- 1Postgraduate Program in Pharmaceutical Science, Department of Biophysical and Pharmacology, Federal University of Rio Grande Norte, Natal, Brazil
- 2Postgraduate Program in Biotechnology /RENORBIO, Department of Biophysical and Pharmacology, Federal University of Rio Grande Norte, Natal, Brazil
- 3Department of Biophysical and Pharmacology, Federal University of Rio Grande Norte, Natal, Brazil
- 4Postgraduate Program in Biochemistry and Molecular Biology, Department of Biophysical and Pharmacology, Federal University of Rio Grande Norte, Natal, Brazil
- 5Postgraduate Program in Oral Sciences, Department of Biophysical and Pharmacology, Federal University of Rio Grande Norte, Natal, Brazil
- 6Postgraduate Program in Health Sciences, Department of Morphology, Federal University of Rio Grande Norte, Natal, Brazil
- 7Postgraduate Program in Functional and Structural Biology UFRN, Department of Morphology, Federal University of Rio Grande Norte, Natal, Brazil
- 8Postgraduate Program in Pharmaceutical Science, Department of Pharmaceutical Science, Federal University of Rio Grande Norte, Natal, Brazil
- 9Department of Dentistry, Federal University of Rio Grande Norte, Natal, Brazil
- 10Department of Morphology, Federal University of Ceará, Fortaleza, Brazil
Background: Intestinal mucositis is one of the most common and important side effects of 5-fluorouracil (5-FU). Currently, there are still no specific and effective protocols for its prevention and treatment. The aim of the present study was to evaluate the effect of oral administration of Lacticaseibacillus casei (L. casei) on the progression of 5-FU-induced intestinal mucositis. Methods: L. casei (1x109 CFU/ml) or saline was orally administered to Swiss mice, beginning 15 days before intestinal mucositis induction by single intraperitoneal 5-FU administration (450 mg/kg). Body weight, number of peripheral leukocytes and fecal lactic acid bacteria were monitored. After euthanasia, on day 18, tissue samples from colon and each small intestine segment were collected for histopathology. Jejunal tissues were collected and evaluated for iNOS and TNF-alpha immunoexpression, IL-1-beta, IL-6 and TNF-alpha levels, malonaldehyde (MDA) accumulation, invertase activity and factor nuclear kappa B (NFkB-P65) gene expression, toll like receptor-4 (TLR-4), mucin-2 (MUC-2), occludin and zonula occludens-1 (ZO-1).
Results: The positive impact of L. casei on 5-FU-induced leukopenia was observed, but not on 5-FU-induced weight loss in mice. L. casei reduced 5-FU-induced inflammation in the colon and small intestine (p<0.05). Decreased TNF-α, IL-1β, IL-6 (p<0.05) and MDA (p<0.05) levels, as well as decreased iNOS and TNF-alpha protein expressions (p<0.05) were found in the jejunum from L casei group. In addition, L-casei down-regulated NFKB-P65 (p<0.05) and TLR-4 (p<0.05) gene expressions and up-regulated MUC-2 and mucosal barrier proteins occludin and ZO-1 gene expressions (p<0.05). Furthermore, greater lactic acid bacteria population (p<0.05) was found in the L. casei group when compared to control groups.
Conclusion: Oral L. casei administration can protect the intestine of Swiss mice from 5-FU-induced intestinal mucositis, thus contributing to overall health.
Introduction
5-Fluorouracil (5-FU) is a widely used anticancer drug. It has played an important role in the treatment of colon cancer and is used for patients with breast and other cancers, like those of head and neck. However, approximately 80% of patients undergoing 5-FU treatment suffer from gastrointestinal mucositis (1).
Mucositis can be characterized by three phases (inflammation, epithelial degradation and ulceration), which leads to rapid loss of bowel structure and functionality (2) and presents a series of clinical manifestations such as pain when swallowing, loss of appetite, vomiting, abdominal distention, diarrhea, in addition to changes in the intestinal barrier, increasing its permeability (3).
At present, there are no well-established therapeutic strategies available for the management of intestinal mucositis. Thus, the use of probiotics as an effective intervention against chemotherapy-induced mucositis has been investigated (4).
Probiotics are “live microorganisms that, when administered in adequate amounts, provide health benefits to the host” (5). They are able to restore the intestinal microbiota balance upon the occurrence of inflammation and also stimulate both specific and non-specific immune responses (6). The investigation of probiotics as a therapeutic approach in 5-FU-induced intestinal mucositis is justified by the fact that 5-FU treatment alters the diversity and the community composition of the gut microbiota (7).
In fact, the literature has reported the positive effects of gram-positive lactic acid-producing bacteria of the genus Lactobacillus on experimental chemotherapy-induced mucositis, preventing weight loss, diarrhea and intestinal ulcers (8). It has been shown that the L. casei BL23 strain is able to reduce inflammation associated to 5-FU-induced mucositis in mice (9). However, further studies are needed to elucidate the mechanisms by which probiotics exert beneficial effects, which include the preservation of the intestinal barrier, as reported in literature (10).
Therefore, in order to investigate a novel strategy to prevent 5-FU-induced-intestinal mucosite, the effect of oral Lacticaseibacillus casei administration on an experimental intestinal mucositis model in mice was investigated.
Materials and methods
Lacticaseibacillus casei preparation
Lacticaseibacillus casei (L. casei) probiotic was provided by Farmafórmula LTDA-Farmácia Manipulação, a recognized Brazilian supplier of medicines and supplements, whose products are regulated by the new legal guidelines for food supplements from the National Health Surveillance Agency (ANVISA). The strain was deposited on the China General Microbial Culture Collection Management Center, CGMCC No 1.5409. The viability of freeze-dried L. casei was evaluated by plating on MRS agar, according to methodology described by Ribeiro et al. (11). For L. casei treatment, freeze-dried L. casei at concentration of 2 x 1010 CFU/g was suspended using sterile water and diluted to obtain concentration of 1 × 109 CFU/mL.
Animals and sample size
Female Swiss mice (Mus musculus), weighing 25–30 g (mean age of 8 weeks) were housed in polypropylene boxes and kept under controlled temperature (24 ± 2 °C) and relative air humidity conditions (50 ± 5%), 12 h light/dark cycle and ad libitum access to food and water. Animals were obtained from the animal facilities of the Biosciences Center - Federal University of Rio Grande do Norte (UFRN), Brazil. All experimental protocols were approved by the Ethics Committee on the use of Animals (CEUA) of the Federal University of Rio Grande do Norte (Protocol No.017/2019) and performed in accordance with the ARRIVE ethical guidelines. All methods were performed in accordance with relevant guidelines and regulations.
The number of animals per group was determined according to the sample size formula n = DF/k + 1, which can be used for three common ANOVA designs applicable to animal studies, where k = number of groups, n = number of subjects per group, and DF= degrees of freedom (12). In view of the ethical considerations that recommend the sample size refinement in studies with animals, we chose to use the minimum number of animals (5 animals/group) to carry out experiments.
Experimental intestinal mucositis
Intestinal mucositis was induced as previously described (8). Briefly, a single dose of 450 mg/kg of 5-Fluorouracil (Libbs Pharmaceuticals Ltd. (São Paulo, Brazil)) was intraperitoneally administered and animals were euthanized 3 days later by overdose of ketamin (240mg/kg) and xylazin (30mg/kg).
Experimental and control groups
To investigate the impact of L. casei on 5-FU-induced intestinal mucositis, a group of 5 animals received once-daily oral administration of L. casei (1x109 CFU/ml), starting 15 days before the 5- FU administration, until euthanasia, 3 days after the 5-FU injection, on day 18 (L. casei group). The control animals were divided into two control subgroups (n=5/group): a group of healthy animals, not submitted to 5-FU-induced intestinal mucositis, that received once-daily administration of saline solution until euthanasia and a single intraperitoneal injection of saline solution on day 15 (saline group) and a group of animals submitted to 5-FU-induced intestinal mucositis that received once-daily administration of saline solution from day 0 to day 18 (5-FU group). All animals were euthanized 4 days after the 5-FU injection, on day 19.
The animals were monitored daily up to 18th day for signs of moribundity and mortality, such as lack of responsiveness to manual stimulation; immobility; and/or an inability to eat or drink.
Body weight and peripheral blood leucocyte counts
In an attempt to detect possible systemic 5-FU-associated toxicity, body weight (measured on days 1 and 18) and white blood cell counts were investigated. Immediately before euthanasia, blood samples (20 μL), collected from heart puncture of anesthetized animals, was diluted in 380 μL of Turk’s solution. The total leukocytes were counted manually using a Neubauer chamber and the results are expressed as the number of white blood cells per mm3 of blood.
Histopathological analysis
Following euthanasia, tissue samples (including the mucosal, submucosal, muscle, and serosa layer) of each small intestine sections (duodenum, jejunum and ileum) and colon were collected and fixed in 10% neutral-buffered formalin, dehydrated, and embedded in paraffin for immunohistochemistry and histopathological analysis. Sections (5 µm thick) were obtained for hematoxylin and eosin staining (H&E) and for subsequent light microscopy examination (200x). The severity of mucositis was evaluated in a single-blinded fashion and was graded using a modification of the Macpherson and Pfeiffer histopathological grading system (13). Score 0: Normal histological findings; Score 1: Mucosa: villus blunting, loss of crypt architecture, sparse inflammatory cell infiltration, vacuolization and edema. Normal muscular layer; Score 2: Mucosa: villus blunting with fattened and vacuolated cells, crypt necrosis, intense inflammatory cell infiltration, vacuolization and edema. Normal muscular layer; Score 3: Mucosa: villus blunting with fattened and vacuolated cells, crypt necrosis, intense inflammatory cell infiltration, vacuolization and edema. Muscular: edema, vacuolization, sparse neutrophil infiltration.
Invertase activity
For the assay of invertase activity in the jejunal tissues, the 3,5-dinitrosalicylic acid (DNS) method was used, as previously described by Araujo et al. (14). To generate a standard curve, a stock solution of 1 g/L glucose was prepared, and 1 mL, 0.8 mL, 0.6 mL, 0.4 mL, and 0.2 mL aliquots of this solution were added to five tubes, followed by the addition of 0 mL, 0,2 mL, 0.4 mL, 0.6 mL, and 0.8 mL distilled water to achieve the following concentrations of glucose: 1 g/L, 0.8 g/L, 0.6 g/L, 0.4 g/L, and 0.2 g/L. In addition, a sixth tube contained 1 mL distilled water. DNS (3,5-dinitro-2-hydroxybenzoic acid, Sigma-Aldrish, São Paulo, Brazil) reagent (0.5 mL) was added to each test tube. The tubes were heated in a 100°C water bath for 5 min, and then were transferred to a cold-water bath. Distilled water (8.5 mL) was added to each tube (total volume, 10 mL), and the percent transmittance values at 540 nm were recorded. The results are expressed as the amount of enzyme required to release one µmol of reducing sugar in one minute.
Malondialdehyde dosage
The content of MDA, a product of lipid peroxidation, was investigated in the jejunal tissue samples (n=5/group) as a marker of oxidative stress, as previously described (15). Briefly, samples were suspended in Trizma 1:5 (w/v) buffer. The material was incubated for 40 minutes at 45°C in a water bath, centrifuged at 2500 G for 5 minutes at 4°C; 300 μL was then removed, read at 586 nm, and interpolated in a standard curve. Supernatants were tested for MDA content and placed in microplates. The absorbance of each sample was measured at 586 nm. The results are expressed as nanomoles of MDA per ml of plasma or mg of tissue.
Immunohistochemical analysis
Sections (4 µm thick) were prepared from parafin-embedded intestinal tissues. After deparaffinization, antigens were recovered by incubating the slides in citrate buffer (pH 6.0) for 20min at 95°C. Endogenous peroxidase was blocked with 3% H2O2 for 10 min to reduce nonspecific binding. Sections were then incubated with TNF-α (ab270264, Abcan) or iNOS (ab3523, Abcan) for 2h. Sections were then incubated for 30 min with polymer (K4061, DAKO). Antibody binding sites were visualized by incubating the samples with diaminobenzidine–H2O2 (DAB, DAKO) solution. Sections incubated with antibody diluent, without a primary antibody were used as negative controls. The amounts of DAB products from immunostaining were estimated from digital images of at least ten different areas of each section (from 4 specimens per group) at 400x magnification using Adobe Photoshop sofware. The results are expressed as the percentage of immunopositive area, calculated by dividing the DAB-positive staining (immunostaining-positive pixels) by the number of pixels per total tissue image multiplied by 100, as previously described (16).
Cytokine assay (IL-1β, IL-6, TNF-α)
The IL-1β, IL-6 and TNF-α levels were determined from jejunum samples (n=5/group), which were stored at − 80 °C until required for this assay. The samples were homogenized and processed as previously described (17). The concentrations of IL-1β, IL-6 and TNF-α in the samples were determined using a commercial ELISA kit (R&D Systems, Minneapolis, MN, USA). Briefly, microtiter plates were coated overnight at 4 °C with antibodies against IL-1β (detection range: 62.5–4000 pg/mL; sensibility or lower limit of detection: 12.5 ng/mL of recombinant mouse IL-1β), IL-6 (detection range:125–8000 pg/mL) and TNF-α (detection range: 62.5–4000 pg/mL; sensibility or lower limit of detection: 50 ng/mL of recombinant mouse TNF-α). After blocking the plates, the samples and standard at various dilutions were added in duplicate and incubate at 4 °C for 24 h. After washing the plates (three times with buffer) biotinylated polyclonal anti-IL-1β or anti-TNF-α, diluted 1:1000 with assay buffer 1% BSA, was added to the wells. After further incubation at room temperature for 1 h, the plates were washed and streptavidin-HRP, diluted 1:5000, was added to each well. The chromogenic reagent O-phenylenediamine was added 15 min later and the plates were incubated in the dark for 15 min. The enzymatic reaction was interrupted with H2SO4 and the absorbance was measured at 490 nm using UV–VIS spectrophotometry. The results are expressed as pg/mL (18).
RT-PCR gene marker analysis
The ribonucleic acid (RNA) was isolated from jejunum segments (n=5/group), using Trizol reagent (Invitrogen, Carlsbad, CA, USA) according to the manufacturer’s instructions. RNA was quantified by NanoDrop and the samples purity was verified by 260/280 ratios >1.8. Five micrograms of isolated total RNA (10ul) was transcribed to cDNA in a reaction mixture containing 2 ul 10X RT buffer, 0.8 ul 25X dNTP Mix, 2ul 10X RT oligo dT, 1ul MultiScribe Reverse transcriptase 4,2ul H20 (High-Capacity cDNA Reverse Transcription Kit, Foster City, CA, USA) in a total volume of 20 μL. The reaction mixture was incubated at 25°C for 10 min, 37ªC for 120 min, 85°C for 5min and 4°C for ∞.
The cDNA was stored at −80°C until further use. qPCR was performed using SYBR Green PCR Master Mix (Applied Biosystems), as described in the manufacturer’s instructions. The sequences of the primers are listed in Table 1. To compare gene expression under different conditions, the expression under each condition (normalized to ACTB, the endogenous control) was quantified relative to the control condition. qPCR amplification was performed in a CFX Connect system (Bio-Rad) under the following conditions: 50°C for 2 min and 95°C for 10 min, followed by 40 cycles of 95°C for 15 s and 60°C for 60 s. The relative expression levels of the genes were calculated using the threshold cycle (2−ΔΔCT) method (19).
Fecal lactic acid bacteria analysis
The population of fecal lactic acid bacteria (LAB) was determined on the 7th and 18th days after the first probiotic administration. Stool samples were collected immediately after defecation on a clean surface. Samples were dispersed in phosphate buffered saline (PBS; pH 7.0) and homogenized using a glass rod for 5 minute (20). Serial decimal dilutions of samples were made in sterile PBS and plated in triplicate on Petri dishes containing MRS agar. The dishes were incubated at 37°C under anaerobic conditions for 72 hours. The results are expressed as a logarithm of Colony Forming Units per gram of a sample (log10 CFU/g), as previously described (11).
Statistical analysis
Data were analyzed using descriptive (mean and standard deviation) and analytical statistics using parametric tests such as ANOVA, followed by a Bonferroni post-test and non-parametric Kruskal–Wallis test at a 5% significance level (Graph Pad Prism 6.01 software).
Results
L. casei protects against 5-FU-induced leukopenia, but not against 5-FU-induced weight loss
No animal exhibited signs of cachexia and no mortality was recorded. The prophylactic use of L. casei did not have an impact on 5-FU-induced weight loss, as illustrated in Figure 1. In addition, Figure 1 also shows that L. casei was able to protect mice against leukopenia (reduction in the number of leukocytes/mm3), a cytotoxic effect of 5-FU (p<0.05).
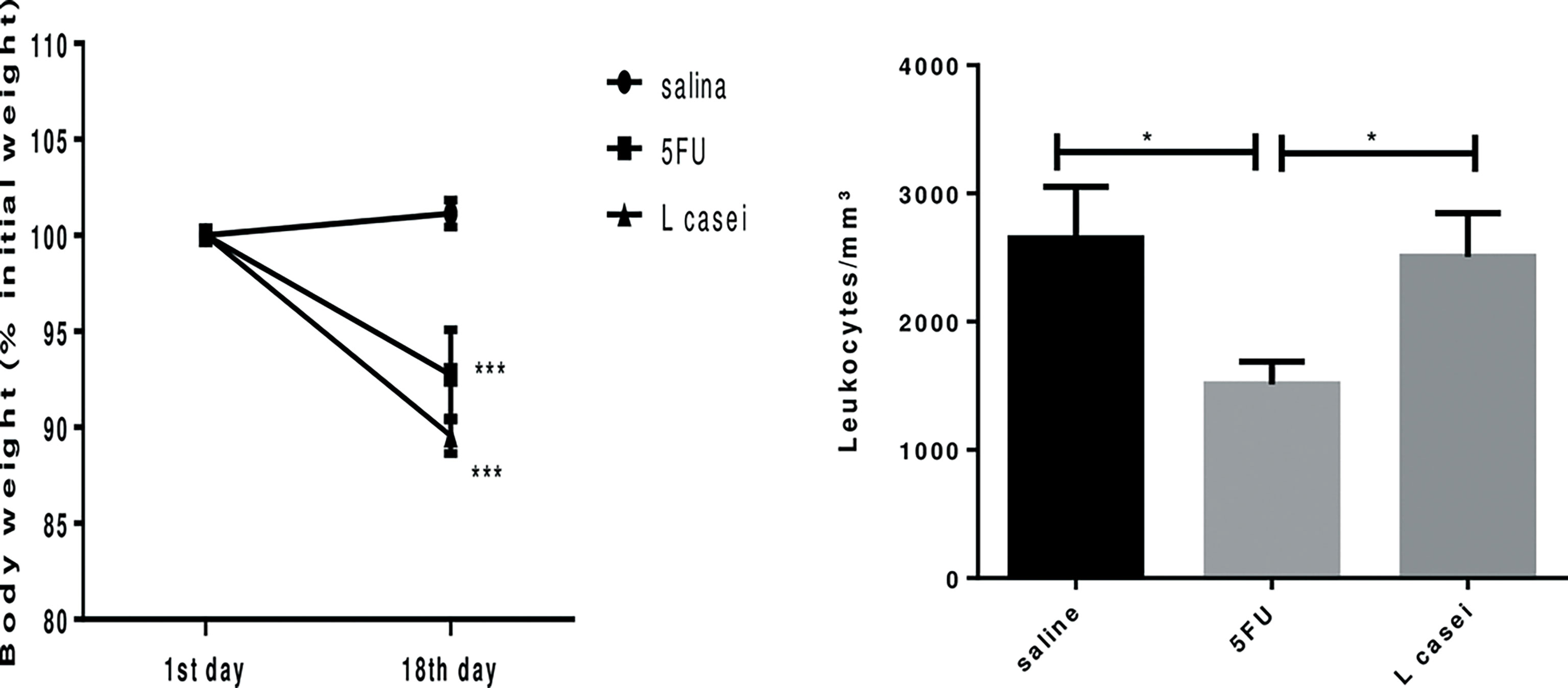
Figure 1 Weight of the animals (1st day and 18th day of treatment) and the leukocyte count (mm³) in the blood after euthanasia. Groups: Saline, 5FU (5-fluorouracil) and L. Casei (Lacticaseibacillus casei). *p < 0.05, ***p < 0.001.
L. casei protects against 5-FU-induced histopathological injury
L. casei protected the colon and all small intestine segments against 5-FU-induced injury (p<0.05), as shown in Table 2 and Figure 2. 5-FU-induced damage resulted in shortened villi, loss of crypt architecture, submucosal edema, and pronounced inflammatory cell infiltrate in the lamina propria (Figures 2B, E, H, K) compared to the saline group (Figures 2A, D, G, J). On the other hand, L. casei pretreatment was able to attenuate these histological changes promoted by 5-FU in all intestine segments, as shown in Figure 2, with preserved villi and crypts in the duodenum (2C), jejunum (2F) and ileum (2I), associated with significantly less edema and less inflammatory cell infiltrate (2C, 2F, 2I and 2L) when compared to the respective 5-FU intestinal segments.
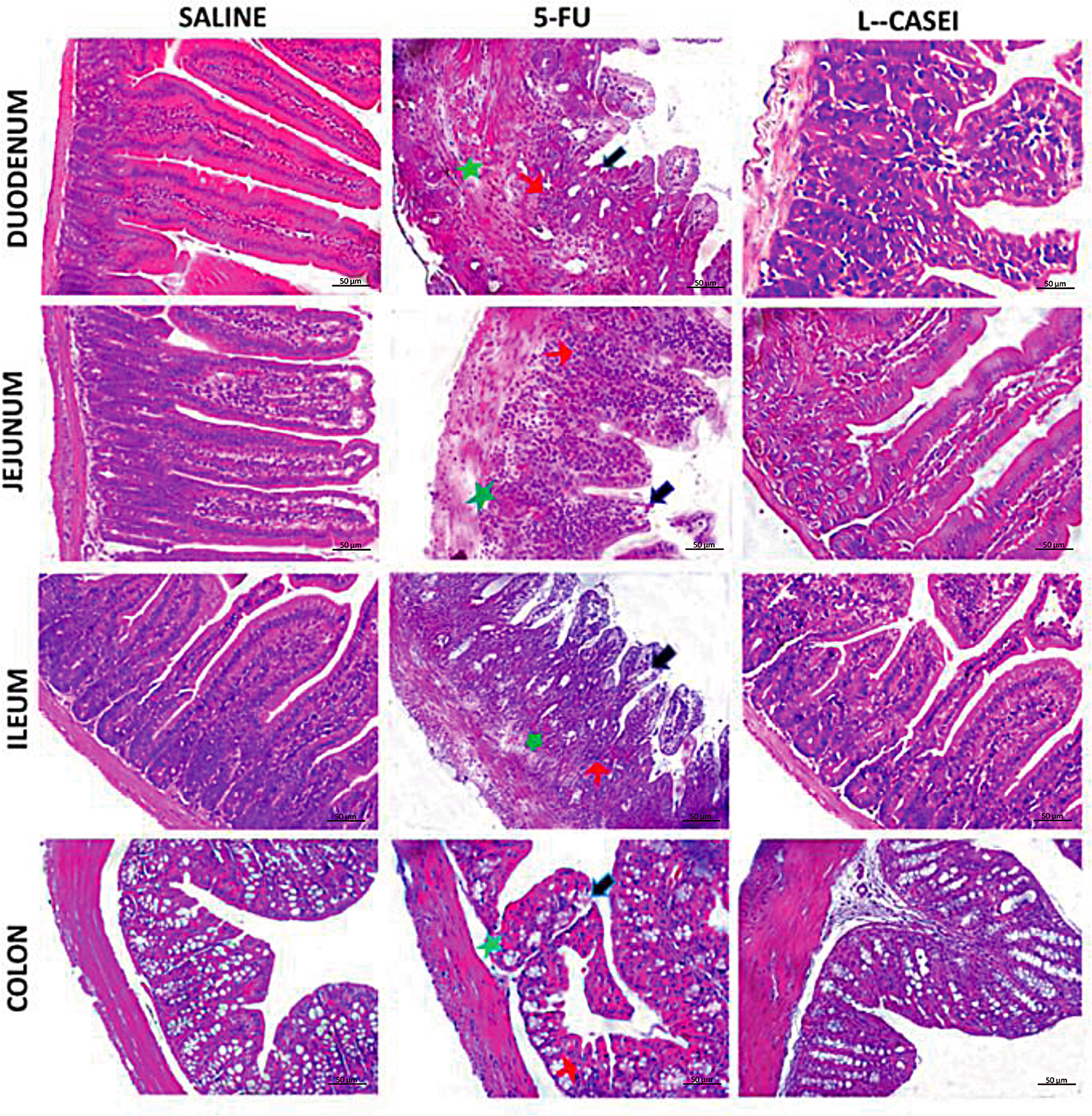
Figure 2 Histophatological analysis. Lacticaseibacillus casei (LC), prevented 5-FU-induced histopathological injuries in duodenum, jejunum, ileum and colon. 5-FU induces villi shortening (black arrows), loss of crypt architecture (green arrows) and intense inflammatory cell infiltrate (red arrows) in the duodenum, jejunum, ileum and colon, and edema (brown arrows) in the colon submucosal layer. H&E; scale bar corresponds to 200 µm.
L. casei protects against 5-FU-induced increase in malonaldehyde (MDA) levels, but not against 5-FU-induced reduction of invertase activity
MDA levels, investigated as a marker of lipid peroxidation oxidative stress in jejunal tissues from the 5-FU group were significant higher when compared to saline group (p < 0.05). L. casei was able to protect against this effect of 5-FU, since a significant reduction in MDA levels was found in jejunal tissues of the L. casei group when compared to the 5-FU group (p < 0.05), as shown in Figure 3.
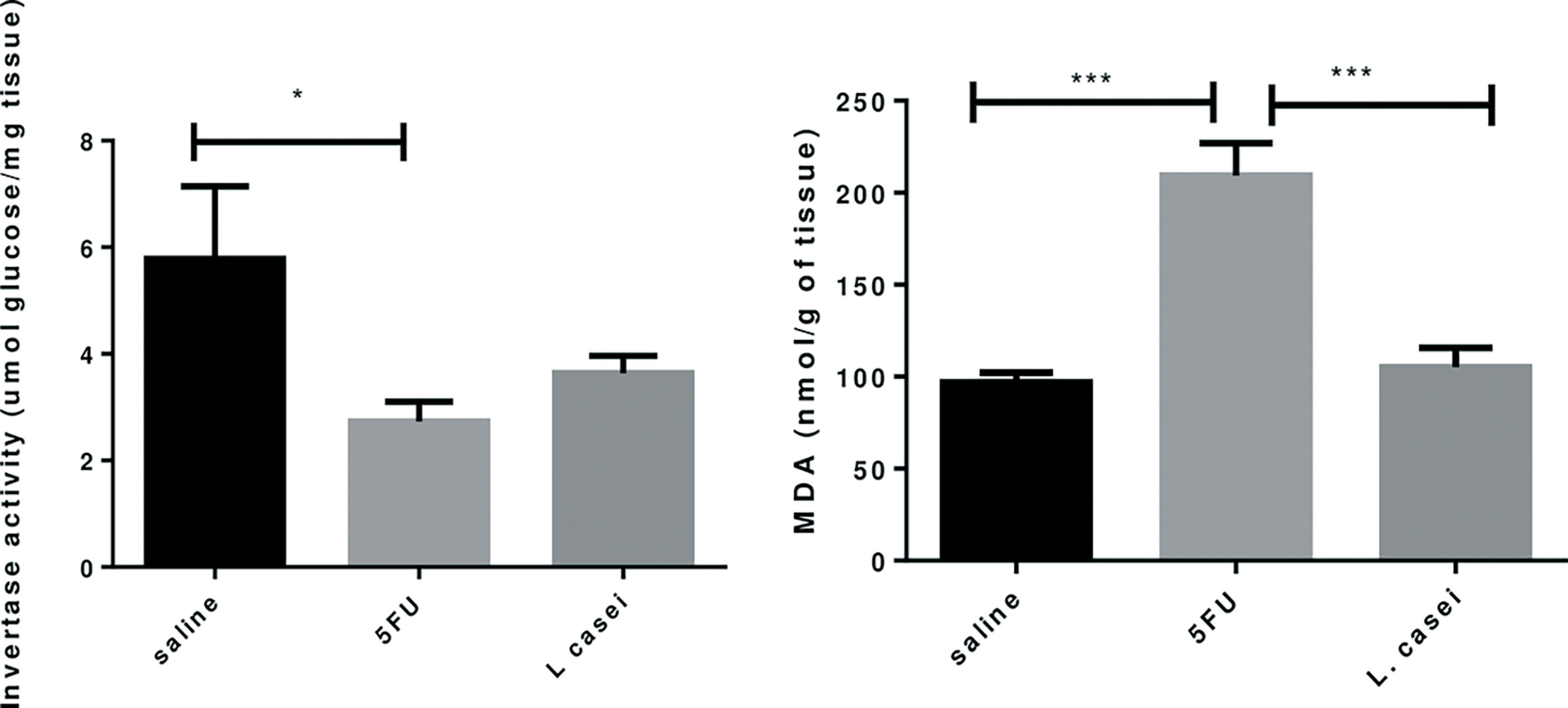
Figure 3 Invertase activity, Malonaldehyde Dosage (MDA). Groups: Saline, 5FU (5-fluorouracil) and L. Casei (Lacticaseibacillus casei). *p < 0.05, ***p < 0.001.
Figure 3 also shows that the invertase activity was significantly reduced in the 5-FU group (p<0.05) compared to the saline group. Pretreatment with L. casei was not able to preserve the invertase activity, since no significant difference was found between 5-FU and L. casei groups (p>0.05), as illustrated in Figure 3.
L. casei protects against 5-FU-induced iNOS and TNF-α overexpression
The jejunal tissues of mice submitted to 5-FU-induced intestinal mucositis showed marked iNOS (Figures 4B, D) and TNF-α immunostaining (Figures 5B, D) on inflamed conjunctive tissue compared to the saline control group (Figures 4A, D, 5A, D). Pretreatment with L. casei significantly reduced (p<0.05) the jejunal iNOS (Figures 4C, D) and TNF-α immunostaining (Figures 5C, D) when compared to the 5-FU group. No significant differences were found between iNOS and TNF-α immunoexpression between L.casei and saline groups (p>0.05).
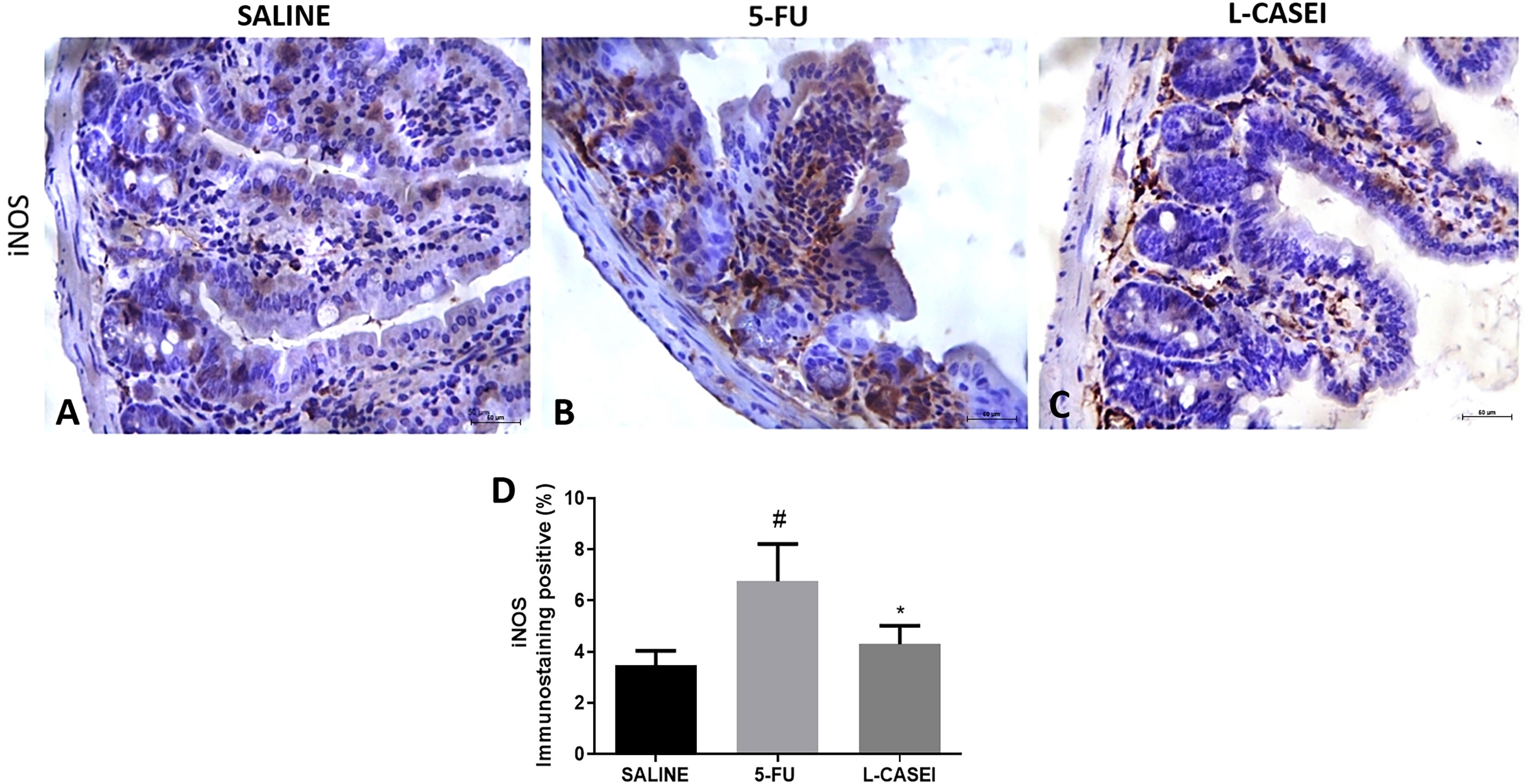
Figure 4 Representing examples of iNOS immunostaining: Control (Saline; A), 5-FU (B); L. casei (LC; C) and percentage of iNOS immunolabeled cells (D). Values were expressed as mean ± SEM. For statistical analysis, one-way ANOVA was used followed by Tukey's test, where #p < 0.05 vs. control group, *p < 0.05 vs. 5-FU group. Magnification x400.
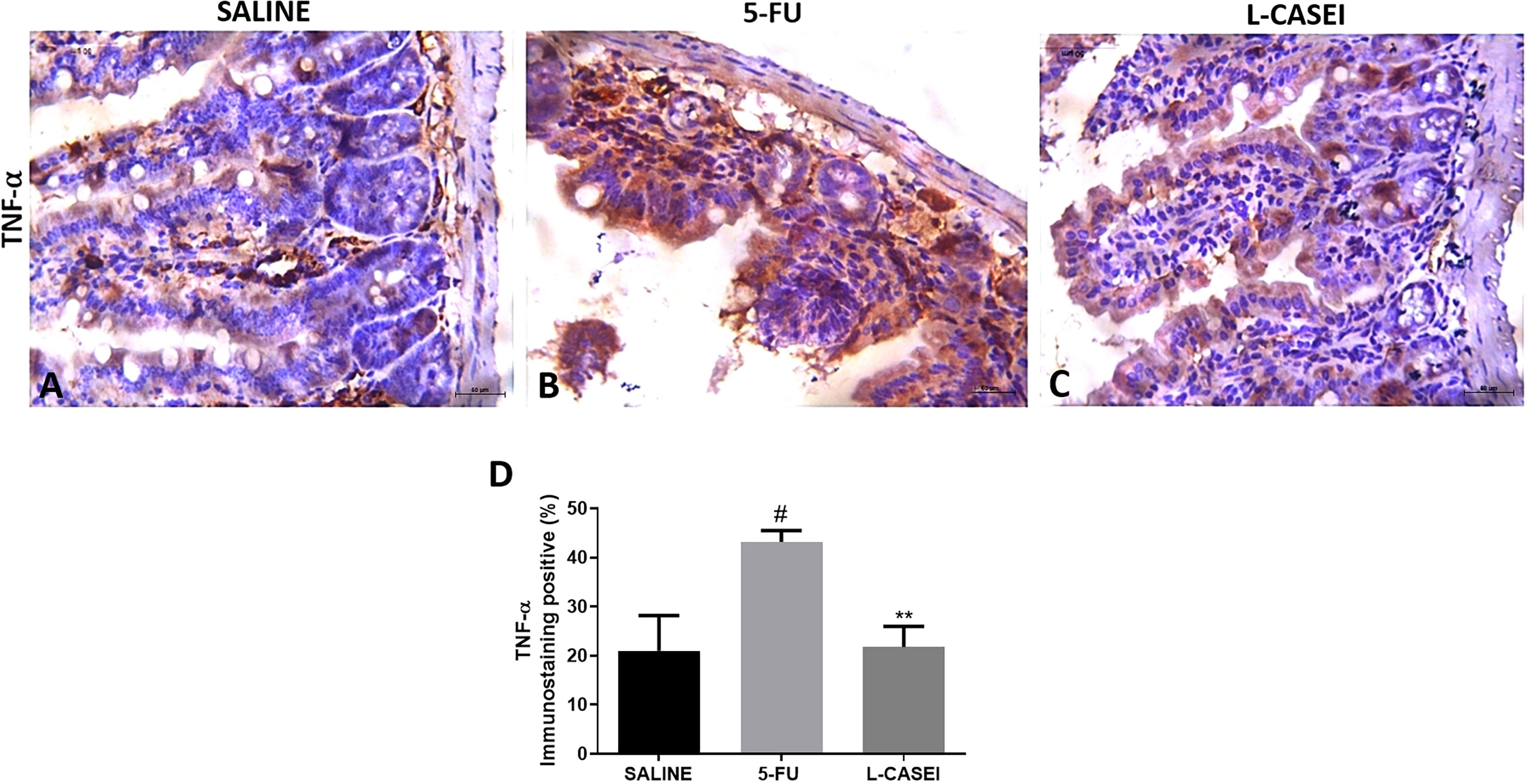
Figure 5 Representing examples of TNF-α immunostaining: Control (Saline; A), 5-FU (B); L. casei (LC; C) and percentage of TNF-α immunolabeled cells (D). Values were expressed as mean ± SEM. For statistical analysis, one-way ANOVA was used followed by Tukey’s test, where #p < 0.001 vs. control group, **p < 0.01 vs. 5-FU group. Magnification x400.
L. casei protects against 5-FU-induced increase in IL-1 beta, IL-6 and TNF-α levels
IL-1 beta, IL-6 and TNF-α levels in the jejunal tissues of animals submitted to 5-FU-induced intestinal mucositis were significantly increased (p<0.05) when compared to the saline group. Pretreatment with L. casei significantly reduced (p<0.05) the 5-FU-induced increase in these inflammatory cytokines, restoring these parameters to the level of their respective controls in the saline group, as shown in Figure 6.
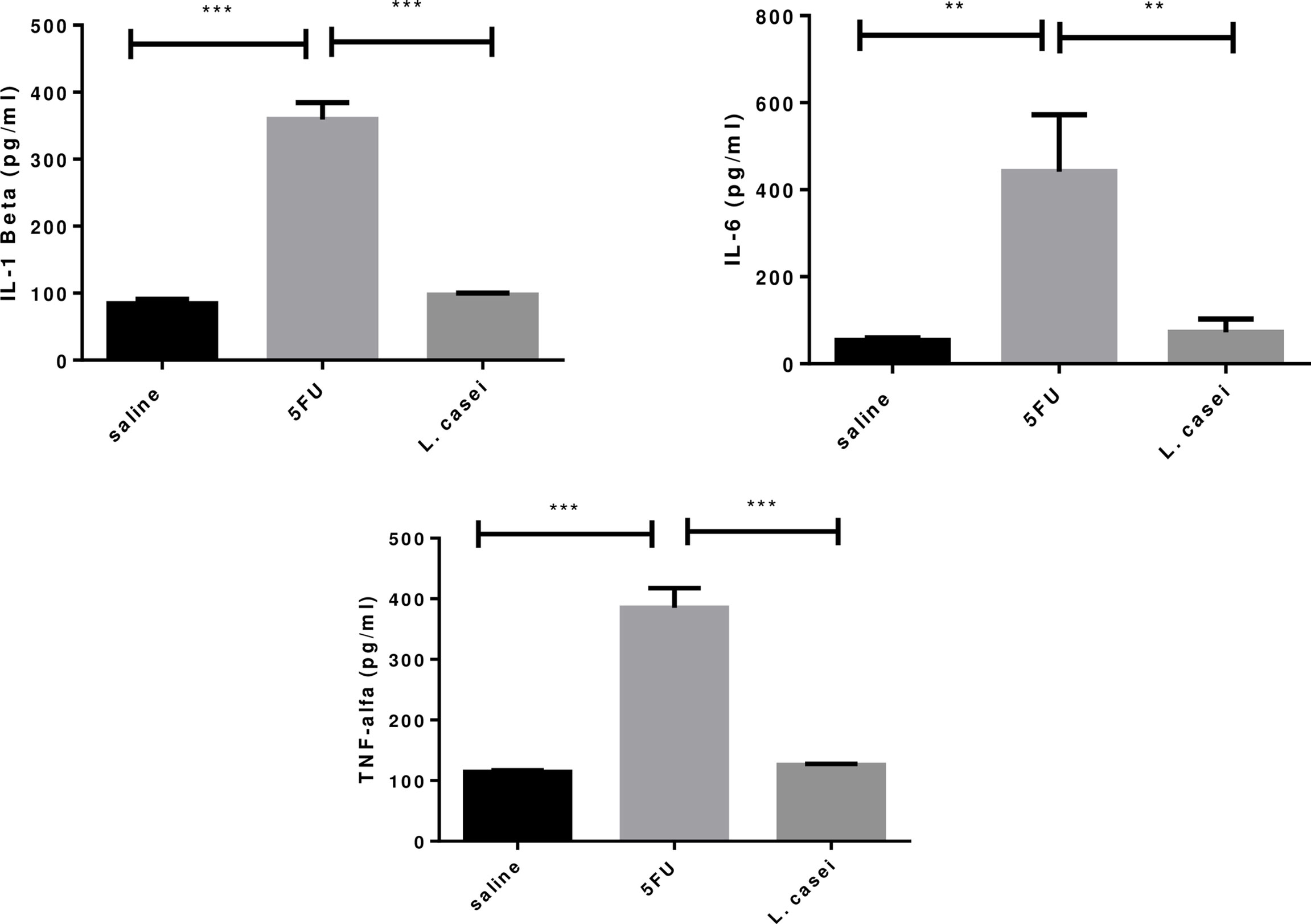
Figure 6 IL-1 beta, IL-6, TNF-α levels. Groups: Saline, 5FU (5-fluorouracil) and L. Casei (Lacticaseibacillus case). **p < 0.01, ***p < 0.001.
L. casei protects against 5-FU-induced upregulation of NFKB-P65 and TLR-4 gene expressions and 5-FU-induced downregulation of MUC-2, occludin and zonula occludin gene expressions
RT-PCR analysis showed significant increase in NFKB-P65 and TLR-4 gene expressions in the 5-FU group when compared to the saline group. Pretreatment with L. casei was able to protect against these 5-FU effects, as illustrated in Figure 7, which shows that L. casei significant down-regulated TLR-4 (p<0.05) and NFKB-P65 gene expressions. Figure 7 also shows that L. casei up-regulated MUC-2 (p<0.05), occludin (p <0.05) and ZO-1 (p<0.05), compared to the 5-FU group.
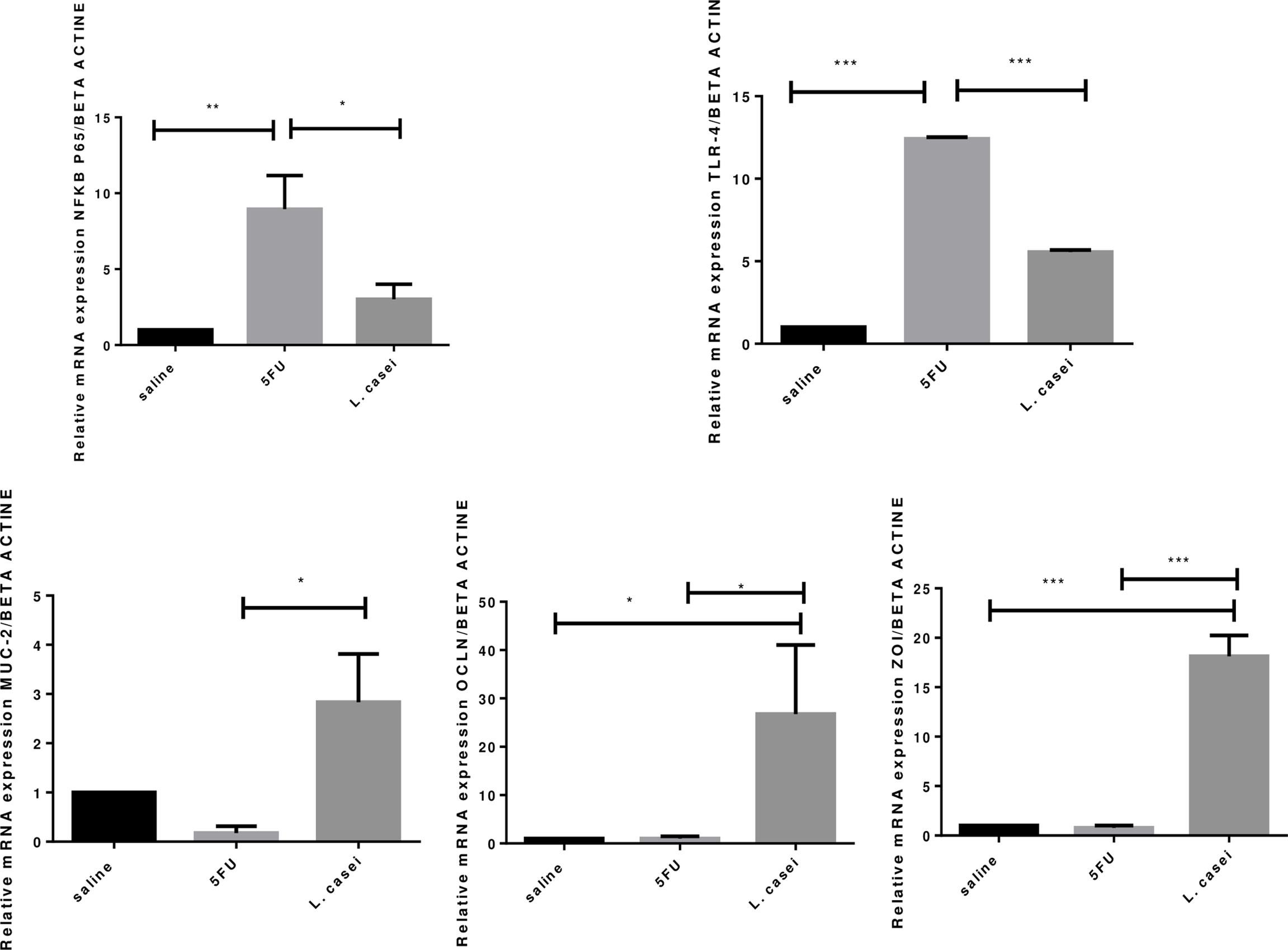
Figure 7 Quantification of gene expression. Beta actin, NFKB-P65, TLR-4, MUC-2 (mucine), OCLN (ocludine), ZO-1 (zonula occluden). Groups: Saline, 5FU (5-fluorouracil) and L. Casei (Lacticaseibacillus casei). *p < 0.05, **p < 0.01, ***p < 0.001.
L. casei protects against the effect of 5-FU, increasing fecal lactic acid bacteria
Lactic Acid Bacteria (LAB) were isolated from feces of saline, 5-FU and L.casei groups, 7 and 18 days after the first L.casei administration. The values, expressed as mean ± SD, are significantly increased (p<0.05) in the L. casei group, on days 7 (8.3 ± 0.3) and 18 (8.3 ± 0.3), when compared to both saline (6.4 ± 0.1 and 6.4 ± 0.2, respectively) and 5-FU (6.9 ± 0.1 and 6.5 ± 0.1, respectively) groups. No significant differences were observed between saline and 5-FU groups at any of the evaluated times.
Discussion
This study provides evidence that L. casei protects mice against intestinal damage induced by 5-fluorouracil (5-FU). Other Lactobacillus strains were found to be protective (21, 22), or partially protective (23) in experimental 5-FU-induced intestinal injury. In contrast, the ineffectiveness of probiotics in preventing the deleterious effects of 5-FU in mice has also been reported. Whitford et al. (24) demonstrated that Streptococcus thermophilus was unable to ameliorate 5-FU induced mucositis (24). Interestingly, their results suggest that it still may have a therapeutic potential effect on oncologic patients, as it was able to decrease mitotic activity and reduce crypt fission, both anti-cancer effects. Mauger et al. (25), using the noninvasive sucrose breath test, reported that Lactobacillus fermentumBR11, Lactobacillus rhamnosusGG, or Bifidobacterium lactisBB12 were not able to reduce the severity of small intestinal mucositis at the tested dose (25). We believe that these conflicting results may be attributed to differences between lactobacilli strains, manufacturing conditions and to the influence of methodological choices adopted across studies.
In the current study, pretreatment with L. casei was unable to protect against 5-FU-induced weight loss, probably because it is a consequence of anorexia and dehydration commonly associated with 5-FU treatment (26). On the other hand, protective effect of L. casei on leukopenia was found, a common side effect of 5-FU. This systemic effect of L. casei may have contributed to alleviate 5-FU-induced intestinal inflammation, since leukocytes represent defense cells and can protect animals against bacterial and fungal infections, commonly associated with mucositis (27). In accordance to our results, previous study (28) has shown that oral L. casei administration for 5 or 15 days reversed cyclophosphamide-induced immunosuppression in mice by increasing blood red and white blood cell levels and splenocyte and bone marrow cell counts. The beneficial effects of Lactobacillus delbrueckii on 5-FU-induced leukopenia in mice has also been demonstrated (21).
The experimental model used in the present study does not allow us assessing whether L. casei interferes with 5-FU efficacy. A recent study, however, demonstrated that Lactobacillus-derived metabolites enhance the antitumor activity of 5-FU and inhibit metastatic behavior in 5-FU-resistant colorectal cancer cells by regulating claudin-1 expression (29). Accordingly, the analysis of cell adhesion-related gene expression shows significant up-regulation of occludin and zonula occludens-1 (ZO-1), important tight junction proteins, in the intestine of the L. casei group. Our results are in agreement with previous studies, showing the role of lactobacilli on the intestinal epithelial barrier regulation (30, 31).
Mechanisms involved in the pathogenesis of mucositis are very complex. Damage to cells due to chemotherapy results in multiple inflammatory events, causing activation of toll like receptors (TLRs), especially TLR4 (32). This elicits upregulation and release of IL-1β, IL-6 and TNF-α via the transcription of nuclear factor-κB (NF-κB) (1). In addition to proinflammatory cytokines, the production of large amounts of nitric oxide by inducible nitric oxide enzyme (iNOS) has shown to play a major role in 5-FU- and radiation-induced mucositis, suggesting the important role of reactive oxygen species in the pathogenesis of oral mucositis (33).
In the present study, L. casei was able to protect against all 5-FU deleterious effects. Notably, pretreatment with L. casei ameliorated the histopathological alterations in the small intestine and colon, associated with reduced IL-1, IL-6 and TNF-α levels, reduced MDA accumulation, an oxidative stress marker, and decreased iNOS and TNF-α protein expressions in jejunal tissues. According to our results, Tang et al. found that a mixture of four probiotic strains ameliorated 5-FU-induced intestinal mucositis and dysbiosis in mice by reducing proinflammatory cytokines, neutrophil infiltration and TLR2 and TLR4 mRNA expression (34). In addition, it has been reported that probiotic bacteria are capable of producing antioxidant compounds that contribute to mitigating the effects of oxidative stress associated with inflammatory bowel disease (21, 35).
Greater lactic acid bacteria population in the feces of the L. casei group was observed, compared to both saline and 5-FU control groups. Lactic acid bacteria is a general term for a class of non-spore forming, gram-positive bacteria, whose main product of fermented sugar is lactic acid, a health-regulating probiotic metabolite (36). A clinical trial has demonstrated that humans consuming Lactobacillus Rhamnousus had increased concentration of the probiotic in feces (37). Another study (38) also observed increase in viable lactobacilli in feces during Lactobacillus acidophilus administration, persisting for at least 7 days after the end of supplementation.
The beneficial effects of L. casei observed in the present study may be associated with normalization of intestine microbiota, previously disrupted by 5-FU (34). It has been well established that intestinal microbiota acts in symbiosis to modulate different functions, such as the stimulation-regulation of epithelial innate immunity and competitive adherence to the mucosa and epithelium, acting as a true barrier to aggressive agents (6).
Microbiota analysis was not performed, which is a limitation for this study, but a significant decrease in the invertase activity was found in the intestine of mice submitted to 5-FU-induced intestinal mucositis. This finding may be associated with 5-FU-induced dysbiosis, since invertase is produced by microorganisms (39). L. casei was not able to preserve the invertase activity. However, significant and greater increase in the mucin gene expression was found in the intestine of L. casei group compared to both saline and 5-FU groups. Strong link between bacteria (intestinal flora) and mucin secretion has been reported, both of which being shown to be affected in chemotherapy-induced mucositis (40).
Mucins, the main components of the intestinal epithelium surface, play an important role in the integrity of the gut microbiota by providing attachment sites for intestinal flora and pathogenic bacteria, as well as simultaneously protecting the mucosa from bacterial overgrowth and/or penetration (40).
There are limitations in this study that should be discussed. Further studies are needed to investigate the gut microbiota variation after 5-FU administration, as well as the impact of L. casei. In addition, although probiotic therapy seems to be safe and risk-free, more animal studies should be developed to assess the safety and efficacy of L. casei, prior to its introduction as a therapy in clinical practice.
The results of this study show that oral Lacticaseibacillus casei administration has beneficial effects on 5-FU-induced mucositis, acting on the modulation of genes related to the barrier function, controlling the levels of pro-inflammatory cytokines and considerably attenuating the damage to the mucosa caused by 5-FU. Thus, new perspectives are opened for the use of Lacticaseibacillus casei as an alternative strategy for the prevention or management of chemotherapy-induced mucositis in the future.
Data availability statement
The original contributions presented in the study are included in the article/supplementary material. Further inquiries can be directed to the corresponding authors.
Ethics statement
The animal study was reviewed and approved by Ethics Committee on the use of Animals (CEUA) of Federal University of Rio Grande do Norte.
Author contributions
SB, MO, GG, FS, AA conceived and planned the experiments. SB, MO, SR, CM, ML, GG, RA, FS, AM, DF, RA, CR, GB, RL, AA carried out the experiments. SB, MO, SR, CM, ML, GG, RA, FS, AM, DF, RA, CR, GB, RL, AA contributed to sample preparation. SB, MO, SR, CM, ML, GG, RA, FS, AM, DF, RA, CR, GB, RL, AA contributed to the interpretation of the results. SB, MO, CM, GG, FS, CR, GB, RL, AA took the lead in writing the manuscript. All authors provided critical feedback and helped shape the research, analysis and manuscript. All authors contributed to the article and approved the submitted version.
Funding
This study was supported by the Conselho Nacional de Desenvolvimento Científico e Tecnológico (CNPQ) Number: 304382/2020-5, PROPLAN Number 184/2020. This study was financed in part by the Coordenação de Aperfeicoamento de Pessoal de Nível superior -Brasil (CAPES)-Finance Code 001.
Conflict of interest
The authors declare that the research was conducted in the absence of any commercial or financial relationships that could be construed as a potential conflict of interest.
Publisher’s note
All claims expressed in this article are solely those of the authors and do not necessarily represent those of their affiliated organizations, or those of the publisher, the editors and the reviewers. Any product that may be evaluated in this article, or claim that may be made by its manufacturer, is not guaranteed or endorsed by the publisher.
References
1. Chang CT, Ho TY, Lin H, Liang JA, Huang HC, Li CC, et al. 5-fluorouracil induced intestinal mucositis via nuclear factor-κB activation by transcriptomic analysis and in vivo bioluminescence imaging. PLos One (2012) 7(3):e31808. doi: 10.1371/journal.pone.0031808
2. Duncan M, Grant G. Oral and intestinal mucositis - causes and possible treatments. Aliment Pharmacol Ther (2003) 18(9):853–74. doi: 10.1046/j.1365-2036.2003.01784.x
3. Bowen JM, Stringer AM, Gibson RJ, Yeoh AS, Hannam S, Keefe DM. VSL3 probiotic treatment reduces chemotherapy-induced diarrhea and weight loss. Cancer Biol Ther (2007) 6(9):1449–54. doi: 10.4161/cbt.6.9.4622
4. Prisciandaro LD, Geier MS, Butler RN, Cummins AG, Howarth GS. Evidence supporting the use of probiotics for the prevention and treatment of chemotherapy-induced intestinal mucositis. Crit Rev Food Sci Nutr (2011) 51(3):239–47. doi: 10.1080/10408390903551747
5. Broadbent JR, Larsen RL, Deibel V, Steele JL. Physiological and transcriptional response of lactobacillus casei ATCC 334 to acid stress. J Bacteriol (2010) 192(9):2445–58. doi: 10.1128/jb.01618-09
6. Bermudez-Brito M, Plaza-Díaz J, Muñoz-Quezada S, Gómez-Llorente C, Gil A. Probiotic mechanisms of action. Ann Nutr Metab (2012) 61(2):160–74. doi: 10.1159/000342079
7. Yuan L, Zhang S, Li H, Yang F, Mushtaq N, Ullah S, et al. The influence of gut microbiota dysbiosis to the efficacy of 5-fluorouracil treatment on colorectal cancer. BioMed Pharmacother (2018) 108:184–93. doi: 10.1016/j.biopha.2018.08.165
8. Justino PF, Melo LF, Nogueira AF, Morais CM, Mendes WO, Franco AX, et al. Regulatory role of lactobacillus acidophilus on inflammation and gastric dysmotility in intestinal mucositis induced by 5-fluorouracil in mice. Cancer Chemother Pharmacol (2015) 75(3):559–67. doi: 10.1007/s00280-014-2663-x
9. Cordeiro BF, Oliveira ER, da Silva SH, Savassi BM, Acurcio LB, Lemos L, et al. Whey protein isolate-supplemented beverage, fermented by lactobacillus casei BL23 and propionibacterium freudenreichii 138, in the prevention of mucositis in mice. Front Microbiol (2018) 9:2035. doi: 10.3389/fmicb.2018.02035
10. Mennigen R, Nolte K, Rijcken E, Utech M, Loeffler B, Senninger N, et al. Probiotic mixture VSL3 protects the epithelial barrier by maintaining tight junction protein expression and preventing apoptosis in a murine model of colitis. Am J Physiol Gastrointest Liver Physiol (2009) 296(5):G1140–9. doi: 10.1152/ajpgi.90534.2008
11. Ribeiro ESS, Damasceno KSFSC, Dantas L, Azevedo W, Leite PIP, Assis C, et al. Fermented yellow mombin juice using lactobacillus acidophilus NRRL b-4495: Chemical composition, bioactive properties and survival in simulated gastrointestinal conditions. PLos One (2020) 15(9):e0239392–e. doi: 10.1371/journal.pone.0239392
12. Arifin WN, Zahiruddin WM. Sample size calculation in animal studies using resource equation approach. Malays J Med Sci (2017) 24(5):101–5. doi: 10.21315/mjms2017.24.5.11
13. MacPherson BR, Pfeiffer CJ. Experimental production of diffuse colitis in rats. Digestion (1978) 17(2):135–50. doi: 10.1159/000198104
14. de Araújo AA, Borba PB, de Souza FHD, Nogueira AC, Saldanha TS, Araújo TEF, et al. In a methotrexate-induced model of intestinal mucositis, olmesartan reduced inflammation and induced enteropathy characterized by severe diarrhea, weight loss, and reduced sucrose activity. Biol Pharm Bull (2015) 38(5):746–52. doi: 10.1248/bpb.b14-00847
15. Siddique YH, Ara G, Afzal M. Estimation of lipid peroxidation induced by hydrogen peroxide in cultured human lymphocytes. Dose Response (2012) 10(1):1–10. doi: 10.2203/dose-response.10-002.Siddique
16. Brey EM, Lalani Z, Johnston C, Wong M, McIntire LV, Duke PJ, et al. Automated selection of DAB-labeled tissue for immunohistochemical quantification. J Histochem Cytochem (2003) 51(5):575–84. doi: 10.1177/002215540305100503
17. Safieh-Garabedian B, Poole S, Allchorne A, Winter J, Woolf CJ. Contribution of interleukin-1 beta to the inflammation-induced increase in nerve growth factor levels and inflammatory hyperalgesia. Br J Pharmacol (1995) 115(7):1265–75. doi: 10.1111/j.1476-5381.1995.tb15035.x
18. Araújo AA, Souza TO, Moura LM, Brito GA, Aragão KS, Araújo LS, et al. Effect of telmisartan on levels of IL-1, TNF-α, down-regulated COX-2, MMP-2, MMP-9 and RANKL/RANK in an experimental periodontitis model. J Clin Periodontol (2013) 40(12):1104–11. doi: 10.1111/jcpe.12160
19. Livak KJ, Schmittgen TD. Analysis of relative gene expression data using real-time quantitative PCR and the 2(-delta delta C(T)) method. Methods (2001) 25(4):402–8. doi: 10.1006/meth.2001.1262
20. A.Oguntoye M, O.Ezekiel O, A.Oridupa O. Viability of lactobacillus rhamnosus GG in provitamin a cassava hydrolysate during fermentation, storage, in vitro and in vivo gastrointestinal conditions. Food Bioscience (2020) 40:386. doi: 10.1016/j.fbio.2020.100845
21. Jesus LCL, Drumond MM, Carvalho A, Santos SS, Martins FS, Ferreira Ê, et al. Protective effect of lactobacillus delbrueckii subsp. lactis CIDCA 133 in a model of 5 fluorouracil-induced intestinal mucositis. J Funct foods (2018) 2018 197–207. doi: 10.1016/j.jff.2018.12.027
22. Ciobanu L, Tefas C, Oancea DM, Berce C, Vodnar D, Mester A, et al. Effect of lactobacillus plantarum ACTT 8014 on 5−Fluorouracil induced intestinal mucositis in wistar rats. Exp Ther Med (2020) 20(6):209. doi: 10.3892/etm.2020.9339
23. Prisciandaro LD, Geier MS, Butler RN, Cummins AG, Howarth GS. Probiotic factors partially improve parameters of 5-fluorouracil-induced intestinal mucositis in rats. Cancer Biol Ther (2011) 11(7):671–7. doi: 10.4161/cbt.11.7.14896
24. Whitford EJ, Cummins AG, Butler RN, Prisciandaro LD, Fauser JK, Yazbeck R, et al. Effects of streptococcus thermophilus TH-4 on intestinal mucositis induced by the chemotherapeutic agent 5-fluorouracil (5-FU). Cancer Biol Ther (2009) 8(6):505–11. doi: 10.4161/cbt.8.6.7594
25. Mauger CA, Butler RN, Geier MS, Tooley KL, Howarth GS. Probiotic effects on 5-fluorouracil-induced mucositis assessed by the sucrose breath test in rats. Dig Dis Sci (2007) 52(3):612–9. doi: 10.1007/s10620-006-9464-y
26. Oliveira MMB, de Araújo AA, Ribeiro SB, de Sales Mota PCM, Marques VB, da Silva Martins Rebouças C, et al. Losartan improves intestinal mucositis induced by 5-fluorouracil in mice. Sci Rep (2021) 11(1):23241. doi: 10.1038/s41598-021-01969-x
27. Cinausero M, Aprile G, Ermacora P, Basile D, Vitale MG, Fanotto V, et al. New frontiers in the pathobiology and treatment of cancer regimen-related mucosal injury. Front Pharmacol (2017) 8:354. doi: 10.3389/fphar.2017.00354
28. Jang S-E, Joh E-H, Ahn Y-T, Huh C-S, Han MJ, Kim D-H. Lactobacillus casei HY7213 ameliorates cyclophosphamide-induced immunosuppression in mice by activating NK, cytotoxic T cells and macrophages. Immunopharmacol Immunotoxicol (2013) 35(3):396–402. doi: 10.3109/08923973.2013.789055
29. An J, Ha EM. Lactobacillus-derived metabolites enhance the antitumor activity of 5-FU and inhibit metastatic behavior in 5-FU-resistant colorectal cancer cells by regulating claudin-1 expression. J Microbiol (2020) 58(11):967–77. doi: 10.1007/s12275-020-0375-y
30. Hou Q, Huang Y, Wang Y, Liao L, Zhu Z, Zhang W, et al. Lactobacillus casei LC01 regulates intestinal epithelial permeability through mIR-144 targeting of OCLN and ZO1. J Microbiol Biotechnol (2020) 30(10):1480–7. doi: 10.4014/jmb.2002.02059
31. Yeung CY, Chiang Chiau JS, Chan WT, Jiang CB, Cheng ML, Liu HL, et al. In vitro prevention of salmonella lipopolysaccharide-induced damages in epithelial barrier function by various lactobacillus strains. Gastroenterol Res Pract (2013) 2013:973209. doi: 10.1155/2013/973209
32. Khan S, Wardill HR, Bowen JM. Role of toll-like receptor 4 (TLR4)-mediated interleukin-6 (IL-6) production in chemotherapy-induced mucositis. Cancer Chemother Pharmacol (2018) 82(1):31–7. doi: 10.1007/s00280-018-3605-9
33. Leitão RF, Brito GA, Oriá RB, Braga-Neto MB, Bellaguarda EA, Silva JV, et al. Role of inducible nitric oxide synthase pathway on methotrexate-induced intestinal mucositis in rodents. BMC Gastroenterol (2011) 11:90. doi: 10.1186/1471-230x-11-90
34. Tang Y, Wu Y, Huang Z, Dong W, Deng Y, Wang F, et al. Administration of probiotic mixture DM1 ameliorated 5-fluorouracil-induced intestinal mucositis and dysbiosis in rats. Nutrition (2017) 33:96–104. doi: 10.1016/j.nut.2016.05.003
35. Wang Y, Wu Y, Wang Y, Xu H, Mei X, Yu D, et al. Antioxidant properties of probiotic bacteria. Nutrients (2017) 9(5):1–15. doi: 10.3390/nu9050521
36. Deng Z, Hou K, Zhao J, Wang H. The probiotic properties of lactic acid bacteria and their applications in animal husbandry. Curr Microbiol (2021) 79(1):22. doi: 10.1007/s00284-021-02722-3
37. Wind RD, Tolboom H, Klare I, Huys G, Knol J. Tolerance and safety of the potentially probiotic strain lactobacillus rhamnosus PRSF-L477: a randomised, double-blind placebo-controlled trial in healthy volunteers. Br J Nutr (2010) 104(12):1806–16. doi: 10.1017/s0007114510002746
38. Mai V, Waugh S, Byrd D, Simpson D, Ukhanova M. Novel encapsulation improves recovery of probiotic strains in fecal samples of human volunteers. Appl Microbiol Biotechnol (2017) 101(4):1419–25. doi: 10.1007/s00253-016-7915-8
39. Lincoln L, More SS. Bacterial invertases: occurrence, production, biochemical characterization, and significance of transfructosylation. J Basic Microbiol (2017) 57(10):803–13. doi: 10.1002/jobm.201700269
Keywords: chemotherapy, 5-fluorouracil, mucositis, probiotic, Lacticaseibacillus casei
Citation: Barbosa SJdA, Oliveira MMB, Ribeiro SB, de Medeiros CACX, Lima MLdS, Guerra GCB, de Araújo Júnior RF, de Sousa Junior FC, Martins AA, Paiva DFF, Andrade RVS, Rebouças CSM, Brito GAdC, Leitâo RFdC and de Araújo A A (2022) The beneficial effects of Lacticaseibacillus casei on the small intestine and colon of Swiss mice against the deleterious effects of 5-fluorouracil. Front. Immunol. 13:954885. doi: 10.3389/fimmu.2022.954885
Received: 27 May 2022; Accepted: 07 October 2022;
Published: 21 October 2022.
Edited by:
Philippe Saas, INSERM U1098 Interactions Hôte-Greffon-Tumeur & Ingénierie Cellulaire et Génique, FranceReviewed by:
Gordon Stanley Howarth, University of Adelaide, AustraliaYaohua Yang, Vanderbilt University Medical Center, United States
Copyright © 2022 Barbosa, Oliveira, Ribeiro, de Medeiros, Lima, Guerra, de Araújo Júnior, de Sousa Junior, Martins, Paiva, Andrade, Rebouças, Brito, Leitâo and de Araújo. This is an open-access article distributed under the terms of the Creative Commons Attribution License (CC BY). The use, distribution or reproduction in other forums is permitted, provided the original author(s) and the copyright owner(s) are credited and that the original publication in this journal is cited, in accordance with accepted academic practice. No use, distribution or reproduction is permitted which does not comply with these terms.
*Correspondence: Renata Ferreira de Carvalho Leitâo, cmVuYXRhLmNhcnZhbGhvQHVmYy5icg==; Aurigena Antunes de Araújo, YXVyaWdlbmEuYW50dW5lc0B1ZnJuLmJy