- 1Department of Biochemistry and Molecular Biology, Hebei Medical University, Shijiazhuang, China
- 2The Second General Surgery, The Fourth Hospital of Hebei Medical University, Shijiazhuang, China
- 3National Research Institute for Family Planning, National Human Genetic Resources Center, Beijing, China
- 4Graduate School, Peking Union Medical College, Beijing, China
- 5Department of Otolaryngology, Henan Province Hospital of Traditional Chinese Medicine, Zhengzhou, China
The tumor microenvironment (TME) is defined as a complex and dynamic tissue entity composed of endothelial, stromal, immune cells, and the blood system. The homeostasis and evolution of the TME are governed by intimate interactions among cellular compartments. The malignant behavior of cancer cells, such as infiltrating growth, proliferation, invasion, and metastasis, is predominantly dependent on the bidirectional communication between tumor cells and the TME. And such dialogue mainly involves the transfer of multifunctional regulatory molecules from tumor cells and/or stromal cells within the TME. Interestingly, increasing evidence has confirmed that exosomes carrying regulatory molecules, proteins, and nucleic acids act as an active link in cellular crosstalk in the TME. Notably, extensive studies have identified non-coding RNAs (ncRNAs), including long non-coding RNAs (lncRNAs), microRNAs (miRNAs), and circular RNAs (circRNAs), that could be encapsulated by exosomes, which regulate the coordinated function within the TME and thus participate in cancer development and progression. In this review, we summarize recent literature around the topic of the functions and mechanisms of exosomal ncRNAs in the TME and highlight their clinical significance.
Introduction
Tumors are stubbornly described as a simply accumulation of oncocytes. However, it is rather a heterogeneous collection of tumor, immune, stromal cells, various signaling molecules, and luxuriant extracellular matrix (ECM), namely the tumor microenvironment (TME) (1). TME refers to the complex and plentiful multicellular environment shaped by tumor cells that is permissive for their survival and growth. During the early stages of neoplasia, a vibrant interactive partnership emerges between tumor cells and constituents of the TME that renders tumor cell local infiltration and distant dissemination possible. In an attempt to conquer hypoxic, a prominent element compared to the natural inherent environment, TME has organized an accelerated angiogenesis operation to maintain the supply of oxygen and nutrients and to clear metabolic waste products. Nowadays, the paradigm of cancer research and treatment has shifted from a tumor-centered model to a TME-centered model.
As a subtype of extracellular vesicles (EVs)—a group of membranous vesicles shed from the plasmalemma with encapsulated particles—exosomes are characterized by a lipid bilayer membrane extracellular structure with an endosomal origin (2). In addition to proteins and DNAs, exosomes generally encompass lipids, mRNAs, as well as non-coding RNAs (ncRNAs) (3). Although exosomes share common characteristics and structures, the molecular constitutions of exosomes secreted by different cell types vary extraordinarily. As a robust messenger of intercellular communication, exosomes play a pivotal role in mediating cancer progression. Exosomes released by tumor cells participate in substance transmission and information exchange in vivo and serve as the benchmark for cancer development and progression (4). Moreover, exosome biology and its multifaceted functions in the TME have drawn increasing attention in recent years. Of note, with the advanced proceedings in ncRNA sequencing methodologies, the special characters of exosomes in the TME are increasingly elicited, which has provoked tremendous interest in this research field.
ncRNAs are mainly subdivided into three main categories, namely: long ncRNAs (lncRNAs), microRNAs (miRNAs), and circular RNAs (circRNAs) (5). Until recently, the prevalence of ncRNAs in intercellular communication has been demonstrated by various investigations, thus shedding light on the complex crosstalk between cancer cells and non-cancer cells. On one hand, ncRNAs represent one of the most informative and significant components of all exosomal cargos (6). On the other hand, ncRNAs are increasingly being recognized as key regulators involved in the TME (7). Furthermore, tumor-derived exosomes (TDEs) contain a considerable proportion of ncRNAs, which are implicated in modulating cellular interplays and communication in the TME. Recent findings have strongly pinpointed that the dysfunction of global exosomal ncRNAs abundance is correlated with cancer immunity, metastasis, angiogenesis, drug resistance, and cancer stem cell (CSC) stemness (8–11). The multiple roles of exosomal ncRNAs in the TME explain their potential emerging clinical implications for developing novel treatment applications. Additionally, the exosomal ncRNAs display a variety of characteristics, including high abundance, relative stability, and cross-species conservation. And these features are critical considering the current worldwide endeavors to determine ncRNA signatures in tumor biopsy tissues using high-throughput sequencing technologies to develop effective diagnostic and prognostic biomarkers.
Nevertheless, the underlying mechanism of exosomal ncRNAs in regulating the TME is still unclear, warranting further exploration. Therefore, uncovering the exact functions and underlying regulatory mechanisms of exosomal ncRNAs in the TME is essential for optimizing current therapeutic strategies. Our previous study concluded the functions and mechanisms of ncRNAs in colorectal cancer (CRC) (12). This review aims to summarize the interactions between exosomal ncRNAs and TME. Also, the promising clinical significance of exosomal ncRNAs in tumor immunotherapy has been discussed in this paper.
Biogenesis and Characteristics of Exosomes
Exosomes are nanoscale vesicles with a size ranging from 30–150 nm (13). Current knowledge has confirmed that exosomes are formed by dynamic multistep endocytosis pathways (14). A natural course of exosome formation is composed of the following procedures (Figure 1). First, the cytoplasmic membrane invaginates to materialize endocytic vesicles, which in turn form early secretory endosomes. Subsequently, with the assistance of the Golgi complex, early endosomes undergo some changes and gradually mature into late endosomes (15). Then, in a series of finely modulated maturation processes, membrane-enclosed ILVs are produced by the inward budding of late endosomal compartments limiting membrane. Different types of cargo are sorted into ILVs during this procedure. Thereafter, this structure is referred to as an MVB, which is then carried to the cell surface along protein tracks. Then, the MVBs fuse with either the lysosome (where the ILVs are disintegrated) or the plasma membrane, activating the discharge of the vesicles inside the MVBs (16). Once these vesicles have been released by fusion with the plasma membrane, they eventually become known as exosomes. Notably, during the period of exosome biogenesis, various surface proteins and molecules (e.g., CD9, CD63, CD81, integrin, and flotillin) that are characteristics of the parent cells are selectively expressed on released exosomes.
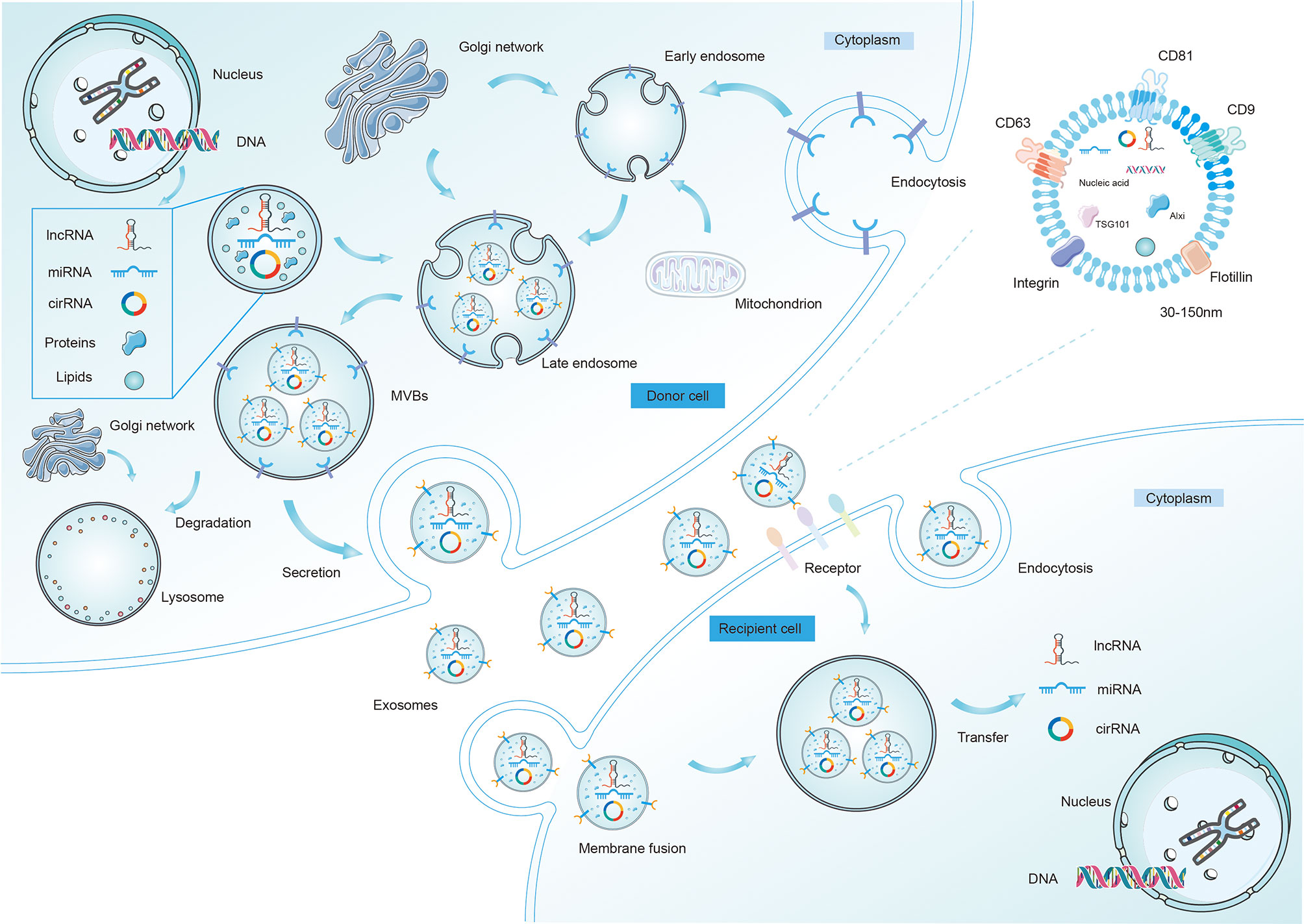
Figure 1 The biogenesis, release, and characteristics of exosomes. The formation and release of exosomes is a multi-step process. First, it begins with early endosome formation through endocytosis of the plasma membrane, followed by formation of the late endosome with different ncRNAs, lipids, and proteins inside. Second, the late endosomes undergo multiple depressions and bud inward to form microvesicles, thus transforming into multi-vesicular bodies (MVBs), which then may be degraded by lysosomes or fuse with the cell membrane and are released into the extracellular space as exosomes. After being secreted from donor cells into extracellular matrix, exosomes can be taken up by recipient cells and regulate the behaviors of the recipient cells by transferring regulatory non-coding RNAs (mainly miRNAs, lncRNAs, and circRNAs). Exosomes enter recipient cells in three ways, which are fusion, endocytosis, and receptor interactions. Several proteins are used as markers for exosomes (CD9, CD63, CD81, flotillin, integrin). Exosomes (30-150nm) also contain different intracellular proteins (such as TSG101 and Alix), RNAs, DNAs, and metabolites.
As previously mentioned, exosomes contain diverse cargos, including proteins and RNA molecules. Most recently, exosomal RNA cargoes have been broadly investigated as to their crucial roles in intercellular communication during TME evolution. Accumulating evidence has indicated a conspicuous discordant enrichment distribution of RNAs in exosomes owing to the intrinsic sorting mechanism that aggressively manipulates the exosomal loading of certain RNAs. And these machineries appear to be based on RNA binding proteins (RBPs) and their affiliated companions, which dispatch RNAs to predetermined locations of exosome formation and shield RNAs from damage or destruction (17). The present research mainly concentrates on the regulatory factors of miRNA partitioning into exosomes. The enrichment of intracytoplasmic miRNAs is likely to be the first layer to bear the brunt since the presence of the miRISCs complex (main components within it are AGOs and GW182), which possesses the ability to drive the RNA-silencing process and affect miRNA pool placement (18). Interestingly, AGO2 can be passively incorporated into exosomes and therefore fine-tune the sorting of specific miRNAs (e.g., let-7a), revealing that AGO2 acts as an imperative transporter for exosomal miRNAs (19). Nonetheless, the biological significance of this voluntary sorting process in lncRNAs and circRNAs is not yet fully understood.
These secretory exosomes can be transported into adjacent or distant cells to mediate intercellular interactions by transferring regulatory ncRNAs or other functional molecules (20). Exosomes that reach other cells can function by binding to protein receptors on the cell surface. For one thing, exosomes directly perceive surface molecules located on the target cell, inducing signal transduction through receptor-ligand interactions. For another, via fusing with the plasma membranes of recipient cells, exosomes can also emit their contents into the cytosol, leading to changes in cellular functions. Additionally, receptor cells are able to take up exosomes through endocytosis. It has been shown that exosomes can preserve their cargo (including three main ncRNA species) from enzymatic degradation in plasma and bile. Correspondingly, exosomal ncRNAs are more stable, so they can exert stronger effects on recipient cells through their actions on target genes and pathways compared to those ncRNAs directly released into the extracellular space. To illustrate, lncUCA1 showed a discordant profile in serum exosomes and homologous cancerous tissues. Specifically, in CRC, the abundance of UCA1 was detected to be downregulated in TDEs while upregulated in CRC biopsies (21). This conflicting finding might be explained by the fact that UCA1 was cherished by CRC cells, which impelled the preservation of this lncRNA by tumor cells by reducing its release through exosomes. Another thing is that such a differential distribution tendency between primary cancers and exosomes might be the result of asymmetric interactions and ncRNA crosstalk among TME cells (22). Despite intensive studies on the biogenesis and characterization of exosomes, however, very little is known about their biological roles in exosome-secreting cells. As such, systematic experimental research is currently needed to elucidate the detailed functions and intrinsic sorting mechanisms of exosomal ncRNAs.
The Inherent Characteristics and Functions of the TME
The TME is a complex and dynamic ecosystem involved in various stages of tumorigenesis. It plays a multifaceted role in driving tumor initiation, promotion, propagation, and metastasis. And such involvement relies on intercellular interactions between cancer and non-cancer cells, where the trajectory of cancer greatly depends on the spatiotemporal communication between various cell types. It is, therefore, essential to more thoroughly deconstruct the complexity of the TME and to identify how TME cells crosstalk with each other to create an environment favorable for tumor progression. The TME is composed of assorted ingredients, including immune cells [e.g., various types of lymphocytes, natural killer cells (NKs), regulatory T cells (Tregs), and dendritic cells (DCs)], stromal constitutions (e.g., fibroblasts and fibers), tumor cells, myeloid-derived suppressor cells (MDSCs), cancer-associated fibroblasts (CAFs), and tumor-associated macrophages (TAMs), as well as other infiltrated biomolecules (23) (Figure 2). It is noteworthy that prior studies predominantly focused on tumor cells. But cancerous cells are not alone, as TME functions as a necessary determinant in altering both cancer cells and the surrounding stroma during cancer occurrence and development (24). This phenomenon gives rise to a mode of conversion in which carcinoma is currently recognized not only as a disorder defined by molecular (epigenetic and inherited) events within the cells, but also as an “ecological disease” manipulated by TME components (2).
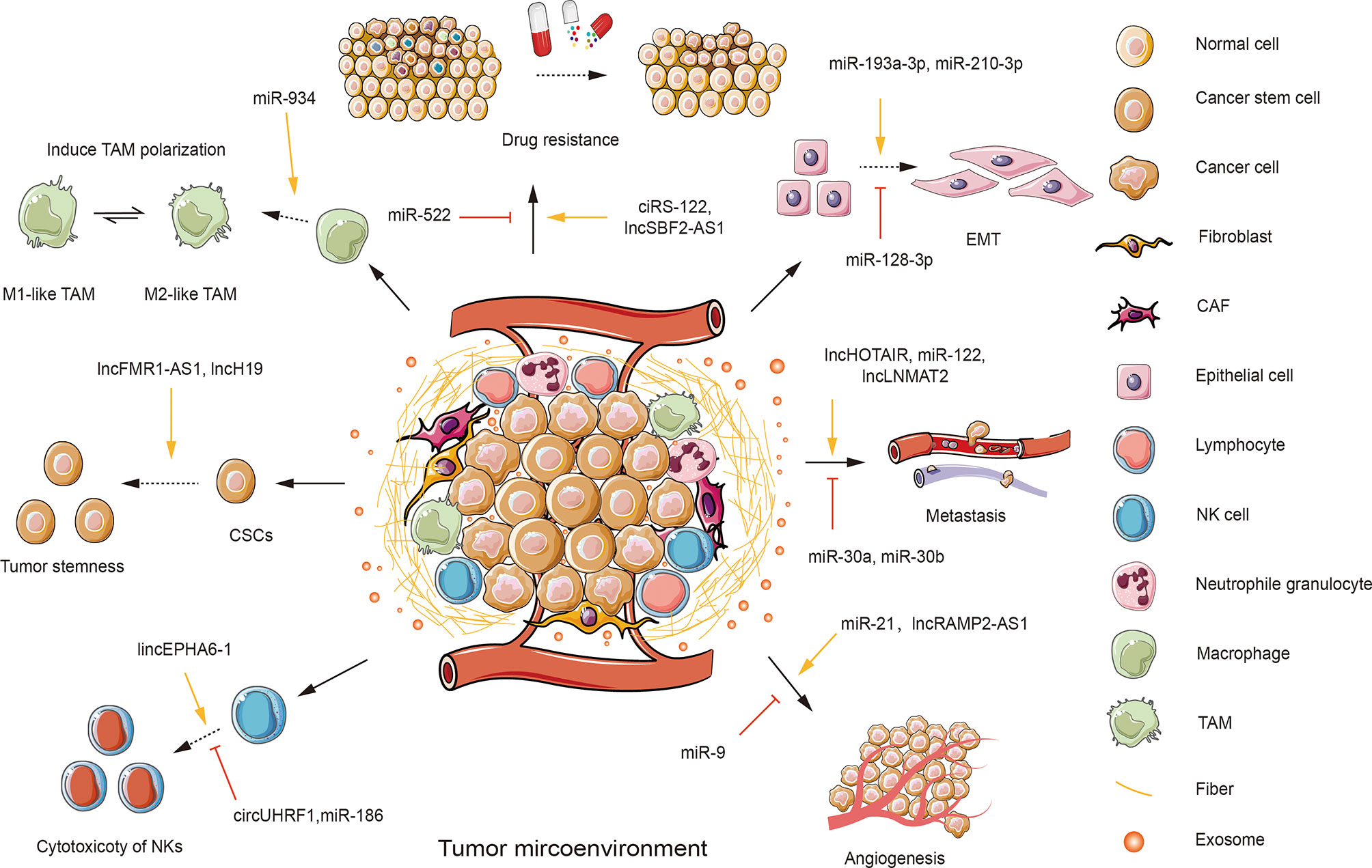
Figure 2 An overview of the roles of exosomal ncRNAs in the tumor microenvironment (TME). TME is composed of various components, including stromal cells, such as fibroblasts; immunity cells, such as T and B lymphocytes, natural killer cells (NKs), neutrophile granulocytes, macrophages; cancer cells; cancer stem cells (CSCs); cancer-associated cells, such as cancer-associated fibroblasts (CAFs) and tumor-associated macrophages (TAMs); and other infiltrated biomolecules, such as exosomes. Tumor cells could transfer functional exosomal ncRNAs to regulate the behaviors of recipient cells in the TME. Tumor-derived exosomal miR-934 can induce the polarization of TAMs. Several exosomal ncRNAs can suppress the cytotoxicity of NKs (e.g., circUHRF1 and miR-186). While some exosomal ncRNAs are shown to enhance the cytotoxicity of NKs (e.g., lincEPHA6-1). Some exosomal ncRNAs (e.g., lncFMR1-AS1 and miR-454) can enhance tumor stemness. Additionally, a few exosomal ncRNAs are involved in the regulation of tumor progression. Some exosomal ncRNAs can promote (e.g., miR-21 and lncRAMP2-AS1) or inhibit tumor angiogenesis (e.g., miR-9). Tumor-derived exosomes can transfer exosomal ncRNAs to induce (e.g., lncHOTAIR and miR-122) or suppress (e.g., miR-30a and miR-30b) tumor metastasis. Many exosomal ncRNAs play a role in facilitating (e.g., miR-193-3p and miR-210-3p) or repressing (e.g., miR-128-3p) epithelial-mesenchymal transition (EMT). Several exosomal ncRNAs are involved in promoting tumor drug resistance (e.g., ciRS-122 and lncSBF2-AS1).
The exchange and interaction of information within the TME have a significant impact on cancer initiation and progression, such as angiogenesis, metastasis, invasion, and other aggressive malignant behaviors of tumors (25). These tumor-influencing factors are largely dependent on TDEs excreted into the tumor stroma at the primary tumor site (26). Simultaneously, TDEs also impact non-cancerous cells, leading to the creation of a more aggressive TME that is conducive to tumor growth and metastatic spread (27). Numerous lines of literature imply that ncRNAs are selectively sorted into exosomes, which can be delivered and transferred between cells and regulate the initiation and development of cancer (4). More importantly, it has been confirmed that exosomal ncRNAs are one of the most decisive factors in cancer progression. They are involved in modulating cancer angiogenesis and metastasis, manipulating cancer chemoresistance, dominating host immune responses, and remodeling the cells in the TME (Figure 2).
TDEs can affect the functions of endothelial cells (ECs), thus inducing tumor angiogenesis and metastasis (28). In addition, TDEs are available to stimulate fibroblasts to differentiate into pro-tumorigenic CAFs and pro-angiogenic fibroblasts (29). Mechanistic studies revealed that it is cancer cell-derived exosomal ncRNAs that control these phenotypic switches. To further complicate matters, TDEs can initially inhibit immune cells, such as T cells, B cells, DCs, NKs, and so on. Subsequently, TDEs shape a T cell-absent immunosuppressive microenvironment to evade surveillance by excluding T cells from the neoplastic site or shifting immune cells into pro-tumorigenic or pro-metastatic phenotypes as the tumor progresses (30). And such phenotypic changes in immune cells are attributed to the transmission of functional oncoproteins from TDEs to recipient cells. Distinctively, these exosomal oncoproteins initiate MAPK and PI3K/AKT/mTOR pathways, facilitating tumorigenesis and metastasis (31). Further functional studies revealed that exosomal miR-934 markedly disturbs or impairs the functions of effector cells, including enhancing the enlargement trend and the immunosuppressive capacity of MDSCs, stimulating macrophages to polarize toward an M2-like phenotype, and inhibiting lymphocyte infiltration through matrix remodeling mediated by CAF (30). Growing evidence has verified that TDEs are indispensable for regulating cancer immunity, either through immune suppression or immune escape (32). For instance, glioma cells can shape and remodel immune cells by secreting exosomes. And these glioma-associated immune cells manage to control host immune responses and create an optimal environment for tumor survival and growth, thereby accelerating tumor development (33, 34).
The Functions of Exosomal ncRNAs in the TME
Adequate comprehension of the molecular mechanisms by which cancer-associated immunity influences the evolution of the TME is one of the priorities of immunological research, which may facilitate the development of more potent immunotherapies. In light of previous studies, cancer immunity is a double-edged sword in cancers, where it either protects the host or promotes tumorigenesis depending on the tissue specificity. Tumor cells create a favorable environment for themselves by releasing a series of regulatory cytokines, chemokines, and other factors that modify or recode the surrounding immune cells. The spatiotemporal dialogue between tumor cells and proximal immune cells eventually culminates in an aggressive TME that accelerates cancer cell local invasion and metastatic spread. The characteristics of exosomes in the immune response during cancer evolution partly depend upon where they come from. Because exosomes from immune cells or tumors have different compositions, which can exert distinct biological effects. Exosomes from immune cells, such as those secreted by DCs, can induce T helper 1 (Th1) cell responses (e.g., IFN-γ production) in culture (35). Exosomes shed by DCs pulsed with ovalbumin (OVA) are more effective than micro-vesicles in stimulating activation of antigen OVA-specific CD8+ T cells (36). Activated T cells have been shown to initiate the host immune system, thereafter generating an anti-tumor immune response capable of limiting tumor growth and metastasis. In general, immune cells actively interact with each other by spontaneously producing exosomes. However, in most investigations, they are stromal cell receivers of TDEs. The ncRNAs-containing TDEs are information carriers that can alter or reprogram the functions of immune cells such as various T cells, DCs, macrophages, NKs, and MDSCs, thereby disrupting the immune responses of the host (37).
The topic of exosomal ncRNAs in cancer immunity has evolved at an impressive rate in the past few decades, rendering it challenging or impractical to collect every single exosomal ncRNA in one review article. In what follows below, we concentrate predominantly on the most recent and paramount examples to expand the landscapes of representative exosomal ncRNAs in the TME. The regulation and mechanisms of several selected exosomal ncRNAs in immune cells are shown in Figure 3. Additionally, in Table 1, we summarize the functions and mechanisms of prominent exosomal ncRNAs in TME.
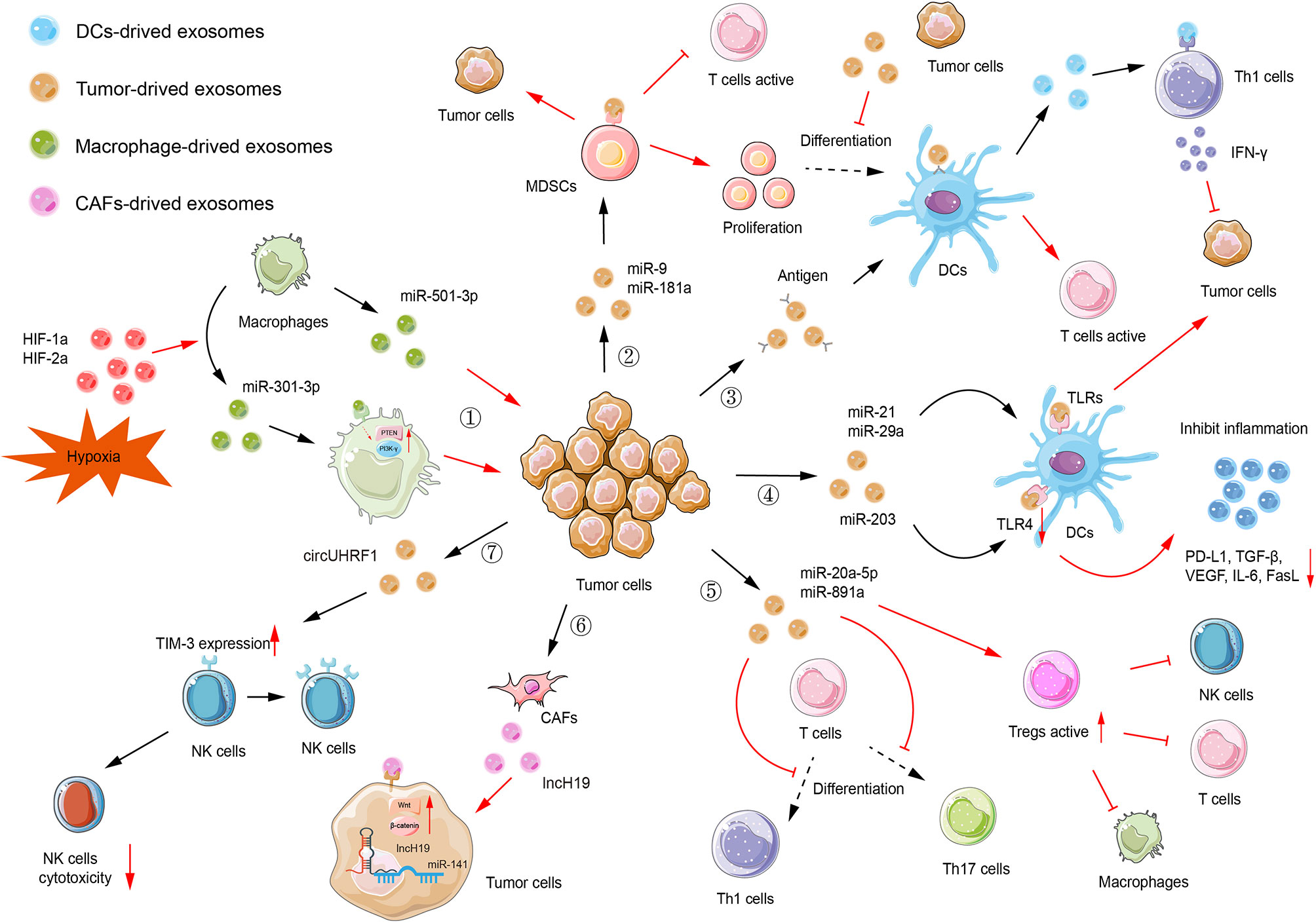
Figure 3 Exosomal ncRNAs-mediated immune regulation. (1) Macrophages-derived exosomal miR-501-3p facilitates tumor invasion and metastasis. Hypoxia can upregulate miR-301a-3p expression by modulating HIF-1a and HIF-2a, thus inhibiting the PTEN/PI3K-γ signaling pathway and promoting tumor invasion, migration, and epithelial-mesenchymal transition (EMT). (2) Tumor-derived exosomal miR-9 and miR-181a consequently affect proliferation of myeloid-derived suppressor cells (MDSCs) to promote tumorigenesis. Also, these MDSCs inhibit the activation of T cells. (3) Antigens can be encapsulated in exosomes and delivered to dendritic cells (DCs). Then, DCs activate T cells to initiate the immune response. Tumor-derived exosomes can inhibit the differentiation of MDSCs into DCs. DCs-derived exosomes facilitate the release of IFN-γ, which subsequently inhibits tumorigenesis. (4) Tumor-derived exosomal miR-21 and miR-29a can target TLRs to provoke pro-tumoral inflammation, rendering tumor growth and metastasis. Tumor-derived exosomal miR-203, targeting TLR4 in DC cells, downregulated the expression of TLR4 and related cytokines, such as PD-L1, TGF-β, and VEGF. (5) Tumor-derived exosomal miR-20a-5p and miR-891a inhibit Th1 and Th17 cell differentiation while increasing the activity of regulatory T cells (Tregs). (6) CAF-derived exosomal lncH19 can be transferred into tumor cells to activate Wnt/β-catenin signaling via serving as a ceRNA for miR-141. (7) Tumor-derived exosomal circUHRF1 can elevate the expression of TIM-3 on the surface of NKs, thus inhibiting the cytotoxicity of NKs.
The Functions of Exosomal ncRNAs in T Cells
T cells form their unique T cell receptor (TCR) and perform a variety of biological functions. Within the TME, in particular, a few crucial T cell populations significantly influence tumorigenesis and progression. Tumor-specific T cells are a vital part of the adaptive immune system, and the vast majority of T cells depletion within the TME will trigger tumor immune envision and immunosuppression (56).
It is well known that programmed death-1 (PD-1) and its ligand PD-L1 are imperative immune checkpoint molecules involved in tumor immune invasion (57). PD-1 is a key receptor displayed on activated T cells and once PD-1 is mobilized, it prevents T cells from directing immune responses by reversibly suppressing T cell activation and proliferation (58). Intriguingly, non-small cell lung cancer (NSCLC) cells inactivate CD8+ T cells via delivering PD-L1-containing exosomes in vitro culture systems. In-depth investigations elucidate the detailed molecular mechanism by which circ-CAP4 inhibits PD-L1 inside and outside of the cell through binding to let-7 miRNA. intracellular PD-L1 governs NSCLC cell proliferation, metastasis, cisplatin resistance, and CSCs properties, while extracellular PD-L1 provokes the deactivation of CD8+ T cells to avoid immune clearance (59). In addition, ER stress is capable of dysregulating anti-tumor immunity via promoting the secretion of miR-23a-3p-rich exosomes from hepatocellular carcinoma (HCC) cells. Exosomal miR-23a-3p decreases PTEN transcriptional level and launches the AKT pathway, therefore elevating PD-L1 abundance in macrophages, and ultimately suppressing T cell functions (60). In CD8+ T cells, it has been identified LIMIT (lncRNA Inducing MHC-I and Immunogenicity of Tumor) is a novel immunogenic lncRNA, which is sensitive to IFN-γ. LIMIT engages in the modulation of IFNγ-induced MHC-I degree without modifying overall IFN-γ signaling (61). Distinctively, MHC-I is markedly ascended by LIMIT, whose abnormal expression is modulated by TAA-specific CD8+ T cell-mediated anti-tumor ability. TDEs are identified as possessing surface MHC-1, and thus potentially directly activate CD8+ T cells against tumors (62). Due to the excellent spatial proximity to GBPs, LIMIT elevates the transcription of GBPs in a cis-regulatory manner, stimulating CD8+ T cell priming and activation (63). Thus, serving as a cis-acting lncRNA, LIMIT fosters MHC-I assembly by energizing GBPs, which are transduced with HSP90 to release HSP90-associated HSF1 and enhance cancer immunity. Although numerous therapy strategies targeting HSP90 have been developed in clinical settings, no effective HSP90 inhibitor has been widely accepted into clinical applications so far. Considering the intimate interaction between GBPs and HSP90 as well as the unleashing of HSP90-induced HSF1 without adjusting HSP90 animation, targeting GBPs appears to be an attractive and potent strategy to modify HSF1 for anti-tumor immunity treatment. Delivery of lncRNA LIMIT-containing exosomes is capable of achieving this goal, and their advantages and convenience further boost the development of GBPs-based therapies (Figure 4A).
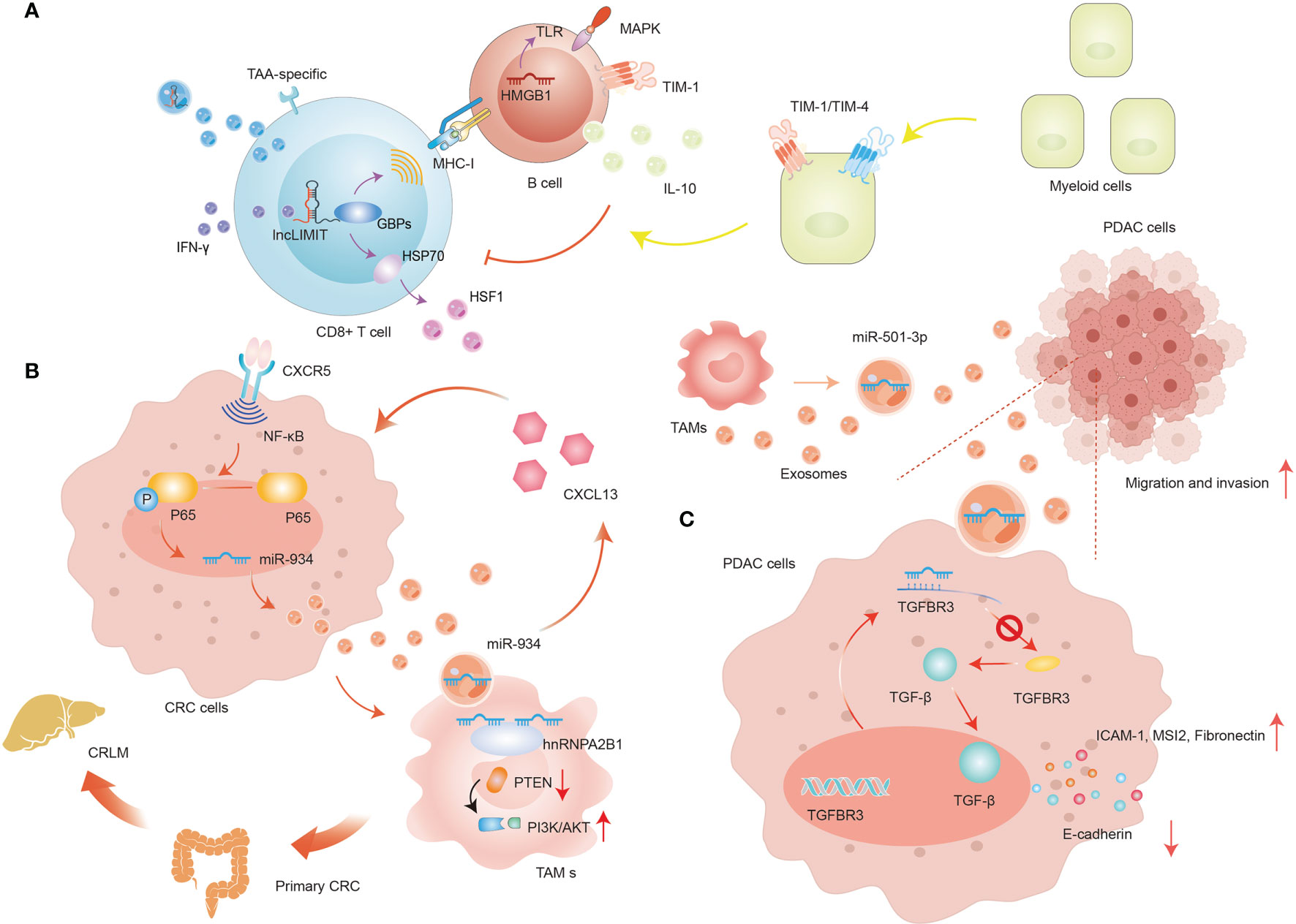
Figure 4 The regulation of exosomal ncRNAs in immune cells. (A) The regulation of exosomal lncRNA LIMIT in T cells. (B) The positive-feedback loop of CXCL13/CXCR5/NF-κB/p65/miR-934 in CRC cells. (C) The role of exosomal miR-501-3p in PDAC cells.
CD8+ T cells have been recognized as effective fighters defending against tumors; in contrast, CD4+ T cells intend to elicit a sustained immune response. Every nascent CD4+ T cell clone possesses the potential to differentiate into one of the separate subtypes (e.g., diverse T helper cells), thereby orchestrating different immune reactions in the context of the TME (64). This is essential because abnormal or inappropriate CD4+ T cell responses may result in the initiation and development of a primary tumor. In nasopharyngeal carcinoma (NPC), miR-24-3p is profoundly upregulated in TDEs that can be delivered into T cells, where it then inhibits the percentage of CD4+ T cells and decreases the production of IFN-γ and IL-17 while provoking the augment of CD4+ Foxp3+ T cells (Tregs) (65). Further investigations indicate that exosomal miR-24-3p has the potential of occupying the 3’-UTR regions of FGF11, which is responsible for followingCD4+ T cell degradation. Interestingly, the abundance of miR-24-3p in NPC is positively related to the frequency of CD4+ and CD8+ T cells and a dismal prognosis. Moreover, hypoxia upregulates miR-24-3p expression in exosomes, thus elevating the transcriptional level of P-ERK, P-STAT1, and P-STAT3, while reducing the abundance of P-STAT5 in CD8+ and CD4+ T cells.
Tregs, characterized by their exclusive cell surface molecules (CD25 and CTLA-4) and nuclear marker FoxP3, are a captivating subpopulation of T cells frequently involved in coordinating autoimmunity and homeostasis (66). CRC cell-derived exosomal miR-208b dramatically facilitates Tregs amplification by directly blockading the 3’-UTR regions of PDCD4 mRNA. PDCD4, a previously disregardful tumor suppressor gene engaged in programmed cell death, is strongly required in negatively administering Tregs expansion (67, 68). Moreover, mass transfer of miR-208b into Tregs shackles PDCD4 abundance and is positively associated with CRC oxaliplatin resistance (69).
Distinguishingly, it has been proposed that γδ T cells can exert immunosuppressive effects on a wide range of cancers (70). With the advancement of immune-oncology, γδ T cells have joined the “T cell arsenal” of anti-cancer. Nevertheless, the accurate subpopulation of γδ T cells and their regulatory mechanisms in BRC are not yet fully understood. Ni et al. found increasing numbers of infiltrating γδ1 T cells and identified CD73+γδ1 T cells are new Tregs in BRC. Further studies revealed that CD73+γδ1 T cells represent the primary Tregs in BRC and play an immunosuppressive role in dampening BRC progression through targeting adenosine (71). lncRNA SNHG16 is available to be delivered into tumor-infiltrating γδ1 T cells via TDEs. Exosomal SNHG16 functions as a competing endogenous RNA (ceRNA) to upregulate CD73 expression in γδ1 T cells by sponging miR-16-5p, resulting in the depression of its downstream gene SMAD5 and the augmentation of the TGF-β1/SMAD5 pathway (71).
The Functions of Exosomal ncRNAs in DCs
DCs, as professional antigen-presenting cells, is responsible for bridging the gap between human innate and adaptive immune responses, and play an anti-tumor role in tumor progression, although it can be reversed in the TME. When DCs are threatened by pathogens or receive activation signals, they tend to initiate the activation of pattern-recognition receptors [e.g., toll-like receptors (TLRs)] and move to secondary lymphoid structures within the lymph nodes where they modify naive B or T cells (72). Once the TLR expressed on DCs is stimulated, DCs are induced to augment the synthesis and release of pro-inflammatory cytokines and the expression of costimulatory molecules, which subsequently activate T cells and initiate immune responses (73). Lewis lung carcinoma (LLC) cells secrete a certain number of miR-21- and miR-29-containing exosomes. And the two exosomal miRNAs directly target TLRs to provoke pro-tumoral inflammation, rendering tumor growth and metastasis (74). A coexistence of miR-29a and cytokeratin has been identified in the tumor vortex. Notably, miR-29a is colocalized with macrophage marker F-11 rather than cytokeratin at the edge of LLC. miR-29a is mainly concentrated in macrophages and can directly target TLR7 and TLR8 of macrophages. Both miR-21 and miR-29a exhibit similar GU features (GUUG of miR-21 and GGUU of miR-29a) in nucleotide fragments 18-21, and the GU pattern is dominant in TLR-activated RNA33. By targeting TLR8, these two exosomal miRNAs dominate the initiation of NF-κB pathway to mediate TNF-α and IL-6 emitting, thus inhibiting tumor propagation and expansion (74). Among LLC cells transfected with LNA anti-miR-21/29a, only miR-29a is not managed to be eliminated and is available to orientate the lung tissues, which implies that exosomal ncRNAs may be employed as potent inhibitors against TLR family members. Interestingly, it has been proven that the expression of TLR4 exerts a significant anti-tumor effect in DC-based immunotherapy. In DCs, tumor-derived exosomal miR-203 reduces TLR4 expression and inhibits the release of downstream cytokines (e.g., TNF-α, crucial to DCs maturation, and IL-12, required for Th1 differentiation), thus promoting cancer immunosuppressive microenvironment (75). In mice, the volume of MHC II and costimulatory molecules in miR-155-deficient DCs have no intensity fluctuations (76). While such DCs are relatively sluggish to the T cell stimulus. Alternatively, in human monocyte-derived DCs (moDCs), exosomal miR-155 is observed to be significantly upregulated during the processing of moDCs maturation. LPS exhibits great suppressive effects on the translation and cohesion of exosomal miR-155 by incompletely occupying its recognition region within the 3’-UTR (77). Further research demonstrates that a number of typical pathways are mutated in miR-155 knockdown LPS-activated moDCs, where IL-1 and its signaling components are involved in most of the detected signaling. Thus, by targeting TAB2, miR-155 can act as an important component of feedback negative regulator that downregulates the synthesis and release of inflammatory cytokine production through TLR4/IL-1 signaling, which also communicates in tandem with the p38 MAPK pathway.
Also, TDEs can serve as vehicles to deliver exogenous miR-155 into DCs, thus enhancing T cell proliferation and elevating LPS expression levels (78). Exosomal miRNA-155 facilitates DC maturation, which in turn enhances T cell proliferation and elevates DC IL-12p70 and IFN-γ expression. Interestingly, these DCs are more capable of activating the proliferative capacity of lymphocytes and mediating naive T cells’ differentiation into Th1 cells (79). miR-148 and miR-152 are overexpressed in TLR-activated DCs that decrease TLR-induced cytokine (e.g., IL-6, IL-12, and TNF-α) production and restrict Ag-specific CD4+ T cell clone and expansion. Also, these two miRNAs can directly inhibit CaMKIIa to mediate the upregulation of MHC II levels and the secretion of related cytokines in DCs (80).
The Functions of Exosomal ncRNAs in Macrophages
Macrophages are one of the most prevalent and influential immune-associated stromal cell types in TME. Broadly, macrophages fail into two major phenotypes depending on the different responses of macrophages to specific stimuli: one is the classical inflammation-induced M1 macrophages, and the other is the alternatively-activated M2 macrophages, which are commonly designated as tumor-associated macrophages (TAMs) (81). Interestingly, TDEs have been demonstrated to induce macrophages differentiation into TAMs, therefore expediting tumor growth, angiogenesis, immune escape, and ECM rebuilding to facilitate locoregional and distant metastasis (82). Pancreatic ductal adenocarcinoma (PDAC) remains a recalcitrant and deadly disease with a dismal prognosis, and its incidence is still rising in recent years (83). However, remarkable progress has been made in understanding how exosome-mediated ncRNAs regulate the intercellular crosstalk in PDAC. For instance, an isolated study disclosed the exact roles of TAM-derived exosomal miR-501-3p during PDAC development (84) (Figure 4B). miR-501-3p decreases TGFBR3 expression by binding to the 3364-3370 bases of the TGFBR3-3’-UTR region. Moreover, it has been documented TGFBR3 plays an integral pro-tumorigenesis role in a wide range of tumors via the TGF-β signaling (85). Then, TGF-β diminishes the abundance of ICAM-1, MSI2, and Fibronectin, while promoting E-cadherin in TAMs in vivo. Knockdown of miR-501-3p in TAMs-derived exosomes partly shackles the transcriptional level of CSCs-related genes, eliciting PDAC metastasis and tube formation regression.
In PC, miR-301a-3p is significantly upregulated in exosomes and can be transferred into macrophages. By enhancing HIF-1a and HIF-2a expression, hypoxia elevates the expression level of miR-301a-3p both in PC cells and PC-derived exosomes. Knocking out miR-301a-3p in PC cells inhibits exosome releasing and polarization of the macrophages to an M2 phenotype by targeting PTEN/PI3K-signaling, ultimately curbing PC cell growth, invasion, migration, and EMT (86). Another recent study showed that, in PC, lncRNA TALC is largely expressed in TDEs. The relocated exosomal TALC in TAMs stimulates the M2 polarization of microglia. M2 polarization is associated with the excretion of the complement components C5/C5a. Exosomal lncRNA TALC stimulates p38 MAPK phosphorylation through targeting downstream ENO1. Functionally, C5 contributes to TMZ-mediated recovery from DNA damage, causing chemoresistance. And TALC-mediated TMZ resistance can be strongly limited by C5a-targeted to improve the effectiveness of immunotherapy (87). Intriguingly, two aberrant upregulated exosomal ncRNAs, miR-21 and miR-1246, which may change microglia and macrophages phenotype to enhance glioma proliferation (88, 89). lncSBF2-AS1 is exceptionally upregulated in glioma tissues, which is closely connected with temozolomide (TMZ) resistance (90). Also, SBF2-AS1 can be assigned into exosomes to relocate from chemotherapy-resistant glioblastoma (GBM) cells to chemotherapy-sensitive GBM cells, thereby transferring the capacity of TMZ resistance to recipient cells.
In CRC, miR-934 is markedly upregulated in tumor tissues and primary cells, in particular, in CRC liver metastasis (CRLM), and positively correlated with unfavorable prognosis (91). hnRNPA2B1 is able to mediate the sorting and wrapping of miR-934 into exosomes and dominate subsequent delivery of miR-934-containing exosomes to macrophages. Moreover, the exosomal miR-934 is implicated in inducing M2 macrophage polarization by regulating the expression level of PTEN and mobilizing the PI3K/AKT signaling pathway. Specifically, miR-934 downregulates PTEN abundance through binding to its 3’-UTR and stimulating the contents of p-AKT and p-PI3K in PMA-induced THP-1 cells. The invasive and liver-metastatic potential of CRC is closely related to the encouragement of TAMs, which are responsible for secreting chemokines and cytokines involved in the malignant transformation and more aggressive behaviors of tumors. And these regulatory molecules are presented in TDEs-polarized M2 macrophages (92). Further, miR-934 can dramatically elevate the release of CXCL13 in Kupffer cells. CXCL13 is a potent chelator that boosts accretion of CXCR5-expressing cells, while silencing CXCL13 and CXCR5 in CRC inhibits TAM-mediated metastasis, suggesting that exosomal miR-934 promotes CRLM progression by targeting the CXCL13/CXCR5 axis. Considering that the CXCL13/CXCR5 axis arouses the traditional NF-κB singling and exacerbates the inflammatory reactions in tumors, there might be a more elaborate regulatory mechanism of miR-934 that seems to be unexplored (93). Indeed, CXCL13 enhances p65 phosphorylation, increasing the level of NF-κB, MMP2, and MMP9 while decreasing IκBα. These alterations of CXCL13 can be reversed by helenalin, which exhibits great suppressive effects on NF-κB/p65 pathway, indicating that miR-934 could also be positively manipulated by NF-κB/p65 pathway via a positive feedback loop. In-deep studies showed that p65 straightforwardly upregulates miR-934 level inside the -2002 and -1500 bp domains by combining a specialized sequence (GGAAATGCCT) between p65 and miR-934 promoter. And the abnormal of the aforementioned specialized sequence eliminates the facilitative effect of CXCL13 and the inhibitory effect of NF-κB/p65 pathway on miR-934 enrichment. Taken as a whole, polarized M2 macrophages trigger pre-metastatic niche arrangement and foster CRLM by excreting CXCL13 and actuating a positive-feedback loop of CXCL13/CXCR5/NF-κB/p65/miR-934 in CRC cells (Figure 4C).
The Functions of Exosomal ncRNAs in NKs
NKs are one of the major subsets of T cells in the innate branch of immunity. NKs commonly patrol the circulatory system, hunting for cancer cells. Among all the immune guardians, NKs are one of the most aggressive, with a potent ability to kill transplanted and spontaneous tumors. They are capable of producing a series of anti-tumor cytokines (e.g., IFN-γ), which not only influences target cells but also mobilizes T cells and macrophages to destroy tumor cells or enhance the anti-tumor vitality of other immune cells (94). To date, the exact roles of exosomal ncRNAs in NK biology have been controversial. TIM-3 is a vital co-inhibitory molecule expressed on NKs. Peripheral NKs from cancer patients tend to exhibit excessive expression of surface TIM-3, which is correlated with dysfunction and impairment of NKs (95). In HCC, circUHRF1 is upregulated in TDEs and it can increase the level of TIM-3, therefore impeding the physiological functions of NKs (38). Previous investigations have confirmed that circRNAs are primarily considered miRNA decoys, which attach to miRNAs and in turn boost the transcription of their target genes. Correspondingly, it has been identified that circUHRF1 can function as a targeting platform for miR-449c-5p, which attenuates the production of IFN-γ and TNF-α in NKs. Interestingly, there appears to be reciprocal regulation between circUHRF1 and miR-449c-5p in NKs. TIM-3 mRNA is a target of miR-449c-5p. It is notable that both permeating NKs and CD8+ T cells within the TME exhibit enrichment of TIM-3 (95, 96). These results show that there exist a circUHRF1/miR-449c-5p/TIM-3 axis in NKs and CD8+ T cells, denoting a novel mechanism of circRNA in the coordination of these two immunocyte types. However, the regulatory axis described above is unique in NKs and not present in CD8+ T cells. Forced exosomal circUHRF1 expression limits the susceptibility of HCC cells to anti-PD1 therapy. For another thing, the TIM-3/Gal-9 pathway is now well-established in NKs, therefore, PD1/PD-L1 and TIM-3/Gal-9 restriction may enhance the efficiency of NKs in immunotherapy (97). circUHRF1 in TDEs promotes TIM-3 expression, triggering NKs exhaustion. As a consequence, the height of circUHRF1 plays a decisive role during the anti-PD1 treatment of HCC. In neuroblastoma patients, miR-186 is lowly expressed in tumor cells and positively associated with clinical severity and prognosis outcome (48). miR-186 directly identifies the target sequence and impedes the translation of TGFBR1 and TGFBR2. Also, miR-186 is observed to be capsulated into NK-derived cytotoxic exosomes and act on MYCN, AURKA, as well as the TGF-β pathway (98). Exosomal miR-186, in particular, inhibits the cytotoxicity of NK by downregulating TGFBR1- and TGFBR2-induced suppression of TGF- signaling. That is, delivering miR-186 to the tumor site not only limits neuroblastoma cell growth but also independently counteracts the TGF-mediated immunosuppressive mechanisms that hinder the efficacy of ADCC-based treatment approaches. Intriguingly, killing molecules (e.g., perforin 1) are significantly reduced in exosomes originated from TGF-β-treated or growth factor-starved NK cells, implying that miR-186 (and possibly other exosomal RNA molecules) may have a unique impact on regulating the cytotoxicity of NK-derived exosomes. In addition, an increase in CD73 expression is detected in NKs, and the frequency of these CD73+ NKs are associated with larger tumor size in BRC patients (99). By acting on actin polymerization-dependent exocytosis, NKs are able to deliver CD73 from intracellular vesicles to cell surfaces and extracellular spaces upon contacting with 4-1BBL on cancer cells. Undergoing transcriptional reprogramming, these CD73+ NKs enhance IL-10 synthesis through STAT3 transcriptional activity, which subsequently inhibits CD4+ T cell proliferation and IFN-γ generation (99). This finding suggests that each immune cell population does not exist in isolation in the TME; instead, abundant information exchange between NK cells and other immune cells in TME. And such interactions largely depend on ncRNAs-containing exosomes shuttled in the TME.
The Functions of Exosomal ncRNAs in CAFs
CAFs account for a considerable proportion of the stromal cells within TME and are an integral contributing factor in the generation of an aberrant TME that facilitates cancer development and progression. According to recent findings, CAFs are capable of regulating the biological properties of tumor cells and other stromal cells through intercellular communication. Specifically, CAFs alter and remodel the structure of the ECM that initiates tumor cell invasion. Also, they can interact with themselves or alternative TME cells by synthesizing and releasing cytokines, growth factors, and chemokines (100). Previous research has indicated that CAFs and tumor cells contact not only via traditional paracrine signaling pathways (such as the previously mentioned cytokines and chemokines) but also via exosomes (51). A study by Dou et al. used miRNA profiling assays and found abnormal expression of miR-92 isolated from CAFs exosomes (101). Exosomes-treated BRC cells exhibit higher levels of miR-92 and PD-L1 (102). miR-92 can directly bind YAP1 to promote YAP1 phosphorylation and consequently block YAP1 nuclear translocation. It is intriguing that YAP1 activity has been purported to modulate PD-L1 expression in certain types of carcinoma (103). Using ChIP, it has been verified that YAP1 occupies the enhancer region of PD-L1 to upregulate PD-L1 expression. Furthermore, CAF-derived exosomes increase PD-L1 expression, significantly enhancing T cell apoptosis and impairing T cell proliferation (101). Ren et al. identified the precise mechanism of Wnt/β-catenin signaling activation through CAF-derived exosomes by transfer of exosomal lncH19 (104).
The Functions of Exosomal ncRNAs in MDSCs
MDSCs are immature, pathologically activated neutrophils and myeloid cells that are phenotypically and morphologically similar to monocytes. The immunosuppressive activity of MDSCs empowers them to modulate the immune response in numerous cancers. In human and mouse peripheral blood, MDSCs have been identified as immature cell populations with the capability to inhibit T cell activation and aggregation of MDSCs in vivo and in vitro (105). Jiang et al. discovered that the progression of early-stage MDSCs (eMDSCs) in BRC with elevated IL-6 expression is closely related to the SOCS3 deficiency-dependent overactivation of the JAK/STAT signaling (106). Both miR-9 and miR-181a are significantly overexpressed in eMDSCs, which not only facilitate the amplification of immature eMDSCs but also suppress the immunity of T cells. In addition, exosomal miR-9 and miR-181a can promote tumorigenesis and immunosuppression by enhancing in situ infiltration of eMDSCs. Nevertheless, the intrinsic mechanisms underlying the two miRNAs are different. miR-9 and miR-181a can induce the development of eMDSCs by diminishing SOCS3 and PIAS3 (two key regulators in JAK/STAT signaling pathway), respectively (106). In glioma, exosomal miR-1246 is shown to stimulate the activation and differentiation of MDSCs in a DUSP3-dependent or ERK-dependent manner. More importantly, the high exosomal miR-1246 in cerebrospinal fluid of postoperative patients is strongly associated with decreased survival and increased tumor recurrence (107). It is generally accepted that hypoxia is a critical feature of the GBM microenvironment. Hypoxia enhances the transcriptional and selective packaging of miR-1246 by upregulating POU5F1 and hnRNPA1, increasing miR-1246 expression in TDEs (108). Furthermore, the mechanism by which 2-methoxyestradiol, a microtubule inhibitor presently enters pre-clinical trials and clinical research in GBM, inhibits MDSC activation by suppressing the expression of the hypoxia-derived exosomal miR-1246 in tumor cells and PD-L1 expression in MDSCs has been identified (107).
The Functions of Exosomal ncRNAs in B Cells
B cells are dedicated immune cells charged with antibody generation, processing of antigens and their presentation, and proinflammatory cytokine release. In general, B cells mainly reside in the periphery of tumor and are normally presented in lymph nodes adjacent to the TME. Similar to formerly discussed T cells, there exists a regulatory subtype in the B cell population known as regulatory B cells (Bregs) that is indispensable for tumor immunomodulatory within the TME (109). The role of T cells in tumor immunosurveillance had received a great deal of research attention. But only a few studies are concerning the functional characteristics of B cells in the TME. For example, an isolated study found that in HCC, miRNA HMGB1 plays a pro-tumorigenic role in infiltrating TIM-1+ Bregs (110). TIM-1 is an important transmembrane glycoprotein displayed on B cells as a unique biological label for Bregs (111). In HCC, Bregs secret large amounts of IL-10 and manifest eminently inhibitory effects on CD8+ T cells (Figure 4A). And such suppressive reaction can be further enhanced by myeloid cells by TIM-1/TIM-4 signaling. In addition, sufficient HMGB1-containing TDEs trigger TIM-1+B cell clones via targeting the TLR/MAPK signaling pathway. Mechanistically, activating TLR2/4 markedly augments the percentage of TIM-1+ B cells and heightens the negative roles of TDEs in CD8+ T cell breeding and TNF-α and IFN-γ procreation (110).
B cells are capable of forming a variety of complex tumor-associated immune aggregates, spanning from disorganized irregular small clusters to regular tertiary lymphoid structures (TLS), which supports further maturation and subtype conversion of tumor-specific B cells and the progression of tumor-specific T cell responses (112). In particular, antigen-specific reciprocal relationships betwixt T and B cells appear to be integral in TLS, and the antitumorigenic effects of T cells in the TME are largely dependent upon collaboration with B cells (113). Apoptotic exosome‐like vesicles (AELVs), emanated in a caspase-3-dependent way, are observed to induce TLS arrangement, thus facilitating autoimmunity and allograft inflammation (114). And such accommodative dysfunction is commonly accompanied by elevated CD20+ B cells density across the vessel wall and augmented CD3+ T cells infiltration in the intimal regions (115). Furthermore, AELVs provoke the accumulation of γδTh17 cells (a subset of CD3+ cells) to the allograft and foster the formation of γδT dependent TLS, while regulating IL-17 generation other CD3+ T cells (except γδTh17 cells) in the allogeneic organs in vivo.
The exosomal ncRNAs that are involved in the TME and determine susceptibility to tumor cells in T cells, DCs, and macrophages have been researched extensively over the past years, while similar studies on B cells are still scarce. One tantalizing research topic concerning exosomal ncRNAs in the TME is their precise regulatory machinery and biological significance in B cells.
The Functions of Exosomal ncRNAs in Cancer Stem Cells
Within the tumor tissue, there exists a small group of cells with extremely high tumorigenic capacity and very low differentiation, called cancer stem cells (CSCs). Similar to the typical undifferentiated cells in tissues, CSCs represent the properties to perform self-regenerate and differentiation (116). They are indispensable for cancer progression and are the root causative factors of tumor recurrence, metastasis, and drug resistance. Nowadays, the presence of CSCs has been demonstrated in tumors of different tissue origins, such as BRC, CRC, and HCC (117).
It has been demonstrated that exosomal ncRNAs emerge as a novel layer affecting CSC characteristics. For example, lncRNA FMR1-AS1 is abundant in exosomes that can be released into the TME. lncFMR1-AS1 can bind to TLR7, activate the TLR7/NF-κB signaling, and elevate c-Myc expression, therefore promoting esophageal squamous cell carcinoma (ESCC) proliferation, anti-apoptosis, and invasive capacity (118). Intra-tumor hypoxia has been shown to be one of the main features of TME (119). Hypoxic bladder cancer cell-derived exosomal lncRNA UCA1 enhances CSCs proliferation and induces EMT to facilitate tumor metastasis (9). Ren et al. found that CAFs promote CRC cell stemness by emitting lncRNA H19-containing exosomes that activate Wnt/β-catenin signaling (104). This observation further confirms that tumor cells preferentially reside in the vicinity of stromal myofibroblasts, which exhibit higher Wnt signaling activity (120).
Emerging evidence implicates that CSC-derived exosomal ncRNAs play an essential role during tumorigenesis and tumor progression. Glioma stem cells (GSCs) are closely related to radiation and chemotherapy resistance as well as high recurrence rates of gliomas (121). An initial study reported that miR-944 levels are significantly decreased in high-grade gliomas, and the ectopic expression of miR-944 was associated with a dismal prognosis (122). Furthermore, the GSC-derived exosomal miR-944 dramatically limits glioma angiogenesis by targeting the 3’UTR of VEGFC, reducing VEGFC expression, and blocking the AKT/ERK signaling. Due to the self-renewal and drug resistance of CSCs within tumor tissues, novel effective therapies currently need to be developed. These new therapeutic interventions should not only have intrinsic anti-tumor activity but also the ability to kill CSC subpopulations. In BRC cells, RAB27B has been observed to facilitate the delivery of exosomes from stromal cells to BRC cells through transferring exosomal 5’-triphosphates. Exosomal 5’-triphosphates eventually stimulates the RIG-I signaling in recipient cells, resulting in the activation of IRDS genes. Simultaneously, activated RIG-I can act on the NOTCH3 signaling to muddy the extension of anti- tumor infiltrating cells in CSCs (123).
Of interest is the fact that the immune components of the TME are centered on the tumor cells and spread along the periphery, dynamically evolving with the stage of tumor progression. The various immune cells within the TME are not isolated from each other, instead, there is a colorful spatio-temporal dialogue among them. In addition, they create this complex network together with tumor cells, and stromal components. Also, exosomal ncRNAs play an indispensable role in the contact among TME compositions. Thus, exploring the underlying mechanisms of the TME and identifying the precise target exosomal ncRNAs of intervention can improve the therapeutic efficacy of cancers.
Exosomal ncRNAs Regulate Tumor Progression in the TME
Exosomal ncRNAs Control Tumor Angiogenesis in the TME
An enormous of research has unveiled that exosome is intrinsically connected with tumor angiogenesis (124). Nowadays, evidence is accumulating that exosome executes its angiogenic regulatory roles partially by delivering exosomal ncRNAs in the TME (125). Therefore, elucidating the underlying molecular mechanisms correlated with tumor angiogenesis is imperative to advance modern anti-angiogenic therapies.
One of the major pathways in which exosomal ncRNAs are engaged in tumor angiogenesis is through shackling the vascular endothelial growth factor (VEGF) and its receptor family. VEGF interacts with tumor angiogenesis by adjusting ECs arranged on blood vessels in an army of ways. In neighboring normal bronchial cells, lung cancer (LC) cell-derived exosomal miR-21 induces VEGF biosynthesis and angiogenesis progression in a STAT3-dependent manner (126). It was observed that miR-21 overexpression augments the expression of HIF-1 and VEGF, which in turn enhances the AKT and ERK1/2 signaling pathways, leading to an intensification of tumor angiogenesis (127). Exosomal circSHKBP1 is notoriously elevated in GC cells and is reported to aberrantly promote VEGF secretion and accelerate angiogenesis through the attachment of miR-582-3p, thereby enhancing HUR expression and improving VEGF mRNA cohesion (128). In chondrosarcoma cells, lncRNA RAMP2-AS1 is rich in TDEs. Simultaneously, exosomal lncRAMP2-AS1 functions as a ceRNA for miR-2355-5p to coordinate the expression of VEGFR2, therefore driving the angiogenic ability of human umbilical vein endothelial cells (HUVECs) (129). Likewise, PC cell-derived exosomal lncUCA1 is reported to be able to be transferred to and internalized by HUVECs, which may provide a novel paradigm for tumor angiogenesis (130).
In addition to targeting VEGF, tumor-derived exosomal ncRNAs can promote angiogenic potential by acting on other membrane and intracellular molecules. miR-9 directly targets MDK and regulates the PDK/AKT pathway to accelerate tumor angiogenesis (131). Hypoxia is another factor engaged in angiogenic proceedings that may influence the movement of various substances and manipulate the expression of exosomal ncRNAs. Under hypoxic conditions, LC cells produce quantities of exosomes in which miR-23a is strongly upregulated and restricts the action of prolyl hydroxylases 1 and 2, leading to the assembly of HIF-1 α in ECs and thus angiogenesis in LC cells is further enhanced (28). circ100338 is abundant in HCC-derived exosomes that can be transferred into recipient ECs to enhance the proangiogenic activity of HCC cells (132). Despite being the subject of intensive research, studies on the explicit functions of exosomal ncRNAs in tumor angiogenesis are yet in their infancy, which drives the need for further investigation.
Exosomal ncRNAs Modulate Tumor Metastasis in the TME
As a hallmark of malignancy, metastatic dissemination is the leading cause of more than 90% of cancer-related deaths and is currently an insurmountable problem for curative therapies (133). Evidence is accumulating that the intercellular interactions among TME cells are a pivotal element of metastatic potential and organ-specific metastasis. However, recent studies are indicative of the vital roles of TDEs in modifying the microenvironment of both local and distant non-tumor cells. Furthermore, the construction of TDEs creates a favorable microenvironment for future metastatic sites, allowing tumor cell growth, expansion, and dissemination through augmented vascular permeability and metastasis of distant organs in a non-random metastatic pattern (134, 135). And the determinants of exosome-mediated organ-specific regulation render a change in the direction of metastasis. Specific integrins expressed on TDEs are distinctive, which defines the adhesion of exosomes to different cell types and ECM fragments in distinct organs (136).
Many groups have demonstrated that exosomal ncRNAs fuel initial tumorigenesis and endow tumor cells with metastatic properties. lncRNA HOTAIR is enriched in LC-derived exosomes. Its anomalous expression dramatically accelerates LC cell growth, invasion, and metastasis (137). It is worth noting that miR-30a-5p is plentiful in exosomes originating from vascular ECs that can be transported into lung adenocarcinoma cells to promote tumor malignant progression by targeting CCNE2 (138). In bladder cancer, exosomal lncLNMAT2 is observed to be capsulated by directly interacting with hnRNPA2B1. LNMAT2 is then recognized and taken up by HLECs, thereafter augmenting the transcription level of PROX1 via recruiting hnRNPA2B1 to its promoter, ultimately leading to lymphangiogenesis and lymphatic metastasis (139). Moreover, research has established that upregulation of circIARS in PC patients was positively correlated with tumor metastasis as well as unfavorable survival (140). IARS specifically targets miR-122 in HUVECs to escalate RhoA, leading to an increase in RhoA expression level and activity, thus further promoting ZO-1 expression and inducing F-actin expression. Subsequently, IRAS is internalized into HUVECs via TDEs, thereby enhancing the penetration of endothelial monolayer and thereby exacerbating tumor infiltration and dissemination.
Exosomal ncRNAs Regulate EMT in the TME
EMT is generally defined as a complex biological process engaged in tissue reconstitution and has been extensively investigated as a promotor of tumor invasion and metastasis. Recently, considerable research efforts have been dedicated to achieving a comprehensive understanding of the exact functions of exosomal ncRNAs in modulating EMT (141, 142). During cancer development, activation of EMT grows the percentage of a mesenchymal phenotype related to highly invasive tumor cells that infiltrate the circulatory and lymphatic systems and reach distant sites for settlement. A recent study identified several miRNAs, including miR-193a-3p, miR-210-3p, and miR-5100, with altered levels in the exosomes of hypoxic bone marrow mesenchymal stem cells (BMSCs) that are observed to be transferred into epithelial cancer cells to activate STAT3 signaling and elevate the expression of mesenchymal-associated molecules, thereby promoting EMT progression and initiating tumor metastasis (143). Moreover, Duo et al. reported that the CRC-derived exosomal miR-27b-3p was upregulated in EMT and that it could increase the permeability of blood vessels by targeting p120 and VE-cad (144). p120 is required in the formation of VE-Cad cytoplasmic territory and expedites the stabilization of VE-Cad, which is indispensable for the establishment of adherent junctions owing to its ability to preserve junctional integrity and limit unrestricted vascular growth (145). miR-27b-3p inhibits ovarian cancer angiogenesis mimicry by acting on VE-Cad. In HUVECs, exosomal miR-27b-3p suppress VE-Cad and p120 expression, which accordingly leads to increased vascular permeability. Exosome-mediated linc02470 and linc00960 in high-grade bladder cancer cells induce tumor EMT by upregulating Notch signaling, β-catenin signaling, and Smad2/3 signaling (146). Mechanistically, Notch signaling stimulates the DNA-binding capacity of NF-κB, which elevates MMP9 expression. The MMP9 can reshape the ECM and promote the extravasation of tumor cells (147). Smad complex binds to regulatory elements and induces transcription of crucial genes correlated with EMT (148). Active Smad2 facilitates invasion of mesenchymal spindle cancer cells while suppressing E-cadherin expression (149).
Exosomal ncRNAs Mediate Tumor Drug Resistance in the TME
Chemotherapy, or chemotherapy-based combination treatment, appears to be one of the most widely used cancer therapies, but the current carcinoma research field remains plagued by a major obstacle: chemotherapy resistance, which restricts the efficacy of the cancer treatment. Various molecular mechanisms have been associated with drug resistance, including markedly ascended levels of drug efflux and target mutations (150). Exosomes, as fundamental communicators of cell-to-cell crosstalk, are engaged in the formation and transmission of drug resistance. As a hot topic of research in recent years, exosomal ncRNAs are emerging as an imperative factor during the transmission of tumor drug resistance. Therefore, identifying the underlying adjustment paths of exosomal ncRNAs in tumor chemoresistance is indispensable for optimizing current therapeutic strategies.
It has been found that several cell membrane transporter proteins facilitate the efflux of drugs as a means of conferring resistance to chemotherapeutics that are commonly used. Most notably, the transmembrane proteins of the ATP-binding cassette (ABC) transporter family regulate the flow of diverse unrelated chemotherapy drugs across the cell membrane (151). The ABC transporter increases drug efflux, which is one of the mechanisms of drug resistance in tumors. Oxaliplatin resistance is currently an insurmountable problem for patients with advanced-stage CRC. Oxaliplatin resistance is currently an insurmountable problem for patients with advanced-stage CRC. Oxaliplatin-resistant CRC cell-derived exosomal circ0005963 (also termed as ciRS-122) can be transferred into oxaliplatin-sensitive cells, where it hastens glycolysis by elevating PKM2 level, resulting in enhanced ATP production (152). It is widely accepted that solid tumor cells frequently bank on anaerobic oxygen glycolysis rather than aerobic oxidation for biosynthesis and ATP requirements despite the low efficiency of such energy production (153). Thus, sufficient energy could confer greater motivation to transporters to pump oxaliplatin out of cancer cells in an ATP-dependent way, reducing the maintenance period of drug efficacy and accelerating drug efflux (154). Cells overexpressing ABC transporters have multiple drug resistance phenotypes after undergoing EMT, which also affects cellular resistance to drug-induced apoptosis and subsequently modulates tumor resistance by influencing drug efflux (155, 156). Liu et al. have investigated the predominant concerning factors of exosomal miR-128-3p in tumor chemoresistance (157). In CRC, overexpression of miR-128-3p is conducive to DNA repair and IC50 reinforcement, as well as low apoptosis rates, illustrating miR-128-3p as a predicted candidate of responsiveness to oxaliplatin. miR-128-3p-loaded exosomes can be assigned to cancer cells, negatively handling the promotor of Bmi1 and MRP5, which participate in oxaliplatin-mediated EMT and chemical agent excretion by targeting TWIST1 and EZH2 respectively. Also, this high degree of 128-exo leads to increased tissue distribution and internalized drug localization, reprogramming destructive DNA-platinum polymer that subsequently eradicates cancer cells.
Drug resistance is also influenced by alterations in drug targets, such as mutations or changed expression levels. As previously discussed, in oxaliplatin-resistant CRC cells, miR-128-3p was able to alleviate chemosensitivity by regulating Bmi1 and MRP5 (157). The miR-522 present in serum is predominantly produced from CAFS-derived exosomes. Interestingly, hnRNPA1 can mediate the packaging of miR-522 into exosomes, and ubiquitin-specific protease 7 (USP7) improves the stability of hnRNPA1 via de-ubiquitination. Cisplatin and paclitaxel are shown to stabilize hnRNPA1 by activating the USP7/hnRNPA1 axis. This promotes the secretion of CAF-derived exosomal miR-522, leading to inhibition of ALOX15 and reduced lipid-ROS aggregation in tumor cells, thereby suppressing tumor chemosensitivity (11). Another study showed that hnRNPA1 can directly bind a specific sequence (UAGGUA) of miR-196a, thus packaging miR-196 into CAFs-derived exosomes. miR-196a is then transmitted into head and neck cancer (HNC) cells, wherein it targets CDKN1B and ING5 to increase cell proliferation and decrease apoptosis (158). These findings have significant implications for the understanding of how exosomal ncRNAs are involved in tumor resistance. Also, more efforts are required to investigate other mechanisms underlying tumor resistance.
The Clinical Significance of Exosomal ncRNAs in the TME
The promising role of exosomes as novel organelles in tumor diagnosis, prognosis, and therapy has attracted a wide range of attention. Exosome-mediated ncRNAs, unlike those unleashed straightforwardly into the circulatory system, are sheltered by a lipid bilayer, which can prevent ribonuclease destruction in a wide range of biological liquids (159). Additionally, they are highly mounted into exosomes in tumor cells and remain stable at different temperatures (160, 161). Given the merits of exosomal ncRNAs and the fact that exosomes are present in multiple bodily fluids (e.g., blood, urine, tears, and breast milk), as well as the specificity of the maternal cell cargo that produces exosomes and the conditions under which they are produced, there is a bright future for diagnostic and prognostic techniques based on exosomal ncRNAs (Figure 5A). An isolated study assessing the expression of GC tissue-specific and pre/post-operative plasma-specific miRNAs found let-7c expression was decreased while miR-21 and miR-106a expression were magnified in plasma. The ratio of miR-106a/let-7a expression serves as a biomarker for distinguishing GC (162). Urinary-specific exosomal circPRMT5 was significantly elevated in patients with bladder urothelial cancer, and high circPRMT5 expression intensifies lymph node metastasis (163). This suggests that urinary exosome-specific circPRMT5 is valuable in cancer diagnosis. Clinical studies have validated the enrichment of exosomal circUSP7 in NSCLC samples and upregulated USP7 predicts unfavorable outcomes and CD8+ T cell dysfunction, suggesting that it might be adopted in clinical arena to harness its clinical potential (39). Furthermore, the abundance of exosomal circUSP7 is not linked with TP53, KRAS, or ALK mutations, which have been confirmed as predictors in anti-PD1/PD-L1 therapy (164). Thus, detecting the level of exosomal circUSP7 appears to be a novel and appealing predictive biomarker in anti-PD1 immunotherapy in NSCLC. These discoveries help to classify patients to identify those who need follow-up for early diagnosis of recurrence and additional treatment.
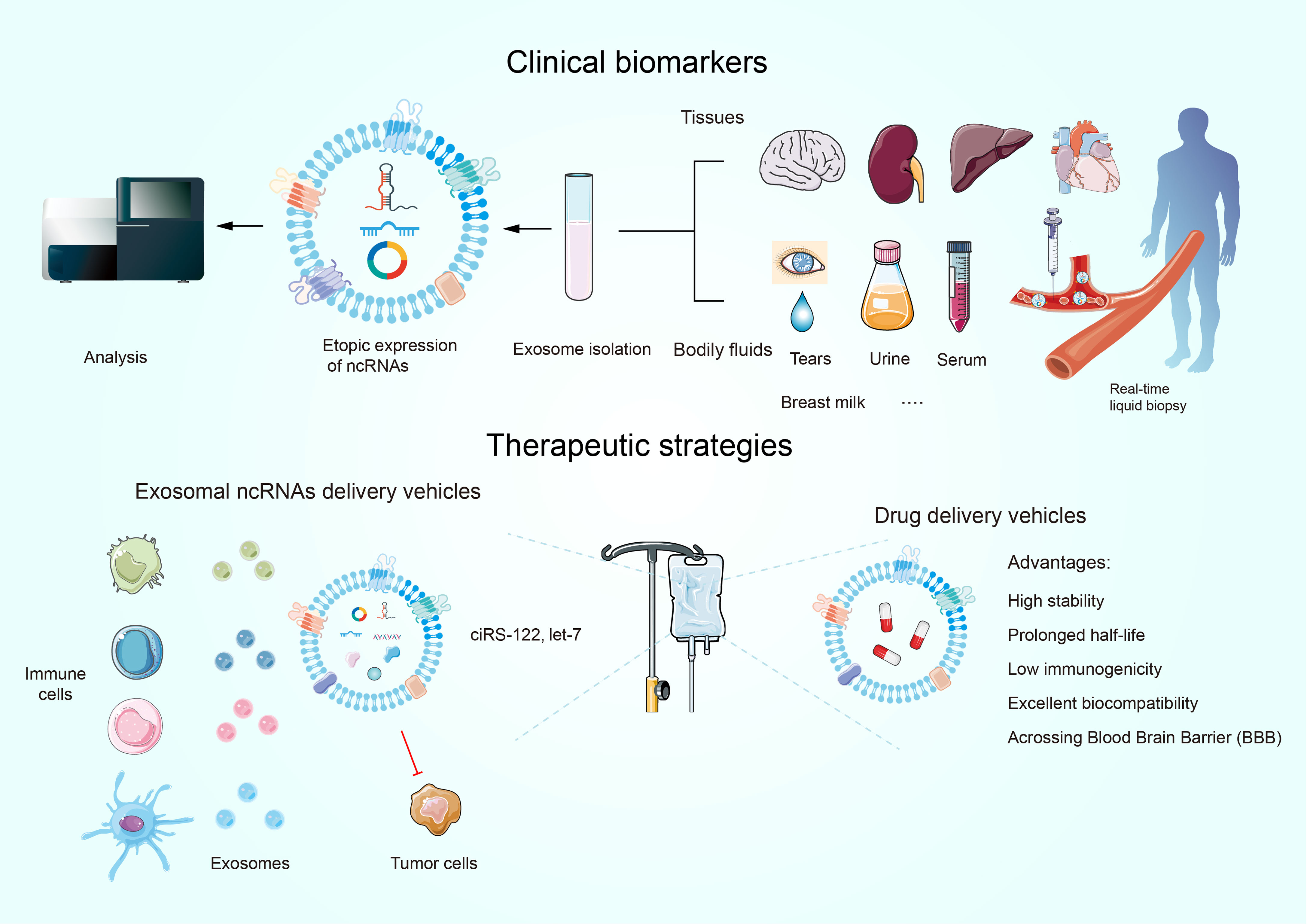
Figure 5 Exosomal ncRNAs as promising biomarkers for the diagnosis and prognosis of human cancers. Exosomes have novel therapeutic applications.
Currently, drug delivery pattern dependent on nanotechnology represents one of the most potent vehicles to attain this intention. It is noteworthy that exosomes display a number of advantages over other nanoparticles owing to their good biocompatibility, high stability, extended half-life, low immunogenicity, precise targetability, and their ability to cross physical barriers (e.g., the blood-brain barrier) (Figure 5B) (165). Furthermore, the utility of exosomal ncRNAs as therapeutic tools calls for the ability of exosomes to deliver boundary ncRNAs to dysfunctional cells at both the primary research and clinical practice layers. For example, let-7a suppresses cancer progression by decreasing the levels of HMGA2 or RAS family members (e.g., KRAS) (166). GE11-positive exosomes, which contain let-7a, are used to inject intravenously into tumor-bearing mice by the tail-vein injection. Although numerous studies have demonstrated that let-7a does not appear to impact the expression of HMGA2 or RAS family members either in vivo or in vitro, GE11-positive exosomes specifically target EGFR-expressing cancer cells and restrain the growth of HCC70 BRC cells in vivo compared to let-7a-free and GE11-free exosomes (167). This suggests that let-7-free GE11 exosomes are a potential tool for delivering drugs to EGFR-expressing cancers such as BRC.
Alternatively, given their functions in mobilizing the migration and mediating the intercellular crosstalk of immunocytes, exosomal ncRNAs are tightly linked to both anti-tumor and pro-tumor immunoreactions, as well as the sensitivity and tolerance to tumor immunotherapies. Studies have shown that KRAS-induced exosomes play multifaceted roles in maintaining lung immunosuppressive metastasis and bring about novel routes for intractable metastasis suppression, especially in chemotherapy-resistant cancers. KRAS-induced exosomes independently drive the reprogramming of SMARCE1/NCOR1 chromatin genes, accelerating the construction of pre-metastatic niches in LC. In patients with metastatic LC, co-cultured with carboplatin primed RIP3/TNF-α-dependent necroptosis concomitant with a distinct abundance of immune inhibitor miR-146/miR-210 (168). It is of great interest to inhibit the level of KRAS-derived exosomes, thus enhancing the immune response to limit LC migration and dissemination. And this therapy might be successful with exogenous administration of exosomal miR-146, a crucial immunosuppressive regulator which is actively involved in RIP-3 dependent necroptosis. Through augmenting the PD-L1 expression in recipient cells, mesenchymal stem cells (MSCs)-derived exosomes (the main cargos are TGF-β, C1q, and semaphorins) dramatically contribute to the aggregation of CD206+ MDSCs and TAMs in the TME, leading to the accumulation of TGF-β and depletion of INFγ+CD8+ T cells (169). In contrast, GW4869-treated MSCs can reverse the alteration of PD-L1 expression and block the polarization of M2 macrophages. It is clear that the peculiar re-sculpturing of MSCs at cancer beds is responsible for the generation and release of exosomes, which are essential for building up the novel immune microenvironment into one that favors the escape of tumor cells from tumor-responsive T cells. This observation provides compelling evidence that targeting MSCs is a promising therapeutic strategy available to reinstate T cell-mediated anti-tumor cytotoxicity in patients with BRC. However, corresponding clinical trials that have not yet been conducted need to be carried out in future studies.
To date, considerable efforts have been devoted to investigating therapeutic strategies of exosomal miRNAs. However, only a few studies have addressed how to develop the therapeutic potential and value of exosomal circRNAs and lncRNAs. Oxaliplatin-sensitive cells respond trickly to the exosomal ciRS-122 from oxaliplatin-resistant cells, which endorses CRC glycolysis via enhancing PKM2 expression (152). Delivery si-ciRS-122 based on exosomes can significantly attenuate CRC glycolysis and rescue cell responsive sensitivity to oxaliplatin in vivo. HCC cells could react with circ0051443-containing exosomes from normal cells and reversely inhibit the self-malignant phenotype (170). Effective molecular inhibitors of cancer exosomal lncRNAs are still nascent and exploiting their specific tertiary structures (hairpin repeats or triple helices) may assart a new territory for the development of cancer treatment.
Conclusion and Perspective
Studies involved in the interplay of exosomal ncRNAs and TME have become a hot spot worldwide. The body of work outlined here demonstrates a clear role for the exosomal ncRNAs in the extrinsic regulation of TME, including T cells, B cells, macrophages, NKs, CAFs, DCs, and MDSCs. Exosomal ncRNAs are crucial signaling molecules and biological messengers for dialogue among TME cells and play an indispensable role in angiogenesis, EMT, and metastasis of the TME. Notably, TDEs are quickly becoming an important element of a tumor-curated information network aimed at promoting tumor immune escape. The immunosuppressive molecules and factors secreted by TDEs affect immune cell functions such as anti-tumor activity. However, their regulatory mechanisms and functional characteristics are still in their infancy and need to be further explored. Indeed, there are a set of challenges that remain. First, stringent isolation of specific exosome populations is required since the numerous types of exosomes from diverse sources pose problems for detection when they sink into body fluids. One tantalizing possibility for the purification of exosomes is the single exosome assay technique to individually label exosomes that could examine fingerprinting characteristic information for each exosome. In turn, tumor-associated specific exosome subpopulations are identified through big data and artificial intelligence analysis and used as biomarkers for tumor warning signals. Second, is the feasibility of loading the desired cargo into exosomes. Although most efforts have been devoted to developing small-molecule inhibitors, exosomal ncRNAs appear to be emerging as a platform with much broader and complementary clinical implementations. For instance, ncRNAs could be skillfully incorporated into exosomes by handling the source cells to produce excessive ncRNAs cargoes. However, this process is difficult to achieve in terms of both technical means and experimental methods. Researchers failed to mount miRNA into HEK-293-derived exosomes with electroporation (171). Also, other methods of obtaining the desired exosomes may exist: i.e., by engineering specific inducers to boost the secretion of the desired exosomes, or by designing powerful agonists to potentiate the effect of the exosomes. Third, considering the large body of studies and clinical investigation work committed to pushing exosomal ncRNA-based immunotherapeutic methods, it is necessary to precisely quantitate exosomal ncRNAs and their underlying clinical toxicity. The fact that exosomal ncRNAs are involved in both pro- and anti-tumor effects reveals the complicatedness of the exosome system, as a given exosomal ncRNA may exert a double response in a temporal- or spatial-dependent manner during tumor progression. One exosomal ncRNA might serve as a tumor suppressor, and transmission of this ncRNA does provide a potential therapeutic strategy. Nevertheless, this ncRNA may also play an integral role in a wide range of biological processes, and its aberrant regulation would entail a destabilization of cellular homeostasis and therefore trigger severe secondary consequences. As a result, the comprehensive exosomal ncRNAs landscape in the TME needs to be taken into consideration in the milieu of the force of cancer immunity to ascertain their functions in the overall response. On the other hand, the distribution trend of specific exosomal ncRNAs could be correlated with susceptibility to immunotherapies and, as such, they are available to be utilized as encouraging biomarkers for surveying and forecasting the response to treatment.
Despite advances in the interaction between exosomal ncRNAs and TME, there is still a long way from basic research to clinical practice: exosomal ncRNAs as biomarkers and therapeutic targets. Therefore, comprehensive and cross-sectional research integrating multiple clinical data sets such as therapeutic significance will be needed to achieve this goal.
Author Contributions
ZL collected the related paper. ZJ wrote the draft and revised it. JJ revised it. LY collected the tables and designed them. All authors contributed to the article and approved the submitted version.
Conflict of Interest
The authors declare that the research was conducted in the absence of any commercial or financial relationships that could be construed as a potential conflict of interest.
Publisher’s Note
All claims expressed in this article are solely those of the authors and do not necessarily represent those of their affiliated organizations, or those of the publisher, the editors and the reviewers. Any product that may be evaluated in this article, or claim that may be made by its manufacturer, is not guaranteed or endorsed by the publisher.
References
1. Anderson NM, Simon MC. The Tumor Microenvironment. Curr Biol (2020) 30(16):R921–5. doi: 10.1016/j.cub.2020.06.081
2. Xu R, Rai A, Chen M, Suwakulsiri W, Greening DW, Simpson RJ. Extracellular Vesicles in Cancer - Implications for Future Improvements in Cancer Care. Nat Rev Clin Oncol (2018) 15(10):617–38. doi: 10.1038/s41571-018-0036-9
3. Sun Z, Yang S, Zhou Q, Wang G, Song J, Li Z, et al. Emerging Role of Exosome-Derived Long Non-Coding RNAs in Tumor Microenvironment. Mol Cancer (2018) 17(1):82. doi: 10.1186/s12943-018-0831-z
4. Fan Q, Yang L, Zhang X, Peng X, Wei S, Su D, et al. The Emerging Role of Exosome-Derived Non-Coding RNAs in Cancer Biology. Cancer Lett (2018) 414:107–15. doi: 10.1016/j.canlet.2017.10.040
5. Tang XH, Guo T, Gao XY, Wu XL, Xing XF, Ji JF, et al. Exosome-Derived Noncoding RNAs in Gastric Cancer: Functions and Clinical Applications. Mol Cancer (2021) 20(1):99. doi: 10.1186/s12943-021-01396-6
6. Cheng J, Meng J, Zhu L, Peng Y. Exosomal Noncoding RNAs in Glioma: Biological Functions and Potential Clinical Applications. Mol Cancer (2020) 19(1):66. doi: 10.1186/s12943-020-01189-3
7. Drak Alsibai K, Meseure D. Tumor Microenvironment and Noncoding RNAs as Co-Drivers of Epithelial-Mesenchymal Transition and Cancer Metastasis. Dev Dyn (2018) 247(3):405–31. doi: 10.1002/dvdy.24548
8. Zhang L, Zhang S, Yao J, Lowery FJ, Zhang Q, Huang WC, et al. Microenvironment-Induced PTEN Loss by Exosomal microRNA Primes Brain Metastasis Outgrowth. Nature (2015) 527(7576):100–4. doi: 10.1038/nature15376
9. Xue M, Chen W, Xiang A, Wang R, Chen H, Pan J, et al. Hypoxic Exosomes Facilitate Bladder Tumor Growth and Development Through Transferring Long Non-Coding RNA-Uca1. Mol Cancer (2017) 16(1):143. doi: 10.1186/s12943-017-0714-8
10. Zeng Z, Li Y, Pan Y, Lan X, Song F, Sun J, et al. Cancer-Derived Exosomal miR-25-3p Promotes Pre-Metastatic Niche Formation by Inducing Vascular Permeability and Angiogenesis. Nat Commun (2018) 9(1):5395. doi: 10.1038/s41467-018-07810-w
11. Zhang H, Deng T, Liu R, Ning T, Yang H, Liu D, et al. CAF Secreted miR-522 Suppresses Ferroptosis and Promotes Acquired Chemo-Resistance in Gastric Cancer. Mol Cancer (2020) 19(1):43. doi: 10.1186/s12943-020-01168-8
12. Jia Z, An J, Liu Z, Zhang F. Non-Coding RNAs in Colorectal Cancer: Their Functions and Mechanisms. Front Oncol (2022) 12:783079. doi: 10.3389/fonc.2022.783079
13. Jeong S, Park J, Pathania D, Castro CM, Weissleder R, Lee H. Integrated Magneto-Electrochemical Sensor for Exosome Analysis. ACS Nano (2016) 10(2):1802–9. doi: 10.1021/acsnano.5b07584
14. Wortzel I, Dror S, Kenific CM, Lyden D. Exosome-Mediated Metastasis: Communication From a Distance. Dev Cell (2019) 49(3):347–60. doi: 10.1016/j.devcel.2019.04.011
15. Robbins PD, Morelli AE. Regulation of Immune Responses by Extracellular Vesicles. Nat Rev Immunol (2014) 14(3):195–208. doi: 10.1038/nri3622
16. Ibrahim A, Marbán E. Exosomes: Fundamental Biology and Roles in Cardiovascular Physiology. Annu Rev Physiol (2016) 78:67–83. doi: 10.1146/annurev-physiol-021115-104929
17. Hu W, Liu C, Bi Z-Y, Zhou Q, Zhang H, Li L-L, et al. Comprehensive Landscape of Extracellular Vesicle-Derived RNAs in Cancer Initiation, Progression, Metastasis and Cancer Immunology. Mol Cancer (2020) 19(1):102. doi: 10.1186/s12943-020-01199-1
18. Gibbings DJ, Ciaudo C, Erhardt M, Voinnet O. Multivesicular Bodies Associate With Components of miRNA Effector Complexes and Modulate miRNA Activity. Nat Cell Biol (2009) 11(9):1143–9. doi: 10.1038/ncb1929
19. McKenzie AJ, Hoshino D, Hong NH, Cha DJ, Franklin JL, Coffey RJ, et al. KRAS-MEK Signaling Controls Ago2 Sorting Into Exosomes. Cell Rep (2016) 15(5):978–87. doi: 10.1016/j.celrep.2016.03.085
20. Bebelman MP, Smit MJ, Pegtel DM, Baglio SR. Biogenesis and Function of Extracellular Vesicles in Cancer. Pharmacol Ther (2018):1–11 188. doi: 10.1016/j.pharmthera.2018.02.013
21. Barbagallo C, Brex D, Caponnetto A, Cirnigliaro M, Scalia M, Magnano A, et al. LncRNA UCA1, Upregulated in CRC Biopsies and Downregulated in Serum Exosomes, Controls mRNA Expression by RNA-RNA Interactions. Mol Ther Nucleic Acids (2018) 12:229–41. doi: 10.1016/j.omtn.2018.05.009
22. Ragusa M, Barbagallo C, Cirnigliaro M, Battaglia R, Brex D, Caponnetto A, et al. Asymmetric RNA Distribution Among Cells and Their Secreted Exosomes: Biomedical Meaning and Considerations on Diagnostic Applications. Front Mol Biosci (2017) 4:66. doi: 10.3389/fmolb.2017.00066
23. Hinshaw DC, Shevde LA. The Tumor Microenvironment Innately Modulates Cancer Progression. Cancer Res (2019) 79(18):4557–66. doi: 10.1158/0008-5472.CAN-18-3962
24. Ma Z, Shuai Y, Gao X, Wen X, Ji J. Circular RNAs in the Tumour Microenvironment. Mol Cancer (2020) 19(1):8. doi: 10.1186/s12943-019-1113-0
25. Le MTN, Hamar P, Guo C, Basar E, Perdigão-Henriques R, Balaj L, et al. miR-200-Containing Extracellular Vesicles Promote Breast Cancer Cell Metastasis. J Clin Invest (2014) 124(12):5109–28. doi: 10.1172/JCI75695
26. He L, Zhu W, Chen Q, Yuan Y, Wang Y, Wang J, et al. Ovarian Cancer Cell-Secreted Exosomal miR-205 Promotes Metastasis by Inducing Angiogenesis. Theranostics (2019) 9(26):8206–20. doi: 10.7150/thno.37455
27. Kahroba H, Hejazi MS, Samadi N. Exosomes: From Carcinogenesis and Metastasis to Diagnosis and Treatment of Gastric Cancer. Cell Mol Life Sci CMLS (2019) 76(9):1747–58. doi: 10.1007/s00018-019-03035-2
28. Hsu YL, Hung JY, Chang WA, Lin YS, Pan YC, Tsai PH, et al. Hypoxic Lung Cancer-Secreted Exosomal miR-23a Increased Angiogenesis and Vascular Permeability by Targeting Prolyl Hydroxylase and Tight Junction Protein ZO-1. Oncogene (2017) 36(34):4929–42. doi: 10.1038/onc.2017.105
29. Webber J, Steadman R, Mason MD, Tabi Z, Clayton A. Cancer Exosomes Trigger Fibroblast to Myofibroblast Differentiation. Cancer Res (2010) 70(23):9621–30. doi: 10.1158/0008-5472.CAN-10-1722
30. Yi M, Xu L, Jiao Y, Luo S, Li A, Wu K. The Role of Cancer-Derived microRNAs in Cancer Immune Escape. J Hematol Oncol (2020) 13(1):25. doi: 10.1186/s13045-020-00848-8
31. Xu J, Xiao Y, Liu B, Pan S, Liu Q, Shan Y, et al. Exosomal MALAT1 Sponges miR-26a/26b to Promote the Invasion and Metastasis of Colorectal Cancer via FUT4 Enhanced Fucosylation and PI3K/Akt Pathway. J Exp Clin Cancer Res CR (2020) 39(1):54. doi: 10.1186/s13046-020-01562-6
32. Greening DW, Gopal SK, Xu R, Simpson RJ, Chen W. Exosomes and Their Roles in Immune Regulation and Cancer. Semin Cell Dev Biol (2015) 40:72–81. doi: 10.1016/j.semcdb.2015.02.009
33. Rooj AK, Mineo M, Godlewski J. MicroRNA and Extracellular Vesicles in Glioblastoma: Small But Powerful. Brain Tumor Pathol (2016) 33(2):77–88. doi: 10.1007/s10014-016-0259-3
34. Chistiakov DA, Chekhonin VP. Extracellular Vesicles Shed by Glioma Cells: Pathogenic Role and Clinical Value. Tumour Biol (2014) 35(9):8425–38. doi: 10.1007/s13277-014-2262-9
35. Tkach M, Kowal J, Zucchetti AE, Enserink L, Jouve M, Lankar D, et al. Qualitative Differences in T-Cell Activation by Dendritic Cell-Derived Extracellular Vesicle Subtypes. EMBO J (2017) 36(20):3012–28. doi: 10.15252/embj.201696003
36. Wahlund CJE, Güclüler G, Hiltbrunner S, Veerman RE, Näslund TI, Gabrielsson S. Exosomes From Antigen-Pulsed Dendritic Cells Induce Stronger Antigen-Specific Immune Responses Than Microvesicles In Vivo. Sci Rep (2017) 7(1):17095. doi: 10.1038/s41598-017-16609-6
37. Han Q, Zhao H, Jiang Y, Yin C, Zhang J. HCC-Derived Exosomes: Critical Player and Target for Cancer Immune Escape. Cells (2019) 8(6). doi: 10.3390/cells8060558
38. Zhang P-F, Gao C, Huang X-Y, Lu J-C, Guo X-J, Shi G-M, et al. Cancer Cell-Derived Exosomal Circuhrf1 Induces Natural Killer Cell Exhaustion and May Cause Resistance to Anti-PD1 Therapy in Hepatocellular Carcinoma. Mol Cancer (2020) 19(1):110. doi: 10.1186/s12943-020-01222-5
39. Chen S-W, Zhu S-Q, Pei X, Qiu B-Q, Xiong D, Long X, et al. Cancer Cell-Derived Exosomal Circusp7 Induces CD8 T Cell Dysfunction and Anti-PD1 Resistance by Regulating the miR-934/SHP2 Axis in NSCLC. Mol Cancer (2021) 20(1):144. doi: 10.1186/s12943-021-01448-x
40. Zhang L, Zhang J, Li P, Li T, Zhou Z, Wu H. Exosomal Hsa_Circ_0004658 Derived From RBPJ Overexpressed-Macrophages Inhibits Hepatocellular Carcinoma Progression via miR-499b-5p/JAM3. Cell Death Dis (2022) 13(1):32. doi: 10.1038/s41419-021-04345-9
41. Lu Q, Wang X, Zhu J, Fei X, Chen H, Li C. Hypoxic Tumor-Derived Exosomal Circ0048117 Facilitates M2 Macrophage Polarization Acting as miR-140 Sponge in Esophageal Squamous Cell Carcinoma. OncoTarg Ther (2020) 13:11883–97. doi: 10.2147/OTT.S284192
42. Chen J, Zhang K, Zhi Y, Wu Y, Chen B, Bai J, et al. Tumor-Derived Exosomal miR-19b-3p Facilitates M2 Macrophage Polarization and Exosomal LINC00273 Secretion to Promote Lung Adenocarcinoma Metastasis via Hippo Pathway. Clin Trans Med (2021) 11(9):e478. doi: 10.1002/ctm2.478
43. Sun J, Jia H, Bao X, Wu Y, Zhu T, Li R, et al. Tumor Exosome Promotes Th17 Cell Differentiation by Transmitting the lncRNA CRNDE-H in Colorectal Cancer. Cell Death Dis (2021) 12(1):123. doi: 10.1038/s41419-020-03376-y
44. Wang L-P, Lin J, Ma X-Q, Xu D-Y, Shi C-F, Wang W, et al. Exosomal DLX6-AS1 From Hepatocellular Carcinoma Cells Induces M2 Macrophage Polarization to Promote Migration and Invasion in Hepatocellular Carcinoma Through microRNA-15a-5p/CXCL17 Axis. J Exp Clin Cancer Res CR (2021) 40(1):177. doi: 10.1186/s13046-021-01973-z
45. Yang K, Zhang J, Bao C. Exosomal Circeif3k From Cancer-Associated Fibroblast Promotes Colorectal Cancer (CRC) Progression via miR-214/PD-L1 Axis. BMC Cancer (2021) 21(1):933. doi: 10.1186/s12885-021-08669-9
46. Deng Z, Rong Y, Teng Y, Zhuang X, Samykutty A, Mu J, et al. Exosomes miR-126a Released From MDSC Induced by DOX Treatment Promotes Lung Metastasis. Oncogene (2017) 36(5):639–51. doi: 10.1038/onc.2016.229
47. Ma Y-S, Wu T-M, Ling C-C, Yu F, Zhang J, Cao P-S, et al. M2 Macrophage-Derived Exosomal microRNA-155-5p Promotes the Immune Escape of Colon Cancer by Downregulating ZC3H12B. Mol Ther Oncolytics (2021) 20:484–98. doi: 10.1016/j.omto.2021.02.005
48. Neviani P, Wise PM, Murtadha M, Liu CW, Wu C-H, Jong AY, et al. Natural Killer-Derived Exosomal miR-186 Inhibits Neuroblastoma Growth and Immune Escape Mechanisms. Cancer Res (2019) 79(6):1151–64. doi: 10.1158/0008-5472.CAN-18-0779
49. Zhao Q, Huang L, Qin G, Qiao Y, Ren F, Shen C, et al. Cancer-Associated Fibroblasts Induce Monocytic Myeloid-Derived Suppressor Cell Generation via IL-6/Exosomal miR-21-Activated STAT3 Signaling to Promote Cisplatin Resistance in Esophageal Squamous Cell Carcinoma. Cancer Lett (2021) 518:35–48. doi: 10.1016/j.canlet.2021.06.009
50. Wang X, Qin X, Yan M, Shi J, Xu Q, Li Z, et al. Loss of Exosomal miR-3188 in Cancer-Associated Fibroblasts Contributes to HNC Progression. J Exp Clin Cancer Res CR (2019) 38(1):151. doi: 10.1186/s13046-019-1144-9
51. Chen B, Sang Y, Song X, Zhang D, Wang L, Zhao W, et al. Exosomal miR-500a-5p Derived From Cancer-Associated Fibroblasts Promotes Breast Cancer Cell Proliferation and Metastasis Through Targeting USP28. Theranostics (2021) 11(8):3932–47. doi: 10.7150/thno.53412
52. Li S, Zhu A, Ren K, Li S, Chen L. Ifnβ-Induced Exosomal Linc-EPHA6-1 Promotes Cytotoxicity of NK Cells by Acting as a ceRNA for hsa-miR-4485-5p to Up-Regulate NKp46 Expression. Life Sci (2020) 257:118064. doi: 10.1016/j.lfs.2020.118064
53. Mi X, Xu R, Hong S, Xu T, Zhang W, Liu M. M2 Macrophage-Derived Exosomal lncRNA AFAP1-AS1 and MicroRNA-26a Affect Cell Migration and Metastasis in Esophageal Cancer. Mol Ther Nucleic Acids (2020) 22:779–90. doi: 10.1016/j.omtn.2020.09.035
54. Zhang F, Sang Y, Chen D, Wu X, Wang X, Yang W, et al. M2 Macrophage-Derived Exosomal Long Non-Coding RNA AGAP2-AS1 Enhances Radiotherapy Immunity in Lung Cancer by Reducing microRNA-296 and Elevating NOTCH2. Cell Death Dis (2021) 12(5):467. doi: 10.1038/s41419-021-03700-0
55. Fan J-T, Zhou Z-Y, Luo Y-L, Luo Q, Chen S-B, Zhao J-C, et al. Exosomal lncRNA NEAT1 From Cancer-Associated Fibroblasts Facilitates Endometrial Cancer Progression via miR-26a/B-5p-Mediated STAT3/YKL-40 Signaling Pathway. Neoplasia (N Y NY) (2021) 23(7):692–703. doi: 10.1016/j.neo.2021.05.004
56. Madden MZ, Rathmell JC. The Complex Integration of T-Cell Metabolism and Immunotherapy. Cancer Discov (2021) 11(7):1636–43. doi: 10.1158/2159-8290.CD-20-0569
57. Song TL, Nairismägi M-L, Laurensia Y, Lim J-Q, Tan J, Li Z-M, et al. Oncogenic Activation of the STAT3 Pathway Drives PD-L1 Expression in Natural Killer/T-Cell Lymphoma. Blood (2018) 132(11):1146–58. doi: 10.1182/blood-2018-01-829424
58. Massard C, Gordon MS, Sharma S, Rafii S, Wainberg ZA, Luke J, et al. Safety and Efficacy of Durvalumab (MEDI4736), an Anti-Programmed Cell Death Ligand-1 Immune Checkpoint Inhibitor, in Patients With Advanced Urothelial Bladder Cancer. J Clin Oncol (2016) 34(26):3119–25. doi: 10.1200/JCO.2016.67.9761
59. Hong W, Xue M, Jiang J, Zhang Y, Gao X. Circular RNA Circ-CPA4/ Let-7 miRNA/PD-L1 Axis Regulates Cell Growth, Stemness, Drug Resistance and Immune Evasion in Non-Small Cell Lung Cancer (NSCLC). J Exp Clin Cancer Res CR (2020) 39(1):149. doi: 10.1186/s13046-020-01648-1
60. Liu J, Fan L, Yu H, Zhang J, He Y, Feng D, et al. Endoplasmic Reticulum Stress Causes Liver Cancer Cells to Release Exosomal miR-23a-3p and Up-Regulate Programmed Death Ligand 1 Expression in Macrophages. Hepatol (Baltimore Md) (2019) 70(1):241–58. doi: 10.1002/hep.30607
61. Li G, Kryczek I, Nam J, Li X, Li S, Li J, et al. LIMIT is an Immunogenic lncRNA in Cancer Immunity and Immunotherapy. Nat Cell Biol (2021) 23(5):526–37. doi: 10.1038/s41556-021-00672-3
62. You L, Mao L, Wei J, Jin S, Yang C, Liu H, et al. The Crosstalk Between Autophagic and Endo-/Exosomal Pathways in Antigen Processing for MHC Presentation in Anticancer T Cell Immune Responses. J Hematol Oncol (2017) 10(1):165. doi: 10.1186/s13045-017-0534-8
63. Sun Q, Hao Q, Prasanth KV. Nuclear Long Noncoding RNAs: Key Regulators of Gene Expression. Trends Genet (2018) 34(2):142–57. doi: 10.1016/j.tig.2017.11.005
64. Ruterbusch M, Pruner KB, Shehata L, Pepper M. In Vivo CD4 T Cell Differentiation and Function: Revisiting the Th1/Th2 Paradigm. Annu Rev Immunol (2020) 38:705–25. doi: 10.1146/annurev-immunol-103019-085803
65. Ye S-B, Zhang H, Cai T-T, Liu Y-N, Ni J-J, He J, et al. Exosomal miR-24-3p Impedes T-Cell Function by Targeting FGF11 and Serves as a Potential Prognostic Biomarker for Nasopharyngeal Carcinoma. J Pathol (2016) 240(3):329–40. doi: 10.1002/path.4781
66. Sakaguchi S, Mikami N, Wing JB, Tanaka A, Ichiyama K, Ohkura N. Regulatory T Cells and Human Disease. Annu Rev Immunol (2020) 38:541–66. doi: 10.1146/annurev-immunol-042718-041717
67. Yin H, Xiong G, Guo S, Xu C, Xu R, Guo P, et al. Delivery of Anti-miRNA for Triple-Negative Breast Cancer Therapy Using RNA Nanoparticles Targeting Stem Cell Marker Cd133. Mol Ther J Am Soc Gene Ther (2019) 27(7):1252–61. doi: 10.1016/j.ymthe.2019.04.018
68. Pan T, Jia P, Chen N, Fang Y, Liang Y, Guo M, et al. Delayed Remote Ischemic Preconditioning ConfersRenoprotection Against Septic Acute Kidney Injury via Exosomal miR-21. Theranostics (2019) 9(2):405–23. doi: 10.7150/thno.29832
69. Ning T, Li J, He Y, Zhang H, Wang X, Deng T, et al. Exosomal miR-208b Related With Oxaliplatin Resistance Promotes Treg Expansion in Colorectal Cancer. Mol Ther J Am Soc Gene Ther (2021) 29(9):2723–36. doi: 10.1016/j.ymthe.2021.04.028
70. Peng G, Wang HY, Peng W, Kiniwa Y, Seo KH, Wang R-F. Tumor-Infiltrating Gammadelta T Cells Suppress T and Dendritic Cell Function via Mechanisms Controlled by a Unique Toll-Like Receptor Signaling Pathway. Immunity (2007) 27(2):334–48. doi: 10.1016/j.immuni.2007.05.020
71. Ni C, Fang Q-Q, Chen W-Z, Jiang J-X, Jiang Z, Ye J, et al. Breast Cancer-Derived Exosomes Transmit lncRNA SNHG16 to Induce CD73+γδ1 Treg Cells. Signal Transduction Targeted Ther (2020) 5(1):41. doi: 10.1038/s41392-020-0129-7
72. Thwe PM, Amiel E. The Role of Nitric Oxide in Metabolic Regulation of Dendritic Cell Immune Function. Cancer Lett (2018) 412:236–42. doi: 10.1016/j.canlet.2017.10.032
73. Gardner A, Ruffell B. Dendritic Cells and Cancer Immunity. Trends Immunol (2016) 37(12):855–65. doi: 10.1016/j.it.2016.09.006
74. Fabbri M, Paone A, Calore F, Galli R, Gaudio E, Santhanam R, et al. MicroRNAs Bind to Toll-Like Receptors to Induce Prometastatic Inflammatory Response. Proc Natl Acad Sci USA (2012) 109(31):E2110–6. doi: 10.1073/pnas.1209414109
75. Zhou M, Chen J, Zhou L, Chen W, Ding G, Cao L. Pancreatic Cancer Derived Exosomes Regulate the Expression of TLR4 in Dendritic Cells. Via miR-203 Cell Immunol (2014) 292(1-2):65–9. doi: 10.1016/j.cellimm.2014.09.004
76. Rodriguez A, Vigorito E, Clare S, Warren MV, Couttet P, Soond DR, et al. Requirement of Bic/microRNA-155 for Normal Immune Function. Science (2007) 316(5824):608–11. doi: 10.1126/science.1139253
77. Ceppi M, Pereira PM, Dunand-Sauthier I, Barras E, Reith W, Santos MA, et al. MicroRNA-155 Modulates the Interleukin-1 Signaling Pathway in Activated Human Monocyte-Derived Dendritic Cells. Proc Natl Acad Sci USA (2009) 106(8):2735–40. doi: 10.1073/pnas.0811073106
78. Asadirad A, Hashemi SM, Baghaei K, Ghanbarian H, Mortaz E, Zali MR, et al. Phenotypical and Functional Evaluation of Dendritic Cells After Exosomal Delivery of miRNA-155. Life Sci (2019) 219:152–62. doi: 10.1016/j.lfs.2019.01.005
79. Seder RA, Gazzinelli R, Sher A, Paul WE. Interleukin 12 Acts Directly on CD4+ T Cells to Enhance Priming for Interferon Gamma Production and Diminishes Interleukin 4 Inhibition of Such Priming. Proc Natl Acad Sci USA (1993) 90(21):10188–92. doi: 10.1073/pnas.90.21.10188
80. Liu X, Zhan Z, Xu L, Ma F, Li D, Guo Z, et al. MicroRNA-148/152 Impair Innate Response and Antigen Presentation of TLR-Triggered Dendritic Cells by Targeting Camkiiα. J Immunol (2010) 185(12):7244–51. doi: 10.4049/jimmunol.1001573
81. Zilionis R, Engblom C, Pfirschke C, Savova V, Zemmour D, Saatcioglu HD, et al. Single-Cell Transcriptomics of Human and Mouse Lung Cancers Reveals Conserved Myeloid Populations Across Individuals and Species. Immunity (2019) 50(5):1317–34. doi: 10.1016/j.immuni.2019.03.009
82. Cheng H, Wang Z, Fu L, Xu T. Macrophage Polarization in the Development and Progression of Ovarian Cancers: An Overview. Front Oncol (2019) 9:421. doi: 10.3389/fonc.2019.00421
83. Elyada E, Bolisetty M, Laise P, Flynn WF, Courtois ET, Burkhart RA, et al. Cross-Species Single-Cell Analysis of Pancreatic Ductal Adenocarcinoma Reveals Antigen-Presenting Cancer-Associated Fibroblasts. Cancer Discov (2019) 9(8):1102–23. doi: 10.1158/2159-8290.CD-19-0094
84. Yin Z, Ma T, Huang B, Lin L, Zhou Y, Yan J, et al. Macrophage-Derived Exosomal microRNA-501-3p Promotes Progression of Pancreatic Ductal Adenocarcinoma Through the TGFBR3-Mediated TGF-β Signaling Pathway. J Exp Clin Cancer Res CR (2019) 38(1):310. doi: 10.1186/s13046-019-1313-x
85. Nishida J, Miyazono K, Ehata S. Decreased TGFBR3/betaglycan Expression Enhances the Metastatic Abilities of Renal Cell Carcinoma Cells Through TGF-β-Dependent and -Independent Mechanisms. Oncogene (2018) 37(16):2197–212. doi: 10.1038/s41388-017-0084-0
86. Wang X, Luo G, Zhang K, Cao J, Huang C, Jiang T, et al. Hypoxic Tumor-Derived Exosomal miR-301a Mediates M2 Macrophage Polarization via PTEN/Pi3kγ to Promote Pancreatic Cancer Metastasis. Cancer Res (2018) 78(16):4586–98. doi: 10.1158/0008-5472.CAN-17-3841
87. Li Z, Meng X, Wu P, Zha C, Han B, Li L, et al. Glioblastoma Cell-Derived lncRNA-Containing Exosomes Induce Microglia to Produce Complement C5, Promoting Chemotherapy Resistance. Cancer Immunol Res (2021) 9(12):1383–99. doi: 10.1158/2326-6066.CIR-21-0258
88. Qian M, Wang S, Guo X, Wang J, Zhang Z, Qiu W, et al. Hypoxic Glioma-Derived Exosomes Deliver microRNA-1246 to Induce M2 Macrophage Polarization by Targeting TERF2IP via the STAT3 and NF-κb Pathways. Oncogene (2020) 39(2):428–42. doi: 10.1038/s41388-019-0996-y
89. Abels ER, Maas SLN, Nieland L, Wei Z, Cheah PS, Tai E, et al. Glioblastoma-Associated Microglia Reprogramming Is Mediated by Functional Transfer of Extracellular miR-21. Cell Rep (2019) 28(12):3105–19. doi: 10.1016/j.celrep.2019.08.036
90. Zhang Z, Yin J, Lu C, Wei Y, Zeng A, You Y. Exosomal Transfer of Long non-Coding RNA SBF2-AS1 Enhances Chemoresistance to Temozolomide in Glioblastoma. J Exp Clin Cancer Res CR (2019) 38(1):166. doi: 10.1186/s13046-019-1139-6
91. Zhao S, Mi Y, Guan B, Zheng B, Wei P, Gu Y, et al. Tumor-Derived Exosomal miR-934 Induces Macrophage M2 Polarization to Promote Liver Metastasis of Colorectal Cancer. J Hematol Oncol (2020) 13(1):156. doi: 10.1186/s13045-020-00991-2
92. Franklin RA, Liao W, Sarkar A, Kim MV, Bivona MR, Liu K, et al. The Cellular and Molecular Origin of Tumor-Associated Macrophages. Science (2014) 344(6186):921–5. doi: 10.1126/science.1252510
93. Allen IC, Wilson JE, Schneider M, Lich JD, Roberts RA, Arthur JC, et al. NLRP12 Suppresses Colon Inflammation and Tumorigenesis Through the Negative Regulation of Noncanonical NF-κb Signaling. Immunity (2012) 36(5):742–54. doi: 10.1016/j.immuni.2012.03.012
94. Dong W, Wu X, Ma S, Wang Y, Nalin AP, Zhu Z, et al. The Mechanism of Anti-PD-L1 Antibody Efficacy Against PD-L1-Negative Tumors Identifies NK Cells Expressing PD-L1 as a Cytolytic Effector. Cancer Discov (2019) 9(10):1422–37. doi: 10.1158/2159-8290.CD-18-1259
95. Zheng Y, Li Y, Lian J, Yang H, Li F, Zhao S, et al. TNF-α-Induced Tim-3 Expression Marks the Dysfunction of Infiltrating Natural Killer Cells in Human Esophageal Cancer. J Trans Med (2019) 17(1):165. doi: 10.1186/s12967-019-1917-0
96. Tang R, Rangachari M, Kuchroo VK. Tim-3: A Co-Receptor With Diverse Roles in T Cell Exhaustion and Tolerance. Semin Immunol (2019) 42:101302. doi: 10.1016/j.smim.2019.101302
97. Guillerey C, Huntington ND, Smyth MJ. Targeting Natural Killer Cells in Cancer Immunotherapy. Nat Immunol (2016) 17(9):1025–36. doi: 10.1038/ni.3518
98. Jong AY, Wu C-H, Li J, Sun J, Fabbri M, Wayne AS, et al. Large-Scale Isolation and Cytotoxicity of Extracellular Vesicles Derived From Activated Human Natural Killer Cells. J Extracell Vesicles (2017) 6(1):1294368. doi: 10.1080/20013078.2017.1294368
99. Neo SY, Yang Y, Record J, Ma R, Chen X, Chen Z, et al. CD73 Immune Checkpoint Defines Regulatory NK Cells Within the Tumor Microenvironment. J Clin Invest (2020) 130(3):1185–98. doi: 10.1172/JCI128895
100. Gaggioli C, Hooper S, Hidalgo-Carcedo C, Grosse R, Marshall JF, Harrington K, et al. Fibroblast-Led Collective Invasion of Carcinoma Cells With Differing Roles for RhoGTPases in Leading and Following Cells. Nat Cell Biol (2007) 9(12):1392–400. doi: 10.1038/ncb1658
101. Dou D, Ren X, Han M, Xu X, Ge X, Gu Y, et al. Cancer-Associated Fibroblasts-Derived Exosomes Suppress Immune Cell Function in Breast Cancer via the miR-92/PD-L1 Pathway. Front Immunol (2020) 11:2026. doi: 10.3389/fimmu.2020.02026
102. Mittal D, Vijayan D, Smyth MJ. Overcoming Acquired PD-1/PD-L1 Resistance With CD38 Blockade. Cancer Discov (2018) 8(9):1066–8. doi: 10.1158/2159-8290.CD-18-0798
103. Kim MH, Kim CG, Kim S-K, Shin SJ, Choe EA, Park S-H, et al. YAP-Induced PD-L1 Expression Drives Immune Evasion in BRAFi-Resistant Melanoma. Cancer Immunol Res (2018) 6(3):255–66. doi: 10.1158/2326-6066.CIR-17-0320
104. Ren J, Ding L, Zhang D, Shi G, Xu Q, Shen S, et al. Carcinoma-Associated Fibroblasts Promote the Stemness and Chemoresistance of Colorectal Cancer by Transferring Exosomal lncRNA H19. Theranostics (2018) 8(14):3932–48. doi: 10.7150/thno.25541
105. Taghikhani A, Farzaneh F, Sharifzad F, Mardpour S, Ebrahimi M, Hassan ZM. Engineered Tumor-Derived Extracellular Vesicles: Potentials in Cancer Immunotherapy. Front Immunol (2020) 11:221. doi: 10.3389/fimmu.2020.00221
106. Jiang M, Zhang W, Zhang R, Liu P, Ye Y, Yu W, et al. Cancer Exosome-Derived miR-9 and miR-181a Promote the Development of Early-Stage MDSCs via Interfering With SOCS3 and PIAS3 Respectively in Breast Cancer. Oncogene (2020) 39(24):4681–94. doi: 10.1038/s41388-020-1322-4
107. Qiu W, Guo X, Li B, Wang J, Qi Y, Chen Z, et al. Exosomal miR-1246 From Glioma Patient Body Fluids Drives the Differentiation and Activation of Myeloid-Derived Suppressor Cells. Mol Ther J Am Soc Gene Ther (2021) 29(12):3449–64. doi: 10.1016/j.ymthe.2021.06.023
108. Cooks T, Pateras IS, Jenkins LM, Patel KM, Robles AI, Morris J, et al. Mutant P53 Cancers Reprogram Macrophages to Tumor Supporting Macrophages via Exosomal miR-1246. Nat Commun (2018) 9(1):771. doi: 10.1038/s41467-018-03224-w
109. Mauri C, Bosma A. Immune Regulatory Function of B Cells. Annu Rev Immunol (2012) 30:221–41. doi: 10.1146/annurev-immunol-020711-074934
110. Ye L, Zhang Q, Cheng Y, Chen X, Wang G, Shi M, et al. Tumor-Derived Exosomal HMGB1 Fosters Hepatocellular Carcinoma Immune Evasion by Promoting TIM-1 Regulatory B Cell Expansion. J Immunother Cancer (2018) 6(1):145. doi: 10.1186/s40425-018-0451-6
111. Ding Q, Yeung M, Camirand G, Zeng Q, Akiba H, Yagita H, et al. Regulatory B Cells Are Identified by Expression of TIM-1 and Can Be Induced Through TIM-1 Ligation to Promote Tolerance in Mice. J Clin Invest (2011) 121(9):3645–56. doi: 10.1172/JCI46274
112. Sautès-Fridman C, Petitprez F, Calderaro J, Fridman WH. Tertiary Lymphoid Structures in the Era of Cancer Immunotherapy. Nat Rev Cancer (2019) 19(6):307–25. doi: 10.1038/s41568-019-0144-6
113. Sharonov GV, Serebrovskaya EO, Yuzhakova DV, Britanova OV. Chudakov DM. B Cells, Plasma Cells and Antibody Repertoires in the Tumour Microenvironment. Nat Rev Immunol (2020) 20(5):294–307. doi: 10.1038/s41577-019-0257-x
114. Dieudé M, Bell C, Turgeon J, Beillevaire D, Pomerleau L, Yang B, et al. The 20S Proteasome Core, Active Within Apoptotic Exosome-Like Vesicles, Induces Autoantibody Production and Accelerates Rejection. Sci Transl Med (2015) 7(318):318ra200. doi: 10.1126/scitranslmed.aac9816
115. Dieudé M, Turgeon J, Karakeussian Rimbaud A, Beillevaire D, Qi S, Patey N, et al. Extracellular Vesicles Derived From Injured Vascular Tissue Promote the Formation of Tertiary Lymphoid Structures in Vascular Allografts. Am J Transplant (2020) 20(3):726–38. doi: 10.1111/ajt.15707
116. Atashzar MR, Baharlou R, Karami J, Abdollahi H, Rezaei R, Pourramezan F, et al. Cancer Stem Cells: A Review From Origin to Therapeutic Implications. J Cell Physiol (2020) 235(2):790–803. doi: 10.1002/jcp.29044
117. Batlle E, Clevers H. Cancer Stem Cells Revisited. Nat Med (2017) 23(10):1124–34. doi: 10.1038/nm.4409
118. Li W, Zhang L, Guo B, Deng J, Wu S, Li F, et al. Exosomal FMR1-AS1 Facilitates Maintaining Cancer Stem-Like Cell Dynamic Equilibrium via TLR7/Nfκb/C-Myc Signaling in Female Esophageal Carcinoma. Mol Cancer (2019) 18(1):22. doi: 10.1186/s12943-019-0949-7
119. Tirino V, Desiderio V, Paino F, De Rosa A, Papaccio F, La Noce M, et al. Cancer Stem Cells in Solid Tumors: An Overview and New Approaches for Their Isolation and Characterization. FASEB J (2013) 27(1):13–24. doi: 10.1096/fj.12-218222
120. Vermeulen L, De Sousa E Melo F, van der Heijden M, Cameron K, de Jong JH, Borovski T, et al. Wnt Activity Defines Colon Cancer Stem Cells and Is Regulated by the Microenvironment. Nat Cell Biol (2010) 12(5):468–76. doi: 10.1038/ncb2048
121. Chan XHD, Nama S, Gopal F, Rizk P, Ramasamy S, Sundaram G, et al. Targeting Glioma Stem Cells by Functional Inhibition of a Prosurvival oncomiR-138 in Malignant Gliomas. Cell Rep (2012) 2(3):591–602. doi: 10.1016/j.celrep.2012.07.012
122. Jiang J, Lu J, Wang X, Sun B, Liu X, Ding Y, et al. Glioma Stem Cell-Derived Exosomal miR-944 Reduces Glioma Growth and Angiogenesis by Inhibiting AKT/ERK Signaling. Aging (2021) 13(15):19243–59. doi: 10.18632/aging.203243
123. Boelens MC, Wu TJ, Nabet BY, Xu B, Qiu Y, Yoon T, et al. Exosome Transfer From Stromal to Breast Cancer Cells Regulates Therapy Resistance Pathways. Cell (2014) 159(3):499–513. doi: 10.1016/j.cell.2014.09.051
124. Wang Y, Wang L, Chen C, Chu X. New Insights Into the Regulatory Role of microRNA in Tumor Angiogenesis and Clinical Implications. Mol Cancer (2018) 17(1):22. doi: 10.1186/s12943-018-0766-4
125. Behera J, Kumar A, Voor MJ, Tyagi N. Exosomal lncRNA-H19 Promotes Osteogenesis and Angiogenesis Through Mediating Angpt1/Tie2-NO Signaling in CBS-Heterozygous Mice. Theranostics (2021) 11(16):7715–34. doi: 10.7150/thno.58410
126. Liu Y, Luo F, Wang B, Li H, Xu Y, Liu X, et al. STAT3-Regulated Exosomal miR-21 Promotes Angiogenesis and Is Involved in Neoplastic Processes of Transformed Human Bronchial Epithelial Cells. Cancer Lett (2016) 370(1):125–35. doi: 10.1016/j.canlet.2015.10.011
127. Wang S, Ma F, Feng Y, Liu T, He S. Role of Exosomal Mir−21 in the Tumor Microenvironment and Osteosarcoma Tumorigenesis and Progression (Review). Int J Oncol (2020) 56(5):1055–63. doi: 10.3892/ijo.2020.4992
128. Xie M, Yu T, Jing X, Ma L, Fan Y, Yang F, et al. Exosomal Circshkbp1 Promotes Gastric Cancer Progression via Regulating the miR-582-3p/HUR/VEGF Axis and Suppressing HSP90 Degradation. Mol Cancer (2020) 19(1):112. doi: 10.1186/s12943-020-01208-3
129. Cheng C, Zhang Z, Cheng F, Shao Z. Exosomal lncRNA RAMP2-AS1 Derived From Chondrosarcoma Cells Promotes Angiogenesis Through miR-2355-5p/VEGFR2 Axis. OncoTarg Ther (2020) 13:3291–301. doi: 10.2147/OTT.S244652
130. Guo Z, Wang X, Yang Y, Chen W, Zhang K, Teng B, et al. Hypoxic Tumor-Derived Exosomal Long Noncoding RNA UCA1 Promotes Angiogenesis via miR-96-5p/AMOTL2 in Pancreatic Cancer. Mol Ther Nucleic Acids (2020) 22:179–95. doi: 10.1016/j.omtn.2020.08.021
131. Lu J, Liu Q-H, Wang F, Tan J-J, Deng Y-Q, Peng X-H, et al. Exosomal miR-9 Inhibits Angiogenesis by Targeting MDK and Regulating PDK/AKT Pathway in Nasopharyngeal Carcinoma. J Exp Clin Cancer Res CR (2018) 37(1):147. doi: 10.1186/s13046-018-0814-3
132. Huang X-Y, Huang Z-L, Huang J, Xu B, Huang X-Y, Xu Y-H, et al. Exosomal circRNA-100338 Promotes Hepatocellular Carcinoma Metastasis via Enhancing Invasiveness and Angiogenesis. J Exp Clin Cancer Res CR (2020) 39(1):20. doi: 10.1186/s13046-020-1529-9
133. Paget S. The Distribution of Secondary Growths in Cancer of the Breast. 1889 Cancer Metastasis Rev (1989) 8(2):98–101.
134. Sun W, Ren Y, Lu Z, Zhao X. The Potential Roles of Exosomes in Pancreatic Cancer Initiation and Metastasis. Mol Cancer (2020) 19(1):135. doi: 10.1186/s12943-020-01255-w
135. Klein CA. Cancer Progression and the Invisible Phase of Metastatic Colonization. Nat Rev Cancer (2020) 20(11):681–94. doi: 10.1038/s41568-020-00300-6
136. Hoshino A, Costa-Silva B, Shen TL, Rodrigues G, Hashimoto A, Tesic Mark M, et al. Tumour Exosome Integrins Determine Organotropic Metastasis. Nature (2015) 527(7578):329–35. doi: 10.1038/nature15756
137. Zhang C, Xu L, Deng G, Ding Y, Bi K, Jin H, et al. Exosomal HOTAIR Promotes Proliferation, Migration and Invasion of Lung Cancer by Sponging miR-203. Sci China Life Sci (2020) 63(8):1265–8. doi: 10.1007/s11427-019-1579-x
138. Tao K, Liu J, Liang J, Xu X, Xu L, Mao W. Vascular Endothelial Cell-Derived Exosomal miR-30a-5p Inhibits Lung Adenocarcinoma Malignant Progression by Targeting CCNE2. Carcinogenesis (2021) 42(8):1056–67. doi: 10.1093/carcin/bgab051
139. Chen C, Luo Y, He W, Zhao Y, Kong Y, Liu H, et al. Exosomal Long Noncoding RNA LNMAT2 Promotes Lymphatic Metastasis in Bladder Cancer. J Clin Invest (2020) 130(1):404–21. doi: 10.1172/JCI130892
140. Li J, Li Z, Jiang P, Peng M, Zhang X, Chen K, et al. Circular RNA IARS (Circ-IARS) Secreted by Pancreatic Cancer Cells and Located Within Exosomes Regulates Endothelial Monolayer Permeability to Promote Tumor Metastasis. J Exp Clin Cancer Res CR (2018) 37(1):177. doi: 10.1186/s13046-018-0822-3
141. Choi P-W, Ng S-W. The Functions of MicroRNA-200 Family in Ovarian Cancer: Beyond Epithelial-Mesenchymal Transition. Int J Mol Sci (2017) 18(6). doi: 10.3390/ijms18061207
142. Li Z, Jiang P, Li J, Peng M, Zhao X, Zhang X, et al. Tumor-Derived Exosomal lnc-Sox2ot Promotes EMT and Stemness by Acting as a ceRNA in Pancreatic Ductal Adenocarcinoma. Oncogene (2018) 37(28):3822–38. doi: 10.1038/s41388-018-0237-9
143. Zhang X, Sai B, Wang F, Wang L, Wang Y, Zheng L, et al. Hypoxic BMSC-Derived Exosomal miRNAs Promote Metastasis of Lung Cancer Cells via STAT3-Induced EMT. Mol Cancer (2019) 18(1):40. doi: 10.1186/s12943-019-0959-5
144. Dou R, Liu K, Yang C, Zheng J, Shi D, Lin X, et al. EMT-Cancer Cells-Derived Exosomal miR-27b-3p Promotes Circulating Tumour Cells-Mediated Metastasis by Modulating Vascular Permeability in Colorectal Cancer. Clin Trans Med (2021) 11(12):e595. doi: 10.1002/ctm2.595
145. Liu W, Lv C, Zhang B, Zhou Q, Cao Z. MicroRNA-27b Functions as a New Inhibitor of Ovarian Cancer-Mediated Vasculogenic Mimicry Through Suppression of Expression. RNA (2017) 23(7):1019–27. doi: 10.1261/rna.059592.116
146. Huang C-S, Ho J-Y, Chiang J-H, Yu C-P, Yu D-S. Exosome-Derived LINC00960 and LINC02470 Promote the Epithelial-Mesenchymal Transition and Aggressiveness of Bladder Cancer Cells. Cells (2020) 9(6). doi: 10.3390/cells9061419
147. Mohamed OA, Clarke HJ, Dufort D. Beta-Catenin Signaling Marks the Prospective Site of Primitive Streak Formation in the Mouse Embryo. Dev Dyn (2004) 231(2):416–24. doi: 10.1002/dvdy.20135
148. Oft M, Akhurst RJ, Balmain A. Metastasis Is Driven by Sequential Elevation of H-Ras and Smad2 Levels. Nat Cell Biol (2002) 4(7):487–94. doi: 10.1038/ncb807
149. Vincent T, Neve EPA, Johnson JR, Kukalev A, Rojo F, Albanell J, et al. A SNAIL1-SMAD3/4 Transcriptional Repressor Complex Promotes TGF-Beta Mediated Epithelial-Mesenchymal Transition. Nat Cell Biol (2009) 11(8):943–50. doi: 10.1038/ncb1905
150. Galluzzi L, Senovilla L, Vitale I, Michels J, Martins I, Kepp O, et al. Molecular Mechanisms of Cisplatin Resistance. Oncogene (2012) 31(15):1869–83. doi: 10.1038/onc.2011.384
151. Holohan C, Van Schaeybroeck S, Longley DB, Johnston PG. Cancer Drug Resistance: An Evolving Paradigm. Nat Rev Cancer (2013) 13(10):714–26. doi: 10.1038/nrc3599
152. Wang X, Zhang H, Yang H, Bai M, Ning T, Deng T, et al. Exosome-Delivered circRNA Promotes Glycolysis to Induce Chemoresistance Through the miR-122-PKM2 Axis in Colorectal Cancer. Mol Oncol (2020) 14(3):539–55. doi: 10.1002/1878-0261.12629
153. Schell JC, Olson KA, Jiang L, Hawkins AJ, Van Vranken JG, Xie J, et al. A Role for the Mitochondrial Pyruvate Carrier as a Repressor of the Warburg Effect and Colon Cancer Cell Growth. Mol Cell (2014) 56(3):400–13. doi: 10.1016/j.molcel.2014.09.026
154. Zhou Y, Tozzi F, Chen J, Fan F, Xia L, Wang J, et al. Intracellular ATP Levels are a Pivotal Determinant of Chemoresistance in Colon Cancer Cells. Cancer Res (2012) 72(1):304–14. doi: 10.1158/0008-5472.CAN-11-1674
155. Dartier J, Lemaitre E, Chourpa I, Goupille C, Servais S, Chevalier S, et al. ATP-Dependent Activity and Mitochondrial Localization of Drug Efflux Pumps in Doxorubicin-Resistant Breast Cancer Cells. Biochim Biophys Acta Gen Subj (2017) 1861(5 Pt A):1075–84. doi: 10.1016/j.bbagen.2017.02.019
156. Du B, Shim JS. Targeting Epithelial-Mesenchymal Transition (EMT) to Overcome Drug Resistance in Cancer. Molecules (2016) 21(7). doi: 10.3390/molecules21070965
157. Liu T, Zhang X, Du L, Wang Y, Liu X, Tian H, et al. Exosome-Transmitted miR-128-3p Increase Chemosensitivity of Oxaliplatin-Resistant Colorectal Cancer. Mol Cancer (2019) 18(1):43. doi: 10.1186/s12943-019-0981-7
158. Qin X, Guo H, Wang X, Zhu X, Yan M, Wang X, et al. Exosomal miR-196a Derived From Cancer-Associated Fibroblasts Confers Cisplatin Resistance in Head and Neck Cancer Through Targeting CDKN1B and ING5. Genome Biol (2019) 20(1):12. doi: 10.1186/s13059-018-1604-0
159. Wang M, Yu F, Ding H, Wang Y, Li P, Wang K. Emerging Function and Clinical Values of Exosomal MicroRNAs in Cancer. Mol Ther Nucleic Acids (2019) 16:791–804. doi: 10.1016/j.omtn.2019.04.027
160. Srivastava A, Filant J, Moxley KM, Sood A, McMeekin S, Ramesh R. Exosomes: A Role for Naturally Occurring Nanovesicles in Cancer Growth, Diagnosis and Treatment. Curr Gene Ther (2015) 15(2):182–92. doi: 10.2174/1566523214666141224100612
161. Ge Q, Zhou Y, Lu J, Bai Y, Xie X, Lu Z. miRNA in Plasma Exosome Is Stable Under Different Storage Conditions. Molecules (2014) 19(2):1568–75. doi: 10.3390/molecules19021568
162. Tsujiura M, Ichikawa D, Komatsu S, Shiozaki A, Takeshita H, Kosuga T, et al. Circulating microRNAs in Plasma of Patients With Gastric Cancers. Br J Cancer (2010) 102(7):1174–9. doi: 10.1038/sj.bjc.6605608
163. Chen X, Chen R-X, Wei W-S, Li Y-H, Feng Z-H, Tan L, et al. PRMT5 Circular RNA Promotes Metastasis of Urothelial Carcinoma of the Bladder Through Sponging miR-30c to Induce Epithelial-Mesenchymal Transition. Clin Cancer Res Off J Am Assoc Cancer Res (2018) 24(24):6319–30. doi: 10.1158/1078-0432.CCR-18-1270
164. Dong Z-Y, Zhong W-Z, Zhang X-C, Su J, Xie Z, Liu S-Y, et al. Potential Predictive Value of and Mutation Status for Response to PD-1 Blockade Immunotherapy in Lung Adenocarcinoma. Clin Cancer Res Off J Am Assoc For Cancer Res (2017) 23(12):3012–24. doi: 10.1158/1078-0432.CCR-16-2554
165. Nam GH, Choi Y, Kim GB, Kim S, Kim SA, Kim IS. Emerging Prospects of Exosomes for Cancer Treatment: From Conventional Therapy to Immunotherapy. Adv Mater (2020) 32(51):e2002440. doi: 10.1002/adma.202002440
166. Boyerinas B, Park S-M, Hau A, Murmann AE, Peter ME. The Role of Let-7 in Cell Differentiation and Cancer. Endocr Relat Cancer (2010) 17(1):F19–36. doi: 10.1677/ERC-09-0184
167. Ohno S-i, Takanashi M, Sudo K, Ueda S, Ishikawa A, Matsuyama N, et al. Systemically Injected Exosomes Targeted to EGFR Deliver Antitumor microRNA to Breast Cancer Cells. Mol Ther J Am Soc Gene Ther (2013) 21(1):185–91. doi: 10.1038/mt.2012.180
168. Petanidis S, Domvri K, Porpodis K, Anestakis D, Freitag L, Hohenforst-Schmidt W, et al. Inhibition of Kras-Derived Exosomes Downregulates Immunosuppressive BACH2/GATA-3 Expression via RIP-3 Dependent Necroptosis and miR-146/miR-210 Modulation. Biomed Pharmacother Biomed Pharmacother (2020) 122:109461. doi: 10.1016/j.biopha.2019.109461
169. Biswas S, Mandal G, Roy Chowdhury S, Purohit S, Payne KK, Anadon C, et al. Exosomes Produced by Mesenchymal Stem Cells Drive Differentiation of Myeloid Cells Into Immunosuppressive M2-Polarized Macrophages in Breast Cancer. J Immunol (2019) 203(12):3447–60. doi: 10.4049/jimmunol.1900692
170. Chen W, Quan Y, Fan S, Wang H, Liang J, Huang L, et al. Exosome-Transmitted Circular RNA Hsa_Circ_0051443 Suppresses Hepatocellular Carcinoma Progression. Cancer Lett (2020) 475:119–28. doi: 10.1016/j.canlet.2020.01.022
Keywords: exosomes, tumor microenvironment, non-coding RNAs, tumor immunity, cancer
Citation: Jia Z, Jia J, Yao L and Li Z (2022) Crosstalk of Exosomal Non-Coding RNAs in The Tumor Microenvironment: Novel Frontiers. Front. Immunol. 13:900155. doi: 10.3389/fimmu.2022.900155
Received: 20 March 2022; Accepted: 22 April 2022;
Published: 19 May 2022.
Edited by:
Peixin Dong, Hokkaido University, JapanCopyright © 2022 Jia, Jia, Yao and Li. This is an open-access article distributed under the terms of the Creative Commons Attribution License (CC BY). The use, distribution or reproduction in other forums is permitted, provided the original author(s) and the copyright owner(s) are credited and that the original publication in this journal is cited, in accordance with accepted academic practice. No use, distribution or reproduction is permitted which does not comply with these terms.
*Correspondence: Zhihan Li, c3RldmVubWVyY3VyeUBoZWJtdS5lZHUuY24=