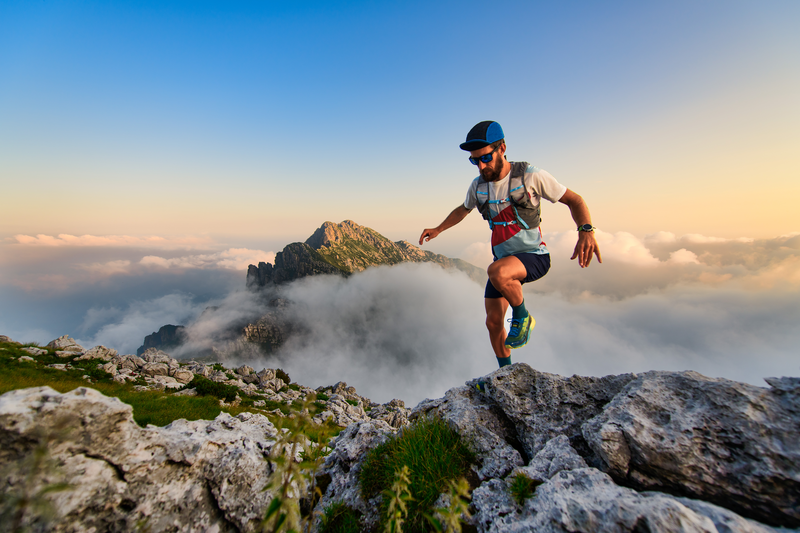
94% of researchers rate our articles as excellent or good
Learn more about the work of our research integrity team to safeguard the quality of each article we publish.
Find out more
REVIEW article
Front. Immunol. , 28 June 2022
Sec. Autoimmune and Autoinflammatory Disorders
Volume 13 - 2022 | https://doi.org/10.3389/fimmu.2022.941071
This article is part of the Research Topic The Role of Monocytes/Macrophages in Autoimmunity and Autoinflammation View all 19 articles
Psoriasis is a chronic inflammatory skin disease characterized by scaly indurated erythema. This disease impairs patients’ quality of life enormously. Pathological findings demonstrate proliferation and abnormal differentiation of keratinocytes and massive infiltration of inflammatory immune cells. The pathogenesis of psoriasis is complicated. Among immune cells, dendritic cells play a pivotal role in the development of psoriasis in both the initiation and the maintenance phases. In addition, it has been indicated that macrophages contribute to the pathogenesis of psoriasis especially in the initiation phase, although studies on macrophages are limited. In this article, we review the roles of dendritic cells and macrophages in the pathogenesis of psoriasis.
Psoriasis is a chronic inflammatory skin disease characterized by scaly indurated erythema. This disease impairs patients’ quality of life enormously. Pathological findings demonstrate proliferation and abnormal differentiation of keratinocytes and massive infiltration of inflammatory immune cells. The pathogenesis of psoriasis is complicated, but it has been revealed by intensive research. Among immune cells, dendritic cells (DC) play a pivotal role in the development of psoriasis in both the initiation and the maintenance phases. In addition, it has been indicated that macrophages contribute to the pathogenesis of psoriasis especially in the initiation phase, although studies on macrophages are limited. In this article, we review the roles of DC and macrophages in the pathogenesis of psoriasis. Since the contributions of DC to the pathogenesis of psoriasis have already been well-described in the previous literature (1, 2), we give a concise overview of the current understanding. Then we review findings on the involvement of macrophages in the pathogenesis of psoriasis.
Previous review articles have provided detailed descriptions of the pathogenesis of psoriasis (3–5). We focus on DC and macrophages (Figure 1). Briefly, in early-phase psoriasis, nucleic acids and a variety of antimicrobial peptides released from damaged keratinocytes activate innate immune cells, including plasmacytoid DC (pDC) and macrophages, which produce interferon (IFN)-α and tumor necrosis factor (TNF)-α. The release of IFN-α causes the maturation of resident dermal DC and the differentiation of monocytes into inflammatory DC (iDC). Mature resident DC and the rapidly increasing numbers of iDC produce interleukin (IL)-23, IL-12, TNF-α and other cytokines, which strongly activate the differentiation of naive T cells into Th1, Th17 and Th22. IL-23 maintains and promotes the proliferation of pathogenic Th17 cells. The release of IL-17 and IL-22 induces proliferation and abnormal differentiation of keratinocytes. Keratinocytes also act as immune cells by producing TNF-α, IL-8, vascular endothelial growth factor (VEGF), antimicrobial peptides, etc., some of which activate DC. This vicious inflammatory cycle causes the plaque to remain and deteriorate in the chronic phase of psoriasis (1, 2, 5, 6).
Figure 1 Overview of the current understanding of the pathogenesis of psoriasis and the roles of dendritic cells and macrophages. DC, dendritic cells; pDC, plasmacytoid DC; iDC, inflammatory DC.
DC are heterogenous and are sub-classified based on location, origin, and function. Detected subtypes of DC are different in the steady state or in the inflammatory state (6). Furthermore, there is a little difference in surface marker expressions between human DC and mouse DC (1).
In human peripheral blood, three main subsets of DC can be identified: plasmacytoid DC (pDC), and two types of conventional DC (cDC), i.e., CD1c(BDCA-1)+ cDC (cDC1) and CD141(BDCA-3)+ cDC (cDC2) (7, 8), as shown in Table 1 (6). Hierarchical clustering of mouse lymph nodes and human blood DC subsets by genome-wide expression profiling revealed clustering of human pDC with mouse pDC, human CD1c+ cDC1 with mouse CD11b+ DC, and human CD141+ cDC2 with mouse CD8α+ DC (9).
Table 1 Three main subsets of dendritic cells in human peripheral blood under steady-state conditions.
In the skin under steady-state conditions, two dermal DC subsets identical to CD1c+ and CD141+ blood cDC have been identified (10, 11) (Table 2). However, pDC are absent during steady-state conditions. Human tissues also harbor migratory CD14+ DC, which do not have an identified murine equivalent (10, 11). Its phenotype and transcriptomic expression profiles show the characteristics of blood monocytes and tissue macrophages (10, 11), which raises the question of the origin of DC. Langerhans cells (LC) which are located in the epidermis, survey the epidermis for foreign antigens as antigen-presenting cells and activate T cells as needed (12).
In psoriatic lesions, pDC and myeloid DC in addition to Th1/17 CD4+ cells are observed in the dermis (13). Dermal DC in lesional skin can be divided into three subsets: CD1c+ DC, CD141+ DC, and CD11c+CD1c−CD141− inflammatory DC (iDC), including Tip-DC and 6-sulfo LacNAc DC (slanDC), as shown in Table 2. In inflammatory skin conditions including psoriasis, in addition to LC, CD1c+ DC, and CD141+ DC that are already present during the steady state, pDC and iDC migrate into the skin. pDC originate in the bone marrow and migrate to the skin under pathological conditions (14). The surface expression of CCR2, a chemokine receptor expressed by monocytes and required for their migration, on iDC indicates that iDC are derived from monocytes (15–17).
Increased infiltration of pDC is observed not only in lesional skin but also in non-lesional skin of psoriasis patients, compared to normal skin from healthy controls (18–21). pDC recognize self-nucleic acids, thereby initiating inflammation of psoriasis through IFN-α production (18). Antimicrobial peptides in the epidermis of psoriasis patients, including LL-37, human β-defensin (hBD)-2, hBD-3 and lysozyme, bind self-DNA/RNA fragments released by stressed or injured keratinocytes, thereby inducing activation of pDC via TLR7/9 (22–27). Furthermore, DNA structures containing the neutrophil serine protease cathepsin G (CatG) and the secretory leukocyte protease inhibitor (SLPI), which are detected in lesional skin of psoriasis patients, induce the production of IFN-α in pDC. pDC play a role in early psoriasis (28).
IFN-α released by pDC activates dermal resident DC, and drives their maturation (29). Moreover, IFN-α induces rapid differentiation of human monocytes into iDC and polarizes CD4+ T cells into Th1 and Th17 cells (30, 31). However, an anti-IFN-α monoclonal antibody failed to ameliorate plaque psoriasis in a phase I clinical trial (32), indicating that IFN-α is not important in the maintenance phase. It rather contributes to the development of psoriasis in the early phase.
IFN-α and TNF-α released by pDC, macrophages, and other cells promote maturation and activation of myeloid DC, which play an important role in the chronic phase of psoriasis. In psoriasis patients, CD11c+ DC are abundant in lesional skin, while there are relatively low numbers of these cells in non-lesional skin (33). Dermal DC derived from lesional skin induce proliferation of Th1 and polarization of Th17, and they are the source of IL-23 (33–40). As stated above, dermal DC in lesional skin can be divided into three subsets: CD1c+ DC, CD141+ DC, and iDC (Table 2). The number of CD1c+ DC was lower in non-lesional and lesional skin of psoriasis patients than in normal skin, whereas the number of CD141+ DC was higher. Lesional skin showed a considerable increase in infiltrating iDC compared with samples obtained from healthy controls (37), which mostly account for the total increase in CD11c+ DC in lesional skin. Both the CD1c+ DC and CD1c− DC populations from psoriatic skin strongly induced T-cell proliferation and production of IFN-γ and/or IL-17 to the same extent (37).
CD11c+CD1c−CD141− dermal iDC, including TNF-α and inducible nitric oxide synthase (iNOS)-producing DC (Tip-DC) and slanDC, have been identified in the dermis of psoriasis patients (41–43), and they seem to play a pivotal role in the pathogenesis of psoriasis (1). These iDC in psoriasis are identified as CD11c+CD1c− DC, distinguishing them from resident cDC, and are assumed to be derived from monocytes (11, 37, 43, 44). Tip-DC express high levels of TNF-α and iNOS. TNF-α induces keratinocytes to express ICAM-1, CXCL8, and also pro-inflammatory cytokines including IL-1β and IL-6. iNOS in inflamed tissues catalyzes the production of nitric oxide (NO), which results in vasodilation of dermal blood vessels in the lesional skin of psoriasis patients (13). In addition, Tip-DC have been shown to produce high levels of IL-23 (6, 45, 46).
Through the expression of CX3CR1 and C5aR, slanDC are recruited by the increased expression CX3CL1 and C5a in psoriatic skin (42). The complete transcriptional overlap of blood slanDC with CD16+ monocytes indicates that skin slanDC are derived from monocytes (11, 44). As with pDC, dermal slanDC are reactive to self-RNA-LL37 complexes (42) and induce Th1/17 cells to produce IL-17, IL-22, TNF-α and IFN-γ (42, 43). In lesional skin of psoriasis patients, dermal slanDC express abundant IL-23-p19 and TNF-α (42, 47). Autocrine TNF-α stimulation of slanDC allows for higher production levels of IL-12, IL-23, IL-1β and IL-6 (48). Treatment with infliximab and dimethyl fumarate rapidly reduced the number of slanDC (1, 49, 50). Their phenotypic signatures suggest that dermal Tip-DC and slanDC represent the same inflammatory DC population although subpopulations may exist (6).
DC3 is a newly identified subset of inflammatory CD5−CD163+CD14+ DC (51). Recently, single-cell analysis of human skin revealed that CD14+ DC3 increased in psoriasis lesional skin, and they produced IL-1β and IL-23 (52), which could contribute to the pathogenesis of psoriasis.
Accumulating dermal iDC play a key role in the progression and sustenance of psoriasis by secreting large amounts of pro-inflammatory factors including iNOS, IL-23, and TNF-α (1, 6).
LC are antigen-presenting cells residing in the epidermis. Once they recognize an antigen, they migrate into regional lymph nodes and present antigens. A Recent study reported identification of two steady-state (LC1 and LC2) and two activated LC subsets in the epidermis of human skin and in LC derived from CD34+ hemopoietic stem cells (53). LC1 are characterized as classical LCs, mainly related to innate immunity and antigen processing. LC2 are involved in immune responses and leukocyte activation. LC1 remain stable under inflammatory microenvironment, whereas LC2 are prone to being activated and demonstrated elevated expression of immuno-suppressive molecules.
In the steady state, LC are continuously replaced from a resident precursor pool (54–56). However, in the inflammatory state, LC are repopulated by blood precursors (6, 57–60).
Their role in psoriasis has not yet been elucidated. The number of LCs in lesional skin of psoriasis patients was reported to be increased (61, 62), decreased (63, 64), or the same as the number of LC in control skin samples in various articles (65, 66).
The migration of LC is impaired in psoriatic patients (67, 68). Impaired LC migration in psoriasis is due to an altered keratinocyte phenotype induced by IL-17 (69).
LC play various roles in psoriasis according to previous studies. Some articles reported that LC play an anti-inflammatory role in psoriasis (53, 62, 70). In contrast, other studies indicated that LC are involved in the development of psoriasis (66, 71–74). Several studies demonstrated that LC produced IL-23 (66, 71, 72).
The discrepant data on LC are possibly due to different LC-deficient models, methods, or other factors (1). Further investigation is necessary to clarify the roles of LC in the pathogenesis of psoriasis.
The diversity of DC populations and different functions in psoriasis may be accounted for by plasticity of DC.
Macrophages are categorized into two types: tissue-resident and infiltrating macrophages (6). Tissue-resident macrophages are long-lived non-migratory cells, and play an essential role in maintaining tissue homeostasis by clearing cell debris and promoting resolution of inflammation and wound healing (75). They are potent promoters of inflammation by producing chemokines, including CCL2, CXCL1, and macrophage inhibitory factor (MIF), and cytokines such as IL-6 and TNF-α, resulting in recruitment and activation of other immune cells (76, 77). Most tissue-resident macrophages are considered to be present from birth and are self-maintaining, independently from monocytes (78–81), except intestinal macrophages (82); however, this is still controversial.
Meanwhile, infiltrating macrophages are recruited to tissues in inflammatory conditions (6). Murine studies revealed that infiltrating macrophages originate from inflammatory monocytes. Infiltrating macrophages are divided into three populations based on function, displaying a pro-inflammatory profile (originally coined “classically activated” or “M1” macrophages), a regulatory profile, or a wound-healing profile (the latter two were originally grouped under the term “alternatively activated” or “M2” macrophages), depending on the tissue context and environmental stimuli (83, 84). According to their cell surface markers, secreted cytokines and biological functions, M2 macrophages are divided into M2a, M2b, M2c, and M2d subcategories (85). M1 polarization occurs in the presence of IFN-γ, LPS, and TNF-α. Cell surface markers of M1 macrophages are CD14++CD16−, CD40, and CD68. M1 macrophages produce IL-1β, IL-6, IL-12, IL-23, MCP-1, and TNF-α. In contrast, M2 polarization occurs in response to IL-4, IL-10, and IL-13. M2 macrophages express CD14+CD16++, CD163, and CD209 on their surface. M2 macrophages produce EGF, IL-10, PDGF, TGF-β, and VEGF (86). Among M2 macrophages, M2a macrophages, activated by IL-4 or IL-13, lead to the increased expression of IL-10, TGF-β, CCL17, CCL18, and CCL22 (85). These macrophages enhance the endocytic activity, promote cell growth and tissue repairing.
Murine studies demonstrated that depletion of macrophages improved psoriasis inflammation (87–89) and reduced the levels of Th1 cytokines, including IL-1α, IL-6, IL-23 and TNF-α to normal levels (90). These results underscore that macrophages contribute to the development and maintenance of psoriatic lesions (86).
Psoriasis patients have an increased level of circulating monocytes in peripheral blood (91, 92), and they favored the M1 phenotype (93). Furthermore, a considerable number of macrophages was observed in lesional skin (94). Immunofluorescence staining revealed that CD68+iNOS+ M1 macrophages were increased and CD68+CD163+ M2 macrophages were decreased in human psoriasis lesional skin compared with skin samples from normal individuals (95). Another study demonstrated accumulation of dermal CD68+ macrophages that expressed TNF-α in human psoriatic plaques (96). Other studies demonstrated that the number of CD163+ macrophages increased in psoriatic lesional human skin, which decreased to non-lesional skin levels after an effective treatment with TNF-α inhibitors (33, 89, 97, 98). Moreover, it was indicated that CD163+ macrophages produce IL-23p19 and IL-12/23p40 in addition to TNF-α and iNOS in human lesional skin (97, 99). Murine experiments revealed that in skin injected with IL-23, monocytes/macrophages characterized by the strong presence of Ly6ChiMHC-IIhi cells were the dominant immune population, particularly late in the model, and showed high expression of TNF-α but not IL-17A (100). In another murine study, when peritoneal macrophages freshly isolated from resting mice were treated with IL-23, they produced large amounts of IL-17A, IL-22 and IFN-γ, and expressed a distinctive gene expression profile compared with those of M1 and M2 macrophages (101). Under the condition of abundant IL-23 in psoriasis lesional skin, some macrophages possibly produce IL-17A, IL-22 and IFN-γ in addition to TNF-α. Since macrophage are highly plastic cells (102), the diversity of macrophage populations in psoriasis may reflect a heightened cellular plasticity.
Recently, the involvement of macrophage NLRP3 inflammasome activation in psoriasis has been reported (103–105).
The ratio of M1 to M2a macrophage polarization was higher in psoriatic patients comparing with that in controls (93). Treatment with TNF-α inhibitors decreased M1 phenotypes according to improvement of their clinical severity scores (88, 93).
Inappropriate and excessive activation of endosomal Toll-like receptors 7, 8, and 9 (TLRs 7-9) at the psoriasis lesion plays a pathogenic role in the onset of psoriasis. Murine experiments showed that treatment with a TLR7 agonist shifted macrophages in the psoriatic lesions to a higher M1/M2 ratio. Both exogenous and endogenous TLR7-9 ligands favored M1 macrophage polarization (106).
Blocking the signaling of 4-1BBL, a member of the TNF superfamily, reduced the expression of hallmark genes of M1 macrophages such as Tnf, Nos2, and Il23 in imiquimod-treated mice. In vitro experiments revealed that deficiency of 4-1BBL resulted in reduced expression of Tnf, Nos2, Il23, Il6, and Cxcl10 in LPS-and-IFN-γ–treated macrophages (M1), whereas the expression levels of Il10, Arg1, Fizz1, Ym1, Egr2, and Mrc1 (Cd206) were increased in IL-4–treated 4-1BBL knock-out cells, suggesting that 4-1BBL favors the M1 polarization of macrophages (107).
Response gene to complement (RGC)-32 is important for M2 macrophage polarization and phagocytic activity, and inhibits the development of M1 macrophages (108). The level of RGCC (the gene encoding RGC-32) mRNA was significantly lower in lesional psoriasis than in samples from normal individuals (95). Furthermore, Rgcc expression was significantly reduced in the lesional skin of imiquimod-induced psoriasiform dermatitis. Considering that RGC-32 participates in M2 macrophage polarization, its reduced expression in psoriatic lesions possibly contributes to skewed macrophage polarization toward the M1 phenotype (95).
IL-35, known as an anti-inflammatory cytokine (109, 110), decreased the total number of macrophages and ratio of M1/M2 macrophages in three psoriasis models: a human keratinocyte cell line (HaCaT), a keratin 14-VEGF A-transgenic mouse model, and an imiquimod-induced psoriasis mouse model (111).
Hsa_circ_0004287, one of circular RNA (circRNA), inhibited M1 macrophage activation in an N 6-methyladenosine-dependent manner in atopic dermatitis and psoriasis (112).
Increased M1 polarization was associated with higher disease severity in psoriasis, returning to baseline after successful treatment by TNF-α inhibitors (93). TNF-α blockage inhibited M1 polarization through STAT1- and IRF-1-independent pathways.
Sphingosine-1-phosphate receptor 4 (S1PR4)-dependent CCL2 production may be involved in macrophage recruitment to the psoriatic lesion (113). In imiquimod-induced psoriasiform dermatitis, psoriasis severity was ameliorated in S1PR4-deficient mice without altered IL-17 production compared with those in psoriatic wild-type mice. Instead, deficiency of S1PR4 attenuated the production of CCL2, IL-6, and CXCL1 and subsequently reduced the number of infiltrating monocytes and granulocytes. Migration assays revealed reduced CCL2 production in murine skin and attenuation of monocyte migration under the conditions lacking S1PR4. S1PR4 signaling synergized with TLR signaling in resident macrophages to produce CCL2. They speculated that S1PR4 activation enhanced the TLR response of resident macrophages to increase CCL2 production, which attracted further macrophages.
Furthermore, the importance of the interaction between CX3CL1 and CX3CR1 has been postulated in psoriasis (114). CX3CR1, a receptor for CX3CL1, mediates migration of inflammatory cells. CX3CR1 deficiency attenuated imiquimod-induced psoriasis-like skin inflammation with decreased M1 macrophages.
Macrophage-specific soluble factors are involved in the pathogenesis of psoriasis. Macrophages produce monocyte chemoattractant protein-1 (MCP-1), which recruits Th1 inflammatory cells (86). MCP-1 and its receptor CCR2, expressed on dermal macrophages (115), are essential for monocyte recruitment from the circulation (116). Increased expression of MCP-1 is observed in psoriatic keratinocytes (115, 117). MCP-1 polymorphisms have been associated with an increased risk of psoriasis, and serum MCP-1 levels are higher in psoriatic patients (118) and in induced psoriatic lesions of murine models (88, 94). Local production of chemotactic MCP-1 correlated with macrophage accumulation in psoriasis, suggesting that MCP-1 dysregulation may contribute to the pathogenesis of psoriasis.
Macrophage migration inhibitory factor (MIF) is another cytokine implicated in the pathogenesis of psoriasis. MIF is produced by macrophages and recruits inflammatory cells (86). MIF polymorphisms have been associated with an increased risk of psoriasis (119–121). MIF drives murine psoriasiform dermatitis (122). Serum MIF levels were higher in psoriasis patients than in healthy controls, and the serum MIF level was positively correlated with the clinical severity score. Peripheral blood mononuclear cells from psoriatic patients spontaneously produced approximately ten-fold more MIF in in vitro culture, indicating an inherent overproduction of this cytokine in psoriatic patients (123). In MIF-null mice, severity of psoriasiform dermatitis was lower and macrophage recruitment was impaired (122). Thus, MIF may be involved in the recruitment of macrophages in psoriasis patients. However, in contrast to elevated serum MIF in psoriasis patients, MIF-positive cells were significantly decreased in the lesional psoriatic epidermis (124). Further studies are needed to clarify the involvement of MIF in the pathogenesis of psoriasis.
Recently, studies on macrophages in the synovial fluid (SF) of arthritic joints in patients with psoriatic arthritis (PsA) have been reported.
PsA SF cells are dominated by monocytes/macrophages. CD14+CD16- classical monocytes/macrophages were lower in PsA SF than in the SF of patients with rheumatoid arthritis (RA), while CD14+CD16+ intermediate monocytes/macrophages were more predominant in PsA SF compared to RA SF (125). Proteinase-activated receptor 2 (PAR2) and its activating proteinases, including tryptase-6, could be important mediators of inflammation in PsA (125).
In the synovial tissues of patients with PsA and RA, synovial tissue stromal cells and CD163+ macrophages are the main source of granulocyte-macrophage colony-stimulating factor (GM-CSF) (126). Synovial tissue CD163+ macrophages express pro-inflammatory polarization markers (activin A, TNF-α, and MMP12) and exhibit a predominantly GM-CSF-dependent pro-inflammatory polarization state.
Expression of the prolactin receptor (PRLR) is higher in synovial tissue from RA and PsA patients than in synovial tissue from osteoarthritis (OA) patients, and prolactin (PRL) cooperates with other pro-inflammatory stimuli such as CD40L and TNF to activate macrophages by increasing the expression of pro-inflammatory cytokines including IL-6, IL-8 and IL-12β (127). Although serum PRL levels were similar in female and male RA patients, PRLR expression was significantly higher in RA and PsA synovial tissue compared with OA synovial tissue. PRLR colocalized with synovial CD68+ macrophages and von Willebrand factor+ endothelial cells. An in vitro study showed that PRLR was prominently expressed in IFN-γ- and IL-10-polarized macrophages. The production of PRL by macrophages was increased by unknown components of RA and PsA SF (128), where PRL could contribute to disease progression.
Tie2 is a tyrosine kinase receptor essential for vascular development and blood vessel remodeling through interaction with its ligands, angiopoietin-1 (Ang-1) and Ang-2 (129). Tie2 and its ligands were expressed in RA and PsA synovial tissue at higher levels than in the synovial tissue of healthy controls and OA patients (130–132). In RA and PsA synovial tissue, Tie2 was expressed by fibroblast-like synoviocytes, endothelial cells and macrophages (131, 133). Kabala et al. revealed that Tie2 signaling enhanced TNF-dependent activation of macrophages in synovial inflammation in RA and PsA patients (134).
Some of the above-mentioned factors are not specific to PsA. The role of macrophages in arthritis might be common in many aspects between RA and PsA. Although the contribution of macrophages to the development of PsA is indicated, the data are limited at present.
pDC play an important role in the early phase of psoriasis by producing IFN-α, which causes the maturation of resident dermal DC and the differentiation of monocytes into iDC. Increased numbers of iDC produce key cytokines of psoriasis, including IL-23, which strongly activate the differentiation of naive T cells into Th17 and Th22. IL-23 contributes to the maintenance and proliferation of pathogenic Th17 cells. The contribution of LC to the pathogenesis of psoriasis is controversial.
M1 macrophages are considered to contribute to the development of psoriasis especially in early-phase psoriasis, by producing TNF-α. Recently, IL-23 production by CD163+ macrophages has been reported. Further investigation is needed to clarify the involvement of macrophages in the pathogenesis of psoriasis.
MK wrote the first draft of the manuscript. YT contributed to conception, review and editing. All authors contributed to manuscript revision, read, and approved the submitted version.
The authors declare that the research was conducted in the absence of any commercial or financial relationships that could be construed as a potential conflict of interest.
All claims expressed in this article are solely those of the authors and do not necessarily represent those of their affiliated organizations, or those of the publisher, the editors and the reviewers. Any product that may be evaluated in this article, or claim that may be made by its manufacturer, is not guaranteed or endorsed by the publisher.
1. Wang A, Bai Y. Dendritic Cells: The Driver of Psoriasis. J Dermatol (2020) 47:104–13. doi: 10.1111/1346-8138.15184
2. Vičić M, Kaštelan M, Brajac I, Sotošek V, Massari LP. Current Concepts of Psoriasis Immunopathogenesis. Int J Mol Sci (2021) 22:11574. doi: 10.3390/ijms222111574
3. Lynde CW, Poulin Y, Vender R, Bourcier M, Khalil S. Interleukin 17A: Toward a New Understanding of Psoriasis Pathogenesis. J Am Acad Dermatol (2014) 71:141–50. doi: 10.1016/j.jaad.2013.12.036
4. Ogawa E, Sato Y, Minagawa A, Okuyama R. Pathogenesis of Psoriasis and Development of Treatment. J Dermatol (2018) 45:264–72. doi: 10.1111/1346-8138.14139
5. Kamata M, Tada Y. Efficacy and Safety of Biologics for Psoriasis and Psoriatic Arthritis and Their Impact on Comorbidities: A Literature Review. Int J Mol Sci (2020) 21:1690. doi: 10.3390/ijms21051690
6. Boltjes A, van Wijk F. Human Dendritic Cell Functional Specialization in Steady-State and Inflammation. Front Immunol (2014) 5:131. doi: 10.3389/fimmu.2014.00131
7. Dzionek A, Fuchs A, Schmidt P, Cremer S, Zysk M, Miltenyi S, et al. BDCA-2, BDCA-3, and BDCA-4: Three Markers for Distinct Subsets of Dendritic Cells in Human Peripheral Blood. J Immunol (2000) 165:6037–46. doi: 10.4049/jimmunol.165.11.6037
8. Ziegler-Heitbrock L, Ancuta P, Crowe S, Dalod M, Grau V, Hart DN, et al. Nomenclature of Monocytes and Dendritic Cells in Blood. Blood (2010) 116:e74–80. doi: 10.1182/blood-2010-02-258558
9. Robbins SH, Walzer T, Dembélé D, Thibault C, Defays A, Bessou G, et al. Novel Insights Into the Relationships Between Dendritic Cell Subsets in Human and Mouse Revealed by Genome-Wide Expression Profiling. Genome Biol (2008) 9:R17. doi: 10.1186/gb-2008-9-1-r17
10. Schlitzer A, McGovern N, Teo P, Zelante T, Atarashi K, Low D, et al. IRF4 Transcription Factor-Dependent CD11b+ Dendritic Cells in Human and Mouse Control Mucosal IL-17 Cytokine Responses. Immunity (2013) 38:970–83. doi: 10.1016/j.immuni.2013.04.011
11. Haniffa M, Shin A, Bigley V, McGovern N, Teo P, See P, et al. Human Tissues Contain CD141hi Cross-Presenting Dendritic Cells With Functional Homology to Mouse CD103+ Nonlymphoid Dendritic Cells. Immunity (2012) 37:60–73. doi: 10.1016/j.immuni.2012.04.012
12. Klechevsky E, Morita R, Liu M, Cao Y, Coquery S, Thompson-Snipes L, et al. Functional Specializations of Human Epidermal Langerhans Cells and CD14+ Dermal Dendritic Cells. Immunity (2008) 29:497–510. doi: 10.1016/j.immuni.2008.07.013
13. Chu CC, Di Meglio P, Nestle FO. Harnessing Dendritic Cells in Inflammatory Skin Diseases. Semin Immunol (2011) 23:28–41. doi: 10.1016/j.smim.2011.01.006
14. Suzuki T, Sakabe J, Kamiya K, Funakoshi A, Tokura Y. The Vitamin D3 Analogue Calcipotriol Suppresses CpG-Activated TLR9-MyD88 Signalling in Murine Plasmacytoid Dendritic Cells. Clin Exp Dermatol (2018) 43:445–8. doi: 10.1111/ced.13397
15. Tamoutounour S, Guilliams M, Montanana Sanchis F, Liu H, Terhorst D, Malosse C, et al. Origins and Functional Specialization of Macrophages and of Conventional and Monocyte-Derived Dendritic Cells in Mouse Skin. Immunity (2013) 39:925–38. doi: 10.1016/j.immuni.2013.10.004
16. Menezes S, Melandri D, Anselmi G, Perchet T, Loschko J, Dubrot J, et al. The Heterogeneity of Ly6C(hi) Monocytes Controls Their Differentiation Into iNOS(+) Macrophages or Monocyte-Derived Dendritic Cells. Immunity (2016) 45:1205–18. doi: 10.1016/j.immuni.2016.12.001
17. Provoost S, Maes T, Joos GF, Tournoy KG. Monocyte-Derived Dendritic Cell Recruitment and Allergic T(H)2 Responses After Exposure to Diesel Particles are CCR2 Dependent. J Allergy Clin Immunol (2012) 129:483–91. doi: 10.1016/j.jaci.2011.07.051
18. Nestle FO, Conrad C, Tun-Kyi A, Homey B, Gombert M, Boyman O, et al. Plasmacytoid Predendritic Cells Initiate Psoriasis Through Interferon-Alpha Production. J Exp Med (2005) 202:135–43. doi: 10.1084/jem.20050500
19. Wollenberg A, Wagner M, Günther S, Towarowski A, Tuma E, Moderer M, et al. Plasmacytoid Dendritic Cells: A New Cutaneous Dendritic Cell Subset With Distinct Role in Inflammatory Skin Diseases. J Invest Dermatol (2002) 119:1096–102. doi: 10.1046/j.1523-1747.2002.19515.x
20. Tohyama M, Yang L, Hanakawa Y, Dai X, Shirakata Y, Sayama K. IFN-α Enhances IL-22 Receptor Expression in Keratinocytes: A Possible Role in the Development of Psoriasis. J Invest Dermatol (2012) 132:1933–5. doi: 10.1038/jid.2011.468
21. Zheng QY, Liang SJ, Xu F, Li GQ, Luo N, Wu S, et al. C5a/C5aR1 Pathway Is Critical for the Pathogenesis of Psoriasis. Front Immunol (2019) 10:1866. doi: 10.3389/fimmu.2019.01866
22. Takagi H, Arimura K, Uto T, Fukaya T, Nakamura T, Choijookhuu N, et al. Plasmacytoid Dendritic Cells Orchestrate TLR7-Mediated Innate and Adaptive Immunity for the Initiation of Autoimmune Inflammation. Sci Rep (2016) 6:24477. doi: 10.1038/srep24477
23. Kopfnagel V, Wagenknecht S, Harder J, Hofmann K, Kleine M, Buch A, et al. RNase 7 Strongly Promotes TLR9-Mediated DNA Sensing by Human Plasmacytoid Dendritic Cells. J Invest Dermatol (2018) 138:872–81. doi: 10.1016/j.jid.2017.09.052
24. Lande R, Gregorio J, Facchinetti V, Chatterjee B, Wang YH, Homey B, et al. Plasmacytoid Dendritic Cells Sense Self-DNA Coupled With Antimicrobial Peptide. Nature (2007) 449:564–9. doi: 10.1038/nature06116
25. Lande R, Chamilos G, Ganguly D, Demaria O, Frasca L, Durr S, et al. Cationic Antimicrobial Peptides in Psoriatic Skin Cooperate to Break Innate Tolerance to Self-DNA. Eur J Immunol (2015) 45:203–13. doi: 10.1002/eji.201344277
26. Morizane S, Yamasaki K, Mühleisen B, Kotol PF, Murakami M, Aoyama Y, et al. Cathelicidin Antimicrobial Peptide LL-37 in Psoriasis Enables Keratinocyte Reactivity Against TLR9 Ligands. J Invest Dermatol (2012) 132:135–43. doi: 10.1038/jid.2011.259
27. Ganguly D, Chamilos G, Lande R, Gregorio J, Meller S, Facchinetti V, et al. Self-RNA-Antimicrobial Peptide Complexes Activate Human Dendritic Cells Through TLR7 and TLR8. J Exp Med (2009) 206:1983–94. doi: 10.1084/jem.20090480
28. Skrzeczynska-Moncznik J, Wlodarczyk A, Banas M, Kwitniewski M, Zabieglo K, Kapinska-Mrowiecka M, et al. DNA Structures Decorated With Cathepsin G/secretory Leukocyte Proteinase Inhibitor Stimulate IFNI Production by Plasmacytoid Dendritic Cells. Am J Clin Exp Immunol (2013) 2:186–94.
29. Lande R, Gilliet M. Plasmacytoid Dendritic Cells: Key Players in the Initiation and Regulation of Immune Responses. Ann N Y Acad Sci (2010) 1183:89–103. doi: 10.1111/j.1749-6632.2009.05152.x
30. Santini SM, Lapenta C, Logozzi M, Parlato S, Spada M, Di Pucchio T, et al. Type I Interferon as a Powerful Adjuvant for Monocyte-Derived Dendritic Cell Development and Activity In Vitro and in Hu-PBL-SCID Mice. J Exp Med (2000) 191:1777–88. doi: 10.1084/jem.191.10.1777
31. Ueyama A, Yamamoto M, Tsujii K, Furue Y, Imura C, Shichijo M, et al. Mechanism of Pathogenesis of Imiquimod-Induced Skin Inflammation in the Mouse: A Role for Interferon-Alpha in Dendritic Cell Activation by Imiquimod. J Dermatol (2014) 41:135–43. doi: 10.1111/1346-8138.12367
32. Bissonnette R, Papp K, Maari C, Yao Y, Robbie G, White WI, et al. A Randomized, Double-Blind, Placebo-Controlled, Phase I Study of MEDI-545, an Anti-Interferon-Alfa Monoclonal Antibody, in Subjects With Chronic Psoriasis. J Am Acad Dermatol (2010) 62:427–36. doi: 10.1016/j.jaad.2009.05.042
33. Zaba LC, Cardinale I, Gilleaudeau P, Sullivan-Whalen M, Suárez-Fariñas M, Fuentes-Duculan J, et al. Amelioration of Epidermal Hyperplasia by TNF Inhibition is Associated With Reduced Th17 Responses. J Exp Med (2007) 204:3183–94. doi: 10.1084/jem.20071094
34. Austin LM, Ozawa M, Kikuchi T, Walters IB, Krueger JG. The Majority of Epidermal T Cells in Psoriasis Vulgaris Lesions Can Produce Type 1 Cytokines, Interferon-Gamma, Interleukin-2, and Tumor Necrosis Factor-Alpha, Defining TC1 (Cytotoxic T Lymphocyte) and TH1 Effector Populations: A Type 1 Differentiation Bias Is Also Measured in Circulating Blood T Cells in Psoriatic Patients. J Invest Dermatol (1999) 113:752–9. doi: 10.1046/j.1523-1747.1999.00749.x
35. Ferenczi K, Burack L, Pope M, Krueger JG, Austin LM. CD69, HLA-DR and the IL-2R Identify Persistently Activated T Cells in Psoriasis Vulgaris Lesional Skin: Blood and Skin Comparisons by Flow Cytometry. J Autoimmun (2000) 14:63–78. doi: 10.1006/jaut.1999.0343
36. Nestle FO, Turka LA, Nickoloff BJ. Characterization of Dermal Dendritic Cells in Psoriasis. Autostimulation of T Lymphocytes and Induction of Th1 Type Cytokines. J Clin Invest (1994) 94:202–9. doi: 10.1172/jci117308
37. Zaba LC, Fuentes-Duculan J, Eungdamrong NJ, Abello MV, Novitskaya I, Pierson KC, et al. Psoriasis is Characterized by Accumulation of Immunostimulatory and Th1/Th17 Cell-Polarizing Myeloid Dendritic Cells. J Invest Dermatol (2009) 129:79–88. doi: 10.1038/jid.2008.194
38. Guttman-Yassky E, Lowes MA, Fuentes-Duculan J, Zaba LC, Cardinale I, Nograles KE, et al. Low Expression of the IL-23/Th17 Pathway in Atopic Dermatitis Compared to Psoriasis. J Immunol (2008) 181:7420–7. doi: 10.4049/jimmunol.181.10.7420
39. Lee E, Trepicchio WL, Oestreicher JL, Pittman D, Wang F, Chamian F, et al. Increased Expression of Interleukin 23 P19 and P40 in Lesional Skin of Patients With Psoriasis Vulgaris. J Exp Med (2004) 199:125–30. doi: 10.1084/jem.20030451
40. Piskin G, Sylva-Steenland RM, Bos JD, Teunissen MB. In Vitro and in Situ Expression of IL-23 by Keratinocytes in Healthy Skin and Psoriasis Lesions: Enhanced Expression in Psoriatic Skin. J Immunol (2006) 176:1908–15. doi: 10.4049/jimmunol.176.3.1908
41. Lowes MA, Chamian F, Abello MV, Fuentes-Duculan J, Lin SL, Nussbaum R, et al. Increase in TNF-Alpha and Inducible Nitric Oxide Synthase-Expressing Dendritic Cells in Psoriasis and Reduction With Efalizumab (Anti-CD11a). Proc Natl Acad Sci U S A (2005) 102:19057–62. doi: 10.1073/pnas.0509736102
42. Hänsel A, Günther C, Ingwersen J, Starke J, Schmitz M, Bachmann M, et al. Human Slan (6-Sulfo LacNAc) Dendritic Cells are Inflammatory Dermal Dendritic Cells in Psoriasis and Drive Strong TH17/TH1 T-Cell Responses. J Allergy Clin Immunol (2011) 127:787–94. doi: 10.1016/j.jaci.2010.12.009
43. Ahmad F, Döbel T, Schmitz M, Schäkel K. Current Concepts on 6-Sulfo LacNAc Expressing Monocytes (slanMo). Front Immunol (2019) 10:948. doi: 10.3389/fimmu.2019.00948
44. Cros J, Cagnard N, Woollard K, Patey N, Zhang SY, Senechal B, et al. Human CD14dim Monocytes Patrol and Sense Nucleic Acids and Viruses via TLR7 and TLR8 Receptors. Immunity (2010) 33:375–86. doi: 10.1016/j.immuni.2010.08.012
45. Lowes MA, Kikuchi T, Fuentes-Duculan J, Cardinale I, Zaba LC, Haider AS, et al. Psoriasis Vulgaris Lesions Contain Discrete Populations of Th1 and Th17 T Cells. J Invest Dermatol (2008) 128:1207–11. doi: 10.1038/sj.jid.5701213
46. Wilson NJ, Boniface K, Chan JR, McKenzie BS, Blumenschein WM, Mattson JD, et al. Development, Cytokine Profile and Function of Human Interleukin 17-Producing Helper T Cells. Nat Immunol (2007) 8:950–7. doi: 10.1038/ni1497
47. Baran W, Oehrl S, Ahmad F, Döbel T, Alt C, Buske-Kirschbaum A, et al. Phenotype, Function, and Mobilization of 6-Sulfo LacNAc-Expressing Monocytes in Atopic Dermatitis. Front Immunol (2018) 9:1352. doi: 10.3389/fimmu.2018.01352
48. Kunze A, Förster U, Oehrl S, Schmitz M, Schäkel K. Autocrine TNF-α and IL-1β Prime 6-Sulfo LacNAc(+) Dendritic Cells for High-Level Production of IL-23. Exp Dermatol (2017) 26:314–6. doi: 10.1111/exd.13134
49. Brunner PM, Koszik F, Reininger B, Kalb ML, Bauer W, Stingl G. Infliximab Induces Downregulation of the IL-12/IL-23 Axis in 6-Sulfo-LacNac (Slan)+ Dendritic Cells and Macrophages. J Allergy Clin Immunol (2013) 132:1184–93.e8. doi: 10.1016/j.jaci.2013.05.036
50. Oehrl S, Olaru F, Kunze A, Maas M, Pezer S, Schmitz M, et al. Controlling the Pro-Inflammatory Function of 6-Sulfo LacNAc (Slan) Dendritic Cells With Dimethylfumarate. J Dermatol Sci (2017) 87:278–84. doi: 10.1016/j.jdermsci.2017.06.016
51. Dutertre CA, Becht E, Irac SE, Khalilnezhad A, Narang V, Khalilnezhad S, et al. Single-Cell Analysis of Human Mononuclear Phagocytes Reveals Subset-Defining Markers and Identifies Circulating Inflammatory Dendritic Cells. Immunity (2019) 51:573–89.e8. doi: 10.1016/j.immuni.2019.08.008
52. Nakamizo S, Dutertre CA, Khalilnezhad A, Zhang XM, Lim S, Lum J, et al. Single-Cell Analysis of Human Skin Identifies CD14+ Type 3 Dendritic Cells Co-Producing IL1B and IL23A in Psoriasis. J Exp Med (2021) 218:e20202345. doi: 10.1084/jem.20202345
53. Liu X, Zhu R, Luo Y, Wang S, Zhao Y, Qiu Z, et al. Distinct Human Langerhans Cell Subsets Orchestrate Reciprocal Functions and Require Different Developmental Regulation. Immunity (2021) 54:2305–20.e11. doi: 10.1016/j.immuni.2021.08.012
54. Merad M, Manz MG, Karsunky H, Wagers A, Peters W, Charo I, et al. Langerhans Cells Renew in the Skin Throughout Life Under Steady-State Conditions. Nat Immunol (2002) 3:1135–41. doi: 10.1038/ni852
55. Deckers J, Hammad H, Hoste E. Langerhans Cells: Sensing the Environment in Health and Disease. Front Immunol (2018) 9:93. doi: 10.3389/fimmu.2018.00093
56. Otsuka M, Egawa G, Kabashima K. Uncovering the Mysteries of Langerhans Cells, Inflammatory Dendritic Epidermal Cells, and Monocyte-Derived Langerhans Cell-Like Cells in the Epidermis. Front Immunol (2018) 9:1768. doi: 10.3389/fimmu.2018.01768
57. Ginhoux F, Tacke F, Angeli V, Bogunovic M, Loubeau M, Dai XM, et al. Langerhans Cells Arise From Monocytes. In Vivo. Nat Immunol (2006) 7:265–73. doi: 10.1038/ni1307
58. Singh TP, Zhang HH, Borek I, Wolf P, Hedrick MN, Singh SP, et al. Monocyte-Derived Inflammatory Langerhans Cells and Dermal Dendritic Cells Mediate Psoriasis-Like Inflammation. Nat Commun (2016) 7:13581. doi: 10.1038/ncomms13581
59. Seré K, Baek JH, Ober-Blöbaum J, Müller-Newen G, Tacke F, Yokota Y, et al. Two Distinct Types of Langerhans Cells Populate the Skin During Steady State and Inflammation. Immunity (2012) 37:905–16. doi: 10.1016/j.immuni.2012.07.019
60. Lee M, Kim SH, Kim TG, Park J, Lee JW, Lee MG. Resident and Monocyte-Derived Langerhans Cells Are Required for Imiquimod-Induced Psoriasis-Like Dermatitis Model. J Dermatol Sci (2018) 91:52–9. doi: 10.1016/j.jdermsci.2018.04.003
61. Fujita H, Shemer A, Suárez-Fariñas M, Johnson-Huang LM, Tintle S, Cardinale I, et al. Lesional Dendritic Cells in Patients With Chronic Atopic Dermatitis and Psoriasis Exhibit Parallel Ability to Activate T-Cell Subsets. J Allergy Clin Immunol (2011) 128:574–82. doi: 10.1016/j.jaci.2011.05.016
62. Terhorst D, Chelbi R, Wohn C, Malosse C, Tamoutounour S, Jorquera A, et al. Dynamics and Transcriptomics of Skin Dendritic Cells and Macrophages in an Imiquimod-Induced, Biphasic Mouse Model of Psoriasis. J Immunol (2015) 195:4953–61. doi: 10.4049/jimmunol.1500551
63. Bos JD, Hulsebosch HJ, Krieg SR, Bakker PM, Cormane RH. Immunocompetent Cells in Psoriasis. In Situ Immunophenotyping by Monoclonal Antibodies. Arch Dermatol Res (1983) 275:181–9. doi: 10.1007/bf00510050
64. Lisi P. Investigation on Langerhans Cells in Pathological Human Epidermis. Acta Derm Venereol (1973) 53:425–8.
65. Guttman-Yassky E, Lowes MA, Fuentes-Duculan J, Whynot J, Novitskaya I, Cardinale I, et al. Major Differences in Inflammatory Dendritic Cells and Their Products Distinguish Atopic Dermatitis From Psoriasis. J Allergy Clin Immunol (2007) 119:1210–7. doi: 10.1016/j.jaci.2007.03.006
66. Martini E, Wikén M, Cheuk S, Gallais Sérézal I, Baharom F, Ståhle M, et al. Dynamic Changes in Resident and Infiltrating Epidermal Dendritic Cells in Active and Resolved Psoriasis. J Invest Dermatol (2017) 137:865–73. doi: 10.1016/j.jid.2016.11.033
67. Wohn C, Ober-Blöbaum JL, Haak S, Pantelyushin S, Cheong C, Zahner SP, et al. Langerin(neg) Conventional Dendritic Cells Produce IL-23 to Drive Psoriatic Plaque Formation in Mice. Proc Natl Acad Sci U S A (2013) 110:10723–8. doi: 10.1073/pnas.1307569110
68. Cumberbatch M, Singh M, Dearman RJ, Young HS, Kimber I, Griffiths CE. Impaired Langerhans Cell Migration in Psoriasis. J Exp Med (2006) 203:953–60. doi: 10.1084/jem.20052367
69. Eaton LH, Mellody KT, Pilkington SM, Dearman RJ, Kimber I, Griffiths CEM. Impaired Langerhans Cell Migration in Psoriasis Is Due to an Altered Keratinocyte Phenotype Induced by Interleukin-17. Br J Dermatol (2018) 178:1364–72. doi: 10.1111/bjd.16172
70. Glitzner E, Korosec A, Brunner PM, Drobits B, Amberg N, Schonthaler HB, et al. Specific Roles for Dendritic Cell Subsets During Initiation and Progression of Psoriasis. EMBO Mol Med (2014) 6:1312–27. doi: 10.15252/emmm.201404114
71. Nakajima K, Kataoka S, Sato K, Takaishi M, Yamamoto M, Nakajima H, et al. Stat3 Activation in Epidermal Keratinocytes Induces Langerhans Cell Activation to Form an Essential Circuit for Psoriasis via IL-23 Production. J Dermatol Sci (2019) 93:82–91. doi: 10.1016/j.jdermsci.2018.11.007
72. Zheng T, Zhao W, Li H, Xiao S, Hu R, Han M, et al. P38α Signaling in Langerhans Cells Promotes the Development of IL-17-Producing T Cells and Psoriasiform Skin Inflammation. Sci Signal (2018) 11:eaa01685. doi: 10.1126/scisignal.aao1685
73. Yoshiki R, Kabashima K, Honda T, Nakamizo S, Sawada Y, Sugita K, et al. IL-23 From Langerhans Cells Is Required for the Development of Imiquimod-Induced Psoriasis-Like Dermatitis by Induction of IL-17A-Producing γδ T Cells. J Invest Dermatol (2014) 134:1912–21. doi: 10.1038/jid.2014.98
74. Xiao C, Zhu Z, Sun S, Gao J, Fu M, Liu Y, et al. Activation of Langerhans Cells Promotes the Inflammation in Imiquimod-Induced Psoriasis-Like Dermatitis. J Dermatol Sci (2017) 85:170–7. doi: 10.1016/j.jdermsci.2016.12.003
75. Davies LC, Jenkins SJ, Allen JE, Taylor PR. Tissue-Resident Macrophages. Nat Immunol (2013) 14:986–95. doi: 10.1038/ni.2705
76. Boltjes A, Movita D, Boonstra A, Woltman AM. The Role of Kupffer Cells in Hepatitis B and Hepatitis C Virus Infections. J Hepatol (2014) 61:660–71. doi: 10.1016/j.jhep.2014.04.026
77. Schiwon M, Weisheit C, Franken L, Gutweiler S, Dixit A, Meyer-Schwesinger C, et al. Crosstalk Between Sentinel and Helper Macrophages Permits Neutrophil Migration Into Infected Uroepithelium. Cell (2014) 156:456–68. doi: 10.1016/j.cell.2014.01.006
78. Schulz C, Gomez Perdiguero E, Chorro L, Szabo-Rogers H, Cagnard N, Kierdorf K, et al. A Lineage of Myeloid Cells Independent of Myb and Hematopoietic Stem Cells. Science (2012) 336:86–90. doi: 10.1126/science.1219179
79. Guilliams M, De Kleer I, Henri S, Post S, Vanhoutte L, De Prijck S, et al. Alveolar Macrophages Develop From Fetal Monocytes That Differentiate Into Long-Lived Cells in the First Week of Life via GM-CSF. J Exp Med (2013) 210:1977–92. doi: 10.1084/jem.20131199
80. Hashimoto D, Chow A, Noizat C, Teo P, Beasley MB, Leboeuf M, et al. Tissue-Resident Macrophages Self-Maintain Locally Throughout Adult Life With Minimal Contribution From Circulating Monocytes. Immunity (2013) 38:792–804. doi: 10.1016/j.immuni.2013.04.004
81. Jenkins SJ, Ruckerl D, Cook PC, Jones LH, Finkelman FD, van Rooijen N, et al. Local Macrophage Proliferation, Rather Than Recruitment From the Blood, Is a Signature of TH2 Inflammation. Science (2011) 332:1284–8. doi: 10.1126/science.1204351
82. Zigmond E, Jung S. Intestinal Macrophages: Well Educated Exceptions From the Rule. Trends Immunol (2013) 34:162–8. doi: 10.1016/j.it.2013.02.001
83. Lichtnekert J, Kawakami T, Parks WC, Duffield JS. Changes in Macrophage Phenotype as the Immune Response Evolves. Curr Opin Pharmacol (2013) 13:555–64. doi: 10.1016/j.coph.2013.05.013
84. Mosser DM, Edwards JP. Exploring the Full Spectrum of Macrophage Activation. Nat Rev Immunol (2008) 8:958–69. doi: 10.1038/nri2448
85. Atri C, Guerfali FZ, Laouini D. Role of Human Macrophage Polarization in Inflammation During Infectious Diseases. Int J Mol Sci (2018) 19:1801. doi: 10.3390/ijms19061801
86. Kuraitis D, Rosenthal N, Boh E, McBurney E. Macrophages in Dermatology: Pathogenic Roles and Targeted Therapeutics. Arch Dermatol Res (2022) 314:133–40. doi: 10.1007/s00403-021-02207-0
87. Clark RA, Kupper TS. Misbehaving Macrophages in the Pathogenesis of Psoriasis. J Clin Invest (2006) 116:2084–7. doi: 10.1172/jci29441
88. Wang H, Peters T, Kess D, Sindrilaru A, Oreshkova T, Van Rooijen N, et al. Activated Macrophages are Essential in a Murine Model for T Cell-Mediated Chronic Psoriasiform Skin Inflammation. J Clin Invest (2006) 116:2105–14. doi: 10.1172/jci27180
89. Lorthois I, Asselineau D, Seyler N, Pouliot R. Contribution of In Vivo and Organotypic 3d Models to Understanding the Role of Macrophages and Neutrophils in the Pathogenesis of Psoriasis. Mediators Inflammation (2017) 2017:7215072. doi: 10.1155/2017/7215072
90. Ward NL, Loyd CM, Wolfram JA, Diaconu D, Michaels CM, McCormick TS. Depletion of Antigen-Presenting Cells by Clodronate Liposomes Reverses the Psoriatic Skin Phenotype in KC-Tie2 Mice. Br J Dermatol (2011) 164:750–8. doi: 10.1111/j.1365-2133.2010.10129.x
91. Nguyen CTH, Kambe N, Yamazaki F, Ueda-Hayakawa I, Kishimoto I, Okamoto H. Up-Regulated Expression of CD86 on Circulating Intermediate Monocytes Correlated With Disease Severity in Psoriasis. J Dermatol Sci (2018) 90:135–43. doi: 10.1016/j.jdermsci.2018.01.005
92. Golden JB, Groft SG, Squeri MV, Debanne SM, Ward NL, McCormick TS, et al. Chronic Psoriatic Skin Inflammation Leads to Increased Monocyte Adhesion and Aggregation. J Immunol (2015) 195:2006–18. doi: 10.4049/jimmunol.1402307
93. Lin SH, Chuang HY, Ho JC, Lee CH, Hsiao CC. Treatment With TNF-α Inhibitor Rectifies M1 Macrophage Polarization From Blood CD14+ Monocytes in Patients With Psoriasis Independent of STAT1 and IRF-1 Activation. J Dermatol Sci (2018) 91:276–84. doi: 10.1016/j.jdermsci.2018.05.009
94. Leite Dantas R, Masemann D, Schied T, Bergmeier V, Vogl T, Loser K, et al. Macrophage-Mediated Psoriasis Can be Suppressed by Regulatory T Lymphocytes. J Pathol (2016) 240:366–77. doi: 10.1002/path.4786
95. Kim HJ, Jang J, Lee EH, Jung S, Roh JY, Jung Y. Decreased Expression of Response Gene to Complement 32 in Psoriasis and Its Association With Reduced M2 Macrophage Polarization. J Dermatol (2019) 46:166–8. doi: 10.1111/1346-8138.14733
96. Marble DJ, Gordon KB, Nickoloff BJ. Targeting TNFalpha Rapidly Reduces Density of Dendritic Cells and Macrophages in Psoriatic Plaques With Restoration of Epidermal Keratinocyte Differentiation. J Dermatol Sci (2007) 48:87–101. doi: 10.1016/j.jdermsci.2007.06.006
97. Fuentes-Duculan J, Suárez-Fariñas M, Zaba LC, Nograles KE, Pierson KC, Mitsui H, et al. A Subpopulation of CD163-Positive Macrophages Is Classically Activated in Psoriasis. J Invest Dermatol (2010) 130:2412–22. doi: 10.1038/jid.2010.165
98. Mahil SK, Capon F, Barker JN. Update on Psoriasis Immunopathogenesis and Targeted Immunotherapy. Semin Immunopathol (2016) 38:11–27. doi: 10.1007/s00281-015-0539-8
99. Yawalkar N, Tscharner GG, Hunger RE, Hassan AS. Increased Expression of IL-12p70 and IL-23 by Multiple Dendritic Cell and Macrophage Subsets in Plaque Psoriasis. J Dermatol Sci (2009) 54:99–105. doi: 10.1016/j.jdermsci.2009.01.003
100. Wang Y, Edelmayer R, Wetter J, Salte K, Gauvin D, Leys L, et al. Monocytes/Macrophages Play a Pathogenic Role in IL-23 Mediated Psoriasis-Like Skin Inflammation. Sci Rep (2019) 9:5310. doi: 10.1038/s41598-019-41655-7
101. Hou Y, Zhu L, Tian H, Sun HX, Wang R, Zhang L, et al. IL-23-Induced Macrophage Polarization and its Pathological Roles in Mice With Imiquimod-Induced Psoriasis. Protein Cell (2018) 9:1027–38. doi: 10.1007/s13238-018-0505-z
102. Das A, Sinha M, Datta S, Abas M, Chaffee S, Sen CK, et al. Monocyte and Macrophage Plasticity in Tissue Repair and Regeneration. Am J Pathol (2015) 185:2596–606. doi: 10.1016/j.ajpath.2015.06.001
103. Gaire BP, Lee CH, Kim W, Sapkota A, Lee DY, Choi JW. Lysophosphatidic Acid Receptor 5 Contributes to Imiquimod-Induced Psoriasis-Like Lesions Through NLRP3 Inflammasome Activation in Macrophages. Cells (2020) 9:1753. doi: 10.3390/cells9081753
104. Syed SN, Weigert A, Brüne B. Sphingosine Kinases are Involved in Macrophage NLRP3 Inflammasome Transcriptional Induction. Int J Mol Sci (2020) 21:4733. doi: 10.3390/ijms21134733
105. Deng G, Chen W, Wang P, Zhan T, Zheng W, Gu Z, et al. Inhibition of NLRP3 Inflammasome-Mediated Pyroptosis in Macrophage by Cycloastragenol Contributes to Amelioration of Imiquimod-Induced Psoriasis-Like Skin Inflammation in Mice. Int Immunopharmacol (2019) 74:105682. doi: 10.1016/j.intimp.2019.105682
106. Lu CH, Lai CY, Yeh DW, Liu YL, Su YW, Hsu LC, et al. Involvement of M1 Macrophage Polarization in Endosomal Toll-Like Receptors Activated Psoriatic Inflammation. Mediators Inflammation (2018) 2018:3523642. doi: 10.1155/2018/3523642
107. Miki H, Han KH, Scott D, Croft M, Kang YJ. 4-1bbl Regulates the Polarization of Macrophages, and Inhibition of 4-1BBL Signaling Alleviates Imiquimod-Induced Psoriasis. J Immunol (2020) 204:1892–903. doi: 10.4049/jimmunol.1900983
108. Tang R, Zhang G, Chen SY. Response Gene to Complement 32 Protein Promotes Macrophage Phagocytosis via Activation of Protein Kinase C Pathway. J Biol Chem (2014) 289:22715–22. doi: 10.1074/jbc.M114.566653
109. Wang RX, Yu CR, Dambuza IM, Mahdi RM, Dolinska MB, Sergeev YV, et al. Interleukin-35 Induces Regulatory B Cells That Suppress Autoimmune Disease. Nat Med (2014) 20:633–41. doi: 10.1038/nm.3554
110. Shen P, Roch T, Lampropoulou V, O'Connor RA, Stervbo U, Hilgenberg E, et al. IL-35-Producing B Cells Are Critical Regulators of Immunity During Autoimmune and Infectious Diseases. Nature (2014) 507:366–70. doi: 10.1038/nature12979
111. Zhang J, Lin Y, Li C, Zhang X, Cheng L, Dai L, et al. IL-35 Decelerates the Inflammatory Process by Regulating Inflammatory Cytokine Secretion and M1/M2 Macrophage Ratio in Psoriasis. J Immunol (2016) 197:2131–44. doi: 10.4049/jimmunol.1600446
112. Yang L, Fu J, Han X, Zhang C, Xia L, Zhu R, et al. Hsa_circ_0004287 Inhibits Macrophage-Mediated Inflammation in an N(6)-Methyladenosine-Dependent Manner in Atopic Dermatitis and Psoriasis. J Allergy Clin Immunol (2021) 149:2021–33. doi: 10.1016/j.jaci.2021.11.024
113. Schuster C, Huard A, Sirait-Fischer E, Dillmann C, Brüne B, Weigert A. S1PR4-Dependent CCL2 Production Promotes Macrophage Recruitment in a Murine Psoriasis Model. Eur J Immunol (2020) 50:839–45. doi: 10.1002/eji.201948349
114. Morimura S, Oka T, Sugaya M, Sato S. CX3CR1 Deficiency Attenuates Imiquimod-Induced Psoriasis-Like Skin Inflammation With Decreased M1 Macrophages. J Dermatol Sci (2016) 82:175–88. doi: 10.1016/j.jdermsci.2016.03.004
115. Vestergaard C, Just H, Baumgartner Nielsen J, Thestrup-Pedersen K, Deleuran M. Expression of CCR2 on Monocytes and Macrophages in Chronically Inflamed Skin in Atopic Dermatitis and Psoriasis. Acta Derm Venereol (2004) 84:353–8. doi: 10.1080/00015550410034444
116. Vanbervliet B, Homey B, Durand I, Massacrier C, Aït-Yahia S, de Bouteiller O, et al. Sequential Involvement of CCR2 and CCR6 Ligands for Immature Dendritic Cell Recruitment: Possible Role at Inflamed Epithelial Surfaces. Eur J Immunol (2002) 32:231–42. doi: 10.1002/1521-4141(200201)32:1<231::Aid-immu231>3.0.Co;2-8
117. Gillitzer R, Wolff K, Tong D, Müller C, Yoshimura T, Hartmann AA, et al. MCP-1 mRNA Expression in Basal Keratinocytes of Psoriatic Lesions. J Invest Dermatol (1993) 101:127–31. doi: 10.1111/1523-1747.ep12363613
118. Wang L, Yang L, Gao L, Gao TW, Li W, Liu YF. A Functional Promoter Polymorphism in Monocyte Chemoattractant Protein-1 Is Associated With Psoriasis. Int J Immunogenet (2008) 35:45–9. doi: 10.1111/j.1744-313X.2007.00734.x
119. Donn RP, Plant D, Jury F, Richards HL, Worthington J, Ray DW, et al. Macrophage Migration Inhibitory Factor Gene Polymorphism Is Associated With Psoriasis. J Invest Dermatol (2004) 123:484–7. doi: 10.1111/j.0022-202X.2004.23314.x
120. Hernández-Bello J, Rodríguez-Puente M, Gutiérrez-Cuevas J, García-Arellano S, Muñoz-Valle JF, Fafutis-Morris M, et al. Macrophage Migration Inhibitory Factor Gene Polymorphisms (SNP -173 G>C and STR-794 CATT5-8) Confer Risk of Plaque Psoriasis: A Case-Control Study. J Clin Lab Anal (2021) 35:e23999. doi: 10.1002/jcla.23999
121. Chhabra S, Banerjee N, Narang T, Sood S, Bishnoi A, Goel S, et al. Single-Nucleotide Polymorphism and Haplotype Analysis of Macrophage Migration Inhibitory Factor Gene and Its Correlation With Serum Macrophage Migration Inhibitory Factor Levels in North Indian Psoriatic Patients With Moderate Disease Severity: A Cross-Sectional Study. Indian J Dermatol Venereol Leprol (2021) 1-7. doi: 10.25259/ijdvl_988_19
122. Bezdek S, Leng L, Busch H, Mousavi S, Rades D, Dahlke M, et al. Macrophage Migration Inhibitory Factor (MIF) Drives Murine Psoriasiform Dermatitis. Front Immunol (2018) 9:2262. doi: 10.3389/fimmu.2018.02262
123. Shimizu T, Nishihira J, Mizue Y, Nakamura H, Abe R, Watanabe H, et al. High Macrophage Migration Inhibitory Factor (MIF) Serum Levels Associated With Extended Psoriasis. J Invest Dermatol (2001) 116:989–90. doi: 10.1046/j.0022-202x.2001.01366.x
124. Shimizu T, Nishihira J, Mizue Y, Nakamura H, Abe R, Watanabe H, et al. Histochemical Analysis of Macrophage Migration Inhibitory Factor in Psoriasis Vulgaris. Histochem Cell Biol (2002) 118:251–7. doi: 10.1007/s00418-002-0435-x
125. Abji F, Rasti M, Gómez-Aristizábal A, Muytjens C, Saifeddine M, Mihara K, et al. Proteinase-Mediated Macrophage Signaling in Psoriatic Arthritis. Front Immunol (2020) 11:629726. doi: 10.3389/fimmu.2020.629726
126. Fuentelsaz-Romero S, Cuervo A, Estrada-Capetillo L, Celis R, García-Campos R, Ramírez J, et al. GM-CSF Expression and Macrophage Polarization in Joints of Undifferentiated Arthritis Patients Evolving to Rheumatoid Arthritis or Psoriatic Arthritis. Front Immunol (2020) 11:613975. doi: 10.3389/fimmu.2020.613975
127. Tang MW, Reedquist KA, Garcia S, Fernandez BM, Codullo V, Vieira-Sousa E, et al. The Prolactin Receptor is Expressed in Rheumatoid Arthritis and Psoriatic Arthritis Synovial Tissue and Contributes to Macrophage Activation. Rheumatol (Oxford) (2016) 55:2248–59. doi: 10.1093/rheumatology/kew316
128. Tang MW, Garcia S, Malvar Fernandez B, Gerlag DM, Tak PP, Reedquist KA. Rheumatoid Arthritis and Psoriatic Arthritis Synovial Fluids Stimulate Prolactin Production by Macrophages. J Leukoc Biol (2017) 102:897–904. doi: 10.1189/jlb.2A0317-115RR
129. Huang H, Bhat A, Woodnutt G, Lappe R. Targeting the ANGPT-TIE2 Pathway in Malignancy. Nat Rev Cancer (2010) 10:575–85. doi: 10.1038/nrc2894
130. Scott BB, Zaratin PF, Colombo A, Hansbury MJ, Winkler JD, Jackson JR. Constitutive Expression of Angiopoietin-1 and -2 and Modulation of Their Expression by Inflammatory Cytokines in Rheumatoid Arthritis Synovial Fibroblasts. J Rheumatol (2002) 29:230–9.
131. Shahrara S, Volin MV, Connors MA, Haines GK, Koch AE. Differential Expression of the Angiogenic Tie Receptor Family in Arthritic and Normal Synovial Tissue. Arthritis Res (2002) 4:201–8. doi: 10.1186/ar407
132. Fearon U, Griosios K, Fraser A, Reece R, Emery P, Jones PF, et al. Angiopoietins, Growth Factors, and Vascular Morphology in Early Arthritis. J Rheumatol (2003) 30:260–8.
133. Krausz S, Garcia S, Ambarus CA, de Launay D, Foster M, Naiman B, et al. Angiopoietin-2 Promotes Inflammatory Activation of Human Macrophages and Is Essential for Murine Experimental Arthritis. Ann Rheum Dis (2012) 71:1402–10. doi: 10.1136/annrheumdis-2011-200718
134. Kabala PA, Malvar-Fernández B, Lopes AP, Carvalheiro T, Hartgring SAY, Tang MW, et al. Promotion of Macrophage Activation by Tie2 in the Context of the Inflamed Synovia of Rheumatoid Arthritis and Psoriatic Arthritis Patients. Rheumatol (Oxford) (2020) 59:426–38. doi: 10.1093/rheumatology/kez315
Keywords: dendritic cell (DC), macrophage - cell, monocyte - macrophage, langerhans cell (LC), psoriasis, psoriatic arthritis (PsA)
Citation: Kamata M and Tada Y (2022) Dendritic Cells and Macrophages in the Pathogenesis of Psoriasis. Front. Immunol. 13:941071. doi: 10.3389/fimmu.2022.941071
Received: 11 May 2022; Accepted: 01 June 2022;
Published: 28 June 2022.
Edited by:
Naoki Iwamoto, Nagasaki University Hospital, JapanReviewed by:
Chunying Xiao, Fourth Military Medical University, ChinaCopyright © 2022 Kamata and Tada. This is an open-access article distributed under the terms of the Creative Commons Attribution License (CC BY). The use, distribution or reproduction in other forums is permitted, provided the original author(s) and the copyright owner(s) are credited and that the original publication in this journal is cited, in accordance with accepted academic practice. No use, distribution or reproduction is permitted which does not comply with these terms.
*Correspondence: Yayoi Tada, eXRhZGEtdGt5QHVtaW4uYWMuanA=
Disclaimer: All claims expressed in this article are solely those of the authors and do not necessarily represent those of their affiliated organizations, or those of the publisher, the editors and the reviewers. Any product that may be evaluated in this article or claim that may be made by its manufacturer is not guaranteed or endorsed by the publisher.
Research integrity at Frontiers
Learn more about the work of our research integrity team to safeguard the quality of each article we publish.