- 1Department of Pathology, University of Arkansas for Medical Sciences, Little Rock, AR, United States
- 2Winthrop P. Rockefeller Cancer Institute, University of Arkansas for Medical Sciences, Little Rock, AR, United States
Vesicular stomatitis virus (VSV), a negative-strand RNA virus of the Vesiculovirus genus, has demonstrated encouraging anti-neoplastic activity across multiple human cancer types. VSV is particularly attractive as an oncolytic agent because of its broad tropism, fast replication kinetics, and amenability to genetic manipulations. Furthermore, VSV-induced oncolysis can elicit a potent antitumor cytotoxic T-cell response to viral proteins and tumor-associated antigens, resulting in a long-lasting antitumor effect. Because of this multifaceted immunomodulatory property, VSV was investigated extensively as an immunovirotherapy alone or combined with other anticancer modalities, such as immune checkpoint blockade. Despite these recent opportunities to delineate synergistic and additive antitumor effects with existing anticancer therapies, FDA approval for the use of oncolytic VSV in humans has not yet been granted. This mini-review discusses factors that have prompted the use of VSV as an immunovirotherapy in human cancers and provides insights into future perspectives and research areas to improve VSV-based oncotherapy.
Introduction
Vesicular stomatitis virus (VSV) is non-pathogenic, enveloped, negative-strand RNA Rhabdovirus with potent vaccine and oncolytic potential (1–6). VSV can infect nearly all cell types but cannot initiate a productive infection in healthy cells due to an antiviral response mediated by type-I interferons (IFNs).11 However, defects in IFN signaling often coincide with tumorigenesis (7, 8). Thus, VSV is capable of infecting and selectively destroying cancer cells with minimal damage to normal cells, making it an attractive therapeutic agent. Furthermore, VSV is particularly appealing as an oncolytic vector (OV) and vaccine agent due to low anti-VSV immunity in the general population (pre-existing immunity to OVs limits their intratumoral spread) and fast replication kinetics in cancer cells (9).
The nonsegmented VSV genome is typical of viruses in the Vesiculovirus genus. The approximately 11-kb genome encodes five structural proteins, including the nucleocapsid protein (N), phosphoprotein (P), matrix protein (M), glycoprotein (G), and the large polymerase protein (L) (10–12). VSV genome encapsidation is facilitated by specific interactions between the N and P proteins (12). The N protein is essential for suppressing the transcription-termination signal during viral replication (13). The P and L proteins function as co-factors of the RNA-dependent RNA polymerase (RdRp); they exert indispensable and versatile functions, including regulating the initiation, elongation, and encapsidation of viral RNAs (14, 15). Specifically, the RdRp binds the encapsidated viral genome at the leader region, then sequentially transcribes each gene (16).
The M protein is involved in virus assembly and budding (17). It has also been shown to inhibit innate antiviral responses and alter host transcriptional machinery, ultimately coercing tumor cells to undergo apoptosis (18). Therefore, viruses with mutant M proteins were developed to restrict viral replication to tumor cells with an altered type-I IFN signaling axis (19).
The G protein forms spike-like structures on the viral particle surface and plays an essential role in the initial stages of infection (20). In the Indiana strain, the G protein was shown to mediate viral attachment via interaction with the low-density lipoprotein receptor (LDL-R) and its family members (21). The VSV-G protein is capable of binding LDL-R via the cysteine-rich LDL-R domains CR2 and CR3, resulting in clathrin-dependent endocytosis and intracellular uptake of the VSV genome (22, 23). However, several other reports showed that isogenic pairs of wild type LDL-R and LDL-R–knockout (-/-) (24, 25) cell lines can be infected efficiently by VSV and other closely related family members, highlighting the potential role of other surface proteins or cell-intrinsic mechanisms in viral entry (22). The broad cellular tropism of VSV is attributable to its G protein; thus, it is often replaced with entry proteins from other viruses to improve the safety and selectivity of VSV-based oncolytic vectors (26).
Numerous studies have shed light on the fundamental mechanisms of VSV–host cell interactions, the dynamics of viral gene expression, and the pathogenesis of viral infection (26). These findings have greatly expanded our understanding of the biology and structure of VSV, informing the design of recombinant VSV (rVSV) vectors with improved safety and selectivity towards a broad range of cancer cells. Despite this progress, VSV-based immunovirotherapy has not lived up to its expectations, and FDA approval has not yet been granted. Thus, we eagerly await the published outcomes of various completed, recruiting, or active cancer treatment trials (clinicaltrials.gov) in the United States using rVSV as an immunovirotherapy platform. In the meantime, it is equally important to review the past and recent developments of VSV vectors in cancer therapy to derive insights into ways to refine and improve the antitumor efficacy of such vectors.
Development of VSV as a Vaccine Platform
The use of reverse genetics enabled researchers to rescue infectious negative-strand RNA viruses from viral genomic cDNAs, leading to significant improvement in our ability to manipulate and study RNA viruses for vaccine development and cancer therapy applications (27, 28). Owing to their ability to prime robust humoral and cellular immunity, VSV vectors have also been used as vaccine agents to generate protective immunity against infections with highly lethal human viruses, including Ebola, HIV, Marburg, Lassa, Zika, and SARS-COV-2 viruses (29–40). Other vaccine candidates using attenuated VSV vectors were evaluated in preclinical models to prevent illnesses due to influenza (41), hepatitis B virus (42), different strains of coronavirus causing respiratory diseases (43, 44), Yersinia pestis (bubonic plague) (45), respiratory syncytial virus (RSV) (46), herpes simplex virus 2 (HSV2) (47), Dengue virus (48), Chikungunya virus (49), Nipah virus (50), and human papillomavirus (HPV) (51).
However, despite abundant evidence of therapeutic efficacy, only one VSV-based vaccine is FDA approved (52). This is mainly due to concerns related to the promiscuous nature of the VSV entry glycoprotein (VSV-G), allowing the virus to infect neurons and induce encephalitis in mice (7, 53, 54). Thereby, questions were raised regarding the potential neurotoxicity of VSV in humans following systemic delivery, limiting its widespread clinical deployment as a vaccine vector in humans. To address this critical concern, several groups have engineered VSV vectors with mutated G proteins or harboring G proteins from other non-neurotropic viruses to ablate interactions with LDL-R, which is highly expressed in neurons (49, 55–57). Many VSV-derived vectors that have progressed to preclinical and clinical testing as vaccine agents also displayed lytic potency and elicited a strong, durable cytotoxic T-cell response in permissive tumors (58, 59). While most oncolytic viruses such as VSV induce robust tumor-cell killing in vitro, recent clinical reports strongly suggest that, in vivo, OVs turn “cold” tumors into hot tumors (60), as discussed below.
Rationale for Developing VSV as an Oncolytic Agent
Wild type VSV causes mild disease in cattle, horses, and swine, causing vesicles (blisters) around the mouth (61). The few reported cases of human VSV infections were limited to agricultural and laboratory workers, characterized by an incubation period between 8 to 48 hours, with mild flu-like symptoms (26, 62, 63). VSV is a highly cytopathic virus that infects nearly all cell types, but its infection and replication are enhanced in tumor cells with a defective IFN signaling pathway (64). This feature makes it an ideal oncolytic virus therapy agent. In addition, VSV has a fast kinetic cycle, does not integrate into the host genome (65), and is a potent inducer of apoptosis in the infected cancer cells—a critical feature of viral therapeutics (66, 67). The VSV genome is also relatively small and can accommodate the insertion of one or more foreign, functional genes (68). Importantly, VSV has demonstrated anticancer activity in a vast array of cancer cells, including osteosarcoma (69), cervical cancer (70), breast cancer (71), melanoma (72), hepatocellular carcinoma (73), pancreatic cancer (57), and glioblastoma (74).
Although VSV-based oncolytic vectors have shown efficacy in mouse models and led to multiple human studies (Table 1), barriers to FDA approval and clinical application remain. These barriers include variability in the efficiency by which VSV kills cancer cells, even among cancers from the same tissue of origin,4 and reports of VSV-induced encephalitis in laboratory animals and humans (87, 88). Furthermore, the heterogeneous therapeutic responses in solid cancers (e.g., pancreatic cancer) are attributed to factors such as a fibrotic and dense extracellular matrix, hypoxia, high interstitial tumor pressure, and low pH in the tumor microenvironment, limiting viral spread and immunogenic cell death in response to oncolytic therapy.13 The fact that VSV is cleared rapidly by the immune system (e.g., via neutralizing antibodies and complement molecules) has further dampened enthusiasm for this vector (7, 53, 54). These obstacles have severely limited the anticancer efficacy of VSV—particularly the inability to administer multiple doses to achieve tumor shrinkage and, most importantly, the inability to bypass the immune system and infect neoplastic cells.
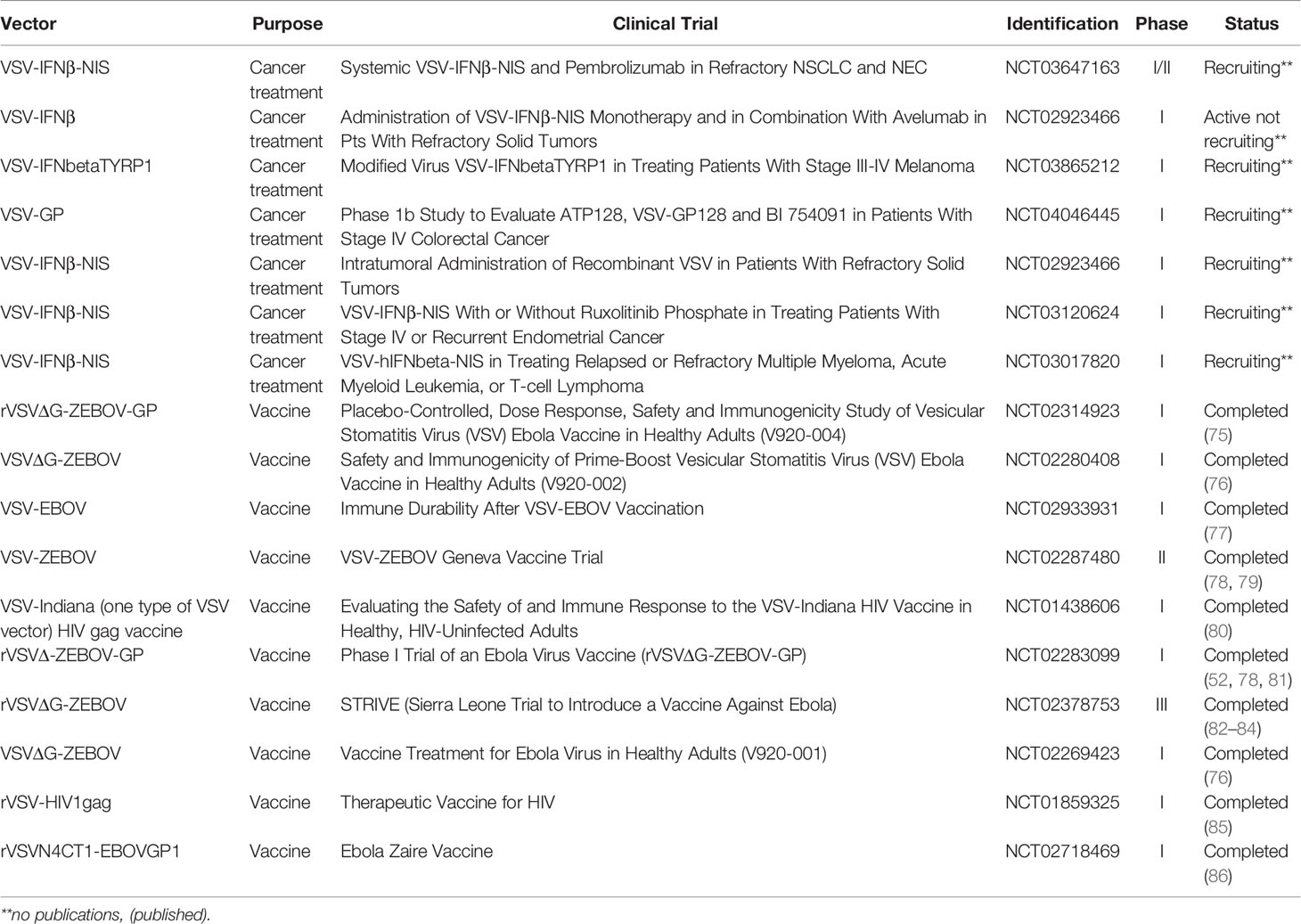
Table 1 Reported VSV-based vaccine and cancer treatment clinical trials (http://clinicaltrial.gov/).
A decade ago, the first VSV trial in human cancers was posted to clinicaltrials.gov; however, no trial results have been disseminated (Table 1). This lack of information raises pertinent questions about whether we can achieve the desired therapeutic outcomes with current VSV vectors. Although it is not clear when these results will be available, the eagerly awaited outcomes of these studies will undoubtedly guide the future development of VSV-based oncotherapy for clinical translation. Nonetheless, several groups developing and testing oncolytic vesiculoviruses have proposed ingenious viral engineering strategies (65, 89, 90) to improve patient safety and vector potency.
Strategies Designed to Enhance VSV Oncolytic Ability
Each genetic modification approach attempted to improve the antitumor activity of rVSV and its safety profile. Although rapid progress in nanotechnologies has enabled the improvement of delivery, pharmacokinetics, bioavailability in the tumor of rVSV vectors, many of these studies are in the early preclinical stages (91). Thus, this section will focus on vector engineering strategies to enhance safety, immunogenic apoptosis, and immune clearance.
Optimizing rVSV Design to Enhance Immunogenic Apoptosis and Reduce Neurotoxicity
Studies have shown that mechanistically, VSV-induced oncolysis results in the release of a series of molecules, including tumor-associated antigens (TAAs), pathogen-associated molecular patterns (PAMPs), and damage-associated molecular patterns (DAMPs) (92–94). The build-up of TAAS in the tumor microenvironment elicits the recruitment and activation of tumor-specific cytotoxic (CD8+) T cells (92–94). PAMPS and DAMPS promote infiltration of neutrophils, natural killer (NK) cells, and dendritic cells (DC) into tumor sites. This simultaneous activation of innate and adaptive immunity is essential for priming a robust and durable antitumor immune response. These earlier works on the ability of rVSV to induce immunogenic cell death or apoptosis have influenced the field in many ways. For example, Wu and colleagues demonstrated that rVSV expressing murine gammaherpesvirus M3 protein (rVSV[M-Δ51]-M3) induced enhanced tumor necrosis and prolonged survival substantially in an animal model compared to parental VSV (19). M3 is a secreted chemokine-binding protein that binds to a broad range of mammalian chemokines with high affinity (19). In addition to decreasing neurotoxicity, delivery of exogenous M3 enhanced rVSV(M-Δ51)-M3 oncolytic activity by curtailing the activation of host innate immunity against oncolytic VSV in the tumor. Similar to the mutant M protein vectors, VSVs harboring mutations in G (95), P, or L proteins (96) with improved oncoselectivity and potency were also developed and evaluated preclinically. Although rVSVs with mutated viral proteins have enabled some safety improvements, as evidenced by no apparent neurovirulence and no visible pathogenesis in animal models, these vectors are often highly attenuated (i.e., reduce viral replication capacity) and thus are not appropriate for clinical deployment. Therefore, Russell and colleagues have adopted a different approach by incorporating microRNA target sequences (e.g., for miR-125) into the viral genome to decrease the ability of the virus to replicate in neurons (97).
Generation of rVSV Vectors With Improved Immunostimulatory Activity
Multiple studies have also attempted to increase VSV safety and oncolytic properties by inserting into the viral genome genes encoding immunostimulatory proteins, chemoattractant molecules, or effectors that induce apoptosis in tumor cells (26, 59). One example is a VSV vector carrying the full-length p53 gene. Tumor protein p53 is a potent activator of apoptosis, and it is the most frequently mutated gene in human tumors (98). Indeed, the reactivation of p53 has been shown to potentiate antitumor immune activity (99). In an animal model, the vector VSV-M(Δ51)-p53, expressing p53, improved antitumor activity and enhanced CD49b+ NK and tumor-specific CD8+ T-cell responses (99, 100).
Immunostimulatory cytokines function in a synergistic or cascade fashion to modulate immune responses. Consequently, combining cytokines (101) with oncolytic viruses was seen as worth investigating for possible additive or synergistic long-term responses in clinical settings. Granulocyte–macrophage colony-stimulating factor (GM-CSF) is a potent immunostimulatory cytokine involved in the maturation and migration of macrophages and dendritic cells, which activate cytotoxic T cells (102). Hence, GM-CSF–expressing VSV (VSV-GM-CSF) vectors were developed, in which the transgene was inserted upstream of the VSV N gene or between the M and G genes (103–105). These vectors were attenuated and well-tolerated in vivo, and they triggered strong cellular and humoral antitumor immune responses (103–105). This work with VSV vectors expressing immunomodulatory cytokines demonstrated that tumor stage and type; immune mechanisms; and timing, dosage, and route of administration are crucial for obtaining the desired therapeutic effect with oncolytic viruses (106).
While IL-15 preferentially stimulates the proliferation of NK and memory CD8+ T cells and increases their antitumor activity (107), IL-12 functions as a “bridging” cytokine, providing an essential regulatory link between innate and adaptive immunity (108). Additionally, IL-23 has been shown to establish stable gene expression for activation of TH17 cells, but it is also crucial to activate innate immune cells, which are scattered across non-lymphoid organs (109). Thus, rVSV expressing IL-15, IL-12, or IL-23 (rVSV-IL-15, rVSV-IL-12, rVSV-IL-23) were generated and considerably improved synergistic antitumor efficacy compared to parental rVSV (110–112). Along the same line, IL-4 (113), thymidine kinase (113), IL-28 (114), Fms-like tyrosine kinase 3 ligand (115), and IFN-β (116) were expressed in VSV vectors, and their oncolytic activities have been documented across various cancer types. Based on encouraging preclinical studies, rVSV expressing human type-I IFN-β and a reporter known as sodium iodide symporter (VSV-IFNβ-NIS) has advanced through early to late phases of clinical testing (Table 1). Although VSV-based cytokine expression promotes superior oncolytic activity, it is essential to note that it can also potentiate viral clearance and impact the overall antitumor efficacy of the vectors.
Addressing Issues With Rapid Immune Clearance, Dense Stroma While Promoting Strong Apoptotic Activity
A plethora of viral engineering strategies has been proposed to enhance the oncolytic ability of VSV. Chimeric VSV displaying fusion (F) and hemagglutinin (H) proteins from Newcastle disease virus or measles virus was shown to abolish VSV-associated neurotoxicity and the effect of virus-neutralization antibody (NAbs) on the bioavailability of viral vectors through the formation of syncytia-like structures (7, 57). The molecular mechanisms by which wild-type VSV and recombinant rVSV vectors induce intrinsic, extrinsic, or endoplasmic reticulum stress-mediated apoptosis have been elucidated in numerous studies (64, 65). This has prompted several authors to employ vectors such as VSV-vCKBPs (117), VSV-UL141 (118),, and rVSV-FAST (119), capable of exerting robust oncolytic activity while resisting rapid viral clearance. It is reasonable to speculate that many viruses in tumor sites could infect tumor cells and induce enough oncolysis to eradicate the tumors on their own. However, virus-mediated oncolysis also provides conditions for priming antitumor immunity by activating tumor-specific cytotoxic T cells (120–131). The most critical aspect of rVSVs in cancer vaccines’ context is their ability to efficiently modulate anti-tumor immune responses. Consequently, current oncolytic viruses, such as rVSV and rVSV-derived vectors, may be applicable to cancer patients with functional immune systems. In addition, the tumor microenvironment is complex, characterized by a sophisticated interplay between tumor cells and many components, including immune cells, extracellular matrix, fibroblasts, and various molecules, such as enzymes. This harsh environment is a known barrier to therapy, including immunotherapy and oncolytic virus therapy.15 It is now evident that rVSV vectors alone have limited long-term antitumor activity and may achieve only a partially curative effect. Combining rVSVs with other therapies, including radiotherapy, T-cell therapies, and immune checkpoint blockades, could serve to unleash their full oncolytic potential (132–138). We enthusiastically await the results of VSV clinical trials and expect novel combinations of VSV vectors with other cancer treatments to emerge in the coming years.
Challenges and Future Directions
Despite evidence of the therapeutic benefits of rVSV-based oncotherapy, most investigations remain in the preclinical stage due to numerous challenges. These limitations include neurotoxicity (e.g., due to the promiscuous nature of the VSV entry glycoprotein [VSV-G]), rapid clearance by the immune system (e.g., via pre-existing VSV antibodies), and hepatotoxicity (e.g., viral interaction with Kupffer cells) (139, 140). Several strategies were proposed to address these obstacles, including modifying the VSV-G protein to achieve optimal therapeutic benefits (7, 53, 54, 141). Moreover, the lack of biomarkers that could be used to select patients who would benefit from oncolytic virus therapy represents a significant hurdle that we must seriously consider in future designs and clinical testing. Despite these challenges, the therapeutic potential of rVSV in cancer treatments is indisputable; indeed, VSV-IFN-β has advanced into late-phase clinical testing, renewing enthusiasm for oncolytic VSV.
Discussion
Early research into the biology of VSV, including genomic structure, immunogenic properties, and pan-tropism, paved the way for developing this promising oncolytic agent and vaccine vector. However, the seamless clinical translation of VSV oncotherapy still faces significant challenges, and VSV has not yet been utilized to its full potential as an oncolytic vector. As the mechanisms of tumor resistance to molecular therapy continue to be elucidated, we fully expect new VSV vectors with enhanced potency and selectivity to be evaluated soon.
Author Contributions
YZ and BN contributed to the study concept, drafting, and critical revision of the manuscript. Editorial support was provided by the Science Communication Group at the University of Arkansas for Medical Sciences. All authors approved the final, submitted version of the manuscript.
Funding
This work was supported by the National Institutes of Health (NIH) through a National Cancer Institute (NCI) grant (CA234324 to BN) and start-up funds from the Winthrop P. Rockefeller Cancer Institute at the University of Arkansas for Medical Sciences to BN. The article contents are solely the responsibility of the authors and do not necessarily represent the official views of the NIH.
Conflict of Interest
The authors declare that the research was conducted in the absence of any commercial or financial relationships that could be construed as a potential conflict of interest.
Publisher’s Note
All claims expressed in this article are solely those of the authors and do not necessarily represent those of their affiliated organizations, or those of the publisher, the editors and the reviewers. Any product that may be evaluated in this article, or claim that may be made by its manufacturer, is not guaranteed or endorsed by the publisher.
References
1. Kuzmin IV, Novella IS, Dietzgen RG, Padhi A, Rupprecht CE. The Rhabdoviruses: Biodiversity, Phylogenetics, and Evolution. Infect Genet Evol (2009) 9(4):541–53. doi: 10.1016/j.meegid.2009.02.005
2. Hayek AM, McCluskey BJ, Chavez GT, Salman MD. Financial Impact of the 1995 Outbreak of Vesicular Stomatitis on 16 Beef Ranches in Colorado. J Am Vet Med Assoc (1998) 212(6):820–3.
3. Abdelmageed AA, Ferran MC. The Propagation, Quantification, and Storage of Vesicular Stomatitis Virus. Curr Protoc Microbiol (2020) 58(1):e110. doi: 10.1002/cpmc.110
4. Luo M. The Nucleocapsid of Vesicular Stomatitis Virus. Sci China Life Sci (2012) 55(4):291–300. doi: 10.1007/s11427-012-4307-x
5. Burton AC. Stomatitis Contagiosa in Horses. Veterinary J (1917) 73:234–42. doi: 10.1016/S0372-5545(17)56190-8
6. Hanson RP. The Natural History of Vesicular Stomatitis. Bacteriol Rev (1952) 16(3):179–204. doi: 10.1128/br.16.3.179-204.1952
7. Ayala-Breton C, Barber GN, Russell SJ, Peng KW. Retargeting Vesicular Stomatitis Virus Using Measles Virus Envelope Glycoproteins. Hum Gene Ther (2012) 23(5):484–91. doi: 10.1089/hum.2011.146
8. Jebar AH, Errington-Mais F, Vile RG, Selby PJ, Melcher AA, Griffin S. Progress in Clinical Oncolytic Virus-Based Therapy for Hepatocellular Carcinoma. J Gen Virol (2015) 96(Pt 7):1533–50. doi: 10.1099/vir.0.000098
9. Barber GN. VSV-Tumor Selective Replication and Protein Translation. Oncogene (2005) 24(52):7710–9. doi: 10.1038/sj.onc.1209042
10. Libersou S, Albertini AA, Ouldali M, Maury V, Maheu C, Raux H, et al. Distinct Structural Rearrangements of the VSV Glycoprotein Drive Membrane Fusion. J Cell Biol (2010) 191(1):199–210. doi: 10.1083/jcb.201006116
11. Gaudier M, Gaudin Y, Knossow M. Crystal Structure of Vesicular Stomatitis Virus Matrix Protein. EMBO J (2002) 21(12):2886–92. doi: 10.1093/emboj/cdf284
12. Green TJ, Macpherson S, Qiu S, Lebowitz J, Wertz GW, Luo M. Study of the Assembly of Vesicular Stomatitis Virus N Protein: Role of the P Protein. J Virol (2000) 74(20):9515–24. doi: 10.1128/JVI.74.20.9515-9524.2000
13. Blumberg BM, Leppert M, Kolakofsky D. Interaction of VSV Leader RNA and Nucleocapsid Protein may Control VSV Genome Replication. Cell (1981) 23(3):837–45. doi: 10.1016/0092-8674(81)90448-7
14. Morin B, Rahmeh AA, Whelan SP. Mechanism of RNA Synthesis Initiation by the Vesicular Stomatitis Virus Polymerase. EMBO J (2012) 31(5):1320–9. doi: 10.1038/emboj.2011.483
15. Bilsel PA, Rowe JE, Fitch WM, Nichol ST. Phosphoprotein and Nucleocapsid Protein Evolution of Vesicular Stomatitis Virus New Jersey. J Virol (1990) 64(6):2498–504. doi: 10.1128/jvi.64.6.2498-2504.1990
16. Whelan SP, Wertz GW. The 5’ Terminal Trailer Region of Vesicular Stomatitis Virus Contains a Position-Dependent Cis-Acting Signal for Assembly of RNA Into Infectious Particles. J Virol (1999) 73(1):307–15. doi: 10.1128/JVI.73.1.307-315.1999
17. Raux H, Obiang L, Richard N, Harper F, Blondel D, Gaudin Y. The Matrix Protein of Vesicular Stomatitis Virus Binds Dynamin for Efficient Viral Assembly. J Virol (2010) 84(24):12609–18. doi: 10.1128/JVI.01400-10
18. Ahmed M, McKenzie MO, Puckett S, Hojnacki M, Poliquin L, Lyles DS. Ability of the Matrix Protein of Vesicular Stomatitis Virus to Suppress Beta Interferon Gene Expression is Genetically Correlated With the Inhibition of Host RNA and Protein Synthesis. J Virol (2003) 77(8):4646–57. doi: 10.1128/JVI.77.8.4646-4657.2003
19. Wu L, Huang TG, Meseck M, Altomonte J, Ebert O, Shinozaki K, et al. rVSV(M Delta 51)-M3 is an Effective and Safe Oncolytic Virus for Cancer Therapy. Hum Gene Ther (2008) 19(6):635–47. doi: 10.1089/hum.2007.163
20. Belot L, Ouldali M, Roche S, Legrand P, Gaudin Y, Albertini AA. Crystal Structure of Mokola Virus Glycoprotein in its Post-Fusion Conformation. PloS Pathog (2020) 16(3):e1008383. doi: 10.1371/journal.ppat.1008383
21. Finkelshtein D, Werman A, Novick D, Barak S, Rubinstein M. LDL Receptor and its Family Members Serve as the Cellular Receptors for Vesicular Stomatitis Virus. Proc Natl Acad Sci U S A (2013) 110(18):7306–11. doi: 10.1073/pnas.1214441110
22. Nikolic J, Belot L, Raux H, Legrand P, Gaudin Y, Albertini AA. Structural Basis for the Recognition of LDL-Receptor Family Members by VSV Glycoprotein. Nat Commun (2018) 9(1):1029. doi: 10.1038/s41467-018-03432-4
23. Johannsdottir HK, Mancini R, Kartenbeck J, Amato L, Helenius A. Host Cell Factors and Functions Involved in Vesicular Stomatitis Virus Entry. J Virol (2009) 83(1):440–53. doi: 10.1128/JVI.01864-08
24. Essletzbichler P, Konopka T, Santoro F, Chen D, Gapp BV, Kralovics R, et al. Megabase-Scale Deletion Using CRISPR/Cas9 to Generate a Fully Haploid Human Cell Line. Genome Res (2014) 24(12):2059–65. doi: 10.1101/gr.177220.114
25. Kravtsova-Ivantsiv Y, Shomer I, Cohen-Kaplan V, Snijder B, Superti-Furga G, Gonen H, et al. KPC1-Mediated Ubiquitination and Proteasomal Processing of NF-Kappab1 P105 to P50 Restricts Tumor Growth. Cell (2015) 161(2):333–47. doi: 10.1016/j.cell.2015.03.001
26. Hastie E, Cataldi M, Marriott I, Grdzelishvili VZ. Understanding and Altering Cell Tropism of Vesicular Stomatitis Virus. Virus Res (2013) 176(1-2):16–32. doi: 10.1016/j.virusres.2013.06.003
27. Schnell MJ, Mebatsion T, Conzelmann KK. Infectious Rabies Viruses From Cloned cDNA. EMBO J (1994) 13(18):4195–203. doi: 10.1002/j.1460-2075.1994.tb06739.x
28. Stobart CC, Moore ML. RNA Virus Reverse Genetics and Vaccine Design. Viruses (2014) 6(7):2531–50. doi: 10.3390/v6072531
29. Munis AM, Bentley EM, Takeuchi Y. A Tool With Many Applications: Vesicular Stomatitis Virus in Research and Medicine. Expert Opin Biol Ther (2020) 20(10):1187–201. doi: 10.1080/14712598.2020.1787981
30. Case JB, Rothlauf PW, Chen RE, Kafai NM, Fox JM, Smith BK, et al. Replication-Competent Vesicular Stomatitis Virus Vaccine Vector Protects Against SARS-CoV-2-Mediated Pathogenesis in Mice. Cell Host Microbe (2020) 28(3):465–74 e4. doi: 10.1016/j.chom.2020.07.018
31. Oa Donnell KL, Clancy CS, Griffin AJ, Shifflett K, Gourdine T, Thomas T, et al. Optimization of Single Dose VSV-Based COVID-19 Vaccination in Hamsters. Front Immunol (2021) 12:788235. doi: 10.3389/fimmu.2021.788235
32. Roberts A, Buonocore L, Price R, Forman J, Rose JK. Attenuated Vesicular Stomatitis Viruses as Vaccine Vectors. J Virol (1999) 73(5):3723–32. doi: 10.1128/JVI.73.5.3723-3732.1999
33. Haglund K, Leiner I, Kerksiek K, Buonocore L, Pamer E, Rose JK. High-Level Primary CD8(+) T-Cell Response to Human Immunodeficiency Virus Type 1 Gag and Env Generated by Vaccination With Recombinant Vesicular Stomatitis Viruses. J Virol (2002) 76(6):2730–8. doi: 10.1128/JVI.76.6.2730-2738.2002
34. Tober R, Banki Z, Egerer L, Muik A, Behmuller S, Kreppel F, et al. VSV-GP: A Potent Viral Vaccine Vector That Boosts the Immune Response Upon Repeated Applications. J Virol (2014) 88(9):4897–907. doi: 10.1128/JVI.03276-13
35. Marzi A, Feldmann F, Geisbert TW, Feldmann H, Safronetz D. Vesicular Stomatitis Virus-Based Vaccines Against Lassa and Ebola Viruses. Emerg Infect Dis (2015) 21(2):305–7. doi: 10.3201/eid2102.141649
36. Yahalom-Ronen Y, Tamir H, Melamed S, Politi B, Shifman O, Achdout H, et al. A Single Dose of Recombinant VSV-G-Spike Vaccine Provides Protection Against SARS-CoV-2 Challenge. Nat Commun (2020) 11(1):6402. doi: 10.1038/s41467-020-20228-7
37. Choi JA, Wu K, Kim GN, Saeedian N, Seon SH, Park G, et al. Induction of Protective Immune Responses Against a Lethal Zika Virus Challenge Post-Vaccination With a Dual Serotype of Recombinant Vesicular Stomatitis Virus Carrying the Genetically Modified Zika Virus E Protein Gene. J Gen Virol (2021) 102(4). doi: 10.1099/jgv.0.001588
38. Rose NF, Marx PA, Luckay A, Nixon DF, Moretto WJ, Donahoe SM, et al. An Effective AIDS Vaccine Based on Live Attenuated Vesicular Stomatitis Virus Recombinants. Cell (2001) 106(5):539–49. doi: 10.1016/S0092-8674(01)00482-2
39. Mire CE, Geisbert JB, Agans KN, Satterfield BA, Versteeg KM, Fritz EA, et al. Durability of a Vesicular Stomatitis Virus-Based Marburg Virus Vaccine in Nonhuman Primates. PloS One (2014) 9(4):e94355. doi: 10.1371/journal.pone.0094355
40. Matassov D, Marzi A, Latham T, Xu R, Ota-Setlik A, Feldmann F, et al. Vaccination With a Highly Attenuated Recombinant Vesicular Stomatitis Virus Vector Protects Against Challenge With a Lethal Dose of Ebola Virus. J Infect Dis (2015) 212 Suppl 2:S443–51. doi: 10.1093/infdis/jiv316
41. Roberts A, Kretzschmar E, Perkins AS, Forman J, Price R, Buonocore L, et al. Vaccination With a Recombinant Vesicular Stomatitis Virus Expressing an Influenza Virus Hemagglutinin Provides Complete Protection From Influenza Virus Challenge. J Virol (1998) 72(6):4704–11. doi: 10.1128/JVI.72.6.4704-4711.1998
42. Cobleigh MA, Buonocore L, Uprichard SL, Rose JK, Robek MD. A Vesicular Stomatitis Virus-Based Hepatitis B Virus Vaccine Vector Provides Protection Against Challenge in a Single Dose. J Virol (2010) 84(15):7513–22. doi: 10.1128/JVI.00200-10
43. Kapadia SU, Rose JK, Lamirande E, Vogel L, Subbarao K, Roberts A. Long-Term Protection From SARS Coronavirus Infection Conferred by a Single Immunization With an Attenuated VSV-Based Vaccine. Virology (2005) 340(2):174–82. doi: 10.1016/j.virol.2005.06.016
44. Padron-Regalado E. Vaccines for SARS-CoV-2: Lessons From Other Coronavirus Strains. Infect Dis Ther (2020) 9:255–274. doi: 10.1007/s40121-020-00300-x
45. Palin A, Chattopadhyay A, Park S, Delmas G, Suresh R, Senina S, et al. An Optimized Vaccine Vector Based on Recombinant Vesicular Stomatitis Virus Gives High-Level, Long-Term Protection Against Yersinia Pestis Challenge. Vaccine (2007) 25(4):741–50. doi: 10.1016/j.vaccine.2006.08.010
46. Kahn JS, Roberts A, Weibel C, Buonocore L, Rose JK. Replication-Competent or Attenuated, Nonpropagating Vesicular Stomatitis Viruses Expressing Respiratory Syncytial Virus (RSV) Antigens Protect Mice Against RSV Challenge. J Virol (2001) 75(22):11079–87. doi: 10.1128/JVI.75.22.11079-11087.2001
47. Natuk RJ, Cooper D, Guo M, Calderon P, Wright KJ, Nasar F, et al. Recombinant Vesicular Stomatitis Virus Vectors Expressing Herpes Simplex Virus Type 2 gD Elicit Robust CD4+ Th1 Immune Responses and are Protective in Mouse and Guinea Pig Models of Vaginal Challenge. J Virol (2006) 80(9):4447–57. doi: 10.1128/JVI.80.9.4447-4457.2006
48. Lauretti F, Chattopadhyay A, de Oliveira Franca RF, Castro-Jorge L, Rose J, Fonseca BA. Recombinant Vesicular Stomatitis Virus-Based Dengue-2 Vaccine Candidate Induces Humoral Response and Protects Mice Against Lethal Infection. Hum Vaccin Immunother (2016) 12(9):2327–33. doi: 10.1080/21645515.2016.1183857
49. van den Pol AN, Mao G, Chattopadhyay A, Rose JK, Davis JN. Chikungunya, Influenza, Nipah, and Semliki Forest Chimeric Viruses With Vesicular Stomatitis Virus: Actions in the Brain. J Virol (2017) 91(6):e02154–16. doi: 10.1128/JVI.02154-16
50. DeBuysscher BL, Scott D, Thomas T, Feldmann H, Prescott J. Peri-Exposure Protection Against Nipah Virus Disease Using a Single-Dose Recombinant Vesicular Stomatitis Virus-Based Vaccine. NPJ Vaccines (2016) 1:16002 . doi: 10.1038/npjvaccines.2016.2
51. Liao JB, Publicover J, Rose JK, DiMaio D. Single-Dose, Therapeutic Vaccination of Mice With Vesicular Stomatitis Virus Expressing Human Papillomavirus Type 16 E7 Protein. Clin Vaccine Immunol (2008) 15(5):817–24. doi: 10.1128/CVI.00343-07
52. Poetsch JH, Dahlke C, Zinser ME, Kasonta R, Lunemann S, Rechtien A, et al. Detectable Vesicular Stomatitis Virus (VSV)-Specific Humoral and Cellular Immune Responses Following VSV-Ebola Virus Vaccination in Humans. J Infect Dis (2019) 219(4):556–61. doi: 10.1093/infdis/jiy565
53. Bourgeois-Daigneault MC, Roy DG, Falls T, Twumasi-Boateng K, St-Germain LE, Marguerie M, et al. Oncolytic Vesicular Stomatitis Virus Expressing Interferon-Gamma has Enhanced Therapeutic Activity. Mol Ther Oncolytics (2016) 3:16001. doi: 10.1038/mto.2016.1
54. Dold C, Rodriguez Urbiola C, Wollmann G, Egerer L, Muik A, Bellmann L, et al. Application of Interferon Modulators to Overcome Partial Resistance of Human Ovarian Cancers to VSV-GP Oncolytic Viral Therapy. Mol Ther Oncolytics (2016) 3:16021. doi: 10.1038/mto.2016.21
55. Kim J, Castellano JM, Jiang H, Basak JM, Parsadanian M, Pham V, et al. Overexpression of Low-Density Lipoprotein Receptor in the Brain Markedly Inhibits Amyloid Deposition and Increases Extracellular A Beta Clearance. Neuron (2009) 64(5):632–44. doi: 10.1016/j.neuron.2009.11.013
56. Ramsburg E, Rose NF, Marx PA, Mefford M, Nixon DF, Moretto WJ, et al. Highly Effective Control of an AIDS Virus Challenge in Macaques by Using Vesicular Stomatitis Virus and Modified Vaccinia Virus Ankara Vaccine Vectors in a Single-Boost Protocol. J Virol (2004) 78(8):3930–40. doi: 10.1128/JVI.78.8.3930-3940.2004
57. Nagalo BM, Breton CA, Zhou Y, Arora M, Bogenberger JM, Barro O, et al. Oncolytic Virus With Attributes of Vesicular Stomatitis Virus and Measles Virus in Hepatobiliary and Pancreatic Cancers. Mol Ther Oncolytics (2020) 18:546–55. doi: 10.1016/j.omto.2020.08.007
58. Wollmann G, Drokhlyansky E, Davis JN, Cepko C, van den Pol AN. Lassa-Vesicular Stomatitis Chimeric Virus Safely Destroys Brain Tumors. J Virol (2015) 89(13):6711–24. doi: 10.1128/JVI.00709-15
59. Felt SA, Grdzelishvili VZ. Recent Advances in Vesicular Stomatitis Virus-Based Oncolytic Virotherapy: A 5-Year Update. J Gen Virol (2017) 98(12):2895–911. doi: 10.1099/jgv.0.000980
60. Lemos de Matos A, Franco LS, McFadden G. Oncolytic Viruses and the Immune System: The Dynamic Duo. Mol Ther Methods Clin Dev (2020) 17:349–58. doi: 10.1016/j.omtm.2020.01.001
61. Rozo-Lopez P, Drolet BS, Londono-Renteria B. Vesicular Stomatitis Virus Transmission: A Comparison of Incriminated Vectors. Insects (2018) 9(4):190. doi: 10.3390/insects9040190
62. Hanson RP, Rasmussen AF Jr., Brandly CA, Brown JW. Human Infection With the Virus of Vesicular Stomatitis. J Lab Clin Med (1950) 36(5):754–8.
63. Johnson KM, Vogel JE, Peralta PH. Clinical and Serological Response to Laboratory-Acquired Human Infection by Indiana Type Vesicular Stomatitis Virus (VSV). Am J Trop Med Hyg (1966) 15(2):244–6. doi: 10.4269/ajtmh.1966.15.244
64. Simovic B, Walsh SR, Wan Y. Mechanistic Insights Into the Oncolytic Activity of Vesicular Stomatitis Virus in Cancer Immunotherapy. Oncolytic Virother (2015) 4:157–67. doi: 10.2147/OV.S66079
65. Bishnoi S, Tiwari R, Gupta S, Byrareddy SN, Nayak D. Oncotargeting by Vesicular Stomatitis Virus (VSV): Advances in Cancer Therapy. Viruses (2018) 10(2):90. doi: 10.3390/v10020090
66. Koyama AH. Induction of Apoptotic DNA Fragmentation by the Infection of Vesicular Stomatitis Virus. Virus Res (1995) 37(3):285–90. doi: 10.1016/0168-1702(95)00026-M
67. Rogers C, Fernandes-Alnemri T, Mayes L, Alnemri D, Cingolani G, Alnemri ES. Cleavage of DFNA5 by Caspase-3 During Apoptosis Mediates Progression to Secondary Necrotic/Pyroptotic Cell Death. Nat Commun (2017) 8:14128. doi: 10.1038/ncomms14128
68. Obuchi M, Fernandez M, Barber GN. Development of Recombinant Vesicular Stomatitis Viruses That Exploit Defects in Host Defense to Augment Specific Oncolytic Activity. J Virol (2003) 77(16):8843–56. doi: 10.1128/JVI.77.16.8843-8856.2003
69. Kubo T, Shimose S, Matsuo T, Fujimori J, Sakaguchi T, Yamaki M, et al. Oncolytic Vesicular Stomatitis Virus Administered by Isolated Limb Perfusion Suppresses Osteosarcoma Growth. J Orthop Res (2011) 29(5):795–800. doi: 10.1002/jor.21307
70. Le Boeuf F, Niknejad N, Wang J, Auer R, Weberpals JI, Bell JC, et al. Sensitivity of Cervical Carcinoma Cells to Vesicular Stomatitis Virus-Induced Oncolysis: Potential Role of Human Papilloma Virus Infection. Int J Cancer (2012) 131(3):E204–15. doi: 10.1002/ijc.27404
71. Ahmed M, Puckett S, Lyles DS. Susceptibility of Breast Cancer Cells to an Oncolytic Matrix (M) Protein Mutant of Vesicular Stomatitis Virus. Cancer Gene Ther (2010) 17(12):883–92. doi: 10.1038/cgt.2010.46
72. Wollmann G, Davis JN, Bosenberg MW, van den Pol AN. Vesicular Stomatitis Virus Variants Selectively Infect and Kill Human Melanomas But Not Normal Melanocytes. J Virol (2013) 87(12):6644–59. doi: 10.1128/JVI.03311-12
73. Ebert O, Shinozaki K, Huang TG, Savontaus MJ, Garcia-Sastre A, Woo SL. Oncolytic Vesicular Stomatitis Virus for Treatment of Orthotopic Hepatocellular Carcinoma in Immune-Competent Rats. Cancer Res (2003) 63(13):3605–11.
74. Cary ZD, Willingham MC, Lyles DS. Oncolytic Vesicular Stomatitis Virus Induces Apoptosis in U87 Glioblastoma Cells by a Type II Death Receptor Mechanism and Induces Cell Death and Tumor Clearance In Vivo. J Virol (2011) 85(12):5708–17. doi: 10.1128/JVI.02393-10
75. Heppner DG Jr., Kemp TL, Martin BK, Ramsey WJ, Nichols R, Dasen EJ, et al. Safety and Immunogenicity of the rVSVG-ZEBOV-GP Ebola Virus Vaccine Candidate in Healthy Adults: A Phase 1b Randomised, Multicentre, Double-Blind, Placebo-Controlled, Dose-Response Study. Lancet Infect Dis (2017) 17(8):854–66. doi: 10.1016/S1473-3099(17)30313-4
76. Regules JA, Beigel JH, Paolino KM, Voell J, Castellano AR, Hu Z, et al. A Recombinant Vesicular Stomatitis Virus Ebola Vaccine. N Engl J Med (2017) 376(4):330–41. doi: 10.1056/NEJMoa1414216
77. Huttner A, Agnandji ST, Combescure C, Fernandes JF, Bache EB, Kabwende L, et al. Determinants of Antibody Persistence Across Doses and Continents After Single-Dose rVSV-ZEBOV Vaccination for Ebola Virus Disease: An Observational Cohort Study. Lancet Infect Dis (2018) 18(7):738–48. doi: 10.1016/S1473-3099(18)30165-8
78. Agnandji ST, Huttner A, Zinser ME, Njuguna P, Dahlke C, Fernandes JF, et al. Phase 1 Trials of rVSV Ebola Vaccine in Africa and Europe. N Engl J Med (2016) 374(17):1647–60. doi: 10.1056/NEJMoa1502924
79. Huttner A, Dayer JA, Yerly S, Combescure C, Auderset F, Desmeules J, et al. The Effect of Dose on the Safety and Immunogenicity of the VSV Ebola Candidate Vaccine: A Randomised Double-Blind, Placebo-Controlled Phase 1/2 Trial. Lancet Infect Dis (2015) 15(10):1156–66. doi: 10.1016/S1473-3099(15)00154-1
80. Fuchs JD, Frank I, Elizaga ML, Allen M, Frahm N, Kochar N, et al. First-In-Human Evaluation of the Safety and Immunogenicity of a Recombinant Vesicular Stomatitis Virus Human Immunodeficiency Virus-1 Gag Vaccine (HVTN 090). Open Forum Infect Dis (2015) 2(3):ofv082. doi: 10.1093/ofid/ofv082
81. Dahlke C, Kasonta R, Lunemann S, Krahling V, Zinser ME, Biedenkopf N, et al. Dose-Dependent T-Cell Dynamics and Cytokine Cascade Following rVSV-ZEBOV Immunization. EBioMedicine (2017) 19:107–18. doi: 10.1016/j.ebiom.2017.03.045
82. Jarrett OD, Seward JF, Fombah AE, Lindblad R, Jalloh MI, El-Khorazaty J, et al. Monitoring Serious Adverse Events in the Sierra Leone Trial to Introduce a Vaccine Against Ebola. J Infect Dis (2018) 217(suppl_1):S24–32. doi: 10.1093/infdis/jiy042
83. Samai M, Seward JF, Goldstein ST, Mahon BE, Lisk DR, Widdowson MA, et al. The Sierra Leone Trial to Introduce a Vaccine Against Ebola: An Evaluation of rVSVG-ZEBOV-GP Vaccine Tolerability and Safety During the West Africa Ebola Outbreak. J Infect Dis (2018) 217(suppl_1):S6–S15. doi: 10.1093/infdis/jiy020
84. Legardy-Williams JK, Carter RJ, Goldstein ST, Jarrett OD, Szefer E, Fombah AE, et al. Pregnancy Outcomes Among Women Receiving Rvsvdelta-ZEBOV-GP Ebola Vaccine During the Sierra Leone Trial to Introduce a Vaccine Against Ebola. Emerg Infect Dis (2020) 26(3):541–8. doi: 10.3201/eid2603.191018
85. Gianella S, Chaillon A, Chun TW, Sneller MC, Ignacio C, Vargas-Meneses MV, et al. HIV RNA Rebound in Seminal Plasma After Antiretroviral Treatment Interruption. J Virol (2020) 94(15):e00415–20. doi: 10.1128/JVI.00415-20
86. Clarke DK, Xu R, Matassov D, Latham TE, Ota-Setlik A, Gerardi CS, et al. Safety and Immunogenicity of a Highly Attenuated Rvsvn4ct1-EBOVGP1 Ebola Virus Vaccine: A Randomised, Double-Blind, Placebo-Controlled, Phase 1 Clinical Trial. Lancet Infect Dis (2020) 20(4):455–66. doi: 10.1016/S1473-3099(19)30614-0
87. Quiroz E, Moreno N, Peralta PH, Tesh RB. A Human Case of Encephalitis Associated With Vesicular Stomatitis Virus (Indiana Serotype) Infection. Am J Trop Med Hyg (1988) 39(3):312–4. doi: 10.4269/ajtmh.1988.39.312
88. Patterson WC, Mott LO, Jenney EW. A Study of Vesicular Stomatitis in Man. J Am Vet Med Assoc (1958) 133(1):57–62.
89. Maroun J, Munoz-Alia M, Ammayappan A, Schulze A, Peng KW, Russell S. Designing and Building Oncolytic Viruses. Future Virol (2017) 12(4):193–213. doi: 10.2217/fvl-2016-0129
90. Pol JG, Atherton MJ, Bridle BW, Stephenson KB, Le Boeuf F, Hummel JL, et al. Development and Applications of Oncolytic Maraba Virus Vaccines. Oncolytic Virother (2018) 7:117–28. doi: 10.2147/OV.S154494
91. Howard F, Muthana M. Designer Nanocarriers for Navigating the Systemic Delivery of Oncolytic Viruses. Nanomed (Lond) (2020) 15(1):93–110. doi: 10.2217/nnm-2019-0323
92. Prestwich RJ, Errington F, Diaz RM, Pandha HS, Harrington KJ, Melcher AA, et al. The Case of Oncolytic Viruses Versus the Immune System: Waiting on the Judgment of Solomon. Hum Gene Ther (2009) 20(10):1119–32. doi: 10.1089/hum.2009.135
93. Hamada M, Yura Y. Efficient Delivery and Replication of Oncolytic Virus for Successful Treatment of Head and Neck Cancer. Int J Mol Sci (2020) 21(19):7073. doi: 10.3390/ijms21197073
94. Bartlett DL, Liu Z, Sathaiah M, Ravindranathan R, Guo Z, He Y, et al. Oncolytic Viruses as Therapeutic Cancer Vaccines. Mol Cancer (2013) 12(1):103. doi: 10.1186/1476-4598-12-103
95. Duntsch CD, Zhou Q, Jayakar HR, Weimar JD, Robertson JH, Pfeffer LM, et al. Recombinant Vesicular Stomatitis Virus Vectors as Oncolytic Agents in the Treatment of High-Grade Gliomas in an Organotypic Brain Tissue Slice-Glioma Coculture Model. J Neurosurg (2004) 100(6):1049–59. doi: 10.3171/jns.2004.100.6.1049
96. Muik A, Dold C, Geiss Y, Volk A, Werbizki M, Dietrich U, et al. Semireplication-Competent Vesicular Stomatitis Virus as a Novel Platform for Oncolytic Virotherapy. J Mol Med (Berl) (2012) 90(8):959–70. doi: 10.1007/s00109-012-0863-6
97. Kelly EJ, Nace R, Barber GN, Russell SJ. Attenuation of Vesicular Stomatitis Virus Encephalitis Through microRNA Targeting. J Virol (2010) 84(3):1550–62. doi: 10.1128/JVI.01788-09
98. Ozaki T, Nakagawara A. Role of P53 in Cell Death and Human Cancers. Cancers (Basel) (2011) 3(1):994–1013. doi: 10.3390/cancers3010994
99. Xue W, Zender L, Miething C, Dickins RA, Hernando E, Krizhanovsky V, et al. Senescence and Tumour Clearance is Triggered by P53 Restoration in Murine Liver Carcinomas. Nature (2007) 445(7128):656–60. doi: 10.1038/nature05529
100. Heiber JF, Barber GN. Vesicular Stomatitis Virus Expressing Tumor Suppressor P53 is a Highly Attenuated, Potent Oncolytic Agent. J Virol (2011) 85(20):10440–50. doi: 10.1128/JVI.05408-11
101. Pearl TM, Markert JM, Cassady KA, Ghonime MG. Oncolytic Virus-Based Cytokine Expression to Improve Immune Activity in Brain and Solid Tumors. Mol Ther Oncolytics (2019) 13:14–21. doi: 10.1016/j.omto.2019.03.001
102. Shi Y, Liu CH, Roberts AI, Das J, Xu G, Ren G, et al. Granulocyte-Macrophage Colony-Stimulating Factor (GM-CSF) and T-Cell Responses: What We do and Don’t Know. Cell Res (2006) 16(2):126–33. doi: 10.1038/sj.cr.7310017
103. Ramsburg E, Publicover J, Buonocore L, Poholek A, Robek M, Palin A, et al. A Vesicular Stomatitis Virus Recombinant Expressing Granulocyte-Macrophage Colony-Stimulating Factor Induces Enhanced T-Cell Responses and is Highly Attenuated for Replication in Animals. J Virol (2005) 79(24):15043–53. doi: 10.1128/JVI.79.24.15043-15053.2005
104. Lemay CG, Rintoul JL, Kus A, Paterson JM, Garcia V, Falls TJ, et al. Harnessing Oncolytic Virus-Mediated Antitumor Immunity in an Infected Cell Vaccine. Mol Ther (2012) 20(9):1791–9. doi: 10.1038/mt.2012.128
105. Bergman I, Griffin JA, Gao Y, Whitaker-Dowling P. Treatment of Implanted Mammary Tumors With Recombinant Vesicular Stomatitis Virus Targeted to Her2/neu. Int J Cancer (2007) 121(2):425–30. doi: 10.1002/ijc.22680
106. Jenner A, Cassidy T, Belaid K. In Silico Trials Predict That Combination Strategies for Enhancing Vesicular Stomatitis Oncolytic Virus are Determined by Tumor Aggressivity (Vol 9, E001387, 2021). J Immunother Cancer (2021) 9(10):e001387. doi: 10.1136/jitc-2020-001387corr1
107. Steel JC, Waldmann TA, Morris JC. Interleukin-15 Biology and its Therapeutic Implications in Cancer. Trends Pharmacol Sci (2012) 33(1):35–41. doi: 10.1016/j.tips.2011.09.004
108. Lasek W, Zagozdzon R, Jakobisiak M. Interleukin 12: Still a Promising Candidate for Tumor Immunotherapy? Cancer Immunol Immunother (2014) 63(5):419–35. doi: 10.1007/s00262-014-1523-1
109. Li J, Zhang L, Zhang J, Wei Y, Li K, Huang L, et al. Interleukin 23 Regulates Proliferation of Lung Cancer Cells in a Concentration-Dependent Way in Association With the Interleukin-23 Receptor. Carcinogenesis (2013) 34(3):658–66. doi: 10.1093/carcin/bgs384
110. Stephenson KB, Barra NG, Davies E, Ashkar AA, Lichty BD. Expressing Human Interleukin-15 From Oncolytic Vesicular Stomatitis Virus Improves Survival in a Murine Metastatic Colon Adenocarcinoma Model Through the Enhancement of Anti-Tumor Immunity. Cancer Gene Ther (2012) 19(4):238–46. doi: 10.1038/cgt.2011.81
111. Shin EJ, Wanna GB, Choi B, Aguila D 3rd, Ebert O, Genden EM, et al. Interleukin-12 Expression Enhances Vesicular Stomatitis Virus Oncolytic Therapy in Murine Squamous Cell Carcinoma. Laryngoscope (2007) 117(2):210–4. doi: 10.1097/01.mlg.0000246194.66295.d8
112. Miller JM, Bidula SM, Jensen TM, Reiss CS. Vesicular Stomatitis Virus Modified With Single Chain IL-23 Exhibits Oncolytic Activity Against Tumor Cells In Vitro and In Vivo. Int J Interferon Cytokine Mediat Res (2010) 2010(2):63–72. doi: 10.2147/ijicmr.s9528
113. Fernandez M, Porosnicu M, Markovic D, Barber GN. Genetically Engineered Vesicular Stomatitis Virus in Gene Therapy: Application for Treatment of Malignant Disease. J Virol (2002) 76(2):895–904. doi: 10.1128/JVI.76.2.895-904.2002
114. Wongthida P, Diaz RM, Galivo F, Kottke T, Thompson J, Pulido J, et al. Type III IFN Interleukin-28 Mediates the Antitumor Efficacy of Oncolytic Virus VSV in Immune-Competent Mouse Models of Cancer. Cancer Res (2010) 70(11):4539–49. doi: 10.1158/0008-5472.CAN-09-4658
115. Leveille S, Goulet ML, Lichty BD, Hiscott J. Vesicular Stomatitis Virus Oncolytic Treatment Interferes With Tumor-Associated Dendritic Cell Functions and Abrogates Tumor Antigen Presentation. J Virol (2011) 85(23):12160–9. doi: 10.1128/JVI.05703-11
116. Naik S, Nace R, Barber GN, Russell SJ. Potent Systemic Therapy of Multiple Myeloma Utilizing Oncolytic Vesicular Stomatitis Virus Coding for Interferon-Beta. Cancer Gene Ther (2012) 19(7):443–50. doi: 10.1038/cgt.2012.14
117. Altomonte J, Wu L, Chen L, Meseck M, Ebert O, Garcia-Sastre A, et al. Exponential Enhancement of Oncolytic Vesicular Stomatitis Virus Potency by Vector-Mediated Suppression of Inflammatory Responses In Vivo. Mol Ther (2008) 16(1):146–53. doi: 10.1038/sj.mt.6300343
118. Altomonte J, Wu L, Meseck M, Chen L, Ebert O, Garcia-Sastre A, et al. Enhanced Oncolytic Potency of Vesicular Stomatitis Virus Through Vector-Mediated Inhibition of NK and NKT Cells. Cancer Gene Ther (2009) 16(3):266–78. doi: 10.1038/cgt.2008.74
119. Le Boeuf F, Gebremeskel S, McMullen N, He H, Greenshields AL, Hoskin DW, et al. Reovirus FAST Protein Enhances Vesicular Stomatitis Virus Oncolytic Virotherapy in Primary and Metastatic Tumor Models. Mol Ther Oncolytics (2017) 6:80–9. doi: 10.1016/j.omto.2017.08.001
120. Kane JR MJ, Young JS, Kanojia D, Kim JW, Lesniak MS. Sui Generis: Gene Therapy and Delivery Systems for the Treatment of Glioblastoma. Neuro Oncol (2015) 17 Suppl 2):ii24–36. doi: 10.1093/neuonc/nou355
121. Martuza RL, Malick A, Markert JM, Ruffner KL, Coen DM. Experimental Therapy of Human Glioma by Means of a Genetically Engineered Virus Mutant. Science (1991) 252(5007):854–6. doi: 10.1126/science.1851332
122. Patel MR JB, Ji Y, Drees J, Tang S, Xiong K, Wang H, et al. Vesicular Stomatitis Virus Expressing Interferon-β is Oncolytic and Promotes Antitumor Immune Responses in a Syngeneic Murine Model of non-Small Cell Lung Cancer. Oncotarget (2015) 6(32):(33165–77). doi: 10.18632/oncotarget.5320
123. Russell SJ, Peng KW, Bell JC. Oncolytic Virotherapy. Nat Biotechnol (2012) 30(7):658–70. doi: 10.1038/nbt.2287
124. Stojdl DF, Lichty B, Knowles S, Marius R, Atkins H, Sonenberg N, et al. Exploiting Tumor-Specific Defects in the Interferon Pathway With a Previously Unknown Oncolytic Virus. Nat Med (2000) 6(7):821–5. doi: 10.1038/77558
125. Zeyaullah M, Patro M, Ahmad I, Ibraheem K, Sultan P, Nehal M, et al. Oncolytic Viruses in the Treatment of Cancer: A Review of Current Strategies. Pathol Oncol Res (2012) 18(4):771–81. doi: 10.1007/s12253-012-9548-2
126. Kevin J Harrington IP J, Hechtb R, Stephen Hodic F, Szabod Z, Murugappane S, Kaufmanf HL. Clinical Development of Talimogene Laherparepvec (T-VEC): A Modified Herpes Simplex Virus Type-1–Derived Oncolytic Immunotherapy. Expert Rev Anticancer Ther (2015) 15(12):1389–403. doi: 10.1586/14737140.2015.1115725
127. Ammour YI, Ryabaya OO, Milovanova AV, Sidorov AV, Shohin IE, Zverev VV, et al. Oncolytic Properties of a Mumps Virus Vaccine Strain in Human Melanoma Cell Lines. Mol Biol (Mosk) (2018) 52(4):659–66. doi: 10.1134/S0026893318040027
128. Kurokawa C, Iankov ID, Anderson SK, Aderca I, Leontovich AA, Maurer MJ, et al. Constitutive Interferon Pathway Activation in Tumors as an Efficacy Determinant Following Oncolytic Virotherapy. J Natl Cancer Inst (2018) 110(10):1123–32. doi: 10.1093/jnci/djy033
129. Msaouel P, Opyrchal M, Dispenzieri A, Peng KW, Federspiel MJ, Russell SJ, et al. Clinical Trials With Oncolytic Measles Virus: Current Status and Future Prospects. Curr Cancer Drug Targ (2018) 18(2):177–87. doi: 10.2174/1568009617666170222125035
130. Son HA, Zhang L, Cuong BK, Van Tong H, Cuong LD, Hang NT, et al. Combination of Vaccine-Strain Measles and Mumps Viruses Enhances Oncolytic Activity Against Human Solid Malignancies. Cancer Invest (2018) 36(2):106–17. doi: 10.1080/07357907.2018.1434539
131. Grossardt C, Engeland CE, Bossow S, Halama N, Zaoui K, Leber MF, et al. Granulocyte-Macrophage Colony-Stimulating Factor-Armed Oncolytic Measles Virus is an Effective Therapeutic Cancer Vaccine. Hum Gene Ther (2013) 24(7):644–54. doi: 10.1089/hum.2012.205
132. Suzuki M. Partners in Crime: Combining Oncolytic Viroimmunotherapy With Other Therapies. Mol Ther (2017) 25(4):836–8. doi: 10.1016/j.ymthe.2017.03.005
133. Udayakumar TS, Betancourt DM, Ahmad A, Tao W, Totiger TM, Patel M, et al. Radiation Attenuates Prostate Tumor Antiviral Responses to Vesicular Stomatitis Virus Containing IFNbeta, Resulting in Pronounced Antitumor Systemic Immune Responses. Mol Cancer Res (2020) 18(8):1232–43. doi: 10.1158/1541-7786.MCR-19-0836
134. Shen W, Patnaik MM, Ruiz A, Russell SJ, Peng KW. Immunovirotherapy With Vesicular Stomatitis Virus and PD-L1 Blockade Enhances Therapeutic Outcome in Murine Acute Myeloid Leukemia. Blood (2016) 127(11):1449–58. doi: 10.1182/blood-2015-06-652503
135. Durham NM, Mulgrew K, McGlinchey K, Monks NR, Ji H, Herbst R, et al. Oncolytic VSV Primes Differential Responses to Immuno-Oncology Therapy. Mol Ther (2017) 25(8):1917–32. doi: 10.1016/j.ymthe.2017.05.006
136. Gebremeskel S, Nelson A, Walker B, Oliphant T, Lobert L, Mahoney D, et al. Natural Killer T Cell Immunotherapy Combined With Oncolytic Vesicular Stomatitis Virus or Reovirus Treatments Differentially Increases Survival in Mouse Models of Ovarian and Breast Cancer Metastasis. J Immunother Cancer (2021) 9(3):e002096. doi: 10.1136/jitc-2020-002096
137. Wongthida P, Diaz RM, Pulido C, Rommelfanger D, Galivo F, Kaluza K, et al. Activating Systemic T-Cell Immunity Against Self Tumor Antigens to Support Oncolytic Virotherapy With Vesicular Stomatitis Virus. Hum Gene Ther (2011) 22(11):1343–53. doi: 10.1089/hum.2010.216
138. Melzer MK, Lopez-Martinez A, Altomonte J. Oncolytic Vesicular Stomatitis Virus as a Viro-Immunotherapy: Defeating Cancer With a “Hammer” and “Anvil”. Biomedicines (2017) 5(1):8. doi: 10.3390/biomedicines5010008
139. Altomonte J, Ebert O. Sorting Out Pandora’s Box: Discerning the Dynamic Roles of Liver Microenvironment in Oncolytic Virus Therapy for Hepatocellular Carcinoma. Front Oncol (2014) 4:85. doi: 10.3389/fonc.2014.00085
140. Zhang L, Steele MB, Jenks N, Grell J, Suksanpaisan L, Naik S, et al. Safety Studies in Tumor and Non-Tumor-Bearing Mice in Support of Clinical Trials Using Oncolytic VSV-IFNbeta-NIS. Hum Gene Ther Clin Dev (2016) 27(3):111–22. doi: 10.1089/humc.2016.061
Keywords: vesicular stomatitis virus, oncolytic virus, genetically modified virus, cancer therapy, immunotherapy
Citation: Zhang Y and Nagalo BM (2022) Immunovirotherapy Based on Recombinant Vesicular Stomatitis Virus: Where Are We? Front. Immunol. 13:898631. doi: 10.3389/fimmu.2022.898631
Received: 17 March 2022; Accepted: 31 May 2022;
Published: 28 June 2022.
Edited by:
Anil Shanker, Meharry Medical College, United StatesReviewed by:
Ma Luo, Public Health Agency of Canada (PHAC), CanadaCopyright © 2022 Zhang and Nagalo. This is an open-access article distributed under the terms of the Creative Commons Attribution License (CC BY). The use, distribution or reproduction in other forums is permitted, provided the original author(s) and the copyright owner(s) are credited and that the original publication in this journal is cited, in accordance with accepted academic practice. No use, distribution or reproduction is permitted which does not comply with these terms.
*Correspondence: Bolni Marius Nagalo, Qk1OYWdhbG9AdWFtcy5lZHU=