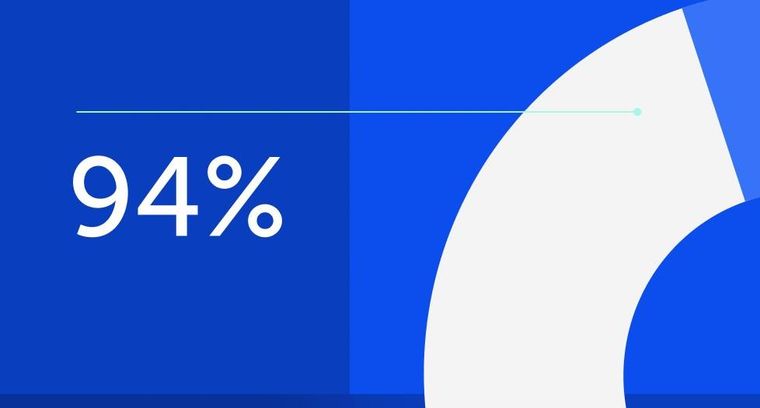
94% of researchers rate our articles as excellent or good
Learn more about the work of our research integrity team to safeguard the quality of each article we publish.
Find out more
REVIEW article
Front. Immunol., 13 June 2022
Sec. Antigen Presenting Cell Biology
Volume 13 - 2022 | https://doi.org/10.3389/fimmu.2022.902567
This article is part of the Research TopicInsights in Antigen Presenting Cell Biology: 2021View all 9 articles
To be, or not to be, that is the question. (William Shakespeare, Hamlet)
Endoplasmic reticulum aminopeptidases 1 and 2 (ERAP1 and ERAP2, respectively) play a role in trimming peptides that are too long to be bound and presented by class I HLA (HLA-I) molecules to CD8+ T cells. They may also affect the HLA-I-presented peptide repertoire by overtrimming potential epitopes. Both enzymes may also be released from the cell to cleave cytokine receptors and regulate blood pressure. Both enzymes are polymorphic, which affects their expression, specificity, and activity, resulting in their role in diseases associated with HLA-I. In this brief review, we concentrate on ERAP2, less investigated because of its lack in laboratory mice and 25% of humans, as well as a lower polymorphism. ERAP2 was found to be associated with several diseases and to influence ERAP1 effects. It was discovered recently that the defective ERAP2 gene, not encoding functional aminopeptidase, may nevertheless, during viral infections, produce a truncated protein isoform of unknown function, possibly interfering with ERAP1 and full-length ERAP2 by heterodimer formation. The disease associations of ERAP2, alone or in combination with ERAP1, are reviewed.
The human body is constantly exposed to infections with viruses, bacteria, fungi, and parasites. It is also confronted with arising neoplastically transformed self-cells. Therefore, it needs a defense that is provided by two branches of immunity: (i) innate, which is fast-acting but much less specific; and (ii) adoptive, which develops slower but is antigen-specific, i.e., focused on the given pathogen without being distracted by other, innocent structures. Both branches of immunity recognize foreign structures using proper receptors: In innate immunity, these are pattern-recognition receptors recognizing pathogen-associated molecular patterns, Toll-like receptors, distributed on several cell types, and killer cell immunoglobulin-like receptors (KIRs), the latter present on natural killer (NK) cells and subsets of T lymphocytes. KIRs recognize a lack or decrease in expression of human leukocyte antigen class I (HLA-I) molecules on the surface of target cells, and HLA-I-bound peptides influence this interaction (1–6). Adoptive immunity uses highly specific T-cell receptors (TCRs) that recognize antigenic peptides (derived from foreign or neoplastic proteins) presented to them by HLA molecules. Class I HLA molecules present intracellular antigens to TCRs of cytotoxic T cells (CD8+) which kill infected or transformed cells producing virus or tumor antigens, respectively. Both virus-infected cells and tumor cells frequently have decreased expression of cell surface HLA-I molecules to escape T-cell killing (7). Thus, both NK and T cells may eliminate a source of infection or malignant tumor, depending on HLA-I expression. HLA-I molecules bind their peptide cargo (8–10 amino acid long) in endoplasmic reticulum (ER). Peptides longer than 10 amino acids are trimmed by endoplasmic reticulum aminopeptidases to fit the peptide-binding groove of the HLA-I molecule. Class II HLA (HLA-II) molecules present extracellular antigens to helper T cells (CD4+). These then secrete cytokines and stimulate B lymphocytes to produce antigen-specific antibodies, recognizing antigens on viruses, bacteria, fungi, and parasites (8).
Aminopeptidases are exopeptidases that hydrolyze amino acid residues from the N-terminus of peptides or protein substrates (9). M1 aminopeptidases (12 enzymes in humans) require Zn2+ for hydrolysis (10). Endoplasmic reticulum aminopeptidases 1 and 2 (ERAP1 and ERAP2, respectively) belong to the oxytocinase subfamily of M1 aminopeptidases together with insulin-responsive aminopeptidase (IRAP)1, the latter being present in intracellular vesicles including endosomes and engaged in cross-presentation, i.e., unconventional presentation of extracellular antigens by HLA-I molecules, and several other functions (11–13). These proteins are composed of four domains. In domain I, they contain an amino acid residue interacting with N-terminal residue of the peptidic substrate. Domain II contains two amino acid motifs important for the enzyme’s function: GAMEN is responsible for exopeptidase specificity by interaction with N-terminal amino acid of the trimmed peptide; Gln181 also contributes to the specificity. HELAH(X)18D is a zinc ligand and is essential for the catalytic activity of the enzymes. Domain III has a β-sandwich structure that links domains II and IV; it rotates in relation to domain II, forming an open and closed conformation of the molecule. Domain IV is a bowl that forms most of the large cavity accommodating the substrate. The enzyme is active in the closed conformation and may release the trimmed peptide and bind a new substrate in the open conformation (10, 14; and references therein).
ERAP1, in addition to the catalytic site, has a regulatory site distinct from but not far from the active site. Binding of the C-terminus of the peptide (in the open conformation) to a regulatory site promotes a change to a closed conformation with increased enzymatic activity. This mechanism provides trimming peptides of 9–16 amino acids in length but not shorter ones, which do not reach the regulatory site (“molecular ruler” model) (15 and references therein; 16). In spite of nearly 50% shared identity and a similar four-domain structure, ERAP2 is different from ERAP1 by a lack of this regulatory site binding the peptide C-terminus. This structural feature enables ERAP2 to digest octamers and shorter peptides but not so efficiently longer ones (15, 17; and references therein). Therefore, the effects of ERAP1 and ERAP2 on immunopeptidomes of particular HLA-I allotypes are different (18 and references therein). Nevertheless, using cells with different ERAP1 allotypes of high or low activity and expressing ERAP2 or not, it was observed that a remarkable part of immunopeptidome remains untouched by these changes (19, 20; and references therein).
Genes for three aminopeptidases are present, in different combinations, in vertebrates. Most mammals express ERAP1 and ERAP2 except for rodents, which are negative for ERAP2; virtually, all mammals have IRAP (21). In humans, ERAP1 and ERAP2 are highly (49% identity) homologous (11) but differ in their specificity: ERAP1 preferentially trims N-terminal hydrophobic amino acid residues, while ERAP2 prefers positively charged residues, particularly arginine (22).
In addition to their role in the endoplasmic reticulum, both enzymes may be released from the cell, where they may (i) perform cleavage of cell surface receptors for many cytokines, thus modulating immune responses (23) and (ii) regulate blood pressure (see ERAPS in the Modulation of Cytokine-Mediated Signaling, in the Regulation of Blood Pressure, and in Sars-Cov-2 and HIV Infection).
ERAP1 is highly polymorphic. Ten allotypes with frequencies at or above 0.4% were described in Europeans (24), and most of them are present also in other populations (25). The frequencies of these allotypes are different in distinct human populations and parallel the differences in HLA-I allele frequencies there. This may reflect the exposure of these populations to distinct pathogens in their environment. Interestingly, for example, homozygotes of ERAP1 allotype No. 10, of the lowest enzymatic activity, are absent or detected in only trace amounts in African, Asian, and Amerindian populations but are present in nearly 5% of Europeans (25). Allotypes of ERAP1 were reproducibly reported to be associated with those human diseases where HLA-I plays a role. These associations were reviewed recently (14, 18, 19, 23, 24, 26) and are out of the scope of the present article. It should be noted here that a remarkable fraction of peptides transported to the endoplasmic reticulum do not require any ERAP1-mediated pretreatment to be bound by HLA-I (20). At least some of them may be trimmed by ERAP2, and this may be a reason for the frequent coexistence of active ERAP2 with low activity ERAP1 in a haplotype (25, 27) (see the next section).
ERAP2 was less extensively examined because it is absent in 25% of humans and in mice, which are an important in vivo model for immunologic studies. However, it is also polymorphic, although to a lower extent (see the next section).
ERAP2 is less polymorphic than ERAP1, and most single nucleotide polymorphisms (SNPs) in this gene are in strong linkage disequilibrium (LD). This leads to two main allotypes differing in rs2248374A>G SNP, distributed at roughly equal frequencies in human populations (allotype A, rs2248374A, and allotype B, rs2248374G), and several minor variants of these two. Individuals homozygotic or heterozygotic for rs2248374A express functional ERAP2 protein, whereas rs2248374G homozygotes produce truncated transcript undergoing nonsense-mediated decay and therefore have no functional ERAP2 enzyme (28). Therefore, roughly every fourth individual in most human populations is devoid of ERAP2 activity. This, in many cases, is compensated by the presence of highly activity ERAP1 and vice versa. For example, in ERAP2 rs2248374GG (no ERAP2) individuals, the ERAP1 allotype No. 2 homozygotes (high ERAP1 activity) are 14 times more frequent than in rs2248374AA homozygotes. On the other hand, the highest ERAP2 expression allotype is found more frequently in individuals that carry an intermediate or low-activity ERAP1 allotype (25). Generally, the higher the activity of ERAP1, the less frequently functional ERAP2 coexists with it in a haplotype and vice versa (27). Thus, a lack of functional ERAP2 apparently brings a need for higher activity of ERAP1. The close localization of both ERAP genes on chromosome 5q15 (29) favors complementing ERAP1–ERAP2 haplotypes to provide at least one active ERAP. In addition, a rs75862629 SNP between both genes regulates mutually their expression in such a way that the rs75862629G allele is associated with high ERAP1 and low ERAP2 expression, and the opposite is observed with the A allele (30).
In most populations, the SNP rs2549782G>C,T (Lys392Asn) is in very strong LD with rs2248374, which decides whether functional ERAP2 is produced (28). The Asn392 variant has about 160 times higher activity than Lys392 (31). However, due to this strong LD, this variant is normally never produced except for very rare recombinants, and even in an exceptional population of Chileans, where such a recombinant is present in 2% of the population, Asn392/Asn392 homozygotes could not be detected (28). By using Asn392-overexpressing transfectants of the trophoblast cell line, Warthan etal. (32) found that these cells were killed by T and NK cells, and Lospinoso etal. (33) observed that such transfection caused downregulation of many genes important for cell survival, which together may explain the lack of Asn392 homozygotes in Chileans.
Other SNPs in ERAP2 are also in very strong LD in humans (34). Therefore, two allotypes prevail in human populations: allotype A (with rs2248374A), a functional enzyme, and allotype B (with rs2234874G), not expressed in normal conditions (see ERAP2 Isoforms).
The lack of functional ERAP2 in roughly every forth human individual is not exceptional in nature. Most rodents do not even possess the ERAP2 gene. Other mammals generally possess both ERAP1 and ERAP2 genes. Interestingly, multiple different combinations of ERAP1 and ERAP2 (and also IRAP) appear in other vertebrates; for example, about 60% of bony fish species possess ERAP2, but only 2% have the ERAP1 gene (21).
Similarly to ERAP1, ERAP2 polymorphisms also have an impact on the immunopeptidome, albeit with different results than the former, as stated in Endoplasmic Reticulum Aminopeptidases. Therefore, the lack of active ERAP2 enzyme in allotype B homozygotes results in changes in the immunopeptidome, as it was shown for HLA-B*27, associated with ankylosing spondylitis (35). However, the association of ERAP2 with this disease appears independent of the presence of HLA-B*27, in contrast to the ERAP1 association in epistasis with HLA-B*27 (36), which may speak to other functions of ERAP2 in addition to epitope production (see ERAPS in the Modulation of Cytokine-Mediated Signaling, in the Regulation Of Blood Pressure, and in Sars-Cov-2 and HIV Infection). Among other conditions where associations of ERAP2 presence versus absence were observed, we may also list psoriasis, Crohn’s disease, hypertension, and birdshot chorioretinopathy (14, 17, 37). Similar associations were also described in gynecological diseases: preeclampsia (31, 38–40) and recurrent implantation failure after in vitro fertilization (41).
Interestingly, addition of recombinant full-length ERAP2 protein to cultures of peripheral blood mononuclear cells (from both allotype A and allotype B donors) infected with HIV-1 reduced viral replication by activation of cytotoxic CD8+ T cells (42). Moreover, even in CD8+ T-cell depleted peripheral blood mononuclear cell cultures, viral replication was reduced due to the activation of monocytes by ERAP2 (43).
Both ERAPs appear in several protein isoforms. Two ERAP1 isoforms resulting from alternative splicing, 19 exons (19E)- and 20 exons (20E)-containing transcript variants were described, whose expression is inversely dependent on the rs7063 SNP genotype (44). As ERAP1 is not the subject of this review, we will not go further here in this direction.
Although ERAP2 rs2248374GG individuals, as stated above, do not produce active aminopeptidase, it was recently found that during influenza infection, a truncated protein devoid of enzymatic activity was detected in these individuals. It has been named isoform 3 (Iso3) in opposition to Iso1, the normal functional ERAP2 present in rs2248374A-possessing individuals, and Iso2 and Iso4, truncated transcripts from rs2248374G undergoing nonsense-mediated decay and therefore giving no protein products (45) (Table 1). Later, Iso3 expression was confirmed also for other viral infections (HIV, human cytomegalovirus, SARS-CoV-2). Iso3 is apparently also present in bacterial infections, as it was also induced in vitro by lipopolysaccharide (46).
It has been suggested that, as ERAP2/Iso3 possesses a domain required for homo- and heterodimer formation, it may possibly interfere with ERAP1 and ERAP2/Iso1 function in virus-infected cells (45, 46). It is not known yet whether such interactions positively or negatively affect antiviral immune responses.
ERAP1 and ERAP2 may act separately, but about 30% of their molecules form heterodimers that may digest substrates faster than individual ERAPs (47, 48). Computational molecular dynamics calculations, based on experimentally determined homo-dimerization interfaces observed in crystal structures of ERAP2 or homologous enzymes, suggested the most likely ERAP1/ERAP2 heterodimerization topology. It involves the exon 10 loop (49), previously implicated in interactions between ERAP1 and the disulfide-bond shuffling chaperone ERp44. This latter interaction was postulated to be responsible for retaining ERAP1 (not possessing the ER retention signal) in the ER (50). The ERAP1/ERAP2 heterodimers produce fewer different peptide sequences than each of them separately, although in larger amounts; they leave only peptides resistant to degradation by each of them (51). Thus, on one hand, ERAPs produce, from longer precursors, peptides which may be bound and presented by HLA-I molecules; on the other hand, they may overtrim peptides, preventing them from being presented. Therefore, ERAP1 and ERAP2 may complement each other in epitope production or, on the contrary, mutually destroy peptides produced by each of them. Some peptides, sensitive to ERAP1-mediated overtrimming, may be protected from it if bound by ERAP2, particularly in the context of an ERAP1 allotype with low activity (27).
As stated above, a simultaneous activity of ERAP1 and ERAP2 (and their heterodimer) may result in a different immunopeptide repertoire than that of each enzyme alone. It is not surprising, then, that ERAP1–ERAP2 haplotypes were described as being associated with several human diseases stronger than each gene separately.
Ankylosing spondylitis is strongly associated with HLA-B*27—90% of patients are positive (52–54), versus less than 10% in the healthy Europeans, e.g., in Poles (55). Nevertheless, only a small fraction of HLA-B*27-positive individuals get the disease. Several groups of researchers examined the role of ERAP1 and ERAP2 as ankylosing spondylitis risk factors in addition to HLA-B*27 (reviewed by 17, 18, 26, 56–58). The role of ERAPs in shaping the HLA-B*27:05 peptide repertoire was examined by the Lopez de Castro group: both highly active ERAP1 allotype and the presence of ERAP2 affected the HLA-B*27 immunopeptidome, but their effects were distinct, suggesting that they act here as separate, independent entities (17, 19, 35). The same haplotype (highly active ERAP1 and the presence of ERAP2) was described as a risk factor, while ERAP1 with low activity and a lack of functional ERAP2 were protective (19, 24, 35). Interestingly, while ERAP1 was associated with ankylosing spondylitis only in HLA-B*27-positive individuals, ERAP2 was associated with this disease independently from HLA-B*27 (17, 18, 26, 56–58), which may suggest it participates in another activity in addition to peptide trimming (see the next section). Moreover, we observed that the same SNP combination in ERAP1 was associated with risk or protection from AS or not, depending on the absence or presence of ERAP2 (59).
Psoriasis, a relatively common skin disease, is associated with HLA-C*06:02, although some 30% of patients do not possess this allele (19, 26). A genome-wide association study of psoriasis identified eight previously unreported regions of the genome, among them ERAP1 (60). Although originally the ERAP1 association (ERAP2 was not examined there) with psoriasis was claimed to be independent of HLA-C*06:02 (61), we recently observed different associations of ERAP1–ERAP2 haplotypes, depending on the presence versus absence of the HLA-C*06:02 gene: namely, low activity ERAP1 together with ERAP2 was protective in HLA-C*06:02-positive individuals but associated with disease risk in HLA-C*06:02-negatives. The same ERAP1 without ERAP2 had no effect in HLA-C*06:02-positive patients but was protective in HLA-C*06:02-negative patients. Another ERAP1 allotype, with high activity, was protective in both HLA-C*06:02-positive and HLA-C*06:02-negative patients if accompanied by the presence of ERAP2, but brought risk in HLA-C*06:02-positive patients and had no effect in HLA-C*06:02-negative patients when ERAP2 was absent (62). This suggests different trimming requirements of HLA-I molecules associated with psoriasis in HLA-C*06:02-positive and HLA-C*06:02-negative patients, respectively. Three different autoantigens have already been implicated in psoriasis: cathelicidin LL37, an antimicrobial peptide (63); ADAMTSL5, a melanocyte-derived autoantigen (64); and keratin 17, homologous to streptococcal M-proteins (65). All three are overexpressed in psoriatic skin. LL37 was recognized by CD8+ T cells in the context of HLA-C*06:02 or HLA-A*11, depending on the HLA background of the patient (63). ADAMTSL5 was HLA-C*06:02-presented (64). Keratin 17 induced stronger CD8+ T-cell responses in HLA-C*06:02-positive patients than in HLA-C*06:02-negative ones (65). Therefore, psoriatogenic autoantigens are generally presented more effectively by HLA-C*06:02 than by other HLA-I allotypes, but the latter may also play a role in this disease, as is obviously the case in HLA-C*06:02-negative individuals. A contribution of other psoriatogenic antigens in HLA-C*06:02-negative cases cannot be excluded.
Another skin disease, atopic dermatitis, as an allergic disease, is mainly associated with HLA class II alleles (66). However, HLA-I alleles may play a role in disease outcome (67), and indeed, we found KIR2DS1 (a receptor of NK cells recognizing HLA-C) was associated with protection against atopic dermatitis (68). We also found an association of rs26618 in ERAP1, a SNP affecting enzyme activity, with this disease (69). Although ERAP2 presence/absence had no effect on disease susceptibility, homozygosity for the ERAP2 presence (i.e., rs2248374A/A) together with rs26618C/C resulted in a higher risk of atopic dermatitis than rs26618 alone (70).
A rare disease, birdshot chorioretinopathy, manifesting as small light-colored fundus spots on the retina, scattered in a pattern like birdshot from a shotgun, is a condition most strongly associated with HLA-I, namely with HLA-A*29 (virtually all patients are HLA-A*29-positive). It affects mostly Europeans or patients of European descent. Although HLA-A*29 is not infrequent in some non-European populations, birdshot chorioretinopathy is much less frequent there. The ERAP1 rs2287987 or ERAP2 rs10044354 SNPs are present in 90% of patients, and the rs2287987-rs10044354 haplotype is in 50% (and twice less frequent in controls). This haplotype is less common in non-Europeans, which may explain why the disease is afflicting mostly people of European descent (27). Both ERAP1 and ERAP2 polymorphisms have an influence on the HLA-A*29-bound immunopeptidome (19), providing an explanation for their association with birdshot chorioretinopathy.
Also, in nonsmall cell lung cancer some ERAP1 allotypes were associated with cancer risk, protection, or without effect, depending on the presence versus absence of ERAP2 (ERAP2 separately was without effect). Interestingly, these effects were frequently different in smokers and never smokers (71).
Thus, in many clinical conditions, the effect of the combined activities of ERAP1 and ERAP2 on susceptibility to disease and its outcome is different from the effects of each enzyme alone. Therefore, typing for polymorphisms of both ERAP1 and ERAP2 may have a predictive value for therapy utilizing ERAP inhibitors.
In addition to their role in trimming HLA-I-presented peptides (including SARS-CoV-2 peptides) in the endoplasmic reticulum, ERAPs also perform other functions after secretion from cells. There, they may trim cell surface receptors, e.g., cytokine receptors such as tumor necrosis factor receptor 1, interleukin 6 receptor alpha, and interleukin 1 receptor II (72, 73) and plasma proteins (74 and references therein). Although soluble receptors do not transmit signals directly, they nevertheless can affect ligand binding and activation of membrane receptors and therefore indirectly modulate cellular signaling (75). Solubilized receptors can affect cytokine signaling far away from their site of origin (56 and references therein). In this way, ERAPs contribute to the regulation of several physiological processes in addition to antigen presentation. Among other substrates, ERAPs cleave angiotensins engaged in keeping the balance between hyper- and hypotension (see below).
Blood pressure is regulated by the renin-angiotensin system using a complicated array of proteins, enzymes cleaving them, and receptors. Briefly, angiotensinogen is cleaved by renin to angiotensin I, which is further converted by angiotensin-converting enzyme (ACE) to angiotensin II. This protein binds the ACE/Ang II/Ang II receptor type 1 (AT1R), resulting in vasoconstriction, hypertension, and inflammation. ACE2 counter-regulates this process via the cleaving of angiotensin I to angiotensin 1–7 and angiotensin II to angiotensin 1–9, which binds the Mas receptor, initiating vasodilation, hypotension, and anti-inflammatory activity (76, 77). ERAPs also contribute to the regulation of blood pressure. Both ERAPs, in addition to their intracellular role in antigen presentation, after secretion regulate hypertension by trimming angiotensin II to angiotensin III (ERAP1) and angiotensin III to angiotensin IV (ERAP1 and ERAP2) which then bind to receptors AT2R and AT4R, respectively, stimulating vasodilation and counteracting hypertension (29, 50, 78–80). These angiotensins consist of eight (angiotensin II: DRVYIHPF), seven (angiotensin III: RVYIHPF), and six (angiotensin IV: VYIHPF) amino acids (77). These lengths seem to be too short for ERAP1 but may still be acceptable for ERAP2. In addition, the sequence of angiotensin II starts with an Asp-Arg dipeptide and that of angiotensin III with Arg, which are not efficiently excised by ERAP1 (18, 22). Therefore, their trimming by ERAP1, although experimentally proven, is hard to explain. Perhaps it is indeed performed, but slowly and with low efficiency. Angiotensin III sequence starts with Arg, which is a favorable substrate for ERAP2 (18, 22), so its conversion to angiotensin IV by this aminopeptidase is understandable.
ERAP2/Iso3, and also ERAP1 low activity allotypes, cannot perform these functions, thus moving equilibrium to stimulation of AT1R which causes vasoconstriction and hypertension (81). It is conceivable that the primary function of ERAPs (and IRAP) was regulation of blood pressure through the renin–angiotensin system and that epitope production by peptide trimming evolved later, paralleling the evolution of the specific immune system, including the major histocompatibility complex (21). Interestingly, however, in a recent review of genetic polymorphisms affecting the regulation of blood pressure, Cuevas etal. (76) do not mention ERAPs at all.
During SARS-CoV-2 infection, the virus uses cell membrane angiotensin I-converting enzyme 2 (ACE2) as a receptor to bind to the cell; the complex of the virus with ACE2 is internalized, viral RNA is released to the cytosol, and the viral particles are produced and released to infect other cells. The decrease of ACE2 from the cell surface by endocytosis and enzymatic degradation results in ACE/ACE2 imbalance and dominance of the ACE-mediated pathway leading to hypertension, acute lung injury, and multiorgan damage. Defective variants of ERAPs (i.e., low activity ERAP1 allotypes and lack of functional ERAP2) exacerbate the impact of SARS-CoV-2 on hypertension and organ damage. On the other hand, active ERAP variants may counter-balance SARS-CoV-2-mediated pathogenesis (81).
It should be noted here that secreted ERAP2 was postulated also to play a role in HIV infection: by trimming N-terminal arginine from extracellular substrates, it increases free arginine concentration, essential for synthesis of nitric oxide, an important biological messenger. Thus, ERAP2 may affect protection against HIV in this way in addition to its role in immunopeptidome production, contribution to the regulation of hypertension, and a possible effect of interactions of truncated ERAP2/Iso3 with ERAP1 or with untruncated ERAP2/Iso1 (42, 43, 46).
The original finding that functional ERAP2 protein is absent in 25% of individuals who mostly remain healthy, and that the immune system of the mouse copes well without ERAP2, suggested that it is basically unimportant and plays only a subsidiary role for ERAP1. However, ERAP2 activity may support effective immune response to a pathogen. On the other hand, the lack of ERAP2 may, in many instances, prevent it from overtrimming antigenic peptides produced by ERAP1, which are necessary to fight infection or contribute to autoimmune disease. In addition, the defective ERAP2 allele is not without function in immune defense as, in viral infections, it produces truncated protein isoform possibly interfering with ERAP1 and functional (allotype A) ERAP2. In addition, ERAPs secreted from the cells may trim cell surface cytokine and growth factor receptors, affecting immune response indirectly, and this activity may also be dependent on their polymorphisms. They may also regulate blood pressure and influence the severity of the coronavirus disease 2019 (COVID19). Therefore, some ERAP1–ERAP2 combinations may be protective against one disease but carry a risk for another. So, for ERAP2, the question “to be or not to be,” i.e., whether it is better to have ERAP2 or not, may depend on the genetic background of an individual (including, first and foremost, his/her HLA genotype) as well as on environmental factors. In some circumstances, the presence of an active ERAP2 variant may be beneficial, but in others, it may be harmful. Some of us, due to bad luck, improper genetic constitution (among it, the presence or absence of ERAP2) and environmental influence, contract serious or even fatal diseases, but our species as a whole survives even the worst pandemics such as cholera, plague, AIDS, or, now, COVID-19. For those less lucky, whose genetic (including ERAP2) and environmental factors predispose them to a disease, ERAP2 inhibitors may in some circumstances, become helpful if the patients possess this enzyme in its active form and if it contributes to an unfavorable condition.
The author confirms being the sole contributor of this work and has approved it for publication.
The author’s work on associations of ERAPs with human diseases was supported by grants 14/2015, 14/2016, 14/2018, 14/2019, 14/2020, 14/2021, and 14/2022 from the Hirszfeld Institute of Immunology and Experimental Therapy, the grants N402685040, OPUS 8 2014/15/B/NZ5/03517, and 2018/29/N/NZ5/00940 from the Polish National Science Centre, and the Medical University of Łódź research study 503/5 064 01/503 1.
The author declares that the research was conducted in the absence of any commercial or financial relationships that could be construed as a potential conflict of interest.
All claims expressed in this article are solely those of the authors and do not necessarily represent those of their affiliated organizations, or those of the publisher, the editors and the reviewers. Any product that may be evaluated in this article, or claim that may be made by its manufacturer, is not guaranteed or endorsed by the publisher.
The author is deeply grateful to all his coworkers and coauthors of refs. 41, 62 and 68–71.
1. Malnati MS, Peruzzi M, Parker KC, Biddison WE, Ciccone E, Moretta A, et al. Peptide Specificity in the Recognition of MHC Class I by Natural Killer Cell Clones. Science (1995) 267:1016–8. doi: 10.1126/science.7863326
2. Peruzzi M, Parker KC, Long EO, Malnati MS. Peptide Sequence Requirements for the Recognition of HLA-B*2705 by Specific Natural Killer Cells. J Immunol (1996) 157:3350–6.
3. Zappacosta F, Borrego F, Brooks AG, Parker KC, Coligan JE. Peptides Isolated From HLA-Cw*0304 Confer Diferent Degrees of Protection From Natural Killer Cell-Mediated Lysis. Proc Natl Acad Sci USA (1997) 94:6313–8. doi: 10.1073/pnas.94.12.6313
4. Mandelboim O, Wilson SB, Vales-Gomez M, Reyburn HT, Strominger JL. Self and Viral Peptides Can Initiate Lysis by Autologous Natural Killer Cells. Proc Natl Acad Sci USA (1997) 94:4604–9. doi: 10.1073/pnas.94.9.4604
5. Rajagopalan S, Long EO. The Direct Binding of a P58 Killer Cell Inhibitory Receptor to Human Histocompatibility Leukocyte Antigen (HLA)-Cw4 Exhibits Peptide Selectivity. J Exp Med (1997) 185:1523–8. doi: 10.1084/jem.185.8.1523
6. Hansasuta P, Dong T, Thananchai H, Weekes M, Willberg C, Aldemir H, et al. Recognition of HLA-A3 and HLA-A11 by KIR3DL2 Is Peptide-Specific. Eur J Immunol (2004) 34:1673–9. doi: 10.1002/eji.200425089
7. Kelly A, Trowsdale J. Genetics of Antigen Processing and Presentation. Immunogenetics (2019) 71:161–70. doi: 10.1007/s00251-018-1082-2
8. Murphy KM, Weaver C, Berg LJ. Janeway’s Immunobiology. Tenth Edition. New York & London: Garland Science, Taylor & Francis Group (2022)
9. Tailor A. Aminopeptidases: Structure and Function. FASEB J (1993) 7:290–8. doi: 10.1096/fasebj.7.2.8440407
10. Tsujimoto M, Aoki K, Goto Y, Ohnishi A. Molecular and Functional Diversity of the Oxytocinase Subfamily of M1 Aminopeptidases. J Biochem (2021) 169:409–20. doi: 10.1093/jb/mvab009
11. Tsujimoto M, Hattori A. The Oxitocinase Subfamily of M1 Aminopeptidases. Biochim Biophys Acta (2005) 1751:9–18. doi: 10.1016/j.bbapap.2004.09.011
12. Weimershaus M, Evnouchidou I, Saveanu L, van Endert P. Peptidases Trimming MHC Class I Ligands. Curr Opin Immunol (2013) 25:90–6. doi: 10.1016/j.coi.2012.10.001
13. Descamps D, Evnouchidou I, Caillens V, Drajac C, Riffaut S, van Endert P, et al. The Role of Insulin Regulated Aminopeptidase in Endocytic Trafficking and Receptor Signaling in Immune Cells. Front Mol Biosci (2020) 7:58355. doi: 10.3389/fmolb.2020.58355
14. Hanson AL, Morton CJ, Parker MW, Bessette D, Kenna TJ. The Genetics, Structure and Function of the M1 Aminopeptidase Oxytocinase Subfamily and Their Therapeutic Potential in Immune-Mediated Disease. Hum Immunol (2019) 80:281–9. doi: 10.1016/j.humimm.2018.11.002
15. Nguyen TT, Chang SC, Evnouchidou I, York IA, Zikos C, Kenneth L, et al. Structural Basis for Antigenic Peptide Prcursor Processing by the Endoplasmic Reticulum Aminopeptidase ERAP1. Nat. Struct Mol Biol (2011) 18:604–13. doi: 10.1038/nsmb.2021
16. Giastas P, Mpakali A, Papakyriakou A, Lelis A, Kokkala P, Margarete Neu M, et al. Mechanism for Antigenic Peptide Selection by Endoplasmic Reticulum Aminopeptidase 1. Proc Natl Acad Sci USA (2019) 116:26709–16. doi: 10.1073/pnas.1912070116
17. Lopez de Castro JA, Stratikos E. Intracellular Antigen Processing by ERAP2: Molecular Mechanism and Roles in Health and Disease. Hum Immunol (2019) 80:310–7. doi: 10.1016/j.humimm.2018.11.001
18. López de Castro JA, Alvarez-Navarro C, Brito A, Guasp P, Martin-Esteban A, Sanz-Bravo A. Molecular and Pathogenic Effects of Endoplasmic Reticulum Aminopeptidases ERAP1 and ERAP2 in MHC-I-Associated Inflammatory Disorders: Towards a Unifying View. Molec Immunol (2016) 77:193–204. doi: 10.1016/j.molimm.2016.08.005
19. López de Castro JA. How ERAP1 and ERAP2 Shape the Peptidomes of Disease-Associated MHC-I Proteins. Front Immunol (2018) 9:2463. doi: 10.3389/fimmu.2018.02463
20. Admon A. ERAP1 Shapes Just Part of the Immunopeptidome. Hum Immunol (2019) 80:296–301. doi: 10.1016/j.humimm.2019.03.004
21. Paladini F, Fiorillo MT, Tedeschi V, Mattorre B, Sorrentino R. The Multifaceted Nature of Aminopeptidases ERAP1, ERAP2, and LNPEP: From Evolution to Disease. Front Immunol (2020) 11:1576. doi: 10.3389/fimmu.2020.01576
22. Hearn A, York IA, Rock KL. The Specificity of Trimming of MHC Class I-Presented Peptides in the Endoplasmic Reticulum. J Immunol (2009) 183:5526–36. doi: 10.4049/jimmunol.0803663
23. Fruci D, Romania P, D’Alicandro V, Locatelli F. Endoplasmic Reticulum Aminopeptidase 1 Function and Its Pathogenic Role in Regulating Innate and Adaptive Immunity in Cancer and Major Histocompatibility Complex Class I-Associated Autoimmune Diseases. Tissue Antigens (2014) 84:177–86. doi: 10.1111/tan.12410
24. Ombrello MJ, Kastner DL, Remmers EF. Endoplasmic Reticulum-Associated Amino-Peptidase 1 and Rheumatic Disease: Genetics. Curr Opin Rheumatol (2015) 27:349–56. doi: 10.1097/BOR.0000000000000189
25. Hutchinson JP, Temponeras I, Kuiper J, Cortes A, Korczynska J, Kitchen JS, et al. Common Allotypes of ER Aminopeptidase 1 Have Substrate-Dependent and Highly Variable Enzymatic Properties. J Biol Chem (2021) 296:100443. doi: 10.1016/j.jbc.2021.100443
26. Tedeschi V, Paldino G, Paladini F, Mattorre B, Tuosto L, Sorrentino R, et al. The Impact of the ‘Mis-Peptidome’ on HLA Class I-Mediated Diseases: Contribution of ERAP1 and ERAP2 and Effects on the Immune Response. Int J Molec Sci (2020) 21:9608. doi: 10.3390/ijms21249608
27. Kuiper JJW, van Setten J, Devall M, Cretu-Stancu M, Hiddingh S, Ophoff RA, et al. Functionally Distinct ERAP1 and ERAP2 Are a Hallmark of HLA-A29-(Birdshot) Uveitis. Hum Mol Genet (2018) 27:4333–43. doi: 10.1093/hmg/ddy319
28. Andrés AM, Dennis MY, Kretzschmar WW, Cannons JL, Lee-Lin SQ, Hurle B, et al. Balancing Selection Maintains a Form of ERAP2 That Undergoes Nonsense-Mediated Decay and Affects Antigen Presentation. PLoS Genet (2010) 6:e1001157. doi: 10.1371/journal.pgen.1001157
29. Hattori A, Kitatani K, Matsumoto H, Miyazawa S, Rogi T, Tsuruoka N, et al. Characterization of Recombinant Human Adipocyte-Derived Leucine Aminopeptidase Expressed in Chinese Hamster Ovary Cells. J Biochem (2000) 128:755. doi: 10.1093/oxfordjournals.jbchem.a022812
30. Paladini F, Fiorillo MT, Vitulano C, Tedeschi V, Piga M, Cauli A, et al. An Allelic Variant in the Intergenic Region Between ERAP1 and ERAP2 Correlates With an Inverse Expression of the Two Genes. Sci Rep (2018) 8:10398. doi: 10.1038/s41598-018-28799-8
31. Lee ED. Endoplasmic Reticulum Aminopeptidase 2, A Common Immunological Link to Adverse Pregnancy Outcomes and Cancer Clearance? Placenta (2017) 56:40–3. doi: 10.1016/j.placenta.2017.03.012
32. Warthan MD, Washington SL, Franzese SE, Ramus RM, Kim K-R, York TP, et al. The Role of Endoplasmic Reticulum Aminopeptidase 2 in Modulating Immune Detection of Choriocarcinoma. Biol Reproduct (2018) 98:309–22. doi: 10.1093/biolre/ioy001
33. Lospinoso K, Dozmorov M, El Fawal N, Raghu R, Chae W-J, Lee ED. Overexpression of ERAP2N in Human Trophoblast Cells Promotes Cell Death. Int J Mol Sci (2021) 22:8585. doi: 10.3390/ijms221685
34. Vanhille DL, Hill LD, Hilliard DSD, Lee ED, Teves ME, Srinivas S, et al. A Novel ERAP2 Haplotype Structure in a Chilean Population: Implications for ERAP2 Protein Expression and Preeclampsia Risk. Mol Genet Genom Med (2013) 1:98–107. doi: 10.1002/mgg3.13
35. Martin-Esteban A, Sanz-Bravo A, Guasp P, Barnea E, Admon A, Lopez de Castro JA. Separate Effects of the Ankylosing Spondylitis Associated ERAP1 and ERAP2 Aminopeptidases Determine the Influence of Their Combined Phenotype on the HLA-B∗ 27 Peptidome. J Autoimmun (2017) 79:28–38. doi: 10.1016/j.jaut.2016.12.008I
36. Robinson PC, Costello M-E, Leo P, Bradbury LA, Hollis K, Cortes A, et al. ERAP2 Is Associated With Ankylosing Spondylitis in HLA-B27-Positive and HLA-B27-Negative Patients. Arch Rheumatol Dis (2015) 74:1627–9. doi: 10.1136/annrheumdis-2015-207416
37. Pepelyayeva Y, Amalfitano A. The Role of ERAP1 in Autoinflammation and Autoimmunity. Hum Immunol (2019) 80:302–9. doi: 10.1016/j.humimm.2019.02.013
38. Johnson MP, Roten LT, Dyer TD, East CE, Forsmo S, Blangero J, et al. The ERAP2 Gene Is Associated With Preeclampsia in Australian and Norwegian Populations. Hum Genet (2009) 126:655–66. doi: 10.1007/s00439-009-0714-x
39. Hill LD, Hilliard DD, York TP, Srinivas S, Kusanovic JP, Gomez R, et al. Fetal ERAP2 Variation Is Associated With Preeclampsia in African Americans in A Case-Control Study. BMC Med Genet (2011) 12:64. doi: 10.1186/1471-2350-12-64
40. Ferreira LC, Gomes CEM, Duggal P, Holanda IDP, de Lima AS, do Nascimento PRP, et al. Genetic Association of ERAP1 and ERAP2 With Eclampsia and Preeclampsia in Northeastern Brazilian Women. Sci Rep (2021) 11:6764. doi: 10.1038/s41598-021-86240-z
41. Piekarska K, Radwan P, Tarnowska A, Wiśniewski A, Radwan M, Wilczyński JR, et al. ERAP, KIR, and HLA-C Profile in Recurrent Implantation Failure. Front Immunol (2021) 12:755624. doi: 10.3389/fimmu.2021.755624
42. Saulle I, Ibba SV, Torretta E, Vittori C, Fenizia C, Piancone F, et al. Endoplasmic Reticulum Associated Aminopeptidase 2 (ERAP2) Is Released in the Secretome of Activated MDMs and Reduces In Vitro HIV-1 Infection. Front Immunol (2019) 10:16482019. doi: 10.3389/fimmu.2019.016482019
43. Saulle I, Marventano I, Saresella M, Vanetti C, Garziano M, Fenizia C, et al. ERAPs Reduce In Vitro HIV Infection by Activating Innate Immune Response. J Immunol (2021) 206:1609–17. doi: 10.4049/jimmunol.2000991
44. Hanson AL, Cuddihy T, Haynes K, Loo D, Morton CJ, Oppermann U, et al. Genetic Variants in ERAP1 and ERAP2 Associated With Immune-Mediated Diseases Influence Protein Expression and Isoform Profile. Arthritis Rheumatol (2018) 70:255–65. doi: 10.1002/art.40369
45. Ye CJ, Chen J, Villani A-C, Gate RE, Subramaniam M, Bhangale T, et al. Genetic Analysis of Isoform Usage in the Human Anti-Viral Response Reveals Influenza-Specific Regulation of ERAP2 Transcripts Under Balancing Selection. Genome Res (2018) 28:812–1825. doi: 10.1101/gr.240390.118
46. Saulle I, Vanetti C, Goglia S, Vincentini C, Tombetti E, Garziano M, et al. A New ERAP2/Iso3 Isoform Expression Is Triggered by Different Microbial Stimuli in Human Cells. Could It Play a Role in the Modulation of SARS-CoV-2 Infection? Cells (2020) 9:1951. doi: 10.3390/cells9091951
47. Evnouchidou I, Weimershaus M, Saveanu L, van Endert P. ERAP1–ERAP2 Dimerization Increases Peptide-Trimming Efficiency. J Immunol (2014) 193:901–8. doi: 10.4049/jimmunol.1302855
48. Evnouchidou I, van Endert P. Peptide Trimming by Endoplasmic Reticulum Aminopeptidases: Role of MHC Class I Binding and ERAP Dimerization. Hum Immunol (2019) 80:290–5. doi: 10.1016/j.humimm.2019.01.003
49. Papakyriakou A, Mpakali A, Stratikos E. Can ERAP1 and ERAP2 Form Functional Heterodimers? A Structural Dynamics Investigation. Front Immunol (2022) 13:863529. doi: 10.3389/fimmu.2022.863529
50. Hisatsune C, Ebisui E, Usui M, Ogawa N, Akio Suzuki A, Nobuko Mataga N, et al. ERp44 Exerts Redox-Dependent Control of Blood Pressure at the ER. Molec Cell (2015) 58:1015–27. doi: 10.1016/j.molcel.2015.04.008
51. Stamatakis G, Samiotaki M, Mpakali A, Panayotou G, Stratikos E. Generation of SARS-CoV-2 S1 Spike Protein Putative Antigenic Epitopes In Vitro by Intracellulr Aminopeptidases. J Proteome Res (2020) 19:4398–406. doi: 10.1021/acs.jproteome.0c00457
52. Australo-Anglo-American Spondyloarthritis Consortium (TASC), Reveille JD, Sims A-M, Danoy P, Evans DM, Leo P, et al. Genome-Wide Association Study of Ankylosing Spondylitis Identifies Non-MHC Susceptibility Loci. Nat Genet (2010) 42:123–7. doi: 10.1038/ng.513
53. Wellcome Trust Case Control Consortium, Australo-Anglo-American Spondylitis Consortium (TASC), Burton PR, Clayton DG, Cardon LR, Craddock N, et al. Association Scan of 14,500 Nonsynonymous SNPs in Four Diseases Identifies Autoimmunity Variants. Nat Genet (2007) 39:329–1337. doi: 10.1038/ng.2007.17
54. Evans DM, Spencer CCA, Pointon JJ, Su Z, Harvey D, Kochan G, et al. Interaction Between ERAP1 and HLA-B27 in Ankylosing Spondylitis Implicates Peptide Handling in the Mechanism for HLA-B27 in Disease Susceptibility. Nat Genet (2011) 43:761–7. doi: 10.1038/ng.873
55. Schmidt AH, Solloch UV, Pingel J, Baier D, Bohme I, Dubicka K, et al. High-Resolution Human Leukocyte Antigen Allele and Haplotype Frequencies of the Polish Population Based on 20,653 Stem Cell Donors. Hum Immunol (2011) 72:558–65. doi: 10.1016/j.humimm.2011.03.010
56. Pedersen SJ, Maksymowych WP. Beyond the TNF-α Inhibitors: New and Emerging Targeted Therapies for Patients With Axial Spondyloarthritis and Their Relation to Pathophysiology for Patients With Axial Spondyloarthritis and Their Relation to Pathophysiology. Drugs (2018) 78:1397–418. doi: 10.1007/s40265-018-0971-x
57. Kavadichanda CG, Geng J, Bulusu SN, Vir Singh Negi VS, Raghavan M. Spondyloarthritis and the Human Leukocyte Antigen (HLA)-B*27 Connection. Front Immunol (2021) 12:601518. doi: 10.3389/fimmu.2021.601518
58. Wordsworth BP, Cohen CJ, Davidson C, Vecellio M. Perspectives on the Genetic Associations of Ankylosing Spondylitis. Front Immunol (2021) 12:603726. doi: 10.3389/fimmu.2021.603726
59. Wiśniewski A, Kasprzyk S, Majorczyk E, Nowak I, Wilczyńska K, Chlebicki A, et al. ERAP1-ERAP2 Haplotypes Are Associated With Ankylosing Spondylitis in Polish Patients. Hum Immunol (2019) 80:339–43. doi: 10.1016/j.humimm.2019.02.004
60. Strange A, Capon F, Spencer CC, Knight J, Weale ME, Allen MH, et al. Genome Wide Association Study Identifies New Psoriasis Susceptibility Loci and an Interaction Between HLA-C and ERAP1. Nat Genet (2010) 42:985–90. doi: 10.1038/ng.694
61. Lysell J, Padyukov L, Kockum I, Nikamo P, Ståhle M. Genetic Association With ERAP1 in Psoriasis Is Confined to Disease Onset After Puberty and Not Dependent on HLA-C*06. J Investig Dermatol (2013) 133:411–7. doi: 10.1038/jid.2012.280
62. Wiśniewski A, Matusiak Ł., Szczerkowska-Dobosz A, Nowak I, Łuszczek W, Kuśnierczyk P. The Association of ERAP1 and ERAP2 Single Nucleotide Polymorphisms and Their Haplotypes With Psoriasis Vulgaris Is Dependent on the Presence or Absence of the HLA-C*06:02 Allele and Age at Disease Onset. Hum Immunol (2018) 79:109–16. doi: 10.1016/j.humimm.2017.11.010
63. Lande R, Botti E, Jandus C, Dojcinovic D, Fanelli G, Conrad C, et al. The Antimicrobial Peptide LL37 Is a T-Cell Autoantigen in Psoriasis. Nat Commun (2014) 5:1–15. doi: 10.1038/ncomms6621
64. Arakawa A, Siewert K, Stöhr J, Besgen P, Kim S-M, Rühl G, et al. Melanocyte Antigen Triggers Autoimmunity in Human Psoriasis. J Exp Med (2015) 212:2203–12. doi: 10.1084/jem.20151093
65. Johnston A, Gudjonsson JE, Sigmundsdottir H, Love TJ, Valdimarsson H. Peripheral Blood T Cell Responses to Keratin Peptides That Share Sequences With Streptococcal M Proteins Are Largely Estricted to Skin-Homing CD8+ T Cells. Clin Exp Immunol (2004) 138:83–93. doi: 10.1111/j.1365-2249.2004.02600.x
66. Gong JJ, Margolis DJ, Monos DS. Predictive in Silico Binding Algorithms Reveal HLA Specifcities and Autoallergen Peptides Associated With Atopic Dermatitis. Arch Dermatol Res (2020) 312:647–56. doi: 10.1007/s00403-020-02059-0
67. Margolis DJ, Mitra N, Kim BS, Duke JL, Berna RA, Hoffstad OJ, et al. HLA Class I Polymorphisms Influencing Both Peptide Binding and KIR Interactions Are Associated With Remission Among Children With Atopic Dermatitis: A Longitudinal Study. J Immunol (2021) 206:2038–44. doi: 10.4049/jimmunol.2001252
68. Niepiekło-Miniewska W, Majorczyk E, Matusiak Ł., Gendzekhadze K, Nowak I, Narbutt J, et al. Protective Effect of the KIR2DS1 Gene in Atopic Dermatitis. Gene (2013) 527:594–600. doi: 10.1016/j.gene.2013.06.015
69. Niepiekło-Miniewska W, Mpakali A, Stratikos E, Matusiak Ł., Narbutt J, Lesiak A, et al. Endoplasmic Reticulum Aminopeptidase 1 Polymorphism Ile276Met is Associated With Atopic Dermatitis and Affects the Generation of an HLA-C Associated Antigenic Epitope In Vitro. J Eur Acad Dermatol Veneorol (2019) 33:906–11. doi: 10.1111/jdv.15449
70. Niepiekło-Miniewska W, Matusiak Ł., Narbutt J, Lesiak A, Kuna P, Wiśniewski A, et al. Synergy of Endoplasmic Reticulum Aminopeptidase 1 and 2 (ERAP1 and ERAP2) Polymorphisms in Atopic Dermatitis: Effects on Disease Prevalence. Hum Immunol (2021) 82:121–3. doi: 10.1016/j.humimm.2020.11.004
71. Wiśniewski A, Sobczyński M, Pawełczyk K, Porębska I, Jasek M, Wagner M, et al. Polymorphisms of Antigen-Presenting Machinery Genes in Non-Small Cell Lung Cancer: Different Impact on Disease Risk and Clinical Parameters in Smokers and Never-Smokers. Front Immunol (2021) 12:6644742021. doi: 10.3389/fimmu.2021.6644742021
72. Cui X, Rouhani FN, Hawari F, Levine SJ. Shedding of the Type II IL-1 Decoy Receptor Requires a Multifunctional Aminopeptidase, Aminopeptidase Regulator of TNF Receptor Type 1 Shedding. J Immunol (2003) 171:6814–9. doi: 10.4049/jimmunol.171.12.6814
73. Cui X, Rouhani FN, Hawari F, Levine SJ. An Aminopeptidase, ARTS-1, Is Required for Interleukin-6 Receptor Shedding. J Biol Chem (2003) 278:28677–85. doi: 10.1074/jbc.M300456200
74. Cifaldi L, Romania P, Silvia Lorenzi S, Franco Locatelli F, Fruci D. Role of Endoplasmic Reticulum Aminopeptidases in Health and Disease: From Infection to Cancer. Int J Mol Sci (2012) 13:8338–52. doi: 10.3390/ijms13078338
75. Kefaloyianni E. Soluble Forms of Cytokine and Growth Factor Receptors: Mechanisms of Generation and Modes of Action in the Regulation of Local and Systemic Inflammation. FEBS Lett (2022) 596:589–606. doi: 10.1002/1873-3468.14305
76. Cuevas S, Villar VAM, Jose PA. Genetic Polymorphisms Associated With Reactive Oxygen Species and Blood Pressure Regulation. Pharmacogenomics J (2019) 19:315–36. doi: 10.1038/s41397-019-0082-4
77. Paz Ocaranza M, Riquelme JA, García L, Jalil JE, Chiong M, Santos RAS, et al. Counter-Regulatory Renin–Angiotensin System in Cardiovascular Disease. Nat Rev Cardiol (2020) 17:116–29. doi: 10.1038/s41569-019-0244-8
78. Yamamoto N, Nakayama J, Yamakawa-Kobayashi K, Hamaguchi H, Miyazaki R, Arinami T. Identification of 33 Polymorphisms in the Adipocyte-Derived Leucine Aminopeptidase (ALAP) Gene and Possible Association With Hypertension. Hum Mutat (2002) 19:251–7. doi: 10.1002/humu.10047
79. Goto Y, Hattori A, Ishii Y, Tsujimoto M. Reduced Activity of the Hypertension-Associated Lys528Arg Mutant of Human Adipocyte-Derived Leucine Aminopeptidase (A-LAP)/ER-Aminopeptidase-1. FEBS Lett (2006) 580:1833–8. doi: 10.1016/j.febslet.2006.02.041
80. Goto Y, Tanji H, Hattori A, Tsujimoto M. Glutamine-181 Is Crucial in the Enzymatic Activity and Substrate Specificity of Human Endoplasmic-Reticulum Aminopeptidase-1. Biochem J (2008) 416:109–16. doi: 10.1042/BJ20080965
Keywords: ERAP2, genetics, peptide trimming, interactions with ERAP1, polymorphism, isoforms, endoplasmic reticulum aminopeptidase, ERAP1 interaction
Citation: Kuśnierczyk P (2022) To Be or Not to Be: The Case of Endoplasmic Reticulum Aminopeptidase 2. Front. Immunol. 13:902567. doi: 10.3389/fimmu.2022.902567
Received: 23 March 2022; Accepted: 09 May 2022;
Published: 13 June 2022.
Edited by:
Peter M. Van Endert, Institut National de la Santé et de la Recherche Médicale (INSERM), FranceReviewed by:
Athanasios Papakyriakou, National Centre of Scientific Research Demokritos, GreeceCopyright © 2022 Kuśnierczyk. This is an open-access article distributed under the terms of the Creative Commons Attribution License (CC BY). The use, distribution or reproduction in other forums is permitted, provided the original author(s) and the copyright owner(s) are credited and that the original publication in this journal is cited, in accordance with accepted academic practice. No use, distribution or reproduction is permitted which does not comply with these terms.
*Correspondence: Piotr Kuśnierczyk, cGlvdHIua3VzbmllcmN6eWtAaGlyc3pmZWxkLnBs
Disclaimer: All claims expressed in this article are solely those of the authors and do not necessarily represent those of their affiliated organizations, or those of the publisher, the editors and the reviewers. Any product that may be evaluated in this article or claim that may be made by its manufacturer is not guaranteed or endorsed by the publisher.
Research integrity at Frontiers
Learn more about the work of our research integrity team to safeguard the quality of each article we publish.