- 1Laboratory of Molecular and Translational Endocrinology, Division of Endocrinology, Federal University of São Paulo, São Paulo, Brazil
- 2Discipline of Internal Medicine and Laboratory Medicine, Federal University of São Paulo, São Paulo, Brazil
- 3Laboratory of Cancer Molecular Genetics, Faculty of Medical Sciences, University of Campinas (Unicamp), Campinas, Brazil
The immune response is remodeled with aging in a process called immunosenescence. Some immunologists conceive immunosenescence as an adaptation of immunity to the aged immune-environment rather than a merely collapsed reactivity of immune cells against microbes and tumor cells. Others believe on an uninterrupted activation of the innate immune system with aging, leading to a low grade, sterile and chronic proinflammatory state called inflammaging. For instance, it is possible that chronic infection by cytomegalovirus leads to persistent production of viral load. This phenomenon offers periodic stimuli to the immune system that ultimately contribute to the remodeling of the immune response. If investigating immunosenescence at the cellular level is already a difficult task, considering the population level is much more complex. However, by studying immunosenescence at the population level, we can extract valuable results with viable applications. While studies with animal models allow scientists to deepen their understanding of the mechanisms of immunosenescence, studying large populations can bring practical innovations to medicine and the health system. Many researchers and funders have dedicated themselves to producing methods for the evaluation of immunosenescence on a large scale, aiming to elucidate new mechanisms by which diseases are established in the elderly. The description of how the immune response is remodeled with aging emerges as a new tool to identify the subset of subjects in which unhealthy aging is a matter of time, to help better individualize clinical management and select patients who may benefit. of early interventions. This review focuses on functional assays as valuable methods for measuring the remodeling of the immune response with aging and discuss their clinical impact. We also recall fundamental concepts for understanding the aging process of the immune response. In addition, we highlight future prospects for immunosenescence research.
Introduction
The increase in maximum life expectancy that humanity has experienced in recent decades has raised concerns related to healthy aging. In this scenario, understanding the modifications that occur in the immune system emerges as a milestone for physicians and scientists. The immune response is fluidly altered with aging (immunosenescence) and results in increased susceptibility to several clinical conditions, such as infectious diseases (1). This essentially negative concept has given way to another broader and evolutionary-based concept. Some immunologists conceive immunosenescence as an adaptation of immunity to the aged immune-environment rather than a collapsed reactivity of immune cells against microbes and tumor cells. Some of these modifications have been shown to have a negative impact on the functioning of the various immune system components and may have a significant impact on patients’ responsiveness to infections, vaccination and diminished immunosurveillance (2).
Both adaptive and innate immunity are influenced by aging. Some subjects experience uninterrupted activation of the innate immune system, leading to a relative increase in activating cytokines and the production of innate cells. Once uncontrolled, the unproportioned activation of innate immunity may be detrimental and associated with a decrease in functionality in a clinical syndrome known as frailty (Figure 1) (3). The pro-inflammatory state occasioned by continuous immune stimulation is called inflammaging (3). The source of this stimulation may be endogenous or exogenous (4–8) and its final product is a low grade, sterile and chronic pro-inflammatory state (3). Inflammaging may disrupt neuro-endocrine and metabolic homeostasis, leading to loss of lean mass and low performance among elderly (9). It is important to highlight that chronic low-grade inflammation is relevant, but not sufficient to lead to frailty. The risk for this multifactorial condition can be influenced by several sociodemographic variables (10).
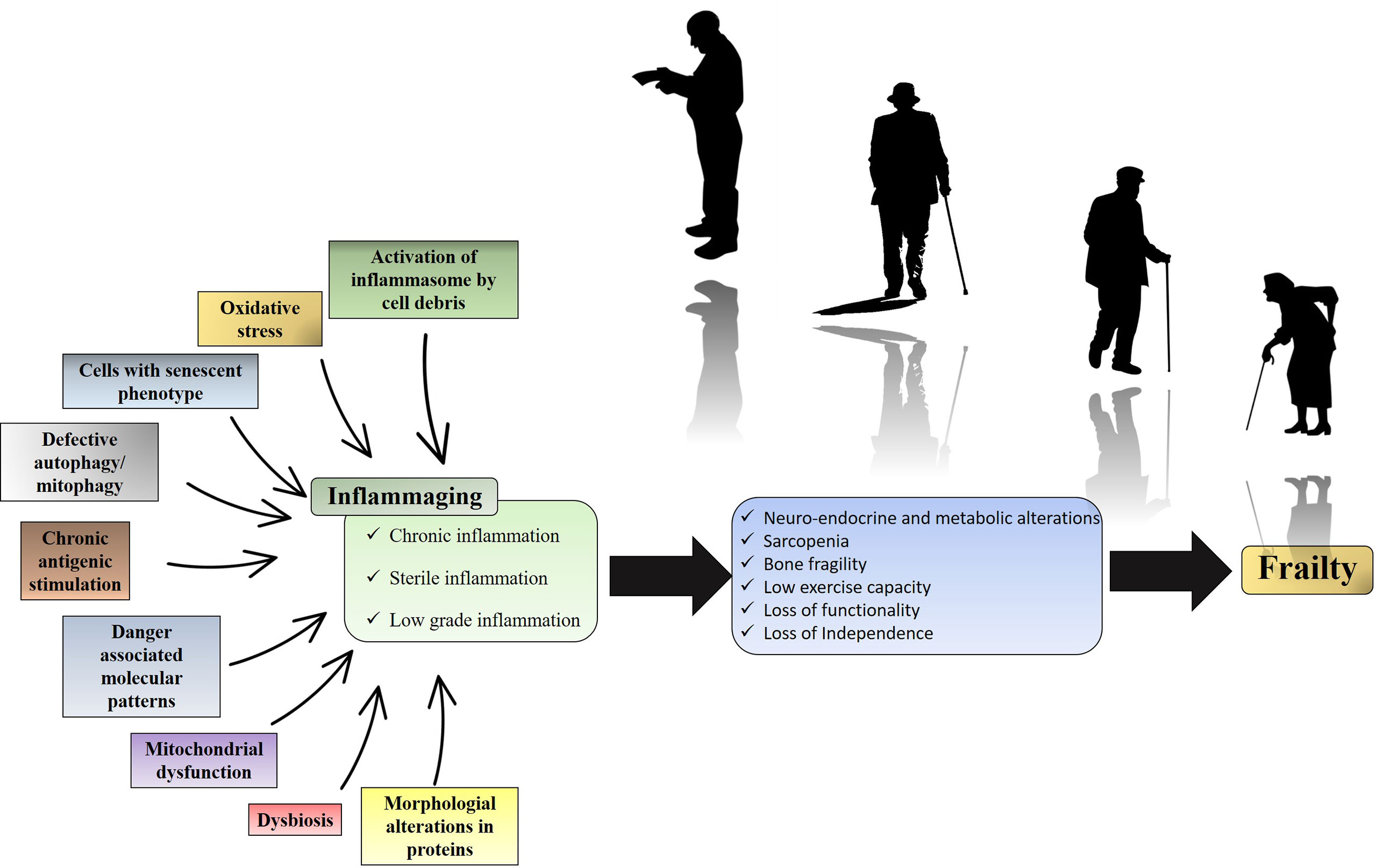
Figure 1 Inflammaging and its clinical impact. Over time, endogenous or exogenous chronic immune stimulation leads to an increase in the pro-inflammatory tone characteristic of inflammaging. The metabolic consequence of this pro-inflammatory state is the biochemical imbalance that culminates in loss of strength, loss of performance and loss of functionality. While the figure summarizes the impact of inflammation on frailty, it is important to note that frailty is also influenced by sociodemographic risk factors.
Likewise, adaptive immunity remarkably changes as age evolves. Bone marrow is reorganized, and the hematopoietic stem cell pool most prominently differentiates into the myeloid lineage, outnumbering the lymphoid compartment (11). To this progenitor disequilibrium should be added thymic involution, which compromises the generation of new naïve T cells. However, the assessment of the functionality of the immune system rather than the absolute number of immune cells alone seems to better reflect the complexity of reshaping process of the immune response with age.
Assessing the functionality of the immune system is a great challenge. In fact, many functional assays have been developed to trace the steps related to the establishment of the immune response. Herein, we discuss the assessment of immunosenescence in human populations. The literature was accessed through international databases where we searched for articles on clinical and experimental research that added new knowledge about the field of immunosenescence. Our aim is to focus on functional assays as valuable methods to measure immune response remodeling with aging and their clinical impact. We discuss fundamental concepts for understanding the aging process of the immune response and present future perspectives in the investigation of immunosenescence. This discussion will bring new insights to the clinical management of the elderly.
Physiology of immunosenescence
Aging is associated with changing patterns in physiological functions. More than a simple state of relative immunodeficiency, immunosenescence must be considered a complex ongoing remodeling of immune cells and their biological microenvironment. Indeed, immunosenescence has been recognized as a more intricate process involving the composition, phenotype and function of cells from both innate and adaptive subsets of immunity (12, 13). Table 1 summarizes the physiological alterations that occur with immune cells.
Age-related remodeling may influence the homeostasis of neutrophils, natural killer (NK) cells, monocytes/macrophages, and dendritic cells, all considered hallmarks of the innate immune response (15). It has been recently reported that the function of innate immunity extends beyond protection against infections (14, 24). NK cells also promote granule exocytosis targeting senescent cells (24). NK cells from elderly individuals exhibit impaired perforin release upon stimulation (14), suggesting that NK cell immunosenescence may be a mechanism that justifies the accumulation of senescent cells in aged tissue. It is noteworthy that human aging is associated with a reduced frequency of NKp46+ NK cells (25). The NKp46 receptor mediates the recognition and elimination of inflammatory cells by NK cells (14). This reinforces the notion that the rates of NK-cell-mediated inflammatory cell apoptosis may be reduced in elderly individuals, explaining the slower resolution of inflammation (26).
The age-related remodeling of polymorphonuclear leukocytes and macrophages may help to explain the delay in wound healing also observed in older patients (16). In addition, aging is associated with a subclinical chronic inflammatory state characterized by elevated levels of proinflammatory cytokines and acute phase proteins, as well as reduced levels of anti-inflammatory cytokines, a state called inflammaging (27). Most likely, elderly individuals sustain a low-grade inflammation by stable antigenic stimulation (4). The source of antigens may be exogenous, such as cytomegalovirus (5, 6), or endogenous, such as posttranslational-modified macromolecules (7).
Cytomegalovirus (CMV) is a beta herpesvirus that completes its cycle in human cells and can be in latency (reservoir cells include hematopoietic progenitors, monocytes, dendritic cells, endothelial cells, and lymphoid vessels) for a long time (28). Figure 2 shows the many steps through which CMV can influence the remodeling of the immune system over time. The first observations linking CMV infection to immunosenescence were reported by Looney et al, who demonstrated that there is a strong and independent association between CMV seropositivity and an increased number of CD28- CD4 or CD8 T cells (29). After an acute infection, CMV elicits a CD8-based T-cell response, as well as CD4 and B lymphocyte activation (30). Persistent production of CMV viral load offers periodic stimuli to the immune system, leading to the maintenance of virus-specific T cells in lymphoid organs and peripheral vessels (31). Thereafter, a subset of CMV-specific CD8+ T cells is generated in each viral reactivation cycle (a phenomenon known as memory inflation) (31, 32). The majority of CMV-specific CD8+ T cells are mature and terminally differentiated (33), and CMV infection is associated with a reduction in the telomere length of the circulating T-cell pool (34). Both these features are typical of immunosenescence. Interestingly, Khan et al. demonstrated that in elderly individuals, CMV positivity leads to the development of oligoclonal populations of CMV-specific CD8 T cells that can constitute up to one-quarter of the total CD8 T-cell population (35). These results suggest that CMV infection could contribute to the acceleration of immunosenescence by promoting the contraction of the CD8 T-cell repertoire with aging. However, there are some contradictory results that indicated no causality with inflammation (36). Some authors failed to show the association between CMV and frailty, and CMV infection was even correlated with improved survival in the elderly, indicating that further investigation is needed to clarify the role of CMV in immunosenescence (37, 38).
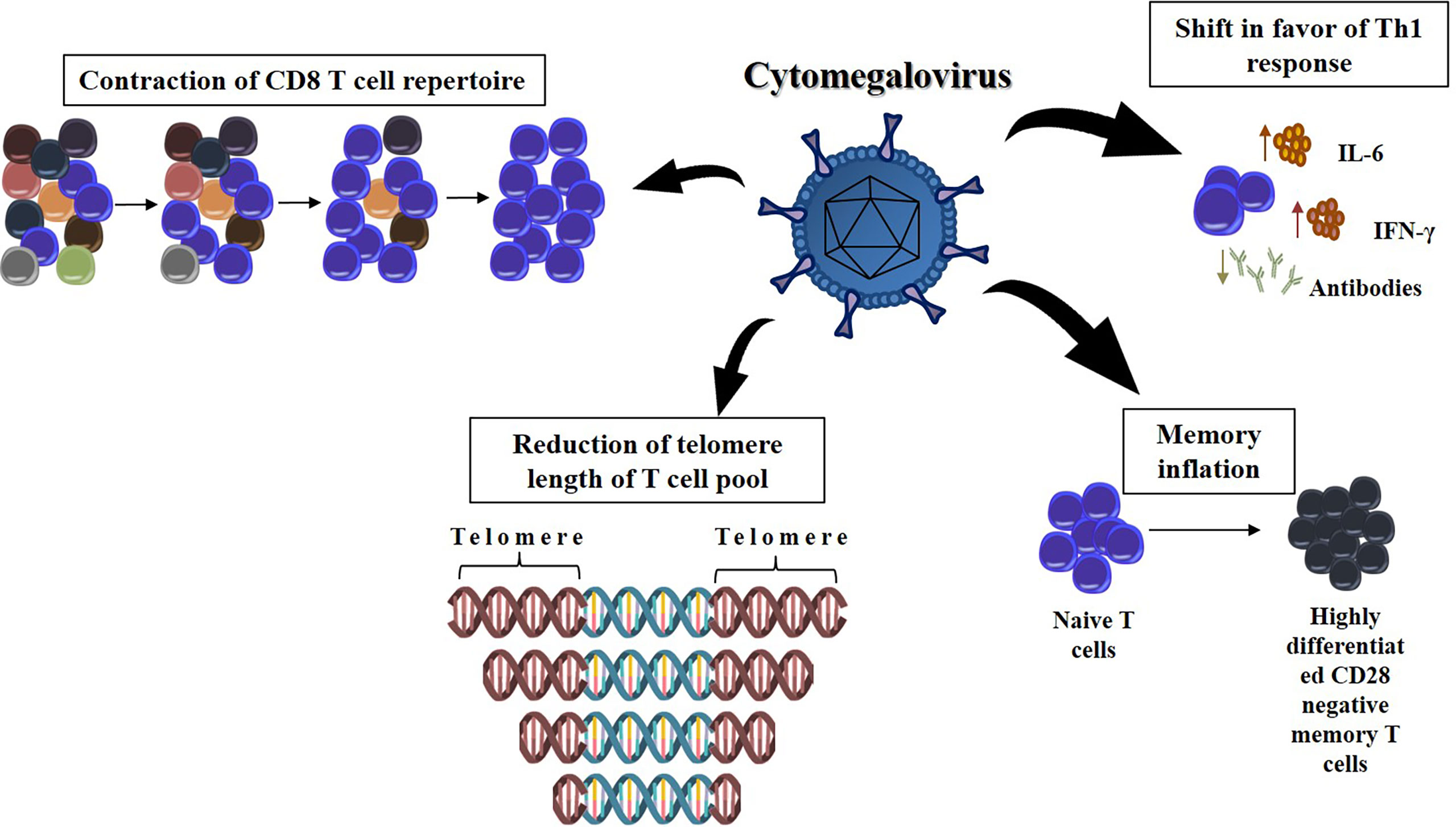
Figure 2 The effect of chronic infection by cytomegalovirus at the remodeling of the immune response with age. The CMV infection can dramatically decrease the T cell repertoire, narrowing the range of new pathogenic bioagents that can be recognized by the immune response. Furthermore, the presence of CMV DNA in peripheral blood monocytes was longitudinally associated with higher serum levels of IL-6, suggesting shift in favor of the Th1 immune response. Each cycle of viral reactivation generates a subset of CMV-specific CD8+ T cells. It causes these terminally differentiated lymphocytes to be overrepresented in immune system (memory inflation). It is also possible that T cells chronically infected with CMV have shortened telomeres, which limits the lifetime these cells are available for an immune response.
The adaptive immune response also changes as age evolves. The adaptive immune response may become impaired with age as a consequence of thymic involution and insufficiency of hematopoietic stem cells (39, 40). The elderly are less able to respond to neoantigens than young individuals because fewer new naïve cells leave the thymus in elderly individuals, although some older people may experience a phenomenon of partial replenishment of the T cell repertoire (22, 23). Indeed, T cells are the most affected compartment of the adaptive immunity response because T cells from aged individuals are usually lower in number and slower than those of young individuals regarding proliferation, telomerase activity, or induction of signaling events (41, 42). Furthermore, almost all adult regulatory T lymphocytes are suppressive and apoptosis-prone populations at a late stage of differentiation (43). In addition, transplant patients at old age may present increased incidence and severity of rejection, as well as increased resistance to tolerance induction due to accumulation of both T and B memory cells (44, 45).
It is suggested that naïve T and B cells become dysfunctional with aging. In contrast, the function of memory T and B cells is relatively maintained (46–48). This can be explained by intrinsic dysfunctions in which T and B cells accumulate over time. When naïve T lymphocytes collected from elderly individuals are stimulated in vitro, they show slower and fainted activation than those obtained from younger individuals (49, 50). The decline in T lymphocyte activation with age may be partially explained by impaired immune synapse construction (51), weakening of the signal transduced into the cytoplasm (52), dysregulation of cytoskeletal function (53), modification in the glycosylation pattern of molecules considered essential for the activation of T cells (54) and insufficient production of IL-2 following T-cell activation (55).
The B cell response in the elderly has intrinsic age-dependent dysfunction. Investigations of vaccine trials suggest that the diversity of the B cell repertoire decreases over time, particularly in frail patients (19, 20). Elderly individuals are deficient in class switch recombination, an immunological phenomenon in which B cells secrete specific antibodies with different effector functions from different classes (21). Class switching is well characterized in the germinal centers of lymph nodes and spleen and can be facilitated by both T-dependent and T-independent stimuli (21, 56). Activation-induced cytidine deaminase is known to induce class switch recombination and immunoglobulin somatic hypermutation (57). Activated old murine B cells also have less activation-induced cytidine deaminase and fewer antibodies exchanged, reinforcing that the effectiveness of humoral immunity is impaired with aging (58, 59).
Functional assessment of immunosenescence
The functional assessment of the aging of the immune system may be of clinical relevance. In fact, some elderly experience some clinical symptoms and signs that suggest a mild impairment of the immune response. Clinicians must be aware of certain patterns such as recurrent infections, chronic diarrhea, malabsorption and coexistence of infection, autoimmunity and malignancy (60). The management of elderly people with possible deficiency in the immune response must include the distinction between a primary and a secondary immunodeficiency (61). Secondary causes (eg, poorly-controlled medical conditions, malnutrition, malignancy, drug induced immunossupression, metabolic diseases, HIV) are more common among older adults (62). After the initial tests for common clinical conditions, the immune response may be assessed by laboratory techniques such as serum immunoglobulins measurement and lymphocyte subsets via flow cytometry (63). In addition, there is some future perspective on the assessment of cytokine production assays that may reveal specific immune defects among adults suspect for immunodeficiency (64).
The functional assessment of the immunosenescence is relevant at a research level. The physiological process leading to immunosenescence essentially begins at the molecular and cellular levels. Many of the alterations responsible for the remodeling of the immune system can be scrutinized through methods that could indirectly reflect the aging of the immune cells. Naturally, none of these methods is flawless, and it is up to the scientist to discern which cellular aspect of immunosenescence is better represented by a specific method. Table 2 lists the main pathways used to assess immune function with age.
Neutrophils are the most prevalent cells among white blood cells. Neutrophils are indispensable cells of the innate immune response, as they act as a fierce trigger of various immune effector mechanisms. One of these mechanisms is the formation of the extracellular neutrophil trap (NET), previously described as the extracellular release of granular proteins linked to a decondensed chromatin mesh that favors the elimination of microbes and parasites (81, 82). As a potent effector mechanism, it must be strictly regulated by the immune system (83). When disturbed, NET formation could result in vascular damage and tissue insult characteristic of autoimmunity (84, 85).
The microbicidal potential of neutrophils is reduced with age (17, 18). A decline in NET generation, degranulation and phagocytosis over time (69, 70) has been documented in both animal models (86) and humans (18). Hazeldine et al. were the first to assess NET formation in the context of immunosenescence. They observed that older adults had a lower ability to generate NET as significantly lower amounts of extracellular DNA were extruded by neutrophils treated with IL-8 or LPS, probably due to impaired signal transduction following IL-8 and LPS stimulation (18).
Reactive oxygen species (ROS) are free radicals fundamental for the microbicidal function of innate immune cells (87). ROS not only directly contributes to bacterial elimination, but can also trigger NET formation (88, 89). The production of free radicals by polymorphonuclear leukocytes has been investigated, and most studies found that the production of free radicals by properly stimulated polymorphonuclear leukocytes was decreased in the elderly (71, 72).
The assessment of immunosenescence at the cellular level has been widely available through the characterization of animal models of premature immunosenescence. Mice with the earlier immunosenescence phenotype (66), when longitudinally investigated, have a shorter life span than control mice. Their peritoneal leukocytes exhibit lower proliferative response to stimuli, a decline in NK activity and increased TNF alpha production compared to control mice (66). In addition, macrophages of premature models are less functional, with a marked loss of antimicrobial capacity (90).
New improved models of immunosenescence have been developed (67, 68). They allowed us to observe that immunosenescence is accompanied by complex neuroimmunoendocrine reshaping that is expressed by neuropsychological deficits, poor neuromuscular coordination, and worse sensorimotor abilities (66, 68, 90). The key phenomenon that seems to be central is oxidative and inflammatory stress, which, not without reason, are associated with several chronic non-communicable diseases prevalent in the elderly (40, 67, 91). Indeed, immune cells harvested from the spleen and thymus of immunosensitive preterm mouse models had lower values of antioxidant defenses and higher values of oxidants and pro-inflammatory cytokines than cells from controls (67). Interestingly, the antioxidant versus oxidant balance of immunosenescent preterm mice was similar to that of cells from aged animals, suggesting a causal relationship between this imbalance and the remodeling of the immune response observed with aging (67, 92).
T-cell function declines with aging, as described above (3). It is noteworthy that the T cell dysfunction observed in the elderly is not the same concept as the dysfunction reported as T cell exhaustion. Indeed, T cell exhaustion is the least responsive state mediated by conditions such as chronic viral infection and cancer (73), as exemplified in Figure 1. Constant antigenic stimulation progressively depletes the T cell, which gradually loses its proliferative and responsive capabilities. Chronic viral infection is an example of chronic stimulation. Persistent exposure to viral particles leads to upregulation of co-inhibitory (93). The co-inhibitory molecules downregulate the TCR-stimulated intracellular signal and T cells become anergic (94). Interestingly, while different in concept, the T-cell dysfunction observed in immunosenescence shares many mechanisms with T-cell exhaustion.
Investigations using a mouse model suggest that there is an accumulation of T cells with an exhausted phenotype over time (52, 74–77). In fact, Shimada et al. demonstrated that both the mRNA and protein expression levels of PD-1 and CTLA-4 are higher in cells from old mice than in cells from young controls (74). The majority of PD-1-positive cells were not activated and had an effector memory phenotype (74). When challenged with anti-CD3 and anti-CD28 antibodies, these PD-1+ T cells failed to proliferate, suggesting that this subset of cells from old mice was hypo responsive (74). Investigations focused on chronic viral infections revealed that Tim-3 is another coinhibitory receptor marker of exhausted T cells (95, 96). Tim-3 interacts with Galectin-9 and leads to T-cell death (97). Lee et al. also investigated T-cell exhaustion in old mice and reported an accumulation of both Tim-3-positive and PD-1-positive T cells with aging (76). The proliferative capacity of both Tim-3-negative PD-1-positive and Tim-3-positive PD-1-positive CD8 T cells was impaired, reinforcing that these immunosenescent and exhausted cells display an anergic phenotype (76). Interestingly, the age-related exhaustion observed by Lee was not exactly the same as that classically reported in chronic infection-induced exhaustion, in which abundant CD160 expression was noted (98). The authors also noted that age-associated Tim-3-positive PD-1-positive CD8 T cells secrete high levels of IL-10 and have the potential to stimulate the expression of IL-10 in normal CD8 T cells, contributing to the increased systemic levels of IL-10 (76). According to Lee´s results, it is reasonable to consider that exhausted and immunosenescent T cells are generated by exposure to many different antigens over a lifespan, rather than by a single specific viral infection (76). This concept is consistent with the previous thought that conceive CMV is one of the triggers of the immunosenescent phenotype.
Song et al. described that immunosenescent T cells from old adults are enriched with TIGIT-positive CD8 T cells (77). T-cell immunoglobulin and immunoreceptor tyrosine-based inhibitory motif (ITIM) domain (TIGIT) are coinhibitory receptors expressed on activated T cells and compete with their costimulatory counterpart CD226 for the same ligands (CD155 and CD112) (99, 100). This close interaction leads to immune suppression in models of tumors and chronic infections (99, 100). Older adults may accumulate TIGIT-positive T cells, which exhibit a terminally differentiated, depleted phenotype (77). TIGIT-positive CD8 T cells from elderly individuals have been shown to retain their proliferative capacity while TNF-alpha, IFN-gamma, and IL-2 are poorly produced when compared to TIGIT-negative CD8 T cells (77). In addition to this dysfunctional feature, exhausted and immunosenescent TIGIT-positive CD8 T cells obtained from the elderly are more susceptible to cell death (77), suggesting that although T cell exhaustion and immunosenescence are different phenomena, they may be different points on the same spectrum of remodeling immunity.
Assessment of immunosenescence at the population level
If investigating immunosenescence at the cellular level is already a difficult task, considering the population level is much more complex. However, by studying immunosenescence at the population level, we can extract valuable results with viable applications. The inference of an immunological age, for example, is only possible by studying human populations. While studies with animal models allow scientists to deepen knowledge of immunosenescence mechanisms, studying large populations can bring practical innovations to medicine and the health care system. It is no wonder that many researchers and funders have devoted themselves to producing methods for the assessment of immunosenescence on a large scale.
Immunogerontological studies have expanded over the last three decades, mainly after the publication of the SENIEUR protocol. The SENIEUR protocol was developed by Ligthart et al. in a working party in the framework of the EURAGE Concerted Action Programme on Aging of the European Community and was designed to provide a reference measurement of immunosenescence in the healthy aged population (101). It consists of the establishment of strict admission criteria for immunogerontological studies, intending to avoid selection bias. This made it possible to conduct investigations that identified that a loss of T-cell homeostasis takes place as the age goes on (102). It was reflected by a decrease in the number of CD4 cells, an increase in the CD8 subset in individuals with an inverted CD4:CD8 ratio, and proliferation of terminally differentiated effector memory T cells (102, 103). In addition, CMV DNA in peripheral monocytes was longitudinally associated with serum IL-6 levels (104). In fact, CMV-dependent T-cell immunosenescence affects healthy aging and may be related to frailty, loss of functionality, morbidity and mortality (105–108). This finding reinforces the idea that immunological age is determined not only by endogenous but also by exogenous factors.
To perform a more real-world characterization of immunosenescence, Nilsson et al. used a SENIEUR-modified protocol to include elderly individuals in the investigation of immune parameters (109). The design of the study allowed them to compare different subgroups defined according to health status (very healthy, moderately healthy, and frail groups). Comparison between the elderly and middle-aged groups suggested an increase in the subset of cells with the immune risk phenotype in the elderly, characterized by both a high CD8 and low CD4 proportion and poor T-cell proliferation in peripheral blood lymphocytes. Interestingly, this difference was independent of the health status, suggesting that phenotypic characterization alone fails to predict healthy aging.
To skew the reductionist approach in the analysis of individual components of the immune system, Longo et al. described a new technology known as single-cell network profiling (SCNP) (78). Single-cell network profiling uses multiparameter flow cytometry and monitors phospho-protein responses to molecular stimuli at the single cell level. By exposing immune cell signaling networks to different inputs, SCNP can discern unique immune cell responses, assessing the signal produced by a phospho-protein mediator. The SCNP requires the definition of the “signaling node” to refer to a specific protein readout in the presence of a given stimulus. Then, the response to IL-4 stimulation can be assessed using p-STAT5 as a readout. Since each signaling pathway is measured in each cell subset, the cell subset is noted as follows, e.g., “IL-4 → p-STAT5 | T helper lymphocytes”. The authors observed the impact of age on the immune signaling responses of four nodes (negative correlation for IFN-α → p-STAT5 | CD45RA+ cytotoxic T cells; negative correlation for IL-27 → p-STAT5 | CD45RA+ cytotoxic T cells; negative correlation for IL-4 → p-STAT6 | CD45RA+ cytotoxic T cells; and positive correlation for IL-2 → p-STAT5 | CD45RA+ Th cells). Interestingly, all age-associated immune signaling nodes occurred within CD45RA+ T (naïve) cells, whose functionality has been reported to be remodeled over time (22). The authors raised an important consideration by noting that only a single age-dependent node involved CD45RA+ helper T cells, which was an increased activation of Stat 5 induced by IL-2. This signaling is necessary for lymphocyte activation and decreases with aging, suggesting that it could be a potential target to reverse helper T-cell immunosenescence (55). It is noteworthy that few elderly people were included in the study, which may make it difficult to extrapolate these results as a model of immunosenescence.
The same group performed the functional analysis of immune cells in an independent cohort and obtained blood samples from 174 healthy individuals (144 over 65 years and 30 between 25 and 40 years) (79). They used a similar approach to the SCNP technique and compared the signaling produced by elderly versus young people. Twenty-four signaling nodes were measured in 12 cell subsets. They confirmed the previous finding of a close association between immune signaling response and age in subsets of CD45RA+ T cells. Fifty-seven nodes showed a pattern of age association, with 51 showing lower responsiveness and 6 showing higher responsiveness in the elderly. Higher responsiveness was observed mostly in B cells, probably in the memory compartment. The authors observed an age association among monocytes, T cells and NK cells, suggesting that the remodeling of immune function virtually splits out throughout all immune cells.
Whiting et al. expanded the measurement of functional immune status by investigating 243 healthy donors aged 40 to 97 years old (80). They described phospho-protein analysis of cytokine signaling, cytokine production, and gene expression from stimulated PBMCs in a stimulation-response manner. This system allowed them to create a public open access platform, encouraging scientists around the world to mine most of the data. The major contribution of this investigation was the evaluation of the functionality of the immune system through different ways, integrating cellular, protein and genomic data at the population level. Age, sex and CMV status may influence immune assays results. For example, phosphoprotein assessment was strongly correlated with CMV status, stimulated cytokine secretion was associated with age, and immune cell phenotype was associated with CMV status and age. Whiting et al. undermined the idea that a single immune system analyte could span immune age, similar to an “immune clock”. They noted a multiple significant association between pairs of analytes. Thereafter, the authors used elastic net regression to establish a model that could predict age. The regression finally listed 14 analytes chosen by the model, including 6 clinical laboratory or morphometric tests, 3 immunophenotyping, cytokines, sex, and CMV status. The model predicted age but overestimated the age of younger participants and underestimated the age of older subjects (80).
Unfortunately, an ideal statistical model that meticulously describes immune system remodeling with age is far from available. Instead, the immune response appears to be best defined as a group of continuous variables closely correlated with each other. Kaczorowski et al. evaluated the immunological phenotype and the functional immune response of 398 individuals and observed that the individuals’ immunotypes are continuously distributed in several healthy individuals (110). Elderly people exhibited greater heterogeneity in their immunotypes than younger people. This finding is consistent with the idea that age alone cannot predict the immune response. How the immune system is remodeled with age depends on an individual’s immunotype.
Even though the previously mentioned studies are endowed with genius, all of them are cross-sectional-based investigations. This imposes severe statistical limitations, as longitudinality becomes an extrapolation. To circumvent this problem, Alpert et al. performed longitudinal screening of various immunological parameters (cellular phenotype, cytokine-stimulation assays and whole blood gene expression) of 135 healthy subjects over 9 years (111). This allowed the authors to follow the cellular and molecular changes that took place in the participants’ immune system year after year to infer a mathematical model that could predict the immune trajectory. They endorsed many previous ratified pieces of confirmed knowledge, for example, that naive CD8 T cells decline with age and the impact of CMV infection on immunosenescence. Interestingly, the data suggested that immune cell phenotype dynamics can be classified into three stages according to convergence at a high-dimensional attractor point. According to the authors, as the immune system ages, the cell dynamics move toward a stable subset´s level, in a steady-state manner. This dynamic could be “slow linear” (e.g. CD85j+ CD8+ T cells), “asymptotic” (e.g. naïve CD4+ T cells) or “fluctuating” (e.g., monocytes), and the cells land to the homeostatic point sequentially. Interestingly, the authors observed that the cytokine response correlated negatively with the immune trajectory model obtained from the collected data. Bringing all these concepts together, the authors defined an IMM-AGE score, which can be valuable in predicting overall survival (111).
Conclusion
The immune response is under continuous and complex adaptation over time, leading to functional and phenotypic changes in the immune response. The consequence of immune remodeling can be recognized in many clinical features that characterize the older subpopulation. In this context, the evaluation of immunosenescence allows scientists to understand how the immune system ages. It may elucidate new mechanisms by which diseases are established in the elderly. By tracking these mechanisms, potential new targets for aging-related disorders may be revealed. As chronological age does not seem to perfectly reflect the set of molecular and cellular changes that occur with aging, population-level investigations of immune system remodeling allow clinicians to estimate the “immune age” parameter.
The description of immunological age emerges as a new tool to identify the subset of subjects in which unhealthy aging is a matter of time, helping to better individualize clinical management and sort out patients who may benefit from early interventions.
Author contributions
LC: conception and design, critical review of the literature and data, composition of the manuscript and final approval. LW: critical review of the literature and data, composition of the manuscript and final approval. All authors contributed to the article and approved the submitted version.
Funding
We thank São Paulo State Research Foundation/FAPESP and Brazilian Society of Endocrinology and Metabology (SBEM) for the investment.
Conflict of interest
The authors declare that the research was conducted in the absence of any commercial or financial relationships that could be construed as a potential conflict of interest.
Publisher’s note
All claims expressed in this article are solely those of the authors and do not necessarily represent those of their affiliated organizations, or those of the publisher, the editors and the reviewers. Any product that may be evaluated in this article, or claim that may be made by its manufacturer, is not guaranteed or endorsed by the publisher.
References
1. Aiello A, Farzaneh F, Candore G, Caruso C, Davinelli S, Gambino CM, et al. Immunosenescence and its hallmarks: How to oppose aging strategically? a review of potential options for therapeutic intervention. Front Immunol (2019) 10:2247. doi: 10.3389/fimmu.2019.02247
2. Crooke SN, Ovsyannikova IG, Poland GA, Kennedy RB. Immunosenescence and human vaccine immune responses. Immun Ageing (2019) 16:25. doi: 10.1186/s12979-019-0164-9
3. Fulop T, Larbi A, Dupuis G, Le Page A, Frost EH, Cohen AA, et al. Immunosenescence and inflamm-aging as two sides of the same coin: Friends or foes? Front Immunol (2017) 8:1960. doi: 10.3389/fimmu.2017.01960
4. Bellon M, Nicot C. Telomere dynamics in immune senescence and exhaustion triggered by chronic viral infection. Viruses (2017) 9(10):1–23. doi: 10.3390/v9100289
5. Pita-Lopez ML, Gayoso I, DelaRosa O, Casado JG, Alonso C, Muñoz-Gomariz E, et al. Effect of ageing on CMV-specific CD8 T cells from CMV seropositive healthy donors. Immun Ageing (2009) 6:11. doi: 10.1186/1742-4933-6-11
6. Pawelec G, Gouttefangeas C. T-Cell dysregulation caused by chronic antigenic stress: The role of CMV in immunosenescence? Aging Clin Exp Res (2006) 18(2):171–3. doi: 10.1007/BF03327436
7. Byun HO, Lee YK, Kim JM, Yoon G. From cell senescence to age-related diseases: Differential mechanisms of action of senescence-associated secretory phenotypes. BMB Rep (2015) 48(10):549–58. doi: 10.5483/BMBRep.2015.48.10.122
8. Biagi E, Candela M, Fairweather-Tait S, Franceschi C, Brigidi P. Aging of the human metaorganism: The microbial counterpart. Age (Dordr) (2012) 34(1):247–67. doi: 10.1007/s11357-011-9217-5
9. Dupont J, Antonio L, Dedeyne L, O'Neill TW, Vanderschueren D, Rastrelli G, et al. Inflammatory markers are associated with quality of life, physical activity, and gait speed but not sarcopenia in aged men (40-79 years). J Cachexia Sarcopenia Muscle (2021) 12(6):1818–31. doi: 10.1002/jcsm.12785
10. He B, Ma Y, Wang C, Jiang M, Geng C, Chang X, et al. Prevalence and risk factors for frailty among community-dwelling older people in China: A systematic review and meta-analysis. J Nutr Health Aging (2019) 23(5):442–50. doi: 10.1007/s12603-019-1179-9
11. Pawelec G, Picard E, Bueno V, Verschoor CP, Ostrand-Rosenberg S. MDSCs, ageing and inflammageing. Cell Immunol (2021) 362:104297. doi: 10.1016/j.cellimm.2021.104297
12. Globerson A, Effros RB. Ageing of lymphocytes and lymphocytes in the aged. Immunol Today (2000) 21(10):515–21. doi: 10.1016/S0167-5699(00)01714-X
13. Pawelec G. Immunosenescence: impact in the young as well as the old? Mech Ageing Dev (1999) 108(1):1–7. doi: 10.1016/s0047-6374(99)00010-x
14. Hazeldine J, Hampson P, Lord JM. Reduced release and binding of perforin at the immunological synapse underlies the age-related decline in natural killer cell cytotoxicity. Aging Cell (2012) 11(5):751–9. doi: 10.1111/j.1474-9726.2012.00839.x
15. Brubaker AL, Rendon JL, Ramirez L, Choudhry MA, Kovacs EJ. Reduced neutrophil chemotaxis and infiltration contributes to delayed resolution of cutaneous wound infection with advanced age. J Immunol (2013) 190(4):1746–57. doi: 10.4049/jimmunol.1201213
16. Swift ME, Burns AL, Gray KL, DiPietro LA. Age-related alterations in the inflammatory response to dermal injury. J Invest Dermatol (2001) 117(5):1027–35. doi: 10.1046/j.0022-202x.2001.01539.x
17. Simell B, Vuorela A, Ekström N, Palmu A, Reunanen A, Meri S, et al. Aging reduces the functionality of anti-pneumococcal antibodies and the killing of streptococcus pneumoniae by neutrophil phagocytosis. Vaccine (2011) 29(10):1929–34. doi: 10.1016/j.vaccine.2010.12.121
18. Hazeldine J, Harris P, Chapple IL, Grant M, Greenwood H, Livesey A, et al. Impaired neutrophil extracellular trap formation: A novel defect in the innate immune system of aged individuals. Aging Cell (2014) 13(4):690–8. doi: 10.1111/acel.12222
19. Jiang N, He J, Weinstein JA, Penland L, Sasaki S, He XS, et al. Lineage structure of the human antibody repertoire in response to influenza vaccination. Sci Transl Med (2013) 5(171):171ra19. doi: 10.1126/scitranslmed.3004794
20. Gibson KL, Wu YC, Barnett Y, Duggan O, Vaughan R, Kondeatis E, et al. B-cell diversity decreases in old age and is correlated with poor health status. Aging Cell (2009) 8(1):18–25. doi: 10.1111/j.1474-9726.2008.00443.x
21. Frasca D, Blomberg BB. Aging affects human b cell responses. J Clin Immunol (2011) 31(3):430–5. doi: 10.1007/s10875-010-9501-7
22. Goronzy JJ, Fang F, Cavanagh MM, Qi Q, Weyand CM. Naive T cell maintenance and function in human aging. J Immunol (2015) 194(9):4073–80. doi: 10.4049/jimmunol.1500046
23. Di Benedetto S, Derhovanessian E, Steinhagen-Thiessen E, Goldeck D, Müller L, Pawelec G. Impact of age, sex and CMV-infection on peripheral T cell phenotypes: Results from the Berlin BASE-II study. Biogerontology (2015) 16(5):631–43. doi: 10.1007/s10522-015-9563-2
24. Sagiv A, Biran A, Yon M, Simon J, Lowe SW, Krizhanovsky V. Granule exocytosis mediates immune surveillance of senescent cells. Oncogene (2013) 32(15):1971–7. doi: 10.1038/onc.2012.206
25. Almeida-Oliveira A, Smith-Carvalho M, Porto LC, Cardoso-Oliveira J, Ribeiro A, Falcão RR, et al. Age-related changes in natural killer cell receptors from childhood through old age. Hum Immunol (2011) 72(4):319–29. doi: 10.1016/j.humimm.2011.01.009
26. Sapey E, Greenwood H, Walton G, Mann E, Love A, Aaronson N, et al. Phosphoinositide 3-kinase inhibition restores neutrophil accuracy in the elderly: Toward targeted treatments for immunosenescence. Blood (2014) 123(2):239–48. doi: 10.1182/blood-2013-08-519520
27. Bueno V, Lord JM, Jackson T. The ageing immune system and health. New York, NY: Springer Science+Business Media (2016) 1:100p.
28. Goodrum F. Human cytomegalovirus latency: Approaching the Gordian knot. Annu Rev Virol (2016) 3(1):333–57. doi: 10.1146/annurev-virology-110615-042422
29. Looney RJ, Falsey A, Campbell D, Torres A, Kolassa J, Brower C, et al. Role of cytomegalovirus in the T cell changes seen in elderly individuals. Clin Immunol (1999) 90(2):213–9. doi: 10.1006/clim.1998.4638
30. Wertheimer AM, Bennett MS, Park B, Uhrlaub JL, Martinez C, Pulko V, et al. Aging and cytomegalovirus infection differentially and jointly affect distinct circulating T cell subsets in humans. J Immunol (2014) 192(5):2143–55. doi: 10.4049/jimmunol.1301721
31. Nikolich-Žugich J, van Lier RAW. Cytomegalovirus (CMV) research in immune senescence comes of age: Overview of the 6th international workshop on CMV and immunosenescence. Geroscience (2017) 39(3):245–9. doi: 10.1007/s11357-017-9984-8
32. Karrer U, Sierro S, Wagner M, Oxenius A, Hengel H, Koszinowski UH, et al. Memory inflation: Continuous accumulation of antiviral CD8+ T cells over time. J Immunol (2003) 170(4):2022–9. doi: 10.4049/jimmunol.170.4.2022
33. Jagannathan P, Osborne CM, Royce C, Manion MM, Tilton JC, Li L, et al. Comparisons of CD8+ T cells specific for human immunodeficiency virus, hepatitis c virus, and cytomegalovirus reveal differences in frequency, immunodominance, phenotype, and interleukin-2 responsiveness. J Virol (2009) 83(6):2728–42. doi: 10.1128/JVI.02128-08
34. van de Berg PJ, Griffiths SJ, Yong SL, Macaulay R, Bemelman FJ, Jackson S, et al. Cytomegalovirus infection reduces telomere length of the circulating T cell pool. J Immunol (2010) 184(7):3417–23. doi: 10.4049/jimmunol.0903442
35. Khan N, Shariff N, Cobbold M, Bruton R, Ainsworth JA, Sinclair AJ, et al. Cytomegalovirus seropositivity drives the CD8 T cell repertoire toward greater clonality in healthy elderly individuals. J Immunol (2002) 169(4):1984–92. doi: 10.4049/jimmunol.169.4.1984
36. Bartlett DB, Firth CM, Phillips AC, Moss P, Baylis D, Syddall H, et al. The age-related increase in low-grade systemic inflammation (Inflammaging) is not driven by cytomegalovirus infection. Aging Cell (2012) 11(5):912–5. doi: 10.1111/j.1474-9726.2012.00849.x
37. Derhovanessian E, Maier AB, Hähnel K, Zelba H, de Craen AJ, Roelofs H, et al. Lower proportion of naïve peripheral CD8+ T cells and an unopposed pro-inflammatory response to human cytomegalovirus proteins in vitro are associated with longer survival in very elderly people. Age (Dordr) (2013) 35(4):1387–99. doi: 10.1007/s11357-012-9425-7
38. Collerton J, Martin-Ruiz C, Davies K, Hilkens CM, Isaacs J, Kolenda C, et al. Frailty and the role of inflammation, immunosenescence and cellular ageing in the very old: cross-sectional findings from the Newcastle 85+ study. Mech Ageing Dev (2012) 133(6):456–66. doi: 10.1016/j.mad.2012.05.005
39. Pawelec G. Hallmarks of human "immunosenescence": adaptation or dysregulation? Immun Ageing (2012) 9(1):15. doi: 10.1186/1742-4933-9-15
40. Cunha LL, Perazzio SF, Azzi J, Cravedi P, Riella LV. Remodeling of the immune response with aging: Immunosenescence and its potential impact on COVID-19 immune response. Front Immunol (2020) 11:1748. doi: 10.3389/fimmu.2020.01748
41. Plunkett FJ, Franzese O, Finney HM, Fletcher JM, Belaramani LL, Salmon M, et al. The loss of telomerase activity in highly differentiated CD8+CD28-CD27- T cells is associated with decreased akt (Ser473) phosphorylation. J Immunol (2007) 178(12):7710–9. doi: 10.4049/jimmunol.178.12.7710
42. Larbi A, Dupuis G, Khalil A, Douziech N, Fortin C, Fülöp T. Differential role of lipid rafts in the functions of CD4+ and CD8+ human T lymphocytes with aging. Cell Signal (2006) 18(7):1017–30. doi: 10.1016/j.cellsig.2005.08.016
43. Taams LS, Smith J, Rustin MH, Salmon M, Poulter LW, Akbar AN. Human anergic/suppressive CD4(+)CD25(+) T cells: a highly differentiated and apoptosis-prone population. Eur J Immunol (2001) 31(4):1122–31. doi: 10.1002/1521-4141(200104)31:4<1122::AID-IMMU1122>3.0.CO;2-P
44. Martins PN, Tullius SG, Markmann JF. Immunosenescence and immune response in organ transplantation. Int Rev Immunol (2014) 33(3):162–73. doi: 10.3109/08830185.2013.829469
45. Seyda M, Quante M, Uehara H, Slegtenhorst BR, Elkhal A, Tullius SG. Immunosenescence in renal transplantation: a changing balance of innate and adaptive immunity. Curr Opin Organ Transplant (2015) 20(4):417–23. doi: 10.1097/MOT.0000000000000210
46. Haynes L, Lefebvre JS. Age-related deficiencies in antigen-specific CD4 T cell responses: Lessons from mouse models. Aging Dis (2011) 2(5):374–81.
47. Lang A, Nikolich-Zugich J. Functional CD8 T cell memory responding to persistent latent infection is maintained for life. J Immunol (2011) 187(7):3759–68. doi: 10.4049/jimmunol.1100666
48. Sadighi Akha AA, Miller RA. Signal transduction in the aging immune system. Curr Opin Immunol (2005) 17(5):486–91. doi: 10.1016/j.coi.2005.07.004
49. Thoman ML, Weigle WO. Lymphokines and aging: interleukin-2 production and activity in aged animals. J Immunol (1981) 127(5):2102–6.
50. Grossmann A, Maggio-Price L, Jinneman JC, Rabinovitch PS. Influence of aging on intracellular free calcium and proliferation of mouse T-cell subsets from various lymphoid organs. Cell Immunol (1991) 135(1):118–31. doi: 10.1016/0008-8749(91)90259-E
51. Krogsgaard M, Huppa JB, Purbhoo MA, Davis MM. Linking molecular and cellular events in T-cell activation and synapse formation. Semin Immunol (2003) 15(6):307–15. doi: 10.1016/j.smim.2003.09.002
52. Decman V, Laidlaw BJ, Doering TA, Leng J, Ertl HC, Goldstein DR, et al. Defective CD8 T cell responses in aged mice are due to quantitative and qualitative changes in virus-specific precursors. J Immunol (2012) 188(4):1933–41. doi: 10.4049/jimmunol.1101098
53. Garcia GG, Miller RA. Age-dependent defects in TCR-triggered cytoskeletal rearrangement in CD4+ T cells. J Immunol (2002) 169(9):5021–7. doi: 10.4049/jimmunol.169.9.5021
54. Garcia GG, Miller RA. Age-related defects in CD4+ T cell activation reversed by glycoprotein endopeptidase. Eur J Immunol (2003) 33(12):3464–72. doi: 10.1002/eji.200324310
55. Haynes L, Linton PJ, Eaton SM, Tonkonogy SL, Swain SL. Interleukin 2, but not other common gamma chain-binding cytokines, can reverse the defect in generation of CD4 effector T cells from naive T cells of aged mice. J Exp Med (1999) 190(7):1013–24. doi: 10.1084/jem.190.7.1013
56. Stavnezer J, Schrader CE. IgH chain class switch recombination: Mechanism and regulation. J Immunol (2014) 193(11):5370–8. doi: 10.4049/jimmunol.1401849
57. Zan H, Casali P. AID- and ung-dependent generation of staggered double-strand DNA breaks in immunoglobulin class switch DNA recombination: A post-cleavage role for AID. Mol Immunol (2008) 46(1):45–61. doi: 10.1016/j.molimm.2008.07.003
58. Frasca D, Landin AM, Lechner SC, Ryan JG, Schwartz R, Riley RL, et al. Aging down-regulates the transcription factor E2A, activation-induced cytidine deaminase, and ig class switch in human b cells. J Immunol (2008) 180(8):5283–90. doi: 10.4049/jimmunol.180.8.5283
59. Frasca D, Diaz A, Romero M, Landin AM, Phillips M, Lechner SC, et al. Intrinsic defects in b cell response to seasonal influenza vaccination in elderly humans. Vaccine (2010) 28(51):8077–84. doi: 10.1016/j.vaccine.2010.10.023
60. Danieli MG, Mezzanotte C, Verga JU, Menghini D, Pedini V, Bilò MB, et al. Common variable immunodeficiency in elderly patients: A long-term clinical experience. Biomedicines (2022) 10(3):1–11. doi: 10.3390/biomedicines10030635
61. Tannou T, Koeberle S, Manckoundia P, Aubry R. Multifactorial immunodeficiency in frail elderly patients: Contributing factors and management. Med Mal Infect (2019) 49(3):167–72. doi: 10.1016/j.medmal.2019.01.012
62. Patel SY, Carbone J, Jolles S. The expanding field of secondary antibody deficiency: Causes, diagnosis, and management. Front Immunol (2019) 10:33. doi: 10.3389/fimmu.2019.00033
63. Oliveira JB, Fleisher TA. Laboratory evaluation of primary immunodeficiencies. J Allergy Clin Immunol (2010) 125(2 Suppl 2):S297–305. doi: 10.1016/j.jaci.2009.08.043
64. Ten Oever J, van de Veerdonk FL, Joosten LA, Simon A, van Crevel R, Kullberg BJ, et al. Cytokine production assays reveal discriminatory immune defects in adults with recurrent infections and noninfectious inflammation. Clin Vaccine Immunol (2014) 21(8):1061–9. doi: 10.1128/CVI.00152-14
65. Rodriguez IJ, Lalinde Ruiz N, Llano León M, Martínez Enríquez L, Montilla Velásquez MDP, Ortiz Aguirre JP, et al. Immunosenescence study of T cells: A systematic review. Front Immunol (2020) 11:604591. doi: 10.3389/fimmu.2020.604591
66. Guayerbas N, Puerto M, Víctor VM, Miquel J, de la Fuente M. Leukocyte function and life span in a murine model of premature immunosenescence. Exp Gerontol (2002) 37(2-3):249–56. doi: 10.1016/S0531-5565(01)00190-5
67. Garrido A, Cruces J, Ceprián N, Vara E, de la Fuente M. Oxidative-inflammatory stress in immune cells from adult mice with premature aging. Int J Mol Sci (2019) 20(3):1–23. doi: 10.3390/ijms20030769
68. Garrido A, Cruces J, Ceprián N, Hernández-Sánchez C, de la Fuente M. Premature aging in behavior and immune functions in tyrosine hydroxylase haploinsufficient female mice. A longitudinal study. Brain Behav Immun (2018) 69:440–55. doi: 10.1016/j.bbi.2018.01.003
69. Wenisch C, Patruta S, Daxböck F, Krause R, Hörl W. Effect of age on human neutrophil function. J Leukoc Biol (2000) 67(1):40–5. doi: 10.1002/jlb.67.1.40
70. Butcher SK, Chahal H, Nayak L, Sinclair A, Henriquez NV, Sapey E, et al. Senescence in innate immune responses: Reduced neutrophil phagocytic capacity and CD16 expression in elderly humans. J Leukoc Biol (2001) 70(6):881–6. doi: 10.1189/jlb.70.6.881
71. Fulop T, Le Page A, Fortin C, Witkowski JM, Dupuis G, Larbi A. Cellular signaling in the aging immune system. Curr Opin Immunol (2014) 29:105–11. doi: 10.1016/j.coi.2014.05.007
72. Fulop T, Larbi A, Douziech N, Fortin C, Guérard KP, Lesur O, et al. Signal transduction and functional changes in neutrophils with aging. Aging Cell (2004) 3(4):217–26. doi: 10.1111/j.1474-9728.2004.00110.x
73. Wherry EJ, Kurachi M. Molecular and cellular insights into T cell exhaustion. Nat Rev Immunol (2015) 15(8):486–99. doi: 10.1038/nri3862
74. Shimada Y, Hayashi M, Nagasaka Y, Ohno-Iwashita Y, Inomata M. Age-associated up-regulation of a negative co-stimulatory receptor PD-1 in mouse CD4+ T cells. Exp Gerontol (2009) 44(8):517–22. doi: 10.1016/j.exger.2009.05.003
75. Channappanavar R, Twardy BS, Krishna P, Suvas S. Advancing age leads to predominance of inhibitory receptor expressing CD4 T cells. Mech Ageing Dev (2009) 130(10):709–12. doi: 10.1016/j.mad.2009.08.006
76. Lee KA, Shin KS, Kim GY, Song YC, Bae EA, Kim IK, et al. Characterization of age-associated exhausted CD8+ T cells defined by increased expression of Tim-3 and PD-1. Aging Cell (2016) 15(2):291–300. doi: 10.1111/acel.12435
77. Song Y, Wang B, Song R, Hao Y, Wang D, Li Y, et al. T-Cell immunoglobulin and ITIM domain contributes to CD8. Aging Cell (2018) 17(2):1–12. doi: 10.1111/acel.12716
78. Longo DM, Louie B, Putta S, Evensen E, Ptacek J, Cordeiro J, et al. Single-cell network profiling of peripheral blood mononuclear cells from healthy donors reveals age- and race-associated differences in immune signaling pathway activation. J Immunol (2012) 188(4):1717–25. doi: 10.4049/jimmunol.1102514
79. Longo DM, Louie B, Ptacek J, Friedland G, Evensen E, Putta S, et al. High-dimensional analysis of the aging immune system: Verification of age-associated differences in immune signaling responses in healthy donors. J Transl Med (2014) 12:178. doi: 10.1186/1479-5876-12-178
80. Whiting CC, Siebert J, Newman AM, Du HW, Alizadeh AA, Goronzy J, et al. Large-Scale and comprehensive immune profiling and functional analysis of normal human aging. PloS One (2015) 10(7):e0133627. doi: 10.1371/journal.pone.0133627
81. Carmona-Rivera C, Khaznadar SS, Shwin KW, Irizarry-Caro JA, O'Neil LJ, Liu Y, et al. Deficiency of adenosine deaminase 2 triggers adenosine-mediated NETosis and TNF production in patients with DADA2. Blood (2019) 134(4):395–406. doi: 10.1182/blood.2018892752
82. Brinkmann V, Reichard U, Goosmann C, Fauler B, Uhlemann Y, Weiss DS, et al. Neutrophil extracellular traps kill bacteria. Science (2004) 303(5663):1532–5. doi: 10.1126/science.1092385
83. Hawez A, Al-Haidari A, Madhi R, Rahman M, Thorlacius H. MiR-155 regulates PAD4-dependent formation of neutrophil extracellular traps. Front Immunol (2019) 10:2462. doi: 10.3389/fimmu.2019.02462
84. Grayson PC, Kaplan MJ. At The bench: Neutrophil extracellular traps (NETs) highlight novel aspects of innate immune system involvement in autoimmune diseases. J Leukoc Biol (2016) 99(2):253–64. doi: 10.1189/jlb.5BT0615-247R
85. Lee KH, Kronbichler A, Park DD, Park Y, Moon H, Kim H, et al. Neutrophil extracellular traps (NETs) in autoimmune diseases: A comprehensive review. Autoimmun Rev (2017) 16(11):1160–73. doi: 10.1016/j.autrev.2017.09.012
86. Tseng CW, Kyme PA, Arruda A, Ramanujan VK, Tawackoli W, Liu GY. Innate immune dysfunctions in aged mice facilitate the systemic dissemination of methicillin-resistant s. aureus. PloS One (2012) 7(7):e41454. doi: 10.1371/journal.pone.0041454
87. Lord JM, Butcher S, Killampali V, Lascelles D, Salmon M. Neutrophil ageing and immunesenescence. Mech Ageing Dev (2001) 122(14):1521–35. doi: 10.1016/S0047-6374(01)00285-8
88. Nishinaka Y, Arai T, Adachi S, Takaori-Kondo A, Yamashita K. Singlet oxygen is essential for neutrophil extracellular trap formation. Biochem Biophys Res Commun (2011) 413(1):75–9. doi: 10.1016/j.bbrc.2011.08.052
89. Palmer LJ, Cooper PR, Ling MR, Wright HJ, Huissoon A, Chapple IL. Hypochlorous acid regulates neutrophil extracellular trap release in humans. Clin Exp Immunol (2012) 167(2):261–8. doi: 10.1111/j.1365-2249.2011.04518.x
90. Guayerbas N, Catalán M, Víctor VM, Miquel J, de la Fuente M. Relation of behaviour and macrophage function to life span in a murine model of premature immunosenescence. Behav Brain Res (2002) 134(1-2):41–8. doi: 10.1016/S0166-4328(01)00449-1
91. da Costa RM, Rodrigues D, Pereira CA, Silva JF, Alves JV, Lobato NS, et al. Nrf2 as a potential mediator of cardiovascular risk in metabolic diseases. Front Pharmacol (2019) 10:382. doi: 10.3389/fphar.2019.00382
92. Viveros MP, Arranz L, Hernanz A, Miquel J, de la Fuente M. A model of premature aging in mice based on altered stress-related behavioral response and immunosenescence. Neuroimmunomodulation (2007) 14(3-4):157–62. doi: 10.1159/000110640
93. Anderson AC, Joller N, Kuchroo VK. Lag-3, Tim-3, and TIGIT: Co-inhibitory receptors with specialized functions in immune regulation. Immunity (2016) 44(5):989–1004. doi: 10.1016/j.immuni.2016.05.001
94. Parry RV, Chemnitz JM, Frauwirth KA, Lanfranco AR, Braunstein I, Kobayashi SV, et al. CTLA-4 and PD-1 receptors inhibit T-cell activation by distinct mechanisms. Mol Cell Biol (2005) 25(21):9543–53. doi: 10.1128/MCB.25.21.9543-9553.2005
95. Jin HT, Anderson AC, Tan WG, West EE, Ha SJ, Araki K, et al. Cooperation of Tim-3 and PD-1 in CD8 T-cell exhaustion during chronic viral infection. Proc Natl Acad Sci U S A (2010) 107(33):14733–8. doi: 10.1073/pnas.1009731107
96. Jones RB, Ndhlovu LC, Barbour JD, Sheth PM, Jha AR, Long BR, et al. Tim-3 expression defines a novel population of dysfunctional T cells with highly elevated frequencies in progressive HIV-1 infection. J Exp Med (2008) 205(12):2763–79. doi: 10.1084/jem.20081398
97. Rodriguez-Manzanet R, DeKruyff R, Kuchroo VK, Umetsu DT. The costimulatory role of TIM molecules. Immunol Rev (2009) 229(1):259–70. doi: 10.1111/j.1600-065X.2009.00772.x
98. Blackburn SD, Shin H, Haining WN, Zou T, Workman CJ, Polley A, et al. Coregulation of CD8+ T cell exhaustion by multiple inhibitory receptors during chronic viral infection. Nat Immunol (2009) 10(1):29–37. doi: 10.1038/ni.1679
99. Johnston RJ, Comps-Agrar L, Hackney J, Yu X, Huseni M, Yang Y, et al. The immunoreceptor TIGIT regulates antitumor and antiviral CD8(+) T cell effector function. Cancer Cell (2014) 26(6):923–37. doi: 10.1016/j.ccell.2014.10.018
100. Chew GM, Fujita T, Webb GM, Burwitz BJ, Wu HL, Reed JS, et al. TIGIT marks exhausted T cells, correlates with disease progression, and serves as a target for immune restoration in HIV and SIV infection. PloS Pathog (2016) 12(1):e1005349. doi: 10.1371/journal.ppat.1005349
101. Ligthart GJ, Corberand JX, Fournier C, Galanaud P, Hijmans W, Kennes B, et al. Admission criteria for immunogerontological studies in man: The SENIEUR protocol. Mech Ageing Dev (1984) 28(1):47–55. doi: 10.1016/0047-6374(84)90152-0
102. Olsson J, Wikby A, Johansson B, Löfgren S, Nilsson BO, Ferguson FG. Age-related change in peripheral blood T-lymphocyte subpopulations and cytomegalovirus infection in the very old: The Swedish longitudinal OCTO immune study. Mech Ageing Dev (2000) 121(1-3):187–201. doi: 10.1016/s0047-6374(00)00210-4
103. Strindhall J, Skog M, Ernerudh J, Bengner M, Löfgren S, Matussek A, et al. The inverted CD4/CD8 ratio and associated parameters in 66-year-old individuals: The Swedish HEXA immune study. Age (Dordr) (2013) 35(3):985–91. doi: 10.1007/s11357-012-9400-3
104. Li H, Weng P, Najarro K, Xue QL, Semba RD, Margolick JB, et al. Chronic CMV infection in older women: Longitudinal comparisons of CMV DNA in peripheral monocytes, anti-CMV IgG titers, serum IL-6 levels, and CMV pp65 (NLV)-specific CD8(+) T-cell frequencies with twelve year follow-up. Exp Gerontol (2014) 54:84–9. doi: 10.1016/j.exger.2014.01.010
105. Schmaltz HN, Fried LP, Xue QL, Walston J, Leng SX, Semba RD. Chronic cytomegalovirus infection and inflammation are associated with prevalent frailty in community-dwelling older women. J Am Geriatr Soc (2005) 53(5):747–54. doi: 10.1111/j.1532-5415.2005.53250.x
106. Wang GC, Kao WH, Murakami P, Xue QL, Chiou RB, Detrick B, et al. Cytomegalovirus infection and the risk of mortality and frailty in older women: A prospective observational cohort study. Am J Epidemiol (2010) 171(10):1144–52. doi: 10.1093/aje/kwq062
107. Roberts ET, Haan MN, Dowd JB, Aiello AE. Cytomegalovirus antibody levels, inflammation, and mortality among elderly latinos over 9 years of follow-up. Am J Epidemiol (2010) 172(4):363–71. doi: 10.1093/aje/kwq177
108. Yang FJ, Shu KH, Chen HY, Chen IY, Lay FY, Chuang YF, et al. Anti-cytomegalovirus IgG antibody titer is positively associated with advanced T cell differentiation and coronary artery disease in end-stage renal disease. Immun Ageing (2018) 15:15. doi: 10.1186/s12979-018-0120-0
109. Nilsson BO, Ernerudh J, Johansson B, Evrin PE, Löfgren S, Ferguson FG, et al. Morbidity does not influence the T-cell immune risk phenotype in the elderly: Findings in the Swedish NONA immune study using sample selection protocols. Mech Ageing Dev (2003) 124(4):469–76. doi: 10.1016/S0047-6374(03)00024-1
110. Kaczorowski KJ, Shekhar K, Nkulikiyimfura D, Dekker CL, Maecker H, Davis MM, et al. Continuous immunotypes describe human immune variation and predict diverse responses. Proc Natl Acad Sci U S A (2017) 114(30):E6097–E106. doi: 10.1073/pnas.1705065114
Keywords: immunosenescence, inflammaging, population, adaptive immunity, innate immunity
Citation: Cunha LL, Valsecchi VAdS and Ward LS (2022) Investigating population-level immunosenescence: From bench to bedside. Front. Immunol. 13:949928. doi: 10.3389/fimmu.2022.949928
Received: 21 May 2022; Accepted: 02 August 2022;
Published: 17 August 2022.
Edited by:
Andre Luis Lacerda Bachi, Universidade Santo Amaro, BrazilReviewed by:
Thibault Teissier, University of Oxford, United KingdomGuilherme Eustaquio Furtado, Instituto Politécnico da Guarda, Portugal
Copyright © 2022 Cunha, Valsecchi and Ward. This is an open-access article distributed under the terms of the Creative Commons Attribution License (CC BY). The use, distribution or reproduction in other forums is permitted, provided the original author(s) and the copyright owner(s) are credited and that the original publication in this journal is cited, in accordance with accepted academic practice. No use, distribution or reproduction is permitted which does not comply with these terms.
*Correspondence: Lucas Leite Cunha, lucas.leite@unifesp.br