- 1Department of Anesthesia and Intensive Care, Istituti di Ricovero e Cura a Carattere Scientifico (IRCCS) San Raffaele Scientific Institute, Milan, Italy
- 2Faculty of Medicine, Vita-Salute San Raffaele University, Milan, Italy
- 3Diabetes Research Institute, Istituti di Ricovero e Cura a Carattere Scientifico (IRCCS) San Raffaele Scientific Institute, Milan, Italy
Introduction: A great number of anti-inflammatory drugs have been suggested in the treatment of SARS-CoV-2 infection. Reparixin, a non-competitive allosteric inhibitor of the CXCL8 (IL-8) receptors C-X-C chemokine receptor type 1 (CXCR1) and C-X-C chemokine receptor type 2 (CXCR2), has already been tried out as a treatment in different critical settings. Due to the contrasting existing literature, we decided to perform the present meta-analysis of randomized controlled trials (RCTs) to investigate the effect of the use of reparixin on survival in patients at high risk for in-hospital mortality.
Methods: We created a search strategy to include any human RCTs performed with reparixin utilization in patients at high risk for in-hospital mortality, excluding oncological patients. Two trained, independent authors searched PubMed, EMBASE, and the Cochrane Central Register of Controlled Trials (CENTRAL) for appropriate studies. Furthermore, references of review articles and included RCTs were screened to identify more studies. No language restrictions were enforced. To assess the risk of bias of included trials, the Revised Cochrane risk-of-bias tool for randomized trials (RoB 2) was used.
Results: Overall, six studies were included and involved 406 patients (220 received reparixin and 186 received the comparator). The all-cause mortality in the reparixin group was significantly lower than that in the control group [5/220 (2.3%) in the reparixin group vs. 12/186 (6.5%) in the control group, odds ratio = 0.33 (95% confidence interval 0.12 to 0.96), p-value for effect 0.04, p for heterogeneity 0.20, I2 = 36%]. In addition, no difference in the rate of pneumonia, sepsis, or non-serious infections was shown between the two groups.
Conclusion: Our meta-analysis of randomized trials suggests that short-term inhibition of CXCL8 activity improved survival in patients at high risk for in-hospital mortality without increasing the risk of infection.
Meta-analysis registration: PROSPERO, identifier CRD42021254467.
Introduction
The coronavirus disease 2019 (COVID-19) pandemic, caused by the severe acute respiratory syndrome coronavirus 2 (SARS-CoV-2), made it necessary to repurpose existing drugs (1). Since an amplified inflammatory response leading to an uncontrolled cytokine release can be a consequence of SARS-CoV-2 infection, several anti-inflammatory drugs (glucocorticoids, non-steroidal anti-inflammatory drugs, interleukin antagonists, and kinase inhibitors) are being evaluated to be repositioned in COVID-19 (2).
Immediately after the start of the pandemic, we had suggested reparixin (also called repertaxin), a non-competitive allosteric inhibitor of the CXCL8 (IL-8) receptors C-X-C chemokine receptor type 1 (CXCR1) and C-X-C chemokine receptor type 2 (CXCR2), as a potentially effective molecule after the successful treatment of four patients with extremely severe COVID-19 (3). The rationale for using reparixin lay in the hypothesis that the systemic and autocrine IL-8–CXCR-1/-2 axis was at the center of neutrophil-driven immunopathology in severe COVID-19. In fact, neutrophil activation is a key pathophysiological feature of the systemic inflammatory response; while neutrophils play a protective role against invading pathogens, their unrestrained activation may lead to tissue injury associated with the release of cytotoxic neutrophil extracellular traps (NETs) (4, 5). Subsequent data confirmed our initial hypothesis (6–13). Elevated levels of NETs, neutrophilia, high neutrophil-to-lymphocyte ratio (NLR), neutrophil activators (CXCL8/IL-8 and granulocyte colony-stimulating factor), and effectors (resistin, the IL-8-inducer lipocalin-2, and hepatocyte growth factor) have been reported as indicators of severe respiratory disease and poor outcomes in COVID-19 patients (14–20). As further confirmation, the administration of reparixin in patients with severe COVID-19 pneumonia improved clinical outcomes and facilitated respiratory recovery in 56 patients enrolled in an open-label, randomized, phase 2 study (21).
Before and independently of the COVID-19 outbreak, evidence was provided in support of the involvement of exaggerated pro-inflammatory activation of neutrophils, accompanied by the release of cytotoxic NETs, in the pathogenesis of clinical derangements present in critically ill patients (4, 22–24). High NLR, neutrophil percentage-to-albumin ratio (NPAR), neutrophil-to-albumin ratio (NAR), neutrophil-derived enzyme myeloperoxidase, IL-8, and NETs have been reported as indicators of severe disease and poor outcomes in cardiogenic and septic shock, in acute lung injury (ALI) and acute respiratory distress syndrome (ARDS), in disseminated intravascular coagulation, and in acute kidney injury (22, 25–42).
Since several RCTs on the use of reparixin patients at high risk for in-hospital mortality were published, we decided to perform a systematic review and meta-analysis of RCTs to investigate the effect of reparixin on survival in these patients.
Method
Search strategy and study selection
Two trained, independent investigators searched PubMed, EMBASE, ClinicalTrials.gov, and the Cochrane Central Register of Controlled Trials (CENTRAL) for appropriate studies. Due to the paucity of studies, the full search strategies simply included the word reparixin or repertaxin. We selected any RCTs ever performed with reparixin in patients at high risk for in-hospital mortality with the exclusion of oncological settings. Furthermore, we contacted international experts and applied backward snowballing to retrieve additional manuscripts (i.e., looking through references of an identified set of articles and reviews).
At first, two investigators independently examined references at a title or abstract level with divergences resolved by mediation of a third author. Relevant references were collected as complete articles.
The inclusion criteria used for potentially pertinent studies were random allocation to treatment (reparixin vs. any comparator without restrictions on dose or time of administration) and studies involving at high risk for in-hospital mortality (patients were considered at high risk for in-hospital mortality if they had at least one organ dysfunction and/or were receiving intensive care or emergency treatments at the time of randomization). The exclusion criteria were oncological settings and non-adult patients. Compliance to selection criteria was assessed by two independent investigators and studies were selected for the final analysis. Divergences were resolved by consensus. Searches are updated on 26 April 2022.
Data extraction
Two investigators individually retrieved data on baseline, procedure, and outcome. They extracted data following the intention-to-treat principle whenever possible. In case of missing data, they contacted the corresponding authors via e-mail. The primary endpoint of the present review was mortality rate at the longest available follow-up. Secondary endpoints were the risk of getting pneumonia, of having sepsis, and the occurrence of a non-serious infection.
Assessment of risk of bias
The risk of bias of randomized studies was appraised according to the Revised Cochrane risk-of-bias tool for randomized trials (RoB 2) (43), and divergences were resolved by consensus. Publication bias was evaluated with visually inspecting funnel plots.
Data analysis
The meta-analysis was accomplished using Review Manager software (RevMan, version 5.4. Copenhagen: The Nordic Cochrane Centre, The Cochrane Collaboration, 2020).
The odds ratio (OR) with a 95% confidence interval (CI) was calculated for dichotomous variables, whereas the risk ratio (RR) with a 95% CI was calculated for common events, defined as the frequency of the event occurring in the control group being >10%. We calculated the proportion of patients with the outcome in each group, and the p-value for the comparison between the groups. A p-value ≤ 0.05 was considered statistically significant. In addition, we also calculated the number needed to treat (NNT).
Heterogeneity was explored using I² statistic and the χ² test, with significance being set at p-values of 0.10. A fixed-effects model for the meta-analysis was used in the presence of low heterogeneity, defined as I² result < 50% and a p-value > 0.10 in the χ² test. If significant heterogeneity was identified, defined as a p-value of ≤0.10, we employed a random-effects model, unless one or two trials were found to dominate the available evidence, or significant publication bias was present.
Sensitivity analyses were performed by analyzing the data with a fixed-effects model versus a random-effects model, and changing the summary statistics (ORs, risk differences, or RRs) or by removing each study in turn.
We performed a fixed-effects model trial sequential analysis with an overall type I error of 5% and a power of 80%. We hypothesized a 20% relative risk reduction (RRR) and a mortality of 10% in the control arm. The meta-analysis monitoring boundaries, required information size (RIS), diversity-adjusted information size (D2), and adjusted 95% confidence intervals were quantified. All data analyses were performed with R version 3.6.1, except trial sequential analysis using TSA software version 0.9.5.10.
This study was registered on PROSPERO (CRD 42021254467) and performed in compliance with The Cochrane Collaboration and Preferred Reporting Items for Systematic Reviews and Meta-Analyses guidelines.
Results
Database searches, contacts with experts, and snowballing yielded a total of 13 articles (Supplementary Figure 1). Six studies were excluded because of our prespecified exclusion criteria: one was not randomized (44), two did not involve patients at high risk for in-hospital mortality (45, 46), two included cancer patients (47, 48), and one was conducted in healthy volunteers (49). A sixth study (50) was excluded because it was a post-hoc analysis of a single-center small cohort derived from a multicentric trial (46). The six manuscripts included in the present meta-analysis (21, 51–55) randomized 406 patients (220 received reparixin and 186 received the comparator).
Trials’ characteristics
Studies were conducted in North America and Europe and were published from 2008 to 2022 (Table 1). Trials were performed in solid organ transplant recipients (three trials, 215 patients) (51, 53, 54), in patients with severe chronic or recurrent acute pancreatitis undergoing total pancreatectomy with islet autotransplantation (55), in patients undergoing on-pump coronary artery bypass grafting (52), and in patients with severe COVID-19 pneumonia (21) (one trial each with 102, 32 and 55 patients, respectively). The most frequently used dose was an intravenous infusion of 2.8 mg/kg/h, and the most frequently used length of infusion was 1 week (Table 2). The most frequent control treatment was placebo (four trials, 149 patients), while the other two trials used standard care as control (Table 2).
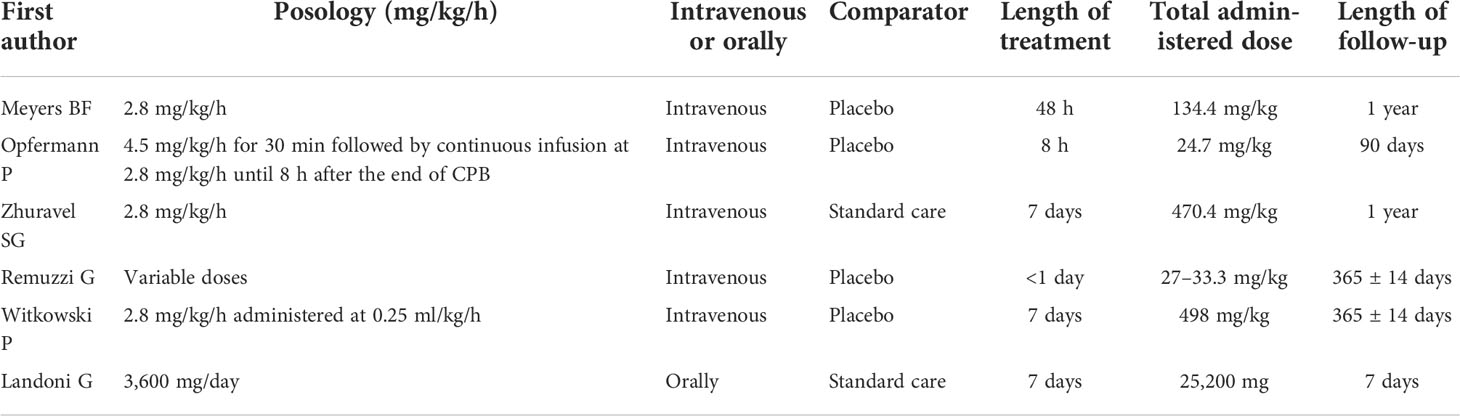
Table 2 Doses and modalities of administration of reparixin in the eight included randomized studies.
Quantitative data synthesis
Primary endpoint
Figure 1 shows the forest plot of the effect of reparixin on mortality according to the six included randomized studies. Thus, mortality of patients treated with reparixin was significantly lower than mortality in controls: 5/220 (2.3%) in the reparixin group vs. 12/186 (6.5%) in the control group, OR = 0.33 [95% CI 0.12 to 0.96], p for effect 0.04, p for heterogeneity 0.20, I2 = 36%, number needed to treat = 24.
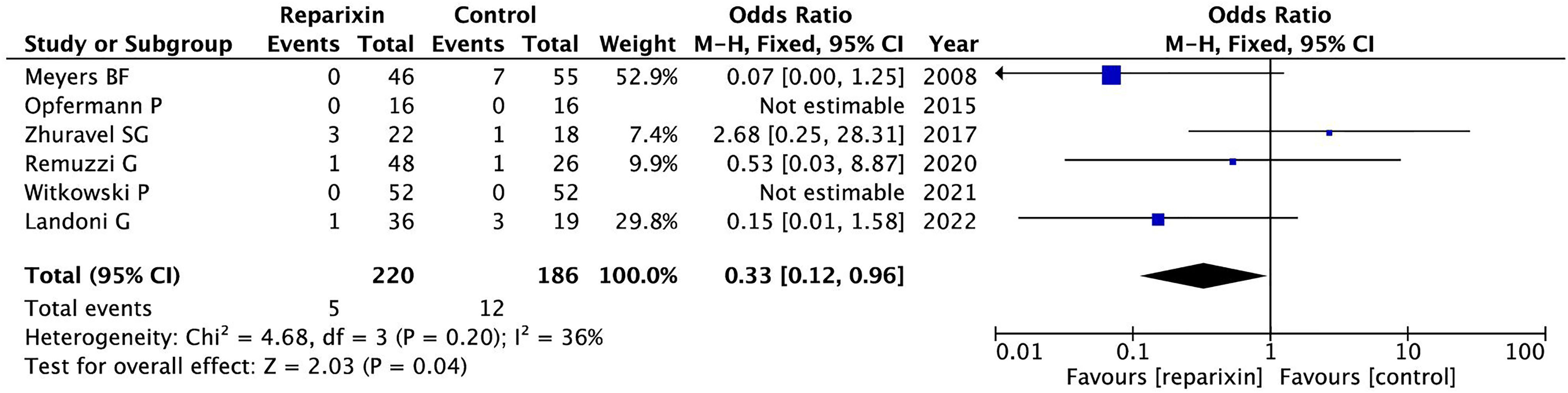
Figure 1 Forest plot of the effect of reparixin on mortality according to the six included randomized studies.
Magnitude and direction of findings were maintained in all sensitivity analyses including those that removed one study in turn (Supplementary Figure 2) and in subgroup perioperative settings (Supplementary Figure 3), transplant patients (Supplementary Figure 4), and length of treatment ≥48 h (Supplementary Figure 5).
Overall, risk of bias analysis showed that four included studies were at low risk of bias (accounting for 311 patients), and two trials were considered at unclear risk of bias (95 patients) (Supplementary Figure 6).
The funnel plot did not reveal the presence of small study bias (Figure 2). Moreover, trial sequential analysis (OR = 0.33; trial sequential analysis-adjusted 95% CI 0.12–0.96; p = 0.04; I2 = 36%) did not indicate that our findings are conclusive. The cumulative Z-curve did not cross the monitoring boundary curve for benefit and did not reach the required information size (n = 742) (Supplementary Figure 7).
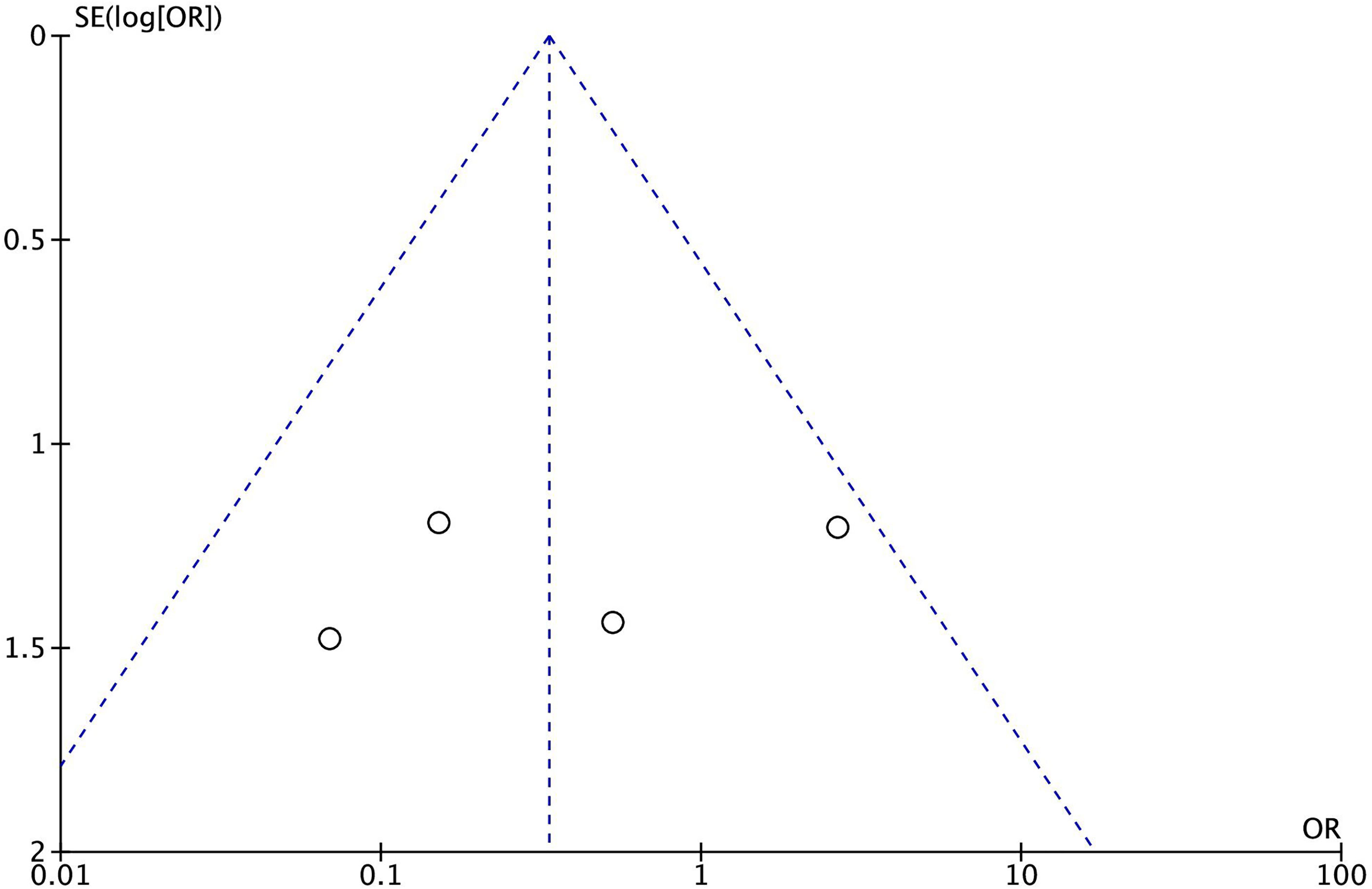
Figure 2 Funnel plot of the effect of reparixin on mortality according to the six included randomized studies.
Mortality reduction was confirmed in the subgroups of perioperative, transplant, and length of treatment ≥48 h (Supplementary Figures 1–3).
Secondary endpoint
We found no difference in the rate of pneumonia (3 of 100 [3.0%] in the reparixin group vs. 6 of 78 [7.7%] in the control group, OR = 0.44 [95% CI 0.12–1.65], p for effect = 0.23, I2 = 23%, two trials included; see Figure 3), sepsis (2 of 100 [2.0%] in the reparixin group vs. 2 of 78 [2.6%] in the control group, OR = 0.76 [95% CI 0.16–3.56] p for effect = 0.73, I2 = 68%, two trials included; see Figure 4), and non-serious infections (13 of 116 [11.2%] in the reparixin group vs. 9 of 94 [9.6%] in the control group, OR = 1.08 [95% CI 0.43–2.73] p for effect = 0.86, I2 = 29%, three trials included; see Figure 5) between reparixin and controls.
Discussion
In this meta-analysis of randomized studies, we found that reparixin, a non-competitive allosteric inhibitor of CXCR1 and CXCR2, is associated with better survival in patients at high risk for in-hospital mortality. This was associated with a similar rate of infective complications. Chemokine receptors are relevant therapeutic targets for the treatment of many human diseases; indeed, more than 100 experimental chemokine receptor antagonists have been advanced for targeting different chemokine receptors (56). Despite this, many of them failed to show sufficient clinical responses. In fact, until now, only three chemokine antagonists have been approved for clinical use (57): (i) the CXCR4 antagonist plerixafor, a small molecule that mobilizes hematopoietic stem cells; (ii) the noncompetitive CCR5 antagonist maraviroc, a small molecule that prevents the binding of HIV envelope glycoprotein to CCR5; and (iii) the CCR4 antagonist mogamulizumab, a defucosylated humanized monoclonal antibody approved for the treatment of mycosis fungoides or Sézary syndrome. Some general reasons could explain the treatment failures associated with chemokine receptor antagonists. First, as diseases are associated with many chemokine receptors, blocking only one may not be enough. Second, chemokine receptors take part in many immune and inflammatory activities, so blocking key chemokine receptors may lead to the occurrence of severe adverse events. Third, an effective dosage of non-toxic antagonists, sufficiently metabolically stable in the circulation, is needed to block the chemokine receptor–ligand interactions.
Aggregating the data of 406 patients treated in the various randomized trials performed so far through our meta-analysis, we suggested, for the first time, a significant efficacy of reparixin on a hard endpoint such as the survival. Of note, trials including severely ill patients (lung and kidney transplant recipients, critically ill COVID-19 patients) contributed most to the result. This is not unexpected as interleukin 8 level and neutrophil activation were both previously associated with acute kidney injury and acute respiratory distress syndrome in critically ill patients (37, 58–61).
The meta-analysis results could allow the opportunity not only to identify reparixin as an agent for the treatment of COVID-19-related ARDS (where it is now under evaluation) (62), but also to suggest it as a new therapeutic to treat ARDS of any origin or cause to modulate the inflammatory response and its clinical consequences (63).
Further supporting this is the fact that no safety issues emerged from our meta-analysis, confirming the excellent tolerability profile of reparixin reported in each trial. This was not taken for granted. In fact, it is not possible to exclude a priori that an interleukin 8 receptor inhibitor does not increase the risk of infections. Neutrophils play a central role in innate immunity acting as the first line of host defense against infection, and CXCL8 activity is required for neutrophil migration and recruitment to inflamed sites during infection (64, 65). The evidence that the risk of infections is not increased by reparixin treatment supports a complex model of neutrophil recruitment during infection characterized by an early phase, mediated by short-lived signals, and by an amplification phase, which is mediated by signaling cascades through leukotriene-B4 and IL-8/CXCR1-2 pathway (66, 67). Similar to AZD5069 (68), another selective antagonist of CXCR2, the net effect of CXCR2 inhibition by reparixin probably allows neutrophil migration without impacting neutrophil-mediated phagocytic and oxidative burst activities, but preventing the excess neutrophil infiltration and activation (69).
Our study has some limitations that are consistent with meta-analyses of highly heterogeneous studies including differences in target populations, targeted effects, survey recruitment, administration methods, and timing of outcome measurements. Moreover, some of the studies included have a small number of participants and a limited range of age within the studied population.
In conclusion, short-term inhibition of CXCL8 activity with the allosteric inhibitor reparixin improved survival in patients at high risk for in-hospital mortality treated in RCTs. This evidence suggests that the role of IL-8 and its receptors is complex and, overall, clinically relevant. Therapeutic interventions targeting IL-8 receptors in the future should be investigated in critically ill patients with hyperinflammatory complications like ARDS.
Data availability statement
The raw data supporting the conclusions of this article will be made available by the authors, without undue reservation. Requests to access the data should be directed to landoni.giovanni@hsr.it, piemonti.lorenzo@hsr.it
Author contributions
All authors participated to ideation, data collection or analysis, and drafting or correcting the manuscript. All authors approved the final version of the manuscript.
Funding
This manuscript was supported by departmental fund only.
Acknowledgments
We thank Yuki Kotani (MD), Tóth Krisztina (MD), and Eros Pilia (MD) for carefully revising the manuscript.
Conflict of interest
The authors declare that the research was conducted in the absence of any commercial or financial relationships that could be construed as a potential conflict of interest.
Publisher’s note
All claims expressed in this article are solely those of the authors and do not necessarily represent those of their affiliated organizations, or those of the publisher, the editors and the reviewers. Any product that may be evaluated in this article, or claim that may be made by its manufacturer, is not guaranteed or endorsed by the publisher.
Supplementary material
The Supplementary Material for this article can be found online at: https://www.frontiersin.org/articles/10.3389/fimmu.2022.932251/full#supplementary-material
References
1. Sultana J, Crisafulli S, Gabbay F, Lynn E, Shakir S, Trifirò G. Challenges for drug repurposing in the COVID-19 pandemic era. Front Pharmacol (2020) 11:588654(1657). doi: 10.3389/fphar.2020.588654
2. Shawki MA, Elsayed NS, Mantawy EM, Said RS. Promising drug repurposing approach targeted for cytokine storm implicated in SARS-CoV-2 complications. Immunopharmacol immunotoxicol (2021) 43(4):395–409. doi: 10.1080/08923973.2021.1931302
3. Piemonti L, Landoni G. COVID-19 and islet transplantation: Different twins. Am J Transpl (2020) 20(11):2983–8. doi: 10.1111/ajt.16001
4. Grégoire M, Uhel F, Lesouhaitier M, Gacouin A, Guirriec M, Mourcin F, et al. Impaired efferocytosis and neutrophil extracellular trap clearance by macrophages in ARDS. Eur Respir J (2018) 52(2):1702590. doi: 10.1183/13993003.02590-2017
5. Hemmat N, Derakhshani A, Bannazadeh Baghi H, Silvestris N, Baradaran B, De Summa S. Neutrophils, crucial, or harmful immune cells involved in coronavirus infection: A bioinformatics study. Front Genet (2020) 11:641. doi: 10.3389/fgene.2020.00641
6. Middleton EA, He X-Y, Denorme F, Campbell RA, Ng D, Salvatore SP, et al. Neutrophil extracellular traps contribute to immunothrombosis in COVID-19 acute respiratory distress syndrome. Blood (2020) 136(10):1169–79. doi: 10.1182/blood.2020007008
7. Kaiser R, Leunig A, Pekayvaz K, Popp O, Joppich M, Polewka V, et al. Self-sustaining interleukin-8 loops drive a prothrombotic neutrophil phenotype in severe COVID-19. JCI Insight (2021) 6(18):e150862. doi: 10.1172/jci.insight.150862
8. Skendros P, Mitsios A, Chrysanthopoulou A, Mastellos DC, Metallidis S, Rafailidis P, et al. Complement and tissue factor–enriched neutrophil extracellular traps are key drivers in COVID-19 immunothrombosis. J Clin Invest (2020) 130(11):6151–7. doi: 10.1172/JCI141374
9. Zuo Y, Yalavarthi S, Shi H, Gockman K, Zuo M, Madison JA, et al. Neutrophil extracellular traps in COVID-19. JCI Insight (2020) 5(11):e138999. doi: 10.1172/jci.insight.138999
10. Silvin A, Chapuis N, Dunsmore G, Goubet A-G, Dubuisson A, Derosa L, et al. Elevated calprotectin and abnormal myeloid cell subsets discriminate severe from mild COVID-19. Cell (2020) 182(6):1401–18.e18. doi: 10.1016/j.cell.2020.08.002
11. Busch MH, Timmermans SA, Nagy M, Visser M, Huckriede J, Aendekerk JP, et al. Neutrophils and contact activation of coagulation as potential drivers of COVID-19. Circulation (2020) 142(18):1787–90. doi: 10.1161/CIRCULATIONAHA.120.050656
12. Schulte-Schrepping J, Reusch N, Paclik D, Baßler K, Schlickeiser S, Zhang B, et al. Severe COVID-19 is marked by a dysregulated myeloid cell compartment. Cell (2020) 182(6):1419–40.e23. doi: 10.1016/j.cell.2020.08.001
13. Meizlish ML, Pine AB, Bishai JD, Goshua G, Nadelmann ER, Simonov M, et al. A neutrophil activation signature predicts critical illness and mortality in COVID-19. Blood Advances (2021) 5(5):1164–77. doi: 10.1182/bloodadvances.2020003568
14. Liu J, Liu Y, Xiang P, Pu L, Xiong H, Li C, et al. Neutrophil-To-Lymphocyte ratio predicts critical illness patients with 2019 coronavirus disease in the early stage. J Trans Med (2020) 18:1–12. doi: 10.1186/s12967-020-02374-0
15. Veras FP, Pontelli MC, Silva CM, Toller-Kawahisa JE, de Lima M, Nascimento DC, et al. SARS-CoV-2–triggered neutrophil extracellular traps mediate COVID-19 pathology. J Exp Med (2020) 217(12):e20201129. doi: 10.1084/jem.20201129
16. Xiong Y, Liu Y, Cao L, Wang D, Guo M, Jiang A, et al. Transcriptomic characteristics of bronchoalveolar lavage fluid and peripheral blood mononuclear cells in COVID-19 patients. Emerging Microbes Infect (2020) 9(1):761–70. doi: 10.1080/22221751.2020.1747363
17. Masso-Silva JA, Moshensky A, Lam MT, Odish M, Patel A, Xu L, et al. Increased peripheral blood neutrophil activation phenotypes and NETosis in critically ill COVID-19 patients: A case series and review of the literature. Clin Infect Dis (2021) 74(10):1889–90. doi: 10.1093/cid/ciab437
18. Li H, Zhang J, Fang C, Zhao X, Qian B, Sun Y, et al. The prognostic value of IL-8 for the death of severe or critical patients with COVID-19. Medicine (2021) 100(11):e23656. doi: 10.1097/MD.0000000000023656
19. Del Valle DM, Kim-Schulze S, Huang H-H, Beckmann ND, Nirenberg S, Wang B, et al. An inflammatory cytokine signature predicts COVID-19 severity and survival. Nat Med (2020) 26(10):1636–43. doi: 10.1038/s41591-020-1051-9
20. Singh K, Mittal S, Gollapudi S, Butzmann A, Kumar J, Ohgami RS. A meta-analysis of SARS-CoV-2 patients identifies the combinatorial significance of d-dimer, c-reactive protein, lymphocyte, and neutrophil values as a predictor of disease severity. Int J Lab Hematol (2021) 43(2):324–8. doi: 10.1111/ijlh.13354
21. Landoni G, Piemonti L, Monforte AD, Grossi P, Zangrillo A, Bucci E, et al. A multicenter phase 2 randomized controlled study on the efficacy and safety of reparixin in the treatment of hospitalized patients with COVID-19 pneumonia. Infect Dis Ther (2022) 26:1–16. doi: 10.1007/s40121-022-00644-6
22. Mikacenic C, Moore R, Dmyterko V, West TE, Altemeier WA, Liles WC, et al. Neutrophil extracellular traps (NETs) are increased in the alveolar spaces of patients with ventilator-associated pneumonia. Crit Care (2018) 22(1):358–. doi: 10.1186/s13054-018-2290-8
23. Millar JE, Fanning JP, McDonald CI, McAuley DF, Fraser JF. The inflammatory response to extracorporeal membrane oxygenation (ECMO): A review of the pathophysiology. Crit Care (2016) 20(1):1–10. doi: 10.1186/s13054-016-1570-4
24. Lenz M, Draxler DF, Zhang C, Kassem M, Kastl SP, Niessner A, et al. Toll-like receptor 2 and 9 expression on circulating neutrophils is associated with increased mortality in critically ill patients. Shock. (2020) 54(1):35–43. doi: 10.1097/SHK.0000000000001467
25. Ham SY, Yoon HJ, Nam SB, Yun BH, Eum D, Shin CS. Prognostic value of Neutrophil/Lymphocyte ratio and mean platelet Volume/Platelet ratio for 1-year mortality in critically ill patients. Sci Rep (2020) 10(1):1–8. doi: 10.1038/s41598-020-78476-y
26. Yan L, Hu Z-D. Red blood cell distribution width, neutrophil-To-Lymphocyte ratio, and in-hospital mortality in dyspneic patients admitted to the emergency department. Dis Markers (2020) 2020:8839506–. doi: 10.1155/2020/8839506
27. Hu Y, Huang K, Ji Z, Wang S, Bai M, Pan S, et al. High neutrophil-to-Lymphocyte ratio is associated with poor clinical outcome in patients with critically ill stroke. Minerva Anestesiologica (2020) 86(9):939–47. doi: 10.23736/S0375-9393.20.14310-4
28. Fan LL, Wang YJ, Nan CJ, Chen YH, Su HX. Neutrophil-lymphocyte ratio is associated with all-cause mortality among critically ill patients with acute kidney injury. Clinica Chimica Acta (2019) 490:207–13. doi: 10.1016/j.cca.2018.09.014
29. Li W, Ai X, Ni Y, Ye Z, Liang Z. The association between the neutrophil-To-Lymphocyte ratio and mortality in patients with acute respiratory distress syndrome: A retrospective cohort study. Shock (2019) 51(2):161–7. doi: 10.1097/SHK.0000000000001136
30. Dilektasli E, Inaba K, Haltmeier T, Wong MD, Clark D, Benjamin ER, et al. The prognostic value of neutrophil-to-Lymphocyte ratio on mortality in critically ill trauma patients. J Trauma Acute Care Surg (2016) 81(5):882–8. doi: 10.1097/TA.0000000000000980
31. Abrams ST, Morton B, Alhamdi Y, Alsabani M, Lane S, Welters ID, et al. A novel assay for neutrophil extracellular trap formation independently predicts disseminated intravascular coagulation and mortality in critically ill patients. Am J Respir Crit Care Med (2019) 200(7):869–80. doi: 10.1164/rccm.201811-2111OC
32. Camicia G, Pozner R, de Larrañaga G. Neutrophil extracellular traps in sepsis. Shock (2014) 42(4):286–94. doi: 10.1097/SHK.0000000000000221
33. Fuchs TA, Brill A, Duerschmied D, Schatzberg D, Monestier M, Myers DD, et al. Extracellular DNA traps promote thrombosis. Proc Natl Acad Sci (2010) 107(36):15880–5. doi: 10.1073/pnas.1005743107
34. Delabranche X, Stiel L, Severac F, Galoisy A-C, Mauvieux L, Zobairi F, et al. Evidence of netosis in septic shock-induced disseminated intravascular coagulation. Shock: Injury Inflamm Sepsis: Lab Clin Approaches (2017) 47(3):313–7. doi: 10.1097/SHK.0000000000000719
35. Lefrançais E, Mallavia B, Zhuo H, Calfee CS, Looney MR. Maladaptive role of neutrophil extracellular traps in pathogen-induced lung injury. JCI Insight (2018) 3(3):e98178. doi: 10.1172/jci.insight.98178
36. Lv X, Wen T, Song J, Xie D, Wu L, Jiang X, et al. Extracellular histones are clinically relevant mediators in the pathogenesis of acute respiratory distress syndrome. Respir Res (2017) 18(1):1–9. doi: 10.1186/s12931-017-0651-5
37. Anderson BJ, Calfee CS, Liu KD, Reilly JP, Kangelaris KN, Shashaty MGS, et al. Plasma Stnfr1 and IL8 for prognostic enrichment in sepsis trials: A prospective cohort study. Crit Care (2019) 23(1):400–. doi: 10.1186/s13054-019-2684-2
38. Miller E, Cohen A, Nagao S, Griffith D, Maunder R, Martin T, et al. Elevated levels of NAP-1/Interleukin-8 are present in the airspaces of patients with the adult respiratory distress syndrome and are associated with increased mortality. Am Rev Respir Dis (1992) 146(2):427–32. doi: 10.1164/ajrccm/146.2.427
39. Yu Y, Liu Y, Ling X, Huang R, Wang S, Min J, et al. The neutrophil percentage-To-Albumin ratio as a new predictor of all-cause mortality in patients with cardiogenic shock. BioMed Res Int (2020) 2020:7458451–. doi: 10.1155/2020/7458451
40. Sun T, Shen H, Guo Q, Yang J, Zhai G, Zhang J, et al. Association between neutrophil percentage-To-Albumin ratio and all-cause mortality in critically ill patients with coronary artery disease. BioMed Res Int (2020) 2020:8137576–. doi: 10.1155/2020/8137576
41. Peng Y, Xue Y, Wang J, Xiang H, Ji K, Wang J, et al. Association between neutrophil-to-Albumin ratio and mortality in patients with cardiogenic shock: A retrospective cohort study. BMJ Open (2020) 10(10):e039860-e. doi: 10.1136/bmjopen-2020-039860
42. Gong Y, Li D, Cheng B, Ying B, Wang B. Increased neutrophil percentage-to-Albumin ratio is associated with all-cause mortality in patients with severe sepsis or septic shock. Epidemiol Infect (2020) 148:e87-e. doi: 10.1017/S0950268820000771
43. Sterne JAC, Savović J, Page MJ, Elbers RG, Blencowe NS, Boutron I. RoB 2: A revised tool for assessing risk of bias in randomised trials. BMJ (2019) 366:l489. doi: 10.1136/bmj.l4898
44. Schott AF, Goldstein LJ, Cristofanilli M, Ruffini PA, McCanna S, Reuben JM, et al. Phase ib pilot study to evaluate reparixin in combination with weekly paclitaxel in patients with HER-2-Negative metastatic breast cancer. Clin Cancer Res (2017) 23(18):5358–65. doi: 10.1158/1078-0432.CCR-16-2748
45. Citro A, Cantarelli E, Maffi P, Nano R, Melzi R, Mercalli A, et al. CXCR1/2 inhibition enhances pancreatic islet survival after transplantation. J Clin Invest (2012) 122(10):3647–51. doi: 10.1172/JCI63089
46. Maffi P, Lundgren T, Tufveson G, Rafael E, Shaw JAM, Liew A, et al. Targeting CXCR1/2 does not improve insulin secretion after pancreatic islet transplantation: A phase 3, double-blind, randomized, placebo-controlled trial in type 1 diabetes. Diabetes Care (2020) 43(4):710–8. doi: 10.2337/dc19-1480
47. Goldstein LJ, Perez RP, Yardley D, Han LK, Reuben JM, Gao H, et al. A window-of-Opportunity trial of the CXCR1/2 inhibitor reparixin in operable HER-2-Negative breast cancer. Breast Cancer Res (2020) 22(1):4. doi: 10.1186/s13058-019-1243-8
48. ClinicalTrial.gov NCT02370238. A double-blind study of paclitaxel in combination with reparixin or placebo for metastatic triple-negative breast cancer (FRIDA). Available at: https://clinicaltrials.gov/ct2/show/NCT02370238.
49. Leitner JM, Mayr FB, Firbas C, Spiel AO, Steinlechner B, Novellini R, et al. Reparixin, a specific interleukin-8 inhibitor, has no effects on inflammation during endotoxemia. Int J Immunopathol Pharmacol (2007) 20(1):25–36. doi: 10.1177/039463200702000104
50. Bachul PJ, Golab K, Basto L, Zangan S, Pyda JS, Perez-Gutierrez A, et al. Post-hoc analysis of a randomized, double blind, prospective study at the university of Chicago: Additional standardizations of trial protocol are needed to evaluate the effect of a CXCR1/2 inhibitor in islet allotransplantation. Cell Transpl (2021) 30:9636897211001774. doi: 10.1177/09636897211001774
51. Meyers BF, Keshavjee S, Zamora MR, Davis RD, Smith MA, McFadden PM, et al. 405: A multicenter prospective, randomized, placebo-controlled trial of a CXCL8 inhibitor (Reparixin) to prevent primary graft dysfunction after lung transplantation. J Heart Lung Transpl (2008) 27:S206–7. doi: 10.1016/j.healun.2007.11.417
52. Opfermann P, Derhaschnig U, Felli A, Wenisch J, Santer D, Zuckermann A, et al. A pilot study on reparixin, a CXCR1/2 antagonist, to assess safety and efficacy in attenuating ischaemia-reperfusion injury and inflammation after on-pump coronary artery bypass graft surgery. Clin Exp Immunol (2015) 180(1):131–42. doi: 10.1111/cei.12488
53. ClinicalTrial.gov NCT03031470. Pilot study of reparixin for early allograft dysfunction prevention in liver transplantation. Available at: https://clinicaltrials.gov/ct2/show/NCT03031470.
54. ClinicalTrial.gov NCT00248040. Reparixin in prevention of delayed graft function after kidney transplantation. Available at: https://clinicaltrials.gov/ct2/show/NCT00248040.
55. Witkowski P, Wijkstrom M, Bachul PJ, Morgan KA, Levy M, Onaca N, et al. Targeting CXCR1/2 in the first multicenter, double-blinded, randomized trial in autologous islet transplant recipients. Am J Transplant (2021) 21(11):3714–24. doi: 10.1111/ajt.16695
56. Solari R, Pease JE, Begg M. “Chemokine receptors as therapeutic targets: Why aren’t there more drugs?” Eur J Pharmacol (2015) 746:363–7. doi: 10.1016/j.ejphar.2014.06.060
57. Miao M, De Clercq E, Li G. Clinical significance of chemokine receptor antagonists. Expert Opin Drug Metab Toxicol (2020) 16:11–30. doi: 10.1080/17425255.2020.1711884
58. Törnblom S, Nisula S, Vaara ST, Poukkanen M, Andersson S, Pettilä V, et al. Neutrophil activation in septic acute kidney injury: A Post hoc analysis of the FINNAKI study. Acta Anaesthesiologica Scandinavica (2019) 63:1390–7. doi: 10.1111/aas.13451
59. Flori H, Sapru A, Quasney MW, Gildengorin G, Curley MAQ, Matthay MA, et al. A prospective investigation of interleukin-8 levels in pediatric acute respiratory failure and acute respiratory distress syndrome. Crit Care (2019) 23:128–8. doi: 10.1186/s13054-019-2342-8
60. Rondina MT, Tatsumi K, Bastarache JA, Mackman N. Microvesicle tissue factor activity and interleukin-8 levels are associated with mortality in patients with influenza A/H1N1 infection. Crit Care Med (2016) 44:e574-e578. doi: 10.1097/CCM.0000000000001584
61. Pike F, Murugan R, Keener C, Palevsky PM, Vijayan A, Unruh M, et al. Biological markers for recovery of kidney study I: Biomarker enhanced risk prediction for adverse outcomes in critically ill patients receiving RRT. Clin J Am Soc Nephrol CJASN (2015) 10:1332–9. doi: 10.2215/CJN.09911014
62. ClinicalTrial.gov NCT05254990. Reparixin as add-on therapy to standard of care to limit disease progression in adult patients with COVID-19. Available at: https://clinicaltrials.gov/ct2/show/NCT05254990.
63. Cesta MC, Zippoli M, Marsiglia C, Gavioli EM, Mantelli F, Allegretti M, et al. The role of interleukin-8 in lung inflammation and injury: Implications for the management of COVID-19 and hyperinflammatory acute respiratory distress syndrome. Front Pharmacol (2022) 12:808797. doi: 10.3389/fphar.2021.808797
64. Lee SK, Kim SD, Kook M, Lee HY, Ghim J, Choi Y, et al. Phospholipase D2 drives mortality in sepsis by inhibiting neutrophil extracellular trap formation and down-regulating CXCR2. J Exp Med (2015) 212:1381–90. doi: 10.1084/jem.20141813
65. Shen X-F, Zhao Y, Cao K, Guan W-X, Li X, Zhang Q, et al. Wip1 deficiency promotes neutrophil recruitment to the infection site and improves sepsis outcome. Front Immunol (2017) 8:1023. doi: 10.3389/fimmu.2017.01023
66. Sadik CD, Luster AD. Lipid-Cytokine-Chemokine cascades orchestrate leukocyte recruitment in inflammation. J Leukoc Biol (2012) 91:207–15. doi: 10.1189/jlb.0811402
67. De Oliveira S, Rosowski EE, Huttenlocher A. Neutrophil migration in infection and wound repair: Going forward in reverse. Nat Rev Immunol (2016) 16:378–91. doi: 10.1038/nri.2016.49
68. Uddin M, Betts C, Robinson I, Malmgren A, Humfrey C. The chemokine CXCR2 antagonist (AZD5069) preserves neutrophil-mediated host immunity in non-human primates. Haematologica (2017) 102:e65-e68. doi: 10.3324/haematol.2016.152371
Keywords: CXCR2 antagonist, Reparixin, CXCL-8, intensive & critical care, COVID-19, SARS-CoV-2
Citation: Landoni G, Zangrillo A, Piersanti G, Scquizzato T and Piemonti L (2022) The effect of reparixin on survival in patients at high risk for in-hospital mortality: a meta-analysis of randomized trials. Front. Immunol. 13:932251. doi: 10.3389/fimmu.2022.932251
Received: 29 April 2022; Accepted: 28 June 2022;
Published: 25 July 2022.
Edited by:
Janos G. Filep, Université de Montréal, CanadaReviewed by:
Piotr Witkowski, The University of Chicago, United StatesFabrizio Martelli, National Institute of Health (ISS), Italy
Anna Rita Migliaccio, Campus Bio-Medico University, Italy
Copyright © 2022 Landoni, Zangrillo, Piersanti, Scquizzato and Piemonti. This is an open-access article distributed under the terms of the Creative Commons Attribution License (CC BY). The use, distribution or reproduction in other forums is permitted, provided the original author(s) and the copyright owner(s) are credited and that the original publication in this journal is cited, in accordance with accepted academic practice. No use, distribution or reproduction is permitted which does not comply with these terms.
*Correspondence: Lorenzo Piemonti, piemonti.lorenzo@hsr.it