an essential driver of macrophage polarization (71). EMT-colorectal cancer (CRC) programmed cells not only stimulate the production of various cytokines, such as IL-4 and CCL2, but also deliver exosomes directly to macrophage activation signaling cascade targets that directly inhibit programmed cell death at the post-transcriptional level, thereby enhancing M2-like polarization (73–75).
TEPs are promoters and protectors of blood metastasis. Entanglements of platelets and fibrin surrounding tumor cells protect CTCs from NK lysis (76). This makes it possible for CTCs to survive within the vasculature (immune evasion) and spread from the bloodstream. Moreover, activated platelets may facilitate vessel growth and maintain vascular integrity during tumor development (77). In addition, TEPs enhance the adhesion between CTCs and vascular endothelial cells through a selectin-dependent pathway to prepare for CTC extravasation (78). The function of TEP, a biomarker trove for liquid biopsy, has been proved, especially RNA (79). Particle-swarm optimization (PSO)-enhanced algorithms diagnose cancer, exploiting selected gene panels from TEP, which has also been demonstrated for accuracy in early and advanced non-small cell lung cancer (NSCLC) diagnosis. Consequently, TEPs possess the potential value as a liquid biopsy for various clinical and investigational applications (22, 80).
Although the role of CTCs as tumor biomarkers of liquid biopsy for research and clinical diagnosis has been widely concerned (81–83), isolating CTCs is a technical challenge owing to the rarity and heterogeneity of CTCs. Nevertheless, microfluidic-based isolation technologies are expected to break this limitation and promote the transformation of cancer clinical diagnosis and treatment mode (84). Technological advances make it feasible to convert from CTC counting to the thorough analysis of the CTC gene panels, transcriptome, protein, epigenome, and various functional characteristics, which can be used to monitor prognosis, anticipate micrometastasis, and act as an auxiliary means of tumor staging (85).
Progression
In the progression phase, micrometastases attract more tumor cells to colonize, directly or indirectly promoting further microenvironment maturation by producing cytokines. This enables metastatic cancer cells to grow, invade, and progress at the site, creating a vicious cycle that culminates in macrometastases. For example, in bone metastases, there are two types of tumors: osteoblastic (bone-forming) and osteolytic (bone-lysing), of which prostate bone metastases are often the former and breast cancer bone metastases are the latter (86, 87). Several studies have shown that in osteolytic bone metastases, bone-derived chemokines and growth factors as chemoattractants, such as monocyte chemoattractant protein 1 and stromal cell-derived factor 1, could attract tumor cells to bone. Likewise, the interaction between bone marrow stromal cells and tumor cells could lead to increased production of growth factors and cytokines, further promoting PMN formation and attracting tumor cell colonization. For instance, the ligand for receptor activator of nuclear factor kappaB or IL-6 could accelerate angiogenesis, bone destruction, and tumor growth (88). This vicious cycle between bone microenvironment and tumor cells leads to osteoclastic lesions evolving, which macroscopically manifests as malignant metastasis of the tumor, a fatal event with a poor prognosis. Therefore, there is a demand for detecting tumor cells or components of the PMN prior to macrometastases, which could be discovered by imaging, to block cancer metastasis before it is incurable.
Clinical Application of Liquid Biopsy in PMN
The heterogeneity of cancer cells within tumors is an essential obstacle to curative effect. Current cancer treatments, such as surgery, radiotherapy, and chemotherapy drugs, often kill healthy cells and poison patients, which cannot overcome tumor heterogeneity well (89). Therefore, it is essential to understand the molecular basis of tumors, such as the formation of PMN and the emergence of new diagnostic techniques. The current trend is to use liquid biopsy technology to obtain cancer cells or cancer-related molecules, which can explore epigenetic changes and oncogene expression based on the molecular level and flexibly apply the relevant results to clinical diagnosis and treatment. The table lists some critical molecular and cellular components in the process of PMN formation that can be detected by liquid biopsy, as well as their clinical applications (Table 1).
Diagnostic Value
Early detection by liquid biopsy for cancer is promising (109). In the early stages of the disease, CTCs are already circulating in the blood prior to clinical evidence of metastasis. 5-Hydroxymethylcytosine signatures in cfDNA are highly predictive for colorectal and gastric cancer as an ideal diagnostic biomarker for human cancers, which are superior to conventional biomarkers, and comparable to the 5-hydroxymethylcytosine biomarker in tissue biopsy (110). Additionally, as a liquid biopsy marker, the urine epigenetic biomarkers have manifested satisfactory sensitivity and specificity in detecting upper tract urinary carcinoma (111). The effect of extracellular vesicles and particles (EVPs) in tumor detection and determination of cancer type has been demonstrated. Analyzing the protein contents of EVPs distinguished tumors from nearby noncancerous tissue and profiling extracellular vesicle proteins obtained from plasma may also reveal cancer type.
Moreover, both tissue-derived and plasma-derived EVPs were detected with high specificity (112). As an emerging biomarker for early and minimal malignancy diagnosis, exosomal microRNA has captured people’s attention because of its stability in multiple body fluids (113). Serum miR-378 levels were analyzed in 60 normal controls and 103 NSCLC patients. In NSCLC patients, exosomal miR-378 was significantly overexpressed, and its upregulation was associated with advanced TNM stage and positive LN metastasis. Additionally, the combination of serum exosomal miR-378 expression and carcinoembryonic antigen (CEA) had a high discriminating power to differentiate NSCLC subjects from controls (114). Similarly, the role of exosome-encapsulated microRNAs as a circulating diagnostic marker for low alpha-fetoprotein hepatocellular carcinoma has been demonstrated (115).
Prognostic Value
The prognostic role of CTC counts as a tool for liquid biopsy can be seen in a variety of cancers (116–118). Previous studies have reported a lack of identification of novel biomarkers associated with breast cancer (119). Research on estrogen receptor-positive breast cancer suggested that independent prognostic information required for late clinical recurrence could be obtained from a single positive CTC assay (120). The presence of CTC is related to adverse prognosis in patients with metastatic CRC. While the presence of CTC weeks after surgery is not noticeably associated with CRC-related survival (CCRS) and recurrence-free survival (RFS) for patients with non-metastatic CRC, the association increases remarkably with time. Similarly, the presence of CTC in patients with optimistic preoperative staging was connected with a significant reduction in RFS and CCRS (121). In a prospective trial, CSF-derived cfDNA copy number variations were used as a surrogate for minimal residual disease (MRD) to detect disease progression (122). These phenomena may demonstrate that highly sensitive liquid biopsy assays can be applied to detect and characterize MRD (123). Blood tests based on CTC phenotype simulations can also assess overall survival and tumor metastasis in pancreatic ductal adenocarcinomas (PDAC) patients. CTC transcriptional profiling can be used not only as an independent prognostic marker but also to determine the emergence of multiple androgen receptor signaling inhibitors resistance mechanisms, which can guide the choice of treatment options (83). Besides the prognostic assessment, liquid biopsy technology can achieve individualized management of clinical patients. For instance, in stage II colon cancer, ctDNA-guided therapy reduces adjuvant chemotherapy use without compromising recurrence-free survival (124).
Therapeutic Application
The clinical utility of CTC as a marker of liquid biopsy for prognosis and monitoring of systemic treatment response was reported a decade ago (125). In recent years, the study of ctDNA, circulating cfRNA, EVs, and TEPs has also attracted much attention (126–128). The serum level of miR-378 in 73 patients with NSCLC decreased significantly after radiotherapy, which can be used as an indicator of the efficacy of radiotherapy for NSCLC (114). The effect of immunotherapy on cancer patients can be evaluated by liquid biopsy. In one study, patients who tested positive for ctDNA showed improvement in disease-free survival and overall survival when receiving adjuvant atezolizumab instead of observation (129).
Additionally, it was attractive that exosomal miRNAs conveyed the drug resistance message. Exosomes miR-3913-5p and miR-184, as biomarkers of osimertinib resistance, are suitable for NSCLC patients to detect their expression. This may be related to the abnormal activation of alternative pathways (PI3K pathway activation and RAS-MAPK pathway abnormality), indicating that miRNAs derived from peripheral blood exosomes are involved in the resistance mechanism of osimertinib through the pathway. In addition, miR-433 can inhibit cisplatin chemoresistance by regulating DNA damage and inactivating the WNT/β-catenin signaling pathway by targeting p24 transporter 5 in NSCLC. These studies suggest that miRNA can provide latent therapeutic targets for patients with NSCLC (130). The homing effect of exosomes on primary tumor cells is promising for targeted therapy. For example, PMN mimics, engineered biomaterials embedded with ovarian cancer exosomes into the peritoneal cavity of mouse models, can effectively recruit and capture free ovarian tumor cells in ascites, thereby arresting colonization in normal pelvic organs, reducing metastasis, and improving patient survival (131, 132). Furthermore, exosomes can also be used as a delivery system to load drugs and improve drug spillover in tumors (133). Generally, therapeutic applications, such as therapeutic response monitoring, targeted therapy, and drug resistance detection, have enriched remedies for cancer and are expected to enhance efficacy.
Opportunities and Challenges of Liquid Biopsy Technology in Clinical Practice
Compared with tissue biopsy and imaging diagnosis, liquid biopsy possesses the advantage of being non-invasive, repeatable, and economical, and having an early diagnosis, which could surmount the temporal and spatial heterogeneity of tumors (134). With the improvement of liquid biopsy technology, its sensitivity and operability have also been significantly strengthened. For example, CAPP-seq personalized cancer analysis technology is an economical and susceptible method to quantify ctDNA. In NSCLC, ctDNA levels are closely associated with tumor volume, distinguishing between treatment-related imaging transformations and residual disease, allowing for earlier response assessment and personalized cancer treatment (135). There are also many other detection techniques such as polymerase chain reaction-based, microfluidic methods, chip-based, next-generation sequencing-based, and fluorescence in situ hybridization-based (136). However, the specificity of liquid biopsy results poses some challenges. Firstly, gene mutations associated with cancer occur with age, even in people who have never experienced cancer. Therefore, while technological advances have made ctDNA testing more specific, false-positive results from its use in cancer screening can cause significant anxiety (137, 138). Secondly, widespread clinical application of liquid biopsy technology remains unrealistic because the standardization and replication of test results are challenged (139). However, advances in the characterization and detection of ctDNA and the application of single-gene and multi-gene detection methods have made the clinical application of targeted therapy possible. In addition, the application of liquid biopsy in the systemic treatment of sufferers with “ctDNA relapse” has also been noted. This is a new concept to detect cancer recurrence by detecting ctDNA after treatment, which is earlier than imaging examination (140). The advanced technology currently used for liquid biopsy is the detection of exosomes derived from cancer with biosensors, with highly specific target selection (125). Single-cell sequencing has increased the understanding of the molecular pathways involved in triggering cancer progression (141). Molecular imaging, especially when combined with liquid biopsy for screening, promises early disease localization because biochemical changes precede anatomical changes (142). The methylation patterns informed by cfDNA sequencing can be used for epigenetic variation assessment, with potential value for early detection of fatal malignancies (143). In conclusion, the emergence and development of these new technologies have contributed to the evolution of precision medicine.
Conclusion and Perspectives
As a non-invasive, reproducible method, liquid biopsy has achieved remarkable success in the early detection and tracking of biomarkers. Furthermore, biological interactions between the tumor microenvironment and PMN are increasingly vital as potential mechanisms of tumor progression. In this process, the role of soluble factors, exosomes, and circulating cells from the tumor microenvironment has been emphasized as neoplastic markers for cancer diagnosis, prediction and prognosis, therapeutic response monitoring, and therapy guidance. However, the liquid biopsy technique demands a breakthrough in clinical practice. For example, the high heterogeneity and nanoscale size of exosomes pose great technical difficulties for the isolation and detection of their molecular information. There are various methods of detecting CTCs and ctDNA, and the diagnostic procedures are not standardized, which requires high enrichment technology of CTC in blood. These problems need to be overcome to achieve widespread clinical application. Early diagnosis and blocking of cancer progression before the formation of micrometastases or even PMN is a promising research direction, which requires liquid biopsy technology to break through the limitations and be flexible for clinical practice. In summary, as the three branches of liquid biopsy, CTC, ctDNA, and exosomes are crucial components for the preparation and biological function of PMN. Therefore, liquid biopsy diagnosis clarifies the biological characteristics of PMN, possibly changing the process at the initial stage of metastasis, and has tremendous potential in precision medicine.
Author Contributions
ZL, XH, and QD provided direction and guidance throughout the preparation of this manuscript. YK, QD, and ZL wrote and edited the manuscript. QD, JL, NL, and YH reviewed and made significant revisions to the manuscript. SW, YZ, YR, and ZL collected and prepared the related papers. All authors read and approved the final manuscript.
Conflict of Interest
The authors declare that the research was conducted in the absence of any commercial or financial relationships that could be construed as a potential conflict of interest.
Publisher’s Note
All claims expressed in this article are solely those of the authors and do not necessarily represent those of their affiliated organizations, or those of the publisher, the editors and the reviewers. Any product that may be evaluated in this article, or claim that may be made by its manufacturer, is not guaranteed or endorsed by the publisher.
Abbreviations
BMDCs, bone marrow-derived cells; CAFs, carcinoma-associated fibroblasts; CCRS, CRC related survival; CEA, carcinoembryonic antigen; CECs, circulating endothelial cells; cfDNA, circulating cell-free DNA; CRC, colorectal cancer; CSF, cerebrospinal fluid; CTCs, circulating tumor cells; ctDNA, circulating tumor DNA; CTECs, circulating tumor-derived endothelial cells; EGF, epidermal growth factor; EMT, epithelial–mesenchymal transformation; EVs, extracellular vesicles; EVPs, extracellular vesicles and particles; LECs, lymphatic endothelial cells; LN, lymph node; MDSCs, myeloid-derived suppressor cells; MIF, migration inhibitory factor; MMP, matrix metalloproteinases; MRD, minimal residual disease; MSCs, mesenchymal stem cells; NSCLC, non-small cell lung cancer; PDACs, pancreatic ductal adenocarcinomas; PMNs, pre-metastatic niches; PSA, prostate-specific antigen; PSO, particle-swarm optimization; RFS, recurrence-free survival; TAM, tumor-associated macrophages; TDEs, tumor-derived exosomes; TDSFs, tumor-derived soluble factors; EPs, tumor-educated blood platelets; TF, transcription factor.
References
1. Lambert AW, Pattabiraman DR, Weinberg RA. Emerging Biological Principles of Metastasis. Cell (2017) 168:670–91. doi: 10.1016/j.cell.2016.11.037
2. Yu XQ, Dasgupta P, Baade P. Quantifying the Absolute Number of Cancer Deaths That Would be Avoided If Cancers Were Diagnosed Prior to Progressing to Distant Metastasis, New South Wales, Australia 1985-2014. Int J Cancer (2022) 150:1760–9. doi: 10.1002/ijc.33931
3. Cortés-Hernández LE, Eslami SZ, Alix-Panabières C. Circulating Tumor Cell as the Functional Aspect of Liquid Biopsy to Understand the Metastatic Cascade in Solid Cancer. Mol Aspects Med (2020) 72:100816. doi: 10.1016/j.mam.2019.07.008
4. Shin SH, Bode AM, Dong Z. Addressing the Challenges of Applying Precision Oncology. NPJ Precis Oncol (2017) 1:28. doi: 10.1038/s41698-017-0032-z
5. Mortezaee K. CXCL12/CXCR4 Axis in the Microenvironment of Solid Tumors: A Critical Mediator of Metastasis. Life Sci (2020) 249:117534. doi: 10.1016/j.lfs.2020.117534
6. Steeg PS. Tumor Metastasis: Mechanistic Insights and Clinical Challenges. Nat Med (2006) 12:895–904. doi: 10.1038/nm1469
7. Peinado H, Zhang H, Matei IR, Costa-Silva B, Hoshino A, Rodrigues G, et al. Pre-Metastatic Niches: Organ-Specific Homes for Metastases. Nat Rev Cancer (2017) 17:302–17. doi: 10.1038/nrc.2017.6
8. Psaila B, Lyden D. The Metastatic Niche: Adapting the Foreign Soil. Nat Rev Cancer (2009) 9:285–93. doi: 10.1038/nrc2621
9. Liu Y, Cao X. Characteristics and Significance of the Pre-Metastatic Niche. Cancer Cell (2016) 30:668–81. doi: 10.1016/j.ccell.2016.09.011
10. Fleming V, Hu X, Weber R, Nagibin V, Groth C, Altevogt P, et al. Targeting Myeloid-Derived Suppressor Cells to Bypass Tumor-Induced Immunosuppression. Front Immunol (2018) 9:398. doi: 10.3389/fimmu.2018.00398
11. Olmeda D, Cerezo-Wallis D, Riveiro-Falkenbach E, Pennacchi PC, Contreras-Alcalde M, Ibarz N, et al. Whole-Body Imaging of Lymphovascular Niches Identifies Pre-Metastatic Roles of Midkine. Nat (2017) 546:676–80. doi: 10.1038/nature22977
12. Zeng Y, Yao X, Liu X, He X, Li L, Liu X, et al. Anti-Angiogenesis Triggers Exosomes Release From Endothelial Cells to Promote Tumor Vasculogenesis. J Extracell Vesicles (2019) 8:1629865. doi: 10.1080/20013078.2019.1629865
13. Seibold T, Waldenmaier M, Seufferlein T, Eiseler T. Small Extracellular Vesicles and Metastasis-Blame the Messenger. Cancers (Basel) (2021) 13:4380. doi: 10.3390/cancers13174380
14. Bibby AC, Dorn P, Psallidas I, Porcel JM, Janssen J, Froudarakis M, et al. ERS/EACTS Statement on the Management of Malignant Pleural Effusions. Eur Respir J (2018) 52:1800349. doi: 10.1183/13993003.00349-2018
15. Ferrara F, Zoupanou S, Primiceri E, Ali Z, Chiriacò MS. Beyond Liquid Biopsy: Toward non-Invasive Assays for Distanced Cancer Diagnostics in Pandemics. Biosens Bioelectron (2022) 196:113698. doi: 10.1016/j.bios.2021.113698
16. Parikh AR, Leshchiner I, Elagina L, Goyal L, Levovitz C, Siravegna G, et al. Liquid Versus Tissue Biopsy for Detecting Acquired Resistance and Tumor Heterogeneity in Gastrointestinal Cancers. Nat Med (2019) 25:1415–21. doi: 10.1038/s41591-019-0561-9
17. Yi X, Ma J, Guan Y, Chen R, Yang L, Xia X. The Feasibility of Using Mutation Detection in ctDNA to Assess Tumor Dynamics. Int J Cancer (2017) 140:2642–7. doi: 10.1002/ijc.30620
18. Zhou H, Zhu L, Song J, Wang G, Li P, Li W, et al. Liquid Biopsy at the Frontier of Detection, Prognosis and Progression Monitoring in Colorectal Cancer. Mol Cancer (2022) 21:86. doi: 10.1186/s12943-022-01556-2
19. Siravegna G, Mussolin B, Venesio T, Marsoni S, Seoane J, Dive C, et al. How Liquid Biopsies can Change Clinical Practice in Oncology. Ann Oncol (2019) 30:1580–90. doi: 10.1093/annonc/mdz227
20. Das J, Kelley SO. High-Performance Nucleic Acid Sensors for Liquid Biopsy Applications. Angew Chem Int Ed Engl (2020) 59:2554–64. doi: 10.1002/anie.201905005
21. Pantel K, Alix-Panabières C. Circulating Tumour Cells in Cancer Patients: Challenges and Perspectives. Trends Mol Med (2010) 16:398–406. doi: 10.1016/j.molmed.2010.07.001
22. Best MG, Sol N, In 't Veld S, Vancura A, Muller M, Niemeijer AN, et al. Swarm Intelligence-Enhanced Detection of Non-Small-Cell Lung Cancer Using Tumor-Educated Platelets. Cancer Cell (2017) 32:238–52.e239. doi: 10.1016/j.ccell.2017.07.004
23. Yu W, Hurley J, Roberts D, Chakrabortty SK, Enderle D, Noerholm M, et al. Exosome-Based Liquid Biopsies in Cancer: Opportunities and Challenges. Ann Oncol (2021) 32:466–77. doi: 10.1016/j.annonc.2021.01.074
24. Cheng ML, Pectasides E, Hanna GJ, Parsons HA, Choudhury AD, Oxnard GR. Circulating Tumor DNA in Advanced Solid Tumors: Clinical Relevance and Future Directions. CA Cancer J Clin (2021) 71:176–90. doi: 10.3322/caac.21650
25. Mehran R, Nilsson M, Khajavi M, Du Z, Cascone T, Wu HK, et al. Tumor Endothelial Markers Define Novel Subsets of Cancer-Specific Circulating Endothelial Cells Associated With Antitumor Efficacy. Cancer Res (2014) 74:2731–41. doi: 10.1158/0008-5472.Can-13-2044
26. Siravegna G, Marsoni S, Siena S, Bardelli A. Integrating Liquid Biopsies Into the Management of Cancer. Nat Rev Clin Oncol (2017) 14:531–48. doi: 10.1038/nrclinonc.2017.14
27. Miller AM, Shah RH, Pentsova EI, Pourmaleki M, Briggs S, Distefano N, et al. Tracking Tumour Evolution in Glioma Through Liquid Biopsies of Cerebrospinal Fluid. Nat (2019) 565:654–8. doi: 10.1038/s41586-019-0882-3
28. Kipps E, Tan DS, Kaye SB. Meeting the Challenge of Ascites in Ovarian Cancer: New Avenues for Therapy and Research. Nat Rev Cancer (2013) 13:273–82. doi: 10.1038/nrc3432
29. Liebs S, Eder T, Klauschen F, Schütte M, Yaspo ML, Keilholz U, et al. Applicability of Liquid Biopsies to Represent the Mutational Profile of Tumor Tissue From Different Cancer Entities. Oncogene (2021) 40:5204–12. doi: 10.1038/s41388-021-01928-w
30. Cheng DT, Mitchell TN, Zehir A, Shah RH, Benayed R, Syed A, et al. Memorial Sloan Kettering-Integrated Mutation Profiling of Actionable Cancer Targets (MSK-IMPACT): A Hybridization Capture-Based Next-Generation Sequencing Clinical Assay for Solid Tumor Molecular Oncology. J Mol Diagn (2015) 17:251–64. doi: 10.1016/j.jmoldx.2014.12.006
31. Heitzer E, Haque IS, Roberts CES, Speicher MR. Current and Future Perspectives of Liquid Biopsies in Genomics-Driven Oncology. Nat Rev Genet (2019) 20:71–88. doi: 10.1038/s41576-018-0071-5
32. Vasconcelos MH, Caires HR, Ābols A, Xavier CPR, Linē A. Extracellular Vesicles as a Novel Source of Biomarkers in Liquid Biopsies for Monitoring Cancer Progression and Drug Resistance. Drug Resist Updat (2019) 47:100647. doi: 10.1016/j.drup.2019.100647
33. Liu W, Vivian CJ, Brinker AE, Hampton KR, Lianidou E, Welch DR. Microenvironmental Influences on Metastasis Suppressor Expression and Function During a Metastatic Cell's Journey. Cancer Microenviron (2014) 7:117–31. doi: 10.1007/s12307-014-0148-4
34. Ordóñez-Morán P, Huelsken J. Complex Metastatic Niches: Already a Target for Therapy? Curr Opin Cell Biol (2014) 31:29–38. doi: 10.1016/j.ceb.2014.06.012
35. Quail DF, Joyce JA. Microenvironmental Regulation of Tumor Progression and Metastasis. Nat Med (2013) 19:1423–37. doi: 10.1038/nm.3394
36. Semenza GL. Hypoxia-Inducible Factors in Physiology and Medicine. Cell (2012) 148:399–408. doi: 10.1016/j.cell.2012.01.021
37. Deep G, Jain A, Kumar A, Agarwal C, Kim S, Leevy WM, et al. Exosomes Secreted by Prostate Cancer Cells Under Hypoxia Promote Matrix Metalloproteinases Activity at Pre-Metastatic Niches. Mol Carcinog (2020) 59:323–32. doi: 10.1002/mc.23157
38. Gallagher FA, Kettunen MI, Day SE, Hu DE, Ardenkjaer-Larsen JH, Zandt R, et al. Magnetic Resonance Imaging of pH In Vivo Using Hyperpolarized 13C-Labelled Bicarbonate. Nat (2008) 453:940–3. doi: 10.1038/nature07017
39. Calcinotto A, Filipazzi P, Grioni M, Iero M, De Milito A, Ricupito A, et al. Modulation of Microenvironment Acidity Reverses Anergy in Human and Murine Tumor-Infiltrating T Lymphocytes. Cancer Res (2012) 72:2746–56. doi: 10.1158/0008-5472.Can-11-1272
40. Logozzi M, Angelini DF, Iessi E, Mizzoni D, Di Raimo R, Federici C, et al. Increased PSA Expression on Prostate Cancer Exosomes in In Vitro Condition and in Cancer Patients. Cancer Lett (2017) 403:318–29. doi: 10.1016/j.canlet.2017.06.036
41. Crane CA, Ahn BJ, Han SJ, Parsa AT. Soluble Factors Secreted by Glioblastoma Cell Lines Facilitate Recruitment, Survival, and Expansion of Regulatory T Cells: Implications for Immunotherapy. Neuro Oncol (2012) 14:584–95. doi: 10.1093/neuonc/nos014
42. Yu H, Kortylewski M, Pardoll D. Crosstalk Between Cancer and Immune Cells: Role of STAT3 in the Tumour Microenvironment. Nat Rev Immunol (2007) 7:41–51. doi: 10.1038/nri1995
43. Hiratsuka S, Watanabe A, Aburatani H, Maru Y. Tumour-Mediated Upregulation of Chemoattractants and Recruitment of Myeloid Cells Predetermines Lung Metastasis. Nat Cell Biol (2006) 8:1369–75. doi: 10.1038/ncb1507
44. Qian BZ, Li J, Zhang H, Kitamura T, Zhang J, Campion LR, et al. CCL2 Recruits Inflammatory Monocytes to Facilitate Breast-Tumour Metastasis. Nat (2011) 475:222–5. doi: 10.1038/nature10138
45. Wang D, Sun H, Wei J, Cen B, DuBois RN. CXCL1 Is Critical for Premetastatic Niche Formation and Metastasis in Colorectal Cancer. Cancer Res (2017) 77:3655–65. doi: 10.1158/0008-5472.Can-16-3199
46. Guo Y, Ji X, Liu J, Fan D, Zhou Q, Chen C, et al. Effects of Exosomes on Pre-Metastatic Niche Formation in Tumors. Mol Cancer (2019) 18:39. doi: 10.1186/s12943-019-0995-1
47. Willms E, Johansson HJ, Mäger I, Lee Y, Blomberg KE, Sadik M, et al. Cells Release Subpopulations of Exosomes With Distinct Molecular and Biological Properties. Sci Rep (2016) 6:22519. doi: 10.1038/srep22519
48. Marcoux G, Duchez AC, Cloutier N, Provost P, Nigrovic PA, Boilard E. Revealing the Diversity of Extracellular Vesicles Using High-Dimensional Flow Cytometry Analyses. Sci Rep (2016) 6:35928. doi: 10.1038/srep35928
49. Haraszti RA, Miller R, Stoppato M, Sere YY, Coles A, Didiot MC, et al. Exosomes Produced From 3D Cultures of MSCs by Tangential Flow Filtration Show Higher Yield and Improved Activity. Mol Ther (2018) 26:2838–47. doi: 10.1016/j.ymthe.2018.09.015
50. Jiang M, Zhang W, Zhang R, Liu P, Ye Y, Yu W, et al. Cancer Exosome-Derived miR-9 and miR-181a Promote the Development of Early-Stage MDSCs via Interfering With SOCS3 and PIAS3 Respectively in Breast Cancer. Oncogene (2020) 39:4681–94. doi: 10.1038/s41388-020-1322-4
51. Guo X, Qiu W, Liu Q, Qian M, Wang S, Zhang Z, et al. Immunosuppressive Effects of Hypoxia-Induced Glioma Exosomes Through Myeloid-Derived Suppressor Cells via the miR-10a/Rora and miR-21/Pten Pathways. Oncogene (2018) 37:4239–59. doi: 10.1038/s41388-018-0261-9
52. Webber JP, Spary LK, Sanders AJ, Chowdhury R, Jiang WG, Steadman R, et al. Differentiation of Tumour-Promoting Stromal Myofibroblasts by Cancer Exosomes. Oncogene (2015) 34:290–302. doi: 10.1038/onc.2013.560
53. Gu J, Qian H, Shen L, Zhang X, Zhu W, Huang L, et al. Gastric Cancer Exosomes Trigger Differentiation of Umbilical Cord Derived Mesenchymal Stem Cells to Carcinoma-Associated Fibroblasts Through TGF-β/Smad Pathway. PloS One (2012) 7:e52465. doi: 10.1371/journal.pone.0052465
54. Bai M, Li J, Yang H, Zhang H, Zhou Z, Deng T, et al. miR-135b Delivered by Gastric Tumor Exosomes Inhibits FOXO1 Expression in Endothelial Cells and Promotes Angiogenesis. Mol Ther (2019) 27:1772–83. doi: 10.1016/j.ymthe.2019.06.018
55. Chen G, Huang AC, Zhang W, Zhang G, Wu M, Xu W, et al. Exosomal PD-L1 Contributes to Immunosuppression and is Associated With Anti-PD-1 Response. Nat (2018) 560:382–6. doi: 10.1038/s41586-018-0392-8
56. Zhang W, Zhong W, Wang B, Yang J, Yang J, Yu Z, et al. ICAM-1-Mediated Adhesion is a Prerequisite for Exosome-Induced T Cell Suppression. Dev Cell (2022) 57:329–43.e327. doi: 10.1016/j.devcel.2022.01.002
57. You Y, Shan Y, Chen J, Yue H, You B, Shi S, et al. Matrix Metalloproteinase 13-Containing Exosomes Promote Nasopharyngeal Carcinoma Metastasis. Cancer Sci (2015) 106:1669–77. doi: 10.1111/cas.12818
58. Achen MG, Stacker SA. Molecular Control of Lymphatic Metastasis. Ann N Y Acad Sci (2008) 1131:225–34. doi: 10.1196/annals.1413.020
59. Farnsworth RH, Karnezis T, Shayan R, Matsumoto M, Nowell CJ, Achen MG, et al. A Role for Bone Morphogenetic Protein-4 in Lymph Node Vascular Remodeling and Primary Tumor Growth. Cancer Res (2011) 71:6547–57. doi: 10.1158/0008-5472.Can-11-0200
60. Tammela T, Alitalo K. Lymphangiogenesis: Molecular Mechanisms and Future Promise. Cell (2010) 140:460–76. doi: 10.1016/j.cell.2010.01.045
61. Saharinen P, Tammela T, Karkkainen MJ, Alitalo K. Lymphatic Vasculature: Development, Molecular Regulation and Role in Tumor Metastasis and Inflammation. Trends Immunol (2004) 25:387–95. doi: 10.1016/j.it.2004.05.003
62. Wakisaka N, Hasegawa Y, Yoshimoto S, Miura K, Shiotani A, Yokoyama J, et al. Primary Tumor-Secreted Lymphangiogenic Factors Induce Pre-Metastatic Lymphvascular Niche Formation at Sentinel Lymph Nodes in Oral Squamous Cell Carcinoma. PloS One (2015) 10:e0144056. doi: 10.1371/journal.pone.0144056
63. Ogawa F, Amano H, Eshima K, Ito Y, Matsui Y, Hosono K, et al. Prostanoid Induces Premetastatic Niche in Regional Lymph Nodes. J Clin Invest (2014) 124:4882–94. doi: 10.1172/jci73530
64. Pucci F, Garris C, Lai CP, Newton A, Pfirschke C, Engblom C, et al. SCS Macrophages Suppress Melanoma by Restricting Tumor-Derived Vesicle-B Cell Interactions. Sci (2016) 352:242–6. doi: 10.1126/science.aaf1328
65. Lee E, Fertig EJ, Jin K, Sukumar S, Pandey NB, Popel AS. Breast Cancer Cells Condition Lymphatic Endothelial Cells Within Pre-Metastatic Niches to Promote Metastasis. Nat Commun (2014) 5:4715. doi: 10.1038/ncomms5715
66. Karnezis T, Shayan R, Caesar C, Roufail S, Harris NC, Ardipradja K, et al. VEGF-D Promotes Tumor Metastasis by Regulating Prostaglandins Produced by the Collecting Lymphatic Endothelium. Cancer Cell (2012) 21:181–95. doi: 10.1016/j.ccr.2011.12.026
67. Nishida Y, Tsukushi S, Urakawa H, Sugiura H, Nakashima H, Yamada Y, et al. High Incidence of Regional and in-Transit Lymph Node Metastasis in Patients With Alveolar Rhabdomyosarcoma. Int J Clin Oncol (2014) 19:536–43. doi: 10.1007/s10147-013-0571-4
68. Francart ME, Lambert J, Vanwynsberghe AM, Thompson EW, Bourcy M, Polette M, et al. Epithelial-Mesenchymal Plasticity and Circulating Tumor Cells: Travel Companions to Metastases. Dev Dyn (2018) 247:432–50. doi: 10.1002/dvdy.24506
69. Kalluri R, Weinberg RA. The Basics of Epithelial-Mesenchymal Transition. J Clin Invest (2009) 119:1420–8. doi: 10.1172/jci39104
70. Kaplan RN, Riba RD, Zacharoulis S, Bramley AH, Vincent L, Costa C, et al. VEGFR1-Positive Haematopoietic Bone Marrow Progenitors Initiate the Pre-Metastatic Niche. Nat (2005) 438:820–7. doi: 10.1038/nature04186
71. Brabletz T, Kalluri R, Nieto MA, Weinberg RA. EMT in Cancer. Nat Rev Cancer (2018) 18:128–34. doi: 10.1038/nrc.2017.118
72. Noy R, Pollard JW. Tumor-Associated Macrophages: From Mechanisms to Therapy. Immun (2014) 41:49–61. doi: 10.1016/j.immuni.2014.06.010
73. Wei C, Yang C, Wang S, Shi D, Zhang C, Lin X, et al. Crosstalk Between Cancer Cells and Tumor Associated Macrophages is Required for Mesenchymal Circulating Tumor Cell-Mediated Colorectal Cancer Metastasis. Mol Cancer (2019) 18:64. doi: 10.1186/s12943-019-0976-4
74. Lin X, Wang S, Sun M, Zhang C, Wei C, Yang C, et al. miR-195-5p/NOTCH2-Mediated EMT Modulates IL-4 Secretion in Colorectal Cancer to Affect M2-Like TAM Polarization. J Hematol Oncol (2019) 12:20. doi: 10.1186/s13045-019-0708-7
75. Yang C, Dou R, Wei C, Liu K, Shi D, Zhang C, et al. Tumor-Derived Exosomal microRNA-106b-5p Activates EMT-Cancer Cell and M2-Subtype TAM Interaction to Facilitate CRC Metastasis. Mol Ther (2021) 29:2088–107. doi: 10.1016/j.ymthe.2021.02.006
76. Placke T, Kopp HG, and Salih HR. Modulation of Natural Killer Cell Anti-tumor Reactivity by Platelets. J Innate Immun (2011) 59:1295–300.
77. Gay LJ, Felding-Habermann B. Contribution of Platelets to Tumour Metastasis. Nat Rev Cancer (2011) 3:374–82. doi: 10.1159/000323936
78. Läubli H, Borsig L. Selectins Promote Tumor Metastasis. Semin Cancer Biol (2010) 20:169–77. doi: 10.1016/j.semcancer.2010.04.005
79. Best MG, Sol N, Kooi I, Tannous J, Westerman BA, Rustenburg F, et al. RNA-Seq of Tumor-Educated Platelets Enables Blood-Based Pan-Cancer, Multiclass, and Molecular Pathway Cancer Diagnostics. Cancer Cell (2015) 28:666–76. doi: 10.1016/j.ccell.2015.09.018
80. Liu L, Lin F, Ma X, Chen Z, Yu J. Tumor-Educated Platelet as Liquid Biopsy in Lung Cancer Patients. Crit Rev Oncol Hematol (2020) 146:102863. doi: 10.1016/j.critrevonc.2020.102863
81. Suvilesh KN, Nussbaum YI, Radhakrishnan V, Manjunath Y, Avella DM, Staveley-O'Carroll KF, et al. Tumorigenic Circulating Tumor Cells From Xenograft Mouse Models of non-Metastatic NSCLC Patients Reveal Distinct Single Cell Heterogeneity and Drug Responses. Mol Cancer (2022) 21:73. doi: 10.1186/s12943-022-01553-5
82. Strati A, Zavridou M, Kallergi G, Politaki E, Kuske A, Gorges TM, et al. A Comprehensive Molecular Analysis of in Vivo Isolated EpCAM-Positive Circulating Tumor Cells in Breast Cancer. Clin Chem (2021) 67:1395–405. doi: 10.1093/clinchem/hvab099
83. Sperger JM, Emamekhoo H, McKay RR, Stahlfeld CN, Singh A, Chen XE, et al. Prospective Evaluation of Clinical Outcomes Using a Multiplex Liquid Biopsy Targeting Diverse Resistance Mechanisms in Metastatic Prostate Cancer. J Clin Oncol (2021) 39:2926–37. doi: 10.1200/jco.21.00169
84. Descamps L, Le Roy D, Deman AL. Microfluidic-Based Technologies for CTC Isolation: A Review of 10 Years of Intense Efforts Towards Liquid Biopsy. Int J Mol Sci (2022) 23:1981. doi: 10.3390/ijms23041981
85. Chauhan A, Kaur R, Ghoshal S, Pal A. Exploration of Circulating Tumour Cell (CTC) Biology: A Paradigm Shift in Liquid Biopsy. Indian J Clin Biochem (2021) 36:131–42. doi: 10.1007/s12291-020-00923-4
86. Logothetis CJ, Lin SH. Osteoblasts in Prostate Cancer Metastasis to Bone. Nat Rev Cancer (2005) 5:21–8. doi: 10.1038/nrc1528
87. Kozlow W, Guise TA. Breast Cancer Metastasis to Bone: Mechanisms of Osteolysis and Implications for Therapy. J Mammary Gland Biol Neoplasia (2005) 10:169–80. doi: 10.1007/s10911-005-5399-8
88. David Roodman G. Role of Stromal-Derived Cytokines and Growth Factors in Bone Metastasis. Cancer (2003) 97:733–8. doi: 10.1002/cncr.11148
89. Zaimy MA, Saffarzadeh N, Mohammadi A, Pourghadamyari H, Izadi P, Sarli A, et al. New Methods in the Diagnosis of Cancer and Gene Therapy of Cancer Based on Nanoparticles. Cancer Gene Ther (2017) 24:233–43. doi: 10.1038/cgt.2017.16
90. Costa-Silva B, Aiello NM, Ocean AJ, Singh S, Zhang H, Thakur BK, et al. Pancreatic Cancer Exosomes Initiate Pre-Metastatic Niche Formation in the Liver. Nat Cell Biol (2015) 17:816–26. doi: 10.1038/ncb3169
91. Liu C, Zeng X, An Z, Yang Y, Eisenbaum M, Gu X, et al. Sensitive Detection of Exosomal Proteins via a Compact Surface Plasmon Resonance Biosensor for Cancer Diagnosis. ACS Sens (2018) 3:1471–9. doi: 10.1021/acssensors.8b00230
92. Preis M, Gardner TB, Gordon SR, Pipas JM, Mackenzie TA, Klein EE, et al. MicroRNA-10b Expression Correlates With Response to Neoadjuvant Therapy and Survival in Pancreatic Ductal Adenocarcinoma. Clin Cancer Res (2011) 17:5812–21. doi: 10.1158/1078-0432.Ccr-11-0695
93. Meng X, Müller V, Milde-Langosch K, Trillsch F, Pantel K, Schwarzenbach H. Diagnostic and Prognostic Relevance of Circulating Exosomal miR-373, miR-200a, miR-200b and miR-200c in Patients With Epithelial Ovarian Cancer. Oncotarget (2016) 7:16923–35. doi: 10.18632/oncotarget.7850
94. Pan B, Qin J, Liu X, He B, Wang X, Pan Y, et al. Identification of Serum Exosomal Hsa-Circ-0004771 as a Novel Diagnostic Biomarker of Colorectal Cancer. Front Genet (2019) 10:1096. doi: 10.3389/fgene.2019.01096
95. Cheng L, Wang Y, Huang L. Exosomes From M1-Polarized Macrophages Potentiate the Cancer Vaccine by Creating a Pro-Inflammatory Microenvironment in the Lymph Node. Mol Ther (2017) 25:1665–75. doi: 10.1016/j.ymthe.2017.02.007
96. Yun JW, Lee S, Kim HM, Chun S, Engleman EG, Kim HC, et al. A Novel Type of Blood Biomarker: Distinct Changes of Cytokine-Induced STAT Phosphorylation in Blood T Cells Between Colorectal Cancer Patients and Healthy Individuals. Cancers (Basel) (2019) 11:1157. doi: 10.3390/cancers11081157
97. Abajo A, Boni V, Lopez I, Gonzalez-Huarriz M, Bitarte N, Rodriguez J, et al. Identification of Predictive Circulating Biomarkers of Bevacizumab-Containing Regimen Efficacy in Pre-Treated Metastatic Colorectal Cancer Patients. Br J Cancer (2012) 107:287–90. doi: 10.1038/bjc.2012.242
98. Bencsikova B, Budinska E, Selingerova I, Pilatova K, Fedorova L, Greplova K, et al. Circulating T Cell Subsets are Associated With Clinical Outcome of Anti-VEGF-Based 1st-Line Treatment of Metastatic Colorectal Cancer Patients: A Prospective Study With Focus on Primary Tumor Sidedness. BMC Cancer (2019) 19:687. doi: 10.1186/s12885-019-5909-5
99. Baccelli I, Schneeweiss A, Riethdorf S, Stenzinger A, Schillert A, Vogel V, et al. Identification of a Population of Blood Circulating Tumor Cells From Breast Cancer Patients That Initiates Metastasis in a Xenograft Assay. Nat Biotechnol (2013) 31:539–44. doi: 10.1038/nbt.2576
100. Ulz P, Perakis S, Zhou Q, Moser T, Belic J, Lazzeri I, et al. Inference of Transcription Factor Binding From Cell-Free DNA Enables Tumor Subtype Prediction and Early Detection. Nat Commun (2019) 10:4666. doi: 10.1038/s41467-019-12714-4
101. Lee JH, Choi JW, Kim YS. Serum TIMP-1 Predicts Survival Outcomes of Invasive Breast Carcinoma Patients: A Meta-analysis. Arch Med Res (2011) 42:463–8. doi: 10.1016/j.arcmed.2011.09.006
102. Park KS, Bandeira E, Shelke GV, Lässer C, Lötvall J. Enhancement of Therapeutic Potential of Mesenchymal Stem Cell-Derived Extracellular Vesicles. Stem Cell Res Ther (2019) 10:288. doi: 10.1186/s13287-019-1398-3
103. Cima I, Kong SL, Sengupta D, Tan IB, Phyo WM, Lee D, et al. Tumor-Derived Circulating Endothelial Cell Clusters in Colorectal Cancer. Sci Transl Med (2016) 8:345ra389. doi: 10.1126/scitranslmed.aad7369
104. Manzoni M, Comolli G, Torchio M, Mazzini G, Danova M. Circulating Endothelial Cells and Their Subpopulations: Role as Predictive Biomarkers in Antiangiogenic Therapy for Colorectal Cancer. Clin Colorectal Cancer (2015) 14:11–7. doi: 10.1016/j.clcc.2014.12.002
105. Rahbari NN, Schölch S, Bork U, Kahlert C, Schneider M, Rahbari M, et al. Prognostic Value of Circulating Endothelial Cells in Metastatic Colorectal Cancer. Oncotarget (2017) 8:37491–501. doi: 10.18632/oncotarget.16397
106. Giussani M, Triulzi T, Sozzi G, Tagliabue E. Tumor Extracellular Matrix Remodeling: New Perspectives as a Circulating Tool in the Diagnosis and Prognosis of Solid Tumors. Cells (2019) 8:81. doi: 10.3390/cells8020081
107. Papachristos A, Kemos P, Katsila T, Panoilia E, Patrinos GP, Kalofonos H, et al. VEGF-A and ICAM-1 Gene Polymorphisms as Predictors of Clinical Outcome to First-Line Bevacizumab-Based Treatment in Metastatic Colorectal Cancer. Int J Mol Sci (2019) 20:5791. doi: 10.3390/ijms20225791
108. Inanç M, Er O, Karaca H, Berk V, Ozkan M, Saraymen R, et al. Prognostic Value of Tumor Growth Factor Levels During Chemotherapy in Patients With Metastatic Colorectal Cancer. Med Oncol (2012) 29:3119–24. doi: 10.1007/s12032-012-0250-8
109. Kaiser J. 'Liquid Biopsy' for Cancer Promises Early Detection. Sci (2018) 359:259. doi: 10.1126/science.359.6373.259
110. Li W, Zhang X, Lu X, You L, Song Y, Luo Z, et al. 5-Hydroxymethylcytosine Signatures in Circulating Cell-Free DNA as Diagnostic Biomarkers for Human Cancers. Cell Res (2017) 27:1243–57. doi: 10.1038/cr.2017.121
111. Xu Y, Ma X, Ai X, Gao J, Liang Y, Zhang Q, et al. A Urine-Based Liquid Biopsy Method for Detection of Upper Tract Urinary Carcinoma. Front Oncol (2020) 10:597486. doi: 10.3389/fonc.2020.597486
112. Hoshino A, Kim HS, Bojmar L, Gyan KE, Cioffi M, Hernandez J, et al. Extracellular Vesicle and Particle Biomarkers Define Multiple Human Cancers. Cell (2020) 182:1044–61.e1018. doi: 10.1016/j.cell.2020.07.009
113. Preethi KA, Selvakumar SC, Ross K, Jayaraman S, Tusubira D, Sekar D. Liquid Biopsy: Exosomal microRNAs as Novel Diagnostic and Prognostic Biomarkers in Cancer. Mol Cancer (2022) 21:54. doi: 10.1186/s12943-022-01525-9
114. Zhang Y, Xu H. Serum Exosomal miR-378 Upregulation is Associated With Poor Prognosis in non-Small-Cell Lung Cancer Patients. J Clin Lab Anal (2020) 34:e23237. doi: 10.1002/jcla.23237
115. Ghosh S, Bhowmik S, Majumdar S, Goswami A, Chakraborty J, Gupta S, et al. The Exosome Encapsulated microRNAs as Circulating Diagnostic Marker for Hepatocellular Carcinoma With Low Alpha-Fetoprotein. Int J Cancer (2020) 147:2934–47. doi: 10.1002/ijc.33111
116. Hugenschmidt H, Labori KJ, Brunborg C, Verbeke CS, Seeberg LT, Schirmer CB, et al. Circulating Tumor Cells are an Independent Predictor of Shorter Survival in Patients Undergoing Resection for Pancreatic and Periampullary Adenocarcinoma. Ann Surg (2020) 271:549–58. doi: 10.1097/sla.0000000000003035
117. Gazzaniga P, Gradilone A, de Berardinis E, Busetto GM, Raimondi C, Gandini O, et al. Prognostic Value of Circulating Tumor Cells in Nonmuscle Invasive Bladder Cancer: A CellSearch Analysis. Ann Oncol (2012) 23:2352–6. doi: 10.1093/annonc/mdr619
118. Antonarakis ES, Lu C, Luber B, Wang H, Chen Y, Zhu Y, et al. Clinical Significance of Androgen Receptor Splice Variant-7 mRNA Detection in Circulating Tumor Cells of Men With Metastatic Castration-Resistant Prostate Cancer Treated With First- and Second-Line Abiraterone and Enzalutamide. J Clin Oncol (2017) 35:2149–56. doi: 10.1200/jco.2016.70.1961
119. Tang Y, Tian W, Xie J, Zou Y, Wang Z, Li N, et al. Prognosis and Dissection of Immunosuppressive Microenvironment in Breast Cancer Based on Fatty Acid Metabolism-Related Signature. Front In Immunol (2022) 13:843515. doi: 10.3389/fimmu.2022.843515
120. Sparano J, O'Neill A, Alpaugh K, Wolff AC, Northfelt DW, Dang CT, et al. Association of Circulating Tumor Cells With Late Recurrence of Estrogen Receptor-Positive Breast Cancer: A Secondary Analysis of a Randomized Clinical Trial. JAMA Oncol (2018) 4:1700–6. doi: 10.1001/jamaoncol.2018.2574
121. van Dalum G, Stam GJ, Scholten LF, Mastboom WJ, Vermes I, Tibbe AG, et al. Importance of Circulating Tumor Cells in Newly Diagnosed Colorectal Cancer. Int J Oncol (2015) 46:1361–8. doi: 10.3892/ijo.2015.2824
122. Liu APY, Smith KS, Kumar R, Paul L, Bihannic L, Lin T, et al. Serial Assessment of Measurable Residual Disease in Medulloblastoma Liquid Biopsies. Cancer Cell (2021) 39:1519–30.e1514. doi: 10.1016/j.ccell.2021.09.012
123. Pantel K, Alix-Panabières C. Liquid Biopsy and Minimal Residual Disease - Latest Advances and Implications for Cure. Nat Rev Clin Oncol (2019) 16:409–24. doi: 10.1038/s41571-019-0187-3
124. Tie J, Cohen JD, Lahouel K, Lo SN, Wang Y, Kosmider S, et al. Circulating Tumor DNA Analysis Guiding Adjuvant Therapy in Stage II Colon Cancer. N Engl J Med (2022) 386:2261–72. doi: 10.1056/NEJMoa2200075
125. Cheng N, Du D, Wang X, Liu D, Xu W, Luo Y, et al. Recent Advances in Biosensors for Detecting Cancer-Derived Exosomes. Trends Biotechnol (2019) 37:1236–54. doi: 10.1016/j.tibtech.2019.04.008
126. Schwarzenbach H, Hoon DS, Pantel K. Cell-Free Nucleic Acids as Biomarkers in Cancer Patients. Nat Rev Cancer (2011) 11:426–37. doi: 10.1038/nrc3066
127. Anfossi S, Babayan A, Pantel K, Calin GA. Clinical Utility of Circulating non-Coding RNAs - an Update. Nat Rev Clin Oncol (2018) 15:541–63. doi: 10.1038/s41571-018-0035-x
128. Kalluri R, LeBleu VS. The Biology, Function, and Biomedical Applications of Exosomes. Sci (2020) 367:eaau6977. doi: 10.1126/science.aau6977
129. Vandekerkhove G, Lavoie JM, Annala M, Murtha AJ, Sundahl N, Walz S, et al. Plasma ctDNA is a Tumor Tissue Surrogate and Enables Clinical-genomic Stratification of Metastatic Bladder Cancer. Nat Commun (2021) 12:184. doi: 10.1038/s41467-020-20493-6
130. Li X, Chen C, Wang Z, Liu J, Sun W, Shen K, et al. Elevated Exosome-Derived miRNAs Predict Osimertinib Resistance in non-Small Cell Lung Cancer. Cancer Cell Int (2021) 21:428. doi: 10.1186/s12935-021-02075-8
131. Wu M, Wang G, Hu W, Yao Y, Yu XF. Emerging Roles and Therapeutic Value of Exosomes in Cancer Metastasis. Mol Cancer (2019) 18:53. doi: 10.1186/s12943-019-0964-8
132. de la Fuente A, Alonso-Alconada L, Costa C, Cueva J, Garcia-Caballero T, Lopez-Lopez R, et al. M-Trap: Exosome-Based Capture of Tumor Cells as a New Technology in Peritoneal Metastasis. J Natl Cancer Inst (2015) 107:djv184. doi: 10.1093/jnci/djv184
133. Liu C, Su C. Design Strategies and Application Progress of Therapeutic Exosomes. Theranostics (2019) 9:1015–28. doi: 10.7150/thno.30853
134. Tellez-Gabriel M, Heymann MF, Heymann D. Circulating Tumor Cells as a Tool for Assessing Tumor Heterogeneity. Theranostics (2019) 9:4580–94. doi: 10.7150/thno.34337
135. Newman AM, Bratman SV, To J, Wynne JF, Eclov NC, Modlin LA, et al. An Ultrasensitive Method for Quantitating Circulating Tumor DNA With Broad Patient Coverage. Nat Med (2014) 20:548–54. doi: 10.1038/nm.3519
136. Chang L, Li J, Zhang R. Liquid Biopsy for Early Diagnosis of non-Small Cell Lung Carcinoma: Recent Research and Detection Technologies. Biochim Biophys Acta Rev Cancer (2022) 1877:188729. doi: 10.1016/j.bbcan.2022.188729
137. Kulasingam V, Diamandis EP. Strategies for Discovering Novel Cancer Biomarkers Through Utilization of Emerging Technologies. Nat Clin Pract Oncol (2008) 5:588–99. doi: 10.1038/ncponc1187
138. Razavi P, Li BT, Brown DN, Jung B, Hubbell E, Shen R, et al. High-Intensity Sequencing Reveals the Sources of Plasma Circulating Cell-Free DNA Variants. Nat Med (2019) 25:1928–37. doi: 10.1038/s41591-019-0652-7
139. Geeurickx E, Hendrix A. Targets, Pitfalls and Reference Materials for Liquid Biopsy Tests in Cancer Diagnostics. Mol Aspects Med (2020) 72:100828. doi: 10.1016/j.mam.2019.10.005
140. Ignatiadis M, Sledge GW, Jeffrey SS. Liquid Biopsy Enters the Clinic - Implementation Issues and Future Challenges. Nat Rev Clin Oncol (2021) 18:297–312. doi: 10.1038/s41571-020-00457-x
141. González-Silva L, Quevedo L, Varela I. Tumor Functional Heterogeneity Unraveled by scRNA-Seq Technologies: (Trends in Cancer 6, 13-19, 2020). Trends Cancer (2021) 7:265. doi: 10.1016/j.trecan.2021.02.001
142. Roll W, Weckesser M, Seifert R, Bodei L, Rahbar K. Imaging and Liquid Biopsy in the Prediction and Evaluation of Response to PRRT in Neuroendocrine Tumors: Implications for Patient Management. Eur J Nucl Med Mol Imaging (2021) 48:4016–27. doi: 10.1007/s00259-021-05359-3
Keywords: pre-metastatic niche, liquid biopsy, tumor microenvironment, molecular mechanism, clinical application
Citation: Liu Z, Kong Y, Dang Q, Weng S, Zheng Y, Ren Y, Lv J, Li N, Han Y and Han X (2022) Liquid Biopsy in Pre-Metastatic Niche: From Molecular Mechanism to Clinical Application. Front. Immunol. 13:958360. doi: 10.3389/fimmu.2022.958360
Received: 31 May 2022; Accepted: 22 June 2022;
Published: 15 July 2022.
Edited by:
Min Xue, University of California, Riverside, United StatesReviewed by:
Jindong Xie, Sun Yat-sen University Cancer Center (SYSUCC), ChinaZheyu Kong, Peking University People’s Hospital, China
Copyright © 2022 Liu, Kong, Dang, Weng, Zheng, Ren, Lv, Li, Han and Han. This is an open-access article distributed under the terms of the Creative Commons Attribution License (CC BY). The use, distribution or reproduction in other forums is permitted, provided the original author(s) and the copyright owner(s) are credited and that the original publication in this journal is cited, in accordance with accepted academic practice. No use, distribution or reproduction is permitted which does not comply with these terms.
*Correspondence: Xinwei Han, ZmNjaGFueHdAenp1LmVkdS5jbg==
†These authors have contributed equally to this work and share the first authorship