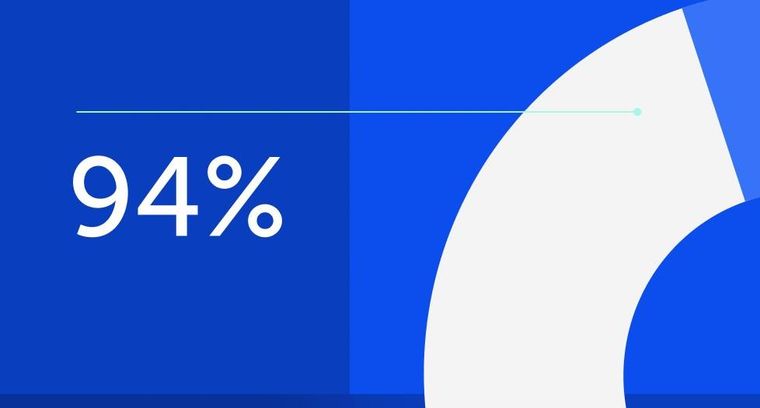
94% of researchers rate our articles as excellent or good
Learn more about the work of our research integrity team to safeguard the quality of each article we publish.
Find out more
REVIEW article
Front. Immunol., 11 August 2022
Sec. Inflammation
Volume 13 - 2022 | https://doi.org/10.3389/fimmu.2022.919973
This article is part of the Research TopicTermination of Pro-Inflammatory Signaling and Its Dysregulation in Autoimmune DiseasesView all 7 articles
Interleukin-18 (IL-18) is a potent pro-inflammatory cytokine involved in host defense against infections and regulates the innate and acquired immune response. IL-18 is produced by both hematopoietic and non-hematopoietic cells, including monocytes, macrophages, keratinocytes and mesenchymal cell. IL-18 could potentially induce inflammatory and cytotoxic immune cell activities leading to autoimmunity. Its elevated levels have been reported in the blood of patients with some immune-related diseases, including rheumatoid arthritis, systemic lupus erythematosus, type I diabetes mellitus, atopic dermatitis, psoriasis, and inflammatory bowel disease. In the present review, we aimed to summarize the biological properties of IL-18 and its pathological role in different autoimmune diseases. We also reported some monoclonal antibodies and drugs targeting IL-18. Most of these monoclonal antibodies and drugs have only produced partial effectiveness or complete ineffectiveness in vitro, in vivo and human studies. The ineffectiveness of these drugs targeting IL-18 may be largely due to the loophole caused by the involvement of other cytokines and proteins in the signaling pathway of many inflammatory diseases besides the involvement of IL-18. Combination drug therapies, that focus on IL-18 inhibition, in addition to other cytokines, are highly recommended to be considered as an important area of research that needs to be explored.
Interleukin 18 belongs to the IL-1 family of cytokines, which is a group comprising 11 member cytokines that promote the activity of the innate immune system (1, 2). IL-18 stimulates both the innate immune and acquired immune responses. It acts on T helper 1 (Th1) cells, macrophages, Natural killer (NK) cells, natural killer T (NKT) cells, B cells, dendritic cells (DCs), and even non-polarized T cells to produce interferon gamma (IFN-γ) in the presence of IL-12. In the absence of IL-12, IL-18 with IL-2, induces type 2 T helper (Th2) cytokines from NK cells, NKT cells with a CD4+ phenotype, and even committed Th1 cells. Additionally, IL-18, in synergy with IL-3, induces basophils and mast cells to produce IL-4 and IL-13 (3, 4). IL-18 displays its pleiotropic action depending on its cytokine milieu suggesting its important pathophysiological role in health and disease (5).
The activity of IL-18 in both the innate and adaptive immune response implicates it in several inflammatory and autoimmune conditions (6). IL-18 levels are usually elevated in psoriasis (7), systemic lupus erythematosus (SLE) (8, 9), hypertension, chronic kidney disease (10), multiple sclerosis (MS) patients (11, 12) and Coronavirus disease 2019 (COVID-19) (13, 14) which correlates with caspase-1 levels (15). In a mouse model of autoimmune diabetes, IL-18 messenger RNA (mRNA) expression strongly correlated with destructive insulitis most likely due to IFN-γ secretion (16, 17). It was also shown that IL-18-/- NOD mice developed less reactive islet cells than NOD wild type mice (18). To further strengthen its role in autoimmune diseases, IL18-/- mice showed better disease outcome in Collagen-induced arthritis model in mice (19). In addition, IL18-/- mice did not develop disease in animal models of experimental autoimmune encephalitis (20, 21) and experimental autoimmune myasthenia gravis (EAMG) (22). Gene expression analysis in pregnant women revealed more than four times higher expression of IL-18 in patients experiencing recurrent miscarriage (23). This clearly shows that IL-18 cytokine has a role in the progression or development of some inflammatory and autoimmune diseases.
Since the introduction of the hybridoma technique in 1975 by Kohler and Milstein (24), more than 550 therapeutic antibodies targeting specific antigens have been studied and at least 70 approved for clinical use (25). Generation of humanized antibodies (26) brought about great advances in this field (27). These antibodies and other biologics can bind to their antigens with high fidelity and affect disease processes or by modulating immune responses (28) and hence, provide powerful tools in the management of chronic conditions such as cancer and autoimmune diseases (29).
In this review, we will provide some information about the production, activation, signaling, and pathophysiology of IL-18. We will also report and discuss the used monoclonal antibodies, inhibitors, and drugs targeting this cytokine.
IL-18 was initially called “IFN-γ‐inducing factor” (30) due to its action to induce IFN-γ secretion in CD3-stimulated Th1 cells leading to liver toxicity. Subsequently, it was refined from mouse liver cells treated with Proprionibacterium acnes and lipopolysaccharides (LPS), with the isolate then renamed IL-18 (31, 32). At first, the liver toxicity resulting from the induction of IFN-γ secretion was attributed to IL-12, but was found to be prevented by an IL-18 antibody. Further research showed that IL-18 deficient mice primed with LPS did not develop liver damage (33, 34). The mechanism for the liver toxicity was found out to be the induction of Fas ligand (FasL) expression and tumor necrosis factor alpha (TNF-α) production in liver NK cells (33–36). Human IL-18 is a 193 amino acid-protein while mouse IL-18 consist of 192 amino acids (30, 37).
Unexpectedly, IL-18 was found to share similarities with IL-1β and the IL-1 family in 4 ways: 1) homology in the amino acid sequence 2) they share a common β-pleated sheet structure, 3) they are all secreted as an inactive precursor, and 4) they have similar signaling pathways (3, 38–40). Apart from these similarities, other features between IL-18 and IL-1β are remarkably distinctive. As the name indicates, IL-1 was the first member of the family to be discovered and it has been extensively studied in various immune processes and disease conditions (1).
The receptors of the IL-1 family contain an extracellular immunoglobulin domain and a Toll/IL-1 receptor (TIR) cytoplasmic domain. Binding of the ligand to the appropriate receptor recruits a second receptor subunit. The receptor heterodimer formed and the alignment of two TIR domains facilitates the recruitment of myeloid differentiation primary response protein 88 (Myd88), IL-1R-associated kinase 4 (IRAK4), TNFR-associated factor 6 (TRAF6), and other signaling molecules. The interaction usually engages the activation of the nuclear factor-κB (NF-κB) and mitogen-activated protein kinase (MAPK) pathways (41–43).
The members of the IL-1 family of cytokines are released in response to Toll like receptor (TLR) signaling to amplify the danger messages to other cells that cannot recognize microbial antigens (due to the lack of certain receptors). Basically, these cytokines stimulate the innate immune system and serve a critical link between innate immune responses and the appropriate adaptive immune response. The members of the IL-1 family, IL-18 inclusive, are produced by neutrophils, monocytes, and macrophages. The IL-1 family members also respond to stimulation by IL-1, IL-18, and IL-33 (44–47).
Unlike members of the IL-1 family of cytokines, IL‐18 gene in humans is located on chromosome 11, and in mice it is found on chromosome 9. The gene contains 7 exons with two distinct promoters on exon 1 and 2 including an interferon consensus sequence binding protein and a PU.1 binding sites (a hematopoietic-specific transcription factor) (3). Another defining feature of the IL-18 gene unlike other cytokine genes, is that it has few RNA‐destabilizing elements, and this translates to an unusually stable cytokine expression. IL‐18 gene encodes for a 193 amino acid-24 kDa inactive precursor localized in the cell cytoplasm. TLR binding of Pathogen associated molecular patterns (PAMPs) and activation of the NF‐kB pathway induces transcription of IL‐18 precursor (48).
Many cell types are capable of producing IL-18 including hematopoietic and non-hematopoietic cells (Figure 1). It was originally thought to be secreted only by Kupffer cells and liver-resident macrophages at resting stage. The IL‐18 precursor is constitutively produced in circulating monocytes, resident macrophages, and DCs, unlike IL‐1β which is not found in healthy individuals (39, 49). The IL‐18 precursor is also found to be released by most endothelial cells, keratinocytes, osteoblasts, most intestinal epithelial cells, and mesenchymal cells (48). IL‐18 can also be discharged in its precursor form from dead cells which can be acted upon by neutrophil proteases such as proteinase 3 (50) into its active form.
As with other members of the IL-1 family, IL-18 cytokine is produced in the cytoplasm as an inactive precursor named pro-IL-18. To be secreted, it requires proteolytic processing into biologically active IL-18 (3, 30, 51). It was further revealed that cleavage of pro-IL-18 (just like pro-IL-1β) into mature IL-18, relied on the action of caspase-1, an intracellular cysteine protease produced in the NACHT-LRR and pyrin domain-containing protein 3 (NLRP3) inflammasome. This complex consists of NLRP3, pro-caspase-1, and apoptosis-associated speck-like protein containing a caspase recruitment domain (adaptor molecule ASC) (52–55). Caspase 1 can be activated by several established inflammasomes and could belong either to the AIM2‐like receptors, Nod‐like receptors, or the TRIM family containing either a PYD or a CARD domain (56). The activation of Caspase 1 results in pyroptosis - a cell‐death program, which prompts the membrane pores formation and the release of mature IL‐1β and IL‐18 (5, 48). This is why many researchers use IL-18 as a marker of inflammasome activation (57)
It is widely believed that other caspase-mediated pathways are involved in IL-18 production because treatment with FasL led to stimulation of Fas-expressing macrophages to produce active IL-18 independent of caspase-1 involvement (58). It was showed that caspase-8 was involved in the Fas mediated noncanonical IL-1β and IL-18 maturation (59). Proteases that can activate IL-18 without the contribution of the inflammasome include proteinase 3 (50), chymase (60), and granzyme B (61). Recently, mitochondrial reactive oxygen species (mROS) have been shown to be critical in T-cell receptor (TCR) independent activation of IL-18 via downstream activation of STAT4 and NFkB which is regulated by Fas/FasL signaling (62)
IL-18 receptor (IL-18R) is requisite for IL-18 signaling. IL-18R is expressed in T cells and NK cells which is essential for IFN-γ production via STAT4 (Signal transducer and activator of transcription 4) signaling (63–66). It is also expressed by non-immune cells such as neurons and epithelial cells but in this case, it is involved in cell differentiation and survival (5).
The IL-18R is composed of 2 subunits - IL-18Rα chain (which is inducible and also called IL-1R-related protein or IL-1R5) and IL-18Rβ chain (which is constitutively expressed and also called IL-1R-associated protein-like or IL-R7) (3, 48). The IL-18Rα and IL-18Rβ chains are constitutive members of the IL-1R family and their cytoplasmic domains contain a TIR domain, which is shared by the TLRs (1, 3, 45, 48, 67). When triggered by IL-18, IL-18Rα forms a high-affinity binding heterodimer with IL-18Rβ that facilitates downstream signal transduction (68, 69). The TIR domains of the formed IL-18R complex (IL-18/IL-18Rα/IL-18Rβ) recruits MyD88, a signal adaptor which contains a TIR domain, thereby creating a TIR–TIR interface (1, 3, 45, 48, 67, 70) which is critical for IL-18 signaling (70). MyD88 binding subsequently recruits IRAK1, IRAK4, and TRAF6 (70). Upon binding to TRAF6, inhibitor of κB (IκB) is deactivated, this allows phosphorylated p65/p50 to translocate into the nucleus (71), which results in the activation of NFkB. Also activated are Extracellular signal-regulated Kinase (ERK), and c-jun N-terminal kinase (JNK), which make up the MAPK cascade. Eventually, these lead to the expression of appropriate genes such as Il-4, Il-13, IFN-γ, and TNF, which facilitates cell differentiation/survival, and FasL which facilitates apoptosis (33, 35, 72).
IL-18 stimulation phosphorylates and activates the members of the phosphatidylinositol-3 kinase (PI3K) family such as mammalian target of rapamycin (mTOR) and the expressions of Bcl-xL and Bcl2 (73, 74). PI3K and its pathway in myeloid cells is known to suppress inflammatory cytokine production via TLR signaling (75), but in this case, IL-18 stimulation increases the proliferation and survival of both immune and non-immune cells such as NK cells, keratinocytes, and neurons (76, 77). In neutrophils, the p38 MAP kinase pathway has been shown to be stimulated by the IL‐18/IL‐18Rα/IL‐18Rβ complex. This complex was also demonstrated to phosphorylate STAT3 in NK and hippocampal cell lines (78–80).
IL-18-dependent cell activation can be inhibited by the naturally occurring IL-18-binding protein (IL-18BP) (81) due to its high affinity for IL-18. Its binding leads to a downregulation of IL-18-induced cell responses, such as IFN-γ production. IL-37, an anti-inflammatory cytokine and a member of the IL-1 family of cytokines is another inhibitor of IL-18 (82), which binds to IL-18Rα with low affinity. This interaction recruits IL-1R8 (SIGIRR), an orphan receptor to form an IL-37/IL-18Rα/IL-1R8 complex. This complex cannot bind to MyD88 and hence cannot recruit IL-18Rβ, thereby obliterating signal transduction via IL-18R, but instead induces an anti-inflammatory signal into the cell (4, 48).
In order to trigger the innate immune system, IL-18 combines with IL-12, stimulating NK cells to respond to cancers and infections. Hence, IL-18 and IL-12 play a key role in enhancing NK cell activities. The vital role played by IL-18 in establishing NK cell activity has been demonstrated in IL-18 deficient mice, which have increased susceptibility to infection and impaired NK cell activity (83). The combined stimulation with IL-18, IL-15, and IL-12 has also been linked with the generation of memory-like NK cells (84). Similarly, IL-18 together with IL-12 can activate macrophages, which can produce IFN-γ (85). Other cell types which are capable of producing IFN-γ via the synergistic action of IL-18 and IL-12 include non-polarized T cells, Th1 cells, DCs, and B cells (4)
In the adaptive immune system, IL-18 promotes activation and the differentiation of T cells (48). IL-18 is able to upregulate the production of IFN-γ, which is required for host defense. To induce marked IFN-γ production, IL-18 requires IL-12 or IL-15 as a surrounding cytokine in synergy since they increase the expression of IL-18Rα. In vitro, the production of IFN-γ by IL-18 in combination with IL-12 occurs by acting on NF-kB in CD4+ and CD8+ T cells (3). Nevertheless, in the absence of IL-12, IL-18 induces the secretion of IL-2 and IL-13 as well as a little amount of IFN-γ in T cells stimulated with anti-CD3 antibody (86). In Th1 cells, IL-18 promotes the expression of IL-12Rβ2 while IL-12 upregulates IL-18R expression (87). IL-18 acts as a co-stimulant to amplify the production of IFN-γ, Granulocyte-Macrophage Colony Stimulating Factor (GM-CSF), IL-2, and IL-2Rα by Th1 cells but not Th2 cells. Hence, IL-18 cannot act on Th2 cells (86).
IL-18 also upregulates the cytotoxic activities of NK and CD8 T cells by killing target cells through cytotoxic molecules such as perforin or by inducing apoptosis using Fas-expressing target cells (88–90). It induces IL-4 production and may be implicated in the induction of allergic inflammation (91). In vivo, IL-18 remains a potent IFN-γ inducing factor (68) and it is highly essential for the development of NK cells but does not play a major role in the development of Th1 cells (33). It has been observed that the IL-18 mediated Th1 cell amplifying actions contributes to microbial resistance (92).
Independent of IL-12 or IL-15, IL-18 also plays a vital role as a principal protective agent in host defense. The complement system and antibodies principally respond to extracellular microbes while T and NK cells eliminate intracellular microbes. Therefore, protection against intracellular microbes is majorly dependent on NK and T cells especially Th1 that produces IFN-γ. IFN-γ is critically important in eliminating microbes via the activation of type 2 nitric oxide synthase (NOS2) (86). In a mouse model of disseminated intracellular bacterium - Mycobacterium avium infection, IL-18 has been shown to be valuable in offering protective immunity to mycobacteria through IFN-γ induction (93). Another study also observed similar expression of IL-18 and IFN-γ in children with severe Mycoplasma pneumoniae pneumonia (94). In another study in mice infected with Leishmania major (an intracellular protozoon), daily administration of IL-12 and IL-18 offered protective immunity against reinfection and inhibited the expansion of L. major infection (92).
In addition, IL-18 may play a potent role in activating CD8+ T cells, which have activity against viral infection. In mouse model infected with Herpes simplex virus (HSV), IL-18 has a protective role against viral infection (95). Epstein-Barr virus (EBV) was also showed to activate mucosal-associated invariant T cell, which were found to be a potent source of IL-18. Its dysregulation has been theorized as a mechanism for EBV associated T/NKT cell lymphoproliferative disease (96). IL-18 in collaboration with IL-12 may be involved in inhibiting IgE production (97) in an IFN-γ-dependent manner but IL-18 alone induces in vivo IgE accumulation (91). In SJL mice, IL-18 suppressed IgE production following helminth infection. The weakened IgE production was restored by the administration of anti-IL-12 and IL-18, signifying active suppression by macrophages that secrete IL-12 and IL-18 (98). Finally, IL-18, independent of IFN-γ or other cytokines, shows the characteristics of other pro-inflammatory cytokines. Some of these activities include increased cell adhesion molecule (CAM), production of chemokines and synthesis of NO (91). IL-18 has also been shown to contribute to host defense and inflammation in the Paneth cells of the intestinal mucosa in an IL-22 dependent manner via STAT3 signaling (99).
IL-18 might also play a role in tumor control and cancer chemotherapy/immunotherapy. It has been shown that cytotoxic drugs like doxorubicin, paclitaxel, topotecan, carboplatin and gemcitabine selectively co-operate with IL-18 to improve anti-tumor effectiveness (100, 101). Moreover, tumor-derived IL-18BP is an immune checkpoint molecule that inhibits IL-18 mediated anti-tumor activity in mouse and human tumors. An engineered decoy-resistant IL-18 restored IL-18 signaling and subsequent anti-tumor activity (102, 103). Not only does the cytokine improve anti-tumor activity; IL-18 and IL-12 transduced DCs also showed better recruitment of CD4 and CD8 T cells into tumor microenvironment in mouse colorectal tumor models leading to inhibition of tumor growth (104, 105)
The broad biological role of the IL-18 cytokine on immune cells have revealed its potential role in inflammatory and autoimmune diseases. IL-18 have been shown to be a diagnostic marker and predictor of inflammatory conditions like myocardial ischemia (106), acute respiratory distress syndrome (107, 108), chronic obstructive pulmonary disease (109), post infectious bronchiolitis obliterans (110) and sepsis-induced multi organ injury (111). IL-18 has also received increase attention in the pathophysiology of neuro-vascular diseases such as in intracerebral hemorrhage (112), Japanese encephalitis (113), ocular Behcet disease (114), abdominal aortic aneurysm (115), amyotrophic lateral sclerosis (116), glioma (117) and most interestingly in cognitive impairment (118) and agitation in severe mental disease (119).
Here, we mentioned the contribution of IL-18 to several autoimmune diseases. Table 1 provides a brief overview of the mechanisms of involvement of IL-18 in some autoimmune diseases and Table 2 shows some clinical studies indicating the pathogenic role of IL-18 in inflammatory diseases.
The autoimmune destruction of the host pancreatic β cells that produce insulin results in a chronic disease named Type 1 diabetes (T1D). It was suggested that inflammatory cells may invade islets, destroy β cells, and release cytokines including TNF-α, IL-1β, and IFN-γ leading to pancreatic β cells apoptosis (132). It has recently been shown that IL-18 actually maintains islet β cells function and homeostasis. IL-18 expressed on islet α cells, IL-18R on acinar cells and Na-Cl co-transporter (NCC) on β cells play a role in this homeostasis. A deficiency in NCC on β cells or IL-18R in acinar cells reduces β cell proliferation and islet size with a concomitant rise in β cell apoptosis and exocrine macrophage accumulation (146). T1D is possibly a Th1 cell-mediated disease (147) that affects millions of people around the world and its etiology is complex with a combination of genetic and environmental pathogenic factors (148, 149). Although the Human leukocyte antigen (HLA) genes are known to have a role in the development of T1D, evidence have continued to indicate that the pro-inflammatory IL-18 contributes to the genetic susceptibility to T1D (150). Studies in both humans and mice have revealed that IL-18 genes are localized in chromosome areas associated with T1D susceptibility (151). Comprising of six exons and five introns, the IL-18 gene is located on chromosome 11q22.2-q22.3 (152). Several IL-18 polymorphisms have been identified, however the genetic relationship between single nucleotide polymorphisms (SNPs) at positions-137, -607 in IL-18 gene promoter and T1D have been of interest and is widely reported in previous studies (153). Conversely, other studies failed to show any association between T1D and these SNPs. A subclinical early report showed that serum IL-18 levels were elevated in first degree relatives of T1D patients, indicating a predictive role of IL-18 in human diseases (131). Many other studies have indicated that increased serum IL-18 levels in patients was associated with elevated glycated hemoglobin (HbA1C), which might indicate a relation between hyperglycemia and IL-18 (132). Higher serum IL-18 levels have also been linked with diabetic ketoacidosis (6, 120) and nephropathy (133). It was found that elevated IL-18 mRNA production by macrophages and a subsequent increase in IFN-γ circulating levels was correlated with an active stage of auto-immune diabetes in non-obese diabetic (NOD) mice (a well-established model for the study of autoimmune diabetes) (154). Furthermore, progression from benign to destructive insulitis have been linked with IL-18 mRNA in NOD mice (16). The administration of IL-18 via IL-18 expressing plasmid delivery to 4 weeks old NOD mice promoted the development of insulitis/diabetes (154). However, when IL-18 was administered to 10 weeks old female NOD mice, exogenously, the mice were protected from diabetes (155). Since IL-18 regulates Th1 and Th2 responses based on the cytokine present in its environment, the contrast aforementioned may be attributed to this dual role of IL-18 (86). Moreover, in another animal model of diabetes, short-term prophylactic treatment with inhibitors of IL-18 (IL-18Bp-Fc-fusion molecule) significantly protected animals from developing overt diabetes, further strengthening the evidence suggesting the role of IL-18 in T1D (156). Similar findings were observed after treatment with HIV-1 protease inhibitors in rats (157). IL-18 has also been shown to be a mediator of polycystic ovarian syndrome – a condition strongly linked to T1D (158, 159). Taken together, the results of these numerous animal and human studies suggest that IL-18 may play a pathogenic role in T1D through its interferon gamma (IFN- γ)-inducing potential. This, therefore, opens the possibility of IL-18 being a potential therapeutic target.
This is a chronic progressive autoimmune disease wherein the immune system attacks and destroys the protective myelin sheaths over the nerve fibers in the central nervous system (CNS). Inflammation plays a vital role in the advancement of MS. It is characterized by neuro-inflammatory and neuro-degenerative processes leading to the activation of auto-immune T cells and “CNS macrophages” - microglia that enhance a pathological immune response called cytokine storm (121). The SNPs- rs1946518, rs360719, and rs187238 have also been implicated in patients with MS patients (122) with high serum IL-18 levels. IL-18 and IL-1β may drive these inflammatory responses. Inflammasome is a complex intracellular receptors and stressor sensors that activate inflammatory signaling pathways vital for host defense (160). However, the dysregulation of the inflammasome can result to auto-inflammatory and auto-immune disorders (121). The NLRP3 inflammasome has been established as a critical contributor of neuro-inflammation and drives the activation of caspase 1 and the processing of IL-1β and IL-18, which subsequently mediates immune cascade responses (161). As in human MS, experimental autoimmune encephalomyelitis (EAE) in mice is also characterized by demyelinating inflammation induced by immunization with antigens (such as myelin basic protein-MBP) which serves an equivalent analogy (162). Blood caspase1 mRNA levels were increased in EAE mice which correlated with the severity of MS. This result corroborated well with the studies in humans, wherein amplified Caspase-1 levels were observed in the cerebrospinal fluid of patients with acute MS (15). In IL-18-/- mice, antibodies blocking IL-18Rα caused mice to be MS-resistant implying the existence of IL-18Rα ligands and IL-18-/- (6). It was reported that spleen cells from IL-18Rα-/- mice yielded considerably greater amount of pro-inflammatory cytokines in comparison to those from wild type or IL-18-/- mice in response to concanavalin A (con A) stimulation (163). Serum levels of IL-18 were the highest levels in patients with chronic MS compared to those relapsing-remitting MS – both acute and stable (11). Similarly, mRNA and protein levels of caspase 1 and IL-18 were identified in peripheral blood mononuclear cells in MS patients who were never administered immunomodulatory drugs (164). In summary, while data from EAE model suggests a role for IL-18R, data from acute relapsing MS patients suggests a role for IL-18 in disease advancement. This may mean that aside from IL-18, other ligands may be involved.
Myasthenia gravis (MG) is an autoantibody-mediated disease affecting the neuro-muscular junctions (NMJs). It is mediated by auto-antibodies against nicotinic AchR (acetylcholine receptor) (123). The EAMG model in mice or rats is a disease model mimicking the clinical and immunopathological characteristics of human MG (165, 166). Disease progression in both humans and mice is dependent on the production of reactive autoantibodies at the NMJ by B cells. Activated T cells help B cells proliferate and differentiate by secreting IL-12 and IFN-γ. Since IFN-γ is involved in the development of EAMG in both acute and chronic MG stages, blocking IL-18 with anti-IL-18 antibodies or disrupting CD40-CD40L interaction was found to suppress MG severity (165). The mechanism appears to be by the regulation of Th1 and CD40L levels and upregulation of TGF-β and CTLA-4 (167). Moreover, in MG patients treated with immunosuppressive drugs, serum IL-18 levels were considerably diminished (123, 168). Overall, there are only a few clinical studies showing the role played by IL-18 in contributing to the pathology of MG. This is an area that still needs to be explored.
Studies have pointed the role played by IL-18 in Inflammatory bowel disease (IBD) by promoting intestinal homeostatic auto-inflammatory responses or protecting against the breach of pathogens through the epithelial barrier (169). The SNP– IL-18 rs1946518 has been shown to be a predisposing factor to IBD development (170). IBD is a chronic complex autoimmune disease characterized by the inflammation of the intestinal mucosa. This disease may be subclassified into Crohn’s disease (CD) and ulcerative colitis which have dissimilar clinical manifestations but similarly characterized by chronic relapsing pathogenic inflammation and intestinal epithelial cell injury (171). The role of IL-18 in IBD may be primarily related to its place in regulating pro-inflammatory responses. Following inflammasome activation, pro-IL-18 promotes the production of IFN-γ, NK cell cytotoxicity and Th1 cell differentiation (172). The mitogen-activated protein kinase 2 (MAP3K2) is necessary for the IL-18-Th1 mediated intestinal inflammation (173) via the IL-18-MAP3K2-JNK axis. It was recently shown that telomere dysfunction drives ataxia-telegientaxia mutated (ATM) activation of the transcriptional factor YAP1 thereby upregulating pro-IL-18 which when stimulated by caspase 1 due to colonic microbiome drives IL-18 signaling (174). Pharmacological reactivation of telomerase activity was able to control the ATM/YAP1/pro-IL-18 axis (175). Interestingly, a deficiency in NLRP6 inflammasome, a known regulator of colonic homeostasis predominantly found in intestinal epithelial cells, is detrimental in dextran sodium sulfate (DSS) induced colitis (DSS is a frequently used mouse model for colitis in which drinking water spiked with DSS injures the intestinal epithelium like IL-18 deficiency) (169). In agreement, the favorable role of IL-18 inhibition using neutralizing anti-IL-18 antibodies or IL-18 Binding Protein have been reported in DSS or Trinitrobenzene sodium (TSNBS) induced models of IBD (176, 177). A study has shown that mice were prevented from developing DSS-induced colitis and mucosa damage when IL-18R was deleted (171). Double knock out of IL-1β and IL-18 cytokines increased transgenic mice protection from TNBS colitis induction compared to deletion of either cytokine (178). In clinical studies, elevated secretion of IL-18 has been linked with IBD severity (179). Serum IL-18 concentration was considerably increased in patients with Crohn’s disease than healthy patients suggesting that infiltrated macrophages in the inflamed intestinal mucosa produced IL-18 which then potentially regulate intestinal mucosa lymphocytes (136, 180). A Mendelian randomization study has positioned anit-IL-18 therapy to be useful for managing IBD (181). This assertion was further strengthened in a study that found that Crohn’s disease patients resistant to anti-TNF therapy had genetically susceptible IL-18 SNP and high serum IL-18 level (182).
Several studies suggest that Rheumatoid arthritis (RA) is a Th1-driven systemic inflammatory disease of the synovial joints. SNP- IL-18 rs1946518 (−607 A > C), and IL-18 rs187238 (−137 G > C) in the IL-18 gene have been correlated with RA in certain populations (183, 184). In cell cultures using synovium from patients who had undergone synovectomy or total knee replacement surgery, results demonstrate the role of IL-18 and the expression of CXC chemokines by fibroblasts via NFκB signaling (185). In RA, IL-18 cytokine may contribute to inflammation by leukocyte extravasation via the upregulation of endothelial cell adhesion molecules, act directly as monocyte, neutrophil chemoattractant or lymphocyte and release chemokines from synovial fibroblasts (186, 187). The administration of IL-18 to mice with collagen induced arthritis (CIA) or incomplete Freund’s adjuvant immunized mice facilitated the development and severity of inflammation of cartilage (188, 189). This result was similar to that obtained when IL-18-/- mice received IL-18 (19). In contrast, low levels of IFN-γ have been detected in RA synovitis (190). This is explained to occur because IL-18 sustains the Th1 phenotype but does not induce levels of IFN-γ production in the presence of elevated expression of inhibitory molecules such as IL-10 and TGF-β. The unique role of IL-18 in inducing the discharge and upregulation of angiogenic factors such as SDF-1α, MCP-1 and VEGF in RA synovial tissues via distinct pathways have been described (191). Similarly, the use of IL-18 to stimulate RA synovial fibroblasts in vitro induced the expression of surface vascular CAM and neutrophil chemoattractant (192); more IL-18 is then produced by synovial fibroblasts and through the action of TNF-α produced by synovial macrophages in a positive feedback mechanism. Significantly higher levels of IL-18 mRNA and protein was detected in RA synovial tissues but not osteoarthritis patients experiencing age related joint disorder (193). In systemic juvenile idiopathic arthritis, elevated IL-18 levels are also a hallmark of the disease. Impaired IL-18 signaling in NK cells were implicated with a dysregulated phosphorylation of the MAPK and NFkB pathways (194, 195).
Although its etiology is unknown, Psoriasis is known to be a chronic inflammatory skin condition suggested to involve a multifaceted plethora of cytokines and chemokines secreted by immune cells and other tissue cells (196, 197). The role of IL-18 in stimulating Th1 cells which produce IFN-γ-mediated inflammation in psoriatic lesions have been described (6). Human keratinocytes are able to produce IL-18. Hence, it is proposed that IL-18 secreted by keratinocytes might be involved in the dermal Th1 immune response involved in psoriatic lesion (126). In an IL-18 knockout mouse model of psoriasis induced by Imiquimod (IMQ), IMQ induced mice manifested larger areas of Munro micro abscesses and had upregulated expression of IL-1β, IL-4, and IL-27 compared to wild type (WT) (196). This indicates that IL-18 may exacerbate psoriatic inflammation and influence its pathology. Similarly, in human studies, skin sections of psoriasis patients showed elevated levels of IL-18 and Caspase-1 compared to healthy subjects (126). Furthermore, psoriasis patients serum revealed high levels of circulating IL-18 (7). The stimulation of the human keratinocyte cell line - HaCaT with ultraviolet B radiation upregulated the production of IL-18 (198). Several studies have strongly indicated that IL-18 is a strong biomarker for clinical psoriasis symptoms (139, 140, 199). Overall, these data may indicate the potential role of IL-18 in psoriasis therapy.
SLE is an autoimmune disease characterized by B cell hyperactivity, antibody secretion, and organ damage (200). Studies have continued to show conflicting results on the relationship between IL-18 and SLE, although more recently accumulating evidence reveal that IL-18 may play a vital role in SLE pathogenesis (128). Lupus disease was exacerbated when exogenous IL-18 was administered to MRL/lpr mice but was suppressed following the treatment with anti-IL 18 (201). Particularly, in MRL-Lpr/lpr mice model of SLE, mice administered with cDNA vector expressing IL-18 developed auto-antibodies to IL-18 and had suppressed IFN-γ, milder kidney damage, and less mortality compared to the control mice (202). In agreement, human studies reveal that patients with lupus nephritis had increased concentration of serum IL-18, and kidney biopsies showed IL-18 positive glomeruli compared to normal subjects (8). Several meta-analyses have validated the claim that circulating levels of IL-18 is higher in SLE patients, which suggests the role of IL-18 in the pathogenesis of SLE (202, 203).
AOSD is an auto-inflammatory systemic disease of unknown etiology and characterized by spiking fever, rash, arthritis, leukocytosis, and other signs (204, 205). IL-18 is one of the likely inflammatory agents involved in the pathogenesis of AOSD. Its overexpression has been linked to driving the inflammatory process among other immunological factors. Particularly, high concentrations of IL-18 have been described in AOSD and were correlated with laboratory markers of the disease (129, 206). It was indicated that free IL-18 serum concentrations are significantly higher in AOSD patients compared to either healthy or disease controls including RA, SLE, axial spondyloarthritis and psoriatic arthritis. The free IL-18 levels correlated with AOSD activity suggesting that IL-18 may represent a potential target for the treatment of AOSD. In another study, the sera levels of IL-18 of 26 Italian patients with AOSD was investigated to assess the role of IL-18 cytokine as a disease marker and compared with that of 21 patients with RA, 21 patients with Sjogren’s syndrome, 20 patients with SLE and 21 healthy controls (205). Herein, IL-18 serum levels were significantly higher in patients with active AOSD than non-active as well as control groups. More so, IL-18 serum levels significantly correlated with disease activities and other laboratory parameters such as ferritin and C-reactive protein suggesting the possible targeting of IL-18 cytokine as a therapeutic option because of the role it plays in the disease state (207–210).
PM and DM are inflammatory myopathies characterized by muscle weakness, suppressed muscle endurance and skin involvement (DM only) (145, 211). Generally, the pathologies of DM and PM are different. DM is thought to arise from CD4+T- and B- cells mediated inflammation while PM is considered to result from autoreactive cytotoxic T cells which may mediate cytotoxic activities against auto-antigens (212, 213). In one study to investigate IL-18 expression in symptomatic and asymptomatic muscle tissues of patients with PM and DM, 2 cohorts of patients were used (145). One cohort consisted of 10 new-onset myositis patients and IL-18 expression levels were compared between the muscle biopsies results of symptomatic and asymptomatic patients before treatment. In the second cohort, 10 patients with repeated muscle biopsies before and 8 months after treatment with immunosuppressive therapy were recruited. Results indicated that the expression of total IL-18 in muscle tissues from new-onset patients (symptomatic and asymptomatic) were significantly higher compared to healthy controls. IL-18 total expression levels were lower in biopsies from patients receiving immunosuppressive treatment compared to other patients. The results indicate that IL-18 is highly expressed in muscle tissues in inflammatory myopathies. In another study to ascertain the involvement of IL-18 in PM and DM inflammation, 33 patients with DM and 16 patients with PM were enrolled in the study (there were some patients with interstitial lung disease in both groups) (130). It was revealed that serum IL-18 levels were significantly higher in DM and PM patients compared with healthy controls. These authors along with others (145, 213, 214) concluded that serum IL-18 levels were strikingly elevated in DM and PM patients and particularly in DM patients complicated with interstitial lung disease. IL-18 levels also indicate clinical severity of dermatomyositis (215) with recent findings reporting that IL-18 containing 5-gene region can be used to differentiate histologically identical dermatomyositis and other skin lesions (216).
Targeting IL-18 appears like an obvious potential therapy due to its role in inflammation. The cytokine IL-18 could be blocked using monoclonal antibodies (mAbs), by inhibiting its production in cells, its secretion from cells or molecular binding blockade using binding proteins or antibodies (5, 217). Table 3 shows some clinical trials targeting IL-18 in the treatment of inflammatory diseases
IL-18 binding protein (IL-18BP) is a natural antagonist of IL-18, belonging to the immunoglobulin like receptor type but is not cleaved on cell surface (221). It is a naturally occurring 18 binding agent was first identified in 1999. In mice, two isoforms of IL-18 BP exist (c and d), while a, b, c and d are the human isoforms (222). The IL-18 BPa is the unique and major splice variant of IL-18BP that controls the biological activity of IL-18 by binding with high affinity. Thus, it acts as a soluble decoy receptor (81). Recently resolved crystal structure of the IL-18:IL-18BP complex reveals a sequestration of the IL-18 by IL-18BP influenced by molecular mimicry and steric competition of binding sites compared to IL-18R. The IL-18:IL-18BP also showed a novel higher order 2:2 binding stoichiometry compared to the standard 1:1 binding of IL-18:IL-18R (223). The mechanisms of Th1 responses to microbes may be blunted by the action of IL-18 BP thus reducing autoimmune responses to an infection. Several studies have shown the potential of targeting IL-18BP against IL-18. The suppression of disease severity has been reported in 33 disease models in which the administration of anti-IL18 antibodies or IL-18 BP led to the inhibition of IL-18 (40). Accordingly, in murine models of inflammation including DSS colitis, recombinant IL-18 BP suppressed disease severity (224). Inflammation in the joints of mice treated with IL-18BP were suppressed and mice also exhibited reduced inflammatory infiltration and cartilage destruction as observed in the histopathology analysis. Furthermore, in vivo studies revealed that short-term supplementation with IL-18 BP Fc prophylactically protected NOD mice from the acceleration of autoimmune diabetes (18). At low dosing of IL-18 BP, inflammation was suppressed in a model of RA (39). Recently, in a clinical trial using human recombinant IL-18 BP in RA and Psoriasis patients, therapeutic efficacy was not achieved (219). Aside from the use of IL-18 BP for binding IL-18, it could also be bound by anti-IL 18 antibodies. In agreement, the severity of Collagen induced arthritis was reduced significantly in the course of treatment with polyclonal anti-IL-18 antibodies and was more effective compared to IL-18 BP (224). In addition, DSS colitis was minimally suppressed in experimental mice using antimurine IL-18 antiserum (225). In MRL/MpJ-Tnfrsf6lpr mice exhibiting lupus like autoimmune syndrome, vaccination with IL-18 cDNA in order to inhibit IL-18 showed that IFN-y production was suppressed and mice showed less glomerulonephritis and renal damage (202). In mice, a neutralizing IL-18Ra antibody showed significant protective effect on a graft-versus-host disease model (226), a condition characterized by systemic inflammation and multiple organ damage.
Caspase 1 appears to be a suitable target to suppress the production of IL-18 and also IL-1β (217). The active form of caspase-1 is a tetramer that cleaves the proform of IL-1β and IL-18 to their mature forms (44), which then leaves the cytosol. A study revealed that IL-1β or IL-18 deficient mice were not completely protected from septic shock unlike caspase-1 deficient mice (227, 228). Similarly, in Caspase-1 deficient mice, the severity of DSS induced colitis was suppressed correlating with IL-18 reduced expression (229). Pralnacasan is an oral caspase 1 inhibitor clinically tested and observed in patients in a phase II RA clinical trial (220). It was well tolerated and suppressed inflammation but was suspected to promote liver toxicity.
Uncontrolled mature IL-18 secretion and IL-1β is responsible for severe autoimmune disorders as they bind to their receptors, initiate several signaling, and ultimately activate NF-kB (230). The antagonism of P2X-7, a purinergic receptor located on the cells of hematopoietic origin, might be a potential target for treating autoimmune diseases with inflammatory origin. This is because of the importance of P2X-7 receptor in the biology and secretion of IL-1β and IL-18. This has been confirmed in P2X-7 deficient mice (217, 231).
Currently, GSK 1070806, a humanized mAb, is in a single-blind randomized placebo-controlled phase 1 trial against IBD. The study aims to investigate the use of the drug in both healthy and obese male subjects for the treatment of IBD as well as its safety, tolerability, pharmacokinetics, and dynamics. In this multicenter, randomized, single-blind, placebo-controlled parallel group phase IIa trial conducted in obese patients of either sex with poorly controlled Type 2 diabetes patients on metformin monotherapy using GSK1070806, although it was well tolerated, IL-18 inhibition did not improve glucose control (218). Recently, a novel anti-human IL-1R7 monoclonal antibody that blocks and suppresses the inflammatory signaling of IL-18 was developed. It acts by reducing IL-18 induced NFkB and IFN-γ activation and IL-6 production in human cell lines. It is important to note that IL-1R7 is a potential virgin therapeutic strategy for the investigation of its clinical potential in treating IL-18 mediated diseases as this area remains to be explored (232).
Another novel development in the generation of IL-18 monoclonal antibodies lies in the identification of a neoepitope that is generated after IL-18 is cleaved by the caspases. This neoepitope - 37YFGKLESK44 can be used to distinguish between physiological and pathological IL-18 (233). This led to the generation of 2 high affinity antagonistic IL-18 antibodies recognizing epitope 63NRPLFEDMT68 of the full-length human IL-18 cytokine or recognizing only the neoepitope - 37YFGKLESK44 in humans (233) or 36NFGRLHCTT44 in mice (234). This strategy of controlling pathogenic IL-18 sounds promising for therapeutic purposes.
Despite abundant evidence showing the role of IL-18 as a biomarker in several inflammatory and autoimmune conditions, it is not being utilized as a target for biologics to control these conditions.
Despite a large number of reports that have indicated the indispensable role of IL-18 in autoimmune diseases, many of them are still elusive. For instance, the definitive steps required in IL-18 signaling and activation triggers in different autoimmune diseases are widely unknown. More clinical trials of IL-18 BP and other antibodies are necessary to properly ascertain the role of the cytokine IL-18 in treating diseases. IL-18 plays a vital pathogenic role in diseases by promoting T cell mediated responses and may be Th1 or Th2 related. Many in vitro studies, animal models, and some clinical studies support the vital role of IL-18 in Psoriasis, MG, and other diseases. Therefore, in order to gain more insight into the place of IL-BP and other drugs targeting IL-18 for the treatment and control of autoimmune conditions, additional research is required. Finally, we recommend the development of combination drug therapies that specifically focus on IL-18 inhibition in addition to the inhibition of other specific cytokines such as TNF-α, IL-1β, IL-6, or IFN-γ which have been indicated to be strongly involved in the pathogenesis of any of the inflammatory diseases.
All authors (SAI, SDA, ZZ, TS, MS, SM, and GA) helped conceptualize the manuscript. SAI and SDA wrote first draft of the manuscript, figures and tables. All authors (SAI, SDA, ZZ, TS, MS, SM, and GA) reviewed the manuscript and contributed to edits. All authors contributed to the article and approved the submitted version.
Authors MS and SM were employed by AryoGen Pharmed Inc.
The remaining authors declare that the research was conducted in the absence of any commercial or financial relationships that could be construed as a potential conflict of interest.
All claims expressed in this article are solely those of the authors and do not necessarily represent those of their affiliated organizations, or those of the publisher, the editors and the reviewers. Any product that may be evaluated in this article, or claim that may be made by its manufacturer, is not guaranteed or endorsed by the publisher.
1. Sims JE, Smith DE. The IL-1 family: regulators of immunity. Nat Rev Immunol (2010) 10(2):89–102. doi: 10.1038/nri2691
2. Sims JE, Nicklin MJ, Bazan JF, Barton JL, Busfield SJ, Ford JE, et al. A new nomenclature for IL-1-family genes. Trends Immunol (2001) 22(10):536–7. doi: 10.1016/S1471-4906(01)02040-3
3. Nakanishi K, Yoshimoto T, Tsutsui H, Okamura H. Interleukin-18 regulates both Th1 and Th2 responses. Annu Rev Immunol (2001) 19:423–74. doi: 10.1146/annurev.immunol.19.1.423
4. Nakanishi K. Unique action of interleukin-18 on t cells and other immune cells. Front Immunol (2018) 9:763. doi: 10.3389/fimmu.2018.00763
5. Yasuda K, Nakanishi K, Tsutsui H. Interleukin-18 in health and disease. Int J Mol Sci (2019) 20(3):649–701. doi: 10.3390/ijms20030649
6. Sedimbi SK, Hagglof T, Karlsson MC. IL-18 in inflammatory and autoimmune disease. Cell Mol Life Sci (2013) 70(24):4795–808. doi: 10.1007/s00018-013-1425-y
7. Gangemi S, Merendino RA, Guarneri F, Minciullo PL, DiLorenzo G, Pacor M, et al. Serum levels of interleukin-18 and s-ICAM-1 in patients affected by psoriasis: preliminary considerations. J Eur Acad Dermatol Venereol (2003) 17(1):42–6. doi: 10.1046/j.1468-3083.2003.00647.x
8. Park MC, Park YB, Lee SK. Elevated interleukin-18 levels correlated with disease activity in systemic lupus erythematosus. Clin Rheumatol (2004) 23(3):225–9. doi: 10.1007/s10067-004-0867-x
9. Rezaieyazdi Z, AkbariRad M, Saadati N, Salari M, Orang R, Sedighi S, et al. Serum interleukin-18 and its relationship with subclinical atherosclerosis in systemic lupus erythematosus. ARYA Atheroscler (2021) 17(6):1–6. doi: 10.22122/arya.v17i0.2126
10. Thomas JM, Huuskes BM, Sobey CG, Drummond GR, Vinh A. The IL-18/IL-18R1 signalling axis: Diagnostic and therapeutic potential in hypertension and chronic kidney disease. Pharmacol Ther (2022) 239:108191. doi: 10.1016/j.pharmthera.2022.108191
11. Nicoletti F, Di Marco R, Mangano K, Patti F, Reggio E, Nicoletti A, et al. Increased serum levels of interleukin-18 in patients with multiple sclerosis. Neurology (2001) 57(2):342–4. doi: 10.1212/WNL.57.2.342
12. Losy J, Niezgoda A. IL-18 in patients with multiple sclerosis. Acta Neurol Scand (2001) 104(3):171–3. doi: 10.1034/j.1600-0404.2001.00356.x
13. Takahashi T, Ellingson MK, Wong P, Israelow B, Lucas C, Klein J, et al. Sex differences in immune responses that underlie COVID-19 disease outcomes. Nature (2020) 588(7837):315–20. doi: 10.1038/s41586-020-2700-3
14. Rodrigues TS, de Sa KSG, Ishimoto AY, Becerra A, Oliveira S, Almeida L, et al. Inflammasomes are activated in response to SARS-CoV-2 infection and are associated with COVID-19 severity in patients. J Exp Med (2021) 218(3):e20201707. doi: 10.1084/jem.20201707
15. Franciotta D, Martino G, Zardini E, Furlan R, Bergamaschi R, Gironi M, et al. Caspase-1 levels in biological fluids from patients with multiple sclerosis and from patients with other neurological and non-neurological diseases. Eur Cytokine Netw (2002) 13(1):99–103. doi: 10.1084/jem.20201707
16. Rothe H, Hibino T, Itoh Y, Kolb H, Martin S. Systemic production of interferon-gamma inducing factor (IGIF) versus local IFN-gamma expression involved in the development of Th1 insulitis in NOD mice. J Autoimmun (1997) 10(3):251–6. doi: 10.1006/jaut.1997.0135
17. Marleau AM, Sarvetnick NE. IL-18 is required for self-reactive T cell expansion in NOD mice. J Autoimmun (2011) 36(3-4):263–77. doi: 10.1016/j.jaut.2011.02.005
18. Zaccone P, Phillips J, Conget I, Cooke A, Nicoletti F. IL-18 binding protein fusion construct delays the development of diabetes in adoptive transfer and cyclophosphamide-induced diabetes in NOD mouse. Clin Immunol (2005) 115(1):74–9. doi: 10.1016/j.clim.2004.11.007
19. Wei XQ, Leung BP, Arthur HM, McInnes IB, Liew FY. Reduced incidence and severity of collagen-induced arthritis in mice lacking IL-18. J Immunol (2001) 166(1):517–21. doi: 10.4049/jimmunol.166.1.517
20. Gutcher I, Urich E, Wolter K, Prinz M, Becher B. Interleukin 18-independent engagement of interleukin 18 receptor-alpha is required for autoimmune inflammation. Nat Immunol (2006) 7(9):946–53. doi: 10.1038/ni1377
21. Chandrasekar B, Patel DN, Mummidi S, Kim JW, Clark RA, Valente AJ. Interleukin-18 suppresses adiponectin expression in 3T3-L1 adipocytes via a novel signal transduction pathway involving ERK1/2-dependent NFATc4 phosphorylation. J Biol Chem (2008) 283(7):4200–9. doi: 10.1074/jbc.M708142200
22. Shi FD, Wang HB, Li H, Hong S, Taniguchi M, Link H, et al. Natural killer cells determine the outcome of b cell-mediated autoimmunity. Nat Immunol (2000) 1(3):245–51. doi: 10.1038/79792
23. Lob S, Ochmann B, Ma Z, Vilsmaier T, Kuhn C, Schmoeckel E, et al. The role of interleukin-18 in recurrent early pregnancy loss. J Reprod Immunol (2021) 148:103432. doi: 10.1016/j.jri.2021.103432
24. Kohler G, Milstein C. Continuous cultures of fused cells secreting antibody of predefined specificity. Nature (1975) 256(5517):495–7. doi: 10.1038/256495a0
25. Kaplon H, Reichert JM. Antibodies to watch in 2019. MAbs (2019) 11(2):219–38. doi: 10.1080/19420862.2018.1556465
26. Jones PT, Dear PH, Foote J, Neuberger MS, Winter G. Replacing the complementarity-determining regions in a human antibody with those from a mouse. Nature (1986) 321(6069):522–5. doi: 10.1038/321522a0
27. Tsurushita N, Hinton PR, Kumar S. Design of humanized antibodies: from anti-tac to zenapax. Methods (2005) 36(1):69–83. doi: 10.1016/j.ymeth.2005.01.007
28. Lefranc MP. IMGT, the international immunogenetics information system. Cold Spring Harb Protoc (2011) 2011(6):595–603. doi: 10.1101/pdb.top115
29. Lu RM, Hwang YC, Liu IJ, Lee CC, Tsai HZ, Li HJ, et al. Development of therapeutic antibodies for the treatment of diseases. J BioMed Sci (2020) 27(1):1. doi: 10.1186/s12929-019-0592-z
30. Okamura H, Tsutsi H, Komatsu T, Yutsudo M, Hakura A, Tanimoto T, et al. Cloning of a new cytokine that induces IFN-gamma production by T cells. Nature (1995) 378(6552):88–91. doi: 10.1038/378088a0
31. Nakamura K, Okamura H, Nagata K, Komatsu T, Tamura T. Purification of a factor which provides a costimulatory signal for gamma interferon production. Infect Immun (1993) 61(1):64–70. doi: 10.1128/iai.61.1.64-70.1993
32. Dinarello CA. IL-18: A TH1-inducing, proinflammatory cytokine and new member of the IL-1 family. J Allergy Clin Immunol (1999) 103(1 Pt 1):11–24. doi: 10.1016/S0091-6749(99)70518-X
33. Takeda K, Tsutsui H, Yoshimoto T, Adachi O, Yoshida N, Kishimoto T, et al. Defective NK cell activity and Th1 response in IL-18-deficient mice. Immunity (1998) 8(3):383–90. doi: 10.1016/S1074-7613(00)80543-9
34. Sakao Y, Takeda K, Tsutsui H, Kaisho T, Nomura F, Okamura H, et al. IL-18-deficient mice are resistant to endotoxin-induced liver injury but highly susceptible to endotoxin shock. Int Immunol (1999) 11(3):471–80. doi: 10.1093/intimm/11.3.471
35. Matsui K, Yoshimoto T, Tsutsui H, Hyodo Y, Hayashi N, Hiroishi K, et al. Propionibacterium acnes treatment diminishes CD4+ NK1.1+ T cells but induces type I T cells in the liver by induction of IL-12 and IL-18 production from kupffer cells. J Immunol (1997) 159(1):97–106.
36. Kawa K, Tsutsui H, Uchiyama R, Kato J, Matsui K, Iwakura Y, et al. IFN-gamma is a master regulator of endotoxin shock syndrome in mice primed with heat-killed propionibacterium acnes. Int Immunol (2010) 22(3):157–66. doi: 10.1093/intimm/dxp122
37. Ushio S, Namba M, Okura T, Hattori K, Nukada Y, Akita K, et al. Cloning of the cDNA for human IFN-gamma-inducing factor, expression in escherichia coli, and studies on the biologic activities of the protein. J Immunol (1996) 156(11):4274–9.
39. Dinarello CA, Novick D, Kim S, Kaplanski G. Interleukin-18 and IL-18 binding protein. Front Immunol (2013) 4:289. doi: 10.3389/fimmu.2013.00289
40. Novick D, Kim S, Kaplanski G, Dinarello CA. Interleukin-18, more than a Th1 cytokine. Semin Immunol (2013) 25(6):439–48. doi: 10.1016/j.smim.2013.10.014
41. O’Neill LA. The interleukin-1 receptor/Toll-like receptor superfamily: signal transduction during inflammation and host defense. Sci STKE (2000) 2000(44):re1. doi: 10.1126/stke.442000re1
42. Dunne A, O’Neill LA. The interleukin-1 receptor/Toll-like receptor superfamily: signal transduction during inflammation and host defense. Sci STKE (2003) 2003(171):re3. doi: 10.1126/stke.2003.171.re3
43. O’Neill LA. The interleukin-1 receptor/Toll-like receptor superfamily: 10 years of progress. Immunol Rev (2008) 226:10–8. doi: 10.1111/j.1600-065X.2008.00701.x
44. Dinarello CA. Biologic basis for interleukin-1 in disease. Blood (1996) 87(6):2095–147. doi: 10.1182/blood.V87.6.2095.bloodjournal8762095
45. Dinarello CA. Immunological and inflammatory functions of the interleukin-1 family. Annu Rev Immunol (2009) 27:519–50. doi: 10.1146/annurev.immunol.021908.132612
46. Smith DE. IL-33: a tissue derived cytokine pathway involved in allergic inflammation and asthma. Clin Exp Allergy (2010) 40(2):200–8. doi: 10.1111/j.1365-2222.2009.03384.x
47. Gabay C, McInnes IB. The biological and clinical importance of the ‘new generation’ cytokines in rheumatic diseases. Arthritis Res Ther (2009) 11(3):230. doi: 10.1186/ar2680
48. Kaplanski G. Interleukin-18: Biological properties and role in disease pathogenesis. Immunol Rev (2018) 281(1):138–53. doi: 10.1111/imr.12616
49. Dinarello CA, Novick D, Puren AJ, Fantuzzi G, Shapiro L, Muhl H, et al. Overview of interleukin-18: more than an interferon-gamma inducing factor. J Leukoc Biol (1998) 63(6):658–64. doi: 10.1002/jlb.63.6.658
50. Sugawara S, Uehara A, Nochi T, Yamaguchi T, Ueda H, Sugiyama A, et al. Neutrophil proteinase 3-mediated induction of bioactive IL-18 secretion by human oral epithelial cells. J Immunol (2001) 167(11):6568–75. doi: 10.4049/jimmunol.167.11.6568
51. Tsutsui H, Matsui K, Okamura H, Nakanishi K. Pathophysiological roles of interleukin-18 in inflammatory liver diseases. Immunol Rev (2000) 174:192–209. doi: 10.1034/j.1600-0528.2002.017418.x
52. Gu Y, Kuida K, Tsutsui H, Ku G, Hsiao K, Fleming MA, et al. Activation of interferon-gamma inducing factor mediated by interleukin-1beta converting enzyme. Science (1997) 275(5297):206–9. doi: 10.1126/science.275.5297.206
53. Yamamoto M, Yaginuma K, Tsutsui H, Sagara J, Guan X, Seki E, et al. ASC is essential for LPS-induced activation of procaspase-1 independently of TLR-associated signal adaptor molecules. Genes Cells (2004) 9(11):1055–67. doi: 10.1111/j.1365-2443.2004.00789.x
54. Imamura M, Tsutsui H, Yasuda K, Uchiyama R, Yumikura-Futatsugi S, Mitani K, et al. Contribution of TIR domain-containing adapter inducing IFN-beta-mediated IL-18 release to LPS-induced liver injury in mice. J Hepatol (2009) 51(2):333–41. doi: 10.1016/j.jhep.2009.03.027
55. Martinon F, Mayor A, Tschopp J. The inflammasomes: guardians of the body. Annu Rev Immunol (2009) 27:229–65. doi: 10.1146/annurev.immunol.021908.132715
56. Jorgensen I, Miao EA. Pyroptotic cell death defends against intracellular pathogens. Immunol Rev (2015) 265(1):130–42. doi: 10.1111/imr.12287
57. Latz E, Xiao TS, Stutz A. Activation and regulation of the inflammasomes. Nat Rev Immunol (2013) 13(6):397–411. doi: 10.1038/nri3452
58. Tsutsui H, Kayagaki N, Kuida K, Nakano H, Hayashi N, Takeda K, et al. Caspase-1-independent, Fas/Fas ligand-mediated IL-18 secretion from macrophages causes acute liver injury in mice. Immunity (1999) 11(3):359–67. doi: 10.1016/S1074-7613(00)80111-9
59. Bossaller L, Chiang PI, Schmidt-Lauber C, Ganesan S, Kaiser WJ, Rathinam VA, et al. Cutting edge: FAS (CD95) mediates noncanonical IL-1beta and IL-18 maturation via caspase-8 in an RIP3-independent manner. J Immunol (2012) 189(12):5508–12. doi: 10.4049/jimmunol.1202121
60. Omoto Y, Tokime K, Yamanaka K, Habe K, Morioka T, Kurokawa I, et al. Human mast cell chymase cleaves pro-IL-18 and generates a novel and biologically active IL-18 fragment. J Immunol (2006) 177(12):8315–9. doi: 10.4049/jimmunol.177.12.8315
61. Omoto Y, Yamanaka K, Tokime K, Kitano S, Kakeda M, Akeda T, et al. Granzyme b is a novel interleukin-18 converting enzyme. J Dermatol Sci (2010) 59(2):129–35. doi: 10.1016/j.jdermsci.2010.05.004
62. Rackov G, Tavakoli Zaniani P, Colomo Del Pino S, Shokri R, Monserrat J, Alvarez-Mon M, et al. Mitochondrial reactive oxygen is critical for IL-12/IL-18-induced IFN-gamma production by CD4(+) T cells and is regulated by Fas/FasL signaling. Cell Death Dis (2022) 13(6):531. doi: 10.1038/s41419-022-04907-5
63. Tomura M, Maruo S, Mu J, Zhou XY, Ahn HJ, Hamaoka T, et al. Differential capacities of CD4+, CD8+, and CD4-CD8- T cell subsets to express IL-18 receptor and produce IFN-gamma in response to IL-18. J Immunol (1998) 160(8):3759–65.
64. Nakahira M, Tomura M, Iwasaki M, Ahn HJ, Bian Y, Hamaoka T, et al. An absolute requirement for STAT4 and a role for IFN-gamma as an amplifying factor in IL-12 induction of the functional IL-18 receptor complex. J Immunol (2001) 167(3):1306–12. doi: 10.4049/jimmunol.167.3.1306
65. Freudenberg MA, Merlin T, Kalis C, Chvatchko Y, Stubig H, Galanos C. Cutting edge: a murine, IL-12-independent pathway of IFN-gamma induction by gram-negative bacteria based on STAT4 activation by type I IFN and IL-18 signaling. J Immunol (2002) 169(4):1665–8. doi: 10.4049/jimmunol.169.4.1665
66. Matikainen S, Paananen A, Miettinen M, Kurimoto M, Timonen T, Julkunen I, et al. IFN-alpha and IL-18 synergistically enhance IFN-gamma production in human NK cells: differential regulation of Stat4 activation and IFN-gamma gene expression by IFN-alpha and IL-12. Eur J Immunol (2001) 31(7):2236–45. doi: 10.1002/1521-4141(200107)31:7<2236::AID-IMMU2236>3.0.CO;2-G
67. Tsutsui H, Nakanishi K. Immunotherapeutic applications of IL-18. Immunotherapy (2012) 4(12):1883–94. doi: 10.2217/imt.12.137
68. Yoshimoto T, Takeda K, Tanaka T, Ohkusu K, Kashiwamura S, Okamura H, et al. IL-12 up-regulates IL-18 receptor expression on T cells, Th1 cells, and b cells: synergism with IL-18 for IFN-gamma production. J Immunol (1998) 161(7):3400–7.
69. Hoshino K, Tsutsui H, Kawai T, Takeda K, Nakanishi K, Takeda Y, et al. Cutting edge: generation of IL-18 receptor-deficient mice: evidence for IL-1 receptor-related protein as an essential IL-18 binding receptor. J Immunol (1999) 162(9):5041–4.
70. Adachi O, Kawai T, Takeda K, Matsumoto M, Tsutsui H, Sakagami M, et al. Targeted disruption of the MyD88 gene results in loss of IL-1- and IL-18-mediated function. Immunity (1998) 9(1):143–50. doi: 10.1016/S1074-7613(00)80596-8
71. Robinson D, Shibuya K, Mui A, Zonin F, Murphy E, Sana T, et al. IGIF does not drive Th1 development but synergizes with IL-12 for interferon-gamma production and activates IRAK and NFkappaB. Immunity (1997) 7(4):571–81. doi: 10.1016/S1074-7613(00)80378-7
72. Hoshino T, Wiltrout RH, Young HA. IL-18 is a potent coinducer of IL-13 in NK and T cells: a new potential role for IL-18 in modulating the immune response. J Immunol (1999) 162(9):5070–7.
73. El-Darawish Y, Li W, Yamanishi K, Pencheva M, Oka N, Yamanishi H, et al. Frontline science: IL-18 primes murine NK cells for proliferation by promoting protein synthesis, survival, and autophagy. J Leukoc Biol (2018) 104(2):253–64. doi: 10.1002/JLB.1HI1017-396RR
74. Deason K, Troutman TD, Jain A, Challa DK, Mandraju R, Brewer T, et al. BCAP links IL-1R to the PI3K-mTOR pathway and regulates pathogenic Th17 cell differentiation. J Exp Med (2018) 215(9):2413–28. doi: 10.1084/jem.20171810
75. Fukao T, Tanabe M, Terauchi Y, Ota T, Matsuda S, Asano T, et al. PI3K-mediated negative feedback regulation of IL-12 production in DCs. Nat Immunol (2002) 3(9):875–81. doi: 10.1038/ni825
76. Hosotani Y, Kashiwamura S, Kimura-Shimmyo A, Sekiyama A, Ueda H, Ikeda T, et al. Interleukin-18 prevents apoptosis via PI3K/Akt pathway in normal human keratinocytes. J Dermatol (2008) 35(8):514–24. doi: 10.1111/j.1346-8138.2008.00513.x
77. Zhou J, Ping FF, Lv WT, Feng JY, Shang J. Interleukin-18 directly protects cortical neurons by activating PI3K/AKT/NF-kappaB/CREB pathways. Cytokine (2014) 69(1):29–38. doi: 10.1016/j.cyto.2014.05.003
78. Kalina U, Kauschat D, Koyama N, Nuernberger H, Ballas K, Koschmieder S, et al. IL-18 activates STAT3 in the natural killer cell line 92, augments cytotoxic activity, and mediates IFN-gamma production by the stress kinase p38 and by the extracellular regulated kinases p44erk-1 and p42erk-21. J Immunol (2000) 165(3):1307–13. doi: 10.4049/jimmunol.165.3.1307
79. Wyman TH, Dinarello CA, Banerjee A, Gamboni-Robertson F, Hiester AA, England KM, et al. Physiological levels of interleukin-18 stimulate multiple neutrophil functions through p38 MAP kinase activation. J Leukoc Biol (2002) 72(2):401–9. doi: 10.1189/jlb.72.2.401
80. Alboni S, Montanari C, Benatti C, Sanchez-Alavez M, Rigillo G, Blom JM, et al. Interleukin 18 activates MAPKs and STAT3 but not NF-kappaB in hippocampal HT-22 cells. Brain Behav Immun (2014) 40:85–94. doi: 10.1016/j.bbi.2014.02.015
81. Novick D, Kim SH, Fantuzzi G, Reznikov LL, Dinarello CA, Rubinstein M. Interleukin-18 binding protein: a novel modulator of the Th1 cytokine response. Immunity (1999) 10(1):127–36. doi: 10.1016/S1074-7613(00)80013-8
82. Nold-Petry CA, Lo CY, Rudloff I, Elgass KD, Li S, Gantier MP, et al. IL-37 requires the receptors IL-18Ralpha and IL-1R8 (SIGIRR) to carry out its multifaceted anti-inflammatory program upon innate signal transduction. Nat Immunol (2015) 16(4):354–65. doi: 10.1038/ni.3103
83. Freeman BE, Raue HP, Hill AB, Slifka MK. Cytokine-mediated activation of NK cells during viral infection. J Virol (2015) 89(15):7922–31. doi: 10.1128/JVI.00199-15
84. Cooper MA, Elliott JM, Keyel PA, Yang L, Carrero JA, Yokoyama WM. Cytokine-induced memory-like natural killer cells. Proc Natl Acad Sci U S A. (2009) 106(6):1915–9. doi: 10.1073/pnas.0813192106
85. Munder M, Mallo M, Eichmann K, Modolell M. Murine macrophages secrete interferon gamma upon combined stimulation with interleukin (IL)-12 and IL-18: A novel pathway of autocrine macrophage activation. J Exp Med (1998) 187(12):2103–8. doi: 10.1084/jem.187.12.2103
86. Nakanishi K, Yoshimoto T, Tsutsui H, Okamura H. Interleukin-18 is a unique cytokine that stimulates both Th1 and Th2 responses depending on its cytokine milieu. Cytokine Growth Factor Rev (2001) 12(1):53–72. doi: 10.1016/S1359-6101(00)00015-0
87. Chang JT, Segal BM, Nakanishi K, Okamura H, Shevach EM. The costimulatory effect of IL-18 on the induction of antigen-specific IFN-gamma production by resting T cells is IL-12 dependent and is mediated by up-regulation of the IL-12 receptor beta2 subunit. Eur J Immunol (2000) 30(4):1113–9. doi: 10.1002/(SICI)1521-4141(200004)30:4<1113::AID-IMMU1113>3.0.CO;2-P
88. Tomura M, Zhou XY, Maruo S, Ahn HJ, Hamaoka T, Okamura H, et al. A critical role for IL-18 in the proliferation and activation of NK1.1+ CD3- cells. J Immunol (1998) 160(10):4738–46.
89. Okamoto I, Kohno K, Tanimoto T, Ikegami H, Kurimoto M. Development of CD8+ effector T cells is differentially regulated by IL-18 and IL-12. J Immunol (1999) 162(6):3202–11.
90. Kagi D, Ledermann B, Burki K, Zinkernagel RM, Hengartner H. Molecular mechanisms of lymphocyte-mediated cytotoxicity and their role in immunological protection and pathogenesis in vivo. Annu Rev Immunol (1996) 14:207–32. doi: 10.1146/annurev.immunol.14.1.207
91. Yoshimoto T, Mizutani H, Tsutsui H, Noben-Trauth N, Yamanaka K, Tanaka M, et al. IL-18 induction of IgE: dependence on CD4+ T cells, IL-4 and STAT6. Nat Immunol (2000) 1(2):132–7. doi: 10.1038/77811
92. Ohkusu K, Yoshimoto T, Takeda K, Ogura T, Kashiwamura S, Iwakura Y, et al. Potentiality of interleukin-18 as a useful reagent for treatment and prevention of leishmania major infection. Infect Immun (2000) 68(5):2449–56. doi: 10.1128/IAI.68.5.2449-2456.2000
93. Kobayashi K, Nakata N, Kai M, Kasama T, Hanyuda Y, Hatano Y. Decreased expression of cytokines that induce type 1 helper T cell/interferon-gamma responses in genetically susceptible mice infected with mycobacterium avium. Clin Immunol Immunopathol (1997) 85(1):112–6. doi: 10.1006/clin.1997.4421
94. Zhu R, Mao S, Shi W, Wu L, Zhang J. A prediction study of IL-18 and IFN-gamma in glucocorticoid treatment response in infants and young children with severe mycoplasma pneumoniae pneumonia. Transl Pediatr (2022) 11(5):738–47. doi: 10.21037/tp-22-139
95. Fujioka N, Akazawa R, Ohashi K, Fujii M, Ikeda M, Kurimoto M. Interleukin-18 protects mice against acute herpes simplex virus type 1 infection. J Virol (1999) 73(3):2401–9. doi: 10.1128/JVI.73.3.2401-2409.1999
96. Ishikawa Y, Yamada M, Wada N, Takahashi E, Imadome KI. Mucosal-associated invariant T cells are activated in an interleukin-18-dependent manner in Epstein-Barr virus-associated t/natural killer cell lymphoproliferative diseases. Clin Exp Immunol (2022) 207(2):141–8. doi: 10.1093/cei/uxab004
97. Yoshimoto T, Okamura H, Tagawa YI, Iwakura Y, Nakanishi K. Interleukin 18 together with interleukin 12 inhibits IgE production by induction of interferon-gamma production from activated b cells. Proc Natl Acad Sci U S A. (1997) 94(8):3948–53. doi: 10.1073/pnas.94.8.3948
98. Yoshimoto T, Nagai N, Ohkusu K, Ueda H, Okamura H, Nakanishi K. LPS-stimulated SJL macrophages produce IL-12 and IL-18 that inhibit IgE production in vitro by induction of IFN-gamma production from CD3intIL-2R beta+ T cells. J Immunol (1998) 161(3):1483–92.
99. Chiang HY, Lu HH, Sudhakar JN, Chen YW, Shih NS, Weng YT, et al. IL-22 initiates an IL-18-dependent epithelial response circuit to enforce intestinal host defence. Nat Commun (2022) 13(1):874. doi: 10.1038/s41467-022-28478-3
100. Alagkiozidis I, Facciabene A, Carpenito C, Benencia F, Jonak Z, Adams S, et al. Increased immunogenicity of surviving tumor cells enables cooperation between liposomal doxorubicin and IL-18. J Transl Med (2009) 7:104. doi: 10.1186/1479-5876-7-104
101. Kim JE, Jang MJ, Jin DH, Chung YH, Choi BS, Park GB, et al. Paclitaxel-exposed ovarian cancer cells induce cancerspecific CD4+ T cells after doxorubicin exposure through regulation of MyD88 expression. Int J Oncol (2014) 44(5):1716–26. doi: 10.3892/ijo.2014.2308
102. Zhou T, Damsky W, Weizman OE, McGeary MK, Hartmann KP, Rosen CE, et al. IL-18BP is a secreted immune checkpoint and barrier to IL-18 immunotherapy. Nature (2020) 583(7817):609–14. doi: 10.1038/s41586-020-2422-6
103. Nakamura K, Bald T, Smyth MJ. Cancer-killing, decoy-resistant interleukin-18. Immunol Cell Biol (2020) 98(6):434–6. doi: 10.1111/imcb.12359
104. Satoh Y, Esche C, Gambotto A, Shurin GV, Yurkovetsky ZR, Robbins PD, et al. Local administration of IL-12-transfected dendritic cells induces antitumor immune responses to colon adenocarcinoma in the liver in mice. J Exp Ther Oncol (2002) 2(6):337–49. doi: 10.1046/j.1359-4117.2002.01050.x
105. Mierzejewska J, Wegierek-Ciura K, Rossowska J, Szczygiel A, Anger-Gora N, Szermer-Olearnik B, et al. The beneficial effect of il-12 and il-18 transduced dendritic cells stimulated with tumor antigens on generation of an antitumor response in a mouse colon carcinoma model. J Immunol Res (2022) 2022:7508928. doi: 10.1155/2022/7508928
106. Zhao G, Zhang H, Zhu S, Wang S, Zhu K, Zhao Y, et al. Interleukin-18 accelerates cardiac inflammation and dysfunction during ischemia/reperfusion injury by transcriptional activation of CXCL16. Cell Signal (2021) 87:110141. doi: 10.1016/j.cellsig.2021.110141
107. Boyle AJ, Ferris P, Bradbury I, Conlon J, Shankar-Hari M, Rogers AJ, et al. Baseline plasma IL-18 may predict simvastatin treatment response in patients with ARDS: a secondary analysis of the HARP-2 randomised clinical trial. Crit Care (2022) 26(1):164. doi: 10.1186/s13054-022-04025-w
108. Yang Y, Li G, Zhang R. Correlation analysis of acute coronary syndrome with serum IL-18, MMP-9, hs-CRP, and plasma FIB. BioMed Res Int (2022) 2022:5984184. doi: 10.1155/2022/5984184
109. Zhao N, Dong C. Correlation of serum il-18, bdnf, and il-1beta with depression and prognosis after acute exacerbation of chronic obstructive pulmonary disease. Comput Math Methods Med (2022) 2022:3555982. doi: 10.1155/2022/3555982
110. Sismanlar Eyuboglu T, Aslan AT, Ramasli Gursoy T, Pekcan S, Kose M, Hangul M, et al. Caspase-1 and interleukin-18 in children with post infectious bronchiolitis obliterans: a case-control study. Eur J Pediatr (2022). doi: 10.1007/s00431-022-04528-2
111. Qu HQ, Snyder J, Connolly J, Glessner J, Kao C, Sleiman P, et al. Circulating LIGHT (TNFSF14) and interleukin-18 levels in sepsis-induced multi-organ injuries. Biomedicines (2022) 10(2):264–76. doi: 10.3390/biomedicines10020264
112. Li H, Tian J, Yin Y, Diao S, Zhang X, Zuo T, et al. Interleukin-18 mediated inflammatory brain injury after intracerebral hemorrhage in male mice. J Neurosci Res (2022) 100(6):1359–69. doi: 10.1002/jnr.25044
113. Zhou Y, Bian P, Du H, Wang T, Li M, Hu H, et al. The comparison of inflammatory cytokines (il-6 and il-18) and immune cells in japanese encephalitis patients with different progression. Front Cell Infect Microbiol (2022) 12:826603. doi: 10.3389/fcimb.2022.826603
114. Celik F, Coteli E, Gul FC, Ozsoy E, Kobat SG, Karagoz ZK, et al. Interleukin 18, soluble cluster of differentiation 40, platelet factor 4 variant 1, and neutrophil gelatinase-associated lipocalin can be used as biomarkers to aid activity and diagnosis in ocular behcet’s disease. Int Ophthalmol (2022). doi: 10.1007/s10792-022-02331-4
115. Liu CL, Ren J, Wang Y, Zhang X, Sukhova GK, Liao M, et al. Adipocytes promote interleukin-18 binding to its receptors during abdominal aortic aneurysm formation in mice. Eur Heart J (2020) 41(26):2456–68. doi: 10.1093/eurheartj/ehz856
116. Eitan C, Siany A, Barkan E, Olender T, van Eijk KR, Moisse M, et al. Whole-genome sequencing reveals that variants in the interleukin 18 receptor accessory protein 3’UTR protect against ALS. Nat Neurosci (2022) 25(4):433–45. doi: 10.1038/s41593-022-01040-6
117. Yang Y, Song YJ, Nie QB, Wang YF, Zhang M, Mao GS. Correlations of IL-18 and IL-6 gene polymorphisms and expression levels with onset of glioma. Eur Rev Med Pharmacol Sci (2022) 26(5):1475–83. doi: 10.26355/eurrev_202203_28211
118. Cheng L, Dong R, Song C, Li X, Zhang L, Shi M, et al. Mediation effects of il-1beta and il-18 on the association between vitamin d levels and mild cognitive impairment among chinese older adults: a case-control study in taiyuan, china. Front Aging Neurosci (2022) 14:836311. doi: 10.3389/fnagi.2022.836311
119. Hjell G, Szabo A, Morch-Johnsen L, Holst R, Tesli N, Bell C, et al. Interleukin-18 signaling system links to agitation in severe mental disorders. Psychoneuroendocrinology (2022) 140:105721. doi: 10.1016/j.psyneuen.2022.105721
120. Dong G, Liang L, Fu J, Zou C. Serum interleukin-18 levels are raised in diabetic ketoacidosis in Chinese children with type 1 diabetes mellitus. Indian Pediatr (2007) 44(10):732–6.
121. D’Angelo C, Reale M, Costantini E, Di Nicola M, Porfilio I, de Andres C, et al. Profiling of canonical and non-traditional cytokine levels in interferon-beta-treated relapsing-remitting-multiple sclerosis patients. Front Immunol (2018) 9:1240. doi: 10.3389/fimmu.2018.01240
122. Jahanbani-Ardakani H, Alsahebfosoul F, Etemadifar M, Abtahi SH. Interleukin 18 polymorphisms and its serum level in patients with multiple sclerosis. Ann Indian Acad Neurol (2019) 22(4):474–6. doi: 10.4103/aian.aian_515_18
123. Jander S, Stoll G. Increased serum levels of the interferon-gamma-inducing cytokine interleukin-18 in myasthenia gravis. Neurology (2002) 59(2):287–9. doi: 10.1212/WNL.59.2.287
124. Naftali T, Novick D, Gabay G, Rubinstein M, Novis B. Interleukin-18 and its binding protein in patients with inflammatory bowel disease during remission and exacerbation. Isr Med Assoc J (2007) 9(7):504–8.
125. Gualberto Cardoso PR, Diniz Lopes Marques C, de Melo Vilar K, Dantas AT, Branco Pinto Duarte AL, Pitta IDR, et al. Interleukin-18 in Brazilian rheumatoid arthritis patients: Can leflunomide reduce it? Autoimmune Dis (2021) 2021:6672987. doi: 10.1155/2021/6672987
126. Ohta Y, Hamada Y, Katsuoka K. Expression of IL-18 in psoriasis. Arch Dermatol Res (2001) 293(7):334–42. doi: 10.1007/s004030100240
127. Pietrzak D, Pietrzak A, Grywalska E, Kicinski P, Rolinski J, Donica H, et al. Serum concentrations of interleukin 18 and 25-hydroxyvitamin D3 correlate with depression severity in men with psoriasis. PloS One (2018) 13(8):e0201589. doi: 10.1371/journal.pone.0201589
128. Xiang M, Feng Y, Wang Y, Wang J, Zhang Z, Liang J, et al. Correlation between circulating interleukin-18 level and systemic lupus erythematosus: a meta-analysis. Sci Rep (2021) 11(1):4707. doi: 10.1038/s41598-021-84170-4
129. Girard C, Rech J, Brown M, Allali D, Roux-Lombard P, Spertini F, et al. Elevated serum levels of free interleukin-18 in adult-onset still’s disease. Rheumatol (Oxford) (2016) 55(12):2237–47. doi: 10.1093/rheumatology/kew300
130. Gono T, Kawaguchi Y, Sugiura T, Ichida H, Takagi K, Katsumata Y, et al. Interleukin-18 is a key mediator in dermatomyositis: potential contribution to development of interstitial lung disease. Rheumatol (Oxford) (2010) 49(10):1878–81. doi: 10.1093/rheumatology/keq196
131. Mironczuk K, Okruszko A, Wawrusiewicz-Kurylonek N, Kretowski A, Kinalska I, Gorska M. Interleukin 18 and sICAM-1 serum levels in families with type 1 diabetes mellitus. Rocz Akad Med Bialymst (2005) 50:151–4.
132. Harms RZ, Yarde DN, Guinn Z, Lorenzo-Arteaga KM, Corley KP, Cabrera MS, et al. Increased expression of IL-18 in the serum and islets of type 1 diabetics. Mol Immunol (2015) 64(2):306–12. doi: 10.1016/j.molimm.2014.12.012
133. Mahmoud RA, el-Ezz SA, Hegazy AS. Increased serum levels of interleukin-18 in patients with diabetic nephropathy. Ital J Biochem (2004) 53(2):73–81.
134. Karakas Celik S, Oz ZS, Dursun A, Unal A, Emre U, Cicek S, et al. Interleukin 18 gene polymorphism is a risk factor for multiple sclerosis. Mol Biol Rep (2014) 41(3):1653–8. doi: 10.1007/s11033-013-3013-5
135. Furuya D, Yagihashi A, Komatsu M, Masashi N, Tsuji N, Kobayashi D, et al. Serum interleukin-18 concentrations in patients with inflammatory bowel disease. J Immunother (2002) 25 Suppl 1:S65–7. doi: 10.1097/00002371-200203001-00010
136. Leach ST, Messina I, Lemberg DA, Novick D, Rubenstein M, Day AS. Local and systemic interleukin-18 and interleukin-18-binding protein in children with inflammatory bowel disease. Inflammation Bowel Dis (2008) 14(1):68–74. doi: 10.1002/ibd.20272
137. Vasilev G, Manolova I, Ivanova M, Stanilov I, Miteva L, Stanilova S. The role of IL-18 in addition to Th17 cytokines in rheumatoid arthritis development and treatment in women. Sci Rep (2021) 11(1):15391. doi: 10.1038/s41598-021-94841-x
138. Joosten LA, Radstake TR, Lubberts E, van den Bersselaar LA, van Riel PL, van Lent PL, et al. Association of interleukin-18 expression with enhanced levels of both interleukin-1beta and tumor necrosis factor alpha in knee synovial tissue of patients with rheumatoid arthritis. Arthritis Rheumatol (2003) 48(2):339–47. doi: 10.1002/art.10814
139. Valenzuela F, Fernandez J, Jimenez C, Cavagnola D, Mancilla JF, Astorga J, et al. Identification of IL-18 and soluble cell adhesion molecules in the gingival crevicular fluid as novel biomarkers of psoriasis. Life (Basel) (2021) 11(10):1000–11. doi: 10.3390/life11101000
140. Forouzandeh M, Besen J, Keane RW, de Rivero Vaccari JP. The inflammasome signaling proteins asc and il-18 as biomarkers of psoriasis. Front Pharmacol (2020) 11:1238. doi: 10.3389/fphar.2020.01238
141. Mende R, Vincent FB, Kandane-Rathnayake R, Koelmeyer R, Lin E, Chang J, et al. Analysis of serum interleukin (il)-1beta and il-18 in systemic lupus erythematosus. Front Immunol (2018) 9:1250. doi: 10.3389/fimmu.2018.01250
142. Italiani P, Manca ML, Angelotti F, Melillo D, Pratesi F, Puxeddu I, et al. IL-1 family cytokines and soluble receptors in systemic lupus erythematosus. Arthritis Res Ther (2018) 20(1):27. doi: 10.1186/s13075-018-1525-z
143. Wu CY, Yang HY, Yao TC, Liu SH, Huang JL. Serum IL-18 as biomarker in predicting long-term renal outcome among pediatric-onset systemic lupus erythematosus patients. Med (Baltimore) (2016) 95(40):e5037. doi: 10.1097/MD.0000000000005037
144. Priori R, Barone F, Alessandri C, Colafrancesco S, McInnes IB, Pitzalis C, et al. Markedly increased IL-18 liver expression in adult-onset still’s disease-related hepatitis. Rheumatol (Oxford) (2011) 50(4):776–80. doi: 10.1093/rheumatology/keq397
145. Helmers SB, Bruton M, Loell I, Ulfgren AK, Gracie AJ, McInnes IB, et al. Expression of interleukin-18 in muscle tissue of patients with polymyositis or dermatomyositis and effects of conventional immunosuppressive treatment. Rheumatol (Oxford) (2018) 57(12):2149–57. doi: 10.1093/rheumatology/key222
146. Zhang X, Luo S, Wang M, Huang Q, Fang W, Li J, et al. IL18 signaling causes islet beta cell development and insulin secretion via different receptors on acinar and beta cells. Dev Cell (2022) 57(12):1496–511 e6. doi: 10.1016/j.devcel.2022.05.013
147. Din AMNEL, Dayem SMAEL, Atwa HA, Rasmy H. Assessment of interleukin 18 in children with type 1 diabetes and their relatives: its relation to autoantibodies. J Med Sci (2006) 6(4):603–8. doi: 10.3923/jms.2006.603.608
148. Li J, Wu S, Wang MR, Wang TT, Li BK, Zhu JM. Association of the interleukin-18 -137 C/G, -607 A/C polymorphisms with type 1 diabetes: A meta-analysis. BioMed Rep (2014) 2(1):57–62. doi: 10.3892/br.2013.186
149. Knip M. Pathogenesis of type 1 diabetes: implications for incidence trends. Horm Res Paediatr (2011) 76 Suppl 1:57–64. doi: 10.1159/000329169
150. Rabinovitch A. An update on cytokines in the pathogenesis of insulin-dependent diabetes mellitus. Diabetes Metab Rev (1998) 14(2):129–51. doi: 10.1002/(SICI)1099-0895(199806)14:2<129::AID-DMR208>3.0.CO;2-V
151. Pearson JA, Wong FS, Wen L. Inflammasomes and type 1 diabetes. Front Immunol (2021) 12:686956. doi: 10.3389/fimmu.2021.686956
152. Sugiura T, Kawaguchi Y, Harigai M, Terajima-Ichida H, Kitamura Y, Furuya T, et al. Association between adult-onset still’s disease and interleukin-18 gene polymorphisms. Genes Immun (2002) 3(7):394–9. doi: 10.1038/sj.gene.6363922
153. Osman AE, Brema I, AlQurashi A, Al-Jurayyan A, Bradley B, Hamza MA. Association of single-nucleotide polymorphisms in tumour necrosis factor and human leukocyte antigens genes with type 1 diabetes. Int J Immunogenet (2021) 48(4):326–35. doi: 10.1111/iji.12535
154. Oikawa Y, Shimada A, Kasuga A, Morimoto J, Osaki T, Tahara H, et al. Systemic administration of IL-18 promotes diabetes development in young nonobese diabetic mice. J Immunol (2003) 171(11):5865–75. doi: 10.4049/jimmunol.171.11.5865
155. Rothe H, Hausmann A, Casteels K, Okamura H, Kurimoto M, Burkart V, et al. IL-18 inhibits diabetes development in nonobese diabetic mice by counterregulation of Th1-dependent destructive insulitis. J Immunol (1999) 163(3):1230–6.
156. Nicoletti F, Di Marco R, Papaccio G, Conget I, Gomis R, Bernardini R, et al. Essential pathogenic role of endogenous IL-18 in murine diabetes induced by multiple low doses of streptozotocin. prevention of hyperglycemia and insulitis by a recombinant IL-18-binding protein: Fc construct. Eur J Immunol (2003) 33(8):2278–86. doi: 10.1002/eji.200323864
157. Lou H, Guo L. Effect of HIV-1 protease inhibitor on IL-18 and IL-1beta in rats with insulinoma. Dis Markers (2022) 2022:1868749. doi: 10.1155/2022/1868749
158. Kabakchieva P, Gateva A, Velikova T, Georgiev T, Yamanishi K, Okamura H, et al. Elevated levels of interleukin-18 are associated with several indices of general and visceral adiposity and insulin resistance in women with polycystic ovary syndrome. Arch Endocrinol Metab (2022) 66(1):3–11. doi: 10.20945/2359-3997000000442
159. Spritzer PM. Inflammatory mediators in polycystic ovary syndrome: the case of interleukin-18. Arch Endocrinol Metab (2022) 66(1):1–2. doi: 10.20945/2359-3997000000455
160. Zheng D, Liwinski T, Elinav E. Inflammasome activation and regulation: toward a better understanding of complex mechanisms. Cell Discovery (2020) 6:36. doi: 10.1038/s41421-020-0167-x
161. Olcum M, Tastan B, Kiser C, Genc S, Genc K. Microglial NLRP3 inflammasome activation in multiple sclerosis. Adv Protein Chem Struct Biol (2020) 119:247–308. doi: 10.1016/bs.apcsb.2019.08.007
162. Milovanovic J, Arsenijevic A, Stojanovic B, Kanjevac T, Arsenijevic D, Radosavljevic G, et al. Interleukin-17 in chronic inflammatory neurological diseases. Front Immunol (2020) 11:947. doi: 10.3389/fimmu.2020.00947
163. Lewis EC, Dinarello CA. Responses of IL-18- and IL-18 receptor-deficient pancreatic islets with convergence of positive and negative signals for the IL-18 receptor. Proc Natl Acad Sci U S A. (2006) 103(45):16852–7. doi: 10.1073/pnas.0607917103
164. Huang WX, Huang P, Hillert J. Increased expression of caspase-1 and interleukin-18 in peripheral blood mononuclear cells in patients with multiple sclerosis. Mult Scler (2004) 10(5):482–7. doi: 10.1191/1352458504ms1071oa
165. Im SH, Barchan D, Maiti PK, Raveh L, Souroujon MC, Fuchs S. Suppression of experimental myasthenia gravis, a b cell-mediated autoimmune disease, by blockade of IL-18. FASEB J (2001) 15(12):2140–8. doi: 10.1096/fj.01-0072com
166. Christadoss P, Poussin M, Deng C. Animal models of myasthenia gravis. Clin Immunol (2000) 94(2):75–87. doi: 10.1006/clim.1999.4807
167. Souroujon MC, Maiti PK, Feferman T, Im SH, Raveh L, Fuchs S. Suppression of myasthenia gravis by antigen-specific mucosal tolerance and modulation of cytokines and costimulatory factors. Ann N Y Acad Sci (2003) 998:533–6. doi: 10.1196/annals.1254.069
168. Lin X, Qi G. Observation on the efficacy of different methylprednisolone regimens in the treatment of myasthenia gravis. Pak J Med Sci (2022) 38(4Part-II):910–5. doi: 10.12669/pjms.38.4.5069
169. Williams MA, O’Callaghan A, Corr SC. IL-33 and IL-18 in inflammatory bowel disease etiology and microbial interactions. Front Immunol (2019) 10:1091. doi: 10.3389/fimmu.2019.01091
170. Su Y, Zhao H. Predisposition of inflammatory bowel disease is influenced by il-8, il-10, and il-18 polymorphisms: a meta-analysis. Int Arch Allergy Immunol (2020) 181(10):799–806. doi: 10.1159/000509110
171. Nowarski R, Jackson R, Gagliani N, de Zoete MR, Palm NW, Bailis W, et al. Epithelial il-18 equilibrium controls barrier function in colitis. Cell (2015) 163(6):1444–56. doi: 10.1016/j.cell.2015.10.072
172. Chaix J, Tessmer MS, Hoebe K, Fuseri N, Ryffel B, Dalod M, et al. Cutting edge: Priming of NK cells by IL-18. J Immunol (2008) 181(3):1627–31. doi: 10.4049/jimmunol.181.3.1627
173. Wu N, Chen D, Sun H, Tan J, Zhang Y, Zhang T, et al. MAP3K2 augments Th1 cell differentiation via IL-18 to promote T cell-mediated colitis. Sci China Life Sci (2021) 64(3):389–403. doi: 10.1007/s11427-020-1720-9
174. Chakravarti D, Hu B, Mao X, Rashid A, Li J, Li J, et al. Telomere dysfunction activates YAP1 to drive tissue inflammation. Nat Commun (2020) 11(1):4766. doi: 10.1038/s41467-020-18420-w
175. Chakravarti D, Lee R, Multani AS, Santoni A, Keith Z, Hsu WH, et al. Telomere dysfunction instigates inflammation in inflammatory bowel disease. Proc Natl Acad Sci USA (2021) 118(29):e2024853118. doi: 10.1073/pnas.2024853118
176. Sivakumar PV, Westrich GM, Kanaly S, Garka K, Born TL, Derry JM, et al. Interleukin 18 is a primary mediator of the inflammation associated with dextran sulphate sodium induced colitis: blocking interleukin 18 attenuates intestinal damage. Gut (2002) 50(6):812–20. doi: 10.1136/gut.50.6.812
177. Ishikura T, Kanai T, Uraushihara K, Iiyama R, Makita S, Totsuka T, et al. Interleukin-18 overproduction exacerbates the development of colitis with markedly infiltrated macrophages in interleukin-18 transgenic mice. J Gastroenterol Hepatol (2003) 18(8):960–9. doi: 10.1046/j.1440-1746.2003.03097.x
178. Impellizzeri D, Siracusa R, Cordaro M, Peritore AF, Gugliandolo E, Mancuso G, et al. Therapeutic potential of dinitrobenzene sulfonic acid (DNBS)-induced colitis in mice by targeting IL-1beta and IL-18. Biochem Pharmacol (2018) 155:150–61. doi: 10.1016/j.bcp.2018.06.029
179. Pizarro TT, Michie MH, Bentz M, Woraratanadharm J, Smith MF Jr., Foley E, et al. IL-18, a novel immunoregulatory cytokine, is up-regulated in crohn’s disease: expression and localization in intestinal mucosal cells. J Immunol (1999) 162(11):6829–35.
180. Kanai T, Watanabe M, Okazawa A, Nakamaru K, Okamoto M, Naganuma M, et al. Interleukin 18 is a potent proliferative factor for intestinal mucosal lymphocytes in crohn’s disease. Gastroenterology (2000) 119(6):1514–23. doi: 10.1053/gast.2000.20260
181. Mokry LE, Zhou S, Guo C, Scott RA, Devey L, Langenberg C, et al. Interleukin-18 as a drug repositioning opportunity for inflammatory bowel disease: A mendelian randomization study. Sci Rep (2019) 9(1):9386. doi: 10.1038/s41598-019-45747-2
182. Bank S, Andersen PS, Burisch J, Pedersen N, Roug S, Galsgaard J, et al. Genetically determined high activity of IL-12 and IL-18 in ulcerative colitis and TLR5 in crohns disease were associated with non-response to anti-TNF therapy. Pharmacogenomics J (2018) 18(1):87–97. doi: 10.1038/tpj.2016.84
183. Pawlik A, Kurzawski M, Drozdzik M, Dziedziejko V, Safranow K, Herczynska M. Interleukin-18 gene (IL18) promoter polymorphisms in patients with rheumatoid arthritis. Scand J Rheumatol (2009) 38(3):159–65. doi: 10.1080/03009740802600748
184. Koper-Lenkiewicz OM, Sutkowska K, Wawrusiewicz-Kurylonek N, Kowalewska E, Matowicka-Karna J. Proinflammatory cytokines (il-1, -6, -8, -15, -17, -18, -23, tnf-alpha) single nucleotide polymorphisms in rheumatoid arthritis-a literature review. Int J Mol Sci (2022) 23(4):2106–30. doi: 10.3390/ijms23042106
185. Morel JC, Park CC, Kumar P, Koch AE. Interleukin-18 induces rheumatoid arthritis synovial fibroblast CXC chemokine production through NFkappaB activation. Lab Invest (2001) 81(10):1371–83. doi: 10.1038/labinvest.3780351
186. Volin MV, Koch AE. Interleukin-18: a mediator of inflammation and angiogenesis in rheumatoid arthritis. J Interferon Cytokine Res (2011) 31(10):745–51. doi: 10.1089/jir.2011.0050
187. Szekanecz Z, Koch AE. Cell-cell interactions in synovitis. endothelial cells and immune cell migration. Arthritis Res (2000) 2(5):368–73. doi: 10.1186/ar114
188. Luross JA, Williams NA. The genetic and immunopathological processes underlying collagen-induced arthritis. Immunology (2001) 103(4):407–16. doi: 10.1046/j.1365-2567.2001.01267.x
189. Schinnerling K, Rosas C, Soto L, Thomas R, Aguillon JC. Humanized mouse models of rheumatoid arthritis for studies on immunopathogenesis and preclinical testing of cell-based therapies. Front Immunol (2019) 10:203. doi: 10.3389/fimmu.2019.00203
190. Dayer JM. Interleukin-18, rheumatoid arthritis, and tissue destruction. J Clin Invest (1999) 104(10):1337–9. doi: 10.1172/JCI8731
191. Amin MA, Mansfield PJ, Pakozdi A, Campbell PL, Ahmed S, Martinez RJ, et al. Interleukin-18 induces angiogenic factors in rheumatoid arthritis synovial tissue fibroblasts via distinct signaling pathways. Arthritis Rheumatol (2007) 56(6):1787–97. doi: 10.1002/art.22705
192. Morel JC, Park CC, Zhu K, Kumar P, Ruth JH, Koch AE. Signal transduction pathways involved in rheumatoid arthritis synovial fibroblast interleukin-18-induced vascular cell adhesion molecule-1 expression. J Biol Chem (2002) 277(38):34679–91. doi: 10.1074/jbc.M206337200
193. Thomson A, Hilkens CMU. Synovial macrophages in osteoarthritis: the key to understanding pathogenesis? Front Immunol (2021) 12:678757. doi: 10.3389/fimmu.2021.678757
194. Vandenhaute J, Wouters CH, Matthys P. Natural killer cells in systemic autoinflammatory diseases: a focus on systemic juvenile idiopathic arthritis and macrophage activation syndrome. Front Immunol (2019) 10:3089. doi: 10.3389/fimmu.2019.03089
195. Ohya T, Nishimura K, Murase A, Hattori S, Ohara A, Nozawa T, et al. Impaired interleukin-18 signaling in natural killer cells from patients with systemic juvenile idiopathic arthritis. ACR Open Rheumatol (2022) 4(6):503–10. doi: 10.1002/acr2.11426
196. Niu XL, Huang Y, Gao YL, Sun YZ, Han Y, Chen HD, et al. Interleukin-18 exacerbates skin inflammation and affects microabscesses and scale formation in a mouse model of imiquimod-induced psoriasis. Chin Med J (Engl) (2019) 132(6):690–8. doi: 10.1097/CM9.0000000000000140
197. Grozdev I, Korman N, Tsankov N. Psoriasis as a systemic disease. Clin Dermatol (2014) 32(3):343–50. doi: 10.1016/j.clindermatol.2013.11.001
198. Cho D, Seung Kang J, Hoon Park J, Kim YI, Hahm E, Lee J, et al. The enhanced IL-18 production by UVB irradiation requires ROI and AP-1 signaling in human keratinocyte cell line (HaCaT). Biochem Biophys Res Commun (2002) 298(2):289–95. doi: 10.1016/S0006-291X(02)02433-6
199. Pourani MR, Abdollahimajd F, Zargari O, Shahidi Dadras M. Soluble biomarkers for diagnosis, monitoring, and therapeutic response assessment in psoriasis. J Dermatolog Treat (2021) 1–8. doi: 10.1080/09546634.2021.1966357
200. Ruiz-Irastorza G, Khamashta MA, Castellino G, Hughes GR. Systemic lupus erythematosus. Lancet (2001) 357(9261):1027–32. doi: 10.1016/S0140-6736(00)04239-2
201. Jacob N, Stohl W. Cytokine disturbances in systemic lupus erythematosus. Arthritis Res Ther (2011) 13(4):228. doi: 10.1186/ar3349
202. Bossu P, Neumann D, Del Giudice E, Ciaramella A, Gloaguen I, Fantuzzi G, et al. IL-18 cDNA vaccination protects mice from spontaneous lupus-like autoimmune disease. Proc Natl Acad Sci U S A. (2003) 100(24):14181–6. doi: 10.1073/pnas.2336094100
203. Liang R, Zheng L, Ji T, Zheng J, Liu J, Yuan C, et al. Elevated serum free IL-18 in neuropsychiatric systemic lupus erythematosus patients with seizure disorders. Lupus (2022) 31(2):187–93. doi: 10.1177/09612033211069853
204. Yamaguchi M, Ohta A, Tsunematsu T, Kasukawa R, Mizushima Y, Kashiwagi H, et al. Preliminary criteria for classification of adult still’s disease. J Rheumatol (1992) 19(3):424–30.
205. Colafrancesco S, Priori R, Alessandri C, Perricone C, Pendolino M, Picarelli G, et al. IL-18 serum level in adult onset still’s disease: a marker of disease activity. Int J Inflam (2012) 2012:156890. doi: 10.1155/2012/156890
206. Mavragani CP, Spyridakis EG, Koutsilieris M. Adult-onset still’s disease: from pathophysiology to targeted therapies. Int J Inflam (2012) 2012:879020. doi: 10.1155/2012/879020
207. Fautrel B, Le Moel G, Saint-Marcoux B, Taupin P, Vignes S, Rozenberg S, et al. Diagnostic value of ferritin and glycosylated ferritin in adult onset still’s disease. J Rheumatol (2001) 28(2):322–9.
208. Gerfaud-Valentin M, Jamilloux Y, Iwaz J, Seve P. Adult-onset still’s disease. Autoimmun Rev (2014) 13(7):708–22. doi: 10.1016/j.autrev.2014.01.058
209. Sfriso P, Bindoli S, Galozzi P. Adult-onset still’s disease: molecular pathophysiology and therapeutic advances. Drugs (2018) 78(12):1187–95. doi: 10.1007/s40265-018-0956-9
210. Girard-Guyonvarc’h C, Harel M, Gabay C. The role of interleukin 18/interleukin 18-binding protein in adult-onset still’s disease and systemic juvenile idiopathic arthritis. J Clin Med (2022) 11(2):430–7. doi: 10.3390/jcm11020430
211. Dalakas MC, Hohlfeld R. Polymyositis and dermatomyositis. Lancet (2003) 362(9388):971–82. doi: 10.1016/S0140-6736(03)14368-1
212. Dalakas MC. Immunopathogenesis of inflammatory myopathies. Ann Neurol (1995) 37 Suppl 1:S74–86. doi: 10.1002/ana.410370709
213. Tucci M, Quatraro C, Dammacco F, Silvestris F. Increased IL-18 production by dendritic cells in active inflammatory myopathies. Ann N Y Acad Sci (2007) 1107:184–92. doi: 10.1196/annals.1381.020
214. Kaser A, Kaser S, Kaneider NC, Enrich B, Wiedermann CJ, Tilg H. Interleukin-18 attracts plasmacytoid dendritic cells (DC2s) and promotes Th1 induction by DC2s through IL-18 receptor expression. Blood (2004) 103(2):648–55. doi: 10.1182/blood-2002-07-2322
215. Chen M, Quan C, Diao L, Xue F, Xue K, Wang B, et al. Measurement of cytokines and chemokines and association with clinical severity of dermatomyositis and clinically amyopathic dermatomyositis. Br J Dermatol (2018) 179(6):1334–41. doi: 10.1111/bjd.17079
216. Tsoi LC, Gharaee-Kermani M, Berthier CC, Nault T, Hile GA, Estadt SN, et al. IL18-containing 5-gene signature distinguishes histologically identical dermatomyositis and lupus erythematosus skin lesions. JCI Insight (2020) 5(16):e139558. doi: 10.1172/jci.insight.139558
217. Jelusic M, Lukic IK, Batinic D. Biological agents targeting interleukin-18. Drug News Perspect (2007) 20(8):485–94. doi: 10.1358/dnp.2007.20.8.1157617
218. McKie EA, Reid JL, Mistry PC, DeWall SL, Abberley L, Ambery PD, et al. A study to investigate the efficacy and safety of an anti-Interleukin-18 monoclonal antibody in the treatment of type 2 diabetes mellitus. PloS One (2016) 11(3):e0150018. doi: 10.1371/journal.pone.0150018
219. Harel M, Fauteux-Daniel S, Girard-Guyonvarc’h C, Gabay C. Balance between interleukin-18 and interleukin-18 binding protein in auto-inflammatory diseases. Cytokine (2022) 150:155781. doi: 10.1016/j.cyto.2021.155781
221. Li X, Cui W, Hull L, Wang L, Yu T, Xiao M. IL-18 binding protein (IL-18BP) as a novel radiation countermeasure after radiation exposure in mice. Sci Rep (2020) 10(1):18674. doi: 10.1038/s41598-020-75675-5
222. Kim SH, Eisenstein M, Reznikov L, Fantuzzi G, Novick D, Rubinstein M, et al. Structural requirements of six naturally occurring isoforms of the IL-18 binding protein to inhibit IL-18. Proc Natl Acad Sci U S A. (2000) 97(3):1190–5. doi: 10.1073/pnas.97.3.1190
223. Detry S, Andries J, Bloch Y, Gabay C, Clancy DM, Savvides SN. Structural basis of human IL-18 sequestration by the decoy receptor IL-18 binding protein in inflammation and tumor immunity. J Biol Chem (2022) 298(5):101908. doi: 10.1016/j.jbc.2022.101908
224. Plater-Zyberk C, Joosten LA, Helsen MM, Sattonnet-Roche P, Siegfried C, Alouani S, et al. Therapeutic effect of neutralizing endogenous IL-18 activity in the collagen-induced model of arthritis. J Clin Invest (2001) 108(12):1825–32. doi: 10.1172/JCI200112097
225. Siegmund B, Fantuzzi G, Rieder F, Gamboni-Robertson F, Lehr HA, Hartmann G, et al. Neutralization of interleukin-18 reduces severity in murine colitis and intestinal IFN-gamma and TNF-alpha production. Am J Physiol Regul Integr Comp Physiol (2001) 281(4):R1264–73. doi: 10.1152/ajpregu.2001.281.4.R1264
226. Li X, Zhang C, Chen W, Pan B, Kong F, Zheng K, et al. Protective effect of neutralizing anti-IL-18alpha monoclonal antibody on a mouse model of acute graft-versus-host disease. Oncol Rep (2015) 34(4):2031–9. doi: 10.3892/or.2015.4176
227. Fantuzzi G, Dinarello CA. The inflammatory response in interleukin-1 beta-deficient mice: comparison with other cytokine-related knock-out mice. J Leukoc Biol (1996) 59(4):489–93. doi: 10.1002/jlb.59.4.489
228. Li P, Allen H, Banerjee S, Franklin S, Herzog L, Johnston C, et al. Mice deficient in IL-1 beta-converting enzyme are defective in production of mature IL-1 beta and resistant to endotoxic shock. Cell (1995) 80(3):401–11. doi: 10.1016/0092-8674(95)90490-5
229. Siegmund B, Lehr HA, Fantuzzi G, Dinarello CA. IL-1 beta -converting enzyme (caspase-1) in intestinal inflammation. Proc Natl Acad Sci U S A. (2001) 98(23):13249–54. doi: 10.1073/pnas.231473998
230. Tsutsumi N, Yokota A, Kimura T, Kato Z, Fukao T, Shirakawa M, et al. An innate interaction between IL-18 and the propeptide that inactivates its precursor form. Sci Rep (2019) 9(1):6160. doi: 10.1038/s41598-019-42661-5
231. Solle M, Labasi J, Perregaux DG, Stam E, Petrushova N, Koller BH, et al. Altered cytokine production in mice lacking P2X(7) receptors. J Biol Chem (2001) 276(1):125–32. doi: 10.1074/jbc.M006781200
232. Li S, Jiang L, Beckmann K, Hojen JF, Pessara U, Powers NE, et al. A novel anti-human IL-1R7 antibody reduces IL-18-mediated inflammatory signaling. J Biol Chem (2021) 296:100630. doi: 10.1016/j.jbc.2021.100630
233. Nariai Y, Kamino H, Obayashi E, Kato H, Sakashita G, Sugiura T, et al. Generation and characterization of antagonistic anti-human interleukin (IL)-18 monoclonal antibodies with high affinity: Two types of monoclonal antibodies against full-length IL-18 and the neoepitope of inflammatory caspase-cleaved active IL-18. Arch Biochem Biophys (2019) 663:71–82. doi: 10.1016/j.abb.2019.01.001
Keywords: inflammatory diseases, autoimmune diseases, interleukin-18, monoclonal antibodies, immunity
Citation: Ihim SA, Abubakar SD, Zian Z, Sasaki T, Saffarioun M, Maleknia S and Azizi G (2022) Interleukin-18 cytokine in immunity, inflammation, and autoimmunity: Biological role in induction, regulation, and treatment. Front. Immunol. 13:919973. doi: 10.3389/fimmu.2022.919973
Received: 14 April 2022; Accepted: 25 July 2022;
Published: 11 August 2022.
Edited by:
Guochang Hu, University of Illinois at Chicago, United StatesReviewed by:
Sylvie Amu, University College Cork, IrelandCopyright © 2022 Ihim, Abubakar, Zian, Sasaki, Saffarioun, Maleknia and Azizi. This is an open-access article distributed under the terms of the Creative Commons Attribution License (CC BY). The use, distribution or reproduction in other forums is permitted, provided the original author(s) and the copyright owner(s) are credited and that the original publication in this journal is cited, in accordance with accepted academic practice. No use, distribution or reproduction is permitted which does not comply with these terms.
*Correspondence: Gholamreza Azizi, YXppemlAYWJ6dW1zLmFjLmly
†These authors have contributed equally to this work
Disclaimer: All claims expressed in this article are solely those of the authors and do not necessarily represent those of their affiliated organizations, or those of the publisher, the editors and the reviewers. Any product that may be evaluated in this article or claim that may be made by its manufacturer is not guaranteed or endorsed by the publisher.
Research integrity at Frontiers
Learn more about the work of our research integrity team to safeguard the quality of each article we publish.