- 1Department of Hematology, National Key Clinical Specialty of Hematology, Yunnan Blood Disease Clinical Medical Center, Yunnan Blood Disease Hospital, The First People’s Hospital of Yunnan Province, Kunming, China
- 2Department of Hematology, The First Affiliated Hospital of Nanjing Medical University, Jiangsu Province Hospital, Collaborative Innovation Center for Cancer Personalized Medicine, Nanjing, China
Poor graft function (PGF) is a life-threatening complication that occurs after transplantation and has a poor prognosis. With the rapid development of haploidentical hematopoietic stem cell transplantation, the pathogenesis of PGF has become an important issue. Studies of the pathogenesis of PGF have resulted in some success in CD34+-selected stem cell boosting. Mesenchymal stem cells, N-acetyl-l-cysteine, and eltrombopag have also been investigated as therapeutic strategies for PGF. However, predicting and preventing PGF remains challenging. Here, we propose that the seed, soil, and insect theories of aplastic anemia also apply to PGF; CD34+ cells are compared to seeds; the bone marrow microenvironment to soil; and virus infection, iron overload, and donor-specific anti-human leukocyte antigen antibodies to insects. From this perspective, we summarize the available information on the common risk factors of PGF, focusing on its potential mechanism. In addition, the safety and efficacy of new strategies for treating PGF are discussed to provide a foundation for preventing and treating this complex clinical problem.
Introduction
Hematopoietic stem cell transplantation (HSCT) is an effective treatment for malignant hematological diseases. However, delayed or incomplete hematopoietic recovery, also known as poor graft function (PGF), limits the success of HSCT. The definition of PGF is currently controversial. The European Society for Blood and Marrow Transplantation (EBMT) defined PGF as two or three episodes of cytopenia lasting for more than 2 weeks, after day +28 in the presence of donor chimerism > 5% (1). Given the chimerism kinetics and potential for confusion with graft failure (GF), most recent studies based on clinical practice proposed that PGF should be defined as the presence of at least two hematopoietic cell count lines that do not meet the engraftment standard (absolute neutrophil count > 1.5 × 109/L, platelet (PLT) count > 30 × 109/L, hemoglobin > 85g/L) lasting for more than two consecutive weeks beyond day +28 post-HSCT, in the presence of full donor chimerism and primary disease in remission without severe graft-versus-host disease (GVHD) and relapse (2). Secondary poor graft function (sPGF) refers to the loss of donor cells after the initial engraftment. Whereas primary PGF is characterized by no initial donor cell engraftment, it is nearly impossible to recover autologous hematopoiesis; thus, patients with this condition are likely to die of infection and/or other complications and urgently require a secondary transplant.
The cumulative incidence of PGF after allogeneic HSCT (allo-HSCT) varies between 5% and 27% (3–5). Differences in underlying diseases and management strategies affect the incidence of PGF. At Peking University Institute of Hematology, the incidence of primary PGF after unmanipulated haploidentical HSCT (haplo-HSCT) was found to be approximately 5.6% (6, 7), and sPGF developed in 5.7% of patients after allo-HSCT (4). In a prospective study, approximately 15% of patients with severe aplastic anemia (AA) who underwent haplo-SCT developed primary PGF (8). Primary PGF shows a very poor prognosis, with a 1-year overall survival (OS) rate of 25.0% (5) and 2-year OS of 6% in patients without hematopoietic recovery (9). Because of persistent leukocytopenia and thrombocytopenia, PGF is often accompanied by complications such as infection and bleeding, thus increasing the mortality rate. As PGF is a life-threatening complication, new prevention and treatment strategies are urgently needed.
The occurrence of PGF is related to numerous factors such as primary disease, quality and quantity of hematopoietic stem cells (HSCs), damaged bone marrow (BM) microenvironment, donor-specific anti-human leukocyte antigen (HLA) antibodies (DSA), and viral infection. Based on this information, we hypothesized that the seed, soil, and insect theories of AA pathogenesis can be applied to PGF (Figure 1). Based on this theory, we review current research progress on the pathogenesis, prevention, and treatment of PGF.
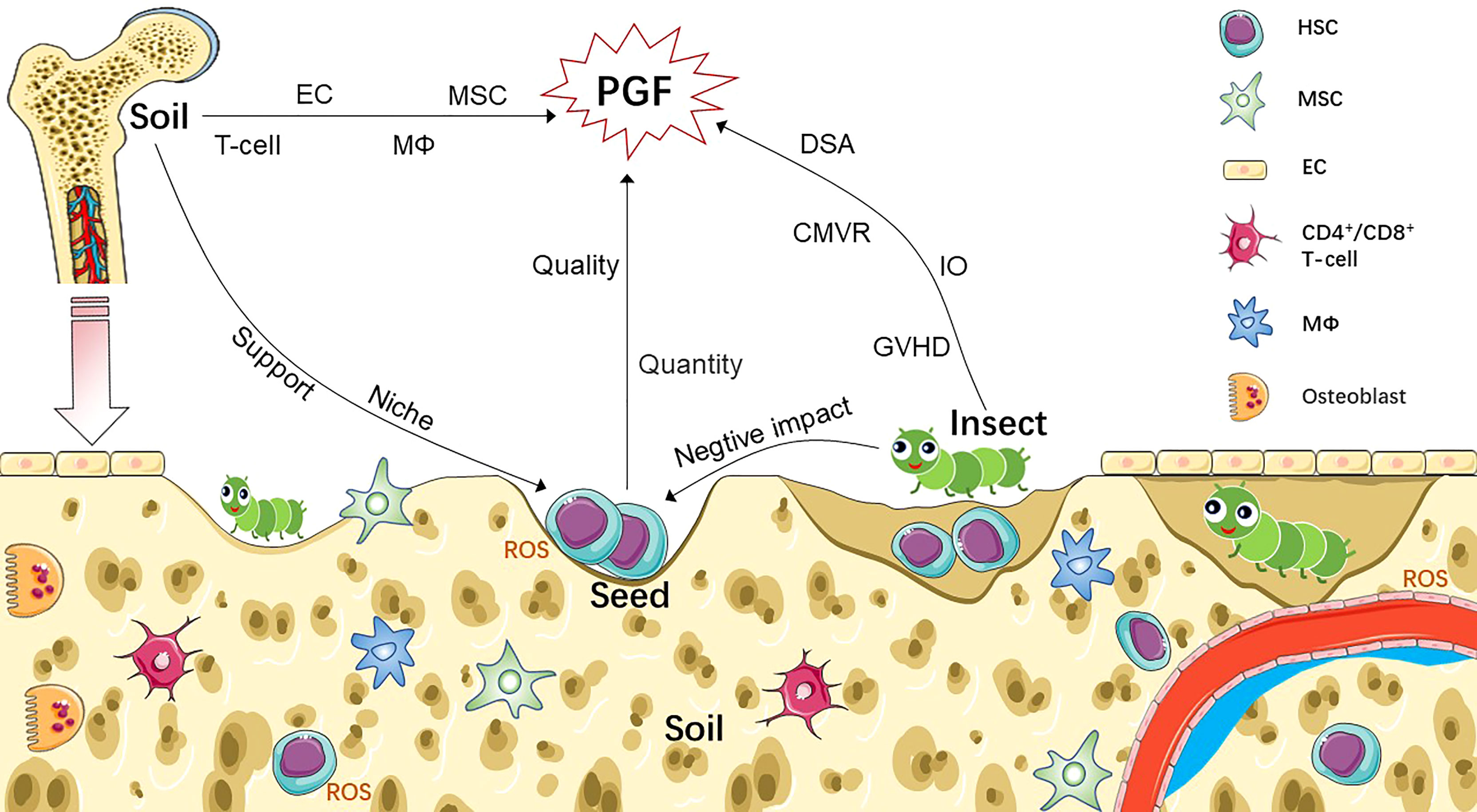
Figure 1 Pathophysiology of PGF based on seed-soil-insect theory. Non-hematopoietic cells (such as MSC, EC) and HSC-derived cells (such as T-cell, macrophages) participate as part of the bone marrow niche, which not only regulate the function of HSC, but also involved in the pathogenesis of PGF after transplantation. Abnormal increased ROS is also contributing to the damage of both HSCs and BM niche of PGF patients. We compare CD34+ cells to seeds; bone marrow microenvironment to soil; and various factors associated with immune imbalance to insects, such as GVHD, DSA, CMVR and IO. Seeds, insects and soil interact to form a complex network that leads to PGF. Sufficient number of seeds with good quality and healthy soil are key to successful transplantation. Invading insects damage seeds and soil through knock-on effects, resulting in the occurrence of PGF. CMVR, cytomegalovirus reactivation; DSA, donor-specific anti-human leukocyte antigen antibody; EC, endothelial cell; GVHD, graft-versus-host disease; HSC, hematopoietic stem cell; IO, iron overload; MSC, mesenchymal stem cell; MΦ, macrophage; PGF, poor graft function; ROS, reactive oxygen species.
The Seed
Similar to seeds, HSCs have the potential of self-renewal and multi-differentiation. Sufficient and functional stem cells have historically been considered key to the success of HSCT. Defects in HSCs, including changes in their quality and quantity, can lead to PGF or GF (Figure 1). The donor’s choice directly determines the seed source. Young people are considered better donors than elders, men are superior to women as donors, and matched sibling donors (MSDs) should be prioritized over haploidentical donors (HIDs). However, an older donor/recipient age, female-to-male transplants, and donor-recipient ABO major-mismatch transplants are major risk factors for transplant-related mortality (10). For patients > 50 years old and with high-risk leukemia, HID-HSCT led to a better prognosis compared to MSD-HSCT, with a reduced relapse rate and/or improved leukemia-free survival and OS (11, 12). Interestingly, HID-HSCT may have a stronger graft-versus-leukemia effect than that of MSD-HSCT (12, 13). Thus, in 2021, the Chinese Society of Hematology recommendations noted that “HIDs are the preferred donor choice over MSDs for patients with high-risk leukemia or elderly patients with young offspring donors in experienced centers” (14). The peripheral blood (PB) and BM are the predominant sources of stem cells. There is evidence that in haplo-HSCT with post-transplantation cyclophosphamide using a PB graft, compared with a BM graft, increased the risk of acute GVHD (aGVHD), whereas the 2-year OS, chronic GVHD, relapse, or non-relapse mortality (NRM) were comparable (15, 16). However, another study showed that patients receiving BM had significantly higher 2-year relapse rates compared to those in the PB cohort (36% vs. 16%) (17). Before 2019, mixed grafts of BM + PB were preferred over PB alone in HID-HSCT, because they achieved longer disease-free survival (18). A recent study reported that mixed grafts or PB alone did not influence clinical outcomes (19). Except for haploidentical grafts, rapidly accessible cord blood is a suitable alternative for pediatric patients without HLA-matched donors. Interestingly, the lower incidence of GVHD was not correlated with an increase in long-term survival. For example, in T-cell depleted PB stem cell transplantation, because of the delayed recovery of immune function, the incidence and severity of GVHD are low but are accompanied by an increased risk of infection, relapse, PGF, and transplantation-related mortality, particularly following transplantation with purified CD34+ cells (20, 21). As a result, different transplant centers have attempted to use transplant protocols without T-cell depleted conditions, suggesting that unmanipulated transplants are an alternative strategy under haploidentical settings (22). Additionally, a study at Peking University showed that haplo-HSCT without T-cell depleted transplantation has a comparable prognosis as MSD-HSCT (23, 24). Currently, the impact of different graft sources on the incidence of PGF is not well-understood.
A high CD34+ cell count is favorable for rapid hematopoietic recovery (8). Cell thresholds were devised to guide whether to carry out further apheresis collection. Currently, most centers use 2 × 106/kg CD34+ cells as the minimum threshold. CD34+ cells ≥ 5 × 106/kg are currently recommended as the optimal dose by the EBMT. Granulocyte colony-stimulating factor, alone or in combination with chemotherapy, is a standard mobilization regimen for collecting larger amounts of CD34+ cells. Plerixafor, a recently approved mobilization agent, is a small-molecule antagonist of CXC chemokine receptor 4 that can effectively block the binding between this chemokine and stromal cell-derived factor-1α, and then release HSCs from the BM to the PB. The combination of granulocyte colony-stimulating factor and plerixafor significantly increased the yield of CD34+ cells without causing adverse reactions (25). CD34 molecules were first identified on the surfaces of human hematopoietic stem and progenitor cells (HSPCs). CD34 is also an established marker of other non-hematopoietic cells, including vascular endothelial progenitor cells (EPCs), mesenchymal stem cells (MSCs), and embryonic fibroblasts (26). Thus, the collected “CD34+ cells” should not be confused with “CD34+ HSPCs.” Introducing new HSPCs markers, such as the signaling lymphocytic activation molecule family (including CD150, CD48, and CD244) (27), may enrich the purer stem cell population. In a nested case-control study of 830 patients, CD34+ cell dose < 5 × 106/kg was an independent risk factor for primary PGF (5). A low CD34+ cell dose (<median, 2.64 × 106/kg) was also an independent risk factor for sPGF (4). In recent decades, the use of CD34+-selected stem cells boosted without preconditioning has significantly improved the prognosis of patients with PGF. In a small-scale study (28), most patients with PGF who received selected CD34+ PB stem cells from matched unrelated or mismatched related donors achieved rapid engraftment; more importantly, the procedure was safe, with a low risk of de novo grade I–III aGVHD (6%), which was resolved completely. In a long-term follow-up study, CD34+-selected infusion without conditioning was feasible in recipients with full donor chimerism and in those with mixed chimerism, whose recovery was similar; patients showing complete recovery had a longer 5-year OS than those with partial recovery (74.4% vs 16.7%) (29). Active infection was considered as the strongest predictor of the efficacy of CD34+-selective infusion (29), possibly because of the impaired immune microenvironment caused by inflammation. Cryopreserved products are viable alternatives when additional fresh stem cells cannot be collected. Although the median selection of CD34+ counts per kilogram of recipient weight was relatively low (1 × 106/kg), this method achieved promising results; five of the eight cryopreserved product recipients (63%) exhibited a complete hematologic response (25).
It is essential to maintain high-quality stem cells at each step of the transplantation process. An increasing number of studies have been performed to optimize the cell handling, freezing, and thawing steps to ensure stem cell quality (30). Although methods for improving the viability and recovery rate of thawed stem cells are continuously being developed, the procedures still have a negative effect on the product quality and potency (31). Colony assays are the gold standard for stem cell proliferation and differentiation potency in vitro, which are used as an additional quality criterion. Watts et al. (32) demonstrated that if the granulocyte-macrophage colony-forming cell dose exceeded 2 × 105/kg after 14 days in culture, total CD34+ cells between 1 and 1.9 × 106/kg were also acceptable. When colony assays cannot be performed, cell doses below the threshold should be declared as inadequate, and remobilization and recollection are necessary. However, few centers routinely perform clonal analysis, as these tests require technical support, laboratory standardization, and higher expenses. A rapid method was recently developed to assess the cord blood unit potency for frozen cord blood based on aldehyde dehydrogenase (33). Further studies are necessary to confirm whether this method can be used as an alternative to clonal analysis. In addition, the detection of blood disease-related mutations in donor stem cells prior to transplantation may be of some significance in ensuring the quality of stem cells.
Accumulating evidence has suggested that excess levels of oxygen species (ROS) are responsible for defective hematopoiesis of HSC in patients with PGF, which may be related to disruptions in the stem cell cycle caused by elevated ROS. After transplantation, transiently elevated oxygen tension is beneficial for the rapid proliferation of engrafted HSCs, because it promotes the regeneration of hematopoiesis during the “engraftment window” in the BM niche (34). The BM microenvironment gradually returns to conditions of hypoxic homeostasis with increased oxygen consumption after hematopoietic reconstitution. In vivo imaging showed that ROS mediated the initial homing and proliferation of HSCs in lethally irradiated mice, but is not indispensable for long-term hematopoietic reconstitution after transplantation (35). More importantly, Cheng et al. (36, 37) found that in transplanted human HSCs, radiation-induced bystander effects increased ROS levels, contributing to HSC damage and a decrease in transplantation efficiency. It has been hypothesized that forkhead homeobox type O transcription factors are key mediators of ROS regulation in HSCs, contributing to stem cell maintenance and the DNA damage repair response (38). As a negative regulator of forkhead homeobox type O transcription factors, the phosphoinositide 3-kinase (PI3K)/AKT pathway is suppressed in HSCs but activated in hematopoietic progenitors. Activated PI3K/AKT signaling induced HSCs re-entry into the cell cycle, and eventually exhaust HSCs through deletion of phosphatase and tensin homologs (39). In BM, elevated ROS levels induce DNA strand breaks and apoptosis, contributing to the exhaustion of CD34+ cells through the p53-p21 pathway in patients with PGF following allo-HSCT, even if the CD34+ cells are functionally normal before transplantation (40). Thus, activated p53 can induce HSC depletion. However, Hainaut et al. (41) demonstrated that p53 can also function against ROS-induced DNA damage through its intrinsic redox dependence. Therefore, p53 as a regulator of ROS, playing a dual role in stem cell maintenance.
The Soil
The BM microenvironment, as the niche for HSC survival, consists of blood vessels, nerves, and a variety of cells that form a complex and precise network to regulate the functional characteristics of HSCs; thus, we compared this microenvironment to soil. In recent years, the mechanisms of various cell and molecular interactions in the BM microenvironment involved in the pathogenesis of PGF have been determined. Huang et al. (42) observed that patients in the sPGF group had marked marrow hypoplasia, and the proportion of CD34+ cells, EPCs, CD146+ perivascular cells, and endosteal cells were significantly lower than those in the good graft function and healthy control groups. Three years later, they demonstrated that the BM microenvironment was equally damaged in both early and late PGF (43). Recently, a series of translational studies demonstrated that defective autophagy regulated by Beclin-1 (44) or abnormal glycolysis induced by PFKFB3 (45) results in damage to BM endothelial cells (ECs), particularly their decreased hematopoiesis-supporting ability, which is involved in the pathogenesis of PGF post-HSCT. Thus, some transplant events, which may trigger an abnormal increase in ROS in the BM microenvironment, may be essential factors contributing to damage to the BM niche in patients with PGF. As described above, a dysfunctional BM microenvironment may contribute to PGF pathogenesis (Figure 1).
Endothelial Cells
ECs play a crucial role in regulating hematopoiesis by secreting stem cell factor and chemokine ligand 12 in the BM microenvironment (46). An unexpected finding regarding the origin of BM ECs was reported by Plein et al. (47) during early embryogenesis, ECs arise from erythro-myeloid progenitors. Thus, HSCs may provide survival and proliferation signals for EPCs. Accumulating evidence has shown that decreased and dysfunctional BM ECs post-HSCT contribute to the development of PGF (43, 48). Huang et al. (49) provided further evidence that pre-HSCT, BM ECs dysfunction was responsible for the pathogenesis of PGF after haplo-HSCT. BM ECs <0.1% pre-HSCT was an independent risk factor for PGF. Defective hematopoiesis caused by damaged BM ECs is positively correlated with ROS levels (49). Radiation therapy is commonly used in anticancer treatment and myeloablative conditioning regimens before HSCT. Irradiation also severely damages the BM vascular system, particularly sinusoidal ECs, leading to elevated ROS in the BM (35). Notably, elevated ROS levels are observed in ECs and recovering bones in the non-hematopoietic state, even at 2 weeks after sub-lethal irradiation (7 Gy) (50). Huang et al. (48) also showed that the intracellular ROS levels of BM EPCs were elevated after transplantation, and these cells had decreased proliferation and migration capacities. Vasculature reconstruction-mediated hematopoietic engraftment after radiotherapy depends on the expression of vascular cell adhesion molecule 1 on ECs (35), vascular endothelial growth factor receptor 2 signaling in apelin+ ECs (51), and vascular endothelial growth factor A provided by transplanted HSPCs (52).
Allogeneic EPCs infusions induced hematopoietic and immune reconstitution in mice, accelerated BM microvascular recovery, and ameliorated GVHD (53, 54). Few studies have reported the infusion of EPCs in humans for clinical treatment, likely because of the limited number of circulating EPCs. In contrast, atorvastatin, a lipid-lowering drug widely used in clinics, was reported to quantitatively improve the impaired function of BM EPCs in vivo by downregulating the p38 MAPK pathway in subjects with PGF (48). The antioxidant N-acetyl-l-cysteine can reduce ROS levels both in vitro and in vivo (49, 55). Prophylactic intervention with oral N-acetyl-l-cysteine not only prevents the occurrence of PGF post-HSCT, but also promotes hematopoietic reconstitution effectively by repairing impaired BM ECs in patients with PGF (49). These results indicate that it is valuable to use antioxidant drugs to improve PGF caused by elevated ROS levels.
Mesenchymal Stem Cells
MSCs are a type of BM stromal cells with multi-directional differentiation potential, immunoregulatory and hematopoietic support capabilities (56). MSCs show potential for use in treating PGF after HSCT. The mechanisms of MSCs in the pathogenesis of PGF are only beginning to be understood. Compared to in patients with good graft function, BM MSCs from patients with PGF exhibited increased intracellular ROS, higher levels of apoptosis and senescence, and a significantly reduced hematopoiesis-supporting ability in vitro (57). Animal experiments and early-phase clinical trials showed that co-infusion of MSCs and HSCs promoted HSC engraftment and improved PGF (58–60). Nevertheless, whether MSCs with immune-suppressive properties increase the incidence of infection and relapse remains controversial. As early as 2007, a clinical study demonstrated that all patients who underwent co-transplantation of ex vivo expanded MSC with HLA-disparate CD34+ cells showed continuous hematopoietic engraftment, without additional infection compared to in the control group (61). Recently, a systematic review and meta-analysis of children and young individuals showed that MSC co-infusion improved the absolute neutrophil count and PLT engraftment, and greatly reduced the risk of chronic GVHD but had a minimal impact on aGVHD and NRM (59). Similarly, in a systematic review and meta-analysis of haplo-HSCT for severe AA, there was no obvious difference in the 2-year OS, incidence of GVHD and cytomegalovirus (CMV) infection between the MSC co-transplantation group and group not transplanted with MSCs (62). Although the effect of MSCs on PGF was not discussed in the article, co-administration of MSCs with HSCs may not be suitable for patients with severe AA undergoing haplo-HSCT. When infusion of MSCs was performed after transplantation, 17 of 20 patients with primary or secondary PGF experienced hematopoietic recovery when the MSCs were from a third-party donor (60). Moreover, some patients also developed varying degrees of CMV or Epstein-Barr virus infection, acute or chronic GVHD of varying degrees, and even relapse or non-relapse death (60). As summarized above, MSC infusion either before or after transplantation is an effective option for improving PGF, possibly because of their hematopoietic support capabilities. Possible risk factors such as long-term treatment with immunoinhibitors and HLA mismatch may affect the susceptibility to infection. Further studies are needed to determine whether MSCs increase the incidence of infection.
The Insects
Immune-mediated destruction of hematopoiesis is well-established in the pathogenesis of AA, most likely in the form of AA, an immune imbalance in abnormal hematopoiesis post-HSCT may be responsible for PGF. We compare various factors potentially associated with immune dysregulation post-transplantation, such as GVHD and CMV infection, to insects. GVHD is a fatal complication of allo-HSCT and occurs when donor immunoreactive cells recognize and attack recipient tissue. Grade III–IV GVHD is significantly associated with PGF development (9). Various factors that lead to GVHD, such as ongoing immune stimulation, may prevent hematopoietic reconstitution and exhaust hematopoietic precursor cells, eventually resulting in PGF development after transplantation.
Both CD4+ and CD8+ T cells are dramatically polarized towards the type 1 immune response in patients with PGF after allo-HSCT (63, 64). Thus, dysregulated T cell responses in the BM immune microenvironment may be involved in the pathogenesis of PGF after HSCT. Luo et al. (65) found that M2 macrophages (MΦs) supported and M1 MФ suppressed HSC self-renewal and expansion in vitro. MΦs derived from patients with PGF exhibited significantly increased M1 and decreased M2 relative to those from patients with good graft function and healthy donors (66). Furthermore, the function of MΦs was impaired, characterized by reduced hematopoiesis-supporting ability, resulting in BM CD34+ cell dysfunction through p38 MAPK pathway upregulation, and aggravated pancytopenia in patients with PGF (66). Moreover, Zhao et al. (67) confirmed the opposing effects of M1 and M2 MФs on megakaryocytes: M1 MФs inhibit whereas M2 MФs promote MK maturation and platelet formation. MФs in patients with prolonged isolated thrombocytopenia also polarized towards M1, and unbalanced MΦs polarization impaired the megakaryopoiesis-supporting ability of BM MФs, which was rescued by activation of the PI3K-AKT pathway. Further studies are needed to determine how these dysfunctional immune cells interact with other cellular elements or directly affect hematopoiesis, which may provide insight into the underlying molecular mechanisms and potential therapeutic strategies for patients with PGF after HSCT.
Thrombocytopenia caused by ongoing immune attacks limits the recovery of PGF. The thrombopoietin (TPO) receptor agonist (TPO-RA), romiplostim and eltrombopag showed promising results for treating immune thrombocytopenia (ITP). Additionally, the combination of eltrombopag with standard immunosuppressive therapy (horse antithymocyte globulin plus cyclosporine) shows great potential for treating severe AA (68), because it improves the rate, rapidity, and strength of hematologic responses in severe AA without causing toxic effects. Moreover, eltrombopag has been successfully used to treat PFG after allo-HSCT (69). Eltrombopag has changed the paradigm of AA treatment, however, some patients do not respond to this treatment. Nakao et al. (70) reported that high-dose romiplostim (20 μg/kg) was highly effective in patients with AA refractory to eltrombopag. Avatrombopag is a second-generation TPO-RA approved for second-line treatment of primary chronic ITP. A multicenter study performed in the United States confirmed that: patients with ITP who previously used other TPO-RAs (eltrombopag or romiplostim) and responded poorly exhibited a high response rate to avatrombopag (71). Thus, TPO-RA may be another option for treating PGF.
Donor-Specific Anti-HLA Antibodies
Antibody-mediated graft rejection is considered to cause GF. Various preformed antibodies are detectable in patients after allo-HSCT (72). DSA refers to specific antibodies corresponding to a mismatched antigen produced in patients after organ/tissue transplantation. Circulating DSA can lead to hyperacute rejection and thus is an important factor affecting HSC engraftment and is related to PGF, particularly primary PGF (7, 73). In a retrospective analysis of 394 patients who underwent haplo-HSCT, DSA with median fluorescence intensity (MFI) ≥1000 was significantly correlated with prolonged isolated thrombocytopenia (hazard ratio 3.262; P = 0.009) (74). DSA with MFI ≥ 1000 was also considered associated with the cumulative incidence of neutrophil engraftment for 60 days after single-unit cord blood transplantation (P = 0.03) (75). Ciurea et al. (76) found that patients with high DSA levels (> 5000 MFI) and complement-binding DSA antibodies (C1q-positive) exhibited graft rejection at the time of transplantation, whereas patients whose C1q became negative after desensitization therapy were successfully engrafted by donor cells. In the absence of effective salvage approaches, the mortality rate of patients with GF is close to 100%, particularly after haplo-HSCT. A second early transplant can often successfully salvage GF (77, 78). The absence of DSA was associated with lower NRM and improved OS (79). These studies support the consensus guidelines from the EBMT, suggesting that DSA and C1q levels must be monitored to further assess the risk of allograft in patients with DSAs ≥ 1,000 MFI (80).
Reducing DSA levels is essential for preventing primary PGF. Plasma exchange, rituximab, PLT transfusions, bortezomib, and immunoglobulin are often used clinically to decrease DSA levels. The rate of granulocyte reconstruction in the DSA-positive group was lower than that in the DSA-negative group after desensitization therapy (81–83). During HLA-mismatched HSCT, a single dose of rituximab was effective for desensitization and prevented the onset of primary PGF in DSA-positive patients, whereas bortezomib and immunoglobulin alone showed a limited ability to rapidly decrease DSA levels (81). Patients were desensitized to a DSA level < 2000 MFI after combination therapy with rituximab and/or plasmapheresis (83). However, DSA may rapidly rebound at any time. In a case report by Hassan et al. (84), during hematopoietic progenitor cell transplantation, DSA unexpectedly rebounded and rapidly increased during desensitization with repeated plasma exchange and immunoglobulin, finally leading to primary PGF. However, the cause of this phenomenon remains unclear. As an IgG-degrading enzyme of Streptococcus pyogenes, imlifidase can inhibit complement-and FcγR-mediated effector functions by cleaving donor-specific IgG into Fc and F (ab’) 2 fragments (85). Endoglycosidase of S. pyogenes (EndoS) reduces the affinity of IgG for FcγRs by specifically hydrolyzing glycans of all subclasses of human IgG (86). Both imlifidase and EndoS partially block DSA’s function. To further reduce the titer and inhibit the effector functions of residual DSA, Anderson et al. (87) demonstrated that a combination of imlifidase and EndoS can be used to inactivate DSA and inhibit DSA-mediated killing of donor BM cells in allogeneic BM transplantation. Further studies are needed to confirm whether enzyme-mediated DSA blocking prevents antibody rebound.
Cytomegalovirus Infection
CMV and Epstein-Barr virus reactivation are independent risk factors for sPGF within the first 100 days of allo-HSCT (4, 88). CMV and Epstein-Barr virus co-reactivation not only leads to a shorter 1-year OS and leukemia-free survival, but also results in poor regulatory T cell reconstitution at day 30 after allo-HSCT (89). CMV reactivation (CMVR) after HSCT can lead to a variety of common life-threatening infectious complications such as pneumonia, retinitis, or sPGF. The prognosis of CMVR and immune reconstitution of CMV-specific T-cells are closely related (90, 91). In the first year post-transplantation, clonal expansion of CMV-specific effector memory T-cells drives the reconstitution of CD4+ and CD8+ T-cells. Furthermore, the heterogeneity and diversity of the remaining T-cell repertoire are impaired in patients who experience reactivation (92, 93).
Previous studies suggested that CMVR after transplantation is strongly associated with aGVHD. Recent evidence demonstrated that mismatches in major or minor histocompatibility antigens promote CMV disease by inducing non-cognate transplantation tolerance, which inhibits the efficient reconstitution of antiviral CD8+ T cells, eventually resulting in cytopathogenic tissue infections (90). CMVR was associated with an increased risk of NRM with or without GVHD; however, the interaction between GVHD and CMVR was not significant (P = 0.326) (94). Therefore, aGVHD does not appear to be necessary for CMVR. However, the results of different studies varied based on the baseline characteristics. Single-center studies reported that CMVR after allo-HSCT is positively correlated with a decreased risk of relapse in acute myelocytic leukemia but not in other hematological malignancies. This benefit is of little significance considering the increased NMR and overall mortality (95). Another study of the Center for International Blood and Marrow Transplant Research database confirmed CMVR as a risk factor for poor prognosis, but showed no benefit of CMVR on the relapse of hematologic disease (96). CMV peak titers, disease stage, and T-cell depletion with antithymocyte globulin, which are associated with immunity, may modulate the impact of CMVR on leukemia relapse (97, 98).
In the era of PCR-based monitoring, universal prophylaxis or preemptive therapy strategy is typically adopted to prevent and treat CMV infections after HSCT (99). In the 1980s and 1990s, high-dose acyclovir and valacyclovir showed limited efficacy in preventing CMV disease (100). Some agents have been used for decades to control CMV infection and lead to significant toxicity. For example, ganciclovir (101, 102) is hemotoxic and frequently leads to secondary bacterial and fungal infections. Additionally, foscarnet (103) and cidofovir (102) exhibit severe renal toxicity. The introduction of letermovir (LMV) is an important advancement. As a CMV DNA terminase complex inhibitor, LMV can be administered orally and intravenously and has no myelotoxicity or nephrotoxicity (102, 104). Co-administration of cyclosporine increased the bioavailability of LMV from 35% to 85%; thus, lower doses are required in patients taking cyclosporine to prevent GVHD. Increasing evidence has shown that prophylactic LMV treatment effectively prevents the development of refractory or resistant CMV infections and ultimately decreases transplant-related mortality (105–107). In addition, Zamora et al. (108) provided initial evidence that compared with ganciclovir preemption, LMV prophylaxis-associated CMV antigen exposure reduction delays CMV-specific T-cell reconstitution after HSCT.
Virological monitoring of CMV in the blood plasma is routinely performed using quantitative PCR, but there is currently no consensus on the plasma viral load threshold when initiating CMV preemptive treatment. Real-time CMV-specific cell-mediated immunity responses were successfully applied to predict clinical CMV events and guide the early discontinuation of antivirals (109). Future strategies may involve vaccination dependent on functional reconstitution of CD4+ T cells and B cells (91, 110), other novel antiviral agents [maribavir (111), CMX001 (112)], antibodies that block cell-to-cell spread and kill latently infected cells (113, 114), and adoptive cell therapy not limited by GVHD and steroids (115).
Iron Overload
Iron is a raw material for hematopoiesis. Long-term blood transfusion and inflammation are the most common factors leading to iron overload (IO) in patients with hematological malignancies. Studies have shown mixed results regarding the impact of IO pre-transplant on PGF and prognosis, possibly because of differences in marker selection and baseline data of the study population. Serum ferritin (SF) is a biomarker of IO. In two prospective studies by Zhao et al. (5) and Malki et al. (116), SF > 2000 ng/mL before HSCT was identified as an independent risk factor for primary PGF and a strong poor prognostic factor. In a subsequent prospective multicenter study, patients with SF > 1500 ng/mL before the start of conditioning with allo-HSCT had an inferior OS (hazard ratio, 2.5, CI = 1.5-4.1, P = 0.0005) and progression-free survival (hazard ratio, 2.4, CI = 1.6-3.8, P < 0.0001) (117). In contrast, in a prospective cohort study using liver magnetic resonance imaging to quantify the liver iron content, there was no significant correlation between IO (liver iron content >1.8 mg/g) before allo-HSCT and the cumulative incidence of multiple complications, OS, or NRM after HSCT (118). Interestingly, using SF or the liver iron content as a marker of IO revealed that IO was not related to the occurrence of acute or chronic GVHD (117, 118). Hepcidin expressed by the liver, it modulates iron absorption and release and is overexpressed when IO decreases these processes, and the erythropoiesis demands can eventually not be met (119). The rates of OS and PLT engraftment were significantly lower in the high hepcidin group than in the low hepcidin group (120). Hepcidin may be an alternative marker of IO to predict delayed PLT engraftment after allo-HSCT; however, there is currently no accepted validated method for evaluating hepcidin. Growth differentiation factor 15 belongs to the transforming growth factor-beta superfamily and has been proposed as an erythroid regulator involved in hepcidin suppression (121). Erythroferrone is a new erythroid regulator of hepcidin produced by erythroid precursors in response to stress erythropoiesis via the Jak2/Stat5 signaling pathway (122).
Zhao et al. (123) reported that IO damaged the erythroid colony-forming capacity of normal HSPCs and reduced the frequency of abnormal HSPCs in MDS mice. Impaired erythroid HSPCs are, at least in part, related to growth differentiation factor 15-induced ROS (123). In addition, IO contributes to MSC damage through the AMPK/MFF/Drp1 pathway, which displays increased cell apoptosis, decreased cell viability, and extensive autophagy, all of which are ROS-dependent (124). Excess iron levels can compromise BM stromal cells, inhibit erythropoietin and thrombopoietin levels, and disrupt hematopoietic function by increasing oxidative stress (125). Thus, the holistic situation during treatment and the link between IO and ROS should be considered. Currently, deferiprone and deferasirox are the most commonly used iron-chelating agents for removing IO in the clinic (126). Eltrombopag is also a powerful iron chelator with intracellular iron mobilization characteristics that can reduce iron-induced ROS and stimulate stem cell hematopoiesis independently of the TPO receptor (127, 128). Tang et al. (69) preliminarily verified the feasibility of treating sPGF post-allo-HSCT with eltrombopag in a retrospective analysis. Co-administration of eltrombopag with clinically available chelators, such as deferasirox, may be an effective means for indirect PGF treatment (127). Additionally, upregulation of ferritin (129) and transferrin infusion (130) improve BM hematopoietic function induced by IO in mice; further studies are required to confirm its clinical feasibility.
Conclusion
The pathogenesis of PGF involves a complex, interlocking network. Future approaches to address PGF should focus on optimizing seeds, improving soil, and killing insects, emphasizing the importance of early detection and treatment to avoid PGF. With regard to seeds, a certain scale of research has focused on improving the quantity; and quality inspection before infusion should be performed to prevent the occurrence of PGF. Emerging research attempts to describe the relationship between the BM microenvironment and PGF, and improve the understanding of how various stromal cells, related factors, and abnormally activated transduction pathways interact to promote the initiation and development of PGF, these may lead to the development of prevention and treatment strategies. From a superficial perspective, different “insects” have different impacts on PGF through different mechanisms. These insects are products of immunodeficiencies. Therefore, new drugs that kill insects and focus on targeted immune modulation are needed. The quality of donor CD34+ cells should be routinely evaluated prior to transplantation. If possible, to predict the incidence of PGF, the content and functional status of donor cells and level of oxidative stress in the recipient’s BM microenvironment should be detected to determine whether to continue transplantation or administer corresponding treatment in advance. These steps may be feasible preventive measures for PGF and require further validation.
Author Contributions
YW and TY conceptualized the outline and topic of the article. YM, XY, and ZL participated in collecting literature and draft manuscripts. ZL designed the figure. YM and XY analyzed the literature and made the figure. YW, TY, and YG coordinated the revision. All authors contributed to the article and approved the submitted version.
Funding
This work was supported by the National Natural Science Foundation of China (82070173, 82060810,81900109), the Basic Applied Research Projects of Yunnan Province (202101AW070017), the Doctoral Research Fund of the First People’s Hospital of Yunnan Province (KHBS-2020-007), the Open Project of Yunnan Blood Disease Clinical Medical Center (2020LCZXKF-XY14).
Conflict of Interest
The authors declare that the research was conducted in the absence of any commercial or financial relationships that could be construed as a potential conflict of interest.
Publisher’s Note
All claims expressed in this article are solely those of the authors and do not necessarily represent those of their affiliated organizations, or those of the publisher, the editors and the reviewers. Any product that may be evaluated in this article, or claim that may be made by its manufacturer, is not guaranteed or endorsed by the publisher.
References
1. Carreras E, Dufour C, Mohty M, Kröger N. The Ebmt Handbook: Hematopoietic Stem Cell Transplantation and Cellular Therapies. In: Carreras E, Dufour C, Mohty M, Kroger N, editors. The Ebmt Handbook: Hematopoietic Stem Cell Transplantation and Cellular Therapies. Cham (CH: Springer) (2019).
2. McLornan DP, Hernandez-Boluda JC, Czerw T, Cross N, Joachim Deeg H, Ditschkowski M, et al. Allogeneic Haematopoietic Cell Transplantation for Myelofibrosis: Proposed Definitions and Management Strategies for Graft Failure, Poor Graft Function and Relapse: Best Practice Recommendations of the Ebmt Chronic Malignancies Working Party. Leukemia (2021) 35(9):2445–59. doi: 10.1038/s41375-021-01294-2
3. Alchalby H, Yunus DR, Zabelina T, Ayuk F, Kroger N. Incidence and Risk Factors of Poor Graft Function After Allogeneic Stem Cell Transplantation for Myelofibrosis. Bone Marrow Transplant (2016) 51(9):1223–7. doi: 10.1038/bmt.2016.98
4. Sun YQ, Wang Y, Zhang XH, Xu LP, Liu KY, Yan CH, et al. Virus Reactivation and Low Dose of Cd34+ Cell, Rather Than Haploidentical Transplantation, Were Associated With Secondary Poor Graft Function Within the First 100 Days After Allogeneic Stem Cell Transplantation. Ann Hematol (2019) 98(8):1877–83. doi: 10.1007/s00277-019-03715-w
5. Zhao Y, Gao F, Shi J, Luo Y, Tan Y, Lai X, et al. Incidence, Risk Factors, and Outcomes of Primary Poor Graft Function After Allogeneic Hematopoietic Stem Cell Transplantation. Biol Blood Marrow Transplant (2019) 25(9):1898–907. doi: 10.1016/j.bbmt.2019.05.036
6. Sun YQ, He GL, Chang YJ, Xu LP, Zhang XH, Han W, et al. The Incidence, Risk Factors, and Outcomes of Primary Poor Graft Function After Unmanipulated Haploidentical Stem Cell Transplantation. Ann Hematol (2015) 94(10):1699–705. doi: 10.1007/s00277-015-2440-x
7. Chang YJ, Zhao XY, Xu LP, Zhang XH, Wang Y, Han W, et al. Donor-Specific Anti-Human Leukocyte Antigen Antibodies Were Associated With Primary Graft Failure After Unmanipulated Haploidentical Blood and Marrow Transplantation: A Prospective Study With Randomly Assigned Training and Validation Sets. J Hematol Oncol (2015) 8:84. doi: 10.1186/s13045-015-0182-9
8. Arcuri LJ, Nabhan SK, Cunha R, Nichele S, Ribeiro AAF, Fernandes JF, et al. Impact of Cd34 Cell Dose and Conditioning Regimen on Outcomes After Haploidentical Donor Hematopoietic Stem Cell Transplantation With Post-Transplantation Cyclophosphamide for Relapsed/Refractory Severe Aplastic Anemia. Biol Blood Marrow Transplant (2020) 26(12):2311–7. doi: 10.1016/j.bbmt.2020.09.007
9. Prabahran A, Koldej R, Chee L, Wong E, Ritchie D. Evaluation of Risk Factors for and Subsequent Mortality From Poor Graft Function (Pgf) Post Allogeneic Stem Cell Transplantation. Leuk Lymphoma (2021) 62(6):1482–9. doi: 10.1080/10428194.2021.1872072
10. Wang Y, Wu DP, Liu QF, Xu LP, Liu KY, Zhang XH, et al. Donor and Recipient Age, Gender and Abo Incompatibility Regardless of Donor Source: Validated Criteria for Donor Selection for Haematopoietic Transplants. Leukemia (2018) 32(2):492–8. doi: 10.1038/leu.2017.199
11. Wang Y, Liu QF, Wu DP, Xu LP, Liu KY, Zhang XH, et al. Improved Survival After Offspring Donor Transplant Compared With Older Aged-Matched Siblings for Older Leukaemia Patients. Br J Haematol (2020) 189(1):153–61. doi: 10.1111/bjh.16303
12. Yu S, Huang F, Wang Y, Xu Y, Yang T, Fan Z, et al. Haploidentical Transplantation Might Have Superior Graft-Versus-Leukemia Effect Than Hla-Matched Sibling Transplantation for High-Risk Acute Myeloid Leukemia in First Complete Remission: A Prospective Multicentre Cohort Study. Leukemia (2020) 34(5):1433–43. doi: 10.1038/s41375-019-0686-3
13. Guo H, Chang YJ, Hong Y, Xu LP, Wang Y, Zhang XH, et al. Dynamic Immune Profiling Identifies the Stronger Graft-Versus-Leukemia (Gvl) Effects With Haploidentical Allografts Compared to Hla-Matched Stem Cell Transplantation. Cell Mol Immunol (2021) 18(5):1172–85. doi: 10.1038/s41423-020-00597-1
14. Zhang XH, Chen J, Han MZ, Huang H, Jiang EL, Jiang M, et al. The Consensus From the Chinese Society of Hematology on Indications, Conditioning Regimens and Donor Selection for Allogeneic Hematopoietic Stem Cell Transplantation: 2021 Update. J Hematol Oncol (2021) 14(1):145. doi: 10.1186/s13045-021-01159-2
15. Ruggeri A, Labopin M, Bacigalupo A, Gulbas Z, Koc Y, Blaise D, et al. Bone Marrow Versus Mobilized Peripheral Blood Stem Cells in Haploidentical Transplants Using Posttransplantation Cyclophosphamide. Cancer (2018) 124(7):1428–37. doi: 10.1002/cncr.31228
16. Yu X, Liu L, Xie Z, Dong C, Zhao L, Zhang J, et al. Bone Marrow Versus Peripheral Blood as a Graft Source for Haploidentical Donor Transplantation in Adults Using Post-Transplant Cyclophosphamide-A Systematic Review and Meta-Analysis. Crit Rev Oncol Hematol (2019) 133:120–8. doi: 10.1016/j.critrevonc.2018.05.017
17. Sharma N, Faisal MS, Zhao Q, Jiang J, Elder P, Benson DM, et al. Outcomes of Bone Marrow Compared to Peripheral Blood for Haploidentical Transplantation. J Clin Med (2021) 10(13):2843. doi: 10.3390/jcm10132843
18. Xu L, Chen H, Chen J, Han M, Huang H, Lai Y, et al. The Consensus on Indications, Conditioning Regimen, and Donor Selection of Allogeneic Hematopoietic Cell Transplantation for Hematological Diseases in China-Recommendations From the Chinese Society of Hematology. J Hematol Oncol (2018) 11(1):33. doi: 10.1186/s13045-018-0564-x
19. Ma YR, Zhang X, Xu L, Wang Y, Yan C, Chen H, et al. G-Csf-Primed Peripheral Blood Stem Cell Haploidentical Transplantation Could Achieve Satisfactory Clinical Outcomes for Acute Leukemia Patients in the First Complete Remission: A Registered Study. Front Oncol (2021) 11:631625. doi: 10.3389/fonc.2021.631625
20. Elfeky R, Shah RM, Unni MNM, Ottaviano G, Rao K, Chiesa R, et al. New Graft Manipulation Strategies Improve the Outcome of Mismatched Stem Cell Transplantation in Children With Primary Immunodeficiencies. J Allergy Clin Immunol (2019) 144(1):280–93. doi: 10.1016/j.jaci.2019.01.030
21. Ciurea SO, Mulanovich V, Saliba RM, Bayraktar UD, Jiang Y, Bassett R, et al. Improved Early Outcomes Using a T Cell Replete Graft Compared With T Cell Depleted Haploidentical Hematopoietic Stem Cell Transplantation. Biol Blood Marrow Transplant (2012) 18(12):1835–44. doi: 10.1016/j.bbmt.2012.07.003
22. Chang YJ, Huang XJ. Haploidentical Bone Marrow Transplantation Without T-Cell Depletion. Semin Oncol (2012) 39(6):653–63. doi: 10.1053/j.seminoncol.2012.09.003
23. Chen H, Liu KY, Xu LP, Chen YH, Han W, Zhang XH, et al. Haploidentical Hematopoietic Stem Cell Transplantation Without in Vitro T Cell Depletion for the Treatment of Philadelphia Chromosome-Positive Acute Lymphoblastic Leukemia. Biol Blood Marrow Transplant (2015) 21(6):1110–6. doi: 10.1016/j.bbmt.2015.02.009
24. Lu DP, Dong L, Wu T, Huang XJ, Zhang MJ, Han W, et al. Conditioning Including Antithymocyte Globulin Followed by Unmanipulated Hla-Mismatched/Haploidentical Blood and Marrow Transplantation Can Achieve Comparable Outcomes With Hla-Identical Sibling Transplantation. Blood (2006) 107(8):3065–73. doi: 10.1182/blood-2005-05-2146
25. Ghobadi A, Fiala MA, Ramsingh G, Gao F, Abboud CN, Stockerl-Goldstein K, et al. Fresh or Cryopreserved Cd34(+)-Selected Mobilized Peripheral Blood Stem and Progenitor Cells for the Treatment of Poor Graft Function After Allogeneic Hematopoietic Cell Transplantation. Biol Blood Marrow Transplant (2017) 23(7):1072–7. doi: 10.1016/j.bbmt.2017.03.019
26. Sidney LE, Branch MJ, Dunphy SE, Dua HS, Hopkinson A. Concise Review: Evidence for Cd34 as a Common Marker for Diverse Progenitors. Stem Cells (2014) 32(6):1380–9. doi: 10.1002/stem.1661
27. Kiel MJ, Yilmaz OH, Iwashita T, Yilmaz OH, Terhorst C, Morrison SJ. Slam Family Receptors Distinguish Hematopoietic Stem and Progenitor Cells and Reveal Endothelial Niches for Stem Cells. Cell (2005) 121(7):1109–21. doi: 10.1016/j.cell.2005.05.026
28. Mainardi C, Ebinger M, Enkel S, Feuchtinger T, Teltschik HM, Eyrich M, et al. Cd34(+) Selected Stem Cell Boosts Can Improve Poor Graft Function After Paediatric Allogeneic Stem Cell Transplantation. Br J Haematol (2018) 180(1):90–9. doi: 10.1111/bjh.15012
29. Cuadrado MM, Szydlo RM, Watts M, Patel N, Renshaw H, Dorman J, et al. Predictors of Recovery Following Allogeneic Cd34+-Selected Cell Infusion Without Conditioning to Correct Poor Graft Function. Haematologica (2020) 105(11):2639–46. doi: 10.3324/haematol.2019.226340
30. Watts MJ, Linch DC. Optimisation and Quality Control of Cell Processing for Autologous Stem Cell Transplantation. Br J Haematol (2016) 175(5):771–83. doi: 10.1111/bjh.14378
31. Purtill D, Antonenas V, Chiappini P, Tong D, O'Flaherty E, Bajel A, et al. Variable Cd34+ Recovery of Cryopreserved Allogeneic Hpc Products: Transplant Implications During the Covid-19 Pandemic. Blood Adv (2020) 4(17):4147–50. doi: 10.1182/bloodadvances.2020002431
32. Watts MJ, Ings SJ, Balsa C, Antonio A, Hack S, Linch DC. Re-Evaluation of Progenitor Thresholds and Expectations for Haematopoietic Recovery Based on an Analysis of 810 Autologous Transplants: Implications for Quality Assurance. Br J Haematol (2016) 175(4):673–6. doi: 10.1111/bjh.14276
33. Shoulars K, Noldner P, Troy JD, Cheatham L, Parrish A, Page K, et al. Development and Validation of a Rapid, Aldehyde Dehydrogenase Bright-Based Cord Blood Potency Assay. Blood (2016) 127(19):2346–54. doi: 10.1182/blood-2015-08-666990
34. Moirangthem RD, Singh S, Adsul A, Jalnapurkar S, Limaye L, Kale VP. Hypoxic Niche-Mediated Regeneration of Hematopoiesis in the Engraftment Window Is Dominantly Affected by Oxygen Tension in the Milieu. Stem Cells Dev (2015) 24(20):2423–36. doi: 10.1089/scd.2015.0112
35. Lewandowski D, Barroca V, Duconge F, Bayer J, Van Nhieu JT, Pestourie C, et al. In Vivo Cellular Imaging Pinpoints the Role of Reactive Oxygen Species in the Early Steps of Adult Hematopoietic Reconstitution. Blood (2010) 115(3):443–52. doi: 10.1182/blood-2009-05-222711
36. Shen H, Yu H, Liang PH, Cheng H, XuFeng R, Yuan Y, et al. An Acute Negative Bystander Effect of Gamma-Irradiated Recipients on Transplanted Hematopoietic Stem Cells. Blood (2012) 119(15):3629–37. doi: 10.1182/blood-2011-08-373621
37. Hu L, Yin X, Zhang Y, Pang A, Xie X, Yang S, et al. Radiation-Induced Bystander Effects Impair Transplanted Human Hematopoietic Stem Cells Via Oxidative DNA Damage. Blood (2021) 137(24):3339–50. doi: 10.1182/blood.2020007362
38. Storz P. Forkhead Homeobox Type O Transcription Factors in the Responses to Oxidative Stress. Antioxid Redox Signal (2011) 14(4):593–605. doi: 10.1089/ars.2010.3405
39. Yilmaz OH, Valdez R, Theisen BK, Guo W, Ferguson DO, Wu H, et al. Pten Dependence Distinguishes Haematopoietic Stem Cells From Leukaemia-Initiating Cells. Nature (2006) 441(7092):475–82. doi: 10.1038/nature04703
40. Kong Y, Song Y, Hu Y, Shi MM, Wang YT, Wang Y, et al. Increased Reactive Oxygen Species and Exhaustion of Quiescent Cd34-Positive Bone Marrow Cells May Contribute to Poor Graft Function After Allotransplants. Oncotarget (2016) 7(21):30892–906. doi: 10.18632/oncotarget.8810
41. Hafsi H, Hainaut P. Redox Control and Interplay Between P53 Isoforms: Roles in the Regulation of Basal P53 Levels, Cell Fate, and Senescence. Antioxid Redox Signal (2011) 15(6):1655–67. doi: 10.1089/ars.2010.3771
42. Kong Y, Chang YJ, Wang YZ, Chen YH, Han W, Wang Y, et al. Association of an Impaired Bone Marrow Microenvironment With Secondary Poor Graft Function After Allogeneic Hematopoietic Stem Cell Transplantation. Biol Blood Marrow Transplant (2013) 19(10):1465–73. doi: 10.1016/j.bbmt.2013.07.014
43. Kong Y, Wang YT, Hu Y, Han W, Chang YJ, Zhang XH, et al. The Bone Marrow Microenvironment Is Similarly Impaired in Allogeneic Hematopoietic Stem Cell Transplantation Patients With Early and Late Poor Graft Function. Bone Marrow Transplant (2016) 51(2):249–55. doi: 10.1038/bmt.2015.229
44. Lyu ZS, Cao XN, Wen Q, Mo XD, Zhao HY, Chen YH, et al. Autophagy in Endothelial Cells Regulates Their Haematopoiesis-Supporting Ability. EBioMedicine (2020) 53:102677. doi: 10.1016/j.ebiom.2020.102677
45. Lyu ZS, Tang SQ, Xing T, Zhou Y, Lv M, Fu HX, et al. Glycolytic Enzyme Pfkfb3 Determines Bone Marrow Endothelial Progenitor Cell Damage Post Chemotherapy and Irradiation. Haematologica (2022). doi: 10.3324/haematol.2021.279756
46. Crane GM, Jeffery E, Morrison SJ. Adult Haematopoietic Stem Cell Niches. Nat Rev Immunol (2017) 17(9):573–90. doi: 10.1038/nri.2017.53
47. Plein A, Fantin A, Denti L, Pollard JW, Ruhrberg C. Erythro-Myeloid Progenitors Contribute Endothelial Cells to Blood Vessels. Nature (2018) 562(7726):223–8. doi: 10.1038/s41586-018-0552-x
48. Shi MM, Kong Y, Song Y, Sun YQ, Wang Y, Zhang XH, et al. Atorvastatin Enhances Endothelial Cell Function in Posttransplant Poor Graft Function. Blood (2016) 128(25):2988–99. doi: 10.1182/blood-2016-03-702803
49. Kong Y, Wang Y, Zhang YY, Shi MM, Mo XD, Sun YQ, et al. Prophylactic Oral Nac Reduced Poor Hematopoietic Reconstitution by Improving Endothelial Cells After Haploidentical Transplantation. Blood Adv (2019) 3(8):1303–17. doi: 10.1182/bloodadvances.2018029454
50. Mesnieres M, Bohm AM, Peredo N, Trompet D, Valle-Tenney R, Bajaj M, et al. Fetal Hematopoietic Stem Cell Homing Is Controlled by Vegf Regulating the Integrity and Oxidative Status of the Stromal-Vascular Bone Marrow Niches. Cell Rep (2021) 36(8):109618. doi: 10.1016/j.celrep.2021.109618
51. Hooper AT, Butler JM, Nolan DJ, Kranz A, Iida K, Kobayashi M, et al. Engraftment and Reconstitution of Hematopoiesis Is Dependent on Vegfr2-Mediated Regeneration of Sinusoidal Endothelial Cells. Cell Stem Cell (2009) 4(3):263–74. doi: 10.1016/j.stem.2009.01.006
52. Chen Q, Liu Y, Jeong HW, Stehling M, Dinh VV, Zhou B, et al. Apelin(+) Endothelial Niche Cells Control Hematopoiesis and Mediate Vascular Regeneration After Myeloablative Injury. Cell Stem Cell (2019) 25(6):768–83.e6. doi: 10.1016/j.stem.2019.10.006
53. Zeng L, Chen C, Song G, Yan Z, Xu S, Jia L, et al. Infusion of Endothelial Progenitor Cells Accelerates Hematopoietic and Immune Reconstitution, and Ameliorates the Graft-Versus-Host Disease After Hematopoietic Stem Cell Transplantation. Cell Biochem Biophys (2012) 64(3):213–22. doi: 10.1007/s12013-012-9387-5
54. Salter AB, Meadows SK, Muramoto GG, Himburg H, Doan P, Daher P, et al. Endothelial Progenitor Cell Infusion Induces Hematopoietic Stem Cell Reconstitution in Vivo. Blood (2009) 113(9):2104–7. doi: 10.1182/blood-2008-06-162941
55. Hu L, Cheng H, Gao Y, Shi M, Liu Y, Hu Z, et al. Antioxidant N-Acetyl-L-Cysteine Increases Engraftment of Human Hematopoietic Stem Cells in Immune-Deficient Mice. Blood (2014) 124(20):e45–8. doi: 10.1182/blood-2014-03-559369
56. Crippa S, Santi L, Berti M, De Ponti G, Bernardo ME. Role of Ex Vivo Expanded Mesenchymal Stromal Cells in Determining Hematopoietic Stem Cell Transplantation Outcome. Front Cell Dev Biol (2021) 9:663316. doi: 10.3389/fcell.2021.663316
57. Song Y, Zhao HY, Lyu ZS, Cao XN, Shi MM, Wen Q, et al. Dysfunctional Bone Marrow Mesenchymal Stem Cells in Patients With Poor Graft Function After Allogeneic Hematopoietic Stem Cell Transplantation. Biol Blood Marrow Transplant (2018) 24(10):1981–9. doi: 10.1016/j.bbmt.2018.06.021
58. Carrancio S, Romo C, Ramos T, Lopez-Holgado N, Muntion S, Prins HJ, et al. Effects of Msc Coadministration and Route of Delivery on Cord Blood Hematopoietic Stem Cell Engraftment. Cell Transplant (2013) 22(7):1171–83. doi: 10.3727/096368912X657431
59. Li T, Luo C, Zhang J, Wei L, Sun W, Xie Q, et al. Efficacy and Safety of Mesenchymal Stem Cells Co-Infusion in Allogeneic Hematopoietic Stem Cell Transplantation: A Systematic Review and Meta-Analysis. Stem Cell Res Ther (2021) 12(1):246. doi: 10.1186/s13287-021-02304-x
60. Liu X, Wu M, Peng Y, Chen X, Sun J, Huang F, et al. Improvement in Poor Graft Function After Allogeneic Hematopoietic Stem Cell Transplantation Upon Administration of Mesenchymal Stem Cells From Third-Party Donors: A Pilot Prospective Study. Cell Transplant (2014) 23(9):1087–98. doi: 10.3727/096368912X661319
61. Ball LM, Bernardo ME, Roelofs H, Lankester A, Cometa A, Egeler RM, et al. Cotransplantation of Ex Vivo Expanded Mesenchymal Stem Cells Accelerates Lymphocyte Recovery and May Reduce the Risk of Graft Failure in Haploidentical Hematopoietic Stem-Cell Transplantation. Blood (2007) 110(7):2764–7. doi: 10.1182/blood-2007-04-087056
62. Li R, Tu J, Zhao J, Pan H, Fang L, Shi J. Mesenchymal Stromal Cells as Prophylaxis for Graft-Versus-Host Disease in Haplo-Identical Hematopoietic Stem Cell Transplantation Recipients With Severe Aplastic Anemia?-A Systematic Review and Meta-Analysis. Stem Cell Res Ther (2021) 12(1):106. doi: 10.1186/s13287-021-02170-7
63. Wang YT, Kong Y, Song Y, Han W, Zhang YY, Zhang XH, et al. Increased Type 1 Immune Response in the Bone Marrow Immune Microenvironment of Patients With Poor Graft Function After Allogeneic Hematopoietic Stem Cell Transplantation. Biol Blood Marrow Transplant (2016) 22(8):1376–82. doi: 10.1016/j.bbmt.2016.04.016
64. Kong Y, Wang YT, Cao XN, Song Y, Chen YH, Sun YQ, et al. Aberrant T Cell Responses in the Bone Marrow Microenvironment of Patients With Poor Graft Function After Allogeneic Hematopoietic Stem Cell Transplantation. J Transl Med (2017) 15(1):57. doi: 10.1186/s12967-017-1159-y
65. Luo Y, Shao L, Chang J, Feng W, Liu YL, Cottler-Fox MH, et al. M1 and M2 Macrophages Differentially Regulate Hematopoietic Stem Cell Self-Renewal and Ex Vivo Expansion. Blood Adv (2018) 2(8):859–70. doi: 10.1182/bloodadvances.2018015685
66. Zhao HY, Lyu ZS, Duan CW, Song Y, Han TT, Mo XD, et al. An Unbalanced Monocyte Macrophage Polarization in the Bone Marrow Microenvironment of Patients With Poor Graft Function After Allogeneic Haematopoietic Stem Cell Transplantation. Br J Haematol (2018) 182(5):679–92. doi: 10.1111/bjh.15452
67. Zhao HY, Zhang YY, Xing T, Tang SQ, Wen Q, Lyu ZS, et al. M2 Macrophages, But Not M1 Macrophages, Support Megakaryopoiesis by Upregulating Pi3k-Akt Pathway Activity. Signal Transduct Target Ther (2021) 6(1):234. doi: 10.1038/s41392-021-00627-y
68. Peffault de Latour R, Kulasekararaj A, Iacobelli S, Terwel SR, Cook R, Griffin M, et al. Eltrombopag Added to Immunosuppression in Severe Aplastic Anemia. N Engl J Med (2022) 386(1):11–23. doi: 10.1056/NEJMoa2109965
69. Tang C, Chen F, Kong D, Ma Q, Dai H, Yin J, et al. Successful Treatment of Secondary Poor Graft Function Post Allogeneic Hematopoietic Stem Cell Transplantation With Eltrombopag. J Hematol Oncol (2018) 11(1):103. doi: 10.1186/s13045-018-0649-6
70. Hosokawa K, Yamazaki H, Tanabe M, Imi T, Sugimori N, Nakao S. High-Dose Romiplostim Accelerates Hematologic Recovery in Patients With Aplastic Anemia Refractory to Eltrombopag. Leukemia (2021) 35(3):906–9. doi: 10.1038/s41375-020-0950-6
71. Al-Samkari H, Jiang D, Gernsheimer T, Liebman H, Lee S, Wojdyla M, et al. Adults With Immune Thrombocytopenia Who Switched to Avatrombopag Following Prior Treatment With Eltrombopag or Romiplostim: A Multicentre Us Study. Br J Haematol (2022) 197(3):359–66. doi: 10.1111/bjh.18081
72. Ruggeri A, Rocha V, Masson E, Labopin M, Cunha R, Absi L, et al. Impact of Donor-Specific Anti-Hla Antibodies on Graft Failure and Survival After Reduced Intensity Conditioning-Unrelated Cord Blood Transplantation: A Eurocord, Societe Francophone D'histocompatibilite Et D'immunogenetique (Sfhi) and Societe Francaise De Greffe De Moelle Et De Therapie Cellulaire (Sfgm-Tc) Analysis. Haematologica (2013) 98(7):1154–60. doi: 10.3324/haematol.2012.077685
73. Ciurea SO, Thall PF, Wang X, Wang SA, Hu Y, Cano P, et al. Donor-Specific Anti-Hla Abs and Graft Failure in Matched Unrelated Donor Hematopoietic Stem Cell Transplantation. Blood (2011) 118(22):5957–64. doi: 10.1182/blood-2011-06-362111
74. Zhao X, Zhao X, Huo M, Fan Q, Pei X, Wang Y, et al. Donor-Specific Anti-Human Leukocyte Antigen Antibodies Predict Prolonged Isolated Thrombocytopenia and Inferior Outcomes of Haploidentical Hematopoietic Stem Cell Transplantation. J Immunol Res (2017) 2017:1043836. doi: 10.1155/2017/1043836
75. Fuji S, Oshima K, Ohashi K, Sawa M, Saito T, Eto T, et al. Impact of Pretransplant Donor-Specific Anti-Hla Antibodies on Cord Blood Transplantation on Behalf of the Transplant Complications Working Group of Japan Society for Hematopoietic Cell Transplantation. Bone Marrow Transplant (2020) 55(4):722–8. doi: 10.1038/s41409-019-0712-0
76. Ciurea SO, Thall PF, Milton DR, Barnes TH, Kongtim P, Carmazzi Y, et al. Complement-Binding Donor-Specific Anti-Hla Antibodies and Risk of Primary Graft Failure in Hematopoietic Stem Cell Transplantation. Biol Blood Marrow Transplant (2015) 21(8):1392–8. doi: 10.1016/j.bbmt.2015.05.001
77. Giammarco S, Raiola AM, Di Grazia C, Bregante S, Gualandi F, Varaldo R, et al. Second Haploidentical Stem Cell Transplantation for Primary Graft Failure. Bone Marrow Transplant (2021) 56(6):1291–6. doi: 10.1038/s41409-020-01183-9
78. Sun YQ, Wang Y, Wang FR, Yan CH, Cheng YF, Chen YH, et al. Graft Failure in Patients With Hematological Malignancies: A Successful Salvage With a Second Transplantation From a Different Haploidentical Donor. Front Med (Lausanne) (2021) 8:604085. doi: 10.3389/fmed.2021.604085
79. Srour SA, Kongtim P, Rondon G, Chen J, Petropoulos D, Ramdial J, et al. Haploidentical Transplants for Patients With Relapse After the First Allograft. Am J Hematol (2020). doi: 10.1002/ajh.25924
80. Ciurea SO, Cao K, Fernandez-Vina M, Kongtim P, Malki MA, Fuchs E, et al. Correction: The European Society for Blood and Marrow Transplantation (Ebmt) Consensus Guidelines for the Detection and Treatment of Donor-Specific Anti-Hla Antibodies (Dsa) in Haploidentical Hematopoietic Cell Transplantation. Bone Marrow Transplant (2019) 54(5):784. doi: 10.1038/s41409-018-0332-0
81. Chang YJ, Xu LP, Wang Y, Zhang XH, Chen H, Chen YH, et al. Rituximab for Desensitization During Hla-Mismatched Stem Cell Transplantation in Patients With a Positive Donor-Specific Anti-Hla Antibody. Bone Marrow Transplant (2020) 55(7):1326–36. doi: 10.1038/s41409-020-0928-z
82. Yoshihara S, Maruya E, Taniguchi K, Kaida K, Kato R, Inoue T, et al. Risk and Prevention of Graft Failure in Patients With Preexisting Donor-Specific Hla Antibodies Undergoing Unmanipulated Haploidentical Sct. Bone Marrow Transplant (2012) 47(4):508–15. doi: 10.1038/bmt.2011.131
83. Choe H, Gergis U, Hsu J, Phillips A, Shore T, Christos P, et al. Bortezomib and Immune Globulin Have Limited Effects on Donor-Specific Hla Antibodies in Haploidentical Cord Blood Stem Cell Transplantation: Detrimental Effect of Persistent Haploidentical Donor-Specific Hla Antibodies. Biol Blood Marrow Transplant (2019) 25(2):e60–e4. doi: 10.1016/j.bbmt.2018.10.018
84. Hassan S, West KA, Ward WW, Kanakry JA, Flegel WA. Rebound and Overshoot of Donor-Specific Antibodies to Human Leukocyte Antigens (Hla) During Desensitization With Plasma Exchanges in Hematopoietic Progenitor Cell Transplantation: A Case Report. Transfusion (2021) 61(6):1980–6. doi: 10.1111/trf.16411
85. Lorant T, Bengtsson M, Eich T, Eriksson BM, Winstedt L, Jarnum S, et al. Safety, Immunogenicity, Pharmacokinetics, and Efficacy of Degradation of Anti-Hla Antibodies by Ides (Imlifidase) in Chronic Kidney Disease Patients. Am J Transplant (2018) 18(11):2752–62. doi: 10.1111/ajt.14733
86. Allhorn M, Briceno JG, Baudino L, Lood C, Olsson ML, Izui S, et al. The Igg-Specific Endoglycosidase Endos Inhibits Both Cellular and Complement-Mediated Autoimmune Hemolysis. Blood (2010) 115(24):5080–8. doi: 10.1182/blood-2009-08-239020
87. Lin J, Boon L, Bockermann R, Robertson AK, Kjellman C, Anderson CC. Desensitization Using Imlifidase and Endos Enables Chimerism Induction in Allosensitized Recipient Mice. Am J Transplant (2020) 20(9):2356–65. doi: 10.1111/ajt.15851
88. Xiao Y, Song J, Jiang Z, Li Y, Gao Y, Xu W, et al. Risk-Factor Analysis of Poor Graft Function After Allogeneic Hematopoietic Stem Cell Transplantation. Int J Med Sci (2014) 11(6):652–7. doi: 10.7150/ijms.6337
89. Zhou JR, Shi DY, Wei R, Wang Y, Yan CH, Zhang XH, et al. Co-Reactivation of Cytomegalovirus and Epstein-Barr Virus Was Associated With Poor Prognosis After Allogeneic Stem Cell Transplantation. Front Immunol (2020) 11:620891. doi: 10.3389/fimmu.2020.620891
90. Reddehase MJ, Holtappels R, Lemmermann NAW. Consequence of Histoincompatibility Beyond Gvh-Reaction in Cytomegalovirus Disease Associated With Allogeneic Hematopoietic Cell Transplantation: Change of Paradigm. Viruses (2021) 13(8):1530. doi: 10.3390/v13081530
91. Degli-Esposti MA, Hill GR. Immune Control of Cytomegalovirus Reactivation in Stem Cell Transplantation. Blood (2021) 139(9):1277–88. doi: 10.1182/blood.2020010028
92. Suessmuth Y, Mukherjee R, Watkins B, Koura DT, Finstermeier K, Desmarais C, et al. Cmv Reactivation Drives Posttransplant T-Cell Reconstitution and Results in Defects in the Underlying Tcrbeta Repertoire. Blood (2015) 125(25):3835–50. doi: 10.1182/blood-2015-03-631853
93. Yeh AC, Varelias A, Reddy A, Barone SM, Olver S, Chilson K, et al. Cmv Exposure Drives Long-Term Cd57+ Cd4 Memory T Cell Inflation Following Allogeneic Stem Cell Transplant. Blood (2021) 138(26):2874–85. doi: 10.1182/blood.2020009492
94. Akahoshi Y, Kimura SI, Inamoto Y, Seo S, Muranushi H, Shimizu H, et al. Effect of Cytomegalovirus Reactivation With or Without Acute Graft-Versus-Host Disease on the Risk of Nonrelapse Mortality. Clin Infect Dis (2021) 73(3):e620–e8. doi: 10.1093/cid/ciaa1871
95. Takenaka K, Nishida T, Asano-Mori Y, Oshima K, Ohashi K, Mori T, et al. Cytomegalovirus Reactivation After Allogeneic Hematopoietic Stem Cell Transplantation Is Associated With a Reduced Risk of Relapse in Patients With Acute Myeloid Leukemia Who Survived to Day 100 After Transplantation: The Japan Society for Hematopoietic Cell Transplantation Transplantation-Related Complication Working Group. Biol Blood Marrow Transplant (2015) 21(11):2008–16. doi: 10.1016/j.bbmt.2015.07.019
96. Teira P, Battiwalla M, Ramanathan M, Barrett AJ, Ahn KW, Chen M, et al. Early Cytomegalovirus Reactivation Remains Associated With Increased Transplant-Related Mortality in the Current Era: A Cibmtr Analysis. Blood (2016) 127(20):2427–38. doi: 10.1182/blood-2015-11-679639
97. Turki AT, Tsachakis-Muck N, Leserer S, Crivello P, Liebregts T, Betke L, et al. Impact of Cmv Reactivation on Relapse of Acute Myeloid Leukemia After Hct Is Dependent on Disease Stage and Atg. Blood Adv (2021) 6(1):28–36. doi: 10.1182/bloodadvances.2021005509
98. Leserer S, Bayraktar E, Trilling M, Bogdanov R, Arrieta-Bolanos E, Tsachakis-Muck N, et al. Cytomegalovirus Kinetics After Hematopoietic Cell Transplantation Reveal Peak Titers With Differential Impact on Mortality, Relapse and Immune Reconstitution. Am J Hematol (2021) 96(4):436–45. doi: 10.1002/ajh.26094
99. Einsele H, Ljungman P, Boeckh M. How I Treat Cmv Reactivation After Allogeneic Hematopoietic Stem Cell Transplantation. Blood (2020) 135(19):1619–29. doi: 10.1182/blood.2019000956
100. Ljungman P, de la Camara R, Milpied N, Volin L, Russell CA, Crisp A, et al. Randomized Study of Valacyclovir as Prophylaxis Against Cytomegalovirus Reactivation in Recipients of Allogeneic Bone Marrow Transplants. Blood (2002) 99(8):3050–6. doi: 10.1182/blood.v99.8.3050
101. Selby PR, Shakib S, Peake SL, Warner MS, Yeung D, Hahn U, et al. A Systematic Review of the Clinical Pharmacokinetics, Pharmacodynamics and Toxicodynamics of Ganciclovir/Valganciclovir in Allogeneic Haematopoietic Stem Cell Transplant Patients. Clin Pharmacokinet (2021) 60(6):727–39. doi: 10.1007/s40262-020-00982-z
102. Imlay HN, Kaul DR. Letermovir and Maribavir for the Treatment and Prevention of Cytomegalovirus Infection in Solid Organ and Stem Cell Transplant Recipients. Clin Infect Dis (2021) 73(1):156–60. doi: 10.1093/cid/ciaa1713
103. Metafuni E, Chiusolo P, Sica S, Laurenti L, Bregante S, Van Lint MT, et al. Foscarnet Treatment of Cytomegalovirus Infection in Haploidentical or Unrelated Donor Transplants. Bone Marrow Transplant (2018) 53(12):1560–7. doi: 10.1038/s41409-018-0200-y
104. Marty FM, Ljungman P, Chemaly RF, Maertens J, Dadwal SS, Duarte RF, et al. Letermovir Prophylaxis for Cytomegalovirus in Hematopoietic-Cell Transplantation. N Engl J Med (2017) 377(25):2433–44. doi: 10.1056/NEJMoa1706640
105. Mori Y, Jinnouchi F, Takenaka K, Aoki T, Kuriyama T, Kadowaki M, et al. Efficacy of Prophylactic Letermovir for Cytomegalovirus Reactivation in Hematopoietic Cell Transplantation: A Multicenter Real-World Data. Bone Marrow Transplant (2021) 56(4):853–62. doi: 10.1038/s41409-020-01082-z
106. Sassine J, Khawaja F, Shigle TL, Handy V, Foolad F, Aitken S, et al. Refractory and Resistant Cytomegalovirus After Hematopoietic Cell Transplant in the Letermovir Primary Prophylaxis Era. Clin Infect Dis (2021) 73(8):1346–54. doi: 10.1093/cid/ciab298
107. Ljungman P, Schmitt M, Marty FM, Maertens J, Chemaly RF, Kartsonis NA, et al. A Mortality Analysis of Letermovir Prophylaxis for Cytomegalovirus (Cmv) in Cmv-Seropositive Recipients of Allogeneic Hematopoietic Cell Transplantation. Clin Infect Dis (2020) 70(8):1525–33. doi: 10.1093/cid/ciz490
108. Zamora D, Duke ER, Xie H, Edmison BC, Akoto B, Kiener R, et al. Cytomegalovirus-Specific T-Cell Reconstitution Following Letermovir Prophylaxis After Hematopoietic Cell Transplantation. Blood (2021) 138(1):34–43. doi: 10.1182/blood.2020009396
109. Kumar D, Mian M, Singer L, Humar A. An Interventional Study Using Cell-Mediated Immunity to Personalize Therapy for Cytomegalovirus Infection After Transplantation. Am J Transplant (2017) 17(9):2468–73. doi: 10.1111/ajt.14347
110. Adler SP, Lewis N, Conlon A, Christiansen MP, Al-Ibrahim M, Rupp R, et al. Phase 1 Clinical Trial of a Conditionally Replication-Defective Human Cytomegalovirus (Cmv) Vaccine in Cmv-Seronegative Subjects. J Infect Dis (2019) 220(3):411–9. doi: 10.1093/infdis/jiz141
111. Maertens J, Cordonnier C, Jaksch P, Poire X, Uknis M, Wu J, et al. Maribavir for Preemptive Treatment of Cytomegalovirus Reactivation. N Engl J Med (2019) 381(12):1136–47. doi: 10.1056/NEJMoa1714656
112. Marty FM, Winston DJ, Rowley SD, Vance E, Papanicolaou GA, Mullane KM, et al. Cmx001 to Prevent Cytomegalovirus Disease in Hematopoietic-Cell Transplantation. N Engl J Med (2013) 369(13):1227–36. doi: 10.1056/NEJMoa1303688
113. Schleiss MR. Recombinant Cytomegalovirus Glycoprotein B Vaccine: Rethinking the Immunological Basis of Protection. Proc Natl Acad Sci USA (2018) 115(24):6110–2. doi: 10.1073/pnas.1806420115
114. De Groof TWM, Elder EG, Lim EY, Heukers R, Bergkamp ND, Groves IJ, et al. Targeting the Latent Human Cytomegalovirus Reservoir for T-Cell-Mediated Killing With Virus-Specific Nanobodies. Nat Commun (2021) 12(1):4436. doi: 10.1038/s41467-021-24608-5
115. Menger L, Gouble A, Marzolini MA, Pachnio A, Bergerhoff K, Henry JY, et al. Talen-Mediated Genetic Inactivation of the Glucocorticoid Receptor in Cytomegalovirus-Specific T Cells. Blood (2015) 126(26):2781–9. doi: 10.1182/blood-2015-08-664755
116. Malki MMA, Song JY, Yang D, Cao T, Aldoss I, Mokhtari S, et al. Iron Overload Is Associated With Delayed Engraftment and Increased Nonrelapse Mortality in Recipients of Umbilical Cord Blood Hematopoietic Cell Transplantation. Biol Blood Marrow Transplant (2020) 26(9):1697–703. doi: 10.1016/j.bbmt.2020.06.002
117. Penack O, Peczynski C, van der Werf S, Finke J, Ganser A, Schoemans H, et al. Association of Serum Ferritin Levels Before Start of Conditioning With Mortality After Allosct - a Prospective, Non-Interventional Study of the Ebmt Transplant Complications Working Party. Front Immunol (2020) 11:586. doi: 10.3389/fimmu.2020.00586
118. Trottier BJ, Burns LJ, DeFor TE, Cooley S, Majhail NS. Association of Iron Overload With Allogeneic Hematopoietic Cell Transplantation Outcomes: A Prospective Cohort Study Using R2-Mri-Measured Liver Iron Content. Blood (2013) 122(9):1678–84. doi: 10.1182/blood-2013-04-499772
119. Dallalio G, Law E, Means RT Jr. Hepcidin Inhibits in Vitro Erythroid Colony Formation at Reduced Erythropoietin Concentrations. Blood (2006) 107(7):2702–4. doi: 10.1182/blood-2005-07-2854
120. Sakamoto S, Kawabata H, Kanda J, Uchiyama T, Mizumoto C, Kitano T, et al. High Pretransplant Hepcidin Levels Are Associated With Poor Overall Survival and Delayed Platelet Engraftment After Allogeneic Hematopoietic Stem Cell Transplantation. Cancer Med (2017) 6(1):120–8. doi: 10.1002/cam4.974
121. Iolascon A, Andolfo I, Russo R. Congenital Dyserythropoietic Anemias. Blood (2020) 136(11):1274–83. doi: 10.1182/blood.2019000948
122. Kautz L, Nemeth E. Molecular Liaisons Between Erythropoiesis and Iron Metabolism. Blood (2014) 124(4):479–82. doi: 10.1182/blood-2014-05-516252
123. Jin X, He X, Cao X, Xu P, Xing Y, Sui S, et al. Iron Overload Impairs Normal Hematopoietic Stem and Progenitor Cells Through Reactive Oxygen Species and Shortens Survival in Myelodysplastic Syndrome Mice. Haematologica (2018) 103(10):1627–34. doi: 10.3324/haematol.2018.193128
124. Zheng Q, Zhao Y, Guo J, Zhao S, Fei C, Xiao C, et al. Iron Overload Promotes Mitochondrial Fragmentation in Mesenchymal Stromal Cells From Myelodysplastic Syndrome Patients Through Activation of the Ampk/Mff/Drp1 Pathway. Cell Death Dis (2018) 9(5):515. doi: 10.1038/s41419-018-0552-7
125. Okabe H, Suzuki T, Uehara E, Ueda M, Nagai T, Ozawa K. The Bone Marrow Hematopoietic Microenvironment Is Impaired in Iron-Overloaded Mice. Eur J Haematol (2014) 93(2):118–28. doi: 10.1111/ejh.12309
126. Tenneti P, Chojecki A, Knovich MA. Iron Overload in the Hct Patient: A Review. Bone Marrow Transplant (2021) 56(8):1794–804. doi: 10.1038/s41409-021-01244-7
127. Vlachodimitropoulou E, Chen YL, Garbowski M, Koonyosying P, Psaila B, Sola-Visner M, et al. Eltrombopag: A Powerful Chelator of Cellular or Extracellular Iron(Iii) Alone or Combined With a Second Chelator. Blood (2017) 130(17):1923–33. doi: 10.1182/blood-2016-10-740241
128. Kao YR, Chen J, Narayanagari SR, Todorova TI, Aivalioti MM, Ferreira M, et al. Thrombopoietin Receptor-Independent Stimulation of Hematopoietic Stem Cells by Eltrombopag. Sci Transl Med (2018) 10(458):eaas9536. doi: 10.1126/scitranslmed.aas9563
129. Gardenghi S, Ramos P, Marongiu MF, Melchiori L, Breda L, Guy E, et al. Hepcidin as a Therapeutic Tool to Limit Iron Overload and Improve Anemia in Beta-Thalassemic Mice. J Clin Invest (2010) 120(12):4466–77. doi: 10.1172/JCI41717
Keywords: hematopoietic stem cell transplantation, poor graft function, prognosis, bone marrow microenvironment (BMME), hematopoietic stem cell (HSC)
Citation: Man Y, Lu Z, Yao X, Gong Y, Yang T and Wang Y (2022) Recent Advancements in Poor Graft Function Following Hematopoietic Stem Cell Transplantation. Front. Immunol. 13:911174. doi: 10.3389/fimmu.2022.911174
Received: 02 April 2022; Accepted: 06 May 2022;
Published: 02 June 2022.
Edited by:
Ying-Jun Chang, Peking University People’s Hospital, ChinaReviewed by:
Ren Lin, Southern Medical University, ChinaYuan Kong, Peking University People’s Hospital, China
Copyright © 2022 Man, Lu, Yao, Gong, Yang and Wang. This is an open-access article distributed under the terms of the Creative Commons Attribution License (CC BY). The use, distribution or reproduction in other forums is permitted, provided the original author(s) and the copyright owner(s) are credited and that the original publication in this journal is cited, in accordance with accepted academic practice. No use, distribution or reproduction is permitted which does not comply with these terms.
*Correspondence: Tonghua Yang, eW5hbmJsb29kQGFsaXl1bi5jb20=; Yajie Wang, a2JiMTY1d3lqQHNpbmEuY29t