- Department of Orthopedics, the Affiliated Cancer Hospital of Zhengzhou University and Henan Cancer Hospital, Zhengzhou, China
There is an urgent clinical need for new therapeutic regimens for the effective treatment of advanced sarcomas. Accumulating evidence suggests that programmed death receptor-1/programmed death protein ligand-1 (PD-1/L1) inhibitors have synergistic effects with chemotherapy and have been approved for treatment of lung cancer, gastroesophageal cancer, and breast cancer. In this review, we reviewed the synergistic mechanism of PD-1/L1 inhibitors plus chemotherapy in the treatment of cancers, and the application of this combined regimen in several cancers, followed by a summary of the current evidence on the application of this combined regimen in the treatment of sarcomas as well as the main clinical trials currently underway. Based on the findings of this review, we believe that this combined approach will play an important role in the treatment of some subtypes of sarcomas in the future.
Introduction
Although they account for only approximately 1% of malignant tumors, there are still numerous new sarcoma cases annually (1). More than half of the newly diagnosed sarcoma cases will eventually reach an advanced stage (2). The conventional first-line treatment for advanced sarcomas is chemotherapy. Doxorubicin-based and docetaxel plus gemcitabine regimens are the main chemotherapy regimens for sarcoma treatment (3). However, due to the high heterogeneity of sarcomas (more than 70 subtypes) (4), the overall response rate (ORR) for any chemotherapy regimen is less than 20% (3, 5). The low efficacy of chemotherapy results in a median overall survival (OS) of only approximately 1 year for advanced sarcomas (1, 6). Therefore, there is a strong need for new therapeutic regimens that can effectively treat advanced sarcomas.
Binding of the programmed death receptor‐1 (PD‐1) to its ligand, programmed death protein ligand‐1 (PD‐L1), triggers inhibitory signals that result in reduced proliferation of many anti-tumor immune cells, thereby preventing effector immune cells from killing cancer cells. Blocking this binding can restore effector T-cell activity and anti-tumor immune response, thereby increasing the anti-tumor effects. PD-1/L1 inhibitors are a class of new immune targeting drugs that can effectively block the binding of PD-1 and PD-L1 (7). PD-1/L1 inhibitors have been widely used in the treatment of many malignancies (7). Although PD-1/L1 inhibitors have shown some efficacy in a few sarcoma subtypes, they have limited efficacy in most sarcoma subtypes (8, 9). To improve the efficacy of PD-1/L1 inhibitors in malignant tumors, other treatment methods have been combined with PD-1 inhibitors to achieve synergistic sensitization (10, 11). Among them, the combination of PD-1/L1 inhibitor and chemotherapy has been studied extensively and has been approved by the FDA for the treatment of some cancers such as lung cancer, gastric cancer, esophageal cancer, and breast cancer (12). Although research on the treatment of sarcomas with PD-1/L1 inhibitor plus chemotherapy is still in its infancy, the combination has shown some synergistic effect, which is expected to improve the treatment of advanced sarcomas (13, 14).
In this review, we first summarize the mechanisms underlying the synergistic anti-tumor effects of PD-1/L1 inhibitor plus chemotherapy and its efficacy in various malignant tumors. Then, the evidence for this combination therapy in sarcoma and the ongoing clinical trials are reviewed. Finally, the research strategy and future direction of PD1/L1 inhibitor plus chemotherapy in the treatment of sarcoma are discussed to provide a reference for studies on the treatment of advanced sarcomas.
Mechanisms of PD-1/L1 inhibitor plus chemotherapy
Anti-tumor immune response is a complex process, involving cellular and humoral immunity. This anti-tumor reaction generally occurs in the tumor microenvironment (TME) (Figure 1). In the TME, tumor cells that generate immune progenitors are first recognized by the natural killer (NK) cells and dendritic cells (DCs) (15, 16). NK cells are activated by recognizing the major histocompatibility complex I (MHC-I) antigen and other tumor antigens expressed on tumor cells. Subsequently, NK cells initiate a cytotoxic reaction to kill the tumor cells directly. Simultaneously, they secrete relevant cytokines as immune helper cells to recruit, stimulate, and regulate DCs, CD4+ T cells, and CD8+ T cells to further activate the anti-tumor immune response (17–19). After DCs are activated by NK cells or tumor antigens, they stimulate CD8+ T cells and induce the anti-tumor immune response of specific cytotoxic T lymphocytes (CTLs). They further regulate the activity and anti-tumor immune response of NK cells and CD4+ T cells via various mechanisms (16, 18). After CD4+ T cells are mainly activated by activated DCs, they can directly recognize and kill MHC-II+ tumor cells as well as recruit and activate more NK cells, DCs, and CD8+ T cells, and enhance the tumor cell-killing ability of CTLs (20, 21). Finally, after being activated by DCs, CD8+ T cells are activated into CTLs to kill tumor cells (22, 23). This anti-tumor immune response process is negatively regulated by immunosuppressive cells including myeloid-derived suppressor cells (MDSCs), regulatory T cells (Tregs), and cancer-associated fibroblasts (CAFs) (23, 24). In addition, tumor-associated macrophages (TAMs) and intestinal microbiota can promote or inhibit the tumor immune response (25, 26).
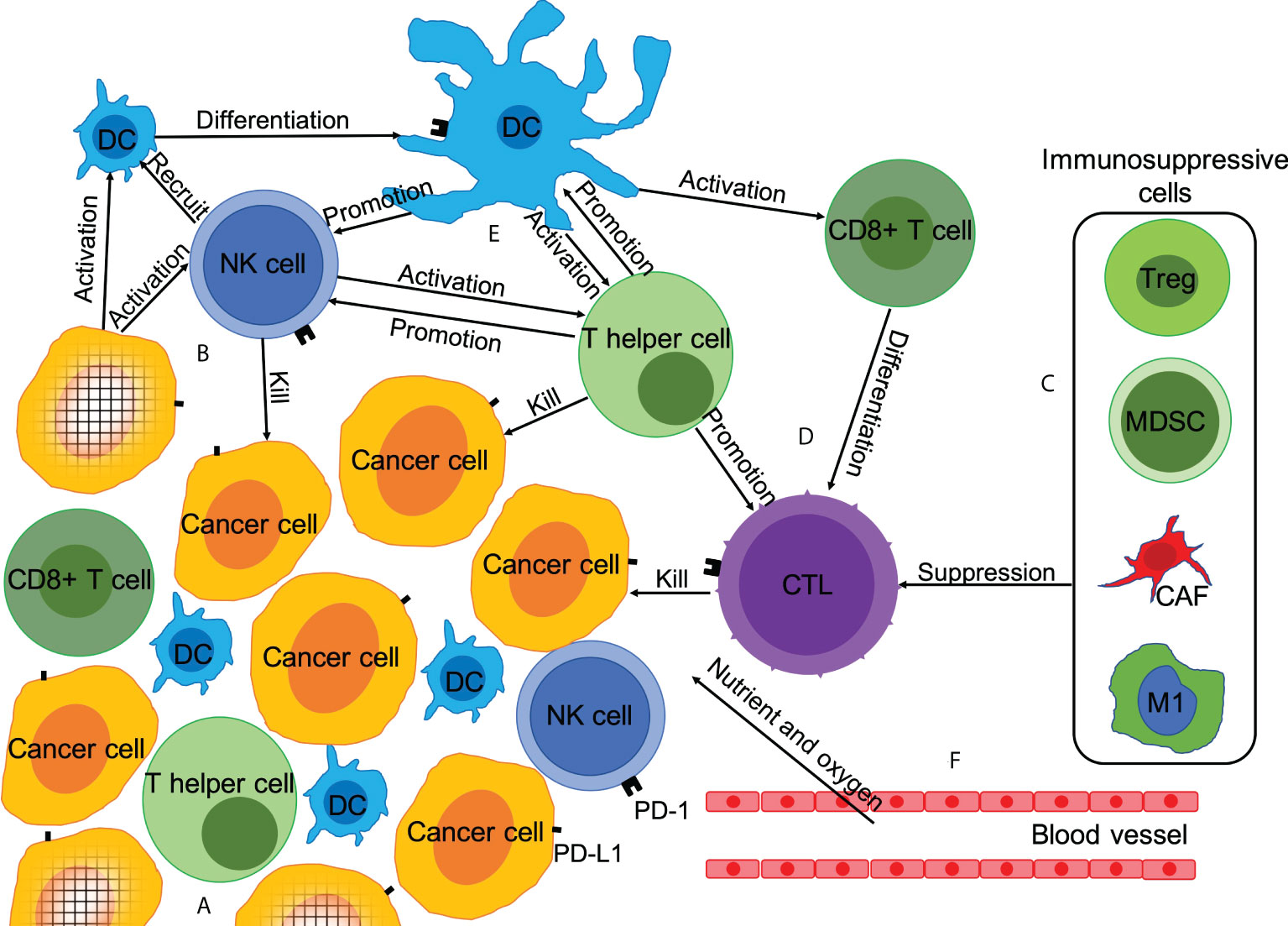
Figure 1 Microenvironment of tumor immunity and mechanism of chemotherapy promoting anti-tumor immune response. Anti-tumor immune response is a complex process, which is affected by the dynamic balance between immune-promoting factors and immunosuppressive factors. Chemotherapy can affect the anti-tumor immune response in at least six ways. (A) Reduction in the number of tumor cells; (B) Promotion of immunogenic tumor cell death; (C) Depletion of immunosuppressive cells; (D) Increase in the number and activity of anti-tumor immune effector T cells; (E) Enhanced expression of PD-L1 and secretion of anti-tumor immune cytokines; (F) Anti-angiogenesis.
The functions of innate immune cells and adaptive immune cells in the TME can be inhibited by various mechanisms. The most famous one is the PD-1/PD-L1 pathway. As a ligand of PD-1, PD-L1 can be overexpressed on tumor cells, inhibiting the activation and function of CD8+ T cells to avoid the anti-tumor immune response (27). PD-1/L1 inhibitors are one of the most promising and attractive anticancer immune checkpoint blockers (7). There is increasing evidence that apart from T cells, PD-1/L1 inhibitors can also directly or indirectly promote the function of other immune cells, thereby inhibiting tumor progression (Figure 1) (28).
Chemotherapeutic drugs have extensive effects on the TME. Numerous studies have confirmed the synergistic mechanism between chemotherapy and anti-tumor immunotherapy (12). We have summarized and listed these mechanisms below (Figure 1), based on the research results of various chemotherapeutic drugs in different malignant tumors. However, it is impossible for any one type of chemotherapeutic drug to have a synergistic mechanism with PD-1 inhibitor that can cover all the aspects listed below. Some chemotherapeutic drugs can even inhibit the effect of PD-1 inhibitors under certain conditions. Since the anti-tumor immune response is highly complex, it is impossible for any mechanism to exist alone. The mechanisms listed below are widely related, and some mechanisms may even be mutually causal.
Reduction in the number of tumor cells
Tumor cells can compete with immune cells for nutrients and key metabolites in the TME, resulting in impaired metabolism, limited proliferation, and weakened anti-tumor immune function of immune cells (29, 30). The reduction in the tumor tissue volume and number of tumor cells caused by chemotherapy can not only reduce the expansion of the immune cells and anti-tumor immune pressure but also reduce the chances of tumor cell variation and immune escape. Additionally, larger tumor lesions can inhibit the function of the systemic immune system, and reduction in the tumor lesions caused by chemotherapy can reduce or relieve this inhibition (31).
Promotion of immunogenic tumor cell death
Chemotherapeutic drugs mainly cause apoptosis of tumor cells. Tumor cells injured by chemotherapy first transmit danger signals to antigen-presenting cells in the form of endogenous damp-associated molecular patterns (DAMPs). DAMPs represent changes in the surface and microenvironment of the cells in response to stress, including that induced by chemotherapy, which is reflected by the expression of new (usually unexpressed) membrane-bound or secreted proteins, such as type I interferon. Antigen-presenting cells (mainly DCs) recognize these changes on the surface and microenvironment of injured tumor cells and activate tumor-specific immune responses (32). The dead tumor cells also release a large number of cytoplasmic and nuclear proteins, cancer cell cytosol, and nucleic acids (including DNA and different forms of RNA), which promote the cross presentation of DC-mediated tumor antigens to CTLs, ultimately promoting the recognition and clearance of residual cancer cells (33).
In conclusion, chemotherapy leads to a stress response and apoptosis, resulting in many new tumor immune antigens on the cell surface and TME. These antigens stimulate an anti-tumor immune response, which can co-operate with the anti-tumor immune response activated by PD-1/L1 inhibitors to kill the residual cancer cells (12).
Depletion of immunosuppressive cells
In the normal human body, there is always a dynamic balance between the activation and inhibition of the immune system. The main immunosuppressive cells associated with the anti-tumor immune response include MDSCs, Treg cells, CAFs, and TAMs (23, 28). Chemotherapy can significantly reduce these cells in the peripheral blood and TME, and reduce the inhibitory factors of the anti-tumor immune response (34, 35). Nevertheless, chemotherapy can lead to the loss and reduction of almost all immune cells (35). If the timing is correct, PD-1/L1 inhibitor can first activate the function of the effector T cells and promote their quantitative expansion, thereby ensuring a more efficient anti-tumor response in the TME lacking immunosuppressive cells (32, 34).
Increased number and activity of anti-tumor immune effector T cells
The various immune cells in the body are in dynamic balance. Reduction in the number of other immune cells can make more space for effector T cells and expose effector T cells to more immune- and growth-promoting cytokines (36). Several studies have confirmed the above hypothesis (37, 38). Furthermore, CD8+ T cells demonstrate relative elasticity to the effects of many cytotoxic chemotherapeutic drugs. Although these drugs may cause a temporary decrease in the number of CD8+ T cells in the blood, in most cases, the CD8+ T cell population recovers well, mainly due to the expansion of the effector memory pool. The chemotherapeutic agents can even induce enhanced activation of the CD8+ T cells (39, 40). Consequently, after a recovery period, the CD8+ T cell pool may actually be enhanced after some chemotherapy regimens, which is due to an increase in the cytotoxic activity and transfer of the cell population from terminal effector cells to effector memory cells (35). If PD-1/L1 inhibitor is administered at this time, the higher proportion of effector T cells are more effective in responding to the effect of the PD-1/L1 inhibitor. However, different doses of chemotherapeutic drugs have significantly different effects on effector T cells. Generally, the higher the dose of the chemotherapeutic drug, the more serious the damage to effector T cells (38). Therefore, when chemotherapy is combined with a PD-1/L1 inhibitor, the dose of chemotherapy drugs should not be too high.
Enhanced expression of PD-L1 and secretion of anti-tumor immune cytokines
Many studies have confirmed that chemotherapeutic drugs can enhance the expression of PD-L1 in tumor tissues (41, 42). A high expression of PD-L1 has been considered an effective marker for PD-1/L1 inhibitor use in various malignant tumors (43, 44). Chemotherapy can also lead to changes in the various cytokine lineages in the TME (41). These alterations are complex and even contradictory, promoting or inhibiting anti-tumor immunity. For example, in some studies, cisplatin has been shown to enhance the release of various chemokines that attract T cells and enhance anti-tumor immunity (38, 45). In other studies, it led to a decrease in tumor necrosis factor α levels or an increase in the pro-inflammatory cytokine levels that promote tumor proliferation (46, 47). Obviously, these changes are related to the different doses of the different chemotherapeutic drugs.
In conclusion, under the influence of appropriate doses of appropriate chemotherapeutic drugs, PD-L1 expression and cytokine lineage in the TME can change in the direction of promoting the efficacy of PD-1/L1 inhibitors.
Anti-angiogenesis
Anti-angiogenesis can lead to tumor vascular degeneration, tumor necrosis, and improve antigen presentation by DC cells (48). The combination of antiangiogenic agent and PD-1 inhibitor also enhanced the presence and activation of CTLs in TME, further enhancing the antitumor effect (49, 50). Existing research has shown that chemotherapeutic drugs can inhibit tumor angiogenesis, even at low doses (51, 52). This can not only make it easier for T lymphocytes to penetrate the vascular endothelial barrier to reach the tumor lesions but also lead to hypoxia and nutrient deprivation of the tumor tissue and TME (53). This is a double-edged sword for tumor immunity. Hypoxia may increase the activity of CTLs and may also lead to a TME-promoting tumor proliferation (54). At present, only a few studies have confirmed the synergistic effect of chemotherapeutic drugs and PD-1 inhibitors in this regard (55). The mechanism of synergy in this area needs more in-depth research.
Efficacy of PD-1/L1 inhibitor plus chemotherapy in different cancers
Although the potential synergistic mechanisms of PD-1/L1 inhibitor plus chemotherapy are numerous, they must achieve better outcomes in clinical practice to be meaningful. At present, PD-1/L1 inhibitor plus chemotherapy has completed phase 3 clinical trials in many cancers (Table 1).
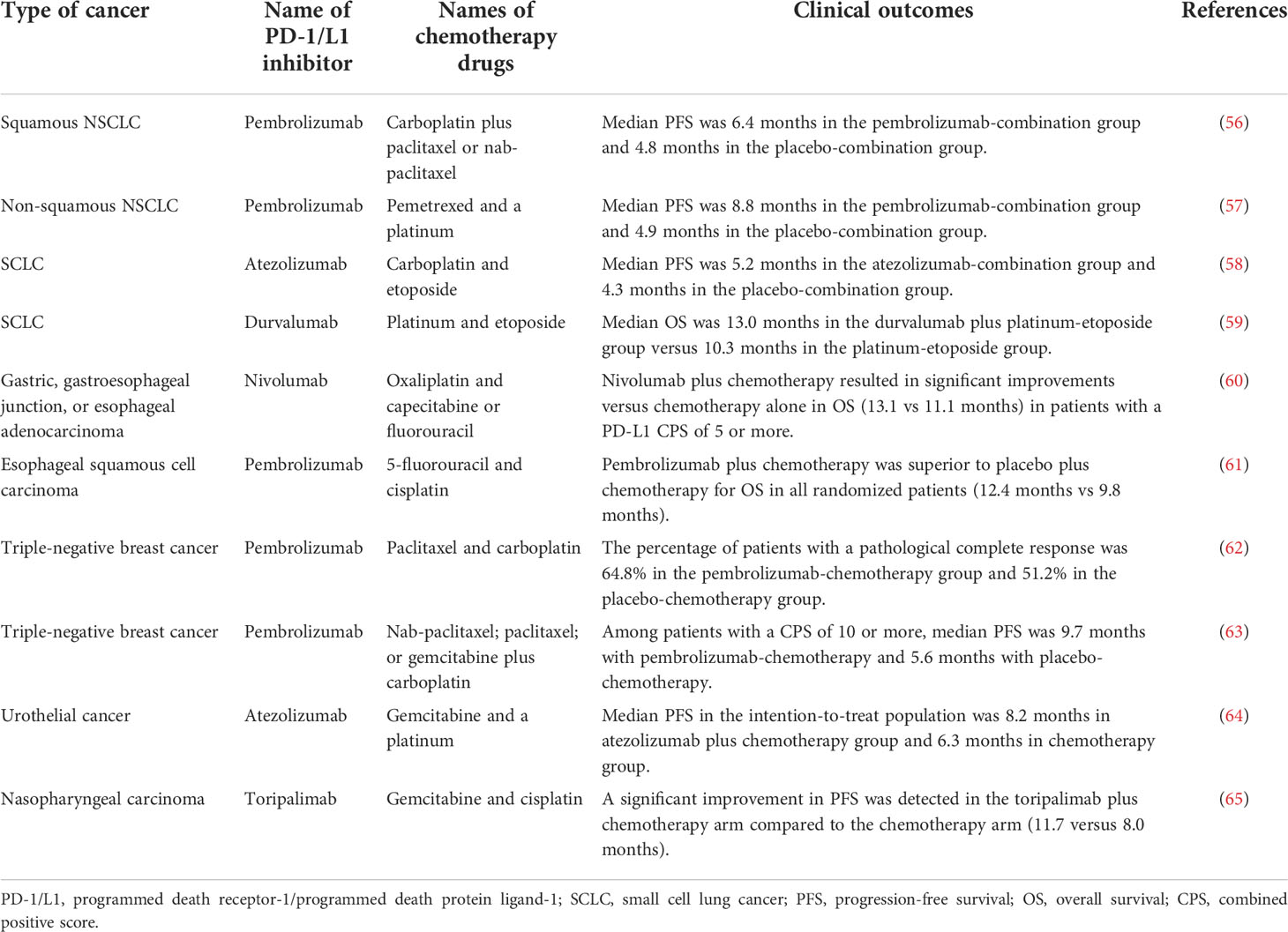
Table 1 Partial phase 3 clinical trials of PD-1/L1 inhibitor plus chemotherapy in different cancers.
PD-1/L1 inhibitor has been proven to have synergistic effects with chemotherapy drugs (platinum, paclitaxel, pemetrexed, and etoposide) with a significant improvement in the efficacy in various types of advanced lung cancer (56–59). Based on these and related studies, pembrolizumab in combination with pemetrexed and platinum-based chemotherapy was approved by the FDA in 2018 as the first-line treatment for metastatic non-squamous non-small-cell lung cancer lacking EGFR or ALK mutations. Nivolumab plus chemotherapy was approved by the FDA for its ability to significantly prolong the OS and progression-free survival (PFS) compared with chemotherapy alone in patients with gastric, gastroesophageal junction, or esophageal adenocarcinoma (60). In esophageal squamous cell carcinoma, combination therapy with pembrolizumab plus 5-fluorouracil and cisplatin was approved by the FDA due to a significantly longer OS and PFS than chemotherapy alone (61). PD-1 inhibitor plus chemotherapy has been approved by the FDA as it provides significant benefits to patients with triple-negative breast cancer compared with chemotherapy alone, both as neoadjuvant therapy and advanced adjuvant therapy (62, 63). Furthermore, PD-1/L1 inhibitor plus chemotherapy significantly extended PFS compared with chemotherapy alone in urothelial and nasopharyngeal cancers (64, 65).
In conclusion, PD-1/L1 inhibitor plus chemotherapy has shown good synergistic efficacy in multiple cancers, which verifies the relevant theoretical research results. At present, many clinical trials are underway in different countries with different PD-1/L1 inhibitors and chemotherapy regimens for different cancers. It is believed that with the completion of these studies, PD-1/L1 inhibitor combined chemotherapy will become the first-line treatment in more and more cancers. However, not all PD-1/L1 inhibitors combined with chemotherapy show synergistic efficacy. In gastric cancer, ovarian cancer, head and neck squamous cell carcinoma, and urothelial carcinoma, some clinical trials have shown no significant advantages of this combined regimen (66–70). The possible reasons are diverse and need further research.
Research evidence in sarcomas
Compared with studies on the effect of chemotherapy on the TME in other cancers, there are few studies on the effect of chemotherapy on the TME in sarcomas. Nevertheless, current studies have initially elucidated the effects of chemotherapeutic drugs (doxorubicin, cyclophosphamide, gemcitabine docetaxel and trabectedin) that commonly used in sarcomas on TME. Doxorubicin can not only stimulate the anti-tumor immune response by inducing immunogenic cell death (71), it also up-regulated the expression of PD-L1 in both the clinical osteosarcoma tissue samples and the osteosarcoma cell lines (72, 73). Cyclophosphamide can selectively inhibit immunosuppressive cells, including Treg cells and MDSCs, and induce interferon-γ-mediated anti-tumor immune response (74). Gemcitabine can effectively increase the proportion of T cells and the total proportion of infiltrating immune cells, reduce the proportion of MDSCs and macrophages, and promote the expression of PD-L1 on tumor cells (75). Docetaxel-based chemotherapy promotes the intratumor infiltration of T cells and upregulates the abundance of PD-1 and PD-L1 in mouse tumor transplantation model, thereby sensitizing tumor to anti-PD1 blockers (76). Trabectedin selectively reduces monocytes and TAMs in treated tumors and can increase the presence and functional activity of T lymphocytes. In fibrosarcoma models with poor response to PD-1 immunotherapy, treatment with Trabectedin prior to anti-PD-1 can improve antitumor efficacy (77, 78). In addition, an earlier study showed that the chemotherapy drug decitabine facilitates immune recognition of sarcoma cells by upregulating cancer/testis antigens, MHC molecules, and intracellular cell adhesion molecule-1 (79). Another study revealed that chemotherapy could significantly increase the total immune cell infiltration in the TME of sarcoma, especially TAM-2 macrophages, B cells, and CD4+ T cells. Upregulation of genes and cytokines associated with antigen presentation was also observed. An increase in monocyte infiltration is associated with a good pathological reaction (necrosis ≥ 90% after chemotherapy) (80). Additionally, chemotherapy combined with local hyperthermia can lead to increased tumor infiltrating lymphocyte and decreased Treg count in the TME, which is significantly related to a better prognosis (81).
Although there are only a few clinical studies on the efficacy of PD-1/L1 inhibitor plus chemotherapy in sarcomas, some studies have reported improved efficacy (Table 2). At present, several retrospective studies have suggested that chemotherapy combined with PD-1 inhibitor has certain efficacy in soft tissue sarcomas (85–87). In these retrospective studies, each sarcoma subtype responded differently to the combination regimen. Overall, undifferentiated pleomorphic sarcomas had the best response. However, the limited nature of retrospective studies limits the level of evidence. In addition to that, several prospective clinical trials have reported promising results. A phase 1/2 non-randomized clinical trial in 2020 showed that pembrolizumab plus doxorubicin was well tolerated. Although the primary end point of ORR was not reached, the PFS and OS compared favorably to those of previous studies (14). The results of another phase 2 clinical trial in 2021 confirmed that the combination of pembrolizumab plus doxorubicin has an efficacy similar to that reported in a previous trial on sarcomas (13). However, some clinical trials did not report synergistic efficacy of PD-1 inhibitor plus chemotherapy in sarcomas. An open label, multi-center phase IB study in 2021 showed that the ORR and PFS in the sarcoma expansion cohort who received durvalumab plus trabectedin treatment were comparable to those in a trabectedin single agent study, and there was no clear evidence of synergistic activity in this unselected population (84). Another clinical trial concluded that pembrolizumab plus cyclophosphamide did not show meaningful activity in sarcoma (82). However, the latest research shows that the efficacy of pembrolizumab plus cyclophosphamide significantly improved in sarcoma patients with tertiary lymphoid structures (83). This topic is worth further research.
In conclusion, PD-1 inhibitor plus chemotherapy has shown promising activity in sarcomas, especially in undifferentiated pleomorphic sarcoma, dedifferentiated liposarcoma, and angiosarcoma. However, there is little research in this field, and some studies did not show the synergistic effect of this combination therapy (Table 2). The possible reasons are diverse and need further research.
Ongoing trials on PD-1/L1 inhibitor plus chemotherapy in sarcomas
At present, at least 20 clinical trials of PD-1/L1 inhibitor plus chemotherapy in the treatment of sarcomas have been registered in clinicaltrials.gov and are recruiting (Table 3). The PD-1 inhibitors used in these clinical trials include camrelizumab, durvalumab, nivolumab, pembrolizumab, retifanlimab, sintilimab, and Toripalimab. While the chemotherapy drugs are commonly recommended for sarcoma treatment, including doxorubicin, gemcitabine, docetaxel, paclitaxel, ifosfamide, trabectedin, and eribulin. Most of these clinical studies are investigating the treatment of the common sarcoma subtypes, and some of the drugs are also used in the treatment of osteosarcoma and retroperitoneal sarcoma. Several clinical trials are attempting to determine the efficacy of PD-1 inhibitor plus chemotherapy as neoadjuvant therapy for sarcomas.
The completion of these clinical trials will further validate the synergistic efficacy of different PD-1/L1 inhibitors plus chemotherapeutic agents in the treatment of sarcoma. This is a necessary step to improve the therapeutic outcome of sarcoma patients. However, these clinical trials are only conducting preliminary verification, and some deep-seated and detailed problems have not been examined. The major problems with these trials include 1) not selecting a specific sarcoma subtype; 2) Many treatment regimens are not based on solid theoretical and preclinical trials; 3) The combination of chemotherapy drugs and immunotherapy drugs is too mechanical, and the influence of dose and timing of administration is not considered; 4) The effect of adjuvant medication was not considered.
Discussion
We first reviewed the synergistic mechanism of PD-1/L1 inhibitor plus chemotherapy in the treatment of cancers and the effectiveness of this combined regimen in many cancers and then summarized the current evidence on the application of this combined regimen in the treatment of sarcomas as well as the main ongoing clinical trials. The clinical efficacy of this combination therapy in lung cancer, gastroesophageal cancer, and breast cancer has been proven, and it has been approved by the FDA. PD-1/L1 inhibitor plus chemotherapy is an important breakthrough in the treatment of many cancers, which requires further research for more progress.
However, the application of PD-1/L1 inhibitor plus chemotherapy in sarcoma is still in the preliminary verification stage. To improve the therapeutic outcome in sarcomas, the research on PD-1/L1 inhibitor plus chemotherapy in sarcomas needs to be more in-depth and detailed (Figure 2). There are various subtypes of sarcomas, and the drug sensitivity of different sarcomas varies greatly (88). Single-agent immunotherapy may be appropriate in some sarcoma subtypes. The first step should be to determine which sarcoma subtypes are more likely to respond to treatment with combination regimens, and validate each sarcoma subtype individually (89). Second, chemotherapeutic drugs suitable for combination with PD-1/L1 inhibitor should be screened. Obviously, the interaction mechanism and effect of each chemotherapeutic drug with the PD1/L1 inhibitor may be different. Some drugs are suitable for combination with PD1/L1 inhibitor, while others are not. For example, it has been demonstrated that the use of glucocorticoids while immunotherapy will lead to poor prognosis (90). Therefore, chemotherapy drugs that need to be accompanied by glucocorticoids may not be suitable for combination with immunotherapy. In addition, different sarcoma subtypes have different sensitivities to different chemotherapy drugs. Therefore, it is necessary to choose different combination chemotherapy agents for different sarcoma subtypes. Another important problem neglected at present is the dose of chemotherapeutic drugs and the timing of administration of PD-1/L1 inhibitor, which can have a great impact on the therapeutic effectiveness (34, 91). Excessive doses of chemotherapeutic drugs inevitably lead to severe immunosuppression and weaken the efficacy of PD-1/L1 inhibitor. If the timing of administration is not appropriate, T cells activated by PD-1/L1 inhibitor will also be reduced by chemotherapy and inhibit the efficacy of anti-tumor immunity. The effects of concomitant medication have also not been considered. There are many concomitant drugs in chemotherapy, many of which have been preliminarily confirmed to inhibit the efficacy of PD-1/L1 inhibitor (92). Therefore, the concomitant medication should be considered in detail in the design of various clinical trials. The safety and efficacy of combination therapy in different application scenarios are also worth exploring, such as in elderly or juvenile patients, or under the setting of neoadjuvant therapy. Finally, efficacy markers require further investigations. At present, high PD-L1 expression, microsatellite instable-high (MSI-H)/mismatch repair deficient (dMMR) phenotype, and tumor mutation burden-high status (TMB-H) have been proposed as a predictive biomarker to predict response to PD-1 inhibitors in many cancers (93). However, sarcomas with one of these three characteristics are rare, and the available evidence has not found that they can predict the response of sarcomas to PD-1 inhibitors (94, 95). Further clinical trials are needed to confirm whether chemotherapy can change the sensitivity of sarcomas with high PD-L1 expression, MSI-H/dMMR phenotype, or TMB-H to immunotherapy.
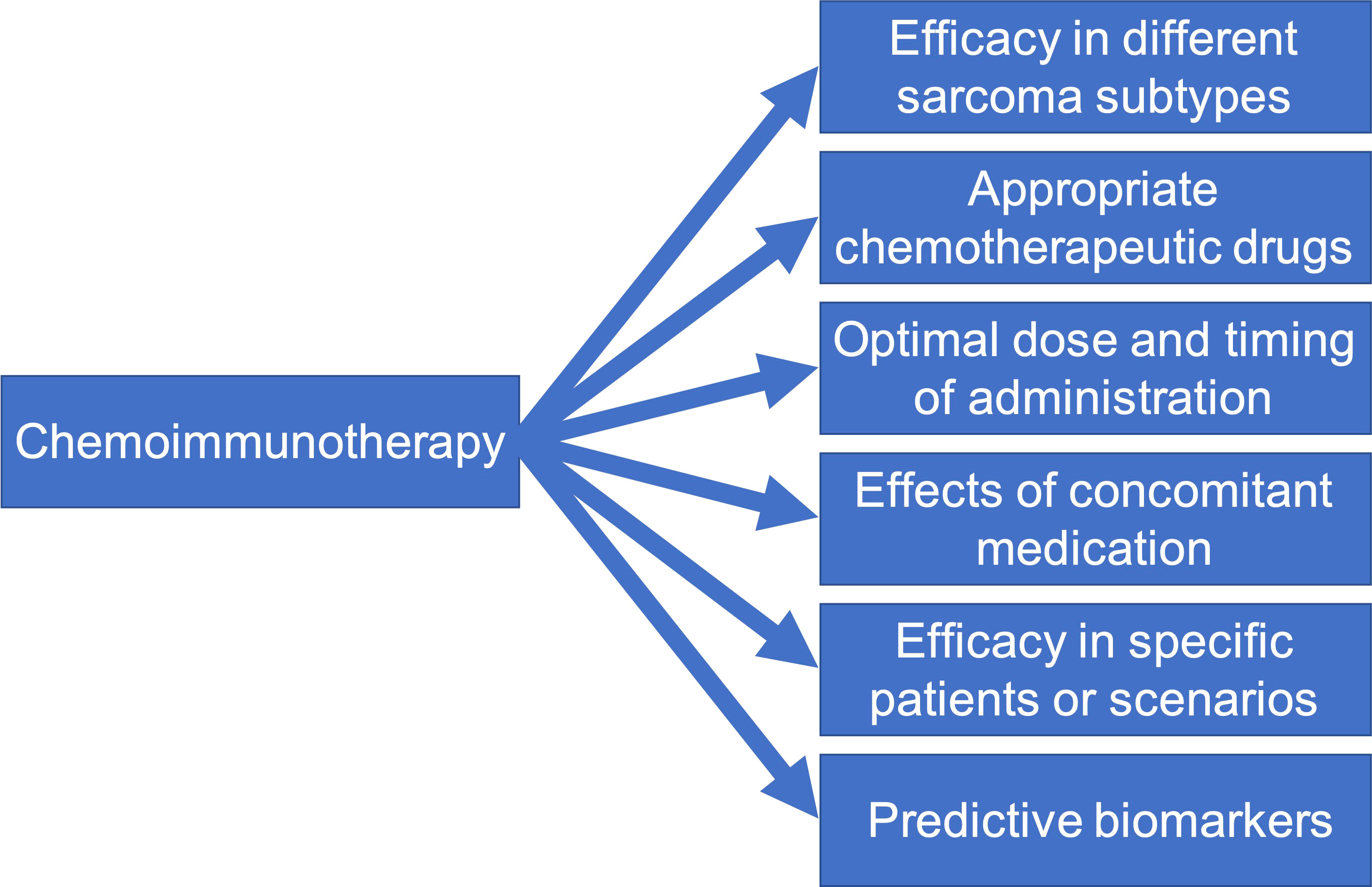
Figure 2 Six future directions for mechanism and clinical studies on PD-1/L1 inhibitor plus chemotherapy in soft-tissue sarcomas.
Although PD-1/L1 inhibitor plus chemotherapy has demonstrated synergistic efficacy in some cancers, there are some problems that cannot be ignored, including chemotherapy toxicity, immunosuppressive effect of chemotherapeutic or concomitant drugs, and ineffective clinical trial results. Obviously, these problems need to be explored by future clinical studies. Despite these doubts, it cannot be denied that PD-1/L1 inhibitor plus chemotherapy is a promising treatment for sarcomas. We believe that this combined approach will play an important role in the treatment of some subtypes of sarcoma in the future.
Author contributions
All authors listed have made a substantial, direct, and intellectual contribution to the work, and approved it for publication.
Conflict of interest
The authors declare that the research was conducted in the absence of any commercial or financial relationships that could be construed as a potential conflict of interest.
Publisher’s note
All claims expressed in this article are solely those of the authors and do not necessarily represent those of their affiliated organizations, or those of the publisher, the editors and the reviewers. Any product that may be evaluated in this article, or claim that may be made by its manufacturer, is not guaranteed or endorsed by the publisher.
References
1. Bessen T, Caughey GE, Shakib S, Potter JA, Reid J, Farshid G, et al. A population-based study of soft tissue sarcoma incidence and survival in Australia: An analysis of 2 6,970 cases. Cancer Epidemiol (2019) 63:101590. doi: 10.1016/j.canep.2019.101590
2. Yang Z, Zheng R, Zhang S, Zeng H, Li H, Chen W. Incidence, distribution of histological subtypes and primary sites of soft tissue sarcoma in China. Cancer Biol Med (2019) 16(3):565–74. doi: 10.20892/j.issn.2095-3941.2019.0041
3. Seddon B, Strauss SJ, Whelan J, Leahy M, Woll PJ, Cowie F, et al. Gemcitabine and docetaxel versus doxorubicin as first-line treatment in previously untreated advanced unresectable or metastatic soft-tissue sarcomas (GeDDiS): A randomised controlled phase 3 trial. Lancet Oncol (2017) 18(10):1397–410. doi: 10.1016/S1470-2045(17)30622-8
4. Kallen ME, Hornick JL. The 2020 WHO classification: What's new in soft tissue tumor pathology? Am J Surg Pathol (2021) 45(1):e1–e23. doi: 10.1097/PAS.0000000000001552
5. Gronchi A, Ferrari S, Quagliuolo V, Broto JM, Pousa AL, Grignani G, et al. Histotype-tailored neoadjuvant chemotherapy versus standard chemotherapy in patients with high-risk s oft-tissue sarcomas (ISG-STS 1001): An international, open-label, randomised, controlled, phase 3, m ulticentre trial. Lancet Oncol (2017) 18(6):812–22. doi: 10.1016/S1470-2045(17)30334-0
6. Hall F, Villalobos V, Wilky B. Future directions in soft tissue sarcoma treatment. Curr Probl Cancer (2019) 43(4):300–7. doi: 10.1016/j.currproblcancer.2019.06.004
7. Chen Y, Pei Y, Luo J, Huang Z, Yu J, Meng X. Looking for the optimal PD-1/PD-L1 inhibitor in cancer treatment: A comparison in basic structure, function, and clinical practice. Front Immunol (2020) 11:1088. doi: 10.3389/fimmu.2020.01088
8. Nakata E, Fujiwara T, Kunisada T, Ito T, Takihira S, Ozaki T. Immunotherapy for sarcomas. Jpn J Clin Oncol (2021) 51(4):523–37. doi: 10.1093/jjco/hyab005
9. Martin-Broto J, Moura DS, Van Tine BA. Facts and hopes in immunotherapy of soft-tissue sarcomas. Clin Cancer Res (2020) 26(22):5801–8. doi: 10.1158/1078-0432.CCR-19-3335
10. Longo V, Brunetti O, Azzariti A, Galetta D, Nardulli P, Leonetti F, et al. Strategies to improve cancer immune checkpoint inhibitors efficacy, other than abscopal effect: A systematic review. Cancers (Basel) (2019) 11(4):539. doi: 10.3390/cancers11040539
11. Kong X, Lu P, Liu C, Guo Y, Yang Y, Peng Y, et al. A combination of PD1/PDL1 inhibitors: The prospect of overcoming the weakness of tumor immunotherapy (Review). Mol Med Rep (2021) 23(5):362. doi: 10.3892/mmr.2021.12001
12. Salas-Benito D, Perez-Gracia JL, Ponz-Sarvise M, Rodriguez-Ruiz ME, Martinez-Forero I, Castanon E, et al. Paradigms on immunotherapy combinations with chemotherapy. Cancer Discov (2021) 11(6):1353–67. doi: 10.1158/2159-8290.CD-20-1312
13. Livingston MB, Jagosky MH, Robinson MM, Ahrens WA, Benbow JH, Farhangfar CJ, et al. Phase II study of pembrolizumab in combination with doxorubicin in metastatic and unresectable soft-tissue sarcoma. Clin Cancer Res (2021) 27(23):6424–31. doi: 10.1158/1078-0432.CCR-21-2001
14. Pollack SM, Redman MW, Baker KK, Wagner MJ, Schroeder BA, Loggers ET, et al. Assessment of doxorubicin and pembrolizumab in patients with advanced anthracycline-naive sarcoma: A phase 1/2 nonrandomized clinical trial. JAMA Oncol (2020) 6(11):1778–82. doi: 10.1001/jamaoncol.2020.3689
15. Wang F, Lau JKC, Yu J. The role of natural killer cell in gastrointestinal cancer: killer or helper. Oncogene (2021) 40(4):717–30. doi: 10.1038/s41388-020-01561-z
16. Murphy TL, Murphy KM. Dendritic cells in cancer immunology. Cell Mol Immunol (2022) 19(1):3–13. doi: 10.1038/s41423-021-00741-5
17. Huntington ND, Cursons J, Rautela J. The cancer-natural killer cell immunity cycle. Nat Rev Cancer (2020) 20(8):437–54. doi: 10.1038/s41568-020-0272-z
18. Long EO, Kim HS, Liu D, Peterson ME, Rajagopalan S. Controlling natural killer cell responses: Integration of signals for activation and inhibition. Annu Rev Immunol (2013) 31:227–58. doi: 10.1146/annurev-immunol-020711-075005
19. Mikelez-Alonso I, Magadan S, Gonzalez-Fernandez A, Borrego F. Natural killer (NK) cell-based immunotherapies and the many faces of NK cell memory: A look into how nanoparticles enhance NK cell activity. Adv Drug Deliv Rev (2021) 176:113860. doi: 10.1016/j.addr.2021.113860
20. Ben Khelil M, Godet Y, Abdeljaoued S, Borg C, Adotevi O, Loyon R. Harnessing antitumor CD4(+) T cells for cancer immunotherapy. Cancers (Basel) (2022) 14(1):260. doi: 10.3390/cancers14010260
21. Richardson JR, Schollhorn A, Gouttefangeas C, Schuhmacher J. CD4+ T cells: Multitasking cells in the duty of cancer immunotherapy. Cancers (Basel) (2021) 13(4):596. doi: 10.3390/cancers13040596
22. Raskov H, Orhan A, Christensen JP, Gogenur I. Cytotoxic CD8(+) T cells in cancer and cancer immunotherapy. Br J Cancer (2021) 124(2):359–67. doi: 10.1038/s41416-020-01048-4
23. Farhood B, Najafi M, Mortezaee K. CD8(+) cytotoxic T lymphocytes in cancer immunotherapy: A review. J Cell Physiol (2019) 234(6):8509–21. doi: 10.1002/jcp.27782
24. Tuccitto A, Shahaj E, Vergani E, Ferro S, Huber V, Rodolfo M, et al. Immunosuppressive circuits in tumor microenvironment and their influence on cancer treatment efficacy. Virchows Arch (2019) 474(4):407–20. doi: 10.1007/s00428-018-2477-z
25. Derosa L, Routy B, Desilets A, Daillere R, Terrisse S, Kroemer G, et al. Microbiota-centered interventions: The next breakthrough in immuno-oncology? Cancer Discovery (2021) 11(10):2396–412. doi: 10.1158/2159-8290.CD-21-0236
26. Malfitano AM, Pisanti S, Napolitano F, Di Somma S, Martinelli R, Portella G. Tumor-associated macrophage status in cancer treatment. Cancers (Basel) (2020) 12(7):1987. doi: 10.3390/cancers12071987
27. He M, Yang T, Wang Y, Wang M, Chen X, Ding D, et al. Immune checkpoint inhibitor-based strategies for synergistic cancer therapy. Adv Healthc Mater (2021) 10(9):e2002104. doi: 10.1002/adhm.202002104
28. Wang Q, Xie B, Liu S, Shi Y, Tao Y, Xiao D, et al. What happens to the immune microenvironment after PD-1 inhibitor therapy? Front Immunol (2021) 12:773168. doi: 10.3389/fimmu.2021.773168
29. Reinfeld BI, Madden MZ, Wolf MM, Chytil A, Bader JE, Patterson AR, et al. Cell-programmed nutrient partitioning in the tumour microenvironment. Nature (2021) 593(7858):282–8. doi: 10.1038/s41586-021-03442-1
30. Rangel Rivera GO, Knochelmann HM, Dwyer CJ, Smith AS, Wyatt MM, Rivera-Reyes AM, et al. Fundamentals of T cell metabolism and strategies to enhance cancer immunotherapy. Front Immunol (2021) 12:645242. doi: 10.3389/fimmu.2021.645242
31. Rabinovich GA, Gabrilovich D, Sotomayor EM. Immunosuppressive strategies that are mediated by tumor cells. Annu Rev Immunol (2007) 25:267–96. doi: 10.1146/annurev.immunol.25.022106.141609
32. Galluzzi L, Humeau J, Buque A, Zitvogel L, Kroemer G. Immunostimulation with chemotherapy in the era of immune checkpoint inhibitors. Nat Rev Clin Oncol (2020) 17(12):725–41. doi: 10.1038/s41571-020-0413-z
33. Galluzzi L, Buqué A, Kepp O, Zitvogel L, Kroemer G. Immunogenic cell death in cancer and infectious disease. Nat Rev Immunol (2017) 17(2):97–111. doi: 10.1038/nri.2016.107
34. Zhu C, Shi Y, Li Q, Luo L, Li X, Luo Z, et al. Rational administration sequencing of immunochemotherapy elicits powerful anti-tumor effect. J Control Release (2022) 341:769–81. doi: 10.1016/j.jconrel.2021.12.022
35. Truong NTH, Gargett T, Brown MP, Ebert LM. Effects of chemotherapy agents on circulating leukocyte populations: Potential implications for the success of CAR-T cell therapies. Cancers (Basel) (2021) 13(9):2225. doi: 10.3390/cancers13092225
36. Chulpanova DS, Kitaeva KV, Green AR, Rizvanov AA, Solovyeva VV. Molecular aspects and future perspectives of cytokine-based anti-cancer immunotherapy. Front Cell Dev Biol (2020) 8:402. doi: 10.3389/fcell.2020.00402
37. Gaudreau PO, Negrao MV, Mitchell KG, Reuben A, Corsini EM, Li J, et al. Neoadjuvant chemotherapy increases cytotoxic T cell, tissue resident memory T cell, and b cell infiltration in resectable NSCLC. J Thorac Oncol (2021) 16(1):127–39. doi: 10.1016/j.jtho.2020.09.027
38. Fu D, Wu J, Lai J, Liu Y, Zhou L, Chen L, et al. T Cell recruitment triggered by optimal dose platinum compounds contributes to the therapeutic effica cy of sequential PD-1 blockade in a mouse model of colon cancer. Am J Cancer Res (2020) 10(2):473–90.
39. Mackall CL, Fleisher TA, Brown MR, Andrich MP, Chen CC, Feuerstein IM, et al. Distinctions between CD8+ and CD4+ T-cell regenerative pathways result in prolonged T-cell subset imb alance after intensive chemotherapy. Blood (1997) 89(10):3700–7. doi: 10.1182/blood.V89.10.3700
40. Coleman S, Clayton A, Mason MD, Jasani B, Adams M, Tabi Z. Recovery of CD8+ T-cell function during systemic chemotherapy in advanced ovarian cancer. Cancer Res (2005) 65(15):7000–6. doi: 10.1158/0008-5472.CAN-04-3792
41. Principe DR, Kamath SD, Korc M, Munshi HG. The immune modifying effects of chemotherapy and advances in chemo-immunotherapy. Pharmacol Ther (2022) 236:108111. doi: 10.1016/j.pharmthera.2022.108111
42. Peng J, Hamanishi J, Matsumura N, Abiko K, Murat K, Baba T, et al. Chemotherapy induces programmed cell death-ligand 1 overexpression via the nuclear factor-kappaB to foster an immunosuppressive tumor microenvironment in ovarian cancer. Cancer Res (2015) 75(23):5034–45. doi: 10.1158/0008-5472.CAN-14-3098
43. Yi M, Niu M, Xu L, Luo S, Wu K. Regulation of PD-L1 expression in the tumor microenvironment. J Hematol Oncol (2021) 14(1):10. doi: 10.1186/s13045-020-01027-5
44. Yamaguchi H, Hsu JM, Yang WH, Hung MC. Mechanisms regulating PD-L1 expression in cancers and associated opportunities for novel small-molecule therapeutics. Nat Rev Clin Oncol (2022) 19(5):287–305. doi: 10.1038/s41571-022-00601-9
45. Hong M, Puaux AL, Huang C, Loumagne L, Tow C, Mackay C, et al. Chemotherapy induces intratumoral expression of chemokines in cutaneous melanoma, favoring T-cell inf iltration and tumor control. Cancer Res (2011) 71(22):6997–7009. doi: 10.1158/0008-5472.CAN-11-1466
46. Reers S, Pfannerstill AC, Rades D, Maushagen R, Andratschke M, Pries R, et al. Cytokine changes in response to radio-/chemotherapeutic treatment in head and neck cancer. Anticancer Res (2013) 33(6):2481–9.
47. Gartung A, Yang J, Sukhatme VP, Bielenberg DR, Fernandes D, Chang J, et al. Suppression of chemotherapy-induced cytokine/lipid mediator surge and ovarian cancer by a dual COX-2/ sEH inhibitor. Proc Natl Acad Sci (2019) 116(5):1698–703. doi: 10.1073/pnas.1803999116
48. Ansari MJ, Bokov D, Markov A, Jalil AT, Shalaby MN, Suksatan W, et al. Cancer combination therapies by angiogenesis inhibitors; A comprehensive review. Cell Commun Signal (2022) 20(1):49. doi: 10.1186/s12964-022-00838-y
49. Hu H, Chen Y, Tan S, Wu S, Huang Y, Fu S, et al. The research progress of antiangiogenic therapy, immune therapy and tumor microenvironment. Front Immunol (2022) 13:802846. doi: 10.3389/fimmu.2022.802846
50. Chambers A, Kundranda M, Rao S, Mahmoud F, Niu J. Anti-angiogenesis revisited: Combination with immunotherapy in solid tumors. Curr Oncol Rep (2021) 23(9):100. doi: 10.1007/s11912-021-01099-7
51. Ueda M, Ueki K, Kumagai K, Terai Y, Okamoto Y, Ueki M, et al. Apoptosis and tumor angiogenesis in cervical cancer after preoperative chemotherapy. Cancer Res (1998) 58(11):2343–6.
52. Hanahan D, Bergers G, Bergsland E. Less is more, regularly: metronomic dosing of cytotoxic drugs can target tumor angiogenesis in mice. J Clin Invest (2000) 105(8):1045–7. doi: 10.1172/JCI9872
53. Fukumura D, Kloepper J, Amoozgar Z, Duda DG, Jain RK. Enhancing cancer immunotherapy using antiangiogenics: Opportunities and challenges. Nat Rev Clin Oncol (2018) 15(5):325–40. doi: 10.1038/nrclinonc.2018.29
54. Labiano S, Palazon A, Melero I. Immune response regulation in the tumor microenvironment by hypoxia. Semin Oncol (2015) 42(3):378–86. doi: 10.1053/j.seminoncol.2015.02.009
55. Otsuka K, Mitsuhashi A, Goto H, Hanibuchi M, Koyama K, Ogawa H, et al. Anti-PD-1 antibody combined with chemotherapy suppresses the growth of mesothelioma by reducing myeloid-derived suppressor cells. Lung Cancer (2020) 146:86–96. doi: 10.1016/j.lungcan.2020.05.023
56. Paz-Ares L, Luft A, Vicente D, Tafreshi A, Gümüş M, Mazières J, et al. Pembrolizumab plus chemotherapy for squamous non-Small-Cell lung cancer. N Engl J Med (2018) 379(21):2040–51. doi: 10.1056/NEJMoa1810865
57. Gandhi L, Rodríguez-Abreu D, Gadgeel S, Esteban E, Felip E, De Angelis F, et al. Pembrolizumab plus chemotherapy in metastatic non-Small-Cell lung cancer. N Engl J Med (2018) 378(22):2078–92. doi: 10.1056/NEJMoa1801005
58. Horn L, Mansfield AS, Szczęsna A, Havel L, Krzakowski M, Hochmair MJ, et al. First-line atezolizumab plus chemotherapy in extensive-stage small-cell lung cancer. N Engl J Med (2018) 379(23):2220–9. doi: 10.1056/NEJMoa1809064
59. Paz-Ares L, Dvorkin M, Chen Y, Reinmuth N, Hotta K, Trukhin D, et al. Durvalumab plus platinum-etoposide versus platinum-etoposide in first-line treatment of extensive-sta ge small-cell lung cancer (CASPIAN): A randomised, controlled, open-label, phase 3 trial. Lancet (2019) 394(10212):1929–39. doi: 10.1016/S0140-6736(19)32222-6
60. Janjigian YY, Shitara K, Moehler M, Garrido M, Salman P, Shen L, et al. First-line nivolumab plus chemotherapy versus chemotherapy alone for advanced gastric, gastro-oesopha geal junction, and oesophageal adenocarcinoma (CheckMate 649): A randomised, open-label, phase 3 tri al. Lancet (2021) 398(10294):27–40. doi: 10.1016/S0140-6736(21)00797-2
61. Sun JM, Shen L, Shah MA, Enzinger P, Adenis A, Doi T, et al. Pembrolizumab plus chemotherapy versus chemotherapy alone for first-line treatment of advanced oesophageal cancer (KEYNOTE-590): A randomised, placebo-controlled, phase 3 study. Lancet (2021) 398(10302):759–71. doi: 10.1016/S0140-6736(21)01234-4
62. Schmid P, Cortes J, Pusztai L, McArthur H, Kümmel S, Bergh J, et al. Pembrolizumab for early triple-negative breast cancer. N Engl J Med (2020) 382(9):810–21. doi: 10.1056/NEJMoa1910549
63. Cortes J, Cescon DW, Rugo HS, Nowecki Z, Im SA, Yusof MM, et al. Pembrolizumab plus chemotherapy versus placebo plus chemotherapy for previously untreated locally recurrent inoperable or metastatic triple-negative breast cancer (KEYNOTE-355): A randomised, placebo-c ontrolled, double-blind, phase 3 clinical trial. Lancet (2020) 396(10265):1817–28. doi: 10.1016/S0140-6736(20)32531-9
64. Galsky MD, Arija JA, Bamias A, Davis ID, De Santis M, Kikuchi E, et al. Atezolizumab with or without chemotherapy in metastatic urothelial cancer (IMvigor130): A multicentre , randomised, placebo-controlled phase 3 trial. Lancet (2020) 395(10236):1547–57. doi: 10.1016/S0140-6736(20)30230-0
65. Mai HQ, Chen QY, Chen D, Hu C, Yang K, Wen J, et al. Toripalimab or placebo plus chemotherapy as first-line treatment in advanced nasopharyngeal carcinoma: A multicenter randomized phase 3 trial. Nat Med (2021) 27(9):1536–43. doi: 10.1038/s41591-021-01444-0
66. Moore KN, Bookman M, Sehouli J, Miller A, Anderson C, Scambia G, et al. Atezolizumab, bevacizumab, and chemotherapy for newly diagnosed stage III or IV ovarian cancer: Place bo-controlled randomized phase III trial (IMagyn050/GOG 3015/ENGOT-OV39). J Clin Oncol (2021) 39(17):1842–55. doi: 10.1200/JCO.21.00306
67. Pujade-Lauraine E, Fujiwara K, Ledermann JA, Oza AM, Kristeleit R, Ray-Coquard IL, et al. Avelumab alone or in combination with chemotherapy versus chemotherapy alone in platinum-resistant or platinum-refractory ovarian cancer (JAVELIN ovarian 200): An open-label, three-arm, randomised, phase 3 study. Lancet Oncol (2021) 22(7):1034–46. doi: 10.1016/S1470-2045(21)00216-3
68. Shitara K, Van Cutsem E, Bang YJ, Fuchs C, Wyrwicz L, Lee KW, et al. Efficacy and safety of pembrolizumab or pembrolizumab plus chemotherapy vs chemotherapy alone for patients with first-line, advanced gastric cancer: The KEYNOTE-062 phase 3 randomized clinical trial. JAMA Oncol (2020) 6(10):1571–80. doi: 10.1001/jamaoncol.2020.3370
69. Burtness B, Harrington KJ, Greil R, Soulières D, Tahara M, de Castro G, et al. Pembrolizumab alone or with chemotherapy versus cetuximab with chemotherapy for recurrent or metastatic squamous cell carcinoma of the head and neck (KEYNOTE-048): A randomised, open-label, phase 3 study. Lancet (2019) 394(10212):1915–28. doi: 10.1016/S0140-6736(19)32591-7
70. Powles T, Csőszi T, Özgüroğlu M, Matsubara N, Géczi L, Cheng SY, et al. Pembrolizumab alone or combined with chemotherapy versus chemotherapy as first-line therapy for advan ced urothelial carcinoma (KEYNOTE-361): A randomised, open-label, phase 3 trial. Lancet Oncol (2021) 22(7):931–45. doi: 10.1016/S1470-2045(21)00152-2
71. Casares N, Pequignot MO, Tesniere A, Ghiringhelli F, Roux S, Chaput N, et al. Caspase-dependent immunogenicity of doxorubicin-induced tumor cell death. J Exp Med (2005) 202(12):1691–701. doi: 10.1084/jem.20050915
72. Wang J, Hu C, Wang J, Shen Y, Bao Q, He F, et al. Checkpoint blockade in combination with doxorubicin augments tumor cell apoptosis in osteosarcoma. J Immunother (2019) 42(9):321–30. doi: 10.1097/CJI.0000000000000281
73. Yati S, Silathapanasakul A, Thakaeng C, Chanasakulniyom M, Songtawee N, Porntadavity S, et al. Extracellular vesicle-mediated IL-1 signaling in response to doxorubicin activates PD-L1 expression in osteosarcoma models. Cells (2022) 11(6):1042. doi: 10.3390/cells11061042
74. Galluzzi L, Senovilla L, Zitvogel L, Kroemer G. The secret ally: Immunostimulation by anticancer drugs. Nat Rev Drug Discovery (2012) 11(3):215–33. doi: 10.1038/nrd3626
75. Wang H, He X, Fang D, Wang X, Guan J, Shi ZW, et al. Gemcitabine-facilitated modulation of the tumor microenvironment and PD-1/PD-L1 blockade generate a synergistic antitumor effect in a murine hepatocellular carcinoma model. Clin Res Hepatol Gas (2022) 46(4):101853. doi: 10.1016/j.clinre.2021.101853
76. Ma Z, Zhang W, Dong B, Xin Z, Ji Y, Su R, et al. Docetaxel remodels prostate cancer immune microenvironment and enhances checkpoint inhibitor-based immunotherapy. Theranostics (2022) 12(11):4965–79. doi: 10.7150/thno.73152
77. Belgiovine C, Frapolli R, Liguori M, Digifico E, Colombo FS, Meroni M, et al. Inhibition of tumor-associated macrophages by trabectedin improves the antitumor adaptive immunity in response to anti-PD-1 therapy. Eur J Immunol (2021) 51(11):2677–86. doi: 10.1002/eji.202149379
78. Kerrison WGJ, Lee ATJ, Thway K, Jones RL, Huang PH. Current status and future directions of immunotherapies in soft tissue sarcomas. Biomedicines (2022) 10(3):573. doi: 10.3390/biomedicines10030573
79. Krishnadas DK, Bao L, Bai F, Chencheri SC, Lucas K. Decitabine facilitates immune recognition of sarcoma cells by upregulating CT antigens, MHC molecules, and ICAM-1. Tumour Biol (2014) 35(6):5753–62. doi: 10.1007/s13277-014-1764-9
80. Goff PH, Riolobos L, LaFleur BJ, Spraker MB, Seo YD, Smythe KS, et al. Neoadjuvant therapy induces a potent immune response to sarcoma, dominated by myeloid and b cells. Clin Cancer Res (2022) 28(8):1701–11. doi: 10.1158/1078-0432.CCR-21-4239
81. Issels RD, Noessner E, Lindner LH, Schmidt M, Albertsmeier M, Blay JY, et al. Immune infiltrates in patients with localised high-risk soft tissue sarcoma treated with neoadjuvant chemotherapy without or with regional hyperthermia: A translational research program of the EORTC 62961-ESHO 95 randomised clinical trial. Eur J Cancer (2021) 158:123–32. doi: 10.1016/j.ejca.2021.09.015
82. Toulmonde M, Penel N, Adam J, Chevreau C, Blay JY, Le Cesne A, et al. Use of PD-1 targeting, macrophage infiltration, and IDO pathway activation in sarcomas: A phase 2 clinical trial. JAMA Oncol (2018) 4(1):93–7. doi: 10.1001/jamaoncol.2017.1617
83. Italiano A, Bessede A, Bompas E, Piperno-Neumann S, Chevreau C, Penel N, et al. PD1 inhibition in soft-tissue sarcomas with tertiary lymphoid structures: A multicenter phase II trial. J Clin Oncol (2021) 39(15_suppl):11507–7. doi: 10.1200/JCO.2021.39.15_suppl.1150
84. Toulmonde M, Brahmi M, Giraud A, Chakiba C, Bessede A, Kind M, et al. Trabectedin plus durvalumab in patients with advanced pretreated soft tissue sarcoma and ovarian carcinoma (TRAMUNE): An open-label, multicenter phase ib study. Clin Cancer Res (2021) 28(9):1765–72. doi: 10.1158/1078-0432.CCR-21-2258
85. Tian Z, Yang Y, Yang J, Zhang P, Zhang F, Du X, et al. Safety and efficacy of PD-1 inhibitors plus chemotherapy in advanced soft tissue sarcomas: A retrospective study. Cancer Manag Res (2020) 12:1339–46. doi: 10.2147/CMAR.S237300
86. Liu Z, Liu C, Yao W, Gao S, Wang J, Zhang P, et al. Efficacy and safety of toripalimab combined with doxorubicin as first-line treatment for metastatic soft tissue sarcomas: An observational study. Anticancer Drugs (2021) 32(9):962–8. doi: 10.1097/CAD.0000000000001088
87. Tian Z, Dong S, Yang Y, Gao S, Yang Y, Yang J, et al. Nanoparticle albumin-bound paclitaxel and PD-1 inhibitor (sintilimab) combination therapy for soft tissue sarcoma: A retrospective study. BMC Cancer (2022) 22(1):56. doi: 10.1186/s12885-022-09176-1
88. Tawbi HA, Burgess M, Bolejack V, Van Tine BA, Schuetze SM, Hu J, et al. Pembrolizumab in advanced soft-tissue sarcoma and bone sarcoma (SARC028): A multicentre, two-cohort, single-arm, open-label, phase 2 trial. Lancet Oncol (2017) 18(11):1493–501. doi: 10.1016/S1470-2045(17)30624-1
89. Siozopoulou V, Domen A, Zwaenepoel K, Van Beeck A, Smits E, Pauwels P, et al. Immune checkpoint inhibitory therapy in sarcomas: Is there light at the end of the tunnel? Cancers (Basel) (2021) 13(2):360. doi: 10.3390/cancers13020360
90. Tian Z, Yao W. Albumin-bound paclitaxel: Worthy of further study in sarcomas. Front Oncol (2022) 12:815900. doi: 10.3389/fonc.2022.815900
91. Yao W, Zhao X, Gong Y, Zhang M, Zhang L, Wu Q, et al. Impact of the combined timing of PD-1/PD-L1 inhibitors and chemotherapy on the outcomes in patients with refractory lung cancer. ESMO Open (2021) 6(2):100094. doi: 10.1016/j.esmoop.2021.100094
92. Cortellini A, Tucci M, Adamo V, Stucci LS, Russo A, Tanda ET, et al. Integrated analysis of concomitant medications and oncological outcomes from PD-1/PD-L1 checkpoint inhibitors in clinical practice. J Immunother Cancer (2020) 8(2):e001361. doi: 10.1136/jitc-2020-001361
93. Cho YA, Lee H, Kim DG, Kim H, Ha SY, Choi YL, et al. PD-L1 expression is significantly associated with tumor mutation burden and microsatellite instability score. Cancers (Basel) (2021) 13(18):4695. doi: 10.3390/cancers13184659
94. Pestana RC, Beal JR, Parkes A, Hamerschlak N, Subbiah V. Impact of tissue-agnostic approvals for patients with sarcoma. Trends Cancer (2022) 8(2):135–44. doi: 10.1016/j.trecan.2021.11.007
Keywords: PD-1 inhibitor, PD-L1 inhibitor, chemotherapy, sarcoma, immunochemotherapy
Citation: Tian Z and Yao W (2022) PD-1/L1 inhibitor plus chemotherapy in the treatment of sarcomas. Front. Immunol. 13:898255. doi: 10.3389/fimmu.2022.898255
Received: 17 March 2022; Accepted: 10 August 2022;
Published: 23 August 2022.
Edited by:
Robin Lewis Jones, Royal Marsden Hospital, United KingdomReviewed by:
Thierry Alcindor, McGill University, CanadaAndrea Napolitano, Royal Marsden Hospital, United Kingdom
Copyright © 2022 Tian and Yao. This is an open-access article distributed under the terms of the Creative Commons Attribution License (CC BY). The use, distribution or reproduction in other forums is permitted, provided the original author(s) and the copyright owner(s) are credited and that the original publication in this journal is cited, in accordance with accepted academic practice. No use, distribution or reproduction is permitted which does not comply with these terms.
*Correspondence: Weitao Yao, ywt00001@163.com