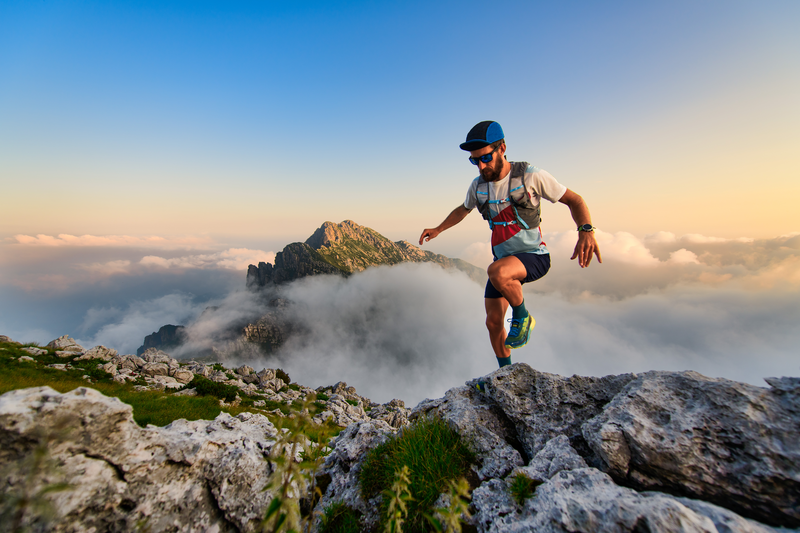
95% of researchers rate our articles as excellent or good
Learn more about the work of our research integrity team to safeguard the quality of each article we publish.
Find out more
MINI REVIEW article
Front. Immunol. , 26 May 2022
Sec. Vaccines and Molecular Therapeutics
Volume 13 - 2022 | https://doi.org/10.3389/fimmu.2022.912529
This article is part of the Research Topic Current Concepts of Cellular and Biological Drugs to Modulate Regulatory T cell Activity in the Clinic, volume II View all 7 articles
Over the past decades, atopic diseases, including allergic rhinitis, asthma, atopic dermatitis, and food allergy, increased strongly worldwide, reaching up to 50% in industrialized countries. These diseases are characterized by a dominating type 2 immune response and reduced numbers of allergen-specific regulatory T (Treg) cells. Conventional allergen-specific immunotherapy is able to tip the balance towards immunoregulation. However, in mouse models of allergy adaptive transfer of Treg cells did not always lead to convincing beneficial results, partially because of limited stability of their regulatory phenotype activity. Besides genetic predisposition, it has become evident that environmental factors like a westernized lifestyle linked to modern sanitized living, the early use of antibiotics, and the consumption of unhealthy foods leads to epithelial barrier defects and dysbiotic microbiota, thereby preventing immune tolerance and favoring the development of allergic diseases. Epigenetic modification of Treg cells has been described as one important mechanism in this context. In this review, we summarize how environmental factors affect the number and function of Treg cells in allergic inflammation and how this knowledge can be exploited in future allergy prevention strategies as well as novel therapeutic approaches.
The prevalence of allergic and of most autoimmune diseases like diabetes, inflammatory bowel disease, multiple sclerosis, and rheumatoid arthritis, has dramatically increased worldwide, reaching epidemic dimensions especially in industrialized countries. The immunological mechanisms of (atopic) allergic diseases, that is, allergic rhinitis, asthma, atopic dermatitis, insect venom allergy, and IgE-mediated food allergy, are characterized by an aberrant type 2 immune response to otherwise harmless allergens and a decrease in allergen-specific regulatory T (Treg) cells that are restored during allergen-specific immunotherapy (AIT), the only causal-oriented treatment known so far. However, AIT is cumbersome and not established for all kinds of allergies. Therefore, the clinical demand for other therapies remains high. In this review article, we discuss novel treatment strategies to restore the function of Treg cells in allergic inflammation by either boosting Treg cell expansion in vivo or by reinfusion of isolated and ex vivo engineered Treg cells.
During sensitization against a specific allergen, several cell types of the innate and adaptive immune system and various ligand-receptor-interactions are triggered (1–3). Initially, the allergens traverse epithelial barriers and are internalized by antigen-presenting cells (APC), especially DC, located in the skin, airways and gut mucosa. According to the epithelial barrier hypothesis, an increase in epithelial barrier-damaging agents due to industrialization like toxins, surfactants and emulsifiers in household cleaning agents or processed food, cigarette smoke, particulate matter, diesel exhaust particles, ozone, nanoparticles and microplastics, is associated with the rise in allergic, autoimmune and other chronic inflammatory diseases. Protease activity of allergens as well as exposure to certain bacteria and viruses was also shown to facilitate disruption of tight junction proteins further leading to epithelial barrier leakiness (4). Damaged epithelial cells release alarmins (IL-25, IL-33, thymic stromal lymphopoietin (TSLP)), which activate DC and group 2 innate lymphoid cells (ILC2). Activated DC migrate to local lymph nodes where they present processed allergen peptides to naive CD4+ T cells on MHC class II molecules. Recognition of these peptide/MHC complexes by the T-cell receptor induces differentiation of T helper cells with a Th2-type cytokine profile (IL-4, IL-5, IL-9, and IL 13) depending on additional costimulatory signals from the DC and the surrounding micromilieu. Furthermore, IL-5, IL-13, and IL-9 are produced by ILC2 (4). Expression of IL-4 and IL-13 together with ligation of suitable costimulatory molecules (CD40 with CD40 ligand, and CD80 or CD86 with CD28) induces an immunoglobulin class switch and the production and secretion of IgE antibodies by B cells. IgE is bound to the surface of effector cells such as mast cells and basophils via the high affinity IgE-receptor (FcϵRI). In sensitized individuals, crosslinking of these IgE-FcϵRI-complexes after re-exposure to the same allergen rapidly leads to release of pro-inflammatory mediators like histamine, tryptase, prostaglandins, leukotrienes, and cytokines, which cause the first early phase response with typical allergic symptoms such as rhinorrhea, airway mucus secretion, broncho-constriction as well as urticaria, vomiting, and diarrhea in the case of food allergies and even leading to anaphylaxis. The late phase response, characterized by the recruitment of further mast cells and eosinophils mainly induced through IL-5, follows within several hours. During the repeated exposure the allergen is also taken up by DC, thereby activating memory Th2 cells to produce cytokines and other mediators, which may further lead to chronic airway inflammation, goblet cell hyperplasia and tissue remodeling (3, 5, 6).
The concept of immune tolerance by specialized lymphocytes to self-antigens has long been suspected but only proven after the identification by Sakaguchi et al. that a small population of CD25-expressing CD4+ T cells could prevent autoimmunity in mice (7). A second breakthrough was the discovery of the transcription factor FoxP3 as master regulator of Treg cell differentiation and function. Mutations or deletion of this gene cause severe autoimmune disease known as scurfy phenotype in mice and IPEX (immune dysregulation, polyendocrinopathy, enteropathy, X-linked) syndrome in humans which suffer from diabetes mellitus, high serum IgE levels, eosinophilia, and food allergy (8–10). CD4+CD25highCD127low Treg cells constitute about 5-7% of circulating CD4+ T cells, and they are either generated in the thymus (tTreg) or induced in the periphery (pTreg), especially in the gut, from conventional T cells in the presence of TGF-ß or retinoic acid (11–13). While tTreg constitutively express the transcription factor FoxP3, which is essential for their differentiation and function, pTreg can lose FoxP3 expression and convert into IL-17- and IFN-γ-producing inflammatory effector T cells (14). In general, Treg cells possess remarkable plasticity in response to a changing cytokine milieu by expressing alternate lineage transcription factors such as T-bet, GATA3 and RORCγt (15, 16). Furthermore, at least in humans, FoxP3 is also transiently expressed by activated effector T cells. Stability of FoxP3 expression relies on DNA hypomethylation of the Treg cell-specific demethylated region (TSDR) within the conserved non-coding sequence 2 (CNS2) in the Foxp3 gene region (17). Helios and neuropilin-1 are also almost exclusively expressed in tTreg compared to pTreg (18, 19). Another population of Treg cells, namely CD49b+LAG-3+ T regulatory type 1 (Tr1) cells, can be induced in the periphery upon antigen exposure under tolerogenic conditions which exert their suppressive capacity independently from FoxP3 mainly via secretion of IL-10 (20–22).
Treg cells play a critical role in maintaining immune tolerance to allergens and all above-mentioned Treg cell subsets have been described to control type 2 immune cells by producing inhibitory cytokines such as IL-10, TGF-ß and IL-35 (Table 1). Furthermore, Treg cells express high-levels of surface markers associated with suppression like CTLA-4, PD-1, GITR, ICOS, LAP, GARP, TIM-3, TIGIT, CD39 and CD73, and suppress effector T cells through cell-cell-contact, cytolysis and metabolic disruption, reviewed in (31). Although we and others have shown that the suppressive capacity of peripheral blood derived Treg cells from allergic individuals is not generally defective compared to healthy controls, it may be reduced under certain conditions (32–34). For example, Treg from grass-pollen allergic donors failed to inhibit proliferation of T effector cells at high allergen doses while Treg cells from non-allergic donors did not fail at these allergen concentrations, probably due to production of high amounts of IL-2 by responder T cells following increased stimulation thereby escaping suppression (35, 36). The fact that only a few soluble proteins from inhaled allergens are Th2 and IgE inducers/targets, while the vast majority of encountered proteins attached to inhaled particles is still tolerated, also argues against a global defect in Treg cell deficiency in allergic patients (37). Nevertheless, on the other hand, recent reports have shown that tissue derived Treg cells are quantitatively reduced and functionally impaired in asthmatic children (38). Furthermore, patients with allergic rhinitis have lower percentages of antigen-specific Tr1 cells in peripheral blood compared to healthy donors, and induced peanut-specific Tr1 cells from allergic patients are functionally defective (39, 40). Importantly, improvement of allergic symptoms in successful AIT, characterized by repeated subcutaneous or sublingual applications of increasing doses of the specific allergen, is associated with an increased number of allergen-specific Treg cells (Table 1) as well as and Breg cells (23, 41, 42). In contrast, the recently defined dysregulated FoxP3+ILT3+ Treg cell subset, occurring in a higher frequency in asthmatic compared to healthy donors, is reduced during AIT (Table 1) (30, 43). Increased antigen-induced regulatory T-cell function has also been observed after peanut or milk oral immunotherapy (OIT) (25, 44). Overall, a decrease in allergen-specific IgE together with an increase in protective IgG4 antibodies is achieved (23, 26, 41, 42). Furthermore, Treg have been demonstrated to directly block mast cell degranulation and ILC2 through OX40-OX40L or ICOS-ICOSL interaction, respectively (45, 46).
Table 1 Treg cell subsets involved in allergic inflammation and increased (green) or decreased (red) during AIT or OIT.
Despite a genetic predisposition or other risk factors like recurrent upper respiratory tract infections in early life, it is becoming increasingly clear that the increased incidence of allergic diseases is related to changes in the mucosal microbiome and to mucosal barrier defects. These changes are due to modern sanitized living with reduced exposure to environmental microorganisms, as suggested by the so-called hygiene hypothesis (47, 48), as well as to the consumption of unhealthy foods, high in fat, sugar and certain food additives, and low in fiber (49). The importance of the microbiota for immune tolerance has been demonstrated in mice raised under germ-free conditions which display strongly reduced Treg cell frequency in the gut and are more susceptible to develop allergies (50, 51). Recolonization of the gut with commensal bacteria such as Firmicutes, Bacteroidetes, Bifidobacterium and Prevotella restored Treg cell numbers via different mechanisms (52). For instance, fiber is fermented to short chain fatty acids (SCFA), and it has been demonstrated in several animal models and in human studies that the most abundant SCFA acetate, propionate and especially butyrate can promote Treg cell differentiation and function, thereby preventing or ameliorating the development of food and inhalational allergy (53–55). Butyrate has also been shown to ameliorate ILC2-driven airway inflammation (56). These effects appeared to be mediated by SCFA-induced G-protein-coupled receptors (GPCR) activation, mainly GPR41, GPR43 and GPR109A, by activation of transcription factors such as aryl hydrocarbon receptor (AhR), or via epigenetic modification through inhibition of histone deacetylases (HDAC). Vitamin A and its metabolite retinoid acid further induce Treg cell development and a combination of both vitamin A and dietary fiber was required to maintain protection against food allergy (53, 57–60). In addition, consumption of unsaturated omega-3 fatty acids present for example in olive oil and fish has also been associated with reduced risk of allergic rhinitis and asthma (61). Molecules like polysaccharide A (PSA) produced by Bacteroides fragilis can also boost Treg cell generation via Toll-like receptor 2 (TLR2) signaling (62). Furthermore, tryptophan metabolites derived from intestinal commensals or catabolized by indoleamine 2,3-dioxygenase (IDO)-expressing tolerogenic DC ameliorate allergic responses (63). In contrast, wheat amylase trypsin inhibitors (ATI), activators of intestinal myeloid cells via TLR4 and promoters of microbial dysbiosis (64, 65), have been shown to enhance allergic intestinal and airway inflammation (66, 67). Importantly, it has recently been reported that high frequencies of RORCγt+ Treg are required to tolerate the intestinal microbiota and to prevent inflammatory type 2 diseases in the gut (68). Furthermore, RORCγt+ Treg possess a protective role in a model of food allergy (69). Altogether, a disturbed microbiota favors intolerances to food-derived antigens, whereas administration of probiotics may promote protection from allergic intestinal and airway inflammation (49, 59, 70).
As already mentioned above, allergen-specific Treg cells are induced during successful AIT. However, AIT is not yet applicable for all kinds of allergies, and did not lead to beneficial results in all patients. Therefore, other therapeutic settings aiming to induce or expand Treg cells are urgently needed (summarized in Figure 1). Using a humanized mouse model of allergy, where PBMC from highly sensitized allergic donors were injected into immunodeficient nonobese diabetic-severe combined immunodeficiency gamma chain knockout (NSG) mice, we have recently shown that Treg cells can be induced by administration of the chemokine CCL18 produced by tolerogenic IL-10 DC (71–73) or soluble GARP (74, 75). Both factors led to inhibition of allergen-specific human IgE production in mouse sera and subsequently to prevention of allergen-driven IgE-dependent airway and intestinal inflammation (73–75). GARP has formerly been described to be up-regulated on activated Treg and to mediate their suppressive function (76, 77). In addition, administration of soluble GARP prevented the onset of a xenogeneic graft-versus-host disease (GvHD) in NSG mice being injected with human PBMC directly after birth also by enhancing Treg cell activity (78). In both models, the suppressive function of Treg could further be enhanced by the CD4-binding HIV-1 surface protein gp120, preventing GvHD or allergic airway inflammation, respectively (79, 80). Recently, it has been reported that low-dose IL-2 or a combination of IL-2/anti-IL-2 restored Treg numbers and function in respiratory and food allergies, thereby improving allergic markers and symptoms (81–83).
Manipulation of the intestinal microbiota associated with induction or expansion of Treg cells as shown in numerous animal models of allergy and asthma may also offer novel strategies for immunomodulation of food allergies. However, only a slight improvement could be observed in clinical studies so far. For example, in a double-blind, placebo-controlled clinical trial, co-administration of the probiotic Lactobacillus rhamnosus reduced peanut-specific IgE levels during peanut oral immunotherapy while peanut-specific IgG4 levels were increased (29). Additionally, colonization of mice and humans with certain Clostridia species has been shown to protect from food allergy via increasing Treg cell numbers (60, 69). In a more recent study, fecal transplantation from healthy but not cow’s milk allergic infants successfully prevented anaphylactic responses in susceptible germ-free mice, thus confirming the importance of intestinal bacteria in promoting tolerance to dietary antigens (84). Importantly, the early-life microbiota established in uterus and during the first months of life plays a crucial role for proper immunological development and overall health status (85). In this respect, an increased risk of the development of food allergy and asthma has been reported for children born by caesarian instead of vaginal delivery. This may be due to a direct impact of the vaginal microbiota being rich in Lactobacilli species on the microbiota development of the baby (86, 87). Perinatal antibiotic prophylaxis after caesarian delivery or the absence of stress factors associated with a vaginal delivery may also contribute to this effect. In general, a strong relationship between increased antibiotic use in the first few years of life and allergy development has been reported (88). Antibiotics not only reduce important protolerogenic bacteria, they also induce metabolic changes and enhance susceptibility to obesity (60, 89). Furthermore, Lactobacilli and Bifidobacteria as well as oligosaccharides in the breast milk are important triggers of a balanced healthy immune system, and longer duration of breastfeeding is associated with Treg cell expansion and reduced allergy development (90–92). In addition, a dietary diversity in the first years of life and early consumption of peanuts or eggs as well as other factors relating to the hygiene hypothesis, like living in a farm environment and drinking farm milk, or exposure to a large number of pets, both associated with an enhanced microbial diversity, have been described to protect against allergy and asthma, particularly via the induction of Treg cells (93–99). For example, chronic exposure to low-dose endotoxin or farm dust has been shown to inhibit developing house dust mite-induced asthma in mice (100). Of note, the allergy protective farm effect was previously imitated by the lipocalin beta-lactoglobulin (BLG) present in cow’s raw milk and farm dust when loaded with iron-flavonoid complexes. Holo-BLG promoted Treg cells through AhR activation and down-regulation of antigen presentation in monocytes and DC via transport of iron (101). In a follow-up clinical pilot trial, the same authors assessed the efficacy of a FSMP (food for special medical purposes) lozenge containing BLG with iron, polyphenols, retinoic acid, and zinc in allergic women and could show that this holo-BLG lozenge improved nasal symptom score after nasal provocation as well as symptom medication score during birch or grass pollen season by more than 40% compared to the placebo group (102).
However, more and larger studies are needed to confirm all these positive results and to develop public health strategies to prevent allergic diseases in the future.
The therapeutic potential of FoxP3+ Treg cells has been demonstrated in many preclinical models and clinical trials aiming to prevent graft-versus-host disease during organ transplantation and in several autoimmune diseases such as type 1 diabetes mellitus, inflammatory bowel disease, systemic lupus erythematosus, multiple sclerosis, and allergy (103). Tr1 cells therapy in the context of allogeneic hematopoietic stem cell transplantation has also been successfully employed (104). Overall, the functional stability of Treg cells is of fundamental importance for the efficacy and safety of Treg cell-based therapies.
Gene editing technologies in medical research are becoming a central player to investigate and treat complex biological diseases. Constant balanced examination of the immune system of autonomous and non-autonomous antigens protects healthy cells and eliminates invading foreign particles. However, failing in recognition of self and non-self antigens leads to various autoimmune and inflammatory diseases such as inflammatory bowel disease, type 1 diabetes, rheumatoid arthritis and multiple sclerosis (105). Standard medication for various autoimmune diseases including allergy required immunosuppression in a form of pharmacological compounds and antibody targeted treatment which subjected patients to other complications such as cancer and opportunistic pathogens. In this regard, cell-based therapy such as adoptive cell transfer of engineered Treg cells could provide an effective therapeutic alternative to combat autoimmune diseases.
Adoptive cell transfer of Treg cells is effective and well tolerated. For instance, in a phase I/IIa clinical trial investigating Treg cell therapy in kidney transplantation, patients engrafted with autologous Treg had similar rejection rates compared to the group receiving the standard immunosuppression with basiliximab (anti-CD25), but displayed reduced infection rates (106). In clinical settings, polyclonal expansion, antigen-specific stimulation and engineered (CAR: chimeric antigen receptor and TCR: predefined T cell receptor) Treg cells are the prime strategies. In polyclonal expansion, Treg cells from donor PBMC were activated in the presence of anti-CD3/28 beads and IL-2 whereas an antigen-specific approach employed allogeneic Treg cells from the recipient stimulated by antigen presenting cells from the graft donor. However, an antigen-specific approach is more efficient with higher specificity than polyclonal Treg cells and suitable for the transplantation. Engineered approaches use genetic modification of polyclonal Treg cells with CAR or TCR to recognize desired antigens and provides abundant antigen-specific Treg cells; Figure 2. The advantage of engineered approaches is the reduction of side effects due to nonspecific suppression by Treg cells (103).
Figure 2 Possible adoptive CAR-Treg cell therapy for allergic asthma. Treg cells are isolated from peripheral blood of healthy and allergic donor, cultured with IL-2 for polyclonal Treg cells (CD3/CD28 beads), antigen-specific cells (antigen presenting cells activate alloreactive Treg cells) and genetically engineered Treg cells. In genetically engineered Treg approach, cells could be transduced with retroviruses, lentiviruses, adenovirus-associated virus (AAV), transcription activator-like effector nucleases (TALEN), Zinc finger nucleases (ZFN) or CRISPR-Cas to express CAR, TCR, BAR and others. With this approach immunological rejection would be avoided (introduction of the non-classical HLA and deletion of donor HLA molecules), in case of off target, suicidal gene system could be activated and co-expression of others such as PDL1, CTLA4, and IL-2R would help in the specific migration, suppression and inactivation of T cells. Further, following GMP regulations, expanded cells could be infused in the patients.
Traditional gene transfer approach employed retroviruses, lentiviruses, adenovirus-associated virus (AAV) and transposases which dispense specific and randomized insertion of targeted genes in Treg cells (107, 108). However, randomized insertion by transposases could raise safety concerns (109). Other gene editing or nucleases approaches such as transcription activator-like effector nucleases (TALEN), Zinc finger nucleases (ZFN) and more recently CRISPR-Cas applications advancing Treg therapy and its product (110, 111).
TCR engineered Treg cells are multi protein complex consisting of α- and β-chain which recognize major histocompatibility complex (intra/extracellular antigen) and trigger CD3 dimer complexes (CD3ϵ, CD3γ, and CD3ζ chains) (103). TCR engineered Treg cells provide bystander and antigen-specific suppression by assembling itself in the targeted tissue during autoimmunity (112). Expression of single antigen in a cell is adequate for TCR engineered Treg cell activation but its recognition capacity is limited over a specific population. In the multiple sclerosis mouse model of experimental allergic encephalomyelitis (EAE), myelin basic protein (MBP) activated human Treg cells control the expression of T-effector cells with enhanced Foxp3 expression (113). Further, human TCR transduced for factor VIII (FVIII) also showed dominance over FVIII-specific T-effector cell proliferation and its cytokine production. Moreover, antibody production was also inhibited in antigen-specific manner in FVIII-deficient mice (114). Additionally, transduced Bet v 1-specific Treg cells were more potent than regular tTreg in suppression of allergen-specific T-effector cell proliferation and cytokine production (115). For the future TCR therapy, TCR should be considered from Treg instead of effector T cells.
CAR are the single protein complex consisting of single chain variable fragment which recognize antigen in the outer part, a hinge and inner part with CD3ζ and CD28/4-1BB domain. Elegant work performed by the group of Eshhar licensed the innovation of CAR (116). Initially based on its stimulatory domain, CAR have been alienated into several generations. First generation poses single stimulatory domain (CD3ζ) followed by second generation which display co-stimulatory domain (CD3ζ and CD28), third generation (CD28, CD27, 4-11BB, OX40, ICOS) and fourth generation encompasses with ScFv-CD3ζ, CD28, and 4-1BB (117).
CAR offer direct and broad-spectrum recognition unlike TCR (MHC restricted) and have limited IL-2 dependency but require a high number of targeted tissue antigens (100–10,000 antigens/cell) (118, 119). They can spot entire proteins, major histocompatibility complexes and extracellular antigens (103). CAR engineered Treg specific to HLA-A2 prevent HLA A2+ skin rejection and graft versus host disease in mouse models (120, 121). However, CAR recognized extracellular antigens, therefore new tools which combined TCR, and CAR have been developed (122). For instance, the modular chimeric antigen receptor approach unveils suppression functions of co-stimulatory molecules (CD137 and CD28) in Treg and limits the T-effector cells (123). In another study, improved pancreatic islet allograft in mAb-directed CAR expressing Treg cells towards MHC-1 peptides and circumvents skin rejection was monitored (124).
CAR T cells have been utilized for B-cells autoantibodies (IgG) in Pemphigus vulgaris (PV) where CAAR expressing Dsg3 (keratinocyte adhesion protein) specifically targeted autoreactive B cells without influencing other cell types and protected through CD137 signaling cascade (125, 126). Since IgE is a major contributor in the pathogenesis of allergic disease, therefore targeting IgE producing memory B-cells possess great potential for allergic and autoimmune diseases. In this regard, CAR-T-IgE containing α-chain of IgE receptor and FCϵRI displayed specificity towards mouse IgE-B-cell and could be adapted to the clinical settings (127, 128). In asthma, autoantibodies for cell-junction, endothelial and epithelial cells have already delineated which could also be targeted in future (129, 130). In asthma mouse model, mobilization of CAR-Treg cells towards lungs and tracheobronchial lymph node diminished airway hyperreactivity, reduced mucus secreting cells, eosinophilic activity, Th2 cytokines and allergen-specific IgE to control asthma (131). Recently, CAR approach was employed in an allergic model where ovalbumin (OVA) antigen was targeted for mast cells (IgE-sensitized) and B-cells, termed as B-cell Antibody Receptor (BAR). Post OVA challenged for anaphylactic reaction, OVA-BAR expressing Treg cells shielded mice from hypothermia (132). However, human clinical studies using CAR-Treg cells in allergic inflammation are missing so far.
Taken together, Treg therapies and its applications are entering into an exciting era but still confronted with some limitations such as engraftment, safety concerns and long term stability without acquiring plasticity. However, adverse effect or cytokine storm from engineered CAR-Treg cells could be controlled with CRISPR-Cas9 suicidal system containing pro-apoptotic suicidal gene (128, 133). Stability and plasticity, post CAR-Treg cells transplantation could likely be resolved with CRISPR applications either by inserting (IL-10, TGF-β) or removal (IL-17, IL-4, IFN-γ, IL-6) of desired genes (134–136). Further, engraftment efficacy to the targeted tissue is potentially enhanced by expressing desired chemokine receptor in CAR-Treg cells in accordance with overexpressed ligand in the targeted tissue as demonstrated in Figure 2 (103, 137). Most of the promising preclinical data demanded further optimization and characterization of genetically modified Treg therapy with higher efficacy targeting asthma and allergic diseases to offer alternative curative options at least for patients with severe or chronic respiratory diseases in future.
All authors listed have made a substantial, direct, and intellectual contribution to the work, and approved it for publication.
This work was supported by Deutsche Forschungsgemeinschaft (DFG), grants BE 4504/3-3.
The authors declare that the research was conducted in the absence of any commercial or financial relationships that could be construed as a potential conflict of interest.
All claims expressed in this article are solely those of the authors and do not necessarily represent those of their affiliated organizations, or those of the publisher, the editors and the reviewers. Any product that may be evaluated in this article, or claim that may be made by its manufacturer, is not guaranteed or endorsed by the publisher.
1. Lambrecht BN, Hammad H. The Immunology of the Allergy Epidemic and the Hygiene Hypothesis. Nat Immunol (2017) 18(10):1076–83. doi: 10.1038/ni.3829
2. Kubo M. Innate and Adaptive Type 2 Immunity in Lung Allergic Inflammation. Immunol Rev (2017) 278(1):162–72. doi: 10.1111/imr.12557
3. Hammad H, Lambrecht BN. The Basic Immunology of Asthma. Cell (2021) 184(6):1469–85. doi: 10.1016/j.cell.2021.02.016
4. Akdis CA. Does the Epithelial Barrier Hypothesis Explain the Increase in Allergy, Autoimmunity and Other Chronic Conditions? Nat Rev Immunol (2021) 21(11):739–51. doi: 10.1038/s41577-021-00538-7
5. Galli SJ, Tsai M, Piliponsky AM. The Development of Allergic Inflammation. Nature (2008) 454(7203):445–54. doi: 10.1038/nature07204
6. Pulendran B, Artis D. New Paradigms in Type 2 Immunity. Science (2012) 337(6093):431–5. doi: 10.1126/science.1221064
7. Sakaguchi S, Sakaguchi N, Asano M, Itoh M, Toda M. Immunologic Self-Tolerance Maintained by Activated T Cells Expressing Il-2 Receptor Alpha-Chains (Cd25). Breakdown of a Single Mechanism of Self-Tolerance Causes Various Autoimmune Diseases. J Immunol (1995) 155:1151–64.
8. Fontenot JD, Gavin MA, Rudensky AY. Foxp3 Programs the Development and Function of Cd4+Cd25+ Regulatory T Cells. Nat Immunol (2003) 4(4):330–6. doi: 10.1038/ni904
9. Hori S, Nomura T, Sakaguchi S. Control of Regulatory T Cell Development by the Transcription Factor Foxp3. Science (2003) 299(5609):1057–61. doi: 10.1126/science.1079490
10. Bennett CL, Christie J, Ramsdell F, Brunkow ME, Ferguson PJ, Whitesell L, et al. The Immune Dysregulation, Polyendocrinopathy, Enteropathy, X-Linked Syndrome (Ipex) Is Caused by Mutations of Foxp3. Nat Genet (2001) 27(1):20–1. doi: 10.1038/83713
11. Shevach EM, Thornton AM. Ttregs, Ptregs, and Itregs: Similarities and Differences. Immunol Rev (2014) 259(1):88–102. doi: 10.1111/imr.12160
12. Shevach EM. Foxp3(+) T Regulatory Cells: Still Many Unanswered Questions-A Perspective After 20 Years of Study. Front Immunol (2018) 9:1048. doi: 10.3389/fimmu.2018.01048
13. Sakaguchi S, Mikami N, Wing JB, Tanaka A, Ichiyama K, Ohkura N. Regulatory T Cells and Human Disease. Annu Rev Immunol (2020) 38:541–66. doi: 10.1146/annurev-immunol-042718-041717
14. Zhou X, Bailey-Bucktrout SL, Jeker LT, Penaranda C, Martinez-Llordella M, Ashby M, et al. Instability of the Transcription Factor Foxp3 Leads to the Generation of Pathogenic Memory T Cells in Vivo. Nat Immunol (2009) 10(9):1000–7. doi: 10.1038/ni.1774
15. DuPage M, Bluestone JA. Harnessing the Plasticity of Cd4(+) T Cells to Treat Immune-Mediated Disease. Nat Rev Immunol (2016) 16(3):149–63. doi: 10.1038/nri.2015.18
16. Ohnmacht C, Eyerich S. Diversity of T Helper and Regulatory T Cells and Their Contribution to the Pathogenesis of Allergic Diseases. Handb Exp Pharmacol (2022) 268:265–96. doi: 10.1007/164_2021_486
17. Floess S, Freyer J, Siewert C, Baron U, Olek S, Polansky J, et al. Epigenetic Control of the Foxp3 Locus in Regulatory T Cells. PloS Biol (2007) 5(2):e38. doi: 10.1371/journal.pbio.0050038
18. Thornton AM, Korty PE, Tran DQ, Wohlfert EA, Murray PE, Belkaid Y, et al. Expression of Helios, an Ikaros Transcription Factor Family Member, Differentiates Thymic-Derived From Peripherally Induced Foxp3+ T Regulatory Cells. J Immunol (2010) 184(7):3433–41. doi: 10.4049/jimmunol.0904028
19. Yadav M, Louvet C, Davini D, Gardner JM, Martinez-Llordella M, Bailey-Bucktrout S, et al. Neuropilin-1 Distinguishes Natural and Inducible Regulatory T Cells Among Regulatory T Cell Subsets in Vivo. J Exp Med (2012) 209(10):1713–22, S1-19. doi: 10.1084/jem.20120822
20. Roncarolo MG, Gregori S, Bacchetta R, Battaglia M, Gagliani N. The Biology of T Regulatory Type 1 Cells and Their Therapeutic Application in Immune-Mediated Diseases. Immunity (2018) 49(6):1004–19. doi: 10.1016/j.immuni.2018.12.001
21. Gagliani N, Magnani CF, Huber S, Gianolini ME, Pala M, Licona-Limon P, et al. Coexpression of Cd49b and Lag-3 Identifies Human and Mouse T Regulatory Type 1 Cells. Nat Med (2013) 19(6):739–46. doi: 10.1038/nm.3179
22. Passerini L, Di Nunzio S, Gregori S, Gambineri E, Cecconi M, Seidel MG, et al. Functional Type 1 Regulatory T Cells Develop Regardless of Foxp3 Mutations in Patients With Ipex Syndrome. Eur J Immunol (2011) 41(4):1120–31. doi: 10.1002/eji.201040909
23. Radulovic S, Jacobson MR, Durham SR, Nouri-Aria KT. Grass Pollen Immunotherapy Induces Foxp3-Expressing Cd4+ Cd25+ Cells in the Nasal Mucosa. J Allergy Clin Immunol (2008) 121(6):1467–72. doi: 10.1016/j.jaci.2008.03.013
24. Palomares O, Ruckert B, Jartti T, Kucuksezer UC, Puhakka T, Gomez E, et al. Induction and Maintenance of Allergen-Specific Foxp3+ Treg Cells in Human Tonsils as Potential First-Line Organs of Oral Tolerance. J Allergy Clin Immunol (2012) 129(2):510–20, 20.e1-9. doi: 10.1016/j.jaci.2011.09.031
25. Syed A, Garcia MA, Lyu SC, Bucayu R, Kohli A, Ishida S, et al. Peanut Oral Immunotherapy Results in Increased Antigen-Induced Regulatory T-Cell Function and Hypomethylation of Forkhead Box Protein 3 (Foxp3). J Allergy Clin Immunol (2014) 133(2):500–10. doi: 10.1016/j.jaci.2013.12.1037
26. Nouri-Aria KT, Wachholz PA, Francis JN, Jacobson MR, Walker SM, Wilcock LK, et al. Grass Pollen Immunotherapy Induces Mucosal and Peripheral Il-10 Responses and Blocking Igg Activity. J Immunol (2004) 172(5):3252–9. doi: 10.4049/jimmunol.172.5.3252
27. Bohle B, Kinaciyan T, Gerstmayr M, Radakovics A, Jahn-Schmid B, Ebner C. Sublingual Immunotherapy Induces Il-10-Producing T Regulatory Cells, Allergen-Specific T-Cell Tolerance, and Immune Deviation. J Allergy Clin Immunol (2007) 120(3):707–13. doi: 10.1016/j.jaci.2007.06.013
28. Francis JN, Till SJ, Durham SR. Induction of Il-10+Cd4+Cd25+ T Cells by Grass Pollen Immunotherapy. J Allergy Clin Immunol (2003) 111(6):1255–61. doi: 10.1067/mai.2003.1570
29. Tang ML, Ponsonby AL, Orsini F, Tey D, Robinson M, Su EL, et al. Administration of a Probiotic With Peanut Oral Immunotherapy: A Randomized Trial. J Allergy Clin Immunol (2015) 135(3):737–44.e8. doi: 10.1016/j.jaci.2014.11.034
30. Boonpiyathad T, Sokolowska M, Morita H, Ruckert B, Kast JI, Wawrzyniak M, et al. Der P 1-Specific Regulatory T-Cell Response During House Dust Mite Allergen Immunotherapy. Allergy (2019) 74(5):976–85. doi: 10.1111/all.13684
31. Boonpiyathad T, Sozener ZC, Akdis M, Akdis CA. The Role of Treg Cell Subsets in Allergic Disease. Asian Pac J Allergy Immunol (2020) 38(3):139–49. doi: 10.12932/AP-030220-0754
32. Bellinghausen I, Klostermann B, Knop J, Saloga J. Human Cd4+Cd25+ T Cells Derived From the Majority of Atopic Donors Are Able to Suppress Th1 and Th2 Cytokine Production. J Allergy Clin Immunol (2003) 111(4):862–8. doi: 10.1067/mai.2003.1412
33. Ling EM, Smith T, Nguyen XD, Pridgeon C, Dallman M, Arbery J, et al. Relation of Cd4+Cd25+ Regulatory T-Cell Suppression of Allergen-Driven T-Cell Activation to Atopic Status and Expression of Allergic Disease. Lancet (2004) 363(9409):608–15. doi: 10.1016/S0140-6736(04)15592-X
34. Grindebacke H, Wing K, Andersson AC, Suri-Payer E, Rak S, Rudin A. Defective Suppression of Th2 Cytokines by Cd4cd25 Regulatory T Cells in Birch Allergics During Birch Pollen Season. Clin Exp Allergy (2004) 34(9):1364–72. doi: 10.1111/j.1365-2222.2004.02067.x
35. Bellinghausen I, König B, Böttcher I, Knop J, Saloga J. Regulatory Activity of Human Cd4+Cd25+ T Cells Depends on Allergen Concentration, Type of Allergen and Atopy Status of the Donor. Immunology (2005) 116:103–11. doi: 10.1111/j.1365-2567.2005.02205.x
36. George TC, Bilsborough J, Viney JL, Norment AM. High Antigen Dose and Activated Dendritic Cells Enable Th Cells to Escape Regulatory T Cell-Mediated Suppression in Vitro. Eur J Immunol (2003) 33(2):502–11. doi: 10.1002/immu.200310026
37. Bacher P, Scheffold A. The Effect of Regulatory T Cells on Tolerance to Airborne Allergens and Allergen Immunotherapy. J Allergy Clin Immunol (2018) 142(6):1697–709. doi: 10.1016/j.jaci.2018.10.016
38. Hartl D, Koller B, Mehlhorn AT, Reinhardt D, Nicolai T, Schendel DJ, et al. Quantitative and Functional Impairment of Pulmonary Cd4+Cd25hi Regulatory T Cells in Pediatric Asthma. J Allergy Clin Immunol (2007) 119(5):1258–66. doi: 10.1016/j.jaci.2007.02.023
39. Akdis M, Verhagen J, Taylor A, Karamloo F, Karagiannidis C, Crameri R, et al. Immune Responses in Healthy and Allergic Individuals Are Characterized by a Fine Balance Between Allergen-Specific T Regulatory 1 and T Helper 2 Cells. J Exp Med (2004) 199(11):1567–75. doi: 10.1084/jem.20032058
40. Pellerin L, Jenks JA, Chinthrajah S, Dominguez T, Block W, Zhou X, et al. Peanut-Specific Type 1 Regulatory T Cells Induced in Vitro From Allergic Subjects Are Functionally Impaired. J Allergy Clin Immunol (2018) 141(1):202–13 e8. doi: 10.1016/j.jaci.2017.05.045
41. Berings M, Karaaslan C, Altunbulakli C, Gevaert P, Akdis M, Bachert C, et al. Advances and Highlights in Allergen Immunotherapy: On the Way to Sustained Clinical and Immunologic Tolerance. J Allergy Clin Immunol (2017) 140(5):1250–67. doi: 10.1016/j.jaci.2017.08.025
42. Celebi Sozener Z, Mungan D, Cevhertas L, Ogulur I, Akdis M, Akdis C. Tolerance Mechanisms in Allergen Immunotherapy. Curr Opin Allergy Clin Immunol (2020) 20(6):591–601. doi: 10.1097/ACI.0000000000000693
43. Ulges A, Klein M, Reuter S, Gerlitzki B, Hoffmann M, Grebe N, et al. Protein Kinase Ck2 Enables Regulatory T Cells to Suppress Excessive Th2 Responses in Vivo. Nat Immunol (2015) 16(3):267–75. doi: 10.1038/ni.3083
44. Zhang Y, Li L, Genest G, Zhao W, Ke D, Bartolucci S, et al. Successful Milk Oral Immunotherapy Promotes Generation of Casein-Specific Cd137(+) Foxp3(+) Regulatory T Cells Detectable in Peripheral Blood. Front Immunol (2021) 12:705615. doi: 10.3389/fimmu.2021.705615
45. Gri G, Piconese S, Frossi B, Manfroi V, Merluzzi S, Tripodo C, et al. Cd4+Cd25+ Regulatory T Cells Suppress Mast Cell Degranulation and Allergic Responses Through Ox40-Ox40l Interaction. Immunity (2008) 29(5):771–81. doi: 10.1016/j.immuni.2008.08.018
46. Rigas D, Lewis G, Aron JL, Wang B, Banie H, Sankaranarayanan I, et al. Type 2 Innate Lymphoid Cell Suppression by Regulatory T Cells Attenuates Airway Hyperreactivity and Requires Inducible T-Cell Costimulator-Inducible T-Cell Costimulator Ligand Interaction. J Allergy Clin Immunol (2017) 139(5):1468–77 e2. doi: 10.1016/j.jaci.2016.08.034
47. Haspeslagh E, Heyndrickx I, Hammad H, Lambrecht BN. The Hygiene Hypothesis: Immunological Mechanisms of Airway Tolerance. Curr Opin Immunol (2018) 54:102–8. doi: 10.1016/j.coi.2018.06.007
48. Strachan DP. Hay Fever, Hygiene, and Household Size. BMJ (1989) 299(6710):1259–60. doi: 10.1136/bmj.299.6710.1259
49. Perdijk O, Marsland BJ. The Microbiome: Toward Preventing Allergies and Asthma by Nutritional Intervention. Curr Opin Immunol (2019) 60:10–8. doi: 10.1016/j.coi.2019.04.001
50. Herbst T, Sichelstiel A, Schar C, Yadava K, Burki K, Cahenzli J, et al. Dysregulation of Allergic Airway Inflammation in the Absence of Microbial Colonization. Am J Respir Crit Care Med (2011) 184(2):198–205. doi: 10.1164/rccm.201010-1574OC
51. Atarashi K, Tanoue T, Shima T, Imaoka A, Kuwahara T, Momose Y, et al. Induction of Colonic Regulatory T Cells by Indigenous Clostridium Species. Science (2011) 331(6015):337–41. doi: 10.1126/science.1198469
52. Kemter AM, Nagler CR. Influences on Allergic Mechanisms Through Gut, Lung, and Skin Microbiome Exposures. J Clin Invest (2019) 130:1483–92. doi: 10.1172/JCI124610
53. Smith PM, Howitt MR, Panikov N, Michaud M, Gallini CA, Bohlooly YM, et al. The Microbial Metabolites, Short-Chain Fatty Acids, Regulate Colonic Treg Cell Homeostasis. Science (2013) 341(6145):569–73. doi: 10.1126/science.1241165
54. Furusawa Y, Obata Y, Fukuda S, Endo TA, Nakato G, Takahashi D, et al. Commensal Microbe-Derived Butyrate Induces the Differentiation of Colonic Regulatory T Cells. Nature (2013) 504(7480):446–50. doi: 10.1038/nature12721
55. Trompette A, Gollwitzer ES, Yadava K, Sichelstiel AK, Sprenger N, Ngom-Bru C, et al. Gut Microbiota Metabolism of Dietary Fiber Influences Allergic Airway Disease and Hematopoiesis. Nat Med (2014) 20(2):159–66. doi: 10.1038/nm.3444
56. Thio CL, Chi PY, Lai AC, Chang YJ. Regulation of Type 2 Innate Lymphoid Cell-Dependent Airway Hyperreactivity by Butyrate. J Allergy Clin Immunol (2018) 142(6):1867–83 e12. doi: 10.1016/j.jaci.2018.02.032
57. Tan J, McKenzie C, Vuillermin PJ, Goverse G, Vinuesa CG, Mebius RE, et al. Dietary Fiber and Bacterial Scfa Enhance Oral Tolerance and Protect Against Food Allergy Through Diverse Cellular Pathways. Cell Rep (2016) 15(12):2809–24. doi: 10.1016/j.celrep.2016.05.047
58. McKenzie C, Tan J, Macia L, Mackay CR. The Nutrition-Gut Microbiome-Physiology Axis and Allergic Diseases. Immunol Rev (2017) 278(1):277–95. doi: 10.1111/imr.12556
59. Berni Canani R, Paparo L, Nocerino R, Di Scala C, Della Gatta G, Maddalena Y, et al. Gut Microbiome as Target for Innovative Strategies Against Food Allergy. Front Immunol (2019) 10:191. doi: 10.3389/fimmu.2019.00191
60. Stefka AT, Feehley T, Tripathi P, Qiu J, McCoy K, Mazmanian SK, et al. Commensal Bacteria Protect Against Food Allergen Sensitization. Proc Natl Acad Sci USA (2014) 111(36):13145–50. doi: 10.1073/pnas.1412008111
61. Venter C, Meyer RW, Nwaru BI, Roduit C, Untersmayr E, Adel-Patient K, et al. Eaaci Position Paper: Influence of Dietary Fatty Acids on Asthma, Food Allergy, and Atopic Dermatitis. Allergy (2019) 74(8):1429–44. doi: 10.1111/all.13764
62. Round JL, Mazmanian SK. Inducible Foxp3+ Regulatory T-Cell Development by a Commensal Bacterium of the Intestinal Microbiota. Proc Natl Acad Sci USA (2010) 107(27):12204–9. doi: 10.1073/pnas.0909122107
63. Van der Leek AP, Yanishevsky Y, Kozyrskyj AL. The Kynurenine Pathway as a Novel Link Between Allergy and the Gut Microbiome. Front Immunol (2017) 8:1374. doi: 10.3389/fimmu.2017.01374
64. Junker Y, Zeissig S, Kim SJ, Barisani D, Wieser H, Leffler DA, et al. Wheat Amylase Trypsin Inhibitors Drive Intestinal Inflammation Via Activation of Toll-Like Receptor 4. J Exp Med (2012) 209(13):2395–408. doi: 10.1084/jem.20102660
65. Pickert G, Wirtz S, Matzner J, Ashfaq-Khan M, Heck R, Rosigkeit S, et al. Wheat Consumption Aggravates Colitis in Mice Via Amylase Trypsin Inhibitor-Mediated Dysbiosis. Gastroenterology (2020) 159(1):257–72.e17. doi: 10.1053/j.gastro.2020.03.064
66. Bellinghausen I, Weigmann B, Zevallos V, Maxeiner J, Reissig S, Waisman A, et al. Wheat Amylase-Trypsin Inhibitors Exacerbate Intestinal and Airway Allergic Immune Responses in Humanized Mice. J Allergy Clin Immunol (2019) 143(1):201–12 e4. doi: 10.1016/j.jaci.2018.02.041
67. Zevallos VF, Raker VK, Maxeiner J, Scholtes P, Steinbrink K, Schuppan D. Dietary Wheat Amylase Trypsin Inhibitors Exacerbate Murine Allergic Airway Inflammation. Eur J Nutr (2019) 58(4):1507–14. doi: 10.1007/s00394-018-1681-6
68. Ohnmacht C, Park JH, Cording S, Wing JB, Atarashi K, Obata Y, et al. Mucosal Immunology. The Microbiota Regulates Type 2 Immunity Through Rorgammat(+) T Cells. Science (2015) 349(6251):989–93. doi: 10.1126/science.aac4263
69. Abdel-Gadir A, Stephen-Victor E, Gerber GK, Noval Rivas M, Wang S, Harb H, et al. Microbiota Therapy Acts Via a Regulatory T Cell Myd88/Rorgammat Pathway to Suppress Food Allergy. Nat Med (2019) 25(7):1164–74. doi: 10.1038/s41591-019-0461-z
70. Mennini M, Dahdah L, Artesani MC, Fiocchi A, Martelli A. Probiotics in Asthma and Allergy Prevention. Front Pediatr (2017) 5:165. doi: 10.3389/fped.2017.00165
71. Azzaoui I, Yahia SA, Chang Y, Vorng H, Morales O, Fan Y, et al. Ccl18 Differentiates Dendritic Cells in Tolerogenic Cells Able to Prime Regulatory T Cells in Healthy Subjects. Blood (2011) 118(13):3549–58. doi: 10.1182/blood-2011-02-338780
72. Vulcano M, Struyf S, Scapini P, Cassatella M, Bernasconi S, Bonecchi R, et al. Unique Regulation of Ccl18 Production by Maturing Dendritic Cells. J Immunol (2003) 170(7):3843–9. doi: 10.4049/jimmunol.170.7.3843
73. Bellinghausen I, Reuter S, Martin H, Maxeiner J, Luxemburger U, Tureci O, et al. Enhanced Production of Ccl18 by Tolerogenic Dendritic Cells Is Associated With Inhibition of Allergic Airway Reactivity. J Allergy Clin Immunol (2012) 130(6):1384–93. doi: 10.1016/j.jaci.2012.08.039
74. Eschborn M, Weigmann B, Reissig S, Waisman A, Saloga J, Bellinghausen I. Activated Glycoprotein a Repetitions Predominant (Garp)-Expressing Regulatory T Cells Inhibit Allergen-Induced Intestinal Inflammation in Humanized Mice. J Allergy Clin Immunol (2015) 136(1):159–68. doi: 10.1016/j.jaci.2015.04.020
75. Meyer-Martin H, Hahn SA, Beckert H, Belz C, Heinz A, Jonuleit H, et al. Garp Inhibits Allergic Airway Inflammation in a Humanized Mouse Model. Allergy (2016) 71(9):1274–83. doi: 10.1111/all.12883
76. Wang R, Kozhaya L, Mercer F, Khaitan A, Fujii H, Unutmaz D. Expression of Garp Selectively Identifies Activated Human Foxp3+ Regulatory T Cells. Proc Natl Acad Sci USA (2009) 106(32):13439–44. doi: 10.1073/pnas.0901965106
77. Shevach EM. Garp as a Therapeutic Target for Modulation of T Regulatory Cell Function. Expert Opin Ther Targets (2017) 21(2):191–200. doi: 10.1080/14728222.2017.1275568
78. Hahn SA, Stahl HF, Becker C, Correll A, Schneider FJ, Tuettenberg A, et al. Soluble Garp Has Potent Antiinflammatory and Immunomodulatory Impact on Human Cd4(+) T Cells. Blood (2013) 122(7):1182–91. doi: 10.1182/blood-2012-12-474478
79. Becker C, Taube C, Bopp T, Becker C, Michel K, Kubach J, et al. Protection From Graft-Versus-Host Disease by Hiv-1 Envelope Protein Gp120-Mediated Activation of Human Cd4+Cd25+ Regulatory T Cells. Blood (2009) 114(6):1263–9. doi: 10.1182/blood-2009-02-206730
80. Martin H, Reuter S, Dehzad N, Heinz A, Bellinghausen I, Saloga J, et al. Cd4-Mediated Regulatory T-Cell Activation Inhibits the Development of Disease in a Humanized Mouse Model of Allergic Airway Disease. J Allergy Clin Immunol (2012) 129(2):521–8. doi: 10.1016/j.jaci.2011.09.038
81. Bonnet B, Vigneron J, Levacher B, Vazquez T, Pitoiset F, Brimaud F, et al. Low-Dose Il-2 Induces Regulatory T Cell-Mediated Control of Experimental Food Allergy. J Immunol (2016) 197(1):188–98. doi: 10.4049/jimmunol.1501271
82. Klein M, Misme-Aucouturier B, Cheminant MA, De Carvalho M, Wauters M, Tranquet O, et al. Engineering a Safe Monoclonal Anti-Human Il-2 That Is Effective in a Murine Model of Food Allergy and Asthma. Allergy (2022) 77(3):933–45. doi: 10.1111/all.15029
83. Smaldini PL, Trejo F, Cohen JL, Piaggio E, Docena GH. Systemic Il-2/Anti-Il-2ab Complex Combined With Sublingual Immunotherapy Suppresses Experimental Food Allergy in Mice Through Induction of Mucosal Regulatory T Cells. Allergy (2018) 73(4):885–95. doi: 10.1111/all.13402
84. Feehley T, Plunkett CH, Bao R, Choi Hong SM, Culleen E, Belda-Ferre P, et al. Healthy Infants Harbor Intestinal Bacteria That Protect Against Food Allergy. Nat Med (2019) 25(3):448–53. doi: 10.1038/s41591-018-0324-z
85. Renz H, Skevaki C. Early Life Microbial Exposures and Allergy Risks: Opportunities for Prevention. Nat Rev Immunol (2021) 21(3):177–91. doi: 10.1038/s41577-020-00420-y
86. Eggesbo M, Botten G, Stigum H, Nafstad P, Magnus P. Is Delivery by Cesarean Section a Risk Factor for Food Allergy? J Allergy Clin Immunol (2003) 112(2):420–6. doi: 10.1067/mai.2003.1610
87. Papathoma E, Triga M, Fouzas S, Dimitriou G. Cesarean Section Delivery and Development of Food Allergy and Atopic Dermatitis in Early Childhood. Pediatr Allergy Immunol (2016) 27(4):419–24. doi: 10.1111/pai.12552
88. Hoskin-Parr L, Teyhan A, Blocker A, Henderson AJ. Antibiotic Exposure in the First Two Years of Life and Development of Asthma and Other Allergic Diseases by 7.5 Yr: A Dose-Dependent Relationship. Pediatr Allergy Immunol (2013) 24(8):762–71. doi: 10.1111/pai.12153
89. Cho I, Yamanishi S, Cox L, Methe BA, Zavadil J, Li K, et al. Antibiotics in Early Life Alter the Murine Colonic Microbiome and Adiposity. Nature (2012) 488(7413):621–6. doi: 10.1038/nature11400
90. Seppo AE, Autran CA, Bode L, Jarvinen KM. Human Milk Oligosaccharides and Development of Cow's Milk Allergy in Infants. J Allergy Clin Immunol (2017) 139(2):708–11.e5. doi: 10.1016/j.jaci.2016.08.031
91. van den Elsen LWJ, Garssen J, Burcelin R, Verhasselt V. Shaping the Gut Microbiota by Breastfeeding: The Gateway to Allergy Prevention? Front Pediatr (2019) 7:47. doi: 10.3389/fped.2019.00047
92. Wood H, Acharjee A, Pearce H, Quraishi MN, Powell R, Rossiter A, et al. Breastfeeding Promotes Early Neonatal Regulatory T-Cell Expansion and Immune Tolerance of Non-Inherited Maternal Antigens. Allergy (2021) 76(8):2447–60. doi: 10.1111/all.14736
93. von Mutius E, Vercelli D. Farm Living: Effects on Childhood Asthma and Allergy. Nat Rev Immunol (2010) 10(12):861–8. doi: 10.1038/nri2871
94. Stein MM, Hrusch CL, Gozdz J, Igartua C, Pivniouk V, Murray SE, et al. Innate Immunity and Asthma Risk in Amish and Hutterite Farm Children. N Engl J Med (2016) 375(5):411–21. doi: 10.1056/NEJMoa1508749
95. Du TG, Roberts G, Sayre PH, Bahnson HT, Radulovic S, Santos AF, et al. Randomized Trial of Peanut Consumption in Infants at Risk for Peanut Allergy. N Engl J Med (2015) 372(9):803–13. doi: 10.1056/NEJMoa1414850
96. Bellach J, Schwarz V, Ahrens B, Trendelenburg V, Aksunger O, Kalb B, et al. Randomized Placebo-Controlled Trial of Hen's Egg Consumption for Primary Prevention in Infants. J Allergy Clin Immunol (2017) 139(5):1591–9 e2. doi: 10.1016/j.jaci.2016.06.045
97. Ege MJ, Mayer M, Normand AC, Genuneit J, Cookson WO, Braun-Fahrlander C, et al. Exposure to Environmental Microorganisms and Childhood Asthma. N Engl J Med (2011) 364(8):701–9. doi: 10.1056/NEJMoa1007302
98. Schroder PC, Illi S, Casaca VI, Lluis A, Bock A, Roduit C, et al. A Switch in Regulatory T Cells Through Farm Exposure During Immune Maturation in Childhood. Allergy (2017) 72(4):604–15. doi: 10.1111/all.13069
99. Depner M, Taft DH, Kirjavainen PV, Kalanetra KM, Karvonen AM, Peschel S, et al. Maturation of the Gut Microbiome During the First Year of Life Contributes to the Protective Farm Effect on Childhood Asthma. Nat Med (2020) 26(11):1766–75. doi: 10.1038/s41591-020-1095-x
100. Schuijs MJ, Willart MA, Vergote K, Gras D, Deswarte K, Ege MJ, et al. Farm Dust and Endotoxin Protect Against Allergy Through A20 Induction in Lung Epithelial Cells. Science (2015) 349(6252):1106–10. doi: 10.1126/science.aac6623
101. Roth-Walter F, Afify SM, Pacios LF, Blokhuis BR, Redegeld F, Regner A, et al. Cow's Milk Protein Beta-Lactoglobulin Confers Resilience Against Allergy by Targeting Complexed Iron Into Immune Cells. J Allergy Clin Immunol (2021) 147(1):321–34 e4. doi: 10.1016/j.jaci.2020.05.023
102. Bartosik T, Jensen SA, Afify SM, Bianchini R, Hufnagl K, Hofstetter G, et al. Ameliorating Atopy by Compensating Micronutritional Deficiencies in Immune Cells: A Double-Blind Placebo-Controlled Pilot Study. J Allergy Clin Immunol Pract (2022). doi: 10.1016/j.jaip.2022.02.028
103. Raffin C, Vo LT, Bluestone JA. Treg Cell-Based Therapies: Challenges and Perspectives. Nat Rev Immunol (2020) 20(3):158–72. doi: 10.1038/s41577-019-0232-6
104. Sayitoglu EC, Freeborn RA, Roncarolo MG. The Yin and Yang of Type 1 Regulatory T Cells: From Discovery to Clinical Application. Front Immunol (2021) 12:693105. doi: 10.3389/fimmu.2021.693105
105. Boardman DA, Levings MK. Emerging Strategies for Treating Autoimmune Disorders With Genetically Modified Treg Cells. J Allergy Clin Immunol (2022) 149(1):1–11. doi: 10.1016/j.jaci.2021.11.007
106. Sawitzki B, Harden PN, Reinke P, Moreau A, Hutchinson JA, Game DS, et al. Regulatory Cell Therapy in Kidney Transplantation (the One Study): A Harmonised Design and Analysis of Seven Non-Randomised, Single-Arm, Phase 1/2a Trials. Lancet (2020) 395(10237):1627–39. doi: 10.1016/S0140-6736(20)30167-7
107. Dull T, Zufferey R, Kelly M, Mandel RJ, Nguyen M, Trono D, et al. A Third-Generation Lentivirus Vector With a Conditional Packaging System. J Virol (1998) 72(11):8463–71. doi: 10.1128/JVI.72.11.8463-8471.1998
108. Amini L, Greig J, Schmueck-Henneresse M, Volk HD, Bezie S, Reinke P, et al. Super-Treg: Toward a New Era of Adoptive Treg Therapy Enabled by Genetic Modifications. Front Immunol (2020) 11:611638. doi: 10.3389/fimmu.2020.611638
109. Hacein-Bey-Abina S, Von Kalle C, Schmidt M, McCormack MP, Wulffraat N, Leboulch P, et al. Lmo2-Associated Clonal T Cell Proliferation in Two Patients After Gene Therapy for Scid-X1. Science (2003) 302(5644):415–9. doi: 10.1126/science.1088547
110. Urnov FD, Miller JC, Lee YL, Beausejour CM, Rock JM, Augustus S, et al. Highly Efficient Endogenous Human Gene Correction Using Designed Zinc-Finger Nucleases. Nature (2005) 435(7042):646–51. doi: 10.1038/nature03556
111. Cortez JT, Montauti E, Shifrut E, Gatchalian J, Zhang Y, Shaked O, et al. Crispr Screen in Regulatory T Cells Reveals Modulators of Foxp3. Nature (2020) 582(7812):416–20. doi: 10.1038/s41586-020-2246-4
112. Wright GP, Notley CA, Xue SA, Bendle GM, Holler A, Schumacher TN, et al. Adoptive Therapy With Redirected Primary Regulatory T Cells Results in Antigen-Specific Suppression of Arthritis. Proc Natl Acad Sci USA (2009) 106(45):19078–83. doi: 10.1073/pnas.0907396106
113. Kim YC, Zhang AH, Yoon J, Culp WE, Lees JR, Wucherpfennig KW, et al. Engineered Mbp-Specific Human Tregs Ameliorate Mog-Induced Eae Through Il-2-Triggered Inhibition of Effector T Cells. J Autoimmun (2018) 92:77–86. doi: 10.1016/j.jaut.2018.05.003
114. Kim YC, Zhang AH, Su Y, Rieder SA, Rossi RJ, Ettinger RA, et al. Engineered Antigen-Specific Human Regulatory T Cells: Immunosuppression of Fviii-Specific T- and B-Cell Responses. Blood (2015) 125(7):1107–15. doi: 10.1182/blood-2014-04-566786
115. Schmetterer KG, Haiderer D, Leb-Reichl VM, Neunkirchner A, Jahn-Schmid B, Kung HJ, et al. Bet V 1-Specific T-Cell Receptor/Forkhead Box Protein 3 Transgenic T Cells Suppress Bet V 1-Specific T-Cell Effector Function in an Activation-Dependent Manner. J Allergy Clin Immunol (2011) 127(1):238–45, 45 e1-3. doi: 10.1016/j.jaci.2010.10.023
116. Gross G, Waks T, Eshhar Z. Expression of Immunoglobulin-T-Cell Receptor Chimeric Molecules as Functional Receptors With Antibody-Type Specificity. Proc Natl Acad Sci USA (1989) 86(24):10024–8. doi: 10.1073/pnas.86.24.10024
117. Esmaeilzadeh A, Tahmasebi S, Athari SS. Chimeric Antigen Receptor -T Cell Therapy: Applications and Challenges in Treatment of Allergy and Asthma. BioMed Pharmacother (2020) 123:109685. doi: 10.1016/j.biopha.2019.109685
118. Krooss SA, Dai Z, Schmidt F, Rovai A, Fakhiri J, Dhingra A, et al. Ex Vivo/in Vivo Gene Editing in Hepatocytes Using "All-In-One" Crispr-Adeno-Associated Virus Vectors With a Self-Linearizing Repair Template. iScience (2020) 23(1):100764. doi: 10.1016/j.isci.2019.100764
119. Ortinski PI, O'Donovan B, Dong X, Kantor B. Integrase-Deficient Lentiviral Vector as an All-In-One Platform for Highly Efficient Crispr/Cas9-Mediated Gene Editing. Mol Ther Methods Clin Dev (2017) 5:153–64. doi: 10.1016/j.omtm.2017.04.002
120. Boardman DA, Philippeos C, Fruhwirth GO, Ibrahim MA, Hannen RF, Cooper D, et al. Expression of a Chimeric Antigen Receptor Specific for Donor Hla Class I Enhances the Potency of Human Regulatory T Cells in Preventing Human Skin Transplant Rejection. Am J Transplant (2017) 17(4):931–43. doi: 10.1111/ajt.14185
121. Dawson NA, Lamarche C, Hoeppli RE, Bergqvist P, Fung VC, McIver E, et al. Systematic Testing and Specificity Mapping of Alloantigen-Specific Chimeric Antigen Receptors in Regulatory T Cells. JCI Insight (2019) 4(6):e123672. doi: 10.1172/jci.insight.123672
122. Akatsuka Y. Tcr-Like Car-T Cells Targeting Mhc-Bound Minor Histocompatibility Antigens. Front Immunol (2020) 11:257. doi: 10.3389/fimmu.2020.00257
123. Koristka S, Kegler A, Bergmann R, Arndt C, Feldmann A, Albert S, et al. Engrafting Human Regulatory T Cells With a Flexible Modular Chimeric Antigen Receptor Technology. J Autoimmun (2018) 90:116–31. doi: 10.1016/j.jaut.2018.02.006
124. Pierini A, Iliopoulou BP, Peiris H, Perez-Cruz M, Baker J, Hsu K, et al. T Cells Expressing Chimeric Antigen Receptor Promote Immune Tolerance. JCI Insight (2017) 2(20):e92865. doi: 10.1172/jci.insight.92865
125. Ellebrecht CT, Bhoj VG, Nace A, Choi EJ, Mao X, Cho MJ, et al. Reengineering Chimeric Antigen Receptor T Cells for Targeted Therapy of Autoimmune Disease. Science (2016) 353(6295):179–84. doi: 10.1126/science.aaf6756
126. Hammers CM, Chen J, Lin C, Kacir S, Siegel DL, Payne AS, et al. Persistence of Anti-Desmoglein 3 Igg(+) B-Cell Clones in Pemphigus Patients Over Years. J Invest Dermatol (2015) 135(3):742–9. doi: 10.1038/jid.2014.291
127. Ward DE, Fay BL, Adejuwon A, Han H, Ma Z. Chimeric Antigen Receptors Based on Low Affinity Mutants of Fcepsilonri Re-Direct T Cell Specificity to Cells Expressing Membrane Ige. Front Immunol (2018) 9:2231. doi: 10.3389/fimmu.2018.02231
128. Roth-Walter F, Adcock IM, Benito-Villalvilla C, Bianchini R, Bjermer L, Boyman O, et al. Immune Modulation Via T Regulatory Cell Enhancement: Disease-Modifying Therapies for Autoimmunity and Their Potential for Chronic Allergic and Inflammatory Diseases-An Eaaci Position Paper of the Task Force on Immunopharmacology (Tipco). Allergy (2021) 76(1):90–113. doi: 10.1111/all.14478
129. Mukherjee M, Nair P. Autoimmune Responses in Severe Asthma. Allergy Asthma Immunol Res (2018) 10(5):428–47. doi: 10.4168/aair.2018.10.5.428
130. Mukherjee M, Bulir DC, Radford K, Kjarsgaard M, Huang CM, Jacobsen EA, et al. Sputum Autoantibodies in Patients With Severe Eosinophilic Asthma. J Allergy Clin Immunol (2018) 141(4):1269–79. doi: 10.1016/j.jaci.2017.06.033
131. Skuljec J, Chmielewski M, Happle C, Habener A, Busse M, Abken H, et al. Chimeric Antigen Receptor-Redirected Regulatory T Cells Suppress Experimental Allergic Airway Inflammation, a Model of Asthma. Front Immunol (2017) 8:1125. doi: 10.3389/fimmu.2017.01125
132. Abdeladhim M, Zhang AH, Kropp LE, Lindrose AR, Venkatesha SH, Mitre E, et al. Engineered Ovalbumin-Expressing Regulatory T Cells Protect Against Anaphylaxis in Ovalbumin-Sensitized Mice. Clin Immunol (2019) 207:49–54. doi: 10.1016/j.clim.2019.07.009
133. Di Stasi A, Tey SK, Dotti G, Fujita Y, Kennedy-Nasser A, Martinez C, et al. Inducible Apoptosis as a Safety Switch for Adoptive Cell Therapy. N Engl J Med (2011) 365(18):1673–83. doi: 10.1056/NEJMoa1106152
134. Hagenstein J, Melderis S, Nosko A, Warkotsch MT, Richter JV, Ramcke T, et al. A Novel Role for Il-6 Receptor Classic Signaling: Induction of Rorgammat(+)Foxp3(+) Tregs With Enhanced Suppressive Capacity. J Am Soc Nephrol (2019) 30(8):1439–53. doi: 10.1681/ASN.2019020118
135. Noval Rivas M, Burton OT, Wise P, Charbonnier LM, Georgiev P, Oettgen HC, et al. Regulatory T Cell Reprogramming Toward a Th2-Cell-Like Lineage Impairs Oral Tolerance and Promotes Food Allergy. Immunity (2015) 42(3):512–23. doi: 10.1016/j.immuni.2015.02.004
136. McGovern JL, Wright GP, Stauss HJ. Engineering Specificity and Function of Therapeutic Regulatory T Cells. Front Immunol (2017) 8:1517. doi: 10.3389/fimmu.2017.01517
Keywords: allergic inflammation, regulatory T cells, allergen-specific immunotherapy, microbiota, Treg engineering, therapeutic application
Citation: Bellinghausen I, Khatri R and Saloga J (2022) Current Strategies to Modulate Regulatory T Cell Activity in Allergic Inflammation. Front. Immunol. 13:912529. doi: 10.3389/fimmu.2022.912529
Received: 05 April 2022; Accepted: 02 May 2022;
Published: 26 May 2022.
Edited by:
Robert Zeiser, University of Freiburg, GermanyReviewed by:
Stefan F. Martin, University of Freiburg Medical Center, GermanyCopyright © 2022 Bellinghausen, Khatri and Saloga. This is an open-access article distributed under the terms of the Creative Commons Attribution License (CC BY). The use, distribution or reproduction in other forums is permitted, provided the original author(s) and the copyright owner(s) are credited and that the original publication in this journal is cited, in accordance with accepted academic practice. No use, distribution or reproduction is permitted which does not comply with these terms.
*Correspondence: Iris Bellinghausen, aXJpcy5iZWxsaW5naGF1c2VuQHVuaW1lZGl6aW4tbWFpbnouZGU=
Disclaimer: All claims expressed in this article are solely those of the authors and do not necessarily represent those of their affiliated organizations, or those of the publisher, the editors and the reviewers. Any product that may be evaluated in this article or claim that may be made by its manufacturer is not guaranteed or endorsed by the publisher.
Research integrity at Frontiers
Learn more about the work of our research integrity team to safeguard the quality of each article we publish.