- 1British Columbia Centre for Excellence in HIV/AIDS, Vancouver, BC, Canada
- 2Faculty of Health Sciences, Simon Fraser University, Burnaby, BC, Canada
- 3Department of Molecular Biology and Biochemistry, Simon Fraser University, Burnaby, BC, Canada
- 4Division of Medical Microbiology and Virology, St. Paul’s Hospital, Vancouver, BC, Canada
- 5Department of Pathology and Laboratory Medicine, Providence Health Care, Vancouver, BC, Canada
- 6Department of Pathology and Laboratory Medicine, University of British Columbia, Vancouver, BC, Canada
- 7Department of Medicine, University of British Columbia, Vancouver, BC, Canada
- 8British Columbia Centre for Disease Control Public Health Laboratory, Vancouver, BC, Canada
SARS-CoV-2 Omicron infections are common among individuals who are vaccinated or have recovered from prior variant infection, but few reports have immunologically assessed serial Omicron infections. We characterized SARS-CoV-2 humoral responses in an individual who acquired laboratory-confirmed Omicron BA.1.15 ten weeks after a third dose of BNT162b2, and BA.2 thirteen weeks later. Responses were compared to 124 COVID-19-naive vaccinees. One month post-second and -third vaccine doses, the participant’s wild-type and BA.1-specific IgG, ACE2-displacement and virus neutralization activities were average for a COVID-19-naive triple-vaccinated individual. BA.1 infection boosted the participant’s responses to the cohort ≥95th percentile, but even this strong “hybrid” immunity failed to protect against BA.2. Reinfection increased BA.1 and BA.2-specific responses only modestly. Though vaccines clearly protect against severe disease, results highlight the continued importance of maintaining additional protective measures to counteract the immune-evasive Omicron variant, particularly as vaccine-induced immune responses naturally decline over time.
Introduction
SARS-CoV-2 infections, predominantly fueled by the Omicron (B.1.1.529) variant, are increasingly common among individuals who are vaccinated and/or have recovered from prior infections (1–3). Globally, the highly transmissible and immune-evasive Omicron variant has rapidly overtaken the previously dominant Delta variant (3–7), and the original Omicron BA.1 strain is being outcompeted by newer Omicron sub-lineages BA.2, BA.3, BA.4 and BA.5 (8, 9). In British Columbia (BC), Canada, Omicron BA.1 had overtaken Delta by December 2021, and BA.2 had largely outcompeted BA.1 by March 2022 (10, 11).
COVID-19 vaccine coverage in BC is relatively high, with 93%, 90% and 57% of individuals aged 12 years or older having received one, two and three COVID-19 immunizations, respectively, by May 2022 (12). Persons at elevated risk of severe COVID-19 were also eligible for fourth doses at this time (13). Despite this, the province experienced fifth and sixth waves of COVID-19, dominated by BA.1 and BA.2, respectively, as public health measures were gradually relaxed (10, 11). Indeed, it is estimated that between December 2021 and March 2022, nearly half of British Columbians experienced a SARS-CoV-2 infection, likely due to Omicron (14, 15).
Several reports have examined post-vaccination Omicron infections, or Omicron reinfections following exposure to prior variants (16–23), but we are aware of only one study that assessed repeat Omicron infection incidence through viral genomic surveillance (24). The prior study however did not measure immune responses (24). In fact, at the time of writing, no studies to our knowledge appear to have investigated vaccine- and infection-induced immune responses after serial Omicron infections. Here, we longitudinally characterize SARS-CoV-2 humoral responses in an individual who experienced serial BA.1 and BA.2 Omicron infections following three-dose COVID-19 mRNA vaccination. Responses were compared to those of 124 COVID-19-naive vaccinees over the same period. Taken together with existing literature, our results suggest that vaccination provides limited protection against infection and/or reinfection by Omicron variants, though the fact that the individual’s symptoms were not serious enough to require hospitalization demonstrates that vaccination was nevertheless effective in its primary goal of preventing severe disease.
Methods
Observational COVID-19 vaccine cohort and SARS-CoV-2 infection monitoring
In December 2020, we established a prospective longitudinal study in Vancouver, Canada, to examine SARS-CoV-2 specific humoral immune responses following vaccination with BNT162b2 (Comirnaty; BioNTech/Pfizer) or mRNA-1273 (Spikevax; Moderna) in a cohort of adults aged 24-98 years [described in (25, 26)]. Serum and plasma were collected longitudinally up to 6 months following the third dose (Figure 1A). At each visit, serum was tested for the presence of SARS-CoV-2 anti-nucleocapsid (N) antibodies, which indicate seroconversion following infection, using the Elecsys Anti-SARS-CoV-2 assay on a Cobas e601 module analyzer (Roche Diagnostics). In addition to the case participant, immune measures from a comparison group of 124 participants of this cohort, who remained anti-N seronegative up until at least one month post-third vaccine dose, are included for context.
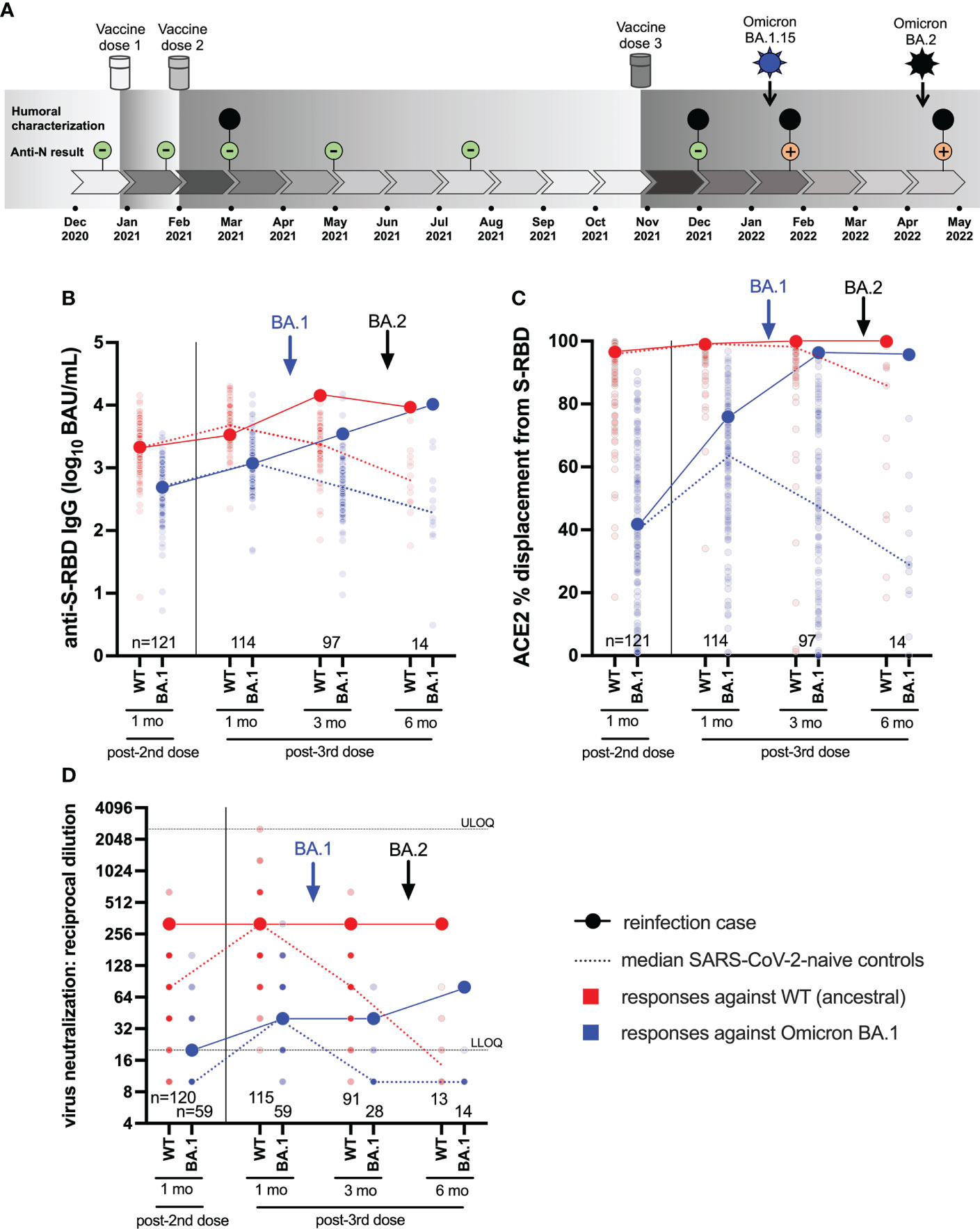
Figure 1 Case participant history and longitudinal humoral responses against wild-type and Omicron BA.1 SARS-CoV-2. Panel (A) Case participant timeline. Immunizations and SARS-CoV-2 Omicron infection history are shown at the top. Longitudinal SARS-CoV-2 anti-N serology results are shown in small green (anti-N negative) or orange (anti-N positive) circles. Large black circles denote time points where additional humoral functions, shown in panels below, were measured. Panel (B) Longitudinal anti-S-RBD IgG concentrations, expressed in log10 BAU/mL, in the case participant (large circles) versus the comparison group of SARS-CoV-2-naive individuals (small circles) at various time points following two- and three-dose COVID-19 vaccination. Wild-type (WT) specific anti-S-RBD responses are shown in red; Omicron BA.1-specific ones are shown in blue. Matching solid lines connect the participant’s longitudinal values, while dotted lines connect the median values for the comparison group. Approximate times of BA.1 and BA.2 infections are shown with arrows. Total Ns are shown at the bottom of the plot. Later time points have smaller Ns because some control participants were censored due to post-vaccination SARS-CoV-2 infection or had not yet completed the visit. Panel (C) same as (B), but for longitudinal ACE2% displacement function from wild-type (red) and BA.1 (blue) S-RBDs. Panel (D) same as (B), but for longitudinal live virus neutralization function against wild-type (red) and BA.1 (blue) strains. ULOQ/LLOQ: upper/lower limit of quantification.
Ethics approval
All participants or their authorized substitute decision makers provided written informed consent. This study was approved by the University of British Columbia/Providence Health Care and Simon Fraser University Research Ethics Boards (protocol H20-03906).
SARS-CoV-2 diagnostics and lineage confirmation
Diagnostic samples from the case participant’s two SARS-CoV-2 infections were tested at the St. Paul’s Hospital Virology Laboratory using the cobas® SARS-CoV-2 Test which targets conserved regions within the Orf1a/b and E genes (Roche Diagnostics) followed by screening using a real-time reverse transcription (RT)-PCR based algorithm for SARS-CoV-2 lineage classification that is frequently updated to detect emerging variants (27, 28). Following this, the diagnostic samples were subjected to full-genome SARS-CoV-2 sequencing in two independent laboratories: the BC Centre for Disease Control, the provincial laboratory that performs all SARS-CoV-2 sequencing for epidemiological surveillance, and the BC Centre for Excellence in HIV/AIDS. Both laboratories use the Illumina platform. The SARS-CoV-2 full genome sequences for the participant’s BA.1.15 and subsequent BA.2 infections are available in GISAID (Accession IDs EPI_ISL_12767799 and EPI_ISL_12662303, respectively) and in Genbank (Accession Numbers OP237526 and OP237527).
Binding antibody assays
We quantified anti-Spike Receptor Binding Domain (RBD) binding IgG concentrations in serum using the V-plex SARS-CoV-2 (IgG) ELISA kit (Panel 22, Meso Scale Diagnostics), which features wild-type and Omicron BA.1 RBD antigens. For a subset of participants, Anti-Spike binding IgG concentrations in serum were also quantified using the V-plex SARS-CoV-2 (IgG) ELISA kit (Panel 25, Meso Scale Diagnostics), which features full-length S antigens from wild-type, Omicron BA.1 and Omicron BA.2. This panel was used because, at the time of analysis, no reagents featuring Omicron BA.2 RBD were offered by the manufacturer. Both assays were performed on a Meso QuickPlex SQ120 instrument, with sera diluted 1:10000. Results are reported as WHO International Standard Units (BAU/mL), using the manufacturer-provided conversion factors.
ACE2 competition assays
We assessed the ability of serum antibodies to block the wild-type and Omicron BA.1 RBD-ACE2 receptor interaction by competition ELISA (Panel 22 V-plex SARS-CoV-2 [ACE2]; Meso Scale Diagnostics). For a subset of 28 participants, we also assessed the ability of serum antibodies to block the wild-type, BA.1 and BA.2 Spike-ACE2 receptor interaction using the same methods (Panel 25 V-plex SARS-CoV-2 [ACE2]). Both assays were performed on a Meso QuickPlex SQ120 instrument, with sera diluted 1:40. Results are reported as % ACE2 displacement.
Live virus neutralization assays
Neutralizing activity in plasma was examined in live SARS-CoV-2 assays using a wild-type isolate (USA-WA1/2020; BEI Resources) and a local Omicron BA.1 isolate (GISAID Accession # EPI_ISL_9805779) on VeroE6-TMPRSS2 (JCRB-1819) target cells. Viral stock was adjusted to 50 TCID50/200 µl in Dulbecco’s Modified Eagle Medium in the presence of serial 2-fold plasma dilutions (from 1/20 to 1/2560), incubated at 4°C for 1 hour and added to target cells in 96-well plates in triplicate. Cultures were maintained at 37°C with 5% CO2 and the appearance of viral cytopathic effect (CPE) was recorded three days post-infection. Neutralizing activity is reported as the reciprocal of the highest plasma dilution able to prevent CPE in all triplicate wells. Samples exhibiting partial or no neutralization at 1/20 dilution were defined as below the limit of quantification (BLOQ).
Data analysis
Data visualization and analysis was conducted in Prism v9.2.0 (GraphPad). As this is a report of a single case where immune response data are contrasted to those observed in a COVID-19-naive comparison group, response magnitudes are characterized in detail, but no formal statistical tests were applied.
Results
Case participant SARS-CoV-2 vaccination and infection timeline
The participant was a frontline health care worker, approximately 30 years of age. The participant had no major health conditions, but did take medications for hypothyroidism and familial hypercholesterolemia, both of which were well-controlled. The participant received three doses of mRNA vaccine (all BNT162b2; 30 mcg) in late December 2020, early February 2021 and late October 2021 (Figure 1A). All blood samples collected up to one month following the third immunization were anti-N seronegative.
In early January 2022, ten weeks after the third immunization, the participant experienced moderate COVID-19 symptoms including sore throat, fatigue, congestion, body aches, severe headaches, loss of taste and smell, coughing, shortness of breath and nausea. Symptoms, primarily cough, intensified in the second week after diagnosis requiring corticosteroid therapy. By the third week, symptoms had subsided except for shortness of breath and fatigue, with minimal improvement from short- and long-acting bronchodilating agents. A saline gargle collected on January 10, 2022 tested positive on the cobas® SARS-CoV-2 Test with a cycle threshold (Ct) value of 21 for both Orf1a/b and E gene targets. Real-time RT-PCR-based molecular screening identified the infection as Omicron BA.1, with subsequent full-genome viral sequencing confirming the specific lineage as BA.1.15.
In early April 2022, 13 weeks following the BA.1 infection (and 23 weeks following the third immunization) the participant experienced a profile of milder COVID-19 symptoms that differed compared to the first infection, consisting of a sore throat, fever, body aches, headaches, and diarrhea. No change in sense of taste or smell was noted. The participant noted persisting weakness, fatigue and mental fog, as well as severe long-term, treatment-resistant shortness of breath triggered by mild activities or exercises. A nasopharyngeal swab collected on April 9, 2022 tested positive on the cobas® SARS-CoV-2 Test with Ct values of 24 (Orf1a/b) and 23 (E). This infection was identified as BA.2 by molecular screening and confirmed by full-genome viral sequencing.
Longitudinal humoral responses to wild-type and Omicron BA.1 variants
We began by investigating the magnitude of the participant’s humoral immune responses following immunization, in context of a control group of 124 COVID-naïve individuals who were vaccinated during the same period. The comparison group was 74% female with a median age of 57 (Interquartile Range [IQR] 38-76) years. We quantified antibody responses to wild-type and Omicron BA.1 strains in the participant and the comparison group at one month after the second and third vaccine doses, as these time points should capture peak responses post-vaccination (Figures 1B–D).
One month post-second dose, the participant’s wild-type and BA.1-specific RBD IgG concentrations were 3.34 and 2.70 log10 BAU/mL respectively, which were equivalent to the 53rd and 50th percentile values of the comparator cohort (Figure 1B). One month post-third dose, the participant’s wild-type and BA.1-specific RBD IgG concentrations had increased to 3.51 and 3.06 log10 BAU/mL respectively, equivalent to the 30th and 50th percentile values of the cohort. Similarly, one month post-second dose, the participant’s ability to disrupt the interaction between the ACE2 receptor and the wild-type and BA.1 RBDs were 97% (57th percentile) and 42% (55th percentile) respectively (Figure 1C). At one month post-third dose, the participant’s wild-type- and BA.1-specific RBD-ACE2 displacement activities had increased to 99% (74th percentile) and 76% (73rd percentile) respectively. Finally, at one month post-second dose, the participant’s plasma neutralized wild-type and BA.1 SARS-CoV-2 at reciprocal dilutions of 320 and 20, which were equivalent to the 97th and 76th percentile values of the cohort. At one month post-third dose, the participant’s wild-type and BA.1 neutralization titers were 320 (78th percentile) and 40 (59th percentile) respectively. These results indicate that the participant’s overall vaccine responses were typical of the cohort, but nevertheless insufficient to prevent infection by BA.1 approximately six weeks later.
Seventeen days after testing positive with BA.1 (which coincided with a three-month post-third-dose study visit), the participant’s wild-type and BA.1-specific responses were boosted substantially, reaching the cohort 95th percentile for most measures at this time when immune responses had begun to decline in the comparator cohort (Figures 1B–D). The participant’s wild-type RBD IgG concentration increased to 4.15 log10 BAU/mL, while their BA.1-specific RBD IgG concentration increased to 3.55 log10 BAU/mL (Figure 1B). For context, these values would have placed the participant in the 94th and 91st percentiles of “peak” cohort values, measured at one month post-third vaccine dose. Similarly, the participant’s wild-type-specific RBD-ACE2 competition activity remained high at 99.9%, while their BA.1-specific RBD-ACE2 competition activity increased to 96.3%. For context, these values would be equivalent to the 99th percentiles of peak cohort values one month post-third vaccine dose (Figure 1C). The participant’s wild-type and BA.1-specific neutralization values held at 320 and 40, respectively, equivalent to the 78th and 59th percentiles of peak cohort values (Figure 1D). These results indicate that BA.1 infection boosted the participant’s humoral response, particularly in terms of binding antibody concentrations and ACE2 displacement activity. Nevertheless, this boost was insufficient to prevent reinfection by BA.2 approximately 10 weeks later.
Sixteen days after testing positive with BA.2 (which coincided with a six-month post-third-dose study visit), the participant’s wild-type-specific responses remained steady or declined slightly (e.g. RBD IgG) from prior measurements (Figures 1B–D). Nevertheless, most of the participant’s wild-type-specific responses remained at the cohort 100th percentile at this time point, which is unsurprising given that vaccine-induced responses had declined substantially over this time in the COVID-19 naive comparison group. For context, the participant’s wild-type-specific responses at this time point would have represented the 84th (RBD IgG), 99th (ACE2 competition) and 78th (neutralization) percentiles of peak cohort values measured at one month post-third vaccine dose.
By contrast, the BA.2 reinfection had mixed effects on BA.1 responses. While the participant’s BA.1-specific RBD IgG concentration rose substantially to 4.01 log10 BAU/mL (whereas the cohort median at this time point was nearly 2 log10 lower), no change was seen for BA.1-specific RBD-ACE2 competition, and BA.1 neutralization increased only modestly (Figures 1B–D). The more pronounced impact of BA.2 reinfection was to extend the duration of BA.1-specific responses in the participant, who maintained an RBD-ACE2 competition activity of 95.7% (compared to the cohort median 29% at this time point) and a neutralization activity of 80 (compared to the cohort median BLOQ at this time point). For further context, the participant’s BA.1-specific responses at this time point would represent the 99th (RBD IgG), 99th (ACE2 competition) and 83rd (neutralization) percentiles of peak cohort values measured at one month post-third vaccine dose. Nevertheless, despite BA.1 infection and BA.2 reinfection, the participant’s virus neutralization activity against BA.1 at this time point, which represented the highest activity measured during the study, remained 4-fold lower compared to that against the wild-type strain one month post-third vaccine dose (Figure 1D). The substantially weaker ability of even “hybrid” (vaccine and dual-infection induced) immune responses to neutralize Omicron compared to wild-type suggests that the participant may remain at risk of additional Omicron infection.
Longitudinal humoral responses to Omicron BA.2
We next characterized BA.2-specific Spike IgG and ACE2 competition activities in the participant, compared to a subset of 28 control vaccinees (79% Female, median age 59 years) beginning one month following the third vaccine dose (Figure 2). As these analyses focus on whole Spike (rather than RBD antigen), the corresponding wild-type and BA.1 Spike-specific responses are also shown for context (Figure 2). We additionally confirmed the (strong) correlations between wild-type- and BA.1-specific RBD and Spike responses in these individuals (all p<0.0001; Figure S1). At one month post-third vaccine dose, the participant displayed wild-type, BA.1 and BA.2-specific Spike IgG concentrations of 3.76, 3.14 and 3.41 log10 BAU/mL respectively (Figure 2A), and ACE2 competition activities of 99.4%, 50.6% and 64.3% respectively (Figure 2B). The participant’s wild-type and BA.2-specific Spike-specific IgG concentrations and ACE2 competition activities were broadly average compared to control vaccinees at this time (54th and 68th percentiles for IgG, respectively; 46th and 54th percentiles for ACE2 competition, respectively). The participant’s values for BA.1-specific IgG and BA.1 Spike-specific ACE2 competition however were slightly lower than the averages of the control group (37th and 39th percentiles, respectively).
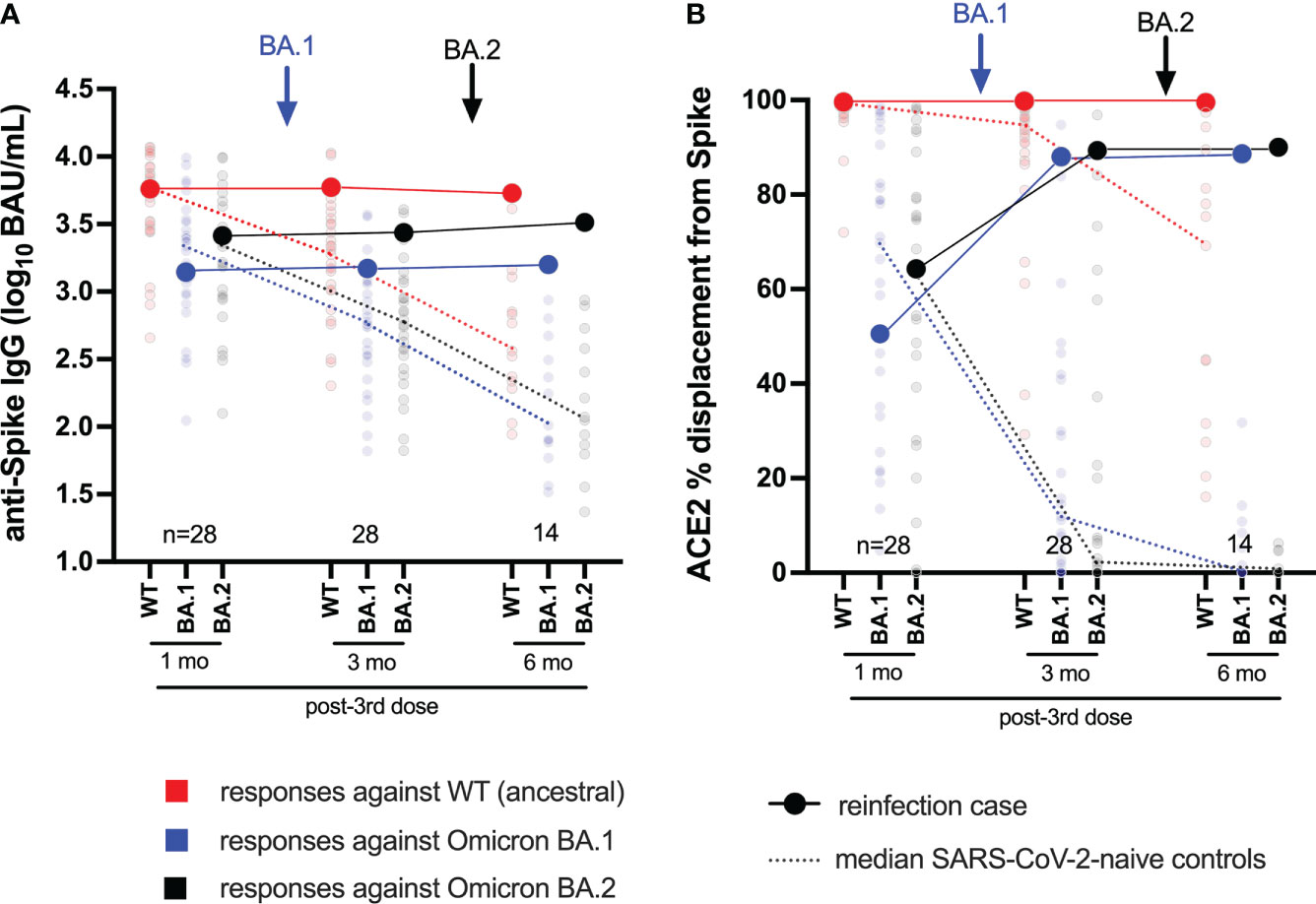
Figure 2 Longitudinal humoral responses against wild-type, BA.1 and BA.2 Spike antigens. Panel (A) Anti-Spike IgG concentrations, expressed in log10 BAU/mL, in the case participant (large circles) versus a subset of the comparison group of SARS-CoV-2-naive individuals (small circles) at one, three and six months following three-dose COVID-19 vaccination. Wild-type-specific (WT) anti-Spike responses are in red; BA.1-specific ones are in blue; BA.2-specific ones are in black. Matching solid lines connect the participant’s longitudinal values; dotted lines connect the median values for the comparison group. Approximate times of BA.1 and BA.2 infections are shown with arrows. Total Ns are shown at the bottom of the plot; the final time point has a smaller N because some control participants were censored due to post-vaccination SARS-CoV-2 infection or had not yet completed the visit. Panel (B) same as (A), but for longitudinal ACE2% displacement function from wild-type (red), BA.1 (blue) and BA.2 (black) Spike protein.
Following BA.1 infection, the participant’s wild-type, BA.1 and BA.2-specific Spike IgG concentrations increased modestly, to 3.77, 3.17 and 3.44 log10 BAU/mL, respectively (Figure 2A). Though the magnitude of these increases was not as pronounced as those observed in the RBD-based assays (shown in Figure 1B), these values nevertheless placed the participant at or above the 85th percentile compared to control vaccinees at this time point, when immune responses had begun to decline in the broader cohort. For context, these values would place the participant in the 57th, 39th, and 68th percentile of peak cohort values measured at one month post-third vaccine dose. Similar to the ACE2 competition activities measured using RBD antigens (shown in Figure 1C), the participant’s wild-type Spike-ACE2 competition activities remained high at 99.7%, while BA.1 and BA.2 Spike-ACE2 activities rose substantially to 87.9% and 89.3%, respectively (Figure 2B); values that represented the 71st, 75th, and 79th percentiles of peak values for the control vaccinees at one month post-third vaccine dose.
Following BA.2 infection, the participant’s wild-type Spike IgG concentration declined slightly to 3.73 log10 BAU/mL, whereas their BA.1 and BA.2-specific values increased slightly to 3.20 and 3.51 log10 BAU/mL, respectively (Figure 2A). These trends were consistent with the anti-RBD IgG concentrations measured in the primary analysis (shown in Figure 1B), though of a smaller magnitude. Similar to the ACE2 competition activities measured using RBD antigens (shown in Figure 1C), the participant’s wild-type Spike-ACE2 competition activity remained high (99.5%) after BA.2 infection. BA.1 and BA.2 Spike-ACE2 activities increased, though only marginally, to 88.6% and 90% respectively (Figure 2B).
Together, these results confirm that the participant’s humoral responses to wild-type and Omicron variants were broadly average for a COVID-19-naive individuals one month post-third vaccine dose. While subsequent BA.1 infection boosted Omicron-specific immune responses (highlighted by an increase in BA.1 and BA.2 Spike-ACE2 competition activities), BA.2 reinfection did not substantially augment these activities further, but rather extended the duration of these responses.
Discussion
This study provides a detailed characterization of humoral responses in a laboratory-confirmed case of serial infection by SARS-CoV-2 Omicron subvariants BA.1 and BA.2 in an individual who had mounted typical immune reactivity to three doses of COVID-19 mRNA vaccine. While data on repeat Omicron infections remain limited, a recent genomics-based study from Denmark identified 47 cases of BA.2 reinfection that occurred between 20 and 60 days following BA.1 infection (24). The authors concluded that such events were rare (<0.1% of cases during the brief window of analysis) and more likely to occur among unvaccinated individuals, but further evaluation of the data indicates that most reinfection cases were due to BA.2 following BA.1. The fact that the present case participant was one of only 151 original enrollees of our observational COVID-19 vaccine study (25, 26), which would translate into an Omicron serial infection prevalence of 0.7%, suggests that the risk of serial infection with Omicron subvariants may be higher than existing estimates. We note however that the participant’s status as a frontline healthcare worker may have resulted in an increased risk of exposure and infection over the general population (29). The potential influence of the participant’s hypothyroidism and familial hypercholesterolemia (though well-controlled by medication) on the symptoms profile following infection is also unknown.
Acknowledging that our ability to generalize from a single case is limited, we note that initial vaccine-induced IgG, ACE2 competition and virus neutralization response magnitudes against wild-type and Omicron BA.1 in the participant were comparable to the median values observed in diverse COVID-19-naive controls who were vaccinated along the same timeline. The observation that average humoral responses to three-dose vaccination failed to protect the participant against Omicron BA.1 infection is consistent with the extremely high rates of community transmission observed in many regions during recent Omicron-driven pandemic waves. Given that third vaccine doses substantially boost humoral responses in individuals of all ages (30–33), the relative risk of Omicron breakthrough infection is likely to be even higher among individuals who have received fewer than three doses (18, 34). Relative risk is also likely to increase with time following vaccination due to natural declines in antibody responses (26, 35–38), which, combined with natural Spike antigenic drift, may lead to ongoing risk of periodic re-infection (39). Additional studies are needed to assess these factors, as well as to investigate the impact of Omicron (re)infections following three-dose vaccination in larger numbers of individuals.
While it is perhaps unsurprising that COVID-19 vaccines based on ancestral SARS-CoV-2 sequences will not generate sterilizing immunity against Omicron strains that have evolved to evade host immune responses (4, 40–44), various lines of evidence suggest that “hybrid” immunity resulting from vaccination plus infection nevertheless provides enhanced protection against SARS-CoV-2 variants (5, 45), due in part to maturation of Spike-specific antibodies (46–48) and expansion of antiviral T cells (49–54). In light of this, we note that symptomatic BA.1 infection boosted the case participant’s vaccine-induced humoral responses against both BA.1 and BA.2. The heightened response nevertheless failed to prevent subsequent symptomatic infection by BA.2 ten weeks later, suggesting that these responses were insufficient to block infection, or that they had already declined to below-protective levels in this relatively short timeframe. Moreover, even after vaccination plus two Omicron infections, the participant’s ACE2 competition and virus neutralization responses against BA.1 (as well as ACE2 competition activity against BA.2) plateaued at levels substantially lower than those seen against the wild-type strain, suggesting that the participant will remain at risk of new Omicron infections. A limitation of our study is that it did not assess T cell responses, which can reduce disease severity but may have less impact on virus transmission (55, 56), and thus we may be underestimating the protection that results from infection and reinfection in this case.
Despite documentation of this case, it is important to keep in perspective that the participant’s symptoms following both infections were not severe enough to require hospitalization. This clearly demonstrates that vaccination was nevertheless effective in its primary goal of preventing severe disease. Results of our study should therefore not be mis-interpreted to suggest that COVID-19 vaccines (including booster doses) are not effective at their primary goal - indeed, substantial evidence clearly indicates that a third COVID-19 dose significantly reduces the risk of severe disease outcomes, including against Omicron (40, 57–59). Rather, our observation that “typical” antibody responses to vaccination failed to prevent Omicron infection in the case participant, and that the resulting enhanced “hybrid” immunity also failed to prevent Omicron re-infection, are consistent with the potentially limited ability of current vaccines to prevent recurrent symptomatic Omicron infections. Our study thus highlights the importance of additional preventive measures to reduce transmission, including masking, pre-exposure prophylaxis [e.g (60)], and potentially variant-specific immunizations (61–64), particularly as vaccine-induced immune responses naturally decline over time.
Data availability statement
The datasets presented in this study can be found in online repositories. The names of the repository/repositories and accession number(s) can be found below: NCBI Genbank, accession numbers OP237526 and OP237527.
Ethics statement
The studies involving human participants were reviewed and approved by University of British Columbia/Providence Health Care and Simon Fraser University Research Ethics Boards (protocol H20-03906). The participants or their authorized substitute decision makers provided their written informed consent to participate in this study.
Author contributions
HL and FM contributed equally as first authors. MR, MB, and ZB obtained project funding and contributed equally as senior authors. HL, FM, MB, and ZB designed the study. HL, FM, PC, YS, FY, RK, SD, RW, GU, SE, LY, WD, DK, and LB contributed to specimen collection and data analysis. HL, YS, VL, DH, MD, JS, NM, JM, CB, NP, MN, CL, MR, MB, and ZB supervised the research, laboratory assays and/or contributed to project or cohort management. HL, MB, and ZB wrote the original manuscript draft. All authors contributed to the article and approved the submitted version.
Funding
This work was supported by the Public Health Agency of Canada through a COVID-19 Immunology Task Force COVID-19 “Hot Spots” Award (2020-HQ-000120 to MR, ZB, MB). Additional funding was received from the Canadian Institutes for Health Research (GA2-177713 and the Coronavirus Variants Rapid Response Network (FRN-175622) to MB) and the Canada Foundation for Innovation through Exceptional Opportunities Fund - COVID-19 awards (to MB, MD, MN, RP, CL, ZB). MD and ZB hold Scholar Awards from the Michael Smith Foundation for Health Research. FM is supported by a fellowship from the CIHR Canadian HIV Trials Network. FY was supported by an SFU Undergraduate Student Research Award. GU holds a Ph.D. fellowship from the Sub-Saharan African Network for TB/HIV Research Excellence (SANTHE), a DELTAS Africa Initiative [grant # DEL-15-006]. The DELTAS Africa Initiative is an independent funding scheme of the African Academy of Sciences (AAS)’s Alliance for Accelerating Excellence in Science in Africa (AESA) and supported by the New Partnership for Africa’s Development Planning and Coordinating Agency (NEPAD Agency) with funding from the Wellcome Trust [grant # 107752/Z/15/Z] and the UK government.
Acknowledgments
We thank the leadership and staff of Providence Health Care for their support of this study. We thank the phlebotomists and laboratory staff at St. Paul’s Hospital, the BC Centre for Excellence in HIV/AIDS, the Hope to Health Research and Innovation Centre, and Simon Fraser University for assistance. Above all, we thank the participants, without whom this study would not have been possible.
Conflict of interest
The authors declare that the research was conducted in the absence of any commercial or financial relationships that could be construed as a potential conflict of interest.
Publisher’s note
All claims expressed in this article are solely those of the authors and do not necessarily represent those of their affiliated organizations, or those of the publisher, the editors and the reviewers. Any product that may be evaluated in this article, or claim that may be made by its manufacturer, is not guaranteed or endorsed by the publisher.
Author disclaimer
The views expressed in this publication are those of the authors and not necessarily those of the funders, including AAS, NEPAD Agency, Wellcome Trust or the UK government.
Supplementary material
The Supplementary Material for this article can be found online at: https://www.frontiersin.org/articles/10.3389/fimmu.2022.947021/full#supplementary-material
Supplementary Figure 1 | Correlations between wild-type (WT) and BA.1-specific anti-S-RBD and anti-Spike humoral responses measured following three COVID-19 vaccine doses using Meso Scale Diagnostics V-plex panels 22 and 25. All participants who were evaluated for BA.2 responses (i.e. those shown in Figure 2) are included in this analysis. Symbols are colored based on post-vaccination time point, though the Spearman’s rho (ρ) and p-value reported are for the combined data.
References
1. Pulliam JRC, van Schalkwyk C, Govender N, von Gottberg A, Cohen C, Groome MJ, et al. Increased risk of SARS-CoV-2 reinfection associated with emergence of omicron in south Africa. Science (2022) 376(6593):eabn4947. doi: 10.1126/science.abn4947
2. Bastard J, Taisne B, Figoni J, Mailles A, Durand J, Fayad M, et al. Impact of the omicron variant on SARS-CoV-2 reinfections in France, march 2021 to February 2022. Euro Surveill (2022) 27(13):pii=2200247. doi: 10.2807/1560-7917.ES.2022.27.13.2200247
3. Altarawneh HN, Chemaitelly H, Hasan MR, Ayoub HH, Qassim S, AlMukdad S, et al. Protection against the omicron variant from previous SARS-CoV-2 infection. N Engl J Med (2022) 386(13):1288–90. doi: 10.1056/NEJMc2200133
4. Cele S, Jackson L, Khoury DS, Khan K, Moyo-Gwete T, Tegally H, et al. Omicron extensively but incompletely escapes pfizer Bnt162b2 neutralization. Nature (2022) 602(7898):654–6. doi: 10.1038/s41586-021-04387-1
5. Smid M, Berec L, Pribylova L, Majek O, Pavlik T, Jarkovsky J, et al. Protection by vaccines and previous infection against the omicron variant of SARS-CoV-2. J Infect Dis (2022). doi: 10.1093/infdis/jiac161
6. Ahava MJ, Jarva H, Jaaskelainen AJ, Lappalainen M, Vapalahti O, Kurkela S. Rapid increase in SARS-CoV-2 seroprevalence during the emergence of omicron variant, Finland. Eur J Clin Microbiol Infect Dis (2022) 41:997–9. doi: 10.1007/s10096-022-04448-x
7. Chaguza C, Coppi A, Earnest R, Ferguson D, Kerantzas N, Warner F, et al. Rapid emergence of SARS-CoV-2 omicron variant is associated with an infection advantage over delta in vaccinated persons. Med (NY) (2022) 3(5):325–34. doi: 10.1016/j.medj.2022.03.010
8. Viana R, Moyo S, Amoako DG, Tegally H, Scheepers C, Althaus CL, et al. Rapid epidemic expansion of the SARS-CoV-2 omicron variant in Southern Africa. Nature (2022) 603(7902):679–86. doi: 10.1038/s41586-022-04411-y
9. Khan K, Karim F, Ganga Y, Bernstein M, Jule Z, Reedoy K, et al. Omicron Sub-lineages Ba.4/Ba.5 escape Ba.1 infection elicited neutralizing immunity. medRxiv (2022). doi: 10.1101/2022.04.29.22274477
10. BC Centre for Disease Control. Weekly update on variants of concern. Available at: http://www.bccdc.ca/health-info/diseases-conditions/covid-19/data.
11. BC Centre for Disease Control. Weekly reporting of covid-19 cases, hospitalizations, and deaths (2022). Available at: http://www.bccdc.ca/health-info/diseases-conditions/covid-19/data.
12. Public Health Agency of Canada. Canadian Covid-19 vaccination coverage report (2022). Available at: https://health-infobase.canada.ca/covid-19/vaccination-coverage/.
13. Government of British Columbia. Get a booster dose - province of British Columbia (2022). Available at: https://www2.gov.bc.ca/gov/content/covid-19/vaccine/booster.
14. Steacy LBC. Covid news: Omicron infected half of British columbians, Henry says Vancouver, Canada (2022). Available at: https://bc.ctvnews.ca/omicron-wave-exposed-infected-50-per-cent-of-british-columbians-henry-1.5850076.
15. Miller A. Almost half of canadians may have caught covid: CBC news (2022). Available at: https://www.cbc.ca/news/health/omicron-immunity-canada-covd19-vaccines-1.6428536.
16. Paterson SBC. Doctors seeing covid-19 reinfection in patients who recently had omicron Vancouver, Canada (2022). Available at: https://bc.ctvnews.ca/b-c-doctors-seeing-covid-19-reinfection-in-patients-who-recently-had-omicron-1.5846079.
17. Chang MR, Ke H, Coherd CD, Wang Y, Mashima K, Kastrunes GM, et al. Analysis of a SARS-CoV-2 convalescent cohort identified a common strategy for escape of vaccine-induced anti-rbd antibodies by beta and omicron variants. EBioMedicine (2022) 80:104025. doi: 10.1016/j.ebiom.2022.104025
18. Curlin ME, Bates TA, Guzman G, Schoen D, McBride SK, Carpenter SD, et al. Omicron neutralizing antibody response following booster vaccination compared with breakthrough infection. medRxiv (2022). doi: 10.1101/2022.04.11.22273694
19. Servellita V, Syed AM, Morris MK, Brazer N, Saldhi P, Garcia-Knight M, et al. Neutralizing immunity in vaccine breakthrough infections from the SARS-CoV-2 omicron and delta variants. Cell (2022) 185(9):1539–48.e5. doi: 10.1016/j.cell.2022.03.019
20. Glatman-Freedman A, Bromberg M, Hershkovitz Y, Sefty H, Kaufman Z, Dichtiar R, et al. Effectiveness of Bnt162b2 vaccine booster against SARS-CoV-2 infection and breakthrough complications, Israel. Emerg Infect Dis (2022) 28(5):948–56. doi: 10.3201/eid2805.220141
21. Seaman MS, Siedner MJ, Boucau J, Lavine CL, Ghantous F, Liew MY, et al. Vaccine breakthrough infection with the SARS-CoV-2 delta or omicron (Ba.1) variant leads to distinct profiles of neutralizing antibody responses. medRxiv (2022). doi: 10.1101/2022.03.02.22271731
22. Evans JP, Zeng C, Carlin C, Lozanski G, Saif LJ, Oltz EM, et al. Neutralizing antibody responses elicited by SARS-CoV-2 mrna vaccination wane over time and are boosted by breakthrough infection. Sci Transl Med (2022) 14(637):eabn8057. doi: 10.1126/scitranslmed.abn8057
23. Kuhlmann C, Mayer CK, Claassen M, Maponga T, Burgers WA, Keeton R, et al. Breakthrough infections with SARS-CoV-2 omicron despite mrna vaccine booster dose. Lancet (2022) 399(10325):625–6. doi: 10.1016/S0140-6736(22)00090-3
24. Stegger M, Edslev SM, Sieber RN, Cäcilia Ingham A, Ng KL, Tang M-HE, et al. Occurrence and significance of omicron Ba.1 infection followed by Ba.2 reinfection. medRxiv (2022). doi: 10.1101/2022.02.19.22271112
25. Brockman MA, Mwimanzi F, Lapointe HR, Sang Y, Agafitei O, Cheung P, et al. Reduced magnitude and durability of humoral immune responses to covid-19 mrna vaccines among older adults. J Infect Dis (2022) 225(7):1129–40. doi: 10.1093/infdis/jiab592
26. Mwimanzi F, Lapointe HR, Cheung PK, Sang Y, Yaseen F, Umviligihozo G, et al. Older adults mount less durable humoral responses to two doses of covid-19 mrna vaccine, but strong initial responses to a third dose. J Infect Dis (2022). doi: 10.1093/infdis/jiac199
27. Matic N, Lowe CF, Ritchie G, Stefanovic A, Lawson T, Jang W, et al. Rapid detection of SARS-CoV-2 variants of concern, including B.1.1.28/P.1, British Columbia, Canada. Emerg Infect Dis (2021) 27(6):1673–6. doi: 10.3201/eid2706.210532
28. Matic N, Lowe CF, Ritchie G, Young M, Lawson T, Romney MG. Omicron (B.1.1.529) SARS-CoV-2 viral load among nasopharyngeal and oral samples compared to other variants of concern and impact on diagnostic testing strategy. Clin Microbiol Infect (2022). doi: 10.1016/j.cmi.2022.04.022
29. Nguyen LH, Drew DA, Graham MS, Joshi AD, Guo CG, Ma W, et al. Risk of covid-19 among front-line health-care workers and the general community: A prospective cohort study. Lancet Public Health (2020) 5(9):e475–e83. doi: 10.1016/S2468-2667(20)30164-X
30. Lustig Y, Gonen T, Meltzer L, Gilboa M, Indenbaum V, Cohen C, et al. Superior immunogenicity and effectiveness of the third compared to the second Bnt162b2 vaccine dose. Nat Immunol (2022) 23:940–6. doi: 10.1038/s41590-022-01212-3
31. Liu X, Munro APS, Feng S, Janani L, Aley PK, Babbage G, et al. Persistence of immunogenicity after seven covid-19 vaccines given as third dose boosters following two doses of Chadox1 ncov-19 or Bnt162b2 in the uk: Three month analyses of the cov-boost trial. J Infect (2022) 84(6):795–813. doi: 10.1016/j.jinf.2022.04.018
32. Moreira ED Jr., Kitchin N, Xu X, Dychter SS, Lockhart S, Gurtman A, et al. Safety and efficacy of a third dose of Bnt162b2 covid-19 vaccine. N Engl J Med (2022) 386(20):1910–21. doi: 10.1056/NEJMoa2200674
33. Yoon SK, Hegmann KT, Thiese MS, Burgess JL, Ellingson K, Lutrick K, et al. Protection with a third dose of mrna vaccine against SARS-CoV-2 variants in frontline workers. N Engl J Med (2022) 386(19):1855–7. doi: 10.1056/NEJMc2201821
34. Goel RR, Painter MM, Lundgreen KA, Apostolidis SA, Baxter AE, Giles JR, et al. Efficient recall of omicron-reactive b cell memory after a third dose of SARS-CoV-2 mrna vaccine. Cell (2022) 185 (11):1875–87. doi: 10.1016/j.cell.2022.04.009
35. Mantus G, Nyhoff LE, Edara VV, Zarnitsyna VI, Ciric CR, Flowers MW, et al. Pre-existing SARS-CoV-2 immunity influences potency, breadth, and durability of the humoral response to SARS-CoV-2 vaccination. Cell Rep Med (2022) 3(4):100603. doi: 10.1016/j.xcrm.2022.100603
36. Tartof SY, Slezak JM, Puzniak L, Hong V, Xie F, Ackerson BK, et al. Durability of Bnt162b2 vaccine against hospital and emergency department admissions due to the omicron and delta variants in a Large health system in the USA: A test-negative case-control study. Lancet Respir Med (2022) 10(7):689–99. doi: 10.1016/S2213-2600(22)00101-1
37. Bonnet B, Chabrolles H, Archimbaud C, Brebion A, Cosme J, Dutheil F, et al. Decline of humoral and cellular immune responses against SARS-CoV-2 6 months after full Bnt162b2 vaccination in hospital healthcare workers. Front Immunol (2022) 13:842912. doi: 10.3389/fimmu.2022.842912
38. Sogaard OS, Reekie J, Johansen IS, Nielsen H, Benfield T, Wiese L, et al. Characteristics associated with serological covid-19 vaccine response and durability in an older population with significant comorbidity: The Danish nationwide enforce study. Clin Microbiol Infect (2022) 28(8):1126–33. doi: 10.1016/j.cmi.2022.03.003
39. Yewdell JW. Antigenic drift: Understanding covid-19. Immunity (2021) 54(12):2681–7. doi: 10.1016/j.immuni.2021.11.016
40. Andrews N, Stowe J, Kirsebom F, Toffa S, Rickeard T, Gallagher E, et al. Covid-19 vaccine effectiveness against the omicron (B.1.1.529) variant. New Engl J Med (2022) 386(16):1532–46. doi: 10.1056/NEJMoa2119451
41. Bjork J, Bonander C, Moghaddassi M, Rasmussen M, Malmqvist U, Inghammar M, et al. Covid-19 vaccine effectiveness against severe disease from SARS-CoV-2 omicron Ba.1 and Ba.2 subvariants - surveillance results from southern Sweden, December 2021 to march 2022. Euro Surveill (2022) 27(18):pii=2200322. doi: 10.2807/1560-7917.ES.2022.27.18.2200322
42. Collie S, Champion J, Moultrie H, Bekker LG, Gray G. Effectiveness of Bnt162b2 vaccine against omicron variant in south Africa. N Engl J Med (2022) 386(5):494–6. doi: 10.1056/NEJMc2119270
43. Yu J, Collier AY, Rowe M, Mardas F, Ventura JD, Wan H, et al. Neutralization of the SARS-CoV-2 omicron Ba.1 and Ba.2 variants. N Engl J Med (2022) 386(16):1579–80. doi: 10.1056/NEJMc2201849
44. Pedersen RM, Bang LL, Madsen LW, Sydenham TV, Johansen IS, Jensen TG, et al. Serum neutralization of SARS-CoV-2 omicron Ba.1 and Ba.2 after Bnt162b2 booster vaccination. Emerg Infect Dis (2022) 28(6):1274–5. doi: 10.3201/eid2806.220503
45. Nordstrom P, Ballin M, Nordstrom A. Risk of SARS-CoV-2 reinfection and covid-19 hospitalisation in individuals with natural and hybrid immunity: A retrospective, total population cohort study in Sweden. Lancet Infect Dis (2022) 22(6):781–90. doi: 10.1016/S1473-3099(22)00143-8
46. Chen Y, Tong P, Whiteman N, Moghaddam AS, Zarghami M, Zuiani A, et al. Immune recall improves antibody durability and breadth to SARS-CoV-2 variants. Sci Immunol (2022):eabp8328. doi: 10.1126/sciimmunol.abp8328
47. Cho A, Muecksch F, Schaefer-Babajew D, Wang Z, Finkin S, Gaebler C, et al. Anti-SARS-CoV-2 receptor-binding domain antibody evolution after mrna vaccination. Nature (2021) 600(7889):517–22. doi: 10.1038/s41586-021-04060-7
48. Muecksch F, Weisblum Y, Barnes CO, Schmidt F, Schaefer-Babajew D, Wang Z, et al. Affinity maturation of SARS-CoV-2 neutralizing antibodies confers potency, breadth, and resilience to viral escape mutations. Immunity (2021) 54(8):1853–68 e7. doi: 10.1016/j.immuni.2021.07.008
49. Rodda LB, Morawski PA, Pruner KB, Fahning ML, Howard CA, Franko N, et al. Imprinted SARS-CoV-2-Specific memory lymphocytes define hybrid immunity. Cell (2022) 185(9):1588–601.e14. doi: 10.1016/j.cell.2022.03.018
50. Riou C, Keeton R, Moyo-Gwete T, Hermanus T, Kgagudi P, Baguma R, et al. Escape from recognition of SARS-CoV-2 variant spike epitopes but overall preservation of T cell immunity. Sci Transl Med (2022) 14(631):eabj6824. doi: 10.1126/scitranslmed.abj6824
51. Keeton R, Richardson SI, Moyo-Gwete T, Hermanus T, Tincho MB, Benede N, et al. Prior infection with SARS-CoV-2 boosts and broadens Ad26.Cov2.S immunogenicity in a variant-dependent manner. Cell Host Microbe (2021) 29(11):1611–9.e5. doi: 10.1016/j.chom.2021.10.003
52. Keeton R, Tincho MB, Ngomti A, Baguma R, Benede N, Suzuki A, et al. T Cell responses to SARS-CoV-2 spike cross-recognize omicron. Nature (2022) 603(7901):488–92. doi: 10.1038/s41586-022-04460-3
53. Gao Y, Cai C, Grifoni A, Muller TR, Niessl J, Olofsson A, et al. Ancestral SARS-CoV-2-Specific T cells cross-recognize the omicron variant. Nat Med (2022) 28(3):472–6. doi: 10.1038/s41591-022-01700-x
54. Goel RR, Painter MM, Apostolidis SA, Mathew D, Meng W, Rosenfeld AM, et al. Mrna vaccines induce durable immune memory to SARS-CoV-2 and variants of concern. Science (2021) 374(6572):abm0829. doi: 10.1126/science.abm0829
55. Pollard AJ, Bijker EM. A guide to vaccinology: From basic principles to new developments. Nat Rev Immunol (2021) 21(2):83–100. doi: 10.1038/s41577-020-00479-7
56. Jeyanathan M, Afkhami S, Smaill F, Miller MS, Lichty BD, Xing Z. Immunological considerations for covid-19 vaccine strategies. Nat Rev Immunol (2020) 20(10):615–32. doi: 10.1038/s41577-020-00434-6
57. Abu-Raddad LJ, Chemaitelly H, Ayoub HH, AlMukdad S, Yassine HM, Al-Khatib HA, et al. Effect of mrna vaccine boosters against SARS-CoV-2 omicron infection in Qatar. N Engl J Med (2022) 386(19):1804–16. doi: 10.1056/NEJMoa2200797
58. Chemaitelly H, Ayoub HH, AlMukdad S, Coyle P, Tang P, Yassine HM, et al. Duration of mrna vaccine protection against SARS-CoV-2 omicron Ba.1 and Ba.2 subvariants in Qatar. Nat Commun (2022) 13(1):3082. doi: 10.1038/s41467-022-30895-3
59. Link-Gelles R, Levy ME, Gaglani M, Irving SA, Stockwell M, Dascomb K, et al. Effectiveness of 2, 3, and 4 covid-19 mrna vaccine doses among immunocompetent adults during periods when SARS-CoV-2 omicron Ba.1 and Ba.2/Ba.2.12.1 sublineages predominated - vision network, 10 states, December 2021-June 2022. MMWR Morb Mortal Wkly Rep (2022) 71(29):931–9. doi: 10.15585/mmwr.mm7129e1
60. Levin MJ, Ustianowski A, De Wit S, Launay O, Avila M, Templeton A, et al. Intramuscular Azd7442 (Tixagevimab-cilgavimab) for prevention of covid-19. N Engl J Med (2022) 386(23):2188–200. doi: 10.1056/NEJMoa2116620
61. Hawman DW, Meade-White K, Clancy C, Archer J, Hinkley T, Leventhal SS, et al. Replicating rna platform enables rapid response to the SARS-CoV-2 omicron variant and elicits enhanced protection in naive hamsters compared to ancestral vaccine. EBioMedicine (2022) 83:104196. doi: 10.1016/j.ebiom.2022.104196
62. Lam JY, Ng YY, Yuen CK, Wong WM, Yuen KY, Kok KH. A nasal omicron vaccine booster elicits potent neutralizing antibody response against emerging SARS-CoV-2 variants. Emerg Microbes Infect (2022) 11(1):964–7. doi: 10.1080/22221751.2022.2053365
63. Ying B, Scheaffer SM, Whitener B, Liang CY, Dmytrenko O, Mackin S, et al. Boosting with variant-matched or historical mrna vaccines protects against omicron infection in mice. Cell (2022) 185(9):1572–87.e11. doi: 10.1016/j.cell.2022.03.037
Keywords: COVID-19, vaccine, Omicron variant, reinfection, humoral immunity
Citation: Lapointe HR, Mwimanzi F, Cheung PK, Sang Y, Yaseen F, Kalikawe R, Datwani S, Waterworth R, Umviligihozo G, Ennis S, Young L, Dong W, Kirkby D, Burns L, Leung V, Holmes DT, DeMarco ML, Simons J, Matic N, Montaner JSG, Brumme CJ, Prystajecky N, Niikura M, Lowe CF, Romney MG, Brockman MA and Brumme ZL (2022) Serial infection with SARS-CoV-2 Omicron BA.1 and BA.2 following three-dose COVID-19 vaccination. Front. Immunol. 13:947021. doi: 10.3389/fimmu.2022.947021
Received: 18 May 2022; Accepted: 16 August 2022;
Published: 06 September 2022.
Edited by:
Edwin Bölke, Heinrich Heine University of Düsseldorf, GermanyReviewed by:
Jean-Pierre Routy, McGill University, CanadaPinkus Tober-Lau, Charité University Medicine Berlin, Germany
Copyright © 2022 Lapointe, Mwimanzi, Cheung, Sang, Yaseen, Kalikawe, Datwani, Waterworth, Umviligihozo, Ennis, Young, Dong, Kirkby, Burns, Leung, Holmes, DeMarco, Simons, Matic, Montaner, Brumme, Prystajecky, Niikura, Lowe, Romney, Brockman and Brumme. This is an open-access article distributed under the terms of the Creative Commons Attribution License (CC BY). The use, distribution or reproduction in other forums is permitted, provided the original author(s) and the copyright owner(s) are credited and that the original publication in this journal is cited, in accordance with accepted academic practice. No use, distribution or reproduction is permitted which does not comply with these terms.
*Correspondence: Zabrina L. Brumme, zbrumme@sfu.ca
†These authors have contributed equally to this work
‡These authors have contributed equally to this work and share senior authorship