- 1Dongguan Key Laboratory of Medical Bioactive Molecular Developmental and Translational Research, Guangdong Provincial Key Laboratory of Medical Molecular Diagnostics, Guangdong Medical University, Dongguan, China
- 2Department of Pathology, Dongguan Hospital Affiliated to Jinan University, Binhaiwan Central Hospital of Dongguan, Dongguan, China
- 3Guangdong Provincial Key Laboratory of Medical Molecular Diagnostics, School of Medical Technology, Guangdong Medical University, Dongguan, China
- 4Key Laboratory of Medical Bioactive Molecular Research for Department of Education of Guangdong Province, School of Basic Medicine, Guangdong Medical University, Dongguan, China
- 5Collaborative Innovation Center for Antitumor Active Substance Research and Development, Department of Biochemistry and Molecular Biology, School of Basic Medicine, Guangdong Medical University, Zhanjiang, China
- 6Dongguan Metabolite Analysis Engineering Technology Center of Cells for Medical Use, Guangdong Xinghai Institute of Cell, Dongguan, China
Inflammatory bowel diseases (IBDs) represent a group of chronic inflammatory disorders of the gastrointestinal (GI) tract including ulcerative colitis (UC), Crohn’s disease (CD), and unclassified IBDs. The pathogenesis of IBDs is related to genetic susceptibility, environmental factors, and dysbiosis that can lead to the dysfunction of immune responses and dysregulated homeostasis of local mucosal tissues characterized by severe inflammatory responses and tissue damage in GI tract. To date, extensive studies have indicated that IBDs cannot be completely cured and easy to relapse, thus prompting researchers to find novel and more effective therapeutics for this disease. Due to their potent multipotent differentiation and immunomodulatory capabilities, mesenchymal stem/stromal cells (MSCs) not only play an important role in regulating immune and tissue homeostasis but also display potent therapeutic effects on various inflammatory diseases, including IBDs, in both preclinical and clinical studies. In this review, we present a comprehensive overview on the pathological mechanisms, the currently available therapeutics, particularly, the potential application of MSCs-based regenerative therapy for IBDs.
1 Introduction
Inflammatory bowel diseases (IBDs) include two major types of disorders in gastrointestinal (GI) tract, ulcerative colitis (UC) and Crohn’s disease (CD) (Table 1), characterized by idiopathic gastrointestinal inflammation and tissue damage with a high recurrent rate (1). According to epidemiology, the prevalence of IBDs in Western countries is significantly higher than that in Eastern countries, but it is also rapidly increasing in Asian countries (2). The clinical signs and symptoms of the IBDs mainly include enteritis, diarrhea, recurrent hemorrhage, abdominal pain, reduced appetite, and weight loss, etc. (3, 4). Currently, there is still no cure for IBDs (5). In the early stages of IBDs, it is treated mainly with medication and surgery therapies, but the former can only control symptoms, while the latter is invasive and carries a high risk of complications (6). The clinical remission rates of IBDs range from 20% to 30% with monotherapy, but the remission rate would be around 50% if a combination of treatments were used (7). If the disease is not treated in time, the protracted course of IBDs can eventually trigger cancer, such as colitis-associated cancer (CAC), caused by external oncogenic factors (8, 9).
In recent years, mesenchymal stem cells (MSCs)-based therapy has emerged as a promising strategy for the treatment of IBDs due to their potent immuno-modulatory and tissue-repair functions (10). MSCs were first described by Friedenstein et al. as a population of bone marrow derived adherent cells, which were fibroblast-like and non-phagocytic cells but could differentiate into adipocytes, osteocytes, and chondrocytes under specific induction conditions in vitro (11). Bone marrow has long been regarded as the main source of MSCs. Nevertheless, isolation of bone marrow-derived MSCs (BM-MSCs) is a highly invasive process that may cause severe morbidity, while the number of BM-MSCs obtained usually decreases significantly with aging (12). In addition to bone marrow, MSCs have also been isolated from a variety of other tissues, such as adipose tissue (AT-MSCs), umbilical cord blood (UCB-MSCs), human amniotic tissue (HA-MSCs), and gingiva tissues (GMSCs) that are easily accessible (13). In order to standardize MSCs of different tissue origins, the International Society of Cell Therapy proposed three minimal standards for MSCs (1): plastic adherence in vitro culture conditions (2); the expression of a panel of phenotypic markers CD73+, CD90+, and CD105+ and the absence of hematopoietic marker CD11b-, CD14-, CD19-, CD34-, CD45-, CD79α-, and HLA-DR- (3); the ability to differentiate into osteoblasts, adipocytes, and chondroblasts in vitro (14) (Figure 1A).
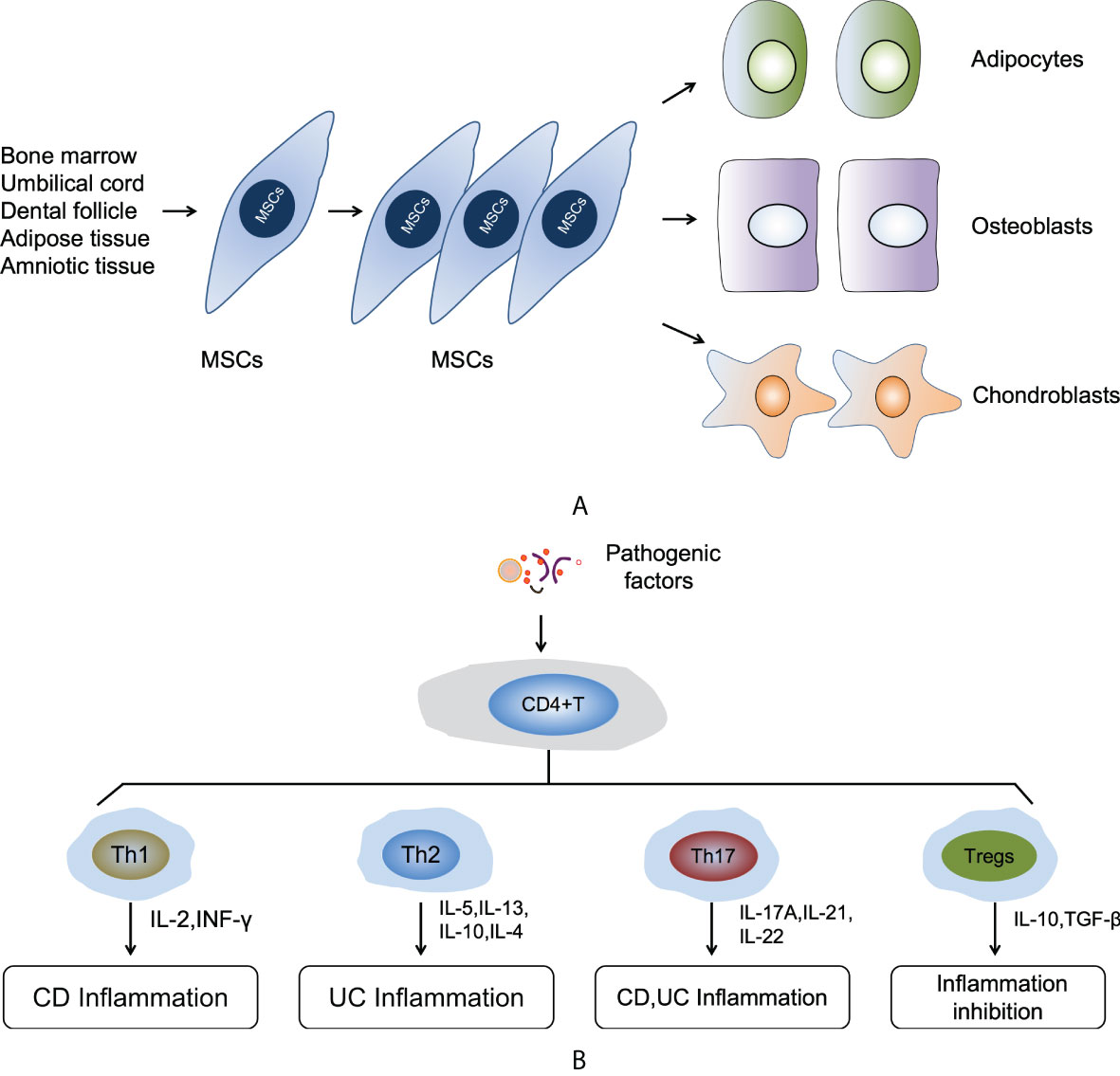
Figure 1 (A) The self-renewal and multipotent differentiation functions of MSCs. (B) The mechanism of immune responses in the pathogenic process of IBDs.
Given their wide existence in almost all tissues and organs of the body and their multifaceted biological functions, MSCs have been implicated to play essential roles in tissue homeostasis, regeneration, and diseases (15–19). Under physiological conditions, they may play an essential role in development, organogenesis, and maintenance of tissue and immune homeostasis through their cross-talks with specialized tissue cells and resident immune cells (15, 18, 19). On the other hand, in response to various insult signals, resident or endogenous MSCs may act as sensors of various insult signals and become activated by secreting a myriad of bioactive mediators that can foster or temper the immune/inflammatory responses, leading to the establishment of a pro-reparatory or regenerative microenvironment (20). However, various factors or pathological conditions, e. g. aging, can dysregulate the property and function of MSCs, thus contributing to the pathogenesis of various diseased conditions, including chronic inflammatory and fibrotic diseases (15–17, 21) and even tumorigenesis (20). In the review article, we also highlighted the latest research progress on the potential role of dysregulated MSCs in the pathogenesis of IBDs.
2 Pathogenic mechanism of IBDs
The etiology of IBDs is very complicated and has not yet been completely understood. To date, it is generally accepted that the pathogenesis of IBDs involves a complex interaction of environmental factors, genetic susceptibility, and dysregulated immune responses (22). Among the environmental factors, smoking, drugs use, diet habits, mental stress, and many other external factors are related to the occurrence of IBDs (23). In particular, smoking increases the risk of CD and is related with an increase in the recurrence rate of postoperative diseases (24). Air pollution can also increase the risk of CD and UC disease (25, 26). At the same time, Bitton et al. (27) also proposed that people with less stress would have less chance of developing IBDs. In addition, IBDs has a strong genetic tendency, especially in the first-degree relatives of patients who are at higher risk for IBDs. Compared with fraternal twins, identical twins have a higher prevalence rate of IBDs (28, 29). Genetic studies have reached a consistent conclusion: genetic factors play an important but non-decisive role in the occurrence of IBDs.
The dysregulated immune responses also play a key role in the pathogenic process of IBDs. The most fundamental pathogenetic patterns of IBDs is the dysregulation of innate and adaptive immunity. However, the adaptive immune responses are considered to be the main driver of IBDs (22). In response to different types of pathologic factors and inflammatory cytokines, naive CD4+ T cells can differentiate into distinct subsets of CD4+ T-helper (Th) cells, such as Th1, Th2, Th17, and CD4+FoxP3+ T regulatory cells (Tregs) (30). Th1 and Th17 cells can secrete a variety of inflammatory cytokines that induce intestinal epithelial inflammatory cells infiltrate and acute or chronic enteritis. However, intestinal inflammation can be suppressed via the differentiation Tregs and the supplementary of Th2 cells (31). Disturbance of immune homeostasis results in an imbalance of various subtypes of T cells as evidenced by increased proinflammatory cytokines derived from Th1 and Th17 cells in CD disease or Th2 cells in UC (32–34), and a decrease in the infiltration of anti-inflammatory Tregs in both CD and UC (35). For a long time, many studies believed that CD was driven by Th1 response, while UC was related to Th2 response. In the CD mucosa, macrophage-derived IL-12, IL-18, and TNF-α are overexpressed, driving the Th1 immune response to increase the production of IL-2 and IFN-ɤ. This response is thought to cause intestinal inflammation. In contrast, UC is characterized by increased expression of IL-5 and IL-13, which are members of the Th2 cytokine family, and this response will induce intestinal inflammation. In addition to the Th1 and Th2 responses, the role of Th17 cells, a subset of inflammatory T cells that expand under the action of pro-inflammatory cytokines, has been the focus research at this current stage Th17 cells are induced by IL-6 and TGF-β and produce IL-17A, IL-21, and IL-22, and those soluble factors will cause inflammation in CD and UC mucosa (36). IL-10 and TGF-β secreted by Tregs create an immunosuppressive microenvironment that is beneficial for repairing gastrointestinal dysfunction and colonic mucosal lesions (Figure 1B).
In recent years, accumulating evidence has highlighted the important role of resident MSCs in the establishment of a unique tissue niche that is essential for tissue and immune homeostasis, while the dysregulated MSCs might contribute to the development of various pathological conditions (15–17, 19, 21). Similarly, recent studies have also implicated the importance of intestinal MSCs in digestive organ development, mucosal tissue, and immune homeostasis, which can provide multiple niche signals to support functional integration of mucosal epithelial cells, immune cells, and gut microbiota (37–39). On the other hand, deficiency or aberrant activation of intestinal MSCs may lead to disturbance in mucosal and immune homeostasis, thus contributing to the pathogenesis of IBDs (40). Most recently, the emerging studies using scRNA-seq have significantly improved our understanding of the heterogeneity and the distinct role of diverse subsets of intestinal MSCs in regulating mucosal homeostasis and immunity by providing different niche signals under both physiological and inflammatory conditions (37, 38). For instance, Jasso et al. recently identified distinct subpopulations of stromal fibroblasts with gene signatures that are differentially regulated by chronic inflammation through scRNA-seq analysis of colon-derived mesenchymal stromal cells, thus providing mechanistic insight into how inflammation affects the function and behavior of intestinal MSCs and their crucial role in orchestrating mucosal tissue remodeling and healing (41).
3 Mechanisms of MSCs-based therapy of IBDs
3.1 MSCs-mediated immunomodulatory modulation
Compared with traditional therapeutics, MSC-based therapy is emerging as a promising platform for the treatment of IBDs. MSCs have the potential ability to restore immune homeostasis in patients with IBDs through turning the adverse pro-inflammatory mucosal immune responses into beneficial anti-inflammatory immune responses (42, 43). Many reported suggest that normal derived-MSCs (N-MSCs) can play a potential role in immunomodulatory or migrated to lesions to perform special functions during disease occurrence (44). However, the research on lesions-derived MSCs (L-MSCs) is still relatively fragmented (45, 46). L-MSCs, including but not limited to tumors, granulomas, oral, cervical, skin, precancerous, and white matter lesions, are the origin of disease occurrence and development (47–49). A few papers described the presence of L-MSCs possess many similarities with N-MSCs. It is noteworthy that L-MSCs may have directly or indirectly promoted the occurrence and development of local inflammatory diseases by increased proinflammatory factors and decreased anti-inflammatory factors (50). For instance, it has been reported that periapical lesions (PL)-MSCs possess similar immunomodulatory functions compared to N-MSCs (51). Dokic et al. reported that PL-MSCs increased the production of IL-6, IL-1β, TNF-α, and TGF-β, but not IL-8, thus exerting an pro-inflammatory role. These PL-MSCs can also inhibit T cell proliferation by suppressing IL-2 production and cell-cycle regulatory proteins. Additionally, TGF-β secreted by PL-MSCs can inhibit both Th1 and Th2 differentiation, and stimulate the expression of RORγt and FoxP3, the master regulators of Th17 and Tregs, respectively (52). Liu et al. (50) also showed that skin lesions-derived MSCs affects the activity of T lymphocytes in local lesions by increasing IL-11 secretion and reduced IL-6 and HGF. However, Galland et al. observed that tumor-associated MSCs (T-MSCs) have stronger immunosuppressive effect than N-MSCs, and affected both NK function and phenotype, such as the expression of CD56. T-MSCs shifted NK cells toward the CD56dim phenotype and differentially regulate the function of a subset of CD56bright/dim. Moreover, T- and N-MSCs both affect degranulation and activating receptor expression in the CD56dim subset, where they predominantly inhibit IFN-γ production to regulates immune function (53). Additionally, T-MSCs may largely rely on PGE2 and to a lesser extent on IL-6 to exert their immunosuppressive effects, and this effect by T-MSCs may be determined by signals derived from the tumor cells or the microenvironment, which may vary from patient to patient (20). Therefore, these studies have indicated that N- and L-MSCs possess immunomodulatory properties, which could make N- and L-MSCs based therapy of IBDs through mechanisms involving the secretion of anti-inflammatory soluble factors, direct cell-to-cell contact, and other regulatory pathways (54, 55).
3.1.1 The secretion of anti-inflammatory soluble factors
The paracrine functions of transplanted MSCs are much complex and controversial under the pathological setting of IBDs. Upon injury, MSCs respond to insult signals and become activated by secreting an array of soluble bioactive factors that can serve as feedback signals to foster the immunomodulatory and tissue repair functions of MSCs (56). It is widely assumed that the property and function of MSCs are determined by the local microenvironment where they reside. Transplanted MSCs can secrete biological factors, e.g. anti-inflammatory or immunosuppressive factors, either “spontaneously” or following stimulation by pro-inflammatory cytokines or other soluble factors produced by local immune cells, such as IL-1β, TNF-α, and IFN-γ (57–59). For instance, Galland et al. demonstrated that T-MSCs exhibit an immunosuppressive phenotype mainly through PGE2 mediated suppression of NK cell function, including inhibition of IFN-γ production, the shift toward the CD56dim phenotype, and downregulation of NK cell activating receptors (53). However, it has been reported that MSCs are not always weaponized with immunosuppressive functions. Fuenzalida et al. showed that MSCs pretreated with TLR3 ligands could secrete extra immunosuppressive cytokines and inhibit active T cells proliferation, while activation of TLR4 could prime MSCs to secrete proinflammatory factors (60, 61).
Under inflammatory conditions, transplanted MSCs stimulated by pro-inflammatory factors undergo a cascade of immune responses (62). Stimulated MSCs release PGE-2 and IL-6 to inhibit the maturation and function of dendritic cells (DCs), which leads to the decrease of TNF-α expression in myeloid DCs and upregulation of IL-1 level in lymphoid DCs (63, 64). MSCs inhibit T cell proliferation by elevating the expression of IDO and the secretion of TGF-β, IL-10, and PGE2, which can increase the expression of IFN- γ and IL- 21 (65). MSCs also could suppress macrophages activation through release of tumor-specific glycoproteins (TSGs) to convert the phenotype of macrophages from pro-inflammatory M1 macrophage characterized by secretion of inflammatory cytokines, e.g. TNF-α and IL-12, to anti-inflammatory M2 macrophages characterized by secretion of anti-inflammatory cytokines such as IL-10 and TGF-β (66). Naive CD4+ T cells differentiate into Th17 cells, which are characterized by the production of high levels of IL-17A, IL-17F, IL-21, and IL-22, upon combined stimulation of IL-6 and TGF-β, while their expansion is sustained by IL-23 secreted by macrophages and DCs (66, 67). It has been shown that MSCs restrain the development and activation of Th1 and Th17 cells by producing anti-proinflammatory factors, such as HLA, IL-10, TGF-β, and PGE2, and boost T cells and suppress B cells proliferation by promoting the expression of CD40, IL-6, IL-10, and TGF-β in colitis (68) (Figure 2A).
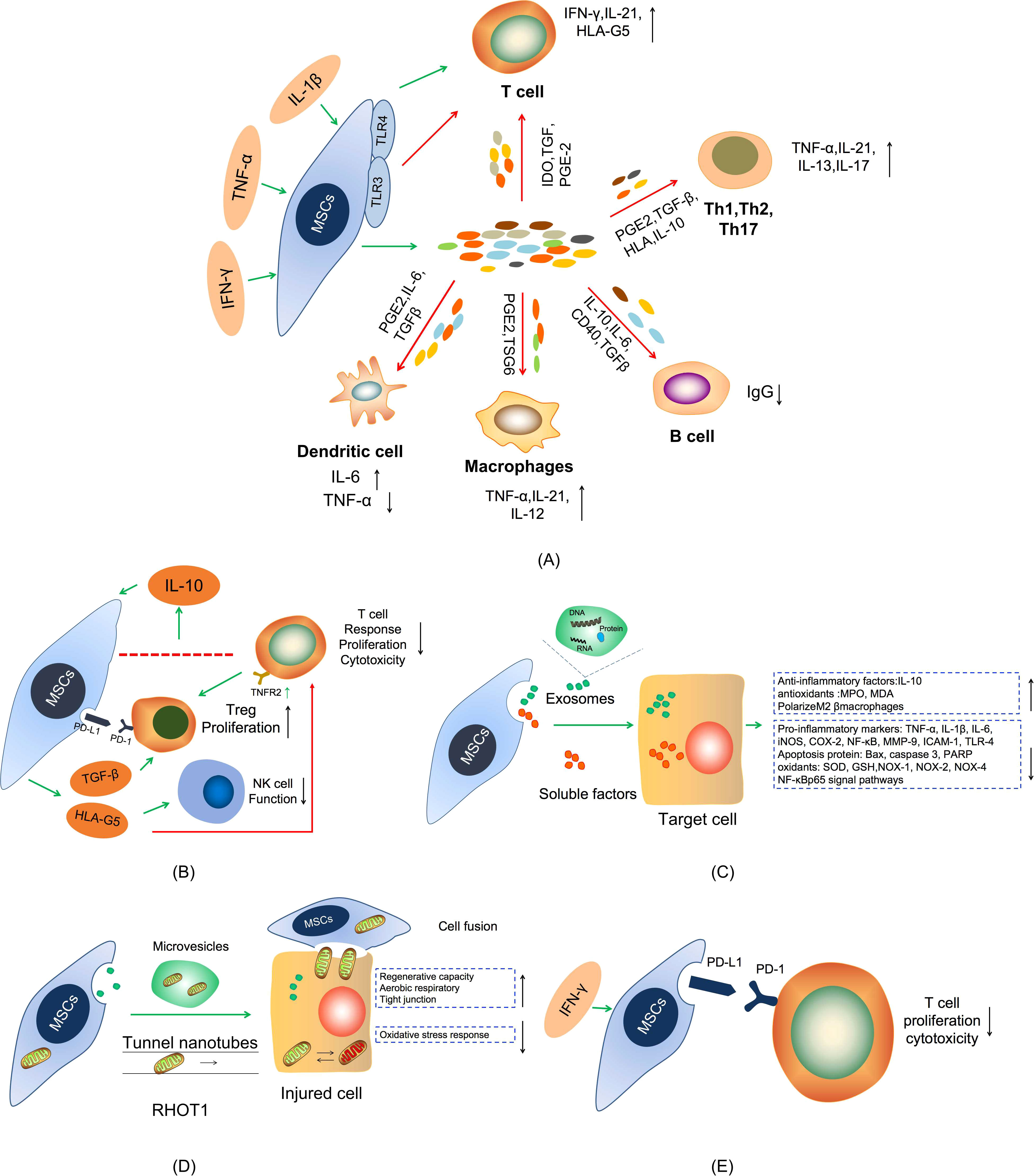
Figure 2 Schematic diagram of the mechanism of MSCs treatment in IBDs. (A) The secretion of anti-proliferative soluble factors. (B) Cell-To-Cell Contact. (C) Exosomes treatment. (D) Mitochondria transfer. (E) MSCs express PD-L1.
3.1.2 Direct cell-to-cell contact mechanisms
In addition to secreting anti-inflammatory factors, research evidence shows that MSCs are also capable of enhancing IL-10 production through a cell-to-cell contact mechanism between activated T cells and MSCs, which in turn stimulates the release of soluble human leukocyte antigen G5 (HLA-G5) (69, 70). HLA-G5 can significantly downregulate naive and memory antigen-specific T cells response, inhibit T cell proliferation and cytotoxicity, and suppresses NK cell function. Meanwhile, MSCs can induce T cells to form functional Tregs through mechanisms involving TGF-β and PD-1/PDL-1 or depends on T cell-dependent expression of TNFR2 (71, 72). This mechanism of cell-to-cell contact reaction suggests that MSCs exert powerful immunosuppressive effects in IBDs (Figure 2B).
3.1.3 MSCs-derived extracellular vesicles
Extracellular vesicles (EVs), including exosomes, are nanoscale microvesicles released by cells that play an important role in intercellular communication via transferring cargos containing various bioactive components (protein, DNA, mRNA, and non-coding RNA) involved in various of physiological and pathological processes (73, 74). There is increasing evidence that MSC-derived EVs display powerful therapeutic effects in several preclinical models of inflammatory diseases, suggesting that MSC-EVs may be a promising cell-free therapy because their properties are relatively stable and the safety risk is lower than that of the cell-producing similar products (75, 76). The mechanism of MSC-EVs in treating IBDs may be through inhibiting the secretion of pro-inflammatory cytokines and promoting the secretion of anti-inflammatory factors, regulating colonic macrophages, inhibiting apoptosis protein, regulating the expression of transcription factors, regulating signaling pathways, etc. (Figure 2C). For instance, Yang et al. found that intravenous injection of bone marrow mesenchymal stem cell-derived extracellular vesicles (BMSC-EVs) reduced the level of iNOS, COX-2, TNF-α, and IL-1β, inhibited apoptosis and NF- κBp65 signal transduction pathway, and regulated the balance between antioxidants (MPO, MDA) and oxidants (SOD, GSH) in IBDs mice (77). Liu et al. found that BMSC-EVs can act on colonic macrophages to produce IL-10, polarize intestinal M2β macrophages, inhibit inflammation and maintain the integrity of the colon barrier through metallothionein-2 (78). In addition to, Chang et al. found that adipose derived mesenchymal stem cell-derived extracellular vesicles (ADMSCs-EVs) effectively suppress acute inflammatory colitis in rats by down-regulating the expression of inflammatory markers (IL-1β, IL-6, TNF-α, NF-κB, COX-2, MMP-9, TLR-4, ICAM-1), oxidative stress markers (NOX-1, NOX-2, NOX-4) apoptotic, proteins (Bax, caspase 3, PARP), and fibrosis markers (Smad3, TGF-β) (79). Recently, a growing body of evidence has shown that EVs released by cells of the intestinal mucosa, immune cells, and gut microbiota play a significant role in maintaining the intestinal mucosal and immune homeostasis and the pathogenesis of IBDs (80). However, little is known about the role of EVs released by intestinal MSCs in both health and disease.
3.1.4 MSCs-mediated mitochondria transfer
MSCs may exert their therapeutic effects through mitochondrial transfer, a process to transfer healthy mitochondria from MSCs to cells with mitochondrial dysfunction through the formation of tunnel nanotubes, gap junctions, microvesicles, cell fusion and separation, or other mechanisms to restore their aerobic respiratory function (81) (Figure 2D). MSCs are able to express high levels of RHOT1, a key RhO GTPase that supports mitochondrial transport from MSCs to adaptor cells (82). The transfer of mitochondria from MSCs with low levels of RHOT1 to injured intestinal epithelial cells is reduced in comparison with that from the MSCs with higher RHOT1. This reduction is not due to the decrease in nanotube formation, but to the decrease in RHOT1 mediated mitochondrial motility through the nanotubes. Mitochondria donated by MSCs can strengthen the tight junction of intestinal epithelial cells, provide sufficient ATP for intestinal epithelial cells, and reduce the oxidative stress response of recipient cells, thus relieving the intestinal symptoms of IBDs (83). In addition, MSCs can recognize mitochondria released from damaged intestinal epithelial cells as danger signals and activate the possibility of regenerative therapy (84). However, there is a need for further studies to determine the specific mechanism and key factors associated with mitochondrial transfer in MSC-based therapy for IBDs.
3.1.5 Involvement of PD-L1 in MSCs-mediated immunosuppression
The regulation of immune checkpoint pathway plays pivotal roles in the treatment of immune system diseases (85). Among them, the programmed cell death-1 (PD-1)/programmed cell death-ligand 1 (PD-L1) checkpoint pathway is one of the important components to inhibit the immune response and maintain immune homeostasis (86). PD-1, a costimulatory molecule, is induced to be expressed on the surface of activated T cells, B cells and NK cells. PD-L1 (also known as B7 homolog 1, or B7-H1) is a ligand of PD-1. It is expressed in T cells, B cells, DCs, macrophages, and some non-hematopoietic cells. The PD-L1 binding with PD-1 prevents immune diseases by inhibiting the activity of T and B cells (87). However, recent studies have shown that MSCs also express PD-L1, PD-L2 that contribute independently to their immunosuppressive effects, which provides a new research direction for the treatment of IBD (88). In the inflammatory environment, the PD-L1 and PD-L2 induced by IFN-γ turned out to be constitutively expressed on MSCs derived from bone marrow, liver, and placenta. Meanwhile, PD-1 and PD-2 overexpression on the surface of T cells influences MSC-mediated inhibitory effects on T cell proliferation and cytotoxicity in vitro (89). Therefore, it is worthwhile to explore whether the expression of PD-L1 and PD-L2 can also contribute to MSC mediated immunosuppression in the treatment IBDs (Figure 2E).
3.1.6 Signaling pathways
Several signaling pathways involved in immune and inflammatory responses have been implicated in MSC-based therapy of IBDs, including Wnt/β-catenin signaling pathway, the NF-κB pathway, the Notch signaling pathway, the PTEN/PI3K/Akt signaling pathway, and the FAS/FASL signaling pathway (90, 91). For instance, several reports have shown that MSCs inhibit the activity of macrophages, DCs, and T cells via TNF-α stimulated gene protein 6 (TSG-6) by activating the NF-κB signaling axis downstream of the CD44 receptor (92, 93). In addition, MSCs are capable of secreting monocyte chemotactic protein-1 (MCP-1) to promote apoptosis of CD4+ T cells via the FAS/FASL signaling pathway, thereby inhibiting T lymphocyte proliferation in IBDs (94).
3.2 MSCs alleviate IBDs via restoration of intestinal mucosal barrier
3.2.1 Repair the intestinal microenvironment
The intestinal microenvironment has an important role in MSC-mediated therapeutic effects on IBDs. Under a normal environment, the sustainable renewal and proliferation of intestinal stem cells promote the continuous renewal of intestinal epithelium. During injury or damage to the intestinal tract, the disrupted microenvironment impairs the ability of intestinal stem cells to self-renewal, proliferate, and differentiate (95). The microenvironment of intestinal stem cells is jointly regulated by Wnt, Notch, and BMP signaling pathways (96). For instance, intestinal Paneth cells and pericryptal fibroblasts are essential components of the niche that mediate major signaling pathways of Wnt, Notch, and BMP to regulate the mechanism of self-renewal, proliferation, and differentiation of intestinal MSCs (97). Constituents of the crypt lumen produced by epithelial cells or bacteria may have potent effects on the intestinal stem cells. Furthermore, intestinal subepithelial myofibroblasts mediate interactions between epithelial and mesenchymal cells via secreting multiple morphogenetic factors involved in stem cell homeostasis. This process will determine the intestinal architecture and the balance between intestinal cell proliferation and differentiation (98).
3.2.2 Repair intestinal epithelial cell
The view of “cell fusion” holds that when the in situ stem cells in tissues and mature cells are seriously damaged and unable to be rebuilt, the mature cells can be re-entered into the stem cell state by nuclear transfer (99). For example, BM-MSCs can differentiate into epithelial cells through “cell fusion” in vitro under certain culture conditions that include HGF, EGF, KGF, and IGF-II (100). In previous studies, Rizvi et al. used double-labeled intestinal epithelial cells with Y-FISH and enhanced green fluorescent protein immunohistochemical method, the former as a recipient source marker and the latter as a donor source marker. Among the tested cells, double-positive epithelial cells were found, which confirmed that donor BM-MSCs were involved in the repair of intestinal epithelial cell injury through the cell fusion mechanism after transplantation (101). However, Ferrand et al. argued that further studies are warranted to explore whether BM-MSCs can acquire epithelial characteristics through “cell fusion” with resident intestinal epithelial cells after engraftment (102).
3.2.3 Tissue homing and tissue regeneration
The homing or recruitment of MSCs to the damaged tissue can also contribute an important role to the therapeutic efficacy of MSCs-based therapy for various inflammatory diseases, including IBDs. Tissue-oriented homing means that MSCs have the capacity to migrate and engraft specifically into damaged tissue sites, where they can differentiate into functional cells to replace damaged or diseased cells (103). Previous studies have shown that the molecular mechanisms underlying tissue homing of MSCs involve the expression of chemotactic receptors, matrix metalloproteinases (MMPs), and adhesion molecules. The chemotactic receptors mainly include CCR1, CCR2, CCR4, CCR5, CCR9, CXCR1, CXCR4, and CXCR5, whereby CCR2 and CCR4 assist the migration of MSCs, and CXCR4/SDF-1 axis can directly promote MSCs migration (104). The receptors expressed on MSCs can specifically bind to their ligands, which are released by certain histiocytes in the enteritis environment, such as CCL5, CCL19, CCL22, CCL25, CXCL8, CXCL13, etc. (105). MSCs may alleviate disease severity by expressing some adhesion molecules, such as CD29, CD44, CD49e, CD54, CD105, CD106, and CD166, which are essential for MSCs’ tissue homing (104). In addition, the adhesion molecules including P-selectin, VCAM-1, ALCAM, and VLA-4 have been demonstrated to promote the adhesion of MSCs to endothelial cells (106). Therefore, the expression of adhesion molecules may promote MSCs to integrate into damaged intestinal tissues to facilitate tissue regeneration through differentiation into intestinal epithelial cells, suppressing inflammation, and promoting angiogenesis (107). Additionally, several MMPs, such as MMP-2 and MT1- MMP, are also indispensable for tissue homing and tissue regeneration of MSCs. Tissue regeneration has been found after MSCs arrive at inflamed tissue (108). It is well accepted that MSCs contribute to tissue repair, mainly due to their ability to stimulate local tissue proliferation and survival by secreting proteolytic enzymes and angiogenic factors, while inhibiting tissue apoptosis and fibrosis (109). Some bioactive molecules, such as NO, IFN-γ, and TNF-α, can also stimulate tissue repair functions of MSCs through altering their migration, differentiation, or immunologic properties (110, 111). In particular, the migration rate and duration of MSCs are decisive factor affecting the efficiency of tissue repair and regeneration. However, there are still few studies on tissue homing and tissue regeneration through MSCs in the treatment of IBDs. Table 2 Summarizes the related mechanisms of MSCs therapy.
4 Route of MSCs-based therapy for IBDs
The administration route can affect the therapeutic efficacy of MSCs for various pathological conditions, including IBDs. Two main approaches have been developed for administration of MSCs for IBDs treatment: local administration of MSCs for treatment of perianal fistulizing CD and systemic administration of MSCs for treatment of luminal inflammatory disease (131). Studies have demonstrated that local administration of autologous or allogeneic BM-MSCs and AT-MSCs achieved obvious clinical efficacy in patients with fistulazing CD by downregulating local immune responses and initiating wound healing (131). The effects of systemic administration of autologous or allogeneic MSCs have been evaluated in clinical trials., indicating that the systemic administration of AT-MSCs significantly improved the clinical outcome and prognosis for IBDs (132). However, there is still no standard reference for selecting the route for MSCs administration, either local or systemic administration, which might be determined according to the specification of diseases to be treated.
5 MSCs-based therapy for IBDs
MSCs can differentiate from other stem cells. Human pluripotent stem cells (hPSCs) include human embryonic stem cells (hESCs) and induced pluripotent stem cells (iPSCs), both of which can differentiate into MSCs (133). MSCs derived from hESCs and iPSCs exhibited similar properties, such as their ability to secrete anti-inflammatory soluble factors, and to restore the intestinal mucosal barrier in IBDs (134). hESCs-MSCs exhibit potent immunosuppressive on the colonic mucosa through preferentially homing to inflamed tissues and secondary lymphoid organs. It has a very effective inhibitory effect on the proliferation of Th1, but not the Th2 (135). However, iPSCs-MSCs can exhibit immunosuppressive directly inhibiting Th2 differentiation and promoting Tregs responses, depending on the mechanism of PGE2 production and cell-cell contact (136). For instance, Xu et al. reported that intravenous injection of hESCs-MSCs alleviated both acute and chronic DSS-induced colitis in mice through increasing endogenous IGF-1 secretion and maintaining colonic epithelial integrity and regenerative (137). Soontararak et al. showed that iPSCs-MSCs ameliorated clinical abnormalities in IBDs by stimulating intestinal epithelial cell proliferation increasing the numbers of Lgr5+ intestinal stem cells, and increasing intestinal angiogenesis, changing the microbiome in colitis and restoring its normal microecology (124).
Due to the self-renewal, multipotency, and immunosuppressive characteristics of MSCs, more clinical trials have been conducted to find a suitable treatment for IBDs using MSCs. In recent years, a growing number of clinical trials have proved the beneficial effects of MSCs on IBDs. Through searching the ClinicalTrials.gov database, 34 clinical trials have been identified on MSC based therapy for IBDs (Table 3). In these data, we found that different sources of MSCs, different injection methods, and doses of IBDs can improve clinical symptoms in different degrees. However, the clinical treatment of IBDs has a large sample size and a long cycle. The therapeutic effect still needs to be further observed.
Besides using MSCs to treat IBDs, it has also been shown that MSCs exhibit a powerful therapeutic function in CAC, which develops from chronic enteritis and frequently occurs in areas of chronic inflammation (31). The research found that hUC-MSCs migrated into the intestinal structure and then moved to the colon to reduce the number of tumors with the reduction of Ki67 by inhibiting chronic inflammation and the Smad2 signaling pathway (138). However, the therapeutic effect of MSCs in colorectal cancer is in dispute due to the potent immunosuppressive properties of MSCs that can contribute to the immunosuppressive tumor microenvironment favoring immune evasion of cancer cells, and thus, negatively affect the therapeutic effect of CAC (139).
MSCs-based therapy for many other diseases has also shown great promise. Numerous basic studies and clinical trials have proved that MSCs exhibit an obvious therapeutic effect on nervous system diseases, cardiovascular system diseases, pulmonary lung diseases, etc., and show potent regenerative potential in diseased liver, lung, kidney, skin, and other organs (140, 141). The regenerative therapeutic potentials of MSCs are mainly attributed to their unique properties, such as self-renewal and multipotent differentiation capability, immunomodulatory/anti-inflammatory function, easy isolation and expansion in vitro, etc. Meanwhile, the lack of expression of the major MHC II molecule and the low expression level of MHC I and co-stimulatory molecule (CD40, CD80, CD86, and CD154) coin MSCs with a hypoimmunogenic and immune tolerant phenotype, which allows MSCs to escape immune recognition and clearance in vivo delivery (142). In addition, MSCs have great application value in tissue engineering, wound repair, gene therapy, cell replacement therapy, etc (143).
6 Unanswered questions and future perspectives
Even though the regenerative and therapeutic potentials of MSCs have been widely studied in both preclinical studies and clinical trials, it remains largely unknown about the cellular and molecular mechanisms underlying MSC mediated therapeutic effects in vivo. There are still numerous issues to be solved when MSCs are widely used in the clinic, such as the suitable source of MSCs, the dosage and modality of administration, the long-term fate of transplanted cells, and the potential side effects e.g. tumorigenicity following transplantation, etc. Similarly, such issues also exist in MSC-based therapy of IBDs. Therefore, more in-depth mechanistic basic and preclinical studies, clinical trials, and long-term follow-up are required to establish optimal treatment modalities for MSC based therapy of IBDs.
6.1 Sources of MSCs
Sources of MSCs will obviously influence the therapeutic effect. For a long time, BM-MSCs are the main source for the acquisition of MSCs, but their isolation is an invasive treatment method (12). Therefore, alternative sources of MSCs, such as umbilical cord blood and adipose tissue, have been aggressively pursued. The biggest advantages of AT-MSCs are that they can be acquired in large numbers and are less invasive procedures. There is a growing body of data showing differences between BM-, AT-, and UCB-MSCs, including their immunomodulatory properties (144). Several reports found that AT- and UCB-MSCs may suppress immune responses more effectively than BM-MSCs in vitro (145). However, there are still few studies on the sources of L-MSC in the treatment of IBDs. Therefore, studies comparing their efficacy in vivo will need to be done to choose the best type of MSCs to use for IBDs treatment.
6.2 Modalities and dosage of administration
There is a general agreement by now that local injection of MSCs is the most suitable route of administration for treating IBDs. In the recent trials, data have shown that local injection of MSCs into the fistula wall itself was appropriate for perianal fistulas, which makes partial healing of the lesion without rejection of the cells and adverse effects. The detailed procedure of the local injection has a significant impact on the observation and needs to be better elucidated (146). Conversely, systemic administration is a better option in luminal CD disease. Because the intravenous injection is easy, minimally invasive, and safe for patients and plays an important role in the attenuation or progression of CD (147). However, accumulating evidence has shown that a small part of MSCs through intravenous injection is easily stuck in the lungs, so the proportion of MSCs reaching the inflamed intestine needs to be further evaluated. Meanwhile, it is vital that the amount of MSCs transplanted to patients is clearly defined, with an eye toward balancing safety with efficacy in MSC-based therapy (148). The dose of MSCs administration were determined by the sources of MSCs, injection method, and the type of disease. A large number of experiments show that lower dose of MSCs seems to have a higher healing rate in perianal fistulizing Crohn’s disease. According to Molendijk et al’s experiments, the higher healing rate was observed in patients that received 3×107 MSCs when compared to patients that received 9×107 MSCs (131). Therefore, the optimal number of MSCs cannot be determined due to the impact of multiple factors, and dose escalation study is required to address this problem under the condition of the same independent variable.
6.3 Combination of MSCs and immunosuppressant
The combination of MSCs and other drugs will alter the therapeutic effect. MSCs have been used together with immunosuppressive drugs due to their shared common targets in clinical studies. As reported by Duijvestein M, incubation of MSCs with physiological concentrations of immunosuppressive drugs, such as azathioprine, mercaptopurine, methotrexate, and anti-TNFα compounds, does not directly alter the phenotypical, functional properties, survival, and inhibitory effects on peripheral blood mononuclear cell growth in vitro. There may even be an additive effect between 6-mercaptopurine and anti-TNFα antibodies (149). However, azathioprine can reduce the proliferation of rat BM-MSCs and increase their apoptosis and necrosis at a higher level in vitro (150). Dexamethasone has been shown to restrain the expression of iNOS and IDO, thus reversing MSC-mediated immunosuppression in vitro and abolishing the therapeutic effects of MSCs in vivo (151). The clinic experiments have also proven that steroids and MSCs should not be administered in combination (152). Nevertheless, there are few studies on the use of L-MSCs in combination with immunosuppressants in IBDs treatment. Therefore, the molecular mechanisms of interaction between MSCs and immunosuppressants should be further studied carefully in order to enable more effective manipulation of MSCs function for clinical applications.
6.4 MSC-related adverse event
The research showed that intravenous MSCs may cause mild and transient fever, headache, insomnia, dysgeusia, and diarrhea, but these symptoms will disappear after a period of time (153). To date, no serious MSCs related adverse effects have been reported in clinical studies, including clinical trials with patients suffering from IBDs or other diseases (154). Nevertheless, the most worrying adverse effect was whether MSCs have the potential to promote tumor growth and mitigate the effectiveness of treating enteritis due to their tumorigenic characteristics activated by oncogenes (155). To date, the clinical adverse effects have not been fully understood and then further studies are warranted in future clinical studies.
7 Conclusion
MSCs based therapy has unique advantages and shows its irreplaceable potential in medical applications nowadays. It represents a novel therapeutic option for IBDs and other diseases, showing durable efficacy, low trauma, and low recurrence rates, even in cases in which healing cannot be achieved with biologics or conventional surgical procedures cannot be performed. However, MSCs based therapy for IBDs is still at an exploratory stage and further basic mechanistic and clinical studies are warranted.
Author contributions
All authors contributed significantly to the drafting and editing of this manuscript. JZ, ZC, and WY conceived the manuscript idea and wrote the manuscript. YL, WY, and JZ revised the manuscript content. ZY, XZ, and BL created the manuscript tables and figures. All authors contributed to the article and approved the submitted version.
Funding
This study was supported by grants from the Dongguan Social Science and Technology Development Project (20211800904532, 201950715025192), Natural Science Foundation of Guangdong Province (2021B1515140004, 2021B1515140066, 2019A1515110042, 2019A1515011713), Characteristic Innovation Experimental Project of Ordinary Universities in Guangdong Province (2020KTSCX044), Discipline Construction Project of Guangdong Medical University, Research Foundation of Guangdong Medical University for Ph.D. Staff (GDMUB2019038, GDMUB2020017), the Medical Science Foundation of Guangdong Province (A2021438, A2020211).
Acknowledgments
This study was supported by Dongguan Key Laboratory of Medical Bioactive Molecular Developmental and Translational Research.
Conflict of interest
The authors declare that the research was conducted in the absence of any commercial or financial relationships that could be construed as a potential conflict of interest.
Publisher’s note
All claims expressed in this article are solely those of the authors and do not necessarily represent those of their affiliated organizations, or those of the publisher, the editors and the reviewers. Any product that may be evaluated in this article, or claim that may be made by its manufacturer, is not guaranteed or endorsed by the publisher.
References
1. Fujino S, Andoh A, Bamba S, Ogawa A, Hata K, Araki Y, et al. Increased expression of interleukin 17 in inflammatory bowel disease. Gut (2003) 52:65–70. doi: 10.1136/gut.52.1.65
2. Rogler G, Vavricka S. Exposome in ibd: Recent insights in environmental factors that influence the onset and course of ibd. Inflammation Bowel Dis (2015) 21:400–8. doi: 10.1097/MIB.0000000000000229
3. Abraham BP. Symptom management in inflammatory bowel disease. Expert Rev Gastroenterol Hepatol (2015) 9:953–67. doi: 10.1586/17474124.2015.1038241
4. Nowakowski J, Chrobak AA, Dudek D. Psychiatric illnesses in inflammatory bowel diseases - psychiatric comorbidity and biological underpinnings. Psychiatr Pol (2016) 50:1157–66. doi: 10.12740/PP/62382
5. Bonaz B, Sinniger V, Pellissier S. Therapeutic potential of vagus nerve stimulation for inflammatory bowel diseases. Front Neurosci (2021) 15:650971. doi: 10.3389/fnins.2021.650971
6. Rubin DT, LoSavio A, Yadron N, Huo D, Hanauer SB. Aminosalicylate therapy in the prevention of dysplasia and colorectal cancer in ulcerative colitis. Clin Gastroenterol Hepatol (2006) 4:1346–50. doi: 10.1016/j.cgh.2006.08.014
7. Hanauer SB, Feagan BG, Lichtenstein GR, Mayer LF, Schreiber S, Colombel JF, et al. Maintenance infliximab for crohn's disease: The accent I randomised trial. Lancet (2002) 359:1541–9. doi: 10.1016/S0140-6736(02)08512-4
8. Desai D, Desai N. Colorectal cancer surveillance in inflammatory bowel disease: A critical analysis. World J Gastrointest Endosc (2014) 6:541–8. doi: 10.4253/wjge.v6.i11.541
9. Gao R, Gao Z, Huang L, Qin H. Gut microbiota and colorectal cancer. Eur J Clin Microbiol Infect Dis (2017) 36:757–69. doi: 10.1007/s10096-016-2881-8
10. da Silva Meirelles L, Chagastelles PC, Nardi NB. Mesenchymal stem cells reside in virtually all post-natal organs and tissues. J Cell Sci (2006) 119:2204–13. doi: 10.1242/jcs.02932
11. Friedenstein AJ, Chailakhyan RK, Latsinik NV, Panasyuk AF, Keiliss-Borok IV. Stromal cells responsible for transferring the microenvironment of the hemopoietic tissues. cloning in vitro and retransplantation in vivo. Transplantation (1974) 17:331–40. doi: 10.1097/00007890-197404000-00001
12. Stenderup K, Justesen J, Clausen C, Kassem M. Aging is associated with decreased maximal life span and accelerated senescence of bone marrow stromal cells. Bone (2003) 33:919–26. doi: 10.1016/j.bone.2003.07.005
13. Kim D, Lee AE, Xu Q, Zhang Q, Le AD. Gingiva-derived mesenchymal stem cells: Potential application in tissue engineering and regenerative medicine - a comprehensive review. Front Immunol (2021) 12:667221. doi: 10.3389/fimmu.2021.667221
14. Dominici M, Le Blanc K, Mueller I, Slaper-Cortenbach I, Marini F, Krause D, et al. Minimal criteria for defining multipotent mesenchymal stromal cells. the international society for cellular therapy position statement. Cytotherapy (2006) 8:315–7. doi: 10.1080/14653240600855905
15. Vizoso FJ, Eiro N, Costa L, Esparza P, Landin M, Diaz-Rodriguez P, et al. Mesenchymal stem cells in homeostasis and systemic diseases: Hypothesis, evidences, and therapeutic opportunities. Int J Mol Sci (2019) 20:3738. doi: 10.3390/ijms20153738
16. Benabid A, Peduto L. Mesenchymal perivascular cells in immunity and disease. Curr Opin Immunol (2020) 64:50–5. doi: 10.1016/j.coi.2020.03.009
17. Sveiven SN, Nordgren TM. Lung-resident mesenchymal stromal cells are tissue-specific regulators of lung homeostasis. Am J Physiol Lung Cell Mol Physiol (2020) 319:L197–210. doi: 10.1152/ajplung.00049.2020
18. Spallanzani RG. Visceral adipose tissue mesenchymal stromal cells in the intersection of immunology and metabolism. Am J Physiol Endocrinol Metab (2021) 320:E512–E19. doi: 10.1152/ajpendo.00341.2020
19. Molina T, Fabre P, Dumont NA. Fibro-adipogenic progenitors in skeletal muscle homeostasis, regeneration and diseases. Open Biol (2021) 11:210110. doi: 10.1098/rsob.210110
20. Galland S, Stamenkovic I. Mesenchymal stromal cells in cancer: A review of their immunomodulatory functions and dual effects on tumor progression. J Pathol (2020) 250:555–72. doi: 10.1002/path.5357
21. Samarelli AV, Tonelli R, Heijink I, Martin Medina A, Marchioni A, Bruzzi G, et al. Dissecting the role of mesenchymal stem cells in idiopathic pulmonary fibrosis: Cause or solution. Front Pharmacol (2021) 12:692551. doi: 10.3389/fphar.2021.692551
22. Caprioli F, Pallone F, Monteleone G. Th17 immune response in ibd: A new pathogenic mechanism. J Crohns Colitis (2008) 2:291–5. doi: 10.1016/j.crohns.2008.05.004
23. Niu J, Miao J, Tang Y, Nan Q, Liu Y, Yang G, et al. Identification of environmental factors associated with inflammatory bowel disease in a southwestern highland region of China: A nested case-control study. PLoS One (2016) 11:e0153524. doi: 10.1371/journal.pone.0153524
24. Yamamoto T, Watanabe T. Strategies for the prevention of postoperative recurrence of crohn's disease. Colorectal Dis (2013) 15:1471–80. doi: 10.1111/codi.12326
25. Thia KT, Loftus EV Jr., Sandborn WJ, Yang SK. An update on the epidemiology of inflammatory bowel disease in Asia. Am J Gastroenterol (2008) 103:3167–82. doi: 10.1111/j.1572-0241.2008.02158.x
26. Tsironi E, Feakins RM, Probert CS, Rampton DS, Phil D. Incidence of inflammatory bowel disease is rising and abdominal tuberculosis is falling in bangladeshis in East London, united kingdom. Am J Gastroenterol (2004) 99:1749–55. doi: 10.1111/j.1572-0241.2004.30445.x
27. Bitton A, Dobkin PL, Edwardes MD, Sewitch MJ, Meddings JB, Rawal S, et al. Predicting relapse in crohn's disease: A biopsychosocial model. Gut (2008) 57:1386–92. doi: 10.1136/gut.2007.134817
28. Samadder NJ, Valentine JF, Guthery S, Singh H, Bernstein CN, Leighton JA, et al. Family history associates with increased risk of colorectal cancer in patients with inflammatory bowel diseases. Clin Gastroenterol Hepatol (2019) 17:1807–13.e1. doi: 10.1016/j.cgh.2018.09.038
29. Silverberg MS, Mirea L, Bull SB, Murphy JE, Steinhart AH, Greenberg GR, et al. A population- and family-based study of Canadian families reveals association of hla Drb1*0103 with colonic involvement in inflammatory bowel disease. Inflammation Bowel Dis (2003) 9:1–9. doi: 10.1097/00054725-200301000-00001
30. Yang F, Wang D, Li Y, Sang L, Zhu J, Wang J, et al. Th1/Th2 balance and Th17/Treg-mediated immunity in relation to murine resistance to dextran sulfate-induced colitis. J Immunol Res (2017) 2017:7047201. doi: 10.1155/2017/7047201
31. Kang J, Zhang L, Luo X, Ma X, Wang G, Yang Y, et al. Systematic exposition of mesenchymal stem cell for inflammatory bowel disease and its associated colorectal cancer. BioMed Res Int (2018) 2018:9652817. doi: 10.1155/2018/9652817
32. Strober W, Fuss IJ. Proinflammatory cytokines in the pathogenesis of inflammatory bowel diseases. Gastroenterology (2011) 140:1756–67. doi: 10.1053/j.gastro.2011.02.016
33. Siakavellas SI, Bamias G. Role of the il-23/Il-17 axis in crohn's disease. Discovery Med (2012) 14:253–62.
34. Bandzar S, Gupta S, Platt MO. Crohn's disease: A review of treatment options and current research. Cell Immunol (2013) 286:45–52. doi: 10.1016/j.cellimm.2013.11.003
35. Chao K, Zhang S, Yao J, He Y, Chen B, Zeng Z, et al. Imbalances of Cd4(+) T-cell subgroups in crohn's disease and their relationship with disease activity and prognosis. J Gastroenterol Hepatol (2014) 29:1808–14. doi: 10.1111/jgh.12592
36. Shimizu H, Suzuki K, Watanabe M, Okamoto R. Stem cell-based therapy for inflammatory bowel disease. Intest Res (2019) 17:311–16. doi: 10.5217/ir.2019.00043
37. Pasztoi M, Ohnmacht C. Tissue niches formed by intestinal mesenchymal stromal cells in mucosal homeostasis and immunity. Int J Mol Sci (2022) 23:5158. doi: 10.3390/ijms23095181
38. Loe AKH, Rao-Bhatia A, Kim JE, Kim TH. Mesenchymal niches for digestive organ development, homeostasis, and disease. Trends Cell Biol (2021) 31:152–65. doi: 10.1016/j.tcb.2020.11.010
39. Ocansey DKW, Wang L, Wang J, Yan Y, Qian H, Zhang X, et al. Mesenchymal stem cell-gut microbiota interaction in the repair of inflammatory bowel disease: An enhanced therapeutic effect. Clin Transl Med (2019) 8:31. doi: 10.1186/s40169-019-0251-8
40. Barnhoorn MC, Hakuno SK, Bruckner RS, Rogler G, Hawinkels L, Scharl M. Stromal cells in the pathogenesis of inflammatory bowel disease. J Crohns Colitis (2020) 14:995–1009. doi: 10.1093/ecco-jcc/jjaa009
41. Jasso GJ, Jaiswal A, Varma M, Laszewski T, Grauel A, Omar A, et al. Colon stroma mediates an inflammation-driven fibroblastic response controlling matrix remodeling and healing. PLoS Biol (2022) 20:e3001532. doi: 10.1371/journal.pbio.3001532
42. English K. Mechanisms of mesenchymal stromal cell immunomodulation. Immunol Cell Biol (2013) 91:19–26. doi: 10.1038/icb.2012.56
43. Zhang Q, Shi S, Liu Y, Uyanne J, Shi Y, Shi S, et al. Mesenchymal stem cells derived from human gingiva are capable of immunomodulatory functions and ameliorate inflammation-related tissue destruction in experimental colitis. J Immunol (2009) 183:7787–98. doi: 10.4049/jimmunol.0902318
44. Trivanovic D, Krstic J, Djordjevic IO, Mojsilovic S, Santibanez JF, Bugarski D, et al. The roles of mesenchymal Stromal/Stem cells in tumor microenvironment associated with inflammation. Mediators Inflammation (2016) 2016:7314016. doi: 10.1155/2016/7314016
45. Liu RF, Wang F, Wang Q, Zhao XC, Zhang KM. Research note mesenchymal stem cells from skin lesions of psoriasis patients promote proliferation and inhibit apoptosis of hacat cells. Genet Mol Res (2015) 14:17758–67. doi: 10.4238/2015.December.21.49
46. Ahn SY. The role of mscs in the tumor microenvironment and tumor progression. Anticancer Res (2020) 40:3039–47. doi: 10.21873/anticanres.14284
47. Sun Z, Wang S, Zhao RC. The roles of mesenchymal stem cells in tumor inflammatory microenvironment. J Hematol Oncol (2014) 7:14. doi: 10.1186/1756-8722-7-14
48. Jo H, Brito S, Kwak BM, Park S, Lee MG, Bin BH. Applications of mesenchymal stem cells in skin regeneration and rejuvenation. Int J Mol Sci (2021) 22:2410. doi: 10.3390/ijms22052410
49. Couto de Carvalho LA, Tosta Dos Santos SL, Sacramento LV, de Almeida VRJ, de Aquino Xavier FC, Dos Santos JN, et al. Mesenchymal stem cell markers in periodontal tissues and periapical lesions. Acta Histochem (2020) 122:151636. doi: 10.1016/j.acthis.2020.151636
50. Liu R, Chang W, Li J, Cheng Y, Dang E, Yang X, et al. Mesenchymal stem cells in psoriatic lesions affect the skin microenvironment through circular rna. Exp Dermatol (2019) 28:292–99. doi: 10.1111/exd.13890
51. Ciavarella C, Pasquinelli G. The dual nature of mesenchymal stem cells (Mscs): Yin and yang of the inflammatory process. Saudi Arabia: Update on mesenchymal and induced pluripotent stem cells (2019).
52. Dokic J, Tomic S, Cerovic S, Todorovic V, Rudolf R, Colic M. Characterization and immunosuppressive properties of mesenchymal stem cells from periapical lesions. J Clin Periodontol (2012) 39:807–16. doi: 10.1111/j.1600-051X.2012.01917.x
53. Galland S, Vuille J, Martin P, Letovanec I, Caignard A, Fregni G, et al. Tumor-derived mesenchymal stem cells use distinct mechanisms to block the activity of natural killer cell subsets. Cell Rep (2017) 20:2891–905. doi: 10.1016/j.celrep.2017.08.089
54. Mishra R, Dhawan P, Srivastava AS, Singh AB. Inflammatory bowel disease: Therapeutic limitations and prospective of the stem cell therapy. World J Stem Cells (2020) 12:1050–66. doi: 10.4252/wjsc.v12.i10.1050
55. Chabannes D, Hill M, Merieau E, Rossignol J, Brion R, Soulillou JP, et al. A role for heme oxygenase-1 in the immunosuppressive effect of adult rat and human mesenchymal stem cells. Blood (2007) 110:3691–4. doi: 10.1182/blood-2007-02-075481
56. Mohammadipoor A, Antebi B, Batchinsky AI, Cancio LC. Therapeutic potential of products derived from mesenchymal Stem/Stromal cells in pulmonary disease. Respir Res (2018) 19:218. doi: 10.1186/s12931-018-0921-x
57. Bernardo ME, Fibbe WE. Mesenchymal stromal cells: Sensors and switchers of inflammation. Cell Stem Cell (2013) 13:392–402. doi: 10.1016/j.stem.2013.09.006
58. Dazzi F, Krampera M. Mesenchymal stem cells and autoimmune diseases. Best Pract Res Clin Haematol (2011) 24:49–57. doi: 10.1016/j.beha.2011.01.002
59. Newman RE, Yoo D, LeRoux MA, Danilkovitch-Miagkova A. Treatment of inflammatory diseases with mesenchymal stem cells. Inflammation Allergy Drug Targets (2009) 8:110–23. doi: 10.2174/187152809788462635
60. Fuenzalida P, Kurte M, Fernandez-O'ryan C, Ibanez C, Gauthier-Abeliuk M, Vega-Letter AM, et al. Toll-like receptor 3 pre-conditioning increases the therapeutic efficacy of umbilical cord mesenchymal stromal cells in a dextran sulfate sodium-induced colitis model. Cytotherapy (2016) 18:630–41. doi: 10.1016/j.jcyt.2016.02.002
61. Sangaran PG, Ibrahim ZA, Chik Z, Mohamed Z, Ahmadiani A. Lipopolysaccharide pre-conditioning attenuates pro-inflammatory responses and promotes cytoprotective effect in differentiated Pc12 cell lines Via pre-activation of toll-like receptor-4 signaling pathway leading to the inhibition of caspase-3/Nuclear factor-kappaappa b pathway. Front Cell Neurosci (2020) 14:598453. doi: 10.3389/fncel.2020.598453
62. Bai Y, Wang J, He Z, Yang M, Li L, Jiang H. Mesenchymal stem cells reverse diabetic nephropathy disease Via lipoxin A4 by targeting transforming growth factor beta (Tgf-Beta)/Smad pathway and pro-inflammatory cytokines. Med Sci Monit (2019) 25:3069–76. doi: 10.12659/MSM.914860
63. Favaro E, Carpanetto A, Caorsi C, Giovarelli M, Angelini C, Cavallo-Perin P, et al. Human mesenchymal stem cells and derived extracellular vesicles induce regulatory dendritic cells in type 1 diabetic patients. Diabetologia (2016) 59:325–33. doi: 10.1007/s00125-015-3808-0
64. J Z, S C, C L, Z Y, B L, Y L, et al. Mesenchymal Stem/Stromal cells-derived il-6 promotes nasopharyngeal carcinoma growth and resistance to cisplatin Via upregulating Cd73 expression. J Cancer (2020) 11:2068–79. doi: 10.7150/jca.37932
65. Sioud M. New insights into mesenchymal stromal cell-mediated T-cell suppression through galectins. Scand J Immunol (2011) 73:79–84. doi: 10.1111/j.1365-3083.2010.02491.x
66. Sala E, Genua M, Petti L, Anselmo A, Arena V, Cibella J, et al. Mesenchymal stem cells reduce colitis in mice Via release of Tsg6, independently of their localization to the intestine. Gastroenterology (2015) 149:163–76 e20. doi: 10.1053/j.gastro.2015.03.013
67. Kyurkchiev D, Bochev I, Ivanova-Todorova E, Mourdjeva M, Oreshkova T, Belemezova K, et al. Secretion of immunoregulatory cytokines by mesenchymal stem cells. World J Stem Cells (2014) 6:552–70. doi: 10.4252/wjsc.v6.i5.552
68. Chao K, Zhang S, Qiu Y, Chen X, Zhang X, Cai C, et al. Human umbilical cord-derived mesenchymal stem cells protect against experimental colitis Via Cd5(+) b regulatory cells. Stem Cell Res Ther (2016) 7:109. doi: 10.1186/s13287-016-0376-2
69. Rahimzadeh A, Mirakabad FS, Movassaghpour A, Shamsasenjan K, Kariminekoo S, Talebi M, et al. Biotechnological and biomedical applications of mesenchymal stem cells as a therapeutic system. Artif Cells Nanomed Biotechnol (2016) 44:559–70. doi: 10.3109/21691401.2014.968823
70. Prevosto C, Zancolli M, Canevali P, Zocchi MR, Poggi A. Generation of Cd4+ or Cd8+ regulatory T cells upon mesenchymal stem cell-lymphocyte interaction. Haematologica (2007) 92:881–8. doi: 10.3324/haematol.11240
71. Naserian S, Shamdani S, Arouche N, Uzan G. Regulatory T cell induction by mesenchymal stem cells depends on the expression of Tnfr2 by T cells. Stem Cell Res Ther (2020) 11:534. doi: 10.1186/s13287-020-02057-z
72. Azevedo RI, Minskaia E, Fernandes-Platzgummer A, Vieira AIS, da Silva CL, Cabral JMS, et al. Mesenchymal stromal cells induce regulatory T cells Via epigenetic conversion of human conventional Cd4 T cells in vitro. Stem Cells (2020) 38:1007–19. doi: 10.1002/stem.3185
73. Sabbagh Q, Andre-Gregoire G, Alves-Nicolau C, Dupont A, Bidere N, Jouglar E, et al. The Von willebrand factor stamps plasmatic extracellular vesicles from glioblastoma patients. Sci Rep (2021) 11:22792. doi: 10.1038/s41598-021-02254-7
74. X Z, Q X, Z Y, Y L, Z C, M H, et al. Mesenchymal stem cells and tuberculosis: Clinical challenges and opportunities. Front Immunol (2021) 12:695278. doi: 10.3389/fimmu.2021.695278
75. Fu Y, Karbaat L, Wu L, Leijten J, Both SK, Karperien M. Trophic effects of mesenchymal stem cells in tissue regeneration. Tissue Eng Part B Rev (2017) 23:515–28. doi: 10.1089/ten.TEB.2016.0365
76. Tsiapalis D, O'Driscoll L. Mesenchymal stem cell derived extracellular vesicles for tissue engineering and regenerative medicine applications. Cells (2020) 9:991. doi: 10.3390/cells9040991
77. Yang J, Liu XX, Fan H, Tang Q, Shou ZX, Zuo DM, et al. Extracellular vesicles derived from bone marrow mesenchymal stem cells protect against experimental colitis Via attenuating colon inflammation, oxidative stress and apoptosis. PLoS One (2015) 10:e0140551. doi: 10.1371/journal.pone.0140551
78. Liu H, Liang Z, Wang F, Zhou C, Zheng X, Hu T, et al. Exosomes from mesenchymal stromal cells reduce murine colonic inflammation Via a macrophage-dependent mechanism. JCI Insight (2019) 4:e131273. doi: 10.1172/jci.insight.131273
79. Chang CL, Chen CH, Chiang JY, Sun CK, Chen YL, Chen KH, et al. Synergistic effect of combined melatonin and adipose-derived mesenchymal stem cell (Admsc)-derived exosomes on amelioration of dextran sulfate sodium (Dss)-induced acute colitis. Am J Transl Res (2019) 11:2706–24.
80. Diaz-Garrido N, Badia J, Baldoma L. Modulation of dendritic cells by microbiota extracellular vesicles influences the cytokine profile and exosome cargo. Nutrients (2022) 14:344. doi: 10.3390/nu14020344
81. Murray LMA, Krasnodembskaya AD. Concise review: Intercellular communication Via organelle transfer in the biology and therapeutic applications of stem cells. Stem Cells (2019) 37:14–25. doi: 10.1002/stem.2922
82. T A, S M, B P, M K, S S, M K, et al. Miro1 regulates intercellular mitochondrial transport & enhances mesenchymal stem cell rescue efficacy. EMBO J (2014) 33:994–1010. doi: 10.1002/embj.201386030
83. Hsu YC, Wu YT, Yu TH, Wei YH. Mitochondria in mesenchymal stem cell biology and cell therapy: From cellular differentiation to mitochondrial transfer. Semin Cell Dev Biol (2016) 52:119–31. doi: 10.1016/j.semcdb.2016.02.011
84. Mahrouf-Yorgov M, Augeul L, Da Silva CC, Jourdan M, Rigolet M, Manin S, et al. Mesenchymal stem cells sense mitochondria released from damaged cells as danger signals to activate their rescue properties. Cell Death Differ (2017) 24:1224–38. doi: 10.1038/cdd.2017.51
85. Wykes MN, Lewin SR. Immune checkpoint blockade in infectious diseases. Nat Rev Immunol (2018) 18:91–104. doi: 10.1038/nri.2017.112
86. Xu F, Fei Z, Dai H, Xu J, Fan Q, Shen S, et al. Mesenchymal stem cell-derived extracellular vesicles with high pd-L1 expression for autoimmune diseases treatment. Adv Mater (2022) 34:e2106265. doi: 10.1002/adma.202106265
87. Sun C, Mezzadra R, Schumacher TN. Regulation and function of the pd-L1 checkpoint. Immunity (2018) 48:434–52. doi: 10.1016/j.immuni.2018.03.014
88. Davies LC, Heldring N, Kadri N, Le Blanc K. Mesenchymal stromal cell secretion of programmed death-1 ligands regulates T cell mediated immunosuppression. Stem Cells (2017) 35:766–76. doi: 10.1002/stem.2509
89. de Mare-Bredemeijer EL, Mancham S, Verstegen MM, de Ruiter PE, van Gent R, O'Neill D, et al. Human graft-derived mesenchymal stromal cells potently suppress alloreactive T-cell responses. Stem Cells Dev (2015) 24:1436–47. doi: 10.1089/scd.2014.0485
90. Qiu Y, Guo J, Mao R, Chao K, Chen BL, He Y, et al. Tlr3 preconditioning enhances the therapeutic efficacy of umbilical cord mesenchymal stem cells in tnbs-induced colitis Via the Tlr3-Jagged-1-Notch-1 pathway. Mucosal Immunol (2017) 10:727–42. doi: 10.1038/mi.2016.78
91. Yu Y, Liao L, Shao B, Su X, Shuai Y, Wang H, et al. Knockdown of microrna let-7a improves the functionality of bone marrow-derived mesenchymal stem cells in immunotherapy. Mol Ther (2017) 25:480–93. doi: 10.1016/j.ymthe.2016.11.015
92. Choi H, Lee RH, Bazhanov N, Oh JY, Prockop DJ. Anti-inflammatory protein tsg-6 secreted by activated mscs attenuates zymosan-induced mouse peritonitis by decreasing Tlr2/Nf- b signaling in resident macrophages. Blood (2011) 118:330–38. doi: 10.1182/blood-2010-12-327353
93. Oh JY, Lee RH, Ji MY, Ko JH, Prockop DJ. Intravenous mesenchymal stem cells prevented rejection of allogeneic corneal transplants by aborting the early inflammatory response. Mol Ther (2012) 20:2143–52. doi: 10.1038/mt.2012.165
94. Yang R, Yu T, Liu D, Shi S, Zhou Y. Hydrogen sulfide promotes immunomodulation of gingiva-derived mesenchymal stem cells Via the Fas/Fasl coupling pathway. Stem Cell Res Ther (2018) 9:62. doi: 10.1186/s13287-018-0804-6
95. Li Z, Han S, Wang X, Han F, Zhu X, Zheng Z, et al. Rho kinase inhibitor y-27632 promotes the differentiation of human bone marrow mesenchymal stem cells into keratinocyte-like cells in xeno-free conditioned medium. Stem Cell Res Ther (2015) 6:17. doi: 10.1186/s13287-015-0008-2
96. Yen TH, Wright NA. The gastrointestinal tract stem cell niche. Stem Cell Rev (2006) 2:203–12. doi: 10.1007/s12015-006-0048-1
97. De Francesco F, Romano M, Zarantonello L, Ruffolo C, Neri D, Bassi N, et al. The role of adipose stem cells in inflammatory bowel disease: From biology to novel therapeutic strategies. Cancer Biol Ther (2016) 17:889–98. doi: 10.1080/15384047.2016.1210741
98. Medema JP, Vermeulen L. Microenvironmental regulation of stem cells in intestinal homeostasis and cancer. Nature (2011) 474:318–26. doi: 10.1038/nature10212
99. Jaenisch R, Young R. Stem cells, the molecular circuitry of pluripotency and nuclear reprogramming. Cell (2008) 132:567–82. doi: 10.1016/j.cell.2008.01.015
100. Paunescu V, Deak E, Herman D, Siska IR, Tanasie G, Bunu C, et al. In vitro differentiation of human mesenchymal stem cells to epithelial lineage. J Cell Mol Med (2007) 11:502–8. doi: 10.1111/j.1582-4934.2007.00041.x
101. Rizvi AZ, Swain JR, Davies PS, Bailey AS, Decker AD, Willenbring H, et al. Bone marrow-derived cells fuse with normal and transformed intestinal stem cells. Proc Natl Acad Sci U.S.A. (2006) 103:6321–5. doi: 10.1073/pnas.0508593103
102. Ferrand J, Noel D, Lehours P, Prochazkova-Carlotti M, Chambonnier L, Menard A, et al. Human bone marrow-derived stem cells acquire epithelial characteristics through fusion with gastrointestinal epithelial cells. PLoS One (2011) 6:e19569. doi: 10.1371/journal.pone.0019569
103. Barker N. Adult intestinal stem cells: Critical drivers of epithelial homeostasis and regeneration. Nat Rev Mol Cell Biol (2014) 15:19–33. doi: 10.1038/nrm3721
104. Gonzalez MA, Gonzalez-Rey E, Rico L, Buscher D, Delgado M. Adipose-derived mesenchymal stem cells alleviate experimental colitis by inhibiting inflammatory and autoimmune responses. Gastroenterology (2009) 136:978–89. doi: 10.1053/j.gastro.2008.11.041
105. Ko IK, Kim BG, Awadallah A, Mikulan J, Lin P, Letterio JJ, et al. Targeting improves msc treatment of inflammatory bowel disease. Mol Ther (2010) 18:1365–72. doi: 10.1038/mt.2010.54
106. Liao W, Pham V, Liu L, Riazifar M, Pone EJ, Zhang SX, et al. Mesenchymal stem cells engineered to express selectin ligands and il-10 exert enhanced therapeutic efficacy in murine experimental autoimmune encephalomyelitis. Biomaterials (2016) 77:87–97. doi: 10.1016/j.biomaterials.2015.11.005
107. Ren G, Zhao X, Zhang L, Zhang J, L'Huillier A, Ling W, et al. Inflammatory cytokine-induced intercellular adhesion molecule-1 and vascular cell adhesion molecule-1 in mesenchymal stem cells are critical for immunosuppression. J Immunol (2010) 184:2321–8. doi: 10.4049/jimmunol.0902023
108. Yuan M, Hu X, Yao L, Jiang Y, Li L. Mesenchymal stem cell homing to improve therapeutic efficacy in liver disease. Stem Cell Res Ther (2022) 13:179. doi: 10.1186/s13287-022-02858-4
109. Chen QQ, Yan L, Wang CZ, Wang WH, Shi H, Su BB, et al. Mesenchymal stem cells alleviate tnbs-induced colitis by modulating inflammatory and autoimmune responses. World J Gastroenterol (2013) 19:4702–17. doi: 10.3748/wjg.v19.i29.4702
110. Fuseler JW, Valarmathi MT. Modulation of the migration and differentiation potential of adult bone marrow stromal stem cells by nitric oxide. Biomaterials (2012) 33:1032–43. doi: 10.1016/j.biomaterials.2011.10.029
111. Castro-Manrreza ME, Montesinos JJ. Immunoregulation by mesenchymal stem cells: Biological aspects and clinical applications. J Immunol Res (2015) 2015:394917. doi: 10.1155/2015/394917
112. Brown JM, Nemeth K, Kushnir-Sukhov NM, Metcalfe DD, Mezey E. Bone marrow stromal cells inhibit mast cell function Via a Cox2-dependent mechanism. Clin Exp Allergy (2011) 41:526–34. doi: 10.1111/j.1365-2222.2010.03685.x
113. Nikolic A, Simovic Markovic B, Gazdic M, Randall Harrell C, Fellabaum C, Jovicic N, et al. Intraperitoneal administration of mesenchymal stem cells ameliorates acute dextran sulfate sodium-induced colitis by suppressing dendritic cells. BioMed Pharmacother (2018) 100:426–32. doi: 10.1016/j.biopha.2018.02.060
114. Cao L, Xu H, Wang G, Liu M, Tian D, Yuan Z. Extracellular vesicles derived from bone marrow mesenchymal stem cells attenuate dextran sodium sulfate-induced ulcerative colitis by promoting M2 macrophage polarization. Int Immunopharmacol (2019) 72:264–74. doi: 10.1016/j.intimp.2019.04.020
115. Froushani SMA, Mashhouri S. The effect of mesenchymal stem cells pulsed with 17 beta-estradiol in an ameliorating rat model of ulcerative colitis. Zahedan J Res Med Sci (2019) 21:e83762. doi: 10.5812/zjrms.83762
116. Hu J, Zhao G, Zhang L, Qiao C, Di A, Gao H, et al. Safety and therapeutic effect of mesenchymal stem cell infusion on moderate to severe ulcerative colitis. Exp Ther Med (2016) 12:2983–89. doi: 10.3892/etm.2016.3724
117. Fei M, Yunbing W, Xudong T, Jingjing K, Bin Z, Yongmin Y, et al. Exosomes derived from human umbilical cord mesenchymal stem cells relieve inflammatory bowel disease in mice. BioMed Res International,2017,(2017-5-15) (2017) 2017:5356760. doi: 10.1155/2017/5356760
118. Wu Y, Qiu W, Xu X, Kang J, Wang J, Tang X, et al. Exosomes derived from human umbilical cord mesenchymal stem cells alleviate inflammatory bowel disease in mice through ubiquitination. Am J Trans Res (2018) 10:2026–36.
119. Wang G, Joel MDM, Yuan J, Wang J, Mao F. Human umbilical cord mesenchymal stem cells alleviate inflammatory bowel disease by inhibiting erk phosphorylation in neutrophils. Inflammopharmacology (2020) 7:603–16. doi: 10.1007/s10787-019-00683-5
Lu Y, Xu Y, Zhang S, Gao J, Gan X, Zheng J, et al. Human gingiva-derived mesenchymal stem cells alleviate inflammatory bowel disease Via il-10 signalling-dependent modulation of immune cells. Scand J Immunol (2019) 90:e12751. doi: 10.1111/sji.12751
121. Xu X, Chen C, Akiyama K, Chai Y, Le AD, Wang Z, et al. Gingivae contain neural-crest- and mesoderm-derived mesenchymal stem cells. J Dent Res (2013) 92:825–32. doi: 10.1177/0022034513497961
122. Yu T, Yan B, Li J, Zhang T, Yang R, Wang X, et al. Acetylsalicylic acid rescues the immunomodulation of inflamed gingiva-derived mesenchymal stem cells Via upregulating fasl in mice. Stem Cell Res Ther (2019) 10:368. doi: 10.1186/s13287-019-1485-5
123. Miyamoto S, Ohnishi S, Onishi R, Tsuchiya I, Hosono H, Katsurada T, et al. Therapeutic effects of human amnion-derived mesenchymal stem cell transplantation and conditioned medium enema in rats with trinitrobenzene sulfonic acid-induced colitis. Am J Transl Res (2017) 9:940–52.
124. Soontararak S, Chow L, Johnson V, Coy J, Wheat W, Regan D, et al. Mesenchymal stem cells (Msc) derived from induced pluripotent stem cells (Ipsc) equivalent to adipose-derived msc in promoting intestinal healing and microbiome normalization in mouse inflammatory bowel disease model. Stem Cells Transl Med (2018) 7:456–67. doi: 10.1002/sctm.17-0305
125. Song WJ, Li Q, Ryu MO, Nam A, An JH, Jung YC, et al. Canine adipose tissue-derived mesenchymal stem cells pre-treated with tnf-alpha enhance immunomodulatory effects in inflammatory bowel disease in mice. Res Vet Sci (2019) 125:176–84. doi: 10.1016/j.rvsc.2019.06.012
126. Lopez-Santalla M, Hervas-Salcedo R, Fernandez-Garcia M, Bueren JA, Garin MI. Cell therapy with mesenchymal stem cells induces an innate immune memory response that attenuates experimental colitis in the long term. J Crohns Colitis (2020) 14:1424–35. doi: 10.1093/ecco-jcc/jjaa079
127. Qi LL, Fan ZY, Mao HG, Wang JB. The therapeutic efficacy of adipose tissue-derived mesenchymal stem cell conditioned medium on experimental colitis was improved by the serum from colitis rats. Front Bioeng Biotechnol (2021) 9:694908. doi: 10.3389/fbioe.2021.694908
128. Watanabe S, Arimura Y, Nagaishi K, Isshiki H, Onodera K, Nasuno M, et al. Conditioned mesenchymal stem cells produce pleiotropic gut trophic factors. J Gastroenterol (2014) 49:270–82. doi: 10.1007/s00535-013-0901-3
129. Yang H, Feng R, Fu Q, Xu S, Hao X, Qiu Y, et al. Human induced pluripotent stem cell-derived mesenchymal stem cells promote healing Via tnf-Alpha-Stimulated gene-6 in inflammatory bowel disease models. Cell Death Dis (2019) 10:718. doi: 10.1038/s41419-019-1957-7
130. Zibandeh N, Gen D, Duran Y, Banzragch M, Sokwala S, Gker K, et al. Human dental follicle mesenchymal stem cells alleviate T cell response in inflamed tissue of crohn's patients. Turkish J Gastroenterol (2020) 31:400–9. doi: 10.5152/tjg.2020.19358
131. Markovic BS, Kanjevac T, Harrell CR, Gazdic M, Fellabaum C, Arsenijevic N, et al. Molecular and cellular mechanisms involved in mesenchymal stem cell-based therapy of inflammatory bowel diseases. Stem Cell Rev Rep (2018) 14:153–65. doi: 10.1007/s12015-017-9789-2
132. Cho YB, Park KJ, Yoon SN, Song KH, Kim DS, Jung SH, et al. Long-term results of adipose-derived stem cell therapy for the treatment of crohn's fistula. Stem Cells Transl Med (2015) 4:532–7. doi: 10.5966/sctm.2014-0199
133. Liu TM. Application of mesenchymal stem cells derived from human pluripotent stem cells in regenerative medicine. World J Stem Cells (2021) 13:1826–44. doi: 10.4252/wjsc.v13.i12.1826
134. Jiang B, Yan L, Wang X, Li E, Murphy K, Vaccaro K, et al. Concise review: Mesenchymal stem cells derived from human pluripotent cells, an unlimited and quality-controllable source for therapeutic applications. Stem Cells (2019) 37:572–81. doi: 10.1002/stem.2964
135. Sanchez L, Gutierrez-Aranda I, Ligero G, Rubio R, Munoz-Lopez M, Garcia-Perez JL, et al. Enrichment of human esc-derived multipotent mesenchymal stem cells with immunosuppressive and anti-inflammatory properties capable to protect against experimental inflammatory bowel disease. Stem Cells (2011) 29:251–62. doi: 10.1002/stem.569
136. Fu QL, Chow YY, Sun SJ, Zeng QX, Li HB, Shi JB, et al. Mesenchymal stem cells derived from human induced pluripotent stem cells modulate T-cell phenotypes in allergic rhinitis. Allergy (2012) 67:1215–22. doi: 10.1111/j.1398-9995.2012.02875.x
137. Xu J, Wang X, Chen J, Chen S, Li Z, Liu H, et al. Embryonic stem cell-derived mesenchymal stem cells promote colon epithelial integrity and regeneration by elevating circulating igf-1 in colitis mice. Theranostics (2020) 10:12204–22. doi: 10.7150/thno.47683
138. Tang RJ, Shen SN, Zhao XY, Nie YZ, Xu YJ, Ren J, et al. Mesenchymal stem cells-regulated treg cells suppress colitis-associated colorectal cancer. Stem Cell Res Ther (2015) 6:71. doi: 10.1186/s13287-015-0055-8
139. Torre LA, Bray F, Siegel RL, Ferlay J, Lortet-Tieulent J, Jemal A. Global cancer statistics, 2012. CA Cancer J Clin (2015) 65:87–108. doi: 10.3322/caac.21262
140. Le Blanc K, Rasmusson I, Sundberg B, Gotherstrom C, Hassan M, Uzunel M, et al. Treatment of severe acute graft-Versus-Host disease with third party haploidentical mesenchymal stem cells. Lancet (2004) 363:1439–41. doi: 10.1016/S0140-6736(04)16104-7
141. Y L, Z Y, W Y, Q Z, J Z. An update on the potential of mesenchymal stem cell therapy for cutaneous diseases. Stem Cells Int (2021) 2021:8834590. doi: 10.1155/2021/8834590
142. Mao F, Tu Q, Wang L, Chu F, Li X, Li HS, et al. Mesenchymal stem cells and their therapeutic applications in inflammatory bowel disease. Oncotarget (2017) 8:38008–21. doi: 10.18632/oncotarget.16682
143. Tan Q, Wu C, Li L, Liang Y, Bai X, Shao W. Stem cells as a novel biomedicine for the repair of articular meniscus: Pharmacology and applications. Front Pharmacol (2022) 13:897635. doi: 10.3389/fphar.2022.897635
144. Bochev I, Elmadjian G, Kyurkchiev D, Tzvetanov L, Altankova I, Tivchev P, et al. Mesenchymal stem cells from human bone marrow or adipose tissue differently modulate mitogen-stimulated b-cell immunoglobulin production in vitro. Cell Biol Int (2008) 32:384–93. doi: 10.1016/j.cellbi.2007.12.007
145. Li X, Bai J, Ji X, Li R, Xuan Y, Wang Y. Comprehensive characterization of four different populations of human mesenchymal stem cells as regards their immune properties, proliferation and differentiation. Int J Mol Med (2014) 34:695–704. doi: 10.3892/ijmm.2014.1821
146. Panes J, Garcia-Olmo D, Van Assche G, Colombel JF, Reinisch W, Baumgart DC, et al. Expanded allogeneic adipose-derived mesenchymal stem cells (Cx601) for complex perianal fistulas in crohn's disease: A phase 3 randomised, double-blind controlled trial. Lancet (2016) 388:1281–90. doi: 10.1016/S0140-6736(16)31203-X
147. Castelo-Branco MT, Soares ID, Lopes DV, Buongusto F, Martinusso CA, do Rosario A Jr., et al. Intraperitoneal but not intravenous cryopreserved mesenchymal stromal cells home to the inflamed colon and ameliorate experimental colitis. PLoS One (2012) 7:e33360. doi: 10.1371/journal.pone.0033360
148. Gregoire C, Lechanteur C, Briquet A, Baudoux E, Baron F, Louis E, et al. Review article: Mesenchymal stromal cell therapy for inflammatory bowel diseases. Aliment Pharmacol Ther (2017) 45:205–21. doi: 10.1111/apt.13864
149. Duijvestein M, Molendijk I, Roelofs H, Vos AC, Verhaar AP, Reinders ME, et al. Mesenchymal stromal cell function is not affected by drugs used in the treatment of inflammatory bowel disease. Cytotherapy (2011) 13:1066–73. doi: 10.3109/14653249.2011.597379
150. Huang HR, Zan H, Lin Y, Zhong YQ. Effects of azathioprine and infliximab on mesenchymal stem cells derived from the bone marrow of rats in vitro. Mol Med Rep (2014) 9:1005–12. doi: 10.3892/mmr.2014.1905
151. Schneider N, Goncalves Fda C, Pinto FO, Lopez PL, Araujo AB, Pfaffenseller B, et al. Dexamethasone and azathioprine promote cytoskeletal changes and affect mesenchymal stem cell migratory behavior. PLoS One (2015) 10:e0120538. doi: 10.1371/journal.pone.0120538
152. Chen X, Gan Y, Li W, Su J, Zhang Y, Huang Y, et al. The interaction between mesenchymal stem cells and steroids during inflammation. Cell Death Dis (2014) 5:e1009. doi: 10.1038/cddis.2013.537
153. Martinez-Montiel Mdel P, Gomez-Gomez GJ, Flores AI. Therapy with stem cells in inflammatory bowel disease. World J Gastroenterol (2014) 20:1211–27. doi: 10.3748/wjg.v20.i5.1211
154. Wakitani S, Okabe T, Horibe S, Mitsuoka T, Saito M, Koyama T, et al. Safety of autologous bone marrow-derived mesenchymal stem cell transplantation for cartilage repair in 41 patients with 45 joints followed for up to 11 years and 5 months. J Tissue Eng Regener Med (2011) 5:146–50. doi: 10.1002/term.299
Keywords: inflammatory bowel diseases, ulcerative colitis, Crohn’s disease, mesenchymal stem/stromal cells, regenerative therapy
Citation: Che Z, Ye Z, Zhang X, Lin B, Yang W, Liang Y and Zeng J (2022) Mesenchymal stem/stromal cells in the pathogenesis and regenerative therapy of inflammatory bowel diseases. Front. Immunol. 13:952071. doi: 10.3389/fimmu.2022.952071
Received: 24 May 2022; Accepted: 12 July 2022;
Published: 04 August 2022.
Edited by:
Yanwen Peng, Third Affiliated Hospital of Sun Yat-sen University, ChinaReviewed by:
Yumi Matsuzaki, Tokyo Medical University, JapanXinhua Li, Shanghai General Hospital, China
Dong Ren, The First Affiliated Hospital of Sun Yat-sen University, China
Copyright © 2022 Che, Ye, Zhang, Lin, Yang, Liang and Zeng. This is an open-access article distributed under the terms of the Creative Commons Attribution License (CC BY). The use, distribution or reproduction in other forums is permitted, provided the original author(s) and the copyright owner(s) are credited and that the original publication in this journal is cited, in accordance with accepted academic practice. No use, distribution or reproduction is permitted which does not comply with these terms.
*Correspondence: Jincheng Zeng, emVuZ2pjQGdkbXUuZWR1LmNu; Yanfang Liang, bHlmaW5lODRAMTI2LmNvbQ==
†These authors have contributed equally to this work