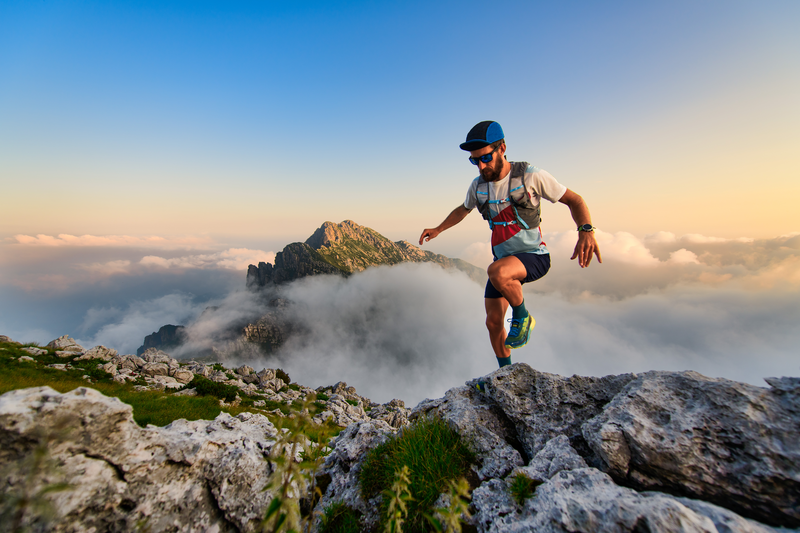
94% of researchers rate our articles as excellent or good
Learn more about the work of our research integrity team to safeguard the quality of each article we publish.
Find out more
REVIEW article
Front. Immunol. , 23 September 2022
Sec. Viral Immunology
Volume 13 - 2022 | https://doi.org/10.3389/fimmu.2022.936235
This article is part of the Research Topic Insights in Viral Immunology: 2022 View all 4 articles
Herpes simplex viruses (HSV) types 1 and 2 are ubiquitous infections in humans. They cause orofacial and genital herpes with occasional severe complications. HSV2 also predisposes individuals to infection with HIV. There is currently no vaccine or immunotherapy for these diseases. Understanding the immunopathogenesis of HSV infections is essential to progress towards these goals. Both HSV viruses result in initial infections in two major sites - in the skin or mucosa, either after initial infection or recurrence, and in the dorsal root or trigeminal ganglia where the viruses establish latency. HSV1 can also cause recurrent infection in the eye. At all of these sites immune cells respond to control infection. T cells and resident dendritic cells (DCs) in the skin/mucosa and around reactivating neurones in the ganglia, as well as keratinocytes in the skin and mucosa, are major sources of cytokines and chemokines. Cytokines such as the Type I and II interferons synergise in their local antiviral effects. Chemokines such as CCL2, 3 and 4 are found in lesion vesicle fluid, but their exact role in determining the interactions between epidermal and dermal DCs and with resident memory and infiltrating CD4 and CD8 T cells in the skin/mucosa is unclear. Even less is known about these mechanisms in the ganglia. Here we review the data on known sources and actions of these cytokines and chemokines at cellular and tissue level and indicate their potential for preventative and therapeutic interventions.
Herpes simplex viruses type 1 and 2 (HSV1 and 2) are members of the Alphaherpesvirinae family and are known for their broad tissue tropism and ability to establish and maintain latency in sensory ganglia (1–3). HSV1 and 2 typically initiate primary infection by gaining access to the deep layers of the epidermis of oropharyngeal and genital mucosal tissue. From these infection sites HSV spreads into the axons of sensory neurons in the epidermis and then travels towards the trigeminal/dorsal root ganglia where it establishes a lifelong latent infection, protected from immune eradication. After recurrence HSV1 & 2 usually cause self-limiting lesions which are primarily found around the mouth, face and eye (HSV1) or genital area (HSV1 and 2), or asymptomatic infection. Occasional severe complications include encephalitis, blindness, predisposition to HIV acquisition, and in severe cases in neonates and immunosuppressed individuals, death. HSV1 and 2 are both highly prevalent in the human population with (as of 2016) 3.7 billion people globally under the age of 50 infected with HSV1 and 491 million people infected with HSV2 (4). Most HSV1 and 2 spread to others occurs through contact with an asymptomatic actively shedding individual through saliva or genital secretions.
HSV1 and 2 reactivate asymptomatically very frequently with occasional noticeable lesions in both oral and genital locations. Reactivation is continually suppressed by the immune system to ensure that reactivation periods are limited. Several types of immune cells and their products function to contain and clear HSV infections such as keratinocytes, which produce chemokines that recruit T cells to the site of infection (5), infiltrating CD4 T cells which secrete interferon-γ (IFN-γ) (6) and effector CD8 T cells that clear infected lesions via direct killing or IFN-γ control of HSV-infected cells (7). These immune responses are initiated by the initial infection of Langerhans cells (LCs) and probably by epidermal type 2 conventional dendritic cells (Epi-cDC2s) in the epidermis (8). LCs undergo apoptosis and migrate to the dermis where they transfer HSV antigen to non-infected dendritic cells (DC) in the dermis in a virus “relay” (9), summarised in Figure 1. All these processes require the secretion and actions of cytokines and chemokines. Cytokines and chemokines are key soluble modulators of immune cells and are secreted initially by resident epidermal and dermal cells – LCs, DCs, macrophages, and keratinocytes; then later by infiltrating immune cells. Some of these communications can be hijacked and modulated by HSV to allow it to further its infection and counteract antiviral immune responses, which will be discussed below. However unlike other Herpesviruses, HSV has not been shown to produce any functional cytokine and chemokine homologs (13).
Figure 1 The Human HSV Viral Relay. As HSV gains access to the epidermis, hypothesised through micro-abrasions, it infects LCs (purple) and Epi-cDC2s (green). LCs then migrate down into the dermis, undergo apoptosis, and cluster with and are taken up by dermal DCs, such as cDC1s (red) and DC-SIGN + DCs/macrophages which are termed CD14 MNPs (mononuclear phagocytes) (orange) (9). Dermal cDC2s (blue) have not yet been shown to cluster with LCs. Epi-cDC2s have been shown to undergo apoptosis but whether they cluster with the dermal DCs is yet to be shown. Subsets of murine dermal DCs carry HSV antigen out of the dermis and have been shown to present the HSV antigen to CD4 and CD8 T cells in the lymph node (10–12). However, this has not yet been shown in human studies.
Cytokines are crucial modulators and communicators between immune cells and non-immune cells, and as such they play a vital role in all functions of the immune system. Cytokines are best known for regulating local and systemic inflammation; however, they can also be involved in cellular migration, cellular proliferation, and wound repair processes (14, 15). As cytokines are mostly involved in regulating inflammation, they are crucial in host defence against disease, and as such there are both pro- and anti- inflammatory cytokines. Cytokines and cytokine receptors can be categorised into several superfamilies depending on their biological activity and molecular structure. Cytokines include interleukins, (denoted by an “IL”), and the superfamilies; interferons (IFN: e.g. IFN-α, β, and γ), tumour necrosis factors (TNF: TNF-α and Lymphotoxin), growth stimulating factors (including granulocyte macrophage-colony stimulating-factor (GM-CSF), and chemokines (16). These superfamilies consist of structurally related but not always functionally similar cytokines.
Chemokines orchestrate cellular migration in the immune system and are an integral part of the immune response. Chemokines are secreted by all the major immune cells into the extracellular space, and remain as either soluble proteins or bind to extracellular matrix components, forming a chemotactic gradient to attract other cells (17). Chemokines are divided into four different families, depending on the location of the first four conserved cysteines in the ligand: CXC, CC, C and CX3C (18–21). Each family of chemokines has corresponding G protein-coupled receptors and ligands (22). Chemokines can also be classified as either inflammatory or homeostatic in their nature (22, 23).
The aim of this review is to examine and collate published data on the cytokines and chemokines produced by immune cells in response to HSV infection and their role in the immune response against HSV. Additionally, the cytokine and chemokine responses to HSV in infected mucosal tissues and the role cytokines and chemokines play in HSV vaccine design will be reviewed. We have specifically compared findings in in vivo murine models, human ex vivo explants and occasionally biopsies of human herpes lesions to help define relevance to human disease and vaccine development.
Primary HSV lesions are an understudied resource as they are rarely obtained. Primary herpetic lesion biopsies can provide unparalleled information of the initial immune response HSV stimulates in human tissue, that cannot be discovered in animal or cell culture models. The role of cytokines and chemokines are yet to be investigated in human primary lesions. The cytokines and chemokines present in recurrent lesions have been studied. However due to the ever-expanding knowledge of immunology and the development of higher parameter technologies, not all cytokines and chemokines were investigated in past studies. Studies of human recurrent lesions showed IL-1α, IL-1β, IL-6, IL-10, IL-12, CCL4, CCL3 and CCL5 were detected on Day 1 post onset. IL-1β and IL-10 levels were highest out of the cytokines, while CCL4 levels were significantly higher than the other chemokines. By Day 3, TNF was detected while levels of IL-1β, IL-6 and CCL4 were decreased compared to Day 1. Levels of IL-10, IL-12 and CCL3 were comparable to those seen on Day 1 (5). Studies of recurrent HSV lesions are still lacking key data, including a comprehensive study of cytokines and chemokines and data for timepoints longer than 3 days. Such work still needs to be conducted, and as such is a major gap in the literature.
Oropharyngeal, genital skin and mucosal tissues are divided into the superficial epidermis and the deeper dermis. Various resident immune cells are found in these tissues, LCs and a new type of conventional dendritic cell, Epi-cDC2s are found in the epidermis, while a wider variety of resident immune cells (e.g. DCs, CD4 and CD8 T cells, macrophages, natural killer (NK) cells, gamma-delta T cells) are found in the dermis (24). All of these cells produce cytokines and chemokines in relation to HSV infection, which will be examined in this section. Figure 2 summarises the main cytokine and chemokine responses from each immune cell and Figure 3 highlights the effects HSV has on initial immune cells and the chemokines and cytokines they produce. Figure 4 summarises each effect of these cytokines and chemokines on HSV replication and disease, while Table 1 highlights the cytokines and chemokines produced by HSV infection and their roles in the immune response.
Figure 2 Cytokines and chemokines and their receptors expressed by key cutaneous and mucosal immune cells. (A) A wide range of chemokine receptors are expressed on cutaneous immune cells in steady state, in both murine and human mucosal tissues. These receptors respond to a variety of differing chemokines, some of which are crucial in HSV infection and immunity. (B) According to mouse and human cell culture models, HSV infected murine LCs produce TNF, while HSV infected MDDCs produce a variety of cytokines and chemokines such as TNF and CXCL10, and uninfected MDDCs alone have been shown to produce a variety of cytokines and chemokines. These models provide a guide to the cytokines and chemokines that human LCs, Epi-cDC2s and dermal DCs may produce in response to HSV infection in the skin. In the epidermis, infected keratinocytes produce many cytokines and chemokines that attract infiltrating cells to the site of infection. In the dermis, murine dermal DCs have been shown to produce IL-6, TNF and Type I IFNs, while resident and infiltrating immune cells such as NK cells, T cells and macrophages produce a plethora of cytokines and chemokines in response to HSV infection of other immune cells. Many of these same cytokines and chemokines have also been found in human HSV vesicular fluid. For further details of which cytokines and chemokines are produced by human and/or murine models please refer to Table 1.
Figure 3 The impact of HSV on initial immune cells production of cytokines and chemokines. In murine models, HSV infection of the initial immune cells, such as LCs, has been shown to lead to impaired migration and apoptosis. This leads to downstream effects seen in dermal DCs where migration and cytokine and chemokine production is inhibited. HSV infection of keratinocytes has also been shown to inhibit apoptosis and the production of IL-1.
Figure 4 Cytokine and chemokine inhibitory and stimulatory effects on HSV replication and disease. HSV infection stimulates the production of various cytokine and chemokines, many of which act to supress viral replication. In some instances, these products have contradictory outcomes based on the tissue site being affected, while others require further study to determine their role. In ocular infection, the stimulated cytokines and chemokines released further HSV pathogenicity instead of resolving infection.
Table 1 Cytokines and chemokines produced during murine or human HSV infection and their subsequent role in immunopathogenesis.
While not usually considered an immune cell, keratinocytes can detect pathogens, including HSV through pattern recognition receptors (PRRs) such as Toll-like receptors (TLRs). Components of HSV can bind to and be detected by TLR2, TLR3 and TLR9, resulting in activation of early innate immune antiviral responses (25, 60). Keratinocytes are the first cells to be infected by HSV, this is initially detected by TLR2 binding of viral glycoproteins (26). TLR2 signalling induction results in the production of pro-inflammatory cytokines including TNF, IL-1, IL-6, and antiviral cytokines such as Type I interferons (25, 28). These cytokines can act on the surrounding keratinocytes and immune cells to reduce viral spread. IFN-α/β induces an antiviral state in surrounding keratinocytes and increases MHC-I expression on the HSV infected keratinocytes allowing them to be more easily targeted and eliminated by CD8 T cells (29, 30). IFN-α/β also stimulates apoptosis. However HSV inhibits apoptosis and opposes this effect in most cell types (31–33). IL-1 is produced by keratinocytes, but its release is hindered by HSV infection. IL-1β, a proinflammatory cytokine, is active in its cleaved form but HSV suppresses this activation. IL-1ɑ is biologically active in its pro form with its release further encouraging inflammation and neutrophil infiltration (61). Another indirect effect of TLR2 stimulation is the enhancement of tight junctions between keratinocytes to reduce virus spread through intercellular interstices (62). Keratinocytes secrete complement factors, C3a and C5a constitutively at low levels, and TNF increases this expression, resulting in chemokine release which recruits innate immune cells (63). Infiltrating T and NK cells produce IFN-γ which also increases TLR3 expression on keratinocytes (64). As HSV infection progresses, keratinocytes have been observed to express HLA-DR after stimulation with IFN-γ released from CD4 T cells. This results in further interaction and subsequent activation of T cells, reducing viral spread (30). Indeed, synergy between the IFN-α, -β and -γ produced from differing cell types may result in synergistic antiviral action which controls HSV spread, particularly after exit from axon termini as seen in recurrent HSV models (65, 66). Keratinocytes also express IL17c during HSV infection, which can protect neurons from infection and is suggested to promote Th17 activity (67, 68). However, in HSV induced stromal keratitis of the eye, Th17 cells were observed to enhance the immunopathologic effect (56).
Keratinocytes produce several chemokines in response to HSV including CCL3, CCL4, CCL5, CXCL9, CXCL10, CXCL5, CCL20 and CCL27 (5, 34–36). CXCL9 and CXCL10 facilitate the recruitment of T cells towards the epidermis. Uninfected keratinocytes produce these mediators at basal levels, but the response to infectious HSV particles and early production of IFN-γ by innate immune cells amplifies their expression (37). In mice a loss of CXCL9 or CXCL10 has been observed to increase viral load and suggests redundancies between CXCR3 ligands (69). TNF and IL-1 together increase the production of CXCL5 and CCL20 (27). CXCL5 acts on CXCR2 which is highly expressed by neutrophils (38). Recruitment of neutrophils to HSV infection sites is well recorded but their benefit is questionable as some studies indicate that neutrophils may enhance damage and worsen disease severity (70). CCL27 production by keratinocytes has been observed, although its expression has not yet been examined in lesions (5, 36, 39). CCL27 acts to recruit T cells to inflamed tissue and has redundancies with CCR4 ligands (34).
Overall, the keratinocyte response to HSV is complex and crucial in early infection. They produce many cytokines upon interaction with HSV, combined with increased production of chemokines released after interaction with other resident and infiltrating immune cells. Cross-signalling between them is critical for optimisation of early host defences and HSV control.
DCs are a crucial part of the immune response to HSV because of their ability to bridge the gap between the innate and adaptive immune system. Their main role in infections is to patrol for pathogens, which upon detection, they engulf and present antigen to naive T cells in lymph nodes.
LCs are one of the first immune cells to encounter HSV, thus making them vital for the HSV immune response (10). As discussed previously, LCs are infected by HSV and migrate down into the dermis where they cluster with dermal DCs, the cells that then present HSV antigens to T cells (9, 71). It is hypothesised that cytokines and chemokines are involved in this process, but little is known about the chemokines and cytokines that could be involved. HSV infected human monocyte derived DCs (MDDCs) have been shown to produce TNF, CXCL10 and IL-8, factors that could be important in the clustering of LCs and DCs. Interestingly, TNF has been shown to upregulate CCR5 on surrounding DCs (and LCs) making them more susceptible to coinfection with HIV (41) and may enhance migration of DCs towards CCR5 ligands produced by HSV infected keratinocytes.
Previously LCs were the only identified immune cell in non-inflamed epidermis, but our recent studies have shown that another immune cell resides in the epidermis, Epi-cDC2s. Epi-cD2s are the predominant cell type in anogenital tissues (e.g. vagina and foreskin). The exact role of Epi-cDC2s in the HSV viral relay is yet to be elucidated, however we have shown them to be highly infected by HSV in tissue explants and in vitro (8). Little is known about which cytokines and chemokines they produce in response to HSV; however, they were shown to produce several cytokines when stimulated with a cocktail of TLR agonists, some of which are key cytokines in the HSV immune response including IL-1β, IL-6 & IL-10 (72).
Human tissue is hard to access, and processing is a complex procedure, meaning most work on HSV infection has been conducted in MDDCs. Cytokine and chemokine responses of skin and mucosal DCs may differ from MDDCs. To-date there has been no comprehensive characterization of the cytokines and chemokines produced and detected by skin DCs in steady state and HSV infection. For MDDCs it has been shown they can express a variety of cytokine and chemokine receptors (i.e., CCR1, CCR2, CCR3, CCR4, CCR5, CCR7, CCR8, XCR1, CX3CR1) (73–77). MDDCs have also been shown to produce a wide variety of cytokines and chemokines including CCL2, CCL3, CCL4, CCL5, CXCL9, CXCL10, CCL17, CCL18, CCL22, under a range of conditions and stimulations (74) (See Table 1 for detailed description of function). At least some of these cytokines and chemokines will be produced or utilised by skin/mucosal DCs in HSV infection as well, specifically in the migration of infected LCs. Although model human MDDCs infected with HSV in vitro show impaired migration in response to CCL19 and to upregulate CCR7 (78–80), this is not complete with authentic epidermal LCs, particularly in humans. In mice a proportion of infected LCs remain trapped in the epidermis but others migrate into the dermis, carrying HSV (10). However in human skin explant models (and biopsied recurrent HSV lesions) HSV infection induces the migration of apoptosing LCs to the dermis where they interact with clusters of dermal DCs and are taken up by them (9). Whether these LCs and also Epi-cDC2s migrate towards particular chemokines expressed during infection or the dermal DCs are attracted to infected LCs via chemokines needs to be investigated.
Several studies conducted in murine models have also been able to elucidate cytokines and chemokines that DCs release in relation to HSV infection. However, some of the data has not been investigated in vivo meaning there might be differences between these seen in vitro. A study by Sato et al. using bone marrow derived DCs showed secretion of IL-6 and IL-12 in response to infection with specific HSV1 KOSTLR2* variants, through either TLR2 or TLR9 stimulation (81, 82). Similar to this, human MDDCs treated with HSV2 dUTPases have also been shown to produce a wide variety of proinflammatory cytokines including IL-6, IL-8 and IL-12, specifically through TLR2 activation (43). Regarding TLR9 another study showed that CD11c+ cDC2s in TLR9 knockout mice produced similar levels of IFN-α, but reduced levels of TNF and IL-6 compared to WT mice (83). These studies show how stimulating various TLRs results in a wide variety of cytokines produced.
Human MDDCs have also been shown to produce cytokines inducing Type I and Type III IFNs (IFN-α, IFN-β and IL-28 or IFN-Λ), TNF and chemokines (CCL5 and CXCL10) in response to HSV1 infection (40). HSV2 infected MDDCs have also been shown to produce TNF and IL-6, Type I and III IFNs, some IL-10 but reduced expression of IL-1β and IL-12 (84). The differences in chemokine and cytokine production in human MDDCs in the reported studies could be due to differences in virus strains used or in experimental designs. Murine CD11c+ cDC2s have also been shown to express Type I and III IFNs, as transgenic CD11c-DTR-tg mice, vaginally infected with HSV2, showed decreased levels of Type I and III IFNs when their CD11c+ DCs were depleted (85).
As well as releasing their own chemokines and cytokines, DCs also stimulate T cells to release cytokines and chemokines. One study in the vaginal tract of HSV2 infected mice showed that inflammatory monocyte derived DCs were required to help Th1 CD4 T cells secrete IFN-γ (86). This process was reliant on Type I IFN signalling and CCR2 mediated migration of the inflammatory monocytes to the vaginal tract (86). Another study showed that vaginal submucosal CD11c+ DCs were important in the stimulation of CD4 T cells to release IFN-γ, when mice were infected with HSV2 (87). Thus, regardless of the origin and type of DC, they are crucial for stimulation of an effective antiviral T cell response.
HSV has a multitude of viral proteins that modulate the immune response and inhibit various functions of model DCs including maturation and production of cytokines and chemokines. One such viral protein is γ134.5. This protein inhibits TLR mediated murine DC maturation by interacting with IKKɑ and IKKβ which leads to NF-κB inactivation, leading also to the inhibition of IL-6, IL-12, IFN-ɑ and IFN-β expression in immature DCs (88, 89). The late viral protein, vhs, has also been shown to decrease IFN-ɑ and IFN-β production by murine bone marrow derived DCs, amongst other DC proteins, as cells infected with a vhs deficient virus show increased levels of IFN-ɑ and IFN-β, compared to those infected with a wild type of virus (90). DCs infected with the wild type virus required endogenous IFNs to activate them compared to the vhs deficient virus (90). These studies need to be repeated in authentic human epidermal LCs and DCs.
Plasmacytoid DCs (pDCs), differing in lineage from myeloid DCs, are the major producer of Type I IFNs, IFN-ɑ more than IFN-β, in the immunological response. In steady states pDCs are only found in blood and lymph nodes (44). Infection with HSV leads to pDCs infiltrating into sites of infection, such as the vagina, where they are seen in the upper dermis/lamina propria, including close to the basement membrane (44). pDCs respond directly to contact with HSV by producing Type I IFNs via endosomal TLR7, although they are not infected. Furthermore, HSV1 stimulation results in IL-18 production which contributes to IFN-γ production by NK cells (45). pDCs have also been shown to produce other cytokines and chemokines such as TNF, IL-6, CXCL10 and CCL3 resulting in recruitment and activation of NK cells and T cells (46).
Macrophages are particularly important for the immune response against HSV contributing to a reduction in viral load in mouse models (91). Macrophages are recruited early into murine primary and human recurrent lesions by chemokines produced by keratinocytes (Figure 2) (6, 91). Resident macrophages are present in skin but their role in human HSV infection has not been sufficiently examined. The main function of macrophages during infection is phagocytosis of cell debris and secretion of cytokines including TNF, IFN-α, IL-6 and IL-12 (47, 92). IFN-α and -γ activate macrophages and modulate their cytokine production (93). Recent studies in the eyes of mice suggest that the major role of macrophages during HSV infection is to contribute to processing of HSV antigens and presentation to T cells, rather than directly degrading viruses, consistent with their deeper location than DCs (94). This is further supported by finding DC-SIGN+ mononuclear phagocytic cells clustering around infected apoptotic LCs in the dermis of human lesions (9, 95). Additionally, the M1/M2 phenotype of macrophages, determined by the cytokine environment, may impact on their role (96). In murine models, using different techniques in the same laboratory, the roles of macrophages appeared to differ (94, 97). In the most recent publication, comparing mice deficient in versus those overexpressing M2 macrophages, overexpression was associated with increased primary HSV replication in mouse eyes, increased latency and increased cytokine production. Yet in mice lacking M2 macrophages there was no difference in these parameters to wild type and no effect on eye disease. These studies seem to suggest the balance of M1/M2 macrophages may affect HSV induced cytokine production and eye disease but needs reproduction in human ex vivo systems.
NK cells contribute to HSV clearance by two main mechanisms; direct cytotoxic effect on infected cells which have downregulated MHC-I expression, principally by the CD56dim CD16+ subset and by releasing IFN-γ from the CD56bright subset (98–100). As discussed above this IFN-γ is a major antiviral effector, enhancing MHC-I and -II expression on infected keratinocytes, activating macrophages and DCs and stimulating B cells. NK cells and γδ T cells are proposed to be the major producers of IFN-γ prior to T cell activation (48). IFN-γ induction in NK cells is also induced by IL-12 secretion by surrounding activated myeloid cells and continues in a powerful feedback loop prior to dampening of the immune response by regulatory T cells (49). The chemokine receptor expression of NK cells varies according to subtype (101, 102). CXCR1 and CXCR2 (receptors of IL-8) are expressed on CD56dim NK cells while CXCR3, CCR5 and CCR7 are expressed on CD56bright NK cells (103). This has important implications for which NK cell subsets home to particular sites during infection and the kinetics of doing so depending on the cascade of cytokines and chemokines produced over time. In addition to NK cells, decreased IFN-γ, IL-6 and IL-12 were observed in symptomatic mice where invariant natural killer T (iNKT) cells were lacking (104). The relative importance of iNKT cells in HSV infection is still poorly understood, although a role for them has been shown in mouse models (104). Therefore the relative roles of NK and iNKT cells in HSV infection should be further explored, partly in human ex vivo model systems.
CD4 and CD8 T cells are a key part of the adaptive immune response against HSV. CD4 T cells are involved as early as 12-48 hours post infection in the immune response to recurrent human HSV infection and are also the most prominent effector cell by 4 days post onset of primary human lesions (6). CD4 T cells help clear HSV by helping DCs to activate CD8 T cells, shaping the immune response, and producing IFN-γ which limits HSV replication and spread and by activating B cells (50–52). The production of IFN-γ by CD4 T cells is crucial and is produced by Th1 CD4 T cells (105). Chemokines, such as CCL5, recruit CD4 T cells to sites of infection, where cytokines, such as IL-12 produced by DCs initiate the differentiation of CD4 T cells towards a Th1 subtype, producing IL-2 and IFN-γ (106, 107). This is crucial as IFN-γ production by CD4 T cells stimulates keratinocytes to secrete CXCL9 and CXCL10, which then recruit CD8 T cells to sites of infection (53, 54). These CD4 T cells can persist for >6 months after infection and are still capable of producing IFN-γ in response to HSV reactivation and release from intraepidermal sensory axons (108, 109). In mouse models of primary HSV1 infection, IFN-γ production has been shown to be essential in preventing vaginal infection (110), and in establishing and maintaining memory responses to HSV2 reinfection (111). These memory responses are driven by HSV specific CD4 Tissue Resident Memory cells (TRMs), as circulating CD4 memory T cells were unable to suppress viral replication (42). The key driver of this retention seems to be CCL5, which is highly upregulated in vaginal tissue following immunisation and infection (42).
In herpes keratitis, CD4 T cells and the cytokines they release are involved in both protection and ocular damage. CD4 T cells may infiltrate the cornea and conjunctiva and can become resident cells (112). As seen in vaginal infection CD4 Th1 cells secrete IFN-γ, as well as IL-2 in initial stages of ocular infection (55). These cytokines regulate neutrophil invasion into the cornea and prohibit CD4 Th17 cells, thus having a protective role (56). CD4 Th2 cells are also involved and secrete IL-10 and IL-4 which is crucial for corneal repair (55). In later stages of infection Th17 cells are observed and secrete IL-17 (56). These cells are involved in primarily stimulating other proinflammatory cytokines and not in limiting viral replication (113). As such these cells lead to the invasion of neutrophils into the cornea, which results in damage to the cornea (56). Mice lacking the IL-17 receptor showed a decreased severity of lesions as well as a decrease in CD4 T cells compared to WT mice (113, 114).
CD8 T cells infiltrate HSV lesions later than CD4 T cells, but their presence is associated with HSV clearance, indicating the vital role they play in the immune response against HSV (6, 7). CD8 T cells eliminate virally infected cells by either inducing apoptosis through Fas-FasL signalling or via perforin and granzyme B release (115). Importantly, CD8 T cells are also able to secrete antiviral IFN-γ in response to HSV infection. This also helps further promote DCs in presenting antigen to CD8 T cells and enhance MHC-I expression on HSV infected target cells (57). After HSV clearance CD8 TRMs remain at the dermo-epidermal junction as sentinels ready for HSV reactivation (116, 117). These cells are primed for encounters and express antiviral, chemotactic, and recruitment genes, and have the ability to produce perforin and granzyme B (118, 119). Studies of HSV lesions in the human female genital tract have shown that there is spatial heterogeneity of the CD8 TRMs and that these cells rely on IFN-γ for their antiviral effects rather than cytotoxicity. This means that HSV can utilise the gaps between the “spheres of IFN-γ” surrounding each of these cells to establish an infection (120, 121). Vaccine studies looking at eliciting CD8 TRMs in tissue have also suggested that IFN-γ is required for vaccine induced protection against HSV2 (122).
Regulatory T cells (Tregs) are a specialised cell of the adaptive immune response, involved in downregulating the immune response. Tregs use several mechanisms to modulate the immune response, such as the release of the immunomodulatory cytokines IL-10, TGF-β and IL-35 (123, 124). Studies of herpes keratitis and herpetic stromal keratitis have shown that Tregs play a key role in minimising damage to the cornea by preventing generation, migration, or effects of pathogenetic T cells to the cornea, shown by worsening conditions of keratitis upon depletion of these cells (125). Indeed at least part of this mechanism Tregs occurs via production of IL-10, which suppresses the proliferation of CD4 and CD8 T cells, and the production of inflammatory cytokines and chemokines (e.g., IL-2, IL-6, CCL3) (58, 59).
In human vaccine studies systemic anti-HSV antibody has been implicated in protection against genital herpes (126) and in murine eye infection models a role for systemic antibody diffusion into the cornea, albeit slow to equilibrate has also been shown (127). The role of locally infiltrating B cells in HSV infection and other sexually transmitted viruses is less clear than that of other infiltrating adaptive immune cells. However recent studies show B cells immigrate to sites of infection by HSV, stimulated by CCL19, CCL21 and CXCL12 and CXCL13 through CCR7 and CXCR4 receptors, respectively. Antibody secreting cells and detectable and increasing levels of neutralising anti-HSV antibodies were present in lesion biopsies and clustered with CD4 T cells suggesting crosstalk (128–131). However circulating high levels of binding and neutralising antibodies within human blood are not proportional to the frequency or severity of recurrent lesions, and vaccine induced antibodies to gD were not protective against genital HSV2 in seronegative subjects (132). Several animal models indicate that local B cell recruitment is necessary for efficient HSV clearance (52, 133, 134). For comparison HIV infected human foreskin also contained antibody secreting B cells close to the dermo-epidermal junction (135), further supporting a local effect of B cell antibody secretion which may contribute to viral clearance in the genital mucosa in humans. In another site, in mouse models of recurrent ocular HSV there were increased frequencies of systemic memory B cells and of antibody secreting plasma cells in the trigeminal ganglion in mice with asymptomatic compared to symptomatic eye infection (136).
Beyond their antibody response, B cells have been observed to secrete IFN-γ, IL-4, IL-6, and a host of other cytokines (137, 138). However, within the context of HSV infection the contribution of these cytokines by activated B cells in lesions is likely to be negligible compared to other immune cells in lesions, considering the limited number of B cells present (6).
The sections above considered the role of individual cell types in producing and responding to cytokines and chemokines in response to HSV infection, especially in lesions. How are these integrated into tissue-wide responses? In this section we will examine and compare specific immune responses to HSV in the eye, usually infected with HSV1, and the vagina, infected by either HSV2 or HSV1, and the roles of cytokines and chemokines. Figure 5 summarises the differences between the responses to cytokines and chemokines in the eye and vagina, while Table 2 presents a summary of the cytokines and chemokines involved in ocular and vaginal HSV infection.
Figure 5 Differences in HSV infection of the Eye and Vagina in relation to immunopathology and protective abilities of cytokines and chemokines. HSV infection pathogenesis is site dependent with tissue-specific immune cell responses influencing this. Ocular infection results in a cytokine storm which results in adaptive and innate immune cell recruitment and further damage. In vaginal infection, similar subsets of immune cells are recruited, however the impact is beneficial and results in resolution of infection. The varying effects of cytokines impacts the outcome at different anatomical regions.
Table 2 Summary of known cytokines and chemokines involved in HSV infections of Eye and Vagina and their functions.
HSV1 infection of the eye is a serious disease, with herpes keratitis as one of the leading causes of infectious blindness in the world (154). Therefore it is crucial to understand how HSV interacts with cells in the eye and the role of cytokines and chemokines in influencing the pathology of herpes keratitis. HSV1 infection of the murine corneal epithelium leads to the production of many cytokines and chemokines, such as IL-8, CCL2, CCL3, CCL4, CCL5, CXCL1, CXCL2 and CXCL10 (141, 155, 156). These signals are key for recruitment of leukocytes into the cornea (142). RNA expression for CCL3, CCL4, CCL5 and CCL2 have been shown to persist into the latent stage of ocular HSV1 infection, i.e., longer than 30 days post infection (143). This prolonged activation of immune cells can cause extensive damage to the eye, resulting in the immunopathology of the disease. This is also reflected in the fact that the RNA expression of some of the corresponding chemokine receptors are still highly expressed in infected corneas, past 30 days post infection (143). Studies have shown that some of these chemokines and their receptors are key drivers of the immunopathology as their reduction or absence leads to a reduction of symptoms. Mice deficient in CCL3 showed a decrease in blindness, reduction in infiltrating CD4 T cells and neutrophils and lower levels of CXCL2 expression when infected with herpes keratitis (157). Other examples of these are CXCR3 and CCR5, where deficiency of these receptors also leads to less severe disease (144).
Most chemokines produced in herpes keratitis are detrimental except it seems for CXCL10. CXCL10 is a homeostatic and protective chemokine that is expressed by epithelial cells (55). CXCL10 controls the inflammatory response through attracting CD8 T cells, NK cells, monocytes and Th1 CD4 T cells (55). Indeed, mice lacking CXCL10 and CXCR3 (the receptor for CXCL10) showed a decrease in CD8 T cells and increased severity of herpes keratitis, compared to WT mice (145, 158). HSV specific CD8 T cell numbers could be restored in the cornea by application of CXCL10 (145). CXCL9, another ligand for CXCR3 produced by corneal cells in the absence of CXCL10, seems to show the opposite function to CXCL10, as it accelerates herpes keratitis progression (139, 140). This immunopathologic effect may be because CXCL9 can aggregate neutrophils to cause an inflammatory response (140) and increase the number of CD4 T cells infiltrating into the cornea. This is supported by experiments showing mice deficient in CXCL9 had a decrease of CD4 T cell infiltration in the cornea (159). These observations highlight the contrasting function of two chemokines which both target CXCR3, with extremely different pathological outcomes. CXCL1, the only chemokine produced by the central corneal epithelium, attracts neutrophils to the cornea but is not involved in clearing HSV (149, 160). CXCL1 is only produced by corneal stromal cells in recurrent herpes keratitis rather than primary infection (150).
Several cytokines are also involved in herpes keratitis immunopathology, such as IL-6 and IL-1α. IL-6 has been seen in mouse HSV infected corneas, and is induced by IL-17 (70). IL-6 has been observed to increase CCL3 and CCL5 expression which supports neutrophil and NK cell infiltration and increased corneal inflammation, without reducing viral load (151). The key role of IL-6 in herpes keratitis immunopathology was demonstrated in mice lacking IL-6; they had decreased corneal opacity, inflammation, and neutrophil recruitment (151). IL-6 regulates the balance of Tregs and Th17 cells, as IL-6 production leads to Th17 differentiation and Treg inhibition, which could lead to more severe HSV infection and ocular damage (154). IL-1α is another cytokine that is produced by HSV infected corneal epithelium (55). IL-1α works together with IL-6 to promote leukocyte infiltration into the cornea (153).
Genital herpes is mainly caused by HSV2 infection of genital mucosal tissues, such as the vagina. However HSV1 is now also a major cause of (initial) genital herpes (152) (126). Chemokines and cytokines play a vital role in the immune response of these tissues, such as mobilising NK cells and T cells to sites of infection where they limit viral spread and disease. Minimal work has been conducted on other genital tissues and human tissues, so this section will mostly focus on HSV infection in the murine vagina.
Many chemokines are produced in the murine vaginal mucosa, some specifically by epithelial cells in the mucosal tissue. CXCL1 is one such chemokine that is produced by epithelial cells of the female genital tract in response to HSV2 infection (161). CXCL1 targets neutrophils, through the CXCR2 receptor, and leads to the migration and activation of these cells (146). Another chemokine constantly produced in the vagina is CXCL17 (147). CXCL17 contributes to the migration of CXCR8 expressing CD44highCD62lowCD8 T Effector Memory cells (TEMs) and establishment of CD103highCD8 TRMs in vaginal tissue infected with HSV1 (147). Other chemokines are also expressed in HSV infected vaginas, such as CCL3 and CCL5 (69, 162). These chemokines are important for recruitment of other immune cells, such as NK cells. CCL5 specifically seems to drive NK cell recruitment during HSV2 vaginal infection, as loss of CCL5 leads to a decrease in infiltrating NK cells and increased viral loads, indicating the importance of NK cells in HSV2 immunity (148). Other important chemokines are CXCL9 and CXCL10. Both chemokines are involved in the mobilisation of NK and cytotoxic T cells (69). Mice deficient in either CXCL9 or CXCL10 had increased viral titres, reduction in immigrating cells (i.e., NKs) increased inflammation and mortality (69). Mice deficient in CXCR3 (the receptor for CXCL9 and CXCL10) are also sensitive to HSV2 infection with increases in viral titres and mortality. Interestingly CD8 T cells are still able to migrate to sites of infection in these mice but there were defects in their effector functions (163).
Cytokines are also important in controlling HSV vaginal infection, especially cytokines produced by epithelial cells. IFN-Ɛ is a Type I IFN that is constitutively expressed by the female reproductive tract (164, 165). Mice lacking IFN-Ɛ showed an increase in HSV2 replication compared to WT mice, indicating that IFN-Ɛ plays a role in HSV2 immunity (165). IFN-λ (Type III IFN) has also been shown to restrict HSV replication in mucosal epithelial cells (166). IL-36γ is an inflammatory mediator at epithelial sites (167). It has also been shown to be expressed in a human vaginal epithelial cell model that was infected with HSV2 (167). In mouse models, pre-treatment with IL-36γ also led to decreased HSV2 replication, limited viral replication, delayed disease onset, decreased severity, and increased survival (167). Pre-treatment also led to the production of proinflammatory cytokines and chemokines including CCL20 and CXCL1 (167) which induce neutrophil infiltration (168). IL-36γ deficient mice develop genital diseases more rapidly, have decreased survival, reduced neutrophil recruitment and CXCL1 and CCL20 expression (168).
Apart from tissue specific cytokines and chemokines, there are many similarities between the cytokines and chemokines produced by the vagina and the eye. However, these similarities are only in terms of production and not in role. For example, CCL3, CCL5 & CXCL9 are found in both eye and vaginal HSV infections, but in the eye these chemokines are known to cause the immunopathology of herpes keratitis, while in the vagina these chemokines have a protective role. Some cytokines are also exclusively produced by the vagina (IFN- Ɛ and IL-36γ), and thus would only play a role in HSV infection in female genital tracts. This highlights that there are differences in how the immune response is shaped against HSV at different sites of infection, and how a ‘one size fits all’ approach to immunotherapy may not work for all areas where HSV infects. This will also need to be considered when developing new vaccines for HSV. Is it also important to note that most of these studies have been conducted in mice, which while an important resource, may not match the cytokines and chemokines produced by humans.
Currently there is still no licensed human prophylactic or therapeutic vaccine for HSV as even the most promising vaccine candidates have only shown partial efficacy in clinical trials. We previously wrote a comprehensive review of HSV vaccine candidates and their current development status and the outcomes of clinical trials (169). All prophylactic vaccines that have so far been trialled have only been able to stimulate neutralising antibodies and CD4 T cell induction. However CD8 T cells are crucial for clearance of HSV from lesions (7).
Incorporation of chemokines and cytokines into DNA vaccines or topical application after vaccination may help attract and stimulate CD4 and CD8 T cells. Indeed, HSV vaccination using the addition of chemokines and cytokines to a DNA vaccine, has been shown to be effective in various murine models. The addition of CCL19 or CCL28 to a HSV2 gD DNA vaccine has been shown to induce long lasting HSV2 specific immunity, such as IgA, IgG and IgM responses, cytokine induction and CCR3+ T cell enrichment in spleens (170), protecting against a lethal HSV2 vaginal challenge in mice (171). Another study also utilised IL-28 in a HSV2 DNA vaccine, leading to increased Th1 and Th2 cytokines, increased neutralising antibodies, decreased viral loads and IFN-γ production from splenocytes, compared to a HSV2 gD DNA vaccine without IL-28 (172). Other studies have utilised cytokine/chemokines adjuvants such as IL-12, IL-21 and CCL3 to increase efficacy. These vaccines have been shown to protect mice from vaginal challenge from HSV2 and were able to induce a strong T cell response to clear the virus in the major site of infection, vagina, and in draining lymph organs (170).
Another vaccine strategy involves using attenuated HSV vaccines and topical chemokine application, in a ‘prime and pull’ method, to recruit and establish a pool of T cells within the peripheral tissue. This approach requires conventional vaccination to elicit systemic T cell responses (prime) followed by the application of topical chemokines to recruit activated T cells to the genital tract and thus establish a pool of long term and protective T cells (pull). In this study mice were vaccinated with an attenuated HSV2 vaccine and then treated with topical vaginal application of CXCL9 and CXCL10. This method led to elevated levels of CD4 and CD8 T cells seen in the vaginal mucosa, with CD8 T cells persisting for over 12 weeks. When presented with a lethal HSV2 challenge, mice had a 100% survival rate, and did not show clinical symptoms (173). Another strategy is to construct a recombinant HSV vaccine where chemokines are expressed by the virus. One study utilised IL-12p35 or IL-12p40, inserted into HSV1 KOS virus as a vaccine for herpes keratitis. Mice immunised with the IL-12p35 insert virus showed decreased viral titres, rapid viral clearance, high neutralising antibody levels, high cytotoxic T cell levels and IFN-γ levels (174). However little quantitative analysis was conducted to determine the efficacy of this vaccine in terms of viral titres in the cornea or ocular health. Therefore the efficacy needs to be more rigorously studied, compared to other vaccines in clinical trials and in other animal models. Nevertheless, these studies indicate that the addition of cytokines or chemokines to vaccines can result in successful protection in mouse models. Such strategies now need to be further developed for and selected for trialling in humans.
In conclusion, cytokines and chemokines are an integral part of the immune response against HSV, as they drive the recruitment and effector functions of many immune cells. However, these responses have been mostly overlooked in vaccine and drug design in the past. Only now is work being conducted on vaccines that specifically utilise cytokines and chemokines to stimulate cells needed for a strong response against HSV. Work on cytokines and chemokines has also sometimes focused on the ligands secreted in tissue infections, but not defined which cells are secreting these factors. This is essential to unravel the key immune cell interactions needed for optimal protection against HSV infection and therefore, to guide development of vaccines and immunotherapies. Since obtaining human samples from key sites of HSV infection such as the vagina and eyes can be difficult, most work has been conducted in murine models or blood derived human cells. Where possible, research must be conducted in human anogenital and corneal tissue samples to ensure the responses seen in mice in vivo and human cells in vitro truly represents the human in vivo setting, particularly as there are anatomical differences in the induced chemokines and cytokines, as reviewed here for the vagina and the eye. However, we acknowledge the fact that murine models and blood derived human cells provide a reasonable guide for work in human anogenital and corneal tissues as these samples are rare and limiting.
JS and JH are equal first authors. AC is the corresponding author. JS, JH, NT, and AC all contributed to editing the manuscript. All authors contributed to the article and approved the submitted version.
Our research was funded by the Australian National Health and Medical Research Council (NHMRC) (APP 1163748 & APP1177942).
All figures were created with Biorender.com.
AC reports other funding to his institution from GSK, Merck and BioCSL/Sequiris, outside the submitted work.
The remaining authors declare that the research was conducted in the absence of any commercial or financial relationships that could be construed as a potential conflict of interest.
All claims expressed in this article are solely those of the authors and do not necessarily represent those of their affiliated organizations, or those of the publisher, the editors and the reviewers. Any product that may be evaluated in this article, or claim that may be made by its manufacturer, is not guaranteed or endorsed by the publisher.
HSV, Herpes Simplex Virus; HIV, Human Immunodeficiency Virus; IFN, Interferon; LC, Langerhans Cell; DC, Dendritic Cell; TNF, Tumour Necrosis Factor; GM-CSF, Granulocyte Macrophage-Colony Stimulating Factor; PRR, Pattern Recognition Receptor; TLR, Toll-Like Receptor; cDC, Conventional Dendritic Cell; MDDC, Monocyte Derived Dendritic Cell; pDC, Plasmacytoid Dendritic Cell; NK, Natural Killer Cell; TRM, Tissue Resident Memory T Cell; Treg, T Regulatory Cell; TEM, T Effector Memory Cell.
1. Kukhanova MK, Korovina AN, Kochetkov SN. Human herpes simplex virus: life cycle and development of inhibitors. Biochem Biokhimiia (2014) 79(13):1635–52. doi: 10.1134/S0006297914130124
2. Agelidis AM, Shukla D. Cell entry mechanisms of HSV: what we have learned in recent years. Future virol (2015) 10(10):1145–54. doi: 10.2217/fvl.15.85
3. Rechenchoski DZ, Faccin-Galhardi LC, Linhares REC, Nozawa C. Herpesvirus: an underestimated virus. Folia Microbiol (2017) 62(2):151–6. doi: 10.1007/s12223-016-0482-7
4. James C, Harfouche M, Welton NJ, Turner KM, Abu-Raddad LJ, Gottlieb SL, et al. Herpes simplex virus: global infection prevalence and incidence estimates, 2016. Bull World Health Organization (2020) 98(5):315–29. doi: 10.2471/BLT.19.237149
5. Mikloska Z, Danis VA, Adams S, Lloyd AR, Adrian DL, Cunningham AL. In vivo production of cytokines and beta (C-c) chemokines in human recurrent herpes simplex lesions–do herpes simplex virus-infected keratinocytes contribute to their production? J Infect Dis (1998) 177(4):827–38. doi: 10.1086/515236
6. Cunningham AL, Turner RR, Miller AC, Para MF, Merigan TC. Evolution of recurrent herpes simplex lesions. an immunohistologic study. J Clin Invest (1985) 75(1):226–33. doi: 10.1172/JCI111678
7. Koelle DM, Posavad CM, Barnum GR, Johnson ML, Frank JM, Corey L. Clearance of HSV-2 from recurrent genital lesions correlates with infiltration of HSV-specific cytotoxic T lymphocytes. J Clin Invest (1998) 101(7):1500–8. doi: 10.1172/JCI1758
8. Bertram KM, Truong NR, Smith JB, Kim M, Sandgren KJ, Feng KL, et al. Herpes simplex virus type 1 infects langerhans cells and the novel epidermal dendritic cell, epi-cDC2s, via different entry pathways. PloS pathogens (2021) 17(4):e1009536. doi: 10.1371/journal.ppat.1009536
9. Kim M, Truong NR, James V, Bosnjak L, Sandgren KJ, Harman AN, et al. Relay of herpes simplex virus between langerhans cells and dermal dendritic cells in human skin. PloS Pathogens (2015) 11(4):e1004812. doi: 10.1371/journal.ppat.1004812
10. Puttur FK, Fernandez MA, White R, Roediger B, Cunningham AL, Weninger W, et al. Herpes simplex virus infects skin gamma delta T cells before langerhans cells and impedes migration of infected langerhans cells by inducing apoptosis and blocking e-cadherin downregulation. J Immunol (Baltimore Md: 1950) (2010) 185(1):477–87. doi: 10.4049/jimmunol.0904106
11. Allan RS, Smith CM, Belz GT, van Lint AL, Wakim LM, Heath WR, et al. Epidermal viral immunity induced by CD8alpha+ dendritic cells but not by langerhans cells. Sci (New York NY) (2003) 301(5641):1925–8. doi: 10.1126/science.1087576
12. Bedoui S, Whitney PG, Waithman J, Eidsmo L, Wakim L, Caminschi I, et al. Cross-presentation of viral and self antigens by skin-derived CD103+ dendritic cells. Nat Immunol (2009) 10(5):488–95. doi: 10.1038/ni.1724
13. Pontejo SM, Murphy PM. Chemokines encoded by herpesviruses. J leukocyte Biol (2017) 102(5):1199–217. doi: 10.1189/jlb.4RU0417-145RR
14. Zhang J-M, An J. Cytokines, inflammation, and pain. Int Anesthesiol Clin (2007) 45(2):27–37. doi: 10.1097/AIA.0b013e318034194e
15. Arango Duque G, Descoteaux A. Macrophage cytokines: Involvement in immunity and infectious diseases. Front Immunol (2014) 5. doi: 10.3389/fimmu.2014.00491
16. Holdsworth SR, Gan P-Y. Cytokines: Names and numbers you should care about. Clin J Am Soc Nephrol (2015) 10(12):2243–54. doi: 10.2215/CJN.07590714
17. Sokol CL, Luster AD. The chemokine system in innate immunity. Cold Spring Harbor Perspect Biol (2015) 7(5):a016303. doi: 10.1101/cshperspect.a016303
18. Zlotnik A, Yoshie O. Chemokines: a new classification system and their role in immunity. Immunity (2000) 12(2):121–7. doi: 10.1016/S1074-7613(00)80165-X
19. Kennedy J, Kelner GS, Kleyensteuber S, Schall TJ, Weiss MC, Yssel H, et al. Molecular cloning and functional characterization of human lymphotactin. J Immunol (Baltimore Md: 1950) (1995) 155(1):203–9.
20. Bazan JF, Bacon KB, Hardiman G, Wang W, Soo K, Rossi D, et al. A new class of membrane-bound chemokine with a CX3C motif. Nature (1997) 385(6617):640–4. doi: 10.1038/385640a0
21. Pan Y, Lloyd C, Zhou H, Dolich S, Deeds J, Gonzalo JA, et al. Neurotactin, a membrane-anchored chemokine upregulated in brain inflammation. Nature (1997) 387(6633):611–7. doi: 10.1038/42491
22. Murphy PM. 10 - chemokines and chemokine receptors. In: Rich RR, Fleisher TA, Shearer WT, Schroeder HW, Frew AJ, Weyand CM, editors. Clinical immunology, Fifth Edition. London: Content Repository Only (2019). p. 157–70.e1.
23. Esche C, Stellato C, Beck LA. Chemokines: key players in innate and adaptive immunity. J Invest Dermatol (2005) 125(4):615–28. doi: 10.1111/j.0022-202X.2005.23841.x
24. Rhodes JW, Botting RA, Bertram KM, Vine EE, Rana H, Baharlou H, et al. Human anogenital monocyte-derived dendritic cells and langerin+cDC2 are major HIV target cells. Nat Commun (2021) 12(1):2147. doi: 10.1038/s41467-021-22375-x
25. Shao Y, Zhang W, Dong X, Liu W, Zhang C, Zhang J, et al. Keratinocytes play a role in the immunity to herpes simplex virus type 2 infection. Acta virol (2010) 54(4):261–7. doi: 10.4149/av_2010_04_261
26. Baker BS, Ovigne JM, Powles AV, Corcoran S, Fry L. Normal keratinocytes express toll-like receptors (TLRs) 1, 2 and 5: modulation of TLR expression in chronic plaque psoriasis. Br J Dermatol (2003) 148(4):670–9. doi: 10.1046/j.1365-2133.2003.05287.x
27. Rauschenberger T, Schmitt V, Azeem M, Klein-Hessling S, Murti K, Grän F, et al. T Cells control chemokine secretion by keratinocytes. Front Immunol (2019) 10:1917. doi: 10.3389/fimmu.2019.01917
28. de Oliviera Nascimento L, Massari P, Wetzler L. The role of TLR2 in infection and immunity. Front Immunol (2012) 3. doi: 10.3389/fimmu.2012.00079
29. Kalali BN, Köllisch G, Mages J, Müller T, Bauer S, Wagner H, et al. Double-stranded RNA induces an antiviral defense status in epidermal keratinocytes through TLR3-, PKR-, and MDA5/RIG-i-mediated differential signaling. J Immunol (Baltimore Md: 1950) (2008) 181(4):2694–704. doi: 10.4049/jimmunol.181.4.2694
30. Cunningham AL, Noble JR. Role of keratinocytes in human recurrent herpetic lesions. ability to present herpes simplex virus antigen and act as targets for T lymphocyte cytotoxicity in vitro. J Clin Invest (1989) 83(2):490–6. doi: 10.1172/JCI113908
31. Aubert M, Blaho JA. The herpes simplex virus type 1 regulatory protein ICP27 is required for the prevention of apoptosis in infected human cells. J virol (1999) 73(4):2803–13. doi: 10.1128/jvi.73.4.2803-2813.1999
32. Jerome KR, Fox R, Chen Z, Sears AE, Lee HY, Corey L. Herpes simplex virus inhibits apoptosis through the action of two genes, Us5 and Us3. J virol (1999) 73(11):8950–7. doi: 10.1128/JVI.73.11.8950-8957.1999
33. Pradhan P, Nguyen ML. Early passage neonatal and adult keratinocytes are sensitive to apoptosis induced by infection with an ICP27-null mutant of herpes simplex virus 1. Apoptosis: an Int J programmed Cell death (2013) 18(2):160–70. doi: 10.1007/s10495-012-0773-7
34. Xiong N, Fu Y, Hu S, Xia M, Yang J. CCR10 and its ligands in regulation of epithelial immunity and diseases. Protein Cell (2012) 3(8):571–80. doi: 10.1007/s13238-012-2927-3
35. Krzyzowska M, Chodkowski M, Janicka M, Dmowska D, Tomaszewska E, Ranoszek-Soliwoda K, et al. Lactoferrin-functionalized noble metal nanoparticles as new antivirals for HSV-2 infection. Microorganisms (2022) 10(1):110. doi: 10.3390/microorganisms10010110
36. Morales J, Homey B, Vicari AP, Hudak S, Oldham E, Hedrick J, et al. CTACK, a skin-associated chemokine that preferentially attracts skin-homing memory T cells. Proc Natl Acad Sci United States America (1999) 96(25):14470–5. doi: 10.1073/pnas.96.25.14470
37. Richmond JM, Bangari DS, Essien KI, Currimbhoy SD, Groom JR, Pandya AG, et al. Keratinocyte-derived chemokines orchestrate T-cell positioning in the epidermis during vitiligo and may serve as biomarkers of disease. J Invest Dermatol (2017) 137(2):350–8. doi: 10.1016/j.jid.2016.09.016
38. Guha D, Klamar CR, Reinhart T, Ayyavoo V. Transcriptional regulation of CXCL5 in HIV-1-Infected macrophages and its functional consequences on CNS pathology. J Interferon Cytokine Res (2015) 35(5):373–84. doi: 10.1089/jir.2014.0135
39. Hensel MT, Marshall JD, Dorwart MR, Heeke DS, Rao E, Tummala P, et al. Prophylactic herpes simplex virus 2 (HSV-2) vaccines adjuvanted with stable emulsion and toll-like receptor 9 agonist induce a robust HSV-2-Specific cell-mediated immune response, protect against symptomatic disease, and reduce the latent viral reservoir. J Virol (2017) 91(9):e02257-16. doi: 10.1128/JVI.02257-16
40. Melchjorsen J, Sirén J, Julkunen I, Paludan SR, Matikainen S. Induction of cytokine expression by herpes simplex virus in human monocyte-derived macrophages and dendritic cells is dependent on virus replication and is counteracted by ICP27 targeting NF-κB and IRF-3. J Gen Virol (2006) 87(5):1099–108. doi: 10.1099/vir.0.81541-0
41. Marsden V, Donaghy H, Bertram KM, Harman AN, Nasr N, Keoshkerian E, et al. Herpes simplex virus type 2–infected dendritic cells produce TNF-α, which enhances CCR5 expression and stimulates HIV production from adjacent infected cells. J Immunol (2015) 194(9):4438–45. doi: 10.4049/jimmunol.1401706
42. Iijima N, Iwasaki A. A local macrophage chemokine network sustains protective tissue-resident memory CD4 T cells. Sci (New York NY) (2014) 346(6205):93–8. doi: 10.1126/science.1257530
43. Ariza ME, Glaser R, Williams MV. Human herpesviruses-encoded dUTPases: a family of proteins that modulate dendritic cell function and innate immunity. Front Microbiol (2014) 5:504–. doi: 10.3389/fmicb.2014.00504
44. Donaghy H, Bosnjak L, Harman AN, Marsden V, Tyring SK, Meng TC, et al. Role for plasmacytoid dendritic cells in the immune control of recurrent human herpes simplex virus infection. J virol (2009) 83(4):1952–61. doi: 10.1128/JVI.01578-08
45. Barr DP, Belz GT, Reading PC, Wojtasiak M, Whitney PG, Heath WR, et al. A role for plasmacytoid dendritic cells in the rapid IL-18-dependent activation of NK cells following HSV-1 infection. Eur J Immunol (2007) 37(5):1334–42. doi: 10.1002/eji.200636362
46. Megjugorac NJ, Young HA, Amrute SB, Olshalsky SL, Fitzgerald-Bocarsly P. Virally stimulated plasmacytoid dendritic cells produce chemokines and induce migration of T and NK cells. J leukocyte Biol (2004) 75(3):504–14. doi: 10.1189/jlb.0603291
47. Malmgaard L, Paludan SR, Mogensen SC, Ellermann-Eriksen S. Herpes simplex virus type 2 induces secretion of IL-12 by macrophages through a mechanism involving NF-kappaB. J Gen virol (2000) 81(Pt 12):3011–20. doi: 10.1099/0022-1317-81-12-3011
48. Marshall JD, Heeke DS, Abbate C, Yee P, Van Nest G. Induction of interferon-γ from natural killer cells by immunostimulatory CpG DNA is mediated through plasmacytoid-dendritic-cell-produced interferon-α and tumour necrosis factor-α. Immunology (2006) 117(1):38–46. doi: 10.1111/j.1365-2567.2005.02261.x
49. Ma X, Chow JM, Gri G, Carra G, Gerosa F, Wolf SF, et al. The interleukin 12 p40 gene promoter is primed by interferon gamma in monocytic cells. J Exp Med (1996) 183(1):147–57. doi: 10.1084/jem.183.1.147
50. Cella M, Scheidegger D, Palmer-Lehmann K, Lane P, Lanzavecchia A, Alber G. Ligation of CD40 on dendritic cells triggers production of high levels of interleukin-12 and enhances T cell stimulatory capacity: T-T help via APC activation. J Exp Med (1996) 184(2):747–52. doi: 10.1084/jem.184.2.747
51. Murphy KM, Stockinger B. Effector T cell plasticity: flexibility in the face of changing circumstances. Nat Immunol (2010) 11(8):674–80. doi: 10.1038/ni.1899
52. Iijima N, Linehan MM, Zamora M, Butkus D, Dunn R, Kehry MR, et al. Dendritic cells and b cells maximize mucosal Th1 memory response to herpes simplex virus. J Exp Med (2008) 205(13):3041–52. doi: 10.1084/jem.20082039
53. Mikloska Z, Kesson AM, Penfold ME, Cunningham AL. Herpes simplex virus protein targets for CD4 and CD8 lymphocyte cytotoxicity in cultured epidermal keratinocytes treated with interferon-gamma. J Infect diseases (1996) 173(1):7–17. doi: 10.1093/infdis/173.1.7
54. Nakanishi Y, Lu B, Gerard C, Iwasaki A. CD8(+) T lymphocyte mobilization to virus-infected tissue requires CD4(+) T-cell help. Nature (2009) 462(7272):510–3. doi: 10.1038/nature08511
55. Wang L, Wang R, Xu C, Zhou H. Pathogenesis of herpes stromal keratitis: Immune inflammatory response mediated by inflammatory regulators. Front Immunol (2020) 11. doi: 10.3389/fimmu.2020.00766
56. Suryawanshi A, Veiga-Parga T, Rajasagi NK, Reddy PBJ, Sehrawat S, Sharma S, et al. Role of IL-17 and Th17 cells in herpes simplex virus-induced corneal immunopathology. J Immunol (Baltimore Md: 1950) (2011) 187(4):1919–30. doi: 10.4049/jimmunol.1100736
57. Zhang J, Liu H, Wei B. Immune response of T cells during herpes simplex virus type 1 (HSV-1) infection. J Zhejiang Univ Sci B (2017) 18(4):277–88. doi: 10.1631/jzus.B1600460
58. Tumpey TM, Cheng H, Yan X-T, Oakes JE, Lausch RN. Chemokine synthesis in the HSV-1-infected cornea and its suppression by interleukin-10. J leukocyte Biol (1998) 63(4):486–92. doi: 10.1002/jlb.63.4.486
59. Sarangi PP, Sehrawat S, Suvas S, Rouse BT. IL-10 and natural regulatory T cells: Two independent anti-inflammatory mechanisms in herpes simplex virus-induced ocular immunopathology. J Immunol (2008) 180(9):6297–306. doi: 10.4049/jimmunol.180.9.6297
60. Iwasaki A, Medzhitov R. Toll-like receptor control of the adaptive immune responses. Nat Immunol (2004) 5(10):987–95. doi: 10.1038/ni1112
61. Milora KA, Miller SL, Sanmiguel JC, Jensen LE. Interleukin-1α released from HSV-1-infected keratinocytes acts as a functional alarmin in the skin. Nat Commun (2014) 5(1):5230. doi: 10.1038/ncomms6230
62. Yuki T, Yoshida H, Akazawa Y, Komiya A, Sugiyama Y, Inoue S. Activation of TLR2 enhances tight junction barrier in epidermal keratinocytes. J Immunol (Baltimore Md: 1950) (2011) 187(6):3230–7. doi: 10.4049/jimmunol.1100058
63. Purwar R, Wittmann M, Zwirner J, Oppermann M, Kracht M, Dittrich-Breiholz O, et al. Induction of C3 and CCL2 by C3a in keratinocytes: A novel autocrine amplification loop of inflammatory skin reactions. J Immunol (2006) 177(7):4444–50. doi: 10.4049/jimmunol.177.7.4444
64. Kajita AI, Morizane S, Takiguchi T, Yamamoto T, Yamada M, Iwatsuki K. Interferon-gamma enhances TLR3 expression and anti-viral activity in keratinocytes. J Invest Dermatol (2015) 135(8):2005–11. doi: 10.1038/jid.2015.125
65. Mikloska Z, Cunningham AL. Alpha and gamma interferons inhibit herpes simplex virus type 1 infection and spread in epidermal cells after axonal transmission. J Virol (2001) 75(23):11821–6. doi: 10.1128/JVI.75.23.11821-11826.2001
66. Torseth JW, Nickoloff BJ, Basham TY, Merigan TC. Beta interferon produced by keratinocytes in human cutaneous infection with herpes simplex virus. J Infect diseases (1987) 155(4):641–8. doi: 10.1093/infdis/155.4.641
67. Peng T, Chanthaphavong RS, Sun S, Trigilio JA, Phasouk K, Jin L, et al. Keratinocytes produce IL-17c to protect peripheral nervous systems during human HSV-2 reactivation. J Exp Med (2017) 214(8):2315–29. doi: 10.1084/jem.20160581
68. Chang SH, Reynolds JM, Pappu BP, Chen G, Martinez GJ, Dong C. Interleukin-17C promotes Th17 cell responses and autoimmune disease via interleukin-17 receptor e. Immunity (2011) 35(4):611–21. doi: 10.1016/j.immuni.2011.09.010
69. Thapa M, Welner RS, Pelayo R, Carr DJJ. CXCL9 and CXCL10 expression are critical for control of genital herpes simplex virus type 2 infection through mobilization of HSV-specific CTL and NK cells to the nervous system. J Immunol (Baltimore Md: 1950) (2008) 180(2):1098–106. doi: 10.4049/jimmunol.180.2.1098
70. Azher TN, Yin X-T, Stuart PM. Understanding the role of chemokines and cytokines in experimental models of herpes simplex keratitis. J Immunol Res (2017) 2017:7261980. doi: 10.1155/2017/7261980
71. Bosnjak L, Miranda-Saksena M, Koelle DM, Boadle RA, Jones CA, Cunningham AL. Herpes simplex virus infection of human dendritic cells induces apoptosis and allows cross-presentation via uninfected dendritic cells. J Immunol (Baltimore Md: 1950) (2005) 174(4):2220–7. doi: 10.4049/jimmunol.174.4.2220
72. Bertram KM, Botting RA, Baharlou H, Rhodes JW, Rana H, Graham JD, et al. Identification of HIV transmitting CD11c(+) human epidermal dendritic cells. Nat Commun (2019) 10(1):2759. doi: 10.1038/s41467-019-10697-w
73. Bachem A, Guttler S, Hartung E, Ebstein F, Schaefer M, Tannert A, et al. Superior antigen cross-presentation and XCR1 expression define human CD11c+CD141+ cells as homologues of mouse CD8+ dendritic cells. J Exp Med (2010) 207(6):1273–81. doi: 10.1084/jem.20100348
74. Sallusto F, Palermo B, Lenig D, Miettinen M, Matikainen S, Julkunen I, et al. Distinct patterns and kinetics of chemokine production regulate dendritic cell function. Eur J Immunol (1999) 29(5):1617–25. doi: 10.1002/(SICI)1521-4141(199905)29:05<1617::AID-IMMU1617>3.0.CO;2-3
75. Beaulieu S, Robbiani DF, Du X, Rodrigues E, Ignatius R, Wei Y, et al. Expression of a functional eotaxin (CC chemokine ligand 11) receptor CCR3 by human dendritic cells. J Immunol (2002) 169(6):2925–36. doi: 10.4049/jimmunol.169.6.2925
76. Colvin BL, Matta BM, Thomson AW. Dendritic cells and chemokine-directed migration in transplantation: where are we headed? Clin Lab Med (2008) 28(3):375–v. doi: 10.1016/j.cll.2008.07.003
77. Johnson LA, Jackson DG. The chemokine CX3CL1 promotes trafficking of dendritic cells through inflamed lymphatics. J Cell Sci (2013) 126(Pt 22):5259–70. doi: 10.1242/jcs.135343
78. Prechtel AT, Turza NM, Kobelt DJ, Eisemann JI, Coffin RS, McGrath Y, et al. Infection of mature dendritic cells with herpes simplex virus type 1 dramatically reduces lymphoid chemokine-mediated migration. J Gen Virol (2005) 86(Pt 6):1645–57. doi: 10.1099/vir.0.80852-0
79. Salio M, Cella M, Suter M, Lanzavecchia A. Inhibition of dendritic cell maturation by herpes simplex virus. Eur J Immunol (1999) 29(10):3245–53. doi: 10.1002/(SICI)1521-4141(199910)29:10<3245::AID-IMMU3245>3.0.CO;2-X
80. Theodoridis AA, Eich C, Figdor CG, Steinkasserer A. Infection of dendritic cells with herpes simplex virus type 1 induces rapid degradation of CYTIP, thereby modulating adhesion and migration. Blood (2011) 118(1):107–15. doi: 10.1182/blood-2010-07-294363
81. Sato A, Linehan MM, Iwasaki A. Dual recognition of herpes simplex viruses by TLR2 and TLR9 in dendritic cells. Proc Natl Acad Sci United States America (2006) 103(46):17343–8. doi: 10.1073/pnas.0605102103
82. Leoni V, Gianni T, Salvioli S, Campadelli-Fiume G. Herpes simplex virus glycoproteins gH/gL and gB bind toll-like receptor 2, and soluble gH/gL is sufficient to activate NF-κB. J virol (2012) 86(12):6555–62. doi: 10.1128/JVI.00295-12
83. Hochrein H, Schlatter B, O’Keeffe M, Wagner C, Schmitz F, Schiemann M, et al. Herpes simplex virus type-1 induces IFN-alpha production via toll-like receptor 9-dependent and -independent pathways. Proc Natl Acad Sci United States America (2004) 101(31):11416–21. doi: 10.1073/pnas.0403555101
84. Stefanidou M, Ramos I, Mas Casullo V, Trepanier JB, Rosenbaum S, Fernandez-Sesma A, et al. Herpes simplex virus 2 (HSV-2) prevents dendritic cell maturation, induces apoptosis, and triggers release of proinflammatory cytokines: potential links to HSV-HIV synergy. J virol (2013) 87(3):1443–53. doi: 10.1128/JVI.01302-12
85. Iversen MB, Ank N, Melchjorsen J, Paludan SR. Expression of type III interferon (IFN) in the vaginal mucosa is mediated primarily by dendritic cells and displays stronger dependence on NF-kappaB than type I IFNs. J virol (2010) 84(9):4579–86. doi: 10.1128/JVI.02591-09
86. Iijima N, Mattei LM, Iwasaki A. Recruited inflammatory monocytes stimulate antiviral Th1 immunity in infected tissue. Proc Natl Acad Sci (2011) 108(1):284–9. doi: 10.1073/pnas.1005201108
87. Zhao X, Deak E, Soderberg K, Linehan M, Spezzano D, Zhu J, et al. Vaginal submucosal dendritic cells, but not langerhans cells, induce protective Th1 responses to herpes simplex virus-2. J Exp Med (2003) 197(2):153–62. doi: 10.1084/jem.20021109
88. Jin H, Yan Z, Ma Y, Cao Y, He B. A herpesvirus virulence factor inhibits dendritic cell maturation through protein phosphatase 1 and ikappa b kinase. J virol (2011) 85(7):3397–407. doi: 10.1128/JVI.02373-10
89. Jin H, Ma Y, Prabhakar BS, Feng Z, Valyi-Nagy T, Yan Z, et al. The gamma 1 34.5 protein of herpes simplex virus 1 is required to interfere with dendritic cell maturation during productive infection. J Virol (2009) 83(10):4984–94. doi: 10.1128/JVI.02535-08
90. Cotter CR, Kim WK, Nguyen ML, Yount JS, López CB, Blaho JA, et al. The virion host shutoff protein of herpes simplex virus 1 blocks the replication-independent activation of NF-κB in dendritic cells in the absence of type I interferon signaling. J virol (2011) 85(23):12662–72. doi: 10.1128/JVI.05557-11
91. Brun P, Scarpa M, Marchiori C, Conti J, Kotsafti A, Porzionato A, et al. Herpes simplex virus type 1 engages toll like receptor 2 to recruit macrophages during infection of enteric neurons. Front Microbiol (2018) 9. doi: 10.3389/fmicb.2018.02148
92. Ellermann-Eriksen S. Macrophages and cytokines in the early defence against herpes simplex virus. Virol J (2005) 2:59–. doi: 10.1186/1743-422X-2-59
93. Hu X, Chakravarty SD, Ivashkiv LB. Regulation of interferon and toll-like receptor signaling during macrophage activation by opposing feedforward and feedback inhibition mechanisms. Immunol Rev (2008) 226:41–56. doi: 10.1111/j.1600-065X.2008.00707.x
94. Lee DH, Ghiasi H. Roles of M1 and M2 macrophages in herpes simplex virus 1 infectivity. J Virol (2017) 91(15):e00578–17. doi: 10.1128/JVI.00578-17
95. McGovern N, Schlitzer A, Gunawan M, Jardine L, Shin A, Poyner E, et al. Human dermal CD14+ cells are a transient population of monocyte-derived macrophages. Immunity (2014) 41(3):465–77. doi: 10.1016/j.immuni.2014.08.006
96. Mosser DM, Edwards JP. Exploring the full spectrum of macrophage activation. Nat Rev Immunol (2008) 8(12):958–69. doi: 10.1038/nri2448
97. Jaggi U, Yang M, Matundan HH, Hirose S, Shah PK, Sharifi BG, et al. Increased phagocytosis in the presence of enhanced M2-like macrophage responses correlates with increased primary and latent HSV-1 infection. PloS pathogens (2020) 16(10):e1008971. doi: 10.1371/journal.ppat.1008971
98. Biron CA, Byron KS, Sullivan JL. Severe herpesvirus infections in an adolescent without natural killer cells. New Engl J Med (1989) 320(26):1731–5. doi: 10.1056/NEJM198906293202605
99. Fehniger TA, Cooper MA, Nuovo GJ, Cella M, Facchetti F, Colonna M, et al. CD56bright natural killer cells are present in human lymph nodes and are activated by T cell–derived IL-2: a potential new link between adaptive and innate immunity. Blood (2003) 101(8):3052–7. doi: 10.1182/blood-2002-09-2876
100. Caligiuri MA. Human natural killer cells. Blood (2008) 112(3):461–9. doi: 10.1182/blood-2007-09-077438
101. Lanier LL, Le AM, Civin CI, Loken MR, Phillips JH. The relationship of CD16 (Leu-11) and leu-19 (NKH-1) antigen expression on human peripheral blood NK cells and cytotoxic T lymphocytes. J Immunol (1986) 136(12):4480.
102. Cooper MA, Fehniger TA, Caligiuri MA. The biology of human natural killer-cell subsets. Trends Immunol (2001) 22(11):633–40. doi: 10.1016/S1471-4906(01)02060-9
103. Lima M, Leander M, Santos M, Santos AH, Lau C, Queirós ML, et al. Chemokine receptor expression on normal blood CD56(+) NK-cells elucidates cell partners that comigrate during the innate and adaptive immune responses and identifies a transitional NK-cell population. J Immunol Res (2015) 2015:839684. doi: 10.1155/2015/839684
104. Dhanushkodi NR, Srivastava R, Prakash S, Roy S, Coulon P-GA, Vahed H, et al. High frequency of gamma interferon-producing PLZF(lo)RORγt(lo) invariant natural killer 1 cells infiltrating herpes simplex virus 1-infected corneas is associated with asymptomatic ocular herpesvirus infection. J virol (2020) 94(9):e00140–20. doi: 10.1128/JVI.00140-20
105. Wuest TR, Carr DJJ. The role of chemokines during herpes simplex virus-1 infection. Front biosci: J virtual library (2008) 13:4862–72. doi: 10.2741/3045
106. VanBenschoten HM, Woodrow KA. Vaginal delivery of vaccines. Advanced Drug Delivery Rev (2021) 178:113956. doi: 10.1016/j.addr.2021.113956
107. Kim HC, Lee HK. Vaccines against genital herpes: Where are we? Vaccines (2020) 8(3):420. doi: 10.3390/vaccines8030420
108. Zhu J, Hladik F, Woodward A, Klock A, Peng T, Johnston C, et al. Persistence of HIV-1 receptor-positive cells after HSV-2 reactivation is a potential mechanism for increased HIV-1 acquisition. Nat Med (2009) 15(8):886–92. doi: 10.1038/nm.2006
109. Schiffer JT, Corey L. Rapid host immune response and viral dynamics in herpes simplex virus-2 infection. Nat Med (2013) 19(3):280–90. doi: 10.1038/nm.3103
110. Lee AG, Scott JM, Fabbrizi MR, Jiang X, Sojka DK, Miller MJ, et al. T Cell response kinetics determines neuroinfection outcomes during murine HSV infection. JCI Insight (2020) 5(5):e134258. doi: 10.1172/jci.insight.134258
111. Chen B, Lee AJ, Chew MV, Ashkar AA. NK cells require antigen-specific memory CD4+ T cells to mediate superior effector functions during HSV-2 recall responses in vitro. J leukocyte Biol (2017) 101(4):1045–52. doi: 10.1189/jlb.4A0416-192R
112. Arnous R, Arshad S, Sandgren K, Cunningham AL, Carnt N, White A. Tissue resident memory T cells inhabit the deep human conjunctiva. Sci Rep (2022) 12(1):6077. doi: 10.1038/s41598-022-09886-3
113. Molesworth-Kenyon SJ, Yin R, Oakes JE, Lausch RN. IL-17 receptor signaling influences virus-induced corneal inflammation. J leukocyte Biol (2008) 83(2):401–8. doi: 10.1189/jlb.0807571
114. Sehrawat S, Suvas S, Sarangi PP, Suryawanshi A, Rouse BT. In vitro-generated antigen-specific CD4+ CD25+ Foxp3+ regulatory T cells control the severity of herpes simplex virus-induced ocular immunoinflammatory lesions. J virol (2008) 82(14):6838–51. doi: 10.1128/JVI.00697-08
115. Dobbs ME, Strasser JE, Chu CF, Chalk C, Milligan GN. Clearance of herpes simplex virus type 2 by CD8+ T cells requires gamma interferon and either perforin- or fas-mediated cytolytic mechanisms. J virol (2005) 79(23):14546–54. doi: 10.1128/JVI.79.23.14546-14554.2005
116. Zhu J, Koelle DM, Cao J, Vazquez J, Huang ML, Hladik F, et al. Virus-specific CD8+ T cells accumulate near sensory nerve endings in genital skin during subclinical HSV-2 reactivation. J Exp Med (2007) 204(3):595–603. doi: 10.1084/jem.20061792
117. Posavad CM, Zhao L, Dong L, Jin L, Stevens CE, Magaret AS, et al. Enrichment of herpes simplex virus type 2 (HSV-2) reactive mucosal T cells in the human female genital tract. Mucosal Immunol (2017) 10(5):1259–69. doi: 10.1038/mi.2016.118
118. Peng T, Zhu J, Phasouk K, Koelle DM, Wald A, Corey L. An effector phenotype of CD8+ T cells at the junction epithelium during clinical quiescence of herpes simplex virus 2 infection. J virol (2012) 86(19):10587–96. doi: 10.1128/JVI.01237-12
119. Zhu J, Peng T, Johnston C, Phasouk K, Kask AS, Klock A, et al. Immune surveillance by CD8alphaalpha+ skin-resident T cells in human herpes virus infection. Nature (2013) 497(7450):494–7. doi: 10.1038/nature12110
120. Schiffer JT. Mucosal HSV-2 specific CD8+ T-cells represent containment of prior viral shedding rather than a correlate of future protection. Front Immunol (2013) 4:209. doi: 10.3389/fimmu.2013.00209
121. Schiffer JT, Swan DA, Roychoudhury P, Lund JM, Prlic M, Zhu J, et al. A fixed spatial structure of CD8(+) T cells in tissue during chronic HSV-2 infection. J Immunol (Baltimore Md: 1950) (2018) 201(5):1522–35. doi: 10.4049/jimmunol.1800471
122. Shin H, Kumamoto Y, Gopinath S, Iwasaki A. CD301b+ dendritic cells stimulate tissue-resident memory CD8+ T cells to protect against genital HSV-2. Nat Commun (2016) 7:13346. doi: 10.1038/ncomms13346
123. Vignali DAA, Collison LW, Workman CJ. How regulatory T cells work. Nat Rev Immunol (2008) 8(7):523–32. doi: 10.1038/nri2343
124. Shevach EM. Mechanisms of Foxp3+ T regulatory cell-mediated suppression. Immunity (2009) 30(5):636–45. doi: 10.1016/j.immuni.2009.04.010
125. Suvas S, Azkur AK, Kim BS, Kumaraguru U, Rouse BT. CD4+CD25+ regulatory T cells control the severity of viral immunoinflammatory lesions. J Immunol (2004) 172(7):4123–32. doi: 10.4049/jimmunol.172.7.4123
126. Belshe RB, Heineman TC, Bernstein DI, Bellamy AR, Ewell M, van der Most R, et al. Correlate of immune protection against HSV-1 genital disease in vaccinated women. J Infect diseases (2014) 209(6):828–36. doi: 10.1093/infdis/jit651
127. Royer DJ, Carr MM, Gurung HR, Halford WP, Carr DJJ. The neonatal fc receptor and complement fixation facilitate prophylactic vaccine-mediated humoral protection against viral infection in the ocular mucosa. J Immunol (Baltimore Md: 1950) (2017) 199(5):1898–911. doi: 10.4049/jimmunol.1700316
128. Egbuniwe IU, Karagiannis SN, Nestle FO, Lacy KE. Revisiting the role of b cells in skin immune surveillance. Trends Immunol (2015) 36(2):102–11. doi: 10.1016/j.it.2014.12.006
129. Ford ES, Sholukh AM, Boytz R, Carmack SS, Klock A, Phasouk K, et al. B cells, antibody-secreting cells, and virus-specific antibodies respond to herpes simplex virus 2 reactivation in skin. J Clin Invest (2021) 131(9):e142088. doi: 10.1172/JCI142088
130. Bernstein DI, Wald A, Warren T, Fife K, Tyring S, Lee P, et al. Therapeutic vaccine for genital herpes simplex virus-2 infection: Findings from a randomized trial. J Infect diseases (2017) 215(6):856–64. doi: 10.1093/infdis/jix004
131. Jiang Y, Patel CD, Manivanh R, North B, Backes IM, Posner DA, et al. Maternal antiviral immunoglobulin accumulates in neural tissue of neonates to prevent HSV neurological disease. mBio (2017) 8(4):e00678–17. doi: 10.1128/mBio.00678-17
132. Gilbert PB, Excler JL, Tomaras GD, Carpp LN, Haynes BF, Liao HX, et al. Antibody to HSV gD peptide induced by vaccination does not protect against HSV-2 infection in HSV-2 seronegative women. PloS One (2017) 12(5):e0176428. doi: 10.1371/journal.pone.0176428
133. Harandi AM, Svennerholm B, Holmgren J, Eriksson K. Differential roles of b cells and IFN-γ-secreting CD4+ T cells in innate and adaptive immune control of genital herpes simplex virus type 2 infection in mice. J Gen Virol (2001) 82(4):845–53. doi: 10.1099/0022-1317-82-4-845
134. Parr EL, Parr MB. Immunoglobulin G is the main protective antibody in mouse vaginal secretions after vaginal immunization with attenuated herpes simplex virus type 2. J virol (1997) 71(11):8109–15. doi: 10.1128/jvi.71.11.8109-8115.1997
135. Lemos MP, Karuna ST, Mize GJ, Fong Y, Montano SM, Ganoza C, et al. In men at risk of HIV infection, IgM, IgG1, IgG3, and IgA reach the human foreskin epidermis. Mucosal Immunol (2016) 9(3):798–808. doi: 10.1038/mi.2015.103
136. Dhanushkodi Nisha R, Prakash S, Srivastava R, Coulon Pierre-Gregoire A, Arellano D, Kapadia Rayomand V, et al. Antiviral CD19+CD27+ memory b cells are associated with protection from recurrent asymptomatic ocular herpesvirus infection. J Virol (2022) 96(5):e02057–21. doi: 10.1128/jvi.02057-21
137. Shen P, Fillatreau S. Antibody-independent functions of b cells: a focus on cytokines. Nat Rev Immunol (2015) 15(7):441–51. doi: 10.1038/nri3857
138. Duddy ME, Alter A, Bar-Or A. Distinct profiles of human b cell effector cytokines: a role in immune regulation? J Immunol (Baltimore Md: 1950) (2004) 172(6):3422–7. doi: 10.4049/jimmunol.172.6.3422
139. Tajfirouz D, West DM, Yin X-T, Potter CA, Klein R, Stuart PM. CXCL9 compensates for the absence of CXCL10 during recurrent herpetic stromal keratitis. Virology (2017) 506:7–13. doi: 10.1016/j.virol.2017.02.022
140. Molesworth-Kenyon SJ, Milam A, Rockette A, Troupe A, Oakes JE, Lausch RN. Expression, inducers and cellular sources of the chemokine MIG (CXCL 9), during primary herpes simplex virus type-1 infection of the cornea. Curr Eye Res (2015) 40(8):800–8. doi: 10.3109/02713683.2014.957779
141. Miyazaki D, Inoue Y, Araki-Sasaki K, Shimomura Y, Tano Y, Hayashi K. Neutrophil chemotaxis induced by corneal epithelial cells after herpes simplex virus type 1 infection. Curr Eye Res (1998) 17(7):687–93. doi: 10.1080/02713689808951244
142. Noisakran S, Carr DJ. Type I interferons and herpes simplex virus infection: a naked DNA approach as a therapeutic option? Immunol Res (2001) 24(1):1–11. doi: 10.1385/IR:24:1:01
143. Cook WJ, Kramer MF, Walker RM, Burwell TJ, Holman HA, Coen DM, et al. Persistent expression of chemokine and chemokine receptor RNAs at primary and latent sites of herpes simplex virus 1 infection. Virol J (2004) 1(1):5. doi: 10.1186/1743-422X-1-5
144. Komatsu K, Miyazaki D, Morohoshi K, Kuo C-H, Kakimaru-Hasegawa A, Komatsu N, et al. Pathogenesis of herpetic stromal keratitis in CCR5- and/or CXCR3-deficient mice. Curr Eye Res (2008) 33(9):736–49. doi: 10.1080/02713680802344716
145. Shen F-H, Wang S-W, Yeh T-M, Tung Y-Y, Hsu S-M, Chen S-H. Absence of CXCL10 aggravates herpes stromal keratitis with reduced primary neutrophil influx in mice. J virol (2013) 87(15):8502–10. doi: 10.1128/JVI.01198-13
146. Moser B, Clark-Lewis I, Zwahlen R, Baggiolini M. Neutrophil-activating properties of the melanoma growth-stimulatory activity. J Exp Med (1990) 171(5):1797–802. doi: 10.1084/jem.171.5.1797
147. Srivastava R, Hernández-Ruiz M, Khan AA, Fouladi MA, Kim GJ, Ly VT, et al. CXCL17 chemokine–dependent mobilization of CXCR8+CD8+ effector memory and tissue-resident memory T cells in the vaginal mucosa is associated with protection against genital herpes. J Immunol (2018) 200(8):2915–26. doi: 10.4049/jimmunol.1701474
148. Thapa M, Kuziel WA, Carr DJ. Susceptibility of CCR5-deficient mice to genital herpes simplex virus type 2 is linked to NK cell mobilization. J virol (2007) 81(8):3704–13. doi: 10.1128/JVI.02626-06
149. Bryant-Hudson KM, Carr DJJ. CXCL1-deficient mice are highly sensitive to pseudomonas aeruginosa but not herpes simplex virus type 1 corneal infection. Invest Ophthalmol Visual science (2012) 53(11):6785–92. doi: 10.1167/iovs.12-10400
150. West DM, Del Rosso CR, Yin X-T, Stuart PM. CXCL1 but not IL-6 is required for recurrent herpetic stromal keratitis. J Immunol (2014) 192(4):1762–7. doi: 10.4049/jimmunol.1302957
151. Fenton RR, Molesworth-Kenyon S, Oakes JE, Lausch RN. Linkage of IL-6 with neutrophil chemoattractant expression in virus-induced ocular inflammation. Invest Ophthalmol Visual science (2002) 43(3):737–43.
152. Belshe RB, Leone PA, Bernstein DI, Wald A, Levin MJ, Stapleton JT, et al. Efficacy results of a trial of a herpes simplex vaccine. N Engl J Med (2012) 366(1):34–43. doi: 10.1056/NEJMoa1103151
153. Biswas PS, Banerjee K, Kim B, Rouse BT. Mice transgenic for IL-1 receptor antagonist protein are resistant to herpetic stromal keratitis: Possible role for IL-1 in herpetic stromal keratitis pathogenesis. J Immunol (2004) 172(6):3736–44. doi: 10.4049/jimmunol.172.6.3736
154. Kimura A, Kishimoto T. IL-6: Regulator of Treg/Th17 balance. Eur J Immunol (2010) 40(7):1830–5. doi: 10.1002/eji.201040391
155. Su YH, Yan XT, Oakes JE, Lausch RN. Protective antibody therapy is associated with reduced chemokine transcripts in herpes simplex virus type 1 corneal infection. J virol (1996) 70(2):1277–81. doi: 10.1128/jvi.70.2.1277-1281.1996
156. Yan XT, Tumpey TM, Kunkel SL, Oakes JE, Lausch RN. Role of MIP-2 in neutrophil migration and tissue injury in the herpes simplex virus-1-infected cornea. Invest Ophthalmol Visual Sci (1998) 39(10):1854–62.
157. Tumpey TM, Cheng H, Cook DN, Smithies O, Oakes JE, Lausch RN. Absence of macrophage inflammatory protein-1α prevents the development of blinding herpes stromal keratitis. J Virol (1998) 72(5):3705–10. doi: 10.1128/JVI.72.5.3705-3710.1998
158. Srivastava R, Khan AA, Chilukuri S, Syed SA, Tran TT, Furness J, et al. CXCL10/CXCR3-dependent mobilization of herpes simplex virus-specific CD8+ TEM and CD8+ TRM cells within infected tissues allows efficient protection against recurrent herpesvirus infection and disease. J Virol (2017) 91(14):e00278–17. doi: 10.1128/JVI.00278-17
159. Wuest T, Farber J, Luster A, Carr DJJ. CD4+ T cell migration into the cornea is reduced in CXCL9 deficient but not CXCL10 deficient mice following herpes simplex virus type 1 infection. Cell Immunol (2006) 243(2):83–9. doi: 10.1016/j.cellimm.2007.01.001
160. Spandau UHM, Toksoy A, Verhaart S, Gillitzer R, Kruse FE. High expression of chemokines gro-α (CXCL-1), IL-8 (CXCL-8), and MCP-1 (CCL-2) in inflamed human corneas in vivo. Arch Ophthalmol (2003) 121(6):825–31. doi: 10.1001/archopht.121.6.825
161. Fernandez S, Gillgrass A, Kaushic C. Differential responses of murine vaginal and uterine epithelial cells prior to and following herpes simplex virus type 2 (HSV-2) infection. Am J Reprod Immunol (New York NY: 1989) (2007) 57(5):367–77. doi: 10.1111/j.1600-0897.2007.00482.x
162. Shao W, Li X, Li M, Shi L, Qin Y, Li K. Macrophage inflammatory protein-1alpha expression plasmid enhances DNA vaccine-induced immune responses against HSV-2. Immunol Cell Biol (2005) 83(6):626–31. doi: 10.1111/j.1440-1711.2005.01380.x
163. Thapa M, Carr DJJ. Chemokines and chemokine receptors critical to host resistance following genital herpes simplex virus type 2 (HSV-2) infection. Open Immunol J (2008) 1:33–41. doi: 10.2174/1874226200801010033
164. Nickodem C, Criscitiello MF, Bazer F, Abiodun-Ojo O, Taylor BD. Interferon epsilon in the reproductive tract of healthy and genital herpes simplex virus-infected pregnant women: Results of a pilot study. Am J Reprod Immunol (2018) 80(3):e12995. doi: 10.1111/aji.12995
165. Fung KY, Mangan NE, Cumming H, Horvat JC, Mayall JR, Stifter SA, et al. Interferon-ε protects the female reproductive tract from viral and bacterial infection. Sci (New York NY) (2013) 339(6123):1088–92. doi: 10.1126/science.1233321
166. Ank N, West H, Bartholdy C, Eriksson K, Thomsen AR, Paludan SR. Lambda interferon (IFN-Λ a type III IFN, is induced by viruses and IFNs and displays potent antiviral activity against select virus infections in vivo. J Virol (2006) 80(9):4501–9. doi: 10.1128/JVI.80.9.4501-4509.2006
167. Gardner JK, Herbst-Kralovetz MM. IL-36γ induces a transient HSV-2 resistant environment that protects against genital disease and pathogenesis. Cytokine (2018) 111:63–71. doi: 10.1016/j.cyto.2018.07.034
168. Gardner JK, Swaims-Kohlmeier A, Herbst-Kralovetz MM. IL-36γ is a key regulator of neutrophil infiltration in the vaginal microenvironment and limits neuroinvasion in genital HSV-2 infection. J Immunol (2019) 203(10):2655–64. doi: 10.4049/jimmunol.1900280
169. Truong NR, Smith JB, Sandgren KJ, Cunningham AL. Mechanisms of immune control of mucosal HSV infection: A guide to rational vaccine design. Front Immunol (2019) 10. doi: 10.3389/fimmu.2019.00373
170. Kim HC, Oh DS, Park JH, Kim H-J, Seo YB, Yoo HJ, et al. Multivalent DNA vaccine protects against genital herpes by T-cell immune induction in vaginal mucosa. Antiviral Res (2020) 177:104755. doi: 10.1016/j.antiviral.2020.104755
171. Yan Y, Hu K, Fu M, Deng X, Luo S, Tong L, et al. CCL19 and CCL28 assist herpes simplex virus 2 glycoprotein d to induce protective systemic immunity against genital viral challenge. mSphere (2022) 6(2):e00058–21. doi: 10.1128/mSphere.00058-21
172. Zhou Y, Wang Z, Xu Y, Zhang Z, Hua R, Liu W, et al. Optimized DNA vaccine enhanced by adjuvant IL28B induces protective immune responses against herpes simplex virus type 2 in mice. Viral Immunol (2017) 30(8):601–14. doi: 10.1089/vim.2017.0033
173. Shin H, Iwasaki A. A vaccine strategy that protects against genital herpes by establishing local memory T cells. Nature (2012) 491(7424):463–7. doi: 10.1038/nature11522
Keywords: HSV, chemokines, cytokines, mucosal, immunology
Citation: Smith JB, Herbert JJ, Truong NR and Cunningham AL (2022) Cytokines and chemokines: The vital role they play in herpes simplex virus mucosal immunology. Front. Immunol. 13:936235. doi: 10.3389/fimmu.2022.936235
Received: 05 May 2022; Accepted: 08 September 2022;
Published: 23 September 2022.
Edited by:
Michael H. Lehmann, Ludwig-Maximilians-Universität München, GermanyReviewed by:
Andreas Wild, Universtätsklinikum Erlangen, GermanyCopyright © 2022 Smith, Herbert, Truong and Cunningham. This is an open-access article distributed under the terms of the Creative Commons Attribution License (CC BY). The use, distribution or reproduction in other forums is permitted, provided the original author(s) and the copyright owner(s) are credited and that the original publication in this journal is cited, in accordance with accepted academic practice. No use, distribution or reproduction is permitted which does not comply with these terms.
*Correspondence: Anthony L. Cunningham, dG9ueS5jdW5uaW5naGFtQHN5ZG5leS5lZHUuYXU=
†These authors have contributed equally to this work and share first authorship
Disclaimer: All claims expressed in this article are solely those of the authors and do not necessarily represent those of their affiliated organizations, or those of the publisher, the editors and the reviewers. Any product that may be evaluated in this article or claim that may be made by its manufacturer is not guaranteed or endorsed by the publisher.
Research integrity at Frontiers
Learn more about the work of our research integrity team to safeguard the quality of each article we publish.