- 1Division of Allergy, Immunology, and Rheumatology, Department of Internal Medicine, Taichung Veterans General Hospital, Taichung, Taiwan
- 2Institute of Clinical Medicine, National Yang-Ming Chiao Tung University, Taipei, Taiwan
- 3Department of Medical Research, Taichung Veterans General Hospital, Taichung, Taiwan
- 4School of Medicine, National Yang-Ming Chiao Tung University, Taipei, Taiwan
- 5Department of Pathology and Laboratory Medicine, Perelman School of Medicine, University of Pennsylvania, Philadelphia, PA, United States
- 6Department of Post-Baccalaureate Medicine, College of Medicine, National Chung Hsing University, Taichung, Taiwan
- 7Department of Industrial Engineering and Enterprise Information, Tunghai University, Taichung, Taiwan
- 8Institute of Biomedical Science and Rong Hsing Research Center for Translational Medicine, National Chung Hsing University, Taichung, Taiwan
- 9Institute of Public Health and Community Medicine Research Center, National Yang-Ming University, Taipei, Taiwan
- 10Department of Medical Education, Taichung Veterans General Hospital, Taichung, Taiwan
- 11Department of Public Health, Fu Jen Catholic University, New Taipei City, Taiwan
- 12Institute of Genomics and Bioinformatics, National Chung Hsing University, Taichung, Taiwan
Objectives: To investigate the differences between the vector vaccine ChAdOx1 nCoV-19/AZD1222 (Oxford-AstraZeneca) and mRNA-based vaccine mRNA-1273 (Moderna) in patients with autoimmune rheumatic diseases (AIRD), and to explore the cell-cell interactions between high and low anti-SARS-CoV-2 IgG levels in patients with rheumatic arthritis (RA) using single-cell RNA sequencing (scRNA-seq).
Methods: From September 16 to December 10, 2021, we consecutively enrolled 445 participants (389 patients with AIRD and 56 healthy controls), of whom 236 were immunized with AZD1222 and 209 with mRNA-1273. The serum IgG antibodies to the SARS-CoV-2 receptor-binding domain was quantified by electrochemiluminescence immunoassay at 4-6 weeks after vaccination. Moreover, peripheral blood mononuclear cells (PBMCs) were isolated from RA patients at 4-6 weeks after vaccination for scRNA-seq and further analyzed by CellChat. ScRNA-seq of PBMCs samples from GSE201534 in the Gene Expression Omnibus (GEO) database were also extracted for analysis.
Results: The anti-SARS-CoV-2 IgG seropositivity rate was 85.34% for AIRD patients and 98.20% for healthy controls. The anti-SARS-CoV-2 IgG level was higher in patients receiving mRNA-1273 than those receiving AZD1222 (β: 35.25, 95% CI: 14.81-55.68, p=0.001). Prednisolone-equivalent dose >5 mg/day and methotrexate use in AIRD patients, and non-anti-tumor necrosis factor-α biologics and Janus kinase inhibitor use in RA patients were associated with inferior immunogenicity. ScRNA-seq revealed CD16-monocytes were predominant in RA patients with high anti-SARS-CoV2-IgG antibodies, and enriched pathways related to antigen presentation via MHC class II were found. HLA-DRA and CD4 interaction was enhanced in high anti-SARS-CoV2-IgG group.
Conclusions: mRNA-1273 and AZD1222 vaccines exhibited differential immunogenicity in AIRD patients. Enriched pathways related to antigen presentation via MHC class II in CD16-monocytes might be associated with higher anti-SARS-CoV2-IgG level in RA patients and further study is warranted.
Introduction
Worldwide, the coronavirus disease (COVID-19) pandemic remains a threat to public health and socioeconomic conditions. Autoimmune rheumatic diseases (AIRD) are chronic systemic inflammatory diseases that disrupt immune responses in approximately 4% of the global population (1, 2). Patients with rheumatic diseases are vulnerable to severe acute respiratory distress syndrome coronavirus 2 (SARS-CoV-2) infection (3, 4). However, not all immunosuppressed patients experience serious illness following SARS-CoV-2 infection, and this may be partly attributed to the differences in the immune disorders and the use of different immunosuppressive agents (5, 6).
The prevention of COVID-19 is a top priority and is vital for restoring normalcy. Previous studies have demonstrated COVID-19 vaccines, mostly mRNA-based vaccines, could elicit adequate immunogenicity in AIRD patients (7, 8). Although a lower percentage of rheumatic disease patients achieved seropositivity and had a relatively lower anti-SARS-CoV-2 immunoglobulin G (IgG) level, compared with healthy controls, these patients did not experience a flaring up of rheumatic diseases nor an increased number of adverse reactions with COVID-19 vaccines (8). The reduced immunogenicity in AIRD patients was probably attributable to the immune disturbance due to rheumatic disease or the immunosuppressive effects of concomitant glucocorticoids, conventional synthetic disease-modifying antirheumatic drugs (csDMARDs), biological DMARDs (bDMARDs), or targeted-synthetic DMARDs (tsDMARDs). Haberman et al. demonstrated that a smaller proportion of rheumatic patients on methotrexate (MTX) achieved adequate immunogenicity compared with non-users (9). Furer et al. showed that patients using glucocorticoids, mycophenolate mofetil (MMF), and non-tumor necrosis factor (TNF)-α bDMARDs, mainly abatacept and rituximab, achieved lower immunogenicity after receiving the BNT162b2 mRNA vaccine (8).
Most of the previous studies focused on the newly developed mRNA-based vaccines. However, there are fewer reports of the immunogenicity of vector vaccines, such as AZD1222, in AIRD patients. Furthermore, differences between mRNA-based and vector vaccines with regard to the immune response and safety profile of these vaccines in patients with rheumatic disease are unclear. Besides, there is a lack of information about the impact of immunosuppressants on immunogenicity in patients with rheumatic disease, especially those who were receiving tsDMARDs. In addition, no previous reports made a thorough exploration of the cellular responses to COVID-19 vaccines according to different immunogenicity in AIRD patients.
Therefore, we aimed to explore the differences in efficacy and safety between the vector vaccine AZD1222 and the mRNA-based vaccine mRNA-1273 as well as the impact of anti-rheumatic medications on vaccine-induced immunogenicity in AIRD patients, and to investigate the cell-cell interactions between high and low anti-SARS-CoV-2 IgG levels in patients with rheumatoid arthritis (RA) by single-cell RNA sequencing (scRNA-seq). The primary objective of this study was to elucidate the immune response induced by COVID-19 vaccination with either AZD1222 (Oxford-AstraZeneca) or mRNA-1273 (Moderna) in a cohort of patients with rheumatic diseases in a tertiary medical center in Taiwan.
Materials and methods
Study participants
From September 16 to December 10, 2021, we consecutively enrolled adult patients (age ≥20 years) with rheumatic diseases and healthy controls who received COVID-19 vaccination at a medical center in Taichung, Taiwan. Rheumatic diseases were diagnosed according to the corresponding classification criteria and confirmed by two experienced rheumatologists (10–16). Patients with a history of SARS-CoV-2 infection were excluded. The date of COVID-19 vaccination was defined as the index date in this study.
This study was conducted in accordance with the Declaration of Helsinki and was approved (CF21297B) by the Institutional Review Board of Taichung Veterans General Hospital, Taiwan. All participants provided written informed consent prior to their enrolment in the study.
Definition of covariates
Demographics and comorbidities were recorded besides the rheumatic disease types and anti-rheumatic medications, which comprised glucocorticoids, csDMARDs, bDMARDs and tsDMARDs such as the Janus kinase (JAK) inhibitors tofacitinib and baricitinib. Drug exposure was defined as treatment received in the 3 months preceding the index date.
The date of vaccination and type of vaccine were documented, and questionnaires were used for the surveillance of vaccine-associated adverse reactions. Moreover, the patient self-reported awareness of disease activities of underlying rheumatic diseases and physician global assessments at 4–6 weeks after the COVID-19 vaccination was recorded.
Anti-SARS-CoV2-IgG detection
At 4–6 weeks after the first or second dose of COVID-19 vaccines, the level of serum IgG antibodies to the SARS-CoV-2 receptor-binding domain (RBD) on spike protein S1 subunit was quantified by electrochemiluminescence immunoassay in accordance with the manufacturer’s instructions (Elecsys Anti-SARS-CoV-2 S assay, Roche Diagnostics, Basel, Switzerland). The interval of analytical measurement was 0.40–250 U/mL; a value <0.8 and ≥0.80 U/mL was recorded as negative and positive, respectively. High immunogenicity following vaccination was defined as anti-SARS-COV2-IgG after vaccination equaled to 250 U/ml; low immunogenicity was defined as anti-SARS-COV2-IgG after vaccination < 10 U/ml.
scRNA-seq datasets of healthy individuals following COVID-19 vaccination
scRNA-seq data of peripheral blood mononuclear cells (PBMCs) from 6 healthy individuals who had received 2 doses of ChAdOx1 (N=2), 2 doses of BNT162b2 (N=2), and heterologous ChAdOx1 and BNT162b2 vaccination were extracted from the National Center for Biotechnology Information (NCBI) in the Gene Expression Omnibus (GEO) Database (GSE201534). The dataset was used for cell proportions and pathway analysis.
Cell preparation and cryopreservation
PBMCs were isolated from RA patients at 4-6 weeks after the first immunization either with AZD1222 or mRNA-1273 in EDTA tubes and isolated by Lymphoprep (STEMCELL, Vancouver, BC, Canada). Briefly, samples were diluted with phosphate-buffered saline (PBS) and layered over Ficoll-Plaque and centrifuged at 800 x g for 20 minutes at room temperature. PBMCs were collected, diluted with PBS and centrifuged again at 300 x g for 15 minutes at room temperature. After one time wash with cold PBS, Cell Freezing Medium (ScienCell, Carlsbad, CA, USA) was used to resuspend cells and transfer to cryogenic storage vials (1x 107 - 1x108 cells/ml). The vials were placed into the Cell Freezing Containers and stored at -80°C overnight. Vials were then stored in liquid nitrogen until use.
Cell thawing
Within the same day before scRNA-seq experiment, frozen cells were removed from liquid nitrogen and thawed slowly in 37°C water bath for 3 minutes. Cells were then transferred to a pre-warmed 15ml falcon with 9ml Roswell Park Memorial Institute (RPMI) and 10% fetal bovine serum (FBS). Samples were incubated at room temperature for 10 minutes and centrifuged at 400 x g for 5 minutes at room temperature. Supernatant was removed and resuspended with 1ml PBS + 2% FBS gently. Cell concentration and viability were determined, and cell concentration was adjusted to approximately 1,000 cells per ml.
ScRNA-seq library preparation and sequencing
The PBMC samples were labeled with CellMultiplex oligos and were mixed following by loading on 10x Genomics Chromium Single Cell Instrument (10x Genomics, Pleasanton, CA, USA). Chromium Next GEM Single Cell 3’Reagent Kits v3.1 (Dual Index) was used for scRNA-seq library preparation according to the manufacturer’s instructions. Gene expression and CellMultiplex libraries was loaded at a ratio of 4:1 and 300pm onto the Illumina NovaSeq 6000 with paired end kits.
ScRNA-seq data analysis
The Fastq files were input into Cell Ranger Suite 6.0.1 for raw reads alignment, demultiplexing of barcodes, and quantification. Cells were filtered if unique feature counts > 9000 or < 200 and were eliminated as noise signals if mitochondrial counts > 10% by Seurat R package (17). SCTransform was used to normalize the post-filter matrix in order to eliminate the batch effect (18). Principal component analysis was used for dimensional reduction, and the k-nearest neighbor (KNN) graph and Louvain algorithm was performed for clustering. CellMarker database and scmap R package were used to annotate every cluster classified (19, 20). CellChat was used to investigate the cell-cell communication signal (21).
Statistical analysis
The chi-square or Mann–Whitney U test was conducted to compare the efficacy and safety between AZD1222 and mRNA-1273. Linear regression analyses were used to examine the immunogenicity and the impact of anti-rheumatic medications on immunogenicity in AIRD patients, expressed as regression coefficient (β) and 95% confidence intervals (95% CI). The Kruskal–Wallis test, followed by the Dunn–Bonferroni test for post hoc analysis, was used to determine immunogenicity in RA patients with or without bDMARDs and tsDMARDs. The Statistical Package for the Social Sciences (SPSS) version 22.0 was used for statistical analysis. Statistical significance was indicated by p<0.05.
Results
Demographics of participants who received COVID-19 vaccination
This study enrolled 445 patients who received COVID-19 vaccination, of whom there were 389 AIRD patients and 56 healthy controls. 236 patients were immunized with AZD1222 and 209 patients were immunized with mRNA-1273. Following vaccination, the anti-SARS-CoV-2 IgG seropositivity rate was 85.34% (332/389) and 98.2% (55/56) in AIRD patients and in healthy controls, respectively. In patients with rheumatic diseases, the seropositivity rate of anti-SARS-CoV-2 IgG was higher after the second doses of COVID-19 vaccine than after the first dose (95.2% vs. 79.6%, p=0.025) (Table 1). Among AIRD patients after the first dose of COVID-19 vaccination, there was markedly lower anti-SARS-CoV-2 IgG level in patients with SLE and RA than in healthy controls (Figure 1A). Nevertheless, there were no significant differences of anti-SARS-CoV-2 IgG level in patients with AIRD than in healthy controls after the second dose of COVID-19 vaccination (Figure 1B).
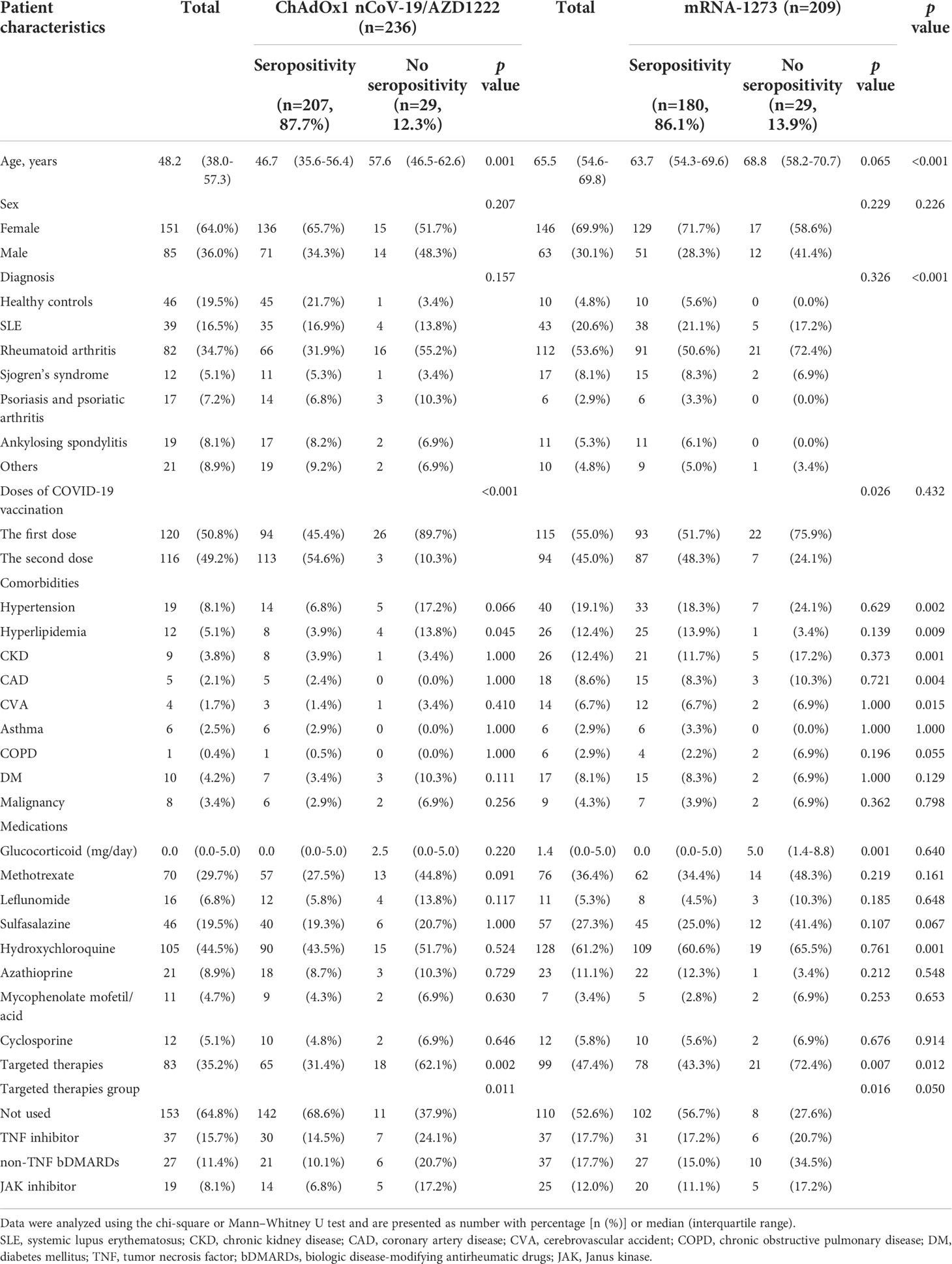
Table 1 Demographics and comorbidities of patients with rheumatic diseases following the first dose of COVID-19 vaccines.
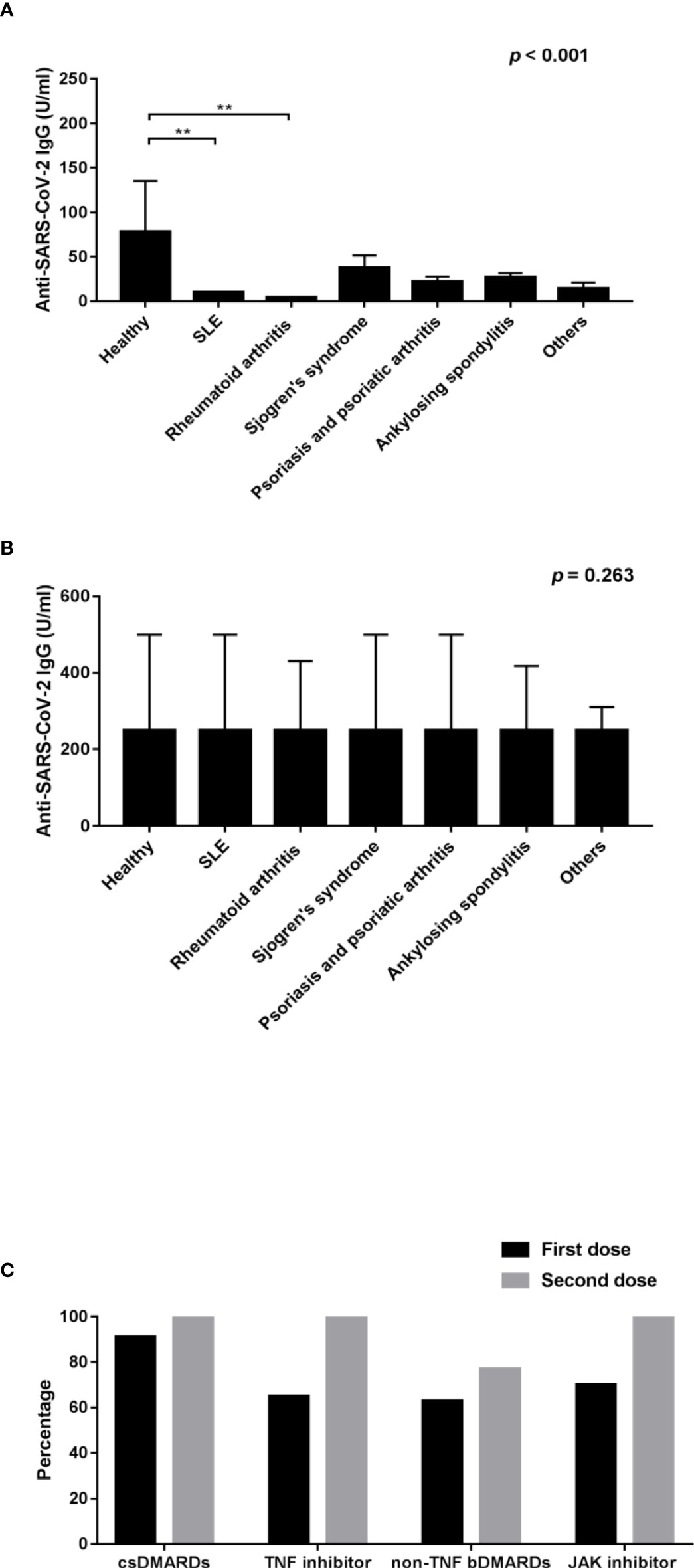
Figure 1 Comparison of the anti-SARS-CoV-2 IgG level after (A) the first dose of COVID-19 vaccines and (B) the second dose of COVID-19 vaccines among healthy controls and patients with individual rheumatic diseases; and (C) comparison of the percentage of seropositivity rate of RA patients using csDMARDs, TNF inhibitors, non-TNF bDMARDs, and JAK inhibitors after the first and second doses of COVID-19 vaccines. SLE, systemic lupus erythematosus; RA, rheumatoid arthritis; csDMARDs, conventional synthetic disease-modifying antirheumatic drugs; TNF, tumor necrosis factor; bDMARDs, biologic disease-modifying antirheumatic drugs; JAK, Janus kinase. **p < 0.001.
Early immunogenicity in rheumatic patients vaccinated against COVID-19
In order to determine early immunogenicity, we analyzed the anti-SARS-CoV-2 IgG level in AIRD patients after the first dose of COVID-19 vaccination. Compared with those receiving the first dose of AZD1222, participants receiving the first dose of mRNA-1273 had a higher anti-SARS-CoV-2 IgG level (Table 2). Compared with non-users, patients using prednisolone-equivalent glucocorticoid dose >5 mg/day, sulfasalazine, and targeted therapies including TNF, non-TNF and JAK inhibitors had a lower anti-SARS-CoV-2 IgG level.
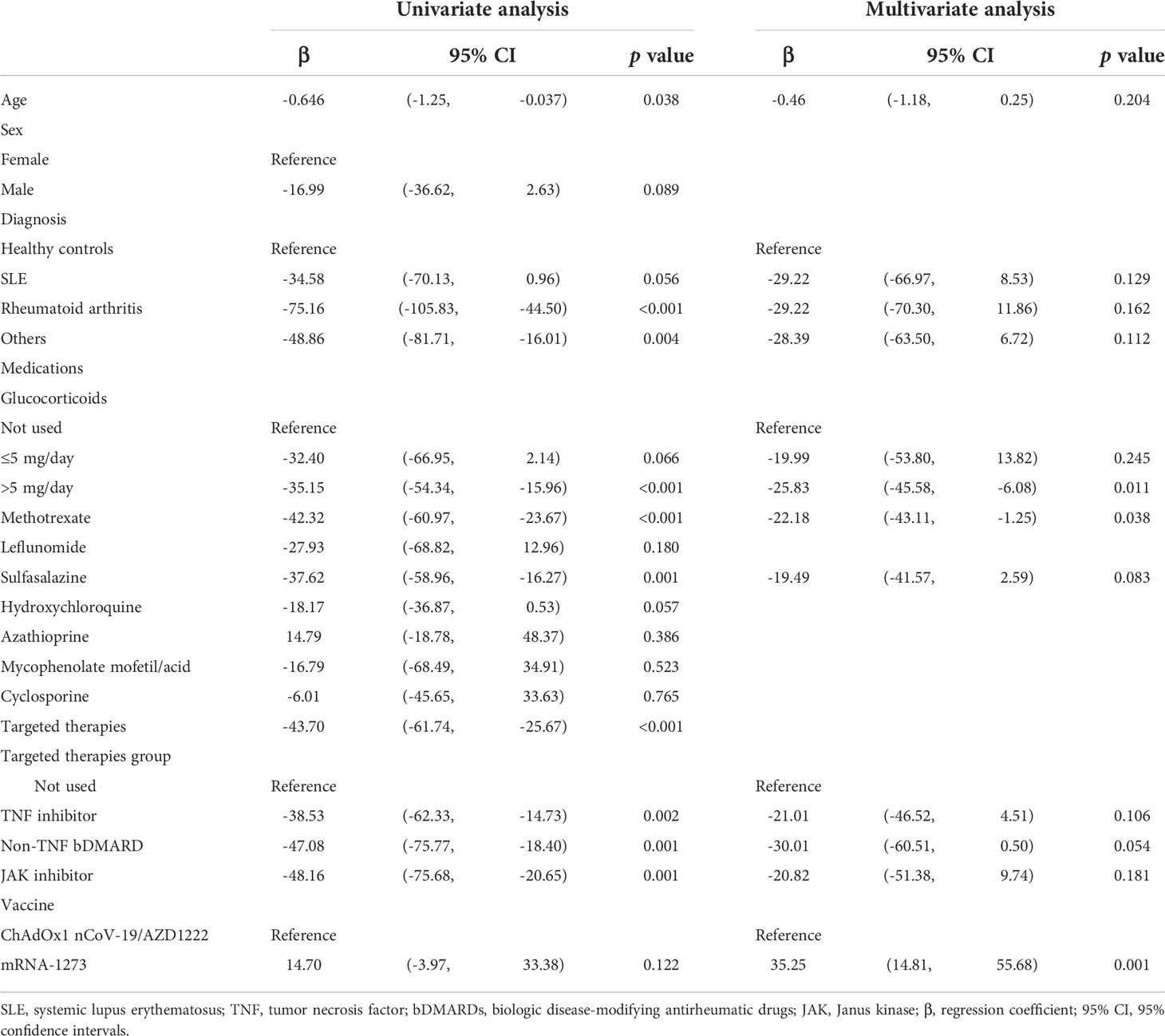
Table 2 Multivariate analysis of anti-SARS-CoV-2 IgG level in patients with rheumatic diseases following the first dose of COVID-19 vaccines.
After adjusting for potential confounders, the serum level of anti-SARS-CoV-2 IgG increased by 35.25 U/mL in patients who received mRNA-1273 than those who received AZD1222. Compared with those without prednisolone use, the anti-SARS-CoV-2 IgG level decreased by 25.83 U/mL on average in patients who used prednisolone >5 mg/day. On average, the anti-SARS-CoV-2 IgG level decreased by 22.18 U/mL in patients treated with MTX compared with those without MTX use.
Immunogenicity of COVID-19 vaccination in RA patients using bDMARDs and tsDMARDs
In order to evaluate the impact of biological agents on immunogenicity of COVID-19 vaccination, we examined the anti-SARS-CoV-2 IgG level in RA patients using different bDMARDs and tsDMARDs. After the first dose of COVID-19 vaccination, the seropositivity rate of anti-SARS-CoV-2 IgG was 90.6%, 65.4%, 62.5%, and 70.0% (p=0.062) (Figure 1C), and the average anti-SARS-CoV-2 IgG level was 27.31 (4.04-59.10), 4.92 (0.40-19.68), 1.43 (0.40-9.20), and 1.99 (0.55-7.49) U/ml in RA patients using csDMARDs, anti-TNF-α bDMARDs, non-anti-TNF-α bDMARDs, and JAK inhibitors, respectively. The anti-SARS-CoV-2 IgG level after the first dose of vaccination was markedly lower in RA patients who received non-anti-TNF-α bDMARDs and JAK inhibitor when compared with participants who received csDMARDs (p=0.004). After the second dose of COVID-19 vaccination, the seropositivity rate and the anti-SARS-CoV-2 IgG level in RA patients was both uplifted to a large extent, except for the seropositivity rate in RA patients receiving non-anti-TNF-α bDMARDs, compared with those receiving csDMARDs (77.4% vs. 100%, p=0.006).
Adverse reactions and patient self-reported disease activities after COVID-19 vaccination
Only non-serious adverse reactions occurred in either of the vaccine groups in AIRD patients. There were less fever, chills and fatigue in rheumatic patients receiving mRNA-1273 than those receiving AZD1222 (Table 3). Disease activities of rheumatic diseases by self-reported and by physician global assessments both remained stable in most patients who received COVID-19 vaccination, and there was no disparity in rheumatic disease activities after either AZD1222 or mRNA-1273 vaccination (Table 3 and Supplementary Table 1).
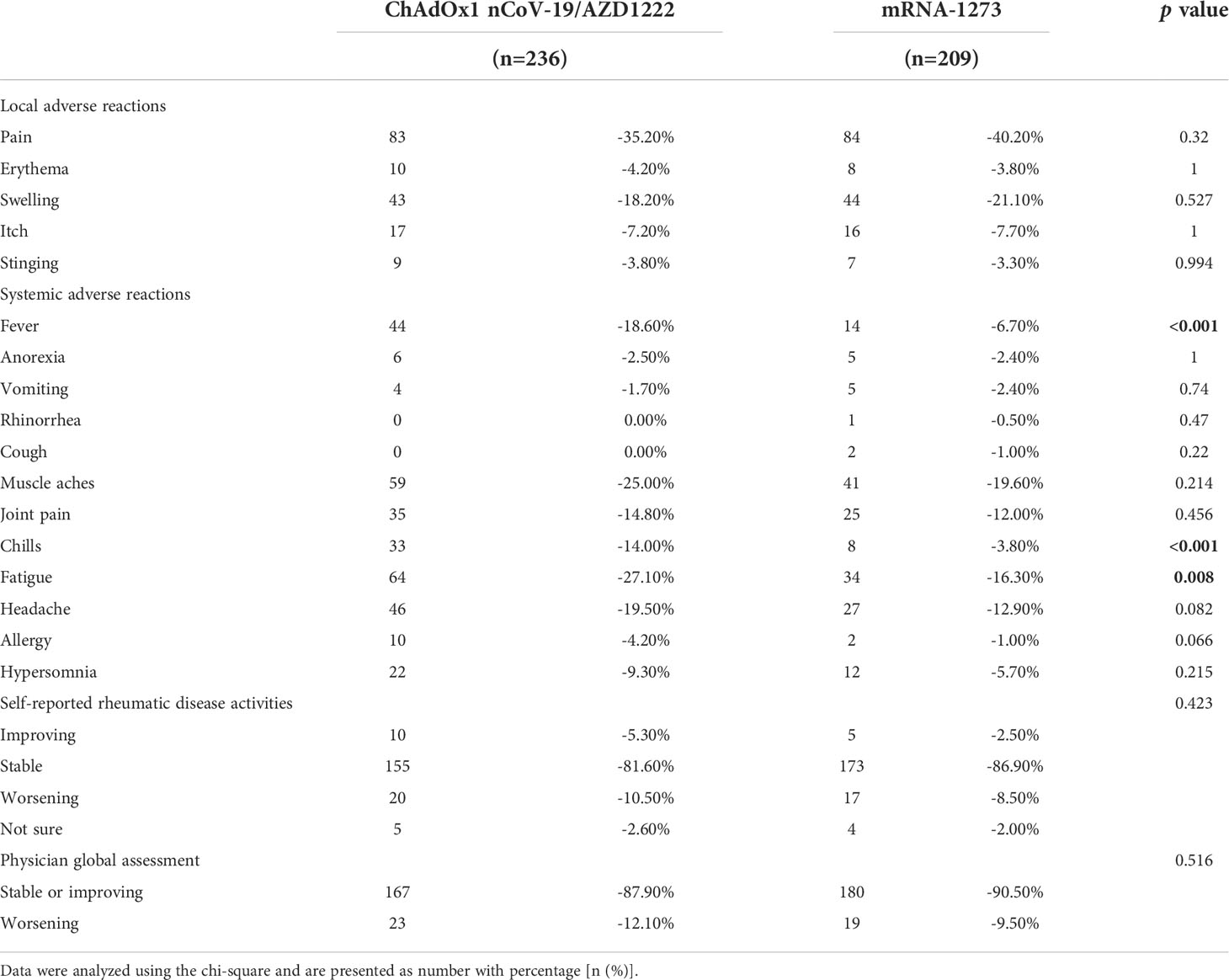
Table 3 Adverse reactions and rheumatic disease activities in rheumatic patients after COVID-19 vaccines.
ScRNA-seq of RA patients following COVID-19 vaccination
We performed scRNA-seq to explore the difference of cell composition using two RA patients with high anti-SARS-CoV2-IgG antibodies (one used no biologics, and one used etanercept) and four patients with low antibodies (one used abatacept, one used rituximab, and two used tofacitinib). The detailed clinical information of these patients was listed in Supplementary Table 2. To identify individual cells, the expression of major phenotype cell markers was analyzed and was shown in Supplementary Figure 1. The frequencies of major identified cell types by scRNA-seq were 25.64% CD16- monocyte, 5.67% CD16+ monocyte, 28.59% T cell, 12.73% B cell, 24.46% natural killer (NK) cell, 1.85% platelet, 0.59% plasmacytoid dendritic cell (pDC) and 0.47% common lymphoid progenitor (CLP) (Figure 2A). The individual cell distribution of each patient was shown in Supplementary Figure 2. The cell proportion of PBMC in RA patients were further confirmed by flow cytometric analysis, which revealed 26.8% T cells, 22.8% CD3-CD56+NK cells, and 26.1% monocytes (22.8% CD16- monocytes and 3.3% CD16+ monocytes) in PBMC from RA patients (Supplementary Figure 3). There were more CD16-monocytes and less NK cells and T cells in high anti-SARS-CoV2-IgG antibody group than low antibody group by scRNA-seq, though there was no statistical significance (Figure 2B).
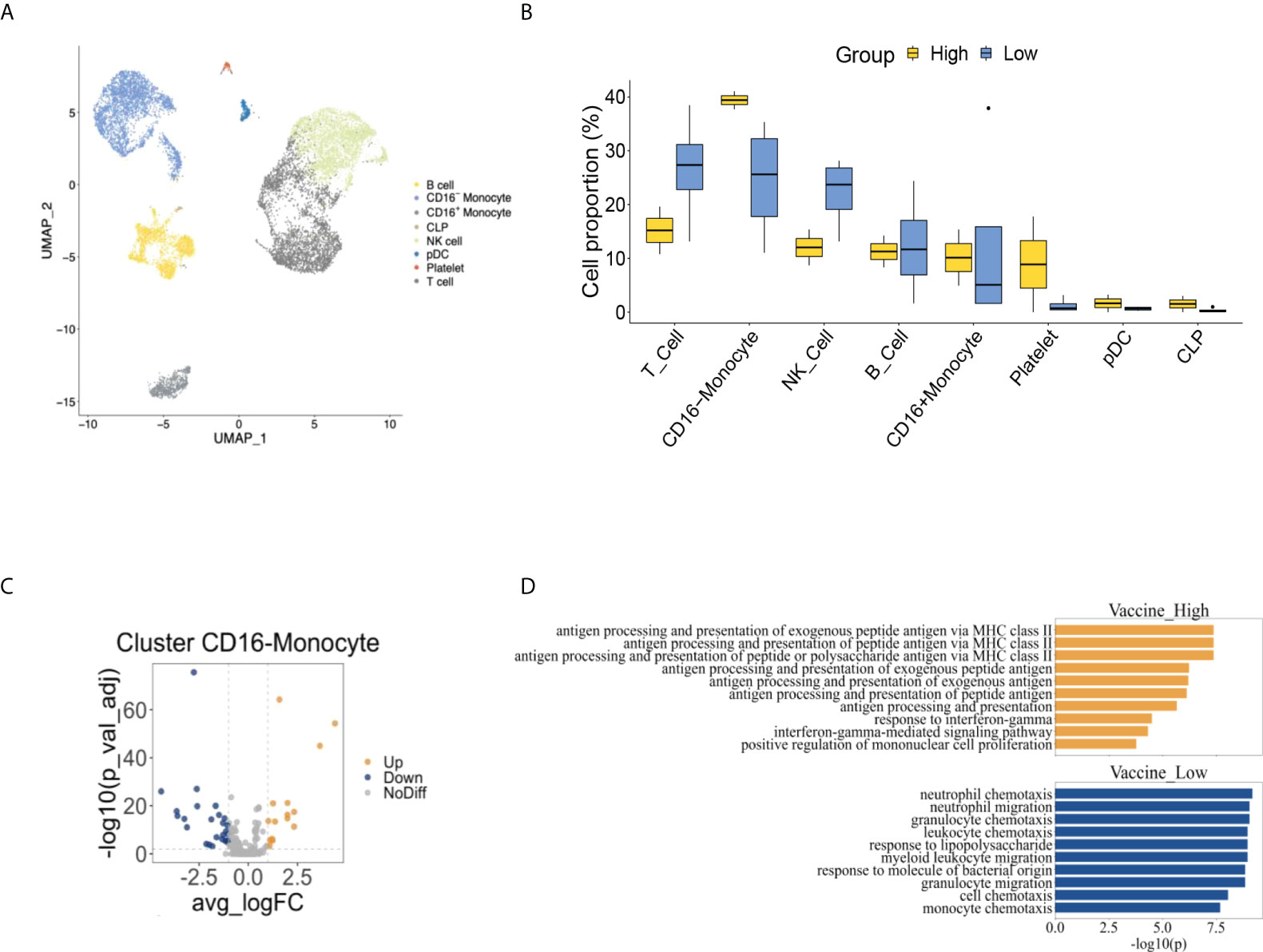
Figure 2 The comprehensive cell atlas of peripheral blood mononuclear cells of rheumatoid arthritis patients with high and low anti-SARS-CoV2-IgG antibodies. (A) UMAP visualization of peripheral blood mononuclear cells from rheumatoid arthritis patients. (B) The proportion of cell types between high and low anti-SARS-CoV2-IgG antibody groups. (C) Volcano plot of CD16-monocyte showed differential expressed genes of high anti-SARS-CoV2-IgG antibody group comparing to low anti-SARS-CoV2-IgG antibody group. (D) Pathway analysis between high and low anti-SARS-CoV2-IgG antibody groups.
Comparisons of the cell composition between healthy controls and RA patients following COVID-19 vaccination by scRNA-seq
To compare the immunogenicity following COVID vaccination between healthy controls and patients with RA, we analyzed scRNA-seq data from public dataset (GSE201534) and our cohort. As depicted in Figures 3A–C, cell atlas and the cell proportions among each participant of our study and GSE201534 demonstrated an increased CD16- monocytes but decreased T cell composition between RA patients and heathy controls.
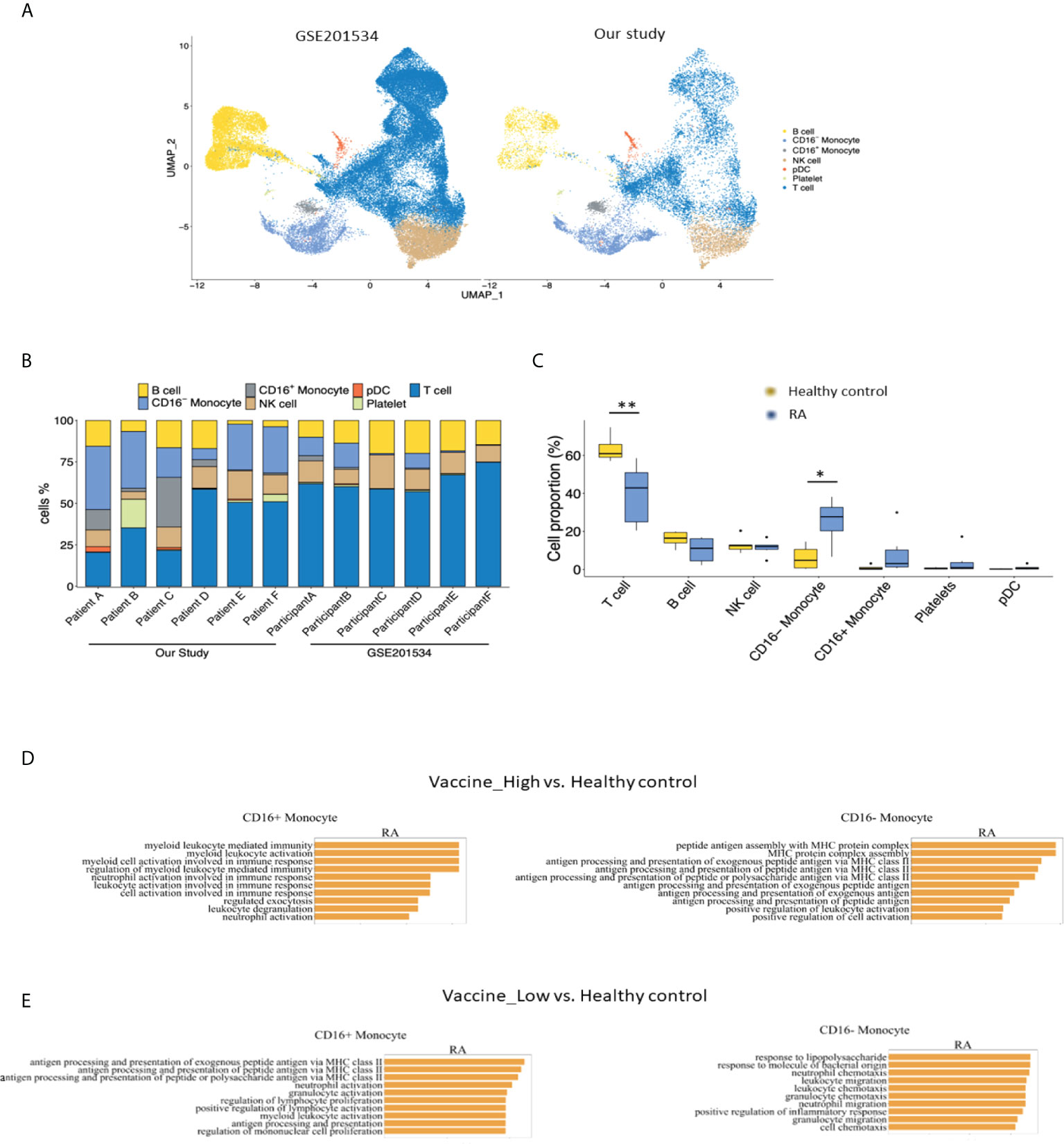
Figure 3 Comparisons of (A) cell atlas of peripheral blood mononuclear cells, (B) the proportions of cell types from each participant and (C) healthy controls from GSE201534 vs. patients with rheumatoid arthritis from our study. Pathway analysis of CD16+ and CD16-monocyte between (D) high anti-SARS-CoV2-IgG antibody group and (E) low anti-SARS-CoV2-IgG antibody group from our study vs. healthy control from GSE201534. .
Enriched pathways between high and low anti-SARS-CoV2-IgG antibody groups in RA patients and healthy controls
We further examined the differentially expressed genes (DEGs) in each major cell population between high and low anti-SARS-CoV2-IgG antibody groups in RA patients and found there were relatively more enriched DEGs in CD16- monocytes than other cells (Supplementary Figure 4). We identified 15 up-regulated and 26 down-regulated DEGs between the two groups in CD16-monocytes (Figure 2C). Through pathway analysis, pathways related to antigen presentation via major histocompatibility complex class II (MHC class II) and response to interferon gamma (IFNγ) were enriched in CD16-monocytes in high anti-SARS-CoV2-IgG antibody group, while those related to chemotaxis were augmented in low anti-SARS-CoV2-IgG antibody group (Figure 2D).
In addition, pathways involved in antigen assembly and processing via MHC class II were enriched in CD16- monocytes from high vaccine immunogenicity RA group in comparisons to healthy controls (Figure 3D). Similarly, pathways related to chemotaxis were differentially enriched in CD16- monocytes from low vaccine immunogenicity RA patients as compared to healthy controls (Figure 3E).
Furthermore, we also performed analysis on B cells since B cells were the main cell type for antibodies production. However, there were no significant cell proportion difference in B cell sub-groups between high and low anti-SARS-CoV-2 IgG groups, and no DEGs could be found between the two groups, either (Supplementary Figure 5).
Different cell-cell interaction between high and low anti-SARS-CoV2-IgG antibody groups in RA patients
Through cell-cell interaction analysis, we found nineteen interactions enriched significantly in AIRD patients with high anti-SARS-CoV-2 IgG level, while nine interactions enriched in those with low anti-SARS-CoV-2 IgG level (Supplementary Figure 6). There were stronger interactions via MHC class II pathway between CD16-monocytes, CD16+monocytes, B cells and pDCs in high anti-SARS-CoV2-IgG antibody group than in low anti-SARS-CoV2-IgG antibody group (Figure 4). HLA-DRA and CD4 interaction was vigorous among all identified MHC class II pathway and was more enhanced in high anti-SARS-CoV2-IgG antibody group than in low antibody group (Figure 4B). The other crosstalks between high and low anti-SARS-CoV2-IgG antibody groups among each cell populations in RA patients via IFNγ and CCL pathway, and the associated ligand-receptor interactions were shown in Supplementary Figure 7.
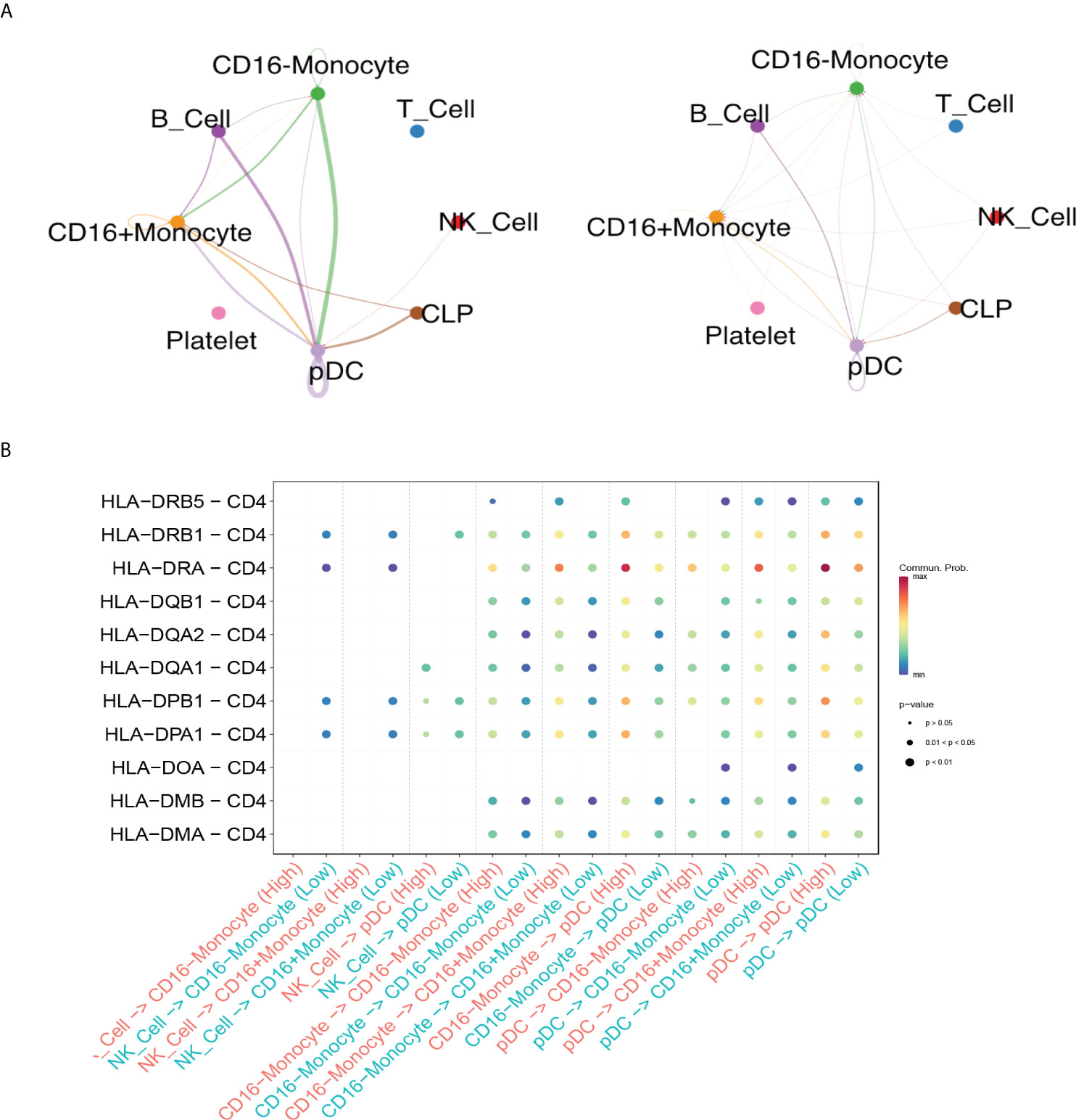
Figure 4 Differential crosstalks between high and low anti-SARS-CoV2-IgG antibody groups among each cell populations in rheumatoid arthritis patients. (A) Circle plot shows the MHC class II pathway outgoing and incoming signaling among high anti-SARS-CoV2-IgG antibody group (left) and low anti-SARS-CoV2-IgG antibody group (right); (B) Bubble plot shows the selected ligand-receptor interactions between NK cells, monocytes and pDC.
Discussion
Our study demonstrated an anti-SARS-CoV-2 IgG seropositivity rate of 85.34% and 98.20% in AIRD patients and in healthy controls, respectively. The serum anti-SARS-CoV-2 IgG level after the first dose of the vaccine was higher in participants who received mRNA-1273 than in those who received AZD1222 vaccine; however, the IgG level was lower in patients who received a prednisolone-equivalent dose >5 mg/day or MTX than in non-users. In RA patients, a markedly lower anti-SARS-CoV-2 IgG level was observed in participants who were treated with non-anti-TNF-α bDMARDs and JAK inhibitors. Higher proportion of CD16- monocytes were observed in RA patients following COVID-19 vaccination in comparison to healthy controls. Pathways related to antigen presentation via MHC class II in CD16- monocytes, especially HLA-DRA and CD4 interaction, might be associated with higher anti-SARS-CoV2-IgG level in RA patients.
In the present study, we found that anti-SARS-CoV-2 IgG levels were remarkably higher after the first dose in rheumatic disease patients who received mRNA-1273 vaccines than in those who received AZD1222. Prendecki et al. demonstrated that, in patients with rheumatic disease, the anti-SARS-CoV-2 IgG levels of those who received BNT2b162 were numerically higher than those of patients who received the vector vaccine AZD1222 (22). Other reports have revealed similar information among the general population. One study in Germany showed that, between weeks 6 and 15, the anti-spike IgG level was higher after the first vaccination with the mRNA-based vaccines mRNA-1273 or BNT162b2, than with the vector vaccine AZD1222 (23). Among individuals who received AZD1222 as their first vaccine, a strong increase in the anti-spike IgG level was observed in those who received a subsequent dose of mRNA-based vaccine than a vector vaccine. However, the correlation between vaccine types and the anti-SARS-CoV-2 IgG level remains controversial, and there is a paucity of data in immunocompromised populations. Furthermore, differences in the neutralization capacities, along with the clinical influence of anti-spike IgG, between different vaccine types are unclear. Further research based on the impact of different vaccine types in patients with rheumatic disease are warranted to provide information for policymakers with regard to vaccine allocation in high-risk populations.
Our results demonstrated that, compared with non-users, the anti-SARS-CoV-2 IgG level was lower in AIRD patients receiving a prednisolone-equivalent glucocorticoid dose >5 mg/day or MTX. The initial analysis of the COVaRiPAD study revealed fewer circulating plasmablasts and a 10-fold reduction of antibody response following mRNA-based vaccination in patients with rheumatic disease receiving glucocorticoids (24). Furthermore, the seropositivity rate decreased to 65% in patients treated with MTX but returned to 92% after discontinuation of prednisolone. Similar findings have been reported from SLE patients following influenza vaccination when a prednisolone dose >20 mg/day was administered (25). We proposed that, in patients with stable underlying rheumatic disease, a pre-vaccination reduction in the glucocorticoid dose could elicit adequate immunogenicity. The dose-dependent effect of glucocorticoids on immunogenicity following COVID-19 vaccination warrants further investigation. Furthermore, Haberman et al. reported that, in a New York cohort, the seropositivity rate in patients with rheumatic disease who were treated with MTX was remarkably lower than that of patients without MTX use (72.0% vs. 92.3%, p=0.023), and the anti-SARS-CoV-2 IgG level tended to be lower in patients with rheumatic disease who were treated with MTX (9). Additionally, MTX use was associated with attenuated CD8+ T-cell activation, which plays a protective role against SARS-CoV-2 infection in a study of rhesus macaques (26). Therefore, impaired antibody production as well as attenuation of CD8+ T-cell response by MTX diminished the immunogenicity of COVID-19 vaccines in patients with rheumatic disease. Nonetheless, it remains unclear whether the temporary discontinuation of MTX could improve the immunogenicity of COVID-19 vaccines, although this possibility is conceivable because higher antibody levels were achieved in RA patients after MTX discontinuation for 2 weeks for influenza vaccination (27). Accordingly, additional vaccine boosters could be considered in patients treated with glucocorticoids or MTX to achieve adequate immunization against SARS-CoV-2.
A notably lower level of anti-SARS-CoV-2 IgG was observed in RA patients treated with JAK inhibitors. However, only a few studies have investigated the impact of JAK inhibitors on the efficacy of COVID-19 vaccination with contradictory results. Deepak et al. suggested that the antibody titers might be reduced 6.6-fold in patients using JAK inhibitors (24). JAK inhibitors target small molecules and are associated with alteration of interferon signaling, change in T- and B-cell counts, and reduction of natural killer cell counts, all of which might lead to decreased immunogenicity after vaccination (25, 28). Nonetheless, Furer et al. reported that there was no difference in the seropositivity rate after BNT162b2 vaccination between rheumatic patients using JAK inhibitors and healthy controls (8). In studies investigating the influenza and pneumococcal vaccine response, immunogenicity was unaltered by JAK inhibitor treatment (29, 30). Further studies are needed to explore the clinical impact of JAK inhibitors on the immunogenicity of COVID-19 vaccination.
Our study revealed that pathways related to antigen presentation via MHC class II in CD16-monocytes, especially HLA-DRA and CD4 interaction, and pathways response to IFNγ might be associated with higher anti-SARS-CoV2-IgG level in RA patients, while pathways related to chemotaxis were kept in those with low anti-SARS-CoV2-IgG antibody. Sureshchandra S et al. demonstrated increasing spike-specific B cells, antigen-specific CD4+ T cells, and CD8+ T cells by scRNA-seq analysis in healthy subjects after mRNA vaccines and those after COVID-19 infection (31). Kramer KJ and the colleagues focused on adaptive immunity and indicated enlarged population of antigen-specific memory CD4+ and CD8+ T cells, and IgA and IgG memory B cells specific to SARS-CoV-2 in healthy participants after BNT162b2 mRNA vaccines (32). Those studies highlighted the importance of antigen presentation and antibodies productions following mRNA vaccines in healthy individuals. In RA patients after COVID-19 vaccination, we found CD16- monocytes might be one of the key players in antigen presentation. Generally, CD16-monocytes were classical monocytes and were considered to be primed for chemotaxis and phagocytosis, while intermediate monocytes were responsible for antigen presentation (33, 34). Nevertheless, our results and analysis of public datasets (GSE201534) might imply that more CD16-monocytes in RA patients with high anti-SARS-CoV-2 IgG level turned to perform antigen presentation via HLA-DRA of MHC class II rather than chemotaxis. Lee J et al. demonstrated that although the intermediate monocytes exhibited the most MHC molecules, classical monocytes might be recruited as antigen presentation cells and express MHC class II to a great extent after stimulation of inflammatory cytokines, such as IFNγ, granulocyte-macrophage colony-stimulating factor (GM-CSF) and IL-4 (35). Since HLA-DRA was one of the MHC class II alpha subunits, which might interact with CD4+ cells and further promote the antibodies production by B cells. Lower level of anti-SARS-CoV-2 IgG and less enriched pathways related antigen presentation in CD6-monocytes were detected in RA patients using non-anti-TNF-α bDMARDs and JAK inhibitors. JAK inhibitors could repress JAK-STAT pathway mediated by GM-CSF in innate immune cells including monocytes. Multiple inflammatory cytokines such as GM-CSF and interferon were direct targets by JAK inhibitors and may possibly suppress the promotion of antigen presentation function in CD16-monocytes (36).
This study has some limitations. First, our study was limited in the sample size, and a selection bias might exist. We are still expanding the case numbers and more studies are underway. Second, most participants in our study received the first dose of COVID-19 vaccine but not the second dose due to a shortage of vaccine supply in Taiwan during the initial period of study enrollment. Third, the neutralizing capacity and T-cell response were not evaluated in our study. As a humoral immune response does not necessarily represent the complete picture of anti-COVID immunity and the efficacy of COVID-19 vaccines, further research is needed to explore the issue.
Conclusion
After the first dose COVID-19 vaccination, the anti-SARS-CoV-2 IgG level in patients with rheumatic diseases receiving mRNA-1273 was higher than that with AZD1222, and mRNA-based vaccines could be prioritized for AIRD patients to elicit adequate humoral immunity against SARS-CoV-2. A prednisolone-equivalent glucocorticoid dose >5 mg/day, MTX use, and RA patients treated with non-anti-TNF-α bDMARDs or JAK inhibitors were risk factors for lower immunogenicity. Moreover, enriched pathways related to antigen presentation via MHC class II in CD16-monocytes might be associated with higher anti-SARS-CoV2-IgG level in RA patients.
Data availability statement
The datasets presented in this study can be found in online repositories. The name of the repository and accession number can be found below: NCBI Gene Expression Omnibus, accession number GSE203081.
Ethics statement
This study was conducted in accordance with the Declaration of Helsinki and was approved (CF21297B) by the Institutional Review Board of Taichung Veterans General Hospital, Taiwan. The patients/participants provided their written informed consent to participate in this study.
Author contributions
Y-MC, T-HH, and Y-JC conceived and designed the study. Y-MC and Y-JC performed the literature search, interpretation of data and drafted the manuscript. P-LC, H-WC, and J-PC conducted data extraction, methodological quality assessments and performed the analysis. P-LC performed the single-cell RNA sequencing experiments. Y-HC, W-NH, H-HC, C-TL, K-TT, W-TH, and T-YH performed critical revision of the manuscript for important intellectual content. All authors read and approved the final version of submitted manuscript.
Funding
This study was financially supported by grants from Taichung Veterans General Hospital (TCVGH-1107302D) and Ministry of Science and Technology (111-2314-B-075A-003 -MY3), Taiwan.
Acknowledgments
The authors thank the Biostatistics Task Force of Taichung Veterans General Hospital for their assistance with the statistical analysis in this study.
Conflict of interest
The authors declare that the research was conducted in the absence of any commercial or financial relationships that could be construed as a potential conflict of interest.
Publisher’s note
All claims expressed in this article are solely those of the authors and do not necessarily represent those of their affiliated organizations, or those of the publisher, the editors and the reviewers. Any product that may be evaluated in this article, or claim that may be made by its manufacturer, is not guaranteed or endorsed by the publisher.
Supplementary material
The Supplementary Material for this article can be found online at: https://www.frontiersin.org/articles/10.3389/fimmu.2022.920865/full#supplementary-material
References
1. Safiri S, Kolahi AA, Hoy D, Smith E, Bettampadi D, Mansournia MA, et al. Global, regional and national burden of rheumatoid arthritis 1990-2017: a systematic analysis of the global burden of disease study 2017. Ann Rheum Dis (2019) 78:1463–71. doi: 10.1136/annrheumdis-2019-215920
2. Mehrmal S, Uppal P, Nedley N, Giesey RL, Delost GR. The global, regional, and national burden of psoriasis in 195 countries and territories, 1990 to 2017: A systematic analysis from the global burden of disease study 2017. J Am Acad Dermatol (2021) 84:46–52. doi: 10.1016/j.jaad.2020.04.139
3. Rutherford MA, Scott J, Karabayas M, Antonelou M, Gopaluni S, Gray D, et al. UK And Ireland vasculitis rare disease group (UKIVAS). risk factors for severe outcomes in patients with systemic vasculitis and COVID-19: A binational, registry-based cohort study. Arthritis Rheumatol (2021) 73:1713–9. doi: 10.1002/art.41728
4. Williamson EJ, Walker AJ, Bhaskaran K, Bacon S, Bates C, Morton CE, et al. Factors associated with COVID-19-related death using OpenSAFELY. Nature (2020) 584:430–6. doi: 10.1038/s41586-020-2521-4
5. Gisondi P, Piaserico S, Naldi L, Dapavo P, Conti A, Malagoli P, et al. Incidence rates of hospitalization and death from COVID-19 in patients with psoriasis receiving biological treatment: A northern Italy experience. J Allergy Clin Immunol (2021) 147:558–560.e1. doi: 10.1016/j.jaci.2020.10.032
6. Brenner EJ, Ungaro RC, Gearry RB, Kaplan GG, Kissous-Hunt M, Lewis JD, et al. Corticosteroids, but not TNF antagonists, are associated with adverse COVID-19 outcomes in patients with inflammatory bowel diseases: Results from an international registry. Gastroenterology (2020) 159:481–491.e3. doi: 10.1053/j.gastro.2020.05.032
7. Geisen UM, Berner DK, Tran F, Sumbul M, Vullriede L, Ciripoi M, et al. Immunogenicity and safety of anti-SARS-CoV-2 mRNA vaccines in patients with chronic inflammatory conditions and immunosuppressive therapy in a monocentric cohort. Ann Rheum Dis (2021) 80:1306–11. doi: 10.1136/annrheumdis-2021-220272
8. Furer V, Eviatar T, Zisman D, Peleg H, Paran D, Levartovsky D, et al. Immunogenicity and safety of the BNT162b2 mRNA COVID-19 vaccine in adult patients with autoimmune inflammatory rheumatic diseases and in the general population: a multicentre study. Ann Rheum Dis (2021) 80:1330–8. doi: 10.1136/annrheumdis-2021-220647
9. Haberman RH, Herati R, Simon D, Samanovic M, Blank RB, Tuen M, et al. Methotrexate hampers immunogenicity to BNT162b2 mRNA COVID-19 vaccine in immune-mediated inflammatory disease. Ann Rheum Dis (2021) 80:1339–44. doi: 10.1136/annrheumdis-2021-220597
10. Arnett FC, Edworthy SM, Bloch DA, McShane DJ, Fries JF, Cooper NS, et al. The American rheumatism association 1987 revised criteria for the classification of rheumatoid arthritis. Arthritis Rheum (1988) 31:315–24. doi: 10.1002/art.1780310302
11. Aletaha D, Neogi T, Silman AJ, Funovits J, Felson DT, Bingham CO 3rd, et al. 2010 Rheumatoid arthritis classification criteria: an American college of Rheumatology/European league against rheumatism collaborative initiative. Arthritis Rheum (2010) 62:2569–81. doi: 10.1002/art.27584
12. Hochberg MC. Updating the American college of rheumatology revised criteria for the classification of systemic lupus erythematosus. Arthritis Rheum (1997) 40:1725. doi: 10.1002/art.1780400928
13. Petri M P, Orbai AM, Alarcón GS, Gordon C, Merrill JT, Fortin PR, et al. Derivation and validation of the systemic lupus international collaborating clinics classification criteria for systemic lupus erythematosus. Arthritis Rheum (2012) 64:2677–86. doi: 10.1002/art.34473
14. Shiboski CH, Shiboski SC, Seror R, Criswell LA, Labetoulle M, Lietman TM, et al. 2016 American college of Rheumatology/European league against rheumatism classification criteria for primary sjögren’s syndrome: A consensus and data-driven methodology involving three international patient cohorts. Ann Rheum Dis (2017) 76:9–16. doi: 10.1136/annrheumdis-2016-210571
15. Lundberg IE, Tjärnlund A, Bottai M, Werth VP, Pilkington C, Visser M, et al. International myositis classification criteria project consortium, the euromyositis register and the juvenile dermatomyositis cohort biomarker study and repository (JDRG) (UK and ireland). 2017 European league against Rheumatism/American college of rheumatology classification criteria for adult and juvenile idiopathic inflammatory myopathies and their major subgroups. Ann Rheum Dis (2017) 76:1955–64. doi: 10.1136/annrheumdis-2017-211468
16. Taylor W, Gladman D, Helliwell P, Marchesoni A, Mease P, Mielants H, et al. Classification criteria for psoriatic arthritis: development of new criteria from a large international study. Arthritis Rheum (2006) 54:2665–73. doi: 10.1002/art.21972
17. Butler A, Hoffman P, Smibert P, Papalexi E, Satija R. Integrating single-cell transcriptomic data across different conditions, technologies, and species. Nat Biotechnol (2018) 36:411–20. doi: 10.1038/nbt.4096
18. Hafemeister C, Satija R. Normalization and variance stabilization of scRNA-seq data using regularized negative binomial regression. Genome Biol (2019) 20:296. doi: 10.1186/s13059-019-1874-1
19. Zhang X, Lan Y, Xu J, Quan F, Zhao E, Deng C, et al. CellMarker: a manually curated resource of cell markers in human and mouse. Nucleic Acids Res (2019) 47:D721–8. doi: 10.1093/nar/gky900
20. Kiselev VY, Yiu A, Hemberg M. Scmap: projection of single-cell RNA-seq data across data sets. Nat Methods (2018) 15:359–62. doi: 10.1038/nmeth.4644
21. Jin S, Guerrero-Juarez CF, Zhang L, Chang I, Ramos R, Kuan CH, et al. Inference and analysis of cell-cell communication using CellChat. Nat Commun (2021) 12:1088. doi: 10.1038/s41467-021-21246-9
22. Prendecki M, Clarke C, Edwards H, McIntyre S, Mortimer P, Gleeson S, et al. Humoral and T-cell responses to SARS-CoV-2 vaccination in patients receiving immunosuppression. Ann Rheum Dis (2021) 80:1322–9. doi: 10.1136/annrheumdis-2021-220626
23. Fabricius D, Ludwig C, Scholz J, Rode I, Tsamadou C, Jacobsen EM, et al. mRNA vaccines enhance neutralizing immunity against SARS-CoV-2 variants in convalescent and ChAdOx1-primed subjects. Vaccines (Basel) (2021) 9:918. doi: 10.3390/vaccines9080918
24. Deepak P, Kim W, Paley MA, Yang M, Carvidi AB, El-Qunni AA, et al. Glucocorticoids and b cell depleting agents substantially impair immunogenicity of mRNA vaccines to SARS-CoV-2. medRxiv [Preprint] (2021):2021.04.05.21254656. doi: 10.1101/2021.04.05.21254656
25. Hodge JA, Kawabata TT, Krishnaswami S, Clark JD, Telliez JB, Dowty ME, et al. The mechanism of action of tofacitinib - an oral janus kinase inhibitor for the treatment of rheumatoid arthritis. Clin Exp Rheumatol (2016) 34:318–28.
26. McMahan K, Yu J, Mercado NB, Loos C, Tostanoski LH, Chandrashekar A, et al. Correlates of protection against SARS-CoV-2 in rhesus macaques. Nature (2021) 590:630–4. doi: 10.1038/s41586-020-03041-6
27. Park JK, Lee YJ, Shin K, Ha YJ, Lee EY, Song YW, et al. Impact of temporary methotrexate discontinuation for 2 weeks on immunogenicity of seasonal influenza vaccination in patients with rheumatoid arthritis: a randomised clinical trial. Ann Rheum Dis (2018) 77:898–904. doi: 10.1136/annrheumdis-2018-213222
28. Winthrop KL, Korman N, Abramovits W, Rottinghaus ST, Tan H, Gardner A, et al. T-Cell-mediated immune response to pneumococcal conjugate vaccine (PCV-13) and tetanus toxoid vaccine in patients with moderate-to-severe psoriasis during tofacitinib treatment. J Am Acad Dermatol (2018) 78:1149–1155.e1. doi: 10.1016/j.jaad.2017.09.076
29. Winthrop KL, Silverfield J, Racewicz A, Neal J, Lee EB, Hrycaj P, et al. The effect of tofacitinib on pneumococcal and influenza vaccine responses in rheumatoid arthritis. Ann Rheum Dis (2016) 75:687–95. doi: 10.1136/annrheumdis-2014-207191
30. Winthrop KL, Bingham CO 3rd, Komocsar WJ, Bradley J, Issa M, Klar R, et al. Evaluation of pneumococcal and tetanus vaccine responses in patients with rheumatoid arthritis receiving baricitinib: results from a long-term extension trial substudy. Arthritis Res Ther (2019) 21:102. doi: 10.1186/s13075-019-1883-1
31. Sureshchandra S, Lewis SA, Doratt BM, Jankeel A, Coimbra Ibraim I, Messaoudi I. Single-cell profiling of T and b cell repertoires following SARS-CoV-2 mRNA vaccine. JCI Insight (2021) 6:e153201. doi: 10.1172/jci.insight.153201
32. Kramer KJ, Wilfong EM, Voss K, Barone SM, Shiakolas AR, Raju N, et al. Single-cell profiling of the antigen-specific response to BNT162b2 SARS-CoV-2 RNA vaccine. Nat Commun (2022) 13:3466. doi: 10.1038/s41467-022-31142-5
33. Ma L, Li H, Lan J, Hao X, Liu H, Wang X, et al. Comprehensive analyses of bioinformatics applications in the fight against COVID-19 pandemic. Comput Biol Chem (2021) 95:107599. doi: 10.1016/j.compbiolchem.2021.107599
34. Noé A, Cargill TN, Nielsen CM, Russell AJC, Barnes E. The application of single-cell RNA sequencing in vaccinology. J Immunol Res (2020) 2020:8624963. doi: 10.1155/2020/8624963
35. Lee J, Tam H, Adler L, Ilstad-Minnihan A, Macaubas C, Mellins ED. The MHC class II antigen presentation pathway in human monocytes differs by subset and is regulated by cytokines. PloS One (2017) 12:e0183594. doi: 10.1371/journal.pone.0183594
Keywords: COVID-19 vaccine, rheumatic disease, autoimmune, anti-rheumatic medications, single-cell RNA sequencing
Citation: Chen Y-J, Cheng P-L, Huang W-N, Chen H-H, Chen H-W, Chen J-P, Lin C-T, Tang K-T, Hung W-T, Hsieh T-Y, Chen Y-H, Chen Y-M and Hsiao T-H (2022) Single-cell RNA sequencing to decipher the immunogenicity of ChAdOx1 nCoV-19/AZD1222 and mRNA-1273 vaccines in patients with autoimmune rheumatic diseases. Front. Immunol. 13:920865. doi: 10.3389/fimmu.2022.920865
Received: 15 April 2022; Accepted: 12 July 2022;
Published: 01 August 2022.
Edited by:
Huji Xu, Tsinghua University, ChinaReviewed by:
Zihan Zheng, Southwest Hospital, Army Medical University, ChinaLiyun Zou, Army Medical University, China
Copyright © 2022 Chen, Cheng, Huang, Chen, Chen, Chen, Lin, Tang, Hung, Hsieh, Chen, Chen and Hsiao. This is an open-access article distributed under the terms of the Creative Commons Attribution License (CC BY). The use, distribution or reproduction in other forums is permitted, provided the original author(s) and the copyright owner(s) are credited and that the original publication in this journal is cited, in accordance with accepted academic practice. No use, distribution or reproduction is permitted which does not comply with these terms.
*Correspondence: Yi-Ming Chen, eW1jaGVuMUB2Z2h0Yy5nb3YudHc=
†These authors have contributed equally to this work and share senior authorship