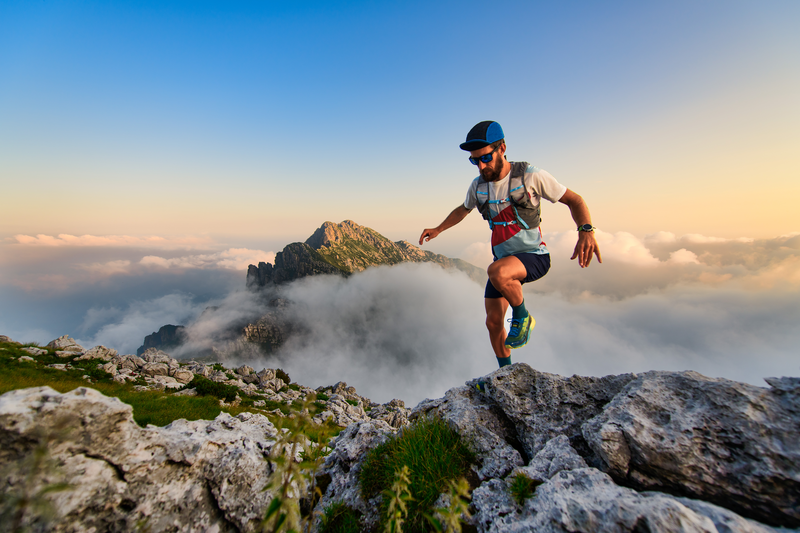
95% of researchers rate our articles as excellent or good
Learn more about the work of our research integrity team to safeguard the quality of each article we publish.
Find out more
MINI REVIEW article
Front. Immunol. , 18 May 2022
Sec. Cancer Immunity and Immunotherapy
Volume 13 - 2022 | https://doi.org/10.3389/fimmu.2022.910704
This article is part of the Research Topic Combinational Immunotherapy of Cancer: Novel Targets, Mechanisms, and Strategies View all 85 articles
With programmed death 1/ligand 1 (PD-1/PD-L1) as the cornerstone, anti-PD antibodies have pioneered revolutionary immunotherapies for malignancies. But most patients struggled to respond to anti-PD owing to primary or acquired resistance or even hyperprogression, pointing to more efforts needed to explore this axis. PD-1 constrains T-cell immunoreactivity via engaging with PD-L1 of tumor/myeloid cells is the canonical PD-1/PD-L1 axis function mode. Studies are increasingly aware of the impact of noncanonical PD-1/PD-L1 expression in various cancers. PD-L1 induced on activated T-cells ligates to PD-1 to mediate self-tolerance or acts on intratumoral myeloid cells and other T-cells, affecting their survival, differentiation and immunophenotyping, leading to tumor immunosuppression. Myeloid PD-1 interferes with their proliferation, differentiation, cytokine secretion and phagocytosis, mediating remarkable pro-tumor effects. Tumor cell intrinsic PD-1 signaling has diverse functions in different tumors, resulting in pro-proliferation or proliferation inhibition. These nonclassical PD-1/PD-L1 functions may be novel anti-PD mechanisms or causes of treatment resistance. This review highlights the nonnegligible role of T-cell-intrinsic PD-L1 and tumor/myeloid PD-1 in the cell interplay network and the complex impact on the efficacy of anti-PD antibodies. Reconsidering and rational utilization of the comprehensive PD-1/PD-L1 axis could cumulate breakthroughs in precision treatment and combination for anti-PD therapies.
Programmed death-1 (PD-1) was discovered in 1992 as an apoptosis-associated gene (1). Subsequent studies identified PD-1 as a negative regulatory immune molecule to maintain self-tolerance, containing cytoplasmic immunoreceptor tyrosine-based inhibitory motif (ITIM) and switch motif (ITSM), and predominantly expressed in T/B-lymphocytes (2–5). Programmed death ligand 1 (PD-L1) was discovered in 1999 as a novel member of the B7 family (6). In 2000, PD-L1 was found to be a ligand for PD-1, which inhibits T-cell proliferation (7). Later studies revealed that PD-L1, abundantly expressed on tumor and myeloid cells, induces T-cell apoptosis and immunosuppression to achieve tumor escape, and is a potential tumor therapeutic target (8–10).
Since 2012, numerous clinical reports (11–19) have shown the unprecedented efficacy of anti-PD antibodies for the treatment of metastatic bladder cancer, renal-cell cancer, colorectal cancer (CRC), non–small-cell lung cancer (NCSLC), and melanoma, etc. Frustratingly, durable responses to PD-1/PD-L1 antibodies are only achieved in about 10-40% of patients, with the majority not benefiting (20). In parallel to the necessity to address resistance and hyperprogression, immune-related adverse events cannot be neglected (21, 22), emphasizing in-depth investigation of the physiological-pathological functions and regulatory mechanisms of the PD-1/PD-L1 axis is paramount.
This review focuses on significant advances in the nonclassical PD-1/PD-L1 axis, summarizes and discusses the roles of T-cell-intrinsic PD-L1 and myeloid/tumor cell-intrinsic PD-1 in cancer progression and the complex implications for anti-PD efficacy, hoping to inspire more rational anti-PD drug design and combination strategies.
Generally, the PD-1/PD-L1 axis is involved in tumor immune escape via antigen-presenting cell (APC) or tumor cell surface PD-L1 mediating suppression of PD-1+ CD8+ T-cells and blocking PD-1/PD-L1 ligation can reinvigorate anti-tumor adaptive immunity (20, 23). The classical PD-1/PD-L1 axis is the main attraction for drug development.
Earlier studies concluded that PD-1 was mainly expressed on thymic and splenic T-cells (3, 24, 25) and that PD-1 ligation on CD8+ T-cells inhibits naive-to-effector differentiation, cytotoxicity, proliferation, and survival during chronic infections and tumor progression (26–32). PD-1/PD-L1 blockade rescues CD8+ T-cells from exhaustion or dysfunction (26, 29, 32). Increased PD-1+ CD8+ T-cells are positively correlated with anti-PD responses (33, 34). Notably, PD-1 expression does not necessarily determine T-cell exhaustion (35). Partial PD-1 intermediate T-cells maintain proliferation and interferon-γ (IFN-γ)/tumor necrosis factor-α (TNF-α) secretion and show well potential for anti-PD reinvigoration (36, 37). PD-1 signaling also affects other T-cell subsets. PD-L1 engagement on CD4+ T-cells affects cytokine secretion and induces differentiation into regulatory T-cells (Tregs) (38–40). Follicular helper T-cells, natural killer T-cells (NKT) and γδ T-cells also reduce antitumor activity or exert regulatory functions due to PD-1 function (41–45). Recent advances demonstrated that PD-1 ligation also regulates the metabolic reprogramming and migration of T-cells (45–47).
PD-L1, abundantly expressed on tumor or myeloid cells, engages on antitumor T-cells to accelerate their apoptosis and malfunction (8, 10). Anti-PD-L1 antibodies are effective in reversing the tumor immunosuppression microenvironment (9, 10). The contribution of host and tumor PD-L1 to the efficacy of anti-PD blockade remains controversial. Recently, researchers suggested that PD-L1 of host myeloid cells mainly determines the efficacy of PD-L1 antibodies (48–51). Tumor cell-derived PD-L1 exosomes were also shown to inhibit the anticancer activity of T-lymphocytes (52). Additionally, complicated membranal protein interactions and intracellular signaling of PD-L1 were revealed, suggesting there are multiple unresolved gaps in PD-L1 function. The cis-CD80/PD-L1 interactions on APCs impede PD-L1/PD-1 and CD80/cytotoxic T-lymphocyte-associated antigen-4 (CTLA-4) inhibitory signaling but do not affect CD28 co-stimulatory signaling (53, 54). Ongoing studies have indicated that PD-L1 as a receptor could transmit signals and impact the anti-apoptosis, chemotaxis, neoantigen presentation, and glycolysis of tumor cells or APCs (55–59). Moreover, cytoplasmic or nuclear translocation of PD-L1 could modulate genomic stability, DNA damage response, pyroptosis, and gene transcription of tumor cells (60–63).
Although the T-cells-intrinsic-PD-L1 has been insufficiently studied, in fact, as early as when PD-L1 was identified, Lieping Chen’s team already found that although PD-L1 was not expressed in freshly isolated human and mouse T-cells, but could be upregulated in activated T-cells (6, 64), especially in CD4+ T-cells and CD45RO+ memory T-cells (65). They also found that autoantibodies against PD-L1 in rheumatoid arthritis patients acted on primary CD4+ T-cells to promote apoptosis of activated CD4+ T-cells in an interleukin-10 (IL-10)-dependent manner. Subsequently, via PD-L1-deficient mice, researchers found that PD-L1 depleting led to increased CD4+ T-cell cytokine production, increased CD8+ T-cell expansion and cytotoxicity, and increased intrahepatic accumulation and survival of CD8+ T-cells, as well as impaired autoimmune tolerance (25, 66, 67). Su-Kil Seo et al. reported that T-cell-associated PD-L1 interacted with PD-1 on T-cells via the alloreactive T–T interaction, resulting in reduced T-cell proliferation and IFN-γ and IL-2 production (68). And Nuriban Valero-Pacheco et al. observed that PD-L1+ CD8+ T-cells were related to a lower T-cell proportion in patients infected with the H1N1 virus (69). These early findings revealed that PD-L1 was expressed on activated T cells and may also act as a receptor to receive signals that affect T-cell activation and self-tolerance maintenance.
Nonetheless, several researches conducted during the same period discussed that T-cell-intrinsic-PD-L1 functions appeared to contradict the preceding conclusions. Oezcan Talay et al. demonstrated that activation and proliferation of PD-L1-/- CD8+ T-cells in the initial phase against influenza virus was impaired and that PD-L1 expressed on naive T-cells was required for T-cell-mediated dendritic cell (DC) maturation (70). Seung-Joo Lee and colleagues also found a significant increase in PD-L1 on CD4+ T-cells during Salmonella infection and that PD-L1-deficiency did not affect specific antibody production and CD4+ T-cell expansion but affected CD4+ T-cell maturation and function (71). For anti-tumor immunity, Vesna Pulko et al. found that PD-L1 upregulation on primed T-cells helped effector T-cells survive the contraction phase, but anti-PD-L1 hindered T-cell survival (72). Their results also showed that PD-L1-deficient CD8+ T-cells were more sensitive to cytotoxicity, whereas adoptive PD-L1-deficient T-cell therapy was ineffective in restraining the growth of B16-OVA tumors. According to Asim Saha et al., upregulated PD-L1 expression in donor T-cells promoted graft-versus-host responses (73). PD-L1-deficient T-cells had fewer gut homing receptors, produced fewer inflammatory cytokines, enhanced apoptosis and multiple bioenergetic pathways.
Additional studies have reported puzzling roles for anti-PD-L1 antibodies in antitumor, anti-infection and anti-autoimmune diseases (74–77). For example, two publications found that Listeria infection enhanced T-cell PD-L1 expression, whereas PD-L1 antibody blockade selectively obstructed the anti-intracellular bacterial responses of CD8+ T-cells (75, 76). Notably, partial anti-PD-L1 antibodies caused apoptosis of PD-L1+ T-cells, even PD-1-knockout T-cells, by activating p38 MAPK, and that such antibodies failed to suppress B16-OVA and RENCA tumor growth in vivo (77). And these PD-L1+ T-cells inhibited the apoptosis of activated CD8+ T-cells via altering phosphorylation of p38 MAPK through intracellular interactions with DNA-PK. These results have sparked a debate about whether T-cell-intrinsic PD-L1 regulates the immune system positively or negatively. Researchers are reminded to focus on the complexity of T-cell-intrinsic PD-L1 function, where the different phases of immune responses, immune cell crosstalks, unexpected protein interactions, and specific anti-PD-L1 functions all require careful exploration.
In recent years, important progresses have been made regarding the expression pattern and immunomodulatory function of T-cell-intrinsic PD-L1 (Figure 1). Donnele Daley and colleagues found that PD-L1 expression switched positive in approximately 50% of γδ T-cells in human and murine pancreatic ductal adenocarcinoma (PDA), and that blocking PD-L1 in γδ T-cells enhanced activation and infiltration of CD4+ and CD8+ T-cells (78). Subsequently, Brian Diskin et al. used extensive experiments to elucidate the regulatory role and mechanisms of T-cell-intrinsic PD-L1 in PDA tumors (79). They detected that PD-L1 was expressed on >50% of intratumoral T-cells in the orthotopic PDA model and increased with progressive oncogenesis. And 63% of T-cells in B16 tumors and 17% of T-cells in MCA38 tumors also expressed PD-L1. Intriguingly, the highest PD-L1 expression in human PDA was found in T-cells, rather than in tumor cells or macrophages as commonly thought. Based on the fact that conditional ablation of PD-L1 in T-cells promoted adaptive anti-tumor responses and activated macrophages, they elucidated that PD-L1+ T-cells reinforce an immune tolerant environment to accelerate carcinogenesis through three ways: (1) PD-L1 engagement on T-cells inhibits Th1 differentiation but promotes Th17 differentiation via a STAT3-dependent manner, while inducing an anergic phenotype in CD8+ T-cells (2) PD-L1+ T-cells deliver inhibitory signals to PD-1+ T-cells; (3) PD-L1+ T-cells engage PD-1+ macrophages to promote M2-preference differentiation. Giorgia Fanelli et al. proved that PD-L1 ligation accompanied by CD3/TCR stimulation tended to transform memory T-cells but not naive T-cells into highly suppressive Tregs by triggering the PD-L1 intracellular pathway as reducing ERK phosphorylation and decreasing AKT/mTOR/S6 signaling (80). And Fabienne Mazerolles et al. suggested that T-cell proliferation was correlated with the PD-L1 expression of activated naive CD4+ effector T-cells regulated by DCs and Tregs (81). Thus, T-cells-intrinsic PD-L1 has bidirectional signaling that affects CD4+ T-cell and macrophage differentiation and attenuates cytotoxic T-cell effects to drive immune tolerance.
Figure 1 Negative regulation of anti-tumor immune responses by T-cell intrinsic PD-L1. (1) Tumor-infiltrating PD-L1+ T-cells act on intratumoral PD-1+ macrophages to induce M2 polarization. M2 can promote tumor progression. (2) PD-1+ cells act on PD-L1+ T-cells to limit the differentiation of Th1 and Th2 and promote the differentiation of Th17 and Tregs, leading to an increase in pro-tumor immunosuppressive factors such as IL-10, IL-17 and TGF-β. (3) PD-L1+ T-cells and PD-1+ T-cells achieve bidirectional signaling through PD-1/PD-L1 interactions, resulting in bidirectional immunosuppression: inhibition of CD4+ T-cells activation, expansion and cytokine secretion; inhibition of CD8+ T-cells activation, expansion and cytotoxicity. PD-L1+ γδ T-cells suppress the activation, intratumoural infiltration and antitumor activity of αβ T-cells via the PD-L1/PD-1 axis.
In addition, numerous evidences have shown peripheral or tumor-infiltrating PD-L1+ T-cell levels have the potential to be served as clinical indicators. Two papers reported that melanoma patients had greater PD-L1+ circulating T-cell levels than healthy volunteers, and PD-L1+ CD8+ T-cells were raised in disease relapsed or disease-related dead patients (82, 83). Furthermore, PD-L1+ circulating CD4+/CD8+ T-cells may be a predictive biomarker for anti-CTLA-4 therapy resistance. Bruktawit A. Goshu et al. demonstrated that anti-PD-L1 (Avelumab) targeting PD-L1+ HIVGag-specific-CD8+ T-cells combined with rhIL-15 enhanced CD8+ T-cell activity during HIV infection (84). Xia Li et al. identified dynamic fluctuations in PD-L1 on CD4+/CD8+ T-cells around the partial mission phase of type 1 diabetes and suggested PD-L1 may be a potential target for prolonging this phase (85). Several analyses (86–91) of patient samples involving ovarian cancer, NSCLC, and chronic lymphocytic leukemia (CLL) suggested an association between low circulating or infiltrating PD-L1+ CD8+ T-cells and prolonged survival, but high PD-L1+ CD8+ T-cell levels predicted a better anti-PD-1/PD-L1 therapy response. Among them, Libin Zhang et al. used a cohort of 378 NSCLC cases to speculate that CD8+ PD-L1+ TILs might indicate a hot but immunosuppressive tumor microenvironment with a high mutation burden (90). Nikolaos Ioannou et al. found that avadomide, via triggering IFN signaling in T-cells to increase PD-L1 expression on T cells, reprogramed patients’ T-cells, which complements PD-L1/PD-1 blockade (91).
Given the predominant biofunction of PD-1 on T-lymphocytes, T-cell-extrinsic PD-1 has been largely neglected. Yet persistent studies focusing on the non-classical PD-1 are shedding further light on previously incomprehensible biological and clinical phenomena. Currently, PD-1 has been identified to be expressed on various clinical tumor cells or tumor cell lines of CRC, melanoma, hepatocellular carcinoma (HCC), NSCLC and PDA (92–102). However, the ramifications of tumor cell-intrinsic PD-1 on oncogenesis have sparked much controversy.
For most oncological diseases, tumor cell-intrinsic PD-1 augmented cancer advancement independently of adaptive immunity (Figure 2A). Sonja Kleffel and colleagues earlier identified that preferential expression of PD-1 by ABCB5+-melanoma cells mediated increased tumorigenic capacity (94). Then they noticed that 3.5% to 16.5% of clinical melanoma cells expressed PD-1, and PD-1 positive frequencies ranged from 11.3% to 29.5% in eight human melanoma cell lines and from 6.6% to 9.4% in two murine melanoma cell lines (95). Through PD-1 knockdown/overexpressing B16 phenotype in NSG, they determined tumor PD-1 on B16 promoted tumorigenesis independently of immunity. By mutating the tyrosine sites of ITIM and ITSM, it was determined that melanoma-PD-1-driven tumorigenesis required the interactions between melanoma-PD-1 and host/melanoma-PD-L1 to initiate the PD-1 intracellular signaling via the mTOR pathway. Hui Li et al. later reported that five HCC cell lines and clinical HCC tissues contained subpopulations upregulating PD-1 (96). PD-1 interacted with and promoted phosphorylation of the mTOR effectors eIF4E and S6 to enhance tumor growth. Ning Pu et al. believed that PD-1 of PDA cells promoted tumor growth and apoptotic resistance via PD-L1 ligation and Hippo signaling (99).
Figure 2 Impact of tumor and myeloid cell-intrinsic PD-1 on tumor progression. (A) The effect of tumor cell-intrinsic PD-1 on melanoma, liver cancer, and other malignancies. PD-L1 expressed by tumor cells or other cells acts on PD-1+ tumor cells to mediate PD-1 signaling in tumor cells via ITIM and ITSM. The Hippo pathway and phosphorylation of mTOR downstream effector molecules eIF4E and S6 can enhance tumor-promoting gene transcription and protein expression. Anti-PD antibodies can block the PD-1/PD L1-mediated tumor promotion independent of adaptive immunity. (B) The role of tumor cell-intrinsic PD-1 in lung cancer. PD-L1 expressed by tumor cells or other cells acts on PD-1+ tumor cells to suppress tumor growth by dampening AKT and ERK signaling. Acetylation of p53 promotes gene transcription of PD-1. Anti-PD antibodies block PD-1/PD-L1-mediated tumor suppression, leading to hyperprogression in immunocompromised patients. (C) Effects of myeloid PD-1 on cell development, differentiation and function. (1) PD-1 expression promotes common myeloid progenitors (CMP) differentiation into granulocyte/macrophage progenitors (GMP), leading to increased MDSCs in granulocyte lineages and suppressing the activity of anti-tumor CD8+ T-cells. (2) PD-1 suppresses M1 polarization by reducing STAT1 and NF-κB phosphorylation and promoted M2 polarization by increasing STAT6 phosphorylation. (3) PD-1 suppresses DC maturation, survival and co-stimulatory molecules expression, consequently downregulating antigen-specific T-cell activity.
These teams also showed that blocking PD-1 inhibited the growth of xenografts in immunodeficient mice, and innovative combination strategies have been proposed and practiced (95, 96, 99). Li Hui et al. tried mTOR inhibitors in combination with anti-PD-1 to accomplish more durable and synergistic tumor regression (96). Ning Pu et al. found that Hippo pathway inhibitors together with anti-PD-1 treatment showed remarkable tumor eradication (99). Besides, the two teams highlighted that tumor cell PD-1 levels were positively correlated with poorer prognosis, further underlining the clinical value of tumor PD-1.
Nevertheless, tumor cell-intrinsic PD-1 has been found to depress malignancies in several lung cancer studies (Figure 2B). Shisuo Du et al. described a NSCLC patient with hyperprogression after palliative radiotherapy and pembrolizumab treatment, and tumor biopsy found PD-1 positive NSCLC cells (97). Increased viability of PD-1+-NSCLC cells M109 following PD-1 blockade was measured in vitro. Anti-PD-1 could significantly promote M109 growth in NSG. Yunlong Zhao et al. reported that PD-1 and PD-L1 were co-expressed in NSCLC subpopulations (98). They found that co-expressed PD-1 bound to PD-L1 in cis and inhibited PD-L1 to bind T-cell-PD-1 in trans to repress canonical PD-1/PD-L1 signaling. Selective blockade of tumor-intrinsic PD-1 could release tumor PD-1 to inhibit T-cell function. Xiaodong Wang et al. identified four lung cancer cell lines and 2/7 NSCLC patients expressing PD-1 protein (100). They demonstrated that in an immune-free condition, knockdown/overexpression of PD-1 in tumor cells altered AKT and ERK1/2 phosphorylation dependent on PD-L1, while Nivolumab and Pembrolizumab administration activated AKT and ERK1/2 signaling to promote the growth of PD-1+ lung cancer cells and even colon cancer cells. This suggests that the anti-tumor function of PD-1 may not be confined to NSCLC. Zhijie Cao et al. unexpectedly identified that the acetylated p53 preferentially recruited the transcriptional co-activator p300/CBP/TIP60 to the promoter region of PD-1 and elevated the accessibility of PD-1 transcription by upregulating the local histones H3K18/27 and H4K16 acetylation (101). PD-1 in cancer cells inhibited NSCLC (H1299) tumor growth, whereas interference with PD-1 transcriptional activation significantly attenuated the p53-dependent tumor suppression, confirming the materiality of the p53-PD-1 axis. These findings imply that anti-PD-1 for PD-1+ NSCLC patients may result in tumor hyperprogression. However, a recent study has suggested that PD-1 expression in lung cancer cell lines (A549, H1975, H1299 and HCC827) can enhance their proliferation and clone formation (102). Therefore, the multifaceted effects of PD-1 on NSCLC still need further research and debate.
Soon after PD-1 was identified, Tasuku Honjo’s team noted that PD-1 was also expressed on myeloid cells (103). Studies have confirmed that PD-1 is expressed on monocytes (104–106), macrophages (79, 107–113), DCs (114–119) and myeloid-derived suppressor cells (MDSCs) (120). Myeloid-PD-1 was markedly upregulated during infections, tumor progression, organ injury and compound induction. Researchers have found that toll-like receptor (TLR) agonists, NOD-like receptor agonists, cytokines, and growth factors all augmented myeloid-PD-1 expression dependent on NF-κB and STAT3, etc. Alexander P. R. Bally and colleagues revealed an NF-κB binding site located in conserved region C upstream of PDCD1 was required for NF-κB-dependent macrophages PD-1 induction (109). Sorim Nam et al. also noticed that PD-1 of MDSCs was regulated by the NK-κB signaling (120). Purushottam Lamichhane et al. found that IL-10 mediated increase in PD-1 of DCs was STAT3 dependent (119). Besides, histone modifications in the PD-1 promoter region are also involved in myeloid-PD-1 induction (109, 112). However, controversy remains in these studies. For example, Sheng Yao et al. reported that PD-1 of spleen DCs was inhibited by TLR9 agonists but not affected by IL-6 and TNF-α (114), but Elias A Said et al. found that TLR9 agonists, IL-6 and TNF-α all promoted PD-1 upregulation in monocytes (104). The differential responses of inducers may be due to cell types and microenvironment, emphasizing that much work remains to be done to investigate the regulatory mechanisms of myeloid-PD-1.
PD-1 engagement affects the differentiation, maturation, survival, metabolism, and effects of myeloid cells (Figure 2C). Myeloid-PD-1 altered the balance of differentiation into monocyte and granulocyte cells (106). PD-1 suppressed M1 polarization by reducing STAT1 and NF-κB phosphorylation and promoted M2 polarization by increasing STAT6 phosphorylation (108, 110, 121). Classical phosphorylation of ITIM and ITSM of PD-1 and recruitment of SHP-2 remained upstream of these signals (110). But PD-1 on DCs suppressed antigen presentation via MHC I expression inhibition dependent on the NF-κB pathway but independent of SHP-2 (117). In addition to host/tumor cells PD-L1 acting on PD-1+ macrophages, T-cell PD-L1 ligation induced M2 differentiation (79). Notably, studies have observed that anti-PD-1 promoted M1 polarization, which may directly function on PD-1+ macrophages besides the indirect effect of PD-1+ T-cells (122–124). PD-1 ligation on myeloid cells hampered glycolysis and cholesterol metabolism (105, 106). In addition, PD-1-deficient DCs exhibited prolonged longevity dependent on increased MAPK1 and CD40 signaling, as well as maturation-promoting and increased cytokines and co-stimulatory molecules expression, consequently promoting antigen-specific T-cells activity (114–116, 118). Similar phenomena have been observed in monocytes/macrophages (104, 107).
Myeloid-PD-1 expression has been shown in ovarian cancer (115), melanoma (125), gastric cancer (121), NSCLC (98), pleural mesothelioma (112), etc, and generally increased with tumor progression. Tumor-infiltrating PD-1+ myeloid cells exhibited immunosuppressive phenotypes with upregulated of PD-L1 and IL-10 and could directly inhibit anti-tumor T-cells infiltration or effects via the PD-1/PD-L1 axis (115, 119). New mechanisms of myeloid-PD-1 involvement in tumor immunity have been unearthed in recent years. Sydney R. Gordon et al. found that tumor-associated macrophages (TAMs) PD-1 expression impeded phagocytic potency against tumor cells, and blockade of PD-1 increased phagocytosis and reduced oncogenesis dependent on macrophages (111). Yunlong Zhao et al. reported that co-expressed PD-1 bound to PD-L1 in cis on APCs to hinder PD-L1 acts on T-cell-intrinsic PD-1 in trans (98). The work of Laura Strauss et al. focused on how myeloid-PD-1 affected myeloid cell differentiation, metabolism and effects, particularly during cancer-driven emergency myelopoiesis (79). They discovered a significant reduction in granulocyte/macrophage progenitors (GMP) in PD-1-deficient mice, and myeloid cells of tumor-bearing mice were skewed toward the LY6C+ monocytic lineage, which was determined by myeloid-PD-1 deletion. PD-1 deficiency or blockade suppressed monocytic immunosuppressive functions. Myeloid-PD-1-knockout was superior to systemic PD-1-knockdout and T-cell PD-1 conditional knockout for tumor inhibition, even in MC38 tumors where T-cells PD-1 knockout functioned slightly but myeloid-PD-1 deletion completely inhibited MC38 growth. Notably, anti-PD-1 antibodies were still effective in mice lacking T-cells.
Thus, the role of myeloid-PD-1 in anti-PD-1 therapy is gaining attention, and several combination strategies have been proposed. Purushottam Lamichhane et al. found that DCs responded to PD-1 blockade by increasing IL-10 production (119). The combination of PD-1 and IL-10 blockade significantly reduced tumor burden. Hirotake Tsukamoto et al. found that blocking PD-1/PD-L1 prompted PD-1+ TAMs to produce IL-6. Depletion of macrophages in melanoma-bearing mice reduced the levels of IL-6 during PD-1/PD-L1 blockade, suggesting that IL-6-neutralizing antibodies are potential candidates for combination with anti-PD-1 antibodies (125). In addition, inhibition of EZH2 methyltransferase was found to promote PD-1 expression on macrophages, and the combination of EZH2 inhibitors and anti-PD-1 antibodies could achieve better anti-tumor efficacy (112).
In addition to the above discussion, unacquainted PD-1/PD-L1 expression in other cell types also requires attention. Taking NK cells as an example, although less studied, available reports have supported that NK cells can express PD-1/PD-L1. PD-L1 engagement can inhibit PD-1+ NK cell-mediated antitumor responses (126, 127). Increased NK cell PD-1 expression is associated with tumor progression and poor prognosis in patients (128, 129). Anti-PD-1 treatment can promote NK cell activation, intratumoral recruitment, and anti-tumor cytotoxicity (130–132). Studies on PD-L1 in NK cells are much rarer. Existing results suggest that the TME can upregulate PD-L1 in NK cells (129, 133). PD-L1 inhibitors can not only block the inhibitory signal of PD-1, surprisingly, also directly activate PD-L1+ NK cells (133).
Furthermore, the expression of PD-1/PD-L1 in other non-immune cells may also affect the efficacy or safety of anti-PD therapy. For example, PD-1 was found to be expressed by primary sensory neurons in the dorsal root ganglion and to affect their signaling, and administration of anti-PD-1 antibodies to mice or non-human primates led to altered opioid-induced antinociception (134).
Shifting the focus of anti-PD therapies from the classical PD-1/PD-L1 axis to noncanonical axis may provide opportunities to broaden the benefits of PD-1/PD-L1 blockade through rational drug design and combination based on the regulatory role of noncanonical PD-1/PD-L1 axis in tumorigenesis.
The involvement of T-cell intrinsic PD-L1 in immunosuppression is increasingly recognized as an additional mechanism for anti-PD-L1 efficacy. Considering several studies emphasized that many anti-PD-L1 antibodies could trigger apoptosis of PD-L1+ T-cells (72, 77), excluding such antibodies via T-cell apoptosis assays and using of Fc with weak effects are spurred. Besides, potential cis-interactions of PD-1/PD-L1 and CD80/PD-L1 on T-cells need to be investigated. They have been shown to contend with PD-1/PD-L1 and CD80/CTLA-4 trans-interactions (54, 98), so that anti-PD-1/PD-L1 antibodies alone lead to the release of inhibitory signals after breaking cis-interactions. Coadministration of anti-PD-1, anti-PD-L1 and anti-CTLA-4 antibodies or treatment of anti-PD-1/PD-L1/CTLA-4 trispecific antibodies may be candidate approaches to completely unleash innate and adaptive immunity to eradicate tumors, which also fits well with cancers with PD-1+ tumor or myeloid cells.
Intracellular signals of tumor-intrinsic PD-1 as accomplices of malignancies are candidate strategies for combination with anti-PD-1 antibodies, such as Hippo and mTOR pathways. Notably, PD-1 of tumor cells has been found to depress NSCLC tumor growth, and anti-PD-1 treatment may even lead to tumor hyperprogression. In general, anti-PD therapies result in significant activation of T-cells in patients to eliminate tumors. However, in immunocompromised patients with low initial activated T-cells, anti-PD antibodies administration could not normalize the intratumoral T-cells function, but may raise the pro-tumor signaling, thus leading to tumor hyperprogression (21). Therefore, for NSCLC or cancers with hyperprogression caused by immunotherapies, caution is needed for anti-PD-1/PD-L1 treatment. Combination with AKT and ERK1/2 inhibitors is an approach to contain the tumor-promoting signaling activated by anti-PD-1 antibodies (100), and combination with innate immune agonists can boost the antitumor responses of patients. Combining them may be a beneficial strategy for patients with hyperprogression.
For myeloid cells, increased secretion of IL-6/IL-10 induced by anti-PD-1 antibodies is also a potential target for combination to further repress the alternative inhibitory molecules (119, 125). In addition, the noncanonical expression of other checkpoint molecules such as lymphocyte activation gene-3 (LAG-3) (135, 136) and T cell immunoglobulin domain and mucin domain-3 (TIM-3) (137) on myeloid cells also needs attention and investigation, and combination with these checkpoint inhibitors holds promise for overcoming antitumor resistance.
In summary, the noncanonical PD-1/PD-L1, represented by T-cell-intrinsic PD-L1, tumor cell-intrinsic PD-1, and myeloid PD-1, exhibits unique protein interactions, signaling and cell crosstalk to regulate cell growth, differentiation, metabolism and effects dependently on immunity or not. But noncanonical signaling contributes to both anti-PD efficacy and resistance, and further studies are needed to resolve, balance, or even exploit these controversies for clinical applications of the noncanonical PD-1/PD-L1 axis. Integrating the classical and non-classical PD-1/PD-L1 axes and revisiting the role of the holistic PD-1/PD-L1 axis on tumor progression in specific cancer types and stages, improved therapeutic efficacy and safety of anti-PD therapies will be achieved through rational drug design and combination.
YL and LG conceived the topic. YL and XY drafted the manuscript and prepared the figures. Others reviewed the manuscript. All authors read and approved the final manuscript. All authors contributed to the article and approved the submitted version.
This work was supported by Foundation of Shanghai Science and Technology Committee (No. 22S11902100), Zhongshan Municipal Bureau of Science and Technology (No. 2020SYF08) and the Strategic Priority Research Program of the Chinese Academy of Sciences (No. XDA 12050305).
The authors declare that the research was conducted in the absence of any commercial or financial relationships that could be construed as a potential conflict of interest.
All claims expressed in this article are solely those of the authors and do not necessarily represent those of their affiliated organizations, or those of the publisher, the editors and the reviewers. Any product that may be evaluated in this article, or claim that may be made by its manufacturer, is not guaranteed or endorsed by the publisher.
All the figures were created with BioRender.com.
1. Ishida Y, Agata Y, Shibahara K, Honjo T. Induced Expression of Pd-1, a Novel Member of the Immunoglobulin Gene Superfamily, Upon Programmed Cell Death. EMBO J (1992) 11(11):3887–95. doi: 10.1002/j.1460-2075.1992.tb05481.x
2. Shinohara T, Taniwaki M, Ishida Y, Kawaichi M, Honjo T. Structure and Chromosomal Localization of the Human Pd-1 Gene (Pdcd1). Genomics (1994) 23(3):704–6. doi: 10.1006/geno.1994.1562
3. Agata Y, Kawasaki A, Nishimura H, Ishida Y, Tsubata T, Yagita H, et al. Expression of the Pd-1 Antigen on the Surface of Stimulated Mouse T and B Lymphocytes. Int Immunol (1996) 8(5):765–72. doi: 10.1093/intimm/8.5.765
4. Finger LR, Pu J, Wasserman R, Vibhakar R, Louie E, Hardy RR, et al. The Human Pd-1 Gene: Complete Cdna, Genomic Organization, and Developmentally Regulated Expression in B Cell Progenitors. Gene (1997) 197(1-2):177–87. doi: 10.1016/s0378-1119(97)00260-6
5. Nishimura H, Nose M, Hiai H, Minato N, Honjo T. Development of Lupus-Like Autoimmune Diseases by Disruption of the Pd-1 Gene Encoding an Itim Motif-Carrying Immunoreceptor. Immunity (1999) 11(2):141–51. doi: 10.1016/s1074-7613(00)80089-8
6. Dong H, Zhu G, Tamada K, Chen L. B7-H1, A Third Member of the B7 Family, Co-Stimulates T-Cell Proliferation and Interleukin-10 Secretion. Nat Med (1999) 5(12):1365–9. doi: 10.1038/70932
7. Freeman GJ, Long AJ, Iwai Y, Bourque K, Chernova T, Nishimura H, et al. Engagement of the Pd-1 Immunoinhibitory Receptor by a Novel B7 Family Member Leads to Negative Regulation of Lymphocyte Activation. J Exp Med (2000) 192(7):1027–34. doi: 10.1084/jem.192.7.1027
8. Dong H, Strome SE, Salomao DR, Tamura H, Hirano F, Flies DB, et al. Tumor-Associated B7-H1 Promotes T-Cell Apoptosis: A Potential Mechanism of Immune Evasion. Nat Med (2002) 8(8):793–800. doi: 10.1038/nm730
9. Iwai Y, Ishida M, Tanaka Y, Okazaki T, Honjo T, Minato N. Involvement of Pd-L1 on Tumor Cells in the Escape From Host Immune System and Tumor Immunotherapy by Pd-L1 Blockade. Proc Natl Acad Sci USA (2002) 99(19):12293–7. doi: 10.1073/pnas.192461099
10. Curiel TJ, Wei S, Dong H, Alvarez X, Cheng P, Mottram P, et al. Blockade of B7-H1 Improves Myeloid Dendritic Cell-Mediated Antitumor Immunity. Nat Med (2003) 9(5):562–7. doi: 10.1038/nm863
11. Brahmer JR, Tykodi SS, Chow LQM, Hwu W-J, Topalian SL, Hwu P, et al. Safety and Activity of Anti–Pd-L1 Antibody in Patients With Advanced Cancer. N Engl J Med (2012) 366(26):2455–65. doi: 10.1056/NEJMoa1200694
12. Topalian SL, Hodi FS, Brahmer JR, Gettinger SN, Smith DC, McDermott DF, et al. Safety, Activity, and Immune Correlates of Anti–Pd-1 Antibody in Cancer. N Engl J Med (2012) 366(26):2443–54. doi: 10.1056/NEJMoa1200690
13. Powles T, Eder JP, Fine GD, Braiteh FS, Loriot Y, Cruz C, et al. Mpdl3280a (Anti-Pd-L1) Treatment Leads to Clinical Activity in Metastatic Bladder Cancer. Nature (2014) 515(7528):558–62. doi: 10.1038/nature13904
14. Borghaei H, Paz-Ares L, Horn L, Spigel DR, Steins M, Ready NE, et al. Nivolumab Versus Docetaxel in Advanced Nonsquamous Non-Small-Cell Lung Cancer. N Engl J Med (2015) 373(17):1627–39. doi: 10.1056/NEJMoa1507643
15. Motzer RJ, Escudier B, McDermott DF, George S, Hammers HJ, Srinivas S, et al. Nivolumab Versus Everolimus in Advanced Renal-Cell Carcinoma. N Engl J Med (2015) 373(19):1803–13. doi: 10.1056/NEJMoa1510665
16. Robert C, Schachter J, Long GV, Arance A, Grob JJ, Mortier L, et al. Pembrolizumab Versus Ipilimumab in Advanced Melanoma. N Engl J Med (2015) 372(26):2521–32. doi: 10.1056/NEJMoa1503093
17. Ferris RL, Blumenschein G Jr, Fayette J, Guigay J, Colevas AD, Licitra L, et al. Nivolumab for Recurrent Squamous-Cell Carcinoma of the Head and Neck. N Engl J Med (2016) 375(19):1856–67. doi: 10.1056/NEJMoa1602252
18. Herbst RS, Baas P, Kim DW, Felip E, Pérez-Gracia JL, Han JY, et al. Pembrolizumab Versus Docetaxel for Previously Treated, Pd-L1-Positive, Advanced Non-Small-Cell Lung Cancer (Keynote-010): A Randomised Controlled Trial. Lancet (Lond Engl) (2016) 387(10027):1540–50. doi: 10.1016/s0140-6736(15)01281-7
19. Kaufman HL, Russell J, Hamid O, Bhatia S, Terheyden P, D'Angelo SP, et al. Avelumab in Patients With Chemotherapy-Refractory Metastatic Merkel Cell Carcinoma: A Multicentre, Single-Group, Open-Label, Phase 2 Trial. Lancet Oncol (2016) 17(10):1374–85. doi: 10.1016/s1470-2045(16)30364-3
20. Zou W, Wolchok JD, Chen L. Pd-L1 (B7-H1) and Pd-1 Pathway Blockade for Cancer Therapy: Mechanisms, Response Biomarkers, and Combinations. Sci Transl Med (2016) 8(328):328rv4. doi: 10.1126/scitranslmed.aad7118
21. Adashek JJ, Subbiah IM, Matos I, Garralda E, Menta AK, Ganeshan DM, et al. Hyperprogression and Immunotherapy: Fact, Fiction, or Alternative Fact? Trends Cancer (2020) 6(3):181–91. doi: 10.1016/j.trecan.2020.01.005
22. Naidoo J, Page DB, Li BT, Connell LC, Schindler K, Lacouture ME, et al. Toxicities of the Anti-Pd-1 and Anti-Pd-L1 Immune Checkpoint Antibodies. Ann Oncol (2015) 26(12):2375–91. doi: 10.1093/annonc/mdv383
23. Sanmamed MF, Chen L. A Paradigm Shift in Cancer Immunotherapy: From Enhancement to Normalization. Cell (2018) 175(2):313–26. doi: 10.1016/j.cell.2018.09.035
24. Yamazaki T, Akiba H, Iwai H, Matsuda H, Aoki M, Tanno Y, et al. Expression of Programmed Death 1 Ligands by Murine T Cells and Apc. J Immunol (2002) 169(10):5538–45. doi: 10.4049/jimmunol.169.10.5538
25. Liang SC, Latchman YE, Buhlmann JE, Tomczak MF, Horwitz BH, Freeman GJ, et al. Regulation of Pd-1, Pd-L1, and Pd-L2 Expression During Normal and Autoimmune Responses. Eur J Immunol (2003) 33(10):2706–16. doi: 10.1002/eji.200324228
26. Barber DL, Wherry EJ, Masopust D, Zhu B, Allison JP, Sharpe AH, et al. Restoring Function in Exhausted Cd8 T Cells During Chronic Viral Infection. Nature (2006) 439(7077):682–7. doi: 10.1038/nature04444
27. Freeman GJ, Wherry EJ, Ahmed R, Sharpe AH. Reinvigorating Exhausted Hiv-Specific T Cells Via Pd-1-Pd-1 Ligand Blockade. J Exp Med (2006) 203(10):2223–7. doi: 10.1084/jem.20061800
28. Petrovas C, Casazza JP, Brenchley JM, Price DA, Gostick E, Adams WC, et al. Pd-1 Is a Regulator of Virus-Specific Cd8+ T Cell Survival in HIV Infection. J Exp Med (2006) 203(10):2281–92. doi: 10.1084/jem.20061496
29. Trautmann L, Janbazian L, Chomont N, Said EA, Gimmig S, Bessette B, et al. Upregulation of Pd-1 Expression on Hiv-Specific Cd8+ T Cells Leads to Reversible Immune Dysfunction. Nat Med (2006) 12(10):1198–202. doi: 10.1038/nm1482
30. Goldberg MV, Maris CH, Hipkiss EL, Flies AS, Zhen L, Tuder RM, et al. Role of Pd-1 and Its Ligand, B7-H1, in Early Fate Decisions of Cd8 T Cells. Blood (2007) 110(1):186–92. doi: 10.1182/blood-2006-12-062422
31. Ahmadzadeh M, Johnson LA, Heemskerk B, Wunderlich JR, Dudley ME, White DE, et al. Tumor Antigen-Specific Cd8 T Cells Infiltrating the Tumor Express High Levels of Pd-1 and Are Functionally Impaired. Blood (2009) 114(8):1537–44. doi: 10.1182/blood-2008-12-195792
32. Ahn E, Araki K, Hashimoto M, Li W, Riley JL, Cheung J, et al. Role of Pd-1 During Effector Cd8 T Cell Differentiation. Proc Natl Acad Sci (2018) 115(18):4749–54. doi: 10.1073/pnas.1718217115
33. Simon S, Labarriere N. Pd-1 Expression on Tumor-Specific T Cells: Friend or Foe for Immunotherapy? Oncoimmunology (2017) 7(1):e1364828. doi: 10.1080/2162402X.2017.1364828
34. Kamphorst AO, Pillai RN, Yang S, Nasti TH, Akondy RS, Wieland A, et al. Proliferation of Pd-1+ Cd8 T Cells in Peripheral Blood After Pd-1-Targeted Therapy in Lung Cancer Patients. Proc Natl Acad Sci USA (2017) 114(19):4993–8. doi: 10.1073/pnas.1705327114
35. Shen Y, Teng Y, Lv Y, Zhao Y, Qiu Y, Chen W, et al. Pd-1 Does Not Mark Tumor-Infiltrating Cd8+ T Cell Dysfunction in Human Gastric Cancer. J Immunother Cancer (2020) 8(2):e000422. doi: 10.1136/jitc-2019-000422
36. Blackburn SD, Shin H, Freeman GJ, Wherry EJ. Selective Expansion of a Subset of Exhausted Cd8 T Cells by αpd-L1 Blockade. Proc Natl Acad Sci USA (2008) 105(39):15016–21. doi: 10.1073/pnas.0801497105
37. Paley MA, Kroy DC, Odorizzi PM, Johnnidis JB, Dolfi DV, Barnett BE, et al. Progenitor and Terminal Subsets of Cd8+ T Cells Cooperate to Contain Chronic Viral Infection. Science (2012) 338(6111):1220–5. doi: 10.1126/science.1229620
38. Francisco LM, Salinas VH, Brown KE, Vanguri VK, Freeman GJ, Kuchroo VK, et al. Pd-L1 Regulates the Development, Maintenance, and Function of Induced Regulatory T Cells. J Exp Med (2009) 206(13):3015–29. doi: 10.1084/jem.20090847
39. Park HJ, Park JS, Jeong YH, Son J, Ban YH, Lee BH, et al. Pd-1 Upregulated on Regulatory T Cells During Chronic Virus Infection Enhances the Suppression of Cd8+ T Cell Immune Response Via the Interaction With Pd-L1 Expressed on Cd8+ T Cells. J Immunol (2015) 195(12):5841–2. doi: 10.4049/jimmunol.1502256
40. Laba S, Mallett G, Amarnath S. The Depths of Pd-1 Function Within the Tumor Microenvironment Beyond Cd8(+) T Cells. Semin Cancer Biol (2021) S1044-579X(21):00153–X. doi: 10.1016/j.semcancer.2021.05.022
41. Hoeres T, Holzmann E, Smetak M, Birkmann J, Wilhelm M. Pd-1 Signaling Modulates Interferon-Gamma Production by Gamma Delta (Gammadelta) T-Cells in Response to Leukemia. Oncoimmunology (2019) 8(3):1550618. doi: 10.1080/2162402X.2018.1550618
42. Chang WS, Kim JY, Kim YJ, Kim YS, Lee JM, Azuma M, et al. Cutting Edge: Programmed Death-1/Programmed Death Ligand 1 Interaction Regulates the Induction and Maintenance of Invariant Nkt Cell Anergy. J Immunol (2008) 181(10):6707–10. doi: 10.4049/jimmunol.181.10.6707
43. Iwasaki M, Tanaka Y, Kobayashi H, Murata-Hirai K, Miyabe H, Sugie T, et al. Expression and Function of Pd-1 in Human Gammadelta T Cells That Recognize Phosphoantigens. Eur J Immunol (2011) 41(2):345–55. doi: 10.1002/eji.201040959
44. Chen Y, Yu M, Zheng Y, Fu G, Xin G, Zhu W, et al. Cxcr5(+)Pd-1(+) Follicular Helper Cd8 T Cells Control B Cell Tolerance. Nat Commun (2019) 10(1):4415. doi: 10.1038/s41467-019-12446-5
45. Shi J, Hou S, Fang Q, Liu X, Liu X, Qi H. Pd-1 Controls Follicular T Helper Cell Positioning and Function. Immunity (2018) 49(2):264–74.e4. doi: 10.1016/j.immuni.2018.06.012
46. Patsoukis N, Bardhan K, Chatterjee P, Sari D, Liu B, Bell LN, et al. Pd-1 Alters T-Cell Metabolic Reprogramming by Inhibiting Glycolysis and Promoting Lipolysis and Fatty Acid Oxidation. Nat Commun (2015) 6:6692. doi: 10.1038/ncomms7692
47. Honda T, Egen JG, Lammermann T, Kastenmuller W, Torabi-Parizi P, Germain RN. Tuning of Antigen Sensitivity by T Cell Receptor-Dependent Negative Feedback Controls T Cell Effector Function in Inflamed Tissues. Immunity (2014) 40(2):235–47. doi: 10.1016/j.immuni.2013.11.017
48. Lau J, Cheung J, Navarro A, Lianoglou S, Haley B, Totpal K, et al. Tumour and Host Cell Pd-L1 Is Required to Mediate Suppression of Anti-Tumour Immunity in Mice. Nat Commun (2017) 8:14572. doi: 10.1038/ncomms14572
49. Lin H, Wei S, Hurt EM, Green MD, Zhao L, Vatan L, et al. Host Expression of Pd-L1 Determines Efficacy of Pd-L1 Pathway Blockade-Mediated Tumor Regression. J Clin Invest (2018) 128(2):805–15. doi: 10.1172/JCI96113
50. Tang H, Liang Y, Anders RA, Taube JM, Qiu X, Mulgaonkar A, et al. Pd-L1 on Host Cells Is Essential for Pd-L1 Blockade–Mediated Tumor Regression. J Clin Invest (2018) 128(2):580–8. doi: 10.1172/jci96061
51. Oh SA, Wu D-C, Cheung J, Navarro A, Xiong H, Cubas R, et al. Pd-L1 Expression by Dendritic Cells Is a Key Regulator of T-Cell Immunity in Cancer. Nat Cancer (2020) 1(7):681–91. doi: 10.1038/s43018-020-0075-x
52. Poggio M, Hu T, Pai CC, Chu B, Belair CD, Chang A, et al. Suppression of Exosomal Pd-L1 Induces Systemic Anti-Tumor Immunity and Memory. Cell (2019) 177(2):414–27.e13. doi: 10.1016/j.cell.2019.02.016
53. Sugiura D, Maruhashi T, Okazaki IM, Shimizu K, Maeda TK, Takemoto T, et al. Restriction of Pd-1 Function by Cis-Pd-L1/Cd80 Interactions Is Required for Optimal T Cell Responses. Science (2019) 364(6440):558–66. doi: 10.1126/science.aav7062
54. Zhao Y, Lee CK, Lin C-H, Gassen RB, Xu X, Huang Z, et al. Pd-L1:Cd80 Cis-Heterodimer Triggers the Co-Stimulatory Receptor Cd28 While Repressing the Inhibitory Pd-1 and Ctla-4 Pathways. Immunity (2019) 51(6):1059–73.e9. doi: 10.1016/j.immuni.2019.11.003
55. Azuma T, Yao S, Zhu G, Flies AS, Flies SJ, Chen L. B7-H1 Is a Ubiquitous Antiapoptotic Receptor on Cancer Cells. Blood (2008) 111(7):3635–43. doi: 10.1182/blood-2007-11-123141
56. Gato-Canas M, Zuazo M, Arasanz H, Ibanez-Vea M, Lorenzo L, Fernandez-Hinojal G, et al. Pdl1 Signals Through Conserved Sequence Motifs to Overcome Interferon-Mediated Cytotoxicity. Cell Rep (2017) 20(8):1818–29. doi: 10.1016/j.celrep.2017.07.075
57. Chang CH, Qiu J, O'Sullivan D, Buck MD, Noguchi T, Curtis JD, et al. Metabolic Competition in the Tumor Microenvironment Is a Driver of Cancer Progression. Cell (2015) 162(6):1229–41. doi: 10.1016/j.cell.2015.08.016
58. Okada M, Shimizu K, Iyoda T, Ueda S, Shinga J, Mochizuki Y, et al. Pd-L1 Expression Affects Neoantigen Presentation. iScience (2020) 23(6):101238. doi: 10.1016/j.isci.2020.101238
59. Lucas ED, Schafer JB, Matsuda J, Kraus M, Burchill MA, Tamburini BAJ. Pd-L1 Reverse Signaling in Dermal Dendritic Cells Promotes Dendritic Cell Migration Required for Skin Immunity. Cell Rep (2020) 33(2):108258. doi: 10.1016/j.celrep.2020.108258
60. Tu X, Qin B, Zhang Y, Zhang C, Kahila M, Nowsheen S, et al. Pd-L1 (B7-H1) Competes With the Rna Exosome to Regulate the DNA Damage Response and Can Be Targeted to Sensitize to Radiation or Chemotherapy. Mol Cell (2019) 74(6):1215–26.e4. doi: 10.1016/j.molcel.2019.04.005
61. Hou J, Zhao R, Xia W, Chang CW, You Y, Hsu JM, et al. Pd-L1-Mediated Gasdermin C Expression Switches Apoptosis to Pyroptosis in Cancer Cells and Facilitates Tumour Necrosis. Nat Cell Biol (2020) 22(10):1264–75. doi: 10.1038/s41556-020-0575-z
62. Zhang W, Jin J, Wang Y, Fang L, Min L, Wang X, et al. Pd-L1 Regulates Genomic Stability Via Interaction With Cohesin-Sa1 in the Nucleus. Signal Transduct Target Ther (2021) 6(1):81. doi: 10.1038/s41392-021-00463-0
63. Gao Y, Nihira NT, Bu X, Chu C, Zhang J, Kolodziejczyk A, et al. Acetylation-Dependent Regulation of Pd-L1 Nuclear Translocation Dictates the Efficacy of Anti-Pd-1 Immunotherapy. Nat Cell Biol (2020) 22(9):1064–75. doi: 10.1038/s41556-020-0562-4
64. Tamura H, Dong H, Zhu G, Sica GL, Flies DB, Tamada K, et al. B7-H1 Costimulation Preferentially Enhances Cd28-Independent T-Helper Cell Function. Blood (2001) 97(6):1809–16. doi: 10.1182/blood.v97.6.1809
65. Dong H, Strome SE, Matteson EL, Moder KG, Flies DB, Zhu G, et al. Costimulating Aberrant T Cell Responses by B7-H1 Autoantibodies in Rheumatoid Arthritis. J Clin Invest (2003) 111(3):363–70. doi: 10.1172/JCI16015
66. Latchman YE, Liang SC, Wu Y, Chernova T, Sobel RA, Klemm M, et al. Pd-L1-Deficient Mice Show That Pd-L1 on T Cells, Antigen-Presenting Cells, and Host Tissues Negatively Regulates T Cells. Proc Natl Acad Sci USA (2004) 101(29):10691–6. doi: 10.1073/pnas.0307252101
67. Dong H, Zhu G, Tamada K, Flies DB, van Deursen JM, Chen L. B7-H1 Determines Accumulation and Deletion of Intrahepatic Cd8(+) T Lymphocytes. Immunity (2004) 20(3):327–36. doi: 10.1016/s1074-7613(04)00050-0
68. Seo SK, Seo HM, Jeong HY, Choi IW, Park YM, Yagita H, et al. Co-Inhibitory Role of T-Cell-Associated B7-H1 and B7-Dc in the T-Cell Immune Response. Immunol Lett (2006) 102(2):222–8. doi: 10.1016/j.imlet.2005.09.007
69. Valero-Pacheco N, Arriaga-Pizano L, Ferat-Osorio E, Mora-Velandia LM, Pastelin-Palacios R, Villasis-Keever MA, et al. Pd-L1 Expression Induced by the 2009 Pandemic Influenza a(H1n1) Virus Impairs the Human T Cell Response. Clin Dev Immunol (2013) 2013:989673. doi: 10.1155/2013/989673
70. Talay O, Shen CH, Chen L, Chen J. B7-H1 (Pd-L1) on T Cells Is Required for T-Cell-Mediated Conditioning of Dendritic Cell Maturation. Proc Natl Acad Sci U S A (2009) 106(8):2741–6. doi: 10.1073/pnas.0813367106
71. Lee SJ, O'Donnell H, McSorley SJ. B7-H1 (Programmed Cell Death Ligand 1) Is Required for the Development of Multifunctional Th1 Cells and Immunity to Primary, But Not Secondary, Salmonella Infection. J Immunol (2010) 185(4):2442–9. doi: 10.4049/jimmunol.1000743
72. Pulko V, Harris KJ, Liu X, Gibbons RM, Harrington SM, Krco CJ, et al. B7-H1 Expressed by Activated Cd8 T Cells Is Essential for Their Survival. J Immunol (2011) 187(11):5606–14. doi: 10.4049/jimmunol.1003976
73. Saha A, O'Connor RS, Thangavelu G, Lovitch SB, Dandamudi DB, Wilson CB, et al. Programmed Death Ligand-1 Expression on Donor T Cells Drives Graft-Versus-Host Disease Lethality. J Clin Invest (2016) 126(7):2642–60. doi: 10.1172/JCI85796
74. Kanai T, Totsuka T, Uraushihara K, Makita S, Nakamura T, Koganei K, et al. Blockade of B7-H1 Suppresses the Development of Chronic Intestinal Inflammation. J Immunol (2003) 171(8):4156–63. doi: 10.4049/jimmunol.171.8.4156
75. Rowe JH, Johanns TM, Ertelt JM, Way SS. Pdl-1 Blockade Impedes T Cell Expansion and Protective Immunity Primed by Attenuated Listeria Monocytogenes. J Immunol (2008) 180(11):7553–7. doi: 10.4049/jimmunol.180.11.7553
76. Seo SK, Jeong HY, Park SG, Lee SW, Choi IW, Chen L, et al. Blockade of Endogenous B7-H1 Suppresses Antibacterial Protection After Primary Listeria Monocytogenes Infection. Immunology (2008) 123(1):90–9. doi: 10.1111/j.1365-2567.2007.02708.x
77. Liu X, Wu X, Cao S, Harrington SM, Yin P, Mansfield AS, et al. B7-H1 Antibodies Lose Antitumor Activity Due to Activation of P38 Mapk That Leads to Apoptosis of Tumor-Reactive Cd8(+) T Cells. Sci Rep (2016) 6:36722. doi: 10.1038/srep36722
78. Daley D, Zambirinis CP, Seifert L, Akkad N, Mohan N, Werba G, et al. Gammadelta T Cells Support Pancreatic Oncogenesis by Restraining Alphabeta T Cell Activation. Cell (2016) 166(6):1485–99.e15. doi: 10.1016/j.cell.2016.07.046
79. Diskin B, Adam S, Cassini MF, Sanchez G, Liria M, Aykut B, et al. Pd-L1 Engagement on T Cells Promotes Self-Tolerance and Suppression of Neighboring Macrophages and Effector T Cells in Cancer. Nat Immunol (2020) 21(4):442–54. doi: 10.1038/s41590-020-0620-x
80. Fanelli G, Romano M, Nova-Lamperti E, Werner Sunderland M, Nerviani A, Scotta C, et al. Pd-L1 Signaling on Human Memory Cd4+ T Cells Induces a Regulatory Phenotype. PloS Biol (2021) 19(4):e3001199. doi: 10.1371/journal.pbio.3001199
81. Mazerolles F, Rieux-Laucat F. Pd-L1 Is Expressed on Human Activated Naive Effector Cd4+ T Cells. Regulation by Dendritic Cells and Regulatory Cd4+ T Cells. PloS One (2021) 16(11):e0260206. doi: 10.1371/journal.pone.0260206
82. Jacquelot N, Roberti MP, Enot DP, Rusakiewicz S, Ternes N, Jegou S, et al. Predictors of Responses to Immune Checkpoint Blockade in Advanced Melanoma. Nat Commun (2017) 8(1):592. doi: 10.1038/s41467-017-00608-2
83. Brochez L, Meireson A, Chevolet I, Sundahl N, Ost P, Kruse V. Challenging Pd-L1 Expressing Cytotoxic T Cells as a Predictor for Response to Immunotherapy in Melanoma. Nat Commun (2018) 9(1):2921. doi: 10.1038/s41467-018-05047-1
84. Goshu BA, Chen H, Moussa M, Cheng J, Catalfamo M. Combination Rhil-15 and Anti-Pd-L1 (Avelumab) Enhances Human Hivgag-Specific Cd8 T-Cell Function. J Infect Dis (2020) 222(9):1540–9. doi: 10.1093/infdis/jiaa269
85. Li X, Zhong T, Tang R, Wu C, Xie Y, Liu F, et al. Pd-1 and Pd-L1 Expression in Peripheral Cd4/Cd8+ T Cells Is Restored in the Partial Remission Phase in Type 1 Diabetes. J Clin Endocrinol Metab (2020) 105(6):1947–56. doi: 10.1210/clinem/dgaa130
86. Liu Y, Zugazagoitia J, Ahmed FS, Henick BS, Gettinger SN, Herbst RS, et al. Immune Cell Pd-L1 Colocalizes With Macrophages and Is Associated With Outcome in Pd-1 Pathway Blockade Therapy. Clin Cancer Res (2020) 26(4):970–7. doi: 10.1158/1078-0432.CCR-19-1040
87. Bekos C, Pils D, Dekan S, Hofstetter G, Horak P, Reinthaller A, et al. Tumor Specimens (Ovarian Tumor Tissues and Intraperitoneal Metastases) of 111 Patients Were Used to Investigate Pd-1 and Pd-L1 Expression on Tils in Peritoneal Metastases Compared to Ovarian Tumor Tissues and Its Associations With Clinical Outcome. Sci Rep (2021) 11(1):6400. doi: 10.1038/s41598-021-85966-0
88. Dart SJ, Cook AM, Millward MJ, McDonnell AM, Chin WL, Hakeem MU, et al. Changes in Expression of Pd-L1 on Peripheral T Cells in Patients With Melanoma and Lung Cancer Treated With Pd-1 Inhibitors. Sci Rep (2021) 11(1):15312. doi: 10.1038/s41598-021-93479-z
89. Li L, Lu G, Liu Y, Gong L, Zheng X, Zheng H, et al. Low Infiltration of Cd8+ Pd-L1+ T Cells and M2 Macrophages Predicts Improved Clinical Outcomes After Immune Checkpoint Inhibitor Therapy in Non-Small Cell Lung Carcinoma. Front Oncol (2021) 11:658690. doi: 10.3389/fonc.2021.658690
90. Zhang L, Chen Y, Wang H, Xu Z, Wang Y, Li S, et al. Massive Pd-L1 and Cd8 Double Positive Tils Characterize an Immunosuppressive Microenvironment With High Mutational Burden in Lung Cancer. J Immunother Cancer (2021) 9(6):e002356. doi: 10.1136/jitc-2021-002356
91. Ioannou N, Hagner PR, Stokes M, Gandhi AK, Apollonio B, Fanous M, et al. Triggering Interferon Signaling in T Cells With Avadomide Sensitizes Cll to Anti-Pd-L1/Pd-1 Immunotherapy. Blood (2021) 137(2):216–31. doi: 10.1182/blood.2020006073
92. Gasser M, Grimm M, Nichiporuk E, Bueter M, Lutz J, Lebedeva T, et al. Pd-1/Pd-L1 Expression in Colorectal Cancer and Its Implications for Apoptosis and Tumor Immune Evasion. Cancer Res (2006) 66(8_Supplement):1118–. doi: 10.1200/jco.2006.24.18_suppl.10046
93. Gasser M, Grimm M, Nichiporuk E, Bueter M, Lutz J, Lebedeva T, et al. Pd-1/Pdl-1 Expression in Colorectal Cancer and Its Implications for Tumor Immune Evasion. J Clin Oncol (2006) 24(18_suppl):10046–. doi: 10.1200/jco.2006.24.18_suppl.10046
94. Schatton T, Schutte U, Frank NY, Zhan Q, Hoerning A, Robles SC, et al. Modulation of T-Cell Activation by Malignant Melanoma Initiating Cells. Cancer Res (2010) 70(2):697–708. doi: 10.1158/0008-5472.CAN-09-1592
95. Kleffel S, Posch C, Barthel SR, Mueller H, Schlapbach C, Guenova E, et al. Melanoma Cell-Intrinsic Pd-1 Receptor Functions Promote Tumor Growth. Cell (2015) 162(6):1242–56. doi: 10.1016/j.cell.2015.08.052
96. Li H, Li X, Liu S, Guo L, Zhang B, Zhang J, et al. Programmed Cell Death-1 (Pd-1) Checkpoint Blockade in Combination With a Mammalian Target of Rapamycin Inhibitor Restrains Hepatocellular Carcinoma Growth Induced by Hepatoma Cell-Intrinsic Pd-1. Hepatology (2017) 66(6):1920–33. doi: 10.1002/hep.29360
97. Du S, McCall N, Park K, Guan Q, Fontina P, Ertel A, et al. Blockade of Tumor-Expressed Pd-1 Promotes Lung Cancer Growth. Oncoimmunology (2018) 7(4):e1408747. doi: 10.1080/2162402X.2017.1408747
98. Zhao Y, Harrison DL, Song Y, Ji J, Huang J, Hui E. Antigen-Presenting Cell-Intrinsic Pd-1 Neutralizes Pd-L1 in Cis to Attenuate Pd-1 Signaling in T Cells. Cell Rep (2018) 24(2):379–90.e6. doi: 10.1016/j.celrep.2018.06.054
99. Pu N, Gao S, Yin H, Li JA, Wu W, Fang Y, et al. Cell-Intrinsic Pd-1 Promotes Proliferation in Pancreatic Cancer by Targeting Cyr61/Ctgf Via the Hippo Pathway. Cancer Lett (2019) 460:42–53. doi: 10.1016/j.canlet.2019.06.013
100. Wang X, Yang X, Zhang C, Wang Y, Cheng T, Duan L, et al. Tumor Cell-Intrinsic Pd-1 Receptor Is a Tumor Suppressor and Mediates Resistance to Pd-1 Blockade Therapy. Proc Natl Acad Sci USA (2020) 117(12):6640–50. doi: 10.1073/pnas.1921445117
101. Cao Z, Kon N, Liu Y, Xu W, Wen J, Yao H, et al. An Unexpected Role for P53 in Regulating Cancer Cell-Intrinsic Pd-1 by Acetylation. Sci Adv (2021) 7(14):eabf4148. doi: 10.1126/sciadv.abf4148
102. Gan F, Zhang C, Xia L, Deng S. Tumor-Endogenous Pd-1 Promotes Cell Proliferation and Predicts Poor Survival in Non-Small Cell Lung Cancer. Transl Cancer Res (2022) 11(1):3–13. doi: 10.21037/tcr-21-1644
103. Nishimura H, Minato N, Nakano T, Honjo T. Immunological Studies on Pd-1 Deficient Mice: Implication of Pd-1 as a Negative Regulator for B Cell Responses. Int Immunol (1998) 10(10):1563–72. doi: 10.1093/intimm/10.10.1563
104. Said EA, Dupuy FP, Trautmann L, Zhang Y, Shi Y, El-Far M, et al. Programmed Death-1-Induced Interleukin-10 Production by Monocytes Impairs Cd4+ T Cell Activation During Hiv Infection. Nat Med (2010) 16(4):452–9. doi: 10.1038/nm.2106
105. Qorraj M, Bruns H, Bottcher M, Weigand L, Saul D, Mackensen A, et al. The Pd-1/Pd-L1 Axis Contributes to Immune Metabolic Dysfunctions of Monocytes in Chronic Lymphocytic Leukemia. Leukemia (2017) 31(2):470–8. doi: 10.1038/leu.2016.214
106. Strauss L, Mahmoud MAA, Weaver JD, Tijaro-Ovalle NM, Christofides A, Wang Q, et al. Targeted Deletion of Pd-1 in Myeloid Cells Induces Antitumor Immunity. Sci Immunol (2020) 5(43):eaay1863. doi: 10.1126/sciimmunol.aay1863
107. Huang X, Venet F, Wang YL, Lepape A, Yuan Z, Chen Y, et al. Pd-1 Expression by Macrophages Plays a Pathologic Role in Altering Microbial Clearance and the Innate Inflammatory Response to Sepsis. Proc Natl Acad Sci U.S.A. (2009) 106(15):6303–8. doi: 10.1073/pnas.0809422106
108. Yao A, Liu F, Chen K, Tang L, Liu L, Zhang K, et al. Programmed Death 1 Deficiency Induces the Polarization of Macrophages/Microglia to the M1 Phenotype After Spinal Cord Injury in Mice. Neurotherapeutics (2014) 11(3):636–50. doi: 10.1007/s13311-013-0254-x
109. Bally AP, Lu P, Tang Y, Austin JW, Scharer CD, Ahmed R, et al. Nf-Kappab Regulates Pd-1 Expression in Macrophages. J Immunol (2015) 194(9):4545–54. doi: 10.4049/jimmunol.1402550
110. Chen W, Wang J, Jia L, Liu J, Tian Y. Attenuation of the Programmed Cell Death-1 Pathway Increases the M1 Polarization of Macrophages Induced by Zymosan. Cell Death Dis (2016) 7:e2115. doi: 10.1038/cddis.2016.33
111. Gordon SR, Maute RL, Dulken BW, Hutter G, George BM, McCracken MN, et al. Pd-1 Expression by Tumour-Associated Macrophages Inhibits Phagocytosis and Tumour Immunity. Nature (2017) 545(7655):495–9. doi: 10.1038/nature22396
112. Hamaidia M, Gazon H, Hoyos C, Hoffmann GB, Louis R, Duysinx B, et al. Inhibition of Ezh2 Methyltransferase Decreases Immunoediting of Mesothelioma Cells by Autologous Macrophages Through a Pd-1-Dependent Mechanism. JCI Insight (2019) 4(18):e128474. doi: 10.1172/jci.insight.128474
113. Triantafyllou E, Gudd CL, Mawhin MA, Husbyn HC, Trovato FM, Siggins MK, et al. Pd-1 Blockade Improves Kupffer Cell Bacterial Clearance in Acute Liver Injury. J Clin Invest (2021) 131(4):e140196. doi: 10.1172/JCI140196
114. Yao S, Wang S, Zhu Y, Luo L, Zhu G, Flies S, et al. Pd-1 on Dendritic Cells Impedes Innate Immunity Against Bacterial Infection. Blood (2009) 113(23):5811–8. doi: 10.1182/blood-2009-02-203141
115. Krempski J, Karyampudi L, Behrens MD, Erskine CL, Hartmann L, Dong H, et al. Tumor-Infiltrating Programmed Death Receptor-1+ Dendritic Cells Mediate Immune Suppression in Ovarian Cancer. J Immunol (2011) 186(12):6905–13. doi: 10.4049/jimmunol.1100274
116. Park SJ, Namkoong H, Doh J, Choi JC, Yang BG, Park Y, et al. Negative Role of Inducible Pd-1 on Survival of Activated Dendritic Cells. J Leukoc Biol (2014) 95(4):621–9. doi: 10.1189/jlb.0813443
117. Karyampudi L, Lamichhane P, Krempski J, Kalli KR, Behrens MD, Vargas DM, et al. Pd-1 Blunts the Function of Ovarian Tumor-Infiltrating Dendritic Cells by Inactivating Nf-Kappab. Cancer Res (2016) 76(2):239–50. doi: 10.1158/0008-5472.CAN-15-0748
118. Lim TS, Chew V, Sieow JL, Goh S, Yeong JP, Soon AL, et al. Pd-1 Expression on Dendritic Cells Suppresses Cd8(+) T Cell Function and Antitumor Immunity. Oncoimmunology (2016) 5(3):e1085146. doi: 10.1080/2162402X.2015.1085146
119. Lamichhane P, Karyampudi L, Shreeder B, Krempski J, Bahr D, Daum J, et al. Il10 Release Upon Pd-1 Blockade Sustains Immunosuppression in Ovarian Cancer. Cancer Res (2017) 77(23):6667–78. doi: 10.1158/0008-5472.CAN-17-0740
120. Nam S, Lee A, Lim J, Lim JS. Analysis of the Expression and Regulation of Pd-1 Protein on the Surface of Myeloid-Derived Suppressor Cells (Mdscs). Biomol Ther (Seoul) (2019) 27(1):63–70. doi: 10.4062/biomolther.2018.201
121. Wang F, Li B, Wei Y, Zhao Y, Wang L, Zhang P, et al. Tumor-Derived Exosomes Induce Pd1(+) Macrophage Population in Human Gastric Cancer That Promotes Disease Progression. Oncogenesis (2018) 7(5):41. doi: 10.1038/s41389-018-0049-3
122. Dhupkar P, Gordon N, Stewart J, Kleinerman ES. Anti-Pd-1 Therapy Redirects Macrophages From an M2 to an M1 Phenotype Inducing Regression of Os Lung Metastases. Cancer Med (2018) 7(6):2654–64. doi: 10.1002/cam4.1518
123. Ho TTB, Nasti A, Seki A, Komura T, Inui H, Kozaka T, et al. Combination of Gemcitabine and Anti-Pd-1 Antibody Enhances the Anticancer Effect of M1 Macrophages and the Th1 Response in a Murine Model of Pancreatic Cancer Liver Metastasis. J Immunother Cancer (2020) 8(2):e001367. doi: 10.1136/jitc-2020-001367
124. Rao G, Latha K, Ott M, Sabbagh A, Marisetty A, Ling X, et al. Anti-Pd-1 Induces M1 Polarization in the Glioma Microenvironment and Exerts Therapeutic Efficacy in the Absence of Cd8 Cytotoxic T Cells. Clin Cancer Res (2020) 26(17):4699–712. doi: 10.1158/1078-0432.CCR-19-4110
125. Tsukamoto H, Fujieda K, Miyashita A, Fukushima S, Ikeda T, Kubo Y, et al. Combined Blockade of Il6 and Pd-1/Pd-L1 Signaling Abrogates Mutual Regulation of Their Immunosuppressive Effects in the Tumor Microenvironment. Cancer Res (2018) 78(17):5011–22. doi: 10.1158/0008-5472.CAN-18-0118
126. Vari F, Arpon D, Keane C, Hertzberg MS, Talaulikar D, Jain S, et al. Immune Evasion Via Pd-1/Pd-L1 on Nk Cells and Monocyte/Macrophages Is More Prominent in Hodgkin Lymphoma Than Dlbcl. Blood (2018) 131(16):1809–19. doi: 10.1182/blood-2017-07-796342
127. Concha-Benavente F, Kansy B, Moskovitz J, Moy J, Chandran U, Ferris RL. Pd-L1 Mediates Dysfunction in Activated Pd-1(+) Nk Cells in Head and Neck Cancer Patients. Cancer Immunol Res (2018) 6(12):1548–60. doi: 10.1158/2326-6066.CIR-18-0062
128. Liu Y, Cheng Y, Xu Y, Wang Z, Du X, Li C, et al. Increased Expression of Programmed Cell Death Protein 1 on Nk Cells Inhibits Nk-Cell-Mediated Anti-Tumor Function and Indicates Poor Prognosis in Digestive Cancers. Oncogene (2017) 36(44):6143–53. doi: 10.1038/onc.2017.209
129. Iraolagoitia XL, Spallanzani RG, Torres NI, Araya RE, Ziblat A, Domaica CI, et al. Nk Cells Restrain Spontaneous Antitumor Cd8+ T Cell Priming Through Pd-1/Pd-L1 Interactions With Dendritic Cells. J Immunol (2016) 197(3):953–61. doi: 10.4049/jimmunol.1502291
130. Trefny MP, Kaiser M, Stanczak MA, Herzig P, Savic S, Wiese M, et al. Pd-1(+) Natural Killer Cells in Human Non-Small Cell Lung Cancer Can Be Activated by Pd-1/Pd-L1 Blockade. Cancer Immunol Immunother (2020) 69(8):1505–17. doi: 10.1007/s00262-020-02558-z
131. Hsu J, Hodgins JJ, Marathe M, Nicolai CJ, Bourgeois-Daigneault MC, Trevino TN, et al. Contribution of Nk Cells to Immunotherapy Mediated by Pd-1/Pd-L1 Blockade. J Clin Invest (2018) 128(10):4654–68. doi: 10.1172/JCI99317
132. Benson DM Jr., Bakan CE, Mishra A, Hofmeister CC, Efebera Y, Becknell B, et al. The Pd-1/Pd-L1 Axis Modulates the Natural Killer Cell Versus Multiple Myeloma Effect: A Therapeutic Target for Ct-011, a Novel Monoclonal Anti-Pd-1 Antibody. Blood (2010) 116(13):2286–94. doi: 10.1182/blood-2010-02-271874
133. Dong W, Wu X, Ma S, Wang Y, Nalin AP, Zhu Z, et al. The Mechanism of Anti-Pd-L1 Antibody Efficacy Against Pd-L1-Negative Tumors Identifies Nk Cells Expressing Pd-L1 as a Cytolytic Effector. Cancer Discov (2019) 9(10):1422–37. doi: 10.1158/2159-8290.CD-18-1259
134. Wang Z, Jiang C, He Q, Matsuda M, Han Q, Wang K, et al. Anti–Pd-1 Treatment Impairs Opioid Antinociception in Rodents and Nonhuman Primates. Sci Transl Med (2020) 12(531):. doi: 10.1126/scitranslmed.aaw6471
135. Workman CJ, Wang Y, El Kasmi KC, Pardoll DM, Murray PJ, Drake CG, et al. Lag-3 Regulates Plasmacytoid Dendritic Cell Homeostasis. J Immunol (2009) 182(4):1885–91. doi: 10.4049/jimmunol.0800185
136. Chiba S, Baghdadi M, Akiba H, Yoshiyama H, Kinoshita I, Dosaka-Akita H, et al. Tumor-Infiltrating Dcs Suppress Nucleic Acid-Mediated Innate Immune Responses Through Interactions Between the Receptor Tim-3 and the Alarmin Hmgb1. Nat Immunol (2012) 13(9):832–42. doi: 10.1038/ni.2376
Keywords: noncanonical PD-1/PD-L1 axis, T-cells, tumor cells, myeloid cells, anti-PD therapy
Citation: Long Y, Yu X, Chen R, Tong Y and Gong L (2022) Noncanonical PD-1/PD-L1 Axis in Relation to the Efficacy of Anti-PD Therapy. Front. Immunol. 13:910704. doi: 10.3389/fimmu.2022.910704
Received: 01 April 2022; Accepted: 21 April 2022;
Published: 18 May 2022.
Edited by:
Xuyao Zhang, Fudan University, ChinaReviewed by:
Yi Lu, Tongji University, ChinaCopyright © 2022 Long, Yu, Chen, Tong and Gong. This is an open-access article distributed under the terms of the Creative Commons Attribution License (CC BY). The use, distribution or reproduction in other forums is permitted, provided the original author(s) and the copyright owner(s) are credited and that the original publication in this journal is cited, in accordance with accepted academic practice. No use, distribution or reproduction is permitted which does not comply with these terms.
*Correspondence: Likun Gong, bGtnb25nQHNpbW0uYWMuY24=
†These authors have contributed equally to this work
Disclaimer: All claims expressed in this article are solely those of the authors and do not necessarily represent those of their affiliated organizations, or those of the publisher, the editors and the reviewers. Any product that may be evaluated in this article or claim that may be made by its manufacturer is not guaranteed or endorsed by the publisher.
Research integrity at Frontiers
Learn more about the work of our research integrity team to safeguard the quality of each article we publish.