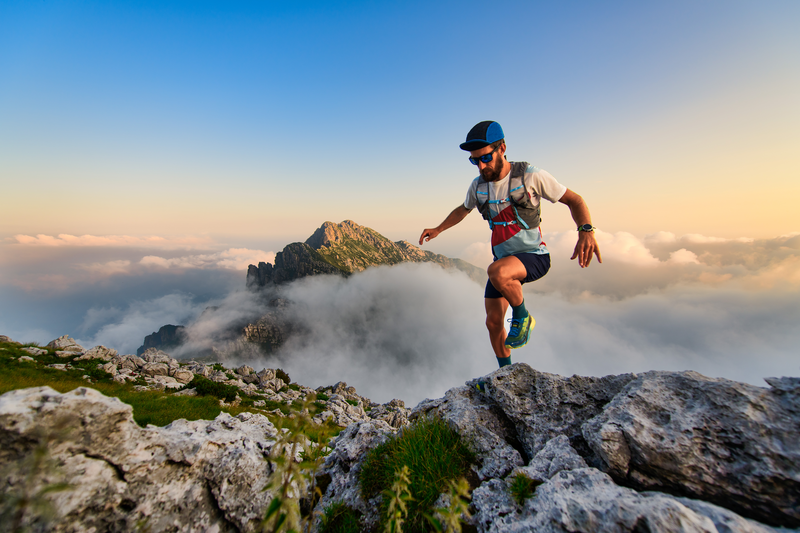
94% of researchers rate our articles as excellent or good
Learn more about the work of our research integrity team to safeguard the quality of each article we publish.
Find out more
REVIEW article
Front. Immunol. , 25 July 2022
Sec. B Cell Biology
Volume 13 - 2022 | https://doi.org/10.3389/fimmu.2022.951385
This article is part of the Research Topic Does Selection Against Autoreactive B Cells Limit Affinity Maturation to Pathogens? View all 5 articles
Antibodies are theoretically limitless in their diversity and specificity to foreign antigens; however they are constrained by the need to avoid binding to self. Germinal centers (GC) allow diversification and maturation of the antibody response towards the foreign antigen. While self-tolerance mechanisms controlling self-reactivity during B cell maturation are well recognized, the mechanisms by which GCs balance self-tolerance and foreign binding especially in the face of cross-reactivity between self and foreign, remain much less well defined. In this review we explore the extent to which GC self-tolerance restricts affinity maturation. We present studies suggesting that the outcome is situationally dependent, affected by affinity and avidity to self-antigen, and the extent to which self-binding and foreign-binding are interdependent. While auto-reactive GC B cells can mutate away from self while maturing towards the foreign antigen, if no mutational trajectories allow for self-reactive redemption, self-tolerance prevails and GC responses to the foreign pathogen are restricted, except when self-tolerance checkpoints are relaxed. Finally, we consider whether polyreactivity is subject to the same level of restriction in GC responses, especially if polyreactivity is linked to an increase in foreign protection, as occurs in certain broadly neutralizing antibodies. Overall, the outcomes for GC B cells that bind self-antigen can range from redemption, transient relaxation in self-tolerance or restriction of the antibody response to the foreign pathogen.
In a world of virtually limitless number of antigenic possibilities, it is unsurprising that a significant overlap of antigenic composition and structural epitopes can be found on those presented on pathogens and those expressed on self-tissues. Whilst B cells have evolved with the ability to undergo B cell receptor (BCR) rearrangements during early development and in the periphery to combat the inevitable antigenic similarities and diversity, the competing interests of balancing self-tolerance while maintaining foreign protection are undeniably complex. Despite this inherent overlap in the majority of instances B cells are able to generate highly specific foreign binding antibodies while avoiding self-reactivity. The exact mechanisms by which these B cells affinity mature and to what extent this avoidance of self-binding is at the expense of reduced binding, is not yet fully elucidated. Complex experimental models and repertoire sequencing datasets in recent times highlight that a variety of factors may be at play, depending on the complexity of foreign antigen and its similarity to self, genetic factors and antibody stability. In some scenarios, in the process of avoiding self-reactivity, B cells may restrict their response to foreign pathogens. However, in other situations there appears to be demonstratable evidence of self-reactivity forming no barrier, or even providing a selective advantage to B cells maturing to foreign antigens near-identical to self. In this review we have sought to explore the mechanisms of B cell tolerance that might affect the response to foreign pathogens and have highlighted some of the complexity and paradoxical evidence provided by experimental models defining the role of B cell response to pathogens mimicking self.
Astonishing diversity within the pre-immune antibody repertoire is achieved by the stochastic recombination of germline immunoglobulin genes; generating an estimated 1012 unique specificities during early B cell development (1–5). The price of such an efficient and random process, however, inevitably results in primary BCR rearrangements with significant reactivity against a variety of soluble, cellular, or structural self-antigens (6). While self-reactive early B cells form a majority of the pre-immune antibody repertoire, nature has evolved elegant counter-selection mechanisms during early B cell development.
Developing B cells in the bone marrow carrying a BCR with high affinity or high avidity binding to surface self-antigens, are either clonally deleted or undergo the process of receptor editing (7–16). Alternatively, potent ligation by self-antigens through the immature self-reactive BCR reactivates the recombinase-activating genes to initiate immunoglobulin V(D)J rearrangements in an attempt to reduce self-reactivity followed by further rounds of clonal selection (17, 18); as a result, the revised self-reactive B cells escape clonal deletion and incidentally contribute to the increasing diversity of the primary B cell repertoire. These central tolerance processes of clonal deletion and receptor editing inhibit strongly self-reactive B cells from escaping into the peripheral circulation.
However, despite multiple brakes cohesively censoring self-reactive B cell clones during B cell development in the bone marrow, between 6-30% of B cells with reactivity to self-antigens enter the periphery (7, 19, 20). For example, the germline IGHV4-34 heavy chain immunoglobulin which confers potentially pathogenic autoantibody reactivity by binding N-linked N-acetyllactosamine expressed by the l/i blood group of self-glycoproteins present on red blood cells and B cells, in fact encodes 5% of the B cell repertoire in a healthy individual (21–23). Instead of being deleted, these self-reactive B cells are maintained in a state of reduced responsiveness or “anergy” triggered by continuous BCR signaling (7, 9, 24–32). The discovery of anergy raised the following question – why would self-reactive B cells, with the potential to produce harmful autoantibodies, be maintained in the circulation? This question is perhaps heightened by the fact that anergy is an active process, requiring ongoing BCR engagement and signaling, and thereby is inherently not infallible (33, 34).
The answer likely lies in the hypothesis that anergy serves a dual purpose, and that anergy is used as a compromise by the immune system to firstly avoid generating “holes” within the B cell repertoire which could be exploited by pathogens and secondly, to retain these cells within the repertoire for defense against foreign antigens that structurally resemble self (35–37). If self-reactive anergic B cells are actively maintained in circulation, it suggests that anergic self-reactive B cells must be available to partake in B cell responses against foreign antigens, at least in some circumstances.
During immune responses to foreign pathogens, activated B cells are recruited to germinal centers (GCs) to facilitate affinity maturation towards the foreign antigen. In order to achieve high affinity foreign binding, GC B cells undergo highly competitive rounds of somatic hypermutation (SHM) of their immunoglobulin variable region genes where they engage with foreign antigens presented on follicular dendritic cells and stimuli delivered by T follicular helper cells (Tfh) (38–43).
The mechanisms driving selection of GC B cell clones that bind the foreign antigen with high affinity have been described in detail in several recent reviews (42, 44–47). In brief, the current prevailing mechanism for selection of B cells with high affinity for the foreign antigen is thought to be driven by competition for limited Tfh cell help. GC B cells that carry a BCR which acquired higher affinity for foreign antigen via random SHM of their BCR become the most efficient at capturing and presenting the foreign antigen to cognate Tfh cells via MHC class II, and as a result, these GC B cells receive a strong survival signal (39, 48). The GC B cells with low affinity for the foreign antigen either submit to apoptosis i.e., “death by neglect” or undergo lower rates of proliferation and eventually become out-competed over time, because they are not able to receive sufficient Tfh-derived stimuli (49, 50). Recent studies have demonstrated that GC positive selection is also driven by isotype class-switching to IgG, which occurs independent of BCR affinity (51, 52). Overall, the final result is that high affinity class-switched GC B cells are selected for differentiation into antibody-producing plasma cells (41, 53–58). In this way, the GC response results in an affinity matured class-switched antibody response against foreign antigens.
While the mechanisms governing GC selection against foreign antigens are well studied, the mechanisms which govern avoidance of self-reactivity in the GC, remain largely undefined. During a GC response, there are two major pathways leading to self-reactivity. The first pathway is the de novo generation of self-reactivity via SHM and the second is through the recruitment and expansion of cross-reactive naïve self-reactive precursor B cells during an anti-foreign response.
In the scenario where a self-reactive B cell is generated de novo in the GC, the acquisition of self-reactivity through SHM can sometimes decrease in foreign antigen binding, triggering their “negative selection” due to reduced competitiveness during affinity-based selection. In instances where de novo acquisition of GC self-reactivity also concurrently increases foreign binding, experimental models have shown self-reactive GC B cells are counter selected (59). Thus, the requirement to maintain foreign-binding is critical during decision-making in GC self-tolerance mechanisms. However, what remains largely unknown, is the mechanisms driving “negative selection” of the GC B cells that increase specificity for foreign but also cross react with self-antigens.
Despite our lack of understanding towards the mechanism, the absence of sustained autoantibody production in most individuals clearly indicates that self-tolerance mechanisms do shape the GC-derived antibody repertoire. This extraordinary capacity of B cells to achieve foreign binding while avoiding self-reactivity of cross-reactive antigens is well evidenced in the antibody response to Campylobacter jejuni. The vast majority of individuals are able to generate antibodies that effectively bind foreign lipooligosaccharide, while effectively avoiding specificity for near identical self-gangliosides that could result in autoimmune Guillian-Barré syndrome (60–62). However the mechanism driving this exquisite self/foreign discrimination of this antigen remains undefined.
It is now recognized that in certain situations this avoidance of self-reactivity can be facilitated through the process known as “autoantibody redemption” (Figure 1). Autoantibody redemption refers to the phenomenon whereby anergic self-reactive B cells enter the GC response and selectively acquire mutations which decrease self-binding. This process was originally described using models which traced the antibody mutation trajectory of HyHEL10 B cells in mice expressing variants of self-antigens Hen Egg Lysozyme (HEL) as model self-antigens immunized with variants of HEL with varying degrees of self-reactivity (36, 63, 64). “Redeemed” self-reactive B cells have since also been found as a component of the antibody response to vaccinia virus and the rhesus D alloantigen (RhD) responses (65), and the response to malaria, whereby a number of B cell clones have shown evidence of a large insertion of LAIR1, which abolishes binding to self-collagen (66, 67).
Figure 1 Schematic illustration of the possible fates of an individual antibody receptor encountering self versus foreign antigen. (A). If a B cell possesses an antibody receptor which binds strongly to the foreign antigen, but exhibits minimal binding of the self-antigen and minimal polyreactivity, there is no restriction of entry into the GC. Within the GC, B cells undergo affinity maturation of its BCR and affinity-based selection. If this B cell acquires mutations that increase self-reactivity de novo it undergoes negative selection by self-tolerance mechanisms. GC B cell with improved anti-foreign binding without detectable increased self-reactivity undergo positive selection to produce high affinity anti-foreign antibodies. (B). If a B cell binds to a foreign antigen but possesses low cross-reactivity or polyreactivity to self-antigens it may still be recruited transiently into the early extrafollicular plasmablast response without entering the GC, releasing unmutated germline polyreactive antibodies. De novo generated GC B cells bearing low self-reactivity or polyreactivity may also leave the GC as terminally differentiated antibody-producing plasma cells, likely in situations where the foreign antigen binding is inherently linked to their polyreactivity properties e.g. broadly neutralizing antibodies. (C). B cell with high cross-reactivity to both self and foreign antigens can enter the GC response but largely by-passes recruitment into the early extrafollicular response. Within the GC, this B cell acquires mutations that reduce its autoreactivity. If mutational trajectories are found which decrease self-binding while concurrently maintaining or enhancing foreign binding, these will undergo positive selection and eventually outcompete mutational trajectories which concurrently decrease self and foreign binding. If no mutational trajectories are possible which reduce autoreactivity while concurrently maintaining foreign binding, B cells with high self-binding will be deleted or outcompeted. Only B cells with diminished binding to both the self and the foreign antigen will be positively selected. The processes that facilitate the selection of GC B cells with reduced foreign and self-binding, are currently undefined.
Interestingly, in some cases, avoidance of self-reactivity by autoantibody redemption during GC responses may actually result in improved antibody responses to the foreign pathogen (Figure 1). In the responses to malaria and RhD alloantigen described above, the pre-immune antibodies showed limited binding to the foreign antigen but were significantly increased in the final post immune antibody repertoire (65–67). The HyHEL10 mouse models have shed further light on this phenomenon, revealing that in certain instances the presence of a self-antigen forced the self-reactive B cells to explore different mutational trajectories to those explored by their non-self-reactive counterparts. The alternative mutational pathways undertaken by the self-reactive precursors were beneficial and resulted in higher affinity outcomes than in the absence of self-antigen (36, 37, 64). This indicates that counterintuitively, in some cases, self-reactive precursors may instead generate antibody responses to the foreign antigen that are improved, rather than restricted. This observation suggests targeting certain anergic self-reactive B cells in the periphery may be the key in designing vaccine strategies to generate broadly neutralizing antibodies for self-mimicking pathogens.
Despite the above evidence that in some situations avoidance of self-reactivity can enhance the foreign binding response, an equivalent body of evidence exists that for certain antigens self-reactivity cannot be removed or “redeemed,” and in these instances, on most occasions self-tolerance prevails over foreign protection (Figure 1). Evidence from the HyHEL10 model antigen system has shown that when there is no pathway to avoid of self-reactivity while increasing foreign binding, the B cell clones mutate away from both self and foreign binding, and thus are referred to as “unredeemable” antibodies (63, 64).
The phenomenon of “unredeemable” antibodies has also been recognized in the human antibody repertoire. When IGHV4-34 B cells paired with IGLV3 light chains, acquire mutations that remove binding to self-poly-N-acetyl-lactosamine, these mutations also simultaneously removed binding to foreign RhD (65, 68). The inability to redeem self-reactivity for foreign binding, is also likely a key factor limiting the production of broadly neutralizing antibodies e.g. broadly neutralizing antibodies to HIV. Despite an extraordinary SHM mutational profile, some broadly neutralizing antibodies to HIV are unable to remove self-binding to N-linked glycans without simultaneously reducing foreign binding (69, 70).
The predominance of self-tolerance over foreign binding is also highlighted by experiments using the HyHEL10 mouse model system. Even when the B cells do not express a pre-existing BCR that binds to self-antigen, they fail to acquire mutations which increase both foreign reactivity and self-binding (59).
In addition to avoidance of self-reactivity, affinity maturation against foreign also appears to be restrained by host mechanisms to avoid antibodies with reduced solubility, at the risk of developing cryoglobulinemia (19). A specific example has been recognized with the CNTO607 monoclonal antibody, which acquires CDR3 mutations which increase solubility, but at the extent of decreasing foreign binding (71, 72). GC B cells that acquired somatic mutations which damage structural immunoglobulin integrity are eliminated via apoptosis (45, 49, 73–75). Hence, antibody responses to foreign pathogens may also be restricted by an attempt to eliminate antibodies with solubility issues which could become pathogenic. However, unlike self-reactivity, it is unlikely that mechanisms that prevent antibody solubility would ever be exploited for vaccine design as the risk of pathogenicity is too high.
Interestingly several studies have shown for certain antigens, anergic self-reactive B cells can be reawakened when immune checkpoints are dysregulated to disrupt self-tolerance, consequently allowing recruitment in the GC and selection of high-affinity and broadly neutralizing anti-foreign B cell clones. Case studies have shown patients with systemic lupus erythematous (SLE) and certain autoimmune mouse models, including the MRL and LPR mice which lack FAS, are more readily capable of generating broadly neutralizing antibodies to HIV (76–79). Similarly, repertoire sequencing data from SLE patients indicates these patients may have advantages in generating broadly neutralizing antibodies to influenza following seasonal influenza vaccination (80). Immunization with HIV antigen in combination with antibodies inhibiting tolerance checkpoints e.g. CTLA-4 blockade or OX40 agonists, increased the GC response and enhanced production of neutralizing antibodies to HIV (81). Recruitment of anergic B cells for broadly neutralizing antibody generation has also shown to occur in non-autoimmune settings using antigen multimerization (82), additional adjuvants or mitogenic signals (70, 83–85) and T cell stimulation (86, 87). It has also been suggested that immune tolerance may become relaxed following severe COVID19, demonstrated by an increase in circulating B cells with autoreactive antibodies specificities in convalescent patients and evidence that anergic B cells may display a heightened state of activation (88). Although much of this immune activation likely results from combinatorial factors including inflammatory cytokines, specific evaluation of self-reactive IGHV4-34 B cells suggests antigen cross-reactivity may contribute to the expansion of autoreactive B cells in COVID19 (89).
In addition to playing a role in the innate-like early response to pathogens, polyreactivity also appears to be a feature of some GC-derived antibodies (Figure 1). Polyreactive antibodies have the ability to bind several different self-antigens with low affinity. This is in contrast to self-reactive autoantibodies that only bind one self-antigen with moderate to high affinity. Since polyreactivity is conflated with self-reactivity in many studies, it can be difficult to interpret and distinguish the outcome of a polyreactive GC B cell compared to a self-reactive GC B cell. Despite this, there is some evidence to suggest that unlike self-reactivity, GC responses may be permissive to polyreactivity, if polyreactivity is exclusively linked to an increase in foreign antigen.
The immune system appears to tolerate some level of polyreactivity. B cells expressing low-affinity polyreactive antibodies e.g. “natural innate-like B1” B cells, splenic marginal zone B cells and extrafollicular, short-lived plasmablasts, are considered important for the first line of defense against pathogens producing a transient wave of polyreactive antibodies (6, 90, 91). The IGHV1-69 immunoglobulin gene is encoded by germline polyreactivity thanks to a hydrophobic motif in CDR2 capable of binding hydrophobic grooves frequently found within antigens of unrelated foreign pathogens e.g. influenza, hepatitis C virus (HCV), Staphylococcus aureus and HIV (92–96). As such germline IGHV1-69 has been proposed to form part of the immediate “SOS” antibody response driving control of certain infections e.g. seasonal influenza, before affinity-matured antibody responses are able provide high affinity protection (97–99).
While polyreactivity is tolerated during short-lived antibody responses, the extent to which polyreactivity is tolerated in GC-dependent long-term antibody responses, is less clear. There is some debate regarding the contribution of the GC response to polyreactivity. Polyreactivity has been reported to occur in up to 25% of IgG+ memory B cells yet only ~6% of naïve B cells (100), which indicates the GC response generates polyreactivity. However, the reverse also been reported. In one study, only ~1-2% of IgG+ memory B cells and plasma cells demonstrated polyreactivity, representing a decrease of roughly 2-4 fold compared to the naïve B cell compartment (19). These conflicting reports may be explained by differences in experimental assays measuring polyreactivity. Alternatively, they may be explained by the observation that early IgG+ memory B cells (typically GC-independent memory B cells) were found to be highly polyreactive (~33%), while late IgG+ memory B cells were devoid of polyreactivity (101). Thus, despite the contradictory reports, it appears that overall, the long-term GC-dependent antibody response is not characterized by polyreactivity. Whether this polyreactivity is actively removed during the GC response via autoantibody redemption similar to self-reactive GC B cells, or indirectly as a consequence of affinity maturation, remains unknown.
While GC responses are generally associated with reduced polyreactivity, there is evidence to suggest polyreactivity may be permitted in GC responses when polyreactivity is linked to foreign binding affinity through “unredeemable” mutations. Polyreactivity is a recurring feature of broadly neutralizing antibodies, especially those directed to HIV-1 and the stalk region of influenza haemagglutinin (102–105). Roughly 95% of anti-haemagglutinin antibodies targeting a broadly neutralizing epitope at the haemagglutinin stalk region, were found to be polyreactive (105). By contrast, anti-influenza antibodies derived from adults either infected with or vaccinated against seasonal influenza, did not demonstrate significant polyreactivity (105). Interestingly, almost a fifth of the broadly neutralizing anti-haemagglutinin stalk antibodies with polyreactivity were encoded by IGHV1-69 versus <3% of non-broadly neutralizing antibodies (105). Most studies indicate the polyreactivity associated with broadly neutralizing antibodies is germline-encoded, meaning polyreactive precursors are recruited, rather than generated by GC responses. Roughly 70% of polyreactive anti-gp140 HIV-1 antibodies maintained their polyreactivity following reversion to germline (102). Similarly, polyreactive anti-haemagglutinin antibodies reverted to germline demonstrated a reduction in affinity for foreign haemagglutinin binding, yet levels of polyreactivity remained unchanged (105). In line with this, SHM is generally associated with a reduction in polyreactivity (19). The high prevalence of polyreactivity amongst broadly neutralizing antibodies demonstrates the GC response can be permissive to polyreactivity, if associated with an increase towards foreign binding.
Thus, this indicates polyreactive GC responses may not be restricted by “unredeemable” mutations like self-reactive GC responses. In support of this, polyreactive antibodies have been shown to demonstrate heteroligation to self and foreign antigen i.e. simultaneous binding to high density self-antigen and low-density foreign antigen (102). One could speculate that polyreactive GC B cells positively selected due to their increased competitiveness for foreign antigen binding, fail to trigger the threshold for self-tolerance that prevails for “unredeemable” self-reactive GC B cells, because affinities for self-antigen are too low. Future studies are needed to elucidate the fate of polyreactive B cells during the GC response including “unredeemable” polyreactive GC B cells, particularly since some of the coveted broadly neutralizing antibodies to influenza and HIV are associated with polyreactivity.
In this review we have sought to explore dualistic and unique challenges faced by B cells combating foreign pathogens that cross-react with self-antigens. We highlight several recent studies in the field that have championed our progress in understanding how GC B cells mitigate antigenic cross-reactivity through the process of autoantibody redemption. However, more studies are required to further address some major outstanding questions in the field, to allow for the adaption of this understanding to benefit vaccine design and pathogen defense.
Autoantibody redemption, at least in experimental mouse model systems, can lead to beneficial and higher affinity outcomes to the foreign antigen. Although it has been shown to occur in both natural infections and vaccination responses it remains currently unknown how frequently these processes occur and whether the process of autoantibody redemption can be harnessed in vaccine design to enhance the broadly neutralizing antibody response. Ongoing research is required to assess the feasibility of recruitment of anergic self-reactive B cell precursors and driving them towards a “redeemable” mutational trajectory via a combination of a germline-targeting and sequential immunization, in the setting of pathogens that thus far remain elusive in universal vaccine development.
Another aspect of the field that remains relatively unexplored, is the fate of polyreactive B cells during GC responses (in clear distinction with self-reactive GC B cells). At a very fundamental level, the field still lacks a clear definition on how much polyreactivity is present in a typical GC-dependent antibody repertoire. Additionally, while it appears polyreactivity is reduced following GC responses, it remains unclear whether polyreactivity is removed via an active mechanism e.g. autoantibody redemption similar to self-reactive GC B cells, or is a passive consequence of SHM during affinity maturation. Since many broadly neutralizing antibodies are inherently polyreactive, understanding how GC responses deal with polyreactivity is also pertinent for the field of vaccine design.
Finally, one major roadblock to understanding of how GC B cell responses to foreign antigens might be inhibited by self-antigens, lies in our complete lack of understanding regarding the mechanisms controlling GC B cell tolerance in the face of “unredeemable” mutations. Without elucidating these mechanisms, we cannot fully understand how GC B cell responses to foreign antigens are shaped by cross-reactivity to self-antigens. Understanding how B cells deal with foreign pathogens that mimic self is important for many aspects of B cell biology and including the fields of autoimmunity and vaccine design.
CY, AL, and DB contributed to conception, design, interpretation and writing of the manuscript. All authors contributed to the article and approved the submitted version.
This work was funded by NHMRC Investigator Grant 1176351. C Young was supported by a University of New South Wales (UNSW) Scientia Scholarship for postgraduate study.
The authors declare that the research was conducted in the absence of any commercial or financial relationships that could be construed as a potential conflict of interest.
All claims expressed in this article are solely those of the authors and do not necessarily represent those of their affiliated organizations, or those of the publisher, the editors and the reviewers. Any product that may be evaluated in this article, or claim that may be made by its manufacturer, is not guaranteed or endorsed by the publisher.
1. Weigert M, Perry R, Kelley D, Hunkapiller T, Schilling J, Hood L. The joining of V and J gene segments creates antibody diversity. Nature (1980) 283(5746):497–9. doi: 10.1038/283497a0
2. Tonegawa S. Somatic generation of antibody diversity. Nature (1983) 302(5909):575–81. doi: 10.1038/302575a0
3. Meffre E, Casellas R, Nussenzweig MC. Antibody regulation of b cell development. Nat Immunol (2000) 1(5):379–85. doi: 10.1038/80816
4. Alberts B JA, Lewis J, Raff M, Roberts K, Walter P. Molecular biology of the cell. New York: Garland Science (2002).
5. Elhanati Y, Sethna Z, Marcou Q, Callan CG, Mora T, Walczak AM. Inferring processes underlying b-cell repertoire diversity. Philos Trans R Soc B: Biol Sci (2015) 370(1676):20140243. doi: 10.1098/rstb.2014.0243
6. Wardemann H, Yurasov S, Schaefer A, Young JW, Meffre E, Nussenzweig MC. Predominant autoantibody production by early human b cell precursors. Science (2003) 301:1374–7. doi: 10.1126/science.1086907
7. Goodnow CC, Crosbie J, Jorgensen H, Brink RA, Basten A. Induction of self-tolerance in mature peripheral b lymphocytes. Nature (1989) 342(6248):385–91. doi: 10.1038/342385a0
8. Nemazee DA, Bürki K. Clonal deletion of b lymphocytes in a transgenic mouse bearing anti-MHC class I antibody genes. Nature (1989) 337(6207):562–6. doi: 10.1038/337562a0
9. Hartley SB, Crosbie J, Brink R, Kantor AB, Basten A, Goodnow CC. Elimination from peripheral lymphoid tissues of self-reactive b lymphocytes recognizing membrane-bound antigens. Nature (1991) 353(6346):765–9. doi: 10.1038/353765a0
10. Gay D, Saunders T, Camper S, Weigert M. Receptor editing: an approach by autoreactive b cells to escape tolerance. J Exp Med (1993) 177(4):999–1008. doi: 10.1084/jem.177.4.999
11. Hartley SB, Cooke MP, Fulcher DA, Harris AW, Cory S, Basten A, et al. Elimination of self-reactive b lymphocytes proceeds in two stages: arrested development and cell death. Cell (1993) 72(3):325–35. doi: 10.1016/0092-8674(93)90111-3
12. Radic MZ, Erikson J, Litwin S, Weigert M. B lymphocytes may escape tolerance by revising their antigen receptors. J Exp Med (1993) 177(4):1165–73. doi: 10.1084/jem.177.4.1165
13. Tiegs SL, Russell DM, Nemazee D. Receptor editing in self-reactive bone marrow b cells. J Exp Med (1993) 177(4):1009–20. doi: 10.1084/jem.177.4.1009
14. Hertz M, Nemazee D. BCR ligation induces receptor editing in IgM+IgD– bone marrow b cells In vitro. Immunity (1997) 6(4):429–36. doi: 10.1016/S1074-7613(00)80286-1
15. Halverson R, Torres RM, Pelanda R. Receptor editing is the main mechanism of b cell tolerance toward membrane antigens. Nat Immunol (2004) 5(6):645–50. doi: 10.1038/ni1076
16. Casellas R, Zhang Q, Zheng N-Y, Mathias MD, Smith K, Wilson PC. Igκ allelic inclusion is a consequence of receptor editing. J Exp Med (2007) 204(1):153–60. doi: 10.1084/jem.20061918
17. Kuwata N, Igarashi H, Ohmura T, Aizawa S, Sakaguchi N. Cutting edge: absence of expression of RAG1 in peritoneal b-1 cells detected by knocking into RAG1 locus with green fluorescent protein gene. J Immunol (1999) 163(12):6355–9.
18. Yu W, Nagaoka H, Jankovic M, Misulovin Z, Suh H, Rolink A, et al. Continued RAG expression in late stages of b cell development and no apparent re-induction after immunizion. Nature (1999) 400(6745):682–7. doi: 10.1038/23287
19. Shehata L, Maurer DP, Wec AZ, Lilov A, Champney E, Sun T, et al. Affinity maturation enhances antibody specificity but compromises conformational stability. Cell Rep (2019) 28(13):3300–3308.e3304. doi: 10.1016/j.celrep.2019.08.056
20. Watanabe A, Su K-Y, Kuraoka M, Yang G, Reynolds AE, Schmidt AG, et al. Self-tolerance curtails the b cell repertoire to microbial epitopes. JCI Insight (2019) 4(10):e122551. doi: 10.1172/jci.insight.122551
21. Li Y, Spellerberg MB, Stevenson FK, Capra DJ, Potter KN. The I binding specificity of human VH4-34 (VH4-21) encoded antibodies is determined by both VHFramework region 1 and complementarity determining region 3. J Mol Biol (1996) 256(3):577–89. doi: 10.1006/jmbi.1996.0110
22. Cappione AJ, Pugh-Bernard AE, Anolik JH, Sanz I. Lupus IgG VH4. 34 antibodies bind to a 220-kDa glycoform of CD45/B220 on the surface of human b lymphocytes. J Immunol (2004) 172(7):4298–307. doi: 10.4049/jimmunol.172.7.4298
23. Boyd SD, Gaëta BA, Jackson KJ, Fire AZ, Marshall EL, Merker JD, et al. Individual variation in the germline ig gene repertoire inferred from variable region gene rearrangements. J Immunol (2010) 184(12):6986–92. doi: 10.4049/jimmunol.1000445
24. Goodnow CC, Crosbie J, Adelstein S, Lavoie TB, Smith-Gill SJ, Brink RA, et al. Altered immunoglobulin expression and functional silencing of self-reactive b lymphocytes in transgenic mice. Nature (1988) 334(6184):676–82. doi: 10.1038/334676a0
25. Cooke MP, Heath AW, Shokat KM, Zeng Y, Finkelman FD, Linsley PS, et al. Immunoglobulin signal transduction guides the specificity of b cell-T cell interactions and is blocked in tolerant self-reactive b cells. J Exp Med (1994) 179(2):425–38. doi: 10.1084/jem.179.2.425
26. Eris JM, Basten A, Brink R, Doherty K, Kehry MR, Hodgkin PD. Anergic self-reactive b cells present self antigen and respond normally to CD40-dependent T-cell signals but are defective in antigen-receptor-mediated functions. Proc Natl Acad Sci United States America (1994) 91(10):4392–6. doi: 10.1073/pnas.91.10.4392
27. Pao LI, Famiglietti SJ, Cambier JC. Asymmetrical phosphorylation and function of immunoreceptor tyrosine-based activation motif tyrosines in b cell antigen receptor signal transduction. J Immunol (1998) 160(7):3305–14.
28. Benschop RJ, Aviszus K, Zhang X, Manser T, Cambier JC, Wysocki LJ. Activation and anergy in bone marrow b cells of a novel immunoglobulin transgenic mouse that is both hapten specific and autoreactive. Immunity (2001) 14(1):33–43. doi: 10.1016/S1074-7613(01)00087-5
29. Ota T, Ota M, Duong BH, Gavin AL, Nemazee D. Liver-expressed igκ superantigen induces tolerance of polyclonal b cells by clonal deletion not κ to λ receptor editing. J Exp Med (2011) 208(3):617–29. doi: 10.1084/jem.20102265
30. Quách TD, Manjarrez-Orduño N, Adlowitz DG, Silver L, Yang H, Wei C, et al. Anergic responses characterize a Large fraction of human autoreactive naive b cells expressing low levels of surface IgM. J Immunol (2011) 186(8):4640–8. doi: 10.4049/jimmunol.1001946
31. Zikherman J, Parameswaran R, Weiss A. Endogenous antigen tunes the responsiveness of naive b cells but not T cells. Nature (2012) 489(7414):160–4. doi: 10.1038/nature11311
32. Yau IW, Cato MH, Jellusova J, Hurtado de Mendoza T, Brink R, Rickert RC. Censoring of self-reactive b cells by follicular dendritic cell–displayed self-antigen. J Immunol (2013) 191(3):1082. doi: 10.4049/jimmunol.1201569
33. Goodnow CC, Brink R, Adams E. Breakdown of self-tolerance in anergic b lymphocytes. Nature (1991) 352(6335):532–6. doi: 10.1038/352532a0
34. Gauld SB, Benschop RJ, Merrell KT, Cambier JC. Maintenance of b cell anergy requires constant antigen receptor occupancy and signaling. Nat Immunol (2005) 6(11):1160–7. doi: 10.1038/ni1256
35. Goodnow CC. Balancing immunity and tolerance: deleting and tuning lymphocyte repertoires. Proc Natl Acad Sci (1996) 93(6):2264–71. doi: 10.1073/pnas.93.6.2264
36. Burnett DL, Langley DB, Schofield P, Hermes JR, Chan TD, Jackson J, et al. Germinal center antibody mutation trajectories are determined by rapid self/foreign discrimination. Science (2018) 360(6385):223–6. doi: 10.1126/science.aao3859
37. Burnett DL, Reed JH, Christ D, Goodnow CC. Clonal redemption and clonal anergy as mechanisms to balance b cell tolerance and immunity. Immunol Rev (2019) 292(1):61–75. doi: 10.1111/imr.12808
38. Allen CD, Okada T, Tang HL, Cyster JG. Imaging of germinal center selection events during affinity maturation. Science (2007) 315(5811):528–31. doi: 10.1126/science.1136736
39. Victora GD, Schwickert TA, Fooksman DR, Kamphorst AO, Meyer-Hermann M, Dustin ML, et al. Germinal center dynamics revealed by multiphoton microscopy with a photoactivatable fluorescent reporter. Cell (2010) 143(4):592–605. doi: 10.1016/j.cell.2010.10.032
40. Heesters BA, Myers RC, Carroll MC. Follicular dendritic cells: dynamic antigen libraries. Nat Rev Immunol (2014) 14(7):495–504. doi: 10.1038/nri3689
41. Ise W, Fujii K, Shiroguchi K, Ito A, Kometani K, Takeda K, et al. T Follicular helper cell-germinal center b cell interaction strength regulates entry into plasma cell or recycling germinal center cell fate. Immunity (2018) 48(4):702–715.e704. doi: 10.1016/j.immuni.2018.03.027
42. Stebegg M, Kumar SD, Silva-Cayetano A, Fonseca VR, Linterman MA, Graca L. Regulation of the germinal center response. Front Immunol (2018) 9:2469. doi: 10.3389/fimmu.2018.02469
43. Crotty S. T Follicular helper cell biology: a decade of discovery and diseases. Immunity (2019) 50(5):1132–48. doi: 10.1016/j.immuni.2019.04.011
44. Shlomchik MJ, Luo W, Weisel F. Linking signaling and selection in the germinal center. Immunol Rev (2019) 288(1):49–63. doi: 10.1111/imr.12744
45. Lau AW, Brink R. Selection in the germinal center. Curr Opin Immunol (2020) 63:29–34. doi: 10.1016/j.coi.2019.11.001
46. Nakagawa R, Calado DP. Positive selection in the light zone of germinal centers. Front Immunol (2021) 12:1053. doi: 10.3389/fimmu.2021.661678
47. Young C, Brink R. The unique biology of germinal center b cells. Immunity (2021) 54(8):1652–64. doi: 10.1016/j.immuni.2021.07.015
48. Meyer-Hermann ME, Maini PK, Iber D. An analysis of b cell selection mechanisms in germinal centers. Math Med Biol: J IMA (2006) 23(3):255–77. doi: 10.1093/imammb/dql012
49. Mayer CT, Gazumyan A, Kara EE, Gitlin AD, Golijanin J, Viant C, et al. The microanatomic segregation of selection by apoptosis in the germinal center. Science (2017) 358(6360):eaao2602. doi: 10.1126/science.aao2602
50. Nakagawa R, Toboso-Navasa A, Schips M, Young G, Bhaw-Rosun L, Llorian-Sopena M, et al. Permissive selection followed by affinity-based proliferation of GC light zone b cells dictates cell fate and ensures clonal breadth. Proc Natl Acad Sci (2021) 118(2):e2016425118. doi: 10.1073/pnas.2016425118
51. Roco JA, Mesin L, Binder SC, Nefzger C, Gonzalez-Figueroa P, Canete PF, et al. Class-switch recombination occurs infrequently in germinal centers. Immunity (2019) 51(2):337–350.e337. doi: 10.1016/j.immuni.2019.07.001
52. Sundling C, Lau AWY, Bourne K, Young C, Laurianto C, Hermes JR, et al. Positive selection of IgG(+) over IgM(+) b cells in the germinal center reaction. Immunity (2021) 54(5):988–1001.e1005. doi: 10.1016/j.immuni.2021.03.013
53. Phan TG, Paus D, Chan TD, Turner ML, Nutt SL, Basten A, et al. High affinity germinal center b cells are actively selected into the plasma cell compartment. J Exp Med (2006) 203(11):2419–24. doi: 10.1084/jem.20061254
54. Chan TD, Gatto D, Wood K, Camidge T, Basten A, Brink R. Antigen affinity controls rapid T-dependent antibody production by driving the expansion rather than the differentiation or extrafollicular migration of early plasmablasts. J Immunol (2009) 183(5):3139. doi: 10.4049/jimmunol.0901690
55. Shulman Z, Gitlin AD, Weinstein JS, Lainez B, Esplugues E, Flavell RA, et al. Dynamic signaling by T follicular helper cells during germinal center b cell selection. Science (2014) 345(6200):1058–62. doi: 10.1126/science.1257861
56. Kräutler NJ, Suan D, Butt D, Bourne K, Hermes JR, Chan TD, et al. Differentiation of germinal center b cells into plasma cells is initiated by high-affinity antigen and completed by tfh cells. J Exp Med (2017) 214(5):1259–67. doi: 10.1084/jem.20161533
57. Luo W, Weisel F, Shlomchik MJ. B cell receptor and CD40 signaling are rewired for synergistic induction of the c-myc transcription factor in germinal center b cells. Immunity (2018) 48(2):313–326.e315. doi: 10.1016/j.immuni.2018.01.008
58. Zhang Y, Tech L, George LA, Acs A, Durrett RE, Hess H, et al. Plasma cell output from germinal centers is regulated by signals from tfh and stromal cells. J Exp Med (2018) 215(4):1227–43. doi: 10.1084/jem.20160832
59. Chan TD, Wood K, Hermes JR, Butt D, Jolly CJ, Basten A, et al. Elimination of germinal-center-derived self-reactive b cells is governed by the location and concentration of self-antigen. Immunity (2012) 37:893–904. doi: 10.1016/j.immuni.2012.07.017
60. Yuki N, Taki T, Inagaki F, Kasama T, Takahashi M, Saito K, et al. A bacterium lipopolysaccharide that elicits Guillain-barre syndrome has a GM1 ganglioside-like structure. J Exp Med (1993) 178(5):1771–5. doi: 10.1084/jem.178.5.1771
61. Bowes T, Wagner ER, Boffey J, Nicholl D, Cochrane L, Benboubetra M, et al. Tolerance to self gangliosides is the major factor restricting the antibody response to lipopolysaccharide core oligosaccharides in campylobacter jejuni strains associated with Guillain-barre syndrome. Infect Immun (2002) 70(9):5008–18. doi: 10.1128/IAI.70.9.5008-5018.2002
62. Wakerley BR, Yuki N. Infectious and noninfectious triggers in Guillain-barre syndrome. Expert Rev Clin Immunol (2013) 9(7):627–39. doi: 10.1586/1744666X.2013.811119
63. Sabouri Z, Schofield P, Horikawa K, Spierings E, Kipling D, Randall KL, et al. Redemption of autoantibodies on anergic b cells by variable-region glycosylation and mutation away from self-reactivity. Proc Natl Acad Sci (2014) 111(25):2567–75. doi: 10.1073/pnas.1406974111
64. Burnett DL, Schofield P, Langley DB, Jackson J, Bourne K, Wilson E, et al. Conformational diversity facilitates antibody mutation trajectories and discrimination between foreign and self-antigens. Proc Natl Acad Sci U.S.A. (2020) 117(36):22341–50. doi: 10.1073/pnas.2005102117
65. Reed JH, Jackson J, Christ D, Goodnow CC. Clonal redemption of autoantibodies by somatic hypermutation away from self-reactivity during human immunization. J Exp Med (2016) 213(7):1255–65. doi: 10.1084/jem.20151978
66. Tan J, Pieper K, Piccoli L, Abdi A, Perez MF, Geiger R, et al. A LAIR1 insertion generates broadly reactive antibodies against malaria variant antigens. Nature (2016) 529(7584):105–9. doi: 10.1038/nature16450
67. Pieper K, Tan J, Piccoli L, Foglierini M, Barbieri S, Chen Y, et al. Public antibodies to malaria antigens generated by two LAIR1 insertion modalities. Nature (2017) 548(7669):597–601. doi: 10.1038/nature23670
68. Thorpe SJ, Boult CE, Stevenson FK, Scott ML, Sutherland J, Spellerberg MB, et al. Cold agglutinin activity is common among human monoclonal IgM Rh system antibodies using the V4-34 heavy chain variable gene segment. Transfusion (1997) 37(11-12):1111–6. doi: 10.1046/j.1537-2995.1997.37111298088038.x
69. Verkoczy L, Kelsoe G, Moody MA, Haynes BF. Role of immune mechanisms in induction of HIV-1 broadly neutralizing antibodies. Curr Opin Immunol (2011) 23(3):383–90. doi: 10.1016/j.coi.2011.04.003
70. Verkoczy L, Chen Y, Zhang J, Bouton-Verville H, Newman A, Lockwood B, et al. Induction of HIV-1 broad neutralizing antibodies in 2F5 knock-in mice: selection against membrane proximal external region-associated autoreactivity limits T-dependent responses. J Immunol (2013) 191(5):2538–50. doi: 10.4049/jimmunol.1300971
71. Wu SJ, Luo J, O'Neil KT, Kang J, Lacy ER, Canziani G, et al. Structure-based engineering of a monoclonal antibody for improved solubility. Protein Eng Des Sel (2010) 23(8):643–51. doi: 10.1093/protein/gzq037
72. Rabia LA, Desai AA, Jhajj HS, Tessier PM. Understanding and overcoming trade-offs between antibody affinity, specificity, stability and solubility. Biochem Eng J (2018) 137:365–74. doi: 10.1016/j.bej.2018.06.003
73. Strasser A, Puthalakath H, Bouillet P, Huang DCS, O'Connor L, O'Reilly LA, et al. The role of bim, a proapoptotic BH3-only member of the bcl-2 family, in cell-death control. Ann New York Acad Sci (2000) 917(1):541–8. doi: 10.1111/j.1749-6632.2000.tb05419.x
74. Danial NN, Korsmeyer SJ. Cell death: critical control points. Cell (2004) 116(2):205–19. doi: 10.1016/S0092-8674(04)00046-7
75. Stewart I, Radtke D, Phillips B, McGowan SJ, Bannard O. Germinal center b cells replace their antigen receptors in dark zones and fail light zone entry when immunoglobulin gene mutations are damaging. Immunity (2018) 49(3):477–489.e477. doi: 10.1016/j.immuni.2018.08.025
76. Liao HX, Lynch R, Zhou T, Gao F, Alam SM, Boyd SD, et al. Co-Evolution of a broadly neutralizing HIV-1 antibody and founder virus. Nature (2013) 496(7446):469–76. doi: 10.1038/nature12053
77. Bonsignori M. Will studies in individuals with systemic lupus erythematosus be the key to future HIV vaccine design? Expert Rev Vaccines (2014) 13(11):1271–3. doi: 10.1586/14760584.2014.938056
78. Schroeder KM, Agazio A, Torres RM. Immunological tolerance as a barrier to protective HIV humoral immunity. Curr Opin Immunol (2017) 47:26–34. doi: 10.1016/j.coi.2017.06.004
79. Schroeder KMS, Agazio A, Strauch PJ, Jones ST, Thompson SB, Harper MS, et al. Breaching peripheral tolerance promotes the production of HIV-1-neutralizing antibodies. J Exp Med (2017) 214(8):2283–302. doi: 10.1084/jem.20161190
80. Kaur K, Zheng NY, Smith K, Huang M, Li L, Pauli NT, et al. High affinity antibodies against influenza characterize the plasmablast response in SLE patients after vaccination. PloS One (2015) 10(5):e0125618. doi: 10.1371/journal.pone.0125618
81. Bradley T, Kuraoka M, Yeh CH, Tian M, Chen H, Cain DW, et al. Immune checkpoint modulation enhances HIV-1 antibody induction. Nat Commun (2020) 11(1):948. doi: 10.1038/s41467-020-14670-w
82. McGuire AT, Gray MD, Dosenovic P, Gitlin AD, Freund NT, Petersen J, et al. Specifically modified env immunogens activate b-cell precursors of broadly neutralizing HIV-1 antibodies in transgenic mice. Nat Commun (2016) 7:1–10. doi: 10.1038/ncomms10618
83. Dosenovic P, Soldemo M, Scholz JL, O'Dell S, Grasset EK, Pelletier N, et al. BLyS-mediated modulation of naive b cell subsets impacts HIV env-induced antibody responses. J Immunol (2012) 188(12):6018–26. doi: 10.4049/jimmunol.1200466
84. Gupta S, Clark ES, Termini JM, Boucher J, Kanagavelu S, LeBranche CC, et al. DNA Vaccine molecular adjuvants SP-D-BAFF and SP-D-APRIL enhance anti-gp120 immune response and increase HIV-1 neutralizing antibody titers. J Virol (2015) 89(8):4158–69. doi: 10.1128/JVI.02904-14
85. Zhang R, Verkoczy L, Wiehe K, Munir Alam S, Nicely NI, Santra S, et al. Initiation of immune tolerance-controlled HIV gp41 neutralizing b cell lineages. Sci Transl Med (2016) 8(336):336ra362. doi: 10.1126/scitranslmed.aaf0618
86. Hanson MC, Crespo MP, Abraham W, Moynihan KD, Szeto GL, Chen SH, et al. Nanoparticulate STING agonists are potent lymph node-targeted vaccine adjuvants. J Clin Invest (2015) 125(6):2532–46. doi: 10.1172/JCI79915
87. Elbahnasawy MA, Donius LR, Reinherz EL, Kim M. Co-Delivery of a CD4 T cell helper epitope via covalent liposome attachment with a surface-arrayed b cell target antigen fosters higher affinity antibody responses. Vaccine (2018) 36(41):6191–201. doi: 10.1016/j.vaccine.2018.08.014
88. Castleman MJ, Stumpf MM, Therrien NR, Smith MJ, Lesteberg KE, Palmer BE, et al. SARS-CoV-2 infection relaxes peripheral b cell tolerance. J Exp Med (2022) 219(6):e20212553. doi: 10.1084/jem.20212553
89. Woodruff MC, Ramonell RP, Saini AS, Haddad NS, Anam FA, Rudolph ME, et al. Relaxed peripheral tolerance drives broad de novo autoreactivity in severe COVID-19. medRxiv (2021). doi: 10.1101/2020.10.21.20216192
90. Griffin DO, Rothstein TL. Human "orchestrator" CD11b(+) B1 cells spontaneously secrete interleukin-10 and regulate T-cell activity. Mol Med (2012) 18:1003–8. doi: 10.2119/molmed.2012.00203
91. Berlin T, Zandman-Goddard G, Blank M, Matthias T, Pfeiffer S, Weis I, et al. Autoantibodies in nonautoimmune individuals during infections. Ann N Y Acad Sci (2007) 1108:584–93. doi: 10.1196/annals.1422.061
92. Kong L, Giang E, Nieusma T, Kadam RU, Cogburn KE, Hua Y, et al. Hepatitis c virus E2 envelope glycoprotein core structure. Science (2013) 342(6162):1090–4. doi: 10.1126/science.1243876
93. Pappas L, Foglierini M, Piccoli L, Kallewaard NL, Turrini F, Silacci C, et al. Rapid development of broadly influenza neutralizing antibodies through redundant mutations. Nature (2014) 516(7531):418–22. doi: 10.1038/nature13764
94. Yeung YA, Foletti D, Deng X, Abdiche Y, Strop P, Glanville J, et al. Germline-encoded neutralization of a staphylococcus aureus virulence factor by the human antibody repertoire. Nat Commun (2016) 7(1):1–14. doi: 10.1038/ncomms13376
95. Boughter CT, Borowska MT, Guthmiller JJ, Bendelac A, Wilson PC, Roux B, et al. Biochemical patterns of antibody polyreactivity revealed through a bioinformatics-based analysis of CDR loops. Elife (2020) 9:e61393. doi: 10.7554/eLife.61393.sa2
96. Khatri I, Berkowska MA, van den Akker EB, Teodosio C, Reinders MJT, van Dongen JJM. Population matched (pm) germline allelic variants of immunoglobulin (IG) loci: Relevance in infectious diseases and vaccination studies in human populations. Genes Immun (2021) 22(3):172–86. doi: 10.1038/s41435-021-00143-7
97. Sui J, Hwang WC, Perez S, Wei G, Aird D, Chen L-m, et al. Structural and functional bases for broad-spectrum neutralization of avian and human influenza a viruses. Nat Struct Mol Biol (2009) 16(3):265–73. doi: 10.1038/nsmb.1566
98. Lerner RA. Rare antibodies from combinatorial libraries suggests an SOS component of the human immunological repertoire. Mol Biosyst (2011) 7(4):1004–12. doi: 10.1039/c0mb00310g
99. Lingwood D, McTamney PM, Yassine HM, Whittle JR, Guo X, Boyington JC, et al. Structural and genetic basis for development of broadly neutralizing influenza antibodies. Nature (2012) 489(7417):566–70. doi: 10.1038/nature11371
100. Tiller T, Tsuiji M, Yurasov S, Velinzon K, Nussenzweig MC, Wardemann H. Autoreactivity in human IgG+ memory b cells. Immunity (2007) 26(2):205–13. doi: 10.1016/j.immuni.2007.01.009
101. Gitlin AD, von Boehmer L, Gazumyan A, Shulman Z, Oliveira TY, Nussenzweig MC. Independent roles of switching and hypermutation in the development and persistence of b lymphocyte memory. Immunity (2016) 44(4):769–81. doi: 10.1016/j.immuni.2016.01.011
102. Mouquet H, Scheid JF, Zoller MJ, Krogsgaard M, Ott RG, Shukair S, et al. Polyreactivity increases the apparent affinity of anti-HIV antibodies by heteroligation. Nature (2010) 467(7315):591–5. doi: 10.1038/nature09385
103. Dennison SM, Anasti K, Scearce RM, Sutherland L, Parks R, Xia S-M, et al. Nonneutralizing HIV-1 gp41 envelope cluster II human monoclonal antibodies show polyreactivity for binding to phospholipids and protein autoantigens. J Virol (2011) 85(3):1340–7. doi: 10.1128/JVI.01680-10
104. Liu M, Yang G, Wiehe K, Nicely NI, Vandergrift NA, Rountree W, et al. Polyreactivity and autoreactivity among HIV-1 antibodies. J Virol (2015) 89(1):784–98. doi: 10.1128/JVI.02378-14
Keywords: somatic hypermutation, autoantibody redemption, B cell tolerance, germinal center, affinity maturation, autoreactivity, anergy
Citation: Young C, Lau AWY and Burnett DL (2022) B cells in the balance: Offsetting self-reactivity avoidance with protection against foreign. Front. Immunol. 13:951385. doi: 10.3389/fimmu.2022.951385
Received: 23 May 2022; Accepted: 29 June 2022;
Published: 25 July 2022.
Edited by:
Laurent Verkoczy, Applied Biomedical Research Institute, United StatesReviewed by:
Eric J. Sundberg, School of Medicine, Emory University, United StatesCopyright © 2022 Young, Lau and Burnett. This is an open-access article distributed under the terms of the Creative Commons Attribution License (CC BY). The use, distribution or reproduction in other forums is permitted, provided the original author(s) and the copyright owner(s) are credited and that the original publication in this journal is cited, in accordance with accepted academic practice. No use, distribution or reproduction is permitted which does not comply with these terms.
*Correspondence: Clara Young, Yy55b3VuZ0BnYXJ2YW4ub3JnLmF1; Deborah L. Burnett, ZC5idXJuZXR0QGdhcnZhbi5vcmcuYXU=
†These authors have contributed equally to this work and share first authorship
Disclaimer: All claims expressed in this article are solely those of the authors and do not necessarily represent those of their affiliated organizations, or those of the publisher, the editors and the reviewers. Any product that may be evaluated in this article or claim that may be made by its manufacturer is not guaranteed or endorsed by the publisher.
Research integrity at Frontiers
Learn more about the work of our research integrity team to safeguard the quality of each article we publish.