- 1Department of Immunology, H. Lee Moffitt Cancer Center & Research Institute, Tampa, FL, United States
- 2Department of Malignant Hematology, H. Lee Moffitt Cancer Center & Research Institute, Tampa, FL, United States
Regulatory T cells (Tregs) are responsible for maintaining immune homeostasis by controlling immune responses. They can be characterized by concomitant expression of FoxP3, CD25 and inhibitory receptors such as PD-1 and CTLA-4. Tregs are key players in preventing autoimmunity and are dysregulated in cancer, where they facilitate tumor immune escape. B-cell lymphoid malignancies are a group of diseases with heterogenous molecular characteristics and clinical course. Treg levels are increased in patients with B-cell lymphoid malignancies and correlate with clinical outcomes. In this review, we discuss studies investigating Treg immunobiology in B-cell lymphoid malignancies, focusing on clinical correlations, mechanisms of accumulation, phenotype, and function. Overarching trends suggest that Tregs can be induced directly by tumor cells and recruited to the tumor microenvironment where they suppress antitumor immunity to facilitate disease progression. Further, we highlight studies showing that Tregs can be modulated by novel therapeutic agents such as immune checkpoint blockade and targeted therapies. Treg disruption by novel therapeutics may beneficially restore immune competence but has been associated with occurrence of adverse events. Strategies to achieve balance between these two outcomes will be paramount in the future to improve therapeutic efficacy and safety.
Introduction
Lymphoid malignancies are a diverse spectrum of diseases including malignancies arising from transformation of B cells, T cells or natural killer (NK) cells (1). Lymphoid malignancies are systemic diseases that can manifest in lymph nodes, bone marrow and peripheral blood. They are among the most common cancers and one of the leading causes of cancer-related death in the United States (2). B-cell non-Hodgkin’s lymphoma (B-NHL) accounts for the majority of lymphoid malignancies and is classified as indolent [grade I/II follicular lymphoma (FL), small lymphocytic lymphoma (SLL), marginal zone lymphoma (MZL)] or aggressive [diffuse large B-cell lymphoma (DLBCL), Burkitt lymphoma (BL)]. SLL cells are immunophenotypically identical to chronic lymphocytic leukemia (CLL) cells, but the patients do not present with lymphocytosis (3). Therefore, both clinical presentations are frequently referred to as the same disease. Although CLL/SLL belongs to the indolent lymphoma group, approximately 3% of patients develop Richter syndrome, undergoing transformation toward aggressive DLBCL (4, 5).
Since the approval of the first anti-CD20 monoclonal antibody (mAb) more than twenty years ago, chemoimmunotherapy has been utilized for first-line treatment of lymphoid malignancies. Rituximab, an anti-CD20 mAb, is often used as monotherapy in grade I/II follicular lymphoma and elicits high overall response (6). First-line treatment with fludarabine, cyclophosphamide and rituximab (FCR) is still an option for CLL patients with mutated immunoglobulin heavy chain gene (IgHV) which is associated with better prognosis (3). However, treatment refractoriness and low rates of response to chemoimmunotherapy are frequently seen in patients with aggressive lymphomas such as DLBCL (7, 8). Targeted therapies such as B-cell receptor (BCR) signaling inhibitors have improved survival of patients with lymphoid malignancies (9–11), but long-term remission is lacking in a subset of patients and most require prolonged treatment (12). Immune checkpoint blockade (ICB) is currently being investigated for use in B-cell malignancies but has produced underwhelming results thus far with the exception of Hodgkin’s disease and mediastinal DLBCL (13). Chimeric antigen receptor (CAR) T-cell therapies has become an option in the third-line setting for patients with B-NHL and has also been recently approved in the second line for DLBCL (14, 15). Despite availability of novel therapeutics, relapsed/refractory (R/R) disease continues to be an unmet need and mechanisms underlying response to treatment need to be further explored.
Immune cell subsets are altered within the tumor microenvironment (TME) of B-NHL and are implicated in malignant cell survival and drug resistance (16). Alterations in T-cell phenotype and function have been broadly observed (17–20). In the FL TME, malignant B cells depend on direct contact with T follicular helper (Tfh) cells, which are essential for the formation and maintenance of the germinal center reaction (21, 22). In CLL and DLBCL, investigators have observed altered CD4+ to CD8+ T cell ratio, inverted T helper (Th)1 to Th2 ratio and increased exhausted T cells expressing TIM-3, LAG-3 and PD-1 (23, 24). T cells from patients with FL, DLBCL and CLL also show impaired immune synapse formation which has been identified as an active immunosuppressive mechanism (19, 20).
Regulatory T cells (Tregs) are conventionally characterized as CD4+ T cells constitutively expressing high surface CD25 (IL-2Rα), low or no CD127 (IL-7Rα) and the transcription factor FoxP3 (25). Tregs are divided into two broad groups based on origin: thymic-derived natural (n)Tregs and peripherally-derived inducible (i)Tregs (26). They are essential for maintaining peripheral self-tolerance and preventing autoimmunity, and loss of the Foxp3 gene results in multiorgan autoimmune disorder in humans and mice (27, 28). Tregs suppress activity of other immune subsets including conventional and effector T cells, B cells, NK cells, NKT cells, monocytes and dendritic cells. Numerous mechanisms of Treg-mediated suppression have been described, including (1) production of immunosuppressive cytokines such as IL-10, TGF-β and IL-35, (2) cytotoxicity by granzymes and perforin, (3) expression of co-inhibitory molecules such as PD-1, CTLA-4, and LAG-3, and (4) interference with metabolism via IL-2 deprivation, adenosine production and cAMP transfer (29, 30).
Tregs are dysregulated in the TME and are key players in tumor immune escape (31). Tregs have been associated with poor prognoses in patients with solid tumors and are thought to exert tumor-promoting roles by directly interfering with cytotoxic T-cell function to suppress antitumor immune responses (31). However, Tregs have been paradoxically correlated with both positive and negative clinical outcomes in B-NHL patients (32). The impact of novel therapies on Treg function, and the role of Tregs in response to therapy and incidence of adverse events have recently become relevant. In this review, we summarize and discuss studies exploring Treg immunobiology in B-NHL, focusing primarily on but not limited to FL, DLBCL and CLL.
Prognostic value
Tregs associate with clinical outcomes in patients with lymphoid malignancies. In the following studies of FL and DLBCL patients, Tregs were detected by immunohistochemistry in biopsy specimens and identified as FoxP3+ unless otherwise indicated. Increased tumor-infiltrating Tregs correlated with better response to therapy and improved overall survival (33), good prognosis defined by follicular lymphoma international prognostic index (FLIPI) score (34), disease-specific and failure-free survival in FL patients (35). Two independent studies found that a diffuse distribution pattern of Tregs in FL specimens correlated with more favorable outcomes than intrafollicular or perifollicular patterns (36, 37).
Other studies similarly reported that tumor-infiltrating Tregs positively associated with overall survival in untreated DLBCL patients (38) and in DLBCL patients treated with chemoimmunotherapy (39–41). Tregs have been examined by flow cytometry in peripheral blood mononuclear cells (PBMCs) of newly diagnosed and treated DLBCL patients (42). Results revealed elevated Tregs present in newly diagnosed patients and patients at remission compared to those with R/R disease (42). Meta-analysis of 23 prior studies including but not limited to B-NHL patients revealed that higher density of tumor-infiltrating Tregs correlated with improved progression-free and overall survival (43). One conflicting report showed that Tregs associated with inferior overall survival in a cohort of DLBCL patients treated with chemoimmunotherapy, but these results were not replicated in a second cohort (23). The overall trend suggests that higher Treg levels correlate with better outcomes in FL and DLBCL patients.
The studies mentioned here examined Tregs by flow cytometry in PBMCs of CLL patients. Elevated Treg levels have been described in untreated CLL patients compared to treated CLL patients and healthy donors (44). However, increased Tregs in CLL patients correlated with worse prognostic factors including Rai and Binet stage, CD38 expression, lactate dehydrogenase (LDH) levels (45–47), and shorter time to first treatment (48, 49). Skewed Treg to Th17 ratio that favored Tregs also correlated with advanced disease (50). None of the studies reviewed here reported association of Tregs with survival, IgHV mutation status or ZAP70 expression in CLL patients. The overall trend therefore suggests that higher Treg levels indicated worse outcomes in CLL patients. Treg associations with clinical outcomes in B-NHL and CLL patients have been summarized in Table 1.
Induction and recruitment to the tumor microenvironment
Mechanisms that increase Tregs in lymphoid malignancies have been widely investigated. Reports indicate that malignant cells directly induce Tregs and that proteins dysregulated by malignant cells facilitate Treg induction (Figure 1). B-NHL cells isolated from patients induced functional Tregs from naïve T cells in a contact-dependent manner (51). Yang et al. showed that CD70+ B-NHL cells induced FoxP3 expression in naïve T cells, and this was abrogated by CD70-CD27 axis blockade (52). Years later, Balsas et al. established that CD70 upregulation in SOX11+ MCL could promote tumor immune evasion via Treg induction (53). Another study revealed a role for IDO1 in B-NHL-mediated Treg induction (54). IDO1 expression positively correlated with FoxP3 expression in B-NHL, and IDO1 inhibition attenuated B-NHL-mediated Treg induction (54). The ICOS-ICOSL axis has been shown to promote FL-mediated Treg induction (55). Results demonstrated that ICOS+ Tregs were induced by coculture with ICOSL+ FL cells, and this was abrogated by ICOS-ICOSL blockade.
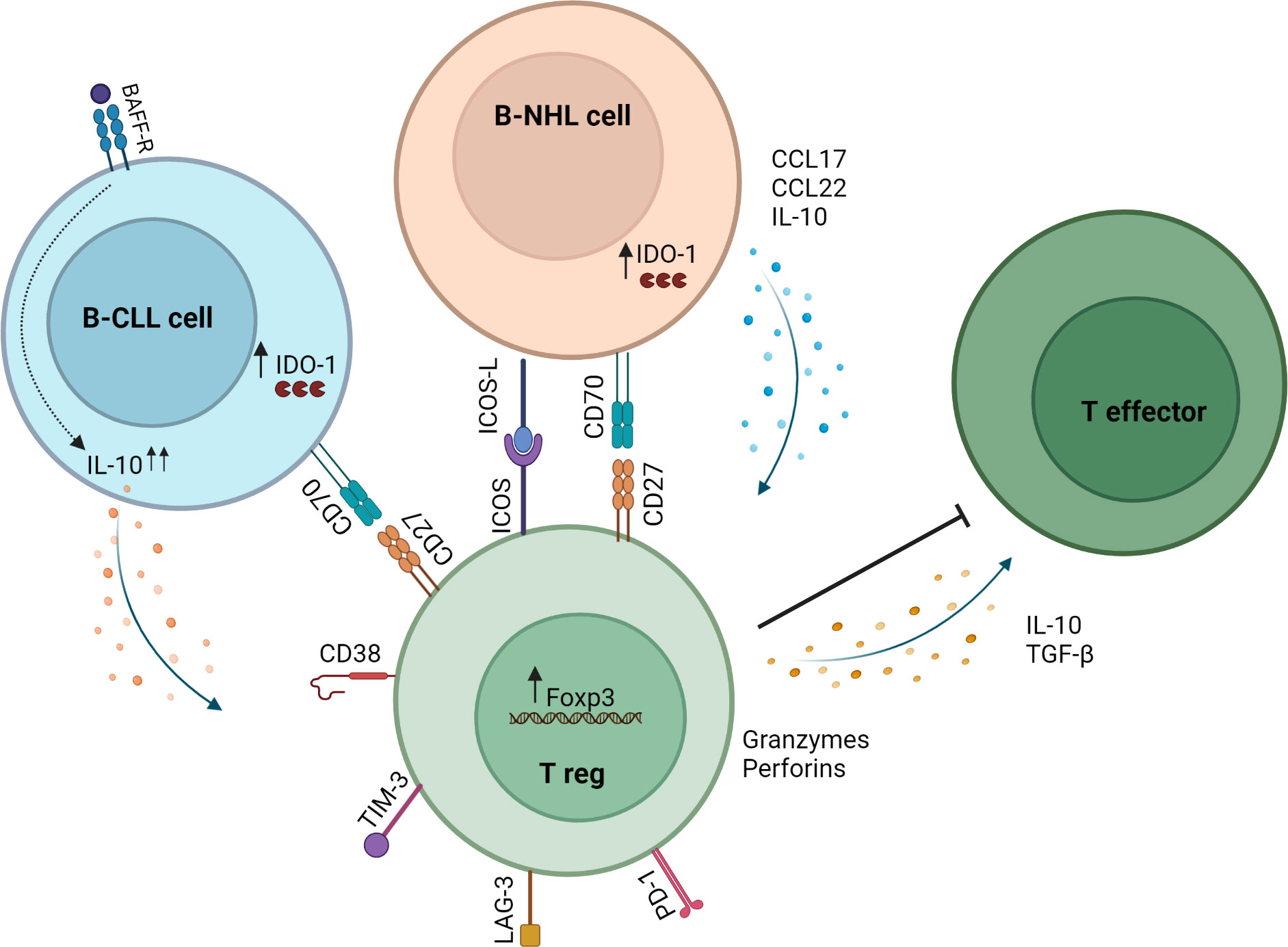
Figure 1 Mechanisms of Treg induction and action in the B-NHL microenvironment. B-NHL and CLL cells can induce Tregs via direct contact with T cells in the tumor microenvironment and through secretion of soluble factors. In B-NHL and CLL, Tregs can inhibit the immune response through the secretion of IL-10 and TGF-β or by killing effector T cells via release of granzymes and perforins. Tumor-infiltrating Tregs in B-NHL and CLL also exhibit high expression of immune checkpoint molecules such as PD-1, LAG-3 and TIM-3.
CLL cells produce IL-10 and share features with normal regulatory B cells referred to as Breg or B10 (56). BAFF is a protein implicated in development and maintenance of CLL cells (57). One study uncovered a key role for BAFF-driven IL-10 production by CLL cells in generating Tregs and enhancing suppressive capacity by upregulating FoxP3 expression (58). Another study demonstrated involvement of IL-10 and TGF-β1 in CLL-mediated induction of Tregs (59). Results showed that CLL cells induced Tregs from naïve T cells, and this was abrogated by blocking IL-10 or TGF-β1 (59). These studies highlight the role of soluble factors in promoting immunosuppression via Treg induction in the CLL microenvironment.
It has been hypothesized that circulating Tregs can be preferentially recruited into the lymphoma microenvironment. A mechanism whereby Tfh cells induce CCL17 and CCL22 production by FL cells to facilitate Treg recruitment has been elucidated (60). In another study, gene expression analysis of Tregs sorted from FL patients revealed a mechanism by which Tregs target to and accumulate within the TME through downregulation of S1PR1, SELL (L-selectin) and CCR7 (61). These data suggest that migratory capacity can be influenced by tumor cells and thus contribute to Treg accumulation in the TME.
Besides malignant cells, other cell subsets within the TME influence Treg biology. Myeloid-derived suppressor cells (MDSCs), which are increased in CLL patient PBMCs, express high levels of IDO and correlate with Treg frequency (62). MDSCs generated from CLL patient samples induced Tregs in coculture experiments. Further, the presence of MDSCs coculture enhanced CLL-mediated Treg induction (62). Collectively, these studies offer mechanistic insight into how lymphoma and CLL cells reshape the TME. Tregs can be directly induced from naïve T cells by tumor cells, or preferentially recruited and retained to the TME. Blocking antibodies or antagonists to inhibit Treg induction and recruitment to shift the balance in favor of antitumor immunity should be further investigated.
Phenotypic and functional complexity
Tregs consist of phenotypically diverse subsets and exhibit specialized functions in lymphoid and non-lymphoid peripheral tissue (63). This diversity suggests that Tregs can alter homeostatic, migratory and suppression programs according to environmental cues (25). Investigators seeking to address the role of Tregs in lymphoma and CLL have described phenotypic and functional complexity. The studies discussed in this section indicate that Tregs harbor altered surface markers and gene expression. Tregs express variable CD25 and CD127 levels, memory, activation, Th and cytotoxicity markers. The heterogeneity of these findings might depend on which Treg subsets are present and influenced by immunoediting effects of tumor cells and microenvironmental cues. Although reports suggest diverse phenotype and function for Tregs, most studies reviewed here suggest that Tregs protect tumor cells by suppressing antitumor T-cell responses.
Tumor-promoting Tregs
Some evidence suggests that Tregs permit tumor immune evasion in B-NHL. Studies have shown that B-NHL-derived Tregs suppressed T-cell proliferation and IFN-γ production, indicating a tumor-protective effect mediated by Tregs (64, 65). Tumor-promoting Tregs in CLL appear to be resistant to apoptosis and exhibit sustained suppressive ability. Both conventional CD4+CD25hiCD127-/lo and unconventional CD4+CD25-/loCD127- FoxP3+ Tregs exhibited lower intrinsic apoptotic rates in CLL patients versus controls (47). Further, expression of anti-apoptotic protein BCL-2 in CLL T cells was associated with an immunosuppressive TME through promotion of Tregs (66). Results of this study revealed that BCL-2+ Tregs harbored increased expression of IL-10 and TGF-β compared to BCL-2- Tregs, indicating superior suppressive capacity (66). These findings suggest that CLL Tregs may be directly targeted by venetoclax, a BCL-2 inhibitor which is approved for treatment of CLL (67).
Mouse models have proven useful in interrogating Treg phenotype and function during leukemia development in ways that are not possible in studies that solely utilize patient samples. Gorgun et al. first noted increased CD4+CD25+FoxP3+CTLA-4+ Tregs occurring alongside leukemia development in the Eμ-TCL1 transgenic mouse model of CLL (68). A comprehensive recent study established a supportive role for Tregs in CLL progression in the adoptive transfer Eμ-TCL1 mouse model (69). In this model, FoxP3+ Treg depletion resulted in expansion of CD8+ effector cells and leukemia clearance. A CD69hiLAG-3hiCD44loCD25lo Treg subset was identified via unbiased clustering analysis of flow cytometry data. This Treg subset exhibited increased expression of genes related to suppression, checkpoints, and chemokines that most likely support leukemia progression. Using the TCL1-ovalbumin (OVA)-expressing mouse model to generate T cells specific for OVA antigen expressed by leukemic cells, this study further demonstrated that Tregs derived from CLL-bearing mice could suppress proliferation of CLL-specific CD8+ T cells (69). Interestingly, these results reflected another study showing that elevated Treg levels in CLL patients correlated with decreased T-cell responses against viral and tumor antigens in functional assays (70). A study in the λ-MYC mouse model of BL similarly demonstrated that Tregs suppressed antitumor immune responses, and Treg depletion delayed disease development (71). Further, Tregs in this model recognized antigens likely to be displayed by tumor cells (71). These reports provide evidence that Tregs can potentiate immune evasion in an antigen-specific manner.
Tregs harboring effector and unconventional phenotypes
Tregs expressing effector T-cell characteristics have been identified in lymphoma and CLL patients. However, there is no standard method of identifying effector-like Tregs at this point. Tregs harboring cytotoxic capacity, expression of Th lineage specific transcription factors besides FoxP3 or memory phenotype have all been referred to as “effector Tregs” in previous literature (29, 72). Tregs can inhibit immune responses by killing effector immune cells via release of granzymes and perforin as well as Fas-FasL interactions in normal and tumor settings (29). Tregs that express characteristics of Th effector cells and Tregs that harbor memory phenotypes have also been described in both settings (73).
Conventional CD4+CD127loFoxP3+ Tregs and unconventional CD4+CD127hiFoxP3+ Tregs expressing cytotoxicity markers CD107 and FasL have been described in lymphoma and CLL patients (73). This study demonstrated that isolated CLL-derived Tregs killed CLL cells in ex vivo assays, indicating the potential to eliminate malignant cells (73). CLL-derived Tregs can switch toward effector Th phenotypes characterized by altered transcriptional and cytokine profiles when compared to healthy donors (74). These effector Tregs co-expressed FoxP3 and T-bet, GATA-3 or RORγt transcription factors resulting in Th1-like, Th2-like or Th17-like Tregs respectively. Th-like Treg subsets were increased in CLL patients compared to healthy donors (74). Other studies have similarly identified Tregs with characteristics of Th cells (75, 76).
It has been proposed that memory Treg differentiation might be a response to inflammatory factors in the TME (77). Chronic antigen stimulation and inflammation occurring in lymphoma and CLL may therefore potentially contribute to the presence of memory Tregs (78). Many investigators studying T-cell subsets in lymphoma and CLL have utilized variations of the widely accepted CD4+CD25hiCD127-/loFoxP3+ flow cytometry gating strategy to identify Tregs, where CD127+/hi cells representing memory T cells are excluded. However, Biancotto et al. reported that CD4+CD25+FoxP3+ Tregs in CLL patient PBMCs could be further divided according to canonical memory surface markers (79). Tregs were identified as naïve (CD45RA+), central memory (CD45RA-CD27+CCR7+), effector memory (CD45RA-CD27+CCR7-) and effector (CD45RA-CD27-CCR7-). The frequencies of these subsets were altered in CLL patients compared to healthy donor controls. CD39 is expressed on exhausted CD8+ T cells and mediates suppressive activity in Tregs (80, 81). CD39+CCR7+ Tregs were identified in CLL patients that were not found in healthy donors, indicating a memory Treg subset with high suppressive capacity (79). The study confirmed that these Tregs suppressed responder cell activity ex vivo. A separate study similarly found intratumoral CD27+CD45RA- memory Tregs in FL patient samples (55).
CD8+ Tregs that express FoxP3 and exhibit suppressive function have been identified, but research on this rare subset is limited (82). CD8+CD25+FoxP3+ Tregs have been noted to be increased in CLL patients compared to healthy donors (79) as well as in progressive versus indolent CLL patients (83). Taken together, these studies suggest that Tregs harboring effector phenotypes are altered in lymphoma and CLL and exhibit variable function. Unconventional CD8+FoxP3+ cells are also altered in CLL, but their existence has not been described in lymphoma. The role of CD8+FoxP3+ cells in normal and tumor settings remains to be established.
Expression of inhibitory receptors and immune checkpoint blockade
Tregs canonically express inhibitory surface receptors that mediate suppression including TIM-3, CTLA-4, TIGIT, LAG-3, PD-1 and NRP-1, among others. Elevated expression of these markers identifies more stable and functional Tregs (84). Earlier correlative studies indicated that CTLA-4+ and TIM-3+ Tregs associated with poorer outcomes in lymphoma patients (85, 86). These findings contradict other studies summarized above that relied on FoxP3 staining alone to identify Tregs. The role of inhibitory receptors, also referred to as immune checkpoints, in T-cell exhaustion has been widely acknowledged (87), and immune checkpoint blockade (ICB) is being tested in lymphoid malignancies (13). The impact of ICB on Tregs in these diseases has therefore become relevant. In this section, we summarize studies addressing the role of Tregs characterized by inhibitory receptor expression and discuss effects of ICB.
TIGIT is frequently expressed by Tregs in FL patients (88). Imbalance of TIGIT and CD226, its competitive co-stimulatory receptor, on CD8+ T cells has been concurrently noted (88). TIGIT+ Tregs are therefore likely to contribute to immunosuppression in FL. Another study found that LAG-3 was highly expressed in DLBCL patient derived-Tregs (89). In these patients, the majority of LAG-3+ Tregs co-expressed PD-1 and TIM-3. These Tregs could be divided into nTregs (defined as CD4+CD25hiCD127lo) and iTregs (defined as CD4+CD25loCD127lo). This study implied that LAG3+ Tregs are involved in complex cellular interactions in the DLBCL microenvironment and suggested that combination PD-1/LAG-3 blockade warrants further investigation (89).
Mouse models have proven useful for examining inhibitory receptor expression on Tregs during disease progression and identifying ICB candidates. In a study utilizing the λ-MYC mouse model of BL, tumor-promoting Tregs were found to express canonical nTregs markers Helios and NRP-1 and recognized antigens expressed by lymphoma cells (71). The same group later observed that Tregs progressively upregulated suppressive phenotypic and functional markers during disease progression in this model, including FoxP3, CD25, CTLA-4, PD-1 and IL-10 (90). Lymphoma-derived Tregs limited responder cell proliferation and IFN-γ production more than WT-derived Tregs, and this suppressive ability was dependent on surface NRP-1, PD-L1 and soluble IL-10. The authors further asked whether ICB could affect Tregs. Combination PD-1/CTLA-4 blockade downregulated FoxP3 and CD25 expression on Tregs, indicating functional disruptions (90). These findings confirmed that Treg changes occurring during lymphoma development could be partially reversed by combination ICB in the λ-MYC model.
CTLA-4 (68) and LAG-3 (69) expression have been observed on Tregs in the Eμ-TCL1 mouse model of CLL that were found to exhibit tumor-promoting characteristics. Through unbiased clustering analysis of high dimensional flow cytometry data, another study found three previously undescribed clusters of Tregs in this model (1) KLRG1+CD69+ Tregs described as terminally differentiated, tumor-infiltrating Tregs (2) FoxP3+CD25loLAG-3+ Tregs that were enriched in leukemic mice and represented a reservoir of suppressive Tregs (3) CD44loCD62L+CD38-KLRG1- Tregs that were not enriched in leukemic mice and represented naïve Tregs (91). KLRG1 has been proposed as an immune checkpoint and KLRG1+ Tregs have been described by other investigators (92, 93). However, KLRG1+ Treg function in the CLL microenvironment is not yet understood. Dual anti-PD-1/LAG-3 treatment restored an immunocompetent response with fewer Treg cells in this model (91). It is unclear if Tregs were directly affected or reduced as a secondary effect of leukemia clearance.
CD38 is expressed on some CLL cells and is a prognostic marker for CLL patients (94). CD38 is also expressed on Tregs and associated with suppressive activity (95). A higher proportion of CD38+ Tregs has been identified in CLL patients compared to healthy donors (59). This study found that CD38+ Tregs were positively associated with CD38+ CLL cells. CD38 blockade ex vivo directly induced cell death in CLL Tregs. Further, daratumumab (anti-CD38) systemic treatment reduced Tregs and expanded antitumor T-cell subsets in a patient-derived xenograft (PDX) CLL mouse model, suggesting that daratumumab provoked a potent antitumor response (59). Collectively, the studies reviewed here imply that ICB can affect Treg subsets that are altered in the context of lymphoma and CLL to enhance antitumor benefit. Blockade of novel checkpoints and combination approaches to modulate Tregs should therefore be investigated.
T follicular regulatory cells
Tfh cells are required in the germinal center reaction where they assist B cells during memory differentiation and generation of high-affinity antibodies (22). T follicular regulatory (Tfr) cells develop from conventional Treg precursors or naïve CD4+ T cells and control germinal center B-cell responses by limiting Tfh function. Tfr cells express markers in common with Tfh cells such as BCL-6, PD-1, CXCR5 and ICOS as well as markers in common with conventional Tregs such as FoxP3, CTLA-4 and GITR (96).
Tfr cells have been identified in FL, DLBCL, and most recently, CLL patients. ICOS+CXCR5+FoxP3+ cells that expressed CTLA-4, GITR and Ki67, consistent with Tfr phenotype, were found to be increased in FL patient tissues compared to controls (55). Elevated Tfr characterized as CD4+CXCR5+FoxP3+ were found in (1) lower stage DLBCL patients compared to higher stage patients and (2) in DLBCL patients who remained in remission following chemoimmunotherapy compared to those who relapsed (97). In ex vivo experiments, stimulated Tfr expressed high levels of anti-apoptotic BCL-6, as well as IL-10 and TGF-β1. Tregs exhibited superior suppressive activity against responder T cells compared to Tfr. Interestingly, Tregs also provided superior support for tumor cell proliferation in coculture assays (97). This study therefore concluded that Tfr were functionally distinct from Tregs.
Another recent study demonstrated that CD4+CXCR5+ T cells from DLBCL patients could be divided into Tfr or Tfh based on whether they were CD25+ or CD25- respectively (98). Tregs and Tfr differed in expression of transcription factors and cytokines, supporting the notion that they exert different functions. This study further described a mechanism whereby IL-21 secreted by Tfh regulated the expression of FoxP3 and IL-10 in Tfr in DLBCL patient samples (98).
Tfr has also recently been investigated in CLL patients, but little is known about their role in CLL. Nurse-like cells represent tumor-associated macrophages in CLL that support CLL survival and proliferation (99). Interestingly, coculture of nurse-like cells with CLL T cells increased Tfr percent and elevated expression of activation and proliferation markers including CD69, PD-1, CTLA-4, TGF-β, IL-10 and Ki67 (98). Collectively these studies indicate that Tfr in lymphoma and CLL are functionally distinct from Tregs. Further studies will be necessary to specifically determine associations of Tfr with clinical outcomes in FL and CLL patients.
T regulatory type 1 cells
T regulatory type 1 (Tr1) cells have been described in other diseases besides lymphoid malignancies and are distinct from CD4+CD25hiFoxP3+ Tregs as they are not characterized by constitutive FoxP3 expression. Tr1 cells produce IL-10 and express coinhibitory receptors, but also harbor cytotoxic activity (100). A single study thus far has investigated Tr1-like cells in CLL and lymphoma (101). The authors observed accumulation of Tr1-like cells in CLL and DLBCL patients’ lymph nodes compared to reactive lymph node controls. Tr1-like cells were identified as CD4+EOMES+PD-1+ T cells expressing IL-10, as well as activation and cytotoxicity markers. In the transgenic and adoptive transfer Eμ-TCL1 mouse models of CLL, Tr1-like cells were found to be essential for CD4+ T cell-mediated control of leukemia development. Further, the study showed that IL-10 signaling was important for the cytotoxic capabilities of these cells (101). As Tr1 cells share phenotypic and functional similarities with both conventional Tregs and effector Tregs, further studies will be essential to define characteristics of this enigmatic subset in the context of lymphoid malignancies.
Impact of targeted therapies
Targeted therapies have emerged as promising alternative treatments options to standard chemoimmunotherapy for B-NHL patients (102). Currently, approved targeted therapies include the immunomodulatory drug (iMiD) lenalidomide, several BTK inhibitors, BCL-2 inhibitor venetoclax, and several PI3K inhibitors (102). Although these inhibitors primarily target malignant B cells, they can directly modulate other immune cells, including T cells, due to cellular homology and off-target activity (103). T-cell modulation by targeted therapies can also occur indirectly due to malignant cell clearance and subsequent restoration of immune competency (103). ICB has demonstrated notable efficacy in several tumor types, including classical Hodgkin’s lymphoma (104). However, studies investigating ICB therapy in B-NHL patients have not yielded similar response rates thus far. The expression of multiple inhibitory receptors on Tregs in B-NHL (discussed in section 5) implies that Tregs could be affected by ICB. The impact of targeted therapies on Tregs has now become a topic of interest. Effects of targeted therapies on Tregs may be beneficial, for example, they may augment antitumor immunity. On the other hand, they can result in T-cell subset imbalance and contribute toward unwanted immune-mediated toxicities. In this section, we discuss studies that describe Treg modulation by approved targeted therapies (Table 2).
Lenalidomide has demonstrated favorable clinical outcomes as a single agent or in combinations, eliciting durable responses in B-NHL (116). Lenalidomide is currently approved for relapsed MCL patients who have progressed after two prior therapies, previously treated MZL and FL patients in combination with rituximab, and R/R DLBCL patients in combination with tafasitamab (116). Prior studies revealed that lenalidomide exerts immunomodulatory effects on CLL and FL T cells (19, 20). Immunophenotyping analysis of clinical trial samples demonstrated Treg modulation after lenalidomide treatment. In CLL patients, Treg percent and number were diminished after lenalidomide single agent treatment comparable to levels in healthy controls (105). Similar results were observed in CLL patients treated with lenalidomide in combination with alemtuzumab (106). In FL patients, lenalidomide treatment triggered T-cell proliferation in PBMCs without compromising Treg suppressive activity (107). Further, a pre-existing Treg signature indicated inferior response to treatment (107). The overall trend suggests that Treg distribution but not function is changed in patients treated with lenalidomide.
Ibrutinib is an irreversible BTK/ITK inhibitor that is currently approved for patients with MCL who have received at least one prior therapy, CLL/SLL including those with 17p deletion, MZL who have received at least one prior anti-CD20 therapy, and Waldenstrom’s macroglobulinemia (WM) (67). Both BTK and ITK belong to the TEC family of kinases (117). While BTK is expressed in B cells and crucial for B-cell receptor signaling (102), ITK is expressed in T cells and necessary for control of TH differentiation (118), including Treg/Th17 balance (119). Accordingly, ibrutinib directly regulates T cells via ITK inhibition (120). Acalabrutinib is a second-generation, irreversible BTK inhibitor with minimal off-target kinase activity against Tec-family kinases compared to ibrutinib that is currently approved for patients with MCL and CLL/SLL (121).
T-cell composition analysis of CLL patients treated with ibrutinib or acalabrutinib demonstrated that ibrutinib exerted immunomodulatory effects on T cells. These effects occurred via inhibition of ITK-mediated, activation-induced cell death (108). Ibrutinib-treated patients harbored reduced Treg to CD4+ ratio compared to baseline, and this occurred due to selective expansion of non-Treg CD4+ subsets. Although acalabrutinib-treated patients also showed a trend in reduced Treg to CD4+ ratio compared to baseline, these differences were not significant (108). Other studies similarly reported lower Treg percent in ibrutinib-treated CLL patients in both first-line and R/R settings (109, 110). Zanubrutinib, another second-generation irreversible BTK inhibitor that is approved for R/R MZL patients, reduced Treg percent and CTLA-4 expression on Tregs in R/R CLL patients (111). Data addressing the effect of BTK inhibitors on Tregs in patients with other types of lymphoid malignancies besides CLL have not yet been reported.
Venetoclax is a selective inhibitor of the anti-apoptotic protein BCL-2 that is currently approved for patients with CLL, and is being investigated for patients with R/R FL, MZL and WM as a single agent or in combination with anti-CD20 antibody (67). BCL-2 expression is constitutively upregulated in several types of B-NHL, conferring anti-apoptotic signals and promoting malignant cell survival (103). T cells also express and depend on BCL-2, but this dependence varies among T-cell subsets (103). As mentioned above, a recent study implied that BCL-2 could be important for Treg function in CLL patients (66). Immune composition was altered in CLL patients after venetoclax treatment compared to baseline (112). Specifically, patients treated with venetoclax/obinutuzumab (anti-CD20) or venetoclax/ibrutinib harbored reduced frequencies of Tregs compared to baseline (112).
The PI3K/AKT pathway is essential for malignant B-cell survival, proliferation, and function (122). PI3K inhibitors targeting one or more class I catalytic subunits (p110α, β, γ, δ) abrogate pro-survival signaling in B cells. Several PI3K inhibitors have been approved for use in B-NHL patients including idelalisib (targeting δ), duvelisib (targeting δ/γ) and copanlisib (targeting α/δ) (123). As T cells also depend on PI3K signaling for differentiation and function (124), the effects of PI3K inhibitors on T cells have been investigated. CLL patients treated with idelalisib exhibit reduced Treg differentiation and function (113, 114). Ex vivo treatment of CLL T cells with idelalisib disrupted Treg function, implying that PI3K inhibitors directly target Tregs (125). Genetic ablation of p110δ or treatment with PI3K inhibitors in the Eμ-TCL1 mouse model of CLL corroborated these findings (126–128).
Treg disruption by PI3K inhibitors has been associated with frequent and severe immune-mediated adverse events (67, 129). A noteworthy study also found that low baseline Tregs in patients treated with duvelisib/FCR predicted immune-mediated toxicities (130). The authors observed that granzyme B+ Tregs were increased after duvelisib/FCR treatment in CLL patients and suggested that this phenotype indicated more activated and apoptotic Tregs (130). PI3K inhibitors are now being developed with strategies in mind to mitigate adverse events by limiting Treg-depleting effects (131, 132). On another note, combinations with PI3K inhibitors are being explored due to their potential ability to restore immune competence. Idelalisib treatment hampered recruitment of Tregs to the FL microenvironment and unleased the activity of venetoclax, indicating potential utility of combining PI3K inhibition with venetoclax (133).
ICB is currently being investigated for treatment of B-NHL. Many trials have focused on antibodies blocking PD-1/PD-L1 and CTLA-4 as single agents or in combinations in B-NHL patients (134). Ipilimumab is an anti-CTLA-4 antibody that is approved to treat metastatic melanoma and several other tumor types (135). Studies in patients with solid tumors indicated that ipilimumab treatment depleted intratumoral Tregs possibly through antibody-dependent cell mediated cytotoxicity (136). Ipilimumab has been tested in patients with R/R B-NHL as a single agent (137), in combination with nivolumab (anti-PD-1) (138), and in combination with rituximab (115), but these treatments elicited modest efficacy rates. Correlative analysis of B-NHL patients treated with rituximab/ipilimumab demonstrated that, after an initial expansion, peripheral blood Tregs and CTLA-4+ Tregs generally decreased over time (115). Additionally, CD45RA- Treg to Treg ratio was elevated in responders compared to non-responders at baseline and following therapy. This data indicated that CD45- Treg to Treg ratio could potentially identify patients who would respond to this combination, however this would need to be validated in larger cohorts (115). Whether antitumor efficacy elicited by ipilimumab in B-NHL patients depends on Treg modulation remains to be further explored.
Taken together, these studies reveal that Tregs are consistently reduced in patients treated with the targeted inhibitors mentioned above. Since Tregs express targets such as ITK, PI3K catalytic subunits and BCL-2, some effects may be direct. Evidence also suggests that Tregs may be affected by ICB therapy. Lower Treg levels could beneficially facilitate restoration of antitumor T-cell responses. In the case of PI3K inhibitors, reduced Tregs appear to associate with increased immune-mediated toxicities, which has hampered the development of this class of inhibitors.
Conclusions and future perspectives
Tregs are key players in the microenvironment of lymphoid malignancies. In this review, we highlighted studies exploring Treg prognostic value, origin, phenotype, function, and the impact of novel therapies. While elevated Tregs indicated better outcomes in FL and DLBCL patients, the opposite trend was observed in CLL patients. Similarly, there are conflicting reports regarding prognostic value of Tregs in solid cancers. While studies in melanoma, lung, ovarian and gastric cancers demonstrated that higher Treg levels in the tumor microenvironment generally correlated with worse prognosis, another study showed that Tregs were correlated with better survival in head and neck, colorectal and esophageal cancers (139). One caveat of studies utilizing FL and DLBCL biopsy specimens is that it is difficult to distinguish Tregs from Tfr cells by immunohistochemistry using FoxP3 staining alone. Inhibitory receptors and CXCR5 could be useful to distinguish these two populations. Further correlative studies to determine if Tregs predict response to novel therapeutics such as targeted therapies, ICB and CAR T-cell therapies in B-NHL patients will be noteworthy.
The studies reviewed here revealed that Tregs can be induced and recruited to the TME, a process facilitated by dysregulated proteins expressed by malignant B cells. Tregs and Tfr are also modulated by other immune subsets in the TME like MDSCs and Tfh, respectively. Tregs comprise phenotypically heterogenous subsets and exhibit features of plasticity. Overall trends suggest that Tregs exert tumor-promoting roles in lymphoma and CLL. Similarly, studies in other cancer types have found that Tregs in the TME exhibit phenotypic heterogeneity and that TME signals can affect Treg differentiation, proliferation and immunosuppressive activity (140). Mouse model studies to further examine Treg stability, function, and response to microenvironmental cues during disease progression could provide deeper insight in the context of lymphoid malignancies.
Tregs expressing increased levels of inhibitory receptors in lymphoma and CLL may be targeted by ICB. As ICB approaches have produced subpar responses in these patients, it would be appealing to determine whether Treg disruption could improve efficacy. In addition, Treg studies could reveal new target candidates and rationale for combinatorial therapies. In contrast, targeted agents have been largely successful in terms of efficacy and tolerability, and therefore they have been incorporated into treatment regimens for lymphoma and CLL patients. Clinical correlatives demonstrated that Tregs are reduced in patients treated with ibrutinib, venetoclax, PI3K inhibitors and ipilimumab. Mouse model studies supported these observations. In studies of patients with other cancer types, reduction of Tregs in tumor tissues have been strongly correlated with clinical efficacy of ipilimumab. In patients with lymphoid malignancies treated with PI3K inhibitors, loss of Tregs have been associated with occurrence of immune-mediated adverse events. Due to expression of molecular targets in Tregs it is likely that these agents exhibit direct immunomodulatory effects on Tregs. However, Treg decrease could also depend on malignant cell clearance. Studies in other tumor types have demonstrated that PI3K inactivation by genetic ablation or pharmacological inhibition disrupts Treg function and Treg-mediated immune tolerance to cancer (141, 142). Functional studies will be required to comprehend the effects of targeted agents on Treg function in the context of lymphoid malignancies.
In the future, it would be interesting to explore optimal methods to modulate Tregs – to strike a balance between restoring antitumor immunity while preventing immune-mediated adverse events. Since most studies characterizing the effects of targeted agents on Tregs were performed in CLL patients, studies on patients with other lymphoid malignancies would enrich our understanding of this area. Future developments could help to devise strategies to optimize therapeutic interventions such as combinatorial approaches with improved efficacy and safety.
Author contributions
KM conceived, wrote, and revised the manuscript. AU wrote and revised the manuscript. ES and JP-I conceived and revised the manuscript. All authors contributed to the article and approved the submitted version.
Funding
We would like to thank Jorge and Silvia Ferioli for the generous financial support they provided to help make this project possible.
Acknowledgments
Figure 1 was created with BioRender.com.
Conflict of interest
JP-I declares consulting and fees from Janssen Pharmaceuticals, Pharmacyclics, AbbVie and AstraZeneca, and research funding from SecuraBio.
The remaining authors declare that the research was conducted in the absence of any commercial or financial relationships that could be construed as a potential conflict of interest.
Publisher’s note
All claims expressed in this article are solely those of the authors and do not necessarily represent those of their affiliated organizations, or those of the publisher, the editors and the reviewers. Any product that may be evaluated in this article, or claim that may be made by its manufacturer, is not guaranteed or endorsed by the publisher.
References
1. Swerdlow SH, Campo E, Pileri SA, Harris NL, Stein H, Siebert R, et al. The 2016 revision of the world health organization classification of lymphoid neoplasms. Blood (2016) 127(20):2375–90. doi: 10.1182/blood-2016-01-643569
2. Teras LR, DeSantis CE, Cerhan JR, Morton LM, Jemal A, Flowers CR. US Lymphoid malignancy statistics by world health organization subtypes. CA Cancer J Clin (2016) 66(6):443–59. doi: 10.3322/caac.21357
3. Hallek M, Al-Sawaf O. Chronic lymphocytic leukemia: 2022 update on diagnostic and therapeutic procedures. Am J Hematol (2021) 96(12):1679–705. doi: 10.1002/ajh.26367
4. Parikh SA, Rabe KG, Call TG, Zent CS, Habermann TM, Ding W, et al. Diffuse large b-cell lymphoma (Richter syndrome) in patients with chronic lymphocytic leukaemia (CLL): a cohort study of newly diagnosed patients. Br J Haematol (2013) 162(6):774–82. doi: 10.1111/bjh.12458
5. Al-Sawaf O, Robrecht S, Bahlo J, Fink AM, Cramer P, VT J, et al. Richter Transformation in chronic lymphocytic leukemia (CLL)-a pooled analysis of German CLL study group (GCLLSG) front line treatment trials. Leukemia (2021) 35(1):169–76. doi: 10.1038/s41375-020-0797-x
6. Cahill KE, Smith SM. Follicular lymphoma: a focus on current and emerging therapies. Oncol (Williston Park) (2022) 36(2):97–106. doi: 10.46883/2022.25920946
7. Coiffier B, Thieblemont C, Van Den Neste E, Lepeu G, Plantier I, Castaigne S, et al. Long-term outcome of patients in the LNH-98.5 trial, the first randomized study comparing rituximab-CHOP to standard CHOP chemotherapy in DLBCL patients: a study by the groupe d'Etudes des lymphomes de l'Adulte. Blood (2010) 116(12):2040–5. doi: 10.1182/blood-2010-03-276246
8. Sarkozy C, Sehn LH. New drugs for the management of relapsed or refractory diffuse large B-cell lymphoma. Ann Lymphoma (2019) 3:10. doi: 10.21037/aol.2019.09.01
9. Coutre SE, Byrd JC, Hillmen P, Barrientos JC, Barr PM, Devereux S, et al. Long-term safety of single-agent ibrutinib in patients with chronic lymphocytic leukemia in 3 pivotal studies. Blood Adv (2019) 3(12):1799–807. doi: 10.1182/bloodadvances.2018028761
10. Wang ML, Rule S, Martin P, Goy A, Auer R, Kahl BS, et al. Targeting BTK with ibrutinib in relapsed or refractory mantle-cell lymphoma. N Engl J Med (2013) 369(6):507–16. doi: 10.1056/NEJMoa1306220
11. Noy A, de Vos S, Coleman M, Martin P, Flowers CR, Thieblemont C, et al. Durable ibrutinib responses in relapsed/refractory marginal zone lymphoma: long-term follow-up and biomarker analysis. Blood Adv (2020) 4(22):5773–84. doi: 10.1182/bloodadvances.2020003121
12. Tambaro FP, De Novellis D, Wierda WG. The role of BTK inhibition in the treatment of chronic lymphocytic leukemia: A clinical view. J Exp Pharmacol (2021) 13:923–35. doi: 10.2147/JEP.S265284
13. Kline J, Godfrey J, Ansell SM. The immune landscape and response to immune checkpoint blockade therapy in lymphoma. Blood (2020) 135(8):523–33. doi: 10.1182/blood.2019000847
14. Jacobson CA, Chavez JC, Sehgal AR, William BM, Munoz J, Salles G, et al. Axicabtagene ciloleucel in relapsed or refractory indolent non-Hodgkin lymphoma (ZUMA-5): a single-arm, multicentre, phase 2 trial. Lancet Oncol (2022) 23(1):91–103. doi: 10.1016/S1470-2045(21)00591-X
15. Wang M, Munoz J, Goy A, Locke FL, Jacobson CA, Hill BT, et al. KTE-X19 CAR T-cell therapy in relapsed or refractory mantle-cell lymphoma. N Engl J Med (2020) 382(14):1331–42. doi: 10.1056/NEJMoa1914347
16. Liu Y, Zhou X, Wang X. Targeting the tumor microenvironment in b-cell lymphoma: challenges and opportunities. J Hematol Oncol (2021) 14(1):125. doi: 10.1186/s13045-021-01134-x
17. Riches JC, Davies JK, McClanahan F, Fatah R, Iqbal S, Agrawal S, et al. T Cells from CLL patients exhibit features of T-cell exhaustion but retain capacity for cytokine production. Blood (2013) 121(9):1612–21. doi: 10.1182/blood-2012-09-457531
18. Tamma R, Ingravallo G, Gaudio F, Annese T, Albano F, Ruggieri S, et al. STAT3, tumor microenvironment, and microvessel density in diffuse large b cell lymphomas. Leuk Lymphoma (2020) 61(3):567–74. doi: 10.1080/10428194.2019.1678154
19. Ramsay AG, Clear AJ, Kelly G, Fatah R, Matthews J, Macdougall F, et al. Follicular lymphoma cells induce T-cell immunologic synapse dysfunction that can be repaired with lenalidomide: implications for the tumor microenvironment and immunotherapy. Blood (2009) 114(21):4713–20. doi: 10.1182/blood-2009-04-217687
20. Ramsay AG, Johnson AJ, Lee AM, Gorgun G, Le Dieu R, Blum W, et al. Chronic lymphocytic leukemia T cells show impaired immunological synapse formation that can be reversed with an immunomodulating drug. J Clin Invest (2008) 118(7):2427–37. doi: 10.1172/JCI35017
21. Dobano-Lopez C, Araujo-Ayala F, Serrat N, Valero JG, Perez-Galan P. Follicular lymphoma microenvironment: An intricate network ready for therapeutic intervention. Cancers (2021) 13(4):641. doi: 10.3390/cancers13040641
22. Fazilleau N, Mark L, McHeyzer-Williams LJ, McHeyzer-Williams MG. Follicular helper T cells: lineage and location. Immunity (2009) 30(3):324–35. doi: 10.1016/j.immuni.2009.03.003
23. Autio M, Leivonen SK, Bruck O, Mustjoki S, Meszaros Jorgensen J, Karjalainen-Lindsberg ML, et al. Immune cell constitution in the tumor microenvironment predicts the outcome in diffuse large b-cell lymphoma. Haematologica (2021) 106(3):718–29. doi: 10.3324/haematol.2019.243626
24. Maharaj K, Sahakian E, Pinilla-Ibarz J. Emerging role of BCR signaling inhibitors in immunomodulation of chronic lymphocytic leukemia. Blood Adv (2017) 1(21):1867–75. doi: 10.1182/bloodadvances.2017006809
25. Chaudhry A, Rudensky AY. Control of inflammation by integration of environmental cues by regulatory T cells. J Clin Invest (2013) 123(3):939–44. doi: 10.1172/JCI57175
26. Adeegbe DO, Nishikawa H. Natural and induced T regulatory cells in cancer. Front Immunol (2013) 4:190. doi: 10.3389/fimmu.2013.00190
27. Fontenot JD, Gavin MA, Rudensky AY. Foxp3 programs the development and function of CD4+CD25+ regulatory T cells. Nat Immunol (2003) 4(4):330–6. doi: 10.1038/ni904
28. Khattri R, Cox T, Yasayko SA, Ramsdell F. An essential role for scurfin in CD4+CD25+ T regulatory cells. Nat Immunol (2003) 4(4):337–42. doi: 10.1038/ni909
29. Li C, Jiang P, Wei S, Xu X, Wang J. Regulatory T cells in tumor microenvironment: new mechanisms, potential therapeutic strategies and future prospects. Mol Cancer (2020) 19(1):116. doi: 10.1158/1557-3125.HIPPO19-B11
30. Schmidt A, Oberle N, Krammer PH. Molecular mechanisms of treg-mediated T cell suppression. Front Immunol (2012) 3:51. doi: 10.3389/fimmu.2012.00051
31. Toker A, Ohashi PS. Expression of costimulatory and inhibitory receptors in FoxP3(+) regulatory T cells within the tumor microenvironment: Implications for combination immunotherapy approaches. Adv Cancer Res (2019) 144:193–261. doi: 10.1016/bs.acr.2019.05.001
32. Wang J, Ke XY. The four types of tregs in malignant lymphomas. J Hematol Oncol (2011) 4:50. doi: 10.1186/1756-8722-4-50
33. Carreras J, Lopez-Guillermo A, Fox BC, Colomo L, Martinez A, Roncador G, et al. High numbers of tumor-infiltrating FOXP3-positive regulatory T cells are associated with improved overall survival in follicular lymphoma. Blood (2006) 108(9):2957–64. doi: 10.1182/blood-2006-04-018218
34. Wahlin BE, Aggarwal M, Montes-Moreno S, Gonzalez LF, Roncador G, Sanchez-Verde L, et al. A unifying microenvironment model in follicular lymphoma: outcome is predicted by programmed death-1–positive, regulatory, cytotoxic, and helper T cells and macrophages. Clin Cancer Res (2010) 16(2):637–50. doi: 10.1158/1078-0432.CCR-09-2487
35. Tzankov A, Meier C, Hirschmann P, Went P, Pileri SA, Dirnhofer S. Correlation of high numbers of intratumoral FOXP3+ regulatory T cells with improved survival in germinal center-like diffuse large b-cell lymphoma, follicular lymphoma and classical hodgkin's lymphoma. Haematologica (2008) 93(2):193–200. doi: 10.3324/haematol.11702
36. Farinha P, Al-Tourah A, Gill K, Klasa R, Connors JM, Gascoyne RD. The architectural pattern of FOXP3-positive T cells in follicular lymphoma is an independent predictor of survival and histologic transformation. Blood (2010) 115(2):289–95. doi: 10.1182/blood-2009-07-235598
37. Nelson LS, Mansfield JR, Lloyd R, Oguejiofor K, Salih Z, Menasce LP, et al. Automated prognostic pattern detection shows favourable diffuse pattern of FOXP3(+) tregs in follicular lymphoma. Br J Cancer (2015) 113(8):1197–205. doi: 10.1038/bjc.2015.291
38. Lee NR, Song EK, Jang KY, Choi HN, Moon WS, Kwon K, et al. Prognostic impact of tumor infiltrating FOXP3 positive regulatory T cells in diffuse large b-cell lymphoma at diagnosis. Leuk Lymphoma (2008) 49(2):247–56. doi: 10.1080/10428190701824536
39. Ahearne MJ, Bhuller K, Hew R, Ibrahim H, Naresh K, Wagner SD. Expression of PD-1 (CD279) and FoxP3 in diffuse large b-cell lymphoma. Virchows Arch (2014) 465(3):351–8. doi: 10.1007/s00428-014-1615-5
40. Nam SJ, Go H, Paik JH, Kim TM, Heo DS, Kim CW, et al. An increase of M2 macrophages predicts poor prognosis in patients with diffuse large b-cell lymphoma treated with rituximab, cyclophosphamide, doxorubicin, vincristine and prednisone. Leuk Lymphoma (2014) 55(11):2466–76. doi: 10.3109/10428194.2013.879713
41. Chang C, Chen YP, Medeiros LJ, Chen TY, Chang KC. Higher infiltration of intratumoral CD25+ FOXP3+ lymphocytes correlates with a favorable prognosis in patients with diffuse large b-cell lymphoma. Leuk Lymphoma (2021) 62(1):76–85. doi: 10.1080/10428194.2020.1817438
42. Dehghani M, Kalani M, Golmoghaddam H, Ramzi M, Arandi N. Aberrant peripheral blood CD4(+) CD25(+) FOXP3(+) regulatory T cells/T helper-17 number is associated with the outcome of patients with lymphoma. Cancer Immunol Immunother (2020) 69(9):1917–28. doi: 10.1007/s00262-020-02591-y
43. Peng F, Qin Y, Mu S, Li J, Ai L, Hu Y. Prognostic role of regulatory T cells in lymphoma: a systematic review and meta-analysis. J Cancer Res Clin Oncol (2020) 146(12):3123–35. doi: 10.1007/s00432-020-03398-1
44. Palma M, Gentilcore G, Heimersson K, Mozaffari F, Nasman-Glaser B, Young E, et al. T Cells in chronic lymphocytic leukemia display dysregulated expression of immune checkpoints and activation markers. Haematologica (2017) 102(3):562–72. doi: 10.3324/haematol.2016.151100
45. D'Arena G, Laurenti L, Minervini MM, Deaglio S, Bonello L, De Martino L, et al. Regulatory T-cell number is increased in chronic lymphocytic leukemia patients and correlates with progressive disease. Leuk Res (2011) 35(3):363–8. doi: 10.1016/j.leukres.2010.08.010
46. Lad DP, Varma S, Varma N, Sachdeva MU, Bose P, Malhotra P. Regulatory T-cells in b-cell chronic lymphocytic leukemia: their role in disease progression and autoimmune cytopenias. Leuk Lymphoma (2013) 54(5):1012–9. doi: 10.3109/10428194.2012.728287
47. Mpakou VE, Ioannidou H-D, Konsta E, Vikentiou M, Spathis A, Kontsioti F, et al. Quantitative and qualitative analysis of regulatory T cells in b cell chronic lymphocytic leukemia. Leukemia Res (2017) 60:74–81. doi: 10.1016/j.leukres.2017.07.004
48. D'Arena G, D'Auria F, Simeon V, Laurenti L, Deaglio S, Mansueto G, et al. A shorter time to the first treatment may be predicted by the absolute number of regulatory T-cells in patients with rai stage 0 chronic lymphocytic leukemia. Am J Hematol (2012) 87(6):628–31. doi: 10.1002/ajh.23170
49. Weiss L, Melchardt T, Egle A, Grabmer C, Greil R, Tinhofer I. Regulatory T cells predict the time to initial treatment in early stage chronic lymphocytic leukemia. Cancer (2011) 117(10):2163–9. doi: 10.1002/cncr.25752
50. Lad DP, Varma S, Varma N, Sachdeva MU, Bose P, Malhotra P. Regulatory T-cell and T-helper 17 balance in chronic lymphocytic leukemia progression and autoimmune cytopenias. Leuk Lymphoma (2015) 56(8):2424–8. doi: 10.3109/10428194.2014.986479
51. Mittal S, Marshall NA, Duncan L, Culligan DJ, Barker RN, Vickers MA. Local and systemic induction of CD4+CD25+ regulatory T-cell population by non-Hodgkin lymphoma. Blood (2008) 111(11):5359–70. doi: 10.1182/blood-2007-08-105395
52. Yang ZZ, Novak AJ, Ziesmer SC, Witzig TE, Ansell SM. CD70+ non-Hodgkin lymphoma b cells induce Foxp3 expression and regulatory function in intratumoral CD4+CD25 T cells. Blood (2007) 110(7):2537–44. doi: 10.1182/blood-2007-03-082578
53. Balsas P, Veloza L, Clot G, Sureda-Gomez M, Rodriguez ML, Masaoutis C, et al. SOX11, CD70, and treg cells configure the tumor-immune microenvironment of aggressive mantle cell lymphoma. Blood (2021) 138(22):2202–15. doi: 10.1182/blood.2020010527
54. Liu XQ, Lu K, Feng LL, Ding M, Gao JM, Ge XL, et al. Up-regulated expression of indoleamine 2,3-dioxygenase 1 in non-Hodgkin lymphoma correlates with increased regulatory T-cell infiltration. Leuk Lymphoma (2014) 55(2):405–14. doi: 10.3109/10428194.2013.804917
55. Le KS, Thibult ML, Just-Landi S, Pastor S, Gondois-Rey F, Granjeaud S, et al. Follicular b lymphomas generate regulatory T cells via the ICOS/ICOSL pathway and are susceptible to treatment by anti-ICOS/ICOSL therapy. Cancer Res (2016) 76(16):4648–60. doi: 10.1158/0008-5472.CAN-15-0589
56. DiLillo DJ, Weinberg JB, Yoshizaki A, Horikawa M, Bryant JM, Iwata Y, et al. Chronic lymphocytic leukemia and regulatory b cells share IL-10 competence and immunosuppressive function. Leukemia (2013) 27(1):170–82. doi: 10.1038/leu.2012.165
57. Haiat S, Billard C, Quiney C, Ajchenbaum-Cymbalista F, Kolb JP. Role of BAFF and APRIL in human b-cell chronic lymphocytic leukaemia. Immunology (2006) 118(3):281–92. doi: 10.1111/j.1365-2567.2006.02377.x
58. Saulep-Easton D, Vincent FB, Quah PS, Wei A, Ting SB, Croce CM, et al. The BAFF receptor TACI controls IL-10 production by regulatory b cells and CLL b cells. Leukemia (2016) 30(1):163–72. doi: 10.1038/leu.2015.174
59. Manna A, Kellett T, Aulakh S, Lewis-Tuffin LJ, Dutta N, Knutson K, et al. Targeting CD38 is lethal to breg-like chronic lymphocytic leukemia cells and tregs, but restores CD8+ T-cell responses. Blood Adv (2020) 4(10):2143–57. doi: 10.1182/bloodadvances.2019001091
60. Rawal S, Chu F, Zhang M, Park HJ, Nattamai D, Kannan S, et al. Cross talk between follicular Th cells and tumor cells in human follicular lymphoma promotes immune evasion in the tumor microenvironment. J Immunol (2013) 190(12):6681–93. doi: 10.4049/jimmunol.1201363
61. Nedelkovska H, Rosenberg AF, Hilchey SP, Hyrien O, Burack WR, Quataert SA, et al. Follicular lymphoma tregs have a distinct transcription profile impacting their migration and retention in the malignant lymph node. PloS One (2016) 11(5):e0155347. doi: 10.1371/journal.pone.0155347
62. Jitschin R, Braun M, Buttner M, Dettmer-Wilde K, Bricks J, Berger J, et al. CLL-cells induce IDOhi CD14+HLA-DRlo myeloid-derived suppressor cells that inhibit T-cell responses and promote TRegs. Blood (2014) 124(5):750–60. doi: 10.1182/blood-2013-12-546416
63. Gratz IK, Campbell DJ. Organ-specific and memory treg cells: specificity, development, function, and maintenance. Front Immunol (2014) 5:333. doi: 10.3389/fimmu.2014.00333
64. Yang ZZ, Novak AJ, Ziesmer SC, Witzig TE, Ansell SM. Attenuation of CD8(+) T-cell function by CD4(+)CD25(+) regulatory T cells in b-cell non-hodgkin's lymphoma. Cancer Res (2006) 66(20):10145–52. doi: 10.1158/0008-5472.CAN-06-1822
65. Yang ZZ, Novak AJ, Stenson MJ, Witzig TE, Ansell SM. Intratumoral CD4+CD25+ regulatory T-cell-mediated suppression of infiltrating CD4+ T cells in b-cell non-Hodgkin lymphoma. Blood (2006) 107(9):3639–46. doi: 10.1182/blood-2005-08-3376
66. Liu L, Cheng X, Yang H, Lian S, Jiang Y, Liang J, et al. BCL-2 expression promotes immunosuppression in chronic lymphocytic leukemia by enhancing regulatory T cell differentiation and cytotoxic T cell exhaustion. Mol Cancer (2022) 21(1):59. doi: 10.1186/s12943-022-01516-w
67. Minson A, Tam C, Dickinson M, Seymour JF. Targeted agents in the treatment of indolent b-cell non-hodgkin lymphomas. Cancers (2022) 14(5):1276. doi: 10.3390/cancers14051276
68. Gorgun G, Ramsay AG, Holderried TA, Zahrieh D, Le Dieu R, Liu F, et al. E(mu)-TCL1 mice represent a model for immunotherapeutic reversal of chronic lymphocytic leukemia-induced T-cell dysfunction. Proc Natl Acad Sci U S A. (2009) 106(15):6250–5. doi: 10.1073/pnas.0901166106
69. Goral A, Firczuk M, Fidyt K, Sledz M, Simoncello F, Siudakowska K, et al. A specific CD44lo CD25lo subpopulation of regulatory T cells inhibits anti-leukemic immune response and promotes the progression in a mouse model of chronic lymphocytic leukemia. Front Immunol (2022) 13:781364. doi: 10.3389/fimmu.2022.781364
70. Giannopoulos K, Schmitt M, Kowal M, Wlasiuk P, Bojarska-Junak A, Chen J, et al. Characterization of regulatory T cells in patients with b-cell chronic lymphocytic leukemia. Oncol Rep (2008) 20(3):677–82. doi: 10.3892/or_00000059
71. Ahmetlic F, Riedel T, Homberg N, Bauer V, Trautwein N, Geishauser A, et al. Regulatory T cells in an endogenous mouse lymphoma recognize specific antigen peptides and contribute to immune escape. Cancer Immunol Res (2019) 7(4):600–8. doi: 10.1158/2326-6066.CIR-18-0419
72. Strauss L, Bergmann C, Whiteside TL. Human circulating CD4+CD25highFoxp3+ regulatory T cells kill autologous CD8+ but not CD4+ responder cells by fas-mediated apoptosis. J Immunol (2009) 182(3):1469–80. doi: 10.4049/jimmunol.182.3.1469
73. Lindqvist CA, Christiansson LH, Thorn I, Mangsbo S, Paul-Wetterberg G, Sundstrom C, et al. Both CD4+ FoxP3+ and CD4+ FoxP3- T cells from patients with b-cell malignancy express cytolytic markers and kill autologous leukaemic b cells in vitro. Immunology (2011) 133(3):296–306. doi: 10.1111/j.1365-2567.2011.03439.x
74. De Matteis S, Molinari C, Abbati G, Rossi T, Napolitano R, Ghetti M, et al. Immunosuppressive treg cells acquire the phenotype of effector-T cells in chronic lymphocytic leukemia patients. J Transl Med (2018) 16(1):172. doi: 10.1186/s12967-018-1545-0
75. Overacre-Delgoffe AE, Chikina M, Dadey RE, Yano H, Brunazzi EA, Shayan G, et al. Interferon-gamma drives treg fragility to promote anti-tumor immunity. Cell (2017) 169(6):1130–41.e11. doi: 10.1016/j.cell.2017.05.005
76. Beriou G, Costantino CM, Ashley CW, Yang L, Kuchroo VK, Baecher-Allan C, et al. IL-17-producing human peripheral regulatory T cells retain suppressive function. Blood (2009) 113(18):4240–9. doi: 10.1182/blood-2008-10-183251
77. Chaudhary B, Elkord E. Regulatory T cells in the tumor microenvironment and cancer progression: role and therapeutic targeting. Vaccines (2016) 4(3):28. doi: 10.3390/vaccines4030028
78. Caligaris-Cappio F. Inflammation, the microenvironment and chronic lymphocytic leukemia. Haematologica (2011) 96(3):353–5. doi: 10.3324/haematol.2010.039446
79. Biancotto A, Dagur PK, Fuchs JC, Wiestner A, Bagwell CB, McCoy JP Jr. Phenotypic complexity of T regulatory subsets in patients with b-chronic lymphocytic leukemia. Mod Pathol (2012) 25(2):246–59. doi: 10.1038/modpathol.2011.164
80. Duhen T, Duhen R, Montler R, Moses J, Moudgil T, de Miranda NF, et al. Co-Expression of CD39 and CD103 identifies tumor-reactive CD8 T cells in human solid tumors. Nat Commun (2018) 9(1):2724. doi: 10.1038/s41467-018-05072-0
81. Zhao H, Bo C, Kang Y, Li H. What else can CD39 tell us? Front Immunol (2017) 8:727. doi: 10.3389/fimmu.2017.00727
82. Mishra S, Srinivasan S, Ma C, Zhang N. CD8(+) regulatory T cell - a mystery to be revealed. Front Immunol (2021) 12:708874. doi: 10.3389/fimmu.2021.708874
83. Jadidi-Niaragh F, Yousefi M, Memarian A, Hojjat-Farsangi M, Khoshnoodi J, Razavi SM, et al. Increased frequency of CD8+ and CD4+ regulatory T cells in chronic lymphocytic leukemia: association with disease progression. Cancer Invest (2013) 31(2):121–31. doi: 10.3109/07357907.2012.756110
84. Lucca LE, Dominguez-Villar M. Modulation of regulatory T cell function and stability by co-inhibitory receptors. Nat Rev Immunol (2020) 20(11):680–93. doi: 10.1038/s41577-020-0296-3
85. Nakayama S, Yokote T, Akioka T, Hiraoka N, Nishiwaki U, Miyoshi T, et al. Infiltration of effector regulatory T cells predicts poor prognosis of diffuse large b-cell lymphoma, not otherwise specified. Blood Adv (2017) 1(8):486–93. doi: 10.1182/bloodadvances.2016000885
86. Zhong W, Liu X, Zhu Z, Li Q, Li K. High levels of Tim-3(+)Foxp3(+)Treg cells in the tumor microenvironment is a prognostic indicator of poor survival of diffuse large b cell lymphoma patients. Int Immunopharmacol (2021) 96:107662. doi: 10.1016/j.intimp.2021.107662
87. Schnell A, Bod L, Madi A, Kuchroo VK. The yin and yang of co-inhibitory receptors: toward anti-tumor immunity without autoimmunity. Cell Res (2020) 30(4):285–99. doi: 10.1038/s41422-020-0277-x
88. Josefsson SE, Huse K, Kolstad A, Beiske K, Pende D, Steen CB, et al. T Cells expressing checkpoint receptor TIGIT are enriched in follicular lymphoma tumors and characterized by reversible suppression of T-cell receptor signaling. Clin Cancer Res (2018) 24(4):870–81. doi: 10.1158/1078-0432.CCR-17-2337
89. Keane C, Law SC, Gould C, Birch S, Sabdia MB, Merida de Long L, et al. LAG3: a novel immune checkpoint expressed by multiple lymphocyte subsets in diffuse large b-cell lymphoma. Blood Adv (2020) 4(7):1367–77. doi: 10.1182/bloodadvances.2019001390
90. Bauer V, Ahmetlic F, Homberg N, Geishauser A, Rocken M, Mocikat R. Immune checkpoint blockade impairs immunosuppressive mechanisms of regulatory T cells in b-cell lymphoma. Transl Oncol (2021) 14(9):101170. doi: 10.1016/j.tranon.2021.101170
91. Wierz M, Pierson S, Guyonnet L, Viry E, Lequeux A, Oudin A, et al. Dual PD1/LAG3 immune checkpoint blockade limits tumor development in a murine model of chronic lymphocytic leukemia. Blood (2018) 131(14):1617–21. doi: 10.1182/blood-2017-06-792267
92. Greenberg SA, Kong SW, Thompson E, Gulla SV. Co-Inhibitory T cell receptor KLRG1: human cancer expression and efficacy of neutralization in murine cancer models. Oncotarget (2019) 10(14):1399–406. doi: 10.18632/oncotarget.26659
93. Cheng G, Yuan X, Tsai MS, Podack ER, Yu A, Malek TR. IL-2 receptor signaling is essential for the development of Klrg1+ terminally differentiated T regulatory cells. J Immunol (2012) 189(4):1780–91. doi: 10.4049/jimmunol.1103768
94. Durig J, Naschar M, Schmucker U, Renzing-Kohler K, Holter T, Huttmann A, et al. CD38 expression is an important prognostic marker in chronic lymphocytic leukaemia. Leukemia (2002) 16(1):30–5. doi: 10.1038/sj.leu.2402339
95. Patton DT, Wilson MD, Rowan WC, Soond DR, Okkenhaug K. The PI3K p110delta regulates expression of CD38 on regulatory T cells. PloS One (2011) 6(3):e17359. doi: 10.1371/journal.pone.0017359
96. Xie MM, Dent AL. Unexpected help: Follicular regulatory T cells in the germinal center. Front Immunol (2018) 9:1536. doi: 10.3389/fimmu.2018.01536
97. Cha Z, Gu H, Zang Y, Wang Z, Li J, Huang W, et al. The prevalence and function of CD4(+)CXCR5(+)Foxp3(+) follicular regulatory T cells in diffuse large b cell lymphoma. Int Immunopharmacol (2018) 61:132–9. doi: 10.1016/j.intimp.2018.05.025
98. Ma X, Zha J, He J, Chen L, Huang J, Wu W, et al. T Follicular helper cell-mediated IL-21 production suppresses FOXP3 expression of T follicular regulatory-like cells in diffuse large b cell lymphoma patients. Hum Immunol (2020) 81(8):452–9. doi: 10.1016/j.humimm.2020.05.008
99. Boissard F, Fournie JJ, Laurent C, Poupot M, Ysebaert L. Nurse like cells: chronic lymphocytic leukemia associated macrophages. Leuk Lymphoma (2015) 56(5):1570–2. doi: 10.3109/10428194.2014.991731
100. Roncarolo MG, Gregori S, Bacchetta R, Battaglia M, Gagliani N. The biology of T regulatory type 1 cells and their therapeutic application in immune-mediated diseases. Immunity (2018) 49(6):1004–19. doi: 10.1016/j.immuni.2018.12.001
101. Roessner PM, Llao Cid L, Lupar E, Roider T, Bordas M, Schifflers C, et al. EOMES and IL-10 regulate antitumor activity of T regulatory type 1 CD4(+) T cells in chronic lymphocytic leukemia. Leukemia (2021) 35(8):2311–24. doi: 10.1038/s41375-021-01136-1
102. Profitos-Peleja N, Santos JC, Marin-Niebla A, Roue G, Ribeiro ML. Regulation of b-cell receptor signaling and its therapeutic relevance in aggressive b-cell lymphomas. Cancers (2022) 14(4):8660. doi: 10.3390/cancers14040860
103. Svanberg R, Janum S, Patten PEM, Ramsay AG, Niemann CU. Targeting the tumor microenvironment in chronic lymphocytic leukemia. Haematologica (2021) 106(9):2312–24. doi: 10.3324/haematol.2020.268037
104. De Goycoechea D, Stalder G, Martins F, Duchosal MA. Immune checkpoint inhibition in classical Hodgkin lymphoma: From early achievements towards new perspectives. J Oncol (2019) 2019:9513701. doi: 10.1155/2019/9513701
105. Lee BN, Gao H, Cohen EN, Badoux X, Wierda WG, Estrov Z, et al. Treatment with lenalidomide modulates T-cell immunophenotype and cytokine production in patients with chronic lymphocytic leukemia. Cancer (2011) 117(17):3999–4008. doi: 10.1002/cncr.25983
106. Winqvist M, Mozaffari F, Palma M, Eketorp Sylvan S, Hansson L, Mellstedt H, et al. Phase I-II study of lenalidomide and alemtuzumab in refractory chronic lymphocytic leukemia (CLL): effects on T cells and immune checkpoints. Cancer Immunol Immunother (2017) 66(1):91–102. doi: 10.1007/s00262-016-1922-6
107. Menard C, Rossille D, Dulong J, Nguyen TT, Papa I, Latour M, et al. Lenalidomide triggers T-cell effector functions in vivo in patients with follicular lymphoma. Blood Adv (2021) 5(8):2063–74. doi: 10.1182/bloodadvances.2020003774
108. Long M, Beckwith K, Do P, Mundy BL, Gordon A, Lehman AM, et al. Ibrutinib treatment improves T cell number and function in CLL patients. J Clin Invest (2017) 127(8):3052–64. doi: 10.1172/JCI89756
109. Solman IG, Blum LK, Hoh HY, Kipps TJ, Burger JA, Barrientos JC, et al. Ibrutinib restores immune cell numbers and function in first-line and relapsed/refractory chronic lymphocytic leukemia. Leuk Res (2020) 97:106432. doi: 10.1016/j.leukres.2020.106432
110. Podhorecka M, Goracy A, Szymczyk A, Kowal M, Ibanez B, Jankowska-Lecka O, et al. Changes in T-cell subpopulations and cytokine network during early period of ibrutinib therapy in chronic lymphocytic leukemia patients: the significant decrease in T regulatory cells number. Oncotarget (2017) 8(21):34661–9. doi: 10.18632/oncotarget.16148
111. Zou YX, Zhu HY, Li XT, Xia Y, Miao KR, Zhao SS, et al. The impacts of zanubrutinib on immune cells in patients with chronic lymphocytic leukemia/small lymphocytic lymphoma. Hematol Oncol (2019) 37(4):392–400. doi: 10.1002/hon.2667
112. de Weerdt I, Hofland T, de Boer R, Dobber JA, Dubois J, van Nieuwenhuize D, et al. Distinct immune composition in lymph node and peripheral blood of CLL patients is reshaped during venetoclax treatment. Blood Advances (2019) 3(17):2642–52. doi: 10.1182/bloodadvances.2019000360
113. Chellappa S, Kushekhar K, Munthe LA, Tjonnfjord GE, Aandahl EM, Okkenhaug K, et al. The PI3K p110delta isoform inhibitor idelalisib preferentially inhibits human regulatory T cell function. J Immunol (2019) 202(5):1397–405. doi: 10.4049/jimmunol.1701703
114. Gadi D, Griffith A, Wang Z, Tyekucheva S, Rai V, Fernandes SM, et al. Idelalisib reduces regulatory T cells and activates T helper 17 cell differentiation in relapsed refractory patients with chronic lymphocytic leukaemia. Br J Haematol (2022) 197(2):207–11. doi: 10.1111/bjh.18053
115. Tuscano JM, Maverakis E, Groshen S, Tsao-Wei D, Luxardi G, Merleev AA, et al. A phase I study of the combination of rituximab and ipilimumab in patients with Relapsed/Refractory b-cell lymphoma. Clin Cancer Res (2019) 25(23):7004–13. doi: 10.1158/1078-0432.CCR-19-0438
116. Ioannou N, Jain K, Ramsay AG. Immunomodulatory drugs for the treatment of B cell malignancies. Int J Mol Sci (2021) 22(16):8572. doi: 10.3390/ijms22168572
117. Horwood NJ, Urbaniak AM, Danks L. Tec family kinases in inflammation and disease. Int Rev Immunol (2012) 31(2):87–103. doi: 10.3109/08830185.2012.670334
118. Boucheron N, Ellmeier W. The role of tec family kinases in the regulation of T-helper-cell differentiation. Int Rev Immunol (2012) 31(2):133–54. doi: 10.3109/08830185.2012.664798
119. Gomez-Rodriguez J, Wohlfert EA, Handon R, Meylan F, Wu JZ, Anderson SM, et al. Itk-mediated integration of T cell receptor and cytokine signaling regulates the balance between Th17 and regulatory T cells. J Exp Med (2014) 211(3):529–43. doi: 10.1084/jem.20131459
120. Dubovsky JA, Beckwith KA, Natarajan G, Woyach JA, Jaglowski S, Zhong Y, et al. Ibrutinib is an irreversible molecular inhibitor of ITK driving a Th1-selective pressure in T lymphocytes. Blood (2013) 122(15):2539–49. doi: 10.1182/blood-2013-06-507947
121. Abbas HA, Wierda WG. Acalabrutinib: A selective bruton tyrosine kinase inhibitor for the treatment of b-cell malignancies. Front Oncol (2021) 11:668162. doi: 10.3389/fonc.2021.668162
122. Pongas G, Cheson BD. PI3K signaling pathway in normal b cells and indolent b-cell malignancies. Semin Oncol (2016) 43(6):647–54. doi: 10.1053/j.seminoncol.2016.11.011
123. Tarantelli C, Argnani L, Zinzani PL, Bertoni F. PI3Kδ inhibitors as immunomodulatory agents for the treatment of lymphoma patients. Cancers (2021) 13(21):5535. doi: 10.3390/cancers13215535
124. Han JM, Patterson SJ, Levings MK. The role of the PI3K signaling pathway in CD4(+) T cell differentiation and function. Front Immunol (2012) 3:245. doi: 10.3389/fimmu.2012.00245
125. Rohrbacher L, Brauchle B, Ogrinc Wagner A, von Bergwelt-Baildon M, Bucklein VL, Subklewe M. The PI3K partial differential-selective inhibitor idelalisib induces T- and NK-cell dysfunction independently of b-cell malignancy-associated immunosuppression. Front Immunol (2021) 12:608625. doi: 10.3389/fimmu.2021.608625
126. Dong S, Harrington BK, Hu EY, Greene JT, Lehman AM, Tran M, et al. PI3K p110delta inactivation antagonizes chronic lymphocytic leukemia and reverses T cell immune suppression. J Clin Invest (2019) 129(1):122–36. doi: 10.1172/JCI99386
127. Maharaj K, Powers JJ, Achille A, Mediavilla-Varela M, Gamal W, Burger KL, et al. The dual PI3Kdelta/CK1epsilon inhibitor umbralisib exhibits unique immunomodulatory effects on CLL T cells. Blood Adv (2020) 4(13):3072–84. doi: 10.1182/bloodadvances.2020001800
128. Hanna BS, Roessner PM, Scheffold A, Jebaraj BMC, Demerdash Y, Ozturk S, et al. PI3Kdelta inhibition modulates regulatory and effector T-cell differentiation and function in chronic lymphocytic leukemia. Leukemia (2019) 33(6):1427–38. doi: 10.1038/s41375-018-0318-3
129. Lampson BL, Kasar SN, Matos TR, Morgan EA, Rassenti L, Davids MS, et al. Idelalisib given front-line for treatment of chronic lymphocytic leukemia causes frequent immune-mediated hepatotoxicity. Blood (2016) 128(2):195–203. doi: 10.1182/blood-2016-03-707133
130. Gadi D, Griffith A, Tyekucheva S, Wang Z, Rai V, Vartanov A, et al. A T cell inflammatory phenotype is associated with autoimmune toxicity of the PI3K inhibitor duvelisib in chronic lymphocytic leukemia. Leukemia (2022) 36(3):723–32. doi: 10.1038/s41375-021-01441-9
131. Chauhan AF, Cheson BD. Copanlisib in the treatment of relapsed follicular lymphoma: Utility and experience from the clinic. Cancer Manag Res (2021) 13:677–92. doi: 10.2147/CMAR.S201024
132. Hus I, Puła B, Robak T. PI3K inhibitors for the treatment of chronic lymphocytic leukemia: Current status and future perspectives. Cancers (2022) 14(6):1571. doi: 10.3390/cancers14061571
133. Serrat N, Guerrero-Hernandez M, Matas-Cespedes A, Yahiaoui A, Valero JG, Nadeu F, et al. PI3Kdelta inhibition reshapes follicular lymphoma-immune microenvironment cross talk and unleashes the activity of venetoclax. Blood Adv (2020) 4(17):4217–31. doi: 10.1182/bloodadvances.2020001584
134. Hatic H, Sampat D, Goyal G. Immune checkpoint inhibitors in lymphoma: challenges and opportunities. Ann Transl Med (2021) 9(12):1037. doi: 10.21037/atm-20-6833
135. Shiravand Y, Khodadadi F, Kashani SMA, Hosseini-Fard SR, Hosseini S, Sadeghirad H, et al. Immune checkpoint inhibitors in cancer therapy. Curr Oncol (2022) 29(5):3044–60. doi: 10.3390/curroncol29050247
136. Sasidharan Nair V, Elkord E. Immune checkpoint inhibitors in cancer therapy: a focus on T-regulatory cells. Immunol Cell Biol (2018) 96(1):21–33. doi: 10.1111/imcb.1003
137. Ansell SM, Hurvitz SA, Koenig PA, LaPlant BR, Kabat BF, Fernando D, et al. Phase I study of ipilimumab, an anti-CTLA-4 monoclonal antibody, in patients with relapsed and refractory b-cell non-Hodgkin lymphoma. Clin Cancer Res (2009) 15(20):6446–53. doi: 10.1158/1078-0432.CCR-09-1339
138. Armand P, Lesokhin A, Borrello I, Timmerman J, Gutierrez M, Zhu L, et al. A phase 1b study of dual PD-1 and CTLA-4 or KIR blockade in patients with relapsed/refractory lymphoid malignancies. Leukemia (2021) 35(3):777–86. doi: 10.1038/s41375-020-0939-1
139. Sobhani N, Tardiel-Cyril DR, Davtyan A, Generali D, Roudi R, Li Y. CTLA-4 in regulatory T cells for cancer immunotherapy. Cancers (2021) 13(6):1440. doi: 10.3390/cancers13061440
140. Gao R, Shi GP, Wang J. Functional diversities of regulatory T cells in the context of cancer immunotherapy. Front Immunol (2022) 13:833667. doi: 10.3389/fimmu.2022.833667
141. Zhang Z, Richmond A. The role of PI3K inhibition in the treatment of breast cancer, alone or combined with immune checkpoint inhibitors. Front Mol Biosci (2021) 8:648663. doi: 10.3389/fmolb.2021.648663
Keywords: Treg, B cell, non-Hodgkin’s lymphoma, chronic lymphocytic leukemia, immune checkpoint blockade, BTK, PI3K, BCL-2
Citation: Maharaj K, Uriepero A, Sahakian E and Pinilla-Ibarz J (2022) Regulatory T cells (Tregs) in lymphoid malignancies and the impact of novel therapies. Front. Immunol. 13:943354. doi: 10.3389/fimmu.2022.943354
Received: 13 May 2022; Accepted: 11 July 2022;
Published: 01 August 2022.
Edited by:
James Badger Wing, Osaka University, JapanReviewed by:
Raheleh Roudi, Stanford University, United StatesWilliam K. Decker, Baylor College of Medicine, United States
Copyright © 2022 Maharaj, Uriepero, Sahakian and Pinilla-Ibarz. This is an open-access article distributed under the terms of the Creative Commons Attribution License (CC BY). The use, distribution or reproduction in other forums is permitted, provided the original author(s) and the copyright owner(s) are credited and that the original publication in this journal is cited, in accordance with accepted academic practice. No use, distribution or reproduction is permitted which does not comply with these terms.
*Correspondence: Javier Pinilla-Ibarz, Javier.pinilla@moffitt.org