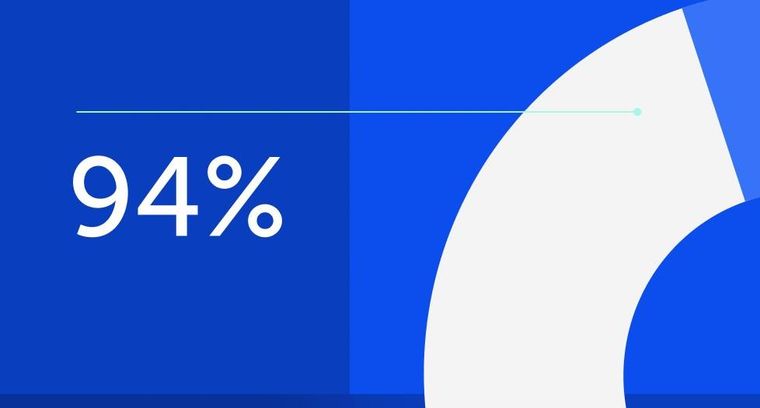
94% of researchers rate our articles as excellent or good
Learn more about the work of our research integrity team to safeguard the quality of each article we publish.
Find out more
REVIEW article
Front. Immunol., 26 July 2022
Sec. Cancer Immunity and Immunotherapy
Volume 13 - 2022 | https://doi.org/10.3389/fimmu.2022.956090
This article is part of the Research TopicCombinational Immunotherapy of Cancer: Novel Targets, Mechanisms, and StrategiesView all 85 articles
LAG-3, a type of immune checkpoint receptor protein belonging to the immunoglobulin superfamily, is confirmed to be expressed on activated immune cells, mainly including activated T cells. LAG-3 can negatively regulate the function of T cells, exerting important effects on maintaining the homeostasis of the immune system under normal physiological conditions and promoting tumor cells immune escape in the tumor microenvironment. Given its important biological roles, LAG-3 has been regarded as a promising target for cancer immunotherapy. To date, many LAG-3 inhibitors have been reported, which can be divided into monoclonal antibody, double antibody, and small molecule drug, some of which have entered the clinical research stage. LAG-3 inhibitors can negatively regulate and suppress T cell proliferation and activation through combination with MHC II ligand. Besides, LAG-3 inhibitors can also affect T cell function via binding to Galectin-3 and LSECtin. In addition, LAG-3 inhibitors can prevent the FGL1-LAG-3 interaction, thereby enhancing the human body’s antitumor immune effect. In this review, we will describe the function of LAG-3 and summarize the latest LAG-3 inhibitors in the clinic for cancer therapy.
A large amount of evidence from literature has manifested that tumor cells can effectively avoid being recognized and killed by the immune system through immune checkpoint receptor proteins, suggesting that blocking immune checkpoint receptors is a new immunotherapy for human cancers (1, 2). The most well-studied immune checkpoint receptors mainly include programmed cell death 1/programmed cell death 1 ligand (PD-1/PD-L1) and cytotoxic T lymphocyte-associated antigen-4 (CTLA-4) (3–6). However, due to the treatment tolerance, low response, or significant increase in toxicity of previously discovered antibody drugs targeting PD-1/PD-L1 or CTLA-4 (7–9), it is very necessary to investigate new targets against immune checkpoint receptor proteins.
Lymphocyte activation gene-3 (LAG-3, also named CD223 or FDC protein), is a new class of immune checkpoint receptors, which was first isolated and reported by French immunologist Frédéric Triebel and colleagues in 1990 (10). LAG-3, as a key member of the immunoglobulin superfamily (IgSF) locating on human chromosome 12, is a type I transmembrane protein containing 498 amino acids, consisting of transmembrane region, the extracellular region, and cytoplasmic region (11). The expression level of LAG-3 is closely related to the prognosis of human tumors. High level of LAG-3 in kidney renal clear cell carcinoma, non-small cell lung cancer (NSCLC), primary central nervous system lymphoma (PCNSL), hepatocellular carcinoma (HCC) and muscle invasive bladder cancer (MIBC) indicates a poor prognosis, whereas in gastric carcinoma and melanoma predicts a better prognosis (12). LAG-3 is detected to be expressed on the surface of effector T cells and regulatory T cells (Tregs) that participate in the regulation of T lymphocytes and antigen-presenting cells (APCs) signaling pathways and play a crucial part in the adaptive immune response (13). Consistent with CTLA-4 and PD-1/PD-L1, LAG-3 is induced on CD8+ and CD4+ T cells upon persistent antigenic stimulation, rather than expressed on naive T cells (14). Since the inhibitory function of LAG-3 is closely associated with its expression level on the activated immune cells, the blockage and inhibition of LAG-3 expression through antibody drugs or small molecule inhibitors are critical. Prolonged infection with viruses, fungus, and bacteria results in sustained exposure to antigens, leading to high levels of persistent expression of LAG-3 and other inhibitory co-receptors on CD8+ and CD4+ T cells (15). These T cells lose powerful effector functions, known as exhausted T cells, resulting in decreased tumor lethality and response rate, and upregulation of Treg immunosuppressive function (15). Studies have shown that blockage or inhibition of LAG-3 can allow T cells to regain cytotoxic activity and reduce the function of regulating T cells to suppress immune responses, thereby enhancing the killing effect on tumors (16, 17). It was observed that simultaneous blockage of LAG-3 activity and anti-PD-1 or PD-L1 in tumor cells has dual inhibitory effects, including inhibiting Treg activity, promoting dendritic cells (DCs) maturation, and rescuing dysfunctional CD4+/CD8+ T cells (18–20). LAG-3 has been regarded as an indicator of tumor prognosis and become a novel tumor immunotherapy target beyond PD-1/PD-L1 and CTLA-4. Herein, we aim to describe the structure and the known ligands of LAG-3 and summarize the immune-regulatory effects on active T cells in tumor microenvironment, as well as the LAG-3 inhibitors which have been evaluated in the clinic.
LAG3 co-localizates with CD4, CD8, and CD3 molecules within lipid rafts. The structure of LAG-3 is essentially different from that of CD3 and CD8, whereas it is highly homologous to CD4. LAG-3 consists of three parts: transmembrane region, extracellular region, and cytoplasmic region. In the transmembrane-cytoplasmic part, LAG-3 breaks away from the cell membrane under the action of metalloproteinases ADAM10/17, which can regulate the function of LAG-3. The extracellular domain is responsible for binding to the ligands and consists of four IgSF domains, namely D1, D2, D3, and D4. The D1 domain contains a loop domain rich in proline (~30) and an in-chain disulfide bond, which is species-specific and is known as the V immunoglobulin superfamily. However, D2, D3, and D4 belong to the C2 family. The cytoplasmic region of LAG-3 consists of three parts: the serine phosphorylation site S454 (substrates for protein kinase C, PKC), the highly conserved “KIEELE” motif, and the glutamate-proline dipeptide repeat motif (EP sequence) (Figure 1) (21, 22). Importantly, KIEELE mutant resulted in complete loss of LAG-3 function, which proved that the “KIEELE” motif was crucial to the function of LAG-3 (23).
Figure 1 LAG-3 structure and ligands. LAG-3 consists of extracellular region, transmembrane region and cytoplasmic region. The extracellular domain is composed of four IgSF domains, namely D1, D2, D3 and D4. The D1 domain contains a loop domain rich in proline and an in-chain disulfide bond. In the transmembrane- cytoplasmic part, LAG-3 breaks away from the cell membrane under the action of metalloproteinases ADAM10/17. The cytoplasmic region of LAG-3 consists of three parts: the serine phosphorylation site S454, the highly conserved “KIEELE” motif and the glutamate-proline dipeptide repeat motif (EP sequence). MHC II, Galectin-3, LSECtin and FGL1 are the confirmed ligands of LAG-3 in tumor microenvironment.
LAG-3 was detected to be mainly expressed on the surface of activated T cells (CD8+ T cells and CD4+ T cells), naturalkiller cells (NK cells), B cells, and DCs under physiological conditions, and negatively regulate T cell function. Researchers also found a small number of LAG-3+ lymphocytes in inflammatory lymphoid tissues, such as tonsils or lymph nodes (24). In pathological state, LAG-3 was reported to be highly expressed on the surface of tumor-infiltrating lymphocytes (TILs), the expression level of which was positively correlated with the occurrence and development of human tumors, such as non-small cell lung cancer (NSCLC) and hepatocellular carcinoma (HCC) (25–27). LAG-3 negatively regulates the function of T cells and plays significant roles in maintaining the homeostasis of immune system under normal physiological conditions and promoting tumor cells immune escape in the tumor microenvironment, indicating a promising target for tumor immunotherapy.
It has been reported four ligands of LAG-3 in tumor microenvironment mainly including galactose lectin-3 (Galectin-3), major histocompatibility complex II (MHC II), fibrinogen-like protein 1 (FGL1), and hepatic sinusoid endothelial cell lectin (LSECtin) (Figure 1). MHC II is the main ligand of LAG-3 (28). Due to the high homology of LAG3 and CD4, MHC II is the common ligand of LAG3 and CD4. However, LGA3 and MHC II shows 100 times higher in binding affinity than CD4, suggesting that CD4 and LAG3 may competitively bind to MHC II, thereby negatively regulating the function of CD4 (29, 30). Studies have shown that although LAG3 mutants unable to bind MHC II exhibit reduced inhibitory function (21), tail mutations in the intracellular domain of LAG3 lead to loss of inhibitory effect, further suggesting that the intracellular domain is critical for inhibiting signal transduction (23). It is possible that LAG3 acts not primarily by interfering with the interaction of MHC II and CD4, but rather by transmitting inhibitory signals via the cytoplasmic domain (23), although the exact character of this signal is unclear.
Galectin-3, another ligand of LAG-3, is a 31-kDa soluble lectin (Figure 1). Studies have shown that LAG3 is highly glycosylated and can interact with Galectin-3, which regulates T cell responses via several mechanisms (28). In vitro experiments showed that LAG3 played important roles in Galectin-3-mediated inhibition of IFN-γ secretion by CD8+ T cells (31). Furthermore, Galectin-3 expressed by a variety of cells in the tumor microenvironment instead of the tumor itself may interact with LAG3 on tumor-specific CD8+ T cells, thus resulting in the modulation of anti-tumor immune responses (32). LSECtin, a potential ligand of LAG-3, belongs to the C-type lectin receptor superfamily and is mainly expressed in liver (33). LSECtin has also been found in human melanoma tissues. The interaction between LSECtin and LAG-3 promotes tumor growth through suppression of anti-tumor T cell response in melanoma cells (34).
Jun Wang et al. found that FGL1 is an immune inhibitory ligand of LAG-3 independent of MHC II (Figure 1). LAG-3 binds with FGL1 through the domains of D1 and D2. The interaction between FGL1 and LAG-3 mutually promotes tumor immune escape through inhibiting the activation of antigen-specific T cell (35). Notably, a recent study has revealed that the binding of LAG-3 to MHC II but not to FGL1 mediated the suppression of T cells (36). Of course, other LAG3 ligands have not yet been discovered. In addition, a study has shown that LAG3 binds preformed fibrils of α-synuclein in the central nervous system, thereby promoting the pathogenesis of Parkinson’s disease in a mouse model (37), suggesting that LAG3 may also have functions outside the immune system.
LAG-3 interacts with its ligands to regulate the function of T cells. The interaction between MHC II and LAG-3 can down-regulate the cytokine secretion level and proliferation ability of CD4+ T cells (Figure 2). The anti-LAG-3 antibody can restore CD4+ T cells activity. Nevertheless, the specific regulatory mechanism remains unknown (38, 39). It is worth noting that LAG-3 selectively binds to antigen peptide-MHC II (pMHC II), thus inhibiting pMHC II-responsive CD4+ T cells (40, 41). LAG-3 was found to negatively regulate the mitochondrial activity in naive CD4+ T cells, restricting the normal metabolism and expansion of naive CD4+ T cells and leading to T cell exhaustion and anti-tumor response (42). In addition, LAG-3 was also observed to be upregulated in CD8+ T cells stimulated with tumor antigens (Figure 2) (43). CD8+ T cells in LAG-3-deficient mice exhibited significantly higher activity than that in normal mice, suggesting that LAG-3 has an inhibitory effect on CD8+ T cells. LAG-3 has been demonstrated to directly inhibit CD8+ T cells via signal transduction, independent of the role of MHC II and CD4+ T cells (44, 45). LAG-3 can also enhance the function of regulatory T cells (Treg cells) (Figure 2). Treg cells play a negative role in immune regulation and can down-regulate T cell activity. Common types of Treg cells include natural regulatory T cells (nTreg cells) and inducible regulatory T cells (iTreg cells). LAG-3 can positively induce Treg cells activation and stimulate their immunosuppressive function (46–48). LAG-3 may synergize with other inhibitory molecules (PD-1, CTLA-4) to improve the inhibitory activity of Treg cells, leading to APC-induced immune tolerance (49).
Figure 2 Roles of LAG-3 in CD+4 cells, CD8+ cells, Treg cells and DC cells in tumor microenvironment.
LAG-3 also plays immune adjuvant roles and participates in the tumor immune escape. LAG-3 can induce the maturation and activation of DC cells through regulation of intracellular protein phosphorylation and promotion of the chemokines and tumor necrosis factor α (TNFα) production (Figure 2) (50). LAG-3 highly expressed on the TILs interacts with ligands located on the surface of tumor cells to cause T cell dysfunction or even exhaustion, promoting tumor immune escape, the phenomenon of which is particularly evident in CD8+ T cells (25, 51, 52). Moreover, it is confirmed that LAG-3 displayed potential roles in activation of NK cells, although its underling mechanisms remains to be further studied.
LAG-3 is confirmed to be highly expressed on TILs of various solid tumors, including colon cancer, NSCLC, head and neck cell cancer, and pancreatic cancer (18, 53–55). LAG-3 has been revealed to play a vital role in regulating T cell activation, proliferation, homeostasis, and T cell-depleted immune microenvironments. LAG-3 was also found to be co-expressed with PD-1 in the tumor microenvironment. LAG-3 and PD-1 induced T cell function inhibition through different signaling pathways, which may synergistically lead to exhaustion of T cells. Studies have shown that co-blockade of PD1 and LAG3 expressed on CD8+ and CD4+ TILs exhibited enhanced antitumor responses in some preclinical mouse models of ovarian cancer, colon adenocarcinoma, and melanoma (52, 56, 57). LAG3 expressed on iTreg cells induces the production of TGF-β1 and IL-10, which contributes to tumor immune escape. The blocking LAG3 antibodies can reduce the inhibitory effect of Tregs, resulting in the restoration of CD8+ TIL activity (57, 58) (Figure 2).
Studies have shown that inhibition of LAG-3 can allow T cells to regain cytotoxic activity and reduce the function of regulating T cells to suppress immune responses, enhancing the killing effect on tumors (59) (Figure 2). Blocking LAG-3 activity and anti-PD-1 or PD-L1 in tumor cells has dual inhibitory effects, including inhibiting Treg activity, promoting DC maturation, and rescuing dysfunctional CD4+/CD8+ T cells (60). LAG-3 has become a novel tumor immunotherapy target beyond CTLA-4 and PD-1/PD-L1. The overall drug types of LAG-3 inhibitors can be divided into monoclonal antibody, double antibody, and small molecule drug, some of which have entered the clinical research stage. More than 80 clinical trials are underway globally to evaluate the drug candidates targeting LAG-3.
LAG-3 inhibitors can directly bind LAG-3 molecules or their ligands, blocking the interaction between ligands and LAG-3, and downregulating the inhibitory efficacy of LAG-3 toward the immune system. LAG-3 antibodies not only restore T cell function, but also inhibit Treg cells activity. In previous studies, antibodies against PD-1 can only activate T cells, but cannot inhibit the activity of Treg cells (61–63). Taken together, LAG-3 inhibitors may have a better therapeutic effect, further demonstrating a novel tumor immunotherapy target of LAG-3 beyond PD-1/PD-L1 and CTLA-4.
As a promising target for cancer immunotherapy, LAG3 has been hotly pursued by academia and pharmaceutical companies. In the past, significant progress has been made in the discovery of many LAG3 modulators and some of them are currently in the clinic as anticancer drugs, which are summarized in Table 1, involving LAG3-targeted cancer immunotherapy that are either completed, ongoing, or recruiting participants (ClinicalTrials.gov). Eftilagimod alpha, developed by Immutep S.A.S. as the initial first-in-class LAG3 modulator, could activate APCs via interacting with canonical ligand (MHC class II), which has been also found to enhance Treg immunosuppression, stimulate the proliferation of DCs, and ameliorate antigen crosspresentation to CD8+ T cells (64). Three clinical trials for Eftilagimod alpha have been completed, and three others are recruiting participants as shown in Table 1. In addition, 10 different LAG3-specific monoclonal antibodies and six bispecific antibodies are currently under investigation at various clinical stages for the treatment of cancer (Table 1). As the first anti-LAG3 human IgG4 monoclonal antibody and novel immune checkpoint inhibitor, relatlimab, discovered by Bristol-Myers Squibb, is currently undergoing 46 different clinical trials for cancer therapy (65). As the first commercially developed anti-LAG-3 antibody, relatlimab entered the clinical trials in 2013 (66). However, due to the limited efficacy of relatlimab alone, it is generally used in combination with other checkpoint inhibitors, including CTLA-4 inhibitors (ipilimumab) or PD-1 inhibitors (nivolumab), to synergistically improve the efficacy (39). Encouragingly, relatlimab in combination with the PD-1 inhibitor nivolumab received FDA approval in March 2022 as the first approved monoclonal antibody to treat unresectable or metastatic melanoma (67).
Table 1 LAG3-modulating candidatesa.
Since its discovery in 1990, LAG3 has gained widespread interest and been regarded as a promising target for cancer immunotherapy. LAG-3 plays an important immunoregulatory role in a variety of human tumors, and blocking LAG-3 can enhance the proliferation of TILs and the secretion of cytokines, and enhance anti-tumor immunity. Many LAG3 inhibitors have been discovered and are currently in the clinic. Single anti-LAG3 therapy was demonstrated to be modest benefit, supporting a potential combination approach with other inhibitory receptors. LAG-3 inhibitors, together with CTLA-4 or PD-1/PD-L1 inhibitors, have been extensively explored in the different clinical trials for cancer therapy, which can not only avoid drug tolerance but also improve the clinical efficacy of LAG-3 inhibitors. No evidence reveals the feasibility of the combination between LAG-3 inhibitors and other immune checkpoint inhibitors. So far, the regulatory mechanism of LAG-3 has not been fully explored and the clinical efficacy of its inhibitors is uncertain. Based on the current clinical data, the early therapeutic effect of LAG-3 monoclonal antibody is not satisfactory. According to the phase I clinical data of LAG-3 monoclonal antibody MK-4280 published by Merck, the objective response rate (ORR) among 18 patients with solid tumors that failed other treatments was only 6%, and the disease control rate is only 17%. Therefore, it is mainly to explore the combination strategy, especially the combination of LAG-3 and PD-1. The bi-functional monoclonal antibody is worthy of attention and exploration. There are only a few interim reports of the combination therapies targeting LAG-3 and PD-1. Evidence revealed that the combination exerted better tolerance and higher ORR, extended progression-free survival, as well as a lower risk of death (13). The exact efficacy of anti-LAG-3 antibodies as monotherapy and the additive effects of anti-LAG-3 antibodies in the combination therapy targeting PD-1 and LAG-3 need to be further explored.
Inevitably, there are still many questions that remain to be resolved regarding the understanding of LAG3 biology, the exact signaling pathway and the potential ligands, as well as the mechanism underlying synergistic effect with other immune checkpoint molecules, although the development of LAG-3 inhibitors is in full swing. If these problems could be solved, the research on LAG-3 and its related drugs will make significant progress for cancer therapy.
J-LH and NL conceptualized the ideas. Y-TW, W-JF, J-LH, and NL drafted the original manuscript. Z-SL revised the manuscript. All the authors approved the final version of the manuscript.
This work was financially supported by the General Program of the National Natural Science Foundation of China General Project (No. 81970633) and National Natural Science Foundation of China Joint project (NO. U21A20348).
The authors declare that the research was conducted in the absence of any commercial or financial relationships that could be construed as a potential conflict of interest.
All claims expressed in this article are solely those of the authors and do not necessarily represent those of their affiliated organizations, or those of the publisher, the editors and the reviewers. Any product that may be evaluated in this article, or claim that may be made by its manufacturer, is not guaranteed or endorsed by the publisher.
1. Villadolid J, Amin A. Immune checkpoint inhibitors in clinical practice: update on management of immune-related toxicities. Transl Lung Cancer Res (2015) 4(5):560–75. doi: 10.3978/j.issn.2218-6751.2015.06.06
2. Darvin P, Toor SM, Sasidharan NV, Elkord E. Immune checkpoint inhibitors: recent progress and potential biomarkers. Exp Mol Med (2018) 50(12):1–11. doi: 10.1038/s12276-018-0191-1
3. Blank C, Gajewski TF, Mackensen A. Interaction of PD-L1 on tumor cells with PD-1 on tumor-specific T cells as a mechanism of immune evasion: implications for tumor immunotherapy. Cancer Immunol Immunother (2005) 54(4):307–14. doi: 10.1007/s00262-004-0593-x
4. Lin C, Chen X, Liu J, Huang Y, Ou-Yang X. Advances of PD-1/PD-L1 signaling pathway in immune escape and treatment for non-small cell lung cancer. Zhongguo Fei Ai Za Zhi (2014) 17(10):734–40. doi: 10.3779/j.issn.1009-3419.2014.10.05
5. Yi M, Jiao D, Xu H, Liu Q, Zhao W, Han X, et al. Biomarkers for predicting efficacy of PD-1/PD-L1 inhibitors. Mol Cancer (2018) 17(1):129. doi: 10.1186/s12943-018-0864-3
6. Rowshanravan B, Halliday N, Sansom DM. CTLA-4: a moving target in immunotherapy. Blood (2018) 131(1):58–67. doi: 10.1182/blood-2017-06-741033
7. Hodi FS, O'Day SJ, McDermott DF, Weber RW, Sosman JA, Haanen JB, et al. Improved survival with ipilimumab in patients with metastatic melanoma. N Engl J Med (2010) 363(8):711–23. doi: 10.1056/NEJMoa1003466
8. Topalian SL, Hodi FS, Brahmer JR, Gettinger SN, Smith DC, McDermott DF, et al. Safety, activity, and immune correlates of anti-PD-1 antibody in cancer. N Engl J Med (2012) 366(26):2443–54. doi: 10.1056/NEJMoa1200690
9. Postow MA, Chesney J, Pavlick AC, Robert C, Grossmann K, McDermott D, et al. Nivolumab and ipilimumab versus ipilimumab in untreated melanoma. N Engl J Med (2015) 372(21):2006–17. doi: 10.1056/NEJMoa1414428
10. Triebel F, Jitsukawa S, Baixeras E, Roman-Roman S, Genevee C, Viegas-Pequignot E, et al. LAG-3, a novel lymphocyte activation gene closely related to CD4. J Exp Med (1990) 171(5):1393–405. doi: 10.1084/jem.171.5.1393
11. Triebel F. LAG-3: a regulator of T-cell and DC responses and its use in therapeutic vaccination. Trends Immunol (2003) 24(12):619–22. doi: 10.1016/j.it.2003.10.001
12. Shi AP, Tang XY, Xiong YL, Zheng KF, Liu YJ, Shi XG, et al. Immune checkpoint LAG3 and its ligand FGL1 in cancer. Front Immunol (2021) 12:785091. doi: 10.3389/fimmu.2021.785091
13. Maruhashi T, Sugiura D, Okazaki IM, Okazaki T. LAG-3: from molecular functions to clinical applications. J Immunother Cancer (2020) 8(2):e001014. doi: 10.1136/jitc-2020-001014
14. Qin S, Xu L, Yi M, Yu S, Wu K, Luo S. Novel immune checkpoint targets: moving beyond PD-1 and CTLA-4. Mol Cancer (2019) 18(1):155. doi: 10.1186/s12943-019-1091-2
15. Anderson AC, Joller N, Kuchroo VK. Lag-3, Tim-3, and TIGIT: Co-inhibitory receptors with specialized functions in immune regulation. Immunity (2016) 44(5):989–1004. doi: 10.1016/j.immuni.2016.05.001
16. Puhr HC, Ilhan-Mutlu A. New emerging targets in cancer immunotherapy: the role of LAG3. ESMO Open (2019) 4(2):e482. doi: 10.1136/esmoopen-2018-000482
17. Ruffo E, Wu RC, Bruno TC, Workman CJ, Vignali D. Lymphocyte-activation gene 3 (LAG3): The next immune checkpoint receptor. Semin Immunol (2019) 42:101305. doi: 10.1016/j.smim.2019.101305
18. Andrews LP, Marciscano AE, Drake CG, Vignali DA. LAG3 (CD223) as a cancer immunotherapy target. Immunol Rev (2017) 276(1):80–96. doi: 10.1111/imr.12519
19. Sato H, Okonogi N, Nakano T. Rationale of combination of anti-PD-1/PD-L1 antibody therapy and radiotherapy for cancer treatment. Int J Clin Oncol (2020) 25(5):801–9. doi: 10.1007/s10147-020-01666-1
20. Yi M, Zheng X, Niu M, Zhu S, Ge H, Wu K. Combination strategies with PD-1/PD-L1 blockade: current advances and future directions. Mol Cancer (2022) 21(1):28. doi: 10.1186/s12943-021-01489-2
21. Huard B, Mastrangeli R, Prigent P, Bruniquel D, Donini S, El-Tayar N, et al. Characterization of the major histocompatibility complex class II binding site on LAG-3 protein. Proc Natl Acad Sci U.S.A. (1997) 94(11):5744–9. doi: 10.1073/pnas.94.11.5744
22. Wang JH, Meijers R, Xiong Y, Liu JH, Sakihama T, Zhang R, et al. Crystal structure of the human CD4 n-terminal two-domain fragment complexed to a class II MHC molecule. Proc Natl Acad Sci U.S.A. (2001) 98(19):10799–804. doi: 10.1073/pnas.191124098
23. Workman CJ, Dugger KJ, Vignali DA. Cutting edge: molecular analysis of the negative regulatory function of lymphocyte activation gene-3. J Immunol (2002) 169(10):5392–5. doi: 10.4049/jimmunol.169.10.5392
24. Huard B, Tournier M, Hercend T, Triebel F, Faure F. Lymphocyte-activation gene 3/major histocompatibility complex class II interaction modulates the antigenic response of CD4+ T lymphocytes. Eur J Immunol (1994) 24(12):3216–21. doi: 10.1002/eji.1830241246
25. Baitsch L, Baumgaertner P, Devevre E, Raghav SK, Legat A, Barba L, et al. Exhaustion of tumor-specific CD8(+) T cells in metastases from melanoma patients. J Clin Invest (2011) 121(6):2350–60. doi: 10.1172/JCI46102
26. Ma QY, Huang DY, Zhang HJ, Wang S, Chen XF. Function and regulation of LAG3 on CD4(+)CD25(-) T cells in non-small cell lung cancer. Exp Cell Res (2017) 360(2):358–64. doi: 10.1016/j.yexcr.2017.09.026
27. Hald SM, Rakaee M, Martinez I, Richardsen E, Al-Saad S, Paulsen EE, et al. LAG-3 in non-small-cell lung cancer: Expression in primary tumors and metastatic lymph nodes is associated with improved survival. Clin Lung Cancer (2018) 19(3):249–59. doi: 10.1016/j.cllc.2017.12.001
28. Baixeras E, Huard B, Miossec C, Jitsukawa S, Martin M, Hercend T, et al. Characterization of the lymphocyte activation gene 3-encoded protein. a new ligand for human leukocyte antigen class II antigens. J Exp Med (1992) 176(2):327–37. doi: 10.1084/jem.176.2.327
29. Huard B, Prigent P, Tournier M, Bruniquel D, Triebel F. CD4/major histocompatibility complex class II interaction analyzed with CD4- and lymphocyte activation gene-3 (LAG-3)-Ig fusion proteins. Eur J Immunol (1995) 25(9):2718–21. doi: 10.1002/eji.1830250949
30. Weber S, Karjalainen K. Mouse CD4 binds MHC class II with extremely low affinity. Int Immunol (1993) 5(6):695–8. doi: 10.1093/intimm/5.6.695
31. Kouo T, Huang L, Pucsek AB, Cao M, Solt S, Armstrong T, et al. Galectin-3 shapes antitumor immune responses by suppressing CD8+ T cells via LAG-3 and inhibiting expansion of plasmacytoid dendritic cells. Cancer Immunol Res (2015) 3(4):412–23. doi: 10.1158/2326-6066
32. Dumic J, Dabelic S, Flogel M. Galectin-3: an open-ended story. Biochim Biophys Acta (2006) 1760(4):616–35. doi: 10.1016/j.bbagen.2005.12.020
33. Liu W, Tang L, Zhang G, Wei H, Cui Y, Guo L, et al. Characterization of a novel c-type lectin-like gene, LSECtin: demonstration of carbohydrate binding and expression in sinusoidal endothelial cells of liver and lymph node. J Biol Chem (2004) 279(18):18748–58. doi: 10.1074/jbc.M311227200
34. Xu F, Liu J, Liu D, Liu B, Wang M, Hu Z, et al. LSECtin expressed on melanoma cells promotes tumor progression by inhibiting antitumor T-cell responses. Cancer Res (2014) 74(13):3418–28. doi: 10.1158/0008-5472.CAN-13-2690
35. Wang J, Sanmamed MF, Datar I, Su TT, Ji L, Sun J, et al. Fibrinogen-like protein 1 is a major immune inhibitory ligand of LAG-3. Cell (2019) 176(1-2):334–47. doi: 10.1016/j.cell.2018.11.010
36. Maruhashi T, Sugiura D, Okazaki IM, Shimizu K, Maeda TK, Ikubo J, et al. Binding of LAG-3 to stable peptide-MHC class II limits T cell function and suppresses autoimmunity and anti-cancer immunity. Immunity (2022) 55(5):912–24. doi: 10.1016/j.immuni.2022.03.013
37. Mao X, Ou MT, Karuppagounder SS, Kam TI, Yin X, Xiong Y, et al. Pathological alpha-synuclein transmission initiated by binding lymphocyte-activation gene 3. Science (2016) 353(6307):aah3374. doi: 10.1126/science.aah3374
38. Zhang J, Liu W, Xie T, Huang L, Hu Y, Wen B, et al. Elevated LAG-3 on CD4(+) T cells negatively correlates with neutralizing antibody response during HCV infection. Immunol Lett (2019) 212:46–52. doi: 10.1016/j.imlet.2019.06.003
39. Yu X, Huang X, Chen X, Liu J, Wu C, Pu Q, et al. Characterization of a novel anti-human lymphocyte activation gene 3 (LAG-3) antibody for cancer immunotherapy. Mabs-Austin (2019) 11(6):1139–48. doi: 10.1080/19420862.2019.1629239
40. Maruhashi T, Okazaki IM, Sugiura D, Takahashi S, Maeda TK, Shimizu K, et al. LAG-3 inhibits the activation of CD4(+) T cells that recognize stable pMHCII through its conformation-dependent recognition of pMHCII. Nat Immunol (2018) 19(12):1415–26. doi: 10.1038/s41590-018-0217-9
41. Hoffmann MM, Slansky JE. T-Cell receptor affinity in the age of cancer immunotherapy. Mol Carcinog (2020) 59(7):862–70. doi: 10.1002/mc.23212
42. Previte DM, Martins CP, O'Connor EC, Marre ML, Coudriet GM, Beck NW, et al. Lymphocyte activation gene-3 maintains mitochondrial and metabolic quiescence in naive CD4(+) T cells. Cell Rep (2019) 27(1):129–41. doi: 10.1016/j.celrep.2019.03.004
43. Ascione A, Arenaccio C, Mallano A, Flego M, Gellini M, Andreotti M, et al. Development of a novel human phage display-derived anti-LAG3 scFv antibody targeting CD8(+) T lymphocyte exhaustion. BMC Biotechnol (2019) 19(1):67. doi: 10.1186/s12896-019-0559-x
44. Grosso JF, Kelleher CC, Harris TJ, Maris CH, Hipkiss EL, De Marzo A, et al. LAG-3 regulates CD8+ T cell accumulation and effector function in murine self- and tumor-tolerance systems. J Clin Invest (2007) 117(11):3383–92. doi: 10.1172/JCI31184
45. Mei Z, Huang J, Qiao B, Lam AK. Immune checkpoint pathways in immunotherapy for head and neck squamous cell carcinoma. Int J Oral Sci (2020) 12(1):16. doi: 10.1038/s41368-020-0084-8
46. Huang CT, Workman CJ, Flies D, Pan X, Marson AL, Zhou G, et al. Role of LAG-3 in regulatory T cells. Immunity (2004) 21(4):503–13. doi: 10.1016/j.immuni.2004.08.010
47. Okamura T, Yamamoto K, Fujio K. Early growth response gene 2-expressing CD4(+)LAG3(+) regulatory T cells: The therapeutic potential for treating autoimmune diseases. Front Immunol (2018) 9:340. doi: 10.3389/fimmu.2018.00340
48. Camisaschi C, Casati C, Rini F, Perego M, De Filippo A, Triebel F, et al. LAG-3 expression defines a subset of CD4(+)CD25(high)Foxp3(+) regulatory T cells that are expanded at tumor sites. J Immunol (2010) 184(11):6545–51. doi: 10.4049/jimmunol.0903879
49. Liang B, Workman C, Lee J, Chew C, Dale BM, Colonna L, et al. Regulatory T cells inhibit dendritic cells by lymphocyte activation gene-3 engagement of MHC class II. J Immunol (2008) 180(9):5916–26. doi: 10.4049/jimmunol.180.9.5916
50. Avice MN, Sarfati M, Triebel F, Delespesse G, Demeure CE. Lymphocyte activation gene-3, a MHC class II ligand expressed on activated T cells, stimulates TNF-alpha and IL-12 production by monocytes and dendritic cells. J Immunol (1999) 16:10072520. doi: 10.1084/jem.171.5.1393
51. Gandhi MK, Lambley E, Duraiswamy J, Dua U, Smith C, Elliott S, et al. Expression of LAG-3 by tumor-infiltrating lymphocytes is coincident with the suppression of latent membrane antigen-specific CD8+ T-cell function in Hodgkin lymphoma patients. Blood (2006) 108(7):2280–9. doi: 10.1182/blood-2006-04-015164
52. Matsuzaki J, Gnjatic S, Mhawech-Fauceglia P, Beck A, Miller A, Tsuji T, et al. Tumor-infiltrating NY-ESO-1-specific CD8+ T cells are negatively regulated by LAG-3 and PD-1 in human ovarian cancer. Proc Natl Acad Sci U.S.A. (2010) 107(17):7875–80. doi: 10.1073/pnas.1003345107
53. Andrews LP, Yano H, Vignali D. Inhibitory receptors and ligands beyond PD-1, PD-L1 and CTLA-4: breakthroughs or backups. Nat Immunol (2019) 20(11):1425–34. doi: 10.1038/s41590-019-0512-0
54. McLane LM, Abdel-Hakeem MS, Wherry EJ. CD8 T cell exhaustion during chronic viral infection and cancer. Annu Rev Immunol (2019) 37:457–95. doi: 10.1146/annurev-immunol-041015-055318
55. Turnis ME, Andrews LP, Vignali DA. Inhibitory receptors as targets for cancer immunotherapy. Eur J Immunol (2015) 45(7):1892–905. doi: 10.1002/eji.201344413
56. Woo SR, Turnis ME, Goldberg MV, Bankoti J, Selby M, Nirschl CJ, et al. Immune inhibitory molecules LAG-3 and PD-1 synergistically regulate T-cell function to promote tumoral immune escape. Cancer Res (2012) 72(4):917–27. doi: 10.1158/0008-5472.CAN-11-1620
57. Chen J, Chen Z. The effect of immune microenvironment on the progression and prognosis of colorectal cancer. Med Oncol (2014) 31(8):82. doi: 10.1007/s12032-014-0082-9
58. Workman CJ, Vignali DA. The CD4-related molecule, LAG-3 (CD223), regulates the expansion of activated T cells. Eur J Immunol (2003) 33(4):970–9. doi: 10.1002/eji.200323382
59. Melaiu O, Lucarini V, Giovannoni R, Fruci D, Gemignani F. News on immune checkpoint inhibitors as immunotherapy strategies in adult and pediatric solid tumors. Semin Cancer Biol (2022) 79:18–43. doi: 10.1016/j.semcancer.2020.07.001
60. Jiang H, Ni H, Zhang P, Guo X, Wu M, Shen H, et al. PD-L1/LAG-3 bispecific antibody enhances tumor-specific immunity. Oncoimmunology (2021) 10(1):1943180. doi: 10.1080/2162402X.2021.1943180
61. Wang X, Bao Z, Zhang X, Li F, Lai T, Cao C, et al. Effectiveness and safety of PD-1/PD-L1 inhibitors in the treatment of solid tumors: a systematic review and meta-analysis. Oncotarget (2017) 8(35):59901–14. doi: 10.18632/oncotarget.18316
62. Jiang Z, Pan Z, Ren X. Progress of PD-1/PD-L1 inhibitors in non-small cell lung cancer. Zhongguo Fei Ai Za Zhi (2017) 20(2):138–42. doi: 10.3779/j.issn.1009-3419.2017.02.09
63. Bagchi S, Yuan R, Engleman EG. Immune checkpoint inhibitors for the treatment of cancer: Clinical impact and mechanisms of response and resistance. Annu Rev Pathol (2021) 16:223–49. doi: 10.1146/annurev-pathol-042020-042741
64. Legat A, Maby-El HH, Baumgaertner P, Cagnon L, Abed MS, Geldhof C, et al. Vaccination with LAG-3Ig (IMP321) and peptides induces specific CD4 and CD8 T-cell responses in metastatic melanoma patients–report of a phase I/IIa clinical trial. Clin Cancer Res (2016) 22(6):1330–40. doi: 10.1158/1078-0432.CCR-15-1212
65. Available at: https://www.fda.gov/drugs/resources-information-approved-drugs/fda-approves-opdualag-unresectable-or-metastatic-melanoma (Accessed Mar 21, 2022).
Keywords: LAG-3, immune checkpoint, cancer immunotherapy, tumor microenvironment, T cell
Citation: Huo J-L, Wang Y-T, Fu W-J, Lu N and Liu Z-S (2022) The promising immune checkpoint LAG-3 in cancer immunotherapy: from basic research to clinical application. Front. Immunol. 13:956090. doi: 10.3389/fimmu.2022.956090
Received: 29 May 2022; Accepted: 07 July 2022;
Published: 26 July 2022.
Edited by:
Xuyao Zhang, Fudan University, ChinaReviewed by:
Lijie Zhao, University of Michigan, United StatesCopyright © 2022 Huo, Wang, Fu, Lu and Liu. This is an open-access article distributed under the terms of the Creative Commons Attribution License (CC BY). The use, distribution or reproduction in other forums is permitted, provided the original author(s) and the copyright owner(s) are credited and that the original publication in this journal is cited, in accordance with accepted academic practice. No use, distribution or reproduction is permitted which does not comply with these terms.
*Correspondence: Nan Lu, nan.lu@ntu.edu.sg; Zhang-Suo Liu, zhangsuoliu@zzu.edu.cn
Disclaimer: All claims expressed in this article are solely those of the authors and do not necessarily represent those of their affiliated organizations, or those of the publisher, the editors and the reviewers. Any product that may be evaluated in this article or claim that may be made by its manufacturer is not guaranteed or endorsed by the publisher.
Research integrity at Frontiers
Learn more about the work of our research integrity team to safeguard the quality of each article we publish.