- 1Laboratory of Immunology, Program of Food and AgriLife Science, Graduate School of Integrated Sciences for Life, Hiroshima University, Higashi-Hiroshima, Japan
- 2Precursory Research for Embryonic Science and Technology, Japan Science and Technology Agency, Saitama, Japan
Group 2 innate lymphoid cells (ILC2s) are typically known for their ability to respond rapidly to parasitic infections and play a pivotal role in the development of certain allergic disorders. ILC2s produce cytokines such as Interleukin (IL)-5 and IL-13 similar to the type 2 T helper (Th2) cells. Recent findings have highlighted that ILC2s, together with IL-33 and eosinophils, participate in a considerably broad range of physiological roles such as anti-tumor immunity, metabolic regulation, and vascular disorders. Therefore, the focus of the ILC2 study has been extended from conventional Th2 responses to these unexplored areas of research. However, disease outcomes accompanied by ILC2 activities are paradoxical mostly in tumor immunity requiring further investigations. Although various environmental factors that direct the development, activation, and localization of ILC2s have been studied, IL-33/ILC2/eosinophil axis is presumably central in a multitude of inflammatory conditions and has guided the research in ILC2 biology. With a particular focus on this axis, we discuss ILC2s across different diseases.
Introduction
Recent expansion in our understanding of innate lymphoid cells (ILCs) began with several epoch‐making reports in 2010 (1–4). The ILCs were originally indicated as interleukin (IL)-25 responsive non-B/non-T lymphocytes (5). ILCs are classified into five distinct cell populations based on their characteristics, including the profile of cytokines produced and the key transcription factors involved in their major immunological functions. These are the natural killer (NK) cells, group 1 ILC (ILC1), ILC2, ILC3, and lymphoid tissue inducer (LTi) cells (6). This classification should be observed with caution because ILCs possess a unique plastic ability to adapt to the surrounding milieu and can undergo transdifferentiation into another group of ILCs (7–9). ILC2s are tissue-resident cells (10), preferentially inhabiting the mucosal organs such as lung and intestine, and display tissue-specific transcriptional features influenced by the surrounding environment (11). The mucosal surface is the first line of defense against infectious pathogens; hence, ILC2s inherently display a prompt response through the secretion of IL-5 and IL-13. Unlike T cells, ILC2s lack antigen-specific receptors and instead express receptors for epithelial-derived cytokines such as thymic stromal lymphopoietin (TSLP), IL-25, and IL-33, thereby ensuring signal recognition from exogenous agents. ILC2s not only participate in acute responses but also in the subsequent antigen-specific adaptive immune responses in cooperation with type 2 T helper (Th2) cells (12, 13). In addition to Th2 cytokines, ILC2s in the lung were found to produce IL-10 (14–16) and IL-10-producing ILCs, so-called ILCreg, have been reported in the intestine (17). Interestingly, like other antigen-presenting cells, ILC2s communicate through major histocompatibility complex class II molecules to activate acquired immune response (18). Thus, ILC2s provide a link between innate and acquired immunity (19).
ILC2s are involved in various immunological disorders and host defense (20). Asthma is a chronic airway inflammatory disease and one of the best-characterized allergic disorders associated with ILC2s (21, 22). ILC2s serve to establish predominant Th2 inflammation synergistically and/or competitively by interacting with other ILC subsets and immune cells (23). ILC2s in respiratory diseases are also evident in humans (24). To eliminate invading parasites, ILC2s mediate Th2 immune response in collaboration with adaptive Th2 cells (25, 26). In anti-viral immunity, although ILC2s exacerbate airway hyperreactivity through IL-13 production (27), they contribute to tissue repair by producing a wound-healing protein, amphiregulin (28). In most cases, IL-33 is considered a central cytokine for such ILC2-mediated immune responses.
Although the functions of IL-33 in allergies are well known (29, 30), the focus has currently shifted to its role in cancer (31–33) and cardiovascular diseases (34–36). IL-33 is one of the most effective cytokines for regulating ILC2s. In a steady state, IL-33 resides in the nucleus and is released by necrotic cells within damaged tissue (29, 37). When a tissue is injured/infected by pathogens, IL-33 acts by alarming the immune cells in the vicinity to mediate immune responses, and is thus called an “alarmin” or damage-associated molecular pattern. The IL-33 receptor comprises ST2 (IL-1 receptor-like 1) and IL-1 receptor accessory protein (38–40) which is expressed on various immune cells (41, 42). The binding of IL-33 to ST2 on the cell surface ensures Th2 responses, whereas soluble ST2 (sST2) in circulation inhibits excess IL-33-mediated responses and protects against disease development (29). In an allergic inflammation, platelets act as reservoirs and suppliers of IL-33 (43) and are capable of boosting ILC2 activities through direct interaction (44). In the lung tissue, platelets are supplied by the resident megakaryocytes (45) and may participate in regulation of ILC2 function.
IL-5 is a homodimeric cytokine and its engagement with its receptor, comprising an IL-5Rα and a common β-chain, plays critical roles in eosinophil biology starting from the early phase of its ontogeny in bone marrow (46, 47). Eosinophils store mediators such as major basic protein (MBP) in granules and are involved in both health and disease (48, 49). Genetic blockade of IL-5 signaling results in severe defects in eosinophil regulation (50, 51), and therefore treatments with anti-IL-5 or anti-IL-5Rα monoclonal antibodies (mAb) have been promising in patients with severe eosinophilic asthma (52–54).
In this review, we will discuss recent findings describing ILC2s in different types of disorders, such as cancer, obesity, and cardiovascular diseases. These findings suggest that roles of ILC2s are pleiotropic and diverse, largely depending on the surrounding environment. An ILC2-targeted therapeutic approach effective for one disease might be deleterious for another. This highlights the requirement for a detailed investigation and verification of the association and mechanisms of ILC2s.
Contradictory Roles of ILC2s in Tumor Immunity
Recent findings have shed light on both anti- and pro-tumor activities of ILC2s (55–60). The anti-tumorigenic activity of ILC2s appears to be largely dependent on the requirement of eosinophils at the site of malignancy. Histological evidence for the involvement of eosinophils in human cancers exists (61–63), however, the findings are controversial (64, 65). The number of infiltrated eosinophils in colonic or colorectal carcinomas significantly correlates with improved prognosis (63, 66–70). Conversely, in cervical cancer (71), nasopharyngeal carcinoma (72), and lymph node metastasis or lymphatic invasion (73), eosinophils were associated with unfavorable prognoses. In addition to the direct cytotoxic activity of the granules containing MBP on tumor cells (74, 75), eosinophils in tumor microenvironment (TME), when activated by interferon (IFN)-γ and tumor necrosis factor (TNF)-α efficiently promote mobilization of CD8+ cytotoxic T cells from circulation (76). Eosinophils, however, display functional and phenotypical heterogeneity and their influence seems to rely on tumor types, TME, and cancer stages (64).
Involvement of IL-5-producing ILC2s in antitumorigenic activities was reported using an IL-5 reporter mouse (77), wherein lung ILC2s were required to retain sufficient number of eosinophils against tumor metastasis, and a blockade of IL-5 signaling resulted in an increased B16F10 metastasis. This is supported by the findings from a study that included three groups of mice deficient in C-C motif chemokine ligand 11 (CCL11), both CCL11 and IL-5, and eosinophils, respectively; all the three groups of mice exhibited increased tumor growth in chemically-induced fibrosarcoma (78). Antitumorigenic ILC2s are primed by their environment modulated by IL-33 (31–33). Mice inoculated with IL-33-expressing tumor cell lines, including EL4, CT26, and B16F10, resulted in a substantial expansion of intertumoral ILC2, which inhibited tumor growth and induced apoptosis of tumor cells through the production of CXC chemokine receptor 2 ligands (79). IL-33-expressing A9, a murine lung tumor cell line, was also reported to augment the antimetastatic functions of ILC2s (80). Although ILC2s were not investigated in mice administered with IL-33, tumor growth and metastasis were inhibited via eosinophil recruitment (81). Mice deficient in ILC2s failed to control the incidence of experimentally induced colorectal cancers, whereas ILC2 infiltration correlated with better overall survival of patients with colorectal cancers (82). TME induces the expression of programmed cell death-1 (PD-1) on CD8+ T cells as well as ILC2s, which results in the inhibition of cytokine production from these cells. Importantly, a blockade of PD-1 on the surface of ILC2s leads to revival of their antitumorigenic properties (83, 84). Interestingly, both serum IL-5 and IFN-γ levels are useful in predicting the efficacy of anti-PD1 mAb treatment in patients with non-small-cell lung and gastric cancer (85).
Accumulating evidence has also suggested pro-tumorigenic roles of ILC2s. In contrast to the previous study (77), IL-5 was reported to facilitate tumor metastasis (86). Additionally, IL-5 was suggested to enhance the migration of bladder cancer cells (87), and esophageal squamous cell carcinoma (88) in humans. Furthermore, IL-5 enhanced metastasis of breast cancer cells in obese mice (89). Consistent with these reports, ILC2s facilitated tumor metastasis in IL-33-treated animals by limiting cytotoxic activity of NK cells (90). Moreover, IL-13 derived from ILC2s promoted differentiation of myeloid-derived suppressor cells and were pro-tumorigenic in acute promyelocytic leukemia (91), bladder cancer recurrence (92), and metastasis of breast cancer (93).
Roles of ILC2s, eosinophils and IL-33 in tumor immunity show contrasting results, which poses a difficulty in understanding the distinct roles of these players in deciding the fate of tumor cells. However, the possibility of environmental cues as a key determinant for ILC2s to be antitumorigenic or pro-tumorigenic can be envisaged. For instance, lactic acid from tumor cells is pro-tumorigenic (94) whereas higher levels of IL-33 in TME are shown to induce antitumorigenic activities of ILC2s (81). This suggests that an assessment of the regulation of ILC2s by TME is essential for therapeutic intervention.
Anti-Inflammatory and Thermogenic Roles of ILC2 in Obesity
Obesity is a highly prevalent condition worldwide in which excess fat accumulates in the body. It is often associated with type 2 diabetes, high blood pressure, hyperlipidemia, and cardiovascular diseases (95). Apart from the roles of ILC2s in typical Th2 immune responses, they also contribute to homeostatic and metabolic regulation in adipose tissues (96, 97). Adipose tissues are categorized into white, brown, and beige. In comparison to white, beige and brown adipose tissues display higher and the highest thermogenic activity, respectively, and are thus specialized in generating heat. Initially, eosinophils were demonstrated to be the major IL-4-producing cells in white adipose tissue involved in inducing anti-inflammatory M2 macrophages (98) which prevents weight gain. Furthermore, ILC2s in adipose tissues were the major sources of IL-5 and IL-13 and recruited eosinophils to produce an anti-obese environment (99). Conversely, ILC2s in the small intestine were reported to induce obesity through the production of IL-2 (100), indicating the importance of the interplay between distal organs.
ILC2s also directly regulate adipocytes and participate in thermogenesis (101, 102). Adipose ILC2s promote beiging, conversion from white to beige, through production of methionine-enkephalin peptide, which can directly affect the adipocytes and upregulate the expression of uncoupling protein 1 (101), which was brought about by IL-33 (103). In response to cold stimuli, ILC2s are responsible for proliferation of platelet-derived growth factor receptors (PDGFR)-α+ adipocyte progenitors and subsequent differentiation to beige adipocytes (102). IL-13 from ILC2s and/or IL-4 from eosinophils have been shown to stimulate PDGFRα+ progenitors through their surface IL-4R.
Cell-cell interaction is important for the activation of adipose ILC2s. Both glucocorticoid-induced TNF receptor (104) and death receptor 3 (105) belong to the TNFR superfamily and are expressed on adipose ILC2s. Post ligand binding, ILC2s accelerate the production of IL-5 and IL-13 and improve glucose tolerance and insulin sensitivity, demonstrating their potential to be used in type 2 diabetes therapy. In contrast, IL-33 in the presence of TNF-α in obese conditions upregulates PD-1 expression on adipose ILC2s and limits their production of IL-5 and IL-13 (106). Recently, regulation of ILC2s by sympathetic nerves via adipose mesenchymal stromal cells was observed (107). Elucidation of the precise regulatory mechanism and knowledge on the specific activators of adipose ILC2s will aid in therapy for obesity or type 2 diabetes.
Reparative Roles of ILC2s in Cardiac Dysfunction
ILC2s are involved in healing cardiac tissue with cooperation from various types of immune cells to recover and regenerate cardiac tissue damage caused by myocardial infarction (MI) (108). ST2 is expressed on cardiomyocytes, and levels of sST2 in serum from animals and humans were elevated after MI (109). Therefore, IL-33 being the only known ligand of ST2 (38), its role in cardiovascular and vascular diseases (34–36) was investigated. In contrast to the known pro-inflammatory functions of IL-33, IL-33/ST2 signaling protected animals from experimentally induced cardiac failure by antagonizing angiotensin II-induced cardiomyocyte hypertrophy (110). Furthermore, IL-33 also dictates healing processes indirectly via ILC2s.
Under physiological conditions, ILCs reside in heart and display a progenitor-like phenotype (111). These heart resident ILCs are evident in biopsy samples from animals and humans with ischemic cardiomyopathy and myocarditis and are fated to differentiate to ILC2s in response to cardiac failure (111). ILC2s in pericardial adipose tissue (PcAT) proliferate in an IL-33 dependent manner in response to MI, and animals deficient in ILC2 exhibited incomplete recovery from heart dysfunction and a worsened mortality rate post-MI (112). Although the precise mechanism of ILC2s is unknown, the recruitment of eosinophils by IL-5 is considered in the recovery of cardiac function. This is supported by the observation that animals deficient in eosinophils failed to ameliorate cardiac functions after MI and that IL-4 from eosinophils was essential for recovery (113). However, the infiltration of eosinophils into heart needs to be regulated in order to avoid eosinophilia which induces inflammatory dilated cardiomyopathy (114).
Interestingly, low-dose IL-2 (aldesleukin) administration in patients with acute coronary syndrome exhibited transient activation of blood ILC2s, with a concomitant increase in serum IL-5 and eosinophil counts, demonstrating recovery of cardiac function (112). Further research on ILC2s in cardiac diseases will provide beneficial insights into developing unprecedented therapies.
Protective Roles of ILC2s in Atherosclerosis
Atherosclerosis is an arterial disease characterized by the deposition of plaques on inner walls; and lipid modifications in plaques result in the generation of non-self-antigens, causing chronic inflammation. Atherosclerosis is the primary cause of most cardiovascular diseases. Administration of cytokines related to ILC2 activation were effective in reducing atherosclerosis in animals (115). TSLP (116), IL-25 (117), and IL-33 showed protective effects, and the effectiveness of IL-33 was largely dependent on IL-5 (118). ILC2s that were experimentally expanded with IL-2/IL-2R complexes protected from the development of atherosclerosis, although, the contribution of IL-5/eosinophils was limited (119). In contrast, ILC1 and NK cells were shown to play etiologic roles in disease development (120). This correlated well with a significantly high ILC1/ILC2 ratio in patients with acute cerebral infarction, commonly caused by rupture of atherosclerotic plaques (121). By selectively depleting ILC2s in an animal model of atherosclerosis, regional ILC2s that were in proximity to atherosclerotic lesions, sufficiently reduced atherosclerosis, possibly through phenotypic alteration of macrophages to anti-inflammatory M2 macrophages (122). Furthermore, transfer of ILC2s into mice that developed atherosclerosis led to an increase in B1 cell-derived atheroprotective IgM antibodies with reduction in plaque deposition (123). Collectively, ILC2s appear to be protective against atherosclerosis.
Etiologic Roles of ILC2s in Pathogenesis of Pulmonary Arteries
In contrast to the protective roles of ILC2s in cardiac failure and atherosclerosis, chronic inflammation in lungs possibly drives ILC2s to act in mediating disorders of blood vessels, including pulmonary arterial hypertension (PAH). PAH is a progressive vascular disease characterized by a severe obstruction such as hypertrophy of small pulmonary arteries with high pulmonary arterial pressure, thereby resulting in right ventricular failure. It is categorized as one of the five groups of clinical classification for pulmonary hypertension (PH) (124). PAH is an intractable rare disease and its development is multifactorial. Although the investigation of causative factors of PAH is ongoing, chronic inflammation may have a plausible role in the underlying mechanism (125). Evidence suggests chronic allergic conditions in mice, concomitant with eosinophilia, lead to the induction of vessel remodeling with remarkable collagen deposition and enhanced proliferation of α-smooth muscle cells (126, 127). Subsequently, Th2 cytokines (128) or IL-5 and eosinophils (129) were reported to be necessary for the initiation of arterial remodeling. In humans, parasitic infection, in which Th2 cytokines such as IL-4 and IL-13 are predominant, is believed to be the most common cause of PAH (130, 131), with Th2 cytokines inducing arterial hypertrophy and other arterial modifications (131, 132).
Pulmonary arterial hypertrophy can also be experimentally induced by prolonged administration of IL-33 in mice (133). Histological examination revealed that perivascular ILC2s and eosinophils were evident around hypertrophied arteries, and this hypertrophy was ameliorated with anti-IL-5Rα mAb that depleted eosinophils (134). The proximity of ILC2s to blood vessels in lungs, as visualized in collagen-rich (135) adventitial niches (136), may facilitate their vascular regulation through eosinophil recruitment. In this region, ILC2s are maintained by IL-33-expressing stromal cells (136) which possibly regulate ILC2s in case of arterial hypertrophy. Thus, elucidation of the precise regulatory mechanism will help to understand the initial phase of disease development.
Because of the lack of histological evidence in humans on initial phase of arterial hypertrophy, animal models of PAH are essential to reveal causative factors. Despite reports of advanced arterial hypertrophy in animal studies, severe PH or right ventricular hypertrophy is not evident (101, 102, 105). The establishment of animal models that are more relevant to human PAH will not only help us to understand the underlying mechanism but is also imperative in developing a therapeutic strategy.
Discussion/Conclusion
Recent advances in ILC2 research have revealed their pleiotropic roles in various diseases (Figure 1). Due to heterogeneity in the function of ILC2s in various disease conditions, their clinical application faces many obstacles. A treatment that targets ILC2s in one disease may be detrimental to another. For example, therapy for obesity by activating ILC2s with low doses of IL-2 may result in excess amounts of IL-5 from the ILC2s and facilitate tumor metastasis (89). These may present a similar effect in related diseases such as atherosclerosis (119) and MI (112). Thus, understanding the precise action of ILC2s in a particular disease and the extent of its effect on other diseases is indispensable. Delicate procedures for regulating ILC2s are required in addition to careful analyses of experimental and clinical observations, which will ultimately lead to efficient therapeutic regimes.
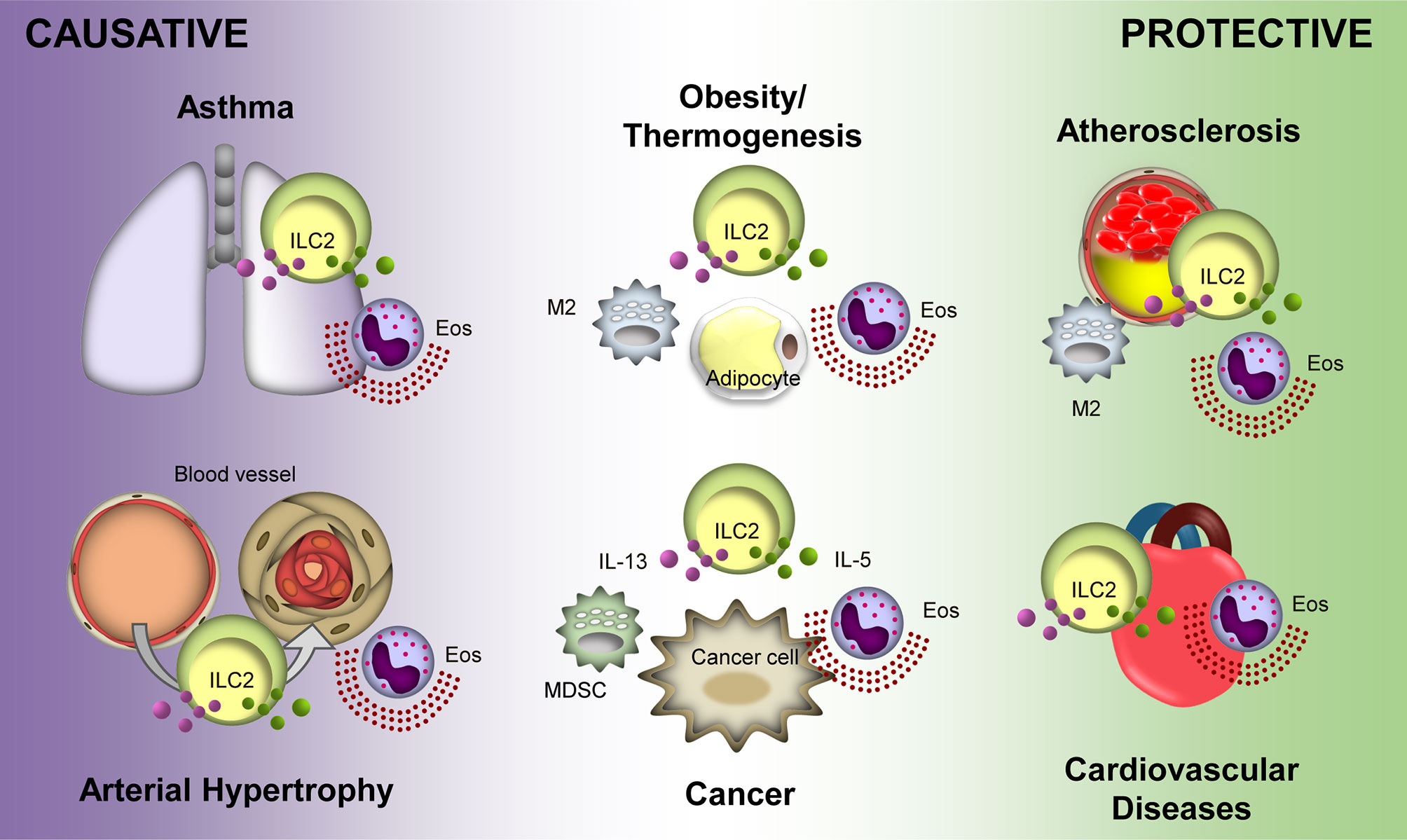
Figure 1 Protective and causative roles of Group 2 Innate Lymphoid Cells (ILC2s) in different diseases. ILC2s display a protective role in atherosclerosis and cardiovascular diseases. Overall, ILC2s is protective in obesity and thermogenesis; however, distal ILC2s may cause obesity. Conversely, ILC2s potentiate asthma and arterial hypertrophy. Their roles in cancer are varied depending on the tumor microenvironment and type of cancer. Purple and green particles depicted in the figure are interluekin-13 (IL-13) and IL-5, respectively. Eos, eosinophil; M2, M2 macrophage; MDSC, myeloid-derived supressor cell.
Author Contributions
MI designed and wrote the manuscript. SN reviewed and revised the manuscript prior to submission. All authors have read and approved the final version of the manuscript.
Funding
This work was supported by a Grant-in-Aid for Scientific Research (C) (Grant Number 19K07632 to MI) and (B) (21H02963 to SN) from the Japan Society for the Promotion of Science, and Precursory Research for Embryonic Science and Technology, Japan Science and Technology Agency (JPMJPR18H6 to SN).
Conflict of Interest
The authors declare that the research was conducted in the absence of any commercial or financial relationships that could be construed as a potential conflict of interest.
Publisher’s Note
All claims expressed in this article are solely those of the authors and do not necessarily represent those of their affiliated organizations, or those of the publisher, the editors and the reviewers. Any product that may be evaluated in this article, or claim that may be made by its manufacturer, is not guaranteed or endorsed by the publisher.
Acknowledgments
We thank all lab members at Hiroshima University for supporting our research.
References
1. Moro K, Yamada T, Tanabe M, Takeuchi T, Ikawa T, Kawamoto H, et al. Innate Production of T(H)2 Cytokines by Adipose Tissue-Associated C-Kit(+)Sca-1(+) Lymphoid Cells. Nature (2010) 463(7280):540–4. doi: 10.1038/nature08636
2. Price AE, Liang HE, Sullivan BM, Reinhardt RL, Eisley CJ, Erle DJ, et al. Systemically Dispersed Innate Il-13-Expressing Cells in Type 2 Immunity. Proc Natl Acad Sci USA (2010) 107(25):11489–94. doi: 10.1073/pnas.1003988107
3. Neill DR, Wong SH, Bellosi A, Flynn RJ, Daly M, Langford TK, et al. Nuocytes Represent a New Innate Effector Leukocyte That Mediates Type-2 Immunity. Nature (2010) 464(7293):1367–70. doi: 10.1038/nature08900
4. Saenz SA, Siracusa MC, Perrigoue JG, Spencer SP, Urban JF Jr., Tocker JE, et al. Il25 Elicits a Multipotent Progenitor Cell Population That Promotes T(H)2 Cytokine Responses. Nature (2010) 464(7293):1362–6. doi: 10.1038/nature08901
5. Fallon PG, Ballantyne SJ, Mangan NE, Barlow JL, Dasvarma A, Hewett DR, et al. Identification of an Interleukin (Il)-25-Dependent Cell Population That Provides Il-4, Il-5, and Il-13 at the Onset of Helminth Expulsion. J Exp Med (2006) 203(4):1105–16. doi: 10.1084/jem.20051615
6. Vivier E, Artis D, Colonna M, Diefenbach A, Di Santo JP, Eberl G, et al. Innate Lymphoid Cells: 10 Years on. Cell (2018) 174(5):1054–66. doi: 10.1016/j.cell.2018.07.017
7. Bal SM, Bernink JH, Nagasawa M, Groot J, Shikhagaie MM, Golebski K, et al. Il-1beta, Il-4 and Il-12 Control the Fate of Group 2 Innate Lymphoid Cells in Human Airway Inflammation in the Lungs. Nat Immunol (2016) 17(6):636–45. doi: 10.1038/ni.3444
8. Silver JS, Kearley J, Copenhaver AM, Sanden C, Mori M, Yu L, et al. Inflammatory Triggers Associated With Exacerbations of Copd Orchestrate Plasticity of Group 2 Innate Lymphoid Cells in the Lungs. Nat Immunol (2016) 17(6):626–35. doi: 10.1038/ni.3443
9. Ohne Y, Silver JS, Thompson-Snipes L, Collet MA, Blanck JP, Cantarel BL, et al. Il-1 Is a Critical Regulator of Group 2 Innate Lymphoid Cell Function and Plasticity. Nat Immunol (2016) 17(6):646–55. doi: 10.1038/ni.3447
10. Gasteiger G, Fan XY, Dikiy S, Lee SY, Rudensky AY. Tissue Residency of Innate Lymphoid Cells in Lymphoid and Nonlymphoid Organs. Science (2015) 350(6263):981–5. doi: 10.1126/science.aac9593
11. Ricardo-Gonzalez RR, Van Dyken SJ, Schneider C, Lee J, Nussbaum JC, Liang HE, et al. Tissue Signals Imprint Ilc2 Identity With Anticipatory Function. Nat Immunol (2018) 19(10):1093–9. doi: 10.1038/s41590-018-0201-4
12. Halim TY, Steer CA, Matha L, Gold MJ, Martinez-Gonzalez I, McNagny KM, et al. Group 2 Innate Lymphoid Cells Are Critical for the Initiation of Adaptive T Helper 2 Cell-Mediated Allergic Lung Inflammation. Immunity (2014) 40(3):425–35. doi: 10.1016/j.immuni.2014.01.011
13. Kamijo S, Takeda H, Tokura T, Suzuki M, Inui K, Hara M, et al. Il-33-Mediated Innate Response and Adaptive Immune Cells Contribute to Maximum Responses of Protease Allergen-Induced Allergic Airway Inflammation. J Immunol (2013) 190(9):4489–99. doi: 10.4049/jimmunol.1201212
14. Bando JK, Gilfillan S, Di Luccia B, Fachi JL, Secca C, Cella M, et al. Ilc2s Are the Predominant Source of Intestinal Ilc-Derived Il-10. J Exp Med (2020) 217(2):e20191520. doi: 10.1084/jem.20191520
15. Morita H, Kubo T, Ruckert B, Ravindran A, Soyka MB, Rinaldi AO, et al. Induction of Human Regulatory Innate Lymphoid Cells From Group 2 Innate Lymphoid Cells by Retinoic Acid. J Allergy Clin Immunol (2019) 143(6):2190–201.e9. doi: 10.1016/j.jaci.2018.12.1018
16. Seehus CR, Kadavallore A, Torre B, Yeckes AR, Wang Y, Tang J, et al. Alternative Activation Generates Il-10 Producing Type 2 Innate Lymphoid Cells. Nat Commun (2017) 8(1):1900. doi: 10.1038/s41467-017-02023-z
17. Wang S, Xia P, Chen Y, Qu Y, Xiong Z, Ye B, et al. Regulatory Innate Lymphoid Cells Control Innate Intestinal Inflammation. Cell (2017) 171(1):201–16.e18. doi: 10.1016/j.cell.2017.07.027
18. Oliphant CJ, Hwang YY, Walker JA, Salimi M, Wong SH, Brewer JM, et al. Mhcii-Mediated Dialog Between Group 2 Innate Lymphoid Cells and Cd4(+) T Cells Potentiates Type 2 Immunity and Promotes Parasitic Helminth Expulsion. Immunity (2014) 41(2):283–95. doi: 10.1016/j.immuni.2014.06.016
19. Symowski C, Voehringer D. Interactions Between Innate Lymphoid Cells and Cells of the Innate and Adaptive Immune System. Front Immunol (2017) 8:1422. doi: 10.3389/fimmu.2017.01422
20. Starkey MR, McKenzie AN, Belz GT, Hansbro PM. Pulmonary Group 2 Innate Lymphoid Cells: Surprises and Challenges. Mucosal Immunol (2019) 12(2):299–311. doi: 10.1038/s41385-018-0130-4
21. Kato A. Group 2 Innate Lymphoid Cells in Airway Diseases. Chest (2019) 156(1):141–9. doi: 10.1016/j.chest.2019.04.101
22. Kubo M. Innate and Adaptive Type 2 Immunity in Lung Allergic Inflammation. Immunol Rev (2017) 278(1):162–72. doi: 10.1111/imr.12557
23. Hsu AT, Gottschalk TA, Tsantikos E, Hibbs ML. The Role of Innate Lymphoid Cells in Chronic Respiratory Diseases. Front Immunol (2021) 12:733324. doi: 10.3389/fimmu.2021.733324
24. van der Ploeg EK, Carreras Mascaro A, Huylebroeck D, Hendriks RW, Stadhouders R. Group 2 Innate Lymphoid Cells in Human Respiratory Disorders. J Innate Immun (2020) 12(1):47–62. doi: 10.1159/000496212
25. Yasuda K, Muto T, Kawagoe T, Matsumoto M, Sasaki Y, Matsushita K, et al. Contribution of Il-33-Activated Type Ii Innate Lymphoid Cells to Pulmonary Eosinophilia in Intestinal Nematode-Infected Mice. Proc Natl Acad Sci USA (2012) 109(9):3451–6. doi: 10.1073/pnas.1201042109
26. Miller MM, Reinhardt RL. The Heterogeneity, Origins, and Impact of Migratory Iilc2 Cells in Anti-Helminth Immunity. Front Immunol (2020) 11:1594. doi: 10.3389/fimmu.2020.01594
27. Chang YJ, Kim HY, Albacker LA, Baumgarth N, McKenzie AN, Smith DE, et al. Innate Lymphoid Cells Mediate Influenza-Induced Airway Hyper-Reactivity Independently of Adaptive Immunity. Nat Immunol (2011) 12(7):631–8. doi: 10.1038/ni.2045
28. Monticelli LA, Sonnenberg GF, Abt MC, Alenghat T, Ziegler CGK, Doering TA, et al. Innate Lymphoid Cells Promote Lung-Tissue Homeostasis After Infection With Influenza Virus. Nat Immunol (2011) 12(11):1045–54. doi: 10.1038/ni.2131
29. Liew FY, Girard JP, Turnquist HR. Interleukin-33 in Health and Disease. Nat Rev Immunol (2016) 16(11):676–89. doi: 10.1038/nri.2016.95
30. Lott JM, Sumpter TL, Turnquist HR. New Dog and New Tricks: Evolving Roles for Il-33 in Type 2 Immunity. J Leukoc Biol (2015) 97(6):1037–48. doi: 10.1189/jlb.3RI1214-595R
31. Andreone S, Gambardella AR, Mancini J, Loffredo S, Marcella S, La Sorsa V, et al. Anti-Tumorigenic Activities of Il-33: A Mechanistic Insight. Front Immunol (2020) 11:571593. doi: 10.3389/fimmu.2020.571593
32. Afferni C, Buccione C, Andreone S, Galdiero MR, Varricchi G, Marone G, et al. The Pleiotropic Immunomodulatory Functions of Il-33 and Its Implications in Tumor Immunity. Front Immunol (2018) 9:2601. doi: 10.3389/fimmu.2018.02601
33. Wasmer MH, Krebs P. The Role of Il-33-Dependent Inflammation in the Tumor Microenvironment. Front Immunol (2016) 7:682. doi: 10.3389/fimmu.2016.00682
34. Altara R, Ghali R, Mallat Z, Cataliotti A, Booz GW, Zouein FA. Conflicting Vascular and Metabolic Impact of the Il-33/Sst2 Axis. Cardiovasc Res (2018) 114(12):1578–94. doi: 10.1093/cvr/cvy166
35. Zhang T, Xu C, Zhao R, Cao Z. Diagnostic Value of Sst2 in Cardiovascular Diseases: A Systematic Review and Meta-Analysis. Front Cardiovasc Med (2021) 8:697837. doi: 10.3389/fcvm.2021.697837
36. Aimo A, Migliorini P, Vergaro G, Franzini M, Passino C, Maisel A, et al. The Il-33/St2 Pathway, Inflammation and Atherosclerosis: Trigger and Target? Int J Cardiol (2018) 267:188–92. doi: 10.1016/j.ijcard.2018.05.056
37. Cayrol C, Girard JP. Interleukin-33 (Il-33): A Nuclear Cytokine From the Il-1 Family. Immunol Rev (2018) 281(1):154–68. doi: 10.1111/imr.12619
38. Schmitz J, Owyang A, Oldham E, Song Y, Murphy E, McClanahan TK, et al. Il-33, an Interleukin-1-Like Cytokine That Signals Via the Il-1 Receptor-Related Protein St2 and Induces T Helper Type 2-Associated Cytokines. Immunity (2005) 23(5):479–90. doi: 10.1016/j.immuni.2005.09.015
39. Ali S, Huber M, Kollewe C, Bischoff SC, Falk W, Martin MU. Il-1 Receptor Accessory Protein Is Essential for Il-33-Induced Activation of T Lymphocytes and Mast Cells. Proc Natl Acad Sci United States America (2007) 104(47):18660–5. doi: 10.1073/pnas.0705939104
40. Chackerian AA, Oldham ER, Murphy EE, Schmitz J, Pflanz S, Kastelein RA. Il-1 Receptor Accessory Protein and St2 Comprise the Il-33 Receptor Complex. J Immunol (2007) 179(4):2551–5. doi: 10.4049/jimmunol.179.4.2551
41. Griesenauer B, Paczesny S. The St2/Il-33 Axis in Immune Cells During Inflammatory Diseases. Front Immunol (2017) 8:475. doi: 10.3389/fimmu.2017.00475
42. Lu J, Kang J, Zhang C, Zhang X. The Role of Il-33/St2l Signals in the Immune Cells. Immunol Lett (2015) 164(1):11–7. doi: 10.1016/j.imlet.2015.01.008
43. Takeda T, Unno H, Morita H, Futamura K, Emi-Sugie M, Arae K, et al. Platelets Constitutively Express Il-33 Protein and Modulate Eosinophilic Airway Inflammation. J Allergy Clin Immunol (2016) 138(5):1395–403.e6. doi: 10.1016/j.jaci.2016.01.032
44. Orimo K, Tamari M, Takeda T, Kubo T, Ruckert B, Motomura K, et al. Direct Platelet Adhesion Potentiates Group 2 Innate Lymphoid Cell Functions. Allergy (2021) 77(3):843–855. doi: 10.1111/all.15057
45. Lefrancais E, Ortiz-Munoz G, Caudrillier A, Mallavia B, Liu F, Sayah DM, et al. The Lung Is a Site of Platelet Biogenesis and a Reservoir for Haematopoietic Progenitors. Nature (2017) 544(7648):105–9. doi: 10.1038/nature21706
46. Takatsu K. Interleukin-5 and Il-5 Receptor in Health and Diseases. Proc Jpn Acad Ser B Phys Biol Sci (2011) 87(8):463–85. doi: 10.2183/pjab.87.463
47. Takatsu K, Nakajima H. Il-5 and Eosinophilia. Curr Opin Immunol (2008) 20(3):288–94. doi: 10.1016/j.coi.2008.04.001
48. Rosenberg HF, Dyer KD, Foster PS. Eosinophils: Changing Perspectives in Health and Disease. Nat Rev Immunol (2013) 13(1):9–22. doi: 10.1038/nri3341
49. Weller PF, Spencer LA. Functions of Tissue-Resident Eosinophils. Nat Rev Immunol (2017) 17(12):746–60. doi: 10.1038/nri.2017.95
50. Kopf M, Brombacher F, Hodgkin PD, Ramsay AJ, Milbourne EA, Dai WJ, et al. Il-5-Deficient Mice Have a Developmental Defect in Cd5+ B-1 Cells and Lack Eosinophilia But Have Normal Antibody and Cytotoxic T Cell Responses. Immunity (1996) 4(1):15–24. doi: 10.1016/S1074-7613(00)80294-0
51. Yoshida T, Ikuta K, Sugaya H, Maki K, Takagi M, Kanazawa H, et al. Defective B-1 Cell Development and Impaired Immunity Against Angiostrongylus Cantonensis in Il-5r Alpha-Deficient Mice. Immunity (1996) 4(5):483–94. doi: 10.1016/S1074-7613(00)80414-8
52. McBrien CN, Menzies-Gow A. The Biology of Eosinophils and Their Role in Asthma. Front Med (Lausanne) (2017) 4:93. doi: 10.3389/fmed.2017.00093
53. Farne HA, Wilson A, Powell C, Bax L, Milan SJ. Anti-Il5 Therapies for Asthma. Cochrane Database Syst Rev (2017) 9:CD010834. doi: 10.1002/14651858.CD010834.pub3
54. McGregor MC, Krings JG, Nair P, Castro M. Role of Biologics in Asthma. Am J Respir Crit Care Med (2019) 199(4):433–45. doi: 10.1164/rccm.201810-1944CI
55. Bald T, Wagner M, Gao Y, Koyasu S, Smyth MJ. Hide and Seek: Plasticity of Innate Lymphoid Cells in Cancer. Semin Immunol (2019) 41:101273. doi: 10.1016/j.smim.2019.04.001
56. Bruchard M, Ghiringhelli F. Deciphering the Roles of Innate Lymphoid Cells in Cancer. Front Immunol (2019) 10:656. doi: 10.3389/fimmu.2019.00656
57. Wagner M, Koyasu S. Cancer Immunoediting by Innate Lymphoid Cells. Trends Immunol (2019) 40(5):415–30. doi: 10.1016/j.it.2019.03.004
58. Huang YK, Busuttil RA, Boussioutas A. The Role of Innate Immune Cells in Tumor Invasion and Metastasis. Cancers (Basel) (2021) 13(23):5885. doi: 10.3390/cancers13235885
59. Roma S, Carpen L, Raveane A, Bertolini F. The Dual Role of Innate Lymphoid and Natural Killer Cells in Cancer. From Phenotype to Single-Cell Transcriptomics, Functions and Clinical Uses. Cancers (Basel) (2021) 13(20):5042. doi: 10.3390/cancers13205042
60. Wagner M, Koyasu S. Innate Lymphoid Cells in Skin Homeostasis and Malignancy. Front Immunol (2021) 12:758522. doi: 10.3389/fimmu.2021.758522
62. Rivoltini L, Viggiano V, Spinazze S, Santoro A, Colombo MP, Takatsu K, et al. In-Vitro Antitumor-Activity of Eosinophils From Cancer-Patients Treated With Subcutaneous Administration of Interleukin-2 - Role of Interleukin-5 (Vol 54, Pg 8, 1993). Int J Cancer (1993) 54(5):887. doi: 10.1002/ijc.2910540103
63. Fernandez-Acenero MJ, Galindo-Gallego M, Sanz J, Aljama A. Prognostic Influence of Tumor-Associated Eosinophilic Infiltrate in Colorectal Carcinoma. Cancer (2000) 88(7):1544–8. doi: 10.1002/(Sici)1097-0142(20000401)88:7<1544::Aid-Cncr7>3.0.Co;2-S
64. Grisaru-Tal S, Itan M, Klion AD, Munitz A. A New Dawn for Eosinophils in the Tumour Microenvironment. Nat Rev Cancer (2020) 20(10):594–607. doi: 10.1038/s41568-020-0283-9
65. Simon SCS, Utikal J, Umansky V. Opposing Roles of Eosinophils in Cancer. Cancer Immunol Immunother (2019) 68(5):823–33. doi: 10.1007/s00262-018-2255-4
66. Pretlow TP, Keith EF, Cryar AK, Bartolucci AA, Pitts AM, Pretlow TG, et al. Eosinophil Infiltration of Human Colonic Carcinomas as a Prognostic Indicator. Cancer Res (1983) 43(6):2997–3000.
67. Mcginnis MC, Bradley EL, Pretlow TP, Ortizreyes R, Bowden CJ, Stellato TA, et al. Correlation of Stromal Cells by Morphometric Analysis With Metastatic Behavior of Human Colonic Carcinoma. Cancer Res (1989) 49(21):5989–93.
68. Harbaum L, Pollheimer MJ, Kornprat P, Lindtner RA, Bokemeyer C, Langner C. Peritumoral Eosinophils Predict Recurrence in Colorectal Cancer. Mod Pathol (2015) 28(3):403–13. doi: 10.1038/modpathol.2014.104
69. Prizment AE, Vierkant RA, Smyrk TC, Tillmans LS, Lee JJ, Sriramarao P, et al. Tumor Eosinophil Infiltration and Improved Survival of Colorectal Cancer Patients: Iowa Women's Health Study. Mod Pathol (2016) 29(5):516–27. doi: 10.1038/modpathol.2016.42
70. Reichman H, Itan M, Rozenberg P, Yarmolovski T, Brazowski E, Varol C, et al. Activated Eosinophils Exert Antitumorigenic Activities in Colorectal Cancer. Cancer Immunol Res (2019) 7(3):388–400. doi: 10.1158/2326-6066.CIR-18-0494
71. vanDriel WJ, Hogendoorn PCW, Jansen FW, Zwinderman AH, Trimbos JP, Fleuren GJ. Tumor-Associated Eosinophilic Infiltrate of Cervical Cancer Is Indicative for A Less Effective Immune Response. Hum Pathol (1996) 27(9):904–11. doi: 10.1016/S0046-8177(96)90216-6
72. Leighton SEJ, Teo JGC, Leung SF, Cheung AYK, Lee JCK, vanHasselt CA. Prevalence and Prognostic Significance of Tumor-Associated Tissue Eosinophilia in Nasopharyngeal Carcinoma. Cancer (1996) 77(3):436–40. doi: 10.1002/(SICI)1097-0142(19960201)77:3<436::AID-CNCR3>3.0.CO;2-I
73. Hu G, Wang S, Zhong K, Xu F, Huang L, Chen W, et al. Tumor-Associated Tissue Eosinophilia Predicts Favorable Clinical Outcome in Solid Tumors: A Meta-Analysis. BMC Cancer (2020) 20(1):454. doi: 10.1186/s12885-020-06966-3
74. Kubo H, Loegering DA, Adolphson CR, Gleich GJ. Cytotoxic Properties of Eosinophil Granule Major Basic Protein for Tumor Cells. Int Arch Allergy Imm (1999) 118(2-4):426–8. doi: 10.1159/000024154
75. Mattes J, Hulett M, Xie W, Hogan S, Rothenberg ME, Foster P, et al. Immunotherapy of Cytotoxic T Cell-Resistant Tumors by T Helper 2 Cells: An Eotaxin and Stat6-Dependent Process. J Exp Med (2003) 197(3):387–93. doi: 10.1084/jem.20021683
76. Carretero R, Sektioglu IM, Garbi N, Salgado OC, Beckhove P, Hammerling GJ. Eosinophils Orchestrate Cancer Rejection by Normalizing Tumor Vessels and Enhancing Infiltration of Cd8(+) T Cells. Nat Immunol (2015) 16(6):609–17. doi: 10.1038/ni.3159
77. Ikutani M, Yanagibashi T, Ogasawara M, Tsuneyama K, Yamamoto S, Hattori Y, et al. Identification of Innate Il-5-Producing Cells and Their Role in Lung Eosinophil Regulation and Antitumor Immunity. J Immunol (2012) 188(2):703–13. doi: 10.4049/jimmunol.1101270
78. Simson L, Ellyard JI, Dent LA, Matthaei KI, Rothenberg ME, Foster PS, et al. Regulation of Carcinogenesis by Il-5 and Ccl11: A Potential Role for Eosinophils in Tumor Immune Surveillance. J Immunol (2007) 178(7):4222–9. doi: 10.4049/jimmunol.178.7.4222
79. Kim J, Kim W, Moon UJ, Kim HJ, Choi HJ, Sin JI, et al. Intratumorally Establishing Type 2 Innate Lymphoid Cells Blocks Tumor Growth. J Immunol (2016) 196(5):2410–23. doi: 10.4049/jimmunol.1501730
80. Saranchova I, Han J, Zaman R, Arora H, Huang H, Fenninger F, et al. Type 2 Innate Lymphocytes Actuate Immunity Against Tumours and Limit Cancer Metastasis. Sci Rep (2018) 8(1):2924. doi: 10.1038/s41598-018-20608-6
81. Lucarini V, Ziccheddu G, Macchia I, La Sorsa V, Peschiaroli F, Buccione C, et al. Il-33 Restricts Tumor Growth and Inhibits Pulmonary Metastasis in Melanoma-Bearing Mice Through Eosinophils. Oncoimmunology (2017) 6(6):e1317420. doi: 10.1080/2162402X.2017.1317420
82. Huang Q, Jacquelot N, Preaudet A, Hediyeh-Zadeh S, Souza-Fonseca-Guimaraes F, McKenzie ANJ, et al. Type 2 Innate Lymphoid Cells Protect Against Colorectal Cancer Progression and Predict Improved Patient Survival. Cancers (Basel) (2021) 13(3):559. doi: 10.3390/cancers13030559
83. Moral JA, Leung J, Rojas LA, Ruan J, Zhao J, Sethna Z, et al. Ilc2s Amplify Pd-1 Blockade by Activating Tissue-Specific Cancer Immunity. Nature (2020) 579(7797):130–5. doi: 10.1038/s41586-020-2015-4
84. Jacquelot N, Seillet C, Wang M, Pizzolla A, Liao Y, Hediyeh-Zadeh S, et al. Blockade of the Co-Inhibitory Molecule Pd-1 Unleashes Ilc2-Dependent Antitumor Immunity in Melanoma. Nat Immunol (2021) 22(7):851–64. doi: 10.1038/s41590-021-00943-z
85. Zhao Q, Bi Y, Sun H, Xiao M. Serum Il-5 and Ifn-Gamma Are Novel Predictive Biomarkers for Anti-Pd-1 Treatment in Nsclc and Gc Patients. Dis Markers (2021) 2021:5526885. doi: 10.1155/2021/5526885
86. Zaynagetdinov R, Sherrill TP, Gleaves LA, McLoed AG, Saxon JA, Habermann AC, et al. Interleukin-5 Facilitates Lung Metastasis by Modulating the Immune Microenvironment. Cancer Res (2015) 75(8):1624–34. doi: 10.1158/0008-5472.CAN-14-2379
87. Lee EJ, Lee SJ, Kim S, Cho SC, Choi YH, Kim WJ, et al. Interleukin-5 Enhances the Migration and Invasion of Bladder Cancer Cells Via Erk1/2-Mediated Mmp-9/Nf-κB/Ap-1 Pathway: Involvement of the P21waf1 Expression. Cell Signal (2013) 25(10):2025–38. doi: 10.1016/j.cellsig.2013.06.004
88. Liu P, Gao Y, Huan J, Ge X, Tang Y, Shen W, et al. Upregulation of Pax2 Promotes the Metastasis of Esophageal Cancer Through Interleukin-5. Cell Physiol Biochem (2015) 35(2):740–54. doi: 10.1159/000369734
89. Quail DF, Olson OC, Bhardwaj P, Walsh LA, Akkari L, Quick ML, et al. Obesity Alters the Lung Myeloid Cell Landscape to Enhance Breast Cancer Metastasis Through Il5 and Gm-Csf. Nat Cell Biol (2017) 19(8):974–87. doi: 10.1038/ncb3578
90. Schuijs MJ, Png S, Richard AC, Tsyben A, Hamm G, Stockis J, et al. Ilc2-Driven Innate Immune Checkpoint Mechanism Antagonizes Nk Cell Antimetastatic Function in the Lung. Nat Immunol (2020) 21(9):998–1009. doi: 10.1038/s41590-020-0745-y
91. Trabanelli S, Chevalier MF, Martinez-Usatorre A, Gomez-Cadena A, Salome B, Lecciso M, et al. Tumour-Derived Pgd2 and Nkp30-B7h6 Engagement Drives an Immunosuppressive Ilc2-Mdsc Axis. Nat Commun (2017) 8(1):593. doi: 10.1038/s41467-017-00678-2
92. Chevalier MF, Trabanelli S, Racle J, Salome B, Cesson V, Gharbi D, et al. Ilc2-Modulated T Cell-To-Mdsc Balance Is Associated With Bladder Cancer Recurrence. J Clin Invest (2017) 127(8):2916–29. doi: 10.1172/JCI89717
93. Zhao N, Zhu W, Wang J, Liu W, Kang L, Yu R, et al. Group 2 Innate Lymphoid Cells Promote Tnbc Lung Metastasis Via the Il-13-Mdsc Axis in a Murine Tumor Model. Int Immunopharmacol (2021) 99:107924. doi: 10.1016/j.intimp.2021.107924
94. Wagner M, Ealey KN, Tetsu H, Kiniwa T, Motomura Y, Moro K, et al. Tumor-Derived Lactic Acid Contributes to the Paucity of Intratumoral Ilc2s. Cell Rep (2020) 30(8):2743–57.e5. doi: 10.1016/j.celrep.2020.01.103
95. Hruby A, Hu FB. The Epidemiology of Obesity: A Big Picture. Pharmacoeconomics (2015) 33(7):673–89. doi: 10.1007/s40273-014-0243-x
96. Painter JD, Akbari O. Type 2 Innate Lymphoid Cells: Protectors in Type 2 Diabetes. Front Immunol (2021) 12:727008. doi: 10.3389/fimmu.2021.727008
97. Chalubinski M, Luczak E, Wojdan K, Gorzelak-Pabis P, Broncel M. Innate Lymphoid Cells Type 2 - Emerging Immune Regulators of Obesity and Atherosclerosis. Immunol Lett (2016) 179:43–6. doi: 10.1016/j.imlet.2016.09.007
98. Wu D, Molofsky AB, Liang HE, Ricardo-Gonzalez RR, Jouihan HA, Bando JK, et al. Eosinophils Sustain Adipose Alternatively Activated Macrophages Associated With Glucose Homeostasis. Science (2011) 332(6026):243–7. doi: 10.1126/science.1201475
99. Molofsky AB, Nussbaum JC, Liang HE, Van Dyken SJ, Cheng LE, Mohapatra A, et al. Innate Lymphoid Type 2 Cells Sustain Visceral Adipose Tissue Eosinophils and Alternatively Activated Macrophages. J Exp Med (2013) 210(3):535–49. doi: 10.1084/jem.20121964
100. Sasaki T, Moro K, Kubota T, Kubota N, Kato T, Ohno H, et al. Innate Lymphoid Cells in the Induction of Obesity. Cell Rep (2019) 28(1):202–17.e7. doi: 10.1016/j.celrep.2019.06.016
101. Brestoff JR, Kim BS, Saenz SA, Stine RR, Monticelli LA, Sonnenberg GF, et al. Group 2 Innate Lymphoid Cells Promote Beiging of White Adipose Tissue and Limit Obesity. Nature (2015) 519(7542):242–6. doi: 10.1038/nature14115
102. Lee MW, Odegaard JI, Mukundan L, Qiu Y, Molofsky AB, Nussbaum JC, et al. Activated Type 2 Innate Lymphoid Cells Regulate Beige Fat Biogenesis. Cell (2015) 160(1-2):74–87. doi: 10.1016/j.cell.2014.12.011
103. Miller AM, Asquith DL, Hueber AJ, Anderson LA, Holmes WM, McKenzie AN, et al. Interleukin-33 Induces Protective Effects in Adipose Tissue Inflammation During Obesity in Mice. Circ Res (2010) 107(5):650–8. doi: 10.1161/CIRCRESAHA.110.218867
104. Galle-Treger L, Sankaranarayanan I, Hurrell BP, Howard E, Lo R, Maazi H, et al. Costimulation of Type-2 Innate Lymphoid Cells by Gitr Promotes Effector Function and Ameliorates Type 2 Diabetes. Nat Commun (2019) 10(1):713. doi: 10.1038/s41467-019-08449-x
105. Shafiei-Jahani P, Hurrell BP, Galle-Treger L, Helou DG, Howard E, Painter J, et al. Dr3 Stimulation of Adipose Resident Ilc2s Ameliorates Type 2 Diabetes Mellitus. Nat Commun (2020) 11(1):4718. doi: 10.1038/s41467-020-18601-7
106. Oldenhove G, Boucquey E, Taquin A, Acolty V, Bonetti L, Ryffel B, et al. Pd-1 Is Involved in the Dysregulation of Type 2 Innate Lymphoid Cells in a Murine Model of Obesity. Cell Rep (2018) 25(8):2053–60.e4. doi: 10.1016/j.celrep.2018.10.091
107. Cardoso F, Klein Wolterink RGJ, Godinho-Silva C, Domingues RG, Ribeiro H, da Silva JA, et al. Neuro-Mesenchymal Units Control Ilc2 and Obesity Via a Brain-Adipose Circuit. Nature (2021) 597(7876):410–4. doi: 10.1038/s41586-021-03830-7
108. Zlatanova I, Pinto C, Silvestre JS. Immune Modulation of Cardiac Repair and Regeneration: The Art of Mending Broken Hearts. Front Cardiovasc Med (2016) 3:40. doi: 10.3389/fcvm.2016.00040
109. Weinberg EO, Shimpo M, De Keulenaer GW, MacGillivray C, Tominaga S, Solomon SD, et al. Expression and Regulation of St2, an Interleukin-1 Receptor Family Member, in Cardiomyocytes and Myocardial Infarction. Circulation (2002) 106(23):2961–6. doi: 10.1161/01.cir.0000038705.69871.d9
110. Sanada S, Hakuno D, Higgins LJ, Schreiter ER, McKenzie AN, Lee RT. Il-33 and St2 Comprise a Critical Biomechanically Induced and Cardioprotective Signaling System. J Clin Invest (2007) 117(6):1538–49. doi: 10.1172/JCI30634
111. Bracamonte-Baran W, Chen G, Hou X, Talor MV, Choi HS, Davogustto G, et al. Non-Cytotoxic Cardiac Innate Lymphoid Cells Are a Resident and Quiescent Type 2-Commited Population. Front Immunol (2019) 10:634. doi: 10.3389/fimmu.2019.00634
112. Yu X, Newland SA, Zhao TX, Lu Y, Sage AS, Sun Y, et al. Innate Lymphoid Cells Promote Recovery of Ventricular Function After Myocardial Infarction. J Am Coll Cardiol (2021) 78(11):1127–42. doi: 10.1016/j.jacc.2021.07.018
113. Liu J, Yang C, Liu T, Deng Z, Fang W, Zhang X, et al. Eosinophils Improve Cardiac Function After Myocardial Infarction. Nat Commun (2020) 11(1):6396. doi: 10.1038/s41467-020-19297-5
114. Diny NL, Baldeviano GC, Talor MV, Barin JG, Ong S, Bedja D, et al. Eosinophil-Derived Il-4 Drives Progression of Myocarditis to Inflammatory Dilated Cardiomyopathy. J Exp Med (2017) 214(4):943–57. doi: 10.1084/jem.20161702
115. Engelbertsen D, Lichtman AH. Innate Lymphoid Cells in Atherosclerosis. Eur J Pharmacol (2017) 816:32–6. doi: 10.1016/j.ejphar.2017.04.030
116. Yu K, Zhu P, Dong Q, Zhong Y, Zhu Z, Lin Y, et al. Thymic Stromal Lymphopoietin Attenuates the Development of Atherosclerosis in Apoe-/- Mice. J Am Heart Assoc (2013) 2(5):e000391. doi: 10.1161/JAHA.113.000391
117. Mantani PT, Duner P, Bengtsson E, Alm R, Ljungcrantz I, Soderberg I, et al. Il-25 Inhibits Atherosclerosis Development in Apolipoprotein E Deficient Mice. PloS One (2015) 10(1):e0117255. doi: 10.1371/journal.pone.0117255
118. Miller AM, Xu D, Asquith DL, Denby L, Li Y, Sattar N, et al. Il-33 Reduces the Development of Atherosclerosis. J Exp Med (2008) 205(2):339–46. doi: 10.1084/jem.20071868
119. Engelbertsen D, Foks AC, Alberts-Grill N, Kuperwaser F, Chen T, Lederer JA, et al. Expansion of Cd25+ Innate Lymphoid Cells Reduces Atherosclerosis. Arterioscler Thromb Vasc Biol (2015) 35(12):2526–35. doi: 10.1161/ATVBAHA.115.306048
120. Wu C, He S, Liu J, Wang B, Lin J, Duan Y, et al. Type 1 Innate Lymphoid Cell Aggravation of Atherosclerosis Is Mediated Through Tlr4. Scand J Immunol (2018) 87(5):e12661. doi: 10.1111/sji.12661
121. Li Q, Liu M, Fu R, Cao Q, Wang Y, Han S, et al. Alteration of Circulating Innate Lymphoid Cells in Patients With Atherosclerotic Cerebral Infarction. Am J Transl Res (2018) 10(12):4322–30.
122. Newland SA, Mohanta S, Clement M, Taleb S, Walker JA, Nus M, et al. Type-2 Innate Lymphoid Cells Control the Development of Atherosclerosis in Mice. Nat Commun (2017) 8:15781. doi: 10.1038/ncomms15781
123. Mantani PT, Duner P, Ljungcrantz I, Nilsson J, Bjorkbacka H, Fredrikson GN. Ilc2 Transfers to Apolipoprotein E Deficient Mice Reduce the Lipid Content of Atherosclerotic Lesions. BMC Immunol (2019) 20(1):47. doi: 10.1186/s12865-019-0330-z
124. Simonneau G, Montani D, Celermajer DS, Denton CP, Gatzoulis MA, Krowka M, et al. Haemodynamic Definitions and Updated Clinical Classification of Pulmonary Hypertension. Eur Respir J (2019) 53(1). doi: 10.1183/13993003.01913-2018
125. Price LC, Wort SJ, Perros F, Dorfmuller P, Huertas A, Montani D, et al. Inflammation in Pulmonary Arterial Hypertension. Chest (2012) 141(1):210–21. doi: 10.1378/chest.11-0793
126. Tormanen KR, Uller L, Persson CG, Erjefalt JS. Allergen Exposure of Mouse Airways Evokes Remodeling of Both Bronchi and Large Pulmonary Vessels. Am J Respir Crit Care Med (2005) 171(1):19–25. doi: 10.1164/rccm.200406-698OC
127. Rydell-Törmänen K, Johnson JR, Fattouh R, Jordana M, Erjefält JS. Induction of Vascular Remodeling in the Lung by Chronic House Dust Mite Exposure. Am J Respir Cell Mol Biol (2008) 39:61–7. doi: 10.1165/rcmb.2007-0441OC
128. Daley E, Emson C, Guignabert C, de Waal Malefyt R, Louten J, Kurup VP, et al. Pulmonary Arterial Remodeling Induced by a Th2 Immune Response. J Exp Med (2008) 205(2):361–72. doi: 10.1084/jem.20071008
129. Weng M, Baron DM, Bloch KD, Luster AD, Lee JJ, Medoff BD. Eosinophils Are Necessary for Pulmonary Arterial Remodeling in a Mouse Model of Eosinophilic Inflammation-Induced Pulmonary Hypertension. Am J Physiol Lung Cell Mol Physiol (2011) 301(6):1801913. doi: 10.1152/ajplung.00049.2011
130. Dos CJC, Fernandes S, Jardim CVP, Hovnanian A, Hoette S, Morinaga LK, et al. Schistosomiasis and Pulmonary Hypertension. Expert Rev Resp Med (2011) 5(5):675–81. doi: 10.1586/Ers.11.58
131. Graham BB, Mentink-Kane MM, El-Haddad H, Purnell S, Zhang L, Zaiman A, et al. Schistosomiasis-Induced Experimental Pulmonary Hypertension: Role of Interleukin-13 Signaling. Am J Pathol (2010) 177(3):1549–61. doi: 10.2353/ajpath.2010.100063
132. Crosby A, Jones FM, Southwood M, Stewart S, Schermuly R, Butrous G, et al. Pulmonary Vascular Remodeling Correlates With Lung Eggs and Cytokines in Murine Schistosomiasis. Am J Respir Crit Care Med (2010) 181(3):279–88. doi: 10.1164/rccm.200903-0355OC
133. Ikutani M, Tsuneyama K, Kawaguchi M, Fukuoka J, Kudo F, Nakae S, et al. Prolonged Activation of Il-5-Producing Ilc2 Causes Pulmonary Arterial Hypertrophy. JCI Insight (2017) 2(7):e90721. doi: 10.1172/jci.insight.90721
134. Ikutani M, Ogawa S, Yanagibashi T, Nagai T, Okada K, Furuichi Y, et al. Elimination of Eosinophils Using Anti-Il-5 Receptor Alpha Antibodies Effectively Suppresses Il-33-Mediated Pulmonary Arterial Hypertrophy. Immunobiology (2018) 223(6-7):486–92. doi: 10.1016/j.imbio.2017.12.002
135. Nussbaum JC, Van Dyken SJ, von Moltke J, Cheng LE, Mohapatra A, Molofsky AB, et al. Type 2 Innate Lymphoid Cells Control Eosinophil Homeostasis. Nature (2013) 502(7470):245–8. doi: 10.1038/nature12526
Keywords: group 2 innate lymphoid cell, interleukin-5, interleukin-33, eosinophil, anti-tumor immunity, obesity, cardiovascular disease
Citation: Ikutani M and Nakae S (2022) Heterogeneity of Group 2 Innate Lymphoid Cells Defines Their Pleiotropic Roles in Cancer, Obesity, and Cardiovascular Diseases. Front. Immunol. 13:939378. doi: 10.3389/fimmu.2022.939378
Received: 09 May 2022; Accepted: 02 June 2022;
Published: 29 June 2022.
Edited by:
Hiroki Kabata, Keio University School of Medicine, JapanReviewed by:
Kiyoshi Hirahara, Chiba University, JapanCopyright © 2022 Ikutani and Nakae. This is an open-access article distributed under the terms of the Creative Commons Attribution License (CC BY). The use, distribution or reproduction in other forums is permitted, provided the original author(s) and the copyright owner(s) are credited and that the original publication in this journal is cited, in accordance with accepted academic practice. No use, distribution or reproduction is permitted which does not comply with these terms.
*Correspondence: Masashi Ikutani, mikutani@hiroshima-u.ac.jp; Susumu Nakae, snakae@hiroshima-u.ac.jp