- 1State Key Laboratory of Oral Diseases, National Clinical Research Center for Oral Diseases, Chinese Academy of Medical Sciences Research Unit of Oral Carcinogenesis and Management, West China Hospital of Stomatology, Sichuan University, Chengdu, China
- 2Chengdu Blood Center, Blood Research Laboratory, Chengdu, China
The immune system has evolved since the birth of humans. However, immune-related diseases have not yet been overcome due to the lack of expected indicators and targeting specificity of current medical technology, subjecting patients to very uncomfortable physical and mental experiences and high medical costs. Therefore, the requirements for treatments with higher specificity and indicative ability are raised. Fortunately, the discovery of and continuous research investigating circular RNAs (circRNAs) represent a promising method among numerous methods. Although circRNAs wear regarded as metabolic wastes when discovered, as a type of noncoding RNA (ncRNA) with a ring structure and wide distribution range in the human body, circRNAs shine brilliantly in medical research by virtue of their special nature and structure-determined functions, such as high stability, wide distribution, high detection sensitivity, acceptable reproducibility and individual differences. Based on research investigating the role of circRNAs in immunity, we systematically discuss the hotspots of the roles of circRNAs in immune-related diseases, including expression profile analyses, potential biomarker research, ncRNA axis/network construction, impacts on phenotypes, therapeutic target seeking, maintenance of nucleic acid stability and protein binding research. In addition, we summarize the current situation of and problems associated with circRNAs in immune research, highlight the applications and prospects of circRNAs in the treatment of immune-related diseases, and provide new insight into future directions and new strategies for laboratory research and clinical applications.
1 Introduction
CircRNAs are molecules belonging to the noncoding RNA family that form ring-like structures with covalent bonds without 5’ caps and 3’ poly (A) tails (1). CircRNAs were first found in pathogens but were regarded as meaningless or even incorrect expression products for decades. In recent years, researchers have begun to realize the importance of circRNAs with the rapid development of specific biochemical and computational methods, such as high-throughput sequencing technology and microarray techniques (2, 3). CircRNAs are generally stable and thought to have unique structural conformations that differ from their linear RNA homology (4). As confirmed, circRNAs are principally formed via the junction of a downstream 3’ site with an upstream 5’ site, generated via back-splicing or exon skipping of premRNAs in general (5, 6). Over years of research, circRNAs have been found to feature four main characteristics (Figure 1). First, circRNAs are connected from end to end to form ring structures, enhancing their stability and resistance to most ribonucleases. Studies have shown that the half-life of circRNAs is longer than that of corresponding linear transcripts, which is beneficial for the transportation, preservation, and analysis of samples. Second, circRNAs are conserved, tissue- and spatiotemporal specific, resulting in acceptable reproducibility and individual differences (7). Third, circRNAs are abundant and almost endogenous (8, 9). The expression level of circRNAs changes accordingly under physiological or pathological conditions. Therefore, the change in the amount of circRNAs can reflect the stage of disease to some extent. Fourth, circRNAs are widely distributed and have high detection sensitivity (8). Currently, circRNAs are commonly divided into the following four categories according to their constituent sequences: exonic circRNAs (ecircRNAs), circular intronic RNAs (ciRNAs), exon–intron circRNAs (EIciRNAs) and tRNA intronic circular RNAs (tricRNAs). However, the circRNAs currently found are mainly derived from exons (5, 9).
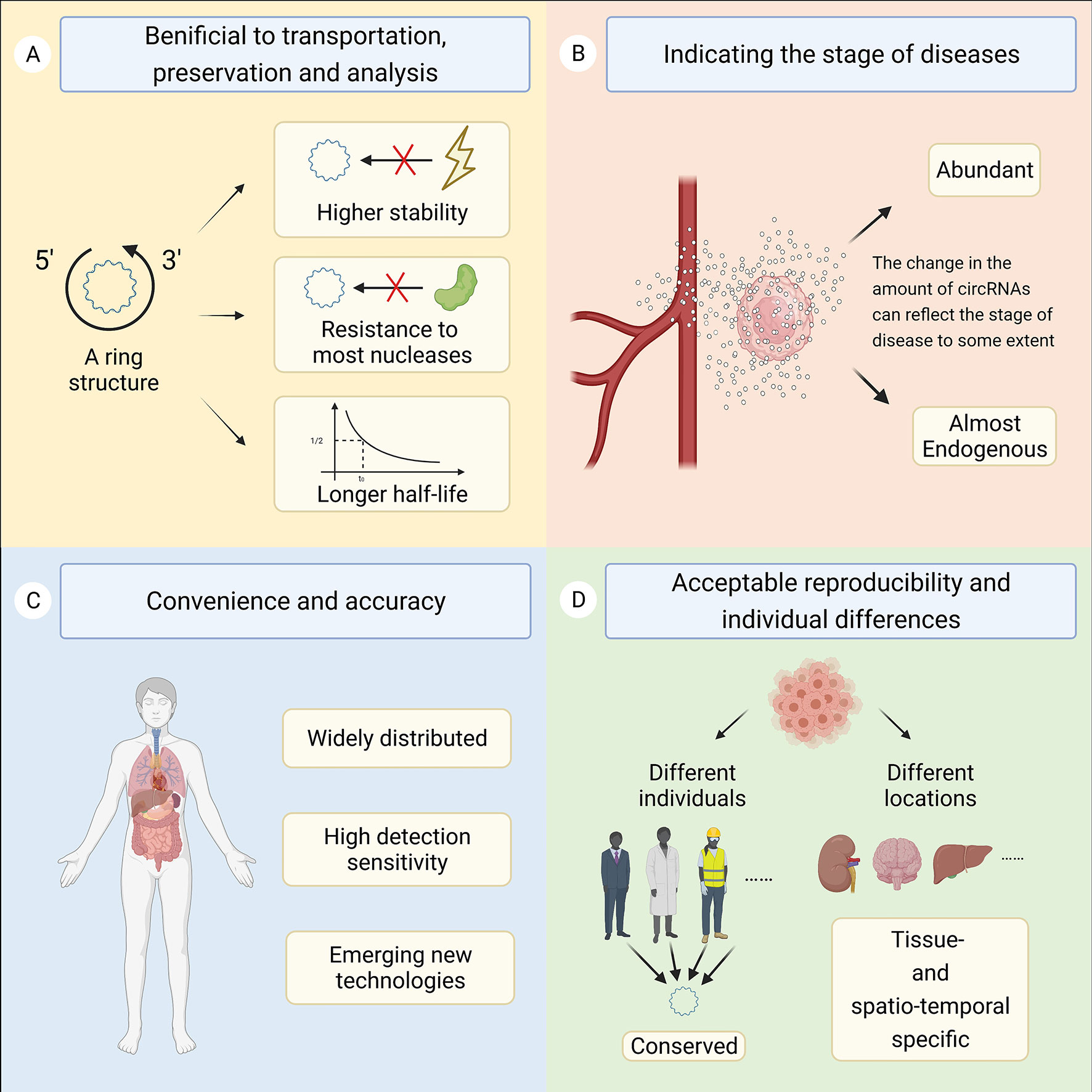
Figure 1 This figure shows the four main characteristics of circRNAs. (A) The ring structure is beneficial for the transportation, preservation, and analysis of samples. (B) The change in the amount of circRNAs can reflect the stage of disease to some extent. (C) CircRNAs are widely distributed and have high detection sensitivity. (D) CircRNAs are conserved, tissue- and spatiotemporal specific, resulting in acceptable reproducibility and individual differences.
Since the advent of empirical immunology, research investigating immunity has lasted for a long time. The immune system plays a dual role in the fight against diseases. On the one hand, the body’s immune barrier acts as a defense against intruders, and a functioning immune system can quickly detect and address abnormal conditions in the body. On the other hand, a dysregulated response of the human immune system may lead to the deterioration of the disease or the emergence of autoimmune diseases. With the development of research, scientists have noticed that circRNAs are of vital importance in human immunity and have potential clinical significance in the diagnosis and treatment of autoimmune diseases, tumor immunity, infectious diseases and other immune-related diseases (9–12).
This review expounds upon the progress and existing problems in this field and provides potential development directions for the future to improve the environment for clinical treatment.
2 Biological Functions of circRNAs
According to current studies, circRNAs participate in different physiological processes of human diseases and perform a wide range of functions as miRNA sponges, transcription templates, special protein binding sites and regulators of host genes. Many current studies have focused on circRNAs in the cytoplasm, and some of those circRNA were reported to act as competing endogenous RNAs (ceRNAs) and usually function as sponges for miRNAs, thereby regulating miRNAs targeting gene expression (13, 14). A typical example is CDR1as, namely, ciRS-7, which contains more than 70 miRNA response elements (MREs) for miR-7 and can combine with miR-7 to downregulate its miRNA actions (15–17). In addition, circRNAs can use their specific regions to interact with proteins (18), function as protein scaffolds (19) and recruit specific proteins to certain locations in cells (20); thus, circRNAs with internal ribosome entry sites (IRESs) and infinite open reading frames (ORFs) can be translated under specific circumstances (21, 22). Moreover, circRNAs correlated with RNA polymerase II (Pol II) in human cells, localizing in the nucleus, could modulate the expression of their host genes (23, 24). However, unfortunately, research concerning the function of circRNAs only focuses on a small fraction of circRNAs that have been found, thereby requiring more specificity. Therefore, there is still more development space for other types of circRNAs that are less studied, rending the future of this field full of uncertainty and promises.
3 Roles of circRNAs in Immune-Related Diseases
Many studies have been performed to uncover the mechanism of immunity with the purpose to solve the problems of immunological diseases. With continuous research development, increasing evidence has emerged showing that circRNAs are able to intervene in the biological processes of assorted immune-related diseases by acting as miRNA sponges, protein interactors, mRNA stability maintainers, potential biomarkers and therapeutic targets via diverse axes and intricate signaling pathways (Table 1). In this section (Figure 2), we describe new experimental progress in circRNAs that participate in organ-specific autoimmune diseases (OSADs), systemic autoimmune diseases (SADs), tumor immunology, and infectious diseases and summarize the roles of circRNAs in other studies.
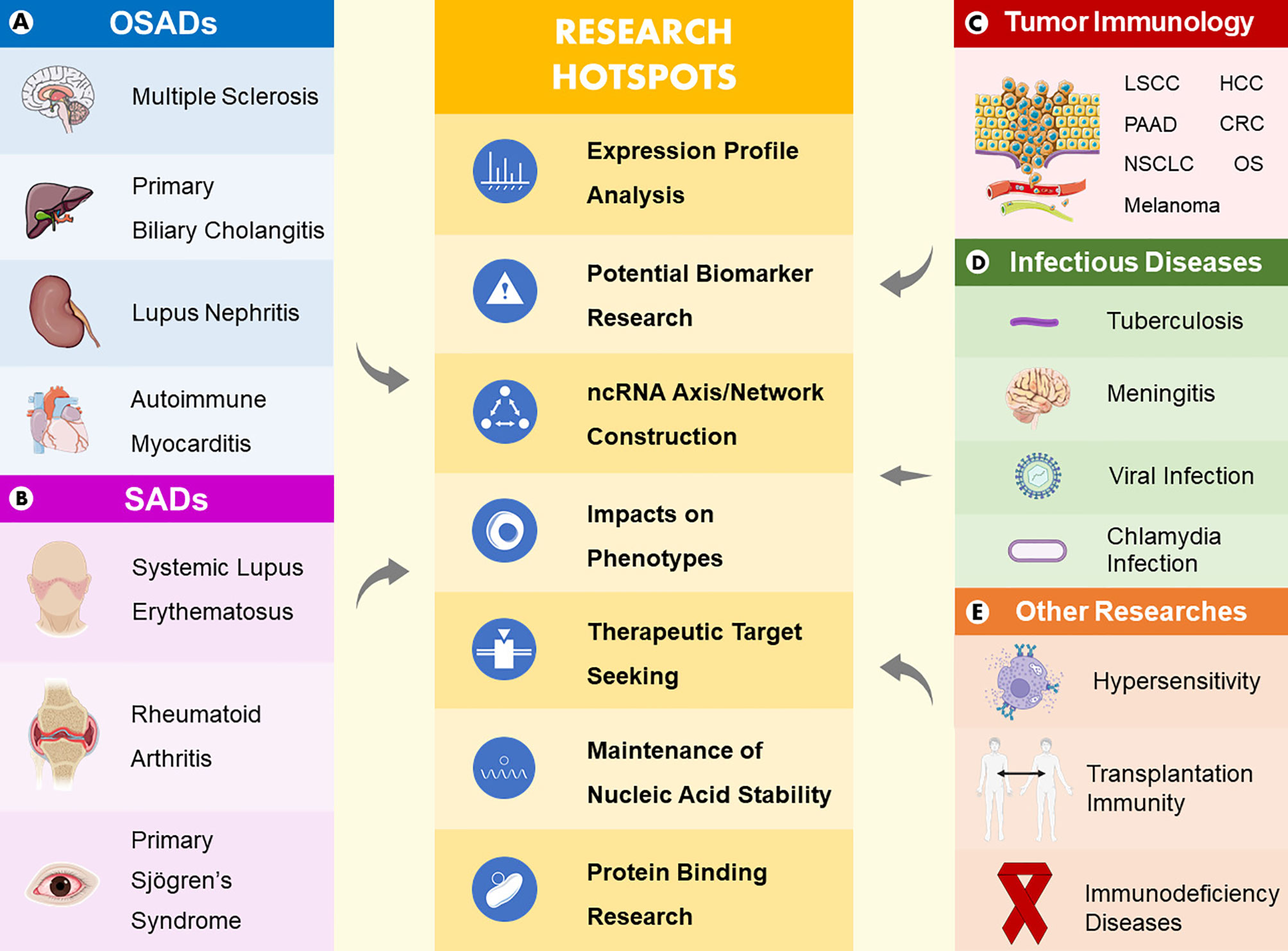
Figure 2 Main areas and research hotspots of the roles of circRNAs in immune-related diseases. Five main areas consist of organ-specific autoimmune diseases (A), systemic autoimmune diseases (B), tumor immunology (C), infectious diseases (D), and other studies (E). In summary, research hotspots of the roles of circRNAs in immune-related diseases include expression profile analyses, potential biomarker research, ncRNA axis/network construction, impacts on phenotypes, therapeutic target seeking, maintenance of nucleic acid stability and protein binding research.
3.1 CircRNAs in Organ-Specific Autoimmune Diseases
In OSADs, autoantigens are a specific component of an organ, and the pathological damage and dysfunction of tissue are limited to the organ targeted by antibodies or lymphocytes. Multiple sclerosis (MS) is an autoimmune disease that demyelinates the white matter of the central nervous system. Although the specific pathogenesis remains unclear, circRNAs have been found to participate in the progression of MS (55). Cardamone et al. indicated that the abnormal metabolism of circRNAs is a potential characteristic of MS (26). Moreover, Iparraguirre et al. found two downregulated circRNAs and considered them potential biomarkers of MS (25). Among ncRNAs, miRNAs and lncRNAs are currently popular issues in MS, while circRNAs are relatively less studied; thus, more research is needed to supplement the regulatory network of ncRNAs in MS (56).
In addition to MS, circRNAs play an important role in many other OSDAs. For example, circRNAs showed promise as candidate biomarkers of primary biliary cholangitis (27), and plasma circRNA_002453 could be used as a novel biomarker of lupus nephritis (28). Researchers also found that circSnx5 control the immunogenicity of dendritic cells as a miRNA sponge, thereby alleviating experimental autoimmune myocarditis (29). Although the participation of circRNAs has further increased the complexity of the mechanism of OSAD, current research is still very simple, and it is difficult to promote the understanding of this type of disease and the therapeutic application of circRNAs.
3.2 CircRNAs in Systemic Autoimmune Diseases
Systemic autoimmune disease is a type of systemic multiple organ damage caused by the extensive deposition of antigen and antibody complexes on the vascular wall, and systemic lupus erythematosus (SLE) is a common disease. The exact cause of SLE is still unclear, but circRNAs have recently been regarded as vital molecules in SLE. Li et al. compared the different circRNA profiles in T cells from healthy and ailing patients and then revealed the biofunction of hsa_circ_0045272 (30). Currently, many circRNAs have been identified as biomarkers of SLE (31–34). Recently, Zhang et al. retrieved the GEO database and obtained a regulatory network, providing novel insight into the role of circRNAs in SLE (57).
Another common type of systemic autoimmune disease is rheumatoid arthritis (RA), whose pathogenesis has not been fully elucidated thus far. Currently, in the research field of circRNAs in RA, research mainly focuses on expression profile analyses, biomarker research and the proliferation and migration of fibroblast-like synovial cells. Wen et al. constructed a network of differentially expressed circRNAs and miRNAs and eventually revealed the expression profile of peripheral blood mononuclear cells (PBMCs) in patients with RA (35). Yang et al. used RNA sequencing technology to uncover the circRNA expression profiles of PBMCs in experimental and control groups and found that circRNAs are novel diagnostic markers of RA (36). Regarding the proliferation and migration of fibroblast-like synovial cells, Zhong et al. found that hsa_circ_0088036 promoted the proliferation and migration of fibroblast-like synovial cells via the miR-140-3p/SIRT1 axis in RA (37). In addition to SLE and RA, circRNAs are of great concern in some SADs. For example, Su et al. reported that hsa_circ_001264 might be a biomarker of primary Sjögren’s syndrome (pSS) (38). Although circRNAs are closely connected to SADs such as SLE, RA and pSS, research concerning SADs, such as scleroderma, dermatomyositis and polymyositis, currently mainly focuses on miRNAs. Consequently, there is substantial untapped potential in research investigating the mechanisms of circRNAs and SADs.
3.3 CircRNAs in Tumor Immunology
Currently, the study of tumor immunology focuses on the body’s immune response to tumors, the mechanism of tumor immune escape tumor immune diagnosis and immune prevention. Over years of research, scientists have discovered that circRNAs are very important molecules in various tumors, playing a variety of immunological functions. The hottest research area is the competing endogenous RNA (ceRNA) network and its regulatory molecules. Sun et al. constructed ceRNA networks based on 133 laryngeal squamous cell carcinoma (LSCC) patients and found that hsa_circ_001569 and hsa_circ_001859 might regulate the expression of CD274, IL-10 and FOXP3, thus intervening in the immune escape of LSCC (39). In another study on ceRNA networks in pancreatic adenocarcinoma (PAAD), Zhao et al. reported that CXCR4 and ZEB1 were regulated by the circUBAP2-mediated ceRNA network, inhibiting antigen presentation and promoting tumor immune escape (40). Among studies investigating ceRNA networks, research focusing on the circRNA/miRNA/mRNA axis is especially plentiful. The effects on cell phenotypes are mainly reflected in the ability to drive tumor immune escape and promote proliferation and metastasis via different axes (41, 42). In addition, studies related to anti-PD-1 therapy are included in these reports (43, 44).
With the deepening of understanding, researchers have discovered the potential of circRNAs as therapeutic targets and biomarkers with abilities to assist with diagnosis and prognosis and their function in the immune regulation of exosomes. Wang et al. showed that circSPARC might conceivably act as a possible biomarker for diagnosis and prognosis and a target for therapy in colorectal cancer (CRC) (45). In another study concerning hepatocellular carcinoma (HCC), scientists reported that the upregulated level of plasma exosomal circUHRF1 decreased NK-cell tumor infiltration, curbing the function of NK cells (44).
Generally, from the laboratory to the clinical level, current research focusing on circRNAs in tumor immunology is proceeding in an orderly manner. Therefore, the application of circRNAs in the future may have a very positive impact on many aspects of tumor immunotherapy, such as diagnosis, treatment, and prognosis.
3.4 CircRNAs in Infectious Diseases
Infectious diseases refer to diseases in which bacteria, viruses, fungi, parasites, and other infectious agents, invade, grow, and reproduce in the body, causing damage to the normal metabolic functions of the tissue structure. Thus far, circRNAs have been found to be used as biomarkers of many infectious diseases in most cases, while emergent corroboration indicates that a small number of circRNAs are verified to directly impact the regulatory network of infectious diseases (58). By regulating the NF-κB pathway, the potential miRNA targets of hsa_circ_001937 exert effectiveness in antibacterial immune responses in patients with tuberculosis (46, 48). In a bioinformatics analysis experiment, Zhuang et al. found that hsa_circ_0005836 could be a novel biomarker for diagnosis and prognosis and a target for therapy of active pulmonary tuberculosis (47). In a similar experiment, Yang et al. performed a circRNA transcription analysis of primary human brain microvascular endothelial cells infected with meningeal Escherichia coli and preliminarily constructed a potential regulatory network that enhanced our understanding of the mechanisms of bacterial meningitis (49). Additionally, in Marinov et al.’s study focusing on an LPS-inducible circRNA called circRasGEF1B, the authors assumed that inducible RasGEF1B circular RNA may play an essential role in protecting cells against microbial infection by preserving the constancy of the mature mRNA of ICAM-1 in LPS-activated cells (53), providing a new idea for antimicrobial infection therapies. CircRNAs are of vital importance to the infection of viruses because the abnormal expression of circRNAs may promote or suppress the infection progress of viruses, and vice versa. Studies have shown that when infected with viruses, circRNAs are rapidly degraded by RNase L, releasing PKR (dsRNA-activated protein kinase) linked to circRNAs and participating in innate cellular immune responses (35, 59). Notably, circRNAs initiate innate immunity by combining with K63, which links ubiquitin chains, and RIG-I (retinoic acid-inducible gene I). Exogenous circRNAs without m6A modification can attach to K63 and RIG-I. This complex can promote the polymerization and activation of RIG-I, affect the aggregation of downstream mitochondrial antiviral signals, guide the dimerization and activation of interaction regulating factor 3 (IRF3), and finally induce the expression of autoimmune-related pathway genes (50, 60, 61). The antiviral dsRNA-binding protein NF90/110 can stabilize the secondary structure of intronic RNA, thereby promoting the biogenesis of circRNAs. NF90/110 can also act as global regulators of circRNA biogenesis by reducing their nuclear levels during viral infection (51, 62).
Since the end of 2019, the world has experienced several rounds of outbreaks caused by variants of severe acute respiratory syndrome coronavirus 2 (SARS-CoV-2) and improper anti-epidemic measures. Omicron, a newly discovered SARS-COV-2 variant with high transmission, is causing unease and uncertainty. Therefore, whether focusing on the present or the future, it is particularly important to develop new treatment technologies on the basis of existing epidemic prevention measures such as drug development and vaccination (63). CircRNAs, molecules closely associated with viral infection, is one option. A differential host circRNA expression profile analysis in human lung epithelial cells infected with SARS-CoV-2 was completed (64), and two circRNA profile analyses revealed abundant and diverse information regarding the identification and characterization of the circRNAs encoded by SARS-CoV-1, SARS-CoV-2 and MERS-CoV (65, 66), facilitating future studies concerning on COVID-19 infection and pathogenesis. Arora et al. identified a circRNA/lncRNA-miRNA–mRNA ceRNA network involving two circRNAs in SARS-CoV-2-infected cells, enhancing the current understanding of the mechanisms associated with coronavirus disease 2019 (COVID-19) (52). Specific segments of the SARS-CoV-2 5’-untranslated region can be expeditiously accessed by particular antisense circRNAs, resulting in bringing an approximately 90% cutback in virus proliferation in cell culture with a minimal duration of 2 days, which is attractive and promising (67). Briefly, relevant research focused on expression profile analyses, ceRNA construction and therapeutic target seeking. Although circRNAs are in the initial stage in the prevention and treatment of novel coronavirus, with the development of cross-discipline and the emergence of more advanced technology, it is believed that there will be opportunities for circRNAs to display their clinical talents in the future.
In addition to bacteria and viruses, circRNAs function in many other infectious diseases, such as chlamydia infection (54). In addition to modulating the human body, circRNAs can play a regulatory role in other organisms during infectious diseases, such as parasite infection. Broadbent et al. identified developmentally regulated lncRNAs and circRNAs by strand-specific RNA sequencing in Plasmodium falciparum malaria (68), but currently, there is no clinical significance.
Overall, current research concerning circRNAs in infectious diseases mostly focuses on viral and bacterial infections, but in addition to research as biomarkers, these results are still a long way from clinical application.
3.5 CircRNAs in Other Immunological Research
Thus far, we mentioned that circRNAs are of great significance in autoimmune diseases, tumors, and bacterial and viral infections. In addition, expanding the perspective to the whole area, circRNAs perform effectively in hypersensitivity, immunodeficiency diseases and transplantation immunity, namely, the pathological changes caused by immune defense function. However, because the contents and categories of current related studies are relatively similar, there are only a few examples, which are no longer explained in detail here. For instance, circHIPK3 was proven to modulate the proliferation of airway smooth muscle cells by the miR-326/STIM1 axis in asthma (69), a group of ample circRNAs and ceRNA networks were found to likely contribute to acquired immune deficiency syndrome (AIDS) (70), and a two-circular RNA signature of donors was thought to be a biomarker of early allograft dysfunction after liver transplantation (71). In addition to the above diseases, circRNAs play vital roles in a variety of immunological diseases and immune cells. Under stimulation by different pathological factors, the way that circRNAs are involved in the activation of macrophages is a large subject (53, 72–74). In addition, a variety of circRNAs have been identified to influence various immune cells, such as intestinal immune cells (75), lung immune cells poisoned by Nd2O3 (76), CD4+ T cells in asthma (77) and immune cells in periodontitis (78). In addition to these effects on different immune cells, there are also some studies focusing on innovative technologies. Recently, Wesselhoeft et al. showed that unmodified exogenous circRNA can bypass cellular RNA sensors, thereby avoiding immune responses in RIG-1- and Toll-like receptor (TLR)-competent cells and mice, suggesting that RNA circularization reduces immunogenicity and can prolong the translation time in vivo (61).
Studies concerning circRNAs in immune-related diseases are miscellaneous, but the core functions and mechanisms are constant. The discovery of circRNAs has further deepened researchers’ understanding of the intricate immune regulatory network. Generally, the immune system has three major functions, namely, immune defense, immune surveillance and immune homeostasis, and circRNAs realize immune-related mechanisms as follows: 1) during immune defense functions, circRNAs can assist the body in removing pathogenic microorganisms and other antigens in various ways; however, hypersensitivity or immune deficiency occurs when the immune response is too high or too low; 2) when immune surveillance operates regularly, circRNAs can help the body remove mutant cells and virus-infected cells through various pathways; if this function is abnormal, it could lead to tumor occurrence and persistent virus infection; and 3) when immune homeostasis occurs naturally, circRNAs can aid the body in removing damaged or senescent cells in various ways, but imbalance could lead to autoimmune diseases. Therefore, the balance between the immune system and circRNAs plays a key role in whether the body is in a healthy or pathological state.
3.6 Regulatory Mechanisms of circRNAs in Immune-Related Diseases
The regulatory mechanism of circRNAs in immune-related diseases can be summarized into the following two aspects: the regulatory effects of circRNAs on immune-related signaling pathways (Figure 3), such as the MAPK signaling pathway, endocytosis signaling pathway, JAK-STAT signaling pathway, mTOR signaling pathway, and Wnt signaling pathway, and the regulatory effects of circRNAs on immune cells, such as the regulation of macrophages, etc.
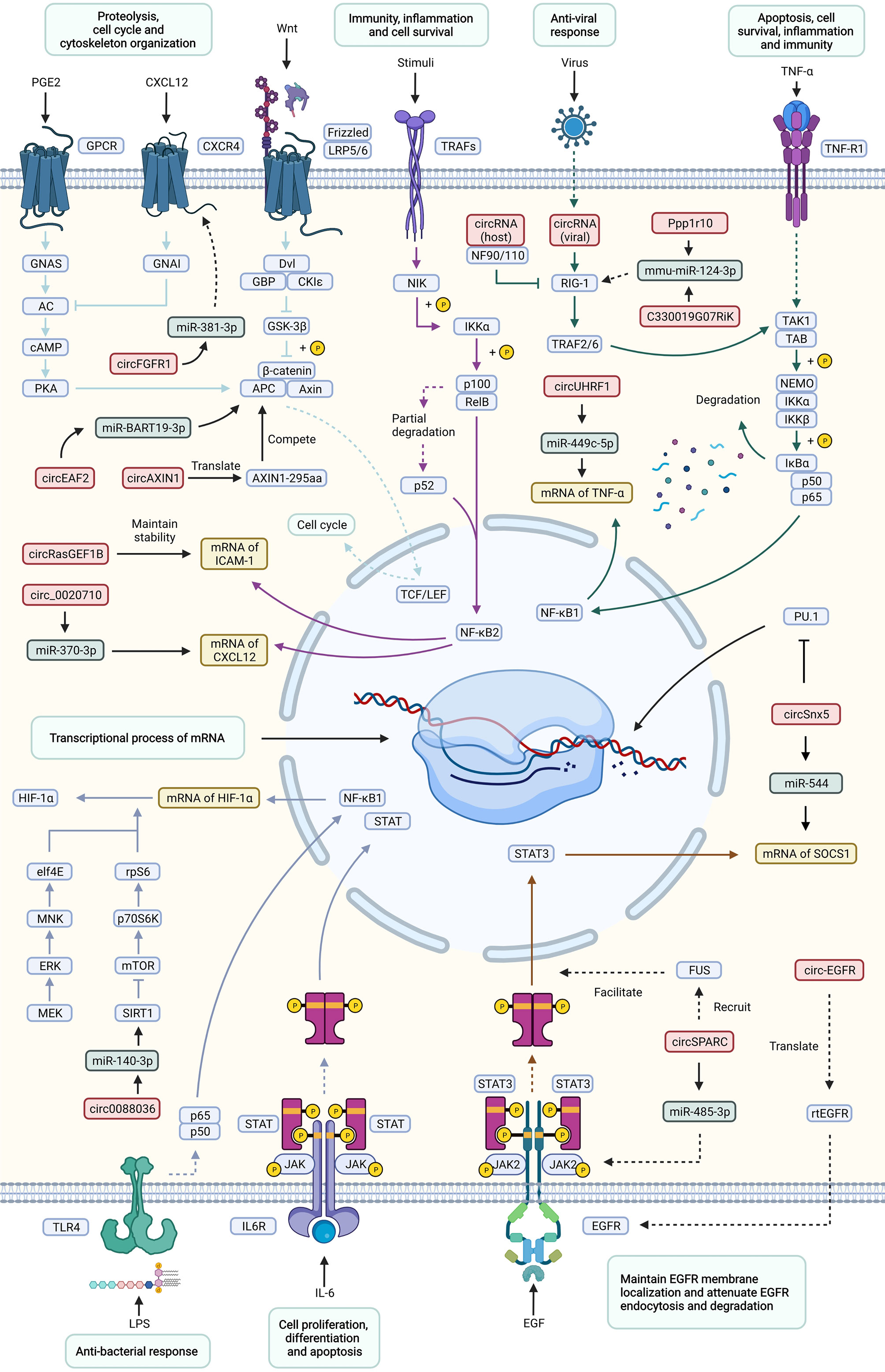
Figure 3 Important signaling pathways of circRNAs involved in the regulation of immune-related diseases. This figure shows how circRNAs influence immune-related diseases via a variety of signaling pathways, including the Wnt, TNF, NF-κB, JAK-STAT, mTOR, antiviral and antibacterial pathways. and the corresponding responses. Effects and processes are shown in light green rectangles, circRNAs are shown in red rectangles, miRNAs are shown in dark green rectangles, mRNAs are shown in yellow rectangles, and proteins are shown in blue rectangles. Different signaling pathways are distinguished by arrows and inhibitors of different colors. Solid lines represent direct interactions between molecules, while dotted lines represent indirect interactions.
3.7 Important Signaling Pathways of circRNAs Involved in the Regulation of Immune-Related Diseases
3.7.1 MAPK Signaling Pathway
The MAPK signaling pathway is a signal transduction system important for eukaryotic cells to mediate extracellular signals in the intracellular response. This pathway transduces extracellular signals in the form of a triple kinase cascade, namely, MAP kinase kinase kinase (MKKK), MAP kinase kinase (MKK) and MAP kinase (MAPK), which regulates a variety of physiological processes, such as cell growth, differentiation, apoptosis and death. There are four main branches of the MAPK pathway as follows: extracellular-signal regulated protein kinase (ERK), c-Jun N-terminal kinase (JNK), p38 mitogen-activated protein kinase (p38 MAPK) and ERK5. Among them, JNK and p38 have similar functions, which are related to inflammation, apoptosis and cell growth; ERK is mainly responsible for cell growth and differentiation, and its upstream signals are the famous Ras and Raf proteins (79, 80). According to current studies, circRNAs mainly play roles as miRNA sponges in the MAPK signaling pathway in immune-related research. For example, Chen et al. found that circSnx5 acted as a sponge of miR-544 to upregulate suppressor of cytokine signaling 1 (SOCS1) (81). Zhang et al. revealed the circUHRF1/miR-449c-5p/TIM-3 axis in HCC (44). Zhao et al. constructed a ceRNA network consisting of 4 DEcircRNAs, 3 DEmiRNAs and 149 DEmRNAs in PAAD (40), which also showed the sponge function of circRNAs. Among the regulated proteins, SOCS1 (82–84), TIM-3 (85–87), ZEB1 (88–90), etc., serve as important regulators in the MAPK signaling pathway.
3.7.2 Endocytosis Signaling Pathway
Endocytosis is the process of transporting extracellular substances into cells through the deformed movement of the plasma membrane. Endocytosis can be divided into phagocytosis, pinocytosis and receptor-mediated endocytosis according to the size and mechanism. According to clathrin dependence, endocytosis can be divided into clathrin-dependent endocytosis (CDE) and clathrin-independent endocytosis (CIE). In terms of trends, the mechanism of the relationship between signal transduction and endocytosis has received increasing attention in studies investigating of the occurrence and development of many diseases. Endocytosis has been proven to be closely related to lipid metabolism, intracellular iron homeostasis, metabolism, immunity and other functions (91–93). On the basis of existing research, circRNAs mainly participate in the endocytosis pathway as miRNA sponges in immune-related research. Abnormally expressed circRNAs were identified in pulmonary tuberculosis (46) and chlamydia infection (54), and all were predicted to be miRNA sponges. Through bioinformatics analyses, these circRNAs were found to be related to the endocytosis signaling pathway. A special study focused on the protein translation function of circRNAs, and verified that circ-EGFR attenuates EGFR endocytosis and degradation (94).
3.7.3 JAK-STAT Signaling Pathway
The JAK-STAT signaling pathway has been revealed to consist of the following four parts: extracellular signaling factors, tyrosine kinase-related receptors, tyrosine kinase called Janus kinase (JAK) that transmits signals, and transducer and activator of transcription (STAT) that exerts effects. When a variety of cytokines and growth factors bind receptors, JAK is activated, and then the activated JAK phosphorylates the receptor and itself. These phosphorylated sites become the binding sites of STAT with an SH2 structure, thus recruiting and phosphorylating STAT and allowing it to enter the nucleus in the form of a dimer to bind to target genes, regulating the transcription of downstream genes and modulating the process of cell proliferation, differentiation and apoptosis (95, 96). In light of research conducted thus far, circRNAs mainly act as miRNA sponges in the JAK-STAT signaling pathway in immune-related research. For instance, Wang et al. uncovered the circSPARC/miR-485-3p/JAK2 axis in CRC (45). In type 1 diabetes mellitus, Yang et al. identified the hsa_circ_0060450/miR-199a-5p/mRNAs axis, which suppressed the JAK-STAT signaling pathway triggered by IFN-I (97).
3.7.4 mTOR Signaling Pathway
Mammalian target of rapamycin (mTOR) is an evolutionarily conserved serine/threonine protein kinase that can regulate a variety of cell functions by phosphorylating its downstream target protein. There are two key complexes in the mTOR signaling pathway called mTOR complex 1 (mTORC1, including mTOR, Raptor, mLST8, etc.) and mTOR complex 2 (mTORC2, including mTOR, Rictor, mLST8, etc.). mTORC1 is activated in the presence of lysosome levels, ER stress, sterols, hypoxia and energy stress to regulate several biological processes, including lipid metabolism, autophagy, protein synthesis and ribosomal biogenesis, while mTORC2 responds to growth factors and controls cytoskeletal organization, metabolism and cell survival (98–100). According to studies, circRNAs mainly exert an influence as miRNA sponges in the mTOR signaling pathway in immune-related research. For example, Zhong et al. revealed the circ0088036/miR-140-3p/silent information regulator 1 (SIRT1) axis in the promotion of RA (37). Wei et al. indicated the importance of the circ_0020710/miR-370-3p/CXCL12 axis in melanoma (41). Regarding the regulated proteins, SIRT1 (101–103), CXCL12 (104–106), etc., served as important regulators in the mTOR signaling pathway.
3.7.5 Wnt Signaling Pathway
The Wnt signaling pathway is a complex regulatory network that has been verified to include at least the following three branches: the classical Wnt signaling pathway, namely, the Wnt/β-catenin signaling pathway, Wnt/planar cell polarity (PCP) pathway and Wnt/Ca2+ pathway activated by Wnt5a and Wnt11. Wnt mainly transmits signals through 7 transmembrane receptors of the Frizzled family and LRP5/6 coreceptors and plays a regulatory role in cells through key molecules such as CK1, Deshevelled, GSK3, APC, Axin, and β-Catenin (107–109). Currently, circRNAs mainly produce marked effects as miRNA sponges in the Wnt signaling pathway in immune-related research. For instance, Zhang et al. stated that the circFGFR1/miR-381-3p/CXCR4 axis promoted NSCLC progression and resistance to anti-programmed cell death 1 (PD-1)-based therapy (43). Zhao et al. proposed that circEAF2 counteracts Epstein–Barr virus-positive diffuse large B-cell lymphoma progression via the miR-BART19-3p/APC/β-catenin axis (110). Regarding the regulated proteins, CXCR4 (111–113), APC (114–116), etc., served as important regulators in the Wnt signaling pathway. Specifically, a study revealed that a novel protein AXIN1-295aa encoded by circAXIN1 activated the Wnt/β-catenin signaling pathway to promote gastric cancer progression (117).
In addition to the pathways highlighted above, circRNAs participate in the regulation of the TNF, AMPK, HIF-1 and NF-κB. signaling pathways, but generally, the mechanisms are similar; thus, circRNAs exert effects on immune function and immune-related diseases mainly by translating proteins and acting as miRNA sponges.
3.7.6 Regulation of circRNAs in Immune Cells
CircRNAs have various regulatory functions and have been detected in different types of immune cells, such as macrophages, dendritic cells (DCs), natural killer cells (NK cells), CD4+ T cells and CD8+ T cells. By inhibiting or promoting the activation or exhaustion of these cells, circRNAs participate in the development of various diseases.
3.7.7 Regulation of circRNAs in Macrophages
CircRNAs affect the activation of macrophages. For instance, mouse macrophages specifically express circ-RasGEF1B in the form of NF-κB after being stimulated by lipopolysaccharide (LPS), which can activate macrophages by positively regulating the expression of intercellular adhesion molecule-1 (ICAM-1) (53, 72). Zhang et al. found that circPPM1F participates in the activation of MI macrophages in diabetic patients (118), while another study showed that hsa_circ_0110102 inhibits macrophage activation via the miR-580-5p/PPARα/CCL2 pathway (119). In addition, SiO2 induces macrophage activation through the circHECTD1/HECTD1 pathway and circZC3H4 RNA and ZC3H4 protein in the process of pulmonary fibrosis (120, 121). In addition, circRNA HIPK3 and circUbe3a can activate macrophages, while the latter participates in the process of myocardial fibrosis (122, 123).
Furthermore, circRNAs can lead to the polarization of tumor-associated macrophages to M1 or M2 macrophages. One study showed that circN4 bp1 could act as a miR-138-5p sponge for the modulation of macrophage polarization through the regulation of the expression of EZH2 (a histone methyltransferase) (124). Moreover, circRNA Cdyl, circPrkcsh and circPPM1F were found to play a role in inducing M1 macrophage polarization (118, 125, 126). Many studies have highlighted the importance of circRNAs in the occurrence and development of tumors, and one effect is the mediation of the polarization of M2 macrophages. For example, tumor-derived extracellular circFARSA was discovered to mediate the polarization of M2 macrophages (127). Additionally, cyclic RNA PLCE1, circITGB6, circ_0001142 and hsa_circ_0074854 were also found to play such a role (128–131).
CircRNAs also play a role in regulating the macrophage-related inflammatory response; for example, hsa_circ_0005567 can promote M2 macrophage polarization via the mir-492/SOCS2 axis (132). Moreover, hsa_circ_0004287 inhibits macrophage-mediated inflammation in an N-methyladenosine-dependent manner in atopic dermatitis and psoriasis (133). Furthermore, circRNAs can also advance the inflammatory response. In gouty arthritis, circHIPK3 was found to be able to activate the macrophage inflammasome (134), as did hsa_circ_0087352, circ_1639 and circ_0001490 (135–137).Significantly, in Mycobacterium tuberculosis infection, circRNAs TRAPPC6B and hsa_circ_0045474 can induce autophagy in macrophages (138, 139). Other studies have found that the circRNA calcitonin gene-related peptide (CGRP) can induce macrophages to express IL-6 (140).
3.7.8 The Regulation of circRNAs on Other Immune Cells
Current research investigating the correlation between circRNAs and immune cells mainly focuses on macrophages, and there are relatively few studies of other cells. Here, we briefly review the regulation of circRNAs in NK cells, DCs, CD4+ T cells and CD8+ T cells.
CircRNAs can promote NK-cell depletion and regulate cytotoxicity. A study found that hsa_circ_0048674 and cancer cell-derived exosome circUHRF1 can induce NK-cell dysfunction (44, 141). Hsa_circ_0007456 regulates NK-cell-mediated hepatocellular carcinoma cytotoxicity through the mir-6852-3p/ICAM-1 axis (131). Moreover, circARSP91 can enhance innate immune surveillance by strengthening the cytotoxicity of NK cells (142). In addition, circrHT1 knockout can aggravate the sensitivity of bladder cancer cells to NK cells, and another study showed that circ_0000977 knockout can enhance the killing effect of NK cells on pancreatic cancer cells through HIF1A and ADAM1 (143, 144). A GO analysis showed that circRNAs were involved in regulating DC differentiation and other biological functions (145). Chen et al. found that circSnx5 controls the immunogenicity of DCs through the miR-544/SOCS1 axis (29). Furthermore, Wang et al. discovered that the knockdown of circFSCN1 could affect the ability of DCs to activate T cells and enhance Treg generation (146). Another study showed that growth differentiation factor 15 induces tolerant DCs (Tol DCs) by inhibiting the circ_malat-1 and NF-κB signaling pathways and upregulating IDO (147).
Research investigating the connection between circRNAs and CD4+ T cells mainly concentrates on systemic lupus erythematosus (SLE) and asthma. Studies have shown that the DNA methylation of CD11a and CD70 in CD4 T cells form patients with SLE is associated with the downregulation of hsa_circ0012919 (148). In addition, the regulatory network among circHIPK3, LncGAS5 and miR-495 can promote Th2 differentiation in allergic rhinitis (149), and hsa_circ_0002594 and hsa_circ_0005519 can affect asthma by regulating CD4+ T cells (77, 150). Moreover, N-methyladenosine-modified circIGF2BP3 was found to inhibit CD8+ T-cell responses and promote tumor immune evasion (151), while exogenous circTRPS1 was proven to be related to CD8+ T-cell exhaustion (152). In addition, Chen et al. noted that the expression of circRNA100783 is affected by time- and CD28-related CD8(+) T-cell aging during antigen exposure (153). Clinically, cancer cell-derived exosomal circUSP7 was proven to induce CD8+ T cell dysfunction and anti-PD1 resistance by regulating the miR-934/SHP2 axis in NSCLC (154).
3.8 Applications and Prospects of circRNAs in the Treatment of Immune-Related Diseases
Immunotherapy refers to a treatment technique that artificially heightens or represses the immune function of the body to treat immune-related diseases in accordance with the low or hyperactive immune state of the body. Because of their unique structure and various functions, circRNAs have broad application prospects in the treatment of immune-related diseases.
At the current stage, most studies investigating the functions of circRNAs are still in the laboratory stage, and only a few theories have been developed for technical applications in clinical treatment, such as gene therapy. Tens of thousands of studies have proven circRNAs to be substantially considerable in the advancement of many immune-related diseases, suggesting the roles of circRNAs as therapeutic agents and targets (50, 62, 147, 155–157). To date, there are four main approaches to realizing gene therapy as follows: inducing or inhibiting the expression of the target circRNA upstream, chemically modifying key molecules, designing analogs of the target circRNA and designing downstream molecular analogs of the circRNA, i.e., miRNA.
In addition to gene therapy, with the discovery of the function of encoding proteins, circRNAs are speculated to have the potential to be novel drug delivery carriers. Wesselhoeft et al. produced a protein with high quality and stable expression in eukaryotic cells after the circularization of mRNA in vitro and indicated that RNA circularization can reduce immunogenicity and extend translational duration in vivo (61, 158, 159), providing insight into the treatment of immune-related diseases. In addition, circRNAs have the potential to function as appropriate biomarkers of immune-related diseases. In case of immune-related diseases, it is usually difficult for patients to determine whether they fell ill by the clinical symptoms in the early stage so that they will not go to the hospital until their symptoms worsen (9). Therefore, circRNAs can function as ideal biomarkers due owing to the four main characteristics mentioned above. Thus far, numerous circRNAs have been found in exosomes, and changes in the content of circRNAs in exosomes can reflect the process of diseases (159). However, the current problem that has blocked the application of circRNAs as biomarkers in the clinic is that with the continuous improvement of the circRNA database in immune-related diseases, the expression of the same circRNA in different diseases may have the same trend, which may interfere with the judgment. Therefore, a more refined database needs to be established.
Unparalleled strides have been made in cancer treatment with the use of immune checkpoint blockade (ICB), but ICB resistance hinders the efficacy of cancer immunotherapies (160). Based on existing research, regulating gene expression at the transcriptional level, acting as miRNA sponges, binding functional proteins and encoding proteins are the four major biological functions of circRNAs, and these functions can play a vital role in regulating immune diseases, such as immune escape, immune tolerance, and antitumor and anti-infection effects, either independently or in combination (7, 12, 161–167). Moreover, circRNAs can achieve cross-cellular regulation via exosomes. Recent studies have certified the potential role of exosomes in tumor immunity and resistance to ICB (160). For instance, Lu et al. suggested that immuno-repression and anti-PD1 resistance were caused by exosomal circTMEM181 by increasing the expression of CD39, and suppressing the ATP-adenosine signaling pathway by targeting CD39 on macrophages could rescue anti-PD1 therapy resistance in HCC (168). Therefore, via exosomes, circRNAs may yield unusually brilliant clinical results in ICB.
Considering that specially designed antisense circRNAs can effectively access the SARS-CoV-2 5’-untranslated region and inhibit the proliferation of most viruses for a time, circRNAs also an option for the clinical treatment of COVID-19, which is a major achievement that uses of the unique structure of circRNAs and artificial assistance for modification, showing many advantages. The best advantage is that the antisense sequence of circRNAs is better than the corresponding linear configuration and modified antisense oligonucleotides, and antisense circRNAs have strong activity against point mutations in the target sequence. This approach manifests the function of circRNAs as nucleic acid binders, starting novel applications for designing circRNAs and hopeful therapeutic strategies for COVID-19 (67). Fortunately, Qu et al. designed a circular RNA vaccine encoding the receptor domain (RBD) of the spike protein of SARS-CoV-2 for the very virus and its mutants and found that the circRNARBD-Delta vaccine designed for the SARS-CoV-2 Delta mutant was a candidate vaccine for COVID-19 with broad-spectrum protection in rhesus monkeys. A series of comparative evaluations showed that compared with mRNA vaccines, circRNA vaccines have higher stability and a higher proportion of neutralizing antibodies, which can effectively reduce the potential side effects of vaccine-associated respiratory diseases (VAERD) (169).
Despite numerous studies, research focusing on circRNAs is still limited, and many problems remain to be solved. Although the structure of circRNAs can help attenuate off-target effects, this problem cannot be avoided. Moreover, a specific circRNA may have different functions in different cells and may cause uncontrollable side effects. In addition, if an exogenous circRNA is synthesized without protein-binding partners, it may be recognized by RIG-I as a virus-derived circRNA and thus induce innate immunity (7–9, 62).
3.9 Discussion and Perspectives
CircRNAs perform the functions of sponging miRNAs, binding specific proteins and regulating gene transcription, and some can even encode proteins. Meanwhile, circRNAs are widely distributed in cells, the internal environment and exosomes, coupled with stable ring structures; thus, they have application potential in the diagnosis, treatment and prognosis of immune-related diseases. However, current research investigating related diseases mainly focuses on tumor immunity, bacterial and viral infections, and some autoimmune diseases, while relatively uncommon diseases are rarely studied. The hotspots of the roles of circRNAs in immune-related diseases include expression profile analyses, potential biomarker research, ncRNA axis/network construction, impacts on phenotypes, therapeutic target seeking, maintenance of nucleic acid stability and protein binding research. In addition, the study of the mechanism of circRNAs in immune regulation only occupies the tip of the iceberg in immunology. Currently, few studies on the regulation of circRNAs in the establishment of the immune system and the regulation of the immune system in the normal physiological state. A representative study showed that the structure and decomposition of circRNAs modulate PKR activation in innate immunity (4). At present, this field also faces some unsolved problems, such as off-target effects and unpredictable side effects. Therefore, continuing to supplement the regulatory network of circRNAs, attempting to explore new mechanisms, and developing new functions will be crucial for the entire field in the future, and the birth of new technologies will further contribute to the complete unveiling of the roles of circRNAs in immunity and immune-related diseases.
Author Contributions
JG, CS and FH wrote the manuscript, designed the figures, collected the related references and edited the manuscript, and JL and ZY provided guidance and revised this manuscript. All authors approved the final manuscript.
Funding
This work was supported by grants from the National Natural Science Foundation of China (81872211 and 82072999), the Sichuan Science and Technology Program (2020YJ0102), the Innovation Research Project of Sichuan University (2022SCUH0029), and the CAMS Innovation Fund for Medical Sciences (2020-I2M-C&T-A-023).
Conflict of Interest
The authors declare that the research was conducted in the absence of any commercial or financial relationships that could be construed as a potential conflict of interest.
Publisher’s Note
All claims expressed in this article are solely those of the authors and do not necessarily represent those of their affiliated organizations, or those of the publisher, the editors and the reviewers. Any product that may be evaluated in this article, or claim that may be made by its manufacturer, is not guaranteed or endorsed by the publisher.
Acknowledgments
The figures were created with Biorender (https://biorender.com) and Servier Medical Art (https://smart.servier.com).
References
1. Zhou Z, Sun B, Huang S, Zhao L. Roles of Circular RNAs in Immune Regulation and Autoimmune Diseases. Cell Death Dis (2019) 10:503. doi: 10.1038/s41419-019-1744-5
2. Patop I, Wüst S, Kadener S. Past, Present, and Future of circRNAs. EMBO J (2019) 38:e100836. doi: 10.15252/embj.2018100836
3. Xie R, Zhang Y, Zhang J, Li J, Zhou X. The Role of Circular RNAs in Immune-Related Diseases. Front Immunol (2020) 11:545. doi: 10.3389/fimmu.2020.00545
4. Liu C, Li X, Nan F, Jiang S, Gao X, Guo S, et al. Structure and Degradation of Circular RNAs Regulate PKR Activation in Innate Immunity. Cell (2019) 177:865–80.e21. doi: 10.1016/j.cell.2019.03.046
5. Geng X, Jia Y, Zhang Y, Shi L, Li Q, Zang A, et al. Circular RNA: Biogenesis, Degradation, Functions and Potential Roles in Mediating Resistance to Anticarcinogens. Epigenomics (2020) 12:267–83. doi: 10.2217/epi-2019-0295
6. Eger N, Schoppe L, Schuster S, Laufs U, Boeckel J. Circular RNA Splicing. Adv Exp Med Biol (2018) 1087:41–52. doi: 10.1007/978-981-13-1426-1_4
7. Li X, Yang L, Chen L. The Biogenesis, Functions, and Challenges of Circular RNAs. Mol Cell (2018) 71:428–42. doi: 10.1016/j.molcel.2018.06.034
8. Holdt L, Kohlmaier A, Teupser D. Circular RNAs as Therapeutic Agents and Targets. Front Physiol (2018) 9:1262. doi: 10.3389/fphys.2018.01262
9. Chen X, Yang T, Wang W, Xi W, Zhang T, Li Q, et al. Circular RNAs in Immune Responses and Immune Diseases. Theranostics (2019) 9:588–607. doi: 10.7150/thno.29678
10. Yang L, Fu J, Zhou Y. Circular RNAs and Their Emerging Roles in Immune Regulation. Front Immunol (2018) 9:2977. doi: 10.3389/fimmu.2018.02977
11. Wang M, Yu F, Wu W, Zhang Y, Chang W, Ponnusamy M, et al. Circular RNAs: A Novel Type of Non-Coding RNA and Their Potential Implications in Antiviral Immunity. Int J Biol Sci (2017) 13:1497–506. doi: 10.7150/ijbs.22531
12. Meng L, Ding P, Liu S, Li Z, Sang M, Shan B. The Emerging Prospects of Circular RNA in Tumor Immunity. Ann Trans Med (2020) 8:1091. doi: 10.21037/atm-19-4751
13. Salmena L, Poliseno L, Tay Y, Kats L, Pandolfi P. A ceRNA Hypothesis: The Rosetta Stone of a Hidden RNA Language? Cell (2011) 146:353–8. doi: 10.1016/j.cell.2011.07.014
14. Thomson D, Dinger M. Endogenous microRNA Sponges: Evidence and Controversy. Nat Rev Genet (2016) 17:272–83. doi: 10.1038/nrg.2016.20
15. Hansen T, Jensen T, Clausen B, Bramsen J, Finsen B, Damgaard C, et al. Natural RNA Circles Function as Efficient microRNA Sponges. Nature (2013) 495:384–8. doi: 10.1038/nature11993
16. Bossi L, Figueroa-Bossi N. Competing Endogenous RNAs: A Target-Centric View of Small RNA Regulation in Bacteria. Nat Rev Microbiol (2016) 14:775–84. doi: 10.1038/nrmicro.2016.129
17. Memczak S, Jens M, Elefsinioti A, Torti F, Krueger J, Rybak A, et al. Circular RNAs Are a Large Class of Animal RNAs With Regulatory Potency. Nature (2013) 495:333–8. doi: 10.1038/nature11928
18. Abdelmohsen K, Panda A, Munk R, Grammatikakis I, Dudekula D, De S, et al. Identification of HuR Target Circular RNAs Uncovers Suppression of PABPN1 Translation by Circpabpn1. RNA Biol (2017) 14:361–9. doi: 10.1080/15476286.2017.1279788
19. Du W, Yang W, Liu E, Yang Z, Dhaliwal P, Yang B. Foxo3 Circular RNA Retards Cell Cycle Progression via Forming Ternary Complexes With P21 and CDK2. Nucleic Acids Res (2016) 44:2846–58. doi: 10.1093/nar/gkw027
20. Chen N, Zhao G, Yan X, Lv Z, Yin H, Zhang S, et al. A Novel FLI1 Exonic Circular RNA Promotes Metastasis in Breast Cancer by Coordinately Regulating TET1 and DNMT1. Genome Biol (2018) 19:218. doi: 10.1186/s13059-018-1594-y
21. Godet A, David F, Hantelys F, Tatin F, Lacazette E, Garmy-Susini B, et al. IRES Trans-Acting Factors, Key Actors of the Stress Response. Neurosci Lett (2019) 20:134952. doi: 10.20944/preprints201901.0081.v1
22. Abe N, Matsumoto K, Nishihara M, Nakano Y, Shibata A, Maruyama H, et al. Rolling Circle Translation of Circular RNA in Living Human Cells. Sci Rep (2015) 5:16435. doi: 10.1038/srep16435
23. Zhang Y, Zhang X, Chen T, Xiang J, Yin Q, Xing Y, et al. Circular Intronic Long Noncoding RNAs. Mol Cell (2013) 51:792–806. doi: 10.1016/j.molcel.2013.08.017
24. Bose R, Ain R. Regulation of Transcription by Circular RNAs. Adv Exp Med Biol (2018) 1087:81–94. doi: 10.1007/978-981-13-1426-1_7
25. Iparraguirre L, Muñoz-Culla M, Prada-Luengo I, Castillo-Triviño T, Olascoaga J, Otaegui D. Circular RNA Profiling Reveals That Circular RNAs From ANXA2 can be Used as New Biomarkers for Multiple Sclerosis. Hum Mol Genet (2017) 26:3564–72. doi: 10.1093/hmg/ddx243
26. Cardamone G, Paraboschi E, Rimoldi V, Duga S, Soldà G, Asselta R. The Characterization of GSDMB Splicing and Backsplicing Profiles Identifies Novel Isoforms and a Circular RNA That Are Dysregulated in Multiple Sclerosis. Int J Mol Sci (2017) 18:576. doi: 10.3390/ijms18030576
27. Jiajia Z, Zhenrong L, Tiancheng W, Yang Z, Yongfeng W. Microarray Expression Profile of Circular RNAs in Plasma From Primary Biliary Cholangitis Patients. Cell Physiol Biochem (2017) 44:1271–81. doi: 10.1159/000485487
28. Ouyang Q, Huang Q, Jiang Z, Zhao J, Shi G-P, Yang M. Using Plasma circRNA_002453 as a Novel Biomarker in the Diagnosis of Lupus Nephritis. Mol Immunol (2018) 101:531–8. doi: 10.1016/j.molimm.2018.07.029
29. Chen Q, Mang G, Wu J, Sun P, Li T, Zhang H, et al. Circular RNA Circsnx5 Controls Immunogenicity of Dendritic Cells Through the miR-544/SOCS1 Axis and PU.1 Activity Regulation. Mol Ther (2020) 28:2503–18. doi: 10.1016/j.ymthe.2020.07.001
30. Li L, Zhu Z, Zhao W, Tao S, Li B, Xu S, et al. Circular RNA Expression Profile and Potential Function of Hsa_Circ_0045272 in Systemic Lupus Erythematosus. Immunology (2018) 155:137–49. doi: 10.1111/imm.12940
31. Guo G, Wang H, Ye L, Shi X, Yan K, Lin K, et al. Hsa_circ_0000479 as a Novel Diagnostic Biomarker of Systemic Lupus Erythematosus. Front Immunol (2019) 10:2281. doi: 10.3389/fimmu.2019.02281
32. Luo Q, Zhang L, Li X, Fu B, Guo Y, Huang Z, et al. Identification of Circular RNAs Hsa_Circ_0044235 and Hsa_Circ_0068367 as Novel Biomarkers for Systemic Lupus Erythematosus. Int J Mol Med (2019) 44:1462–72. doi: 10.3892/ijmm.2019.4302
33. Miao Q, Zhong Z, Jiang Z, Lin Y, Ni B, Yang W, et al. RNA-Seq of Circular RNAs Identified Circptpn22 as a Potential New Activity Indicator in Systemic Lupus Erythematosus. Lupus (2019) 28:520–8. doi: 10.1177/0961203319830493
34. Zhang M, Wang J, Zhu Z, Li L, Liu R, Yang X, et al. Differentially Expressed Circular RNAs in Systemic Lupus Erythematosus and Their Clinical Significance. Biomed Pharmacother = Biomed Pharmacother (2018) 107:1720–7. doi: 10.1016/j.biopha.2018.08.161
35. Wen J, Liu J, Zhang P, Jiang H, Xin L, Wan L, et al. RNA-Seq Reveals the Circular RNA and miRNA Expression Profile of Peripheral Blood Mononuclear Cells in Patients With Rheumatoid Arthritis. Biosci Rep (2020) 40:BSR20193160. doi: 10.1042/BSR20193160
36. Yang X, Li J, Wu Y, Ni B, Zhang B. Aberrant Dysregulated Circular RNAs in the Peripheral Blood Mononuclear Cells of Patients With Rheumatoid Arthritis Revealed by RNA Sequencing: Novel Diagnostic Markers for RA. Scand J Clin Lab Invest (2019) 79:551–9. doi: 10.1080/00365513.2019.1674004
37. Zhong S, Ouyang Q, Zhu D, Huang Q, Zhao J, Fan M, et al. Hsa_circ_0088036 Promotes the Proliferation and Migration of Fibroblast-Like Synoviocytes by Sponging miR-140-3p and Upregulating SIRT 1 Expression in Rheumatoid Arthritis. Mol Immunol (2020) 125:131–9. doi: 10.1016/j.molimm.2020.07.004
38. Su L, Xu W, Liu X, Fu L, Huang A. Altered Expression of Circular RNA in Primary Sjögren's Syndrome. Clin Rheumatol (2019) 38:3425–33. doi: 10.1007/s10067-019-04728-6
39. Sun J, Lian M, Ma H, Wang R, Ma Z, Wang H, et al. Competing Endogenous RNA Network Analysis of CD274, IL−10 and FOXP3 Co−Expression in Laryngeal Squamous Cell Carcinoma. Mol Med Rep (2018) 17:3859–69. doi: 10.3892/mmr.2017.8307
40. Zhao R, Ni J, Lu S, Jiang S, You L, Liu H, et al. CircUBAP2-Mediated Competing Endogenous RNA Network Modulates Tumorigenesis in Pancreatic Adenocarcinoma. Aging (2019) 11:8484–501. doi: 10.18632/aging.102334
41. Wei C, Zhu M, Lu N, Liu J, Yang Y, Zhang Y, et al. Circular RNA Circ_0020710 Drives Tumor Progression and Immune Evasion by Regulating the miR-370-3p/CXCL12 Axis in Melanoma. Mol Cancer (2020) 19:84. doi: 10.1186/s12943-020-01191-9
42. Pei X, Chen S, Long X, Zhu S, Qiu B, Lin K, et al. circMET Promotes NSCLC Cell Proliferation, Metastasis, and Immune Evasion by Regulating the miR-145-5p/CXCL3 Axis. Aging (2020) 12:13038–58. doi: 10.18632/aging.103392
43. Zhang P, Pei X, Li K, Jin L, Wang F, Wu J, et al. Circular RNA Circfgfr1 Promotes Progression and Anti-PD-1 Resistance by Sponging miR-381-3p in Non-Small Cell Lung Cancer Cells. Mol Cancer (2019) 18:179. doi: 10.1186/s12943-019-1111-2
44. Zhang P, Gao C, Huang X, Lu J, Guo X, Shi G, et al. Cancer Cell-Derived Exosomal Circuhrf1 Induces Natural Killer Cell Exhaustion and may Cause Resistance to Anti-PD1 Therapy in Hepatocellular Carcinoma. Mol Cancer (2020) 19:110. doi: 10.1186/s12943-020-01222-5
45. Wang J, Zhang Y, Song H, Yin H, Jiang T, Xu Y, et al. The Circular RNA circSPARC Enhances the Migration and Proliferation of Colorectal Cancer by Regulating the JAK/STAT Pathway. Mol Cancer (2021) 20:81. doi: 10.1186/s12943-021-01375-x
46. Zhang X, Zhu M, Yang R, Zhao W, Hu X, Gan J. Identification and Comparison of Novel Circular RNAs With Associated Co-Expression and Competing Endogenous RNA Networks in Pulmonary Tuberculosis. Oncotarget (2017) 8:113571–82. doi: 10.18632/oncotarget.22710
47. Zhuang Z, Zhang J, Luo H, Liu G, Lu Y, Ge N, et al. The Circular RNA of Peripheral Blood Mononuclear Cells: Hsa_circ_0005836 as a New Diagnostic Biomarker and Therapeutic Target of Active Pulmonary Tuberculosis. Mol Immunol (2017) 90:264–72. doi: 10.1016/j.molimm.2017.08.008
48. Huang Z, Yao F, Xu J, Deng Z, Su R, Peng Y, et al. Microarray Expression Profile of Circular RNAs in Peripheral Blood Mononuclear Cells From Active Tuberculosis Patients. Cell Physiol Biochem (2018) 45:1230–40. doi: 10.1159/000487454
49. Yang R, Xu B, Yang B, Fu J, Liu L, Amjad N, et al. Circular RNA Transcriptomic Analysis of Primary Human Brain Microvascular Endothelial Cells Infected With Meningitic Escherichia Coli. Mol Ther Nucleic Acids (2018) 13:651–64. doi: 10.1016/j.omtn.2018.10.013
50. Chen Y, Chen R, Ahmad S, Verma R, Kasturi S, Amaya L, et al. N6-Methyladenosine Modification Controls Circular RNA Immunity. Mol Cell (2019) 76:96–109.e9. doi: 10.1016/j.molcel.2019.07.016
51. Li X, Liu C, Xue W, Zhang Y, Jiang S, Yin Q, et al. Coordinated circRNA Biogenesis and Function With NF90/NF110 in Viral Infection. Mol Cell (2017) 67:214–27.e7. doi: 10.1016/j.molcel.2017.05.023
52. Arora S, Singh P, Dohare R, Jha R, Ali Syed M. Unravelling Host-Pathogen Interactions: ceRNA Network in SARS-CoV-2 Infection (COVID-19). Gene (2020) 762:145057. doi: 10.1016/j.gene.2020.145057
53. Ng W, Marinov G, Liau E, Lam Y, Lim Y, Ea C. Inducible RasGEF1B Circular RNA Is a Positive Regulator of ICAM-1 in the TLR4/LPS Pathway. RNA Biol (2016) 13:861–71. doi: 10.1080/15476286.2016.1207036
54. Liu Y, Hu C, Sun Y, Wu H, Chen X, Liu Q. Identification of Differentially Expressed Circular RNAs in HeLa Cells Infected With Chlamydia Trachomatis. Pathog Dis (2019) 77:ftz062. doi: 10.1093/femspd/ftz062
55. Zurawska A, Mycko M, Selmaj K. Circular RNAs as a Novel Layer of Regulatory Mechanism in Multiple Sclerosis. J Neuroimmunol (2019) 334:576971. doi: 10.1016/j.jneuroim.2019.576971
56. Ghafouri-Fard S, Taheri M. A Comprehensive Review of Non-Coding RNAs Functions in Multiple Sclerosis. Eur J Pharmacol (2020) 879:173127. doi: 10.1016/j.ejphar.2020.173127
57. Zhang J, Liu Y, Shi G. The circRNA-miRNA-mRNA Regulatory Network in Systemic Lupus Erythematosus. Clin Rheumatol (2020) 40(1):331–9. doi: 10.1007/s10067-020-05212-2
58. Xi WD CHEN. Research Progress on circRNA and Infectious Diseases. Surg Res N Tech (2020) 9:110–3.
59. Gal-Ben-Ari S, Barrera I, Ehrlich M, Rosenblum K. PKR: A Kinase to Remember. Front Mol Neurosci (2018) 11:480. doi: 10.3389/fnmol.2018.00480
60. Chen Y, Kim M, Chen X, Batista P, Aoyama S, Wilusz J, et al. Sensing Self and Foreign Circular RNAs by Intron Identity. Mol Cell (2017) 67:228–38.e5. doi: 10.1016/j.molcel.2017.05.022
61. Wesselhoeft R, Kowalski P, Parker-Hale F, Huang Y, Bisaria N, Anderson D. RNA Circularization Diminishes Immunogenicity and Can Extend Translation Duration In Vivo. Mol Cell (2019) 74:508–20.e4. doi: 10.1016/j.molcel.2019.02.015
62. Cadena C, Hur S. Antiviral Immunity and Circular RNA: No End in Sight. Mol Cell (2017) 67:163–4. doi: 10.1016/j.molcel.2017.07.005
63. Omicron is Bad But the Global Response Is Worse. Nature (2021) 600:190. doi: 10.1038/d41586-021-03616-x
64. Yang M, Qi M, Xu L, Huang P, Wang X, Sun J, et al. Differential Host circRNA Expression Profiles in Human Lung Epithelial Cells Infected With SARS-CoV-2. Infect Genet Evol (2021) 93:104923. doi: 10.1016/j.meegid.2021.104923
65. Yang S, Zhou H, Cruz-Cosme R, Liu M, Xu J, Niu X, et al. Circular RNA Profiling Reveals Abundant and Diverse circRNAs of SARS-CoV-2, SARS-CoV and MERS-CoV Origin. BioRxiv Preprint Server Biol (2020). doi: 10.1101/2020.12.07.415422
66. Cai Z, Lu C, He J, Liu L, Zou Y, Zhang Z, et al. Identification and Characterization of circRNAs Encoded by MERS-CoV, SARS-CoV-1 and SARS-CoV-2. Briefings Bioinf (2021) 22:1297–308. doi: 10.1093/bib/bbaa334
67. Pfafenrot C, Schneider T, Müller C, Hung L, Schreiner S, Ziebuhr J, et al. Inhibition of SARS-CoV-2 Coronavirus Proliferation by Designer antisense-circRNAs. Nucleic Acids Res (2021) 49(21):12502–16. doi: 10.1093/nar/gkab1096
68. Broadbent K, Broadbent J, Ribacke U, Wirth D, Rinn J, Sabeti P. Strand-Specific RNA Sequencing in Plasmodium Falciparum Malaria Identifies Developmentally Regulated Long Non-Coding RNA and Circular RNA. BMC Genomics (2015) 16:454. doi: 10.1186/s12864-015-1603-4
69. Lin J, Feng X, Zhang J. Circular RNA Circhipk3 Modulates the Proliferation of Airway Smooth Muscle Cells by miR-326/STIM1 Axis. Life Sci (2020) 255:117835. doi: 10.1016/j.lfs.2020.117835
70. Zhang Y, Zhang H, An M, Zhao B, Ding H, Zhang Z, et al. Crosstalk in Competing Endogenous RNA Networks Reveals New Circular RNAs Involved in the Pathogenesis of Early HIV Infection. J Trans Med (2018) 16:332. doi: 10.1186/s12967-018-1706-1
71. Wang K, Wei X, Wei Q, Lu D, Li W, Pan B, et al. A Two-Circular RNA Signature of Donor Circfoxn2 and Circnectin3 Predicts Early Allograft Dysfunction After Liver Transplantation. Ann Trans Med (2020) 8:94. doi: 10.21037/atm.2019.12.132
72. Ng W, Marinov G, Chin Y, Lim Y, Ea C. Transcriptomic Analysis of the Role of RasGEF1B Circular RNA in the TLR4/LPS Pathway. Sci Rep (2017) 7:12227. doi: 10.1038/s41598-017-12550-w
73. Xu M, Xie F, Tang X, Wang T, Wang S. Insights Into the Role of Circular RNA in Macrophage Activation and Fibrosis Disease. Pharmacol Res (2020) 156:104777. doi: 10.1016/j.phrs.2020.104777
74. Holdt L, Stahringer A, Sass K, Pichler G, Kulak N, Wilfert W, et al. Circular Non-Coding RNA ANRIL Modulates Ribosomal RNA Maturation and Atherosclerosis in Humans. Nat Commun (2016) 7:12429. doi: 10.1038/ncomms12429
75. Zhu P, Zhu X, Wu J, He L, Lu T, Wang Y, et al. IL-13 Secreted by ILC2s Promotes the Self-Renewal of Intestinal Stem Cells Through Circular RNA Circpan3. Nat Immunol (2019) 20:183–94. doi: 10.1038/s41590-018-0297-6
76. Hua Q, Chen Y, Liu Y, Li M, Diao Q, Xue H, et al. Circular RNA 0039411 Is Involved in Neodymium Oxide-Induced Inflammation and Antiproliferation in a Human Bronchial Epithelial Cell Line via Sponging miR-93-5p. Toxicol Sci (2019) 170:69–81. doi: 10.1093/toxsci/kfz074
77. Huang Z, Cao Y, Zhou M, Qi X, Fu B, Mou Y, et al. Hsa_circ_0005519 Increases IL-13/IL-6 by Regulating Hsa-Let-7a-5p in CD4 T Cells to Affect Asthma. Clin Exp Allergy (2019) 49:1116–27. doi: 10.1111/cea.13445
78. Li J, Xie R. Circular RNA Expression Profile in Gingival Tissues Identifies Circ_0062491 and Circ_0095812 as Potential Treatment Targets. J Cell Biochem (2019) 120:14867–74. doi: 10.1002/jcb.28748
79. Fang J, Richardson B. The MAPK Signalling Pathways and Colorectal Cancer. Lancet Oncol (2005) 6:322–7. doi: 10.1016/S1470-2045(05)70168-6
80. Yaeger R, Corcoran R. Targeting Alterations in the RAF-MEK Pathway. Cancer Discov (2019) 9:329–41. doi: 10.1158/2159-8290.CD-18-1321
81. Chen Q, Mang G, Wu J, Sun P, Li T, Zhang H, et al. Circular RNA Circsnx5 Controls Immunogenicity of Dendritic Cells Through the miR-544/SOCS1 Axis and PU.1 Activity Regulation. Mol Ther (2020) 28:2503–18. doi: 10.1016/j.ymthe.2020.07.001
82. Souma Y, Nishida T, Serada S, Iwahori K, Takahashi T, Fujimoto M, et al. Antiproliferative Effect of SOCS-1 Through the Suppression of STAT3 and P38 MAPK Activation in Gastric Cancer Cells. Int J Cancer (2012) 131:1287–96. doi: 10.1002/ijc.27350
83. Zhou H, Miki R, Eeva M, Fike F, Seligson D, Yang L, et al. Reciprocal Regulation of SOCS 1 and SOCS3 Enhances Resistance to Ionizing Radiation in Glioblastoma Multiforme. Clin Cancer Res (2007) 13:2344–53. doi: 10.1158/1078-0432.CCR-06-2303
84. Khalaf H, Demirel I, Bengtsson T. Suppression of Inflammatory Gene Expression in T Cells by Porphyromonas Gingivalis Is Mediated by Targeting MAPK Signaling. Cell Mol Immunol (2013) 10:413–22. doi: 10.1038/cmi.2013.23
85. Yoon S, Lee M, Shin D, Kim J, Chwae Y, Kwon M, et al. Activation of Mitogen Activated Protein Kinase-Erk Kinase (MEK) Increases T Cell Immunoglobulin Mucin Domain-3 (TIM-3) Transcription in Human T Lymphocytes and a Human Mast Cell Line. Mol Immunol (2011) 48:1778–83. doi: 10.1016/j.molimm.2011.05.004
86. Liu Y, Cai P, Wang N, Zhang Q, Chen F, Shi L, et al. Combined Blockade of Tim-3 and MEK Inhibitor Enhances the Efficacy Against Melanoma. Biochem Biophys Res Commun (2017) 484:378–84. doi: 10.1016/j.bbrc.2017.01.128
87. Kataoka S, Manandhar P, Lee J, Workman C, Banerjee H, Szymczak-Workman A, et al. The Costimulatory Activity of Tim-3 Requires Akt and MAPK Signaling and its Recruitment to the Immune Synapse. Sci Signal (2021) 14:eaba0717. doi: 10.1126/scisignal.aba0717
88. Lim E, Kim S, Oh Y, Suh Y, Kaushik N, Lee J, et al. Crosstalk Between GBM Cells and Mesenchymal Stemlike Cells Promotes the Invasiveness of GBM Through the C5a/p38/ZEB1 Axis. Neuro-Oncology (2020) 22:1452–62. doi: 10.1093/neuonc/noaa064
89. Sheng W, Shi X, Lin Y, Tang J, Jia C, Cao R, et al. Musashi2 Promotes EGF-Induced EMT in Pancreatic Cancer via ZEB1-ERK/MAPK Signaling. J Exp Clin Cancer Res CR (2020) 39:16. doi: 10.1186/s13046-020-1521-4
90. Richard G, Dalle S, Monet M, Ligier M, Boespflug A, Pommier R, et al. ZEB1-Mediated Melanoma Cell Plasticity Enhances Resistance to MAPK Inhibitors. EMBO Mol Med (2016) 8:1143–61. doi: 10.15252/emmm.201505971
91. Sorkin A, von Zastrow M. Endocytosis and Signalling: Intertwining Molecular Networks. Nat Rev Mol Cell Biol (2009) 10:609–22. doi: 10.1038/nrm2748
92. Khan I, Steeg P. Endocytosis: A Pivotal Pathway for Regulating Metastasis. Br J Cancer (2021) 124:66–75. doi: 10.1038/s41416-020-01179-8
93. Doherty G, McMahon H. Mechanisms of Endocytosis. Annu Rev Biochem (2009) 78:857–902. doi: 10.1146/annurev.biochem.78.081307.110540
94. Liu Y, Li Z, Zhang M, Zhou H, Wu X, Zhong J, et al. Rolling-Translated EGFR Variants Sustain EGFR Signaling and Promote Glioblastoma Tumorigenicity. Neuro-Oncology (2021) 23:743–56. doi: 10.1093/neuonc/noaa279
95. O'Shea J, Plenge R. JAK and STAT Signaling Molecules in Immunoregulation and Immune-Mediated Disease. Immunity (2012) 36:542–50. doi: 10.1016/j.immuni.2012.03.014
96. Villarino A, Kanno Y, O'Shea J. Mechanisms and Consequences of Jak-STAT Signaling in the Immune System. Nat Immunol (2017) 18:374–84. doi: 10.1038/ni.3691
97. Yang L, Han X, Zhang C, Sun C, Huang S, Xiao W, et al. Hsa_circ_0060450 Negatively Regulates Type I Interferon-Induced Inflammation by Serving as miR-199a-5p Sponge in Type 1 Diabetes Mellitus. Front Immunol (2020) 11:576903. doi: 10.3389/fimmu.2020.576903
98. Shimobayashi M, Hall M. Making New Contacts: The mTOR Network in Metabolism and Signalling Crosstalk. Nat Rev Mol Cell Biol (2014) 15:155–62. doi: 10.1038/nrm3757
99. Laplante M, Sabatini D. mTOR Signaling at a Glance. J Cell Sci (2009) 122:3589–94. doi: 10.1242/jcs.051011
100. Saxton R, Sabatini D. mTOR Signaling in Growth, Metabolism, and Disease. Cell (2017) 168:960–76. doi: 10.1016/j.cell.2017.02.004
101. Zhang H, Wang F, Wang Y, Zhao Z, Qiao P. lncRNA GAS5 Inhibits Malignant Progression by Regulating Macroautophagy and Forms a Negative Feedback Regulatory Loop With the Mir−34a/mTOR/SIRT1 Pathway in Colorectal Cancer. Oncol Rep (2021) 45:202–16. doi: 10.3892/or.2020.7825
102. Wang J, Song X, Tan G, Sun P, Guo L, Zhang N, et al. NAD+ Improved Experimental Autoimmune Encephalomyelitis by Regulating SIRT1 to Inhibit PI3K/Akt/mTOR Signaling Pathway. Aging (2021) 13:25931–43. doi: 10.18632/aging.203781
103. Yao Q, Wu Q, Xu X, Xing Y, Liang J, Lin Q, et al. Resveratrol Ameliorates Systemic Sclerosis via Suppression of Fibrosis and Inflammation Through Activation of SIRT1/mTOR Signaling. Drug Design Dev Ther (2020) 14:5337–48. doi: 10.2147/DDDT.S281209
104. Gao D, Tang T, Zhu J, Tang Y, Sun H, Li S. CXCL12 has Therapeutic Value in Facial Nerve Injury and Promotes Schwann Cells Autophagy and Migration via PI3K-AKT-mTOR Signal Pathway. Int J Biol Macromol (2019) 124:460–8. doi: 10.1016/j.ijbiomac.2018.10.212
105. Li S, Fan Y, Kumagai A, Kawakita E, Kitada M, Kanasaki K, et al. Deficiency in Dipeptidyl Peptidase-4 Promotes Chemoresistance Through the CXCL12/CXCR4/mTOR/Tgfβ Signaling Pathway in Breast Cancer Cells. Int J Mol Sci (2020) 21:805. doi: 10.3390/ijms21030805
106. Yang F, Takagaki Y, Yoshitomi Y, Ikeda T, Li J, Kitada M, et al. Inhibition of Dipeptidyl Peptidase-4 Accelerates Epithelial-Mesenchymal Transition and Breast Cancer Metastasis via the CXCL12/CXCR4/mTOR Axis. Cancer Res (2019) 79:735–46. doi: 10.1158/0008-5472.CAN-18-0620
107. Parsons M, Tammela T, Dow L. WNT as a Driver and Dependency in Cancer. Cancer Discov (2021) 11:2413–29. doi: 10.1158/2159-8290.CD-21-0190
108. Rim E, Clevers H, Nusse R. The Wnt Pathway: From Signaling Mechanisms to Synthetic Modulators. Annu Rev Biochem (2022). doi: 10.1146/annurev-biochem-040320-103615
109. Zhou Y, Xu J, Luo H, Meng X, Chen M, Zhu D. Wnt Signaling Pathway in Cancer Immunotherapy. Cancer Lett (2022) 525:84–96. doi: 10.1016/j.canlet.2021.10.034
110. Zhao C, Yan Z, Wen J, Fu D, Xu P, Wang L, et al. CircEAF2 Counteracts Epstein-Barr Virus-Positive Diffuse Large B-Cell Lymphoma Progression via miR-BART19-3p/APC/β-Catenin Axis. Mol Cancer (2021) 20:153. doi: 10.1186/s12943-021-01458-9
111. Zhao S, Wang J, Qin C. Blockade of CXCL12/CXCR4 Signaling Inhibits Intrahepatic Cholangiocarcinoma Progression and Metastasis via Inactivation of Canonical Wnt Pathway. J Exp Clin Cancer Res CR (2014) 33:103. doi: 10.1186/s13046-014-0103-8
112. Yu T, Liu K, Wu Y, Fan J, Chen J, Li C, et al. MicroRNA-9 Inhibits the Proliferation of Oral Squamous Cell Carcinoma Cells by Suppressing Expression of CXCR4 via the Wnt/β-Catenin Signaling Pathway. Oncogene (2014) 33:5017–27. doi: 10.1038/onc.2013.448
113. Hu T, Yao Y, Yu S, Han L, Wang W, Guo H, et al. SDF-1/CXCR4 Promotes Epithelial-Mesenchymal Transition and Progression of Colorectal Cancer by Activation of the Wnt/β-Catenin Signaling Pathway. Cancer Lett (2014) 354:417–26. doi: 10.1016/j.canlet.2014.08.012
114. Hankey W, Frankel W, Groden J. Functions of the APC Tumor Suppressor Protein Dependent and Independent of Canonical WNT Signaling: Implications for Therapeutic Targeting. Cancer Metastasis Rev (2018) 37:159–72. doi: 10.1007/s10555-017-9725-6
115. Deng S, Zhang X, Qin Y, Chen W, Fan H, Feng X, et al. miRNA-192 and -215 Activate Wnt/β-Catenin Signaling Pathway in Gastric Cancer via APC. J Cell Physiol (2020) 235:6218–29. doi: 10.1002/jcp.29550
116. Borowsky J, Dumenil T, Bettington M, Pearson S, Bond C, Fennell L, et al. The Role of APC in WNT Pathway Activation in Serrated Neoplasia. Mod Pathol (2018) 31:495–504. doi: 10.1038/modpathol.2017.150
117. Peng Y, Xu Y, Zhang X, Deng S, Yuan Y, Luo X, et al. A Novel Protein AXIN1-295aa Encoded by Circaxin1 Activates the Wnt/β-Catenin Signaling Pathway to Promote Gastric Cancer Progression. Mol Cancer (2021) 20:158. doi: 10.1186/s12943-021-01457-w
118. Zhang C, Han X, Yang L, Fu J, Sun C, Huang S, et al. Circppm1fcircular RNA Modulates M1 Macrophage Activation and Pancreatic Islet Inflammation in Type 1 Diabetes Mellitus. Theranostics (2020) 10:10908–24. doi: 10.7150/thno.48264
119. Wang X, Sheng W, Xu T, Xu J, Gao R, Zhang ZJA. CircRNA Hsa_Circ_0110102 Inhibited Macrophage Activation and Hepatocellular Carcinoma Progression via miR-580-5p/Pparα/CCL2 Pathway. Aging (Albany NY) (2021) 13:11969–87. doi: 10.18632/aging.202900
120. Zhou Z, Jiang R, Yang X, Guo H, Fang S, Zhang Y, et al. circRNA Mediates Silica-Induced Macrophage Activation Via HECTD1/ZC3H12A-Dependent Ubiquitination. Theranostics (2018) 8:575–92. doi: 10.7150/thno.21648
121. Yang X, Wang J, Zhou Z, Jiang R, Huang J, Chen L, et al. Silica-Induced Initiation of Circular ZC3H4 RNA/ZC3H4 Pathway Promotes the Pulmonary Macrophage Activation. FASEB J (2018) 32:3264–77. doi: 10.1096/fj.201701118R
122. Lian C, Sun J, Guan W, Zhang L, Zhang X, Yang L, et al. Circular RNA Circhipk3 Activates Macrophage NLRP3 Inflammasome and TLR4 Pathway in Gouty Arthritis via Sponging miR-561 and miR-192. Inflammation (2021) 44:2065–77. doi: 10.1007/s10753-021-01483-2
123. Wang Y, Li C, Zhao R, Qiu Z, Shen C, Wang Z, et al. CircUbe3a From M2 Macrophage-Derived Small Extracellular Vesicles Mediates Myocardial Fibrosis After Acute Myocardial Infarction. Theranostics (2021) 11:6315–33. doi: 10.7150/thno.52843
124. Zhao D, Wang C, Liu X, Liu N, Zhuang S, Zhang Q, et al. CircN4bp1 Facilitates Sepsis-Induced Acute Respiratory Distress Syndrome Through Mediating Macrophage Polarization via the miR-138-5p/EZH2 Axis. Mediators Inflamm (2021) 2021:7858746. doi: 10.1155/2021/7858746
125. Song H, Yang Y, Sun Y, Wei G, Zheng H, Chen Y, et al. Circular RNA Cdyl Promotes Abdominal Aortic Aneurysm Formation by Inducing M1 Macrophage Polarization and M1-Type Inflammation. Mol Ther (2022) 30:915–31. doi: 10.1016/j.ymthe.2021.09.017
126. Li X, Kang J, Lv H, Liu R, Chen J, Zhang Y, et al. CircPrkcsh, a Circular RNA, Contributes to the Polarization of Microglia Towards the M1 Phenotype Induced by Spinal Cord Injury and Acts via the JNK/p38 MAPK Pathway. FASEB J (2021) 35:. doi: 10.1096/fj.202100993R
127. Chen T, Liu Y, Li C, Xu C, Ding C, Chen J, et al. Tumor-Derived Exosomal circFARSA Mediates M2 Macrophage Polarization via the PTEN/PI3K/AKT Pathway to Promote Non-Small Cell Lung Cancer Metastasis. Cancer Treat Res Commun (2021) 28:100412. doi: 10.1016/j.ctarc.2021.100412
128. Yi B, Dai K, Yan Z, Yin ZJB. Circular RNA PLCE1 Promotes Epithelial Mesenchymal Transformation, Glycolysis in Colorectal Cancer and M2 Polarization of Tumor-Associated Macrophages. Bioengineered (2022) 13:6243–56. doi: 10.1080/21655979.2021.2003929
129. Li H, Luo F, Jiang X, Zhang W, Xiang T, Pan Q, et al. CircITGB6 Promotes Ovarian Cancer Cisplatin Resistance by Resetting Tumor-Associated Macrophage Polarization Toward the M2 Phenotype. J Immunother Cancer (2022) 10:e004029. doi: 10.1136/jitc-2021-004029
130. Lu C, Shi W, Hu W, Zhao Y, Zhao X, Dong F, et al. Endoplasmic Reticulum Stress Promotes Breast Cancer Cells to Release Exosomes Circ_0001142 and Induces M2 Polarization of Macrophages to Regulate Tumor Progression. Pharmacol Res (2022) 177:106098. doi: 10.1016/j.phrs.2022.106098
131. Wang Y, Gao R, Li J, Tang S, Li S, Tong Q, et al. Downregulation of Hsa_Circ_0074854 Suppresses the Migration and Invasion in Hepatocellular Carcinoma via Interacting With HuR and via Suppressing Exosomes-Mediated Macrophage M2 Polarization. Int J Nanomed (2021) 16:2803–18. doi: 10.2147/IJN.S284560
132. Zhang J, Cheng F, Rong G, Tang Z, Gui BJB. Circular RNA Hsa_Circ_0005567 Overexpression Promotes M2 Type Macrophage Polarization Through miR-492/SOCS2 Axis to Inhibit Osteoarthritis Progression. Bioengineered (2021) 12:8920–30. doi: 10.1080/21655979.2021.1989999
133. Yang L, Fu J, Han X, Zhang C, Xia L, Zhu R, et al. Hsa_circ_0004287 Inhibits Macrophage-Mediated Inflammation in an N-Methyladenosine-Dependent Manner in Atopic Dermatitis and Psoriasis. J Allergy Clin Immunol (2021) S0091-6749(21)02685-3. doi: 10.1016/j.jaci.2021.11.024
134. Katopodi T, Petanidis S, Domvri K, Zarogoulidis P, Anestakis D, Charalampidis C, et al. Kras-Driven Intratumoral Heterogeneity Triggers Infiltration of M2 Polarized Macrophages via the Circhipk3/PTK2 Immunosuppressive Circuit. Sci Rep (2021) 11:15455. doi: 10.1038/s41598-021-94671-x
135. Ma X, Xu J, Lu Q, Feng X, Liu J, Cui C, et al. Hsa_circ_0087352 Promotes the Inflammatory Response of Macrophages in Abdominal Aortic Aneurysm by Adsorbing hsa-miR-149-5p. Int Immunopharmacol (2022) 107:108691. doi: 10.1016/j.intimp.2022.108691
136. Lu X, Liu Y, Xuan W, Ye J, Yao H, Huang C, et al. Circ_1639 Induces Cells Inflammation Responses by Sponging miR-122 and Regulating TNFRSF13C Expression in Alcoholic Liver Disease. Toxicol Lett (2019) 314:89–97. doi: 10.1016/j.toxlet.2019.07.021
137. Deng Q, Huang J, Yan J, Mao E, Chen H, Wang CJT. Circ_0001490/miR-579-3p/FSTL1 Axis Modulates the Survival of Mycobacteria and the Viability, Apoptosis and Inflammatory Response in Mycobacterium Tuberculosis-Infected Macrophages. Tuberculosis (Edinb) (2021) 131:102123. doi: 10.1016/j.tube.2021.102123
138. Luo H, Pi J, Zhang J, Yang E, Xu H, Luo H, et al. Mycobacterium Tuberculosiscircular RNA TRAPPC6B Inhibits Intracellular Growth While Inducing Autophagy in Macrophages by Targeting microRNA-874-3p. Clin Transl Immunol (2021) 10:. doi: 10.1002/cti2.1254
139. Wu M, Liu Z, Zhang S. Down-Regulation of Hsa_Circ_0045474 Induces Macrophage Autophagy in Tuberculosis via miR-582-5p/TNKS2 Axis. Innate Immun (2022) 28:11–8. doi: 10.1177/17534259211064285
140. Deng T, Yang L, Zheng Z, Li Y, Ren W, Wu C, et al. Calcitonin Gene−Related Peptide Induces IL−6 Expression in RAW264.7 Macrophages Mediated by Mmu_circRNA_007893. Mol Med Rep (2017) 16:9367–74. doi: 10.3892/mmr.2017.7779
141. Li S, Chen Z, Zhou R, Wang S, Wang W, Liu D, et al. Hsa_circ_0048674 Facilitates Hepatocellular Carcinoma Progression and Natural Killer Cell Exhaustion Depending on the Regulation of miR-223-3p/PDL1. Histol Hisphatol (2022) 18440. doi: 10.14670/HH-18-440
142. Ma Y, Zhang C, Zhang B, Yu H, Yu Q. circRNA of AR-Suppressed PABPC1 91 Bp Enhances the Cytotoxicity of Natural Killer Cells Against Hepatocellular Carcinoma via Upregulating UL16 Binding Protein 1. Oncol Lett (2019) 17:388–97. doi: 10.3892/ol.2018.9606
143. Ou Z, Luo Z, Wei W, Liang S, Gao T, Lu Y. Hypoxia-Induced Shedding of MICA and HIF1A-Mediated Immune Escape of Pancreatic Cancer Cells From NK Cells: Role of Circ_0000977/miR-153 Axis. RNA Biol (2019) 16:1592–603. doi: 10.1080/15476286.2019.1649585
144. Ke H, Zhang J, Wang F, Xiong Y. ZNF652-Induced Circrhot1 Promotes SMAD5 Expression to Modulate Tumorigenic Properties and Nature Killer Cell-Mediated Toxicity in Bladder Cancer via Targeting miR-3666. J Immunol Res (2021) 2021:7608178. doi: 10.1155/2021/7608178
145. Luan J, Jiao C, Kong W, Fu J, Qu W, Chen Y, et al. circHLA-C Plays an Important Role in Lupus Nephritis by Sponging miR-150. Mol Ther Nucleic Acids (2018) 10:245–53. doi: 10.1016/j.omtn.2017.12.006
146. Wang B, Zhou Q, Li A, Li S, Greasley A, Skaro A, et al. Preventing Alloimmune Rejection Using Circular RNA FSCN1-Silenced Dendritic Cells in Heart Transplantation. J Heart Lung Transpl (2021) 40:584–94. doi: 10.1016/j.healun.2021.03.025
147. Zhang Y, Zhang G, Liu Y, Chen R, Zhao D, McAlister V, et al. GDF15 Regulates Malat-1 Circular RNA and Inactivates Nfκb Signaling Leading to Immune Tolerogenic DCs for Preventing Alloimmune Rejection in Heart Transplantation. Front Immunol (2018) 9:2407. doi: 10.3389/fimmu.2018.02407
148. Zhang C, Wang X, Chen Y, Wu Z, Zhang C, Shi W. The Down-Regulation of hsa_circ_0012919, the Sponge for miR-125a-3p, Contributes to DNA Methylation of CD11a and CD70 in CD4 + T Cells of Systemic Lupus Erythematous. Clin Sci (Lond) (2018) 132:2285–98. doi: 10.1042/CS20180403
149. Zhu X, Wang X, Wang Y, Zhao Y. The Regulatory Network Among CircHIPK3, LncGAS5, and miR-495 Promotes Th2 Differentiation in Allergic Rhinitis. Cell Death Dis (2020) 11:216. doi: 10.1038/s41419-020-2394-3
150. Huang Z, Fu B, Qi X, Xu Y, Mou Y, Zhou M, et al. Diagnostic and Therapeutic Value of Hsa_circ_0002594 for T Helper 2-Mediated Allergic Asthma. Int Arch Allergy Immunol (2021) 182:388–98. doi: 10.1159/000511612
151. Liu Z, Wang T, She Y, Wu K, Gu S, Li L, et al. N-Methyladenosine-Modified Circigf2bp3 Inhibits CD8 T-Cell Responses to Facilitate Tumor Immune Evasion by Promoting the Deubiquitination of PD-L1 in Non-Small Cell Lung Cancer. Mol Cancer (2021) 20:105. doi: 10.1186/s12943-021-01398-4
152. Yang C, Wu S, Mou Z, Zhou Q, Dai X, Ou Y, et al. Exosome-Derived Circtrps1 Promotes Malignant Phenotype and CD8+ T Cell Exhaustion in Bladder Cancer Microenvironments. Mol Ther (2022) 30:1054–70. doi: 10.1016/j.ymthe.2022.01.022
153. Wang Y, Yu X, Luo S, Han H. Comprehensive Circular RNA Profiling Reveals That Circular RNA100783 is Involved in Chronic CD28-Associated CD8(+)T Cell Ageing. Immun Ageing (2015) 12:17. doi: 10.1186/s12979-015-0042-z
154. Chen S, Zhu S, Pei X, Qiu B, Xiong D, Long X, et al. Cancer Cell-Derived Exosomal Circusp7 Induces CD8 T Cell Dysfunction and Anti-PD1 Resistance by Regulating the miR-934/SHP2 Axis in NSCLC. Mol Cancer (2021) 20:144. doi: 10.1186/s12943-021-01448-x
155. Li M, Chi C, Zhou L, Chen Y, Tang X. Circular PVT1 Regulates Cell Proliferation and Invasion via miR-149-5p/FOXM1 Axis in Ovarian Cancer. J Cancer (2021) 12:611–21. doi: 10.7150/jca.52234
156. Sekar S, Cuyugan L, Adkins J, Geiger P, Liang W. Circular RNA Expression and Regulatory Network Prediction in Posterior Cingulate Astrocytes in Elderly Subjects. BMC Genomics (2018) 19:340. doi: 10.1186/s12864-018-4670-5
157. Xia P, Wang S, Ye B, Du Y, Li C, Xiong Z, et al. A Circular RNA Protects Dormant Hematopoietic Stem Cells From DNA Sensor cGAS-Mediated Exhaustion. Immunity (2018) 48:688–701.e7. doi: 10.1016/j.immuni.2018.03.016
158. Wesselhoeft R, Kowalski P, Anderson D. Engineering Circular RNA for Potent and Stable Translation in Eukaryotic Cells. Nat Commun (2018) 9:2629. doi: 10.1038/s41467-018-05096-6
159. De-lai K, Yi-fan Z, Wen-jun Z, Ai-zhen G, Ying-yu C. Research Progress on Circular RNA. Chin Vet Sci (2021) 51(03):1–7. doi: 10.16656/j.issn.1673-4696.2021.0027
160. Morad G, Helmink B, Sharma P, Wargo J. Hallmarks of Response, Resistance, and Toxicity to Immune Checkpoint Blockade. Cell (2021) 184(21):5309–37. doi: 10.1016/j.cell.2021.09.020
161. Song H, Liu Q, Liao Q. Circular RNA and Tumor Microenvironment. Cancer Cell Int (2020) 20:211. doi: 10.1186/s12935-020-01301-z
162. Li W, Liu J, Chen M, Xu J, Zhu D. Circular RNA in Cancer Development and Immune Regulation. J Cell Mol Med (2020) 26(6):1785–98. doi: 10.1111/jcmm.16102
163. Shang Q, Yang Z, Jia R, Ge S. The Novel Roles of circRNAs in Human Cancer. Mol Cancer (2019) 18:6. doi: 10.1186/s12943-018-0934-6
164. Xu Z, Li P, Fan L, Wu M. The Potential Role of circRNA in Tumor Immunity Regulation and Immunotherapy. Front Immunol (2018) 9:9. doi: 10.3389/fimmu.2018.00009
165. Hegde P, Chen D. Top 10 Challenges in Cancer Immunotherapy. Immunity (2020) 52:17–35. doi: 10.1016/j.immuni.2019.12.011
166. Billan S, Kaidar-Person O, Gil Z. Treatment After Progression in the Era of Immunotherapy. Lancet Oncol (2020) 21:e463–e76. doi: 10.1016/S1470-2045(20)30328-4
167. Wang R, Wang H. Immune Targets and Neoantigens for Cancer Immunotherapy and Precision Medicine. Cell Res (2017) 27:11–37. doi: 10.1038/cr.2016.155
168. Lu J, Zhang P, Huang X, Guo X, Gao C, Zeng H, et al. Amplification of Spatially Isolated Adenosine Pathway by Tumor-Macrophage Interaction Induces Anti-PD1 Resistance in Hepatocellular Carcinoma. J Hematol Oncol (2021) 14:200. doi: 10.1186/s13045-021-01207-x
Keywords: circRNAs, immunity, immune-related diseases, autoimmune diseases, tumor, infectious diseases
Citation: Gu J, Su C, Huang F, Zhao Y and Li J (2022) Past, Present and Future: The Relationship Between Circular RNA and Immunity. Front. Immunol. 13:894707. doi: 10.3389/fimmu.2022.894707
Received: 12 March 2022; Accepted: 28 April 2022;
Published: 25 May 2022.
Edited by:
Damjan Glavač, University of Ljubljana, SloveniaCopyright © 2022 Gu, Su, Huang, Zhao and Li. This is an open-access article distributed under the terms of the Creative Commons Attribution License (CC BY). The use, distribution or reproduction in other forums is permitted, provided the original author(s) and the copyright owner(s) are credited and that the original publication in this journal is cited, in accordance with accepted academic practice. No use, distribution or reproduction is permitted which does not comply with these terms.
*Correspondence: Jing Li, bGlqaW5nMTk4NEBzY3UuZWR1LmNu; Yuwei Zhao, Y2RiY3p5d0AxNjMuY29t
†These authors have contributed equally to this work